1.1-I1
Marc T.M. Koper is Professor of Surface Chemistry and Catalysis at Leiden University, The Netherlands. He received his PhD degree (1994) from Utrecht University (The Netherlands) in the field of electrochemistry. He was an EU Marie Curie postdoctoral fellow at the University of Ulm (Germany) and a Fellow of Royal Netherlands Academy of Arts and Sciences (KNAW) at Eindhoven University of Technology, before moving to Leiden University in 2005. His main research interests are in fundamental aspects of electrocatalysis, proton-coupled electron transfer, theoretical electrochemistry, and electrochemical surface science.
The electrocatalytic reduction of CO2 is very sensitive to the nature of the electrolyte near the electrode surface. The presence of cations and anions, and how they are indirectly affected by the (local) pH, play a crucial role, both for CO2 reduction and for the competing hydrogen evolution reaction. In my talk, I will demonstrate these effects for CO2 reduction on flat and nanoporous gold electrodes, and show how mass transport regulates them. I will discuss the effect of monovalent cations, multivalent cations, bicarbonate concentration (and how bicarbonate responds to the presence of cations), and varying mass transport rates as regulated by a rotating disc electrode.
1.1-I2
Prof. Beatriz Roldán Cuenya is currently Director of the Interface Science Department at the Fritz Haber Institute of the Max Planck Society in Berlin (Germany). She is an Honorary Professor at the Technische Universität Berlin, at the Freie Universität Berlin, and at the Ruhr-University Bochum, all in Germany. Also, she serves as a Distinguished Research Professor at the University of Central Florida (USA).
Prof. Roldán Cuenya began her academic career by completing her M.S./B.S. in Physics with a minor in Materials Science at the University of Oviedo, Spain in 1998. Afterwards she moved to Germany and obtained her Ph.D. from the Department of Physics of the University of Duisburg-Essen with summa cum laude in 2001. Subsequently, she carried out her postdoctoral research in the Department of Chemical Engineering at the University of California Santa Barbara (USA) until 2003.
In 2004, she joined the Department of Physics at the University of Central Florida (UCF) as Assistant Professor where she moved through the ranks to become a full professor in 2012. In 2013 Prof. Roldan Cuenya, moved to Germany to become Chair Professor of Solid State Physics in the Department of Physics at Ruhr-University Bochum until 2017.
During her academic career, Prof. Roldan Cuenya received an Early CAREER Award from the US National Science Foundation (2005) and the international Peter Mark Memorial award from the American Vacuum Society (2009). In 2016 she became Fellow of the Max Planck Society in Germany and also received the prestigious Consolidator Award from the European Research Council. In 2020, she became a member of the Academia Europaea (Academy of Europe). She received the AVS Fellow Award (2021), the International Society of Electrochemistry-Elsevier Prize for Experimental Electrochemistry (2021), the 2022 Paul H. Emmet Award of the North American Catalysis Society, and the Röntgen Medal of the City of Remscheid (2022).
Prof. Dr. Beatriz Roldan Cuenya is the author of 245 peer-reviewed publications, 6 book chapters and 6 patents. She has been supervising 74 postdoctoral fellows and 36 PhD students. She has given 245 invited talks, with 13 plenary talks and 33 keynote lectures since 2017. Her H-factor is 74 (Google Scholar) and her work has received over 21,500 citations.
She presently serves on the editorial advisory boards of the Journal of Catalysis and Chemical Reviews. In addition, she also contributes to a number of advisory committees, including the Liquid Sunlight Alliance (USA), the Advanced Research Center Chemical Building Blocks Consortium (Utrecht, the Netherlands), the Spanish Synchrotron Facility ALBA (Barcelona, Spain), the German Synchrotron DESY (Hamburg, Germany), the Helmholtz-Zentrum Berlin for the strategic development of BESSY II (Berlin, Germany), the Institute of Chemical Research of Catalonia (ICIQ in Tarragona, Spain), the UK Catalysis Hub and the Ertl Center for Electrochemistry & Catalysis (South Korea).
Prof. Roldan Cuenya’s research program explores physical and chemical properties of nanostructures, with emphasis on advancements in nanocatalysis based on operando microscopic and spectroscopic characterization.
In the quest for developing a sustainable energy economy, the electrocatalytic reduction of carbon dioxide (CO2RR) into value-added chemicals and fuels offers the potential to close the anthropogenic carbon cycle and store renewable energy into chemical bonds. It is therefore of particular interest to develop efficient and selective electrocatalysts, which reduce the reaction overpotential and steer the reaction toward hydrocarbons and multicarbon oxygenates. Nonetheless, in order to tailor the chemical reactivity of CO2RR nanocatalysts at the atomic level, fundamental understanding of their structure and surface composition under reaction conditions must be obtained. The latter requires a synergistic experimental approach taking advantage of a variety of cutting-edge microscopy (EC-AFM, EC-TEM, LEEM) and spectroscopy (XPS, XAS, MS/GC, XPEEM, Raman spect.) methods.
This talk will provide new insights into the CO2RR and CORR, with special focus on: (i) the evolution of the surface structure of well-oriented single crystal Cu catalysts [Cu(100), Cu(111), Cu(310)]; (ii) the design of size- and shape-controlled catalytically active nanoparticle pre-catalysts (Cu, Cu2O, Ag-Cu2O cubes, Au-Cu2O cubes, ZnO/Cu2O cubes), and (iii) the correlation between the dynamically evolving structure and composition of the electrocatalysts under operando reaction conditions, including pulse electrolysis treatments, and their activity and selectivity. The results are expected to open up new routes for the reutilization of CO2 through its direct conversion into valuable chemicals and fuels such as ethylene and ethanol.
1.1-I3
Marc Robert was educated at the Ecole Normale Supérieure (Cachan, France) and gained his Ph.D. in 1995 from Paris Diderot University under the guidance of Claude Andrieux and Jean-Michel Savéant. After one year as a postdoctoral fellow at Ohio State University (USA), working alongside Matt Platz, he joined the faculty at Paris Diderot University as Associate Professor. He was promoted to full Professor in 2004, and distinguished Professor in 2019 at Université de Paris. He became a junior Fellow of the University Institute of France (IUF) in 2007 and a senior Fellow in 2017. He was a JSPS (Japan Society for the Promotion of Science) research Fellow (2015). Among various distinctions, Marc Robert received the first International Prize Essential Molecules Challenge from Air Liquide (2016) and the Chemistry and Energy Research Prize from the French Chemical Society (2019). His interests include electrochemical, photochemical, and theoretical approaches of electron transfer reactions and reactivity in chemistry, as well as catalytic activation of small molecules, mainly CO2 and N2.
Electrochemical reduction of carbon dioxide (CO2) to carbon monoxide (CO) and other C1 products under mild conditions opens a way to produce fuels and chemical feedstock. Molecular catalysts made of Earth-abundant metals may be operated in homogeneous conditions or as supported heterogeneous systems.
Co and Fe complexes demonstrate excellent conversion of CO2 to CO with a selectivity reaching 99 %, in organic solvent as well as in water. By setting proper conditions, we recently discovered that formaldehyde and methanol (Co complex) as well as methane (Fe complex) could be obtained. In other words, CO2 could be reduced with 2, 4, 6 and 8 electrons with molecular catalysts. Our most recent results will be presented.
1.2-T1
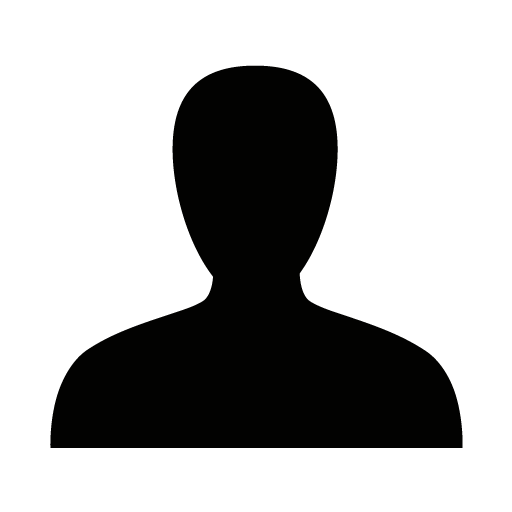
In this work, we present a mechanistic study of the electrochemical CO2 reduction catalyzed by a highly active fac‐[MnI(CO)3(bis‐MeNHC)MeCN]+ complex (Mn-MeCN+).[1] By combining in-situ IR-SEC, chemical synthesis of intermediates and DFT calculations, we have identified and fully characterized Mn-CO+ (under CO2) and Mn-H (under Ar) as key intermediates in the CO2 reduction mechanism. Our observations confirm that, in the presence of low water concentrations, the CO2 reduction to CO occurs through a protonation-first pathway. However, at higher concentrations of water, Mn-H is formed and easily inserts CO2 to give the corresponding Mn-O2CH intermediate. The formate release from the latter intermediate is a slow process which explains the decrease of the catalytic activity in the presence of high concentrations of added acid.
1.2-T2
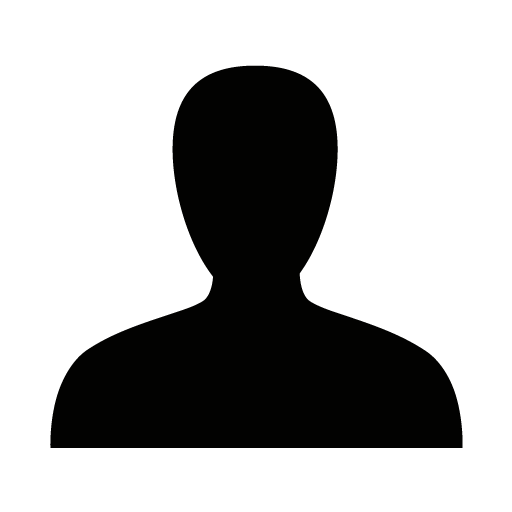
Greenhouse gases have maintained the conditions on Earth habitable. Nonetheless, the
atmospheric level of these gases, in particular carbon dioxide (CO2), has increased considerably in
the latest years. One way to cope with this increase is by electrochemically reduce CO2 into other
building blocks or fuels. However, the kinetic inertness and the thermodynamic stability of this
molecule makes its activation rather difficult, requiring the use of catalysts. Molecular catalysts,
such as iron porphyrins, are among the most efficient, robust, and selective catalysts towards the
reduction of CO2 to CO, by also being optimal catalysts in both organic and aqueous solutions.1,2 In
order to consider the reduction of CO2 by iron porphyrins as an interesting path to re-use and take
advantage of a waste product, fundamental knowledge is a pre-requisite to better understand this
reaction, improve their performance and orient their selectivity.
Even if electrochemical methods have allowed the determination of kinetic parameters,3,4 a
complete picture of the mechanism is still missing due to the lack of spectroscopic evidence. By
coupling electrochemical techniques with X-Ray absorption spectroscopy, we aimed at obtaining
a complete view of the modifications undergone by the catalyst during electroreduction and
catalysis. In this context, we have recently developed a spectroelectrochemical set-up for in situ
CO2RR studies by iron porphyrins in organic media. We present here the spectral changes
observed at the pre-edge and XANES region. This has allowed to give insights on the structural
and electronic changes that occur at the iron center and its interactions with the CO2, proposing
some possible intermediate species which are crucial to describe the CO2 reduction mechanism.
References:
(1) Costentin, C.; Robert, M.; Savéant, J.-M.; Tatin, A. Proc Natl Acad Sci USA 2015, 112 (22), 6882–6886.
(2) Costentin, C.; Drouet, S.; Robert, M.; Savéant, J.-M. Science 2012, 338 (6103), 90–94.
(3) Bhugun, I.; Lexa, D.; Savéant, J.-M. J. Am. Chem. Soc. 1996, 118 (7), 1769–1776.
(4) Costentin, C.; Passard, G.; Robert, M.; Savéant, J.-M. J. Am. Chem. Soc. 2014, 136 (33), 11821–11829.
1.2-T3
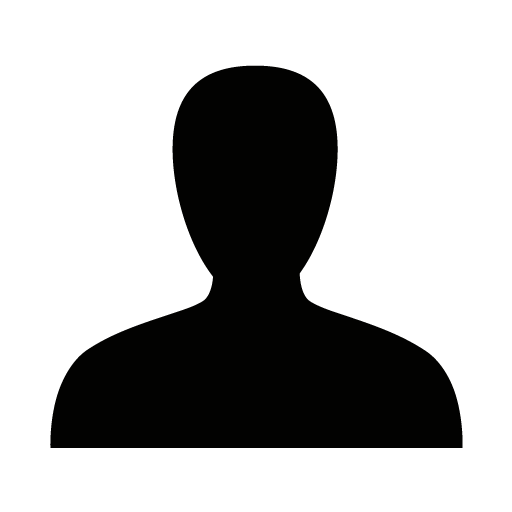
Effective large-scale CO2 conversion to fuels or value-added chemicals using renewable energies is critical to reduce our environmental impact [1]. To this end, better understanding of the CO2 mechanism is needed to develop efficient and selective catalysts that operates in water controlling H2 evolution. Covalent Organic Frameworks (COFs) are reticular materials, which can be used to combine the advantages of the well-defined molecular catalysts and the heterogeneous ones [2]. In this work, we present the first COF based on tricarbonyl Mn units (COFbpyMn), that by π-π stacking is attached to MWCNTs form electrocatalytic electrodes active for CO2 reduction in neutral water. The activity of the catalyst was evaluated by electrochemical techniques with good stability in aqueous solution. The COFbpyMn shows a low CO2RR onset potential (η = 190 mV) and high current densities (>12 mA·cm–2, at 550 mV overpotential) in water. TOFCO and TONCO values are as high as 1100 h–1 and 5800 (after 16 h), respectively, which are more than 10-fold higher than those obtained for the equivalent manganese-based molecular catalyst. The encapsulation of tricarbonyl Mn single-atom centers within the reticular covalent organic structure plays an important role by favouring the electrocatalytic CO2 reduction over competitive H2 evolution reaction. The spectroelectrochemical studies evidence the formation of five-coordinate species in the catalytic cycle for CO formation. The COF imposes mechanical constraints on the {fac-Mn(CO)3S} centers.
1.3-I1
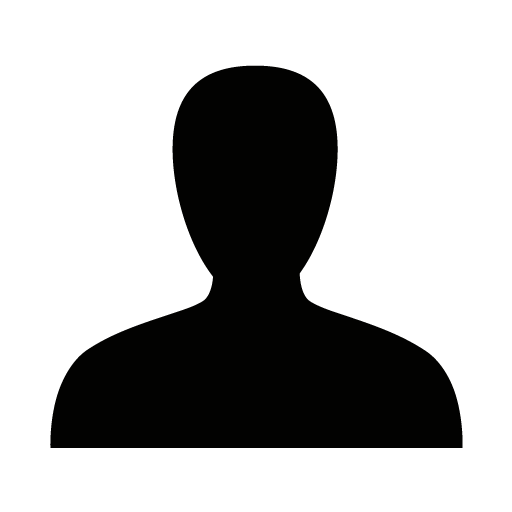
Cytochrome c nitrite reductase (CcNiR) is a multi-heme enzyme that catalyzes the six-electron, seven-proton reduction of nitrite to ammonia as part of the global nitrogen cycle. In this talk, the activity of iron and cobalt complexes that perform the multielectron reduction of nitrite, mimicking the activity of CcNiR, will be described. One complex is a small cobalt-peptide complex (CoGGH) that catalyzes the complete reduction of nitrite to ammonium with an onset potential at -0.65 V vs Ag/AgCl (1 M KCl) at pH 7.2. CoGGH also catalyzes the reduction of proposed intermediates nitric oxide and hydroxylamine to ammonium. The second catalyst is an iron complex featuring a macrocyclic redox-active ligand (FeN5H2), which catalyzes nitrite reduction with an onset potential of -0.90 V to form hydroxylamine. FeN5H2 is unusual for being an iron-based catalyst for multielectron nitrite reduction that functions near neutral pH. CoGGH also catalyzes proton reduction to H2; the study of this reaction yields insights into proton handling by this catalyst relevant to understanding the nitrite reduction mechanism.
1.3-I2
Mediated electrochemistry complements conventional electrocatalysis and introduces flexibility in catalyst design by enabling electrode-driven reactions to take place off-electrode under more conventional thermochemical conditions.[1] This talk will highlight the development and application of electron-proton transfer mediators that support redox processes relevant to fuel cells, emphasizing the oxygen reduction reaction (ORR).[2-4] Off-electrode mediated oxygen reduction proceeds effectively with non-precious-metal M-N-C (M = Fe, Co) catalysts that are similar or identical to those commonly used for direct electrocatalytic ORR. Mechanistic studies have been conducted to probe the mechanism of the thermochemical ORR with a hydroquinone mediator. In principle, this mediated ORR could proceed on the catalyst surface via the coupling of two independent half-reactions (IHR), consisting of hydroquinone oxidation and O2 reduction, or by direct inner-sphere reaction (ISR) between O2 and the hydroquinone. Data have been obtained that support the inner-sphere reaction pathway,[5] and these results have important implications for mediated electrochemical processes. Different mechanisms have different linear-free energy relationships (i.e., Tafel slopes) that offer potential advantages in overpotentials and/or rates of (net) electrochemical reactions.
2.1-I1
Prof. Magalí Lingenfelder is a PI with an excellent track record and a passion for atomically controlled interfaces. Her work contributes to the design of new materials by elucidating chemical processes by Scanning Probe Microscopies and Surface Sensitive Spectroscopies, including dynamic (bio) molecular recognition processes at the liquid/solid interface.
She created and led for over 10 years the Max Planck-EPFL laboratory for Molecular Nanoscience at EPFL campus in Switzerland, and is currently leading the Helvetia Institute for Science and Innovation.
She studied physical and biological chemistry at the National University of Córdoba in Argentina. In 2003, she finished her MSc thesis at the Max Planck Institute for the Solid State Research (MPI-FKF in Stuttgart, Germany) with seminal contributions to the field of metal-organic coordination networks on solid surfaces. She continued with her doctoral studies in Physics, and received the Otto Hahn medal of the Max Planck Society in 2008 for the microscopic understanding of the chiral recognition process with submolecular resolution. In her quest to study molecular recognition going from 2D to 3D complex systems, she made postdoctoral stays at the Institute of Materials Sciences in Barcelona, and at the Molecular Foundry of the Lawrence Berkeley National Lab in the US.
She is a committed mentor who directed 4 MSc theses, 5 PhD theses, and 5 postdocs. She advocates for problem-oriented interdisciplinary research, by pioneering the emerging field of BioNanoarchitectonics. She led 5 international research consortiums, delivered over 50 invited presentations, and organized 9 conferences and 4 doctoral schools. She and her team had received multiple awards and international recognitions for their creative and rigurous work on molecular recognition, chirality and operando studies at catalytic interfaces. In 2018, the Royal Society of Chemistry included her work in the first collection “Celebrating Excellence in Research: 100 Women of Chemistry”.
In this famous quote that most surface scientists love and hate in equal proportions, Pauli said: „God made the bulk; surfaces were invented by the devil“.
In this talk, I will show you how even the most well designed and atomically controlled 2D catalysts, and even the most common metal surfaces suffer from dynamic structural transformations when you look at them at the nanoscale, under electrochemical reaction conditions. The „devil“ face of electrocatalysts can affect the product selectivity trends in detrimental ways, but it can also be tuned to increase their performance in key reactions in artificial photosynthesis schemes. I will show you a few examples on the surface dynamics of Cu electrodes involved in CO2 electroreduction (CO2RR), and 2D transition metal-based materials that catalyze oxygen evolution reactions (OER). I will discuss the use of scanning probe microscopy and solid state spectroscopy to track the surface dynamics of electrocatalysts in-situ, and present a few unconventional strategies to reach significant boosts in the catalytic activity of Earth-abundant 2D materials (using spin-selective mechanisms).
2.1-I2
I will present the most recent advances on the role of reconstructions and electrolytes and how they affect the modeling of electrocatalytic systems.
2.1-I3
Catalysis is key in the future development of green chemistry and in the renewable production of chemicals and fuels. However, this transition requires a new approach to catalysis, as central chemical reactions cannot be catalyzed with existing materials. The grand challenge is to discover new catalyst materials, which are both stable and active. There are several energy conversion reactions, which are corner stones for the green transition and in urgent need of new catalysts.
High Entropy Alloys are solid solutions where five or more elements are mixed randomly together. The realization of HEAs has opened for a vast composition space with a practically infinite number of new not yet explored catalyst materials. We can tune their properties by smoothly change their composition. This has led to the statement that: HEA is a shift of paradigm “from using the materials we have, to engineer the materials we need”. I the talk I will present a simulation approach to discover new catalysis materials based on HEAs.
2.2-T1
Hydrogen plays a critical role in the energy transformation to a sustainable future, due to its widespread applications as a fuel for transport, feedstock to chemical industries and a heat source for buildings. Low temperature water electrolysis can enable hydrogen production renewably and at scale, but device efficiencies are currently limited by poor kinetics of water oxidation at the anode [1]. The low operating pH and high oxidizing conditions experienced at the anode in proton exchange membrane water electrolyzers limits the choice of materials to catalyse the oxygen evolution reaction to oxides of iridium, which is highly scarce [1],[2]. Mechanistic understanding of the water oxidation reaction on iridium oxides can enable rational development of more active catalysts with lesser precious metal loading.
Iridium oxides exhibit complex redox chemistry, which cannot be understood using electrochemical measurements alone. Particularly, the redox transitions on iridium oxides can exhibit non-Nernstian behavior, resulting in the redox transitions being dependent on pH and cations present in the electrolyte. In this talk, I will demonstrate the power of optical spectroscopy to track potential-dependent electroabsorption changes as a function of electrolyte pH from 1 to 13 on iridium based oxides. These measurements enable a direct comparison of the nature of redox active species in acidic and alkaline solution and also provide insights about the electrolyte environment on the redox potential, and consequently binding energetics of oxygenated species on IrOx. Using complementary stepped potential spectroelectrochemical measurements, the density of these redox active sites can also be computed as a function of potential [3] and the kinetics of water oxidation in acid and base can be linked directly to the density of oxidized species [4], [5]. Furthermore, in addition to quantitative detection of redox states, measurements of the kinetics of charge accumulation in natural and deuterated electrolyte provides insights into the role of ion transport within the oxide and the degree of bulk iridium redox. Therefore, based on our spectroscopic results on iridium-based oxides, we can experimentally probe the effect of interfacial pH and ions on charge accumulation and its impact on water oxidation kinetics.
2.2-T2
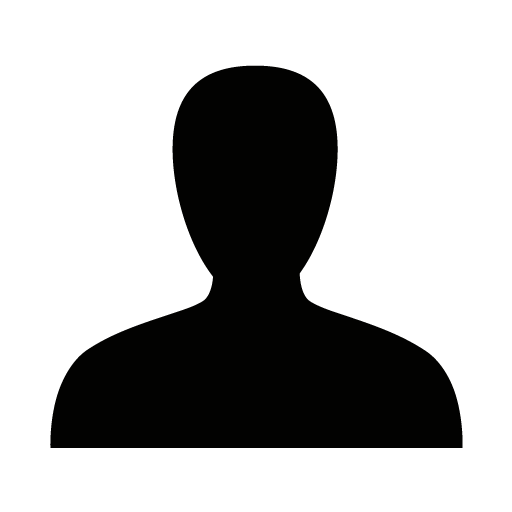
Iridium oxide is the state-of-the-art electrocatalysts for water oxidation in polymer electrolyte membrane (PEM) electrolysers for green hydrogen production. Hydrous, amorphous iridium oxides (IrOx) show far higher activity than the rutile crystalline iridium oxides (IrO2), but the reason why they are more active is still under intense debate. Various differences between IrOx and IrO2, including the differences in active surface area, chemical state of Ir, fractional coverage of -OH groups and the range of ordered structure, renders it difficult to identify the origin of the differing activity.[1], [2] Thus far, ambiguities in measuring the number of sites participating in the reaction have prevented the accurate measurement of intrinsic catalytic activity, or turnover frequency, for amorphous iridium oxides and rutile iridium oxide.
In this talk, I will present the results from a range of different spectroscopic techniques and electrochemical measurements to identify the redox species present in IrOx and IrO2 and establish how these control the reaction kinetics. The techniques include (i) time-resolved UV-vis absorption spectroscopy (ii) on chip electrochemical mass spectrometry (iii) time of flight secondary ion mass spectrometry.
We have identified the same short-lived and long-lived oxidised species for both IrOx and IrO2 at potentials from 1.4 V to 1.55 V. We have correlated these species to the O2 evolution kinetics. We attribute the lower activity of rutile IrO2 to a lower concentration of the species responsible for driving water oxidation. The electrochemical mass spectrometry allows us to separate capacitive currents from O2 evolution on the two catalysts. Combining TOF-SIMs with a paraffin wax cover method,[3] the depth of proton penetrating into the bulk catalysts was also revealed.
By comparing the concentration of oxidised species to reaction rates, we can directly measure turnover frequency (TOF) for IrOx and IrO2 as a function of applied potential. In summary, this study quantitively measured the intrinsic activity of amorphous and crystalline iridium oxides without any assumption of surface area and therefore shines insights into the origin of activity difference between amorphous and rutile iridium oxides.
2.2-T3
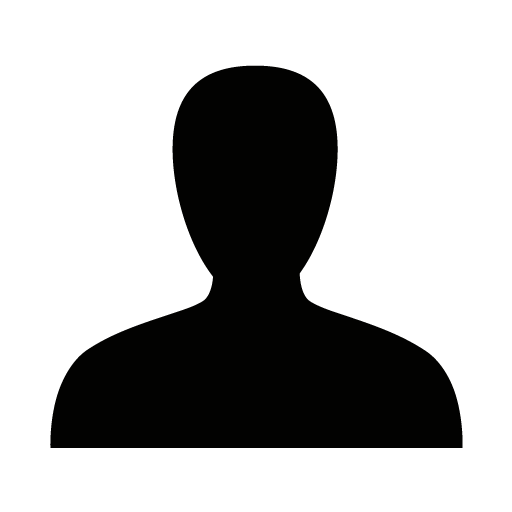
A carbon-neutral and sustainable future requires advanced electrocatalysis systems[1]. Magnetism-induced enhancement of electrocatalytic reactions has been gaining increasing attention [2],[3],[4]. A simple and efficient way to achieve an enhancement is to apply an external magnetic field to the reaction[5]. Various mechanisms concerning different aspects of the reactions, e.g., reaction kinetics[5],[6] and mass transfer processes[7], have been introduced. However, the enhancement is likely a result of a synergistic effect of at least two mechanisms. Here, using key electrocatalytic reactions, i.e., oxygen evolution reaction (OER), oxygen reduction reaction (ORR) and hydrogen evolution reaction (HER), and homogeneous magnetic fields provided by an electromagnet, we demonstrate the importance of experimentally decoupling the mechanisms affecting the electrocatalytic reactions at electrodes with different magnetic properties. Our results show that the effect on the reaction kinetics and selectivity is exclusive to ferromagnetic catalysts and electron spin-dependent reactions under sufficient magnetic field strength; however, an enhancement of the mass transfer is universal. We provide a versatile strategy to optimize the magnetic enhancement that can be implemented to boost electrocatalytic reactions.
2.2-T4
Ben was awarded his PhD in 2020, working in the group of James Durrant, studying photocatalytic materials for water splitting using transient diffuse reflectance at Imperial College London. Following post-doctoral work developing automated optical spectroscopies for electrochemistry with Prof. Ifan Stephens, Ben was awarded a Schmidt AI in Science Fellow at the iX institute at Imperial College London, working with Prof. Aron Walsh to develop new algorithms to analyse operando spectroscopies In the Mantiram group, Ben is a Lindeman postdoctoral fellow focusing on combining interpretable machine learning algorithms with high throughput operando spectroscopies. Outside of this research, Ben is committed to the development of open-source hardware for spectroscopies, providing his designs at www.opensourcespectroscopy.com.
The conversion of renewable energy into storable, high value fuels is a key outstanding challenge in the transition to a carbon neutral economy. The primary source of electrons for the production chemically reduced fuel compounds is from the electrocatalytic oxidation of water. However, large quantities of energy are currently lost in the form of catalytic overpotential during water oxidation. This creates a challenge in scaling up electrolysis technology. To surmount these challenges, water oxidation activity must be improved using earth abundant catalysts. However, progress towards this goal is stymied by the complex ways in which applied voltage can drive water oxidation. This complexity is a result of the cooperative effects arising from interactions between oxidized species when large fraction of an electrocatalytic surface becomes oxidised. Competing, but not mutually exclusive, hypotheses, point to changes in activation energy1 and changes in the catalytic mechanism involving the coupling of multiple oxidising equivalents during catalysis2 as driving changes in rate constant and Tafel slope as a function of potential.
Cobalt compounds are used as water oxidation electrocatalysts for water oxidation across a wide rage of pH. However, the convolution of overlapping, non-Nernstian redox processes and changes in catalytic mechanism complicate interpretation of Tafel slopes. In-situ spectroelectrochemistry can be used to separate redox driven changes in surface electronic structure from catalytic effects3. In this talk I demonstrate the construction and capabilities of a new potential and time operando spectroelectrochemical system to help resolve these questions in two model cobalt catalysts. First, cobalt-iron hexacyanoferrate4, in which catalytic Co sites should be physically isolated from one another,5,6 and secondly, amorphous cobalt phosphate oxide, in which Co centres interact during catalysts by oxo coupling and also via non-nernstian effects.7,8 Using global analysis of a rich, 3d spectreoelectrochemial data set, the potential dependence of the density of catalytically active states in both materials can quantified and compared. Time resolved spectral measurements as the catalysts relax from an operating potential to rest under open circuit conditions are used to access the kinetics of water oxidation. These results show that contrast to cobalt phosphate oxide, catalysis in cobalt hexacyanoferrate shows negligible cooperative effects, both in terms of an enthalpic change in surface reactivity and multi-metallic catalysis, indicating a simple connection between the density of catalytic states and the Tafel slope.
2.3-I1
Vincent Artero was born in 1973. He is a graduate of the Ecole Normale Supérieure (Ulm; D/S 93) and of the University Pierre et Marie Curie (Paris 6). He received the Ph.D. degree in 2000 under the supervision of Prof. A. Proust. His doctoral work dealt with organometallic derivatives of polyoxometalates. After a postdoctoral stay at the University of Aachen (Aix la Chapelle) with Prof. U. Kölle, he joined in 2001 the group of Prof. M. Fontecave in Grenoble with a junior scientist position in the Life Science Division of CEA. Since 2016, he is Research Director at CEA and leads the SolHyCat group. His current research interests are in bio-inspired chemistry including catalysis related to hydrogen energy and artificial photosynthesis.
Vincent Artero received the "Grand Prix Mergier-Bourdeix de l'Académie des Sciences" in 2011 and has been granted with a Consolidator Grant from the European Research Council (ERC, photocatH2ode project 2012-2017). He's a member of the Young academy of Europe (YAE). He currently acts as Chair of the Scientific Advisory Board of the ARCANE Excellence Laboratory Network (LABEX) for bio-driven chemistry in Grenoble and as co-head of the French network (CNRS-Groupement de recherche) on Solar Fuels. Since 2016, Vincent Artero is associate editor of the Royal Society of Chemistry journal "Sustainable Energy and Fuels". From January 2018 onward, he actsas associate editor of the Royal Society of Chemistry flagship journal "Chemical Science"
Hydrogen is now confirmed as a key component of a CO2-neutral economy, we need to transition towards. The production of large quantities of hydrogen now requires breakthroughs in finding new catalysts that are efficient, stable and cheap, i.e. based on abundant elements. Indeed fuel formation involves multi-electron multi-proton reactions that are inherently kinetically sluggish. Efficient catalysts can be found in living micro-organisms producing or metabolizing hydrogen thanks to hydrogenases. Catalysis in these enzymes only requires Earth-abundant metal centers, the reactivity of which is enhanced thanks to the presence of basic sites acting as proton relays [1] at their vicinity. We will show how such active sites can be used as an inspiration to design new synthetic catalysts for H2 evolution [2-4] and oxidation [5-6] and how the introduction of proton relays significantly impacts the catalytic properties of such catalytic platforms, opening in some cases the possibility for bidirectional and even reversible catalysis.
2.3-I2
Electrosynthesis strategies have seen a resurgence over the last decade, although most electrocatalysts for electrosynthesis are either traditional metal catalysts (i.e. Pt, Pd, or alloy catalysts) or molecular electrocatalysts. However, biocatalysts can be utilized for improving the selectivity of electrosyntheses. This talk will discuss the use of microbial and enzymatic bioelectrocatalysis for electrosynthesis. It will compare and contrast microbial and enzymatic bioelectrocatalysis. Then, the talk will show the use of enzymes and microbes for mediated and direct bioelectrosynthesis of ammonia and value-added chemical products (i.e. chiral amines). Finally, the talk will discuss the future directions in bioelectrocatalysis for electrosynthesis.
2.3-I3
Boettcher is a Professor in the Department of Chemistry and Biochemistry at the University of Oregon. His research is at the intersection of materials science and electrochemistry, with a focus on fundamental aspects of energy conversion and storage. He has been named a DuPont Young Professor, a Cottrell Scholar, a Sloan Fellow, and a Camille-Dreyfus Teacher-Scholar. He was included as an ISI highly cited researcher (top 0.1% over past decade) over the past two years. In 2019, he founded the Oregon Center for Electrochemistry and in 2020 launched the nation’s first targeted graduate program in electrochemical technology.
Heterogeneous electrocatalysts for the oxygen evolution reaction (OER) are complicated materials with dynamic structures. They exhibit potential-induced phase transitions, potential-dependent electronic properties, variable oxidation and protonation states, and disordered local/surface phases. These properties make understanding the OER, and ultimately designing higher-efficiency catalysts, challenging. Measurements of intrinsic activity show that, by far, the most-active phases for OER under alkaline conditions are Fe-containing mixed-metal oxyhydroxides, but exactly how they function remains controversial. I will discuss our work to understand the key properties of these catalysts, including morphology, composition, and molecular/electronic structure, and how they evolve and are dynamic under active catalytic conditions. Specifically, I will highlight evidence for the critical role of “surface” Fe-O clusters on CoOOH and NiOOH porous supports as the key active site motif for OER. These concepts inform design strategies for higher-performance catalyst architectures and for their incorporation into practical electrolyzer devices to make clean hydrogen fuel from inexpensive renewable electricity for green long-distance transportation and long-duration energy storage.
Session-K1
Prof. Qing Shen received her Bachelor’s degree in physics from Nanjing University of China in 1987 and earned her Ph.D. degree from the University of Tokyo in 1995. In 1996, she joined the University of Electro-Communications, Japan and became a full professor in 2016. In 1997, she got the Young Scientist Award of the Japan Society of Applied Physics. In 2003, she got the Best Paper Award of the Japan Society of Thermophysical Properties and the Young Scientist Award of the Symposium on Ultrasonic Electronics of Japan. In 2014, she got the Excellent Women Scientist Award of the Japan Society of Applied Physics. She has published nearly 140 peer-reviewed journal papers and book chapters. Her current research interests focus on solution processed nano-materials and nanostructures, semiconductor quantum dot solar cells and perovskite solar cells, and especially the photoexcited carrier dynamics (hot carrier cooling, multiple exciton generation, charge transfer at the interface) in perovskite solar cells, quantum dot and dye sensitized solar cells, organic-inorganic hybrid solar cells.
Perovskite quantum dots (QDs) as a new type of colloidal nanocrystals (NCs) have gained significant attention for both fundamental research and applications of optoelectronic devices owing to their appealing optoelectronic properties and excellent chemical processability. For their wide range of potential applications, synthesizing colloidal QDs with high crystal quality and stability is of crucial importance. However, like most common QD systems, those reported perovskite QDs still suffer from a certain density of trapping defects, giving rise to detrimental non-radiative recombination centers and thus quenching luminescence. Very recently, we have suceeded in synthesis of phase stable and less defect preovksite QDs, including APbX3 NCs (A: FA, MA, Cs; X: I, Br, Cl) and Sn-Pb alloyed NCs [1-5]. We have demonstrated that a high room-temperature photoluminescence quantum yield (PL QY) of close to 100% can be obtained in the APbX3 perovskite QDs, signifying the achievement of ignorable less trapping defects in the APbX3 QDs. Ultrafast kinetic analysis with time-resolved transient absorption spectroscopy evidences the negligible electron or hole trapping pathways in our QDs, which explains such a high quantum efficiency. In addtion, photoexcited hot and cold carrier dynamics as well as charge transfer at the heterojunction of QD/metal oxide were systematically investigated [4]. Solar cells based on these high-quality perovskite QDs exhibit power conversion efficiency of over 12%, showing great promise for practical application. On the other hand, through incorporation of alkali ion Na+, we have realized for the first time efficient near-infrared emission from highly defective Sn-Pb perovskite QDs with substantially improved PL QY from ~0.3% to 28% [5]. Our findings provide new insights into the materials design strategies for improved optoelectronic properties of Sn-containing perovskites. We anticipate their use in near-infrared devices is very promising if issues of the sustainability of PL QY can be fully addressed in the near future.
1.1-I1
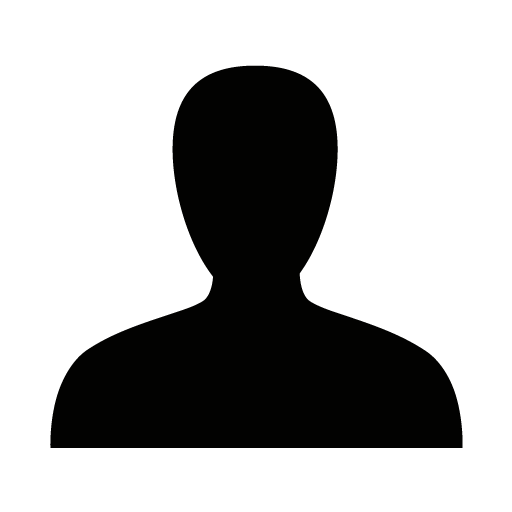
Over the past years we have developed various approaches to fabricate materials with sophisticated chemical and structural complexity. We have focused on synthesis in non-aqueous solution since this approach is not limited to one particular class of materials. Thus, it gives us flexibility to tailor the composition and properties of materials in respect to the application, for examples carbon dioxide sensors and photo-electrochemical devices.
In this talk, I will present how X-ray synchrotron methods, far from merely providing new tools, are extending the ways we study, understand and design such complex structures. A combination of spectroscopic, scattering and microscopic X-ray methods and rapid data acquisition help to uncover the complex chemical world behind the synthesis of functional materials. It gives complementary information about chemical reaction in solution and nucleation, growth and crystal phase transition of nanoparticles. [1-4] Moreover, on the selected examples, I will discuss how the possibility to select with high-energy resolution the incident and emission hard X-ray energies offers unprecedented site selectivity and give access to determine structure – function relationship of photo-, electroactive materials and devices. [5-6] Finally, will discuss the advantages and the pitfalls of the synchrotron methods for in situ and operando studies.
1.1-I2
Marco Favaro is the deputy head of the Institute for Solar Fuels at the Helmholtz Zentrum Berlin (HZB), Germany. After his PhD at the University of Padua (Italy) and Technical University of Munich (Germany), concluded in 2014, he spent two years as a Post-doctoral fellow at the Joint Center for Artificial Photosynthesis in Berkeley, USA, in the group of Dr. Junko Yano. He moved to Germany in 2017 to join the HZB. Here, his research activity focuses on understanding chemical composition/electronic-structural properties/performance interplay in photoelectrocatalysts by coupling operando multimodal spectroelectrochemical investigations with synchrotron-based in situ/operando spectroscopies.
Solar-assisted carbon dioxide reduction reaction (CO2RR) offers the possibility to use atmospheric CO2
and sunlight to produce highly-valuable hydrocarbons such as methane, ethylene, and ethanol. So far, the
majority of the CO2RR investigations were carried out in water environments. Although this approach is
environmental-friendly and constitutes a promising strategy for future implementation with solar electro-
lyzers, three main drawbacks exist: the limited solubility of CO2 in water (~ 35 mmol/L at room tempera-
ture) (i), the competitive hydrogen evolution reaction (HER) occurring when performing CO2RR in water
that limits the overall Faradaic efficiency (ii), and the difficulty to use molecular homogeneous (pho-
to)catalysts that boost the unless poor selectivity of the reaction (iii). Therefore, the mid-term strategy is
to develop a double-compartment photoelectrochemical device where the anolyte and catholyte sides are
separated by polymeric membranes. Here, solar-driven oxygen evolution reaction (OER) occurs at the
water-based anolyte side, while CO2RR undergoes at the non-aqueous catholyte side of the cell. In this talk, we will present our recent in situ hard X-ray ambient pressure photoelectron spectroscopy
(AP-HAXPES) investigations of electrified solid/liquid interfaces. The experiments were performed at the
new SpAnTeX (Spectroscopic Analysis with Tender X-rays) end-station operating at the BESSY II syn-
chrotron facility. The seminar will focus on three activities performed at the SpAnTeX end-station:
• First, we will show how the chemistry of BiVO4 photoanode surfaces can be tuned when the sem-
iconducting material is in contact with an aqueous phosphate buffer solution;
• Second, we will present the results obtained on a gold polycrystalline surface/N,N-
dimethylformamide interface, as a function of the applied overpotential. At such an interface, io-
dine electrooxidation and CO2RR were studied under in situ conditions;
• Third, we will show some recent results obtained on newly-developed in situ cell for multimodal
in situ investigations, equipped with a gas diffusion layer and a polymeric membrane. We will conclude this contribution by discussing about future perspectives and technical implementations
for multimodal in situ investigations of (photo)electrocatalytic processes.
1.1-I3
Dr. Christopher Kley leads the Helmholtz Young Investigator group "Nanoscale Operando CO2 Photo-Electrocatalysis" at the Helmholtz Center Berlin for Materials and Energy and the Fritz Haber Institute of the Max Planck Society. Previously, he was working at the Department of Chemistry at the University of California, Berkeley (Postdoc), Max Planck Institute for Solid State Research and École Polytechnique Fédérale de Lausanne (PhD), Max Planck Institute for Chemical Energy Conversion (master), and Karlsruhe Institute of Technology (study of physics). His research group focuses on deciphering the structural, electrical and catalytic properties of materials under liquid phase reaction conditions by in situ scanning probe microscopy based approaches paired with electrochemical and spectroscopic characterization as well as material synthesis.
While the performance of catalysts for electrochemical CO2 reduction reaction (CO2RR) strongly depends on the nature of electrode-electrolyte interfaces, it remains a key challenge to spatially resolve interfacial physical-chemical properties under liquid phase reaction conditions. In this talk, recent advances in electrochemical atomic force microscopy (EC-AFM) to decipher in situ an electrocatalyst’s morphology, structure and reaction-rate determining local charge transfer variations will be discussed. Selected results include deciphering the potential-dependent morphology of copper-based electrodes during CO2RR. Distinct surface morphologies are revealed for a copper(100) electrode over wide potential ranges upon electrode exposure to electrolytes and during CO2RR, which can be understood by surface mobility and specific adsorption. Decisively different behavior of copper electrocatalysts in the presence of halides compared to plain electrolytes is observed. Conductive AFM will be demonstrated as a correlative tool for revealing simultaneously the nanoscale electric current, morphology and friction force of electrocatalysts in relevant aqueous electrolytes. The broader relevance of implemented in situ EC-AFM methods will be discussed in the context of establishing reliable structure-property relationships for the rational design of advanced catalysts and electrochemical interfaces.
1.2-I1
Hydrogen production through water splitting has attracted great interest in recent years due to its potential of generating energy without causing pollution. One of the best candidates for water splitting is nickel oxyhydroxide with iron content (Ni 1− x FexOOH) that has excellent efficiency at alkaline conditions and is now studied widely. But pure NiOOH has poor efficiency unless doped with Fe and Co. We explore the influence of co-doping on the efficiency of NiOOH in the process of water oxidation by using Density Functional Theory +U (DFT+U). We also test the effect of strain on catalytic efficiency by modeling water oxidation on expanded and contracted surfaces of NiFeCoOOH. We find that several doping locations of Co have a similar result as if NiFeOOH had no Co content. Iron is responsible for the high activity at the Fe active site due to the low energy required for charge extraction. Yet, the valence band edge includes Fe, Co and Ni states hybridized which allows better charge extraction during deprotonation. The valence band edge position is higher upon Co-doping, which should allow better hole transport toward the surface. Hence, the presence of Ni and Co atoms surrounding the active site is vital for better efficiency. Moreover, we found that applying strain does not improve the efficiency and therefore a substrate with minimal mismatch should be used for NiFeCoOOH electrocatalysis.
1.2-I2
Machine learning accelerated catalyst discovery efforts have seen much progress in the last few years. Datasets of computational calculations have improved, models to connect surface structure with electronic structure or adsorption energies have gotten more sophisticated, and active learning exploration strategies are becoming routine in discovery efforts. However, there are several large challenges that remain: to date, models have had trouble generalizing to new materials or reaction intermediates and applying these methods requires significant training. I will briefly introduce the Open Catalyst Project and the Open Catalyst 2020 dataset, a collaborative project to span surface composition, structure, and chemistry and enable a new generation of deep machine learning models for catalysis. I will then discuss initial results for state-of-the-art deep graph convolutional models and significant recent progress from others in the community, many of which are likely to improve models in related materials science areas.
1.3-I1
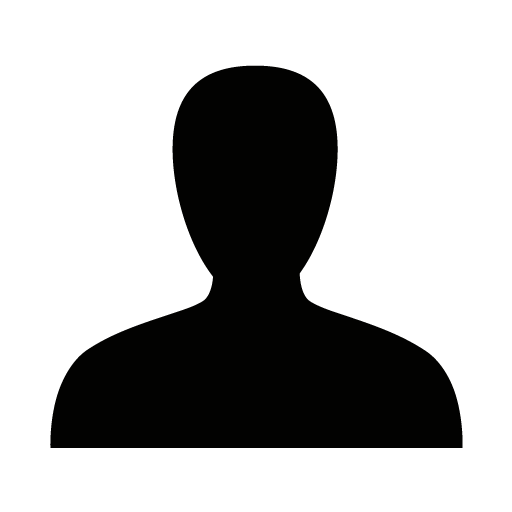
Transition metal oxides are promising photoelectrode materials for solar-to-fuel conversion applications. However, their performance is limited by the low carrier mobility (especially electron mobility) due to the formation of small polarons. Recent experimental studies have shown improved carrier mobility and conductivity by atomic doping; however the underlying mechanism is not understood. A fundamental atomistic-level understanding of the effects on small polaron transport is critical to future material design with high conductivity. In this talk, we will discuss the effect of small polaron formation on optical and carrier transport properties of transition metal oxides from first-principles calculations.
First, we resolve the conflicting findings that have been reported on the optical gap of a well-known catalysis Co3O4 as an example[1]. We confirm that the formation of small hole polarons significantly influences the optical absorption spectra and introduces extra spectroscopic signature below the intrinsic band gap, leading to a 0.8 eV transition that is often misinterpreted as the band edge that defines the fundamental gap.
Then we discuss the formation of small polarons' effect on carrier concentration, by resolving the controversy of nature of "shallow" or "deep" impurities of intrinsic oxygen vacancies in BiVO4 as an example[2], i.e. how to unify different experiments with the correct definition of ionization energy in polaronic oxides. We further discuss why certain dopants can have very low optimal concentrations (or very early doping bottleneck) in polaronic oxides such as Fe2O3, through a novel "electric-multipole" clustering between dopants and polarons[3]. These multipoles can be very stable at room temperature and are difficult to fully ionize compared to separate dopants, and thus they are detrimental to carrier concentration improvement. This allows us to uncover mysteries of the doping bottleneck in hematite and provide guidance for optimizing doping and carrier conductivity in polaronic oxides toward highly efficient energy conversion applications. In addition, we show the importance of synthesis condition such as synthesis temperature and oxygen partial pressure on dopant and polaron concentrations, and how to optimize the synthesis condition based on theoretical predictions.
At the end, we show different theoretical models for polaron mobility calculations from a macroscopic dielectric continuum picture with an example of spin polarons in CuO[4] and a microscopic polaron hopping picture by combining generalized Landau-Zener theory and kinetic Monte-Carlo samplings for doped oxides[5].
Our first-principles calculations provide important insights and suggest design principles for optimal optical and transport properties of polaronic oxides.
1.3-I2
Interfaces play an essential role in nearly all aspects of life and are critical for electrochemistry. Electrochemical systems ranging from high-temperature solid oxide fuel cells (SOFC) to batteries to capacitors have a wide range of important interfaces between solids, liquids, and gases which play a pivotal role in how energy is stored, transferred, and/or converted. This talk will focus on our use of ambient pressure XPS (APXPS) to directly probe the solid/gas and solid/liquid electrochemical interface. APXPS is a photon-in/electron-out process that can provide both atomic concentration and chemical-specific information at pressures greater than 20 Torr. Using synchrotron X-rays at Lawrence Berkeley Nation Laboratory, the Advanced Light Source has several beamlines dedicated to APXPS endstations that are outfitted with various in situ/operando features such as heating to temperatures > 500 °C, pressures greater than 20 Torr to support solid/liquid experiments and electrical leads to support applying electrical potentials support the ability to collect XPS data of actual electrochemical devices while it's operating in near ambient pressures. This talk will introduce APXPS and provide several solid/gas and solid/liquid interface electrochemistry examples using in situ and operando APXPS including the probing a Pt metal electrode undergoing a water-splitting reaction to generate oxygen[1], utilization of theory and experiment to understand CO2’s interaction with Cu and Ag metal surfaces [2], [3], [4], and the ability to probe the electrochemical double layer (EDL) [5]. Gaining new insight to guide the design and control of future electrochemical interfaces.
1.3-I3
Dr. Tyler Mefford is a Senior Staff Research Scientist in the Department of Materials Science & Engineering at Stanford University. He has a B.S. in Chemistry from Stanford University (2012), a Ph.D. in Analytical Chemistry from the University of Texas at Austin (2016), and did postdoctoral research in the Department of Materials Science and Engineering at Stanford University (2016-2020). His research is focused on developing advanced electrocatalysts for green chemical production through atomically-controlled synthesis, operando electrochemical scanning probe, X-ray, and electron microscopies, and first principles/microkinetic modeling approaches.
The properties of emerging non-precious metal (oxy)hydroxide electrocatalysts for the oxygen evolution reaction (OER) evolve dynamically with voltage during operation. Understanding how material properties govern the kinetics of electron and ion transfer during the OER requires integrating electrochemical cells into advanced characterization methods to study reactivity during operation. Further, the sub-particle spatial heterogeneity of electrocatalysts during operation requires that these operando methods must be able to image the local chemical, physical, and electronic structure at the nanoscale and link these properties to the local electrochemical activity.
In this talk, I will describe a suite of correlative operando microscopy techniques we have developed to study the OER on faceted Co (oxy)hydroxide nanoplatelets.[1] Operando Scanning X-Ray Transmission Microscopy (STXM) and Electrochemical Atomic Force Microscopy (EC-AFM) are used to map the local Co oxidation state and particle morphology across voltages spanning the bulk ion insertion reactions and OER regimes. These results are correlated to local electrochemical activity maps obtained through Scanning Electrochemical Cell Microscopy (SECCM) providing insight into where and why oxygen is evolved on the Co (oxy)hydroxide platelets. These experimental material properties and local reactivity measurements are integrated into a computational model that combines thermodynamic adsorption energetics of OER surface intermediates and microkinetic modeling to derive the experimental electrochemical Tafel behavior through first principles.[2] The results from our multi-modal correlative approach alter long standing assumptions about the OER-active properties of the Co (oxy)hydroxide system and provide an improved methodology towards developing predictive electrocatalytic theories based on material properties that govern reactivity away from open circuit conditions.
Session-K1
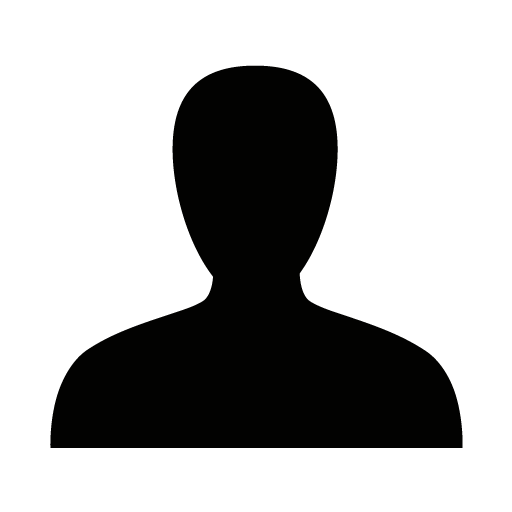
Some Cu(I)-based oxides with delafossite structure have attracted attention as promising photocathode materials for photoelectrochemical water splitting due to their intrinsic p-type conductivity and good stability against photo-corrosion. Beside CuFeO2, which has been intensely studied and found to have some limitations, CuRhO2 is also of interest for its considerable stability and favorable energy gap. To obtain insight into the fundamental bulk and surface properties of CuRhO2 that are relevant to such application, we have studied the bulk and majority (001) surface of CuRhO2 in vacuum and electrochemical environment using first principles calculations. We examine the bulk electronic structure and intrinsic defects, the surface stability diagram and band alignment at the oxide-water interface, and the energetics of the hydrogen evolution reaction at the interface. Based on these results, a comparative analysis of the properties of CuRhO2 and CuFeO2 is presented.
Session-K2
Prof. Pozzo’s research interests are in the area of colloids, polymers and soft-matter systems. Her research group focuses on controlling and manipulating materials structure for applications in health, clean energy and separations. Her group also develops and utilizes new advanced measurement techniques based on neutron and x-ray scattering. Prof. Pozzo obtained her B.S. from the University of Puerto Rico at Mayagüez and her PhD in Chemical Engineering from Carnegie Mellon University in Pittsburgh PA. She also worked in the NIST Center for Neutron Research as a post-doctoral fellow and is currently the Boeing-Roundhill Professor of Chemical Engineering at the University of Washington where she has served since 2007. She is also chairs the Department of Materials Science and Engineering at UW. In addition to her research activities, she is also dedicated to improving engineering education with course development in areas of entrepreneurship and service-oriented global engagement.
Polymer blends, which harness desirable properties from two or more complementary materials, have numerous applications in aerospace, pharmaceuticals, medical devices, electronics, and clean energy. In this work we investigate how polymer-polymer and polymer-solvent interactions affect the phase morphology and performance of polymer composites comprised of a majority non-conjugated polymer matrix component and a dispersed minority conjugated (semi-conductive) polymer additive. These blends are of interest for numerous organic electronic device applications as the matrix component can add mechanical durability, environmental stability and, in some cases, ionic conductivity. Meanwhile the minority conjugated component has been shown to retain optoelectronic performance at loading fractions as low as 1-10 wt%. Small angle and ultra-small angle neutron and X-ray scattering are particularly useful to characterize these composite blends and the self-assembled conjugated polymer components over length scales spanning from micrometers to fractions of a nanometer. We use small angle X-ray and neutron scattering and electrochemical analysis to develop a deep understanding of how blend morphology affects performance as a function of processing conditions and composition. This talk first provides a review of past work focused on blends of commodity homopolymers (i.e. polystyrene) and conjugated polymers (i.e. polythiophene derivatives). It will then cover more recent work focused on structured composite blends where the non-conjugated component is a block copolymer capable of self-assembly into mesoscale structures with long range structural order. These new structured composites include thermoplastic elastomers with high elasticity as well as water soluble block copolymers capable of forming templated hydrogel organic mixed ionic electronic conductors (OMIECs). In these cases, scattering experiments demonstrate that the block-copolymer templates can induce the organization of the conjugated polymer component over long length scales at significant loadings (up to 5wt%).
Session-K1
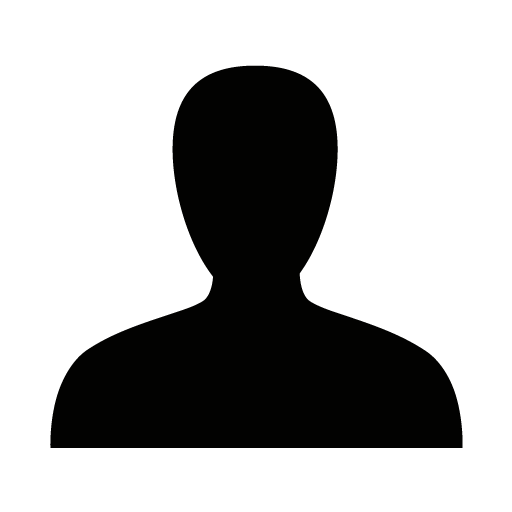
The emergence of electrically conductive metal-organic frameworks (MOFs) has been one of the most paradoxical developments in the field in the last few years. Indeed, how can one transport charges through a material that is mostly “empty” space? In this sense, MOFs made from layers of organic ligands connected by (typically) square-planar metal ions have shown particularly good electrical conductivity. However, a precise mechanism for charge transport is still the subject of debate, with various experimental and computational reports describing these materials as metals, semiconductors, semimetals, or even borderline insulators. Most of the discussion on this point has been focused on the effects of in-plane metal-ligand conjugation and the efficiency of in-plane transport. This lecture will describe the latest efforts from our group to understand the intrinsic properties of these materials, especially as related to single-crystal electrical measurement studies, and will discuss in particular the unexpectedly large influence of out-of-plane transport. Time allowing, I will discuss unexpected results stemming from the behavior of these materials as 1D metals, and applications in energy storage and conversion.
1.1-I1
Antoni Llobet was born in Sabadell (Barcelona) in 1960.
He obtained his PhD at the Universitat Autònoma de Barcelona (UAB) with Prof. Francesc Teixidor in July 1985, and then moved to the University of North Carolina at Chapel Hill for a postdoctoral stay with Prof. Thomas J. Meyer, until the end of 1987.
After a short period again at UAB and at University of Sussex-Dow Corning (UK) he then become a Scientific Officer for the Commission of the European Communities, based in Brussels, Belgium (1990-1991).
Then he was appointed Senior Research Associate at Texas A&M University in College Station (USA) from 1992 till 1993, working with the groups of Prof. Arthur E. Martell and Donald T. Sawyer. From 1993 till 2004 he joined the faculty of the Universitat de Girona where he was promoted to Full Professor in 2000. At the end of 2004 he joined the faculty of UAB also as Full Professor.
In September 2006, he was appointed as Group Leader at the Institute of Chemical Research of Catalonia (ICIQ) in Tarragona.
His research interests include the development of tailored transition metal complexes as catalysts for selective organic and inorganic transformations including the oxidation of water to molecular dioxygen, supramolecular catalysis, the activation of C-H and C-F bonds, and the preparation low molecular weight complexes as structural and/or functional models of the active sites of oxidative metalloproteins.
In 2000 he received the Distinction Award from Generalitat de Catalunya for Young Scientists. In 2011 he was awarded the Bruker Prize in Inorganic Chemistry from the Spanish Royal Society of Chemistry (RSEQ) and in 2012 he has been awarded with the “Hermanos Elhuyar-Hans Goldschmidt” lecture jointly by RSEQ and the German Chemical Society (GDCh).
At present he is a member of the Editorial Advisory Board of “Catalysis Science and Technology” from the Royal Society of Chemistry, “Inorganic Chemistry” from the American Chemical Society and “European Journal of Inorganic Chemistry” from Wiley-VCH.
The replacement of fossil fuels by a clean and renewable energy source is one of the most urgent and challenging issues our society is facing today, which is why intense research has been devoted to this topic recently. Nature has been using sunlight as the primary energy input to oxidize water and generate carbohydrates (a solar fuel) for over a billion years. Inspired, but not constrained by nature, artificial systems[1] can be designed to capture light and oxidize water and reduce protons, CO2 or other compounds to generate useful chemical fuels and feedstocks. In this context, this contribution will describe the preparation of efficient molecular water oxidation catalysts both in homogeneous phase and confined into solid conductive or semiconductive supports. Further the nature of the anchoring strategy on the performance of these molecular (photo)anode will be further discussed as well their implications for the generation of solar fuels.[2]
[1] (a) Berardi, S.; Drouet, S.; Francàs, L.; Gimbert-Suriñach, C.; Guttentag, M.; Richmond, C.; Stoll, T.; Llobet, A. Chem. Soc. Rev., 2014, 43, 7501-7519. (b) Matheu, R.; Ertem, M. Z.; Gimbert-Suriñach, C; Sala, X.; Llobet, A. Chem. Rev. 2019, 119, 3453–3471. (c) Matheu, R.; Garrido Barros, P.; Gil Sepulcre, M.; Ertem, M. Z.; Sala, X; Gimbert-Suriñach, C; Llobet, A. Nat. Rev. Chem. 2019, 3, 331–341.
[2] (a) Matheu, R.; Gray, H. B.; Brunschwig, B. S.; Llobet, A; Lewis, N. S. et al., J. Am. Chem. Soc. 2017, 139, 11345-11348. (b) Garrido-Barros, P.; Gimbert-Suriñach, C.; Llobet, A. et al., J. Am. Chem. Soc. 2017, 139, 12907–12910. (c) Hoque, Md. A.; Gil-Sepulcre, M.; Llobet, A. et al. Nat. Chem. 2020, 12, 1060–1066. (d) Schindler, D.; Gil‐Sepulcre, M.; Llobet, A.; Würthner, F. Adv. Energy Mater. 2020, 2002329. (e) Gil-Sepulcre, M.; Lindner, J. O.; Schindler, D.; Velasco, L.; Moonshiram, D.; Rüdiger, O.; DeBeer, S.; Stepanenko, V.; Solano, E.; Würthner, F.; Llobet, A. J. Am. Chem. Soc. 2021, 143, 11651–11661.
1.1-I2
In a world that is running out of natural resources, there is a growing need to design and develop sustainable and green energy resources. In that respect, photo-electrocatalytically driven reactions for the production of alternative fuels (such as water splitting or CO2 reduction) hold the potential to provide a route for future carbon neutral energy economy. Nevertheless, the slow kinetics of those catalytic reactions demands the development of efficient catalysts in order to drive it at lower overpotentials. Indeed, a variety of molecular catalysts based on metal complexes are capable of electrochemically reducing CO2 and/or protons. Yet, despite the significant progress in this field, practical realization of molecular catalysts will have to involve a simple and robust way to assemble high concentration of these catalysts in an ordered, reactant-accessible fashion onto a conductive electrode.
Our group utilizes Metal-Organic Frameworks (MOFs) based materials as a platform for heterogenizing molecular electrocatalysts. Their unique properties (porosity and flexible chemical functionality), enables us to use MOFs for integrating all the different functional elements needed for efficient catalysts: 1) immobilization of molecular catalysts, 2) electron transport elements, 3) mass transport channels, and 4) modulation of catalyst secondary environment. Thus, in essence, MOFs could possess all of the functional ingredients of a catalytic enzyme.
In this talk, I will present our recent study on (photo)-electrocatalytically active MOFs incorporating molecular catalysts for solar fuel reactions.
1.2-I1
Maria Wächtler studied Chemistry at the Friedrich Schiller University in Jena where she also received her PhD in 2013. After a postdoctoral period at Leibniz Institute of Photonic Technology Jena (Leibniz-IPHT), she was appointed head of work group Ultrafast Spectroscopy in the department Functional Interfaces in 2015 and since 2020 she is head of work group Quantum Confined Nanostructures at Leibniz-IPHT. Her research focuses on the design of systems for light-driven water splitting based on colloidal semiconductor nanostructures and the investigation of function determining interactions and light-driven processes by (time-resolved) spectroscopy.
A long-standing challenge is the development of artificial photosynthetic approaches for capturing and storing the energy of sunlight in the chemical bonds of a fuel, e.g. molecular hydrogen, offering a clean, sustainable and abundant source of energy. In this respect, colloidal nanostructured semiconductor materials are explored extensively as photosensitizers and photocatalysts. To push forward the development of functional materials based on semiconductor nanocrystals, function immanent exciton and charge-separation/recombination dynamics in relation to structural parameters need to be understood. Factors like composition, structure and dimensions of the semiconductor particles, the nature of cocatalysts and the type of surface ligands are severely influencing the efficiencies for solar to hydrogen conversion. By applying time-resolved transient absorption and photoluminescence spectroscopy we strive to reveal the connections between structure, charge carrier dynamics and the targeted function. To illustrate this approach, I will give a brief overview on the results of our studies on CdSe@CdS seeded nanorods functionalized with metal particles of varying composition and morphology as cocatalyst and the insights gained on the connection between charge separation at the nanosized semiconductor/metal interface and activity for hydrogen evolution.[1, 2] Besides the transfer of electrons to the cocatalyst, a key step in these heterostructures is hole localization in the CdSe seed which enables the formation of long-lived charge separation supporting charge accumulation at the catalytic reaction centers necessary to drive multi-electron redox reactions. Latest results revealed the influence of the nature of the surface ligands on the efficiency of the hole localization process.[3] Finally, interfacing semiconductor nanocrystals with molecular reaction centers presents an attractive alternative to metal particles and equally high charge transfer rates as at semiconductor/metal interfaces can be reached in principle.[4] Nevertheless, anchoring strategies with sufficient stability and supporting efficient transfer of multiple charge carriers and reducing unwanted charge recombination, e.g. by anchoring units serving as electron relay, still need to be established. First results indicate that bioinspired redox-active polymer matrices could play an important role in the future design of photocatalytically active inorganic/organic hybrid materials.[5]
1.2-T1
Transparent conductive oxides are well-known inorganic materials that have found wide use in various fields of both research and industry. In particular, doped metal oxides have aroused great interest in power generation applications thanks to a very convenient combination of optical and electronic properties. To the effective absorption of light usually in the ultraviolet frequency range and to the typical optical transparency window in the visible, a tunable feature is added that enriches the versatility of these materials. It is the plasmon resonance in the infrared. This property is the result of the aliovalent doping of the oxide, which induces the typical conductive character thanks to the excess of extrinsic charges [1]. Further advantages in terms of preparation, costs and applications derive from processing in the nanoscale and, among the nanocrystalline forms of these oxides, one of the most widely used species is indium tin oxide (ITO) [2]. More recently, the possibility of a further increase in charge density through a zero environmental impact post-synthetic treatment has renewed the attraction for these materials and intensified their study. Many metal oxides nanocrystals have in fact demonstrated an exponential increase in charges through photo-doping induced by multiple events of light absorption [3]. This phenomenon encourages even more the use of these semiconductors in the field of solar energy conversion, but also in related fields such as photoelectrochemistry and photocatalysis. In each of these applications, one of the most current research lines in fact concerns the analysis of the multiple charges accumulated in the light-driven process and the possibility of accessing processes involving multiple charge transfers [4]. The consequences would concern both the increase in efficiency and the widening of the application window of these materials. In this work,[5] we report a first evidence, in the case of photodoped ITO nanocrystals, of the possible transfer process of more than one electron by means of a gradual oxidative treatment using an electron-acceptor molecule, the F4TCNQ. The spectroscopic analysis of the titration allows shedding light on the reactivity of the nanocrystals induced by light and on the potential transfer of multiple charges.
1.2-I2
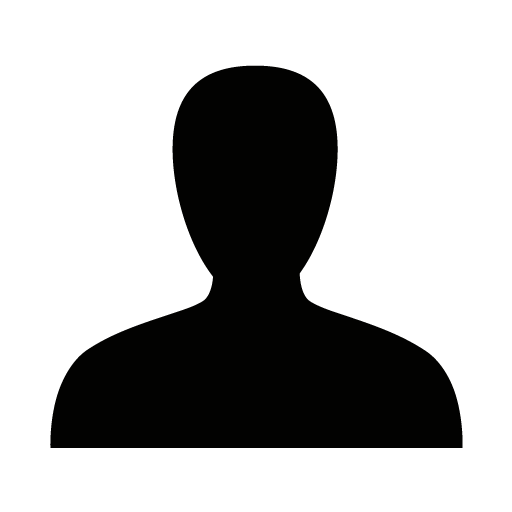
Nanocarbon in Photochemistry and Electrochemistry
Artificial photosynthesis, a process in which the light energy is converted into the chemical bond, represents an important area of research aimed toward generation of Sun-derived fuels and value-added chemicals. To successfully utilize photons from Sun, such solar reactors need to contain light-absorbing chromophores that efficiently and rapidly channel the absorbed energy toward desired catalytic sites where useful chemistry can takes place with high selectivity and low kinetic barriers. The Glusac group investigates molecular chromophores and catalysts for artificial photosynthesis. We utilize advanced time-resolved laser spectroscopy techniques to investigate mechanisms of energy and charge migration in molecular excited states and evaluate the parameters that control undesired energy losses through fast charge recombination. We also explore molecular electrocatalysts that are able to receive electrons and holes from excited chromophores and covert then into desired products.
Three projects are currently under investigation in our labs: (i) Bio-inspired CO2 reduction using metal-free NAD+/NADH analogs, where we look for strong hydride donors that can selectively reduce CO2 to methanol and that can be recycled photochemically; (iii) Light-harvesting by graphene quantum dot assemblies, where we explore excited-state energy and charge redistributions in chromophore-catalyst assemblies using advanced time-resolved laser spectroscopy methods; (iii) Carbon-based platforms for electrocatalysis. In this project, we investigate methods to decorate carbon electrodes with molecular catalytic motifs that can perform useful chemistry.
2.1-I1
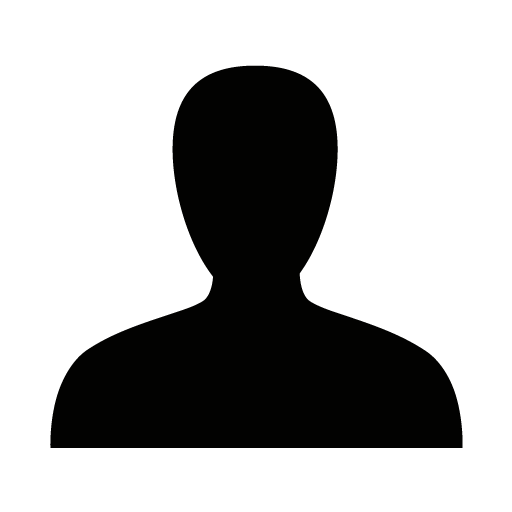
Roland A. Fischer, Prof. Dr. rer. nat., Dr. phil. h.c., holds the Chair of Inorganic and Metal-Organic Chemistry at the Technical University Munich (TUM) and is Director of the TUM Catalysis Research Centre. Previously he was Professor of Inorganic Chemistry at Ruhr-University Bochum (1997-2015) and Heidelberg University (1996-1997). He has been elected Vice President of the Deutsche Forschungsgemeinschaft (DFG) in 2016. He is member of the Award Selection Committee of the Alexander von Humboldt Foundation, member of the Scientific Advisory Board of the German Chemical Industry Fund and was elected member of the European Academy of Sciences. His research interest focuses on functional molecular materials for advanced applications in energy conversion, catalysis, gas storage and separation, chemical sensing, photonics and microelectronics. To illustrate, metal-rich complexes, atomic precise clusters, nanoparticles and nanocomposites can substitute rare noble metals for important catalytic transformation of small molecules. In addition, the combinatorial building-block principle of coordination network compounds such as metal-organic frameworks (MOFs) yields ample opportunities for the manipulation and design of the chemistry of coordination space in pores and channels accessible to guest molecules. The goal is to integrate chemical and physical multifunctionality in photo-active, electrical conductive, catalytic and stimuli-responsive MOFs. Currently, he is steering the DFG Priority Program 1928 “Coordination Networks: Building Blocks for Functional Systems”.
Syngas, an industrially relevant mixture of CO and H2, is a high-priority intermediate resource for producing several commodity chemicals, e.g., ammonia, methanol, and synthetic hydrocarbon fuels. Accordingly, parallel photocatalytic conversion of CO2 and protons to syngas is a key step in fostering a sustainable energy cycle. State-of-the-art catalytic systems often fall short as concurrent CO and H2 evolution requires challenging reaction conditions which can hamper stability, selectivity, and efficiency under application-oriented conditions. Here a light-harvesting metal-organic framework and two molecular catalysts are associated to design colloidal, water-stable, versatile nanoreactors for photocatalytic syngas generation with highly controllable product ratios. The host provides efficient directional light-harvested energy transport to the active sites, yielding sustained CO2 reduction and H2 evolution with incident photon conversions up to 36% and turnover numbers setting a benchmark, thus paving the way for application in solar energy-driven syngas generation. System bottlenecks, such as limited light absorption and kinetics, were identified, and proven addressable upon modularly co-hosting molecular additives owing to the system’s intrinsic versatility. Based on our findings, we highlight the uniqueness of MOF materials’ coordination space for rational designing and directing of such multicomponent supramolecular assemblies including antenna chromophores, auxiliary photosensitizers, and several catalysts of orthogonal photo-physical and chemical properties to minimize energy loss. This opens perspectives to tackle scalability, synthesize value-added products from the full redox cycle, harness the full solar spectrum energy, and precisely implement active sites with co-factors to mimic natural enzyme functionalities.[1-3]
2.1-I2
Shengqian Ma obtained his B.S. degree from Jilin University, China in 2003, and graduated from Miami University (Ohio) with a Ph.D. degree under the supervision of Hong-Cai Joe Zhou (currently at Texas A&M University) in 2008. After finishing two-year Director’s Postdoctoral Fellowship at Argonne National Laboratory, he joined the Department of Chemistry at University of South Florida (USF) as an Assistant Professor in August 2010. He was promoted to an Associate Professor with early tenure in 2015 and to a Full Professor in 2018. In August 2020, he joined the Department of Chemistry at University of North Texas (UNT) as the Robert A. Welch Chair in Chemistry.
Metal–organic frameworks (MOFs) represent a new class of materials, and one of their striking features lies in the tunable, designable, and functionalizable nanospace. The nanospace within MOFs allows the designed incorporation of different functionalities for targeted applications, such as gas storage, gas separation, sensing, drug delivery, environmental remediation, heterogeneous catalysis; and it has also provided plenty of opportunities for photocatalysis application. We will discuss a series of strategies to tailor MOFs as photocatalysts for photocatalytic small molecule activation under visible light irradiation including hydrogen production from water and water oxidation coupled carbon dixoide reduction reminiscent of photosynthesis in nature.
2.2-I1
Amanda Morris is a Professor of Inorganic and Energy Chemistry at Virginia Tech. Her research education conducted at Penn State University (B.S.), Johns Hopkins University (Ph.D.), and Princeton University (Postdoctoral) has been focused on addressing critical environmental issues with fundamental science including water remediation, solar energy harvesting and storage, and carbon dioxide conversion. As her publication record shows, Morris is a classically trained photo-electrochemist with demonstrated success utilizing various techniques (cyclic voltammetry, spectroelectrochemistry, and pulsed-laser spectroscopy) to explore new frontiers in renewable energy. Her research group’s current focus is on light-matter interactions and catalysis. She has received numerous awards for her research pursuits listed below. In addition to her academic pursuits, Morris has a demonstrated record in service including the recruitment and retention of minority chemists. In recognition of this work, she has received the Alan F. Clifford Service Award and College of Science Diversity Award. She currently serves as an American Chemical Society Expert in the area of Sustainable Energy and through this effort has worked to communicate science to the broader national audience with interviews on NPR, newspaper editorials, and press conferences. She also serves as an Associate Editor of Chemical Physics Reviews and sits on the Editorial Advisory Boards for ACS Applied Energy Materials and EnergyChem.
Carbon dioxide reduction by porphrynic metal-organic frameworks has been widely studied. Changes in porphyrin moiety (non-metallated, different central metal atoms) and three-dimensional structures result in putative changes in mechanism and resulting products, namely carbon monoxide and formate. There are two prevailing mechanisms that are debated in the literature (1) the formation of a porphyrin-node charge-separated state, where the node acts as the catalytic site, (2) porphyrin driven chemistry where the linker acts as both the photo-active species and catalytic site with the nodes simply serving as structural supports. Our collaborators have systematically explored the reduction rates and products for different non-metallated and metallated MOF-525. To support the mechanistic implications, we conducted photophysical studies, including transient absorption spectroscopy. Interestingly, the results do not support either of the prevailing mechanisms. Transient absorption spectroscopy eliminates the possibility for the formation of a charge-separated state. EPR spectroscopy confirms that the photogenerated Fe(II) species is not capable of carbon dioxide activation, consistent with previously published molecular mechanisms. Therefore, we turned to theory to provide insight. The computational results support a mechanism where the sacrificial donor plays a key role in the catalytic mechanism. Other recent mechanistic studies on molecular carbon dioxide reduction catalysts have put forth similar stories with sacrificial donors playing multiple roles in catalysis. The implications of sacrificial reducing agents in the field will be mentioned, as well as potential pathways for future research.
1.1-I1
Since 2019, Yana Vaynzof holds the Chair for Emerging Electronic Technologies at the Technical University of Dresden. Prior to that (2014-2019), she was a juniorprofessor in the Department of Physics and Astronomy, Heidelberg University (Germany). She received a B.Sc degree (summa cum laude) in electrical engineering from the Technion - Israel Institute of Technology (Israel) in 2006, and a M.Sc. degree in electrical engineering from Princeton University, (USA) in 2008. She pursued a Ph.D. degree in physics under the supervision of Prof. Sir. Richard Friend at the Optoelectronics Group, Cavendish Laboratory, University of Cambridge (UK), and investigated the development of hybrid polymer solar cells and the improvement of their efficiency and stability. Upon completing her PhD in 2011, she joined the Microelectronics group at the University of Cambridge as a Postdoctoral Research Associate focusing on the research of surfaces and interfaces in organic and hybrid optoelectronics. Yana Vaynzof was the recipient of a number of fellowships and awards, including the ERC Starting Grant, Gordon Y. Wu Fellowship, Henry Kressel Fellowship, Fulbright-Cottrell Award and the Walter Kalkhof-Rose Memorial Prize.
Despite a decade of research, much remains unknown about the role that grain boundaries play in impacting the photophysics of lead halide perovskites and influencing their performance in solar cells. In this talk, I will describe how by combining experimental studies with theoretical device simulations, we find that the recombination at grain boundaries is diffusion limited and depends on the grain area with small grains acting as recombination hot spots. We show that the distribution of grain sizes not only influences the overall performance of perovskite solar cells, but also leads to significant current exchange between small and large grains at open-circuit conditions.
1.1-I2
On the wave of their success in strategic fields of application, as energy conversion and light-emission, great effort was devoted to better understand and clarify the native semiconducting properties of halide perovskite materials. As a result, the electronic properties of these systems are nowadays well understood, with clear identification of the influence of quantum confinement and chemical tuning on the corresponding band structure, as relevant in the case of dimensionally tailored materials,[1] and in mixed halide or double perovskites,[2] respectively. The situation however becomes more complex when one goes beyond the single particle picture. The Coulomb interaction between the photogenerated electron-hole pairs in fact may result in the formation of stable excitons, with distinct properties (energetics, polarization, degeneracy, etc.) with respect to the corresponding single particle transitions.
Here, we aim to provide a general frame for the discussion of the exciton properties of metal halide perovskite frames, which encompasses effects like quantum confinement and chemical composition. Symmetry-based, group theory analysis will provide a sounded ground for the discussion, with clear indication about the expected splitting of the excitonic features in the materials, when going from 3D, to 2D and to other systems of practical relevance.[3] These results will be then referred to recent spectroscopic experiments[4-6] and to cutting edge first-principle calculations,[7] so demonstrating the possibilities of modern first-principle simulations tools in supporting experimental investigations and predicting the excitonic properties of halide perovskite materials. Excitons dominate the optical response of two-dimensional layered perovskite materials, where quantum and dielectric confinement enhance the electron-hole interactions;[1] still, they are shown to play a fundamental role also for double perovskites,[8] with serious impact on the relative role of dimensional confinement.[9] In addition, also for 3D lead-based halide perovskites, featuring exciton binding as small as 14-25 meV at room temperature,[10] the clear understanding and assignment of the spectral signatures must rely on an excitonic picture.[4-5]
1.1-I3
Luisa De Marco received her PhD in Nanoscience from Università del Salento in 2010 working on nanostructured semiconductors for photovoltaics. Since 2016 she is researcher at CNR NANOTEC leading a 6-person team working on the development of low-dimensional inorganic and hybrid nanomaterials. She is author of more than 70 papers that collectively have received more than 2600 citations, with an h-index of 31. Among the publications stand out Advanced Materials, Nature Nanotechnology, Energy & Environmental Science, ACS Nano and Science Advances.
Her research interests focus on the development and engineering of hybrid and inorganic low-dimensional semiconductors having specifically tailored functional properties and on design and fabrication of optoelectronic devices.
Two-dimensional (2D) hybrid organic-inorganic perovskites are among the most promising materials for optoelectronic applications thanks to the great synthetic versatility that allows tailoring their structural and photophysical properties. The alternation between organic ligands and inorganic layers creates a natural multiple quantum well (MQW) structure where the inorganic layers act as potential ‘wells’ while organics serve as energetic ‘barriers’. This structure provides strong electronic confinement and, as a consequence, creates stable excitons with high binding energy at room temperature. [1]
In this talk I will show that large single-crystal flakes of 2D perovskite are able to establish strong light-matter coupling with the generation of exciton−polaritons. These half-light half-matter quasi-particles have unique properties: they possess strong intrinsic nonlinearities, inherited from their excitonic component and extremely small effective mass and long coherence length, inherited from their photonic component.
By comparing different hybrid perovskites with the same inorganic layer but different organic interlayers, it is shown how the nature of the organic ligands controllably affects the out-of-plane exciton–photon coupling. [2]
Moreover, 2D single-crystals perovskite are able to sustain strong polariton nonlinearities at room temperature, with exciton-exciton interaction energies remarkably similar to the ones known for inorganic quantum wells at cryogenic temperatures and more than one order of magnitude larger than alternative room temperature polariton devices reported so far. [3]
Because of their easy fabrication, large dipolar oscillator strengths, and strong nonlinearities, these materials ideal candidate for integrated photonic circuits and electro-optical devices working at room temperature. [4 - 6]
1.2-T1
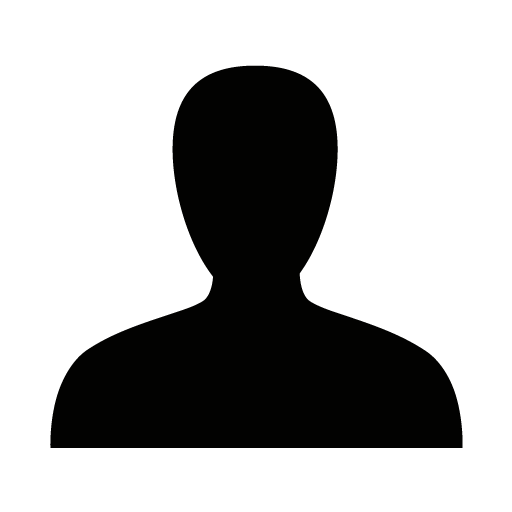
Hybrid perovskites have generated a lot of interest in the field of photovoltaics during the past decade. In recent years, perovskite solar cells regularly output over 20 % of photoconversion efficiency, approaching conventional Silicon based devices.
Even though, remarkable optoelectronic properties of hybrid perovskites and easy fabrication routs have enabled fast development of devices, the common fabrication methods still result in formation of substantial density of defect states in hybrid perovskite thin films [1]. These defects when act as charge trapping centers, have been known to induce non-radiative losses and limit the maximum photoconversion efficiency of perovskite solar cells [2]. The progress in fundamental understanding of these defects, in particular about their formation sites and their exact impact on device efficiency, has been slower. Such knowledge, however, is critical to design successful passivation strategies and improve performance and viability of perovskite photovoltaic devices.
Here we elucidate the very origin of nanoscale defects that form in state-of-the-art solution processed triple cation mixed halide perovskite thin films, and evaluate their roles in charge trapping processes. We employed photoemission electron microscopy to directly image the distribution of defects on nanoscale [3] and revealed their spatial arrangement with respect to surface morphology. By adding the time resolution to our photoemission experiments, we accessed the exact roles of these defects in performance of perovskite thin films by monitoring charge trapping processes that occur at defect sites. We thus uncovered the presence of multiple types of nanoscale defect clusters, and found that depending on their origin, they play very different roles in photoexcited hole trapping – from very detrimental to relatively benign [4]. Our results highlight the need to develop targeted approaches to remove each undesired type of defect.
1.2-T2
Spatial photoluminescence heterogeneity is a frequently recognized phenomenon in lead halide perovskite thin films meant for efficient photovoltaic devices. Deep trap states associated with local variation in structure, composition and strain are the main players dictating this photoluminescence heterogeneity and the device performance. These trap states can appear as nanoscale clusters and majorly reside in the grain boundaries.1 Despite notable progress in understanding the fundamental aspects of these traps such as origin, location, and distribution, real time response of these trap states remains elusive. Temporal photoluminescence heterogeneity or intensity fluctuation introduced by the traps can be a major loss channel in the film and would require detailed investigation. In this context several questions that can arise are: (i) Are these photoluminescence quenching traps static or metastable? (ii) what are the quenching domains of these traps? (iii) Do they have any correlation with grain size and grain boundaries? (iv) Do they have any working timescale? (v) How does the surrounding environment influence formation and annihilation of these traps?
We observed large photoluminescence fluctuation in MAPbI3 thin films when investigated in real time. The fluctuation varies as a function of grain size (500 nm-several µm) and surrounding environment. This fact indicates presence of metastable traps which can deplete significant amount of photogenerated carriers even in good quality films. Unlike spatially isolated crystals, proximity of the grains and crystallites in thin films provide a mixed photoluminescence signal making the trapping dynamics and carrier recombination more complex for individual grains. We have developed an advanced photoluminescence mapping technique based on correlation of the intensity fluctuation which divide the image into microscale clusters having high intra-cluster and low inter-cluster correlation, respectively. Size of the clusters changes as the grain size or the surrounding environment changes from air to inert. By correlating these clusters with electron microscopy image, we obtain important insight about the microscale quenching domain of these metastable traps. Power spectral density estimation of the intensity fluctuation of each cluster reveals existence of different types of metastable traps with timescales ranging from hundreds of milliseconds to tens of seconds.2 Distinctly different response time of these quenching traps are noted when grains size is varied, and porosity is introduced into the sample. This approach demonstrates a methodology to understand several structural (quenching domain, grain size dependence) and dynamical aspects (metastability and working timescale) of the photoluminescence quenching traps in any thin films.
1.3-I1
Paulina Plochocka, Directrice de recherché de 2e classe (DR2) in Laboratoire National des Champs Magnétiques Intenses (LNCMI), CNRS in Toulouse.
P. Plochocka obtained her PhD cum-laude in 2004 at the University of Warsaw working on the dynamics of many-body interactions between carriers in doped semi-magnetic quantum wells (QW). During her first post doc at Weizmann Institute of science, she started working on the electronic properties of a high mobility 2D electron gas in the fractional and integer quantum Hall Effect regime. She continued this topic during second post doc in LNCMI Grenoble, where she was holding individual Marie Curie scholarship. At the same time, she enlarged her interest of 2D materials towards graphene and other layered materials as TMDCs or black phosphorus. In 2012 she obtained permanent position in LNCMI Toulouse, where she created the Quantum Electronics group, which investigates the electronic and optical properties of emerging materials under extreme conditions of high magnetic field and low temperatures. Examples include semiconducting layer materials such as transition metal dichalcogenides, GaAs/AlAs core shell nanowires and organic inorganic hybrid perovskites.
High environmental stability and surprisingly high efficiency of solar cells based on 2D perovskites have renewed interest in these materials. These natural quantum wells consist of planes of metal-halide octahedra, separated by organic spacers. Remarkably the organic spacers play crucial role in optoelectronic properties of these compounds. The characteristic for ionic crystal coupling of excitonic species to lattice vibration became particularly important in case of soft perovskite lattice. The nontrivial mutual dependencies between lattice dynamics, organic spacers and electronic excitation manifest in a complex absorption and emission spectrum which detailed origin is subject of ongoing controversy. First, I will discuss electronic properties of 2D perovskites with different thicknesses of the octahedral layers and two types of organic spacer. I will demonstrate that the energy spacing of excitonic features depends on organic spacer but very weakly depends on octahedral layer thickness. This indicates the vibrionic progression scenario which is confirmed by high magnetic fields studies up to 67T. Furtheremore, I will show that in 2D perovskites, the distortion imposed by the organic spacers governs the effective mass of the carriers. As a result, and unlike in any other semiconductor, the effective mass in 2D perovskites can be easily tailored. Finally I will discuss the exciton fince structure. Optically inactive dark exciton states play an important role in light emission processes in semiconductors because they provide an efficient nonradiative recombination channel. Understanding the exciton fine structure in materials with potential applications in light-emitting devices is therefore critical. Our studies of the exciton fine structure in the family of two-dimensional (2D) perovskites show that in-plane magnetic field mixes the bright and dark exciton states, brightening the otherwise optically inactive dark exciton. The bright-dark splitting increases with increasing exciton binding energy. Hot photoluminescence is observed, indicative of a non-Boltzmann distribution of the bright-dark exciton populations. We attribute this to the phonon bottleneck, which results from the weak exciton–acoustic phonon coupling in soft 2D perovskites. Hot photoluminescence is responsible for the strong emission observed in these materials, despite the substantial bright-dark exciton splitting.
1.3-I2
Quasi-two-dimensional (Q2D) organic-inorganic halide perovskites are an exciting family of heterogeneous layered semiconductors, which combine chemical and structural versatility of 3D organic-inorganic metal-halide perovskites with the ability to design light-matter interactions driven by structural and dielectric confinement [1]. They are a broad and versatile playground not only for materials discovery, but also for exploring fundamental excited state physics and development of novel optoelectronic applications. In particular, the structural and chemical diversity of this family of materials offers a unique opportunity to understand the role of chemistry and structure on photoexcited states of complex semiconductors in a systematic way; first principles computational modeling takes a key role in this context.
In this talk, I will present our recent work understanding optical excitations complex Q2D organic-inorganic halide perovskites using state-of-the-art first principles computational modeling techniques, such as the GW method [2] and the Bethe-Salpeter equation [3]. I will present our first principles calculations of optical excitations in single-layered Q2D halide perovskites, revealing the role of the organic cations in enhancing the dielectric screening of these complex systems, and show our recent work on understanding the optoelectronic properties of self-assembled perovskite-non-perovskite self-assembled interfaces [4].
References:
[1] Smith, Crace, Jaffee & Karunadasa, Annu. Rev. Mater. Res., 48, 111-136 (2018).
[2] Hybertsen & Louie, Phys. Rev. B 34, 5390 (1986).
[3] Rohlfing & Louie, Phys. Rev. Lett. 81, 2312 (1998).
[4] Aubrey, Valdes, Filip, Connor, Lindquist, Neaton & Karunadasa, Nature, 597, 355-359 (2021).
1.3-I3
Carlos Silva earned a PhD in chemical physics from the University of Minnesota, with the late Professor Paul Barbara. His graduate research focused on ultrafast polar solvation dynamics, probed by transient absorption spectroscopy on the solvated electron and transition-metal mixed-valence complexes. Following his graduate degree in 1998, he was Postdoctoral Research Fellow with Professor Sir Richard Friend at the Cavendish Laboratory, University of Cambridge, where he developed an ultrafast spectroscopy laboratory to investigate the photophysics of conjugated polymers and related organic semiconductors. In 2001, he began his independent academic career as Advanced Research Fellow of the UK Engineering and Physical Science Research Council at the Cavendish Laboratory, and simultaneously became Research Fellow in Darwin College, University of Cambridge. He moved to the Université de Montréal with a Canada Research Chair in 2005, where he developed an ultrafast spectroscopy laboratory for the study of electronic processes in organic semiconductor materials. In recognition of his rising international leadership, he was awarded the 2010 Herzberg Medal and the 2016 Brockhouse Medal by the Canadian Association of Physicists. Since 2017, Carlos’s research career at Georgia Tech has built on his previous research experiences to bring innovative optical probes of organic and hybrid organic-inorganic semiconductor materials. His research program exploits a range of spectroscopic techniques, including nonlinear ultrafast spectroscopies such as two-dimensional coherent excitation spectroscopies, and quantum spectroscopy, in which quantum properties of light are exploited to unravel light-matter interactions with intricate detail. These techniques are applied to understand key electronic processes in a wide range of materials, with many target applications in optoelectronics, on timescales ranging from femtoseconds to milliseconds. He is a Fellow of the Royal Society of Chemistry.
Two-dimensional Ruddlesden-Popper metal halides (2D-RPMHs) are materials composed of quasi-2D layers of metal-halide octahedra separated by long (~1nm) organic cationic layers. The latter facilitate electron and hole quantum confinement within the metal-halide layers resulting in a quantum-well like structure. Properties of excitons (i.e., the electron-hole bound states) in such structures are characterized by strong binding energy (>200 meV) arising from the dynamically screened Coulomb interactions [1]. We have experimentally observed that polaronic effects arising from the lattice dressing of the carriers, are not only active but that they fundamentally define excitons in 2D-RPMHs [2]. We thus refer to such excitons as the exciton-polarons, with properties that are measurably distinct than those of free excitons in semiconductors [1]. In this talk, I will discuss the quantum dynamics of exciton-polarons and provide spectroscopic insights into the peculiar phonon-phonon [3], exciton-phonon and exciton-exciton [4] interactions. I will present our perspective on how the coherent optical response of 2D perovskites can be effectively rationalized within the “exciton-polaron” framework, in which lattice dressing of photo-carriers constitute an integral component of excitonic wavefunction [1], with consequences on exciton recombination dynamics and diffusion.
References
[1] A. R. Srimath Kandada and C. Silva, J. Phys. Chem. Lett., 11, 3173-3184 (2020).
[2] F. Thouin, D. Valverde-Chavez, C. Quarti, D. Cortecchia, I. Bargigia, D. Beljonne, A. Petrozza, C. Silva and A. R. Srimath Kandada, Nature Materials, 18, 349-356 (2019).
[3] E. Rojas-Gatjens, C. Silva-Acuna and A. R. Srimath Kandada, Peculiar anharmonicity of Ruddlesden Popper metal halides: Temperature dependent dephasing, Materials Horizons (2022).
[4] A. R. Srimath Kandada, H. Li, F. Thouin, E. R. Bittner and C. Silva, Stochastic scattering theory for excitation-induced dephasing: Time dependent nonlinear coherent exciton lineshapes, J. Chem. Phys., 153, 164706 (2020).
1.4-T1
Dr. Beatriz Martín-García received her Ph.D. in Chemical Physics (Cum Laude) from University of Salamanca (Spain) in 2013. Then, she joined Istituto Italiano di Tecnologia (Italy) under the Graphene Flagship project working during almost 6 years on the modulation of optoelectronic properties of different materials (nanocrystals, 2D materials and hybrid metal-halide perovskites) by chemical-design and surface-functionalization strategies for their integration in solar cells, photodetectors and memories. She is currently an Ikerbasque researcher and Ramón y Cajal fellow at CIC nanoGUNE BRTA, leading a research line developing tailor-made low-dimensional materials and studying them by Raman and photoluminescence spectroscopy techniques to drive the selection of desired properties for their integration in optoelectronic and spintronic devices.
Metal halide double perovskites, especially the Cs2AgBiBr6, have attracted the attention of researchers in the search for less toxic candidates as active materials in optoelectronic devices.[1,2] From this 3D structure, low-dimensional double perovskites are fabricated by introducing large organic cations, resulting in organic/inorganic architectures with one or more inorganic-octahedra layers separated by organic cations.[3] Here, we synthesize a series of layered double perovskites from Cs2AgBiBr6 that consist of double (2L) or single (1L) inorganic octahedra layers, using ammonium cations of different size and chemical structure. By carrying out temperature-dependent Raman spectroscopy measurements, we highlight phase transition signatures in both inorganic lattice and organic moieties by detecting changes in the position and linewidth of the vibrational modes. Variations in the conformational arrangement of the organic cations from an ordered to disordered state match with a phase transition in the 1L systems with propyl- and butyl-ammonium moieties. Density functional theory calculations of the band structure reveal that the bandgap is direct only for the 1L crystal structures, and it becomes indirect in 2L systems. The direct bandgap character in 1L compounds stems from an extremely flat lowest conduction band, which enables small octahedral distortions to produce significant changes in the band structure across the bandgap. This translates to the optical properties, where we observe significant changes of photoluminescence intensity around the transition temperature as result of octahedral tilting rearrangement emerging at the phase transition.[4] Our work provides novel insights into the structure-optical properties relationship in layered double perovskites, and demonstrates how the proper selection of the organic cations in the layered perovskites is relevant in terms of thermal stability or the use of the phase transition for active switching in future applications.
1.4-T2
Solution processable semiconducting perovskites hold great promise for demanding applications involving light emission, such as printable lasers or quantum light sources. A case example is that of fully inorganic CsPbBr3 quantum wells (CQWs) which display high quantum yield at room temperature. Less studied than their organic-inorganic counterparts, these CQWs sustain high single exciton binding energies and luminescence quantum yields, yet little is known on the nature of the exciton and multi-excitons states required for advanced applications. Here, we show that charge carriers in fully inorganic 2D perovskites exist as stable exciton - polarons, a complex between a charge neutral exciton and a lattice deformation. Next, we show that these unique species can fuse together to form a hereto unexplored bi-exciton polaron state, i.e. a two-particle complex bound by attractive Coulomb attraction whilst simultaneously being strongly coupled to the lattice. Finally, we show that net stimulated emission occurs through radiative recombination from this unique bi-exciton polaron state to a single free exciton polaron, showing the stability of the newly found species. Consequences of the polaronic character are identified as a low threshold for stimulated emission but with limited optical gain coefficients, both of which we can fully reproduce using a thermodynamic gain model. As such, our results provide a general framework to understand and predict the behavior of not only single, but also multi-exciton polaron states in perovskite materials.
1.4-T3
Two-dimensional (2D) hybrid organic−inorganic perovskites are attracting interest thanks to their wide range of applications for solar energy conversion and beyond. Among their advantages, the tunability of their structural and optical properties is unique. For example, as the structural features of the organic spacers vary, the distancing between the inorganic layers can be affected – which affects the quantum confinement and hence the bandgap of the 2D perovskite. To experimentally elucidate the effect of the organic spacer on the optical and structural properties of perovskites, we applied hydrostatic pressures up to 375 MPa onto 2D materials with different spacers - e.g. butylammonium, benzylammonium, phenylehtylammonium, naphthalene-O-propylammonium, and perylene-O-ehtylammonium. In addition to a different degree of rigidity and extent of bandgap shift of the 2D materials for different spacers, we found that when butylammonium is used as the spacer, the 2D material undergoes a first-order phase transition at 350 MPa. By studying the specific effect of aromaticity and alkyl chain length of the organic spacers on the overall rigidity (hence, bandgap shift) of the 2D material and finding a pressure-dependent phase transition in one of the studied materials, our work is essential to designing 2D perovskites with finely tuned optical and structural properties.
1.4-T4
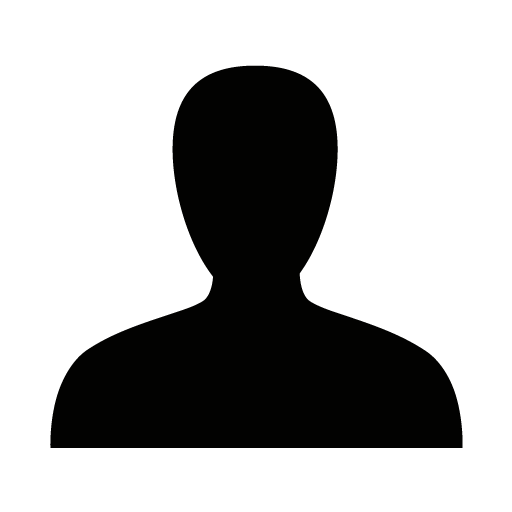
Halide perovskites are the new wonder material of the optoelectronics community due totheir outstanding photoluminescence quantum yield, tunable emission wavelength and simplesolution or vapor-phase deposition. At the same time, their facile ion migration andtransformation under optical, electrical and chemical stress are seen as a major limitation fordevice implementation. Mixed halide perovskites are particularly problematic sinceoptical excitation can cause changes in the band gap that are detrimental for solar cell and light-emitting diode efficiency and stability. In this work, instead of preventing suchchanges, we exploit photo-induced halide segregation in perovskites to enable responsive,reconfigurable and self-optimizing materials. We show how a mixed halide perovskite film can betrained to give highly directional light emission using a nanophotonic microlens: through a self-optimized process of halide photosegregation, the system mimics the training stimulus. Longertraining leads to more highly directional emission, while the different halide migration kineticsin the light (fast training) and dark (slow forgetting) allow for material memory. This self-optimized material performs significantly better than lithographically aligned quantum dots[1], because it eliminates lens-emitter misalignment and automatically corrects for lens aberrations.Our system shows a combination of mimicking, improving over time, and memory, which make itcompatible with the basic requirements for learning,[2,3] and give the intriguing prospectof intelligent optoelectronic materials
2.1-I1
Keshav Dani is currently an Associate Professor at the Okinawa Institute of Science and Technology (OIST), Graduate University in Okinawa, Japan. He joined OIST in Nov. 2011 as a tenure-track Assistant Professor after completing a Director’s Postdoctoral Fellowship at the Center for Integrated Nanotechnologies at Los Alamos National Laboratory. Keshav graduated from UC Berkeley in 2006 with a PhD in Physics, where he explored the nonlinear optical response of the quantum Hall system under the supervision of Daniel Chemla at LBNL. Prior to his PhD, he obtained a BS from Caltech in Mathematics with a senior thesis in Quantum Information Theory under John Preskill and Hideo Mabuchi. His current research interests lie in using novel time-resolved photoemission techniques (PEEM and ARPES) to understand the properties of photoexcited perovskite photovoltaic materials and two-dimensional semiconductor heterostructures.
Hybrid perovskite photovoltaic devices have rapidly emerged as promising contenders for next generation, low-cost solar cell technology. Yet, the presence of defect states critically impacts device operation, including device efficiency and potentially long-term stability. Understanding the nature of these defects and their role in photocarrier trapping, requires techniques that are capable of probing ultrafast photocarrier dynamics at the nanoscale.
In this talk, I will discuss the development of time-resolved photoemission electron microscopy (TR PEEM) techniques in my lab [1], [2], applied to hybrid perovskite solar materials. Thereby, we directly visualize the presence of the performance limiting nanoscale defect clusters and elucidate the role of diffusion in the charge carrier trapping process [3]. By correlating PEEM measurements with other spatially resolved microscopies, we identify different types of defects that form, and study how passivation strategies may have a varied impact on them [4].
2.1-I2
Lead-halide perovskite APbX3 (A=Cs or organic cation; X=Cl, Br, I) quantum dots (QDs) are subject of intense research due to their exceptional properties as both classical1 and quantum light sources.2-4 Here5,6 we present perovskite-type (ABO3) binary nanocrystal superlattices, created via the shape-directed co-assembly of steric-stabilized, highly luminescent cubic CsPbBr3 nanocrystals (which occupy the B and/or O lattice sites), assembled in combination with spherical Fe3O4 or NaGdF4 nanocrystals (A sites). These ABO3 superlattices, as well as the binary NaCl and AlB2 superlattice structures that we demonstrated, exhibit a high degree of orientational ordering of the CsPbBr3 nanocubes which preserve their high oscillator strength and long exciton coherence time in the assembly. Such superlattices exhibit superfluorescence—a collective emission that results in a burst of photons with ultrafast radiative decay (22 picoseconds) that could be tailored, by structural engineering of the nanoparticle assembly, for use in ultrabright (quantum) light sources. Our work paves the way for further exploration of complex, ordered and functionally useful perovskite mesostructures.
References
[1] Akkerman et al., Genesis, challenges and opportunities for colloidal lead halide perovskite nanocrystals. Nat. Mater. 17, 394–405 (2018).
[2] Becker et al., Bright triplet excitons in caesium lead halide perovskites. Nature 553, 189–193 (2018).
[3] Rainò et al., Superfluorescence from lead halide perovskite quantum dot superlattices. Nature 563, 671–675 (2018).
[4] Utzat et al., Coherent single-photon emission from colloidal lead halide perovskite quantum dots. Science 363, 1068–1072 (2019).
[5] Cherniukh et al., Perovskite-type superlattices from lead halide perovskite nanocubes. Nature 593, 535–542 (2021).
[6] Cherniukh et al., Shape-Directed Co-Assembly of Lead Halide Perovskite Nanocubes with Dielectric Nanodisks into Binary Nanocrystal Superlattices ACS Nano 15, 10, 16488–16500, (2021).
2.1-I3
Lead halide perovskites have emerged as promising new semiconductor materials for high-efficiency photovoltaics, light-emitting applications and quantum optical technologies. Their luminescence properties are governed by the formation and radiative recombination of bound electron-hole pairs known as excitons, whose bright or dark character of the ground state remains debated.
Spectroscopically resolved emission from single lead halide perovskite nanocrystals at cryogenic temperatures provides unique insight into physical processes that occur within these materials. At low temperatures the emission spectra collapse to narrow lines revealing a rich spectroscopic landscape and unexpected properties, completely hidden at the ensemble level and in bulk materials.
In this talk, I will discuss how magneto-photoluminescence spectroscopy provides a direct spectroscopic signature of the dark exciton emission of single lead halide perovskite nanocrystals. The dark singlet is located several millielectronvolts below the bright triplet, in fair agreement with an estimation of the long-range electron hole exchange interaction. Nevertheless, these perovskites display an intense luminescence because of an extremely reduced bright-to-dark phonon-assisted relaxation. Spectral multiplets assigned to the biexciton recombination are also revealed at the single nanocrystal level, which directly provides the biexciton binding energy and evidence for extremely slow spin relaxation between the exciton fine structure sublevels.
2.2-T1
Dr. Galian received her Ph.D in Chemistry at the National University of Cordoba, Argentina in 2001. Then, she was a postdoc researcher at the Polythecnic University of Valencia, University of Valencia and University of Ottawa. During those years, she has studied photosensibilization processes by aromatic ketones using laser flash photolysis techniques and was involved in photonic crystal fiber/semiconductor nanocrystal interaction projects. In 2007, Dr. Galian came back to Spain with a Ramon y Cajal contract to study the surface chemistry of quantum dots and since 2017 she has a permanent position as Scientist Researcher at the University of Valencia. Her main interest is the design, synthesis and characterization of photoactive nanoparticles and multifunctional nanosystems for sensing, electroluminescent applications and photocatalysis.
Lead halide perovskites (LHP) nanomaterials have emerged as an exciting new class of semiconductor materials with outstanding electronic and optical properties, easy synthesis, and high photoluminescence quantum yield. Beyond their solar cell application, they have been extensively exploited in diverse fields such as LED technology, laser, and more recently in photocatalysis. [1]
They respond to the general formula APbX3, where A is an organic cation such as methylammonium, formamidinium or inorganic cation such as Cs+, and X is the halide anion (Cl-, Br- or I-). The key role of the organic ligands for surface passivation and colloidal dispersion has been demonstrated in the preparation of the first CH3NH3PbBr3 nanocrystals in 2014, using octylammonium bromide and oleic acid as capping agents. [2] The nature of the organic ligands can determine the dimensionality of the inorganic framework and the dimension of the perovskite material, and therefore their optical properties.
The synthesis of colloidal low dimensional perovskites with deep blue-emissive will be presented. In particular, ultrathin [CsPbBr3]PbBr4 nanoplatelets and pure two-layered L2[CH3NH3PbX3]PbX4 perovskite nanosheets were synthesized by thermal/ultrasound methodology and ligand assisted techniques, respectively. [3, 4] Their emissive properties and chemical/photochemical stability will be discussed. The long-term stability in the solid-state together with the high processability of the colloidal nanosheets enabled the preparation of blue-emissive solid films, which may be suitable for assembling on a large area on-demand and, consequently, be useful for preparing high-quality films for flexible and ultrathin optoelectronic devices, or they could be combined with other types of 2D materials.
2.2-T2
Ivan Scheblykin obtained Ph.D. in 1999 from Moscow Institute of Physics and Technology and Lebedev Physical Institute of Russian Academy of Sciences on exciton dynamics in J-aggregates. After a postdoctoral stay in the KU Leuven, Belgium, he moved to Sweden to start the single molecule spectroscopy group at the Division of Chemical Physics in Lund University where he became a full professor in 2014. His interests cover fundamental photophysics of organic and inorganic semiconductors and, in particular, energy transfer, charge migration and trapping. The general direction of his research is to comprehend fundamental physical and chemical processes beyond ensemble averaging in material science and chemical physics using techniques inspired by single molecule fluorescence spectroscopy and single particle imaging.
Are all important non-radiative recombination centres in perovskites metastable?
Ivan G. Scheblykin
Chemical Physics and Nano Lund, Lund University, Sweden
Despite of very substantial efforts, it is still difficult to quantitatively account for charge dynamics in metal-halide perovskites over a broad range of excitation condition by the standard theoretical approaches of semiconductor physics.1
One aspect which has never been taken into account in any modelling of this kind is defect metastability. A defect state is metastable if, for example, it undergoes transition from its active state, in this state it induces strong non-radiative (NR) recombination, to its passive state where it does not influence charge dynamics at all.2 Obviously, defect metastability must influence charge carrier dynamics substantially, especially if switching of the defects is light or/and charge carrier concentration dependent. Evidence for the defect metastability is overwhelming:
1. Photoluminescence (PL) blinking of individual microcrystals and even grains of polycrystalline films.3
2. Reversible PL enhancement and PL bleaching4,5
3. Self-healing property of metal-halide perovskites in general terms6
I will discuss how we can see the metastable NR centers in action by observation of PL blinking. I will argue, that most probably all important defect states causing NR recombination, which scientists from all fields from fundamental to applied care about, are, in fact, metastable and see then in PL blinking experiments. So far, I do not see any experimental observations which would disprove to this hypothesis.
Despite that PL blinking in perovskites is known from 2015,7 so far there had been no real connection drawn from the results obtained on individual crystals and properties of films and devices. If all important defects are metastable, understanding this metastability becomes very crucial with PL micro spectroscopy as an ideal tool.
2.2-T3
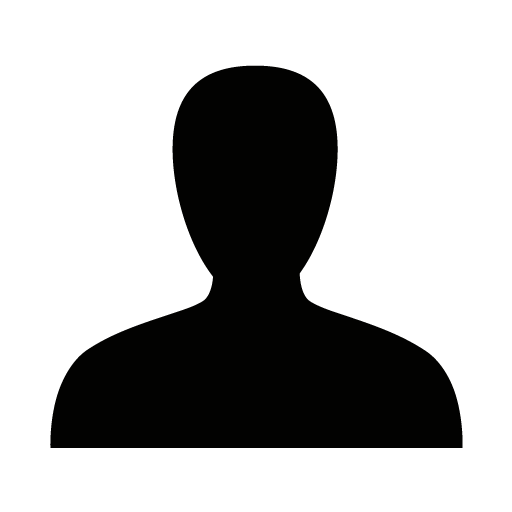
Metal-halide perovskites (MHPs) hold great potential for next-generation optoelectronic technologies due to their remarkable characteristics. While the influence of strain on the intrinsic properties of MHPs is gaining interest, its combined effect with an external electric field has been largely overlooked. Here we perform an electric-field-dependent PL intensity study on heteroepitaxially strained surface guided CsPbBr3 nanowires. We reveal an unexpected linear coupling between the alternating field and the PL intensity with a significant modulation depth (up to 40%), stemming from an induced internal dipole. Low-frequency polarized-Raman spectroscopy shows structural modifications under an external field, associated with the observed polarity. These results reflect the important interplay between an external field and strain in MHPs and offer new insight into the design of novel MHP-based nano-optoelectronics.
2.2-T4
Perovskite solar cells, with solution-based, cheap synthesis methods and a rapid increase in power conversion efficiency, are a promising candidate for future solar cells. However, a major hurdle for commercialization remains, namely the intrinsic instability of these systems. Ion migration, the process by which the A, B and ions of the ABX3 structure become mobile in the perovskite layer, represents a key challenge to tackle.
MAPbBr3 was shown to be more stable under environmental conditions when compared to MAPbI3. Using transient ion drift, we show that this stems from key changes in ion migration when going from MAPbI3 to MAPbBr3: methylammonium migration is suppressed, while bromide migration is reduced. Nowadays, state-of-the-art perovskite devices combine multiple ions: we therefore extend our study to ion migration in mixed-halide perovskites with varying ratios of iodide to bromide, and find interesting dynamics regarding the phase segregation phenomenon. In order to benefit both from the high efficiency of the 3D perovskites and from the stability of the 2D perovskites, new device architectures composed of a 2D layer on top of a 3D layer have emerged. We quantify the ion migration dynamics in these mixed-dimensionality perovskites, and find that ion migration is hindered in all systems incorporating a 2D layer. The specific hindering mechanism is however dependent on the 2D spacer molecule. Finally, we investigate the evolution of ion migration in different MAPbBr3 solar cells as a function of the grain size of the active perovskite film. We show that beyond composition engineering, crystallinity can be another effective tool to control ion migration.
2.3-T1
Dr Luis Lanzetta is a Postdoctoral Fellow at King Abdullah University of Science and Technology (KAUST, KSA). He obtained his PhD in Chemistry at Imperial College London (UK) in 2020, where he focused on developing eco-friendly, tin-based perovskites for photovoltaic and light-emitting applications. His research focuses on next-generation materials for energy harvesting. Specifically, his expertise lies in the chemical degradation and stabilisation mechanisms of halide perovskite solar cells, aiming to provide design rules towards more efficient and stable technologies. He is additionally interested in molecular doping approaches for narrow-bandgap perovskites, as well as the spectroscopic and surface characterisation of this class of materials.
Google Scholar: https://scholar.google.com/citations?user=OcCV1VUAAAAJ&hl=es
The broad implementation of halide perovskites into electronic applications beyond photovoltaics relies on effectively tuning free carrier concentrations in this class of semiconductors. However, doping in these materials remains difficult and is far from being fully understood. Specifically, detailed knowledge on the perovskite-dopant chemical interactions is key to enable effective doping strategies. This talk will cover our recent work on carrier compensation in inherently p-type MASnxPb1-xI3 (where MA is methylammonium) by employing an n-type molecular dopant, i.e. n-DMBI. We observe a decrease in free hole concentration of nearly one order of magnitude, an increase in Seebeck coefficient and a shallower perovskite Fermi level, in consistence with electron donation from n-DMBI. This is shown to occur via dopant hydride loss and subsequent radical transfer to perovskite. We then find that only oxidised molecular dopant binds onto perovskite surface Sn sites via Lewis acid-base interactions, suggesting that charge transfer is facilitated by dopant/perovskite chemical coordination. We expect the detailed chemical insight on charge transfer doping provided herein to give design guidelines towards future molecular dopants for perovskite-based technologies.
2.3-T2
In this work we show that thin polycrystalline films of spin-coated formamidinium tin triiodide (FASnI3) perovskite demonstrate efficient random lasing (RL) possessing distinguished optical properties such as high mode stability, very high quality factor (~ 104) and low threshold (≈ 2 and ≈ 25 microJ/cm2 for 15 and 300 K, respectively). While usually RL is characterized by chaotic changes of the narrow lines spectral positions, the found in our case RL mode stability is a unique property, which is observed here for the first time for polycrystalline semiconductor systems. We demonstrate that the efficient random lasing and mode stability are due to the high efficiency of light scattering by FASnI3 grains, which is a result of high refractive index of the material (higher than in the case of Pb-based halide perovskites) as well as of optimized grain size distribution (average size is close to the emission wavelength). We demonstrate also that different excitation conditions are required for generation of ultra-narrow RL lines and rather broad-band Amplified Spontaneous Emission (ASE): RL lines dominate when excitation spot size is a few tens of micrometers, while ASE dominates in case of large size excitation spot of a few hundreds of micrometers. From the above, it follows that the stability in time of narrow RL lines is ensured by the strong space localization of RL modes and the absence of interaction between them [1].
[1] Chirvony, V. S.; Suárez, I.; Sanchez-Diaz, J.; Sánchez, R. S.; Rodríguez-Romero, J.; Mora-Seró, I.; Martínez-Pastor, J. P. Stable non-chaotic high Q near-infrared random lasing in thin polycrystalline films of lead-free formamidinium tin triiodide perovskite. To be submitted.
2.3-T3
Transient photoluminescence (trPL) is a powerful technique to study charge carrier dynamics and identify carrier recombination channels in luminescent thin films and devices. It has been widely used to quantify carrier radiative and non-radiative lifetimes,[1] to compute the charge extraction velocity [2], and to study the influence of the interfaces on the recombination mechanisms.[3]
Data fitting helps you extract this information from experimental trPL decays, but considering the number and nature of involved variables, the set of extracted values is most of the time not unique. Indeed, the trPL decay is ruled by the radiative and non-radiative recombination processes which follow, respectively, a quadratic and a linear dependence on the photogenerated carriers. The concentration of these carriers depends on several parameters, such as the injection level, the doping of the emitting material, the radiative recombination coefficient, and the trap density. Because of this interdependence, it is easy to obtain comparable trPL decay fits by using different combinations of values and, hence, interpreting incorrectly the recombination events. To obtain reliable information, it is better to use a robust simulation approach where the parameters are distinctly assessed and recursively optimized according to experimental data from the literature.
We demonstrate how to simulate experimental trPL curves of a halide perovskite on glass by fitting the data with a fully-coupled 1D optoelectronic simulation. The validity of the simulated trPL curves is also verified by fitting the decay with a bi-exponential function, which allows estimating the lifetimes of the different recombination mechanisms. We will present a robust routine to ascertain the reliability of the obtained results. This routine is based on experimentally measured material properties and on the analysis of the band diagram evolution during the PL transient. Our approach is not limited to the analysis of bare perovskites. We will show also a first analysis of the influence of the absorber interfaces on the trPL. The mentioned routine for trPL simulation can be further extended with the inclusion of photon recycling.[4]
2.4-I1
The renaissance of interest in halide perovskites, triggered by the discovery of their unprecedented performance in opto-electronic applications, elicited worldwide efforts to uncover a variety of intriguing physical properties, with a special interest about spin-orbit and Rashba/Dresselhaus effects. The current work presents for the first-time magneto-optical experimental evidence for non- uniaxial Rashba field with surplus contribution by a Dresselhaus effect arising from the bulk of MAPbBr3. Magneto-photoluminescence (MPL) spectra, monitored along several different crystallographic directions, were dominated by dual exciton emission peaks, while each exhibited a highly non-linear response to a magnetic field, with distinguished behavior at extreme points. Moreover, these plots depicted anisotropy from-B0 to +B0, with strong dependence on the axis of observations. Furthermore, ground state electron spin resonance spectroscopy, illustrated resonances bands coinciding with the extreme points in the MPL spectrum. Those resonance appeared only after illumination and disappeared a few hours later, designating an association with electron-nuclear coupling (the Overhauser effect. A theoretical model corroborating the experimental results, implementing anisotropic Rashba/Dresselhaus terms, anisotropy in the Landé g-factors, and a lesser contribution from an Overhauser effect, corroborated the experimental results. The origin of the Rashba/Dresselhaus effects in the bulk of halide perovskites is assumed to be attributed to the presence of grain boundaries. The research discoveries put a basic ground for expansion of possible applications of the halide perovskites toward spin-base devices.
2.4-I2
During recent years, the picture of dynamic charge carrier screening in lead halide perovskites (LHPs) evolved to explain long carrier lifetimes and diffusion lengths, leading to the nowadays famous optoelectronic properties of this material class. However, the most important prerequisite for such anticipated structural screening, the direct observation of related lattice- or organic cation motion within the estimated polaron formation time (<1ps), remains elusive. By developing two-dimensional optical Kerr effect (2D-OKE) spectroscopy, we unveil that the ultrafast polarization response to near-bandgap excitation pulses is overwhelmed by the instantaneous electronic polarizability [1]. In this case, the nonlinear mixing of highly anisotropic and dispersive light propagations is responsible for oscillatory Kerr-type modulations at terahertz (THz) frequencies [1, 2]. Therefrom-arising 2D-OKE fingerprints can be harnessed to trace lattice anisotropy across non-trivial phase transitions or in multi-cation alloyed LHPs [3]. Despite this useful characterization of static lattice distortions, the dynamic lattice response seems elusive for the OKE with excitation pulses in the visible spectral range.
Ultimately, to study the ultrafast lattice response to a transient electric field, we move the excitation to the THz spectral region, resonant to the optical modes of the lead halide lattice, by employing intense phase-stable THz fields. Conceptually, this close to single-cycle electric field with a rise-time below 1 ps, can be seen as proxy for ultrafast electron-hole separation during optical excitation and consecutive thermalization. By probing the THz-induced Kerr effect (TKE), we observe a strong THz polarizability in both inorganic CsPbBr3 and in the hybrid MAPbBr3 materials. Also here, we show that it is crucial to account for dispersion and optical anisotropy in interpreting the transient responses via rigorous four-wave mixing simulations. Nevertheless, we finally observe a contribution from the lattice polarizability by witnessing the nonlinear excitation of coherent phonons, in full agreement with static Raman spectra. Moreover, we pinpoint a single phonon mode which dominates the lattice polarizability, providing another stepping stone towards a better understanding of how lattice dynamics enable charge carrier protection in this class of defect tolerant semiconductors.
References:
[1] S.F. Maehrlein et al., PNAS, 118,7, e2022268118 (2021)
[2] L. Huber et al., J. Chem. Phys., 154, 9, p. 094202 (2021)
[3] F. Wang et al., J. Phys. Chem. Lett., 12, 20, 5016–5022 (2021)
1.1-I1
Maria Antonietta Loi studied physics at the University of Cagliari in Italy where she received the PhD in 2001. In the same year she joined the Linz Institute for Organic Solar cells, of the University of Linz, Austria as a post doctoral fellow. Later she worked as researcher at the Institute for Nanostructured Materials of the Italian National Research Council in Bologna Italy. In 2006 she became assistant professor and Rosalind Franklin Fellow at the Zernike Institute for Advanced Materials of the University of Groningen, The Netherlands. She is now full professor in the same institution and chair of the Photophysics and OptoElectronics group. She has published more than 130 peer review articles in photophysics and optoelectronics of nanomaterials. In 2012 she has received an ERC starting grant.
Highly performing mixed Sn/Pb-perovskite solar cells (PSCs) are among the most promising options to reduce Pb content in perovskite devices and enable the fabrication of all-perovskite tandem solar cells owing to their reduced band gap. Here, I will show that the introduction of 2,3,4,5,6-pentafluorophenethylaminium cations in a perovskite active layer of composition (FASnI3)0.5(MAPbI3)0.5 enhances the crystallinity of the active layer, reduces the voltage losses and increases the material stability. The addition of the fluorinated cations allows the fabrication of highly oriented films with improved thermal stability. Moreover, the treated films exhibits merged grains with no evidence of 2D structures, which we believe leads to the passivation of trap states at the grain boundaries. Solar cells fabricated adding to the active layer the fluorinated cation display reduced trap-assisted recombination losses and lower background carrier density, which led to enhanced open-circuit voltage than the reference sample using phenethylammonium cations. The best perovskite solar cell showed an efficiency of 19.13%, with an open-circuit voltage of 0.84 V, which is substantially improved respect to the reference sample which exhibits 17.47% efficiency and 0.77 V as open-circuit voltage. More importantly, the fluorinated cations' addition help to improve the device's thermal stability, maintaining 90.3 % of its initial efficiency after 90 min of thermal stress at 85 C° in Nitrogen atmosphere.
1.1-I2
Nowadays, overcoming the stability issue of perovskite solar cells (PSCs) while keeping high efficiency has become an urgent need for the future of this technology. We will show that getting stable PSC begins with the preparation of high-quality perovskite layers and that employing additives in the perovskite precursor solution allows to reach this objective. Two families of additives will be developed in the talk: halides and nanoparticles. We have highlighted the effect of chloride additives on the formation mechanism of methylammonium-free Cs0.1FA0.9PbI3 films upon annealing. By composition profile evolution tracking, two formation steps are distinguished: first the superficial residual solvent is eliminated, second, the solvent in the depth of the film is evaporated. The elimination profile signs the final morphology of the layer. The downward (top-down) growth has been encountered for the pristine and potassium chloride additive cases. It led to the formation of multiple boundaries and middle-sized grain morphology. We unveil that employing both potassium chloride and ammonium chloride additives forces the homogeneous elimination of the solvent across the layer, and then the lateral growth of the grains. It results in large size, monolithic and defect-poor grains with good coverage of the substrate which are the targeted properties for high efficiency and stability. Similar film formation steps and growth direction control have been highlighted by comparing the formation mechanism of MAPbI3 films with and without gold nanoparticles. The pristine layer grows in the top-down direction, while the presence of nanoparticles results in a lateral growth and formation of a monolithic structure.
1.2-T1
Organic-inorganic hybrid perovskite solar cells (PSCs) have attracted much attention due to their high power conversion efficiency (>25%) and low-cost fabrication. Yet, improvements are still needed for more stable and higher performing solar cells. In this presentation, three engineering approaches are proposed to enhance the photovoltaic efficiency and stability of PSCs: (1) On the first method, a series of highly oriented vertical TiO2 nanocolumn electron-transporting photonic structures were intentionally fabricated on half of the compact TiO2-coated fluorine-doped tin oxide substrate by glancing angle deposition with magnetron sputtering. These vertically aligned nanocolumn arrays were then applied as the electron transport layer into triple-cation lead halide perovskite solar cells based on Cs0.05(FA0.83MA0.17)0.95Pb(I0.83Br0.17)3. (2) On the second method, we investigated the effect of removing the excess PbI2 at the interface between the triple-cation Cs0.05(FA0.83MA0.17)0.95Pb(I0.83Br0.17)3 perovskite and the Spiro-OMETAD hole-transport layer. For this purpose, four different organic salts, including methylammonium iodide (MAI), formamidinium iodide (FAI), methylammonium bromide (MABr) and methylammonium chloride (MACl) were applied and compared. (3) The last aspect that will be presented involves the application of the downshifting optical property of colloidal carbon quantum dots to enhance the stability of perovskite solar cells against UV degradation.
On the above-mentioned engineering methods, different characterization methods, including far-field and near-field optical experiments, structural and spectroscopic investigations, impedance spectroscopy, together with solar cell efficiency and in particular device stability measurements are presented together in order to understand the underlying origins of the efficiency and stability enhancement observed in triple-cation perovskite solar cells.
1.2-T2
While organic-inorganic (hybrid) perovskites achieved competitive power conversion efficiency within an impressive short time [1], they still suffer from insufficient stability [2]. Instability of hybrid perovskite thin films under thermal stress and/or in humid air is one of the major obstacles for the commercialization of perovskite-based solar modules [2,3]. One of the most promising approaches to improve the long-term stability is the incorporation of bulky, hydrophobic molecules into the perovskite layer [4]. While the beneficial effects of these post-deposition treatments are generally accepted, there is an ongoing discussion about the mechanism of this passivation effect. Particularly, it is under debate when optimum surface passivation is reached using different concentrations of bulky molecules where a transition is supposed to happen from the surface attached bulky molecule itself to a 2D layer formation with general structure R2An−1BnX3n+1 [5], which forms via intermixing of the bulky molecules and PbI2 from the 3D perovskite. Here, A denotes the cation, B the metal ion, and X the halogen anion of the 3D perovskite, R is the bulky organic cation.
Here, we use in situ photoluminescence (PL) measurements during spin-coating and annealing to probe the dynamic deposition of 2-thiophenemethylammonium iodide (2-TMAI) and phenylethylammonium (PEAI) with varied concentration on 3D triple cation perovskites. For both molecules, we find the transition from molecular passivation to the formation of an R2An−1BnX3n+1 layer at concentrations around 4-10 mmol/L. Using higher concentrations, we see the formation of a distinct surface layer. During spin-coating and annealing of these higher concentrated solutions, we furthermore monitor the transition from a single inorganic layer spaced by the bulky cations (n=1) to mixed 2D layers (n=2 and higher) and, in the case of 2-TMAI, to the formation of a mixed disordered phase [6]. The latter may negatively affect the electronic properties of the perovskite layer [7]. Our results illustrate how in situ PL can be used to gain mechanistic understanding on the 2D layer formation, its interaction with the 3D perovskite, and its transformation to the disordered phase. Therefore, it can be utilized to deliberately optimize the annealing sequence targeting an ideal 2D/3D interface satisfying enhanced charge transport and stability.
Combining abovementioned results with in situ GIWAXS measurements, we propose a model for the surface passivation mechanism either via molecular passivation or 2D layer formation, compare the mechanisms for both investigated molecules, and correlate them with device stability proposing an optimized stabilization treatment.
1.3-I1
Lead halide perovskite absorber materials have attracted enormous attention from academia and industry due to their fascinating optoelectronic characteristics featuring multiple possible applications. In particular, the solution-processed perovskite solar cells demonstrated certified power conversion efficiencies of >25.5% thus coming very close to the best crystalline silicon solar cells. Low operational stability of perovskite solar cells is currently the main obstacle to their practical implementation.
Overcoming stability issues requires a very deep understanding of the compositional and structural dynamics of the perovskite absorber materials under the action of stress factors such as light, heat and electric field. Furthermore, the impact of defects and impurities on such dynamics is of crucial importance. These aspects remain insufficiently investigated, whereas many of the proposed aging pathways are still controversial.
In this lecture, we will discuss compositional and structural inhomogeneities in solution-cast thin films of classical 3D and mixed-dimensional 2D/3D perovskites as revealed by advanced microscopy: SEM, AFM, KPFM and IR s-SNOM. A particular focus will be made on the light-induced phase segregation effects induced by both anion and cation (de)mixing. Furthermore, we will consider typical impurities present in the perovskite absorber films, their localization, effects on the grain boundaries and impact on the operational stability of solar cells.
1.3-I2
Prof. Mónica Lira-Cantú is Group Leader of the Nanostructured Materials for Photovoltaic Energy Group at the Catalan Institute of Nanoscience and Nanotechnology (www.icn.cat located in Barcelona (Spain). She obtained a Bachelor in Chemistry at the Monterrey Institute of Technology and Higher Education, ITESM Mexico (1992), obtained a Master and PhD in Materials Science at the Materials Science Institute of Barcelona (ICMAB) & Autonoma University of Barcelona (1995/1997) and completed a postdoctoral work under a contract with the company Schneider Electric/ICMAB (1998). From 1999 to 2001 she worked as Senior Staff Chemist at ExxonMobil Research & Engineering (formerly Mobil Technology Co) in New Jersey (USA) initiating a laboratory on energy related applications (fuel cells and membranes). She moved back to ICMAB in Barcelona, Spain in 2002. She received different awards/fellowships as a visiting scientist to the following laboratories: University of Oslo, Norway (2003), Riso National Laboratory, Denmark (2004/2005) and the Center for Advanced Science and Innovation, Japan (2006). In parallel to her duties as Group Leader at ICN2 (Spain), she is currently visiting scientist at the École Polytechnique Fédérale de Lausanne (EPFL, CH). Her research interests are the synthesis and application of nanostructured materials for Next-generation solar cells: Dye sensitized, hybrid, organic, all-oxide and perovskite solar cells. Monica Lira-Cantu has more than 85 published papers, 8 patents and 10 book chapters and 1 edited book (in preparation).
Single-junction halide perovskite solar cells (PSCs) have already achieved a certified power conversion efficiency (PCE) above 25 %, making them one of the most promising emerging photovoltaic technologies. One of the main bottlenecks towards their commercialization is their long-term stability, which should exceed the 20-year mark. Many are the strategies applied to extend device lifetime, among them are the use of additives, the optimization of the fabrication process of perovskite thin films or the replacement of unstable organic transport layers such as Spiro-OMeTAD. Although most of these approaches can effectively improve device efficiency, they frequently fail at providing stable PSCs as defined as those able to display less than 10 % degradation after 1000 h of continuous illumination under 1 sun. In this respect, the understanding of defects is of paramount importance for the development of stable halide perovskite solar cells (PSCs). However, isolating their distinctive effects on the device efficiency and stability is currently a challenge. In this talk, we will show our most recent results on additive engineering to enhance the stability of highly efficient PSCs. We demonstrate that adding the organic molecule 3-phosphonopropionic acid (H3pp) to the halide perovskite results in unchanged overall optoelectronic performance while having a tremendous impact on the device stability. We obtained PSCs with ~21 % efficiency that retain ~100 % of the initial efficiency after 1000 h at the maximum power point under simulated AM1.5 illumination. The strong interaction between the perovskite and the H3pp molecule through two types of hydrogen bonds (H…I and O…H), results in shallow point defect passivation that has a significant impact on the device stability but not on the nonradiative recombination and device efficiency. We expect that our work will have important implications for the current understanding and advancement of operational PSCs.
2.1-I1
Professor Anita Ho-Baillie is the John Hooke Chair of Nanoscience at the University of Sydney, an Australian Research Council Future Fellow and an Adjunct Professor at University of New South Wales (UNSW). Her research interest is to engineer materials and devices at nanoscale for integrating solar cells onto all kinds of surfaces generating clean energy. She is a highly cited researcher since 2019. In 2021, she was an Australian Museum Eureka Prize Finalist and was named the Top Australian Sustainable-Energy Researcher by The Australian Newspaper Annual-Research-Magazine. She won the Royal Society of NSW Warren Prize in 2022 for her pioneering work in the development of next generation solar cells. She has been a finalist for the Australian Space Awards for various categories in 2023 and 2024. In 2024, she is the recipient of the Australian Academy of Science Nancy Millis Medal. She is a Fellow of the Australian Institute of Physics, the Royal Society of New South Wales and the Royal Society of Chemistry.
I will talk about our work on understanding intrinsic stability of perovskites and meta-stability of perovskite solar cells and strategies for boosting perovskite solar cells’ durability against thermal extremes and humidity. Our perovskite solar cells encapsulated by a polymer-based encapsulation scheme were the first to exceed the strict requirements of International Electrotechnical Commission standards for thermal cycling damp heat and humidity freeze. I will then review some of the glass-to-glass bonding technologies that can be carried out at low-temperatures suitable for perovskite solar cells. This will be followed by our recent results of developing polymer-free glass-to-glass bonding technique for encapsulating perovskite solar cells.
2.1-I2
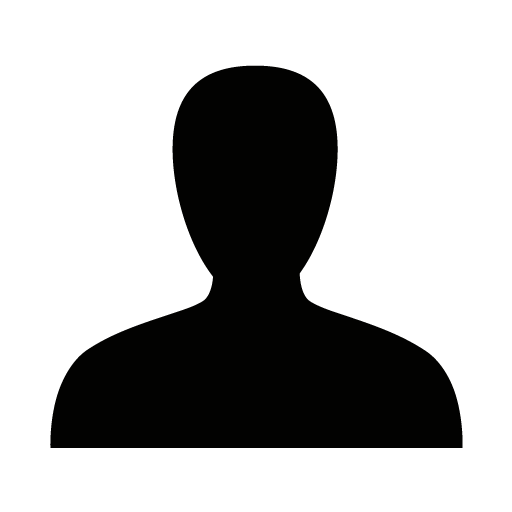
Nanometer-sized colloidal metal halide perovskite semiconductors have emerged and brought unique opportunities for photovoltaic application due to the high defect tolerance of perovskite and many features that emerge at the nanoscale. Perovskite quantum dots (QDs) or more broadly, nanocrystals (NCs), show high photoluminescence (PL) quantum yields, spectrally tunable bandgap, flexible compositional control, and crystalline strain benefits. Metal halide perovskite nanocrystals are readily synthesized with exceptional optoelectronic quality, opening a route for next generation light emitters, as well as exploring LHP physics at the nanoscale. CsPbX3 (X=Cl-, Br-, I-, or mixed halide) QDs exhibit PL tunable from ultraviolet to near-infrared wavelengths by adjusting the halide composition and/or QD size. In 2016, CsPbI3 QDs also became a point of interest in PV research. Currently CsPbI3 holds the record efficiency for QD solar cells (16.6%) proving better than any previous QD material composition. This talk will highlight the unique potential of perovskite QD (PQD) solar cells, from synthesis to devices. We will discuss current state of the art and lay out many open opportunities in perovskite QD solar cells, and the related present and future pursuits in QD preparation and device architecture.
Reference:
Protesescu, L. et al. Nanocrystals of cesium lead halide perovskites (CsPbX3, X = Cl, Br, and I): Novel optoelectronic materials showing bright emission with wide color gamut. Nano Lett. 15, 3692-3696 (2015).
Swarnkar, A. et al. Quantum dot-induced phase stabilization of α-CsPbI3 perovskite for high-efficiency photovoltaics. Science 354, 92-95 (2016).
Yuan, J. et al. Band-aligned polymeric hole transport materials for extremely low energy loss α-CsPbI3 perovskite nanocrystal solar cells. Joule 2, 2450-2463 (2018).
2.2-T1
Mixed-dimensional perovskites containing mixtures of organic cations hold great promise to deliver highly stable and efficient solar cells. When used in small amounts, the low-dimensional species can act as a dopant to passivate defects at the grain boundaries of the 3D counterpart, leading to improved performance and stability. However, although a plethora of relatively bulky organic cations have been reported for such purpose, a fundamental understanding of the materials' structure, composition, and phase, along with their correlated effects on the corresponding optoelectronic properties and degradation mechanism remains elusive. Herein, we systematically engineer the structures of bulky organic cations to template the formation of low-dimensional perovskites with contrasting inorganic framework dimensionality (2D to 0D), connectivity (flat vs. corrugated), and coordination deformation. By combining X-ray single-crystal structural analysis with various spectroscopic techniques, such as depth-profiling XPS, solid-state NMR, and femtosecond transient absorption, it is revealed that not all low-dimensional species work equally well as dopants. Instead, it was found that inorganic architectures with lesser structural distortion tend to yield a less disordered energetic and defect landscape in the resulting mixed-dimensional perovskites, augmented in materials with higher PLQY (up to 11%), better solar cell performance (PCE more than 19%) and improved thermal stability (T70 up to 1000 hrs, unencapsulated). Our study highlights the importance of designing templating organic cations that yield low-dimensional materials with much less structural distortion profiles to be used as additives in stable and efficient perovskite solar cells.
2.2-T2
Recently, organometallic hybrid perovskite materials are experiencing a real progress for solar cell applications. Due to particularly interesting properties: adaptable band gap, high crystallinity, high charge transport capacity and high thin film efficiency, these materials have the potential to exceed the performance limits of current technologies. They also combine a low cost and processing versatility. Among alternative device structures, carbon-based perovskite solar cells (C-PSCs) look highly promising due to their low cost and abundantly available materials (TiO2, ZrO2, carbon black and graphite powders), cost-efficient scalable fabrication methods and the inherent high stability. A one step (CH3NH3)x(AVA)1-xPbI3 perovskite solution (with AVA= ammonium valeric acid additive) was pipetted to infiltrate mp-TiO2/mp-ZrO2 through a thick porous carbon layer [1][2]. In order to reveal their maximum photovoltaic performance, these devices should be first matured under humidity and temperature. This step lasts of approximately 100-150 h and improves of the cell’s performance. To further investigate their stability, aging campaigns at 85°C/85%RH have been conducted during 1000 h. The macroscopic observations show an inhomogeneous degradation of the perovskite layer, the interfaces and the electrodes, mainly located at the edges. This inhomogeneity probably results from the pipetting process used to infiltrate the perovskite. This was confirmed by the variation of PV parameters during aging, which showed an important decrease in performance close to 50% after 1000 h of aging. In this study, a basic encapsulated system based on glass and a surlyn gasket was used, enabling the humidity permeation up to solar cells and inducing probably an accelerated degradation of devices. Thanks to dedicated characterization techniques, such as laser beam induced current (LBIC) measurements and photoluminescence imaging, the local performances have been correlated to the degradation inhomogeneity. The modifications of the perovskite layer have been evaluated with others more common techniques (X-Ray diffraction, UV-visible absorption and photoluminescence spectroscopy). Thanks to this multiscale approach, a degradation mechanism could be proposed highlighting the role playing by the prior maturation step. Today, others technological solutions are tested such as the inkjet printing for the perovskite infiltration and more advanced encapsulation systems, to improve the stability of these PV devices.
2.3-T1
Abstract: Despite of huge success in photovoltaic community, Pb based halide perovskites have energy band gap higher than the optimal band gap required for single junction solar cells, governed by the Shockley–Queisser radiative limit. Sn based perovskites emerge as an alternative with rapidly improving efficiencies. Due to comparable optoelectronic properties as the Pb counterpart and their ability to lead to lower band gaps, Sn based perovskites open a new door to all-perovskite tandem solar cells. Along with efforts for efficiency, it is worth analysing the in-depth recombination dynamics for further development of Sn based perovskite solar cells (PSCs). The long photo-excited charge carrier lifetime (τ) is often attributed for the high performance of PSCs. Herein, we study the effect of ‘B’ cation in ABX3 (A = MA+, FA+, and Cs+; B = Pb2+, Sn2+, and X = I−) perovskite structure on the charge carrier recombination dynamics to understand the possible recombination mechanism. We fabricated PSCs with p-i-n configuration based on FA0.95Cs0.05PbI3 (pure Pb), MA0.20FA0.75Cs0.05SnI3 (pure Sn), and (MAPbI3)0.4(FASnI3)0.6 (Pb-Sn mixed) perovskitres. We optimized the Sn based perovskite thin film (pure Sn) in terms of moisture and thermal stability in order to minimize the error due to perovskite degradation. Further, we compared the charge carrier recombination dynamics of the three devices through transient photovoltage (TPV) measurement.1 TPV is used to establish the relation between charge carrier density (n) and recombination rate constant (k) under different DC background intensities.2 This study establish the rate law of charge carrier decay in the three devices and reveals the nonlinear dependence of k on n in Sn based PSCs, which will be correlated to the slow relaxation lifetime of the charge carrier and their recombination through the defect states in the perovskite thin films.
Keywords: Pb-free perovskite, rate law of charge carrier decay, transient photovoltage, defects, bimolecular recombination
2.3-T2
M. C. López González received the B.S. degree in Electrical Industrial Engineering in 2010 from the University of Malaga and the B.S. degree in Materials Engineering in 2014 from the University of Seville. In the latter institution she also received the M.S. degree in Science and Technology of New Materials in 2016.
She is currently working as a Predoctoral Researcher in the Electronics Technology Area of the Universidad Rey Juan Carlos, within the Photonic and Electronic Organic Devices gorup (DELFO). Her work focuses on the study of hybrid perovskite solar cells, through the electro-optical characterization of the devices by impedance and degradation spectroscopy techniques, as well as their fabrication.
One of the most promising third generation photovoltaic technologies is Perovskite Solar Cells (PSC). This perovskite family presents outstanding properties such as high absorption coefficient, long diffusion lengths, low-cost technology and ease of scalability. Currently, the efficiency record is set to 25.6 % [1]. On the other hand, the development of new materials combining multiple cations and multiple halides is one of the main research strategies to achieve high performance.
In this work, we have analysed the temperature behaviour of perovskite based solar cells with structure FTO/c-TiOx/m-TiOx/RbCsFAMAPbIBr/Spiro/Au. We have obtained the temperature dependence of the solar cell parameters (Voc, Isc, FF and efficiency) from -20 ºC up to 50 ºC. Impedance Spectroscopy (IS) has been carried out at room temperature and Cole-Cole spectra have been fitted using the Matryoshka circuit. Ideality factor has been obtained both from Voc vs light and from the recombination resistance extracted from IS measurements. In order to determine the dominant recombination mechanism, the activation energy has been estimated from the extrapolation of Voc down to 0 K [2, 3].
2.3-T3
Dr Luis Lanzetta is a Postdoctoral Fellow at King Abdullah University of Science and Technology (KAUST, KSA). He obtained his PhD in Chemistry at Imperial College London (UK) in 2020, where he focused on developing eco-friendly, tin-based perovskites for photovoltaic and light-emitting applications. His research focuses on next-generation materials for energy harvesting. Specifically, his expertise lies in the chemical degradation and stabilisation mechanisms of halide perovskite solar cells, aiming to provide design rules towards more efficient and stable technologies. He is additionally interested in molecular doping approaches for narrow-bandgap perovskites, as well as the spectroscopic and surface characterisation of this class of materials.
Google Scholar: https://scholar.google.com/citations?user=OcCV1VUAAAAJ&hl=es
Hybrid tin halide perovskite solar cells stand as the most promising alternative to their toxic lead-based counterparts, although addressing their poor ambient stability remains as the main challenge to make this technology competitive. Hence, detailed knowledge of their chemical degradation pathways is essential to mitigate their decomposition. This talk will cover our recent findings on the degradation mechanism of tin perovskite thin films (i.e. (PEA)0.2(FA)0.8SnI3, where PEA is phenylethylammonium and FA is formamidinium) via a combination of spectroscopy, diffraction and ab initio simulation techniques [1]. We find that SnI4, a product that forms as a result of the oxygen-mediated decomposition of perovskite, reacts further to form iodine via the combined action of atmospheric water and oxygen. Iodine is then shown to be a highly aggressive species that rapidly leads to further perovskite degradation to give more SnI4, establishing a cyclic degradation mechanism. We then find the ambient stability of tin perovskite films to be highly dependent on the hole transport layer chosen as the substrate, which is used to tackle the oxidative degradation of the material and increase its ambient stability. We expect the findings presented herein to provide key design rules towards stable, lead-free tin perovskite solar cells.
2.4-I1
Philip Schulz holds a position as Research Director for Physical Chemistry and New Concepts for Photovoltaics at CNRS. In this capacity he leads the “Interfaces and Hybrid Materials for Photovoltaics” group at IPVF via the “Make Our Planet Great Again” program, which was initiated by the French President Emmanuel Macron. Before that, Philip Schulz has been a postdoctoral researcher at NREL from 2014 to 2017, and in the Department of Electrical Engineering of Princeton University from 2012 to 2014. He received his Ph.D. in physics from RWTH Aachen University in Germany in 2012.
In the past decade, metal halide perovskite (MHP)-based solar cells marked a breakthrough in photovoltaic technologies and reach power conversion efficiencies exceeding 25%. While MHPs exhibit a remarkable defect tolerance, film degradation will eventually deteriorate the optoelectronic properties and hence device performance. A key strategy to substantially enhance the stability is to tailor the interfaces in the device.1
Here, I will discuss the impact of interface formation on device performance also considering the effect of chemical reactions on interface energetics and durability,2 particularly for our recent research activities on oxide buffer- and transport layers.3 In particular, I will describe our use of surface-sensitive photoemission spectroscopy (PES) as a primary tool to provide guidelines for controlling the chemistry and optimize the electronic properties of MHP interfaces. In combination with further advanced characterization techniques, such as Kelvin probe force microscopy (KPFM) to determine surface photovoltage of the investigated layer stacks or quantitative photoluminescence imaging for the analysis of bulk optical properties, we specifically use PES methods to put the role of charge selective contacts in the focus of our research.4
2.4-I2
Perovskite solar cells promise to yield efficiencies beyond 30% by further improving the quality of the materials and devices. Electronic defect passivation and suppression of detrimental charge-carrier recombination at the different device interfaces has been used as a strategy to achieve high performance perovskite solar cells. However, the mechanisms that allow for carriers to be transferred across these interfaces are still unknown.
In this presentation, I will discuss the role of crystal surface structural defects on optoelectronic properties of lead halide perovskites through synchrotron-based techniques. The importance of interfaces and their contribution to detrimental recombination will also be discussed. As a result of these contributions to better understanding 2D and 3D defects, the perovskite solar cell field has been able to improve device performance. Albeit the rapid improvements in performance, there is still a need to understand how these defects affect long term structural stability and thus optoelectronic performance over the long term.
1.1-I1
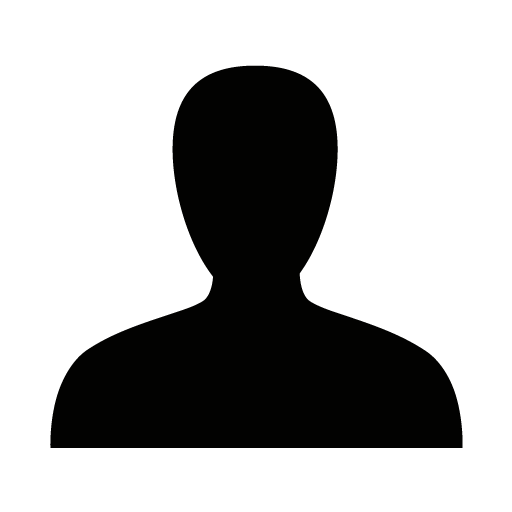
Perovskite/silicon tandem is a new high-efficiency photovoltaic platform that converts sunlight into electricity with excellent performance. In few years, this driving factor have pushed the tandem technology already at the entrance of the photovoltaic market. However, to succeed towards their commercialization, the tandems need to secure the stability of the performances, in line with the warranty certificates of commercial crystalline silicon modules. Enhancing the stability while preserving record performances is a challenge that can be addressed with different approaches. In this direction, bifacial tandem solar cells are a potential solution. In this configuration, light is absorbed both at the sun-side and at the rear-side of the device by exploiting the albedo - the scattered and reflected photons from the ground. Thanks to the additional photons arising from the albedo, the bifacial configuration enhances its current above the conventional configuration, resulting in even better performances. The current enhancement is achieved by tailoring the perovskite bandgap, resulting in bromide-free composition. This composition significantly improve the stability of the performances, by suppressing the mechanism of halide segregation. Overall, the bifacial configuration combine together enhanced efficiency and better stability, promising the technology at the utility scale, towards a carbon-sustainable economy.
1.1-I2
The usage of self-assembled monolayers (SAMs) as hole-selective layers was a key advancement for achieving >29%-efficient monolithic perovskite/silicon and >24%-efficient perovskite/CIGSe tandem solar cells. The SAMs also enabled model systems for a systematic study of charge extraction by transient methods.
This talk covers a short introduction into the development of SAMs for perovskite solar cells (PSCs), shows their application in perovskite tandem solar cells and shows which design guidelines could be concluded for a high-performance hole-selective interface. A combination of time-resolved and absolute photoluminescence spectroscopy, together with solar cell characterization identified how non-radiative recombination losses were minimized at the hole-selective interface, leading to high open-circuit voltages. The SAM model system further allowed an analysis of the influence of hole extraction speed on the fill factor of the PSCs. We found that hole extraction is around 100-times slower than electron extraction in p-i-n PSCs, which still leaves room for further fill factor gains. The dissection of charge extraction and non-radiative recombination in a perovskite absorber deposited on hole-selective layers was further detailed by a transient surface photovoltage study, confirming and completing the photoluminescence and solar cell analysis.
Finally, an overview of the wide variety of use cases for the SAMs in perovskite solar cells is shown, underlining their versatility, simplicity and robustness.
1.1-I3
Perovskite-based multijunction photovoltaics are about to surpass power conversion efficiencies of 30% on a laboratory scale and thereby have irrevocably developed into one of the most promising next-generation photovoltaic technologies with enormous market potential. Unfolding this potential requires solving scientific and technological challenges related to high-efficiency device architectures, the development of stable perovskite materials, light management, and scalable fabrication processes of the perovskite top solar cell. In this contribution, our recent progress on novel passivation strategies using 2D/3D heterostructures, optimization of light management textures, and scalable fabrication processes (evaporation, lamination, and solution-based coating) for perovskite-based tandem photovoltaics will be presented. Prototype perovskite/Si and perovskite/CIGS tandem solar cells in 4-terminal architecture, as well as 2-terminal architecture, will demonstrate the maturity of these developments.
1.2-T1
Tandem solar cells based entirely on thin-film metal halide perovskite absorbers hold the potential of high efficiencies at low cost and large flexibility.[1] Prototypes of such technology have been fabricated successfully, and efficiencies exceeding 26% have been reached.[2] However, compared to the more established combination of metal halide perovskite top cells with high-efficiency silicon bottom cells, the performance potential and limitations of all-perovskite tandems are less explored. One major difference with respect to the perovskite-silicon reference is the relevance of wave optical effects and of photon recycling for both, top and bottom absorbers. Hence, the optical coupling of the subcells needs to be considered rigorously in a simulation-based assessment of the performance potential.
Here, we present an extension of our recently introduced model for photon recycling in thin-film perovskite absorbers[3] to the situation of all-perovskite tandem solar cells, including a consistent consideration of luminescent coupling due to re-absorption in the bottom perovskite cell of the light emitted by the top perovskite absorber. The impact of photon-recycling and luminescent coupling on the photovoltaic device performance is assessed in the limit of ideal transport – using detailed balance – and under consideration of realistic charge transport based on a drift-diffusion model. The latter includes also losses due to both non-idealities in the subcell connection via the recombination junction and non-radiative recombination in the bulk and at interfaces.
This fully coupled optoelectronic approach enables an in-depth analysis of subcell performance issues and their propagation to the performance of the tandem device.[4]
1.2-T2
Solution-processed all-perovskite tandem solar cells have the potential to surpass the efficiency limits for single-junction devices by using complementary ~1.8 eV and ~1.2 eV absorbers, thereby reducing thermalization and transmission losses. However, both absorber categories, mixed-halide wide-bandgap and lead-tin narrow-bandgap, suffer from significant non-radiative recombination losses that can limit the overall open-circuit voltage of the multijunction device. Additionally, the complex optical stack in a tandem device can introduce optical losses due to parasitic absorption and reflection of incident light. This work presents an integrated all-perovskite tandem where the sub-cells use surface passivation strategies to reduce non-radiative recombination at the perovskite-fullerene charge-selective interfaces, yielding a high open-circuit voltage. Further, by using optically benign transparent electrode and charge-selective layers, the external quantum efficiency in the narrow-bandgap sub-cell is improved leading to reduced current-mismatch between sub-cells. Cumulatively, these strategies allow the development of a monolithic tandem solar cell exhibiting a power-conversion efficiency of over 23%.
1.3-I1
Dr Laura Miranda Perez is the Head of Materials Research and Characterisation at Oxford PV, a spin-out of Oxford University that is commercialising perovskites for photovoltaic applications. Laura has a strong background in materials synthesis and characterisation. Prior to joining Oxford PV she was a fellow at the University of Oxford, where her work focused on perovskites and carbon materials. Before this, Laura held a fellowship in perovskite thin film materials at the College du France in Paris. Laura undertook her PhD in Madrid, Spain and Sheffield, UK, in the screening of new families of hexagonal perovskite materials.
Meeting the ambitious challenge of net-zero greenhouse gas emissions by 2050 and holding the average increase of global temperature below 1.5°C necessitates the upscaling of readily available renewable energy sources, the window of time to achieve this goal is closing fast, so it is key to accelerate the decarbonisation of the global energy system by increasing the power output of solar cells. In this presentation, I will show how the integration of perovskites into the well-established silicon production infrastructure can raise the rate of solar deployment. Perovskite/silicon tandems deliver a technological disruption in efficiency while maintaining compatibility with the present photovoltaics industry, making it the fastest route to enhance the silicon market and rapidly address climate change.
1.3-I2
Tandem solar cells based on Perovskite with silicon or another perovskite can further reduce the cost of photovoltaics due to their high efficiency, and low cost (including low material and fabrication cost). To realize their full potential, the perovskites need to be deposited by scalable fabrication process, and the efficiency of each subcells need to reach their maximum. In this talk, I will present our recent progress in understanding the issues that limit open circuit voltage in wide bandgap perovskites and solutions to address them. I will also discuss the progress in stabilize narrow bandgap Sn-based narrow bandgap perovskites based on the understanding of its degradation mechanism. Finally, I will present the demonstrate of large area perovskite/silicon and perovskite/perovskite minimodules with high efficiency.
1.3-I3
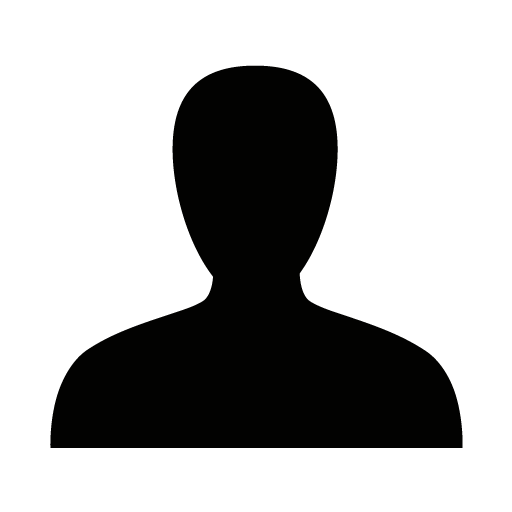
Tomas obtained his PhD at Oxford for his work understanding degradation mechanisms and photophysical processes in dye sensitized and perovskite solar cells. He was a Marie Curie fellow at Stanford, where he co-developed the first all-perovskite tandem solar cells and helped develop the perovskite-silicon tandem solar cells that became the first points on the NREL chart for these tandems. He then carried that research further at the National Renewable Energy Laboratory as a Staff Scientist. He is a co-founder and the chief technical officer of Swift Solar, which is developing and commercializing perovskite tandem PV.
Tandem solar cells made of perovskite semiconductors offer unique advantages over existing PV technologies. Specifically exciting are opportunities to integrate high tandem PV into novel form factors and enable integration of PV into new areas. Here, we will give an update on R&D progress at Swift, focusing on developing scalable fabrication methods well suited to all kinds of perovskite tandem solar cell manufacturing. We will discuss the development of flexible perovskite tandems and show some early product prototypes and highlight manufacutirng and stability challenges unique to applications in the consumer electronics space as well as for integration into electric vehicles.
2.1-I1
Hybrid halide perovskites are excellent building-blocks for multi-junction architectures, that provide the prospect to overcome fundamental efficiency limits of single-junctions. While perovskite/silicon or all-perovskite tandem cells have shown some remarkable progress, as of yet, perovskite/organic tandem cells show subpar efficiencies of ~20 per cent, limited by the low open circuit voltage of wide-gap perovskite cells, and serious optical/electrical losses introduced by the interconnect between the sub-cells [1].
Organic and perovskite semiconductors share similar processing technologies, which makes them attractive partners in multi-junction architectures. More importantly, the introduction of non-fullerene acceptors has boosted the efficiency of organic solar cells to levels beyond 18 per cent [2].
Here, we demonstrate perovskite/organic tandem cells with an efficiency up to 24 per cent, setting a new milestone for perovskite/organic tandem devices, which now for the first time outperform the most efficient single junction perovskite cells in p-i-n architecture [3].
This achievement draws from progress in all parts of the tandem:
Firstly, our ternary organic sub-cells (PM6:Y6:PC61BM) provide an enhanced efficiency in the near infrared spectral region and complement the perovskite cell. Most strikingly, under the filtered illumination conditions in the tandem, where excitons are solely generated on the Y6 acceptor, they are outstandingly stable even under long-term continuous operation of more than 5000 hours. This result is also in notable contrast to all-perovskite tandems, where stability issues of Sn-based narrow‑gap perovskite cells are a very serious issue.
Secondly, we managed to overcome interfacial losses, that are the predominant factors limiting the performance of wide-gap perovskite cells with high Br content. This allows us to access previously unreached territory of combined high open circuit voltage and fill factor.
Finally, we introduce a novel recombination interconnect for the two sub-cells, that is based on an ultra-thin (~1.5 nanometers) ALD grown indium oxide layer, that shows metallic properties and offers unprecedented low optical and electrical losses.
In an optimistic scenario, we envision that perovskite/organic tandem architectures bear a realistic prospect to reach efficiencies above 31 per cent.
2.1-I2
Dr. Fan Fu is a group leader at Empa-Swiss Federal Laboratories for Materials Science and Technology. He received his bachelor's and master's degrees in materials science from the Wuhan University of Technology in 2010 and 2013, respectively. He joined Prof. Ayodhya N. Tiwari's group as a Ph.D. student in 2014 and earned his Ph.D. degree from ETH Zürich with distinction in 2017. His doctoral thesis on perovskite-CIGS thin-film tandem solar cells was awarded ETH Medal. From January 2018 to May 2019, he worked as a postdoc researcher in Prof. Christophe Ballif's group at EPFL. In June 2019, he joined Empa as a group leader. He is currently leading a research team investigating novel perovskite semiconductors for energy and optoelectronics applications. In particular, his group's recent research efforts focus on upscaling high-performance perovskite-based tandem solar cells and mini-modules on flexible substrates.
Metal halide perovskite solar cells have gained tremendous attention due to their high power conversion efficiencies and potential for low‐cost manufacturing. Their wide and tunable bandgap makes perovskites ideal candidates for tandem solar cells with narrow bandgap photovoltaic technologies, such as crystalline silicon, Cu(In,Ga)Se2, and low-bandgap perovskite, to go beyond the efficiency beyond the Shockley–Queisser single-junction limit. The past 5 years have witnessed unprecedented advancements in perovskite-based tandems, with lab-scale devices (up to around 1cm2) approaching or even surpassing efficiency records of their single-junction building blocks. Currently, the majority of the perovskite-based tandems are fabricated on rigid glass substrates. It is highly desirable to develop perovskite tandem solar cells on flexible and lightweight substrates. This would open up the possibilities for high throughput roll-to-roll manufacturing and various attractive applications including the portable and wearable electronics, smart buildings, transportations, etc., where a combination of high efficiency, lightweight, and flexibility are important considerations. In this contribution, we will present our progress on developing highly efficient flexible wide-bandgap perovskite solar cells with high near-infrared transmittance and flexible narrow-bandgap CIGS and Pb-Sn perovskite solar cells. We demonstrate over 22% and 24% in all-perovskite and perovskite-CIGS flexible thin film tandems, respectively.
2.1-I3
Organic-inorganic halide perovskites have received widespread attention thanks to their strong light absorption, long carrier diffusion lengths, tunable bandgaps, and low temperature processing. Single-junction perovskite solar cells (PSCs) have achieved a boost of the power conversion efficiency (PCE) from 3.8% to 25.5% in just a decade. With the continuous growth of PCE in single-junction PSCs, exploiting of monolithic all-perovskite tandem solar cells is now an important strategy to go beyond the efficiency available in single-junction PSCs. However, their actual efficiencies today are diminished by the subpar performance of mixed lead-tin narrow-bandgap subcells. We firstly reported a strategy to reduce Sn vacancies in mixed Pb-Sn perovskites that use metallic tin to reduce the Sn to Sn via a comproportionation reaction. We increased thereby the charge carrier diffusion length in narrow-bandgap perovskites from sub-micrometer to 3 micrometers. We further reported simultaneous enhancements in the efficiency, uniformity and stability of narrow-bandgap subcells using strongly-reductive surface-anchoring zwitterionic molecules. The zwitterionic antioxidant inhibits Sn oxidation and passivates defects at the grain surfaces in mixed lead-tin perovskite film, enabling an efficiency of 21.7% (certified 20.7%) for single-junction solar cells. We obtained a certified efficiency of 24.2% in 1-cm -area all-perovskite tandem cells, and in-lab PCEs of 25.6% and 21.4% for 0.049 and 12 cm devices, respectively. We further obtained record-performing, 26.4%-efficient tandem devices by increasing the thickness of narrow-bandgap subcell without sacrificing the electrical performance. We recently also achieved a certified PCE of 21.7% for 20-cm2 all-perovskite tandem solar modules fully using scalable processing techniques. The encapsulated tandem devices retain 88% of their initial performance following 500 hours of operation under one-sun illumination in ambient conditions.
2.2-I1
November 2021, Jan Christoph Goldschmidt has started as professor of Physics of Solar Energy Conversion at the University of Marburg, Germany.
Before, he has been Head of Group "Novel Solar Cells Concepts" at Fraunhofer ISE, Freiburg, Germany since 2010. In 2012/2013 he visited Imperial College, London, UK and the MCC Berlin, Germany for research stays.
He received his PhD from the University of Konstanz, Germany for his work at Fraunhofer ISE. He studied Physics at the Albert-Ludwigs University Freiburg and the UNSW, Sydney, Ausstralia.
Resource limitations are becoming increasingly important for Terawatt-scale photovoltaics. Reducing the CO2-emissions from processing, substantially reducing the silver demand and ramping up flat-glass production capacity must become key priorities. Furthermore, high-efficiencies obtainable by perovskite-silicon tandem solar cells are one strong leverage to reduce overall resource demand. Here, we report on record high currents for planar perovskite silicon tandem devices by more transparent TCOs and adapted layer thicknesses. Using a TCO stack design, soft damage free processing could be combined with low parasitic absorption. We outline how further layer and interface optimization, as well as the application of textured bottom solar cells can lead to over 30% efficiency. We discuss, how usage of critical materials and costs can be reduced. First by the implementation of TOPCon-based bottom solar cells, with a PERC-like rear structure and a silicon tunnel junction front. Second, by the first-time successful demonstration of copper plating on perovskite solar cells.
2.2-I2
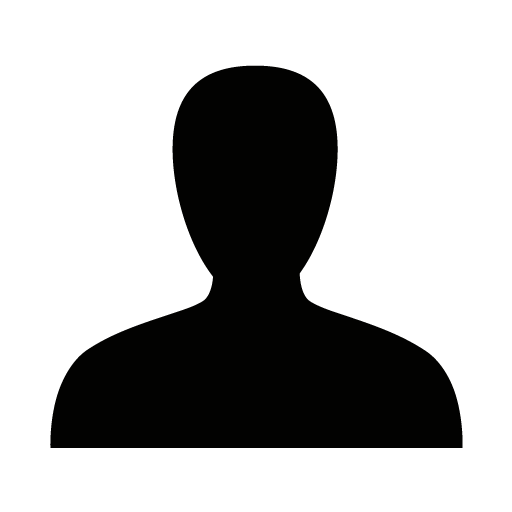
As the efficiency of 2-terminal PK/Si tandem devices continues to increase towards 30% in the laboratory, the question of manufacturability of these devices must be addressed. This is important as the efficiency gap between what is being currently made in laboratory and what is possible using more scalable methods and materials is a critical issue for the future of this technology. Specifically, the integration of these devices into established PV process flows must be investigated to understand where adaption is possible and where new processes must be made. To this end, we present a proposed process flow for the fabrication of large area 2-terminal PK/Si tandems based on a heterojunction bottom cell. Methods to adapt chemical etch, metallization, and encapsulation are presented. Additionally, a high throughput meniscus coating approach for the perovskite layer will be demonstrated and the integration challenges discussed. Using this process flow, we demonstrate that PK/Si tandem solar cells based on commercially available CZ M2 wafers can be fabrication with efficiencies of over 22% on an active area of 100 cm2.
1.1-I1
Alexander S. Urban studied Physics at the University of Karlsruhe (Germany) obtaining an equivalent to an M.Sc. degree (German: Dipl. Phys.) at the University of Karlsruhe (Germany) in 2006. During his studies he spent a year at Heriot Watt University (UK), where he obtained an M.Phys. in Optoelectronics and Lasers in 2005. He then joined the Photonics and Optoelectronics Chair of Jochen Feldmann at the Ludwig-Maximilians-University (LMU) Munich (Germany) in 2007 where he worked on the optothermal manipulation of plasmonic nanoparticles, earning his Ph.D. summa cum laude in 2010. He expanded his expertise in the fields of plasmonics and nanophotonics in the group of Naomi J. Halas at the Laboratory for Nanophotonics at Rice University (Houston, TX, USA), beginning in 2011. He returned to the LMU in 2014 to become a junior group leader with Jochen Feldmann, where he led the research thrusts on optical spectroscopy, focusing on hybrid nanomaterials such as halide perovskite nanocrystals and carbon dots. In 2017 he was awarded a prestigious Starting Grant from the European Research Council and shortly after that in 2018 he received a call as a Full Professor of Physics (W2) at the LMU. Here, he now leads his own research group working on nanospectroscopy in novel hybrid nanomaterials.
Halide perovskite nanocrystals (NCs) have emerged as an intriguing material for optoelectronic applications, most notably for light-emitting diodes (LEDs), lasers, and solar cells. Their emission wavelength depends not only on material composition but also on size and dimensionality, as in the case of two-dimensional (2D) nanoplatelets (NPLs). These colloidal quantum wells have additional appeal for light emission, as the one-dimensional quantum confinement enhances their radiative rates and enables directional outcoupling. On top of this, due to a monolayer-precise control over their thickness, they constitute an intriguing system for spectroscopic studies on their fundamental optical, phononic, and energetic properties.
In this talk, I will explore our recent results on halide perovskite NPLs, including their synthesis. I will focus on their interesting excitonic properties, such as the energetic fine structure1 with a strong thickness-dependent bright-dark exciton splitting and on exciton-phonon coupling.2 Further, I will highlight their advantages and disadvantages for integration into optoelectronic applications.
1.1-I2
The urgency for affordable and reliable detectors for ionizing radiation in medical diagnostics, nuclear control and particle physics is generating growing demand for innovative scintillator devices combining efficient scintillation, fast emission lifetime, high interaction probability with ionizing radiation, as well as mitigated reabsorption to suppress losses in large volume/high-density detectors. Prized for their solution processability, strong light-matter interaction, large electron-hole diffusion length and tunable, intense luminescence at visible wavelengths, lead halide perovskite nanocrystals (LHP-NCs) are attracting growing attention as highly efficient emitters in artificial light sources and as high-Z materials for next generation scintillators and photoconductors for ionizing radiation detection. Nonetheless, several key aspects, such as the trapping and detrapping mechanisms to/from shallow and deep trap states involved in the scintillation process and the radiation hardness of LHP NCs under high doses of ionizing radiation are still not fully understood, leaving scientists without clear indications of the suitability of LHP-NCs in real world radiation detectors or design strategies for materials optimization. In this talk I will present on our recent strategies for high performance radiation detection schemes and will report recent spectroscopic results of the scintillation process and its competitive phenomena, ultimately offering a possible path to the realization of highly efficient and extremely radiation hard LHP-NCs.
1.1-I3
Over the last few years, halide perovskite nanocrystals (NCs) have received great attention in many fields ranging from chemistry to physics and engineering owing to their extremely interesting properties such as defect tolerance, high photoluminescence quantum yield (PLQY), tunable optical bandgap not only by their dimensions but also by composition, enhanced stability compared to thin-film perovskites, and ease of synthesis. In general, ion exchange reactions offer access to NCs with precise chemical compositions that are inaccessible by direct synthetic routes. While anion (halide)-exchange in halide perovskite NCs has been greatly exploited to tune their optical properties, cation exchange has been less explored. This talk will be focused on post-synthetic cation-composition tuning in lead halide perovskite NCs for fine-tuning their optical properties.
1.2-T1
Semiconducting lead halide perovskites are excellent candidates for realizing low threshold light amplification due to their tunable and highly efficient luminescence, ease of processing and strong light-matter interactions. Indeed, several solution processable lasers have been demonstrated, even operating under nanosecond and continuous wave optical pumping. All of these examples use perovskite architectures with little to no confinement, for example using bulk films, nanowires or quantum dots (QDs) with average sizes well above the Bohr diameter. As such, there is no clear picture whether the use of perovskites in the strong 3D confinement regime would be beneficial, nor is there any insight in how optical gain would develop under those conditions. Here, we show through a combination of quantitative transient absorption and femtosecond fluorescence spectroscopy, that optical gain in strongly confined perovskite 0-dimensional QDs develops at remarkably low average exciton numbers per nanocrystal (<N> << 1). The gain magnitude is however smaller compared to bulk-like perovskite systems and the lifetime is capped by rapid Auger recombination. We are able to explain our observations using a 3-level model that takes both the strong exciton-exciton repulsion and Stokes shift due to electron-phonon coupling into account of strongly confined QDs. The enhanced repulsions and shifts act as a double edge sword, reducing the gain threshold but decreasing gain magnitude and lifetime. The concepts shown here provide a rational approach to look for low threshold optical gain materials based on an optimal interplay between electronic and vibrational degrees of freedom.
1.2-T2
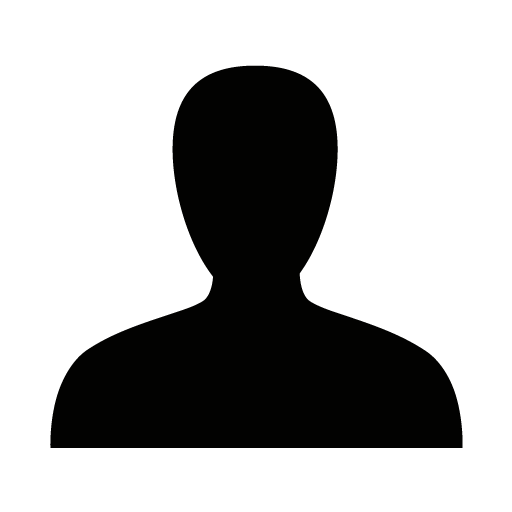
Self-assembly of colloidal nanocrystals into long-range ordered superlattices holds great promise in the multiscale engineering of solid-state materials with controlled and programmed functionalities which result not only from combination and enhancement of size-dependent properties of constituent building blocks but also from synergistic effects and novel interactions between neighboring nanocrystals. Thus far, the reports had mainly focused on single-component and binary systems of spherical NCs, yielding SLs isostructural with the known atomic lattices [1]. Far greater structural space is anticipated from combining nanocrystals of various shapes. Caesium lead halide perovskite nanocrystals, possessing unique optoelectronic properties (narrow-band tunable bright emission, high oscillator strength of bright triplet excitons, slow dephasing) and being synthetically available as uniform, monodisperse cubes, are promising building blocks for creating superlattice structures that exhibit collective optical properties.
We show that broad structural diversity enabling effective tuning of the relative position and orientation of sub-10 nm CsPbBr3 nanocubes can be achieved by their co-assembly with spherical, truncated cuboid and disk-shaped building blocks into long-range ordered multicomponent superlattices. CsPbBr3 nanocubes combined with spherical Fe3O4 or NaGdF4 nanocrystals and truncated cuboid PbS nanocrystals form binary SLs of six structure types, namely, NaCl-, AlB2-, CuAu- as well as uncommon to all-sphere assemblies novel AB2-, quasi-ternary ABO3- and ABO6-types. In these structures, nanocubes preserve orientational coherence. Co-assembly of CsPbBr3 nanocudes with larger disk-shaped LaF3 NCs (1.6 nm in thickness) results in the formation of seven columnar and lamellar structures with A2B, AB, AB2, AB4 and AB6 stoichiometry, not observed before for systems comprising spheres and disks. We rationalize the effect of the cubic shape on assembly outcome using packing-density calculations. In the systems with comparable dimensions of nanocubes (8.6 nm) and nanodisks (6.5–12.5 nm), other, non-columnar structures are observed, such as ReO3-type SL, featuring intimate intermixing and face-to-face alignment of disks and cubes.
1.2-T3
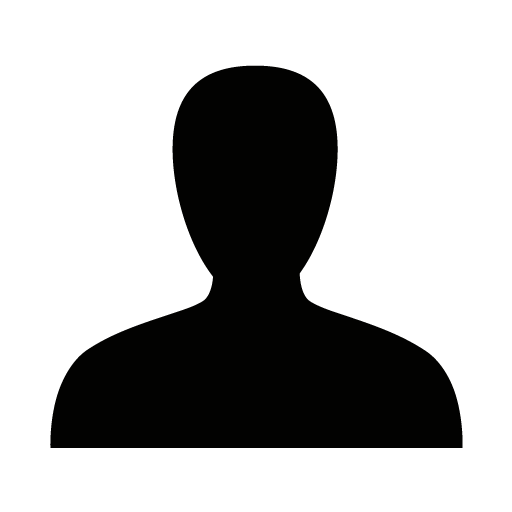
Recently, lead halide perovskites (LHP) have attracted a lot of attention in the field of optoelectronics, inter alia, the application in light emitting devices (LED) is of enormous interest.[1,2] One promising approach to increase the efficiency is the utilization of nanocrystals (NC) due to their ability of tailoring the ligand shell to specific needs.[3]
Therefore, the ligand dynamics at the NC surface was examined on soy-lecithin covered LHPs and a highly dynamic surface equilibrium was discovered. It was found that depending on the surface ligand density the lateral diffusion, i.e., the movement of the ligand along the NC surface can be tuned. This behavior can be rationalized by the zwitterionic nature of lecithin and the binding situation at the surface. Precisely, as more ligand is added, less surface area per ligand is available and, thus, the molecules can only attach with one functional group rather than both, which results in significantly faster dynamics.
In a subsequent step, ligand exchange on CsPbBrI2@SiOx LHPs was performed utilizing organic semiconductors, namely metal derivatives of mono-carboxy tetraphenylporphyrin (mMTPP). By tethering the novel ligand to the NCs, the electronic conductivity could be increased whilst simultaneously suppressing ionic currents. The underlying phenomenon of this effect was the decrease of the charging energy. Upon examining the NCs in electroluminescent devices it was found that the current efficacy and turn-on voltage was improved by the semiconducting ligand almost fivefold.[4]
However, an especially important downside of perovskite-based devices is the stability of the material against environmental influences. From the temperature-dependent conductivity of CsPbBrI2 NCs an increased phase stability was found by introducing mZnTPP as a ligand.[4] Upon exposing the system to X-ray radiation, we found a radiation-induced, isotropic contraction of the crystal lattice which in turn resulted in an altered electrostatic potential. This could be correlated to a characteristic shift in X-ray photoelectron spectroscopy signals, i.e., the electronic structure. Furthermore, these structural changes could be identified as a very likely driving force for the decomposition, which appears to be ligand dependent. For an oleic acid / oleylamine ligand shell, the decomposition into the corresponding salts was found, whereas the exchanged system exhibited a novel, significantly slower decay in the form of a disproportionation of Pb2+ to Pb0 and Pb3+.
1.3-T1
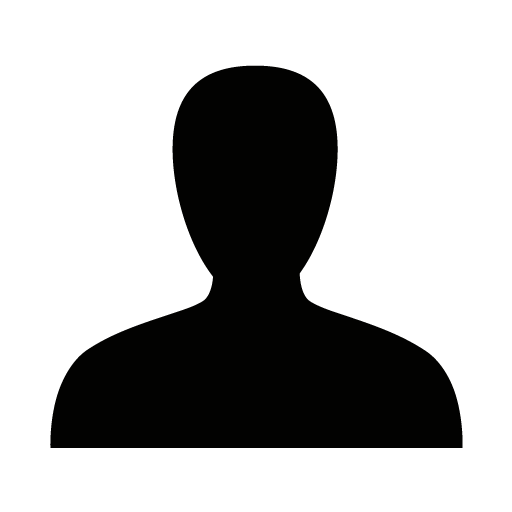
Metal halide perovskites are one of the most investigated semiconductor materials, with the 3D CsPbX3 structure being renowned for its enhanced optoelectronic performance. Multiple studies are currently attempting to substitute lead with other elements while still retaining the original properties of this material. This effort has led to the fabrication of metal halides of lower dimensionality. Recently, the layered perovskite structures have captured attention for their potential as an emerging class of colloidal semiconductors.
Here we report the colloidal synthesis of the pure Ruddlesden – Popper phase Cs2CdCl4:Sb3+, using a facile hot injection approach under atmospheric conditions. Through strict adjustment of the synthesis parameters with emphasis on the ligand ratio, we obtained nanoplatelets with a well-defined size and morphology. The particles underwent extensive structural characterization through synchrotron X-ray diffraction, pair distribution function analysis and transmission electron microscopy. Rietveld Refinements assigned a 14/mmm tetragonal space group and confirmed the aforementioned phase. Bright field imaging revealed nanoplatelets with a natural tendency to stack along the ab surface, self-assembling into long chains. Size distribution analysis assigned a length of 22.9 ± 3.8 nm and a thickness of 3.7 ± 0.8 nm, which were consistent with the dimension predictions given by the texture analysis from the x-ray diffraction data [1].
Spectroscopic characterization revealed an intense cyan emission, centered at 510 nm, and with a measured absolute PLQY of 20 ± 5% [1]. The emission is ascribed to the doped Sb3+ within the structure and is attributed to an s-p transition that originates from self-trapped excitons from the Sb3+ hosts [1][2]. Time-resolved photoluminescence measurements gave a double exponential decay, characterized by a fast (∼1.3 ns) and a long (∼1626 ns) component [1]. The existence of these two components is thought to result from two different recombination routes taking place from the excited Sb3+ states. A similar behaviour was previously reported in Sb3+ doped double-layered perovskites and is consistent with the findings from the respective bulk phase [2] [3] [4].
This work proves as a confirmation that colloidal synthetic approaches can give access to a new generation of RP phase nanocrystals through halides tuning, metal alloying, the replacement of Cs+ by alternative counterions and the introduction of various dopants, such as Bi3+ or Mn2+.
1.3-T2
Despite the increased efforts to replace the Pb in the lead halide perovskites-based solar cells with Sn analogs materials, in the nanocrystal field, limited progress is reported. The intrinsic chemical instability of the Sn2+ hinders a clear understanding of the origin of the chemical instability and poor optical performance.
Here we present the Sn halide perovskites nanomaterials with tunable size and dimensionality (3D - Sn halide perovskites: ASnX3, A=Cs+, FA+, X=I-, Br- and 2D - Ruddlesden-Popper (R-NH3+)2Csn-1SnnX3n+1), and characteristic optical properties. The formation mechanism of the perovskite nanocrystals is based on the pre-formation of the 1D chained of [SnI6]2- octahedra. Similar to Pb-based perovskites, the stability of the NCs is increased for 10 nm particles while the optical performance remains inferior to the Pb cousins.
The relation between the kinetic parameters during the synthesis processes and the chemical and optical stability of the obtained 3D and 2D perovskites nanostructures will be discussed.
1.3-T3
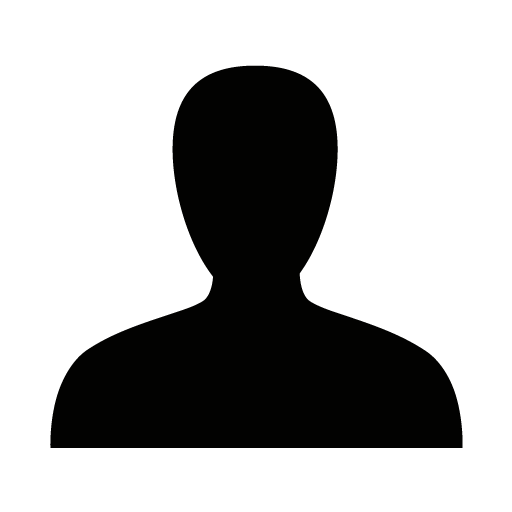
A common strategy for downshifting luminescence is based on a trivalent lanthanide (Ln3+) ion as emission center and a sensitizer as light absorption center. Lead halide perovskites can be used as sensitizer to meet the demands for downshifting applications. However, perovskite sensitizers suffer from low visible absorption, poor stability, and toxicity. In this study, we demonstrate a downshifting configuration based on lead-free cesium manganese bromide nanocrystals enabling efficient transfer of high energy absorbed photons to the low energy emission centers in the near-infrared spectral region. For that, we obtained phase-pure CsMnBr3 and Cs3MnBr5 nanocrystals via a controlled synthesis and successfully doped them with Er3+, Yb3+, Tm3+ and Nd3+. The correlation of the CsMnBr3 broadband visible absorption to the lanthanides emission was confirmed with steady-state excitation spectra and time-resolved photoluminescence measurements. This work provides a lead-free material as an efficient sensitizer, which can help to develop and design visible to NIR downshifters.
1.4-I1
A new synthetic method for colloidal perovskite nanocrystals has been designed, which offers slow thermodynamic control [1] instead of conventional kinetic growth [2]. The reaction time is increased up to 30 minutes while a wide size range of nanoparticles, some even reaching the strong confinement regime, is obtained with high level control of size and shape [1]. The synthesized quantum dots (QDs) turn out to have a spheroidal shape on average with remarkably well-separated higher absorption peaks. For the first time, this allows for a direct comparison between theory and experimental data related to the transitions beyond the lowest absorption line. Using empirical modelling with second-order many body perturbation theory, we are able to predict the energy positions as well as the oscillator strength of not only the lowest 1s-1s exciton but also of the higher excitonic transitions [3]. The calculated values are in fair agreement with the experimental data. Besides, by taking into consideration the spherical and cuboidal confining potentials, our theory offers an explanation for the well-defined higher transitions in the spheroidal QDs compared to cuboidal ones obtaining from more standard synthetic approaches [4]. The accuracy of the theoretical methods will be also critically discussed
1.4-I2
Lead-halide perovskite (LHP) thin-films and nanocrystals have advanced to the forefront of materials research for a wide array of applications from solar-cells and related optoelectronic devices, near unity quantum yield light sources, to coherent single-photon emitters for quantum information processing. Electron-phonon coupling (EP-coupling) plays a critical role in LHPs, proposed to both enhance performance metrics in some cases, e.g. ‘polaronic protection’ of charge carriers, and limit them in others, e.g. exciton coherence loss and broadened emission in perovskite nanocrystals. At the root of EP-coupling is a shift of the equilibrium atomic coordinates of the atoms in a material, a lattice reorganization, upon a change of the electronic configuration. While various time-resolved spectroscopies have shed light on the phonons involved, the nature of the lattice reorganization, and therefore the mechanisms underlaying EP-coupling in LHPs, remains unclear. Valuable insight can be provided through physical characterization of the inherent excited-states structural dynamics of these materials. In principle, EP-coupling-driven lattice reorganizations can be directly measured through time resolved diffraction, and NCs are an attractive system to probe these dynamics. Here, we perform femtosecond-resolved optical pump diffraction probe measurements to quantify the suprisingly large lattice reorganization occurring as a result of exciton-phonon coupling to the interband transition in formamidinium-lead-bromide (FAPbBr3, FA = CH5N2) NCs. A variety of Ab Intio techniques coupled with modelling are employed to understand the observed effect. Our findings provide an intuitive explanation for the origin of lower energy optical phonon coupling, and provide insight into both the excited state and equilibrium structure of LHPs.
1.4-I3
Two-dimensional (2D) lead halide perovskite nanoplatelets are particularly promising for display light sources and single-photon emitters given the large exciton binding energies and the phenomenon of giant oscillator transition strength expected in these confined systems. Here, we describe the thickness-dependent level structure and fine structure of excitons in colloidal 2D lead halide perovskite nanoplatelets. We examine the effects of dielectric and quantum confinement on the exciton binding energy in individual and stacked nanoplatelets while accounting for quantum-confinement-induced effective mass anisotropy. We calculate the exciton fine structure and optical selection rules in an electron-hole exchange model, elucidating the roles of intrinsic crystal field splitting [1] and quantum-confinement-induced Bloch function mixing [2] in conjunction with the effects of shape anisotropy and image charges via the long range exchange interaction.
2.1-I1
Metal halide perovskites have attracted substantial interest due to their accessible fabrication process, promising properties for optoelectronic applications. The doping of cations in lattice of perovskite can therefore endow host many novel properties. However, the post-synthetic treatment of perovskite ABX3 nanocrystals (e.g., FAPbI3 or CsPbCl3) for doping remains elusive. The straightforward doping method for both A and B sites are rarely reported, which hinders the further application of optimized doping materials for optoelectronic devices. Herein, employing hybrid water-oil phase (water-hexane), we have partially replaced of Cs or Pb with FA and Mn by similar method for the first time, respectively. Taking advantage of in-situ photoluminescence tracking, we have distinguished the doping process and proposed a model to provide better understanding for the doping process. What’s more, this method could not only be use for doping, but also for synthesis of new materials. This study hence provides a controllable way for doping metal halide perovskites or even synthesis new materials to meet more complexed and specific requirements for different applications.
2.1-I2
Lead (Pb) halide based perovskites nanocrystals show outstanding optoelectronic properties. But Pb-toxicity remains an issue for their widespread application. Therefore, search for Pb-free metal halide perovskites is natural. The fundamental question is how to identify alternative metal halides that could replicate optoelectronic properties of the Pb-halide perovskites.[1,2] I will address this question in the first part of my talk. Unfortunately, till date, Pb-free perovskites could not compete with optoelectronic properties Pb-halide perovskites. Why so? This question will also be discussed in the talk. Finally, in the last 3-4 years, we changed the goal post of our research. Instead of attempting to replicate the optoelectronic properties of Pb-halide perovskites, we started to explore newer directions. For example, we dope lanthanides like Yb3+ and Er3+ in Cs2AgInCl6 double perovskites to develop short-wave-infrared (SWIR) light emitting materials.[3] Furthermore, doping and/or codoping ions like Bi3+ and Sb3+ (that has two electrons in the outermost s-orbiatl) tailor both optical excitation and emission in the UV-visible region.[4,5] In the last part of my talk, I will discuss about such exploration.
2.1-I3
Magnetic doping in halide perovskite semiconductors is of timely interest with prospective for their use in opto-electronic and spin-related devices. Here, we report a thorough investigation of the optical and magneto-optical properties of Ni2+-doped caesium lead halide perovskite with a chemical formula CsPb(Br1-xClx)3, implementing steady-state and transient polarized photoluminescence (PL), and optically detected magnetic resonance (ODMR) spectroscopies. ODMR measurements probe magnetic resonance transitions of photo-generated electrons and holes in these emissive excited states, exposing phenomenological g-factors that deviate from those of band-edge charge carriers. Simulations of the ODMR spectra suggest carrier trapping in shallow traps with a slight anisotropic surrounding and with weak electron-hole exchange coupling. Furthermore, we observe substantial broadening of the hole resonance, associated with magnetic exchange coupling between Ni2+ unpaired spins and the trapped hole spin. Overall, these ODMR measurements uncover the role of the dopant in localizing photo-generated carriers while stiffening the crystal structure, and for the first time, provide a direct observation of carrier-dopant spin exchange interactions in metal-halide perovskite nanocrystals. These results offer insight into the influence of magnetic dopants on the electronic structures of metal-halide perovskites, with a view toward emerging spin-based devices made from perovskites.
2.2-T1
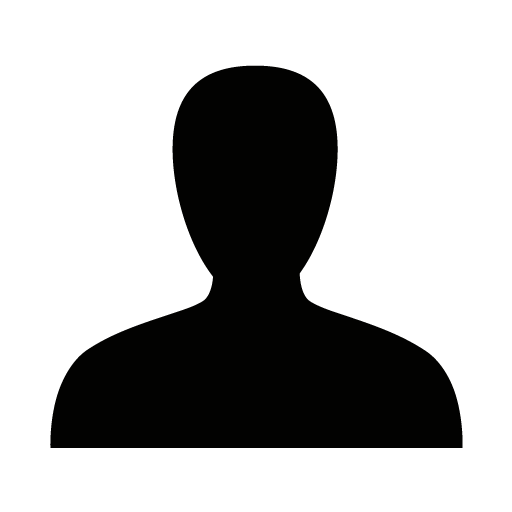
Despite extensive reports on red and green perovskite-based LEDs (PeLEDs), development of white PeLEDs remains limited by the low photoluminescence quantum yield of white-emitting perovskites and the undesired energy-transfer (ET) process occurring in multidomain Ruddlesden–Popper perovskites. While ET is beneficial for achieving efficient monochromatic emissions, the broadband spectrum required for white electroluminescence makes this phenomenon undesirable. Processing-induced physical separation of emitters has been proposed as an effective way to curb ET. Here, it is shown that by adopting a bilayered emitter configuration, achieved through a facile antisolvent-assisted spin-coating process, an increase in spatial separation between the blue perovskite and red emitting organic species employed can be realized. This, in turn, has allowed for effective reduction of ET efficiency, leading to a record efficiency of 1.3%, the highest achieved to date from a perovskite-based white electroluminescent device.
2.2-T2
The unique properties of lead halide perovskite nanocrystals (NCs) have drawn major attention of researchers over the last decade. However, the high toxicity of the lead in the composition and the relatively low phase stability are delaying their commercialization, therefore, diminishing their advantages [1]. These challenges are one of the major focuses of the community leading to the ongoing search for new stable lead-free compositions. However, up to date, there are only a few promising candidates to surpass the characteristics of lead halide perovskite NCs [2].
So far, no attention to cesium manganese chloride (CsMnCl3) NCs was given likely due to the absence of promising properties [3]. We are the first ones to synthesize and characterize emissive colloidal CsMnCl3 NCs through the conventional hot-injection route. The optical, structural, and morphological properties of CsMnCl3 NCs were studied by the means of steady-state-, time-resolved, and low-temperature spectroscopy, transmission electron microscopy (TEM), inductively coupled plasma mass spectrometry (ICP-MS), and X-ray diffractometry (XRD) respectively. The synthesized CsMnCl3 NCs display photoluminescence at 670 nm, large Stokes shift (250 nm), broad emission (FWHM = 97 nm), lifetime decay in the millisecond range, and fairly high quantum yield of 25%.
Moreover, Rietveld structural refinement was carried out to confirm the adopted structure of the NCs and investigate the relationship between synthesis conditions, crystal structure, and photoluminescence. It was found that when the synthesis temperature was tuned, the CsMnCl3 adopt different crystal structures (i.e. cubic and rhombohedral). By optimizing the temperature and the precursors' ratio, a pure rhombohedral structure is formed. It is worth nothing, that up to date, there is no work showing the luminescent rhombohedral CsMnCl3 as well as the dependence between the synthesis conditions, structure, and optical properties.
In addition, the synthesized NCs show moderate phase and optical stability (more than 2 weeks) under the ambient conditions (dark, RH 40-60%, RT). Together with its unique optical properties, CsMnCl3 is a promising candidate to substitute lead-halide perovskite NCs. The results of our work provide important insights into the photochemistry of manganese-based materials.
2.3-I1
Abstract:
The fast rising demand for the ultralow detection limit of ionizing radiation in medical radiography, high-energy physics and security screening, has led to extensive research on X-ray imaging scintillators and detections. However, high-performance scintillators consist mainly of ceramic that needs harsh and costly preparation conditions. Therefore, searching for new scintillation materials is of great interest to material scientists, chemists, and engineers. Organic emitters and perovskites, are excellent candidates as scintillation materials due to their good processability and low-fabrication cost. In this talk, we will present the room-temperature synthesis of a colloidal scintillator comprising CsPbBr3 nanosheets of large concentration (up to 150 mg/mL). We found that the CsPbBr3 colloid exhibits a light yield (∼21000 photons/MeV) higher than that of the commercially available Ce:LuAG single-crystal scintillator (∼18000 photons/MeV). Interestingly, we reveal that the energy transfer process inside those stacked thin and thick nanosheet solids is indeed responsible for their superb scintillation performance. Moreover, we will present a highly efficient energy transfer strategy between the interfaces of these CsPbBr3 perovskite nanosheets and thermally activated delayed fluorescence (TADF) to obtain an efficient and reabsorption-free organic X-ray imaging scintillator with excellent performance. More specifically, the fabricated nanocomposite scintillators exhibit a high X-ray imaging resolution of around 100 μm and a low detection limit of 38.7 nGy/s. This detection limit is about 142 times lower than a typical dose of X-ray medical imaging, making this composite an excellent candidate for X-ray radiography.
2.3-I2
Lead halide perovskites (LHP) are long-known crystalline materials with ABX3 general formula (where A=Cs+, MA:CH3NH3+ or FA:CH(NH2)2+, B=Pb2+ and X=Cl-,Br-,I-), characterized by a three-dimensional [PbX6]4- framework and a large A cation residing the cuboctahedra cavities. These materials in form of nanocrystals (NCs) are considered ideal candidates to be integrated in television displays and LEDs.[1] Due to the dynamic nature of the perovskite lattice, preventing the charge carriers from trapping, LHP NCs are highly tolerant to structural defects and surface states, which are considered benign with respect to their electronic and optical properties.[2]
The flexible nature of the perovskite framework, very prone to structural defectiveness, coupled with the reduced size of crystalline domains, makes these materials unsuitable for conventional crystallographic methods. At this purpose total scattering techniques based on the Debye Scattering Equation (DSE), have been established as effective methods for characterizing nanoscale materials and taking into account size-induced structural defects, emerging upon downsizing.[3] Through the DSE-based method developed by some of us,[4] starting from real space atomistic models, structural and microstructural information on NCs can be simultaneously derived within a unified approach, with all the well-known advantages associated to the use of reciprocal space methods.
In this talk, experimental and modelling aspects, related to the DSE approach and applied to provide atomic-to-nanometer scale insights on key nanoscale features of LHP NCs, will be presented.
A broad spectrum of structural and morphological features of LHP NCs, unveiled through a synergic combination of reciprocal space methods based on the DSE, will be analysed, from their peculiar defectiveness,[5] to faceting and surface termination,[6] to the formation of self-organized superstructures.
2.3-I3
Lead-halide perovskite nanocrystals (NCs) have emerged as attractive nano-building blocks for photovoltaics and optoelectronic devices. Optimization of perovskite NC-based devices relies on a better knowledge of the fundamental electronic and optical properties of the band-edge exciton, whose fine structure has long been debated. This talk will give an overview of our recent magneto-optical spectroscopic studies [1-5] revealing the entire excitonic fine structure and relaxation mechanisms in these materials, using a single-NC approach to get rid of the inhomogeneities in the NC morphologies and crystal structures. It will highlight the prominent role of the electron-hole exchange interaction in the order and splitting of the bright triplet and dark singlet exciton sublevels and discuss the effects of size, shape anisotropy and dielectric screening on the fine structure. The spectral and temporal manifestations of the thermal mixing between bright and dark excitons allows extracting the specific nature and strength of the exciton-phonon coupling, which sheds light on the remarkable photovoltaic properties of these materials and provides an explanation for their remarkably bright photoluminescence at low temperature although the ground exciton state is optically inactive. These findings make single perovskite NCs attractive for a potential use as quantum light sources.
- Fu, M ; Tamarat, P. ; Huang, H. ; Even, J. ; Rogach, A. L. ; Lounis, B. Neutral and charged exciton fine structure in single lead halide perovskite nanocrystals revealed by magneto-optical spectroscopy, Nano Letters (2017) 17, 2895-2901
10.1021/acs.nanolett.7b00064
- Fu, M ; Tamarat, P. ; Trebbia, J.-B. ; Bodnarchuk, M. I. ; Kovalenko, M. V. ; Even, J. ; Lounis, B. Unraveling exciton-phonon coupling in individual FAPbI3 nanocrystals emitting near-infrared single photons, Nature Communications (2018), 9, 3318
10.1038/s41467-018-05876-0
- Tamarat, P. ; Bodnarchuk, M. I. ; Trebbia, J.-B. ; Erni, R. ; Kovalenko, M. V. ; Even, J. ; Lounis, B. The ground exciton state of formamidinium lead bromide perovskite nanocrystals is a singlet dark state, Nature Materials (2019) 18, 717-724.
10.1038/s41563-019-0364-x
- Tamarat, P. ; Hou, L. ; Bodnarchuk, M. I. ; Trebbia, Swarnkar, A. ; Biadala, L. ; Louyer, Y. ; Bodnarchuk, M. I. ; Kovalenko, M. V. ; Even, J. ; Lounis, B. The dark exciton ground state promotes photon-pair emission in individual perovskite nanocrystals, Nature Communications (2020) 11, 6001.
10.1038/s41467-020-19740-7
- Hou, L. ; Tamarat, P. ; Lounis, B. Revealing the Exciton Fine Structure in Lead Halide Perovskite Nanocrystals, Nanomaterials (2021) 11, 1058.
10.3390/nano11041058
1.1-I1
Christoph J. Brabec is holding the chair “materials for electronics and energy technology (i-MEET)” at the materials science of the Friedrich Alexander University Erlangen-Nürnberg. Further, he is the scientific director of the Erlangen division of the Bavarian research institute for renewable energy (ZAE Bayern, Erlangen).
He received his PhD (1995) in physical chemistry from Linz university, joined the group of Prof Alan Heeger at UCSB for a sabbatical, and continued to work on all aspects of organic semiconductor spectroscopy as assistant professor at Linz university with Prof. Serdar Sariciftci. He joined the SIEMENS research labs as project leader for organic semiconductor devices in 2001 and joined Konarka in 2004, where he was holding the position of the CTO before joining university.
He is author and co-author of more than 150 papers and 200 patents and patent applications, and finished his habilitation in physical chemistry in 2003.
Perovskite photovoltaics based on metal halide perovskites is a most promising photovoltaic
technology, but further advance demands improved device stability. Here we show the importance of
perovskite composition engineering by demonstrating long-term stable perovskite solar cells. A high-
throughput robotic approach is used to screen 160 mixed-cation mixed-halide perovskites based on
several optical characterizations, such as UV-vis absorption and photoluminescence spectra. Such
automated big data approaches allow to uniquely identify the most photo-thermal-stable perovskites
under elevated temperature and 1-Sun illumination. Most interestingly, while several perovskite
compositions are found to be stable against high temperatures of up to 140 C and are capable of
passing the damp/heat test without being packaged, the situation is more complex in working devices,
as a stable device requires both - a stable semiconductor layer and stable interfacesi. A p-type interface
consisting of polymeric multi-layers with variable doping is introduced as a robust anode interfaceii.
By integrating the most stable perovskite into this structure, we achieve stable devices that attain
about 100% of their initial efficiency after > 1000 hrs of continuous mpp operation at low
temperatures of 30-40 degrees Celsius. Increasing the temperature to beyond 65 °C caused interface
corrosion, as clearly seen by the formation of s-shaped jV curves. Meticulous engineering of the
dopand / host interactions allowed to overcome this instability, and most recent devices pass up to
2000 hrs under 1 sun @ 65 C without notable degradationiii. Nevertheless, degradation is still
observable when the operation temperature is further raised towards 85 C and above, which
highlights that the microscopic degradation mechanisms in perovskite devices are still not fully
understood. This work introduces into the fundamentals how to accelerate the screening for stable
perovskites layer and devices for operation at elevated temperatures.
REFERENCES:
i Y. Zhang et al and C. J. Brabec , Nature Communication 2021.
ii Y. Hou et al and C. J. Brabec, Science 2017.
iii Y. Zhang et al and C. J. Brabec, Nature Energy 2021, in print.
1.1-I2
Giulia is Associate Professor at Physical Chemistry Unit at University of Pavia, leading the PVsquared2 team, and running the European Grant ERCStG Project “HYNANO”aiming at the development of advanced hybrid perovskites materials and innovative functional interfaces for efficient, cheap and stable photovoltaics. Within this field, Giulia contributed to reveal the fundamental lightinduced dynamical processes underlying the operation of such advanced optoelectronic devices whose understanding is paramount for a smart device development and for contributing to the transition of a green economy.
Giulia received an MS in Physical Engineering in 2008 and obtained her PhD in Physics cum laude in 2012 at the Politecnico of Milan. Her experimental thesis focused on the realisation of a new femtosecond-microscope for mapping the ultrafast phenomena at organic interfaces. During her PhD, she worked for one year at the Physics Department of Oxford University where she pioneered new concepts within polymer/oxide solar cell technology. From 2012-2015, she was a post-doctoral researcher at the Italian Institute of Technology in Milan. In 2015, she joined the Ecole Polytechnique Fédérale de Lausanne (EPFL) with a Co-Funded Marie Skłodowska-Curie Fellowship. From 2016 to 2019, she has been awarded by the Swiss Ambizione Energy Grant providing a platform to lead her independent research group at EPFL focused on the developemnt of new generation hybrid perovskite solar cells.
She is author of 90 peer-reviewed scientific papers bringing her h-index to 44 (>13’000 citations), focused on developement and understanding of the interface physics which governs the operation of new generation solar cells.
Recently, she received the USERN prize in Physical Science, the Swiss Physical Society Award in 2018 for Young Researcher and the IUPAP Young Scientist Prize in Optics. She is currently USERN Ambassador for Italy and board member of the Young Academy of Europe.
More can be found at https://pvsquared2.unipv.it.
Weblink: https://people.epfl.ch/giulia.grancini?lang=en
Engineering interfaces in perovskite solar cells is nowadays paramount in the optimization of multilayer perovskite device stack. This stem true for multi-dimensional (2D/3D) perovskite based solar cells, where high efficiency can be combined with promising device durability. However, the exact function of the 2D/3D interface in controlling the device behaviour and the interface physics therein are still vague.
Here I will discuss the 2D/3D functions which can simultaneously act as surface passivant, electron blocking layer, and driving efficient and selective charge extraction. In particular, I will demonstrate that the exact knowledge on the interface energetics is crucial to obtain for a smart interface engineering. As an example, I will discuss the case of thiophene-based 2D perovskite/ 3D perovskite interfaces forming a p-n junction. This leads to a reduction of the electron density at the hole transport layer interface and ultimately suppress the interfacial recombination. As a consequence, we demonstrate that photovoltaic devices with enhanced fill factor (FF) and open-circuit voltage (VOC) of 1.19V which approaches the potential internal Quasi-Fermi Level Splitting (QFLS) voltage of the perovskite absorber, nullifying the interfacial losses. We thus identify the essential parameters and energetic alignment scenario required for 2D/3D perovskite systems in order to surpass the current limitations of hybrid perovskite solar cell performances. This knowledge turns fundamental for device design, opening a new avenue for perovskite interface optimization. In addition, I will discuss the role of 2D cations versus 2D cation passivation to state the exact role of having a 2D perovskite layer on top if necessary – or maybe not – to push device performances.
1.1-I3
Aldo Di Carlo is Director of the Institute of Structure of Matter of the National Research Council and Full Professor of Optoelectronics and Nanoelectronics at the Department of Electronics Engineering of the University of ROme "Tor Vergata". His research focuses on the study and fabrication of electronic and optoelectronic devices, their analysis and their optimization. Di Carlo founded the Center for Hybrid and Organic Solar Cells (CHOSE) which nowadays involve more than40 researchers dealing with the development of III generation solar cells (DSC, OPV and Perovskite) and on scaling-up of these technologies for industrial applications. CHOSE has generated 6 spin-off companies and a public/private partnership. Di Carlo is author/coauthor of more than 500 scientific publications in international journals, 13 patents and has been involved in several EU projects (three as EU coordinator)
The halide perovskite photovoltaic technology can be scaled to large area modules and panels using printing processes and laser patterning. Here, we will present the progresses made to scale up from small area solar cells to modules and panels up to a dimension of 0.5 sqm. By working in controlled atmosphere (Glove Box with nitrogen) and apply conventional spin-coating technique it Is possible to easily scale up from 9 to 140 cm2 active area with efficiencies above 20% for the smallest modules and 14.7% for the largest. Specific efforts have been devoted to developing a deposition process out of the glove box (GB) in conventional ambient air. We transfer out of the GB several coating technologies, including blade coating and slot-die. To do this without penalizing efficiency and stability, a specific formulation of perovskite absorber and doping strategies of transporting layer have been formulated together with specific quencing techniques based on air, vacuum and solvents. These optimizations permitted to realized perovskite solar modules with an efficiency of > 17% on an active area of 43 cm2, keeping above 90% of the initial efficiency after 800 h thermal stress at 85 °C. One of the critical issues scaling the cell to module size is the control of interface properties. We demonstrated that tuning of interface properties can be successfully obtained by applying two-dimensional (2D) materials, such as graphene, functionalized MoS2, MXenes as well as 2D Perovskite. This permitted also to increase the stability of the cell (T80) well beyond 1000h under light soaking and thermal stress tests.
1.2-T1
The discovery of a family of semiconductor materials based on the complex halides of group 14 elements opened a big research arena and led to the emergence of a new perovskite photovoltaic technology. Impressive photovoltaic performances were demonstrated for perovskite solar cells based on lead halides, whereas their practical implementation is still severely impeded by the low device operational stability. Most importantly, complex lead halides were found sensitive to both light and heat, which are unavoidable satellites under the realistic solar cell operational conditions. Suppressing these intrinsic degradation pathways requires a thorough understanding of their mechanistic aspects. Herein, we explored the temperature effects in the light-induced decomposition of the model systems represented by MAPbI3 and PbI2 thin films under well-controlled anoxic conditions. We show that decreasing the sample temperature from 55 oC to 30 oC can extend the perovskite lifetime spectacularly by a factor of >10-100 and also alter the material decomposition pathway. The analysis of the aging kinetics revealed that MAPbI3 and PbI2 photolysis have quite high effective activation energies of ~85 and ~106 kJ mol-1, respectively, which explain the observed strong effect of the temperature on the rate of the material photodecomposition. These findings suggest that controlling the temperature of the perovskite solar panels might be a key factor for reaching their long operational lifetimes (>20 years) required for the practical implementation of this promising technology.
1.2-T2
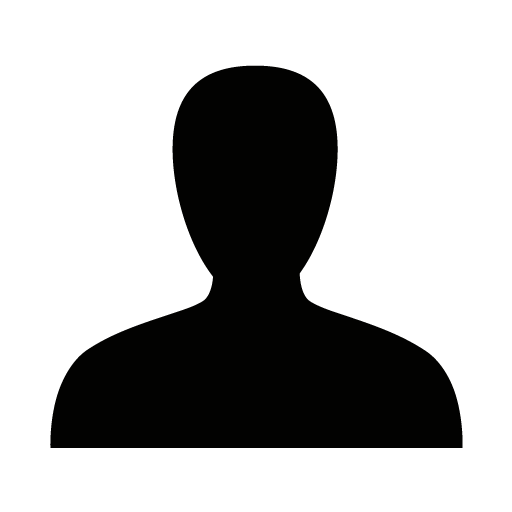
Despite the tremendously rapid development of perovskite photovoltaics (PV) in terms of power conversion efficiency (PCE) the stability of such devices typically still does not fulfill the requirements for commercialization of this technology. Up to date, PV devices with carbon-based back-electrodes (CBEs) have demonstrated the longest operational stability, surpassing several IEC 61215 standards [1]. Moreover, recently we reported that such modules could withstand requirements of the IEC hot-spot test (evaluating the stability of PV devices against reverse-bias degradation),[2] which is one of the most crucial hurdles not only for perovskite PV, but even for such well-established PV technology as c-Si. [3] Thus, devices with CBEs represent a promising method for up-scalable manufacturing of stable and low-cost perovskite PV devices.[4,5]
However, besides cost and stability, we must also consider greenhouse gas (GHG) emissions coming from PV manufacturing, which are projected to grow beyond national emissions of countries like France or Germany in the next 10 years.[6] Although the production of perovskite PV with CBEs has the potential to reach the lowest CO2-footprint limit possible for large scale PV applications,[4] one of the methods to reduce the GHG emissions of PV industry further is the development of effective recycling strategies, where perovskite materials offer unique advantage of liquid processing.
Up to now, only studies on the recyclability and life-cycle-assessments of non-encapsulated devices have been demonstrated. To show that perovskite PV devices with CBEs can not only be stable but also recyclable, we manufactured such solar cells and modules, encapsulated with thermoplastic polyolefin and polyisobutylene edge-seal, which provide sufficient protection against moisture, passing the IEC damp-heat test. [7] Through life-cycle assessment, we show that most of the negative environmental impact comes from the layer deposition, rather than the environmental footprint of material itself, making the re-use of as many layers as possible the most preferable option. We demonstrate an effective mechanochemical method to remove the edge-seal and encapsulant, as well as degraded perovskite and CBEs, leaving metal oxide layers intact making them available for re-use. Our novel recycling method of such devices results in minimal performance loss, which helps to reduce the negative environmental impact of such devices (global warming potential in kg CO2-eq./kWp) by > 40%. Such strong reduction in the global-warming potential of perovskite solar modules with CBEs qualifies them as truly sustainable PV technology meeting all requirements for its introduction to the PV market.
1.2-T3
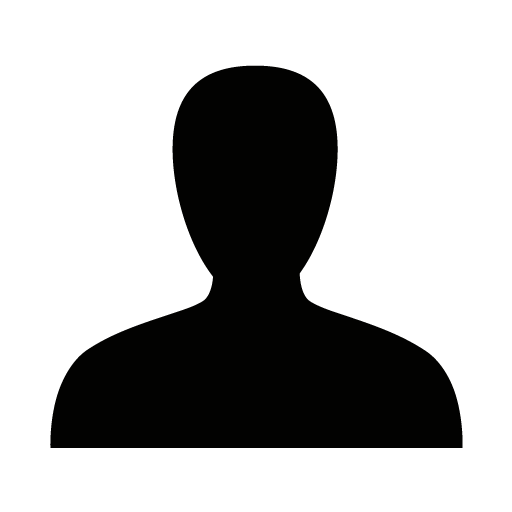
Organic-inorganic perovskites have the strong potential to replace the mainstream silicon photovoltaic technology due to their extensive research. The power conversion efficiency (PCE) of perovskite solar cells of just below 26% is advancing to that of silicon photovoltaics. The advancements make it a highly viable technology for broad applications especially building-integrated PVs and Tandems. Nevertheless, the efficiency of these cells is recorded on a small area. Scaling the small area cells into modules with minimal loss is a major challenge to be addressed. We will here, present our work on the optimization strategies of the semitransparent modules to achieve a power conversion efficiency slightly below 15% scaled from the semitransparent cell of 19.7%. The transparent electrode plays a major role in the performance of the semitransparent module. Our work addresses the challenges in reducing the loss in PCE between small area cells and modules.
1.3-I1
Dr. Md. K. Nazeeruddin received M.Sc. and Ph. D. in inorganic chemistry from Osmania University, Hyderabad, India. His current research focuses on Dye-sensitized solar cells, Hydrogen production, Light-emitting diodes and Chemical sensors. He has published more than 400 peer-reviewed papers, nine book chapters, and inventor of 49 patents. The high impact of his work has been recognized with invitations to speak at over 100 international conferences. He appeared in the ISI listing of most cited chemists, and has more than 10000 citations with an h-index of 93. He is directing, and managing several industrial, national, and European Union projects on Hydrogen energy, Photovoltaics (DSC), and Organic Light Emitting Diodes. He was awarded EPFL Excellence prize in 1998 and 2006, Brazilian FAPESP Fellowship in 1999, Japanese Government Science & Technology Agency Fellowship, in 1998, Government of India National Fellowship in 1987-1988. Recently he has been appointed as World Class University (WCU) professor for the period of March 1, 2009 ~ December 31, 2012 by the Korea University, Jochiwon, Korea.
Deposition of two-dimensional perovskite layers between perovskite absorber and hole-transporting layer is considered to be an essential strategy to reduce defects in state-of-the-art perovskite solar cells (PSCs). This strategy, however, suffers from the inevitable formation of in-plane favoured two-dimensional (2D) perovskite layers with impaired charge transport, especially under thermal conditions, impeding photovoltaic performance and device scale-up. Therefore, to overcome this limitation, we designed and investigated various cations that form 2D perovskite layers on top of the 3-dimensional perovskite layer. The ensuing PSCs achieve an efficiency of over 24% with long-term operational stability (over 1000 hours). Notably, a record efficiency of 23% for the perovskite module with an active area of 26 cm2 was achieved using compositionally engineered perovskite. In this talk, we will present strategies to enhance PSC's power conversion efficiency and stability.
1.3-I2
Prof. Mónica Lira-Cantú is Group Leader of the Nanostructured Materials for Photovoltaic Energy Group at the Catalan Institute of Nanoscience and Nanotechnology (www.icn.cat located in Barcelona (Spain). She obtained a Bachelor in Chemistry at the Monterrey Institute of Technology and Higher Education, ITESM Mexico (1992), obtained a Master and PhD in Materials Science at the Materials Science Institute of Barcelona (ICMAB) & Autonoma University of Barcelona (1995/1997) and completed a postdoctoral work under a contract with the company Schneider Electric/ICMAB (1998). From 1999 to 2001 she worked as Senior Staff Chemist at ExxonMobil Research & Engineering (formerly Mobil Technology Co) in New Jersey (USA) initiating a laboratory on energy related applications (fuel cells and membranes). She moved back to ICMAB in Barcelona, Spain in 2002. She received different awards/fellowships as a visiting scientist to the following laboratories: University of Oslo, Norway (2003), Riso National Laboratory, Denmark (2004/2005) and the Center for Advanced Science and Innovation, Japan (2006). In parallel to her duties as Group Leader at ICN2 (Spain), she is currently visiting scientist at the École Polytechnique Fédérale de Lausanne (EPFL, CH). Her research interests are the synthesis and application of nanostructured materials for Next-generation solar cells: Dye sensitized, hybrid, organic, all-oxide and perovskite solar cells. Monica Lira-Cantu has more than 85 published papers, 8 patents and 10 book chapters and 1 edited book (in preparation).
Halide perovskite solar cells (PSCs) have emerged as a competitive photovoltaic technology with power conversion efficiencies (PCEs) surpassing the 22 % mark. One of the main bottlenecks of the technology is their long-term stability. Understanding the different degradation mechanisms of the constituent materials, as well as interface instabilities, is of crucial importance for commercialization. Semiconductor oxides (SO) constitute a fundamental part of highly efficient photovoltaic technologies such as PSCs. Electron transport semiconductor oxides, like TiO2, are characterized by an oxygen vacancy (Ovac)-mediated conductivity caused by a deviation in stoichiometry, the presence of impurities, or both. In oxygen-containing atmospheres, and especially under UV light, holes generated at the nonstoichiometric oxide surface react with the oxygen adsorbed at an Ovac increasing charge recombination and degradation of the solar cell. Different methods have been employed to passivate or eliminate these Ovac. For example, the application of organic interfacial modifiers with anchoring groups specifically selected to bond with oxides, or the application of less reactive SnO2 which results in less hygroscopicity, fewer Ovac at its surface, and less UV-damage. Another possibility is the application of a coating of secondary oxides, like Al2O3, applied to supress surface defects, avoid interfacial recombination, and enhance device stability. A less-explored option is the application of complex oxides with singular properties, such as ferroelectric, multiferroic, magnetic, etc. In this talk, we report our most recent studies on the application of classic oxides (binary, doped, nanostructured) and complex oxide compounds (ternary, ferroelectric, etc.) as transport layers in Halide Perovskite Solar Cells. We will discuss their effect on the long-term stability of complete solar cell devices.
1.3-I3
Senol Öz obtained his diploma in chemistry in 2013 at the University of Cologne
(Germany). Completing his PhD under supervision of Prof. Sanjay Mathur in 2018 at
University of Cologne (Merck KGaA PhD scholarship). In 2019 he joined Prof.
Tsutomu Miyasaka`s group as a post-doctoral fellow at Toin University of Yokohama
under a JSPS scholarship. His research interests include the synthesis, chemical
engineering, and solution processing of inorganic-organic hybrid metal halide
perovskite materials for photovoltaic application. He is currently a senior R&D project
leader at Saule Technologies and managing director of Solaveni GmbH.
Since the emergence of perovskite photovoltaics in 2009 the scientific community has witnessed steep progress of the technology in terms of solar to electric power conversion efficiency 25.5% accompanied by advancement in addressing stability issues and developing a deeper understanding of fundamental structure-processing-property relationships.
The technology transfer from lab to fab has proven challenging, however merely a decade after discovery the commercialization of organic-inorganic metal halide perovskites in terms of photovoltaic devices is just around the corner.
Many issues had to be tackled to meet industrialization, among them large area deposition techniques, safety standards and material wastage without compromising efficiency and stability. Thus, a cost-effective, reliable fabrication process capable of delivering highly efficient and durable large-area perovskite modules is vital for success of the technology. Saule Technologies has been developing a fully scalable inkjet printing process of perovskite solar cell modules on lightweight flexible substrates. This talk will focus on recent advancements in the technology and the product development process required to bring inkjet printed perovskite modules closer to commercial utility. Our perovskite solar cell solutions offer high power density at low light intensity conditions and at the same time exhibit long lifetime to guarantee autonomous operation for IoT devices far beyond typical battery lifetime. It has been a long but exciting way from the first laboratory samples to the first commercial perovskite solar cell production line. The first applications of hybrid perovskite solar cells will include powering IoT devices and many more will follow soon.
2.1-I1
Tsutomu (Tom) Miyasaka received his Doctor of Engineering from The University of Tokyo in 1981. He joined Fuji Photo Film, Co., conducting R&Ds on high sensitivity photographic materials, lithium-ion secondary batteries, and design of an artificial photoreceptor, all of which relate to electrochemistry and photochemistry. In 2001, he moved to Toin University of Yokohama (TUY), Japan, as professor in Graduate School of Engineering to continue photoelectrochemistry. In 2006 to 2009 he was the dean of the Graduate School. In 2004 he has established a TUY-based company, Peccell Technologies, serving as CEO. In 2005 to 2010 he served as a guest professor at The University of Tokyo.
His research has been focused to light to electric energy conversion involving photochemical processes by enhancing rectified charge transfer at photo-functional interfaces of semiconductor electrodes. He has contributed to the design of low-temperature solution-printing process for fabrication of dye-sensitized solar cells and solid-state hybrid photovoltaic (PV) cells. Since the discovery of the organic inorganic hybrid perovskite as PV material in 2006 and fabrication of high efficiency PV device in 2012, his research has moved to R&Ds of the lead halide perovskite PV device. He has promoted the research field of perovskite photovoltaics by organizing international conferences and by publishing many papers on enhancement of PV efficiency and durability, overall citation number of which is reaching more than 5,000 times. In 2009 he was awarded a Ministry of Science & Education prize on his achievements of green sustainable solar cell technology. In 2017 he received Chemical Society of Japan (CSJ) Award. He is presently directing national research projects funded by Japan Science and Technology Agency (JST) and Japan Aerospace Exploration Agency (JAXA).
Perovskite solar cells are still improving their high performance in J-V characteristics in various compositions of perovskites including all inorganic materials. The most important element in the J-V performance is open-circuit voltage (Voc) which reflects suppression of charge recombination losses at the interfaces. We achieved high levels of Voc for all inorganic CsPbI2Br (bandgap 1.9eV) with 1.42 V 1 and for mixed cation Cs-FA-MAPb(I,Br)3 perovskite cells (bandgap 1.51eV) with 1.19V.2 The CsPbI2Br device was fabricated by using amorphous SnOx layer that passivate the perovskite and meso-SnO2 interface, and enables Voc exceeding 1.1V even under week indoor illumination (200 lx) with power conversion efficiency (PCE) >34%. The mixed cation perovskite cells, working with conversion efficiency >22%, shows Voc close to its SQ limit (ca.1.21V) as a result of interfacial modification with phenyethylamine bromide as a dipole-inducing layer. 2 These works show that the successful passivation of the junction interfaces is essential for improving Voc toward application of perovskite photovoltaic cells to circumstances of large light intensity variation. Technologies based on interfacial molecular engineering are expected to improve the device performance of lightweight flexible plastic devices. We employed an organic peroxide, artemisinin (water-insoluble anti-malarial drug), to modify the interface of SnO2 ETL and multi-cation perovskite (Rb-Cs-FA-MA)Pb(I,Br)3).3 This redox-active molecule is considered to interreact with the oxide surface and lead cation. The artemisinin-based passivation was applied to the SnO2-perovskite interface of a plastic film flexible device. The device showed decreased hygroscopicity due to the effect of artemisinin, leading to an increase in shelf life. Its effects of enhancing Voc improved the device efficiency up to 21%. Organic passivators should be chemically and thermally stable non-ionic materials and inactive against their diffusion. Our challenges to design durable passivation materials for Voc improvement are underway.
2.1-I2
Ion migration is a well-known problem in perovskite materials. It causes baseline drift, lowers imaging resolution, accelerated decomposition and device performance degradation. In particular in X-ray detectors, the effect of ion migration is more obvious under working bias. The first principals study reveals that the 0D structure perovskite would show effectively reduced ion migration between neighbouring unit cells compared with the popular 2D and 3D perovskites. A nucleation-controlled strategy is developed to grow superior inch-sized high-quality 0D-structured lead-free (CH3NH3)3Bi2I9 perovskite single crystals (MA3Bi2I9 PSCs) with significantly lower ion migration, much reduced dark current and better environmental stability compared to other perovskite materials, enabling us to design and fabricate a new type of 0D-structured lead-free perovskite X-ray detector. It is found that the X-ray detectors show surprisingly high sensitivity, 15 times more than that of the state-of-the-art commercial α-Se detectors, with very low detection limit that is desired for medical diagnostics, material inspection, etc. Furthermore, their response time is as short as 0.98 ms, the shortest among all X-ray detectors reported in literature, which may allow us to develop an X-ray screening system with reduced X-ray dose and improved resolution.
2.1-I3
Since 2019, Yana Vaynzof holds the Chair for Emerging Electronic Technologies at the Technical University of Dresden. Prior to that (2014-2019), she was a juniorprofessor in the Department of Physics and Astronomy, Heidelberg University (Germany). She received a B.Sc degree (summa cum laude) in electrical engineering from the Technion - Israel Institute of Technology (Israel) in 2006, and a M.Sc. degree in electrical engineering from Princeton University, (USA) in 2008. She pursued a Ph.D. degree in physics under the supervision of Prof. Sir. Richard Friend at the Optoelectronics Group, Cavendish Laboratory, University of Cambridge (UK), and investigated the development of hybrid polymer solar cells and the improvement of their efficiency and stability. Upon completing her PhD in 2011, she joined the Microelectronics group at the University of Cambridge as a Postdoctoral Research Associate focusing on the research of surfaces and interfaces in organic and hybrid optoelectronics. Yana Vaynzof was the recipient of a number of fellowships and awards, including the ERC Starting Grant, Gordon Y. Wu Fellowship, Henry Kressel Fellowship, Fulbright-Cottrell Award and the Walter Kalkhof-Rose Memorial Prize.
The emergence of metal halide perovskites has revolutionized the field of emerging photovoltaics. Typically, the active layers of perovskite solar cells are deposited either from solution, or alternatively by thermal evaporation. In this talk, I will describe how the two methods can be combined to fabricate highly efficient all-inorganic CsPbI3 perovskite solar cells. Specifically, half of the active layer is deposited by solution processing, following by thermally evaporating the second half. While devices fabricated by each method separately show a reasonable performance of 13-15%, the combination of the two methods leads to perovskite solar cells with improved open-circuit voltage, short-circuit current and fill factor, leading to a maximum photovoltaic performance >20%. Moreover, devices fabricated by this hybrid approach exhibit a significantly reduced hysteresis. We show that the improvement in performance and decrease in hysteresis are associated with a reduced density of defects in the active layers fabricated by the hybrid approach, thus demonstrating its high potential for the fabrication of efficient perovskite solar cells.
2.2-T1
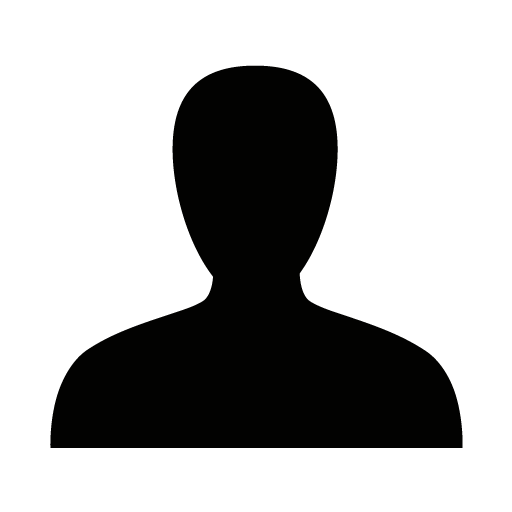
Hybrid organic-inorganic perovskites are highly promising absorber materials for third generation photovoltaic technologies. These materials have low production costs and enable solar cell efficiencies comparable to the best crystalline silicon PV cells [1]. The main obstacle for their widespread practical implementation is the low operational stability defined by both external and internal factors. External factors such as the action of oxygen and moisture from the atmosphere can be suppressed by encapsulating the photoactive layer. Intrinsic factors causing degradation of the materials include thermal and photochemical aging effects. In particular, the light-induced decomposition of MAPbI3 leads to the formation of metallic lead and molecular iodine due to photolysis of PbI2 [2].
Although the degradation mechanisms are usually considered as a bulk property, they are more likely to occur at the scale of individual grains and between the boundaries. Along with this, infrared scattering scanning near-field microscopy (IR s-SNOM) techniques are being actively developed to visualize the cation dynamics in lead halide perovskites [3]. Since changes in the concentration and localization of organic cations have a strong influence on the material optoelectronic characteristics and the solar cells performance, the IR s-SNOM provides great opportunities for experimental investigation of the photochemical degradation processes in these materials with nanoscale spatial resolution. Herein, we applied IR s-SNOM technique for the first time to follow the nanoscale dynamics of the light-induced decomposition of MAPbI3 films under well-controlled anoxic conditions inside the glove box.
The obtained results revealed that the light-induced aging of the MAPbI3 films has a spatially heterogeneous character. The perovskite decomposition is started at the grain boundaries and is accompanied by the formation of core-shell structures, where the perovskite grains are covered with a “skin” of PbI2, which is the MAPbI3 main aging product. It is shown that lead iodide does not decompose further into metallic lead and molecular iodine under the used experimental conditions (low temperature, LED light). On the contrary, PbI2 recrystallization with the formation of big flat crystallites is observed.
To summarize, we demonstrated a high potential of using IR s-SNOM as a method to study the photodegradation of perovskite films with nanoscale local spatial resolution. The application of this technique in combination with other complementary methods represents a promising approach for identifying the most stable absorber material compositions.
2.2-T2
Long-term operational stability is a prerequisite for the commercialization of perovskite solar cells. Inorganic perovskite solar cells exhibit a high thermal stability and efficiencies of 20.8 %, which corresponds to 72 % of the Shockley-Queisser limit for the band gap of 1.72 eV [1]. However, the reported PCE is still lower than for organic-inorganic perovskite solar cells, mainly due to lower open-circuit voltages (VOC). The VOC of the record inorganic perovskite solar cell with CsPbI3-xBrx composition and 1.72 eV band gap is 1.23 V, while organic-inorganic cells of the same band gap reach a VOC of 1.31 V [2].
This study investigates state-of-the-art inorganic CsPbI2Br perovskite solar cells to reveal the efficiency potential and the losses induced by each layer. This is achieved by measuring intensity-dependent photoluminescence (PL) of each layer stack. This technique enables us to construct potential JV curves, revealing not only the potential VOC, but also the potential fill factor [3]. Based on realistic assumptions of the photocurrent, we can therefore calculate the efficiency potential of the solar cell or stack which could be reached by avoiding any transport losses.
For the present study, CsPbI2Br solar cells in n-i-p and p-i-n configuration are fabricated using an air-annealing procedure specifically developed for this composition. The CsPbI2Br films have an efficiency potential of 21.6 % when deposited on glass, 20.7 % on MeO-2PACz and 19.9 % on mesoporous TiO2, suggesting a very low defect density in the CsPbI2Br perovskite.
In the n-i-p CsPbI2Br solar cells, losses in QFLS are caused in almost equal parts by the electron transport layer (ETL) and hole transport layer (HTL). In the p-i-n solar cells, the main part of the losses is caused by the ETL. Importantly, both configurations show a strong mismatch between the quasi Fermi level splitting (QFLS) in the perovskite layer and the measured VOC at the contacts. The inorganic p-i-n solar cell has a QFLS-e∙VOC mismatch of 170 meV. The organic-inorganic reference solar cell with the same band gap shows a QFLS- e∙VOC mismatch of only 10 meV. Using ultraviolet photoelectron spectroscopy (UPS) measurements, we examine possible reasons for this striking difference and discuss potential solutions.
Overall, this study helps to identify the most promising layer stacks for inorganic n-i-p and p-i-n perovskite solar cells and reveals the contribution of each interface to the voltage and fill factor losses.
2.2-T3
APbX3 lead perovskites, where A is either an organic (methylammonium MA+ and formamidinium FA+) or an inorganic (Cs+) species, are a highly promising photovoltaic materials, due to the exceptionally high power conversion efficiency (> 25%) demonstrated recently in the case of mixed-cation and mixed-halide perovskites. However, the poor intrinsic stability of complex lead halides remains a major hindrance in the commercialization of this emerging photovoltaic technology. Experimentally it is known, and has been demonstrated by our group that bromide-containing mixed halide perovskites have much lower photostability when compared to the equivalent iodide-based materials. The light-induced photochemical aging produces metallic lead as one of the final decomposition products in the case of all the experimentally studied complex lead halides (MA0.15FA0.85PbI2.55Br0.45, Cs0.1MA0.15FA0.75PbI2.55Br0.45, Cs0.15FA0.85PbI2.55Br0.45, MA0.15FA0.85PbI3, Cs0.1MA0.15FA0.75PbI3 ) except for Cs0.15FA0.85PbI3, which demonstrated outstanding stability under white light exposure. To check the effect of the halide substitution our theoretical calculations compared FAPbI3 with the bromide-containing mixed-halide perovskite FAPbBr0.45I2.55 and indicated that hole-coupling drives the formation of interstitial-vacancy halide pair defects to become more thermodynamically favorable, thus leading to the accelerated degradation of the halide-mixed perovskites. It is notable that oxidation of this type is possible also without illumination; however, our calculations demonstrate that illumination greatly promotes it. Our calculations also showed the relative insensitivity of the halide vacancy towards the composition of the perovskite.
2.2-T4
Perovskite solar cells (PSCs) have achieved tremendous progress in terms of power conversion efficiencies (PCE) during the last decade which features a high commercialization potential of this PV technology. To achieve high PCE, significant efforts have been made to design and investigate new hole-transport layer (HTL) materials. Along with the spiro-OMeTAD, polytriarylamines (PTAAs) represent one of the most commonly used families of HTL materials [1]. However, even the most promising HTL materials delivering high PCEs will not bring PSCs towards commercialization if their high cost is not taken into consideration [2]. Unfortunately, both spiro-OMeTAD and PTAA are prohibitively expensive materials with the current price of ~500 and ~2000 $/g respectively [3].
Spiro-OMeTAD is expensive due to its multistep synthesis that requires low temperature, sensitive and aggressive reagents, and costly sublimation purification. The high cost of PTAA is driven by both complexity of the utilized synthetic methods, which often require several synthetic steps, and the high cost of metal-based catalysts, including noble metal complexes. For example, Pd-catalyzed Suzuki reaction has been actively utilized as the method of polycondensation of triarylamines with halogen and boron-based functional groups [4]. Unstable and pricey Ni(COD)2 has also been utilized for the polymerization of brominated triarylamines. Although the oxidative polymerization of arylamines has been actively utilized for the synthesis of materials for electroluminescent devices [5], the application of this approach for the synthesis of HTL materials for perovskite solar cells remains still unexplored.
Herein, we report a straightforward synthesis of a series of polytriarylamines using an efficient and cheap FeCl3-assisted oxidative polymerization. This simple yet efficient synthetic approach allowed us to obtain a series of polytriarylamines. These polymers outperformed commercial PTAA, which was utilized as a reference, when used as HTL materials in MAPbI3-based n-i-p perovskite solar cells. In particular, reproducible PCEs of >18% were reached for ITO|SnO2|PCBA|MAPbI3|HTL|VOx|Ag device configuration.
This work was supported by Russian Science Foundation (project 19-73-30020).
2.3-I1
Dr. Annalisa Bruno is an Associate Professor Nanyang Technological University (ERI@N), coordinating a team working on perovskite solar cells and modules by thermal evaporation. Annalisa is also a tenured Scientist at the Italian National Agency for New Technologies, Energy, and Sustainable Economic Development (ENEA). Previously, Annalisa was a Post-Doctoral Research Associate at Imperial College London. Annalisa received her B.S., M.S., and Ph.D. Degrees in Physics from the University of Naples Federico II. Her research interests include perovskite light-harvesting and charge generation properties and their implementation in solar cells and optoelectronic devices.
Metal-halide perovskites have recently emerged as one of the most promising low-cost promising materials for photovoltaic and light-emitting technologies, due to their excellent optoelectronic properties and fabrication versatility. Since the advent of the first perovskite solar cells (PSCs) in 2009, their power conversion efficiency (PCE) has now reached 25.6% [1], for active areas smaller than 1 cm2. Moreover, their operational stability is also constantly improving [2-3]. The interest in transferring the existing technology into large-area perovskite modules using industrial compatible techniques, necessary for industrial development is exploding.
Lately, we have demonstrated highly efficient, large area, planar PSCs where the MAPbI3 perovskite layer has been deposited by thermal co-evaporation of PbI2 and MAI. The high-quality co-evaporated perovskite thin films are uniform over large areas showing low surface roughness, and a long carrier lifetime. The high-quality perovskite thin films together with vacuum processed charge transport layers PSCs with PCE above 20% in both n.i.p [4, 5] and p.i.n [6] configurations. The co-evaporated MAPbI3 guarantee an impressive thermal and environmental stability maintaining over ≈95% and ≈80% of their initial PCE after 1000 and 3600 h respectively under continuous thermal aging at 85 °C without encapsulation. TE_MAPbI3 PSCs demonstrate remarkable structural robustness, absence of pinholes, or significant variation in grain sizes, and intact interfaces with the HTM, upon prolonged thermal aging.
The first co-evaporated mini-modules achieved record PCEs of 18.13% and 18.4% for active areas of 20 cm2 [7] and 6.4 cm2 [8].
Moreover, looking forward to tandem integration and building-integrated photovoltaics we have also developed coloured semi-transparent PSCs and mini-modules for all the range of colours realized.
These results represent a significant step towards the development of high-quality large-area perovskite solar cells and mini-modules, one of the main requirements for the commercialization of the technology.
2.3-I2
Sjoerd Veenstra - Program Manager Perovskite Solar Cells and Modules at TNO, partner in Solliance.
Sjoerd has a passion for photovoltaics (PV). He received his PhD from the University of Groningen (2002). Sjoerd stayed at UCSB (intern) and Cornell University (visiting scientist). He started as a researcher working on organic solar cells at the Energy research Center of the Netherlands (ECN, 2002). In 2011 he moved to Eindhoven (NL) when ECN joined the thin film PV activities of Solliance. He started working on perovskite solar cells in 2014. In 2018 ECN and TNO merged and since he works for TNO and leads the perovskite team.
Mixed metal-halide perovskite solar cells (PSC) are advancing in power conversion efficiency (PCE) faster than any other photovoltaic technologies, reaching over 25% [1]. However, the reported record efficiencies are obtained with lab-scale devices. Promoting this technology to the market level with efficient and stable devices manufactured with cost-effective, industrially scalable processes is the persisting challenge. Here we report on bifacial PIN perovskite photovoltaic modules processed on glass, passing the 1000 hour damp-heat test by retaining 94% of its initial PCE. The same device stack was transferred to a flexible substrate. This resulted in the first flexible, bifacial PIN modules with a state of the art efficiency and passing the 1000 hour damp-heat test by retaining 100% of its initial PCE.
Figure 1: Image of the flexible, semi-transparent bifacial PIN perovskite module with monolithic series laser interconnection.
REFERENCES:
[1] https://www.nrel.gov/pv/assets/pdfs/pv-efficiency-chart.20200203
2.3-I3
Sam Stranks is Professor of Energy Materials Optoelectronics in the Department of Chemical Engineering & Biotechnology and the Cavendish Laboratory, University of Cambridge. He obtained his DPhil (PhD) from the University of Oxford in 2012. From 2012-2014, he was a Junior Research Fellow at Worcester College Oxford and from 2014-2016 a Marie Curie Fellow at the Massachusetts Institute of Technology. He established his research group in 2017, with a focus on the optical and electronic properties of emerging semiconductors for low-cost electronics applications.
Sam received the 2016 IUPAP Young Scientist in Semiconductor Physics Prize, the 2017 Early Career Prize from the European Physical Society, the 2018 Henry Moseley Award and Medal from the Institute of Physics, the 2019 Marlow Award from the Royal Society of Chemistry, the 2021 IEEE Stuart Wenham Award and the 2021 Philip Leverhulme Prize in Physics. Sam is also a co-founder of Swift Solar, a startup developing lightweight perovskite PV panels, and an Associate Editor at Science Advances.
Halide perovskite solar cells are on the brink of commercialisation with exciting efficiency trajectories for both single junction and tandem configurations. Critical to their commercial success is demonstration of long-term stability, yet our fundamental understanding of failure modes is still lacking. Here, I will show our recent work investigating how nanoscale heterogeneity plays a key role in performance and stability [1]. I will show how nanoscale phase impurities, even at trace levels, lead to carrier traps [2] and critical photo-instabilities in devices. Finally, I will show how phase engineering will be essential to remove these problematic inclusions, ultimately leading to extremely phase and operational stable halide perovskite absorbers and devices.
1.1-I1
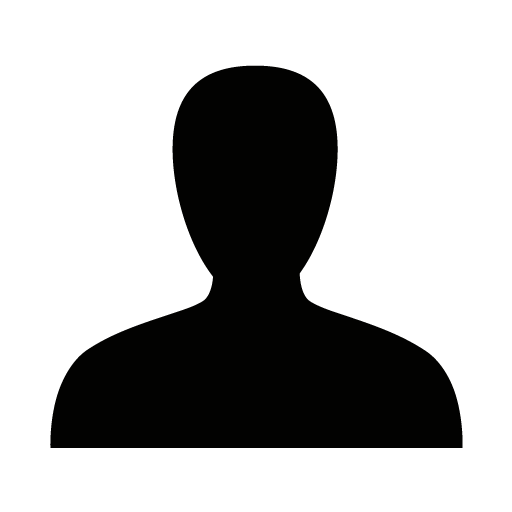
Deterministic GHz-rate single photon sources at room-temperature would be essential components for various quantum applications. However, both the slow intrinsic decay rate and the omnidirectional emission of typical quantum emitters are two obstacles towards achieving such a goal which are hard to overcome simultaneously. We solve this challenge by a hybrid approach, using a complex monolithic photonic resonator constructed of a gold nanocone responsible for the rate enhancement, enclosed by a circular Bragg antenna for emission directionality [1,2]. A repeatable process accurately binds colloidal quantum dots to the tip of the antenna-embedded nanocone. As a result we achieve simultaneous 20-fold emission rate enhancement and record-high directionality leading to an increase in the observed brightness by a factor as large as 450 (80) into an NA = 0.22 (0.5). We project that these miniaturised on-chip devices can reach photon rates approaching 1.4´108 single photons/second thus enabling ultrafast light-matter interfaces for quantum technologies at ambient conditions.
We also demostrate a significant progress towards a practical plug-and-play single photon source using giant colloidal quantum dots that show blinkingless emission which is stable over hours, on an antenna device which allows back optical pumping of the quantum dots and a front directional single photon emission with high single photon and an almost unity colllection efficiency [3,4].
Keywords: Single photon sources, quantum dots, quantum optics, quantum information, plasmonics, colloidal nanocrystals
References:
[1] Hamza Abudayyeh and Ronen Rapaport, Quantum Sci. Technol. 2 034004 (2017)
[2] Abudayyeh et al., ACS Nano 15, 11, 17384 (2021)
[3] Abudayyeh, et al., APL Photonics 6, 036109 (2021)
[4] Abudayyeh, et al., ACS Photonics 6, 446 (2019)
1.1-I2
Lead-halide perovskite APbX3 (A=Cs or organic cation; X=Cl, Br, I) quantum dots (QDs) are subject of intense research due to their exceptional properties as both classical1 and quantum light sources.2-4 Here, we report a comprehensive investigation of the room temperature single QD optical properties. The results reveal the origin of the QD homogeneous PL linewidths, and the peculiar size-dependent exciton photoluminescence line broadening and the exciton and multi-excitons recombination dynamics. Experimental results are corroborated by ab-initio molecular dynamics.
Such findings guide the further design of robust single photon sources operating at room temperature.
References
[1] Akkerman et al., Genesis, challenges and opportunities for colloidal lead halide perovskite nanocrystals. Nat. Mater. 17, 394–405 (2018).
[2] Becker et al., Bright triplet excitons in caesium lead halide perovskites. Nature 553, 189–193 (2018).
[3] Rainò et al., Superfluorescence from lead halide perovskite quantum dot superlattices. Nature 563, 671–675 (2018).
[4] Utzat et al., Coherent single-photon emission from colloidal lead halide perovskite quantum dots. Science 363, 1068–1072 (2019).
1.1-I3
Multiply-excited states in semiconductor nanocrystals feature intriguing physics and play a crucial role in nanocrystal-based technologies. While photo-luminescence provides a natural probe to investigate these states, room temperature single-particle spectroscopy of their emission has so far proved elusive due to the temporal and spectral overlap with emission from the singly excited and charged states. In this work, we perform heralded spectroscopy of single quantum dots by incorporating the rapidly developing technology of single-photon avalanche diode arrays in a spectrometer setup. This allows us to directly observe the biexciton-exciton emission cascade and to measure the biexciton binding energy of single nanocrystals at room temperature, even though it is well below the scale of thermal broadening of the transitions due to finite temperature and that of spectral diffusion, the shift of the transition energy due to fluctuating electric fields. Single-particle heralded spectroscopy enables us to identify correlations of the biexciton binding energy with both charge-carrier confinement and fluctuations of the local electrostatic potential, which are masked in ensemble measurements, and to overcome artifacts due to inhomogeneous broadening [1]. Time-resolved spectrometry, as demonstrated here, has the potential of greatly extending our understanding of charge carrier dynamics in multielectron systems and of parallelization of quantum optics protocols.
1.2-T1
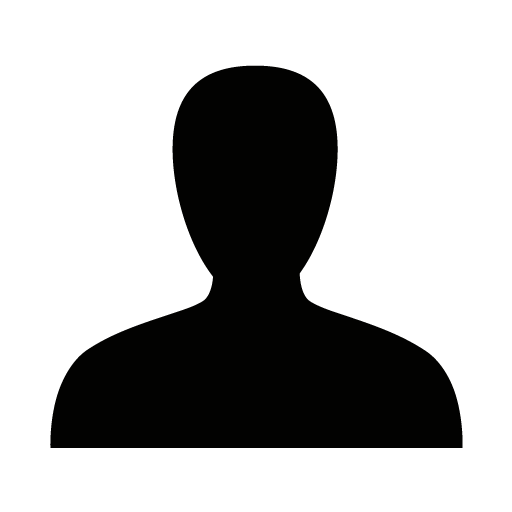
Periodic arrays of nanoholes are patterned by electron beam lithography (EBL) upon a 170 µm thick glass coverslip, after the deposition of a sputter coated Indium Tin Oxide (ITO) and a spin coated polymethyl methacrylate (PMMA) electronic resist thin films. Colloidal semiconductor giant core/shell CdSe/CdS nanocrystals, shielded by an additional silica layer, are then trapped with remarkable efficiency into the PMMA holes by a capillary assembly method based on a finely tuned drop casting process. The resulting planar solid state arrays of semiconductor quantum dots demonstrate single photon emission properties, studied in detail by photoluminesce and second order photon correlation measurements.
1.2-T2
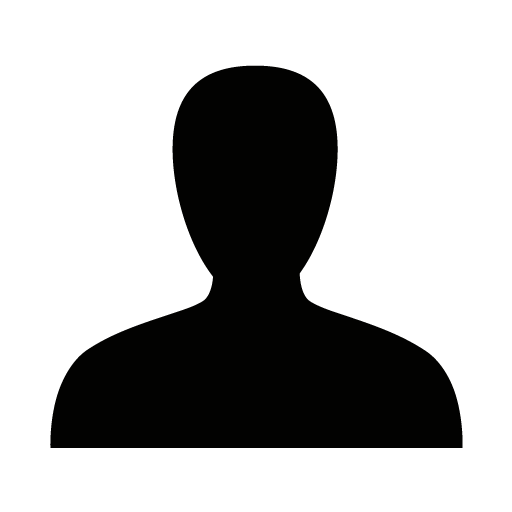
Multiply-excited states in semiconductor nanocrystals (NCs) exhibit intriguing physics and are key in nanocrystal-based quantum technologies. While simultaneous multiple photon emission is typically quenched in quantum dots, in nanoplatelets (NPLs) its probability can be tuned according to size and shape. In particular, multiple excitons strongly bound in just one dimension are free to re-arrange in the lateral plane, reducing the probability for multi-body collisions. In this work, we analyze multi-exciton dynamics in two-dimensional CdSe/CdS core/shell NPLs of various sizes through the measurement of second-, third-, and fourth-order photon correlations.1 Thus, for the first time, we could directly probe the dynamics of the two, three, and four exciton states at the single NC level. Remarkably, although higher orders of correlation vary substantially among a NPLs sample, they strongly correlate with the value of second-order antibunching. The scaling of the higher-order moments with the degree of antibunching presents a small yet clear deviation from the accepted model of Auger recombination through binary collisions. This deviation suggests that many-body contributions are present already at the level of tri-excitons.
In addition, multi-exciton interactions can be visually expressed in the emission patterns of different excitonic states. Type-II ZnSe/CdS dot-in-rod NCs, presenting a dipole radiation pattern due to anisotropy, can be used to demonstrate this phenomenon. A defocused imaging setup coupled to a novel single-photon avalanche detector (SPAD) array containing 23 pixels makes it possible to directly observe and analyze the out-of-focus dipole emission patterns of single excitons and bi-excitons in a single NC, allowing a deeper understanding of the underlying multi-exciton dynamics in semiconductor NCs.
1.2-T3
Colloidal Quantum Dot (CQD) Molecules represents a new class of artificial molecules where the electron wavefunction in two neighboring CQD artificial atoms hybridizes within the connected nanocrystals. Like naturally occurring molecules, the electronic coupling strength is expected to manifest widely varied properties in CQD molecules, altering not only single-particle characteristics but also complex many-body interactions in CQDs. Herein, we discuss in detail the signatures of single CQD molecules at different coupling strengths, as reflected on their emitted photon statistics at room temperature. The neck diameter in CdSe/CdS core/shell CQD homodimer molecules allowed to tune the hybridization of the electron wavefunction at a fixed center-to-center distance. At small neck diameter, the electronic between two CQDs are weak, which increases progressively with neck filling. We show that the photophysical properties of CQD molecules at weak coupling regime are governed by strong localization of electron wavefunction at individual CQD atoms, whereas in the strong coupling regime, due to facile delocalization the CQD molecules resemble the properties of single artificial atoms. A radiative multi-exciton configuration is preferred in the photo-excited weakly coupled molecules mostly governed by dipole-interactions, leading to strong photon bunching. In the strong coupling regime, electron tunneling activates a unique Auger process leading to single-photon emission via dissociation of biexciton, from a system consisting of two CQDs. This study validates the analogy of CQD molecules with the bonding nature of naturally occurring molecules that exhibit distinct ionic and covalent types of bonding in different coupling regimes, manifesting different properties. A detailed spectroscopic signatures following the time-tagged-time-resolved data will be discussed. The demonstration of multitude opto-electronic configuration tuning the extent of hybridization brands the CQD molecules as novel building blocks for many applications such as in low-threshold lasing and single-photon emitter in quantum applications.
1.3-I1
The spectrum of multiexciton emission from colloidal quantum dots at room temperature is important for their use in high-power applications, but an in-depth characterization has not been possible until now. We present and apply a novel spectroscopic method to quantify the biexciton linewidth and biexciton binding energy of single CdSe/CdS/ZnS colloidal QDs. In our method, we select photons emitted by a biexciton emission cascade and reconstruct their spectrum. We find a biexciton linewidth of 86 meV on the single-QD scale, similar to that of the exciton. Variations in the biexciton energy are correlated with but are more narrowly distributed than variations in the exciton energy. Using a simple quantum-mechanical model, we conclude that inhomogeneous broadening in our sample is primarily due to variations in the CdS shell thickness.
1.3-I2
Jennifer A. Hollingsworth is a Los Alamos National Laboratory (LANL) Fellow and Fellow of the American Physical Society, Division of Materials Physics, and The American Association for the Advancement of Science. She currently serves as Councilor for the Amercan Chemical Society Colloid & Surface Chemistry Division. She holds a BA in Chemistry from Grinnell College (Phi Beta Kappa) and a PhD degree in Inorganic Chemistry from Washington University in St. Louis. She joined LANL as a Director’s Postdoctoral Fellow in 1999, becoming a staff scientist in 2001. In 2013, she was awarded a LANL Fellows’ Prize for Research for her discovery and elaboration of non-blinking “giant” quantum dots (gQDs). In her role as staff scientist in the Center for Integrated Nanotechnologies (CINT; http://www.lanl.gov/expertise/profiles/view/jennifer-hollingsworth), a US DOE Nanoscale Science Research Center and User Facility, she endeavors to advance fundamental knowledge of optically active nanomaterials, targeting the elucidation of synthesis-nanostructure-properties correlations toward the rational design of novel functional materials. Her gQD design has been extended to multiple QD and other nanostructure systems, and several are being explored for applications from ultra-stable molecular probes for advanced single-particle tracking to solid-state lighting and single-photon generation. A recent focus of her group is to advance scanning probe nanolithography for precision placement of single nanocrystals into metasurfaces and plasmonic antennas.
Recent and nearly simultaneous developments in the fields of nanocrystal semiconductors, nanoplasmonics and nanophotonics may conspire to address deficiencies of available single-photon sources (SPSs). Namely, SPSs may now be accessible that operate in the telecommunications bands at room temperature, that are bright, and that can be easily integrated into quantum-advantaged communications, networking and sensing technologies. I will discuss our efforts to understand, design and synthesize heterostructured colloidal semiconductor quantum dots (QDs) that can meet the rigorous requirements for single-photons-on-demand in the infrared (IR) and at room-temperature.1-4 In general, QD emitters, especially those that emit in the infrared, are slow to cycle photons. In order to dramatically enhance the rate of spontaneous emission, such emitters can be combined with nanoscale plasmonic materials or antennas. Traditionally, nanoscale noble metals such as gold and silver have been used to achieve the targeted enhancements in light-matter interactions that result from the presence of localized surface plasmons (LSPs). However, interest has recently shifted to intrinsically doped semiconductor nanocrystals (NCs) for their ability to display LSP resonances (LSPRs) over a much broader spectral range, including the IR. Among semiconducting plasmonic NCs, spinel metal oxides (sp-MOs) are an emerging material with distinct advantages in accessing the telecommunications bands in the IR and affording useful environmental stability. Here, I will discuss the plasmonic properties of several sp-MO NCs, known previously only for their magnetic functionality, and demonstrate their ability to modify the light-emission properties of telecom-emitting QDs, achieving Purcell enhancement factors up to ~50-fold for telecom-emitting QDs in simple plasmonic-spacer-emitter sandwich structures or significantly higher radiative rate enhancement aided by more sophisticated plasmonic nanoantenna architectures. Finally, in collaborative work,5 we have demonstrated prospects for on-chip integration and addressing losses in brightness associated with the omnidirectionality of free-standing emitters. In particular, using a scanning-probe method we directly place QDs into hybrid metal-dielectric antenna that cause their photons to emit in a highly focused, directional stream, dramatically enhancing collection efficiency and utility as a SPS. Taken together, these advances in nanomaterial chemistry, nanointegration and hybrid-material design are showing the way to practical utilization of nano-enabled SPSs for quantum applications.
2.1-T1
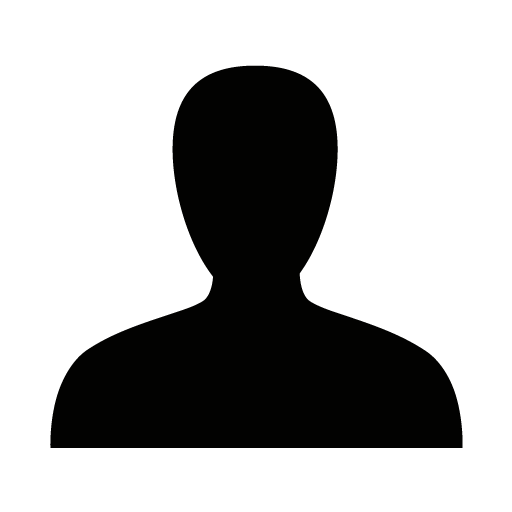
Luminescent colloidal CdSe quantum dots (QDs) provide an opportunity to cover a wide range of emission wavelengths across the visible spectrum. Their high-temperature synthesis procedures offer crystalline materials with precise dimensions and well-defined optoelectronic properties. However, the core-only CdSe QDs show low photoluminescence (PL) quantum efficiency (QE) due to inefficient surface passivation by organic ligands, leading to charge trapping. Core/shell CdSe/CdS nanostructures with wurzite CdSe cores are known to improve the PL QE and long-term stability. A small lattice mismatch of 4% between the crystal structures of CdSe and CdS offers to grow a thick or giant CdS shell. Due to the electron delocalization in CdSe/CdS giant shell nanocrystals (NCs), the PL lifetime can be tuned from a few nanoseconds to microseconds. In this project, we studied a straight forward approach to synthesize high quality CdSe/CdS NCs with near-unity PL QE. Design of experiments (DOE), a statistics supported approach, is used to define the influence of reaction parameters in order to achieve a better synthesis procedure. Thirteen experiments were performed according to the DOE and monitored at 13 respective points for each experiment. PL QE and CdS shell thickness were analyzed with respect to all the variables used in DOE. PL QE up to 97 % has been achieved. Positive and negative effects were analyzed and optimal condition for the factors has been discussed.
Keywords Colloidal quantum dots, core/shell nanostructures, near unity PL QY, Design of experiments
2.1-T2
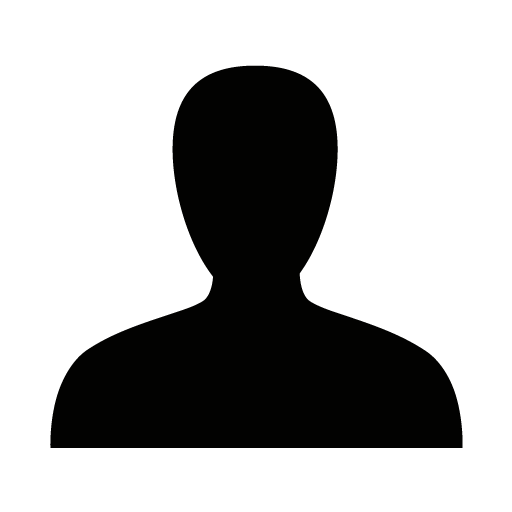
The low-temperature colloidal production of II-VI nanoplatelet heterostructures has stimulated the interest of researchers due to the possible uses of these materials in various optoelectronic devices. Here, we report a room-temperature coating by CdS or ZnS dots of pre-prepared CdSe nanoplatelets. The dot coating process made use of a synthesis developed for the formation of free-standing CdS and ZnS species, involving injection of a metal precursor into reactive sulfur-amine solutions at room temperature. CdSe structured nanoplatelets with 1.75 nm thickness were used as the core constituent. The structural properties were investigated using Fourier transform infrared spectroscopy and advanced electron microscopy, while the elemental mapping was verified using high-angle annular dark field transmission electron microscopy. The results showed a dots-on-plate structure, resembling an intermediate configuration between core/crown or core/shell heterostructures. A thorough study of optical properties demonstrated a dramatic spectral shift of the absorption edge to lower energies, regardless of the uniformity of the coating layer, maintaining spectral stability over two months while stored at ambient conditions. Other optical measurements included examination of temperature-dependent photoluminescence and transient photoluminescence decay, from room temperature down to ~ 4 K. These measurements revealed excitons, trions, and trapped carrier recombination emission with variable relative intensities following the temperature change. The dots-on-plate structures studied displayed a short photoluminescence decay (< nanosecond), compatible with that of core/shell (crown) structures prepared at high temperatures. As a result, the presented techniques are alternate pathways that eliminate the requirement for excessive temperatures and/or multi-step processes, instead providing rapid, inexpensive, and scalable procedures with practical advantages.
2.2-T1
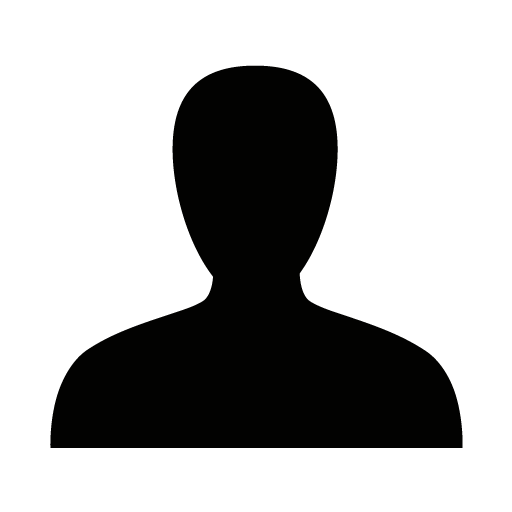
This talk will address the influence of doping on the optical and magneto-optical properties of lead halide perovskites, revealing possibilities of their implementation in various spin-based applications. The Ni2+ ions were embedded into cesium lead halide-perovskite via a combined cation/anion exchange process. This study uses a thorough investigation of Ni2+ doping effects on the magneto-optical properties of CsPb(BrxCl1-x)3 nanocubes, monitored by extensive spectroscopic methods such as steady-state, transient and magneto photoluminescence (PL) spectroscopy which recorded at various temperatures. The PL decay curve showed evidence for charge carrier trapping. The magneto-PL measurements revealed the occurrence of multiple recombination events with different degrees of circular polarization (DCP), with an apparent contrasting behavior in the undoped and doped samples. These observations unfold energy splitting of the bright triplet states (ms = ±1 components) and the existence of emission processes related to delayed fluorescence by carrier localization at trapping sites (also confirmed by ODMR). The study reveals the role of the dopant in stiffening the entire crystal to the degree that enabled resolving the identification of carriers' localization sites.
2.2-T2
Layer-by-layer (LBL) assembly is an appealing approach for the processing of nanoparticles and nanocolloids into thin films suitable for various applications1. One of the major advantages of this method is its simplicity: the process requires neither sophisticated hardware nor high-purity components. This simplicity is combined with the high quality of the resulting coatings, whose thicknesses can be controlled at the nanometer level. In the field of light-emitting diodes (LEDs), thickness of the emissive material film is very important to assure the copious recombination of the opposite charges injected with resulting high photoemission2. In addition, for Quantum Dot-based LEDs it’s crucial to have a good conductivity within the thin layer of emissive nanocrystals to facilitate the carrier mobility2. Therefore, to merge these two important requirements, we developed a ligand exchange process on CsPbX3 perovskite nanocrystal films which enables Layer-by-layer deposition.
We replaced, in solid state, oleate and oleylamine ligands (long insulant chains generally used for the synthesis of CsPbX3 nanocrystals), with Didodecyldimethylammonium Bromide, Ammonium Thiocyanate and other ligands that enhance the photoluminescence quantum yield and improve the performances of LEDs. The exchange has been carried out through a spin-coating technique, using solvents with strategic polarity to avoid NCs dissolution or damaging. Maintaining the quick and simple method of spin-coating, we deposited an increasing number of layers and, through AFM measurements, we noticed considerable thickening of material.
The easy handling of this twofold process makes it very attractive for a laboratory-to-industry scale-up transition and the potential applicability to many nanoscale materials opens the door to a large variety of layer permutations.
2.2-T3
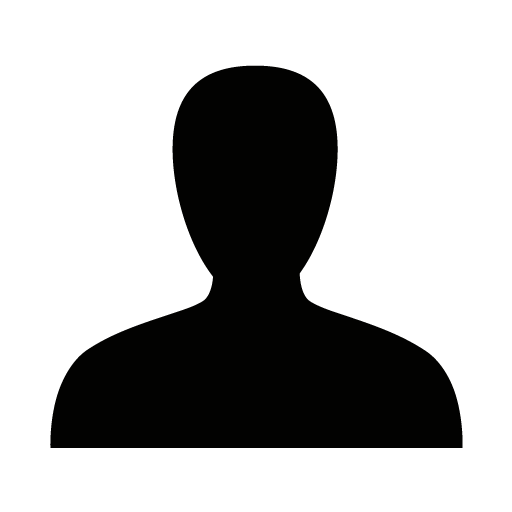
Transition metal dichalcogenide (TMD) nanosheets have become an intensively investigated topic in the field of 2D nanomaterials, due to their semiconductor nature, the direct band gap transition and the broken inversion symmetry going from bulk to monolayer. These properties makes TMDs suitable for different technological applications such as photovoltaics, valleytronics, or hydrogen evolution reactions (HER), or transistors. Among them, MoX2 (X = S, Se) are only direct-gap semiconductors when their thickness is reduced to a monolayer, hence an important effort is devoted to obtain single layer TMDs. Colloidal synthesis of TMDs has been developed in recent years as it provides a cost-efficient and scalable way to produce few-layer TMDs having homogenous size and thickness, yet obtaining a monolayer has proved challenging. Here we present a general method for the colloidal synthesis of mono- and few-layer MoX2 (X = S, Se) using elemental chalcogenide and metal chloride as precursors. Using a synthesis with slow injection of the MoCl5 precursor under nitrogen atmosphere, and optimizing the synthesis parameters with a Design Of Experiments (DOE) approach, we obtained a monolayer MoX2 sample with the required semiconducting (2H) phase, a band gap of 1.96 eV for 2H-MoS2 and 1.67 eV for 2H-MoSe2, respectively, both displaying fluorescence at cryogenic and elevated temperatures. A correlation between the blue shifted absorption spectrum and the spectral difference between the Raman modes was established and confirmed that a single-layer thickness was obtained.
2.3-I1
The recent advances in the colloidal synthesis of strongly emitting lead halide perovskite nanocrystals (NCs) with precise size and composition control open new possibilities for the fabrication of optoelectronic devices. Yet, the physics of the band-edge exciton fine structure of perovskites is still a subject of discussion [1], despite its central impact on the optical properties of these materials and therefore on their potential use in various applications, especially as quantum light sources. At cryogenic temperatures, we show that single cesium lead halide (CsPbX3) perovskite NCs display bright photoluminescence even though their band-edge exciton fine structure presents a dark ground singlet state. We introduce resonant PL excitation of the excitonic sublevels to investigate the indistinguishability character of the photons emitted by these NCs. Importantly, we demonstrate that the presence of a long-lived ground exciton state favors the formation of biexcitons and thus the emission of pairs of correlated photons. We show that this is a general behavior that can be observed for perovskite NCs as well as for conventional CdSe quantum dots. This property makes single CsPbI3 NCs versatile bright, quantum light sources with photon statistics that can be tuned from bunching to antibunching, using magnetic coupling or thermal mixing between dark and bright exciton states [2].
Finally, I’ll describe a recent study [3] of the photophysical properties of copper indium sulfide quantum dots which are emerging as promising alternatives to cadmium- and lead-based chalcogenides systems in various applications and for which the nature of the emission pathways remains a subject of debate.
2.3-I2
Agnès Maître obtained in 1994 the grade of Doctor of the Ecole Polytechnique for her work performed in the domain of non linear optics and atomic physics: in Laboratoire Kastler Brossel, she used to study “self generated transverse optical instabilities in rubidium vapor”. She became in 1995 assistant professor of University Denis Diderot and laboratoire Kastler Brossel. She used to work in the fields of continuous variable quantum optics and quantum imaging. She used to study temporal and spatial quantum correlations for beams emitted by an optical parametric oscillator. Since 2005, she has a position of professor at Sorbonne university and works in the Institut des NanoSciences de Paris. She is now involved in nanophotonics. More specifically, she is studying plasmonic nanoantenna, single photon sources, and emission of a single emitter in a highly confined nanostructured environment. She is author of over 70 publications in peer reviewed international journals and co-inventor of 2 patents.
Nanometric semi-conductor colloidal nanocrystals, like CdSe/CdS ones, are excellent single photon sources. Their emission is stable and bright, with a spectral bandwidth of the order of Dl=20-30nm. In specific conditions of high excitation or high confinement, their emission is dramatically changed. They lose their single photon source quality, the dynamic of the emission is accelerated. the spectrum becomes very large and can reach a few 100nm. Such condition can be achieved either by exciting at high power the emitters or by coupling them to antennas [1], increasing optical confinement.
By in situ optical lithography using high order Laguerre Gaussian mode, we deterministically positioned a single colloidal CdSe/CdS emitter inside a plasmonic patch antenna. The single nanocrystal embedded in a thin dielectric medium, is sandwiched between a thick gold layer and thin gold patch. We achieve inside plasmonic antennas a high interaction between the emitters and the confined field excited inside the antenna. We observe large modification of emission for quantum inside antennas illustrated by directive emission, high brightness and increased efficiency. We evidence a dramatic increase of absorption cross-section of emitters inside these antennas. At higher excitation, multi excitation recombination becomes radiative and the emission intensity becomes non linear.
[1] Amit Raj Dhawan, Cherif Belacel, Juan U. Esparza-Villa, Michel Nasilowski, Zhiming Wang, Catherine Schwob, Jean-Paul Hugonin, Laurent Coolen, Benoît Dubertret, Pascale Senellart, Agnès Maître, Extreme multiexciton emission from deterministically assembled single emitter subwavelength plasmonic patch antennas, Light: science and application, 9, 33 (2020)
[2] A. R. Dhawan, M. Nasilowski, Z. Wang, B. Dubertret, A. Maitre , sunder review, ,https://hal.archives-ouvertes.fr/hal-03376851, Efficient single-emitter plasmonic patch antenna fabrication by deterministic in situ optical lithography using spatially modulated light (under review)
1.1-I1
II-VI semiconductors have been known to favour three-dimensional crystal structures. It is therefore a surprise that they form two-dimensional platelets at the few monolayers limit. Considering nanoplatelets of CdSe, we examine both the bulk limit where a zinc-blende crystal structure is favoured as well as the few monolayers limit considering nanoplatelets with varying thicknesses, within density functional theory based calculations. In agreement with experiment, we find that the formation energy is less for the nanoplatelets for few layers than for the bulk structure, indicating that it is energetically more favourable for them to form. Different surface terminations for the nanoplatelets as well as different atoms at the surface are explored in order to understand the energetics. Here again, we find the Cd-terminated (100) surface facets to be most stable. The possible energetics allowing the nanoplatets to form as well as their electronic structure will be discussed.
1.1-I2
The anion and cation exchanges in nanocrystals are common synthetic strategies to tune their optoelectronic properties. The process of ion exchanges often leads to the formation of heterostructures inside the nanocrystals - that may control their stability and other properties. A fundamental understanding of ion exchange processes and ion exchange induced heterostructures is necessary.
In this talk, we will be discussing the anion exchange in CsPbX3 nanocrystals and how it can lead to the formation of internal heterostructures. It is generally believed that the anion-exchanged nanocrystals possess a homogeneously alloyed composition. We have employed variable energy hard X-ray photoelectron spectroscopy to determine the internal heterostructure of anion-exchanged nanocrystals - which has a gradient-alloyed heterostructure. We will also discuss the mechanism of the anion exchange in pre-synthesized CsPbX3 nanocrystals.
1.1-I3
Celso de Mello Donega is an Associate Professor in the Chemistry Department of the Faculty of Sciences at Utrecht University in the Netherlands. His expertise is in the field of synthesis and optical spectroscopy of luminescent materials. His research is focused on the chemistry and optoelectronic properties of nanomaterials, with particular emphasis on colloidal nanocrystals and heteronanocrystals.
Colloidal semiconductor heteronanocrystals (HNCs) exhibit unique optoelectronic properties that are inaccessible to single-component NCs, making them promising materials for a wide range of applications. The optoelectronic properties of HNCs are determined not only by the bandgap and band alignment of its constituent materials but also by their morphology and heteroarchitecture [1]. Applications requiring efficient charge separation (e.g., photovoltaics and photocatalysis) greatly benefit from anisotropic morphologies, such as heteronanorods. Most of the work on heteronanorods has focused on Cd-chalcogenide-based HNCs. However, given the toxicity of Cd, the potential of these materials for large scale applications is limited.
Copper-chalcogenide based NCs have attracted increasing attention as promising alternatives for Cd- and Pb-chalcogenide NCs due to their low toxicity, large absorption cross-sections across a broad spectral range, composition-dependent band gaps in the 1 to 2.5 eV range, and photoluminescence tunability, spanning a spectral window that extends from the UV to the NIR depending on the NC size and composition [2,3].
Several synthetic strategies have been used in the quest for high-quality colloidal copper chalcogenide based HNCs. The most promising ones are based on the multistage approach, which allows the combination of different synthesis techniques (e.g., cation exchange [4,5] or seeded growth [6-9]) in a sequential manner in order to achieve the targeted preparation of colloidal HNCs. In this talk, I will discuss a selection of recent examples, chosen in order to provide an overview of the current status of the quest for Cd- and Pb-free HNCs and to illustrate specific synthesis strategies: CuInSe2/CuInS2 dot core/rod shell heteronanorods [4], Cu1.8S-based multicomponent axially segmented heteronanorods [5], CuInS2/ZnS dot core/rod shell heteronanorods [6], and Janus-type Cu2‒xS/CuInS2 and Cu2−xS/ZnS heteronanorods [8,9]. I will show that by combining the design principles of post-synthetic heteroepitaxial seeded growth and nanoscale cation exchange into multistage synthesis strategies one can potentially gain access to a plethora of Cu-chalcogenide-basedmulticomponent heteronanorods with diameters in the quantum confinement regime.
1.2-T1
Benefiting from their large compositional space, direct bandgap nature, and outstanding structural stability,[1–4] vacancy-ordered layered double perovskites with formula Cs4M(II)M(III)2X12 (M(II): Cu2+, Mn2+; M(III): Bi3+, Sb3+, In3+; X: Cl-, Br-, I-), have recently attracted increasing attention as substitutive materials of lead-based halide perovskites for potential commercial optoelectronic applications. The layered double perovskite structure comprises one layer of [M(II)X6]4- octahedra inserted in between two layers of [M(III)X6]3- octahedra. As a representative, Cs4CuSb2Cl12 has been successfully synthesized both in the form of single-crystalline powder[2] and NCs,[3,5] exhibiting a narrow direct bandgap (1.0–1.8 eV) and impressive stability, which however still suffers from the high toxicity of Sb element and the absence of emission at room temperature. To overcome these drawbacks, Cs4CuIn2Cl12, with toxic Sb3+ substituted by relatively non-toxic In3+, could be a promising candidate to fulfill the requirement of compositional engineering and optical tunability particularly in the UV range. Yet, to date, the synthesis of Cs4CuIn2Cl12 layered double perovskites has not yet been reported for neither bulk film nor NCs.
Herein, we report the first-ever colloidal synthesis of lead-free Cs4CuIn2Cl12 layered double perovskite NCs using a modified hot-injection method.[5,6] The synthetic details are described in the Supporting Information (SI). While a standard hot-injection reaction in moisture-free environment resulted in Cs4CuIn2Cl12 with a very low photoluminescence quantum yield (PLQY) of 0.12%, we found that the handling of synthesis precursors in the presence of moisture (RH~40%) enhances PLQY by more than one order of magnitude up to 1.70 %. Water-assisted in-situ synthesis has been recognized as an effective strategy to tune the optical properties and stability for both lead-based[7,8] and lead-free halide perovskite NCs[9,10] via the controlling of NCs size, shape, and crystallinity. Nevertheless, there is still a lack of deep understanding of how water influences NCs growth regime and corresponding PL property, especially for lead-free double perovskite NCs. The introduction of moisture in the precursor (namely “wet” precursor conditions) of Cs4CuIn2Cl12 NCs (w-Cs4CuIn2Cl12) induces the morphological transformation from 3D nanocubes (NCus) to 2D nanoplatelets (NPLs), driven by the ionized H3O+ and OH- from the water content as additional capping ligands. The ultrafast transient absorption studies suggest a strengthened self-trapped exciton (STE) effect for w-Cs4CuIn2Cl12 NCs compared to the NCs synthesized in “dry” conditions (d-Cs4CuIn2Cl12 NCs), resulting in the conversion of dark transitions into radiative transitions, which directly contributes to the PLQY.
1.2-T2
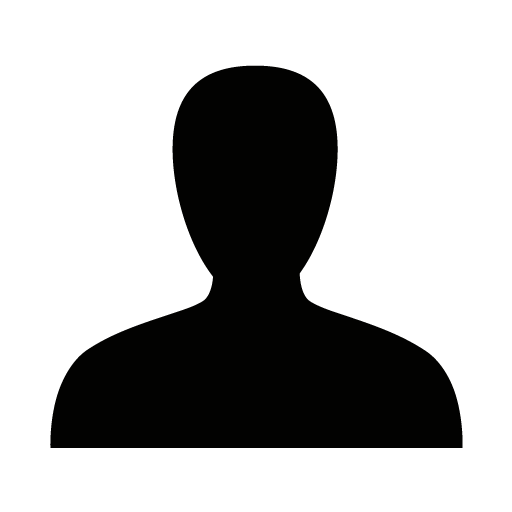
Investigating halide perovskites’ optoelectronics properties for stimuli-responsive materials (SRMs) opens new avenues in smart chromic windows, switchable optoelectronics, and information display applications. Here, we carried out an in-depth study of the structural transformation of FAn+2PbnBr3n+2 (2≤ n ≤∞) layered halide perovskite using excess organic cation (FA/Pb>1), strain, optical and thermal input. Initially as FA/Pb ratio increases, three-dimensional (3D, FA/Pb=1, n=∞) (FAPbBr3) perovskite transform to two-dimensional (2D, FA/Pb=1, n=2) (FA2PbBr4) layered halide perovskite and is aligned in <110> orientation. The intermediate n phases (2≤ n ≤∞) in FAn+2PbnBr3n+2 system stabilize in metastable states and are accessible via strain, optical or thermal input. These results were supported by X-ray diffraction, solid-state nuclear magnetic response (ssNMR), in-situ temperature-dependent Raman spectroscopy, optical spectroscopy, and ab initio simulation techniques. Furthermore, upon photo-decomposition of FABr, the <110> oriented 2D perovskite transforms to 3D perovskite. Therefore, a metal oxide mesoporous substrate has been introduced to prevent FA decomposition and impart reversibility (2D to 3D and vice versa), which facilitate as a reservoir for FA cations. Shuttling of FABr between the reservoir and the HP film under light and moisture ensure reversible chromism. We realised that the transformation in the FAn+2PbnBr3n+2 structure is governed by the H-bonds strength of the intermediate n phase (lower n phases to higher n phases or vice versa). Additionally, the remnant organic solvent in the film (observed using proton transfer reaction-mass spectroscopy (PTR-MS)) is crucial to realize structural transformation and, therefore, the reversible chromism. Finally, we relate photo-induced reconfigurability with photo-adaptable intelligent sensors. The realization of structural configurability using just one cation is not only employable in solar cells and LEDs but simultaneously can be utilized in artificially intelligent sensors by optimizing their operating parameters according to the ambient environment.
1.2-T3
Ternary I-III-VI2 NCs, such as CuInS2 and AgInS2 are receiving attention as heavy-metals-free materials for solar cells, luminescent solar concentrators (LSCs), LEDs, and bio-imaging. The origin of the optical properties of NCs are however not fully understood. A recent theoretical model suggests that their characteristic Stokes-shifted and long-lived luminescence arises from the structure of the valence band (VB) and predicts distinctive optical behaviors in defect-free NCs: the quadratic dependence of the radiative decay rate and the Stokes shift on the NC radius. If confirmed, this would have crucial implications for LSCs as the solar spectral coverage ensured by low-bandgap NCs would be accompanied by increased re-absorption losses. In this work, we performed spectroscopic studies on stoichiometric CuInS2 and AgInS2 NCs, and in the process it was revealed for the first time, the spectroscopic signatures predicted for the free band-edge exciton, thus supporting the VB-structure model. At very low temperatures, we also observed dark-state emission from these NCs likely originating from enhanced electron-hole spin interaction. The impact of the observed optical behaviors on LSCs was evaluated by Monte Carlo ray-tracing simulations. Based on the emerging device design guidelines, optical-grade large-area (30×30 cm2) LSCs with optical power efficiency (OPE) as high as 6.8% were fabricated, corresponding to the highest value reported to date for large-area devices.
1.3-T1
Prof. Z. Hens received his PhD in applied physics from Ghent University in 2000, worked as a postdoctoral fellow at Utrecht University and was appointed professor at the Ghent University department of inorganic and physical chemistry in 2002. His research concerns the synthesis, processing and characterization of colloidal nanocrystals.
While initial theories on quantum confinement in colloidal quantum dots (QDs) led to analytical band-gap/size relations or sizing functions, numerical methods proofed more accurate to describe size quantization. However, for lack of reliable sizing functions, researchers fit experimental band-gap/size datasets using models with redundant, physically meaningless parameters that break down upon extrapolation. Here, we propose a new sizing function based on a proportional correction for non-parabolic bands. Using known bulk semiconductor parameters, we accurately predict size quantization for group IV, III-V, II-VI, IV-VI and metal halide perovskite semiconductors, including straightforward adaptations for negative-gap semiconductors and non-spherical QDs. Refinement with respect to experimental data is possible using the Bohr diameter as a fitting parameter, by which we show a statistically relevant difference in band-gap/size relation for wurtzite and zinc blende CdSe. The general sizing function proposed here unifies QD size calibration, and enable researchers to assess bulk semiconductor parameters and predict size quantization in unexplored materials
1.3-T2
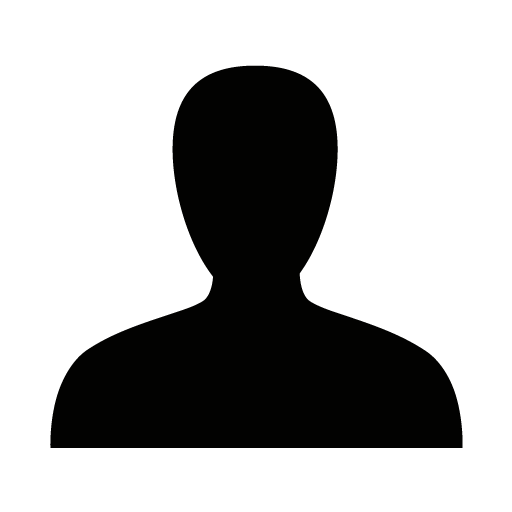
Two-dimensional nanoplatelets (NPLs) of II-VI semiconductors exhibit the narrowest optical features among nanocrystals. Despite the great maturity of cadmium based NPLs, their optical properties are limited by the band gap of the bulk material. To push toward infra-red wavelength, mercury-based NPLs are good candidates as they keep narrow optical properties[1].
To obtain such material, only cation exchange is yet available. However, this procedure leads to the creation of structure defects and to the degradation of the optical properties. In this context, we developed an optimized procedure for cation exchange and rationalized the role of the solvent, the temperature and the ligands during this process. This optimized procedure has then been applied to Cd-based alloys of CdSexTe1-x to obtain HgSexTe1-x NPLs of 3MLs with photoluminescence for every composition. Using UV-visible and X-ray spectroscopy, the evolution of the band alignment according to the composition has been established revealing a crossover from n- to p-type behavior as the Te content increases in HgSexTe1-x NPLs. In parallel, this trend has also been observed in time-resolved photoemission measurements.
1.3-T3
Quasi-Type II CdSe@CdS dot-in-rod nanorods (NRs) exhibit high absorption coefficients (~107 M-1 cm-1) in the UV/Vis and efficient charge-separation following photoexcitation, with the photogenerated hole localizing to the CdSe core and the electron delocalized over the entire structure. These properties render them attractive materials as photosensitizers in photocatalytic reactions such as light-driven water splitting, especially when coupled to a reaction centre such as metal nanoparticles.1 On the other hand, some drawbacks of these structures have to be considered when designing a catalytic system. For instance, molecular functionalization of NRs is limited to specific anchoring groups such as thiols and amines.2 Additionally, the catalytic efficiency is limited by slow (> 100 ps) hole removal, whereas the electron transfer steps are usually very fast (<10s of ps). Last, these structures are prone to photo-oxidation, which limits their long-term usage.
Recently, we demonstrated a photocatalytically able system consisting of polydopamine (PDA) coated NRs.3 The PDA-coating tried to counteract the above-mentioned drawbacks of NRs: it served as a scaffold for a molecular catalyst and acted as a charge-mediator between NRs and catalyst, potentially improving long-term stability. However, the underlying charge transfer processes have not been fully resolved yet.
Here, the photoinduced charge transfer processes between NRs and PDA are investigated. First, as a model system, the interaction between water-soluble NRs and molecular dopamine is investigated at different pH values and dopamine concentrations using steady-state and time-resolved absorption and photoluminescence spectroscopies. Insights gained from these quenching experiments serve as a basis for explaining the charge transfer processes in PDA-coated nanorods. Last, implications of these fundamental processes on photocatalysis are considered.
1.3-T4
Prof. R. Robinson received his PhD in Applied Physics from Columbia University. After his PhD, Prof. Robinson was awarded a postdoctoral fellowship at University of California, Berkeley/LBNL in the research group of Paul Alivisatos. There, he worked on nanoparticle synthesis, chemical transformations of nanoparticles, and advanced property characterizations of nanoparticles. In 2008 Richard began a faculty position at Cornell University in the Materials Science Department, and is currently an associate professor. His primary research interests are: (I) Synthesis and chemical transformations in nanocrystals, (II) Nanocrystals in energy applications, and (III) Synchrotron x-ray characterization of nanomaterials.
therobinsongroup.org/
Chemical transformations reactions, such as the cation exchange reaction, have been one of the most exciting means of studying and post-synthetically modifying nanocrystals. These reactions can create atomic arrangements that are impossible to reach in bulk materials due to kinetic limitations, and these reactions are believed to enhance diffusion beyond bulk-derived limits. In this talk I’ll first discuss cation exchange induced accelerated diffusion and then cation exchange to form a dual-interfaced heteroepitaxy.
The phenomenology of solid-state transformations and diffusion at short length scales remains poorly understood but is increasingly important for nanostructured devices that utilize “nano properties”. Using in-situ synchrotron x‑ray diffraction (XRD), we directly interrogate the structure and reaction kinetics of lead sulfide (PbS) nanocrystals transforming into cadmium sulfide (CdS) through cation exchange. The epitaxial relationship of zincblende CdS to rocksalt PbS breaks the overall symmetry of the core-shell nanocrystal without requiring the loss of unit cell symmetry, leading to anomalous peak shifts in the diffraction pattern. The magnitude of the interdiffusion coefficient, D̃, is larger by four orders of magnitude or more compared to the slowest diffusing species in our system (self-diffusion of Cd in CdS). This surprising result suggests interdiffusion is enhanced in nanocrystals. These results illustrate that the distinction between chemical diffusion in a potential gradient and diffusion at thermodynamic equilibrium has not been fully appreciated.
Additionally, through a cation exchange reaction on copper sulfide nanoparticles we have created dual interface Cu2-xS-ZnS heterostructures, with a metastable Cu2-xS layer. The copper sulfide phase region can be tuned to form two-dimensional (2D), single atomic layers (<1 nm). As the nanoparticles transform, we observe a solid-solid phase transformation of the copper sulfide phase from the initial low-copper phase Cu1.8S into a higher copper phase djurleite (Cu1.94S), but as the epitaxial strain increases a second phase transformation back to roxbyite Cu1.8S occurs to minimize strain energy. This work demonstrates novel routes to metastable phases through strain stabilization. The copper sulfide can be etched with phosphines in oxidizing conditions. Importantly, this etching reaction is capable of removing Cu2-xS from Cu2-xS-ZnS epitaxial heterostructures with perfect selectivity, that is, the phosphines completely remove the Cu2-xS without disturbing the ZnS. The etching reaction is preceded by abstraction of sulfur from the particles, destabilizing the Cu1.81S roxbyite phase.
2.1-I1
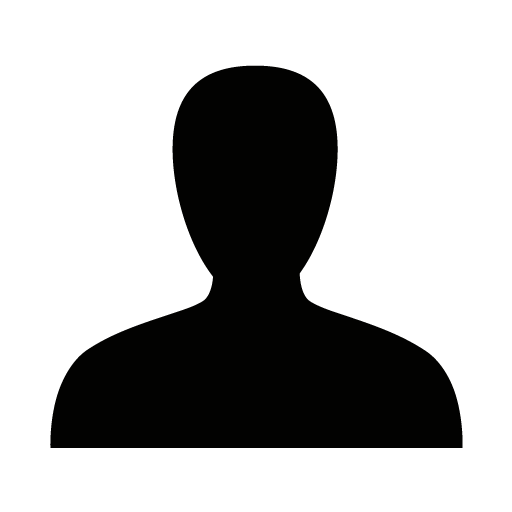
Heterojunction nanocrystals (HNCs) consisting of two or more semiconductor components contain junctions at the interface of the constituent blocks. Unlike the electronic property tuning by changing the size or shape of mono-component nanocrystal, HNCs rely on controlling energy states via band-offset engineering at the material interface. We demonstrate the usefulness of band-offsets by designing component size modulated type-II coupled quantum dots (CQDs-HNCs) composed of a fixed sized ZnSe quantum dots and size-tuned CdS quantum dots. The interface of single CQDs-HNCs is probed by using scanning tunneling microscopy (STM) at the local scale. Furthermore, we demonstrate fabrication of a differentiator using a single Cu2S-CdS HNC. The differentiator is capable for conversion of variety of waveforms demonstrating the possibility of fabrication of single HNC electronic component.
2.1-I2
Professor Uri Banin is the incumbent of the Larisch Memorial Chair at the Institute of Chemistry and the Center for Nanoscience and Nanotechnology at the Hebrew University of Jerusalem (HU). Dr. Banin was the founding director of the Harvey M. Kreuger Family Center for Nanoscience and Nanotechnology (2001-2010) and led the program of the Israel National Nanotechnology Initiative at HU (2007-2010). He served on the University’s Executive Committee and on its board of managers and was a member of the board of Yissum. He served on the scientific advisory board of Nanosys. In 2009 Banin was the scientific founder of Qlight Nanotech, a start-up company based on his inventions, developing the use of nanocrystals in display and lighting applications. Since 2013, Banin is an Associate Editor of the journal Nano Letters. His distinctions include the Rothschild and Fulbright postdoctoral fellowships (1994-1995), the Alon fellowship for young faculty (1997-2000), the Yoram Ben-Porat prize (2000), the Israel Chemical Society young scientist award (2001), the Michael Bruno Memorial Award (2007-2010), and the Tenne Family prize for nanoscale science (2012). He received two European Research Council (ERC) advanced investigator grant, project DCENSY (2010-2015), and project CoupledNC (2017-2022). Banin’s research focuses on nanoscience and nanotechnology of nanocrystals and he authored over 180 scientific publications in this field that have been extensively cited.
Colloidal semiconductor Quantum Dots (CQDs) that contain hundreds to thousands of atoms manifest size dependent tunable properties and have reached an exquisite level of control, leading to their technological applications in optoelectronics and bioimaging. Considering them as artificial atoms, CQD molecules connected with molecular linkers such as DNA strands were studied. Yet, in these structures the presence of a high potential barrier limits coupling between the quantum states of neighboring CQDs limiting their full potential as coupled systems. Although coupled quantum dots were prepared by means of molecular beam epitaxy (MBE), their typical dimensions limit coupling to small energy scales suitable to low temperature operation. Furthermore, while MBE grown structures are inherently buried within a host semiconductor, CQDs are free in solution and accessible for wet-chemical manipulations.
Herein, we introduce a facile and powerful strategy for programmed synthesis of coupled CQDs molecules with precise control over the composition and size of the barrier in between the artificial atoms to allow for tuning the electronic coupling characteristics and their optical properties.1 Our approach entails fusing two CdSe/CdS core/shell CQDs via constrained oriented attachment.2 This yields a dimer with a tailored barrier dictated by the shell composition, thickness and fusion reaction conditions. The fusion reaction also enables tuning the neck barrier width.3 The possible nanocrystal facets in which such fusion takes place are analysed with atomic resolution revealing the distribution of possible crystal fusion scenarios. Coherent coupling is revealed by ensemble and single particle spectroscopic signatures, in agreement with quantum mechanical simulations.4 Cryogenic single nanocrystal spectroscopy accompanied by the simulations, unravels the complete tracking of fluorescence and charging events in such coupled colloidal quantum dot molecules.
This sets the stage for nanocrystals chemistry to yield a diverse selection of coupled CQD molecules utilizing the rich collection of ubiquitous artificial atom core/shell CQD building blocks. Such CQD molecules are of direct relevance for numerous applications including in displays, sensing, biological tagging and emerging quantum technologies.
2.1-I3
Peter Reiss is researcher at the Interdisciplinary Research Institute of Grenoble (IRIG), France, and Head of the Laboratory Synthesis, Structure and Properties of Functional Materials (STEP). He graduated from University of Karlsruhe (Germany), and earned his PhD in Inorganic Chemistry under the supervision of Prof. Dieter Fenske (2000). His research activities focus on the synthesis and properties of colloidal semiconductor quantum dots and metal halide perovskites (nanoparticles and thin films). The studied applications range from biological imaging / detection over LEDs and displays to new strategies for energy conversion (photovoltaics, thermoelectrics, photocatalysis) and storage. Dr. Reiss acts as Associate Editor for Nanoscale Research Letters and Frontiers in Materials - Energy Materials, and is Editorial Board Member of Scientific Reports. He co-organizes the biennial conference NaNaX – Nanoscience with Nanocrystals (cf. http://nanax.org).
Lead halide perovskite nanocrystals (NCs) have drawn tremendous research interest in the past five years fueled by their outstanding optical and electronic properties.[1, 2] In particular CsPbBr3 NCs exhibit excellent photoluminescence with a very narrow peak and high quantum yield. They can be conveniently synthesized in high yield by means of hot-injection methods.[3-5] However, due to their ionic nature, perovskite NCs are unstable in polar solvents, and when dispersed in water they quickly lose their luminescence properties and chemical integrity.
Many efforts have been undertaken to enhance aqueous stability, encompassing changes in stoichiometry (synthesis under Pb-poor conditions)[6] and encapsulation with appropriate organic compounds or oxides (e.g., block copolymers,[7] SiO2[8]). On the other hand, the growth of an inorganic shell, as generally applied in the case of conventional nanocrystals/quantum dots, has turned out to be challenging: upon prolonged heating of perovskite NCs, uncontrolled ripening leads to a variety of sizes and shapes. Furthermore, classic shell materials crystallize in different structures and exhibit large lattice mismatches.
Here, we present novel approaches for the growth of metal sulfide and metal oxide shells on CsPbBr3 nanocrystals, keeping a low size distribution and high fluorescence quantum yield. In the case of metal sulfide shells, aqueous phase transfer of the core/shell NCs could be achieved while maintaining the photoluminescence in water for extended periods. The improved stability of the core/shell perovskite NCs paves the way for their use in various applications such as single-photon emitters and photocatalysis.
2.2-T1
Semi-conducting nanoplatelets (NPL) exhibit outstanding optical properties but contain toxic heavy metals such as cadmium, lead or mercury. Indium sulfide is believed to be a credible alternative for semi-conductor based applications. We report the synthesis of ultrathin indium sulfide In2S3 nanoplatelets which display a giant aspect ratio using a simple and fast solvothermal method. We show that these NPL display a thickness controlled at the atomic level below the nanometer, a width of around 9 nm and a length which can reach several micrometers. We determined the atomic composition of the inorganic core by Rutherford backscattering spectrometry (RBS) and measured by X-ray photoelectron spectrometry (XPS) an oleylamine surface coverage of 2.3 ligands per nm2. X-ray diffraction experiments and simulations as well as high-resolution dark-field STEM point towards a trigonal crystallographic structure (gamma phase). Depending on the dispersion solvent, these long ribbon-like nanoplatelets can form well dispersed colloids or bundles in which they stack face to face. Their large aspect ratio induces the formation of gels. Their lateral dimensions can be tuned by the amount of water present in the reaction media: anhydrous synthesis conditions lead to hexagonal nanoplates while controlled addition of water induces a symmetry break yielding long NPL with a rectangular
2.2-T2
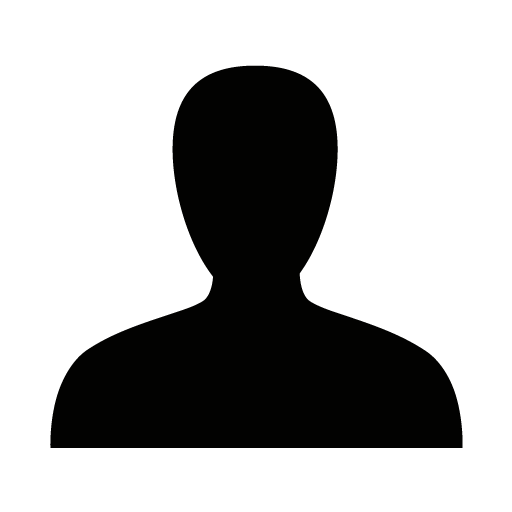
Transition Metal Dichalcogenide (TMDC) monolayers exhibit a direct band gap in their semiconducting crystal phase and have great potential for future photonics through spin- and valleytronics and their ultrafast response to external stimuli, which is essential for fast optoelectronic components [1, 2]. The materials are typically obtained by exfoliation and chemical vapor deposition methods that yield samples of high quality for fundamental research but lack potential scalability. Colloidal wet-chemistry represents a challenging yet promising approach to synthesize TMDCs from precursors in a solution-processed and flexible manner. We show how fundamental properties of MoS2 nanosheets including the layer count, the lateral dimension and the crystal phase is controlled. The initially forming a metallic crystal phase, attractive for catalytic hydrogen evolution, is kinetically preferred and transforms into the thermodynamically stable semiconducting crystal phase throughout the reaction. In order to show that the colloidal approach is able to compete with conventional manufacturing methods we investigate photoluminescence of ultrathin colloidal WS2 nanosheets synthesized with WCl6 and elemental sulfur in oleic acid and oleylamine at 320 °C, finding photoemission for the first time [3]. We observe mono- as well as multilayer photoluminescence exhibiting comparable characteristics to exfoliated TMDC monolayers and underpinning the high quality of colloidal WS2 nanosheets. In addition, the observed monolayer emission has a narrow linewidth, which qualifies colloidal WS2 as a potential single photon emitter complementing epitaxially grown WS2 quantum emitters [4]. Our results render colloidal WS2 as straightforwardly synthesized and highly promising 2D semiconductors with optical properties competitive with conventionally fabricated ultrathin WS2.
2.2-T3
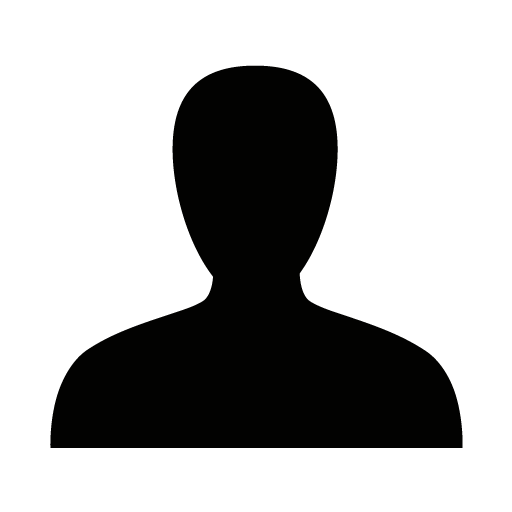
Among semiconductor colloidal nanocrystals, 2D nanoplatelets (NPLs) are geometrically seen as well-defined flexible substrates for the self-assembly of molecules. In the presence of stress brought by surface stabilizers, helical structures are formed according to the parameters of the initial material [1,2].
Here, we demonstrate the control of the NPLs helices radii through the organic ligands, described as an anchoring group and an aliphatic chain of a given length. A perfect control in surface chemistry allows the tuning of the morphological feature of these nanohelices. Nonetheless, their optical properties are well-preserved upon surface modification. Structural studies done on these anisotropic nanoparticles unveil a preferential orientation effect on the resulting X-ray scattering patterns.
A mechanical model accounting for the misfit strain between the inorganic core and the surface ligands, enables to predict the nanohelices radii. The model treats the substrate layer, anchoring group and aliphatic chain contributions individually and demonstrates good agreement for all studied homo- and hetero-structure NPLs. It reveals ultimately that the self-assembly of organic ligands is equivalent to a layer, of Young modulus in lateral compression estimated close to 0.9 GPa. Furthermore, the chirality of the nanohelices shown in this work can be tuned by the ligands anchoring group and inverted from one population to another.
2.2-T4
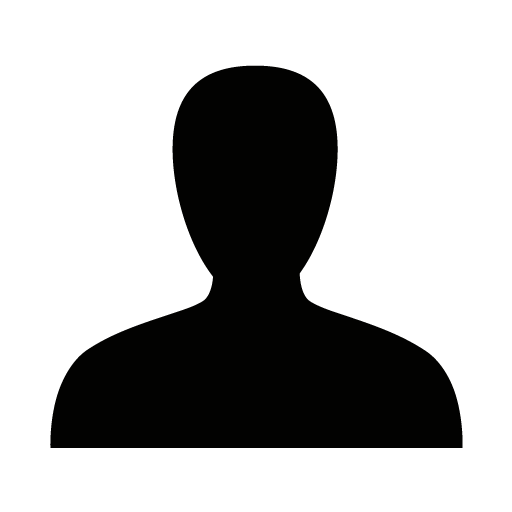
2.3-T1
Colloidal nanocrystals (QDs) have been developed as excellent opto-electronic materials over the past 3 decades. Being used in applications as diverse as light emission, conversion and detection, they found their way mostly in those requiring interaction with visible or near-infrared radiation. Going further into the mid-infrared spectrum however, a plethorum of applications await in fields as defense, security, trace gas detection, imaging, etc. Over the past decade, giant leaps have been made using inter – and intraband processes in QDs to address this exciting wavelength range, resulting in solution processable photo-detectors with state-of-the-art performance at a fraction of the cost. [1]
As important as light detection is the ability to develop light emitting devices in the mid-infrared. Sources with broadband or coherent narrowband radiation would be disruptive in several fields. However, many properties of excitons and multi-excitons that enable these devices remain unexplored for these narrow gap materials, such as exciton cooling, multi-exciton interactions (shifts, Auger processes, …) and the fate of excitations in an environment full of quenching sites, i.e. non-radiative transfer of energy to surface ligands.
In this contribution, we show our recent work on applying ultra-broadband 1D mid-infrared spectroscopy to n-doped HgSe QDs. These advanced measurements provide for the first time a complete overview of the ultrafast time dynamics of these materials over the full spectral range, revealing intraband cooling, photo-induced absorption and multi-exciton shifts. Our results pave the way towards a full understanding of the (multi-) exciton physics of intraband colloidal nanomaterials.
2.3-T2
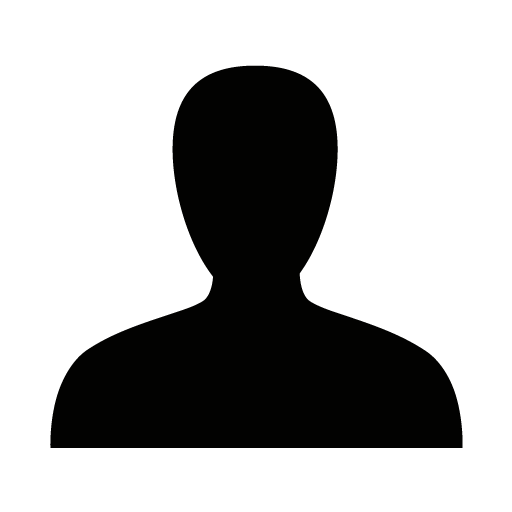
During the past decade, nanocrystals (NCs) have shown that they were an efficient and low-cost photodetection technology to address the infrared range. In particular, in the short-wave infrared by using interband transitions in narrow band gap semiconductors such as HgTe NCs [1]. Here, to go beyond and access the mid-wave infrared range, we use an interesting alternative that are intraband transitions of HgSe doped NCs [2][3]. Throughout a dye sensitized approach which couples an intraband absorber to an undoped material driving the charge conduction, the limitations observed from intraband materials (high dark current, slow response, and low activation energy) are ruled out [3]. Using mid-infrared transient reflectivity (TR) measurement, we unveil the coupling between both material in this heterostructure. The latter displays a unique feature in the TR signal which dynamic matches the hopping time and therefore is an evidence of a charge transfer. Moreover, we develop a strategy to enhance the hybrid material’s photodetection performance by coupling it to an optical nano-resonator. Finally, we demonstrate that the final structure has an enhanced absorption of a factor 4 allowing for an 80 K increase of the operating temperature. The resulting device has among the highest intraband-based photodetection performances.
2.3-T3
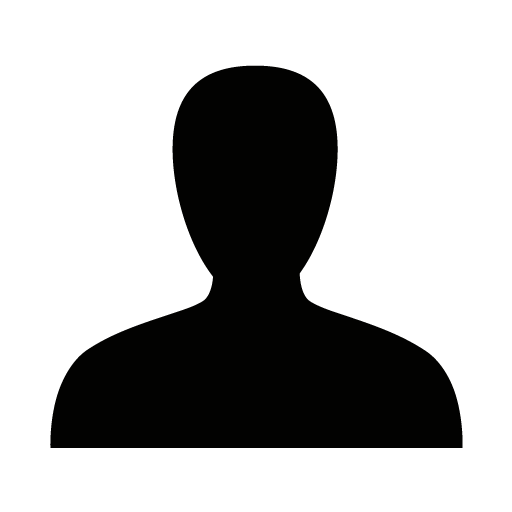
Nanostructured semiconductors are heavily investigated for their applications in light emission such as light emitting diodes and, more challenging, lasers[1-4]. Using quantum confined Cd-based QDs, several groups have shown light amplification and ensuing lasing action in the red part of the spectrum. Although further work is necessary to reduce gain threshold densities for efficient lasing action, there has been some push toward moving away from the current red gain band region, toward green and near-infrared stimulated emission.
In this work, we take a look at weakly confined “giant” CdS and CdSe Quantum Dots which display disruptive optical gain metrics in the green and near-infrared spectrall region. While showing similar gain thresholds compared to state-of-the-art materials, the gain window, amplitude (up to 50000/cm) and gain lifetime (up to 3ns) outpace other materials in the same spectral region.
These remarkable results are explained by using a bulk semiconductor gain model, which can be done due to the large size of the quantum dots (8-12 nm). We can quantitatively reconstruct the gain spectrum with this model, yet only by including large bandgap renormalizations (up to 70 meV). This inclusion helps us to understand the gain mechanism in these particles. Our results indicate a paradigm shift towards weakly confined photo-physics as a means to push quantum dots towards efficient solution processable lasers.
2.3-T4
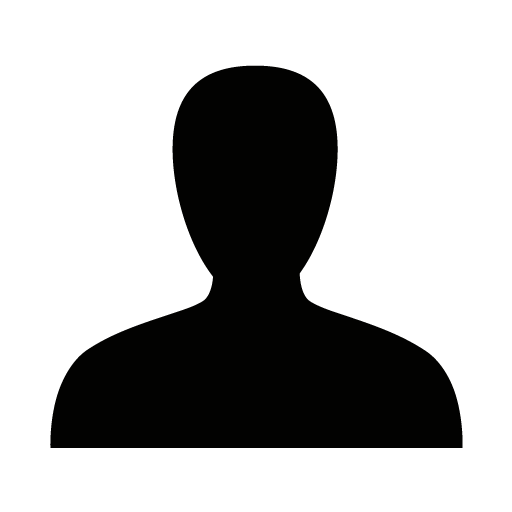
Optical properties tunability is one of the essential characteristics of nanocrystals (NCs). Nonetheless, obtaining reconfigurable spectral response in NC-based optoelectronic devices remains a hardly explored domain. This is because the conventional strategies, such as exploiting Stark effect or integrating NCs into MEMS structures, are not straightforward to apply for NC-based devices due to high electrostatic field requirement and fabrication incompatibility. Here, we demonstrate optical tunability with bias in a device coupling HgTe1 NCs with a plasmonic structure. Bias tunability can be obtained thanks to the interplay between absorption inhomogeneity due to the plasmonic cavity2 and the NC hopping transport. As a result, 15 meV blueshift can be observed under the application of 3 V bias voltage for a device working in the extended short-wave infrared3. We show that hopping transport, which is usually seen as a limitation of NCs, can be combined with inhomogeneous absorption to obtain an active photonic NC-based device.
2.3-T5
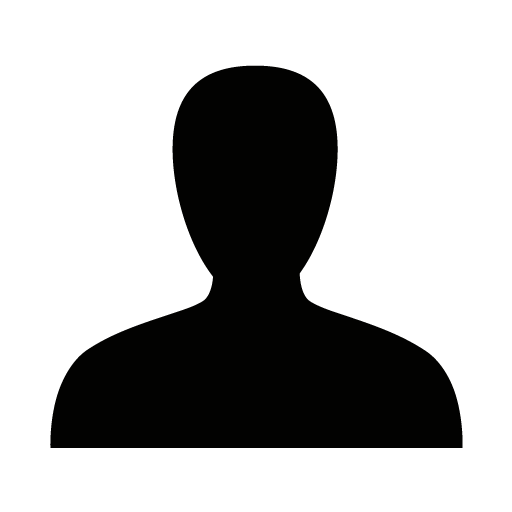
Metal oxide (MO) doped semiconductor nanocrystals (NCs) are characterized by tunable optoelectronic properties, which can be modified by appropriate engineering of the NCs geometry, structure and carrier density profile [1]. Their versatility makes them tailorable for specific applications, ranging from electronic [2] and optical devices [3] to energy storage[4].
We report a semi-classical multi-layer optical model that can effectively describe the response of colloidal NCs with different doping profiles in core-shell structures. In particular, we applied our model to the investigation of the multi-peak absorption of Sn-doped Indium Oxide (ITO) NCs. We demonstrate that our model is suitable for predicting the experimentally observed absorption peak splitting, both with variable NCs doping profiles and, post-synthetically, via photodoping. In both cases, we found that depletion layer is fundamental in order to correctly describe the spectrum evolution. We foresee that our model can be employed as a useful tool to design the optoelectronic properties of core-shell NCs systems in the framework of energy band and depletion layer engineering.
2.4-I1
Ferry Prins is a tenure-Track Group leader at the Condesed Matter Physics Center (IFIMAC) of the Universidad Autonoma de Madrid. Ferry obtained an MSc in Chemistry from Leiden University (2007) and a PhD in Physics from the Kavli Institute of Nanoscience at Delft University of Technology (2011). After completion of his PhD, he joined the the group of Prof. Will Tisdale at Massachusetts Institute of Technology (MIT). There, he started exploring the optical properties of nanomaterial assemblies with an emphasis on excitonic energy-transfer interactions. In 2014 he moved to ETH Zurich for a postdoc with Prof. David Norris at the Optical Materials Engineering Laboratory. With support from the Swiss National Science Foundation, he started an independent group at ETH in 2015. In Spring 2017 he joined he Condensed Matter Physics Center (IFIMAC) at the Autonoma University of Madrid where he directs the Photonic Nanomaterials and Devices Lab. His group specializes in the development of light-management strategies for semiconductor nanomaterials.
Efficient transport of excited state energy in nanocrystal thin-films is essential in the operation of light harvesting and light emitting devices. Transient Photoluminescence Microscopy has emerged as a powerful tool to characterize energy transport, allowing for a direct visualization of the transport in both space and time.[1]
In this talk, I will present past and current work of our use of Transient Photoluminescence Microscopy in characterizing energy transport in different nanocrystal solids.[2,3] I will specifically highlight the role of energetic disorder in these materials, which greatly impacts the transport characteristics. For example, size-polydispersity in colloidal quantum-dot solids leads to inhomogeneous energy landscapes that cause disitinct non-equilibrium effects in the transport of energy carriers. Similarly, statistical fluctuations in the doping levels of perovskite nanocrystals casue local variations in the energy landscape. Using Transient Photoluminescence Microscopy, we provide a direct visualization of the impact of disorder on the transport characteristics in these materials, and allow us to start formulating desing-rules for optimized energy landscapes.
2.4-I2
Understanding exciton transport in quantum dot (QD) solids is crucial to their broad applications in emerging devices. Here we reveal the early-time (femtosecond) dynamics of exciton in QD solids by transient absorption microscopy [1]. We find unusually high exciton diffusivities (~102 cm2 s-1 ) in lead chalcogenide QDs within the first few hundred femtoseconds after photoexcitation, followed by a transition to a slower transport regime (10-1~1 cm2 s-1). Counterintuitively, the initial diffusivity is higher in QD solids with larger interdot distances, and the transport phase also lasts longer. This initial fast transport only occurs in materials with exciton Bohr radii much larger than the QD sizes, suggesting this regime is based on delocalized excitons, and the transition to slower transport is related to the process of exciton localization. Both higher QD packing density and heterogeneity accelerate the transition. These results suggest new principals to control the optoelectronic properties of QD solids.Understanding exciton transport in quantum dot (QD) solids is crucial to their broad applications in emerging devices. Here we reveal the early-time (femtosecond) dynamics of exciton in QD solids by transient absorption microscopy. We find unusually high exciton diffusivities (~102 cm2 s-1 ) in lead chalcogenide QDs within the first few hundred femtoseconds after photoexcitation, followed by a transition to a slower transport regime (10-1~1 cm2 s-1). Counterintuitively, the initial diffusivity is higher in QD solids with larger interdot distances, and the transport phase also lasts longer. This initial fast transport only occurs in materials with exciton Bohr radii much larger than the QD sizes, suggesting this regime is based on delocalized excitons, and the transition to slower transport is related to the process of exciton localization. Both higher QD packing density and heterogeneity accelerate the transition. These results suggest new principals to control the optoelectronic properties of QD solids.
[1] Zhang et al., Nature Materials, 2022, In Press
2.4-I3
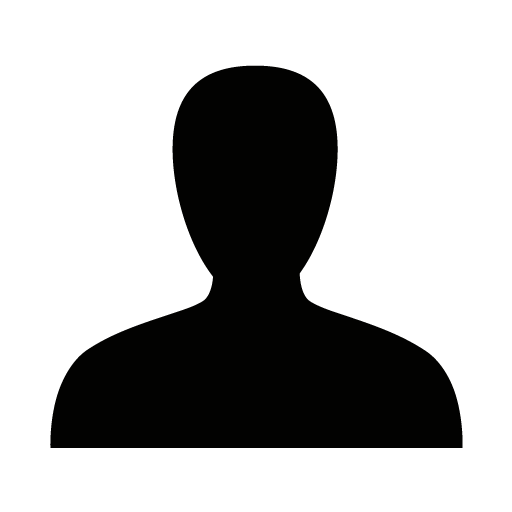
In the past 30 years, material scientists have largely capitalized on the grand appeal of utilizing quantum confinement to obtain size-tunable inter-band optical transitions and implement colloidal quantum dots (CQDs) in optoelectronic applications throughout the electromagnetic spectrum. The infrared region is particularly exciting with applications in telecommunications, night-time surveillance, and satellite imaging for agricultural water conservation. While most progress with CQDs in the infrared (IR) has been achieved using inter-band transitions in Pb- and Hg-based heavy metal compounds, intra-band optical transitions originating from external- or self- dopants can potentially expand the library of materials to generate IR-optoelectronic devices with non-toxic materials. In this talk, I will focus on my group’s work on silver chalcogenide (Ag2Se) quantum dots that exhibit distinct optical absorption in the mid-wave IR wavelength spectrum. These CQDs demonstrate a narrow bandgap metastable tetragonal phase, not available in bulk, and contain excess electrons in the lowest level of the conduction band. This allows for intra-band optical transitions between the first and the second conduction energy level which can potentially decrease Auger recombination rates and avoid the need for cryogenic cooling. I will present a detailed study of the size-dependent inter-band to intra-band optical transition and compare the competing effects of quantum confinement, environmental Fermi level and particle stoichiometry to provide guidelines for stable electron occupation of the 1Se state and obtaining tunable mid-wave IR absorption. Finally, I will also discuss some of the challenges with Ag2Se quantum dot devices and potential strategies to overcome these issues.
3.1-I1
Colloidal 2D semiconductors (nanosheets (NSs) and nanoplatelets (NPLs)) are only a few atom layers thick and strongly quantum-confined in their thickness dimension. This leads to increased exciton binding energies in the structures and optical properties that are wet-chemically tunable from visible to infrared wavelengths. Synthetic fine-tuning of the NS and NPL to control their narrow absorption and efficient emission is highly interesting for photonic and optoelectronic applications.[1,2]
In the first part of my talk, I will touch on our recent results on the synthesis of colloidal 2D transition metal dichalcogenides (WS2 and MoS2). By adjusting the Mo- and W-precursor concentration and reaction times, a control on the formation of the semiconducting vs. the metallic TMDC crystal phase during the reaction is gained and followed by XPS. We applied micro-photoluminescence spectroscopy to study semiconducting WS2 mono- and multilayer photoluminescence comparable to exfoliated WS2 for the first time.[3]
The second part of my talk is dedicated to our results on the direct synthesis of infrared-emitting 2D PbSe NPLs. By synthetically tuning the lateral size of the NPLs through the addition of small amounts of octylamine to the reaction, we obtain efficient emission of the NPLs covering the telecom O-, E- and S-band, respectively.[4] Infrared emitting NPLs are highly interesting for emerging photonic quantum technologies, e.g. single photon emission at technologically relevant wavelengths.
3.1-I2
Carriers confined in colloidal nanoplatetelets feel strong Coulomb interactions, enhanced by dielectric confinement and quasi-planar geometry. We review from a theoretical perspective how these interactions make the optoelectronic response diverge from that of quantum dots and even that of quantum wells, thus providing nanoplatelets with characteristic properties. Large exciton[1] (and trion[2]) binding energies, Giant (and Dwarf) Oscillator Strength[3] and radiative Auger processes[4] are some of the effects that can be observed with due material engineering. Special attention is paid to the role of Coulomb repulsions, which make biexcitons behave differently from simpler species, and stimulate the formation of spontaneous magnetic phases in few-electron nanoplatelets.
Further, prospects of exploiting topological effects in colloidal systems are addressed in two systems:
(i) Core/crown nanoplatelets, where carriers localized in the crown are shown to be susceptible of displaying Aharonov-Bohm phenomena;
(ii) Mercury chalcogenide nanoplatelets. Using multi-band k·p theory, we explain why recent experiments with such structures show absorption spectra which are reminiscent of cadmium-based ones, in spite of the inverted band gap these materials present in bulk. Predictions are made on the structural conditions which will permit the formation of topological (surface) states in such systems.
3.1-I3
Maria Ibáñez was born in La Sénia (Spain). She graduated in physics at the University of Barcelona, where she also obtained her PhD in 2013, under the supervision of Prof. Dr. Cabot and Prof. Dr. Morante. Her PhD thesis was qualified Excellent Cum Laude and awarded with the Honors Doctorate by the University of Barcelona. Her PhD research was funded by a Spanish competitive grant (FPU) which supported her to conduct short-term research stays in cutting-edge laboratories. In particular she worked at CEA Grenoble (2009), the University of Chicago (2010), the California Institute of Technology (2011), the Cornell University (2012) and the Northwestern University (2013). In 2014, she joined the group of Prof. Dr. Kovalenko at ETH Zürich and EMPA as a research fellow where in 2017 she received the Ružička Prize. In September 2018 she became an Assistant Professor (tenure-track) at IST Austria and started the Functional Nanomaterials group.
Thermoelectricity enables the direct and reversible conversion between heat and electricity through solid-state devices. However, despite many potential applications, their extended use has been seriously hampered by the relatively high production cost and low efficiency of thermoelectric materials. The problem is that thermoelectric materials require high electrical conductivity (s), high thermopower (S), and low thermal conductivity (k), three strongly interdependent properties.
Thermoelectric materials are often dense, polycrystalline inorganic semiconductors. Among the various strategies to produce them, the use of solution-processed nanoparticles as precursors allows their production with mild synthesis conditions, inexpensive equipment, and the possibility of high-throughput processing. However, solution synthesis generally involves the presence of additional molecules or ions belonging to the precursors or added to enable solubility and/or regulate nucleation and growth. These molecules or ions can end up in the particles either as impurities within the crystal lattice or as surface adsorbates and, therefore, interfere in the material properties.
Herein, we demonstrate the unavoidable but generally overlooked presence of ionic adsorbates in solution-processed surfactant-free synthesis and their importance in the transport properties. Furthermore, we explain the rationale behind its presence based on the fundamentals of colloidal science. These findings highlight the importance of evaluating possible unintentional impurities and their origin to i) establish the proper structure-property relationships and ii) redefine synthetic protocols to tune material properties controllably [1].
1.1-I1
A limitation for polymeric mixed ionic/electronic conductors (MIECs) is the trade-off between ionic and electronic conductivity; changes made that improve one typically hinder the other. In order to address this fundamental problem, we investigated a common oligoethylene glycol side chain polymer by adjusting the oxygen atom content and position, providing structural insights for materials that better balanced the two conduction pathways. The investigated polymer series showed the prototypical conflict between ionic and electronic conduction for oxygen atom content, with increasing oxygen atom content increasing ionic conductivity, but decreasing electronic conductivity; however, by increasing the oxygen atom distance from the polymer backbone, both ionic and electronic conductivity could be improved. Following these rules, we show that poly(3-(methoxyethoxybutyl)thiophene), when blended with lithium bistrifluoromethanesulfonimide (LiTFSI), matches the ionic conductivity of a comparable MIEC [poly(3-(methoxyethoxyethoxymethyl)thiophene)], while simultaneously showing higher electronic conductivity, highlighting the potential of this design strategy. We also provide strategies for tuning the MIEC performance to fit a desired application, depending on if electronic, ionic, or balanced conduction is most important. These results have implications beyond just polythiophene-based MIECs, as these strategies for balancing backbone crystallization and coordinating group interconnectivity apply for all semicrystalline conjugated polymers.
1.1-I2
Jenny Nelson is a Professor of Physics at Imperial College London, where she has researched novel varieties of material for use in solar cells since 1989. Her current research is focussed on understanding the properties of molecular semiconductor materials and their application to organic solar cells. This work combines fundamental electrical, spectroscopic and structural studies of molecular electronic materials with numerical modelling and device studies, with the aim of optimising the performance of plastic solar cells. She has published around 200 articles in peer reviewed journals, several book chapters and a book on the physics of solar cells.
Conjugated polymers with polar side chains are capable of mixed electronic and ionic conduction, making them attractive candidates for electrodes in electrochemical devices. Their electrochemical and redox properties can be tuned through choice of polymer backbone and side chain [1, 2] while the processible nature of the materials facilitates both manufacture and recycling. When applied as electrodes in electrochemical energy storage devices, conjugated polymer electrodes show excellent charging and discharging rates, high coulometric efficiency and compatibility with simple salt-water electrolytes, whilst specific capacity and stability in ambient environments need to be improved [3]. In this work, we investigate the impact of the of side-chain and backbone structure on the redox properties, charging rate, specific capacity, mass uptake and cycling stability of the electrodes. We show that small changes of the side chain composition can significantly influence the degree of water uptake (and thereby the mechanical stability of the electrodes), the redox-stability of the materials in aqueous electrolytes, and the electrodes’ specific capacity. We use molecular dynamics simulations to understand how the side chain structure controls the internal microstructure of the polymer electrode and its response to water and ion uptake. We also explore differences in the mechanisms of charging in n and p-type polymers. Finally, we consider different strategies for enhancing specific capacity. The findings can help to develop chemical designs for improved conjugated polymer electrodes for aqueous environments.
1.1-I3
-
Organic electrochemical transistors (OECTs) are in a stage of rapid development as novel applications that use these versatile devices continue to emerge. OECTs are characterized by the coupling of both ionic and electronic inputs to modulate transistor channel conductance, which makes them ideal for interfacing electronics with biology. However, the current performance mismatch between p-type (hole-transporting) and n-type (electron-transporting) OECTs hinders the development of power-efficient complementary devices/circuits, essential to many (bio-)electronic applications. Here, we will summarize our effort to develop n-type mixed ionic-electronic conducting polymers for OECTs. We will discuss the impact of polymer backbone rigidity and molecular weight on the OECT performance. We will show large-area printing/integration of these devices and demonstrate neurosynaptic circuits capable of Hebbian learning.
1.2-T1
David Ohayon is a Postdoctoral Fellow in Biological and Environmental Science and Engineering at King Abdullah University of Science and Technology (KAUST). He received his M.Sc. and B.Sc. degrees in chemistry and physics from the Graduate School of Chemistry, Biology and Physics of Bordeaux in France in 2016, focusing on the patterning of conjugated polymers for controlled cell adhesion. He completed his Ph.D. degree in Biosciences at KAUST in 2020, focusing in the development of n-type conjugated polymers for organic bioelectronics and point-of-care applications. His research interests cover advanced and functional materials for early, rapid, and accurate disease and pathologies diagnosis, (bio)actuation, and the development of biomedical devices.
Organic bioelectronics has experienced tremendous growth over the past two decades thanks to the expansion of the library of organic electronic materials available. Electron conducting (n-type) polymers are particularly suitable to translate biological events that involve the generation of electrons. However, n-type polymers that are stable when addressed electrically in aqueous media are relatively scarce, and the performance of existing ones lags behind their hole conducting (p-type) counterparts. Here, we report a new family of donor-acceptor type polymers based on naphthalene-1,4,5,8-tetracarboxylic-diimide-bithiophene (NDI-T2) backbone where the NDI unit always bears an ethylene glycol (EG) side chain. We study how small variations in the side chains tethered to the acceptor as well as the donor unit affect the performance of the polymer films in the state-of-the-art bioelectronic device, the organic electrochemical transistor (OECT). First, we show that substitution of the T2 core with an electron-withdrawing group (i.e., methoxy) or an EG side chain leads to ambipolar charge transport properties and causes significant changes in film microstructure revealed by ex-situ X-ray scattering studies, which overall impairs the n-type OECT performance. We thus find that the best n-type OECT performer is the polymer that has no substitution on the T2 unit. Next, we evaluate the distance of the oxygen from the NDI unit as a design parameter by varying the length of the carbon spacer placed between the EG unit and the backbone. We find that the distance of the EG from the backbone affects the film order and crystallinity, and thus, the electron mobility. As such, we develop the best performing NDI-T2 based n-type OECT material to date. Our work provides new guidelines for the side chain architecture of n-type polymers for OECTs and insight on the structure-performance relationships for mixed ionic-electronic conductors, crucial for devices where the film operates at the aqueous electrolyte interface.
1.2-T2
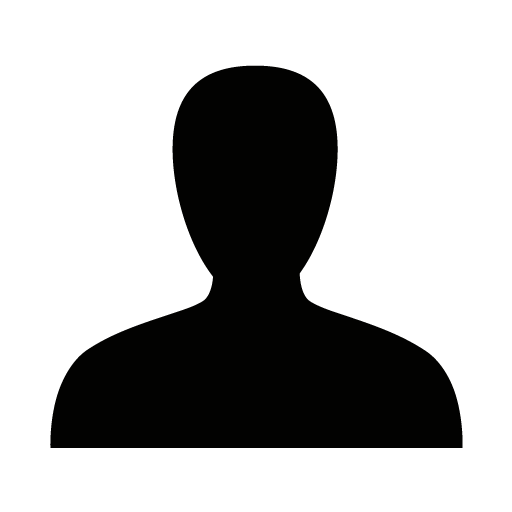
A very promising strategy to design highly efficient materials for organic mixed-ionic-electronic conductors, is based on the idea of using the backbone structure of “more traditional” conjugated polymers – already designed and optimised specifically for electronic transport – and to modify the chemical composition of their side-chains in order to achieve efficient ionic transport. However, it is well-known that side-chains play an essential role in determining the microstructure of polymer thin films, which in turns deeply affects the charge carrier mobilities of the material and the efficiency in devices. As a consequence, understanding the structural effects of exchanging alkyl for glycol side chains in conjugated polymers is of central importance in determining accurate structure-function relationships for this new class of materials.
In this talk I will demonstrate that by using our recently developed combination of vacuum electrospray deposition (ESD) and high-resolution scanning tunnelling microscopy (STM) [1], it is possible to image conjugated polymers used in organic electronic and bioelectronic applications at the (sub-)molecular scale, thereby revealing their conformation and assembly with unprecedented spatial resolution [2,3]
I will present results on the prototypical semicrystalline polythiophene polymer pBTTT and directly compare these with analogous results on the mixed-ionic-electronic conductors obtained by substituting the alkyl side-chains of pBTTT with ethylene glycol (EG) side-chains. Our high-resolution images identify clear differences in the tendency of assembling between the two types of polymers and show that this depends on the local polymer density. I will further demonstrate that glycol side-chain polymers are capable of interdigitating and use the observed structural characteristics to interpret X-ray diffraction measurements of 3D thin films [4].
Finally, I will show that our observations can be explained based on a simple model that accounts for the different intermolecular interactions between alkyl and EG chains and propose this as a general framework to rationalise the assembly of conjugated polymers with EG side-chains. Moreover, I will show that atomistic molecular dynamic simulations (Nelson group, Imperial College London) can reproduce very closely the experimentally observed polymer conformations and assembly patterns.
1.2-T3
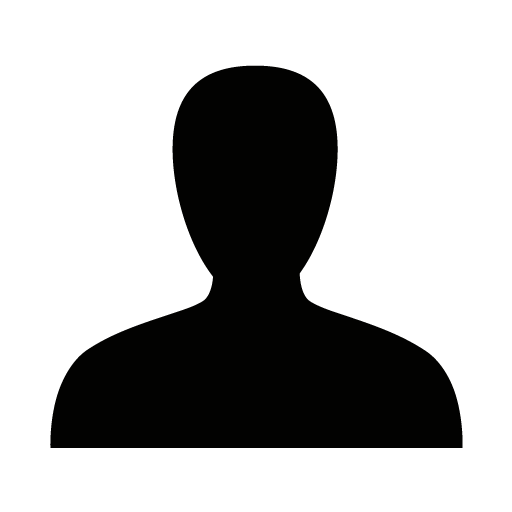
When combined with oxidase enzymes, the NDI-T2 based electron transporting (n-type) polymer led to high performance metabolite sensors, yet their working mechanism has been poorly understood.[1], [2] By monitoring oxygen, hydrogen peroxide, and pH changes in the electrolyte surrounding the n-type channel and gate as well as the potential of each electrical contact in the transistor, we shed light on the catalytic events occurring at the polymer-enzyme interface. We show that in its doped sate, the n-type film performs oxygen reduction reaction and that the n-OECT characteristics are sensitive to oxygen. We find a correlation between the amount of dissolved oxygen and the n-OECT sensor current generated during the metabolite oxidation and that using the n-type polymer at the gate electrode is critical for sensor operation. Our results show the importance of in operando analysis for understanding polymer-catalytic enzyme activity, as well as the importance of ambient oxygen in the operation of n-type devices.
1.2-T4
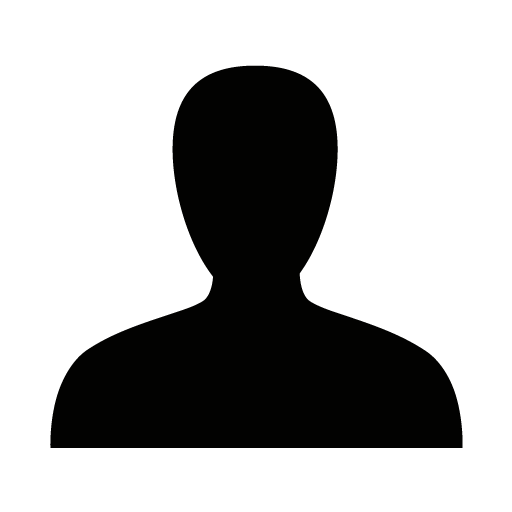
Successful adoption of defect management and carrier confinement strategies in Ruddlesden−Popper (RP) perovskites has driven the impressive improvements to performance of perovskite-based light-emitting diodes (PeLEDs) seen to date. Although functional additives have been advantageous in mitigating defects, their influence over crystallization behavior of RP (L2Am−1PbmX3m+1) perovskites has yet to be fully studied. This is especially important for blue-emitting monohalide RP perovskites, where stringent control over m domain distribution is needed for efficient PeLEDs. Herein, we investigate the effect of triphenylphosphine oxide (TPPO) on crystallization behavior of blue RP (PBA2Csm−1PbmBr3m+1) perovskites. Despite TPPO addition, its absence in the resulting film eliminates its role as a passivating agent. Instead, TPPO acts as crystallization and phase distribution modulator promoting the formation of a narrow distribution of higher m domains with higher Br content. In doing so, an enhancement of ∼35% was noted with the champion device yielding efficiency of 3.8% at λ of 483 nm.
1.3-I1
Organic mixed ionic/electronic conductors (OMIECs) have gained considerable interest in bioelectronics, power electronics, circuits and neuromorphic computing. These organic, often polymer-based, semiconductors rely on a combination of ionic transport, electronic transport, and high volumetric charge storage capacity. Growth in this field has produced a vast library of new materials and an expanding suit of new or improved device implementation strategies. In this talk, we highlight recent synthetic and processing approaches used to tailor electrochemical device properties and stability, as well as new device concepts enabled by such advances. Control of ionic transport and trapping for example presents a promising avenue towards the development of non-volatile electrochemical transistors. We show that additives in prototypical conducting polymers can lead to neuromorphic function which can mimic basic neural function. The need for deeper fundamental studies to solve persistent barriers in OMIECs is also addressed.
1.3-I2
The field of organic neuromorphic electronics has been recently emerged for biology-oriented applications as well as for the implementation of on-chip learning circuitry. Various neuromorphic devices will be presented that are based on organic-mixed conductors (ionic-electronic), materials that are traditionally used in organic bioelectronics. A prominent example of a device in bioelectronics that exploits mixed conductivity phenomena is the organic electrochemical transistor (OECT). Devices based on OECTs show volatile, non-volatile and tunable dynamics suitable for the emulation of synaptic plasticity functions. Finally, small-scale organic neuromorphic circuits allow for local learning in robotics and the realization of on-chip neuronal dynamics that are biophysically realistic.
1.3-I3
Darren J. Lipomi earned his bachelor’s degree in chemistry with a minor in physics as a Beckman Scholar at Boston University in 2005. He earned his PhD in chemistry at Harvard University in 2010, with Prof. George M. Whitesides, where he was supported by a fellowship from the ACS Organic Division. From 2010 – 2012, he was an Intelligence Community Postdoctoral Fellow in the laboratory of Prof. Zhenan Bao at Stanford University. He is now a Professor in the Department of NanoEngineering and Program in Chemical Engineering at the University of California, San Diego. His research interests include the chemistry of organic materials, especially the mechanical properties of pi-conjugated polymers for flexible solar cells, biomechanical sensors, and phenomena that occur at the intersection of materials chemistry with human perception and cognition. He is the recipient of the NSF BRIGE award, the AFOSR Young Investigator Program award, the NIH Director’s New Innovator Award, and the Presidential Early Career Award for Scientists and Engineers. He hosts a podcast, “Molecular Podcasting with Darren Lipomi” and associated YouTube channel (Darren_Lipomi) that together have >10,000 subscribers. These venues serve as a resource to students, postdocs, and other early-career researchers. His research website is lipomigroup.org.
Mechanical deformability underpins many of the advantages of semiconducting and stimuli-responsive polymers in applications from flexible solar cells to wearable devices for healthcare and virtual touch. The mechanical properties of these materials are, however, diverse, and the molecular characteristics that permit deformability while retaining function remain poorly understood. In this talk, I describe the ways in which molecular structure and solid-state packing structure govern the mechanical properties of organic semiconductors, especially of π-conjugated polymers. In particular, I describe how low modulus, good adhesion, and absolute extensibility prior to fracture enable robust performance. I will also present my group’s recent work on the intersection between the science of soft materials and the science of touch. This field, which we have named “organic haptics,” combines active polymers, contact mechanics, and psychophysics. We are beginning to understand the ways in which stick slip friction, adhesion, and capillary forces between planar surfaces and human skin affect the ways materials produce tactile objects in consciousness as mediated by the sense of touch. This work, which combines human subject experiments, laboratory mockups of human skin, and analytical models accounting for friction, has led to several important observations. In particular, we have elucidated the mechanism by which humans can differentiate hydrophilic from hydrophobic surfaces when bulk parameters such as hardness, roughness, and thermal conductivity are held constant. We have taken the insights from these psychophysical experiments to design new electroactive and ionically conductive materials to produce haptic biomaterials whose goal is to produce realistic sensations for applications in tactile therapy, instrumented prostheses, education and training, and virtual and augmented reality.
2.1-I1
H2O2 plays a significant role in a vast range of physiological processes in aerobic environments where it is produced by cells and performs vital tasks in redox signaling. The sensitivity of many biological signaling pathways to H2O2 opens up a unique direction in the development of bioelectronics devices to control levels of reactive-oxygen species (ROS). Here we present a microfabricated ROS modulation device which relies on controlled faradaic reactions. We report a concentric pixel arrangement of a peroxide-evolving cathode surrounded by an anode ring which decomposes the peroxide, resulting in localized peroxide delivery. We exploit the conducting polymer (poly(3,4-ethylenedioxythiophene), PEDOT, as the cathode. PEDOT selectively catalyzes the oxygen reduction reaction resulting in the production of hydrogen peroxide (H2O2) via a two-electron pathway. To complete the pixel, a palladium anode is used to catalytically consume peroxide. Using electrochemical and optical assays, combined with computational modeling, we benchmark the performance of the devices. The concentric pixels generate tunable gradients of peroxide and oxygen concentrations. We prototype the faradaic pixel devices by successfully modulating human H2O2-sensitive Kv7.2/7.3 (M-type) channels expressed in a single-cell model (Xenopus laevis oocytes). The Kv7 ion channel family is responsible for regulating action potential firing and neuronal excitability in various tissues as the heart, brain, and vascular smooth muscles, making it an ideal testing platform for faradaic ROS stimulation. We present also a wireless, light-driven version of this device. Our results demonstrate the potential of PEDOT to act as a H2O2 delivery system, paving the way to novel ROS-based organic bioelectronics.
2.1-I2
Though electrochemistry of conducting polymers is a rather old topic[1], only recently conducting polymers have received renewed attention as inherently mixed-ionic-electronic conductors for a number of emerging switchable technologies including actuators, electrochromic displays and electrically switchable metasurfaces.
One of the work-horses of the community remains poly(ethylenedioxythiophene) :poly(styrenesulfonate) (PEDOT:PSS). While typically known as “synthetic metal” with application as transparent flexible electrodes, the material is a mixed conductor[2] and shows ionic conductivity which is strongly affected by humidity.[3] The humidity dependence of the PSS polyelectrolyte phase together with the electroactive nature of the PEDOT can be used to create multifunctional and multiresponsive materials. A recent example from my group is the preparation of “intelligent” humidity-triggered bilayer actuators whose bending behavior (curvature) can be nicely explained by the humidity-dependent mechanical behavior of the constituents.[4] In collaboration with nanooptics experts the electrochemical stimuli were further used to switch nanoantennas “on” and “off” between the metallic and the insulating state.[5] In this context advanced electrochemical techniques based on coupling of cyclic voltammetry with in-situ transmission spectroscopy, spectroscopic ellipsometry and conductivity measurements help to shed light on the dependence of optical constants, conductivity and swelling behavior as function of the redox state.
2.1-I3
Jodie L. Lutkenhaus is holder of the Axalta Chair and Professor in the Artie McFerrin Department of Chemical Engineering at Texas A&M University. Lutkenhaus received her B.S. in Chemical Engineering in 2002 from The University of Texas at Austin and her Ph.D in Chemical Engineering in 2007 from Massachusetts Institute of Technology. Current research areas include polyelectrolytes, redox-active polymers, energy storage, and composites. She has received recognitions including World Economic Forum Young Scientist, Kavli Fellow, NSF CAREER, AFOSR Young Investigator, 3M Non-tenured Faculty Award. She is the past-Chair of the AICHE Materials Engineering & Sciences Division. Lutkenhaus is the Deputy Editor of ACS Applied Polymer Materials and a member of the U.S. National Academies Board of Chemical Sciences & Technology.
Conjugated polymers have promising applications in electronics and energy storage due to the polymer’s tunable conductivity and redox activity. For example, the conductivity of poly(3-hexylthiophene) (P3HT) is heavily dependent upon the doping level and the dopant type. This feature becomes especially important when considering P3HT or similar conjugated polymers for devices that require switching between electronic states (conductive vs insulating). In this study, the mechanism of mixed ion-electron transfer studied using electrochemical quartz crystal microbalance with dissipation monitoring (EQCM-D) is discussed. During cyclic voltammetry and galvanostatic charge-discharge experiments, the mass change of a P3HT film is monitored in real time. Distinct mass transfer regions are quantified as a function of doping level and potential, which are then correlated to changes with in situ conductance and spectroelectrochemical response. To identify the time scale at which the doping reaction transitions from kinetic to diffusion control, electrochemical impedance spectroscopy is coupled with EQCM-D. This work gives valuable insight into the nature of mixed ion-electron transfer, including its time scale, as it relates to the electronic properties of P3HT
1.1-I1
Professor Wendy L. Queen received her Ph.D. from Clemson University (USA) in 2009. Afterwards, she accepted a postdoctoral fellowship from the National Research Council, which was carried out at the NIST Center for Neutron Research (USA). In 2012, she took a scientific position at the Molecular Foundry at Lawrence Berkeley National Laboratory, and in 2015 she accepted an Assistant Professorship in the Institute of Chemical Sciences and Engineering at École Polytechnique Fédérale de Lausanne (EPFL) in Switzerland. Her research is focused on the synthesis and characterization of novel porous adsorbents, namely metal-organic frameworks, that are of interest in a number of host-guest applications. The ultimate goal of her research is to contribute knowledge towards solving globally relevant problems, like reducing energy consumption, cutting CO2 emissions, and water purification. Her desire is to help train a new generation of researchers that have the knowledge and scientific skill set necessary to become future frontrunners in energy-related research.
Among several classes of porous materials, metal-organic frameworks (MOFs) are particularly attractive due to their unprecedented internal surface areas (up to 7800 m2/g),[1] easy chemical tunability, and strong, selective binding of a host of guest species. Through judicious selection of MOF building blocks, which include metal ions and organic ligands, one can readily modify their properties for a variety of potential applications. Despite these attractive features, there are still challenges in the field that limit our ability to use MOFs as a solution for a wide range of industrial problems. For instance, some MOFs have limited mechanical and chemical stability, particularly in highly humid, acidic or basic environments. Overcoming this problem could lead to extended lifetimes and hence increased feasibility for their use in areas where such conditions are required.
In response to these needs, we have recently begun to combine MOFs and polymers in an effort to boost MOF performance and extend their stability. Our recent work demonstrates that selected polymers can significantly enhance MOF performance in a number of important liquid and gas separations[2-4] as well as extend catalyst lifetimes in selected reactions.[5] In addition to this, controlled polymerization processes were employed to enhance the mechanical stability[6] of large pore frameworks and extend the chemical stability of a number structurally diverse MOFs not only in humid environments, but also in acidic and basic media.[7] We hope such work can help bring these frameworks a few steps closer to their deployment into a range of ecologically and economically important applications. In this presentation, our recent work, devoted to the development of novel MOF polymer composites, will be outlined.
1.1-I2
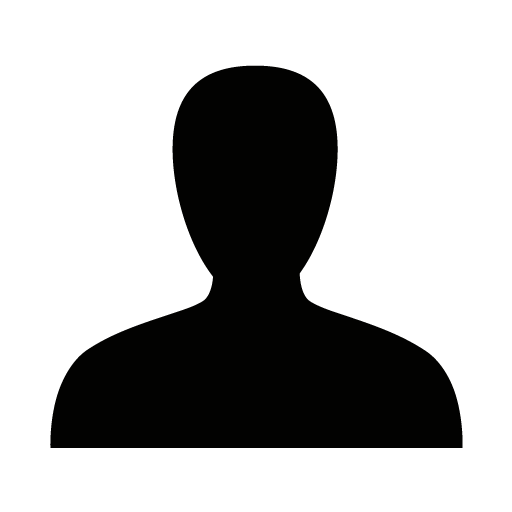
A naive comparison between nature and chemists on production capacities, e.g. biopolymers and plastics productions, respectively, marks nature as clear winner. The same accounts for the required feedstocks, because nature uses CO2 directly from air whereas the chemist relies on crude oil. Although both parties have been independent actors for such a long time, the development of Green Chemistry has helped chemists to learn nature´s biodegradation strategies in materials design and to synthesize novel bio-inspired materials. Within this respect, I will demonstrate how the chemist can dress chemistry in green on examples covering metal-free catalysts1-2, sustainable methods for Li-ion batteries recycling (Figure 1)3-4, functional lignin-based coatings5-6, electrocatalytic denitrification7, and bio-inspired fibres8.
Figure 1. Green MOF for sustainable separation of cobalt and nickel from leaching solutions.
(1) T.Zhang, A.Slabon, S.Das et al. ACS Catalysis 2021 in print
(2) I. Szewczyk, A. Slabon, P. Kuśtrowski et al. Chem. Mater. 2020, 32, 7273
(3) J. Piątek, A. Slabon et al. Adv. Energ. Mater. 2021 in print
(4) J.Piątek, A.Slabon et al. ACS Sustainable Chem. Eng. 2021, 9, 9770
(5) A.Moreno, A.Slabon, M.H.Sipponen et al. Angew. Chem. Int. Ed. 2021, 60, 20897
(6) T.M. Budnyak, A. Slabon et al. ACS Sustain. Chem. Eng. 2020, 8, 16262
(6) Z. Ma, A. Slabon et al. ACS Sustain. Chem. Eng. 2021, 9, 3658
(7) W.F. de Deus, A. Slabon, B.V.M. Rodrigues et al. ACS App. Mater. Interfaces 2021, 13, 24493
1.2-T1
Modern syntheses of colloidal nanocrystals yield extraordinarily narrow size distributions that are believed to result from a rapid “burst of nucleation” [1] followed by diffusion limited growth and size distribution focusing [2] Using a combination of in situ X-ray scattering, optical absorption, and 13C nuclear magnetic resonance (NMR) spectroscopy, we monitor the kinetics of PbS solute generation, nucleation, and crystal growth from three thiourea precursors whose conversion reactivity spans a 2-fold range. In all three cases, nucleation is found to be slow and continues during > 50% of the precipitation. A population balance model based on a size dependent growth law (1/r) fits the data with a single growth rate constant (kG) across all three precursors. However, the magnitude of the kG and the lack of solvent viscosity dependence indicates that the rate limiting step is not diffusion from solution to the nanoparticle surface. Several surface reaction limited mechanisms and a ligand penetration model that fits data our experiments using a single fit parameter are proposed to explain the results.
1.2-T2
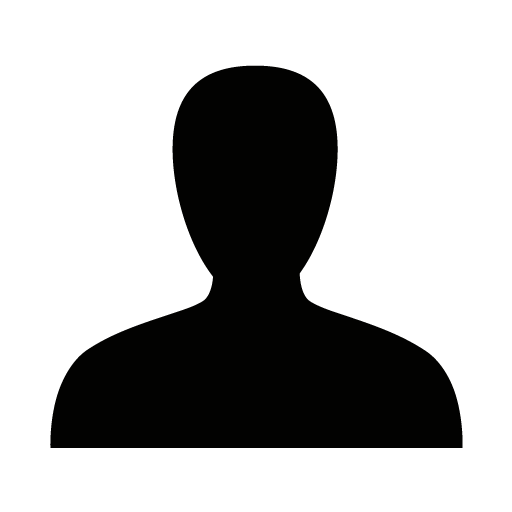
With the extremely fast growth of electronics and optoelectronics market for devices with a transparency window in the visible range, the need for proper electrodes and the related raw materials has increased exponentially1. State of the art devices for photovoltaics, lighting and energy-related applications make use, in many cases, of Transparent Conductive Oxides (TCOs) thin films2. These materials present peculiar optical and electronic properties, tailorable to meet specific requirements.
Even more desirable is the use of TCOs in the form of Nanocrystals (NCs), considering for example their solution processability. This enables a wider range of low cost and versatile implementations, such as the use of flexible, polymeric substrates3.
Nonetheless, to consider them as valid alternative materials in optoelectronics, it’s essential to have a firm control over synthesis parameters4. Process scalability holds a crucial value, considering future applications and greater production volumes.
In this contribution, we investigated methods for composition control and dopant placement in the NCs structure through colloidal synthesis. In particular, we analyzed the effects of the variation of precursor concentration injected overtime. This applies to a wide variety of doped and undoped TCOs. Among them, we focused on Indium Tin Oxide (ITO) NCs, with different composition profiles. Moreover, we present some preliminary analysis on Zinc Iron Oxide (ZIO) NCs. Both materials are interesting in energy storage applications, owing to light-driven charge accumulation.
1.2-T3
The self-assembly of nanocrystals into binary superlattices enables the targeted integration of orthogonal physical properties, like photoluminescence and magnetism, into a single superstructure, unlocking a vast design space for multifunctional materials. Yet, the formation of binary nanocrystal superlattices remains poorly understood, restricting the use of simulation to predict structure and properties of the final superlattices. Here, we use in situ scattering experiments to unravel the time-dependent self-assembly of nanocrystals into 3D binary superlattices, and molecular dynamics simulations to obtain interparticle interactions consistent with experimental observations. We show definitively that short-ranged, attractive interparticle forces are necessary to obtain the binary crystalline phases observed in experiment. The short-ranged attraction stabilizes these crystalline phases relative to fluid phases, dramatically enhancing their formation kinetics over the purely repulsive interactions of the hard-sphere model. In these conditions, the formation of binary nanocrystal superlattices proceeds through homogeneous nucleation in the absence of intermediate ordered structures. These results establish a robust correspondence between experiment and theory, paving the way towards a priori prediction of binary nanocrystal superlattices.
1.2-T4
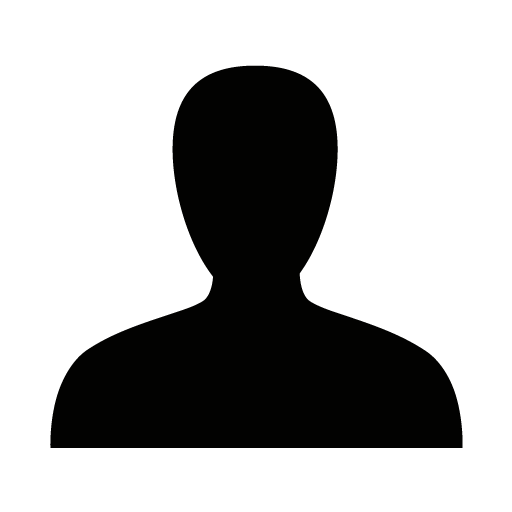
Nanoparticles and their assemblies offer unique properties, which are highly related to their composition, size, shape[1] and - for assemblies - to their nature of connection[2],[3]. By connecting CdSe/CdS (NR) dot-in-rod semiconductor nanoparticles into network structures, excited electrons can travel through the CdS shell while holes are trapped in the CdSe core region. In our work, we present an experimental approach to evaluate the spatial extent of fluorescence quenching due to the separation of the excited electrons and holes in the connected NR networks.[4] To extract this information, we perform photoluminescence spectroscopy (such as fluorescence lifetime and photoluminescence quantum yield measurements) on different types of materials, namely colloidal mixtures, hydrogels, lyogels and aerogels consisting of the semiconductor and a noble metal component. The ratio of the NRs and the Au nanoparticles (NP) attached to the NR semiconductor network is varied in a wide range. Thus, the scale of the electron travelling distances within the connected NR backbone upon using internal standards can be extracted from the macroscopic optical measurements.[4] One Au NP is able to quench the photoluminescence of several semiconductor NRs and can reach a number of nine NRs (roughly 400 nm connected CdS shell) quenched in the interconnected network.[4]
1.3-I1
Giuseppe Portale is associate professor at the Zernike Institute for Advanced Materials, University of Groningen, the Netherlands. He received his Ph.D. degree in Chemistry from the University La Sapienza, Rome and he carried out postdoc research at the ESRF in Grenoble. From 2009 to 2015 he was beamline responsible at the ESRF and in 2015 he was appointed as professor at the University of Groningen. He is the head of the Polymer Physics group, focusing on the study of structure-property relationship in polymer-based materials and on the influence of processing conditions on the final structure of polymer specimens and devices.
Nanoparticle thin films and polymer/nanoparticle composites show a wide range of applications in catalysis, electrochemistry, optoelectronics, sensors and nanomedicine. In all these applications, detailed knowledge of the nanoparticle size and aggregation behavior is necessary to understand the properties of the produced systems.
Metallic and metal oxide nanoparticles can be produced chemically first and later deposited in thin films or included inside a polymeric matrix. Despite the chemical route allows great control of the nanoparticle shape and the size dispersity, the use of insulating ligands to passivate the nanoparticle surface and enhance nanoparticle stability and solubility is not advantageous for electronic applications. Alternatively, ligand-free nanoparticles can be produced by physical methods in the gas phase and be transported via aereosol to a substrate to form thin films or composites.
In this talk, the production of different metallic nanoparticles (Au, Pt and Sn) using spark ablation technique is discussed. Using X-ray scattering, information on the nanoparticle synthesis and their deposition in thin and ultra-thin films are obtained. Quantitative analysis is demonstarted, allowing the measurement of size polydispersity and the aggregation behavior when deposited on silicon substrated. The quantitative analysis can be conducted on well-defined nanoparticles (Pt and Au) as well as on reactive (Sn/SnOx) nanoparticles.
A recent example of the use of these aereosol-generated nanoparticles in conducting polymer nanocomposites is also illustrated. Low energy deposition methods was employed to gentle deposite nanoparticle on a thin films of a conducting, thermoelectric polymer such as PEDOT:PSS. Own to the naked nanoparticle nature and the interaction between the nanoparticle and the polymer, the thermoelectric properties of PEDOT:PSS can be drastically enhanced.[1]
1.3-I2
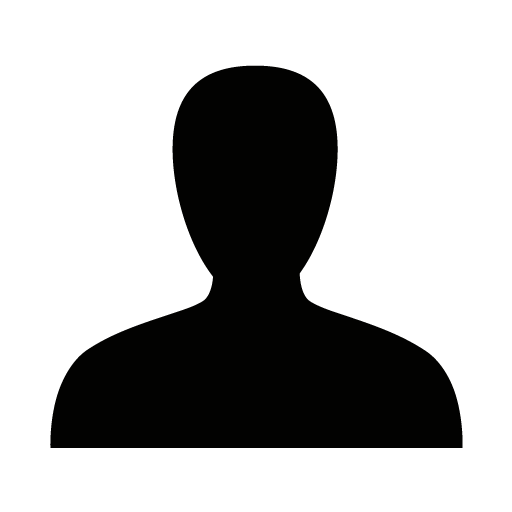
The ability to organize functional nanoscale components into the targeted architectures promises to enable a broad range of nanotechnological applications, from designed biomaterials to photonic devices and information processing systems. However, we are currently lacking an adaptable and broadly applicable methodology for the 3D bottom-up nanomaterials fabrication with ability to prescribe a structure of organizations and to integrate different types of components. The talk will discuss our progress in establishing a versatile platform for the fabrication of designed large-scale and finite-size nano-architectures from diverse nanocomponents through the DNA-programmable assembly. The recent advances in creating periodic and hierarchical organizations from inorganic nanoparticles, proteins and enzymes will be presented. The use of the developed assembly approaches for generating functional nanomaterials with nano-optical, electrical, mechanical and biochemical functions will be demonstrated.
2.1-I1
Our team works on developing ultrathin optoelectronic devices for biomedical implants that can stimulate biophysical processes. All of these devices rely on near infrared irradiation in the tissue transparency window to actuate nanoscale organic semiconductor components. Our motivation is to provide a minimalistic wireless implant which can perform the duty of standard implantable electrodes, but without invasive wiring. The devices we fabricate are not only wireless, but also 100-1000 times thinner than most existing technologies. Making implants have as small as possible mechanical footprint improves the efficacy of bioelectronic medical treatments by minimizing the risk for inflammation and making surgical implantation less invasive.
Organic semiconductor thin films can afford charging of electrolytic double layers or faradaic reactions. The magnitude of these two effects will depend on the thermodynamics of the materials used in the devices, in particular the nature of the cathodic and anodic components of the device, as well as the capacitance. Through judicious selection of materials one can obtain high photovoltages which can either drive efficient charging of double layer capacitors or faradaic reactions. The former is used to generate displacement currents which can capacitively couple with the cell membrane potential of nearby cells – this can be used to stimulate action potentials. Our experiment and model converge to create a detailed picture of how such devices, known as organic electrolytic photocapacitors, work to affect the gating of ion channels. This device can mimic biphasic current-pulse neurostimulation and thus transduces an optical signal into directly-evoked action potentials in neurons. On the other hand, the other block of our research efforts is directed at devices which, when stimulated with light, perform faradaic chemistry. We focus on the delivery of controlled amounts of reactive oxygen species (mostly peroxide). We aim to study the effects of photoelectrochemically-generated peroxides on physiological processes, with the hope of developing novel therapeutic approaches to neurodegenerative diseases.
2.1-I2
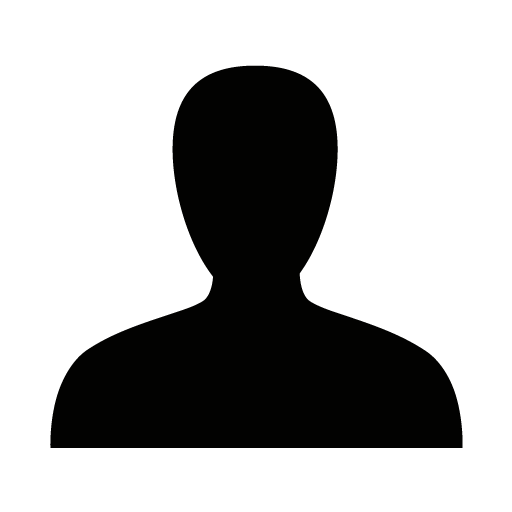
Breaking the symmetry: Designing colloidal microswimmers
Breaking symmetry is at the very core of achieving propulsion at the microscale, where viscous forces dominate. Nature has perfected a range of different strategies to reach this goal for swimming microorganisms, which scientists have taken inspiration from to produce artificial micro-swimmers [1]. A common way to achieve propulsion at the colloidal scale is to produce artificial particles that have asymmetric shapes and surface properties. I will show a new fabrication strategy to create microswimmers with full control on their geometrical and compositional asymmetry. The method is based on the sequential deposition of microspheres on topographical templates, where we independently define the swimmers’ shape by defining the shape of template, and we program their composition by fixing the deposition sequence [2]. I will show how we can use this fabrication strategy to design and obtain particles that translate, rotate, switch between these two modes of motion and even perform drag-and-drop tasks in crowded environments, propelled by uniform AC electric fields [3,4]. These results show how the design of microswimmers can enable the development of active components for the realization of autonomous systems working in complex environments.
2.2-T1
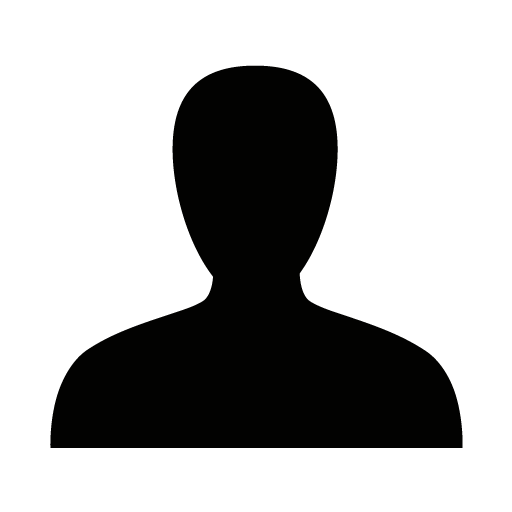
Nanocrystals (NCs) of intermetallic compounds (IMCs, long-range ordered alloys) are a large family of emerging materials. They offer attractive characteristics originating from well-defined crystallinity, synergistically combined properties of the incorporated metals and unique size- and surface-related physical and chemical phenomena exhibited by NCs. Intermetallic NCs have recently acquired a large amount of research interest due to their extensive applications in catalysis, electronics, e.g. thermoelectric devices and memory technologies, superconductivity, energy storage and conversion technologies, photonics and in life sciences and medicine. Nevertheless, a general synthetic method towards uniform intermetallic nanocrystals was still lacking.
We have developed a wet-chemical colloidal synthesis method towards uniform intermetallic nanocrystals based on the amalgamation of monometallic nanocrystal seeds with low-melting point metals [1]. We use this approach to achieve crystalline and compositionally uniform intermetallic nanocrystals of Au-Ga, Ag-Ga, Cu-Ga, Ni-Ga, Pd-Ga, Pd-In and Pd-Zn compounds. We demonstrate both compositional tunability across the phase spaces (e.g. AuGa2, AuGa, Au7Ga2 and Ga-doped Au), size tunability (e.g. 14.0, 7.6 and 3.8 nm AuGa2) and size uniformity (e.g. 5.4 % size deviations). In summary, the developed amalgamation seeded growth approach makes it possible to systematically achieve size and composition controlled intermetallic nanocrystals of excellent quality, opening up a multitude of possibilities for these materials.
2.2-T2
Intermetallic nanoparticles (NPs), defined by an atomically ordered structure with high stability, have shown enhanced catalytic properties as compared to their disordered alloy counterparts.1,2 The enhancement in catalytic properties is partially attributed to changes in the nature of available surface sites, which are induced by changes in the crystal structure.3,4, To advance green energy solutions and pave the way for new improved catalytic materials comprised of intermetallic NPs, it is therefore crucial that we understand what controls the formation of intermetallic NPs to synthetically promote these structures. By carefully selecting the additives used in a PdCu NP synthesis, we show that monodisperse, intermetallic PdCu NPs can be synthesized in a controllable manner. In this synthesis, the additives iron(III) chloride and ascorbic acid are used to control both the morphology and polymorph of the intermetallic NPs. Ascorbic acid provides a fast reduction of the ionic metal precursor species, while iron(III) chloride facilitates ligand exchange with the metal ion complexes and can assist in oxidative etching. Combined, ascorbic acid and iron(III) chloride provide a synergetic effect resulting in precursor reduction and defect-free growth; ultimately leading to monodisperse intermetallic PdCu NPs. Using in situ X-ray total scattering and pair distribution function analysis, we follow the disorder-order transformation all the way from the initial precursor structure to the formation of intermetallic PdCu NPs. We report a hitherto unknown transformation pathway that diverges from the commonly reported co-reduction disorder-order transformation. A Cu-rich structure initially forms, followed by incorporation of Pd(0) into the structure to obtain the disordered alloy PdCu structure. The formation of a Cu-rich structure suggests that it is not essential that the metallic species reduce at the same rate to ultimately form stoichiometric intermetallic PdCu NPs, as previously believed. These findings underpin the importance and strength of performing further combined multi-technique studies to uncover the driving force of intermetallic NP formation. When we understand how intermetallic NPs are formed, these mechanistic insights might open new opportunities to expand our library of intermetallic NPs by exploiting synthesis by design.
2.2-T3
Colloidal heterostructure nanocrystals (NCs) exhibit enhanced physical and electronic properties due to the synergistic effect of chemically distinguished domains.[1], [2] Among them, multi-pods are more active in electrochemical processes than core-shell structures accredited to the more accessible core with adequate shielding from electrochemical reaction-related adverse structural deformations.3 However, the synthesis and development of colloidal multipods lack a direct growth approach. Here, we synthesize colloidal Bi-Cu2-xS multipods, and single pod NCs in a solution-liquid-solid (SLS) growth of Cu2-xS on insitu formed Bi NCs (Figure 1). We reveal that controlling the state (liquid or solid) of Bi seed and influx rate of Cu+ cations can alter the number of pods formation during hetero-nucleation of Bi seeded Cu2-xS heterostructures. The ex-situ mechanistic investigation of the growth process reveals that the equivalent amount of diphosphonic acid (DPA) stabilizes the Cu-thiolate complex, thus lowering the free Cu+ concentration and increasing the induction time. Therefore, Bi NCs transition into solid faceted NCs, forming a single Cu2-xS pod. In contrast, a low DPA (0 to 0.5 mmol) concentration cannot stabilize the Cu-thiolate complex, resulting in high free Cu+ concentration and multipods of Cu2-xS on Bi. Furthermore, we studied the electrochemical performance of Bi-Cu2-xS as anode material for potassium ion batteries.
2.3-T1
Loren Deblock is a PhD researcher in chemistry, based in the Department of Chemistry of Ghent University. He specializes in inorganic chemistry but is also particularly interested in organic synthesis and the usage of inorganic nanomaterials for biomedical applications.
Iron oxide and hafnium oxide nanocrystals are two of the few successful examples of inorganic nanocrystals used in a clinical setting. Although crucial to their application, their aqueous surface chemistry is not fully understood. The literature contains conflicting reports regarding the optimum binding group. To alleviate these inconsistencies, we set out to systematically investigate the interaction of carboxylic acids, phosphonic acids and catechols to metal oxide nanocrystals in polar media. Here [1], we use Nuclear Magnetic Resonance spectroscopy and Dynamic Light Scattering to map out the pH-dependent binding affinity of the ligands towards hafnium oxide nanocrystals (an NMR compatible model system). Carboxylic acids easily desorb in water from the surface and only provide limited colloidal stability from pH 2 – 6. Phosphonic acids on the other hand provide colloidal stability over a broader pH range but also feature a pH-dependent desorption from the surface. They are most suited for acidic to neutral environments (pH < 8). Finally, nitrocatechol derivatives provide a tightly bound ligand shell and colloidal stability at physiological and basic pH (6-10). While dynamically bound ligands (carboxylates and phosphonates) do not provide colloidal stability in phosphate buffered saline, the tightly bound nitrocatechols provide long term stability. We thus shed light on the complex ligand binding dynamics on metal oxide nanocrystals in aqueous environments. Finally, we provide a practical colloidal stability map, guiding researchers to rationally design ligands for their desired application.
2.3-T2
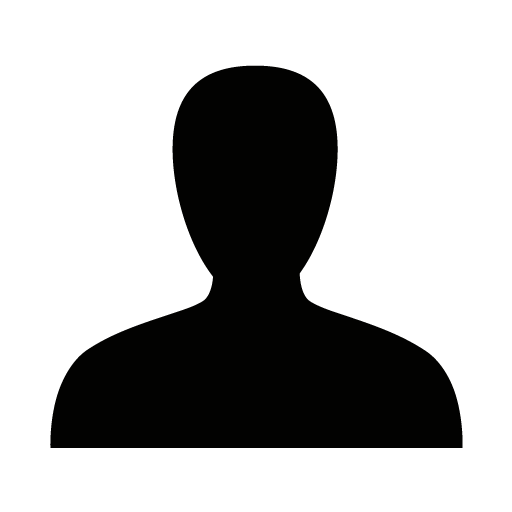
Colloidal InAs quantum dots (QDs) are considered as an infrared material candidate for many applications. Yet, there is not enough realization of the surface chemistry of these QDs. Here, 1H NMR has been used to analyze the surface chemistry of recently reported InAs QDs produced using In(I)Cl as both the reducing agent and the precursor. After exposing QDs surface to acids, we realize that Oleylammonium Chloride salt is removed from the surface, suggesting InAs QDs are terminated by Oleylamine used as the sole coordinating solvent and Cl anions. In fact, there is only a partial displacement between the initially present Oleylamine and the added acid. Comparison of initial and final concentrations of bound Oleylamine results an exchanged fraction of about 56% after titration with excess carboxylic acid. Furthermore, addition of excess alkylthiol proved to result in a similar ligand exchange process in which it binds as a thiolate together with the desorption of Oleylammonium Chloride salt proved by H NMR and X-ray Photoelectron Spectroscopy analyses.
2.3-T3
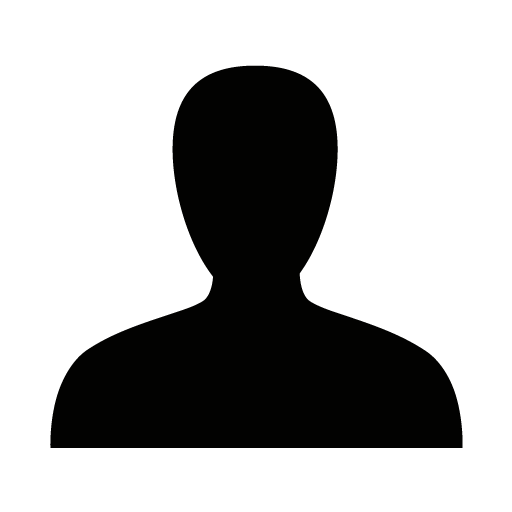
Hybrid organic–inorganic perovskite semiconductors have triggered a paradigm shift in the field of photovoltaics (PVs). Low dimensional 2D hybrid organic–inorganic lead halide semiconductors have recently attracted major attention [1] owing to the emergence of new photo-physical properties compared to the 3D perovskites, including exciton-phonon coupling, edge state based charge dissociation at grain boundaries and photon confinement [2]. The ionic nature of these layered materials also make them ideal candidates for solution processing into both thin films and nanostructured crystals [3]. Studying the wide range of process parameters in different solution processing techniques like spin coating [4], drop casting [5,6], meniscus coating [7] is important as they greatly influence the crystallization of 2D perovskites. However, this plethora of parameters in solution processing of 2D perovskites are not understood as well as their 3D counterparts.
Here we report the effects of different lead precursors in the crystallization process of 2D perovskite during a simple drop-cast based synthesis. We study the role of lead iodide (PbI2), lead carbonate (PbCO3) and lead dioxide (PbO2) in the crystallization of BA2PbI4 perovskite in IPA-based solvent system. Our in-situ observations during the drop-casting process reveal the different crystallization kinetics based on the initial lead precursor. We also observe crystallization of the perovskite either on the substrate or in solution as floating micro-plates or both depending on the type of the precursor. We attribute this behavior to the formation of different co-ordinate complexes based on the initial lead precursor. Upon structural and optical investigation of the synthesized perovskite crystals (on substrate and in solution), we find them to be highly crystalline when compared against the traditionally grown and exfoliated 2D perovskites.
2.4-I1
i
Semiconductor nanoplatelets have attracted interest due to their exceptionally narrow optical features. However, the direct synthesis of semiconductor nanoplatelets is limited to nanoparticles with thicknesses from 2 to 6 monolayers (N monolayers NPLs present N+1 planes of cations alternated with N planes of chalcogens in the [001] direction of the zinc blende crystal structure). It thus limits the wavelength range reached by such materials. Here, we show that it is possible to synthesize thicker NPLs by carefully tuning the NPLs surface energy. The native carboxylate ligands are exchanged with halides ligands which induce a further dissolution of the NPLs and a recrystallization of the released monomers on the top and bottom wide facets. This versatile method is applied to all three cadmium chalcogenide semiconductor. Thus, in NPLs, where the surface represents a high ratio of the particles, the surface chemistry is a key factor. In the second part of the presentation, we will show that the control of surface chemistry is also a way to tune the shape of the NPLs.
2.4-I2
Maria Ibáñez was born in La Sénia (Spain). She graduated in physics at the University of Barcelona, where she also obtained her PhD in 2013, under the supervision of Prof. Dr. Cabot and Prof. Dr. Morante. Her PhD thesis was qualified Excellent Cum Laude and awarded with the Honors Doctorate by the University of Barcelona. Her PhD research was funded by a Spanish competitive grant (FPU) which supported her to conduct short-term research stays in cutting-edge laboratories. In particular she worked at CEA Grenoble (2009), the University of Chicago (2010), the California Institute of Technology (2011), the Cornell University (2012) and the Northwestern University (2013). In 2014, she joined the group of Prof. Dr. Kovalenko at ETH Zürich and EMPA as a research fellow where in 2017 she received the Ružička Prize. In September 2018 she became an Assistant Professor (tenure-track) at IST Austria and started the Functional Nanomaterials group.
The conversion of thermal energy to electricity and vice versa through solid-state thermoelectric devices is extremely appealing for many applications. Greater than 60% of all the energy produced is lost as waste heat. However, thermoelectric materials have high production costs and low efficiency, limiting their use to niche applications. The problem is that thermoelectric materials require high electrical conductivity (s), high thermopower (S), and low thermal conductivity (k), three strongly counteracting properties.
Thermoelectric materials are often dense, polycrystalline inorganic semiconductors. Solution synthesis of nanoparticles emerged as an alternative to prepare thermoelectric materials with less demanding processing conditions than conventional solid-state synthetic methods. Moreover, it provides opportunities to synthesize nanoparticles with well-defined structures (size, shape, composition, and crystal structure), offering a broad range of tunable properties to target the desired polycrystalline material.
One of the most remarkable properties of a polycrystalline material, especially if the crystal domains go down to the nanoscale, is the high density of grain boundaries/interfaces and related defects. Such structural properties determine the material transport properties and, therefore, the thermoelectric performance. However, defect engineering in polycrystalline materials suffers the loss of control by the tendency of the crystals to grow and defects to annihilate, especially during high-temperature processes and operations. This limitation is particularly concerning for the bottom-up engineering of nanostructured thermoelectric materials. To date, very little attention has been paid to the grain growth of the original nanostructured powder during thermal processing or even during operation.
The work presented here report two important advances [1]:
- Crystalline grain growth inhibition in nanocomposites at high processing and operating temperatures obtained through a particle surface treatment.
- Scalable, simple, and economical method to produce high-performance polycrystalline thermoelectric materials through defect engineering.
1.1-I1
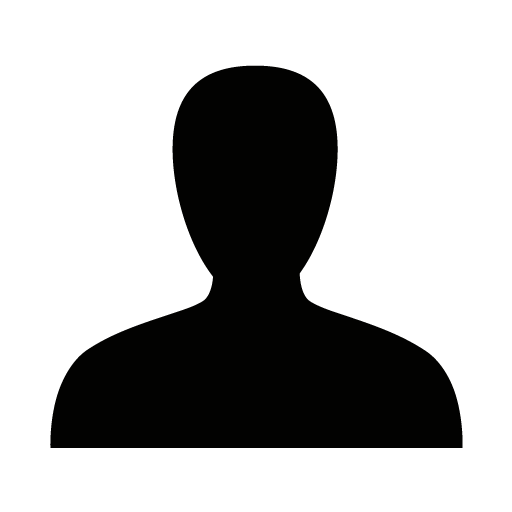
Graphene quantum dots (GQDs) and carbon dots (CDs) have been attracting research attention as carbon-based and environmentally friendly alternative of the inorganic semiconductor quantum dots. Similar to their inorganic counterpart, GQDs and CDs demonstrate promising optical properties with emission wavelength dependent on their sizes. However, their precise chemical structures are unknown and uncontrollable in most of the cases, which makes it difficult to fine-tune the properties or establish the structure-property relationship. To this end, the methods of synthetic organic chemistry provide the bottom-up approach to obtain GQDs with atomically precise structures, namely large polycyclic aromatic hydrocarbons (PAHs) that are also called nanographenes [1]. We have synthesized dibenzo[hi,st]ovalene (DBOV) as a molecular GQD with a combination of armchair and zigzag edges, which demonstrated strong red emission and stimulated emission [2]. Regioselective bromination of DBOV could be established, enabling the edge-functionalization to install various functional groups for the fine-tuning of the optical and electronic properties [3]. For example, fluoranthene imide groups were attached to DBOV, which led to red-shifted emission with larger Stokes shift [4]. The π-extension of DBOV was achieved through Pd-catalyzed alkyne benzannulation, affording circumpyrene as GQD with multiple zigzag edges [5]. On the other hand, nitrogen-doped DBOV showed acid- and metal-sensitive fluorescence, indicating its potential for sensing applications [6]. We have more recently also synthesized a wider variety of functionalized DBOVs as well as novel GQDs, providing further insights into the structure-property relationship. These results highlight the high potential of molecular GQDs for a wide range of applications in the optoelectronics and photonics.
1.1-I2
Nonlinear optics is of paramount importance in several fields of science and technology: it is commonly used for frequency conversion, self-referencing of frequency combs, sensing, and ultra-short pulse generation and characterization. Large efforts have been devoted in the last years to realizing electrical and all-optical modulation of the nonlinear optical response of atomically thin materials, which are easy to integrate on photonic platforms and thus ideal for novel nanoscale devices [1][2]. In this talk, I will present different approaches to achieve large modulation of the harmonic generation in graphene and in transition metal dichalcogenides (TMDs). The first method is based on the electrical control of the graphene’s Fermi energy. This allows to tune the nonlinear multi-photon resonances occurring within the Dirac cone and thus to achieve a large electrical modulation of the third harmonic generation [3]. In addition, third harmonic generation in graphene can be modulated by tuning the electronic temperature in an all-optical approach [4]. The second example regards broadband all-optical modulation of the second harmonic generation. The concept is based on symmetry considerations and thus it is applicable to any material of the D3h symmetry group and with deep sub-wavelength thickness, such as all monolayer TMDs. With this approach we demonstrated a 90° rotation of the second harmonic polarization on a time-scale limited only by the fundamental pulse duration. In addition, this ultrafast polarization switch can be immediately applied to realize all-optical second harmonic amplitude modulation [5].
1.1-I3
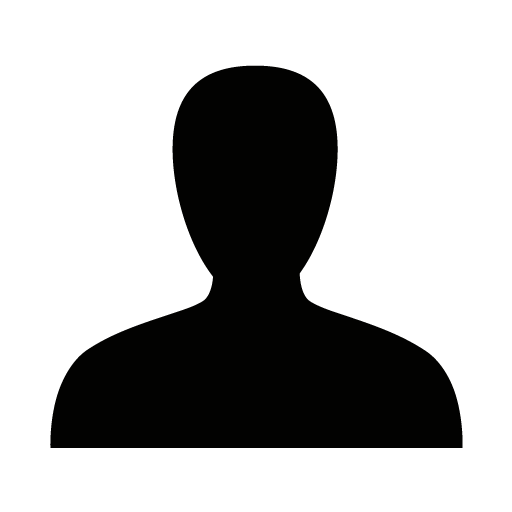
The development of industrial-scale, reliable, inexpensive production processes of graphene and related two-dimensional materials (GRMs)[1,2] is a key requirement for their widespread use in several application areas,[1-6] providing a balance between ease of fabrication and final product quality. In particular, in the energy sector, the production of GRMs in liquid phase [2,6] represents a simple and cost-effective pathway towards the development of GRMs-based energy devices, presenting huge integration flexibility compared to other production methods.
In this presentation, I will first briefly introduce the key properties of GRMs. Then, I will present the strategy of BeDimensional in the production of GRMs by wet-jet milling [7] and the Industrial scale up. Afterward, I will provide a brief overview on some key applications of the as-produced GRMs, for anticorrosion coatings and energy conversion and storage devices. [3,8-16]
REFERENCES:
[1] F. Bonaccorso, et. al., Adv. Mater. 28, 6136-6166 (2016).
[2] F. Bonaccorso, et al., Materials Today, 15, 564-589, (2012).
[3] F. Bonaccorso, et. al., Nature Photonics 4, 611-622, (2010).
[4] E. Pomerantseva, F. Bonaccorso, et al., Science 366 (6468) eaan8285 (2019).
[5] G. Iannaccone F. Bonaccorso, et al., Nature Nanotech 13, 183, (2018).
[6] A. C. Ferrari, F. Bonaccorso, et al., Nanoscale, 7, 4598-4810 (2015).
[7] A. E. Del Rio Castillo et. al., Mater. Horiz. 5, 890 (2018).
[8] F. Bonaccorso, et. al., Science, 347, 1246501 (2015).
[9] L. Najafi et al., Advanced Energy Materials 8 (16), 1703212 (2018).
[10] E. Lamanna et al., Joule 4, 865-881 (2020).
[11] S. Bellani, et al., Chem. Soc. Rev. DOI: 10.1039/D1CS00106J (2021)
[12] M. Garakani, et al. Energy Storage Materials 34, 1-11 (2020).
[13] S. Bellani, et al. Nano Lett. 18, 7155-7164 (2018).
[14] A. E. Del Rio Castillo, et al., Chem. Mater. 30, 506-516 (2018).
[15] S. Bellani, et al. Nanoscale Horizons 4, 1077 (2019).
[16] S. Bellani, et al. Adv. Funct. Mater. 29, 1807659 (2019).
"This project has received funding from the European Union's Horizon 2020 research and innovation programme under grant agreement GrapheneCore3 - 881603"
1.2-T1
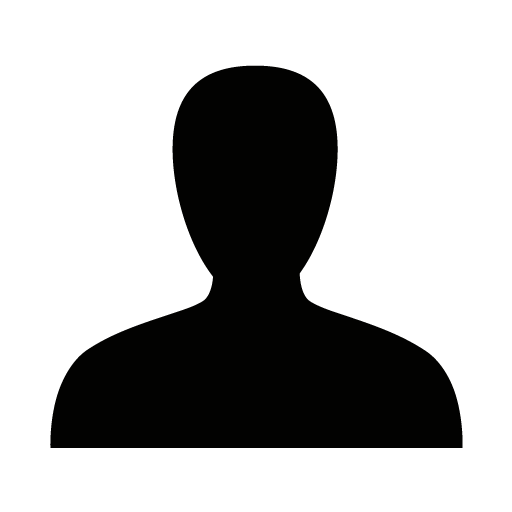
Lasers based on organic active media have attracted great attention for many years, mainly because they enable wavelength tuning across the visible spectrum. The development of thin film organic lasers brought additional advantages of compactness, mechanical flexibility and low-cost production.[1] Among such devices, distributed feedback (DFB) lasers have demonstrated potential for applications in different areas, such as spectroscopy, optical communications and chemical and biological sensing. For some of these applications, materials operating in the near-infrared (NIR) region, where only a few organic lasers have been demonstrated up to date, are pursued. The principal reason for this lack of NIR emitters is the detrimental effect of fast non-radiative decay following the energy gap law, concentration quenching mechanisms and intra-gap absorption of charge-transfer and triplet states.[2] Hence, a proper understanding of such mechanisms and how to prevent them might lead to a new generation of NIR organic emitters. In this regard, nanographenes (NGs) are emerging materials which offer a great promise for light-emitting devices and integration with other technologies.[3]
In this presentation, the photophysical and laser properties of two series of NGs dispersed in thermoplastic polymer films will be described, both appertaining to the same family of [n,m]peri-acenoacenes in which a number m of [n]acenes are fused into a rhombic nanoflake (see figure). These four-zigzag edged NGs are an excellent platform for investigating the effect of size increase and zigzag edge elongation upon their photophysical and lasing properties.[4,5] Getting insights into such mechanisms provide useful information for the design of materials with emission in the NIR region. In particular, electronic wave function delocalization over the zigzag edge contributes to the red-shift improvement.[6] Following this approach, light amplification in the NIR has been demonstrated with two different NGs of the peri-acenoacene family.
Additionally, the suitability of these compounds for lasing applications has been proved with the manufacture of DFB lasers consisting of active films with top-layer resonators (1D relief gratings fabricated by holographic lithography).[7] The excellent control that provides the lithographic method over the resonator parameters and the quality of the laser architecture enable the manufacture of operational laser devices across the visible spectrum and entering into the NIR region by using the different NGs.
1.2-T2
I was born and raised in Uberlandia, Brazil. I studied Physics and Mathematics at the Federal University of Uberlandia, Brazil, and I did my PhD in Physics in the Sao Carlos Institute of Physics at the University of Sao Paulo, Brazil. Afterwards, I spent nearly 4 years as an Assistant Professor of Physics at the University of Alfenas, Brazil, and I held researcher positions at the University of California-Davis, USA, University of Chicago, USA, and University of Duisburg-Essen, Germany. In August 2019 I was appointed Lecturer in Physics in the School of Chemical and Physical Sciences at Keele University, England.
Combining two-dimensional (2D) materials with organic materials can be very attractive for applications that require flexibility and where size and weight are important parameters to be considered, such as in wearable, portable and mobile applications. Organic materials usually exhibit excellent optical absorption efficiency and photo- and temperature-induced conformational changes, while 2D materials often show relatively high carrier mobility, superior mechanical flexibility, and tunable electronic and optical properties. Combining both systems can stabilize the organic materials and lead to heterostructures with both high carrier mobility and high optical absorption efficiency, which is promising for solar energy conversion. In this work we investigate, by means of density-functional-theory calculations, heterostructures composed of pentacene molecules and transition metal dichalcogenides (TMD) for application in photovoltaic devices. We examine the interaction between the molecules and monolayer TMDs as well as the band alignment of the heterostructures, considering effects of the molecular coverage and dielectric screening. Our results show that the band edge positions of pentacene change significantly when going from the isolated molecule to the monolayer coverage, affecting the band alignment with a TMD monolayer.
1.2-T3
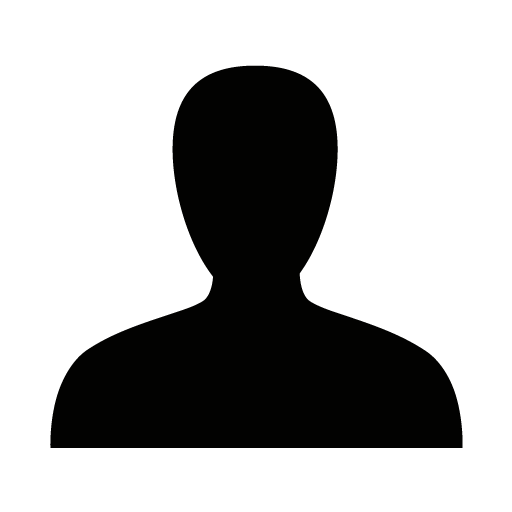
Several works focused on studying the properties of 2D materials[1],[2] and on trying to optimize their production[3],[4], in order to employ them as active components for different applications. However, the use of pristine 2D materials is often not enough to target specific uses and therefore chemical functionalization can come into play as a versatile method to tune and bring additional properties to the nano sheets.
In this contribution, we will report on our work carried out on the functionalization and cross-linking of liquid-exfoliated graphene and 2D MoS2 to produce tridimensional networks, using polymers as cross-linkers between the different nano-sheets. The chemical functionalization is the key to the construction of such networks: for graphene we employ the Tour reaction[5] to directly bind ad-hoc decorated aryl moieties on the basal plane that are then exploited to connect the sheets to each other through a polymerization reaction. For 2D MoS2, instead, bifunctional thiols are employed to both functionalize and cross-link the material in a one pot reaction. The formation of hydrogels is facilitated by the use of cross-linkers well-known for their gelation properties such poly-acrylates and poly ethylene glycols. A subsequent step of freeze-drying allows to obtain the corresponding aerogels, composite materials characterized by high surface areas available for exchange processes, like catalysis or charge-discharge.
The resulting networks are characterized by a combination of techniques to infer the structural and electronic properties. The electrochemical characterization further allows to infer the potential for use of this materials in electrocatalysis and in energy storage applications.
1.2-T4
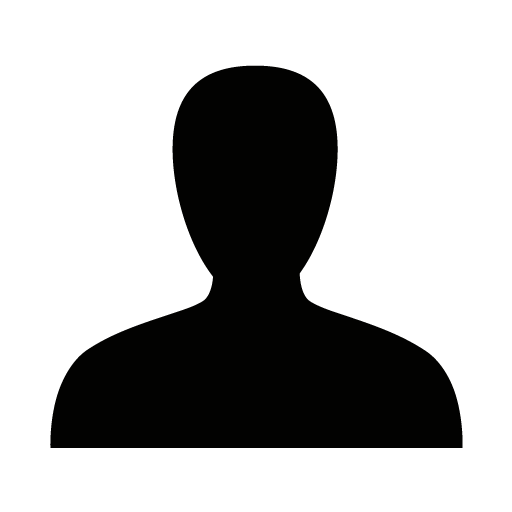
The efficient harvesting and subsequent storage of solar radiation is now becoming one the main challenges for the development of clean and sustainable energy sources. The ever increasing demand to reduce the usage of rare and toxic materials as well as the need to overcome current limitations in solar energy storage calls for environmentally-friendly approaches based on completely new concepts. In this context, hybrid inorganic/organic nanosystems employing metal oxides nanocrystals (MO NCs) and graphene quantum dots (GQDs) are emerging as potential candidates for both harvesting and storage of solar radiation due to the ability of MO NCs to accumulate electrons upon UV light absorption.[1] Here, by means of chemical titration combined with optical spectroscopic tools we investigate the possibility for efficient multiple charge transfers in this type of hybrid nanosystems. Exploiting multiple charge transfer processes together with the combination of energy conversion and storage in a single set of materials will open new routes toward the development of efficient and compact light driven energy storage devices.
1.3-T1
Ultrathin 2-D van der Waals (vdW) semiconductor materials have procured scientific and technological interest since the discovery of single layered graphene in 2004.1 Similar to graphene, transition metal trichalcogenides, with the general chemical formula MPX3 (M= 1st row transition metals, X = chalcogenides), possess fast electron transport and strong spin orbit coupling without the drawback of no bandgap. These vdW inorganic lamellar compounds are characterized by strong intralayer covalent bonding and weak vdW interaction between adjacent layers. Furthermore, they possess weak vdW interlayer interactions meaning that cleaving few- or single-layers from the bulk material is relatively simple using either mechanical or liquid exfoliation techniques. Furthermore, the transition metal atoms endow these materials with magnetic (either ferromagnetic or antiferromagnetic) and magneto-optical properties which can be utilized for new generation 2-D magnets and opto-spintronic devices. Yet, the fundamental understanding of these materials as well as the manipulation of their intrinsic magnetism via external stimuli remains to be an unexplored endeavor.
MPX3 offers a large range of chemical compositions tunable via the M and X elements, consequently forming semiconductors with varying band gap energies ranging from UV to near- infrared. In this way the intrinsic magnetic properties that originate from the M atoms in the MPX3 materials can also be tuned. Depending on the M atoms within the MPX3 layers, they can be endowed with magnetic (either ferromagnetic or antiferromagnetic) and magneto-optical properties. In the case of FePS3 antiferromagnetic Ising magnetic ordering is exhibited. Investigations of bulk and few layer FePS3 have shown optical linear dichroism believed to be attributed to its zigzag antiferromagnetic ordering and anisotropic crystal structure. However, the intrinsic magnetic properties of FePS3 and their effect on the material’s linear dichroism has yet to be explored. Furthermore, the anisotropic crystal structure of FePS3 gives rise to inequivalent K(K’) points in the brilloiun zone. This suggests the possibility of valleytronic properties as seen in transition metal dichalcogenides (TMDs).2–4 To date, these properties have not being shown in TMTCs such as FePS3.
In this talk, evidence of optical linear dichroism will be shown as a function of external magnetic field and temperature. Furthermore, the possibility of valleytronic properties of FePS3 will be shown using circularly polarized excitation as a function of external magnetic field.
1.3-T2
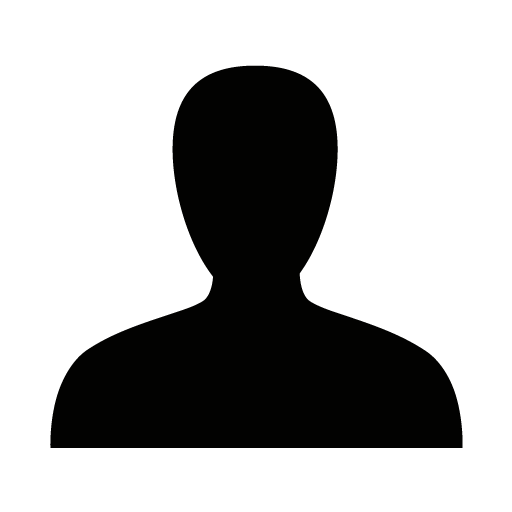
In this work, we combine experimental and theoretical studies of the excitation transfer in heterostructures consisting of two different van der Waals materials: a monolayer transition metal dichalcogenide (TMD) and a 2D perovskite. Our results show that the band alignment inhibits the electron transfer. We present evidence for the excitation transfer and show that it is dominated by either non-radiative energy transfer or by hole transfer, depending on the alignment of exciton states.
Due to the unprecedented flexibility offered by the total relaxation of lattice matching requirements, stacks of van der Waals semiconductors are currently the focus of intense investigations with view of applications in ultrathin optoelectronics. Such heterostructures (HSs) exhibit novel properties, absent from the constituent materials. Excitation transfer between the stacked layers can occur via a charge [1] or energy [2] transfer. Design of future devices requires a thorough understanding, which of the mechanisms dominates. This knowledge is, at present, lacking.
Here, we present a combined spectroscopic and density functional theory (DFT) studies of three samples: PEA2PbI4 /MoSe2 (PEPI/MoSe2), PEA2PbI4 /WS2 (PEPI/WS2) stacks (where PEA stands for phenylethylammonium) and BA2PbI4 /MoSe2 (BAPI/MoSe2, where BA is butylammonium). For all three HSs, the DFT calculations have shown that the obtained stacks exhibit a type II band alignment with valence band edge (VBE) in the perovskite and conduction band edge (CBE) in the TMD layer. However, the electron transfer between materials is hindered by the presence of organic spacer layers in-between slabs of PbI4 octahedra.
Low temperature photoluminescene (PL) mapping, PL excitation, and time-resolved PL measurements provided compelling evidence for the excitation transfer in all the studied HSs. However, the nature of the transfer depends on the energy alignment of the exciton states. Namely, in PEPI/WS2, where the PEPI exciton is in resonance with the B-exciton of WS2, a non-radiative resonant energy transfer together with hole transfer, are observed. On the other hand, in PEPI/MoSe2 and BAPI/MoSe2, where the B-exciton lies lower in energy, the resonant transfer is inhibited and a hole transfer dominates in the systems. It leads to an appearance of a long lived interlayer exciton, demonstrated for the first time in these HSs.
1.3-T3
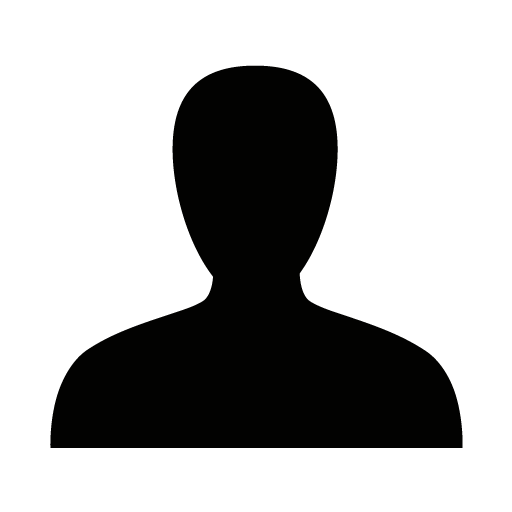
The growing demand for renewable energy and self-powered electronic devices is fueling the research on novel energy storage solutions. Some of the main limitations to the diffusion of photovoltaic energy are linked to the intermitting nature of this source, as well as to stability issues, conversion losses, and limitations related to the size of the devices. Here, we report a hybrid nanostructure based on Metal Oxide Nanocrystals (MO NCs) and MoS2 monolayers capable of light-driven charging, combining in a unique system both the energy conversion and energy storage aspects.[1-3] We achieve the direct storage of photons energy as extra positive and negative charges, separated by nanometric distances within the ultra-thin heterostructure. Photo-generated holes are quenched at the semiconductor's surface and injected into the MoS2 monolayer, while the photo-excited electrons accumulate in the ITO nanocrystal (with an average of 75 per NC). The careful design of similar heterostructures opens the path towards all-solid-state solutions for the direct storage of the solar energy.
1.3-T4
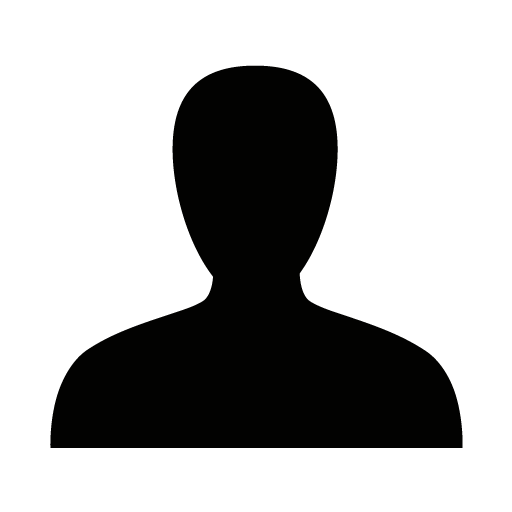
Recent developments have shown that chemical synthesis is a powerful tool for controlling the size, shape, and composition of two-dimensional (2D) crystals with broad implications for their implementation in optoelectronic and quantum devices. However, the gas-phase reactions carried out by most researchers are not conducive to precise manipulation of the 2D crystal edge structure. Here we demonstrate a salt-assisted low-pressure chemical vapor deposition method, which enables growth of 2D WSe2 monolayers whose edge morphology can be tuned from straight, to sawtooth, to fractal-like by adjusting the ratio of WO3 to NaCl. We discuss the volatility of the metal precursor and its role in dictating the edge structure, defect density, and excitonic environment within the as-grown crystals. These studies provide important insight into new 2D crystal growth modes and synthetic strategies for producing crystals with unique structures and optoelectronic properties.
1.4-I1
Bismuth-halide-based semiconductors have gained increasing attention for optoelectronics, owing to their low toxicity, high environmental stability under ambient conditions, and easy processability by a wide range of scalable methods. In particular, bismuth-halide-based materials have been proposed to replicate key features of the electronic structure of lead-halide perovskites that may give rise to defect tolerance, but without the toxicity limitations of the latter [1]. This talk will examine in detail the case of bismuth oxyiodide (BiOI; a layered compound), focussing on its carrier dynamics, defect tolerance and charge transport. Through density functional theory calculations, we show that the most common point defects in BiOI are resonant within the bands, or shallow within the bandgap [2]. To experimentally probe the defect tolerance of BiOI, we intentionally introduce defect states, and probe how these influence the electronic structure (as measured by photoemission spectroscopy) and charge-carrier lifetime (as measured by transient absorption spectroscopy) [3]. We develop an all-inorganic device structure, and devise a route to control the preferred orientation of the vapour-deposited BiOI films to achieve photovoltaics with external quantum efficiencies reaching up to 80% at 450 nm wavelength [2,4]. Further, we demonstrate the strong potential of BiOI for indoor light harvesting to power Internet of Things electronics [5]. We finish with a discussion of the key factors that will need to be addressed in order to further improve the performance of this material in optoelectronics, focussing especially on the role of carrier-phonon coupling on charge-carrier transport and dynamics.
1.4-I2
Hernán Míguez (born in Buenos Aires, Argentina, 1971) is Research Professor of the Spanish Research Council (CSIC) in the Institute of Materials Science of Seville. He studied Physics in the Universidad Autónoma de Madrid and did his PhD in the Institute of Materials Science of Madrid. After a postdoctoral stay at the University of Toronto in the group of Prof. Ozin, he returned to Spain and joined the CSIC in 2004. He leads the group of Multifunctional Optical Materials, whose activities are devoted to the development, characterization and modeling of new photonic architectures for applications in different fields, among them solar energy conversion and light emission. He has received an ERC starting grant (2012, Consolidator Modality) and the “Real Sociedad Española de Física-Fundación BBVA 2017” Prize in the modality of “Physics, Innovation and Technology”.
Optical quality films of lead halide perovskite nanocrystals displaying quantum confinement effects can be achieved by in situ preparation and processing within the void space of insulating porous matrices.[1] In this talk, the main photophysical properties of embedded quantum dots will be reviewed, with emphasis on the possibilities that the absence of organic ligands offer to achieve control over the optical and charge transport properties. Evidence of efficient dot-to-dot transport,[2] fast photoactivation,[3] high photoluminescence quantum yield (>85%) and enhanced durability,[4] with respect to their bulk counterparts, will be provided for different films made of MAPbI3, MAPbBr3, CsPbI3 and FAPbBr3 quantum dots embedded in porous matrices. Also, the absence of ligands implies an excellent opportunity to analyse fundamental interactions, such electron-phonon coupling[5] and the response to the environment,[6] without the interference of organic capping layers. Overall, these results demonstrate that adequately designed networks of ligand-free perovskite quantum dots can be used as both light harvesters and photocarrier conductors, in an alternative configuration to those employed in previously developed QD optoelectronic devices.
1.5-T1
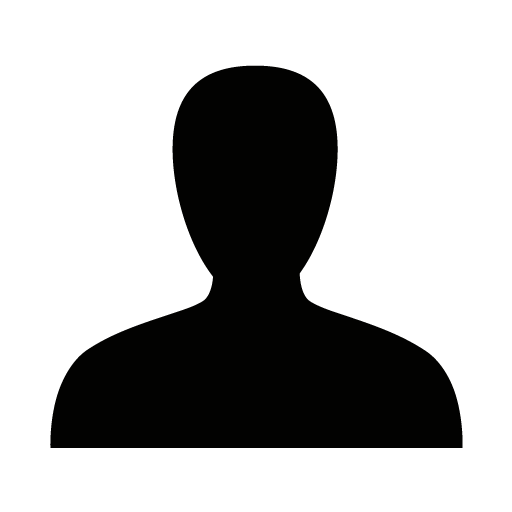
Transition metal dichalcogenide (TMDC) is a class of material with a layered structure in bulk with an indirect bandgap, which becomes direct at the K point in momentum space if only a monolayer is present. This bandgap transition leads to a strong light-matter interaction such as enhanced photoluminescence (PL), compared to the bulk. Monolayer sheets of TMDC with large interaction area can easily be coupled to other low dimensional materials such as 0D nanocrystals, 1D nanowires, or other 2D-2D heterostructures. Halide perovskites are a class of materials with a structural formula ABX3 where A and B are cations such as Cs, Pb, etc and X is halogen. Halide perovskites in both bulk and low dimension show excellent photon absorption and emission properties. Due to its large range of visible light absorption, halide perovskites have made their way and have significantly improved in the field of photovoltaics. A heterostructure combination of the two systems (0D-2D) can show new electronic properties. A plethora of options available in these two classes of materials in terms of band offsets and bandgaps can lead to both type I and II systems.
In this contribution, we present the large exciton energy funneling in a 0D-2D heterostructure of CsPbBr3 (di-dodecyl, dimethyl ammonium as ligand) and MoSe2 monolayer. MoSe2 and CsPbBr3 heterostructure form a type I structure with MoSe2 being the lower bandgap material. We use steady-state and time-resolved µ-PL spectroscopy techniques to probe the photo-induced energy transfer between a set of different CsPbBr3 NCs and MoSe2. Spectroscopy results will be discussed in detail in the frame of exciton energy transfer and its efficiency will be compared to literature.
1.5-T2
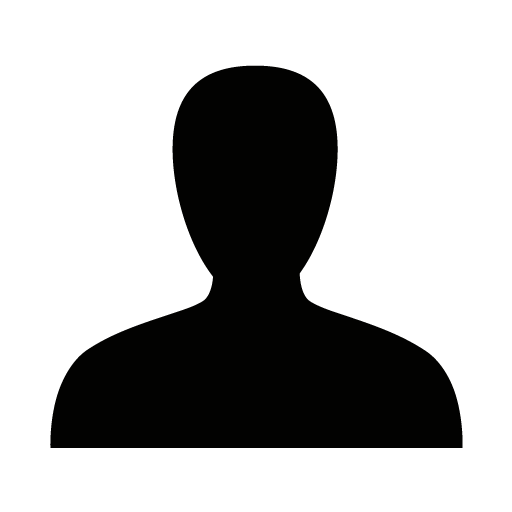
High performance microscale photodetectors which provide fast and efficient optical-to-electrical signal conversion are critical components for next-generation light-sensing applications. Two-dimensional (2D) metal halide hybrid perovskites are an emerging attractive 2D system that combine appealing optoelectronic properties, i.e. strong optical absorption, high carrier mobility, with high stability, easy-processing and low-cost manufacturing. However, photodetectors based on 2D metal halide hybrid perovskites usually exhibit low responsivity if compared to their 3D counterpart.
Herein, we exploit the improved photostability and suitable optoelectronic properties of high-quality 2D fluorinated-phenethylammonium lead iodide perovskite (F-PEA) single crystals to demonstrate the fabrication of lateral metal-F-PEA-metal junction photodetectors. These devices exhibit larger sensitivity and faster time response than reported to date in 2D perovskites. Finally, we discuss the use of these devices for high-resolution light-sensing applications.
1.5-T3
Carbon electrode based perovskite solar cells (C-PSC) are promising candidates for commercialization of perovskite devices considering their low processing costs and extraordinary stability. However, due to the lack of hole selective layers, this device architecture still suffers from severe performance losses at the perovskite/carbon electrode interface. [1]
Recent advances in interface engineering by low dimentional 2D perovskites have proven to effectively passivate the surface of the 3D perovskite absorber.[2] We introduce a 2D perovskite passivation layer as an electron blocking layer at the perovskite/carbon interface in hole selective layer free carbon electrode based perovskite solar cells. The successful passivation of the interface was assessed through X-ray diffraction, X-ray photoemission spectroscopy, and an advanced spectrally resolved photoluminescence (PL), revealing the formation of a high band gap 2D perovskite layer. We confirm the electron blocking characteristic of the 2D perovskite through electrochemical impedance spectroscopy and illumination intensity dependent JSC-VOC measurements of carbon electrode based perovskite devices implementing the 2D perovskite at the 3D perovskite/carbon interface. We show a substantial reduction in charge extraction and interfacial recombination yielding a record efficiency of 18.5% with an improved stability over 500 hours of continuous illumination.
We thus employ a 2D-perovskite as an electron blocking layer in hole selective layer free carbon electrode based perovskite solar cells with printable low temperature carbon electrode. We demonstrate its electron blocking characteristic at the perovskite/carbon interface effectively allowing for less charge recombination losses leading to highly efficient devices.
2.1-I1
Andrea Toma has a long-standing experience in the fabrication and characterization of 3D nanostructures, facing cutting-edge issues in light-matter interaction and nanophotonics. He is staff scientist at the Italian Institute of Technology where he coordinates the Clean Room Facility and the ERC CoG grant "REPLY - Reshaping Photocatalysis via Light-Matter Hybridization in Plasmonic Nanocavities".
Andrea Toma is Adjunct Professor at the University of Genova and, since 2012, member of the Proposal Study Panel at the Lawrence Berkeley National Laboratory. He published more than 100 scientific papers in some of the most impacting Journals of the field, with an h-index of 41. In 2017 he has been awarded with a Visiting Scientist - Full Professorship position by the Chinese Academy of Sciences (under the President's International Fellowship Initiative) with both research and training responsibilities.
He works as referee for many international journals and international funding agencies, serving as program committee member/organizer of conferences on light-matter interaction and nanofabrication.
Light-matter interaction pervades our everyday life and typically involves the exchange of energy between electromagnetic (EM) field and quantum states of matter. When the interaction strength is high enough to promote an exchange rate of energy faster than any other competing relaxation process, the overall light-matter system undergoes a drastic change in its pristine properties. This results into the formation of new hybrid states within the so called “strong coupling” (SC) regime [1].
In this view, hybrid systems composed by optical nanocavities and quantum dots (QDs) represents a key approach to acquire/induce new and distinctive physico-chemical properties with significant implications in fields ranging from cavity quantum electrodynamics and condensed matter physics to polariton chemistry [2], [3].
Here, we report on the SC interaction between surface plasmon polaritons (SPPs) and excitons in CdSe QDs, investigated by steady-state spectroscopic method and transient absorption measurements [4], [5], [6].
In particular the dispersion of the exciton–plasmon hybrid states revealed the typical signature of SC, i.e. anticrossing behavior. Concurrently, the presence of two distinctive bleaching signals appeared in the transient absorption spectra, whose relaxation dynamics underlined a decay of the hybrid states only slightly slower than the lifetime of bare CdSe nanoparticles, and much longer than the SPP damping time.
In addition to the exciton-plasmon coupling the very same hybrid platform was used to impart control over the vibrational energy landscape of semiconductor QDs. Specifically, we demonstrated phonon mode hybridization both in THz and Raman spectroscopies, thus confirming the possibility of altering the intrinsic phonon response of a nanomaterial using properly tailored optical nanoresonators [7].
2.1-I2
Prof. R. Robinson received his PhD in Applied Physics from Columbia University. After his PhD, Prof. Robinson was awarded a postdoctoral fellowship at University of California, Berkeley/LBNL in the research group of Paul Alivisatos. There, he worked on nanoparticle synthesis, chemical transformations of nanoparticles, and advanced property characterizations of nanoparticles. In 2008 Richard began a faculty position at Cornell University in the Materials Science Department, and is currently an associate professor. His primary research interests are: (I) Synthesis and chemical transformations in nanocrystals, (II) Nanocrystals in energy applications, and (III) Synchrotron x-ray characterization of nanomaterials.
therobinsongroup.org/
Because magic-sized clusters (MSCs) are smaller than nanoparticles, they can mimic and provide insight into molecular-level processes and assume novel properties. In this talk I will highlight two recent discoveries on MSCs. The first is on inorganic isomerizations. Structural transformations are ubiquitous at all length scales, spanning from isomerization reactions of small molecules to solid-solid transformations in bulk crystals. Despite attempts to merge understanding of these disparate regimes by reducing the domain size down to nanocrystals (~2 nm), previous work found that bulk-like solid-solid transformation behavior still predominates at nanocrystal length scales. Here we show that MSCs—where, at (~1.5 nm) are smaller than nanocrystals but larger than small molecules—can exhibit a reversible isomeric transformation, and possess essential characteristics of both solid-solid transformations and molecular isomerization reactions. The diffusionless reconfiguration of the inorganic core is evidenced by our reconstruction of the atomic pair distribution function (PDF) from total x-ray scattering. The first order kinetics of the transformation are driven by a distortion of the ligand binding motifs. This reversible transformation of MSCs presents a missing bridge between molecular isomerization and solid-solid transformations.
The MSCs small size and high ligand/core ratio, gives them “softer” inter-particle interactions, with access to a richer phase diagram beyond the classical close packed structures seen with larger particles. We have recently found remarkable hierarchical assembly behavior of these MSC nanomaterials. These CdS MSCs can self-assemble into highly aligned structures, which span over six orders of magnitude in length scale. The MSCs assemble into filaments with hexagonal interparticle geometry, which bundle into larger fibers, and into centimeter-length superstructures of highly ordered thin films patterns. The thin films have long-range periodicity and interesting optical properties that emanate from the MSC core and/or the organic interconnections. The multiscale self-organization behavior of these MSC patterned films displays similarities to biosystems, providing a new platform for the design and study of materials.
References: JACS 140, 3652 (2018), Science 363, 731 (2019), Nat. Mat. (accepted 2022)
2.1-I3
Bio Professional Preparation M.S. in Chemistry, with Honours, University of Bari, Italy, 1996 Ph.D. in Chemistry, University of Bari, Italy, 2001 Research interests Prof. L. Manna is an expert of synthesis and assembly of colloidal nanocrystals. His research interests span the advanced synthesis, structural characterization and assembly of inorganic nanostructures for applications in energy-related areas, in photonics, electronics and biology.
Halide perovskite semiconductors can merge the highly efficient operational principles of conventional inorganic semiconductors with the low‑temperature solution processability of emerging organic and hybrid materials, offering a promising route towards cheaply generating electricity as well as light. Following a surge of interest in this class of materials, research on halide perovskite nanocrystals (NCs) as well has gathered momentum in the last years. While most of the emphasis has been put on CsPbX3 perovskite NCs, more recently the so-called double perovskite NCs, having chemical formula A+2B+B3+X6, have been identified as possible alternative materials, together with various other metal halides structures and compositions, often doped with various other elements. This talk will also discuss the research efforts of our group on these materials. I will highlight how for example halide double perovskite NCs are much less surface tolerant than the corresponding Pb-based perovskite NCs and that alternative surface passivation strategies will need be devised in order to further optimize their optical performance.
2.2-T1
Organic-inorganic group 14 metal-halide hybrids have received extraordinary attention recently, owing to the remarkable electronic and photonic properties that three-dimensional (3D) lead-halide perovskites display, which have revolutionized the field of photovoltaics and light emission. This talk would explore our findings beyond the focus of the 3-D systems, highlighting instead the outstanding synthetic versatility of the more diverse low-dimensional (i.e. 2-D, 1-D, and 0-D) related family of the materials. In particular, the importance of the judicial choice of organic species in determining the formation of specific inorganic lattice architectures and/or supramolecular frameworks would be emphasized. The effects of molecular tuning on the physical properties of the overall materials, which manifest in the tailored performances of the corresponding solution-processed optoelectronic devices, such as solar cells, light-emitting diodes, and memory resistors would be covered. Future direction and opportunity in which the materials can play a role in technologically-relevant applications would also be discussed.
2.2-T2
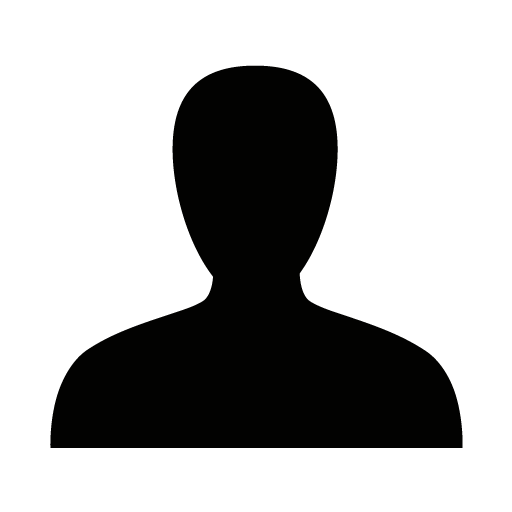
Lead halide perovskites (LHPs) have emerged as one of the most important class of materials for potential optoelectronics. Creative choice of ‘A’ site cation leads to the realization of structures having intriguing properties. LHPs with reduced dimensionality exhibit a strong light-matter interaction due to stronger quantum and dielectric confinements. We have investigated linear and non-linear optical properties in face-shared one-dimensional pyridinium lead iodide (PyPbI3) single crystals. An efficient third-harmonic generation (THG) with a high laser-induced damage threshold (LIDT) is the highlight of this system. We observe a selective enhancement in THG for excitation at optical communication wavelength (~1.5 microns) corresponding to bandgap resonance. Strong exciton-phonon interaction results in highly Stokes-shifted self-trapped excitonic (STE) emission at the temperature range of 5-300 K. Temperature-dependent photoluminescence (PL) study reveals an interplay between anharmonicity and dynamic disorder leading to complex emission properties, which gets further complicated by a phase transition at 170 K. Our results shed light on the fundamental physics behind the complex carrier recombination process and provide an encouraging beginning to exploring similar 1D metal halides for potential application in non-linear optical photonic devices.
2.2-T3
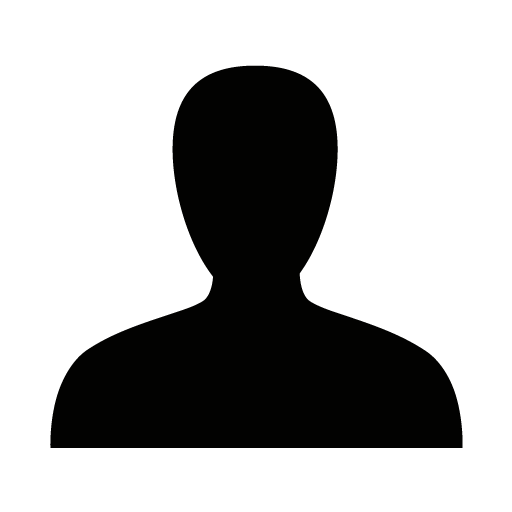
Colloidal semiconductor nanocrystals (NCs) are a class of nanomaterials that exhibit intriguing physical properties for application in optoelectronic devices such as solar cells, photodetectors, lasers, and light-emitting diodes (LEDs). Colloidal NCs are typically synthesized with wet-chemistry approaches that require further post-synthesis treatments to tune the properties of the obtained nanomaterial for the desired application. For example, the organic ligands that passivate the NC surface can be replaced by careful surface-engineering via ligands exchange reactions, and ad-hoc purification procedures must be employed to remove impurities in the NC solution which can tamper with the material in solid-state. Post-synthesis treatments are particularly relevant in the case of perovskite NCs CsPbBr3 [1] to improve the photoluminescence quantum yield [2] and device performance [3]. Nevertheless, other types of colloidal NCs benefit from post-synthesis treatments leading to tailored device performance and increased stability [4].
Here, I will show how post-synthesis treatments can be employed to improve the performance of LEDs based on colloidal semiconductor NCs. In the case of perovskite NCs, I will discuss a novel purification process based on fractional freezing of solutions. In fact, we exploited the solubility differences between ligands and NC at low temperatures by precipitating the excess of organics freezing the NC dispersion at -20°C. The obtained LEDs demonstrated a noticeable improvement in performance upon fractional freezing of impurities, achieving an external quantum efficiency up to 8.9%, which is 3 times the maximum EQE obtained with the classical antisolvent approach (EQEmax = 2.4%).
2.2-T4
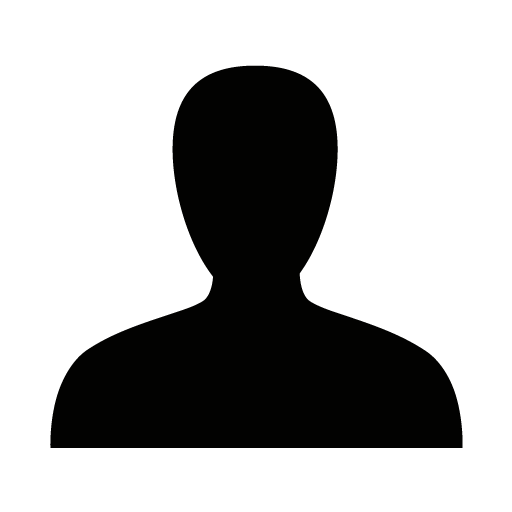
Optical and electronic properties of metal oxide nanocrystals (MO NCs) strongly depend on the presence of depletion layers derived from the presence of surface states. In addition, MO NCs exhibit a localized surface plasmon resonance (LSPR), offering tunable features enabled by doping, both via electrochemical or photochemical charging. [1] Dynamic control over the LSPR makes MO NCs promising in optoelectronics and storage devices. [2] By manipulating the NCs depletion layer, it is possible to control their electronic properties. However, the mechanism behind this phenomenon is very complex, and not yet fully understood. [3] In particular, the tuning of several parameters, including the material under consideration, the size of the NCs, and the presence of multiple core-shell systems, enable the depletion layer engineering. To do this, it is possible to calculate the band and carrier density profiles for NCs with different features. In this work, a new framework has been introduced that can predict the behavior and physics under the MO NC photodoping process, revealing that the charging mechanism is unexpectedly based on the electronic rearrangement of the energy bands. Numerical simulations were experimentally supported by studying the case of a core-shell structure of Sn:In2O3/In2O3 NCs, by tuning the thickness of the shell, as well as post-synthetically, both by photodoping and reversible chemical reactions. The engineering of the depletion layer and the consequent manipulation of the electronic structure allows to significantly increase the sensitivity of LSPR and to target specific properties in MO NCs. The fine-tuning of the NCs band structure has enabled an improvement in charge storage capacity, which represents a step towards fully light-driven energy storage devices.
2.3-T1
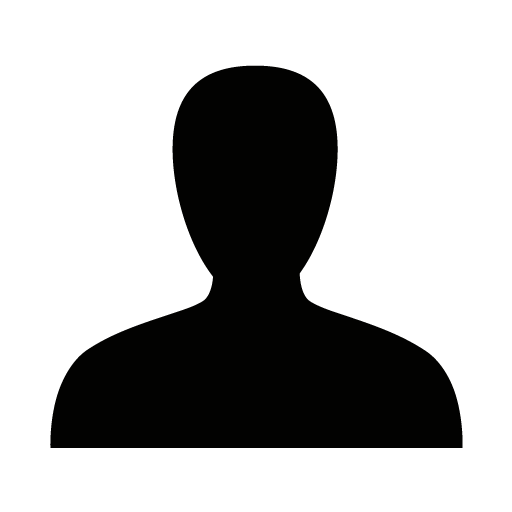
CoSe is considered as a promising non-noble-metal electrocatalyst for hydrogen evolution reaction (HER) in acid media. However, there is still space for improvement on this electrocatalyst, and one of the possible strategies is to combine it with small amount of noble metal. Therefore, we synthesized CoSe nanocrystals (NCs) decorated with Ru clusters. The Ru-CoSe nanocomposite shows better performance than the pristine CoSe NCs, and is even found to outperform the benchmark Pt/C catalyst under high current conditions. To find the reason behind the outstanding electrocatalytic performance of Ru-CoSe nanocomposite, we discover that this Ru−CoSe system undergoes chemical and structural transformations under hydrogen evolution conditions in acid media: the hexagonal CoSe NCs are converted into CoSe2 NCs which have a cubic structure. The Co that is extracted from CoSe during this process combines with oxide and hydroxide species and forms a Co oxide/hydroxide layer; the Ru clusters aggregate and form Ru NCs. Such modifications result in a final Ru−CoOx/Co(OH)x−CoSe2 nanocomposite that exhibits an enhanced hydrogen evolution activity.
2.3-T2
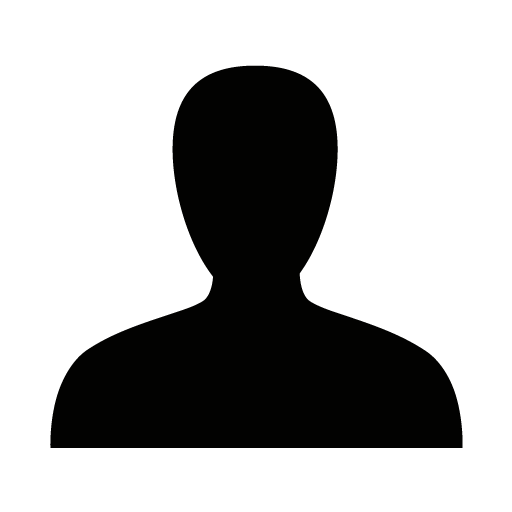
Photonic crystals (PhCs) are defined as composites made of media bearing different dielectric constant or refractive index ordered in a mono, bi or tridimensional periodic structure, and whose lattice constant is comparable to the wavelength of visible or near-infrared light. PhCs are nowadays exploited in functional architecture, enhancement of photon absorption for photovoltaic cells, emission control, lasing, and sensing.[1]
Recently, fabrication of polymer planar photonic crystals from polymer solutions has been receiving particular attention thanks to the ease of tuning of their properties and of scaling their fabrications on large areas.[2] Although major improvements of their performances were made through sophisticated engineering of their structure and properties, solution processed polymer structures still cannot compete with vacuum-deposited inorganic systems which are capable of reaching higher dielectric contrasts, strongly sought-after for their applicability.[3] On the other hand, these fabrications are hardly scalable and extremely costly.
We will demonstrate an alternative way to prepare highly inorganic transparent hybrid thin films of titania (TiO2) and silica (SiO2) in presence of a polymer stabilizer via spin-coating including comparison with similar structures from literature. Fabrication of films is achieved by exploiting sol-gel like in situ reactions between inorganic matrices from alkoxide precursors and polymer additive. The new titania based hybrid shows the highest refractive index reported in the literature for solution processed films leading to the largest value of dielectric contrast for solution processable planar PhCs. Moreover, the design of the hybrids allows for mutual processability with 0D colloidal nanocrystals solutions in the fabrication of optical microcavities for emission control and lasing applications.[4] Some examples will be reported and discussed.
2.3-T3
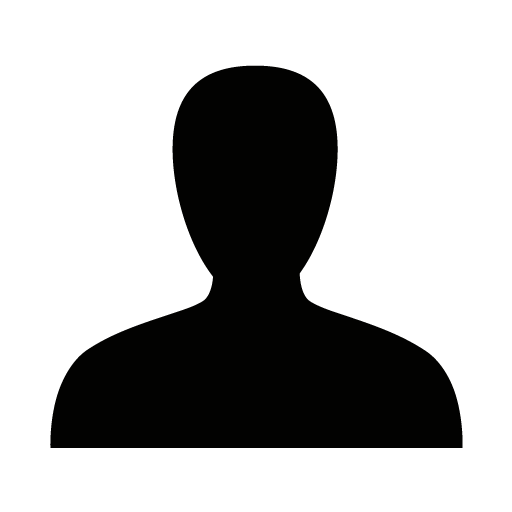
CdSe/CdS core-crown nanoplatelets display minimal shifts in optical features relative to their respective CdSe core, but yield higher stability and photoluminescence quantum efficiencies,[1] imperative for cutting-edge photonic applications including lasing. In the present work, using femtosecond pump-probe spectroscopy we assessed the influence of crown (CdS) lateral area on the optical gain threshold, gain lifetime and gain bandwidth of CdSe/CdS core-crown nanoplatelets. Our results demonstrate that thin CdS crowns lowers the gain threshold twofold compared to the CdSe core, achieving gain close to 1 exciton per nanoplatelet. The lower gain thresholds for the thin-crown nanoplatelets is likely a consequence of efficient surface trap passivation, as we also observed an increasing gain lifetime of 700 ps, nearly threefold longer than CdSe cores for similar exciton densities. Further increase of the crown lateral area increased the gain threshold to an exciton density similar to the core nanoplatelets, yet the threshold is obtained at a fourfold lower photon flux, as a result of higher absorption cross section. The gain lifetime of 400 ps is also still twofold longer than core nanoplatelets. Furthermore, the thick crown broadened the gain bandwidth to 105 nm. The maximum material gain obtained from the core and core-crown nanoplatelets are similar and reach 15000 cm-1. Our studies confirm that core-crown nanoplatelets are promising materials for light amplification.
2.3-T4
Superparticles made from colloidal nanocrystals have recently shown great promise in bridging the nanoscale and mesoscale, building artificial materials with properties designed from the bottom-up. As these properties depend on the dimension of the superparticle, there is a need for a general method to produce monodisperse nanocrystal superparticles. Here, we demonstrate an approach that readily yields spherical nanocrystal superparticles with a polydispersity as low as 2%. We show that this strategy is general and rapid, yielding monodisperse superparticles with controllable sizes and morphologies, including core/shell structures, within a few minutes. The superparticles show a high optical quality that results in lasing through the whispering gallery modes of the spherical structure, with an average quality factor of 1600. Assembling superparticles into small clusters selects the wavelength of the lasing modes, demonstrating an example of collective photonic behavior using these artificial solids.
2.4-I1
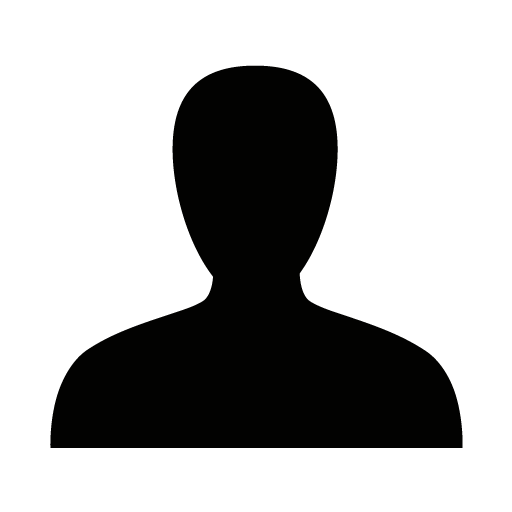
2D materials such as chemically modified graphenes, transition metal dichalcogenides, layered double hydroxide to name only a few, are having a huge impact on electrocatalysis providing materials with outstanding activity for a variety of reactions.[1] However, despite the intense research efforts in this field, a clear identification of the real active sites in many reactions remains a great challenge, given the necessity to employ spatially and structurally sensitive techniques in operando conditions (i.e. during the application of an electrochemical potential in the presence of an electrolyte). Recently, we have developed an innovative approach to the study of 2D materials by using electrochemical Scanning tunneling microscopy. As demonstrated by a seminal paper,[2] this technique allows identifying catalytic processes at the nanoscale by observing a typical noise in the tunneling current, which is due to instantaneous variations of the tunneling junction. Starting from here, we have introduced a new quantity, the tunneling current roughness, which allowed us to acquire quantitative measurements of the electrocatalytic activity with subnanometric precision.
By using special model systems consisting of CVD grown transition metal dichalcogenides thin films (MoSe2 and WSe2), and iron ultrathin films covered by graphene, we achieved even atomic resolution in operando during the hydrogen evolution reaction. This allowed us to identify and quantitatively compare the chemical activity of several chemical and morphological features such as single atom vacancies, Fe-C4 defects, step edges, and even exotic line defects such as metallic twin boundaries.[3] In particular we could determine that iron single atoms trapped within the graphene basal plane are even more active than platinum, the benchmark catalyst for the hydrogen evolution reaction.[4]
2.4-I2
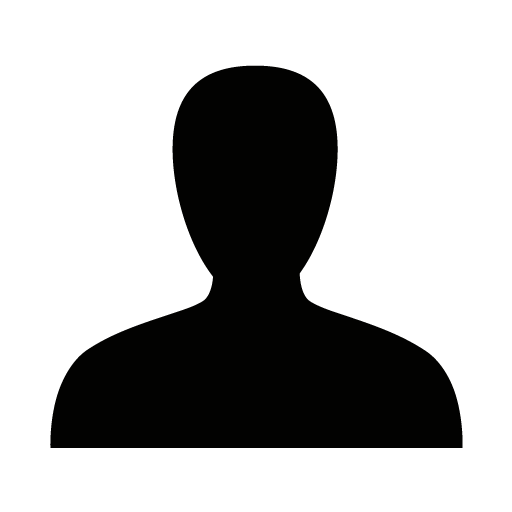
In recent years, a plethora of material systems have been designed and prepared to increase the performance of light harvesting and light-emitting technologies, and to develop new and attractive applications. Limitations of state-of-the-art devices based on organics (both conjugated polymers or small molecules/oligomers) derive largely from material stability issues after prolonged operation. This challenge could be tackled by leveraging the enhanced stability of carbon nanostructures, including carbon nanotubes, nanoribbons and the large family of graphene based materials, in carefully designed nano-hybrid or nano-composite architectures to be integrated within photo-active layers, paving the way to the exploitation of these materials in contexts in which their potential has not been yet fully revealed. In this talk, we discuss the theoretical background behind carbon nanomaterials hybridization with other materials for the establishment of novel optoelectronic properties. By retrieving to a multiscale computational protocol, it is possible to assess the opto-electronic, transport and transfer properties of the assembly and its components, in order to optimize the absorption of light and the transfer of energy/charges at the interface. In particular, we will focus on 0D/2D interfaces in which graphene is coupled with small organic molecules, and how the creation of an hybrid system is beneficial for the enhancement of the optoelectronic and transport properties of the materials.
2.4-I3
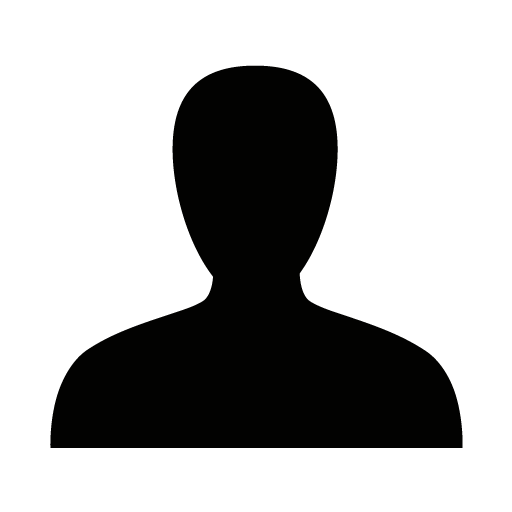
Dr. Ji Ma is the research group leader in the Chair of Molecular Functional Materials at Faculty of Chemistry and Food Chemistry, Technische Universität Dresden. He received his Master's degree in Polymer Chemistry in July 2015 from Fudan University, China. In August 2015, he joined the group of Prof. Xinliang Feng in Technische Universität Dresden as a PhD student, and received his doctorate degree in Synthetic Chemistry in November 2019. After that, he was appointed as a research group leader for the synthetic carbon subgroup in the Chair.
Curved π-conjugated polycyclic hydrocarbons (or nanographenes) has become an important research targets owing to their fascinating intermolecular packing and extraordinary chiraloptical properties resulting from their contorted conformation. In general, two distinct approaches have been established for the synthesis of curved nanographenes: one is the incorporation of steric strain in their periphery, the other is to introduce the non-hexagonal rings (i.e. pentagon, heptagon, octagon) in their skeleton which induce the nonplanar nature. The resultant curvature in a π-conjugated system often yields an unusual electronic structure and unprecedented physical properties. Here, I will talk the reasonable synthesis of several curved nanographenes and graphene nanoribbons with different topologies, such as saddle-shaped and wavy-shaped open-shell radicaloids,[1], [2] azulene-embedded helical nanographenes,[3] and curved graphene nanoribbons with multiple edge structures.[4],[5] Apart from the synthetic strategies, the structure-property relations of these π-systems as well as their optical, electronic and magnetic properties will be also presented. Our work provides a new insight into the synthesis of functional curved aromatics as well as their potential applications in nanoelectronic and spintronic devices.
1.1-I1
Herein the morphology and exciton/charge carrier dynamics in bulk heterojunctions (BHJs) of various donor polymers and molecular acceptors are investigated. The impact of polymer-NFA blend composition upon morphology, energetics, charge carrier recombination kinetics, and photocurrent properties are studied. By changing film composition, morphological structures are varied from consisting of highly intermixed polymer-NFA phases to consisting of both intermixed and pure phase. Transient absorption spectroscopy reveals the importance of an energetic cascade between mixed and pure phases in the electron–hole dynamics in order to well separate spatially localized electron–hole pairs. It appears that the increase in NFA electron affinity in pure phases relative to mixed phases is correlated with a transition from a relatively planar backbone structure of NFA in pure, aggregated phases, to a more twisted structure in molecularly mixed phases. For high crystalline blends, transient absorption data indicate exciton separation leads to the formation of two spectrally distinct species, assigned to interfacial charge transfer (CT) states and separated charges. CT state decay is correlated with the appearance of additional separate charges, indicating relatively efficient CT state dissociation, attributed to the high crystallinity of this blend. The results emphasize the potential for high material crystallinity to enhance charge separation and collection in OSCs, but also that long exciton diffusion lengths are likely to be essential for efficient exciton separation in such high crystallinity devices.
1.1-I2
The currently best organic solar cells suffer from relatively large voltage losses due to non-radiative recombination as compared to inorganic or perovskite solar cells. Further enhancement of the power conversion efficiency to values over 20% will require a reduction of these losses, inevitably corresponding to an increase in the electroluminescence quantum efficiency of the devices.[1] For a large number of donor-acceptor combinations, we have observed that non-radiative voltage losses decrease with increasing charge-transfer-state energies, consistent with non-radiative decay being facilitated by a common high frequency molecular vibrational mode.[2] We further identify small molecule donor-acceptor blends with an optical gap in the visible spectral range, with strongly reduced non-radiative losses as compared to systems with a gap in the near infrared (NIR).[3] This highlights the possibility of a simultaneous occurrence of a high photovoltaic quantum efficiency as well as a high electroluminescence quantum efficiency, occurring in a single organic donor-acceptor blend. For photovoltaic blends with strong absorption in the NIR, we show that the lowest non-radiative decay rates correspond to systems with the narrowest emission linewidths and steepest absorption tails.[4]
1.1-I3
Recent advances in organic solar cells (OSCs) based on non-fullerene acceptors (NFAs) come along with reduced non-radiative voltage losses. We show that the non-radiative voltage losses in these state-of-the-art donor:NFA OSCs show no correlation with the energies of charge-transfer electronic states at donor:acceptor interfaces, different from conventional fullerene-based OSCs. Based on a combined temperature-dependent electroluminescence experiments and dynamic vibronic simulations, we have been able to rationalize the low voltage losses in these devices, where we highlight the critical role of the thermal population of local exciton states in decreasing the non-radiative losses. An important finding is that the molecular photoluminescence properties of the pristine materials define the limit of non-radiative voltage losses in OSCs, indicating that it is critical to design high-luminescence-efficiency donor and acceptor materials with complementary optical absorption bands extending into the near-infrared region. We further demonstrate that there is no intrinsic limit for efficient charge separation in OSCs with small non-radiative voltage losses.
1.2-I1
We present a variety of ultrafast spectroscopic techniques in the visible and terahertz (THz) range, showing how they can be optimized to study excited states and light-induced processes in organic semiconductors. We discuss case studies, such as the charge dynamics from generation to extraction in highly efficient organic photovoltaic systems containing non-fullerene acceptors, the short-range transport properties evaluated by THz spectroscopy of doped organic thin films, or time-resolved processes in organic electrochemical transistors. We emphasize the depth of understanding that can be gained by exploring such systems from multiple spectroscopic angles, and the relevance to applications such as solar cells or bioelectronics.
1.2-I2
James Durrant is Professor of Photochemistry in the Department of Chemistry, Imperial College London and Ser Cymru Solar Professor, University of Swansea. His research addresses the photochemistry of new materials for solar energy conversion targeting both solar cells (photovoltaics) and solar to fuel (i.e.: artificial photosynthesis. It is based around employing transient optical and optoelectronic techniques to address materials function, and thereby elucidate design principles which enable technological development. His group is currently addressing the development and functional characterisation of organic and perovskite solar cells and photoelectrodes for solar fuel generation. More widely, he leads Imperial's Centre for Processable Electronics, founded the UK�s Solar Fuels Network and led the Welsh government funded S�r Cymru Solar initiative. He has published over 500 research papers and 5 patents, and was recently elected a Fellow of the Royal Society
Many studies have reported that charge transfer (CT) states are important for the function of organic solar cells, impacting both on charge photogeneration and on the recombination pathways limiting device open circuit voltage. Such states can be most readily observed optically as CT state absorption or emission, allowing direct observation of CT state energetics. Indeed measurement of CT state energies has been central to many analyses of organic solar cell function. However recent several studies of high performance, low energy offset organic solar cells employing non-fullerene solar cells have reported that CT state absorption and emission is no longer clearly observable. This raises the question of how to probe CT states in such devices, and indeed whether CT states are important for function of these devices. In my talk, I will address the charge carrier dynamics which underlie the performance of organic solar cells employing non-fullerene acceptors. I will consider the impact of charge transfer states on these dynamics, in particular for low energy offset, high performance devices, and analyses of the function of such devices when CT state absorption and emission is not easily measurable.
1.2-I3
Processes taking place at contacts are of particular importance in organic and perovskite solar cells where selective contacts that can efficiently collect majority carriers, simultaneously blocking minority carriers are desired. The surface recombination velocity SR is a key-parameter in describing the dynamic processes at interfaces.
We have extended the analytical framework of the charge extraction by linearly increasing voltage (CELIV) theory taking the effect of built-in voltage, diffusion and band-bending into account [1-4]. By doing so we have been able to extend the CELIV into obtaining new regimes, namely for metal-insulator-metal structures, doped semiconductors and for metal-insulator-semiconductor structures. We have used the new regimes of CELIV as in-device characterization techniques to clarify important device physical parameters. We have derived analytical expressions describing the effective reduction of the built-in voltage, the (effective) open-circuit voltage providing means to quantify and distinguish various loss-mechanisms occurring at contacts in thin-film solar cells.
We show how to use CELIV to directly determine surface recombination velocities at selective and/or blocking contacts in thin-film devices, allowing us to directly estimating the dynamics at selective contacts.
2.1-I1
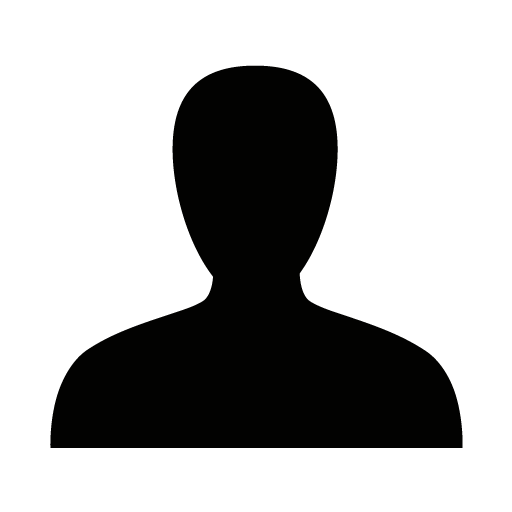
Modest exciton diffusion lengths dictate the need for nanostructured bulk heterojunctions in organic photovoltaic (OPV) cells, however, this morphology compromises charge collection. Here, we reveal rapid exciton diffusion in films of a fused-ring electron acceptor that, when blended with a donor, already outperforms fullerene-based OPV cells. Temperature-dependent ultrafast exciton annihilation measurements are used to resolve a quasi-activationless exciton diffusion coefficient of at least 2 ×10-2 cm2 / s – substantially exceeding typical organic semiconductors, and consistent with the 20-50 nm domain sizes in optimized blends.[1] Enhanced 3-dimensional diffusion accounted for computationally [2] and is shown to arise from molecular and packing factors; the rigid planar molecular structure is associated with low reorganization energy, good transition dipole moment alignment, high chromophore density, and low disorder – all enhancing long-range resonant energy transfer. Relieving exciton diffusion constraints has important implications for OPVs; large, ordered, and pure domains enhance charge separation and transport, and suppress recombination, thereby boosting fill factors. Further enhancements to diffusion lengths may even obviate the need for the bulk heterojunction morphology [3].
2.1-I2
Ivan Kassal is an Associate Professor in the School of Chemistry at the University of Sydney. He graduated from Stanford University in 2006 and completed his PhD in Chemical Physics at Harvard University in 2010. He is a theorist working at the intersection of quantum science, chemistry, biophysics, and materials science. He pioneered some of the first applications of quantum computers to chemistry, showing they could dramatically accelerate difficult chemical calculations. He has also unravelled ways that photosynthetic organisms use quantum effects to improve their light harvesting, and is using those lessons to better understand next-generation materials, especially organic solar cells. He is a recipient of a DECRA fellowship, a Westpac fellowship, and the Le Fèvre Medal of the Australian Academy of Science for “outstanding basic research in chemistry”.
The transport of charges and excitons is well understood in two extremes: in highly ordered materials, transport is by band conduction, while in highly disordered ones, it is by hopping. Many organic semiconductors fall in the intermediate regime between band transport and hopping, making either set of approximations inaccurate. In particular, intermolecular couplings mean that there is usually some delocalisation across multiple molecules (or segments of polymers), while disorder ensures that this effect is spatially limited. Theoretically describing the movement of partially delocalised carriers and excitons is difficult, because it depends on a complicated interplay of energetic disorder, quantum-mechanical couplings, and polaron formation.
We report delocalised kinetic Monte Carlo (dKMC), a new computational method that is able to describe the motion of partially delocalised charges and excitons in all regimes of disorder [1]. We implement numerical innovations that allow us to work in three dimensions, a regime that had proven too complicated for all comparable approaches. dKMC reveals new, basic physics of transport in organic semiconductors and explain why mobilities predicted by traditional kinetic Monte Carlo are usually too low. In particular, delocalisation over just a few molecules can increase mobilities by orders of magnitude.
We also extend dKMC to describe charge separation at a heterojunction [2], the first approach to do so that includes all the necessary ingredients: delocalisation, disorder, and polaron formation. We show that delocalisation can play a decisive role in charge separation, with even modest delocalisation able to double internal quantum efficiencies.
[1] Balzer, Smolders, Blyth, Hood, and Kassal, Chem. Sci. 12, 2276 (2021).
[2] Balzer and Kassal, arXiv:2108.05032 (2021).
2.1-I3
Efficient charge photogeneration in conjugated polymers typically requires the presence of a second component to act as electron acceptor. Here, we report a novel low band-gap conjugated polymer with a donor / orthogonal acceptor motif, referred to as PCPDT-sFCN [1]. The role of the orthogonal acceptor is to spatially isolate the LUMO from the HOMO, allowing for negligible exchange energy between electrons in these orbitals and minimising the energy gap between singlet and triplet charge transfer states. We employ ultrafast and microsecond transient absorption spectroscopy to demonstrate that, even in the absence of a separate electron acceptor, PCPDT-sFCN shows efficient charge photogeneration in both pristine solution and film. This efficient charge generation is a result of an isoenergetic singlet/triplet charge transfer state equilibrium acting as a reservoir for charge carrier formation.
Furthermore, clear evidence of enhanced triplet populations, which form in less than 1 ps, is observed in PCPDT-sFCN. Using group theory, we show that this ultrafast triplet formation is due to highly efficient, quantum mechanically allowed intersystem crossing between the bright, initially photoexcited local singlet state and the triplet charge transfer state. Remarkably, the free charges that form via the charge transfer state are extraordinarily long-lived with millisecond lifetimes, due to the stabilisation imparted by the spatial separation of PCPDT-sFCN’s donor and orthogonal acceptor motifs. The efficient generation of long-lived charge carriers in a pristine polymer paves the way for single-material applications such as organic photovoltaics and photodetectors.
2.2-T1
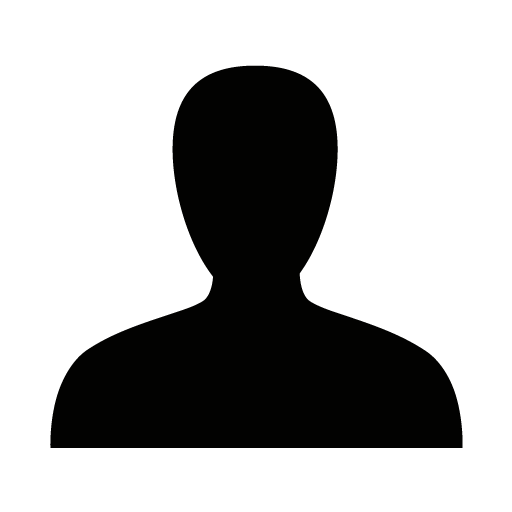
The photogeneration of free charges in light-harvesting devices is a multi-step process, which can be challenging to probe due to the complexity of the involved inter-and-intramolecular energetic states and the competitive character of various driving mechanisms. Here, we present a technique which allows the measurement of photogenerated charge carrier densities sensitively and to probe charge generation processes in thin-film solar cells. Our technique is based on the integral time-of-flight method of sandwich-type thin-film devices but extended to the low intensity regime (LIITOF) and combined with voltage dependent device capacitance measurements. The theoretical framework of LIITOF is verified by drift-diffusion simulations and the applicability of our method is demonstrated on thin-film solar cells based on organic and perovskite semiconductors by examining the voltage dependence of charge generation efficiency. Our experimental results are compared to those obtained via Time Delayed Collection Field (TDCF) measurements conducted on the same devices and found to be in excellent agreement.
2.2-T2
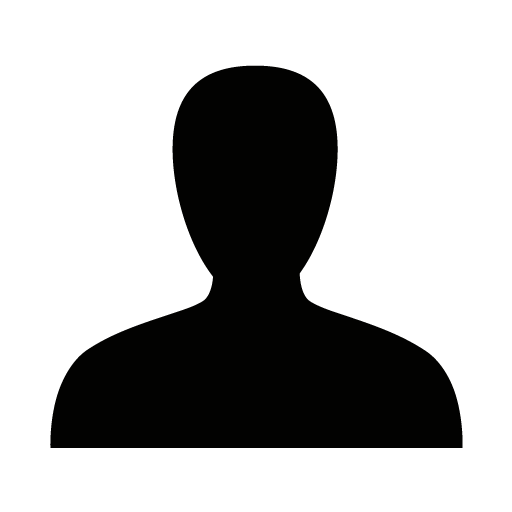
Organic photovoltaics have been developed in the concept of bulk heterojunctions (BHJs) by blending donor and acceptor materials because of the short exciton diffusion length of classic organic semiconductors. While non-fullerene acceptors (NFAs) have recently demonstrated long-range exciton diffusion, most of studies focus yet on blended polymer:NFA systems. Here, we calculate the long exciton diffusion length of a NFA (~40 nm) by ultrafast spectroscopy measurements and fabricate NFA/polymer planar heterojunctions (PHJs) as a function of the thickness of the NFA. From thick to thin NFA layer, close to the exciton diffusion length of the NFA, additional hole transfer and photocurrent was observed, indicating that more excitons created away from the NFA/polymer interface can diffuse to the interface. Field-dependent charge generation is observed by exciton diffusion from the neat NFA. Further, due to smaller acceptor/donor interfacial area, the PHJ devices exhibit less bimolecular recombination losses and suppressed dark leakage current than the corresponding BHJ device. By employing the NFA/polymer PHJ to photodetectors, we demonstrate 3.6 times higher detectivity at -2 V bias than that of the BHJ photodetector.
2.2-T3
The device performance of organic bulk heterojunction (BHJ) photovoltaic cells is ultimately derived from processes taking place between excitons, charge-transfer states, and free charge carriers. In fullerene-acceptor-based BHJ systems, characterized by large donor-acceptor energy level offsets, the photovoltage is critically limited by the energetics and kinetics of CT states [1]. However, this picture has been challenged with the emergence of nonfullerene-acceptor-based systems with low energy offsets, where the role of excitons becomes important [2]. In this work, factors limiting key device parameters in organic BHJ solar cells based on low-offset systems are investigated. We find that photovoltaic parameters are not only limited by the energetics but also critically determined by the relative kinetics between different species [3,4]. Finally, based on these considerations, the corresponding power conversion efficiency limits are predicted. These findings provide insights into the operation of state-of-art non-fullerene organic photovoltaic cells with low offsets, paving the way for organic solar cells with power conversion efficiencies exceeding 20%.
2.3-I1
Prof. Dieter Neher studied physics at the University of Mainz. In 1990 he gained his PhD with Prof. G. Wegner. From 1990-1992 he was a research associate at the Optical Sciences Centre, Tucson, Arizona and at the Centre for Research in Electrooptics and Lasers, Orlando, Florida with Prof. G. Stegeman. 1992 he joined again Prof. G. Wegner at the MPI-P, heading the group Electrooptical Phenomena in Polymers. Following his habilitation in November 1998, he became Professor of Soft Matter Physics at the Institute for Physics and Astronomy at the University of Potsdam. Current research interests are electrical, optical and optoelectronic processes in conjugated materials.
Organic solar cells (OSCs) are currently experiencing a second golden age thanks to the development of novel non-fullerene acceptors (NFAs) [1]. One particularly successful type of NFAs are Y-type moleucles such as Y6 [2]. In blends with PM6 and other donor polymers, high efficiency (>18 %) have been recently achieved in single junction devices, with the perspective to approach the commercially relevant 20 %. Here, we summarizes our recent understanding of the processes governing the performance of OSCS based on Y6 and related compounds. For PM6:Y6, we find that free charge generation is essentially barrierless [3] and that the fill factor of the device is essentially limited by the diffusion length of the charges, which is smaller than the active layer thickness [4]. This puts the understanding of the recombination processes at the focus of the further understanding. By studying the emission properties of such cells, we conclude that the radiative recombination of free charges in Y6-based cells proceeds almost entirely through the re-occupation and decay of the Y6 singlet, but that this pathway contributes to less than 1 % of the total recombination current [5]. As such, the open-circuit voltage of the PM6:Y6 blend is almost entirely determined by the energetics and kinetics of the charge transfer (CT) state, irrespective of the position and emission properties of the singlet state. Most recently, we addressed the role of energetic disorder in the competition between charge extraction and recombination [6] By performing temperature dependent charge transport and recombination studies, we come to a consistent picture of the the density of state distributions for free charges, which allows us to analytically describe the dependence of the open-circuit voltage on temperature and illumination intensity. We conclude that energetic disorder of charge separated states has to be considered in the analysis of the photovoltaic properties, even for the more ordered PM6:Y6 blend.
2.3-I2
With the advent of non-fullerene acceptors, organic solar cells have made impressive improvements in terms of power conversion efficiency, so that breaking the 20 % limit is within close reach. Understanding the efficiency-limiting processes remains important to optimize the solar cells effectively.
The impact of the most important loss mechanisms for state-of-the-art organic solar cells is schematically shown in the Figure. We will present our recent findings on two of them, nongeminate recombination of charge carriers, and a loss in fill factor due to the transport resistance.
Nongeminate recombination takes place via charge transfer complexes, and we discuss different models for describing them, in particular their absorption and emission, and how they relate to the open circuit voltage. Concerning the transport resistance, it is a major loss mechanism in non-fullerene based solar cells. We will discuss our findings on how the related voltage drop is related to the active layer conductivity.
1.1-I1
In the view of a rapid increase in efficiency of organic solar cells, reaching their long-term operational stability represents one of the main challenges to be addressed on the way toward commercialization of this photovoltaic technology. However, intrinsic degradation pathways occurring in organic solar cells under realistic operational conditions remain poorly understood. The light-induced dimerization of fullerene-based acceptor materials discovered recently is considered to be one of the main causes for burn-in degradation of organic solar cells. In this work, we reveal the mechanism of the light-induced dimerization of the fullerene derivatives and establish important correlations with their molecular structure and electronic properties.
We also show that conjugated polymers and small molecules undergo similar light-induced crosslinking regardless of their chemical composition and structure. In case of conjugated polymers, crosslinking leads to a rapid increase in their molecular weight and consequent loss of solubility, which can be revealed in a straightforward way by gel permeation chromatography analysis via a reduction/loss of signal and/or smaller retention times.
Our results, thus, shift the paradigm of research in the field toward designing a new generation of organic absorbers with enhanced intrinsic photochemical stability in order to reach practically useful operation lifetimes required for successful commercialization of organic photovoltaics.
1.1-I2
Professor of Materials Physics at Karlstad University, Sweden, since 2011. Research interests: morphology of conjugated polymer thin films, photodegradation of OPV materials, energy level allignment in organic and perovskite multilayer structures. Employed at Karlstad university since 2000. Previously Research Scientist at Cambridge Display Technology in Cambridge,UK, and Research Assistant at University of Cambridge. Post-doc at EPFL Lausanne (1996-98) and TU Delft (1995-1996). PhD degree from the Weizmann Institute of Science in Rehovot, Israel.
The fine-structured film morphology in the active layer of all-polymer solar cells is the key to their high performance. Polymeric non-fullerene acceptors offer the potential to restrict the self-aggregation that is typical for small molecule non-fullerene acceptors. But what limits the phase separation that otherwise dominates in polymer-polymer blends? In this study, we employed a blend of the polymeric acceptor PF5-Y5 and the donor polymer PBDB-T to investigate the molecular interactions in solution in a joint experimental-theoretical spectroscopy study. Solar cells prepared of this blend have reached power conversion efficiencies of over 14%.(1) From absorption spectroscopy of the PBDB-T:PF5-Y5 blend solutions at increasing temperatures, combined with concentration-dependent fluorescence spectroscopy and excitation spectroscopy, we could conclude that in addition to temperature-induced disaggregation of both donor and acceptor polymers, donor-acceptor complexes are formed in dilute blend solutions of PBDB-T and PF5-Y5. The formation of the donor-acceptor complexes competes with the donor and acceptor self-aggregation and the solvent environment is found to influence these interactions. Our results show also that the donor-acceptor polymer complexes are stabilized in more polar solvents. The near IR-region of the absorption spectrum could be matched with the calculated electronic excitations of donor-acceptor complexes of PBDB-T and PF5-Y5 oligomers. The results corroborate that van der Waals interaction between segments of the donor and acceptor polymer chains favours the formation of donor-acceptor charge transfer complexes, stabilized by hybridization of the molecular orbitals, which reduces the electronic energy. These pre-formed donor-acceptor complexes in solution can be expected to have important consequences on the resulting film morphology. These insights are also expected to direct the future design of compatible donor-acceptor polymer pairs for high-performance all-polymer solar cells.
1.1-I3
Christoph J. Brabec is holding the chair “materials for electronics and energy technology (i-MEET)” at the materials science of the Friedrich Alexander University Erlangen-Nürnberg. Further, he is the scientific director of the Erlangen division of the Bavarian research institute for renewable energy (ZAE Bayern, Erlangen).
He received his PhD (1995) in physical chemistry from Linz university, joined the group of Prof Alan Heeger at UCSB for a sabbatical, and continued to work on all aspects of organic semiconductor spectroscopy as assistant professor at Linz university with Prof. Serdar Sariciftci. He joined the SIEMENS research labs as project leader for organic semiconductor devices in 2001 and joined Konarka in 2004, where he was holding the position of the CTO before joining university.
He is author and co-author of more than 150 papers and 200 patents and patent applications, and finished his habilitation in physical chemistry in 2003.
OPV cells have a proven efficiency of over 18 % while OPV modules have a proven record efficiency of 13.5 %. Both values are still increasing towards > 20 % for small area cells and > 15 % for large scale modules. With these performance values, OPV is reaching out to applications that are going beyond the typical niche markets. The first generation of commercially available OPV modules shows lifetimes in the order of 5 years and more under outdoor conditions. Independent of the application, operational lifetime of organic solar cells is not fully understood. Few publications highlight operational lifetimes of over 25000 hrs under lab conditions. Organic solar cell materials being stable under light and oxygen are reported as well. We recently demonstrated solar cells that canbe operated in water and under 1 sun for hundreds of hours – unpackaged. However, all these “best you can do” lifetime values are reported for different material and interface systems.
This talk will analyze the most common degradation mechanisms and outline, how they are overcome in modern OPV materials. Bulk degradation will be distinguished from interface degradation, and, not unexpected, interface degradation or interface related degradation is found to be the currently leading degradation mechanism. All these findings indicate that we can expect a significant improvement in operational stability of OPV in the next few years.
1.2-T1
The transition from fullerene to non-fullerene acceptors (NFAs) has dramatically increased the power conversion efficiency of organic solar cells and re-ignited the interest in these devices. Even though, the efficiency of NFA based devices has surpassed 18 % [1], these devices are still challenged by poor device stability. It has been shown, that the popular electron transport layer (ETL), ZnO, causes a photoinduced degradation of the NFA molecule ITIC, which contributes to a poor device stability under continuous light soaking in PCE12:ITIC devices with ZnO as an ETL [2]. Recently, we have shown that replacing ZnO with sputtered TiOx increases the device stability of high performing PCE12: ITIC devices significantly [3], when the sputtered TiOx is optimized in terms of composition and microstructure. Photoemission and spectrophotometry measurements have shown that the sputtered TiOx films possess less surface defects, which to a large extend mitigates the photoinduced degradation of ITIC as opposed to conventional ZnO ETLs. To better understand the mechanisms behind the improved stability of devices that are based on the sputtered TiOx, electronic structure and the full energy level alignment between TiOx (ETL) and ITIC (NFA) has been studied using synchrotron-based X-ray photoelectron spectroscopy, X-ray absorption spectroscopy and resonant X-ray photoelectron spectroscopy to uncover any charge transfer, chemical bonding, trap states and interfacial states at the ETL/acceptor interface. These results will be presented during this talk.
[1] Q. Liu et al. 18 % efficiency organic solar cells, Science Bulletin, 65, 272-275, (2020).
[2] S. Park et al. Intrinsic photo-degradation and mechanism of polymer solar cells: the crucial role of non-fullerene acceptors, J. Mater. Chem. A., 7, 25830-25837, (2019).
[3] M. Ahmadpour et al., in preparation, (2021).
1.2-T2
Organic solar cells (OSCs) have lately received a lot of interest as power sources to drive low power consumption devices for the Internet of Things application by harvesting the energy from indoor artificial light.[1,2] In this work, an enhanced power conversion efficiency (PCE) in indoor LED illuminated OSCs was observed, 60% higher than those of AM1.5 G illuminated OSCs by a cathode interface engineering strategy. Moreover, the photostability study under indoor artificial light, which is rarely investigated, is critical for the practical applications of this emerging technology. Along with the performance enhancement, we observed that OSCs illuminated under indoor LED light had better photostability than OSCs illuminated under 1 Sun scenario. Impedance analysis with the 3RC equivalent circuit models elucidates the mechanisms behind the enhanced performance and improved photostability of indoor OSCs.
1.2-T3
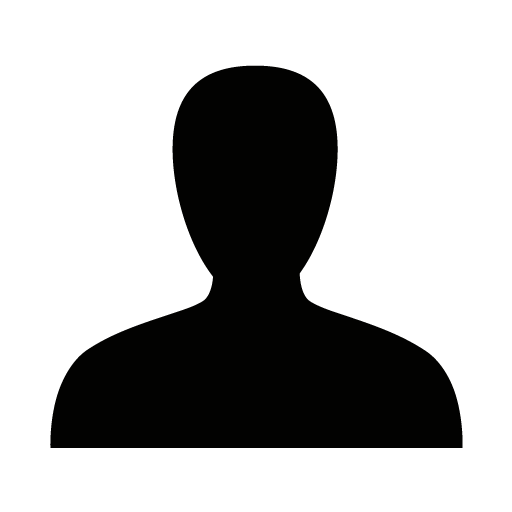
Implementation of 2D materials in organic photovoltaic (OPV) cells has emerged as a promising route to modify fundamental device properties, and potentially improve device efficiency and stability. Recently, the 2D material MXene has attracted huge attention and demonstrated a large potential for next-generation solar cells due to exciting optical and electronic properties, and especially the ability to tune work function via surfaces termination routes which is highly desirable in OPV device interlayers. In this work, we employ such 2D MXene, Ti3C2Tx, in conventional ETL to develop composite 2D based electron transport layers (2D-ETL), and demonstrate their high performance for non-fullerene acceptor (NFA) based inverted OPV processed with the non-halogenated solvent o-xylene. The PM6:N3 OPV based on the composite 2D-ETL exhibited power conversion efficiencies (PCE) of around 14%, and importantly, a superior device lifetime when compared to conventional 2D-free ETL. In this work, the integration of such 2D interlayers in 2D-ETL is investigated in terms of morphology and optical as well as electrical properties, while the degradation and stability mechanisms are studied by optical spectroscopy techniques and ISOS-L device lifetimes measurements. Here, the usage of 2D MXene is shown to possess a great potential for the development of ambient stable and efficient flexible NFA OPV devices in the future.
1.2-T4
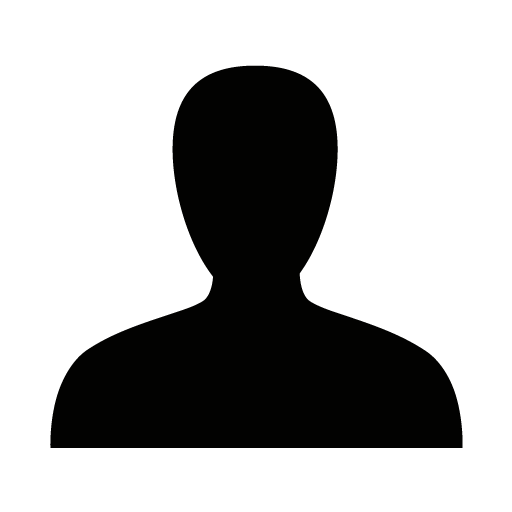
The introduction of non-fullerene acceptors (NFA) has provided several recent record efficiencies in organic photovoltaic (OPV) cells, reaching now above 18%1 for single-junction devices. While these developments have provided a strong boost to the OPV field, more efforts have to be devoted to their application, such as their use in windows to lower the carbon footprint of buildings. To improve the performance of such semi-transparent NFA OPV, a highly reflective Bragg mirror can be formed to reflect selective parts of the sunlight spectrum that match specific parts of the absorption spectrum of the active layer, e.g. the contribution from the near-infrared absorbing NFA molecules. With the progress in the film formation using reactive sputtering, it is possible to tune the thickness, composition, transparency, and uniformity of alternating low and high refractive index oxide thin films, which is needed to form well-performing DBR stacks, making it an ideal technique for this application.
Here, recent progress in adjusting the reflectance of thin film oxide based DBR, e.g. by fine-tuning composition and thickness of the individual layers in order to match the absorption region of specific high performance non-fullerene acceptor molecules, as well as their integration in efficient semi-transparent NFA OPV devices with low visible transmission loss, is demonstrated. Supported by a variety of surface science characterization studies, the importance of the detailed thin film composition and microstructure on the optical properties2 and intrinsic stability of these DBR is discussed. To meet the requirements on scalable OPV development, the up-scaling of these new DBRs is discussed, considering recent results on industrially compatible OPV device development3,4. This includes Roll-to-Roll (R2R) processing of OPV cells and modules using combined solution and vacuum-based techniques including also the reactive sputtering process on R2R scale utilized for DBR development.
1.3-I1
In recent years, organic solar cells (OSCs) have shown a tremendous increase in power conversion efficiencies (PCEs) to over 18% thanks to the development of novel non-fullerene acceptors (NFAs) and corresponding donor polymers. While the performance of OSCs has reached the level of commercial applications, the stability of the devices needs to be improved and is now the focus of research. This presentation will review recent progress in evaluating and understanding the intrinsic photostability of high-efficiency NFAs including ITIC derivatives, IDTBRs, Y family, and the resulting blends with PBDB-T (PCE12) or PBDB-T-2F (PM6) as the donor polymer by addressing impact of film crystallinity and molecular structure.
1.3-I2
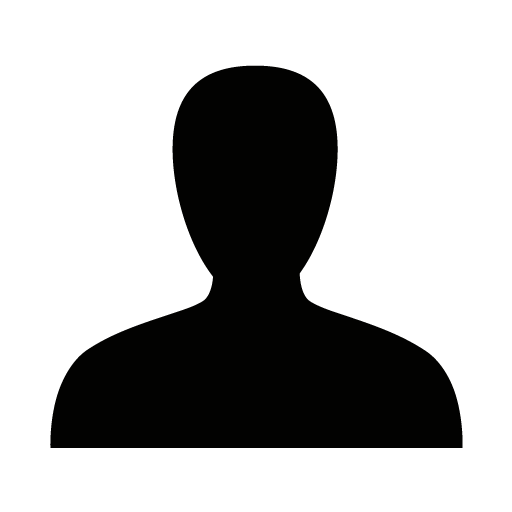
Organic solar cells have witnessed a rapid improvement in device performance over the past few years, now achieving an exceptional power conversion efficiency of over 18% under standard solar irradiation (over 30% under indoor illumination) in a single junction device, rapidly closing the performance gap with potentially competing technologies such as crystalline silicon and halide perovskite solar cells. This upsurge in performance is primarily driven by the emergence of non-fullerene organic small molecular and polymeric acceptors, surpassing conventional fullerene acceptors due to stronger optical absorption, optimal energy levels and potentially lower cost in synthesis and purification. This is further coupled with major advances in device design (e.g. ternary bulk-heterojunction blends, novel device interlayers), placing organic solar cells in an unprecedentedly promising position for potential large scale commercialisation in multiple application areas.
A further key factor to realise the full commercialisation potential of organic solar cells is stability, that is, a PV device must have a sufficiently long lifespan that exceeds the required operational period for a particular application. For example, it is desirable for a PV device lifespan of >20 years for building integrated applications, whereas a shorter lifespan of ~5-10 years may be sufficient for powering indoor autonomous sensors. The modest device stability has been a widely-recognised and long-standing challenge for conventional fullerene-based organic solar cells, with multiple degradation mechanisms already identified that result in rapid losses of device performance under illumination, ambient air and thermal stress conditions. Nevertheless, the recent transition of organic solar cells from fullerene acceptors to non-fullerene acceptors, as well as the major advances in their molecular and device design, has brought exciting opportunities to fully overcome this challenge.
In this talk I will give a summary of the recent research progress of my group in understanding the degradation mechanisms of non-fullerene organic solar cells. I will highlight the distinct roles of the donor and acceptor materials as well as the device organic/inorganic interfaces in the degradation, and propose potential strategies to overcome these degradation mechanisms.
1.3-I3
Thuc-Quyen Nguyen is a professor in the Center for Polymers and Organic Solids and the Chemistry & Biochemistry Department at University of California, Santa Barbara (UCSB). She received her Ph.D. degree in physical chemistry from the University of California, Los Angeles, in 2001 under the supervision of Professor Benjamin Schwartz. Her thesis focused on photophysics of conducting polymers. She was a research associate in the Department of Chemistry and the Nanocenter at Columbia University working with Professors Louis Brus and Colin Nuckolls on molecular self-assembly, nanoscale characterization and molecular electronics. She also spent time at IBM Research Center at T. J. Watson (Yorktown Heights, NY) working with Richard Martel and Phaedon Avouris. Her current research interests are structure-function-property relationships in organic semiconductors, sustainable semiconductors, doping in organic semiconductors, interfaces in optoelectronic devices, bioelectronics, and device physics of OPVs, photodetectors, and electrochemical transistors. Recognition for her research includes 2005 Office of Naval Research Young Investigator Award, 2006 NSF CAREER Award, 2007 Harold Plous Award, 2008 Camille Dreyfus Teacher Scholar Award, the 2009 Alfred Sloan Research Fellows, 2010 National Science Foundation American Competitiveness and Innovation Fellows, 2015 Alexander von Humboldt Senior Research Award, 2016 Fellow of the Royal Society of Chemistry, 2015-2019 World’s Most InfluentialScientific Minds; Top 1% Highly Cited Researchers in Materials Science by Thomson Reuters and Clarivate Analytics, 2019 Fellow of the American Association for the Advancement of Science (AAAS), 2023 Wilhelm Exner Medal from Austria, 2023 Fellow of the US National Academy of Inventors, 2023 de Gennes Prize in Materials Chemistry from the Royal Society of Chemistry, 2023 Elected Member of the US National Academy of Engineering, 2024 Fellow of the European Academy of Sciences, and 2025 ACS Henry H. Storch Award in Energy Chemistry.
Organic solar cells (OSCs) using non-fullerene acceptors (NFAs) have garnered a lot of attention during the last years and showed dramatic increases in the power conversion efficiency (PCE). PCEs higher than 19% for single-junction systems were achieved, but these high-performance organic photovoltaic cells are often processed with halogenated solvents. To accelerate the mass fabrication of OSCs, green solvent processing is crucial to reduce the harmful effect of halogenated solvents to human health and our environment. In this talk, I will discuss the design, synthesis, and performance of organic semiconductors processed from green solvents such as xylene and 2-methyl tetrahydrofuran (2-MeTHF). 2-MeTHF can be obtained from furfural, which is derived from agricultural by-products. It fits within the class of solvents sought from renewable resources and the concept of capitalizing on waste to generate useful chemicals. Moreover, the toxicity of 2-MeTHF is lower than that of commonly used halogenated and aromatic solvents, such as chlorobenzene and chloroform. A combination of characterization methods were employed to gain insight into the film morphology and solar cell performance.
2.1-I1
Organic conjugated materials have many favourable properties that make them interesting for a variety of electronic applications. The aim of my group is to understand the fundamental processes underlying their functionality. We use ultrafast spectroscopic techniques, such as transient absorption (TA) and time-domain terahertz (TD-THz) spectroscopies, to investigate charge carriers in organic semiconductors. While femtosecond TA measurements bring insights to the nature and evolution of the photoexcited species, we use TD-THz spectroscopy to gain information about the charge transport properties on the nanoscale. After presenting an overview of our experimental techniques, I will show results about charge generation in highly efficient solar cell materials based on organic polymer:fullerene [1] polymer:non-fullerene blends [2] with negligible driving force for interfacial charge transfer. Efficiencies beyond 18% have recently been achieved by combining low bandgap conjugated polymers with small-molecule non-fullerene acceptors (NFAs). I will discuss how charge generation and recombination processes depend on parameters such as the charge transfer driving force, the short-range charge mobility and the morphology.
2.1-I2
Jenny Nelson is a Professor of Physics at Imperial College London, where she has researched novel varieties of material for use in solar cells since 1989. Her current research is focussed on understanding the properties of molecular semiconductor materials and their application to organic solar cells. This work combines fundamental electrical, spectroscopic and structural studies of molecular electronic materials with numerical modelling and device studies, with the aim of optimising the performance of plastic solar cells. She has published around 200 articles in peer reviewed journals, several book chapters and a book on the physics of solar cells.
In a molecular photovoltaic device, charge separation and energy conversion result from the evolution of a photogenerated exciton into a charge separated state, in competition with recombination to ground. The efficiency of charge separation is a function of the molecular packing and energy level alignment near the interface, and of disorder in these properties. We need to understand and isolate the effects of chemical structure, molecular packing, energetics and disorder on the competition between charge separation and recombination in order to identify the factors controlling device efficiency. Electro- and photo-luminescence have proved to be valuable tools to probe the energy and dynamics of excited states involved in photoinduced charge separation, and to identify structural and energetic disorder at molecular interfaces. Here, we use luminescence and other spectroscopic probes along with transient electrical measurements to study charge generation and photovoltage in molecular donor: acceptor solar cells. We explore how the properties of the intermediate charge-transfer state influence recombination losses and show, with the aid of a numerical models how control of these molecular properties could benefit performance [1]. We study the effect of hybridisation of charge-transfer and local exciton states [2] and of disorder in CT state energies [3] on non-radiative voltage losses. We then develop an integrated modeling framework in which excited state dynamics are combined with a one-dimensional device model that accounts for spatial variations in charge density. The integrated model allows different experimental measurements to be reconciled within a single picture and helps to show how the energies and dynamics of interfacial states influnce the overall device performance. We use our results to consider the ultimate limitations placed on solar to electric conversion by the molecular nature of the materials.
2.1-I3
René Janssen is university professor at the Eindhoven University of Technology (TU/e). He received his Ph.D. in 1987 from the TU/e for a thesis on electron spin resonance and quantum chemical calculations of organic radicals in single crystals. He was lecturer at the TU/e since 1984, and a senior lecturer in physical organic chemistry since 1991. In 1993 and 1994 he joined the group of Professor Alan J. Heeger (Nobel laureate in 2000) at the University of California Santa Barbara as associate researcher to work on the photophysical properties of conjugated polymers. Presently the research of his group focuses on functional conjugated molecules and macromolecules as well as hybrid semiconductor materials that may find application in advanced technological applications. The synthesis of new materials is combined with time-resolved optical spectroscopy, electrochemistry, morphological characterization and the preparation of prototype devices to accomplish these goals. René Janssen has co-authored more than 600 scientific papers. He is co-recipient of the René Descartes Prize from the European Commission for outstanding collaborative research, and received the Research Prize of The Royal Institute of Engineers and in The Netherlands for his work. In 2015 René Janssen was awarded with the Spinoza Prize of The Dutch Research Council.
In the past two decades the power conversion efficiency of organic solar cells has increased significantly and is now closing the gap to crystalline silicon. This remarkable advancement finds its origin in the design and synthesis of novel organic and polymer semiconductors with electron donating and electron accepting properties, next to the optimization of the solar cell device architecture. The organic semiconductors used in these devices have to meet several chemical, optical, electronic, and morphological requirements to provide such high efficiencies. The chemical structure, intrachain defects, optical band gap, redox potentials, molecular weight, processing conditions, charge transport layers, and device architectures all exert important roles to reach the intrinsic limits of these materials. Recent progress on developing new donor and acceptor polymers and molecules, combined improved device layouts, will be presented that result in organic solar cells with power conversion efficiencies close to 18%. Detailed analysis of these devices reveals that the feature low energy losses and relatively low energetic disorder at the optical band gap. This analysis provides directions for further advancements.
2.2-T1
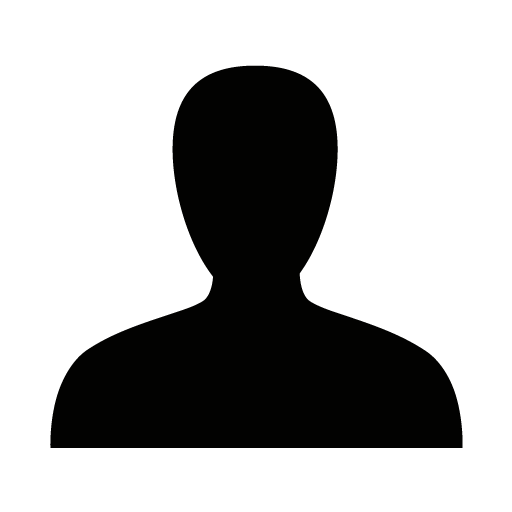
Polymer solar cells (PSCs) are a promising candidate as a future photovoltaic technology due to their lightweight, flexible and solution processable features. Development of non-fullerene acceptors (NFAs) allows significantly improved power conversion efficiencies (PCEs) of PSCs over 18% with their stronger light absorption properties and a wider absorption ranges than the fullerene acceptors. However, achieving highly efficient and highly stable PSCs is still challenging due to intrinsic disadvantages in the NFA based PSCs such as photochemically active molecular moiety in the photoactive materials and poor miscibility of donor and acceptor. Besides the features of the photoactive layer, interfacial layers are also critical to determine the stability of PSCs. Zinc oxide (ZnO) has been widely employed as an electron transport layer (ETL) in the inverted device structure because of their transparency in the visible spectral range, good electronic conductivity and solution processability. However, ZnO shows strong photocatalytic activity under illumination with UV light and induces the decomposition at the ETL/photoactive layer interface. In this work, we introduce an effective strategy to improve stability at the ZnO/photoactive layer interface by adding fullerene-based self-assembled monolayer(C60-SAM) in the photoactive layer as an additive. It is found that the C60-SAM in the photoactive layer can be spontaneously formed at the surfaces of the ZnO during spin-coating process due to the carboxylic acid group in the C60-SAM. PSCs without and with C60-SAM were fabricated and the devices with C60-SAM show efficiency enhancements of ~4-14% compared to the PSCs without C60-SAM additive. Furthermore, PCE drops of PSCs with C60-SAM are improved of ~26-30% under 1 sun illumination. The C60-SAM in the photoactive layer acts multiple functions to improve the molecular ordering of the photoactive materials and to decrease the photo bleaching rate of the photoactive materials by UV light.
2.2-T2
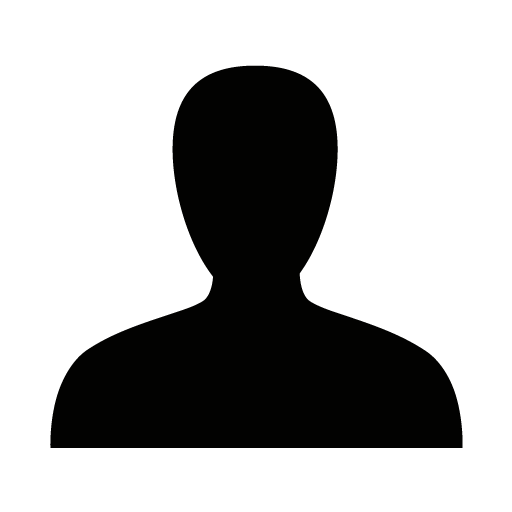
The long-time operational stability is a central challenge for developing organic solar cells (OSCs) to market readiness. However, many state-of-the-art OSCs based on the bulk-heterojunction concept suffer from stability problems caused by severe morphological changes upon thermal or illumination stress [1]. Single-component materials, enabled by the covalently-bonded structure with donor and acceptor in one molecule, present attractive advantages such as a simplification of device fabrication and stabilization of microstructure [2]. Recently, with the rapid improvement of efficiency from 2-3% to 11.3% for single-component organic solar cells (SCOSCs), this class of materials is getting into the research focus of the OPV community. However, reports on their operational stability are still scanty.
In this work, we systematically investigated for the first time the stability under thermal and illumination stress for a series of SCOSCs based on polymeric (SCP3, PBDBPBI-Cl) and molecular (dyad 1, 2, 3, and 4) materials. Under significant thermal stress, double-cable polymer-based SCOSCs exhibited excellent thermal stability with no degradation at 90 oC for 3000 hours. Furthermore, in order to compare polymeric with molecular single component materials, we studied the thermal stability among a series of SCOSCs based on D-A small molecules (dyad 1, 2, and 3) with the same donor and acceptor units but differently long alkyl space linkers. Since macroscopic diffusion of molecules is excluded in these dyads, the length of the spacer can only provide the necessary flexibility for sub-nm rearrangements caused by thermal stress. Interestingly, the single dyads showed a distinctly different behavior: dyad 1 with the shortest linker exhibited the highest thermal stability, while dyad 3 with the longest linker showed the relatively lowest thermal stability [3]. This highlights the need for further in-depth studies on optimizing the spacer length in parallel for performance and stability. Moreover, dyad 1-based SCOSCs exhibited exceptional illumination stability, retaining 98% of the initial PCE under concentrated light equal to 7.5-suns for over 1000 hours, which is among the best values for solution-processed OSCs. Based on the outstanding stability, SCOSCs could be an ideal candidate to study the ultimate stability under extremely rugged conditions such as high temperature and concentrated light. Since the morphological evolution is excluded, SCOSCs could serve as a model system to selectively study interface degradation. Shortly, SCOSCs are predicted to see a prospective renaissance with efficiencies over 10% and a lifetime of over 20 years, thus closing the gap towards industrial applications.
2.2-T3
Organic solar cells (OPV) impress with their low energy consumption during production and their low raw material requirements [1]. Since the layer thicknesses are only around 100 nm, around 1 g of an organic semiconductor is sufficient to produce a solar cell area of 10 m2. In the future, the energy payback times of organic solar cells will ideally be in the range of just a few days [2]. Over 18% power conversion efficiencies have now been achieved on a laboratory scale [3], however, OPV suffers from limited photostability and hence relatively short lifetimes, which remains a great challenge on the way to the widespread use of this technology.
The lifetime of OPV devices is negatively influenced by light, oxygen and humidity initiated degradation, and also by the morphological rearrangements accelerated by elevated temperatures. The additive assisted photooxidative stabilization of OPV by implementation of a third component into the active layer is one of the most promising strategies to overcome degradation. Recently, our group has reported stabilization of OPV devices by antioxidants which can simultaneously enhance the mechanical properties of OPV. Introduction of naturally abundant carotenoid compounds as photooxidative stabilizers has resulted in drastic improvement of accumulated power generation of the devices, attributed to their singlet oxygen as well as singlet oxygen precursor (e,g, fullerene triplet states) quenching capabilities [4,5]. Our newest results demonstrate the successful application of this approach to NFA-based systems, and we elucidate the dominating stabilizing mechanism via advanced microscopic and spectroscopic measurements.
2.3-I1
In the first part of the talk I will present current upscaling activities at Fraunhofer ISE, i.e., from small cells processed by means of spin-coating from chlorinated solvents over larger area cells processed from non-chlorinated solvents to large area roll-to-roll processed modules. Furthermore, this includes also the transition from an Indium Tin Oxide (ITO) based rigid architecture to an ITO-free flexible cell stack.
In the second part I will present results of electro- and photoluminescence studies of high-efficiency donor:acceptor blends as well as of complete solar cells. Using both steady-state and transient techniques, we try to elucidate the principal working mechanisms of organic solar cells. A strong focus is on the relation between charge carrier density, the (internal) quasi Fermi level separation and the (outer) voltage measurable between the terminals. Our aim is to contribute to the answer of the question whether organic solar cells differ from their crystalline inorganic counterparts in more than the fact that in organic solar cells, charge carrier generation is mostly achieved via electron transfer at donor/acceptor interfaces.
2.3-I2
Throughout his career, Jens has worked with successfully designing and developing complex experiments for in situ structural studies in the fields of polymer solar cells and functional organic materials, catalysis and hydrogen storage using synchrotron radiation, in fact since the beginning of his PhD studies. From 2001-2010 his focus was mainly on synchrotron radiation scattering techniques and their use for in situ experiments and for determining structure-property relations in functional thin films.
In 2011, Jens shifted his focus towards synchrotron-based 3D imaging of energy materials, particularly the very demanding case of polymer solar cells, where the low-contrast soft matter constitute a specific challenge. Through a dedicated effort of synchrotron experiments this led from 3D ptychographic imaging of roll to roll coated polymer solar cell active layers to 3D imaging of a complete polymer tandem solar cell. The scope of applications is all the time broadening, and Jens is now developing new tools for 3D imaging and organizing training and teaching in these.
In the same period, he developed a new setup for fast mapping of nano-structure, crystallinity and texture in R2R coated thin-films, which is still being improved and extended. Most recently, he has taken up research in ultrafast X-ray scattering and spectroscopy applied to solar energy materials, using X-ray free electron lasers.
In parallel with the studies of nano-structure in energy materials, Jens is running a small group that works with upscaling of organic solar cells, in order to maintain research into the entire development of this technology, from the fundamental understanding of charge generation and transport, over mesoscale structure formation to the performance of the final devices.
With the recent substantial leap in power conversion efficiency of organic solar cells, partly driven by formulations with new non-fullerene acceptors, new challenges has arisen as regards the fundamental understanding of the connection between mesoscale structure and the active layer performance. Unless this connection is understood and controlled, it will not be possible to overcome the recurring lab-to-fab challenge, or the scaling lag, as it is sometimes referred to. Typically, no less than 50% efficiency is lost when scaling up from minute, spin-coated devices to the large areas that are required for commercially viable modules.
We have identified three crucial focus points for overcoming the lab-to-fab challenge: (i) dual temperature control, i.e. simultaneous control of the ink and substrate temperatures during deposition, (ii) systematic in situ morphology studies of active layer inks with new, green solvent formulations during continuous deposition, and (iii) development of protocols for continuous solution processing of smooth, transparent interfacial layers with efficient charge transfer to the active layer. Combining these efforts and in general accompanying such studies with stability analyses and fabrication of large-area, scalably processed devices are believed to accelerate the relevance of organic solar cells for large-scale energy supply [1].
In this presentation, I focus on our efforts in developing an in-line methodology that is supported by molecular dynamics simulation [2] in order to disentangle the scattering fingerprints that may eventually be used to steer mesoscale structure formation to achieve the optimal photovoltaic performance of a bulk heterojunction. As will be shown, the methodology may be applied both on laboratory scale setups [3] as well as on synchrotron beam lines [4], and may even be used in conjunction with coating conditions far removed from ambient, i.e. with fully heated solution, coating head and substrate to handle materials that otherwise quickly aggregate and gelate. In combination with optical probes and machine learning techniques, the methodology is expected to close the lab-to-fab gap that continues to hold back the commercial breakthrough of organic solar cells.
1.1-I1
Prof. Adachi obtained his doctorate in Materials Science and Technology in 1991 from Kyushu University. Before returning to Kyushu University as a professor of the Center for Future Chemistry and the Department of Applied Chemistry, he held positions as a research chemist and physicist in the Chemical Products R&D Center at Ricoh Co., a research associate in the Department of Functional Polymer Science at Shinshu University, research staff in the Department of Electrical Engineering at Princeton University, and an associate professor and professor at Chitose Institute of Science and Technology. He became a distinguished professor at Kyushu University in 2010, and his current posts also include director of Kyushu University’s Center for Organic Photonics and Electronics Research (OPERA) since 2010 and program coordinator of Kyushu University’s Education Center for Global Leaders in Molecular Systems for Devices and director of the Fukuoka i3 Center for Organic Photonics and Electronics Research since 2013.
hrough the extensive R&D of organic light-emitting diodes (OLEDs) for more than 30 years, plenty of well-elaborated novel organic optoelectronic materials and device architectures have been extensively developed, resulted in the unique commercial utilization of OLEDs for cutting-edge smartphones, large-area TVs, and further new future display applications by taking advantage of light-weight and flexibility. From the aspect of materials science, the creation of novel light-emitting materials in OLEDs has been the central issue aimed for high electroluminescence quantum efficiency (EQE). Starting from the development of conventional fluorescence materials (1st generation) during 1990-2000th, the room-temperature phosphorescence (2000-) (2nd generation) and thermally activated delayed fluorescence (TADF) (2012-) (3rd generation) continuously pioneered the novel possibilities of organic emitters, resulted in not only high-performance OLEDs but also enriched organic photochemistry. In recent days, there have been a wide variety of studies on TADF-OLEDs because of the unlimited possibilities of TADF molecular design. Further, hyperfluorescence (HP)-OLEDs have been developed since they can realize the compatibility of high efficiency and narrow spectral width, which is ideal for practical display applications. Here we report our recent cutting-edge HP-OLEDs demonstrating high OLED performance by optimizing host, TADF, and terminal emitter (TE) molecules1-3). In particular, we focus on the blue-emission, which is capable of showing narrow FWHM and high EL quantum yield. Blue HP-OLEDs based on two new TEs are fabricated, resulting in high external quantum efficiency (EQE) of over 20%, high color purity, and high brightness. By analyzing the transient PL characteristics of the HP-OLEDs, we found that the presence of efficient FRET between TADF-assistant dopant (TADF-AD) and TE molecules. Further, transient EL analysis confirmed that a smaller EHOMO difference between TADF-AD and TE efficiently helps to decrease hole trapping inside the emitting layer, hence resulting in a lower efficiency rolloff and a longer operational device lifetime. This report provides a designing principle for a TADF and TE in HP-OLEDs with well-matched energy levels, leading to efficient FRET and no significant carrier trapping.
References:
[1] C-Y. Chan et al., Nature Photonics, 15, 203 – 207 (2021).
[2] Y-T. Lee et al., Advanced Electronic Materials, 7, 4, 2001090 (2021).
[3] M. Tanaka et al., ACS Applied Materials & Interfaces, 12, 45, 50668 – 50674 (2020).
1.1-I2
Thermally Activated Delayed Fluorescence (TADF) process has appeared as the most popular design strategy towards reaching 100% internal quantum efficiency for Organic Light-Emitting Diodes (OLEDs). TADF consists in promoting upconversion of triplet excited states into emissive singlet ones through Reverse InterSystem Crossing (RISC), a process driven by spin-orbit coupling (SOC) and requiring a small singlet-triplet gap DEST. The advancement of the TADF field occurred essentially through materials design, the first strategy, as proposed by C. Adachi and co, consisting in connecting electron donating and accepting units to decrease the DEST. However, in doing so, the lower-lying singlet and triplet excited states bear a dominant charge transfer character that translates into a broad emission spectrum.
In this contribution, we will discuss two different topics based on computational considerations:
How doped triangleshaped molecules can lead to (i) concomitant narrow emission, high quantum yield of emission and small DEST resulting in a whole new generation of TADF emitters, the multiresonant TADF emitters and to (ii) a new family of compounds with an inverted singlet-triplet gap and potentially, a downwards energy RISC. To do so, we rely on high level quantum chemical calculations and show that an accurate description of electron correlation effects is key to correctly predict the excited states ordering as well as the optical properties of these compounds.
How the interactions in the solid state can turn RISC from a SOCdriven to a hyperfine interaction (HFI)-driven mechanism. Combining time-resolved and transient electron paramagnetic resonance spectroscopies as well as (time-dependent) density functional theory calculations, we demonstrated that HFI-RISC occurs through delocalized charge transfer states in a curcuminoid derivative.
1.1-I3
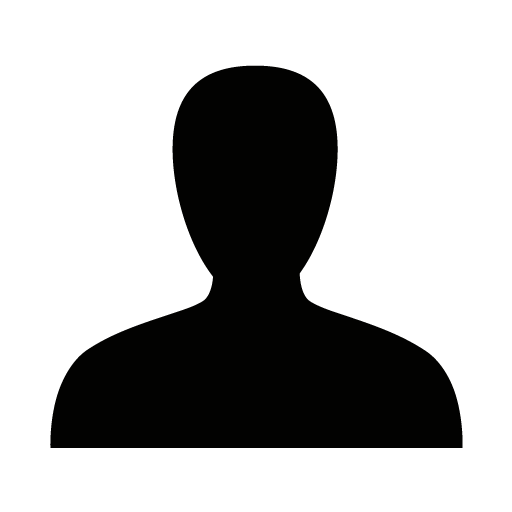
Organic diradicals based on p-conjugated molecules have been proposed to fulfill the thermodynamic requirements for singlet exciton fission (SEF), S1 ≈ 2 T1. This is based on the particular stabilization of the first triplet excited state (i.e., T1) regarding the singlet ground electronic state (i.e., S0) due to the mitigation of exchange repulsion in the open-shell form. In the case of diradicals made of quinoidal organic cores, the energy distribution of the excited state is controlled by the gaining of aromaticity in the ground electronic state. This provides with a powerful tool to design organic molecules with varying diradical character to be applied as chromophores in SEF. SEF is proposed as a way to increase the charge generation efficiency of organic photovoltaics devices. In this talk, the conceptual basis of the electronic structure of diradicals will be presented together with the implications in the design of molecules for SEF as well as a few examples on organic molecules showing this phenomenon.
1.2-T1
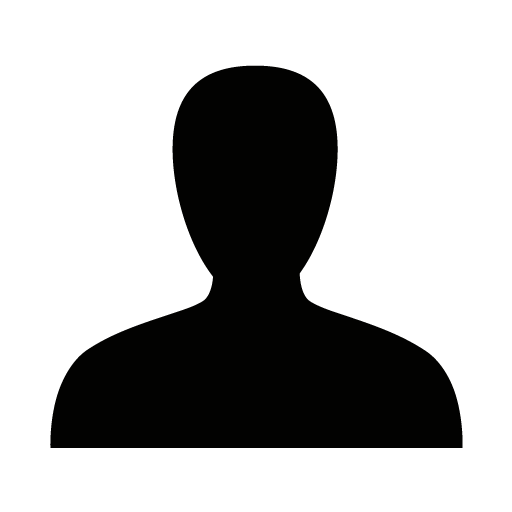
Organic radicals have emerged as the basis of more efficient organic light-emitting diodes (OLEDs) with higher performance limits than typical molecular emitters. This is achieved by doublet fluorescence with nanosecond emission in contrast to standard singlet-triplet photophysics found in organic optoelectronics. Here direct charge recombination at radical sites is considered the primary emission process. However, due to the low-energy radical orbitals, severe electron trapping occurs in devices with narrow emission zones at the interface of emitting layers and electron transport layers, causing significant efficiency roll-off, low radiance and poor stability. To improve the optoelectronic performance of organic radical electroluminescence (EL) devices, the device structure is optimised by tuning charge transport layers and utilising an ambipolar host. The concept is tested by transient EL measurements and single-carrier device analysis that show the limiting emission process. We demonstrate highly efficient organic radical light-emitting diodes using tris(2,4,6-trichlorophenyl)methyl (TTM)-based radicals with higher than 15% EQE at 700 nm, including substantially improved efficiency roll-off and maximum radiance over 100,000 mW sr-1 m-2 in an important step to practical organic radical light-emitting diodes.
1.2-T2
The light harvesting properties of a solar absorber can be modified by the effect of exciton-photon coupling, as a result of the reconfiguration of the electronic structure of the molecules, that can be achieved when the exciton state of the molecule is in resonance with confined electromagnetic field of an optical cavity. Whenever strong or ultra-strong coupling regime is reached, two new hybrid light-matter states, also known as polaritons, are formed with an energy separation that is proportional to the coupling strength.[1] This form of tailoring of the eigenstate of the system represents a compelling tool in photovoltaics, being conversion efficiency, light harvesting directionality and charge transport direct consequences of such modification.
Recently, subphthalocyanine derivatives have been integrated in a polaritonic organic solar cell acting as an optical resonator in order to modify the absorption onset and tune the optoelectronic properties of the devices.[2] In view of this, a comprehensive analysis on how the different electronic transitions of an absorber can be coupled to the resonant modes of a solar polaritonic device is compelling.
Herein, the light harvesting properties of broad band light harvesting molecules operating under weak, strong and ultra-strong coupling regimes are presented. The effect of directional and spectral response, as well as the effect of polaritons on unproductive absorption due to the presence of metallic films in the structure, are discussed in detail.[3,4] These results allow to determinate the optimum configuration to exploit the potential of solar cells devised as optical resonators.
1.2-T3
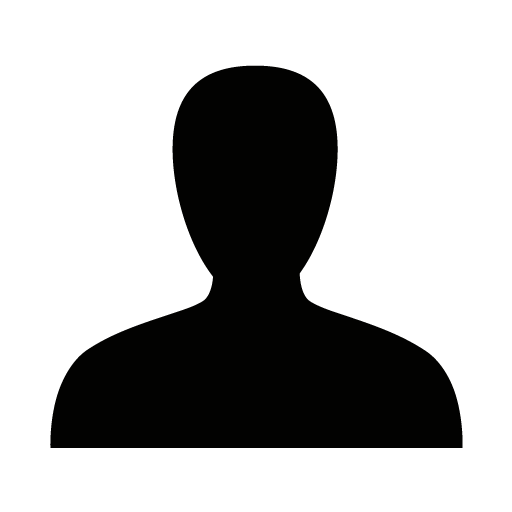
The great progress in organic photovoltaics (OPV) over the past few years was achieved largely by the development of non-fullerene acceptors (NFAs) with power conversion efficiencies now approaching 20%. To achieve this result and further enhance device performance, loss mechanisms must be identified and minimized. Especially triplet excitons are known as being detrimental to device performance, since they can form energetically trapped states, responsible for non-radiative losses or even device degradation. Using the complementary spin-sensitive methods of photoluminescence detected magnetic resonance (PLDMR), transient electron paramagnetic resonance (trEPR) and transient absorption (TA) corroborated by quantum-chemical calculations, we reveal exciton pathways and identify energetically trapped triplet excitons in OPV blends employing the polymer donors PBDB-T, PM6 and PM7 together with NFAs Y6 and Y7. Thereby, all blends reveal long-lived triplet excitons on the NFA via non-geminate hole back transfer. Further, we detect additional triplet generation on the NFA via spin-orbit coupling induced intersystem crossing in blends with the best performing halogenated polymers PM6 and PM7. In conclusion, we identify triplet formation in all tested solar cell absorber films, which underlines that there is an untapped potential for improved charge collection efficiency and elimination of device degradation caused by triplet excitons.
1.3-I1
Singlet exciton fission (SF), and its reverse, triplet–triplet annihilation (TTA), represent two promising ways of manipulating photons in optoelectronic devices via energetic down- or up-conversion, respectively. They are also fascinating physical processes, the study of which offer insights into the fundamental physics of organic semiconductors and photoactive proteins.
In this talk, I will focus on some recent work from my group on understanding the fundamentals of SF and TTA [1] in organic molecular thin films [2,3], photoactive proteins [4] and microcavities [5]. Our recent work shows that SF and TTA both proceed via a real, emissive triplet-pair state in endo- and exothermic singlet fission materials. We find that in microcavities in the strong coupling regime, delayed emission from triplet-triplet annihilation is enhanced compared with bare films and that the spin-statistics governing up-conversion yield can be modified through molecular an intermolecular design [6].
1.3-I2
Michael R. Wasielewski is currently the Clare Hamilton Hall Professor of Chemistry at Northwestern University, Executive Director of the Institute for Sustainability and Energy at Northwestern, and Director of the Center for Molecular Quantum Transduction, a United States Department of Energy Energy Frontier Research Center. His research has resulted in over 730 publications and focuses on light-driven processes in molecules and materials, artificial photosynthesis, molecular electronics, quantum information science, ultrafast optical spectroscopy, and time-resolved electron paramagnetic resonance spectroscopy. His honors and awards include membership in the National Academy of Sciences and the American Academy of Arts and Sciences; the Bruker Prize in Electron Paramagnetic Spectroscopy (EPR); the Josef Michl American Chemical Society Award in Photochemistry; the International EPR Society Silver Medal in Chemistry; the Royal Society of Chemistry Physical Organic Chemistry Award; the Chemical Pioneer Award of the American Institute of Chemists; the Royal Society of Chemistry Environment Prize; the Humboldt Research Award; the Arthur C. Cope Scholar Award of the American Chemical Society; the Porter Medal for Photochemistry; and the James Flack Norris Award in Physical Organic Chemistry of the American Chemical Society. He received his B.S., M.S., and Ph.D. degrees from the University of Chicago.
Multiexciton quintet states, 5(TT), photogenerated in organic semiconductors using singlet fission (SF) consist of four quantum entangled spins, promising to enable new applications in quantum information science. However, the factors that determine the spin coherence of these states remain underexplored. Here, we engineer the packing of tetracene molecules within single crystals of 5,12-bis(tricyclohexylsilylethynyl)tetracene (TCHS-tetracene) to demonstrate a 5(TT) state that exhibits promising spin qubit properties, including a coherence time, T2, = 3 μs at 10 K, a population lifetime, Tpop, = 130 μs at 5 K, and stability even at room temperature. The single crystal platform also enables global alignment of the spins and, consequently, individual addressability of the spin sublevel transitions. Decoherence mechanisms, including exciton diffusion, electronic dipolar coupling, and nuclear hyperfine interactions, are elucidated, providing design principles for increasing T2 and the operational temperature of 5(TT). By dynamically decoupling 5(TT) from the surrounding spin bath, T2 = 10 μs is achieved. These results demonstrate the viability of harnessing singlet fission to initiate multiple electron spins in a well-defined quantum state for next-generation molecular-based quantum technologies.
1.3-I3
Thuc-Quyen Nguyen is a professor in the Center for Polymers and Organic Solids and the Chemistry & Biochemistry Department at University of California, Santa Barbara (UCSB). She received her Ph.D. degree in physical chemistry from the University of California, Los Angeles, in 2001 under the supervision of Professor Benjamin Schwartz. Her thesis focused on photophysics of conducting polymers. She was a research associate in the Department of Chemistry and the Nanocenter at Columbia University working with Professors Louis Brus and Colin Nuckolls on molecular self-assembly, nanoscale characterization and molecular electronics. She also spent time at IBM Research Center at T. J. Watson (Yorktown Heights, NY) working with Richard Martel and Phaedon Avouris. Her current research interests are structure-function-property relationships in organic semiconductors, sustainable semiconductors, doping in organic semiconductors, interfaces in optoelectronic devices, bioelectronics, and device physics of OPVs, photodetectors, and electrochemical transistors. Recognition for her research includes 2005 Office of Naval Research Young Investigator Award, 2006 NSF CAREER Award, 2007 Harold Plous Award, 2008 Camille Dreyfus Teacher Scholar Award, the 2009 Alfred Sloan Research Fellows, 2010 National Science Foundation American Competitiveness and Innovation Fellows, 2015 Alexander von Humboldt Senior Research Award, 2016 Fellow of the Royal Society of Chemistry, 2015-2019 World’s Most InfluentialScientific Minds; Top 1% Highly Cited Researchers in Materials Science by Thomson Reuters and Clarivate Analytics, 2019 Fellow of the American Association for the Advancement of Science (AAAS), 2023 Wilhelm Exner Medal from Austria, 2023 Fellow of the US National Academy of Inventors, 2023 de Gennes Prize in Materials Chemistry from the Royal Society of Chemistry, 2023 Elected Member of the US National Academy of Engineering, 2024 Fellow of the European Academy of Sciences, and 2025 ACS Henry H. Storch Award in Energy Chemistry.
Solution processed doped layers are of extreme importance for high throughput production of organic electronic devices via roll-to-roll or ink-jet printing. In this talk, I will discuss tuning the conductivity of conjugated polymers containing Lewis basic sites by Lewis acids. Addition of the Lewis acid effectively p-dopes the hole transport in the parent polymer, leading to increases in the free hole density and conductivity. This methodology is advantageous since the polymer and Lewis acid have excellent solubility in organic solvents, negating the need for co-solvents that uses in molecular dopant such as F4TCNQ. We use a combination of techniques including electrical measurements, optical absorption, XPS, UPS, IPES, and electron paramagnetic resonance (EPR), electron-nuclear double resonance (ENDOR) and nuclear magnetic resonance (NMR), and gas chromatography techniques in conjunction with density functional theory, to gain insight into the doping mechanism of 4,4-dihexadecyl-4H-cyclopenta[1,2-b:5,4-b′]dithiophene (CPDT) and 2,1,3-benzothiadiazole (BT)-based (PCPDTBT) by Lewis acids, BCF, and to explain why the doping efficiency is much higher for BCF than for F4TCNQ.
2.1-I1
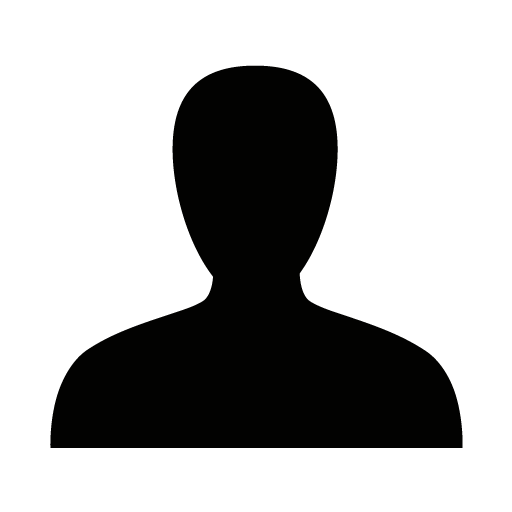
Over the past five years, the solar power conversion efficiencies of organic solar cells (OSC) have greatly improved from 11% to 18%, closing the gap with their inorganic and hybrid counterparts. The major breakthrough behind the rapid efficiency improvement is the development of non-fullerene acceptor molecules, replacing the traditional fullerene molecules as electron-accepting materials. Understanding the photophysical processes underlying these high-performance materials is crucial to OSC research. In this talk, I will present transient optical spectroscopy results on non-fullerene OSC blends with small interfacial energy offsets. By optically probing the time evolution of excited states, we show that free charges are generated via thermal activation of interfacial charge-transfer (CT) states on a hundred picosecond timescale. Reduced charge separation rate is observed at lower temperatures, leading to increasing charge recombination either directly at the donor-acceptor interface or via the emissive singlet exciton state. A kinetic model is used to rationalize the results, showing that although photogenerated charges have to overcome a significant Coulomb potential to generate free carriers, OSC blends can achieve high photocurrent generation yields even at reduced temperatures given that the thermal dissociation rate of charges outcompetes the recombination rate.
2.1-I2
Solar cells fabricated from organic semiconductors possess several benefits over traditional inorganic technologies. Organic solar cells are lightweight and flexible, whilst also offering the possibility of semi-transparent modules and superior performance in low-light intensity applications. However, whilst the power conversion efficiencies of organic solar cells have exceeded 18% under sunlight, this is still lower than inorganic technologies, where efficiencies of >20% are commonplace. Of relevance to solar cell operation, organic semiconductors have a reduced ability to screen the Coulomb interaction between two charge carriers of opposite signs compared to their inorganic counterparts. Consequently, organic semiconductors are excitonic materials, where the strong Coulombic interactions between electrons and holes results in a large exciton binding energy (~0.2-0.5 eV) and localised excited states. In these excitonic states, the spins of the paired electrons interact strongly, resulting in the formation of distinct spin-singlet (electron spins anti-parallel) and spin-triplet (electron spins parallel) states. As singlet and triplet excitons possess dramatically different optical and electronic properties, exciton spin becomes a key consideration in the operation of optoelectronic devices fabricated from organic semiconductors, such as solar cells and light emitting diodes.
In this talk, I will present our recent work on spin management in organic solar cells[1]. We find that in benchmark organic solar cells fabricated using non-fullerene electron acceptors, up to 90% of the charge carrier recombination proceeds via low energy triplet exciton states. As the radiative decay of triplet excitons back to the spin-singlet ground state is spin-forbidden, recombination via dark triplet excitons constitutes a significant voltage loss pathway in organic solar cells. Through the identification of systems where recombination via triplet excitons is suppressed, we demonstrate a novel tactic to turn off this loss pathway that involves the strong electronic coupling between the electron donor and acceptor components in the organic solar cell. Consequently, we propose molecular design rules to engineer-out recombination via triplet excitons in future systems. Therefore, our findings provide a framework to further reduce the performance gap between organic and inorganic solar cell technologies.
2.1-I3
Jess is an Imperial College Research Fellow investigating spin selective charge transport through chiral systems in the Department of Materials. She currently works in SPIN-Lab at Imperial, which is led by Professor Sandrine Heutz. She previously worked as a postdoctoral researcher in the Fuchter group at Imperial College London, where she optimised these chiral systems such that can absorb/emit circularly polarised (CP) light for CP OLEDs and OPDs. For her PhD Jess concentrated on organic photovoltaics and the development of advanced characterisation techniques to better understand molecular packing under the supervision of Dr Ji-Seon Kim. Outside of the lab, Jess is involved with several science communication and outreach initiatives. She is committed to improving diversity in science, both online and offline, and since the start of 2018 has written the Wikipedia biographies of women and people of colour scientists every single day.
Organic thin films that emit and absorb circularly polarised (CP) light have been demonstrated with the promise of achieving important technological advances; from efficient, high-performance displays, to 3D imaging and all-organic spintronic devices. As a result, interest in the identification of materials that can emit CP light has surged in recent years. Unfortunately, the selectivity in absorption or emission (so-called dissymmetry or g-factor) of left-handed versus right-handed CP light is low for many molecular systems. Conjugated polymer thin films can give rise to very large dissymmetry (|gabs| >1).The precise origins of the large chiroptical effects in such films had, until recently, remained elusive. We have investigated the emergence of such phenomena in achiral polymers blended with chiral small-molecule additives and intrinsically chiral-sidechain polymers using a combination of spectroscopic methods and structural probes. We show that – under conditions relevant for device fabrication – the large chiroptical effects are caused by magneto-electric coupling (natural optical activity), not structural chirality as previously assumed, and may occur because of local order in a cylinder blue phase-type organisation and increased delocalisation of their chromophore/excitonic coupling. We show that optimised chiral polymer systems can be used as the active layers of CP Organic Photodetectors (OPDs) and CP Organic Light Emitting Diodes (OLEDs) with exceptional device performance. For example, CP OLEDs based on the widely studied π-conjugated polymer F8BT can achieve current efficiencies of 16.4 cd/A, power efficiency of 16.6 lm/W, a maximum luminance of over 28,500 cd/m2, and a high electroluminescence dissymmetry of 0.57, whilst CP OPDs achieve photocurrent dissymmetries of 0.85, low dark currents (10 pA) and ultrafast response times. Our disruptive mechanistic insight into chiral polymer thin films will offer new approaches towards the development of chiroptical materials and devices.
2.2-T1
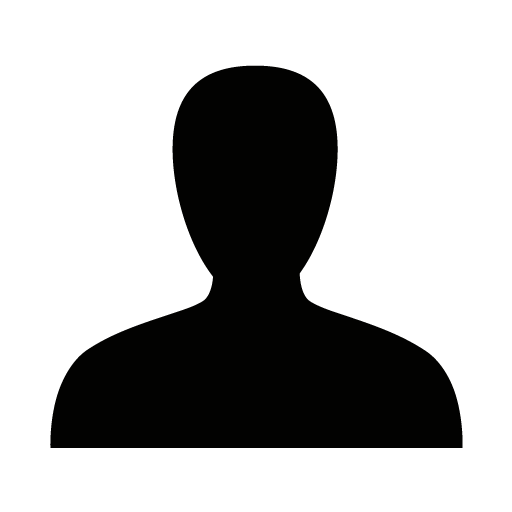
Single-component organic solar cells (SCOSCs) have witnessed great improvement during the last few years with the champion efficiency jumping from the previous 2-3% to currently 6-11% for the representative material classes. However, the photophysics in many of these materials has not been sufficiently investigated, lacking essential information regarding charge carrier dynamics as a function of microstructure, which is highly demanded for a better understanding and potential guidance to further improvements.
In this work, for the first time, the charge carrier dynamics on different time scales of a representative double-cable polymer, which achieves efficiencies of over 6% as an active layer in SCOSCs, has been investigated across 7 orders of magnitude in time scale, from fs-ps TAS and ps-ns TRPL for probing charge generation to ns-µs TAS for charge recombination. Specific emphasis is placed on understanding the evolution of charge generation, transport as well as charge recombination behind the gradually improved photovoltaic performance upon thermal annealing treatment of the representative double-cable polymer PBDBPBI-Cl. By increasing the thermal annealing temperature, geminate recombination is reduced accompanied by more efficient charge dissociation and suppressed bimolecular recombination. Annealing the photoactive layer at 230 oC results in the highest photovoltaic performance correlating well with the findings from transient studies. This work successfully presents a complete picture of the charge carrier dynamics in SCOSCs.
2.2-T2
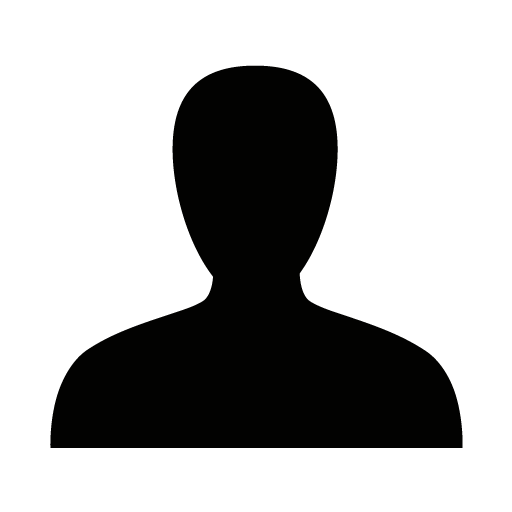
Spin doublet radical organic semiconductors can show near unity luminescence yield from their lowest energy excited state and are attractive as the emissive component in organic light-emitting diodes (OLEDs). [1]
Here I will present our recent measurements of direct, rapid, spin-allowed energy transfer from triplet excitons generated within a closed-shell organic host to a doublet chromophore. We use a carbene-metal-amide (CMA-CF3) as a model host, since following photoexcitation it undergoes extremely fast intersystem crossing to set up a population of triplet excitons within a few picoseconds. We track the subsequent energy transfer to the TTM-3PCz radical using transient absorption and temperature-dependent transient photoluminescence spectroscopies. These show that direct triplet-to-doublet energy transfer is the dominant channel that accounts for over 90% of all radical emission. OLEDs based on the CMA-CF3:TTM-3PCz blend show improved device characteristics compared to TTM-3PCz radical OLEDs without triplet-enhanced energy transfer.
Our design overcomes triplet-imposed performance limits for optoelectronics by activating spin-allowed triplet-doublet transfer on picosecond–nanosecond timescales, with light emission obtained orders of magnitude faster than derived from conventional triplet(-singlet) management technologies.
This method allows photophysical studies to reflect the mechanisms "Behind the Device" by mimicking spin statistics present under electrical charge injection, which may be a powerful tool for the wider organic electronics community.
2.2-T3
In 2023, Drew earned his PhD Sêr SAM group at Swansea University. His focus is on the disentangling of various relaxation pathways in disordered semiconductor systems including organics and perovskites. He is the resident Ultrafast expert and has been involved in the construction of many apparatuses the group currently uses. Drew’s interests lie in Ultrafast and Semiconductor Physics, specifically the relaxation mechanisms in disordered semiconductors. Throughout his career, Drew has worked with start-up companies, lectured to undergraduate students, tutored and taught at the undergraduate level, and volunteered with various science outreach groups. Before completing his eduction, Drew worked as a pastry chef. He is an avid musician, surfer, and traveller.
Excitonic transport in organic semiconductors underpins charge generation in all manner of organic electronic devices[1]. In this presentation I will discuss the role that exciton diffusion length plays in organic photovoltaic devices and introduce a steady-state technique to measure the diffusion length in organic semiconductors through exciton-exciton annihilation (EEA), named pulsed-PLQY. Previously, accurate measurements of exciton diffusion length through EEA required highly specialized time-resolved equipment[2]. I will show that by measuring in steady-state the required equipment specialization, cost, and time can be reduced, while the accuracy is improved.
Specifically, a Kinetic Monte-Carlo hopping model is used to simulate the exciton dynamics in an organic semiconductor. These dynamics are analysed using traditional and steady-state EEA techniques. It is found that pulsed-PLQY is less sensitive to the choice of initial density and has increasing confidence with increasing densities used. These simulations are validated by preforming both steady-state and traditional EEA experiments on organic semiconductors, including technologically relevant non-fullerene acceptors (NFAs). Overall, it is found that NFAs show an increase in diffusion length, driven primarily by an increases in diffusivity, when compared to the benchmark fullerene acceptor [3].
2.3-I1
Conjugated polymers and molecular semiconductors are emerging as a viable semiconductor technology in industries such as displays, electronics, renewable energy, sensing and healthcare. A key enabling factor has been significant scientific progress in improving the charge transport properties and carrier mobilities of these materials, which has been made possible by better understanding of the molecular structure-property relationships and the underpinning charge transport physics. Here we aim to present a coherent review of how we understand the unique charge transport physics in these van-der Waals bonded semiconductors, that can be understood within the framework of transient localisation which is intermediate between classical hopping and band transport. Specific questions of interest include a discussion of the coupling between charge and structural dynamics, how the transport physics of conjugated polymers and small molecule semiconductors are related and how the incorporation of a high concentration of counterions in doped films, as used for example in thermoelectric devices, affects the electronic structure and charge transport properties and how these processes can be studied by spectroscopic tools.
2.3-I2
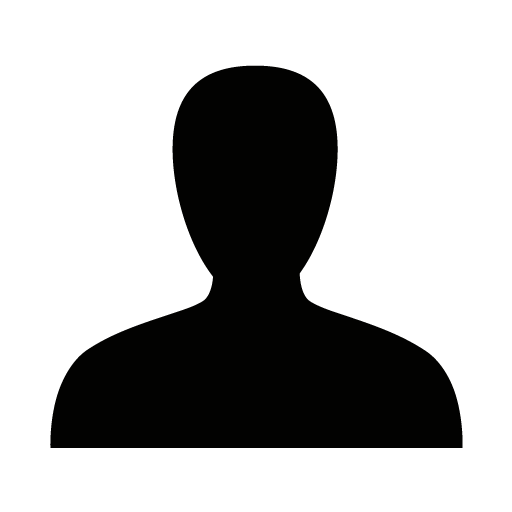
The function of essentially all present and future quantum devices, from quantum computers over quantum sensors to photocatalytic systems or solar cells, relies on the motion of charges and spins on ultrafast time and exceedingly short length scales. Usually these dynamics are governed by such a complex interplay between electronic and nuclear motion that their understanding is quite limited and we rely on particle-like transport models for describing the dynamics and function of those systems.
In my talk, I will introduce and discuss several systems in which this classical, particle-like transport regime breaks down and the wave-like coherent transport of energy and charge becomes dominant, even in disordered nanostructures and at room temperature. I will try to demonstrate this for different transport processes and in quite diverse systems, ranging from artificial light-harvesting molecules to technologically-relevant materials such as halide perovskite crystals. Specifically, I will discuss coherent intramolecular charge transport processes in donor/acceptor molecules (1) and organic thin films (2), and coherent intermolecular charge transfer in P3HT/PCBM solar cell layers (3). More recently, we have found strong evidence for the role of intermolecular conical intersections (CoIns) in the energy transport in aggregated donor/acceptor thin films (4). Using an advanced spectroscopic method, two-dimensional electronic spectroscopy (2DES), we have followed the coherent wavepacket motion across such a CoIn (4). Finally, I will discuss what 2DES can tell us about electron-phonon couplings in halide perovskites (5). Our studies of charge transport in organic materials give unexpected evidence for long-lived vibronic quantum coherence and outline strategies for molding the flow of charge in nanostructures by tailoring and controlling their coherent coupling to vibrational modes of the materials. These advances became possible by probing the optical properties of nanostructures with a time resolution of few femtoseconds only, faster than any of the functionally relevant vibrational modes of the material, and by comparing the experimental results to advanced theoretical modelling..
1.1-I1
Luisa Torsi received her Laurea degree in Physics from the University of Bari in 1989 and a Ph.D. in Chemical Sciences from the same institution in 1993. She was a post-doctoral fellow at Bell Labs from 1994 to 1996. In 2005 and 2006 she was invited professor at the University of Anger and Paris 7, respectively. Since 2005 she is a full professor of chemistry at the University of Bari and since 2017 she is an adjunct professor at the Abo Academy University in Finland.
In 2010 she has been awarded the Heinrich Emanuel Merck prize for analytical sciences, this marking the first time the award is given to a woman. Prof. Luisa Torsi is also the winner of the Wilhelm Exner Medal 2021 (https://www.wilhelmexner.org/en/). The medal has been awarded since 1921 by the Austrian Association of Industries to celebrate excellence in research and science and as many as 23 Nobel prize winners have been awarded too. She is also the recipient, at the British Library in London, of the 2015 main overall platinum prize of the Global-Women Inventors and Innovators Network. The IUPAC - International Union of Pure and Applied Chemistry awarded her with the 2019 Distinguished Women in Chemistry or Chemical Engineering. The analytical chemistry division of the European Chemical Society (EuChemS) conferred her the Robert Kellner Lecturer 2019.
Since 2020 she has been appointed National Representative for the Marie Skłodowska-Curie Action of Horizon Europe by the Italian Minister for Education and Research. She is also past president of the European Material Research Society being the first women to serve on this role. She has been also elected 2017 Fellow of the Material Research Society, for pioneering work in the field of organic (bio) electronic sensors and their use for point-of-care testing.
Awarded research funding for over 26 million € in thirteen years, comprises several European contracts as well as national and regional projects. She is coordinating the “Single molecule bio-electronic smart system array for clinical testing – SiMBiT” a H2020-ICT-2018-2020 research and innovation action financed with over 3 M€. The PRIN 17 national project “ACTUAL: At the forefront of Analytical ChemisTry: disrUptive detection technoLogies to improve food safety (2017RHX2E4)” is also coordinated by Torsi. She has also coordinated a “European Industrial Doctorate” Marie Curie project in collaboration with Merck and was principal investigator in a Marie Curie ITN. She has also coordinated a Marie Curie ITN European network, several national PRIN projects, and was principal investigator in an ICT STREP proposal. She has also been the scientific coordinator of a Structural Reinforcement PON Project awarded to UNIBA for 2012-2014 and is engaged with a number of other Structural Reinforcement PON projects.
Torsi has authored almost 230 ISI papers, including papers published in Science, Nature Materials, Nature Communications, PNAS, Advanced Materials, Scientific Reports, and is co-inventor of several international awarded patents. Her works gathered almost 13.500 Google scholar citations resulting in an h-index of 55. She has given more than 170 invited lectures, including almost 50 plenary and keynotes contributions to international conferences.
Prof. Torsi is committed to the role of model for younger women scientists. She has been giving a number of talks on this topic such as a TEDx talk. Prof. Torsi is one of the 100Experts (https://100esperte.it) a project led by Fondazione Bracco comprising an online databank with the names and CVs of female experts in STEM, a sector historically underrepresented by women but a strategic one for the economic and social development of Italy. In a recent campaign to foster the idea of gender equality in Science among children, prof. Torsi was featured in a story of TOPOLINO (Italian comic digest-size series of Disney comics), as “Louise Torduck”, a successful female scientist of the Calisota valley.
Nanosized bioelectronic detecting interfaces have been the privileged pathway to single-molecule detections so far. However, while giving access to rarer events, this near-field approach is unsuited to detect at concentrations lower than nanomolar because of the diffusion-barrier issue. Namely, it will be statistically extremely improbably for a nanometric interface to encounter the target analyte if the solution is too diluted.
At the same time, biomarkers are becoming the preferred highway toward early diagnosis of progressive diseases, such as tumors or neurodegenerative syndromes. When it comes to infective diseases the immunometric direct detection of a pathogen (instead of its genome) is a faster way, as it requires no sample pretreatment. The possibility of detecting a marker (proteins, genomic strands as well as whole viruses or bacteria) in a peripheral biofluid such as blood or even saliva, makes the process also minimally invasive. The sooner the detection is, the earlier the diagnosis will be, the easier for a clinician to fight the battle against a disease, is. Nowadays there is the possibility to detect a single strand of a mutated gene for the early diagnosis of tumours or to detect a single copy of a viral DNA. Still a commercial system that can reliably detect a single-proteins in a sample of 0.1 ml of a real biofluid, is not available.
Large-area (μm^2 - mm^2 wide) bioelectronic transistors are perceived as unsuited due to the irrelevant footprint of a single molecule on a much larger detecting interface. Indeed, detecting such an event would be like spotting the wave generated by a single droplet of water falling on a one-kilometer-wide pond. However, many field-effect large-area biosensors have been shown to detect at limit-of-detection below femtomolar, being also naturally suited for point-of-care applications. In this lecture the field is reviewed, illustrating device architectures, materials used, and target analytes that can be selectively detected. The sensing mechanisms and the amplification effects enabling the large-area bioelectronic sensor to detect at the physical limit are also detailed.
1.1-I2
Use of light for selective and spatio-temporally resolved control of cell functions (photoceutics) is emerging as a valuable alternative to standard electrical and chemical methods. We propose the use of organic semiconductors as efficient and biocompatible optical transducers, and we focus in particular on breakthrough applications in the field of regenerative medicine. Devices able to selectively and precisely modulate the fate of living cells upon visible light will be presented and critically discussed. Examples of practical applications include optical modulation of the activity of both excitable and non-excitable cells, control of essential cellular switches like transient receptor potential channels and other cationic channels, as well as effective modulation of intracellular calcium signalling for precise control of cell metabolic processes.
As a representative example with a high translational potential, we describe here in detail a biointerface to gain optical control of Endothelial Colony Forming Cells, which represent the only known Endothelial Progenitor Cell subset and are valuable candidates to induce revascularization of ischemic tissues. We demonstrate that polymer-mediated optical excitation induces a robust enhancement of lumen formation in vitro. We identify the pathways leading to this effective enhancement in Endothelial Colony Forming Cells network formation, as due to light-induced activation of the non-selective Transient Receptor Potential Vanilloid 1 (TRPV1) cation channel. Moreover, we show that polymer-mediated optical excitation induces a long-lasting increase in intracellular calcium ions concentration, [Ca2+]i. Pharmacological and genetic manipulation reveals that the Ca2+ response to light is triggered by extracellular Ca2+ entry through TRPV1, whose activation requires the non-toxic production of reactive oxygen species at the interface between the photoactive material and the cell membrane.
Our results represent, to the best of our knowledge, the first report of use of polymer-based optical modulation to restore cardiac function in vitro, by modulating cellular activities of one of the main characters involved in cardiac repair.
1.1-I3
Achilleas Savva is an Assistant Professor in the Bioelectronics group at Delft University of Technology, in The Netherlands. He received his B.Sc. and M.Sc. in chemical engineering from Aristotle University of Thessaloniki in Greece, in 2010. He then obtained his PhD in Materials Science and Engineering from Cyprus University of Technology in 2014. His PhD research was focused on organic optoelectronics for renewable energy. In 2017 he joined the group of Professor Sahika Inal in KAUST, Saudi Arabia, as a postdoc, and expanded his research on organic bioelectronics. In 2019, he joined the group of Professor Róisín Owens at the University of Cambridge where he secured the Marie Skłodowska-Curie Postdoctoral Fellowship. He developed several novel organic bioelectronic devices such as biosensors, light sensitive devices for photo-stimulation of neurons, 3D in vitro human stem cell models, among others. Achilleas was born in Limassol, Cyprus.
Organic bioelectronics is an emerging interdisciplinary field, encompassing organic electronic interfaces operating in physiological conditions hand-in-hand with biological systems. These interfaces are mostly based on conjugated polymers that have emerged as ideal materials due to their biocompatibility, tissue-like mechanical properties, and their ability to simultaneously conduct electronic and ionic currents. Importantly, these properties can be tailored to meet the requirements of different types of human tissue with relatively easy methods – e.g. by chemical synthesis or by simply mixing wet solutions of conjugated polymers with other additives. These profound advantages enabled the development of organic bioelectronic devices that push the boundaries in a range of applications, from brain interfaces to in vitro diagnostics and tissue engineering. Here we will present the development of multifunctional bioelectronic interfaces to monitor and control cellular activity with conventional electrical measurements, in vitro. First, the development of a free standing, photosensitive platform based on a combination of conjugated polymers will be presented. This platform is able to control wirelessly the activity of primary cortical neurons with white light at low intensity. Later on, the development of 3D mesoporous polymer scaffolds for growing human stem cells tissue will be presented. These structures are made from composites of conjugated polymers and are able to recapitulate 3D tissue-like environments as well as exhibit multifunctional properties (i.e low young modulus, good electrical conductivity and photo-sensitivity). The pore size/network inside the scaffold can be also tailored by changing the amount and the nature of the cross-linker, which can in turn control stem cell growth rates and size. These biomimetic, multifunctional 3D structures are addressed electrically to form 3D electrodes that allow for monitoring human stem cell proliferation rates with electrical impedance measurements. Finally we will present preliminary results on how we can leverage the properties of multifunctional scaffolds to control cell fate and differentiate “naïve” stem cell tissue to neurons via chemical, electrical and light stimulation.
1.2-T1
In vitro monitoring of electrogenic cells represents a very interesting approach in different scientific fields such as neuroengineering, pharmacology, and potentially also translational medicine. One of the key aspects of all cellular cultures, and this is particularly true for standard electrogenic cell cultures, as well as for cardiac organoids and neurospheroids, is their complexity, mainly due to the different kinds of both chemical and electrical signals that characterize their response to external stimuli and that ultimately govern their behavior. During the past 50 years, this extraordinary complexity pushed the research community to develop increasingly complex devices and tools with the idea of fully exploiting all the different aspects of these multiparametric systems. To the aim of providing a simple and integrated approach for the study of in vitro electrogenic cultures, and thus offer a potentially simpler solution to the monitoring of different cellular features, we present here an organic transistor-based device capable of measuring two of the most important cellular parameters, namely the electrical and the metabolic activity, using the ultra-sensitive Organic Charge Modulated FET (OCMFET). The OCMFET, thanks to its peculiar structure and outstanding versatility, can be conveniently engineered in order to meet the desired sensing needs, ultimately allowing to obtain multisensing and multisite tools capable of simultaneously recording the aforementioned parameters using a single type of organic transistor, differently from other existing approaches. The device, called Micro OCMFET Array (MOA), has been tested using primary cardiac rat myocytes, and allowed to evaluate the metabolic and electrical variations that occur upon the administration of different drugs. Although preliminary, these results demonstrate the very interesting potentials of organic transistors in this highly complex and multifaceted scientific field, thus laying the basis for the development of a multi-sensing tool for the in vitro monitoring of cell aggregates. As a future perspective, the possibility of obtaining MOA devices with 3D recording sites will also be addressed. This capability, together with the already-mentioned versatility of the system, may represent a potentially game-changing feature within the rising field of in vitro organoid studies.
1.2-T2
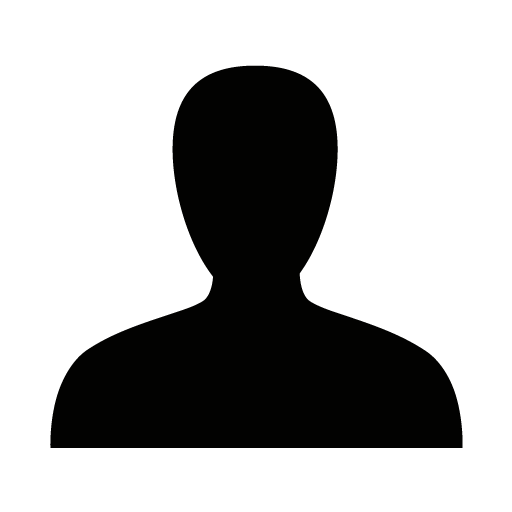
The ability to operate in aqueous environments makes poly(3,4-ethylenedioxythiophene):poly(styrenesulfonate), PEDOT:PSS, based organic electrochemical transistors (OECTs) excellent candidates for a variety of biological applications. Current research in PEDOT:PSS based-OECTs is primarily focused on improving the conductivity of PEDOT:PSS film to achieve high transconductance (gm). The improved conductivity and electronic transport are attributed to the formation of enlarged PEDOT-rich domains and shorter PEDOT stacking, but such a change in morphology sacrifices the ionic transport and, therefore, the doping/de-doping process. Additionally, little is known about the effect of such morphology changes on the gate bias that makes the maximum gm, threshold voltage, and transient behavior of PEDOT:PSS based OECTs. Here, we tune the molecular packing and nanostructure of PEDOT:PSS films using ionic liquids as additives, namely, 1-Ethyl-3-methylimidazolium (EMIM) as cation and anions of chloride (Cl), trifluoromethanesulfonate (OTF), bis(trifluoromethylsulfonyl)imide (TFSI), and tricyanomethanide (TCM). We demonstrate that an optimal morphology is realised using EMIM OTF ionic liquids that generate smaller fibril-like PEDOT-rich domains with relatively loose structures. Such optimal morphology improves ion accessibility, lowering the gate bias required to completely de-dope the channel, and thus enabling to achieve high transconductance, fast transient response and at lower gate bias window simultaneously.
1.2-T3
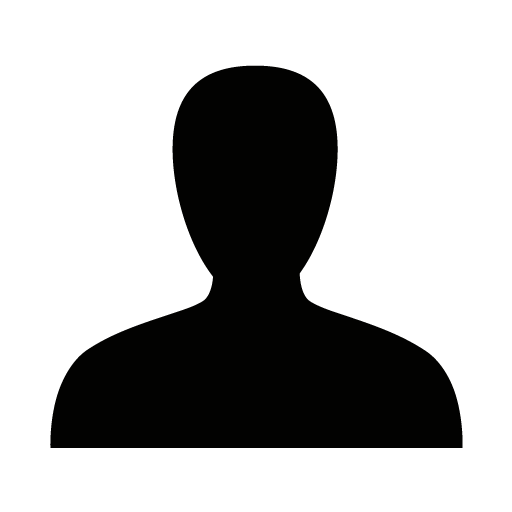
Interfacing electronics and biology opens the need for materials having suitable electrical and mechanical properties, as transporting both ions and electrons and soft mechanical properties. Among all materials, polythiophene conjugates emerged for their higher biocompatibility, ionic as well as electronic conduction, and optical properties in the visible range. Remarkable results have been obtained interfacing polythiophenes-based transistors and actuators with mammalian cells, as well as plants, to a lower extent. However, the phenomena occurring at the interface with aqueous environment are yet to be characterized and understood. In particular, electrochemical doping/de-doping results in volume change, which can diminish device performance and reduce stability. However, the possibility to electrically address and modulate on-demand the volume can pave the way for a new class of devices, where both mechanical stimulation and mixed conduction play together. Here, we investigate the doping/de-doping behaviour of a new class of polythiophene based materials, conjugated to ethylene glycol side chain of different lengths. According to previous publications, our findings show that the electrochemical doping involves materials volume change in aqueous environment. Additionally, we demonstrate that the amount of volume change depends on the ionic strength of the solvent, thus further investigations are being carried out to elucidate the fundamental processes involved in the recorded large volume changes. The ionic intercalation is also influencing the mechanical properties of the doped as well as the de-doped material, as the mechanism is not fully reversible. We report also the mechanical characterization of the material family as a function of the ionic strength of the solvent.
Our findings deterministically correlate the mechanical properties, the volume changes and the doping state of the material, laying the basis for the development of electrically addressable devices. This class of material and devices has a great potential interest for bioelectronic applications, as it accounts for ionic/electronic conduction and on-demand modification of stiffness, emerging as a smart platform for mammalian and plant cell stimulation and monitoring.
1.3-I1
Conjugated polymers have found a fertile field of application in bioelectronics. Their ability to change doping state after injection of ions from an electrolyte (the so-called electrochemical doping process) makes them versatile transducers of ionic-to-electronic conduction. This process is at the heart of most bioelectronic devices. I will present a device-level description of this process, where electronic and ionic carrier injection/extraction is followed by bipolar transport and compensation, and discuss the role of extrinsic processes including electrolysis and charge transfer to species in the electrolyte. Experiments and simulations will show the relative importance of these processes in typical materials systems.
1.3-I2
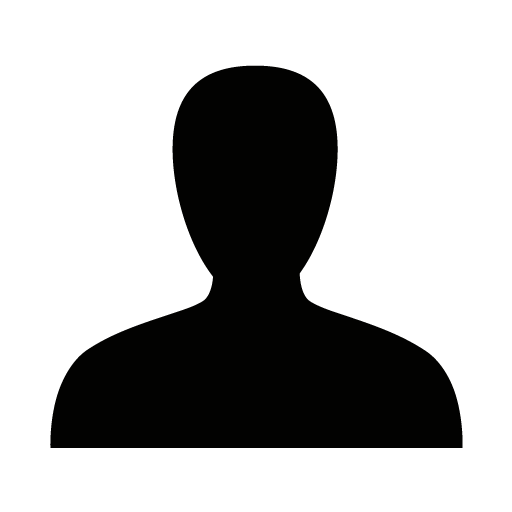
A cornerstone of clinical management for many neurological disorders is recording and manipulating pathologic electrical brain signals. However, limitations in the types and resolution of neural activity that can be acquired continue to exist, and use of current neural interface devices often carries the risk of significant side effects. Here, we demonstrate the capacity for soft, organic electronics to facilitate large-scale recording and closed loop neuromodulation of brain signals. We are able to record high spatiotemporal resolution intracranial and scalp electroencephalography (EEG) from human subjects. We have innovated devices to acquire such signals from the developing rodent brain, tracking physiologic developmental trajectories and identifying networks involved in emergence of epileptic activity. We have leveraged this data to create fully implantable closed-loop devices that can suppress pathologic activity in epileptic networks. These applications of organic electronics highlight the potential of such materials and devices to benefit diagnosis and therapy of neurological disorders.
1.3-I3
Alberto Salleo is currently an Associate Professor of Materials Science at Stanford University. Alberto Salleo graduated as a Fulbright Fellow with a PhD in Materials Science from UC Berkeley in 2001 working at Lawrence Livermore National Laboratory on laser-induced optical breakdown in fused silica. From 2001 to 2005 Salleo was first post-doctoral research fellow and successively member of research staff at Xerox Palo Alto Research Center, where he worked with Bob Street on device and materials physics of disordered and polymeric semiconductors. In 2005 Salleo joined the Materials Science and Engineering Department at Stanford as an Assistant Professor. While at Stanford, Salleo won the NSF Career Award, the 3M Untenured Faculty Award, the SPIE Early Career Award and the Tau Beta Pi Excellence in Undergraduate Teaching Award. Salleo is an Associate Editor of the Journal of Electronic Materials and a Principal Editor of MRS Communications.
Conjugated mixed conductors have attracted much attention lately as soft materials with applications in bioelectronics, energy storage and brain-like computing. In addition to the well-known PEDOT:PSS blend, new families of polymers with glycolated side-chains have been recently developed with promising performance. Interestingly, details of how charge transport occurs and how it’s related to ionic transport are still not well understood in both PEDOT:PSS blends and newer materials. This lack of understanding hinders the rational design of the next generation of high-performance materials. In this talk I will show the result of experiments that suggest that while its role in ion transport is well understood, the insulating PSS phase also plays an unexpected role in electronic transport in PEDOT:PSS blends, suggesting new design rules to control electronic transport in these materials. Furthermore, I will show how the microstructure of glycolated polymers must show a balance between aggregates and disordered regions in order to ensure optimal electrochemical performance. Finally, I will show how understanding these materials properties is instrumental in understanding how they operate and the limits of their performance.
2.1-I1
Magnus Berggren received his MSc in Physics in 1991 and graduated as PhD (Thesis: Organic Light Emitting Diodes) in Applied Physics in 1996, both degrees from Linköping University. He then joined Bell Laboratories in Murray Hill, NJ in the USA, for a one-year post doc period focusing on the development of organic lasers and novel optical resonator structures.
In 1997 he teamed up with Opticom ASA, from Norway, and former colleagues of Linköping University to establish the company Thin Film Electronics AB (ThinFilm). From 1997 to 1999 he served Thin Film as its founding managing director and initiated the development of printed electronic memories based on ferroelectric polymers.
After this, he returned to Linköping University and also to a part time manager at RISE Acreo. In 1999, he initiated the research and development of paper electronics, in part supported by several paper- and packaging companies. Since 2002, he is the professor in Organic Electronics at Linköping University and the director of the Laboratory of Organic Electronics, today including close to 90 researchers.
Magnus Berggren is one of the pioneers of the Organic Bioelectronics and Electronic Plants research areas and currently he is the acting director of the Strategic Research Area (SFO) of Advanced Functional Materials (AFM) at LiU. In 2012 Magnus Berggren was elected member of the Royal Swedish Academy of Sciences and in 2014 he received the Marcus Wallenberg Price. He is also the co-founder of 7 companies: ThinFilm, Invisense, DP Patterning, Consensum Prodcution, OBOE IPR, OBOE Players and Ligna Energy.
Thiophene-based trimers is a new class of organic electronic molecular units, which enable the organization and polymerization of conjugated structures within living biological systems and inside operating electronic devices and systems. The trimers can be equipped with side groups that targets and promotes coupling to specific surfaces and (bio-)chemical cues making self-organization and -assembly of organic bioelectronics possible in a novel manner. The chemical and physical fundamentals of the trimers, the route of polymerization, and their performance while operating in neuromorphic and in vivo-manufactured bioelectronics will be reported. Specifically, neuromorphic systems based on organic electrochemical transistors including the trimers will be described along with bioelectronic systems formed inside living plants, cells and animal models. Our findings promise for radically new ways of forming bioelectronic systems in living systems, which mimicks the structures and functions of the signaling of biology.
2.1-I2
Electronically controlled drug delivery offers the potential for precisely controlled release of pharmaceuticals when and where they are needed. This concept could lead to more effective therapies with minimal side effects and minimal requirements for user input. This talk will overview recent advances in electronic drug delivery devices that leverage the favorable mixed conducting properties of organic electronics. This will include advances in engineering and applcations as well as fundamental insights into device physics and optimal material properties. The talk will conclude with an perspective on criticals areas for future developments in order to realize the full potential for electronic drug delivery.
2.1-I3
The necessity for quick, reliable, easy-access and low-cost devices for healthcare assessment is currently attracting a great deal of interest and the market for such products is growing rapidly. This contribution deals with a novel class of Point-of-Care devices based on poly(3,4-ethylenedioxythiophene):polystyrene sulfonate (PEDOT:PSS) Organic Electrochemical Transistors (OECTs).
OECTs are promising electronic platforms that have recently attracted increasing interest, since they can provide intrinsic signal amplification without the need of a freestanding reference electrode, they can operate at low power (< 100 μW) and can be easily miniaturized and adapted to non-flat, flexible and even textile substrates. In addition, providing high sensitivity together with biocompatibility and low-cost, could be proposed as analytical tools for the reliable detection of a wide range of low concentration analytes even in biological fluids.
All PEDOT:PSS OECTs can be used as chemical sensor for the detection of redox analytes able to undergo oxidation at PEDOT:PSS, such as ascorbic acid, dopamine, adrenaline or uric acid. The redox molecules react with PEDOT:PSS by extracting charge carriers from the transistor channel, and consequently an increase of analyte concentration leads to a decrease of the absolute value of the drain current that is used as the analytical signal[1].
On the other hand, the selectivity issue must be addressed to allow the widespread use in real-life applications, through a proper functionalization of the gate electrode with molecules able to selectivity interact with the target analyte [2]. In this regard glucose and lactate biosensors have been fabricated by immobilizing the enzyme glucose oxidase or lactate oxidase on the gate surface; moreover pH or chloride sensors have been described, entrapping iridium oxide or silver/silver-chloride nanoparticles on the PEDOT polymer, thus developing a two terminal sensor able to work without an external gate electrode.
As an example of application, a smart bandage has been realized for the real-time monitoring of wound pH, which has been reported to correlate with the healing stages, thus potentially giving direct access to the wound status without disturbing the wound bed. The fully textile device is realized by integrating a sensing layer, including the two-terminal pH sensor made of a semiconducting polymer and iridium oxide particles, and an absorbent layer ensuring the delivery of a continuous wound exudate flow across the sensor area[3].
2.2-T1
I am a PostDoc in the research group of Rainer Schindl. Together with the lab of Daniel Simon (LiU) and the neurosurgery department at Med. Univ. Graz we work on developing tools for local tumor treatment using organic electronics.
Many chemotherapeutic agents are limited in their success in cancer treatment due to poor delivery and systemic toxicity. A way to efficiently interfere with cancer growth and to reduce tumor recurrence is local chemotherapy. This is especially of interest for the treatment of high grade brain tumors, which remain an unmastered clinical challenge due to high recurrence rates and frequently occurring resistance to standard chemotherapeutics.
We present miniature organic electronic devices for drug delivery able to administer chemotherapeutics via electric control with high spatiotemporal precision.1 Incorporated in these devices are anionic hyperbranched polyglycerol membranes, forming an ion selective matrix of multiple fixed negative charges.2 Through this polymeric membrane, drugs electromigrate in an electric field towards a target of choice. Here, a free-standing capillary prototype was used for proof-of-principle experiments.
The performance of these bioelectronic devices, called chemotherapeutic ion pumps (chemoIPs), was characterized with voltammetric and amperometric measurements and tested in different brain tumor models with increasing complexity (cell culture to short-term in vivo model). ChemoIP-mediated drug delivery was compared to computer simulations. Additionally, the influence of Gem on neural and cancerous brain cultures was analyzed with whole proteome analysis. The treatment efficiency was analyzed based on cell death, suppression of tumor growth and drug distribution.
ChemoIPs enable drug delivery with pmol*min-1 precision at currents in the nano-ampere range. The further application of this electrical and temporal control was shown in the cell monolayer and 3D cell culture, triggering the disintegration of targeted brain tumor spheroids among chemoIP treatment. Via whole proteome and cell viability analysis we confirmed that Gem efficiently kills brain tumor cells, while neuronal cultures are unaffected. Additionally, we show preliminary results indicating that the chemoIP treatment significantly induces tumor shrinkage in a short-term in vivo brain tumor model.
The here exemplified electrically-driven drug delivery via chemoIPs is a drug administration method that can serve as basis for further implant development, which has the potential to increase the efficacy of chemotherapy due to highly-targeted and locally-controlled drug delivery.
2.2-T2
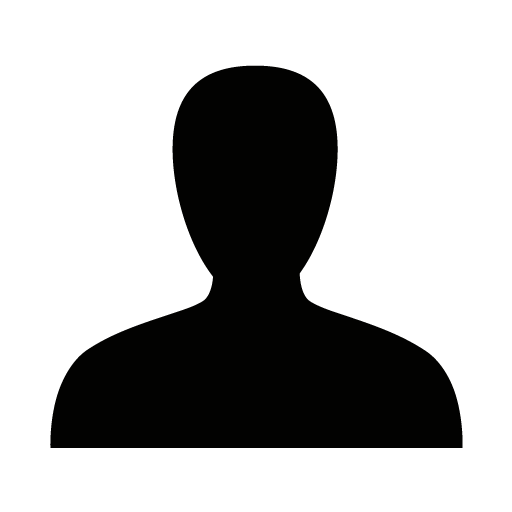
Recently emerged on-skin electronics with applications in human-machine interfaces and on-body healthy monitoring call for the development of high-performance skin-like electrodes and semiconducting polymers. The development of waterproof and breathable membranes that can provide a high level of protection for human skins and a comfortable contact between electronics and human skin are the pressing demands for on-skin electronics. However, major challenges remain, such as the limited mechanical durability and permeability of gas and liquid, hindering long-term stability and reusability. Therefore, it is highly desirable to develop a new type of skin-breathable and waterproof on-skin electronics. Herein, we report a fibrous electrolyte containing polymer matrix and ionic liquid by electrospinning method, which is highly robust, breathable, waterproof, and conformal with human skin, after bonding a parylene layer between fibers. The improvement of electrical conductivity of both electrodes and organic semiconducting polymer, and ionic conductivity of fibrous electrolyte were demonstrated. Serving as fibrous substrate and electrolyte of organic electrochemical transistors (OECTs), a high transconductance of ~0.8 mS, fast response time of 60 ms and stability over pulsing and time (~1000 cycles and 30 days) were achieved. The waterproof and breathable capabilities of fibrous OECTs enable comfortable contact after attaching to human skin, which can also reduce the interfacial impedance to achieve local amplification of the high-quality electrocardiography signals (SNR of 21.7 dB) even in skin squeezed state or after one week. These results indicated that our fibrous OECTs have huge potential for versatile on-skin electronics such as non-invasive medical monitoring, soft sensors, and textile electronics.
2.3-T1
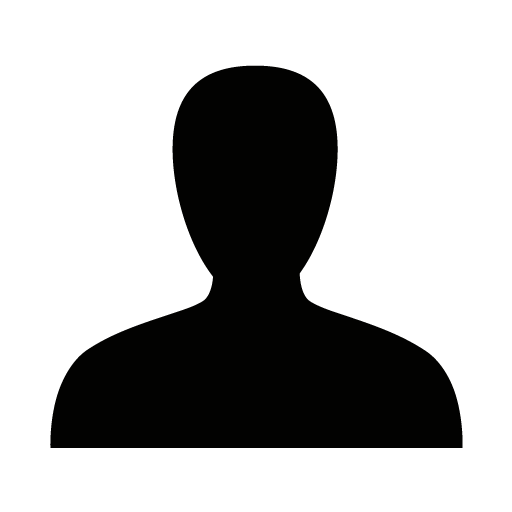
Wearable and Internet of Things technologies represent a potentially high-impact breakthrough for next future strategies targeting improved occupational safety and health management. In particular, they could enable the real-time monitoring of health-related and environmental information to the wearer, emergency responders and inspectors regarding air pollution, indoor air quality, aerosol exposure and detection of biothreat agents in the workplace. Among hazardous gaseous compounds causing severe health issues, ammonia shows a high solubility in aqueous environment and the contact with its vapours immediately causes irritation to eyes, mucous membranes and whole respiratory system, while exposure to high concentrations can lead to life-threatening conditions. This contribution describes the development of a wearable gas sensor for NH3 detection at room temperature [1] based on the organic semiconductor poly(3,4-ethylenedioxythiophene): poly(styrenesulfonate) (PEDOT:PSS), electrochemically deposited iridium oxide particles (IrOx Ps) and a hydrogel film based on agarose that assists the sensing mechanism, which relies on electrochemical gating [2-5]. The working principle significantly differs from most of the state-of-the-art NH3 gas sensors and originates from the potentiometric response of IrOx Ps, which are embedded within the organic semiconductor and respond to local pH variations in the hydrogel, thus modulating the doping state of the semiconductor. The hydrogel interface, where the gaseous analyte reversibly absorbs and dissolves producing pH variations, is actually the key component of the sensor structure for the achievement of a reversible and selective response. Its composition, acid-base properties and morphology were finely optimized to obtain self-healing properties, as well as the desired porosity, adhesion to the substrate and stability to humidity variations. Thanks to the reliability of the analytical response, the simple two-terminal configuration and the low power consumption (around 0.1 mW), the PEDOT:PSS/IrOx Ps/hydrogel sensor was realised on a flexible plastic foil and successfully tested in a wearable configuration with wireless connectivity to a smartphone. The wearable sensor showed stability to mechanical deformations and good analytical performances, with a sensitivity of 60 ± 8 μA decade-1 in a wide concentration range (17 – 7899 ppm), which includes the safety limits set by law for NH3 exposure, e.g. 25 ppm averaged over an eight-hour workday and 35 ppm for short-term exposure (according to the National Institute for Occupational Safety and Health).
2.3-T2
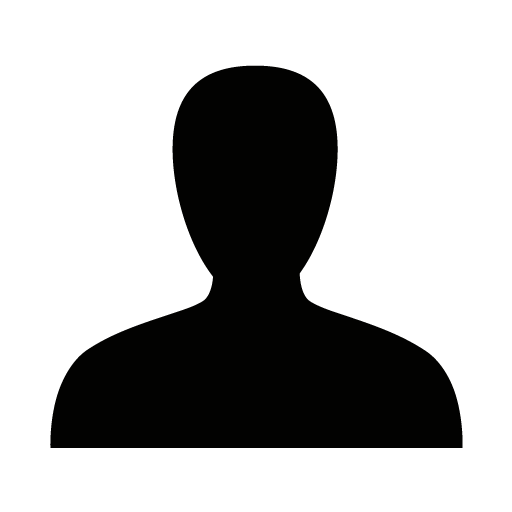
Organic electrochemical transistors (OECTs) have recently attracted attention due to their high transconductance, low operating voltage, and compatibility with aqueous solutions for broad bio-sensing applications. Poly-3,4-ethylenedioxythiophene:poly-4-styrenesulfonate (PEDOT:PSS) is a typical material acting as the active channel layer in OECTs. However, the poor electrical conductivity of pristine PEDOT:PSS diminish the lifetime and restrict the application scope of the electronic device systems. Although some additives in PEDOT:PSS have been proposed to enhance the conductivity, those additives are either toxic or not proved to be biocompatible. Herein, a biocompatible ionic liquid is proposed as an effective additive to enhance the performance of PEDOT:PSS based OECTs. The influence of the ionic liquid on the conductivity, morphology, and the redox process of PEDOT:PSS during electrochemical doping/de-doping processes are explored. The PEDOT:PSS/the ionic liquid (PEDOT:PSS mixed with the ionic liquid) film as OECT channel layer exhibits enhanced transistor characteristics, such as higher transconductance [the normalized gm/(Wd/L) are 22.3 ± 4.5 mS μm-1], a high μC* product [283.80 ± 29.66 F cm-1 V-1 s-1], fast response times [40.57 μs for the device (W/L = 100 μm/10 μm, d = 69 nm)] and excellent switching cyclical stability [> 95% retention after 5000 cycles]. In addition, flexible and biocompatible OECTs are designed using the PEDOT:PSS/the ionic liquid for electrophysiological (ECG) signals acquisition. These OECTs showed robust performance against physical deformation and successfully recorded ECG signals from the heart of a volunteer. Furthermore, an on-skin electronic device consisting of PEDOT:PSS/the ionic liquid based OECT and sensing component is demonstrated to monitor full-range human motions. In conclusion, PEDOT:PSS/the ionic liquid based devices exhibit promising potential for skin-based physiological signals monitoring.
2.4-I1
Dion Khodagholy is an assistant professor in the Department of Electrical Engineering at Columbia University in New York City.
He received his Master’s degree from the University of Birmingham (UK) in Electronics and Telecommunication Engineering. This was followed by a second Master’s degree in Microelectronics at the Ecole des Mines. He attained his PhD in Microelectronics at the Department of Bioelectronics (BEL) of the Ecole des Mines (France). His postdoctoral research at New York University, Langone Medical Center was focused on large-scale cortical acquisition and analysis.
His research explores the interface of electronics and the brain in the context of both applied and discovery sciences, with the ultimate goal of new innovations in device engineering and neuroscience methods to improve diagnosis and treatment of neuropsychiatric disease.
As our understanding of the brain’s physiology and pathology progresses, increasingly sophisticated technologies are required to advance discoveries in neuroscience and develop more effective approaches to treating neuropsychiatric disease. To facilitate clinical translation of advanced materials, devices, and technologies, all components of bioelectronic devices have to be considered. Organic electronics offer a unique approach to device design, due to their mixed ionic/electronic conduction, mechanical flexibility, enhanced biocompatibility, and capability for drug delivery. We design, develop, and characterize conformable organic electronic devices based on conducting polymer-based electrodes, particulate electronic composites, high-performance transistors, conformable integrated circuits, and ion-based data communication. These devices facilitate large-scale neurophysiology experiments and have led to discovery of a novel cortical oscillation involved in memory consolidation as well as elucidated patterns of neural network maturation in the developing brain. The biocompatibility of the devices also allowed intra-operative recording from patients undergoing epilepsy and deep brain stimulation surgeries, highlighting the translational capacity of this class of neural interface devices. In parallel, we are developing the high-speed conformable implantable integrated circuits and embedded acquisition and storage systems required to make high channel count, chronic neurophysiological recording from animals and human subjects possible. This multidisciplinary approach will enable the development of new devices based on organic electronics, with broad applicability to the understanding of physiologic and pathologic network activity, control of brain-machine interfaces, and therapeutic closed-loop devices.
2.4-I2
Susan Daniel is the Fred H. Rhodes Professor of Chemical Engineering and the William C. Hooey Director of the Robert Frederick Smith School of Chemical and Biomolecular Engineering at Cornell University. Her research team strives to understand phenomena at biological interfaces and chemically patterned surfaces that interact with soft matter – liquids; polymers; and biological materials, like cells, viruses, proteins, and lipids. Her team pioneered “biomembrane chips” to conduct cell-free, biophysical studies of mammalian, bacterial, and plant cell membranes, and recently merged this technology with organic electronic devices for expanded sensing capabilities.
Transmembrane proteins (TMPs) are Nature’s biorecognition, sensing, and transduction elements and critical components in biomimetic sensing. However, they are among the most challenging biomolecules to integrate into biosensors because they require a lipid bilayer to remain functional. Successful bilayer and TMP integration with bioelectronic devices would enable new capabilities in in vitro biosensing and biological actuation. To date, reconstitution of lipid bilayers onto sensing surfaces requires significant optimization of the abiotic/biotic interface. Nonetheless, current hybrid biotic-abiotic devices show great promise in biosensing, but even more so if arrays of TMPs tailored to sense specific targets could be produced. Such scale up is necessary for rapidly identifying the most sensitive protein sensors, providing a myriad of sensing elements that can monitor a variety of targets simultaneously, or producing enough data to enable machine learning approaches that can extend beyond biosensing into bioanalytical applications.
Our team has developed several approaches to integrate TMPs into supported lipid bilayers that functionalize organic bioelectronic devices. In this presentation, I will cover two methods. First, I will describe a technique to harvest membrane vesicles from live cells and using them to coat a surface of a bioelectronic device. Once formed, they serve as an authentic replica of the plasma membrane from a variety of cell types. I will describe two sensing examples using this approach: 1) measurements of ion channel activity for drug screening applications and 2) sensing of host-pathogen interactions, in particular, virus entry processes that lead to host cell infection. For the second approach, we use cell-free synthesis of proteins, in which cellular extracts are used to synthesize transmembrane proteins directly into lipid bilayers that coat the sensing surface. I will provide an example of ion channel sensing using TMPs formed by this approach.
Our goal is to build TMP arrays on bioelectronic devices that sense their functions and activity levels using the dual modality of electronic and optical means. The criticality of dual mode data collection is that, for transporters and ion channels, electrical means can be used label-free to read out flux of material across the membrane. Optical approaches offer the possibility to simultaneously obtain confirmation of folding with protein folding reporters, as well as obtaining structural information. Combining all these features into one platform offers a wealth of possibilities for many biosensing and bioanalytical applications and could be used to understand how the properties of the membrane influence the activity of TMPs.
2.4-I3
Thuc-Quyen Nguyen is a professor in the Center for Polymers and Organic Solids and the Chemistry & Biochemistry Department at University of California, Santa Barbara (UCSB). She received her Ph.D. degree in physical chemistry from the University of California, Los Angeles, in 2001 under the supervision of Professor Benjamin Schwartz. Her thesis focused on photophysics of conducting polymers. She was a research associate in the Department of Chemistry and the Nanocenter at Columbia University working with Professors Louis Brus and Colin Nuckolls on molecular self-assembly, nanoscale characterization and molecular electronics. She also spent time at IBM Research Center at T. J. Watson (Yorktown Heights, NY) working with Richard Martel and Phaedon Avouris. Her current research interests are structure-function-property relationships in organic semiconductors, sustainable semiconductors, doping in organic semiconductors, interfaces in optoelectronic devices, bioelectronics, and device physics of OPVs, photodetectors, and electrochemical transistors. Recognition for her research includes 2005 Office of Naval Research Young Investigator Award, 2006 NSF CAREER Award, 2007 Harold Plous Award, 2008 Camille Dreyfus Teacher Scholar Award, the 2009 Alfred Sloan Research Fellows, 2010 National Science Foundation American Competitiveness and Innovation Fellows, 2015 Alexander von Humboldt Senior Research Award, 2016 Fellow of the Royal Society of Chemistry, 2015-2019 World’s Most InfluentialScientific Minds; Top 1% Highly Cited Researchers in Materials Science by Thomson Reuters and Clarivate Analytics, 2019 Fellow of the American Association for the Advancement of Science (AAAS), 2023 Wilhelm Exner Medal from Austria, 2023 Fellow of the US National Academy of Inventors, 2023 de Gennes Prize in Materials Chemistry from the Royal Society of Chemistry, 2023 Elected Member of the US National Academy of Engineering, 2024 Fellow of the European Academy of Sciences, and 2025 ACS Henry H. Storch Award in Energy Chemistry.
Organic electrochemical transistors (OECTs) have been demonstrated in a wide range of applications such as analyte detection, neural interfacing, impedance sensing and neuromorphic computing. Majority of OECTs use PEDOT:PSS and liquid electrolytes. In this talk, I will discuss the development of conjugated polyelectrolytes (CPEs) as semiconductors for OECTs and the working mechanism of CPE-based OECTs. CPEs are materials that comprise a conjugated polymer backbone and charge-functionalized alkyl side chains and counterions. The anionic CPE poly[2,6-(4,4-bis-potassium butanylsulfonate-4H-cyclopenta-[2,1-b;3,4-b’]- dithiophene)-alt-4,7-(2,1,3-benzothiadiazole)] (PCPDTBTSO3K, or CPE-K) is part of a unique class of organic semiconducting polymers that are soluble in water and become doped in the presence of a proton source. CPE-based OECTs can operate in either the accumulation or depletion mode. We investigate the impact of the alkyl chain lengths and the conjugated backbone on the optical property, the film morphology, the electronic and ionic conductivity and the transconductance in OCETs.