Download here the latest version of the conference program. (Updated: 10 January. 2025)
Kihada-K1
Maksym Kovalenko has been a tenure-track Assistant Professor of Inorganic Chemistry at ETH Zurich since July 2011 and Associate professor from January 2017. His group is also partially hosted by EMPA (Swiss Federal Laboratories for Materials Science and Technology) to support his highly interdisciplinary research program. He completed graduate studies at Johannes Kepler University Linz (Austria, 2004-2007, with Prof. Wolfgang Heiss), followed by postdoctoral training at the University of Chicago (USA, 2008-2011, with Prof. Dmitri Talapin). His present scientific focus is on the development of new synthesis methods for inorganic nanomaterials, their surface chemistry engineering, and assembly into macroscopically large solids. His ultimate, practical goal is to provide novel inorganic materials for optoelectronics, rechargeable Li-ion batteries, post-Li-battery materials, and catalysis. He is the recipient of an ERC Consolidator Grant 2018, ERC Starting Grant 2012, Ruzicka Preis 2013 and Werner Prize 2016. He is also a Highly Cited Researcher 2018 (by Clarivate Analytics).
This lecture will span the discovery of colloidal lead halide perovskite nanocrystals (LHP NCs), as well as our latest work on their synthesis, self-organization, and optical properties, including unpublished work. LHP NCs are of broad interest as classical light sources (LED/LCD displays) and as quantum light sources (quantum sensing and imaging, quantum communication, optical quantum computing). The current development in LHP NC surface chemistry, using designer phospholipid capping ligands, allows for increased stability down to single particle level [1]. The brightness of such a quantum emitter is ultimately described by Fermi’s golden rule, where a radiative rate proportional to its oscillator strength (intrinsic emitter property) and the local density of photonic states (photonic engineering, i.e. cavity). With perovskite NCs, we present a record-low sub-100 ps radiative decay time for CsPb(Br/Cl)3, almost as short as the reported exciton coherence time, by the NC size increase to 30 nm [2]. The characteristic dependence of radiative rates on QD size, composition, and temperature suggests the formation of giant transition dipoles, as confirmed by effective-mass calculations for the case of the giant oscillator strength. Importantly, the fast radiative rate is achieved along with the single-photon emission despite the NC size being ten times larger than the exciton Bohr radius. When such bright and coherent QDs are assembled into superlattices, collective properties emerge, such as superradiant emission from the inter-NC coupling [3,4].
[1] V. Morad, A. Stelmakh, M. Svyrydenko, L.G. Feld, S.C. Boehme, M. Aebli, J. Affolter, C.J. Kaul, N.J. Schrenker, S. Bals, Y. Sahin, D.N. Dirin, I. Cherniukh, G. Raino, A. Baumketner, M.V. Kovalenko. Nature, 2024, 626, 542–548
[2] C. Zhu, S.C. Boehme, L.G. Feld, A. Moskalenko, D.N. Dirin, R.F. Mahrt, T. Stöferle, M.I. Bodnarchuk, A.L. Efros, P.C. Sercel, M.V. Kovalenko, G. Rainò. Nature, 2024, 626, 535–541
[3] I. Cherniukh, G. Rainò, T. Stöferle, M. Burian, A. Travesset, D. Naumenko, H. Amenitsch, R. Erni, R.F. Mahrt, M.I. Bodnarchuk & M.V. Kovalenko. Nature 2021, 593, 535–542
[4] T.V. Sekh, I. Cherniukh, E. Kobiyama, T.J. Sheehan, A. Manoli, C. Zhu, M. Athanasiou, M. Sergides, O. Ortikova, M.D. Rossell, F. Bertolotti, A. Guagliardi, N. Masciocchi, R. Erni, A. Othonos, G. Itskos, W.A. Tisdale, T. Stöferle, G. Rainò, M.I. Bodnarchuk, and M.V. Kovalenko. ACS Nano 2024, 8423–8436
Kihada-I1
Our research group focuses on the development of functional polymers and hybrid perovskites for use in various optoelectronic devices, including thin-film transistor (TFT), (photo-)memory, light-emitting diode (LED), and solar cell devices. We pay particular attention to the structure-property relationships of polymers and perovskites. In addition to advances in the controlled synthesis of these solution-processable semiconductors, we also explore innovative interface and device engineering to optimize device performance (https://chuehslab2016.wixsite.com/ccchueh). In this presentation, we will share our recent works in exploring the potential of pseudohalide-based 2D perovskites and Sn-based 2D perovskites for optoelectronic applications, especially transistor memory. We will first discuss a comprehensive study that combines composition tuning, process engineering, and morphological analyses to explore the promise of these 2D perovskites will be first discussed, and then discuss their device applications and optimization. Finally, we will specifically showcase the potential of 2D Sn-based perovskites in memory and synaptic applications.
Kihada-I1
Prof. Qing Shen received her Bachelor’s degree in physics from Nanjing University of China in 1987 and earned her Ph.D. degree from the University of Tokyo in 1995. In 1996, she joined the University of Electro-Communications, Japan and became a full professor in 2016. In 1997, she got the Young Scientist Award of the Japan Society of Applied Physics. In 2003, she got the Best Paper Award of the Japan Society of Thermophysical Properties and the Young Scientist Award of the Symposium on Ultrasonic Electronics of Japan. In 2014, she got the Excellent Women Scientist Award of the Japan Society of Applied Physics. She has published nearly 140 peer-reviewed journal papers and book chapters. Her current research interests focus on solution processed nano-materials and nanostructures, semiconductor quantum dot solar cells and perovskite solar cells, and especially the photoexcited carrier dynamics (hot carrier cooling, multiple exciton generation, charge transfer at the interface) in perovskite solar cells, quantum dot and dye sensitized solar cells, organic-inorganic hybrid solar cells.
Colloidal quantum dots (QDs) have gained significant attention for both fundamental research and applications of optoelectronic devices owing to their appealing optoelectronic properties and excellent chemical processability. For their wide range of potential applications, synthesizing colloidal QDs with high crystal quality and stability is of crucial importance. One key is how to reduce the surface defects of QDs which give rise to detrimental non-radiative recombination centers and thus quenching luminescence. In recent years, we have suceeded in synthesis of less defect QDs such as PbS QDs and preovksite QDs including APbX3 QDs, Sn-Pb alloyed QDs and Sn-based QDs[1-10]. On the other hand, QD solar cell has attracted much interest as a next-generation solar cell because of its low cost and potential to surpass the Shockley–Queisser limit. However, the current power conversion efficiency (PCE) of QD solar cells remains significantly below its theoretical limit, highlighting the need for fundamental research. The main challenges in improving the PCE include inefficient charge transport and extraction, as well as high non-radiative recombination at the device interfaces. In this talk, recent breakthroughs in enhancing QD solar cell PCE through interface engineering and charge carrier management will be presented.
Kihada-I2
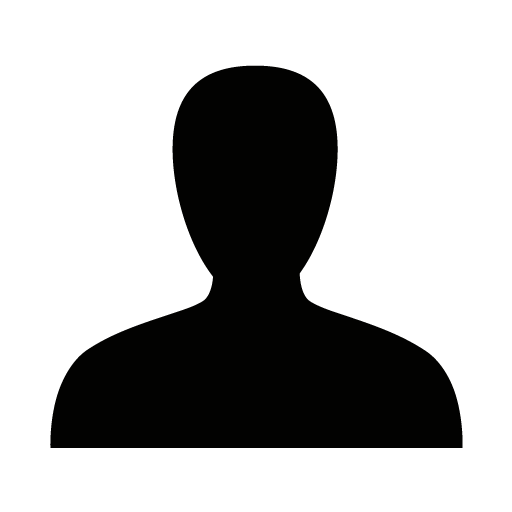
Halide perovskites hold immense potential for the future of optoelectronics. Although there is still much to uncover and harness in terms of their capabilities, it's evident that perovskites can outperform established semiconductors in many applications, offering similar performance at a fraction of the production cost. Over the past decade, photovoltaics have emerged as the most appealing application, with perovskites displaying remarkable adaptability to work alongside silicon as the most efficient single-junction for both outdoor and indoor applications. Undoubtedly, perovskites will play a pivotal role in the future of photovoltaics, prompting considerations about the environmental and health impact of large-scale production. The mainstream perovskite for photovoltaics contains lead, a highly toxic and water-soluble element, posing significant environmental and health risks. Addressing the toxicity risk is crucial, and proposing in situ quenching of the lead in case of water ingress in the photovoltaic module can mitigate this issue. This solution avoids the risk of dispersing lead into the environment, but challenges remain, including disposal or recycling. The ultimate solution lies in replacing lead with a non-toxic element like tin.
Tin-based perovskites have the potential to outperform lead-based perovskites for solar cells, albeit being more sensitive to external degradation factors linked to oxygen and water. I will present the most recent progress in enhancing the stability of tin-based perovskites for photovoltaics. Sn-based perovskites have achieved a record efficiency of 17%, compared to 26% for their lead counterparts. However, lead-based perovskites suffer from intrinsic instability issues due to the oxidation of iodide. In contrast, tin-based perovskites exhibit no inherent instability. Once isolated from water and oxygen, tin-based perovskites prove to be more stable than lead-based perovskites. With this in mind, it is imperative to focus on developing tin as a lead-free alternative for the future of perovskites.
Kihada-S1
Tokyo Chemical Industry (TCI) is a global supplier of laboratory chemicals and specialty materials, and also leading the next-generation solar technology by providing high-quality and reliable materials, including metal halide perovskite precursors and materials for carrier transporting.
Organic-Inorganic Perovskite Precursors
https://www.tcichemicals.com/assets/brochure-pdfs/Brochure_F2035_E.pdf
Perovskite Precursor for Solar Cell Purified Lead(II) Iodide
https://www.tcichemicals.com/assets/brochure-pdfs/Brochure_FF031_E.pdf
High Purity Perovskite Precursor Purified Lead(II) Bromide
https://www.tcichemicals.com/assets/brochure-pdfs/Brochure_FF088_E.pdf
Perovskite Precursor Lead Acetate Anhydrous
https://www.tcichemicals.com/assets/brochure-pdfs/Brochure_FF070_E.pdf
Perovskite Precursors Tin(II) Iodide, Tin(II) Bromide
https://www.tcichemicals.com/assets/brochure-pdfs/Brochure_FF075_E.pdf
n-Type SAM Forming Agent Enabling Efficient Perovskite Solar Cell: PANDI (New)
https://www.tcichemicals.com/assets/brochure-pdfs/Brochure_FF190_E.pdf
Hole Selective Self-Assembled Monolayer (SAM) Forming Agents: PACzs
https://www.tcichemicals.com/assets/brochure-pdfs/Brochure_FF092_E.pdf
SAM Formation Reagent with Face-on Orientation to Substrate Surface: 3PATAT-C3
https://www.tcichemicals.com/assets/brochure-pdfs/Brochure_FF169_E.pdf
Hole Transport Materials For Stable and Practical Perovskite Solar Cells: TOP-HTMs
https://www.tcichemicals.com/assets/brochure-pdfs/Brochure_FF090_E.pdf
High Quality Hole Transport Material: Spiro-OMeTAD
https://www.tcichemicals.com/assets/brochure-pdfs/Brochure_FF158_E.pdf
Dopants for Organic Electronics Research
https://www.tcichemicals.com/assets/brochure-pdfs/Brochure_FF194_E.pdf
Solar Cell Materials (PSC, OPV, DSSC Materials)
https://www.tcichemicals.com/assets/brochure-pdfs/Brochure_F2033_E.pdf
In this short talk, these highly useful materials will be introduced including most recently commercialized new products.
Kihada-I3
Hendrik (Henk) Bolink obtained his PhD in Materials Science at the University of Groningen in 1997 under the supervision of Prof. Hadziioannou. After that he worked at DSM as a materials scientist and project manager in the central research and new business development department, respectively. In 2001 he joined Philips, to lead the materials development activity of Philips´s PolyLED project.
Since 2003 he is at the Instituto de Ciencia Molecular (ICMol )of the University of Valencia where he initiated a research line on molecular opto-eletronic devices. His current research interests encompass: inorganic/organic hybrid materials such as transition metal complexes and perovskites and their integration in LEDs and solar cells.
The benefits of vacuum processed perovskite solar cells will be discussed focusing on thermal co-sublimation but also using close-space sublimation methods. The performance of these cells under accelerated stress conditions and under outdoor stressing will be presented. Adding co-sublimation additives to the perovskite leads to improvements in performance and power conversion efficiencies around 20 % for perovskites with a bandgap around 1.68 eV can be achieved. Benefits of passivation molecules on the photoluminescence quantum yield and open circuit voltage will be described.
I will also discuss the benefit of using these perovskites in substrate configuration as well as semitransparent cells for bifacial operation and for building integrated PV. The use of sublimed perovskite in perovskite-silicon tandem cells using fully textured Si bottom cells will be presented, including methods to identify the shortcomings of these tandem cells such as selective Sun-Voc measurements allowing to identify the open circuit voltage of the perovskite and silicon sub cells and selective external quantum efficiency measurements.
Kihada-I1
The growth of high quality perovskite film is critical for the realization of high performance devices. However, currently, the growth kinetics of perovskite film remains to be elusive. In this talk, I will present our recent work of controlling perovskite growth kinetics by controlling precursor supersaturation, surface energy regulation, coordination number optimization, and reagent variation etc. We developed a supersaturation controlled strategy to balance the nucleation and crystal growth speeds. By this strategy, we are able to find an ideal supersaturation region to realize a balance of nucleation and crystal growth, which yields highly crystallized perovskite films with micrometer-scale grains. A propeller‐shaped halogenated tertiary ammonium is synthesized, showing high binding energy on the perovskite surface and large steric hindrance. This molecule can significantly reduce the barrier of high surface energy that suppresses the growth of the α‐phase CsxFA1−xPbI3 structure. We explored formamidine acetate (FAAc) and ammonium iodide (NH4I) to replace the generally used formamidinium iodide (FAI) in the growth of a tin perovskite film. This triple reagent (FAAc + NH4I + SnI2) method prolongs the reaction path for the growth of the tin perovskite film. It impedes the growth of a three-dimensional (3D) perovskite structure at room temperature while having less impact on the growth of a low-dimensional structure. As a result, the low-dimensional structure formed at room temperature serves as a seed structure for 3D structure growth in subsequent annealing.
Kihada-S1
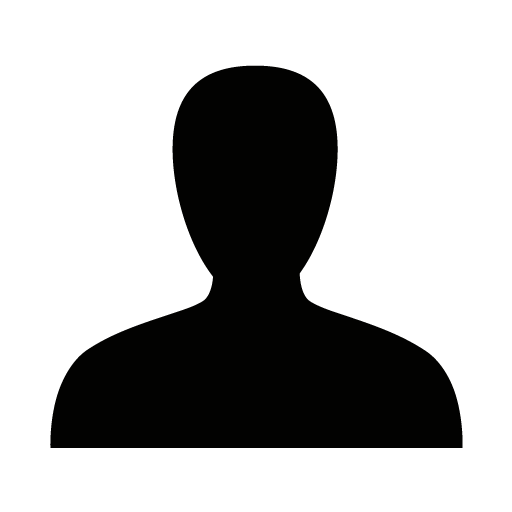
KOREA KIYON has more than 40 years of history to develop R&D and Production System in the fields of Chemical Test, Perovskite, Battery, Display and Nuclear. Our solutions help researchers to facilitate their research with both Dry Vacuum Chamber and Wet Process solutions which consist with Thermal Evaporator, Sputter, E-Beam, ALD, Customs Glovebox, Slot Die and Inkjet Printing System.
Kihada-I2
The application of solar cells is one of the most promising solutions for satisfying the ever-increasing global energy demand. Recently, organic-inorganic hybrid lead halide perovskite solar cells (PSCs) are receiving considerable attention and have emerged as an extremely promising photovoltaic technology due to their remarkable photovoltaic performance and potentially low production cost. To date, progress has been made on each layer, with major emphasis on perovskite film processing and relevant material design. Consequently, the power conversion efficiency of lead halide perovskite based thin-film photovoltaic devices has already surpassed 26 % [1].
Charge (both, hole and electron) transporting material (CTM) is one of the main factors of PSCs, which determines the cost, energy conversion effectiveness, and stability of the device. However, most CTMs have many disadvantages, including complicated and expensive synthesis, the tendency of self-aggregation, which degrade the quality of the charge transporting layer and reduce device stability and efficiency. Therefore, considerable and continuous efforts have been made to develop more ideal CTMs, which advantages should be as follows: matching well with the valence band and conduction band of the perovskite to enable hole or electron extraction and blocking, minimal absorption in the visible and near infrared regions, sufficient charge mobility, excellent thermal and photochemical stability, high processability, and low cost.
This lecture will cover results of our investigations in the field of molecular engineering of low molecular charge transporting materials for perovskite solar cells. Our group has been successful in creating several classes of novel organic hole and electron transporting materials, which are competitive both in the conventional and in the inverted PSCs. The molecularly engineered new charge transporting materials were synthesized via facile preparation procedures from commercially available and relatively inexpensive starting reagents, resulting in up to several fold cost reduction of the final product compared with well-known reference CTMs.
Kihada-IS1
State-of-the-art organic photovoltaics (OPVs) using π-conjugated polymers and small molecules as electron donors (D) and acceptors (A) exhibit power conversion efficiencies exceeding 19% with external quantum efficiency approaching 90%, and fill factors as high as 0.80. However, this success applies only to a limited set of materials and their combinations; many OPV material systems have inefficient charge generation efficiency. It is still crucial to understand which factors determine the charge generation efficiency of the systems.
We have investigated the charge generation process and its electric field dependence in OPVs. Detailed investigations of OPVs using bulk-heterojunction (BHJ) and planar-heterojunction (PHJ) structures revealed the efficiency-limiting factors of the charge generation process in OPVs. We have found that the charge generation efficiency primarily depends on the energetic difference between the singlet-excited and charge-transfer state at the D/A interface. Insufficient energetic offset causes less efficient, field-dependent charge generation. Second, the relative molecular orientation of acceptor material to donor domains strongly affects charge generation efficiency. For efficient, electric-field independent charge generation, end-on orientation of the acceptor molecules relative to the donor domains is better than face-on packing.
Kihada-O1
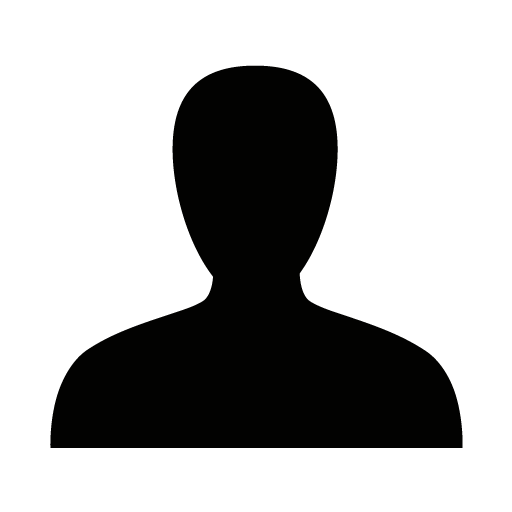
This study aims to expand the practical applications of large-area flexible organic photovoltaics (OPV), such that they retain their high stability and efficiency even under various weather conditions and external stresses. By developing polyethyleneimine derivative-based cathode interfacial layer (CIL) and non-fullerene acceptor (NFA) material in OPVs, the efficiency and stability decrease rapidly due to an undesirable interaction when these two materials are concomitantly applied. In this study, we designed a novel low-cost metal-mediated cross-linked non-conjugated polymer interfacial layer (c-PEIE) and improved the device performance and stability of flexible OPVs from cell-to-module scale. c-PEIE CIL based flexible OPV cell achieves one of the highest power conversion efficiencies (PCE) of 16.45% and remarkable photostability, retaining 77.58% of its initial PCE for 110 h under continuous light illumination, while the conventional PEIE based cells realize a PCE of 12.58% and poor device stability. Accordingly, a 50 cm2, large-area, flexible OPV module was also fabricated with an excellent PCE of 13.12% which is ~80% as efficient as small-area flexible cells. Notably, c-PEIE based OPV devices maintain high output power on cloudy days, which is a particular point of interest to efficiently harvest sunlight energy constantly. The tunable energy barrier and significant low-leakage current of CIL plays a critical role in reducing open-circuit voltage and fill factor losses under low-light environments. Such energy source can provide power efficiently all day long in low-light or cloudy environment for Internet of Things wireless networks integrated with battery independent photovoltaics.
Kihada-O2
Atomic layer deposition of aluminum oxide (ALD-AlOx) layers has been extensively studied for stabilizing perovskite solar cells (PSCs) against environmental stressors, such as humidity and oxygen. [1,2,3] In addition, the ALD-AlOx layer acts as a protective barrier, mitigating the pernicious halide ion migration from the perovskite toward the hole transport interface. However, its effectiveness in preventing the infiltration of ions and additives from the hole-transport layer into perovskites remains insufficiently understood. Herein, we demonstrate the in-situ growth mechanism of a compact ultrathin (~0.75 nm) ALD-AlOx layer using in-situ ellipsometry and conductive-atomic force microscopy. Our result highlights the conformal growth of <1 nm of the ALD-AlOx layer follows the morphology of a triple-cation perovskite film over a large area. This promotes effective mechanical adhesion of the spiro-OMeTAD layer on top of the perovskite. The electronic and chemical structure of the ALD-AlOx is explored via in situ XPS and reflective electron energy loss spectroscopy uncovering the underlying reasons for improved charge carrier collection between these two layers. Upon systematically investigating the layer-by-layer structure of the PSC stack, we discovered that ALD-AlOx also acts as a diffusion barrier layer for the degraded species from the adjacent transport layer into the perovskite. In addition to all protection capabilities, ALD-AlOx impedes the transition of crystalline perovskites to an undesired amorphous phase instead of a yellow delta phase. Consequently, the dual functionality (i.e., enhanced mechanical adhesion and diffusion barrier) of the ALD-AlOx protection enhanced the device performance from 19.1% to 20.5%, retaining 85% of its initial power conversion efficiency (PCE) compared to 10% for pristine devices after 180 days of shelf-aging, followed by 1000 min of maximum power point tracking under ambient conditions. In conclusion, our outdoor testing (ISOS-O-2 protocol) for 3000 hrs of our encapsulated devices shows the ALD-AlOx device maintains up to 85% of its initial as compared to 20% for the pristine devices. Finally, this study deepens our understanding of the mechanism of ALD-Al2O3 as a two-way diffusion barrier, highlighting the multifaceted role of buffer layers in interfacial engineering for the long-term stability of PSCs.
1+2-IS1
Perovskite solar cells (PSCs) are expected to become one of the next-generation solar cells owing to their advantages such as low cost, flexibility, and high efficiency. Much research has been carried out to improve device performance and the dominant factors governing the device performance have been elucidated. However, not enough research has been conducted from a microscopic viewpoint at the molecular level [1,2]. In this study, solar-cell devices and thin-film samples were fabricated using perovskite with mixed cations and mixed halogens, and the charge states including charge diffusion and charge formation at the interfaces between hole- or electron-transport layers (HTL or ETL) and perovskite layers were investigated by a microscopic method with high sensitivity, electron spin resonance (ESR). We have utilized the ESR method to study the charge states and charge transfer in organic and perovskite solar cells and their materials under not only dark conditions but also under device operation [3-10]. The ESR investigation under device operation is called as an operando ESR method [4,7,8]. In the dark state, charge diffusion occurs in stacked films of HTL or ETL and perovskite. This causes a vacuum level shift at the interfaces between HTL or ETL and perovskite, forming band bending at the perovskite interface. This band bending has been shown to dominate device performance during device operation. The results of lead- and tin-based PSCs will be presented.
1+2-O1
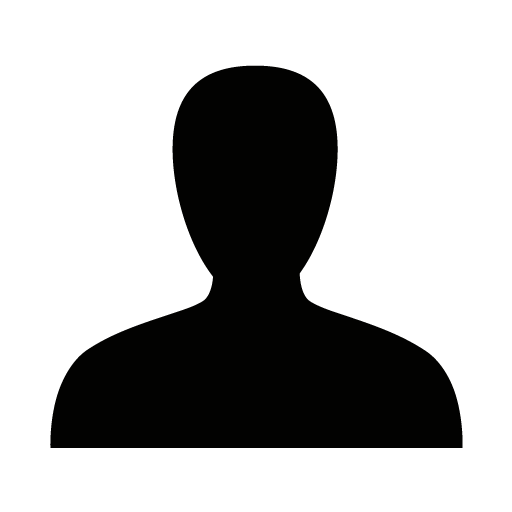
Perovskite solar cells (PSCs) have attracted worldwide attention due to their high power conversion efficiency (PCE) and low-cost solution processing. Besides the film and interfacial engineering of the perovskite layer, the development of hole-collecting materials (HCMs) is a critical key to boosting the performance of PSCs.
In inverted PSCs, the use of chemically adsorbed monolayers that can efficiently collect photo-generated holes from the perovskite layer and transport them to a transparent conducting oxide (TCO) electrode as the hole-collecting layer (HCL) has become one of the mainstream directions.[1]
Recently, we developed a tripodal molecule composed of a triazatruxene core connected with three phosphonic acid anchoring groups (PATAT).[2] We demonstrated that after being chemically adsorbed on the TCO surface, PATAT molecules tend to form a monolayer with a face-on orientation, resulting in improved hole-collection compared to their monopodal counterpart, and the corresponding inverted PSCs using the PATAT monolayer as HCL exhibited PCEs up to 23%. However, PATAT still has its own drawbacks that need to be improved, such as its difficulty in further functionalization and the hydrophobicity of its monolayer.
In this work, we designed a series of isotriazatruxene derivatives bearing three phosphonic acid anchoring groups, iso-PATAT and its tribromo-substituted analogue, iso-PATAT-Br, as hole-collecting monolayer materials. Isotriazatruxene is an isomer of triazatruxene, which has two indole moieties facing each other, leading to a steric hindrance when bulky substituents such as alkyl phosphonic acid groups were introduced into –NH positions. Taking advantage of this point, after being chemically adsorbed, instead of binding to the TCO surface, some phosphonic acid groups would point upward, leading to a hydrophilic monolayer and improved surface wettability.
Inverted PSC devices using these iso-PATAT molecules as hole-collecting monolayers were fabricated and evaluated. Iso-PATAT-Br-based PSCs exhibited superior performances with PCEs up to 25.2%, which is higher than the devices fabricated with our previously reported PATAT (PCE = 25.0%) under the same condition.
In this presentation, molecular design, characterization, and device evaluation will be discussed in detail.
1+2-O2
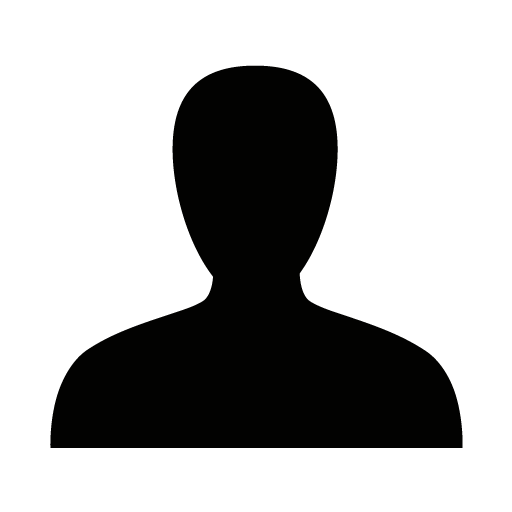
The development of large-area all-perovskite tandem solar modules presents a promising advancement in photovoltaic (PV) technology, offering significant potential for improving efficiency and cost-effectiveness in renewable energy. This work provides an overview of the scalable manufacturing processes and challenges associated with producing both rigid and flexible large-area two-terminal (2T) and four-terminal (4T) all-perovskite tandem modules, towards scalable sheet-to-sheet and roll-to-roll manufacturing of efficient solar devices.
In our research, efficient and stable wide band-gap (WBG) semi-transparent perovskite solar cells (ST-PSCs) were manufactured through optimization of the device stack, reducing optical and electrical losses. This led to ST-PSCs efficiencies of 20.7% for spin-coated (1.6 eV) and 18.1% for blade-coated (1.7 eV) small area (0.09 cm2) devices with high near infra-red (NIR) transparency for tandem application. Tandem solar cells offer a way to higher PCEs by stacking cells with different bandgaps on top of each other, which results in a more optimal utilization of the solar spectrum. A dual passivation approach, targeting both bulk and perovskite/electron transport layer (ETL) interfaces, successfully minimized non-radiative recombination losses. Additionally, reference narrow band-gap (NBG) ST-PSCs (1.25 eV) with 17.3% efficiency were developed for fabricating of bifacial all-perovskite tandem solar cells and modules. Both the optimized WBG and NBG ST-PSCs retained 95% of their initial stabilized power conversion efficiency (PCE) after 1000 hours of aging at 85°C in a nitrogen atmosphere, while control devices degraded within a few days. As a reference, for all-perovskite tandem devices, spin-coated and blade-coated small area WBG ST-PSCs were coupled with a silicon heterojunction (SHJ) bottom solar cell leading to 31.2% and 28.5% stabilized PCE respectively, with more than 6.4% and 3.8% absolute efficiency gain with respect to the standalone bottom Si cell. Importantly, we successfully transferred the technology from small-area device manufacturing to scalable processes for potential large-volume production. As a result, semi-transparent WBG, NBG, and monolithic 2T all-perovskite tandem modules with an aperture area of 100 cm² were fabricated using slot-die coating, evaporation, sputtering, and spatial atomic layer deposition (sALD) scalable techniques. Encapsulated control ST WBG and NBG modules demonstrated a PCE of 10.0% under monofacial conditions and retained nearly 100% of their initial stabilized PCE after 1000 hours of aging under 85% RH/85°C damp-heat stress conditions. The results of optimized bifacial ST WBG, NBG, and tandem devices will be presented during the conference after proper characterization. To our knowledge, this is the first global report on the upscaling of NBG and all-perovskite tandem devices in 100cm2 area via slot-die coating perovskite layers. We believe these findings represent a crucial step towards the commercialization of all-perovskite tandem solar technologies.
1+2-O3
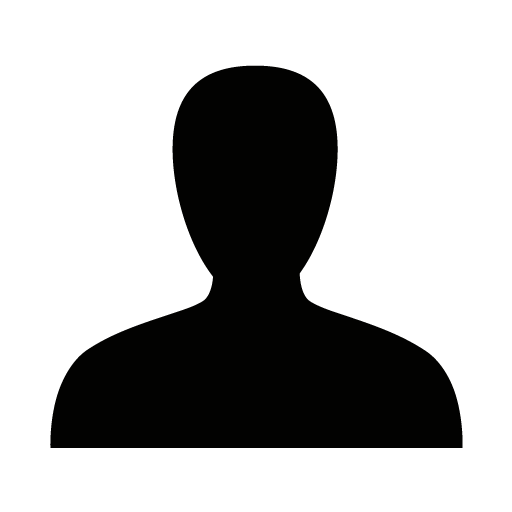
Currently, there is a significant need to increase the spatial resolution of medical X-ray imaging while maintaining high detection efficiency to provide high-quality images at low radiation doses [1]. This pushes research for the development of novel detector materials and architectures. Besides diverse state-of-the-art detector materials such as Si a-Se, and CdTe, recently, a novel class of semiconductors - metal halide perovskites - emerged as promising materials for radiography and computed tomography applications. However, existing studies lack persuasive examples of fully integrated perovskite detectors that would combine a high spatial resolution of multi-pixel detectors together with high detection efficiency (DE) [2], for which direct integration of single crystals on the readout substrates would be required. Here we show a method of fabricating high-quality, thick large-grain polycrystalline CsPbBr3 films by melt growth directly on pixelated glass interposers. The obtained detector arrays show a remarkable 20 lp mm-1 spatial resolution with a DE of 75.4% and low noise equivalent dose (NED) of ca. 28 photons for 22 keV X-rays under a low reverse bias voltage. The combination of these characteristics results in unprecedently, for charge-integrating mode, high values of 20% for detective quantum efficiency (DQE) at the Nyquist frequency. Single-pixel devices demonstrate single-photon counting performance for γ-radiation with an energy-resolved peak of 241Am. Melt-grown CsPbBr3 films, featuring a unique combination of detection efficiency, scalable fabrication, and cost-effectiveness, are promising for the development of high spatial resolution, low-dose X-ray imaging systems.
1+2-O4
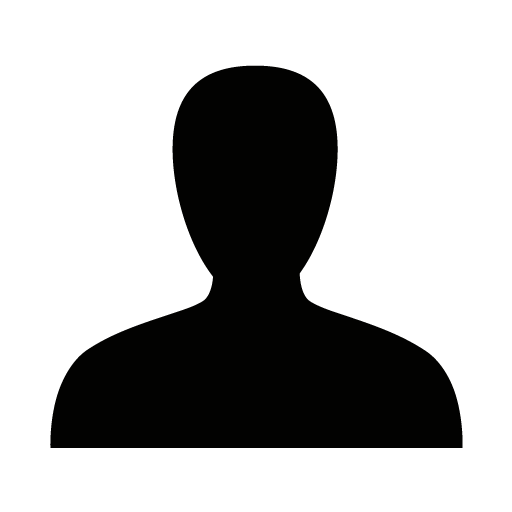
Tin (Sn)-based perovskites have garnered significant interest for their applications in perovskite transistors. Despite significant progress, Sn-based perovskites are still plagued by a propensity for rapid oxidation from Sn2+ to Sn4+, leading to unwanted self p-doping and reduced stability. In particular, two-dimensional (2D) Sn-based perovskites have higher stability than three-dimensional (3D) counterparts. In our study, we demonstrated that the strategic incorporation of porphyrin like additives, especially, effectively mitigates Sn oxidation in 2D perovskite films, thereby reducing defect density. Consequently, devices modified with these additives show improved ambient and electrical stability, enhanced photoresponse, and higher mobility, with film mobility increases of up to 5 cm2 V−1 s−1. Furthermore, the perovskite transistors exhibit non-volatile photomemory characteristics. Also, we use several different wavelength of light to stimulate our devices and tried many stimulate time. These enhancements, especially in photoresponse and air stability, render the modified devices as promising candidates for future photomemory technology applications.
4+5-IS1
Akinori Saeki received BS and MS degrees in nuclear engineering from Osaka University in 1999 and 2001, respectively. He received Dr of engineering in applied chemistry from Osaka University in 2007. He had been an assistant professor at The Institute of Scientific and Industrial Research (ISIR), Osaka University in 2003-2009, an assistant professor (tenure-track) in 2010-2014, and an associate professor in 2014-2019 at the Graduate School of Engineering, Osaka University. He had joined in JST-PRESTO research programs of "Photoenergy conversion systems and materials for the next generation solar cells" in 2009-2013 and "Materials Informatics" in 2015-2019. He is currently a professor at Graduate School of Engineering, Osaka University (2019-present). His research interest is in nanometer-scale dynamics of chemical intermediates in condensed matters such as organic semiconductors, organic liquids, and organic-inorganic hybrid materials.
Solution-processed inorganic solar cells with less toxic and earth-abundant elements are emerging as viable alternatives to high-performance lead-halide perovskite solar cells. However, the wide range of elements and process parameters impedes the rapid exploration of vast chemical spaces. Here, we developed an automated robot-embedded measurement system that performs photoabsorption spectroscopy, optical microscopy, and white-light-flash time-resolved microwave conductivity (TRMC). We tested 576 films of quaternary element-blended wide-bandgap Cs–Bi–Sb–I semiconductors with various compositions, organic salt additives (MACl, FACl, MAI, and FAI, where MA and FA represent methylammonium and formamidinium, respectively), and thermal annealing temperatures. Among them, we found that the maximum power conversion efficiency (PCE) was 2.36%, which is significantly higher than the PCE of 0.68% of a reference film without an additive. Machine learning (ML) and statistical analyses revealed significant features and their relationships with TRMC transients, thereby demonstrating the advantages of combining ML and automated experiments for high-throughput exploration of photovoltaic materials.
4+5-O1
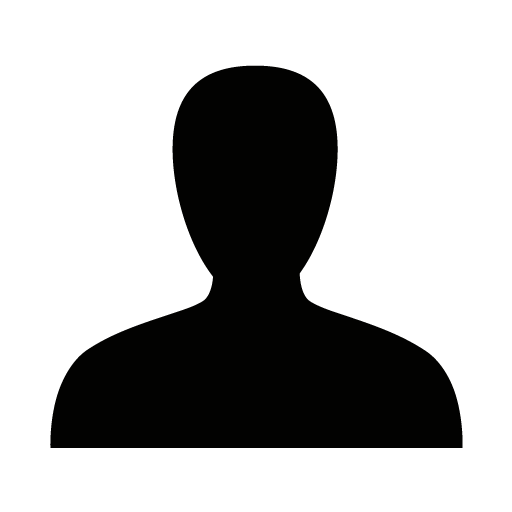
The introduction of self-assembled monolayer (SAM) hole transporting materials (HTMs) made a significant contribution towards development of p-i-n architecture perovskite solar cells (PSCs). Carbazole-based derivative V1036 was one of the first SAM HTMs to be successfully used instead of standard PTAA. Although it demonstrated slightly lower efficiency (17.8 %) compared to PTAA (19.2 %), it was evident that monolayer can be efficient compared to the polymeric films of regular thickness [1]. Not long after, V1036 structure was optimized, simplified and its analogues 2PACz and MeO-2PACz were introduced. These small but important structural changes resulted in an increase in p-i-n PSC performance, as 2PACz and MeO-2PACz exhibited PCEs of 20.8 % and 20.2 % respectively, higher than that of PTAA (18.9 %) [2]. Combined with conformal coverage, minimal parasitic absorption and material consumption, SAM HTMs became an important research subject for optoelectronic applications, such as organic photovoltaics, perovskite/silicon tandems or organic light-emitting diodes [3-5]. In this research, new SAM HTMs were synthesized and investigated as 2PACz alternatives to further broaden the scope of possible applications. Two main strategies for structural modifications were applied: selection of different chromophores and incorporation of different functional groups. New SAM materials have been successfully used to construct devices such as p-i-n PSCs, bulk heterojunction organic photovoltaics, organic light-emitting diodes and sensors.
4+5-O2
Perovskite solar cells (PSCs) have become a major object of interest in the field of photovoltaics during the last decade. Over time this new technology has managed to demonstrate superior results in the power conversion efficiency (PCE) exceeding 26 % certified by the NREL. An essential component for PSC fabrication is hole transporting layer (HTL), being responsible for the hole extraction at the perovskite/HTM interface. Recently introduced self-assembling materials (SAMs), which form dense and uniform monolayers on top of ITO by forming strong bonds between anchoring groups and the oxide surface, help to reduce the usual quantity of HTMs to a minimum, allow to avoid doping procedures, ensuring the longevity of devices, and provide opportunities for a large-scale PSC production because of the convenient deposition techniques.[1-2] However, the effect of differently anchored SAMs on the performance parameters of PSCs is not completely understood. Therefore, further research is necessary to assess how various structural modifications affect surface wettability, alignment of energy levels, hole-collection properties and overall photovoltaic performance.
In the present study, a new series of enamine-based self-assembling hole transporting materials containing different anchors, such as carboxyl, acetic acid, cyanoacrylic acid, phosphonic acid, methylene phosphonic acid, cyanovinyl phosphonic acid groups, were functionalized via one-, two- or three-step synthetic procedure. Contact angle measurements were carried out to evaluate surface properties of the synthesized SAMs. Contact angles of water droplets on the ITO/SAM films containing carboxyl group were found to be more hydrophilic and similar to that of MeO-2PACz, compared to the monolayers with phosphonic acid. Inverted (p-i-n) solar cells were fabricated to test novel materials as SAMs. Initial studies on the performance of devices demonstrated that the most efficient PSCs with SAMs containing carboxyl group showed efficiency exceeding 22%, furthermore monolayers with phosphonic acid group allowed devices to reach similar results. This work confirms that enamine-based monolayers can provide necessary characteristics to achieve high efficiency results in PSCs.
4+5-O3
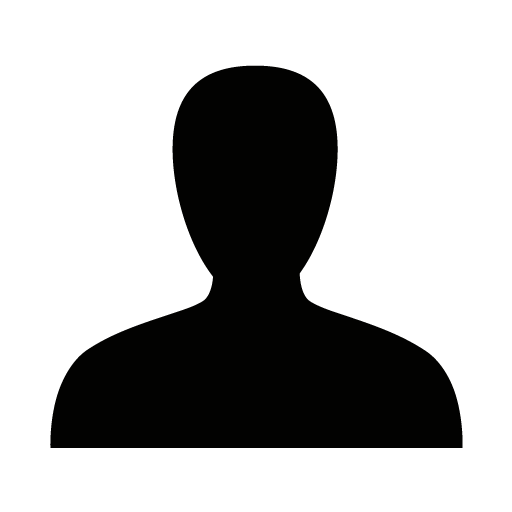
Tin perovskite solar cells (PSCs) are emerging as the most sustainable lead-free alternative in the field of thin film photovoltaics. DMSO-free processed tin PSCs are gaining interest due to the adverse effects of DMSO on tin oxidation reducing device stability. However, replacing DMSO with other solvents is challenging due to the rapid crystallization dynamics during tin perovskite film formation, which are even more pronounced in DMSO-free systems. In this study, we modulate the colloidal formation and interaction within perovskite precursors using additive engineering to optimize the crystallization process. Specifically, we employed piperazinium diiodide (PDAI) as a colloidal interaction modulator to arrange larger clusters in perovskite precursor suspension.[1] At the same time we used 4-tert-butyl pyridine (tBP) as a colloidal stabilizer to retard the aggregation process by interacting with Sn-X units, achieving a balanced crystallization rate synergistically.[2] This synergistic approach achieves a balanced crystallization rate, leading to tin perovskite films with higher crystallinity and improved microstructure, as directly observed by in-situ photoluminescence (PL) during real-time monitoring of crystallization during spin-coating. Devices treated with tBP and PDAI exhibited a champion power conversion efficiency of 7.8% and excellent dark stability without any degradation for 3000 hours in N2 glovebox. Our findings provide an advancement in understanding and managing crystallization in DMSO-free solvent-processed tin perovskite solar cells, paving the way for further performance enhancements and broader application in optoelectronic devices.
Kihada-K1
Polymer-based organic photovoltaics (OPVs) have been intensively investigated in the last few decades since they can be lightweight, flexible, and semi-transparent. Recently, owing to the development of π-conjugated polymers as the electron donor and non-fullerene acceptors, the power conversion efficiency of OPVs has significantly improved. One of the important strategies for developing conjugated polymers is coplanarization of the backbone, which leads to higher crystallinity and thereby higher photovoltaic performance. However, coplanarization often decreases solubility of the polymer and miscibility with the acceptor materials, which are detrimental to the fabrication and thereby photovoltaic performance. Therefore, achieving high coplanarity and high solubility at the same time is a critical issue for conjugated polymers. On the other hand, with the increase in the efficiency, the chemical structures of the conjugated polymers have become significantly more complex compared to traditional materials. Therefore, how to synthesize them efficiently and how to design their molecular structure simpler are important issues. In this presentation, I will introduce our strategy for the design and synthesis of coplanar and soluble polymers and easily accessible polymers.
Kihada-I1
Considering the technical standards required for wearable electronics, such as mechanical robustness, the development of intrinsically-stretchable OSCs (IS-OSCs) should be accelerated. Concurrently, IS-OSCs offer an intriguing platform for testing the mechanical and electrical properties of new polymeric materials. This presentation will discuss key studies aimed at making each layer of IS-OSCs both stretchable and efficient, with an emphasis on strategies to simultaneously enhance the photovoltaic and mechanical properties of the active layer. I will outline material design strategies to enhance the mechanical robustness of the PSCs as well as their power conversion efficiencies (PCEs). These strategies include; i) incorporating a high-molecular weight polymer acceptor as a tie molecule into active layers, ii) developing new electroactive polymers consisting of hard and soft segments and iii) developing new materials that improve molecular miscibility in the donor-acceptor blends. With these contributions, the IS-OSCs achieving over 14% PCE and high stretchability have been developed.
References
(1) Lee, J. W. et al. Joule 2024, 8, 204
(2) Lee, J. W. et al. Energy Environ. Sci. 2022, 15, 4672
(3) Park, J. S. et al. Adv. Mater. 2022, 34, 2207544
(4) Cheng, S. et al. Joule 2023, 7, 416
(5) Wan, Q. et al. JACS 2023, 145, 11914
Kihada-I1
Kenjiro Fukuda received his Ph.D. from the Department of Applied Physics at the University of Tokyo in 2011. From 2011 to 2015, he worked at Yamagata University as an assistant professor, and then joined RIKEN, where he is currently a Senior research scientist in the thin-film device laboratory and emergent soft system research team, Center for Emergent Matter Science. From 2014 to 2018, he has also been a PRESTO researcher of the Japan Science and Technology Agency. His current research interests include organic transistors, flexible electronics, and printed electronics.
Extreme thinness can reduce the weight of electronics, which dramatically reduces discomfort when wearing. Furthermore, it also improves their mechanical robustness to bending because applied strain is determined by the material's softness and device thickness. In the simplified model, the film thickness is inversely proportional to the strain value, such that a thinner thickness means that the device experiences less strain for the same bending radius [1]. Furthermore, intrinsically stretchability enables attachment onto movable parts such as body joints. These advantages have inspired research into ultra-thin and stretchable organic solar cells and related integrated electronics.
We are focusing on to improvement both power conversion efficiency (PCE) and environmental stability of flexible/stretchable organic solar cells. We achieved water proof and high-performance ultrathin organic solar cells that can be operated underwater [1]. An innovation on the structures enabled much improved water stability of flexible organic solar cells. Additionally, we developed a self-powered wearable sensor by integrating all-solution processed trilayer structure with organic optoelectronics [2]. With such ultrathin organic solar cells, we enabled rechargeable wearable electronic systems for living small insects using ultra-thin organic solar cells [3]. The ultimate thinness and lightweight properties enable secured basic motion abilities of insects even with our solar cell modules attached to their abdominal surface.
Kihada-I2
Xiaozhang Zhu graduated from the Department of Chemistry, Jilin University with a bachelor's degree in 2001, and from the Institute of Chemistry, Chinese Academy of Sciences with a doctor's degree in 2006. From 2006 to 2012, he worked as a postdoctoral researcher in University of Ulm in Germany with Prof. Peter Baeuerle and University of Tokyo in Japan with Prof. Eiichi Nakamura. In 2012, he joined the Key Laboratory of Organic Solids School of Chemistry as an independent investigator. Since 2015, he has also served as a professor at the University of Chinese Academy of Sciences. He is currently the associate editor of Organic Materials, Thieme Publishing House in Germany, the young editorial board member of Chemical Journal of Chinese Universities, and the member of the Young Chemical Researchers Committee of Chinese Chemical Society. His main research interest is organic/polymer optoelectronic materials and devices. Since 2012, As the corresponding author, he has published more than 120 papers in academic journals including Nat. Energy, Nat. Commun., Sci. Adv., Joule, Chem, J. Am. Chem. Soc., Angew. Chem. Int. Ed., Adv. Mater., Energy Environ. Sci., Chem. Soc. Rev., Acc. Chem. Res., of which 12 have been selected as ESI highly cited papers. He was awarded with the fellowships from the Alexander von Humboldt Foundation and the Japan Society for the Promotion of Science. He is a recipient of the National Science Found for Distinguished Young Scholars.
Organic solar cells (OSCs) have been regarded as a promising technology for converting renewable solar energy to electricity because of their outstanding advantages such as low cost, light weight, high flexibility, controllable transparency, and large-area solution processability. During the last decade, we focused on the development of N-type near-infrared organic photovoltaic materials to break the efficiency bottleneck of OSCs.[1] We proposed a new design concept, “enhancing the quinoidal resonance of electron donor (D)-electron acceptor (A) systems”, and developed a series of N-type photovoltaic materials (ATT and AQx series) featuring highly adjustable electronic structure by convenient modular synthesis.[2] By developing the precise regulation strategies on electronic[3] and aggregation[4] structures of bulk-heterojunction active layer, we achieved the continuing breakthroughs on the OSC efficiency, and currently a record efficiency over 20% has been realized for single-junction organic solar cells.[5] In order to achieve both high average visible-light transmittance and high power conversion efficiency in semi-transparent solar cells, we proposed the strategy,“near-infrared complementary absorption of electron donor and acceptor materials”, and developed ultra-small bandgap electron acceptor materials, leading to record-high light utilization efficiencies.[6]
Kihada-S1
Kihada-I3
Perovskite solar cells have come to the forefront of solar research in the last decade with
certified efficiencies of now >26%. This is approaching rapidly the Shockley-Queisser limit for
single-junction solar cells, implying that the main breakthroughs for perovskites were
achieved with relatively narrow bandgaps.[1a,1b] Less progress, however, was made for
wider bandgap perovskites, which are of interest for multijunction photovoltaics, detector
applications, or water splitting. These wide bandgap perovskites are often comprised of fully
inorganic components, which are hard to dissolve in conventional solvent systems and
require more sophisticated synthesis as well as crystallisation techniques.
In this talk, I will discuss strategies to address these challenges by providing a library of
hitherto unexplored wide bandgap perovskites using combinatorics. Unfortunately, the
newly formulated liquid precursors often exhibit complex crystallization behaviour struggling
to expel the typically used DMSO solvent. To delay the crystallization time, two strategies
are proposed to remove the strongly complexating DMSO molecules through a) modified
processing of the liquid thin-film[2] and b) a coordination solvent with a high donicity and a
low vapor-pressure[3] leading to a marked improvement in the overall film quality.
Lastly, interface manipulation, especially on top of the formed perovskite, is becoming a
central topic to advance further. Typically, this involves chemical surface treatments with a
complex interaction. Here, light annealing is introduced as a universal, non-chemical
approach to modify the perovskite surface resulting in a reduced surface recombination.[4]
[1a] Saliba et al. Energy & Environmental Science (2016), [1b] Turren-Cruz, Hagfeldt, Saliba; Science (2018)
[2] Byranvand,…, Saliba; One‐Step Thermal Gradient‐and Antisolvent‐Free Crystallization of All‐Inorganic
Perovskites for Highly Efficient and Thermally Stable Solar Cells, Advanced Science (2022)
[3] Zuo,…, Saliba; Coordination Chemistry as a Universal Strategy for a Controlled Perovskite Crystallization,
Advanced Materials (2023)
[4] Kedia,…, Saliba; Light Makes Right: Laser Polishing for
Kihada-I1
Semiconductor materials based on metal halide perovskites have attracted enormous attention in both research community and industry sectors in the past decade owing to their impressive performance demonstrated in the area of solar cells and other optoelectrical devices such as light emitting diodes, transistors etc. Currently the most widely used methods for synthesis of perovskite films is based on solution processing. The standard protocols of synthesis of the perovskite materials involves usage of large amount of hazardous, highly coordinating, aprotic polar solvent such as dimethylformamide (DMF) or carcinogenic N-methyl-2-pyrrolidone (NMP). The hazardous nature of these solvent raises concern for health, safety and environmental impact of these chemicals to humans and some of these solvent (e.g. DMF) has been banned to be used in manufacture in Europe. This restricts the commercial production of metal halide perovskite materials for various applications in practice.[1] Therefore it is highly critical to develop alternative sustainable solution processing for synthesis of metal halide perovskite using environmentally friendly, green solvent systems. In my talk, I will present our research on development of green processing methods for synthesis of metal halide perovskite using environmentally friendly solvent such as water, protic ionic liquids and biomass-derived solvent.[2] I will show that how the solvent system used in the material synthesis influence the physicochemical (morphology, optical and electrical) properties of the perovskite materials and the choice of precursor materials, which in turn determines the material’s performance in applications such as solar cells and light emitting diodes.
Kihada-I2
Inverted perovskite solar cells (PSCs) show great promise both as standalone technology and in tandem with silicon. However, the commercialization of cutting-edge inverted PSCs faces two major challenges: interface recombination losses and degradation. To tackle these issues, we have developed novel interface materials and innovative device design concepts. Our recent research represents a breakthrough, with our inverted PSCs achieving record-breaking performance. Specifically, we have reached a certified steady-state efficiency of over 24.0% for a 1 cm² active area, surpassing conventional designs.
In this presentation, I will explore the rationale behind the inverted perovskite design and discuss our strategies for unlocking its full potential in various tandem configurations. I will highlight the advantages of this technology and its future prospects, including the design of wide-bandgap perovskites to achieve record performances in perovskite/CIGS (~30%, 4T) and triple-junction perovskite/perovskite/Si tandems (>27%, NREL certified). I will also explain how perovskite-based tandem solar cells have achieved high efficiencies and demonstrate the vast potential of integrating traditional PV and perovskite technologies in tandem devices.
Kihada-IS1
Prof. Satoshi Uchida is a professor (born in 1965) in Research Center for Advanced Science and Technology (RCAST), The University of Tokyo. He received his PhD from Tohoku University in 1995 and moved to current position in 2006. For more than 15 years his research focused on the field of dye-sensitized solar cells (DSSCs), specifically cell assembling technique such as full-plastic, light-weight, film type as a ubiquitous power source. He is now also showing strong activity of Perovskite Solar Cells research based on the crystallography, surface engineering and electronic simulation.
Perovskite solar cells have emerged as a cutting-edge research field in the realm of new-generation photovoltaic technologies, owing to their remarkable performance potential and the promise of cost-effective production. As of 2024, these cells have achieved an impressive power conversion efficiency exceeding 26%, surpassing conventional solar cell technologies like CIGS or amorphous Si. To push their performance further beyond the Shockley–Queisser limit, which hovers around 30%, a deep understanding of the physical chemistry rooted in crystallography becomes imperative.
Here in this research, we directly confirmed that specific grain boundaries in organometal halide perovskites impede charge carrier flow. To address this, we introduced a new fabrication technique: rapid cooling (quenching) of the crystallographic phase of the organometal halide perovskite, which effectively minimizes microscale defects such as grain boundaries. To further investigate the details and impact of grain boundaries in organometal halide perovskites, we performed TEM and in situ current density−voltage (J−V) analyses. These analyses revealed that a significant physical gap, originating from the grain boundaries of MAPbI3 perovskite, severely obstructs charge carrier flow. As a result, we achieved a power conversion efficiency (PCE) of 20.23% with a single-cation MAPbI3 PSC. The restricted crystallographic transition was demonstrated through scanning electron microscopy (SEM) images and confirmed by X-ray diffraction (XRD) analyses.
Kihada-O1
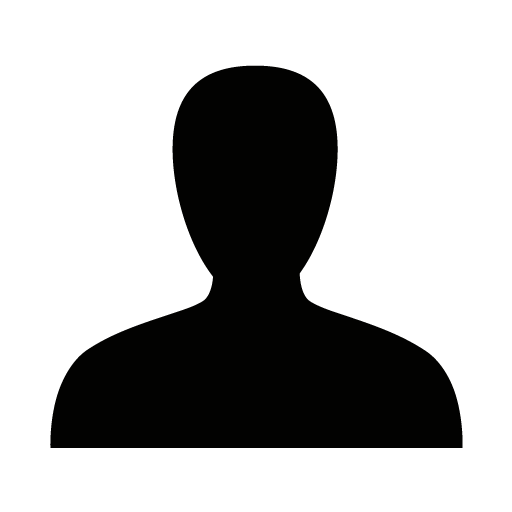
Tin halide perovskites, with the general formula ABX3 [A = Formamidinium (FA), B = Sn, X = Br, I], offer a narrower bandgap (~1.3 eV) than their lead counterparts, making them suitable for the light-absorbing component in single-junction photovoltaic devices. Moreover, this attribute makes tin halide perovskites integral to all-perovskite tandems, with the best-performing cells comprising a 50-50 Sn-Pb composition in the low bandgap component. Nevertheless, record-breaking pure-Sn and Sn-Pb devices still exhibit severe losses in both open-circuit voltage (Voc) and short-circuit current (Jsc), keeping power conversion efficiencies well below theoretical limits.
Non-radiative voltage loss is directly linked to lower photoluminescence quantum yield (PLQY), and thus the prevalence of non-radiative recombination. [1] This picture is complicated in Sn-containing perovskites given that the presence of Sn4+ impurities in the precursor solution leads to both the introduction of background hole dopants (serving to increase the PLQY) and non-radiative recombination centers (serving to decrease the PLQY).[2]
We use the observation of pseudo-first order photoluminescence (PL) decay kinetics in Sn-containing perovskite films to establish a method for characterizing the hole dopant level and non-radiative recombination rate constant with combined time-correlated single photon counting (TCSPC) and photoluminescence quantum yield (PLQY) measurements. We find that untreated films exhibit large hole doping concentrations as high as p0 ~ 1 x 1019 cm-3, which can be reduced to p0 ~ 1016 cm-3 after precurosor sublimation and SnF2 treatments. While it is well known that the radiative recombination rates are increased with p0, we reveal that the non-radiative rate can also be increased. Using correlated microscopy, we find that microscale p-type regions are also centers for non-radiative recombination. We find significant PL heterogeneity even in films with moderate dopant levels, suggesting that new strategies to eliminate deleterious defects in Sn-containing perovskites should be developed. [3]
Kihada-O2
Despite perovskite solar cells (PSCs) have demonstrated a promising power conversion efficiency, their long-term operational stability is still widely recognized as the key bottleneck to next-step development and commercialization [1]. Specifically, mixed-cation perovskites, which push the record efficiency up to more than 26%, suffer from intrinsic instability, especially under thermal stress [2]. However, for outdoor operation, solar cells must face with extreme and harsh conditions during their service lifetime, which has important implications for real-world energy yields. Up to now, most reported lifetimes are within thousands of hours, significantly lagging behind silicon photovoltaics with a 25 years' lifetime [3].
Regarding this stability concern, in this work we employ a novel deposition method to achieve state-of-the-art efficiency and excellent long-term stability under thermal and illumination stress, fundamentally overcoming the intrinsic instability challenge for mixed-cation perovskite materials. We found that the as-fabricated PSCs can maintain its power output for over 2,000 hours without any sign of degradation under continuous max power point tracking (MPPT) at room temperature. To further explore the full potential of the stability of our devices, we elevated to an unprecedently high temperature of 110°C and found that it can maintain 80% of initial efficiency (T80) for more than 520 hours under MPPT. This performance drives us to conduct accelerated aging test to investigate the degradation mode and predict the lifetime of our devices under real-world operations. Here, we set temperature as the key stress factor and monitor indoor operational output of PSCs at different high temperatures ranging from 65 to 110°C. The data from accelerated tests is then fitted with Arrhenius model, returning an estimation of the field lifetime. As a result, the projected operational lifetimes of different temperatures converge at 45,000 hours, which aligns with the extrapolated T80 lifetime derived from room-temperature measurement. Furthermore, considering different local global horizontal irradiation worldwide, the converted lifetime of PSCs can sufficiently ensure 25 years of outdoor exposure in Singapore, Japan and most areas in US. This result is the best-to-date stability performance of mixed-cation PSCs reported so far in literature. More importantly, it's the first time that PSCs is proved to realize such a long lifespan comparable to silicon solar cells, paving the way to future development and commercialization of perovskite-based photovoltaics.
Kihada-O3
Iodide and bromide integration facilitate broad bandgap tunability in wide-bandgap perovskites [1], yet high concentrations of bromide incorporation lead to notorious halide phase segregation phenomenon [2], which will adversely affect the efficiency and stability of solar cell devices [3]. Herein [4], 2-amino-4,5-imidazoledicarbonitrile (AIDCN), with highly polarized charge distribution, compact molecular configuration and designed functional groups, is incorporated into a 1.86 eV wide-bandgap perovskite to effectively suppress photoinduced iodine escape and halide phase segregation by inducing specific chemical interactions with perovskite. Hyperspectral photoluminescence microscopy reveals that AIDCN mitigates halide phase segregation under continuous laser exposure. Concurrent in situ grazing-incidence wide-angle X-ray scattering and X-ray fluorescence measurements further validate suppressed iodine escape, evidenced by a notable slowing down of lattice shrinkage and a well-maintained overall chemical composition of the perovskite under continuous illumination. These findings, for the first time, provide experimental evidences that correlate the initiation of halide phase segregation to the photoinduced iodine escape process. Ultimately, by applying AIDCN, we achieve a power conversion efficiency (PCE) of 18.52% in 1.86 eV wide-bandgap perovskite solar cells with a T80 lifetime of 271 hours under continuous tracking at the maximum power point. By integrating this perovskite subcell with a PM6:BTP-eC9 organic subcell, the perovskite-organic tandem solar cell attains a maximum PCE of 25.13%, with a Japan Electrical Safety & Environment Technology Laboratories-certified stabilized PCE of 23.40% included in the “best research-cell efficiency chart” by National Renewable Energy Laboratory of US. These results underscore the efficacy of our approach in strategically designing the molecular structure of additives to induce specific chemical interactions, which not only mitigate halide phase segregation and photoinduced iodine escape but also significantly enhance the efficiency and stability of perovskite-based tandem solar cells.
Kihada-O4
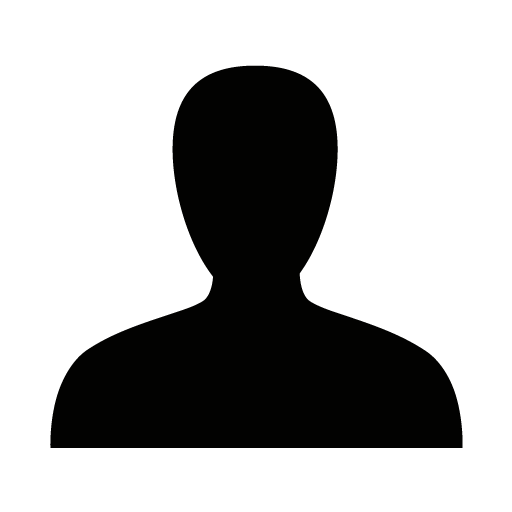
Before any perovskite solar cells (PSCs) are released for commercial use, their outdoor behaviour must be measured. This is an incredibly time consuming process that for relevant and slow degrading devices can last several months. In this work we demonstrate a flexible machine learning driven pipeline that can accurately determine the lifetime and degradation behaviour of any device, given short accelerated indoor tests. Additionally, by training our algorithm on different indoor tests, we are able to automatically determine the most relevant aging protocols, a process which so far relied heavily on intuition. Lastly, by identifying the most relevant stress factors, we can shed light on the outdoor degradation pathways. Our model has been tested on sollar cells fabricated in nip. Configuration of FTO/c-TiO2/m-TiO2/CsMAFAPb(IBr)3/Spiro-OmeTAD/Au in 6 different annealing temperatures. We verified the results by incorporating two a-priori known facts, namely that indoor aging under air will bear little relevance to outdoor degradation of encapsulated panels and aging under 1.4 sun and nitrogen is highly correlated to aging under 1.0 sun and nitrogen. Both effects were succesfully reconstructed by the model, therefore increasing the certainty that it can be applied at a larger scale. Finally, it must be noted that our methodology is not specific to PSCs and can be extended to other PV technologies where degradation and its mechanisms are a crucial element of their widespread adoption.
1+2-IS1
Optimization of a photovoltaic device is a typical example of optimizing a complicated chemical network system. Herein, two chemical networks are involved: one consists of component materials, and a series of optimizations of each component does not ensure the overall device optimization. Another network consists of a series of experimental procedures after choosing the component materials, where each is pertinent to the morphologies of the materials fabricated therein. The number of combinations in materials and experimental procedures is infinite and may be a typical example of a “combinatorial explosion”. Although a variety of impressive and successful design principles have been introduced so far and resulted in rapid and steady improvements have been achieved, we are still challenging the problem of finding “a needle in a haystack”. While continuous updates of the device fabrication processes and materials are strongly demanded in such orthodox and deductive optimizations, which may be called “a normal design”, recent progress in computational techniques seems to provide new opportunities in the system optimizations through "inverse designs" or "virtual (i.e., computational) screenings". In this presentation, we will introduce and discuss our recent efforts toward optimizing the perovskite solar cell through applications of various computational techniques, including device simulations, atomistic calculations, generative AI models, and image regression to predict device performance.
1+2-O1
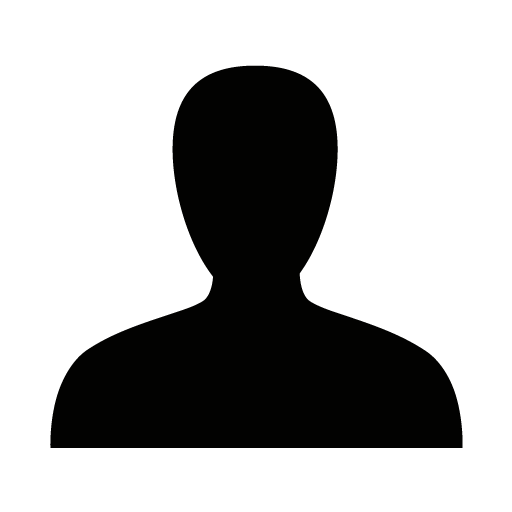
Vacancy defects in perovskites are a significant problem that leads to the degradation of perovskite solar cells (PSCs). Strategies of introducing excessive ionic iodide has been employed to compensate for these vacancies. However, these excessive ionic iodides cannot effectively reduce defects during the perovskite crystallization process, as their similar chemical properties as the original ionic iodide in the precursor solution. Moreover, these iodide ions present in the perovskite films can act as interstitial defects, which are detrimental to the stability of the perovskite. We find that vacancy defects can be effectively suppressed by inclusion of α-iodo ketone during the perovskite crystallization. This suppression is achieved by a rapid collective transformation of lead polyhalides through halides ions release of Kornblum oxidation reaction. More importantly, excessive iodides within formed perovskite film can be removed by a subsequent elimination reaction. Based on this strategy, the PSC achieves significantly improved thermal stability, which maintain 82% of initial PCE for 2800 h at 85 °C.
1+2-O2
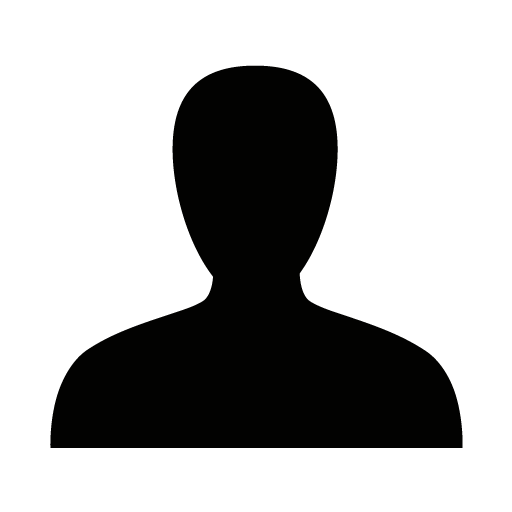
The efficiency of lead perovskite solar cells (Pb-PVK-PV) has now exceeded 26%. Tandem cells consisting of Pb-PVK-PV as the top cell and Si solar cell as the bottom cell have attracted much attention as solar cells with higher efficiency than Pb-PVK-PV. The certified efficiency has now reached 32.5%. In addition, especially from the perspective of flexible tandem solar cells, all-perovskite tandem solar cells have advantages over tandem cells consisting of Pb-PVK-PV (top)/inorganic solar cell (bottom) because both the top and bottom cells are manufactured using a low-temperature printing process. The efficiency of all-perovskite tandem solar cells is reported to be 28%. However, the toxicity of Pb has long been criticized, and for this reason, people have developed various Pb substitutes: Sn, Ge, etc. Among them, Sn-based perovskite solar cells have developed rapidly and have now exceeded 15%. Since it is very difficult to further improve the efficiency of single-cell Sn-based perovskite solar cells, in order to further manufacture non-toxic perovskite solar cells, we need to develop new device structures. Our research focuses on the efficiency breakthrough of wide-bandgap Sn-based perovskite solar cells. This is to prepare for our subsequent GIGS/Sn-PVK tandem cells. In order to further improve the efficiency of wide-bandgap solar cells, we introduced ferrocene derivatives at the PEDOT:PSS/PVK interface. By repeatedly optimizing the concentration of the modifier, we successfully increased the efficiency of the WBG Sn PSC to more than 11%. At the same time, we successfully prepared a CIGS/WBG-Sn-PVK tandem solar cell with an efficiency of more than 14%. This is the world's first report on all-lead-free perovskite tandem solar cells, and it also provides guidance and help for further improving the efficiency of Sn-based solar cells.
1+2-O3
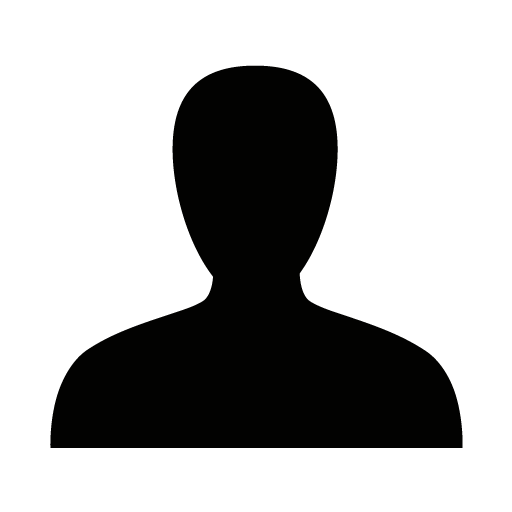
Optimizing the interface between the perovskite and transport layers, and modifying electron transport layer (ETL) are critical for enhancing the performance of perovskite solar cells. Herein, we introduced three conjugated polymers (DPPF-BDD, DPPT-BDD and DPPSe-BDD) as antisolvent additive and ETL blending. After polymer treated, PSCs displayed maximum PCE of 23.48%, 22.85% and 23.35%, respectively, surpassing the control device (22.83%), which was benefited from the perovskite near-infrared (NIR) region absorption[1], surface passivation and the electron transport layer (ETL) charge extraction improvement. Finally, we performed the long-term stability test for DPPF-BDD, DPPT-BDD and DPPSe-BDD based PSCs stability at 85oC in glovebox. The control device degraded to 39.8% of its initial PCE after 240 h. The DPPF-BDD, DPPT-BDD and DPPSe-BDD treated devices still remained 74.8%, 57.6% and 43.0% of the initial PCE after 430 h respectively. The stability improvement is attributed to the passivation effect, which effectively smoothed perovskite surface and reduced defects.
1+2-O4
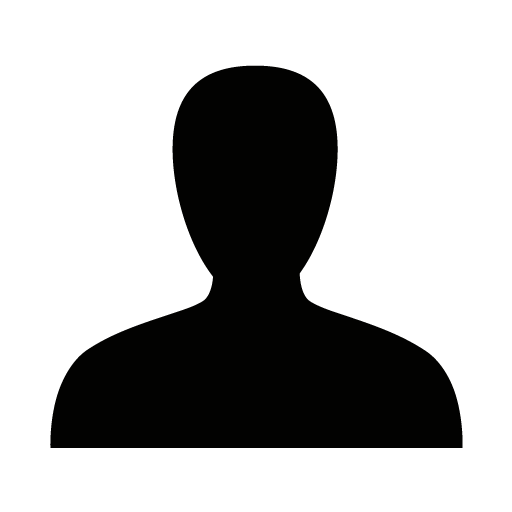
Transparent photovoltaics are garnering significant interest for power generation in applications in building integrated photovoltaics (BIPV), including solar windows and other shading elements. Metal halide perovskites have emerged as one of the most lucrative material classes for such device architectures due to their exceptional optoelectronic properties, compositional versatility enabling a wide range of transparency levels, and low power to weight ratio.[1] Traditionally, research has primarily focused on semi-transparent (ST) solar cell architectures, where transparency is managed by either adjusting the perovskite layer thickness or by increasing the bandgap, yet their coloured appearance as well as efficiency and stability limitations hinder their practical applicability. Here, we focus on an alternative approach to realize both efficient and colour-neutral, transparent perovskite solar cells (PSC) by utilising a fully opaque device which is partially covering the surface, i.e. semi-opaque (SO). This approach is especially appealing since under ideal conditions the generated power by a SO cell is linearly proportional to the aerial coverage, and therefore the efficiency limits are derived from the fully opaque device performance.
We begin by conducting a computational comparative analysis between ST-PSC and SO-PSC configurations based on their predicted performance by means of the trade-off between power conversion efficiency (PCE) and average visual transmittance (AVT), as well as their aesthetic appearance through the CIE colour coordinates and colour rendering index (CRI).[2] Our results show that based on practical literature limits, SO devices are predicted to yield superior performance compared to ST-PSCs at transparencies below ~60% AVT.
One of the biggest challenges to realize efficient SO-PSCs is the fabrication of multi-layered patterned perovskite architectures within the best performing device configurations. This key hurdle stems mostly from the sensitivity of organo-metallic perovskites to conventional lithographic processes.[3] Motivated by this gap, we developed a scalable patterning method that allows a controlled self-assembly of the perovskite solution via a simple stamping step. Through micro-contact printing of the substrate with a hydrophobic template comprising octadecylphosphonic acid molecules, the perovskite solution is constrained to crystallize only in the desired regions, thus allowing for a quick fabrication of patterned films without requiring any post deposition processing.[4]
Utilising this approach, SO-PSCs were fabricated with varying levels of transparency between 20-50%. Our preliminary results demonstrate minimal loss per active area relative to fully opaque devices, indicating the potential of this patterning approach. Moreover, we show that by applying an antireflective coating on both sides, the overall transparency is significantly improved through both reduced reflection from the front side and increased light outcoupling from the back side. Compared to literature benchmarks, these devices are well on-par with the best performing ST and SO devices reported to date, while exhibiting the highest efficiency for color-neutral PSC over a wide transparency range.
4+5-IS1
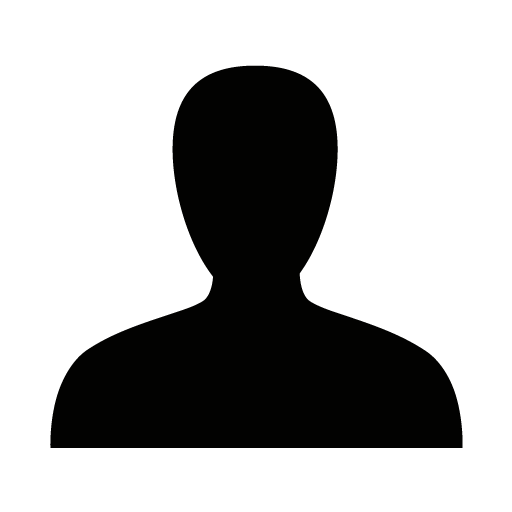
Perovskite solar cells have been intensively examined with a rapid increase in power conversion efficiency (PCE) up to 26.7% [1]. To overcome Shockley-Queisser limit of a single-junction solar cell, tandem-type solar cells are developed to utilize a wide range of the solar spectrum. One of the most interesting tandem solar cells is the perovskite/silicon (Si) tandem solar cell. Presently, there are two principal intriguing tandem structures: two-terminal (2T) and four-terminal (4T) configurations. The 2T connection requires current matching, whereas the 4T configuration does not. The top cell and bottom cell in a 4T tandem can be developed separately. The 4T tandem is thus interesting. Currently, the record PCE of rigid 4T perovskite/Si tandem solar cell is 30.9% under 1 SUN [2]. It is additionally known that lightweight flexible photovoltaic (PV) modules are intriguing since they are readily applied on rooftops and also important for car automotive applications. In this work, flexible and semi-transparent perovskite solar cells are developed as the top cell of 4T perovskite/Si tandem solar cell, which should have not only high PCE but also high transmittance in the long wavelength region (infrared) from 800 nm to 1100 nm to increase JSC of the Si bottom cell, resulting in a high PCE (>30%) of 4T tandem solar cell.
The flexible, semi-transparent, and inverted perovskite solar cells with an area of 0.1 cm2 is fabricated, where its structure is composed of flexible substrate/ITO/thin transparent conductive oxide (TCO)/hole transport layer (HTL)/passivation layer/perovskite absorber/electron transport layer/buffer layer/TCO. Here, hole-collecting monolayer material was used as HTL. In this work, flexible substrate was used for a flexible and semi-transparent perovskite solar cell. However, the sheet resistance of flexible substrate is too high to obtain high fill factor (FF). Consequently, thin TCO was proposed and added on flexible substrate to act as the layer adjusting the sheet resistance of the substrate to increase FF.
In the result, the sheet resistance was decreased by the insertion of a thin TCO on the flexible substrate, thus increasing FF of the device. High transmittance of the flexible and semi-transparent perovskite solar cells with a thin TCO is still realized with average transmittance in a wavelength region from 800 nm to 1100 nm (Averaged T800-1100 nm) of 80.9% in Figure (a). The high PCE of 22.4% (VOC of 1.18 V, JSC of 23.1 mA/cm2, and FF of 0.82) for the flexible and semi-transparent perovskite solar cell is demonstrated with high Averaged T800-1100 nm of 80.9% in the infrared, which is suitable as top cell of 4T tandem. Under a semi-transparent perovskite cell filter, which has all the layers similar to the active semi-transparent perovskite cell, the PCE of the Si HIT bottom cell under the perovskite filter is 8.0%. Ultimately, a PCE of 30.4% for the 4T perovskite/Si tandem solar cell is achieved, as shown in Figure (b) and Table. This is the first report to use the flexible and semi-transparent perovskite top cell and realize the high PCE of over 30% for 4T perovskite/Si tandem solar cell, promising for lightweight flexible PV modules.
4+5-O1
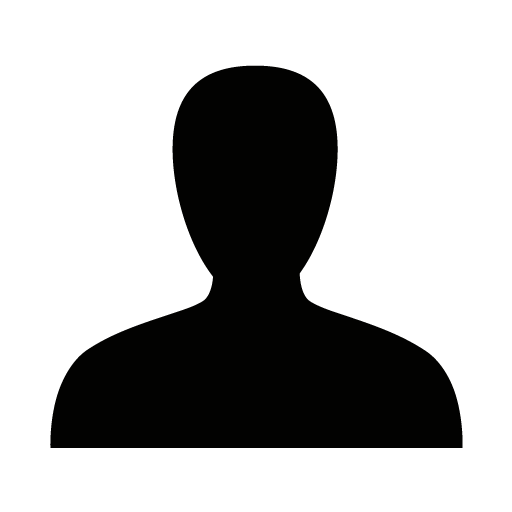
CsPbBr3 single crystal X-ray and gamma-ray detectors match state-of-the-art performance with a few exceptions, particularly the detection speed is insufficient for photon counting medical imaging. The slow perovskite response is intrinsically limited by the low charge carrier mobility (µ), reported in CsPbBr3 to be 10-30 times smaller than that of CdTe. By using Time of Flight (ToF) technique with alternative voltage biasing (AC) mode CsPbBr3 crystals grown by the modified Bridgman method demonstrated two component hole mobility with median high mobility of 300 cm2V-1s-1 (while the best achieved - 520 cm2V-1s-1) and low mobility of 30 cm2V-1s-1. The fast component is quenched due to ionic migration and the reaction of the Bi electrode with Br- ions, while applying DC bias mode, commonly used in perovskite hard radiation detectors. Additionally, we examine the influence of temperature on charge carrier mobility. The reported mobility is the highest among lead-based perovskites and shows promise for applications with fast response requirements.
4+5-O2
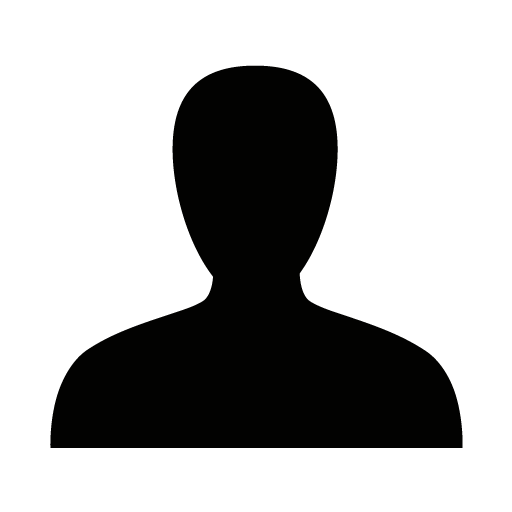
All-inorganic CsPbBr3 perovskite solar cells (PSCs) have recently drawn significant attention due to their superior thermal stability. However, fabricating high-quality CsPbBr3 films through conventional one-step solution processing is quite challenging because of the poor solubility of CsBr in polar solvents. To overcome this limitation, we developed a Thiourea (T) -assisted one-step spin deposition method to enhance the crystallinity, phase purity, and grain size of CsPbBr3 films. By incorporating different amounts of Thiourea (T-10/T-20/T-30/T-40/T-50 mg/mL) into the precursor solution, T-30 led to a remarkable enhancement in power conversion efficiency (PCE), increasing it from 3.25% to 5.18%, representing a 59.4% improvement. This improvement is attributed to enhanced crystallinity and the reduction of trap states in the perovskite layer.
The optimized device architecture (FTO/c-TiO2/m-TiO2/CsPbBr3/Spiro/Au) with T-30 showed a JSC of 10.77 mA/cm2, a VOC of 0.70 V, and a fill factor (FF) of 0.68. XRD analysis revealed pronounced diffraction peaks at 15.6°, 22.08°, and 31.15°, corresponding to the CsPbBr3 phase, with the high intense peaks for the T-30 film, particularly at the (100) and (200) lattice orientations. Scanning electron microscopy (SEM) images demonstrated that Thiourea facilitates the formation of larger perovskite grains, resulting in compact films with minimal defects and smooth surfaces. UV-visible spectroscopy showed that the T-30 film exhibited the highest absorbance and a bandgap (Eg) of 2.3 eV. Photoluminescence (PL) measurements also indicated increased peak intensity, confirming reduced recombination. FTIR analysis revealed a shift in the C=S stretching vibration from 738 cm-1 to 745 cm-1, indicating strong interactions between Thiourea and PbBr2. The quantum efficiency (QE) spectra further supported the enhanced photocurrent response in the T-30 device. Importantly, the unencapsulated device maintained over 90% of its initial efficiency after 15 days under ambient conditions. This Thiourea-assisted strategy presents a promising route for fabricating stable, high-performance inorganic PSCs.
4+5-O3
Nuclear batteries present an appealing energy source characterized by an exceptionally long operational lifespan and a remarkable energy density, finding prominent application for equipment requiring sustained, autonomous operation over protracted durations, encompassing spacecraft, cardiac pacemakers, subaqueous systems and automated scientific stations. Except of radiation safety issues, the primary constraint hindering the extensive adoption of nuclear batteries is attributed to the limited efficiency and intricate fabrication processes of energy-conversion materials. Most widespread nuclear batteries are radioisotope thermoelectric generators which exhibit an energy conversion efficiency of merely 5%. In the quest for augmented efficiency, research endeavors have shifted towards direct conversion of gamma and beta radiation through radiovoltaic cells, where the best efficiency is so far achieved with costly and non-scalable diamond battery technology. In this study, we address the issue of efficiency, reporting 10% power conversion efficiency for the direct conversion of high-energy photons up to 20 keV with solution-grown methylamonium-formamidinium lead iodide (MAFAPbI3) perovskite single-crystal radiovoltaic cells. Our experimental investigation determined the electron-hole pair creation energy for MAFAPbI3 single crystals to be 5.05 eV, substantiating that the short-circuit current closely approaches the theoretical upper limit, as defined by the Klein model. Additionally, the devices exhibited a high open-circuit voltage of 600 mV even under low irradiation power density conditions of 69 nW mm-2 . Furthermore, the perovskite radiovoltaic cells demonstrated remarkable stability, maintaining their performance for a continuous 24-hour X-ray irradiation at a power density of 100 nW mm-2. These findings underscore the promising potential of highly efficient and low-cost perovskite-based nuclear batteries.
4+5-O4
Perovskite solar cells (PSCs) have experienced significant global advancements, and are at a pivotal point in the scale-up from laboratory to factory. However, there is limited understanding of the harmful reactions at the heterointerfaces between charge transport layers (CTLs) and the perovskite absorber, which are affecting both the initial PCE and the long-term stability of PSCs. Meanwhile, along with progress of self-assembled monolayers (SAMs), now the bilayer structure of SAM/metal-oxide is a promising industrial CTL architecture for achieving high-performance PSCs. Considering the wide temperature fluctuations in real-world settings, striking a balance between robust mechanical bonding and preventing adverse degradation reactions at the perovskite/CTL interface is especially important for the longevity of these PSCs. However, achieving thermal cycling stability according to IEC/ISOS standard remains a challenge among most efficient and state-of-the-art PSCs. Finding ways to enhance solar cell stability with high efficiency is crucial for transitioning perovskite technology from laboratory research to commercial fabrication.
Our research provides new insights into these critical chemical and mechanical interactions that undermine the long-term stability of perovskite solar cells (PSCs). For the first time, we have developed a balanced strategy that achieves high efficiency while also meeting or exceeding industrial standards for stability. The main findings and contributions of our study include:
1. Implementation of an innovative bonding-debonding technique to universally examine interfacial interactions and degradation mechanisms at perovskite/CTL heterointerfaces, contributing to a deeper understanding of PSC fabrication and aging processes.
2. Establishment of a direct correlation between interface bonding strength, interfacial proton-transfer interactions, and the degradation processes of perovskite/metal-oxide heterointerfaces.
3. Introduction of the 'competing anchoring concept' to enhance interface bonding and mitigate adverse reactions, leading to optimized PSCs (D4P/NiOx-based) with a PCE of up to 25.6%, 1.3% absolutely higher than conventional Me-4PACz/NiOx-based PSCs.
4. We emphasize our use of an extended dwell time (30 minutes) in thermal cycling tests, a method that exceeds IEC 61215 standard (ISOS-T-3I) practices and effectively simulates real industrial conditions. Our device, with a longevity of over 500 cycles (T95 > 500 cycles), stands out as the best example of a perovskite device that optimally balances PCE with durability. Furthermore, it has demonstrated exceptional resilience in a variety of standard photo- and thermal-related stability tests according to ISOS protocols.
Kihada-I1
Tsutomu (Tom) Miyasaka received his Doctor of Engineering from The University of Tokyo in 1981. He joined Fuji Photo Film, Co., conducting R&Ds on high sensitivity photographic materials, lithium-ion secondary batteries, and design of an artificial photoreceptor, all of which relate to electrochemistry and photochemistry. In 2001, he moved to Toin University of Yokohama (TUY), Japan, as professor in Graduate School of Engineering to continue photoelectrochemistry. In 2006 to 2009 he was the dean of the Graduate School. In 2004 he has established a TUY-based company, Peccell Technologies, serving as CEO. In 2005 to 2010 he served as a guest professor at The University of Tokyo.
His research has been focused to light to electric energy conversion involving photochemical processes by enhancing rectified charge transfer at photo-functional interfaces of semiconductor electrodes. He has contributed to the design of low-temperature solution-printing process for fabrication of dye-sensitized solar cells and solid-state hybrid photovoltaic (PV) cells. Since the discovery of the organic inorganic hybrid perovskite as PV material in 2006 and fabrication of high efficiency PV device in 2012, his research has moved to R&Ds of the lead halide perovskite PV device. He has promoted the research field of perovskite photovoltaics by organizing international conferences and by publishing many papers on enhancement of PV efficiency and durability, overall citation number of which is reaching more than 5,000 times. In 2009 he was awarded a Ministry of Science & Education prize on his achievements of green sustainable solar cell technology. In 2017 he received Chemical Society of Japan (CSJ) Award. He is presently directing national research projects funded by Japan Science and Technology Agency (JST) and Japan Aerospace Exploration Agency (JAXA).
Achieving power conversion efficiency (PCE) of 26%, the current R&Ds of perovskite solar cells (PSCs) have focused on durability improvement by interfacial engineering using organic molecules, for defect passivation at grain boundaries and heterojunction interfaces in PSCs.1 Our recent study also focuses on the method of interfacial passivation with polarizable organic molecules enable high voltage output (close to theoretical limit) in photovoltaic performance.2 In compositional engineering, all-inorganic perovskites, which have high thermal stability, are targets of interfacial modification. CsPbX3 (X=I, Br) was combined with dopant-free polymer hole transport material (HTMs) with high thermal stability.2 By modifying the interface of electron transport material (ETM, SnO2) and CsPbI2Br (bandgap 1.9eV) with an amorphous SnOx layer (<5 nm), PSC achieved high Voc over 1.4V with PCEs of >17%. Under indoor LED illumination, the device works at PCE >34% being supported by high Voc >1.2V. Further, passivation of iodine defects with 2,5-thiophenedicarboxylic acid (TDCA) achieved Voc of 1.54V for CsPbIB2.3 These studies demonstrate that the development of heat-resistant all-inorganic PSCs is promising through proper modification of the heterojunction interface. Interfacial engineering is a more serious challenge for lead-free perovskites which undergo a large recombination loss in voltage generation. Among lead-free compositions, Ag-Bi iodide materials are environmentally benign solution-processible materials and highly stable against heat and moisture (H2O). By introducing an SnOx layer on a surface of SnO2 ETM, a device using perovskite-like Ruddorfite material, Ag2BiI5 (bandgap 1.88eV), was improved to have a high Voc close to 0.9V.4 Molecular passivation and modification of junction interfaces and grain surfaces are pivotal to ensure high Voc performance of all-inorganic lead-based and lead-free perovskite materials.
Kihada-K1
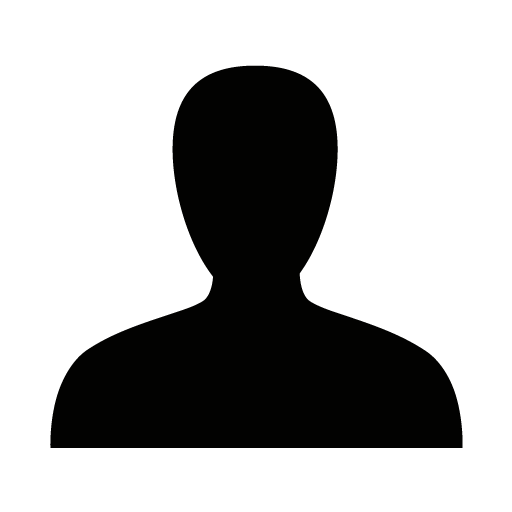
Tin-based perovskite solar cells (lead-free tin perovskite solar cells and tin-lead alloyed perovskite solar cells) follow the lead perovskite solar cell. The research trends of these tin-based perovskite solar cell are reviewed. The efficiency of the lead-free tin perovskite solar cells and that of tin-lead alloyed provskite soalr cells are over 15-16% and 23-24%, respectively. By suppressing the Sn4+ concentration, decreasing the defect density by the surface passivation, decreasing the band-offset of the conduction bands and the balence bands, relaxing the lattice strain and so on, these efficiencies have been enhanced. We have reported 15.3% efficiency of lead-free tin perovskie solar cells and 23.3% efficiency of tin-lead alloyed perovskite solar cells by using Ge 2+ addition, the passivation with ethylene diamine, the direct deposition of tin-metal, the incorporation of inorganic layers, the introduction of spike band structure and mixed SAM, and A site engineering relaxing the lattice strain1,2). The interface between the tin perovskite layer and these inorganic nanoparticles are discussed from the view point of charge transfers increasing carrier densities under dark and decreasing photovoltaic performances3). Thermal stability of the thin-lead alloyed perovskite solar cells (85 ℃, 1000 h) was improved by covering the grain-boundary with GeOx and inserting the SnOx layers in the electron transporting layer4). The importance of the position of inserting ALD SnOx is discussed, together with modified electron-transporting layer enhanceing the thermal stability. All-perovskite tandem solar cells are composed of the wide bandgap lead-perovskite solar cells, the narrow bandgap tin-lead alloyed perovskite solar cells and the interconnecting layer. The efficiency of all-perovskite solar cells is now over 30%. We have reported 26-27% efficiency5, 6). The research focused on the interconnecting layer for enhancing the efficiency are reviewed.