G1-K1
James Durrant is Professor of Photochemistry in the Department of Chemistry, Imperial College London and Ser Cymru Solar Professor, University of Swansea. His research addresses the photochemistry of new materials for solar energy conversion targeting both solar cells (photovoltaics) and solar to fuel (i.e.: artificial photosynthesis. It is based around employing transient optical and optoelectronic techniques to address materials function, and thereby elucidate design principles which enable technological development. His group is currently addressing the development and functional characterisation of organic and perovskite solar cells and photoelectrodes for solar fuel generation. More widely, he leads Imperial's Centre for Processable Electronics, founded the UK�s Solar Fuels Network and led the Welsh government funded S�r Cymru Solar initiative. He has published over 500 research papers and 5 patents, and was recently elected a Fellow of the Royal Society
The kinetics of charge generation, separation and recombination are critical to the function of both organic and perovskite solar cells. In my talk, I will address some of the factors determining these kinetics in both organic and perovskite solar cells, and how these impact upon device performance, discussing lessons learnt in particular from transient optical and optoelectronic measurements. I will start by introducing some of the key current challenges and opportunities for these devices. In organic solar cells, I will then go on to focus on a comparison of the kinetics of fullerene and non-fullerene acceptors, and the role of interfacial charge transfer states in limiting performance. For perovskite solar cells, I will focus on interfacial charge transfer and recombination in planar p-i-n MAPI3 based devices. The impact of tail states on charge carrier recombination, and thus on device performance, will be addressed for both devices. Throughout my talk, I will try to highlight the similarities and difference between these two classes of solar cells.
G1-O1
Juan Bisquert (pHD Universitat de València, 1991) is a Professor of applied physics at Universitat Jaume I de Castelló, Spain. He is the director of the Institute of Advanced Materials at UJI. He authored 360 peer reviewed papers, and a series of books including . Physics of Solar Cells: Perovskites, Organics, and Photovoltaics Fundamentals (CRC Press). His h-index 95, and is currently a Senior Editor of the Journal of Physical Chemistry Letters. He conducts experimental and theoretical research on materials and devices for production and storage of clean energies. His main topics of interest are materials and processes in perovskite solar cells and solar fuel production. He has developed the application of measurement techniques and physical modeling of nanostructured energy devices, that relate the device operation with the elementary steps that take place at the nanoscale dimension: charge transfer, carrier transport, chemical reaction, etc., especially in the field of impedance spectroscopy, as well as general device models. He has been distinguished in the 2014-2019 list of ISI Highly Cited Researchers.
The development of organic-inorganic lead halide perovskites with very large efficiency requires us to understand the operation of the solar cell. This class of semiconductors presents remarkable bulk electronic and optical properties, but the contacts to the device are a key aspect of the operation and show important dynamic interactions. We describe the results of analysis of kinetic phenomena using frequency modulated techniques. First with impedance spectroscopy we provide an interpretation of capacitances as a function of frequency both in dark and under light, and we discuss the meaning of resistances and how they are primarily related to the operation of contacts in many cases [1]. The capacitance reveals a very large charge accumulation at the electron contact, which has a great impact in the cell measurements, both in photovoltage decays, recombination, and hysteresis. We also shows the identification of the impedance of ionic diffusion by measuring single crystal samples [2]. Working in samples with lateral contacts, we can identify the effect of ionic drift on changes of photoluminescence, by the creation of recombination centers in deffects of the structure [3]. We also address new methods of characterization of the optical response by means of light modulated spectroscopy. The IMPS is able to provide important influence on the measured photocurrent. We describe important insights to the measurement of EQE in frequency modulated conditions, which shows that the quantum efficiency can be variable at very low frequencies [4].
G1-I1
Recently, metal halide perovskite semiconductors are being intensively studied with respect to both fundamental physics and implementation in solution-processed functional devices. While solution-grown bulk single crystals have been employed to reveal the intrinsic optical properties of perovskites [1-4], colloidal synthesis promises further technological advances since this enables preparation of novel perovskite nanocrystals, combining excellent optoelectronic properties with quantum confinement effects. The highly efficient luminescence of the perovskite nanocrystals has a narrow bandwidth and its wavelength can be tuned over the entire visible spectrum. These properties make them particular interesting for display phosphors, light-emitting diodes, lasers, and single photon sources. The successful design of such new optoelectronic devices depends on the detailed knowledge about excitons and excitonic complexes in the nanocrystals. Here, we report on the optical responses of organic-inorganic hybrid and all-inorganic perovskite nanocrystals. Their luminescence quantum yields and the lifetimes of excitons, charged excitons, and biexcitons [5-7] were evaluated by employing femtosecond transient-absorption and single-dot spectroscopy [8-10]. It is shown that both nonradiative Auger ionization and charged surface defects can contribute to the charged exciton generation in perovskite nanocrystals. We discuss the photophysics of the charged excitons, because they determine the device performance of nanocrystal-based light emitters.
G1-I2
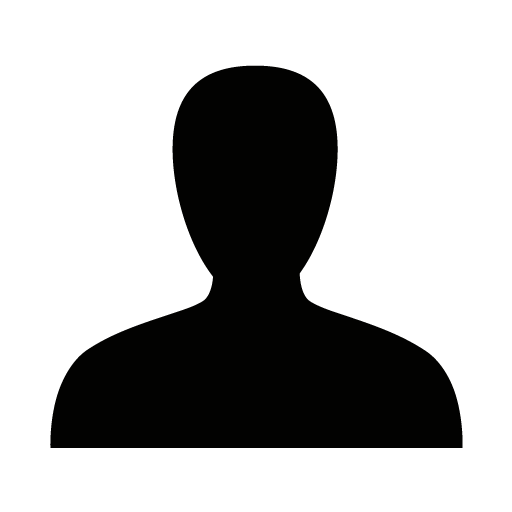
Nano-size semiconductor structures have a reach spectrum of properties related to quantum confinement which are not available in the corresponding bulk materials. Size-tuneable spectrum is, perhaps, the best known and understood such property. Here we investigate dissipation and transport dynamics of various semiconductor nanosystems and their composites. We have studied colloidal quantum dots, plasmonic metal nanoparticles, hybrid and all inorganic perovskite nanocrystals and Ruddlesden–Popper 2D perovskites. We apply a set of modern ultrafast techniques like photocurrent and fluorescence detected coherent 2D spectroscopy, transient terahertz and absorption spectroscopies revealing detailed information about photoexcitation dynamics.
As an example [1]. Photo-generated charge carrier dynamics in Ruddlesden-Popper 2D perovskites with linear (n-BA) and branched (iso-BA) butylamine as spacing cations were studied by using transient absorption and time-resolved photoluminescence spectroscopies. Both n-BA and iso-BA perovskites consist of mixed-phase 2D quantum wells with various layer thicknesses, where the photo-generated charges undergo inter-phase charge transfer from thinner quantum wells to thicker ones. By shortening the spacer from n-BA to iso-BA, the transfer rates are significantly increased, which can also diminish the charge accumulation in thin quantum wells induced by the unbalanced electron and hole charge transfer rates. Under high excitation intensity, the shorter spacing cation is found to further facilitate transport, which can compete with fast high-order carrier recombination and consequently improve the charge transfer efficiency. Intriguingly, we observe the existence of extra bulk 3D phases embedded within iso-BA perovskites, which can efficiently collect the confined charges within 2D phases and then transport them with faster carrier mobility and slower recombination rates.
G1-I3
Thuc-Quyen Nguyen is a professor in the Center for Polymers and Organic Solids and the Chemistry & Biochemistry Department at University of California, Santa Barbara (UCSB). She received her Ph.D. degree in physical chemistry from the University of California, Los Angeles, in 2001 under the supervision of Professor Benjamin Schwartz. Her thesis focused on photophysics of conducting polymers. She was a research associate in the Department of Chemistry and the Nanocenter at Columbia University working with Professors Louis Brus and Colin Nuckolls on molecular self-assembly, nanoscale characterization and molecular electronics. She also spent time at IBM Research Center at T. J. Watson (Yorktown Heights, NY) working with Richard Martel and Phaedon Avouris. Her current research interests are structure-function-property relationships in organic semiconductors, sustainable semiconductors, doping in organic semiconductors, interfaces in optoelectronic devices, bioelectronics, and device physics of OPVs, photodetectors, and electrochemical transistors. Recognition for her research includes 2005 Office of Naval Research Young Investigator Award, 2006 NSF CAREER Award, 2007 Harold Plous Award, 2008 Camille Dreyfus Teacher Scholar Award, the 2009 Alfred Sloan Research Fellows, 2010 National Science Foundation American Competitiveness and Innovation Fellows, 2015 Alexander von Humboldt Senior Research Award, 2016 Fellow of the Royal Society of Chemistry, 2015-2019 World’s Most InfluentialScientific Minds; Top 1% Highly Cited Researchers in Materials Science by Thomson Reuters and Clarivate Analytics, 2019 Fellow of the American Association for the Advancement of Science (AAAS), 2023 Wilhelm Exner Medal from Austria, 2023 Fellow of the US National Academy of Inventors, 2023 de Gennes Prize in Materials Chemistry from the Royal Society of Chemistry, 2023 Elected Member of the US National Academy of Engineering, 2024 Fellow of the European Academy of Sciences, and 2025 ACS Henry H. Storch Award in Energy Chemistry.
Organic solar cells are the subject of an extensive research effort due to their potential application as cheap, light-weight and flexible energy sources. An important step towards the realization of commercialization is attainment of higher efficiencies and improvements of the scale-up process. Both objectives are possible only through a more complete understanding of factors limiting the device performance. In this talk, I will discuss the nature and origin of the charge recombination mechanisms that govern the J-V characteristics of several blend systems. We employ impedance-photocurrent device analysis (IPDA) to quantitatively characterize the competition between charge extraction and charge recombination under steady state operational conditions. Results from the IPDA technique exhibit significantly improved reliability and self-consistency compared to the open-circuit voltage decay technique (OCVD). IPDA measurements also reveal a significant negative electric field dependence of the bimolecular recombination coefficient in high fill factor devices. Combined with morphological characterization of blend films using TEM, GIWAXS, and resonant soft X-ray scattering (R-SoXS), these results shed light on the morphological and electrical properties that can reduce recombination losses in solution-processed BHJ solar cells. This comprehensive investigation provides insight into factors that govern BHJ organic solar cell performance. The IPDA method can also be applied to investigate charge recombination in perovskite solar cells.
G1-I4
A crucial issue facing polymer-based solar cells is how to maximize short-circuit current density (Jsc) and open-circuit voltage (Voc) at the same time and thus the power conversion efficiency. A key to resolving the issue is to reduce the relatively large photon energy loss, which is defined by the difference between the optical bandgap of the material and Voc. Here, I will show that a semiconducting polymer based on naphthobisoxadiazole (PNOz4T) [1], having a narrow bandgap of 1.52 eV, can give high Voc of approximately 1 V with relatively high efficiencies of more than 8%, when combined with PC71BM. As a result, the photon energy loss was found to be as small as 0.52 eV, which is much smaller than that of typical high performance polymer/fullerene systems and approaches the values of inorganic and perovskite solar cells. It is also interesting to note that the efficiency of the PNOz4T system is one of the highest values among the polymer/fullerene cells with such small photon energy loss.[2] I will also show ternary blend system with well controlled energetic alignment can give small photon energy loss of less than 0.5 eV.
talk-S1
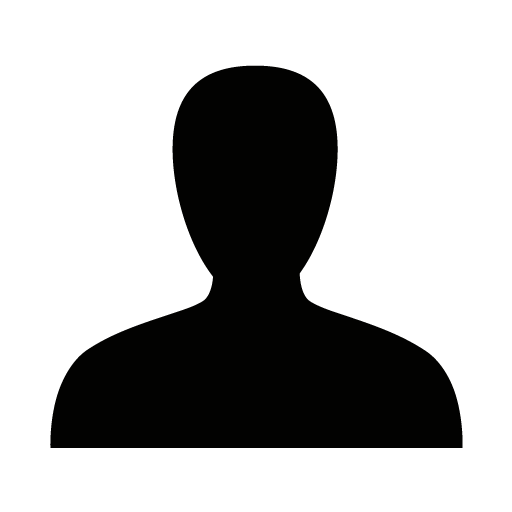
TCI is a leading global manufacturer of fine chemicals for research and commerce. We manufacture more than 28,000 organic laboratory chemicals using our own original techniques. Many of these chemicals are highly specialized including a number of reagents available only through TCI. Our manufacturing techniques have been developed over the course of more than 60 years of synthesis experience.
We are able to supply a wide selection of Fine and Specialty Chemicals by drawing upon our unrivaled expertise and sophisticated manufacturing techniques. With proven production experience and varied reaction capabilities, which rank us among the best in the industry, TCI can supply most compounds in sizes ranging from milligram to ton quantities.
For materials not commercially available, TCI is ready to discuss how we can perform a timely and efficient Custom Synthesis to meet your requirements. We have more than 60 years of custom synthesis experience behind us, ready to work for you. We can synthesize or scale-up using your methodology, or our own proven skills and experience.
In pursuit of meeting customer demands throughout the world, we have laid out an infrastructure which enables us to deliver our high quality reagents rapidly to customers worldwide by establishing overseas offices and plants in North America, Europe, China and India.
G2-O1
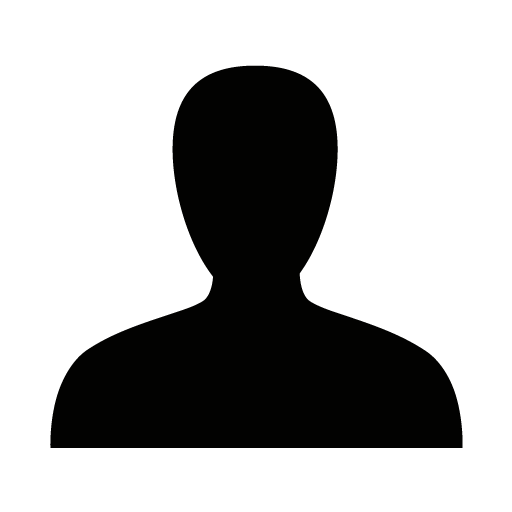
Despite the high-efficiency of these lead-based perovskite (PVK) solar cells, the problem associated with the toxic nature of lead has open a new research direction focusing on lead-free perovskite materials. Tin has been proposed to replace lead. The highest efficiency obtained with Sn only perovskite was 9 % which was based on 2D and 3D mixture of FASnI3. We previously reported mixed metal SnPb-PVK solar cells with several % efficiency. The efficiency has been enhanced now up to 17-20%2. In this study, Pb of SnPb-PVK was replaced with Ge. The use of germanium-based perovskite in solar cell was realized by Krishnamoorthy et. al. The solar cell performance was 0.11 % for CsGeI3 and 0.20 % for MAGeI3. A theoretical study showed that a stable SnGe-PVK material absorbing the sunlight spectrum can be realized. The new type of SnGe mixed metal perovskite solar cells are reported with enhanced efficiency and stability. FA0.75MA0.25Sn1-xGexI3 (abbreviated as SnGe(x)-PVK) were used for the mixed metal SnGe-PVK3. The structure of Ge-doped Sn perovskite was discussed from the view point of band gap, conduction and valence band level, XPS analysis, and the Urbach energy. It can be concluded that many Ge atoms are located at the both of hetero-interfaces, which decreased hetero-interface resistivity and contributed to the enhancement of Jsc. For SnGe(0)-PVK device, the average Jsc was 17.61 mA/cm2 with 0.46 V of VOC, 0.41 of FF, and 3.31% of PCE. By doping with 5 wt% of Ge, the JSC increased to 19.80 mA/cm2 and FF was improved up to 0.55, leading to enhanced efficiency of 4.48 %. After optimization, 7.9% of SnGe(5)-PVK device is reported. In addition, the stability of solar cells in air has been improved significantly with the Ge doping, which would be brought about by passivation effect of GeOx on the PVK layer. The electrical properties including carrier density, carrier mobility and carrier diffusion length of GeSn-PVK were compared with those of Sn-PVK and Pb-PVK, and the efficiency for the GeSn-PVK in future study are discussed with the comparison of those of Pb-PVK solar cells.
A1-IS1
Recently, perovskite solar cells have received much attention due to their rapid progress in efficiency with certified efficiency of 23.3%. On the other hand, one of the biggest concerns for commercialization remains stability. Especially, compatibility of high efficiency and thermal stability is one of the biggest challenges since stability at high temperature frequently suffers significant reductions in efficiency due to limited choice of the materials or device structure. Here, we achieved 92% retention at 85 ̊C /85% RH stress test, which is specified in international standard (IEC 61215), with exceeding 20% power conversion efficiency (certified efficiency of 20.8% at 1 cm2) by engineering perovskite compositions. Furthermore, we demonstrated the world highest level of 12.6% power conversion efficiency at 20cm×20cm size module (aperture area of 354.45cm2, 35 cells in series). The latest result of module development will be reported in the conference. These results show great feasibility for practical use of perovskite solar cells.
A1-O7
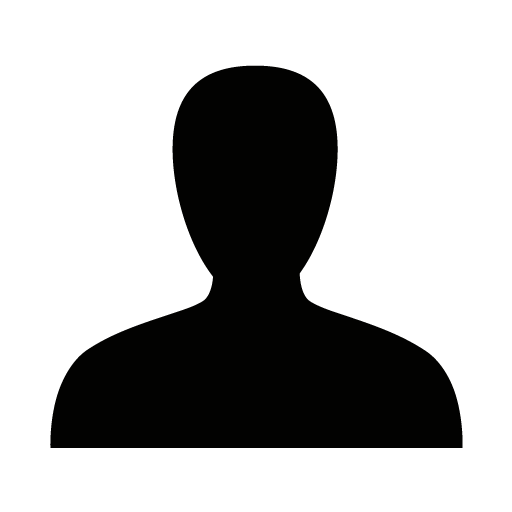
Over the past few years, organic–inorganic hybrid perovskites have attracted signicantly attention as light absorbers in efficient photovoltaics. While impressive power conversion efficiencies exceeding 23% have been attained within a period of only a few years, concerns have been raised about the viability of this class of photovoltaics as a scalable and long-term reliable energy source. In our group the printable perovskite solar cells was developed with triple mesoscopic layers. The conjugated or non-conjugated bifunctional molecules were introduced into the perovskite materials to enhance its stability and efficiency. The results indicated that the interface engineering plays a key role of directing the crystal formation and growth during the infiltration and precipitation of the perovskite within the mesoporous oxide scaffold. The fully printable mesoscopic perovskite solar cell presents no obvious decay within over 10000h light soaking and high efficiency of more than 16%. TIn addition, the crystals of the perovskite films are reconstructed by post-treating with methylamine gas and allows the regeneration of the photodegraded PSCs via the crystal reconstruction and the PCE can recover to 91% of the initial value after two cycles of the photodegradation-recovery process. Meanwhile, the characterization of the mesoscopic perovskite solar cells under the UV light soaking condition was performed. A 110 m2 mesoscopic perovskite solar system was exhibited in WonderSolar Co., Ltd. These results offer a promising prospect for its commercial application.
A1-O6
Udo Bach is a full professor at Monash University in the Department of Chemical Engineering; the Deputy Director of the ARC Centre of Excellence in Exciton Science and an ANFF-VIC Technology Fellow at the Melbourne Centre of Nanofabrication (MCN). He received his PhD from the Swiss Federal Institute of Technology (EPFL, Switzerland) working in the research group of Prof Michael Grätzel and worked for 3 years in a technology start-up company in Dublin (Ireland). Subsequently he spent 15 months as a postdoc in the group of Prof. Paul Alivisatos in UC Berkeley (USA) before moving to Monash University in November 2005 to establish his own research group.
Prof Bach has a strong background in the area of photovoltaics and nanofabrication. He is involved in fundamental and applied research in the area of perovskite and dye-sensitized solar cells. He has additional research activities in the area of nanofabrication, DNA-directed self-assembly, nanoprinting, plasmonics for sensing, photovoltaic applications and combinatorial photovoltaic materials discovery.
Back-contact concepts are well established in the field of silicon solar cells, where their implementation has resulted in significant efficiency gains, compared to conventional contacting architectures. The main advantage of back-contact concepts is that optical transmission losses can be avoided, arising from the top charge collection electrode. Charge collection in these devices is typically facilitated by a set of two interdigitated finger electrode arrays, co-located on the backside of the silicon wafer. Here we discuss the applicability of back-contact charge collection concepts to thin-film perovskite solar cells. We introduce two novel back-contact architectures, namely the quasi-interdigitated and honeycomb back-contact designs. Charge diffusion lengths in single-crystalline silicon are in the order of millimeters while for perovskite solar cells they are in the micron range. This requires the fabrication of back-contact electrode arrays with micron or ideally sub-micron dimensions to achieve efficient charge collection. In the second part of this presentation we will analyse how dipole-fields generated by self-assembled molecular monolayers can be used in solar cells to generate built-in fields and facilitate charge extraction.
A1-O5
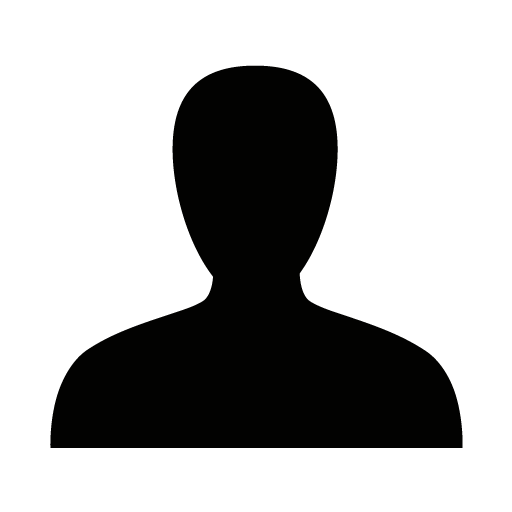
Perovskite solar cells (PSCs) have been attracting much attention from researchers and engineers due to their high performance and low cost. As the research on PSCs proceeds, the structure has gradually evolved from the Graetzel structure to a sandwich structure which is the most widely used structure for high-performance PSCs. Behind the scenes, improvement of the perovskite crystallinity has played an important role in promoting the structural evolution. In the beginning, ultra-small perovskite crystal could only be used as a sensitizer in Graetzel structure.[1] It was not until crystal size matched film thickness that the sandwich structure became the main structure for PSCs. Recently, crystal size in perovskite thin films already reached several tens of micrometers, much larger than the thickness of the film.[2] How can we take advantage of the enlarged crystal size and further boost the efficiency of PSCs?
Here, we propose that the integrated-back-contact (IBC) structure, which has been widely used in Si photovoltaics, maybe the next-generation structure for PSCs. The IBC structure can largely reduce light loss by eliminating reflection and absorption caused by substrates. The Large crystal size of the perovskite film can help to reduce charge recombination between the two electrodes of the IBC structure. By using a numerical simulation method, we demonstrated that the IBC-PSCs would outperform sandwich-PSCs by 11%.[3] In the presentation, I will discuss detailed requirements to maximize the performance of IBC-PSCs.
A1-O4
Perovskite Solar Cells (PSC) have been one of the most promising current alternative to Silicon-based photovoltaics and big efforts have been devoted to the development of new efficient materials and innovative architectures for this class of devices. Their outstanding properties in terms of easy processability, transport and low non-radiative recombination rate led to very fast development of research activities both on the device implementation and on the characterization sides. This material has recently aroused great interest in the scientific community, as it enabled power-conversion efficiencies of over 23%. Deposition methods and morphological study have also been developed in the common effort of the scientific community to control the crystallization and correlate structure and energy conversion properties in these devices. To this extend the control of these properties is mandatory for a proper optimization of the system. Here a control of the morphology and perovskite crystal growth is presented by the use of different additives introduced in the conventional perovskite layer, aiming to highlight the profound dependence in the growth mechanisms of perovskite introduced by materials of different nature as molecules or nanoparticles. A particular attention is devoted to the study of intermolecular interactions occurring in solution deeply modifying the self-assembly process, and their relationship with the properties of the deposited thin film. The interaction among perovskite and the introduced additives in PSC is found to be crucial in influencing crystalline order, morphology and photo-physical properties of the final material. Experimental characterization and photovoltaic applications of different perovskite-based nanocomposites is presented.
A1-O3
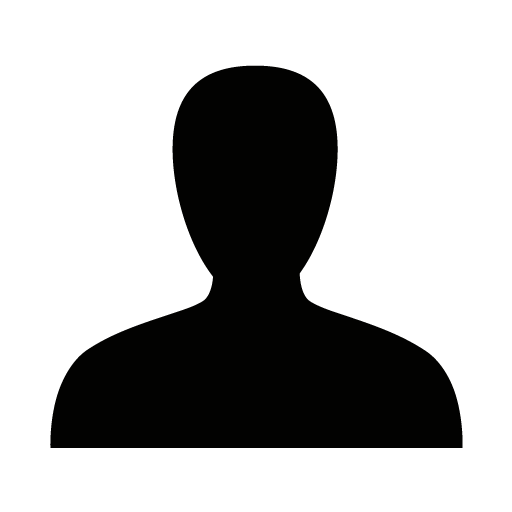
Organometal Halide Perovskites Template Growth for Highly Efficient Light-Emitting and Photovoltaic Devices
Andrea Listorti
The control of Organometal Halide Perovskites (OHPs) formation is a fundamental requirement foreseeing the exploitation of these outstanding, eclectic materials in optoelectronics. OHPs, extensively used in solar cells[1] and light emitting diodes (PeLEDs)[2] are formed from solution, throughout a self-assembly process of their chemical precursors. The resulting polycrystalline film shows often a far from ideal behaviour, due to unsuitable morphology or to an elevated density of electronic defects, direct consequences of a scarcely controllable assembly. Based on our previous findings on the interaction between perovskite precursors and additives,[3-4] and particularly on the hydrogen bond effect upon such interactions, here we explore the use of a tailored biopolymer, the starch, as templating agent for the growth of formamidinium (FA)- and methylammonium (MA)-based tri-iodide perovskite films. We prove how the presence of the macromolecule leads to a fundamental technological advantage allowing a one-step deposition method of an optimum perovskite film, avoiding the complex solvent dripping or sequential two-steps method. Furthermore, it allows a fine tuning of the i) solution viscosity (making it compatible to different large area deposition techniques), of the ii) perovskite grain size and of the iii) film thickness, by simply adjusting the polymer:perovskite relative concentration. We validated our approach by embedding these composites in photovoltaic (PV) and light-emitting (PeLED) devices. As result we obtained an inverted, planar, low-temperature processed solar cell showing a remarkable 17% efficiency and a highly efficient LED (EQE of ~5%) exhibiting outstanding radiance values above 200 W/srm2obtained at very high currents (about 1000 mA/cm2) which are among the highest reported radiances for NIR PeLEDs.[5]
The nano and micro-structural changes, as a function of starch concentration, and their influence on optoelectronic properties were studied with SEM, XRD and advanced spectroscopic analyses, confirming that the device performances are strongly related to the composite (starch:perovskite) structure. The overall picture that emerged form the combined investigations is that the presence of starch influences: i) the interface properties within the device stack, ii) the crystallographic behaviour of the perovksite material, iii) the dielectric landscape surrounding the perovskite crystals, leading in case of the PeLED, to an overall enhancement of the perovskite light emission, a minimization of Auger losses at high current regimes, thus an improvement of the device capability in sustaining high carriers densities.[5]
A1-O2
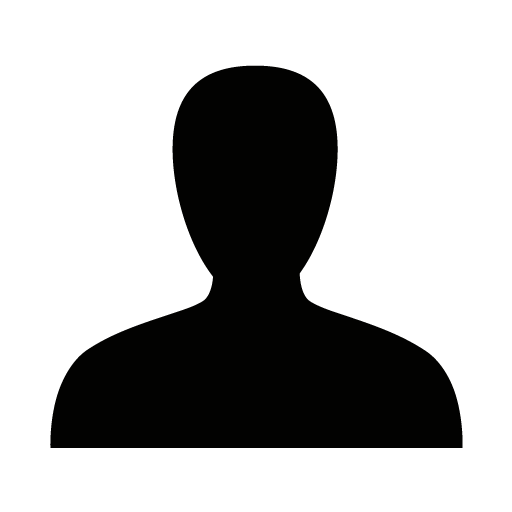
High sensitive photodetection with large amplified photocurrent (gain) has been generally achieved by photoelectron emission or avalanche effect in inorganic photodetectors such as Si and GaAs, which needs a sufficiently strong external electric field (ca. 100 V). Here, we demonstrate a high-gain and low-voltage photodetector with an organic-inorganic hybrid structure composed of an interfacial perovskite layer between an organic compound coordinating Europium (Eu) and TiO2 mesoporous film. The devise achieves significantly high incident photon to current efficiency of 2.9 × 105% (gain value of 2900) with the highest level of responsivity up to 1289 A/W even under low applied bias -0.5 V and low irradiation light (< 1 mW/cm2), which is more than four orders of magnitude larger than those of inorganic photodetectors. Such high performance of the detector is caused by photomultiplication phenomena at the specific interface composed of the perovskite and Eu complex thin-film layers. The perovskite thin layers are excited by visible light irradiation, and their electrons transfer to the conduction band of TiO2 under the applied reverse bias, whereas the holes are trapped at the interface between perovskite and Eu complex layers. The trapped holes are accumulated at the interface between perovskite and Eu-terpy complex, which results in buildup of a high electric field at the interface. Finally, a large external tunneling injection of electrons occurs from the Ag electrode, and the incident photon to current efficiency of the device exceeds 105% even under low applied bias -0.5 V.
A1-O1
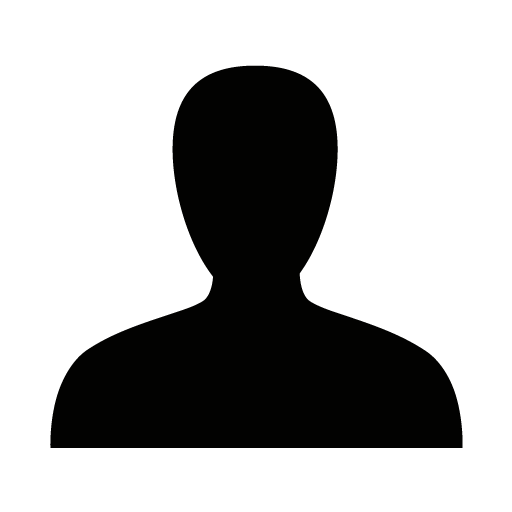
In May 2016, IEC (International Electrotechnical Commission ) TC82(Photovoltaic ) launched TR project on performance measurement protocol of emerging PV (OPV, DSC and PSC), closed the discussion in September 2018 and will publish TR document shortly. This TR(Technical Report) project is intended to bring relevant information of currently available standards and recent technologies in order to start formal discussion toward world standard. The team members consist of 30 scientists from 13 countries.
The discussion is limited to performance measurement only and it does not include anything about long time durability. Both basic performance measurement and measurement method for applications such as indoor and tandem, are discussed, but main focus remains on basic performance measurement. In order to address the new materials to which currently available standards cannot cover rightly, it is recommended, in TR, to introduce new definitions on steady state condition and stabilized state.
Exact description of the definition is carefully avoided in TR, because it should be made in formal standardization process. It is expected to have good start toward world standard based on the discussion and recommendation in this TR, with variety of people’s involvement. In order to have good standard, it is crucial for both material/device scientists and metrology experts to sit and discuss together.
B1-IS1
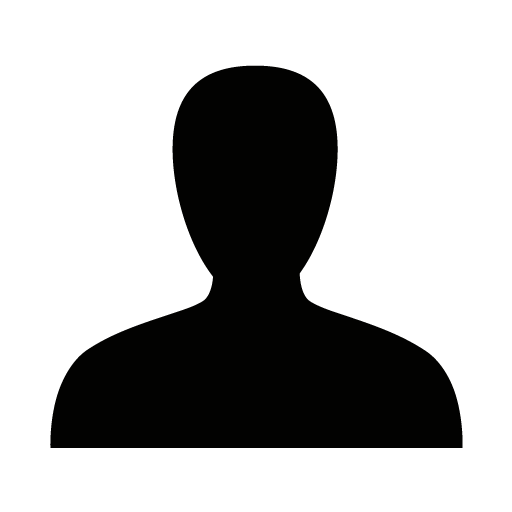
Three-dimensional (3D) methylammonium lead iodide perovskite solar cells are undoubtedly leading the photovoltaic scene with their power conversion efficiency (PCE) >22%, holding the promise to be the near future solution to harness solar energy [1]. Tuning the material composition, i.e. by cations and anions substitution, and functionalization of the device interfaces have been the successful routes for a real breakthrough in the device performances [1]. However, poor stability (= device lifetime), mainly due to material decomposition upon contact with water, is now the bottleneck for the widespread of this technology. Diverse technological approaches have been proposed delivering appreciable improvements, but still failing by far the market requirements demanding 25-years lifetime. In this talk, I will show a new concept by using a different class of perovskites, arranging into a two-dimensional (2D) structure, i.e. resembling natural quantum wells. 2D perovskites have demonstrated high stability, far above their 3D counterparts. However, their narrow band gap limits their light-harvesting ability, compromising their photovoltaic action. Combining 2D and 3D into a new hybrid 2D/3D heterostructure will be discussed as a new way to boost device efficiency and stability, together. The 2D/3D composite self-assembles into an exceptional gradually organized interface where the 2D perovskite anchors on the TiO2 substrate, templating the growth of a highly ordered 3D perovskite on top. This results in mesoporous solar cells leading to 12.9% PCE [2]. Aiming at the up-scaling of this technology, we realize 10x10 cm2 large-area solar modules using a fully printable, hole conductor free device configuration (i.e. where a carbon electrode is used to replace the organic hole transporter and gold). The module delivers 11.2% efficiency stable for more than 10,000 hours with no degradation under accelerated testing conditions, leading to a record one-year stability (see results in Figure 1). On the other side a 3D/2D interface will be also presented, where 2D layer lies on top of the 3D as a mean to physically protect the 3D underneath while also blocking the electron hole recombination at the perovskite/hole transporter interface. This results in enhanced stability without compromising the efficiency, leading to PCE=20% stable for 1000 h[3, 4].
B1-O7
Dr Ma is a professor of chemistry and PhD supervisor at Kyushu Institute of Technology, in Japan Since 2013-present. She works at the Graduate School of Life Science and Systems Engineering. Japan. Professor Ma spent 25 years researching new concept solar cell and photocatalyst and energy storage in Japan and abroad. Professor Ma is also a council member of the following organizations: Japan Society for Chemistry: Materials Research Society US, China Energy Society, China Solar Energy, and so on. She leads her research teams studying dye-sensitized solar cells and perovskite solar cells, also including development of electrochemistry catalysts, hydrogen production, fuel cell, and metal batteries. She has published over 300 papers and 10 books.
Perovskite solar cells (PSCs) have attracted much attention due to their high-energy conversion efficiency and low production cost. However, the problems of stability and toxicity for PSCs are still unresolved.
Recently, MXene, a newly intriguing family of two-dimensional (2D) materials, have recently attracted considerable attention owing to their excellent properties such as high electrical conductivity and mobility, tunable structure and termination groups. In this work, the Ti3C2TxMXene is incorporated into the perovskite absorber layer for the first time, which aims for enhancement of efficiency and stability. Results show that the termination groups of the 2D materials can retard the crystallization rate, thereby increasing the crystal size of MAPbI3. We found that the high electrical conductivity and mobility of MXene can accelerate the charge transfer. After optimizing the key parameters, 12% enhancement in device performance is achieved by adding the MXene. We also used MXene as back electrode to replace the Au electrode. The obtained perovskite solar cell showed good performance. The results will also be presented. These works unlock opportunities for the use of MXene as potential materials in PSCs applications.
References:
[1] Z. Guo, L. Gao, Z. Xue, S, Teo, . Y. Kamada, T. Ma, Small, in press, 2018.
[2] K. Wang, Y. Shi, Q. Dong, Y. Li, S. Wang, X. Yu, M. Wu, T. Ma, J. Phys. Chem. Lett. 2015, 6, 755.
[2] K. Wang, Y. Shi, Y. Li, S. Wang, X. Yu, M. Wu, T. Ma, Adv. Mater 2016. 28, 1891–1897
B1-O6
Organic-inorganic lead halide perovskite solar cells (PSCs) have attracted a great deal of attention owing to the high absorption coefficient, low fabrication cost, and flexible applications. The high absorption arises from a suitable bandgap of perovskite materials, which is close to the Shockley–Queisser limit of 1.4 eV. FAPbI3 is potentially more suitable as an active material than MAPbI3 for PSCs since FA-based lead iodide perovskite (FAPbI3) has been reported to possess an ideal band gap of 1.4 eV, which translate to the sunlight absorption up to ca.886 nm. However, pure FAPbI3 has been reported to exhibit low stability for its trigonal α-phase is sensitive to humidity, and readily turns into a non-photoactive hexagonal δ-phase at room temperature. Although more thermally and structurally stable FAPbI3 has been realized through partial substitution of FA by Cs (FACsPbI3), the stability should be improved further. Recently, we reported 2D perovskite-added FACsPbI3 (2D/3D FACsPbI3) where the 2D PEA-based perovskite protects the 3D grains from α to δ phase transition. 2D/3D FACsPbI3-based PSCs in a normal-type structure gave a certified efficiency of PCE of 19.8%with a remarkable stability.
Even though normal-type PSCs give higher PCEs, inverted-type PSCs provides a wider selection of flexible substrates along with reduced hysteresis and improved device stability under UV illumination. Thus far, a limited study on FAPbI3–based inverted-type PSCs has been reported. We investigated 2D/3D FACsPbI3 in inverted-type PSCs and discovered that they show higher stability and reproducibility than the normal-type devices. The higher stability came from reduced trap-charge at interface, hydrophobicity of poly(triarylamine) (PTAA), and exclusion of 2,2',7,7'-tetrakis[N,N-di(4-methoxyphenyl)amino]-9,9'-spirobifluorene (spiro-MeOTAD). The higher reproducibility came from the elastic nature of PTAA charge carrier layer, onto which brittle 2D/3D FACsPbI3 film is being spin-coated, compared to hard and brittle SnO2 for normal-type PSCs. The strain caused by both 2D perovskites at grain boundaries and the big size of FA led to 2D/3D FACsPbI3 being liable to forming cracks. We investigated the relationship between the mechanical property the films with the device reproducibility, the energetics of the 2D/3D FACsPbI3 films in inverted-type PSCs, and the origin of the phase stability of 2D/3D FACsPbI3. The inverted-type 2D/3D FACsPbI3 PSCs gave a PCE of 18.2% with a greater stability and higher reproducibility than the normal type PSCs.
B1-O5
Prof. Qing Shen received her Bachelor’s degree in physics from Nanjing University of China in 1987 and earned her Ph.D. degree from the University of Tokyo in 1995. In 1996, she joined the University of Electro-Communications, Japan and became a full professor in 2016. In 1997, she got the Young Scientist Award of the Japan Society of Applied Physics. In 2003, she got the Best Paper Award of the Japan Society of Thermophysical Properties and the Young Scientist Award of the Symposium on Ultrasonic Electronics of Japan. In 2014, she got the Excellent Women Scientist Award of the Japan Society of Applied Physics. She has published nearly 140 peer-reviewed journal papers and book chapters. Her current research interests focus on solution processed nano-materials and nanostructures, semiconductor quantum dot solar cells and perovskite solar cells, and especially the photoexcited carrier dynamics (hot carrier cooling, multiple exciton generation, charge transfer at the interface) in perovskite solar cells, quantum dot and dye sensitized solar cells, organic-inorganic hybrid solar cells.
Perovskite quantum dots (QDs) as a new type of colloidal nanocrystals have gained significant attention for both fundamental research and commercial applications owing to their appealing optoelectronic properties and excellent chemical processability[1]. For their wide range of potential applications, synthesizing colloidal QDs with high crystal quality is of crucial importance. However, like most common QD systems, those reported perovskite QDs still suffer from a certain density of trapping defects, giving rise to detrimental non-radiative recombination centers and thus quenching luminescence. Very recently, we have proposed an improved synthetic protocol that involves introducing organolead compound trioctylphosphine-PbI2 (TOP-PbI2) as the reactive precursor, which also leads to a significantly improved stability for the resulting perovskite CsPbI3 QD and Sn-Pb alloyed QD solutions[2-4]. We have demonstrated that a high room-temperature photoluminescence quantum yield (PL QY) of up to 100% can be obtained in CsPbI3 perovskite QDs, signifying the achievement of almost complete elimination of the trapping defects. Ultrafast kinetic analysis with time-resolved transient absorption spectroscopy evidences the negligible electron or hole trapping pathways in our QDs, which explains such a high quantum efficiency. Solar cells based on these high-quality perovskite QDs exhibit power conversion efficiency of 12%, showing great promise for practical application. We expect the successful synthesis of the “ideal” perovskite QDs will exert profound influence on their applications to both QD-based light-harvesting and -emitting devices in the near future.
B1-O4
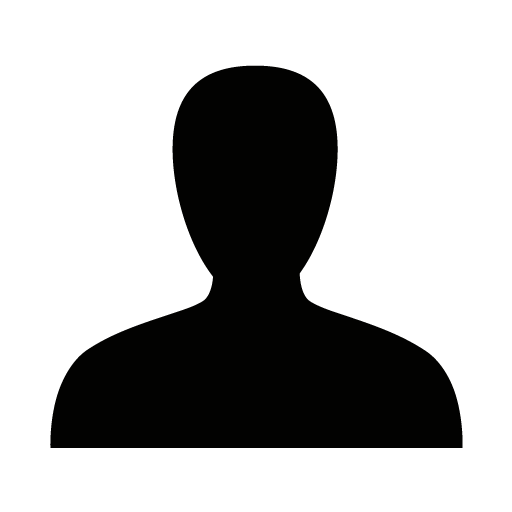
The organic–inorganic hybrid organometal halide perovskites achieved a remarkable power conversion efficiency (PCE) of 22.1% in less than five years due to their superior photonic properties, and facile solution-processed fabrication of active layer perovskite. In this report, we successfully demonstrated the efficient perovskite solar cells with all-inorganic metal oxide as selective contact layers. For the p-i-n structure, we employed microwave-assisted solution process to synthesize metal oxide particles for inorganic selective contacts. The perovskite solar cells fabricated with mesoporous nickel oxide (NiO) and as-synthesized metal oxides (IZO, IGZO) as hole-transporting layer (HTL) and electron-transporting layer (ETL), respectively. Using IZO to replace C60 and PCBM can solve the stability issue of fullerene (derivative) degeneration and their reaction with bottom electrode or perovskite layer. The IZO-based perovskite solar cells demonstrate a PCE of over 16% and impressive long-term stability under air or light-soaking conditions. With encapsulation, our device retained 85% of the initial power conversion efficiency after 460 hours of light soaking. For the n-i-p structure, a recyclable monolithic substrate applying metal oxide selective contact with a nanoporous (np) Au:NiOx counter electrode is successfully demonstrated for efficient perovskite solar cells, of which the perovskite active layer is deposited in the final step for device fabrication. Through annealing of the Ni/Au bilayer, the nanoporous NiO/Au electrode is formed in virtue of interconnected Au network embedded in oxidized Ni. With mp-TiO2/mp-Al2O3/np-Au:NiOx as a template, the original perovskite solar cell with PCE of 10.25% can be rejuvenated by rinsing off the perovskite material with dimethylformamide (DMF) and refilling with newly deposited perovskite. The application of all-inorganic metal oxide selective contacts for both p-i-n and n-i-p structure has potential for commercializing cost-effective mass production.
B1-O3
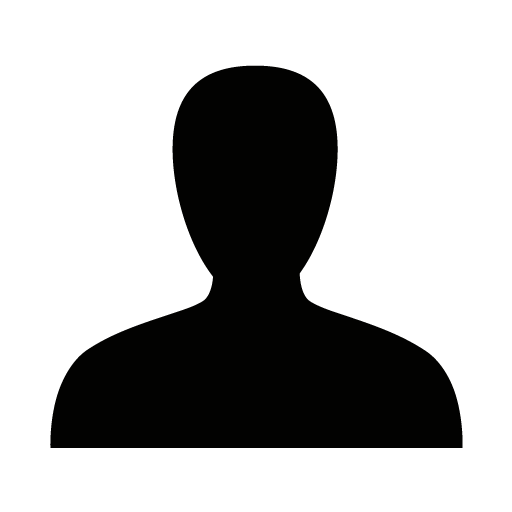
Although organic-inorganic hybrid perovskites like MAPbI3 (MA = methylammonium), FAPbI3 (FA= formamidinium) and the mixed cation based perovskites (FA/MA, MA/Cs, FA/Cs, FA/MA/Cs, etc.) are leading in terms of efficiency, they have been facing a huge challenge of long-term stability. It is the organic cation part (MA or FA), which is believed to be responsible for poor thermal and environmental stability of these materials. For instance, MAPbI3 degrades easily at temperature as low as 120 oC, even at 80 oC if heated for long time. Therefore, replacement of organic cation with an inorganic cation is considered as a good strategy to improve stability. Cesium-based perovskite structures like CsPbX3 and CsSnX3 (X = Cl, Br, I) are indeed the first halide perovskites that were studied by Wells et al. in 1983. With their band gap controlled by the halide ions (CsPbI3; 1.77eV and CsPbBr3; 2.38eV), CsPbX3 perovskite have been considered as a model compound among all all-inorganic perovskites. However, the main challenge with such inorganic perovskites (CsPbI3) is stabilization/formation of the “black” photoactive phase (α-CsPbI3) at room temperature and ambient conditions because CsPbI3 usually crystallizes in a photo-inactive yellow phase (δ-CsPbI3) at room temperature. For application in solar cells, the black phase (absorbing visible light) is required. In the present work, we show how Eu (Eu2+ and Eu3+) inclusion could stabilize the black phase of CsPbI3 and the solar cells using these Eu-doped CsPbI3 showed a power conversion efficiency of ~ 7% in best cases. The study also reveals stability issues of the cells related to additives in the hole transport material.
B1-O2
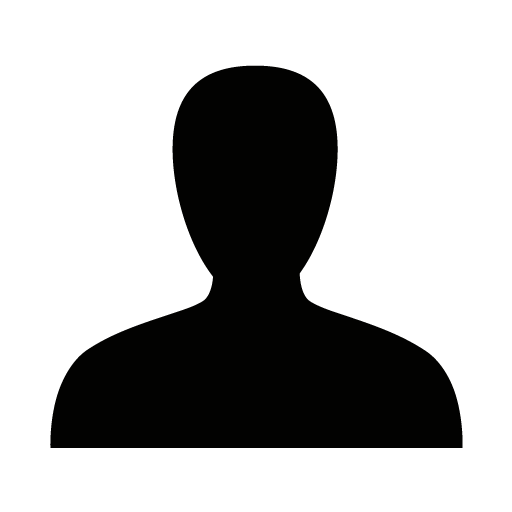
Although the fabrication techniques for halide perovskite solar cells have made startling progress with device efficiency 23% exceeding, the underlying device physics is not still well understood.[1,2] Since the carrier transport layers (CTLs) influence on the performance and stability of perovskite solar cells (PSCs).[3,4] It is imperative to investigate the characteristic impacts on HaPSC induced by the CTL to shade light on some of the key factors underlying the device physics. In this work, we will discuss the impacts of nature of the hole transport layer (HTL) (organic/inorganic HTLs) in PSCs by analyzing the elemental distribution, current-voltage characteristics, and capacitance spectroscopy. The organic HTL device exhibits a lower activation energy which indicates a dominant interface mediated recombination.[5] The admittance analysis reveals that the device with the inorganic HTL induces rather deep trap and higher trap densities. This is found to be induced from the diffusion of metal cations into the perovskite during its crystallization upon the inorganic HTLs. Our analysis suggests that the passivation of deep defect and suppression of trap densities in the HaP either using ideal CTLs or optimizing the fabrication route is crucial to improving the device parameters approaching the theoretical limit.
B1-O1
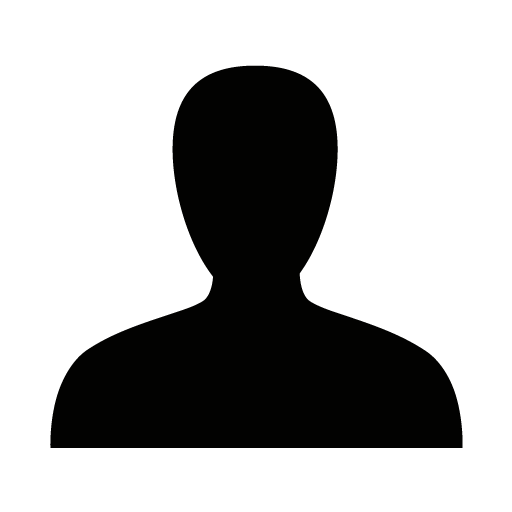
Designing organic molecules efficient for charge extraction and transport when integrated into optoelectronic devices remains a great challenge for many advanced applications. In perovskite solar cells (PSCs), the hole extraction/transport and the device stability are strongly dependent on the molecular structure of the hole transporting material (HTM). Herein we have engineered a dendritic core carbazole-based HTM, which combines the advantages of both small molecules and polymeric materials. We have investigated in-depth the relationship between the chemical structure of the HTM and both the photovoltaic efficiency and the device stability. The development of new HTMs alternative to Spiro-OMeTAD and the understanding of the role of doping agents on these layers are also important research axes in the field. It requires the use of appropriate characterization tools enabling to discriminate the bulk and interface effects. In the present work, we fully analyze the effect of HTM doping and of the material on the impedance response of PSCs. It has been shown that the dendritic core is a promising approach leading to both enhanced device performance and stability. The new HTM has been proved to act as a good barrier and protect satisfactorily the perovskite surface. The power conversion efficiencies (PCE) increase from 11.5% for the simple model compound to a promising 14.6%.Additionally, the normalized PCE of carbazole-based PSC decreased by only 5% after more than three weeks of storage under ambient conditions meanwhile the cell using the most popular HTM (Spiro-OMeTAD)dropped off by more than 40%. The presented results demonstrate that introducing dendritic concept is a simple strategy to design HTM for efficient and stable PSC (1). We also show that the impedance spectroscopy is of high practical interest for the development of new HTMs and for the optimization of the layer doping (2).
C1-IS1
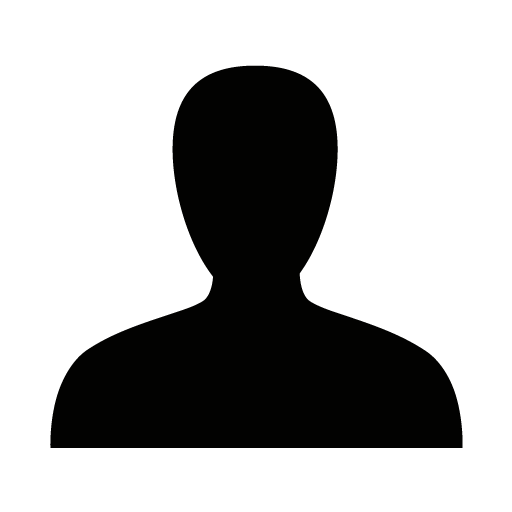
Colorful, semitransparent organic photovoltaic cells (OPVs) are increasing in demand due to their applicability in aesthetically-fashioned power-generating windows. The traditional method of generating different colors in OPVs has been through employing different active materials exhibiting distinct absorption spectra. This can complicate fabrication processes for production and cause deviations in device performance among differently colored OPVs. Herein, we demonstrate semitransparent and colorful OPVs with a single broadband-absorbing active material of fixed thickness using Ag-TiOx-Ag color filters (CFs). The CF enables the OPV to transmit spectrally pure colors with peak transmission efficiencies surpassing 25%, and allows the colors to be freely tuned without disturbing the charge transport properties such that the device performance stays consistent. The CF not only functions as an effective electrode but as a mirror for light outside of the spectral transparency window. We show that when compared to a transparent OPV consisting of the same active layer material and thickness, CF-integrated OPVs generate 32.38(±0.03) ~ 34.10(±0.02)% more short circuit current. Such results point to a simple and convenient solution to achieving spectrally pure colors, moderate transmission efficiencies, decoupled optical and charge transport properties, and enhanced charge generation relative to a transparent OPV in semitransparent, colorful power-generating windows.
C1-O6
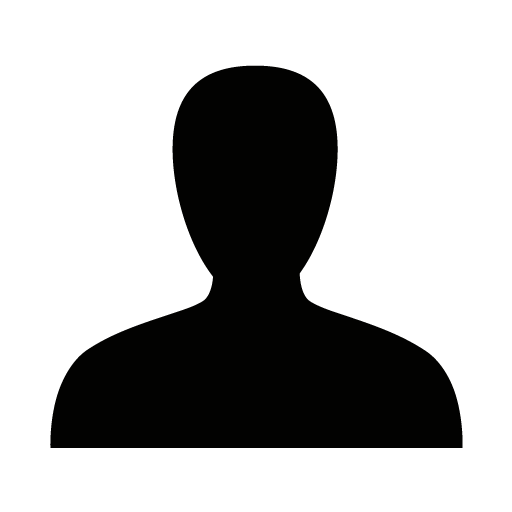
It is often a great challenge to achieve optimal performance in organic photovoltaic devices (OPVs) because of the limited control over the arrangement of constituent materials in the active layer. In this connection, we are exploring the potential of a unique “photoprecursor approach” for controlled deposition of small-molecule organic semiconductors [1–3]. This approach employs α-diketone (DK)-type derivatives of acenes as solution-processable precursors that can be cleanly converted to corresponding acene compounds upon visible-light irradiation. The conversion of DK-type photoprecursors is generally accompanied with a considerable decrease in solubility, and when the solubility of resulting acene is sufficiently low, one can deposit another material on top of it via a solution process for constructing a multilayer structure. We have applied this sequential solution deposition to the preparation of ternary OPV active layers with a controlled vertical composition profile [4]. The resulting devices showed an approximately two times higher power-conversion efficiency as compared to the corresponding binary bulk-heterojunction system. This work opens up new possibilities in designing materials and active layers for solution-processed OPVs and other organic electronic devices.
C1-O5
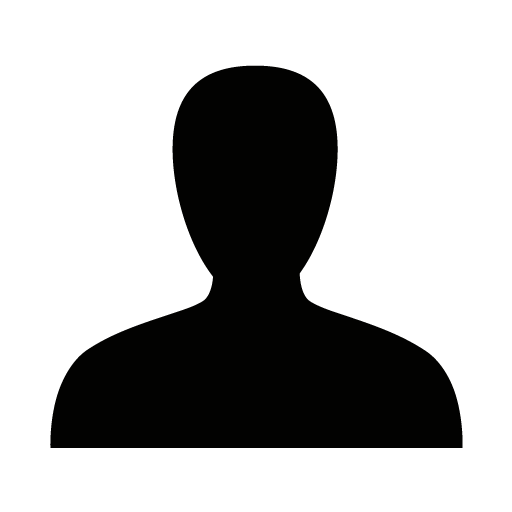
While inorganic solar cells enjoy a level of success, high manufacturing costs and bulky modules limit their broad applicability. In contrast, organic photovoltaic (OPV) devices employ flexible thin-films of organic small molecules or polymers as the photoactive layer. OPV could provide a low-cost alternative to traditional PV, and a system of solution processing coupled with roll-to-roll printing of lightweight, flexible solar cells is envisioned. However, current OPV technology relies on precise control of the domains and orientations of the organic molecules in the active layer, and these are difficult to achieve and maintain with processing techniques during printing. Furthermore, many high-performance OPV materials rely on toxic solvents for processing which is not environmentally appealing.
In this research we address these shortcomings through the use of amphiphilic block copolymers as active layer materials. Block copolymers are well-known for their ability to adopt regular morphological structures in thin-film, controllable by varying the structure of the polymer components.1 Careful design can ensure that desired morphologies are thermodynamically favoured, leading the polymers to readily adopt and maintain the ideal conformations. Amphiphilic block copolymers, in which hydrophilic moieties are incorporated into one block, are thermodynamically more likely to undergo phase separation into well-defined morphologies. Additionally, the suitably chosen hydrophilic moieties can increase the solubility of the active layer material in green solvents. In this work, we demonstrate the benefit of the amphiphilic block copolymer systems and their spontaneous adoption of thermally stable morphologies when deposited from industrially relevant solvents.2,3 We then use X-ray scattering techinques to examine the interplay between phase separation, crystallinity, and device performance in two novel block copolymer materials.
C1-O4
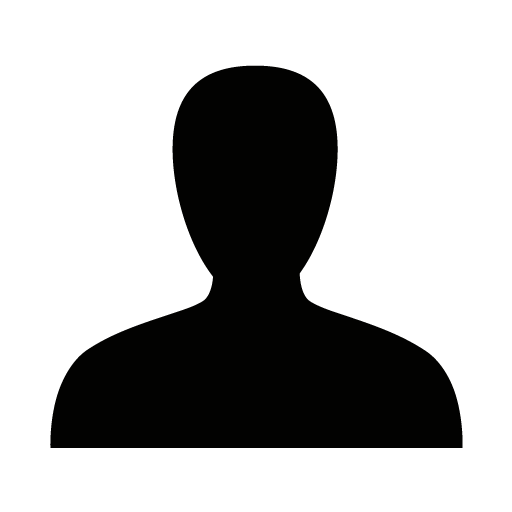
Precise control of the molecular arrangements at the donor/acceptor interfaces in mixed bulk heterojunctions (BHJs) remains challenging, despite its vital importance for achieving high efficiency in organic photovoltaics (OPVs). This study reveals that the patterns of linear and branched alkyl side chains on electron-donating/-accepting alternating copolymers (D-A copolymers) can control the positions of fullerene acceptor molecules around the polymer main chains in mixed BHJs. Two-dimensional 1H-13C heteronuclear correlation solid-state NMR (2D HETCOR SS-NMR) results show a clear difference in the location of fullerene molecule between the BHJ films for twin polymers BDTP-1 and BDTP-2. A copolymer (BDTP-1) with an electron-accepting unit positioned in close proximity to fullerene molecule shows higher OPV performance. This is the first report to clearly demonstrate the molecular arrangements at the donor/acceptor interface in mixed BHJs. Our polymer design offers precise control over the interfacial molecular structure, thereby paving the way for overcoming the current limitations of OPVs based on mixed BHJs.
C1-O3
Tomokazu Umeyama was born in 1976 and studied chemistry at Kyoto University. He received his BS (1999), MS (2001), and PhD (2004) in polymer chemistry under the guidance of Prof. Y. Chujo. He was also a fellow of the Japan Society for the Promotion of Science (JSPS) in 2003-2004. He moved to the Department of Molecular Engineering in the same institute and has been an Assistant Professor (2004−2013) and an Associate Professor (2013−) in the group of Prof. Imahori. His current interests involve development of photofunctional organic and nanocarbon materials.
[70]Fullerene derivatives are preferentially employed in organic photovoltaic (OPV) devices compared with [60]fullerene derivatives. A C70 derivative of [6,6]-phenyl-C61 butyric acid methyl ester ([60]PCBM), i.e. [70]PCBM, and its analogues are widely used in high-performance OPV devices in combination with low-bandgap conjugated polymers. However, an important aspect of C70 derivatives, which is usually overlooked in photovoltaic applications, is that as-prepared C70 mono-adducts consist of regioisomers due to the lower symmetry of rugby-ball shaped C70 over spherical C60. As contrasted with C60, C70 has four distinct types of [6,6]-bonds (a-, b-, e-, and k-type bonds), and thereby [70]fullerene derivatives including [70]PCBM have been used as a regioisomer mixture in OPV devices. Considering that the miscibility with conjugated polymer donors and the molecular arrangement of [70]fullerene derivatives in the active layer have a large impact on charge separation and charge-transporting properties, pure isomers of [70]fullerene derivatives rather than the isomer mixtures would yield a more desirable donor-acceptor network toward highly efficient OPVs.
In this study, by using a high-performance liquid chromatography (HPLC) suitable for the separation of fullerenes, we separated as-purchased [70]PCBM (denoted as mix-[70]PCBM) into isomerically pure ones and investigated the isomer separation effects on the photovoltaic properties [1-4]. For instance, in combination with a high-performance conjugated polymer donor (PffBT4T-2OD), one of the diastereomers of the b-isomer (b1-[70]PCBM) showed an extraordinary cohesion nature in the blend film, deteriorating the OPV device performance (0.4%). OPV devices based on the other isomers, i.e. a-[70]PCBM (8.4%) and b2-[70]PCBM (8.4%), rival or slightly surpass the device with the as-synthesized isomer mixture (mix-[70]PCBM, 8.2%).
C1-O2
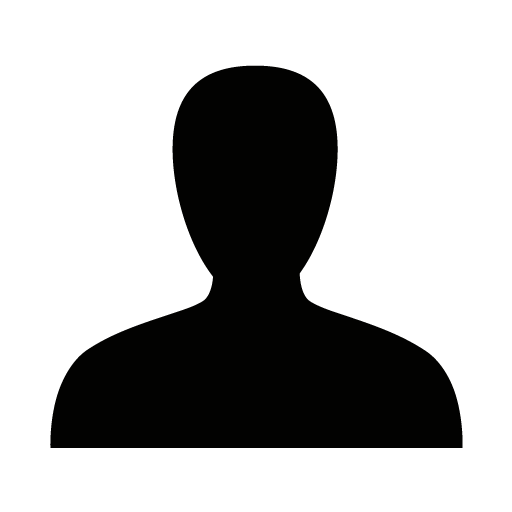
To improve power conversion efficiencies (PCEs) of organic solar cells (OCSs), it is critical to reduce the relatively large photon energy loss (Eloss) which is defined by Eex−eVOC, where Eex and Voc are the optical bandgap (the exciton energy) and the open-circuit voltage (VOC), respectively. The Eloss is typically 0.7–1.0 eV in high-efficiency OSCs, while those of ca. 0.6 eV have been recently reported by several groups [1]. However, the mechanism of the energy loss has not yet been fully understood.
In the operation of OSC, the exciton generated by the photo-absorption in an organic layer is separated through the charge-transfer (CT) state at the donor/acceptor interface to the free carriers of the hole in the donor and the electron in the acceptor, called the charge-separation (CS) state. The free carriers are collected by the electrodes where the difference in the quasi Fermi levels at the electrode is Voc. Thus, the energy of each step is necessary to understand the mechanism of the energy loss. Compared with the energies of the excition and CT states as well as Voc, the energy of the CS state has been only roughly evaluated. Basically, the CS energy is the sum of the ionization energy of donor ID and the electron affinity of acceptor AA. These values are usually estimated from the oxidation potential of the donor and the reduction potential of the acceptor measured using cyclic voltammetry, respectively. Recently, it has been pointed out that the energy levels around the donor and acceptor interface can depend on the molecular orientation (or the orientation of polymer backbone in polymers) and roughness at the bulk-heterojunction [2]. Such variation of energy levels is explained by the electrostatic interaction between the molecular ion and the permanent charge of the surrounding molecules which depends on the molecular orientation and the macroscopic shape of the film. Thus the CS energy should be determined by ID and AA of the film with the bulk-heterojunction structure instead of ID and AA of the individual materials measured in the solution.
In the previous work, we have demonstrated that the energy levels of hole and electron states can be precisely determined by use of the ultraviolet photoelectron spectroscopy (UPS) and low-energy inverse photoelectron spectroscopy (LEIPS) [3,4], respectively. Further, the electrostatic energy has been evaluated from the UPS and LEIPS data [5,6]. In this work, extending this idea, we demonstrate a procedure to determine the energy of the CS level in the bulk-heterojunction. We apply this method to the low-energy loss OSC, the bulk-heterojunction of PNOz4T:PCBM [1] and the result was compared with that of the high-efficiency OSC with the ordinal Eloss, PNTz4T:PCBM. The determined CS energy is found to be higher by 0.1-0.2 eV than the CT energy in each D-A combination indicating that difference in the energy loss between the ordinary and the low energy loss OCSs mainly occurs at the process from the exciton to the CT states. The resultant energy increase between the CT and CS states will also be discussed in terms of a role of the entropy [7].
C1-O1
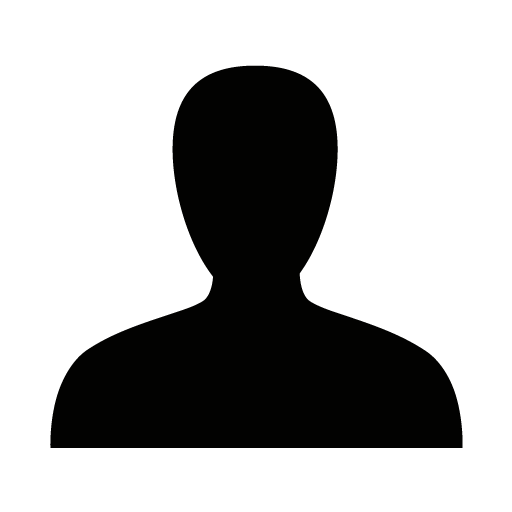
During the last two years, the power conversion efficiencies (PCEs) of polymer solar cells (PSCs) could be increased beyond 13% for single and 17.3% for tandem heterojunctions due to the discovery of novel non-fullerene acceptor (NFA) [1, 2]. In the field of NFAs, we have recently studied ternary blend approaches to increase open circuit voltage [3] as well the determination of charge transfer state energies from luminescence spectra in solar cells [4]. As now the performance of NFA based PSCs makes it highly promising as photovoltaic technology for a large number of niche markets, it is important to study aspects such as processing of PSCs with industrial relevant materials and printing techniques. Ink-jet or digital printing is one of the promising techniques. Indeed it allows to generate PSCs controlled in shape and size for niche markets demanding personalized engineering of solar cells. Recently ink jet printing was used to print PSC with efficiencies [5] over 4 % and tunability in shape [6]. Improvements in the efficiency can be expected by the usage of novel NFAs family of the ITIC based on indacenodithieno-[3, 2-b]-thiophene, (IDT), usually end-capped with 2-(3-oxo-2, 3-dihydroinden-1-ylidene)-malononitrile [1] in combination with more efficient printable interfacial layers and electrodes. We recently demonstrated that specific formulations of PEDOT:PSS allow to print highly efficient PSC using fullerene based acceptor with efficiency over 6.5 % [S. Ben Dkhil, P. Pierron, O. Margeat, J. Ackermann, patent application submitted 2018, paper in preparation] revealing the high potential of PEDOT:PSS for ink jet printing.
In order to go beyond this efficiency and to benefit from the unique properties of the new generation of non fullerene acceptors, we studied here two ITIC derivatives in combination with PBDB-T as well as PTB7-Th polymers towards stable fully solution processed high efficiency OSCs. First we optimized interfacial layers for fully solution processing of PCSs based on NFAs. We show that replacement of MoOx as HTL by PEDOT:PSS in an inverted structures introduce only performance losses from 9.4% for PBDB-T:ITIC to 7.7% making fully printed high efficiency PSCs possible. Finally, we present fully ink jet printed ITIC based solar cells processed in air and evaluate their stability under storage conditions and under illumination compared to devices that were processed by spin coating under Argon.
G3-K1
Sang Il Seok is a Distinguished Professor in the Department of Energy and Chemical Engineering at the Ulsan National Institute of Science and Technology (UNIST) in Korea. He obtained his Ph.D. in the Department of Inorganic Materials Engineering from Seoul National University in Korea. After completing his Ph.D., he worked as a postdoctoral researcher at Cornell University in the USA, where he investigated defects and transport in the Fe-Ti-O Spinel structure. He also experienced a visiting scholar at the University of Surrey in UK in 2003 and École Polytechnique Fédérale de Lausanne (EPFL) in Switzerland in 2006. Prior to joining UNIST in 2015, he served as the principal investigator of the Korea Research Institute of Chemical Technology (KRICT) and as a professor at the Department of Energy Science at Sungkyunkwan University. He is a highly cited researcher, selected by Clarivate Analytics since 2018, and has published over 200 peer-reviewed papers, including three Nature and seven Science articles as a corresponding author. He has received several awards for his excellence, such as the "Korean Scientist Award" from the Korean government in 2017, and the Kyung-Ahm Prize in 2019. In 2022, he was awarded the Rank Prize, recognized as one of the seven scientists pioneering in perovskite solar cells. His research focuses on functional inorganic-organic hybrid materials and devices, particularly on perovskite solar cells.
Over the last several years, inorganic-organic hybrid perovskites have shown dramatic achievements in photovoltaic performance and device stability via additive engineering. In addition to the role of antisolvents in solvent engineering processes to control nucleation and crystal growth, precursor-coordinating molecule interactions in solution are also affected by various additives. Therefore, additives contribute not only to the formation of uniform and dense cotings but also to efficiency and stability improvement due to defect concentration and passivation effect of the resultant perovskite film. Furthermore, as far as the fabrication of perovskite solar cells by the solution process is concerned, it is necessary to consider not only the chemical factors of the precursor solution but also the physical properties of the coating solution such as viscosity, evaporation rate of solvents, the wettability and penetration onto substrates. Fundamentals understanding of precursor solution chemistry with additives will be able to achieve further improved photovoltaic performance and device stability. Various additives such as a cation or an anion are capable of functioning as active species in improving the quality of perovskite films. In this presentation, structural evolution and function by additives such as a long alkylammonium halide and metal halide etc. will be presented.
G3-I1
Christopher Case is the Chief Technology Officer at Oxford PV, a spin-out of Oxford University (UK) that is commercialising perovskites for tandem solar cell applications. Most recently, he was the Chief Technology Officer for Linde Electronics, a gas and equipment supplier and the former Chief Scientific Officer of The BOC Group (UK). A long time chair of the International Technology Roadmap for Semiconductors, he spent 10 years at AT&T Bell Labs in Murray Hill, NJ (US). He was an assistant professor of engineering at Brown University and director of the Thin Film Institute. He was a Fulbright-Hays scholar at the Université de Bordeaux and holds a Ph.D. degree in materials science from Brown University where he studied thin film chalcopyrite photovoltaic materials.
Single junction silicon PV technology has reached the extremely impressive feat of 26.7% power conversion efficiency but is forever shackled by the thermodynamic limits of Schockley and Queisser to efficiencies below 30%. Multijunction technology is the roadmap for mass deployment of photovoltaic technology beyond 30% - raising the fundamental limit to 45% for silicon tandem and 49% for triple junction. In a few short years the perovskite technology has reached 23.3% in single junction and in tandem with silicon achieved 27.3% - exceeding for the first time the very best silicon single junction. Theoretical combination of existing best-in-class single junctions predict obtainable tandem and triple junction efficiencies of 34% and 39% respectively. So what is between us and the 34% efficient perovskite-silicon tandem? We discuss the specific challenges to overcome and our progress on the tandem roadmap beyond 30%, addressing fundamental loss mechanisms in the perovskite absorber and its contact heterojunctions as well as aspects of optical design and deployment in mono or bifacial format. We will address how these solutions can be delivered at the full wafer-scale in a production environment at rates of tens of thousands of wafers/hr.
G3-I2
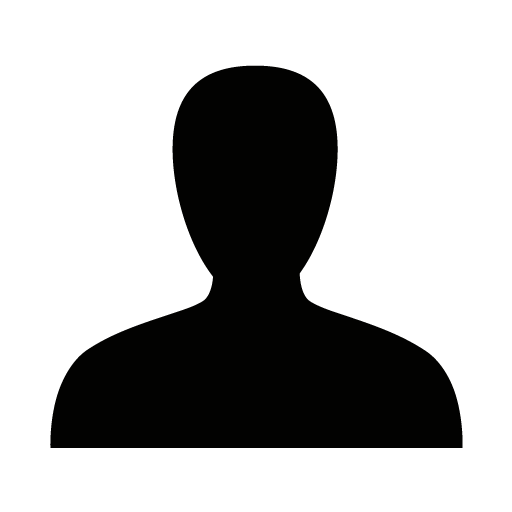
Perovskites have emerged as low-cost, high efficiency photovoltaics with certified efficiencies of 22.1% approaching already established technologies. The perovskites used for solar cells have an ABX3 structure where the cation A is methylammonium (MA), formamidinium (FA), or cesium (Cs); the metal B is Pb or Sn; and the halide X is Cl, Br or I. Unfortunately, single-cation perovskites often suffer from phase, temperature or humidity instabilities. This is particularly noteworthy for CsPbX3 and FAPbX3 which are stable at room temperature as a photoinactive “yellow phase” instead of the more desired photoactive “black phase” that is only stable at higher temperatures. Moreover, apart from phase stability, operating perovskite solar cells (PSCs) at elevated temperatures (of 85 °C) is required for passing industrial norms.
Recently, double-cation perovskites (using MA, FA or Cs, FA) were shown to have a stable “black phase” at room temperature.(1,2) These perovskites also exhibit unexpected, novel properties. For example, Cs/FA mixtures supress halide segregation enabling band gaps for perovskite/silicon or perovskite/perovskite tandems.(3) In general, adding more components increases entropy that can stabilize unstable materials (such as the “yellow phase” of FAPbI3 that can be avoided using the also unstable CsPbI3). Here, we take the mixing approach further to investigate triple cation (with Cs, MA, FA) perovskites resulting in significantly improved reproducibality and stability.(4) We then use multiple cation engineering as a strategy to integrate the seemingly too small rubidium (Rb) (that never shows a black phase as a single-cation perovskite) to study novel multication perovskites.(5)
One composition containing Rb, Cs, MA and FA resulted in a stabilized efficiency of 21.6% and an electroluminescence of 3.8%. The Voc of 1.24 V at a band gap of 1.63 eV leads to a very small loss-in-potential of 0.39 V, one of the lowest measured on any PV material indicating the almost recombination-free nature of the novel compound. Polymer-coated cells maintained 95% of their initial performance at 85°C for 500 hours under full illumination and maximum power point tracking. This is a crucial step towards industrialisation of perovskite solar cells.
Lastly, to explore the theme of multicomponent perovskites further, molecular cations were revaluated using a globularity factor. With this, we calculated that ethylammonium (EA) has been misclassified as too large. Using the multication strategy, we studied an EA-containing compound that yielded an open-circuit voltage of 1.59 V, one of the highest to date. Moreover, using EA, we demonstrate a continuous fine-tuning for perovskites in the "green gap" which is highly relevant for lasers and display technology.
The last part elaborates on a roadmap on how to extend the multicatio to multicomponent engineering providing a series of new compounds that are highly relevant candidates for the coming years.
(1) Jeon et al. Nature (2015)
(2) Lee et al. Advanced Energy Materials (2015)
(3) McMeekin et al. Science (2016)
(4) Saliba et al., Cesium-containing triple cation perovskite solar cells: improved stability, reproducibility and high efficiency. Energy & Environmental Science (2016)
(5) Saliba et al., Incorporation of rubidium cations into perovskite solar cells improves photovoltaic performance. Science (2016).
G3-I3
Professor Kwanghee currently leads research and development program of organic solar cells in Gwangju Institute of Science and Technology (GIST) as a director of the Research Institute of Solar and Sustainable Energies (RISE). He is also appointed as a “Distinguished Professor” of the School of Materials Science and Engineering of GIST. Dr. Lee started his professorship at Pusan National University in 1997 after finishing his Ph.D. and Post-Doc at the University of California Santa Barbara (UCSB). Then he moved to GIST in 2007 and have organized and acted as a co-director of the Heeger Center for Advanced Materials (HCAM) together with the director, Professor Alan J. Heeger, who is a 2000 year Nobel Laureate in Chemistry. Now Dr. Lee is a leading scientist in the area of “plastic electronics” including organic solar cells, polymer LEDs, and organic FETs. Dr. Lee finished his B.S. in Nuclear Engineering at Seoul National University and M.S. in Physics at KAIST. Then he earned his Ph.D. in Physics at UCSB (USA) under the guidance of Prof. Heeger with a subject of metallic and semiconducting polymers.
Realizing industrial-scale, large-area photovoltaic modules without any considerable performance losses compared with the performance of lab-scale, small-area perovskite solar cells (PSCs) has been a challenge for practical applications of PSCs. Highly sophisticated patterning processes for achieving series connections, typically fabricated using printing or laser-scribing techniques, cause unexpected efficiency drops and require complicated manufacturing processes. In this presentation, we report high-efficiency, large-area PSC modules fabricated via a new electrochemical patterning process. The intrinsic ion-conducting features of perovskites enabled us to create metal-filamentary nanoelectrodes to facilitate the monolithic serial interconnections of PSC modules. By fabricating planar-type PSC modules through low-temperature annealing and all-solution processing, we demonstrate a notably high module efficiency of 14.0% (a certified value of 14.1%) for a total area of 9.06 cm2 with a high geometric fill factor of 94.1%.
We also report our recent achievement of burn-in loss-free and high-performance PSCs with a power conversion efficiency (PCE) of 18.8% maintaining over 80% and 90% of their initial PCEs even after 1,000 hours of real-operation (under A.M 1.5G irradiation) and heat-damp (at 85°C in the dark) test under nitrogen gas atmosphere, respectively.
G3-I4
Maksym Kovalenko has been a tenure-track Assistant Professor of Inorganic Chemistry at ETH Zurich since July 2011 and Associate professor from January 2017. His group is also partially hosted by EMPA (Swiss Federal Laboratories for Materials Science and Technology) to support his highly interdisciplinary research program. He completed graduate studies at Johannes Kepler University Linz (Austria, 2004-2007, with Prof. Wolfgang Heiss), followed by postdoctoral training at the University of Chicago (USA, 2008-2011, with Prof. Dmitri Talapin). His present scientific focus is on the development of new synthesis methods for inorganic nanomaterials, their surface chemistry engineering, and assembly into macroscopically large solids. His ultimate, practical goal is to provide novel inorganic materials for optoelectronics, rechargeable Li-ion batteries, post-Li-battery materials, and catalysis. He is the recipient of an ERC Consolidator Grant 2018, ERC Starting Grant 2012, Ruzicka Preis 2013 and Werner Prize 2016. He is also a Highly Cited Researcher 2018 (by Clarivate Analytics).
We review the important differences that exist in the chemistry and physics of colloidal lead halide perovskite nanocrystals (APbX3, NCs, A=Cs+, FA+, FA=formamidinium; X=Cl, Br, I) as compared to conventional semiconductor NCs made of metal pnictides and chalcogenides. We survey the the synthesis methods, optical properties and prospects of these NCs for optoelectronic applications [1, 2, 3].
The absorption spectral, sponaneous and stimulated emission spectra of these NCs are readily tunable over the entire visible spectral region of 400-800 nm by composition as well as by the NC size and shape [4-5]. Post-synthestic chemical transformations of colloidal NCs, such as ion-exchange reactions, provide an avenue to compositional fine tuning or to otherwise inaccessible materials and morphologies [6]. The photoluminescence of these NCs is characterized by narrow emission line-widths of <100 meV (12-45 nm from blue-to-near-infrared), wide color gamut covering up to 140% of the NTSC color standard, high quantum yields of up to 100%. Cs- and FA-based perovskite NCs are highly promising for luminescence downconversion (bright and narrow emission at 530 and 640 nm; backlighting for displays), for light-emitting diodes and as precursors/inks for perovskite solar cells. In particular, high purity colloids are ideal for further engineering as needed for photochemical/photocatalytic applications. Towards these applications, a unique feature is that perovskite NCs appear to be trap-free without any electronic surface passivaiton, making photogenerated electrons and holes readily availably for surface chemical reactions.
The processing and optoelectronic applications of perovskite NCs are, however, hampered by the loss of colloidal stability and structural integrity due to the facile desorption of surface capping molecules during isolation and purification. To address this issue, we have developed a new ligand capping strategy utilizing common and inexpensive long-chain zwitterionic molecules, resulting in much improved chemical durability [7].
References:
M. V. Kovalenko et al. Science 2017, 358, 745-750
Q.A. Akkerman et al. Nature Materials, 2018, 17, 394–405
M. A. Becker et al, Nature, 2018, 553, 189-193
L. Protesescu et al. Nano Letters 2015, 15, 3692–3696
L. Protesescu et al. ACS Nano 2017, 11, 3119–3134
G. Nedelcu et al. Nano Letters 2015, 15, 5635–5640
F. Krieg et al. ACS Energy Letters 2018, 3, 641–646
G. Raino et al. Nature 2018, in print
G3-I5
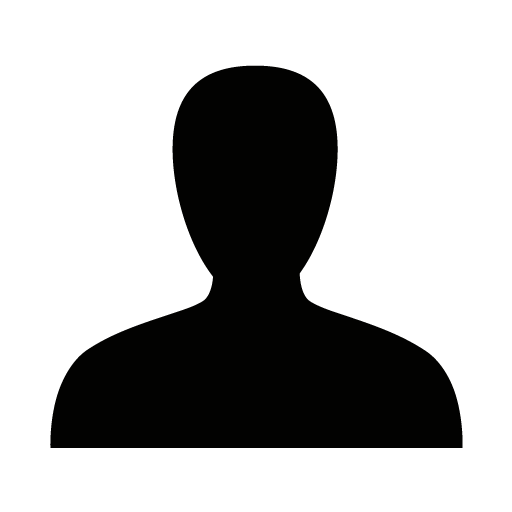
The A-D-A architecture has been proved quite successful for oligomer-like molecules used for both donor and acceptors in OPV. In this talk, the recent process from our group will be presented for these molecules used for both single junction and tandem cells. Our understanding for the reasons behind the success of the A-D-A architecture and recent modelling for the practical OPV performance limit will also be presented. With both material and device optimization, a PCE of 17.4% of tandem cell has been achieved. These result indicate that OPV has much more potential than previously thought.
Lingxian Meng, Yamin Zhang, Xiangjian Wan*, Chenxi Li, Xin Zhang, Yanbo Wang, Xin Ke, Zuo Xiao, Liming Ding*, Ruoxi Xia, Hin-Lap Yip, Yong Cao, Yongsheng Chen*, Science, 2018, 361, 1094-1098.
Miaomiao Li†, Ke Gao†, Xiangjian Wan*, Qian Zhang, Bin Kan, Ruoxi Xia, Feng Liu,Xuan Yang, Huanran Feng, Wang Ni, Yunchuang Wang, Jiajun Peng, Hongtao Zhang, Ziqi Liang, Hin-Lap Yip, Xiaobin Peng*, Yong Cao and Yongsheng Chen* , Nature Photon., 2017, 11, 85-90
talk-S1
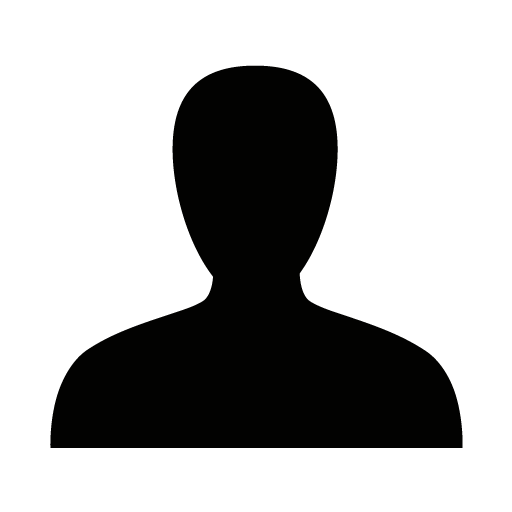
Greatcell Solar Limited (GSL) was listed on ASX as Dyesol Limited (DYE) in July 2005 and is headquartered in Queanbeyan, NSW, in Australia. The Company was founded to develop, scale-up and commercialise the rapidly emerging 3rd generation solar photovoltaic (PV) technology known as Dye Solar Cells (DSC), a technology invented by Grätzel and O’Regan at the École Polytechnique Fédérale de Lausanne in the early 1990s. Greatcell Solar is an original licensee of the EPFL core technology in perpetuity and also has an extensive patent portfolio of its own which extends across materials, processes and PV design.
DSC PV technology has lower embodied energy, is cheaper to produce and is more versatile than 1st and 2nd generation PV technology. It is particularly suited to building integrated photovoltaics (BIPV), often considered to be the end-point or ‘holy grail’ for PV development. DSC technology encountered technical challenges, though, as scientific investigation and development uncovered further potential for the 3rd generation of PV technology. Some of the materials it used were relatively expensive and the use of a liquid electrolyte raised issues as to its long-term durability. The Sun can be very destructive and long-term durability is a key attribute in successful commercialisation of PV panels.
A2-IS1
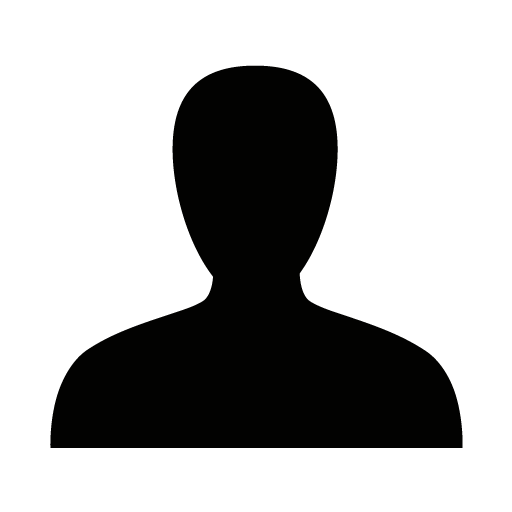
Organic lead halide perovskite possesses desirable optical and electronic properties for solar cell application resulting in rapid progress in device performance. The ease of fabrication and versatilities in cell design and cell processing and the many facets of material properties make metal halide perovskite solar cells the most researched solar technology in the last few years. I will be talking about some of the work in my research group that seeks to improve performance and stability and to reduce the reliance on lead for perovskite solar cells.
One of the stability issues is hysteresis observed in the current-voltage characteristic. In one of our work, full perovskite cells (not test structures) have been adapted to be compatible with photoluminescence characterizations. By analyzing the dynamic PL lifetime and intensity under different light soaking conditions and correlating these results with the shape of the voltage current curve measured under similar light soaking conditions, we explain the different scenarios of ion migration and accumulation at the interface and in the bulk that result in different hysteresis behaviors.
We have also been part of an inter-comparison of measurement methods for perovskite solar cells. I will present recommendations from this work for identifying the most appropriate measurement method for a given cell, depending on its stabilization and degradation behavior. This allows for more accurate and meaningful efficiency measurements of perovskite solar cells.
In terms of perovskite fabrication, the roles of bulky cations in organic lead halide perovskites are further understood. Depending on how these cations are employed, they can provide surface passivation or grain boundary passivation improving cell performance and stability. Their roles in assisting perovskite formation and their segregation behaviors will be presented.
In terms of deposition method, some of the new insights on two-step sequential solution process will be presented.
Work on inorganic cesium-based perovskites have provided many insights into the different film formation mechanisms when films are fabricated by different deposition methods. The role of lead-substituting-dopants i) on self-assembled surface passivation layer and ii) on engineering colloidal cluster size in the precursor solution and their subsequent effect of film morphology will be discussed.
We have also developed an electrode design that allows lower resistivity transparent conductive oxide to be used on the substrate while still achieving high efficiency at 19.63% certified on 1.02cm2.
Recent developments in encapsulation methods allowing perovskite cells to pass the IEC61646 standard damp heat, thermal cycling and humidity freeze tests will be presented.
Finally, details of recent work demonstrating >21% large area (>10cm2)monolithic perovskite/silicon tandem solar cell will be presented. The simplicity of the design (interface layer-free), the ease of scale-up to large area and the use of homo-junction-silicon as the bottom cell make the tandem cell design appealing for commercialization.
A2-O1
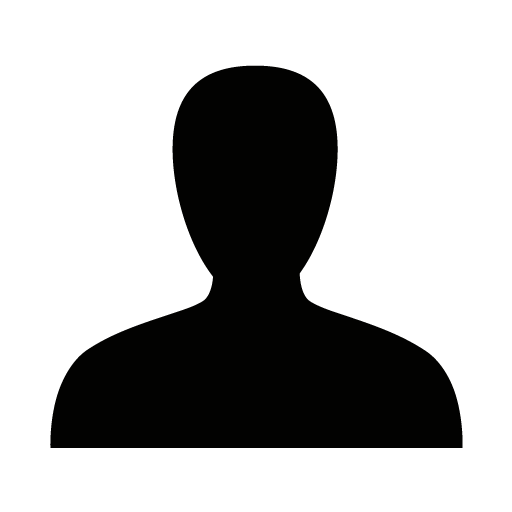
Semi-transparent perovskite solar cells (ST-PSCs) are highly attractive to improve the efficiency of commercial silicon (Si) and CIGS (copper indium gallium selenide) photovoltaic modules in tandem architectures. However, the commercial deployment of ST-PSCs is limited by stability and the development requires high IR transparency requirements.
In this work, efficient rigid and flexible semi-transparent perovskite solar cells (ST-PSCs) were prepared in inverted (light incidence through hole transport layer) configuration. All layers were deposited by a combination of low-temperature solution-based, sputtering and spatial atomic layer deposition (sALD) techniques, which can be potentially up-scalable for sheet to sheet and roll to roll manufacturing lines. The rigid and flexible opaque PSCs reach stabilized power conversion efficiency (PCE) of 19% and 16% [1], while rigid ST-PSC reaches 16.4% stabilized PCE. Besides those results, we have developed our current flexible opaque stack to the flexible semi-transparent stack using top TCO electrode and sALD buffer layer. We also highlight that the inverted configuration exhibited extremely high infrared transparency [2] and a remarkable stability during the light and thermal stress. The encapsulated ST-PSCs retained 93% of their initial stabilized PCE after 3000h aging at 85°C in a nitrogen atmosphere. Interestingly, using highly transparent transport layers, rigid and flexible ST-PSCs show comparable efficiency by illumination from both rear and front TCO sides, resulting in a bifacility of 99%.
In order to use the ST-PSCs in 4-terminal tandem architecture in combination with bottom c-Si and CIGS cells, the high near-infrared transmission (average 93%) of the ST-PSCs was optimized by improving TCO quality and tuning the thickness of component layers in cells [2]. The ST-PSC with optimized IR transparency was coupled with 6-inch ECN part of TNO (silicon heterojunction) SHJ c-Si bottom cell leading to 26.3% efficiency (with a possibility to further improvement by increasing the transparency of TCO electrodes and tuning the perovskite bandgap). Furthermore, using flexible ST-PSCs in combination with flexible CIGS cells, we created efficient flexible 4T tandem devices. The 4T tandem cells efficiencies are determined with the tandem measurement procedure as described by Werner et al. [3].
In conclusion, the stability, efficiency, IR transparency and scalability of PSCs developed for hybrid tandem applications, is reported. Further improvement on transparency and efficiency of our ST-PSCs and consequently on the enhancement of our tandem results will be reported during the conference. By combining the rigid and flexible ST-PSCs with c-Si and CIGS bottom cells state-of-the-art results are obtained.
A2-O2
Perovskite solar cells have shown impressive power conversion efficiency (PCE) over the last five years. To reduce the cost and overcome the Shockley-Queisser limit of the single junction solar cells, the tandem structure becomes an emerging technology. In this study, organic/perovskite two-terminal hybrid tandem solar cells, which made from an organic solar cell of P3HT:ICBA and the state-of-the-art perovskite solar cell of MAPbI3, were successfully air fabricated. The perovskite sub-cell and organic sub-cells are made from solution-processed method. An open circuit voltage (VOC) of 1.7 V of tandem solar cells is achieved, which sums the VOC of organic and perovskite sub-cells, 0.8 V and 0.9 V respectively. The current-voltage hysteresis of hybrid tandem solar cells mainly comes from the perovskite sub-cell, while that of organic sub-cells is zero. It was greatly suppressed by a modified interface of ZnO and perovskite layers with a buffer layer of PCBM. The results offer a facile and attractive way to the cost-efficient organic/perovskite tandem solar cells.
A2-O3
To date, halide perovskites are extraordinary photovoltaic materials that enabled to prepare solar cells of more than 23% power conversion efficiency which surpasses commercialised PV technologies based on CIGS and CdTe [1]. Nonetheless, the operational long-term stability is one of the most pressing challenges that may hinder their commercialization. The devices lose initial efficiency because of the convolution of external factors such as moisture, heat, light, oxygen, as well as intrinsic instability of the perovskite. Recent studies have shown that many degradation pathways start from the defects of the material. The films with a large defect concentration are more prone to degradation. Hence, a key pathway towards the long-term stability and high efficiency of halide perovskite solar cells is to reduce the defects concentration presented in the material. Incorporating small amounts of metal cations into the perovskite precursors has been shown to improve device performance and stability. For example, monovalent cations such as rubidium, and potassium are particularly effective to boost efficiency in state-of-the-art devices [2].
More recently, divalent cations especially alkaline earth metals are attracting growing attention. It has been speculated that the divalent cations such as strontium can passivate the surface of perovskite and significantly improves device’s performance [3]. It seems that the small amount of foreign ions in the precursor has a different working mechanism compared to the classical doping of inorganic semiconductor in which the charge carrier concentration is changed due to dopants.
Herein, we propose a metal cation doping mechanism of halide perovskite to passivate defects. In this work, we explore the effect of strontium and magnesium on methylammonium lead iodide. We use advanced characterisation technique based on synchrotron high energy X-ray source to understand the impact of foreign ions on the chemical and electronic environment of the material. Furthermore, X-ray Diffraction patterns refinement creates an opportunity to identify the interaction of the dopants with methylammonium lead iodide lattice. From the experimental results, we speculate that the dopants affect the defect chemistry of perovskite which is supported by the theoretical calculation. Then, we prepare perovskite solar cells to prove the mechanism in the state-of-the-art devices. As a result, we are able to demonstrate more than 1.15 V open circuit voltage for doped methylammonium lead iodide which is much higher than commonly reported 1 V for this type of perovskite solar cells.
A2-O4
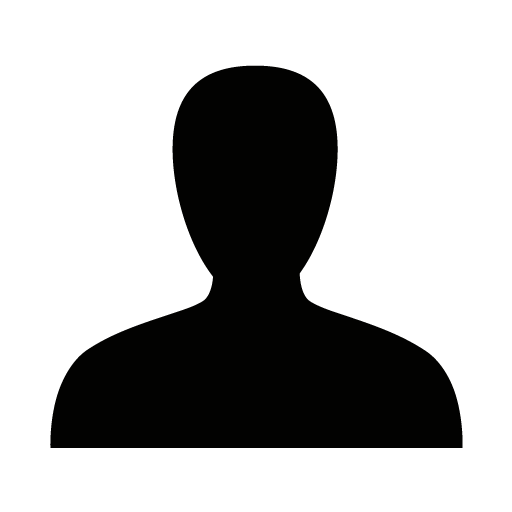
Solution-processed organic–inorganic perovskites based on metal halides have recently gained considerable interest as one of the most promising materials for next-generation photovoltaic devices. This is because of their excellent optoelectronic properties such as direct bandgap with high absorption coefficient, high charge carrier mobility, long diffusion length of charge carriers, Wannier–Mott exciton, and low trap densities. Since the pioneering work of Miyasaka coworkers in 2009, the power conversion efficiency (PCE) of lead-based perovskite solar cells has steeply increased from 3.8 to more than 23%. A further improvement in PCE is still possible by considering the Shockley–Queisser (SQ) limit (≈30%) for photovoltaic efficiency with an optical bandgap (Eg) of absorber (≈1.6 eV). On the other hand, environmentally friendly perovskite solar cells without lead(ΙΙ) element have been in the spotlight from a commercial point of view. More recently, several research groups have successfully fabricated lead-free perovskite solar cells by replacing lead(ΙΙ) element with tin(ΙΙ) element. In most cases, however, the PCE was as low as less than 10%, which still lag far behind that of lead-based counterparts. This is mainly ascribed to a small open-circuit voltage (VOC) (0.2–0.5 V) for tin-based devices. In other words, there is a difference in photon energy loss Eloss (= Eg − qVOC) between the two devices.
Herein, we studied the origin of Eloss in both lead-based and tin-based perovskite solar cells. By measuring the temperature dependence of VOC, we discussed the difference in Eloss between them in terms of charge recombination mechanism. As a result, we found that an activation energy of the diode (Ea), evaluated from a linear extrapolation at 0 K on the basis of qVOC = Ea – kBTln(J00/JSC), is almost the same as the Eg of perovskites for lead-based perovskite solar cells. In contrast, it is substantially smaller than the Eg of perovskites for tin-based devices.[1,2] This suggests that the charge recombination mechanism underlying Eloss is different. In other words, the main loss mechanism is the bulk recombination in a perovskite layer for lead-based perovskite solar cells while that is the surface recombination at the interface for tin-based perovskite solar cells. We further discuss the potential strategies for further improvement of VOC in both devices.
A2-O5
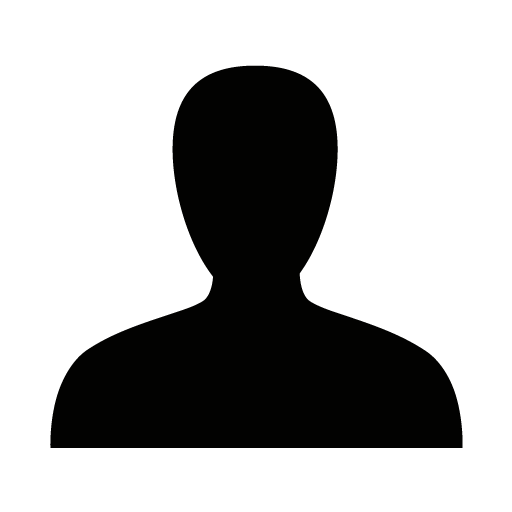
After the seminal work from our group,1 the power conversion efficiency (PCE) of organic inorganic lead halide perovskite solar cells have surpassed 20%.2 Such steep rise in PCE is attributed to perovskite exceptional optoelectronic properties such as ambipolar charge mobility, long carrier diffusion length, low exciton binding energy etc. Despite of several advantages, lead perovskites suffer from long term stability and lead toxicity issue.2, 3 The organic component in lead-perovskite is hygroscopic and gets eliminate from the crystal structure upon exposure to moisture, heat and continuous light illumination leaving behind the lead iodide (non-photoactive component). The exposure of toxic lead to human environment can cause severe damage to nervous and reproductive system and is believed to be an obstacle for commercialization.4 Therefore, development of Pb-free light is vitally important. In this regards, three dimensional (3D) silver-bismuth halide (SBH) materials such as AgBi2I7 have emerged as a promising candidate. However, AgBi2I7 crystallizes at 150 OC and this leads to loss of BiI3 from the crystal structure. Hence obtaining phase pure AgBi2I7 is of critical importance to further evaluate its potential for photovoltaic applications. In the present work, we show solvent engineering approach to obtain phase pure AgBi2I7 thin film at relatively low temperature (90 OC). As a result, AgBi2I7 device incorporating dopant-free HTM demonstrated 2% efficiency with high reproducibility. Moreover the best performing device shows exceptional stability against 60% relative humidity for 70 days and continuous 1 Sun illumination (at maximum power point tracking) for 1 h. This study also reveals an important correlation between solvent-mediated adduct and performance and stability of AgBi2I7 based solar cells and can help in enhancing the performance and stability further, paving a way towards non-toxic lead-free solar cells.
References
A. Kojima, K. Teshima, Y. Shirai, and T. Miyasaka, J. Am. Chem. Soc., A.B Author and C.D. Author, Journal of Materials, 131 (2009), 6050.
T. Miyasaka, Chem. Lett., 44 (2015), 720.
A. Kulkarni, T. Singh, M. Ikegami and T. Miyasaka, RSC Adv., 7 (2017), 9456.
A. Babayigit, A. Ethirajan, M. Muller, B. Conings, Nature Materials, 15 (2016), 247.
B2-IS1
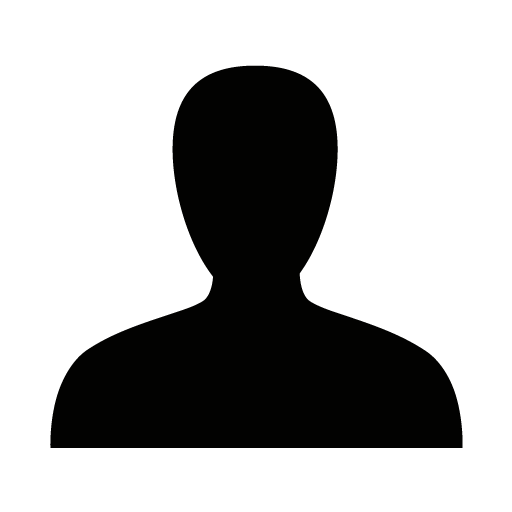
The remarkable performance of perovskite solar cells (> 20%) is hindered by the fact that this material present dynamic optical and electrical responses, which can lead to degradation. Specifically, identifying and controlling the effect of water, oxygen, temperature, bias, and light on their physical behavior is a pressing topic of research. To unfold the contribution of each intrinsic and extrinsic parameter on materials’ properties and devices’ performance, we combine advanced scanning probe methods based on photoluminesce (PL) microscopy and atomic force microscopy (AFM). We investigate a series of hybrid perovskites, including MAPbI3, MAPbBr3, CsxFA1−xPb(IyBr1−y)3 , and triple cation Cs-mixed. Through environmental PL microscopy we identify a humidity-induced PL hysteresis that strongly depends on the Cs/Br ratio [1]. Using fast Kelvin-probe force microscopy we quantify a dynamic open-circuit voltage (Voc) response as a function of perovskite chemical composition and illumination treatment, as will be discussed in details during the presentation [2,3]. The individual and collective effects of the five abovementioned parameters on perovskites ability to recover are further investigated using a machine learning (ML) approach. Our functional imaging platform [4,5], combined with ML, can be expanded to test the stability of emerging perovskites, including Pb-free options, and novel perovskites for multijunction solar cells.
B2-O1
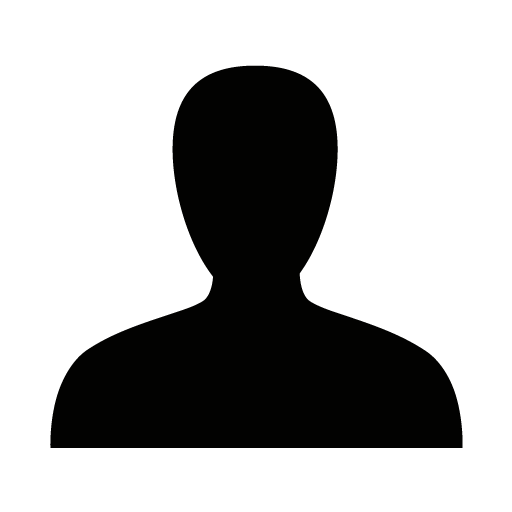
Organic inorganic hybrid perovskites (OIHP) is the most promising material to achieved high power conversion efficiency (PCE) at low cost. The high-quality optoelectronic properties in combination with solution-based preparation methods are responsible for the currently certified PCE record of 23.3%, which is close to the PCE of single crystal silicon solar cells (26.1%). OIHP is generally labelled as an ABX3compound, where A is a monovalent cation such methylammonium (MA), formamidinium (FA), or cesium (Cs), B is a divalent metal, such lead (Pb) or tin (Sn), and X is a halide anion, bromide (Br), iodide (I-). The properties of the perovskite film are direct related to film morphology, composition and crystalline structure, thus a clear understanding of how and when the intermediate and the perovskite phases are forming, as well the distribution of these multiple phases in the bulk and grains boundaries are important questions to be addressed in order to improve perovskite film properties and consequently the PCE of the devices. In this presentation, we will summarize our most recent results using in situtime-resolved grazing incidence wide angle x-ray scattering (GIWAXS) and synchrotron infrared nanospectroscopy (nano-FTIR). GIWAXS experiments allowed us to understand the influence of the relative humidity, type of solvent and time to drop the antisolvent during the preparation of mixed cation perovskite films. We also identified intermediates formed before and during the spin coating process. Nano-FTIR technique was applied for the first time on OIHP. Our results revealed a spatial heterogeneity of the vibrational signal, which are associated to different chemical composition. The nano-FTIR permitted to access grain-to-grain chemistry of OIHP and the identification of PbI2and hexagonal phases which are distributed randomly in a background formed by cubic (black phase) perovskite.
B2-O2
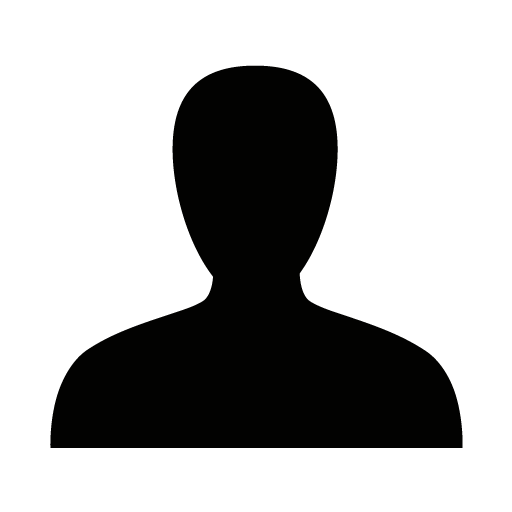
Perovskite solar cells have been attracting increasing attention during half a decade. The control of the crystal structure and analysis of crystallization dynamics of perovskite is very important research topic from the both standpoint of fundamental study and device application. We have been focusing on the fabrication control of perovskite in vacuum by laser deposition method [1] and crystallization dynamics analysis of perovskite by grazing incident X-ray diffraction (GIXD) at SPring-8 [2]. In this presentation, we would like to report our recent research results of crystallization dynamics analysis during laser deposition process or perovskite.
Using laser deposition method, material-supply rate of CH3NH3I and PbI2 was well controlled, which is beneficial for the crystallization control of perovskite. We succeeded in the epitaxial growth of CH3NH3PbI3 on rubrene single crystal, where CH3NH3PbI3 oriented commensurate along with the rubrene crystal direction and atomically flat surface was constructed. The benefit of laser deposition method for perovskite fabrication was demonstrated. We designed special vacuum chamber where laser deposition and GIXD measurement can be conducted simultaneously. The chamber was installed at BL46XU in SPring-8 and crystallization dynamics of the deposition process of perovskite was analyzed. Firstly, PbI2 film was deposited on glass substrate and c-axis oriented crystal was observed. CH3NH3I was supplied on the film and CH3NH3PbI3 perovskite was constructed, where its [1 1 0] axis was oriented perpendicular to the substrate. Based on this analysis, transition of crystal orientation can be discussed.
B2-O3
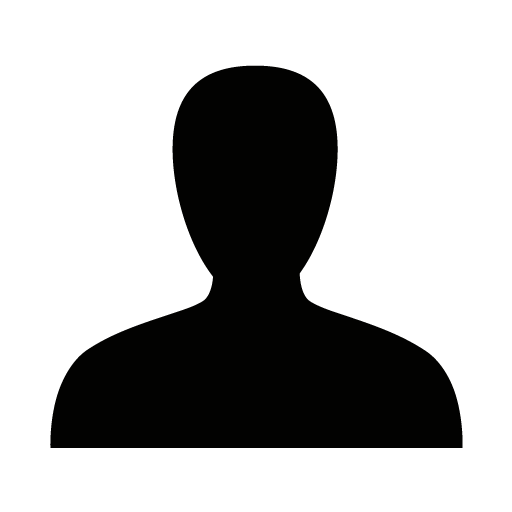
Perovskite semiconductors have shown great promise for making high-efficiency solar cells at low cost[1]. Since their first use in solar cells[2]. The power conversion efficiency (PCEs) of perovskite solar cells (PSCs) have speedily increased. The chemical structure can be tailored by combining various halides or utilizing different aliphatic ammonium ions, leading to tunable band gaps and charge transport properties. [3] The certified power conversion efficiency has reached 22.3%[4]. Despite this rapid increased in power conversion efficiency of perovskite solar cells the maximum current is till upto 25.0 mA/cm2. The efficiency limit of perovskite cells without the angular restriction is about 31%, which approaches to Shockley-Queisser limit (33%) achieved by gallium arsenide (GaAs) cells. [5] Moreover, theoretically the Shockley-Queisser limit could be possible to reached with only a 200 nm thick perovskite solar cell, through integrating a wavelength dependent angular restriction design with a textured light-trapping structure[5] Alkylammonium lead (II) trihalide perovskite act as ambipolar charge transport characteristics and function well in solar cells with an inverted structure. For this device architecture, the perovskite forms a heterojunction with an organic charge transport material, such as fullerene (C60), {6,6}-phenyl C61-butyric acid methyl ester (PCBM) or conjugated polymer. The inverted structure (ITO/EEL/Perovskite/HEL/electrode) can be fabricated with reversed electrode polarity. In general, the inverted structure has better ambient stability and compatibility to all solution roll-to-roll application. Perovskite shows high intrinsic carrier mobility and high photon absorption[6] due to thickness of ~1000 nm there are severe charge recombination and losses[7a,b,c] Therefore, hampering the resultant current8.
Delicate optical interlayer management is utilized to enhance the current. Inorganic interlayers due to their superior electronic properties and environmental stability compared to their organic counter parts and are considered very good candidates for interface engineering. The transition metal oxides with decent transparency across visible and infrared spectra have been widely proven to form good ohmic contact with absorbers due to their high conductivity and appropriate WF [9 (a),(b),(c) & (d)] For an ideal Hole extraction layers (HELs), its HOMO level should align with the Ef,h of CH3NH3PbI3 layer while its LUMO level sits above the Ef,e of CH3NH3PbI3. In this direction we show that introduction of an additional buffer HTL of high work function metal oxide layer on NiOx decreases the concentration of deep-level defects. The interface engineering of this additional buffer layer helps collecting holes effectively hindering electrons this enable the fabrication of PSCs with power conversion efficiency of 21% under standard AM1.5 G conditions. with the highest ever recorded current of 27.8 mA/cm2 which is well matched with the current of 27.7 mA/cm2 obtained from EQE.
References
(1) (a) Nature 2013, 501,395. (b) Nature 2013, 499,316 (c) Sci. Rep. 2012,2,591 (d) Science 2013, 342,344.
(2) J. AM. Chem. Soc. 2009, 131, 6050
(3) (a) J. H. Noh, S.H. Im, J. H. Heo, T.N. Mandal, S.I. Seok, Nano Lett., 2013, (b) M. I. Dar, N. Arora, P. Gao, S. Ahmad, M. Gratzel, M. K. Nazurridin, Nano Lett., 2014
(4) (a) Science 2014, 345,542 (b) Nature 2015, 517,476 (c) Science 2015, 348,1234 (d) Science 2015, 350, 944 (e)
(5) Applied Physics Letters 2015, 106 (22): 221104
(6) Phys. Chem. Chem. Phys., 2015,17,11516-11520
(7) (a) J. AM. Chem. Soc. 2013, 135, 4656-4659 ; (b) Adv. Mater., 2013,25,6642-6671 ; (c) Nat. Photonics, 2013,7,825-833
(8) J. Phys. Chem. Lett., 2013,4,1821-1828
(9) (a) Nanoscale, vol. 8, no. 22, pp. 11403-11412, 2016 ; (b) Chemical Communications, vol. 52, no. 52, pp. 8099-8102, 2016 ;
B2-O4
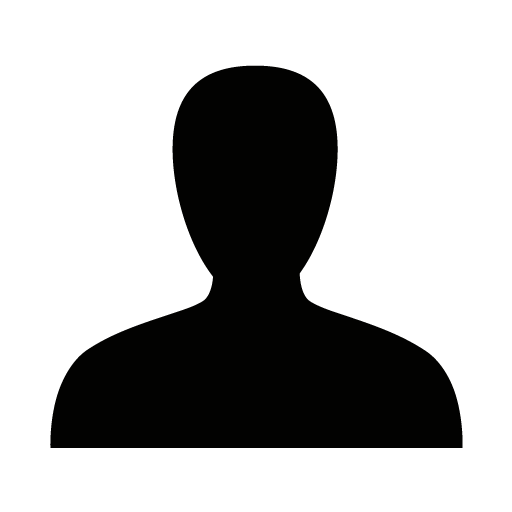
Organic-inorganic hybrid halide perovskites are promising photovoltaic (PV) materials with power conversion efficiencies (PCE) exceeding 23%. Although the toxic lead-based perovskite materials used in the leading perovskite solar cells (PSCs) are a significant impediment to commercialization, obtaining comparable performance using more environmentally friendly metals in place of lead has proven challenging. We are looking at Sn as a replacement for Pb, addressing key issues potentially holding back performance such as reagent purity and film morphology. The higher reactivity of SnI2 compared to PbI2 places additional importance on the purity of the reagents, and in the present work our perovskite films were fabricated from tin perovskite precursor materials such as solvent-coordinated tin halide complexes e.g. [SnI2(dmf)] (Figure 1a)[1] which we confirmed to have significantly higher purity than commercially available SnI2. The growth of smooth, high coverage perovskite films with high crystallinity is also important for high efficiency PSCs and we confirm that Sn-based perovskite films require modified fabrication methods compared to their lead analogs. We have developed two simple methods to improve tin halide perovskite film quality.[2] First, a hot antisolvent treatment (HAT) was found to increase the film coverage and prevent electric shunting in the photovoltaic device. Second, a solvent vapor annealing (SVA) step was noted to increase the average crystallite size. Both the morphology and the electrical properties of the perovskite films are improved as a result of the combined treatments, facilitating the fabrication of Sn-based perovskite solar cell devices with power conversion efficiencies of over 7% (Figure 1b).
B2-O5
Environmental friendly Sn-based perovskite solar cells (PSCs) have emerged as one of the most promising Pb-free photovoltaic materials due to their superior photoelectric property in comparison to Pb-based perovskites. [1-3] In spite of the superior photovoltaic properties, the poor ambient and light soaking stability of Sn-based film still seriously restricts the development of Sn-based PSCs. The reason for the poor stability of Sn-based PSCs is the tendency of oxidation from Sn2+ to Sn4+ state of Sn-based film, which leads to an unwanted p-type doping and damages the suitable semiconducting photovoltaic properties of the Sn-based film. Here in this work, we proposed
an additive engineering strategy in the precursor solution of Sn-based PSCs, significantly increased the crystal quality of the Sn-based film. The high crystal quality of the Sn-based film was clarified by its surface morphology, crystallography, and optical properties. Benefiting from high crystal quality, the oxidation rate of Sn2+ to Sn4+ state of the Sn-based film was greatly slowed down. A power conversion efficiency of over 7% for our Sn-based devices can be achieved. More importantly, the unencapsulated device can maintain over 85% of its initial efficiency for 700 min in ambient, and the encapsulated device can maintain over 90% of its initial efficiency for over 800 h under continuous AM1.5 light soaking.
C2-IS1
Perovskite solar cells have been recognized as a newly emerging solar cell with the potential of achieving high efficiency with a low cost fabrication process. In particular, facile solution processed cell fabrication facilitated rapid development of optimum cell structure and composition. Over the last few years, the cell efficiency has exceeded 22%.
A typical perovskite solar cell employs a perovskite layer sandwiched by p-type semiconductor (such as spiro-OMeTAD, PEDOT or NiO) and n-type semiconductor (such as TiO2, ZnO or PCBM) layers. Following light absorption, an electron and a hole are separated at the perovskite film interface, and are collected at the back electrodes. Choice of the most suitable solar cell structure is crucial to improve the performance further. In this presentation, we will present parameters controlling charge separation and recombination dynamics at the perovskite interfaces employing a series of transient absorption and emission spectroscopies. Nanosecond transient emission spectroscopy (Vis-ns-TES) clarifies charge separation processes, while Vis-NIR submicrosecond-millisecond transient absorption spectroscopies (NIR-smm-TAS) identify charge separation efficiency and charge recombination rates. Correlation of the dynamics results with the solar cell performance will be discussed [1]. An optimum cell structure for methylammonium lead iodide (MAPbI3) perovskite sandwiched by TiO2 and spiro-OMeTAD layers, among planar heterojunction, mesoporous structure and extremely thin absorber structure will be identified.[2]
C2-O4
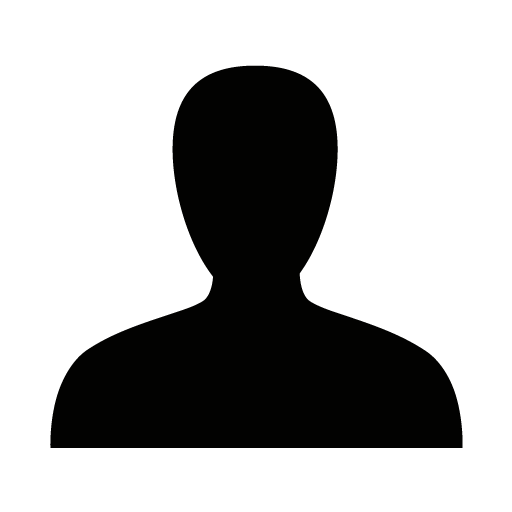
We report on the recurrent photo-bleaching and photo-recovery cycles of photoluminescence (PL) in free-standing polycrystalline films of methyl-ammonium lead iodide, CH3NH3PbI3 (MAPbI3), which were monitored under intermittent illumination with strong fluxes of the visible light. To this end, the PL responses of MAPbI3 thin films, coated onto glass substrates and protected with a thin layer of Poly(methyl-methacrylate) (PMMA), were acquired under repeatable illuminations at two different wavelengths of 470 nm and 546 nm. Specifically, the same spot of the MAPbI3 deposit was exposed for several times to multiple cycles of ‘light (470 nm) – dark - light (546 nm)’. The emission PL spectra were acquired using a custom-modified epi-fluorescent microscope. During the subsequent illumination cycles, the exposure times were of ~2 min and ~10 min, for the excitation wavelengths of 470 nm and 546 nm, respectively. Each short exposure to the wavelength of 470 nm repeatedly resulted in a rapid diminishment of the PL intensity (by ~50%). This marked PL decrease could also be followed as a function of time under the continuous excitation at 470 nm. Interestingly, after switching the excitation wavelength to 546 nm, the PL intensity could be partially recovered on a time scale of ~10 min. Overall, the recurrent exposures to 470 nm light resulted in a relatively rapid photo-bleaching, whereas exposures to 546 nm light lead to a somewhat slower recovery of the PL intensity. Moreover, since the photo-recovery of the PL intensity was not entirely complete, after the recurrent photo-bleaching events, a net decrease in the PL intensities was also observed as a function of illumination time.
In summary, our results point to marked wavelength-dependent mechanisms of light-induced changes in the PL intensity of free-standing polycrystalline films of MAPbI3. Taking into account the time scales of these partially reproducible variations of the PL emission, we infer the occurrence of two types of light-induced processes: (i) rapid ‘photo-bleaching’ at excitation at 470 nm due to the interband transitions within the split valence and conduction bands [1,2], and (ii) partially reversible structural transformation and degradation of polycrystalline deposits of MAPbI3 under excitation at wavelengths from 460 to 480 nm [3].
C2-O5
Akinori Saeki received BS and MS degrees in nuclear engineering from Osaka University in 1999 and 2001, respectively. He received Dr of engineering in applied chemistry from Osaka University in 2007. He had been an assistant professor at The Institute of Scientific and Industrial Research (ISIR), Osaka University in 2003-2009, an assistant professor (tenure-track) in 2010-2014, and an associate professor in 2014-2019 at the Graduate School of Engineering, Osaka University. He had joined in JST-PRESTO research programs of "Photoenergy conversion systems and materials for the next generation solar cells" in 2009-2013 and "Materials Informatics" in 2015-2019. He is currently a professor at Graduate School of Engineering, Osaka University (2019-present). His research interest is in nanometer-scale dynamics of chemical intermediates in condensed matters such as organic semiconductors, organic liquids, and organic-inorganic hybrid materials.
Photon upconversion is an interesting nonlinear process that converts low-energy photons to high-energy photons, contrary to the normal energy relaxation process. This unique phenomenon has been utilized in bio-imaging and fabrication of nano-micro structures, and is potentially applicable in enhancing power conversion efficiency in a solar cell by converting inaccessible near-infrared (IR) sunlight to a photon energy above the bandgap energy. Two-photon absorption (TPA) and triplet-triplet annihilation (TTA) are the major processes, and many organic molecules and metal complexes have reportedly exhibited photon upconversion processes, such as green to blue and red to yellow.
Here, we report a TPA cascade process in inorganic perovskite quantum dots (PQDs) of CsPbBr3 and TTA in an organic molecule (9,10-diphenylanthracene, DPA) mediated by a octaethyl-porphyrinatoplatinum(II) (PtOEP) sensitizer[1]. This sequential energy transfer enables upconversion from four photons from a near-infrared femtosecond laser at 800 nm to one photon at 430 nm with a large anti-Stokes shift of ~1.3 eV. We characterize the energy transfer from PQDs to PtOEP by picosecond lifetime spectroscopy and a Stern-Volmer plot of the steady-state photoluminescence while considering dynamic and static quenching as well as trivial absorption and fluorescence resonant energy transfer (FRET). The serial connection of TPA and TTA achieved in a simple system opens up an attractive avenue in nonlinear photonics and harvesting of low-energy photons.
C2-O1
Dr. Chen received Ph. D. from the Photonic Program in EPFL Switzerland at 2009 under the supervision of Prof. Michael Graetzel. His research topic was focused on solid-state dye sensitized solar cells. Then he moved to Monash University in Australia as a post-doctoral research fellow with Prof. Udo Bach. Dr. Chen joined the Dept. of Photonic in National Cheng Kung University (NCKU, Tainan, Taiwan) in 2010 and became associate Professor and full Professor in 2014 and 2017 respectively. He was the director of the research and education division in the Center for Micro/Nano Science and technology (CMNST) in NCKU between Aug. 2017~ Jan. 2019. Currently his research interests are in the area of various photovoltaic materials and devices including dye-sensitized solar cells (DSCs), hybrid organic-inorganic perovskite-based solar cells (HOIPs) and novel semiconductor compounds. Meanwhile, he also involved in developing synthetic and characterization methods for TCO material, thin film, and semiconductor materials.
The halide perovskites materials have received intensive attention for various photonics applications due to their unique properties. In this talk, we will present various halide perovskites thin film made of low-pressure vapor assisted method to form 2D/3D mixture with the help of large size cation. Their characteristics in photovoltaic and nonlinear optical properties will be discussed. Such new families of organic-inorganic hybrid halide perovskite semiconductors have great potential for next generation photonic devices and applications such as solar cells, light-emitting diode, and lasers. In general, the slightly 2D doped perovskite demonstrated larger grain size and better photovoltaic performances in both power conversion efficiency and stability. While highly 2D doped perovskite thin film, we observed multiple PL emission spectra with significant nonlinear absorption characteristics, which implies the co-existence of multiple n-layered perovskites in one film. The nonlinear optical effects are characterized by multiphoton absorption spectroscopy and TR-PL and PL mapping.
C2-O2
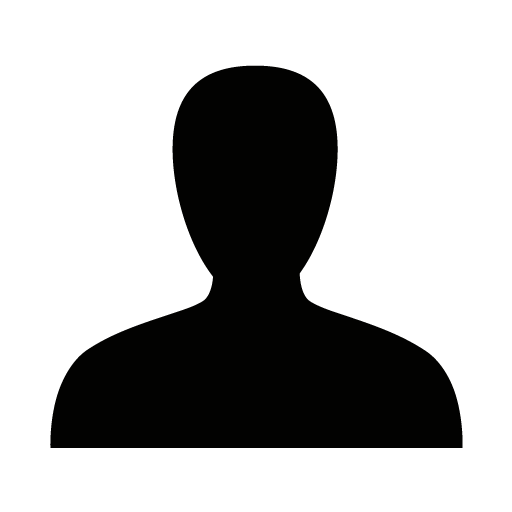
Optoelectronic devices based on hybrid organic-inorganic perovskites (HOIP) have exhibited a meteoric rise in the last years. Current large area solar cells (156 mm × 156 mm) with power conversion efficiencies surpassing 20% in combination with their low production costs make them suitable for the next generation of photovoltaic devices [1]. So far, the photo-physical parameters such as carrier mobility, charge diffusion and recombination dynamics of perovskites have been subjected to an intense scrutiny using several methods [2]. However, a consensus concerning the underlying photophysical properties and charge transport in these materials has still not been reached as all measurements have been performed around temperatures relevant for applications (mostly room temperature) where the thermal energy is high hence making it practically impossible to distinguish between different scattering mechanisms.
To address these issues, we investigated single crystals of different perovskite groups using temperature-dependent photoluminescence, magneto-transport under steady-state illumination [3] and thermal expansion between 4.2 K and room temperature [4]. The latter techniques are beyond conventionally employed characterization techniques. We demonstrate that magneto-transport under steady-state illumination is a powerful tool to unravel the fundamental charge carrier dynamics and underlying carrier scattering mechanisms at different temperatures and show that in contrast to MAPbI3 and MAPbBr3 in which acoustic phonon scattering is the dominant scattering mechanism, optical phonon scattering plays a significant role in CsPbBr3.
By means of a high-resolution capacitive dilatometer, we scrutinize the phase transitions in a temperature range between 4.2 and 280 K. Depending on the material, we observe abrupt changes in the linear thermal expansion ΔL(T)/L that we associate with structural phase transitions. The overall background in linear thermal expansion is positive and varies smoothly within the specific phases. The linear thermal expansion observed for FAPbI3 and FAPbBr3 is by far more complex showing several features attributed to several orientation of the organic group within different symmetry groups where is under investigation via single crystal XRD.
While FAPbBr3 exhibits several sharp so far unidentified but most likely tetragonal phases separated by sharp transitions between 120 and 160 K, FAPbI3 is distinct from all other materials due to two wide regions of negative thermal expansion below 173 and 50 K, i.e. the material expands upon decreasing the temperature. We also show that thermal expansion is a technique to determine the crystal quality [4].
Furthermore, these phase transitions also appear in transport under steady-state illumination. We present the results on MAPbBr3 (see figure 1 as an example), FAPbBr3 and MAPbI3 [4]. The latter exhibits strong increase in resistance of the overall temperature dependence. Results are corroborated with temperature-dependent photoluminescence to unravel the electron-phonon coupling constants and the dominant scattering mechanisms for each of the above mentioned crystals.
These combined techniques can be applied to novel HOIP materials and devices with a different architecture such as the double or triple perovskites where the structure is by far more complicated.
Figure 1. Structural phases of cubic, the tetragonal phases and the low temperature orthorhombic for MAPbBr3 in agreement with transport (Rxx) and normalized photoluminescence as a function of the temperature. The abrupt changes indicate the phase transitions (n is the carrier concentration).
C2-O3
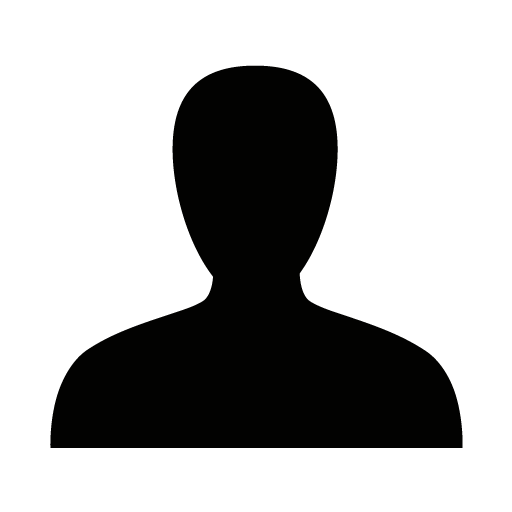
The room-temperature charge carrier mobility and excitation-emission properties of metal halide perovskites are governed by the electronic band structure and intrinsic lattice phonon scattering mechanisms. Establishing how charge carriers interact within this scenario will have far-reaching consequences for developing high-efficiency materials for optoelectronic applications [1]. In this presentation, we evaluate the charge carrier scattering properties and conduction band environment of the double perovskite Cs2AgBiBr6 through a combinatorial approach; single crystal X-ray diffraction, optical excitation and temperature dependent emission spectroscopy, resonant and non-resonant Raman scattering, further supported by first principles calculations. We identify deep conduction band energy levels and that scattering from longitudinal optical phonons – via the Frӧhlich interaction – dominates electron scattering at room temperature, manifesting within the nominally non-resonant Raman spectrum as multiphonon processes up to the fourth order (Figure 1). We measure a Frӧhlich coupling constant nearing 230meV[2], which is inferred from a temperature dependent emission linewidth analysis. When compared to other more popular lead halide perovskites (40 - 60 meV [3]), the value measured here is giant, highlighting the fundamentally different nature of the two “single” and “double” perovskite materials branches.
G4-O1
Tsutomu (Tom) Miyasaka received his Doctor of Engineering from The University of Tokyo in 1981. He joined Fuji Photo Film, Co., conducting R&Ds on high sensitivity photographic materials, lithium-ion secondary batteries, and design of an artificial photoreceptor, all of which relate to electrochemistry and photochemistry. In 2001, he moved to Toin University of Yokohama (TUY), Japan, as professor in Graduate School of Engineering to continue photoelectrochemistry. In 2006 to 2009 he was the dean of the Graduate School. In 2004 he has established a TUY-based company, Peccell Technologies, serving as CEO. In 2005 to 2010 he served as a guest professor at The University of Tokyo.
His research has been focused to light to electric energy conversion involving photochemical processes by enhancing rectified charge transfer at photo-functional interfaces of semiconductor electrodes. He has contributed to the design of low-temperature solution-printing process for fabrication of dye-sensitized solar cells and solid-state hybrid photovoltaic (PV) cells. Since the discovery of the organic inorganic hybrid perovskite as PV material in 2006 and fabrication of high efficiency PV device in 2012, his research has moved to R&Ds of the lead halide perovskite PV device. He has promoted the research field of perovskite photovoltaics by organizing international conferences and by publishing many papers on enhancement of PV efficiency and durability, overall citation number of which is reaching more than 5,000 times. In 2009 he was awarded a Ministry of Science & Education prize on his achievements of green sustainable solar cell technology. In 2017 he received Chemical Society of Japan (CSJ) Award. He is presently directing national research projects funded by Japan Science and Technology Agency (JST) and Japan Aerospace Exploration Agency (JAXA).
Since our start of the photovoltaic cell using organo lead halide perovskites in 2005,1 cell performance recorded a rapid boost in efficiency that has been never experienced by other photovoltaic semiconductors. Although power conversion efficiency (PCE) achieved high value (23.3%), to reap the benefit of low cost perovskite photovoltaic devices is to establish an ambient air-based fabrication of a high performance and stable device by a simple solution process without use of dry oxygen-free chamber and high temperature treatments. We have established ambient atmosphere solution processes to fabricate glass-based triple cation perovskite solar cells (PCE>21%)2 and plastic-film based perovskite solar cells (PCE 18%) both of which maintain good performance under weak incident light (1 mA/cm2). However, a drawback of perovskite device is due to use of thermally unstable hole transporter such as spiro-OMeTAD. To improve this P3HT is one of thermally stable materials. In our collaboration with JAXA, P3HT-based perovskite devices showed high durability by exposure to impacts of high (100oC) and low (-80oC) temperatures and high tolerance to large fluence proton and electron radiations, which is rare advantage of defect tolerant nature of thin film perovskite absorbers.3 We could improve the performance of highly durable P3HT perovskite solar cells to have efficiency above 15% by modifying the quality of P3HT film to have moisture-resistant surface. To reduce the process cost, low temperature coating of metal oxide electron collectors have been extensively studied in our group. This method was applied to the use of MA-free perovskite composition (CsFAPbI3, CsPbI3, etc.)4 that have high thermal stability. Direction of such challenges is to realize a perovskite photovoltaic device that has benefits of low cost, high performance, and high thermal and anti-moisture durability in practical use. The on-going studies on perovskite photovoltaics in our group will be summarized in the talk.
G4-I1
Hiroshi Segawa (born 1961) is a professor at Research Center for Advanced Science and Technology (RCAST), The University of Tokyo, Japan. He obtained his Ph.D. in Molecular Engineering from Graduate School of Engineering of Kyoto University in 1989 and was Research Associate (1989-1995) at the division of Molecular Engineering of Graduate School of Engineering at Kyoto University. He held an additional researcher post (1994-1997) of PRESTO project of Japan Science and Technology Agency (JST). In 1995 he joined the University of Tokyo as Associate Professor of Department of Chemistry at Graduate School of Arts and Sciences. From 1997 he has also been in charge of Department of Applied Chemistry at Graduate School of Engineering. In 2006 Professor Segawa joined the three faculties of RCAST, Department of Chemistry at Graduate School of Arts and Sciences, and Department of Applied Chemistry at Graduate School of Engineering at the University of Tokyo. In 2010, he was appointed director of Academic-Industrial Joint Laboratory for Renewable Energy of RCAST. Currently he is one of core researcher of FIRST Program (Funding Program for World-Leading Innovative R&D on Science and Technology) which is selected top 30 researchers with highest potential from various science fields by Cabinet office, Government of Japan. He is one of the experts in the field of electrochemical solar cells. His research group are focused on construction of photo-energy conversion system. Currently the object is the efficiency enhancement of the meso-structured solar cells. Additionally, he is developing an energy-storable dye-sensitized solar cell.
Organometal halide perovskites have captured wide interest as a promising material for low-cost and high-efficiency solar cells [1, 2].
Through recent studies of organometal halide perovskite solar cells (PSCs), the composition of organometal halide perovskites is recognised as one of the key factors in the improvement of the PCE. In this study, we investigated cation-doping into the perovskite absorber. [3, 4, 5] The results revealed that incorporating a small amount of K+ into the double organic cation perovskite absorber (FA0.85MA0.15Pb(I0.85Br0.15)3) improved the photovoltaic performance of PSCs significantly, and K+ incorporation diminished I-V hysteresis. Consequently, the PSCs of more than 20% power conversion efficiency (PCE) without I-V hysteresis were constructed. The crystal lattice of the organometal halide perovskite was expanded with increasing of the potassium ratio, where both absorption and photoluminescence spectra shifted to the longer wavelength, suggesting that the optical band gap decreased. It is concluded that stagnation-less carrier transportation could minimise the I-V hysteresis of PSCs.
On the other hand, the microstructural aspects within the organometal halide perovskite are still unknown, even though it belongs to a crystal system. In this study, direct observation of the microstructure of the thin film organometal halide perovskite using transmission electron microscopy was investigated [6]. Unlike previous reports, it is identified that the tetragonal and cubic phases coexist at room temperature, and it is confirmed that superlattices composed of a mixture of tetragonal and cubic phases are selforganized without a compositional change. The organometal halide perovskite self-adjusts the configuration of phases and automatically organizes a buffer layer at boundaries by introducing a superlattice. These results shows the fundamental crystallographic information for the organometal halide perovskite and demonstrates new possibilities toward high performance perovskite solar cells.