#H2Future25-I1
Sanjay Mathur is a Chair Professor and Director of the Institute of Inorganic Chemistry at the University of Cologne in Germany. He is also the Director of the Institute of Renewable Energy Sources at the Xian Jiao Tong University, Xian, China and a World Class University Professor at the Chonbuk University in Korea. He is a Visiting Professor in the Institute of Global Innovation Research at TUAT, Japan and a SPARC Faculty at IIT Madras, India. His research interests focus on application of nanomaterials and advanced ceramics for energy technologies. He holds 11 patents and has authored/ co-authored over 500 original research publications (h index, 63) and has edited several books. He serves as the Editor for Journal of Electroceramics, and for NanoEnergy. He is an Academician of the World Academy of Ceramics and Fellow of the American Ceramic Society and ASM International. He was awarded the Honorary Doctorate of the Vilnius University in 2016. He is an Academician of the World Academy of Ceramics and Fellow of the American Ceramic Society. Since 2018, he chairs the Academic Affairs Committee of the Materials Research Society. He was awarded the R C Mehrotra Lifetime Achievement Award of Indian Science Congress Association in January 2020. He is the President-elect of the American Ceramic Society. He was elected to the European Academy of Science in 2020.
Advanced materials are driving innovation across all fields of technology, ranging from construction and mechanical engineering, automotive and electromobility, to medical technology, energy storage and conversion technologies, and microelectronics. Given their technological impact, functional materials represent an essential segment of industrial technologies with significant value creation potential for both established markets and emerging technologies. Especially in the context of sustainable production techniques, substitution of critical raw materials, and energy- and resource-efficient manufacturing, tailored surfaces and interfaces are gaining increasing importance in the future. In this context, chemical processing of nanostructured ceramics in tuning the functional and interfacial properties for better charge transport, higher corrosion protection and enhanced performance. The examples will include the role of functionalized inorganic surfaces in electrolysers for hydrogen production, and advances in photon-harvesting technologies for perovskite-based photovoltaics. This talk will emphasize the power of chemical synthesis in designing new materials for energy transition.
#H2Future25-O1
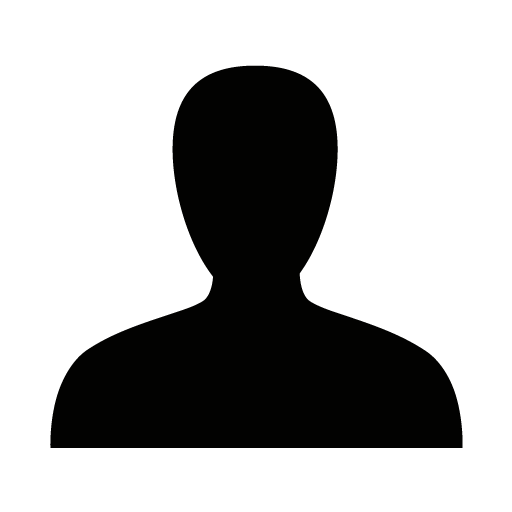
Metal oxide-based photoelectrodes for solar water splitting often utilize nanostructures to increase the solid-liquid interface area. This reduces charge transport distances and increases the photocurrent for materials with short minority charge carrier diffusion lengths. While nanostructuring is well established, the effect of surface order on the photocurrent and carrier recombination has not yet received much attention in the literature. To evaluate the impact of pore ordering on the photoelectrochemical properties, mesoporous CuFe2O4 (CFO) thin film photoanodes were prepared by dip-coating and soft-templating. Here, the pore order and geometry can be controlled by addition of copolymer surfactants poly(ethylene oxide)-block-poly(propylene oxide)-block-poly(ethylene oxide) (Pluronicâ F-127), polyisobutylene-block-poly(ethyleneoxide) (PIB-PEO) and poly(ethylene-co-butylene)-block-poly(ethylene oxide) (Kraton liquidTM-PEO, KLE). The non-ordered CFO showed the highest photocurrent density of 0.2 mA/cm2 at 1.3 V vs. RHE for sulfite oxidation, but the least photocurrent density for water oxidation. Conversely, the ordered CFO present the best photoelectrochemical water oxidation performance. These differences can be understood on the basis of the high surface area which promotes hole transfer to sulfite (a fast hole acceptor), but retards oxidation of water (a slow hole acceptor) due to electron-hole recombination at the defective surface. This interpretation is confirmed by intensity-modulated photocurrent (IMPS) and vibrating Kelvin probe surface photovoltage spectroscopy (VKP-SPS). The lowest surface recombination rate is observed for the ordered KLE-based mesoporous CFO, which retain spherical pore shapes at the surface resulting in fewer surface defects. Overall, this work shows that the photoelectrochemical energy conversion efficiency of copper ferrite thin films is not just controlled by the surface area, but also by surface order. These findings will be of importance for the fabrication of improved metal oxide photoelectrodes and address the issue that nanostructuring of photoanodes with low minority charge carrier diffusion lengths are not generally beneficial for solar water oxidation.
#H2Future25-O2
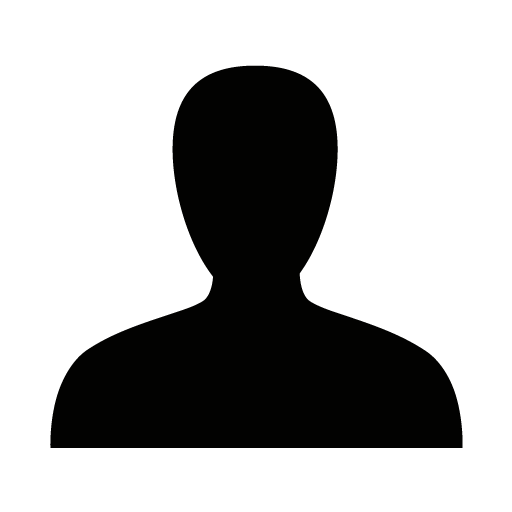
The development of efficient and stable photocathodes is crucial for advancing solar energy harvesting and sustainable chemical fuel production. In this context, selenium (Se) emerges as a promising material due to its favorable band alignment for hydrogen evolution reaction (HER), intrinsic p-type conductivity, and compatibility with scalable fabrication techniques such as co-evaporation. Additionally, Se offers advantages such as relative abundance, low toxicity, and tunable optoelectronic properties. Despite being the first photovoltaic material demonstrated in 1883, Se-based photoelectrochemical (PEC) devices remain largely underexplored, with significant potential for optimization.
This study presents a comprehensive investigation into Se-based photocathodes, focusing on optimizing key parameters to enhance activity, efficiency, stability, and sustainability. The effects of Se crystallization and thickness are systematically examined to balance photon absorption and charge extraction. Additionally, protective layers are studied to improve charge tunneling and overall device performance. Different back contacts and electrolyte formulations are explored to minimize series resistance and accelerate reaction kinetics.
Through these optimizations, this work achieves both the highest-ever efficiency and photocurrent density for a Se-based photocathode, setting a new benchmark in the field. Notably, these performances were achieved without the use of expensive nanoparticles, marking a significant step toward cost-effective and scalable PEC technology. Specifically, an all-time highest HC-STH efficiency of 2.76% and a photocurrent density of 11.35 mA/cm² at 0V(RHE) were obtained using SLG/Mo/Se-based devices (previous literature benchmarks were 0.39% efficiency and J of 7.2mA/cm² at 0V(RHE) [1]). Further advancing the concept of sustainability and reduced toxicity, similar results were achieved in a H₂SO₄-free system, with SLG/Mo/Se/TiO₂ devices in phosphate buffer electrolyte, enhancing both environmental sustainability and device safety.
These findings provide deeper insight into selenium-based photocathodes and highlight their potential for scalable PEC applications. By improving not only performance but also material sustainability and device safety, this study aligns with ongoing efforts to develop next-generation inorganic materials for solar-driven fuel production.
#H2Future25-I2
During my lecture, I will present examples of combining molecular solar cells with high voltage and suitable photocurrent with several catalysts to produce hydrogen catalysis. Combining materials is key to achieving sufficient current and voltage to drive the catalysis. SAMs (Self Assembled Molecules) are key to achieving the desired device performance. The stability of the catalysts under operation conditions is also evaluated.
In this work, a single perovskite solar cell is coupled to a 2-electrode electrochemical cell employing hybrid electroanodes functionalized with Ruthenium-based molecular catalysts. This study is the first illustration of a heterogenised anode used in a photovoltaic-electrochemical (PV-EC) application. The stability of the perovskite cell combined with the tunability of molecular catalysts is a promising strategy for PV-EC applications in ammonia conversion.
Ammonia is an attractive fuel due to its relatively high energy density and very low flammability. It is easy to liquefy and can, therefore, be transported through an existing global logistics infrastructure. Additionally, ammonia is compelling as it is a CO2-free and environmentally friendly fuel. Consequently, catalysts capable of efficiently and selectively performing the ammonia oxidation reaction are required to design useful technological devices. Solar-driven ammonia splitting offers a pathway to distributed hydrogen with minimal energy inputs.1
#H2Future25-I1
The transition toward sustainable energy systems is increasingly driven by innovations in energy materials, which form the cornerstone of modern energy conversion, storage, and harvesting technologies. This talk will provide an overview of recent advances in energy materials research, highlighting breakthroughs in catalyst design, semiconductor development, and nanostructured hybrid systems. We will discuss how these innovations contribute to enhanced performance and durability across the next-generation solar fuels/chemistry technologies.
Solar driven technologies represent a promising strategy for converting solar energy directly into chemical fuels, thereby offering a sustainable alternative to fossil fuels. We will explore the latest developments catalyst engineering that are driving progress in solar-to-chemical energy conversion. The search for new photoactive materials able to efficiently produce solar fuels is a matter of growing interest due to the current global energetic crisis. In response to this situation, the generation of solar fuels has appeared as a sustainable alternative. In this way, photo(elecro) catalytic conversion of H2O and biomass derived products is an interesting route to produce fuels and chemicals. Despite the advances that have been made in this line, it is still necessary to develop new materials and cell configurations to take this technology to a higher scientific level.
Herein, we report different strategies and modifications of photo(electro) catalysts to increase process performance. Among them, an interesting approach to improve charge separation in photocatalytic systems is the use of heterojunctions. In this line, the combination of different semiconductors with noble metal nanoparticles or organic semiconducting polymers leads to a separation of the photogenerated charge carriers to increasing their life time, facilitating charge transfer to adsorbed molecules.
In this way, organo-inorganic hybrid materials show interesting in solar fuels productions. In our studies, conjugated porous polymers (CPPs), COFs and MOFs [1-5], have been shown to interesting photocatalytic properties [3-4] with dramatic reactivity improvement in solar fuels production. However, reaction pathways are not well defined for this reaction and several uncertain are still unsolved. To explain this behavior a combination of in-situ NAP-XPS, FTIR, TAS spectroscopies and theoretical tools has been used, showing a more efficient light absorption and charge transfer in the hybrid photocatalyst compared with bare materials.
#H2Future25-O1
Francisco Fabregat Santiago (B.Sc. in Physics at Universitat de Valencia and University of Leeds in 1995 , Ph.D. from Universitat Jaume I in 2001) joined Universitat Jaume I in 1998 where he is currently full Professor at Physics Department and active member Institute of Advanced Materials (INAM). Among others he made several research stays at Uppsala University, Imperial College, École Polytechnique Fédérale de Lausanne. He authored more than 100 peer reviewed papers and 5 book chapters, that accumulate more than 11000 cites with an h-index of 54. Prof. Fabregat-Santiago is an expert in electro-optical characterization of devices and particularly known by his works in the use of the impedance spectroscopy to model, analyze and interpret the electrical characteristics (charge accumulation, transfer reactions and transport) of films and devices including ZnO and TiO2 nanostructured films (nanocolloids, nanorods and nanotubes), dye sensitized solar cells, perovskite solar cells, electrochromic materials and liquid and solid state hole conductors. His current interests are focused in the in the analysis of the fundamental properties of nano and bio materials for their application in solar cells, water decontamination, bio-energy, sensors and in the (photo)electrochemical production of added value chemicals.
Electrochemical conversion of biomass into added value products is gaining interest in the scientific and industrial community as it offers a real alternative path to avoid the use of fossil fuels as the base of the chemical industry. Thus, either through reduction or oxidation of biomass derivatives it is possible to obtain chemical building blocks of interest for the industry.[1, 2]. To optimise these reactions, it is needed to understand the mechanisms that control the electrochemical process. Impedance spectroscopy is a technique that has been extensively used for the characterization and modeling of the physico-chemial properties of materials and electrodes. In this work we will focus on the analysis of capacitance to unveil the mechanisms of oxidation and reduction of hydroxymethyl furfural and the reduction of nitrobenzene.[3-5] Our results highlight the importance of the coverage of the electrode by the substrate and also shows the activation of the surface absorption and charge transfer, which are key to maximize the performance of these reactions. Finnally we will combine the oxidation and reduction reactions, including hydrogen production, to show their sustainability in industrial processes.
#H2Future25-O2
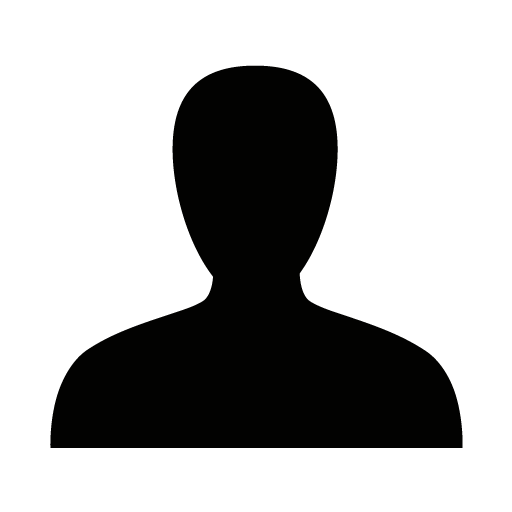
Revolutionizing Water Electrolysis: A Membraneless Approach for Hydrogen
Abstract
Electrochemical hydrogen production from water and renewable energy is promising for decarbonization, but traditional alkaline electrolyzers face efficiency issues, while proton exchange membrane electrolyzers have high material and operating costs. Herein, we design a membraneless electrolyzer leveraging Tesla valve geometry and fluidic forces for efficient hydrogen generation, offering a cost-effective alternative to traditional membrane-based electrolyzers. The Tesla valve-based electrolyzer operates at a 30 mL h⁻¹ flow rate and 300 mA cm⁻² current density. this is the lowest pumping power reported in the literature for the mentioned current density. Computational fluid dynamics (CFD) simulation validates its design, indicating how diodicty optimizes bubble separation and minimizes gas crossover. Notably, by utilizing the diodicity effect, the hydrogen production frequency increases by an average of 13% when the cathode is positioned in the lower channel. This work highlights the potential of integrating electrolyzer geometries and fluidic forces to enhance water splitting, paving the way for sustainable hydrogen production.
#H2Future25-I2
Carolina Gimbert Suriñach obtained her PhD from the Autonomous University of Barcelona (UAB) under the supervision of Prof. A. Vallribera, working on the development of organocatalytic processes. After one year as assistant professor at the same university, she moved to the University of New South Wales (UNSW) to undertake postdoctoral research in the field of bioinorganic chemistry with Prof. S. B. Colbran. Afterwards, she started a second postdoctoral position at the Institute of Chemical Research of Catalonia (ICIQ) in Prof. A. Llobet group. During this time, she developed hydrogen evolution and water oxidation (photo)catalytic systems. Three years later she was promoted to scientific group coordinator in the same group and her research focused on implementing molecular catalysis into water splitting devices. After a short stay at the University of Barcelona (UB) as Serra Húnter professor, she moved back to the Chemistry Department of UAB as Ramón y Cajal fellow and CatSyNanoMat Group co-leader, where she recently promoted to Associate Professor. Her scientific interests are in the field of photocatalysis as well as organic and hybrid materials with application to artificial photosynthesis.
Light induced water splitting (Li-WS) is an attractive way of generating green hydrogen that can be achieved by using devices with different configurations. One example is the straightforward PV-EC design, composed of a photovoltaic cell (PV) connected to an electrolyser (EC), in which light harvesting is separated from the electrolyte chamber where the chemical reactions take place. When more compact designs are envisioned, the process becomes more challenging involving the synchronization of several key phenomena and processes including light absorption, charge separation and chemical bond breakage and formation. Two chemical reactions are to be considered when dealing with Li-WS, namely the hydrogen evolution reaction (HER) and the oxygen evolution reaction (OER) that are usually triggered by hydrogen evolution catalysts (HEC) and water oxidation catalysts (WOC), respectively. This presentation will take you on a journey across key HEC and WOC catalytic centers in Li-WS, emphasizing their mechanistic pathways and interactions with conducting supports and semiconductor materials of different nature, from graphitic materials to inorganic and organic semiconductors.
#H2Future-I1
Sophia Haussener is a Professor heading the Laboratory of Renewable Energy Science and Engineering at the Ecole Polytechnique Federale de Lausanne (EPFL). Her current research is focused on providing design guidelines for thermal, thermochemical, and photoelectrochemical energy conversion reactors through multi-physics modelling and experimentation. Her research interests include: thermal sciences, fluid dynamics, charge transfer, electro-magnetism, and thermo/electro/photochemistry in complex multi-phase media on multiple scales. She received her MSc (2007) and PhD (2010) in Mechanical Engineering from ETH Zurich. She was a postdoctoral researcher at the Joint Center of Artificial Photosynthesis (JCAP) and the Lawrence Berkeley National Laboratory (LBNL) between 2011 and 2012. She has published over 70 articles in peer-reviewed journals and conference proceedings, and 2 books. She has been awarded the ETH medal (2011), the Dimitris N. Chorafas Foundation award (2011), the ABB Forschungspreis (2012), the Prix Zonta (2015), the Global Change Award (2017), and the Raymond Viskanta Award (2019), and is a recipient of a Starting Grant of the Swiss National Science Foundation (2014).
The design and implementation of complete, operational photo-electrochemical devices requires a careful consideration of material requirements and their interplay in an integrated device. The operation of the devices usually is based on constant-intensity simulated solar irradiation. A variety of photo-electrochemical device designs exists and operated at constant intensity for extended time (ten to hundreds of hours). They have achieved competitive efficiencies, but improvement and innovation is still needed for practical implementation and economic competitiveness. Multi-physical, multi-dimensional and multi-scale modeling tools are essential for the investigation of feasibility, optimization and implementation of such devices. Such models can help to come up with novel design ideas and alternative operational scenarios. I will discuss unconventional reactor designs for photocatalytic solar fuel generation based on micro-droplets and show unconventional photo-electrochemical reactor operation, based on reversible operation, pulsed operation and grid-electricity-supported operation. I will show what these innovations bring to the table and how they can help making solar fuels a more competitive technology.
#H2Future-I2
Sixto Giménez (M. Sc. Physics 1996, Ph. D. Physics 2002) is Associate Professor at Universitat Jaume I de Castelló (Spain). His professional career has been focused on the study of micro and nanostructured materials for different applications spanning from structural components to optoelectronic devices. During his PhD thesis at the University of Navarra, he studied the relationship between processing of metallic and ceramic powders, their sintering behavior and mechanical properties. He took a Post-Doc position at the Katholiek Universiteit Leuven where he focused on the development of non-destructive and in-situ characterization techniques of the sintering behavior of metallic porous materials. In January 2008, he joined the Group of Photovoltaic and Optoelectronic Devices of University Jaume I where he is involved in the development of new concepts for photovoltaic and photoelectrochemical devices based on nanoscaled materials, particularly studying the optoelectronic and electrochemical responses of the devices by electrical impedance spectroscopy. He has co-authored more than 80 scientific papers in international journals and has received more than 5000 citations. His current h-index is 31.
The energy crisis and climate change are among the most pressing challenges facing society today. To address the global warming caused by human activities, it is essential to transition from fossil fuels to clean, renewable energy sources like solar and wind. (Photo)electrocatalysis is considered as a promising technology to decarbonize key sectors as energy, industry and transport, providing sustainable energy vectors and added-value chemicals, with low environmental impact. In the present talk, we will focus on BiVO4 photoanodes, which is a very attractive materials for photoelectrochemical oxidation, given its relatively Earth-abundant constituent elements, robust stability, favorable band structure for efficient charge separation and low processing cost. Yet it suffers from slow reaction rates for water oxidation, rapid recombination of photo-generated electron-hole pairs, coupled with poor charge carrier mobility and short diffusion lengths (~70 nm) of the carriers optical properties.
With this overall context, we will discuss on recent studies at our lab, aimed at maximizing the performance of this material by different heterostructing strategies, like deposition of organic hole transport layers and catalytic materials, which lead to excellent photoactivity and stability. Furthermore, scalable solutions can be obtained by using a flow-synthetic approach, achieving photoelectrode sizes of 50 cm2, which achieve state-of-the-art performance. A central focus of our work is the fundamental understanding of the processes that govern device operation, and we will show how we approach carrier dynamics by a comprehensive suite of spectroscopic tools to probe the underlying operation mechanisms.
#H2Future-O1
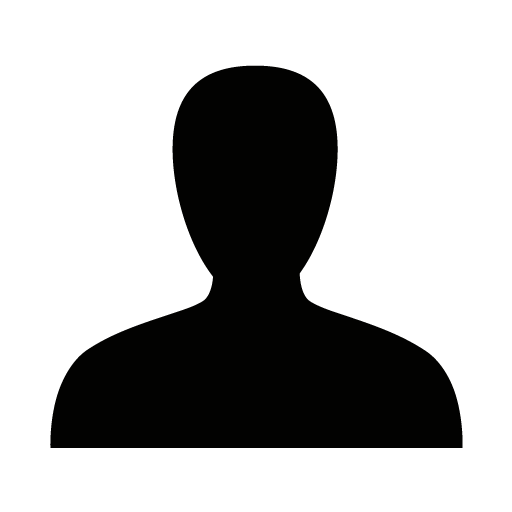
The production of H2 from renewable sources, ideally water and organic molecules coming from waste resources, is one of biggest challenge that the scientific community is facing nowadays. Implementing a catalytic protocol for H2 production is an attractive strategy for increasing efficiency and scalability and reducing energy demand.
In our group we are developing catalysts for hydrogen evolution reaction [1] and reactions strictly related to H2 production, such as water oxidation [2], dehydrogenation of formic acid [3], regeneration of the “natural form” of hydrogen, i.e. NADH [4], dehydrogenation of platform molecules, which are wastes or by-products of industrial processes [5]. We mainly focus on single-site catalytic systems of different nature including, homogeneous, heterogenized and heterogenous [6].
In this contribution, our recent results concerning with the rational development of iridium organometallic (electro-)catalysts for NADH regeneration approaching the performance of enzymes will be reported. Furthermore, it will be shown how decorating organoiridium catalysts with moieties that mimic the functional group of the substrate to activate, is a successful strategy to selectively dehydrogenate glycerol to produce renewable H2 and lactate. Finally, some examples of the utilization of layered materials as catalysts for electrolytic water oxidation will be presented.
#H2Future-I3
Palladium supported on ceria (Pd/CeO2) has recently raised strong interest as an alternative catalyst to platinum on the anode electrode in anion exchange membrane fuel cells1,2. The enhanced activity of Pd/CeO2 catalysts for hydrogen oxidation reaction (HOR) activity in alkaline media has notably been attributed to strong metal-support interactions, but the exact mechanism is still under debate.
In this work, we investigate Pd/CeO2 thin films with well-defined compositions and structures to gain fundamental understanding of the metal-support interface and its influence on HOR activity in alkaline media. We develop an approach combining in situ electrochemical quartz crystal microbalance (E- QCM), ex situ microscopy and spectroscopy, and theoretical calculations to describe the interface between Pd and CeO2.
Pd/CeO2 leads to expected enhancement of HOR in alkaline media compared to pure Pd (Fig 1). Using E-QCM, we provide new fundamental understanding of the adsorption, absorption, physisorption and desorption phenomena occurring during HOR. We show that on pure Pd thin films, absorption of hydrogen dominates while on Pd/CeO2 the oxidation/reduction of ceria and adsorption of hydroxyl groups are the dominating phenomena (Fig 1)3. One of the hypotheses for enhanced reactivity of Pd/CeO2 in HOR is that CeO2 – at the interface with Pd - serves as a source of OH groups which react with adsorbed H to form water4. In this work, we provide new quantitative evidence that this hypothesis is valid. Strong metal-support interactions are also confirmed by TEM, XPS and DFT calculations. An important finding is that Pd atoms embedded in CeO2 are present on the prepared thin films and could be active sites for hydrogen activation.
This work provides strong evidence that the interface between Pd and ceria must be carefully designed to provide synergies and to lead to enhanced reactivity in HOR. Finally, this study underlines the importance of model thin films as appropriate tools to study the interface between metal and supports in situ, during electrochemical reactions.
#H2Future25-I1
Green hydrogen is expected to become a major pillar for the decarbonization of the energy and industrial landscapes. Most initiatives worldwide in this direction, from purely scientific projects to small size industrial pilot plants, aim to combine renewable energy with water electrolysis, are yielding thousands of results with promising results and open questions. Arguably, the major challenge remains to improve the efficiency of the processes while limiting costs and avoiding the use of critical raw materials. In addition, two divergent approaches are competing: centralized and decentralized. On the former, solar power would be transformed into electricity (by photovoltaic or wind farms) and then grid distributed for the production of (green) hydrogen from water electrolysis at industrial hubs.[1,2] On the later, the integration of solar energy capture and water splitting in a single device may offer significant advantages, although through higher complexity. In all cases, catalysis is crucial to speed-up the chemical reactions to proceed at industrially relevant conditions, while minimizing energy loses.[3,4]
In this talk, we will discuss some of the most representative achievements in water electrolysis for (green) hydrogen production, highlighting their advantages, and addressing their current limitations, if any. Many successful catalysts are based on noble metals or critical raw materials, far away from practical applications. When discussing green hydrogen as a plausible contribution to decarbonization, energy security and environmental mitigation plans, sustainability and scaling are additional constrains that must be taken into account. There are many hurdles between a promising lab-scale discovery and a technology, many of them involving economic and societal considerations.
We will address the different challenges in the development of cost and energy efficient processes, starting from the design of the electrocatalysts, and their validation in industrially-ready electrolyzer architectures. We will describe the major problems looking at the activity/stability paradigms, along the successful (or not) strategies that we are exploring to further improve their electrochemical performance: nanostructuration, doping effects, external magnetic fields, coordination frameworks, etc.
[1] Xia, R.; Overa, S.; Jiao, F. Emergung electrochemical processes to decarbonize the chemical industry. JACS Au 2022, 2, 1054–1070.
[2] Xu, Q.; Zhang, L.; Zhang, J.; Wang, J.; Hu, Y.; Jiang, H.; Li, C. Anion exchange membrane water electrolyzer: Electrode design, Lab-scale testing system and performance evaluation. EnergyChem 2022, 4, 100087.
[2] Romano, V.; D'Angelo, G.; Perathoner, S.; Centi, G. Current density in solar fuel technologies. Energy Environ. Sci. 2021, 14, 5760–5787.
[3] H. Nishiyama, H.; Yamada, T.; Nakabayashi, M.; Maehara, Y.; Yamaguchi, M.; Kuromiya, Y.; Nagatsuma, Y.; Tokudome, H.; Akiyama, S.; Watanabe, T.; Narushima, R.; Okunaka, S.; Shibata, N.; Takata, T.; Hisatomi, T.; Domen, K. Photocatalytic solar hydrogen production from water on a 100 m2 scale. Nature 2021, 598, 7880.
#H2Future25-O1
Water electrolysis is a promising approach for converting renewable energy into clean hydrogen fuel. However, its overall efficiency is hindered by the sluggish kinetics and high overpotential of the oxygen evolution reaction (OER). Traditionally, precious metal-based catalysts have been employed due to their high catalytic activity, but their high cost and limited long-term stability significantly restrict large-scale applications. In this context, MXenes have emerged as attractive alternatives, owing to their high electrical conductivity, large surface area, and abundance of active sites. For instance, Ti₃C₂Tx has demonstrated performance comparable to noble metal catalysts in hydrogen evolution reactions (HER). Nevertheless, MXenes face challenges related to long-term operational stability, primarily due to the tendency of their sheets to stack and agglomerate. To address these limitations, hybrid architectures that integrate MXenes with other materials have been developed. These composites enhance both the catalytic activity and structural stability, leading to improved performance in both OER and HER. Such strategies position MXenes as a cost-effective and sustainable alternative to traditional catalysts, opening new avenues for efficient hydrogen production via water electrolysis. In this context, metal doped Mo₂TiC₂ MXene was investigated as a multifunctional electrocatalyst for water splitting. The material was doped with 2.5 wt% of transition metals—Ni, Fe, and Co—to enhance its catalytic activity toward oxygen evolution reaction (OER). Comprehensive catalyst characterization was carried out using scanning electron microscopy (SEM), energy-dispersive X-ray spectroscopy (EDX), and X-ray diffraction (XRD) to confirm successful doping and to analyze the morphological and structural features. Electrochemical measurements were then performed to evaluate HER and OER performance, allowing a comparative analysis of the doped samples. The results demonstrated that metal doping improved electrocatalytic activity, highlighting the potential of metal doped Mo₂TiC₂ as an efficient catalyst for oxygen evolution reaction.
#H2Future25-O2
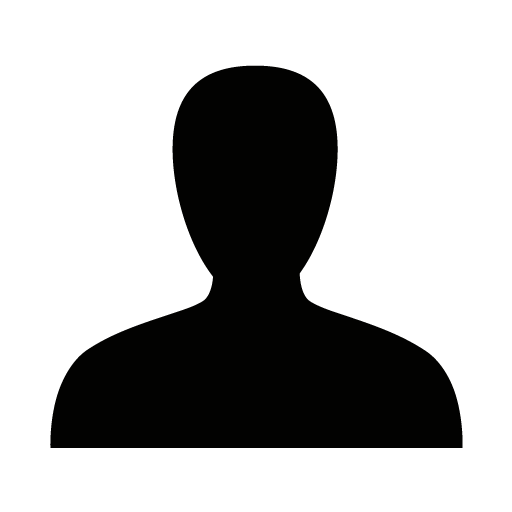
The global climate crisis requires immediate action to cut emissions and transition to sustainable energy sources. Water splitting for green hydrogen production offers a promising solution to decarbonize industries, strengthen energy security, and support the shift to a low-carbon economy. While much research has focused on the photoanodic side of photoelectrochemical cells, there remains significant potential to further develop high-performance and stable photocathode materials.1 Perovskite oxides have been identified as strong candidates for water splitting due to their inherent stability in air and aqueous solutions, along with their favourable bandgaps for visible light absorption. 2
This study explores the synthesis of a novel material, praseodymium iron oxide (PrFeO3), using a straightforward spin coating method and investigates the impact of calcium (Ca) doping on its photoelectrochemical performance as photocathodes.3
Polymer-templated sol-gel spin coating of PrFeO3 based materials produced thin films with uniform morphology and porosity, improving semiconductor/electrolyte interactions, as confirmed by scanning electron microscopy. Transient photocurrent response analysis revealed that doping PrFeO3 with 5 at% calcium (Ca) significantly boosted its photoelectrochemical activity, achieving a peak photocurrent of -124 µA cm⁻² at +0.43 VRHE under simulated sunlight. This enhancement also included an incident photon-to-current efficiency of 3.8% at +0.43 VRHE and 350 nm, along with an onset potential of +1.1 VRHE.3
These findings emphasize the critical role of dopants in enhancing the photocurrent performance of stable perovskite oxides, showcasing their potential to advance photon conversion technologies and, ultimately, green hydrogen production.
#H2Future25-I2
Water electrolysis enables sustainable alternatives to power large industries such as transport, manufacturing and agriculture through green hydrogen. These prospects rely on achieving sufficient performance metrics (e.g., energy efficiency, rate, and stability) in scalable processes. Conventionally, this has been pursued by innovation at the catalyst level, for example, designing materials with target physicochemical properties. This is challenging, since catalysts change substantially during reaction, which precludes a predictive catalyst design. On the other hand, while water is arguably the main ingredient in water electrolysis, its role at the catalyst environment and in the electrolyte remains underexplored.
I will first present a new strategy to program catalyst reconstruction. Starting from the same precatalyst, this can either lead to unpredictable composition and structure or enable predictable catalysts with improved reliability. I will then focus on the liquid-side of the reaction. I will show examples on how control over water at catalyst interfaces can enable achieving distinct physicochemical properties leading to improved stability and activity during water electrolysis.
#H2Future-I1
I will discuss recent advances in our lab with cuprous oxide for solar water splitting. I will mainly focus on thin film Cu2O but will touch briefly on particulate systems as well. The use of selective contacts enables our high quality thermally oxidized p-type Cu2O to be used as both a photocathode and a photoanode. For Cu2O photocathodes, we can achieve > 8 mA/cm2 at +0.2 V vs. RHE for the hydrogen evolution reaction, and for Cu2O photoanodes, we can achieve > 8 mA/cm2 at +1.1 V vs. RHE for the oxygen evolution reaction. Artificial leaf-type architectures will be presented that carry out one of the water splitting reactions coupled with a value-added reaction. We are translating our thin film architectures to particulate systems. We have combined Cu2O hydrogen evolving particles with oxygen evolving particles composed of other materials and we achieve overall water splitting with only light input, albeit with low efficiency and stability for now. An outlook on further development with this material will be presented.
#H2Future-T1
The main objective of a solar cell is the generation of energetically and physically separated electrons and holes. These charge carriers will recombine in the external circuit providing electrical power. This separation of charge carriers is achieved by incorporating charge-selective contacts, called Hole Transport Layer (HTL) and Electron Transport Layer (ETL) respectively. The use of HTL and ETL is already widely established in almost all solar cell technologies.
The principle of operation of a photocatalytic system dedicated to the generation of hydrogen can also be described in an analogous way to a photovoltaic solar cell. Despite this analogy, the use of HTL and ETL is not well established in the manufacture of photocatalytic systems.
In this presentation we will describe the parallelism between the operating principle of a solar cell and a photocatalytic system and we will show that the incorporation of selective contacts improves the efficiency of photocatalytic systems based on Titanium oxide.
#H2Future-T2
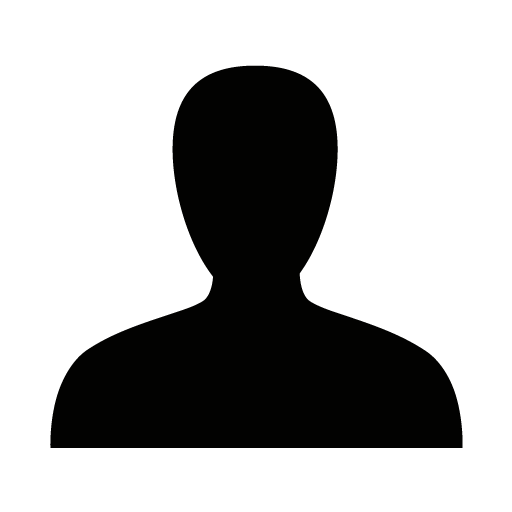
Generation of hydrogen through water splitting using visible-light irradiation in a photoelectrochemical cell (PEC) configuration has been a well-known topic since the 1970’s. Initially, inorganic semiconductor materials such as TiO2 were exploited[1,2] thanks to their suitable electronic configuration and stability under photochemical conditions. However, limitations in solar to hydrogen efficiency has hampered their implementation into high performing devices. On the other hand, organic semiconductors have more recently emerged as promising materials to be used in this field. Prominent examples of this family of semiconductors are 2D-covalent organic frameworks (COFs), which are reticular materials with high crystallinity and porosity.[3,4] They have been applied to photocatalysis including visible light-induced HER with great success.[5,6] One of the advantages of COF materials is their versatility to control chemical and electronic structure, allowing to rationally design better photocatalytic materials by enhancing solar light harvesting, charge separation, interaction with co-catalysts or dispersibility in water.[7-11]
In this work, we have developed a new catalytic system for the photogeneration of hydrogen, based on an enamine linked 2D-COF containing thiazolo[5,4-d]thiazole moieties together with isolated metallic co-catalysts and ascorbic acid as sacrificial electron donor (SED), which resulted in benchmarking values in terms of catalytic rate for hydrogen generation. The catalytic system offers significant advantages compared to the known strategies –which rely on the in-situ formation of the catalytic species–,since it allows to tune and change each component separately and study its effect independently of one another. In this line, we have used advanced spectroscopic techniques to ascertain key aspects of the charge transfer processes between the different components that allowed us to understand preferred mechanistic pathways and to push the photocatalysis performance to the limit.
#H2Future-T3
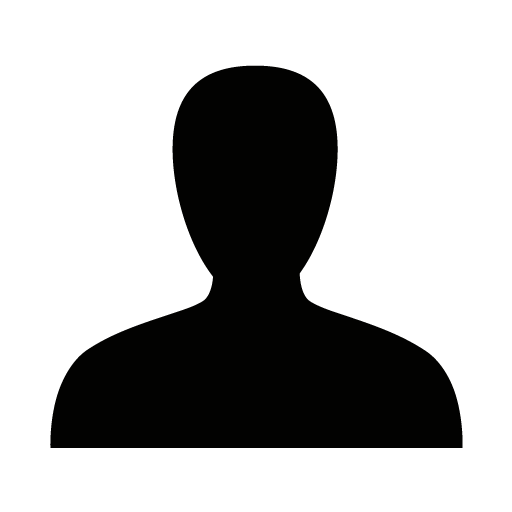
Developing effective electrocatalysts for large-scale seawater splitting that can prevent anodic corrosion while efficiently driving oxygen evolution is a major challenge. Surface engineering plays a pivotal role in advancing electrocatalysts, bridging the gap between basic research and the real-world needs of industrial water-splitting technologies. In this study, we introduce the CoZnCr@MXene heterostructure, which achieves a remarkable cell voltage of 1.55 V at a current density of 50 mA cm⁻², surpassing the performance of RuO₂ in alkaline seawater electrolytes. This outstanding performance is attributed to the combined benefits of compositional optimization, surface modification, and the integration of conductive supports, all of which contribute to significant reductions in overpotentials for both the oxygen evolution reaction (OER) and the hydrogen evolution reaction (HER). The CoZnCr@MXene catalyst shows exceptional stability and selective oxidation in alkaline seawater, with the MXene incorporation effectively preventing chloride-induced corrosion and improving charge transfer efficiency. Additionally, when used in an anion exchange membrane electrolyzer, the CoZnCr@MXene catalyst delivers a current density of 500 mA cm⁻² at an operating voltage of 1.72 V at 60 °C, achieving a cell efficiency of 77.8%. This work marks a significant step forward in the development of durable, noble-metal-free electrodes for industrial-scale alkaline seawater electrolysis.
#H2Future-T4
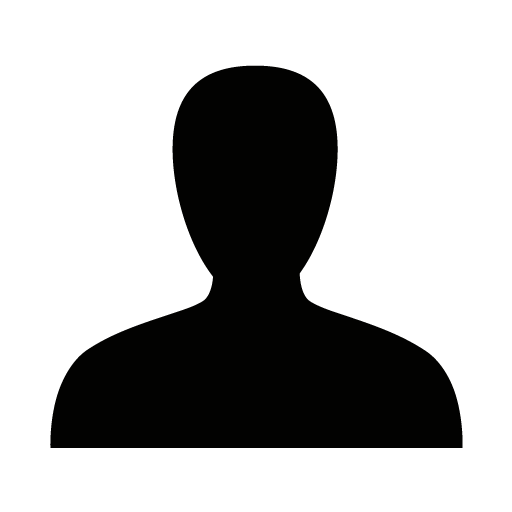
Several rare-earth-based nanocrystalline high-entropy oxides (HEOs) with fluorite type of structure and average crystallite sizes between 6 and 8 nm were prepared and their photocatalytic behaviour towards photocatalytic CO2 reduction and photoelectrochemical water splitting for hydrogen generation was examined [1], [2]. The cationic site in the fluorite lattice consists of five equimolar elements selected from the group of rare-earth elements including La, Ce, Pr, Eu, and Gd and second-row transition metals, Y and Zr. Studied HEOs exhibit bandgaps in the range from 1.91 eV to 3.0 eV and appropriate valence and conduction bands for water splitting. They reveal high photocatalytic activity that is mostly attributed to the accessibility of more photocatalytic active sites. The materials successfully produce hydrogen by photocatalytic water splitting, suggesting the potential of HEOs as new photocatalysts. The photocatalytic performances of all studied HEOs outperform the single fluorite oxides or equivalent mixed oxides. The Ce0.2Zr0.2La0.2Pr0.2Y0.2O2 (CZLPY) engender hydrogen in 9.2 µmolmg–1 per hour that is much higher content than for pristine CeO2 material which amounts to 0.8 µmolmg–1 per hour. Systematic tests showed the effect of a high–entropy system compared to mid–entropy oxides. XPS, in–situ DRIFTS as well as DFT calculation elucidate the synergistic impact of Ce, Zr, La, Nd, and Sm, resulting in an optimal Ce3+/Ce4+ ratio. The observed formate–routed mechanism and a surface with high affinity to CO2 reduction offer insights into the photocatalytic enhancement.
#H2Future-T5
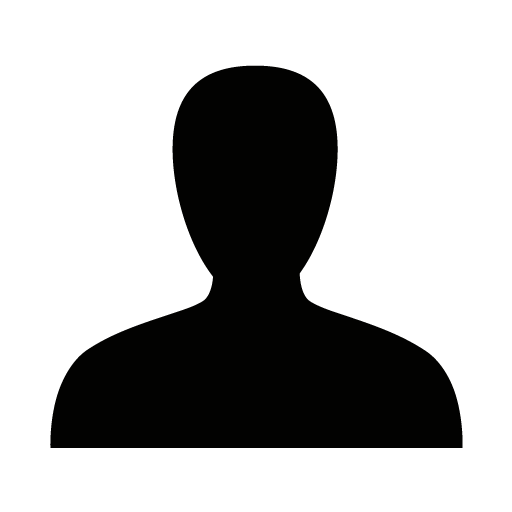
Green hydrogen is emerging as a vital energy vector for the decarbonization of industrial and mobility sectors. In the context of the H2ENRY initiative, our work focuses on advancing three innovative technologies for hydrogen production: Solid Oxide Electrolysis Cells (SOEC), Photoelectrochemical (PEC) water splitting, and integrated Bioelectrochemical Systems combined with dark fermentation (FO).
New perovskite-based nanofibers (PBMO) have been synthesized and characterized to be used as catalysts for SOEC, providing enhanced performance when used at low SOEC temperatures and with transport properties with lower dependency on the temperature. In parallel, promising results have been obtained in the fabrication of photoelectrode materials using scalable techniques such as screen printing. This approach paves the way for cost-effective, high-performance PEC systems that can be readily expanded to meet scalability demand. Additionally, our FO approach leverages bioelectrochemical processes coupled with dark fermentation to generate hydrogen from organic substrates, offering a complementary route that aligns with circular economy principles.
To ensure that the hydrogen produced meets stringent industrial purity requirements, we have also developed advanced purification strategies using polymeric membrane technologies. This integrated approach not only enhances overall process efficiency but also paves the way for scalable and sustainable green hydrogen production.
In this work, we will detail the experimental results, material innovations, and system integration strategies that underpin these breakthroughs. Our findings illustrate a comprehensive pathway toward cost-effective and environmentally sustainable hydrogen production, reinforcing the critical role of advanced electrochemical, photoelectrochemical and bioelectrochemical technologies within the H2ENRY framework.
The program is in CEST Time, check the time converter to know your local time.