1.1-I1
Conversion of carbon dioxide into hydrocarbons (methane, ethylene) and alcohols (ethanol, propanol) using renewable electricity as an energy source is an attractive strategy for storing renewable energies (solar and wind energy) into the form of chemical energy (a fuel) and using carbon dioxide as a raw material for the synthesis of chemical products. However, carbon dioxide activation is a complex process which implies multiple electron and proton transfers, resulting in the need for stable, efficient and selective catalysts in order to make this strategy a practical industrial option. Here we discuss our ongoing research using original Cu-based catalytic materials and addressing several issues associated with on carbon dioxide electrolysis, such as activity, selectivity, carbonate formation and carbon loss. This led us to investigate the effect of molecular modification of catalyst surfaces [1,2,3], optimization of electrolysis under acidic conditions [3,4] as well as carbon monoxide electroreduction into ethylene and alcohols [5,6].
1.1-I2
Using electrical or solar power for CO2 and CO conversion into valuable chemical feedstocks presents a promising solution for storing energy from intermittent renewable sources in chemical bonds. Despite significant efforts in this field, CO2 and CO reduction reactions still face challenges in achieving the desired performance in selectivity, activity, energy efficiency, and stability. This report explores the critical roles of catalyst sites in influencing reaction selectivity and discusses strategies for in situ stabilization of the active sites at reaction interfaces. These approaches enhance performance and stability up to levels relevant to industrial applications. For example, in an electrochemical MEA electrolyzer, we realize a combined 91±2% CO2-to-C2+ Faradaic efficiency, 31±2% full-cell CO2-to-C2+ energy efficiency, and 24 ± 1% single-pass CO2 conversion at a commercially relevant current density of 150 mA cm−2 over 150 h. In a photothermal fix-bed reactor, we achieved a 473mmol g-1 h−1 at a gas hourly space velocity of 80,000 ml g−1 h−1, ~100% CH4 selectivity, ~75% single-pass CO2 conversion and excellent durability. Our findings provide insights into catalysts and systems, advancing sustainable technology for chemical and fuel production using renewable energy.
1.2-I1
Design of photoelectrochemical reactors for solar water splitting brings together science and engineering. It is a truly fascinating interdisciplinary challenge, requiring the understanding of semiconductor physics, (photo-)electrochemistry (including catalysis and corrosion), chemical engineering (including fluid dynamics and mass and heat transfer) and optics, which are all crucial to the development of an efficient and robust device.
I shall discuss the principal factors which constrain device design and present the work we have done on several different systems. I will also present our multiphysics models, which are required for device optimisation to avoid overall performance loss.[1 – 4] Losses in terms of hydrogen production rate and solar-to-hydrogen conversion efficiency could be caused by: severe potential and current distributions across poorly conducing electrodes, overheating, product crossover, optical losses due to light blockage by evolving hydrogen/oxygen bubbles and electrode corrosion.
In general, I am an interested in discovering the answer to the question ‘What might an industrial scale photoelectrochemical reactor system ultimately look like?’. I will talk about the steps taken to answer this question thus far and I will also discuss the research carried our in my research group: Electrochemical Systems Laboratory.
1.2-I2
The electrochemical reduction of CO2 is a valuable strategy to convert this gas into value-added, carbon-containing chemicals. C1 products, such as carbon monoxide (CO), formaldehyde (HCHO) or methanol (CH3OH) can find applications in the industry or as fuels, but their economic value is limited. C2 or C3 products, such as ethylene (C2H4) or propylene (C3H6), are used in such high amounts in the industry (millions of tons per year), that their production from CO2 feedstocks is key to valorizing this gas.
The electrocatalytic reduction of CO2 suffers from one major drawback: the selectivity towards a given product. Decades of research on catalyst development have only been able to achieve high selectivity towards CO or formic acid (HCOOH). Amongst catalysts, transition metal macrocycles have emerged as economical, robust, and effective catalysts for the electroreduction of CO2. Unfortunately, they can only reduce CO2 into C1 products such as CO [1] or methanol [2]. The formation of C2+ products upon electroreduction of CO2 have only been observed, up to now, for copper catalysts.
We have found that a commercially available molecular macrocycle, iron phthalocyanine, is able to convert CO2 into small quantities of methane (CH4), but also C2, C3 and C4 products. Control experiments show that both CO2 and the catalyst are required to observe these products. Gas chromatography and mass spectrometry were used, together with 13C labelled CO2 substrate, to demonstrate the origin of the carbon atoms in the products. X-ray absorption spectroscopy performed under operating conditions confirmed the molecular nature of the catalyst throughout the experiment. Catalytic experiments were performed with alternate substrates, which allowed us to propose a mechanistic hypothesis for this reaction.
1.2-I3
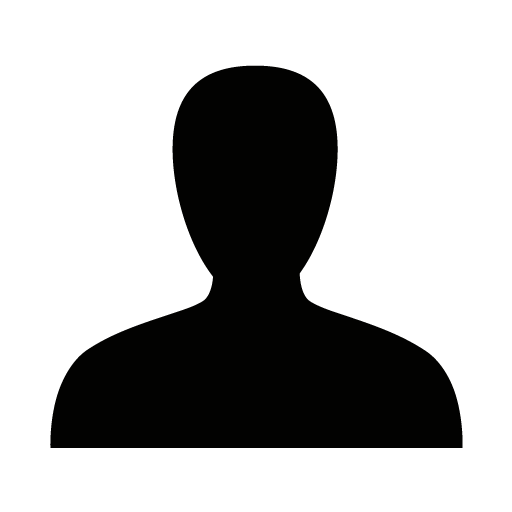
Electrocatalytic carbon dioxide reduction provides a way to generate useful fuels and feedstocks from a waste stream. Historically there has been a strong focus on the use of metal electrodes for carbon dioxide reduction, often Au, Ag and Cu. These have achieved impressive selectivity’s and current densities but they tend to work only when the local pH is high. This gives rise to an issue – it is well understood that the reaction of carbon dioxide with hydroxide lowers the available carbon dioxide for conversion and can cause substantial purification costs. Molecular electrocatalysts based on transition metal catalysts may in principle be tuned to control selectivity by modification of the ligand structure. This gives the possibility of developing catalysts that can operate in challenging environments, such as at low pH, conditions where conventional metal electrodes produce mainly hydrogen. Here I will describe the ongoing efforts by our group to study and utilise molecular electrocatalysts for carbon dioxide reduction. We will discuss both fundamental studies and also work on gas diffusion electrodes in complete electrolysers. We will focus in particular on the application within bipolar membrane electrolysers and explore the challenges in taking the approach forward to real-world application.
1.3-O1
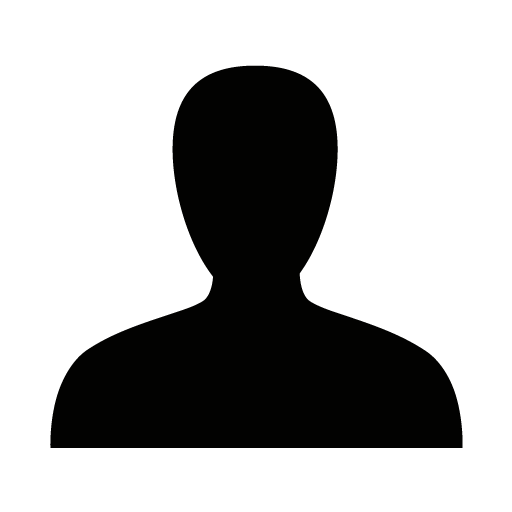
Electrochemical reactions in general, and the CO2 reduction reaction (CO2RR) in particular, are commonly studied at room temperature. However, it is important to elucidate the effect of temperature on the product distribution and activity of CO2RR as practical electrolyzers will operate at elevated temperatures. We studied the effect of temperature on various metals. Gold as it is a relatively simple electrode material only producing CO and H2, copper as it is an unique catalyst for CO2RR capable of producing a variety of products including multi-carbon products such as ethylene and propanol, and Ni because it is capable of producing longer hydrocarbon chains via a Fischer-Tropsch like mechanism.
On Au, we show that increasing temperature leads to both an increase in activity and selectivity of the CO2RR towards CO. However, at higher temperatures mass transport limitations due to the lower CO2 availability and higher local pH become more important. [1]
We show that at ambient pressures Cu has two distinct temperature regimes. From 18 up to ∿ 48 °C, C2+ products are produced with higher Faradaic efficiency, while methane and formic acid selectivity decreases, and hydrogen selectivity stays approximately constant. From 48 to 70 °C, we observe that hydrogen evolution dominates and the activity of CO2RR decreases. Moreover, the CO2RR products produced in this higher temperature range are mainly the C1 products CO and HCOOH. We show that CO surface coverage increases with temperature, which could explain the increase in C2+ products with temperature. Moreover, local pH and kinetics probably also play an important role in the first regime. The trends in the second regime appear most likely to be related to changes in the copper surface, while also a too high local pH will negatively influence the CO2RR. The decreasing CO2 solubility with increasing temperature does not explain the trends observed. [2]
Ni shows increasing activity with temperature at ambient pressures, but the chain growth probability is intrinsically not much affected by the temperature. This means that the product distribution does not significantly change with temperature. However, we do observe that at higher temperatures, faster degradation takes place, probably due to coking of the catalyst.
Further experiments will be performed on both Cu and Ni at elevated pressures to study the effect of pressure and its combined effect with temperature. At higher pressures a larger temperature range can be studied. Moreover, the CO2 solubility increases with increasing pressure, which makes it possible to study the temperature effect without the limitation of CO2 bulk concentration.
1.3-O2
Motivated and enthusiastic researcher at the Austrian Institute of Technology (AIT) Vienna. AIT is Austria’s largest Research and Technology Organisation (RTO) and an international key player in many of the research areas we cover. Proven experience in materials design, electrocatalysis, solar fuels, polymers design and LCA. Research areas of interest are designing novel and efficient electrocatalysts for carbon dioxide reduction and water splitting, electrocatalysis. I am interested in finding the connections and understand the relation between materials properties and design and their activity for reaction of interest using a combination of electrochemical, spectroscopic, and physical characterization techniques. Writing of European and national projects with leading tasks. Public engagement, and project management. Strong research professional with a Double degree PhD from the University of Namur (BE)and the University of Pau (FR) within a MSCA european project. Master Degree (MsC) in Industrial Chemistry from the University of Turin (IT).
Electroreduction of carbon dioxide (CO2) is one of the most promising ways to valorise CO2 as a source of carbon. The development of novel, efficient and scalable catalysts for CO2 electroreduction within electrolysers is still a big challenge. CO2 reduction reaction (CO2RR) can lead to different products. Among them, CO is the most versatile and used in large scale industrial processes. While various metals have been shown to efficiently reduce CO2 to CO, gold (Au) is still the benchmark for this process due to the high selectivity and efficiency. However, silver (Ag) is considered the best candidate as active metal for scaling up the CO2 reduction reaction toward CO and the main alternative to Au. Previous studies have shown how to improve CO selectivity with different Ag morphologies, particle size, surface modifications etc. However, studies for an efficient, fast, scalable, and economically sustainable method to prepare Ag electrode are still scarce.
Here, we report the preparation of sustainable, efficient and stable gas diffusion electrodes utilizing Ag as catalyst for the CO2 electroreduction to CO. These cathodic materials are prepared by sputter deposition and subjected to post-deposition modification using dry, reactive processes. The catalyst is uniformly deposited as a thin layer on the porous structure of PTFE minimizing the amount of Ag required. Our approach allows for fine tuning of the morphology, chemical composition and loading of Ag and their impact on the CO2RR. These electrodes have been evaluated for the CO2 electroreduction and for electrocatalytic studies in a flow reactor with gas-fed CO2. Optimized electrodes show high activity, with current densities > 20 mA*cm-2 at -1,15 V vs. RHE, and faradaic efficiency for CO > 90 %. The stability is tested over periods up to 24 hours showing a significant impact of the post-deposition modification. Indeed, higher porosity, roughness and electrochemical surface area have been achieved after the modification. Noteworthy, our approach allows for outstanding performances using a minimal amount of metal, significantly lower than commercial Ag-loaded GDEs, while using the processing advantages of sputtering as an industrial state-of-the-art, high-throughput, and roll-to-roll compatible technique. Moreover, this route enables the deposition of different metals and alloys of tailored composition for the electrocatalytic CO2 reduction beyond CO.
1.3-O3
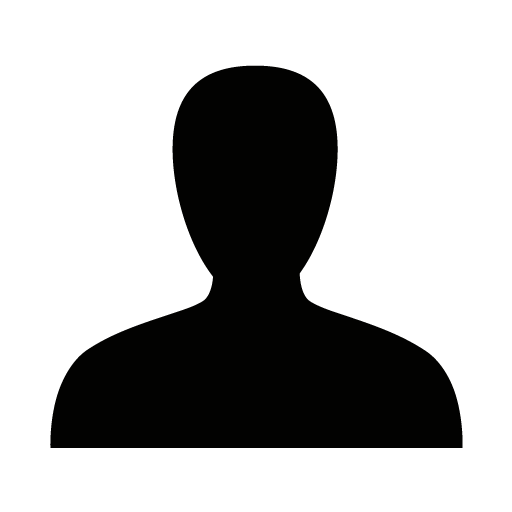
The electrochemical reduction of CO2 into feedstocks, such as carbon monoxide (CO), methane, or higher molecular weight hydrocarbons, is a promising alternative for offsetting greenhouse gas emissions.[1] The transformation, generally mediated by a catalyst of metallic nature under electrochemical conditions, has attracted considerable interest from the scientific community. Since the pioneering work of Hori, the properties of copper, gold or silver have been well studied. While silver and gold have an intrinsic propensity for efficiently reducing CO2 into CO with high selectivity, copper has shown very variable selectivity, producing a mixture of gaseous hydrocarbons and a variety of different alcohols. Importantly, aside from these selectivity considerations, the viability of a catalytic system on the industrial scale is subjected to the implementation of the latter to bulk electrolysis. In such systems, e.g. flow cells or membrane electrode assembly electrolyzers, the mass transport limitations resulting from the poor solubility of CO2 in aqueous media, is overcome using a porous gas diffusion layer (GDL). The GDL allows the formation of a gas/electrolyte/catalyst interface where the local concentration of CO2 rises far above its solubility limit, thus allowing the CO2RR to perform at industry relevant rates. The gases diffuse in and out of the catalytic centre through the diffusion layer’s cross-section, requiring therefore that the catalyst be deposited on the catholyte side of the layer. This is a significant limitation to the types of catalysts that can be adapted to GDLs, since the microporous nature of carbon-based or Teflon based GDLs commonly used in CO2 electroreduction is not compatible with the deposition of metal catalyst as dense homogeneous layers. Consequently, studies based on flow cells are still scarce in the literature, despite the general consensus that catalysts should be assessed under industrially relevant reaction rates.[2]
In the aim of tackling the above setbacks, herein we describe a novel type of hybrid material, which combines attributes of both metallic and molecular catalysts presenting themselves as nanocrystalline powders that can readily immobilized on GDLs. Their chemical structure consists of silver clusters whose main core is composed of several covalently linked silver atoms in a polymer-like fashion, with organic “ligands” orderly distributed in the outer shell. While The molecule, essentially known as silver acetylide involves stable alkyne-silver covalent bond allowing for the direct modulation of the silver-cluster’s electronic properties by tailoring the chemical structure of the organic moiety. The versatility of these entities as catalysts, is further enhanced by the fact that their synthesis involves only one, extremely reproducible, synthetic step from an alkyne precursor and a silver salt, that can be carried out on a multigram scale without any purification step. The availability of an extremely wide selection of commercially available alkyne precursors at a competitive price allows for the synthesis of large libraries of catalysts.
Herein we demonstrate the ability of two silver-acetylide clusters, to efficiently catalyze the CO2RR under high reaction rates in a flow cell electrolyzer. Electrolysis current higher than 200 mA/cm2 were achieved with very high selectivity towards CO (95%).
1.3-O4
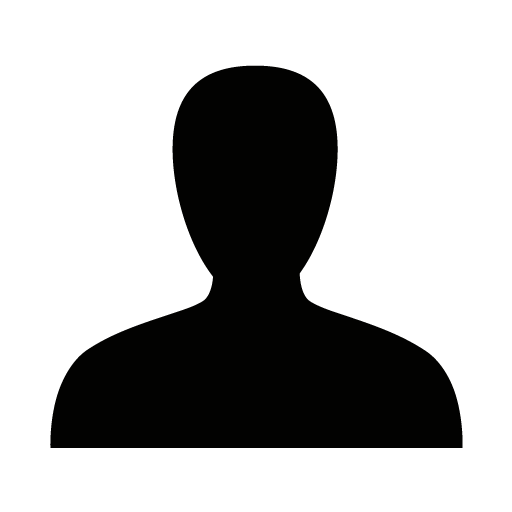
Professor Eileen Yu holds a Chair of Electrochemical Engineering in the Department of Chemical Engineering, Loughborough University. After obtained her PhD from Newcastle University pioneering on the development of direct methanol alkaline fuel cells, she worked as a research fellow at Max Planck Institute for Dynamics of Complex Technical Systems, Germany before she returned to Newcastle University to take a prestigious EPSRC Research Fellowship (Life Science Interface). This fellowship enabled her to extend her research into the biosciences, from which she has developed a multidisciplinary research profile.
She has a wide range of experience in various fields in electrochemical and bioelectrochemical systems for energy, environmental and biomedical applications. She has attracted more than £20m funding from various funding organisations. Her current research includes understanding fundamentals and engineering applications of electrocatalysis and microbial electrosynthesis for CO2 utilisation, resource recovery from wastes, bioremediation and environment monitoring with bioelectrochemical systems.
She is the Editor in Chief of Fuel Cells (Wiley), and Associate editor for several journals including Journal of Colloids and Surfaces C: Environmental Aspects (Elsvier)
Frontiers in Energy Research, Biosensors etc..
Carbon dioxide (CO2) is a major greenhouse gas which is driving the climate change. CO2 capture at the emission sources and from air followed by conversion to fuels/chemicals can achieve carbon neutral energy cycle. Electrochemical CO2 reduction (eCO2R) is one of the most promising methods for the direct production of fuels and chemicals from waste CO2 and water. eCO2R on copper electrodes favours the production of multi-carbon products including hydrocarbons and oxygenates [1]. The nanostructure engineering and alloying to create a variety of interfaces is a potential route for producing materials with higher catalytic activity and selectivity. Gas diffusion electrode further improved mass transfer of CO2 to reaction interface resulted in enhanced selectivity for carbonaceous products. High alkalinity with OH groups around catalyst surface improved the reaction kinetics and moreover stabilize the catalyst surface oxygen during the reduction process. Stability of GDE was improved by tuning hydrophobicity of catalyst and gas diffusion layers. Multi-carbon products, C2 and C3, were synthesised with bi-metallic Cu-Ag prepared by electrochemical spontaneous deposition of Ag on Cu2O nanoparticles [2,3]. From the density functional theory (DFT) analysis, Ag promotes Cu atoms migration towards the surface of the electrode, which seems to adsorb generated CO for the further reduction process to produce higher carbonaceous products (Figure 1). GDE is a promising technology for Sustainability development.
1.3-O5
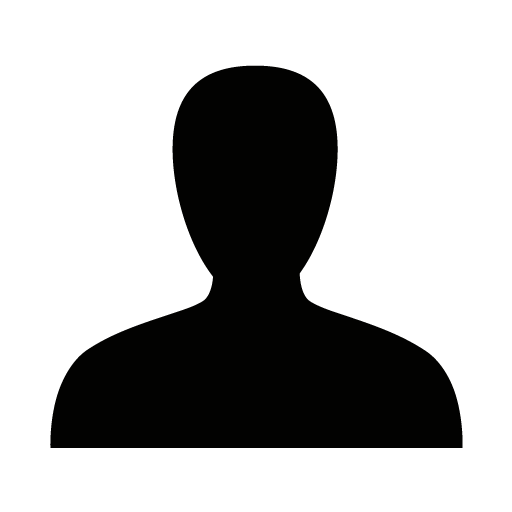
It is becoming likely that a net carbon-zero future will rely on the recycling of atmospheric CO2 to produce sustainable fuels and chemicals. Nevertheless, current processes for CO2 utilization use concentrated CO2 streams and are not integrated with the capture of dilute CO2 sources. As a result, the integration of carbon capture with utilization technologies is paramount toward developing processes for a future net-zero carbon economy. At the same time, recycling of waste plastics is critical to protect our environment from irreversible damages. In this regard, the integration of a carbon capture and utilization (CCU) process with plastic valorization technologies represents a unique opportunity to enable the recycling of two separate waste streams (CO2 and plastic) in a unified process, ideally driven by a renewable energy source.
This talk will discuss our developed integrated CO2 capture and photoelectrochemical (PEC) utilization reactor that captures CO2 from dilute streams and directly converts it into synthesis gas (a precursor for industrial liquid fuels and chemicals syntheses) using sunlight as the sole energy input. The reactor operates by combining CO2-to-fuel reduction at a photocathode with selective oxidation of waste plastic-derived ethylene glycol (EG) to glycolic acid (GA) at the anode, which has applications in the pharmaceutical, food, and textile industries. The reactor captures CO2 in an aqueous amine or glycolic hydroxide solution and the subsequent PEC conversion occurs in a two-compartment, two-electrode setup with a triple cation perovskite-based photocathode. Captured CO2 reduction is enabled by an immobilized molecular Co-phthalocyanine catalyst at the photocathode. A bimetallic Cu26Pd74 alloy anode completes the circuit by catalyzing EG oxidation. Replacing anodic water oxidation with EG oxidation makes the demanding captured CO2 reduction feasible with only sunlight, enabling the system to function even with a single visible-light absorber without any externally applied voltage. The overall process uses flue gas/air as a carbon source, discarded plastic waste as an electron donor, and sunlight as the sole energy input, opening avenues for future carbon-neutral/negative solar fuel and waste upcycling technologies.[1]
2.1-I2
Professor Erwin Reisner received his education and professional training at the University of Vienna (PhD in 2005), the Massachusetts Institute of Technology (postdoc from 2005-2007) and the University of Oxford (postdoc from 2008-2009). He joined the University of Cambridge as a University Lecturer in the Department of Chemistry in 2010, became a Fellow of St. John’s College in 2011, was appointed to Reader in 2015 and to his current position of Professor of Energy and Sustainability in 2017. He started his independent research programme on artificial photosynthesis (solar fuels) with the support of an EPSRC Career Acceleration Fellowship (2009-2015), which also received substantial early support by the Christian Doppler Laboratory for Sustainable SynGas Chemistry (2012-2019). In 2016, he received a European Research Council (ERC) Consolidator Grant to develop the field of semi-artificial photosynthesis (biohybrid systems for solar fuel synthesis) and has recently been awarded an ERC Advanced Grant (now funded by the UKRI underwrite scheme) on semi-biological domino catalysis for solar chemical production. He is the academic lead (PI) of the Cambridge Circular Plastics Centre (CirPlas; since 2019), where his team develops solar-powered valorisation technologies for the conversion of solid waste streams (biomass and plastics) to fuels and chemicals. He has acted as the academic lead of the UK Solar Fuels Network, which coordinates the national activities in artificial photosynthesis (2017-2021) and is currently a co-director of the Centre for Doctoral Training in Integrated Functional Nano (nanoCDT) in Cambridge as well as a member of the European research consortia ‘Sofia’ and ‘solar2chem'.
Solar panels are well known to produce electricity, but they are also in early-stage development for the production of sustainable fuels and chemicals. These panels mimic plant leaves in shape and function as demonstrated for overall solar water splitting to produce green hydrogen.1,2 This presentation will give an overview of our progress to construct prototype solar panel devices for the direct conversion of carbon dioxide and solid waste streams into fuels and higher-value chemicals through molecular surface-engineering of solar panels with catalysts. Specifically, a standalone ‘photoelectrochemical leaf’ based on an integrated lead halide perovskite-BiVO4 tandem light absorber architecture has been constructed for the solar CO2 reduction to produce syngas (CO and H2).3 Further manufacturing advances have enabled the reduction of material requirements to fabricate such devices and make the leaves sufficiently light weight to float on water, thereby enabling application on open water sources.4 The tandem design also allows for the integration of biocatalysts and the selective and bias-free conversion of CO2-to-formate has been demonstrated using enzymes.5 Recent progress in catalyst-development has allowed us to demonstrate carbon-carbon bond formation and the direct production of liquid multicarbon alcohol fuels directly from CO2.6 The versatility of the integrated leaf architecture has been shown by replacing the perovskite light absorber by BiOI for solar water and CO2 splitting with week-long stability.7
An alternative solar carbon capture and utilisation technology is based on co-deposited semiconductor powders on a conducting substrate.2 Modification of these immobilized powders with a molecular catalyst provides us with a photocatalyst sheet that can cleanly produce formic acid from aqueous CO2.8 CO2-fixing bacteria grown on such photocatalyst sheets enable the production of multicarbon products through clean CO2-to-acetate conversion.9 The deposition of a single semiconductor material on glass gives panels for the sunlight-powered conversion plastic and biomass waste into hydrogen and organic products, thereby allowing for simultaneous waste remediation and fuel production.10,11 The concept and prospect behind these integrated systems for solar energy conversion,12 related approaches,13 and their relevance to secure and harness sustainable energy supplies in a fossil-fuel free economy will be discussed.
References
[1] Reece et al., Science, 2011, 334, 645–648
[2] Wang et al., Nat. Mater., 2016, 15, 611–615
[3] Andrei et al., Nat. Mater., 2020, 19, 189–194
[4] Andrei et al., Nature, 2022, 608, 518–522
[5] Moore et al., Angew. Chem. Int. Ed., 2021, 60, 26303–26307
[6] Rahaman et al., Nat. Energy, 2023, 8, 629–638
[7] Andrei et al., Nat. Mater., 2022, 21, 864–868
[8] Wang et al., Nat. Energy, 2020, 5, 703–710
[9] Wang et al., Nat. Catal., 2022, 5, 633–641
[10] Uekert et al., Nat. Sustain., 2021, 4, 383–391
[11] Bhattacharjee et al., Nat. Synth., 2023, 2, 182–192
[12] Andrei et al., Acc. Chem. Res., 2022, 55, 3376–3386
[13] Wang et al., Nat. Energy, 2022, 7, 13–24
2.1-I1
Efficient artificial photosynthesis systems are currently developed for photoelectrochemical (PEC) water oxidation while simultaneously recycling CO2 and generating hydrogen as a solar fuel for storable renewable energy. These systems comprise e.g., integrated semiconductor-electrocatalyst devices which offer several benefits such as high system tunability with respect to the electrocatalyst integration and a directly controllable electron flux for the electrocatalytic process through the adjustability of incoming irradiation. These characteristics could also represent a significant advantage for electrocatalytic dinitrogen reduction, although PEC devices remain little explored for this reaction. Here, we will discuss recent developments in fabricating and designing integrated semiconductor-electrocatalyst systems for small molecule activation - in particular dinitrogen fixation - by focusing on the design principles of the electrocatalyst.
In a second part, we will discuss the possbility of specially engineering the electrocatalyst nanotopography for enhanced gas bubble detachment - a common obstacle in gas producing solar fuel devices, but also in traditional electrolyser systems. Reporting on experimental results obtained from the Bremen Drop Tower where microgravity can be achieved in 9.3 s of free fall, we outline possibilities for designing electrocatalytically active surfaces for efficient gas bubble detachment even in the absence of buoyancy, resulting in significant device performances increases and lower overall energy inputs.
2.2-I1
Photocatalytic water splitting and CO2 reduction are promising reactions to solve resources, energy, and environment issues, and achievement of carbon neutral. These reactions are regarded as artificial photosynthesis, because light energy is converted to storable chemical energy. In the present paper, various metal oxide and sulfide photocatalysts for water splitting and CO2 reduction using water as an electron donor are introduced and discussed.
(1) Photocatalytic water splitting for green H2 production
Rh0.5Cr1.5O3/AgTaO3 (BG 3.4 eV) of a valence-band-controlled metal oxide photocatalyst gave 40% of an apparent quantum yield at 340 nm and 0.13% of a solar to hydrogen conversion efficiency. However, this photocatalyst responds to only UV light because of the wide band gap. It is a challenging topic to develop photocatalysts responding to light with long wavelength. Rh and Sb-codoped SrTiO3 photocatalyst loaded with IrO2 was active for water splitting into H2 and O2 under visible light and simulated sunlight irradiations as a single particle type photocatalyst. This photocatalyst responds to 520 nm of visible light. Moreover, we recently developed Ir-doped SrTiO3 that showed the activity for water splitting up to 600 nm of visible light. On the other hand, SrTiO3:Rh and several metal sulfides of a H2-evolving photocatalyst and BiVO4 of an O2-evolving photocatalyst constructed various type of Z-schematic photocatalyst systems with Fe3+/Fe2+, [Co(bpy)3]3+/2+, [Co(phen)3]3+/2+, and a conductive reduced graphene oxide (RGO) as an electron mediator and even without an electron mediator. Various types of Z-scheme systems for water splitting under visible light irradiation were also successfully developed by employing Rh- and Ir-doped metal oxide powdered materials with relatively narrow energy gaps (EG) to utilize wide range of visible light.
(2) Photocatalytic CO2 reduction using water as an electron donor
Ag cocatalyst-loaded ALa4Ti4O15 (A = Ca, Sr, and Ba) and tantalates photocatalysts such as NaTaO3:Ba with 3.79–4.1 eV of band gaps showed activities for CO2 reduction to form CO and HCOOH in an aqueous medium without any sacrificial reagents. CO is the main reduction product rather than H2 even in an aqueous medium. Especially, the Ag/NaTaO3:Ba photocatalyst gave ca. 90% of the selectivity for the CO2 reduction. When Rh-Ru cocatalyst is used instead of Ag, CH4 was formed with ca. 10% of the selectivity. Thus, an uphill reaction of CO2 reduction accompanied with water oxidation was achieved using the Ag- and Rh-Ru-loaded metal oxide photocatalysts with wide bandgaps. We also constructed a Z-scheme system consisting of (CuGa)0.5ZnS2 of a CO2-reducing photocatalyst with BiVO4 of an O2-evolving photocatalyst and RGO of an electron mediator for CO2 reduction using water as an electron donor under visible light irradiation. The Z-scheme system gave H2, O2, and CO simultaneously under visible light irradiation. When the Z scheme system was modified with Co(dmbpy), the selectivity for CO formation reached higher than 90% accompanied with O2 evolution under visible light irradiation even in an aqueous solution.
2.2-I2
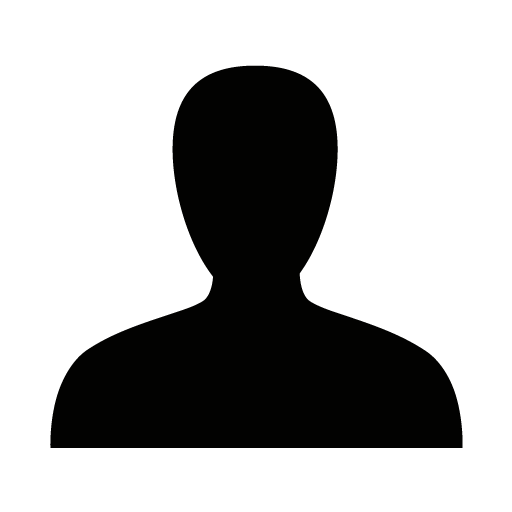
Ammonia is an attractive medium for renewable hydrogen storage. Synthesis of ammonia via the Haber-Bosch process is already the second-largest chemical industrial process in the world, and as a result the infrastructure for ammonia storage and transport already exists. One significant bottleneck limiting the use of ammonia for hydrogen storage is the inability to efficiently extract hydrogen back from ammonia. Electrolysis offers a convenient route to decompose ammonia into nitrogen and hydrogen, which potentially only requires ca. 0.1 V. However, in practice, the overpotential of the ammonia oxidation (nitrogen evolution) half reaction is prohibitively large. We previously reported a transition metal complex, [Ru(tpy)(dmabpy)NH3]2+ (tpy = 2,2′:6′,2′′-terpyridine, dmabpy = 4,4'-dimethylamino-2,2'-bipyridine), which exhibited electrocatalytic activity in presence of ammonia at room temperature and ambient pressure in an attempt to lower the overpotential. In this talk, recent efforts aimed at understanding the mechanism of the ammonia oxidation reaction using a variety of spectroscopic and electrochemical methods will be presented. Interestingly, reactions [Ru(tpy)(dmabpy)NH3]3+ with various Lewis bases are shown to trigger a proposed redox disproportionation reaction, which was followed using variable temperature NMR. An intermediate species was identified as a dinitrogen bridged complex using 15N NMR and Raman spectroscopy on isotopically labeled complexes. This intermediate is proposed to derive from coupling of nitridyl species formed upon sequential redox disproportion reactions. Acetonitrile displaces the dinitrogen bridge to yield free N2. DFT calculations support this lower energy pathway versus that previously reported for ammonia oxidation by the parent [RuIII(tpy)(bpy)NH3]3+ complex. These experimental and computational results are consistent with the interpretation of redox disproportionation involving sequential hydrogen atom transfer reactions by an amide/aminyl intermediate, [Ru(NH2)]+, formed upon deprotonation of the parent complex. Analogous methylamine complexes, [Ru(NH2CH3)]2+/3+, were also prepared to test the proposed mechanism. Treating [Ru(NH2CH3)]3+ with a Lewis base was found to cleanly yield two products [Ru(NH2CH3)]2+ and [Ru(CN)]+ in ~3:1 ratio, fully consistent with our proposed mechanism. In addition, preliminary results of next-generation catalyst systems will be presented.
2.2-I3
Qian Wang is currently an Associate Professor at Nagoya University, Japan. She obtained her Ph.D. in 2014 at the University of Tokyo, Japan. She then worked as a postdoctoral researcher for the Japan Technological Research Association of Artificial Photosynthetic Chemical Processes (ARPChem) project at the University of Tokyo. In 2018, she became a Marie Sklodowska-Curie Research Fellow at the University of Cambridge. She joined Nagoya University as an Associate Professor in May 2021 and established her research group, which is currently developing new materials, approaches, and technologies for solar energy storage in the form of renewable fuels via artificial photosynthesis.
The development of sunlight-driven water splitting or CO2 reduction systems with high efficiency, scalability, and cost-competitiveness is a central issue in the mass production of fuels and energy-rich chemicals. We designed and developed all-solid-state devices, that is, photocatalyst sheets for photocatalytic water splitting and CO2 reduction. These photocatalyst sheets produced hydrogen via overall water splitting with high efficiency or reduced CO2 into formate/acetate with high selectivity, setting new benchmarks in the field of photocatalytic solar fuel production. Moreover, the photocatalyst sheet design is well-suited to large-scale applications. Our study offers a novel and versatile strategy toward sustainable and practical solar fuel production.
2.3-O1
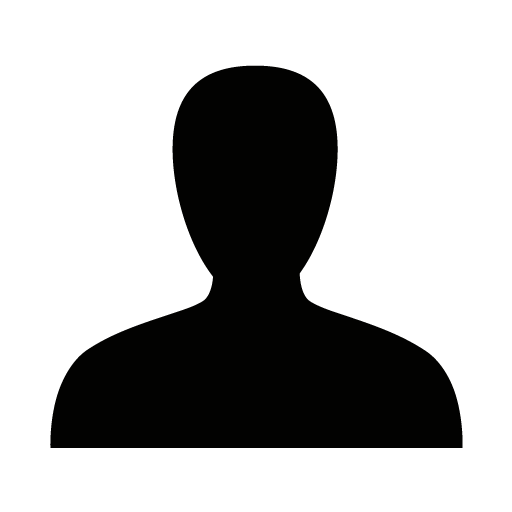
Organic semiconductors have come a long way in recent years and found application in OLEDs, organic photovoltaics, flexible electronics, and even photocatalysis. In this work, we showcase a proof-of-concept study of the previously reported [1,2] and newly designed organic semiconductor nanoparticles as visible-light active photocatalysts for selective carbon dioxide (CO2) conversion to solar fuels, specifically carbon monoxide (CO) and methane (CH4).
Heterojunction nanoparticles with core-shell morphology are fabricated by combining different donor polymers with fullerene and non-fullerene small molecule acceptors. This water-processable catalytic system demonstrates photocatalytic CO2 reduction activity comparable to prototypical materials (e.g., TiO2 (P25), g-C3N4). We achieve CO production rate of 4.7 ± 1.2 mmol g-1 h-1 and CH4 production rate ranging from 3.6 to 4.2 mmol g-1 h-1 depending on the organic photocatalyst employed in the CO2 reduction reaction. Moreover, the integration of different metallic co-catalysts, such as silver or gold, onto the organic nanoparticles provides the necessary control over C1 reaction products while suppressing the competing process of H2 evolution.
Additionally, we aim 1) to address the challenges and limitations of the proposed photocatalytic systems, which include but are not limited to the meticulous preparation process and particle fragility, as well as the need for careful optimisation of reaction conditions, 2) to shine some light on the photophysics behind CO2 photoconversion using advanced steady-state and time-resolved spectroscopy techniques, and 3) to discuss possible future steps towards a more educated nanoparticles’ design which would improve photocatalyst performance and allow upscaling.
2.3-O2
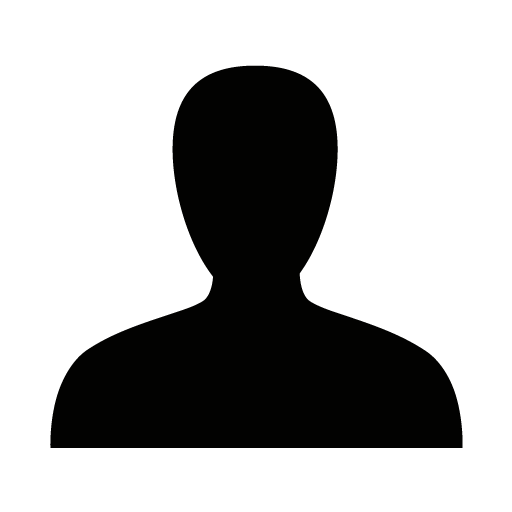
Solar energy conversion plays a very important role in the transition to ore suitable energy system. In this sense, so many materials have been proposed to drive artificial photosynthesis, most of them based on inorganic semiconductors, and the achievements performance continue every day. However most of these materials presents well known short comings such as low light absorption, fast charge recombination, and lack of tunability which limiting their efficiency.[1] The use of conjugated Porous Polymers (CPPs) and their hybrid thereof open the door to multitude of possibilities when it comes to select and design of suitable photocatalyst. [2] Thus our group has been pioneering in the use of hybrid based on inorganic semiconductor and CPPs for hydrogen production and CO2 photoreduction processes[3]. However, when we tried to translate the acquired knowledge to photoelectrocatalysis devices we found difficulties related with the processability of this kind of hybrid materials. In order to design photoelectrocatalysts the main premise is to develop thin film photoelectrodes. But the CPPs synthesized by bulk techniques are big particles (from micrometres to millimetres) which the processing as thin films with enough optical and electrical quality is not possible. In this talk we will show our advance in the implementation of 1) high throughput microfluidic techniques through miniemulsion process; 2) electropolymerization; as well as 3) interfacial synthesis to the synthesis of nanostructured CPPs and how this is a key aspect to improve their performance in the energy field.
[1] Mariam Barawi, Laura Collado, Miguel Gomez-Mendoza, Freddy E. Oropeza, Marta Liras, Victor A. de la Peña O’Shea Adv. Energy Mater, 2021, 2101530
[2] M.Liras, M. Barawi, V. A. de la Peña O´Shea, Chem. Soc. Rev. 2019, 48, 5454-5487.
[3] L. Collado, T. Naranjo, M. Gomez-Mendoza, C. G. López-Calixto, F. E. Oropeza, M. Liras, J. Marugán, V. A. de la Peña O’Shea, Adv. Funct. Mater. 2021, 2105384.
2.3-O3
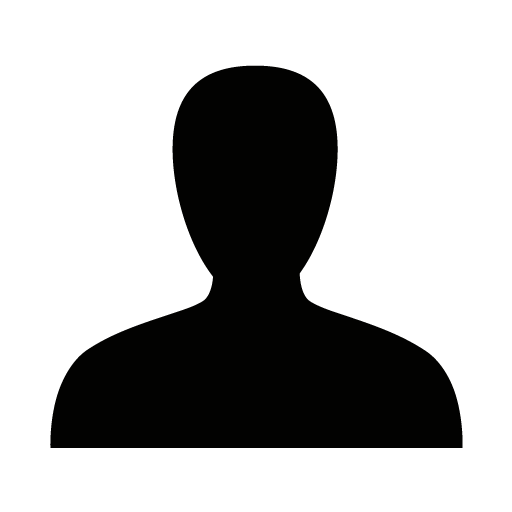
Metal nanoparticles (NPs) are widely used for electrocatalytic applications due to their large surface-to-volume ratio. Their structural features largely affect electrochemical activity, selectivity, and stability [1].
In this study, we report on the design and fabrication of nanostructured electrodes by solid-state dewetting (SSD), i.e., the heat-induced transformation of thin metal films into a “monolayer” of defined, spaced metal NPs (Fig. 1a) with tunable loading, composition, size and structure [2, 3]. Notably, dewetting occurs along with exposure of the substrate, and hence formation of the Pt-substrate-electrolyte interface, i.e., the triple-phase boundary (TPB). We investigate the role of the Pt structure and TPB on the hydrogen evolution reaction (HER) as model system.
For this, Pt films of various thicknesses were deposited by magnetron sputtering on fluorine-doped tin oxide (FTO) followed by heat treatment (500°C) to induce dewetting. As deposited and dewetted electrodes were characterized in view of their electrochemical performance and by various techniques such as X-ray diffraction (XRD), Scanning electron microscopy (SEM), Transmission electron microscopy (TEM), X-ray photoelectron spectroscopy (XPS) and X-ray absorption spectroscopy (XAS).
As-deposited Pt films as thin as 5 nm are closed and polycrystalline, with grain size in the range of the film thickness (i.e., 4-5 nm). Dewetting of these thin films leads to the formation of crystalline, faceted NPs with a mean size of 50 nm (Fig. 1b), and average crystalline domain size of 50 nm, indicating that NPs are mostly single crystals or twins. For 5 nm-thick films, the electrochemical surface area (ECSA, measured by hydrogen underpotential deposition) significantly decreases upon dewetting due to Pt agglomeration as the FTO becomes exposed. Interestingly, despite the decrease in ECSA, dewetted NP electrodes are clearly more active (> 3 times) in HER than as-deposited films (Fig. 1c, specific current density at – 50 mV vs. RHE). A similar increase in activity is also observed upon dewetting thinner Pt films into NPs. Differently, Pt films as thick as 30 and 50 nm do not agglomerate into NPs when heat-treated, show a minor change in grain size and surface area, and expose the FTO substrate to a small extent (i.e., the Pt film forms only few holes). Such films show a minor increase of HER activity after heat-treatment (Fig. 1c).
We rationalize these results proposing that while ECSA and grain size have a minor effect, a key factor governing the HER activity is the extension of the triple-phase boundary, that is, in this case the Pt-FTO-electrolyte triple interface. Heat-treated Pt films on diverse substrates, e.g., carbon paper, boron-doped diamond or Nb electrodes, shows similar activity trends as observed for Pt/FTO electrodes (Fig. 1d), suggesting that crucial for the increase in HER activity is the formation of the TPB due to exposure of the substrate upon dewetting.
In ongoing work, we are investigating further the role of NP faceting, and are quantifying with accuracy the extension of the TPB as a function of the Pt NPs size and how it affects the HER performance.