1A-K1
Richard Friend holds the Cavendish Professorship of Physics at the University of Cambridge. His research encompasses the physics, materials science and engineering of semiconductor devices made with carbon-based semiconductors, particularly polymers. His research advances have shown that carbon-based semiconductors have significant applications in LEDs, solar cells, lasers, and electronics. His current research interests are directed to novel schemes – including ideas inspired by recent insights into Nature’s light harvesting – that seek to improve the performance and cost of solar cells.
We and others have recently reported that certain classes of radical organic semiconductors can be used as efficient light emitters in LED devices. Excitation within the doublet manifold can avoid the formation of non-emissive higher spin states and therefore allow efficient radiative electron-hole recombination [1]. We have developed this primarily for use in light-emitting applications, but the avoidance of ‘dark’ low energy excitations (ordinarily triplets) is also very desirable for improved organic photovoltaic devices.
have found that structures where the lowest double excited state involves promotion of an electron from a HOMO level associated with a donor moiety such as carbazole to the radical SOMO level can show very high luminescence yield [2], and we have optimised structures so that it is possible to electrically excite this doublet state, by sequential charge transfer of electron and hole, taking account of the Coulomb charging energy for double occupancy of the SOMO [3]. have also explored the use the radical semiconductor as the emissive ‘guest’ in a regular organic LED emissive layer ‘host’ where the radical semiconductor is able to harvest both singlet and triplet excitons formed in the host material [4]. I will also report on recent studies of intermolecular charge photogeneration in guest-host radical-donor systems.
1A-I1
Abstract
The recent successes of emerging photovoltaics such as organic and perovskite solar cells are largely driven by innovations in material science. However, closing the gap to commercialization still requires significant innovation to match contradicting requirements such as performance, longevity and recyclability in one and the same material. In this perspective, we start with the notion that the learning curve in innovation must be substantially increased to achieve commercialization in the presence of a mature competitor. Then, we show that the learning curve, as of today, is limited by a lack of design principles linking detailed chemical structure to microscopic structure, and by an incapacity to experimentally access microscopic structure from investigating macroscopic devices. Both limitations in turn are caused by an individualist approach to learning, not being able to produce datasets large enough to find patterns leading us to breakthrough innovations. In this Perspective, we propose a layout of a Digital Twin For PV Materials able to remove both limitations.
Microscopic design principles are required to get handles for a true multi-objective optimization leading to PV devices with unseen properties. We argue that such microscopic design principles can principally not be achieved by either a pure knowledge based or a pure data-driven approach. To solve the problem, our digital twin layout combines ideas from acceleration platforms in material science with digital twin concepts from the engineering world. To tackle the specific challenges in material science, we propose to use ad-hoc trained local surrogates of complex solid state models to achieve self-calibration of simple proxy experiments onto the underlying physics. This allows to build fast but still detailed predictive models across scales, so that inverse design, from the desired performance of a working device to the chemical structure, becomes possible. We show the building blocks that are already available and comment on active research closing the remaining gaps. Finally we propose to overcome the individualist learning approach by adopting the novel paradigm of a federated learning approach, able to protect investments and IP aspects while at the same time maximizing the learning rate for all stakeholders.
1A-I2
The objective of my group is to use ultrafast spectroscopic techniques, such as transient absorption (TA) and time-domain terahertz (TD-THz) spectroscopies, to investigate charge carriers in organic photovoltaic (OPV) materials. While femtosecond TA measurements bring insights to the nature and evolution of the photoexcited species, we use TD-THz spectroscopy to gain information about the charge transport properties on the nanoscale. In this talk, I will present an overview of our results obtained in recent years about the different steps of charge generation in OPVs. After presenting our experimental techniques, I will show results about charge generation in highly efficient solar cell materials based on organic polymer:fullerene [1] and polymer:non-fullerene blends [2] with negligible driving force for interfacial charge transfer, as well as on evaporated dilute solar cells [3]. I will discuss how charge generation and recombination processes depend on parameters such as the charge transfer driving force, the short-range charge mobility and the morphology. The combination of our results allows to visualize all relevant processes that lead to efficient charge extraction in full devices.
1B-I1
Integrating metal halide perovskite top cells with bottom cells formed by crystalline silicon, CIGS or low band gap perovskites into monolithic tandem devices has recently attracted increased attention due to the high efficiency potential and application relevance of these cell architectures. Here we present our recent results on monolithic tandem combinations of perovskite top-cells with crystalline silicon, and Sn-Pb perovskites as well as tandem relevant aspects of perovskite single junction solar cells.
In 2020, we have shown that self-assembled monolayers (SAM) could be implemented as appropriate hole selective contacts. The implementation of new generation SAM molecules enabled further reduction of non-radiative recombination losses with high open circuit voltages and fill factor. By fine-tuning the SAM molecular structure even further, the photostability of perovskite composition with tandem-ideal band gaps of 1.68 eV could be enhanced by reduction of defect density and fast hole extraction. That enabled a certified efficiency for perovskite/silicon tandems at 29.15%.
By optical optimizations, we could further improve this value to 29.80% in 2021. Periodic nanotextures were used that show a reduction in reflection losses in comparison to planar tandems, with the new devices being less sensitive to deviations from optimum layer thicknesses. The nanotextures also enable a greatly increased fabrication yield from 50% to 95%. Moreover, the open-circuit voltage is improved by 15 mV due to the enhanced optoelectronic properties of the perovskite top cell on top of the nanotexture.
In the end of 2022, we enabled a new world record for perovskite/silicon tandem solar cells at 32.5% efficiency. We demonstrate that an additional surface treatment strongly reduces interface recombination and improves the band alignment with the C60 electron transporting material. With these modifications, single junction solar cells show high open circuit voltages of up to 1.28 V in a p-i-n configuration, and we achieve 2.00 V in monolithic tandem solar cells. A comparable surface treatment was also applied to 1.80 eV band gap perovskites to enable high open circuit voltages of 1.35 V and these were integrated into monolithic all-perovskite tandem solar cells enabling a certified efficiency of 27.5%.
In addition to the experimental material and device development, also main scientific and technological challenges and empirical efficiency limits as well as advanced analysis methods will be discussed for perovskite based tandem solar cells. In addition, first results for upscaling of these industrial relevant tandem solar cells by thermal evaporation and slot-die coating will be shown.
1B-S1
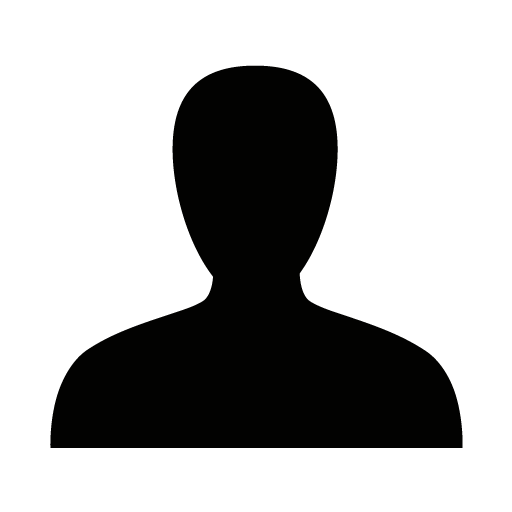
Tokyo Chemical Industry (TCI) is a global supplier of laboratory chemicals and specialty materials, and also leading the next-generation solar technology by providing high-quality and reliable materials, including metal halide perovskite precursors and materials for carrier transporting.
Metal Halide Perovskite Precursors with High Purity and Product Variety
https://www.tcichemicals.com/BE/en/c/12969
Hole Selective Self-Assembled Monolayer (SAM) Forming Agents: PACzs
https://www.tcichemicals.com/BE/en/product/topics/hole-selective_self-assembled_monolayer-forming_agents
Hole Transport Materials For Stable and Practical Perovskite Solar Cells: TOP-HTMs
https://www.tcichemicals.com/BE/en/product/pick/hole_transporting_materials_for_stable_perovskite_solar_cells
Dopants for Organic Electronics Research
https://www.tcichemicals.com/BE/en/product/topics/dopants_for_organic_electronics_research
In this short talk, these highly useful materials will be introduced including most recently commercialized new products.
1B-I2
In hyper-productive research communities in applied research areas such as halide perovskite photovoltaics, often incremental and sometimes serendipitous minor changes lead to continuous optimization and improvement of devices in multiple performance dimensions. Research progress has been primarily reflected in the increase in reported power conversion efficiency. The sheer amount of publications reporting progress in perovskite solar cells is becoming overwhelming and hence difficult to keep track of and to a large degree the data sets generated are by no means findable, accessible, interoperable, and reusable - FAIR.
For this reason, we started a database project in 2019 in an effort to collect data based on "key performance indicators" reported in the domain of perovskite solar cells primarily as a tool to keep track of progress made in the published peer-reviewed literature.[1] In the initial data collection campaign, we collected data from over 42,400 individual perovskite photovoltaic devices that can be viewed, filtered, analyzed, and downloaded dynamically from the database.[2] A year after the launch of the project, we find that despite providing the tools and means to also upload new data, this is not being made use of to a large extent.
In this presentation, I will advocate for the need of our research community to make all their research data - from failed attempts to make small area test devices to long-term performance studies of perovskite modules outdoors - available through central secondary dissemination platforms to accelerate the technological exploitation of perovskites for large-scale solar energy conversion. This will also require expanding the data ontology and data infrastructure further to capture all performance dimensions of PV materials and device exploration and optimization on different technology readiness levels. What will be increasingly valuable for the industrial exploitation of perovskite PV will be a more systematic collection of stability data based on unified testing protocols such as the ISOS protocols [3] and a focus on large area devices to identify current challenges in the scale-up of the device technology. Equally relevant is the dedicated collection of fundamental material properties that enables the prediction of the efficiency and stability of photovoltaic materials to enable the theory and AI-assisted identification of novel materials that could revolutionize the next generation photovoltaics.
1B-I3
Prof. Aron Walsh holds the Chair in Materials Design at Imperial College London. He received his PhD in Chemistry from Trinity College Dublin and later worked at the National Renewable Energy Laboratory, University College London, and the University of Bath. His research combines technique development and applications at the interface between solid-state chemistry and physics. He was awarded the EU-40 prize from the Materials Research Society for his work on the theory of solar energy materials, and is an Associate Editor for the Journal of the American Chemical Society.
Lead halide perovskites have demonstrated impressive improvement in photovoltaic power conversion efficiency over the past decade. This progress have motivated significant efforts, across multiple disciplines, to find low-toxicity and stable alternatives that could mimic the ability of the halide perovskites to achieve high performance with low temperature and scalable fabrication methods. The atomistic origins of the extraordinary performance exhibited by lead-halide perovskites in photovoltaic devices will be addressed, drawing from multi-scale materials theory and simulation, [1] including quantum chemical and machine learning approaches. I will also discuss the progress made and key obstacles remaining in discovering and engineering alternative, next-generation materials including those based on the lone pair cations Sb and Bi, as well as Cu [e.g. 2,3]. The chemical space of interest includes metal halides, metal chalcogenides, and their combinations in chalcohalide semiconductors such as BiSI, where the anisotropic crystal structures and physical properties pose interesting challenges for performance optimisation.
Optimisation-IS1
Despite record-breaking devices, interfaces in perovskite solar cells are still poorly understood, inhibiting further progress. The mixed ionic-electronic nature of lead-halide perovskites results in compositional variations at the interfaces, depending on the history of externally applied biases. This makes it difficult to measure critical parameters accurately, for example, the band energy alignment of charge extraction layers. As a result, the field often resorts to a lengthy trial-and-error process to optimise these interfaces. While techniques to measure interfacial energy level alignment exist, they are typically carried out in a vacuum and on incomplete cells, hence values may not reflect those found in complete device stacks. To address this, we have developed a pulsed measurement technique that can characterise the electrostatic potential energy drop across the perovskite layer in a functioning device. Our method reconstructs the JV curve for a range of stabilisation biases which hold the ion distribution ‘static’ during subsequent rapid voltage pulses. We observe two different regimes: at low applied biases, the reconstructed JV curve is ‘s-shaped’, whereas, at high applied biases, typical diode-shaped curves are returned. Using drift-diffusion simulations, we demonstrate that the intersection of the two regimes changes based on the band offsets at the transport layer interfaces. This approach effectively allows measurements of interfacial energy level alignment in a complete device under illumination and without the need for expensive vacuum equipment.
Optimisation-O1
Lioz Etgar obtained his Ph.D. (2009) at the Technion–Israel Institute of Technology and completed post-doctoral research with Prof. Michael Grätzel at EPFL, Switzerland. In his post-doctoral research, he received a Marie Curie Fellowship and won the Wolf Prize for young scientists. Since 2012, he has been a senior lecturer in the Institute of Chemistry at the Hebrew University. On 2017 he received an Associate Professor position. Prof. Etgar was the first to demonstrate the possibility to work with the perovskite as light harvester and hole conductor in the solar cell which result in one of the pioneer publication in this field. Recently Prof. Etgar won the prestigious Krill prize by the Wolf foundation. Etgar’s research group focuses on the development of innovative solar cells. Prof. Etgar is researching new excitonic solar cells structures/architectures while designing and controlling the inorganic light harvester structure and properties to improve the photovoltaic parameters.
In this work I will present two new concepts related to hybrid perovskite synthesis and devices.
Chiral molecules were implemented into hybrid perovskite forming 2D hybrid perovskite with chirality properties. We used the two enantiomers R)-(+)-α-Methylbenzylamine (R-MBA) and, (S)-(-)-α-Methylbenzylamine (S-MBA). The chirality is manifested at low n values and pure 2D structure measured by circular dichroism (CD). The anisotropy factor (gabs) decreased by an order of magnitude when decreasing the n value achieving 0.0062 for pure 2D. Ab initio many-body perturbation theory successfully describes the band gaps, absorbance and CD measurements. For the first time these quasi 2D chiral perovskites were integrated into the solar cell. Using circular polarization (CP) and cut off filter we were able to distinguish the chirality effect from the solar cells photovoltaic response.
In the second part we developed unique fully printable mesoporous indium tin oxide (ITO) perovskite solar cell. In this structure, the perovskite is not forming a separate layer but fills the pores of the triple-oxide structure. One of the advantageous of this solar cell structure is the transparent contact (mesoporous ITO) which permit the use of this cell structure in bifacial configuration without the need for additional layers or thinner counter electrode. We performed full characterizations on both sides (i.e. ITO-side and glass-side) and elucidate the solar cell mechanism, where the glass side show 15.3% efficiency compare to 3.8% of the ITO-side. Further study of the mechanism shows that the dominant mechanism when illuminating from the glass-side is Shockley-Read-Hall recombination in the bulk, while illuminating from the ITO-side show recombination in multiple traps and inter gap defect distribution which explain the poor PV performance of the ITO-side. Electrochemical impedance spectroscopy shed more light on the resistance and capacitance. Finally, we demonstrate 18.3% efficiency in bifacial configuration. This work shows a fully printable solar cell structure which can function in bifacial configuration.
Optimisation-O2
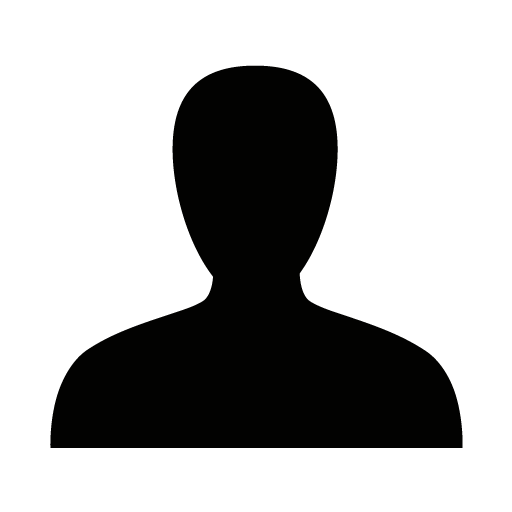
Formamidinium lead iodide (FAPbI3) presents outstanding properties to be applied in different semiconductor applications. Its bandgap is ideal for achieving the highest solar-to-photocurrent power conversion efficiency in solar cells [1] and it is the material of choice for the development of near-infrared perovskite LEDs [2]. The design of effective passivation strategies requires a thorough understanding of defect-assisted recombination to fully exploit its potential.
Here, we explore the role of bromine (Br) introduction into FAPbI3-based perovskites, an effective strategy previously used for solar cells [3], to promote their optoelectronic properties. Previous studies suggest that adding small amounts of Br into pure iodine perovskites results in a reduction of non-radiative recombination, either through deactivation of the detrimental short-lived hole-trapping [4], or the preferred orientation of the formamidinium cation towards the Br positions [5][6]. Thus, FAPbI3-based perovskites would exhibit enhanced optoelectronic properties as a result of improved radiative recombination.
As a first step, we examined the effects of different amounts of Br on the morphology and optical properties of perovskite. Then, using a set of advanced spectroscopic techniques, including photo-emission electron microscopy, time-resolved photoluminescence, and ultrafast transient absorption spectroscopy, we visualized the reduction of the iodine-related defect states. Introducing Br in FAPbI3 results in longer photoluminescence and carrier lifetimes, with improved photoluminescence quantum yields. Last, we confirmed the above findings by implementing Br containing FAPbI3 in working LED devices, obtaining devices with reduced roll-off and increased radiances.
The use of small amounts of Br in formamidinium-based lead halide perovskites can thus be a promising alternative to address iodine-related defects, leading to improved radiative recombination properties.
Optimisation-O3
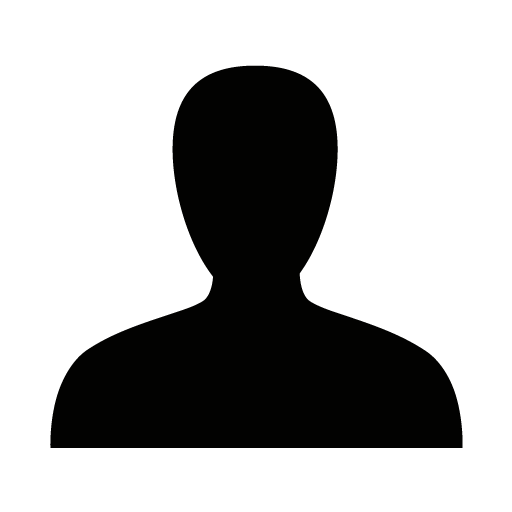
Since lead-based perovskite solar cells still suffer several drawbacks such as a rather low stability against ambient conditions and severe toxicity of lead, lead-free double perovskite have emerged as new potential light-absorbing materials for solar cell applications. Among these materials, Cs2AgBiBr6 has so far been realized in solar cells exceeding a power conversion efficiency of 3 %.[1] To further optimize the solar cell performance, research focuses not only on the absorbing material itself but also on improving the charge-carrier transporting layers.[2] State-of-the-art perovskite solar cells often employ expensive organic hole transporting materials (HTM) such as Spiro-OMeTAD, motivating the search for more economical and better performing alternative HTMs.
Herein we report the crystal structure of EDOT-Amide-TPA from single crystal measurements as well as the first utilization of EDOT-Amide-TPA as a low-cost alternative to Spiro-OMeTAD as HTM in lead-free perovskite solar cells and link its stuctural properties to its superior charge carrier properties. It crystallizes in the triclinic space group P−1 with short intermolecular distances and very dense molecular packing, in contrast to the well-established HTM Spiro-OMeTAD. A comparison of EDOT-Amide-TPA with Spiro-OMeTAD shows an improved efficiency with the former when employed in lead-free double perovskite solar cells. Interestingly, in contrast to lead-based perovskites,[3] the better performance of EDOT-Amide-TPA cannot be attributed to higher VOC-values but rather to an improved charge carrier extraction, leading to higher JSC-values. This is confirmed by PL and EQE measurements and was explained by decreased recombination losses in the lead-free perovskite solar cells compared to the lead-based perovskites. The improved current densities were explained by the rather thin and compact HTM layer that is sufficient for EDOT-Amide-TPA, which is in line with the dense molecular packing in the solid state achieved by EDOT-Amide-TPA. The dense molecular packing of the HTM not only enables the formation of a thinner HTM layer but also increases the reproducibility of solar cell manufacturing.
Optimisation-O4
Prof. Satoshi Uchida is a professor (born in 1965) in Research Center for Advanced Science and Technology (RCAST), The University of Tokyo. He received his PhD from Tohoku University in 1995 and moved to current position in 2006. For more than 15 years his research focused on the field of dye-sensitized solar cells (DSSCs), specifically cell assembling technique such as full-plastic, light-weight, film type as a ubiquitous power source. He is now also showing strong activity of Perovskite Solar Cells research based on the crystallography, surface engineering and electronic simulation.
Recently, perovskite solar cells have become the latest research field among various new generation photovoltaic technologies due to their high performance and potentially low-cost production. The power conversion efficiency has already reached over 25% in 2020 much beyond another solar cells such as CIGS or amorphous Si. The further performance still looks promising toward the Shockley–Queisser limit at around 30%. For that purpose, physical chemistry understanding based on the crystallography must be essential to design the good light harvesting, good charge separation and good charge transfer.
Recently we reported the scientific revelation that the crystal phase of thin film CH3NH3PbI3 consists of the mixture of tetragonal phase and cubic phase. Moreover, bold zebra pattern with d-spacing with 14.2Å (2θ=6.22° for CuKα by XRD) was clearly observed by high resolution TEM with FIB processing that consists with tetragonal and cubic phase superlattice. The typical high magnification In the TEM observation characteristic identity of perovskite can be seen as (1) Phase coexistence (2) Superlattice (3) Nano size domain (10~20nm).
To make more high performance as a solar cell, the crystal phase control with liquid nitrogen quenching just after the hot plate heating was newly examined. The resulting cell performance was impressive, about 2% more than that without Liq. N2 treatment. Details on TEM observations are also provided for such procedures.
Optimisation-O5
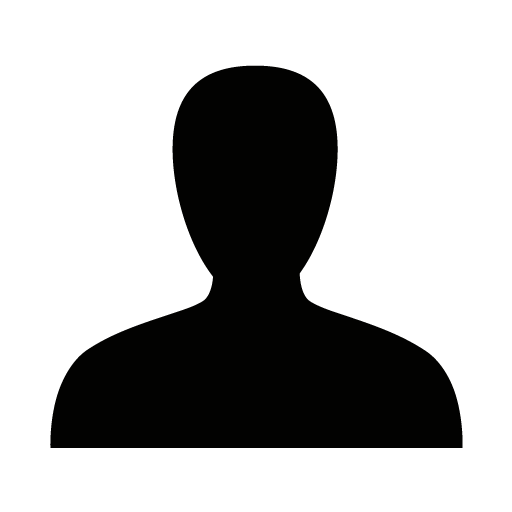
In a perovskite solar cell, the charge transport layers on either side of the light-absorbing perovskite layer provide the asymmetry necessary to extract electrons and holes through opposite sides of the device. As the fabrication of high-quality thin films of metal halide perovskite matures, the performance losses are increasingly dominated by the properties of these charge transport layers (CTLs) and their interfaces [1]. In this work, some of the most widely used CTLs were deposited on 3D lead halide perovskite layers and then studied using a range of time-resolved spectroscopies, the results of which were compared to a numerical simulation.
Using contactless optical techniques allowed the study of bilayers in which only a single interface was present. Bilayers were formed by depositing the electron transport layers (ETLs) PCBM and C60, or the hole transport layer (HTL) Spiro-OMeTAD, on top of a high performance triple cation [2] perovskite layer (FA0.79MA0.16Cs0.05)Pb(I0.83Br0.17)3. Time-resolved terahertz (THz) photoconductivity and transient absorption (TA) spectroscopies were used to track the perovskite carrier population from femtosecond to nanosecond timescales, whilst time resolved photoluminescence (TRPL) followed the population decay on nanosecond to microsecond timescales. Bilayers incorporating fullerene-based ETLs (PCBM and C60) were found to have significantly different carrier dynamics to the bilayers using the Spiro-OMeTAD HTL.
The carrier dynamics were then analysed using a numerical simulation of the spatially- and temporally-dependent carrier density, including the Poisson equation to account for charge separation across the interface, which is usually ignored. This charge separation was found to have a significant effect on the simulated carrier dynamics. To provide additional constraints for the comparison between numerical simulation and experimental data, different initial carrier distributions were generated by exciting the bilayers with different wavelengths and on alternate sides, adjacent to or away from the CTL. This comparison suggests that the PCBM and C60 layers have significant interface recombination on sub-nanosecond timescales, whereas Spiro-OMeTAD may have much slower hole extraction and interface recombination. We discuss the significant ramifications of these findings for device performance.
Optimisation-O6
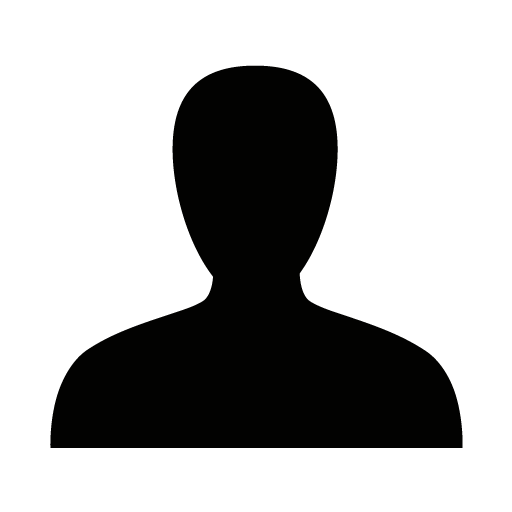
Perovskite solar cells (PSC) have shown an unrivalled increase in performance within the past few years. Key to high performing PSC is interface engineering and defect passivation of the perovskite absorber layer. Passivating the surface of the perovskite by an additional layer on top has shown high efficacy, and strategies of mixing the materials into the perovskite precursor or adding a layer underneath have also been successfully demonstrated. Nevertheless, there is still room for improvement.
To achieve further progress and gain a more profound understanding of how passivation works in PSC, deeper analysis is needed. Time-of-flight secondary ion mass spectrometry (ToF-SIMS) is a powerful tool to address some of the open questions. In addition to the excellent detection limit for relevant elements (e.g. lead, alkali metals, iodine, bromine, chlorine, indium, etc. ), ToF-SIMS can also detect molecules (e.g. phenethylammonium iodide (PEAI) or formamidinium). Moreover, ToF-SIMS depth profiling provides valuable information about the distribution of the elements within the layer stack.
In this work, we compare different passivation strategies such as surface passivation, bulk passivation or the combination of both based on the use of phenethylammonium iodide (PEAI) in highly efficient PSC. While all passivation approaches lead to a more or less pronounced increase in device efficiency, the distribution of the PEA-molecules within the perovskite absorber or at the interfaces between the absorber and the charge transport layers differ significantly. Furthermore, the choice of the used electron transport layer (ETL) as well as the deposition or post-treatment parameters (e.g. annealing) are crucial for the performance of the complete device. ToF-SIMS reveals that residual solvent in the ETL can play a beneficial role regarding the annealing behaviour and the efficiency of the solar cells.
Our findings demonstrate the feasibility of our fabrication approach for opaque and semitransparent PSC, and the ToF-SIMS measurements reveal new insights into the fabrication process, guiding the way for further steps towards commercialization of PSC.
Characterisation-IS1
Hideo Ohkita is a Professor in the Department of Polymer Chemistry at Kyoto University. He obtained a Doctoral degree in 1997 at Kyoto University. He became an Assistant Professor in 1997, was promoted to Associate Professor in 2006, and to Professor of Department of Polymer Chemistry at Kyoto University in 2016. He concurrently worked as an academic visitor with Professor Durrant at Imperial College London from 2005 to 2006, and as a researcher in the Precursory Research for Embryonic Science and Technology (PRESTO) program “Photoenergy Conversion Systems and Materials for the Next Generation Solar Cells”, Japan Science and Technology Agency (JST), from 2009 to 2015. His research interests include studying photophysics and photochemistry in polymer systems. His current research focuses on spectroscopic approach to polymer solar cells.
Organic and metal halide perovskite solar cells have attracted increasing attention as one of the most promising photovoltaic devices. Currently, the power conversion efficiency (PCE) has exceeded 19% for organic solar cells and 25% for perovskite solar cells. In both cases, interfaces in the device would have great impact on photovoltaic performances, especially on open-circuit voltage (VOC) because charge carriers would recombine bimolecularly at the interface. In this talk, I will focus on the relationship between VOC and interface in polymer solar cells and perovskite solar cells. First, I will talk about how interfacial charge transfer (CT) states impact on VOC in polymer solar cells. More specifically, we employed two crystalline polymers (PTzBT-BOHD and PTzBT-12OD), which are based on the same backbone with different side chains (BOHD: butyloctyl and hexyldecyl, 12OD: dodecyl and octadecyl), and a fullerene derivative PCBM. Interestingly, two crystalline polymer cells exhibit different VOC even though BOHD and 12OD neat films exhibit the same ionization energy. By analyzing the interfacial CT state, we found the CT state energy is higher in BOHD/PCBM than in 12OD/PCBM blend films. This is because HOMO level of BOHD is deeper than that of 12OD in disordered mixed phase in blend films, which results from twisted backbone of BOHD [1]. Second, I will talk about how aging and passivation impact on VOC in perovskite solar cells. Interestingly, VOC is further improved after ambient storage even for the passivated device where surface recombination is effectively suppressed by the passivation layer. By analyzing electronic properties of each layer, we found that the HOMO level of spiro-OMeTAD is deepened after ambient storage, resulting in energy matching between perovskite and spiro-OMeTA layers and hence reduced surface recombination [2,3]. These findings indicate that interface engineering is crucial for further improvement in VOC of not only polymer solar cells but also perovskite solar cells.
Characterisation-O1
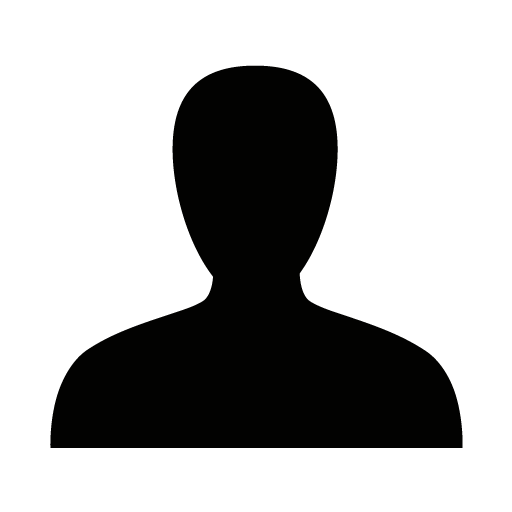
Improvements in the molecular design of non-fullerene acceptors (NFAs) has almost doubled the power-conversion efficiency of organic photovoltaics in the last 5 years, from 11 to 19%.1 However, despite numerous studies, the exact molecular reasons behind why some acceptors (e.g. Y-series) perform better than others (e.g. ITIC-series) remain unclear.
Interestingly, recent studies have shown that some the most efficient non-fullerene acceptors (e.g. Y-series) can achieve relatively high charge-generation efficiency in the absence of a donor-acceptor interface.2 This challenges the current understanding of how photogenerated excitons dissociate into free charges in organic semiconductors. Understanding this phenomenon would raise interesting questions on whether a donor-acceptor interface is necessary for achieving high-efficiency solar cells, or whether a much simpler single-component system would suffice for efficient charge-generation, and what molecular design criteria might be required to synthesise NFAs with high charge-generation efficiency in such a single-component device. Uncovering these questions could have significant implications for the simplified design of organic photovoltaics, as well as photodetectors, solar fuel cells, and light-emitting diodes.
Here, we study the charge-generation processes in a series of NFA molecules in single-component devices, including A-DA′D-A-type acceptors (e.g. Y6) and A-D-A type acceptors (e.g. ITIC) using optoelectronic and spectroscopy characterisation methods under strong applied fields and at different temperatures. By combining experimental results with molecular and device-level calculations, we link exciton and charge dissociation efficiency in NFA films to molecular parameters such as reorganisation energy and electronic coupling and suggest molecular design rules for higher single-component as well as heterojunction device performance.
Characterisation-O2
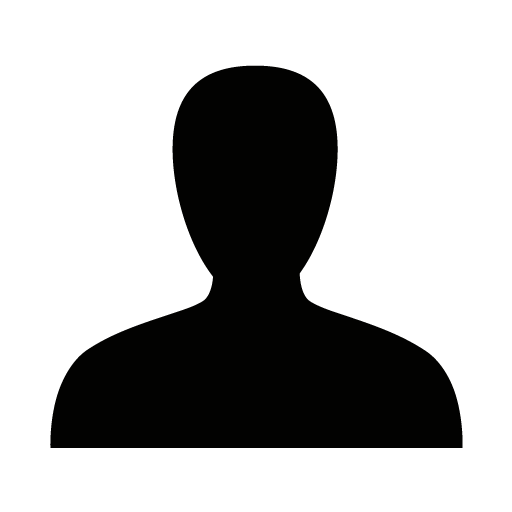
Organic photovoltaic devices employing bulk heterojunctions (BHJs) of polymer donors and small molecular nonfullerene acceptors have recently demonstrated high performance, with strong visible and near-infrared absorption and low energy losses. Such junctions are promising candidates for solar-driven water splitting. Here, we investigate the role of a PM6 polymer overlayer on the photoexcited carrier dynamics in a Y6:PM6 BHJ photoanode undergoing ascorbic acid oxidation. With the additional polymer layer, the hole lifetime is increased in the solid state BHJ film. When the photoanode is electrically coupled to a hydrogen-evolving platinum cathode, hole polaron states are observed on the timescale of seconds under operational conditions. More importantly, we demonstrate that the organic photoanode with the polymer overlayer shows enhanced performance, reaching over 6 mA cm-2 at 1.23 VRHE without a co-catalyst. An EQE of 18% was observed using 850 nm excitation. We propose that the use of an organic overlayer can be an effective design strategy for generating longer charge carrier lifetimes in organic photoanodes for efficient oxidation catalysis.
Characterisation-O3
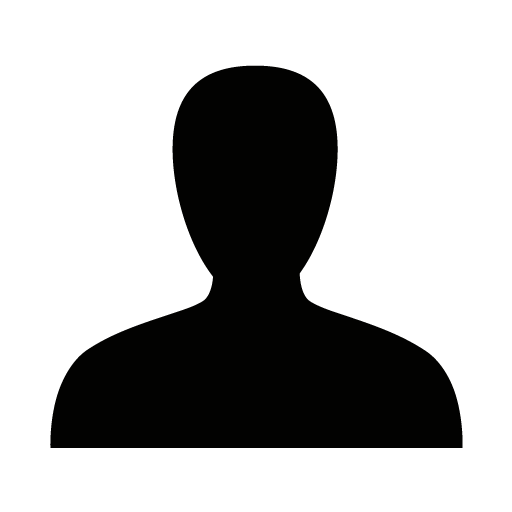
Performance improvement of organic solar cells through fluorination (or, in more general terms, halogenation) of the donor and/or non-fullerene acceptor (NFA) is an effective method. The end-group fluorination of the well-known NFA ITIC yields further extension of the absorption spectrum to the near-infrared, which results in an increment of the device’s photocurrent as compared to the non-fluorinated version. Herein, ITIC and two fluorinated variants of ITIC (ITIC-2F* and ITIC-4F) were synthesized and systematically investigated the influence of end-group fluorination physicochemical properties, optical properties, and photovoltaic performance. Density functional calculations also show that fluorination increases the electron affinity of the acceptor and therefore reduces the open circuit voltage. On the other hand, the molecular quadrupole moment increases with the degree of fluorination, which leads to more efficient dissociation and reduced recombination of charge transfer states at the donor-acceptor interface. At the same time, ionization energy deepens, increasing the driving force for CT state formation. Both processes contribute to the improvement of the internal quantum efficiency upon fluorination. All the results shed light on the importance of the energetic landscape and the quadrupole moment of acceptor beyond the underlying donor-acceptor interface.
Characterisation-O4
The ternary blend approach has been shown to improve spectral coverage and enhance the power conversion efficiency of the single junction organic bulk hetrojunction solar cells. Here, we introduce a high band gap n-type perylene dimer to PM6:Y6 binary blend as a third light absorber to enhance the short circuit current density (JSC) and hence the overall PCE of the organic solar cells (OSCs). Due to strong absorption in the visible region, perylene acts as an antenna to capture high-energy photons and readily transfer them into the primary donor and acceptor molecules via energy and charge transfer processes. A systematic study was conducted to compare charge and energy transfer dynamics and orientational dependence nanomorphology of ternary blends and were compared with their binary counterparts. Femtosecond transient absorption measurements reveal enhanced hole transfer efficiency in the finally tuned ternary mixtures. In addition, we show from the GIWAXS measurements that the incorporation of TPDI enhances lamellar stacking in the PM6 nanodomains along with enhanced crystallization in the Y6 nanodomains, hence decreasing structural disorder. Our study provides insight into employing non-fullerene acceptors (NFA) having complementary absorption to alternatively harvest the photons via resonant energy transfer process to realize improved photovoltaic performance in OSCs.
Characterisation-O5
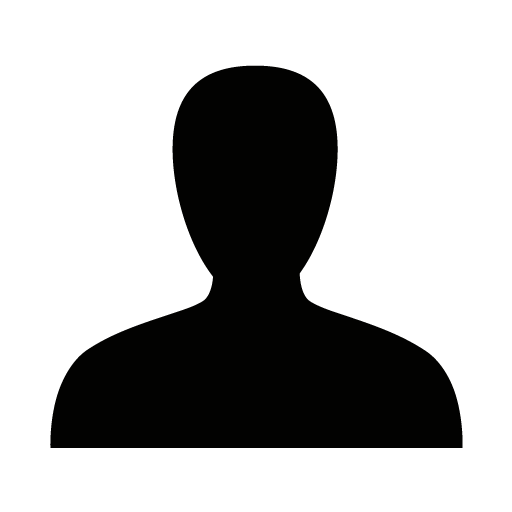
With the advent of non-fullerene acceptors (NFAs) and their record power conversion efficiencies, focus of the academic organic photovoltaics (OPV) research community shifted towards solution processed polymer : non-fullerene solar cells. These performance improvements could not be mirrored in evaporated OPVs since no evaporable NFAs enabling low voltage losses and efficient charge extraction have been introduced to date.[1] Despite this, evaporated small molecule donor : fullerene solar cells continue to be at the forefront of industrial research due to many practical advantages like an easy route to upscale, high reproducibility, the possibility of multijunction systems and intrinsically high thermal stability.[2] Also the commercial success of OLEDs provides important lessons for the upscale of evaporated OPVs. In 2016, Heliatek demonstrated evaporated tandem OPVs with 13.2 %. Further improvements in performance will strenghten the prospects of commercial success for evaporated OPVs.
This presentation combines results from optical spectroscopy, transient optoelectronic techniques, and drift-diffusion simulations to elucidate the performance limitations of a state-of-the-art evaporated OPV system compared to its solution processed counterparts. The donor molecule with an acceptor-donor-acceptor structure was paired with C60 and the morphology and active layer thickness were optimised. Through Grazing-Incidence Wide-Angle and Resonant Soft X-Ray Spectroscopy, we connect the device characteristics to the active layer morphology.
We find that heating the substrate during evaporation enhances the crystallinity and phase purity of the active layer and greatly improves performance due to an enhanced absorption, charge separation and charge collection efficiency. Despite the modest improvements in charge collection efficiency with improved crystallinity, its impact on the fill factor remains the main bottleneck compared to solution processed OPVs. This in turn limits the optimum device thickness and absorption. Measurements of the energetic disorder reveal a broad tailstate density in the evaporated OPVs in contrast to the highest performing Y6 based NFA devices but comparable to other solution processed systems.[3] This disorder leads to highly charge carrier density dependent mobility and recombination as demonstrated by a combination of charge extraction and transient photovoltage measurements. The observations are best described by a recombination mechanism that is limited by the encounter of spatially localised trapped carriers with free carriers of the opposite sign in addition to free-to-free recombination. Further combining current transient measurements with drift diffusion simulations we investigated the role of imbalanced transport and deep traps on the recombination mechanisms. While the recombination rate under device operating conditions is comparable or even lower than in the fullerene and non-fullerene based solution processed references, respectively, the order of magnitude lower effective mobility and the mobility imbalance result in the poor charge collection efficiency even for thin active layers around 50 nm. Using the simulation parameters extracted from a global fit of the experimental data, we give an outlook for possible performance improvements by better transport enabling enhanced absorption for thicker active layers.
Solution-processed blends like the small molecule BTR mixed with PCBM maintain a high fill factor even at active layer thicknesses well beyond 200 nm.[4] This illustrates that neither a polymeric backbone nor highly crystalline NFAs are prerequisites for good hole or electron transport, respectively. Indeed other evaporated blends have demonstrated better transport properties while suffering from poor absorption and large voltage losses.[1] Better morphological control and efforts in synthesizing more crystalline evaporable donor molecules while maintaining the demonstrated high absorption coefficients and good charge generation properties of the blend presented herein are instrumental for further performance improvements. Power conversion efficiencies beyond 10 % for single junction evaporated solar cells are well within reach.
Characterisation-O6
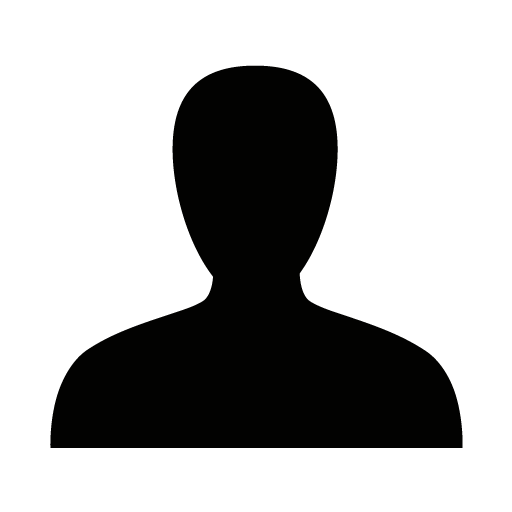
Ternary organic solar cells (TOSCs) employing two donors and an acceptor is a low-cost approach to enhance device performance without the need for complex tandem cells. Organic dyes are promising for incorporation into organic solar cells as the third component as they have been used as co-sensitisers in dye-sensitised solar cells (DSSCs) due to their excellent molar absorption coefficient and high photostability. [1][2]
In this study, an organic dye Rhodamine B (RhB) has been added to a binary blend of PTB7-Th:PC70BM, fabricated with an inverted device architecture under ambient conditions. RhB exhibits a complementary absorption range to the binary blend in the active layer; thus, the ternary blend of RhB:PTB7-Th:PC70BM can maximize photon harvesting across the whole visible light region. This has contributed to enhanced short-circuit current (Jsc)in the ternary blend device. The different weight content of RhB has been optimised to achieve a power conversion efficiency (PCE) of 7.4% which is 27% higher than in the binary blend without RhB. The Jsc and fill factor (FF) have improved by 12.9% and 10.9% respectively, while the open-circuit voltage (Voc) remains consistent with the addition of RhB. Despite the higher Jsc, the FF still increase mainly from a significant decrease in series resistance, likely from improved morphology in the ternary blend that enabled better charge separation and transport in the ternary blend active layer. This is corroborated by the higher values of incident photon-to-current conversion efficiency (IPCE) across 530 - 750 nm, despite RhB mainly absorbing around 530 - 580nm. Further insight into charge transport and recombination in the ternary blend has been studied with J-V curves under different light intensities as well as electrochemical impedance spectroscopy (EIS). Based on steady-state photoluminescence (PL) and transient absorption spectroscopy (TAS) measurements, Förster resonance energy transfer (FRET) has been observed to take place from RhB to PTB7-Th, followed by charge dissociation at PTB7-Th/PC70BM interface. Furthermore, the operational stability of the ternary blend device is significantly enhanced with the addition of RhB. A molecular fluorescence probe for singlet oxygen has also been employed to investigate photochemical degradation in the binary and ternary blend films. Overall, adding RhB as the third component in TOSCs has boosted device performance and lifetime.
Scale-up-IS1
Dr Tom Aernouts is R&D leader of the Thin Film Photovoltaics group at imec. Over the last few years this activity has grown steadily with state-of-the-art work in organic solar cells and recently also perovskite-based photovoltaics, next to inorganic materials like Kesterites for future replacement of the currently strongly growing CIGS thin film solar cells. Also the lab environment was drastically improved with setting-up the O-line infrastructure in 2009 at imec, allowing the processing and characterization of thin film solar cells and modules with area up to 15 x 15 cm². A next upgrade in 2018 enabled to extend the device size to 35x35cm². Dr Aernouts earned his Master of Science and PhD degree in Physics (in 2006) at the Catholic University of Leuven, Belgium. Firstly, he worked on organic oligomer-based diode structures, afterwards continuing his research on organic photovoltaics at imec. There, his work focused on the processing and characterization of polymer-based organic solar cells and monolithic modules, introducing techniques like screen and inkjet printing. He has authored or co-authored more than 80 journal publications, book chapters and conference contributions. Also, his research group participates on a regular basis in a broad range of local and international projects, with the most recent example the coordination of the European H2020 project ESPResSo.
The unprecedented fast rise of power conversion efficiency (PCE) of perovskite-based solar cells (PSC) in recent years has created a vast worldwide research activity in this material class for photovoltaic and other opto-electronic applications. Several materials compositions and device architectures have been described and best reported PCE’s yield recently more than 25%. Also improved stability under specific conditions has been shown for specific architectures. Whereas all these results indicate a high potential for this novel solar technology, further steps must be taken to convince industry and even the whole PV community that perovskite-based photovoltaics can really emerge from the lab into industrially applicable solar module processing. Our R&D program works actively on the upscaling of perovskite solar modules with scalable processes up to sizes of 35x35 cm2.
Similarly, the perovskite PV technology has boosted the tandem research whereby perovskite cells and modules are placed on top of other PV devices like Si or CIGS solar cells. Impressive lab scale results exceeding 30% PCE have been reported. New challenges arise when this needs to be upscaled to full wafer or module size. It will be discussed how we approach these challenges.
Scale-up-O1
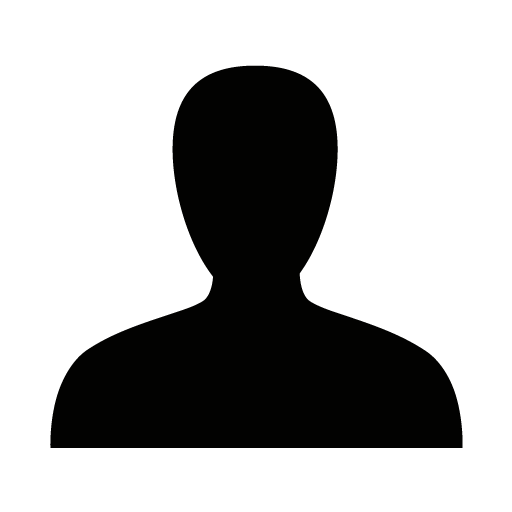
The recent achievement of certified power conversion efficiencies (PCEs) of over 25% in perovskite solar cells (PSCs) highlights their potential in cheap and efficient energy harvesting.[1] While these impressive results have been obtained using spin-coating on small sample sizes (<1cm2), the development of large-scale deposition techniques that are compatible with industrial processes is essential to bring PSCs closer to commercialization.
This study focuses on the slot-die coating method as a deposition technique for perovskite. This method allows for large-scale perovskite deposition with exceptional control over film uniformity, while minimizing material waste. Crystallization is a critical step during the evaporation of the deposited wet film, and various drying methods, such as vacuum drying or N2-blade quenching, are employed to achieve the desired surface morphology and layer quality. This investigation focuses on a sequential slot-die deposition method, which involves depositing a lead iodide intermediate phase followed by conversion to perovskite using a second slot-die coating step. The perovskite film quality is analyzed using hyperspectral photoluminescence imaging techniques as well as STEM experiments to probe the elemental composition in the device cross-section.[2]
Furthermore, chemical bath deposition (CBD) is discussed as a simple and cost-effective technique for the large-scale deposition of tin oxide (SnO2) as an electron extraction layer. Optimization of the CBD solution, deposition conditions, and number of CBD cycles allows for the uniform and reproducible deposition of SnO2.[3] The combination of CBD SnO2 with slot-die coated perovskite has led to the fabrication of perovskite devices (0.09cm2) with over 20% PCE and mini-modules (40cm2) with PCEs of up to 17% (18% on active area). In addition, the latest results on 64 cm2 modules will be presented as well as strategies for going to even larger sample sizes involving fully up-scalable device architectures.
Scale-up-O2
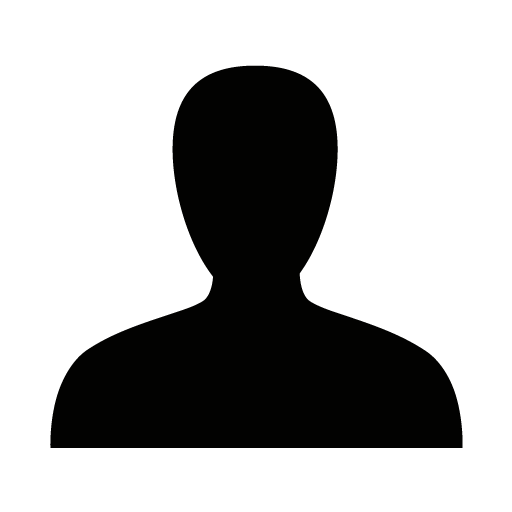
The high-throughput fabrication of PSCs cannot be realized until the costly, low-throughput evaporated metal electrode is replaced by roll-to-roll (R2R) printable (vacuum-free) electrodes. From our understanding, the highest reported power conversion efficiencies (PCEs) for R2R processed PSCs with an evaporated Au electrode and printed back electrode are 13.8% and 4.9%, respectively.[1, 2] In the present work, we introduce a vacuum-free, solvent-free, scalable, and R2R-compatible method to fabricate, and deposit printed electrodes based on electrically conductive pastes. Using this method we avoid potential losses of PSC performance due to solvent migration from the pastes, and additional annealing steps during the electrode deposition. Flexible, R2R-fabricated PSCs with record power conversion efficiencies (PCE) of up to 16.7% were produced by vacuum-free deposition of all active layers, apart from the transparent conductive electrode. This performance compares very favorably with control flexible PSCs comprising an evaporated gold electrode which displayed record PCEs of up to 17.4%. The flexible devices comprising the printed electrode demonstrate outstanding operational and mechanical stability, with negligible loss of PCE after 24 hours of continuous 1-sun illumination and retention of more than 90% of their initial PCE after 3000 cyclic bends. The devices and modules (100 cm2) were completely fabricated in ambient air using readily up-scalable printing and coating technologies.
Furthermore, we have developed a means to deposit the printed electrodes onto rigid, glass-based PSCs to achieve PCEs of over 20% (0.16 cm2 active area), only marginally lower than the 20.7% achieved for the control evaporated gold devices. This readily scalable method provides a pathway forward to substantially improve the production throughput as the electrodes can be deposited on potentially hundreds of cells within a matter of minutes using industrially available equipment. In addition, we significantly reduce (almost halve) the total manufacturing cost of the PSCs by removing the time-consuming and expensive gold evaporation process, all while still retaining exceptional photovoltaic performance. This novel electrode deposition method can be readily adapted to demonstrate record-breaking PSCs incorporating low-cost, printed electrodes.
Scale-up-O3
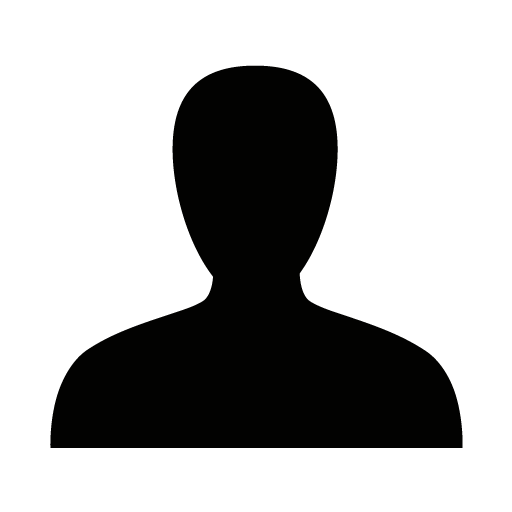
The lab-scale highly efficient perovskite solar cells (PSC) are outperforming many conventional alternatives due to their outstanding optoelectronic properties; however, lab scale fabrication still suffers from high material wastage and scaling challenges [1]. Along with high efficiency perovskite photovoltaics are solution processable and therefore have the potential commercially relevant high-volume manufacture through printing processes.
Scaling up of PSC layers has been demonstrated in recent years through a variety of printing methods yet Roll-to-Roll coating with the slot die coating method shows the greatest promise. Slot-die coating is a cost-effective coating method that allows significant control in coating and can deposit layers with little material wastage, high control on film thickness, and flexibility to coat layers with 2-dimensional patterning. The transition of PSC from lab scale spin coating to industrial scale slot die coating is not trivial with consideration of solution compatibility, rheology and process modelling needed for individual layers [2]. Combining these methods roll to roll slot die coating of active layers including HTL, Perovskite and ETL in combination has resulted in device performances of 12.2% [3] at pilot scale under ambient atmosphere processing.
However, unlocking the potential of industrial scale, high volume, and continuous manufacture of perovskite solar cells requires all layers to be sequentially coated, including the back electrode instead of the high value evaporated metal contacts employed as a post process.
Recently, we demonstrated the Roll-to-Roll slot-die coating of a full n-i-p device stack completed with a sequentially slot die coated carbon electrode [4]. Two developments were critical in achieving this world’s first, formulation of carbon ink electrode and introduction of a low temperature stable interlayer. The formulation of the carbon ink was through two stages, the compatible, non-toxic, low boiling point solvent system was proved through stencil coating on spin coated samples. The carbon ink was then re-formulated for slot die coating before the full stack was sequentially coated. The low-temperature curing PEDOT interlayer between perovskite and carbon electrode analysed by EIS and PL measurements overcomes interlayer incompatibilities and recombination losses achieving 13-14% at small scale retaining over 80% efficiency over 1000hrs stability testing. XPS elemental lead mapping and EL emission photography of R2R coated SnO2/MAPI/PEDOT are also indicate that the coverage is effective and covers high points so as to prevent pinhole/shorting defects. Our work introduces the fabrication pathway, challenges, and achievements of the very first all-printed Roll-to-Roll perovskite device achieving over 10% efficiency, a game-changer in the commercialization of this generation of solar cells.
Keyword: Roll-to-Roll, carbon electrode, perovskite, PEDOT
Scale-up-O4
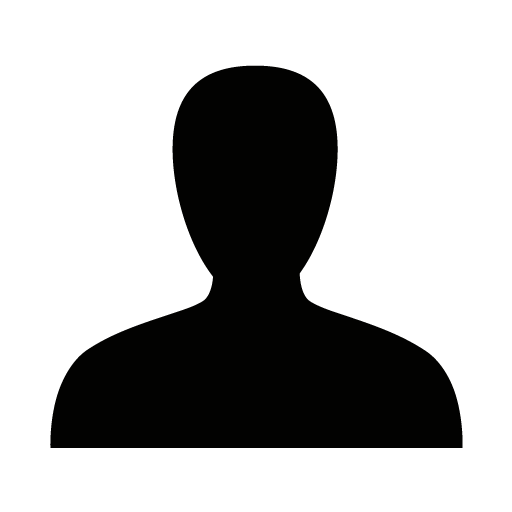
The growing market of connected machines and intelligent devices are becoming more and more important. These technologies are the origin of the development of the field of internet-of-things (IoT).
As a result, Indoor photovoltaics have attracted considerable interest thanks to their potential to power small and portable electronics devices.
Following this, the need of organic photovoltaic (OPV) devices are highly desirable for indoor applications because of their suitable characteristics of light weight, flexibility, and coloration.
In this context, high power conversion efficiency up to 20 % are already achieved with OPV module. further, excellent stability under indoor conditions have been obtained.
In terms of research on developing deposition techniques, Inkjet printing has attracted more and more attention as a printing electronic technology for large-scale printed flexible and stretchable electronics.
In fact, Inkjet printing has the advantage to provide freedom of forms and design on various substrates with low material usage comparing to other deposition techniques. This special characteristic has attracted a lot of attention of researchers on functional devices such as photovoltaic solar cells (PV).
However, inkjet printing still presents many challenges such as the stability of inks to avoid the nozzle clogging, the wetting behavior, compatibility of viscosity, surface tension with printheads.
So, herein, in this work, we present ITO-free all inkjet printed organic photovoltaic modules with high efficiency for indoor application with freedom of shape and design fabricated by Dracula Technologies company.
Scale-up-IS2
Dr. Andreas Hinsch holds a Fellow position at the Fraunhofer Institute for Solar Energy Systems in Freiburg. In 1992 he made his PhD in physics at University of Freiburg. He has worked as post-doc, project leader and senior scientist in Switzerland (EPFL-Lausanne, Glas Trösch), Japan (NIRIN) and the Netherlands (ECN) on dye and organic solar cells. In 2001 he had established a group at Fraunhofer ISE on the topic and has been the coordinator in several European and national projects in the field. From 2007 on he has been involved in the development of building integrated dye solar modules. Since 2013 he is coordinating activities in national and international projects on perovskite solar cells. His scientific interest is highly interdisciplinary research on emerging new types of solar cells and solar converters based on nanostructured materials. He regards the reduction of the energy pay-back time of solar technologies as most essential for the sustainable installation of solar energy sources in the future.
Since the start of perovskite solar cell research, the concept of printed mesoporous, monolithic cells with graphite and carbon-based porous electrode layers (c-PSC) has been intensively investigated by various groups on cell and small to medium-sized module scale. The potential advantage of graphite based electrodes over frequently applied organic hole conductors and metallized electrodes can be seen in the high chemical and electrochemical stability of the material. Recently we reported a certified, stabilized solar efficiency of 15.4% for c-PSC on cell level [1]. Further improvement of the efficiency can be predicted by improving the electron blocking characteristic at the interface between the perovskite and the carbon-graphite which to date reduces the achievable photovoltage to only 75% of that of the theoretical limit. Using an 3D-2D approach here, we reached an efficiency of 18.5 % [2]. Another challenge consists in the long-term stable sealing of c-PSC. For this purpose, we developed a method, in which a gas assisted liquified perovskite precursor [3] is crystallized “in-situ” as the final step of the module manufacturing. This allows the use of glass solder sealing material which is applied by printing and then the two glass substrates are fused together at a temperature above 600 oC resulting in a high-quality sealed prefab-module and a homogenous plate distance of 10 µm. Such a module fabrication concept, which benefits from the cost-effective technologies of the glass industry, has been successfully demonstrated by us for large sized dye solar cell modules in the past [4], and since several years is now transferred to the perovskite technology [5]. In this presentation, we give an overview over the processing steps implemented at Fraunhofer ISE with a focus on industrial scalability and report about the inverse temperature crystallization step as monitored by time dependent and potentiostatic photoluminescence imaging [6]. Several methods are currently studied to control the perovskite seeding process in the m-TiO2 layer. We also report on a novel design of microfluidic channels which are generated via laser assisted glass etching.
Techniques-IS1
Bachelor in Chemistry from University of São Paulo (USP) in 1996, Master's Degree in Chemistry from University of Campinas (UNICAMP) in 1998 and Doctorate in Chemistry from UNICAMP in 2001 under the guidance of Prof. Marco-Aurelio De Paoli. Performed an internship during the Doctorate at Imperial College in London under the supervision of Prof. James R. Durrant. After completing his doctorate he also held a post-doctorate position at Imperial College in the same research group. In 2003, he held another postdoctoral program at USP under the supervision of Prof. Henrique Toma. He is currently Professor of the Chemistry Institute of UNICAMP. He has experience in the field of Chemistry, with emphasis in the application of nanomaterials in Solar Energy Conversion, working mainly in the following subjects: inorganic nanoparticles of chalcogenides and perovskite (quantum dots) in light emitting diodes (LED); photocatalytic oxide / graphene nanocomposites for the generation of hydrogen and direct conversion of CO2 into solar fuels; emerging solar cells (in particular TiO2 / dye cells and perovskite solar cells). In 2017 he held a sabbatical at SLAC-Stanford in the field of application of Synchrotron light in the characterization of materials for energy conversion. Published more than 115 papers, 3 patents, 1 book and 7 book chapters. She is the leader of the reserach on emerging photovoltaics in Latin America.
Metal halide perovskite solar cells have reached the recent efficiency breakthrough of 25.6%, higher than silicon polycrystalline photovoltaics. Such fantastic result was only possible due to a precise control and engineering of the morphology, interfaces, defects, and the use of multiple cations in perovskite A-site, as Rb, Cs, MA (methylamonnium), FA (formamidinium) and long alkyl cations as phenylethylammonium (PEA). Dimensionality of perovskite materials can be easily controlled by the choice of the cation in the A site, providing structures from zero (OD), one (1D), two (2D) and three-dimensions (3D), amplifying the use of these materials in lighting, lasers and sensors.
In this presentation, we will summarize important results using in situ experiments to probe the formation of 2D perovskite materials with different organic cations. Dynamics of the formation of these structures and interfaces in solution or solid state, their stability under thermal stress and aggregation, were studied by in situexperiments probing the samples with both X-rays and/or visible radiation. For that, we employed time-resolved grazing incidence wide angle X-ray scattering (GIWAXS), small angle X-Ray scattering (SAXS), high-resolution XRD and PL spectroscopy taken at the Brazilian Synchrotron National Laboratory and Lawrence Berkeley National Laboratory.
Techniques-O3
Juan Bisquert (pHD Universitat de València, 1991) is a Professor of applied physics at Universitat Jaume I de Castelló, Spain. He is the director of the Institute of Advanced Materials at UJI. He authored 360 peer reviewed papers, and a series of books including . Physics of Solar Cells: Perovskites, Organics, and Photovoltaics Fundamentals (CRC Press). His h-index 95, and is currently a Senior Editor of the Journal of Physical Chemistry Letters. He conducts experimental and theoretical research on materials and devices for production and storage of clean energies. His main topics of interest are materials and processes in perovskite solar cells and solar fuel production. He has developed the application of measurement techniques and physical modeling of nanostructured energy devices, that relate the device operation with the elementary steps that take place at the nanoscale dimension: charge transfer, carrier transport, chemical reaction, etc., especially in the field of impedance spectroscopy, as well as general device models. He has been distinguished in the 2014-2019 list of ISI Highly Cited Researchers.
Recent advancements in computational systems have shown that it is possible to respond instantly to environmental stimuli with minimal energy consumption, making it necessary to develop miniature elements that emulate natural cognitive processes. One key area of focus is the brain, which provides a model for information transmission, learning, computation, and signal processing. Synapses and neurons, in particular, rely on ionic currents and electrochemical potentials to facilitate these processes. Halide perovskite is a material that has garnered attention for its optoelectronic properties, as well as its switchable behavior that mimics biophysical systems. In this study, we explore how the unique properties of halide perovskite can be harnessed for effective neuromorphic elements that rely on the physical properties of the device. We use impedance spectroscopy and equivalent circuits to characterize highly nonlinear models and behaviors that elicit neuron-style behavior. By controlling the exaggerated hysteresis in memristor devices, we can obtain domain characteristics such as spiking and potentiation that are essential for establishing edge computing in systems that operate with minimal power consumption.1-3
(1) Bisquert, J. Hopf bifurcations in electrochemical, neuronal, and semiconductor systems analysis by impedance spectroscopy, Appl. Phys. Rev. 2022, 9, 011318.
(2) Bisquert, J.; Guerrero, A. Chemical Inductor, J. Am. Chem. Soc. 2022, 144, 5996–6009.
(3) Bisquert, J. Negative inductor effects in nonlinear two-dimensional systems. Oscillatory neurons and memristors, Chemical Physics Reviews 2022, 3, 041305.
Techniques-O4
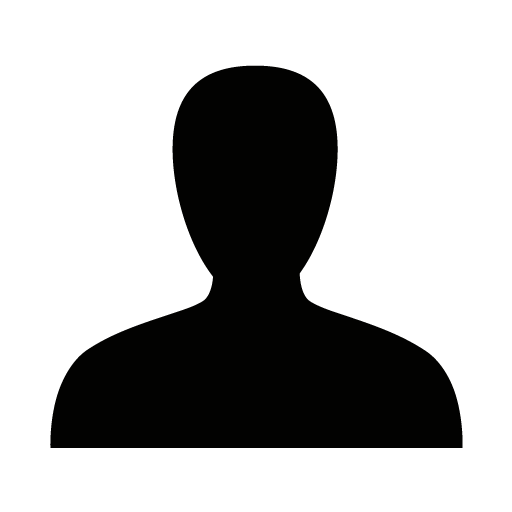
While progress has been made in the design of new organic semiconductors (OSCs) with improved transport properties (i.e., high mobilities), our understanding of the mechanisms involved is still limited and hinders further development, due in large part to the inherent difficulty when comparing OSCs with different molecular structure and morphologies. For silicon, enhanced understanding finally came once comparisons could be made with variations in morphology from single crystals to fully amorphous films. In this work, we achieve a similar feat in one single organic molecular system and using an ultra-sensitive Hall measurement technique.
In particular, rubrene is employed as the OSC as it spans transport mechanisms from thermally activated hopping in its amorphous form to band-like transport in highly ordered crystals. Various transport characterizations including variable temperature conductivity, advanced Hall effect and magnetoresistance measurements are performed on rubrene films with varying levels of order (polycrystalline vs amorphous), crystal phase (orthorhombic vs triclinic) and morphologies (platelet-like vs spherulitic grains). We find that conductivity can be tuned over four orders of magnitude when changing the level of order in the film from fully amorphous to polycrystalline with a few high-quality grains. Our results show that transport in polycrystalline orthorhombic films is limited by grain boundaries, as observed in polycrystalline silicon. The use of advanced Hall measurement, for the first time performed on OSC thin films without the use of “gating” (gate voltage applied through addition of a dielectric and gate electrode), provides access to the intrinsic properties of the semiconductor. Despite the very high resistivity of amorphous and triclinic rubrene, we are able to probe a Hall signal, pointing to the existence of a marginal density of delocalized carriers. Overall, our Hall and magnetoresistance measurements suggest a gradual transition from predominantly hopping transport to predominantly band-like transport as order is increased and crystal phase optimized.
In summary, through this work we provide a comprehensive understanding of the interplay between order, molecular packing, morphology and charge transport in OSCs, akin to research on silicon decades ago. Our results point toward the application of a unified transport model with varying contributions of delocalized and localized carriers. More importantly, our study highlights that order alone is insufficient whereas intermolecular coupling is paramount for optimal transport, providing guidelines for the design of new molecules.
Techniques-O1
The crystallisation pathway of metal halide perovskites from solution significantly impacts optoelectronic quality, defect formation and stability in the formed materials. While many crystalline intermediates - such as solvate and polytype phases - have been identified for typical Pb-based and I-rich compositions, much remains to be uncovered about the effect of cation, metal and halide composition on phase growth. Here we present a holistic understanding of perovskite crystallisation across a wide range of compositions and bandgaps relevant for tandem applications, and also address the role of additives in secondary phase formation.[1][2] Principally we investigate perovskite materials deposited by blade-coating and monitored using synchrotron-based in situ grazing-incidence wide-angle X-ray scattering (GIWAXS). By means of a selection of solution characterisation methods, including nuclear magnetic resonance (NMR) spectroscopy, we establish a clear understanding of the bridge between solution chemistry, precursor phase formation and resulting material properties across the entire range of PV-relevant perovskite compositions. Our work provides important insights into the controlled growth of stable perovskite materials by understanding and manipulating the crystallisation pathway.
Techniques-O2
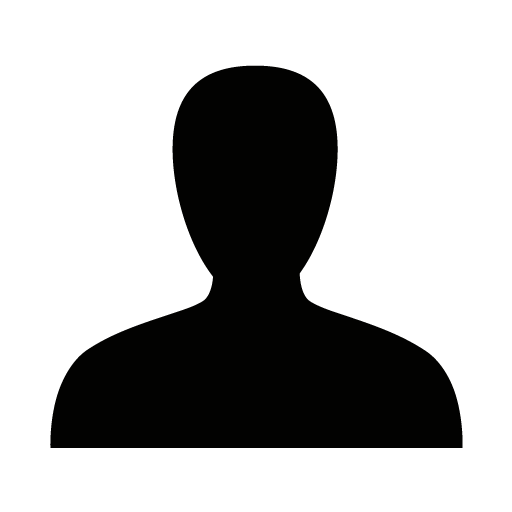
Over the past decade, hybrid halide perovskites have emerged as highly promising semiconducting materials for thin-film photovoltaics. Yet, continuing fundamental research is required to understand properties of these materials to unlock their full potential and to achieve durable perovskite photovoltaics. In this regard, employing of microscopy techniques is a promising approach to study fundamental properties of hybrid perovskites [1]. Microscopy techniques enable direct visualization of mesoscale disorder of hybrid perovskites that impacts the macroscale properties of these materials [2]. Among variety of microscopy techniques, photoemission electron microscopy (PEEM) is a powerful technique that provides valuable insights into the properties of hybrid perovskites on micro- and nanoscale. By employing PEEM, we have successfully imaged nanoscale surface defects in spin-coated perovskite films and demonstrated their roles in trapping of photoexcited holes [3]. We further uncovered the varied nature of these defects [4] and understood their roles in photo-degradation of hybrid halide perovskites [5].
In this talk, I will discuss in detail the principles of PEEM and will explain how this technique can be applied to study hybrid perovskites. I will introduce requirements for PEEM experiments, variety of possible measurements and will highlight considerations for imaging of surface defects of perovskite thin films. I will next discuss how to add time resolution to PEEM measurements and how to employ time-resolved PEEM (TR-PEEM) to study charge trapping dynamics of hybrid perovskites in space and time.
Techniques-O5
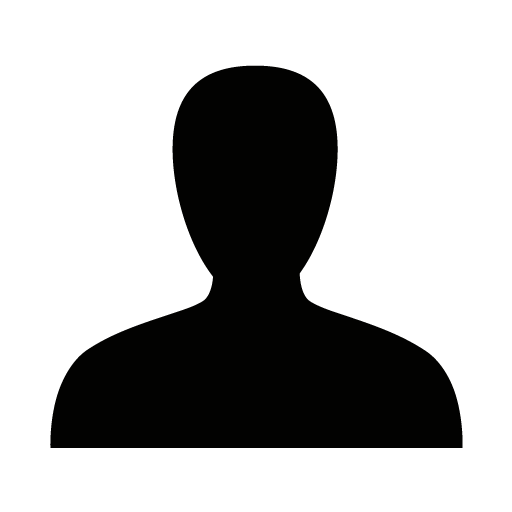
Understanding where the photovoltage is generated in a solar cell and where energy losses occur is a key aspect for the development of any solar cell technology.
In time-resolved photoelectron spectroscopy, photo-induced core level shifts can be measured as a function of pump-probe delay time and used to determine how the electric field between sample surface and substrate changes upon illumination. This gives insight how the photovoltage in the cell rises and decays over time. We have previously used this technique to follow the charge separation and recombination between a lead sulphide quantum dot layer and an electron transport layer from pico- to microsecond timescales.1
Here, I will present how we have extended our previous study to investigating the photovoltage generation in different parts of the quantum solar cell through sample design. In addition to investigating charge separation to an electron transport layer, we also investigated the photovoltage generation at the junction between p- and n-type quantum dot layers. The results are then compared to the photovoltage generation in a full quantum dot solar cell, where a gold contact is present. The highest photovoltage was generated in this latter case, and the presence of the gold contact also led to a decrease in the charge recombination rate.
Finally, I will discuss how this technique could be applied to other solar cell technologies and what potential the technique has for giving insights into the development of solar cells.
Techniques-O6
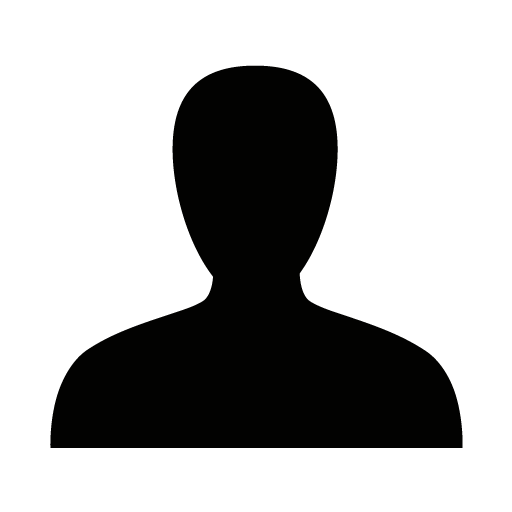
Single junction perovskite solar cells have rapidly become the most promising emerging photovoltaic technology, with record efficiencies now on-par with established silicon technology. A logical next step in the development of perovskite photovoltaic technology is the all-perovskite tandem solar cell. By harvesting a broader range of the solar spectrum more efficiently, tandem solar cells can achieve power conversion efficiencies exceeding those of single junction solar cells. Record efficiencies for all-perovskite tandem solar cells have recently overtaken single junction perovskite solar cell records, but are still far from their theoretical maximum. Better understanding of device properties is essential to systematically increase the performance of all-perovskite tandem solar cells. However, the increased complexity of tandem solar cells complicates the study of device properties.
This work explores the use of electrochemical impedance spectroscopy to study recombination and ion migration processes in all-perovskite solar cells. It is shown that interpreting results is challenging due to the overlapping signal of the tandem sub-cells. However, magnitude and frequency of the impedance spectrum change significantly with light intensity. By precisely controlling how much light is absorbed in each sub-cell by adjusting the emission spectrum of an LED solar simulator, we show that it is possible to selectively enhance or suppress impedance signals arising from each individual sub-cell. This enables the investigation of individual sub-cells within the tandem device stack. We show that our method even works for tandems in which the impedance signal of both sub-cells strongly overlaps, allowing us to extract recombination and ionic motion time constants for the individual sub-cells. Studying individual sub-cells will make it easier to find bottlenecks and allows targeted improvement strategies. The methodology developed in this work makes electrochemical impedance spectroscopy a powerful tool to improve the performance of all-perovskite (and other) tandems, and will allow these solar cells to unlock their full potential.
2A-K1
Thuc-Quyen Nguyen is a professor in the Center for Polymers and Organic Solids and the Chemistry & Biochemistry Department at University of California, Santa Barbara (UCSB). She received her Ph.D. degree in physical chemistry from the University of California, Los Angeles, in 2001 under the supervision of Professor Benjamin Schwartz. Her thesis focused on photophysics of conducting polymers. She was a research associate in the Department of Chemistry and the Nanocenter at Columbia University working with Professors Louis Brus and Colin Nuckolls on molecular self-assembly, nanoscale characterization and molecular electronics. She also spent time at IBM Research Center at T. J. Watson (Yorktown Heights, NY) working with Richard Martel and Phaedon Avouris. Her current research interests are structure-function-property relationships in organic semiconductors, sustainable semiconductors, doping in organic semiconductors, interfaces in optoelectronic devices, bioelectronics, and device physics of OPVs, photodetectors, and electrochemical transistors. Recognition for her research includes 2005 Office of Naval Research Young Investigator Award, 2006 NSF CAREER Award, 2007 Harold Plous Award, 2008 Camille Dreyfus Teacher Scholar Award, the 2009 Alfred Sloan Research Fellows, 2010 National Science Foundation American Competitiveness and Innovation Fellows, 2015 Alexander von Humboldt Senior Research Award, 2016 Fellow of the Royal Society of Chemistry, 2015-2019 World’s Most InfluentialScientific Minds; Top 1% Highly Cited Researchers in Materials Science by Thomson Reuters and Clarivate Analytics, 2019 Fellow of the American Association for the Advancement of Science (AAAS), 2023 Wilhelm Exner Medal from Austria, 2023 Fellow of the US National Academy of Inventors, 2023 de Gennes Prize in Materials Chemistry from the Royal Society of Chemistry, 2023 Elected Member of the US National Academy of Engineering, 2024 Fellow of the European Academy of Sciences, and 2025 ACS Henry H. Storch Award in Energy Chemistry.
Organic solar cells (OSCs) potentially can offer low cost, large area, flexible, light-weight, clean, and quiet alternative energy sources for indoor and outdoor applications. OSCs using non-fullerene acceptors (NFAs) have garnered a lot of attention during the past few years and shown dramatic increases in the power conversion efficiency (PCE). PCEs higher than 19% for single-junction systems have been achieved, but the device lifetime is still too short for practical applications. Thus, understanding the degradation mechanisms in an OSC is crucial to improve its long-term stability. In this talk, I will discuss the degradation mechanisms in organic solar cells based on PM6:Y6. We investigated different device structures on the device lifetime. A combination of characterization methods such as solid state Nuclear Magnetic Resonance (NMR), resonant soft X-ray scattering (RSoXS), AFM, X-ray photoelectron spectroscopy (XPS), Electron paramagnetic resonance (EPR) spectroscopy, and capacitance spectroscopy are employed to gain insight into the device degradation.
2A-I1
The currently best organic solar cells suffer from relatively large voltage losses due to non-radiative recombination as compared to alternative technologies. Further enhancement of the power conversion efficiency to values over 20% will require a reduction of these losses, inevitably corresponding to an increase in the electroluminescence quantum efficiency of the devices.[1] For a large number of donor-acceptor combinations, we have observed that non-radiative voltage losses decrease with increasing charge-transfer-state energies, consistent with non-radiative decay being facilitated by a common high frequency molecular vibrational mode.[2] We further identify small molecule donor-acceptor blends with an optical gap in the visible spectral range, with strongly reduced non-radiative losses as compared to systems with a gap in the near infrared (NIR).[3] This highlights the possibility of a simultaneous occurrence of a high photovoltaic quantum efficiency as well as a high electroluminescence quantum efficiency, occurring in a single organic donor-acceptor blend. For photovoltaic blends with strong absorption in the NIR, we show that the lowest non-radiative decay rates correspond to systems with the narrowest emission linewidths and steepest absorption tails.[4] In this presentation, I will summarize our recent understanding of free carrier recombination in organic solar cells and provide design rules to minimize the associated voltage losses.
2A-I2
Maria Antonietta Loi studied physics at the University of Cagliari in Italy where she received the PhD in 2001. In the same year she joined the Linz Institute for Organic Solar cells, of the University of Linz, Austria as a post doctoral fellow. Later she worked as researcher at the Institute for Nanostructured Materials of the Italian National Research Council in Bologna Italy. In 2006 she became assistant professor and Rosalind Franklin Fellow at the Zernike Institute for Advanced Materials of the University of Groningen, The Netherlands. She is now full professor in the same institution and chair of the Photophysics and OptoElectronics group. She has published more than 130 peer review articles in photophysics and optoelectronics of nanomaterials. In 2012 she has received an ERC starting grant.
Electron and hole transport layers are of outmost importance for thin film solar cells, determining not only their efficiency but also their stability. When considering the necessary steps to bring one of these thin film technologies towards commercialization, more factors besides efficiency and stability become important, for example the ease of deposition in a scalable manner and the cost of the different material’s layers. Herein, highly efficient organic solar cells (OSCs), in the so-called inverted structure (n-i-p), are demonstrated by using as electron transport layer (ETL) tin oxide (SnO2) deposited by atomic layer deposition (ALD) but also by nanocrystals. ALD is an industrial grade technique which can be applied both at the wafer level but also in a roll-to-roll configuration. A champion efficiency of 17.26% and a record FF of 79% is shown by PM6:L8-BO OSCs when using ALD-SnO2 as ETL. These devices outperform not only solar cells with SnO2 nanoparticles casted from solution (PCE 16.03%, FF 74%) but also those utilizing the more common sol-gel ZnO (PCE 16.84%, FF 77%). The outstanding results are attributed to a reduced charge carrier recombination at the interface between the ALD SnO2 and the active layer.
While SnO2 nanocrystals seems to have large problems of light soaking and stability, I will report a very simple method to increase performances of this solution processable ETL making devices become more stable and reaching efficiencies up to 16.26 for PM6:L8-BO OSCs.
2B-I1
Angus Hin-Lap Yip joined the Department of Materials Science and Engineering (MSE) and the School of Energy and Environment (SEE) at the City University of Hong Kong as Professor in 2021. He has been the associate director of the Hong Kong Institute for Clean Energy (HKICE) since 2022. He was also elected as a member of the Hong Kong Young Academy of Sciences. From 2013-2020, he was a Professor at the State Key Laboratory of Luminescent Materials and Devices (SKLLMD) at the South China University of Technology (SCUT). He got his BSc (2001) and MPhil (2003) degrees in Materials Science from the Chinese University of Hong Kong (CUHK) and completed his PhD degree in MSE in 2008 at the University of Washington (UW), Seattle. His research combines materials, interface, and device engineering to improve polymer and perovskite solar cells and other optoelectronic devices. He has published more than 270 scientific papers with citations over 36000 and an H-index of 99. He was also honoured as ESI“Highly Cited Researcher” in Materials Science 9 times from 2014-2022.
The emergence of solution-processed organic and metal halide perovskite solar cells can transform the landscape of photovoltaic technology in delivering scalable and high-performance solar cells to provide sustainable green energy. While the power conversion efficiencies (PCEs) of both single-junction organic solar cells (OSCs) and perovskite solar cells (PSCs) are rapidly ascending to >19% and >25%, respectively, their maximum efficiency is limited to ~33% accordingly to the Shockley-Queisser model for single-junction devices. However, it is possible to significantly increase the efficiency of solar cells by constructing a tandem device that consists of multiple light absorbers with considerably different bandgaps to reduce the solar cells' overall transmission and thermalization losses.
In this talk, I will discuss our work on developing high-performance monolithic perovskite/organic tandem solar cells comprising a wide bandgap perovskite (WBG) front cell and a narrow bandgap (NBG) organic rear cell connected through a recombination junction. The WBG (Eg: 1.7-1.85 eV) PSCs are chosen for the front cell due to their strong and broad absorption for visible light, smaller voltage loss, and higher photoresponse compared to their organic counterparts with approximate bandgap. While NBG (Eg: 1.1-1.25 eV) OSCs can offer better near-infrared absorption tunability and stability compared with the Sn-based NBG perovskites, making them favorable candidates for the rear cell.[1,2] Moreover, the advantage of the perovskite and organic light absorbing layers being processed from orthogonal solvents imposes fewer constraints on the choice of the materials for constructing the recombination junction and provides better flexibility on the device design of tandem solar cells.
To demonstrate state-of-the-art perovskite/organic tandem cells, an integrated strategy combining materials, interface, optical, and process engineering was adopted to optimize the two subcells and the interconnect junction simultaneously.[3,4,5] In addition, a comprehensive optoelectronic model is being developed to simulate the electrical and optical properties of the tandem solar cells and to provide guidelines to optimize their device performance. The successful development of perovskite/organic tandem cells will have far-reaching impacts on producing high-efficiency, low-cost and scalable PV cells for clean energy production.
2B-S1
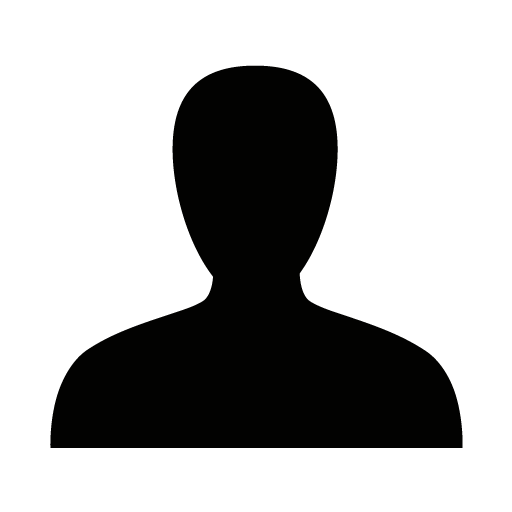
Greatcell Energy, new perspectives and products for Perovskites Greatcell Energy, new perspectives and products for Perovskites Greatcell Energy, new perspectives and products for Perovskites Greatcell Energy, new perspectives and products for Perovskites Greatcell Energy, new perspectives and products for Perovskites Greatcell Energy, new perspectives and products for Perovskites Greatcell Energy, new perspectives and products for Perovskites Greatcell Energy, new perspectives and products for Perovskites Greatcell Energy, new perspectives and products for Perovskites Greatcell Energy, new perspectives and products for Perovskites Greatcell Energy, new perspectives and products for Perovskites Greatcell Energy, new perspectives and products for Perovskites Greatcell Energy, new perspectives and products for Perovskites Greatcell Energy, new perspectives and products for Perovskites Greatcell Energy, new perspectives and products for Perovskites Greatcell Energy, new perspectives and products for Perovskites Greatcell Energy, new perspectives and products for Perovskites Greatcell Energy, new perspectives and products for Perovskites Greatcell Energy, new perspectives and products for Perovskites Greatcell Energy, new perspectives and products for Perovskites Greatcell Energy, new perspectives and products for Perovskites Greatcell Energy, new perspectives and products for Perovskites Greatcell Energy, new perspectives and products for Perovskites Greatcell Energy, new perspectives and products for Perovskites
2B-I2
Joseph J. Berry is a Senior Research Fellow at the National Renewable Energy Laboratory and Associate Professor of Physics a the University of Colorado Boulder working on metal halide perovskite based materials and devices. His Ph.D. for work was on spin transport and physics in semiconductor heterostructures from Penn State University. His efforts at NREL emphasize relating basic interfacial properties to technologically relevant device level behaviors in traditional and novel semiconductor heterostructures including oxides, organics, and most recently hybrid metal halide semiconducting materials. He also leads the DOE SETO “Perovskite Enabled Tandems” Project at NREL and is a PI for the NREL lead Center for Hybrid OrganicInorganic Semiconductors for Energy (CHOISE), an Energy Frontier Research Center financed by the Office of Basic Energy Sciences.
This talk will cover recent advances on metal halide perovskite (MHP) enabled solar cells and modules at the National Renewable Energy Laboratory (NREL). The talk will highlight efforts at NREL across projects to advance these technologies with attention paid to insights that have enabled advances in MHP-PV performance broadly defined. Work undertaken at NREL to understand current limitations in device stability/reliability axis of performance at the cell and module level will be discussed. Similarly, understanding of the basic material, their interfaces and process considerations to enable robust large area modules with single junction efficiencies above 20% and tandems above 30% will be touched upon. Specific advances to understand better practical considerations across composition and band gap, for processing of high performance devices will be discussed.[1-2] Studies providing Initial insights into how the confluence of considerations linking interfaces, defect chemistry, and process creates both challenges and opportunities will also be presented.[3-4] The science underpinning these development in MHP-PV technologies, will also be discussed in the context of sustainability goals that dictate the relevant performance metrics.
2B-I3
Annamaria Petrozza received her PhD in Physics from the University of Cambridge (UK) in 2008 with a thesis on the study of optoelectronic processes at organic and hybrid semiconductors interfaces under the supervision of Dr. J.S. Kim and Prof Sir R.H. Friend. From July 2008 to December 2009 she worked as research scientist at the Sharp Laboratories of Europe, Ltd on the development of new market competitive solar cell technologies (Dye Sensitized Solar cells/Colloidal Quantum Dots Sensitized Solar cells). Since January 2010 she has a Team Leader position at the Center for Nano Science and Technology -IIT@POLIMI. She is in charge of the development of photovoltaic devices and their characterization by time-resolved and cw Photoinduced Absorption Spectroscopy, Time-resolved Photoluminescence and electrical measurements. Her research work mainly aims to shed light on interfacial optoelectronic mechanisms, which are fundamental for the optimization of operational processes, with the goal of improving device efficiency and stability.
Bandgap tuning is a crucial characteristic of metal-halide perovskites, with benchmark lead-iodide compounds having a bandgap of 1.6 eV. To increase the bandgap up to 2.0 eV a straightforward strategy is to partially substitute iodine with bromine in so-called mixed-halide lead perovskites. Such compounds are prone, however, to defect-induced halide segregation resulting in bandgap instabilities, which limits their application in tandem solar cells and a variety of optoelectronic devices. The optimization of the perovskite composition and surface passivating agents can effectively slow down, but not completely stop, such light-induced instabilities. Here I will show how we identify the defect and the relative intra-gap electronic state/charge carrier dynamics that triggers the material transformation and bandgap shift. It allows us to engineer the perovskite band edge energetics by engineering the chemical composition of the perovskite crystalline unit to radically deactivate the photo-activity of such electronic states and stabilize the perovskite bandgap over the entire spectral range above 1.6 eV. Overall, I will show how the photo-instability of lead based single and mixed halide perovskites, which can be seen as photo-degradation and bandgap photo-destabilization, has the same root.
Spectroscopy-IS1
Most technologically-relevant lead halide perovskites are direct-bandgap semiconductors (acknowledging there is some debate on this matter) but are often not as emissive as one should expect. This impacts the application of lead halide perovskites in light emitting diodes and also gives rise to non-radiative voltage loss in solar cells. That said, a small number of very specific compositions have exhibited highly efficient photo-and-electro-luminescence and thus are used in LEDs and solar cells with large open circuit voltages.
It is now believed and partially evidenced that the non-radiative recombination in perovskites is mainly associated with Shockley-Read-Hall (SRH) recombination. In this talk I will show that the inverse of this process occurs in both perovskite and organic semiconductors too, that is, the mid-gap trap states – responsible for SRH recombination – are partially radiative and their signature can show up in the external quantum efficiency spectrum, if measured sensitively enough. This trap-induced signature can be used as an indicator for probing the extent of non-radiative loss caused by mid-gap traps. We show that these traps are mainly at the interface between the perovskite and fullerene layer, while in organic semiconductors are present in the bulk of the material. Implications of these mid-gap traps on photodetector and solar cells operation will be discussed.
Spectroscopy-O1
Christopher Petoukhoff received his PhD in 2017 from Rutgers University in Materials Science and Engineering under the supervision of Prof. Deirdre O’Carroll. During his PhD, he was awarded with a Corning, Inc. fellowship, an NSF-IGERT traineeship, and an NSF-EAPSI – JSPS Summer fellowship. He transitioned to Okinawa Intitute of Science and Technology (OIST) as a postdoc in 2017 to undertake research on ultrafast spectroscopy of energy materials, with a focus on organic-2D heterojunctions. Dr. Petoukhoff’s research interests range from nanophotonics and plasmonics to applications of 2D materials for solar energy harvesting, to semiconducting conjugated polymers for optoelectronic applications. Dr. Petoukhoff uses a combination of materials characterization, ultrafast spectroscopy, and electromagnetic simulations to design nanophotonic architectures to improve efficiencies and stabilities in organic solar cells. Since January 2022, Dr. Petoukhoff has started a posdoctoral fellowship in the King Abdullah University of Science and Technology (KAUST) Solar Center, where he will be investigating liquid-phase exfoliated 2D materials as hole transport layers in high-efficiency organic solar cells.
Solar cells formed from metal halide perovskites (MHPs) have reached remarkably high power conversion efficiencies over the past several years, with nearly 26% in single junction devices and over 27% in all-MHP tandem devices. To achieve such high-efficiency tandem devices, stacking of MHPs with different bandgap energies is a necessity. Bandgap engineering in MHPs can be achieved by varying the stoichiometry of the components; for example, changing the halide ratio in CsFAPb(BrxI1-x)3 can continuously tune the bandgap across a wide range, from 1.6-2.2 eV. While this halide mixing is critical towards developing tandem devices, there is also a drawback: photo-induced phase segregation occurs within these materials, in which different halides separate into iodide-rich and bromide-rich perovskite phases, embedded within the remaining well-mixed phase.
Recently, surface modification of transparent conducting oxides with self-assembled monolayers (SAMs) have emerged as novel hole transport layers (HTLs) in MHP solar cells. The presence of SAMs has been shown to mitigate defect formation at metal oxide/MHP interfaces. Additionally, SAMs benefit from their ability to bond covalently to and tune the work function of transparent electrodes, their vanishingly low parasitic absorption, and their strong dipole moments. One SAM in particular, (2-(9H-carbazol-9-yl)ethyl)phosphonic acid (i.e., 2PACz), and its derivatives have stood out as leading to the highest improvement in device efficiencies. While there have been numerous studies on the improved device performance when incorporating 2PACz-derivatives as HTLs, the interplay between charge extraction and recombination at SAM/MHP interfaces has not yet been fully explored.
In this work, using a combination of time-resolved and steady-state optical spectroscopies, we investigate hole extraction across SAM/MHP interfaces. We explore the use of 2PACz and its derivatives interfaced with MHPs of different bandgap energies. We reveal the competition between hole extraction and recombination through systematic transient absorption (TA) and time-resolved photoluminescence (PL) spectroscopic measurements. We demonstrate that certain 2PACz-derivatives can help suppress halide segregation, by monitoring the rise of the iodide-rich phase photobleach signal at longer pump-probe delay times in TA measurements, and the growth of the iodide-rich phase emission in steady-state and time-resolved PL measurements. Understanding the photophysical processes at SAM/MHP interfaces will help to facilitate more efficient MHP solar cells with greater phase stabilities.
Spectroscopy-O2
Tomas Edvinsson is professor in Solid State Physics at the
Department of Materials Science and Engineering, Uppsala
University, Sweden. He received his Ph.D. 2002 at Uppsala
University, performed post-doctoral work at the Royal Institute
of Technology, Stockholm, on dye-sensitized solar cells and organic-inorganic materials systems, and research for BASF AG until
2007. He is the project leader for several national projects from
the Swedish research council, the Swedish Energy Agency, and
acts as reviewer for several national and international grant
organizations. His research focus on fundamental investigations
of low dimensional materials and their utilization
in sustainable energy applications.
Lead halide perovskites have been in the lime light of emerging photovoltaic materials the last decade, due to their high absorption coefficient, high defect tolerance and charge mobility, and power conversion efficiency exceeding 25% in solar cell devices. The photoexcited charge density response is here important to describe lead halide perovskites under operation, and is in turn related to the material structure [1,2], photoinduced response, and subsequent electronic and lattice relaxation in the system. In this contribution, we present investigations of the photoinduced ion migration mechanism and nature of the excited state [3-6] and their relation to pathways for electronic and lattice relaxations with both experimental and theoretical probes. In particular, we present how the A-site cation and type of halide affect the chemical bonding and photoinduced ion movement in the system. Experiments from photoinduced Stark-effects and Raman spectroscopy are presented as well as corroborating theoretical investigations using both ground state and time-dependent density-functional theory (TDDFT). We show that the excess energy after thermalization into phonons under blue-light illumination is large enough to overcome the activation energy for iodide displacement, and can thus trigger vacancy formation and ion movement in contrast to red-light illumination [4,5]. In addition, we briefly discuss the role of mixed (Cs, FA) monovalent A-site cations in the view of their excited state response and subsequently enhanced optoelectronic properties [6]. The results form a basis for a fundamental understanding of the excited-state properties of halide perovskite material to reveal the underlying mechanism for vacancy formation, photoinduced halide segregation, excitation energy dependent hysteresis effects, and reported defect tolerance when using organic or mixed A-site cations. In an extension, the results give rationale for using dipolar A-site cations and mixed halide perovskites to decrease halide migration, and the mechanistic origin of photoinduced vacancy formation, excitation energy dependent hysteresis, and reported stability issues under blue and UV-light illumination.
Spectroscopy-O3
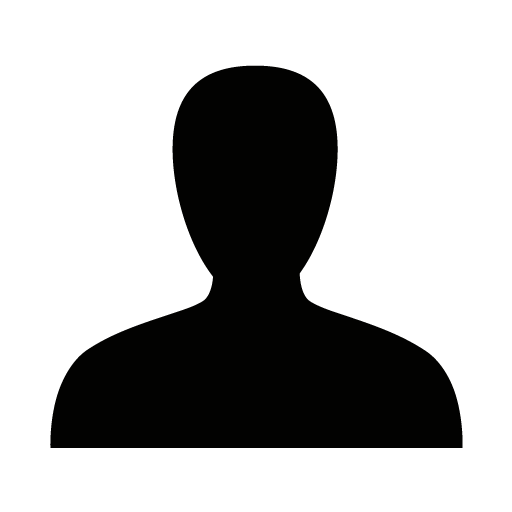
Metal halide perovskites have shown remarkable optoelectronic properties and have been employed in a range of emerging applications including photovoltaics (PV), spintronics and X-ray detectors. Optimising and understanding charge and spin transport properties in perovskite materials has been one of the key research areas to push their performance. In this talk, I will discuss a few examples on how we have used ultrafast spectroscopy techniques including Terahertz time-domain spectroscopy (THz TDS) and transient absorption spectroscopy (TAS) to study the spin and charge transport properties of hybrid perovskite materials.
Firstly, I will discuss the spintronic properties of two-dimensional (2D) perovskites. Theoretical calculations have shown large spin-orbit interaction and layered Rashba states in 2D perovskites, suggesting 2D perovskites are promising candidates for spintronic THz emitters. Herein, our polarization resolved THz emission spectroscopy results indicate that there is an ultrafast spin to charge conversion at the FM/perovskite interface, resulting in asymmetrical THz emission. Further pump polarization dependent THz emission shows that such THz emission can be coherently tuned.
Secondly, I will discuss improving the mechanical reliability of perovskite PV, particularly beneficial for space application. Specifically, I will show that incorporating a long alkly chain molecule (n-octylammonium iodide, OAI) into the perovskite active layer can release its residual stress. This incorporation greatly enhanced the thermal cycling performance of perovskite PV devices in both n-i-p and p-i-n configuration. Femstosecond-nanosecond TAS shows that devices with OAI have good charge transport properties at the perovskite/transport layer interface, whereas devices without OAI suffered from surface delamination and thus hindered charge transport at the interface.
Spectroscopy-O4
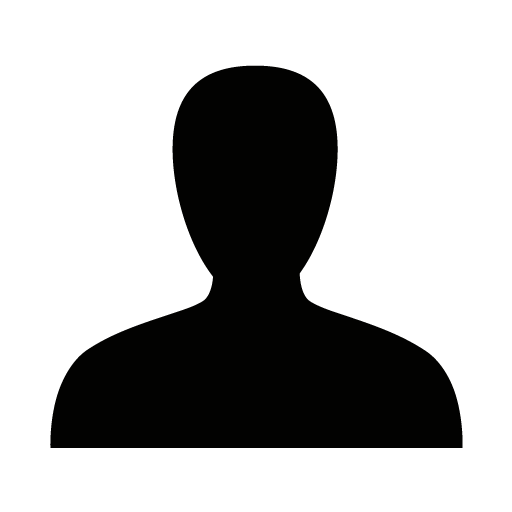
Hot-carrier solar cells are a promising candidate to exceed the detailed balance limit of 33%. One key requirement of the active material in this technology is that the cooling of hot carriers is in the order of nanoseconds to enable charge extraction. Metal halide perovskites are a promising candidate for hot-carrier materials as they show exceptionally slow cooling, often attributed to a hot-phonon bottleneck. A breakthrough was made with FASnI3 (FA being formamidinium) that shows hot-carrier emission on the nanosecond time scale.[1] However, it is unknown how the Sn-based composition affects the carrier cooling time and how the phonon lifetime is correlated with the observed hot-phonon bottleneck. In this work, we exchange FA with Cs and show that both materials show a blueshift and asymmetric broadening of the photoluminescence with increasing laser fluence, indicative of hot-carrier photoluminescence. Interestingly, the carriers in the hybrid compound cool in approximately 3 ns, much slower than sub-nanosecond cooling in the Cs system, suggesting that the organic cations play a crucial role in the cooling dynamics. By doing impulsive stimulated Raman scattering spectroscopy, we identify two coherent phonon modes (25 and 133 cm-1) in FASnI3. The 133 cm-1 mode shows a fast decay (< 1 ps) either by increasing excitation fluence or photon energy. We further show that the phonons in FASnI3 decay much faster compared to CsSnI3. These measurements correlate the pronounced hot-phonon bottleneck in hybrid compounds to the short lifetime of phonons and provide new insights into the cooling dynamics, highly relevant for the further development of hot-carrier materials.
Spectroscopy-O5
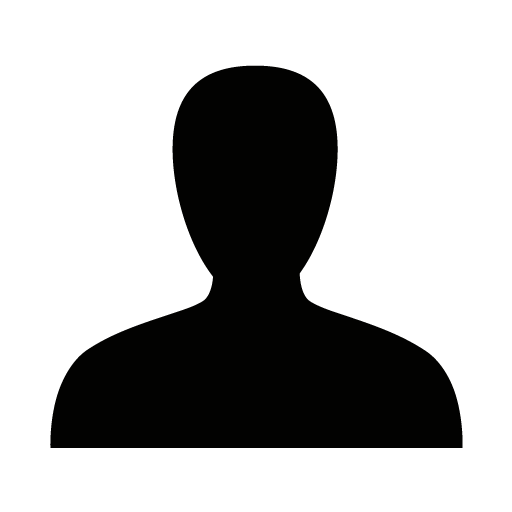
Time-resolved optical spectroscopic techniques typically monitor the evolving population of energy states after photoexcitation; and carrier mobility measurements often require the careful fabrication of full-stack electronic devices. Here, we study carrier dynamics in single-crystal CsPbBr3 using ultrafast THz spectroscopy, a method which is not only sensitive to carrier density but is also a non-contact probe of carrier mobility in bare films. We show that the charge mobility in this highly crystalline material is considerably higher than perovskite thin-films grown by conventional methods. The temperature-dependent photoconductivity reveals strongly non-Drude behaviour in the frequency domain, with strong phonon resonances that support the polaron picture of the soft crystal lattice. We further extend the time-resolved measurements to three-pulse ‘pump-push-probe’ spectroscopy to study hot carrier cooling dynamics which depend on a rich interplay of carrier-phonon and carrier-carrier interactions. Finally, the steady-state fluence-dependent photoluminescence spectra in these controlled-growth lead halide perovskite materials are found to contain features attributed to optical gain.
Spectroscopy-O6
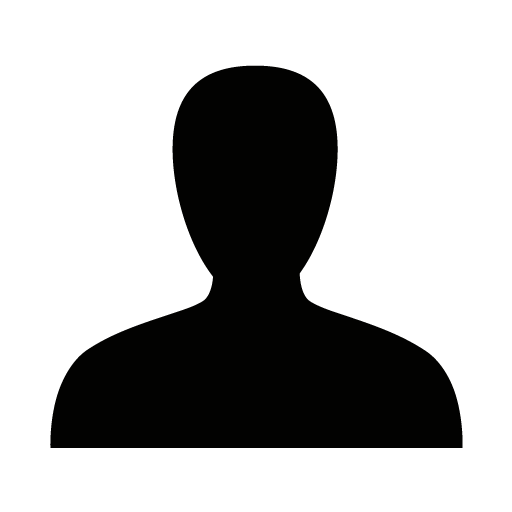
Photovoltage losses in perovskite solar cells are associated with an insufficient understanding of interfacial recombination dynamics. For wide band-gap (2.3 eV) materials, these loses still represent around 0.4 eV with respect to the theoretical voltage at the radiative limit1 which makes them excellent candidates to study these processes in depth. On the other hand, the interest in improving the performance of these materials has grown enormously, due to their potential applications in tandem cells, LEDs technologies and even in electrochemical reactions.2 In this work is presented the role of cationic additives in both electronic and ionic charges distribution and how they can modulate them to reach high photovoltages.3 Using them as sentinels, we find the common origin of two features corresponding to unwanted recombination processes: (i) inverted hysteresis during current-voltage measurements and (ii) inductive processes detectable through impedance spectroscopy, (i.e; negative capacitance).4 The cationic additives can reduce these processes leading to an outstanding record value of 1.65 V for a methylammonium lead bromide perovskite solar cell. Through time-of-flight secondary ion mass spectrometry, X-ray photoelectron spectroscopy and impedance spectroscopy, we have revealed the interaction behind this reduction, unveiling an ionic modulation effect as responsible for this achievement.
morphology-IS1
Ji-Seon Kim is Professor of Solid State Physics and Director of the Plastic Electronics Centre for Doctoral Training (https://www.imperial.ac.uk/plastic-electronics/) at Imperial College London. She has previously taken up an EPSRC Advanced Research Fellowship at the University of Cambridge, obtained a PhD in Physics in 2000. Her research focuses on the basic science and technology of Nanoscale Functional Materials such as organics, organic/ inorganic hybrids, nanomaterials and their related applications, as well as developing novel Nanometrology for these functional materials (http://www.imperial.ac.uk/nanoanalysis-group).
Organic photovoltaic (OPV) devices are attracting significant attention due to their potential to be lightweight, flexible, non-toxic, and compatible with large-scale manufacturing. In particular, the development of the new small molecule-based non-fullerene acceptors (NFAs) has enabled OPVs to show remarkable improvements in device efficiency. Although promising, there is still a lack of clear understanding of the impact of molecular structure and orientation of NFAs on photophysical processes critical for device performance.
In this talk, I will discuss the two key NFA molecular perspectives for high performance OPVs. First, I will show the molecular-structure dependent photostability, with a particular focus on NFA molecular planarity, rigidity, and end groups [1-3]. Second, I will show the molecular orientation-dependent energetic shifts in NFAs, demonstrating the impact of NFA quadruple moments and molecular orientation on material energetics and thereby on the OPV efficiency [4]. Compared to sublimed small molecules where the molecular orientation control is relatively easy [5], there has been no report, to the best of our knowledge, demonstrating the orientation control of solution-processed NFA molecules leading to an energetic shift large enough to impact exciton separation for free charge generation. As such, it is now critical to understand the molecular origins of OPV performance in much deeper detail than before to direct synthesis of organic semiconductors in more promising directions.
References
[1] “Strong Intermolecular Interactions Induced by High Quadrupole Moments Enable Excellent Photostability of Non‐Fullerene Acceptors for Organic Photovoltaics”, Luke J. et al., ADVANCED ENERGY MATERIALS, (2022), 2201267. doi:10.1002/aenm.202201267
[2] “A Commercial Benchmark: Light-Soaking Free, Fully Scalable, Large-Area Organic Solar Cells for Low-Light Applications”, Luke J. et al., ADVANCED ENERGY MATERIALS, (2021), 11(9), doi:10.1002/aenm.202003405
[3] “Twist and Degrade-Impact of Molecular Structure on the Photostability of Nonfullerene Acceptors and Their Photovoltaic Blends”, Luke J. et al., ADVANCED ENERGY MATERIALS, (2019), 9(15), doi:10.1002/aenm.201803755
[4] “Molecular orientation-dependent energetic shifts in solution processed non-fullerene acceptors and their impact on organic solar cell performance”, Fu Y. et al., NATURE COMMUNICATIONS (in press)
[5] “Orientation dependent molecular electrostatics drives efficient charge generation in homojunction organic solar cells”, Dong Y. et al., NATURE COMMUNICATIONS, (2020),11(1), doi:10.1038/s41467-020-18439-z
morphology-O1
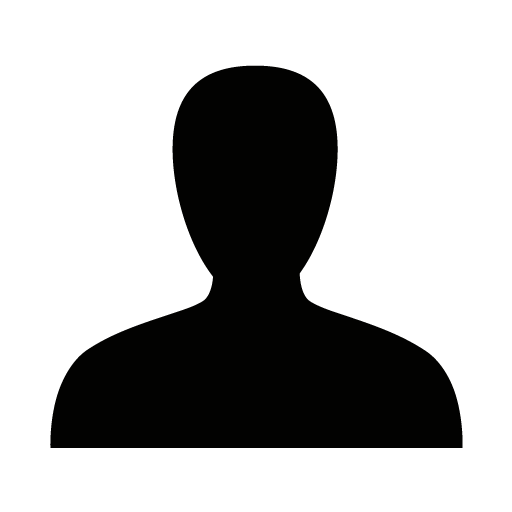
The overall efficiency of organic photovoltaic devices has made impressive progress in recent years. However, in many cases the active materials now require complex, multi-step synthesis, potentially limiting their synthesis at large scale. Here I will discuss approaches to readily synthesise monomeric building blocks in just one or two steps. Such approaches allow the preparation of (donor) polymers of low synthetic complexity which can be readily upscaled. I will highlight how this approach can be used to readily build libraries of monomer of which a variety of different sidechains. Co-polymerisation of such monomers allows the preparation of polymer libraries with systematic variations. Using one such library we investigate how sidechains influence a variety of polymer properties, such as solubility, surface energy, self-assembly and device performance. This allows the identification of a donor which can be readily synthesis and affords a device efficiency greater than 15% when combined with a low band gap non-fullerene acceptor.
morphology-O2
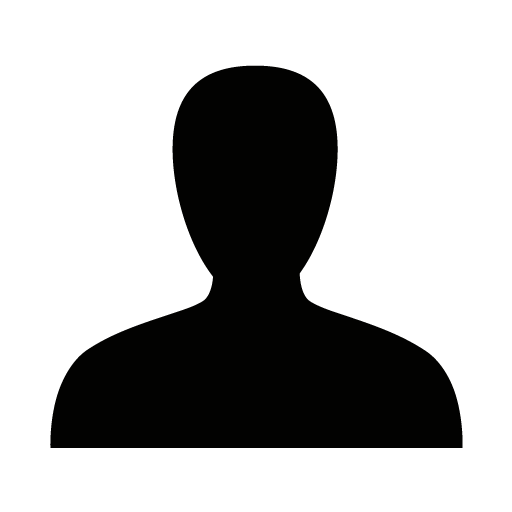
Intimate pi-stacking in organic semiconductors is known to form aggregates, which drive dissociation of photogenerated excitons through wavefunction delocalization. In particular, the onset of non-fullerene acceptors (NFAs) such as Y6 has seen a dramatic rise in discussion of exciton delocalization and its relation to (acceptor-donor) hole transfer. Here, we revisit the concept of donor exciton delocalization and show that such treatment is necessary to accurately describe the (donor-acceptor) electron transfer channel in high performance OPV blends. Specifically, we evaluate how pi-interactions in donor polymer aggregates contribute to delocalization strength, revealing that the formation of a luminescent, delocalized exciton in strongly p-interacting donor materials opens a pathway for free carrier generation. As a result, the electron transfer pathway partially bypasses the formation of performance-limiting singlet charge-transfer states during electron transfer in OPV blends. Moreover, we observe that such aggregation induced delocalization leads to a reduction of the triplet charge transfer state density. These mechanisms improve the internal quantum efficiency in OPVs to realize a power conversion efficiency of 19.2%. Ultimately, we provide insight into overcoming the fundamental limits of OPVs associated with intrinsic material properties. Designing materials with more pronounced delocalization character should maximize the exciton dissociation efficiency and minimize terminal back recombination, pushing OPVs closer to theoretical efficiency limits.
morphology-O3
Dr. Sang Pham joined the University of Leeds in February 2022 as a Postdoctoral Research Fellow working with Dr. Sean Collins on a project aimed at linking atomic structure and optoelectronic properties at defects and interfaces in polymer, small organic molecule, and hybrid semiconductors under active investigation for optoelectronic applications. He is an active and independent user of the advanced electron microscopy facilities at SuperSTEM (the EPSRC National Research Facility for Advanced Electron Microscopy), and the Electron Physical Sciences Imaging Centre (ePSIC) at the Diamond Light Source. Dr. Sang Pham completed his PhD research in Australia at the University of Wollongong in the School of Mechanical, Materials, Mechatronic and Biomedical Engineering where he focused on the development of advanced in-situ TEM experiments, aberration-corrected STEM-EELS, and high-temperature TOF-SIMS imaging characterization techniques for elucidating the structure-property relationship of materials from the nanoscale to the macroscale with the hierarchical structures (e.g. multi-layered tribofilms and double-shell microcapsules). He was awarded a PhD degree with Examiners’ Commendation for an Outstanding Thesis on February 2022. and was nominated as a school nominee for the best 2021 PhD thesis of the Faculty of Engineering and Information Sciences, University of Wollongong. His current research interests are structure-function-property relationships in organic semiconductors, interfaces in optoelectronic devices, charge transport in organic semiconductors, and advanced 4D-STEM analysis of beam-sensitive materials/systems.
Disorder in organic semiconductors (OSCs) plays a determining role in energy transport properties underpinning optoelectronic device performance [1]. Both energetic disorder native to perfect crystals as well as deviations from crystalline order control transport properties [2,3]. Alongside crystallographic defects like stacking faults and grain boundaries, dislocations that distort molecular packing can introduce exciton- or charge-carrier traps that significantly hamper intermolecular energy transport [4]. Electron microscopy has been a mainstay for probing these crystallographic defects in inorganic semiconductors. Recent progress in atomically resolved electron microscopy has enabled imaging of individual defects in hybrid perovskites [5]. But while hybrid perovskites show structural degradation on the order of <200 e–Å-2 before [6], small molecule OSCs may undergo comparable loss of structure under exposures <30 e–Å-2 [7,8]. Methods that enable the crystallographic analysis of dislocations in beam-sensitive OSCs are therefore a necessary first step to establish their performance effects.
A dislocation is described crystallographically in terms of a displacement vector in the lattice, termed a Burgers vector b. Most attempts to characterize dislocations in organic molecular crystals have relied on techniques at low spatial resolution, including etch pit imaging [9] and scanning probe techniques [10]. These approaches are unable to directly record the crystallography of dislocations or access the nanometre spatial resolution required to isolate individual defects. In contrast, electron microscopy combines the necessary spatial resolution to image the dislocation line as well as the crystallographic detail from electron diffraction to retrieve the Burgers vector. Typically, such an analysis is carried out by many repeated electron beam exposures and sample rotations aimed at identifying the crystal planes associated with a diffraction vector g that are not distorted by the dislocation, a so-called ‘invisibility criterion’ at g.b = 0. Here, we advance this approach for OSCs to carry out unambiguous analysis of the dislocation Burgers vector and type (screw, edge, or mixed) using four-dimensional scanning transmission electron microscopy (4D-STEM) now in a single exposure at a fluence of ~10 e–Å-2.
Thin films of p-terphenyl and anthracene were prepared by solution crystallization as a set of benchmark organic optoelectronic materials [11]. On transfer to a lacey carbon support film for electron microscopy, the draping of the OSC crystals on the support film introduces a small amount of sample bending. This bending defines a set of diffraction conditions that produce ribbons of bright intensity running across images of the film referred to as bend contours. These bend contours exhibit an abrupt shift or break on crossing dislocations unless they satisfy the invisibility criterion. Our 4D-STEM approach specifically supports simultaneous analysis of many lattice planes approximately parallel to the crystal direction perpendicular to the film, i.e. [001] for p-terphenyl and [101] for anthracene. Plotting and fitting the magnitude of breaks in the bend contours as a function of the corresponding diffraction vectors (g) for each plane determines the Burgers vector. For instance, this analysis establishes mixed-type b = [010] dislocations in p-terphenyl and anthracene. These generalizable methods make an analysis of the Burgers vectors of dislocations in beam-sensitive OSC films a routine process. The capability to measure the character and type of dislocations will provide experimental input for models of distortions at these structural defects and enables assessing methods for inhibiting dislocation formation during crystal growth and reducing or removing their deleterious contributions to device performance.
morphology-O4
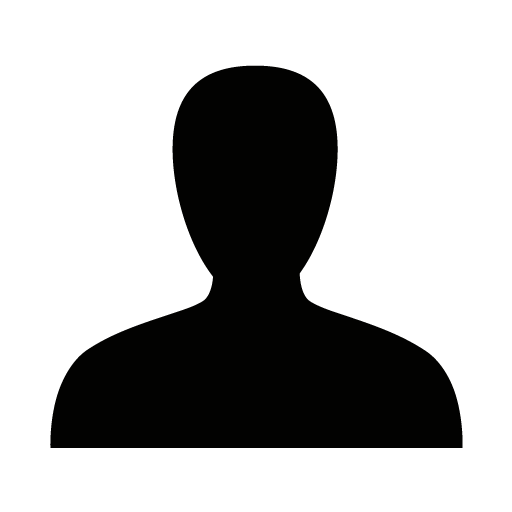
The emergence of nonfullerene acceptors (NFAs) has triggered a rapid advance in the performance of organic solar cells (OSCs), endowing OSCs to arise as a promising contender for 3rd generation photovoltaic technologies. Meanwhile, the ultimate goal of OSCs is to deliver cheap, stable, efficient, scalable, and eco-friendly solar-to-power products contributing to global carbon neutrality. However, simultaneously balancing these five critical factors of OSCs toward commercialization is extremely challenging. In this presentation, I will show the self-assembly strategy we developed to reduce the gap of high power conversion efficiency (PCE), long-term stability, green-solvent-processibility, scalability, and low cost of OSCs and demonstrate our green-solvent-processable and open-air-printable OSCs with simultaneously simplified device architecture and enhanced PCE, shelf, thermal as well as light illumination stability. Further, I will demonstrate a comparison of self-assembled and traditional devices and unveil the enhancement of self-assembled devices on photovoltaic performance and stability in detail with in-situ and ex-situ multimodal characterizations. Finally, I will present our results on scale-up fabrication and perspective to apply our self-assembly strategy to close the lab-to-fab gap of OSCs toward commercialized cheap, stable, efficient, scalable, and eco-friendly OSCs.
morphology-O5
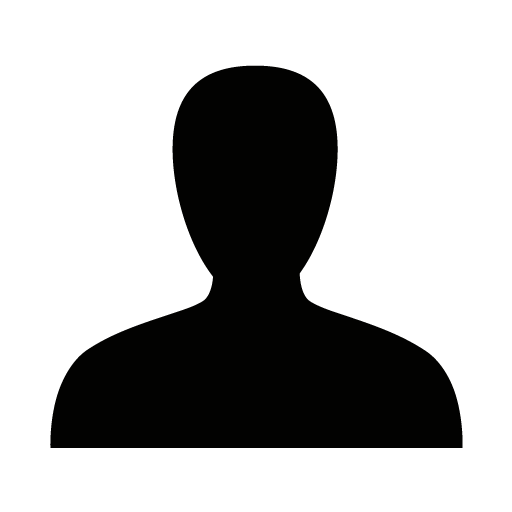
The efficiencies of organic photovoltaics (OPVs) - while lower than the conventional silicon solar cells - have seen a sharp increase in recent years thanks to the development of new non-fullerene acceptors. However, achieving long-term performance stability in the higher efficiency OPVs is still challenging. This is because best performing bulk heterojunction devices are based on blends of different materials that demix when exposed to light and heat, leading to a strong reduction in conversion efficiency after as little as a few hours in some cases (Mateker & McGehee, 2017). A promising approach to tackle the stability issue is the use of single-component macromolecular semiconductors (Review by Roncali, 2021), which have recently showed significant improvements in conversion efficiency reaching >11% in a block co-polymer system while maintaining good performance stability (Wu et al., 2021). By combining the state-of-the art polymer donors and non-fullerene acceptors into block co-polymers, we hope to gain a deeper physical understanding of how charge generation and transport works in those systems. For this we are investigating block co-polymer systems based on the PBDB-T and PYT material groups. On the one hand we are using chemical modifications to the building blocks of the polymers to tune the energetics in the system in order to understand the interplay between through-space and through-bond charge transfer. On the other hand, we are modifying the large-scale structure of the polymers by tuning the length of the donor and acceptor segments to make a comparison to the polymer-polymer bulk heterojunction. These new material systems are analysed using optoelectronic measurements and modelling to better understand the morphological ordering in block co-polymers and the impact the morphology has on the device properties. Combining the advances in efficiency thanks to novel chemical design of donors and acceptors with the long-term stability of the block co-polymer structure will hopefully lead to more industrially viable alternatives to the popular bulk heterojunction devices (He et al., 2022).
morphology-O6
Polymer solar cells (PSCs) have seen rapid progress in recent years, whereby the mixture of polymer donors and small-molecule acceptors (SMAs) are fine-tuned to realize a favorable kinetically trapped morphology and thus a commercially viable device efficiency. However, the thermodynamic relaxation of the mixed domains within the blend raises concerns related to the long-term operational stability of the devices, especially in the record-holding A-DA'D-A type SMAs (typically identified as Y6). In addressing this challenge, we report a new class of dimeric Y6-based SMAs tethered with differential flexible spacers to regulate their aggregation and relaxation behavior. In their polymer blends with PM6, we find that they favor an improved structural order relative to that of Y6 counterpart as evidenced by their shorter facial π-π stacking distance and larger crystal coherence length, leading to higher and more balanced charge transport in device. Most importantly, with flexible spacers to restrict the motion of individual SMAs, the tethered SMAs show large glass transition temperatures to suppress the thermodynamic relaxation in mixed domains, which is also evidenced by the larger Flory–Huggins interaction parameter with the polymer donor. The high performing dimeric blend dominates a conversion efficiency of 17.85%, while those of regular Y6-base devices only 16.9%, respectively. Most importantly, the dimer-based device possess substantially reduced burn-in efficiency loss, retaining more than 80% of the initial efficiency after operating at the maximum power point under continuous illumination for 700 hours. Our tethering approach provides a new direction to develop PSCs with high efficiency and excellent operating stability.
Scale-up-IS1
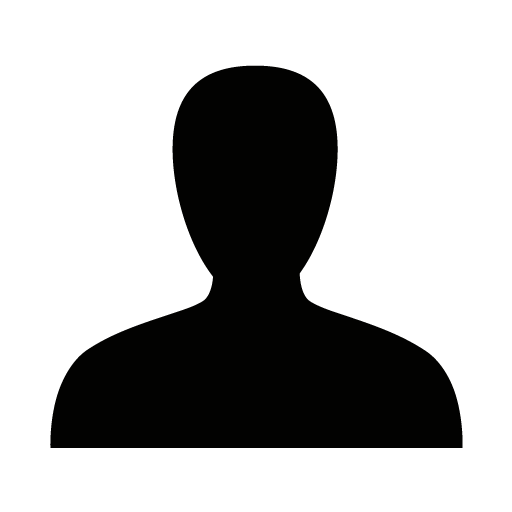
With the advent of non-fullerene acceptors, breaking the 20 % power conversion efficiency limit is within close reach for organic solar cells. Understanding the efficiency-limiting processes remains important.
I will discuss how losses in the fill factor can be due to the transport resistance, a voltage loss because of a low conductivity in the active layer. Its relevance for organic solar cells was only shown a half dozen years ago [1]. I will present transport resistance limiting different organic solar cell types, and then focus on fresh and thermally degraded PM6:Y6 solar cells (heated to 85°C, in the dark, under nitrogen atmosphere), a state-of-the-art system based on the non-fullerene Y6. The increasing fill factor losses on this degradation path are because of the transport resistance [2]. The reason seems to be trap formation in the tail states, which decrease the active layer conductivity. I will show that the transport resistance as fill factor loss is also prominent in other organic solar cells types, not just degraded but also fresh ones.
Scale-up-O1
Solution-processed perovskite solar cells have very high efficiencies, but scaling up this technology requires re-thinking of the fabrication process to suit commercially viable printing techniques. Here, we focus on the slot-die coating technique and develop an anti-solvent treatment process which achieves high quality perovskite films by utilising in-situ optical spectroscopy analysis. Photoluminescence (PL) and transmittance spectroscopy probes were customised to monitor the changes in perovskite crystallisation during and after coating. Using methylammonium lead iodide as a proof of concept material, we investigated several antisolvents with different miscibility to the host solvents and observed, from the PL kinetic analysis that diethyl ether was the slowest acting antisolvent and produced the highest PL peak intensity from intermediate perovskite phases. In-situ transmittance data enabled the analysis of perovskite crystallisation after the transfer of the film to the hot plate, and also showed diethyl ether is the slowest acting solvent. The PL peak position and intensity before transferring the film to the hot plate were found to be the most important parameters in optimising the final perovskite film quality. Electron microscopy and x-ray diffraction confirmed that the highest quality crystals and crystal coverage is achieved by the diethyl ether antisolvent bath.
Using the optimised antisolvent treatment, devices were made from ITO substrate, SnO2 (slot die coating), MAPbI3 (slot die coating), PTAA (spin coating) and gold (thermal evaporation), all in ambient conditions. The champion device showed near 18% power conversion efficiency. These results demonstrate the clear potential of implementing the antisolvent bath treatment in the printing of large area high quality perovskite films. They also demonstrate that in-situ optical analysis is very effective in optimising the deposition processes in the fabrication of large area devices, which can benefit industrial operations.
Scale-up-O2
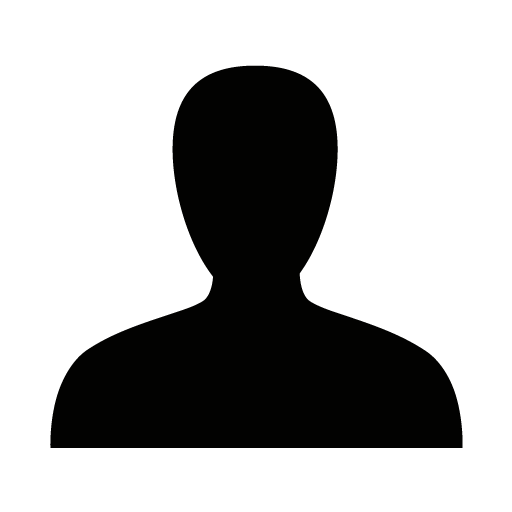
In recent years, organic solar cells based on Y-family NFAs have surpassed 18% efficiency. However, these are typically demonstrated for lab-scale devices processed with halogenated solvents such as chloroform and chlorobenzene, with limited compatibility with scalable fabrication methods due to their environmental toxicity. Considering the latter and owing to the importance of processing solvents in modulating the blend microstructure, a key strategy is to develop solar cells that can be processed from greener solvents, by using molecules designed for increased solubility.
Moreover, there is still a large performance gap between spin-coated, lab-scale devices and large-scale modules. This is due in part to the challenges in maintaining an optimal active layer morphology when switching to industrial fabrication techniques. Contributing factors include thickness constraints, drastically different drying kinetics, and temperature-dependent aggregation. To bridge this gap, it is paramount to understand the interplay among processing, microstructure, thin film properties, and charge carrier kinetics of devices made with scalable techniques, such as blade coating and slot-die coating, and comparing them to their spin-coated counterparts.
In this work, we demonstrate highly efficient organic solar cells fabricated with spin-coating, blade coating, and slot-die coating techniques, using PM6 and Y12 in o-xylene as electron donor and electron acceptor materials, respectively. In particular, we obtain above 14% PCE in slot-die coated devices, comparable to lab-scale devices.
We then investigate the differences and similarities between these techniques with a variety of optoelectronic techniques, including transient photovoltage and charge extraction, to compare charge carrier dynamics.
Scale-up-O3
The photovoltaics (PV) industry is about to enter the terawatt (TW) scale, which is an essential milestone to climate change mitigation. Industry roadmaps predict that this will be accomplished by tandem technologies, likely based on perovskites. Perovskite solar cells (PSC) are often referred to as manufacturable by “abundant materials”. In this contribution, we present a comprehensive quantitative assessment of this statement with the perspective of TW scale perovskite PV. We compare the projected demand of inorganic and synthetic materials to current production (mining and synthesis) and assess the scalability of current material production, in order to identify potential supply risks. For researchers who want to make a contribution to climate change mitigation with their research in sustainable and scalable perovskite PV, the results of this study provide useful selection criteria for materials and designs.
We find that, despite annual material demands in the kilo-ton range, for most materials the supply will likely not become critical. However, indium commonly used in TCOs must urgently be replaced. Cesium used as A-side cation, especially in fully inorganic perovskite, is not sufficiently available for TW-scalable technologies. For several synthetic hole transport materials like spiro-OMeTAD or PTAA, fundamental research yielding scalable synthesis is necessary if they should be implemented in large scale perovskite PV. Other synthetic materials like fullerenes or self-assembled monolayers require roadmaps for industrially scalable synthesis. Solvent supply is found to be not critical. Finally, the foreseeable massive material waste streams mandate that researchers adapt a design-for-recycling thinking already in early stages of technology development.
Scale-up-O4
Javier E. Sebastian Alonso graduated in 2022 with an MSc in Chemistry for Renewable Energy with a joint project between Uppsala University (Sweden) and the University of Groningen (The Netherlands) on Carbazole Based Self – Assembled Monolayers as Hole Transport Layer for Efficient and Stable Cs0.25FA0.75Sn0.5Pb0.5I3 PSCs. His current work at MOED focuses on the Scalability of highly efficient PSCs via vacuum co-evaporation with improved Stability. He brings his work focused on speeding up the deposition of co-evaporated PSCs to enable Lab-to-Fab transition.
Metal Halide Perovskites (HaP) represent the most promising alternative to further develop the photovoltaic industry due to properties such as low exciton binding energy, high absorptivity, tunable band gap or lightweight. Currently, most of the research in Perovskite Solar Cells (PSCs) relies on solution based synthetic pathways of the photoactive HaP layer lidding to inhomogeneities on rough/ textured surfaces. Thus, fabrication of PSCs via vacuum co-evaporation of the precursor salts (i.e. for MAPbI3 we co-sublime PbI2 and MAI) relies on a reproducible procedure for the co-sublimation of PbI2 and MAI based on an evaporator chamber setup with only two quartz crystal microbalances (QCMs) to control their deposition rates. Furthermore, vacuum co-evaporation for thin-film fabrication has been extensively used in industry enabling Lab-to-Fab transition. Nonetheless, for efficient solar cells, vacuum co-evaporation of MAPbI3 can be time consuming (i.e. the usual deposition rate in our lab is 0.65 Å/s, taking around 2 h for the deposition of a 500 nm thick perovskite. Herein, we show that it is possible to speed up these deposition rates four to six times quicker still achieving solar cells with power conversion efficiencies (PCE) close to 20%. Thus, fabricating HaP layers of more than a micron thick yielding an increase in short circuit current (Jsc, ~ 23 mA/cm2) for HaP with a 1.58 eV bandgap without losing on open circuit voltage (Voc).
Scale-up-O5
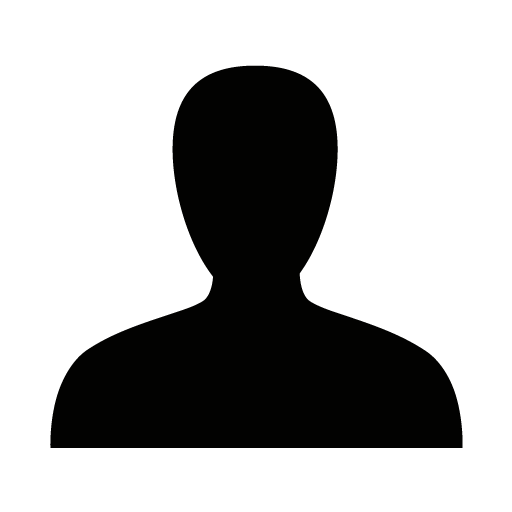
For the consolidation of organic photovoltaics (OPV), it is crucial to create market pull through the identification and target of strategic niches, where this technology can exploit its fundamental differentiators.1 For instance, materials engineering has enabled wavelength-selective harvesting with transparent OPV for power-generating windows2 and building-integrated photovoltaics.3 Therein, a simultaneous high efficiency and high transparency are needed. While the community has made relevant developments to maximize the optoelectronic properties of OPV devices, little attention has been paid to their structural properties. High-volume manufacturing technologies such as plastic thermoforming and injection moulding can help expand the opportunities, the capabilities, and the seamless integration of OPV.
In this work we demonstrate, for the first time, the feasibility of fabricating OPV cells and modules embedded into structural plastic parts through injection moulding. This process yields lightweight OPV devices with enhanced device robustness and durability, thanks to the hermetical and conformable encapsulation resulting from the plastic injection. We discuss the interplay between the plastic processing conditions and the OPV device performance and stability, as well as highlight relevant optomechanical and physico-chemical material properties, including recyclable thermoplastic polymeric materials that might facilitate material reuse. Finally, we also show how plastic processing can be used to fabricate low-cost, three‑level hierarchically organized micro/nanometric surface textures that provide additional functionalities, such as light management or self-cleaning.4
Scale-up-O6
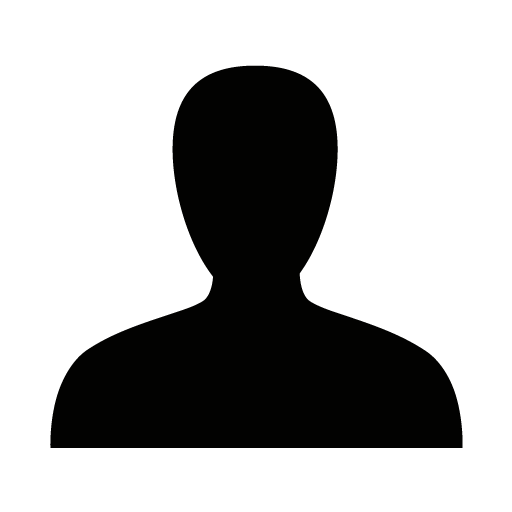
Power conversion efficiencies (PCEs) of bulk heterojunction (BHJ) organic solar cells (OSCs) continue increasing towards the 20% milestone mainly thanks to the emerging non-fullerene acceptors (NFAs). Nevertheless, important factors for industrial application are mostly neglected, such as photostability and cost potential. Single-component organic solar cells (SCOSCs) employing materials with donor and acceptor moieties chemically bonded within one molecule or polymer, successfully overcome the immiscibility between donor and acceptor as well as the resultant self-aggregation under external stress [1]. To inspire a broader interest, in this work, the industrial figure of merit (i-FOM) of OSCs is calculated and analyzed, which includes PCE, photostability, and synthetic complexity (SC) index [2]. Based on the notable advantages of SCOSCs over the correspondent BHJ OSCs, especially the enhanced stability and simplified film processing, we systematically compare the i-FOM values of BHJ OSCs and the corresponding SCOSCs.
SCMs exhibit overall much higher i-FOM values compared with the BHJ OSCs, and the highest value reaches 0.3, which is even higher than the famous PM6:Y6, even though the PCE (8%) is only half of PM6:Y6. With the increase in efficiency, SCOSCs possess the further potential for a higher i-FOM value. Among all factors, the synthetic complexity of SCOSCs is slightly higher than that of the corresponding BHJ OSCs due to the extra synthetic step for connecting donor and acceptor moieties. This feature however overcomes the large-scale phase separation and stability issue for the corresponding BHJ systems [3]. SCOSCs based on dyad 1 exhibit surprisingly high photostability under concentrated light (7.5 suns and 30 suns), corresponding to almost unchanged device stability up to 10,000 hours under 1-sun illumination. For realizing industrial application, SCOSCs have to give a high efficiency comparable to the current high-efficiency BHJ OSCs, while BHJ should be developed in the direction of less complicated synthesis [4]. With joint efforts of researchers from multidiscipline, SCOSCs will see continuing progress in efficiency, reaching an i-FOM value enough for industrial application.
Materials-IS1
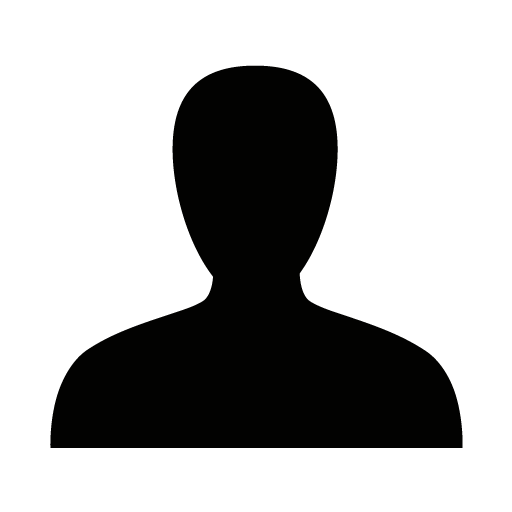
Minimising the regeneration driving force for electron transfer between oxidised dyes attached to semiconductor surfaces and redox mediators in electrolytes (regeneration) is a promising pathway to increased open circuit voltage of regenerative-type photoelectrochemical solar energy conversion devices. Cu1+/2+ complexes dissolved in electrolytes have been claimed to show fast regeneration at only -100 meV driving force. Here we show using transient absorption spectroscopy that regeneration using a heteroleptic Cu1+ complex with a high oxidation potential (dye regeneration driving force -170 meV) is slow. On the other hand, the typically slow (millisecond) recombination between electrons injected into TiO2 and the oxidised dyes is accelerated by three orders of magnitude in the presence of a high redox potential Cu2+ complex. When using the typical Cu1+/2+ complex electrolyte mixture, the acceleration of TiO2 – dye+ recombination can easily be misinterpreted as fast dye regeneration. The accelerated recombination uncovered by selectively measuring TA decays in the presence of Cu1+ and Cu2+ dominant species electrolyes is suggested to be the origin of the significant photocurrent decrease of solar cells using high redox potential Cu1+/2+ complexes with high "apparent" regeneration yield. The presentation will focus on the possible origin of the acceleration of recombination kinetics, updated transient absorption experimental procedures to measure electron recombination and strategies to deccelarate the recombination reaction. Finally, applications where such unprecedented acceleration of interfacial electron transfer kinetics could be beneficial will be presented.
Materials-O1
Dye-sensitised solar cells have recently attracted increasing interest as devices for ambient light harvesting, with reported efficiencies now reaching 37% under indoor light [1]. A remaining challenge however is the comparatively poor stability of devices containing a liquid electrolyte. One approach to overcome this involves drying of the Cu-complex based electrolyte to give a solid-state, so-called “zombie”, cell [2]. Although these can reach good efficiencies, the drying process is currently slow and poorly reproducible, thus impractical for real-world use.
We have fabricated solid-state DSSCs by simply drying out the common liquid I-/I3- electrolyte [3], and have demonstrated power-conversion efficiency for the solid-state cells over 5%, similar to that of the parent liquid cell before drying. In indoor light conditions (1000 Lux), power-conversion efficiencies were around 20%. We observed negligible degradation of efficiency after 12 months dark storage without encapsulation. Furthermore, we have developed a rapid process for cell fabrication, enabling larger area devices made on an open bench [4]. We believe these findings establish a unique new approach to making very simple, stable, solid-state DSSCs of potential practical application, with efficiency that might be optimisable up to around 10%.
Materials-O2
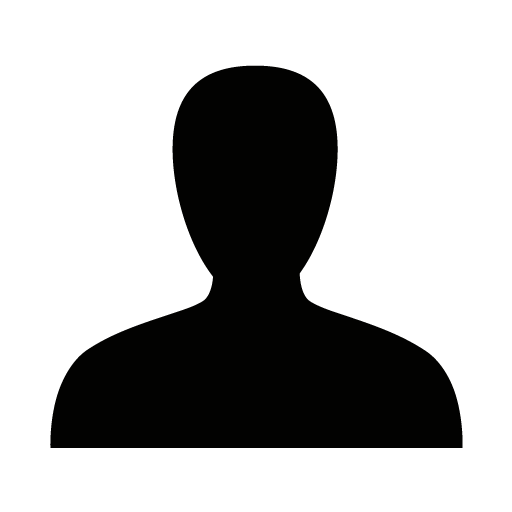
Uniquely designed by controlling the flow of light, photonic crystals (PC) have attracted considerable interest because of their attractive aesthetical aspects. Therefore, following an approach combining both optical modeling and experimental approach in the present work, we report the designing and fabrication of highly reflective photonic crystals incorporating in perovskite solar cells (PvSCs). To start with, two dielectric materials having contrasting refractive index values were chosen as the alternating layers. Variable angle spectroscopic ellipsometry was performed to find the accurate optical constants of each layer which works as the input parameter for the transfer matrix method-based optical modeling. In this work, the radio frequency sputtering technique was used to deposit alternate layers of 1D-PC. For the fabrication of a colored perovskite solar cell (c-PvSC), the PC was deposited on the back side of the ITO-coated glass substrate. The deposition of PC was performed prior to the fabrication of the solar cell to prevent any damage to the PvSC. Using this approach, we successfully fabricated an 11.2% efficient blue-colored PvSC with the power conversion efficiency reaching 94.1% to the conventional opaque PvSC. Hence, this way of fabricating 1D-PC can integrate routes for revolutionizing applications of colored photovoltaics in diverse fields, including building integrated photovoltaics, automobile technology, etc.
Materials-O3
I will discuss the promising use of tellurium-based materials in optoelectronics and how we have developed a new technique, 125Te Magic Angle Spinning (MAS) Nuclear Magnetic Resonance (NMR), to study their atomic-level structure. The focus of our study is on A2TeX6 compounds, where A is Cs and MA, and X is I, Br, and Cl.
I will first provide an overview of how these materials have been applied in optoelectronics to date, including the various synthetic protocols used in the field [1,2]. Then, I will try to answer the following question: why is it important to study both their long-range and local structure? [3] We have discovered that 125Te NMR is highly sensitive to the halide composition and provides a unique insight into the tellurium coordination environments. Consequently, we were able to study halide mixing in situ and found that it occurs on the timescale of seconds to minutes at elevated temperatures. By studying 125Te NMR relaxation and its underlying physics, we were able to quantify halide diffusion and determine its activation energy in these materials. I will discuss how those activation barriers compare to other important, lead- and tin-based, classes of halide perovskite materials.
Our study showcases the versatility of solid-state 125Te NMR spectroscopy in characterizing the local structure of tellurium-based optoelectronic materials at the atomic level. The information gained from our approach provides a valuable tool for understanding the structure-property relationships of these materials and paves the way for studying more complex compositions. By gaining deeper insights into the halide mixing process, which is critical in phenomena such as J-V hysteresis, we can guide the design of new tellurium-based materials with improved properties.
Materials-O4
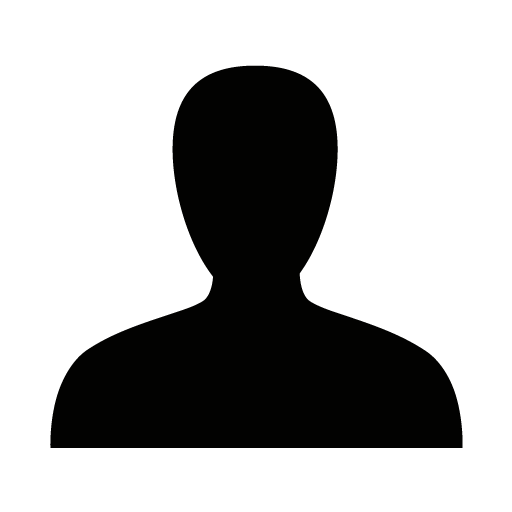
Organic semiconductors have shown an improvement in their molecular design resulting in a rapid increase in their efficiencies in organic photovoltaic and solar-to-fuel production.[1-3] The synthetic tunability of these semiconductors allows the preparation of low-cost and solution-processable materials with broad light absorption. Organic semiconductors heterojuction nanoparticles, prepared from a blend of conjugated polymer donor and non-fullerene small molecules acceptor, are currently among the most efficient visible light-active hydrogen evolution photocatalysts with an external quantum efficiency (EQE) for hydrogen evolution up to 7 % at a wavelength > 600 nm.[2] In this presentation, I will discuss the previusly unexplored charge carrier dynamics of such efficient donor/acceptor heterojunction nanoparticles photocatalysts for hydrogen evolution. Transient and operando photoinduced absorption spectroscopies, on timescales ranging from femtoseconds to seconds after light absorption, were employed to track the charge carrier dynamics of selected heterojunction nanoparticles, aimed to undestand their photophysycal properties and how this differs from those of organic solar cell devices.
Materials-O5
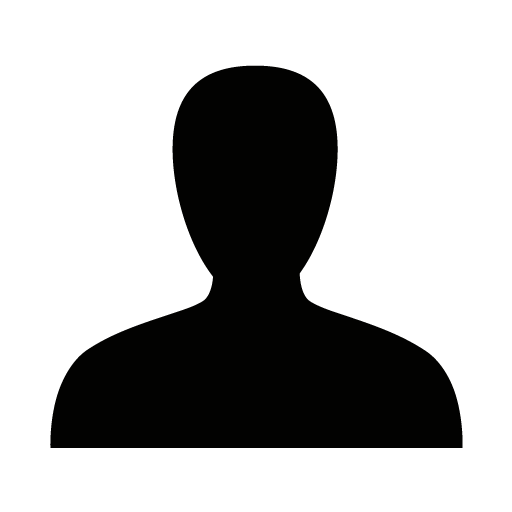
Polymeric photocatalysts made of Earth-abundant elements have been extensively developed over the past decade to take advantage of their synthetic tunability.[1] Within this family, carbon nitrides (CNx) are emerging as exciting photocatalysts because of their high photocatalytic performance combined with good stability and facile synthesis. However, significant gaps remain in our knowledge of the photophysical properties of these organic polymeric materials. Determining the pathways and mechanism of photoinduced processes will greatly aid our efforts to engineer better CNx photocatalysts for solar fuel production.
Time-resolved spectroscopies, particularly transient absorption spectroscopy (TAS), enable us to determine the nature of short lived photoexcited states and determine the kinetics of competing processes. For example, our previous combined TAS and time-resolved photoluminescence (tr-PL) study of CNx provided important insight into the role of charge trapping on timescales ranging from femtoseconds to seconds.[2]
We are taking the next step to develop a full picture of the charge carrier dynamics by expanding our spectroscopic capabilities to transient absorption microscopy (TAM). Notably, our first-of-its-kind TAM system monitors the microsecond – second timescales relevant to the complex multi electron redox reactions that occur to produce solar fuels. Spatial mapping of the charge carrier dynamics on the micron scale provides novel insights into the heterogeneity in individual CNx particles. These new insights into the heterogeneity of charge carrier dynamics in CNx particles can push the field into uncovering the optimal structure and local environment in defect-rich organic semiconductors such as CNx.
Materials-O6
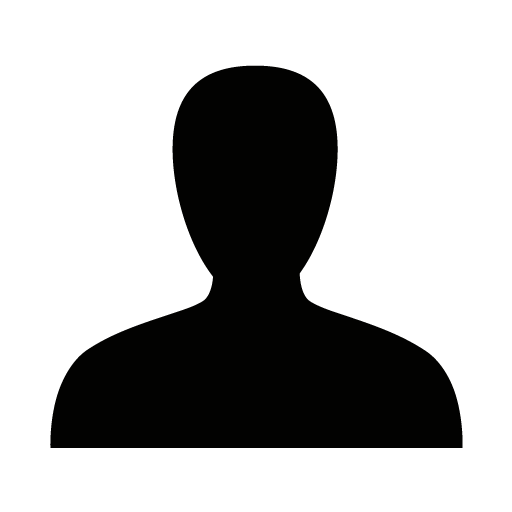
The worldwide increasing number of electronic devices working at the same time supposes a huge demand for on-site power that cannot be supplied by conventional batteries. So, the development of environmental energy harvesters is critical to prompt the self-powered actuation of small, portable-wearable, and wireless electronic devices. In this context, the nanogenerators arise as efficient nanoelectrodes that avoid energy losses and enhance multifunctionality.
The nanoscale design of conductive and transparent materials for these nanoelectrodes is a crucial step for different applications that include optoelectronics and photovoltaics devices and energy harvesters and it is essential in the management of light harvesting under low-intensity conditions. One of the main interests in this direction is the use of new transparent conducting nanoelectrodes consisting of a wide range of materials with different compositions and texturing such as metal nanowires networks, carbon nanotubes, graphene, transparent conducting oxides (TCOs) nanostructures, etc.
In this work, we have synthesized 1-dimensional (1D) and hierarchical Indium Tin Oxide (ITO) nanoelectrodes by a soft-template multistep method that combines vacuum and plasma techniques in a “one-reactor/chamber” configuration.[1] Here, we combined magnetron sputtering and thermal evaporation, two industrially spread deposition techniques. In particular, we have synthesized high-quality ITO nanotubes with resistivities on single-nanotube comparable with single-crystal nanowires reported by other authors. These nanoelectrodes present desirable optical properties in the visible spectra for enhancing light trapping in energy harvesting applications.
The implementation of these nanostructures in Dye-Sensitized Solar Cells (DSSCs) has been carried out following a standard architecture of a photovoltaic device. The photoanode was prepared in two steps to cover the ITO nanostructures with anatase-TiO2: 1) a conformal shell by Plasma Enhanced Chemical Vapor Deposition for then 2) embedding in a mesoporous matrix by screen-printing. The multi-layered system has been sensitized with the YKP-88 dye. [2] To complete the DSSC, we have used a platinum counter electrode on FTO glass (fluorine tin oxide) and two electrolytes based on the iodide/tri-iodide redox pair. The difference between the electrolytes lies in the viscosity: 1) liquid-based and 2) ionic liquid-based, the former having lower viscosity.
The photovoltaic performance of these devices has been measured under a solar simulator (100 mW/cm2) as well as under indoor sources such as white LED (6500 K) over a wide range of intensities (1.77 mW/cm2 to 0.014 mW/cm2). The main results of this study indicate an outstanding increment of the DSSC efficiency incorporating 1D nanoelectrodes for low light intensity conditions. This is a behavior that does not happen with the reference samples (without 1D nanoelectrodes). Such is the increase in efficiency of the 1D nanostructured DSSCs, that they show the highest performance values at lower intensities, above the reference samples.
3A-K1
Sang Il Seok is a Distinguished Professor in the Department of Energy and Chemical Engineering at the Ulsan National Institute of Science and Technology (UNIST) in Korea. He obtained his Ph.D. in the Department of Inorganic Materials Engineering from Seoul National University in Korea. After completing his Ph.D., he worked as a postdoctoral researcher at Cornell University in the USA, where he investigated defects and transport in the Fe-Ti-O Spinel structure. He also experienced a visiting scholar at the University of Surrey in UK in 2003 and École Polytechnique Fédérale de Lausanne (EPFL) in Switzerland in 2006. Prior to joining UNIST in 2015, he served as the principal investigator of the Korea Research Institute of Chemical Technology (KRICT) and as a professor at the Department of Energy Science at Sungkyunkwan University. He is a highly cited researcher, selected by Clarivate Analytics since 2018, and has published over 200 peer-reviewed papers, including three Nature and seven Science articles as a corresponding author. He has received several awards for his excellence, such as the "Korean Scientist Award" from the Korean government in 2017, and the Kyung-Ahm Prize in 2019. In 2022, he was awarded the Rank Prize, recognized as one of the seven scientists pioneering in perovskite solar cells. His research focuses on functional inorganic-organic hybrid materials and devices, particularly on perovskite solar cells.
The power conversion efficiency (PCE) of perovskite solar cells (PSCs) has increased rapidly over the past ten years, and this has been primarily achieved through optimizing several key aspects of the device. Device architecture optimization involves designing the various layers and interfaces of the PSC to maximize the absorption of light, minimize losses due to recombination, and optimize charge transport. The electron transporting layer in nip architecture is also important component in the fabrication of high-efficiency PSCs. Another important aspect is the uniform thin film deposition process. The quality and uniformity of the perovskite layer can greatly affect the device performance, and optimizing the deposition process can help to ensure a high-quality perovskite layer. For example, the addition of methylammonium chloride (MACl) can significantly enhance the crystallinity and homogeneity of the perovskite film, leading to improved device performance. The mechanism behind the improved performance is thought to be related to the role of MACl in controlling the crystallization process of the perovskite. We have focused on research to further expand the role of MACl. In this presentation, I would like to mainly introduce the results of our recent work on the eco-friendly coating of FAPbI3 perovskite thin films using alkylammonium chlorides and their role in stabilizing the a-phase and improving crystallinity.
3A-I1
Aldo Di Carlo is Director of the Institute of Structure of Matter of the National Research Council and Full Professor of Optoelectronics and Nanoelectronics at the Department of Electronics Engineering of the University of ROme "Tor Vergata". His research focuses on the study and fabrication of electronic and optoelectronic devices, their analysis and their optimization. Di Carlo founded the Center for Hybrid and Organic Solar Cells (CHOSE) which nowadays involve more than40 researchers dealing with the development of III generation solar cells (DSC, OPV and Perovskite) and on scaling-up of these technologies for industrial applications. CHOSE has generated 6 spin-off companies and a public/private partnership. Di Carlo is author/coauthor of more than 500 scientific publications in international journals, 13 patents and has been involved in several EU projects (three as EU coordinator)
The potential to continuously adjust the band gap of halide perovskite materials across a wide range from near-infrared to near-ultraviolet wavelengths presents an opportunity to develop semitransparent perovskite solar cells and modules that offer high visual transmittance. By optimizing the band gap of perovskite cells, it is possible to construct a tandem configuration with near-infrared organic solar cells to improve efficiency without compromising the average visual transmittance (AVT). Numerical simulations indicate that an optimized tandem architecture utilizing suitable photonic crystals and materials can achieve a power conversion efficiency of 15% with an AVT of 50%.[1] The EU CITYSOLAR consortium has devised specific strategies to achieve this objective, including material optimization for both perovskite and organic solar cells, as well as light management and new characterization strategies.[2] In this presentation, I will highlight the efforts of the consortium and their progress in surpassing the state of the art showing several strategies ranging from solution processes to physical deposition for the fabrication of see-through photovoltaics. Such endeavors have expanded beyond solar cells and include module-level developments. With respect to perovskite solar cell (PSC) modules, a low-temperature, full blade-coating technique has been devised in air to deposit semi-transparent FAPbBr3-based perovskite modules on 300 cm2 substrates.[3]
3A-I2
Henry Snaith undertook his PhD at the University of Cambridge, working on organic photovoltaics, then spent two years at the EPFL as a post-doc working on dye-sensitized solar cells. Since 2007 he has held a professorship at the University of Oxford Clarendon Laboratory where his group researches organic, hybrid and perovskite optoelectronic devices. Professor Snaith was elected as a Fellow of the Royal Society in 2015, he is a 2017 Clarivate Citation Laureate, and among his awards are the 2017 Royal Society James Joule Medal and Prize. In 2010 he founded Oxford Photovoltaics Ltd. which is commercializing the perovskite solar technology transferred from his laboratory.
In this talk, after presenting an introduction to perovskite solar cells, I will highlight some recent work understanding and mitigating losses in p-i-n perovskite solar cells. Specifically, p-i-n cells generally exhibit lower current density and lower open-circuit voltage than n-i-p cells. It transpires that the lower current density originates from a very rapid (sub-second) short-circuit current loss, which itself arises from a redistribution of ions from the bulk of the perovskite absorber layer to the interfaces. The lower open-circuit voltage has been known to originate from severe non-radiative recombination losses at the perovskite/electron transport layer interface. I will present a range of approaches which are successful in mitigating these losses. Solution processing is the main route employed in the academic field for perovskite thin-film fabrication. However, thermal evaporation (or sublimation) under vacuum is a very useful, highly scalable method which has received comparably little attention. I will present some recent advances in vapor deposited solar cells and insights into enhancing the long-term stability of the devices. I will finish will an industrial outlook towards recent progress and the pathway towards delivering real perovskite PV product into the market.
3B-I1
Professor Kwanghee currently leads research and development program of organic solar cells in Gwangju Institute of Science and Technology (GIST) as a director of the Research Institute of Solar and Sustainable Energies (RISE). He is also appointed as a “Distinguished Professor” of the School of Materials Science and Engineering of GIST. Dr. Lee started his professorship at Pusan National University in 1997 after finishing his Ph.D. and Post-Doc at the University of California Santa Barbara (UCSB). Then he moved to GIST in 2007 and have organized and acted as a co-director of the Heeger Center for Advanced Materials (HCAM) together with the director, Professor Alan J. Heeger, who is a 2000 year Nobel Laureate in Chemistry. Now Dr. Lee is a leading scientist in the area of “plastic electronics” including organic solar cells, polymer LEDs, and organic FETs. Dr. Lee finished his B.S. in Nuclear Engineering at Seoul National University and M.S. in Physics at KAIST. Then he earned his Ph.D. in Physics at UCSB (USA) under the guidance of Prof. Heeger with a subject of metallic and semiconducting polymers.
The past two decades of vigorous interdisciplinary approaches has seen tremendous breakthroughs in both scientific and technological developments of next-generation solar cells, bulk-heterojunction organic photovoltaics (OPVs) based on nanocomposites of π-conjugated organic semiconductors and perovskite solar cells (PSCs) utilizing hybrid organometal halide perovskite materials. Because of their unique functionalities, both solar cells are expected to enable innovative photovoltaic applications that can be difficult to achieve using traditional inorganic solar cells: they are printable, portable, wearable, disposable, biocompatible, and attachable to curved surfaces. The ultimate objective of this field is to develop cost-effective, stable, and high-performance photovoltaic modules fabricated on large-area flexible plastic substrates via high-volume/throughput roll-to-roll printing processing and thus achieve the practical implementation of both OPVs and PSCs.
Recently, intensive research efforts into the development of both photo-active materials, processing techniques, interface engineering, and device architecture have led to a remarkable improvement in power conversion efficiencies, approaching almost 20% for OPVs and exceeding 25% for PSCs, which have finally brought both photovoltaics close to commercialization. Current research interests are expanding from academic to industrial viewpoints to improve device stability and compatibility with large-scale printing processes, which must be addressed to realize viable applications. Here, both academic and industrial issues are reviewed by highlighting historically monumental research results and recent state-of-the-art progress of our group (GIST) in the development of printed modules of OPVs and PSCs. Moreover, perspectives on five core technologies that affect the realization of the practical use of these photovoltaics are presented, including device efficiency, device stability, flexible and transparent electrode, module designs, and printing techniques.
3B-I2
Organic photovoltaic cells (OPVs) have reached power conversion efficiencies of 20.2%[1], with a path to exceeding 25% in the near future. Thus, the potential for serving as a significant renewable energy resource depends on whether the requirements of low cost, high efficiency and prolonged lifetime are simultaneously fulfilled. Even with the fulfillment of these properties, OPVs have to serve applications that are qualitatively different than those filled by the incumbent Si-based PV technology. An application where OPVs have a unique opportunity for ubiquitous deployment is in power generating windows since they can be semitransparent (ST) in the visible while strongly absorbing in the infrared. After giving an overview of the status of OPVs, I will discuss strategies explored in our laboratory to fabricate ST-OPVs with transparencies approaching ~50% and efficiencies of 10% or higher. Further, I will describe structures that show extrapolated intrinsic lifetimes of >30 years[2], and short cost payback times.[3] Approaches to scaling the devices into prototype modules whose performance is only reduced by 5% from discrete, small test cells will also be described. Thus, non-fullerene-acceptor-based OPVs can have performance characteristics that meet many of the demands required in building integrated and applied solar energy harvesting applications.
3B-I3
With the emergence of high-performance non-fullerene electron acceptors (NFA), organic photovoltaics (OPV) are particularly appealing as their power conversion efficiencies (PCEs) are approaching 20%. Understanding the fundamentals and identifying the sources of device degradation in photovoltaic devices, while considering green processing, is as crucial as improving the PCEs from the manufacturing and commercialization point of view. In this presentation, I will first talk about the energy level conundrum in organic semiconductors and how to precisely determine the energy levels in order to evaluate and optimise the performance of new materials in OPV. Further, I will share a green solvent identification and processing strategy from renewable feedstocks that can be used for fabricating high performing stable organic electronicsWith the emergence of high-performance non-fullerene electron acceptors (NFA), organic photovoltaics (OPV) are particularly appealing as their power conversion efficiencies (PCEs) are approaching 20%. Understanding the fundamentals and identifying the sources of device degradation in photovoltaic devices, while considering green processing, is as crucial as improving the PCEs from the manufacturing and commercialization point of view. In this presentation, I will first talk about the energy level conundrum in organic semiconductors and how to precisely determine the energy levels in order to evaluate and optimise the performance of new materials in OPV. Further, I will share a green solvent identification and processing strategy from renewable feedstocks that can be used for fabricating high performing stable organic electronics
Optimisation-IS1
Single-junction perovskite solar cells have reached efficiencies larger than 25%. Despite these great advancements, some drawbacks remain such as the fact that the material contains lead. Questions related to transient effects are not conclusively solved in perovskite optoelectronic devices either.
In this talk, I will discuss how in-situ and in-operando measurements can help to relate various measurement data to device performance. Measurements refer to photo- and electroluminescence, optoelectronic transients, impedance spectroscopy, and AFM-based characterization on the nano- and microscale. Materials under investigation are lead-halide perovskite compositions that show photoinduced phase segregation and lead-free double perovskites. Discussed devices are solar cells, also under reverse bias, and light-emitting diodes under pulsed operation. The characterization experiments are accompanied by device simulations and data-based modelling. Focus is on the role the mixed ionic-electron conductivity plays on the working principle of devices and how performance-limiting processes can be identified. The presented insights are of relevance for both, scientists working on device fabrication and researcher looking into the device physics.
Optimisation-O1
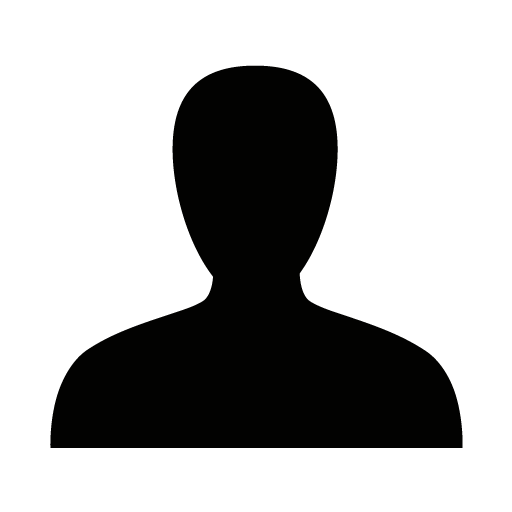
Stability of lead halide perovskites (formula ABX3) is very frigile due to a weak balance between the conditions to be satisfied for the B-X and A-B / A-X bonds that are allowed by the perovskite crystal structure. For all known organic perovskites, the A cation molecule is rotating in the BX3 frame on a picosecond scale. The molecular A cations interact with the BX3 frame only electrostaticly and via the weak hydrogen bonds. Thus, the ion vacancies and ion migration dominate in the mechanisms of the structural destablization.
In this presentation, the new A cations with very much different properties than the known A cations are theoretically proposed. These molecules contain the elements that share the electrons with the Pb corner and X anions of the inorganic frame. In the consequence, the proposed molecular A cations are immobile, and further stabilize the X anions, preventing anions migration. The calculated cohesive energy for the structures with the A cations attached to the PbX3 corner is around 1.5 eV lower than for the geometries with the same A cations positioned in the center of the inorganic octahedral box. As implications, the valence band structure shows the hybridization between the A cation states and PbX3 states. The nature of the chemical bonds is studied with the energy decomposition analysis (EDA). The optical spectra are computed with the ab initio many-body perturbation theory.
Optimisation-O2
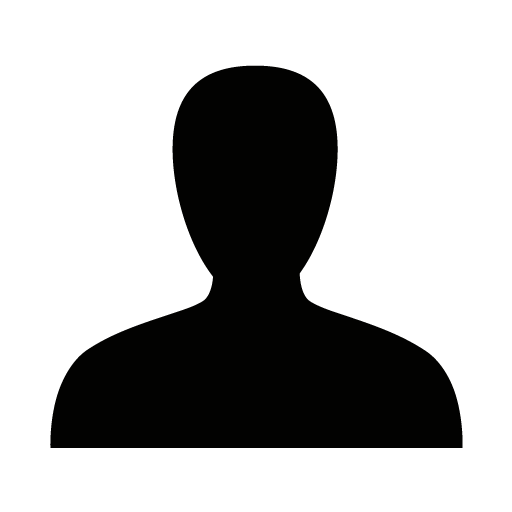
The goal of third-generation PV is to go beyond the Shockley–Queisser limit, the
theoretical limit of 33 % for a single p-n junction solar cell. A hot carrier solar cell
(HCSC) has been proposed to overcome this limit, by harvesting carriers before they
have lost their surplus energy. Theoretically the prevented thermal losses could
boost the efficiency of a HCSC up to 66%
One promising type of materials for these purposes is halide perovskite. Next to
having excellent PCE, being cheap and solution processable, HP’s have shown very
long cooling times, the key parameter for a HCSC. We wish to shed light on why
relaxation times are found to be so long in HP’s.
By using an Ensemble Monte Carlo (EMC) simulation we are able to simulate the
trajectories of charge carriers and model their interactions with their environment. In
the EMC random free flight times are generated and interrupted by scattering events
with desired scattering mechanisms. It is this freedom of choice which makes the
EMC an excellent tool in order to investigate what exactly causes charge carriers in
halide perovskites to cool
In this contribution we identify the roles of electron-phonon and electron-electron
scattering in the thermalisation and cooling process, and show how these processes
depend on several material parameters. Furthermore, we zoom in on how cooling
times are impacted by
the degree of background doping. We show how an ensemble of background
carriers can have a detrimental effect on the cooling time. Our results are important
for the discussion on whether or not tin perovskites are suitable candidates for
HCSCs.
Optimisation-O3
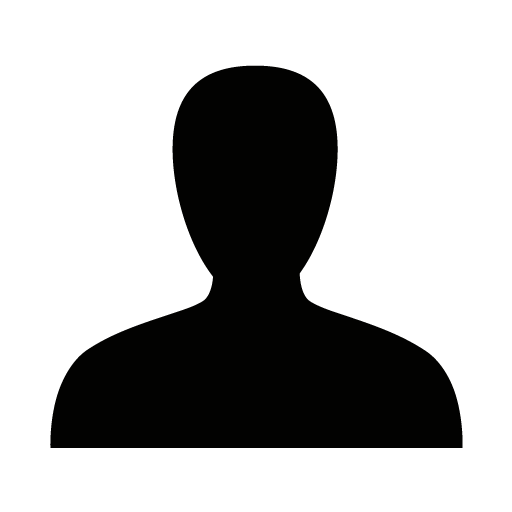
Halide perovskite solar cells have recently emerged as potential candidates for indoor light harvesting with high power conversion efficiencies (PCE). Almost all of the reported studies on indoor light harvesting solar cells utilize white light in the visible wavelength. [1-3] Low wavelength near-ultra-violet lights, also called black lights, are commonly used under indoor environments as decorative lights e.g. for bars, pubs, aquariums, parties, clubs, body art studios, Christmas and Halloween decorations. Despite their high photon energy, such near-UV lights have not been explored for indoor light harvesting.
We have for the first time demonstrated near-UV black light harvesting using perovskite solar cells based on the commonly used methyl ammonium lead iodide absorber. UV stable solar cells fabricated with modified electron transport layer (SnO2 + PCBM-BPhen) delivered a PCE of 26.19 % and power output approaching 1 mW/cm2, when measured under near-UV (395-400 nm) illumination of 3.76 mW/cm2. The devices retained 95.53% of their initial PCE after 24 hours near-UV exposure. [4]
This work marks the beginning of new ear of indoor near-UV harvesting solar cells that are promising for powering modern electronics integrated with IoT sensors located in UV environments such as health care, horticulture and places with near-UV black light decorations.
Optimisation-O4
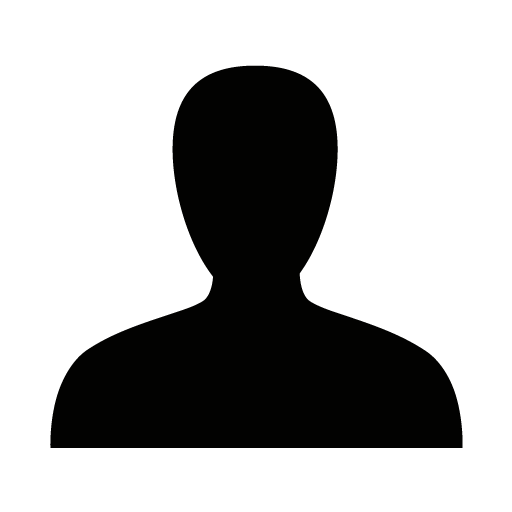
Perovskite solar cells (PSCs) are a promising technology for use in next generation photovoltaics. A unique aspect of PSCs is the presence of mobile ionic species in the active layer, which can significantly impact device performance in ways that are not yet fully understood [1-3]. Furthermore, the efficiency of PSCs is also strongly affected by the choice of electron transport material (ETM), with recombination at the perovskite/ETM interface often thought to limit device performance [4]. Thus, understanding the combined effects of mobile ionic charge and ETM properties on the carrier recombination and extraction dynamics in PSCs under operation is crucial to the further development of these devices. In this talk, we demonstrate the use of operando photoluminescence (PL) to investigate these dynamics in typical p-i-n PSCs with four different ETMs. Operando PL is a method which allows for the measurement of real-time PL spectra during current density-voltage (J-V) scans under 1-sun equivalent illumination. This allows direct comparison between the internal performance (recombination currents and quasi-Fermi-level-splitting (QFLS)) and the external performance (J-V) of a PSC [5]. When combined with the results of device simulations, this technique provides novel insights into the processes occuring during PSC operation.
Under short circuit conditions, significant charge accumulation was observed in all four devices, including our ~20% efficient champion device. That this was the case in all four PSCs, regardless of the properties of the ETM, suggests that this phenomenon is linked to an intrinsic property of the perovskite. By combining the results of operando PL with device simulations, we conclude that short-circuit charge accumulation is mainly due to field screening caused by ion migration in the perovskite layer. Moreover, by comparing different ETMs, we quantify the impact of energetic alignment at the perovskite/ETM interface on extraction and recombination kinetics and thus device efficiency. Whilst a deep LUMO is found to enhance electron transfer from the perovskite to the ETM, it results in a higher electron density on the ETM. This is observed to increase non-radiative recombination at the perovskite/ETM interface, limiting the PSC’s open circuit voltage (VOC) and fill factor. On the contrary, a shallow ETM LUMO is observed to impede electron extraction which leads to a larger QFLS in the bulk perovskite. Although this is beneficial for the VOC, the retarded charge extraction under low voltage conditions (when V < VOC) results in a device with a low fill factor and short circuit current density (JSC). Additionally, our simulation results suggest that the presence of mobile ions increases VOC relative to the case without mobile ions. However, the reduction to both JSC and fill factor due to ionic field screening outweigh this postive effect, meaning that the net effect mobile ions is to lower device efficiency.
Overall, our results demonstrate that, in addition to optimising the energetic alignment at the perovskite/ETM interface, mitigating the effects of ion migration in the perovskite layer is necessary to maximise the efficiency of charge extraction and JSC in PSCs.
Optimisation-O5
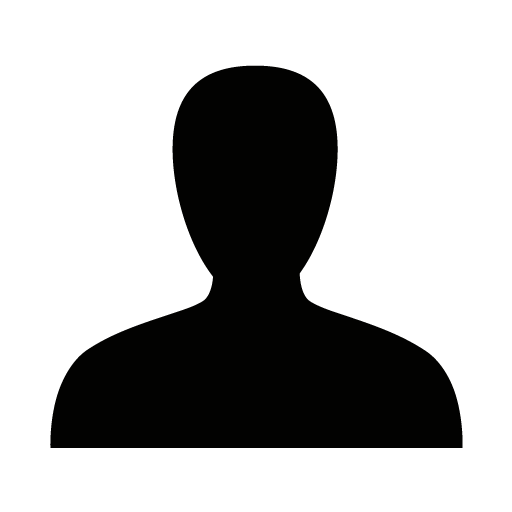
Interface engineering at the interface between the perovskite layer and the charge transport layer (CTL), or CTL and metal electrodes, is critical for demonstrating the efficient and stable perovskite solar cells (PSCs) [1, 2]. Herein, we demonstrate the efficient and stable PSCs by introducing the organic electrolytes (OEs) as a “dual-side passivation layer” in both p-i-n and n-i-p configuration of PSCs. Firstly, a newly synthesized bathocuproine (BCP)-based nonconjugated polyelectrolyte (poly-BCP) is introduced between the tin oxide (SnO2) CTL and the perovskite layer in the n-i-p configuration. Poly-BCP effectively passivate oxygen-vacancy defects of the SnO2 side and simultaneously scavenges ionic defects of perovskite side, suppressing both bulk and interfacial nonradiative recombination in PSCs [3]. As a result, the modified PSCs exhibited a high power conversion efficiency (PCE) of 24.4% and a high open-circuit voltage of 1.21 V. Furthermore, the non-encapsulated PSCs show excellent long-term stability by retaining 93% of the initial PCE after 700 h under continuous 1-sun irradiation in nitrogen atmosphere conditions. Secondly, amine-functionalized small molecule electrolytes (SMEs) are introduced as passivation layer between the PCBM CTL and metal electrode (here, Cu) in the p-i-n configuration [4]. A strong coordination bond of Cu─N forms at the Cu/SMEs interface, leading to the layer–layer growth mode for the dense formation of Cu electrodes with a strong adhesion to the CTL. Thus, this modified electrode prevents the ingress of moisture into the PSCs, resulting in outstanding moisture stability; the efficiency of non-encapsulated PSCs retains 90% of the initial PCE after 200 days of exposure to atmospheric air (25 ℃, relative humidity [RH] ~20–40%). Under harsher conditions (e.g., 25 ℃/RH65%, 25 ℃/RH85% and immersion in water) for a considerable time period, the modified PSCs manifest relatively no degradation compared with the pristine PSCs.
Optimisation-O6
Udo Bach is a full professor at Monash University in the Department of Chemical Engineering; the Deputy Director of the ARC Centre of Excellence in Exciton Science and an ANFF-VIC Technology Fellow at the Melbourne Centre of Nanofabrication (MCN). He received his PhD from the Swiss Federal Institute of Technology (EPFL, Switzerland) working in the research group of Prof Michael Grätzel and worked for 3 years in a technology start-up company in Dublin (Ireland). Subsequently he spent 15 months as a postdoc in the group of Prof. Paul Alivisatos in UC Berkeley (USA) before moving to Monash University in November 2005 to establish his own research group.
Prof Bach has a strong background in the area of photovoltaics and nanofabrication. He is involved in fundamental and applied research in the area of perovskite and dye-sensitized solar cells. He has additional research activities in the area of nanofabrication, DNA-directed self-assembly, nanoprinting, plasmonics for sensing, photovoltaic applications and combinatorial photovoltaic materials discovery.
Lead acetate is a promising precursor to produce ultrasmooth perovskite thin films, without the need for antisolvent quenching or the presence of strongly complexing solvents such as DMSO. Unfortunately, the originally developed lead acetate route failed to produce phase-pure mixed-A-cation perovskites. In our recent work we were able to identify the chemical side reactions in the precursor solution which caused this incompatibility. Furthermore we propose the use of ammonium iodide as alternative halide source to inhibit those unwanted reactions, allowing us to produce phase-pure formamidinium caesium mixed-A-cation perovskite films through our adapted lead acetate synthesis route. Solar cells fabricated from these films exhibited energy conversion efficiencies of up to 21%. The ruggedness of the film formation process of the lead acetate route lends itself to industrial scalable fabrication processes. Based on our work it is now also applicable to high-performing and industrially relevant perovskite compositions. We demonstrate the industrial relevance of our new synthesis route by producing 10 cm2 active area solar cell sub-modules via a blade-coating in air process, yielding solar cells with efficiencies of up to 18.8% and no evidence of efficiency loss after 3300 hours storage at 65 °C. [1]
Optimisation-IS1
Organic solar cells (OSCs) have rapidly advanced, reaching state of the art power conversion efficiency (PCE) of above 19 % for single junction solar cells [1]. The energetic offset between the highest occupied molecular orbital (HOMO) levels of the donor and acceptor components of organic photovoltaic (OPV) blends is well-known to affect the device PCE. It is well established that decreasing the energy offset increases the open circuit voltage (VOC) but decreases the short circuit current (JSC). The latter has been explained by insufficient charge generation. However, the effect of the offset on the recombination and fill factor (FF) and on the underlying processes is less clear. Here, we study free charge generation and recombination in different non-fullerene acceptors, Y6, TPT10, ITIC, Y5 and o-IDBTR, blended with the same donor polymer PM6. We demonstrate that a diminishing HOMO-HOMO energy offset results in field-dependent charge generation. On the other hand, reformation of excitons from free charges is identified as an additional channel for free carrier recombination, which goes along with a substantial rise in the bimolecular recombination coefficient. In combination of these two effects, the FF drops condiserably with decreasing energy offset. We show that bulk properties such as morphology and carrier mobilities can not fully explain the observed difference in the device performance, highlighting again the importance of interfacial kinetics and thermodynamics in controlling the OPV efficiency, both through generation and recombination of charge carriers.
[1] Y. Cui, Y. Xu, H. Yao, P. Bi, L. Hong, J. Zhang, Y. Zu, T. Zhang, J. Qin, J. Ren, Z. Chen, C. He, X. Hao, Z. Wei, and J. Hou, Adv. Mater. 33, (2021).
[2] D. Qian, Z. Zheng, H. Yao, W. Tress, T.R. Hopper, S. Chen, S. Li, J. Liu, S. Chen, J. Zhang, X.-K. Liu, B. Gao, L. Ouyang, Y. Jin, G. Pozina, I.A. Buyanova, W.M. Chen, O. Inganäs, V. Coropceanu, J.-L. Bredas, H. Yan, J. Hou, F. Zhang, A.A. Bakulin, and F. Gao, Nat. Mater. 17, 703 (2018).
[3] X.-K. Chen, D. Qian, Y. Wang, T. Kirchartz, W. Tress, H. Yao, J. Yuan, M. Hülsbeck, M. Zhang, Y. Zou, Y. Sun, Y. Li, J. Hou, O. Inganäs, V. Coropceanu, J.-L. Bredas, and F. Gao, Nat. Energy 6, 799 (2021).
[4] L. Perdigón-Toro, L.Q. Phuong, S. Zeiske, K. Vandewal, A. Armin, S. Shoaee, and D. Neher, ACS Energy Lett. 6, 557 (2021).
Optimisation-O1
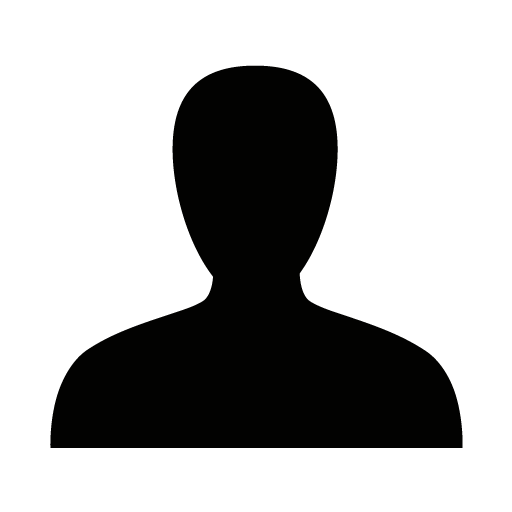
Organic solar cells have made large strides with power conversion efficiencies exceeding 18% and the milestone of 20% well within sight1. The emergence of non-fullerene acceptors (NFAs) has played a vital role in these advancements2. Particularly, there have been extensive studies focusing on the process of free charge generation in organic blends comprising of NFAs with polymeric donors having low energy offset3,4. Adding to this discussion, we systematically explore the role of offset on charge transfer dynamics at bulk heterojunction interfaces between polymeric donor PM6 and two structurally similar NFAs from the Y-series acceptor family, namely Y5 and Y6. Herein, Y5 is found to have a relatively lower offset between the highest occupied molecular orbitals (HOMO) in PM6:Y5 as compared to PM6:Y6. The low offset in case of PM6:Y5 leads to enhancement in open circuit voltage as compared to the optimized blends of PM6:Y6, however this enhancement comes at cost of reduced short circuit current densities and poor fill factors. Here, PM6:Y5 blends are found to show strong voltage dependence of free charge generation as seen through time delayed collection field (TDCF) measurements along with its complete anticorrelation with the bias-dependence of steady state photoluminescence quenching of the blend. Using transient absorption spectroscopy (TAS), we show that the poor free charge generation in optimized PM6:Y5 (1:1.2) blend as compared to PM6:Y6 originates due to the differences in interfacial kinetics which contributes towards poor exciton dissociation yields. We verify the role of interfacial energetics using blends with low acceptor (Y5/Y6) content to avoid contributions from morphology-dependent exciton diffusion. This work underlines the importance of energetic offset on charge generation and in contrast to the earlier reports, indicates that vanishing HOMO-
Liu, F., Zhou, L., Liu, W., Zhou, Z., Yue, Q., Zheng, W., Sun, R., Liu, W. Y., Xu, S., Fan, H., Feng, L., Yi, Y., Zhang, W., Zhu, X., Organic Solar Cells with 18% Efficiency Enabled by an Alloy Acceptor: A Two-in-One Strategy. Adv. Mater. 2021, 33, 2100830
Armin, A., Li, W., Sandberg, O. J., Xiao, Z., Ding, L., Nelson, J., Neher, D., Vandewal, K., Shoaee, S., Wang, T., Ade, H., Heumüller, T., Brabec, C., Meredith, P., A History and Perspective of Non-Fullerene Electron Acceptors for Organic Solar Cells. Adv. Energy Mater. 2021, 11, 2003570.
Bertrandie, J., Han, J., De, C. S. P., Yengel, E., Gorenflot, J., Anthopoulos, T., Laquai, F., Sharma, A., Baran, D., The Energy Level Conundrum of Organic Semiconductors in Solar Cells. Adv. Mater. 2022, 34, 2202575
Zhong, Y., Causa’, M., Moore, G.J. et al. Sub-picosecond charge-transfer at near-zero driving force in polymer:non-fullerene acceptor blends and bilayers. Nat Commun. 2020, 11, 833.
HOMO offset can be detrimental to overall device performance.
Optimisation-O2
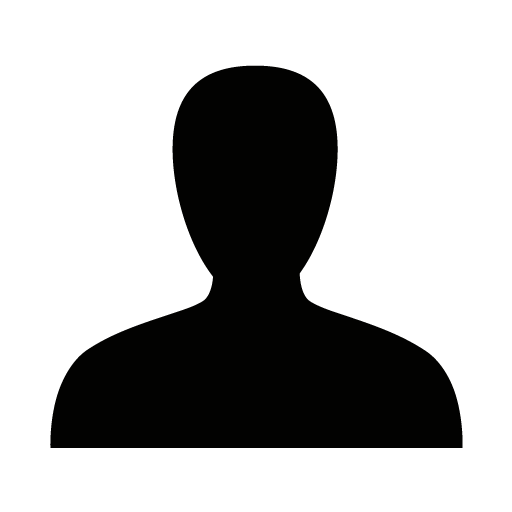
Organic solar cells (OSCs) have enabled a high power conversion efficiency (PCE) of around 20%[1]. The ever-increasing efficiency has been accompanied by a deeper understanding of the dominant performance parameters, including the open-circuit voltage (VOC), short-circuit current (JSC) and fill factor (FF). However, the relationship between these parameters is complex and often involves trade-offs. In particular, the FF in OSCs appears to compete with the VOC, which differs from other semiconductors[2,3].
For an efficient solar cell, the requirements of VOC and FF are consistent in terms of reducing recombination losses. Previous analytical expressions have shown that a higher FF is achievable with a higher VOC. Indeed, for solution-processed perovskite solar cells, suppressed VOC losses are associated with increased FF[4,5]. It seems conceivable that reducing the VOC loss is always beneficial to the maximum FF available in a solar cell. However, this does not apply to OSCs. Reports show that increasing VOC can be detrimental to FF in OSCs, contradicting what has been observed in inorganic and hybrid semiconductors. This raises two important questions: i) what causes this limit and ii) how it can be avoided.
In this work, we explore the FF limit in OSCs. We analyze over 100 sets of OSCs based on two classical NFAs: rylene-diimide-based and linear-fused-ring electron acceptors. These OSCs have voltage loss from 0.5 eV to 1.1 eV and FF from 0.27 to 0.8. We find that transport limit cannot explain the unique FF limit in organics. We select four representative systems based on Y6-series NFAs with low VOC loss. We investigate the charge generation process in these systems and observe an emissive bound state that is suggested to be a hybrid state of local excited (LE) and charge transfer (CT) states. This weakly bound state shows field-dependent charge generation, which is a unique feature in OSCs that causes FF loss besides transport-limit. Moreover, the weakly bound state can influence the transport-limit by reseparating into free charge carriers. The reseparation would reduce recombination coefficient, and its reduction degree is proportional to the dissociation probability of the bound state.
Optimisation-O3
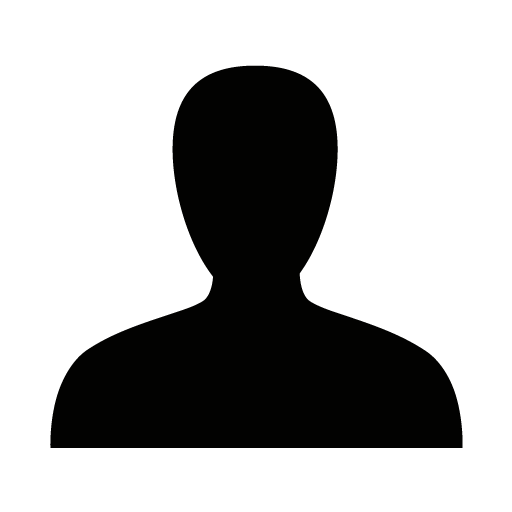
Organic donor:acceptor solar cells have been reestablished as a serious contender among alternative photovoltaic technologies thanks to the emergence of fullerene-free bulk heterojunctions. Record efficiencies have been reported for combinations of polymer donors and small molecule acceptors, which show both a high fill-factor and photocurrent and comparably small voltage losses. In the past, much effort has been made to reveal the role of the interfacial charge transfer states in typical polymer:fullerene blends in order to reduce energetic losses while maintaing high initial exciton separation yields; with non-fullerene acceptors, however, the efficiency gain might at least in part be driven by an effective intra-phase charge separation. At the same time, incorporation of a donor remains necessary to reach good photovoltaic efficiencies.
Under on these circumstances, we need to rethink the role of the donor:acceptor interface, including charge transfer state energetics, and its contribution to charge separation and recombination. At the same time, we are intrigued to have a closer look into photophysics which were deemed less significant with fulleren acceptors to continue increasing the photogeneration yield. Therefore, we have focused on the contributions from several spectral regimes, both below and above the absorption edge, to the photocurrent of organic solar cells in the steady-state and close to working conditions via applied electrical and light biases. By using ultra-sensitive electro-optical quantum efficiency spectroscopy, we are able to resolve their respective field dependency of the photogeneration yield. Our results show that the internal quantum efficiency includes distinct spectral variations in highly effective donor:acceptor blends, indicating that the charge separation efficiency can be optimized even further.
Optimisation-O4
In 2023, Drew earned his PhD Sêr SAM group at Swansea University. His focus is on the disentangling of various relaxation pathways in disordered semiconductor systems including organics and perovskites. He is the resident Ultrafast expert and has been involved in the construction of many apparatuses the group currently uses. Drew’s interests lie in Ultrafast and Semiconductor Physics, specifically the relaxation mechanisms in disordered semiconductors. Throughout his career, Drew has worked with start-up companies, lectured to undergraduate students, tutored and taught at the undergraduate level, and volunteered with various science outreach groups. Before completing his eduction, Drew worked as a pastry chef. He is an avid musician, surfer, and traveller.
Suppression of bi-molecular recombination in organic solar cells is highly correlated with increases in fill-factor and high short-circuit currents. The origins of this non-Langevin recombination are debated and mechanisms related to charge-transfer (CT) state and free-carrier encounter dynamics have been proposed. [1] Further, it is expected that acceptor exciton dynamics play an important role in mediating the CT-state dynamics. [2,3]
In this presentation the dynamics of exciton diffusion, transfer, and dissociation will be explored. Specifically, the methodology known as pulsed-PLQY will be introduced and used to measure diffusion lengths in neat acceptor materials and acceptor domain sizes in bulk-heterojunction films.[4] It will be shown that state-of-the art non-fullerene acceptors (NFA) show enhanced diffusion lengths and domain sizes compared to fullerene predecessors. However, this is not enough to explain the highly suppressed recombination observed in NFA-blends. The ratio of diffusion length to domain size, known as the characteristic length ratio, is shown to correlate with the suppression of bi-molecular recombination, suggesting that the processes engendering non-Langevin recombination are enabled by efficient exciton dynamics.
Optimisation-O5
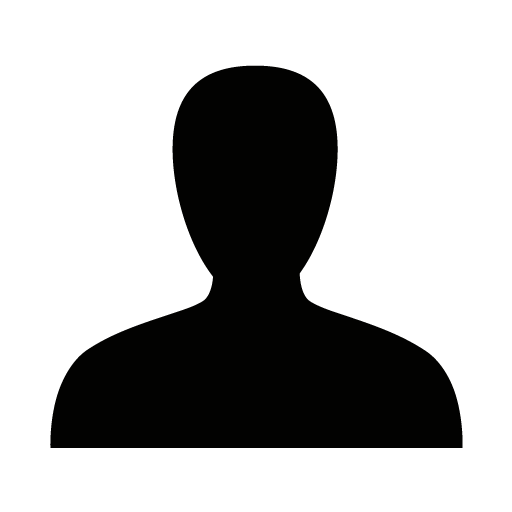
The time-delayed collection field technique (TDCF) is widely used to characterise charge carrier recombination and mobility in organic solar cells.
By using drift diffusion simulations in the time domain we investigate the influence of trap states on charge carrier mobility and recombination rate determination. Reconstructing the TDCF-experiment with additional insight into the physical processes at each time step allow us to better understand the causes for distinct features in TDCF transients.
We find that deep trap states can highly impact the extraction time of carriers and therefore result in an error in mobility. On the other hand the relaxation of charge carriers into deep trap states can cause high initial recombination rates, that are often attributed to surface recombination.
Therefore we advise caution when interpreting TDCF-experiments. A quick initial test is proposed to determine weather deep trap states could impact the results of TDCF experiments on a given device.
Optimisation-O6
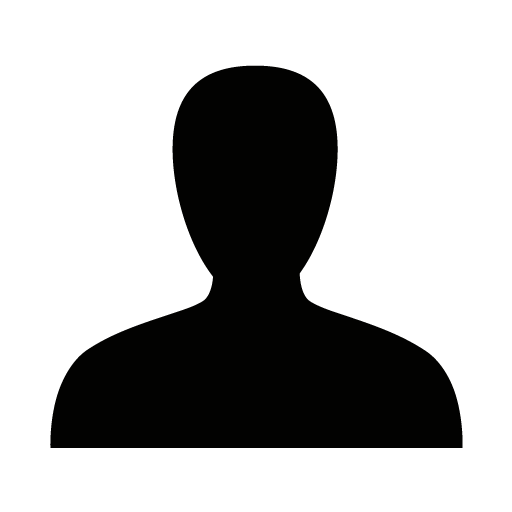
Organic solar cells are highly promising for sustainable energy conversion with power conversion efficiencies already surpassing 19%. [1] One approach to further increase the performance of organic photovoltaics is to reduce the substantial energy loss in organic solar cells stemming from the low‑permittivity nature of the organic absorbers by applying high-permittivity (high-ε) active layer materials. [2] However, despite the increase in permittivity, many of the high-ε materials typically reveal lower efficiencies, which is generally explained with a non-ideal bulk heterojunction morphology.
In this work, we introduced polar sulfone side chains to perylene-based non-fullerene acceptors with the aim of increasing their dielectric permittivity. The novel acceptors PMI-[F-OS] and PMI-[C-OS], exhibited a permittivity increase by 56% (εr from 1.86 to 2.90) and by 44% (εr from 1.91 to 2.76) at 105 Hz and high solubility in THF resulting from the sulfone modification. [3] This provides the possibility of a layer-by-layer processing using only non-halogenated solvents (o-xylene for the donor polymer PTQ10 and THF for the acceptor). By applying the resource-saving design of experiment (DoE) method, a still underrepresented approach in solar cell research, for the optimization of the processing parameters of the solar cells, the PCE maximum could be determined after performing only a comparably small number of experiments. The optimized solar cells revealed a PCE of 5.5% with a high VOC of 1.3 V, surpassing the efficiency of solar cells containing the alkylated PMI-analog [4] of PMI-[F-OS], with the additional benefit of the non-halogenated solvent processing.
These findings clearly demonstrate that layer-by-layer processing can prevent efficiency losses typically stemming from unfavorable donor–acceptor phase separation in bulk heterojunction organic solar cells due to the distinctly changed surface free energies and solubilities of high-ε materials.
Furthermore, we extended this approach to the popular Y-series acceptors and synthesized Y6-derivatives with flexible glycol side chains. In one compound the functionalization was done on the outer thiophene position, the second acceptor bears glycol chains on the pyrrole unit. These compounds exhibit significantly higher permittivities (4.73 and 5.24) compared to Y6 (2.39), while maintaining the beneficial properties of this acceptor family. Besides a detailed morphology investigation of blends of the high-ε Y6-derivatives with PM6, we also present first results of their photovoltaic properties.
Simulations-IS1
He studied electrical engineering in Stuttgart and started working on Si solar cells in 2004 under the guidance of Uwe Rau at the Institute for Physical Electronics (ipe) in Stuttgart. After finishing his undergraduate studies in 2006, he continued working with Uwe Rau first in Stuttgart and later in Juelich on simulations and electroluminescence spectroscopy of solar cells. After finishing his PhD in 2009 and 1.5 years of postdoc work in Juelich, Thomas Kirchartz started a three year fellowship at Imperial College London working on recombination mechanisms in organic solar cells with Jenny Nelson. In 2013, he returned to Germany and accepted a position as head of a new activity on hybrid and organic solar cells in Juelich and simultaneously as Professor for Photovoltaics with Nanostructured Materials in the department of Electrical Engineering and Information Technology at the University Duisburg-Essen. Kirchartz has published >100 isi-listed papers, has co-edited one book on characterization of thin-film solar cells whose second edition was published in 2016 and currently has an h-index of 38.
The efficiency of halide perovskite solar cells has been continuously rising over the past decade to values above 25%. Future technological development will have to deal with issues of device stability but also thrive to further minimize efficiency-limiting loss processes in the bulk and at interfaces within the cell stack. The identification and understanding of electrical losses will require the ability to characterize solar cells and multilayer stacks with a variety of steady-state, time-domain and frequency-domain techniques that are sensitive to the transport and recombination of charge carriers. Especially, time- and frequency-domain techniques offer a large amount of information on dynamic processes in the solar cell, while posing a substantial challenge in terms of the complexity of data analysis.[1]
Here, we discuss three novel and relevant aspects related to transient photoluminescence (TPL) and photovoltage spectroscopy (TPV) applied to halide perovskites. We show that by using extremely low repetition rates and a gated CCD camera, we can obtain high dynamic range TPL data with continuously changing decay times that exceed 100µs. Furthermore, we show that by changing the repetition rate, basically any decay time can be extracted from one sample, whereby the extracted decay time is approximately the inverse repetition rate. We explain why this is the case both mathematically and physically. Whenever, higher order recombination due to e.g. band to band or band to shallow trap transitions affects the decay, the decay time will correlate with the time range of the measurement which is typically limited by the inverse repetition rate. Finally, we show how to separate recombination from extraction by using TPV data combined with a novel analysis approach based on the determination of eigenvalues of a 2 × 2 matrix. The model provides two time constants (the inverse eigenvalues), one for the rise and one for the decay of the voltage after the pulse. These two time constants can be experimentally determined as a function of light intensity. By comparison of model and experimental data, we can then derive a time constant for recombination and one for charge extraction, whereby the ratio of these two time constants is directly correlating with solar cell efficiency.
Simulations-O1
Ivan Kassal is an Associate Professor in the School of Chemistry at the University of Sydney. He graduated from Stanford University in 2006 and completed his PhD in Chemical Physics at Harvard University in 2010. He is a theorist working at the intersection of quantum science, chemistry, biophysics, and materials science. He pioneered some of the first applications of quantum computers to chemistry, showing they could dramatically accelerate difficult chemical calculations. He has also unravelled ways that photosynthetic organisms use quantum effects to improve their light harvesting, and is using those lessons to better understand next-generation materials, especially organic solar cells. He is a recipient of a DECRA fellowship, a Westpac fellowship, and the Le Fèvre Medal of the Australian Academy of Science for “outstanding basic research in chemistry”.
Jumping kinetic Monte Carlo: Easy-to-use description of delocalisation in organic semiconductors
J Willson, D Lalwani, W Liu, D Balzer, I Kassal
In organic semiconductors, even small amounts of delocalisation can considerably enhance charge and exciton transport as well as charge separation. However, models for describing quantum-mechanical delocalisation in disordered materials are either difficult to use and computationally expensive or phenomenological models with unpredictable parameters. Here, we describe jumping kinetic Monte Carlo (jKMC), a transport model that approaches the accuracy of quantum-mechanical treatments with a computational cost only slightly above conventional hopping models [1]. jKMC reproduces quantum-mechanical results where those are known, and allows extrapolation into previously unexplored regimes of delocalisation, allowing us to show that realistic amounts of delocalisation—neglected by conventional hopping—can increase charge mobilities by as much as two orders of magnitude. The functional form of jKMC is a simple and easily understood modification of ordinary Marcus hopping; it includes a correction for allowing charges to jump over multiple sites, which can be included in any existing kinetic Monte Carlo code. The low computational cost of jKMC also allows us to carry out the first calculations of recombination rates that take into account delocalisation, finding that this process is also significantly affected by even modest delocalisation [2].
[1] J Willson, W Liu, D Balzer, I Kassal, arXiv:2211.16165 (2022).
[2] D Lalwani, I Kassal, in preparation (2022).
Simulations-O2
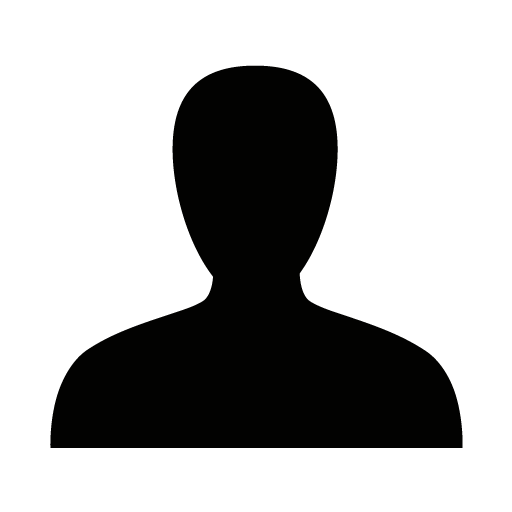
The field of organic photovoltaics research has witnessed a renaissance in recent years.
On the materials side, two recent developments have invigorated, if not revolutionized photoconversion
in organic materials. First, a new generation of highly light-absorbing
organic semiconductors (OS), often referred to as non-fullerene acceptors (NFAs), have propelled
device efficiencies of organic heterojunction solar cells to new levels, >18%[1], up from 11% for
fullerene based solar cells. Second, charge generation has recently been demonstrated for a number
of single component organic systems, or homojunctions.[2] This is significant because homojunctions
have a number of advantages compared to heterojunctions, e.g., simpler materials design and higher
morphological stability.
New regimes of photophysics are reached in these new materials that are currently not well understood
suggesting that more powerful experimental measurements and theoretical and computational models
are urgently needed to rationalize, explain and further build on these advances. This concerns in
particular the development of improved spectroscopic methods, with unprecedented, for device measurements,
time resolution as well as novel scale-bridging computational tools from the nanoscale, on which we
focus in the present contribution, to the device level and their integration with device measurements.
Here we present a powerful (non-adiabatic) molecular simulation method that we have developed
to propagate electronic excitations (excitons)[3,4] as well as charge carriers (electrons, holes)[5,6] through
nanoscale materials by solving the time-dependent Schrödinger equation coupled to intramolecular
and lattice vibrations. Our simulations give a ``first-principles" view on these processes
that is free of the many assumptions that strongly limit the applicability of traditional theoretical models
(band theory, polaronic hopping, Onsager-Brown,...). In particular, our methodology takes into
account the electronic quantum dynamics of delocalization/localization of excitons and charge carriers and allows
us to understand how the quantum dynamics is affected by the dynamic and static disorder that is so
characteristic for these materials.
Particular focus will be placed on discussing our recent non-adiabatic molecular dynamics simulation of exciton diffusion
in molecular organic crystals[3]. We find that in materials featuring some of the highest diffusion
lengths to date, e.g. the non-fullerene acceptor Y6, the exciton propagates via a transient quantum
delocalization mechanism, reminiscent to what was previously proposed for exciton transport in P3HT-based
nanofibres [7]. Yet, the extent of quantum delocalization of excitons is rather modest compared to charge carriers,
even in Y6, and found to be limited by the relatively large exciton reorganization energy.
Based on these simulations, we will chart out a path for rationally improving exciton transport in organic
optoelectronic materials that could remove some of the intrinsic limitations of present-day organic
optoelectronic devices.
Simulations-O3
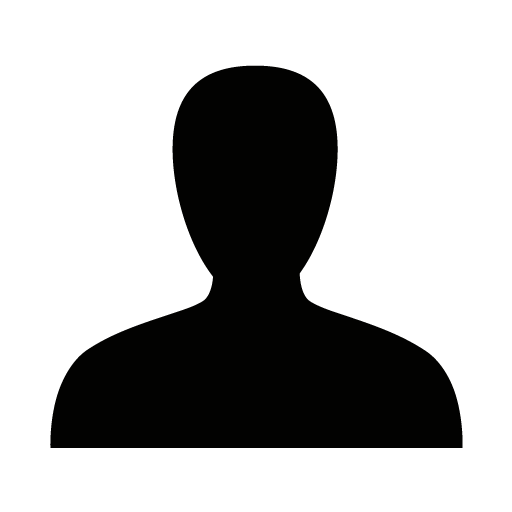
Organic-inorganic metal halide perovskites have attracted a considerable interest in the photovoltaic scientific community demonstrating a rapid and unprecedented increase in conversion efficiency in the last decade. Besides the stunning progress in performance, the understanding of the physical mechanisms and limitations that govern perovskite solar cells are far to be completely unravelled. In this work, we study the origin of their hysteretic behaviour from the standpoint of fundamental semiconductor physics by means of technology computer aided design (TCAD) electrical simulations. More in detail, we explain the hysteresis in current density-voltage (J-V) characteristic of perovskite solar cells (PSCs) in terms of charge carrier accumulation due to slow charge dynamics occurring at energy states near the interfaces with the perovskite absorber.
Our simulations reveal that hysteretic behaviour can be caused by shallow energy states near the interface with ETL (or HTL) with particular properties as: (i) density Nt > 1018 cm-3, (ii) capture cross-section σ ≈ 10‑23 cm2, and (iii) defect energy Et ≈ 0.25 eV with respect to VBE (or Et ≈ 0.2 eV with respect to CBE). Interestingly, such values are consistent with experimental findings reported in literature.
Furthermore, stronger hysteresis at higher defect densities near the ETL or HTL reveals that the hysteretic behaviour is due to recombination processes. We provide an explanation of the observed phenomena in terms of slow charge capture and emission times at interface energy states with capture cross-sections lower than 10-22 cm2. Moreover, the effect is most apparent when the energy states exhibit energies close to the quasi-Fermi levels.
Finally, J-V simulations at different scan rates demonstrate realistic behaviour in the analysis of hysteresis for different scan rates. In particular, we observe that there is a scan rate that maximizes hysteresis as observed in experimental studies. Furthermore, our simulation platform allow us to relate the profile of energy states (energy and position) to interface passivation and crystallinity of absorber material in PCSs. In this regard, we observe that independently improving perovskite material or quenching interface defects is not sufficient to eliminate the hysteresis. Rather, only concurrently pursuing high quality perovskite material and surface passivation yields highest efficiency with negligible hysteresis. Our model can be easily extended and adapted to different device architectures, defect distributions and device life-time for a custom optimization that addresses different fabrication sequence, absorber material formulations, choice of supporting layers and stability.
In conclusion, our results show that hysteresis can be explained using fundamental semiconductor physics alone with realistic defect distributions in both the spatial and energetic domains. This supports the claim that interface defects play a crucial role in the formation of hysteresis. This fact is of particular relevance, because it links defects dynamics with hysteresis and stability of PSCs. Therefore, a deep study of such defects should lead to new insights for solving the reliability issue of PCSs, because defects can be created or disappear in particular conditions such as temperature, illumination and bias potential.
Simulations-O4
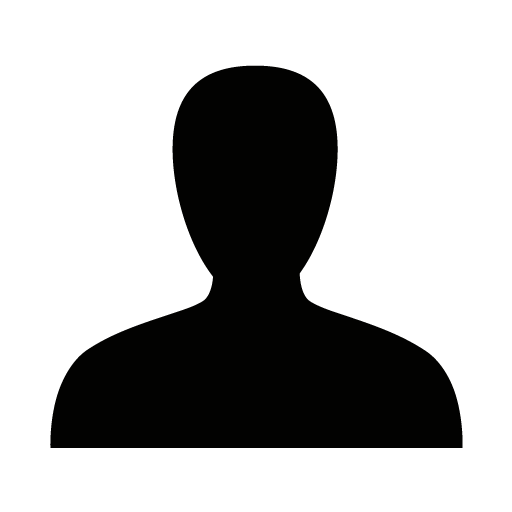
Surfaces and grain boundaries (GBs) are likely the sources of energy losses and degradation in metal-halide perovskites (MHPs). Efficient perovskite solar cells (PSCs) make use of surface passivation layers to improve energy alignment, enhance stability and reduce trap states on MHP surfaces [1], while the local GB structures are more difficult to control. Still, PSCs reach excellent power conversion efficiencies of >25% despite the presence of GBs in their polycrystalline MHP absorber [2]. To date, our understanding of GBs is still far behind, and the literature remains quite controversial. On the one hand, GBs appear benign on charge transport and light absorption but, on the other hand, come along with enhanced defect densities and structural instabilities [3]. To gain control over GBs in MHPs, we must derive a deeper understanding of their structural dynamics and electronic properties under finite temperatures.
We performed ab initio molecular dynamics (AIMD) to shed light on the structural dynamics and electronic properties at the grain boundaries of MHPs using cesium lead iodide, CsPbI3, as our model system [4]. We observe halide-driven structural healing of the GB due to a facile migration of iodine ions in GBs as a response to the lattice strain in the GB region. Density functional theory (DFT) calculations reveal a substantial reduction of hole trap states upon healing of the GB, while shallow electron trap states form by strain-induced Pb–Pb dimers in the GB, likely being a source of open-circuit voltage losses by enhanced non-radiative recombination. On a longer timescale, our AIMD simulations reveal the spontaneous formation of iodine Frenkel defects near lead-iodine-rich GB regions. DFT calculations show a gradual reduction of the defect formation energy from bulk (1 eV) > GB(0.5 eV) > surface (0.1 eV). Overall, our simulations reveal a competition between moderate impact on the electronic properties by structural healing and a detrimental impact on the point defect densities, both connected to the facile migration of iodine ions in GBs. Based on these insights on an atomistic resolution, tailored passivation strategies are derived to mitigate detrimental defect formation at GBs.
Simulations-O5
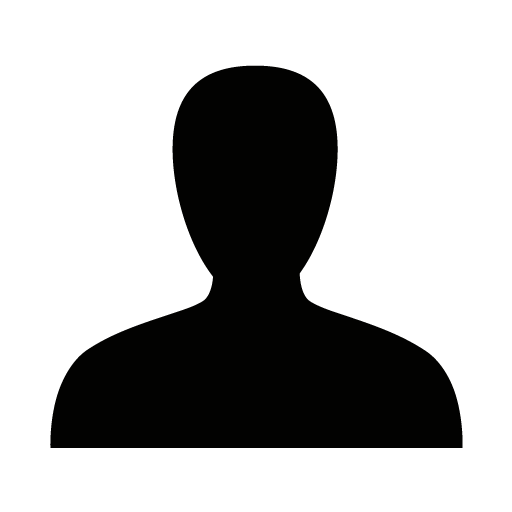
The processing conditions strongly impact the morphology of the photoactive layers in perovskite (PSC) and organic solar cells (OSC), and thus their performances. However, the process-structure relationship remains insufficiently understood. In this talk, we will show how the absorber layer morphology formation of solution-processed solar cells can be simulated, using a recently developed theoretical model. For the first time, this allows to investigate the interplay between all the relevant physical processes (evaporation, crystal nucleation and growth, liquid demixing, composition-dependent kinetic properties), within a single coherent framework.
The approach will be illustrated for OSC based on simulations of the morphology formation for a well-documented polymer-small molecule bulk-heterojunction. The comparison with previously reported in-situ characterization of the drying structure is very convincing: the morphology formation pathways, crystallization kinetics, and final morphology are in line with experimental results. The final morphology is a subtle mixture of pure crystalline donor and acceptor phases, pure and mixed amorphous domains, which depends on the process parameters and material properties. For PSC, simulations showing the impact of the evaporation rate on the final crystalline structure will be shown. The results regarding substrate coverage, film roughness, crystals sizes are in excellent agreement with experimental results, and the conditions for obtaining high-quality films will be discussed.
The major innovative value of the work is that the process-structure relationship in solution-processed photovoltaics can now be investigated with comprehensive computer simulations instead of costly trial and error experiments. This is a worldwide unique approach that represents an outstanding opportunity for the PV-community in order to identify new design rules for ink formulation and processing conditions.
Simulations-O6
Docent Moyses Araujo received his PhD degree, in Condensed Matter Physics, from Uppsala University (UU). Thereafter, he has held a postdoc position at the Royal Institute of Technology (KTH) in Stockholm with a distinguished scholarship from the Swedish Research Council (VR). As a recognition of his work in Sweden, he has won three research awards, viz. Benzelius prize (from the Royal Society of Sciences in Uppsala), Ångstrom Premium (UU), and Bjurzon’s Premium (the highest award for PhD thesis at UU). In 2011, he has moved for a postdoc in USA, at Yale University, with a prestigious scholarship from the Yale Climate and Energy Institute (YCEI). In 2012, he has returned to Sweden as researcher at UU and in 2014 he has started his independent research group in the same institution with support from VR through the Young Researcher Grant. In 2018 he has become Docent in Physics at Uppsala University. From September 2020, he has joint Karlstad University as universitetslektor/Associate Professor in condensed matter theory.
The development of new organic photovoltaic materials based on non-fullerene acceptors (NFAs) has led to a significant increase in the power conversion efficiency of organic photovoltaics (OPV) in the last years. However, the fundamental understanding of the charge photogeneration mechanism at the molecular level is still lacking, a scientific challenge whose solution could be the watershed in the discovery of novel OPV materials. To contribute to this end, we are developing a multi-scale method that combines Quantum Mechanics (QM) calculations and Molecular Dynamics (MD) simulations within the scope of a sequential-QM/MD approach to assess the electronic and optical properties of organic-polymeric photovoltaic materials. Despite being a well-established method to study small molecules in solution, it has not yet been developed to investigate polymer films cast from solution. Our methodology starts with the simulation of film formation through solvent molecules evaporation using classical molecular dynamics simulations. Then additional MD simulations are carried out on the obtained film to generate uncorrelated configurations to be used on the properties’ calculations. The latter is assessed through an electronic embedding scheme where a pre-defined molecular region, of the generated configuration, is treated at the QM level, incorporating explicit effects of the environment. For the QM calculations, density functional theory (DFT) and time-dependent DFT have been employed. The quality of the force field parameters adopted in the MD simulations have been carefully analyzed. We have focused the study on the PF5-Y5 polymer. Given the flexibility of the computational approach, we have been able to study this system both in solution (chlorobenzene) and film. First, we have analyzed the structure of the films with focus, for instance, on the tendency to stabilize pi-pi stacking conformations. Then, the dynamics and molecular environment effects on the electronic transitions have been quantified with an improved description of the optical absorption. Finally, through the calculation of the fundamental and optical gaps the exciton binding energies have been estimated. Here, we have considered both the singlet and triplet excitons. The comparisons with experimental results confirm the suitability of the developed s-QM/MD approach, highlighting the importance of properly describing the dynamics and molecular environment effects in the modeling of the electronic properties of OPV materials.
Architectures-IS1
The ternary blend approach has been shown to improve spectral coverage and enhance power conversion efficiency (PCE) of the single junction organic bulk heterojunction solar cells. However, several parameters, such as domain morphology, exciton lifetime, energy, and charge transfer, influence the resulting PCE. In this regard, we conducted a systematic study to compare charge and energy transfer dynamics and orientational dependence nanomorphology of ternary blends and were compared with their binary counterparts. In this talk, I will discuss our findings into employing non-fullerene acceptors (NFA) having complementary absorption to alternatively harvest the photons via resonant energy transfer process to realize improved photovoltaic performance in ternary blend. In addition, I will also discuss the GIWAXS measurements that the incorporation of third component enhances lamellar stacking in the PM6 nanodomains along with increased crystallization in the Y6 nanodomains, hence decreasing structural disorder. Our study provides insight into employing non-fullerene acceptors (NFA) having complementary absorption to alternatively harvest the photons via resonant energy transfer process to realize improved photovoltaic performance in OSCs.
Architectures-O1
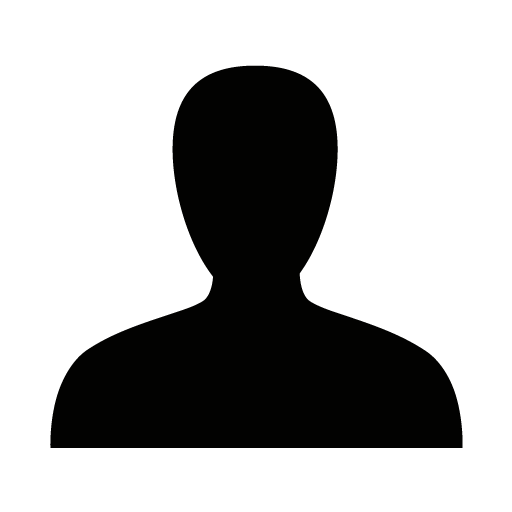
Organic photovoltaic technologies are getting more and more mature. Photoconversion efficiencies are now reaching more than 18 % at laboratory scale with binary systems. However, interesting results can be missed due to the quality of organic semiconductors. Those materials are commonly prepared from palladium-catalyzed polycondensation reaction. With a lack of purification, impurities present in bulk heterojunction, in particular in polymers, can induce severe loss of performances. For example, Bracher et al. demonstrates that remaining metallic nanoparticles from catalyst can induce current shunts within the device [1]. Liu et al. reported polymer degradation assisted by palladium [2]. Moreover, Schopp et al. recently proved that catalyst remaining traces can affect organic photovoltaic devices by affecting charge carriers [3]. Here we want first to present that not only palladium catalysts but also their ligands can affect solar cells efficiency and stability. For this purpose, a series of reaction residues have been investigated and were classified as detrimental or inert towards the device performances. In a second time, to improve our bench mark, we added interfacial buffer layers as recommended by Li et al. [4], i.e. IC‑SAM and C70, to protect our active layer. As a consequence, we observed, first, an improvement of the performances and better stability and second, low impurity concentration effects that were hidden before are now observable in this improved architecture.
Architectures-O2
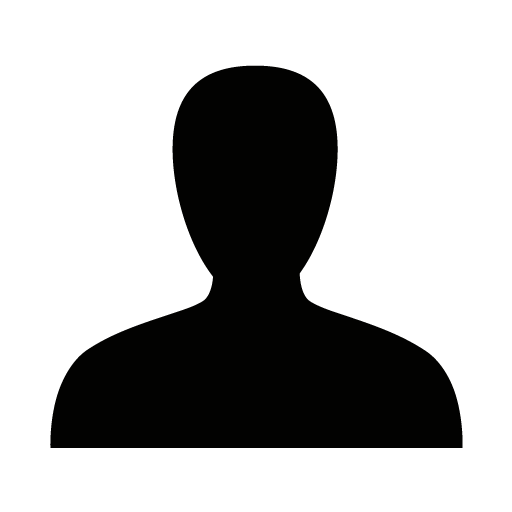
Although ternary blends (consisting of one host binary blend and one guest component) deliver record efficiencies for organic solar cells (OSCs), their performance still lags behind other next-generation photovoltaic technologies, mainly due to the significant voltage losses. The fundamental understanding of how the added guest component affects the open-circuit voltage (VOC ) in ternary organic solar cells (TOSCs) remains controversial, limiting the rational screening and/or design of the guest component. In this study, we systematically investigate the VOC of a series of TOSCs based on the detailed balanced principle and paint a comprehensive picture of how the guest component affects the radiative and non-radiative related parts of VOC in TOSCs. We highlight that the thermal population of charge-transfer and local exciton states provided by the guest component based binary system has a significant influence on the non-radiative voltage losses. Ultimately, we provide two design rules for enhancing the VOC in TOSCs: 1) high emission yield for the guest component based binary blend and similar charge-transfer state energies for the two binary blends; 2) high miscibility of the guest component with the low-optical-gap component in the host binary blend.
Architectures-O3
In the future production of energy will strongly rely on direct light-to-electricity transformation. For the foreseeable future Si-based technologies could be adequate, however, for the further progress higher efficiencies, simpler fabrication and lower costs are needed. Recently significant progress was made in perovskite-based and organic photovoltaics which promise benefits of low cost, high efficiency and large versatility and can potentially be manufactured in a cost-effective fashion. One of the possible pathways to boost the performance and stability of solar cells is improvement in the efficiency of carrier extraction by corresponding charge-transport layers, key components in modern optoelectronic devices.
In our work we have proposed a new way to form a charge selective layer by utilizing the ability of certain organic materials to spontaneously form a monolayer on various surfaces. Use of the carbazole chromophore provides good selectivity for holes, while phosphonic acid group ensures strong binding to the substrate, for example metal oxides.
While initially this idea was mostly driven by scientific curiosity, soon it became clear that it can compete with conventional polymeric and small molecule hole transporting materials. The full potential was demonstrated in various tandem technologies, where world records were achieved (Si/Perovskite [1], CIGS/Perovskite [2], Perovskite/OPV [3]). In addition, this technology allowed to achieve one of the highest efficiencies for OPV [4].
Architectures-O4
The most promising technological application of perovskite solar cells (PSCs) relies on the implementation of single junction perovskite photovoltaic devices in tandem architectures, either as Si-perovskite or all-perovskite. Notoriously, wide-gap perovskites (⁓1.7-1.8 eV) required for such solar cell design are reported to suffer from larger open-circuit voltage (VOC) losses compared to the narrower gap counterparts. We recently demonstrated that these energy losses are associated with strong interface recombination due to an energy misalignment between the perovskite and charge transport layers (CTL), resulting in the external VOC being lower compared to the internal quasi-Fermi level splitting (QFLS) of the same device. While at the p-interface there is a large variety of transport materials that can be used to mitigate this problem (metal oxides, polymers, and self-assembled monolayers), at the n-interface, fullerenes have been the only successful option so far. Due to this limitation, the non-radiative recombination losses at the n-interface are one of the major limitations of wide-gap perovskite solar cells. We also showed that interface passivations (such as GuaBr and ImBr) can reduce non-radiative recombination at this interface and close the QFLS-VOC mismatch. However, perovskite surface passivation methods can be highly sensitive to the type of perovskite and potentially hard to scale up. In this study, instead of using surface passivations or replacing the fullerenes ETL with other electron transport materials, we propose a simple approach to specifically tune the alignment of the electron-transport layer (ETL) by blending different fullerene derivatives in various ratios. We find that fine-tuning the blend ratio of the fullerenes allows for targeting preferential energetic alignment between the ETL and the perovskite layer, drastically reducing the VOC losses while keeping excellent charge transport. By inserting a thin interlayer of this blend between perovskite and C60 in 1.8 eV pin MA-free perovskite devices, we achieve VOCs up to 1.32 V and FF close to 84%, resulting in PCEs approaching 19%, among the highest reported for this bandgap. Importantly, by utilizing a PLQY spectral imaging technique on full devices, we find that the energetic mismatch between QFLS and VOC is effectively reduced when the fullerenes blend ratio is fully optimized. To confirm this point, drift-diffusion simulations modelling devices implementing these different blends show the same QFLS-VOC mismatch reduction when the perovskite and ETL are energetically aligned. We also demonstrate that our approach is fully compatible with perovskite tandem solar cells and potentially allows us to provide ideal alignment for a large variety of perovskite bandgaps. Our study shows that with appropriate interface design, it is possible to access the full QFLS potential of the perovskite material achieving high VOCs. As such, we demonstrate a simple and flexible method to optimize the solar cell architecture for perovskite with different bandgaps without the need of finding specific ETL materials for each perovskite bandgap.
Architectures-O5
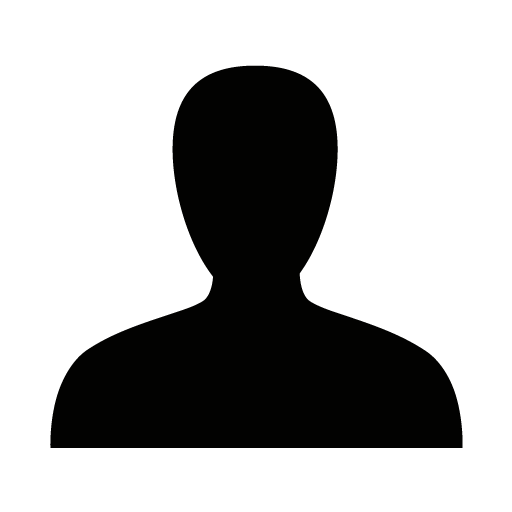
Multi-junction solar cells containing hybrid halide perovskites are often considered to be the next generation photovoltaic (PV) systems, due to the strongly enhanced power conversion efficiency (PCE) and, thus, reduced area-related module costs. Moreover, tandem devices based on combination of perovskite materials with different constituents allow fabrication of ultra-thin and lightweight perovskite-perovskite tandem modules, which could be produced at even lower cost. In either case of tandem applications, the wide energy band gap (>1.7 eV) perovskite absorbers inherently suffer from poor stability mainly due to halide segregation phenomenon in which perovskite halides de-mix and create I-rich and Br-rich domains, leading to spatial variations in energy bandgap and inferior performance. To solve this notorious issue, we have added an alkylammonium molecule to the perovskite precursor solution, which tailors perovskite crystallization, passivates surface states and inhibits halide segregation. From 1H nuclear magnetic resonance (NMR) spectroscopy we show that nucleophilic alkylammonium interacts with Formamidinium (FA) to form a bulky cation releasing ammonia. From grazing incidence X-ray diffraction (GIXRD) measurements we clearly identify the creation of low dimensional perovskite enabled by the long aliphatic part of the created bulky cation. Combining top-view images from scanning electron microscopy (SEM) and microscopically resolved photoluminescence (µ-PL), we demonstrate that addition of the alkylammonium-based additive has a direct impact on the perovskite surface morphology and opto-electronic heterogeneity. From absolute PL quantum yield measurements, we show that moderate quantities of the alkylammonium-based additive passivate surface defects and increase the quasi-Fermi level splitting. Remarkably, perovskite samples produced via the alkylammonium doped solution, which are placed under continuous illumination exhibit exceptional photostability. In stark contrast, the PL peak of perovskite without an addition of the alkylammonium passivant splits into two distinct peaks which is a common signature of halide segregated regions with different bandgaps. Lastly, we show that the addition of the alkylammonium additive results not only in the enhancement of photostability but also in the PCE by 2%. We believe the proposed stabilization method is a prominent and reproducible technique which brings perovskite-based PV closer to commercialization.
Architectures-O6
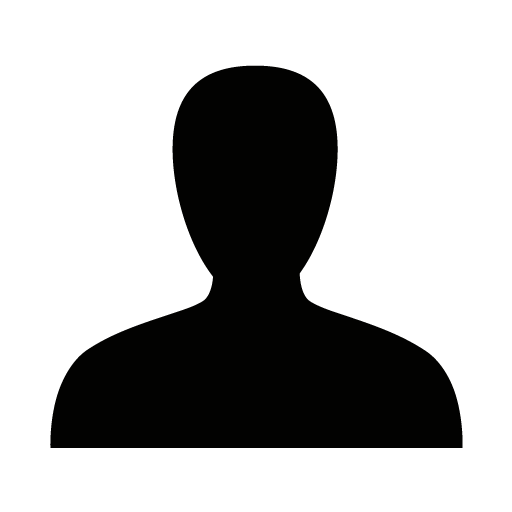
Lead halide perovskites are considered one of the largest breakthroughs in the optoelectronic research field in recent years due to their versatility and their multiple applications including solar cells, LEDs and X-ray detectors among others. All perovskite based optoelectronic devices are made by contacting different materials to the perovskite itself. Therefore, a detailed understanding of the surface and interface properties of lead halide perovskites is crucial for creating more stable and efficient devices. Due to the difficulty of obtaining clean perovskite surfaces for study, up to date, most of surface and interfaces research was done on thin-films, which are more prone to defects and variations in their structure due to more grain boundaries.[1,2] This makes single crystals better suited for studying the intrinsic properties of materials. In our previous work, we investigate the surface properties and electronic structure of in-situ cleaved perovskite single crystals with photoelectron spectroscopy at the FlexPES beamline at MAX IV.[3]
In this work, we go one step further and after generating and characterizing a clean surface of perovskite single crystals, we in-situ evaporate transport materials and follow the chemical changes and band structure evolution with different thicknesses. The use of synchrotron-based soft X-ray photoelectron spectroscopy enables high surface sensitivity and nondestructive depth-profiling. In particular we study several thicknesses of an in-situ formed interface of 6,13-Bis(triisopropylsilylethynyl)pentacene (TIPS-Pentacene, C44H54Si2) with 4 different in situ cleaved perovskite single crystals (MAPbI3, MAPbBr3, FAPbBr3 and CsxFA1-xPbBryI3-y). We found a large band bending of about 0.6 eV to higher binding energies on the TIPS-Pentacene side with no influence on the perovskite energy levels regardless of its composition. Results were reproducible at two different beamlines placed at different synchotron radiation facilities (MAX IV, Sweden and BESSI II, Germany) [4]
We believe that this detailed study of surface and interfaces between in-situ cleave perovskite single crystals and several thicknesses of in-situ evaporated TIPS-Pentacene transport material will provide the scientific community with a model system to contribute on the interfacial understanding of perovskite and transport materials and therefore helping on the way to improve the efficiency and stability of perovskite-based optoelectronic devices.