1A-I1
Flexible perovskite solar cells (f-PSCs) have recently reached power conversion efficiency (PCE) as high as 25.09 % [1] which is reaching their rigid counterparts on glass, which in very short time have achieved 26.1% of certified efficiency. The use of flexible substrates however, thanks to the high power/to weight ratio generated which is the range of 29.4 W/g compared to 8.31 W/g for amorphous silicon and 0.254 W/g for ultra-thin CdS / CdTe [2-3], opens up to a wide range of applications, from sensors for the Internet of Things, to the retrofitting of existing buildings to improve their energy efficiency (building-applied PV), to space.
The investigation of perovskite solar cells for use in space began in 2015, when Miyazawa et al. irradiated their experimental cells with protons and electrons, and found the perovskite cells retained a larger portion of their photovoltaic parameters than the silicon and III-V cells tested on the same experiment [4]. After this initial finding, more irradiation experiments were run, showing similarly high resilience of PSCs to particle radiation, consolidating the interest for space applications of this technology and as the focus broadened to other damage sources present in the space environment, such as atomic oxygen [5] , vacuum, [6], or gamma radiation [7].
Considering particle radiation ageing tests have also started to include resilience to neutrons, which unlike protons and electrons are not electrically charged, and therefore do not directly cause ionization effects in an irradiated material. However, the mass of a neutron however is still significant enough to cause elastic collisions with atoms in the targeted material [8], which can displace nuclei, leaving defects in the lattice [9]. Characterizing the entity of this damage in perovskite solar cells aimed at space applications therefore is important as neutrons are present in various space environments, such as around spacecrafts, whether in orbit or interplanetary travel , or other planets atmospheres [10-11].
In this talk we will give an overview on the effects of neutron irradiation on flexible perovskite solar cells focussing on the role of the hole transporting layers in a NIP configuration [12]. In particular, we will show how the substitution of standard spiro-OMeTAD with P3HT-modified and PTAA-modified polymers affects the overall performances of the unencapsulated devices revealing an higher resilieance of the polymer based system respect to spiro-OMeTAD under neutron irradiation.
1A-I2
Joseph M. Luther obtained B.S. degrees in Electrical and Computer Engineering from North Carolina State University in 2001. At NCSU he began his research career under the direction of Salah Bedair, who was the first to fabricate a tandem junction solar cell. Luther worked on growth and characterization high-efficiency III-V materials including GaN and GaAsN. His interest in photovoltaics sent him to the National Renewable Energy Laboratory (NREL) to pursue graduate work. He obtained a Masters of Science in Electrical Engineering from the University of Colorado while researching effects of defects in bulk semiconductors in NREL�s Measurements and Characterization Division. In 2005, He joined Art Nozik�s group at NREL and studied semiconductor nanocrystals for multiple exciton generation for which he was awarded a Ph.D. in Physics from Colorado School of Mines. As a postdoctoral fellow, he studied fundamental synthesis and novel properties of nanomaterials under the direction Paul Alivisatos at the University of California and Lawrence Berkeley National Laboratory. In 2009, he rejoined NREL as a senior research scientist. His research interests lie in the growth, electronic coupling and optical properties of colloidal nanocrystals and quantum dots.
Perovskite photovoltaics have been shown to recover, or heal, after radiation damage. Here, we deconvolve the effects of radiation based on different energy loss mechanisms from incident protons which induce defects or can promote efficiency recovery. We design a dual dose experiment first exposing devices to low-energy protons efficient in creating atomic displacements. Devices are then irradiated with high-energy protons that interact differently. Correlated with modeling, high-energy protons (with increased ionizing energy loss component) effectively anneal the initial radiation damage, and recover the device efficiency, thus directly detailing the different interactions of irradiation. We relate these differences to the energy loss (ionization or non-ionization) using simulation. Dual dose experiments provide insight into understanding the radiation response of perovskite solar cells and highlight that radiation-matter interactions in soft lattice materials are distinct from conventional semiconductors. These results present electronic ionization as a unique handle to remedying defects and trap states in perovskites.
1A-O1
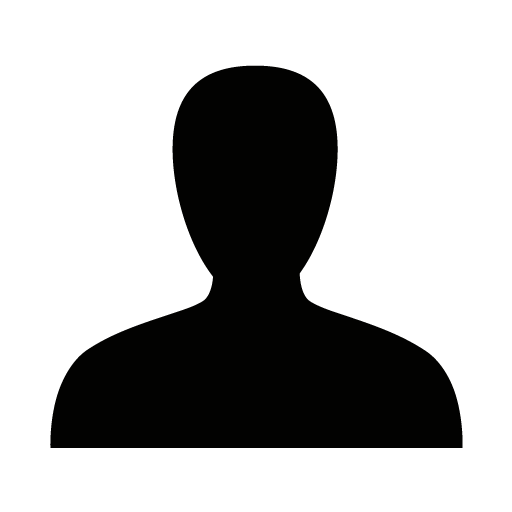
Metal halide perovskites are a groundbreaking family of semiconductors finding applications on several areas of the optoelectronics field. They have attracted attention for their bandgap tunability, long carrier lifetimes, high defect tolerance and solution processable fabrication [1, 2]. Specifically for radiation detection, their large absorption coefficient allows efficient beam absorption even for low thicknesses, associated with the large-Z elements in their composition (Sn, I, Cs, Pb, Bi). In fact, they have already overcome the state-of-the-art X-ray detectors becoming a serious candidate for the next generation of radiation detectors [3].
From the possible architectures, we focus on lateral photoconductors, which excel in their simple and low-cost integration with flexible electronic backplanes. Besides, they have the remarkable ability to decouple the absorber thickness (usually hundreds of μm to 1 mm) [4] from the distance between electrodes, allowing for an operation at low bias voltage. However, relatively to the common vertical photoconductor architecture of radiation detectors, the planar type suffers from lower sensitivity due the unfavourable electric field distribution, and higher dark current from the absence of selective blocking layers.
To tackle this, we printed and micro-structured in-planar electrodes, improving the carrier collection uniformity over the whole volume of the absorber layer. The perovskite absorber was blade coated on the electrodes, from a microparticle ink synthesized with non-toxic solvents [5]. The all-printed devices showed more stable dark current and better sensitivity to white light in the intensity range 10-4 mW/cm2 to 2.5 mW/cm2 than non-printed devices with the same architecture. This resulted in an ON OFF ratio of 4×102 for the most intense light conditions and 8 V of applied bias, a comparable value to reported flexible photodiodes and vertical photoconductors [6].
1B-I1
As a Chief Operating Officer (COO), Silke Traut brings more than 20 years of industrial experience in technology development and production. Her teams translate the latest developments of the worlds fastest X-ray cameras into industrial production. After her Masters Degree in Applied Physics at UMass Lowell she worked in micro-optics and thin-film technology for a few years. She then joined the semiconductor GaAs laser industry for 13 years to work on process technology development. Since 2011 she is with DECTRIS, leading different teams and projects to develop and manufacture X-ray and electron cameras to spark scientific breakthroughs around the world.
DECTRIS emerged from one of the largest and most significant experiments in particle physics: the CMS experiment, which proved the existence of the Higgs Boson. With PSI's visionary efforts and the transfer of the technology to a company, hybrid photon counting was made available to a much broader scientific community. X-ray and electron detectors supplied by DECTRIS today have made an impact in a variety of applications, including structural analysis in life sciences like protein crystallography and materials science, as well as plasma spectroscopy in energy science.
Last year, DECTRIS launched the world's fastest electron detector for 4D STEM, an emerging structural method in materials science. This method enables novel contrast mechanisms that ultimately allow identifying and resolving atomic-level structures that were previously not visible to researchers. To provide such a detector, multiple technologies must be developed, including fast data pipelines, ASIC pixel designs, software infrastructure, and Micro-Nanotechnologies. We partner with various universities, start-ups, and companies on projects continuously to understand, master, and integrate new technologies into the next-generation detector. In this way, and driven by specific requirements, we can bring back detectors with unique new properties to the scientific community to advance their research.
In my talk I will review current sensor types and materials for X-ray and electron detection, their specific requirements for different applications as well as their current limitations. I will elaborate on the evolution of these materials and their properties also from an industrial point of view. With that in mind, I will conclude with our expectation for properties and availability of perovskite materials for X-ray and electron detection in the future.
1B-I2
Obtained PhD degree from National Academy of Sciences of Ukraine in 2007. 2008 - 2013 years Sergii is a PostDoc in JKU Linz, Austria in Prof. Wolfgang Heiss group. In 2013 he joined the group of Prof. Maksym Kovalenko group in ETH Zurich, Switzerland where he is a Senior Research Associate (Oberassistent) since 2018.
The main achievements are for the discovering of perovskite hard radiation and full-colour photo-detectors, optical gain and lasing in perovskite nanocrystal films.
Over the past decade, metal halide perovskites (MHPs) have emerged[1] as revolutionary materials for ionising radiation detection and have captivated the X-ray detector development community. These materials offer an unprecedented combination of ease of growth from precursor solutions at moderate temperatures and properties on par with the best existing detector materials.
The remarkable efficiency of MHPs is attributed to their high heavy atom content, fast charge transport, optimal bandgap and versatility of deposition and growth techniques. Early experiments with polycrystalline solution-grown films showed significant X-ray sensitivity[2]. Subsequent advances in single-crystal fabrication have enabled single-photon counting capabilities and energy resolution, the possibilities which were previously unattainable with solution-grown materials[3].
Despite these successes, optimal solution-grown MHP compositions have faced significant thermodynamic challenges, some of which have been mitigated by strategic modification of cations and anions[4]. The durability of perovskite detectors under external fields, required for efficient charge extraction, has been problematic due to the ionic structure of the crystal lattice, which promotes ion migration. Nevertheless, recent innovations[5] in the confined growth of high-quality single crystals on substrates have led to zero-bias operation of X-ray detectors, achieving near-ideal performance and significantly improved stability[6].
However, the path to commercialisation is hampered by issues such as ion migration and the relatively slow response of the detectors, as well as the need for novel integration strategies with CMOS chips or TFT displays due to the unique mechanical and chemical properties of MHPs.
1B-O1
Metal-halide perovskites are revolutionizing the world of X-ray detectors, due to the development of sensitive, fast, and cost-effective devices. Self-powered operation, ensuring portability and low power consumption, has also been recently demonstrated in both bulk materials and thin films. However, the signal stability and repeatability under continuous X-ray exposure has only been tested up to a few hours, often reporting degradation of the detection performance. Here it is shown that self-powered direct X-ray detectors, fabricated starting from a FAPbBr3 submicrometer-thick film deposition onto a mesoporous TiO2 scaffold, can withstand a 26-day uninterrupted X-ray exposure with negligible signal loss, demonstrating ultra-high operational stability and excellent repeatability. No structural modification is observed after irradiation with a total ionizing dose of almost 200 Gy, revealing an unexpectedly high radiation hardness for a metal-halide perovskite thin film. In addition, trap-assisted photoconductive gain enabled the device to achieve a record bulk sensitivity of 7.28 C Gy−1 cm−3 at 0 V, an unprecedented value in the field of thin-film-based photoconductors and photodiodes for “hard” X-rays. Finally, prototypal validation under the high-energy (up to 6 MeV) X-ray beam produced by a medical linear accelerator (LINAC) for cancer treatment is also introduced: excellent linearity is found between the cumulative charge collected by the detector and the dose delivered to the patient, which is an essential requirement for radiotherapy dosimetry.
1C-I1
Metal halide perovskite solar cells are of interest for high altitude and space applications due to their lightweight and versatile form factor. However, their resilience toward the particle spectrum encountered in space is still of concern. For space cells, the effect of these particles is condensed into an equivalent 1 MeV electron fluence. The effect of high doses of 1 MeV e-beam radiation up to very high accumulated fluence is probed. By using substrate and encapsulation materials that are stable under the high energy e-beam radiation, its net effect on the perovskite film and solar cells can be studied. The quartz substrate-based perovskite solar cells are stable under the high doses of 1 MeV e-beam irradiation.We will present the latest finding on the radiation hardness of single junction perovskite solar cells, using pure iodide as well as mixed cation and mixed halide formulations, demonstrating that perovskite solar cells are promising candidates for space applications.
1C-O1
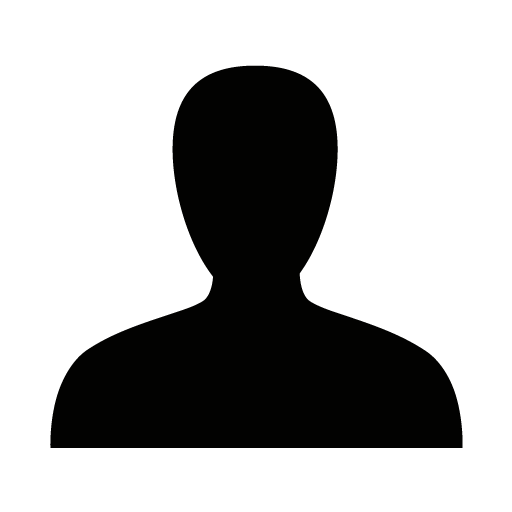
Emerging PV technologies offer promising features for outer space applications: Flexibility, low weight and high specific power. However, stability at space conditions has only been tested on few organic materials used in next-generation solar cell architectures. In this work, the material discovery for this application is accelerated by combining the strengths of high-throughput, lab automation and machine learning. This way, a large material library of more than 120 organic hole transport materials are automatically processed, degraded and measured. The materials are degraded under UVC light in a nitrogen atmosphere, trying to approximate part of the conditions in space. The differential quantum yield is extracted from the changing UV-Vis spectra over time and used as a stability target. Combining Gaussian Process Regression based on predictors from structural fingerprints and manual filtering of the materials by features, design rules for UVC stable materials could be found: High aromaticity, low number of non-nitrogen heteroatoms and lack of vinylene groups. As a further step, some of the materials were also degraded under gamma radiation, showing considerable stability and similar changes to the UV-Vis spectra as for UVC radiation. Gamma radiation is a great benchmark for outer space radiation as it both displaces and ionizes atoms. Finding a correlation factor between gamma and UVC radiation would make predicting radiation hardness from a simple proxy experiment possible. Overall, this work provides great insights into which present and future material candidates to choose for emerging PV technologies having to endure high energy radiation.
1D-O1
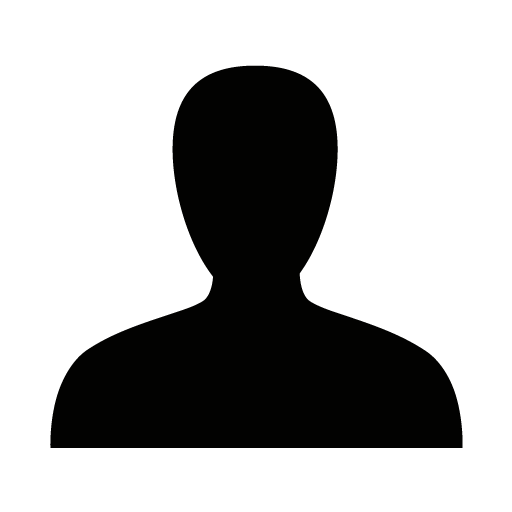
In this study, we examine the use of perovskite photodetectors as a potential replacement for silicon photodiodes in modern cameras. Perovskite photodetectors have high sensitivity to visible light and can be integrated into existing electronics, but their use in CMOS image sensors requires the application of reverse bias. Unfortunately, this leads to unstable detector performance due to ion migration effects. To address this issue, we propose using forward voltage pulses to attenuate ion migration effects while still capturing photoresponse under reverse bias. To demonstrate the feasibility of this approach, we present a proof-of-concept single-pixel readout system that simulates the operation of a real image sensor. We show that applying forward bias pulses after each integration frame allows for stable operation of perovskite photodetectors for over 180 hours, whereas constant reverse bias leads to degradation of detector performance within 10 minutes. Additionally, we demonstrate stable imaging using the read-out with alternating voltage pulses and 8x8 cross-bar arrays of perovskite photodetectors. Our readout scheme is an important step towards the development of highly sensitive perovskite image sensors and cameras that are resistant to ion migration effects.
1D-O2
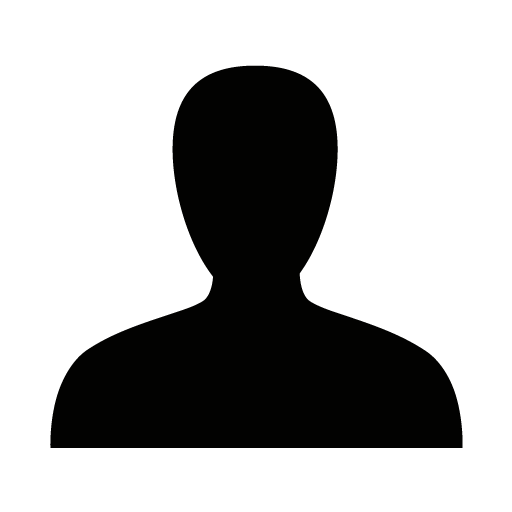
Photoconductors are a type of photodetector consisting in a semiconductor in direct contact with two electrodes. They are typically built in a lateral configuration which, however, limits their performance due to the large electrode spacing. In this work, we evaluate vertical vacuum-deposited p-i-p perovskite photoconductor, where organic hole transport layers (HTLs), both intrinsic and doped, are employed at the perovskite/electrode interface. The effect of the HTL on the performance of the photoconductors is studied, and both the HTL thickness and dopant concentration are analysed. When the perovskite active layer is between intrinsic HTLs the dark current is drastically reduced, especially when these layers are made thicker. Importantly, when a combination of intrinsic and doped HTLs are used, the photoconductors exhibit the benefit from both layers, i.e. reduction of dark currents and enhanced charge extraction/transport.
1D-O3
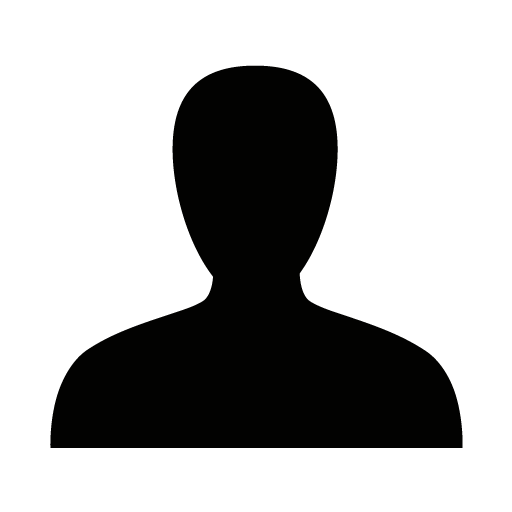
< Abstract >
Transient Photo-response of Halide
Perovskite on Optoelectronic Devices
So-Yeon Kim, Juan Bisquert*
Instituto de Tecnología Química. Universitat Politècnica de València- Consejo Superior de
Investigaciones Científicas (UPV-CSIC).
Perovskite and Organic Semiconductors for Next-Generation Photodetectors and Space
Application (NextPDs 2024)
Dubrovnik, Croatia, 2024 June 10th - 12th
Halide perovskites (HPs) have been attracted as potential materials with their superior opto-
electronic properties such as high absorption coefficient, long diffusion length of charge carrier,
tunable bandgap, low exciton binding energy, and so on. HPs are applied to various fields such
as solar cells, photodetectors, sensors, LED, photonic memory devices and neuromorphic
devices using their characteristic properties that exhibit electrical properties in response to light
sources. Among them, HPs-based photodetectors, which can convert light sources of various
wavelength into electrical signals, have been actively reported in various HP materials and
devices structures until recent research and extensively applied to optoelectronic memories and
synapses. It is important to understand the driving principle and characteristic hysteresis effects
of the HPs-based optoelectronic device to efficiently evaluate devices’ performance and apply
various fields. HPs-based photodetectors are divided into photodiodes, photoconductors, and
phototransistors according to the vertical or lateral stacked structure of the device, in which the
devices run with various working mechanisms in each structure. We focus on investigating the
photoreaction and transient response in the simple symmetrical photodetector structures with
various HPs to identify ionic and electronic transport embedded in HPs. The various HPs-based
photodetectors we have covered exhibit several transient photocurrent responses depending on
a variety of specific variables, such as the composition and structure of active halide
perovskites, the channel length within the device, response time, etc. These characteristic
transient current responses are also seen not only in photodetectors but also in devices in
various fields such a memristors and neuromorphic devices. Modeling and analyzing these
current responses can help effectively design and evaluate next-generation multi-functional
optoelectronic fields and will open up great possibilities.
References
[1] Bisquert, J.; Gonzales, C.; Guerrero, A., Transient On/Off Photocurrent Response of Halide
Perovskite Photodetectors. The Journal of Physical Chemistry C 2023, 127 (43), 21338-21350.
[2 ] Bisquert, J., Hysteresis, Impedance, and Transients Effects in Halide Perovskite Solar Cells
and Memory Devices Analysis by Neuron-Style Models. Advanced Energy Materials n/a (n/a),
2400442.
2A-I1
The use of scintillators for the detection of ionising radiation is critical in many fields, including medicine, nuclear monitoring and homeland security. Lead halide perovskite nanocrystals (LHP-NCs) embedded in plastic matrices are emerging as promising scintillator materials that overcome the drawbacks of conventional types of scintillator materials (namely inorganic crystals and plastic scintillators) and capitalise on their strengths. Importantly, the use of LHP-NCs as nanoscintillators in polymer waveguides not only offers a solution to overcome the scalability limitations of conventional materials, but also potentially enhances the scintillation performance. This is due to the unique photophysics of quantum-confined materials, which provide size-tunable emission spectra that perfectly match the spectral sensitivity of light detectors, and ultrafast sub-nanosecond scintillation kinetics resulting from the recombination of multi-excitons generated upon interaction with ionising radiation.
However, significant challenges remain to affordably upscale synthesis to the multi-gram level, embed LHP-NCs in optical-grade nanocomposites without compromising their optical properties, and deeply understand the fundamental aspects of nanoscale scintillation mechanisms.
In this talk, I will present our recent progress in the fabrication of nanocomposite scintillators based on LHP-NCs. Several approaches for effective integration into plastic waveguides will be disclosed, including NC functionalisation strategies, defect passivation and advanced polymerisation routes. The interplay between NCs and different matrices will be elucidated and fundamental aspects of scintillation photophysics will be discussed, including particle size and fine structure effects towards highly efficient and ultrafast scintillation.
2A-I2
In this presentation the potential of metal halide perovskite solar cells for space power applications will be discussed. This will focus on our recent work on the feasibility of metal halide perovskites for space, which includes the assessment of the systems in low-intensity-low-temperature (LILT) conditions for outer planetary missions, in addition to exposure to high temperatures and variable radiation conditions that directly and independently impact the absorber and transporting layers of the devices. Here, the discussion will include a number of perovskite systems such as solution processed mixed Pb-Sn systems and the FAMACs family of metal halide perovskites, as well as blade-coated architectures. It will be shown that perovskites display remarkable tolerance to high radiation exposure and that while measurements do suggest high energy radiation negatively affects the transporting layers and interfaces in the devices: the perovskite absorber does not appear to be affected in any significant way. Moreover, these systems are observed to self-heal under ambient conditions in the dark demonstrating the unique behavior of perovskite solar cells and their potential for future space applications. This is further substantiated by high temperature measurements that indicate specific triple cation perovskites displays no appreciable or permanent degradation up to 500 K, supporting in particular their potential as candidate systems for future lunar missions.
2A-O1
In recent years, the X-ray detection research community has been motivated by the emergence of new semiconductor materials, particularly lead-based perovskites [1] and other metal halides. As the focus shifted to synthesizing these novel compounds, the absence of a widely accepted characterization approach for X-ray detectors has led to potentially misleading performance assessments. This Comment proposes guidelines to establish figures-of-merit for low-dose X-ray imagers, enabling accurate comparisons between detectors based on both direct conversion semiconductors and scintillators.
Emerging semiconductors, including lead halide perovskite single crystals, polycrystalline films, nanocrystals, and organic and metal halide scintillators, show promise for developing next-generation low-cost, high-sensitivity X-ray detectors. These materials offer strong stopping power to X-rays and high sensitivity either through high luminescence quantum yield with fast emission as scintillators or large carrier mobility and lifetime product for efficient charge extraction and low thermal noise as semiconductor detectors[2]. However, there is a lack of consensus regarding measurable performance metrics correlating with low-dose X-ray imaging requirements.
To address this issue, we present a set of figures-of-merit based on established Detective Quantum Efficiency (DQE), including Noise Equivalent Dose (NED), Detection Efficiency (DE), half absorption energy, spatial resolution, and a synopsis characteristic: Detective Quantum Efficiency index (DQEi). These guidelines will facilitate the reliable determination of device characteristics for both direct conversion detector materials and indirect scintillators, ultimately supporting the development of novel X-ray medical imaging detectors with improved sensitivity, speed, and spatial resolution.
2C-I1
The solar array is one of the most exposed subsystem on the satellite. It is subject to severe environmental loads. Temperature variations in excess of 200 K occur, due to the fact that during eclipse phases the radiative balance between frontside solar flux and rearside emission into space is disturbed. Charged particles, mainly electrons and protons, trapped in the earth magnetic field or originating from the sun directly, produce non-ionizing damage and consequently carrier lifetime degradation in the cell as well as ionizing damage in all shielding materials. Short wavelength UV light is of concern for the transparency of all optical materials. The interaction with space plasma results in charging and the risk of damage due to electrostatic discharge effects. Lastly the mechanical loads experienced during launch and deployment are of key concern for the structural integrity of the solar array.
These environmental boundary conditions will be reviewed in detail, together with an explanation how they are managed in the existing state of the art solar array design, which consist of rigid carbon fiber sandwich panels combined with 30% efficient III-V multijunction cells. This then sets the stage for a review of the current challenges for solar array design. The move from individual high value satellites to large constellations of low-cost satellites puts a strong focus on arrays with a decreased cost. To enable this, new deployable structures, able to provide deployed surface are in space at a lower cost, combined with terrestrial solar cell are the only viable pathway. Thin-film solar cells will have some unique advantages that could be exploited in this context. Areas of development focus are suggested, both for the cells as well as for the photovoltaic assembly of cells integrated into a photovoltaic blanket.
2C-I2
Eric Gros-Daillon joined CEA-Leti in 2006 as a research engineer after a Master degree in detection physics and a Ph.D. on gamma-ray semiconducting detectors for SPECT. His research covered a large range of detectors technologies such as semiconductor detectors working in spectrometric and counting mode (CdTe and GaAs) and scintillator detectors (LYSO and CsI). He also worked on the CMOS readout circuits which are coupled to these detectors. He has supervised 14 PhD and post-doctorant students and is the author of 24 scientific papers and holds 10 patents. Two detectors developed by Dr Gros-Daillon have been successfully transferred to the industry. His present scientific focus is on perovskite detectors for X-ray medical radiography and he is the coordinator of the European project Peroxis.
Cesium lead bromide (CsPbBr3), a promising direct X-ray detector material, faces challenges due to the instability of its dark current, often attributed to the movement of mobile ions under electric field. However, the direct measurement of the movement of ions in the perovskite has been seldom reported. Employing grazing incidence X-ray fluorescence (GIXRF) on CsPbBr3 polycrystalline thick films (~200µm) grown by close-space sublimation [1], we directly investigate the accumulation of ions under the electrode under bias. A quasi monochromatic X-rays beam with energy of the Mo Kα line, i.e. 17.5 keV excite the material at low angle (0.5°) to probe the sample surface (~200nm). The X-ray fluorescence is monitored from a normal angle and a XRF spectrum is recorded each 30s. The dark current is registered during the experiment. Our findings uncover ionic migration of both Cs and Br, accumulating under or moving away the electrode according to the electric field direction. Over a few hours, accumulation rates exhibit a linear relationship with the electric field, with coefficients of (1.7±0.5) × 10-3 % h-1V-1mm-1 for Cs and (-2.0±0.3) × 10−3 % h-1V-1mm-1 for Br. Interestingly, dark current does not appear directly linked to ion accumulation. Overall, this study provides valuable insights into ion movement within CsPbBr3, potentially aiding development of more stable X-ray detectors.
2C-O1
Javier E. Sebastian Alonso graduated in 2022 with an MSc in Chemistry for Renewable Energy with a joint project between Uppsala University (Sweden) and the University of Groningen (The Netherlands) on Carbazole Based Self – Assembled Monolayers as Hole Transport Layer for Efficient and Stable Cs0.25FA0.75Sn0.5Pb0.5I3 PSCs. His current work at MOED focuses on the Scalability of highly efficient PSCs via vacuum co-evaporation with improved Stability. He brings his work focused on speeding up the deposition of co-evaporated PSCs to enable Lab-to-Fab transition.
There are several parameters to be considered when fabricating solar cells for space applications. Flexible space solar cells are usually packed before launching them into orbit where they unfold to harvest light. A flexible substrate widely used in space applications is Kapton, which that stands space radiation but is not suited for photovoltaics due to its low transparency. Additionally, thin-film lightweight flexible solar cells for space use expensive and fragile semiconductors [i.e. GaInP/GaAs/Ge (1.82/1.42/0.67 eV)]. Lately, investigation on perovskite solar cells for space applications is growing rapidly due to their radiation hardness and self-healing behaviour. In this work, we observed that it is possible to fabricate lightweight flexible perovskite solar cells with a mid-bandgap composition (FA0.7MA0.3PbI3 – 1.5 eV) via co-evaporation of the perovskite on polyether ether ketone (PEEK) substrates, which is very transparent in the UV-Vis range. Moreover, vacuum processing allows for more flexibility in the design of the device structure, so that bifacial and substrate (bottom metal – top illumination) configurations can be readily achieved. In this work, we observed that bifacial solar cells can withstand electron beam doses up to 1014 e-/cm2, although degradation is observed for higher doses of 1016 e-/cm2. Aiming to tackle such instability, we worked on substrate configuration cells using sublimed phosphonic acid containing molecules (MeO-2PACz) as hole transport layers, with promising thermal and illumination stability. Test under ionizing radiation being performed.
2C-I1
TBC
2C-O1
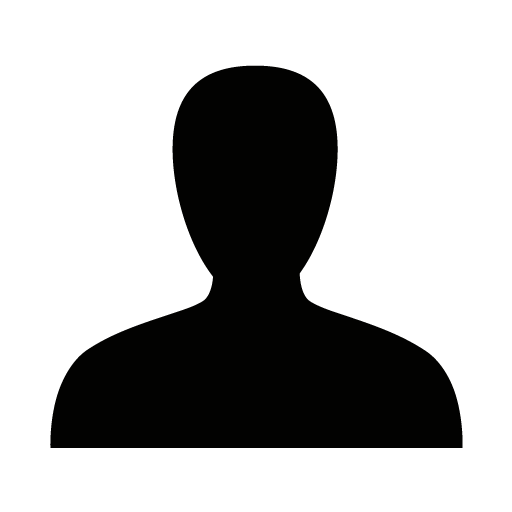
Metal halide perovskite photodiodes have garnered extensive attention owing to their favorable optoelectronic properties, rendering them attractive for visible, near infrared and X-ray sensors. Due to this, they are being implemented in a wide range of applications such as as image sensing, optical communications, gesture recognition or biomedical sensing, amongst others.
However, their predominant reliance on solution-processing deposition techniques poses challenges for seamless integration into existing industrial processes, as they are often restricted to small area and use steps that are difficult to scale up, such as anti-solvent processing. Here, we address this limitation by developing fully vacuum-processed perovskite photodetectors with varying hole transport layers (HTL), that were characterized in the visible and X-ray spectrum. Our findings underscore the critical role of HTL selection in influencing the performance of the devices, in particular in the dark and noise current characteristics of the diodes. To optimize performance, we highlight the significance of minimizing the offset between the HTL transport level (its highest occupied molecular orbital, HOMO) and the perovskite valence band. With an optimized HTL, we obtain photodiodes with low noise current (~3·10-14 A·Hz-1/2) and high specific detectivity (~1012 Jones at 710 nm at -0.5 V). The perovskite photodiodes are also used to detect X-ray radiation up to 150kVp, resulting in an excellent linear response, for both high and low X-ray doses, with a sensitivity of 7.8 ± 0.6 µC/Gy/cm2 and a low limit of detection of 2.0 µG/s.
2D-O1
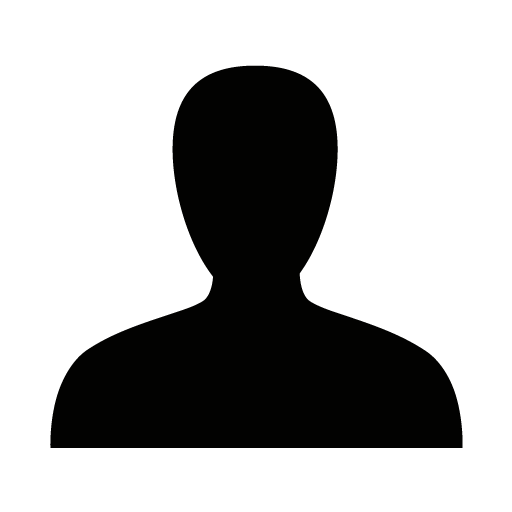
Lead halide perovskites (LHPs) have emerged as promising semiconductors for next-generation optoelectronic devices such as photodetectors (PDs), solar cells, and radiation detectors. LPHs in single crystals (SCs) showed much better stability and optoelectronic properties than the polycrystalline thin-film counterparts due to the absence of grain boundary, lower surface defects, and lower ion migration. Among next-generation LHP SCs, Methylammonium lead bromide (MAPbBr3) has become the centre of research for the last five years due to its feasible crystal growth and stability under ambient conditions.[1,2] Nowadays, self-powered photodetectors (SPPDs) are paramount in various applications because they can operate in remote locations without any external power sources. Photodiodes are one such device that can be use as SPPDs. MAPbBr3 SCs-based photodiodes can be formed by doping various metal ions into the host perovskite structure.
In this work, a planar SPPD was developed from metal-doped p-type MAPbBr3 / n-type MAPbBr3. At first, separately synthesised p-type Ag+ doped MAPbBr3 and n-type Sb3+ doped MAPbBr3 and optimised their doping concentration for better photovoltaic performance. The planar PD with p-type 0.1% Ag+ doped shows favourable photodetection properties, such as responsivity (R) of 3.95 A W-1 and external quantum efficiency of 1093%, almost 20% better than the undoped.[3] Similarly, n-type 0.1% Sb3+ doped PD exhibits improved optoelectronic properties because of the combined effect of high electron concentration and low Schottky barrier height (SBH) between the Ag metal electrode and SC. Based on these results, ultimately, a p-n junction photodiode was developed by growing 0.1% Ag+ doped MAPbBr3 SC epitaxially on the facet of 0.1% Sb3+ doped MAPbBr3 SC. Finally, SPPD was fabricated using MAPbBr3 SC photodiode by depositing asymmetric electrodes to use the combined effect of the built-in electric field in the p-n junction depletion region and the electric field formed by the asymmetric Schottky barrier height to maximise the performance. This p-n junction device incorporated with asymmetric electrodes (Ag and Pt) shows typical photovoltaic behaviour with an excellent open circuit voltage of 0.95 V. This device shows great sensitivity towards the 530 nm illumination without any external bias. Under the mentioned conditions, our device shows responsivity up to 0.41 A W-1 and a specific detectivity of 6.39 × 1011 Jones. These values are the highest reported for any MAPbBr3-based SPPDs. Additionally, this device retained 80% performance after 12 hours of continuous illumination at ambient conditions and restored 94% of initial performance after 36 hours of storage under the dark, dry box. This work on p-n junction photodiode can pave the way for designing new potential perovskite-based SPPDs.
2D-I1
The development of detectors for protons and X-rays is a long-lasting research topic not only for fundamental applications, but more recently, in the medical field for hadron- and radio-therapy of cancer. In this application, ion beams are used for the controlled treatment of cancer by focusing them onto small volumes, to avoid the spreading of the radiation to healthy tissues. For this reason, there is an increasing demand of systems optimized for the accurate in-situ, real-time recording and mapping of the dose delivered during a treatment plan. Metal halide perovskites are rapidly emerging as active materials in lowc-ost high performing ionizing radiation detectors thanks to strong absorption of ionizing radiation, high charge carrier mobilities, long exciton diffusion, long charge carrier lifetime, and excellent optical properties. [1] Further, they combine the high performance of traditional inorganic semiconductors with the lowcost, large area scalable, deposition methods (i.e., printing technologies) typical of organic semiconductors. However, the direct proton beam detection or dose-monitoring by perovskite based devices has scarcely explored yet.
In this work, we propose novel flexible proton detectors based on 2D and mixed 3D and 2D perovskites films deposited from solution [2]. Mixed 3D-2D perovskites are formed by mixing 3D (based on methylammonium (MA) cations) and 2D (based on larger organic ammonium (OA) cations) structure perovskites. Their employment has been reported as an effective strategy to retain the exceptional transport properties of 3D perovskites and the high stability induced by the layered structure of 2D perovskites. By adding the 3D phase (MAPbBr3) we here aim to enhance the radiation absorption of protons by the perovskite film, thanks to the higher density of MAPbBr3 and to the higher thickness of the active layer for mixed compounds.On the other side, the high resistivity of the pure 2D perovskite active layer lowers the dark current of about 2 orders of magnitude with respect to the 2D/3D mixed perovskite improving the Signal to Noise ratio of the detector
The here proposed devices demonstrate an accurate monitoring of proton dose with instant feedback and low limit of detection, and provide a stable response even after hard and long-lasting proton irradiation. The performance of this novel tool is here compared with the one offered by standard commercial large-area technology, namely radiochromic sheets. We demonstrate the great potential of this class of devices by successfully mapping in real-time a 5 MeV proton beam at fluxes between 108 H+ s-1 cm-2 and 1010 H+ s-1 cm-2, confirming the capability to operate in a radiation harsh environment without output signal saturation issues. The versatility and scalability of here proposed detecting system are demonstrated by the development of a multipixel array able to map in real-time a X-ray beam spot.
The presented results provide an effective solution to the challenge of identifying novel functional materials and portable devices for real-time accurate monitoring of proton dose, addressing the quest for radiation hardness, low-cost scalability over large areas and mechanical flexibility, still unsolved for a range of application which span from personal dosimetry to large area and lightweight detectors for large accelerators facilities and space missions.
2D-O2
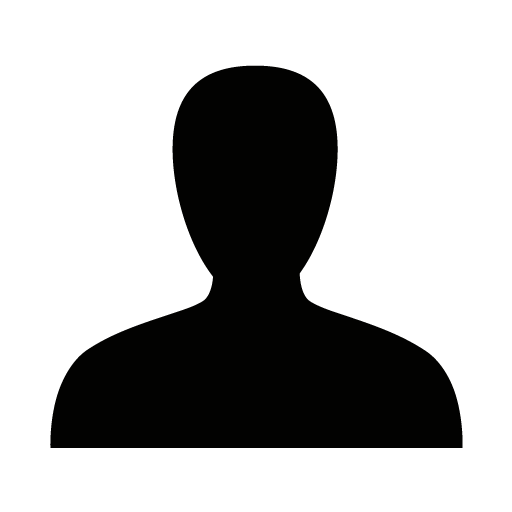
Lead halide perovskites (LHPs) have emerged in a variety of thin film optoelectronic applications such as light-emitting diodes, solar cells, and lasing owing to high photoluminescence quantum yield (PLQY), bandgap tunability, defect tolerance, and facile synthesis routes. LHP materials have also recently gained interest in the scintillator community due, in part, to comparatively low bandgap energies because of the inverse relationship between charge carrier generation in the hot electron impact ionization cascade process and the bandgap energy of the scintillator crystal.[1] Despite the corresponding high theoretical light yield, empirical data has remained marginal at non-cryogenic temperatures due to the thermal quenching of LHP excitons and self-absorption of excitonic emission.[2] Since scintillators must be sufficiently thick to attenuate high-energy photons, low self-absorption is critically important to extract scintillation light efficiently. Therefore, much like in traditional scintillator material systems, extrinsic luminescent dopants such as lanthanide ions are advantageous for increasing the stokes shift and obtaining high PLQY. In particular, work on the quantum cutting (i.e., two-photon emitting) CsPbCl3:Yb3+ system, originally sought for applications in ultraviolet down-conversion for photovoltaic (PV) light harvesting,[3] has recently been translated to scintillator applications due to the large stokes shift, near 200% PLQY in thin films,[4] high light yield, and energy-efficient conversion of thermalized charge carriers to NIR photons.[5], [6]
An as-of-yet unexplored application for this promising optoelectronic material platform is in an integrated, indirect gammavoltaic or X-ray-voltaic device.[7] In such a device, a high-energy incident photon (e.g., radioisotope characteristic γ-rays or an X-ray source) is attenuated by a thick scintillator crystal and is converted to a large multiplicity of near-monochromatic, low-energy photons, characteristic of the luminescent Yb3+ dopant. A bandgap-tuned PV cell is optically coupled to absorb the emitted low-energy scintillation light. The absorbance onset of the PV cell is in resonance with the scintillation emission energy to minimize spectral losses and achieve a high power conversion efficiency.
Here we report preliminary work on the synthesis of polycrystalline CsPbCl3:Yb scintillator pellets and their application in an X-ray-voltaic device. We showcase the optoelectronic properties of this scintillator material and their dependence on Yb3+ dopant concentration. We also show a Shockley-Queisser theoretic analysis of the corresponding PV performance characteristics as a function of the PV bandgap energy, radiation intensity, and scintillation emission spectra. Lastly, we discuss the next steps for precise energy and dose rate-dependent light yield measurements, further optimization of the quantum cutting dynamics, and demonstration of an optimized prototype gammavoltaic device.