S.1.1.-I1
In this talk I will speak about hydrogen generation via photoelectrochemical and photocatalytic water splitting. I will mainly focus on copper oxide (Cu 2 O), synthesized through the thermal oxidation of copper foils. Through the utilization of capacitance-based methods, we investigate the effects of an etching treatment on cuprous oxide, resulting in a noteworthy performance improvement and setting a new performance record. The orientation of millimeter-sized single crystal grains, discernible to the naked eye due to selective etching and optical effects on various facets, facilitates the evaluation of crystal orientation's impact on the photocathodes. The results reveal that high index crystal orientations consistently demonstrate statistically superior performance compared to low index facets, and the underlying reasons for this enhancement will be discussed. To conclude, I will discuss how studies on thin films can contribute to the advancement of photocatalytic particles, presenting disruptive potential in the green hydrogen domain.
S.1.1.-I2
Funneling sunlight to perform chemical reactions by means of photoelectrochemical (PEC) devices opens an intriguing avenue to manufacture a wide range of chemicals in a sustainable manner. Amongst the many semiconductor photoanodes described in the literature, BiVO4 stands out for its early photocurrent onset potential and a relatively high saturation photocurrent, in some cases, reaching close-to-theoretical values. In recent years, the role of the surface composition of BiVO4 photoanodes at controlling the PEC oxygen evolution reaction (OER) has drawn increasing attention [1]. In fact, several reports have pointed out the critical role that the surface Bi:V ratio plays at controlling the performance and delivering benchmarking values [2]. However, to date, there exists a very limited portfolio of strategies to engineer the atomic termination in a controlled manner, while the actual mechanism whereby the surface composition governs the performance remains to be elucidated.
In this talk, a new methodology to tailor the surface termination of BiVO4 will be discussed, while the comprehensive characterization of the electrodes will unravel how the surface Bi:V ratio dictates the solar water oxidation performance [3]. Briefly, annealing in the presence of ammonium metavanadate affords to accurately control the surface Bi:V ratio. Subsequent analyses revealed that the improved response with the decreasing Bi:V ratio originated from the passivation of vanadium vacancies-related recombination centers. In addition, in an attempt to explore the prospects of chemical manufacturing using PEC devices, BiVO4 photoanodes were used to carry out the halogenation of various organic substrates, gaining new insights on the impact of the chemical environment and the reaction mechanism.
S.1.2.-I1
Dr. Teresa Andreu is lecturer professor at the University of Barcelona since 2020. She received the degree in Chemistry (1999) and PhD in Materials Science (2004) from the University of Barcelona. After a period in industry and academia, she joined IREC in 2009 as senior researcher and the Institute of Nanoscience and Nanotechnology at UB in 2020. Her research is focused on the development of materials and reactors for emerging technologies for hydrogen generation and carbon dioxide conversion (photoelectrochemistry, heterogeneous catalysis and plasma-catalysis). She is the author of more than 130 scientific publications and 4 patents.
One of the main problems that slows down the implementation of the green hydrogen (H2) economy is the cost of water electrolysis. While part of this cost is associated to the price of electricity, a significant part relies on the parts of the electrolyzers. Despite their advantages, Proton Exchange Membrane Water Electrolyzers (PEMWE) still have to overcome some drawbacks to reduce its hydrogen production cost, while maintaining high efficiencies.
According to IRENA report 53% of the cost of the electrolyzer stack lies on the bipolar plates (BPPs) and 24% on the catalyst-coated membrane (CCM). INTELEC project aims at developing new highly conductive components to reduce the cost of the green-hydrogen generation sensibly.
For decades, thermal spraying has been used for the production of coatings all over the world because of its versatility in industry for machinery and tools preservation, surface protection and corrosion prevention. This study demonstrates the possibilities of Cold Gas Spray (CGS) for the cost-reduction production of a component of PEMWEs, the Bipolar Plates (BPPs), by metal 3D printing. In this process, the incorporation of a mask between the nozzle exit and the substrate will drastically transform the BPP production to a very fast and automatic bottom-up process where material is deposited layer-by-layer for building up the three-dimensional flow field patterns from a flat surface. Microstructure and topography of 3D printed BPPs were inspected by microscopic techniques. For evaluating the fulfilment of BPPs requirements, interfacial contact resistance and corrosion resistance of the new BPPs were characterized following David’s methods and with potentiodynamic tests in O2-saturated H2SO4 solutions, respectively.
Concerning catalyst coated membranes, significant improvements in the research of highly active electrocatalysts by alloying iridium with ruthenium and supporting it on highly porous substrates such as TiO2 nanoparticles, allowing the demonstration of functional devices below 0.2 mg cm-2. TiO2, as n-type semiconductor is commonly used as electron transport layer (ETL) in devices to extract negative charges, and may compromise the long term stability of CCMs by increasing its resistivity due to the filling of oxygen vacancies during operation. INTELEC project demonstrates the feasibility to modify TiO2 with p-type dopants as a highly stable support for iridium oxide electrocatalysts. The electrochemical characterization in a rotating disc elèctrode demonstrates a mass activity over 700 A gIr-1 at 1.6 VRHE and no degradation after 1000 cycles of accelerated electrochemical test.
S.1.2.-O1
Cost-effective and efficient photoelectrochemical (PEC) water splitting stands out as one of the most promising strategies to address sustainable energy supply in the form of green H2. However, large-area photoelectrodes featuring precise chemical and morphological control are needed for a practical solar-to-hydrogen conversion. In this regard, it is necessary to find efficient ways to up-scale laboratory synthesis to an industrial relevant scale. The emergence of continuous flow technologies offers an alternative strategy for preparing advanced materials in a highly controlled, reproducible, and scalable fashion,[1] influencing the synthesis of nanoparticles (NPs). Semiconductor NPs have attracted a great deal of interest in the last decades due to the increasingly range of applications. Among n-type semiconductors, Bismuth vanadate (BiVO4) is one of the most attractive materials used as photoanode for water oxidation due to its low cost, stability in aqueous solutions and moderate band gap (2.4 eV).[2] However, the large-scale synthesis of this material is still limited to the use of conventional batch processes, and it is necessary to achieve high-production yields due to the increasing demand in the field of energy materials.
In this communication we show the preparation of BiVO4 NPs by using a simple continuous-flow method. The proposed system allows to up-scale the synthesis through a microreactor and provides an affordable methodology for the fabrication of cost-effective, large-scale BiVO4 photoanodes with areas up to 50 cm2 and competitive performance.
S.1.2.-O2
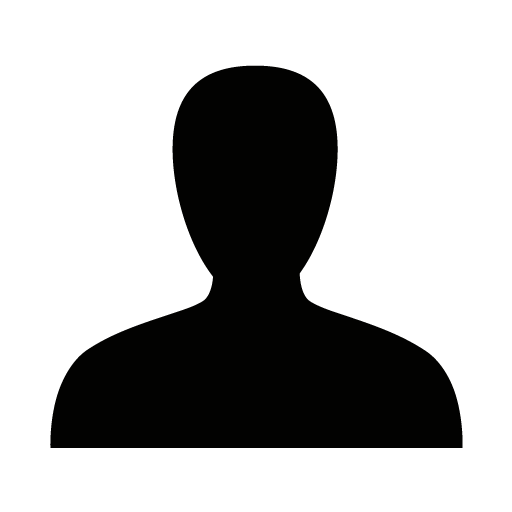
Photovoltaic (PV) systems commonly include maximum power point trackers for optimal power delivery to the connected load or storage device. In this work, a simple design with direct coupling of battery and water electrolyser to PV is explored. A key performing metric of directly coupled PV systems is the coupling factor, or coupling efficiency, which is the ratio of the output power of the PV to its power at maximum power point [1]. With the production of green hydrogen from PV being the focus of the study, system components such as the PV device, the electrochemical (EC) cell and the battery (B) needed for this operation are characterized and the performance of the system in direct coupling is investigated.
In our study we compare performance of two systems, two direct-coupled devices: (i) PV directly coupled to EC referred to as PV-EC, and (ii) PV directly coupled to EC and battery referred to as PV-EC-B. Previous theoretical and experimental studies [2, 3] show that the systems with batteries have potential for higher solar-to-hydrogen efficiency (STH). In this work we strive to demonstrate this potential experimentally at high overall efficiency level. The first step towards enhanced STH is aimed at by using high efficiency components. Therefore, we use Gallium arsenide (GaAs) PV modules with PV efficiency of approx. 29 % under 1 sun (1000 W/m2) and efficiency of up to 34.5 % reached at higher solar concentration of 17.3 sun. The PV operation is achieved using emulated current-voltage (I-V) characteristics with an advanced PV emulator. The emulator is based on an algorithm dynamically controlling the output of a source measuring unit (SMU) in such a way that the output reproduces an I-V characteristic of any required PV device with precision and accuracy on par with class A+ solar simulator. A commercially available EC is used with Pt/Ti cathode for hydrogen evolution reaction (HER) and Ru/Ir anode for oxygen evolution reaction (OER) and operated in acidic electrolyte. To simulate real electrolyzer operation conditions, the acidic electrolyte is kept at an elevated temperature of 80oC with a stable concentration of 0.5 M sulfuric acid. This yields an EC efficiency of about 76% at a current density of 10 mA/cm2. Lithium Titanium Oxide (LTO) batteries with a nominal capacity of 6 Ah and nominal voltage of 2.4 V for each battery are used.
For the PV-EC experiments, a high STH value of approx. 21% is achieved as marked by the orange star in Fig. 1 (TOC graphic). An STH value of approx. 25% is achieved for the PV-EC-B system as marked by blue star in Fig. 1 which is higher than results for the PV-EC and confirms the role of adding a battery to the system in terms of STH gain. The addition of the battery to the PV-EC system allows for lower overpotential operation of the system favourable for high STH [2, 3]. Considering different PV efficiencies, we also present the theoretical limit of STH for the PV-EC system using already established methods [4] as shown with red line in Fig. 1. The STH limit of the PV-EC is therefore obtained to be 29.3% pointing towards optimization directions in terms of obtainable STH when compared to an experimental value of 21%.
We will present detailed analysis of both systems in diurnal operations, discuss the energy efficiencies, and show directions to achieve more optimized performance.
S.1.2.-O3
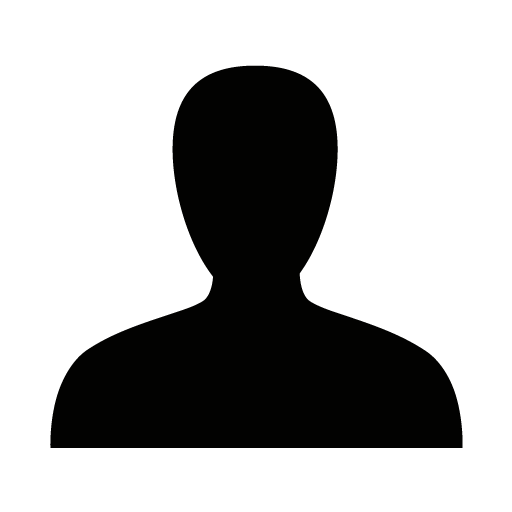
Efficient conversion of solar energy into molecules such as hydrogen is one of the most important challenges for sustainable energy systems of the future. Photovoltaic (PV) driven electrochemical (EC) water splitting particularly in direct-coupled design offers highest solar-to-hydrogen efficiency. In most practical cases, PV and EC devices are developed separately and then tested in PV-EC combination at a later stage. While state-of-the-art PV devices are represented by a range of standardized commercial modules, the electrolyzers are much less established. Large number of materials, approaches, and designs are constantly tested for water electrolyzers. Enormous efforts of many groups produce increasing number of new catalysts every year. In this situation, it is desirable to predict performance of a specific electrolyzer in combination with PV devices of various technologies and sizes. It is useful to evaluate potential efficiency of a PV-EC system from the electrolyzer point of view without actually building and optimizing an experimental device. To address this issue, we developed method for fast assessment of the maximal solar-to-hydrogen efficiency (STH limit) attainable by the specific electrolyzer via the “reverse analysis” of its polarization curve [1]. We show that despite the complexity of the parameter space, there is a surprisingly simple way to estimate the limit of efficiency in any PV-assisted electrolyzer system.
Principle of the reverse analysis is presented in Figure 1. The maximum STH for a specific electrolyzer is achieved when the current-voltage (IV) characteristics of a PV device intersects polarization curve of an EC device at maximum power point (MPP). Conversely, each point on the EC polarization curve can be considered as the MPP of the PV device optimally coupled to the EC device, Figure 1 (a). Therefore, at each point on the polarization curve, the minimum PV efficiency and maximum EC efficiency can be calculated for a specific irradiance, Figure 1 (b). Product of both efficiencies generates the STH limit value attainable at that specific point on the polarization curve, Figure 1 (b). By plotting the STH limit versus PV efficiency, the resulting dependence is achieved, Figure 1 (c). It is the STH limit attained with PV device of any efficiency at the target irradiance and size. The solution is not analytic. It is a shortcut avoiding solving transcendental equations of PV and EC IVs. Therefore, finding STH limit at specific PV efficiency requires numerical interpolation of the dependence in Figure 1 (c). In return this "reverse analysis" is performed with elementary conversion of the EC polarization curve and does not involve any modeling or analysis of PV IV characteristics. It provides quick and simple way to quantify the potential of the electrolyzer as for the STH efficiency limit in combination with any PV device.
In our paper we present the principle of the reverse analysis, show how to identify, and quantify losses in experimental PV-EC combinations. We present an extended set of results of reverse analysis applied to a variety of PV-EC combinations described in the literature. We discuss how the method can be used to analyze mutual scaling of PV and EC devices to address design of practical systems in the field. Finally, we show how the analysis can be used to analyze systems with indirect coupling, involving DC-DC converters or other power management electronics between PV and EC parts.
Session-T3
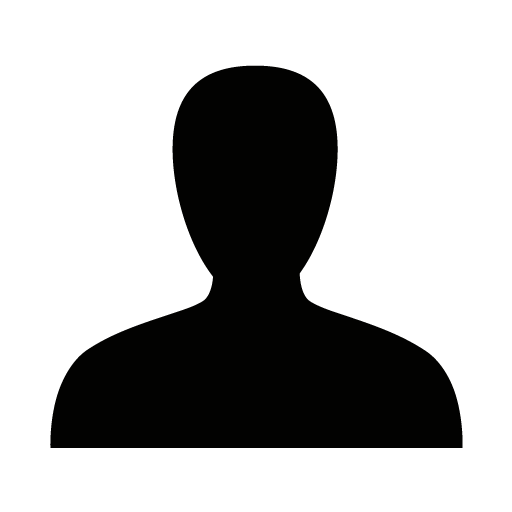
Generation of hydrogen through water splitting under visible-light irradiation has been a known topic for a long time now.1,2 Photochemical water splitting is a complex process in which different components are required to fulfill the overall reaction, each of them having a key role including (i) harvesting the light, (ii) separation of charges, (iii) breaking and (iv) formation of chemical bonds.
Within the context of the hydrogen evolution half reaction (HER), a large range of inorganic semiconductor materials such as TiO2 have been used,1,3 thanks to their suitable electronic configuration and stability under photochemical conditions. However, limitations in solar to hydrogen efficiency hamper their implementation. On the other hand, organic semiconductors have more recently emerged as promising materials to be used in this field. Prominent examples of this family of semiconductors are 2D-covalent organic frameworks (COFs), which are reticular materials with high crystallinity and porosity.4,5 They have been applied to photocatalysis including visible light-induced HER with great success.6,7 One of the advantages of COF materials is their versatility to control chemical and electronic structure, allowing to rationally design better photocatalytic materials by enhancing solar light harvesting, charge separation, interaction with co-catalysts or dispersibility in water.8
In this work we have prepared and tested two families of 2D-COF materials under light-induced HER in combination with different co-catalysts. The organic skeleton of the organic semiconductors has been modified by adding moieties with different electron donor-acceptor properties to tune their light absorption capacities. This work also highlights the importance of having a well-known structure of the co-catalyst to improve the hydrogen production rate.
Session-T1
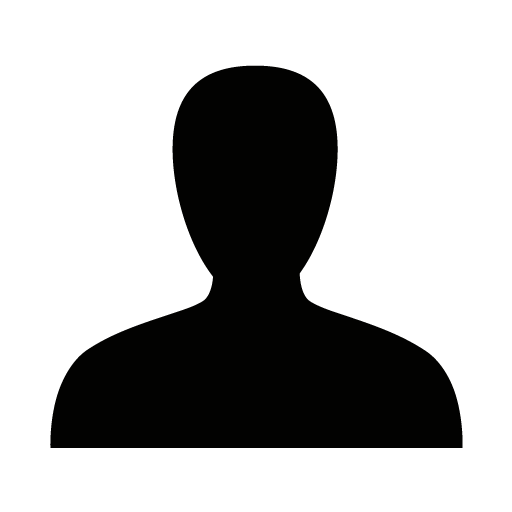
Artificial Photosynthesis is a promising strategy for conversion of solar energy into chemical feedstocks. In this context, Dye-Sensitized Photoelectrochemical Cells (DS-PECs) have achieved a key role as devices able to drive solar light-promoted water splitting. Indeed, due to its abundance and low toxicity, water is identified as the most appealing substance to be exploited in large-scale devices.
The core target of these modular devices is the photo-driven reduction of naturally abundant water to H2, the most appealing energy-dense vector. The Hydrogen Evolution Reaction (HER, E0H+/H2 = 0 V vs the Reversible Hydrogen Electrode, RHE) is typically coupled to the oxidation of water to dioxygen, providing the reducing equivalents to feed H2 fuel synthesis. A key design principle to maximize photon-to-fuel output therefore relies on effective water oxidation catalysts (WOCs), able to overcome the thermodynamically demanding (E0O2/H2O = 1.23 V vs RHE) and kinetically limiting 4e–/4H+ Oxygen Evolution Reaction (OER) from water, requiring competent management of proton-coupled electron transfer (PCET) steps to selectively funnel photogenerated holes into multi-electron WOC activation at low overpotentials.[1,2]
The essential structure of DSPEC photoanodes is composed of a semiconductor, often a nanostructured n-type semiconducting metal oxide (SCO) porous film, interfaced with a transparent conducting oxide (TCO) and integrating a WOC. The SCO is a high-band gap semiconductor (typically SnO2 or TiO2), incorporating a molecular light harvesting unit (i.e., a dye) that extends its light absorption by direct or indirect excited state sensitization.[2,3]
We studied photoactive hybrid dyads composed by organic dyes and Co3O4 nanoparticles as WOC, with the goal of applying them on meso-SnO2 photoanodes. The dyes belong to the unique class of KuQuinones (KuQ), polyquinoid chromophores characterized by a wide absorption in the visible and a highly oxidizing excited state (E1*KuQ/KuQ•– > 2 V vs RHE), able to manage PCET events.[4–6] KuQ was bound through a phosphonate anchor to Co3O4heptOH, 3 nm spherical particles stabilized by a 1-heptanol shell.[7] Co3O4heptOH particles, synthesized via a finely controllable organometallic approach, have been reported as a rare metal-free WOC in electro- and photocatalytic systems.[8,9] The ability of the dyads to evolve O2 under visible light irradiation was associated with fast singlet excited state (1*KuQ) emission quenching via electron transfer to Co3O4, owing to the highly oxidizing character of 1*KuQ and to the direct chemical bond between the dye and the WOC. The hybrid dyads were then studied under visible light irradiation upon deposition on meso-SnO2 films. The photocurrent response was associated to selective O2 evolution through generator-collector chronoamperometric experiments. Indeed, the hybrid nanomaterials have been proven competent in photoelectrochemical OER, with Faradaic efficiency (FEO2) close to 90%. As a comparison, “unbound” photoanodes based on KuQ-sensitized SnO2 and Co3O4heptOH, lacking a direct dye-WOC interaction, displayed similar photocurrent densities, albeit with a poorly reproducible and lower (30–50%) FEO2. Furthermore, the photoaction spectrum, reaching 0.44% incident photon-to-current conversion efficiency (IPCE) at 510 nm, confirmed the role of KuQ as light-harvesting molecular component.
Finally, ex-situ resonance Raman and X-ray Absorption Spectroscopy (XAS) experiments allowed to map the evolution of the hybrid dyads under photoelectrochemical stress. While an expected overall oxidation state increase of the Co3O4 catalytic units was observed, indicating CoIIIOOH as steady-state intermediate in the OER, overall chemical stability of the dyads was also assessed. Remarkably, KuQ was proven unaffected with respect to phosphonate bond hydrolysis and oxidative damage to the chromophore core.
These findings support the applicability of the novel dyads to DS-PECs and reinforce the superior approach of managing the directionality of the electron transfer chain by means of chemical bonds as a key design principle for Artificial Photosynthesis.
Session-T2
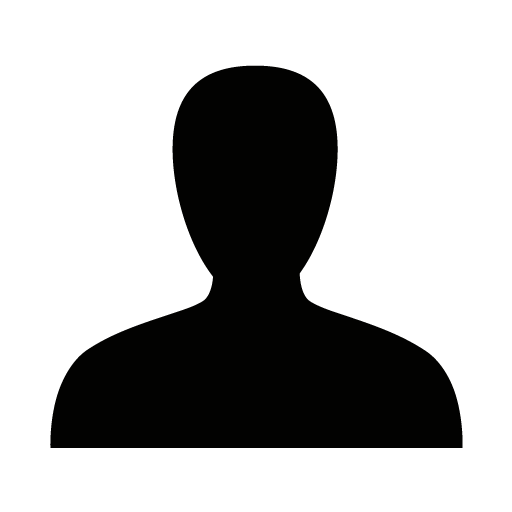
The production of benign, storable, and transportable hydrogen fuel from clean and renewable sources of energy is a stimulating goal.[1,2] In this context, the scientific community draws inspiration from nature to devise green production of hydrogen as an alternative energy carrier by the design of artificial photosynthetic systems.[3]
Artificial photosynthesis (AP) aims to achieve the light-induced water splitting reaction (2H2O → O2 + 2H2O). This reaction can be separated in two half reactions: the hydrogen evolution reaction, HER (4H+ + 4e- → 2H2), for which protons and electrons are supplied by the oxygen evolution reaction, OER (2H2O → O2 + 4H+ + 4e-). For that, thermodynamically (E0O2/H2O = 1.23 V vs RHE) and kinetically demanding water oxidation calls for effective water oxidation catalysts (WOCs), able to overcome the activation barrier of water oxidation reaction to dioxygen.[4,5,6]
The best WOCs reported today are made of Ru and Ir. However, it is highly desirable to use more earth-abundant transition metals such as Mn, Fe, Co, Ni or Cu, whether in form of metal complexes (MC) or metal-oxide nanoparticles (MO-NPs). The latter are generally more stable electrocatalysts but are more challenging to study through mechanistic investigation protocols and pose greater demands when seeking custom optimization. On the other hand, the mechanisms in molecular catalysts are easier to study and understand, resulting in routine optimization via ligand modification. However, first-row transition MCs in OER are still a challenge because they often get deactivated by the hydrolytic behaviour and by the oxidation of the organic ligands.[7]
In this project, we design an anodic catalytic layer to be incorporated in a water splitting electrochemical cell based on a hybrid thin film of SiO2 containing WOC units based on first-row transition metal catalysts, whether in form of MO-NPs or MCs. This approach aims to achieve i) protection against electrodegradation, and ii) the covalent attachment of an active WOC capable of sustain operational current at low overpotentials, ensuring iii) high conductivity.[8] Herein, we present two candidates to achieve optimal performance activity, a family of MO-NPs and a MC based on a Cu macrocyclic unit (Cu-MAC) known to be an efficient WOC at different values of pH.[9] The MC catalytic center has been modified with silylated groups to be incorporated into stabilizing SiO2 matrixes (Cu-MACSiOx).
S.2.1.-I1
I am working at the Institute of Advanced Materials of the University Jaume I. My research is focused in the development of advanced hybrid materials for energy conversion and storage based on catalytic transformations. The hybrid materials are developed from well-defined organometallic complexes. The approach for such applications is divided on three different research lines: i) Organometallic chemistry: design, characterisation and properties of new catalysts ii) Catalytic applications in processes related to hydrogenation and dehydrogenation. iii) New materials: study of the properties and applications of organometallic compounds and metal nanoparticles supported in graphene derivatives for energy conversion and storage. Research Lines:
Ad. Mat. for catalysis
Rationale design of catalytic materials derived from organometallic complexes.
Development of stable metal nanoparticles as improved catalytic systems.
Ligand design for the immobilization of metal complexes and nanoparticles.
Fundamental and applied study of catalytic hydrogenation and dehydrogenation processes.
Ad. Mat. for energy storage
Development of systems for the storage of hydrogen in the liquid form using “Liquid Organic Hydrogen Carriers (LOHCs).
Development of Hydrogen Storage technologies for transport and uses of hydrogen.
Industrial Innovation and Technology Transfer
The group is involved in industrial projects on Hydrogen Storage, depolymerization processes and catalyst development in connection with a regional funding program (AVI).
Green hydrogen is a promising energy vector for many reasons but still there are two limitations that should be addressed for large implementation. These two main limitations are affordable production of green hydrogen and practical storage. In this talk, we will focus on hydrogen storage and in particular in the storage in the liquid form at room temperature. Green hydrogen can be store using different technologies but among them, the storage forming chemical bonds represents an unexplored technology that will have an important impact in handling, management and transporting hydrogen. Our aim is to develop efficient systems for hydrogen storage using Liquid Organic Hydrogen Carriers (LOHCs) [1].
The key point of efficient LOHCs lies in the design of suitable catalysts and processes for the conversion and reconversion of hydrogen into chemical bonds. In this work, we describe the state of the art in the field and our last results in for hydrogen storage in the form LOHCs.[2] [3] [4]
S.2.1.-I2
Prof. Laurent Billon gained his PhD in 1996 from the Université de Pau et des Pays de l’Adour, France (UPPA) and his habilitation in 2005. He is a Professor of exceptional class and was deputy director of the Institute of Analytical Sciences and Physico-Chemistry for Environment and Materials IPREM (CNRS UMR 5254) from 2028 to 2022. He is now leader of the Bio-inspired Materials Group at the UPPA.
Prof. Billon is the coordinator and principal investigator of a large series of national and international research projects such as the coordinator of the H2020 ITN EJD eSCALED Project (european SChool on Artificial Leaf: Electrodes & Devices) with 10 partners from 8 European countries. He has authored 155 scientific publications in highly impact journals, 4 book chapters & 1 book editor, 35 invited lectures at international conferences and 20 patents. From 2016 to 2022, he was invited Professor at the University Immersion Program of Sichuan University (Chengdu, China).
His research activities cover 1) Synthesis of macromolecular designs by controlled radical polymerizations (NMP, RAFT and ATRP) or "click" chemistry; 2) Synthesis of organic colloids in a dispersed medium; 3) Surface chemistry and functionalization; 4) Development of macromolecular systems 5) Stimuli-triggered self-assembly in aqueous solution and self-assembly in bulk and thin film directed by the process for hierarchically structured materials; 6) Multi-scale characterization: from the local scale to the macroscopic scale, through the mesoscopic scale and the (sub) micrometric scale and 7) Biomimicry and bio-inspired materials/processes. The main applications of his research are dedicated to bio-inspired materials for cosmetic/healthcare (Christian DIOR, L’OREAL, CHANEL, Pierre FABRE, Yves ROCHER, SEPPIC) and since many years to energy solutions (CO2 reduction and green H2 evolution)
Encapsulation of molecular catalysts inside polymeric scaffolds has gained substantial attention over the recent years, as it provides a path towards generating systems which mimic the metallo-enzyme morphology as “synthetic hydrogenases”. By conjugating the “active sites” within macromolecular scaffolds, i.e. polymers, an efficient second sphere of coordination with enhanced stability and enzyme-like morphologies and properties is then created. In the context of solar fuels research and chemical energy conversion, this approach has been found to improve both rates and energy efficiency of the catalysis. As proof of concept, we have focused on the encapsulation of a biomimetic azadithiolate (–CH2NHCH2S–, adt2–) bridged {Fe2(μ-S2)(CO)6} based active site (“[2Fe2S]”), inspired by the catalytic cofactor of [FeFe] hydrogenases, within a synthetic pH-sensitive polymeric scaffold bearing hydrophobic stacking group for heterogenization onto Carbon NanoTubes. Such metallo-enzymes were found to be active for electrochemical H2production in neutral aqueous media.[1], [2], [3]
This concept was also extended to the encapsulation of molecular catalyst for CO2 reduction[4],[5],[6] and more recently with water oxidation catalysts. A similar approach with the aim to modify the sphere of coordination of metallic benchmarked electrode demonstrated the impact of a thin polymeric layer on the CO2 reduction efficiency and selectivity.[7], [8]
However, system performance still needs to be improved to reach technologically relevant currents and stability, parameters that are heavily influenced by the nature of the incorporated molecular catalyst or the design of the electrode.
[1] A. Zamader, B. Reuillard, P. Marcasuzaa, A. Bousquet, L. Billon, J. Espi Gallart, G. Berggren, V. Artero ACS Catalysis, 2023, 13, 1246–1256.
[2] A. Zamader, B. Reuillard, J. Pécaut, L. Billon, A. Bousquet, G. Berggren, V. Artero Chem. Eur. J. 2022, e202202260.
[3] A. Zamader, B. Reuillard, L. Billon, G. Berggren, V. Artero Sustainable Energy & Fuels, 2023, 7, 4967-4976.
[4] D. Grammatico, H.N. Tran, Y. Li, S. Pugliese, L. Billon, B-LSu, M. Fontecave ChemSusChem, 2020, 13, 6418-6425.
[5] D. Grammatico, P. Marcasuzaa A. Viterisi, A. Bousquet, B-L. Su, L. Billon ChemComm, 2023, 59, 2279 – 2282.
[6] D. Grammatico, A.J. Bagnall, L. Riccardi, M. Fontecave, B.L. Su, L. Billon, Angewandte Chemie, 2022, 61, e2022063.
[7] A. Fortunati, S. Hernandez, A. Viterisi, P. Marcasuzaa, L. billon European Patent, 2023, EP23306210.8.
[8] F. Vieira, P. Marcasuzaa, L. Billon, A. Viterisi, E. Palomares to be submitted asap, 2024.
S.2.1.-O1
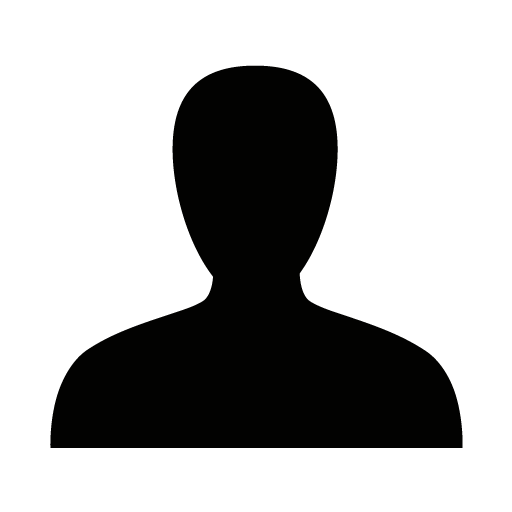
Introduction
Hydrogen is expected to play a key role in a future sustainable energy system. Essential for the use of hydrogen as an energy carrier is the development of the technologies used for its storage and transportation. In this respect, liquid organic hydrogen carriers (LOHC) represent a very promising alternative [1,2]. LOHC technology enables high H2 storage densities in a liquid at ambient conditions [3]. In order to make this process truly cyclic and sustainable, a high selectivity of the catalytic dehydrogenation reaction and a high catalyst stability is necessary. This requires further research, to understand in more detail the processes taking place in the interphase between the solid catalyst and the liquid LOHC.
Materials and Methods
Here we are studying the dehydrogenation reaction of perhydro benzyl-toluene with model Pt catalysts. For understanding the processes contributing to the catalyst degradation at the atomic level, we use high energy X-Ray diffraction (HEXRD) and X-Ray absorption spectroscopy (XAS) in operando mode under realistic conditions (300 °C, 1-5 bar).
We use Pt catalysts synthetized by PVD over single crystal Al2O3 support, that allows us to follow the evolution of the Pt nanoparticles with grazing incidence wide angle X-Ray scattering (WAXS) and small angle X-Ray scattering (SAXS). While we use model catalysts as a simplified system for X-Ray measurements, we replicate the real chemical environment in a large reactor filled with commercial Pt/Al2O3 egg-shell catalyst (Fig.1). The measurements are performed at the ID31 beamline of the ESRF.
Results and Discussion
In this presentation we will report on the design of a semi-industrial reactor adapted for both model and commercial catalysts suitable for operando HEXRD studies at large scale X-ray facilities. We will also discuss the representativeness of model catalysts for these studies, and the importance of the chemical potential and the overall physical conditions in the reactor.
Furthermore, we will present the results from our operando HEXRD measurements, where we can follow the changes of the nanoparticles morphology and, through the lattice parameter change, also the chemical state of the catalyst. In these results, we are able to compare the influence of different sample treatments, such as the selective poisoning of Pt with S, as well as the different results obtained at various positions in the catalytic bed.
We will also report the design of a model cell adapted to study the reaction at small scale using XAS, and show the first results acquired with this technique.
The X-Ray studies have been also complemented with ex-situ characterization (XPS, AFM) to understand the full picture of the processes both structurally and chemically.
Significance
Further research of the dehydrogenation reaction in LOHC technology is a prerequisite for the development of better and fully recyclable catalysts, making this technology more competitive.
S.2.1.-O2
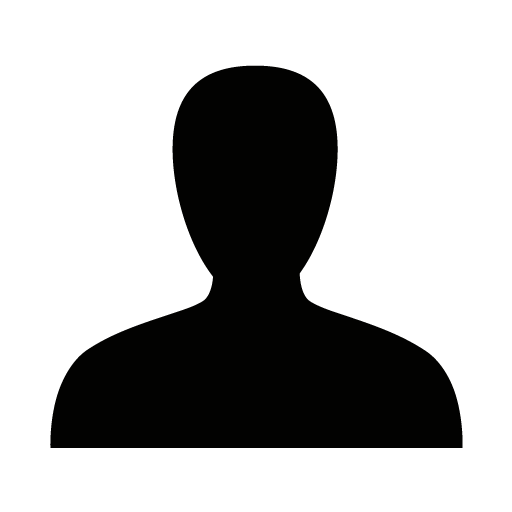
Water splitting stands out as a highly promising avenue for powering our planet without the risk of environmental pollution. Despite its potential, the process faces a thermodynamic uphill battle. Researchers are actively addressing this challenge to enhance energy efficiency by focusing on the development of catalysts that can efficiently drive the hydrogen evolution reaction (HER) and oxygen evolution reactions (OER) [1].
In this regard, 2D layered materials such as transition metal phosphorus trichalcogenides (MPX3; X=S, Se) have recently got copious attention. Their remarkable properties, including atomic-scale thickness, a direct band gap, cost-effective synthesis, and exceptional electronic and mechanical properties, position them as promising candidates for diverse fundamental studies such as electrocatalysis, photocatalysis and hydrogen storage [2].
In this presentation, we will discuss two impactful strategies aimed at boosting the efficiency of our innovative catalyst: i) Confinement and ii) controllable selenium enrichment. Confinement ensures selectivity and stability by averting surface deterioration and particle aggregation of the catalyst [3]. Furthermore, incorporating additional active sites within the 2D layer of the catalyst can favour the overall water splitting mechanism, amplifying the system's performance. Then, controllable Se enrichment on the surface of a catalyst enhances the charge transfer process during HER and optimize the catalyst surface to become thermodynamically/kinetically favourable to produce H2 gas [4].
In this comprehensive exploration, we delve into the development of a versatile array of Mo-confined Se-enriched manganese-based 2D structures. From MnPSe3 to Ex-MnPSe3 (exfoliated MnPSe3), Mo-Se-MnPS3 (Mo-confined Se-enriched MnPS3), and Mo-MnPS3 (Mo confined MnPSe3) our research unfolds the potential of these structures for applications in the field of energy conversion. Extensive chemical-physical and optical characterisation was carried out. SEM and HR-TEM, XRD, EDX, Raman, and Synchrotron based XPS analyses provide a detailed understanding of these innovative structures.
Beyond characterization, our research highlights the exceptional electrocatalytic properties of these materials in the hydrogen evolution reaction (HER) via electrochemical water splitting, as evidenced by their high electroactive surface area, low Tafel slope and impressive performance in linear sweep voltammetry (LSV) measurements. This samples also exhibit robust results in stability test performed by cyclic voltammetry.
These results allowed us to state that by using confined catalysis it is possible to increase the performance and stability of a system by increasing its current delivery and the resulting production of green hydrogen. Moreover, the controllable Se-enrichment enhances the efficient conversion of H+ into H2, offering an exciting avenue for energy conversion.
This study significantly contributes to advancing the understanding of MPX3 nanomaterials and confined catalysis, offering transformative insights for future applications in the field of energy conversion and green hydrogen production.
S.3.1.-I1
In the contemporary era, the interlinked challenges of energy sustainability and environmental stewardship are of paramount importance. The quest for innovative materials capable of catalyzing a transition towards sustainable energy practices is at the heart of this challenge. The development of such materials is not merely an academic endeavor but a necessity for enabling the technological advancements required for a future where energy is both sustainable and abundantly available. This drive stems from an imperative need to not only generate and utilize energy more efficiently but also to ensure its storage and secure its supply in a manner that aligns with environmental conservation principles. Given that the efficiency and cost-effectiveness of energy conversion and storage solutions are predominantly constrained by the materials employed, the pursuit of novel functional materials for the ensuing generation of energy technologies is urgent.
Our research endeavors have illuminated the potential of manipulating the accessible surface area of electrocatalysts via structural and compositional adjustments to bolster their activity and refine their selectivity towards the production of desired chemical products. Remarkably, through meticulous morphological optimization of electrocatalysts, we have achieved a Faradaic efficiency exceeding 95% for formic acid production.[1] Furthermore, our studies have heralded the successful synthesis of C4 products utilizing copper catalysts, marking a significant milestone in electrocatalytic research. [2] Our investigations are geared towards surmounting prevalent challenges in catalyst selectivity, stability, and activity by engineering active sites and fabricating smart, multifunctional nanomaterials. These materials are distinguished by their unique physical and chemical attributes, which significantly amplify catalytic efficacy. A cornerstone of our approach is to elucidate the mechanisms underpinning key energy conversion reactions, thereby informing the design of more potent catalysts. Presently, our research is concentrated on the synthesis of biomass-derived carbon materials and MXene-based hybrids, targeting their application in water splitting and CO2 reduction processes. We have innovatively integrated polyoxometalates (POMs) with MXenes, employing them as electrocatalysts. For hydrogen generation, Cobalt and Tungsten-based POMs were incorporated, demonstrating low overpotential, particularly with Cobalt-based POMs. In electrochemical CO2 reduction, the integration of Copper-based POMs led to the successful production of C2 products.
This research trajectory not only contributes to the advancement of material science but also holds the promise of propelling us towards a more sustainable and environmentally harmonious energy landscape.
S.3.1.-I2
Our group focus on physical chemistry, materials science, and the application of materials for energy production, studying the synthesis-structure-property relationship of functional materials for energy production. We emphasize developing novel syntheses for advanced materials and devices for solar energy into useful forms of sustainable energy & fuels. Our research lies at the intersection between innovative approaches, fundamental studies, and applying advanced materials for solar energy conversion.
Unique insights into the synthesis-structure-properties dependencies of α-SnWO4 photoanodes will be presented, enabled by synthesis conditions far from thermodynamic equilibrium (i.e., non-equilibrium, NE) based on plasma deposition processes combined with rapid thermal processing (RTP).[1]
Recently, we have shown that a highly controlled NE synthesis approach based on pulsed laser deposition (PLD) combined with RTP had significant effects on the crystallinity, morphology, crystallographic orientation, and sulfite oxidation performances of α-SnWO4, culminating in photocurrents ~ 70 % higher than PLD-grown photoanodes annealed via conventional furnaces (furnace heating, FH).[2] In a follow-up study of the structural and electronic properties of α-SnWO4 films annealed via RTP and FH, we utilized X-ray diffraction texture analysis, electron backscatter diffraction in scanning electron microscopy, and atomic force microscopy modes of Kelvin probe, electrical conductivity, and tapping mode for surface morphology.
Our results show that the local (micrometer-scale) crystallographic orientation is very homogenous in the RTP-treated films, both in-plane and perpendicular to the substrate. Considering their reported higher crystallinity and the anisotropic charge transport nature of orthorhombic α-SnWO4,[2–4] the RTP-treated films demonstrate significant changes in contact potential difference (CPD) and conductivity. The CPD between α-SnWO4 and platinum-coated silicon tip was ~ 0.35 eV lower than the CPD of the FH-treated films, indicating increased band bending. This suggests that the RTP treatment strongly contributes to an increased driving force for charge injection. In addition, the local conductivity of the RTP-treated films was higher by more than two orders of magnitude than that of the FH-treated films.
Our results can lead toward broader tunable materials synthesis and design pathways and more scalable discovery and development of new chemical spaces of multinary and multifunctional metal-oxide photoelectrodes inaccessible through conventional solid-state reactions.[5]
S.3.2.-O1
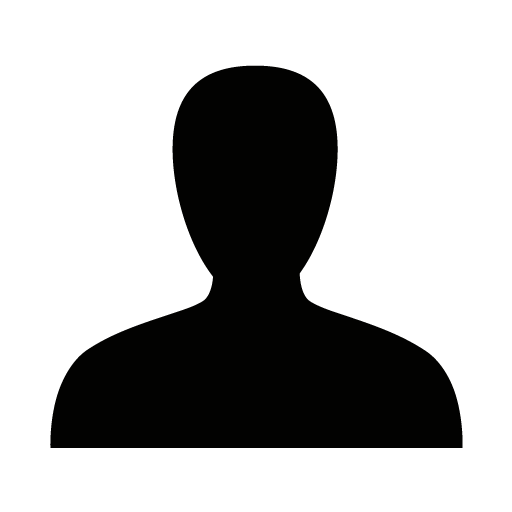
In the quest for effective and sustainable photoelectrochemical devices, BiVO4 has emerged as a promising candidate for use as a photoanode material, owing to its favorable band structure for facilitating water oxidation. Addressing challenges such as poor charge transport and sluggish kinetics inherent in BiVO4 photoanodes, SnO2 films have conventionally been utilized as electron transport layers due to their capability to block holes. However, this approach has faced obstacles, including the presence of high defect concentrations at the SnO2/BiVO4 interface and the occurrence of pinholes in SnO2 layers, which can result in charge recombination. To tackle these limitations and bolster the efficacy of SnO2 as a hole-blocking layer, we investigated the impact of ZrCl4 treatment on BiVO4 photoanodes. Through comprehensive characterizations and performance assessments, our findings reveal that ZrCl4 treatment substantially enhances the hole-blocking properties of SnO2, thereby leading to a significant improvement in the overall efficiency of the photoanode. Notably, the implementation of the ZrCl4 treatment method resulted in a 37% increase in photocurrent density (measured at 1.23 V vs. the reversible hydrogen electrode under 1 sun illumination) and a 40 mV shift in the onset voltage.
S.3.2.-O2
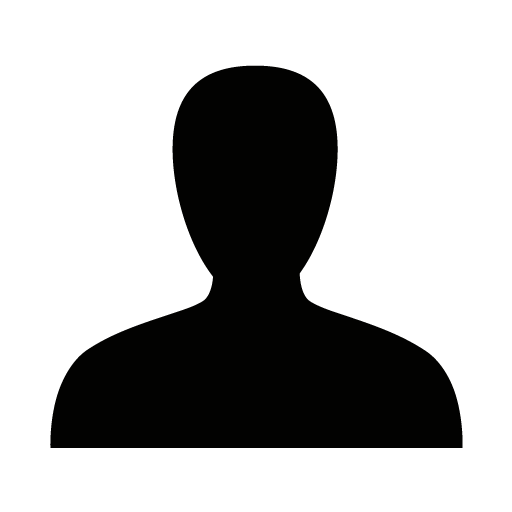
Nanostructured WO3 is a widely studied, attractive material for photocatalysis applications, ranging from degradation of organic contaminants to water photooxidation, related to its relatively small bandgap, good stability, and adequate charge transport and charge transfer efficiencies [1]. It should be considered that the performance of the photoelectrode depends on the morphology of the nanostructured material [2]. In catalysis, a large surface-to-volume ratio generally improves activity; however, this is not always the case for photoelectrochemical systems. In this work, we compare the photocatalytic and photoelectrochemical performance of commercial WO3 nanoparticles with that of WO3 nanofibers prepared by centrifugal spinning [3]. To elucidate the complex charge carriers dynamics, intensity-modulated photocurrent spectroscopy (IMPS) is used. The photocatalytic dye degradation kinetics are faster for the nanofibers, due to their larger specific surface area related to the smaller size of the particles that constitute the fibers. On the other hand, the photoelectrochemical performance is essentially the same for both materials with one loop in the 4th quadrant of the IMPS spectra. This fundamental difference in performance is demonstrated to be due to the influence of trap-limited electron transport [4] on the collection efficiency of photoelectrons, rather than hole transfer to the solution, in the interconnected and nanostructured films, required for the observation of photocurrent in the external circuit, whereas photocatalysis is more surface-driven. These results illustrate the intricacies of photocatalytic processes and indicate that the nanomaterial morphology needs to be optimized for each specific application, keeping in mind the charge carrier dynamics that govern performance [5].
S.3.2.-O3
I am a University Lecturer (Assistant Professor) in Energy Materials in the Institute for Materials Discovery, the faculty of Mathematical and Physical Sciences at the University College London (UCL). I graduated from Sharif University of Technology in 2012 with a BSc (Materials Science and Engineering) and I received my MSc in Materials Science and Engineering in 2014 from École Polytechnique Fédérale de Lausanne (EPFL) in Switzerland. I completed my PhD in Physics at Cavendish Laboratory, University of Cambridge receiving the 2018 Semiconductor Physics Thesis Prize from Institute of Physics. From 2018-2020, I was a Junior Research Fellow at Cambridge University and Wolfson College, Cambridge, where I set up a spin-out company to develop energy harvesting devices based on emerging semiconductors. I established my research group in UCL in 2020, with a focus on material and electronic properties of emerging semiconductors such as halide perovskites and organic semiconductors for low-cost electronics applications including solar-photovoltaics and lighting. My research aims to develop and incorporate new inexpensive materials in optoelectronic devices to alter the energy landscape by reducing the cost of both energy production and consumption.
You can find my publications, media activities and details of ongoing projects on my University webpage (https://iris.ucl.ac.uk/iris/browse/profile?upi=ABDIJ40).
The production of green hydrogen through visible-light-driven water splitting represents an appealing avenue for sustainable and environmentally friendly hydrogen generation[1]. However, the efficiency of photoelectrocatalysis in solar-to-hydrogen conversion is constrained by the limited light absorption of most available semiconductor materials, which typically have wide bandgaps, restricting them to the UV range[2]. Consequently, there is a need to develop catalysts capable of absorbing visible light. In this context, we propose a strategy to enhance the photoelectrocatalytic activity of CeO2 by doping it with transition metals such as Ni and Co. This doping extends the material's visible light absorption and introduces d-states within the forbidden bandgap. Verification of bandgap narrowing and extended visible light absorption was conducted through UV-vis diffuse reflectance, revealing that Ni and Co doping reduced the bandgap of CeO2 from 3.0 eV to 2.7 eV and 2.6 eV, respectively. Density functional theory (DFT) calculations supported these findings, confirming bandgap narrowing and the presence of d-states. Moreover, photoelectrochemical measurements demonstrated superior photoelectrocatalytic activity in the Ni-doped sample compared to Co-doped CeO2. This enhanced catalytic performance is attributed to the introduction of d-states near the Fermi level through Ni doping, while Co doping introduced d-states located near the conduction band of CeO2. Our surface Slab calculations further revealed that Ni-doped CeO2 reduced the Gibbs energy for oxygen evolution reaction (OER) intermediate adsorption compared to pure and Co-doped CeO2. This suggests that doping plays a pivotal role in modulating the electronic environment of the catalyst, facilitating charge transfer through defect d-states near the Fermi level and accelerating reaction kinetics. In summary, our study underscores that doping CeO2 with transition metals like Ni and Co can enhance its photoelectrocatalytic activity for green hydrogen production. Ni doping, in particular, leads to the creation of d-states near the Fermi level, resulting in improved catalytic performance. These findings contribute significantly to the development of efficient catalysts for visible-light-driven water splitting and pave the way for sustainable hydrogen production.
S.3.2.-O4
Nilesh Manwar obtained his BSc and MSc degrees from Sant Gadge Baba Amravati University, India, in 2009 and 2011 respectively. In 2012, he joined the CSIR-National Environmental Engineering Research Institute, Nagpur. After submission his PhD in 2016, he continued the postdoctoral research at Indian Institute of Petroleum, Dehradun (2017-2020), Indian Association for the Cultivation of Science, Kolkata (2020-2021), and the Institute of Chemical Technology, Mumbai (2021-2022). Presently, he has joined the Institute of Physical Chemistry of the Polish Academy of Sciences as an assistant professor under the PASIFIC Programme.
The demand for clean and sustainable energy resources, such as hydrogen and CO2-to-solar fuels, is intensifying to decarbonize future global energy demands. This trend has sparked interest in utilizing renewable hydrogen production methods. Among various approaches, biomass photo-reforming using carbon nitride (CN)-based materials has emerged as a promising avenue due to its potential for efficient and environmentally friendly hydrogen generation.[1] The present study investigates the potential application of CN-based materials as catalysts for biomass photo-reforming to hydrogen, aiming to elucidate their catalytic performance, mechanism, and practical implementation.
Carbon nitrides possess essential properties such as low cost, chemical stability, and tuneable electronic structure, along with N-rich active sites, making them attractive catalysts for solar-driven hydrogen production. Harnessing the unique properties of two-dimensional carbon nitride, researchers have developed innovative approaches to enhance the efficiency and selectivity of the photo-reforming process.[2], [3] Surface modification strategies including heteroatom doping and hybridization with other materials have been employed to tailor the catalytic activity and enhance the stability of CN-based materials for biomass conversion.
Experimental details have demonstrated the effectiveness of CN-based materials in promoting the photoreforming of various biomass aqueous solutions, including sugars, alcohols, and organic acids, into hydrogen gas. By elucidating the underlying mechanisms and kinetics of the photo-reforming process, researchers have gained insights into CN-based catalysts' role in facilitating hydrogen generation from biomass-derived precursors.
Efforts have also been directed toward optimizing the operating conditions and reactor configurations to maximize the hydrogen production yield while minimizing energy consumption and environmental impact. Integrating CN-based photocatalysts into advanced reactor designs, such as photo-flow-reactor systems, holds promise for scalable and sustainable hydrogen production from biomass aqueous solutions.
In conclusion, using CN-based materials for photo-reforming biomass aqueous solutions into hydrogen represents a promising approach toward achieving carbon-neutral energy conversion. Continued research and development in this field are essential to further enhance the efficiency, stability, and practical viability of CN-based photocatalytic systems for biomass-to-hydrogen conversion, contributing to the transition towards a clean and sustainable energy future.
Keywords: Carbon nitride, Biomass, Photoreforming, Hydrogen Production, Renewable Energy