1.1-I1
Victor Mougel completed his Bachelor's and Master's degree in Chemistry at the ENS of Lyon, and obtained his PhD at the University of Grenoble under the supervision of Prof. Marinella Mazzanti. He then joined ETH Zürich as an ETH/Marie Skłodowska-Curie Fellow before starting his independent career as a CNRS associate researcher at Collège de France in 2016. Since December 2018, he is a tenure track assistant professor at the Department of Chemistry and Applied Biosciences at ETH Zürich.
Selective electrochemical reduction of CO2 into energy-dense organic compounds is a promising strategy for using CO2 as a carbon source. However, efficient and selective earth abundant metal catalysts for the two reactions typically required for efficient overall CO2 electrolysis, namely the oxygen evolution reaction (OER) and CO2 reduction, are still scarce. We will present here an array of strategies inspired from biological systems to promote these reactions with high selectivity and efficiency. The importance of tackling these challenging reaction at multiple scale will be illustrated by a series of molecular and heterogeneous catalysts replicating enzymatic features, from their active sites including secondary features such as hydrophilic and hydrophobic domains to the overall shape of organs involved in gas trapping.[1-7]
1.1-I2
The direct CO2 electrochemical reduction reaction (CO2RR) is a potential technology to convert waste CO2 streams into valuable chemicals, using renewable electricity as a driving force. During this process a variety of carbon-based products such as CO, HCOOH and hydrocarbons can be formed. The selectivity of the CO2RR is determined by the nature of the catalyst interphase, which is dependent on the catalyst material, its morphology and the working electrolyte, among other factors. Therefore, it is necessary to get a holistic approach to fully understand the CO2RR and to get optimal catalytic results.
For this contribution we look at the influence of proton concentration on the selectivity of carbon based catalysts. Unlike hydrogen or methane generation, CO production has been shown to be independent of pH concentration on the NHE scale. Therefore, the proton concentration can be used to tune the CO/H2 ratio. In aqueous electrolytes, for instance, the selectivity towards CO on Fe nitrogen-doped carbon is clearly enhanced at neutral pH whereas acidic conditions favored methane and hydrogen production. [1]
For this contribution we studied the CO2RR in aprotic media aiming to suppress the competing hydrogen evolution reaction (HER). We first studied a pure carbon electrode which in aqueous electrolyte selectively produced H2, yet traces of CO were also obtained. By contrast, in an aprotic electrolyte (0.1M NBu4PF6 in acetonitrile) CO was obtained with a faradaic efficiency higher than 90%. Density functional theory (DFT) simulations confirmed this is attributed to the absence of protons, showing that certain carbon defects can reduce CO2 into CO in both media. Nevertheless, carbon sites are predicted to be more active towards the HER and thus in aqueous media the formation of H2 is the predominant process.
While it is remarkable that the pure carbon can selectively reduce CO2, the process takes place at high over potentials, this can be improved by intruding dopants. Metal nitrogen doped carbons, in particular, have been shown to be highly active towards the CO2RR in aqueous media. [2] Working in a non-protic electrolyte, resulted in suppression of both the HER and the CO2RR, as both reactions require protons. Nevertheless, the presence of small amounts of water resulted in a clear enhancement of the CO2RR while keeping a low HER activity. These results highlight the importance of proton concentration on determining the reaction selectivity. On one hand H+ concentration has to be limited to suppress the competing process of the HER. However, protons are also necessary to carry out the reduction of CO2 into CO and methane.
1.1-I3
I am currently an Full Professor at the University of Bonn (Germany), Department of Chemistry. My overarching motivation is to discover and implement the chemistry necessary to transition to a sustainable energy-based society. Specifically, I am developing materials to convert electrical energy to fuels and chemicals.
Energy-intensive thermochemical processes within chemical manufacturing are a major contributor to global CO2 emissions. With the increasing push for sustainability, the scientific community is striving to develop renewable energy-powered electrochemical technologies in lieu of CO2-emitting fossil-fuel-driven methods. However, to fully electrify chemical manufacturing, it is imperative to expand the scope of electrosynthetic technologies, particularly through the innovation of reactions involving nitrogen- and sulfur- based reactants as products from water/CO2 electrolysis do not cover the full scope of industrial needs.
To this end, this talk focuses on my lab’s efforts in the co-electrolysis of CO2 with additional small molecule reactants (NH3, NO3-, SO32-…) in generating products with C-N and C-S bonds like amides, urea, and sulfonates that are important as commodity and fine chemicals in the chemical industry. In particular, I will discuss several new reaction pathways discovered towards several of the above-mentioned products and the application of operando techniques to understand the key C-N/C-S coupling steps in the reaction process.
1.2-T1
Electrocatalytically driven CO2 reduction reaction (CO2RR) to produce alternative fuels and chemicals is a useful means to store renewable energy in the form of chemical bonds. in recent years there has been a significant increase in research efforts aiming to develop highly efficient CO2RR electrocatalysts. Yet, despite having made significant progress in this field, there is still a need for developing new materials that could function as active and selective CO2RR electrocatalysts.
In that respect, Metal–Organic Frameworks (MOFs), are an emerging class of hybrid materials with immense potential in electrochemical catalysis. Yet, to reach a further leap in our understanding of electrocatalytic MOF-based systems, one also needs to consider the well-defined structure and chemical modularity of MOFs as another important virtue for efficient electrocatalysis, as it can be used to fine-tune the immediate chemical environment of the active site, and thus affect its overall catalytic performance.
Our group utilizes Metal-Organic Frameworks (MOFs) based materials as a platform for imposing molecular approaches to control and manipulate heterogenous electrocatalytic systems. In this talk, I will present our recent study on electrocatalytic CO2RR schemes involving MOFs, acting as: a) electroactive unit that incorporates molecular CO2RRelectrocatalysts, or b) non-electroactive MOF-based membranes coated on solid CO2RR catalysts (see illustrative TOC figure).
1.2-T2
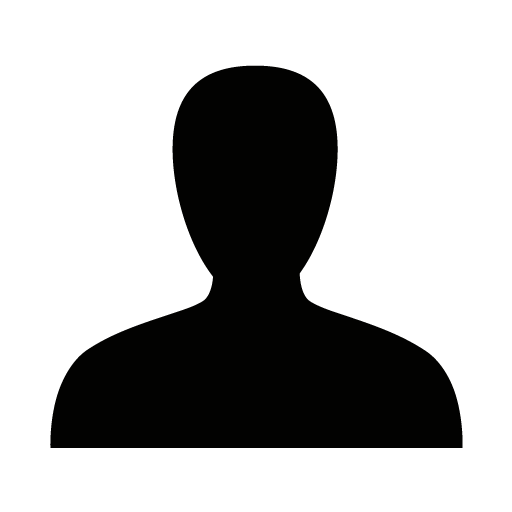
The electrochemical CO 2 reduction reaction (CO 2 RR) is an attractive method to produce renewable fuel
and chemical feedstock using clean energy sources. Although copper (Cu) is a well-known electrocatalyst
for the electroreduction of CO 2 to multiple hydrocarbons, it suffers from poor selectivity, efficiency, and
stability. In this work, a novel bimetallic Cu-Pd alloy anchored on two-dimensional Ti 3 C2 Tx -MXene
nanosheets was further converted to highly conductive three-dimensional Cu-Pd/MXene aerogel, which
acts as an excellent electrocatalyst to reduce CO 2 to formate with high selectivity of 93% and current
density of 150 mA/cm 2 . Compared with Cu-Pd aerogel, the newly designed Cu-Pd/MXene aerogel could
effectively decrease the overpotential and enhance selectivity and current density with great stability
during a long-term reaction. This simple strategy represents an important step toward experimental
demonstration of 3D-MXenes-based electrocatalysts for CO 2 RR application and opens a new platform for
the fabrication of macroscale aerogel MXene-based electrocatalysts that can be used for a range of
applications, including nanocomposites, electronic devices, and all-liquid microfluidic devices.
1.2-T3
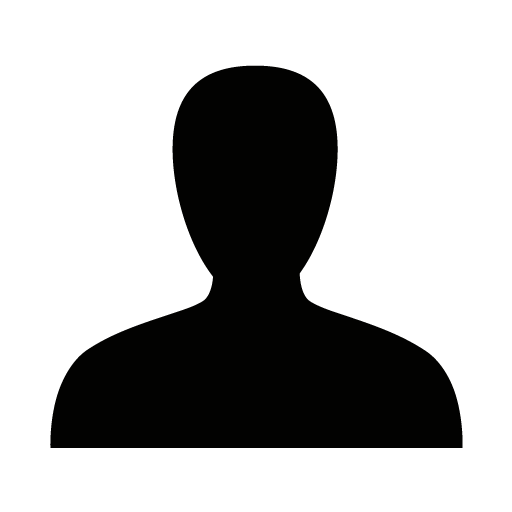
The electrochemical reduction of CO2 (CO2RR) with Cu-based catalysts has a rather poor selectivity towards C2+ molecules, such as ethylene and ethanol. Up to 16 different products can typically be formed during the process. [1] However, copper is the only known element with the ability to make C-C bonds and produce the desired C2+ products.[2] Therefore, it is key to gain a better understanding of the active sites of Cu-based electrocatalysts in order to improve the design and consequently the selectivity towards molecules such as ethylene and ethanol. This can be done, for example, by using well defined surfaces such as single crystals, providing insights into the reactivity of different exposed planes. It has beed shown that ethylene is mainly produced on Cu (111) facets while a higher selectivity for methane was observed for Cu (100) facets. [3],[4]
In this study, cubic and octahedral Cu2O nanocrystals predominantly exposing (100) and (111) facets were synthesized, respectively. Polyvinylpyrrolidone (PVP) was added during the synthesis as a capping agent to delicately control the ratio of (111) over (100) planes, yielding different shape Cu2O nanostructures. [5] The presence of preferential cubic or octahedral shapes on the different synthesis was also confirmed by X-ray poweder diffraction (XRD), Moreover, the ratio of diffraction intensity for the (111) and (200) reflections showed an increase for the octahedral structure. Additionally, the synthesis procedure allowed the control of the size of the nanostructures between 400 and 800 nm as confirmed by SEM. Chronoamperometric measurements revealed that the cubic Cu2O nanocrystals are more selective towards ethylene compared to the octahedral shaped particles. The cubic Cu2O nanocrystals achieved a Faradaic efficiency for C2+ products of approximately 60% at a potential of -1.0 V vs. RHE. These results are in agreement with the observations on Cu single crystals, showing that the surface structure dependence for CO2RR is maintained for Cu2O catalysts.
1.2-T4
Our sustainable future requires finding affordable and green routes to prepare nanostructured materials for more efficient energy conversion reactions. Compared to bulk materials, nanocatalysts show improved electrocatalytic activity due to their high electrochemically active surface area (ECSA) combined with reduced loadings of noble metals. Metal electrodeposition in deep eutectic solvents (DES) has emerged as a versatile, green and affordable alternative to prepare bimetallic nanostructures. DES are non-toxic solvents which show wide potential limits, enough conductivity, high solubility of the majority of the metals and do not require surfactant agents or additives for controlled growth of the nanostructures.[1] In this work, we investigate the preparation of high surface area nanostructures of Cu-Au[2] and Pd-Au[3] with tunable structure and composition by electrodeposition in choline chlorine plus urea DES. We combine electrochemical methods with microscopy and spectroscopy techniques to characterize the electrodeposited nanostructured materials. We assess the increase of the electroactive surface area through the analysis of metal underpotential deposition (UPD) on the prepared films and, CO stripping on the Pd-Au bimetallic films, to investigate the effect of the Pd loading on the electrochemical response. Finally, Pd and Pd-Au nanostructures were tested for formic acid oxidation in comparison to extended polycrystalline surfaces. Overall, I show a simply and eco-friendly method to prepare mono and bimetallic nanostructures for different electrocatalytic reactions.
1.2-T5
Global energy demand is increasing exponentially and the main source of energy is still based on fossil fuels, which are scarce and responsible for the majority of carbon dioxide (CO2) emissions into the atmosphere. Green hydrogen has emerged as a promising energy vector to respond to this increasing energy demand and to decarbonize transportation, heating and fine chemicals sectors. On the other hand, electrochemical CO2 conversion into energy-rich fuels and chemicals has gained significant interest as a potential strategy for simultaneously mitigating increasing global CO2 concentration.
Here we present a cost-effective copper-based electrocatalyst to evolve hydrogen with faradaic efficiencies close to 100%. Moreover, this electrocatalyst in presence of CO2 is able to reduce it in formic acid with faradaic efficiencies close to 70%. Beyond the easy synthesis of this electrocatalyst and its high efficiencies for hydrogen evolution (HER) and CO2 reduction reaction (CO2RR), what really makes this material interesting is its behavior during the electrocatalysis. This copper-derived electrocatalyst enhance its currents as a function of time when evolves hydrogen increasing too the active surface area almost proportionally. While in its application for CO2 reduction, its activity was studied when the electrode is reused several times, showing an increasing selectivity trend toward formic acid in each use.
1.2-T6
Integrating the alkaline capture of CO2 from the air with the electrochemical conversion of the obtained (bi)carbonate solution in one and the same system is among the most promising strategies in the field of Carbon Capture & Utilization (CCU) technologies. Thus far this approach has received little or no attention as a consequence of the challenging conversion of CO2 from bicarbonate solutions as it mostly results in low Faradaic Efficiency (FE) and partial Current Density (CD) towards carbon products during the electrolysis and owing to the parasitic hydrogen evolution reaction (HER). Very recently, thanks to the advances in reactor design and in the understanding of the mechanism of bicarbonate electrolysis, promising results were obtained in terms of performance (i.e., >60% FE towards formate or CO at >50 mA cm-2) [1] and as such provided us with the required knowhow to for the first time, construct and validate a proof-of-concept experimental setup where CO2 is captured from the air with KOH, in the form of a 0.7 M (bi)carbonate solution, through Direct Air Capture and then converted to formate or CO in a zero-gap flow electrolyzer using a bipolar membrane in a Membrane-Electrode Assembly (MEA). The presented results provide a new opportunity towards upscaling the electrochemical conversion of CO2, since integrating the capture and the conversion steps is a crucial step to enhance the economic feasibility of the CCU technology (energy-intensive CO2 separation can be avoided) and thus increase its chances of industrial implementation.
1.2-T7
After completing his bachelor of Materials Engineering at Eskisehir Technical University (EST), Erdem acquired the competitive Erasmus Mundus Joint Master Scholarship (MESC) to work on energy storage technologies at European Institutions (France, Spain & Poland). Then with Fl-Agaur Early-Stage Researcher Grant, he conducted a doctorate on solar fuels in Catalonia Institute for Energy Research (IREC) in Barcelona (Spain), building a proof-of-concept photoelectrochemical flow cell device for artificial photosynthesis in collaboration with Repsol S.A. His Ph.D. thesis was nominated for the Premis Pioneer Award for "Best Doctoral Research of the Year" in 2017 by the Centres de Recerca de Catalonia (CERCA). Between 2017-2020, he focused on the advanced electrolyzer design for water treatment technologies such as sulfide removal and denitrification from waste/ground water in The Catalan Institute for Water Research (ICRA), as well as the scale-up efforts of CO2 processes for the electrification of the chemical industry in University of Antwerp (ELCAT). By joining to TU Delft’s MECS group in 2020, Dr. Erdem Irtem has been working on enhanced electrolysis devices for Selective Electrochemical Reduction of CO2 to High Value Chemicals (SelectCO2 Project) and supporting the objectives of e-Refinery on building a pilot scale 100 kW electroyzer.
The electrochemical reduction of carbon dioxide (CO2) to hydrocarbons provides storage of renewable electricity into energy dense molecules while also neutralizing CO2 emissions. Last decade has shown an unprecedented development towards its industrial application by reaching CO2 reduction reaction efficiencies (>95 % for CO and 87 % for C2H4) thanks to the fine engineering of novel catalyst and membrane types. In chorus, the gas diffusion electrodes (GDE) and flow-type reactors have played a key role to overcome mass transfer limitation and steer the current densities up to industrially relevant values, e.g. ~1 A/cm2.
Currently, most of the gas diffusion layers forming the backbone of the GDEs have been adopted from the proven processes such as fuel-cell and chlor-alkali systems. However they are not ideal for the large scale implementation and long term stability of CO2 electrolysis due to the diverse nature of the reaction. In this work, we will show the main advantages and limitations of the three most common gas diffusion layers employed in the field of CO2 electrolysis which are; nonwoven-, paper and cloth-GDEs with an identical catalyst layer obtained by sputtering of 150 nm thin copper film. By using an operando IR-thermography technique (filed patent), we mapped the temperature distribution profile of the GDEs until their failure by flooding. Thermal imaging results showed a smaller gradient and uniform temperature profile along the Cloth-GDL, which was attributed to the continuous fiber structure forming a straight electron path. On the other hand, the agglomerated PTFE flakes, large pockets and cracks of Paper-GDL were found as failure points to flooding by electrowetting and displayed a resistance to the electron path, evident from the higher potential and temperature value at elevated current densities. Conversely, very compact form of carbon nanoparticle and fiber structure of Nonwoven-GDL underwent premature flooding by the salt deposits and blocked CO2 diffusion to the catalyst layer. Coupled with an advanced ex-situ electron microscopy for post-mortem analysis , we have localized and matched the failure mechanisms of each GDE with a focus on the changes observed in the operando analysis during an hour long electrolysis at 250 mA/cm2. Our results will provide the strategies for inspection and improvement for better performing GDEs which would be beneficial not only to CO2 electrolysis but also to the upscaling of electrical energy conversion and storage devices.
1.3-I1
The field of CO2 electrolysis witnessed rapid development in recent years. At the same time, the role of the anodic half-reaction has received considerably less attention. Iridium is almost exclusively used as the anode catalyst in polyelectrolyte membrane water electrolyzers. This practice quickly became a standard in CO2 electrolysis due to its similarity to water electrolysis (oxygen evolution - OER is the anode process in both cases). An alkaline electrolyte solution is typically recirculated in the anode compartment to ensure a high reaction rate during CO2 electrolysis. However, iridium is known to slowly but continuously dissolve in alkaline solutions. Additionally, while using iridium as the anode catalyst on a laboratory scale is acceptable, economic reasons urge the exploration of non-noble alternatives.
In my presentation, I am going to discuss alternative anode electrocatalysts that have been tried already in CO2 electrolyzers. A closer look will be taken at Ni, which should be stable at least under the initial process conditions. We found that while Ni showed high activity (similar cell voltage to that with iridium) at the beginning of our experiments, it instantaneously started to decrease over time. If the anolyte is recirculated (typical scenario), the continuous carbonate transport through the anion exchange membrane decreases the bulk pH of the anolyte. In the case of the zero-gap electrolyzer cells employed in our study, the carbonate ion flux directly hits the anode catalyst layer, causing a high carbonate ion concentration in the close vicinity of the electrode. In parallel, H+ ions formed at the anode as the result of OER are not neutralized instantly (unlike in water electrolysis), which leads to an acidic surface pH. These two effects together alter the local chemical environment at the surface of the anode electrocatalyst leading to a gradual dissolution of Ni.[1] Based on our measurements, a set of criteria will be described that have to be fulfilled by an anode catalyst to achieve high performance.
In the second part of my talk, I am going to provide an outlook on replacing OER at the anode with various alternative anode reactions (such as electrocatalytic alcohol oxidation) to deliver high-value products in parallel with increasing the energy efficiency for the whole process.[2] A closer look will be taken at issues regarding the selectivity and stability of these systems along with discussing possible mitigation strategies and future research directions.
1.3-I2
Cu is the only monometallic catalyst that can reduce CO producing a large variety of valuable compounds. However, the efficiency and selectivity towards specific products is highly affected by the structure properties of the catalyst and the composition of the electrolyte.[1]
Herein we have investigated the effect of the pH and the anion specifically adsorbed on the surface, on the structure and properties of the Cu(111) and Cu(100)-aqueous electrolyte interface.[2–4] Experiments under potential control were conducted on well-defined Cu single crystalline electrodes, and in presence of CO, to assess the electrolyte and surface orientation effect on the onset potential for the CO reduction.[3] We specifically used cyclic voltammetry in combination with other electrochemical approaches such as the CO displacement technique and laser induced temperature technique, in order to shed lights into the distribution of the surface charge and adsorbed electrolyte species across the whole potential window.[2–4] We experimentally showed that the structure of the Cu-electrolyte interface, at different pH and in presence of different anions, controls the onset potentials of the CO reduction. Lastly, we have investigated lead underpotential deposition to assess the different facets and domains in nanostructured copper, which is relevant to design more efficient electrocatalysts for the CO reduction. [5]
Figure 1 shows the cyclic voltammogram (CV) of Cu(111) in contact with 0.1 M phosphate buffer solution at pH 5 and in presence of CO, recorded at a short potential window (blue line) and long potential window (red line), 50mV/s.
2.1-T1
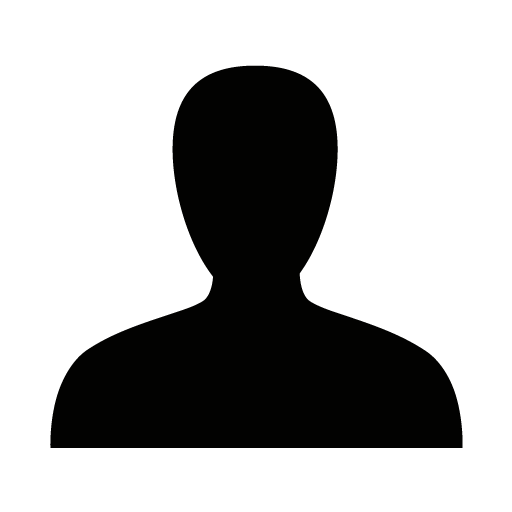
Efficient conversion of solar energy into molecules for energy storage and other purposes is one of the most important challenges for sustainable energy systems of the future. Particularly directly coupled photovoltaic (PV) assisted electrochemical (EC) processes, like e.g., PV-assisted water splitting, are of interest. In most practical cases, PV and EC devices are developed separately and then merged at a later stage to verify their performance as a PV-EC system. Enormous efforts of many groups in catalyst development produce increasing number of new catalysts every year. In turn photovoltaic community continues optimization of variety of PV technologies. In this situation, it would be useful to be able evaluate potential of one or other catalyst pair in combination with different PV technologies without building and optimizing an experimental device. To address this issue, we have recently developed a simple method to assess limit of solar-to-hydrogen efficiency (STH) of a specific electrolyzer based on the “reverse analysis” of its polarization curve [1]. We show that despite the complexity of the parameter space, there is a surprisingly simple way to estimate the limit of efficiency in any PV-assisted electrolyzer system.
The maximum STH for a specific electrolyzer is achieved when the current-voltage (IV) characteristics of the PV device crosses polarization curve of the EC device at maximum power point (MPP). Conversely, each point on the EC polarization curve can be considered the MPP of a PV device optimally coupled to the EC device. Therefore, at each point on the polarization curve, the minimum PV efficiency and maximum EC efficiency can be calculated for a specific irradiance. The product of both efficiencies generates the STH limit that can be attained at that specific point on the polarization curve. The result of the transformation is the dependence of the STH limit on the PV efficiency at the assumed irradiance level and optionally ratio of the active areas of PV and EC devices. This "reverse analysis," carried out with elementary math, does not involve any modeling or analysis of PV IV characteristics and provides a quick simple way to quantify the potential of any electrolyzer.
In our paper we present the principle of the reverse analysis using an example NiMo/NiFeOX catalyst pair. Next, we show how using this analysis losses in an experimental PV-EC combination can be identified and quantified. We present extended set of results of reverse analysis applied to a variety of PV-EC combinations described in the literature. Finally, we discuss how this analysis can be applied to different electrochemical devices coupled to PV like e.g., PV-assisted CO2 reduction.
TOC Figure. Principle of the reverse analysis: (a) at each point on the polarization curve, minimum PV efficiency and maximum EC efficiency are calculated; (b) the product of both efficiencies generates the STH limit at the specific point; (c) the result of the transformation presented as STH limit vs. PV efficiency.
2.1-T2
Energy surplus, if not utilized, are dumped as waste energy in the form of heat, not to mention adding carbon footprint to our daily operations. Yet, developing countries struggle to meet their demand and distribution schedule of energy leading to rotational brownouts. Research on energy generation, storage, and release has been continuously rolling. Hydrogen, generated from water splitting, is postulated as one of the most promising alternatives to fossil fuels. In this context, direct hydrogen generation by electrolysis and fixation to graphene oxide (GO) in an aqueous suspension could overcome storage and distribution problems of gaseous hydrogen. Herein, approaches of hydrogenating graphene oxide were studied primarily by time-resolved Raman spectroscopy, and Fourier transform infrared spectroscopy (FTIR).
GO films, utilized as electrode for hydrogen evolution reaction with dynamic modulation of the potential difference, favoured 160% increase of C-H bond formation. Epoxide ring opening leading to hydroxyl groups suggests that these groups play a key role in hydrogenation. FTIR revealed characteristic -CH2 and -CH3 vibrations. This shows that hydrogenation is significantly also occurring in defective sites and edges of the graphene basal plane, rather than H-C(sp3). Partial reversibility was observed by in-situ Raman when applying a cyclic voltammetry caused by a reversible reaction present in the 0.34 to -1 VRHE range.
Photocatalytic hydrogenation was also explored. Metal organic frameworks (MOF) of graphene oxide, Ni2+, Cu2+ were found to acquire -CH2 and -CH3 moieties upon irradiation by a 75 W xenon lamp with TiO2 photocatalyst. The dispersing media and hole scavengers (water, methanol, and sulphite) were also found to affect the fixation of hydrogen in the (MOF). This method has demonstrated successful evolution of H-C(sp3) in the GO MOF whereas graphene-like structure (loss of oxygen functional groups) is produced when GO was subjected to the same irradiation technique.
2.1-T3
I am working at the Institute of Advanced Materials of the University Jaume I. My research is focused in the development of advanced hybrid materials for energy conversion and storage based on catalytic transformations. The hybrid materials are developed from well-defined organometallic complexes. The approach for such applications is divided on three different research lines: i) Organometallic chemistry: design, characterisation and properties of new catalysts ii) Catalytic applications in processes related to hydrogenation and dehydrogenation. iii) New materials: study of the properties and applications of organometallic compounds and metal nanoparticles supported in graphene derivatives for energy conversion and storage. Research Lines:
Ad. Mat. for catalysis
Rationale design of catalytic materials derived from organometallic complexes.
Development of stable metal nanoparticles as improved catalytic systems.
Ligand design for the immobilization of metal complexes and nanoparticles.
Fundamental and applied study of catalytic hydrogenation and dehydrogenation processes.
Ad. Mat. for energy storage
Development of systems for the storage of hydrogen in the liquid form using “Liquid Organic Hydrogen Carriers (LOHCs).
Development of Hydrogen Storage technologies for transport and uses of hydrogen.
Industrial Innovation and Technology Transfer
The group is involved in industrial projects on Hydrogen Storage, depolymerization processes and catalyst development in connection with a regional funding program (AVI).
One of the major limitations of renewables is their intermittent character which depends on climate conditions. The problem is a complete disconnection between energy production and demand. Consequently, the storage of energy surpluses from renewables becomes a necessity. To tackle this problem, in this work we propose an efficient way of energy storage based on using green hydrogen as an energy vector. The aim is to develop efficient systems for hydrogen storage in the form of chemical bonds based on Liquid Organic Hydrogen Carriers (LOHCs) [1].
From a chemical view, the key point of efficient LOHCs lies in the design of suitable catalysts and processes for the conversion and reconversion of hydrogen into chemical bonds. In this work, we describe our preliminary results in the field [2][3] and the last results in electrocatalytic dehydrogenation of amines and the catalytic hydrogenation of nitriles as efficient systems for hydrogen storage in the form LOHCs.
2.1-T4
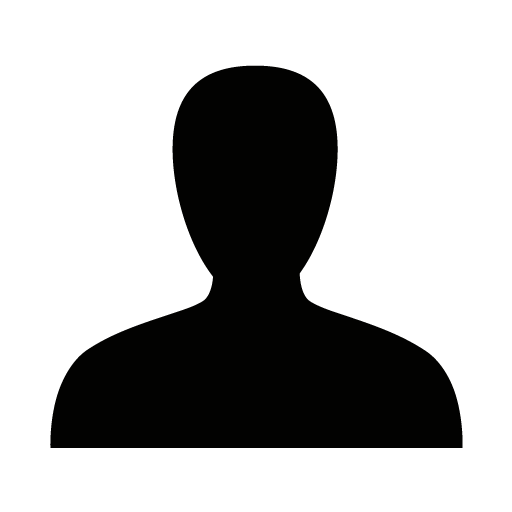
The production of green hydrogen for water electrolysis is very promising for the development of a zero-carbon-emission energy structure. However, proton exchange membrane (PEM) electrolyzers, which operate in acidic media, are limited by the slow kinetics of the oxygen evolution reaction (OER) at the anode. Additionally, the anode requires high amounts of scarce precious metals like Ir as catalysts1. An inherent need for developing preparation methods to decrease mass loadings and enhance activity and stability has therefore arisen. We introduce a feasible and easy way to prepare IrOx catalysts by galvanic displacement of electrodeposited Co and Ni on polycrystalline Au surfaces. We performed the electrodeposition of Co and Ni on gold beads in a deep eutectic solvent (DES) – a non-toxic solvent with a broad potential window and high solubility of many metals2. We fabricated Ir nanoparticles by employing galvanic displacement of Co and Ni in aqueous solutions of IrCl4, and then electrochemically oxidized the metallic Ir. Our preparation of IrOx catalysts replaces complicated plating methods by the galvanic displacement technique and replaces the use of surfactant agents during electrodeposition by a green DES electrolyte. We evaluated the activity and stability of the IrOx catalysts with state-of-the-art Ir-based catalysts. The IrOx-Ni displayed enhanced activity and stability in relation to the IrOx-Co in line with previous reports3.
2.1-T5
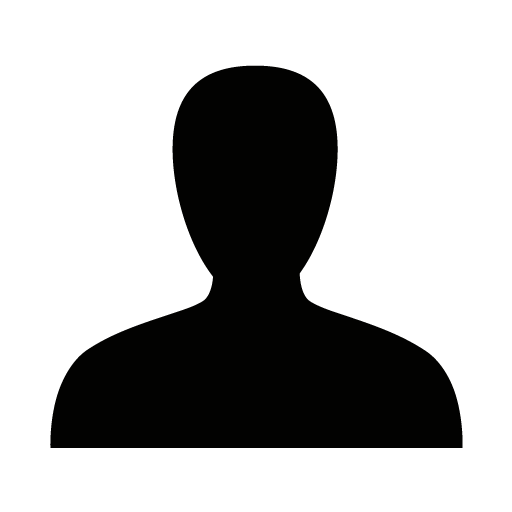
The requirement of the scarce element iridium for PEM electrolyzer anodes is a potential bottleneck in the transition to a fossil-free society. Replacing iridium is difficult due to the harsh conditions at the PEM anode of high potential and low pH. Only a handful of elements are stable as a solid phase in the region of their Pourbaix diagram corresponding to these conditions, most of which are either not conductive and/or not active for water oxidation. Ruthenium, though less stable and almost as rare as iridium, is an interesting candidate because its oxides (RuOx) have higher activity for water oxidation than those of iridium (IrOx). This talk will describe trends in the activity and stability of ruthenium dioxide at low overpotential. We use in-situ detection of O2 to distinguish water oxidation from charging currents [1] and isotope-labeling to probe the mechanistic coupling of water oxidation and catalyst degradation [2]. Ruthenium and iridium are also interesting in that both form rutile oxides, like most of the other elements that are stable (but inactive) under PEM anode conditions. Insights from IrO2 and RuO2 can therefore be transferred to novel solid-solution rutile oxides of the form AxB1-xO2. This talk will also provide a perspective on this approach to noble-metal-free PEM anode electrocatalysts.
2.1-T6
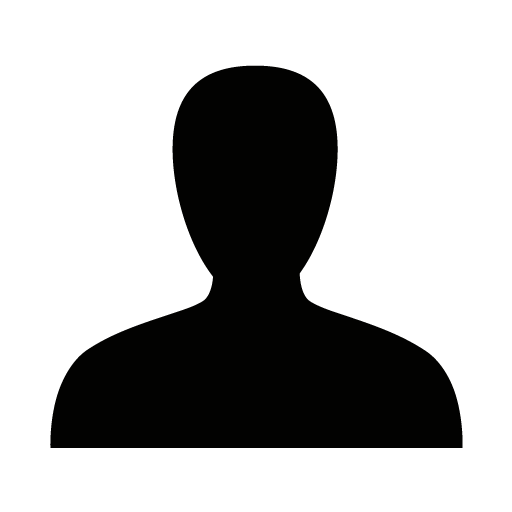
Producing electrodes that comprise highly active but low-cost catalysts for water splitting reactions is still a great challenge for developing new technologies for green energy. In the last few years, transition metal chalcogenides such as NiSe and CoSe were studied as electrochemical catalysts, displaying good electrocatalytic activity. Combining Ni and Co to form ternary compounds allows tuning the active sites for the reaction. In addition, NiSe and CoSe are photothermal materials, where irradiating with light leads to a substantial increase in their temperature.[1] Combining these two functionalities, these materials can be bifunctional, such that their heating contributes to the apparent catalytic reaction rate.
Ternary (NixCoy)Se nanoparticles were synthesized via a solvothermal method. The resulting nanoparticles were 15-30 nm in size, with a hexagonal crystallographic structure. The ternary nanoparticles demonstrated enhanced electro-catalytic activity towards the hydrogen evolution reaction in acidic conditions. (Ni0.25Co0.75)Se exhibited a significantly improved HER performance relative to the binary compounds with an overpotential of 190 mV at 10 mA cm-2 and a Tafel slope of 53 mV dec-1. As for the photothermal performance, (Ni0.9Co0.1)Se proved to be the most efficient photothermal material in the set. Future work will focus on utilizing them for photo-thermal assisted electrochemistry and evaluating the added value of such setups.
2.2-T1
Nitrogen fixation has shaped our society over the past two centuries, providing ammonia derived fertilizers through the Haber-Bosch process to now feed more than half the world population. However, its production is responsible for >1% of our greenhouse gases emissions.[1] It is therefore essential to find alternative synthesis methods sourced by renewable energies.
The electrochemical Li-mediated nitrogen reduction to ammonia (NRR) provides a more sustainable alternative to this challenging reaction.[2,3] This system, operating in a non-aqueous electrolyte analogous to the ones used in Li-ion batteries, provides tangible selectivity towards NH3.[3–5] Just like in Li-ion batteries, a passivating Solid-Electrolyte Interphase (SEI), made of electrolyte degradation products, forms at the cathode of the NRR system. This SEI likely plays a role in controlled proton activity at the electrode surface, limited hydrogen evolution and thus the observed selectivity.[6–8] Consequently, characterisation of this interface (and of the whole system) is vital.
The work presented here expresses guidance for reliable and in-depth electrochemical characterisation of the NRR system and its SEI, all through the lens of a new non-aqueous electrochemical cell design. Drawing further inspiration from batteries, considerations on the cell’s three-electrode geometry,[9–11] construction of a true reference electrode[10,12–14] (as opposed to quasi-reference electrodes in current setups in the field),[3,5,15] and cathode/anode processes separation will be under investigation. As a result, we propose and demonstrate an ideal setup for potential-controlled NRR electrochemistry, capable of measuring accurately the different electrochemical processes occurring over the course of this elusive reaction. Improvements in the accuracy, consistency and reproducibility of the electrochemical measurements resulting from this cell design will be illustrated through the study of a variety of electrolyte architectures.
2.2-T2
Antonio Guerrero is Associate Professor in Applied Physics at the Institute of Advanced Materials (Spain). His background includes synthesis of organic and inorganic materials (PhD in Chemistry). He worked 4 years at Cambridge Dispaly Technology fabricating materiales for organic light emitting diodes and joined University Jaume I in 2010 to lead the fabrication laboratory of electronic devices. His expertise includes chemical and electrical characterization of several types of electronic devices. In the last years he has focused in solar cells, memristors, electrochemical cells and batteries.
Amines are compounds very sensitive to oxidation, and a wide array of products may be generated depending on the oxidant.1 A particularly challenging oxidation is the conversion of a primary amine to a nitrile. In this work, the electrochemistry of primary amines to nitriles is explored using porous Ti/Ni alloys electrodes. These electrodes were recently developed in our group and proved very active in the water splitting oxidation achieving very low overpotentials.2 Here, the electrodes have been used for the oxidation of amines to nitriles. A selection of primary amines have been evaluated to understand formation of secondary reactions that reduced their yield. For example, attachment of the amines to the electrodes and degradation in the reaction media has been reported frequently.3 Several conditions were screened to reduce these secondary reactions such as the use of solvents and their mixtures or modification of pH. Under optimized conditions quantitative conversions and high yields are obtained in the electrochemical reaction of primary amines to nitriles.4
2.2-T3
Upgrading processes are required to improve the quality of raw bio-oil and thereby increase their viability of bio-based fuel usage. Electrochemical upgrading of bio-oil can be an appealing alternative to current upgrading technologies, as they operate at moderate reaction conditions and can employ the expected surplus in electricity due to increasing capacities of renewable energy installations. One way to lower the acid content in bio-oil and thereby improving the quality of the bio-oil is by Kolbe electrolysis. In Kolbe electrolysis, acids are converted to (non)-Kolbe products and carbon dioxide. The reaction conditions strongly influence the product distribution. The influence of electrolyte pH is studied in literature, however its effect on the product outcome is not yet fully resolved. Contradictory observations and conclusions with respect to performance stability have been reported. Therefore, this work investigates the influence of electrolyte pH on the reaction-time and pH dependent product selectivity during Kolbe electrolysis of acetic acid on platinum.
In this study Kolbe electrolysis of acetic acid on a platinum anode in aqueous electrolytes is addressed with a particular focus on electrolyte composition. We will first disclose that a pH similar to, or larger than the pKa of acetic acid is required to favor formation and homocoupling of methyl radicals towards the Kolbe product ethane. However, extended duration of electrolysis of acetate at basic pH results in loss of Faradaic efficiency to the Kolbe product (ethane), compensated by the formation of the Hofer-Moest product (methanol). We postulate that the observed change in selectivity is caused by the dissolution of CO2, resulting in enlarged concentration of (bi)carbonate near the electrode electrolyte interface. During the presentation we present our current understanding of the electrode-electrolyte interface and we will provide evidence that the spatial spacing between two methyl radicals is increased which lowers the dimerization rate. Finally, we will discuss the implications of our observations for practical applications, like bio-oil upgrading.
2.2-T4
Nowadays, the search and development of economic and environmentally friendly synthetic procedures, alternative to traditional ones energetically fed by fossil fuels, is essential to avoid not only climate change, but also the inexorable depletion of such energy resource. Within this context, electrocatalysis has emerged as a suitable synthetic methodology that avoids the use of harsh reaction conditions and fossil fuels. Besides, in electrochemical approaches, the application of an electric potential difference allows performing chemical reactions that in normal atmospheric conditions would not take place.
The main chemical procedures studied in electrochemistry are water splitting and CO2 reduction. In both cases, the oxygen evolution reaction (OER) takes place at the anode, providing the electrons and protons needed in the cathode for the generation of H2 in water splitting or the conversion of CO2 into valuable species, such as CH4 or CH3OH. The OER produces O2 that despite being a very important compound, its price in the market is very low, which limits the economic viability of the process and ultimately reduces the interest in this technology.[1] Furthermore, this reaction exhibits large overpotentials when using catalysts based on earth abundant materials, limiting energy conversion efficiency.[2] For these two reasons, there is a growing interest to find alternative reactions to OER at the anode that, by one side, reduce the overpotentials needed and by the other, produce compounds with higher added-value and interest for the chemical industry.[1,3] In this framework biomass valorization, has emerged as an attractive substitute to the oxidation of H2O.[4] Herein we present our studies in the electrochemical oxidation of 5-hydroxymethylfurfural, a species derived from biomass, as an alternative reaction to OER that allows obtaining 2,5-furandicarboxylic acid (FDCA), a useful building block in the pharmaceutical and polymer industry.[5,6]
2.2-T5
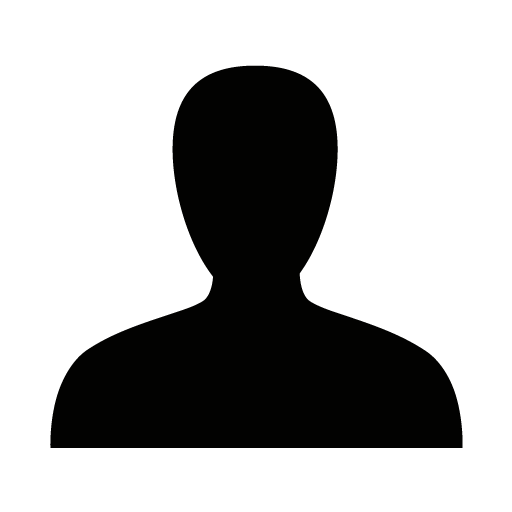
‘Water-in-salt’ type of electrolytes are highly explored in the recent past for its applications in aqueous batteries. Such electrolytes decreases the hydrogen evolution reaction (HER) by extending the water stability window which can be useful for other electrochemical reactions like CO2 reduction reaction or N2 reduction reaction (NRR) as HER is the main competitor reaction, where such processes are highly important in chemical industry. For example, development of methods for economically feasible greener ammonia (NH3) production is gaining tremendous scientific attention due to its importance in fertilizer industry and it is envisaged as a safer liquid hydrogen carrier for futuristic energy resources. In this aspect, electrochemical reduction reaction of nitrogen to ammonia in aqueous electrolyte is a promising way. In our research, an aqueous electrolysis based NH3 production in ambient conditions is discovered, which yields high faradaic efficiency (~12%) NH3 via NRR at lower over potentials (~ -0.6V vs. RHE or -1.1V vs. Ag/AgCl) on polycrystalline copper (Cu) bypassing HER.1 Li+ based aqueous electrolyte is used in varying Li+ concentration as electrolyte, where the role of Li+ in tuning the heterogeneous reaction is established by theory and experiments.2-4 It is observed that the HER activities of metals such as Pt, Ir, and Pd are suppressed by increasing Li+ concentration whereas that of Au, Fe, and Ni augmented with increasing Li+ concentration. Here the tunability in the metal-hydrogen (M-H) bonding energy with Li+ is experimentally and theoretically established, and the studies show that tunability in the HER properties of both noble and non-noble metals can be achieved irrespective of the pH (0 and 13) and counter ions (TFSI-, Cl-, ClO4-, NO3-and OH-) by tuning the M-H bond energy using Li+.
2.2-T6
Transition metal phosphides (TMP) are a promising materials family that has been studied extensively. Still, synthesizing complex and ternary phosphides in a reproducible manner is a challenge, due to the various available oxidation states and crystallographic phases. The various reactivities of the precursors and phase segregation often produce structure of diverse morphologies and compositions. For elucidating the intrinsic properties of these structures as catalysts, such synthetic control is crucial. Here, I will describe a few methods to produce complex ternary structures and their electro-catalytic activity towards hydrogen evolution (HER), oxygen evolution (OER) and alcohol oxidation.
Specifically, I will present a comparative study that illustrates the catalytic activity of three Ni-P phases towards hydrogen production through electrochemical water reduction as well as hydrogen retrieval by hydrolysis of hydrogen storage materials (ammonia-borane and NaBH4). I will show that Ni12P5 was recognized as a suitable platform for the electrochemical production of γ-NiOOH—a particularly active phase—because of its matching crystallographic structure. An additional incorporation of tungsten by doping produces surface roughness, increases the electrochemical surface area (ESCA), and reduces the energy barrier for electron-coupled water dissociation (the Volmer step for the formation of Hads). We explored the three different phases of nickel phosphide also for the electro-oxidation of methanol, ethanol, isopropanol, ethylene glycol, and glycerol. Ni12P5 exhibits excellent activity (210 mA cm-2 at 1.72 V vs RHE), durability, and mass activity (~4.2 A mg-1), outperforming the state-of-the-art catalysts. The high selectivity of the reaction and the large suppression of further oxidation to CO2 are a marker of a preferred bidentate adsorption configuration that conveys a specific O–H activation reaction path. The catalysts show excellent activity towards alcohol oxidation and durability, likely thanks to the mild conditions required for the process, which allow the formation of a regenerating thin active layer of oxidized nickel.
S2.3-I1
Ifan is Professor in Electrocat Imperial College London. Prior to Ifan's appointment at Imperial in 2017, he was Asssociate Professor and Leader of the Electrocatalysis Group at the Technical University of Denmark (DTU).In 2015, Massachusetts Institute of Technology (MIT) appointed Ifan as the Peabody Visiting Associate Professor.
Ifan leverages the insight from fundamental electrochemistry experiments to discover new catalyst materials with unprecedented performance. Ifan’s research ultimately aims to enable the large-scale electrochemical conversion of renewable energy to fuels and valuable chemicals and vice versa. He has 62 peer reviewed publications, 2 patents, 4 patent applicaitons and is cofounder of the spinout company, HPNow.
Current ammonia production via the Haber Bosch process requires high pressures, high temperatures and consumes >1% of our current fossil fuel production. To the contrary, nitrogenase in nature catalyses N2 reduction at room temperature and atmospheric pressures. The translation of the activity and selectivity of nitrogenase to a solid inorganic surface would enable the efficient on-site on demand synthesis of ammonia, powered by renewable electricity.
Amongst solid electrodes, thus far, only lithium based electrodes in organic electrolytes can catalyse N2 reduction to NH3.[1,2] Significant improvements are required to bring the lithium mediated system to an industrial reality; nonetheless, the field is progressing quickly, as we documented in a recent commentary.[3] Nonetheless, we lack comprehensive insight into the factors controlling the reaction.
In this talk, I will discuss why the lithium mediated system is unique amongst solid electrodes in its ability to reduce N2 to NH3. Our data using a combination electrochemical experiments, operando and ex-situ characterisation, and density functional theory calculations.[4] I will draw from concepts from enzymes, homogeneous catalysis and battery science. On this basis, I will discuss the most promising avenues towards more efficient N2 reduction.
S2.3-I2
Electro-organic synthesis has attracted many attentions in the last years due to the possibility of using renewable feedstocks like CO2, CO and N-based compounds to generate valuable products. Among many possible reactions, C-C and C-N bond forming reactions represent an important synthetic class because C2+ products and amine derivatives, respectively, play crucial roles in various applications in synthetic chemistry.
CO2 emitted in the atmosphere has been used as a feedstock for the synthesis of chemicals and fuels, although C2+ products are more attractive because of they have wider applicability and higher energy density [1], while NH3 derivatives are used to generate amines and amino acids [2] .
Here we studied both C-C and C-N coupling reactions using reductive electrochemical transformations.
The synthesis of C2+ products from the electrochemical reduction of CO2 was investigated on Cu-oxide derived nanoparticles. Our results showed the reaction is dependent on the electrode surface structure and proton concentration. Using online GC, ethylene and propanol were detected as products on Cu cubic nanoparticles, whereas on Cu polycrystalline, such C2+ products were not detected.
In order to enhance the spectrum of products, CO2 has been also considered in the coupling reaction with other organic molecules, i.e. aldehydes, to make carboxylic acids via electrocarboxylation. Our initial results showed CO2 reacts with butanal via C-C coupling to yield the corresponding carboxylic acid, in absence of CO2, the electrochemical reduction of butanal leads only to dimers.
We have also investigated the electro-reductive amination of benzyl alcohols to benzylamines in aqueous solvent. Using TEMPO-immobilised graphite electrode, benzyl alcohol was selectively oxidized to benzaldehyde (Figure 1), while in the cathode, t-butylamine was converted into imine, which acts as the main intermediate to the C-N bond formation. Using the electrochemical conditions displayed in scheme 1, MS and NMR data analyses showed faraday efficiency (FE) of 12% towards benzylamine.Despite the low FE, this work opens an opportunity to the synthesis of important fine chemical by using simple renewable feedstocks.
References:
[1] da Silva, A. H. M.; Raaijman, S. J.; Santana, C. S.; Assaf, J. M.; Gomes, J. F.; Koper, M. T. M. Electrocatalytic CO2 Reduction to C2+ Products on Cu and CuxZny Electrodes: Effects of Chemical Composition and Surface Morphology. Journal of Electroanalytical Chemistry 2021, 880, 114750.
[2] Jeong Eun Kim, Seungwoo Choi, Mani Balamurugan, Jun Ho Jang, Ki Tae Nam. Electrochemical C–N Bond Formation for Sustainable Amine Synthesis. Trends in Chemistry, November 2020, 2, 11.
S2.3-I3
Marta Hatzell is an Associate Professor of Mechanical Engineering at Georgia Institute of Technology. Prior to starting at Georgia Tech in August of 2015, she was a Post-Doctoral researcher in the Department of Material Science and Engineering at the University of Illinois - Urbana-Campaign. During her post doc, she worked in the Braun Research group on research at the interface between colloid science and electrochemistry. She completed her PhD at Penn state University in the Logan Research Group. Her PhD explored environmental technology for energy generation and water treatment. During graduate school she was an NSF and PEO Graduate Research Fellow.
Currently her research group focuses on exploring the role photochemistry and electrochemistry may play in future sustainable systems. She is an active member of the American Chemical Society, the Electrochemical Society, ASEEP, AICHE, and ASME. Dr. Hatzell has also been awarded the NSF Early CAREER award in 2019, the Alfred P. Sloan Fellowship in Chemistry in 2020, the ONR Young Investigator Award in 2020, the ECS Toyota Young Investigator award in 2021, and the Moore Inventor Fellow in 2021.
Nitrate (NO2-) is the world’s most widespread surface and ground water contaminant that causes adverse effects on human health such as methemoglobinemia (“blue baby syndrome”) and cancer. Most abiotic nitrate removal strategies center on the use of ion exchange resins. Ion exchange resins are effective, yet are not sustainable the process generates a large amount of waste. Biological approaches are inapplicable for removal of high nitrate ions and large scale applications as well as possibility of bacterial contamination in the drinking water. Electrocatalytic NO3- remediation; however, is one emerging approach for nitrate removal which does not produce waste as NO3- is converted directly to inert dinitrogen (N2) gas. The main challenge with electrocatalytic NO3- reduction is the low NO3- conversion yield, poor N2 selectivity, and lack of understanding regarding catalyst stability. The production of equally harmful contaminant intermediate species such as nitrite (NO2-) and ammonium (NH4+), has also limited the applicability of electrochemical routes for nitrate remediation. Electrocatalytic NO3- valorization is another emerging approach for nitrate removal. Here, NO3- is converted directly to ammonium (NH4+). The main challenge with valorization of NO3- is the low activity and selectivity of the NO3- to NH4+ and the structure sensitivity studies are insufficient. Here, we will describe an investigation on the electrocatalytic properties of palladium (Pd) and copper (Cu) which contains structured surfaces and coatings. The primary aim is to identify which facets and surfaces are highly active and selective for nitrate and nitrite reduction. Engineering the structure of Pd and Cu that enhances the NO3- removal efficiency and enables to steer the selectivity toward N2 and NH4+. To achieve the real-life applications, we also verify the long-term operations for the developed electrocatalysts.Nitrate (NO2-) is the world’s most widespread surface and ground water contaminant that causes adverse effects on human health such as methemoglobinemia (“blue baby syndrome”) and cancer. Most abiotic nitrate removal strategies center on the use of ion exchange resins. Ion exchange resins are effective, yet are not sustainable the process generates a large amount of waste. Biological approaches are inapplicable for removal of high nitrate ions and large scale applications as well as possibility of bacterial contamination in the drinking water. Electrocatalytic NO3- remediation; however, is one emerging approach for nitrate removal which does not produce waste as NO3- is converted directly to inert dinitrogen (N2) gas. The main challenge with electrocatalytic NO3- reduction is the low NO3- conversion yield, poor N2 selectivity, and lack of understanding regarding catalyst stability. The production of equally harmful contaminant intermediate species such as nitrite (NO2-) and ammonium (NH4+), has also limited the applicability of electrochemical routes for nitrate remediation. Electrocatalytic NO3- valorization is another emerging approach for nitrate removal. Here, NO3- is converted directly to ammonium (NH4+). The main challenge with valorization of NO3- is the low activity and selectivity of the NO3- to NH4+ and the structure sensitivity studies are insufficient. Here, we will describe an investigation on the electrocatalytic properties of palladium (Pd) and copper (Cu) which contains structured surfaces and coatings. The primary aim is to identify which facets and surfaces are highly active and selective for nitrate and nitrite reduction. Engineering the structure of Pd and Cu that enhances the NO3- removal efficiency and enables to steer the selectivity toward N2 and NH4+. To achieve the real-life applications, we also verify the long-term operations for the developed electrocatalysts.
3.1-I1
Electrochemistry plays a focal role in the development of better and more efficient catalytic processes for the use of electricity to form or break chemical bonds.
This discipline is closely associated with the development of new renewable energy solutions, such as energy storage (electrolysers, ammonia and other renewable fuels) and chemical production (from CO or biomass). In particular, electrocatalysis has allowed in recent years to achieve significant development on material development and reaction optimization for processes aiming at the synthesis of chemicals and renewable fuels. With this symposium, we want to highlight the scientific excellence in this field.
This discipline is closely associated with the development of new renewable energy solutions, such as energy storage (electrolysers, ammonia and other renewable fuels) and chemical production (from CO or biomass). In particular, electrocatalysis has allowed in recent years to achieve significant development on material development and reaction optimization for processes aiming at the synthesis of chemicals and renewable fuels. With this symposium, we want to highlight the scientific excellence in this field.
Materials and electrochemistry of N-containing compounds
Materials and electrochemistry of carbon dioxide reduction
Electrocatalytic synthesis of high-value compounds: new routes and materials
Electrochemistry for the valorization of biomass
3.1-I2
Density functional theory (DFT) calculations at level of the generalized gradient approximation (GGA) are often used in computational electrocatalysis models [1]. This is because DFT-GGA functionals provide fair descriptions of metals at reasonable computational expenses. In this talk, I will first show that the common practice of using DFT-GGA functionals usually entails large numerical errors for the description of molecules, particularly those with multiple bonds, and how to mitigate them by means of a simple and intuitive semiempirical method [2, 3].
Furthermore, I will show that molecular errors have an impact on calculated equilibrium potentials and free-energy diagrams of electrocatalytic reactions, as observed for CO2 reduction to CO, O2 reduction to H2O and H2O2, O2 evolution, and reactions within the nitrogen cycle [2, 4-6]. Finally, I will show that molecular errors appreciably modify Sabatier-type activity plots [6], such that different guidelines for catalyst design are obtained depending on whether or not molecular errors are corrected. The main conclusion is that it is generally advisable to use gas-phase corrections in screening routines for electrocatalytic materials.
3.1-I3
To further expand fuel cell technology, a full understanding of the processes taking place at the surface of the electrodes is needed. After decades of investigation, platinum (Pt) is still the single metal with the highest electrocatalytic activity for the reactions taking place within a fuel cell. It has been shown that its catalytic properties can be further exploited by tuning the surface structure and composition. Therefore, preparing and understanding bimetallic surfaces is of paramount importance if one is to improve fuel cell performance [1].
Bi-metallic catalysts with well-defined surface structure can be prepared by depositing adatoms on Pt single crystal electrodes [2], through the formation of surface alloys by co-deposition and gently annealing [3], or, as in this study, by preparing bulk alloy single crystal surfaces [4]. Here, the preparation of Pt–Pd bulk alloy single crystal electrodes following a modification of the Clavilier bead method has been revised. The surface composition and structure of the electrodes have been analyzed by X-ray photoelectron spectroscopy (XPS) and low-energy electron diffraction (LEED), respectively, pointing out that the surfaces prepared by this methodology are well-defined and give rise to surface compositions close to the nominal ones. Subsequent correlation between surface structure and composition and the voltammetric response has been assessed by performing cyclic voltammetry in different supporting electrolytes, displaying a characteristic and reproducible response for each surface.
Reportedly, very few studies have been accomplished for fuel cell reactions on well-defined bulk Pt-Pd alloys. Here, we will also show the electrocatalytic behavior of a collection of Pt-Pd single crystal surfaces for certain reactions of interest, such as formic acid oxidation and oxygen reduction reaction. Therefore, this contribution is a step forward towards the understanding of the combined role of surface structure and composition of Pt-Pd bulk single crystal electrodes in their catalytic behavior.
3.2-T1
Electrochemical reduction of NOx, CO2, N2 and combinations hold the promise to be a cornerstone for sustainable production of fuels and chemicals. Importantly, all reactions share a direct competition with hydrogen, and furthermore, several products are formed from each reactant of these reactants.
For electrochemical CO2, a complex reaction, it has been shown to give multiple different products depending on the metal catalyst [1]. The unique copper catalyst, as the only metal catalyst has the ability to bind *CO without the coadsorption of *H, which results in a high-value multiple-carbon product distribution [2].
For electrochemical NOx, also multiple products are formed; N2O, N2 and NH3[3]. Uniquely again the copper catalyst stands out with the ability to bind *NO without the coadsorption of *H, which enables copper to produce ammonia [4].
For electrochemical N2 reduction to ammonia (NH3) the interest at ambient conditions is burgeoning [5-6]. Most interesting for the direct electrochemical N2 reduction in aqueous there is not a “copper” catalyst [7], and instead, the reaction is limited to the non-aqueous lithium-mediated system.
In this talk I will give a unified approach to these reduction reactions versus hydrogen:
► Show an original and simplistic view of reduction reactions versus hydrogen by investigating the *NO, *CO and *N2 binding energies versus the *H binding energies. This gives direct insight into the product formation for NOx and CO2 reduction, and the challenge of the direct N2 reduction reaction.
► Show how one can use these molecular binding energies versus hydrogen in combination to form products beyond the typical reduction reaction products, which reduction reactions are possible based on this framework and how to challenge it [8].
3.2-T2
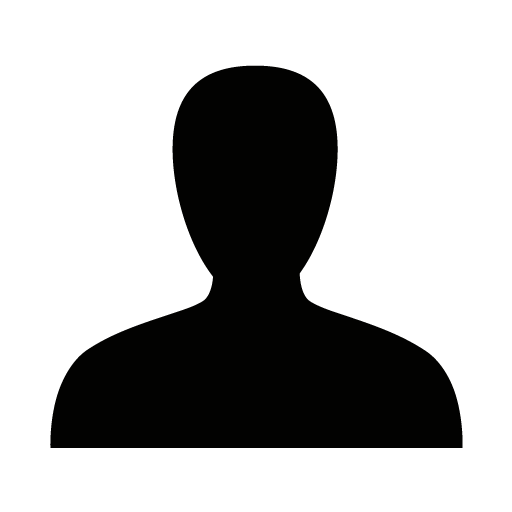
Electrochemical reduction of CO2 to fuels has attracted a great deal of attention in recent years as a potential solution to close the carbon cycle [1]. In search for novel catalyst materials, we present in this study density functional theory-based screening of heteroatom-doped transition metal nitrides [2]. We first examine zinc blend and rock salt polymorphs of transition metal nitrides and then consider doping the surface with 25% B, P, Sb, Bi, and C heteroatoms [3]. We fully assess the stability and activity of the examined catalysts. The catalytic activity is measured by the calculated limiting potential, and the stability is assessed against hydroxyl (*OH) poisoning as well as dissolution under CO reduction relevant potentials. Of the screened nitrides, many are predicted to be active for the CO reduction reaction but only a few are stable under electrochemical conditions. In particular, on nearly all of the metal nitrides with a desired catalytic activity for CO reduction, either the competing hydrogen evolution reaction prevails over CO reduction or *OH poisoning occurs at CO reduction onset potentials. We identify five promising candidates including P-doped NbN, C-doped VN, P-doped VN, TiN, and Sb-doped TiN. Ultimately, this study emphasizes the relevance of stability under electrochemical conditions and the importance of taking into account the competition between the CO reduction, hydrogen evolution, and *OH reduction reactions under electrochemical conditions.
3.2-T3
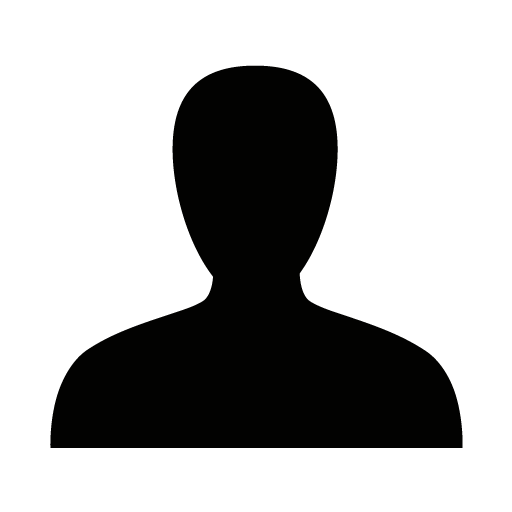
The insertion of heteroatoms with different electronegativity into a carbon network can greatly tune its electronic, optical, and electrochemical properties along with its chemical reactivity. The introduction of nitrogen and sulfur that serve as electron donors or boron as an electron withdrawing group, into a carbon structure will alter its electronic states, (electro)catalytic properties, and chemical stability to oxidation and to high temperatures. The traditional methods of synthesizing heteroatom-modified carbon matrices are carried out by solid state reactions, chemical vapor deposition (CVD), and functionalization of the carbon materials. Although an impressive wide range of materials were synthesized by these methods, there are still some drawbacks that limit the progress in this field.
Herein we show a new, scalable, and easy way to synthesize heteroatom-incorporated carbon materials by means of molten-state intermediate. The molten-state intermediate is achieved by using molten precursors, polycyclic aromatic hydrocarbons as carbon source and other molten precursors for the various heteroatoms e.g. elemental sulfur or ammonia-borane complex (NH3BH3). These precursors once heated above their melting point form the above-mentioned molten-state intermediate which induces strong covalent/coordination bonds between the heteroatoms and the sp2 carbon skeleton. This method enables the synthesis of BNCO, CS materials, transition-metal incorporated carbon/carbon-sulfur-oxide materials. The elemental composition is easily controlled within these materials (up to 30 wt.% boron, 20 wt.% sulfur, 30 wt.% nitrogen, while the transition metal ratio is kept within relevant materials) resulting in fine tuning of the materials properties. These materials show promising performance towards electrochemical OER, Na-ion and Li-ion batteries while illustrating the structure-activity relations.
1.1-T1
Sixto Giménez (M. Sc. Physics 1996, Ph. D. Physics 2002) is Associate Professor at Universitat Jaume I de Castelló (Spain). His professional career has been focused on the study of micro and nanostructured materials for different applications spanning from structural components to optoelectronic devices. During his PhD thesis at the University of Navarra, he studied the relationship between processing of metallic and ceramic powders, their sintering behavior and mechanical properties. He took a Post-Doc position at the Katholiek Universiteit Leuven where he focused on the development of non-destructive and in-situ characterization techniques of the sintering behavior of metallic porous materials. In January 2008, he joined the Group of Photovoltaic and Optoelectronic Devices of University Jaume I where he is involved in the development of new concepts for photovoltaic and photoelectrochemical devices based on nanoscaled materials, particularly studying the optoelectronic and electrochemical responses of the devices by electrical impedance spectroscopy. He has co-authored more than 80 scientific papers in international journals and has received more than 5000 citations. His current h-index is 31.
The development of sustainable strategies for the production of added-value chemicals and fuels using renewable resources is particularly attractive to promote a transition towards a more sustainable energetic landscape, overcoming the dependence of fossil fuels at a global scale.[1] One of the most promising alternatives involves the use of renewable electricity (wind, solar, hydropower, etc…) to power electrochemical conversion processes, which convert abundant molecules (e.g., water, carbon dioxide, and nitrogen) into higher-value products (e.g., hydrogen, hydrocarbons, oxygenates, and ammonia). In all these processes, (photo)-electrocatalytic water oxidation stands out as the preferred reaction to provide the protons and electrons needed for the target reduction reactions. In this context, metal oxides of earth-abundant elements (Fe, Ni, Co, etc…) are identified as excellent candidates, since these materials can fulfil most of the needed requirements, although in some cases their performance needs to be improved for a more realistic technological assessment. On the other hand, a clear mechanistic understanding of the physical-chemical processes taking place during operation is essential. In the present contribution, we will describe our efforts to understand the operation mechanisms of different metal oxides (mainly NiOx)[2-5] and other systems (CoFe prussian blue derivatives) for electrochemical water oxidation, as well as other electrochemical processes, [6-7] with particular emphasis on enhancing their performance. Our studies focus on the correlation of the (photo)electrochemical response of the materials with a detailed structural, optoelectronic and photoelectrochemical characterization carried out by different microscopic and spectroscopic tools.
1.1-T2
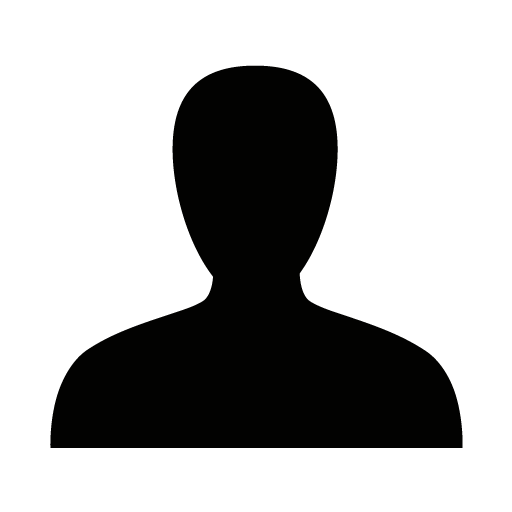
Iridium oxide is the state-of-the-art electrocatalyst for water oxidation in polymer electrolyte membrane (PEM) electrolysers for green hydrogen production. Amorphous iridium oxides (IrOx) show far higher activity than the rutile crystalline iridium oxides (IrO2), but the underlying origin behind the discrepancy in activity for IrOx and IrO2 remains poorly understood. Density functional theory (DFT) calculations are usually used to explain the kinetic difference based on the thermodynamic barrier for forming *OH, *O, and *OOH intermediates on a single Ir site of well-defined model catalysts.[1, 2] However, unambiguously correlating the theoretical free energy with experimentally observed kinetic is very challenging, especially for disordered and non-well defined nano-particle systems, such as amorphous IrOx. In addition, the ambiguities in measuring the number of active states for determining the intrinsic activity per states has further rendered it difficult to understanding the correlation between activity and the observed physical/chemical discrepancies on IrOx and IrO2.
In this talk, I will present our results on using time-resolved UV-vis absorption spectroscopy to experimentally probe the energetics of intermediate states and correlating them with water oxidation kinetics on rutile and amorphous iridium oxides. We have identified the same oxidised species for both IrOx and IrO2 and quantified their concentrations as a function of potential. By comparing the concentration of oxidised states to water oxidation reaction rates, we can directly measure turnover frequency (TOF) for IrOx and IrO2 and correlated them with the experimentally measured energetics of the states. Therefore, this study shines insights into the origin of activity difference between amorphous and rutile iridium oxides.
1.1-T3
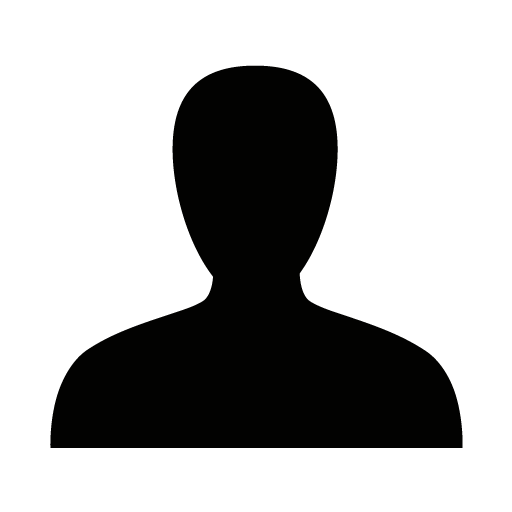
The electrocatalysis systems are very sensitive to electrode surface structure as well as the surface composition. A detail investigation of the nature of electrocatalysts is essential not only for the identification of reaction active sites or components, but also for the clarification of mechanism of electrocatalysts deactivation/activation, as the case of oxygen evolution reaction (OER)1-2. X-ray photoelectron spectroscopy (XPS) coupled with electrochemical methods is a powerful technique for this purpose. However, it is still quite challenging to combine the conventional electrochemical measurement under ambient conditions with XPS measurement under ultra-high vacuum (UHV) conditions. The in situ electrochemical XPS methods can overcome the shortness of sample change during the transfer as it is commonly encountered in ex situ studies3. However, due to presence of both electrolyte and vapor, synchrotron technique is usually required. In addition, the electrochemical signals are usually distorted by poor mass transfer. We have developed a quasi in situ XPS which consist of electrochemical treatment at ambient pressure, air free transfer from ambient pressure to UHV, and detection under UHV conditions. It has great advantages in: 1) acquiring electrochemical signal of good quality that can be directly compared with those in conventional cells; 2) avoiding oxidation of electrocatalysts due to air exposure; 3) collecting high intensity XPS signals by only commercial X-ray source. In this presentation, I will introduction the detailed setup, benchmark practice, and the application of a nearly in situ XPS on obtaining information on oxygen evolution reaction on Ru based catalysts.
1.1-T4
Photoelectrocatalysis has emerged as a promising process to store solar energy into fuels and high added-value chemicals to decarbonise the energy and fine chemical sectors. In this process the generation of H2, carbon-based chemicals or NH3 from H+, CO2 and N2 reduction, is usually limited by the oxidation reaction taking place at the photoanode, in particular when using metal-oxide photoanodes. The photoelectrochemical performance of these photoanodes vary depending on their synthetic route and post-synthesis treatment that can lead to crystal defects such as oxygen vacancies. However, the chemical nature of such oxygen vacancies and their role in photoelectrochemical oxidation of water or organic substrates to produce high added-value chemicals is still in debate.
In this talk, I will present a spectroscopic, microscopic and electrochemical analysis of the chemical nature of light-induced oxygen vacancies in one of the most studied photoanodes such as BiVO4. Oxygen vacancies in these BiVO4 photoanodes were produced by light exposure treatments and are associated with the migration of Bi towards the surface forming nanoparticles.[1] Additionally, I will show the role of oxygen vacancies in the photoelectrochemical behaviour of BiVO4, WO3[2] and a-Fe2O3[3] photoanodes and their role in the water oxidation mechanism as example.
1.1-T5
Growing environmental concerns, as a result of fossil fuel utilization, constitute a driving force towards the development of sustainable technologies to produce chemicals. The electroreduction of CO (CORR) or CO2 (CO2RR) to fuels are part of these technologies as C1 (methane, methanol) and C2+ products (ethylene, propylene, ethanol, propanol etc.) can be obtained [1]. CO is a particularly interesting reactant because it is present in CO-rich waste streams of common industries such as steel manufacture. For instance, it may be valuable to convert CO contained in blast furnaces’ flue gases, considered an irreducible source of COx, into chemical building blocks such as olefins or alcohols. Converting CO to light hydrocarbons and oxygenates by means of renewable electricity could help reducing our dependence on fossil resources. Moreover, CO can also be obtained from the widely studied CO2RR. CO constitutes a key intermediate in C2+ products formation in the aforementioned reaction [2]. Alternative to classical CO hydrogenation (Fischer-Tropsch synthesis), we considered here an electrochemical variant to obtain products with C-C bonds (C2+ products).
In this study, we focused on the electrosynthesis of C2+ products from CO on Cu-based catalysts. We studied the influence of catalyst particle size on the product distribution obtained during CO electroreduction. Particles ranging from smaller than 7 nm up to 45 nm were investigated. Intermediate sizes (20 nm – 30 nm) and a Cu foil have been reported to be the most selective for the formation of C-C bonds (ethanol, ethylene, propanol, etc..) during electroreduction of CO2 [3]. Our results show a different selectivity trend when using CO as reactant. The chronoamperometry data show a clear dependency of the selectivity on the particle size of the Cu electrocatalyst (Figure 1) with intermediate sizes (11 to 27 nm) showing the highest selectivity towards C2+ products while smaller particles favor hydrogen evolution. Among the used characterization methods, in situ XRD was employed to investigate stability of the particles under electrochemical conditions.
1.1-T6
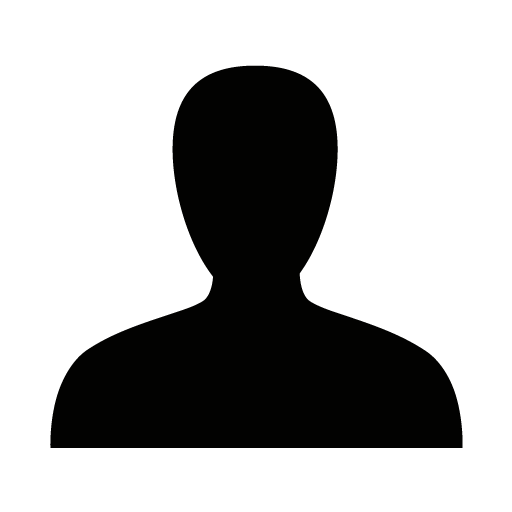
Electrolysis of water, CO2 and nitrogen-based compounds presents the opportunity of generating fossil-free fuels and chemical feedstocks at an industrial scale. These devices are complex in operation, and their activity and efficiency metrics are usually reported as averaged quantities across an electrode. This heat generation, of usually 30-50% of the inputted power, is due to cell inefficiencies. In this work we seek to use these inefficiencies as a measure of spatial electrochemical activity by taking advantage of the link between heat generation and the activity-dependent transport of electrons and ions. To this end we use data from an infrared camera positioned at the backbone of a gas-diffusion electrode in an attempt to develop a 'thermal potentiostat' which provides spatial resolution of electrochemical activity. After a proof-of-concept is displayed for the technique, we present several applications of the technology including catalyst screening, spatial-temporal catalyst measurements, catalyst-defect and ageing detection, and the impact of exothermic CO2-hydroxide interactions during CO2 electrolysis. Combined, we find that a spatial-thermal potentiostat has numerous uses for both novel and established electrochemical reactions towards the production of renewable fuels and feedstocks.
1.2-I1
Interfaces play an essential role in nearly all aspects of life and are critical for electrochemistry. Electrochemical systems ranging from high-temperature solid oxide fuel cells (SOFC) to batteries to capacitors have a wide range of important interfaces between solids, liquids, and gases which play a pivotal role in how energy is stored, transferred, and converted. This talk will focus on using ambient pressure XPS (APXPS) to directly probe the solid/liquid electrochemical interface of ion exchange membranes and composite water electrolysis electrodes. APXPS is a photon-in/electron-out process that can provide both atomic concentrations, chemical-specific, and local electric potential information at pressures greater than 20 Torr. Using synchrotron X-rays at Lawrence Berkeley Nation Laboratory, the Advanced Light Source has several beamlines dedicated to APXPS endstations to probe a wide range of interfaces ranging from solid/gas to solid/liquid and liquid/liquid. To enable our approach to investigating electrochemical solid/liquid interfaces, we leverage tender x-rays to support investigations at pressures greater than 20 Torr. Electrical leads support applying electrical potentials to enable the ability to collect XPS data of actual electrochemical devices while operating near ambient pressures. This talk will introduce APXPS and share how we can use this technique to directly probe the Donnan potential of an ion exchange membrane at the solid/liquid interface for the first time. In addition, I will share the chemical information we can reveal when probing a composite water electrolysis electrode undergoing electrochemical water splitting, gaining new insight to guide the design and control of future electrochemical interfaces.
1.2-I2
Dr. Chris Baeumer is Assistant Professor (Tenure Track) for Electrochemistry of Nanostructures in the Inorganic Materials Science group at the University of Twente and associate research group leader at Forschungszentrum Jülich, Germany. His research focuses on model electrochemical materials and interfaces with precisely controlled, single-crystalline surfaces, and their operando X-ray characterization to reveal the atomic details of complex oxide electrode materials at the solid/liquid interface. For this work, he was awarded the ERC Starting Grant. Before moving to the University of Twente, he was a Marie Skłodowska Curie Fellow at Stanford University and RWTH Aachen University.
Energy storage through the electrocatalytic generation of chemical fuels such as hydrogen is an attractive pathway for storing intermittent renewable energies, and perovskite oxides are among the most attractive candidate materials to catalyze the kinetically limiting half reaction, the oxygen evolution reaction (OER). OER activity is typically correlated to electronic and atomic structure parameters. But the catalyst surface – i.e. where the reaction happens – changes during the reaction. To design next-generation electrocatalysts, a detailed operando understanding of the relationships between catalytic activity, stability and atomic-level surface properties during the reaction is required.
In my talk I will address two essential ingredients to achieve this next-level understanding: controllable atomic-level surface properties and the development of surface-sensitive operando characterization routes.
Epitaxial thin films are a direct route for single crystalline model electrocatalysts that can be fabricated with atomically-tailored surface composition. These offer the ideal platform to derive structure-property-function relationships, track the evolution of the surface properties with applied potential and enable direct comparison to the surfaces investigated in density functional theory. I will summarize our recent findings for the role of surface termination, surface contamination, point defects and transformation pathways during the reaction and the role of solid/solid interfaces in proximity of the solid/liquid interface.
Next, I will summarize recent advances in surface-sensitive operando characterization in a liquid medium and with specific attention to studies with atomically-defined thin film model electrode surfaces. A focus will be the current development of meniscus photoelectron spectroscopy, a discussion about utmost surface- or interface-sensitivity using a standing-wave approach1,2 and a pathway to extract surface-sensitive information from nominally bulk-sensitive techniques such as UV-Vis spectroscopy.1,3
The key example throughout the talk will be LaNiO3 thin films, which are atomically flat both before and after application as electrocatalysts for the OER during water electrolysis. We selectively tuned the surface cationic composition in epitaxial growth. The Ni-termination is approximately twice as active for the OER as the La-termination. Using a suite of ex situ, in situ and operando spectroscopy tools, we found that the Ni-rich surface undergoes a surface transformation towards a catalytically active Ni hydroxide-type surface.1 If LaNiO3 surfaces are exposed to the ambient, however, surface carbonate groups form, which prohibit the formation of the active phase, leading to an activity decrease compared to the clean surfaces.4
Our work thus demonstrates tunability of surface transformation pathways by modifying a single atomic layer at the surface and it shows that active surface phases only develop for select as-synthesized surface terminations, highlighting the instructional value of epitaxial model electrocatalysts. It also highlights the need of and summarizes pathways for the exploration of the three-step relationship between as-prepared surface, transformation under applied potential, and electrocatalytic activity.
1.2-I3
I am currently a joint project researcher between Malmö University and the Nanomax beamline at MAX IV, my current projects focus on Bragg Coherent Diffraction Imaging (BCDI) of nanoparticles in the electrochemical environment and in situ grazing incidence XAFS. I am also actively involved in the development of in situ high-energy surface x-ray diffraction, performing experiments and writing software for data treatment.
I got my Ph.D. in 2016 from the University of Liverpool where my thesis focused on using surface x-ray diffraction to investigate fundamental electrocatalysis. Afterwards I was a postdoc at Lund university, where I focused on combining various synchrotron techniques with anodisation, corrosion, and electrodeposition. I then continued another two years at Lund and worked on in situ high-energy surface x-ray diffraction and some in-situ AP-XPS measurements. Afterwards I did another postdoc at the University of Copenhagen, Denmark, in the Nano-electrochemistry group.
One of my goals is to use synchrotron-based techniques to establish structure-function relationships for model electrocatalysts that will hopefully lead to the development of new catalysts, and a sustainable future. Also it's fun to learn new things, "stupid" experiments sometimes take us to unexpected places.
The performance of an electrocatalyst (its activity, selectivity, and stability) is strongly dependent on the electrode structure and composition, particularly in the near surface region. A successful approach in trying to understand the impact of structure is the use of well-defined model electrodes such as single crystals, to isolate how various changes in structure and composition impact upon the catalyst behavior. Surface x-ray diffraction gives the average surface structure of an isolated facet, whereas real catalysts often contain multiple facets and edge sites. This contribution will discuss two recent advances in the determination of electrocatalyst structure:
a) The application of high energy surface x-ray scattering to quickly map out large volumes of 3D reciprocal space and then extract crystal truncation rods. These truncation rods can then be used to determine atomic coordinates of surface atoms, in operando. I will present examples during methanol oxidation on both Pt(111) and Au(111) surfaces.
b) I will then discuss how we can complement single crystal studies by using coherent Bragg diffraction imaging (and some of the challenges) to look at single nanoparticle electrocatalysts and ceate strain maps to identify important facets and sites.
2.1-I1
The search for commercially viable catalysts for the oxygen evolution reaction (OER), which is typically the bottleneck for electrochemical water splitting, is a key challenge in the worldwide transition to renewables-based electricity generation. Transition-metal oxides in general and cobalt (hydro-)oxides in particular are of great interest in this regard, as they show promising catalytic properties, are stable in alkaline and neutral electrolytes under ambient conditions, and can be easily prepared from earth-abundant materials [1]. A wide variety of different catalysts, based on oxides of Co, Fe, and Ni, have been synthesized and studied, exhibiting rather diverse nanoscale morphologies and usually an unknown or poorly defined surface structure. This makes it difficult to compare their reactivity and correlate it with ab initio theoretical studies, which hinders the development of clear structure-reactivity relationships and unambiguous determination of the OER reaction mechanism.
We here present studies of structurally well-defined Co3O4 films of 10-30 nm thickness, prepared by electrodeposition or molecular beam epitaxy (MBE) on Au(111), Au(100), and Ir(100) substrates. In all cases a well ordered epitaxial Co3O4(111) arrangement results, but the film morphology differs substantially, as shown by AFM and X-ray diffraction measurements. The oxide structure under reaction conditions was studied by detailed operando surface X-ray diffraction [2,3] and in situ X-ray absorption measurements. These techniques allow characterization of the surface and bulk oxide structure over a wide potential range, including the OER regime, and can be performed simultaneously with electrochemical measurements, enabling direct correlations of structure and reactivity.
We find that the films prepared by molecular beam epitaxy are perfectly stable whereas all electrodeposited Co3O4 films exhibit reversible changes in the oxide structure and oxidation state, in agreement with a previous study of polycrystalline Co3O4 catalysts [3]. Specifically, we observe the formation of an ultrathin X-ray-amorphous CoOx(OH)y skin layer above 1 V vs. RHE and the gradual buildup of tensile lattice strain with increasing potential. These structural changes occur for all electrodeposited samples, albeit to a different extent, depending on the film morphology. They were found at pH values between 7 and 13 and in the presence of different cations. Potential step experiments show that the structural transformation proceeds within seconds and is highly reversible. Despite the similar structure, differences in the OER overpotential of up to 150 mV are observed for these samples, with the structurally stable MBE-prepared films having a larger overpotential than the electrodeposited films. This indicates a beneficial effect of the CoOx(OH)y skin layer on the OER activity of Co oxide catalysts.
[1] C. C. L. McCrory et al., Journal of the American Chemical Society 2015, 137, 4347.
[2] F. Reikowski, et al., ACS Catalysis, 2019, 9, 3811.
[3] T. Wiegmann, et al., ACS Catalysis, 2022, 12, 256.
[4] A. Bergmann, et al., Nature Communications, 2015, 6, 8625.
2.1-I2
Doing my BSc/MSc in Physics and PhD in an interdisciplinary program crossing the disciplines like Chemical Engineering, Nanotechnology, and Electrochemistry made me who I am today – a scientist who enjoys the challenge of multifaceted research.
I enjoy doing basic research in order to solve applied tasks. This explains my research interest in fundamental physical chemistry, e.g. oxidation and dissolution of metals and semiconductors, electrocatalysis, and electrochemistry at modified interfaces but also electrochemical engineering, e.g. development and optimization of catalyst layers in fuel cells and water electrolyzes.
Progress in basic research is often a direct outcome of previous achievements in experimental instrumentation. Hence, a significant part of my interest is in the development of new tools, e.g. electrochemical on-line mass spectrometry, gas diffusion electrode approaches, and high-throughput screening methods.
Platinum dissolution and a resulting decrease in the electrocatalyst active surface area is the main cause of the efficiency decrease of the proton exchange membrane fuel cell (PEMFC) with time. It has been extensively investigated on both the device and simplified model catalyst levels for many years, as summarized in our review [1]. In order of complexity increase, the systems can be ordered as follows: Pt low indexes single crystals; polycrystalline Pt; Pt nanoparticles; supported Pt nanoparticles in thin and thick catalyst layers, where the last one closely reminds the catalyst layer of PEMFC.
Over the last years, all these systems were investigated in our group to understand the complex mechanism of Pt dissolution. In most of these studies, an inductively coupled plasma mass spectrometer (ICP-MS) directly connected to an electrochemical cell was used for time- and potential-resolved Pt dissolution analysis. Scanning flow cell (SFC)- and gas diffusion electrode (GDE)-based setups were developed and successfully applied to study different Pt/electrolyte interfaces [2-4].
In this talk, I will present the most recent results of this research. Two systems: Pt/aqueous electrolyte and Pt/ionomer electrocatalytic interfaces, will be compared and contrasted [4, 5]. The main focus will be the understanding of the influence of system complexity and experimental parameters, simulating cell operational conditions, on Pt dissolution, with a final goal to clarify how the results on Pt dissolution from model catalysts can be extrapolated to PEMFCs. Moreover, mitigating approaches to stabilize platinum electrocatalytic interfaces will be discussed [6].
2.1-I3
The quest to design active and stable (electro)catalysts hinges on the ability to identify the reaction mechanism under realistic operating conditions. The past few decades of research have focused on developing a range of spectroscopic and imagining tools to enable the study of the chemical and structural changes at the solid/liquid interface.
This talk will bring the most recent understanding of the electrochemical interface during the energy conversion and storage reactions. The molecular-level understanding of the interfacial electrochemical processes on metal surfaces by operando surface-enhanced infrared absorption spectroscopy (SEIRAS) will be particularly discussed. We will also show that integrating those experimental approaches and density functional theory (DFT) calculation further strengthens the understanding of the reaction mechanism. It will also be demonstrated that the holistic information about the nature of the adsorbed species can provide additional knobs to tune the energetics of key reaction intermediates, leading to further improvements in electrocatalytic activity for energy conversion and storage reactions.
2.2-I1
Prof. Magalí Lingenfelder is a PI with an excellent track record and a passion for atomically controlled interfaces. Her work contributes to the design of new materials by elucidating chemical processes by Scanning Probe Microscopies and Surface Sensitive Spectroscopies, including dynamic (bio) molecular recognition processes at the liquid/solid interface.
She created and led for over 10 years the Max Planck-EPFL laboratory for Molecular Nanoscience at EPFL campus in Switzerland, and has recently joined the Basque Center for Materials as Ikerbasque Professor.
She studied physical and biological chemistry at the National University of Córdoba in Argentina. In 2003, she finished her MSc thesis at the Max Planck Institute for the Solid State Research (MPI-FKF in Stuttgart, Germany) with seminal contributions to the field of metal-organic coordination networks on solid surfaces. She continued with her doctoral studies in Physics, and received the Otto Hahn medal of the Max Planck Society in 2008 for the microscopic understanding of the chiral recognition process with submolecular resolution. In her quest to study molecular recognition going from 2D to 3D complex systems, she made postdoctoral stays at the Institute of Materials Sciences in Barcelona, and at the Molecular Foundry of the Lawrence Berkeley National Lab in the US.
She is a committed mentor who directed 4 MSc theses, 5 PhD theses, and 5 postdocs. She advocates for problem-oriented interdisciplinary research, by pioneering the emerging field of BioNanoarchitectonics. She led 5 international research consortiums, delivered over 50 invited presentations, and organized 9 conferences and 4 doctoral schools. She and her team had received multiple awards and international recognitions for their creative and rigurous work on molecular recognition, chirality and operando studies at catalytic interfaces. In 2018, the Royal Society of Chemistry included her work in the first collection “Celebrating Excellence in Research: 100 Women of Chemistry”.
A sustainable future requires highly efficient energy conversion processes. I will discuss two unconventional strategies to reach significant boosts in the electrocatalytic activity of Earth-abundant materials using spin control:
-At the interfacial level, magnetic fields affect the mass transfer of charged reactants (to) and products (from) the electrolyte. This effect is universal on both magnetic and non-magnetic electrodes. I will demonstrate this phenomenon operando using Pt and transition metal oxides as catalysts for the oxygen evolution reaction (OER), oxygen reduction reaction (ORR) and hydrogen evolution reaction (HER).
-At the local level, we use chiral molecules to induce spin selective pathways on the catalyst’s surface. As a case study, I will show the use of functionalized helicenes to boost the oxygen evolution reaction (OER) by ca. 131 % (at the potential of 1.65 V vs. RHE) at state-of-the-art 2D catalysts via a spin-polarization mechanism) [1].
By decoupling the effects involved in interfacial vs local spin control, we provide a versatile strategy that can be easily implemented to boost electrocatalytic reactions on different materials.
2.2-I2
Surface and interface sensitive operando characterization methodologies are required to unlock electrochemical and electrocatalytic processes. Liquid phase electrochemical transmission electron microscopy (TEM) can provide the sensitivity and selectivity at single particle level. Herein, we investigate oxygen-evolving cobalt-based oxides in alkaline solution and we retrieve morphological, structural, chemical information during cyclic voltammetry measurement. We compare the highly active Ba0.5Sr0.5Co0.8Fe0.2O3−δ (BSCF) to the spinel Co3O4 and rocksalt CoO. The potential-dependent variation of the local contrast is associated to the modification of the wettability from hydrophobic to hydrophilic character at the oxide surfaces through interfacial capacitance phenomena. Molecular oxygen is probed in real-time during its evolution which results to further dewetting of the surfaces. Our work on catalysts exemplifies the crucial role that surface sensitive and electrochemical microcell TEM techniques can play in detailing the mechanism of electrocatalytic processes and can provide a new characterization framework aiding development of novel design routes for targeted catalyst preparation.
2.2-I3
For over 50 years, Raman spectroscopy has remained a key tool in the arsenal of the electrochemists investigating structural and ionic (both solid-state and liquid electrolyte) dynamics in battery and electrocatalytic systems. However, imaging of evolving Raman signals has remained challenging due to the small signals (and hence slow acquisition speeds) of spontaneous Raman. Here, we overcome these limitations and develop and apply high-resolution (sub-300 nm) computational Raman imaging for real-time, chemically specific imaging of operating electrocatalysts and batteries.
Firstly, we track the OER from IrO2 and Li-IrOx electrocatalysts, demonstrating at low overpotentials that oxygen is evolved by the combination of an electrochemical-chemical mechanism and classical electrocatalytic adsorbate mechanism, whereas at high overpotentials only the latter occurs. We then examine electrochemical degradation in LCO and NMC811 electrodes operating at high voltages (above 4.2 V with LP30 electrolyte). For the LCO system, we observe a build-up of degraded electrolyte through the P-F bond intensity and oxidised Co along the edge of the particles.
Our results demonstrate the power of high-resolution Raman microspectroscopy for non-invasive operando, tracking of electrochemical dynamics and reveal new insights into the operation of state-art-of-the-art battery materials and electrocatalysts.
1.1-I1
Corina Andronescu received her B.Sc. and M.Sc. from the University Politehnica of Bucharest (Romania) in 2009 and 2011, respectively. Her Ph.D. title she received from the same university in 2014. In 2016 she joined the group of Prof. W. Schuhmann (Ruhr University Bochum, Germany) first as postdoctoral researcher and later as group leader. December 2018, she was appointed Junior Professor at the University of Duisburg-Essen, where she is currently leading the group of Electrochemical Catalysis in the Faculty of Chemistry. Her research interests include development of hybrid electrocatalysts for the CO2 electroreduction reaction, alcohol electrooxidation as well as investigation of electrocatalysts at nanoscale using Scanning Electrochemical Cell Microscopy.
The field of electrocatalysis experienced an intensely increased interest in the last years due to the urgency to move from a fossil fuel-based industry to one based on green energy. While new electrocatalyst materials are urgently needed, their rational design is still largely hindered by the lack of knowledge about the nature and structure of active centers in differently synthesized catalyst materials and the understanding of operational parameters that influence the catalysis.
Recently, high entropy alloys (HEA) or complex solid solutions (CSS) emerged as new promising materials in the field of electrocatalysis. A minimum of 5 different elements are mixed together, leading to a HEA material stabilized by entropy. Theoretical studies indicate that such materials, which possess many surface atom arrangements with different binding energies, could generate surfaces with an increased density of optimal or close to the optimal binding energy.[1] This may lead to increased activity compared with the already established catalyst and this was recently shown to be the case for the oxygen reduction reaction.[2]
One of the big challenges in the CSS research field arises from the multitude of possible surface atoms arrangement which could result from mixing five different elements. Exploring millions of possible combinations requires the use of high-throughput methods as well as new strategies to explore the space. Besides, the experimental probing and the identification of the surface atom arrangements with increased activity is impossible using classical electrochemical techniques.
Here we will show the potential of two high-throughput techniques: scanning droplet cell (SDC) [3,4] and scanning electrochemical cell microscopy (SECCM) to evaluate the multitude of active sites present in a complex solid solution (CSS).[5] By combining the two techniques and using a zooming-in approach from macro to the nanoscale, we can see an increase in the distribution of active sites in a HEA material.
1.1-I2
Professor Emma Kendrick, CChem FIMMM FRSC FIMMM - Chair of Energy Materials, School of Metallurgy and Materials, University of Birmingham.
Prof Kendrick’s career to date has included industrial and academic roles leading to her current role as Chair of Energy Materials, where in addition to group lead of the energy materials group (EMG), she is co-director of the Centre for Energy Storage (BCES) and part of Birmingham Energy institute (BEI) and Birmingham Centre for Strategic Elements and Critical Materials (BCSECM). The EMG investigates sustainability in novel battery technologies from materials, manufacturing, performance and parameterisation, and recycling. Her recent work has led to a 2021 joint UoB - Imperial College London (ICL) spin out company, based around the methods of experimental parameterisation of applied multi-physics cell models, called About:Energy, for which she is founder and director.
Prior to UoB, she spent two years as Reader in WMG, University of Warwick. Before academia, she led innovations in the battery industry, latterly as Chief Technologist in Energy Storage at SHARP Laboratories of Europe Ltd (SLE) and prior to that for two lithium-ion battery SMEs, Fife Batteries Ltd and Surion Energy Ltd.
She is fellow of the Royal Society of chemistry (RSC) and Institute of Metals, Mining and Materials (IoM3). Recently, she has been recognised through several awards; 2021 Faraday Institution (FI) Researcher Development Champion, RSC 2021 Environment, Sustainability and Energy Division Mid-Career Award, and the 2019 Hothersall Memorial Award for outstanding services to Metal Finishing.
Prof Kendrick holds a PhD from Keele University, obtained as part of a postgraduate transfer partnership (PTP) scheme with CERAM Research, a MSc in new materials from the University of Aberdeen and a BSc in chemistry from the University of Manchester.
Sustainability in batteries needs to be considered at a holistic level, from materials source, manufacturing, lifetime and if there is value in the materials what happens to the batteries at end of life. In this work we discuss three different aspects of sustainable considerations within a sodium-ion technology development. Materials developments, cell lifetime and optimisations, and design for recycling.
The cell is comprised of a nickel based layered oxide material cathode with hard carbon anode. To maximise energy density and life-time degradation of the bulk and surface of the O3-type oxides require stabilisation.1 We discuss a facile method for manufacturing a stabilised O3- type layered oxide via simultaneous doping and surface coating. A higher average voltage is obtained, which stabilised the high voltage phase transition to higher voltages, and results in longer-cycle life.2
To improve the materials life-cycle lifetime of the battery is also considered, and methods to improve the 1st lifetime and use discussed. The formation of the interface layers is key, and in addition to the stabilisation of the cathode surface stabilisation of the negative electrode is required. This is initially achieved with formation processes, and can be modified with different electrolyte additives.3,4 It is hypothesised that water causes significant damage, and removing these small molecules, for example using ZSM-5, in both an electrode and an electrolyte results in significant improvement in lifetime of the cell and the electrodes with less SEI build up over time.
Finally design for remanufacture is also discussed, utilising water-based binder systems we show differences in delamination and material recovery. Utilising different recycling schemes through shredding and physical processing through to disassembly processes improvements in recovery rates are required. Particularly for low value materials, where the techno-economics of the processes do not currently work. Direct recycling of materials reclaimed from the batteries, wherever possible allows value within the material design to be maintained, rather than relying directly upon elemental value. Aspects of direct loop recycling and short loop recycling are discussed with respect to current lithium-ion technologies, and the ability to directly translate this to sodium-ion.
In conclusion three aspects for sustainability considerations are discussed with respect to the materials life-cycle; active material optimisation, longevity and life-time when in use and electrode developments for recycling.
1. Song, T. & Kendrick, E. Recent Progress on Strategies to Improve the High-Voltage Stability of Layered-Oxide Cathode Materials for Sodium-ion Batteries. J. Phys. Mater. 4, 32004 (2021).
2. Song, T. et al. High-Voltage Stabilization of O3-Type Layered Oxide for Sodium-Ion Batteries by Simultaneous Tin Dual Modification. Chem. Mater. 34, 4153–4165 (2022).
3. Chen, L., Kishore, B., Walker, M., Dancer, C. E. J. & Kendrick, E. Nanozeolite ZSM-5 electrolyte additive for long life sodium-ion batteries. Chem. Commun. 56, 11609–11612 (2020).
4. Kishore, B., Chen, L., Dancer, C. E. J. & Kendrick, E. Electrochemical formation protocols for maximising the life-time of a sodium ion battery. Chem. Commun. 56, 12925–12928 (2020).
1.1-I3
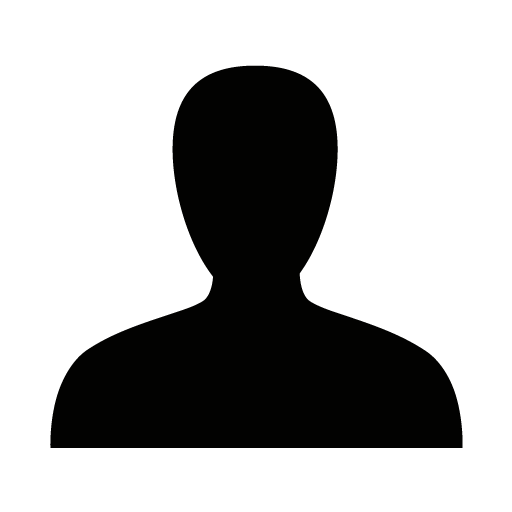
One of the major hindrances to mass commercialization of low-temperature proton-exchange-membrane fuel cells (PEMFCs) is the considerable amount of expensive Pt required, especially at the cathode side, where the oxygen reduction reaction (ORR) takes place.1, 2
In the last decade, great efforts have been made to develop efficient Platinum-Group-Metals (PGM) free catalysts for the ORR, especially metal-nitrogen-doped carbons (M-N-C, with M = Fe, Co), due to the abundance and inexpensiveness of their constituent elements and their atomic dispersion. The activity gap towards Pt has successfully been narrowed, now reaching the activity requirements for practical applications.3-5 Due to the metastability of the ORR active M-N4 sites at the temperature of their pyrolytic formation, the final transition metal loading is currently limited and significant amounts of inorganic by-products are formed. Although synthesis protocols have been successfully optimized, multiple processing steps are required, making the preparation time-consuming.
In our previous work, we showed that via an active-site imprinting strategy followed by a transmetalation reaction, Mg-N-C and Zn-N-C containing Mg-N4 and Zn-N4 sites respectively, can be transformed into active Fe-N-C electrocatalysts, avoiding the formation of elemental iron, or iron carbide side phases.6-8
In this work, we present how Zn−N4 sites in tailor-made Zn−N−C materials are utilized as an active-site imprint for the preparation of the corresponding Fe−N−C catalysts with a high loading of atomically dispersed Fe. The current state of this class of materials is discussed in terms of synthetic methods, activity and stability, including both rotating disk electrode (RDE) and single-cell PEMFC testing.
A. Kongkanand and M. F. Mathias, J. Phys. Chem. Lett., 2016, 7, 1127-1137.
S. T. Thompson and D. Papageorgopoulos, Nature Catalysis, 2019, 2, 558-561.
M. Lefèvre, E. Proietti, F. Jaouen and J.-P. Dodelet, Science, 2009, 324, 71-74.
R. Bashyam and P. Zelenay, Nature, 2006, 443, 63.
H. A. Gasteiger, S. S. Kocha, B. Sompalli and F. T. Wagner, Appl. Catal., B, 2005, 56, 9-3
A. Mehmood, J. Pampel, G. Ali, H. Y. Ha, F. Ruiz-Zepeda and T.-P. Fellinger, Advanced Energy Materials, 2018, 8, 1701771.
D. Menga, F. Ruiz-Zepeda, L. Moriau, M. Šala, F. Wagner, B. Koyutürk, M. Bele, U. Petek, N. Hodnik, M. Gaberšček and T.-P. Fellinger, Advanced Energy Materials, 2019, 9, 1902412.
8. D. Menga, J. L. Low, Y.-S. Li, I. Arčon, B. Koyutürk, F. Wagner, F. Ruiz-Zepeda, M. Gaberšček, B. Paulus and T.-P. Fellinger, Journal of the American Chemical Society, 2021, 143, 18010-18019.
1.2-T1
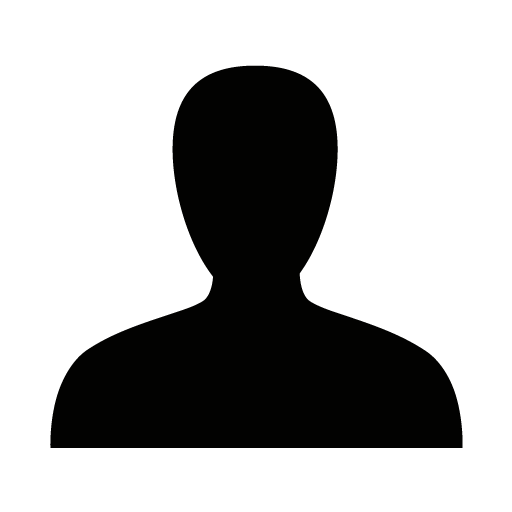
2D halide-perovskites as multifunctional photobattery materials – Fundamental investigations regarding stability, lithium intercalation and light induced processes
Jan Büttner, Taisiia Berestok, Stephan Burger, Michael Daub, Harald Hillebrecht, Ingo Krossing, Anna Fischer
Autonomous photo-rechargeable energy storage systems have received growing attention over the past years. [1–7] Among these highly integrated photobatteries are especially promising systems: they offer grid independent storage of energy to power small devices required for industry 4.0 or IOT applications. To achieve the highest possible level of integration in such photobatteries, multifunctional materials that can both convert sunlight and store energy at the same time and place, are required.
2-(1-cyclohexenyl)ethyl ammonium lead iodide (CHPI), a 2D organic-inorganic lead halide perovskite belonging to the Ruddlesden-Popper (RP) phases, has been reported to be such a type of multifunctional material, i.e. combining photo absorber properties with the ability to intercalate and especially photo-deintercalate lithium ions in combination with a carbonate based polar liquid electrolyte. [8]
In the present work, we investigated CHPI for its stability against dissolution, its Li+-intercalation and photo-assisted deintercalation properties and its behaviour under illumination in combination with carbonate based electrolytes and a newly developed electrolyte based on ortho-difluorobenzene (o-DFB), that has much lower polarity.
We show that (i) CHPI dissolves in carbonate-based electrolytes and that (ii) Li+ does not intercalate from non dissolving low polarity electrolytes and entirely capacitive behavior is displayed. As such, we could not confirm any photo-assisted Li+-deintercalation taking place in these materials.
In addition, we could reveal that under illumination, while being in contact with the non dissolving electrolyte, oxidative photo-corrosion and dissolution of the perovskite occurs. These results are in line with the absence of a reversible redox system in CHPI otherwise found in intercalation battery materials. Indeed only a hypothetical Pb+/Pb2+ system would actually be able to receive electron density, underlining the inability of CHPI to intercalate lithium ions in a significant amount, i.e. higher than just doping.
The chemical instability against polar solvent of any perovskite that can be synthesized by dissolving its educts in a polar solvent for e.g. spin coating prevents their application with standard polar lithium ion battery electrolytes in battery systems. Further electrochemical and photochemical instabilities add to the limitations of this material class towards their useage as truly multifunctional materials for higly integrated photobatteries. Finally their inability to store large amounts electron density prevents any relevant Li+-intercalation.
[1] H. Wei, D. Cui, J. Ma, L. Chu, X. Zhao, H. Song, H. Liu, T. Liu, N. Wang, Z. Guo, J Mater Chem A 2017, 5, 1873–94, 10.1039/C6TA09726J.
[2] A. Gurung, Q. Qiao, Joule 2018, 2, 1217–30, 10.1016/j.joule.2018.04.006.
[3] V. Vega-Garita, L. Ramirez-Elizondo, N. Narayan, P. Bauer, Prog Photovoltaics Res Appl 2018, 27, 346–70, 10.1002/pip.3093.
[4] D. Lau, N. Song, C. Hall, Y. Jiang, S. Lim, I. Perez-Wurfl, Z. Ouyang, A. Lennon, Mater Today Energy 2019, 13, 22–44, 10.1016/j.mtener.2019.04.003.
[5] Z. Fang, X. Hu, D. Yu, Chempluschem 2020, 85, 600–12, 10.1002/cplu.201900608.
[6] Q. Zeng, Y. Lai, L. Jiang, F. Liu, X. Hao, L. Wang, M.A. Green, Adv Energy Mater 2020, 10, 1–30, 10.1002/aenm.201903930.
[7] A. Hauch, A. Georg, U.O. Krašovec, B. Orel, J Electrochem Soc 2002, 149, A1208, 10.1149/1.1500346.
[8] S. Ahmad, C. George, D.J. Beesley, J.J. Baumberg, M. De Volder, Nano Lett 2018, 18, 1856–62, 10.1021/acs.nanolett.7b05153.
1.2-T2
Organic-inorganic metal halides (Perovskites/non-Perovskites phases) are emerging as a next generation optoelectronic material in both nanoscale and bulk form. Besides its novel applications in energy, light emitting diodes etc. these materials are also showing fascinating results in other appealing fields like battery research and sensors. [1]
Meanwhile Ionic liquids (ILs) have gained tremendous attention in various research fields over the last decades. Within melting points generally below 100 °C (much lower than conventional inorganic salts), ILs have been used in several applications, like as an electrolyte in fuel cells or in photovoltaic modules for the ion transport etc. Due to their low vapor pressure and low flammability ILs are quite safe and easy to handle. Even by altering the functional groups ILs or replacing the anions or cations, both the chemical and physical properties can be drastically changed. [2,3]
In this oral presentation, I will talk about organic-inorganic transition metal-halide structures, where ionic liquids act as organic cations. Besides its synthetic and structural details, we have discussed general optical, thermal and conductivity properties. These organic-inorganic metal-halides form 1D structures, which are confirmed by scanning electron microscopy and single crystal analysis. Like other ionic liquid mediated metal-halide structures, these compounds also have a low melting point, which are confirmed through DSC measurements. Most importantly, the here presented compounds show a high conductivity of 10-4 Scm-1 at room temperature. With elevation of temperature the conductivity increases and it reaches to 10-2 Scm-1 at 70 0C. In general, this is the very first report of superior ion conductivity of an organic-inorganic metal-halide structure that could be a very important milestone for future solid-state battery research. [4]
1.2-T3
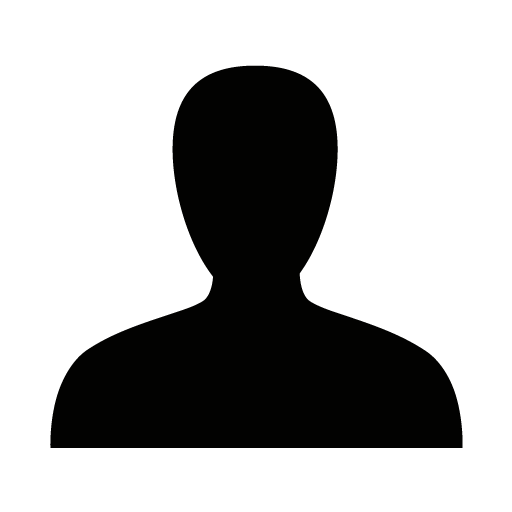
The energy transition to Zero carbon emissions started, and hydrogen is playing a very important role as replacement of the conventional fossil fuels, together with the large research and development in portable and stationary electrochemical energy storage devices as Li and Redox Flow batteries (RFB). An important aspect for these technologies is the materials used; RFBs store the energy in the electrolyte, requiring the use of porous electrodes with high surface are to maximise the electrolyte utilisation. For the Li Batteries, we will focus on the emerging Lithium Sulfur (Li-S), a carbon porous material is required in the cathode, which can accommodate the sulfur expansion during the charge and discharge, and as well avoid the polysulfides shuttle effect. An interesting material that can tackle this issue are carbon aerogels (CAGs), which are hierarchical carbon materials with tuneable porosity. The porosity and structure of these materials can be very easily tuned, changing the synthetic conditions, for each application.
In RFBs, to improve the performance and utilisation of the electrolyte can be done by reducing the thickness of the electrodes. However, some challenges need to be addressed, as mechanical properties, a good porosity and surface area. The CAGs, can be easily growth over different supports as carbon papers and carbon cloths, being in both cases, around 10 times thinner, compared with the standard graphite felts used in RFB. We had studied a series of these thinner electrodes, using carbon paper as support in all-Vanadium RFB, having a very interesting results in the behaviour of the different electrodes prepared [1].
The flexibility in the synthesis of these materials, give us a chance to use them as cathodes for Li-S batteries. In this case, we growth the CAGs in thinner electrodes successfully. In addition, we modify the CAGs doping them with Nitrogen and as well with Fe, trying to obtain a supported high porous single atom catalyst. We studied the electrodes in coin cell batteries to understand the performance and cyclability. In parallel, we started to analyse the CAGs powders and single atom catalyst with different metals in RDE and RRDE [2], trying to get a protocol to benchmark catalyst and materials for the sulfur reactions and understanding his kinetics in the oxidation and reduction.
Other important actor for the zero-carbon energy system pointed out at the beginning is the H2. A very important aspect is to develop materials for an efficient hydrogen production using renewable energies (Green H2), which requires the use of electrolysers to split the water. The major problem for the electrolysers is within the anode, as they use Iridium (Ir), being not very abundant, restricting the large-scale production. However, there is other alternative, the alkaline electrolysers, in which the Ir can be replaced by other metals, more abundant on Earth. A very interesting materials to explore for the alkaline electrolysers are the transition metal phosphides, as they have a very interesting activity for the oxygen reactions with a good corrosion resistance characteristic [3].
Using H2 for energy conversion, we need to mention Fuel Cells, focusing on the low temperature PEMFC, they are electrochemical devices that convert H2 and O2 in electricity and water. This system cannot be reversible due to the slow and complicated kinetics in the O2 reactions. But replacing it with a fast and reversible species used in RFB, give us a very interesting and flexible electrochemical energy storage system, known as hybrid redox flow batteries. For these devices the cathode side it is quite flexible, having the possibility to use an organic molecule [4], vanadium [5] or manganese [6].
1.2-T4
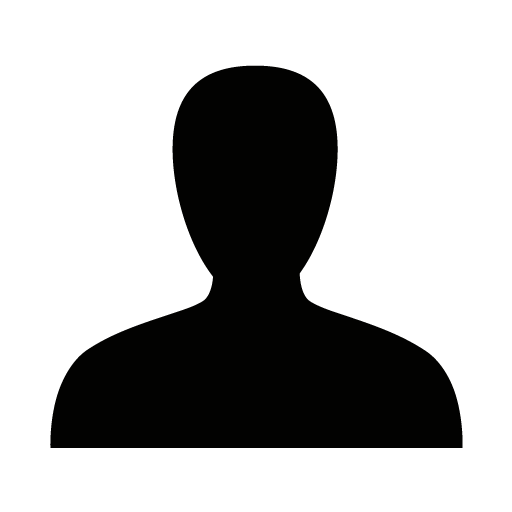
Finding new materials for different electrocatalytic reactions for renewable energy conversion is essential to build a sustainable future. High entropy alloys (HEAs), in which 5 or more elements are mixed forming a new crystallographic structure, have emerged as a new class of materials with interesting properties for electrocatalysis1. There are innumerable possibilities of combining the metals, which allows tailoring the electrocatalytic properties by tuning electronic and geometric effects in the HEA. In addition, investigating these multifunctional electrocatalysts can be used as a platform for the discovery of novel sustainable materials. However, there is a lack of information regarding how the elements distribute on the surface and their stability and structure under different applied potential conditions, which is crucial for the rationalization of energy conversion reactions. Thus, potential-controlled experiments and benchmarking with model well-known monometallic electrode surfaces are necessary to assess catalytic and interfacial properties of HEAs2. In this work, we investigate the oxidation of carbon monoxide on an extended high entropy alloy electrocatalyst over a wide range of pH3. We combine electrochemical characterization with surface-sensitive techniques including x-ray photoelectron spectroscopy and ion scattering spectroscopy to gain an understanding on the structure-reactivity relations.
1.2-T5
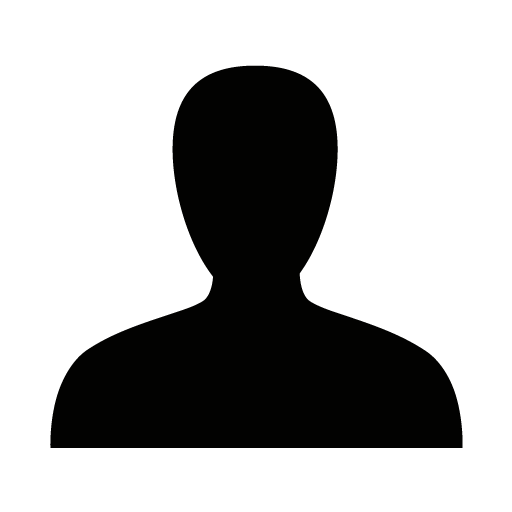
PhD student investigating Fe/N/C electrocatalysts for fuel cells and electrolysers.
Part of Magda Titirici group at Imperial College London.
Low temperature proton exchange membrane fuel cells powered by green hydrogen provide a means to sustainable energy production for stationary and transport applications, such as back-up power and fuel cell vehicles, respectively. Their widespread commercialisation is limited by the cost of the platinum catalyst at the cathode, where oxygen reduction occurs. Atomic FeNx sites within carbon offer a cheap and sustainable alternative, exhibiting the most promising non-precious metal activity for oxygen reduction. However, atomic Fe loading typically cannot exceed >2 wt.% without unstable and inactive FeC and Fe0 formation due to high pyrolysis temperatures (700-1000oC) required during synthesis of the conductive catalyst support. Recent progress has identified the successful use of a decoupled two-step procedure whereby Fe is incorporated at low temperature, following the high temperature pyrolysis, which has enabled >2 wt.% Fe.[1–3] In our work, we adapt a Zn active site imprinting and subsequent low temperature (170oC) Fe (trans-)metalation process,[1] instead using a zeolitic imidazolate framework-8 precursor to yield Fe >5 wt.% (ICP-MS) with oxygen reduction active FeNx sites. The ex-situ atomic nature of the FeNx active site is elucidated by aberration corrected high-angle annular dark field scanning transmission electron microscopy, x-ray absorption spectroscopy, and electron paramagnetic resonance. The high Fe loading also enables novel characterisation by time-of-flight secondary ion mass spectrometry.
1.2-T6
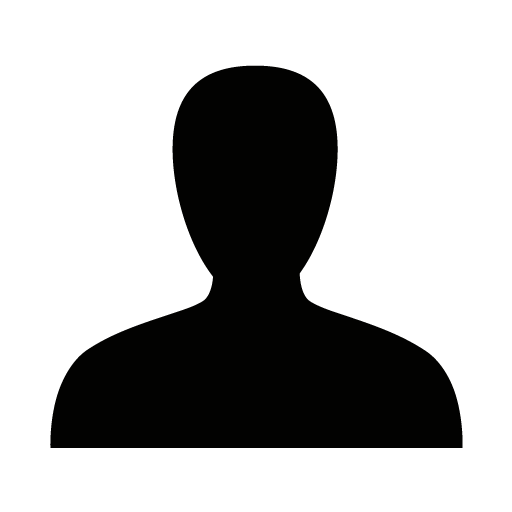
The electrochemical reduction of CO2 (eCO2RR) is a promising eco-friendly alternative to fossil-fuel-based processes used for the production of chemicals. Currently, the conversion of CO2 to CO is a more industrially appealing process than the eCO2RR to multicarbon products, as it requires lower energy consumption and presents high product selectivity.[1] Also, the concomitant hydrogen evolution reaction (HER) occurring at the same potential window is not a serious setback in this case since the mixture of H2 and CO (known as syngas) is a valuable product, which can be directly utilized in established industrial processes such as the Fischer-Tropsch to produce energy-dense chemicals. [2] Silver- and gold-based materials are hitherto considered the state-of-the-art of CO-forming catalysts but their cost and scarcity impede their industrial application. In this regard, composite materials based on transition metal oxide nanoparticles supported on metal-nitrogen-doped carbons (MOx/M-N-C) have been recently explored as inexpensive and efficient electrocatalysts for the eCO2RR-to-syngas process. Several works in literature have reported the synergistic effect between MOx nanoparticles and M-N-Cs, which results in an enhanced eCO2RR electrocatalysis with respect to each one of the materials separately. [3,4] The research of MOx/M-N-Cs for eCO2RR is still in its infancy and, therefore, further development is needed to reach the application of these materials in industrial CO2 electrolyzers.
In this contribution, we introduce several approaches for the preparation of effective MOx/M-N-C-based GDEs. On the one hand, we demonstrate the potential of polybenzoxazine (pBO) resins to synthesize active MOx/M-N-Cs materials, most likely due to the unique properties of the resins. In doing so, we report that gas diffusion electrodes (GDEs) prepared with pBO-derived MOx/M-N-Cs (M= Ni, Fe) exhibited higher activity and CO selectivity in 1 M KOH than the pBO-free ones. On the other hand, we explore the optimization of GDEs in terms of eCO2RR electrocatalysis by the addition of binders into the catalyst layer (CL). The addition of PTFE to the catalyst layer was proved to favor the production of CO over HER, disclosing the essential role of hydrophobicity in the CL for eCO2RR. The GDE fabricated with pBO-derived NiOx/Ni-N-C and PTFE displayed suitable syngas production at industrial current densities, with an H2/CO ratio of ~0.9 at -200 mA cm-2 and ~-0.6 V vs RHE. Finally, we also conclude that the combination of PTFE and Sustainion in the CL has a synergistic effect that confers stability to the pBO-derived NiOx/Ni-N-C-based GDE while keeping intact the hydrophobicity, activity, and CO selectivity, with respect to the GDE with only PTFE. The results of this work provide relevant insight into both the development of MOx/M-N-C materials as syngas-forming electrocatalysts and the engineering of eCO2RR-to-CO GDEs aiming for the industrial implementation.
1.3-I1
Metal-Nitrogen-Carbon catalysts are being developed and studied as alternative catalysts to platinum for catalyzing the O2 reduction reaction (ORR), in both acidic and alkaline media.1, 2 The beginning-of-life activity and performance of optimized M-N-C materials is now sufficiently high to raise interest at the application level in fuel cells (proton-exchange membrane fuel cells, PEMFC, and anion exchange membrane fuel cells, AEMFC), but this class of materials typically suffers from poor stability in acidic medium while the stability in operating environment in alkaline medium is still under-investigated.
This presentation will discuss the most recent understanding on the deactivation/degradation of M-N-C catalysts, highlighting in particular the negative effect of combined electrochemical potential and O2 observed in acidic medium, leading to a loss of active sites but also an oxidation of the carbon matrix. The different effects that Fenton reactions have in different pH conditions will also be shown, and how this explains very different stability trends during operation of such catalysts. Tracking of the fate of the single-atom Fe-N4 sites after operation in fuel cells will be shown to be possible with 57Fe Mössbauer spectroscopy acquired at low temperature, distinguishing clearly nano-Fe-oxides from Fe-N4 sites.3 Finally, an overview of the recent approaches taken towards improving the stability of M-N-C materials will be given.
References
(1) Osmieri, L.; Park, J.; Cullen, D.A.; Zelenay, P.; Myers, D.J.; Neyerlin, K.C.; Current Opinion in Electrochemistry 2021, 25, 100627.
(2) Firouzjaie, H.A.; Mustain, W.E.; ACS Catalysis 2020, 10, 225-334.
(3) Li, J. et al, Nature Catalysis 2021, 4, 10-19
1.3-I2
Ana was born in the Canary Islands, Spain. After finishing her degree in Chemistry (2004), she moved to Barcelona to conduct a PhD in Materials Science at the Autonomous University of Barcelona and the Institute of Materials Science, being awarded a Prize for an outstanding PhD thesis (2009). From 2011 to 2016 she worked as a PDRA at UCL in Chemistry and Chemical Engineering Departments, where she researched metal-free energy materials for photocatalysis, batteries and water electrolysers. In 2016, she took up a position as Academic Fellow at QMUL. In 2019 Ana became a Lecturer and shortly after, Senior Lecturer in 2019 and Reader in Sustainable Energy Materials in 2021. Ana's group studies the design of high-performing sustainable electroactive materials using easy-to-scale processing techniques for application in energy storage and conversion. She is a member of the UK Redox Flow Battery Committee, STFC Battery Steering Committee and Director of the London Energy Materials & Devices Hub.
The development of noble metal-free electrocatalysts for their application in alternative energy conversion and storage technologies, including fuel cells, water electrolysers and batteries is an important step towards a sustainable economy based on renewable energies. Recent studies in electrocatalysts containing abundant metals such iron (II) phtahlocyanine (FePc) and NiFe layered double hydroxide have proved the remarkable potential of these materials as electrocatalysts for the oxygen reduction and evolution reactions, respectively [1-3], key processes governing the working principle of fuel cells, electrolysers and metal-air batteries. In order to implement these electrocatalysts in energy devices, efficient deposition in a conducting matrix is key. Here we present the use of electrospinning to produce freestanding carbon-based non-woven fibres with excellent conductivity that also enable a homogeneous deposition of electrocatalyst. Moreover, lignin, a by-product of the paper industry, was used as carbon source. The resulting materials have exhibited remarkable performance in alkaline environment. The synergetic effect between matrix and electrocatalysts will also be explored.
2.1-I1
Platinum group metal free (PGM-free) electrocatalysts represent an attractive low-cost alternative to PGM-based catalysts for several reactions of fundamental importance for electrochemical energy conversion and storage. Of various proposed PGM-free catalysts, the atomically dispersed transition metal-nitrogen-carbon (M-N-C, M = Fe, Co, Ni, or Cu, etc.) materials have been found to be especially promising for oxygen reduction reaction (ORR), as potential replacement for Pt-based cathode catalysts in low-temperature polymer electrolyte fuel cells (PEFCs), and more recently as catalysts for electrochemical reduction of carbon dioxide (CO2RR). In this presentation, we will summarize recent progress in the development of M-N-C catalysts for these two important reactions.
The research effort in the development of M-N-C ORR electrocatalysts at Los Alamos dates back to early 2000s and has since yielded catalysts with substantially enhanced ORR activity and, lately, also with much improved durability [1-3]. Using advanced Fe‑N-C catalysts, we have achieved an activity of 38 mA/cm2 at 0.90 V (iR-free) in an H2-air PEFC, approaching the DOE 2025 current density target of 44 mA/cm2. We have also demonstrated Fe-N-C catalysts with excellent durability, with no more than 30% loss of their initial activity at 0.70 V in a 600-hour PEFC test. Our Fe-N-C catalysts have also shown promise for anion exchange membrane fuel cells (AEMFCs), achieving 48 mA/cm2 at 0.90 V (iR-free) and peak power density of 0.83 W/cm2.
The development of sustainable carbon-neutral energy technologies to mitigate greenhouse gas emissions has become imperative and urgent. Of special interest and importance in this context is the use of electricity from intermittent renewable energy sources for electrochemical conversion of CO2 to easily storable and transportable value-added products. Recently, M-N-C materials have emerged as promising CO2RR catalysts thanks to their structure and high selectivity for certain reduction products, e.g., CO and HCOOH [4,5], though so far at lower current densities achieved with well-established Cu nanoparticle catalysts [6,7]. In this presentation, we will summarize our study of the activity, selectivity, and stability of M-N-C catalysts for CO2RR in both an H-cell and at a rotating disk electrode (RDE). The CO2RR activities have been improved by increasing metal loading, optimizing local M-N coordination environment, and enhancing metal-carbon substrate interactions. We will also demonstrate the impact of mass transport on performance of M-N-C catalysts in the H-cell and review methods of combatting the formation of hydrogen bubbles on stationary CO2RR cathodes. Finally, we will discuss the use of M‑N-C catalysts in a tandem configuration to generate high energy-density CO2RR products such as C2H4 and C2H3OH.
2.1-I2
Philipp Adelhelm is a physical chemist and works at the interface between the research disciplines of materials science and electrochemistry. His current main interest is research on sustainable batteries.
After studying materials science at the University of Stuttgart, he moved to the Max Planck Institute of Colloids and Interfaces in Potsdam (Department of Prof. Antionetti / Smarsly, 2005-2007) for his doctoral project. This was followed by a 2-year postdoctoral stay at the University of Utrecht (Prof. de Jongh) and then a position as a junior research group leader at the Institute of Physical Chemistry of the Justus Liebig University in Giessen (Prof. Janek, 2009-2015). From 2015-2019 he was a professor at the Institute for Technical Chemistry and Environmental Chemistry at the Friedrich Schiller University Jena.
He has been a professor at the Institute for Chemistry at Humboldt-University since 2019 and heads a joint research group on operando battery analysis at the Helmholtz Zentrum Berlin (HZB).
The rising demand of rechargeable batteries for electric vehicles and grid storage applications sparks a lot of interest on alternatives to “standard Li-ion battery technology”. The size of these markets is so large that great efforts are currently undertaken towards using more cost-effective materials that will not run into supply and/or resource constraints. Here, sodium-ion batteries are one important option that primarily aim at realizing high energy batteries based on sodium and other abundant elements such as carbon, iron or manganese.[1] On the other hand, solid-state batteries (SSBs) are considered as promising option for electric vehicles. In these types of batteries, a solid electrolyte replaces the flammable organic liquid electrolyte, which improves safety. At the same time, SSBs might enable energy densities exceeding conventional lithium-ion technology.
This talk gives an overview on materials aspects on sodium-ion and solid-state batteries and how they compare to lithium-ion batteries. Specific examples on inorganic materials will be discussed, including high capacity metal/carbon negative electrodes[2], layered oxides of the type Na[MnxFeyTMz]O2[3], solvent co-intercalation reactions (graphite)[4] and metal sulfides (CuS, Cu3PS4, NaTixTMyS2)[5] (TM = transition metal).
[1] aI. Hasa, S. Mariyappan, D. Saurel, P. Adelhelm, A. Y. Koposov, C. Masquelier, L. Croguennec, M. Casas-Cabanas, Journal of Power Sources 2021, 482; bY. Li, Y. Lu, P. Adelhelm, M. M. Titirici, Y. S. Hu, Chemical Society Reviews 2019, 48, 4655-4687; cP. K. Nayak, L. Yang, W. Brehm, P. Adelhelm, Angewandte Chemie - International Edition 2018, 57, 102-120.
[2] aT. Palaniselvam, C. Mukundan, I. Hasa, A. L. Santhosha, M. Goktas, H. Moon, M. Ruttert, R. Schmuch, K. Pollok, F. Langenhorst, M. Winter, S. Passerini, P. Adelhelm, Advanced Functional Materials 2020, 30; bT. Palaniselvam, M. Goktas, B. Anothumakkool, Y. N. Sun, R. Schmuch, L. Zhao, B. H. Han, M. Winter, P. Adelhelm, Advanced Functional Materials 2019, 29.
[3] L. Yang, J. M. L. del Amo, Z. Shadike, S. M. Bak, F. Bonilla, M. Galceran, P. K. Nayak, J. R. Buchheim, X. Q. Yang, T. Rojo, P. Adelhelm, Advanced Functional Materials 2020, 30.
[4] aI. Escher, Y. Kravets, G. A. Ferrero, M. Goktas, P. Adelhelm, Energy Technology 2021, 9; bM. Goktas, C. Bolli, E. J. Berg, P. Novák, K. Pollok, F. Langenhorst, M. V. Roeder, O. Lenchuk, D. Mollenhauer, P. Adelhelm, Advanced Energy Materials 2018, 8; cB. Jache, P. Adelhelm, Angew. Chem. Int. Ed. 2014, 53, 10169-10173.
[5] aA. L. Santhosha, N. Nazer, R. Koerver, S. Randau, F. H. Richter, D. A. Weber, J. Kulisch, T. Adermann, J. Janek, P. Adelhelm, Advanced Energy Materials 2020, 10; bW. Brehm, A. L. Santhosha, Z. Zhang, C. Neumann, A. Turchanin, A. Martin, N. Pinna, M. Seyring, M. Rettenmayr, J. R. Buchheim, P. Adelhelm, Advanced Functional Materials 2020, 30; cF. Klein, B. Jache, A. Bhide, P. Adelhelm, Physical Chemistry Chemical Physics 2013, 38, 15876-15887.
2.1-I3
Dr. Maria Gimenez is Principal Investigator at CiQUS, Ramon y Cajal at the University of Santiago de Compostela and Honorary Associate Professor at the University of Nottingham. In 2006 she received her PhD from the University of Valencia working under the supervision of Prof Eugenio Coronado and Dr. Francisco M. Romero on multifunctional materials of interest in molecular magnetism. She then joined the Supramolecular Chemistry and Chemical Nanosciences Group of Prof. Neil Champness working as postdoctoral research fellow for almost three years. In 2009 she was awarded with a two-year Marie Curie Intra-European Fellowship in the Nanocarbon Group of Prof. Andrei Khlobystov at the University of Nottingham. In 2011, she started her independent career as Royal Society Research Fellow and in 2015 she became Assistant Professor of Materials Chemistry in Nottingham. In 2018 she joined the CiQUS, launching her project ERC-STG NANOCOMP supported by the Oportunius Program (Xunta Galicia). She is currently coordinator of a strategic research group on Condensed Matter & Functional Materials (MAT2). The research in her group is currently focused on the development and functional characterisation of hybrid metal-carbon nanostructures for spintronics and energy-related applications.
In the last 10 years her studies have included a number of firsts, including (i) the first demonstrations of a molecular rhombus tiling (Nat. Chem. 2012), a supramolecular bilayer at a surface (Nat. Chem. 2011) and unusual nanoribbons inside carbon nanotubes (Nat. Mater. 2011); (ii) the encapsulation of single molecule magnets (Nat. Commun. 2011); (iii) the controlled assembly of preformed magnetic nanoparticles (Angew. Chem., 2013,) and exploitation of electrochemical nanoparticles (Adv. Mater. 2016, ChemSusChem 2021) in hollow carbon nanostructures. She has discovered a new type of supramolecular fluid (PCT/ES2021/070659, ES2797556 Angew.Chem. 2021) and established a new catalyst technology (PCT/ES2021/070649, ES2796448) offering a unique opportunity to address recyclability & sustainability for cost-effective electrochemical technologies. She has directed four doctoral thesis at the University of Nottingham and 12 MSc theses. She has participated in 15 research projects (11 as PI).
During these years she has been granted different fellowships and awards: Spanish Ministry of Education and Science Undergraduate Fellow, Extraordinary award for highest Degree in Chemistry, Regional Government Fellowship for Doctoral Studies, Marie Curie Intra-European Research Fellowship, Royal Society DH Research Fellowship, ERC Starting Grant-NANOCOMP, Ramon y Cajal contract and a ERC PoC Grant-ZABCAT. In recognition of her multidisciplinary achievements, Maria was awarded in 2012 with a very prestigious prize (Emerging Investigator Award 2012) by the Spanish Royal Society of Chemistry for outstanding and novel research (covered in Angew. Chem. Int. Ed., 2012, 51, 51). In 2016 and 2017 she became Emerging Talent SRUK/CERU Award finalist for the impact of her studies on the development of materials chemistry using carbon nanostructures.
Electrocatalyst materials that drive fuels cells and other fossil fuel-free energy technologies have shown to play an important role on the production of clean and sustainable energy. However, those electrocatalysts containing precious metals, such as Pt, are currently hindered by their short-term durability. Recyclability and re-use of highly active nanocatalysts is still an outstanding global challenge of increasing importance in energy conversion and heterogeneous catalysis[1]. As these precious elements are rapidly diminishing, the research community is forced to urgently address this major issue until more abundant efficient electrocatalysts are put forward. In this respect, hollow carbon nanostructures can provide an excellent mean for the fabrication of highly durable electrocatalyst materials through nanocatalyst confinement [2-4], allowing their sustainable use in a whole variety of electrochemical processes. Thus, these hybrid nanocatalysts have shown performance comparable to commercial electrocatalysts (Pt/carbon black), but most importantly they exhibit outstanding durability, retaining most of the electrocatalytic activity even after 50,000 and 30,000 cycles of the ORR and HER, and thus significantly outperforming all existing electrocatalytic systems under these conditions. The observed behaviours are directly linked to interactions between the metal nanoparticles and the graphitic step-edges within the carbon nanoelectrodes. These surprising and remarkable properties of the reported hybrid electrocatalyst materials have opened up a new strategy for the sustainable use of precious metals in electrocatalysis and other technological applications that require stabilization of metal nanoparticles under harsh conditions (Figure 1).
2.2-T1
Jesús Barrio Hermida received his Bachelor of Science in Chemistry from the Universidad Autónoma de Madrid (Madrid, Spain) in 2014, where he got in touch for the first time with chemical research whilst working in the synthesis and characterization of Fe and Cu coordination polymers in the Inorganic Chemistry department.
In 2016, he obtained his Master in Nanoscience and Molecular Nanotechnology from the same institution. His Master Thesis, carried out at the IMDEA Nanoscience Institute entailed the formation of controlled assemblies of plasmonic building blocks and was directed by Dr. Beatriz H. Juárez and Prof. Félix Zamora.
Due to a scholarship in the Erasmus program, he moved to the Max Planck Institute for Colloids and Interfaces (Potsdam, Germany) for pursuing his doctoral studies, and in September 2016, he joined the Ben-Gurion University of the Negev (Beer-Sheva, Israel) along with his PhD supervisor, Prof. Menny Shalom, where he obtained his PhD in May 2020. His doctoral thesis focused on the design of metal-free carbon nitride materials for photo-electrocatalytic applications.
In June 2020 he joined the Materials Department at Imperial College as a Research Associate for working along with Dr. Ifan Stephens and Prof. Magda Titirici in the design of hybrid metal-carbon composites for different electrochemical applications. In August 2023 he started his independent career as an Imperial College Research Fellow at the Chemical Engineering Deaprtment of Imperial. His research covers the synthesis of carbon-based materials for different energy-related scenarios.
Fe single atoms in nitrogen doped carbon materials (Fe-NC) have attracted plenty of attention during the last decades in the field of electrocatalysis for oxygen reduction and carbon dioxide conversion amongst others. In the cathode of proton exchange membrane fuel cells Fe-NC are the most promising solution to scarce and expensive Platinum-group-metal catalysts;[1] In CO2 reduction, they have achieved similar performance to that of nanostructured Au and Ag. However, their controlled synthesis and stability for practical applications remains challenging. Approaches to enhance their catalytic performance include increasing the loading of Fe single atoms, for example by decoupling high temperature pyrolysis and Fe coordination atoms, or enhancing the intrinsic activity of the FeNx sites through engineering of the coordination environment or by creation of dual atom catalysts.[2,3]
Currently, the utilization of Fe within these materials remains very low owing to the lack of C-N scaffolds that combine adequate micro- and mesoporosity. In this work we employ inexpensive 2,4,6-Triaminopyrimidine (TAP) with MgCl2.6H2O as porogen to prepare a highly porous N-doped carbon material.[4] The hydrogen bonding between nitrogen moieties of TAP and the water molecules of the Mg salt allows an optimal interaction during pyrolysis that leads to remarkable porosity in the nitrogen-doped material (~3300 m2 g-1) and very available N sites for Fe coordination. The subsequent low temperature Fe coordination results in a highly active O2 reduction to electrocatalyst with a mass activity 4.0 A g-1 at 0.8 VRHE in acid electrolyte. Additionally, the material shows near 100% Faradaic Efficiency for the CO2 reduction to CO (FEco = 93.5% at -0.55 V vs RHE) with one of the highest TOF reported up to date (4.5 s-1)
Aberration corrected high-angle annular dark field scanning transmission electron microscopy with energy dispersive x-ray spectroscopy of the catalyst confirms the solely atomic Fe and N distribution pre- and post-accelerated degradation tests. In-situ nitrite stripping reveals a high active site density of 2.54×1019 sites g-1; the remarkable porosity of the graphitic material and hierarchal structure ensures remarkably high electrochemical active site utilisation of 42%. Ex-situ X-ray absorption extended fine structure suggests the presence of penta-coordinated FeN5 sites, potentially enabling the stability of the active site for O2 and CO2 reduction.[5]
2.2-T2
Photoelectrochemical (PEC) solar water splitting could be a potential route to large-scale production of green hydrogen. However, systems based on earth-abundant metal oxides do not yet achieve the high efficiencies required for economically feasible applications. One obstacle to the realization of higher efficiency devices is the appropriate design of the semiconductor-electrolyte interface. In this context, we point out a common misconception that the built-in electric field at the solid-liquid interface is essential for charge separation [1]. A similar discussion, controversial at the time, regarding the role of the built-in field took place in photovoltaic (PV) research community. The purpose of this talk is to present the state-of-the-art knowledge in this field and to seek common ground for an interpretation valid for both photovoltaics and PEC. We hope to provide a more detailed understanding of the energy loss mechanisms and the driving forces that determine charge separation, transport, and recombination of electron and hole pairs in PEC devices. We believe this will be critical for selecting the most appropriate design routes. We emphasize the well-established viewpoint in the photovoltaic research community that the gradient of electrochemical potential is the main driving force for charge separation and efficient solar energy conversion [2]. Based on this insight, we argue that improved (selective) contact design in PEC devices should be one of the most important research directions in PEC device development. To address this challenge, we take a closer look at how optimized contacts have been designed to date and provide examples of potential approaches that may be implement to further improve the performance of PEC devices [3].
Keywords: Water splitting, PEC, charge selective contacts, charge separation, charge transport, interfaces, drift and diffusion, quasi fermi level gradients
2.2-T3
The electrocatalytic reduction of CO2 to formic acid can be performed with promising faradaic efficiencies.[1] It is one of the first reduction products of CO2 and H2O having a relatively high added value among the reported products.[1] Nevertheless, for practical applications, several requirements need to be met. For example, the current density needs to surpass 100 mA/cm2, and the catalyst should be stable and active for thousands of hours.[1] To improve this, researchers have devoted significant efforts to understand the active phase of promising electrocatalysts such as In2O3 or Bi2O3. Several reports suggest that the presence of an oxide surface state of the catalyst is necessary for a high faradaic efficiency towards formate.[2],[3] Such studies were performed for relatively short times (<5 h) and at low current densities (<5 mA/cm2), meaning that the involved oxide phases might not be stable during prolonged use.
Here we report on the use of In2O3 nanoparticles in a gas diffusion electrode (GDE) configuration for CO2 reduction under practical conditions (high current density, long reaction time). Flame spray pyrolysis (FSP) was used to synthesize In2O3 nanoparticles with precise size and crystal structure as shown by TEM and XRD. These particles show good faradaic efficiencies (FE > 80%) at current densities up to 200 mA/cm2. Different GDE configurations were prepared and compared in CO2 electroreduction to understand its influence on overall performance. The active phase was characterized by XPS and in situ Raman spectroscopy. The results show that, under the applied conditions, the initial indium oxide phase is readily reduced, yet remains active for CO2 reduction to formate. By tuning the carbon support and hydrophobicity in the catalyst layer of the GDE, the electrode maintains its activity during 50h CO2 electroreduction.
2.2-T4
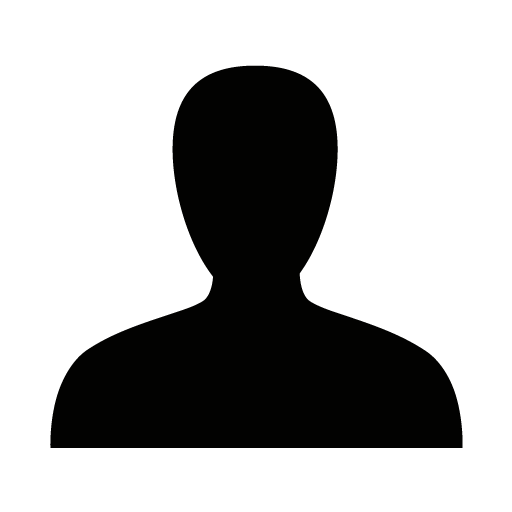
All-perovskite tandem solar cells promise high photovoltaic performance at low cost. So far however, their efficiencies cannot compete with traditional inorganic multi-junction solar cells and they generally underperform in comparison to what is expected from the isolated single junction devices. Understanding performance losses in all-perovskite tandem solar cells is a crucial aspect that will accelerate advancement. Here, we perform extensive selective characterization of the individual sub-cells to disentangle the different losses and limiting factors in these tandem devices. We find that non-radiative losses in the high-gap subcell dominate the overall recombination losses in our baseline system as well as in the majority of literature reports. We consecutively improve the high-gap perovskite subcell through a multifaceted approach, allowing us to enhance the open-circuit voltage (VOC) of the subcell by up to 120 mV. Due to the (quasi) lossless indium oxide interconnect which we employ for the first time in all-perovskite tandems, the VOC improvements achieved in the high-gap perovskites translate directly to improved all-perovskite tandem solar cells with a champion VOC of 2.00 V and a stabilized efficiency of 23.7%. The efficiency potential of our optimized all-perovskite tandems reaches 25.2% and 27.0% when determined from electro- and photo-luminescence respectively, indicating significant transport losses as well as imperfect energy-alignment between the perovskite and the transport layers in the experimental devices. Further improvements to 28.4% are possible considering the bulk quality of both absorbers measured using photo-luminescence on isolated perovskite layers. Our insights therefore not only show an optimization example but a generalizable evidence-based strategy for optimization utilizing optical sub-cell characterization.
2.2-T5
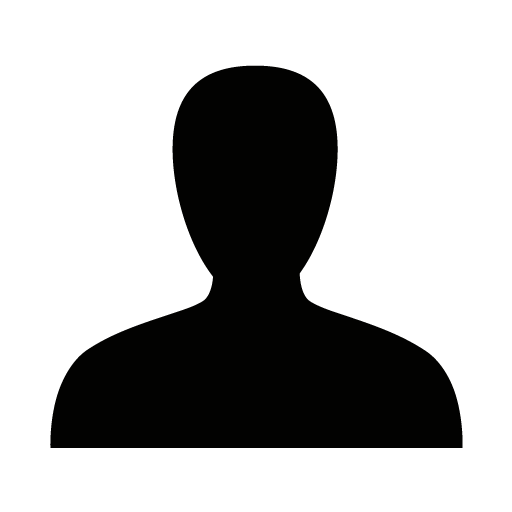
Porous carbons with tuneable functionalities and morphologies have extensively been employed as electrode materials in a variety of electrochemical energy conversion and storage systems for instance in fuel cells and electrolysers as active catalysts and catalyst supports, and in secondary batteries as anode materials. Amorphous carbons with well-developed pore structures are of particular interest due to their superior mass-transport characteristics and remarkable charge storage capacities. The salt-templating method with its advantage of combined soft and hard templating effects provides a sustainable way to synthesize nano- and mesoporous carbons with tailored porosities via in-situ ionothermal template transformation [1]. In this work, we utilized a MgCl2-based salt melt to prepare nitrogen doped carbons (N-C) with different morphologies and porosities, which were evaluated as anode materials in sodium ion batteries. Simultaneously, use of MgCl2 salt leads to the formation of Mg-N4 moieties in those carbons by means of a pyrolytic template-ion effect (active site imprinting) [2]. Porous carbon frameworks with imprinted Mg-N4 sites are interesting particularly for electrocatalysis applications as they offer an ideal platform to prepare M-N-C catalysts (where M= Co, Fe, Ni etc.) by ion-exchange reactions at low temperatures. The resultant M-N-C catalysts consist purely of M-N4 active sites and high porosity of carbon framework facilitates efficient mass-transport of reacting species.
We utilized Mg-N4 imprinted carbons to synthesize morphologically equivalent Ni-N-Cs and Co-N-Cs, containing phase pure Ni-N4 and Co-N4 sites, for electrochemical reduction of carbon dioxide (CO2RR). In electrochemical tests, Ni-N-Cs exhibited an excellent CO2 reduction activity with considerably higher CO selectivity and mass activity as compared to Co-N-C. The faradic efficiency value of Ni-N-C for CO formation was 95% at U= -0.5 to -0.8 VRHE (vs reversible hydrogen electrode) and a mass activity of 23 A g-1. The performance stability test carried out at -0.65 VRHE demonstrated above 90 % retention of the current density and CO selectivity after 100 h of continuous operation, reflecting the structural robustness of the Ni-N-C catalyst.
Finally, these ionothermal carbons with two different morphologies (but without any Ni or Co incorporation) were employed as the anode materials in sodium-ion batteries to evaluate the effects of carbon morphology and functionalization on sodium storage capacities. Compared to the reference carbon material, substantially higher reversible sodium storage capacities were reached with these high porosity carbons that were in the range of 300-500 mAh g-1 [3]. Although the reversible capacity was obtained only after extensive SEI formation, our results reveal the potential for much higher reversible capacities than usually observed using carbons with a tailored porosity in sodium-ion batteries. The talk will include greater details of the structural analysis and sodium storage and CO2 reduction results of these ionothermal carbons.
2.2-T6
Iron-based catalysts represent one of the most promising alternatives to platinum for oxygen reduction. However, the mechanism of oxygen reduction on Fe-based catalysts is still debated and the challenge for pyrolyzed catalysts is to unambiguously identifying the active sites.
Iron macrocycles can assist in understanding the mechanism of oxygen reduction by providing well-defined and reproducible active sites. A first indication of the difference in ORR kinetics among different macrocycles can be obtained by observing the cyclic voltammetry (CV) peaks. Most Fe macrocycles present two peaks in the cyclic voltammetry curve. The conventional wisdom is that the peak at more positive potentials is due to the desorption of *OH and consequent reduction of iron from FeIII to FeII, and the peak at more negative potentials is due to the further reduction of iron to FeI.1 The position of the peak due to *OH adsorption provides a measure of the binding energy of this species; which, in turn, is controlled by the electron-withdrawing or donating nature of the ring.2 This causes a previously reported correlation between the position of the OH adsorption peak and the ORR activity.3,4 However, other macrocycles only present one potential peak in the CV and the activity is inconsistent with its position
In this work we provide a systematic study of oxygen reduction on selected Fe-macrocycles, combining DFT calculations, electrochemical measurements, and in-situ characterization, including operando UV-Vis spectroscopy and x-ray absorption spectroscopy. Our investigations shows that the OH adsorption peak is not accompanied by a change in Fe oxidation state, while the peak at more negative potentials results from the reduction of iron from FeIII to FeII. Furthermore, our analysis indicates the reason why some macrocycles do not show a second voltametric peak in the experimental CV. The insight gained by studying these model molecules will allow the interpretation of more commercially relevant pyrolyzed catalysts and to understand the nature or redox activity in molecular catalysts.
2.3-I1
Carbon is a key electrode material in energy-related applications. A large number of studies have revealed the importance of designing carbon electrodes in terms of their porous morphology. In most cases, porous carbons in a powdery form are produced, which are fixed on an electrode substrate by mixing with binders and conductive agents, resulting in so-called composite electrodes. These additives make it difficult to correlate physicochemical characteristics of an electrode material with electrochemical properties of a composite electrode thereof. In addition, it is impossible to control the porous morphology of a composite electrode because of the uncontrollable pore properties of interparticle gaps. In this context, free-standing and binder-free monolithic electrodes have advantages over the composite electrodes in the controllability of electrodes.
Sol–gel process is a powerful tool to tailor various porous structures at different length scales in a monolithic gel. Several strategies such as supramolecular self-assembly, hard templating, and phase separation have been developed to date. Among them, the sol–gel process accompanied by phase separation offers three-dimensionally interconnected macroporous structures with narrow pore size distribution [1], which contribute to efficient mass transport in a monolith. In addition, the combination of the phase separation method with other techniques allows us to introduce smaller pores in macropore frameworks resulting in a hierarchically porous structure [2,3].
In our research group, we have developed porous resorcinol-formaldehyde (RF) gels based on the phase separation method. Carbon monoliths with controlled pore properties can be obtained by carbonization of the porous RF gels, which are available as free-standing and binder-free electrodes for energy storage devices, such as supercapacitors [3] and batteries [4,5]. The details about the syntheses, pore controls and electrochemical investigations of the monolithic carbon electrodes will be presented [6].
2.3-I2
Dr. Camélia Matei Ghimbeu is a Research Director at Material Science Institute in Mulhouse (IS2M), CNRS, France. She received in 2007 her PhD from University of Metz, France and TU Delft, The Netherlands and her Habilitation in 2015 from University of Haute Alsace, France. She was awarded in 2017 the CNRS Bronze Medal, in 2018 the Award "Solid-State Chemistry Division" (French Chemistry Society) and in 2019 the award Guy Ourisson (Gutenberg Cercle), for her research works devoted to the design of carbon-based materials with controlled characteristics for energy storage and environmental applications. Author of more than 100 articles and about 150 communications, she is leading the “Carbon and Hybrid Materials” group at IS2M, and she is member of French network of Electrochemical Storage of Energy (RS2E).
Sodium ion batteries (SIBs) emerged as alternative energy systems to lithium ion batteries (LIB) owing to the large abundance and availability of sodium resources, which might afford a long term and low cost solution to satisfy continuous growing demands. Hard carbon (HC) materials become the most appealing anodes due to their large interlayer distance and disordered structure facilitating Na-ion insertion-extraction. Many precursors were explored, leading to the design of hard carbons with different features and electrochemical performances. The aim of this work is to give a general overview on how the synthesis conditions (precursors, temperature, process) impact the hard carbon characteristics (porosity, surface chemistry, morphology and structure) [1-2]. Further, the relationship between the HC properties and the performance (initial coulombic efficiency, capacity and cycle stability) will be emphasized [3,4]. Lastly, insights on the sodium storage mechanism in hard carbon will be provided [3, 5] along with some challenges that need to be still addressed [6].
1.1-I1
The advent of solid-state batteries has spawned a recent increase in interest in lithium conducting solid electrolytes. However, many open questions remain when trying to optimize electrolytes and understand solid state battery chemistries.
In this presentation, we will explore the current focus of halide-based ionic conductors in solid state batteries and discuss stability limitations in solid state battery cells at the anode as well as the cathode composites.
In a second part, we show the influence of Si type anode materials on the effective transport and behavior of solid-state batteries
Finally, we will discuss that it is not only important to find fast ionic conductors but that for an effective thermal battery management the thermal transport properties of solid ionic conductors need to be explored and understood. Here we will show the diffusive thermal transport nature of solid electrolytes and their different scaling relations that put in question the assumption of Bruggeman transport in solid state batteries.
1.1-I2
Solid polymer electrolytes based on poly(ethylene oxide) (PEO) are of considerable interest in lithium metal batteries. Electrolytes with high modulus are essential for enabling high specific energy batteries with lithium metal anodes [1]. One approach for obtaining high modulus electrolytes is through the use of microphase separated block copolymers comprising a structural block to provide mechanical rigidity (block A) and a soft conducting block to provide avenues for ion transport (block B) [2]. Block copolymers self-assembly into different morphologies such as lamellae, bicontinuous gyroid networks, cylinders arranged on a hexagonal lattice, and spheres arranged on a body-centered-cubic lattice. The ionic conductivity of a block copolymer electrolyte should increase with the volume fraction of the conducting phase. Herein, we explore the impact of several polymers (PS, PMA-POSS, etc.), lithium salts (LiTFSI, POSS-LiTFSI, etc.) and volume fractions of the conducting phase on morphology, mechanical and electrochemical properties of nanostructured block copolymer electrolytes [3,4].
References
1 Soo, P.P; Huang, B.; Jang, Y.; Chiang, Y.M.; Sadoway, D.R.; Mayes. A.M. Rubbery block copolymer electrolytes for solid‐state rechargeable lithium batteries. J. Electrochem. Soc. 1999, 146, 32.
2 Hallinan, D.T.; Villaluenga I.; Balsara N.P. Polymer and Composite Electrolytes. MRS Bulletin, 2018, 43, 759.
3 Sethi, G.; Jiang, X.; Chakraborty, R.D.; Villaluenga, I.; Balsara, N.P. Anomalous Self-Assembly and Ion Transport in Organic-Inorganic Block Copolymer Electrolytes. ACS Macro Letters, 2018, 7, 1056.
4 Villaluenga, I.; Inceoglu, S.; Jiang, X.; Chen,X.C.; Chintapalli, M.; Wang, D.R.; Devaux, D.; Balsara, N.P. Nanostructured Single-Ion-Conducting Hybrid Electrolytes based on Salty Nanoparticles and Block Copolymers. Macromolecules, 2017, 50, 1998.
1.1-I3
Montse Casas-Cabanas is the scientific coordinator of the Electrochemical Energy Storage Area and group leader of the Advanced Electrode Materials group at CIC energiGUNE. Her research interests focus on the design of battery materials and the understanding of phenomena that occur in energy storage devices through a multidisciplinary approach, with a focus in crystal chemistry.
She is also author of >75 scientific publications in peer reviewed journals and has been PI of several national and european projects. She has co-authored the FAULTS software for the refinement of X-ray data of crystalline structures with planar defects. She is also actively involved in the MESC+ Erasmus Mundus master course and has recently received the 2021 Young Researcher award ("Group Leader" category) from the Spanish Royal Society of Chemistry.
The possibility of developing practical solid-state batteries (SSBs) has gained momentum in recent years thanks to striking advances at the material level, such as the discovery of new highly-conducting solid-state electrolytes. However, the development of SSBs still faces formidable challenges, such as the integration of the various components, the functionality at full cell level, and the scalability of the fabrication processes.[1] Inorganic solid electrolytes stand out for their high Li-ion conductivity but exhibit drawbacks such as low electrochemical stability (sulfides) or rigidity (oxides, phosphates). Indeed, oxide and phosphate-based electrolytes usually require temperature-assisted densification methods that, when applied to composite cathodes, can induce chemical reactivity among the different cathode components resulting in resistive interfaces and by-products.
In this talk we will discuss our recent work regarding oxide and phosphate-based ion-conductors, including the recent synthesis and characterization of a new form of crystalline LiPON,[2] processing challenges of rigid ceramic materials (in particular regarding high temperature material compatibility in composite cathodes), and low-temperature densification strategies, which in turn allow to shed light into grain-boundary contributions to the total ionic conductivity of the studied materials.
1.2-T1
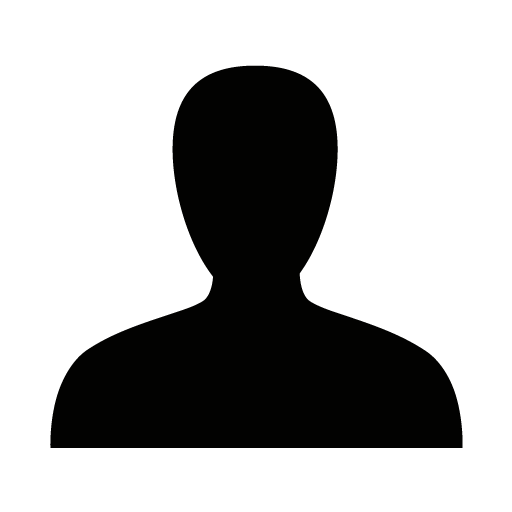
Solid-state batteries pairing a ceramic solid electrolyte with an alkali metal anode promise to improve the safety and energy density of cells. However, cycling solid-state cells at practical current densities in the mA/cm2 range can lead to cell failure. While much attention has been devoted to understanding dendrite penetration when plating the anode (charging), the formation of voids when stripping the metal anode (discharging) also has an important role in failure. In this study, stripping of lithium and sodium metal anodes has been investigated as a function of current density, stack-pressure and temperature, revealing the importance of creep in the alkali metal anode on void formation. To explore this, we have used a combination of 3-electrode cells, scanning electron microscopy and X-ray tomography. We show that above a critical current density on stripping, voids will accumulate on cycling at the metal anode/solid electrolyte interface, eventually leading to failure of the cell. It is therefore necessity to strip the metal anode below this limiting current density, as discharging above this critical rate leads to high local currents that can trigger dendrite penetration into the solid electrolyte on subsequent charges. However, we demonstrate that cycling solid-state batteries under moderately elevated stack-pressures and temperatures increases the critical current for voiding by promoting creep in the metal anode, enabling stable discharge at higher rates.
1.2-T2
Solid-state batteries with polymer electrolytes (PEs) are at the forefront of candidates to boost energy density and improve the safety of conventional Li-ion batteries. PEs require a wide electrochemical stability window to withstand high-voltage positive electrodes (i.e. LiNi0.6Mn0.2Co0.2O2, NMC622) and offer stability with the negative electrode (typically Li-metal).1 However, solid PEs still suffer from low electrochemical stability against highly oxidative and reductive potentials simultaneously. The Double Layer Polymer Electrolyte (DLPE) approach overcomes this issue from the choice of appropriate polymers for the positive electrode and separator respectively, combining them within the same electrochemical device. In this work, polyethylene oxide (PEO), offering stability against Li metal, and polypropylene carbonate (PPC), offering stability at high potentials, were used to prepare DPLE cells with NMC622 and Li-metal electrodes. Nevertheless, the use of a conventional dual-ion conductor lithium-salt (e.g. lithium bis(trifluoromethanesulfonyl)imide, LiTFSI) was found to play a key role on the thermodynamical compatibility between the polymer phases due to ion diffusion from PPC to the more solvating PEO, leading ultimately to cell failure. By modifying the lithium-ion conductive salt we successfully overcome those hurdles and provide guidelines on the design of improved performance cells with DLPE technology.2 Ultimately, this allows to assemble cells with high loading NMC622 (1 mAh cm–2) delivering 160 mAh g–1 and overcoming the performance of ethylene oxide -based cells, which rapidly degrade in the presence of high cut-off voltage active materials.3 This study provides a detailed understanding on the transport properties and ageing mechanisms taking place in SSLMBs, inspiring further the rational design of new DLPE technology with robust electrochemical performance.
1.2-T3
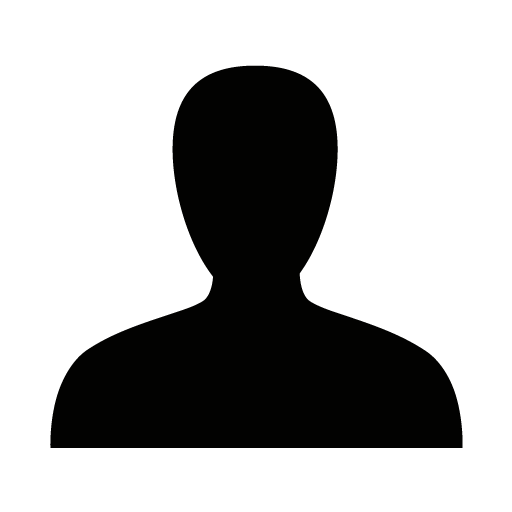
Many efforts in the field of Li-ion batteries are focusing on the development and implementation of solid electrolytes in order to overcome the drawback related to their liquid counterparts such as: flammability, complex encapsulation requirements, high cost and complexity manufacturing processes. Li-conducting glass-based materials gained attention in the last years as solid state electrolytes for lithium batteries, thanks to their good ionic conductivity at room temperature (10-3-10-4 S cm-1) and their wide electrochemical stability windows.
In the present work, Li1.5Al0.5Ge1.5P3O12 (LAGP) glass was 3D-printed by robocasting and stereolitography (SLA) in free-form robust self-standing structures with the main target to obtain 3D batteries with high active area (allowing high specific energy and power per unit volume). The use of 3D printing techniques allowed the fabrication of simple as well as complex architectures, with enhanced contact area with the electrodes. The inks and the printing processes were both optimized in order to reach an accuracy up to ≈100 µm. The printed structures demonstrated an excellent densification after the firing/sintering treatment, as a result of the optimization of the inks formulation, printing process and sintering conditions. Furthermore, ionic conductivity of 3D-printed LAGP electrolyte resulted to be 1.8 10-4 S cm-1 at room temperature, in accordance with the values measured on the same material processed with conventional methods. No detrimental reaction products or degradation phenomena were detected by chemical analyses after the sintering.
3D-printed LAGP was successfully cycled in contact with metal-Li. The interface with the anode was previously engineered by depositing 200nm of metallic Ge in order to prevent detrimental reactions between the two materials. A symmetrical cell Li/Ge coated LAGP/Li was successfully cycled for more than 100h.
The successful implementation of 3D printing techniques in LAGP processing represents an innovative approach that will push further the development of all solid state Li-ion batteries with enhanced energy density, thanks to the easy fabrication of 3D structured solid electrolytes.
1.2-T4
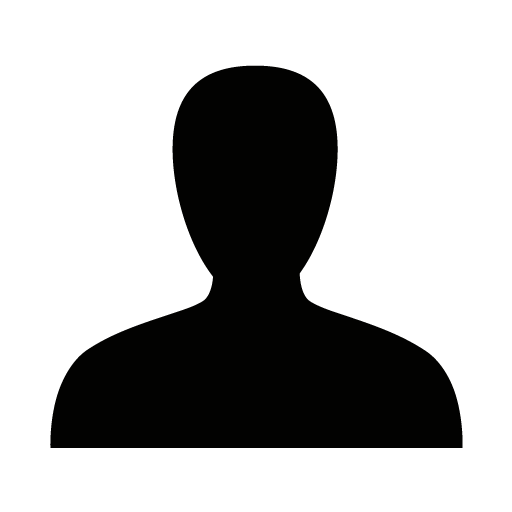
Self-diffusion in polycrystalline Li1+xTi2-xAlx(PO4)3 (0.2 ≤ x ≤ 0.4) samples followed by 7Li PFG (Pulse Field Gradient) NMR spectroscopy
Isabel Sobrados, Virginia Diez-Gómez, Cristina Ruiz-Santaquiteria, Wilmer Bucheli,
Ricardo Jiménez, Jesús Sanz.
Short and long range lithium motions are discussed in powder Li1+xTi2-xAlx(PO4)3 (LTAP) NASICON compounds prepared by ceramic (x= 0.2 and 0.4) and sol-gel (x= 0.3 and 0.4) routes.
Self-diffusion coefficients were determined with the PFG (pulse field gradient) technique. In these experiments, the stimulated echo π/2-t1-π/2-t2-π/2 sequence was used, in which two field gradient pulses of δ width and g intensity were applied between the two first π/2 pulses and after the third π/2 radiofrequency pulse. From the echo-signal attenuation, induced by the increment of exponent parameters, self-diffusion coefficients (DPFG) were deduced, using the Stejskal and Tanner expression [1]
A(2t1+t2)/A0(2t1+t2)=exp[-ɣ2.g2.δ2.(Δ-δ/3)·DPFG]=exp(-b·DPFG)
where A and A0 stand for echo signal intensity at (2t1+t2) with and without field gradient pulses, ɣ is the nuclear gyro-magnetic ratio, and Δ is the diffusion time used in experiments.
In PFG experiments, time scales differ considerably from those involved in 1/T2 and 1/T1 measurements, however, DPFG values deduced for short Δ times are similar to those deduced by NMR relaxometry. In all analyzed samples, diffusion coefficients measured at short Δ times, are between 5x10-12 and 1x10-11 m2 s-1. At increasing Δ, diffusion coefficients decrease due to restriction. In ceramic LTAP02-C sample, Li diffusion is less restricted than in LTAP04-C sample, where DPFG values increase and the particle size decreases. The analysis of DPFG coefficients in sol-gel LTAP03-SG and LTAP04-SG samples, show strong restriction effects that considerably reduce DPFG values when Δ increases, suggesting that Li diffusion is strongly restricted when the LTAP particles are smaller than 1µm. The restricted diffusion inside NASICON particles is compared to "free" diffusion processes.
From the temperature dependence of conductivity and diffusion coefficients, the activation energy and charge carrier concentrations were determined. In this work, PFG-NMR results show that diffusion coefficients rise with the amount of lithium and temperature.
To reduce restriction effects, denser samples should be prepared. In future works, the PFG technique will permit a better optimization of transport properties in fast ion conductors prepared with different conditions.
1. J.E. Tanner, E.O. Stejskal. J Chemical Physics. 49, 1768- 1777 (1968).
1.2-T5
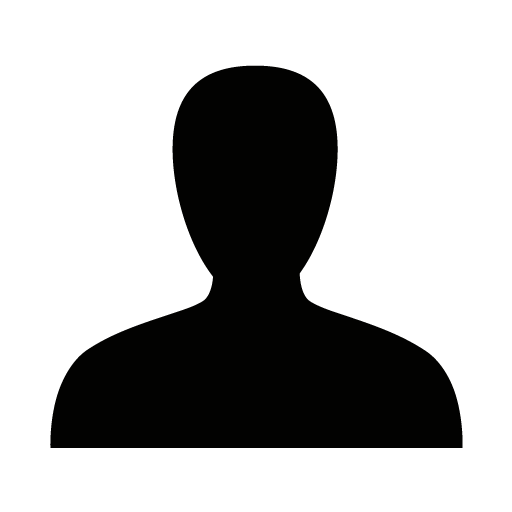
The development of high performance all-solid-state batteries relies on the development of solid electrolytes that have high conductivity, wide chemical, electrochemical and mechanical stability and are easy to process at low temperatures. Halide-type solid electrolytes are a relatively new class of solid electrolytes being studied owing to their compatibility with high voltage cathodes and processability at low temperatures however few structures are known that possess high ionic conductivities within the halide family. Li2ZrCl6 is one such material that demonstrates moderate ionic conductivity of 10-6 S/cm while Cu2ZrCl6 exhibits conductivities several orders of magnitude higher. Understanding the factors affecting lithium mobility in A2ZrCl6 can aid the design of novel solid-halide electrolytes with tailored frameworks for high ionic conductivity.
In this work, we investigate the composition A2ZrCl6 where A is a monovalent cation and the role the A site element has on the crystal structure, ionic conductivity and the associated migration pathways. We demonstrate via long timescale dynamics i.e. kinetic Monte Carlo (KMC) and molecular dynamics (MD) that the A site element does affect the A+ ionic conductivity of the material. In general, the more covalent character the A site element possesses the higher ionic conductivity the material exhibits. These results provide a basis to understanding the origin of fast ion transport in solid halide materials.
1.2-T6
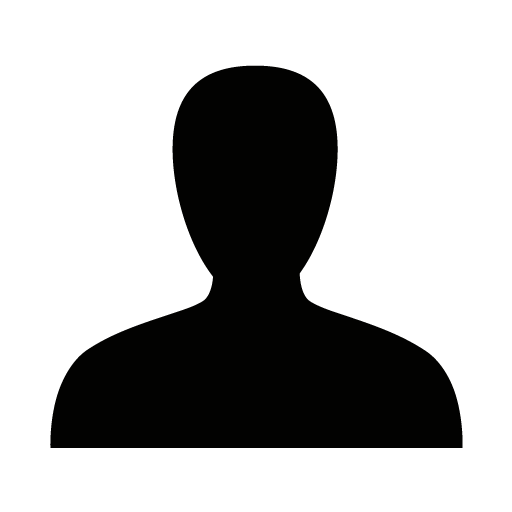
The solid-state electrolyte field knows a hard competition to provide an ideal composition which needs to fulfill numerous criteria such as, high Li+ ionic conductivity, low electronic conductivity, and a high level of mechanical, chemical, electrochemical and air stability as well as interface stability against active electrode materials. Several compositions and chemistries have been investigated, including: oxides, sulfides, halides, polymers; each of them getting their own strengths and weaknesses.[1]
Dense thin-film solid–state electrolyte separator are interesting to offer (a) a model system to investigate interfaces, and more importantly, (b) a promising pathway to increase the energy density of solid-state cells. The reference of thin-film solid-state electrolyte, for a while, is the lithium phosphate oxynitride LiPON, due to its easy way of deposition/synthesis via reactive RF-Magnetron sputtering. However, LiPON has a ceiling ionic conductivity of ~10-6 S.cm-1.[2]
For a decade, the new family of Lithium Rich Antiperovskite (LiRAP) Li3-xOHxHal (Hal = Br,Cl) has been investigated and exhibits low raw material costs, low temperature of synthesis and a bulk ionic conductivity of ~10-3 S.cm-1.[3][4] In addition, previous studies report the possibility to use PVD method to obtain thin layers of the Li3OCl composition with an acceptable Li+, and electronic conductivity.[5][6]
Here, we explore different ways to synthesize thin layers of the Li3OCl solid-state electrolyte by using RF-Magnetron Sputtering or e-Beam with a post annealing treatment. We will presents the structural, chemical and physical characterization, for LiRAP films prepared on glass and platinum coated sapphire substrates, via grazing incident x-ray diffraction, scanning electron microscopy, Fourier-transform infrared spectroscopy, impedance spectroscopy and chronoamperometry. These findings might accelerate the development of thin film electrolyte as a potential pathway to further increase the energy density of solid-state cells.
1.3-I1
In this lecture I discuss a digital twin devoted to the accelerated optimization of the manufacturing process of Lithium Ion Batteries (LIBs). This digital tool is developed by us within the context of the ERC-funded ARTISTIC project.1 It is supported on a hybrid approach encompassing experimental characterizations, a physics-based multiscale modeling workflow and machine learning models.2 Different steps along the LIB cells manufacturing process are simulated, such as the electrode slurry, coating, drying, calendering and electrolyte infiltration. The physics-based modeling workflow encompasses a sequential coupling between Coarse Grained Molecular Dynamics, Discrete Element Method and Lattice Boltzmann Method. It allows predicting the impact of the process parameters on the final electrode microstructure in three dimensions. The predicted electrode microstructures are injected in a performance simulator capturing the influence of the pore networks and spatial location of carbon-binder within the electrodes on the electrochemical response. Machine learning models are used to accelerate the physical models’ parameterization, to mimic their working principles and to unravel manufacturing parameters interdependencies from the physical models’ predictions and experimental data, and for inverse design. The predictive and optimization capabilities of this digital twin, coupling physical models with machine learning models, are illustrated with results for different electrode formulations in the context of LIBs. I also demonstrate the applicability of our approach to Sodium Ion and Solid State Batteries. Finally, the free online battery manufacturing simulation services offered by the project3 to optimize battery electrodes are illustrated through several examples.
1.3-I2
Francesco Ciucci works as an Associate Professor at the Hong Kong University of Science and Technology. He received his M.Sc. and Ph.D. in Mechanical Engineering at the California Institute of Technology where he was supported by a Rotary Ambassadorial Scholarship and a Bechtel Fellowship. Francesco’s current research centers on solid-state energy technologies, including solid-state batteries, reversible fuel cells, and electrolyzers, with a particular emphasis on the modeling of these systems and the development of new functional materials. Francesco is also interested in the probabilistic analysis of electrochemical impedance spectroscopy through the distribution of relaxation methods. In that context, he developed several pieces of software that can be found at https://github.com/ciuccislab.
Solid-state electrolytes with fast lithium conduction are the core of the all-solid-state Li-battery technology. By substituting the organic electrolyte with a piece of non-flammable ceramic material, we can achieve better safety, higher specific capacity, and a higher energy density. To date, the major bottleneck for this technology is the slow Li diffusion in the solid-state electrolyte and the interfacial incompatibility between the electrolyte and electrodes. To resolve these issues, several families of fast ionic conductors have been developed. Understanding Li diffusion in these materials is essential to the development of novel family fast ionic conductors. To this end, atomistic modeling provides us with a unique tool to obtain comprehensive information on atom motion, which is difficult to access with experimental techniques. In this talk, we showcase our group’s atomistic simulations regarding a novel family of superionic conductors, Li-rich antiperovskites (LiRAPs)
LiRAPs are a promising family of solid electrolytes, which exhibit ionic conductivities above 1 mS/cm at room temperature, among the highest reported values to date. Here, we report on the defect chemistry and the associated lithium transport in Li3OCl, a prototypical LiRAP, using DFT calculations and classical MD simulations [1]. We studied these materials’ phase, interfacial, and voltage stability [2,3] with DFT, showing good agreement with experiments, further proposing low-dimensional superionic antiperovskites [3]. In addition, the interfacial properties were studied for both protonated and fluorinated materials [4]. Analogous simulations were also carried out for Na-rich antiperovskites [5].
1.3-I3
Recent proof-of-concept of electrolytes enabling successful plating and stripping of calcium metal catalyzed research exploring the feasibility of a rechargeable battery technology based on calcium.[1] Estimates of prospective figures of merit at the cell level using open energy-cost models[2] indicate that performances comparable to state-of-the art LIB could be achieved with positive electrodes exhibiting moderate potential (2.5V) and a capacity of 200 mAh/g, and that volumetric energy densities above 1000 Wh/l would be possible considering the same capacity but operation at 3V.
In spite of such nice expectations, the investigation of suitable positive electrode materials has been plagued with a number of hurdles. On one hand, the lack of standard electrolytes and protocols may result in side reactions inducing misinterpretation of the electrochemical response achieved, which makes real bulk activity difficult to elucidate unless complementary characterization techniques are used. On the other, testing of materials similar to those used in the Li-ion battery field which would enable reversible intercalation/de-intercalation of calcium has to date led to the identification of few active compounds with diverse success.[3] Electrochemical extraction of calcium in some ternary transition metal ions is feasible but the reversibility of the process is more difficult to achieve, which is likely related to strong solvation of calcium ions, with reactions sometimes involving solvent co-intercalation and high cell overpotential.
Overall, there is a long and winding road to follow before reliable proof-of-concept can be achieved and technological prospects evaluated. Development of reliable experimental setups, including reference and counter electrodes, coupled to complementary characterization techniques, as well as computational tools, is mandatory if steady progress is to be achieved.
2.1-I1
Dr. Hatzell is an assistant professor at Princeton university in the Andlinger Center for Energy and Environment and department of Mechanical and aerospace engineering. Hatzell’s group primarily work on energy storage and is particularly interested at using non-equilibrium x-ray techniques to probe batteries during operando experimentation.
Dr. Hatzell earned her Ph.D. in Material Science and Engineering at Drexel University, her M.S. in Mechanical Engineering from Pennsylvania State University, and her B.S./B.A. in Engineering/Economics from Swarthmore College. Hatzell’s research group works on understanding phenomena at solid|liquid and solid|solid interfaces and works broadly i9n energy storage and conversion. Hatzell is the recipient of several awards including the ORAU Powe Junior Faculty Award (2017), NSF CAREER Award (2019), ECS Toyota Young Investigator Award (2019), finalist for the BASF/Volkswagen Science in Electrochemistry Award (2019), the Ralph “Buck” Robinson award from MRS (2019), Sloan Fellowship in Chemistry (2020), and POLiS Award of Excellence for Female Researchers (2021).
Solid-state batteries (SSBs) offer the opportunity to leverage energy dense metallic anodes and high voltage cathodes to achieve safe, durable, and affordable secondary energy storage systems1-3. However, the intrinsic thermodynamic, electrochemical and mechanical instabilities at the buried interfaces limits their performance. Unfavorable electro-chemo-mechanical dynamics can contribute to non-optimal material utilization, mechanical degradation, and poor ion transport. These mechanisms occur at various time- and length- scales and are not fully understood. Lithium metal anodes undergo a lithium deposition reaction which involves the reduction reaction of Li/Li+, and a lithium stripping reaction which involves the oxidation of surface Li atoms. Deposition processes are surface driven mechanisms and have been widely studied in order to understand dendrite formation. Stripping processes are sub-surface driven mechanisms and involve charge transfer at the interface between lithium and the solid electrolyte (Li|SE). Li stripping can alter the morphology and structure of the lithium metal interface and affect the subsequent deposition process. Non-uniform stripping can lead to sub-surface/interfacial void formation and decrease interfacial contact area between the Li anode and solid electrolyte. Smaller interfacial surface area can lead to high local current densities and decrease the cells threshold for dendrite formation (cell failure). While there are numerous theoretical studies that provide mechanistic hypotheses for pore formation, directly probing lithium stripping is challenging because the phenomena of interest occurs at buried interfaces. Multi-scale characterization of these materials and their electro-chemo-mechanical responses are pivotal towards engineering solid electrolytes for high power and energy applications. Herein, we combine bench top experiments with synchrotron techniques to resolve chemo-mechanics across multiple length scales which impact electrodeposition and dissolution mechanisms (grain, pore, interface).
2.1-I2
Pieremanuele Canepa is an Assistant Professor in the Department of Materials Science and Engineering at the National University of Singapore (NUS). He received his bachelor’s and master’s degrees in Chemistry from the University of Torino (Italy) and a PhD from the University of Kent (UK). Prior NUS, he was a Postdoctoral fellow at the Lawrence Berkeley National Laboratory and the Massachusetts Institute of Technology under the guidance of Prof. Gerbrand Ceder. His research contributes to the rational design of materials for clean energy technologies, including electrode materials for batteries, and electrolytes for sustainable energy storage devices. In 2021, Pieremanuele was elected as fellow of the Royal Society of Chemistry.
Lithium and sodium (Na) mixed polyanion solid electrolytes for all-solid-state batteries display some of the highest ionic conductivities reported to date. However, the effect of polyanion mixing on ion transport properties is still debated. Here, we focus on Na1+ xZr2SixP3− xO12 (0≤ x≤ 3) NASICON electrolyte to elucidate the role of polyanion mixing on Na-transport properties. Although there is a large body of data available on this NASICON system, transport properties extracted from experiments or theory vary by orders of magnitude, signifying the need to bridge the gap between different studies. Here, more than 2,000 distinct ab initio-based kinetic Monte Carlo simulations have been used to map the statistically vast compositional space of NASICON over an unprecedented time range and spatial resolution and across a range of temperatures. We performed impedance spectroscopy of samples with varying Na compositions revealing that the highest ionic conductivity (~ 0.1 S cm–1) is achieved in Na3.4Zr2Si2.4P0.6O12 , in line with our predictions (~ 0.2 S cm–1). Our predictions indicate that suitably doped NASICON compositions, especially with high silicon content, can achieve high Na+ mobilities. Our findings are relevant for the optimization of mixed polyanion solid electrolytes and electrodes, including sulfide-based polyanion frameworks, which are known for their superior ionic conductivities.
2.1-I3
Dr Jordi Jacas obtained his PhD in Materials at Sheffield University, working on the electrical and electrochemical characterisation of electrode materials for Li-ion batteries. During his postdoc at ISIS neutron spallation source and Stockholm University, he developed new tools to characterise batteries in-situ using neutron diffraction. Dr Jacas is currently a leading researcher for the battery section at the Catalonia Institute for Energy Research. His research aims to develop new electrode materials for next-generation batteries, including Co-free, Li-S and solid-state batteries. Since 2020, he has been the coordinator of the COBRA project (H2020-875568) devoted to fabricating generation 3b Li-ion batteries for electric vehicles.
Developing high energy density and fast charge/discharge batteries requires new coatings strategies to overcome interfacial impedances between battery cell components. Silicon is a promising anode material for next-generation batteries due to its high volumetric capacity of 2190 mAhl-1 and low discharge potential of ~ 0.3 V vs Li/Li+. In this paper, we report the fabrication, characterisation and electrochemical performance of nano Si electrodes coated with titanicone (TiGL) using a combination of Atomic Layer Deposition (ALD) and Molecular Layer Deposition (MLD). The optimised coated electrode delivers a capacity of 1200 mAhg−1 at 1C for 350 cycles with a capacity retention of 93 % and a high C-rate performance of 150 mAhg−1 at 20C. The improved discharge capacity, efficiencies, rate capability and electrochemical stability are related to enhanced lithium kinetics, stable SEI formation, and better structural integrity of the TiGL coated electrode compared to bare silicon. The use of titanicone as an interface modifier is discussed in the frame of high capacity electrode materials and solid state batteries.
2.2-I1
Dr. Yaroslav Romanyuk is a scientific group leader at the Laboratory for Thin Films and Photovoltaics since 2008. He received his Ph.D. from the Swiss Federal Institute of Technology, Lausanne in 2005 and completed his postdoctoral education stay at the University of California, Berkeley. His research interests include novel materials for thin-film solar cells, oxide electronics, and lately all-solid-state batteries fabricated with vacuum and printing methods. He supervised 11 completed Ph.D. theses with two in progress. He holds several patents and has co-authored more than 150 research articles (h-factor = 40 as of April 2022).
All-solid-state thin-film batteries (TFBs) combine several features of solid-state batteries, such as long lifetime, high charge/discharge rates, and safety with the potential of large-scale manufacturing using physical vapor deposition (PVD) methods. The talk will review several research highlights from our group on how to build an efficient TFB. These include flash-lamp annealing of layered oxide cathodes that enable thin and flexible aluminum foils to be used as conductive substrates [1], engineering a cathode/electrolyte interface with Nb2O5 interlayers for a fast ionic transport [2], exploiting ultra-thin amorphous LLZO electrolytes to block lithium dendrites [3], and inserting amorphous carbon interlayers at the Cu/LIiPON interface to facilitate homogeneous Li plating and stripping in an anode-free configuration [4]. At last, we will present the concept of monolithic multi-cell (so-called bipolar) TFBs fabricated with PVD methods, which could resolve the principal handicap of the low specific and areal capacity in TFBs and lead to a new generation of high-power solid-state batteries.
2.2-I2
Solid-state batteries offer great potential for large improvements in safety and lifetime, as well as higher energy and power densities. However, the interfacial composition and structure between solid electrolytes and electrode materials often present major deviations from those of the bulk materials. Elucidating the nature of the involved interfaces is required to establish a rational approach towards the successful combination of materials in a new generation of solid-state cells. Controlled interfaces between a solid-state electrolyte, cathode and anode have been realized in 2D-planar and 3D-vertical thin film geometries by applying pulsed laser deposition. The thin film epitaxial model geometries enable full control over the material combination, crystal orientation and elemental termination at all interfaces to explore their evolution during battery operation. Epitaxial engineering is used to realize crystalline thin film model systems, which enables an unique insight into the relation between electrochemistry and structural ordering of the layers and interfaces, not obtainable in conventional polycrystalline battery architectures. Here, I will show the latest results on several different thin film battery architectures, 2D as well as 3D, involving various different cathodes (LiMn2O4, LiCoO2, NMC111, NMC622, NMC811), anodes (Li4Ti5O12, Nb18W16O93) and solid electrolytes (Li0.33La0.57TiO3, Li7La3Zr2O12, Li3PO4).
2.2-I3
All-solid-state batteries are seen as next generation energy storage systems and heavily researched worldwide. Within the three major clases of solid electrolytes, oxide-ceramic Li-ion conductors attract significant attention due to their intrinsic safety. In case of the garnet type Li7La3Zr2O12 (LLZO), the possibility to directly use Li-metal anodes paves the way for high energy densities on cell and battery level. However, the widespread application of such an oxide-based all-solid-state cells depends on the development of scalable synthesis and manufacturing processes while increasing the cell capacity to competitive levels.
While oxides are the most stable solid electrolytes when compared to poylmers or sulfides, there is still some sensitivity to air and protic solvents due to the Li+/H+-exchange. However, with careful tailoring of the manufacturing process due to mechanistic understanding allowed for the development of a new, tailored tape-casting process for LLZO components. 70 µm thick, free-standing LLZO separators can now be fabricated via water based tape casting and all-ceramic free-standing LiCoO2/LLZO mixed cathodes show high active materials utilization. Microstructural engineering by introduction concentration gradients for the active material and the electrolyte allowed to boost discharge capacities to 2.8 mAh cm-2 utilizing 99% of the theoretical capacity. Additionally, the obtained free-standing cathodes have sufficient mechanical stability to allow the application of hybrid and ultra-thin separators to further increase the energy density on the full cell level to industrially relevant levels.
2.3-T1
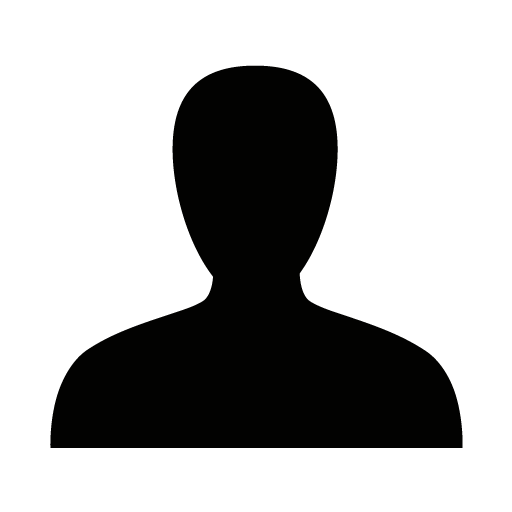
Solid-state lithium metal battery has a huge potential to be a more energy-dense architecture, safety and fast charging capability relative to a current lithium-ion battery. In particular, the cell interface made of oxide-based electrolyte and cathode is believed to withstand more tightly than any other ‘soft’ solid electrolytes (polymer, sulfide electrolytes) once optimized. Sintering at high temperature is generally required to produce dense interfaces for oxide-based electrochemical devices; however, the poor chemical compatibility of the oxide electrolyte with common cathode active materials limits a wide range of material choice, processing and microstructure-performance relationship. Here, potential processing strategies to fabricate oxide-based cathode composite are presented based on an infiltration of LiCoO2 active material and Li7La3Zr2O12 catholyte through the porous scaffolds or a co-firing of both. Discussion is aimed toward opportunities to meet important target parameters including low interfacial resistance, high-loading capability and specific capacity for high energy and power Li-metal battery.
2.3-T2
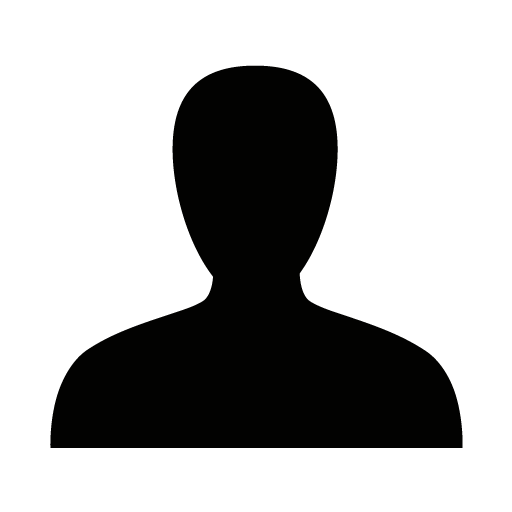
Solid-state lithium-ion batteries are a promising energy storage solution which promise greater safety with energy and power density comparable to current Li-ion technology. Although many advances have been made recently on the materials level, there still remains a need to understand how the properties of individual parts of the cell (e.g. electrolyte, active material, interfaces) combine to determine device performance on the cell level [1]. Research has focussed on improving solid-state battery performance by tailoring individual properties, such as the electrolyte’s ionic conductivity κ, the cathode active-material conductivity σ, and interface resistances or capacitances. When several materials are brought together to form a porous electrode, the cell performance does not always reflect individual properties in straightforward ways. Optimization of cell designs to achieve satisfactory performance requires an understanding of how the properties of various cell constituents interact. We believe that at this early stage of solid-state battery development, broad and simple design principles are preferable to finely resolved mechanistic models.
To that end, in this work we present a simple model of a planar solid-state battery cell based on the Newman–Tobias theory [2], which illustrates the roles of cathode porosity, interfacial contact, electrolyte ionic conductivity, and active-material electronic conductivity. We show that the current distribution in the porous cathode is governed by a few key dimensionless parameters, including the fraction of the conductivities of the two phases s=σ/(σ+κ) , and the ratio of the kinetic resistance to the bulk resistances. The results show – somewhat counterintuitively – that balancing the bulk conductivities of the interpenetrating cathode phases can lead to improved cell performance, as well as a higher electrode utilization. Insights provided by this approach provide useful guidelines for optimizing solid-state battery design.
2.3-T3
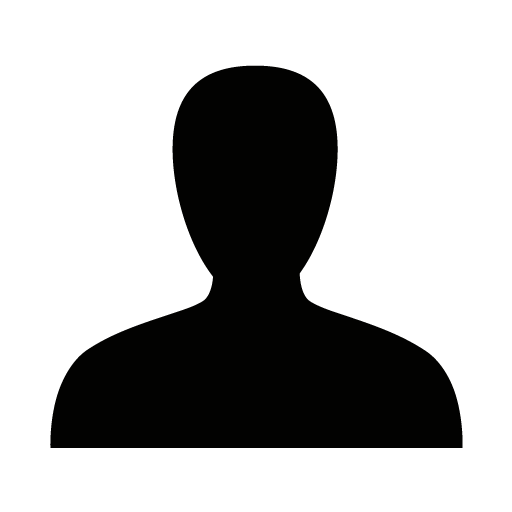
LiNi0.8Mn0.1Co0.1O2 (NMC811) is among the cathode materials most discussed for high-performance Li-ion batteries thanks to its capacity of approximately 200 mAh g-1 and its low Co content. Currently, NMC811 is considered for implementation in next-generation electric vehicles, but the high Ni content (> 60 %) still poses challenges, such as oxygen loss at voltages above 4.3 V, impedance rise, transition metal dissolution, and finally, a loss of active lithium in the electrode. These challenges can be mitigated by utilizing appropriate coating and doping strategies and limiting the voltage to standard cycling conditions (2.8 - 3.0 V to 4.3 - 4.5 V). NMC811 can be overlithiated to form Li2NMC811, in analogy to pure LiNiO2, which can form Li2NiO2 [1]. Such a high lithiation degree increases the initial discharge capacity but leads to a fast voltage loss, especially during the first cycle [2]
In this study, we address two intriguing research questions: (i) Can we control the interface of Ni-rich cathode material with the electrolyte and prevent the formation of detrimental solid electrolyte interphase (ii) Is it possible to take advantage of multi-electron cycling by introducing additional lithium into the host NMC811 structure? To address these questions, Li-rich NMC811 thin-film cathodes were prepared by sputtering from a Li2O-NMC811 target. Li-rich NMC811 cathodes were tested with LiPON as a solid electrolyte in a thin-film architecture, which offers a simplified 2D model with direct access to the cathode-electrolyte interface. The solid-state electrolyte helped to stabilize the interface and prevented capacity fading, voltage decay, and interface resistance growth. In combination with LiPON, cycling at extended voltage ranges of 1.5-4.7 V was possible without increasing interfacial resistance. It was possible to reversible cycle Li2NMC811 by lowering the applied potential to 1.5 V. resulting in a discharge capacity of up to 350 mAh g-1 due to multi-electron cycling. Overall, the all-solid-state cells with a lithium metal anode can cycle in the range of 1.5 V to 4.3 V for 1000 cycles with an average coulombic efficiency of 98.79 %. Our results demonstrate how solid electrolytes that are stable against NMC811 cathodes can unlock the full potential of this Ni-rich cathode class.
2.3-T4
Juan Carlos Gonzalez-Rosillo obtained holds a M.Sc. in Materials Science and Nanotechnology and a PhD in Materials Science from the University Autonomous of Barcelona. He performed his MSc and PhD research (2011-2017) at the Materials Science Institute of Barcelona (ICMAB-CSIC), where he studied the relation of the resistive switching properties of metallic perovskite oxides with their intrinsic metal-insulator transitions for memristive devices and novel computation paradigms. He also was a visiting researcher at the University of Geneva (CH) and Forschungszentrum Jülich (DE). Then he joined the Massachusetts Institute of Technology (USA) for a postdoctoral position (2017-2020) working on the memristive properties of lithium-based oxides for neuromorphic computing and processing of next-generation solid-state electrolyte thin films for All-Solid-State Batteries and Microbatteries. Juan Carlos has been awarded with a Tecniospring postdoctoral fellowship to join IREC and to develop thin film microbattery architectures to power micro- and nanodevices for the Internet of Things revolution
Glassy-type solid state electrolytes such as state-of-the-art LiPON avoid grain boundaries and prevent Li-dendrite propagation leading to extremely good cyclability and the commercialization of planar thin-film LiPON-based microbatteries. However, its reduced ionic conductivity (~1 μS/cm) limits its performance at high/discharge rates and the severe degradation of its electrochemical and structural properties upon their exposure to ambient conditions and high temperatures (> 300 ºC) hinders scalability and manufacturability. Among the next generation solid-state electrolytes, NASICON superionic solid electrolyte Li(1+x)AlxTi(2-x)(PO4)3 (LATP) with 0.3 ≤ x ≤ 0.5 remains one of the most promising solid electrolytes thanks to its good ionic conductivity (~0.5-1 mS·cm-1) and outstanding stability in ambient air. Despite the intensive research for bulk systems, there are only very few studies of LATP in thin film form. In particular, the only successful reports of relatively high ionic conduction (~10-5 S·cm-1) have been achieved through amorphous sputtered films.
In this talk, we will explore the properties of high performance LATP thin films fabricated by Large-Area Pulsed Laser Deposition. The as-deposited thin films exhibit an ionic conductivity around 0.5 μS·cm-1 at room temperature (comparable to the state-of-the-art of LiPON) which increases to remarkably high values of 0.1 mS cm-1 after an additional annealing at 800 ºC. We will discuss the formation of a glassy, intergranular phase connecting highly conducting LATP grains as a possible cause for the significant enhancement in ionic conductivity by two orders of magnitude. The performance of both as-deposited and annealed LATP films makes them suitable as solid electrolytes, which opens the path to a new family of stable and highly performing thin solid-electrolytes. We will discuss its integration with other battery components, with special attention to interfacial coatings matching the electrochemical window at low potentials.
2.3-T5
The development of energy storage and conversion devices demanded to generate clean and sustainable energy relies on the design, synthesis, and characterisation of novel materials with suitable properties in terms of ionic or electronic conductivity, ionic insertion capabilities, redox activity, catalytic properties, etc. The structure of the materials used in energy may condition these properties and their overall good performance, and its knowledge is essential for proper understanding and optimising of these materials. To define the crystalline structure, Neutron powder diffraction (NPD) is a powerful tool that, combined with the Rietveld refinement method, is able to provide detailed structural information. This way, properties closely related to the structure and its evolution with external factors (temperature, pressure, cycling in in-operando systems, etc.) can be assessed with greater certainty. Well-known applications of neutron diffraction are the location of oxygen atoms and oxygen vacancies in oxide ion conductors, Li ions in solid electrolytes or Li-ion battery cathodes, protons in fast H+ conductors, etc. The ionic conductivity of these diverse materials is partly conditioned by the structure, composition, atomic radii, and formal charges of each atom, among others. An inexpensive and relatively efficient way to exploit these data in order to determine properties such as ionic percolation activation energy is the use of Bond‑Valence Energy Landscape (BVEL), which relies on structural data that can be determined by NPD. In this way, NPD and BVEL form a synergistic tandem that is useful in the assessment of ionic conductors. In this talk, we will present some recent results obtained for different types of energy-related materials, including Li-ion battery electrodes, electrolytes in Na batteries, and H+ conductors.
As Li cathode example, an N-doped LiFePO4-type material, where nitrogen partially replaces an oxygen atom of the olivine tetrahedra lattice at 4c Wyckoff site in the Pnma space group. The structure was correctly determined by the Rietveld method from NPD data, and a subsequent calculation by BVEL confirms the decrease in activation energy (Ea) by about 6 % with respect to the pristine structure upon N-doping. As a solid electrolyte example, collaborative work is presented, in which the structures of Mg-doped NZSP NASICON-type electrolytes were determined at different temperatures from neutron data. A decrease in the activation energy concerning the undoped sample and its temperature dependency is assessed by BVEL. Finally, an example of a proton conductor used as a catalyst is presented, the so-called antimonic acid, where the presence and location of two different H+ are verified and identified by means of Fourier density difference maps. In this talk, a brief review of these different materials will be made, with emphasis on the specific structural features determined by the neutrons that explain the desired properties of the materials applied to energy.
1.1-I1
Maksym Kovalenko has been a tenure-track Assistant Professor of Inorganic Chemistry at ETH Zurich since July 2011 and Associate professor from January 2017. His group is also partially hosted by EMPA (Swiss Federal Laboratories for Materials Science and Technology) to support his highly interdisciplinary research program. He completed graduate studies at Johannes Kepler University Linz (Austria, 2004-2007, with Prof. Wolfgang Heiss), followed by postdoctoral training at the University of Chicago (USA, 2008-2011, with Prof. Dmitri Talapin). His present scientific focus is on the development of new synthesis methods for inorganic nanomaterials, their surface chemistry engineering, and assembly into macroscopically large solids. His ultimate, practical goal is to provide novel inorganic materials for optoelectronics, rechargeable Li-ion batteries, post-Li-battery materials, and catalysis. He is the recipient of an ERC Consolidator Grant 2018, ERC Starting Grant 2012, Ruzicka Preis 2013 and Werner Prize 2016. He is also a Highly Cited Researcher 2018 (by Clarivate Analytics).
Colloidal lead halide perovskite (LHP) nanocrystals (NCs), with bright and spectrally narrow photoluminescence (PL) tunable over the entire visible spectral range, are of immense interest as classical and quantum light sources. Severe challenges LHP NCs form by sub-second fast and hence hard-to-control ionic metathesis reactions, which severely limits the access to size-uniform and shape-regular NCs in the sub-10 nm range. We show that a synthesis path comprising an intricate equilibrium between the precursor (TOPO-PbBr2 complex) and the [PbBr3-] solute for the NC nucleation may circumvent this challenge [1]. This results in a scalable, room-temperature synthesis of monodisperse and isolable CsPbBr3 NCs, size-tunable in the 3-13 nm range. The kinetics of both nucleation and therefrom temporally separated growth are drastically slowed, resulting in total reaction times of up to 30 minutes. The methodology is then extended to FAPbBr3 (FA = formamidinium) and MAPbBr3 (MA = methylammonium), allowing for thorough experimental comparison and modeling of their physical properties under intermediate quantum confinement. In particular, NCs of all these compositions exhibit up to four excitonic transitions in their linear absorption spectra, and we demonstrate that the size-dependent confinement energy for all transitions is independent of the A-site cation.
We then show that this synthesis – relying on the labile ligand capping with TOPO-phosphinic acid mixture – makes for a convenient platform for the subsequent surface functionalization with diverse capping ligands [2]. Robust surface functionalization of highly ionic surfaces, as is the case of LHP NCs, has remained a formidable challenge due to the inherently non-covalent weak surface bonding. Leveraging the vast and facile molecular engineering of phospholipids, we present their efficacy as surface capping ligands for LHP NCs. Molecular dynamics simulations and solid-state NMR confirm that the surface affinity of these zwitterionic molecules is primarily governed by the geometric fitness of their anionic and cationic moieties. Judicious selection of the ligands yielded colloidally robust FAPbBr3 and MAPbBr3 NCs and enabled colloids in a variety of solvents, from n-hexane to acetone. Robustness of the surface capping is also reflected in optical properties: NCs exhibit PL quantum yield (QY) above 96% after numerous purifications. NCs are essentially blinking-free at a single particle level.
1. Q. Akkerman et al. submitted
2. V. Morad et al. submitted
1.1-I2
The flexibility of lead-halide perovskites plays a crucial role in their functional response, but the exact structural distortions and their effects on physical properties are still unclear [1]. Here, we provide for the first time the description of the photoinduced and thermally-activated structural distortions in CsPbBr3 perovskite nanocrystals with atomic-level precision. We combine time-resolved X-ray absorption spectroscopy (TR-XAS) and ab initio simulations to show that the photoexcitation of CsPbBr3 nanocrystals (NCs) leads to well-defined polaronic lattice changes, rather than photoinduced structural phase transitions. Additionally, our analysis rules out thermal effects in the photoactivation of the system [2]. We also investigated the purely thermal response of CsPbBr3 with temperature-dependent XAS and first principles molecular dynamics (MD) simulations across its phase diagram. We show that the thermally-activated lattice cannot be reduced to a cubic average structure, because of the presence of dynamically distorted local configurations and lattice anharmonicity [3].
Our comprehensive investigation demonstrates that the structural changes of CsPbBr3 NCs induced by light and thermal functional triggers have fundamentally different physical origins. Even though both effects are related to the flexibility of the perovskite lattice, the photoexcitation is selectively driven by electron-phonon coupling, while the thermal activation drives lattice phonon anharmonicity. The latter leads to significant distortions from the CsPbBr3 space- and time- average lattice symmetry, and it is well rationalized by the soft-mode model in the framework of displacive thermal phase transitions.
These finding clarify the underlying mechanisms of the lattice response under functional activation and offers strategies to control the perovskite nuclear degrees of freedom with different external stimuli. Understanding the thermal processes acting at the atomic-level represents the first step toward a rational design of perovskite-based devices with improved stability.
1.1-I3
Traditionally defects have been considered detrimental for the performance of photoactive devices. For example, in photocatalysts point defects introduce trap states that act as recombination centres lowering quantum yields. However, recent advances in spectroscopy, microscopy and modelling, have opened the door to ground-breaking studies which are challenging our views of disorder. New evidence suggests that defects could play a much more active role than anticipated by controlling parameters as important as the reaction selectivity. The role of disorder is particularly critical in photocatalysis where the act of photoexcitation can, in itself, generate dynamical defects in the active phase. In this talk I will discuss the many different shapes and forms in which disorder can emerge in photo-electrocatalysis. In particular, I will focus on the formation of electronic defects and structural reconstructions during catalytic operation. I will present examples of how disorder-associated phenomena can be monitored in situ and operando and how it can be correlated with catalytic function. Moreover, by drawing parallelisms with the biological world I will discuss if disorder is unavoidable to achieve high performance. Finally, I will contrast the role of disorder in photocatalysis with observations made in other photoactive materials in order to highlight potential routes to use disorder in our favor.
1.2-T1
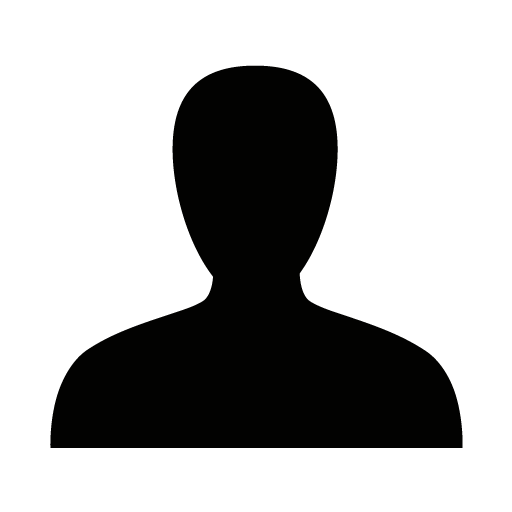
Photocatalytic solar fuel production provides a potential alternative to sustainable energy production. Whilst attention to date has focused on inorganic photocatalysts, carbon-based materials and organic semiconductors have emerged as potential low cost and efficient photocatalyst for hydrogen evolution, mainly due to the tunability of their properties through synthetic control.[1,2] This allow the design of families of materials with tuned opto-electronic properties by incorporating different building blocks.[1] The best performing systems are bulk heterojunctions nanoparticles prepared from a blend of conjugated polymer donor and non-fullerene small molecules acceptor, particularly due to their improved light absorption in the visible range.[2,3] Despite the efficient performance of the donor/acceptor bulk heterojunction photocatalysts for hydrogen evolution, the fundamental understanding of the photophysical processes that determine their performance remain limited. In this presentation, I will discuss the charge carrier dynamics of donor/acceptor heterojunction nanoparticles photocatalysts with different hydrogen evolution activity.[2,3] Transient and operando photoinduced absorption spectroscopies, on timescales of femtoseconds to seconds after light absorption, were employed to monitor the kinetics of photogenerated charges and their correlation with photocatalytic performance.[3] Differences between the function of Donor/Acceptor bulk heterojunction photocatalysts and single conjugated polymers photocatalyst will be discussed. These results can provide design guidelines towards efficient organic semiconductors photocatalyst.
1.2-T2
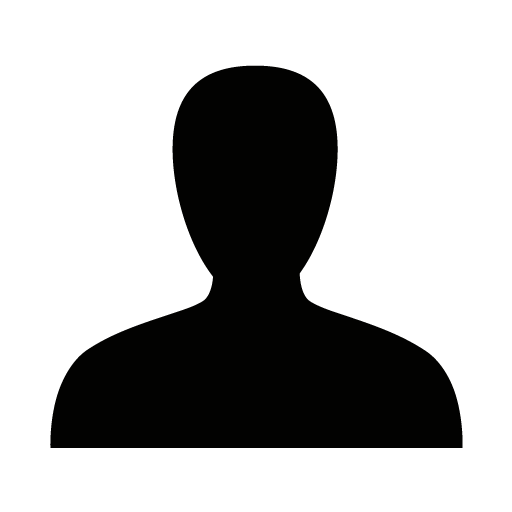
Semiconductor nanoparticles (NPs) have attracted a great deal of interest in the last decades due to the increasingly range of applications. However, NPs were produced usually in batch processes and the production on a larger scale is still limited. Among n-type semiconductors, Bismuth vanadate (BiVO4) has emerged as one of the most promising metal oxides as photoanode for water oxidation due to its moderate band gap (2.4 eV), adequate band positions, low-cost, good chemical stability in aqueous medium and environmental inertness.[1] In this regard, our group designed and studied different methodologies for the preparation of this type of photoelectrodes.[2] However, the large-scale synthesis of this material is still limited to the use of conventional batch processes, and it is necessary to achieve high-production yields due to the increasing demand in the field of energy materials. Since the automatization of this process has significant advantages with respect to cost, safety and more precise control of the physical and chemical properties, the emergence of continuous flow technology offers an alternative for preparing inorganic nanoparticles (NPs).[3]
In this communication we show the preparation of BiVO4 NPs by using a simple continuous-flow method based on two liquid peristaltic pumps. The proposed system allows to up-scale the synthesis through a microreactor and contributes to the possible production of large-scale photoelectrodes.
1.2-T3
Junyi Cui is a Ph.D. student in Dr. Salvador Eslava's group at Imperial College London. She received her MRes degree of Green Chemistry in 2019 from Imperial and B.Eng. of Renewable Energy Materials and Engineering in 2017 from the University of Electronic Science and Technology of China. Her research experience focuses on renewable energy, i.e., lithium-ion batteries, redox flow batteries, and currently on solar-driven water splitting using transition metal oxides.
Two-dimensional (2D) VA group materials, including black phosphorene, bismuthene, and antimonene, attract considerable attention in energy devices because of their unique properties, such as tunable bandgap and superhigh carrier mobility. However, their application in photoelectrochemical devices is rarely reported. In this presentation, we present the successful design of semiconductor /2D VA material/ co-catalyst composite photoanodes, such as BiVO4/bismuthene/NiFeOOH, BiVO4/bismuthene/CoPi, and BiVO4/Sb/NiFeOOH. Specifically, the BiVO4/bismuthene/NiFeOOH photoanode that achieves 3.7 mA cm-2 at 0.8 VRHE are deeply investigated. Comprehensive (photo)electrochemical techniques and surface photovoltage were comprehensively employed to identify the roles of bismuthene and NiFeOOH on BiVO4. We found that bismuthene increases the density of VO of BiVO4 that are beneficial for the oxygen evolution reaction via the formation of oxy/hydroxyl-based water oxidation intermediates. Moreover, bismuthene increases interfacial band bending and fills the VO-related electron traps with charges, leading to more efficient charge extraction. The NiFeOOH significantly increase the surface hole concentration by passivating the r-ss. The use of 2D VA group materials and co-catalysts as functional layers opens new avenues to tune the surface properties of photoanodes for water oxidation.
1.2-S1
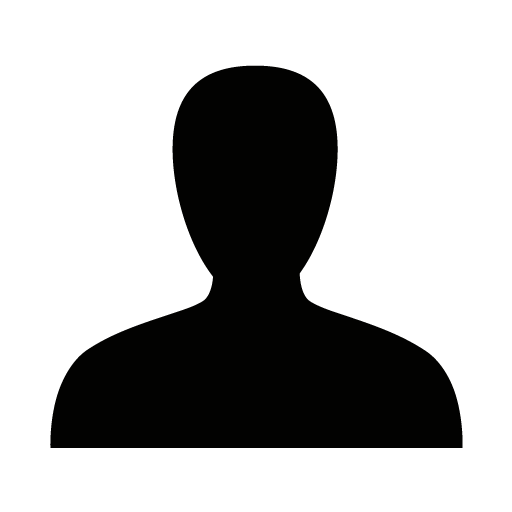
How does the publishing process work behind the scenes? Learn more about what editors look for when they assess manuscripts and hear how the Royal Society of Chemistry is supporting open access publishing.
How does the publishing process work behind the scenes? Learn more about what editors look for when they assess manuscripts and hear how the Royal Society of Chemistry is supporting open access publishing.
How does the publishing process work behind the scenes? Learn more about what editors look for when they assess manuscripts and hear how the Royal Society of Chemistry is supporting open access publishing.
How does the publishing process work behind the scenes? Learn more about what editors look for when they assess manuscripts and hear how the Royal Society of Chemistry is supporting open access publishing.
How does the publishing process work behind the scenes? Learn more about what editors look for when they assess manuscripts and hear how the Royal Society of Chemistry is supporting open access publishing.
1.2-T4
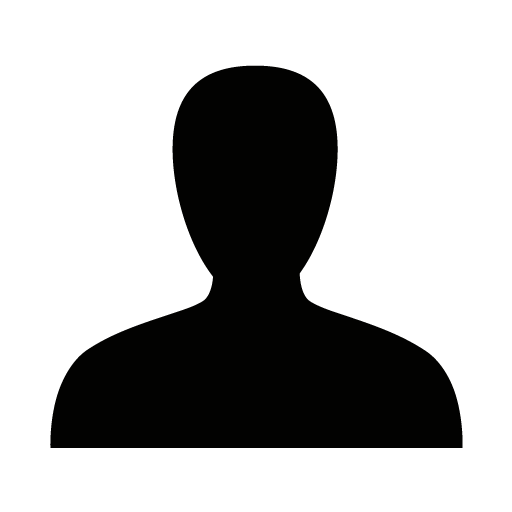
Carles Ros is currently working as senior postdoctoral researcher with the fellowships Juan de la Cierva (JdC-F) and Severo Ochoa Excellence Program in the Organic Nanostructured Photovoltaics group in ICFO research institute lead by Prof. Jordi Martorell in the fields of Photonics, Photovoltaics and Photoelectrochemistry.
He has research experience in the fields of advanced materials science, (photo)electrochemistry, nanostructured synthesis, semiconductor physics and photovoltaics. In particular, (photo)electrocatalysis and light management in metal-oxide-organic-perovskite tandems and protective metal-oxide layers for photoelectrodes for applications in H2, CO2RR, hydrogen storage and release in graphene, solar fuels and photobatteries. His highly interdisciplinary background based on applied physics has helped him find its place in a group that is applying solid light management physics into the field of renewable synthetic fuels.
Photoelectrochemical energy conversion from sunlight directly into chemical bonds is a promising path to fabricate alternative solar fuels. But a single semiconductor is not capable to provide enough voltage for the chosen electrochemical reaction and/or to do so with significant productivities. Tandem PEC/PV monolithic devices can generate the necessary voltage meanwhile increasing overall productivity.
BiVO4, one of the most promising large bandgap (~2.5 eV) photoanode candidates, has been fabricated in tandem with non-fullerene semi-transparent organic (OPV) based thin-film third generation photovoltaics. Although OPVs allow for fine-selection of absorber composition and thus, absorption spectra, the balance is not optimal. Nanophotonic structures are necessary to balance the photon influx to maximize the overall series current in the device.
In this talk we will explain how the many-layers device has been computationally modelled and the nanophotonic structures incorporated, and how the full device has been fabricated and tested at ICFO. The light path for the blue part of the solar spectra has been maximized in the BiVO4, and the PV part has been adequately selected so that the device provides the necessary voltage for either water splitting or CO2 reduction. Several parameters have been taking into account in this model, such as the photovoltage loss of the solar cells under reduced photon influx due to the light management.
This many-layers tandem PEC/PV structure can provide >2.5 V of photovoltage and the 1D light management structures have demonstrated 2x increase of the bias-free photocurrent.
1.2-T5
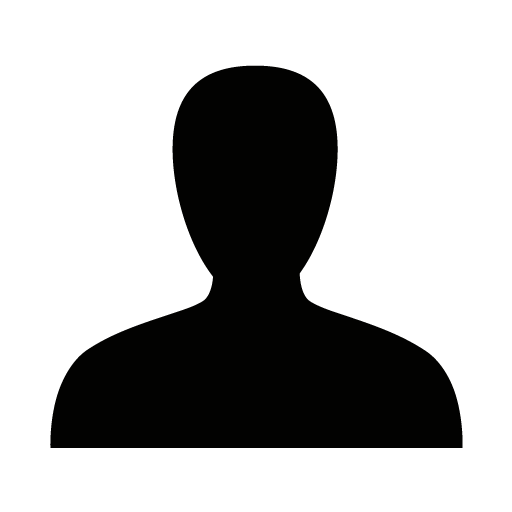
In recent years, bismuth-based photocatalysts have been receiving increasing attention in photocatalysis, due to their appropriate bandgap and tunable surface structure, which make them suitable also for the photocatalytic reduction of CO21. Their performances, however, are still limited by the fast charge carriers recombination. Recently, the exploitation of piezo/ferro-electric potentials in photo-active semiconductors has been adopted as an effective strategy to modulate the charge transfer properties both in the bulk phase and at the surface of semiconductors (i.e. piezo-phototronic effect)2. In this perspective Bismuth-based Aurivillius compounds, owing to their usually strong spontaneous ferroelectric polarization represent a promising option as cell photo-electrodes, allowing the increase of cell efficiency by electrically polarizing the materials. In addition, thanks to their unique layered structure, this peculiar class of perovskites allows the migration of photo-generated holes and electrons within different areas of the materials, thus intrinsically facilitating charge separation.
In this work, the utilization of different Aurivillius compounds (i.e. Bi4Ti3O12 – BiTO, and Bi2MoO6 - BiMO) as photo-electrode materials for solar conversion has been studied in detail. The use of BiTO and BiMO for the photocatalytic reduction of CO2 has recently been reported, however, the main strategies to develop optimized ferroelectric-enhanced photo-electrodes of these materials and their utilization for the ferroelectric potential-assisted CO2 reduction has never been fully investigated. BiTO and BiMO photo-electrodes were fabricated and accurately optimized to obtain both hierarchically oriented nanostructures with different morphologies (i.e. nanosheet/nanorod arrays), and thin-film layers via in situ hydrothermal deposition and through a sol-gel/spin coating coupled process respectively. The effect of the ferroelectric potential on the photo-electrochemical performances of the optimized photo-electrodes with different architectures was therefore accurately studied. Density current increments and enhanced charge transfer ability were registered under the optimal ferroelectric polarization which was directly reflected in the CO2 photo-electrochemical reduction performances. This work, therefore, demonstrates the possibility to adopt ferroelectric polarization coupled with an external bias to effectively control the migration of photo-generated charges in bismuth-based Aurivillius semiconductors for the CO2 photo-electrochemical reduction.
1.2-T6
Photoelectrochemical cells (PECs) have been developed as environmentally friendly systems that can directly utilize photogenerated electron-hole pairs for water splitting, fuel production, conversion of carbon dioxide, and pollutant degradation. Most reports on the photocatalytic or PEC hydrogen (H2) evolution via water splitting have focused on the H2 reduction half-reaction by generating on the photoanode oxygen or using sacrificial agents to consume the generated h+. Lately, much effort has been invested into synthesizing valuable chemicals on the photoanode while retaining the production of H2 on the cathode.
Over the past few years, polymeric carbon nitrides (CN) have attracted widespread attention due to their outstanding electronic properties, which have been exploited in various applications, including photo- and electro-catalysis, heterogeneous catalysis, CO2 reduction, water splitting, light-emitting diodes, and PV cells. CN comprises only carbon and nitrogen, and it can be synthesized by several routes. Its unique and tunable optical, chemical, and catalytic properties, alongside its low price and remarkably high stability to oxidation (up to 500 °C), make it a very attractive material for photoelectrochemical applications. However, few reports regarded CN utilization in PECs due to the difficulty in acquiring a homogenous CN layer on a conductive substrate and our lack of a basic understanding of the intrinsic layer properties of CN.
This talk will introduce new approaches to growing CN layers with altered properties on conductive substrates for photoelectrochemical applications. The growth mechanism and their chemical, photophysical, electronic, and charge transfer properties will be discussed. I will show the utilization of PEC with a CN-based photoanode as a stable and efficient platform for the oxidation of water or organic molecules to oxygen or added-value chemicals, respectively, with hydrogen co-production.
1.3-T1
Many metal oxide semiconductors, such as TiO2, require UV excitation in order to drive photocatalytic reactions. However, UV photons account for only 3-5% of the solar spectrum, and thus strategies to extend the range of harvested photons into the visible wavelength range are required. One potential way is to use triplet-triplet annihilation photon up-conversion (TTA-UC). TTA-UC systems generate higher energy photons from lower energy, low intensity, and non-coherent excitation. In this work, we synthesized several 9,10-diphenylanthracene derivatives and attached them to different wide-bandgap metal oxide nanoparticles, TiO2, ZrO2, and CeO2, which were then dispersed in solutions containing triplet sensitizers. Triplet energy transfer leading to triplet-triplet annihilation photon up-conversion and charge generation were probed through steady-state and transient spectroscopy techniques such as photo-induced absorption (PIA), time-resolved photoluminescence (trPL), and transient absorption (TA) spectroscopy. We characterized these TTA-UC systems as films and suspensions and applied them in different photocatalytic processes as proof of concept.
1.3-T2
Dr. Daniel Grave is an assistant professor in the Department of Materials Engineering at Ben Gurion University, Israel.
Transition metal-oxides are promising photoelectrode materials for photoelectrochemical water splitting and solar fuel conversion. Several materials with empty or filled d-shell configurations (e.g. TiO2, BiVO4) have shown high internal quantum efficiencies, but their band gaps are too large for practical purposes. Metal-oxides with suitable bandgaps generally have open d-shell configurations and suffer from poor photoconversion efficiencies. We have recently introduced spatial collection efficiency analysis, based on optical and external quantum efficiency measurements, as a non-destructive method to probe losses in thin film semiconductor photoelectrodes under operando conditions. [1,2,3] Using hematite as a case study for strongly correlated electron materials, we show that in addition to the well-known recombination losses arising from poor charge transport properties, some of the photon absorption leads to localized excited states rather than to generation of mobile electrons and holes that contribute to the photocurrent. We extract the wavelength-dependent mobile charge carrier photogeneration yield spectrum of hematite and show that it is less than unity across the entire absorption range, fundamentally limiting the attainable photocurrent to roughly half that predicted based on above bandgap absorption. I will discuss our recent advancements on this front, and the implications of our findings on photoconversion efficiency in open d-shell transition metal-oxides.
1.3-I1
Sixto Giménez (M. Sc. Physics 1996, Ph. D. Physics 2002) is Associate Professor at Universitat Jaume I de Castelló (Spain). His professional career has been focused on the study of micro and nanostructured materials for different applications spanning from structural components to optoelectronic devices. During his PhD thesis at the University of Navarra, he studied the relationship between processing of metallic and ceramic powders, their sintering behavior and mechanical properties. He took a Post-Doc position at the Katholiek Universiteit Leuven where he focused on the development of non-destructive and in-situ characterization techniques of the sintering behavior of metallic porous materials. In January 2008, he joined the Group of Photovoltaic and Optoelectronic Devices of University Jaume I where he is involved in the development of new concepts for photovoltaic and photoelectrochemical devices based on nanoscaled materials, particularly studying the optoelectronic and electrochemical responses of the devices by electrical impedance spectroscopy. He has co-authored more than 80 scientific papers in international journals and has received more than 5000 citations. His current h-index is 31.
All-Inorganic Halide Perovskite Quantum Dots (QDs) have emerged as a new class of fascinating nanomaterials with outstanding optoelectronic properties, with promise to revolutionize different disciplines like photovoltaics, lasing and emission. In the present talk, we will describe our efforts towards the application of these materials for solar-driven processes spanning from photocatalysis, environmental remediation,[1] H2 production and organic transformations.[2] We will discuss on the rational design of these fascinating materials towards photocatalytic and photoelectrochemical processes, and the importance of extracting basic electronic and optical information to understand the carrier dynamics,[3] the influence of defect states and to define adequate defect passivation strategies to maximize the performance and stability of these materials.[4] Moreover, we emphasise the need for proper interrogation tools to validate their photoelectrocatalytic activity and selectivity. Our results also highlight the urgent need for stabilization strategies to move beyond the proof-of-concept stage to relevant technological developments.
2.1-T1
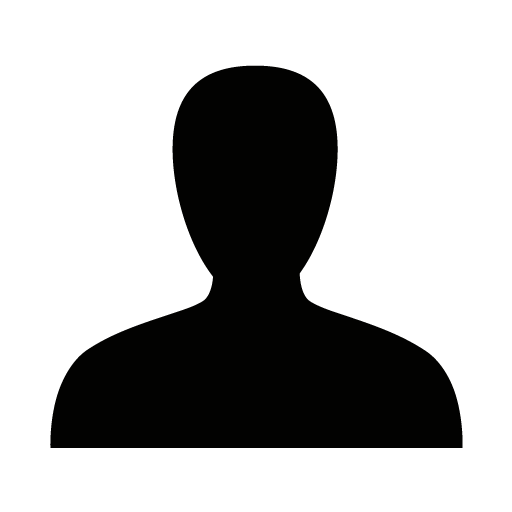
Since their discovery cadmium chalcogenide nanoplatelets (NPLs) attracted immense attention due to the fact that they exhibit optoelectronic properties, which are superior to the properties of counterpart nanocrystals of other dimensionalities with the same composition. In addition, due to their shape, they can be arranged into oriented assemblies further extending control over the optical and electronic features. One of the particular features of such NPLs is atomic smoothness of their surface and strong one-dimensional quantum confinement in the thickness direction, which can be controlled down to one monolayer. On the other hand, the ability to change thickness only in integer steps becomes a severe disadvantage preventing precise tuning of spectral position of absorption and photoluminescence bands.
To some extent, spectral tunability was achieved by varying the lateral size of NPLs, and their surface modification via ligand exchange or shell growth. However, these approaches are considerably limited and still not nearly as flexible as the simple size-tuning of quantum dots. In addition, they generally result in a red-shift of optical bands of NPLs, thus highlighting another longstanding challenge for NPLs – the lack of bright emitters in the UV-blue region.
Another more powerful approach consists in the modification of nanocrystal composition through the synthesis of alloyed nanoparticles. Although some advances in this direction have been already achieved by modifying existing protocols for the synthesis of CdSe and CdS NPLs, the synthesized nanoparticles either suffered from poor photoluminescence quantum yields of < 5 % or a limited range of available compositions.
In this work, we demonstrate that both of these issues can be overcome by employing highly reactive stearoychalcogenides, which rapidly react with cadmium carboxylate yielding small polydisperse nanocrystals. At later reaction stages, these nanocrystals serve as a monomer source for the expansion of NPLs in the lateral direction in a slow Ostwald-like ripening process guided by acetate ions. The separation of these stages essentially decouples the precursor conversion step and lateral growth of NPLs thus affording much better control over the lateral dimensions of NPLs, which in turn is a significant factor for achieving brightly emitting nanocrystals. Conveniently, unlike in previously reported procedures, the Se/(Se+S) ratio in the synthesized NPLs is essentially the same as in the starting reaction mixture which enables straightforward control over the nanocrystal composition. Moreover, we demonstrate that by using essentially the same procedure and changing the reaction temperature it is possible to control the thickness of NPLs thus further expanding the spectral range of the synthesized nanocrystals.
Optical spectroscopy measurements show that the increase of selenium content in the alloy results in a steady red shift of absorption and photoluminescence bands consistent with the shrinkage of the material bandgap. Due to their relatively small lateral size enabled by controlling the lateral growth, obtained alloyed CdSexS1-x NPLs exhibit bright band-edge emission covering the blue-green region from 380 to 520 nm with quantum yields of around 30–50 %, which are ca. 10 and 2 times higher than previously reported for respectively 3.5 and 4.5-monolayer thick NPLs with similar composition.
2.1-T2
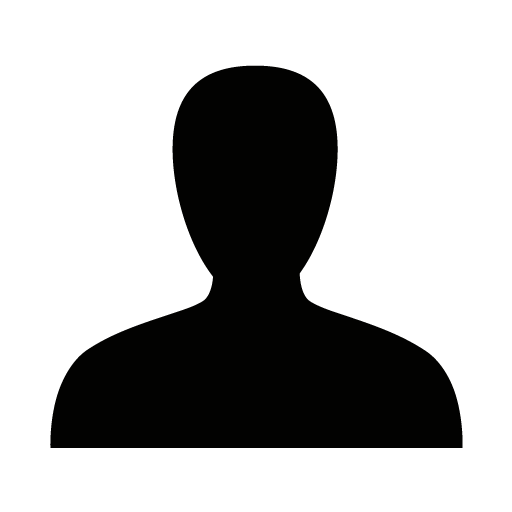
Halide perovskite materials have attracted great scientific interest due to their solution-processability and excellent optoelectronic properties. Applying these perovskites photoactive layers for direct solar water splitting has the potential to provide efficient and inexpensive photoelectrodes for solar hydrogen generation. In this presentation all-inorganic CsPbBr3 perovskite (2.3 eV bandgap) photoelectrodes will be presented, where the photoactive layer is protected from direct contact with the aqueous electrolyte by a graphite sheet and a low-temperature printed carbon layer. The critical role of controlling 2-dimensional (CsPb2Br5) and 0-dimensional (Cs4PbBr6) perovskite phase formation and their effect on stability and efficiency will be discussed. The low annealing temperature carbon layer enables the deposition of an organic hole transport layer, while the graphite sheet allows for electrodeposition of nanoscale NiFeOOH co-catalyst on its surface. It will be shown that all these developments lead to all-inorganic halide perovskite photoanodes with remarkable days-long stability and photocurrent generation (~10 mA cm-2) close to the theoretical efficiency limit of CsPbBr3. Finally, the potential for scaling up these devices will be demonstrated by presenting photoanodes with an area above 1 cm2.
2.1-T3
Ferry Prins is a tenure-Track Group leader at the Condesed Matter Physics Center (IFIMAC) of the Universidad Autonoma de Madrid. Ferry obtained an MSc in Chemistry from Leiden University (2007) and a PhD in Physics from the Kavli Institute of Nanoscience at Delft University of Technology (2011). After completion of his PhD, he joined the the group of Prof. Will Tisdale at Massachusetts Institute of Technology (MIT). There, he started exploring the optical properties of nanomaterial assemblies with an emphasis on excitonic energy-transfer interactions. In 2014 he moved to ETH Zurich for a postdoc with Prof. David Norris at the Optical Materials Engineering Laboratory. With support from the Swiss National Science Foundation, he started an independent group at ETH in 2015. In Spring 2017 he joined he Condensed Matter Physics Center (IFIMAC) at the Autonoma University of Madrid where he directs the Photonic Nanomaterials and Devices Lab. His group specializes in the development of light-management strategies for semiconductor nanomaterials.
The efficient transport of energy carriers plays an essential role in all of optoelectronic technologies. Energy transport characteristics have traditionally been derived from time-resolved spectroscopy or device-level techniques. While such techniques work well for the characterization of the spatially homogeneous lattices of crystalline semiconductors, they fail to capture the spatially varying complexity of nanostructured semiconductors. To tackle this issue, a range of time-resolved microscopy techniques have emerged, capable of directly visualising energy transport with sub-nanosecond and few-nanometer resolution.[1]
In this talk, I will give an overview of the most recent work of my group on improving our understanding of the various forms of anomalous transport phenomena that can be encountered in semiconductors nanomaterials.[2-5] In the first part of the talk, I will discuss the extensive models that we have developed using Brownian dynamics in which we make use of the detailed spatiotemporal information obtained from time-resolved microscopy. In a second part of the talk, I will present recent work in which we use Mn-doped layered perovskites as highly versatile test-bed to understand the role of carrier trapping in energy transport, with Mn sites acting as deep traps for diffusing excitons. By controlling the doping level, we can controllably modify the trap landscape and relate these changes to the observed spatiotemporal dynamics.
Our measurements and the resulting models provide valuable information for the development of optimized nanostructured semiconductors for optoelectronics, including light harvesting and light emitting devices.
2.1-T4
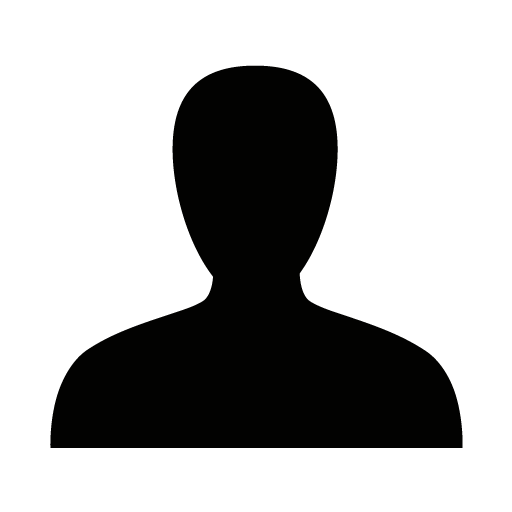
The solution−liquid−solid (SLS) mechanism is a well-established method for forming one-dimensional (1D) nanostructures in a solution. Herein, an SLS mechanism is explored for the formation of metal oxides for the first time. Two key synthetic achievements allow this synthesis: (i) the design of a tailored catalyst with a low melting point and high stability and (ii) control over the reactivity and the oxidation of the precursors. Once these conditions are achieved, the SLS growth of indium and tin oxides ensues. Structural characterization of the products at various stages of the growth confirms the formation of 1D In2O3 and SnO2 nanoscale heterostructures using AuIn2 and Au7Sn3 as catalysts. Both metal oxides showed photocatalytic abilities by the photodegradation of 2-mercaptobenzothiazole (MBT). Furthermore, SLS growth was easily adopted to insert SnO2 rods selectively between two domains of an Au/ZnO rod heterodimer, demonstrating the potential of achieving highly complex multi-component metal oxide nanostructures.
2.1-T5
Perovskite solar cells currently demonstrate more than 25% efficiency, however many fundamental processes still remain unclear. In particular, charge carrier mobility and diffusivity are still poorly characterized and understood. The reported mobility values differ by many orders of magnitude depending on the materials preparation, device architecture and measurement techniques. Therefore, carrier motion in real perovskite solar cells still remains far from clear and the lack of suitable carrier mobility investigation techniques is one of the major problems. Moreover, evaluation of the actual electric field strength in perovskite layer of solar cells is also not a trivial task. Therefore, the charge carrier transport in real operating perovskite solar cells still remains a controversial, heavily disputed question. Carrier motion is expected to be particularly complex in case of archetypical perovskite solar cell architecture, where majority of the perovskite is embedded into mesoporous TiO2 (m-TiO2) layer.
We investigated charge carrier motion and extraction from archetypical methylammonium lead iodide (MAPI) perovskite solar cell. We used an ultrafast electric field-modulated transient absorption technique enabling to directly visualize the carrier motion with subpicosecond time resolution by evaluating electric field dynamics from the time-resolved electroabsorption spectra. We demonstrate that photogenerated holes drift across the mesoporous TiO2/perovskite layer during hundreds of picoseconds, however their extraction to the Spiro-OMeTAD hole transporting layer takes place during tens of nanoseconds suggesting that the hole extraction is limited by the perovskite/Spiro-OMeTAD interface rather than by the hole transport through the perovskite layer. Additionally, we use the ultrafast time-resolved fluorescence technique which reveals fluorescence decay during tens of ps, which we attribute to the spatial electron and hole separation.
2.1-T6
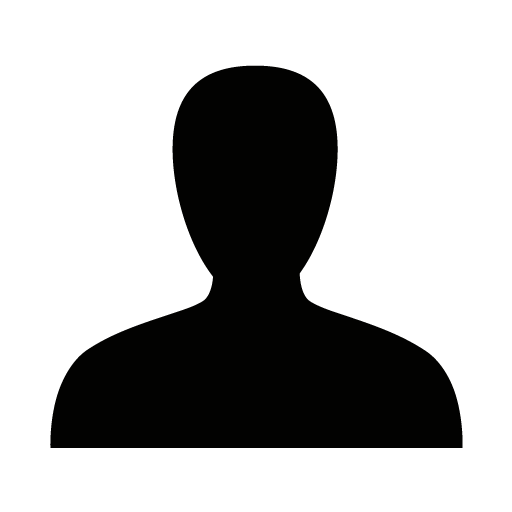
Hybrid halide perovskites show heterogeneity at multiple length scales.[1] Cathodoluminescence (CL) in a scanning electron microscope (SEM) can investigate semiconductor materials by scanning an electron beam over a semiconductor. By collecting the optical properties of the material during scanning, CL-SEM can elucidate structure-property relations of halide perovskites at sub-micrometer spatial resolution.
While most CL studies on perovskites have focused on the inorganic compositions, the characterization of hybrid (organic-inorganic) halide perovskites - including the highest-performing compositions - is limited due to their lower stability under the electron beam. To reduce beam damage, we used a pulsed electron-beam (PM) to measure hyperspectral maps of hybrid perovskite compositions.[2] When PM was used, the CL spectra strongly resembled that of pristine perovskite emission, suggesting the use of PM for more robust high spatial resolution spectral mapping of beam-sensitive hybrid halide perovskite materials.
In this presentation, a series of examples will be presented whereby PM and low-current CL aided in the study of luminescence of several different perovskite compositions. For example, the use of CL was crucial to understand the effect that focused ion beam milling has on the perovskite emission, generally considered to be a rough processing step for TEM specimen preparation. Using CL-SEM in PM, the perovskite layer in the lamellae was found to remain optically active, yet with a small blue-shift due to surface amorphization.[3]
Going beyond conventional high-current CL setups, used for traditional semiconductors, enables CL to start to play a role in resolving the complex heterogeneity of hybrid halide perovskites, and it could also enable the elucidation of many other novel beam sensitive “soft” semiconductors.
2.2-I1
Simply prepared chalcogenide materials have been investigated as light absorbers for photoelectrochemical water splitting. I will begin the talk with our work on antimony selenide, a promising quasi-1D material whose efficiency has rapidly increased in the past ten years. Thin films of antimony selenide are easily prepared by selenization of antimony metal deposited by electrodeposition or sputtering. The effect of a low temperature sulfurization treatment was investigated with time resolved microwave conductivity, photoluminescence, and low frequency Raman spectroscopy, and the effect on both bulk and surface recombination will be discussed. The water splitting photocathodes were characterized under operando conditions using electrochemical impedance spectroscopy. We will then explore the related compound antimony sulfide and its band alignment with atomic layer deposited titanium dioxide, which serves both as an electron extracting contact and corrosion protection layer. Finally, a simple solution processing method for phase pure cuprous sulfide will be presented, where the nanostructure can be controlled with the appropriate solvent mixture of the precursor solution.
2.2-I2
Joseph M. Luther obtained B.S. degrees in Electrical and Computer Engineering from North Carolina State University in 2001. At NCSU he began his research career under the direction of Salah Bedair, who was the first to fabricate a tandem junction solar cell. Luther worked on growth and characterization high-efficiency III-V materials including GaN and GaAsN. His interest in photovoltaics sent him to the National Renewable Energy Laboratory (NREL) to pursue graduate work. He obtained a Masters of Science in Electrical Engineering from the University of Colorado while researching effects of defects in bulk semiconductors in NREL�s Measurements and Characterization Division. In 2005, He joined Art Nozik�s group at NREL and studied semiconductor nanocrystals for multiple exciton generation for which he was awarded a Ph.D. in Physics from Colorado School of Mines. As a postdoctoral fellow, he studied fundamental synthesis and novel properties of nanomaterials under the direction Paul Alivisatos at the University of California and Lawrence Berkeley National Laboratory. In 2009, he rejoined NREL as a senior research scientist. His research interests lie in the growth, electronic coupling and optical properties of colloidal nanocrystals and quantum dots.
The interconversion of spin, charge and light are being exploited in perovskite materials leading to efficient, new, and unique device concepts. In this talk, we present the design rules which control and explain the mechanism behind chiral transfer from chiral molecules to the HOIS framework in nanostructured embodiments. Chirality can be imparted by chiral ligands attached during synthesis or in post-synthetic ligand exchanges where the chirality can be studied as a function of the nanocrystal size and surface to volume ratio. We show chirality induced properties from halide perovskite nanocrystals of different sizes and in films and then construct multiple types of prototype devices using heterostructure concepts that harness and display these fascinating properties. Some examples include heterostructured LEDs which emit spin-controlled circularly polarized light at room temperature without external magnets, detectors which couple perovskite nanocrystals and carbon nanotubes to detect and discern circularly polarized photons, neuromorphic devices which exhibit persistent photoconduction lasting thousands of seconds beyond a weak light pulse and can also display read/write capabilities.
2.2-I3
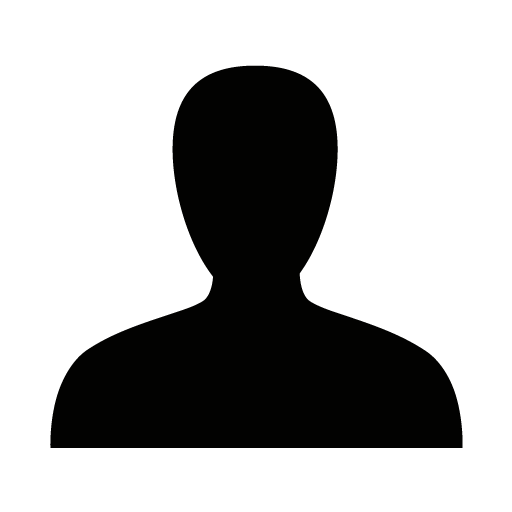
Halide perovskite nanocrystals (NCs) have emerged as an intriguing material for optoelectronic applications, most notably for light-emitting diodes (LEDs), lasers, and solar cells. Their emission wavelength depends not only on material composition but also on size and dimensionality, as in the case of two-dimensional (2D) nanoplatelets (NPLs). These colloidal quantum wells have additional appeal for light emission, as the one-dimensional quantum confinement enhances their radiative rates and enables directional outcoupling. On top of this, due to a monolayer-precise control over their thickness, they constitute an intriguing system for spectroscopic studies on their fundamental optical, phononic, and energetic properties.
In this talk, I will explore our recent results on halide perovskite NPLs, including their synthesis and stabilization. I will focus on their interesting excitonic properties, such as the energetic fine structure[1] with a strong thickness-dependent bright-dark exciton splitting and exciton diffusion in nanocrystal films.[2] Further, I will highlight their advantages and disadvantages for integration into optoelectronic applications.
2.2-I4
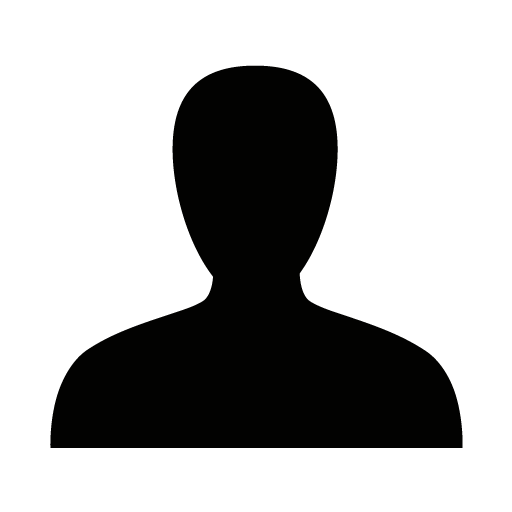
As halide perovskites and their derivatives are being developed for numerous optoelectronic applications, controlling their electronic doping remains a fundamental challenge. We have recently discovered a novel strategy of using redox-active organic molecules as stoichiometric electron acceptors in new expanded perovskite analogs. Compressing the metal-halide framework drives up the valence band relative to the acceptor orbitals of the organic molecules. Thus, the material’s electronic conductivity increases by a factor of 105 with pressure, reaching 50(17) S cm–1 at 60 GPa, exceeding the high-pressure conductivities of most halide perovskites. This conductivity enhancement is attributed to an increased hole density created by reduction of the redox-active molecules. This work elevates the role of organic cations in 3D metal-halides, from templating the structure to serving as charge reservoirs for tuning the carrier concentration.
Redox-active organic cations can act as charge reservoirs in the expanded perovskite analog (dmpz)[Sn2I6]. Material compression increases electronic conductivity by five orders of magnitude. This conductivity rise is attributed to an increased hole density in the valence band, caused by electron transfer to the redox-active molecules
1.1-I1
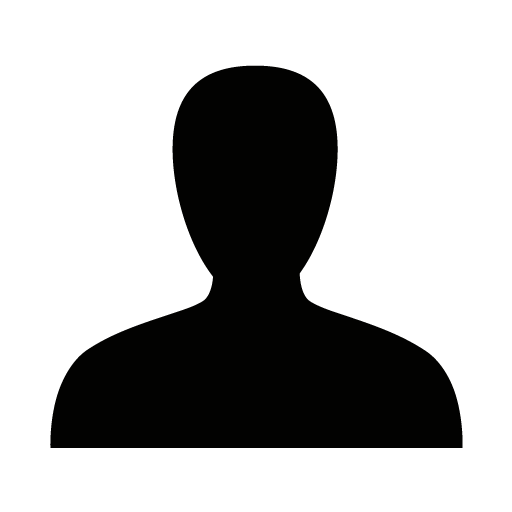
Carbon capture, conversion and utilization (CCU) is gaining attention from the worldwide community for its ability to minimize CO2 accumulation in the atmosphere. Electroreduction of CO2 (eR-CO2) in combination with renewable electricity sources can be one of routes to achieve the target. Economic viability of eR-CO2 relies on improved performance accompanied with scalable system design. Membranes are commonly used for the separation of reduction and oxidation products as well as to provide a suitable micro-environment for CO2R. Commercial membranes often address only one of the key challenges in CO2R: either they offer suitable micro-environment for CO2R (e.g., anion exchange membrane) or suppress carbonate cross-over (e.g., cation exchange membrane and bipolar membrane). Here, we present a cation-infused ultrathin (~3 µm) solid polymer electrolyte (CISPE) that concomitantly addresses both of these challenges via bidirectional ion transport mechanism and suppressed anolyte diffusion. The CISPE was directly deposited into the copper cathode catalyst, eliminating the requirement of a standalone membrane. Our demonstrated system offers high selectivity towards CO2 electrolysis (~90%) and a single-pass CO2 utilization of ~18% at 200 mA/cm2 with the primary product being ethylene (faradaic efficiency of 65%). Our directly-deposited CISPE enabled record low energy consumption of 294 GJ/ton C2H4 along with ~110 hours of stable operation with C2H4 as the primary product. The present work offers a versatile design paradigm for functional polymer electrolyte, opening doors towards stable, and efficient electrolysis for high-value feedstock chemicals and fuels production using low-cost catalysts.
1.1-I2
Dr. Christine Gabardo is the Co-Founder and Director of Technology for CERT Systems Inc. She received her B.Eng ('11) and PhD ('16) from McMaster University, then went on to complete an NSERC Postdoctoral Fellowship at the University of Toronto focused on developing efficient and scalable electrochemical CO2 reduction devices. In 2019, she co-founded CERT Systems Inc. and led the research team in the finals of the NRG COSIA Carbon XPRIZE competition. She has published over 30 scientific articles in the field of CO2 electrocatalysis and was recently recognized with a 2022 Clean50 Emerging Leader Award.
Rising atmospheric carbon dioxide (CO2) levels have motivated the development of alternative chemical production technologies to reduce emissions. The electrochemical CO2 reduction reaction (CO2RR) is one of several promising strategies that have been proposed for the utilization of CO2. Through CO2RR, CO2 can be converted into renewable fuels and valuable chemical feedstocks using clean electricity as an input. Of the possible CO2RR productions, multicarbon hydrocarbons and oxygenates, such as ethylene and ethanol, are promising commercial targets due to their large market sizes and high potential impact for emissions reduction.
CERT Systems Inc. is a carbontech start-up focused on the development of CO2RR to multicarbon product technology. Here we present an overview of CERT’s scale up journey through the NRG COSIA Carbon XPRIZE, a $20M global competition to develop and scale CO2 utilization technologies. We will summarize the scale up process from the laboratory to CERT’s pilot demonstration during the finals of the competition. The key learnings and challenges of scaling up CO2RR to multicarbon products will be discussed. Finally, we will identify next steps for the technology and outlook for electrochemical CO2 utilization.
1.1-I3
Electrochemical power-to-gas and power-to-liquid technologies are promising chemical energy conversion approaches to be coupled with intermittent renewable energy sources to balance the grid while utilizing cheap excess energy at peak times. Production of CO from CO2 reduction seems to be feasible even at pilot-scale, but the selective production of high-value multi-carbon products is challenging. CO reduction is a possible second step in the cascade electrochemical valorization of CO2. Gas-phase electrolyzers offer a suitable platform for this process, but the low solubility of CO still poses notable challenges. This can be tackled by tailoring the microenvironment of the catalyst particles.
Here we demonstrate that a commercially available polymeric pore sealer can be used as catalyst binder, ensuring a high rate and selective CO reduction. Under optimal conditions, we achieved above 70% Faradaic efficiency for C2+ products formation at j = 500 mA cm−2 current density. As no specific interaction between the polymer and the CO reactant was found, we attribute the stable and selective operation of the electrolyzer cell to the controlled wetting of the catalyst layer, due to the homogeneous polymer coating on the catalyst particles’ surface. These findings point out that polymers that form thin films on the catalyst surface (instead of forming nanoparticles that are incorporated among the catalyst particles, e.g., PTFE) might be suitable binders for CORR. In addition, sophistically designed surface modifiers are not necessarily required for CO electrolysis, hence the capital costs can be significantly decreased.
At the end of my talk I will discuss some general differences and similarities of electrochemical CO2 and CO conversion processes, mostly focusing on the role of the reaction environment, provided by the cell components and the cell architecture.
1.2-T1
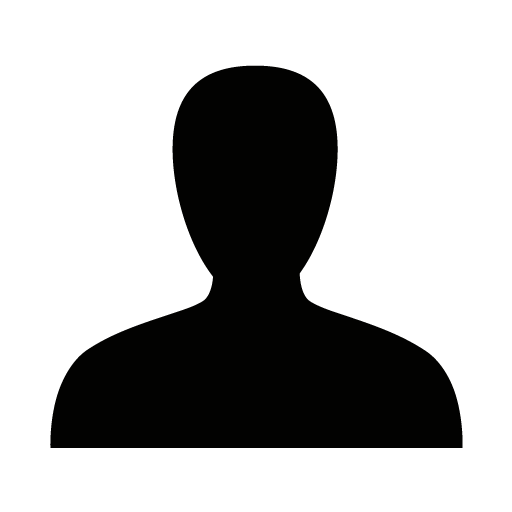
Energy- and cost-efficient CO2 capture is of crucial importance for its subsequent viable utilization. Ionic liquids (ILs) have emerged as a promising material for reducing carbon dioxide emissions from large-scale sources due to their properties and versatility. ILs are considered green solvents with high thermal stability, tunable structure, low volatility, and a strong affinity for CO2 molecules. These properties of ILs make them a potential alternative to amine-based CO2 capture processes. The challenges of using ILs come with their high viscosity, which causes unfavorable transport barriers. Encapsulated ionic liquid has been proposed to overcome mass transfer limitations, increasing the contact between gas and liquid, and improving the mass transfer rate. This study showed the development of new materials, combining efficiency, safety, and sustainable processes. Water-based nanocapsules of Emim[BF4], using poly(ionic liquid) Poly(diallyldimethylammonium tetrafluoroborate) as shell, were successfully obtained by Nanospray dryer B-90. Capsules were characterized and have the potential for CO2 capture evaluated. The combination of these promising materials showed great potential for CO2 capture and CO2/N2 separation (53.4 mg CO2/g; CO2/N2 selectivity: 4.58). Thermal stability and the regeneration of this material after successive sorption–desorption cycles were also successfully demonstrated, emphasizing this as a potential alternative.
1.2-T2
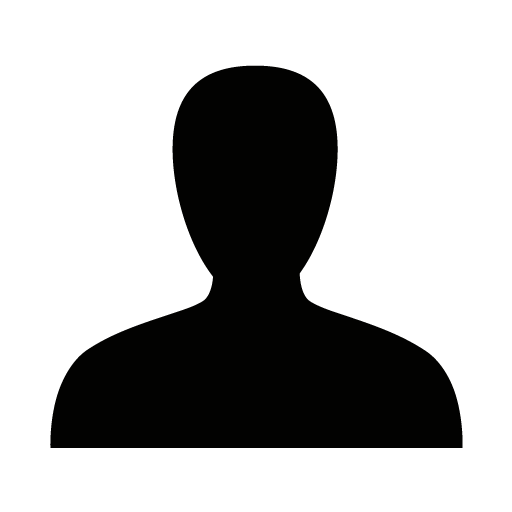
Progressive replacement of fossil fuels by sustainable and renewable energy sources is one of the most actual contemporary challenges. In this context, the solar-based electroconversion of water has become a valuable concept for production of an alternative fuel based on “green” hydrogen generation. Transition metal nanoparticles, such as nickel, are the benchmark catalysts for hydrogen evolution reaction, since they are highly active, stable and earth-abundant.
One of the emerging fields of solar energy harvesting is enhancing the catalytic reaction through the local surface plasmon resonance (LSPR) effect of the catalyst. Most studied mechanisms of the energy transfer from plasmonic nanoparticles are based on hot electron injection and plasmon-induced resonant energy transfer. However, the photothermal effect of the plasmon in transition metal nanoparticles is still often considered detrimental and is severely underestimated in the literature.
In this work, we have synthetized Ni nanoparticles with an elongated average shape by wetness impregnation of the hard silica template. The high distribution of sizes results in a broad plasmonic absorption in the visible range, which leads to a significant photothermal effect under solar illumination, reaching 93°C after 5 min under incident power of 0.5 W cm-2.
Irradiation of the Ni-NPs cathode with concentrated solar light under HER cathodic conditions leads to the increase of the hydrogen production up to 27%. On the other side, by applying galvanostatic conditions to the electrode we were able to decrease the overpotential needed for the hydrogen formation by 185 mV with negligibly small overall heating of the cell.
Pulsed illumination has resulted a three-staged electrochemical response to the illumination, reflecting i) breaking of the nickel/electrolyte equilibrium towards adsorption predominance, ii) blocking of the active sites and iii) establishing of the new balance between the electronic and ionic subsystems. The transient study revealed two main processes in the mechanism of light-induced reaction enhancement at low current densities: i) enhanced electron transfer due to strong plasmonic heating and ii) thermal energy dissipation from the electrode to the electrolyte.
Based on the obtained results, we have shown that the illumination of the nanostructured nickel catalyst with solar light can lead to the enhanced HER rate, and, as a result, to improved hydrogen production. We expect the LSPR photothermal approach to pave way to a more efficient photoelectrochemical water splitting process, taking advantage of visible and near-IR illumination.
1.2-T3
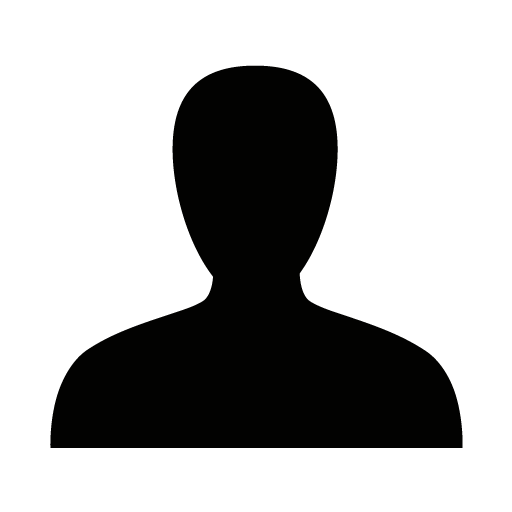
The direct conversion of greenhouse gases into green fuels and valuable chemicals is of paramount interest to achieve a decarbonized future [1]. The use of carbon dioxide and methane are of special interest as they are the main greenhouse gases emitted from anthropogenic sources, commonly considered industrial waste. In terms of fundamental research, the electrochemical carbon dioxide reduction to valuable chemicals has achieved great progress in the last few decades. Notwithstanding, few advances have been achieved in the electrochemical oxidation of methane [2].
Direct electrochemical methane activation strategies focus on tuning the electrode surface structure and composition [2]. The materials selected for the partial oxidation of methane need to activate methane and stabilize the intermediates involved to avoid the formation of carbon dioxide. Theoretical studies have shown that metal oxides are promising catalysts for the direct electrochemical activation of methane through the water discharge mechanism [3]. According to theoretical calculations, iridium oxide (IrOx) catalysts present a low overpotential for the oxygen evolution reaction (OER) and lower free energies of methane activation and *O formation compared to *OOH formation in the OER. Thus, these calculations suggest IrOx should be an interesting catalyst for the electrochemical conversion of methane into methanol. However, the experimental work published in this regard is very limited [4].
In this presentation, we show the results we acquired on the electrochemical methane activation using an IrOx surface as a catalyst in HClO4 electrolyte. For this purpose, we used an Ir polycrystalline oxidized electrochemically. The results aim to bring further understanding of the methane activation using metal oxides and discuss the purposed mechanisms reported in the literature.
1.2-T4
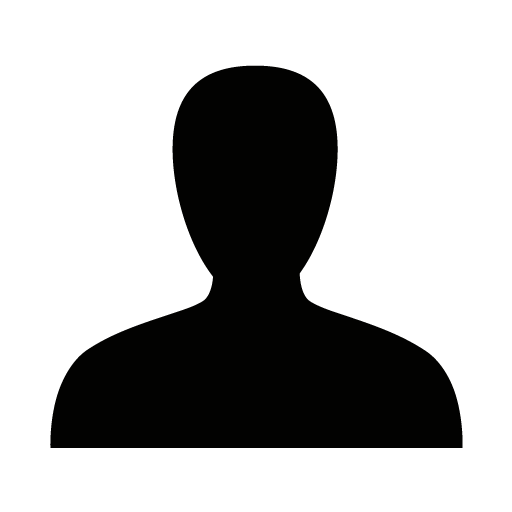
The chemical instability of hybrid halide perovskites in protic solvents remains the main obstacle for their application including solar fuels generation. In our work, we successfully stabilize 2D perovskite PEA2PbI4 in aqueous solutions of phenylethyl ammonium iodide (PEAI). XPS and XRD spectroscopy allow us to determine the 2D perovskite changes before and after the water immersion. Additionally, inductive coupled mass spectroscopy (ICP-MS) reveals the compositional changes of the electrolyte after 2D perovskite immersion. Photoelectrochemical characterization was used in a three electrode setup to measure photocatalytic activity of this material toward the hydrogen evolution reaction. Furthermore, IMPS/IMVS and impedance spectroscopy was implemented to characterize the photoelectrochemical process involved in this reaction. This result denotes the possibility to use layered perovskite absorbers for solar fuels generation in aqueous media, providing valuable information on the general understanding of the degradation of perovskites and opening an exciting window to novel applications of perovskite absorbers.
1.2-T5
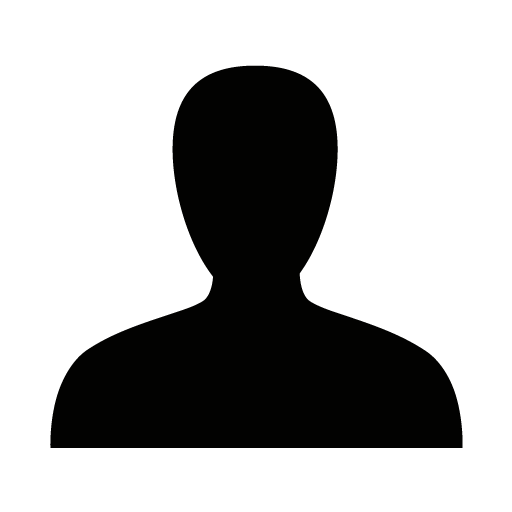
We report the use of a silver sputtered gas diffusion electrode (GDE) for electrochemical CO2 reduction in a membrane electrode assembly and probe the factors affecting catalyst utilization at high current densities. Catalyst utilization is significantly affected by the gas flow channel design at the cathode which distributes the reactant to the catalyst sites. The high pressure drop present in a serpentine flow channel enables a partial current density of 205 mA/cm2 for CO production at a cell voltage of 2.76V. Double layer capacitance measurements reveal significant differences in catalyst wetting between the flow patterns due to changes in pressure drop, salt precipitation at the cathode and reactant distribution at the surface of the GDE. Modelling of the gas flow channel and GDE reveals inhomogeneity in reactant distribution affecting catalyst utilization significantly. These findings can be used to formulate design rules for maximizing catalyst utilization in zero gap CO2 electrolyzers.
1.2-T6
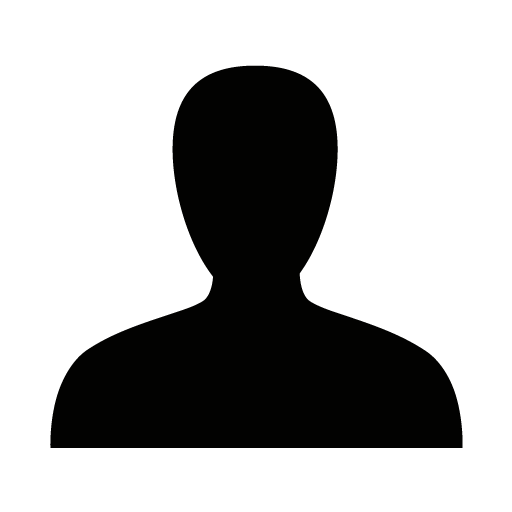
Carbon dioxide (CO2) capture and electrolysis is a promising route to produce valuable chemicals from CO2. Coupling the capture and electrolysis has the potential to intensify the process and reduce the overall energy cost. Taking amine scrubbing process as an example, the CO2 electrolysis can displace the energy-intensive amine recovery of the capture and produce valuable chemicals (e.g., carbon monoxide, CO) to offset the overall carbon abatement cost. Our recent process modelling results[1] found that the integrated route could save up to 42% overall energy if the integrated electrolysers perform similarly to the state-of-the-art gas-fed electrolyser.
However, the reported integrated electrolysers show inferior performance to the gas-fed electrolysers. Understanding the catalytically active species for CO2 conversion in the capture media is essential to rationally design an efficient integrated electrolysis system, but remains underexplored. This talk aims to uncover the dominant active species for the CO2 conversion to CO in a commonly studied ethanolmaine aqueous capture medium from our recent experimental findings. We will first discuss the potential CO2-related species available in the amines. The following part will show how the chemical species can change locally under CO2 electrochemical conditioning, and discuss their role in determining CO2-to-CO conversion efficiency. This talk will conclude with potential research directions that can further boost the performance of the integrated electrolysis.
2.1-I1
The electrochemical partial oxidation of methane to methanol is a promising approach to the transformation of stranded methane resources into a high-value, easy-to-transport fuel and chemical. The direct transformation of methane to methanol in remote locations requires selective catalysts capable of operating at near ambient temperatures inside a modular device. Over the last decade, transition metal oxides have been shown as potential electrocatalysts for this transformation. However, a comprehensive and systematic study of the dependence of methane activation rates and methanol selectivity on catalyst morphology and experimental operating parameters during testing has not been realized.
In this contribution, we describe an electrochemical method for the deposition of a family of thin-film transition metal (oxy)hydroxides as catalysts for the partial oxidation of methane. CoOx, NiOx, MnOx and CuOx are discovered to be active for the partial oxidation of methane to methanol in a carbonate electrolyte. TiOx and FeOx oxides show no selectivity for methanol at the oxidative potentials tested. Taking CoOx as a prototypical methane partial oxidation electrocatalyst, we systematically study the dependence of activity and methanol selectivity on catalyst film thickness, overpotential, temperature and electrochemical cell hydrodynamics in a gas-tight rotating cylinder electrode cell. Optimal conditions of low catalyst film thickness, intermediate overpotentials and intermediate temperatures are identified to favor methanol accumulation in the electrochemical cell. The rate of transport of methane and methanol in and out of the electrocatalyst surface is also shown to be an important design parameter for future methane to methanol electrochemical cells. Through a combination of control experiments and DFT calculations, we show that the oxidized form of the as-deposited (oxy)hydroxide catalyst films are active for the thermal oxidation of methane to methanol even without the application of a bias potential, demonstrating that high valence transition metal oxides are intrinsically active for the activation and oxidation of methane to methanol at ambient temperatures.
2.1-I2
I am currently an Full Professor at the University of Bonn (Germany), Department of Chemistry. My overarching motivation is to discover and implement the chemistry necessary to transition to a sustainable energy-based society. Specifically, I am developing materials to convert electrical energy to fuels and chemicals.
The conversion of biomass platforms to value-added chemicals represents an exciting opportunity to extract value from the anodic half reaction in water/CO2 electrolysis while also holding the potential to lower the cell voltage required to carry out the full reaction. Despite this promise, catalysts still do not exist that can operate at sufficiently low overpotentials with high rates and selectivity and a comprehensive understanding of many of these reactions is still lacking. Against this backdrop, my lab’s work in recent years has focused on the use of operando spectroscopy (carried out as the reaction progresses) to probe reactions such as the oxidation of hydroxymethylfurfural (HMF) to furandicarboxylic acid (FDCA). Further, we translate the insights gained into the rational design of next-generation catalysts with enhanced performance, thus creating an iterative feedback cycle. This talk will focus on research that spans from our first efforts on simple gold nanoparticles all the way to recent complex materials such as metal-organic frameworks (MOFs) that operate in unique ways to electrochemically convert biomass platforms to value-added products.
2.1-I3
One promising technology to decarbonise our economy is to use carbon dioxide (CO2) from a point source or air as a starting feedstock to produce chemicals, while leveraging the rapidly-growing renewable energy as a driving force. Such an approach relies traditionally relies on separate capture and conversion processes. Both processes are energy costly and face their own technical challenges. For example, amine-based capture media requires a typical cost of US$50-150 to capture one tonne of CO2. The recovery of capture media (e.g., amines) and CO2 cost up to 90% of the overall energy for the capture process. Meanwhile, CO2 conversion by electrochemical processes takes substantial energy for the conversion, separation and electrolyte recovery steps.
As a means to reduce energy input and reduce implementation costs, coupling the capture and electrolysis conversions steps into an integrated process has been proposed. Here the conversion process uses the CO2-saturated capture media, instead of free gaseous CO2. The new integrated CO2 electrolysis process can then theoretically displace the energy-intensive amine regeneration of the capture and produce valuable chemicals (e.g., carbon monoxide) to offset the overall carbon abatement cost. However, at present the integrated electrolysers shows inferior performance to the gas-fed electrolysers. Hence, an important question needs to be answered: will the integrated capture and conversion route fulfill its promise to be more energy-efficient than the sequential route?
This talk attempts to answer this question by discussing our recent process energy system modelling results.[1] Firstly, our results find that the electrochemical CO2 conversion is the dominant energy contributor to the overall capture and conversion, and its energy cost must be minimized. If the integrated electrolysis process is of the same energy cost to the state-of-the-art gas-fed electrolyser, the integrated route could then save up to 42% energy as compared to sequential capture and conversion steps. However, this energy advantage quickly diminishes if future gas-fed electrolyser remains more energy efficient, or if bicarbonate formation in the gas-fed system is avoided. This talk will conclude with challenges and opportunities for the development of integrated capture and electrolysis.
2.2-I1
Dr. Teresa Andreu is lecturer professor at the University of Barcelona since 2020. She received the degree in Chemistry (1999) and PhD in Materials Science (2004) from the University of Barcelona. After a period in industry and academia, she joined IREC in 2009 as senior researcher and the Institute of Nanoscience and Nanotechnology at UB in 2020. Her research is focused on the development of materials and reactors for emerging technologies for hydrogen generation and carbon dioxide conversion (photoelectrochemistry, heterogeneous catalysis and plasma-catalysis). She is the author of more than 130 scientific publications and 4 patents.
Glycerol is a residue generated in biodiesel production that has a low economic value due to its overproduction. Recently, different oxidation processes have been developed for glycerol oxidization towards added value products such as dihydroxyacetone or formic acid, one of them being the photoelectrochemical route that improves the process efficiency using solar light. In this work we have synthetized and studied BiVO4 and TiO2 semiconductor electrodes with the objective of evaluating their activity as photocatalysts using different experimental conditions (electrolyte and pH). The studied support electrolytes are sodium sulphate and sodium phosphate at pH 2 and 7. The results show that in an acidic Na2SO4 0,5 M medium the BiVO4 electrode presents its maximum photocurrent and in a neutral Na2SO4 0,5 M medium TiO2 electrode reaches its best performance. It has been assessed that faradaic efficiencies towards glycerol oxidation follows an opposite trend to water oxidation, the latter being more favoured using TiO2 than BiVO4 electrodes. The oxidation product analysis by HPLC and H-NMR shows that BiVO4 presents better faradaic efficiency for glycerol oxidation than TiO2 but with a lower selectivity towards a given product. Furthermore, in all cases, it has been proved the beneficial role of illumination with respect to dark electrolysis. It has been observed that the use of phosphate ions as supporting electrolyte is harmful for the glycerol oxidation due to the peroxide generation, besides the instability of BiVO4 in acidic phosphate electrolyte. On the contrary, using sulphate anions as the supporting electrolyte it has been achieved faradaic efficiencies over 80 % with a constant selectivity at different reaction times.
2.2-I2
Electrochemical CO2 reduction reaction (CO2RR) holds promise for the conversion of green electricity (e.g., solar electricity) into fuels and CO2 mitigation, to address the global warming issue. Biomass, on the other hand, is an abundant renewable energy resource with a potential to provide sustainable source of fuel. Nevertheless, CO2RR suffers from high applied potential mainly due to the inefficient oxygen evolution reaction (OER) in the counter electrode, as well as low selectivity—impeding its commercialization; and the biomass upgrading process emits significant amount of greenhouse gases—hindering its employment. Here in this work, we first design and synthesize active and selective electrocatalysts for the CO2 reduction and biomass oxidation. Combining these two reactions within one unified electrochemical system is proposed in literature to further decrease the total applied potential and improve the energy efficiency, however often the attained current density in biomass oxidation reactions (i.e., few mA/cm2) is not comparable to the commonly used OER and subsequently not suitable for the required current densities in CO2RR systems (i.e., > 100 mA/cm2). Thus, in this work, we demonstrate a feasible approach to attain an energy efficient combined electrochemical system that works at current density > 100 mA/cm2. In this talk, I will report some of our recent achievements in catalyst design for both reactions, their performance, energy efficiency improvements, and molecular understanding of the reaction mechanism. The talk will be mostly focused on CO2RR to formate and methane, and hydroxymethylfurfural (HMF) oxidation to furandicarboxylic acid (FDCA).
2.2-I3
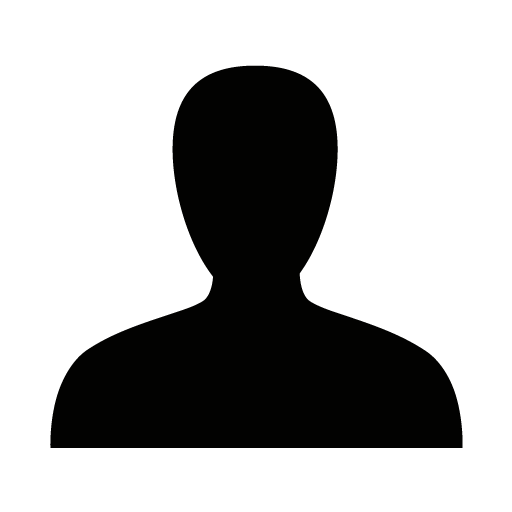
Once solar fuels are accessible economically and widely, we need a device to convert them into useful energy. In this aspect, fuel cells (FCs) are more appealing than conventional internal combustion engine in terms of energy efficiency because of no entropy energy waste. However, mass deployment of FCs demands better electrode-electrolyte interfaces to catalyze oxygen reduction reaction (ORR, especially in acidic environments), the kinetics of which depends on the energetics of surface adsorption as well as on the electrolyte environment. While the former has been well established using experimental and theoretical work, the effect of the electrolyte remains relatively unexplored. In this talk, I will first show an unanticipated effect of nonspecifically adsorbing (NSA) anions on the ORR kinetics on a Pt(111) electrode in acid. The electrolyte-related trends do not follow the usual ORR descriptor, i.e. *OH binding energy. Instead, a new voltammetry-accessible descriptor, namely the reversibility of the *O↔*OH transition on the (111) terrace was proposed to successfully track the dependence of ORR rates on electrolyte properties, including the concentration/identity of NSA anions in acidic media, cations in alkaline media, and the effect of the presence of ionomers, as observed in this study and in the literature. An enhanced model that relates the ORR rate on Pt(111) to the rate of the *O to *OH transition, in addition to the traditional “thermodynamic” *OH binding energy descriptor, was established, suggesting that there is more than one descriptor for the overall ORR rate. Our updated picture of the ORR can help in rationalizing the different trends for the ORR rate on stepped Pt surfaces in acidic vs. alkaline media. This study paves an avenue towards a more complete understanding of the various factors that determine the ORR rate on Pt surfaces.
1.1-I1
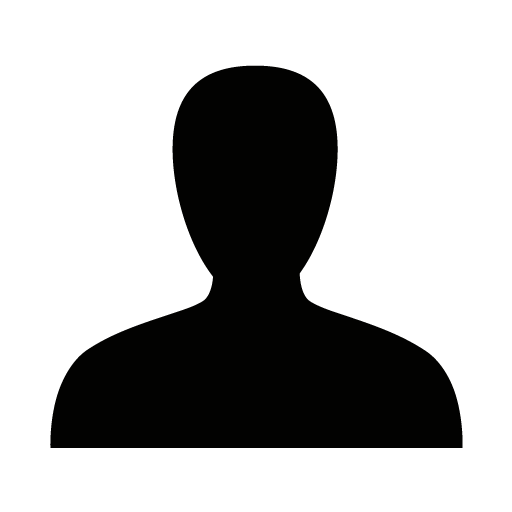
The operational stability of organic solar cells is influenced by several factors including microstructure stability, the electrochemical stability of electrodes, and the photochemical degradation of the materials. Whilst some materials appear to perform better than others, there is as yet insufficient information on the material chemistry or device structure and degradation pathways to design better materials. We investigate the specific chemical structure – degradation relationship for a series of chemically similar materials. For the study we select isolated isomers of the bis-adduct of PCBM, given that the small differences in chemical structure between the different isomers are known exactly and the common degradation pathways for solar cells containing fullerenes are also known. We investigate degradation in the presence and absence of air and seek correlation between the chemical structure (i.e. side chain positions) and different mechanisms involving oxygen radicals and fullerene interactions. We consider more general criteria for improving the photochemical and microstructural stability.
1.1-I2
Eva Herzig’s research interest focuses on the possibilities and limitations in the characterization of nanostructures in functional materials as well as how such nanostructures form and change as functions of external parameters. The examined materials range from organic molecules to nanostructured hybrid and inorganic systems. We examine processing-property relationships and the influence of external fields to investigate how the fundamental self-assembly processes influence the final material performance. To this end we exploit various scattering techniques to observe and control structure and function relationships in the examined materials in-situ. Using grazing incidence x-ray scattering we are particularly sensitive to nanostructures on flat surfaces and within thin films.
The stability of perovskite solar cells is still an unresolved issue and must be addressed for making perovskites a valid option for industrial products, e.g. in the form of enhancing the performance of silicon solar cells in tandem cells. As an initial step for the systematic investigation of structural changes, it is therefore beneficial to enable us to track structural changes within hybrid perovskite thin films. Furthermore, we would like to have the ability to determine the dynamics of such changes as well as the responsible driving forces.
We are using in-situ time-resolved x-ray scattering to resolve structural changes that active layers of hybrid perovskite solar cells undergo during processing [1,2] and conditions necessary for operation. Systematically examining the resulting structural changes while deliberately accelerating the ageing process [3] can help on the route to discover options that help to suppress these structural changes in the end.
1.1-I3
Organic solar cells (OSCs) are one of the most promising cost-effective options for utilizing solar energy in high energy-per-weight or semi-transparent applications. Recently, the OSC field has been revolutionized through synthesis and processing advances, primarily through the development of numerous novel non-fullerene small molecular acceptors (NFA) with efficiencies now reaching >19% when paired with suitable donor polymers. The device stability and mechanical durability of these non-fullerene OSCs have received less attention and developing devices with high performance, long-term morphological stability, and mechanical robustness remains challenging, particularly if the material choice is restricted by roll-to-roll and benign solvent processing requirements and desirable ductility requirements. Yet, morphological and mechanical stability is a prerequisite for OSC commercialization. Here, we discuss our current understanding of the phase behavior of OSC donor:acceptor mixtures and the relation of phase behavior and the underlying hetero- and homo-molecule interactions to performance, processing needs (e.g., kinetic quenches), and morphological and mechanical stability. Characterization methods range from SIMS and DSC measurements to delineate phase diagrams and miscibility to x-ray scattering to determine critical morphology parameters and molecule packing and dynamic mechanical analysis (DMA) to assess specifically the hetero-interactions. The results presented and its ongoing evolution are intended to uncover fundamental molecular structure-function relationships that would allow predictive guidance on how desired properties can be targeted by specific chemical design. Comparative studies show that the molecular hetero-interactions between the donor and NFA are not always the geometric mean of the homo-interactions. This underscores the limited success often encountered when Hanson Solubility Parameters and surface energies are used to estimate molecular interactions.
1.2-T1
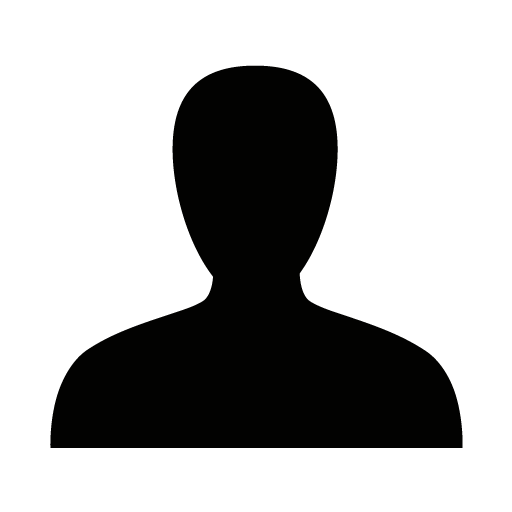
Humankind is living in a climate emergency that requires immediate action. However, in the meantime, the world’s energy demand is continuously increasing; thus, making clean energy generation of paramount importance. Photovoltaics is at the forefront of this clean energy movement with organic photovoltaics emerging as a promising candidate to address this issue and meet this demand. Having said that, organic semiconductors came a long way from fullerene-based solar cells to state-of-the-art non-fullerene acceptor-based (NFAs) cells that show promise as next-generation photovoltaic devices. Organic solar cells employing such materials have already surpassed 20% in power conversion efficiencies (PCEs) after less than a decade of research, excluding initial attempts to outclass their fullerene counterparts in the 90s.[1] Much is done to realize high PCEs by the research community; nonetheless, the urgency to unravel the underlying mechanisms crippling the stability of the photovoltaic devices based on such material systems picked up only in recent years.
Even so, a thorough understanding or a guideline is still lacking to tackle this limitation once and for all. In this work, we implement a novel strategy, which we refer to as donor dilution, to improve the operational and outdoor stability of NFA-based systems in an archetypal donor:acceptor blend, namely, PTB7-Th:IEICO-4F.[2,3] Organic solar cells exploiting this material system with decreasing polymer content in the polymer:small molecule blend progressively perform superior both in operational and outdoor stability tests. Unencapsulated donor-diluted devices retain 85% of their initial PCE under continuous 1 sun equivalent metal halide lamp illumination at 40⁰C after 1000 hours. Furthermore, the in-house developed encapsulation procedure enables these devices to endure the harsh outdoor conditions (~23⁰C and ~64% relative humidity on average throughout winter) for an extended period in the Kingdom of Saudi Arabia.
These findings underpin the universal effort to improve the organic photovoltaic device stability, provide a practical strategy as a proof-of-concept to enhance the lifetime of organic photovoltaic devices, and bring this technology a step closer to commercialization. Future work consists of further examination of the universality of the donor dilution approach and is underway by testing various donor:acceptor blends with different classes of non-fullerene acceptors, such as state-of-the-art Y-series molecules.
1.2-T2
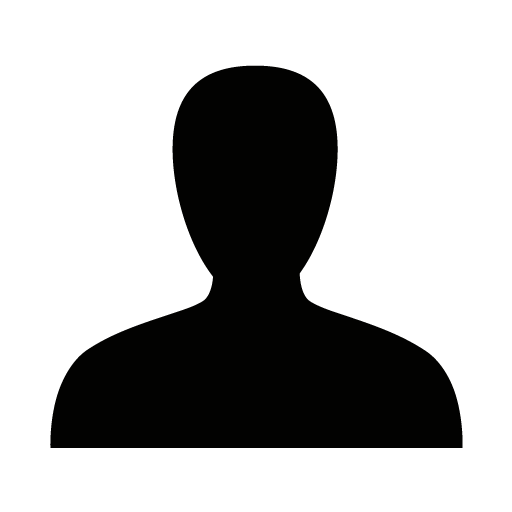
With the advent of recent state-of-the-art blend materials, organic solar cells have become competitive to established technologies by achieving power conversion efficiencies of more than 18~\%. On the path to commercialization, device stability is the next challenge needing to be overcome. We researched the degradation of inverted devices of the high-performance material system PM6:Y6 and found two distinct degradation pathways. While the first one is marked by a reduction of short-circuit current and requires presence of both illumination and oxygen, we focused our research on the second one, which is induced thermally and shows losses of open-circuit voltage and fill factor. We find that, while bulk and interface properties remain overall stable, defect state formation is the primary cause for thermal degradation. The increased trap density reduces charge carrier mobility and leads to increased non-radiative recombination limiting the open-circuit voltage. In addition, we find an aging-induced transport resistance to be the cause for the reduced fill factor. Our findings show that device stability could be increased by suppressing trap formation.
1.2-T3
Recently, organic photovoltaics (OPV) achieved efficiencies close to 20 % under AM1.5G. [1] In parallel OPV for harvesting artificial indoor light approaching efficiencies close to 30 % under 1000 lux warm white LED light. [2] In both cases the development of new donor and acceptor molecules as well as new interlayer materials was in the focus of interest, while the stability of OPV devices has drawn less attention. However, together with high performance the long-term stability of solar cells and modules is one of the key factors for a successful market entry. For indoor OPV the requirements are typically less harsh: much lower irradiation (UV light free), moderate and relatively constant temperature and moisture. Despite, the intrinsic stability of the materials, the stability of the full cell stack and the packaging have to be investigated.
For rigid devices a glass/glass encapsulation is probably the best choice, for flexible photovoltaics however, other solutions have to be found. Challenging are high barrier properties against moisture and oxygen while maintaining high transmission rates, UV resistance and mechanical flexibility (bendability without delamination).
We present stability data for different organic absorber materials in indium tin oxide (ITO)-based and ITO-free cell architectures on rigid and flexible substrates. [3] Our main focus is the long-term stability of devices for indoor usage, thus we performed accelerated (light) aging experiments under typical and elevated indoor light conditions.
For PV-X plus based ITO-free rigid cells with an efficiency of 17% under 500 lux, we could achieve remarkable stability with no losses observedduring more than 3000 h at 50.000 lux. This photon dose corresponds to almost 50 years at 500 lux which is much more than required for typical internet-of-things purposes. For flexible ITO-free devices promising preliminary data is obtained.
While elevated temperatures are typically not present in indoor PV, however for lamination processes temperatures of about 120 °C for shorter periods are required. We performed detailed analysis of the processes involved during thermal aging.
1.2-T4
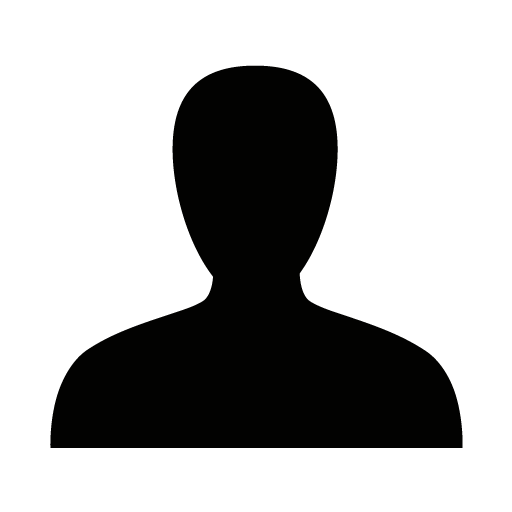
Organic solar cells (OSCs) have attracted great attention owing to their unique advantages such as lightweight, flexibility and solution processability for further commercial applications[1]. In recent years, the efficiency of OSC has grown rapidly with the help of burgeoning Y-series electron acceptors[2]. Benefits from structural modification, well-adjusted energy levels, absorption property, crystallinity and mobility have all attributed to the efficiency enhancement[2]. However, as another critical factor for commercialization, stability, especially photostability change generated from such structural modification remains elusive. To unveil the relationship, four Y-series acceptors with different terminal groups, internal and outer alkyl chains were selected to compare the photo-induced degradation from both device and structural perspectives. Significant increased trap-assisted recombination was observed after continuous illumination, which could be related to the structure change of the acceptors exhibited in NMR and Raman spectra. The energy barriers of happening the light-triggered reactions on acceptors are further evaluated through density functional theory (DFT) calculation. As a result, acceptors with fluorinated terminal groups and long internal alkyl chains will enhance the photostability of devices. This trend is further shown on additional two Y-series acceptors based devices. Finally, encapsulated devices with Y-series acceptors are tested under real-world conditions, showing a similar conclusion as photostability results. Our results provide an in-depth understanding of the light-soaking and outdoor stability of Y-series acceptors, and important guidelines for the designation acceptor materials to achieve stable OSCs.
1.2-T5
With the increasing efficiency of organic solar cells, it is now imperative to focus investigation on the improvement of device stability and the understanding of the degradation mechanisms. It is known that the interface between the active layer and the charge transport materials, such as metal-oxides, can strongly influence the device stability, and many passivation strategies have been reported recently [1]. Herein, we have identified a major source of instability when using nanoparticles of SnO2, which is determined by the presence of ionic species at the nanoparticle’s surface. The induced degradation mechanism is unveiled by means of photophysical and electrical measurements. Furthermore, we discover that with a very simple washing of the metal oxide nanoparticle layer we are able to reduce the device degradation. After the washing procedure, several non-fullerene acceptor based solar cells show a boost of the T80 device lifetime under illumination up to hundreds of hours.
1.2-T6
Juan Bisquert (pHD Universitat de València, 1991) is a Professor of applied physics at Universitat Jaume I de Castelló, Spain. He is the director of the Institute of Advanced Materials at UJI. He authored 360 peer reviewed papers, and a series of books including . Physics of Solar Cells: Perovskites, Organics, and Photovoltaics Fundamentals (CRC Press). His h-index 95, and is currently a Senior Editor of the Journal of Physical Chemistry Letters. He conducts experimental and theoretical research on materials and devices for production and storage of clean energies. His main topics of interest are materials and processes in perovskite solar cells and solar fuel production. He has developed the application of measurement techniques and physical modeling of nanostructured energy devices, that relate the device operation with the elementary steps that take place at the nanoscale dimension: charge transfer, carrier transport, chemical reaction, etc., especially in the field of impedance spectroscopy, as well as general device models. He has been distinguished in the 2014-2019 list of ISI Highly Cited Researchers.
The dynamic response of metal halide perovskite devices shows a variety of physical responses that need to be understood and classified for enhancing the performance and stability and for identifying physical behaviours that may lead to developing new applications. Beyond the well-established characteristics of regular impedance arcs, we address the appearance of inductor effect at high voltage in perovskite solar cell. We present a physical model in terms of delayed recombination current that explains the evolution of impedance spectra and the evolution of current-voltage curves. A multitude of chemical, biological, and material systems present an inductive behavior that is not electromagnetic in origin. Here, it is termed a chemical inductor. We show that the structure of the chemical inductor consists of a two-dimensional system that couples a fast conduction mode and a slowing down element. Therefore, it is generally defined in dynamical terms rather than by a specific physicochemical mechanism. The impedance spectra announce the type of hysteresis, either regular for capacitive response or inverted hysteresis for inductive response. We apply the dynamic picture based on a few neuron-like equations to the characterization of halide perovskite memristors. It has a resistance that depends on the history of the system, and the states can be switched by applied voltage. Memristors show an intense hysteresis that can be characterized in terms of the emergence of inductive components.1-5
(1) Bisquert, J.; Guerrero, A. Dynamic Instability and Time Domain Response of a Model Halide Perovskite Memristor for Artificial Neurons, Jounal of Physical Chemistry Letters 2022, 13, 3789-3795.
(2) Bisquert, J.; Guerrero, A. Chemical Inductor, J. Am. Chem. Soc. 2022, 10.1021/jacs.1022c00777.
(3) Berruet, M.; Pérez-Martínez, J. C.; Romero, B.; Gonzales, C.; Al-Mayouf, A. M.; Guerrero, A.; Bisquert, J. Physical model for the current-voltage hysteresis and impedance of halide perovskite memristors, ACS Energy Lett. 2022, 7, 1214–1222.
(4) Bisquert, J.; Guerrero, A.; Gonzales, C. Theory of Hysteresis in Halide Perovskites by Integration of the Equivalent Circuit, ACS Phys. Chem Au 2021, 1, 25-44.
(5) Guerrero, A.; Bisquert, J.; Garcia-Belmonte, G. Impedance spectroscopy of metal halide perovskite solar cells from the perspective of equivalent circuits, Chemical Reviews 2021, 121, 14430–14484.
1.2-T7
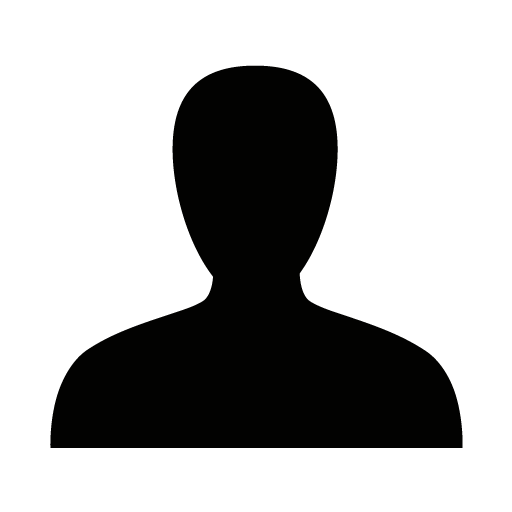
The use of layered, 2D perovskites can greatly improve the stability of metal halide perovskite thin films and devices. However, the charge carrier transport properties in layered perovskites are still not fully understood. We investigated the sum of the electron and hole mobilities (Σμ) in thin films of the 2D perovskite PEA2PbI4, through transient electronically contacted nanosecond-to-millisecond photoconductivity measurements, which are sensitive to long-time, long-range (micrometer length scale) transport processes. After careful analysis, accounting for both early-time recombination and the evolution of the exciton-to-free-carrier population, a long-range mobility of 8.0 +/− 0.6 cm2 (V s)–1, which is ten times greater than the long-range mobility of a comparable 3D material FA0.9Cs0.1PbI3 is determined. These values are compared to ultra-fast transient time-resolved THz photoconductivity measurements, which are sensitive to early-time, shorter-range (tens of nm length scale) mobilities. Mobilities of 8 and 45 cm2 (V s)–1 in the case of the PEA2PbI4 and FA0.9Cs0.1PbI3, respectively, are obtained. This previously unreported concurrence between the long-range and short-range mobility in a 2D material indicates that the polycrystalline thin films already have single-crystal-like qualities. Hence, their fundamental charge carrier transport properties should aid device performance, while keeping the benefits for stability as well.
Adapted from [1]
1.3-I1
Abstract: With the emergence of high-performance nonfullerene electron acceptors (NFA), organic solar cells (OSCs) are particularly appealing as their power conversion efficiencies (PCEs) approaching 20%. Identifying and eliminating the sources of degradation in photovoltaic devices is as crucial as improving the PCEs from the manufacturing and commercialization point of view. In this talk, I will show the strategies we developed to improve the thermal stability of NFA OSCs. Further, I will share the photo degradation behavior of the state-of-the-art NFA OSCs in both indoor and outdoor conditions with multimodal characterizations and in-operando transient electro-optical spectroscopy. Their outdoor operational stability will be assessed under real-world conditions (in a hot and sunny climate in Saudi Arabia). Finally, I present a comparison of indoor and outdoor performance of Y‑series OSCs to demonstrate the importance of materials stability and device longevity for balancing the efficiency, stability, and cost potential of organic photovoltaics in real-world outdoor applications.
1.3-I2
Christoph J. Brabec is holding the chair “materials for electronics and energy technology (i-MEET)” at the materials science of the Friedrich Alexander University Erlangen-Nürnberg. Further, he is the scientific director of the Erlangen division of the Bavarian research institute for renewable energy (ZAE Bayern, Erlangen).
He received his PhD (1995) in physical chemistry from Linz university, joined the group of Prof Alan Heeger at UCSB for a sabbatical, and continued to work on all aspects of organic semiconductor spectroscopy as assistant professor at Linz university with Prof. Serdar Sariciftci. He joined the SIEMENS research labs as project leader for organic semiconductor devices in 2001 and joined Konarka in 2004, where he was holding the position of the CTO before joining university.
He is author and co-author of more than 150 papers and 200 patents and patent applications, and finished his habilitation in physical chemistry in 2003.
OPV cells have a proven efficiency of over 18 % while OPV modules have a proven record efficiency of 13.5 %. Both values are still increasing towards > 20 % for small area cells and > 15 % for large scale modules. With these performance values, OPV is reaching out to applications that are going beyond the typical niche markets. The first generation of commercially available OPV modules shows lifetimes in the order of 5 years and more under outdoor conditions. Independent of the application, the lifetime limitations of organic solar cells are not fully understood. Few publications reported operational lifetimes of over 25000 hrs. Organic solar cell materials being stable against photooxidation were as well reported, and, most recently, we demonstrated solar cells that canbe operated in water and under 1 sun for hundreds of hours – unpackaged. However, all these “best you can do” lifetime values are reported for different combinations of materials and interface systems.
This talk will discuss a degradation mechanism that has been overlooked so far but probably is decisive to understand for longlived operation. The community is well aware of the impact of the light source on the degradation mechanism. Blocking the UV part of the solar spectrum till 380 nm became an unwritten law for outdoor as well as indoor testing. However, one has to critically ask whether a 380 nm cutoff is indeed sufficient to stabilize a solar cell. We have investigated degradation as a function of the spectral distribution, and found degradation mechanisms that have not been reported yet. Most specifically, the combination of the interface material, the semiconductor and the spectrum of the light source dominate degradation kinetics. The spectral excitation spectrum for degradation does not coincide with the EQE of the solar cell, indicating interface induced mechanisms. All these findings indicate that OPV has to overcome another hurdle before further improving the operational stability.
2.1-I1
The modest stability of perovskite solar cells (PSCs) in ambient conditions represents a major impediment to their widespread commercialization. The interface between the hole-transport material (HTM) and perovskite is known to significantly affect the stability of PSCs.[1] Recently, the idea of combining the HTM function with the passivation of the perovskite surface via ad-hoc chemical interaction between the HTM and perovskite has been successfully exploited.[2] As a result, these HTMs anchored to the perovskite surface led to compact and ordered interfaces and to PSCs with a remarkable increase in both the efficiency and stability. In this talk, I will summarize key recent examples reported by us of low-cost HTM designs that can effectively interact with the surface of a triple cation (CsFAMA) perovskite promoting the interfacial charge transfer dynamics and the stability of PSCs. These examples include halogen-bonded[3], sulfonated[4], hydrogen-bonded, and fluorene-based HTM molecular designs.
Furthermore, I will highlight the doping engineering of traditional HTMs (e.g., Spiro-OMeTAD) as an additional valid strategy when aiming at competitive long-term stability. When the widely used hygroscopic dopants (e.g., LiFTSI) of Spiro-OMeTAD are replaced with a single molecular dopant, i.e., F4-TCNQ, a remarkable stability of mesoporous PSCs in air is achieved[5]. We demonstrate that the high uniformity of F4-TCNQ doping in Spiro-OMeTAD and reduced dopant aggregation and dopant migration towards the anode are the main reasons for the increased stability of PSCs in air, beyond the well-known hydrophobic protection of the perovskite induced by the F4-TCNQ dopant.
2.1-I2
Leite is an Associate Professor in Materials Science and Engineering at UC Davis. Her group investigates materials for energy harvesting and storage, from their nano-scale structural, electrical, and optical properties to their implementation in devices. Before joining UC Davis, Leite was an associate professor at the University of Maryland, she worked for two years at NIST and was a post-doctoral scholar at Caltech (Department of Applied Physics and Materials Science). She received her PhD in physics from Campinas State University in Brazil and the Synchrotron Light Source Laboratory. Leite's work has been recognized on the cover of ~30 scientific journals, by the presentation of >140 invited talks, by the 2016 APS Ovshinsky Sustainable Energy Fellowship from the American Physical Society (APS) and the 2014 Maryland Academy of Sciences Outstanding Young Scientist Award. Leite’s research has been funded by the National Science Foundation (NSF), the Army Research Office (ARO), the Defense Advanced Research Projects Agency (DARPA), etc.
Quantifying the role of extrinsic (humidity and oxygen) and intrinsic (light, bias, and temperature) environmental stressors on the degradation of halide perovskites is key for the further development of stable optoelectronic devices. In this talk, I will present a suite of optical and electrical complementary tools that provide a detailed description of the dynamic responses within these materials, from the macro to the nanoscale. At the macroscopic scale, we unravel the influence of relative humidity on charge carrier radiative recombination in CsxFA1−xPb(IyBr1−y)3 perovskites through in situ PL, where we temporally and spectrally measure light emission within loops of critical relative humidity (rH) levels. Then, we apply machine learning algorithms to forecast the material optical response once exposed to distinct temperature and humidity levels. At the nanoscale, we realize state-of-the-art scanning probe microscopy methods to image and quantify ion motion within and between grains, as a function of illumination and relative humidity. Combined, the macro- and nanoscale environmental measurements performed provide an ample framework for tracking, in real-time, the relevant changes that can lead to degradation. Additionally, they exemplify trustworthy diagnosis tools that can be expanded to any perovskite chemical composition.
2.1-I3
Record efficiencies of perovskite solar cells (PSCs) are obtained with spiro-OMeTAD as the hole transport layer. Spiro-OMeTAD is conventionally doped by hygroscopic lithium salts with the assistance of volatile 4-tert-butylpyridine, which, however, brings a time-consuming doping process as well as poor device stability. We successfully develop an instantly efficient and clean doping strategy to replace the conventional spiro-OMeTAD doping. As demonstrated by experimental and theoretical investigations, the radical dopant leads to significant increase of the conductivity through efficient hole polarons generation. The ionic salts can further modulate the work function with negligible effects on the film conductivity, critical for reaching optimal VOC values by a favorable energetic level alignment. Spiro-OMeTAD based on our new doping strategy enables PSCs with a high power conversion efficiency over 25% and an excellent stability against moisture, heat and illumination. Our findings pave the way for achieving PSCs with high efficiencies and excellent stability at the same time. In addition, our doping strategy goes beyond traditional organic semiconductor doping, providing new understanding of organic doping mechanisms which can inspire further optimizations of different optoelectronic devices.
2.2-I1
Halide perovskites quickly overrun research activities in new materials for cost-effective and high-efficiency photovoltaic technologies. Since the first demonstration from Kojima and co-workers in 2009, several perovskite-based solar cells have been reported and certified with rapidly improving power conversion efficiency. Recent reports demonstrated that perovskites could compete with the most efficient photovoltaic materials. At the same time, they still allow processing from solutions as a potential advantage to delivering a cost-effective solar technology.
The most stable and efficient perovskite contains lead, among the most toxic elements on earth. The lead from perovskite is more dangerous than other lead contaminants since it is absorbed rapidly from plants, and thus passed to the food chain, if dispersed in the environment. Lead-free alternatives have been reported with impressive progress in power conversion efficiency for tin-based (lead-free) perovskites. However, the stability of tin-based perovskite solar cells is still unexplored. In the present talk, we will focus on the strength of tin-based (lead-free) perovskite solar cells.
2.2-I2
Iván Mora-Seró (1974, M. Sc. Physics 1997, Ph. D. Physics 2004) is researcher at Universitat Jaume I de Castelló (Spain). His research during the Ph.D. at Universitat de València (Spain) was centered in the crystal growth of semiconductors II-VI with narrow gap. On February 2002 he joined the University Jaume I. From this date until nowadays his research work has been developed in: electronic transport in nanostructured devices, photovoltaics, photocatalysis, making both experimental and theoretical work. Currently he is associate professor at University Jaume I and he is Principal Researcher (Research Division F4) of the Institute of Advanced Materials (INAM). Recent research activity was focused on new concepts for photovoltaic conversion and light emission based on nanoscaled devices and semiconductor materials following two mean lines: quantum dot solar cells with especial attention to sensitized devices and lead halide perovskite solar cells and LEDs, been this last line probably the current hottest topic in the development of new solar cells.
Formamidinium lead iodide (FAPbI3) is the 3D lead perovskite with the highest theoretical efficiency due to its narrower band gap in comparison with Cs or methylammonium perovskites. Unfortunately, the black phase allowing this narrow bandgap is not the most stable one at room temperature. Here, we shown as the fabrication conditions and the interaction with semiconductor quantum dots can boost significantly the stability of FAPbI3. The interaction of halide perovskite and colloidal semiconductor nanostructures (quantum dots or nanoplatelets) can produce interesting synergistic interactions. We show that the interaction of PbS quantum dots and nanoplatelets can produce the stabilization of FAPbI3and FACsPbI3 perovskite black phase and also the increase of the efficiency, stability and reproducibility of the photovoltaic devices prepared with these halide perovskites. Incorporation of PbS QDs allows the dramatic decrease of the annealing temperature for the formation of black FAPbI3 phase perovskite thin film, from the 170ºC required without QDs to 85ºC when QDs are present. In addition, stability of these systems including embedded nanostructures is extended not just for samples prepared in the glove box but fabricated in ambient conditions. In addition, fabrication of devices under air condition can further increase the stability of FAPbI3. We have also verified the synergic combination of different additives for a significant increase of device stability. These result points the interest of Perovskite-Quantum Dot Nanocomposites, for further development of advanced optoelectronic devices. Stabilization of tin based perovskite solar cells will be analyzed. Eventually, stabilization of halide perovskite nanoparticles is a necessary step for the development of high performance Halide Perovskite LEDs. Control of post synthetic washing processes allows the preparation of LEDs with enhanced performance and stability.
2.2-I3
Perovskite solar cells (PSCs) have created much excitement in the past years and attract spotlight attention. This talk will provide an overview of the reasons for this development highlighting the historic development as well as the specific material properties that make perovskites so attractive for the research community.[1-3]
The current challenges are exemplified using a high-performance model system for PSCs (multication Rb, Cs, methylammonium (MA), formamidinium (FA) perovskites).[2,3] The triple cation (Cs, MA, FA) achieves high performances due to suppressed phase impurities. This results in more robust materials enabling breakthrough reproducibility.
Through multication engineering, usually not-considered alakali metals, such as Rb, can be studied[5] resulting in one of the highest voltages compared to the bandgap. Polymer-coated cells maintained 95% of their initial performance at elevated temperature for 500 hours under working conditions, a crucial step towards industrialisation of PSCs.
To explore the theme of multicomponent perovskites further, molecular cations were re-evaluated using a globularity factor. With this, we calculated that ethylammonium (EA) has been misclassified as too large. Using the multication strategy, we studied an EA-containing compound that yielded a high open-circuit voltage of 1.59 V. Moreover, using EA, we demonstrate a continuous fine-tuning for perovskites in the "green gap" which is relevant for lasers and display technology. [6]
The last part elaborates on a roadmap on how to extend the multication to multicomponent engineering providing a series of new compounds that are highly relevant candidates for the coming years, also in areas beyond photovoltaics, for example for medical scintillation detectors.[6,7]
2.2-I4
Perovskite semiconductors differ from most inorganic and organic semiconductors due to the presence of mobile ions in the active layer. Although the phenomenon is intensively investigated, important questions remain, such as the dominant species and overall density of the mobile ions and their exact impact on the device stability and steady-state power conversion efficiency (PCE). In this talk, we propose a simple method to estimate the efficiency loss due to mobile ions via “fast-hysteresis” measurements by preventing the perturbation of mobile ions out of their equilibrium position at fast scan speeds (1000 V/s) and choosing a suitable prebias condition.[1,2] The ”ion-free” PCE of fresh pin-type cells with low levels of apparent hysteresis at typical scan speeds (100 mV/s) is between 1.5-3% higher than the steady-state PCE. However, with device aging, e.g. under elevated temperatures or continuous light illumination, the ionic losses increase substantially. This represents the dominant degradation loss of a triple cation based pin-type perovskite cell. The enhanced ionic losses with aging are also accompanied by a significant increase of the mobile ion density. Finally, we will discuss how the stoichiometry and in particular the PbI2 excess influence the ionic losses and how these losses vary with the charge transport properties. Finally, an outlook is presented on how these losses affect the degradation of solution processed perovskite based tandem cells. Overall, the proposed methods to quantify the ion-induced field screening and PCE losses allow for a better understanding of several key phenomena in perovskite solar cells and shed light on the complex device degradation process.
References:
1 Le Corre, V. M. et al. Sol. RRL 2100772 (2022), DOI: 10.1002/solr.202100772
2 Thiesbrummel, J. et al. Adv. Energy Mater. 11, 2101447 (2021), DOI: 10.1002/aenm.202101447
2.3-I1
Sam Stranks is Professor of Optoelectronocs and Royal Society University Research Fellow in the Department of Chemical Engineering & Biotechnology and the Cavendish Laboratory, University of Cambridge. He obtained his DPhil (PhD) from the University of Oxford in 2012. From 2012-2014, he was a Junior Research Fellow at Worcester College Oxford and from 2014-2016 a Marie Curie Fellow at the Massachusetts Institute of Technology. He established his research group in 2017, with a focus on the optical and electronic properties of emerging semiconductors for low-cost electronics applications.
Sam received the 2016 IUPAP Young Scientist in Semiconductor Physics Prize, the 2017 Early Career Prize from the European Physical Society, the 2018 Henry Moseley Award and Medal from the Institute of Physics, the 2019 Marlow Award from the Royal Society of Chemistry, the 2021 IEEE Stuart Wenham Award and the 2021 Philip Leverhulme Prize in Physics. Sam is also a co-founder of Swift Solar, a startup developing lightweight perovskite PV panels, and an Associate Editor at Science Advances.
Halide perovskite semiconductors are generating enormous excitement for next-generation photovoltaics in both single junction and tandem device forms. Their performance is striking given that these absorber layers are rife with heterogeneity on the nanoscale in their structural, chemical and optoelectronic properties. Here, I will describe how this heterogeneity impacts not just performance but also operational stability of halide perovskite solar cells. Nanoscale trap clusters are present in the absorber layers that act as unwanted non-radiative recombination sites [1] and sites of instability [2] -- and thus must be removed. These trap clusters relate to phase impurities [3] and form in regions that are not stabilised by octahedral tilt [4]. Furthermore, interfaces impact local recombination and instability pathways, both on a local scale and on a macroscopic scale. I will outline how appropriate passivation and compositional control can mitigate these issues, and provide a pathway to realise highly efficient and stable perovskite solar cells.
2.3-T1
In recent years perovskite solar cells (PSCs) have attracted attention due to their promising electro-optical properties leading to high power conversion efficiencies of 25.7% in single-junction devices [1]. However, the stability of PSCs is still limited and thus the bottleneck on their way to commercialisation. One of the main causes of the poor stability is the presence of mobile ions in the perovskite absorber, facilitated by their soft ionic crystal structure. Interestingly, the density of mobile ions reported in literature spans multiple orders of magnitude reaching from 1015 - 1019 cm-3 [2, 3]. One technique that has been previously applied to quantify the activation energy, diffusion coefficient, and density of mobile ions is transient ion drift (TID). In TID, the modulation of the device capacitance due to redistribution of mobile ions after applying a voltage pulse is measured. However, so far, the interpretation of TID was based on an analytical model that assumed a p-doped perovskite and did only account for the drift but not for the diffusion of mobile ions [3, 4]. In this work, we provide an updated interpretation of TID, also accounting for low doping densities in the perovskite, and show what it can teach us about the activation energy, diffusion coefficient, and density of mobile ions.
2.3-T2
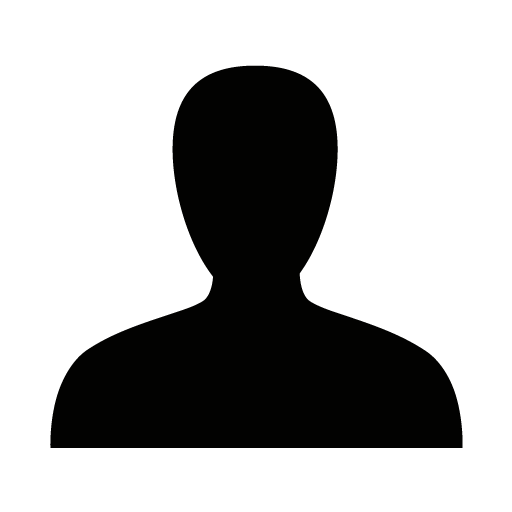
Achieving long-term stability of perovskite solar cells is arguably the most important challenge required to enable widespread commercialization. Understanding the perovskite crystallization process and its direct impact on device stability is critical to achieve this goal. Surprisingly, we find that intermediate phases that occur during the crystallization process strongly influence the long-term perovskite device stability. The commonly employed “dimethyl formamide/dimethyl sulfoxide” (DMF/DMSO) solvent system preparation method results in poor crystal quality and microstructure of the polycrystalline perovskite films. In this work, we introduce a high-temperature “DMSO-free” processing method that utilizes dimethylammonium chloride (DMACl) as an additive to accurately control the perovskite intermediate precursor-phases. By precisely controlling the 2H to 3C perovskite phase crystallization sequence, we tune the grain size, texturing, orientation (corner-up vs face-up) and crystallinity of the formamidinium (FA)yCs1‑yPb(IxBr1-x)3 perovskite system. A population of encapsulated devices showed significantly improved operational stability, with a median T80 lifetime, for the steady-state PCE, of 1190 hours and a champion device showed a T80 of 1410 hours, under simulated sun light at 65 °C in air, under open‑circuit conditions. Our work introduces an innovative processing method that allows higher overall perovskite device stability, by controlling the intermediate phase domains during the perovskite formation. This work highlights the importance of material quality in order to achieve long-term operational stability of perovskite optoelectronic devices.
2.3-T3
Zafar Iqbal is a post-doctoral researcher at Helmholtz-Zentrum Berlin (HZB), working with Professor Antonio Abate. His research focuses on modifying interfaces to achieve energy level alignment in inorganic halide perovskites, aiming to enhance device efficiency and stability. Zafar earned his M.Phil in Physical Chemistry from Quaid-i-Azam University, Islamabad, and subsequently engaged in research and teaching at the Department of Chemistry and Chemical Engineering, LUMS, Lahore. In 2019, he was awarded with Deutscher Akademischer Austauschdienst (DAAD) PhD Scholarship, for his doctoral studies at HZB. He completed his Ph.D. in Physical Chemistry. Additionally, he served for a year on the University Senate Commission for Research and Young Scientists (FNK) for the University of Potsdam, Potsdam. In 2022, he was selected to attend the 71st Lindau Nobel Laureate Meeting as a young researcher.
Here we report a detailed study at the interface for dipole molecule treated inorganic perovskite CsPbI3 based solar cells. An energy level band bending at the passivated CsPbI3 perovskite and hole selective contact interface was introduced by a dipole molecule, trioctylphosphine oxide. X-ray photoelectron Spectroscopy (XPS) study shows binding energy shifts towards lower value, which indicating the acceptance of electrons from perovskite to TOPO, similar to the role as a Lewis acid. The upward shifts in work function in TOPO-treated samples, as compared to the control sample, has been revealed by Ultraviolet Photoelectron Spectroscopy (UPS) and Kelvin Probe measurements independently. Steady-state Photoluminescence (PL) and Time-resolved Photoluminescence Spectroscopy (trPL) measurements show that the dipole molecule contributes marginally to defects passivation of perovskite films. Charge dynamics at the interface was characterized by using transient Surface Photovoltage Spectroscopy (trSPV) at varied photon energies. The charge selectivity at the interface is increased by over six times for TOPO treated samples. Finally, a champion efficiency of 18.7% has been achieved for CsPbI3 perovskite solar cells with an increase of 100 mV in average in open-circuit voltage. Influence of dipole treatment on long-term stability of these devices were characterized by maximum power point tracking of over 500 hours under 1 sun intensity at room temperature with cycled illumination. It has been seen that due to better energy alignment at the interface, dipole molecule treated samples shows a better stability as compared to control CsPbI3 based devices.
2.3-T4
Loreta Angela Muscarella was born in Palermo, Italy. In 2012, she moved to Rome where she started a bachelor in chemistry at Sapienza - University of Rome. During her Master’s studies, she spent seven months at the University of Amsterdam (UvA) under the supervision of Dr. René Williams to write her thesis on the effect of metallic ions in mixed-halide perovskites to improve the stability and optoelectronic properties. She received her MSc degree in inorganic and physical chemistry cum laude (with honors). In 2018, Loreta joined the group of Prof. Dr. Bruno Ehrler at AMOLF as a PhD student. Here, she investigated the relation between structure and optoelectronic properties of 3D and layered 2D lead-halide perovskites by monitoring the optoelectronic properties of mechanically compressed perovskites. In 2022, she joined the group of Dr. Eline Hutter (Utrecht University) as a postdoc to study photochemistry processes using lead-free perovskites. Since January 2024, she is assistant professor at the Vrije Universiteit Amsterdam where her group will combine spectroscopy and compositional engineering of perovskite-based materials to investigate on the external stimuli response of the emerging perovskite-based materials.
A striking difference between lead-halide perovskites and conventional semiconductors (e.g., silicon, III-V’s) is the dual ionic-covalent bond nature within the anionic inorganic framework. This weaker bond nature compared to the purely covalent bond of conventional semiconductors results in the mechanically soft and dynamically disordered perovskite lattice whose alteration affects the optoelectronic properties and the stability of these solids. Thus, metal-halide perovskites are particularly sensitive to variations in composition, fabrication and external stimuli that can induce strain in the material, interesting for developing next-generation memory devices, sensors, and energy-storage technologies. Layered Dion–Jacobson (DJ) and Ruddlesden–Popper (RP) hybrid perovskites are promising materials for optoelectronic applications due to their modular structure. To fully exploit their functionality, mechanical stimuli can be used to control their properties without changing the composition. However, the responsiveness of these systems to pressure compatible with practical applications and industrial fabrication methods (<1 GPa) remains unexploited. Hydrostatic pressure is used to investigate the structure–property relationships in representative iodide and bromide DJ and RP 2D perovskites based on 1,4-phenylenedimethylammonium (PDMA) and benzylammonium (BzA) spacers in the 0–0.35 GPa pressure range. Pressure-dependent X-ray scattering measurements reveal that lattices of these compositions monotonically shrink and density functional theory calculations provide insights into the structural changes within the organic spacer layer. These structural changes affect the optical properties; the most significant shift in the optical absorption is observed in (BzA)2PbBr4 under 0.35 GPa pressure, which is attributed to an isostructural phase transition. Surprisingly, the RP and DJ perovskites behave similarly under pressure, despite the different binding modes of the spacer molecules. This study provides important insights into how the manipulation of the crystal structure affects the optoelectronic properties of such materials, whereas the reversibility of their response expands the perspectives for future applications in e.g., sensors, actuators.
1.1-I1
Bismuth-halide-based semiconductors have gained increasing attention for optoelectronics, owing to their low toxicity, high environmental stability under ambient conditions, and easy processability by a wide range of scalable methods. These materials have also been proposed to replicate key features of the electronic structure of lead-halide perovskites that may give rise to defect tolerance, but without the toxicity limitations of the latter [1]. This talk will examine in detail the case of bismuth oxyiodide (BiOI). Through density functional theory calculations, we show that the most common point defects in BiOI are resonant within the bands, or shallow within the bandgap [2]. We develop an all-inorganic device structure, and devise a route to control the preferred orientation of the vapour-deposited BiOI films to achieve photovoltaics with external quantum efficiencies reaching up to 80% at 450 nm wavelength [2]. Further, we demonstrate the strong potential of BiOI for indoor light harvesting to power Internet of Things electronics [3], as well as for bias-free solar water and CO2 splitting [4]. We finish with a discussion of the key factors that will need to be addressed in order to further improve the performance of this material in optoelectronics, focussing especially on the role of carrier-phonon coupling on charge-carrier transport and dynamics.
1.1-I2
Lorenzo obtained his PhD in Chemistry in 2003 and since 2008 is Assistant Professor at the Chemistry Department of the University of Pavia. In 2021 he was appointed Full Professor in the same department. He was the recipient of the Young Scientist Award for outstanding work in the field of perovskites at the International Conference on Perovskites held in late 2005 in Zürich, of the “Alfredo di Braccio” Prize for Chemistry 2008 of Accademia Nazionale dei Lincei awarded to distinguished under 35-year-old chemists and contributed the Journal Materials Chemistry and Chemical Communications“Emerging Investigator” issues in 2010 and 2011. He is working in several areas of solid state chemistry with particular interest in the investigation of structure–properties correlation in different kinds of functional materials, in particular electrolyte materials for clean energy, hybrid organic-inorganic perovskites and catalysis materials. He is author of more than 200 papers on international peer-reviewed journals. Since 2018 he is member of Academic Senate and Vice-Director of the Chemistry Department. He is Director of the INSTM Reference Center “PREMIO” devoted to the synthesis of innovative materials and member of the Directive Board of INSTM. Since 2014 he is member of the Academic Board of the PhD in Chemistry of Pavia University. He is Editor of Journal of Physics and Chemistry of Solids.
The toxicity of lead, the leaching problems, and the current regulations designed to limit or eliminate substances that are dangerous to the environment and to people, impose to move towards lead-free metal halide perovskites (MHPs). In addition, while MHPs have been thoroughly investigated for photovoltaics (PV) applications, it is now evident that their superior optical properties could be advantageously exploited in several other fields ranging from photodetectors to photocatalysis. Replacing lead with tin or germanium in 3D or 2D perovskites brings well-known stability issues, and the exploration of all-inorganic perovskite-derivatives containing bismuth or antimony may lead to problems of precursors solubility in wet-chemistry film deposition. Notwithstanding these possible obstacles, the PV application of tin-based MHPs has impressively increased in terms of efficiencies in the last few years. In order to further extend and exploit more recent applications of lead-free perovskites, a thorough experimental and computational structure-property correlation investigation should be provided with the aim of designing more stable and optimized materials. In this contribution we will present some recent studies devoted to the design, synthesis, and application of lead-free MHPs with a specific focus to fields beyond PV such as photocatalysis or photodetectors. Among other examples, we will show how, through a careful materials chemistry design, we could improve the water stability of tin and germanium-based MHPs perovskites and apply these characteristics to photocatalytic applications. The combined use of experimental and computational tools allowed to gain a significant comprehension on the mechanisms underpinning such features. At the basis of the results there is the possible manipulation of the compositional space provided for example by low-dimensional perovskites which can be beneficially used to modulate not only the optoelectronic properties but also the stability of these materials. Further examples will be focused on the application of physical vapor deposition techniques to afford the preparation of challenging all-inorganic lead-free perovskites and exploit their use in photodetectors.
1.1-I3
Filippo De Angelis is senior research scientist and a deputy director at the CNR Institute of Molecular Sciences and Technology, in Perugia, Italy. He is the founder and leader of the Computational Laboratory for Hybrid/Organic Photovoltaics. He earned a BS in Chemistry in 1996 and a PhD in Theoretical Inorganic Chemistry in 1999, both from the University of Perugia. He is an expert in the development and application of quantum mechanical methods to the study of hybrid/organic photovoltaics and materials for energy applications. He is Fellow of the European Academy of Sciences. He has published >270 papers with > 17000 citations.
The outstanding opto-electronic properties of metal-halide perovskites (MHPs) have contributed several breakthroughs in photovoltaics and optoelectronics. These materials would be ideally exploited also in heterogeneous photocatalysis, but the poor MHPs stability in aqueous environment has for long inhibited their application to this field. In the last two years, however, a growing family of water-stable and photoactive MHPs have been reported, thus paving the way towards the development MHP-based photocatalysts.
Surprisingly, tin-halide perovskites (THPs), which are notoriously unstable in photovoltaics, have received a large attention because of their suitable band gap, tunable electronic energy levels, and their low toxicity coupled to a superior stability in water environment compared to their lead counterparts. Here, we present high-level ab initio calculations to unveil the key factors determining the reactivity of THPs towards photocatalytic hydrogen production at the perovskite/water interface. Our results highlight that the occurrence of electron polarons at the surface of THPs is paramount in determining the efficiency of the reaction. The stabilization of localized electrons stems from the energy of the conduction band edge and from the peculiar THP defect chemistry, largely centered on tin. Band edge tuning is governed by the interplay between the A-site cation and nature of the halogen, thus fine-tuning the THPs energy levels can be achieved by varying the chemical composition, providing a successful strategy to boost the photo-reactivity of these materials.
Session1.2-T1
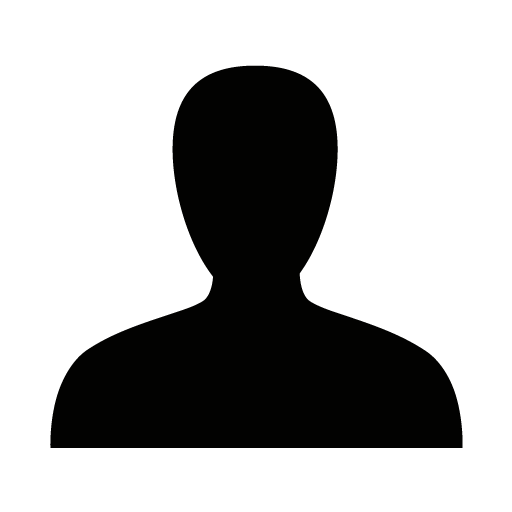
Halide double perovskites are a chemically diverse class of materials featuring a vast range of thermodynamically stable compounds with widely tunable optoelectronic properties [1, 2]. Dimensional reduction of 3D lattices is routinely used for band structure and exciton engineering by exploiting the effects of quantum and dielectric confinement. Excitons in quasi-2D derivatives of Pb-based halide perovskites have been explored extensively in the last years. However, the impact of dimensional reduction on the nature of excitons in halide double perovskite is not yet well-understood.
In this contribution, we discuss the optoelectronic properties of 3D and quasi-2D derivatives of Ag/Bi-based halide double perovskites based on first principles calculations using many-body perturbation theory within the GW approximation [3] and the Bethe-Salpeter equation approach [4]. In the 3D double perovskite Cs2AgBiBr6, non-hydrogenic and strongly localized resonant excitons arise due to the chemical heterogeneity of alternating AgBr6 and BiBr6 octahedra and lead to anisotropic effective masses and pronounced local field effects [5]. We show that dimensional reduction of Cs2AgBiBr6 to mono- and bilayer Ruddlesden-Popper and Dion-Jacobson structures has striking effects on the band structure and excitons of these quasi-2D materials. Our results are in line with the experimentally reported optical properties of these materials [6] and demonstrate that structural distortions, quantum confinement effects, and layer stacking can be used to tune exciton localization and binding energies in chemically complex quasi-2D materials.
Session1.2-T2
Lead-free double halide perovskites AIMIMIIIX6 (A,M – metal cations, X – halide anions) offer unprecedented variability of structures and compositions, with all four positions variable independently resulting in many thousands of possible compounds for the same set of constituent elements. A detailed screening of the properties of these huge families of compounds requires application of a combination of high-throughput synthetic (HTP) approaches with HTP characterization and HTP testing for possible applications related to light conversion and emission.
We have developed a “green” protocol for a HTP synthesis of brightly luminescent lead-free microcrystalline Cs2AgxNa1-xBiyIn1-yCl6 (CANBIC) perovskite phosphors absorbing strongly in UV range and emitting broadband photoluminescence (PL) in the visible range with PL quantum yields reaching 98±2% for specific compositions with x = 0.35-0.40 and y = 0.01-0.02. The CANBIC perovskites revealed high stability of spectral properties during many months of ambient storage, thermal stability at open air till 200-250 oC and photochemical stability under UV illumination, making these compounds highly promising for applications in luminescent solar light concentrators and down-shifters. Our HTP approach to CANBIC perovskites includes robot-assisted automated synthesis with any desirable x and y steps combined with HTP spectral charcterization which includes absorption, PL, PL excitation, and Raman spectroscopy as well as time-resolved PL characterization, allowing many hundreds of samples to be produced and characterized in a single working session.
This HTP approach was expanded to other CANBIC-like perovskites, produced either by substituting InIII with SbIII, or by substituting BiIII with FeIII. In the first case we developed a HTP protocol for the synthesis of Cs2AgxNa1-xBiySb1-yCl6 (CANBSC) perovskites with x and y fractions varied independently from 0 to 1. The CANBSC perovskites can be converted into Cs2AgxNa1-xBiySb1-yBr6 (CANBSB) and Cs3Bi2ySb2(1-y)I9 (CBSI) perovskites by a single-step anionic exchange with NaBr and NaI, respectively. These compounds reveal a band-bowing effect, the bandgaps of mixed perovskites being typically lower than those of corresponding individual BiIII- and SbIII-based perovskites. For CANBSC and CANBSB compounds the band-bowing effect was found to increase with an increase of the silver fraction. The lowest bandgaps reached were 2.47 eV for CANBSB and 2.08 eV for CBSI both at x = y = 0.50.
We found that BiIII in CANBIC perovskite can be partially or completely substituted by FeIII resulting in the latter case in Cs2AgxNa1-xFeyIn1-yCl6 (CANFIC) perovskites showing strong absorbance in the visible spectral range and a high environmental stability. A special feature of CANFIC compounds is a structure-directing influence of In on the formation of perovskite phase. While for y = 1.00 only a multi-phase mixture can be obtained in our conditions, introduction of mere 1% InIII steers the precipitation exclusively to the formation of cubic perovskite phase with no other phases detectable. The lowest bandgap reached so far is ca. 2.0 eV for x = 1.00 and y = 0.95-0.99. Anionic exchange of CANFIC did not result in stable single-phase bromide and iodide compounds. A similar structure-directing effect was also found for BiIII and SbIII introduced instead of InIII. Our preliminary results show the feasibility of introducing simultaneously three MIII metals, for example, BiIII, InIII, and FeIII, into the chloride perovskite while preserving the single-phase solid-solution character of the final products, opening broad possibilities for the compositional design of the optical properties. The unique variability of the MIII site of these double perovskites will be addressed in future using the combined HTP approach to the synthesis and characterization developed earlier for CANBIC perovskite phosphors.
Session1.2-T3
Dr Luis Lanzetta is a Postdoctoral Fellow at King Abdullah University of Science and Technology (KAUST, KSA). He obtained his PhD in Chemistry at Imperial College London (UK) in 2020, where he focused on developing eco-friendly, tin-based perovskites for photovoltaic and light-emitting applications. His research focuses on next-generation materials for energy harvesting. Specifically, his expertise lies in the chemical degradation and stabilisation mechanisms of halide perovskite solar cells, aiming to provide design rules towards more efficient and stable technologies. He is additionally interested in molecular doping approaches for narrow-bandgap perovskites, as well as the spectroscopic and surface characterisation of this class of materials.
Google Scholar: https://scholar.google.com/citations?user=OcCV1VUAAAAJ&hl=es
Electrical doping enables meticulous tuning of the electronic properties in novel hybrid perovskite semiconductors, which is critical for their successful impelmentation as optoelectronic applications. Nevertheless, the use of substitutional/interstitial impurities as dopants remains difficult, mainly due to dopant phase segregation and defect compensation. In contrast, molecular doping stands as a promising pathway to modulate charge carrier density via charge transfer without altering the perovskite crystal structure. However, the underlying processes facilitating molecular doping in perovskites (e.g. host-dopant interactions) remain highly underexplored. In this talk, we will present our recent work on the molecualr doping mechanism of p-type methylammonium tin-lead iodide films by using an n-type molecule, namely 4-(1,3-Dimethyl-2,3-dihydro-1H-benzoimidazol-2-yl)phenyl)-N,N-dimethylbenzenamine (n-DMBI-H). By employing a combination of experimental and first principles simulation techniques, we identify preferential dative bonding between amino moieties in n-DMBI-H and Sn atoms in perovskite surfaces as a dominant host-dopant interaction that mediates charge transfer. We will discuss the dopant localization within films and deternime that n-DMBI-H is located at perovskite surfaces and grain boundaries, allowing charge carrier density tuning of nearly one order of magnitude. The talk will conclude with details on the incorporation of n-DMBI-H in p-i-n tin-lead perovskite solar cells, which results in lower charge carrier recombination and higher charge selectivity at perovskite/transport layer interfaces. This work elucidates important insights of perovskite molecular doping that are expected to inspire strategies towards next-generation perovskite optoelectronic devices.
Session1.2-T4
Over the past few years, mixed Sn-Pb perovskites came to the fore as promising absorbing materials for single- and multi-junction solar cells due to the possibility of manipulating the bandgap by changing the Sn to Pb ratio [1]. However, the opto-electronic properties of tin-containing perovskites are adversely affected by intrinsic and extrinsic factors leading to doping [2][3]. In this work, we produced different Sn-Pb perovskites thin-films of composition Cs0.25FA0.75SnxPb1-xI3 with varying tin content between x=0 and x=0.6 via antisolvent spin-coating. In order to improve the properties of the perovskite layers, 20 mol% SnF2 was added to the tin precursor solution. We studied the effect of light on the background conductivity by means of microwave conductivity techniques. In particular, we looked at the light-induced carge-carriers dynamics before and after exposing the perovskite thin-films to AM1.5 illumination. For the pristine layers, we found charge-carriers mobilities reaching μ ~ 25 cm2/V·s and lifetimes t > 1 μs, which are only marginally dependent on the Sn to Pb ratio. Exposure to external factors, such as ambient air, leads to a severe increase in background conductivity and shortened charge-carriers lifetimes. Lastly, a potential explanation of the degradation mechanism is proposed on basis of defect chemistry.
Session1.2-T5
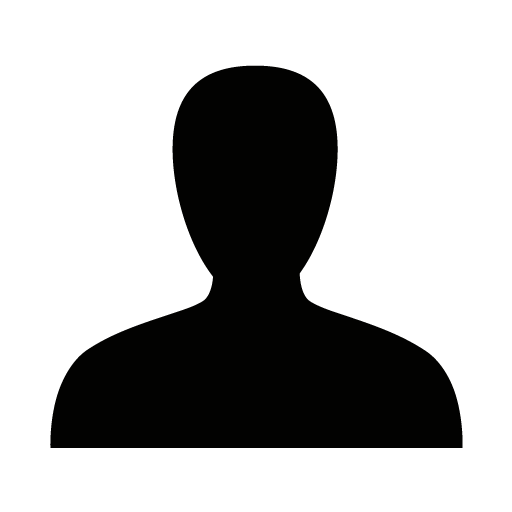
The performance of single-junction solar cells in thermal equilibrium is limited by the conversion of excess photon energy to heat through hot-carrier—phonon interactions [1]. However, hot-carrier photovoltaic devices, a future-generation technology for solar power conversion, could overcome this limitation. If the temperature of the charge-carrier gas is elevated compared to that of the crystal lattice, extraction of the photoexcited charge carriers with above-bandgap energies may be possible, boosting the energy conversion efficiency. Metal-halide perovskites, and in particular tin-iodide perovskite semiconductors, have been investigated in recent years as potential candidates for such technology, owing to the long hot-carrier cooling times observed in these materials [2]. Reported nanosecond-long cooling dynamics could allow for efficient extraction of hot carriers high above the bandgap, pushing the efficiency limit up to 66% PCE [3,4].
Unfortunately, tin-iodide perovskites suffer from tin-vacancy formation. These hole-donating point defects lead to significant electrical doping of the materials. The ocean of cooled, dopant holes could lead to rapid lowering of hot-carrier temperatures through cold-carrier—hot-carrier scattering events, hindering the application of these materials in hot carrier devices operating under solar illumination [5].
In this talk, we present the results of our investigation of carrier cooling dynamics in tin-iodide perovskites, performed using a novel pump-push-probe terahertz spectroscopic technique [6]. We show that, when applied to high-mobility materials, this technique has high sensitivity which enables the investigation of hot-carrier dynamics at lower excitation densities (below hot-phonon bottleneck threshold) than alternative spectroscopic methods. Our study, performed at these low hot-carrier densities, reveal ultrafast, sub-picosecond cooling dynamics in doped tin-iodide perovskite semiconductors, originating from cold-carrier—hot-carrier scattering. Our results are directly relevant to the applicability of these materials in hot-carrier devices, for which the fast thermalisation of the photoexcited carriers with cold ocean of dopant holes could hinder the efficiency of above-bandgap extraction, highlighting the need for further doping suppression methods in lead-free perovskites.
Session1.2-T6
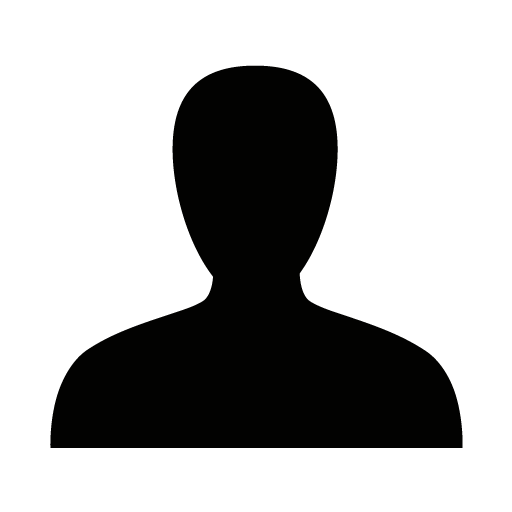
In photochemistry light is used to induce redox reactions, such as CO2 reduction or H2 evolution. To effectively harvest the incident photons, the photoactive material, typically a semiconductor, must have a suitable optical bandgap. A common problem of widely-studied photoactive materials, such as TiO2, is the large bandgap energy which results in a poor conversion efficiency.
An interesting class of materials for photochemistry are elpasolites, also known as double perovskites, due to the tunable bandgap. In particular, Cs2AgBiBr6 shows great promise because of its good photo- and chemical stability and relatively low toxicity. Absorption of visible light is, however, limited because of the indirect nature of the bandgap. The bandgap of such silver-bismuth materials can be manipulated by substituting the Bi3+ cation with another cation.
In this talk we discuss a mechanochemical synthesis approach to (partly) substitute Bi3+ in the Cs2AgBiBr6 crystal structure. Due to the high force and pressure created in a ball mixer, we are able to synthesize phase-pure Cs2AgBi1-xMxBr6 compositions. By controlling the Bi:M ratios we can manipulate the bandgap energy and thus the optical properties. We show that the incorporation of Fe3+ strongly red-shifts the absorption onset of this material, which increases the photochemical activity.
Session1.2-T7
Halide perovskites are well known for their good light absorption properties, tuneable electronic band structures, and good charge carrier mobility. Cs3Bi2Br9 is a lead-free ternary halide perovskite with the ability to absorb light in the UV and visible ranges with a band structure that allows it to be used for photocatalytic CO2 reduction. However, the low surface area, poor CO2 adsorption, and high charge recombination of Cs3Bi2Br9 prevents it from obtaining high production rates of solar fuels. Therefore, the purpose of this work is to design a novel heterojunction between templated g-C3N4 and Cs3Bi2Br9 with Pt nanoparticles as a co-catalyst. SiO2 nanospheres with a diameter of 250 nm were synthesised and used as a template to form homogeneous and well-ordered g-C3N4 inverse opal structures. Templated g-C3N4 were successfully obtained and proven to show a 52% increase in BET surface area with an improvement in CO2 adsorption compared to bulk g-C3N4. Gas-phase photocatalytic reactions were performed and a selectivity shift towards CO production was observed with an increase from 2.23 to 6.28 µmol CO g-1 h-1 using templated g-C3N4. The incorporation of 1 wt% Pt nanoparticles induced the evolution of 71.83 µmol H2 g-1 h-1 in addition to 7.21 µmol CO g-1 h-1 and 1.57 µmol CH4 g-1 h-1. A preliminary assessment of the effectiveness of Cs3Bi2Br9 perovskite showed a production of 5.5 µmol CO g-1 h-1 as well as a good electronic band structure alignment with g-C3N4. Current stages of this project entail optimising the band structure alignment of the two materials to synthesise a heterojunction that further boosts solar fuels production as well as exploring the effects of different cocatalysts on the efficiency of the process.
2.1-T1
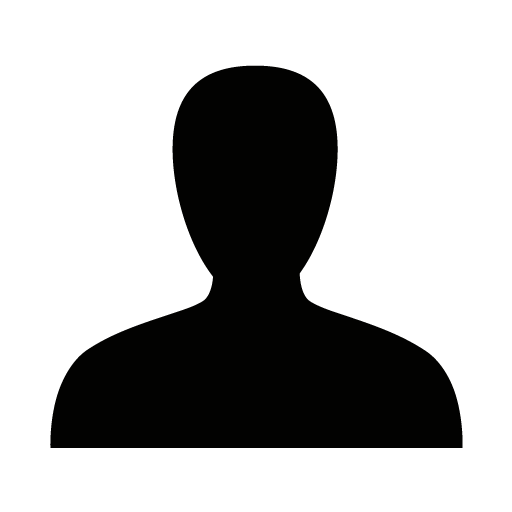
Lead halide perovskite materials now play a pivotal role as an emerging class of semiconductors for photovoltaic applications, with a certified record efficiency of 25.7% in 2022. However, their commercialization has been hindered so far by the toxicity of lead and by their instability. Vacancy-ordered halide perovskites, with general formula A2B+4X6, are one of the alternative structures that have been designed to replace Pb2+ with non-toxic tetravalent cations. Ti, Zr, Pd, Sn, Te and Pt-based vacancy-ordered perovskites have already been synthesized but those containing tin and titanium have shown the best performances in solar cells. We used colloidal synthesis as a low-cost, scalable solution method for the preparation of cesium titanium halide and cesium tin halide nanocrystals [1]. Moreover, with this method we prepared mixed titanium-tin cesium iodide perovskites, with different amounts of tin and titanium and bandgaps suitable for solar cell applications. We investigated the structural and optical properties of these materials, their stability in air, and their feasibility for solar cells applications.
2.1-T2
Daniela Marongiu is associate professor at the Department of Physics of University of Cagliari (Italy). She obtained a PhD in Nanostructure and Nanotechnology in 2011 from the University of Milano-Bicocca, then she moved to University of Sassari and later in 2013 to Cagliari where she has been an associate professor since 2021. She studied a variety of nanomaterial-related topics and now her main scientific interests involve the growth and characterization of hybrid and all-inorganic halide perovskites in the form of thin films and single crystals for energy applications. Recently she also focused on the synthesis of inorganic double-perovskite micro and nanocrystals with a high photoluminescence quantum yield in the visible range including the doping with rare earths such as Yb and Er for highly efficient NIR emitters and stable phosphors.
Double perovskites have been developed to alternatively replace the divalent cations Pb2+ in single perovskite with a combination of a monovalent and trivalent cation, forming structure as A2BB’X6. Among them, the compounds Cs2Na1-xAgxIn1-yBiyCl6 can emit warm white light with almost unity quantum efficiency and are thus among the most promising materials for solid-state lighting(1). The emission spectrum is attributed to self-trapped excitons (STEs), the emission efficiency is, however, sensitive to the material composition since it is strictly related to the simultaneous presence of a small percentage of Ag and Bi.
Whether Bi atoms cure extrinsic defects or provide natural recombination centers is still a wide-open question while the addition of Ag has been linked to parity breaking in valence band orbitals, increasing the oscillator strength of the interband optical transitions(2,3).
A comprehensive understanding of the microscopic mechanisms producing the rules for efficient emission is fulfilled by a systematic study of the structural and optical properties as a function of the composition to understand the role of Ag and Bi in the high efficiency emission. Photoluminescence quantum yield (PLQY) measurements are complemented with photoluminescence excitation (PLE) and ultrafast spectroscopy in the form of differential transmission (DT/T) and time-resolved photoluminescence (TRPL). The dynamic of optical excitation is studied to investigate the formation of STEs and their decay times.
Bi and Ag substitutions at 0.1% levels are sufficient to promote the formation of STEs with a nearly radiative-recombination-limited dynamics, while effectively curing STE trapping in long-lived dark states. An Ag-induced self-trapping polaronic behavior is also suggested as a potential mechanism for formation of bright STEs thus achieving emission efficiency near unity with a warm white light spectrum in a wide variety of compositions.
Double perovskites realize therefore an almost ideal platform for solid state lighting and these results provide guidance for rational optimization of such compounds in view of the use as phosphors and active materials for LEDs and displays.
2.1-I1
Iván Mora-Seró (1974, M. Sc. Physics 1997, Ph. D. Physics 2004) is researcher at Universitat Jaume I de Castelló (Spain). His research during the Ph.D. at Universitat de València (Spain) was centered in the crystal growth of semiconductors II-VI with narrow gap. On February 2002 he joined the University Jaume I. From this date until nowadays his research work has been developed in: electronic transport in nanostructured devices, photovoltaics, photocatalysis, making both experimental and theoretical work. Currently he is associate professor at University Jaume I and he is Principal Researcher (Research Division F4) of the Institute of Advanced Materials (INAM). Recent research activity was focused on new concepts for photovoltaic conversion and light emission based on nanoscaled devices and semiconductor materials following two mean lines: quantum dot solar cells with especial attention to sensitized devices and lead halide perovskite solar cells and LEDs, been this last line probably the current hottest topic in the development of new solar cells.
Halide perovskite solar cells have revolutionized the photovoltaic field in the last decade. In a decade of intensive research it has been a huge improvement in the performance of these devices. However, the two main drawbacks of this system, the use of hazardous Pb and the long term stability, still to be open questions that have not been fully addressed. The photoconversion performance of perovskite solar cells containing alternative metals to Pb is significantly lower than the reported for devices containing Pb, where Sn-based perovskite solar cells is the alternative reporting higher photovoltaic performance close to 14%. Nevertheless, Sn-based perovskite solar cells exhibit a long term stability lower than their Pb containing counterparts, making stability their main problem. In this talk, we highlight how the use of proper additives and light soaking for defect engineering can increase significantly the stability of formamidinium tin iodide (FASnI3) solar cells, and discuss about the different mechanism affecting this stability, beyond the oxidation of Sn2+, and how they can be countered. Effect in Sn-based perovskite LEDs will be also analyzed. Additives can play an important role in stabilization of both Sn-based solar cells and LEDs.
2.1-I2
The versatile characteristics of halide perovskites have led to diverse optoelectronic applications. However, control over their intrinsic charge transport behavior such as electrical conductivity and Seebeck has received limited attention. In addition, doping halide perovskites has been difficult due to their self-compensating nature. In this talk, a wide range of tunability (semiconducting, metallic, p-type, n-type) strategies are demonstrated in halide perovskites leveraging their self-doping behavior and polymorphism. I will show that controlling the A-site cation in tin perovskites offers tuning the electrical conductivity, which is in contrast to general assumption that A-site cation does not play significant role in determining the electronic properties. Finally, I will discuss strategies such as molecular doping and additive engineering in tuning the charge transport in this class of (Sn based) materials. Control over the transport behavior of halide perovskites serves as a viable approach for extending their functionality to other energy conversion applications such as thermoelectrics.
2.2-I1
Christoph J. Brabec is holding the chair “materials for electronics and energy technology (i-MEET)” at the materials science of the Friedrich Alexander University Erlangen-Nürnberg. Further, he is the scientific director of the Erlangen division of the Bavarian research institute for renewable energy (ZAE Bayern, Erlangen).
He received his PhD (1995) in physical chemistry from Linz university, joined the group of Prof Alan Heeger at UCSB for a sabbatical, and continued to work on all aspects of organic semiconductor spectroscopy as assistant professor at Linz university with Prof. Serdar Sariciftci. He joined the SIEMENS research labs as project leader for organic semiconductor devices in 2001 and joined Konarka in 2004, where he was holding the position of the CTO before joining university.
He is author and co-author of more than 150 papers and 200 patents and patent applications, and finished his habilitation in physical chemistry in 2003.
The progress in semiconducting lead-halide perovskite compounds within an unprecedentedly short time period drove their advancement for optoelectronic applications, among them tandem perovskite-based photovoltaic materials, low-dimensional (2D, 0D) quantum confined emitters. With the emergence of lead-halide perovskites, the necessity for a sustainable material and process strategy is arising. Recycling and closed cycle processes gain importance, especially in high priced segments using rare or precious raw materials.
Attempts to develop “green” perovskite compounds are currently concentrating on “lead-free” halide perovskites, with the Sn based perovskites as one leading structure. An alternative approach are double-cation perovskites AIMIMIIIX6, where A is an alkali cation, X is a halide, and a couple of MI+MIII represents an isovalent substitution of two PbII cations in the lead-halide (APbX3)2 perovskite structure. The double lead-free compounds combine the variability of A and X sites typical for APbX3 compounds with new possibilities of independent variation of both MI and MIII sites from corresponding pools of MI = Na+, K+, Ag+, Tl+, Au+, etc. and MIII = In3+, Bi3+, Sb3+, Fe3+, Au3+, etc. Among the double halide perovskites, compounds based on Bi and In with a general formula Cs2AgxNa1-xBiyIn1-yCl6 (or CANBIC by first letters of elements) have started to occupy an outstanding position due to the combination of compositional variability, stability, opportunities for sustainable material combinations and promising photoluminescence quantum yields. Along with variable parameters x and y, both Cs and Cl sites can also be varied and potentially substituted with analogs (for example, Cs for Rb or methylammonium, Cl for Br and I).
Recently, we reported on a relatively “green” single-step synthesis of luminescent CANBIC perovskites in 2-propanol:water mixtures and were able to demonstrate a semi-automatic synthesis on a robot-based pipetting platform. That opens the opportunity to systematically the huge parameter space of lead-free, multinary metal, cation and halide double perovskites is a systematic way. First data libraries are presented and characterized according to their optical properties. The talk will be concluded with an outlook, whether the synthesis platform can be made compatible for a Bayesian optimization process looking for the most promising optoelectronic lead-free perovskites.
2.2-I2
A great challenge in today’s research on perovskites is finding stable, lead-free alternatives for photovoltaic applications. One interesting route is the replacement of divalent lead with a combination of monovalent and trivalent cations, as in Cs2AgBiBr6. Despite showing higher stability, the photovoltaic performance of Cs2AgBiBr6 based devices is inferior to lead perovskite based devices. The indirect bandgap, high exciton binding energies, and presence of trap states have been discussed as limiting factors of the power conversion efficiency. This work aims to provide new insight into the interplay of excitons and trap states on the charge carrier dynamics in Cs2AgBiBr6 thin films. To do so, we investigated Cs2AgBiBr6 thin films and bilayers of Cs2AgBiBr6 with a transport layer (TL) by means of time-resolved photoluminescence (TRPL), transient absorption (TA) measurements and time-resolved microwave conductivity (TRMC). In addition, an entirely novel technique i.e. double pulse excitation time-resolved microwave conductivity (DPE-TRMC) has been developed. Basically, in DPE-TRMC the sample gets illuminated by two laser pulses arriving with a short time delay. By comparing the photoconductance traces induced by the second pulse in presence and absence of the first pulse, we are able to examine the effect of the long-lived species on the charge carrier dynamics. For these experiments we used identical excitation wavelengths for both laser pulses but varying intensities and delay times. We modelled the results introducing a comprehensive model, which accounts for the free carrier generation yield, localization of free carriers, electron trapping by color centers, and shallow trap states for holes. The iterative analysis of the DPE-TRMC experiments with different intensities and delay times reveals the presence of a high concentration of both electron (1015 cm-3) and hole (1016 cm-3) trap states. Slow (partially) radiative decay of trapped electrons with their countercharges is in agreement with the TRPL measurements. Holes are rapidly trapped in shallow states, from where they get slowly released explaining the complementary TA measurements. These results are compared to results obtained on alloys of Cs2Ag(Bi1-xSbx)Br6. Finally, we also looked into the charge collection in double layers of Cs2AgBiBr6 and various transport layers including TiO2 and C60. In our opinion, the long-lived charge carriers in Cs2AgBiBr6 as observed in this work can offer suitable, lead-free alternatives for many other applications, including X-ray detectors or photocatalysis.
2.2-I3
Last update: 31/07/2022
Born on January 7, 1988.
During my undergraduate studies I had the chance to carry out two short-term research projects on nanomaterials at Tohoku University (Japan; 2010) and Université de Sherbrooke (Canada; 2011). I then obtained my Master of Science in Nanoscale Engineering and my PhD on Materials Science from Ecole Centrale de Lyon (France; 2011 and 2014). From 2015 until December 2017 I was a post-doctoral researcher in the Nanochemistry Department of the Istituto Italiano di Tecnologia of Genova (Italy). In 2017 I was awarded a "Marie Sklodowska Curie Actions" fellowship to develop my project PerovSAMs in the Instituto de Ciencia Molecular (ICMol) at the Universidad de Valencia, where I continued working with a "Juan de la Cierva incorporación" fellowship until 2021. In 2022 I joined Universidad Politécnica de Cartagena (UPCT) as a "Ramón y Cajal" fellow.
Throughout my career I have worked in the fields of materials' chemistry, colloidal inorganic nanocrystals, surface analysis and halide perovskites' optoelectronic devices among others. My publications and bibliometric indicators can be found elsewhere (e.g. Google Scholar or Scopus).
Aside from research I have also maintained a teaching activity throughout my career with lectures and practical courses in chemistry and chemical engineering at undergraduate level (Ecole Centrale de Lyon, 2011-2014 and 2020-2021; Universidad de Valencia and Universidad Politécnica de Valencia, 2018-2019; Universidad Politécnica de Cartagena, 2022-present) as well as specific courses in surface analysis techniques for PhD students (Istituto Italiano di Tecnologia; 2015-2017). I have supervised one Master of Science thesis, one PhD thesis and I currently supervise two other PhD theses.
Eventually, I am also involved in the "Federación de Jóvenes Investigadores" where we strive for a better spanish scientific and academic system, especially fighting against the precarity of young or junior researchers.
Replacing toxic Pb2+ cations in halide perovskites is one of the major challenges to make this technology viable for its widespread implementation. A lot of effort has been, and still is, focused on finding alternative B2+ cations such as Sn2+ or combinations of B+ and B3+ cations such as in Cs2Ag+Bi3+X6 double perovskites.
Nevertheless, there exists another option that has been so far mostly overlooked which consists in ABX3 compounds that are isostructural to perovskites but with opposite charges: A-B2-X+3. These compounds are named antiperovskites and several of them have been already demonstrated experimentally.
Here, I will focus on Ag3SX (X=I or Br), which can also be written as XSAg3 to highlight the analogy with perovskites. I will show how these antiperovskites can be synthesized by simple and dry methods based on mechanochemistry and moderate thermal annealing. Based on the nature of the X halide, the optical absorption within the visible range can be tuned which makes these semiconductors especially relevant for photovoltaics.
Thin films have also been deposited by pulsed laser deposition, paving the way to the implementation of these antiperovskites in solar cells.
2.3-I1
Pulsed Laser Deposition (PLD) has offered unique options for the development of complex materials thin film growth, allowing stoichiometric transfer and multi-compound deposition independent of the relative volatility of the elements, and ultimate control of interfaces. In the field of complex perovskite oxides, PLD opened the way to high-Tc superconducting films requiring stoichiometric transfer of multiple (4 to 5) cations. In this presentation we discuss the rather unexplored but huge potential of PLD for the controlled formation and study of single and double halide perovskite thin films. More specifically we present the unique single-source deposition of multi-compound halide perovskites in-vacuum.
We demonstrate PLD of a variety of compounds from CsSnI3, Cs2SnI6 and Cs2AgBiI6 as well as Pb-based compounds. The critical role of the PLD target quality, formed by mechano-synthesis of the individual precursors and pressed into a single solid pellet will be discussed. Moreover, the control of the PLD parameters to achieve near-stoichiometric transfer from a single source target to high quality films presenting the photoactive phases will be demonstrated. This work represents an important step forward in the development of controlled growth and future scalability of halide perovskites for efficient optoelectronic devices.
2.3-I2
Laura Herz is a Professor of Physics at the University of Oxford. She received her PhD in Physics from the University of Cambridge in 2002 and was a Research Fellow at St John's College Cambridge from 2001 - 2003 after which she moved to Oxford. Her research interests lie in the area of organic and organic/inorganic hybrid semiconductors including aspects such as self-assembly, nano-scale effects, energy-transfer and light-harvesting for solar energy conversion.
Organic-inorganic metal halide perovskites have emerged as attractive materials for solar cells with power-conversion efficiencies now exceeding 25%. However, challenges and opportunities remain relating to material microstructure, ionic migration and toxicity. While tin halide perovskites offer lead-free alternatives to the currently best-performing lead halide perovskites, their prevalence towards tin vacancy formation and oxidation makes these materials particularly unstable [1].
We have recently investigated ultrafast charge-carrier dynamics in lead-free silver-bismuth semiconductors[2-4] which promise lower toxicity and potentially higher barriers against ion migration than their more prominent lead-halide counterparts. We examined the evolution of photoexcited charge carriers in the double perovskite Cs2AgBiBr6 using a combination of temperature-dependent photoluminescence, absorption and optical pump−terahertz probe spectroscopy.[2] We observe rapid decays in terahertz photoconductivity transients that reveal an ultrafast, barrier-free localization of free carriers on the time scale of 1.0 ps to an intrinsic small polaronic state. While the initially photogenerated delocalized charge carriers show bandlike transport, the self-trapped, small polaronic state exhibits temperature-activated mobilities, allowing the mobilities of both to still exceed 1 cm2V−1s−1 at room temperature. Self-trapped charge carriers subsequently diffuse to color centers, causing broad emission that is strongly red-shifted from a direct band edge. Overall, our observations suggest that strong electron−phonon coupling in this material induces rapid charge-carrier localization which may inhibit the use of this material as an efficient light harvester in photovoltaic devices.
We further demonstrate the novel lead-free semiconductor Cu2AgBiI6 which exhibits several advantages over Cs2AgBiBr6, namely a low exciton binding energy of ~29 meV and a lower and direct band gap near 2.1 eV,[3,4,5] making it a significantly more attractive lead-free material for photovoltaic applications. However, charge carriers in Cu2AgBiI6 are found to exhibit similarly strong charge-lattice coupling strength[3] to that in Cs2AgBiBr6, suggesting a link with the presence of AgBi. Tuning such charge-lattice interactions therefore emerges as a serious challenge for this class of materials.
2.3-S1
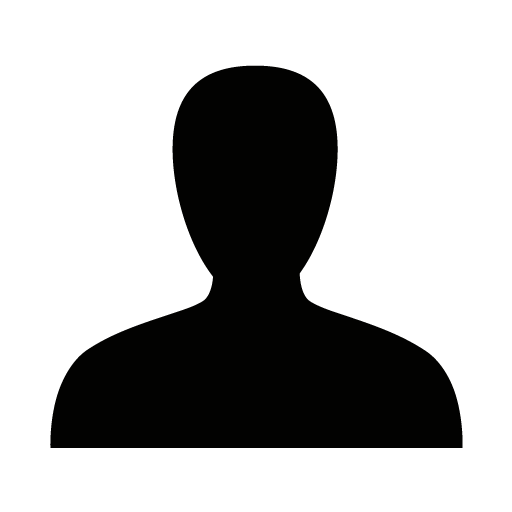
The RSC recently launched Energy Advances, EES Catalysis and RSC Sustainability – three new journals themed around energy & sustainability, as part of our ongoing commitment to support the chemical sciences in facing up to global sustainability challenges. Come along and learn how these journals will accelerate progress to achieving the UN Sustainable Development Goals (SDGs) by 2030.
The RSC recently launched Energy Advances, EES Catalysis and RSC Sustainability – three new journals themed around energy & sustainability, as part of our ongoing commitment to support the chemical sciences in facing up to global sustainability challenges. Come along and learn how these journals will accelerate progress to achieving the UN Sustainable Development Goals (SDGs) by 2030.
The RSC recently launched Energy Advances, EES Catalysis and RSC Sustainability – three new journals themed around energy & sustainability, as part of our ongoing commitment to support the chemical sciences in facing up to global sustainability challenges. Come along and learn how these journals will accelerate progress to achieving the UN Sustainable Development Goals (SDGs) by 2030.
2.3-I3
Silver-bismuth double perovskites (Ag-Bi DPs) are promising candidates for less toxic and highly stable metal halide perovskites, but their optoelectronic performances still lag behind those of the lead halide counterpart, due to the indirect nature of the bandgap and the strong electron-phonon coupling.[1] To overcome these limitations and enable use in photovoltaics and in other applications beyond photovoltaics,[2] researchers are investigating a large plethora of structural variations on these compounds, which might improve their properties and even reveal the emergence of unexected ones.
In this contribution, we will report on our recent efforts addressed at the tuning of the Ag-Bi DP scaffold optical properties, through the doping with lanthanide ions [3] and the variation of the dimensionality into 2D monolayer species.[4,5] We will also show some very recent results on the preparation of highly sustainable photovoltaic devices suitable for indoor applications such as those connected to the powering of the Internet of Things ecosystem,[6] based on Ag-Bi DP photoactive layers and carbon electrodes obtained from the recovery of waste (submitted work).
References
[1] Z. Jin, Z. Zhang, J. Xiu, H. Song, T. Gatti, Z. He "A critical review on Bismuth and Antimony-based halide perovskites for photovoltaics and optoelectronics" J. Mater. Chem. A 8, 16166-16188 (2020).
[2] L. A. Muscarella, E. M. Hutter "Halide double perovskite semiconductors beyond photovoltaics" ACS Energy Lett. 7, 2128–2135 (2022)
[3] F. Schmitz, K. Guo, J. Horn, R. Sorrentino, G. Conforto, F. Lamberti, R. Brescia, F. Drago, M. Prato, Z. He, U. Giovanella, F. Cacialli, D. Schlettwein, D. Meggiolaro, T. Gatti "Lanthanide induced photoluminescence in lead-free Cs2AgBiBr6 bulk perovskite: insights from optical and theoretical investigations" J. Phys. Chem Lett. 11, 8893-8900 (2020)
[4] F. Schmitz, J. Horn, N. Dengo, A. E. Sedykh, J. Becker, E. Maiworm, P. Bélteky, A. Kukovecz, S. Gross, F. Lamberti, K. Müller-Buschbaum, D. Schlettwein, D. Meggiolaro, M. Righetto, T. Gatti "Large cation engineering in two-dimensional silver-bismuth bromide double perovskites" Chem. Mater. 33, 4688-4700 (2021)
[5] F. Schmitz, R. Neisius, J. Horn, J. Sann, D. Schlettwein, M. Gerhard, T. Gatti "Tuning the optical properties of 2D monolayer silver-bismuth bromide double perovskite by halide substitution" Nanotechnology 33, 215706 (2022)
[6] V. Pecunia, L. Occhipinti, R. Z. Hoye "Emerging indoor photovoltaic technologies for sustainable Internet of Things" Adv. Energy Mater. 11, 2100698 (2021)
1.1-T1
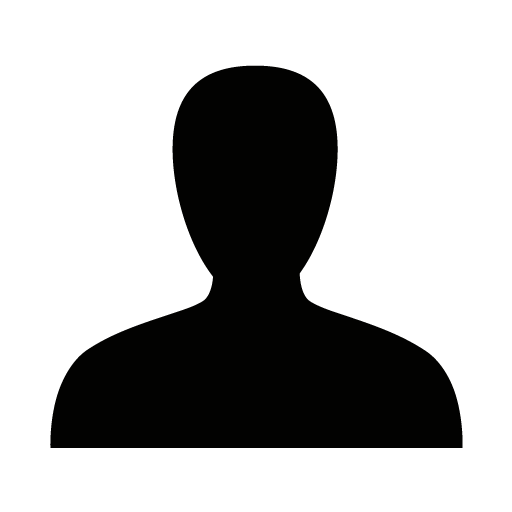
Integrating storage technologies in the future renewable energy system is one of the most important nowadays problems. Recent developments in small scale consumer electronics, trend for implementing “Internet of Things” and smart house smart cities concepts make it imperative to have cheap, wireless power solution for all-time operation electronics. The combination of photovoltaic (PV) devices with rechargeable batteries represents a viable strategy for powering such low power electronic devices. With the increasing use of indoor LED (light emitting diode) lighting worldwide, the matching LED output spectrum with the absorption spectrum of lead halide perovskite solar cells (PSC) affords an opportunity to reuse emitted light with high efficiency to feed low power electronics. Recently, lead halide perovskite cells and modules have demonstrated efficiencies under artificial lighting in excess of 30 % [1] with a record of 40.1 % achieved with an extra thick absorber layer [2]. The main reason bringing forward PSCs is very close overlap of the external quantum efficiency in lead halide perovskite solar cells with the emission spectrum of an LED lamp. However, there is not many publications showing a combination of perovskite-battery devices working efficiently under low light LED illumination intensities.
AIM AND APPROACH
The aim of the work is to demonstrate a successful and highly efficient energy harvesting and storage under a wide range of light emitting diode (LED) illumination intensities by applying lead halide perovskite solar module directly coupled to a high-rate capable next generation sodium ion battery. Direct coupling of PV and batteries require fabrication of perovskite solar module with tailored current-voltage (IV) characteristic to match battery voltage under target irradiance conditions. A 3-cell perovskite module with CH3NH3Pb(I0.8Br0.2)3 absorber layer and fullerene electron transport layer [3] was fabricated with a conversion efficiency of 17.8 %, with a fill factor of 81.3 %, short-circuit current density of 5.91 mAcm-2 and open-circuit voltage of 3.71 V under AM.1.5 illumination. To test the PSC modules for indoor battery charging under LED lighting a sodium ion battery with metallic sodium anode and cathode made from sodium titanium phosphate (NaTi2(PO4)2) coated onto sheets of carbon Nano felt (NTP@CNF) was chosen due to its high charge rate capability, low charge-discharge overpotential and distinct charge and discharge voltage plateau [4]. LED illumination intensity was attenuated by using neutral density filters.
CONCLUSION
High efficiency indoor charging of advanced sodium ion battery based on sodium titanium phosphate (NaTi2(PO4)2) coated onto sheets of carbon Nano felt using a perovskite solar module with CH3NH3Pb(I0.8Br0.2)3 absorber layer under LED illumination was demonstrated. We have directly coupled both devices without any power electronics and achieved overall PV-Battery efficiency of 24.3% under LED illumination. Under target LED illuminance of 300 lux, the perovskite solar cell shows PCE of 29.4%, and coupling factor of 0.87, and roundtrip battery efficiency of 94.9 % [5].
[5] Kin L.C-., Joule, ‘Efficient indoor light harvesting with MAP(I0.8Br0.2)3 solar modules and Na-ion battery’ 2022 submitted.
1.1-T2
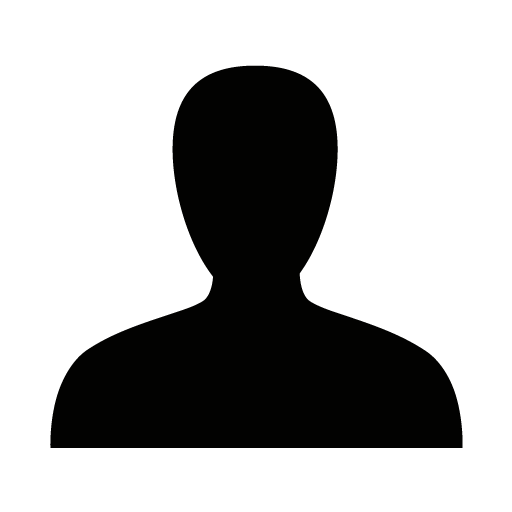
Perovskite solar cells have emerged as a promising and highly efficient solar technology. Despite efficiencies continuing to climb, reaching a new record of 25.7%1, the prospect of industrial manufacture is in part hampered by concerns regarding the safety and sustainability of the solvents used in lab scale manufacture. Here, we aim to present a methodology for green solvent selection informed by EHS considerations from the CHEM-21 solvent guide for succesful methylammonium lead triiodide (MAPbI3) precursor dissolution2. Through the use of this methodology we present a N,N-dimethylformamide (DMF)-free alternative solvent system for deposition of MAPbI3 precursors (MAI and PbI2) consisting of dimethyl sulfoxide (DMSO), dimethylpropyleneurea (DMPU), 2-methyltetrahydrofuran (2-MeTHF) and ethanol (EtOH). Perovskite films cast from the three candidate solutions show improved crystallinity, higher fluorescence emission, and improved crystal size uniformity than those cast from DMF/DMSO and similar photovoltaic performance (16.2% for DMF/DMSO, 16.1% for candidate A solvent system2). We will cover the key solvent parameters which determine effective MAPbI3 precursor dissolution; provide a set of criteria for appropriate alternative solvent selection; and demonstrate the application of green chemistry principles to solvent selection for perovskite photovoltaic manufacturing. Due to significant advancement in the perovskite research sphere, more thermally stable and efficient perovskite compositions have risen to prominence. This includes the now ubiquitous ‘triple cation’ perovskite (Cs0.05(MA0.17FA0.83)0.95Pb(I0.83Br0.17)3)3, providing increased impetus to study solvent interactions in these more complex colloidal dispersions. We will discuss recent progress towards ‘green’ solvent engineering strategies specifically tailored towards these compositions, further highlighting key parameters requiring control to potentially improve the optoelectronic properties of this promising material.
1 Best Research-Cell Efficiency Chart | Photovoltaic Research | NREL, https://www.nrel.gov/pv/cell-efficiency.html, (accessed 7 April 2020).
2 A. J. Doolin, R. G. Charles, C. S. P. De Castro, R. G. Rodriguez, E. V. Péan, R. Patidar, T. Dunlop, C. Charbonneau, T. Watson and M. L. Davies, Green Chem., 2021, 23, 2471–2486.
3 M. Saliba, J.-P. Correa-Baena, C. M. Wolff, M. Stolterfoht, N. Phung, S. Albrecht, D. Neher and A. Abate, Chem. Mater., 2018, 30, 4193–4201.
1.1-T3
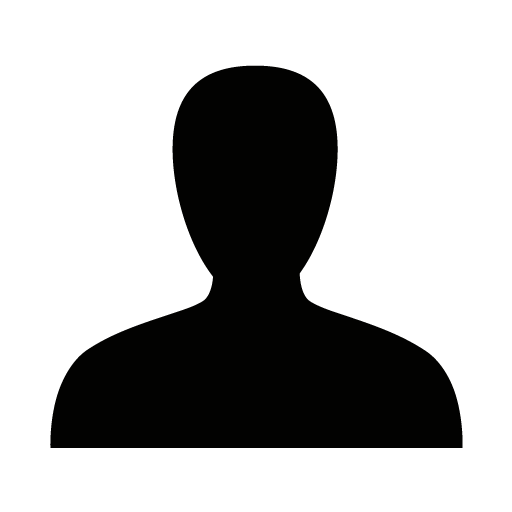
Thermal processing is critical in preparing the functional layers for perovskite solar cells (PSCs), such as metal oxides (TiO2 and SnO2) electron transport layers (ETLs), and perovskite layer. The conventional thermal processing method using a furnace or hotplate with a time-consuming heating and cooling period impedes the possibility of achieving a rapid and in-line production for the commercialization of PSCs.
Laser processing is an advanced manufacturing method that owns various advantages over conventional thermal processing, including being rapid, scalable, contact-free, area-selective, and causing minimal thermal damage.
In this presentation, I will summarize our recent studies carried out at The University of Manchester using lasers to fabricate a range of ETLs, including compact and mesoporous TiO2, Ta-doped TiO2, SnO2, and ZnO for efficient rigid and flexible PSCs [1-3]. In addition, I will introduce our latest study using the laser-induced graphene decorated with the NiOx nanoparticles as the back electrode for hole-transport-layer-free PSCs.
1.1-T4
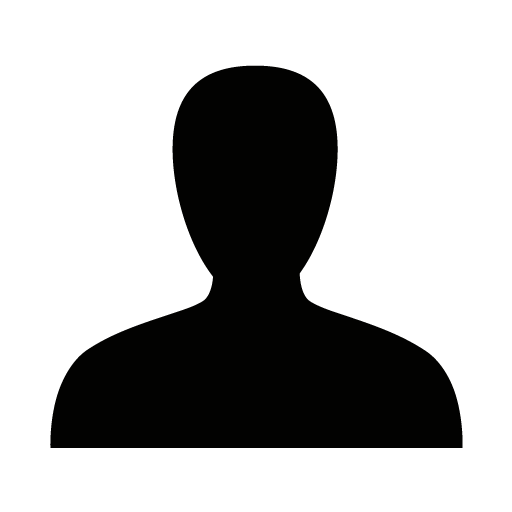
The intensive research on perovskite solar cells (PSC) in the last years has allowed the technology to reach exceptional results, with a record power conversion efficiency (PCE) of 25.5% [1] for single junction cells. Due to low temperature processing, band gap tunability and flexible composition, this class of materials is also highly employed in multijunction, or tandem, photovoltaic applications. With so many demonstrated advantages, one of the remaining challenges for the industrialization and commercialization of the technology is the upscaling of fabrication of perovskite films. Large-area deposition methods such as (ultrasonic) spray coating, inkjet printing, slot dye coating, and blade coating provide great avenues for high throughput fabrication, but often come with a significant decrease in PCE when compared to spin coated devices [2].
This study proposes a design of experiment approach, aiming to optimize the fabrication of perovskite thin films by ultrasonic spray coating. Initially the entire process was reviewed, from substrate cleaning and solution preparation to deposition and post treatment of the film, and key parameters were drawn out. Based on this analysis, and previous studies [3], experiments were designed to gain further insight on two important steps of the process: the deposition of a wet layer of precursor solution by ultrasonic spray coating, and the gas-quenching of the solvent for perovskite crystallization.
The deposition step was examined by a one factor at a time (OFAT) approach, with the establishment of a set of standard parameters and the variation and analysis of each parameter individually. By doing so it was possible to verify that the spray coating parameter are paramount to the formation of a homogeneous wet layer and good coverage of the substrate, yet the fine tuning of the perovskite crystallization, and consequentially the layer quality, depends mostly on the evaporation step.
The gas quenching-assisted evaporation was then thoroughly studied by a full factorial analysis of three key parameters: gas (nitrogen) pressure, distance between nitrogen gun and substrate, and solvent volatility, determined by the ratio between a low volatile and a high volatile solvent. The produced films were then characterized in regard to their morphology and performance as the active layer of a solar cell.
This full factorial analysis provided insight on the complex mechanism of perovskite crystallization in a thin film. It also allowed the assessment of interactions between the parameters and shed light on how they influence the evaporation of solvent in different regimes. Moreover, the use of design of experiments resulted in higher efficiencies than the previously trial-and-error approach, with the best performing cell reaching a PCE of 18.1%, which is in line with spin coated perovskite solar cells with similar architecture, i.e. nickel oxide (NiOx) as hole transport layer, no additives on the perovskite solution, and no anti-reflection or passivation layers.
1.1-T5
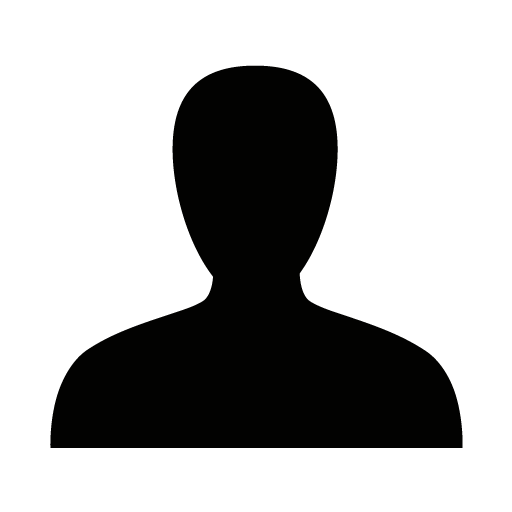
Perovskite-based solar cells have shaken the photovoltaic sector achieving more than 25% efficient laboratory-scale solar cells in around 10 years. Now, they are on the way to breaking the 30% efficiency threshold by being coupled with established technologies such as Silicon and CI(G)S forming a multijunction configuration.
As demand for a more sustainable and decentralized electricity system increases, low-cost and lightweight thin-film based multijunction are an ideal match for integrated PV requirements. On the other hand, urban environments increase stress-induced losses due to partial shading and weather alterations on PV strings. Most importantly, both thin-film technologies (CIGS and Perovskite) have shown lower reverse breakdown voltages[1-2], which increases the need for bypass diodes and in some cases reverse bias-induced degradation [3]. It is therefore essential to consider alternative module designs to optimize the desired power outputs and increase the long term reliability of BIPV/VIPV systems.
In this study, we designed an improved SPICE simulation[4] of a PV module to assess the impact of material’s and design’s parameters under partial shadow conditions, with a focus on alternative designs for thin-film based integrated photovoltaics applications. To guarantee safe real life installation and operation, realistic limitations are also taken into consideration as boundary conditions. As a result of our research, we present suitable alternative designs based on different absorber materials, sizes, and interconnection schemes and inclusive of 2 terminal, current matched multijunction CIGS/Perovskite devices. For each configuration, we also report the local impact of shadow-induced thermal losses based on a SPICE equivalent thermal circuit.
1.1-T6
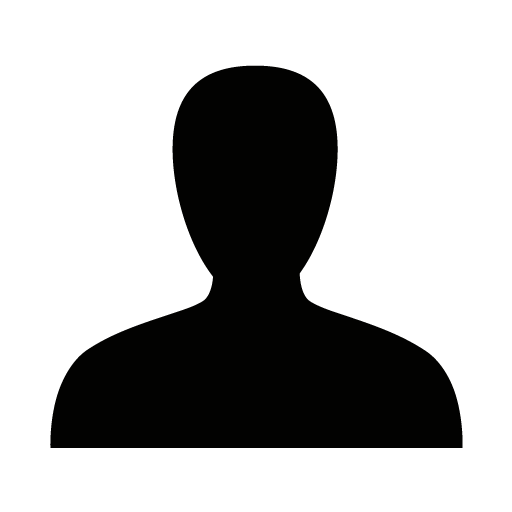
A solar cell is an energy conversion device from natural to renewable energy. Since the supplying energy from a solar cell fluctuates, energy storage is required for a user-on-demand energy supply system. The energy needs to change into the storable form of a battery and of hydrogen. The battery can respond to rapid power change, but the cost is proportional to the storage size. Therefore, it is suitable for a relatively small amount of energy storage. Hydrogen is good for relatively large energy storage because the storage cost is not so expensive. However, it is not suitable for a rapid reaction because the water electrolyzer and fuel cell respond slowly. Thus, the hybrid system of the battery and hydrogen storage is needed for the user-on-demand and especially for the independent energy supply system. The system optimization is, however, still obscure due to its complexity. Suitable device characteristics are also needed for system optimization.
The first discussion point is the device size and efficiency of the system. The direct use of the generated energy from the solar cells is the most efficient, but the user needs the energy independent of the solar power generation period. Thus, the capacity of the storage and the conversion efficiency of devices must be considered in the design of the energy supply system. The design is complicated especially for the battery and hydrogen hybrid storage system due to the multiple storage devices existing in a system. The system design procedure was evaluated using the independent self-controlled energy supply system [1]. It was clarified that the energy storage methods and the generated power by solar cells or the user demand are essential to determine the system size.
The second discussion point is that the device response affects the system's operation. The DC/DC converter is used in the system to convert the voltage between the DC bus line and the device operations [1]. A typical DC/DC converter focuses on the current stability and not on the instantaneous over- or under-voltage existing at the step-like current change. Especially, small-sized DC/DC converters tend to be observed these voltages. These instantaneous voltages affect the system operation and make the system operation unstable in some cases. The response without these voltages was found to be important for stable system operation.
1.2-I1
Solution-processed organic, and perovskite solar cells have reached promising power conversion efficiency (PCE) records in recent years on cells with active area <1 cm2. Transferring the performance from laboratory scale to real modules is typically associated with losses in PCE. The origin of such upscaling losses is manifold and includes potential drops in the electrodes due to a non-optimized electrode design or an increase of defects and inhomogeneities of the active layers. To minimize those losses, the first step is to quantify the significance of each loss channel. In the first part, we use a combination of straightforward steady-state measurement techniques on lab-scale devices and mini-modules with a photoactive area of ~37 cm2 to set up a reliable digital twin of the device in the FEM-based simulation tool Laoss. This digital twin allows us to understand the existing performance limitation of the fabricated device. In a second step, the model is used to optimize performance by sequentially switching off various losses, varying the geometry of the module and combining this to obtain a thorough loss diagram. For the specific module, it is found that 50% of the PCE is lost due to an internal series resistance when moving from lab to module scale. It is attributed to a modified interface when partially fabricated in ambient conditions. This could be further evidenced by evaluating the performance of small cells as well as by electro- (EL) and photoluminescence imaging. In the second part, we use imaging techniques (EL and dark lock-in thermography) to detect different impurities and defects in perovskite solar cells. We find features that can be related to manufacturing issues but also point-like defects that occur during storage. With simulations, we can quantify the latter and eventually follow its evolution during storage time.
1.2-I2
Organic semiconductor-based photovoltaic (OPV) devices have many properties that make them attractive for indoor applications, such as tailorable light absorption, low embodied energy manufacturing and cost, structural conformality, and low material toxicity. Compared to their use as organic solar cells for standard outdoor solar harvesting, indoor OPV (IOPV) devices operate at low light intensities and thus demonstrate different area-scaling behaviour. In particular, the performance of large-area IOPV devices is much less affected by the sheet resistances of the transparent conductive electrodes (a major limit in organic solar cells), but instead by factors such as their shunt resistance at low light intensities. Herein, we review physical insight into IOPV systems using drift-diffusion and finite element modelling and compare this to real devices measured under indoor lighting conditions. Measurement considerations for IOPV devices are reviewed to accurately compare figures of merit necessary for the systems integration into the internet of things (IoT) and embedded sensor applications.
2.1-I1
René Janssen is university professor at the Eindhoven University of Technology (TU/e). He received his Ph.D. in 1987 from the TU/e for a thesis on electron spin resonance and quantum chemical calculations of organic radicals in single crystals. He was lecturer at the TU/e since 1984, and a senior lecturer in physical organic chemistry since 1991. In 1993 and 1994 he joined the group of Professor Alan J. Heeger (Nobel laureate in 2000) at the University of California Santa Barbara as associate researcher to work on the photophysical properties of conjugated polymers. Presently the research of his group focuses on functional conjugated molecules and macromolecules as well as hybrid semiconductor materials that may find application in advanced technological applications. The synthesis of new materials is combined with time-resolved optical spectroscopy, electrochemistry, morphological characterization and the preparation of prototype devices to accomplish these goals. René Janssen has co-authored more than 600 scientific papers. He is co-recipient of the René Descartes Prize from the European Commission for outstanding collaborative research, and received the Research Prize of The Royal Institute of Engineers and in The Netherlands for his work. In 2015 René Janssen was awarded with the Spinoza Prize of The Dutch Research Council.
A unique property of metal halide perovskite solar cells materials is their widely tunable optical bandgap, which enables their use in multijunction solar cells. However, both mixed-halide wide-bandgap perovskites and lead-tin narrow-bandgap perovskites suffer from non-radiative recombination due to the formation of bulk traps and interfacial recombination centers which limit the open-circuit voltage of sub-cells and consequently of the integrated tandem. Additionally, the complex optical stack in a multijunction solar cell can lead to losses stemming from parasitic absorption and reflection of incident light which aggravates the current mismatch between sub-cells, thereby limiting the short-circuit current density of the tandem. Here, we present an integrated all-perovskite tandem solar cell that uses surface passivation strategies to reduce non-radiative recombination at the perovskite-fullerene interfaces, yielding a high open-circuit voltage. By using optically benign transparent electrode and charge-transport layers, absorption in the narrow-bandgap sub-cell is improved, leading to an improvement in current-matching between sub-cells. Collectively, these strategies allow the development of a monolithic tandem solar cell exhibiting a power-conversion efficiency of over 23%.
2.1-I2
Jenny Nelson is a Professor of Physics at Imperial College London, where she has researched novel varieties of material for use in solar cells since 1989. Her current research is focussed on understanding the properties of molecular semiconductor materials and their application to organic solar cells. This work combines fundamental electrical, spectroscopic and structural studies of molecular electronic materials with numerical modelling and device studies, with the aim of optimising the performance of plastic solar cells. She has published around 200 articles in peer reviewed journals, several book chapters and a book on the physics of solar cells.
Achieving deep impacts on greenhouse gas emissions and efficient energy use through use of renewable technologies such as photovoltaics will require the thoughtful integration of the solar module into a final application. The overall efficiency of solar energy utilisation can be improved if the module is integrated with another function, such as photochemical energy conversion, solar thermal conversion, plant growth or energy storage. Organic photovoltaic technology brings potential advantages to some applications where spectral sharing or flexible form factor are needed. In other cases, the choice of PV technology may be much less important than other components or overall system design. Optimising the integrated system requires understanding of the optical and electrical response of the solar module in different conditions, control of those properties and suitable models of the whole system performance. We will present examples of the integration of PV with other functions, including photochemical energy conversion, and consider how system design and operation influence overall performance.
2.1-I3
Christoph J. Brabec is holding the chair “materials for electronics and energy technology (i-MEET)” at the materials science of the Friedrich Alexander University Erlangen-Nürnberg. Further, he is the scientific director of the Erlangen division of the Bavarian research institute for renewable energy (ZAE Bayern, Erlangen).
He received his PhD (1995) in physical chemistry from Linz university, joined the group of Prof Alan Heeger at UCSB for a sabbatical, and continued to work on all aspects of organic semiconductor spectroscopy as assistant professor at Linz university with Prof. Serdar Sariciftci. He joined the SIEMENS research labs as project leader for organic semiconductor devices in 2001 and joined Konarka in 2004, where he was holding the position of the CTO before joining university.
He is author and co-author of more than 150 papers and 200 patents and patent applications, and finished his habilitation in physical chemistry in 2003.
OPV efficiency values are moving towards > 20 % for small area cells and > 15 % for large scale modules. With these performance values, OPV is reaching out to applications that are going beyond the typical niche markets. Integration of OPV into functional objects is attractive for building integrated / attached PV, window integrated PV, vehicle integrated PV, textile PV or agri-/horti PV. Most interestingly, all these application scenarios are related to flexibility and light weight. However, besides few demonstrations of ultra-flexible and foldable organic solar cells, the limitations of lightweight OPV that is compatible to mass production are not fully understood.
OPV devices require encapsulation by barrier films to reduce the degradation driven by extrinsic factors, which in turn limits their flexibility and leads to lower specific power values. In this talk, we analyze the packaging requirements for light weight cells and modules. Fully solution-processed and semitransparent organic solar cells performing comparable with conventional indium tin oxide-based devices are processed directly onto different barrier films of varying thicknesses. Direct cell fabrication onto barrier films leads to the elimination of the additional PET substrate and one of the two adhesive layers. In addition to the increase of the specific power to 0.38 W/g, which is more than four times higher than sandwich-encapsulated devices. These organic solar cells exhibit better flexibility and survive 5000 bending cycles with 4.5 mm bending radius, show comparable stability as conventionally encapsulated devices under constant illumination (1 sun) in ambient air for 1000 h. A further step to increase the W/g Figure of Merit is to replace the top laminated barrier by a printed barrier, reducing the weight by another factor of 2. In combination with high performance NFA based composites, these architectures have the potential to overcome the 1 W/gr milestone while still being compatible to roll to roll production.
2.2-I1
Intel corporation predict that by the end of 2020, up to 200 billion connected Internet of Things (IoT) devices came online and this is predicted to consume over 1000 TWh yr-1 by 2025. To put this in perspective, this is over 1300 x the energy output of EU’s largest solar plant, Núñez de Balboa. The increasing digital interconnectivity of our everyday lives means that this energy burden will only increase in the coming decades. In addition, many portable IoT devices incorporate primary batteries, which consume valuable materials. One solution to this growing demand is the coupling of IoT nodes with energy harvesting devices, such as photovoltaics cells which could provide power to IoT nodes and avoid the need to replace primary batteries.
Hybrid solar cells such as perovskites, OPVs and DSSCs, have all shown promising performance in ambient light [1], meaning that any of these technologies could be candidates to power future IoT devices. Despite the promise of these PV technologies, work needs to be done especially around standards for testing. There is currently no consensus on what constitutes “ambient light” both in terms of spectral and power output and it is difficult to compare results from one laboratory to the next. We can predict the path of the Sun for any given point on Earth, but predicting how much light power is available in any given ambient scenario is difficult and reliant on human factors such as available light sources (e.g. CFT, LED, natural, or combination) and position of the solar cell relative to the light source.
This presentation will explore these issues, discussing what we mean when we say low or ambient light, and showing the results of maximum power measurement in real and simulated scenarios and how in some cases, an IV curve measured at single lux value is not an accurate predicator of real-world performance. Examples of IoT devices powered by organic and perovskite PVs will be shown and real-World performance discussed.
2.2-I2
Semi-transparent Organic Solar Cells (ST-OSCs) have unparalleled ability to tailor the optical transmission to a targeted application such as their integration into buildings, where color-neutrality is generally favored, and integration into greenhouses, where the transmision might be matched to the light needs of plants. Plants have a complex response to light quality (the spectral distributions) and light quantity (the total intensity) and have often adopted to a particular envionment. For example, lattice is a low light plant that can get severely stressed above 20% of solar radiation, at which point photosynthesis plateaus and excess energy if converted into protective molecules such as anthocyanines or shunted. In contrast, tomatoes are high light intensity plants. We will discuss the status of work by a team at NCSU to energy balances, economics, and the impact of filtered light on plant growth. We find that the heating and colling requirements can be significantly effected by ST-OSCs and that greenhouses with net-zero energy balance can be operated in all but the coldest climates in the US [1]. Additionally, studies on lettucie indicate no negative impact on yield [2]. This energy balance in yield translates into favorouble economics when comparing ST-OSC greenhouses to conventional greenhouses across many different climate classes [3]. Interestingly, the use ST-OSC can also impact genetic expression and alter for example the plant’s nitrogen utilization [4]. All these factors bode well for this application of ST-OSC technology and the use-case is rather stong. Yet, the problems regarding lifetime of the devices and fabrication costs of properly encapsualted and integrated modules have yet to be solved.
2.2-I3
Organic solar cells allow for unobtrusive integration into surfaces of consumer electronics that are hardly feasible with established technologies. Alongside with excellent low-light performance, both opaque and semi-transparent solar cells can fit arbitrary shapes and match various colors.[1] To date, the main application for photovoltaics is power generation for the electric grid and accordingly the development of photovoltaics focuses on lowering production costs and maximizing power conversion efficiencies under direct sun illumination. Yet, emerging applications for autarkic IoT devices demand rethinking of what the optimization parameters for photovoltaics are.
Here we demonstrate fascinating properties of self-sufficient “Solar Glasses” – sunglasses that feature semi-transparent organic solar cells instead of shaded lenses.[2]
Quite literally hidden in plain sight, the solar cells serve both as light sensors and live power supply for integrated electronics and LC displays simply by harvesting ambient light. This talk dives into the special requirements and novel opportunities for mobile sensor applications and indoor photovoltaics that are remarkably different from classical power harvesting solar cells.
2.3-I1
Organic-inorganic hybrid perovskite photovoltaics have attracted tremendous attention due to rapid progress in terms of power conversion efficiency in the last few years, from 3.8% to present record values in excess of 25%. However, fundamental problems, such as the toxicity of hybrid lead halide perovskites, hysteresis and structural instability remain to be solved for their application and commercialization. Indeed, the low-temperature solution-processing of perovskite films inevitably causes formation of a certain amount of defects on the surface and at the grain boundaries, which lead to serious trapping, charge accumulation, and recombination problems as well as stability issue. We pursue the interface engineering, defects passivation, and dimensional tunability approaches via introducing various types of multifunctional conjugated organic compounds to improve the stability and performance of perovskite photovoltaics.
In this presentation, we will discuss our achieved results in details for the above-mentioned approaches with a central focus on the fundamental complexity of microstructure and charge transport mechanisms and their correlation to the device performance, stability and indoor application.
2.3-I2
In the recent years, Perovskite solar cells (PSCs) have achieved impressive efficiencies and have progressed on the scale of technological availability close to becoming a potential reality for the market. However, many challenges still need to be addressed to enable full industrial production and commercialization.
Basic research is continually developing and understanding the interactions of materials to increase efficiency and lifetime but most of the time do not taken in consideration the need in a large-scale production, such as solvent toxicity, materials degradetion, the establishment of the supply chain, costs and CAPital expenditure of the production line (CAPEX). Therefore, the technological scale-up, from small laboratory cells to real-size modules and panels, involves several steps and peculiarities.
In the field of PSC, the best results currently obtained on small devices are found on chlorinated or halogen based inks processed in an inert atmosphere, which favor a better crystallization of the active layer and translate into higher performance. Furthermore, lead-based perovskites are the most ready option for a potential market in terms of performance and relative ease of manufacture. However, those formulations are not the best choice for scale-up due to health and safety concerns and best practices, requiring the development of safety control protocols that are not an issue in small-scale or laboratory research.
In this work, we present the main challenges of increasing PSC from rigid laboratory-scale devices to flexible cells fabricated with scalable processing techniques. The transfer from doctor blade devices prepared under controlled conditions (RH <35%) to roll-to-roll solt-die (R2R) coated films manufactured in a laboratory setting (ISO-7 cleanroom) is reported, paving the way for future industrialization of this promising photovoltaic technology.
1.1-T1
Prof. Dieter Neher studied physics at the University of Mainz. In 1990 he gained his PhD with Prof. G. Wegner. From 1990-1992 he was a research associate at the Optical Sciences Centre, Tucson, Arizona and at the Centre for Research in Electrooptics and Lasers, Orlando, Florida with Prof. G. Stegeman. 1992 he joined again Prof. G. Wegner at the MPI-P, heading the group Electrooptical Phenomena in Polymers. Following his habilitation in November 1998, he became Professor of Soft Matter Physics at the Institute for Physics and Astronomy at the University of Potsdam. Current research interests are electrical, optical and optoelectronic processes in conjugated materials.
The introduction of Y-series non-fullerene acceptor such as Y6 have helped to push the power conversion efficiency of organic solar cells above 18 % in single and 19 % in multiple junction organic solar cells. While the wide absorption spectrum of state of the art organic blends guarantees high short circuit currents (Jsc), the fill factor (FF) and in particular the open circuit voltage (Voc) lack well behind the Shockley Queisser limit.
Here we present the results of detailed investigations of Y-series NFAs blended with the donor polymer PM6. Regarding free charge recombination, we find that the reoccupation of the highly emissive Y6 singlet exciton causes almost all photon emission from of the solar cell, but that this pathway contributes to less than 1 % of the total recombination current [1]. On the other hand, while the decay of the decay of the CT state entirely dominates charge recombination, its optical properties remain hidden under the strong Y6 singlet exciton absorption and emission. Interestingly, it is only for very low CT state absorption combined with a fairly high CT radiative efficiency that the solar cell benefits from the radiative properties of the singlet excitons. We also find that despite the high structural order of the PM6:YY6 blend, energetic disorder causes a substantial loss in the Voc [2]. Further studies suggest that the same energetic disorder accelerates the CT decay and with this non-geminate recombination, being the main cause for the non-ideal FF. We conclude that major performances losses in such blends are connected to the CT state properties and energetic disorder, both being difficult to disclose by conventional spectroscopic technqiues
1.1-T2
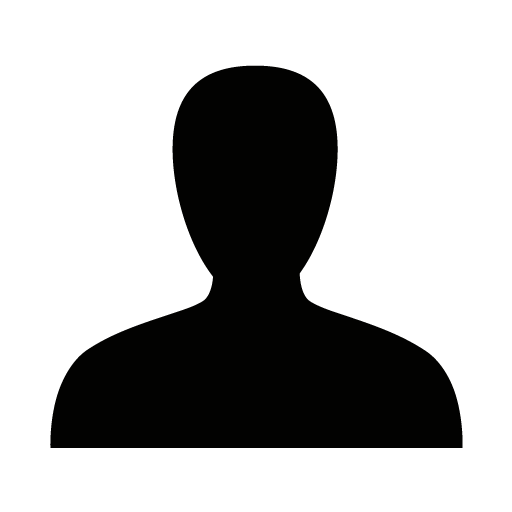
The design and operation of organic photovoltaic devices (OPVs) rely primarily on the electronic structure of organic semiconductors (OSCs). For instance, the dissociation of the exciton at the donor-acceptor (D-A) interface is determined by the energy level offset between the highest energy occupied molecular orbital (HOMO) and the lowest energy unoccupied orbital (LUMO) between the donor and the acceptor. Consequently, the measurement technique and its accuracy to determine the frontier molecular energies of OSCs are critical for designing efficient devices.
Several techniques, such as cyclic voltammetry (CV), ultraviolet photoelectron spectroscopy (UPS), low-energy inverted photoelectron spectroscopy (LE-IPES), or photoelectron spectroscopy in air (PESA), are used to measure the ionization energy (IE) and the electron affinity (EA) of OSCs. CV is more commonly used in OPV field for its accessibility and its simple operation. However, differences between the absolute energy levels measured by CV and photoelectron spectroscopy (PES) have been reported. The range of energy level values for identical material measured with CV shows the uncertainties and the inconsistency of the measurement protocol of this method. Moreover, direct measurement of the EA can be challenging when measured with CV, and indirect methods are used to estimate it by calculation using the IE and the optical gap. Since the optical gap been usually lower than the transport gap (difference between the IE and EA of a same material), that kind of approximation can lead to contradictory conclusion. Several high performing blends have been reported to have negligeable energy offset, but other studies are showing that an IE offset of 0.5eV is necessary for efficient charge transfer.
In this work, we highlight the differences in the IE and EA values for different commonly used OSCs between CV and UPS/LE-IPES measurements. The work on OPV devices made with different D-A blends shows correlation between the energetic properties of the pristine donor and non-fullerene acceptor (NFA) and the OPV devices’ parameters. When measured with PES methods, the photovoltaic gap Epv, difference between the IE of the donor and the EA of the acceptor, corelate better with the open-circuit voltage (Voc), compared to CV measurements. This led to a good path to predict the maximum Voc of OPV devices. A further study on blends like PM6:Y6 reported as high-performing blends with low IE offset, based on CV measurement, reveals a bigger energy offset when measured with PES techniques. In contrast, devices with low IE offset measured with PES methods demonstrate a non-efficient charge generation which can be observed with their low short-circuit current (Jsc). This may bring the community to rethink the behaviors and the correct designs of OPVs. This work establishes a solid base for reliably evaluating material energetics and can be relevant to a broader community working on OSCs for electronic applications.
1.1-T3
Probing the photoluminescence of free charge carriers in organic solar cells: derivation of the separation of the quasi Fermi levels
Uli Würfel1,2
1 Fraunhofer Institute for Solar Energy Systems ISE, Heidenhofstr. 2, 79110 Freiburg, Germany
2 Freiburg Materials Research Center FMF, University of Freiburg, Stefan-Meier-Str. 21, 79104 Freiburg
Photoluminescence (spectroscopy) is an important characterization method in photovoltaics and it has proven to be very valuable for all types of crystalline inorganic solar cells. This is because in these types of devices, the luminescence signal originates from radiative recombination of free electrons and holes and is therefore a direct measure for the product of their concentrations within the photoactive layer. However, in the case of organic solar cells, the interpretation of photoluminescence is much more complex as the absorption of a photon generates a rather strongly bound exciton in the donor or the acceptor phase. Although most photogenerated excitons dissociate into free charge carriers at donor/acceptor interfaces some of them will decay before this happens. In addition, the probability for their decay to be radiative is much larger than for free charge carriers that recombine via charge transfer (CT) states at the donor/acceptor interface. As a consequence, the PL signal of an organic solar cell is dominated by the contribution from photogenerated singlet excitons in the respective material phases and is therefore not directly correlated to the separation of the quasi Fermi levels.
We have developed a new method of how to separate the PL signals of the photogenerated singlet excitons from the one of the free charge carriers in organic solar cells. This paves the way for gathering deeper insight into the working principles of these devices. Moreover, this particular PL method has the advantage of being applicable not only to complete solar cells but also to half cells and even pure absorber films.
Our results from highly efficient organic solar cells show excellent agreement between the quasi Fermi level separation as derived from the PL intensity of free charge carriers and the electrical voltage as measured between the terminals of the devices.
1.1-T4
The major fill factor loss in organic solar cells is caused by the transport resistance, which arises due to low effective conductivity in these systems. Until recently, this loss mechanism has received little experimental attention, hence is not yet well understood. Using the temperature and illumination intensity dependent Suns-Voc and current-voltage measurements, we have determined the effective conductivity in a set of organic donor-acceptor systems, such as solution processed fullerene and non-fullerene acceptor devices, along with thermally evaporated solar cells based on small molecules. We show that the temperature dependence of the conductivity in these solar cells is closely related to the ideality factor. Furthermore, we demonstrate that the transport resistance can be described analytically in the framework of the multiple trapping and release model. Consequently, the shape of the energetic distribution of localised states, determined from the temperature dependence of the ideality factor, plays a key role in understanding the fill factor losses in organic solar cells.
1.1-I1
Pascal is an early-career researcher in the Department of Physics at the University of Oxford, UK. He holds an EPSRC postdoctoral and David Clarke fellowship, which allows him to conduct his own research independently. Pascal currently investigates All-Small-Molecule Organic Solar Cells, processed from solution or in vacuum. He applies a range of optoelectronic and microstructural characterization techniques to understand and improve organic photovoltaics.
Organic solar cells (OSCs) based exclusively on small molecules have distinct advantages over polymer-based OSCs for commercial applications. Unlike polymers, small molecules have a defined molecular weight and can be purified easily, resulting in a simpler, more reproducible synthesis with controlled quality. Solution-processed All-small-molecule (ASM) OSCs have reached 17% power conversion efficiency, and ASM-OSCs fabricated via vacuum thermal evaporation (VTE) are leading OSC commercialization. However, ASM-OSCs remain less investigated than polymer-based OSCs in terms of their semiconducting and microstructure properties.
Here, we perform extensive optoelectronic characterization on high-performing ASM-OSC deposited from solution and in vacuum to quantify absorption, voltage losses and charge transport – probed via ellipsometry, sensitive EQE and CELIV, respectively. Example systems include VTE DCV5T-Me:C60, and solution-processed BTR-Cl:Y6. We find that certain VTE ASM-OSC achieve strong absorption, decent charge carrier mobility, or voltage losses matching corresponding polymer-based OSCs but no VTE blend combines all these favourable properties. We further investigate the blend microstructure in terms of phase separation. Interdiffusion experiments offer a thermodynamic perspective while the actual phase separation in probed via soft X-rays. This talk gives an overview over prospects and challenges of All-Small-Molecule organic solar cells and provides an outlook on open research questions.
1.2-I1
In bulk heterojunction organic solar cells, the energetic landscape at the donor-acceptor interface provides the driving force for charge separation. The mechanism leading to efficient charge separation in fullerene-based blends has been intensively investigated, however with the recent advent of high-efficiency non-fullerene acceptors (NFAs) now surpassing 19% power conversion efficiency, the previous findings have to be revisited for NFA-based systems. In this presentation, I will discuss our latest insights into the photophysical processes governing charge separation, recombination, and energetic (voltage) losses in novel NFA-based systems studied by steady-state and advanced transient spectroscopy techniques. I will address the question, how the interfacial energy offsets control exciton dissociation and charge separation in binary and ternary blends of polymer or small molecular donors with novel NFAs, including photoactive layers using state-of-the-art Y-type acceptors. Generally, it appears that it is primarily the ionization energy (IE) offset that limits the exciton-to-charge transfer (CT) state conversion in many low-bandgap NFA-based systems, while the subsequent separation of the CT state into free charges is barrier-less. Sizeable IE offsets of 0.4-0.5 eV are required to ensure quantitative exciton-to-CT state conversion. The underlying reasons of this limitation, their implications for future donor and acceptor material design strategies, and novel computational (in-silico) approaches to material design will be discussed.
1.2-I2
In recent years, the power conversion efficiency of organic bulk heterojunction solar cells has increased rapidly to values exceeding 18% and can largely be attributed to the development of non-fullerene acceptors, especially the ones with A-D-A structure. Many reports have highlighted the critical role of the photoactive layer nanoscale morphology in determining the power conversion efficiency. Therefore, this work takes a holistic approach to correlate the intrinsic molecular structure of each component in a ternary blend of an organic polymer donor with two non-fullerene acceptors (NFAs), to the nanoscale morphology, and together to the phototophysical processes in these blend systems, in order to gain deeper insights as to the requirements for a more efficient device. We employed resonance Raman (RR) spectroscopy as a sensitive probe of molecular structure to a) identify the effect of blending on the conformation of each component, b) to recognize any interactions that evolve between them, c) to probe the effect of NFA side chain substitution, and d) to assess the effect of thermal annealing treatment. Grazing-incidence wide-angle X-ray scattering (GIWAXS) experiments revealed the extent of crystallinity of each material and the impact of blending and thermal annealing on the film macromolecular structure, complemented by atomic force microscopy (AFM) imaging. We find that blending leads to loss of crystallinity of all the components in the films, which is, however, recovered with thermal annealing, but only for the polymer, leading to phase separation, and affecting the photoexcited species formed as probed by ultrafast transient absorption spectroscopy.
1.2-I3
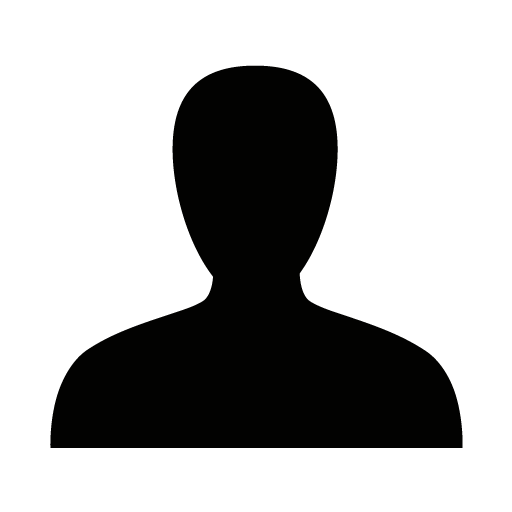
Organic solar cells utilize an energy-level offset between the electron donor and acceptor material to generate free charge carriers. The offset provides the driving force to overcome the Coulomb attraction of the initially formed bound electron-hole pair (exciton). However, this driving force constitutes an internal energy loss. To maximize the open circuit voltage and the short circuit current at the same time, the necessary driving force must be pushed to a minimum without penalizing the free carrier yield. We have shown that in donor-acceptor systems with small driving force for charge separation, a Boltzmann stationary state equilibrium causes a residual population of excitons of the lower bandgap material throughout their lifetime [1]. This highlights the importance of minimizing nonradiative recombination in the pristine non-fullerene acceptor materials.
Recently, several groups, including us, have demonstrated barrierless separation of the coulombically bound intermediate charge transfer state into free charge carriers. We show that if neutral and charged excitations are in a Boltzmann equilibrium, there is an optimum energy of the charge transfer state to maximize both open circuit voltage and short circuit currents [2].
Finally, we will report on recent progress to understand and control electrical performance loss in organic solar cells, and to fine control and interrogate the energetics and dynamics at the interface between donor materials and non-fullerene acceptors.
2.1-I1
Mariano Campoy Quiles´s research is devoted to the understanding and development of solution processed semiconductors for energy and optoelectronic applications. He and his team have built substantial research efforts in two application areas, solar photovoltaic (light to electric) and thermoelectric (heat to electric) energy conversion based on organic and hybrid materials. He studied physics at the Univesity of Santiago de Compostela, obtained his PhD in experimental physics from Imperial College London, and since 2008 he leads his team at the Institute of Materials Science of Barcelona.
Single-junction organic solar cells (OSC) nowadays have reached promising power conversion efficiencies around 19% [1]. Besides new materials, going beyond the current efficiencies could, in principle, be achieved by multi-junction devices, which promise a reduction in thermalization losses. Nevertheless, state-of-the-art multi-junction OSC, leaded by the tandem approach in which two single junction devices are stacked on top of each other, exhibit, thus far, similar efficiency values.[3], [4] This is attributed to the challenges that arise when depositing subsequent layers via solution processing, as well as the need for either a current matching or an extra transparent electrode.[4]
In this talk, we will present a new multi-junction in-plane spectral splitting geometry that we call Rainbow solar cells. In this geometry, a series of sub-cells are placed next to each other laterally, and illuminated through an optical component that splits the incoming white beam into its spectral components, thus matching local spectrum and absorption for each sub-cell. The fabricated n-terminal devices are capable of extracting the maximum power of each sub-cell without the need for any current matching nor processing challenges. After presenting the Rainbow OSCs concept, we use device simulations to provide design rules for increased efficiency in a Rainbow configuration. Then, we will show experimental results for PM6:IO-4Cl and PTB7-Th:COTIC-4F blends, as high and low band-gap sub-cells, respectively. In agreement with simulations, we demonstrate an efficiency increase of around 30% of the Rainbow geometry with respect to our best single junction device.
2.1-I2
Organic semiconductor-based photovoltaic (OPV) devices have many advantageous properties including tailorable light absorption, low embodied energy manufacturing, structural conformality, and low material toxicity. Apart from outdoor solar energy harvesting, these properties also make OPVs attractive for indoor applications which operate at considerably lower light intensities. However, owing to their low charge carrier mobilities, the competition between charge carrier extraction and recombination is an important factor limiting the performance of OPV devices [1]. This competition is known to be strongly dependent on both the applied voltage and the prevailing light intensity, however, the role of the contacts has remained elusive [2],[3]. In this work, we investigate processes limiting the collection of photogenerated charge carriers in OPV devices. We derive analytical expressions describing the current-voltage characteristics of thin-film devices based on low-mobility semiconductors at different light intensity conditions. The theoretical framework is further substantiated by numerical drift-diffusion simulations. Based on these findings, the light intensity and voltage dependence of the photogenerated current, and the impact of different loss mechanisms and contact-related effects, in OPV devices is clarified. This work provides intriguing insights into the differences between OPV devices operating under indoor and outdoor conditions.
2.1-I3
Eva Herzig’s research interest focuses on the possibilities and limitations in the characterization of nanostructures in functional materials as well as how such nanostructures form and change as functions of external parameters. The examined materials range from organic molecules to nanostructured hybrid and inorganic systems. We examine processing-property relationships and the influence of external fields to investigate how the fundamental self-assembly processes influence the final material performance. To this end we exploit various scattering techniques to observe and control structure and function relationships in the examined materials in-situ. Using grazing incidence x-ray scattering we are particularly sensitive to nanostructures on flat surfaces and within thin films.
Nanostructure plays an important role in organic active layers for solar cell applications. The way in which individual material components are arranged with respect to each other is decisive for electronic transport properties and hence for device performance.
In many of these material systems, we have nanostructures that are arrested out-of-equilibrium during a fast processing quench. Therefore the route for structure formation, the provision of energy to the system in form of light and heat or other external stimuli like electric fields [1] can induce structural changes during processing and in the final solar cell [2]. Systematically examining these structural changes as a function of the environmental parameters helps us to identify options for the improvement of structural quality necessary for optimized electronic properties. Using time-resolved optical in-situ investigations as well as x-ray scattering experiments we investigate the quality of nanostructures as well as the effect of accelerating and slowing down such nanostructural changes. This approach allows us to learn more about how to systematically control nanostructure formation and stability.
2.2-I1
Ergang Wang was appointed as a Professor in the Department of Chemistry and Chemical Engineering at Chalmers in 2019. He has been an Associated Professor in 2016-2019 and Assistant Professor in 2012-2016. Previously, he has been a postdoc fellow in the same department in 2008-2011. He obtained his PhD degree in Materials Science in 2008 and Docentship in 2015.
The focus of his research is on the development of new conjugated polymers, 2D materials and Graphene-like materials. The main applications of the materials are organic solar cells, photodetectors, light-emitting diodes, light-emitting electrochemical cells, electrochromics, field effect transistors and supercapacitors.
High efficiency and mechanical robustness are both crucial for the practical applications of all-polymer solar cells (all-PSCs) in stretchable and wearable electronics.[1] In this regard, a series of new polymer acceptors (PAs) is reported by incorporating a flexible conjugation-break spacer (FCBS) to achieve highly efficient and mechanically robust all-PSCs. Incorporation of FCBS affords the effective modulation of the crystallinity and pre-aggregation of the PAs, and achieves the optimal blend morphology with polymer donor (PD), increasing both the photovoltaic and mechanical properties of all-PSCs. [2] In particular, an all-PSC based on PYTS-0.3 PA incorporated with 30% FCBS and PBDB-T PD demonstrates a high power conversion efficiency (PCE) of 14.68% and excellent mechanical stretchability with a crack onset strain (COS) of 21.64% and toughness of 3.86 MJ m-3, which is significantly superior to those of devices with the PA without the FCBS (PYTS-0.0, PCE = 13.01%, and toughness = 2.70 MJ m-3). In a follow up work, we have investigated the influence of the length of flexible spacer. It was found that the polymer acceptor with shorter spacer achieved the highest performance. These results reveal that the introduction of FCBS into the conjugated backbone is a highly feasible strategy to simultaneously improve the PCE and stretchability of PSCs.[3]
2.2-I2
Over the past few decades, organic solar cells (OSCs) have made a significant progress, showing their great potential for low-cost, flexible, lightweight, portable and large-area energy-harvesting devices. Although PC61BM and/or PC71BM structures have been exploited successfully in OSC devices, efforts to modify the fullerene structures for further improving the device performance have been tried recently because fullerene derivatives have the inflexibility in molecular design, difficult purification, poor morphological stability, and limited light absorption in the visible region, etc. In recent years, nonfullerene acceptors have emerged as an alternative candidate of n-type materials to overcome the difficulties of fullerene derivatives in tuning optical and electronic properties. The strong and easily adjustable absorption characteristics of nonfullerene acceptors have been considered as a strong point compared to fullerene-type structures, showing a photovoltaic efficiency over ~18%. To further optimize the OSCs for next generation green energy sources, several important points need to be considered carefully. Here we discuss the fundamental correlations between molecular structure, blend morphology and device performance in new nonfullerene acceptor-based OSCs. The photovoltaic properties of semi-transparent OSCs and sequential layer-by-layer OSCs will be also discussed in detail.
2.2-I3
Thuc-Quyen Nguyen is a professor in the Center for Polymers and Organic Solids and the Chemistry & Biochemistry Department at University of California, Santa Barbara (UCSB). She received her Ph.D. degree in physical chemistry from the University of California, Los Angeles, in 2001 under the supervision of Professor Benjamin Schwartz. Her thesis focused on photophysics of conducting polymers. She was a research associate in the Department of Chemistry and the Nanocenter at Columbia University working with Professors Louis Brus and Colin Nuckolls on molecular self-assembly, nanoscale characterization and molecular electronics. She also spent time at IBM Research Center at T. J. Watson (Yorktown Heights, NY) working with Richard Martel and Phaedon Avouris. Her current research interests are structure-function-property relationships in organic semiconductors, electronic properties of conjugated polyelectrolytes, interfaces in optoelectronic devices, charge transport in organic semiconductors and biological systems, and device physics. Recognition for her research includes the 2005 Office of Naval Research Young Investigator Award, the 2006 NSF CAREER Award, the 2007 Harold Plous Award, the 2008 Camille Dreyfus Teacher Scholar Award, the 2009 Alfred Sloan Research Fellows, the 2010 National Science Foundation American Competitiveness and Innovation Fellows, the 2015 Alexander von Humboldt Senior Research Award, the 2016 Fellow of the Royal Society of Chemistry, and the 2015, 2016, and 2017 World’s Most InfluentialScientific Minds; Top 1% Highly Cited Researchers in Materials Science by Thomson Reuters and Clarivate Analytics. Her current research interests are electronic properties of conjugated polyelectrolytes, doping in organic semiconductors, charge transport in organic semiconductors and biofilms, bioelectronics, and device physics of organic solar cells, ratchets, transistors, and photodetectors.
Organic solar cells (OSCs) using non-fullerene acceptors (NFAs) have garnered a lot of attention during the last years and showed dramatic increases in the power conversion efficiency (PCE). PCEs higher than 19% for single-junction systems were achieved, but these high-performance organic photovoltaic cells are often processed with halogenated solvents. To accelerate the mass fabrication of OSCs, green solvent processing is crucial to reduce the harmful effect of halogenated solvents to human health and our environment. In this talk, I will discuss the design, synthesis, and performance of organic semiconductors processed from green solvents such as xylene and 2-methyltetrahydrofuran (2-MeTHF). 2-MeTHF is a biomass-derived (furfural or levulinic acid) and environmentally friendly solvent that is widely used in organic synthesis, which can be produced from low-cost and renewable agriculture feedstock. A combination of characterization methods were employed to gain insight into the film morphology and solar cell performance.
2.3-I1
Non-radiative recombination is currently still limiting the efficiency of organic solar cells. A reduction of these losses, inevitably leads to an increase in the electroluminescence quantum efficiency of the devices.[1] We have recently identified small molecule donor-acceptor blends with an optical gap in the visible spectral range, with strongly reduced non-radiative losses and high electroluminescence quantum efficiencies (> 1%) as compared to high efficiency systems with a gap in the near infrared (NIR).[2] As a significant fraction of free carrier recombination in these devices results in the emission of a photon, we have identified time resolved emission spectroscopy at nanosecond to millisecond timescale as an excellent probe for the recombination dynamics and interplay between free carriers, charge transfer states and triplet states. In this talk I will discuss the molecular and morphological factors which are responsible for the efficient charge generation and strongly reduced non-radiative recombination in these high gap systems, and will provide guidelines for future high efficiency devices with reduced voltage losses.
2.3-I2
Iain McCulloch holds positions as Professor of Chemical Science within the Division of Physical Sciences and Engineering of KAUST, and a Chair in Polymer Materials within the Chemistry Department at Imperial College. He is also a co-founder and director of Flexink Limited. He is co-inventor on over 60 patents and co-author on over 300 papers with a current h-index of 68. His papers have been cited over 19000 times, including two papers with over 1000 citations. He was cited in Thompson Reuters “Global Top 100 Materials Scientists, 2000-10, Ranked by Citation Impact” at number 35 globally and number 2 in the UK, and was listed on ISI Highly Cited Researchers List 2014, based on ESI Highly Cited Papers 2002-2012. He was awarded the 2009 Royal Society of Chemistry, Creativity in Industry Prize, the 2014 Royal Society of Chemistry Tilden Prize for Advances in Chemistry and a 2014 Royal Society Wolfson Merit Award.
Green hydrogen, produced from water using renewable energy, is expected to become a prominent renewable fuel of the future, providing clean, carbon neutral energy for a wide range of industrial applications. It can also provide complementary energy storage in combination with intermittent solar energy. However, competitive economic solar generated hydrogen production on a large scale remains challenging. One promising approach is photochemical water splitting, using lght absorbing nanoparticle semiconductors that can drive redox reactions on their surface. The more light the photocatalytic nanoparticle absorbs, the more efficiently it can split water into hydrogen and oxygen. Traditionally, wide bandgap inorganic semiconductors have been used for photocatalytic applications. However, these materials almost exclusively absorb UV light which only carries a small fraction (<5%) of solar energy, limiting their efficiency. In this presentation, the development of photocatalysts fabricated from organic semiconductors, chemically tuned to absorb strongly throughout the UV-visible spectrum will be discussed. We demonstrate a larger solar to hydrogen efficiency than traditional inorganic photocatalysts with the potential to achieve solar hydrogen production at a lower levelized cost. We have developed organic semiconductor nanoparticles that contain an internal donor/acceptor heterojunction between two organic semiconductors with a type II energy level offset. The donor/acceptor heterojunction greatly improves charge generation within the nanoparticles, which in turn greatly improves their hydrogen production efficiency. We demonstrate a substantial increase in the H2 production efficiency by tuning the nanoparticle composition. We also observe that the high efficiency of these nanoparticles originates from their ability to generate exceptionally long-lived reactive charges upon illumination, increasing their likelihood to participate in a photocatalytic reaction.
2.3-I3
In the recent years, the development of new non-fullerene-based organic acceptor (NFA) materials have strongly contributed to increasing the efficiency of organic photovoltaics (OPV), going from an average efficiency of about 12% to over 18%. More often than not, these excellent and fundamental developments, in terms of efficiency, are not compatible with potential production applications as well as still having severe limitations in lifetime and stability in a practical context.
It is therefore essential to improve the intrinsic characteristics of the devices, such as organic semiconductor, adapting formulations or manipulation of the interlayers for a fully compatibility with a productive process like slot-die by roll-to-roll (R2R). In the other side it is equally fundamental to have a clear vision of their behavior with the extrinsic stability of the panels themselves, such as both physical and chemistry compatibility with adhesives and barrier properties maintaining reasonable compromise between costs, final application, and context.
In this talk we will analyze some fundamental points required by a real scale-up, practical problems in their applications and give an analysis on the motivation of why these materials still take time to be used commercially.
1.1-I1
Event-based sensor are bio-inspired vision sensors, encoding visual information in the form of sparse asynchronous events. Each event encodes a change in the log-luminosity intensity at a given pixel location. As a consequence, event sensors capture information at extremely high temporal resolution and with high dynamic range, while keeping data and power consumption small.
Converting a sparse events stream to a dense representation allows the use of classical AI methods, such as convolutional neural networks, to event data. This is a practical solution to leverage the well-established AI ecosystem and results in outperforming classical frame-based cameras methods in latency-critical and high dynamic range scenarios. However, the low power and low data property of the camera are lost.
Neuromorphic AI will be the cornerstone of the next generation event-based vision pipelines. In fact, the asynchronous and ultra-low power computation paradigms of neuromorphic architectures are the perfect fit to process event-based data. However, several research directions, both from the algorithmic side and hardware implementation side, are still open and need to be explored.
1.1-I2
Event cameras mimic the workings of the human bio visual pathway by sending image intensity
change pulses to the neural system. They are a promising alternative to conventional frame-based
cameras for detecting ultra fast motion with low latency, robustness to changes in illumination
conditions, and low power consumption. These characteristics make them ideal for mobile robotic
tasks. However, exploiting to its full capacity their unconventional sparse and asynchronous spatio-
temporal data flow efficiently still challenges the computer vision community.
Deep Artificial Neural Networks (ANN), especially, the recent architecture of visual transformers
(ViT) have achieved state-of-the-art performance for various visual tasks [1]. However, the straight-
forward use of ANNs on event input data needs a preprocessing step that constraints its sparse and
asynchronous nature. Inspired by computational neuroscience, Spiking Neural Networks (SNNs) turn
out to be a natural match for event cameras due to their sparse event-driven and temporal processing
characteristics. SNNs have been applied mostly for classification tasks [2]. Some other works involve
regression tasks for optical flow estimation [3], [4], depth estimation [5] angular velocity estimation [6],
and video reconstruction [7]. However, limited work has been done to incorporate SNNs for full 3D
ego-motion estimation.
We first present an optimization-based ego-motion estimation framework that exploits the event-based
optical flow outputs of a trained SNN model [8]. Our method successfully estimates pure rotation and
pure translation motion from input events only and shows the potential of using SNNs for continuous
ego-motion estimation tasks. Secondly, we show our Hybrid RNN-ViT architecture for optical flow
estimation which uses ViT to learn global context. We further present preliminary results for its SNN counterpart which combines SNNs to directly process the event data.
1.1-I3
Amirreza received his BSC. and M.Sc. degrees in electrical engineering from the Tehran Polytechnic (2010) and Sharif University of Technology (2013), Tehran, Iran. In 2014, he was awarded an F.P.I scholarship from Spanish Research Council (CSIC). He received his Ph.D. in the field of neuromorphic engineering at the Instituto de Microelectronica de Sevilla (IMSE-CNM-CSIC), University of Seville, Seville, Spain in 2018. He has been visiting scholar/Postdoctoral Fellow at the University of Manchester (UK), Brain and Mind Institute (CerCo, CNRS, Toulouse), National University of Singapore, imec (Ghent), BrainChip (Toulouse) and GrAI-Matter-Labs (Eindhoven). Since 2020, he is a neuromorphic architect researcher in IMEC (Eindhoven, Netherlands) with a focus on designing low-power neuromorphic processor architecture.
This talk is about the challenges and trade-offs in the design of digital scalable neuromorphic processing architectures. We will talk about IMEC's achievements in neuromorphic sensory and event-based processing technologies. We specifically focus on the IMEC RISC-V-based neuromorphic processor (SENeCA) in comparison to the other state-of-the-art architectures.
SENeCA is a RISC-V-based digital neuromorphic processor to accelerate bio-inspired Spiking Neural Networks for extreme edge application (from 1M to 100M neurons) in /near-sensors where ultra-low power and adaptivity features are required. SENeCA is optimized to exploit unstructured spatio-temporal sparsity in computations and data transfer. It improves the available solutions by 1) addressing the flexibility issue in neuromorphic processors, 2) improving the area efficiency by employing a 3-level memory hierarchy, 3) efficient deployment of advanced learning mechanisms and optimization algorithms by accelerating neural operations in three data types: int4, int8 and BrainFloat16 and 4) efficient event communication by using a novel Network-on-Chip with multicasting, compression mechanism and source-based routing.
The last section of the talk will be about "why we think neuromorphic event-based processing is the future of data-driven computing", "why it is not yet on the market" and the "IMEC roadmap to address the main challenges and trade-offs both in software and hardware in the neuromorphic processing domain".
1.2-I1
To truly understand how the brain processes sensory information into decisions on actions to take, one needs mappings between measurable quantities to the elementary units of computation. While much is measurable, there is also much debate about what constitutes the elementary unit of computation in the brain: artificial neural networks represent only one such abstract mapping and imply fundamental choices on neural coding and functioning. Trained and large-scale ANNs also map to certain aspects of brain functioning. Still, data from experimental neuroscience is strongly suggesting that the ANN abstraction is too simple and omits important computational principles of real neuronal processing, such as the spiking nature of neural communication and the diversity and function of neuronal morphology. To investigate these computational principles, we need to be able to train large and complex networks of spiking neurons for specific tasks. In this talk, I will show how effective online learning rules enable the supervised training of large-scale networks of detailed spiking neuronal models, and how these models can be integrated with brain-derived decision-making circuits to operate continuously. As I will argue, this approach opens up the investigation of both network and neuronal architectures based on functional principles rather than imputed connectivity patterns.
1.2-I2
Our brain relies on spiking neural networks for rapid and ultra-low-power information processing. To build artificial intelligence that leverages spiking networks with comparable efficiency requires instantiating vast spiking network models on neuromorphic hardware accelerators. However, direct end-to-end training of spiking neural networks remains challenging due to the non-differentiability of spiking neuron models.
Surrogate gradients have emerged as a widespread solution to this problem. In my talk, I will briefly introduce the notion of surrogate gradient learning, showcase its robustness, and illustrate its self-calibration capabilities on analog neuromorphic hardware. I will further discuss the importance of network initialization on deep spiking neural network training and introduce effective bio-inspired initialization strategies. Finally, I will sketch how biologically plausible online learning rules naturally emerge through local approximations of surrogate gradients that exploit block-sparse Jacobians. This step is essential for learning from long temporal sequences and paves the way for exciting future on-chip online learning applications.
1.2-I3
Bernabé Linares-Barranco received the B. S. degree in electronic physics in June 1986 and the M. S. degree in microelectronics in September 1987, both from the University of Seville , Sevilla , Spain . From September 1988 until August 1991 he was a Graduate Student at the Dept. of Electrical Engineering of Texas A&M University. He received a first Ph.D. degree in high-frequency OTA-C oscillator design in June 1990 from the University of Seville, Spain, and a second Ph.D deegree in analog neural network design in December 1991 from Texas A&M University , College-Station, USA.
Since June 1991, he has been a Tenured Scientist at the "Instituto de Microelectrónica deSevilla" , (IMSE-CNM-CSIC) Sevilla , Spain , which since 2015 is a Mixed Center between the University of Sevilla and the Spanish Research Council (CSIC). From September 1996 to August 1997, he was on sabbatical stay at the Department of Electrical and Computer Engineering of the Johns Hopkins University . During Spring 2002 he was Visiting Associate Professor at the Electrical Engineering Department of Texas A&M University , College-Station, USA. In January 2003 he was promoted to Tenured Researcher, and in January 2004 to Full Professor. Since February 2018, he is the Director of the "Insitituto de Microelectrónica de Sevilla".
He has been involved with circuit design for telecommunication circuits, VLSI emulators of biological neurons, VLSI neural based pattern recognition systems, hearing aids, precision circuit design for instrumentation equipment, VLSI transistor mismatch parameters characterization, and over the past 20 years has been deeply involved with neuromorphic spiking circuits and systems, with strong emphasis on vision and exploiting nanoscale memristive devices for learning. He is co-founder of two start-ups, Prophesee SA (www.prophesee.ai) and GrAI-Matter-Labs SAS (www.graimatterlabs.ai), both on neuromorphic hardware.
Dr. Linares-Barranco was corecipient of the 1997 IEEE Transactions on VLSI Systems Best Paper Award for the paper "A Real-Time Clustering Microchip Neural Engine", and of the 2000 IEEE Transactions on Circuits and Systems Darlington Award for the paper "A General Translinear Principle for Subthreshold MOS Transistors". He organized the 1995 Nips Post-Conference Workshop "Neural Hardware Engineering ". From July 1997 until June 1999 he has been Associate Editor of the IEEE Transactions on Circuits and Systems Part II , and from January 1998 until December 2009 he was also Associate Editor for IEEE Transactions on Neural Networks . Since April 2010 he is Associate Editor for the new journal "Frontiers in Neuromorphic Engineering", as part of the open access "Frontiers in Neuroscience" journal series (http://www.frontiersin.org/). Since Jan. 2021 he is Specialty Chief Editor of "Frontiers in Neuromorphic Engineering".
He is co-author of the book "Adaptive Resonance Theory Microchips ". He was Chief Guest Editor of the IEEE Transactions on Neural Networks Special Issue on 'Hardware Neural Networks Implementations '. He is an IEEE Fellow since January 2010. He is listed among the Stanford top 2% most world-wide cited scientist in Electrical and Electronic Engineering (top 0.62% world-wide, 8th in Spain, 2nd in Andalucía, 1st in CSIC).
We will briefly give an overview of vision with Dynamic Vision Sensor (DVS) cameras, processing with spiking-based hardware processing modules, and link it with emerging nanoscale synaptic-like devices which can exploit on-line bio-inspired learning. DVS cameras are frame-free strongly bio-inspired vision sensors that result in highly energy efficient visual information encoding, very well suited for processing with spiking neural networks. We will present techniques to process such signals with spiking neural network hardware that can be modularly expanded to scaled-up systems. Spike Timing Dependent Plasticity (STDP) is one type of learning rule for Spiking Neural Networks (SNN). We will present how STDP can be implemented by exploiting novel nano-scale memristor devices, used as synapses, whose resistance changes as correlated spiking signals appear at their terminals. We will show experimental results from a CMOS chip with 4k monolithically integrated nanoscale memristors performing spiking computation and recognition of spiking patterns.
1.2-I4
The recent discovery of surrogate gradient learning (SGL) has been a game changer for the more biology inspired spiking neural networks (SNNs). In short, by solving non-differentiability issues, it reconciles SNNs with backpropagation, THE algorithm that caused the deep learning revolution. SNNs and conventional artificial neural networks (ANNs) can now be trained using the same algorithm and the same auto-differentiation enabled tools (e.g. PyTorch or TensorFlow). This bridges the gap between SNNs and ANNs, and makes the comparison between them fairer.
In this talk, I will review recent works in which we show that SNNs trained with SGL can solve a broad range of problems, just like ANNs, but possibly with orders of magnitude less energy, once implemented on event-based hardware. These problems include image and sound classification, depth and optic flow estimation from event-based cameras, encrypted Internet traffic classification, epileptic seizure detection from electro-encephalograms, etc.
1.1-I1
Aldo Di Carlo is Director of the Institute of Structure of Matter of the National Research Council and Full Professor of Optoelectronics and Nanoelectronics at the Department of Electronics Engineering of the University of ROme "Tor Vergata". His research focuses on the study and fabrication of electronic and optoelectronic devices, their analysis and their optimization. Di Carlo founded the Center for Hybrid and Organic Solar Cells (CHOSE) which nowadays involve more than40 researchers dealing with the development of III generation solar cells (DSC, OPV and Perovskite) and on scaling-up of these technologies for industrial applications. CHOSE has generated 6 spin-off companies and a public/private partnership. Di Carlo is author/coauthor of more than 500 scientific publications in international journals, 13 patents and has been involved in several EU projects (three as EU coordinator)
The large library of two-dimensional materials can be exploited to master interface properties of perovskite solar cells. Here, I will present the results of the use of 2D materials in perovskite cells, modules and panels. In particular I will focus on a new class of 2D, namely Titanium Carbide MXenes (such as Ti3C2). Beside exceptional chemical and mechanical properties, MXenes offer a wide tunabilty of work function (WF) by varying their surface termination. WF can ranges from ≈2 eV (for OH-termination) to ≈6 eV (for O-termination). In particular, by producing well exfoliated Ti3C2Tx MXenes with a relatively low WF (~3.7eV) we demonstrate the capability to tune both perovskite absorber and electron transporting layer (ETL) WFs.[1] This strategy has been applied to nip [1] and pin [2] cells structure and exploited on large area modules [3]. We show that MXene interface engineering used on the n side of pin cell (NiO/perovskite/C60/BCP/Cu) permitx to increase enormously the stability of the cell with a T90 exceeding the 2000 h under continuous light soaking at Maximum Power Point (in ambient conditions) and T80>1000h for thermal stress (85 °C). [4]
The use of a combination of 2D materials to improve performance and stability of perovskite technology has been extended to panels (9 panels of 0.5 sqm each) that have been tested for more than a year in a Solar Farm in Crete.[5] The results of this outodoor test in a real environment will be presented and performance and stability will be discussed.
1.1-I2
Using green energy or increasing energy efficiency is the key to a sustainable world. 2D materials can be used to build a sustainable world in terms of low-cost H2 generation, low-power-consuming devices, and IA-enabled optimization of novel materials. In this talk, I will briefly discuss how we use 2D materials as an ideal platform to explore the mechanism of semiconducting catalysis.
It is known that semiconducting catalysts are great candidates to replace noble metals to make green energy such as H2 due to their low cost. Using 2D materials as the model system, we revisited the semiconductor-electrolyte interface and unraveled a universal self-gating phenomenon through micro-cell-based measurements. [1] We unveiled a surface conductance mechanism that dominates the charge transport in semiconductor electrocatalysts. Based on this, we provided a guideline on how to design a high-performance semiconductor electrocatalyst. We also demonstrate the synthesis of amorphous PtSex and its use in high-performance electrocatalyst. [2]
1.1-I3
He has more than 15 years research experience in the academic sector working on nanoelectronics, spintronics and optoelectronics. He possesses extensive hands-on experience on emerging low-dimensionality electronic systems including nanowire transistors, GaAs single spin quantum-bits, as well emerging phenomena in functional oxide and superconductive/ferromagnetic interfaces towards beyond CMOS technologies. He has served at various academic research positions in high reputation European institutions including the Foundation of Research and Technology in Greece, the Institut Néel CNRS in France and the London centre for Nanotechnology – University College of London in United Kingdom. He obtained his PhD in Nanoelectronics from Grenoble Institute of Technology in France, in 2009. He is currently Researcher (Grade C) in the i-EMERGE Research Institute of the Hellenic Mediterranean University (HMU) and the Team Leader of Innovative Printed Electronics at the Nanomaterials for Emerging Devices research group. His current research interests include 2D materials engineering in various printed device concepts suc as high performing solar cells, functional sensors as well as neuromorhic computation architectures towards energy efficient, smart Internet of Intelligent Things and wearable systems.
Breakthrough discoveries in high-throughput formulation of abundant materials and advanced engineering
approaches are both in utter need as prerequisites for developing novel large-scale energy conversion
technologies required to address our planet’s rising energy demands. Nowadays, the rapid deployment of
Internet of Things (IoT) associated with a distributed network of power-demanding smart devices,
concurrently urges for miniaturized systems powered by ambient energy harvesting. Graphene and other
related two-dimensional materials (GRM) consist a perfect fit to drive this innovation owing to their
extraordinary optoelectronic, physical and chemical properties that emerge at the limit of two-dimensions.
In this review, after a critical analysis of GRM’s emerging properties that are beneficial for power generation,
novel approaches are presented for developing ambient energy conversion devices covering a wide range
of scales. Notable examples vary from GRM-enabled large-scale photovoltaic panels and fuel cells, smart
hydrovoltaics and blue energy conversion routes, to miniaturized radio frequency, piezoelectric, triboelectric,
and thermoelectric energy harvesters. This presentation will focus on GRM-enabled energy
harvesters that have the potential to revolutionize the way that grid-electricity is provided in the cities of the future. At the end of the discussion, perspectives on the trends, limitations and commercialisation potential of these emerging, up-scalable energy conversion technologies are provided.
1.2-T1
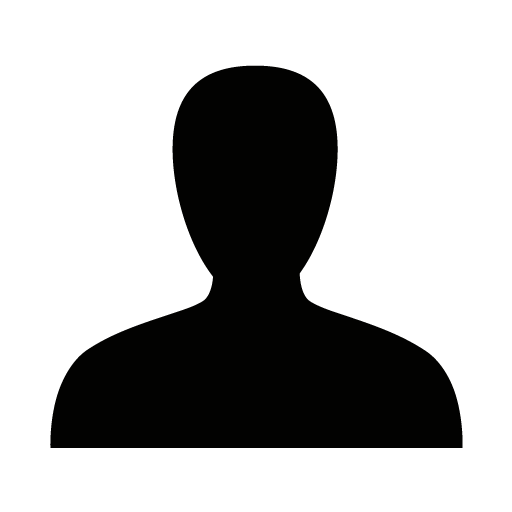
In the present study the analytical modelling of graphene/PMMA nanocomposite under hygro-thermo-mechanical loading is performed. The 2D analytical method consists of the use of the stress-function variational method, which was validated with experimental data in our previous paper [1]. Three cases of loading – heating, cooling and mixed (hygro-thermo-mechanical) are considered and compared with mechanical one. It was found, that all resultant stresses for heating in the layers show the same behavior like mechanical ones with slight increase in amplitude. At cooling the stresses look as a mirror image in respect to the heating case. Also, at heating, the axial stress in graphene increases with increasing the temperature, but the axial stress in the substrate PMMA decreases at the same time. At cooling the stresses show an opposite behavior. The parametric analysis for the influence of external mechanical, temperature and moisture loading, is performed. It was found, that mechanical loading influences significantly the mixed interface shear stress. There is a critical mechanical load of 350 MPa, after that the delamination takes place for mixed case. Without this loading, delamination is not possible nevertheless of applied thermal and/or moisture load. The interface delamination appears from the left and from the right length side of the structure simultaneously.
1.2-T2
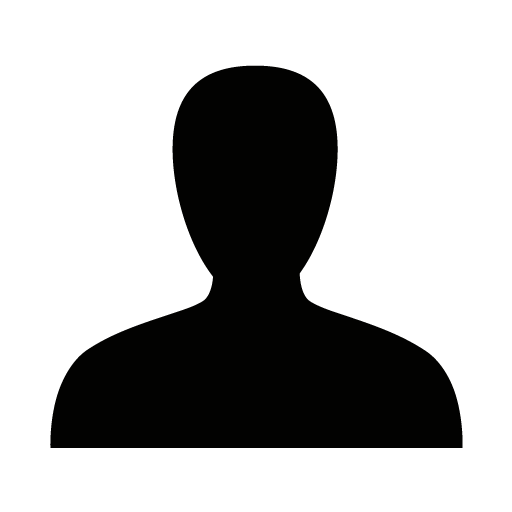
Electrochemical reduction of CO2 to valuable chemical fuels provides a promising pathway for reducing the continuously growing global carbon footprint. One of the critical components of an efficient electrochemical CO2 reduction system is the catalyst which accelerates the reaction’s kinetics.
Metal-Organic Frameworks (MOFs) are a class of crystalline coordination polymers with high surface area, consisting of metal clusters and organic multi-topic linkers. MOFs were highly useful in chemical catalysis because of their unique physical properties, such as high surface area and porosity. These unique properties allow us to use MOFs for integrating the fundamental functional elements needed for the efficient electrocatalytic system: 1) immobilization of high concentration of the molecular catalysts, 2) installation of the redox shuttles for charge transport to and from the catalytic sites, 3) optimization of the mass transport channels through the MOFs pores, and 4) modulation of the catalyst's secondary chemical environment. The notion of using MOF to immobilize high concentrations of molecular electrocatalysts to drive electrochemical reactions was demonstrated. Yet, the modulation of the active-site’s immediate chemical environment to boost electrocatalysis rate and selectivity has rarely been shown..
Herein we demonstrate that in a FeTCPP-Based 2D MOF, using an heterogeneous incorporation of ligands bearing a fixed cationic charge, one can electrostatically-stabilize FeTCPP-bound COO- intermediate, and thus systematically tune its CO2-to-CO selectivity up to practically 100%. As such, we believe that these results will widen our understanding of MOF-based electrocatalytic systems and accelerate their implementation is energy-conversion schemes.
1.2-T3
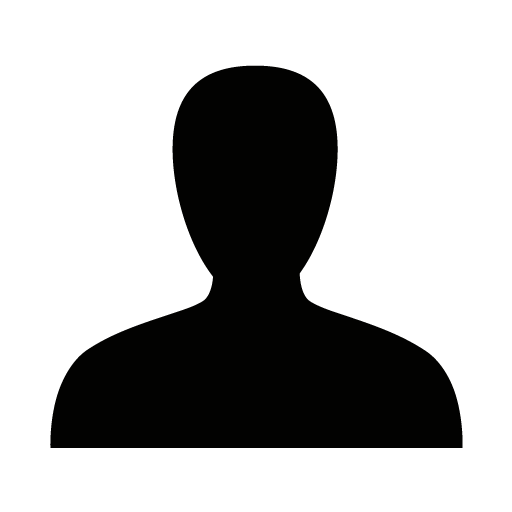
Photoelectrocatalytic hydrogen evolution from water is a promising topic for producing H2 efficiently and environmentally friendly. However, the efficiency of hydrogen production in these photoelectrocatalysis systems is still low. Therefore, the discovery of more efficient phototelectrocatalysts has been considered as one of the important directions in the field of clean and renewable energy. 2D BiOBr is emerging as an interesting photoelectrocatalysts because of its unique internal electric field and band structure that facilitate the separation and mobility of the charge carriers[1,2]. However, pure 2D BiOBr suffers from low catalytic efficiency and photocorrosion from the light source. In order to optimize the catalytic efficiency and stability of 2D BiOBr, a 2D BiOBr/MoS2 heterojunctions with 1 wt%, 5 wt%, 10 wt% and 50 wt% of MoS2 were fabricated by a simple liquid-phase exfoliation method. Raman spectra prove the existence of strong interactions between the MoS2 and BiOBr 2D nanosheets in the heterojunctions. The heterojunction containing 1 wt% of MoS2 shows the best performance and stability in photoelectrocatalytic hydrogen evolution than the other samples. The calculation of the heterojuntions shows a better charge transfer inside the heterojunction than the pure 2D materials as well.
1.2-T4
Researcher (Staff scientist), Lab Manager, and Teacher at the Department of Chemistry at the Ben-Gurion University of the Negev, Israel.
Semiconductor polymeric carbon nitride (CNs) are a family of 2D materials that exhibit excellent photocatalytic properties for diverse chemical transformations thanks to their tunable band gap, suitable energy-band position, high stability under harsh chemical conditions, and low cost. Unfortunately, despite progress in the last decade, their utilization as the photoactive layer in photoelectrochemical (PEC) cells has yet to reach the performance of state-of-the-art metal-oxide-based systems. The main challenges thus far have been the difficulty in depositing high-quality and homogenous CN layers of controlled thickness on substrates, the wide band gap of ‘intrinsic’ CN ca. 2.7 eV, poor charge-separation efficiency, and low electronic conductivity.[1]
We present some noteworthy progress we have recently achieved in both stability and performance towards water-splitting as a result of tackling some of these limitations, mainly through variation of precursor deposition methods,[2–6] externally adding CN-precursor vapor during thermal polymerization,[5] incorporation of conductive carbons,[6,7] and successful incorporation of a NixFe1–xOyHz oxidation co-catalyst.[7] The latter is unique due to the difficulty of forming stable organic–inorganic heterojunctions in porous materials. This was accomplished by in-situ electrochemical transformation inside the porous CN matrix of a solvothermally-deposited pre-catalyst—a Ni/Fe-MIL-53 metal-organic framework (MOF)—into the co-catalyst. This configuration reaches photocurrents of 472 ± 20 µA cm–2 with faradaic efficiency towards O2 and H2 > 80% in 0.1 M KOH solution at 1.23 V vs. RHE. We show stable operation up to 35 h, where degradation occurs due to slow leaching of the co-catalyst.
1.2-T5
Masoud Karimipour received his Ph.D. at 2011 in Solid State Physics from the faculty of science at Ferdowsi University of Mashhad, Iran. He was appointed as an Assistant professor at 2011 and promoted to associate professor in 2016 at Vali-e-Asr University of Rafsanjan, Rafsanjan, Iran. He has published more than 90 papers in nanoscience wit current H-index 19. He worked on the stability of perovskite solar cells as a researcher at the department of physical chemistry at Uppsala University during 2020. Currently, he is working as a Postdoctoral researcher with Prof. Monica Lira Cantu at Institut Català de Nanociència i Nanotecnologia (ICN2).
In this study, we present interface modification of lead halide perovskite solar cells in normal configuration by means of deposition of functionalized Mxene (Ti3C2) nanosheets. H3PP ligand as used as functionalizing and passivation agent molecule at the interface of perovskite-Spiro OMeTAD layers. MXene nanosheets were prepared by delamination of MXene bulk powers with a novel method then dispersed in a pre-dissolved H3pp (0.5 mg/ml) solution. JV measurements showed a PCE of 20.03% for modified device while the reference device PCE was 19.63%. Impedance Spectroscopy analyses and Mott-Schottky analysis revealed the increase of recombination resistance and reduction of trap state densities, respectively, for modified device. Stability studies by means of MPP tracking under N2 atmosphere for 1000 h showed enhanced stability for devices modified by pure MXene nanosheets and H3PP modified MXene nanosheets devices. In addition to that, MPP tracking of encapsulated devices under outdoor conditions showed clear indication of higher stability in performance of H3PP/Mxene modified devices.In this study, we present interface modification of lead halide perovskite solar cells in normal configuration by means of deposition of functionalized Mxene (Ti3C2) nanosheets. H3PP ligand as used as functionalizing and passivation agent molecule at the interface of perovskite-Spiro OMeTAD layers. MXene nanosheets were prepared by delamination of MXene bulk powers with a novel method then dispersed in a pre-dissolved H3pp (0.5 mg/ml) solution. JV measurements showed a PCE of 20.03% for modified device while the reference device PCE was 19.63%. Impedance Spectroscopy analyses and Mott-Schottky analysis revealed the increase of recombination resistance and reduction of trap state densities, respectively, for modified device. Stability studies by means of MPP tracking under N2 atmosphere for 1000 h showed enhanced stability for devices modified by pure MXene nanosheets and H3PP modified MXene nanosheets devices. In addition to that, MPP tracking of encapsulated devices under outdoor conditions showed clear indication of higher stability in performance of H3PP/Mxene modified devices.
1.3-I1
Two-dimensional van der Waals layered materials possess strong Coulomb interactions, giving rise to large exciton binding energies, and weak exciton-phonon coupling, allowing lower carrier cooling rates. Tuning the interface is paramount to pushing the device's performance including its optoelectrical properties. Engineering the interface with various 2D-materials (Transition Metal Dichalcogenides, carbon nitride, and Mxenes) at hole transport layers and perovskite, can eliminate defective charge build-up and suppress the charge carrier recombination rate to induce accelerated photo-induced charge transfer. This in turn minimizes voltage deficit. In some cases, where inorganic hole transport layers are used it also avoids direct contact with metal oxide (eg. NiOx with perovskite, overcoming the possible instability of the active layer via iodide oxidation and deprotonation of cationic amines). The use of 2D materials will serve as an effective interfacial layer for reliability in photovoltaics. We will show examples to optimize the interface and band alignment for efficient charge extraction, push the performance and reliability of the perovskite solar cells, and will decipher device kinetics.
1.3-I2
Dr. Nagore Ortiz-Vitoriano (https://cicenergigune.com/en/nagore-ortiz-vitoriano) is an Ikerbasque Research Associate, who has been spearheading metal-air research at CIC energiGUNE (Spain) since 2016, of which she became research line manager in 2018.
She obtained her doctorate in 2011 for her work on solid oxide fuel cells (University of the Basque Country, UPV/EHU, Spain), during the course of which she undertook research stays at Risø DTU (Denmark) and Imperial College London (UK). In 2013 she was awarded a Marie Curie International Outgoing Fellowship from the European Union, enabling her to join the Department of Mechanical Engineering at the Massachusetts Institute of Technology (MIT) in Cambridge (USA) where she worked with both lithium and sodium-air batteries. In 2015, she continued this fellowship at CIC energiGUNE, where she conducted research stays at Oak Ridge National Laboratory (USA), Deakin University (Australia) and Chalmers University (Sweden). Recently, she has been promoted to Ikerbasque Research Associate and granted the Ramon y Cajal fellowship financed by the European Commission's European Social Fund through the Spanish Ministry of Science and Innovation.
Dr. Ortiz-Vitoriano has focused on both rational design of electrode and electrolyte materials for energy storage (e.g., solid oxide fuel cells, electrocatalysis, Na-ion and metal-air batteries), as well as fundamental research focused on elucidating key processes (by establishing relevant physiochemical models) in order to facilitate rapid future developments at both the material and system levels.
Rechargeable Na-O2 batteries have received a great deal of attention as an alternative to current lithium-ion batteries, mainly due to their potential to provide higher energy densities. Na–O2 batteries are still in an early stage of development, with several challenges that need to be addressed, including limiting kinetics at the air cathode. In this regard, the use of suitable cathode materials is a point of major concern as they are responsible for achieving efficient deposition/redissolution of the solid discharge products formed during battery cycling.
Carbon materials have been widely used as air cathodes due to their low cost, high surface area, chemical and mechanical stability, high electrical conductivity, well-developed porosity and intrinsic catalytic activity towards ORR/OER. In particular, graphene has gained attention due to its superior electrical conductivity and highly accessible 2D area. The real integration of graphene as electrode material in energy storage devices, however, requires the development of sustainable and scalable approaches for its production and processing.
In this talk, graphene aerogels prepared by the graphite oxide route and the electrochemical exfoliation of graphite will be discussed. First, graphene aerogels with different pore sizes obtained by the graphite oxide route revealed a trade-off between pore texture and battery performance. This route, however, is not environmentally friendly and involves multiple steps, including a reduction step to tune the sheet conductivity. As an alternative, we have used a simpler, faster and more eco-friendly route to access high-quality graphene by electrochemical exfoliation of graphite, resorting to natural nucleotides as both exfoliating electrolytes and colloidal stabilizers. An aerogel prepared from this graphene suspension delivered a large discharge capacity and longer cycle life than those cathodes prepared by the graphene oxide route. The origin of such good performance is attributed to the participation of the nucleotide molecules in key chemical processes taking place at the battery cathode, including oxygen electrocatalysis and nucleation of the discharge products.The present work highlights not only the interest on this electrochemical method over more traditional routes when it comes to manufacturing graphene, but also the direct potential benefits of the resulting graphene in novel electrochemical energy storage technologies.
REFERENCES
M. Enterría, C. Botas, J.L. Gómez-Urbano, B. Acebedo, J.M. López del Amo, D. Carriazo, T. Rojo, N. Ortiz-Vitoriano, Journal of Materials Chemistry A, 42 (2018) 20778.
M. Enterría, J.L. Gómez‐Urbano, J.M. Munuera, S. Villar‐Rodil, D. Carriazo, J.I. Paredes, N. Ortiz‐Vitoriano, Small, 17 (2021) 2005034.
1.3-I3
Over the last years, models have evolved to allow us to address the properties
of materials in energy. I will revise some of the local effects for the most relevant
applications including the oxygen evolution reaction and the CO2 reduction with
showing where the most important bottlenecks are present.
1.1-I1
The exact molecular interactions at the interface of an organic heterojunction are key to the development of efficient optoelectronic devices. Due to the difficulty in characterizing these buried and (likely) disordered heterointerfaces the exact interfacial structure in most systems remains a mystery. Here, we demonstrate a novel synthetic strategy to design and control model interfaces, allowing for their detailed study in isolation from the bulk material. This is achieved and highlighted through the synthesis of a donor polymer – non-fullerene acceptor through-space linked system, where the exact position and orientation of the components is completely controlled. By placing the acceptor above different portions of the conjugated polymer, drastic effects on the CT state properties are observed. We observe that through synthetic control the oscillator strength of the CT state and the rate of its formation can be controlled by several orders of magnitude. These results have signficant implications for the design and operation of OPV devices
1.1-I2
Dr Matthew Griffith develops bio-functional electronic inks and crafts new tools to print functional devices with applications in biosensing, energy and interact with human body. He has created soft functional materials that can replace traditional hard electronics, solving longstanding biocompatibility problems that restrict adoption of electronic devices in healthcare.
His achievements include creating biocompatible inks for a printed artificial retina with the potential to restore colour vision, flexible X-ray detectors that are revolutionizing radiotherapy treatment for cancer, and a series of material and technological developments that enabled the first commercial installation of printed solar cells in Australia. His work is published in the top 5% of journals in materials science and has been commercialised through multiple industry partnerships. The bioprinting tools he developed enable a globally unique translation of innovation from the lab to industrial scale roll-to-roll manufacture, leading to their inclusion in the Australian National Fabrication Facility.
Matthew has been awarded $6M+ in prestigious grants and fellowships, half secured as lead researcher. He has authored 45 publications, cited in 160 journals by authors in 68 countries across 19 different subject areas. His outreach includes direct patient engagement, creating a podcast for Australia’s leading chemistry society, and frequent media interactions.
Implantable neurostimulation devices play a key role in treating many injuries and diseases by providing a direct therapeutic link to the nervous system. This enables brain stimulation for treatment of Parkinson’s disease and epilepsy, nerve guidance and regeneration to remedy spinal cord injury, and retinal prosthetic devices that could cure blindness.[1] To address such issues, new bioelectronic systems that can deliver electrical stimulation to nerve cells are required. Although silicon microelectronics and metal electrodes have been the historic gold standard for bioelectronic interfaces, the main obstacles to further translation of these devices include a low biocompatibility that reduces in vivo lifetimes, a mechanical rigidity that is poorly matched with soft tissue, causing inflammation and ineffective electrical contact, and a requirement for costly external power supplies to deliver current.[2] These issues result in indiscriminate tissue activation, with a consequent lack of spatial selectivity.[3]
In this work, we report our recent efforts to simultaneously address these issues by combining soft carbon-based organic semiconductors and nanoscale science to build bioelectrodes that allow optical neurostimulation without external power. Our approach creates bioelectronic interfaces from organic semiconductors that can be formed into customized nanoparticles with established solution-based chemistry methodologies. This approach enables the stimulating electrodes to be combined with targeted pharmaceutical factors in the fabrication procedure, which subsequently optimise connections to the neural network when released in-vivo.[4]
We will discuss how we tuned the optoelectronic properties of the organic nanoparticles to cover red, green, and blue wavelengths, allowing spectrally selective platforms for neurostimulation. These semiconductors are turned into electroactive inks, and subsequently fabricated into pixelated arrays using inkjet printing. This approach establishes a new low-cost manufacturing methodology that is applicable to other organic materials and can be used for a variety of bioelectronic devices, creating a new manufacturing paradigm for healthcare.
We demonstrate both the anatomical and functional biocompatibility of neural tissue with our organic bioelectronic systems using immunolabelling with neuronal marker MAP2 and visualisation with epifluorescence microscopy to detect neurons cultured on the organic conductors. We demonstrate the controlled release of drugs from the organic nanoparticles, aiding in precise spatial delivery of pharmaceutical factors. Finally, we employ whole-cell patch clamp electrophysiology recordings to demonstrate an exciting result; purely optical neurostimultation of dorsal root ganglion nerve cells. We demonstrate that the organic conductors can trigger changes in the nerve cell membrane potentials via a capacitive coupling mechanism, the efficacy of which can be improved by judicious selection of the device architecture.[5]
References:
1.1-I3
Detection of trace levels of hazardous chemicals is an important challenge for environmental monitoring, homeland security and humanitarian landmine clearance. Organic semiconductor sensors, which exploit a fluorescence quenching mechanism in the presence of certain target analyte molecules, offer an attractive approach for extremely sensitive detection. In this presentation we will describe how molecular design and thin-film structure influence both the microscopic physics, and the sensor performance including their sensitivity, speed of response and recovery, and potential for discrimination between target analytes.
We will also present progress and potential in developing applications of these sensors in-field conditions for humanitarian demining and environmental monitoring. For example, the ability to detect the presence of explosives across an area of interest would be of particular use in technical surveys of suspected minefields. We show that these sensors can be used to detect nano-gram level quantities of nitroaromatic (TNT-like) molecules, and combine them for the first time with a novel preconcentration approach to detect buried explosives. Initial field trials on a test minefield will be presented, in which the sensors are used to detect trace explosives collected by colonies of foraging honeybees. We will also describe how these sensors can provide a new approach for early warning of environmental pollution events in water bodies.
1.2-I1
Oana Jurchescu is a Baker Professor of Physics at Wake Forest University (WFU) and a Fellow of the Royal Scoety of Chemistry. She received her PhD in 2006 from University of Groningen, the Netherlands, and was a postdoctoral researcher at the National Institute of Standards and Technology in Gaithersburg, MD, until 2009, when she joined the Physics Department at Wake Forest University as an Assistant professor. Her expertise is in charge transport in organic and organic/inorganic hybrid semiconductors, device physics and semiconductor processing. She published over 100 peer-reviewed articles, 4 invited book chapters, 3 patents and gave over 50 invited or plenary talks at conferences. She won the National Science Foundation CAREER award, the 2022 Pegram Award from APS Southeastern Section (SESAPS) for excellence in teaching and mentoring, several university awards for excellence in research, teaching and mentoring. She served in a variety of capacities, including program chair and co-chair, for over 30 international conferences and workshops such as MRS, APS, SPIE, etc.
The growing demands for smaller, faster, yet less expensive and more versatile electronics, have led to a surge of efforts focused on the development of new componentry technologies. Molecular-scale devices could address this challenge and are being touted to become a core technology of the 21st century, complementing the conventional silicon-based industry with applications currently not possible. One of the simplest configurations is the molecular diode, which consists of a self-assembled monolayer (SAM) sandwiched between two electrodes and its function is to introduce an asymmetry between the current measured under positive and negative bias regimes, respectively. The efficiency of current rectification, quantified as the rectification ratio, is dependent on many factors, including the molecular structure of the SAM, its density and degree of order, as well as coupling to the electrodes. In this talk I will address these topics by discussing first the dependence of the rectification on the strength of the dipole moment of the molecules, followed by an example of manipulating the properties by exploiting the charge-transfer that results from co-assembling molecules with strong electron donor and acceptor termini.1 We further show that the molecule/electron coupling resulting from the delocalization of electrons lone pairs controls the position of the molecular orbitals participating in transport, making it efficient for one bias polarity and inefficient for the opposite polarity. In the case of a strong coupling, this led to rectification ratios greater than 2500.2 This performance was obtained in molecular diodes fabricated on silicon, which has a very low natural roughness, and thus require no additional processing. Our molecules are obtained from a simple, robust, and high-yield synthetic procedure and may yield hybrid systems when integrated with other more mature silicon technologies that are currently included in consumer electronics to expand its use toward novel functionalities governed by the molecular species grafted onto its surface. One example is that of a water sensor, which transduces the ambient moisture absorbed into the device through the porous electrode to electrical signal. By monitoring these changes, we demonstrate clear and reproducible changes in rectification ratio that are reversible upon multiple cycles.
1.2-I2
Mariano Campoy Quiles´s research is devoted to the understanding and development of solution processed semiconductors for energy and optoelectronic applications. He and his team have built substantial research efforts in two application areas, solar photovoltaic (light to electric) and thermoelectric (heat to electric) energy conversion based on organic and hybrid materials. He studied physics at the Univesity of Santiago de Compostela, obtained his PhD in experimental physics from Imperial College London, and since 2008 he leads his team at the Institute of Materials Science of Barcelona.
Most advanced optoelectronic devices rely on the spatial patterning of the relevant properties of the active components. An example of this would be the RGB pixels sitting side by side in LED based screens. Interestingly, between a homogeneous thin film, and a highly complex pixelated board, there is a whole parameter space that can be navigated: that of samples with gradual changes in the properties of interest.
In this talk, I will first describe a number of methods that we have developed to produce organic semiconductors based gradients in film thickness, composition, doping level, molecular orientation and microstructure. Then, I will briefly mention how these samples, containing a wealth of information, can be employed for fundamental studies (such as deducing the phase diagram) or combinatorial optimization of solar cells. And then, in the last part of the talk, I will describe new device concepts based on samples with gradients, such as polarimeters without moving parts based on spherullitic macroscopic alignment, position sensitive photodetectors, and monolithic miniature spectrometers based on microcavities with thickness wedge active layers.
1.3-I1
The rapid development of charge transport and light‐emission in organic materials in the last decades has advanced the field of organic optoelectronics, highlighting the high potential of light‐emitting devices for industrial applications. Demonstrated for the first time over 15 years ago, light-emitting field-effect transistors (LEFETs) have transformed from an optoelectronic curiosity to a serious competitor in the race for cheaper and more efficient displays, also showing promise for injection lasers. Thus, what is a LEFET, how does it work, and what are the current challenges for its integration into mainstream technologies? The talk will shed some light on these questions. The fundamental working principle of LEFETs, materials that have been used, and device physics and architectures involved in the progression of LEFET technology for displays will also be discussed. Finally, the state‐of‐the‐art development of LEFETs will be presented as prospect avenues for the future of research and applications in this area.
1.3-T1
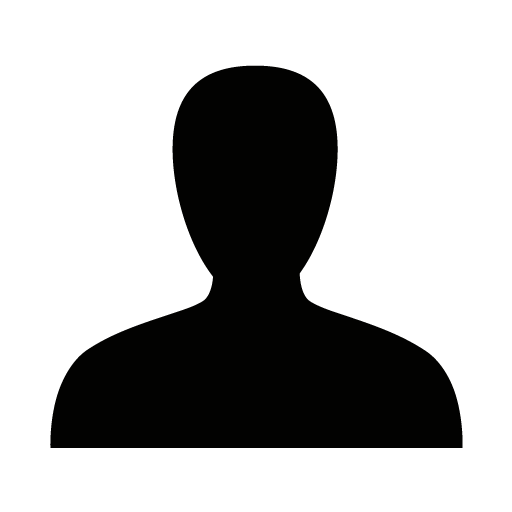
Inherently narrowband near-infrared organic photodetectors (NIR-OPD) are highly desired for many applications, including biological imaging and surveillance. To obtain a narrowband response, one of the most used approaches is to integrate a photodiode in an optical cavity configuration. With manipulating the cavity length, tuneable wavelength-selective NIR-OPDs were realized[1]. However, they suffer from a low external quantum efficiency (EQE) and strongly rely on the used material or on a nano-photonic device architecture. Here, we demonstrate a general and facile approach towards wavelength-selective NIR-detection through intentionally n-doping 500-600 nm-thick nonfullerene blends. We show that a electron-donating amine-interlayer can induce n-doping, resulting in a localized electric field near the anode and selective collection of photo-generated carriers in this region. As only weakly absorbed photons reach this region, the devices have a narrowband response at wavelengths close to the absorption onset of the blends with a high spectral rejection ratio. These spectrally selective NIR-OPDs exhibit EQEs of ~20-30% at NIR wavelengths of 900-1100 nm, with a full-width-at-half- maximum of ≤50 nm, as well as detectivities of >1012 Jones (to be published in Nature communications soon).
1.3-T2
Efficient, sensitive and wavelength-selective light detection has become central to modern consumer electronics, and also in science and technology. Photodetectors based on crystalline inorganic elemental materials such as silicon and compound semiconductors are the core of today’s photodetectors. However, a new trend has begun: next generation semiconductors such as organics, perovskites, and nanocrystals are now becoming increasingly interesting candidates for low noise, color-selective, efficient photodetection. As a promising candidate for next-generation photodetectors, solution-processed organic-based photodetectors (OPDs) provide the opportunity to develop innovative, low cost, and large-area imaging technologies for industrial applications. Thanks to their advantages as a large range of available materials, a tunable spectral response range, compatibility with lightweight flexible substrates and device architectures, they have gained a continuous increasing interest.
OPDs have drawn extensive research efforts due to their tailorable spectral response but to detect specific signals under low illumination intensities, OPD devices should deliver a low dark current and a relatively high sensitivity. The spectral response can be either broadband or narrowband. Benefiting from advanced material synthesizing techniques, narrowband OPDs with many improved properties have been emerging in recent years, with promising features like high selectivity of the target wavelength compared to broadband counterparts. In addition and to avoid specific synthesis of the photo-absorbing materials, the charge collection narrowing (CCN) can be applied.[1] Starting from a broadband absorbers often used in organic solar cells (OSCs), narrowband OPDs are obtained by the realization of thick layers (700 nm), which flattened the spectral response, reduced the dark current and decreased performance variations.
In the present study, broadband and narrowband OPDs were realized with an active layer composed of PM6/ITIC-4F bulk heterojunction blend. During the past years, the development of non-fullerene acceptors (NFA) led to an impressive improvement of the OSC power conversion efficiency to above 18%.[2] This new family of electron accepting materials with absorption edges up to 1100 nm has great potential for both broadband and narrowband OPDs. Depending on the structure of the OPD device, normal or inverted, the switch between broadband and narrowband OPDs has been controlled by the donor:acceptor mass ratio, the final blend concentration, the film thickness or most notably by the post-annealing temperature. The impact and role of these parameters were studied by absorption and water contact angle measurements. The morphology and microstructure of thin films made by liquid deposition techniques were analyzed by AFM, Raman and X-ray diffraction.