1.1-I1
Organic electrochemical transistors (OECTs) have recently been investigated intensively due to their switching and sensing functionality in an electrolytic environment which is compatible with biological systems. The working principle of OECTs differs significantly from the classical electrostatically gated field-effect transistors (FET) due the volumetric capacity control of charge carrier concentration in the channel by ionic motion. The mixed electron-ion transport of the devices has consequence for many parameters of the devices including dynamics and hysteresis. In this talk, our recent work on a better understanding of OECT operation is reviewed. We discuss the properties of a wide variety of devices including solid state electric devices and vacuum-processed devices. It turns out that entropic terms play a major role in the operation of the devices due to the entirely different statistical properties of the ions in the channel. The results show that the often observed hysteresis of OECT can be separated in an intrinsic bistable behavior and a conventional hysteresis behavior related to ion dynamics and other effects. The bistability of the devices offers interesting device applications.
1.1-I2
Doping of organic semiconductor films enhances their conductivity for applications in organic electronics, thermoelectrics and bioelectronics. However, much remains to be learnt about the properties of the conductive charges in order to optimize the design of the materials. Electrochemical doping is important for organic electrochemical transistors (OECTs) used in biomedical sensors. Benefits of doping via electrochemistry include controllable doping levels, reversibility and high achievable carrier densities. We introduce a new technique, applying in-situ terahertz (THz) spectroscopy directly to electrochemically doped polymers in combination with time-resolved spectro-electrochemistry, chronoamperometry and OECT device measurements. We evaluate the intrinsic short-range transport properties of the polymers (without the effects of long-range disorder, grain boundaries and contacts), while precisely tuning the doping level via the applied oxidation voltage. Moreover, temperature-dependent measurements allow to extract the thermodynamic and activation parameters of the electrochemical processes. Results will be shown for a variety of polymers based on polythiophene backbones with different sidechains, aligned polymer chains and novel 2D organic films.
1.1-I3
Organic mixed ionic-electronic conductors (OMIECs) have recently risen as a promising material choice for bioelectronic devices due to their low impedance, soft mechanical properties, and ability to transduce ionic signals to electronic currents. These properties have enabled the development of high-performance devices for electrophysiological recordings, chemical sensing, cell monitoring, and neuromorphic devices. The unique behaviour of OMIECs arises from “electrochemical doping” where ion intercalation through the bulk of the material modifies the oxidation state, and therefore charge carrier concentration, of the conjugated polymer. However, the current understanding of electrochemical gating remains limited. To address this knowledge gap, we use a newly developed hyperspectral differential transmission microscopy technique to probe electrochemical gating in operando.
First, I will present a comprehensive drift-diffusion model to describe mixed ionic-electronic transport in OMIECs using the data collected from in operando microscopy which captures the complex behaviours of electrochemical doping. The proposed model captures several features observed for electrochemical (de)doping of OMIECs including diffusion-like rather than drift-like ion transport and the voltage-dependence of ion kinetics. The results suggest that diffusion of electronic carriers (holes) down electrochemical potential gradients plays a significant role in operation of OMIEC-based devices. In the second part of the presentation, I will show that electrochemical doping consists of two distinct kinetic regimes. Generally, electrochemical doping is assumed to be limited by motion of ions due to their large mass compared to electrons/holes. However, in a state-of-the-art polythiophene, electrochemical doping speeds can be limited by poor hole transport at low doping levels, leading to substantially slower switching speeds than expected. We show that the timescale of hole-limited doping can be controlled by the degree of microstructural heterogeneity, enabling the design of OMIECs with improved electrochemical performance. The framework for understanding the driving forces and kinetics of mixed ionic-electronic transport provides guidance for device design as well as optimization of new materials for improved performance in bioelectronic devices.
1.2-I1
Jenny Nelson is a Professor of Physics at Imperial College London, where she has researched novel varieties of material for use in solar cells since 1989. Her current research is focussed on understanding the properties of molecular semiconductor materials and their application to organic solar cells. This work combines fundamental electrical, spectroscopic and structural studies of molecular electronic materials with numerical modelling and device studies, with the aim of optimising the performance of plastic solar cells. She has published around 200 articles in peer reviewed journals, several book chapters and a book on the physics of solar cells.
Organic semiconductors with polar side groups are capable of mixed electronic and ionic conduction, making them candidates for electrodes in electrochemical devices. In the case of conjugated polymers, electrochemical and redox properties can be tuned through choice of polymer backbone and side chain [1, 2]. Conjugated polymer electrodes can show excellent charging and discharging rates, high coulometric efficiency, efficient charge transport and reversible charging in simple aqueous electrolytes, making them interesting for different applications including energy storage. However, the specific capacity and operational stability need to be improved [3]. We use molecular modelling together with electrochemical measurements to investigate the relationship between polymer chemical structure and the redox properties, charging behaviour and cycling stability of the polymer electrodes. We use molecular dynamics simulations to explore how the side chain structure influences polymer packing, film swelling and stability [4]; how structure influences polymer-ion interactions; and how these interactions influence electronic transport properties. Finally, we use simulations to explore how chemical structure influences specific capacity. The findings can help to develop chemical designs for improved conjugated polymer electrodes for aqueous environments.
1.2-I2
The charge-transport properties of conjugated polymers have been studied intensively for both electronic and optoelectronic device applications. Some polymer semiconductors not only support the ambipolar transport of electrons and holes, but do so with comparable carrier mobilities. This opens the possibility of gaining deeper insight into the charge-transport physics of these complex materials via comparison between electron and hole dynamics while keeping other factors, such as polymer microstructure or vibrational fluctuations, equal. Field-induced electron spin resonance spectroscopy has been recently used to compare the spin relaxation behavior of electron and hole polarons in three ambipolar conjugated polymers, showing three spin relaxation regimes as a function of temperature. We interpret these findings on the basis of calculated electronic structure calculations and state-of-the-art numerical propagation of the hole and electron carrier wavefunctions coupled to nuclear motion in these polymers.
In the second part of the talk, we will report on recent simulations of ion intake and diffusion in crystalline microstructures of conjugated polymers equipped with glycolated side chains for organic electrochemical transistor applications. All-atom simulations support a crystal phase transition upon doping that occurs via a zipping mechanism. We will also describe preliminary phenomenological calculations aiming at describing the changes in electrical conductivity with increasing carrier concentration and that take into account the electrostatic interactions with the ions.
1.2-O1
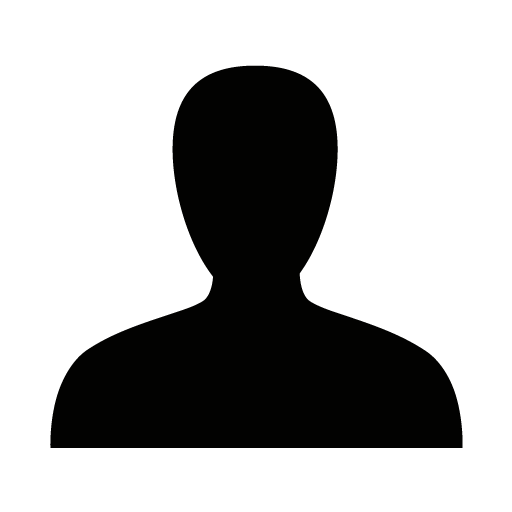
Engineering conjugated polymers with glycol-based side chains has been the main strategy used to improve the performance of mixed ionic-electronic conductors (OMIECs), a promising material class for interfacing biological systems with electronics. Organic electrochemical transistors (OECTs) are a solution for this task and are often used to benchmark OMIECs performance. Different studies have showed that polymers designed with hydrophilic solubilizing chains show better OECT performance, mostly due to their higher ionic uptake and stability in aqueous environments. [1]
In this work, we explore how side chain engineering in poly(3-hexylthiophene) (P3HT) and in indacenodithiophene-co-benzothiadiazole (IDTBT) impact their ionic transport properties. We used time-resolved spectroelectrochemistry to measure the kinetics of doping in these materials with high time resolution. We observe that for thicker films (around 100-200 nm), the films with more glycol content are doped faster, confirming that the ion transport is better in more polar polymers. However, for thinner films (around 20nm), we observe that the kinetics becomes independent of glycol content. We hypothesize that the kinetics in thin films are less dependent on the ion transport and closer to the electrochemical limit. Therefore, the polar content does not affect the intrinsic electrochemical reactions rate and the doping favorability, which remain controlled by the polymer backbone.
1.2-O2
Organic electrochemical transistors (OECTs) are a rapidly advancing technology that plays a crucial role in the development of next-generation bioelectronic devices. Recent advances in p-type/n-type organic mixed ionic-electronic conductors (OMIECs) have enabled power-efficient complementary OECT technologies for various applications, such as chemical/biological sensing, large-scale logic gates, and neuromorphic computing. However, ensuring both high performance and long-term operational stability remains a significant challenge that hinders their widespread adoption. Ladder-type conjugated polymers, with their rigid backbone structure composed of double-strand chains linked by condensed π-conjugated units, can sustain high electrochemical doping levels without any conformational disorder, which leads to exceptional operational stability, high charge carrier mobility, and large volumetric capacitance. In this report, we demonstrate our recent development on ladder-type conjugated polymers, with p-type/n-type high mixed ionic-electronic conducting performance and high stability in long-term OECT operation. Reasonable molecular design and improvement of synthesis methods have enabled the synthesis of high-performance p-type/n-type ladder polymers with mobility increased by more than 10 times. Their unique ladder-type structure enables them to have long-term stability within >90% current remain after 6 hours continues operation, and enables them to accommodate more carrier injections with of up to two charges per repeat unit. Density of states filling and opening of a hard Coulomb gap around the Fermi energy at high electrochemical doping levels enable the ion-tunable antiambipolarity in these ladder polymers. The development of ladder-based polymer ink formulations has also enabled printed electronics. With these ladder-type polymers, we developed complementary inverters with a record-high DC gain of 194 V/V and excellent stability. We report the first organic electrochemical neurons (OECNs) with ion-modulated spiking, based on all-printed complementary organic electrochemical transistors. We demonstrate facile bio-integration of OECNs with Venus Flytrap (Dionaea muscipula) to induce lobe closure upon input stimuli. We report a biorealistic conductance-based organic electrochemical neuron (c-OECN) using a mixed ion–electron conducting ladder-type polymer with stable ion-tunable antiambipolarity. The latter is used to emulate the activation/inactivation of sodium channels and delayed activation of potassium channels of biological neurons. These c-OECNs can spike at bioplausible frequencies nearing 100 Hz, emulate most critical biological neural features, demonstrate stochastic spiking and enable neurotransmitter-/amino acid-/ion-based spiking modulation, which is then used to stimulate biological nerves in vivo.
1.2-O3
Conductive polymers combine high ionic and electronic conductivities with soft mechanical properties, and achieve the conversion of electrochemical processes in liquid environment to mechanical formalization.[1] The resulting material electroactivity has been exploited in electrochemical actuators with low-voltage drive and nanoscale precision, leading to the development of bioelectronics soft actuators.[2] On the other hand, the electrically-induced swelling of conductive polymer layers can be detrimental for applications such as thin film neuromodulation devices, where shear stresses with the substrate can lead to delamination and device failure.[3] During volume change, electroactive polymers translate an external stimulus to a change of the physical properties on a nanometer scale, but the intrinsic mechanism of actuation is not completely understood. In this work, we address this gap of knowledge introducing quantitative measurements of electroswelling using atomic force microscopy (AFM). We exploit AFM as a local probe for volume changes and interface forces that provides transient data on dynamical effects related to electroactuation. We combine electroswelling measurements in the frequency domain with electrochemical impedance spectroscopy on a model conductive polymer, poly(3,4-ethylenedioxythiophene) polystyrene sulfonate (PEDOT:PSS) to correlate morphological changes with information on ionic uptake and local electrochemical potential. Through the development of a mathematical theory, we describe electroswelling in terms of transport and accumulation of hydrated ions, and we achieve multimodal AFM experiments mapping electroswelling amplitude and phase on soft polymer thin films. Our findings highlight the physicochemical mechanisms limiting actuation width and timescales and can be crucial to develop functional materials with enhanced electroactive properties.
1.3-I1
The polyelectrolyte complex of poly(3,4-ethylene dioxythiophene) with poly(styrene sulfonate), PEDOT:PSS, is ubiquitous in the field of organic electronics. Yet, its complex structure in water and in the solid state make it challenging to understand structure-property relationships for optimization or functionalization. Our group focuses on developing a better understanding of PEDOT:PSS, particularly for using it as a mixed ionic-electronic conductor for organic electrochemical transistors (OECTs). In this talk, I will share two recent projects. The first project will describe how to increase the OECT performance of common formulations of PEDOT:PSS without sacrificing stability in water by using a surface functionalization method instead of blending with a crosslinker. For a simple formulation of PEDOT:PSS with ethylene glycol and a dodecylbenzene sulfonic acid (DBSA) surfactant, we found that surface functionalization with (3-glycidyloxypropyl)trimethoxysilane (GOPS) led to a [μC*] value of 376 ± 74 F cm‒1 V‒1 s‒1 instead of 104 ± 20 F cm‒1 V‒1 s−1 when blended with GOPS (the most commonly used approach to maintain water stability). In the second part, I will describe the use of polyethylene oxide (PEO) as either a polymeric additive to commercial formulations or as a block copolymer with PSS to increase the volumetric capacitance of PEDOT:PSS, up to 200 F cm−3 (after washing the films in water) and 120 F cm−3 respectively. When using a relatively low molecular weight PEO, 400 Da, the PEDOT:PSS:PEO blend led to a [μC*] value up to 632 F cm‒1 V‒1 s‒1 after washing with water, close to that obtained for crystalline PEDOT but without having to use sulfuric acid. For both projects, I will describe our approach for the preparation of different formulations, how the additives or block copolymers affect the chemical composition, and detailed microstructure characterization to tease out structure-property relationships in PEDOT:PSS-based mixed conductors.
1.3-I2
Organic mixed ionic/electronic conductors (OMIECs) have gained considerable interest in bioelectronics, power electronics, circuits and neuromorphic computing. These organic, often polymer-based, semiconductors rely on a combination of ionic transport, electronic transport, and high volumetric charge storage capacity. Despite recent progress and a rapidly expanding library of new materials, a full understanding of fundamental processes of OMIECs remains largely unexplored. Critically, useful studies require us to probe these systems in device-relevant conditions, fully considering the effects of ions and solvent on microstructure and transport. To this end, we report on recent efforts towards structure/composition-property relations in high performance organic mixed conductors using ex-situ, in-situ, and operando scattering and spectroscopic techniques. We use a combination of GIWAXS, GISAXS, XPCS, E-QCMd, UV-Vis, XRF, as well as device measurements to explore kinetics, composition, and structural evolution during electrochemical operation. Importantly, these findings provide insights into materials design for enhancing device performance and stability.
1.3-O1
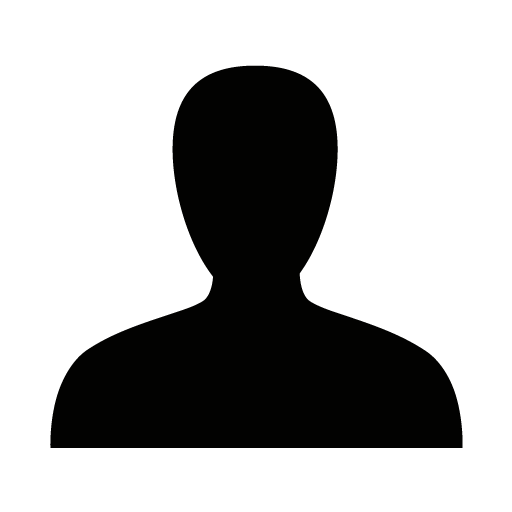
Conjugated polymers (CPs) with hydrophilic side chains are capable of simultaneously conducting electronic and ionic charges, making them interesting materials for applications in bioelectronics, electrochromics, electrocatalysis and energy storage. Whilst numerous p-type mixed-conducting polymers have shown excellent stability and performance, n-type mixed-conducting polymers usually suffer from poor stability in aqueous environments and in air. To date, most reported solution processable n-type mixed conducting polymers that show good stability, high electron mobility and high specific capacity, involve the naphthalenediimide (NDI) unit. [1-3] However, the electrochemical stability of NDI-based mixed-conducting polymers varies with the specific chemical structure and choice of electrolyte. The underlying mechanisms by which ionic charging/discharging deteriorates the electrochemical stability are not properly understood. In this work, we study several NDI-based n-type mixed conducting polymers with different side chain and backbone structures operating in aqueous electrolytes of systematically varying concentrations to control the electrochemical stabilities. We observe that increasing electrolyte concentration and replacing a small fraction of hydrophilic side chains with hydrophobic ones largely improve the electrochemical stability of the NDI-based n-type mixed conducting polymers. To understand the phenomena, we use spectroelectrochemical and electrogravimetric characterisation tools and molecular dynamic (MD) simulations to explore the impact of side chains and electrolytes on the electrochemical stabilities of the polymers. We show that the improvement in stability can be tentatively attributed to a reduction in swelling, which avoids excessive electrolyte uptake, thereby improving the environment for ion transport and maintaining the intermolecular connections. We propose design rules for both material and electrolyte to maximise reversible multi-electron charging of n-type mixed conducting polymers.
1.3-I3
The development of organic electrochemical transistors (OECTs) capable of maintaining their high amplification, fast transient speed, and operational stability in harsh environments will advance the growth of next‐generation wearable electronics, robotics and biological electronics. In this study, a high-performance solid-state OECT (SSOECT) is successfully demonstrated, showing a recorded high transconductance of 220 ± 59 S cm−1, ultrafast device speed of ≈10 kHz with excellent operational stability over 10 000 switching cycles, and thermally stable under a wide temperature range from −50 to 110 °C. The developed SSOECTs are successfully used to detect low-amplitude physiological signals, showing a high signal-to-noise-ratio of 32.5 ± 2.1 dB. For the first time, the amplifying power of these SSOECTs is also retained and reliably shown to collect high-quality electrophysiological signals even under harsh temperatures. The demonstration of high-performing SSOECTs and its application in harsh environment are core steps toward their implementation in next-generation wearable electronics and bioelectronics. We also will present our recent work on developing fibrous electrolyte containing polymer matrix and ionic liquid, which is highly robust, breathable, waterproof, and conformal with human skin is reported. Serving as fibrous substrate and electrolyte of OECTs, a high transconductance of ≈0.8 mS, stability over pulsing and time (≈1000 cycles and 30 days) are achieved. The softness of fibrous OECTs enables a comfortable contact after attaching to human skin, which can reduce the interfacial impedance to achieve a high-quality local amplification of the electrocardiography signals (signal-to-noise ratio of 21.7 dB) even in skin squeezed state or after one week. These results indicated that our fibrous OECTs have huge potential for versatile on-skin electronics such as non-invasive medical monitoring, soft sensors, and textile electronics.
2.1-I1
The presentation will give an overview over recent studies in my group about design and processing of polymeric mixed-ionic-electronic conductors for opto-electronic and switchable technologies including actuators, electrochromic displays and electrically switchable metasurfaces.
In the first part, electrochemistry will be shown as versatile tool to control the doping level of semiconducting polymer films. Data on tunable absorption properties, electrical conductivities and wetting behavior will be presented for p-type materials.[1] Both, in-situ as well as ex-situ electrochemical techniques will be presented and correlations to the morphology of the films will be drawn. For energy level determination within fully organic solar cells particularly in-situ spectroelectrochemistry of blend films proved to be highly useful.[2]
In the second part, poly(ethylenedioxythiophene):poly(styrenesulfonate) (PEDOT:PSS) will be presented as multifunctional material. Electrochemical switching in organic and in aqueous electrolytes results in different degrees of volume swelling as characterized by in-situ spectroscopic ellipsometry.[3] The humidity dependence of the PSS polyelectrolyte phase further allows to prepare volume changes by water uptake. The bending behavior of “intelligent” humidity-triggered bilayer actuators with poly(dimethylsiloxane) (PDMS) passive layers can be explained by the humidity-dependent mechanical behavior of PEDOT:PSS.[4]
2.1-I2
Chemical and electrochemical doping are widely used to modulate the electrical properties of conjugated polymers. Doping of thin films facilitates fundamental spectroscopic studies and, ultimately, is important for the operation of devices from transistors to solar cells. However, some applications such as thermoelectrics and wearable electronics require bulk materials, which complicates doping processes and mass transport, limiting the performance of devices. This talk will explore some of the fundamentals of doping of both thin films and bulk materials. The impact of doping on the electrical as well as mechanical properties will be discussed, and it is shown how doping can be used to tune both the conductivity and stiffness of conjugated polymers. Finally, strategies are explored that permit to decouple the effect of doping on the electrical and mechanical properties, which can be used to prepare a variety conducting bulk materials, from stiff composites and foams to hydrogels and stretchable fibers.
2.1-I3
Gabriel Gomila has got a PhD in Physics from the University of Barcelona (1997) with a thesis based on the theoretical modelling of electron transport at semiconductor interfaces. Later on, he was post-doctoral researcher at three different universities in Italy, France and Spain where he specialized in the theoretical modelling of nanoescale electronic devices. In 2001 he moved to the Department of Electronics at the University of Barcelona thanks to a Ramon y Cajal fellowship, where he expanded his research interests towards the merge of electronics and biological fields, thus focusing on microsystems for biological applications on-a-chip and on Atomic Force Microscopy for the electrical study of biological samples. In 2005 he became Associate Professor at the University of Barcelona and in 2008 Group Leader at the Institute for Bioengineering of Catalunya (IBEC). In 2014 and 2022 he was awarded with the ICREA Academia prize, which recognizes and promotes the research excellence of the university staff of Catalonia. Since 2017 he is Full Professor at the Department of Electronics of the University of Barcelona. His current research interests are centred on the understanding of the bioelectrical phenomena at the nanoscale. He combines research activities with teaching on Nanobiotechnology, Scanning Probe Microscopy, Bioelectricity and Nanomedicine at the University of Barcelona.
Electrolyte-Gated Transistors (EGTs) have emerged as an integral part of numerous applications in biosensing and bioelectronics, owing to their remarkable ability to efficiently transduce biological events into amplified electronic signals while stably operating in aqueous electrolytes. EGT are three-terminal devices consisting of a semiconducting channel between source and drain electrodes capacitively coupled with the gate electrode through ions in the electrolyte. Understanding these devices at the nanoscale is paramount in order to leverage their respective, or combined, functionality for various applications. An optimized level of crystallinity or a balance between ionic and electronic conduction within the semiconductor might be desired, which directly relates to the physical and chemical nature of the semiconducting material and its response to applied electric fields. However, probing the nanoscale properties under operating conditions has been challenging due to the complications arising from the electrolyte environment. In this communication, I will review the progress made in our research group towards developing an advanced scanning probe microscopy technique able to probe different functional properties of the semiconductor materials (morphological, electrical and mechanical) at the nanoscale in operating electrolyte-gated transistors (EGTs). The technique is based on in-Liquid Scanning Dielectric Microscopy (in-Liquid SDM) to which we added automated functionalities and multiparametric characterization capabilities for comprehensive and simultaneous probing of the nanoscale electrical, mechanical and morphological properties in operating EGTs. Examples of applications to Electrolyte Gated Organic Field Effect Transistors (EGOFETs) [1] and Organic Electrochemical Transistors (OECTs) will be presented.
2.2-I1
Juan Bisquert (pHD Universitat de València, 1991) is a Professor of applied physics at Universitat Jaume I de Castelló, Spain. He is the director of the Institute of Advanced Materials at UJI. He authored 360 peer reviewed papers, and a series of books including . Physics of Solar Cells: Perovskites, Organics, and Photovoltaics Fundamentals (CRC Press). His h-index 95, and is currently a Senior Editor of the Journal of Physical Chemistry Letters. He conducts experimental and theoretical research on materials and devices for production and storage of clean energies. His main topics of interest are materials and processes in perovskite solar cells and solar fuel production. He has developed the application of measurement techniques and physical modeling of nanostructured energy devices, that relate the device operation with the elementary steps that take place at the nanoscale dimension: charge transfer, carrier transport, chemical reaction, etc., especially in the field of impedance spectroscopy, as well as general device models. He has been distinguished in the 2014-2019 list of ISI Highly Cited Researchers.
We explore the concept of constructing microscopic electronic neurons that mimic natural brain systems in order to create brain-inspired computational artificial systems. We discuss the essential material and device properties required for these spiking neurons, which can be characterized using impedance spectroscopy and small perturbation equivalent circuit elements. We identify the structural conditions necessary for smooth oscillations, which depend on the dynamics of a conducting system with internal state variables. We emphasize the significance of detecting the Hopf bifurcation, a critical point for achieving spiking behavior, through spectral features of the impedance. We focus on the mixed ionic-electronic conductors in which the slow ionic motion introduces a internal state variable that controls the electronic conductivity. Our findings reveal that the minimal neuron system requires a capacitor, a chemical inductor, and a negative resistance, which can be integrated into the physical response of the device rather than being built from separate circuit elements. Thus, we propose a method to quantify the physical and material properties of devices in order to produce the dynamic properties of neurons that are necessary for a specific computational scheme. We present a novel approach towards building brain-inspired artificial systems and provides insights into the important material and device considerations for achieving spiking behavior in electronic neurons [1].
2.2-O1
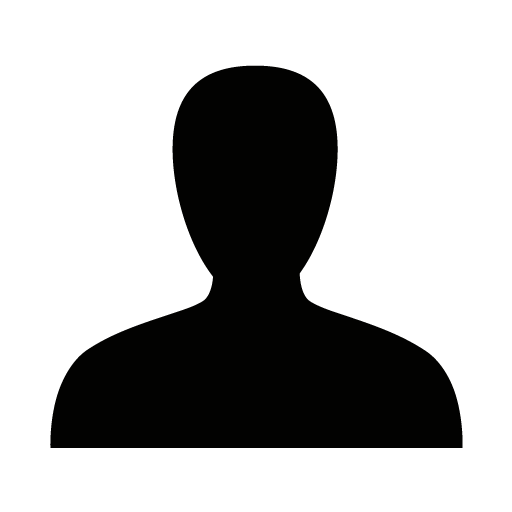
In recent years, there has been continuous progress in the development of n-type organic thermoelectrics (OTE) due to the synthesis of new conjugated polymers and dopants. The primary focus of most designs has been to enhance doping efficiency and increase electrical conductivity, resulting in values surpassing 102 S cm-1. Although effective, doping encounters limitations because of the inherent inverse relationship between the properties that determine the figures of merit in thermoelectric materials. To advance the field further, alternative approaches are needed beyond optimizing doping efficiency and the host:dopant ratio. In this study, we propose the concept of self-induced anisotropy as a solution to overcome the inverse coupling between electrical conductivity and Seebeck coefficient in n-type OTEs. Previous research has suggested methods like rubbing and drawing to induce anisotropy in p-type OTEs based on thiophenes. In our work, we exploit the interactions between solvent, host, and dopant to induce a preferential orientation. This orientation increases the in-plane delocalization length, thereby improving electrical conductivity whitout hindering the Seebeck coefficient. By adopting this approach, we have achieved promising results in the 2DPP-2CNTVT:N-DMBI system. At room temperature, we obtained a Power Factor of 115 μW m-1 K-2 and a figure of merit of 0.17, which further increased to 188 μW m-1 K-2 and 0.36, respectively, at 105 ºC. To explain the origin of self-induced anisotropy, we utilized Hansen Solubility Parameters and established guidelines for formulating solvent-host-dopant systems that can achieve a similar effect. This discovery opens new possibilities for advancing n-type OTEs.
2.2-O2
Dr. Hakan Bildirir obtained his BSc and MSc degrees from Istanbul Technical University (Turkey) and PhD from the Technical University of Berlin (Germany). He worked at Advent Technologies (Greece), TUBITAK (Turkey), Chalmers University of Technology (Sweden) before starting at IMDEA Energy. He developed materials ranging from small molecules to highly crosslinked porous polymeric networks, which were used for separation, latent heat storage, photocatalysis, and organic electronics.
He joined IMDEA Energy in 2021, and is working jointly for the Electrochemical Processes and Photoactivated Processes units to develop materials for energy production and storage.
Conjugated porous polymers (CPPs) are solely organic members of porous materials family. They are very promising compounds for photocatalytic and electronic applications thanks to their the electron rich and porous backbone offering high accessible surface areas and advanced photophysical properties.[1,2] Moreover, their high dimensionality make them particularly interesting for organic electronic devices due to the possibility of orientation independent electron/hole mobility through their 2D/3D skeleton.[3,4] Additionally, the open voids along the porous backbone are perfectly separated phases to introduce guest molecules to form charge-transfer complexes (i.e. doping), which leads improved electrical conductivities.[4]
We have previously reported formation of charge-transfer complexes of electron rich CPPs after iodine treatment.[5–7] In a particular example, we showed that a thiophene based CPP became more conductive after iodine exposure when compared to its linear analogue whereas the latter outperformed the former before the doping.[7]
In this communication, chemical doping an electron rich CPP by using different techniques (i.e. mixing dopant+CPP in solution or vacuum impregnation) will be presented. The spectral (e.g. UV, IR, NMR, EDX, XPS) and physical characterizations (thermal elemental analysis, TGA, and porosity measurements) of the pristine and doped forms indicated the successful incorporation of the guest molecules in the pores. Furthermore, effect of the doping on the electrical impedance and conductivity will also be discussed.
1.1-I1
We present a novel concept for cuff electrodes that greatly simplifies the insertion procedure on nerves as small as 100 µm. Cuff electrodes serve as peripheral nerve interfaces for neural stimulation or recording, with various applications including chronic pain management, sleep apnea treatment, and high blood pressure control. Existing methods for securing cuff electrodes on larger nerves in the millimeter range rely on surgical threading, zip tie-like closing mechanisms, or prefolded spiral cuff geometries. While these approaches are effective for larger nerves containing multiple fibers branching out to different target regions, they present challenges when targeting smaller nerves with diameters of 100 µm or below. The fragility of such nerves and the difficulties in handling small probes make interfacing with typical cuff electrode systems a challenging task.
To overcome these challenges, our approach leverages 3D and 4D printing techniques, in combination with flexible and superabsorbent materials. These materials readily conform to the shape of the nerve upon contact with body fluid, allowing the electrodes to wrap around the nerve without requiring manual deformation [1]. This approach reduces the risk of nerve damage during implantation. Notably, we have successfully implanted these devices in a locust model and demonstrated their efficacy in recording and stimulating neural activity as well as associated muscle activation. The geometric design of our cuff electrodes features electrodes distributed along both the axial and circumferential directions, enabling enhanced spatially selective sensing and stimulation capabilities.
In summary, our nerve cuff electrode concept offers a transformative solution for the implantation of electrodes on ultra-small nerves, streamlining the procedure and minimizing potential harm. Through the utilization of state-of-the-art rapid fabrication techniques and adaptable materials, we have developed a simple procedure for recording and stimulating of neural activity. The incorporation of electrodes distributed along multiple directions further enhances the potential for selective sensing and stimulation.
1.1-I2
Biomaterial scaffolds have emerged as a tool to build 3D cultures of cells which better resemble biological systems, while advancements in bioelectronics have enabled the modulation of cell proliferation, differentiation, and migration.
Here, we first describe a porous conductive hydrogel with the same mechanical modulus and viscoelasticity as neural tissue. Electrical conductivity is achieved by incorporating low amounts (<0.3% weight) of carbon nanomaterials in an alginate hydrogel matrix, and then freeze-drying to self-organize into highly porous networks. The mechanical and electrical properties of the material can be carefully tuned and used to modulate the growth and differentiation of neural progenitor cells (NPCs). With increasing hydrogel viscoelasticity and conductivity, we observe the formation of denser neurite networks and a higher degree of myelination.
To investigate the functionality of neurite networks in 3D, we begin by placing a polydimethylsiloxane (PDMS) microstructure on an underlying multielectrode array (MEA). We then explore different materials and techniques to integrate hydrogels into the PDMS microstructures, such that the hydrogel can facilitate neurons to form 3D networks while still confined by the PDMS. This platform can be used to support the growth of human iPSC-derived sensory neurons, with and without a co-culture of human Schwann cells. Additionally, our platform is compatible with various methods to assess neuronal functionality (e.g. MEA electrical recordings), and can be used to understand the effect(s) of hydrogel properties on the resulting neuronal networks. Both described biomaterial systems can support the growth of neuronal cells for over 12 weeks to investigate neuronal development and disease progression. Further, we have demonstrated the use of these materials for the fabrication of implantable surface electrode arrays which can seamlessly interface with electrically active tissues such as the brain and heart.
1.1-I3
Maria Asplund is professor in Bioelectronics at Chalmers University of Technolology. Her research expertise is in bioelectronics, flexible microtechnology, tissue-device interaction and electronic biomaterials. After completing her PhD at the Royal Institute of Technology (Stockholm, 2009) she led her own research group at the University of Freiburg, Germany (2011-22). Her work has resulted in new technologies which contributes to smaller, more energy efficient and durable bioelectronics in the future. She currently holds ERC starting and proof of concept grants, is a Visiting Professor at Luleå University of Technology (2019-23) and an editorial board member of Scientific Reports. Maria Asplund is furthermore the scientific secretary for the Swedish Society for Medical Engineering.
Bioelectronic medicine have enormous potential not only to replace lost body function but also for guiding and encouraging regenerative processes, helping the body to self-repair. The ability of our skin to self-heal is something so integral to our everyday life that we barely reflect on how remarkable this is. Other parts of our bodies have very limited healing capability, especially the very complex structures of brain and spinal cord. Furthermore, while most of us take our self-repairing skin for granted, old age and certain diseases can drastically reduce this ability, greatly increasing the risk that seemingly uncomplicated wounds develop into chronic ones.
Together wiht my team I have shown how electrical field stimulation can have a guiding effect on skin cell migration, which could be of great significance to speed up wound closure and reduce the risk for chronic wounds. Together with collaboration partners we now explore how similar principles can be used to promote more constructive repair after traumatic injury. The common denominator for these therapeutic concepts is electrical field stimulation, in other words direct current injected into the tissue. This places special constraints on the electrode materials used to ensure biocompatible and reversible direct current injection mechanisms. My talk will outline some material concepts that we have developed for this and which electrochemical boundary conditions that apply to ensure that direct current stimulation can be sustained in tissue even with fully implantable systems. I will showcase how we employ conducting polymer hydrogels for direct current stimulation, and how such technology allows for new discoveries as well as potential future therapies.
1.2-I1
From extracellular matrices (ECM) in connective tissues, to silks spun by spiders, and textile fibers weaved in fabrics, small diameter fibers widely exist in nature and have been closely associated with our daily life. These individual fibers have quasi-one-dimensional flexible structures, with diameters smaller than ~100 µm, and length to width aspect ratios greater than ~100. Orderly assembling fibers into arrays and three-dimensional architectures with designable chemical properties and sensing capabilities could open a range of bioelectronics and biointerface applications. In this presentation, I will demonstrate biofabrication and fiber printing techniques that enable the patterning of functional fiber architectures in three dimensions. These fiber printing techniques extend the materials libraries and device designs, which underpin technological capabilities from enabling fundamental studies in cell migration, to customizable and eco-friendly fabrication of wearable sensors. Finally, I will provide an outlook on the strategic pathways for developing next-generation bioelectronics using fibers as architectural building blocks.
1.2-I2
Use of light for selective and spatio-temporally resolved control of cell functions (photoceutics) is emerging as a valuable alternative to standard electrical and chemical methods. Here, we propose the use of smart materials, and in particular of organic semiconductors, as efficient and biocompatible optical transducers in the field of regenerative medicine.
Devices able to selectively and precisely modulate the fate of living cells, from adhesion to proliferation, from differentiation up to specific function, upon visible light will be presented. Examples of practical applications, recently reported by our group, include optical modulation of the activity of both excitable and non-excitable cells, control of essential cellular switches like transient receptor potential channels and other cationic channels, as well as effective modulation of intracellular calcium signaling for precise control of cell metabolic processes. We describe fabrication and optimization of micro- and nano-structured polymeric interfaces, in the form of beads and 3D scaffolds, with different cell models. As representative examples, we report on (i) functional interaction with intracellular proteins, leading to non-toxic modulation of the cell redox balance [1,2]; (ii) a novel strategy to gain optical control of Endothelial Progenitor Cell (EPC) fate and to optically induce angiogenesis in vitro [3]; (iii) optical modulation of mesenchymal stem cells and human-induced pluripotent stem cells physiological pathways [4]; (iv) effects of light-sensitive 3D scaffolds on neurogenesis [5]. Current knowledge about the photo-activated processes occurring at the conjugated polymer/living cell interface, obtained by complementing several physical/chemical/biological characterization techniques, is also critically discussed. The above mentioned study-cases represent, to the best of our knowledge, first reports on use of organic semiconductors for optical modulation of the cell fate, with disruptive perspectives in cell-based therapies. Future opportunities and perspective applications in regenerative medicine will be critically evaluated in the conclusions.
1.2-O1
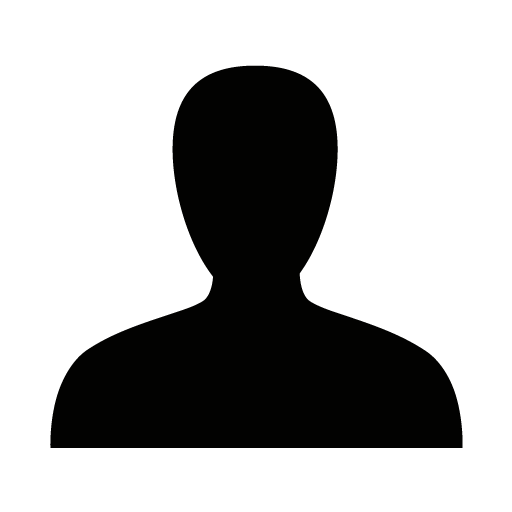
Photovoltaic implants introduce a new wireless method for precise electrical stimulation of tissue using the conversion of light into electrical energy, employing thin layers of organic semiconductors on a flexible substrate. With their remarkable potential for biomedical applications, these implants offer a versatile platform capable of delivering targeted electrical stimuli in the brain, facilitating the development of neuronal networks, and fostering regeneration and neuroprotection following traumatic brain injury [1]. Applications include devices that can be placed on the surface of the brain, or in the form of cuffs wrapped around nerves, which have already been showcased in numerous studies [2,3].
However, these principles may be applied in many other areas. They hold promise for use in electrical nerve conduits. By simultaneously providing guidance and stimulation to regenerating nerves, these conduits can help promote axonal regrowth, reconnection, and functional recovery. The precise control over electrical parameters through light enables tailored interventions, allowing for targeted treatment strategies in cases of nerve damage, peripheral neuropathies, or even spinal cord injuries [4]. On the other hand, the use of optoelectronic devices as capacitive sensors opens up avenues for monitoring tissue properties, neuronal activity and nerve growth, and for investigating brain-diseases in both research and clinical settings.
Our studies emphasize general essential requirements for these different clinical applications. Notably, our focus lies on fabrication methods of new devices, biocompatibility, the distribution of the electric field in biological tissue, and their ability to influence cellular growth.
To assess biocompatibility, Chick Chorioallantoic Membrane (CAM) assays were conducted and tissue was sampled for immunohistochemical studies. Furthermore, colorimetric LDH cytotoxicity assays were used to quantify possible adverse effects of the devices when in contact with primary cell cultures of cortical neurons [5].
In addition to quantifying the biocompatibility of these photovoltaic implants, investigating their generated electric field and long-term stability is imperative to optimize their performance. Therefore, a custom measurement system was employed to characterize the spatial distribution of the electric field in an aqueous solution during stimulation over the span of several hours to several days. By precisely mapping the electric field, we gained insights into the long-term stability of the device, and efficacy and intensity of the stimulation to optimize precise targeting of specific areas with low light intensities while minimizing potential damage to the surrounding tissue.
Furthermore, we utilized in vitro experiments to observe the effects of photovoltaic stimulation on the intrinsic electrical activity, signal transmission and morphology of neuronal cell cultures. Voltage-sensitive dyes provide real-time feedback on the neuronal response to optoelectronic stimulation. Long-term observations of morphological changes using a microscopy setup were used to investigate the phenomenon of electrotropism to give insights into directional cell growth patterns and structural alterations in response to electrical stimulation, to further support the potential of these electrodes to guide and manipulate cellular growth [6].
Through our studies encompassing biocompatibility assessments, electric field mapping, and in vitro experiments, we aim to optimize fabrication steps, increase their performance and promoting research on optoelectronics in biomedical engineering.
1.2-O2
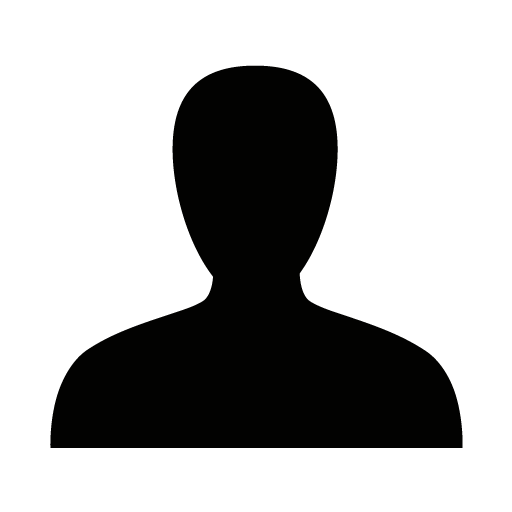
All throughout life, from early embryonic development through the operation and interaction of cells and tissues in the adult body up to the process of regeneration, biophysical signals, particularly mechanosensation, play a crucial role. Extracellular mechanical stimuli cause tissue regeneration, which includes processes including cell division, differentiation, migration, and ejection [1,2,3]. Age causes the body's ability to rejuvenate to decline, which negatively impacts on our lives.
The techniques for modulating or controlling mechanical stimulation of cells that are currently available frequently lack the effectiveness, dependability, reversibility, and spatial sensitivity needed for in vitro and in vivo applications. Here, we demonstrate a cutting-edge method for manipulating mechanosensitive ion channels using exogenous organic semiconductors. Materials of this class may be readily processed into a variety of forms, such as thin films, microstructured devices, or nanoparticles, and are totally biocompatible, providing the potential for in vivo use. However, their particular opto-electrical characteristics stand out the most, offering exceptional visible light responsivity as well as electron and ion conductivity.
In this study, we investigate the potential for accurate and efficient regulation of the activation of mechanosensitive ion channels using organic semiconductors, particularly conjugated polymers. We critically examine the outcomes produced by various techniques, such as microstructured devices and polymer thin films.
Our findings may pave the way for the development of cutting-edge smart materials for tissue regeneration triggered by physical stimuli.
1.2-O3
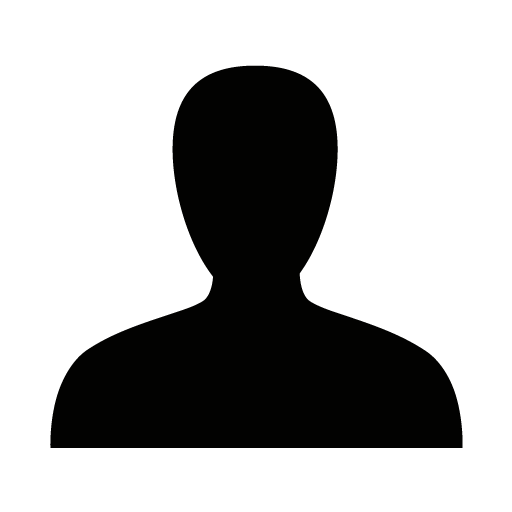
An organic photoelectrochemical transistor (OPECT) is an organic electrochemical transistor (OECT), where the output current is controlled by light. OPECT has promising performance in biosensing with transconductance improved up to the physical limit, enhancing sensor sensitivity. However, to render an OECT photosensitive, photoactive materials should be integrated into the device, relying on additional metals, sophisticated nanostructures, and tedious synthetic approaches. This study developed an all-in-one OPECT structure, where a single photosensitive polymeric mixed ionic-electronic conductor was used as the photoactive gate and the channel material. A range of n-type polymeric mixed conductors was studied to understand the material requirements. The OPECT performance was investigated by evaluating the photoelectrochemical characteristics in electrode configuration and inherent OECT properties in dark conditions. We find that the photovoltage induced by light mainly controls the OECT output, the extent of which is controlled by the lifetime of photoinduced species. Using photosensitive polymers with long exciton lifetimes is key to maximizing OPECTs performance for light-gated bioelectronic devices.
1.3-I1
The field of Bioelectronics aims to integrate electronics and biology, offering promising opportunities in various domains. However, a major hurdle in this field is the mechanical mismatch between rigid electronics and the soft nature of living tissues. Bioelectronic devices based on soft and flexible materials, developed by using microfabrication and printing techniques, aim to bridge the mismatch between the two worlds. In contrast, biological processes polymerize small molecules to create intricate micromachines. Inspired by biology, we utilize enzymatic processes and thiophene-based oligomer building blocks to in vivo generate organic conductors. By harnessing the advantages of this approach, we successfully achieved the in-situ formation of conducting polymer gels within living organisms. These gels exhibited soft mechanical properties that closely resemble those of natural tissues. Furthermore, we demonstrated the enzymatic construction of organic conductors in various tissues and their application as active materials in organic transistors. Our alternative approach overcomes the limitations of conventional methods, providing opportunities for the development of novel, soft, and bio-compatible electronic interfaces. This paradigm shift in the development of organic bioelectronics opens avenues for healthcare, bioengineering, and beyond.
1.3-I2
Husam Alshareef is a Professor of Materials Science and Engineering at King Abdullah University of Science and Technology (KAUST). He obtained his Ph.D. at NC State University followed by a post-doctoral Fellowship at Sandia National Laboratories, USA.
He spent over 10 years in the semiconductor industry where he implemented processes in volume production for chip manufacturing. He joined KAUST in 2009, where he initiated an active research group focusing on the development of nanomaterials for electronics and energy applications. His work has been recognized by over 20 awards including the SEMATECH Corporate Excellence Award, two Dow Sustainability Awards, the Kuwait Prize for Sustainable and Clean Technologies, and the KAUST Distinguished Teaching Award. He has published over 600 papers and 80 issued patents. He is a Fellow of the American Physical Society, IEEE, Institute of Physics (UK), Royal Society of Chemistry, and US National Academy of Inventors. He is a Clarivate Analytics Highly-cited Researcher in Materials Science (2019, 2020, 2021, 2022, 2023).
2D MXenes exhibit a range of interesting properties that make them suitable for healthcare-related applications. These include efficient electronic and ionic charge transport, hydrophilicity, redox activity, biocompatibility, antimicrobial effects, vis-NIR plasmon resonance, photothermal effect (light to heat conversion), tunable surface terminal groups, and structural and chemical tunability. In addition, MXenes can be synthesized using solution processing in water providing a simple method for low-cost processing of 2D flakes, thin and thick films, aerogels, and hydrogels at scale. Recently, the applications of MXene in healthcare and biomedical applications have seen tremendous activity covering areas such as biosensing, electrophysiology, tissue engineering, antimicrobial and antiviral treatments, and therapeutics.
In this talk, I will discuss the use of MXenes in several devices for biomedical applications. This includes using MXene-based hydrogels as physical and electromechanical sensors (e.g., physical motion, body artifacts, muscle fatigue) including wearable and stand-alone sensors. The talk will also cover using MXene-based composites as wearable sweat sensors with a unique modular design that enabled a simple exchange of the specific sensing electrode to tailor to the desired analytes to detect multiple biomarkers in sweat. The talk also includes leveraging the ability of MXene hydrogels to absorb ultrasound energy to develop an implantable battery that can be charged remotely from medical ultrasound probes. Promising new directions for MXenes and related composition will be discussed.
2.1-I1
Our understanding of the brain and general neural systems is based on models of increasing complexity, from 2D cell culture to organoids and organotypic slices to animal models. These models provide significant insight into neuronal information processing in the brain, but require advanced electrophysiological measurement technologies to achieve long-term stable recordings with single-cell and millisecond space-time resolution. Thus, challenges remain to study information processing between neurons with high spatiotemporal resolution and high signal-to-noise ratio (SNR). These challenges are overcome by well-established planar multichannel devices, but at the expense of signal-to-noise ratio. Without sufficient electrode-cell coupling, planar microelectrode arrays (MEAs) provide low-amplitude signals that are difficult to correctly assign by spike sorting algorithms. Furthermore, the unresolved subthreshold signals lose valuable information that is essential for direct estimation of synaptic weights and correct generation of connectivity matrices in neural networks.
To yield better cell-electrode coupling, numerous vertical nanostructures and nanoelectrodes have been developed by several groups. Here we present the concept of nanocavity (NC) MEAs with vertical nanostraws. High aspect ratio nanostraws (NS) were engineered to initiate tight cell-structure coupling, while the nanocavity reduces the electrode impedance. This combination yields a spontaneous tight mechanical coupling and results in long-term recordings with increased signal amplitude, with no poration-inducing external forces or surface functionalization [1]. Moreover, simultaneous patch-clamp and MEA recordings of the coupled neuron directly demonstrated the capability of our device to record post-synaptic potentials. Here we show that PSP resolution persisted throughout the measurements, indicating a stable and long-term subthreshold amplitude sensitivity.
Simultaneous electrical recordings with good spatial and temporal resolution from 3D neuronal structures (organoids or organotypical slices) is also technological limited. Here we present our recent developments to overcome this limitations by 3D MEA which allow the recording from inside the neuronal tissue [Shihada et al, submitted].
2.1-I2
Georgios Spyropoulos (a.k.a George D. Spyropoulos) joined Ghent University as an assistant professor in the Department of Information Technology, Faculty of Engineering and Architecture. He received his B.Sc. and M.Sc. in Materials Science and Technology from the University of Crete (Greece). He joined the nanomaterials & organic electronics group (Greece) of Prof. Kymakis to work on organic electronics for his bachelor and master thesis. He then moved to Prof. Christoph J. Brabec’s group at Materials for Electronics and Energy Technology (i-MEET), focusing on smart device fabrication strategies for solution-processed solar cells to pursue his Ph.D. in Materials Science and Engineering at the Friedrich Alexander University Erlangen-Nürnberg (Germany). He is the recipient of the Cross-disciplinary postdoctoral fellowship awarded by the Human Frontier Science Program Organisation. His postdoctoral research at Prof. Khodagholy's Translational Neuro-Electronics lab of Columbia University (USA) aimed at the development of neural interface devices based on organic electronics.
His multidisciplinary research is focused on innovating neural interfaces that can address fundamental questions regarding the auditory-neurological pathways and the neurobiology of the brain, as well as conduct diagnostics and interventions to mitigate relevant disorders. Prof. Spyropoulos is the principal investigator of the Neural Waves (NeW) lab.
Understanding and modulating neural networks requires high-resolution acquisition of neural activity over time, real-time analysis, and minimally invasive stimulation methods with high specificity. Such procedures are particularly needed for treatment of sensory disfunction (e.g. hearing loss), and certain neurological diseases (e.g. epilepsy). The lack of soft, biocompatible, hybrid and smart neural interfaces hinders our capacity to study complex neural dynamics and efficiently apply responsive neuromodulation therapy. Here, I am presenting the vision of Neural Waves lab towards designing and developing materials and novel fully implantable, contained and responsive neural interfaces that will allow long-term acquisition and closed-loop manipulation of neural circuits with high spatiotemporal resolution over extended period of time to reveal neural dynamics in different neurological pathways and alleviate disfunctions and diseases. I will cover our studies on i) creating artificial basilar membrane based on acousto-sensitive ion-based transistors and soft electronics, ii) innovating electroencephalography interfaces, and iii) utilizing organic perforated multielectrode arrays to investigate the effect of photopharmacological interventions on Cortical Seizures.
2.1-I3
Minimally-invasive neuromodulation using low-intensity focused ultrasound (LIFU) holds great promise for treating various neurological disorders. It has been demonstrated to modulate neural activity, promoting both excitatory and inhibitory effects. This capability allows for restoring aberrant neural circuitry or suppressing hyperactive regions, thereby alleviating symptoms associated with conditions such as Parkinson's disease, epilepsy, chronic pain, and depression.
One of the key advantages of LIFU neuromodulation is its combination of high spatial resolution, minimal invasiveness, and extensive coverage of the nervous system. Unlike traditional electrical, magnetic, or optical neuromodulation techniques, which can achieve high-spatial resolution at the expense of high invasiveness and reduced brain coverage, ultrasound waves benefit from low wavelengths, scattering, and absorption in brain tissue and hence, can be focused virtually anywhere in the brain, with high-spatial-resolution and without requiring brain surgery. This reduces the risk of infection, tissue damage, and other complications associated with invasive procedures, making it a safer option for patients, and also allows for accessing a broader range of neural circuits with high precision.
Despite the abovementioned promise, there is still a knowledge and technological gap in LIFU neuromodulation to maximize its potential. LIFU neuromodulation is achieved by means of an ultrasound transducer. These transducers generate low-intensity focused ultrasound waves that can penetrate deep into tissues while maintaining spatial specificity. By adjusting the parameters of the ultrasound waves, specific regions of the brain or peripheral nerves can be targeted with precision. However, similar to their use in medical diagnostic imaging, conventional ultrasound transducers used in LIFU neuromodulation have hand-held form factors and require off-the-shelf equipment. These bulky setups lead to two severe limitations: in pre-clinical research, the mismatch of size between bulky transducers and in-vitro/in vivo models (mice, rats) impose severe limitations in understanding the effects of ultrasound on the nervous system and how to better apply LIFU neuromodulation for different disease models; secondly, in clinical applications, the use of LIFU neuromodulation can only be applied in a bed-side scenario, where the patient either would visit the clinic once or twice per week to receive a treatment or would operate a LIFU neuromodulation system at home.
This talk will describe the recent research in the field of ultrasound microsystems towards developing the next generation of LIFU neuromodulation systems that can be seamlessly integrated into in vitro/in vivo experimental setups in pre-clinical settings and used as wearable and minimally-invasive devices for clinical treatments. This description will answer two questions: how to massively integrate all the necessary LIFU neuromodulation functionality into a single-miniaturized and battery-powered microsystem? How can ultrasound microsystems be designed to be mechanically flexible to better adjust to the body's natural curvature?
To answer these questions, the talk will focus on novel ultrasound microfabrication and microsystem integration methods and the next-generation ultrasound electronics, while showing a few examples of ongoing projects featuring in vitro and in vivo validation showcasing the potential of LIFU microsystems for minally invasive and precise neuromodulation for the treatment of neurological diseases.
2.2-I1
Rainer SCHINDL is a biophysicist with strong interest in bioelectronic medicine and electrophysiology. His research combines in vivo studies on live-cells and in silico simulations. He has done pioneering work in organic light-triggered semiconductors for neuronal stimulation. Currently, he focuses on optoelectronic neuro-stimulation and electronically controlled local chemotherapy.
The effectiveness of many chemotherapeutic agents in treating cancer is limited by poor delivery efficiency and systemic toxicity. Local chemotherapy approaches utilizing iontronic devices present a promising solution for efficient interference with cancer growth and tumor size reduction. We use iontronic devices with incorporated membranes to enable the continuous delivery of chemotherapeutics with high spatiotemporal resolution. We operate freestanding iontronic pumps with the the chemotherapeutics Gemcitabe (Gem) named GemIPs to treat a GBM model on the chicken chorioallantoic membrane. In addition, we compare results with a conventional topical daily treatment. We see that both administration techniques induce G2-phase cell cycle arrest and apoptosis. Remarkably, growth inhibition was achieved only with the steady Gem dosing with GemIPs, but not with daily topical drug administration at the maximum dosage amounts that was not lethal for the chick embryo. I will discuss how the different transient concentration profiles between the techniques generates this difference in outcome.
2.2-I2
The generation of in vitro platforms capable of mimicking the in vivo situation as an alternative to animal models and/or monitoring cellular processes is necessary for medicine and drug discovery [1]. In this sense, Smart Bioelectronics arise from the combination of smart functional materials, bioelectronics and microfluidics, making Smart Bioelectronics a powerful tool to control cellular microenvironments.
Smart functional materials such as poly(N-isopropylacrylamide) (pNIPAAm) can undergo structural changes due to their inherent lower critical solution temperature (LCST) phase transition in water at 32 ºC. On its side, poly(3,4-ethylenedioxythiopene):poly(styrene sulfonate) (PEDOT:PSS) is a widely used conducting polymer in the bioelectronics field, due to its mixed ionic and electronic conduction properties. When mixing both polymers, the developed PEDOT:PSS/pNIPAAm co-polymer modulates cellular adhesion/detachment of cancer cells and the electrochemical monitoring of the process [2-3].
Moreover, PEDOT can be tailored biochemically and mechanically to replicate a specific tissue. PEDOT polymers made in combination with biopolymers and glycosaminoglycans such as collagen and hyaluronic acid can be used in the generation of 3D bioelectronic interfaces with physiologically relevant conditions [4-5]. On the other hand, microfluidic devices offer optical transparency, miniaturization, and controlled media perfusion required in organ-on-a-chip models. The interface between 3D bioelectronics and microfluidic devices enables the real-time electrical and optical monitoring of cellular processes in a controlled microenvironment.
Here, we present an overview of different 2D and 3D Smart Bioelectronic interfaces for the simultaneous electrical and optical monitoring of cancer cell migration.
2.2-O1
Research on electrolyte-gated and organic electrochemical transistor (OECT) architectures is motivated by the prospect of a highly biocompatible interface capable of amplifying bioelectronic signals at the site of detection. Despite many demonstrations in these directions, a quantitative model for OECTs as impedance biosensors is still lacking. We overcome this issue by introducing a model experiment where we simulate the detection of a single cell by the impedance sensing of a dielectric microparticle. The highly reproducible experiment allows us to study the impact of transistor geometry and operation conditions on device sensitivity. With the data we rationalize a mathematical model that provides clear guidelines for the optimization of OECTs as single cell sensors, and we verify the quantitative predictions in an in-vitro experiment. In the optimized geometry, the OECT-based impedance sensor allows to record single cell adhesion and detachment transients, showing a maximum gain of 20.2±0.9 dB with respect to a single electrode-based impedance sensor.
2.2-O2
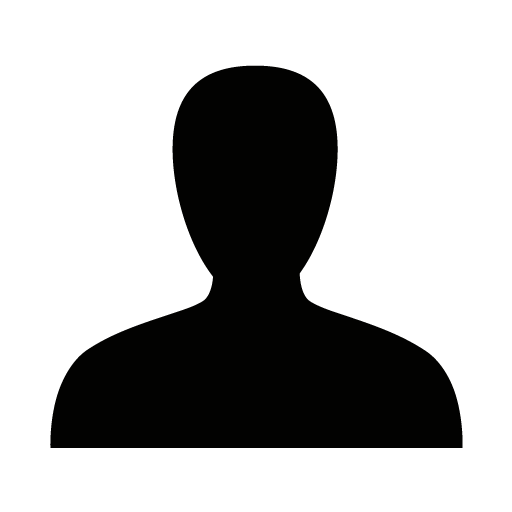
The common methods for in-vivo delivery of biomolecules are strongly limited by invasiveness and/or lack of quantitative delivery control. These limitations can be overcome by the Organic Electronic Ion Pump (OEIP), that electrophoretically delivers ions without fluid flow and ions backflow from tissue, enabling quantitative studies. The capillary-based OEIPs have been demonstrated to be minimally invasive, however, the micro-scale glass capillaries are brittle, thus tend to break during insertion in harder tissues. In this work, we developed flexible and robust OEIPs based on polyimide-coated glass capillaries, enabling their insertion in a wide range of biological tissues. We provide evidence that sufficient intensity of blue light penetrates via the polyimide coating, although it is commonly accepted that polyimide’ high optical absorption prevents polymerization using high energy light. Using low cost, water soluble photoinitiators and blue light, we established a cheap and environmentally safe system for photo-crosslinking via polyimide coating.
The resulting OEIP devices can reliably deliver abscisic acid (ABA), the so-called plant stress hormone, one of the most challenging substances delivered using iontronic devices. The developed OEIP was applied to deliver ABA directly into the petiole of intact Arabidopsis leaves, establishing an advanced petiole feeding tool. In contrary to the conventional petiole feeding assays, OEIP enables ion delivery to intact plants with a high control of the delivered dose and elimination of the disturbances induced by convective delivery. By delivering ABA to the petiole, we triggered immediate and long-lasting stomata closure, without observable wound effect, demonstrating the high potential of the developed bioactuator. The mechanism of the induced stomata closure was studied by delivering endogenously absent deuterium-labeled ABA, confirming that the closure was induced by the delivered ABA ions that were distributed to the leaf blade by the plant vasculature.
2.2-O3
Hi! I am an organic chemist currently working as a postdoctoral researcher at Istituto Italiano di Tecnologia in Italy. I work as a bridge between smart materials and translational pharmacology with my main focus on bioelectronics for drug delivery and wound healing!
Bacterial infections are one of the major threats to public health, food safety and development which makes it is necessary develop materials and strategies that limit or prevent these bacterial proliferations and biofilm infections.[1] Although, medical implants have led to dramatic improvement in patient's health and well-being, they are accompanied by drawbacks that include surgical risks during placement or removal, implant failure and more specifically microbial infections. These implant-associated infections are mainly caused by the bacterial bio-films in which bacteria are more recalcitrant towards treatments. Indeed, implant surfaces are non-vascularized abiotic materials rendering the common strategies inappropriate and ineffective.[2] In this context we have designed and developed innovative and smart interfaces based on phosphonium self-assembled monolayers (SAMs) that can be electrically activated on-demand for eradicating bacterial infections on solid surfaces. Hence, upon electroactivation, a successful eradication of gram-positive and gram-negative bacterial strains has been clearly highlighted on SAM-modified titanium surfaces. Subsequently, we observed 95% and 90% antibacterial efficiencies against Staphylococcus aureus and Klebsiella pneumoniae. More importantly, no cytotoxicity has been observed towards eukaryotic cells which clearly demonstrates the biocompatible character of these novel surfaces for further implementation.
Additionally, in our ongoing work, we have explored the synergistic relationship between electrical stimulus for drug delivery and wound healing. Our bodies naturally generate endogenous electrical fields in order to facilitate the migration of fibroblasts, keratinocytes, macrophages, epithelial cells and ions to the wound site to speed up the healing process.[3] In this regard, we have designed and developed electrochemically polymerized polypyrrole films deposited on ITO substrates encapsulated with antimicrobial peptides, which can be released in a controlled manner upon electrical application. Apart from exploring the phenomenon of electrotaxis in wound healing as a consequence of applying an exogenous electrical field, the bioelectric effect is also being studied in depth to understand the mechanisms by which applied electrical fields render the surface antimicrobial. We highlight the advantages of using electrochemical deposition as a technique for film formation as it allows for precise control over film thickness, surface roughness and homogeneity, and can expand the application for these films to not only as electrically responsive wound healing patches, but also as coatings for food packaging and other wearable electronics. These films have been characterized and their properties have been thoroughly studied using various techniques which further support the successful antimicrobial encapsulation throughout its internal framework. Finally, the biocompatible nature of these films supports their potential in various applications.
1.Tacconelli, E.; Carrara, E.; Savoldi, A.; Harbarth, S.; Mendelson, M.; Monnet, D. L.; Pulcini, C.; Kahlmeter, G.; Kluyt-mans, J.; Carmeli, Y.; Ouellette, M.; Out-terson, K.; Patel, J.; Cavaleri, M.; Cox, E. M.; Houchens, C. R.; Grayson, M. L.; Hansen, P.; Singh, N.; Theuretzbacher, U.; Magrini, N. (2018) Lancet Infect. Dis.18, 318-327.
2. Carrara, S.; Rouvier, F.; Auditto, S.; Brunel, F.; Jeanneau, C.; Camplo, M.; Sergent, M.; About, I.; Bolla, J.-M.; Raimundo, J.-M. Int. J. Mol. Sci. 2022, 23, 2183. https://doi.org/10.3390/ijms23042183
3. Farber, P. L., Isoldi, F. C., & Ferreira, L. M. (2021). Electric Factors in Wound Healing. Advances in wound care, 10(8), 461–476. https://doi.org/10.1089/wound.2019.1114
1.1-I1
Inherited retinal dystrophies and late-stage age-related macular degeneration, for which treatments remain limited, are among the most prevalent causes of legal blindness. Retinal prostheses have been developed to stimulate the inner retinal network; however, lack of sensitivity and resolution, and the need for wiring or external cameras, have limited their application. Here I report on the use of conjugated polymer nanoparticles (P3HT NPs), showing that they mediate light-evoked stimulation of retinal neurons and persistently rescue visual functions when subretinally injected in a rat model of retinitis pigmentosa. In particular I will report on recent results showing that the organic liquid prosthesis is effective also in retinas that have undergone a process of rewiring caused by the loss of photoreceptor function. I will discuss a numerical model that trys to capture the essential nature of the photostimulation mechanism by numerically solving the Poisson-Nernst-Planck (drift-diffusion) equation with proper boundary conditions taking into account theelectron transfer processes occuring at the interface.
1.1-I2
Currently, on-skin wearable or on-skin devices mainly rely on power sources such as non-rechargeable batteries, which are often toxic and harmful to the environment. Therefore, there is a strong need to develop new self-powered energy sources that possess human- and eco-friendly characteristics, including biocompatibility, degradability, and breathability. In this context, biofriendly and sustainable triboelectric nanogenerators (TENGs) emerge as excellent candidates for the next generation of green energy sources. TENG devices harness mechanical energy from various activities and environments, such as biomechanical motions and convert it into electrical energy. They are are cost-effective, easy to fabricate, and highly efficient, making them suitable for self-powered electronics and independent power source modules. Although there is a wide range of triboelectric materials available, many TENGs still rely on fluorinated polymers, which are known to be non-environmentally friendly and may cause discomfort and skin infections when used in on-skin wearables. To address these challenges, we demonstrate a cellulose-based tribopositive layer that enhances the TENG power output by introducing different surface functional groups through nano-coating or chemical/mechanical reduction to form nano-crystals or fibers. Additionally, we report on a new bio-polymer-based tribonegative layer, mimicking the chemical structure of conventional non-biodegradable materials. In the pursuit of a completely biodegradable TENG, we replace the substrate with a biopolymer film and employ carbon-based electrodes. These bio-TENGs offer flexibility, biocompatibility, and breathability, making them suitable for use as wearable devices that can harvest energy from biomechanical motions. Notably, these devices exhibit high performance with output voltage values surpassing 1 kV. This represents a twofold increase compared to conventional substrates and electrode materials employing polyimide as a negative triboelectric layer. Additionally, in the case of all-biopolymers-baed TENGs, the device output exceeds 500 V, which outperforms other reported bio-TENG devices in terms of voltage output generation.
1.1-I3
Onur Parlak earned his PhD in Bioelectronics from Linköping University in 2015. He then received a Fellowship from The Knut and Alice Wallenberg Foundation (KAW) and started postdoctoral research at Stanford University, focusing on wearable bioelectronics. After spending three years, he turned back to Sweden and joined the Karolinska Institutet to translate his engineering skills into medical settings with KAW starting grant.
He has been recently awarded by KI Strategic Funding as an Assistant Professor and research group leader as a part of the KI investment program to recruit and support leading junior researchers with particularly outstanding scientific merits and future potential. Since 2021, Dr. Parlak has been acting as Assistant Professor at the Department of Medicine, Solna, Dermataology and Venereology Unit in Karolinska Institutet where he specializes in personalized diagnostics and wearable bioelectronics.
Bioelectronic devices show great promise in healthcare and environmental sensing due to their ability to provide longitudinal monitoring to maintain optimal status and evaluate physical and (bio)chemical changes. Electrochemical (bio)sensors are at the centre of this effort and offer a vast potential to revolutionize conventional diagnostics and environmental sensing methods that uses traditional laboratory tests-based evaluations, that are slow and mainly require in-person visits or frequent sampling if the long-term analysis is necessary.
In this presentation, I will give a brief overview of our recently developed electrochemical methodologies targeting of sensing and monitoring of various metabolites, hormones and microorganisms to acquire better knowledge on diagnosis and disease progression of diseases and environmental changes. This talk will summarize how to design sustainable sensors, and integrated electronics and how to use them in clinical and environmental settings with our unique access to patient materials and environmental samples, which creates an unprecedented opportunity to address fundamental questions in medical and environmental monitoring.
1.2-I1
Susan Daniel is the Fred H. Rhodes Professor of Chemical Engineering and the William C. Hooey Director of the Robert Frederick Smith School of Chemical and Biomolecular Engineering at Cornell University. Her research team strives to understand phenomena at biological interfaces and chemically patterned surfaces that interact with soft matter – liquids; polymers; and biological materials, like cells, viruses, proteins, and lipids. Her team pioneered “biomembrane chips” to conduct cell-free, biophysical studies of mammalian, bacterial, and plant cell membranes, and recently merged this technology with organic electronic devices for expanded sensing capabilities.
The plant cell is protected by a complex polysaccharide cell wall composed primarily of cellulose, hemicellulose and pectin. This wall stabilizes a semi-permeable barrier, the plasma membrane (PM) that serves two main roles: (1) transport of essential molecules in and out of the cell, and (2) sensory transduction of environmental stimuli. Considerable progress has been made over the past two decades in understanding plant membrane proteins from a genetic perspective, but complementary tools for physiological, electrophysiological, and biochemical characterization necessary for studying protein functions are lacking. This work promotes a new cell-free, bioelectronic platform that captures the properties of green plant PMs and enables the measurement of plant transporter functions.
Monitoring the flux of metal ions across plant cell membranes through protein transporters embedded within them is a significant challenge today, but is fundamental for assigning function to unknown transporter genes; tackling transporter substrate specificities and modes of regulation; and eventually linking metabolic pathways to cellular compartments, plant growth and development, and bridging the genotype to phenotype gap. Today's technologies are inadequate for a number of reasons, including low throughput and lack of sensitivity, especially for transporters, which have fluxes several orders of magnitude lower than ion channels.
In this presentation, I will share a new technology that combines planar green plant membranes with transparent, electrically conducting polymer electrodes for the dual-mode (optical or electrical) measurement of plant protein functions. Organic electrochemical transistors (OECT) based on the conductive polymer PEDOT:PSS offer biocompatibility, high-quality electrical signals, and optical monitoring due to the transparency of PEDOT:PSS thin films. We demonstrate here the application of the OECT to the measurement of copper transporter 1 (COPT1), which maintains plant copper homeostasis, but its function has not been wholly characterized. We formed a supported lipid bilayer (SLB) with membranes from transient GFP and GFP-COPT1-transfected Arabidopsis thaliana mesophyll protoplasts on the surface of the OECT. We measure copper flux through COPT1 in a specific and concentration-dependent manner. After data analysis, we obtain kinetic parameters of transport for COPT1 and report on the coupling of COPT1 with another protein, a copper reductase.
This new kind of plant-derived sensor device is amenable to scale up and we anticipate that it can be highly multiplexed for the collection of large data sets on plant transporter systems in a way that has not been possible before. With this capability, such large data sets can be fed into big data science approaches for enabling discoveries and breakthroughs in our understanding of how plants adapt to genetic perturbations, extreme weather conditions, pathogen pressures, and other critical aspects important for flourishing ecosystems.
1.2-I2
The possibility to control living matter with exogenous stimuli can have tremendous impact on synthetic biology, medicine and materials science, among others. For instance achieving control over cells behaviour remains a challenge at the interface between living and non-living matter,[1] and would enable the development of new bio-mimetic and bio-enabled materials able to perform tasks.[2] Within this context, bacteria have arisen as “active and actively-controllable materials”, exhibiting neuro-like behaviour, extended bioelectric signalling[3,4] and tunable assembly properties.[5] In the last decade, it has been observed that the regulatory element of such an active behaviour is the electrical potential across the membrane, which governs bacteria electrophysiology, metabolisms and bioenergetics.[6] Light can be a powerful tool in these regards, as one can control the membrane potential and, thus, cell function and behaviour remotely and with relatively high spatiotemporal precision.
Here, I will show that a membrane-targeted azobenzene can be used to photo-modulate precisely the membrane potential in cells of the Gram-positive bacterium Bacillus subtilis. We found that upon exposure to blue- green light, the isomerization reaction in the bacteria membrane induces hyperpolarisation of the potential (ΔV = 20 mV), within a bio-mimetic mechanism reproducing the initial fate of retinal. Apart from being promising results in the view to photocontrol bacterial motion and assembly behavior in consortia, this approach also highlights the role of previously uncharacterized ion channels in bacteria electrophysiology.[7]
1.2-I3
The interface between biological cells and non-biological materials has profound influences on cellular activities, chronic tissue responses, and ultimately the success of medical implants and bioelectronic devices. For instance, electroactive materials in contact with cells can have very different composition, surface topography and dimensionality. Dimensionality defines the possibility to have planar (2D), pseudo-3D (planar with nano-micropatterned surface) and 3D conductive materials (i.e., scaffolds) in bioelectronics devices. Their success for both in vivo and in vitro applications lie in the effective coupling/adhesion of cells/tissues with the devices’ surfaces. It is known how a large cleft between the cellular membrane and the electrode surface massively affects the quality of the recorded signals or ultimately the stimulation efficiency of a device. In fact, the shape and the composition of these newly designed electrodes recalls those features of neuronal cells to ultimately induce biomimetic recognition. Furthermore, conductive materials that exhibit dynamic properties and organic composition might better recapitulate the native environment of tissue. However, engineering such new platforms still require materials synthesis, integration into microfabricated platforms and stable cell interfacing. Here, we propose a new class of organic conductive materials which resemble the biomimetic architecture of neurite and dendritic spines, can undergo dynamic reshaping and can be functionalized with artificial membrane to enhance cell coupling and outgrowth. The cell response can be monitored and eventually is adapting to the substrate changes over time. These new electrodes can pave the way to adaptive microdevices of use in bioelectronic applications such as electrophysiology and sensing.
1.2-S1
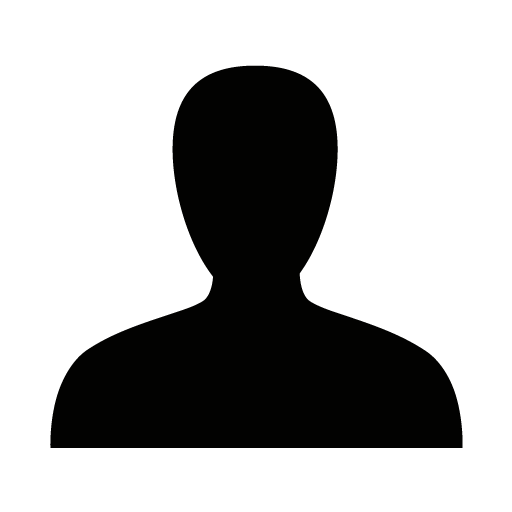
1.2-O1
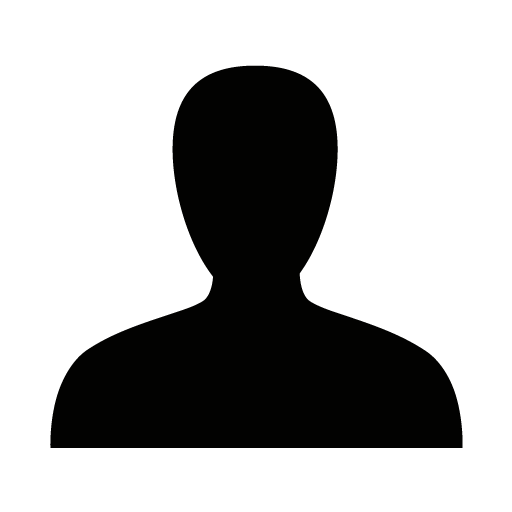
Traditional electronic devices are made of non-renewable and often toxic materials, which can lead to serious environmental contamination upon their disposal. A promising strategy for producing sustainable electronics is the direct writing of laser-induced graphene (LIG) electrically conductive patterns on biological and bio-based substrates. However, high ablation rates, the need to use controlled atmosphere, toxic fire retardants and multiple lasing steps limit the applicability of conventional LIG processes to produce sustainable electronic devices.
We have introduced iron-catalyzed laser-induced graphitization (IC-LIG) as an innovative technique enabling to engrave large-scale electrically conductive patterns on thermally sensitive substrates such as wood and wood-derived materials [1]. Our approach makes use of an aqueous bio-based coating, inspired by the historical iron-gall ink, which protects the wood surface from laser ablation and thermal damage while preserving its mechanical properties. Thanks to our approach, it is possible to engrave highly conductive (up to 2500 S m-1) patterns even on thinnest wood veneers (> 400 µm) and cellulose paper within a single lasing step in ambient atmosphere using a conventional CO2 laser setup.
Here, we explore further the possibilities offered by our IC-LIG approach by elucidating the catalytic effect of iron under different lasing conditions. We can control the iron-carbon composite structure at the nano- and microscale level, e.g., by tuning the concentration of iron in the ink. This, in turn, allows us to tune the iron phases in the resulting IC-LIG materials. By precisely controlling the laser parameters and setup we are able to fabricate bio-inspired surface patterns at the meso- and macroscale, controlling the structure as well as the electrical properties on a multiscale level, allowing us to fabricate sustainable electrodes for prospective uses as energy storage and electrochemical devices.
[1] C. H. Dreimol et al., Nat. Commun. 2022, 13, 3680 (12 pp.).]
1.2-O2
In vitro studies have a significant impact on the progression of novel therapies, cancer and stem cell research, and the drug discovery process. However, the current standard for in vitro models primarily relies on two-dimensional (2D) cell cultures, which fail to fully replicate the intricate cellular architecture and response to microenvironmental cues. To address this limitation, three-dimensional (3D) cell cultures have emerged as more accurate representations of the in vivo cellular environment. Tissue-engineered scaffolds are particularly promising in this regard. However, existing technologies for assessing 3D cell cultures are predominantly limited to endpoint assays, offering only a snapshot of cellular behavior. In this study, our goal is to overcome this challenge by employing continuous electrical assessment of 3D cell cultures cultivated on electroactive composite scaffolds composed of poly(3,4-ethylenedioxythiophene):polystyrene sulfonate (PEDOT:PSS) and MXenes. The scaffold architectures are created using the freeze-drying technique, and we investigate the influence of different scaffold compositions on electrochemical performance, morphology, swelling, biocompatibility. By combining the electroactive properties of PEDOT:PSS with the distinctive characteristics of MXenes, we aim to develop scaffolds that not only support cell growth but also enable real-time, non-invasive monitoring of cellular behavior. Our study surpasses conventional optical-based assessments by introducing continuous electrical measurements as a means of tracking the dynamics of 3D cell cultures. The electroactive composite scaffolds facilitate the detection of electrical signals associated with crucial cellular activities like attachment, proliferation, and differentiation. To evaluate the performance of the electroactive composite scaffolds, we culture them with Human Dermal Fibroblasts and assess parameters such as cell attachment, viability, and growth. By integrating the benefits of 3D cell culture and continuous electrical assessment, our study aims to comprehensively characterize scaffold-cell interactions and their potential applications in tissue engineering and regenerative medicine.
2.1-I1
Biofilm, the predominant lifestyle of all bacterial species, is a form of bacterial growth that enhances survival in wetted natural and artificial environments. The accompanying resilience, including decreased susceptibility to disinfectants and antibiotics, increases the need for prevention of biofilm formation, particularly on implanted medical devices and on food contact surfaces. This has prompted a revision of traditional methods and practices, re-evaluating them in relation to bacterial adherence and growth. The metabolism of bacteria growing in biofilms compared to those undergoing planktonic growth differs vastly. One important determinant is the redox state of the microenvironment in which bacteria exist. Sensing of the environmental redox state controls the switch between aerobic and anaerobic metabolism as well as bacterial virulence. We showed previously that electrically charged surfaces with high charge storage capacity, like PEDOT-based conducting polymers, can be used to modulate biofilm formation by altering the redox states of the surfaces [1, 2]. To gain better understanding of this finding, we performed an in-depth analysis of the process of biofilm formation on redox-challenged PEDOT:PSS surfaces using GFP-expressing Salmonella and the optotracer EbbaBiolight 680. The latter is a non-toxic fluorescent tracer that allowed us to monitor and quantify the production of extracellular matrix components (ECM) in real-time by semi-quantitative spectroscopy and microscopy [3]. Redox challenge performed in a fast-charging electrochemical setup promoted bacterial production of higher amounts of cells and ECM on oxidized PEDOT:PSS surfaces compared to their reduced counterpart. When electrodes were separated and charging was carried out with respect to a Pt electrode, the slower charging process resulted in increased cell numbers as well as increased amount of ECM on the reduced surfaces. When isogenic Salmonella mutants unable to produce the ECM components curli and/or cellulose were tested, none of them responded to the redox challenge. This demonstrates a novel use of surface redox potential to modulate ECM curli production and accordingly biofilm formation. Anti-biofouling surfaces directly affecting ECM production may find use in sensitising biofilm forming strains to the effect of antibiotics and antimicrobial compounds.
2.1-I2
Eleni Stavrinidou is an Associate Professor and leader of the Electronic Plants group at Linköping University. She received a PhD in Microelectronics from EMSE (France) in 2014. She then did her postdoctoral training at Linköping University (Sweden) during which she was awarded a Marie Curie fellowship. In 2017 Eleni Stavrinidou became Assistant Professor in Organic Electronics at Linköping University and established the Electronic Plants group. She received several grants including a Swedish Research Council Starting Grant and she is the Coordinator of the HyPhOE-FET-OPEN project. In 2019 she received the L’ORÉAL-UNESCO For Women in Science prize in Sweden. In 2020 she became Associate Professor and Docent in Applied Physics. The same year she was awarded the Future Research Leaders grant of the Swedish Foundation for Strategic Research. Her research interests focus on organic electronics for plant monitoring and optimization, energy applications and bio-hybrid systems.
Leveraging the biocatalytic machinery of living organisms for in-vivo fabrication of functional bioelectronic interfaces enables seamless integration of devices in tissue and formation of biohybrid systems. Previously we have demonstrated that plants can polymerize conjugated oligomers in-vivo forming conductors within their structure. We showed that the polymerization is enzymatically catalyzed by endogenous peroxidases, and we developed a series of conjugated oligomers that can be enzymatically polymerized in physiological conditions. The conjugated polyelectrolytes integrate within the plant cell wall structure adding electronic functionality into the plant that is then explored for energy storage. Recently we demonstrated intact plants with electronic roots that continue to grow enabling plant-biohybrid systems that maintain fully their biological processes. The electronic roots are used to build supercapacitors and biohybrid circuits to power low power electrochemical devices. Furthermore, we have extended this concept into an animal model system. We demonstrated that Hydra, an invertebrate animal, can polymerize intracellularly conjugated oligomers in cells that expresses peroxidase activity. The conjugated polymer forms electronically conducting and electrochemically active domains in the µm range integrated within the hydra tissue. Our work paves the way for self-organized electronics in plant and animal tissue for modulating biological functions and in-vivo bio-fabrication of hybrid functional materials and devices.
2.1-I3
Advancements in synthetic biology have pushed the frontier of materials science into the realm of engineering living materials. This presentation focuses on bioengineering strategies for coupling the electronic metabolisms of living cells with electrodes. Building on recent discoveries in the extracellular electron transfer mechanisms of S. oneidensis, our research focuses on the heterologous expression of key cytochromes in a facultative anaerobe (E. coli) [1-2]. We employ a combination of colorimetric and electrochemical techniques to characterize extracellular electron transfer in these engineered microbes. Interestingly, the bioengineered strains exhibit enhancements in both mediated and non-mediated extracellular electron transfer, with the extent varying with the specific combinations of expressed proteins. This platform was used to demonstrate enhanced electricity production for applications such as waste water treatment. Importantly, this work represents the first demonstration of the complete electron conduit from S. oneidensis fo bioeletcricity genertaion in a foreign cell. This demonstartion significantly broadens the range of exoelectrogenic microbes, unlocking new applications in microbial electronics across various fields such as biotechnology, environmental science, chemical synthesis, and energy conversion.
2.2-O1
Inspired by biocatalysts in nature, artificial catalyst systems with behavior similar to or exceeding the activity of biocatalysts have been widely studied for different healthcare and environmental applications. In this work, a hybrid organic-inorganic approach is used to combine conducting polymer with inorganic materials for biocatalysis. Three different MoS2 nanosheets (MSNSs) were synthesized based on different methodologies. Methodology 1: ammonium tetra thiomolybdate (NH4)2MoS4 and hydrazine hydrate (N2H4·4H2O) were sonicated and transferred to an autoclave for the hydrothermal process. Methodology 2: MoS2 nanosheets were synthesized through a hydrothermal method using sodium molybdate Na2-MoO4.2H2O and L-cysteine. Methodology 3: MoS2 nanosheets were prepared through exfoliating of bulk MoS2 in N, N-Dimethylformamide (DMF) by sonication process. The morphology, and structural information of MSNSs were analyzed by TEM, SEM, XRD and Raman spectroscopy. Initial results show excellent catalytic performance of the MSNs with different concentrations of hydrogen peroxide ranging from 1µM to 10M. The catalytic behavior of the MSNSs were then compared with the performance of hydrogen peroxidase within the same range of concentration values.
2.2-O2
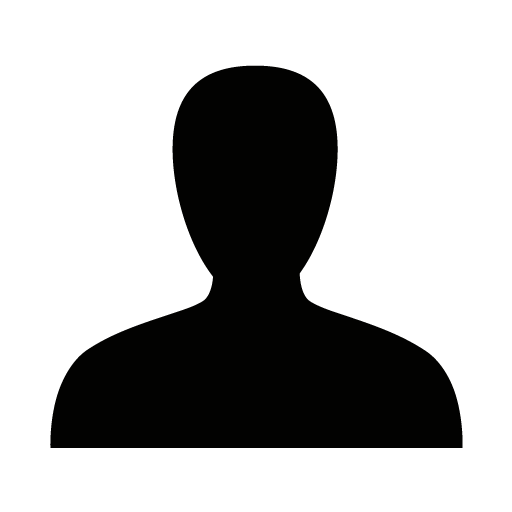
Eman Aljawi, Akbota Kurmangaliyeva, Muhammad Jamali, Bushara Fatma, Waqas Waheed, Dean Everett, Charalampos Pitsalidis, Anas Alazzam, and Anna Maria Pappa.
“Bioelectrocatalytic lab on chip device for infection biomarker monitoring”
Viruses can easily mutate, as evidenced with SARS-Cov2, hence prevention and early detection of infection seems to be the most reliable strategies to cope with pandemics. The current available diagnostics tools, such as real-time polymerize chain reaction (RT-PCR), are not compatible with early detection as they are time-consuming, expensive, don’t offer multiplexing, and don’t offer on-site detection. To tackle these issues, Lab-on-Chip (LoC) biosensors have emerged, overcoming the limitations of conventional methods. Electrochemical biosensors mainly consist of electrodes integrated into a platform to offer portable, on-site, cost-effective, specific, and fast detection. This project introduces a multiplexing LoC biosensor for the simultaneous detection of 4 infection biomarkers; glucose, lactate, hydrogen peroxide, and C-reactive protein (CRP) all in one device using biocatalytic enzymes for the three metabolies and an antigen for CRP as biorecognition elements. Optimization on the electrode materials in terms of bioelectrocatalytic performance leads to the optimum sensing capabilities while allowing for multiplexing on a chip. This work compbines LoC technologies based on microfluidics and the recent advancements in materials science, combining conducting polymers with 2D electronic materials for the electrode fabrication.
1.1-I1
The assembly of supramolecular structures within living systems is an innovative approach for introducing artificial constructs and developing bio-materials capable of influencing and/or regulating the biological responses of living organisms. By integrating chemical, photophysical, morphological, and structural characterizations, we show that the cell-driven assembly of DTTO into fibers results in the formation of a 'biological assisted' polymorphic form, hence the term bio-polymorph. Indeed, X-ray diffraction revealed that cell-grown DTTO fibers present a unique molecular packing leading to specific morphological, optical, and electrical properties. Monitoring the process of fibers formation in cells with time-resolved photoluminescence, we established that cellular machinery is necessary for fibers production and postulated a non-classical nucleation mechanism for their growth. These biomaterials may have different applications for the stimulation and sensing of living cells, but more crucially, the study of their genesis and properties broadens our understanding of life beyond the native components of cells.[GL1]
[GL1]Questa frase è bella, ma va oltre il contributo che stiamo dando, mi pare. Lascio a te decidere, ma noi la toglieremmo.
1.1-I2
Signaling in physiological environments relies on physicochemical cues and biomolecular that are all processed by the cellular machinery to execute a specific response. In this presentation, designer functional peptides will be discussed as synthetically engineered materials that could enable the transduction of light to cellular cues for controlling the behavior and anisotropy of excitable cells such as cardiomyocytes. First, the structural tunability of the assembly behavior of peptides bearing optoelectronic π-conjugated units in aqueous solutions and on surfaces to generate nano- and micropatterns are discussed. While the supramolecular assemblies of these peptides can have dynamic structures in physiologically relevant solutions, on surfaces that have anisotropic topography, the resulting self-assembled optoelectronic peptides can be directed to form 1-D nanostructures with ordered molecular interactions between π-units. Additionally, fabrication approaches that allow for the generation of micropatterns of π-conjugated peptides that are stable under electrolytic cell culture media will be shown. The distinct capability of these patterned π-conjugated peptides to align cardiomyocytes interfaced with these materials will also be discussed. This property provides the potential opportunity for directional stimulation of excitable cells with spatiotemporal control, especially when the stimulation is initiated using light. Finally, material design insights that allow for enhanced photoconduction by these designer peptides will also be presented. Overall, we envision these functional peptides as transducer biomaterials that can offer a new paradigm for having systematically controlled cell-material interactions towards tissue engineering and in vitro modeling applications for excitable cells.
1.1-I3
Alberto Scaccabarozzi is a Postdoctoral Fellow at the Center for Nanoscience and Technology (CNST) of the Istituto Italiano di Tecnologia in Milan (Italy). He received his PhD from Imperial College London (UK) in 2017 under the supervision of Prof. Natalie Stingelin, followed by postdoctoral appointments at CNST in Mario Caironi’s group and at King Abdullah University of Science and Technology (KAUST, Saudi Arabia) in Prof. Thomas Anthopoulos’ group. His research interests encompass the broad field of organic electronics, in particular the study of structure-processing-property relationships of organic semiconductors for a wide range of devices, especially Organic Field-Effect Transistors (OFETs).
The employment of organic semiconductors (OSCs) promises to widen the realm of electronics to countless new applications, thanks to the advantageous properties of organics. Effectively, the field of organic electronics has been forging ahead from its initial proof-of-concept devices towards increasingly diverse materials and applications.
State-of-the-art high performance OSCs are derived from petrochemicals. Nevertheless, this class of materials can also be based on renewable, natural resources. Indeed, nature offers an extraordinary variety of systems that exhibit diverse opto-electronic functionalities. For instance, colours can vary in plants depending on the specific function, weather conditions and health/life cycle stage. Molecules responsible for this property are often dyes and pigments that show π-electron delocalization within their molecular structure and hence, that are potentially prone to showing optimal charge carrier transport properties and frontier energy levels compatible for charge injection.
Effectively, different natural molecules have been reported to display semiconducting properties and few of them have been used as active layers for electronic devices, e.g., organic thin film transistors (OTFTs). For instance, carotenoids are an interesting class of molecules, owing to their widespread availability in a large range of plants, algae, bacteria and fungi. They are hence compatible with a circular economy model, unravelling novel sustainable scenarios in organic electronics, besides being edible, thus contributing to the growth of the rising field of edible electronics. In this work we will show how a fine microstructural tuning of these materials can lead to the fabrication of efficient OTFTs with reasonable stability. Such results indicate that options for sustainable, edible semiconductors are available and can be exploited towards the integration into logic circuits.
1.2-I1
I am a physiologist with more than 10 years of experience in cardiovascular research. I graduated in Biotechnology in 2007 and received my PhD in Physiology and Neuroscience at the University of Pavia in 2012. I then joined IRCCS ICS Maugeri in Pavia and later the Center for Nano Science and Technology (IIT@PoliMI) based in Milan. Currently, I act as an associate professor in Physiology at the University of Milano-Bicocca. I have developed a strong interest in biophysics and acquired a hands-on experience in both basic and translational research. My main goal is to solve problems in physiology and medicine of the cardiovascular system through multidisciplinary and ground-breaking approaches.
The use of light to control the activity of different cell-types has recently come at the forefront of the scientific community thanks to a series of key-enabling features (i.e. spatial/temporal selectivity, and lower invasiveness). Optogenetics is probably the prime example of this approach and since its initial development in neuroscience has been extended to other fields. However, the need of viral gene transfer, required to induce light-sensitivity in the target organism, strongly impairs the clinical translation of optogenetics-based systems. To overcome this issue, non-genetic (geneless) optostimulation is a growing and multidisciplinary field of research at the border among physics, material science and biology that aims to achieve light sensitivity with photoactive actuators. I will present promising photoactuators for cardiac applications, focusing in particular on excitation-contraction coupling modulation. Future clinical perspectives in contexts where the possibility of obtaining precise control of cardiac cell activity could offer new insights (e.g. resynchronization and arrhythmia termination) will also be discussed.
1.2-I2
Nature has evolved in a hierarchical manner to achieve outstanding material properties and complex organismal behaviours. Hierarchy is a ubiquitous organizing and functional principle of natural systems, which are controlled in size, shape and pattern. The properties of skeletal muscles mainly rely on their hierarchical structure, composed of fibrous actuators organized in different levels of aggregation (from cm down to nm). This strong hierarchical organization gives muscles their remarkable time-dependent viscoelastic properties, and their efficient hierarchical actuating organization at different levels, from the single sarcomere to the muscle fascicle.
Muscles are an optimized mechanism over a million years of evolution, and have inspired artificial actuators [1]. Despite that, current actuation technologies are not able to recapitulate the features of natural skeletal muscles. The possibility to grow fully functional living muscles in vitro and use them to power soft machines has the potential to revolutionize robotics, in the long term.
Biohybrid actuation is born as a new and extremely promising actuation method, which combines a live entity, like muscle cells, with an artificial substrate to produce a mechanical movement generated by the contraction of muscle cells. Attractive advantages feature this kind of biological actuator as performance invariance with scalability (from nm to mm), high transduction efficiency, and high power-to-weight ratio. Biohybrid actuators have the potential to deliver remarkable performance with life-like movements at the macroscale to artificial devices. Biohybrid actuators usually rely on a bottom-up approach and include systems based on cardiomyocytes, insect self-contractile tissues, and engineered skeletal muscle tissues (from both mammals and insects), which can convert chemical energy from the environment into mechanical energy. Skeletal muscle cells are the muscle cell types that have generated the most interest because they may be regulated to contract in response to specific stimuli, such as chemical, electrical, or optical signals, following suitable optogenetic changes [2]. There are several examples of bio-hybrid actuators and robots in literature, as the bio-bots can walk or swim [3]. Despite that, the use of a bio-hybrid actuator to actuate a medical device as a catheter has never been explored, so far.
Catheterization is one of the most promising approaches for the targeted treatment of several diseases, including cancer. Indeed, the local administration of medications or chemicals in the area of interest may maximize the therapy efficacy, reducing side effects. Here, we report the concept and an early investigation of a novel intravascular steerable catheter powered by an on-board biohybrid actuator on its tip to navigate deep and tortuous regions within the cardiovascular systems. The catheter performance has been analyzed by analytical and numerical analyses, assessing the influence of the catheter geometrical (e.g., inner and outer radii, wall thickness) and physical (e.g., stiffness) parameters, the actuator geometrical parameters (e.g., length and positioning over the catheter tip) and contractile force. The findings demonstrate the necessity to lower the outer diameters and decrease wall thickness to maximize the catheter deflection. Also, we assessed the influence of the positioning of the biohybrid actuator as the applied forces. These preliminary results hold a lot of promise in light of future experiments using this type of actuation to drive microcatheters through the cardiovascular network, even though the performance of this concept still needs to be improved to match relevant anatomical targets, as the radius of curvature of the inner branches of organs.
1.2-I3
The vast expansion of available synthetic biology tools has led to explosive developments in the field of materials science. The increased accessibility of these tools has pushed the frontier of materials science into the field of engineering biological and even living materials. By coupling the tunability of nanomaterials with the prospect of re-programming living devices, one can re-purpose biology to fulfil needs that are otherwise intractable using traditional engineering approaches.
Optical technologies in particular stand to benefit from the untapped potential in coupling the optical properties of nanomaterials with the specificity and scalability of biological materials. This presentation highlights applications in sensing and energy technologies that exploit the synergistic coupling of nanobio-hybrid materials. This talk will focus on the development of living electronics, such as photovoltaics based on photosynthetic organisms with augmented capabilities. The development include advancements in electrode engineering as well as biological engineering of living organisms. The talk will also discuss nanobionic approaches that focus oin infusing synthetic nanoparticle with living cells.
1.2-O1
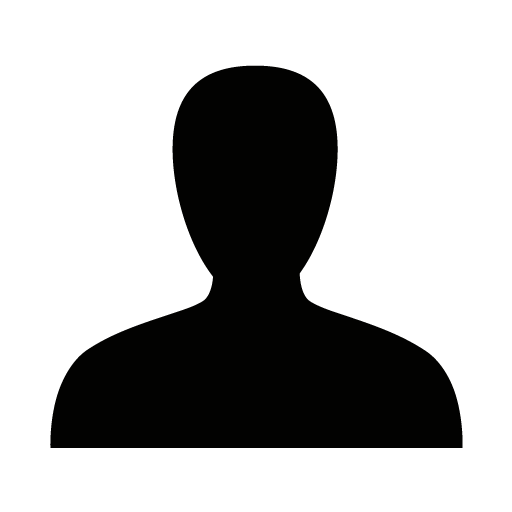
The control of biological functions is crucial for the in-depth understanding of physiological/pathogenic processes, as for the development of novel, ad hoc therapeutic modalities to fight specific diseases. In this regard, light-induced cell control is characterized by lower invasiveness, better space and time resolution, with respect to more traditional electrical-based methods. Exogenous inorganic and organic semiconducting materials have attracted considerable interest, since they can be employed as photoactive transducers to trigger the biological activity, without any need for viral transfection [1]. In particular, semiconducting polymers offer great biocompatibility and stability together with geometrical adaptability. Among them, the green light-absorbing poly(3-hexylthiophene) (P3HT) was successfully employed for the modulation of the physiological activity of different cell models [2-4], including the boosting of both proliferation and tubulogenesis of endothelial cells [5]. The latter result is particularly appealing in view of the regeneration of the cardiovascular tissue for application in cardiovascular disease therapies.
Here, we realize smart biointerfaces between red-light absorbing conjugated polymers and cardiovascular cells. The aim of our work is to study the cellular response both to the polymers alone and in combination with red light excitation. The latter is particularly favorable in view of in vivo applications, given the higher penetration of red light within living tissues, as compared to lower visible wavelengths. We show that conjugated polymers in form of nanoparticles lead to either enhancement or reduction of the angiogenic response of model endothelial cells, depending on the material type and the presence/absence of the light stimulus. Furthermore, we observe that semiconducting polymer thin films efficiently modulates, upon red light photoexcitation, the physiological properties of Cardiomyocytes. In particular, we demonstrate that polymer-mediated photostimulation modulates both the Ca2+ dynamics and the electrical properties of the cells. Very interestingly, we observe an anti-arrhythmogenic effect, unequivocally triggered by polymer photoexcitation.
Overall, our results support the possibility to employ red light-responsive conjugated polymers to regulate cardiovascular functions, in a drug-free, touchless, repeatable, and spatio-temporally controlled manner.
1.1-I1
Dr. Jasna Jankovic is a Professor in the Materials Science and Engineering Department at the University of Connecticut (UConn) since 2018. Prior to joining UConn, she completed her Ph.D. at the University of British Columbia, Department of Chemical Engineering, followed by a 7 years employment as a Senior Research Scientist at the Automotive Fuel Cell Cooperation in Burnaby, Canada, a joint venture between Ford Motor Company and Daimler. Dr. Jankovic’s research focus is in advanced characterization of fuel cells, electrolyzers and batteries using microscopy and spectroscopy techniques, fabrication of novel electrodes for electrochemical devices, as well as Science, Technology, Engineering and Mathematics (STEM) and clean energy education. She has more than 25 years of experience in clean energy sector, more than 50 publications and 2 patents. Dr. Jankovic is a recipient of several Natural Sciences and Engineering Research Council (NSERC) awards in Canada, and a number of National Science Foundation (NSF) awards and Department of Energy (DOE) sub-awards in the US.
With the recent trends in hydrogen technologies, scale-up fabrication of membrane electrode assemblies (MEAs) for the electrochemical systems such as fuel cells and electrolyzers is gaining significant attention. Companies and researchers are focusing on diverse large-scale electrode fabrication processes, such as roll-to-roll and screen-printing. However, optimizing such processes is not trivial, and a number of parameters, including but not limited to catalyst type, solvent, ink mixing, electrode coating and drying, play a role in the quality of the final product. Correlations between the fabrication parameters and resulting electrode microstructure, properties and performance are important to understand, in order to better control the processes. Advanced imaging and spectroscopy techniques, together with image and data processing to quantify important structural and compositional parameters, play an important role in this understanding. Information about catalyst distribution, composition, and surface chemistry can be correlated to ink characteristics, and finally to electrode structures, component distribution and properties, and their effect on MEA performance can be investigated. This talk will offer a plethora of examples of these advanced characterization and quantification techniques within a collaborative project on Overall Research on Electrode Coating Processes (OREO) between four institutions: University of Connecticut, Colorado School of Mines, National Renewable Energy Lab and Fraunhofer ISE.
1.1-I2
The extended commercialization of electrochemical energy conversion devices (i.e., fuel cells and (co-)electrolyzers) relies on the development of improved catalysts for the reactions taking place in their electrodes (i.e., O2-reduction/evolution, H2-oxidation/evolution or CO2-reduction). This in turn passes by deepening our understanding of these reactions’ mechanisms and of the parameters that determine the performance and stability of the electrocatalysts speeding up their kinetics. As a result, great efforts are being devoted to investigate these reactions and materials under operation-relevant conditions. Such in situ / operando studies are often carried out using X-ray absorption spectrocospy (XAS), which is a bulk-sensitive technique realizable at atmospheric pressure and that can provide insight on an analyte’s oxidation state, electronic properties and coordination environment. Moreover, recent developments in fast X-ray energy scanning and spectral acquisition have resulted in a surge of interest in time-resolved XAS studies that can yield additional information on the changes undergone by the catalysts in the course of the reaction [1]. However, the large majority of these works are performed using electrochemical cells and/or experimental conditions (e.g., convective properties, catalyst loadings) optimized to assure the successful completion of the spectroscopic measurements, but that may significantly differ from those encountered by these materials in the real devices. Most importantly, little is known about how these differences can affect the results derived from such spectroelectrochemical studies, as well as on the extent to which their conclusions are amenable to application-relevant operative conditions.
With this motivation, this contribution will start by discussing how XAS-results may be affected by the thickness of the studied catalyst layers (CLs). This was achieved by studying the formation of palladium hydride on electrodes with similar Pd-loadings but ≈ 5-fold different CL-thicknesses, which were prepared using an unsupported Pd-aerogel vs. a carbon-supported Pd-nanoparticle catalyst [2]. In a subsequent step, we will show how this CL-thickness and the intensity of the incident X-ray beam can caused the accumulation of evolved O2-bubbles within the pores of an Ir-oxide-based CL, leading to a localized loss of potential control that can ultimately result in an erroneous assignment of the catalyst’s oxidation state [3]. Finally, we will present our recent efforts at developing a novel electrochemical cell allowing operando spectroscopic studies with ultra-low catalyst loadings, enhanced mass transport properties and the online analysis of the reaction products.
In summary, this contribution will provide novel insight on the impact of various experimental parameters on operando XAS measurements, while providing guidelines for the successful completion of such experiments and the subsequent interpretation of their results.
1.1-I3
One of the main challenges to tackle for the widespread adoption of proton exchange membrane fuel cells (PEMFCs) is minimise the use of PGM catalysts while keeping high activity and durability.
Great advances have been made in the fundamental understanding and experimental development of electrocatalysts [1]. One strategy to address this challenge consists in nano-engineering morphology and composition of the catalysts to increase their ORR mass activity and durability upon prolonged cycling. Another approach consists in the modification of the support material, which can bring enhanced stability to corrosion as well as promote electrocatalysis via interactions with the metal catalyst.
Our group is developing novel electrocatalysts and support materials to enhance performance and durability of PEMFC cathodes.
A range of chemical and electrochemical synthesis techniques to prepare Pt nanoparticles and thin films [2] are investigated to prepare highly active ultra-low PGM loaded electrodes. A solid-phase synthesis is used to prepare Pt-rare earth metal alloys of controlled stoichiometry [3, 4].
In parallel, we are developing functionalised carbons and metal oxide-based support materials with high corrosion resistance combined with electronic electrocatalyst-support interactions beneficial for activity and durability [5, 6]. The morphology of the supports also plays a crucial role. Nanostructured electrodes are prepared by electrospinning allowing control over structure and porosity [2, 5].
In this work, the advances in understanding the role of materials composition and architecture on electroactivity and durability of PEMFC cathodes will be presented.
1.2-I1
Virgil Andrei obtained his Bachelor and Master of Science degrees in chemistry from Humboldt-Universität zu Berlin, where he studied thermoelectric polymer pastes and films in the group of Prof. Klaus Rademann (2014-2016). He then pursued a PhD in chemistry at the University of Cambridge (2016-2020), where he developed perovskite-based artificial leaves in the group of Prof. Erwin Reisner, working closely with the Optoelectronics group of Prof. Richard Friend at the Cavendish Laboratory. He was recently a visiting Winton fellow in the group of Prof. Peidong Yang at University of California, Berkeley, and is currently a Title A Research Fellow at St John's College, Cambridge. His work places a strong focus on scalability, material design, complementary light harvesting and synthesis of added-value carbon products, introducing modern fabrication techniques towards low-cost, high-throughput solar fuel production.
Metal halide perovskites have emerged as promising alternatives to commonly employed light absorbers for solar fuel synthesis, enabling unassisted photoelectrochemical (PEC) water splitting[1,3] and CO2 reduction to syngas.[2,4] While the bare perovskite light absorber is rapidly degraded by moisture, recent developments in the device structure have led to substantial advances in the device stability. Here, we give an overview of the latest progress in perovskite PEC devices, introducing design principles to improve their performance and reliability. For this purpose, we will discuss the role of charge selective layers in increasing the device photocurrent and photovoltage, by fine-tuning the band alignment and enabling efficient charge separation. A further beneficial effect of hydrophobicity is revealed by comparing devices with different hole transport layers (HTLs).[1,3] On the manufacturing side, we will provide new insights into how appropriate encapsulation techniques can extend the device lifetime to a few days under operation in aqueous media.[1,2] To this end, we replace low melting alloys with graphite epoxy paste as a conductive, hydrophobic and low-cost encapsulant.[3,5] These design principles are successfully applied to an underexplored BiOI light absorber, increasing the photocathode stability towards hydrogen evolution from minutes to months.[6] Finally, we take a glance at the next steps required for scalable solar fuels production, showcasing our latest progress in terms of device manufacturing. A suitable choice of materials can decrease the device cost tenfold and expand the device functionality, resulting in flexible, floating artificial leaves.[4] Those materials are compatible with large-scale, automated fabrication processes, which present the most potential towards future real-world applications.[7,8] Similar PEC systems approaching a m2 size can take advantage of the modularity of artificial leaves,[9] whereas thermoelectric generators can further bolster water splitting by utilizing waste heat to provide an additional Seebeck voltage.[10,11]
1.2-I2
Fe-N-C catalysts have made considerable advancements in their initial activity. However, their stability in acidic mediums presents a challenge that must be addressed to replace Pt in fuel cell cathodes. Unfortunately, the complicated phenomena present in fuel cells make it difficult to understand the deactivation mechanisms of Fe-N-C cathodes, hindering the development of prolonged stability solutions. Herein, we show time-resolved changes in active site density (SD) and turnover frequency (TOF) of Fe-N-C catalysts. These changes occur simultaneously with a decrease in oxygen reduction reaction (ORR) current in a temperature/gas controllable gas-diffusion electrode (GDE) flow cell. We identified a strong dependence of SD changes on operating parameters by conducting in operando diagnosis of Fe leaching. Furthermore, we drew a lifetime-dependent stability diagram that reveals a shift in the prime degradation mechanism during operations. We propose a stabilizer concept utilizing ORR-inactive site-isolated Pt ions to suppress undesirable Fe loss during ORR. Through this proof-of-concept strategy, we verified enhanced fuel cell stability with reduced Fe dissolution, offering a new design principle for durable Fe-N-C catalysts.
1.2-O1
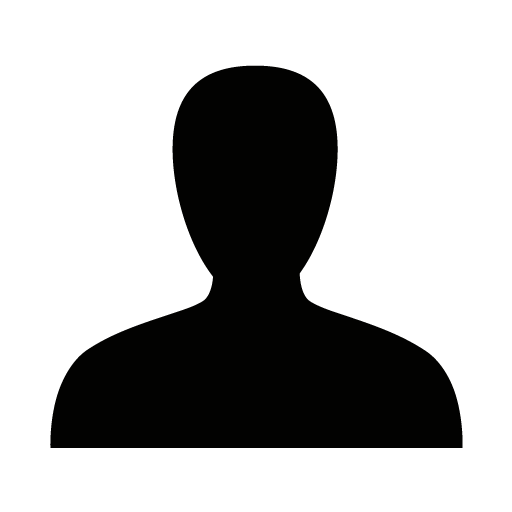
PhD student investigating Fe/N/C electrocatalysts for fuel cells and electrolysers.
Part of Magda Titirici group at Imperial College London.
Low temperature proton exchange membrane fuel cells (PEMFCs) powered by green hydrogen provide a means to sustainable energy production for stationary and transport applications. Their widespread commercialization is limited by the cost of the platinum catalyst at the cathode, where oxygen reduction occurs. Atomic Fe sites within nitrogen-doped carbon (Fe-N-C) offer a cheap and sustainable alternative, exhibiting the most promising non-precious metal activity for oxygen reduction. However, their stability is still below commercial realization owing to several degradation routes. Steps can be taken to minimize these pathways, [1,2] however, demetallation of FeNx active sites is still suggested as a primary performance degradation mechanism in PEMFCs. [3]
To shed new light into Fe demetallation, in this work we focus on monitoring Fe dissolution in 0.1 M HClO4 from our recently reported highly porous and >50% FeNx utilization Fe-N-C catalyst. [4] We compare operando Fe dissolution under O2 (active) and Ar (inert) conditions in both room temperature flow cell (FC) and gas diffusion electrode (GDE) setups, coupled to online inductively-coupled plasma mass spectrometry (ICP-MS). We also monitor the impact of high temperature on Fe dissolution using ex situ rotating disc electrode (RDE) and operando GDE measurements.
Fe dissolution was detected under both atmospheres in FC-ICP-MS configuration, with the amount dependent on potential, electrolyte, and gas atmosphere. After aging test performed at 80oC in O2-saturated electrolyte in RDE post-mortem X-ray absorption spectroscopy and transmission electron microscopy (TEM) analysis show the formation of Fe2O3 nanoparticles. Operando GDE-ICP-MS measurement show reduced Fe dissolution under room temperature O2, but small increase or negligible change at 70oC. Meanwhile, under Ar at room temperature, high Fe dissolution is detected.. Post-mortem TEM and high angle annular dark field scanning TEM shows formation of FexOy nanoparticles under room temperature O2, and limited formation under Ar, in GDE setup. We propose under O2 reduction GDE conditions a degradation mechanism of Fe dissolution from FeNx sites and subsequent reprecipitation into FexOy, which we explore with microkinetic modelling.
1.2-O2
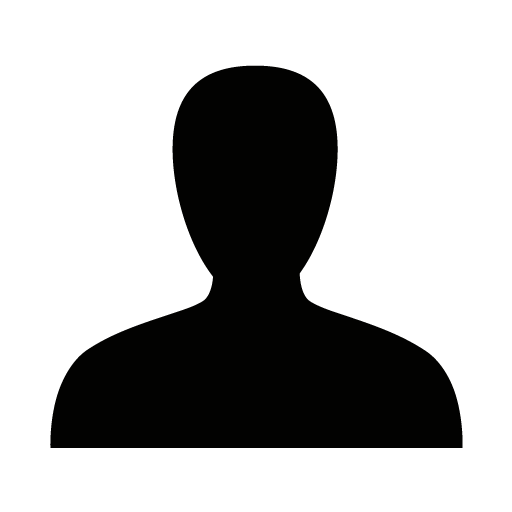
Hydrogen is an important energy vector and plays a key role in global plans to achieve net zero targets and low temperature water electrolysis is a viable technology to produce hydrogen at scale. The efficiency of water electrolysers, however, is limited by the sluggish kinetics of the oxygen evolution reaction (OER) at the anode, which contributes to a significant potential loss [1]. The search for new catalysts, has been largely focussed on optimising the binding energetics of the reaction intermediates on the surface by altering the materials chemistry. However, the role of the interfacial solvent on the OER kinetics is a largely unexplored lever to tune catalytic activity.
In this work, we investigate the influence of the nature of cations in the electrolyte on the OER kinetics on an iridium oxide catalyst in alkaline condition, in order to gain fundamental insight into the polarised solid-liquid interface. The activity in 0.1 M MOH (M = Li, Na, K, Cs) shows marked differences depending on the nature of the cation, with the OER activity being higher for larger cations. We performed operando uv-vis spectroscopy to quantify the potential-dependent redox transitions on the surface from 0.35 VRHE to 1.55 VRHE, and identify the binding energetics and the degree of interaction between surface adsorbates. In order to rationalise the trends in redox kinetics observed, laser-induced current transient measurements were employed to study the behavior of the interfacial water at the electrode surface. In particular, the potential of maximum entropy (PME) was determined for the different electrolyte compositions. This work provides a holistic picture of the electrified interface and allows us to determine how the nature of the electrolyte modulates the OER kinetics.
1.2-O3
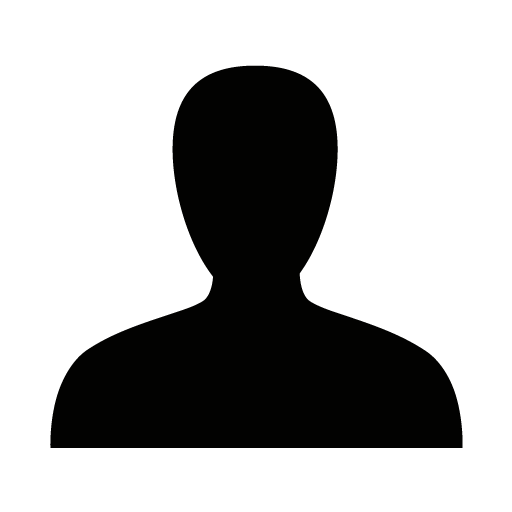
Iridium oxide is the state-of-the-art electrocatalyst for water oxidation in polymer electrolyte membrane (PEM) electrolysers for green hydrogen production. Understanding what controls the reaction rate on such benchmark metal oxides is central to designing more active and stable electrocatalysts for water oxidation in PEM electrolysers.[1]
In this talk, I will present our work on probing active sites and the intrinsic water oxidation rate on iridium-based catalysts using a combination of time-resolved operando optical spectroscopy, X-ray absorption spectroscopy (XAS), and electrochemical mass spectrometry (ECMS). This talk will focus on comparing two state-of-the-art iridium oxides structures- amorphous IrOx versus crystalline rutile IrO2. I will first discuss the intermediate states and catalytically active states on iridium oxides and show how we can use optical spectroscopy to identify and quantify these states . The nature of these states, including Ir oxidation state and surface absorbates on iridium sites, will be elucidated using a combination of XAS and Density Function Theory (DFT). The interaction between these states and its effect on controlling the free energy of elementary reaction steps in water oxidation will also be discussed. Finally, based on the resulting molecular level understanding, I will present a modified Sabatier volcano model to describe trends in OER activity that takes into account the effect of absorbate-absorbate interactions on binding energetics.
1.2-O4
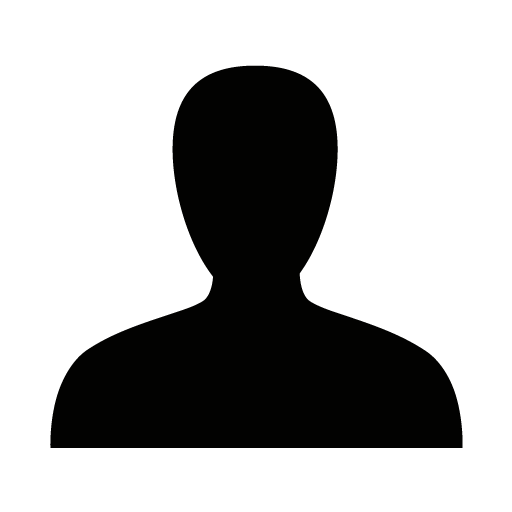
Electrocatalytic water splitting is a topic of investigation of numerous research groups for decades. Oxygen evolution reaction (OER), as a more complex and more demanding reaction, is more frequently studied, despite of the fact that the simpler hydrogen evolution reaction (HER), is generally not well understood. Nowadays, recurrent approach to electrocatalysis is conquest for more active and more stable electrode materials, very often lacking significant input to better understanding of reaction mechanisms or understanding of what are the drivers of electrocatalytic activity [1].
Importantly, if one asks the key question from the conceptual point of view, and that is: what are the origins of electrocatalytic activity? - the answer will be, in the predominant majority of cases, like 70 years ago. Namely, paradigm of electrocatalysis is Sabatier principle, that suggests optimal (“not too strong, not too weak”) binding of intermediates as main precondition of high reaction rate [2]. Conventional wisdom suggests that confirmation of this should be relatively simple. Namely, Brönsted-Evans-Polanyi (BEP) relations suggest linear relationship between adsorption energy of key intermediates and activation energy. In other words, if we have reliable values of adsorption energies relevant for the electrochemical environment [3], they should form linear dependence with experimentally obtained activation energies.
However, high-temperature hydrodynamic experiments on HER indicate that reducing of activation energy by tuning of adsorption energy of intermediates is not necessarily beneficial for enhancement of HER rate. Namely, HER rate is strongly influenced by preexponential frequency factor. Therefore, we propose discussion that will analyse several key aspects of HER electrocatalysis: 1) interplay of activation energy and preexponential factor as a driver of electrocatalytic activity 2) are BEP relations relevant for electrocatalysis 3) can we assess adsorption energies experimentally 4) what are relevant descriptors that shed light on HER activation process, 5) what else is important beyond Sabatier principle for rate of HER [1-5].
1.3-I3
Academic Career
Since May 2020: W3 Associate Professor at the Department of Physics, TUM.
2014 - 2020: W2 Assistant Professor at the Department of Physics, TUM.
2010 - 2014: Group leader ("Adsorption and Electrocatalysis") at the Center for Electrochemical Sciences (CES) at Ruhr University Bochum, Germany.
2008 - 2010: Post-doc, Department of Physics, Technical University of Denmark.
2006 - 2008: Post-doc, Faculty of Science and Technology, University of Twente, the Netherlands.
2002 - 2005: PhD in Physical and Solid State Chemistry, Belarusian State University, Minsk, Belarus.
Awards
• The German National Ernst Haage Award for the research in the field of chemical energy conversion (2016)
• Hans-Jürgen Engell Award of the International Society of Electrochemistry (ISE Prize for Electrochemical Materials Science) for the research on electrocatalysis and in situ characterisation of the electrode–electrolyte interface (2013)
The key procedure in designing and understanding of electrocatalysts is identifying active sites with optimal adsorption properties towards reaction intermediates. However, such identification under reaction conditions is often very complicated and indirect. Normally, ex-situ experiments are used. In alternatives, the available resolution today is rather low, hiding the true structural origin of the activity at the atomic level. Therefore, the nature of active centers is currently known for only a few electrocatalytic reactions and a limited number of catalyst surfaces. In the presentation, I will explain the principles of identification of active sites using electrochemical scanning tunneling microscopy under reaction conditions for several reactions essential for PEMFCs and electrolysers [1-3]. The working principles are based on the analysis of the fluctuations of the tunneling current at different sites when the reaction cannot take place or is enabled due to the right electrode potential being applied. Examples will include metal, metal oxide, and non-metallic (e.g. HOPG and MoS2) electrocatalysts.
1.3-I1
Despite fossil fuels having brought forth the dawn of civilisation as we know it, they have also left an enduring effect on the Earth’s climate. To address this, it is imperative that we devise renewable technologies to decarbonise our economy. For example, one could envision using sunlight to drive (photo)electrochemical water splitting devices to produce H2, a highly energy-dense fuel when compressed and stored. A major roadblock in realizing this, however, is the lack of affordable and efficient catalysts for the oxygen evolution reaction (OER).
In this talk I will describe our recent efforts to rationalize the activity of OER electrocatalysts[1,2] by means of reaction descriptors and how to utilize this knowledge to guide the design of more efficient materials. In addition, I will discuss how this approach can be translated to molecular systems[3] and present a series of catalyst design principles to accelerate the discovery of “ideal” molecular OER catalysts via high-throughput[4] and machine learning studies.[5] Finally, I will present a new platform that we have developed to automate the generation of bottom-up molecular OER databases to boost the discovery of molecular systems which can be ultimately immobilized onto solid supports to design hybrid OER materials.
1.3-I2
Complex oxides have evolved as a major class of functional energy materials applied in a wide range of energy conversion and storage approaches which harvest the ability to precisely tailor and combine oxides on the nanoscale. Heterogeneous interfaces of oxides typically possess distinctly different material properties as compared to the bulk [1] and allow tailoring and tuning of ionic-electronic properties by intentional design of interfaces. Here, we will discuss how dedicated design and understanding of interfacial space charge phenomena can be used to tailor electronic and ionic charge transport along and across electrochemically active oxide interfaces and surfaces, with particular focus on the role of space charge at solid-liquid interfaces operating in alkaline water splitting. We will discuss materials engineering strategies that allow to overcome the limitations of ‘bulk catalyst’ owing to intrinsic scaling relations and the typically observed inverse relationship of catalyst activity and long term stability. [2] As we elaborate the choice and combination of select oxides in from of defined multilayers and superlattices can lead to superior stability compared to the parent materials. [3,4] In this way, the control of space charge and electronic structure can be used to realize hybrid catalysts that attempt to break classical relations of electrochemical activity and stability.
1.3-O1
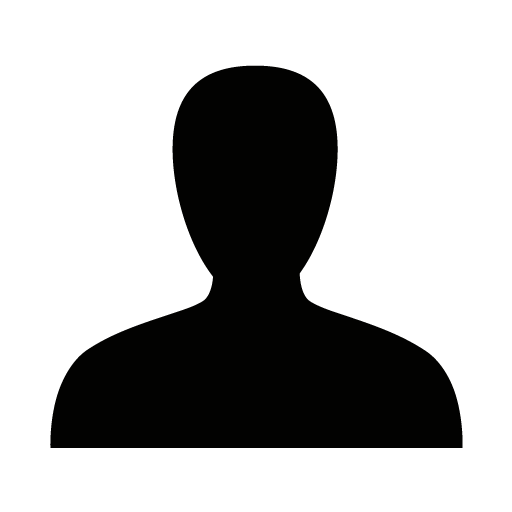
In the future energy scenario, hydrogen is seen as a promising alternative to currently used fossil fuels. Proton exchange membrane (PEM) electrolysis is recognized as the best technology for the sustainable production of hydrogen, however, its widespread utilization is jeopardized by the fact that the catalyst, used for the anodic oxygen evolution reaction, is based on scarce iridium. One of the strategies to reduce its loading in the electrolyzer is the dispersion of Ir-based nanoparticles on ceramic supports, which can withstand harsh conditions in the electrolyzer.
The newly designed materials for electrocatalytic applications, pursuing this goal, are generally first tested on the laboratory scale. The most critical parameters that reveal their viability are their activity, stability, and selectivity. The best metric, indicating the activity of the catalyst is the so-called turnover frequency (TOF), which is in reality difficult to obtain. Instead, normalization of the current by the electrochemically active surface area (ECSA) is used. Unfortunately, there are currently no methodologies for its determination in the case of supported Ir-based catalysts. A promising methodology was recently proposed by Watzele et al. [1], which suggested the use of electrochemical impedance spectroscopy (EIS) to measure the so-called adsorption capacitance of reaction intermediates, which is directly related to the active surface area. In their work, the authors showcased this methodology on thin films.
Here, we applied this methodology to a commercial catalyst Ir on Vulcan (Premetek) to test its suitability for the measurement of ECSA of supported nanostructured catalysts. We prepared electrodes with different loadings of the investigated material and performed a detailed analysis of the impedance spectra. Results showed significant discrepancies between expected and measured values of obtained parameters, which cannot be attributed solely to the experimental errors. Based on the results, we discuss possible reasons for the anomalies and confirm the main hypotheses by impedance simulations using the full physical model ‐ instead of the simplified equivalent circuit.
1.3-O2
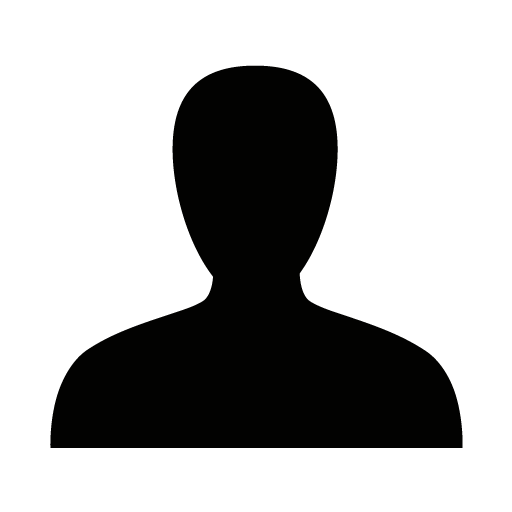
The scarcity of iridium has been the bottle neck of the large-scale implementation of proton exchange membrane (PEM) electrolysers, where high loading of iridium oxide is used to catalyse water oxidation and to sustain an integrated conductive layer. The loading of iridium at the anode can be reduced by introducing a catalyst support.1,2 However, finding materials that are stable and conductive under the oxidative and acidic conditions of the oxygen evolution reaction (OER) is extremely challenging. Most materials suffer from oxidation, and subsequent passivation and/or dissolution during the OER.3 Titanium-based materials have shown excellent corrosion-resistant properties. Titanium metal has been employed as a porous transport layer (PTL) in the PEM electrolysers, and titanium nitride is predicted to be stable in a wide range of pH.4,5 These features indicate that titanium-based materials have potential applicability as catalyst supports for iridium oxide water oxidation catalysts.
Herein, a series of titanium-based thin films (titanium oxide, titanium metal, titanium nitride and titanium-niobium alloy) were synthesised by reactive sputtering and their performance as a support for iridium oxide OER catalysts was examined by electrochemistry mass spectrometry (EC-MS) to measure the O2 evolved in real time. The dissolution of both the catalyst and the supports, i.e., iridium, titanium and niobium, were tested by an inductively coupled plasma mass spectrometry (ICP-MS). The supports’ capability of remaining conductivity was found to directly correlate with catalytic activity of iridium oxide. The activity and stability of iridium oxide was strongly dependent on the support. Therefore, through this study, we couple EC-MS and ICP-MS to rigorously benchmark the performance of the support and catalysts for the OER reaction in acidic electrolyte.
2.1-O1
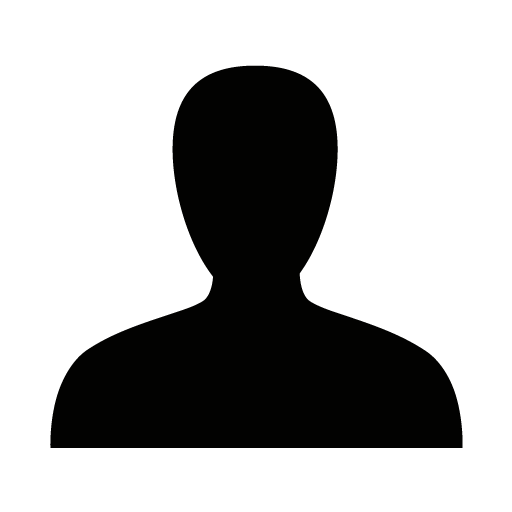
Efficient and long-lasting electrocatalysts for the oxygen reduction reaction (ORR) play a crucial role in ensuring the continuous functionality of advanced energy technologies like proton-exchange membrane fuel cells (PEMFCs). Among the elements, platinum stands out as the most effective and stable electrocatalyst for the ORR so far. However, even platinum is not entirely resistant to degradation under a hostile electrochemical environment of the cathode leading to an irreversible detrimental effect on performance during fuel cell operation. Studies of the degradation mechanisms of ORR catalysts and their underlying principles pointed out that the inhibition of Pt dissolution is crucial for suppressing their deterioration.
Targeting that aim, we investigated Pt–Au alloy model catalysts with various compositions (Pt95Au5, Pt90Au10, and Pt80Au20) prepared by magnetron sputtering. The promising stability improvement of the Pt–Au catalyst, manifested in suppressed platinum dissolution with increasing Au content, was documented over an extended potential range up to 1.5 VRHE. On the other hand, at elevated concentrations, Au showed a detrimental effect on ORR activity.
A systematic study involving multiple complementary characterization techniques such as Synchrotron Radiation Photoelectron Spectroscopy (SRPES), Energy-dispersive X-ray spectroscopy (EDX), X-Ray Diffraction (XRD), Atomic Force Microscopy (AFM), Rotation Disc Electrode (RDE), Scanning Flow Cell coupled to an Inductively Coupled Mass Spectrometer (SFC-ICP-MS), and Monte Carlo (MC) simulations based on Density Functional Theory (DFT) data enabled us to gain a comprehensive understanding of the composition–activity–stability relationship to find optimal Pt–Au alloying for maintaining the activity of monometallic platinum and improving its resistance to dissolution.
The results showed that Pt–Au alloy with 10% gold represents the most promising composition retaining the activity of monometallic Pt while suppressing Pt dissolution by 50% at the upper potential limit of 1.2 VRHE and by 20% at devastating 1.5 VRHE.
2.1-O2
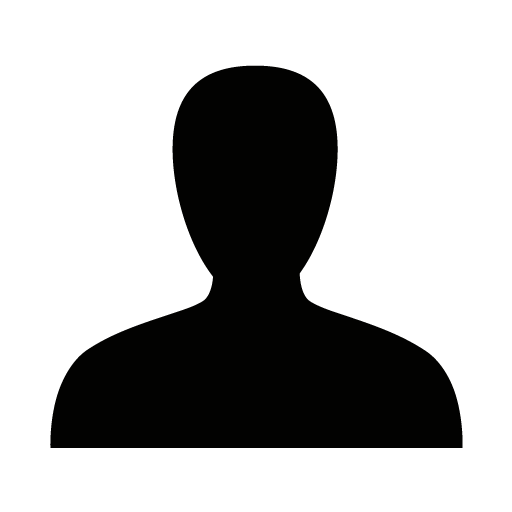
Decarbonisation is one of the main current goals of humanity. One of the most promising ways to achieve this is the establishment of the so-called hydrogen circular economy. Namely, while abundant and renewable energy from the sun and wind can be used to run the water electrolysers to produce the hydrogen, on the other hand, this hydrogen can be used to produce electricity for almost every end-use energy requirements. In particular, the conversion of hydrogen into electricity in fuel cells can be used for a wide range of applications, including light-duty and heavy-duty vehicles. However, there are still challenges that make massive adoption of these vehicles unfeasible. In addition to hydrogen fuel storage and transportation, the electrocatalyst issues are also an important obstacle to building a hydrogen-powered transportation system. Although the very high cost of platinum-based electrocatalysts can be solved by alloying platinum (Pt) with a less noble and less expensive metal (e.g. Co, Cu, Fe, Ni) thus enabling even higher oxygen reduction reaction (ORR) activity, the stability of these Pt-nanoalloy systems is still an open question, usually inappropriately addressed. Hence, a deeper insight into stability of Pt-nanoalloy electrocatalysts is essential.1,2
Here, the latest findings on the stability of the carbon supported intermetallic Pt-alloy nanoparticles (Pt-M/C, where M = Co, Cu or Ni) obtained using advanced, in-house designed methodologies such as the high-temperature accelerated degradation tests (HT-ADTs) and the high-temperature electrochemical flow cell coupled to an inductively coupled plasma mass spectrometry (HT-EFC-ICP-MS), will be presented.2,3 Whereas the former enables ADT to be performed in a liquid electrolyte half-cell by utilisation of a standard rotating disc electrode at temperatures of up to 75 °C, the latter allows for precise (ppb range) time-temperature-and-potential resolved measurements of dissolution of metals. By simulation of close-to-real operational conditions, these technologies enabled to prove that in addition to the carbon corrosion, which follows the Arrhenius law and increases exponentially with temperature,2,4 also Pt dissolution, as well as less noble metal dissolution, increases with increasing temperature.2,3 This applies not only to the operational potential window (0.6-1.0 V) where the metal dissolution is expected to be dominant but also to a wide potential window such as 0.4-1.2 V where both degradation mechanisms (carbon corrosion as well as metal dissolution) can be expected.2–4 Essentially, expanding the potential window results in an exponential growth of temperature impact on metals dissolution.2,3 Nevertheless, with an increase in temperature, not only the dissolution of Pt, but also the rate of its re-deposition (due to the Ostwald ripening) is increased at the same time, resulting in concealment of Pt dissolution.2,3,5 These previous findings about the dependence of the stability of Pt and less noble metal on the temperature and potential window3 will be upgraded with guidelines on how to control stability of Pt-alloy nanoparticles. In other words, the improvement of the stability of Pt-alloy nanoparticles via adjusting non-intrinsic (i.e. potential window) as well as intrinsic properties (i.e. electrocatalyst composition and structure) will be discussed.
2.1-O3
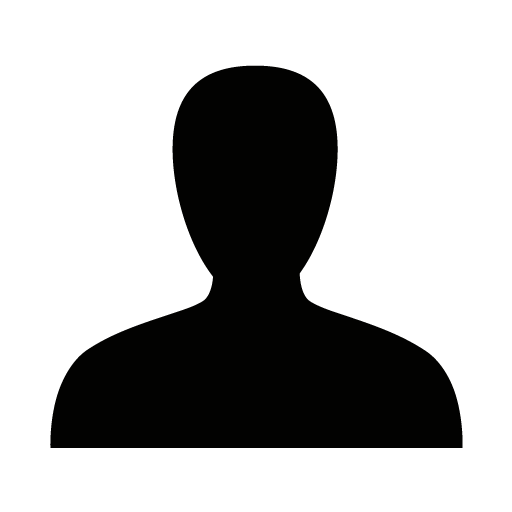
Pt/C and PtRu/C catalysts are widely used in fuel cells and electrolysers in acidic and alkaline media. Considering only anode reactions, both catalysts can be used to oxidise hydrogen or other organic fuels in fuel cells. 1-3 Looking at their application in electrolysers, on the other hand, some organic molecules can also be oxidised on the anode in an electrolyser resulting in value-added products and competitive reaction to oxygen evolution. 4, 5 This widespread use and potential of these catalysts lead to a question of long-term stability in real conditions. Therefore, in this work, we focus on the fundamental investigation of dissolution and degradation of Pt/C and PtRu/C catalysts using a rotating disc electrode and scanning flow cell coupled to ICP-MS. To cover the broad application of these catalysts, we applied different upper potential limits of CVs in 24h accelerated stress tests. The aspect of real conditions was addressed by elevated temperature in these studies. Post-Morten catalyst analysis was carried out in order to understand the catalyst degradation in described conditions. Activity decrease was also evaluated. The obtained data can be used to estimate the stability window of Pt/C and PtRu/C and minimize the degradation of these catalysts in real applications.
2.1-O4
Surface modification using nanoparticles has been widely applied to enhance catalytic activity and stability of the oxygen electrode in solid oxide electrochemical cells, whereas there is still a long way to go for preparing uniform and scalable nanoparticles. Herein, an ultrasonic spray infiltration technique was introduced to produce electrocatalytically reactive nano-sized ceria or double perovskite oxide decorated oxygen electrodes. The uniform nano-sized infiltrates prepared improved oxygen catalytic activity by increasing oxygen vacancies and enlarging reaction sites on the electrode surface. The solid oxide electrochemical cells with tailored oxygen electrodes revealed a performance enhancement during reversible operation in fuel cell and electrolysis cell modes. In addition, ultrasonic spray infiltration-based nanoparticles were applied to a large-area cell (100 cm2), which indicated increased power output and stable performance for long-term operation. Thus, this study proved a scalable and feasible method to tailor bifunctional oxygen electrodes using uniform infiltrates for solid oxide electrochemical cells.
2.1-O5
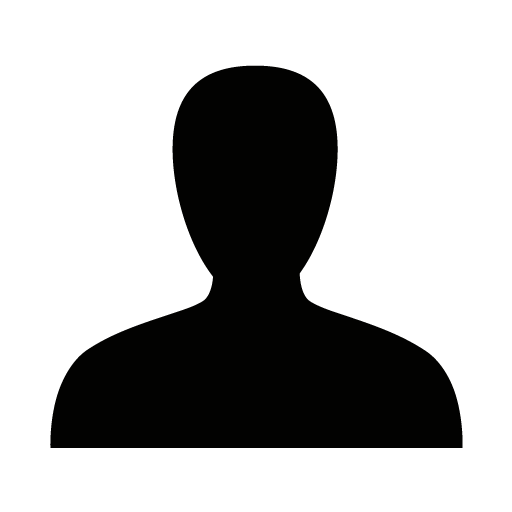
Polymer electrolyte membrane fuel cells (PEMFCs) offer a promising zero-emission energy conversion solution for various applications, including automotive. However, the widespread adoption of PEMFC technology faces challenges that must be addressed. One significant performance bottleneck is the oxygen reduction reaction (ORR), which has led to the use of Pt-alloy nanoparticles on high surface area (HSA) carbon black (CB) supports as state-of-the-art electrocatalysts. Achieving high carbon support and the catalyst layer durability remains a major challenge, particularly for heavy-duty vehicle (HDV) applications that aim to meet the ambitious U.S. Department of Energy (DoE) goal of 30,000 hours of system lifetime. Two research trends focus on improving carbon durability: (i) enhancing existing HSA CB supports and (ii) exploring alternative carbon candidates with desirable properties. Graphene derivatives (GDs) such as reduced graphene oxide (rGO), and graphene nanoribbons (GNRs) have shown potential as alternative carbon supports with improved durability against carbon corrosion.
This presentation investigates the correlation between carbon support properties of graphene derivatives (rGOs, GNRs) and carbon black in Pt-based fuel cell electrocatalysts. Nanoparticulate intermetallic-based electrocatalysts were analyzed, revealing comparable metallic components but notable variations in the carbon support. Specially designed electrochemical accelerated degradation tests (HT-ADTs) demonstrated that rGO-supported catalysts exhibited superior electrochemical durability compared to carbon black-supported counterparts. The results were further validated through direct online measurements using electrochemical cell-mass spectrometry (EC-MS). This study provides valuable insights into the relationship between carbon support properties and electrocatalyst durability, offering guidance for developing more stable carbon supports in line with the Department of Energy (DoE) objectives. The findings contribute to the advancement of fuel cell technology by enhancing the understanding of carbon support properties and their impact on electrocatalyst performance.
2.1-O6
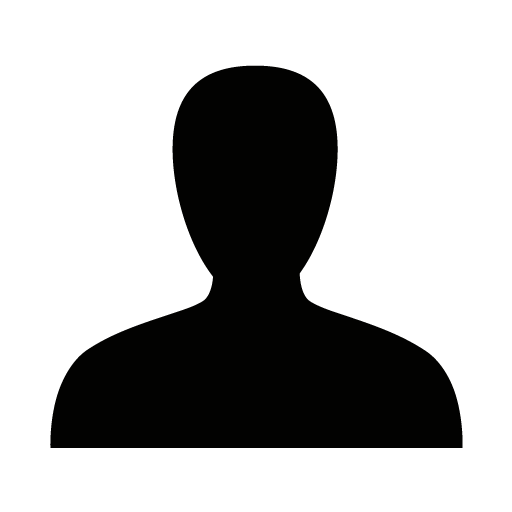
Proton exchange membrane fuel cells are a viable alternative to conventional combustion engines running on fossil fuels. Using hydrogen as fuel, they do not contribute carbon emissions during operation, and can thus make the mobility sector a lot cleaner. However, the electrocatalyst cost still poses an issue, as those advanced materials commonly contain scarce noble metals, like platinum. To catalyze the oxygen reduction reaction inside the fuel cell, supported Pt-alloy nanoparticles are now commonly used to lower the amount of Pt. However, such nanoparticle ensembles are far from uniform, and the effect of certain structural features remains unexplored.
In this contribution, I will present a recent study focusing on carbon-supported platinum-copper nanoparticles containing anti-phase boundaries. Those planar defects, contributing to chemical disorder, were previously described for a bulk alloy, but not how they manifest themselves in a nanoscale catalyst. Experimental and simulated X-ray powder diffraction was used to determine the defect placement, and in-situ high-temperature scans were used to observe their temperature-dependent evolution. Electron diffraction and atomically resolved scanning transmission electron microscopy were used to confirm the defect presence locally, and the oxygen reduction reaction performance was evaluated for several platinum-copper analogs with and without anti-phase boundaries. This study is a step towards a more detailed understanding of the structure-property relationship of nanoparticulate electrocatalysts.
2.2-I1
Investigating the water oxidation mechanism using operando spectroelectrochemistry
Efficient (photo)electrochemical oxidation of water is central to the production of energy carriers such as hydrogen. Hydrogen plays a critical role in the energy transformation to a sustainable future, due to its widespread applications as a fuel for transport, feedstock to chemical industries and a heat source for buildings and industry. Low temperature water electrolysis can enable hydrogen production renewably and at scale; however, the efficiencies of these technologies are currently limited by poor kinetics of the water oxidation reaction at the anode.
State-of-the-art catalysts such as iridium-based oxides in acidic electrolyte and nickel oxyhydroxides in alkaline electrolyte exhibit complex redox chemistry, which cannot be understood using electrochemical measurements alone. Particularly, the redox transitions on these catalysts can exhibit non-Nernstian behaviour, resulting in the redox transitions being dependent on pH. In this talk, I will demonstrate how optical spectroscopy and X-ray absorption spectroscopy can be used to identify and quantify the density of potential-dependent intermediates, the interaction between them, and the intrinsic rate of water oxidation, as a function of pH. In addition to determining the changes in the catalyst, I will also show how the nature of interfacial water can be probed using surface enhanced infrared absorption spectroscopy. Therefore, using a combination of operando spectroscopic techniques, I will elucidate the factors controlling water oxidation kinetics on these materials.
2.2-I2
Hydrogen is regarded as a future energy vector powering society needs fully from renewable sources. In this respect, proton exchange membrane hydrogen fuel cells (PEMFC) are key devices, where energy of hydrogen molecules is transformed to electricity with relatively high efficiency. Pt based catalysts, used at the cathode to speed up the oxygen reduction reaction (ORR), is one of the most expensive parts of the device and in the last two decades scientists are finding a way to limit Pt loading without compromising the performance. This effort led to deep understanding of ORR electrocatalysis and development of a new class of catalysts, where various schemes (alloying, morphology) are used to facilitate the reactions. In spite of these efforts, incorporation of these catalysts in the MEA and optimizing device operation remains a challenge, mainly because of low catalyst stability and various structural and chemical inhomogeneities. In this contribution we will assess the stability issues of bimetallic PtNi catalysts during its lifetime, from the cradle to the grave, using high energy X-rays as an universal in-situ and operando probe.
2.2-I3
Alexandr N. Simonov is a physical chemist specialising in (photo)electrochemistry and (photo)electrocatalysis. Research in his group is aimed at understanding and designing new effective ways to generate and use renewable electricity for the sustainable chemistry technologies. His major research focuses on the development of catalysts, electrode architectures and electrolytic devices for generation of hydrogen through splitting of water (including seawater), reduction of nitrogen to ammonia, as well as selective oxidation of ammonia and nitrogen to nitrates for fertiliser generation. He collaborates with Australian and German industry on several projects aiming to develop new cost-effective water electrolysers. He is a co-founder of a spin-out company Jupiter Ionics Pty Ltd. working on the commercialisation of the Monash technologies for ammonia synthesis and oxidation.
Water electrolysers (WE) with proton-conducting electrolytes, especially those based on the polymer electrolyte membranes (PEM), present many important technological advantages over the traditional alkaline-based electrolyte systems. On a negative side, efficient and practically robust operation of the PEMWEs is challenging to achieve without the use of the oxygen evolution reaction (OER) catalysts based iridium, typically introduced to the anodes at mg cm-2 levels of loading. The key limitation here is not just the very high cost of Ir, but also the availability of this very scarce metal that is mined on a scale of only few tonnes per annum.
Aiming to resolve this challenge, our work has focused on the development of iridium-free OER catalysts capable of robust operation at low pH and elevated temperatures on an extended timescale. Towards this aim, we have implemented a “catalyst-in-matrix” design concept, which is based on combining the catalytically active oxide species, e.g. cobalt or manganese, with an electrically conductive matrix that is thermodynamically stable under the target operating conditions, e.g. oxides of lead and antimony. The talk will present several examples of such systems based on various “catalyst” and “matrix” elements, with a specific focus on the durability of the materials in operation. Integration of some of the most promising catalyst, including those operating in a self-healing mode, into the anodes of the devices for the low-pH water electrolysis will be highlighted.
2.2-I4
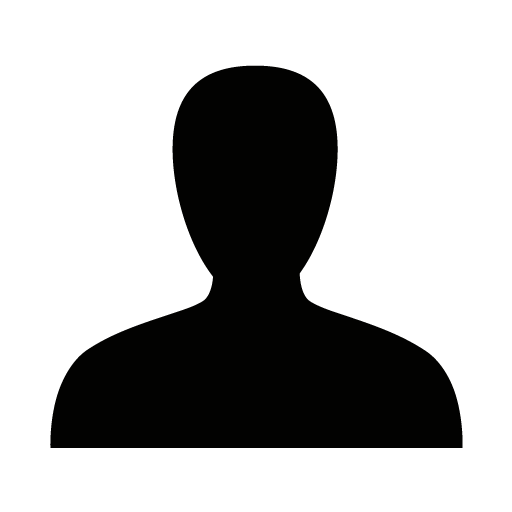
In order to enable mass production and global commercialization of automobiles powered by hydrogen fuel cells it is imperative to reduce the platinum loading from the current 25-30gPt stack, which translates to 0.315 mg Pt/cm2 cathode loading.1 The use of alloy cathode catalysts is currently considered one of the few viable paths towards decreasing the PGM content in fuel cell stacks that can get very close to the loadings used in internal combustion engines (2-8 g Pt / Veh). However, their performance and durability at high current densities (i.e. > 1.0 W/cm2) under real world driving conditions is still not sufficient.
In this presentation we will show recent strategies that will document the challenges of Pt/C and Pt-alloy/C cathodes for high power operation, via investigations of catalyst and layer design. Results will document the importance of tailoring alloy catalyst composition, surface area as well as particle location on existing commercial supports. Advanced characterization and diagnostics techniques will be presented that will determine key differences between different Pt-alloy variants in terms of performance and durability against state of the art Pt/C catalyst. Emphasis will be given to the role of dissolved metal ions during ink formulation and their impact on performance for automotive applications. These results have helped identify the mechanisms that compromise catalyst stability as well as activity and allowed the synthesis of new materials and layers that could be capable of thrifting Pt loading to levels that will help mass commercialization and advance the technology in order to reach the challenging target of 1.8 W/cm2.
1. R. Borup, K. More, A. Weber, DOE FC135: FC-PAD Fuel Cell Performance and Durability
2.3-O1
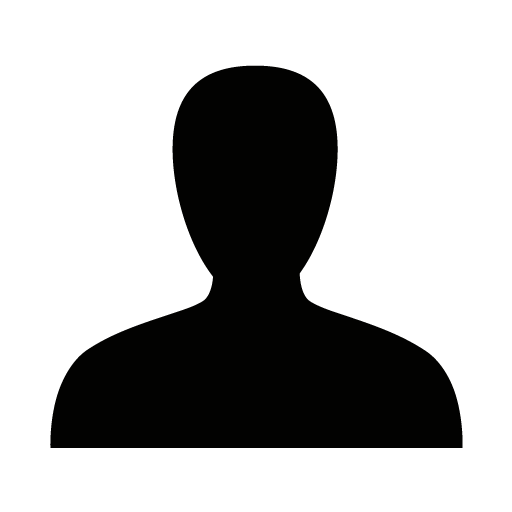
The conversion of renewable energy into valuable chemicals and fuels requires water and CO2 electrolysis. To make these technologies more accessible and affordable worldwide, we need to find catalysts that are cheap, abundant, active, and stable. The catalysts’ performance depends not only on their intrinsic properties but also on the electrolyzer’s design and the type of electrolyte used. However, in CO2 electrolysis, the catalyst may exhibit rapid degradation in a certain electrolyte because of the pH change over time due to ion movement across the membrane.1 Therefore, the anode materials should resist corrosion in a wide range of pH values and/or in electrolytes rich in carbonate. Most of the non-noble transition metals and their compounds are very stable at high pH, but they are less durable than Ir or Pt at low and (near-) neutral pH.2 Interestingly, some recent studies have shown that oxides based on Co can maintain good stability even at low pH.3, 4 However, we still do not understand how this stability works and how non-noble metal oxides catalyze the oxygen evolution reaction (OER) at low or near-neutral pH. In this work, we tried to answer some of the questions. We use a scanning flow cell (SFC) combined with inductively coupled plasma mass spectrometry (ICP-MS) and differential electrochemical mass spectrometry (DEMS) to study the stability of cobalt oxide under different electrochemical conditions at low and mild pH. With these techniques, we can monitor the dissolution of Co online and identify possible narrow stability windows before and during the OER. Moreover, going one step further toward the industrial application of such materials, we tested various Co3O4-based electrodes as anodes in CO2 electrolyzers. Based on our results, we suggest and discuss the dissolution of cobalt oxide and provide perspectives on improving its stability.
2.3-O2
Benjamin Moss is a final year PhD student working under the supervision of Professor James Durrant at Imperial College London. His interests lie in the interplay between electronic stucture and charge carrier dynamics, and their role in determining overal effciency of semiconducting devices for energy coversion. A complete list of publications can be found at: https://scholar.google.co.uk/citations?user=_OZMM_8AAAAJ&hl=en
Extracting electrons from water through the oxygen evolution reaction (OER) is a crucial element of carbon neutral fuel generation and other energy storage technologies.1–3 Large overpotential losses in the OER arise from kinetic barriers associated with multiple proton and electron transfer steps.3 Lowering this barrier is key to improving performance, however a general understanding of the fundamental factors controlling OER kinetics has not yet been reached.
Broadly, two general classes of OER mechanism link applied potential to the rate of catalysis. Electrochemical mechanisms, where the rate determining step (RDS) involves an electron leaving the catalytic intermediate and surface site to be conducted away by the electrode, and chemical mechanisms where no net electron transfer occurs during the RDS.4 In the electrochemical mechanism, the current (rate) scales exponentially with the applied potential, as electrode potential is directly correlated to activation energy; whereas in a purely chemical mechanism, voltage only drives the accumulation of catalytic intermediates.4 In the simplest case, the rate of a chemical RDS would scale with the coverage of the rate limiting intermediate to the power of its stoichiometric coefficient.4 However, more complex behaviour may also arise through interactions between adsorbates changing with coverage, leading to a dependence of activation energy on coverage.5
Beyond fundamental considerations, mechanistic understanding of the OER is challenging due to the complexity of many OER catalysts. For example, despite decades of study, the phase transitions, active oxidation states, active sites and mechanism of cobalt hydroxide/oxyhydroxide – one of the most intensely studied electrocatalysts - remain controversial.6-9 The position, degree of oxidation and chemical nature of the active sites in CoOOH remains a topic of intense debate - obscuring considerations of the fundamental mechanism of operation in this material.
Such conflicting results reflect a crucial unmet technical challenge in electrocatalysis: there does not exist a widely accessible method of simultaneously measuring populations of catalytic intermediates and proving that the measured intermediate drive current through direct measurement of OER kinetics. Herein, we demonstrate the power of spectroelectrochemical analysis built around employing a multi-channel detector to measure continuous spectral changes during the polarisation curve at all points of the polarisation curve to overcome this challenge. We use the example of cobalt oxyhydroxide (hereafter CoOOH) and cobalt-iron Prussian Blue (hereafter CoFe-PB) – another cobalt based catalyst with contrasting behaviour to CoOOH, This capacity is combined with time resolved measurement of the kinetics of implicated catalytic intermediates as they decay under open circuit conditions to verify turnover frequencies. The richness of the resulting dataset enables us to extract quantitative, operando coverages and turnover frequencies of intermediates, reconstruct the polarisation curve, and prove that the intermediates measured turn over quickly enough to support the measured current. Using this approach, we quantify the extent of energetic destabilisation of the rate limiting intermediate of both catalysts as a function of coverage. Supported by molecular level insight gained from density functional theory calculations we show that the interacting chemical mechanism contributes significantly to the current in both catalysts, despite disperate kinetics and electroadsorpative properties. In light of our conclusions, we examine the contribution this destabilisation makes to OER current as well as examine the validity of simple mechanistic interpretations of Tafel slopes.
2.3-O3
Graduate researcher specialising in the design, optimisation and characterisation of electromaterials and electrolytic cell designs within renewable energy and renewable fuel technologies. Senior PhD student (final months of candidature) within the MacFarlane and Simonov Solar Fuels team, with a core motivation towards the technoeconomic feasibility of water electrolysis for large-scale hydrogen production.
Water electrolysis remains the most promising pathway towards the sustainable provisioning of H2 at the terawatt scale. Unfortunately, a competitive hydrogen pricing against that derived from fossil fuels remains limited by the capital and ongoing expenditures of current state-of-the-art water electrolyser technologies. It is therefore necessary for future sustainable hydrogen economies that the development of noble-metal-free electromaterials be made to withstand the harsh electrolytic conditions imposed by efficient proton exchange membrane water electrolysis (PEMWE). However, the advancement of cost-effective anode catalysts for industrially relevant PEMWE has thus far been infrequent and relatively unsuccessful. Of the trivial sum of sufficiently stable modes of the oxygen evolution reaction (OER) within low-pH electrolytes, self-healing systems demonstrate a potential towards earth-abundant OER under industrially relevant PEMWE conditions. Self-healing mechanisms are defined by a quasi-equilibrated state between surface and dissolved catalytic components, whether added intentionally or corroded from the catalyst itself, and can therefore provide a perceptible means for long-lifetime electrolytic operation. From this perspective, detailing such a mode of electrocatalysis proves highly useful within a range of electrolytic applications including but not limited to water oxidation, as a large sum of electromaterials and electrochemical pathways often rely on the equilibration between surface and dissolved electroactive species. Here at Monash University, Swinburne University of Technology and Max-Planck-Institut for Chemical Energy Conversion, operando X-ray spectroscopic and voltammetric techniques have been developed in order to track a self-healing water oxidation mechanism.1-2 Using a highly stable and high performance Co-based OER catalyst within low-pH conditions,3 utilisation of in situ Co K and L3-edge X-ray absorption spectroscopy (XAS) in conjunction with electrochemical Quartz Crystal Microbalance (eQCM) and Fourier Transformed alternating current Voltammetry (FTacV) has allowed the successful tracking of the oxidative changes within the catalysts electronic structure. From a predominant Co(II) ground state to its catalytically relevant Co(III) oxidative structure under operation, the charge- and mass-transfer processes have also been compared against the redox events occurring during the self-healing OER mechanism, providing in detail, the potentials at which both the electron- and mass-transfers begin and the effect of catalytic loading on cobalt selective process. Further details of the mechanistic insights into both static and dynamic electro- and spectroelectrochemical techniques will be elaborated on through both poster submission and oral presentation.
2.3-O4
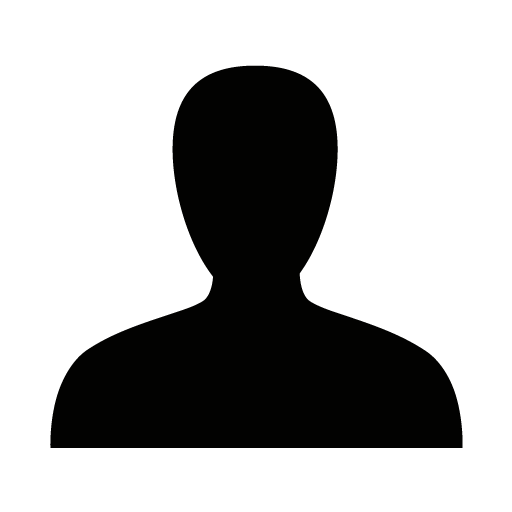
The Oxygen Reduction Reaction (ORR) serves as the fundamental reaction in electrocatalysis systems like fuel cells, metal-air batteries, and hydrogen peroxide (H2O2) electro-generation. The ORR reaction mechanism encompasses two potential pathways: a two-electron (2e-) process and a four-electron (4e-) process, which respectively result in the reduction of oxygen to H2O2 or water (H2O). While fuel cells ideally prefer a direct 4e- ORR pathway, a sequential pathway involving the exchange of 2e- can be an environmentally friendly approach for decentralized H2O2 production [1, 2]. H2O2 holds significant importance for various applications and has been recognized as one of the top 100 most significant chemicals. Current methods for H2O2 production, such as the anthraquinone process or direct synthesis, face challenges due to their complex and hazardous nature. Therefore, electrochemical H2O2 synthesis via 2e- ORR presents a more environmentally friendly, safer, and faster alternative to existing technologies. Carbon-based materials modified with heteroatoms, specifically nitrogen-doped carbon (N-C), are considered promising substitutes for expensive noble metal catalysts like Pt for ORR in fuel cells and Au for ORR to H2O2 [1, 3]. The synthesis of N-C typically involves a carbonization process performed at temperatures ranging from 500-1200°C for a duration of one to tens of hours, under various gas atmospheres such as air, nitrogen, argon, ammonia, etc. [4]. The thermal carbonization process is characterized by high energy consumption and long preparation time.
Alternatively, microwave (MW) irradiation offers a more energy-efficient and environmentally sustainable method for synthesizing porous carbon-based materials, making it economically viable as well. This study investigates the impact of both thermal and microwave heating on the properties of porous carbon derived from polyaniline. Additionally, the electrocatalytic performance of these porous carbons in alkaline media for the ORR is examined. The objective is to identify the optimal carbonization conditions during MW heating, resulting in a structure with a chemical composition that complements that obtained through conventional thermal pyrolysis methods.
Under optimal conditions of 450 W power and 140 seconds of exposure, a one-step MW synthesis yields disordered graphite structures with nitrogen and oxygen functionalities. This sample exhibits a comparable chemical structure to the polyaniline sample thermally carbonized at a temperature of 700-800°C, as confirmed by Fourier-transform infrared spectroscopy, X-ray photoelectron spectroscopy, and Raman spectroscopy. Both the MW (450 W, 140 s) and thermally (700°C, 1 min) prepared samples demonstrate catalyzed electrochemical ORR, with a selectivity of approximately 58±1% towards H2O2.
By replacing thermal carbonization with microwave-based methods, the overall synthesis time and energy consumption for porous carbon derived from polyaniline can be reduced by a factor of 71 and 93, respectively.
The results indicate that the C-450W_140s sample demonstrates comparable performance in alkaline ORR to the thermally prepared samples, highlighting the effectiveness of the MW-carbonization synthesis approach. These findings pave the way for expanding the application of MW carbonized samples in diverse fields such as electrocatalysis, supercapacitors, and zinc-air batteries, thereby complementing sustainable synthesis methods.
2.3-O5
Pyrolized iron-based catalysts are one of the most promising alternatives to platinum, for the oxygen reduction reaction (ORR) taking place at the cathode of fuel cells. However, the pyrolysis step leads to the formation of a plethora of iron sites. Characterizing these sites under reaction conditions is challenging, and thus complicates fundamental studies of the catalyst. In turn, this hinders the rational optimization of pyrolized Fe-based catalysts.
On the other hand, iron macrocycles, with their well-defined structures, offer a platform for the study of the oxygen reduction reaction on FeN4 active sites. These macrocycles exhibit one or two distinctive peaks in their cyclic voltammograms, the origin of which is still debated. There have also been reports suggesting that the position of the high-potential peak correlates with the activity of the motif, making it a powerful performance descriptor and a tool to obtain structure-property relationships of FeN4 sites.
in this study, several iron macrocycles with different ligands and active sites were investigated, using electrochemistry, operando optical spectroscopy and DFT simulations. Optical spectroscopy results (shown in the TOC for the molecule FePC) show potential-dependent spectral changes in concomitance with the CV peaks. This, with the help DFT simulations, allowed us to identify the origin of the spectral change and to propose the existence of two families of macrocycles, with drastically different ORR activity. One group is characterized by two CV peaks, high ORR activity and low peroxide yield, while the other features a single CV peak, poor catalytic performance and higher peroxide production.
This study provides a first step in understanding what controls the activity of FeN4 sites and offers a tool to unravel fundamental properties of pyrolized FeNx catalysts.
2.3-O6
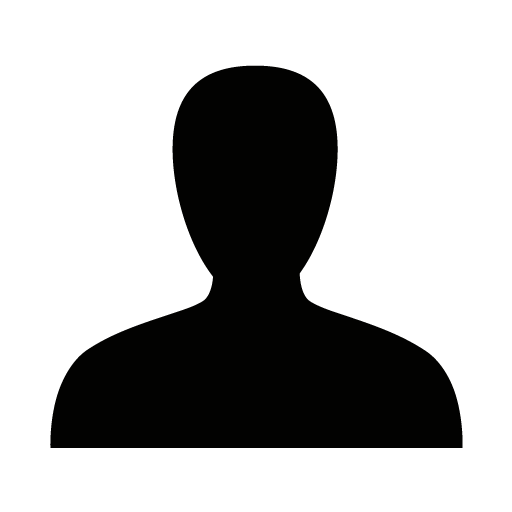
The extensive application of proton exchange membrane fuel cells (PEMFC) is conditioned by the limited supply and high cost of the Pt used in commercial PEMFC catalysts. To make PEMFC more widely available, new electrocatalysts with low Pt loading and high Pt efficiencies must be developed.
An important, but little explored aspect of this, is the interaction between the carbon support, proton conductive ionomer and catalyst. Catalyst support with optimal porosity is suggested to protect the Pt particles from direct ionomer poisoning but still provide the particles with good accessibility to the protons and oxygen.
In our project, carbon nanospheres with highly ordered mesoporous channels have been synthesised sustainably from biomass. Pt nanoparticles were deposited via polyol method onto the as synthesised mesoporous carbon sphere (MCS) and another two commercially widely used carbon substrates (porous Ketjenblack and non-porous Vulcan). Characterization of the catalysts were carried out with SEM/TEM, BET, XPS, XRD, revealing that the Pt nanoparticles are same in geometric and electronic structure while the difference of these catalysts solely arises from the different support porosity thus the different local environment of Pt NPs in the catalysts. All the Pt/C catalysts were tested under a gas diffusion half cell configuration for their oxygen reduction performance. Pt/MCS shows supreme performance where the Pt NPs sit inside the mesoporous channels of the carbon sphere.
To understand the outstanding activity of Pt/MCS, operando X-ray Absorption Spectroscopy was used to evaluate the ionomer coverage on the Pt surface by monitoring the Pt electronic structure change in the potential range of 0.1-1 V (vs RHE). Together with comprehensive nanostructure analysis via gas sorption, we proved that the oxygen reduction performance was boosted via the ideal interaction of Pt and Nafion ionomer, where direct contact in-between these two is eliminated without sacrificing the accessibility of Pt active sites. This will then guild the design strategy for future electrocatalysts development.
2.3-O7
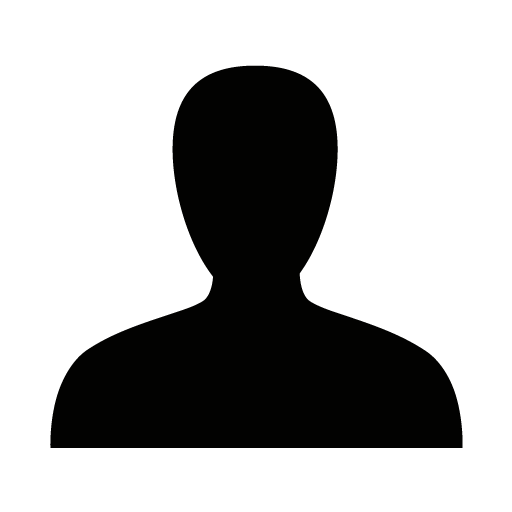
Alkali metal cation (AM+) is commonly used as counterions for anions in aqueous solutions due to its high solubility and ionic conductivity. This cation is typically situated near the electrode and constitute a key component of the electric double layer (EDL) structure, which is traditionally perceived as spectator species in electrochemical reactions. However, recent studies have shown the modulation of aqueous electrocatalysis depending on the identity and concentration of AM+. Several hypotheses have been proposed to explain the role of AM+ in electrocatalysis, while it is still under discussion. Here, we demonstrate that AM+s can directly participate on the model reaction of oxygen reduction reaction (ORR), forming chemical bonds with reactions intermediates and governing kinetics. This conclusion is corroborated by observing the NaO2 intermediate via advanced in situ spectroscopic analysis during the ORR on carbon surface. The Nernstian shift of polarization curve with varying AM+ concentrations and the significantly diminished ORR activity without free AM+, further demonstrate the pivotal role of AM+ in directly interacting with reaction intermediates in aqueous environments.
1.1-I1
Activating of molecular Nitrogen is an extremely important process as it supplies in the form of fertilizer the nitrogen that it is a prerequisite for building all amino acids and nucleic acids essential for life. After brief review of the history of activating nitrogen[1] I shall concentrate on the ammonia synthesis and motivate why an alternative route to the current commercial Haber-Bosch could be attractive Recently we found that a method published by Tsuneto et al. 25 years ago worked and proved that by simultaneous depositing Li in an N2 atmosphere it was indeed possible to activate N2 to synthesize ammonia[2]. We have subsequently followed up on this process and a very simple model for the synthesis has been proposed. Based on this insight we devised experiments that significantly improved the Faradaic and energy efficiency[3] by oscillating the potential. Further improvements have been gained by controlling the oxygen content[4] and by synthesizing of high area electrodes[5] and design of the SEI layer leading to Faradaic efficiency of ~80% and current densities towards 1A/cm2 [6]. Despite excellent recent progress there are still substantial outstanding problems. All data so far were relying on using the electrolyte as a sacrificial proton conductor. This issue was solved in a fuel cell type setup, but now we are back to 6 mA/cm2 and 60 % Faradaic efficiency on the other hand we can now talk about energy efficiencies of some 15% [7]. Further progress is under way and will be discussed
1.1-I2
Alexandr N. Simonov is a physical chemist specialising in (photo)electrochemistry and (photo)electrocatalysis. Research in his group is aimed at understanding and designing new effective ways to generate and use renewable electricity for the sustainable chemistry technologies. His major research focuses on the development of catalysts, electrode architectures and electrolytic devices for generation of hydrogen through splitting of water (including seawater), reduction of nitrogen to ammonia, as well as selective oxidation of ammonia and nitrogen to nitrates for fertiliser generation. He collaborates with Australian and German industry on several projects aiming to develop new cost-effective water electrolysers. He is a co-founder of a spin-out company Jupiter Ionics Pty Ltd. working on the commercialisation of the Monash technologies for ammonia synthesis and oxidation.
Over only few years of intense research, the lithium redox-mediated nitrogen reduction reaction (NRR) has proven to enable electrosynthesis of ammonia at practical yield rates and faradaic efficiencies approaching 100 %. Today, it remains the one and only practical approach towards sustainable electrosynthesis of ammonia. However, further significant research and development efforts are required to translate the redox-mediated NRR into a technology of applied significance, in the first place by addressing the two current major challenges: (i) insufficient stability in operation, and (ii) energy efficiency that is thermodynamically limited to approximately 33% (under standard conditions).
Both of these limitations stem from the need for the operation under strongly reductive conditions defined by the reversible potential of the Li+ + N2 / Li3N redox couple. Under unoptimised conditions, continuous and uncontrollable degradation of the electrolyte solution components results in progressive poisoning of the N2-reducing cathode surface, rapid loss in the performance, and impossibility of passing charge more than few hundred C cm-2 in typically reported experiments. Our research aims to resolve this through understanding the mechanism of operation of the NRR cathodes and design of the electrolyte solutions that prevent reductive degradation pathways while sustaining high rates and faradaic efficiencies of the ammonia production.
The talk will present some of our recent findings in this domain, in particular outcomes of the studies exploring the effects of the chemical nature of the electrolyte, proton carriers and mass-transport on the NRR performance. Feasibility of increasing the energy efficiency of the process will be briefly highlighted.
1.1-I3
Electrochemical reduction of NOx, CO2, N2, and combinations hold the promise to be a cornerstone for the sustainable production of fuels and chemicals. Importantly, all reactions share a direct competition with hydrogen, and furthermore, several products are formed from each reactant of these reactants.
For electrochemical N2 reduction to ammonia (NH3) the interest at ambient conditions is burgeoning [1-3]. Most interesting for the direct electrochemical N2 reduction in aqueous, there is no working catalyst similar to “copper” for CO2 reduction catalyst [4-6]. Instead, the electrochemical reaction is today limited to the non-aqueous lithium-mediated system.
In this talk, I will give a unified approach to these reduction reactions versus hydrogen in aqueous and discuss what it takes to reduce nitrogen electrochemically.
[1] Andersen, Čolić, Yang, Schwalbe, Nielander, McEnaney, Enemark-Rasmussen, Baker, Singh, Rohr, Statt, Blair, Mezzavilla, Kibsgaard, Vesborg, Cargnello, Bent, Jaramillo, Stephens, Nørskov, Chorkendorff, Nature, 2019, 570, 504-508.
[2] H.-L. Du, M. Chatti, R. Y. Hodgetts, P. V. Cherepanov, C. K. Nguyen, K. Matuszek, D- R. MacFarlane, A. N. Simonov. Nature, 2022, 609, 722–727
[3] M. Spry, O. Westhead, R. Tort, B. Moss, Y. Katayama, M.-M. Titirici, I. E. L. Stephens, and A. Bagger, ACS Energy Letters, 2023, 8 (2), 1230-1235
[4] Y. Hori et. al., Journal of the Chemical Society, Faraday Transactions, 1989, 85, 2309-2326.
[5] A. Bagger, W. Ju, AS Varela, P. Strasser, J. Rossmeisl., ChemPhysChem, 2017, 18, 3266–3273.
[6] A. Bagger, H. Wan, I.E.L. Stephens, J. Rossmeisl, ACS Catalysis, 2021, 11 (11), 6596-6601
1.2-I1
While the fixation of dinitrogen to ammonia at ambient conditions has undergone remarkable advances in recent years, the direct use of dinitrogen for the synthesis of other nitrogenous products, such as amines, nitriles or nitro compounds, is a challenging task. Furthemore, catalytic protocols remain unknown.
The reductive splitting of dinitrogen into transition metal nitride complexes represents a potential entry towards N2 fixation beyond ammonia. Over the past years, we have examined the mechanisms of chemically, photochemically, and electrochemically driven reductive N2 splitting by group 6 and 7 metal complexes as a path for the direct conversion of N2 into several products beyond ammonia, such as nitriles, amides and N2O. In this contribution, the reductive splitting of dinitrogen will be presented. Electro- and photochemically driven pathways are discussed that are based on detailed mechanistic studies and electronic structure/reactivity relationships of key intermediates. Synthetic strategies for selectivity control that are guided by N–H proton-coupled electron transfer thermochemistry of intermediates are presented.
1.2-I2
I am currently an Full Professor at the University of Bonn (Germany), Department of Chemistry. My overarching motivation is to discover and implement the chemistry necessary to transition to a sustainable energy-based society. Specifically, I am developing materials to convert electrical energy to fuels and chemicals.
Energy-intensive thermochemical processes within chemical manufacturing are a major contributor to global CO2 emissions. With the increasing push for sustainability, the scientific community is striving to develop renewable energy-powered electrochemical technologies in lieu of CO2-emitting fossil-fuel-driven methods. However, to fully electrify chemical manufacturing, it is imperative to expand the scope of electrosynthetic technologies, particularly through the innovation of reactions involving nitrogen-based reactants as products from water/CO2 electrolysis do not cover the full scope of industrial needs.
To this end, this talk focuses on my lab’s efforts nitrogen fixation to ammonia through the use of innovative electrochemical interfaces. I will proceed to detail new efforts in nitrogen fixation through the co-electrolysis of CO2 with N-reactants (N2, NO32-…) in generating products with C-N bonds like amides, urea that are important as commodity and fine chemicals in the chemical industry. In particular, I will discuss several new reaction pathways discovered towards C-N products and the application of operando techniques to understand the key C-N coupling steps in the reaction process.
1.2-O1
Nitrogen reduction to ammonia stands amongst the hardest processes to decarbonise, with the Haber-Bosch process dominating the market. In that regard, electrochemical ammonia synthesis can meet this unmet need, towards sustainable and decentralised production of fertilisers and carbon-neutral fuel.
So far, the lithium-mediated synthesis is the sole process to unambiguously generate ammonia from nitrogen on a solid electrode.1,2 Tremendous progress has been made in just a few years, both in terms of the underpinning fundamentals,3,4 and in terms of device engineering.5,6 However, all these improvements are burdened with the need to operate at lithium plating potential. This results in enormous overpotentials (>3.2 V) and energy losses (>70% due to lithium plating).7,8 A solution is to identify new catalytic systems with lower intrinsic overpotential, which is highly challenging.9
In this talk, I will walk us through the periodic table of elements, bringing together physical descriptors obtained from theoretical calculations and literature meta-analysis. This exploration will aim at unifying the comparison of electrochemical systems for nitrogen reduction to ammonia, and pinpoint candidate elements which I will investigate experimentally. Electrochemical testing, ex situ / operando characterisation and model experiments will be performed to explore promising catalytic systems. Learning from those, I will provide fundamental explanations to the unique features of lithium and suggest pathways to breach the bottleneck of the lithium chemistry, opening the discussion for more energy efficient and sustainable chemistries.
1.2-O2
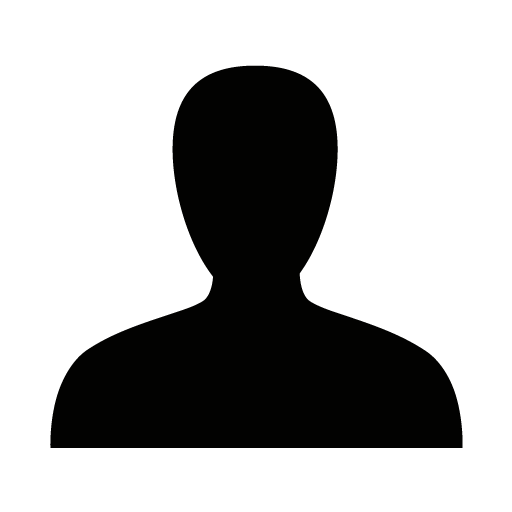
Ammonia (NH3), being the basis of all nitrogen fertilizers, constitutes one of the pillars of modern society, without which nearly half of the population would not be present on Earth.1 Furthermore, thanks to its properties (high energy content and density), it is recently emerging as a backbone fuel towards decarbonisation.2 Up-to-date, NH3 is mainly produced via the energy-intensive Haber-Bosch process and, to meet the future NH3 demand, new green synthesis techniques need to be developed.3
To this end, electrochemical nitrogen and nitrate reduction reactions (E-NRR and E-NO3RR) have received considerable attention since, among other advantages, they permit the utilization of electricity from renewables. Also, taking into account that molybdenum and sulphur play key roles in nitrogenase-based nitrogen fixation, molybdenum disulphide (MoS2) is expected to be active toward E-NRR, even if it has already demonstrated good catalytic activity toward hydrogen evolution reaction (HER, the main competitor of E-NRR).4
In this work, MoS2 has been tested for E-NRR and E-NO3RR in combination with different formulated electrolytes (LiSO4 and K2SO4 at different concentrations and pH values). A flow cell with a gas diffusion electrode, on which the catalyst is immobilized through air-brushing technique, has been used to perform all the experiments. Regarding E-NRR, encouraging results were obtained when employing Li+ as an additive in the electrolyte, leading to Faradaic efficiencies between 5 and 10%, as well as 85-301 μmol g-1 h-1 yield at -0.6 V vs. RHE. Further, since NH3 was detected not only in the cathodic site, but also in the anodic one, as well as absorbed within the cationic membrane, the interactions between the Nafion membrane and NH3 were also studied, observing that NH3 adsorption decreased when the size of the cation raised (around 60% of NH3 was absorbed when employing Li2SO4, ca. 20% when employing K2SO4).
Finally, in the case of NO3RR, design of the experiment and surface response methodology (DoE/RSM) were employed to gain further insight onto the influence of operational conditions (potential, catalyst loading and salt concentration in the electrolyte) on the Faradaic efficiency and NH3 yield of the NO3RR, as well as the possible interaction between those parameters.
1.2-O3
Prof. Kyriaki Polychronopoulou is currently a Full Professor of Mechanical Engineering at Khalifa University, and Visiting Professor at ETH-Zurich. She is also the Founding Director of the Catalysis and Separation Center (CeCaS) at KU, the first of its kind in the United Arab Emirates. CeCaS is actively supporting the vision of the UAE towards alterative fuels (hydrogen, biofuels), decarbonization though CO2 conversion to useful fuels and hydrocarbon exploitation. She is regular member of the Mohammed Bin Rashid Academy of Scientists (MBRAS, https://mbras.ae).
She holds a Ph.D. in Chemistry from the University of Cyprus (2005). Before her appointment at Khalifa University she was a Postdoctoral Fellow at Northwestern University (IL, USA) and University of Illinois at Urbana-Champaign (IL, USA). During her independent career, she has also worked as a Research Fellow in the National Physical Laboratory (UK), Texas A&M (USA), and KAIST (Korea).
Dr. Polychronopoulou's research contribution is focused on experimental and computational catalysis both from fundamental and applied perspective. She focuses her research on unlocking the reaction mechanisms and understanding of surface phenomena and their association with catalytic material microstructure. Processes of primary focus are: hydrogen (H2) production, CO2 conversion, biofuels production.
The Haber-Bosch (HB) reaction is definitely one of the most important reaction of the 20th century with tremendous impact on significant, for mankind, sectors, such as fertilizers, chemical industry, medicine, bio-fuels and ammonia production [1]. It is now well accepted that the relative activity of metallic HB catalysts can be correlated to their binding energies with N-containing species in terms of a volcano-shaped relationship. Metals that bind nitrogen too strongly or too weakly are on either side of the volcano [2]. Thus, it is important to devise a knowledge-based catalyst design that could potentially replace the Haber-Bosch method, while achieving high efficiency and energy saving towards sustainable NH3 production under ambient conditions. In this regard, herein we present two approaches for understanding the N2 acivation and conversion: (a) density functional theory (DFT) calculations are used in order to screen the N2 activation capacity and the accompanied electronic interactions for a portfolio of bimetallic alloys based on Mo, Ru and Fe metals, while using those metals as reference ones. (b) the role of defects in a new family of ceria-based oxide catalysts is explored from both experimental and computational point of view. These new type of oxide systems quickly enhance the ability to form and eliminate oxygen through redox reactions.
1.3-O1
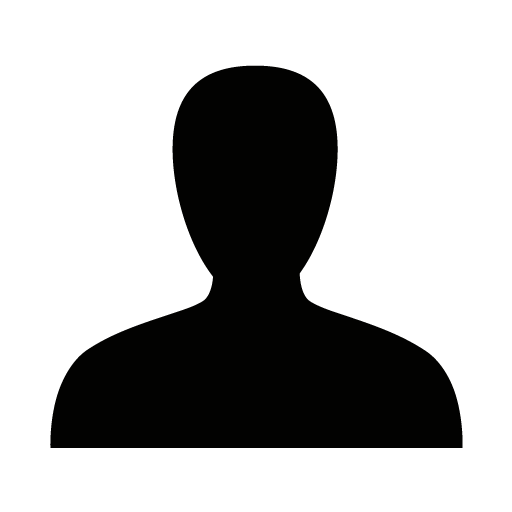
Over the last decade there has been a growing interest in generating ammonia from nitrate with the aim of reducing eutrophication and acidication of water sources that has been exacerbated by anthropogenic activities.[1] In nature, the reduction of nitrate and nitrite ions is governed by Mo- and Fe-containing enzymes as a part of the nitrogen cycle. The key steps in the reduction of nitrate to nitrite are driven by nitrate reductase (Nars) enzymes involving the parent Mo(VI) oxo active site.[2] Subsequently, reduction of the nitrite to ammonium can be catalyzed by cytochrome c nitrite reductase (NrfA) enzymes, which features an Fe heme active site.[3] Inspired by this process, in the present work we introduce an Fe-substituted, two-dimensional molybdenum carbide of the MXene family (Mo2CTx:Fe, where Tx = O, OH, or F surface termination groups) for the electrochemical reduction of nitrate to ammonia.
Recent studies have demonstrated that MXenes efficiently enable various electrocatalytic reactions due to a high degree of control over the structure of their surface sites.[4] In this case, Mo2CTx:Fe contains isolated Fe sites in Mo positions of the host MXene (Mo2CTx), and as discussed above, the combination of these metals is known to facilitate the nitrate reduction reaction in naturally occurring reductase enzymes. Mo2CTx:Fe is shown here to outperform monometallic Mo2CTx for the electrochemical reduction of nitrate in both acidic and neutral electrolytes. Close examination of the electronic and local structure via operando X-ray absorption spectroscopy (Mo K-edge) reveals that substitution of Mo with Fe in the Mo2CTx lattice facilitates the defunctionalization of surface Tx groups upon reduction. This is shown to generate Tx vacancies, which then bind nitrate ions that subsequently fill the vacancies with O* via oxygen transfer. In accordance with experimental observations, density functional theory calculations provide further evidence that Fe sites promote the formation of Tx vacancies, which are thus identified as active sites and function in NO3RR in a close analogy to the mechanism of the natural Mo-based nitrate reductase enzymes.
1.3-O2
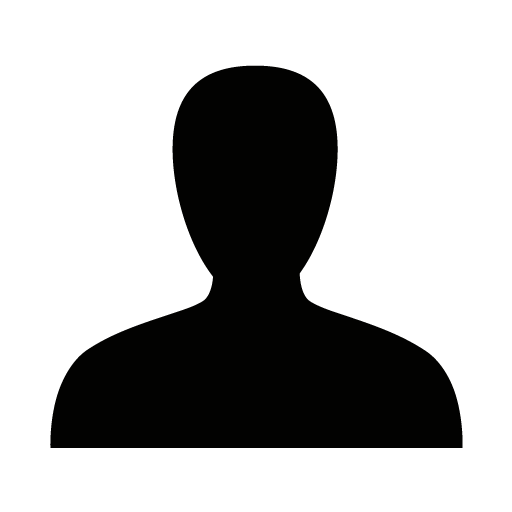
The intermittent availability of renewable energy poses a significant challenge that must be overcome to fully harness its potential. One promising solution is the storage of excess renewable energy in the form of carbon-free chemical fuels like hydrogen (H2) and ammonia (NH3). These energy-dense alternatives provide the means to transport renewable energy independently, without relying on traditional power grids. However, the current production of NH3 primarily relies on the costly Haber-Bosch process, limiting its use as a chemical fuel. To address this limitation, there is a need for a sustainable production method, such as the electrochemical reduction of nitrogen gas (N2) into NH3. Among various electrochemical processes, the lithium-mediated nitrogen reduction reaction (Li-NRR) currently stands out as the sole method capable of achieving this conversion.1
At Monash University, we have made significant advancements in Li-NRR, achieving close to 100% faradaic efficiency for NH3 selectivity.2,3 However, our focus now lies on reducing the energy demands and enhancing the overall sustainability of the Li-NRR process. This calls for the intelligent design of catalysts and electrolytes, which can be facilitated by expanding our knowledge of the solid electrolyte interface (SEI) which forms atop the reactive lithium deposit. Similar SEI layers have been extensively demonstrated to play a critical role in enhancing cell efficiency and lifespan in lithium-ion batteries. However, in the context of the lithium-mediated nitrogen reduction reaction (Li-NRR) system, the SEI may also hold the key to achieving NH3 selectivity and preventing the undesired hydrogen evolution reaction.
This presentation covers the preliminary efforts of our ongoing work using in situ neutron reflectometry to gain insight into the Li-NRR mechanism occurring at the electrode interface and characterize the SEI environment that contributes to achieving exceptional faradaic efficiency.
Using NR, we have been able to observe dynamic surface changes in the scattering length density (SLD) profile of the catalytic deposit during Li-NRR, operating at a modest 10% faradaic efficiency, limited by the experimental constraints of the NR cell. To further deepen our understanding, we are utilizing operando galvanostatic electrochemical impedance spectroscopy to establish correlations between these surface changes and the electrochemical response of the cell. This multidimensional approach allows us to unlock crucial insights into the intricate processes taking place at the electrode interface, paving the way for more efficient and sustainable ammonia production through Li-mediated electrochemical reduction of nitrogen gas.
1.3-O3
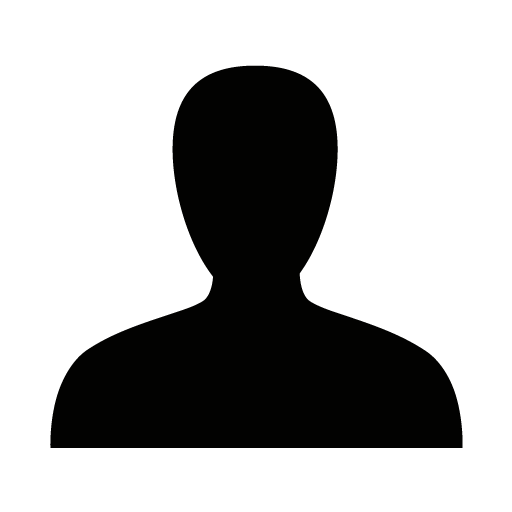
With 170 MT produced in 2020, ammonia is one of the most produced chemicals in the world [1]. However, it is currently produced using the Haber-Bosch process which contributes >1 % of global CO2 emissions. Continuous electrochemical nitrogen Reduction Reaction may not be able to completely replace the Haber-Bosch process but it has numerous advantages which may secure its place in green ammonia production. Continuous electrochemical nitrogen reduction reaction can be done at ambient pressures and temperatures, run directly from (renewable) electricity and enables ammonia production in smaller decentralised facilities. However, research into this field is still relatively new, performance is unoptimised and many important scientific questions remain unanswered. In particular, efficiency and current density are too low for commercial application and the key mechanisms by which the reaction occurs are still unknown. Recently, there have been significant advances which have focused on electrolyte optimisation [2-3] and cell design [4].
Optimising performance through the use of dynamic operating conditions is attractive because it can be done at little extra cost while achieving substantial improvements. Dynamic operating conditions have already been demonstrated to increase activity and selectivity towards desired products in the electrochemical CO2 Reduction Reaction [5-6] and increase electrode stability in electrochemical [7].
In this work, dynamic operating conditions are investigated in the continuous electrochemical nitrogen reduction system using Electrochemistry-Mass Spectrometry, a technique which allows subsecond detection of gases and volatile species in real-time. This work builds on Krempl et al.’s work by improving detection times reducing noise which allows dynamic conditions to be investigated [8]. By monitoring H2 evolution (a key parasitic product) at different voltages, the point at which it is minimised was found. However, operating at this new optimal led to the accumulation of a reaction intermediate (lithium) which was not reacting quickly enough to form ammonia. Dynamic operating conditions were utilised to alternate between this H2 evolution minimum and an ammonia forming maximum. With this combination, ammonia selectivity was found to increase substantially from 7.9 % to 18 %.
1.3-O4
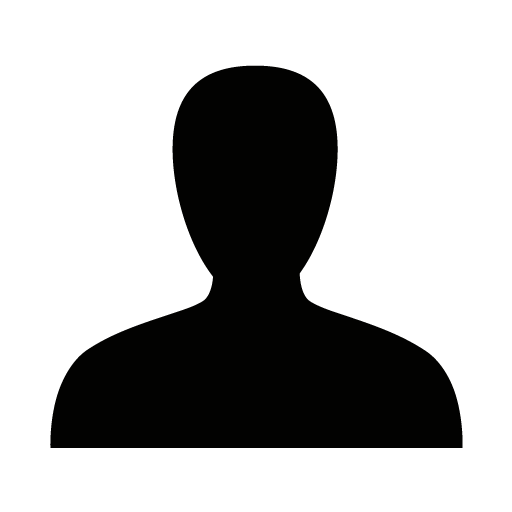
Lithium-mediated nitrogen reduction reaction (Li-NRR) is recognised as a high rate and selectivity pathway for green ammonia synthesis.[1] However, practical implementation requires further advancements in yield rate and stability.[1-3] Our previous study established a stable phosphonium cation/ylide proton shuttling cycle in a LiBF4 electrolyte, achieving a faradaic efficiency (FE) of 69 ± 1%.[4] Recently, the phosphonium-based proton shuttle in our robust high-concentration LiNTf2 electrolyte outperformed LiBF4, with doubled yield rate and improved FE of 75 ± 4%.[5],[6] Despite the performance of the ethanol proton shuttle is still better, which is near 100 % FE and ca. 0.5 μmol s-1 cm-2 yield rate,[5] the phosphonium cation is beneficial over the ethanol for no side products through tetrahydrofuranylation.[6] This motivated us to deeply investigate the paramteres affecting the phosphonium cation shuttle’s performance in the high-concentration LiNTf2 electrolyte.
Herein, we explored the relationship between the mass-transport characteristics of the electrolyte solution and the Li-NRR performance with three smaller relatives of the originally studied phosphonium cation, [P6,6,6,14]+, specifically [P1,2,2,2]+, [P1,4,4,4]+, [P4,4,4,8]+ where the subscripted numbers represent the number of carbons in each of the four alkyl chains. An increase in the phosphonium alkyl chain length results in a noticeable decrease in the ionic conductivity and increase in the viscosity of the electrolyte solution. Furthermore, the diffusion coefficient of phosphonium cations measured by pulsed field gradient NMR techniques decreases as the alkyl chain length increases, with [P6,6,6,14]+ having half the diffusion coefficient (0.93 × 10-12 m2 s-1) of [P1,2,2,2]+. The Li+ diffusion coefficient follows a similar, but less pronounced trend as it is moderately reduced from 0.74 × 10-12 m2 s-1 in the [P1,2,2,2]+ containing solution to 0.67 × 10-12 m2 s-1 when using [P6,6,6,14]+ shuttle. Interestingly, the type of phosphonium cations has minimal impact on the NTf2- diffusion coefficient. Based on these transport behaviours, one might expect that the longer phosphonium alkyl chain would adversely affect the Li-NRR performance because of the lower lithium diffusion. However, contrary to this expectation, the ammonia yield rate and the FE of the process increases steadly in the order [P1,2,2,2]+ < [P1,4,4,4]+ < [P4,4,4,8]+ < [P6,6,6,14]+. This suggests that the size of phosphonium cation directly affects a delicate balance of transport rates of the Li+, the proton shuttle and the N2 transport at the electrolyte|electrode interface. Moreover, differential capacitance analysis demonstrated that the longer phosphonium alkyl chain, the more stable and compact ionic layer on the electrode surface. This well-structured ionic assembly acts as a protective layer that defines the structure of the solid electrolyte interphase and controls the Li+ electroreduction.
Optimising the Li-NRR performance with the best-performing [P6,6,6,14]+ shuttle requires a decrease in the LiNTf2 concentration from 2 to 1.5 M, which leads to an increase in the Li+ diffusion coefficient equal to that of the [P1,2,2,2]+ system. Under this condition, the process is remarkably stable over 68 h with an invariable 80 ± 1% FE and an average yield rate of 300 ± 10 μmol s-1 cm-2.
Our study emphasises the complexity of the Li-NRR relationship to the mass-transport processes through correlations with bulk electrolyte properties, and to the ordering of the ionic species at the electrode surface. To achieve a 100 % FE target with the robust phosphonium cation proton shuttles, future investigations will utilise hydrodynamic and computational modeling techniques.
1.3-O5
Electrocatalytic NOx reduction is a promising way to help restore the natural balance of the nitrogen cycle [1]. Various works agree that nitrate reduction to NH4+/NH3 involves NO as an intermediate, and NO hydrogenation is the potential-limiting step of NO reduction. However, debates on whether *NO hydrogenation produces *NHO or *NOH hamper catalyst optimization and rational design for NOx electroreduction [2,3].
In this contribution, I will provide a simple classification of late transition metals for *NO hydrogenation: Cu, Ag, and Au (group 11 of the periodic table) primarily yield *NHO; Ni, Pd, and Pt (group 10) prefer to form *NOH or a combination of *NOH and *NHO. In turn, Co, Rh, and Ir (group 9) predominantly produced *NHO, particularly on adsorption sites with undercoordinated and/or square symmetry. Additionally, Cu and other group 11 elements prove very active for *NO hydrogenation and NO reduction to NH4+/NH3, while the (100) terraces of various transition metals should display reasonable activities. Statistical analysis enabled qualitative and quantitative conclusions through the use of “catalytic matrices”. These matrices identify that active catalysts for *NO electroreduction statistically favor *NHO over *NOH and possess undercoordinated sites [4].
To conclude, the matrices help uncover that highly active materials for NO reduction should typically contain undercoordinated Cu sites and be mediated by *NHO. The power of the matrices lies in their ability to rapidly extract information and use it to identify active sites with enhanced NOx electroreduction.
1.1-I1
Peter Strasser is the chaired professor of �Electrochemistry for energy conversion and storage� at the Chemical Engineering Division of the Department of Chemistry at the Technical University of Berlin. Prior to his appointment, he was Professor at the Department of Chemical and Biomolecular Engineering at the University of Houston. Before moving to Houston, Prof. Strasser served as Senior Member of staff at Symyx Technologies, Inc., Santa Clara, USA. In 1999, Prof. Strasser earned his doctoral degree in Physical Chemistry and Electrochemistry from the �Fritz-Haber-Institute� of the Max-Planck-Society, Berlin, Germany, under the direction of the 2007 Chemistry Nobel Laureate, Professor Gerhard Ertl. In the same year, he was awarded the �Otto-Hahn Research Medal� by the Max-Planck Society. In 1996, Dr. Strasser was visiting scientist with Sony Central Research, Yokohama, Japan. He studied chemistry at Stanford University, the University of Tuebingen, and the University of Pisa, Italy. Professor Strasser is interested in the fundamental Materials Science and Catalysis of electrified liquid solid interfaces, in particular for renewable energy conversion, energy storage, production of fuels and chemicals.
The rising share of renewable electricity is testament to the increasing importance of solar/wind-electric routes to harvest sun light in form of potential differences and free electrons. While some electricity is used directly or stored capacitively, an increasing portion calls for direct conversion into valuable molecular solar fuels or chemicals. This “dark” e-conversion is made possible by heterogeneous electrocatalysis on the surface of solid electrodes coupled to mass and charge transport processes. Sustainable materials synthesis pathways coupled to novel advanced characterization techniques result in a deeper understanding of the origin of reaction kinetic barriers and the origin of transport limitations. This is critically needed for the design of more efficient, electrochemical materials, interfaces, and electrodes for practical electrolytic devices for the production of e-fuels and e-chemicals.
In this presentation, I will report on recent advances in our design and understanding of electrocatalytic materials, interfaces and electrodes relevant to the electrochemical conversion and valorization of CO2 into value-added molecular compounds. Focus will be placed on CO and ethylene as key target e-compounds in conventional liquid cells and Gas Diffusion Electrodes.
1.1-I2
Many industrial chemical processes involve a high-energy demand (often still derived from fossil fuels), Urea is an important chemical for the agricultural industry, which accounts for 70% of the global nitrogen-containing fertilizer usage. Currently, urea is produced by the reaction of liquid NH3 and CO2[1] in a process known as the Haber-Meiser process. Despite its importance, this process suffers from high energy demand and CO2 emissions, mainly due to the NH3 synthesis step (Haber- Bosch process), which relies on fossil fuel resources. Making urea with electrochemical methods has recently gained interest from the scientific community as this approach provides a way to reduce the high CO2 emissions currently associated with urea production. Among the various nitrogen sources being explored for urea electrosynthesis, nitrate (NO3-) is an attractive one because of its high solubility in water, low dissociation energy and potential to mitigate NO3− contaminations in water. Even though the mechanism of urea synthesis via CO2 and NO3- coupling is still controversial and not broadly studied, most reports implicate CO* and NH2* surface intermediates in urea synthesis.[2] Cu is an attractive electrocatalyst as it can reduce CO2 to CO and further products and it is also an active catalyst for the reduction of nitrate to ammonia Previous reports on Cu and Cu-based materials showed that urea can be obtained from NO2-.[3,4] Early research by Shibata et al. with various catalysts demonstrated that the highest faradaic efficiency towards urea from NO3- was achieved with a Zn catalyst.[5] In this presentation, I will show our latest results on the development of Cu bimetallic catalysts (CuZn and CuRh) for urea electrosysnthesis from CO2 and NO3-.
References:
[1] P. N. Cheremisinoff, Waste Minimization Cost Reduct. Process Ind. 1995, 222–284.
[2] X. Liu, Y. Jiao, Y. Zheng, M. Jaroniec, S. Z. Qiao, Nat. Commun. 2022, 13, 1–9.
[3] S. Liu, S. Yin, Z. Wang, Y. Xu, X. Li, L. Wang, H. Wang, Cell Reports Phys. Sci. 2022, 3, 100869.
[4] N. Cao, Y. Quan, A. Guan, C. Yang, Y. Ji, L. Zhang, G. Zheng, J. Colloid Interface Sci. 2020, 577, 109–114.
[5] M. Shibata, K. Yoshida, N. Furuya, J. Electrochem. Soc. 1998, 145, 595–600.
1.1-I3
Carbon dioxide (CO2) electrolysis to produce hydrocarbons and oxygenates using copper (Cu) based catalysts has attracted substantial interest due to the direct production of versatile C2+ feedstocks . Inside the reactor, however, CO2 electrolyzers produce C2+ compounds occur via a two-step tandem CO2 to CO and CO to C2+ steps. Such knowledge has been utilized in catalyst and cathode-to-anode reactor design, but sparingly in the in-plane design of the system. Here we use the knowledge that CO2 reduction on copper is primarily a tandem reaction, and through modulation of the reactor flow rate achieve C2+ selectivity 84% at CO2 utilizations of 31%, exceeding theoretical CO2 utilization efficiencies of 25% for C2+ products. We show that higher utilizations are possible when a subset of the reactor performing only CO reduction, instead of CO2 reduction, preventing excess CO2 conversion to carbonates. Through use of varied flow field (serpentine, parallel, interdigitated) and pure CO-fed electrolysis, we link these our results to CO residence time. Notably we find that while ethylene production is constant with flow rate (~40%), oxygenates increase substantially at lower flow rates, reaching 45% at 10 SCCM. Finally, we posit that researchers should switch to combined ethylene + ethanol selectivity as a qualifying metric due to the ease of dehydrating ethanol to form ethylene and a demonstrated inability to fully control ethylene:oxygenate branching pathways. Efforts should then shift to the removal of ethanol from membrane electrode assembly systems and downstream recovery through existing commercial processes.
1.1-O1
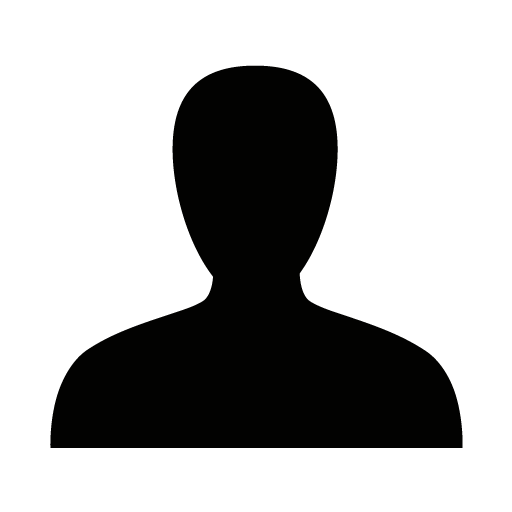
The synthetic methane from hydrogen derived from renewable energy and captured CO2, so-called e-methane, is one of the promising ways to decarbonize gaseous fuel necessary for realizing carbon neutrality. The e-methane can utilize existing city gas infrastructure, and is particularly effective for high-temperature heat demand of 200°C or higher, which is difficult to replace with electricity. To meet the above-mentioned requirement, Tokyo Gas has started developing electrochemical CO2 reduction technology using a polymer electrolyte membrane to synthesize e-methane with Osaka University under the “Green Innovation Fund project” in Japan.
Since the CO2 electrochemical reduction yields chemical species such as CO, ethylene, formic acid, and hydrogen in addition to methane, one of the most important technical challenges is improving methane selectivity.
To solve this challenge and optimize the cell structure, the copper-based catalysts, known to have a potential for methane synthesis, have been designed and evaluated. The progress of catalyst and cell development for improved methane selectivity will be discussed.
2.1-I1
In this talk, I will summarize various results on CO electroreduction to the C2 species ethylene, ethanol and acetaldehyde on Cu-based catalysts.
I will start presenting a pathway for CO electroreduction to ethylene, ethanol and acetaldehyde [1]. An early intermediate in this pathway can be spectroscopically detected, and DFT helps elucidate its structure [2].
Moreover, the pathway predicts that the late stages of ethanol production correspond to the reduction of acetaldehyde [3], while the late stages of ethylene production coincide with the reduction of ethylene oxide [4]. Because these two reactions have different structural sensitivity, one can elucidate the structure of ethanol-producing sites on the rough surface of oxide-derived Cu [5].
Finally, previous work postulated that a *CHO dimer is part of the CO reduction pathway to ethanol [6]. We put that idea to the test by electroreducing glyoxal and determined that the *CHO dimer is unlikely to be a key intermediate of that pathway [7].
Time permitting, I will show that Ag-Cu tandem catalysts open an alternative, ethanol-selective pathway by virtue of their large surface coverage of *CO [8, 9], the structure sensitivity of which can be capitalized on to design active catalysts [10].
2.1-I2
A multi-scale first-principles reaction-transport model is derived for the electrochemical reduction of CO2 to fuels and chemicals on polycrystalline copper electrodes. The model utilizes a continuous stirred-tank reactor (CSTR) approximation that captures the relative timescales for mesoscale stochastic processes at the electrode/electrolyte interface
that determine product selectivity. The model is built starting from a large experimental dataset obtained under a broad range of well-defined transport regimes in a gastight rotating cylinder electrode cell. Product distributions under different conditions of transport, applied potential, bulk electrolyte concentration, temperature and catalyst porosity are rationalized by introducing dimensionless numbers that reduce complexity and capture relative time scales for mesoscopic and microscopic dynamics of electrocatalytic reactions on copper electrodes of any porosity. This work demonstrates that one CO2 reduction mechanism can explain differences in selectivity reported for copper-based electrocatalysts when mass transport, concentration polarization effects, and primary and secondary current distributions are taken into account. The reaction-transport model presented in this talk should enable the rational design of CO2 electrolyzers.
2.1-I3
Prof. Beatriz Roldán Cuenya is currently Director of the Interface Science Department at the Fritz Haber Institute of the Max Planck Society in Berlin (Germany). She is an Honorary Professor at the Technische Universität Berlin, at the Freie Universität Berlin, and at the Ruhr-University Bochum, all in Germany. Also, she serves as a Distinguished Research Professor at the University of Central Florida (USA).
Prof. Roldán Cuenya began her academic career by completing her M.S./B.S. in Physics with a minor in Materials Science at the University of Oviedo, Spain in 1998. Afterwards she moved to Germany and obtained her Ph.D. from the Department of Physics of the University of Duisburg-Essen with summa cum laude in 2001. Subsequently, she carried out her postdoctoral research in the Department of Chemical Engineering at the University of California Santa Barbara (USA) until 2003.
In 2004, she joined the Department of Physics at the University of Central Florida (UCF) as Assistant Professor where she moved through the ranks to become a full professor in 2012. In 2013 Prof. Roldan Cuenya, moved to Germany to become Chair Professor of Solid State Physics in the Department of Physics at Ruhr-University Bochum until 2017.
During her academic career, Prof. Roldan Cuenya received an Early CAREER Award from the US National Science Foundation (2005) and the international Peter Mark Memorial award from the American Vacuum Society (2009). In 2016 she became Fellow of the Max Planck Society in Germany and also received the prestigious Consolidator Award from the European Research Council. In 2020, she became a member of the Academia Europaea (Academy of Europe). She received the AVS Fellow Award (2021), the International Society of Electrochemistry-Elsevier Prize for Experimental Electrochemistry (2021), the 2022 Paul H. Emmet Award of the North American Catalysis Society, and the Röntgen Medal of the City of Remscheid (2022).
Prof. Roldan Cuenya is the author of more than 200 peer-reviewed publications, 6 book chapters, and 6 patents. She has given 245 invited talks, with 13 plenary talks and 33 keynote lectures since 2017. Her H-factor is 74 (Google Scholar) and her work has received over 21,500 citations.
She presently serves on the editorial advisory boards of the Journal of Catalysis and Chemical Reviews. In addition, she also contributes to a number of advisory committees, including the Liquid Sunlight Alliance (USA), the Advanced Research Center Chemical Building Blocks Consortium (Utrecht, the Netherlands), the Spanish Synchrotron Facility ALBA (Barcelona, Spain), the German Synchrotron DESY (Hamburg, Germany), the Helmholtz-Zentrum Berlin for the strategic development of BESSY II (Berlin, Germany), the Institute of Chemical Research of Catalonia (ICIQ in Tarragona, Spain), the UK Catalysis Hub and the Ertl Center for Electrochemistry & Catalysis (South Korea).
Prof. Roldan Cuenya’s research program explores physical and chemical properties of nanostructures, with emphasis on advancements in nanocatalysis based on operando microscopic and spectroscopic characterization.
Climate change concerns have spurred a growing interest in developing environmentally friendly technologies for energy generation, and the electrochemical reduction of CO2 (CO2RR) into value-added chemicals offers a possibility to store renewable energy into chemical bonds. It is therefore of particular interest to develop efficient, selective and durable electrocatalysts. The latter requires fundamental understanding of their structure and surface composition under reaction conditions.
This talk will illustrate how of a multi-technique in-situ/operando experimental approach is able provide in depth mechanistic insights into CO2RR. A synergistic combination of LC-TEM, EC-AFM, NAP-XPS, XAS, XRD, GC/MS, and Raman Spectroscopy, coupled with machine learning-based data analysis, has been employed to investigate the evolution of the structure/composition of mono (Cu2O) and bimetallic (ZnO@Cu2O) cubic nanoparticles and single atom (M-N-C, with M=Fe, Sn, Cu, Co, Ni, Zn) CO2RR electrocatalysts under reaction conditions.
A main aspect that I will discuss is the use of periodic potential pulses to alter the product selectivity toward desired high order hydrocarbons and alcohols. By screening a variety of product-steering pulse-length conditions for pre-reduced Cu2O nanocubes, we identified a critical role of the formation of Cu-Oad or CuOx/(OH)y species as well as the importance of having an optimal OHad versus COad catalyst surface coverage during CO2RR. For the ZnO@Cu2O system, we have further unveiled the role of the dynamic interplay between Zn Oxide, CuZn alloy, and metallic Zn formation, the evolution of Cu crystallites, and the adsorption behavior of CO and OH species. These findings pave the road toward improved catalyst design for non-conventional dynamic CO2RR reaction conditions.
In addition, metal-nitrogen-doped carbons (M-N-C) will be discussed as emerging cost-effective CO2RR catalysts, with emphasis in the study of their stability during operation. Here operando XAS data revealed drastic differences in the structural evolution of the different M-N-C materials, including reversibly formation of metallic clusters for some of the M-N-Cs studied that can be used to rationalize their distinct electrocatalytic performance and durability.
Finally, our studies are expected to open up new routes for the reutilization of CO2 through its direct conversion into industrially valuable chemicals and fuels.
2.2-O1
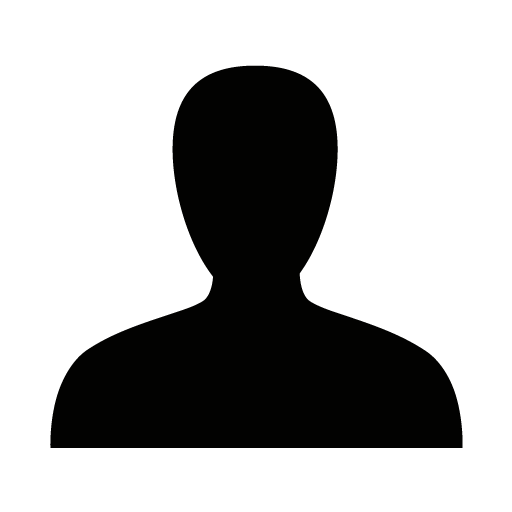
Gas diffusion electrodes (GDEs) improve the performance of CO2 reduction by enhancing the gaseous species transport. GDEs also increase the accessibility of the catalyst and the reaction surface area. A membrane electrode assembly (MEA) electrolyzer is advantageous due to its ease of assembly and low ohmic loss. However, the impact of operational conditions on cell performance and the local variations of mass and charge transfer in the GDE MEA electrolyzer is not well understood, and studies typically focus on individual components rather the complete cell. This study aims to investigate CO2 electrolysis in a GDE MEA configuration using a porous Ni-based single-site catalyst through a combined computational and experimental approach.
The approach followed here was: i) we developed a model based on the assembly structure and geometry of the MEA cell to represent the experimental setup; ii) we extracted morphological information of the catalyst layer and the diffusion layer by pore-level investigations utilizing the exact mesostructured obtained from tomography, as well as kinetics parameters extracted by a boundary layer model that was fit to the H-cell experiments with the same catalytic material as the one used in the MEA cell; iii) we fed these parameters into the MEA cell model that accounts for the three relevant phases (gas phase for reactant/product transport, membrane phase for ion transport, and solid phase for electron transport) and predicted the cell performance; and iv) we compared the predicted results with the experimental data obtained with the MEA cell to validate our model.
The computational model of the GDE MEA cell was able to predict the behavior of the experiments with less than 15% error. The computed potential was about 0.2 V to 0.4 V lower than the potential in the experiment at the same current, mainly attributed to contact resistances in the system. The model provides local distributions of gaseous species and current density at the catalyst layer and diffusion layer interface. At 15 sccm flow rate, the total current density decreased along the gas flow direction and reached 100 mA/cm2 at 50% downstream of the channel. CO2 concentration depleted after 50% of the channel length. To operate at more negative cell potentials, it was necessary to increase the flow rate or reduce the gas channel length to avoid the competing hydrogen evolution reaction becoming the dominant reaction. For a cell running at 15 sccm, the conversion was approaching 99% at a cell potential of -2.7 V. This was also near the point (-2.6 V) where maximum Faradaic efficiency was reached. Beyond this potential, the additional charge will not contribute to CO evolution but rather hydrogen evolution, because of the depletion of CO2.
This work offers a practical methodology to provide first insights into the performance-significant design and operational choices of GDE-MEA CO2 reduction devices. Differences between the model and the experiment are attributed to: i) Competitive adsorption between reactant CO2 and H2O on catalytic sites in the experiment; ii) bypass of flow along the GDE, especially at high flow rate, and iii) contact resistance induced potential drops in the cell in between each component (cathode, membrane, anode, current collector, etc). Future work will account for these factors.
2.2-O2
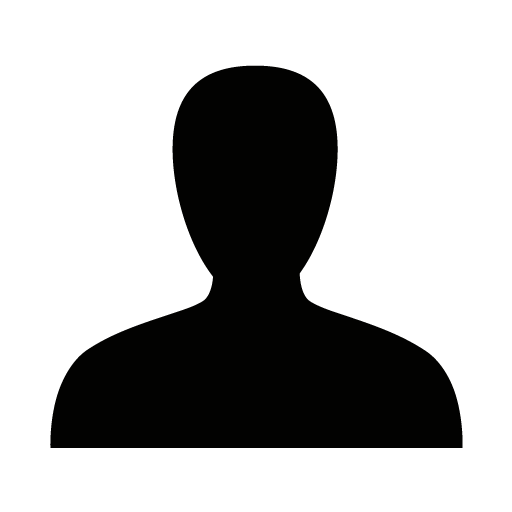
CO2 electroreduction into valuable hydrocarbons with high selectivity is of high market interest yet challenging. Cu-based catalysts have a unique catalytic activity towards hydrocarbon (C2+) products in the CO2 electroreduction reaction and are widely studied to promote the corresponding selectivity and activity. The control of the oxidation state of the catalyst is essential in controlling the desired hydrocarbon production. Herein, we steer CO2 electroreduction towards ethylene production with high selectivity in an alkaline flow cell configuration from a copper phosphate catalyst. An optimum faradaic efficiency of 55% for ethylene production was obtained in a 7 M KOH electrolyte for a total current density of 150 mA.cm-2. A high faradaic efficiency for C2+ products of 60% was also obtained. The investigation of the mechanism shows that Cu nanoparticles are derived during electrolysis which may favour the dimerization of *CO to ethylene formation. This work provides new insights into designing catalysts for high-selectivity hydrocarbon formation.
2.2-O3
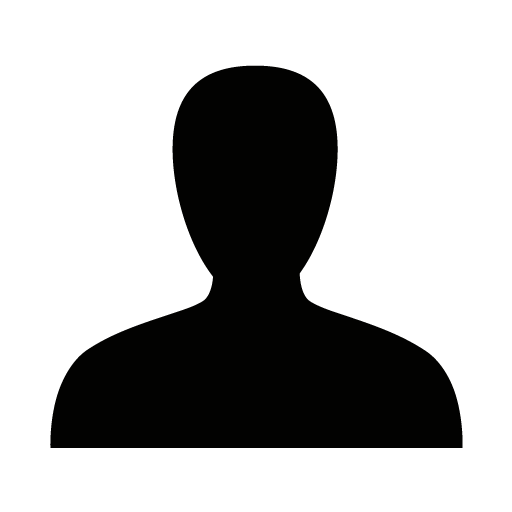
CO2 reduction provides a carbon neutral means of converting waste CO2 and surplus electricity into valuable fuels and chemicals to reach the net zero global goal. Only Cu can yield valuable high energy C2+ products, due to its unique binding energy with *CO and *H intermediate [1], but only at the Faradaic Efficiencies of up to 52% for ethanol [2] and 87% for ethylene [3], and under high over-potentials and harsh conditions. Single crystal studies have shown that Cu(100) with surface defects (under-coordinated atoms) favours such C2+ products even oxygenated products, because of both the correct binding and low barriers for protonation and C-C coupling. Therefore, we hypothesise that by synthesizing nano-porous Cu with (100) orientation, its catalytic activity will be maximised [4 – 6].
Nano-porous materials have many potential applications as sensors, actuators, catalysis, etc, because of their large surface-to-volume ratio and high surface distortion. These structures can be produced by (electro)chemically dealloying a bimetallic alloy, in a regime where one of the components is much more chemically active than the other. As the active species are removed, the remaining material forms a nano-porous ‘sponge’ that has a bi-continuous structure, of which the amount of under-coordinated atoms is significant [7 – 8]. Inspired by the work from Chattot et al [9], where a linear relationship between the surface distortion of Pt and its oxygen reduction reactivity was found, we hypothesise that the CO2 reduction reactivity of Cu surface could also be tailored by introducing surface distortion via dealloying.
Herein, we systematically studied the corrosion behaviour of Cu20Zn80 brass, using cryo-atomic probe tomography (cryo-APT) and in situ synchrotron X-ray diffraction (XRD), as shown by Figure 1. We successfully synthesised a series of nano-porous Cu materials with different ligament sizes, ranging from tens of nanometres to micrometres, via chemical dealloying by just changing temperatures. Synchrotron XRD measurement indicates that there is a huge but different micro-strain on these dealloyed nano-porous Cu, which is caused by the under-coordinated atoms. Electrochemical CO2 and CO reduction measurement was then conducted, showing their significantly different catalytic activities, which correlated well with their micro-strains measured.
2.2-O4
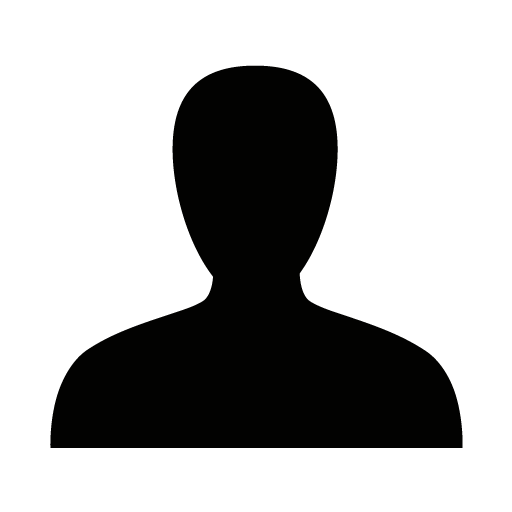
Electrochemical CO2 reduction is a candidate for reduced CO2 concentration in the atmosphere and changes to usable compounds. Zero-gap reactor for CO2 reduction is expected to realization due to its low applied voltage and high current density.
The zero-gap reactor for CO2 reduction requires an anion exchange membrane and an anolyte. These requirements are different from the polymer electrolyte electrochemical cell for water electrolysis. Although the membrane is an anion exchange, cations in anolyte pass through from anode to cathode. The existence of a cation in the cathode is also known to be essential for CO2 reduction, thus, the cation in the cathode has a possibility to affect the properties of CO2 reduction. This report discusses the effects of the anolyte of KHCO3 concentration.
The KHCO3 concentration was 0.01, 0.1, and 1.0 mol/L, the catalysts of the cathode for CO2 reduction and the anode for water oxidation were Cu and IrOx, respectively. The electrode area was 5 cm2. Since the catalyst for the cathode was Cu, the products in the cathode were mainly CO, CH4, and C2H4, with the satellite reaction product of H2 from water reduction.
The applied voltage under a constant current density of 200 mA/cm2 was lowest at the KHCO3 concentration of 1.0 mol/L. The maximum faradaic efficiencies of C2H4 around 35% were obtained at 4.2, 3.6, and 3.2 V for 0.01, 0.1, and 1.0 mol/L, respectively. From these results, the higher anolyte concentration shows the lower applied voltage at the same current density and at the highest faradaic efficiency of C2H4. The reliability was, however, the best at the concentration of 0.1 mol/L because the CO2 gas flow was strangled by the precipitation of the salt at 1.0 mol/L and the current density increased at 0.01 mol/L. The anolyte concentration affects the CO2 reduction and the 0.1 mol/L KHCO3 was the best considering all the results.
2.2-O5
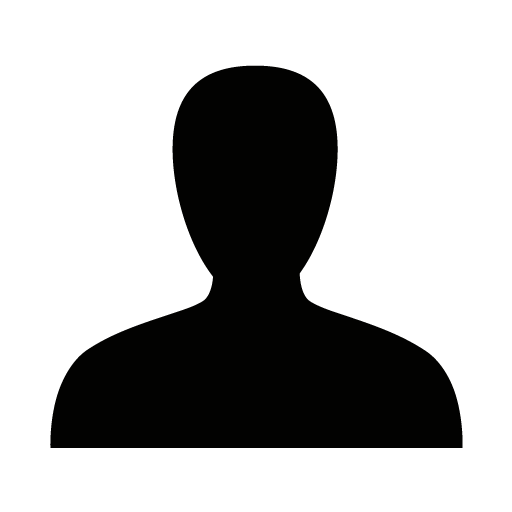
Electrochemical CO reduction (ECOR) can act as a potential bridge between CO2-to-CO technologies and renewable production of C2+ chemicals. Along with the development of catalyst materials for selective and efficient CO reduction, it is imperative to optimize electrolysis conditions and cell parameters to efficiently reduce CO at industrially relevant current density and produce pure concentrated product streams. In this work, we study ECOR is three different cell configurations, firstly, microfluidic configuration, secondly, a hybrid configuration with anode zero gap, and lastly, a zero gap configuration. We establish the optimal working conditions for each configuration and compare the fundamental differences in each configuration.
The zero gap configuration is optimal for producing a stable product stream of ethylene, acetate, ethanol and propanol for current densities upto 1 Acm-2 at reasonable cell potentials and can sustain long term C2+ production of >70% for upto 10 hours at a current density of 200 mAcm-2. The catalytic activity in this configuration is insensitive to anolyte pH and the cell can run at relatively lower alkaline concentrations. On the other hand, the product selectivity in hybrid cell configuration changes with catholyte concentrations. The catholyte ensures a high local pH near the cathode and therefore the product selectivity can be altered by changing alkaline concentration. This hybrid configuration works stable with anode catalyst coated membranes for current densities of upto 0.8 Acm-2, however the flowing catholyte makes the long term operation difficult. It is interesting to note the substantial consequence on catalytic activity by altering cell configuration and electrolysis conditions. These results stress on the importance of optimising cell configuration and measurement parameters for attaining optimum working conditions for reaching industrially relevant current densities.
2.2-O6
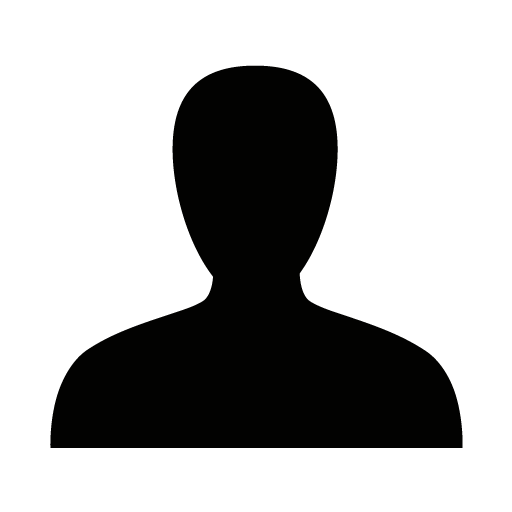
Electrochemical reduction of CO2 presents an attractive way to store renewable energy in chemical bonds in a potentially carbon-neutral way. However, current electrolyzers suffer from intrinsic problems, like flooding and salt accumulation, that must be overcome to industrialize the technology. To resolve flooding and salt precipitation issues, researchers have used ultra-hydrophobic electrodes based on either polytetrafluoroethylene (PTFE) gas-diffusion layers (GDL’s), or carbon-based GDL’s with added PTFE. While the PTFE backbone is highly-resistant to flooding, the non-conductive nature of PTFE means that without additional current collection the catalyst layer itself is responsible for electron-dispersion, which penalizes system efficiency and stability. In this work, we present operando results that illustrate the poor current/potential distribution in thin catalyst layers (~50 nm) deposited onto PTFE GDL’s. We then compare the effects of thicker catalyst layers (~500 nm) and a newly developed non-interfering current collector (NICC). The NICC can maintain even current distribution with 10-fold thinner catalyst layers while improving stability towards ethylene (≥ 30%) by approximately two-fold.
2.2-O7
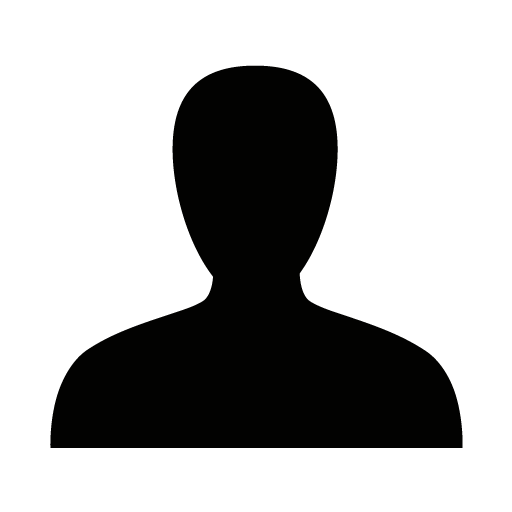
CO2 electrolysis using silver (Ag) and copper (Cu) based catalysts have been widely studied due to their ability to produce CO and multi-carbon products respectively. In industrially relevant membrane electrode assembly configurations, long term operational stability is hampered due to (bi) carbonate precipitation at the cathode triggered by alkali metal cation crossover from the anode. In this work, we investigate the role of cation crossover for Ag and Cu based catalysts by varying cation concentrations and the cation identity. We find that cation crossover from the anode is essential for CO2 activation and cation identity (K+, Na+) affect CO2RR selectivities significantly as shown in a number of previous studies. Further, we find that cation concentration do not alter product selectivity for a Ag catalyst producing CO, but alter product distribution significantly for a Cu catalyst, showing that C-C coupling rates are significantly affected by local cation concentration at the cathode. In contrast, cation crossover is detrimental for long term operation due to (bi) carbonate precipitation at the electrolyte free cathode, that induces flooding of the gas diffusion electrode over time. These results reveal that a proper management of local cation and water concentrations are essential in order to acheive long term operational stability in zero gap CO2 electrolyzers.
2.2-O8
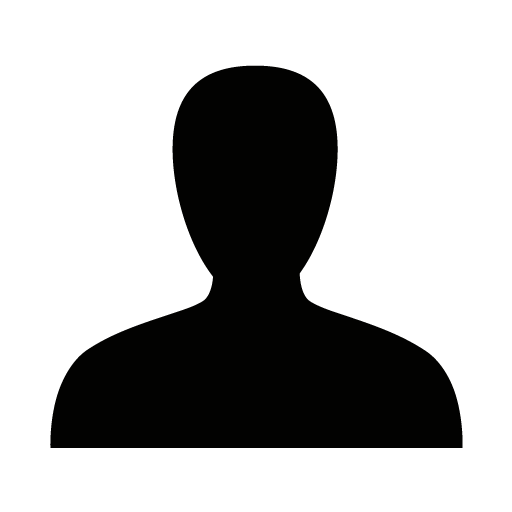
The membrane-electrode assembly (MEA) approach appears to be the most promising technique to realize the high-rate CO2/CO electrolysis, however there are major challenges related to the crossover of ions and liquid products from cathode to anode via the membrane and the concomitant anodic oxidation reactions (AORs). In this perspective, by combining experimental and theoretical analyses, we elaborate on several impacts of anodic oxidation of liquid products in terms of performance evaluation. Firstly, we analyzed the crossover behavior of several typical liquid products through an anion-exchange membrane. Subsequently, two instructive examples (introducing formate or ethanol oxidation during electrolysis) reveals that the dynamic change of the anolyte (i.e., pH and composition) not only brings a slight shift of anodic potentials (i.e., change of competing reactions), but also affects the chemical stability of the anode catalyst. Anodic oxidation of liquid products can also cause either over- or under-estimation of the faradaic efficiency, leading to an inaccurate assessment of overall performance. To comprehensively understand fundamentals of AORs, we further develop a theoretical guideline with hierarchical indicators to predict and regulate the possible AORs in an electrolyzer. We conclude by giving some suggestions on rigorous performance evaluations for high-rate CO2/CO electrolysis in the MEA-based setup.
2.3-I1
This talk will analyze CO2 electrolysis from a device perspective to provide insight into what are the bottlenecks in the field and how to resolve them. In particular we will focus on our results using synchrotron based wide angle x-ray scattering to observe CO2 electrolysis devices in real time while concomitantly measuring anode and cathode product distributions. During higher current density (>100 mA/cm2) CO2 electrolysis devices are prone to cathodic ‘flooding’ leading to greatly enhanced hydrogen production. We show that during this degradatory issue there is chaotic oscillatory fluctuations in potential, and product distribution.1 By analyzing these fluctuations in the presence of a synchrotron, we can monitor salt deposition and even electrolyte penetration by a shift in the background scattering signal. From the comprehensive analysis we have developed a hypothesis of why we both see the oscillations as well as flooding. Interestingly, salt solubility and precipitation has a strong role in flooding issues.
We then analyzed various salts (Li, Na, K, Cs) of differing solubility while doing CO2 electrolysis.2 The synchrotron analysis clearly demonstrates the importance of salt solubility on stable operation. We then switched to CO electrolysis as this allowed for more soluble hydroxides compared to the carbonates used during CO2 electrolysis. While this allowed for more stable hydration management, it did corrode the anode, as evidenced by IrO2 appearing on the cathode. This was resolved by switching to a Ni anode, though long term acetate build-up caused pH issues. The acetate issues was resolved by simply removing the acetate from the anolyte during testing. This allowed for multiple tests with over 100 hour stability.3
2.3-I2
Kazuhide Kamiya received his Ph.D. degree from the University of Tokyo and became an assistant professor at the same university in 2013. He then joined Research Center for Solar Energy Chemistry of Osaka University as an assistant professor in April 2016. He was promoted to associate professor at the same center in 2018. He was a researcher of the PRESTO project of JST from 2014 to 2018. His current research interests include the design of efficient electrocatalysts and electrolysis systems for CO2 valorization.
CO2 electrolysis to value-added products is a promising technology to close the carbon cycle and sequester anthropogenic CO2 into chemical feedstocks; increasing the current density for multi-carbon products is one of the requirements for practical implementation. [1] The use of gas diffusion electrodes (GDEs) that allow the CO2 reduction reactions (CO2RR) to occur at the solid-catalyst/liquid-electrolyte/gaseous-CO2 interface, effectively accelerates the CO2RR by solving the problem of the mass transport limitation due to the inherently low diffusion and solubility of CO2 in water. However, CO2RR at the three-phase interface is complex, and guidelines for catalyst design toward its high activity have not been fully established. Since macrostructure significantly influences activity, it is challenging to distinguish between surface reactivity at the microscale (molecular level) and material transport properties at the macroscale (three-phase interface level). This presentation summarises our recent studies on high-rate CO2RR from the point of view of both novel electrocatalyst designs and appropriate electrode assembly.
We successfully applied various metal-doped covalent triazine frameworks (M-CTFs) as platforms for CO2RR electrocatalysts on GDEs [2-5]. In addition, we conducted systematic first-principles calculations and found that this reaction selectivity correlates with the adsorption energy of the intermediates. As M-CTFs possess the same framework, it is possible to vary only the metal center (catalytic active center) with the identical macrostructure of the catalyst layer. Thus, as we can directly compare the catalytic activity of the metal centers, M-CTFs serve as a model catalyst for establishing design guidelines for gaseous CO2 electrocatalysts consisting of single metal centers.
We also achieved the ultra-high-rate CO2 electrolysis to multicarbon products (C2+) by designing the triple phase interface composed of ordinary materials. We successfully increased the partial current density for C2+ over cupric oxide (CuO) nanoparticles on gas diffusion electrodes in neutral electrolytes to a record value of 1.7 A/cm2 [6,7]. Specifically, we highlighted that the thickness of the catalyst layers is a crucial parameter that impacts the maximum current density for C2+. Although the GDE and electrocatalyst used in this case are not unique, the optimized assembly elicits their potential.
3.1-O1
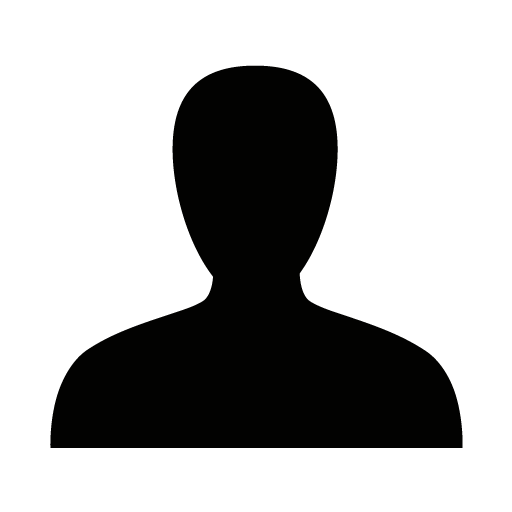
Electrochemical CO2 reduction reaction, is the conversion of CO2 to products through the application of charge in an electrolysis cell. If CO2 could be selectively converted to a particular high value product at low overpotential and high current density, CO2 would be turned into an energy storage molecule and/or a chemical feedstock, driving society closer to closing the carbon loop.
Since the early-mid 90s, research into CO2 reduction reaction materials has primarily centred around copper1-3. Copper has the unique ability to bind to key intermediates *CO and H* moderately4, improving the chance of hydrogenation or coupling reaction steps5. Incremental improvements in cell design and nano structuring have improved the partial current density towards high value C2+ products. Size control6, oxide derived copper7 and facets8 among others effects have been tested, but questions remain whether the intrinsic activity towards the CO2 reduction reaction on copper has been improved. A possible work around, is to alter the localized concentration of key intermediates. Therefore, multiple groups have paired Cu with a secondary, CO producing active site, to form a tandem mechanism9-11.
In this talk I will be highlighting the advantages of using atomic layer deposition (ALD) in electrocatalysis and how it can form a method towards altering selectivity for the CO2 reduction reaction. I will initially present the requirement for altering copper activity through relating activity towards CO2 reduction reaction to surface roughness of nanostructured copper and oxide-derived copper. Subsequently, trends in selectivity with different metal oxide nanoclusters atop copper nanoparticles are shown towards the CO2 reduction reaction, with CO reduction reaction being used as a mechanistic tool. Further characterization techniques both ex and in situ will be presented to shed light on the changes in selectivity including XPS, in-situ contact angle measurements and FTIR amongst others.
3.1-O2
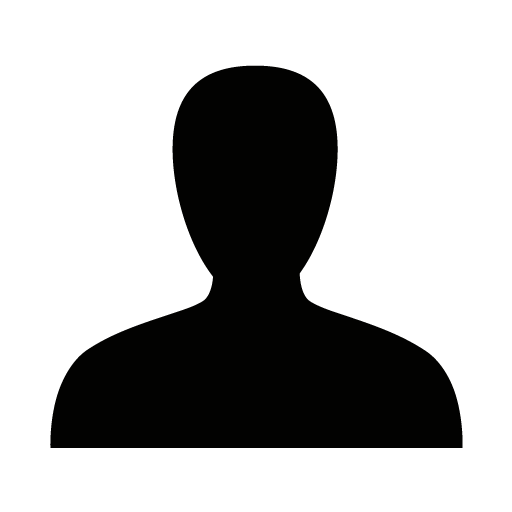
The electrochemical conversion of CO2 (CO2RR) into value-added chemicals using renewable energy sources is seen as one of the most promising approaches to achieve defossilization of the chemical industry.[1] The main targets are the direct production of multicarbon products such as ethylene and ethanol and the generation of syngas (CO+H2), a feedstock in the Fischer Tropsch process. However, even though the CO2RR process has been extensively investigated for the last 30 years,[2] its industrial application still faces significant challenges. To meet the industrial requirements, many operational parameters must be optimized besides the catalyst material such as the electrode configuration, the reaction microenvironment, and the electrolyzer design.[3] The state-of-the-art CO2RR setups, which consist of gas diffusion electrodes (GDEs) in flow cells and membrane electrode assembly (MEA) electrolyzers, already achieve relevant current densities (≥ 200 mA/cm2) but further research is needed to enhance the GDEs stability during long-term operation.
In this contribution, we present the synthesis of Ni and Cu-based electrocatalysts with high conversion to syngas and multicarbon products, respectively. These materials are synthesized via a single pyrolysis using a benzoxazine monomer (BO) as a precursor,[4] which leads to more active and stable catalyst materials than those produced with just the metal precursor. Besides the catalyst synthesis, we will discuss how the gas diffusion layer, and the hydrophobic/ion conductivity properties of the catalyst layer, strongly influence the GDEs’ stability and suppress the parasitic hydrogen evolution reaction. We show that, upon optimization, the Ni-BO-based GDE exhibits syngas production at -200 mA/cm2 with a minimum stability of 2 h while the Cu-BO-based GDE is able to reach current densities up to -600 mA/cm2 with high selectivity for ethanol and ethylene. The present work provides insight into important parameters that must be considered to fabricate stable GDEs for the conversion of CO2 into valuable products at industrially-relevant current densities.
3.1-O3
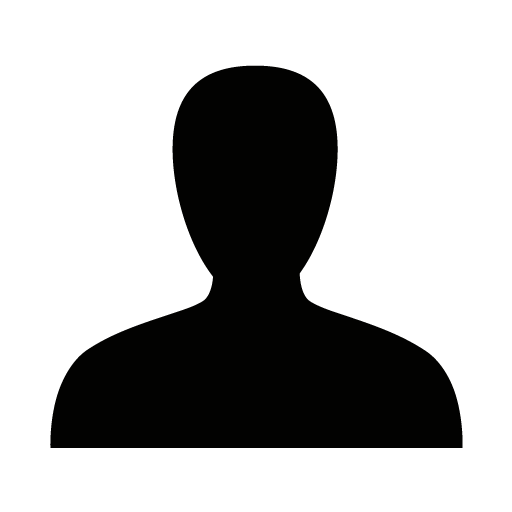
The Single-site MNC catalysts offer a ground-breaking non-precious-metal platform for CO2 electrolysis, demonstrating exceptional faradaic efficiency towards CO (> 90% FECO) at industrial-relevant current densities (> 250 mA cm-2) in regular membrane electrode assembly electrolyzer.[1] However, achieving such performance requires a cell potential (ECell) above 3 V, resulting in energy efficiency (EE) below 40% (EE = ECO2-to-CO * FECO / ECell) [2, 3]. To address this challenge, a crucial technical solution involves further enhancing the catalyst material to minimize catalytic potential loss (for reduced ECell).
The metal center highly influences the intrinsic activity-potential relationship of the MNC catalysts. FeNx exhibits superior binding energy to the crucial intermediate, *COOH, for CO formation, compared to other metal centers. However, the FeNC ones suffer poisoning during the reaction, restricting their practical application in large-scale electrolyzers at high current densities.[4] Hence, to address this limitation, we designed a novel experimental approach to deactivate and reactivate the FeNC catalyst under CO2 electrolysis conditions using pulsed potentials. Interestingly, coupled with a millisecond-resolution differential electrochemical mass spectrometer, we defined an uncommon but potential-dependent recoverable CO2 poisoning phenomenon on the FeNC catalyst. This comprehensive investigation unravels the mechanisms of CO2 poisoning and recovery of the FeNC catalyst at the molecular level. Also, it offers practical guidance for mitigating the poisoning issue in large-scale applications.
3.1-O4
The CO2 reduction reaction (CO2RR) has shown promise for producing C1-based feedstocks, including formic acid, CO, and syngas, under ambient conditions. A great challenge in this effort is to achieve control over the reaction path in order to define the product selectivity. In this work, we exploit the well-defined atomic structure and tunable coordination environment of Bi-based single atom catalysts (SACs)[1] embedded in carbon-nitrogen frameworks to show that the coordination environment of a single metallic species can be used to tune product selectivity. Bi is well-known to be an efficient CO2RR catalyst to produce formic acid,[2, 3] but has also recently been reported for CO generation.[4] While it has been hypothesised that this differing CO2RR product selectivity may arise from different Bi coordination environments, these different products stem from dissimilar catalytic systems. Here, we aim to test this hypothesis on the same catalyst system to exclude other influences on selectivity.
Using Bi SACs within a carbon-nitrogen framework, we find that the CO2RR selectivity can be tuned towards formic acid or syngas production by choosing tailored annealing treatments. Bi SACs anchored on commercially available carbon black were synthesized via a solution-based chemical method followed by inert atmosphere annealing. The single-atomic nature of Bi is confirmed by both scanning transmission electron microscopy and X-ray absorption spectroscopy. Low-temperature (300 °C) annealing of these samples results in oxygen-coordinated Bi SACs and promotes formic acid generation, while high-temperature annealing (800 °C) favours formation of nitrogen-coordinated Bi SACs and syngas production. Since the versatility of a single CO2RR catalyst system for producing two different major products has rarely been reported, our work opens up a new direction of tuning the CO2RR C1 product selectivity using SACs.
3.1-O5
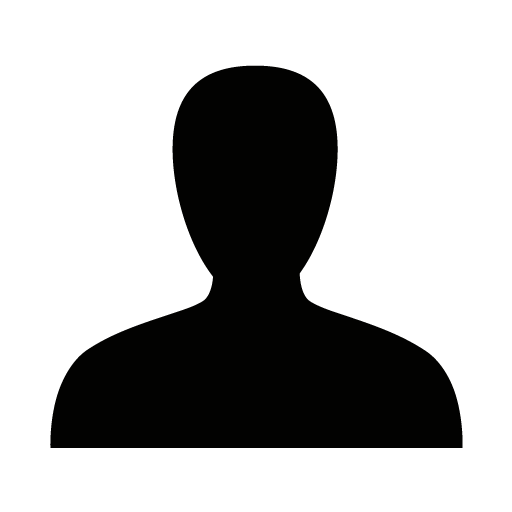
Dr. Lei Zhai is a professor in the Department of Chemistry and the Director of NanoScience Technology Center at the University of Central Florida (UCF). He received his Ph.D. in Chemistry from Carnegie Mellon University, and worked as a postdoctoral researcher at Massachusetts Institute of Technology before he joined UCF in 2005. His research at UCF focuses on the self-assembly of polymers, nanocomposites, polyelectrolyte nanostructures and polymer derived ceramic composites. He has more 20 years experience in materials development and characterization. He has published than 119 peer reviewed scientific articles and 11 granted patents. His publication has been cited for more than 18,000 times according to Google Scholar. Dr. Zhai is a recipient of NSF CAREER Award and a Scialog Fellow (Research Corporation for Science Advancement). He received the Outstanding Chemist Award at American Chemical Society (ACS) Orlando Section in 2013 and serve as the associate editor of Materials.
Nanoparticle composites are widely used as catalysts in various chemical transformations. However, large-scale production of these materials is crucial for their practical implementation, and more efficient methods are needed. This presentation highlights recent advancements in the production of metal nanoparticles on carbon nanofibers through microwave heating. To achieve this, metal ions were deposited onto the carbon nanofiber surfaces using ethanol solutions, which were subsequently exposed to hexane, an antisolvent for metal ions. The carbon fibers were then rapidly and locally heated via Joule heating, resulting in the instantaneous formation of metal nanoparticles on the substrate surfaces in just a few seconds of microwave exposure, all in an ambient environment.
Furthermore, if nickel salt was used to produce nickel nanoparticles, carbon nanotubes (CNTs) were also produced on the carbon fibers during the process. This was due to several factors, including the vaporization of solvent residue that created a local environment for the reduction of metal salt, the decomposition of carbonaceous precursors on nanoparticle catalysts, and the fast cooling related to the condensation of hexane. We are exploring using the system to conver carbon dioxide to CNTs. Overall, this technique holds great promise for the scalable production of supported nanoparticle composites with potentially significant applications in the field of catalysis.
3.1-O6
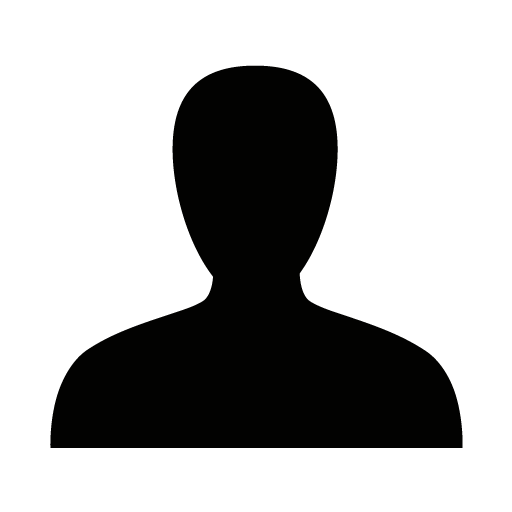
The intermittent photovoltaic (PV) power generation requires long term storage to stabilize energy system. Conversion of the excess PV power into valuable chemicals (products of CO2 reduction, for example) represents an attractive solution for the problem. We address this challenge via ‘artificial leaf’ approach – photovoltaic driven conversion of carbon dioxide (CO2) and water (H2O) into valuable chemicals. In our experiment an electrochemical cell (EC), responsible for the CO2 reduction, was directly connected to the highly efficient silicon heterojunction PV module that generates the sunlight-based electricity.
In this work, we study the performance of the directly coupled PV-driven CO2 reduction cell with emphasis on the effects of realistic temperatures (25-65°C) and irradiances (0.2 to 1 sun) for the PV module. During the experiment we characterized coupling efficiency in the system and product selectivity of the electrolyzer, catalyst stability and finally solar-to-chemical (STC) efficiency. Coupling efficiency in the directly connected PV-EC system is an important performance parameter and is characterized by the coupling factor CPV-EC, the ratio between the operating power and the maximum power deliverable by the PV device. The current-voltage parameters of both PV and EC system components were preselected to ensure high power coupling factor under standard test conditions of PV (1 sun irradiance A.M 1,5G and 25°C). A 5-cells silicon heterojunction PV module with maximum power point voltage of 3.2V, current of 383.7 mA, aperture area of 51.7 cm2, and power conversion efficiency of 23.82% was connected to a commercially available EC cell with an 8.8 cm2 CO-targeted silver catalyst [1].
The directly connected PV-EC device withstands realistic temperature and irradiance variation of the PV module maintaining high coupling factor CPV-EC for the PV-EC device between 0.89 and 1.00. Furthermore, while temperature minimally affects PV-EC working points (VWP, IWP), the variations in irradiance between 0.2 and 1 sun significantly influence the voltage at working point (between 2.55 V and 3.44V respectively) and therefore affect electrolyzer products selectivity. The effect of operating voltage on the electrolyzer product selectivity is studied in a separate experiment with constant voltage steps with 1 hour duration using a potentiostat. As the voltage is increased between 2.6 to 3.4V the product selectivity for CO increases from 80% to 95%. In the operation stability study, we have observed high CO selectivity of approximately 90% over 7 hours of experiment at 3.4V and 330 mA. Performance of the EC cell characterized with the potentiostat suggests that under proper coupling, the PV-EC system can operate with solar to chemical efficiency for CO of approximately 10% over the operating voltage range.
In the ongoing experiments we investigate the CO-selectivity, operating stability and solar to CO efficiency with the directly connected PV-EC device in realistic irradiance and temperature conditions. In addition to that, the catalyst degradation rate on microstructure scale is studied using electron microscopy.
3.2-I1
Prof. Magalí Lingenfelder is a PI with an excellent track record and a passion for atomically controlled interfaces. Her work contributes to the design of new materials by elucidating chemical processes by Scanning Probe Microscopies and Surface Sensitive Spectroscopies, including dynamic (bio) molecular recognition processes at the liquid/solid interface.
She created and led for over 10 years the Max Planck-EPFL laboratory for Molecular Nanoscience at EPFL campus in Switzerland, and has recently joined the Basque Center for Materials as Ikerbasque Professor.
She studied physical and biological chemistry at the National University of Córdoba in Argentina. In 2003, she finished her MSc thesis at the Max Planck Institute for the Solid State Research (MPI-FKF in Stuttgart, Germany) with seminal contributions to the field of metal-organic coordination networks on solid surfaces. She continued with her doctoral studies in Physics, and received the Otto Hahn medal of the Max Planck Society in 2008 for the microscopic understanding of the chiral recognition process with submolecular resolution. In her quest to study molecular recognition going from 2D to 3D complex systems, she made postdoctoral stays at the Institute of Materials Sciences in Barcelona, and at the Molecular Foundry of the Lawrence Berkeley National Lab in the US.
She is a committed mentor who directed 4 MSc theses, 5 PhD theses, and 5 postdocs. She advocates for problem-oriented interdisciplinary research, by pioneering the emerging field of BioNanoarchitectonics. She led 5 international research consortiums, delivered over 50 invited presentations, and organized 9 conferences and 4 doctoral schools. She and her team had received multiple awards and international recognitions for their creative and rigurous work on molecular recognition, chirality and operando studies at catalytic interfaces. In 2018, the Royal Society of Chemistry included her work in the first collection “Celebrating Excellence in Research: 100 Women of Chemistry”.
The electrochemical reduction of CO2 (CO2R) holds tremendous potential as a pivotal component in future energy systems reliant on renewable fuels. CO2R proceeds through a complex reaction scheme consisting of multiple proton-electron steps with numerous possible intermediates and products, out of which many have been detected only recently. An additional level of complexity arises from the surface dynamics of the Cu catalysts undergoing structural transformations under potential, with rapid changes in size, shape, and activity. By combining electrochemical measurements with high-resolution imaging, we identify and track dynamic active sites on the surface of electrodes during electrocatalysis.
In this talk, I will show you examples on the surface dynamics of Cu, Cu oxides and Cu electrodes covered by 2D materials during CO2 electroreduction. I will discuss the use of scanning probe microscopy and solid state spectroscopy to track the surface dynamics of electrocatalysts in-situ, and present a few unconventional strategies to reach significant boosts in the catalytic activity of 2D materials, using spin-selective mechanisms.
3.2-I2
In the presentation I will go throught the latest developments that we have been developing in the group on one side on the type of materials and how they reconstruct under reaction conditions and how the relationships with the electrolyte can affect the chemistry taking place. The model systems investigated will allow to understand the particularities of highly reconstructed materials under relevant reaction conditions, this is a very crucial point when trying to go beyond the standard traditional models materials. Additionally the electrolytes can change the complete picture of the reactivity due to the generation of low energy paths, alternative paths that might contribute to the reactivity and more importantly new ways that reaction can be driven in different ways. The relevance of the results indicate that there are ways to allow some of the constraints that are limiting the technical implementation of CO2 reduction technologies in a short time.
1.1-O1
Concentrated irradiation enables the production of solar fuels using smaller and inexpensive devices, resulting in decreased costs for solar fuel production. Additionally, by carefully selecting optimal operating conditions, it might be possible to enhance the performance of photoelectrochemical (PEC) devices [1]. A large proportion of the research on semiconducting materials for solar-driven water splitting has focused on their behaviour at room temperature and under 1 sun illumination. Hence, the utilization of high photon fluxes presents new possibilities for solar fuel production, as it has been demonstrated for integrated PV+EC devices [2]. Nevertheless, many obstacles hinder the design and testing of PEC cells intended for operation under concentrated irradiation. The potentially advantageous synergy between higher temperatures and irradiation makes the system complex and difficult to study.
In that regard, the effect of temperature alone on the properties of photoelectrodes has been reported recently for α-Fe2O3 [3], showing increased photocurrent densities at high potentials and increased recombination rates at low electrode potentials. In a similar study, BiVO4 photoelectrodes have shown substantial increase in photocurrent densities across all electrode potentials [4]. The behaviour of photoelectrodes under high irradiations has been performed under relatively low irradiances (<30 kW m‑2, c.f. 1 sun ≈ 1 kW m-2) and usually for very stable photoelectrodes, e.g. Fe2O3 [5].
The combined effects of temperature and high irradiations on the properties of photoelectrode materials has been rarely investigated experimentally. With the aim to tackle this challenge, we designed and developed a PEC cell for operation under high irradiations (30 – 360 kW m-2) complemented with a multiphysics model to predict the surface temperature, and the effects of ohmic drop due to bubble evolution, substrate resistance, and current density distributions. The High-Flux PEC cell (HF-PEC) comprises a stainless body steel acting as counter electrode, a high transmission quartz window and a modified Luggin capillary for the reference electrode. To avoid extreme temperatures at the surface of the photoelectrode, the electrolyte was recirculated at the back of the substrate to keep it at lower temperatures. Two photoelectrode materials were investigated: Sn-doped α-Fe2O3 and BiVO4, both deposited on FTO or Ti substrates via spray pyrolysis.
In the case of Ti|α-Fe2O3 photoelectrodes, it was found that at high temperatures under 1 sun illumination, the charge transfer efficiencies decreased with temperature at low potentials (< 1.2 V vs. RHE), but increased at higher potentials; in contrast, impedance spectroscopy under high irradiations indicated that charge transfer efficiencies increased for all electrode potentials, even when the surface of the photoelectrode was at higher temperatures. This suggests, that although e-h recombination kinetics increased with temperature, the increased charge transport kinetics and higher exciton concentration could ultimately favour the charge transfer at the semiconductor-electrolyte interface. For FTO|BiVO4 photoelectrodes, it was found that photocurrent densities do not scale proportionately with irradiance, and that degradation rates for this material are the result of complex interactions between increased transfer efficiencies, high temperatures and high photocurrent densities; it was found that the highest (irradiance-normalized) current density for BiVO4 was achieved at ca. 120 kW m-2.
1.1-O2
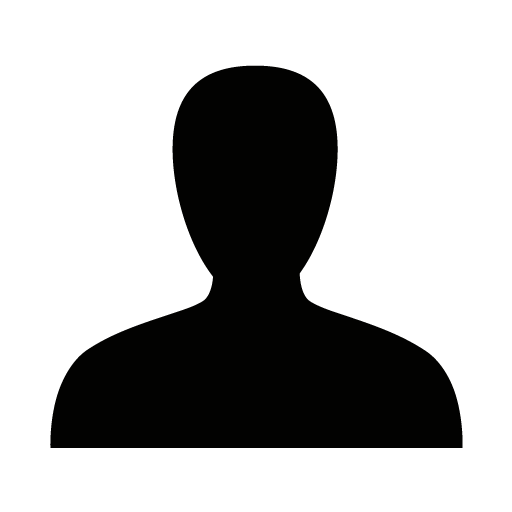
Photoelectrochemical water splitting is a promising way of solar hydrogen generation. However, in order to achieve inexpensive, efficient, and stable photoelectrodes significant further improvements are needed. Perovskite and organic photoactive materials have attracted great scientific interest by reaching record high single-junction solar cell efficiencies, but their photoelectrode performance is currently limited by their instability in an aqueous environment. In this presentation, we will show a cost-effective way of protecting halide perovskite, as well as organic photoactive layers used for solar water splitting to reach both stable (>100 hours) and remarkably high photocurrents (>8 mA cm‑2 and >25 mA cm‑2 at 1.23 VRHE, respectively). The perovskite photoelectrodes were developed using a low annealing temperature carbon paste with tuned energy level CsPbBr3 photoactive layer. The effect of 2-dimensional (CsPb2Br5) and 0-dimensional (Cs4PbBr6) perovskite phases on efficiency and stability will be discussed including ways to control their formation. The organic photoanodes applied a ternary bulk heterojunction blend (non-fullerene acceptor and polymer donor) and the interlayers were optimised for stability under 1 sun illumination. Importantly, the lifetime of the photoelectrodes was increased by applying a protective graphite sheet functionalised with electrodeposited oxygen evolution catalyst (NiFeOOH), allowing to reach remarkable stability of several days – instead of the often-reported few hours – at high photocurrent densities, far beyond the current state-of-the art levels.
1.1-O3
Verena Streibel studied Materials Science at the Technical University of Darmstadt (2007-2013). She completed her doctoral studies at the Fritz Haber Institute of the Max Planck Society, focusing on in situ X-ray spectroscopy during electrochemical water splitting (2016). For her postdoctoral studies, she joined the SUNCAT Center for Interface Science and Catalysis at Stanford University (2018-2020), specializing in density functional theory-based microkinetic modeling of heterogeneous catalysis. In 2021, she joined the Walter Schottky Institute of the technical University of Munich, where she has been leading a BMBF Junior Research Group on artificial photosynthesis since 2024.
Verena's research focuses on surface and interface investigations to elucidate dynamic material changes during (photo)electrochemical processes for energy conversion. To this end, she combines (X-ray) spectroscopy methods under reaction conditions with theoretical modeling. With her research group, she develops thin-film photoelectrode materials and couples them to catalyst systems for solar fuels synthesis.
Transition metal (TM) nitrides are an emerging class of catalytic and photoelectrocatalytic materials.[1],[2] In general, nitrogen-poor TM nitrides are usually refractory materials with metallic character while nitrogen-rich nitrides often possess semiconducting character.[3] Hence, while the former are potential electrocatalyst candidates, the latter may qualify as photoelectrode absorber materials. For example, ZrN has recently been proposed as an electrocatalyst for both the electrochemical nitrogen[4] and oxygen[5] reduction reactions (ORR and NRR), while Zr3N4 and also Zr2N2O have been suggested as potential photoanode materials in photoelectrochemical water oxidation.[2] In this contribution, we test these hypotheses regarding the (photo)electrochemical characteristics of Zr-based (oxy)nitrides by experiment. To this end, we investigate reactively sputtered thin films for the electrochemical NRR/ORR and the photoelectrochemical oxygen evolution reaction (OER). Previous experiments on Ta-based nitrides have shown that addition of oxygen during the reactive sputter process is necessary to access higher metal oxidation states.[6] Indeed, as we introduce controlled amounts of oxygen at otherwise fixed deposition conditions, we observe a transition from metallic ZrN to a disordered nitrogen-rich ZrxNy to a crystalline bixbyite-type Zr2N2O to nitrogen-doped cubic ZrO2. Crystalline Zr3N4 was not accessible under the used experimental conditions. While we observe a lack of electrocatalytic activity for ZrN in NRR and ORR and instabilities of the disordered nitrogen-rich ZrxNy in the photoelectrochemical OER, introducing more oxygen into the structure leads to a more stable crystalline structure (Zr2N2O), the opening of a band gap in the visible range, and the emergence of photoelectrochemical activity for oxidation reactions. Based on chopped linear sweep voltammetry measurements, we show that Zr2N2O films are photoactive for the OER in alkaline electrolyte with low onset potentials, indicating an overall favorable band alignment of the material with respect to the water oxidation and reduction potentials. While the observed photocurrents are still significantly lower than for the benchmark oxynitride TaON, further material optimization could potentially close this gap and provide a materials system functioning as sustainable photoanode.
1.1-O4
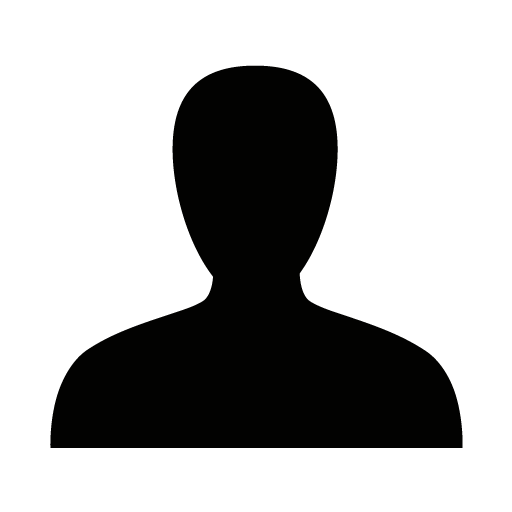
Harnessing solar energy through photoelectrochemical (PEC) water splitting approach offers a promising and sustainable pathway to produce green hydrogen (H2). However, to ensure the viability, cost-effectiveness, and long-term stability of this approach, fabricating an abundant and durable photoelectrode is crucial. Barium stannate (BaSnO3) has not been considered promising material for PEC water splitting applications due to its wide band gap (˃3 eV) and limited solar absorption characteristics.[1] In this study, we optimized a spray pyrolysis method to fabricate phase pure BaSnO3 photoanodes with a smaller optical gap of ∼2.2 eV. By annealing these photoanodes in a mild H2 gas environment, we observed a significant decrease in the optical gap to ~1.5 eV. This led to an enhanced photoelectrochemical performance, with a 5-fold increase in the photocurrent density reaching ~0.5 mAcm-2 at 1.23 V vs. RHE and an improved onset potential of ~0 V vs. RHE. To understand the reason behind this enhancement, we have used a combination of spectroscopy techniques, including photoluminescence (PL), time-resolved surface photovoltage analysis (TR-SPV), and time-resolved microwave photoconductivity (TRMC) measurements. We find that mild H2 annealing of BaSnO3 generates a set of mid-gap defect states associated with oxygen vacancies and Sn2+ centres.[2] Increasing the population of these mid-gap states, shifts the optical onset of photocurrent collection and mobile charge carrier generation to photon energies as low as ~1.5 eV. Furthermore, the transient signals of charge carriers show the extended lifetime after H2 treatment and provides the evidence that carriers are transported via tunnelling through delocalized defect states. We show here that BaSnO3 is a promising material with tunable optical properties and electronic structure.
1.1-O5
The recent emergence of ultrathin oxide layers in catalysis has led to more corrosion-resistant photoelectrodes and enhanced catalytic selectivity – suppressing side reactions and catalyst poisoning [1] Silica nanolayers for example demonstrated enhanced proton transfer [2] and electron transfer [3] across solid-solid interfaces when embedding molecular wires. The nanoscale integration of incompatible catalytic environments of CO2 reduction and H2O oxidation – similar to a thylakoid membrane – can potentially be achieved by such ultrathin oxide layers, provided we can maintain directional electronic and protonic communication across the layer.
Here, we investigate the proton conductivity and O2 impermeability of ultrathin, dense, and amorphous Al2O3 layers and find that the diffusivity of protons is strongly dependent on the flux due to induced morphological changes in the alumina layer over time. Dense amorphous alumina layers were prepared via pulsed laser deposition (PLD; 2.5 nm, 3 nm, and 5 nm) and the porosity was varied via the oxygen background pressure of the chamber. We used electrochemical impedance spectroscopy to determine diffusion coefficients and charge transfer resistances, and complementary electrochemical FT-Infrared reflection-absorption spectroscopy was applied to analyze the dynamic structural changes of alumina over time in-situ (Figure 1b). In fact, we observe that initially, 2.5 nm of alumina can block both protons and oxygen, whereas over time the proton permeation improves while we can exclude dissolution of the Al2O3 layer (Figure 1c)
These discoveries facilitate the integration of incompatible catalytic functions on the nanoscale, thereby opening a new design space for developing macroscale systems. The core–shell nanotube array geometry for developing an artificial photosystem with the goal of placing CO2 reduction inside the tubes and H2O oxidation outside of them is just one among many opportunities to pursue.
2.1-I1
During my talk I will discuss four topics, over which I have felt concern over the past few years:
- The concept of applied bias photon-to-current efficiency (ABPE).
The ABPE is described as ‘a diagnostic measurement in materials development’. However, the widely accepted ABPE equation is not formulated as a true energetic efficiency. Furthermore, negative ABPEs are obtained for applied potentials > 1.23 V, resulting in increasingly negative efficiencies for increasingly high photocurrents, which is non-sensical. Both of these issues invalidate the ABPE equation for the very condition it is designed to represent. It is also noticeable that a lot of recent reviews and systematic studies of photoelectrode materials in biased systems do not use the ABPE as an effective way to compare their performances. I will discuss the issues of the currently accepted ABPE formulation and suggest a more rigorous formulation.
- The perpetual reporting of incident radiation on photoelectrochemical cells as AM 1.5 G and 100 mW cm-2
The accepted basic requirement for the characterisation of solar water splitting systems is the same as for benchmarking the performances of solar cells: the incident spectral irradiance has to match that of the Air Mass 1.5 Global (AM 1.5 G) spectrum of natural sunlight, amounting to 100 mW cm-2 when integrated. The spectra of light sources is rarely an exact match to the AM 1.5 solar spectrum, even if an AM 1.5 filter is used. I will discuss the importance rigorous calibration and the need to report the actual spectra of light sources used during the characterization of photoactive materials and assessment of photoelectrochemical devices.
- The construction of Pourbaix diagrams to predict photoelectrode and catalyst stability – example of BiVO4 degradation
There are some puzzling aspects in the Pourbaix diagrams being used for demonstrating the reactions responsible for light-induced BiVO4 degradation. I will present the published Pourbaix diagrams for the combined aqueous bismuth and vanadium systems and discuss why some postulated reactions that are presented as electrochemical (i.e. involving a change in oxidation state) cannot actually be so. I will also consider why chemical speciation in different electrolytes can contribute to electrode degradation.
- Effect of temperature on (photo)electrolyser performance
Solar cells must be maintained at 25 °C during characterisation and here the characterisation of water splitting systems ceases to follow the same standards. The question is whether this matters. The deviation of photoabsorber temperature from 25°C in solar water splitting systems is not necessarily unreasonable or preventable, since the presence of electrolyte makes it harder to fix the photoabsorber temperature to one specific constant value; photoelectrochemical cells are not usually jacketed or designed to be immersed in water baths and so the electrolyte (and hence photoabsorber) temperature could readily vary by > 5 °C, depending on the ‘room temperature’ of a laboratory at the start of the experiment, and can increase by > 10 °C relative to the starting temperature, depending on the photocurrent density, size of the absorber relative to the volume of electrolyte and the duration of the experiment. It therefore seems more important for the electrolyte temperature to be measured, rather than controlled, since temperature affects Gibbs free energy of formation of the water splitting reaction and the volumetric molar densities of evolving gases. I will show, from the thermodynamic viewpoint, the importance of electrolyte temperature in (photo)electrolysis.
2.1-I2
Jong Hyeok Park is professor at Department of Chemical and Biomolecular Engineering in Yonsei university, Republic of Korea. His research focuses on solar-to-hydrogen conversion devices, Li & Na ion batteries, perovskite solar cells.
He received his Ph.D. in chemical engineering from KAIST, Republic of Korea, in August 2004. Then, he joined University of Texas at Austin, USA, as a postdoctoral researcher in 2004 (under Prof. Allen J. Bard). He is an author and a co-author of 320 papers and 100 patents (h-index: 78).
He has received various prestige awards such as PBFC Award (2012) from The Korean Electrochemical Society, SKKU Young Fellowship (2012) from SKKU, Horace G. Underwood Fellowship (2018) from Yonsei University, Award of Excellence (2017) from Korean Academy of Science and Technology, S-Oil Next Generation Researcher Award (2021).
Millions of families around the world remain vulnerable to water scarcity and have no access to drinking water. Advanced oxidation processes (AOPs) are an effective way towards water purification with qualified reactive oxygen species (ROSs) while are impeded by the high-cost and tedious process in either input of consumable reagent, production of ROS, and the pre-treatment of supporting electrolyte. Herein, we couple solar light-assisted H2O2 production from water and photo-Fenton-like reactions into a self-cyclable system by using artificial leaf, achieving an unassisted H2O2 production rate of 0.77 μmol/(min·cm2) under 1 Sun AM 1.5 illumination. Furthermore, a large (70 cm2) artificial leaf was used for an unassisted solar-driven bicarbonate-activated hydrogen peroxide (BAP) system with recycled catalysts for real-time wastewater purification with requirements for only water, oxygen and sunlight. This demonstration highlights the feasibility and scalability of photoelectrochemical technology for decentralized environmental governance applications from laboratory benchtops to industry.
2.1-O1
Nitriles are interesting class of organic compounds included in a wide variety of natural products and industrial processes, that have been explored as potential Liquid Organic Hydrogen Carriers (LOHCs) due to their high hydrogen capacity and stability. Conventional methods for nitrile synthesis suffer from low atom economy, the use of toxic reagents, and limited selectivity. An alternative approach involves the oxidation of primary amines through organic electrosynthesis, which offers a safer and more environmentally friendly alternative by utilizing electric current and reducing hazardous solvents. Our research focuses on developing a green, selective, and gentle method for synthesizing nitriles in aqueous media, based on electrochemical procedures that allow eliminating the need for harsh reaction conditions. To that end, we have devised a facile alternative process for synthesizing electrodes through the electrodeposition of nickel on various supports for the electrooxidation of amines to nitriles.
In the electrooxidation of primary amines to nitriles with Ni based electrodes, hydrogen in produced at the cathode, whereas de amine is oxidized at the anode due to the redox par Ni2+/Ni3+ formed at the anode. To evaluate the effectiveness of different nickel-based electrodes, we conducted screening tests involving the electrooxidation of different aromatic and aliphatic primary amines. The electrodes tested include Ni foil (NiF), Ni45[1], Ni foam (NF), Ni electrodeposited on NF (NieNF), and Ni electrodeposited on a graphite pencil rod (NieGPR). Among these, the NieNF electrode demonstrated the most favorable results in terms of high yields (>95%) and substrate tolerance. Notably, our proposed electrodes exhibit a significant advantage over Ni/Se electrodes reported in the literature[2] , enabling a reduction of the reaction time for complete amine oxidation by half.
To sum up, the current project paves the way to produce both nitriles and H2 with low cost and high efficiency, thus grasping great potentials in the future energy conversion and storage technologies, sustainable environmental issues and beyond.
2.1-O2
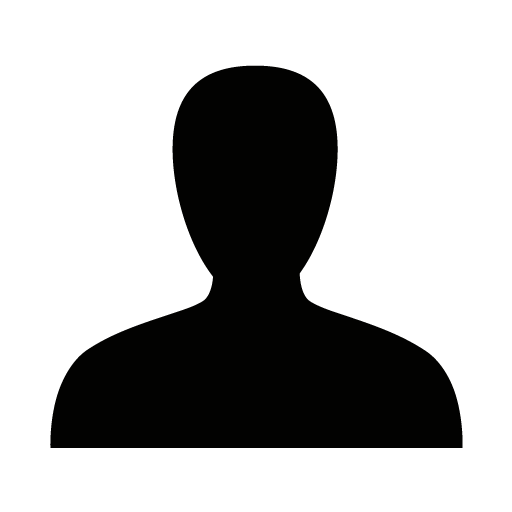
Solar hydrogen generation by photoelectrochemical (PEC) water splitting allows for direct photon-to-molecule conversion in a standalone system. Although it is one of the most promising approaches to generate renewable hydrogen, it is also the most challenging because of the multitude of processes occurring concurrently and at very short time scales.
Bismuth vanadate (BiVO4) is an ideal photoanode material because of its favourable band gap of 2.4 eV [1], which allows it to absorb photons in the visible region of the solar spectrum and has a theoretical solar to hydrogen (STH) efficiency of ~ 9.2%. It has suitable band edges for the water redox process, is durable in aqueous solution, good crystallinity and made up of earth abundant materials. However, BiVO4 photoanodes suffer from poor charge separation in the bulk of the material and at the electrolyte interface during the PEC water splitting process [2]. Doping of BiVO4 is a common strategy used to improve charge separation [3]. Doping by electron donors can improve the electrical conductivity by increasing the electron density of BiVO4 [4]. Doping of BiVO4 with hexavalent Molybdenum (Mo6+) has been shown to enhance photocurrent generation due to more efficient charge separation [5].
In this work, we compare the charge separation efficiency of a homogenous doping concentration profile to a gradient doping concentration profile of Mo-doped BiVO4. Gradient doping induces an upward band bending which amplifies charge separation within the bulk of the BiVO4 film. Mo-doped BiVO4 thin films are deposited on FTO-coated glass substrates by ultrasonic spray pyrolysis (USP). Morphological characterisations reveal controlled successive layered films, wherein the number of layers correspond to the number of cycles using our USP deposition process. Gradient doping is achieved by depositing controlled successive Mo-doped BiVO4 layers that contain increasing dopant concentrations using this method. Their improvement in optical absorption and PEC performance is elucidated by material, optical, Mott-Schottky characterisations and electrochemical impedance spectroscopy.
2.2-I1
Kevin Sivula obtained a PhD in chemical engineering from UC Berkeley in 2007. In 2011, after leading a research group in the Laboratory of Photonics and Interfaces at EPFL, he was appointed tenure track assistant professor. He now heads the Laboratory for Molecular Engineering of Optoelectronic Nanomaterials (http://limno.epfl.ch) at EPFL.
Gas diffusion electrodes are essential components of common fuel and electrolysis cells that use gas phase reactants and products, but are typically made from graphitic carbon or metallic materials, which do not allow light transmittance, and thus limit the development of photoelectrode membrane assemblies for gas-phase solar fuel production. In this presentation, the application of solar H2 production from humid air is motivated and the simple and scalable preparation of transparent gas-diffusion layers using F-doped SnO2 (FTO) coated SiO2 interconnected fiber felt substrates is introduced[1]. The resulting substrates have a porosity of 90 %, a roughness factor of 15.8 and a Young’s Modulus of 0.2 GPa. A sheet resistivity of 20 ± 3 Ω sq−1 and a loss of incident light of 41% at an illumination wavelength of 550 nm is found with FTO coating. The application of various semiconductors on the substrates was established including Fe2O3 (chemical bath deposition), CuSCN and Cu2O[2] (electrodeposition ), and semiconducting polymers[3] (dip coating ) and the liquid-phase photoelectrochemical performance commensurate with flat FTO substrates was confirmed. Finally, gas phase H2 production was demonstrated with a polymer semiconductor photocathode membrane assembly at 1-Sun photocurrent density on the order of 1 mA cm–2 and Faradaic efficiency of 40%.
2.2-I2
A fundamental understanding of processes occurring at the electrode-electrolyte interface is essential for the development of optimized materials in sustainable energy production. In this talk, I will present two examples that highlight the relevance of atomistic studies on model electrocatalytic systems in understanding existing structure-function relationships and reaction-mechanism.
Firstly, I will introduce our custom experimental setup, which enables a comprehensive characterization of structural, chemical, and electrochemical properties on the same sample. This setup facilitates the transfer of electrodes from ultra-high vacuum conditions, ideal for surface science tools, to an electrochemical cell in an inert atmosphere. By employing this approach, we investigated potential-induced changes on Au(111) surfaces in acidic media. We monitored chemical transformations using ex-situ X-ray photoemission spectroscopy (XPS), providing insights into the electrochemical response, including oxide formation during the onset of the oxygen evolution reaction. Additionally, I will discuss the influence of various electrolyte anions on surface electro-oxidation.
Secondly, I will provide an overview of the local properties of point defects in transition metal dichalcogenide semiconductors (MX2, M = transition metal, X = chalcogen) that have been proposed as active sites for the hydrogen evolution reaction (HER). By combining 4K scanning tunneling microscopy and spectroscopy (STM/STS), and non-contact atomic force microscopy (nc-AFM), we achieved atomic-scale correlation between the morphology and electronic properties of different types of structural defects in MoSe2 and WS2 monolayers. Our investigations unveiled the predominance of substitutional oxygen as the point defect in these semiconductors, effectively suppressing deep in-gap states associated with chalcogen vacancies [1, 2], which have been discussed to mediate the HER. These findings highlight the critical role of local properties in determining the functionality of materials for hydrogen production, ultimately governing the underlying reaction mechanism.
2.2-O1
Photoelectrochemical (PEC) water splitting is a promising approach for generating solar hydrogen. To realize inexpensive PEC devices, TiO2 has been considered a potential photoanode material because of its abundance, stability, and suitable valence-band energy edge for water oxidation. In this work, we will present results on post-treatment strategies to extend the high efficiencies of these photoanodes to the visible region of the solar spectrum. Our research paper details the successful introduction of different point defects (VO and VTi) in TiO2 photoanodes using a chemical treatment process. We utilized a range of advanced techniques, including surface photovoltage signal measurement (SPV), electron paramagnetic resonance measurement (EPR), and proton magic angle spinning nuclear magnetic resonance (1H MAS NMR) to investigate the intrinsic band energy structure and surface state of synthesized TiO2 photoanodes. Our finding indicates that VO and VTi have favorable effects on photoanodes, leading to enhanced PEC performance. The enhancement is attributed to the interplay of collective and localized effects of point defects on TiO2. The increased point defects narrow the bandgap and enhance donor density in TiO2, which increases light absorption, conductivity, and photovoltage, as well as reducing the flat-band potential of TiO2 photoanodes. Additionally, our results show that the presence of localized surface VTi surrounded by O- increases the amount of surface hydroxyls and creates a more basic environment for Ti-OH species. This facilitates the water oxidation process by serving as trapping sites and self-reduction sites during OER. Our study on OER kinetics, using photoelectrochemical impedance spectroscopy (PEIS) and intensity modulated photocurrent spectroscopy (IMPS), demonstrates that the interaction between collective and localized effects of point defects can decrease the charge transfer resistance on the TiO2-electrolyte junction and increase the concentration of interface hole flux on TiO2 photoanodes. This is consistent with the observed higher photovoltage after chemical treatment. Our study has revealed that the interaction between VO and VTi has a significant impact on the PEC performance of TiO2 photoanodes. We believe that these findings will make a valuable contribution to the development of efficient and stable TiO2 photoanodes and provide valuable insights into the development of higher performance photoanodes for sustainable energy conversion applications.
2.2-O2
Water oxidation photoanodes based on earth-abundant metal oxide semiconductors are actively studied due to their limited environmental impact, the stability to photo-corrosion and the tunable optoelectronic properties [1]. BiVO4/WO3 heterostructures are now emerging as one of the most promising photoanode, but the activity is limited by surface recombination and sluggish charge transfer kinetics. Cobalt-iron mixed compounds, such as CoFeOx and CoFe hexacyanoferrate, a Prussian blue analogue, proved to effectively enhance photocurrent when coupled to BiVO4/WO3 photoanodes as water oxidation co-catalyst. The origin of the increased efficiency is still debated, but recent studies suggest an enhancement of charge separation efficiency within the semiconductor [2], rather than improved water oxidation kinetics, underlying possible charge accumulation on the co-catalyst leading to transient modification of the local structure. X-ray Absorption Spectroscopy (XAS) provides a unique view of the structural features of ultrathin catalyst layers, however often limited to a steady state ex-situ characterization of the catalyst local structure and valence state. To achieve structural understanding of the catalyst activity in the actual PEC operating conditions, a custom PEC-XAS cell was specifically designed to allow for operando monitoring of Co-Fe based co-catalysts deposited on BiVO4/WO3 photoanodes, upon bias and illumination stimuli fully replicating the ex-situ conditions. The developed setup (Fig 1a), providing limited x-ray photons interaction path with the controlled-flow electrolyte (<100 μ m), prevents bubbles formation and enhances mass transfer (Fig 1a), allowing for stable, real-time correlation of the structural features with the photoelectrochemical response. The activity of CoFeOx and CoFe-PB was investigated by either performing fixed potential spectroscopy (Fig 1b) at Co K-edge, or fixed X-ray energy absorption voltammograms [3] (FEXRAV, Fig 1c). The resulting characterization displayed a drastic modification of the structure between operando and ex-situ analysis, as well as a dependence on bias and illumination. In addition, despite the apparent similarities between CoFeOx and CoFe-PB in terms of composition and PEC activity, their operando-XAS behaviour displays a different enhancement mechanism, further correlated to the charge dynamics characteristics, investigated by IMPS and PEIS.
2.2-O3
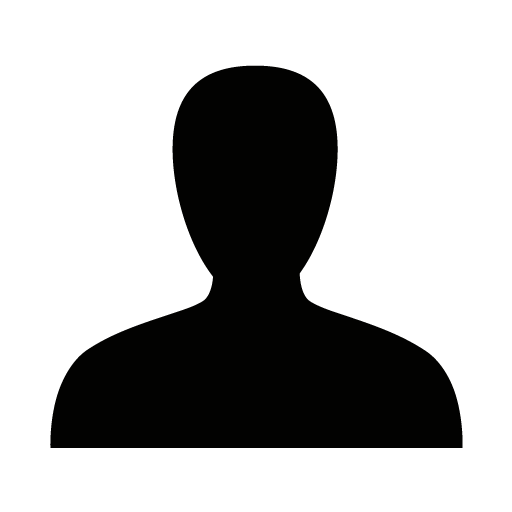
Organic semiconductors heterojunctions have recently gained significant interest in solar fuel production. In contrast to widely studied inorganic photocatalysts, the synthetic tunability of organic semiconductors, such as conjugated polymers and covalent organic framework (COF), allows the design of materials with tuned bandgaps for photocatalysts that can absorb a wider proportion of solar spectrum. Recently, heterojunctions nanoparticles prepared from a blend of conjugated polymer and non-fullerene small molecules, as those used in the organic photovoltaic field, have shown promising efficiency for hydrogen production in the visible. [1,2] Our previous transient and operando studies have shown remarkably long-lived charge generation within the donor:acceptor heterojunction nanoparticles on timescales useful for photocatalysis (microsecond-millisecond).[3] Using a similar approach, 2D templated polymer heterojunctions were explored as a photocatalyst for hydrogen production, prepared by combining donor and acceptor 2D polymers in a templated growth method. Transient absorption spectroscopy on timescales ranging from picoseconds to seconds were employed to monitor the kinetics of photogenerated charges in the 2D heterojunction and single 2D polymer components and their correlation with hydrogen production.[4] In this talk, the charge carrier dynamics of the novel 2D templated polymer photocatalyst with improved hydrogen evolution rates will be discussed, including the effect of charge trapping and metal cocatalyst on charge extraction.
2.3-I1
Ludmilla is an Associate Professor of Inorganic Chemistry at the Univeristy of Oxford. She obtained her B.Sc and M.Sc. degrees from the University of Siegen (Germany). During her undergraduate studies she developed an interest in electrochemistry and semiconductor physics driving her to pursue a M.Sc. project on dye-sensitized solar cells in the group of Professor Michael Grätzel at the École Polytechnique Fédérale de Lausanne (EPFL, Switzerland). Staying in the same group, Ludmilla worked on oxide thin film photoelectrodes applied in photoelectrochemical water splitting and perovskite solar cells during her Ph.D. degree which she obtained in 2016. She then joined the group of Professor James Durrant at Imperial College London to study photochemical and photophysical processes in semiconductors using time-resolved spectroscopy and shortly after was awarded the Marie Skłodowska-Curie Fellowship (2017-2019). Ludmilla began her independent research career as Imperial College Research Fellow (2019-2021) before moving to Oxford in October 2021. Her research at Oxford aims at the design of atomically defined photo- and electrocatalysts that convert CO2, water and other “waste products” to energy-rich fuels and chemicals with high conversion efficiency, selectivity and long operational stability.
The pursuit of efficient, earth-abundant and stable photocatalysts has been the holy grail since TiO2 was discovered as a water-splitting photocatalyst more than 50 years ago.[1,2] Ever since, wide bandgap absorbers such as TiO2, SrTiO3 or Ga2O3 have dominated the field of particulate photocatalyst development predominantly due to their deep valence bands driving water oxidation and remarkable stability.[3] However, with less than 5% UV light in our solar spectrum, the overall solar energy conversion efficiency of such wide-bandgap systems is by default limited to efficiencies below 1%. Even with the most outstanding Al:SrTiO3 photocatalyst achieving one of the highest quantum yields reported, the overall solar-to-hydrogen conversion efficiency was only 0.65%.[4]
Tremendous efforts have been undertaken to extend the absorption into the visible to maximise the light-harvesting efficiency. On this quest, countless oxide and nitride as well as some oxynitride and oxysulfide materials with smaller bandgaps have been intensively studied with varying success.[3,5] In the novel visible light absorber (La,Sr)(Rh,Ti)O3 employed in the Z-scheme photocatalyst sheet device from Profs. Wang and Domen, the controlled A and B site substitutions led to a record 1% solar-to-hydrogen efficiency.[6]
Substitution on A and B-sites of the perovskite ABX3 crystal structure creates interesting defect chemistry widely studied in photo- and electrocatalysis. However, as our recent work on the La,Rh-co-doped SrTiO3 showed, attention to the formation of undesired trap states is particularly important when attempting to induce visible light absorption into wide-bandgap semiconducting photocatalysts.[7]
In this talk, I will dive into defect engineering and chemistry of a small series of oxide perovskites that we employ in photo- and electrocatalysis and discuss challenges and ambiguities in such defect engineering approaches as well as considerations when operating under reducing conditions.
2.3-I2
A key challenge in photocatalysis is to generate sufficiently long-lived excited states capable of driving the desired transformations but with a minimal energy cost. In Photosystem II, extending lifetimes is achieved with a series of redox co-factors. This enables the oxygen evolution reaction to take place, but at a high energy penalty (approx. loss of 40% of the energy of a red photon). Similarly, in artificial systems recombination reactions are suppressed by applying external biases or by using sacrificial reagents. This enables carriers to live long enough to react but lowers the overall energy efficiency of the system. While many photo-absorbers can be prepared, there is still no clear blueprint for the design of materials with intrinsically long-lived carriers that maximise photo-conversion efficiencies. In this talk, I will present an experiment systematically characterising the excited state lifetime and recombination dynamics in a series of energy conversion materials. Our data reveals a correlation between the achievable lifetime and the solid’s electronic configuration pointing towards intrinsic factors limiting performance. I will discuss the implications and opportunities that such intrinsic behaviour has in our ability to generate long-lived excited states for catalysis and other energy conversion systems.
2.3-O1
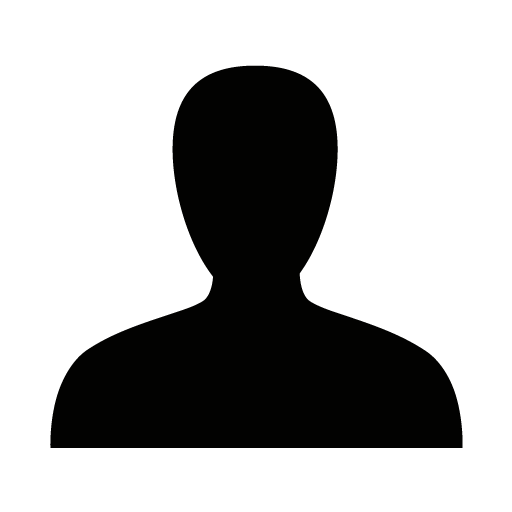
High performance, light absorbing semiconductors are of intense interest for the solar-driven generation of sustainable fuels in photocatalytic and photoelectrochemical systems. Whilst metal oxides are the most-studied photoactive materials for the evolution of hydrogen from water and methanol from carbon dioxide and water, organic semiconductors can advantageously be synthesised from abundant sources; offer control over microstructure, light harvesting properties and redox potentials; and have the potential to be used in technologically simple devices. Despite substantial increases in the activities of organic photo-driven systems over the last five years, few studies[1] have managed to deconvolute the many factors (structural, optical, and electronic) that define the performance of these materials.
In this talk, I will primarily use transient absorption spectroscopy to demonstrate that generating charges which can live for microseconds is critical to the performance of a wide variety of different carbon nitride and linear conjugated polymer photocatalyst and photoelectrode systems [2-5]. However, generating large yields of these long-lived charges is a serious challenge in organic materials as excitons and charges typically recombine on the picosecond-nanosecond timescale. I will present several design strategies which can be employed to retard these processes, with particular focus on the timescales at which sacrificial agents and water influence the photophysics of the polymer system.
I will first use a series of novel sulfone-containing linear conjugated polymer photocatalysts to demonstrate that excitonic hole transfer from the polymer to the sacrificial donor can occur on the picosecond timescale, strongly competing with exciton recombination [2]. Crucially, the ability of the best-performing polymer to generate long-lived electrons is dependent on the thermodynamic driving force for hole transfer to the sacrificial donor.
Alternatively, excitons can be separated using an organic heterojunction. However, whilst a PM6:Y6 photoanode can efficiently separate excitons on the picosecond timescale with no applied bias, long-lived active charges are only observed when spatially separated by an applied bias [3]. We similarly find that low charge mobility in carbon nitride photoanodes limits their ability to physically separate charges, and can be improved by introducing a conductive network into the electrode [4]. Building on these observations, we demonstrate that the lifetime and yield of PM6 hole polarons can be improved in the PM6:Y6 photoanode by adding a PM6 overlayer on top of the bulk heterojunction. The PM6 overlayer aids the spatial separation of charges, retarding bimolecular recombination [3]. Finally we show that the inclusion of oligo(ethylene glycol) side chains to a polymer photocatalyst also extends the lifetime and yield of the active electrons relative to its alkylated analogue [5]. In this case, the hydrophilicity of the side chains causes substantial swelling, with the resulting spatial separation again reducing recombination.
Overall, I aim to show that a wide range of photo(electro)chemical organic materials and device structures can be improved through several common design themes.
2.3-O2
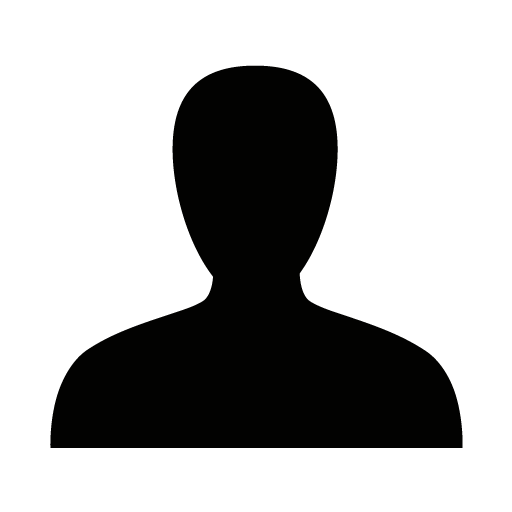
Intensity Modulated Photocurrent Spectroscopy (IMPS) has been largely employed in semiconductors characterization, to probe the operando behavior with widely available facilities. However, the implementation of IMPS data analysis to complex structures, such as the semiconductor-electrolyte interface or buried heterojunctions, is generally limited to qualitative evaluation of the steady-state conversion efficiency. In this work, a new algorithm for the analysis of IMPS data is developed, providing unprecedented insights into the time resolved charge transfer/recombination paths in semiconductors for solar energy conversion, from photoelectrochemical (PEC) water splitting to photovoltaics. The algorithm, based on the previously developed Distribution of Relaxation Times (DRT) analysis, here modified with Lasso regression method, is validated on different PEC systems, such as Ti modified hematite photoanode for PEC water oxidation, identified as a standard platform in the field, but also CoPi modified hematite for valorization of biomass derivated and CIGS based photocathodes for water reduction. The application of the L-DRT algorithm to IMPS data provides several advantages compared to the conventional kinetic analysis, including straightforward identification of multiple charge transfer and recombination paths, improved time resolution, providing precise and reliable description of the characteristic lifetime of each process and extension of the potential window suitable to kinetic analysis due to the deconvolution of subtle recombination peaks [1], [2].
1.1-I3
Sixto Giménez (M. Sc. Physics 1996, Ph. D. Physics 2002) is Associate Professor at Universitat Jaume I de Castelló (Spain). His professional career has been focused on the study of micro and nanostructured materials for different applications spanning from structural components to optoelectronic devices. During his PhD thesis at the University of Navarra, he studied the relationship between processing of metallic and ceramic powders, their sintering behavior and mechanical properties. He took a Post-Doc position at the Katholiek Universiteit Leuven where he focused on the development of non-destructive and in-situ characterization techniques of the sintering behavior of metallic porous materials. In January 2008, he joined the Group of Photovoltaic and Optoelectronic Devices of University Jaume I where he is involved in the development of new concepts for photovoltaic and photoelectrochemical devices based on nanoscaled materials, particularly studying the optoelectronic and electrochemical responses of the devices by electrical impedance spectroscopy. He has co-authored more than 80 scientific papers in international journals and has received more than 5000 citations. His current h-index is 31.
The development of sustainable strategies to produce added-value chemicals and fuels using renewable resources is particularly attractive to promote a transition towards a more sustainable energetic landscape, overcoming the dependence of fossil fuels at a global scale. One of the most promising alternatives involves the use of renewable electricity (wind, solar, hydropower, etc…) to power electrochemical conversion processes, which convert abundant molecules (e.g., water, carbon dioxide, and nitrogen) into higher-value products (e.g., hydrogen, hydrocarbons, oxygenates, and ammonia). In this context, the use of materials based on earth-abundant elements (Fe, Ni, Cu, etc…) is key to ensure the scalability and large-scale implementation. We will discuss the use of some earth-abundant (photo)electrocatalysts based on these elements for different reactions of wide technological interest, also focusing on the mechanistic understanding of the physical-chemical processes taking place during operation.
1.1-I1
Photoelectrochemical (PEC) water splitting is an attractive method for the production of hydrogen from renewable energy sources. We have in recent years focused on both oxygen evolution reaction and hydrogen evolution reaction materials. In this talk, we want to summarize photoelectrode and surface engineering paths to high-performance electrodes for water splitting.
Firstly, we will report on BiFeO3 (BFO) which has recently been identified as a promising photocathode material due to its light absorption and photo-electrochemical properties [1-3]. For practical applications, however, the PEC performance of BFO needs to be improved, which requires understanding of the aspects that limit its activity. We will present the effect of the ratio of Bi to Fe in the precursor solution of the sol-gel synthesis on the properties of BFO thin films [4]. Thin films with a stoichiometric ratio of Bi:Fe and thin films with 10% excess of Bi are prepared on fluorine-doped tin oxide. While bulk characterization techniques (XRD, RBS) show the formation of phase-pure BFO, surface characterization techniques (XPS, LEIS, TEM, ToF-SIMS) indicate Bi enrichment on the surface. The light absorption and band gap do not change upon adding 10% excess Bi in the precursor solution. However, the current density of the excess Bi samples is nearly two times larger than that of the stoichiometric BFO film at 0.6 V vs RHE. Electrochemical impedance spectroscopy explains this improved performance in terms of a lower recombination rate and a lower charge transfer resistance in Bi excess films. The lower recombination rate is attributed to less defects in the thin films, i.e. less Bi and O vacancies (XPS, TOF-SIMS). The low charge transfer resistance is attributed to a distinct Bi-oxide top layer that was detected on all samples (XPS, TOF-SIMS, LEIS, TEM). In summary, we can conclude that adjusting the Bi:Fe ratio is an effective manner to enhance the PEC performance of BFO thin films for hydrogen evolution reaction.
Secondly, we will discuss a series of papers where we use WO3 as oxygen evolution material for photo-electrochemical water splitting [5-8]. We start with discussing physical and chemical defects in WO3 thin films and their impact on photo-electrochemical water splitting. We then transfer the thin film to scalable substrates, such as Si wafers, and discuss how the performance is boosted with WO3/n-Si heterostructures. The role of Si and its impact on interface engineering is investigated. Then, we extend the study to the third dimension by investigating micro-pillars and nanowires and the impact of the three dimensional structure through surface area and light management on the performance.
We will conclude with sketching our further efforts to high-performance electrodes via photoelectrode and surface engineering using and developing further paths to scalable methods.
1.1-I2
Doing my BSc/MSc in Physics and PhD in an interdisciplinary program crossing the disciplines like Chemical Engineering, Nanotechnology, and Electrochemistry made me who I am today – a scientist who enjoys the challenge of multifaceted research.
I enjoy doing basic research in order to solve applied tasks. This explains my research interest in fundamental physical chemistry, e.g. oxidation and dissolution of metals and semiconductors, electrocatalysis, and electrochemistry at modified interfaces but also electrochemical engineering, e.g. development and optimization of catalyst layers in fuel cells and water electrolyzes.
Progress in basic research is often a direct outcome of previous achievements in experimental instrumentation. Hence, a significant part of my interest is in the development of new tools, e.g. electrochemical on-line mass spectrometry, gas diffusion electrode approaches, and high-throughput screening methods.
Moving from materials to devices is unthinkable without a preceding thorough assurance of the durability of all of their constituent components. Considering the crucial green energy technologies, photovoltaic cells for renewable electricity generation and water electrolyzers for hydrogen production using renewables already demonstrate the required operational stability over years, if not decades. Unfortunately, the same cannot be said for the cells used for the photoelectrochemical water splitting. As for now, with numerous efforts toward optimizing the activity, the more significant challenge of tailoring the durability of photoelectrodes to meet industrially relevant levels remains. Considerable research efforts are necessary in order to improve our understanding of the degradation processes and develop mitigation strategies. Dissolution (as part of corrosion) is one of the degradation processes. This talk will present our most recent results on the dissolution of representative photoelectrodes (photoabsorbers with or without co-catalysts) during water splitting.
Over the last years, the dissolution stability of different electrocatalysts has been investigated in our group using an inductively coupled plasma mass spectrometer (ICP-MS) directly connected to an electrochemical cell [1]. In this configuration, time- and potential-resolved dissolution analysis is possible. Recently, such a cell was equipped with a solar simulator, Air Mass 1.5 G filter, and monochromator, allowing standardized photoelectrochemical measurements [2]. The dissolution stability of representative WO3, BiVO4, and Fe2O3 photoabsorbers was investigated in different electrolytes with or without a co-catalyst overlayer [3-5]. With the help of electrolyte and interface engineering, stabilization of the photoabsorbers was demonstrated. The gained insights can then be utilized to advance synthesis and operation approaches of novel materials with improved photostability.
Besides dedicated fundamental studies on well-defined electrodes, flow cell setups are well-suited for operation in a high-throughput manner [6]. This potential was realized in a proof-of-concept study on Fe–Ti–W–O thin film materials libraries using fully automated activity-stability measurements [7]. Such a platform would enable material discovery, which is tailored to search not only for the most active but also for the most stable material.
1.2-O1
Photovoltaic-coupled photoelectrochemical (PV-PEC) water splitting devices offer a route to high efficiency solar-to-hydrogen (STH) conversion with low-cost and non-toxic materials, in particular given the recent advances in efficient and earth-abundant PEC (e.g. metal-oxide) and PV (e.g. organic, perovskite) materials. [1][2][3] However, achieving STH efficiencies relevant for commercialisation (~15-20%) requires careful optimisation of the electronic and optical properties of the constituent materials, as well of the device configuration to minimise parasitic optical and electrical losses.
Here, we present a optical-electronic model for simulating the current-voltage curves of PV-PEC tandem cells, and which includes descriptions of important (optical and electronic) loss mechanisms. PV operation is modelled using diode equations that incorporate loss estimates due to radiative and non-radiative recombination. For describing PEC operation, we extend the Gärtner formulism for PEC photocurrent generation to treat surface recombination due to charge trapping and correct for Fermi level pinning due to an overpotential drop over the Helmholtz layer. We then study the effect of changing semiconductor properties, including conduction and valence band levels, electron and hole masses, charge-diffusion length, Fermi level and trap densities, as well as optical considerations (e.g. parasitic light absorption due to electrolyte and bubble formation) on PV-PEC operation. This allows us to determine the PEC and PV semiconductor properties and device configurations that are required to reach STH efficiencies relevant for commercialisation, in particular using state-of-the-art metal-oxide, organic and perovskite semiconductors.
1.2-O2
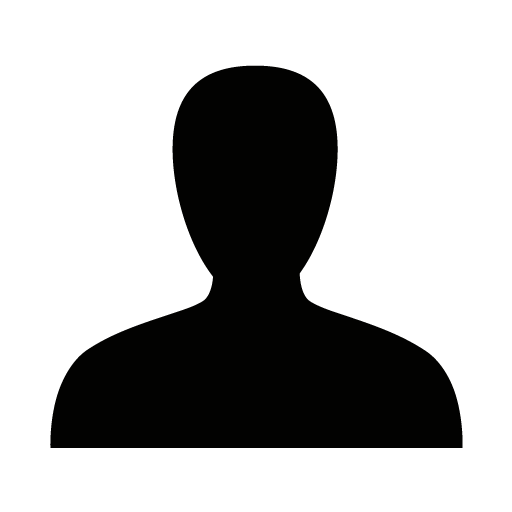
Hydrogen generated from solar-driven water-splitting process has the potential to be a clean, sustainable and abundant energy storage option [1,2]. The devices implemented in an integrated design approach, i.e., submerged in the electrolyte, pose a significant limitation when it comes to up-scaling [2]. The performance decreases due to added ohmic losses in the electrode or electrolyte and local pH gradients represents a significant challenge to overcome. The presence of the hydrogen gas bubbles at electrolyte/photoelectrode interface may assist in managing low pH gradients, if engineered properly. Beyond the state of the art, introducing a porosity to the photoelectrodes has been proposed as a shortcut to minimize the losses associated with up-scaling [2,3]. To study the optimal porous configuration of the water splitting device, mathematical modeling simulations were performed for various pore size (dpore = 10–400 μm) and pitch of 1mm. The overall impact of tailoring the dpore indicates that increasing porosity of the decreases the ohmic loss but increases the product bubble crossover (see Figure below). For the device to be operable with acceptable voltage loss of ~100 mV, the pore size should be less than 200 µm in diameter. These investigations are expected to contribute to advancing the development of large-scale water-splitting technology.
Figure 1 (a) Color map of total voltage losses for 10 cm long electrode with different size of pores. (b) Percentage of H2 crossovers due to porous structures of electrodes.
1.2-O3
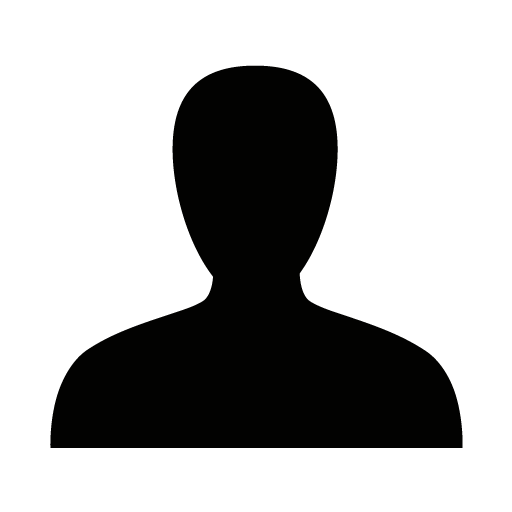
Halide perovskites received significant attention in the photovoltaic community owing to its higher power conversion efficiency, high absorption coefficient, and bandgap tunability. However, their stability in aqueous medium is poor making them unsuitable to employ for direct water splitting. Various protective layers coupled with halide perovskite are utilized to prevent the exposure of the absorber to aqueous medium. However, these protective layers are susceptible to failure with prolonged operation. So, employing a water stable absorber material is found to be the key for successful photoelectrochemical (PEC) water splitting.
Vacancy ordered double perovskites with the formula A2BX6 are a promising alternative to conventional ABX3 perovskites due to excellent stability, comparable absorption coefficient and bandgap tunability. This class of materials have their alternate octahedra being vacant enabling a wider range of B-site elements due to rotational freedom. Here, we have reported the most stable vacancy ordered halide perovskite Cs2PtCl6 and Cs2PtBr6 which showed extraordinary stability in air and even in extreme acidic and basic mediums. Furthermore, these materials exhibit absorption properties that encompass a substantial portion of visible light. The capability of the material to undergo anion exchange from Cs2PtCl6 to Cs2PtBr6 via core-shell mechanism to form Type-II heterostructure is demonstrated. These materials are successfully employed as photoanode for PEC water oxidation which displayed photocurrent density of >0.2 mA/cm2 at 1.23 V vs. RHE.
1.2-O4
While hydrogen production via photoelectrochemical water splitting has been demonstrated on a small scale, developing an industrial scale device is a challenge that brings together researchers from a range of disciplines, including engineers, material scientists and chemists. Development of photoelectrochemical water splitting devices requires an understanding of semiconductor physics, optics, (photo)electrochemistry (including catalysis and corrosion) and chemical engineering (e.g. fluid mechanics, heat and mass transport). These are all critical to the development of efficient and robust devices.
I will discuss our in-house developed photoelectrochemical (PEC) device, with a total photo-absorbing area in excess of 100 cm2. The device operates with photoanodes fabricated by chemical vapour deposition, a prevalent and scalable method, of sequential layers of WO3 nanorods and BiVO4, to form a staggered heterojunction on FTO. The 2.4 to 2.5 eV bandgap of BiVO4 enables light absorption up to 517 nm in wavelength and a theoretical solar-to-hydrogen efficiency (ɳSTH) of up to 9.2 %. The WO3/BiVO4 heterojunction system is one of the most promising in terms of performance, cost and durability. Combined with a Ni mesh cathode and an externally mounted homojunction Si PV, and operated in a pH neutral phosphate buffer solution, this creates a cost-effective and scalable photoelectrochemical-photovoltaic (PV-PEC) device with a commercially viable fabrication method. In initial experiments, we have achieved a spontaneous solar-to-hydrogen efficiency (ɳSTH) of 4.0 %.
Scale-up of PEC devices comes with numerous challenges which must be addressed by both computational and experimental approaches. I will discuss the ways in which we have combined these approaches in our research and initial progress to-date. I will also show strategies we have been developing towards mitigating performance losses within our device, including reducing severe potential and current gradients across poorly conducting photoelectrodes. I will discuss other aspects critical to the scale-up of photoelectrochemical devices, including heat and mass transfer, fluid dynamics and safety challenges associated with increasing product and precursor volumes.
Despite the urgent need for engineering progress in this area to accelerate this type of solar hydrogen technology, there are relatively few academic publications that address this topic. With a lack of demonstration prototypes, it remains difficult to envisage what an industrial-scale installation for clean hydrogen production might look like. Ultimately, availability of green hydrogen is expected to result in the hydrogen market expansion. This research seeks to elucidate engineering challenges of developing large-scale water splitting devices, to facilitate the pathway to commercially viable photoelectrochemical hydrogen production.
Key words
Photoelectrochemistry, hydrogen, scale-up, electrochemical engineering, BiVO4
1.2-O5
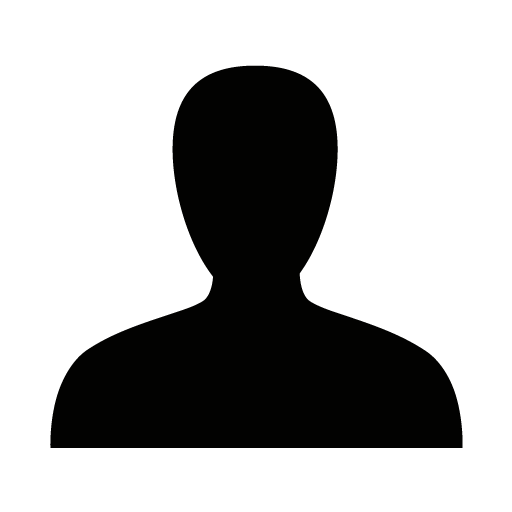
BiVO4 is considered one of the most promising metal oxide semiconductors for photoelectrochemical water oxidation. Many studies focus on addressing the inherent material limitations, such as charge carrier mobility and slow water oxidation kinetics. For example, it has been shown that doping with some metals and non-metals increases the charge carrier mobility and the addition of a co-catalyst improves the water oxidation kinetics. These techniques are effective in such a way that ninety percent of the theoretical AM1.5 photocurrent of BiVO4 has been achieved. This progress has shifted some focus towards scaling up, in which it has unfortunately been reported that the scale-up process significantly impacts the photocurrent and stability. Identifying and addressing the scale-up losses and optimizing the associated overpotential is therefore crucial. In this study, we scaled-up sulfur incorporated BiVO4 photoelectrodes using a screen printing technique. Photoelectrodes with area up to 100 cm2 were fabricated uniformly, and the performance was evaluated using a continuous flow photoelectrochemical cell. Specifically, the effect of scale-up on the overall overpotential of the cell, which includes ohmic and concentration overpotentials, was considered. Strategies to minimize these losses, such as increasing the substrate conductivity and electrolyte engineering (modifying the concentration and the flow rate of electrolyte), were implemented. As a result, we successfully minimize the photocurrent loss to only ~12% when the photoelectrode area is increased by more than two orders of magnitude, from 0.24 to 100 cm2. Further optimization of the ionic and concentration overpotential associated with scale-up is ongoing and will be discussed.
1.2-O6
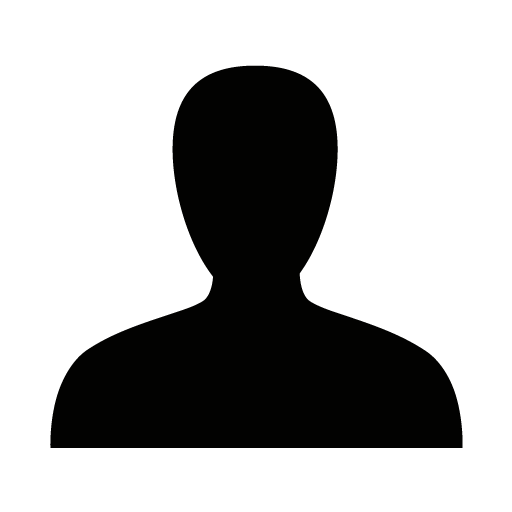
Direct connection of PV devices to electrochemical (EC) water-splitting cells or batteries (B) is a highly efficient and material-saving solution to stabilize intermittent PV generation on long- and short-time scales. In our study, we address a hybrid device with EC water splitting cell in parallel connection to a battery. The first obvious advantage of this PV-EC-B system is the much more stable operating conditions of the electrolyzer. A sufficiently scaled battery keeps the EC cell running overnight and shaves peak load during the daytime. In our works, we have shown the feasibility of the self-sustained operation of the PV-EC-B system [1, 2]. Besides the foreseeable stabilizing effects, we have found theoretically [1] and confirmed experimentally [2] that batteries can improve solar-to-hydrogen efficiency (STH) in PV-EC-B devices as compared to the PV-EC reference. Batteries can boost STH in PV-EC-B systems because, under periodic irradiance conditions, the battery transfers part of the daytime PV energy to the night, reducing EC cell power and suppressing related overpotential (kinetics) losses. The gain is synergistic – even despite additional potential losses in the battery, the EC-B combination has lower losses than the EC cell operating alone [1, 2]. From the point of view of the system design the reduction of operating power and its stability allows for the use of a smaller electrolyzer at the same nominal PV capacity.
In our report we will present details of the PV-EC-B operation, principles behind the gain in STH attained with battery, and our recent experimental study. An increased average STH efficiency per cycle (11.4 % vs. 10.5 % without the battery) has been observed in the PV-EC-B system of a Si heterojunction PV module, bifunctional NiFeMo electrolyzer, and a commercial Li-ion NMC battery. The results are discussed in the context of the generalized STH limit analysis developed earlier for PV-EC combinations [3].
Applying our analysis to different literature sources using PV-EC without batteries we present an analysis of what could be achieved if a battery is applied to these systems in the figure below. We calculate the STH limit with and without a battery for each experimentally reported system in literature as well as the STH gain with a battery.
Each system reported in the literature is represented by four symbols: filled blue circles show the reported experimental STH of each PV-EC system; open blue circles indicate the STH limit calculated for each system using the reverse analysis [3]; horizontal dashes represent the estimate for the STH limit in this system if the battery is included; finally, the red circles show the estimate of the STH gain for each system.
A separate set of points denoted “this work” represents the experiment on the PV-EC-B system in this work.
For approximately half of the reported systems STH gain of 0.5%abs – 1%abs can be expected, several systems can gain 1%abs – 2%abs, while several systems can gain 2%abs – 4%abs. There is a general trend for higher STH gain at higher PV efficiencies, but the data presented in Figure 4 does not constitute any dependence.
1.3-I1
Suitable photo-electrode materials are known to be the main bottleneck for large scale deployment of devices for solar fuel production. These semiconducting materials must be efficient, stable and scalable, although, usually two out three of these qualities are met. Hematite is an extensively researched photoelectrode material, known for its long-lasting properties and facile synthesis. However, the position of its band edges and high recombination rates, resulting in low efficiencies, preclude hematite to be used in real applications. On the other hand, complex photo-electrodes with laborious synthesis, e.g. III-V tandems absorbers (GaInP/GaAs), coupled with RuOx and PtRu catalysts, have proven to be highly efficient for water splitting [1].
With the aim to improve scalability, concentrated light sources allow the use of smaller photoelectrodes, provided they are stable and efficient at operating conditions. However, the use of higher irradiation (>100 kW m-2) unlocks additional constraints related to heat management, ohmic drop due to inordinate current densities, and unknown electron/hole transfer kinetics under high photon flux and at higher temperatures. It is expected that these effects could be alleviated by careful reactor design, and choosing suitable substrates and operating conditions. However, these issues have been seldom investigated and only a few studies on photoelectrochemical cells for irradiations typically below 30 suns have been reported [2].
Here, we present the model and preliminary experimental validation of a photo-electrochemical cell operating under concentrated irradiation (>100 kW m-2), using metal oxides (Fe2O3 and BiVO4) as photoelectrode materials, which were selected due to their well-known and predictable photoelectrochemical behavior [3,4]. The effects of the substrate material (Ti or transparent conductive oxides deposited on glass), electrode configuration, electrolyte flow and spectrally resolved photon intensities on the performance of the cell will be presented. Initial predictions indicate that conductive glass is not a suitable substrate due to its low thermal conductivity, resulting in undesirable high temperatures (>80 °C) when operating under irradiations of 600 kW m-2.
References:
[1] Young, J., Steiner, M., Döscher, H. et al. Nat Energy 2017, 2, 17028.
[2] Vilanova, Mendes, A. Journal of Power Sources 2020, 454, 227890.
[3] Bedoya-Lora, F. Hankin, A. Kelsall, G. J. Mater. Chem. A, 2017, 5, 22683-22696
[4] Gaudy, Y. Haussener, S. et al. J. Mater. Chem. A, 2018, 6, 17337-17352
1.3-I2
In my talk I will revise the recent efforts done in my group regarding the integration of first principles studies into more advanced multiscale simulations. I will show the main difficulties and bottlenecks for the implementation of a more robust computational framework that can be used in a more general manner and that should give us access to more adequate models in the field. For instance I will address the main difficulties in the modeling of excited states and how they are more ubiquotous and diverse than previously considered and how modeling can address some of the challenges ahead of us to obtain proper representations. Also I will revise the types of approaximations when attempting multiscale approaches and how they need to be tuned and supported by machine learning techniques in some of the cases to reduce the dimensionality problems and the low energy events that typically consume the simulation times.
1.3-I3
Fatwa Abdi is an Associate Professor at the School of Energy and Environment, City University of Hong Kong. Until July 2023, he was a group leader and the deputy head of the Institute for Solar Fuels, Helmholtz-Zentrum Berlin (HZB). He obtained his PhD (cum laude) in Chemical Engineering from TU Delft, the Netherlands, in 2013. He was the recipient of the Martinus van Marum prize from the Royal Dutch Society of Sciences and Humanities. His research focusses on the development of novel (photo)electrode materials as well as engineering and scale-up of devices for solar fuels and chemicals conversion.
Green H2 has been recognized as an important element in efforts to decarbonize our fossil fuel-dependent society. One approach to produce green H2 is solar water splitting in a photoelectrochemical (PEC) device. Solar-to-hydrogen (STH) efficiencies of up to 30% have been demonstrated[1] but studies have shown that this approach still results in a relatively high levelized cost of hydrogen (LCOH) of ~10 US$ per kg of H2.[2-3] This is ca. one order of magnitude higher than that of hydrogen produced via steam methane reforming (SMR), which forms the bulk of the currently produced H2. A possible solution is to incorporate an upgrading process of biomass feedstock that generates valuable chemicals into the solar water splitting device. This is expected to not only decrease the overall LCOH but also introduce an alternative renewable pathway in chemical manufacturing. In this study, we propose the concept of solar-driven hydrogenation of biomass-derived feedstock. Photoelectrochemically generated H2 in our solar water splitting device is coupled in situ with the homogenously catalyzed hydrogenation of itaconic acid (IA) to methyl succinic acid (MSA). IA has been identified by the US Department of Energy as one of the twelve building blocks that possess the potential to be transformed subsequently into several high-value bio-based chemicals or materials. MSA is a valuable chemical compound with an estimated global market size of up to ~15,000 tonnes, whose derivatives are ubiquitously used as solvents in cosmetics, polymer synthesis, binders in powder coatings, and organic synthesis, especially for pharmaceutical synthesis.[4-5] Our coupled hydrogenation approach—performed in the PV-electrolyzer and PEC configurations using III-V PV cells and BiVO4-based photoelectrode, respectively—successfully demonstrate solar-driven H2-to-MSA conversion as high as 60%. In comparison to the non-coupled approach (i.e., direct hydrogenation), our coupled system offers synergistic benefits in terms of prolonged durability and a higher degree of flexibility toward other important chemical transformation reactions. In addition, life-cycle net energy assessment and technoeconomic analysis results show that adding the coupled hydrogenation process significantly lowers the energy payback time and the LCOH, respectively, to a point that is competitive even with SMR-produced H2.[6] Further implications and optimization potentials of the coupled PEC hydrogenation approach, also beyond the demonstrated hydrogenation of IA to MSA, will be discussed.
1.1-I1
Green hydrogen is a key future energy resource, and its generation through electrochemical water splitting is a prominent option for industrial applications. This calls for robust and low-cost electrocatalyst to facilitate the kinetically and thermodynamically demanding oxygen evolution reaction (OER) which remains a challenging bottleneck of water splitting. In order to use the full potential of abundant transition metal-based catalysts, their structural design is only one part of the development process. In addition, their dynamic behavior and restructuring over longer operational periods during water splitting needs to be understood.[1]
Over the past years, we have brought forward a broad spectrum of 3d transition metal-based OER catalysts covering a wide range of compound types: from single atom catalysts [2] over molecules and coordination polymers [3, 4] to nanostructured and chalkogenide/phosphide-derived catalysts.[5] Selected examples of these catalyst types will be presented and their operando monitoring through X-ray absorption spectroscopy (XAS) and complementary methods will be discussed to provide in-depth insights into the dynamics of their active sites. This information is vital for the rational design of high-performance and robust electrocatalysts.
We performed a series of studies on Ni/Fe-based electrocatalysts to elucidate the interaction and optimal coordination environments of this highly active metal combination. We first constructed dual-site NiFe single atom catalysts (SACs) via a convenient synthesis protocol using g-C3N4 and glucose as precursors.[2] Their OER performance was superior to single site Ni-, Fe- and Co-SACs and the underlying structural reconstruction of the dual-site NiFe catalysts was investigated with a combination of operando XAS, high resolution HAADF-STEM investigations and DFT calculations. These results revealed that the Ni environment mainly underwent reconstruction towards active Ni-O-Fe moieties wherein both metal centers participate on the *OH deprotonation process, resulting in the formation of bridging O2 species. The improved OER performance of dual-site NiFe SACs is assisted by the formation of spin channels via the Ni-O-Fe bonds. We furthermore designed ultra-thin NiFe-based coordination polymer derivatives for high OER performance.[3] Upon mild reduction with NaBH4, the emerging reduced R-Ni8Fe2-CPs with sub-2 nm layered morphologies excelled through optimal exposure of active sites and fast electron transfer. The performance or their Ni-O-Fe active sites was further enhanced through the targeted incorporation of O and Ni deficiencies. In our next study, these materials were strategically optimized through controlled introduction of sulfur into the oxygen deficiencies around the Ni centers to generate S-R-NiFe-CPs with superior OER performance.[4] Their investigation through operando XAS revealed that sulfur tuning facilitates the formation of active NiIV-O-FeIV moieties, while their reconstruction was hindered in the sulfur-free materials, thereby confirming the benefits of anionic engineering strategies for the OER performance.
For overall water splitting, we developed a Fe-doped cobalt phosphide (Co@CoFe–P) catalyst for both hydrogen evolution reaction (HER) and OER. Low HER overpotentials at 10 mA/cm2 were observed over a wide pH range (0~14), such as 83 mV in 0.5 M H2SO4 or 104 mV in 1.0 M KOH and a good OER activity with a low overpotential of 266 mV for 10 mA/cm2 was obtained in 1.0 M KOH.[5] Operando XAS investigations demonstrated that partial Fe doping promoted the formation of HER active P–Co–O–Fe–P configurations in Co@CoFe–P with a lower energy barrier for water dissociation and H* intermediate adsorption. Under OER conditions, a reconstruction process into OER active intermediates with CoIV–O–FeIV moieties was observed, while the formation of these active unites was hindered in P-free reference Co-FeOOH, thereby underlining the efficiency of anionic substitution strategies.
The talk will be concluded with an outlook on molecular and related Co-based OER catalysts to bridge the design perspectives between molecular and heterogeneous catalysts.
1.1-I2
Antoni Llobet is Professor of Chemistry at the Universitat Autònoma de Barcelona (UAB) and Group Leader at Catalan Institute for Chemical Research (ICIQ) in Tarragona, Spain. He carried out his PhD at UAB on coordination chemistry of first raw transition metals. He then did one post-doct at the University of North Carolina with Thomas J. Meyer on redox properties of Ru complexes and second post-doct at Texas A&M University with Arthur E. Martell and Donald T. Sawyer on redox catalysis. He has now established a group at ICIQ that deals broadly on topics related to artificial photosynthesis with special focus on light harvesting and on oxidative and reductive catalysis. He has published over 125 research papers. In 2000 he received the Distinction Award from Generalitat de Catalunya for Young Scientists and recently he has been awarded the Bruker-Inorganic Chemistry prize of the Spanish Royal Chemical Society.
The replacement of fossil fuels by a clean and renewable energy source is one of the most urgent and challenging issues our society is facing today, which is why intense research is devoted to this topic.[1] Nature has been using sunlight as the primary energy input to oxidize water and generate carbohydrates (a solar fuel) for over a billion years. Inspired but not constrained by nature, artificial systems can be designed to capture light and oxidize water and reduce protons or other compounds such as CO2 to generate useful chemical fuels. One of the key aspects for the efficient design of useful devices for the making solar fuels is the understanding and mastering of the anodic reaction involving the oxidation of water to dioxygen. The talk will describe the initial developments up to the state of the art, of molecular water oxidation catalysts and their anchoring on conductive and semiconductive surfaces. The latter is crucial for the generation of powerful hybrid molecular anodes for the production of solar fuels.[2]
[1] (a) Matheu, R.; Llobet, A. et al. Nat. Rev. Chem. 2019, 3, 331–341.
(b) Vereshchuk, N.; Llobet, A. et al. Chem. Soc. Rev. 2023, 52, 196-211
[2] (a) Hoque, Md. A.; Gil-Sepulcre, M.; Llobet, A. et al. Nat. Chem. 2020, 12, 1060–1066. (b) Schindler, D.; Gil‐Sepulcre, M.; Llobet, A.; Würthner, F. et al. Adv. Energy Mater. 2020, 2002329. (c) Gil-Sepulcre, M.; Llobet, A. et al. J. Am. Chem. Soc. 2021, 143, 11651–11661. (d) Gil-Sepulcre, M.; Llobet, A. Nat. Catal. 2022, 5, 79-82.
1.1-I3
Molecular catalysts play a significant role in chemical transformations, utilizing changes in redox states to facilitate reactions. In the broadening field of carbon dioxide (CO2) electrolysis to value-added products, catalyst choice strongly impacts product formation. To date molecular electrocatalysts have efficiently produced single-carbon products from CO2 but have struggled to achieve the carbon-carbon coupling step needed to reach highly valued multi-carbon products. Conversely, copper acts as the only reliable bulk metal that enables carbon-carbon coupling, but leads to broad C2+ product spectrums.
Here we designed a molecular electrocatalyst system that subverts the traditional redox-mediated reaction mechanisms of organometallic compounds, facilitating electrochemical CO2-to-ethanol yields of 96% at optimal conditions with trace methanol and C3 products. By coupling iron tetraphenylporphyrin (Fe-TPP) with a nickel electrode, we fixed the iron oxidation state during electrocatalytic CO2 reduction to enable further reductions and coupling of *CO intermediates. This represents a marked behavioural shift compared to the same metalloporphyrin deposited onto carbon-based electrodes. Extending the approach to a 3D porous nickel support with adsorbed Fe-TPP, we attain ethanol faradaic efficiencies of 68% +/- 3.2% at -0.3 V vs a reversible hydrogen electrode (pH = 7.7) with partial ethanol current densities of -21 mA cm-2. Separately we demonstrate maintained ethanol production over 60 hours of operation. Further consideration of the wide parameter space of molecular catalyst and metal electrodes shows promise for additional novel chemistries and achievable metrics.1
1.2-O1
Electrocatalysis has emerged as a promising process to store renewable energy into fuels and high added-value chemicals to decarbonise the energy and fine chemical sectors. As such, the water splitting process to generate H2 and O2 is of particular interest, even though both hydrogen and oxygen evolution reactions (HER and OER, respectively) are kinetically challenging, especially when using Earth-abundant metal oxide and chalcogenide catalysts. The efficiency of these catalysts does not only depend on the nature of the metal oxide/chalcogenide, but also on their physical characteristics such as composition, magnetic susceptibility and doping variations, amongst others. However, the reaction mechanism of the HER and OER on metal oxides/chalcogenides as well as the nature of the efficiency loses remains elusive. Obtaining such detailed mechanistic and kinetic knowledge requires the use of operando spectroscopic techniques that can probe the system under operating conditions. In this talk, I will present the characterisation of a Cu2-xS cathode for HER that increases its ECSA as a function of time and a NiFeOx anode for OER that changes its reaction mechanism when a magnetic field is applied to the system.
1.2-O2
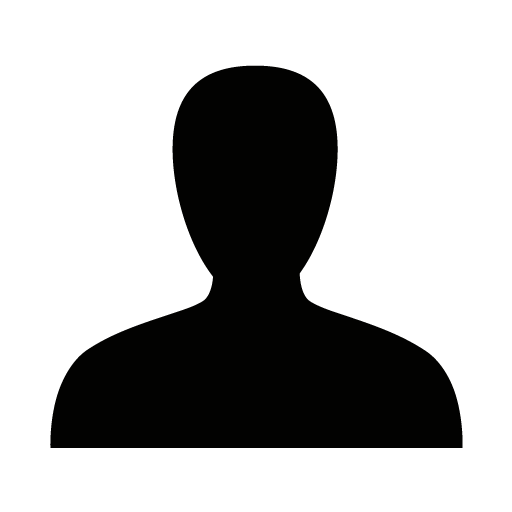
The search for highly efficient carbo-catalysts with novel compositions usually follows a two-step process. Initially, proof of concept is established using conventional fine powders. Subsequently, the focus shifts to the feasibility of scaling up the material and shaping it into practical catalyst forms. This last aspect, often overlooked, plays nonetheless a vital role in determining which catalysts have the potential for industrial application.[1] Previous works already settled the interest of phosphorus-doped carbons for alcohol oxidation reactions, acid catalysis biomass upgrade or as support for electrocatalysis.[2-3] However, little attempts have been made thus far in the direction of a technical catalyst.
We present here a straightforward production of P-doped carbon porous pellets on a multi-gram scale by simply using phytic acid ( and cellulose, both extracted from biomass and combined with a noodle pasta machine for extrusion. The so-formed spaghetti undergo a carbonization at 800 °C yielding a carbonaceous material in the shape of pellets. These latter exhibit good mechanical stability and a high phosphorus content (13 wt.%), predominantly in the form of surface phosphoric acid groups (-OPO3H2). Upon calcination, the carbonization of cellulose leads to the evolution of gases, creating extensive macroporosity beneficial for mass transport. Additionally, the release of phosphoric acid also generates micro- and mesopores (pores < 3 nm, SBET = 1000 m2/g), thereby increasing the density of available reactive sites. These characteristics would ultimately allow for the development of a flow-through catalytic structure.
The so-formed material was studied as a metal-free acid solid catalyst for the pyrolysis of waste cooking oil into biofuel. The resulting organic liquid fraction exhibits an interesting balance between alkanes and aromatics, suitable for an application as a jet fuel. Although the interest of the porous monoliths was demonstrated here in the context of thermo-catalysis, we envision to employ a similarly prepared material as a porous self-standing electrode. This approach would leverage the electronic conductivity of the pellets and the presence of abundant surface chelating groups.
1.2-O3
Dr Hui Luo is an independent academic fellow in the School of Mechanical Engineering Sciences at the University of Surrey, UK. She is also a Fellow of the Institute for Sustainability, member of the Royal Society of Chemistry (MRSC) and member of the Institute of Materials, Minerals and Mining (MIMMM).
Dr. Luo obtained her PhD in Queen Mary University of London in 2019, working on carbon materials for solar hydrogen conversion. in Oct 2019 she moved to Imperial College London working as a research associate, developing biomass electrolyser for green hydrogen and bio-chemical co-production. In Sep 2022 she worked as a senior test engineering at Ceres, before taking the Surrey Future Fellowship and join Surrey in May 2023.
Her research interests include developing and up-scaling efficient electrolysis technologies to convert biomass and plastic wastes into green hydrogen and high-value commodity chemicals. Her expertise includes nanomaterials synthesis and characterisation, water electrolysis and fuel cell technologies, in operando Synchrotron X-ray absorption, surface enhanced Raman and FTIR spectroscopy, as well as gas and liquid chromatography.
In the transition towards Net-Zero, there is significant interest in phasing out fossil fuels as both the energy source and precursor for petrochemicals. Biomass is recognised as an ideal CO2 neutral, abundant, and renewable resource substitute for fossil fuels.1 The rich proton content in most biomass-derived materials endows it to be an effective hydrogen carrier. The inherent chemical structure allows them to be easily catalysed to produce valuable commodity chemicals that can be used in applications such as biodegradable polymers and pharmaceuticals. Although historically biomass has been regarded as waste stream, recent years have seen increasing attention in valorising it into useful products.2
In this talk, I present biomass electrolysis, specifically glycerol (the waste by-product from the bio-diesel industry), as an alternative route to producing hydrogen and value-added chemicals.3 The process resembles water electrolysis, with H2 produced on the cathode via a hydrogen evolution reaction. On the anode, however, instead of oxidising water, partial oxidation of glycerol takes place, with a much lower thermodynamic requirement that can cut the electricity input by half. Here I present the fundamental knowledge on the general reaction mechanisms, acquired through advanced material characterisations and DFT calculation. At the same time, details on catalyst requirements and recent advances for the future strategic design of the processing system will be provided.
Looking beyond, besides utilising biomass, an even higher urgency lies in recycling the accumulating plastic waste into useful products. Mechanochemistry has emerged as a safe, efficient, and green technique. Using just mechanical energy, this process can cleave chemical bonds and depolymerise long-chain molecules such as cellulose and plastics, revealing huge potential in industrial applications.4 By transforming a liquid process into a solid-state reaction, this technique can also significantly reduce solvent consumption. Therefore, this talk will also cover the recent advances in mechanochemical catalysis for plastic recycling, and present the possibility of coupling mechanochemistry with electrochemistry for valorising waste into green hydrogen and value-added chemicals.
1.3-I1
Charles Machan (muh-hahn) was born in Madison, WI and grew up in Wauwatosa, WI where he attended Marquette University High School before going to Washington University in St. Louis (WashU). While at WashU he played football for four years as a defensive tackle and majored in Chemistry and German (B.A. 2008). Charles attended Northwestern University for graduate school and completed a Ph.D. in Inorganic Chemistry (2012) under the supervision of Chad A. Mirkin. At Northwestern he served as President of the Alpha Gamma Chapter of Phi Lambda Upsilon, a co-ed chemistry honors fraternity, and received the Edmund W. Gelewitz Award for Outstanding Senior Graduate Student (2012). From 2013-2016 he was a postdoctoral researcher with Clifford P. Kubiak at the University of California, San Diego. He is currently an Associate Professor in the Department of Chemistry at the University of Virginia.
The steady increase in anthropogenic carbon dioxide (CO2) emissions and atmospheric concentration continues to generate interest in using CO2 as a precursor for fuels and commodity chemicals. The conversion of CO2 has the dual benefit of addressing its associated negative environmental effects and the diminishing supply of petrochemical feedstocks. Likewise, the electrocatalytic reduction of dioxygen (O2), has relevance to the development of more efficient fuel cells and chemical oxidations, as well as our understanding of how bioinorganic systems convert energy-rich molecules during respiration. At the heart of efficient reductive transformations are proton-coupled electron transfer (PCET) reactions, where electrons and protons move in a concerted way to mitigate kinetic and thermodynamic penalties. Mechanistic understanding of these reactions can inform the design of optimized catalyst structures with improved activity and selectivity for specific products. Molecular systems are well-positioned to provide a better understanding of these reactions because of the relative fidelity with which they can be characterized, as well as the possibility for systematic testing of structure-function relationships through iterative molecular design. In addition to developing new Co-, Fe-, Mn-, and Cr-based molecular electrocatalysts for these reactions, we are exploring the use of redox mediators and flow-based electrochemical reactors to understand how these reactions can be scaled relative to comparable heterogeneous systems.
1.3-I2
Head of the Laboratory of Solar Fuels at the Centrum Nowych Technologii Uniwersytetu Warszawskiego. She obtained a PhD in Biological sciences in 1999 from the University of Warwick, UK. Postdoctoral research conducted in the group of James Barber at Imperial College London, UK led to several discoveries of novel molecular mechanisms of photosynthetic adaptation to changing environment (e.g. dissecting the molecular components of state transitions) and refining the crystallographic structure of the PSII oxygen evolving complex. Habilitation in 2009 from the University of Warsaw (UW). Since 2011 Associate Professor having established an independent research group at the UW. In 2011 Prof. Kargul established a node for solar fuels research in Poland and has led several projects on application of robust natural light-harvesting molecular nanomachines for construction of biohybrid solar cells and solar-to-fuel devices. She has extensive experience and success in leading several national and international initiatives (e.g. Founding Partner of ESF EuroSolarFuels and H2020 SUNRISE consortia; Member of Scientific Executive Board of SUNERGY large-scale R&I initiative) as well coordinating the international projects (e.g. bilateral Polish-Turkish consortium POLTUR/GraphESol and Polish/German/French/Turkish consortium Solar driven chemistry 2/SUNCOCAT) which have been focused on natural and semi-artificial solar energy conversion systems. She serves as the International Ambassador of the British Biochemical Society and serves on several editorial and strategic executive boards, e.g., as member of the Scientific Advisory Board of European Society for Photobiology, as Senior Editor of the International Journal of Biochemicstry and Cell Biology, member of the Grants Committee of the Biochemical Society (UK), expert of the NAWA programme of the Polish Ministry of Science and Higher Education, expert in NZ1 Panel of the National Science Centre, member of the Advisory Board of the European Green Deal, member of KIS4 Workgroup of Poland’s Ministry of Economic Development and Technology. Prof. Kargul’s highly interdisciplinary research spans structural biology, biochemistry, and plant physiology with electrochemistry, biophysics and material science. In her current research she focuses on structural and mechanistic aspects of the function and adaptation of the natural photosynthetic apparatus in extremophilic biophotocatalysts. She and her group apply this fundamental knowledge for the rational construction of biomolecular solar-to-fuel devices for optimised solar conversion, incorporating photoenzymes and various materials decorated with plasmonic nanoparticles.
Making fuels and chemicals directly from the sun is a highly promising approach to provide high economic efficiency by complete integration of the working modules. Directions taken include both synthetic and biomolecular or biohybrid photoelectrochemical devices characterised by various degrees of integration. Implementation of the synthetic artificial photosynthetic systems (APS) is often hampered by the necessity to apply harsh conditions for the catalysis in each half-cell to occur efficiently, photocorrosion of electrode materials, often-limited product selectivity and catalyst instability [1]. Moreover, the best performing inorganic and molecular catalysts usually encompass rare/toxic elements, which precludes such APS systems from large-scale implementation. Therefore, the field of biomolecular and biohybrid artificial photosynthesis has emerged through combining the biotic components, which have been evolutionary optimised in their photocatalytic performance, and non-toxic and cost-effective synthetic materials for selective production of target chemicals at ambient conditions [1].
Here, I present the bottom-up rational design that can yield the increased solar conversion efficiency and stability in biomolecular systems based on the robust photoenzyme, photosystem I (PSI). The PSI biophotocatalyst in these devices is interfaced with various cost-efficient, transparent electrode materials for production of green electricity and fuel. The performance of PSI-based devices can be greatly improved by tailoring the structure of the organic conductive interface, based on pyrene-NTA, terpirydine or diazonium salt ligands, to ensure the generation of unidirectional electron flow and minimisation of wasteful back reactions [2-5]. Specifically, incorporating transitional metal redox centres together with plasmonic nanoparticles in the bio-organic molecular wires significantly improves not only the light-harvesting functionality of the PSI photoenzyme but also increases its photostability and the overall photoconversion performance [2-7]. Such rational design paves the way for generation of viable and sustainable technologies for solar energy conversion into fuel and other carbon-neutral chemicals.
1.3-I3
Peter Strasser is the chaired professor of �Electrochemistry for energy conversion and storage� at the Chemical Engineering Division of the Department of Chemistry at the Technical University of Berlin. Prior to his appointment, he was Professor at the Department of Chemical and Biomolecular Engineering at the University of Houston. Before moving to Houston, Prof. Strasser served as Senior Member of staff at Symyx Technologies, Inc., Santa Clara, USA. In 1999, Prof. Strasser earned his doctoral degree in Physical Chemistry and Electrochemistry from the �Fritz-Haber-Institute� of the Max-Planck-Society, Berlin, Germany, under the direction of the 2007 Chemistry Nobel Laureate, Professor Gerhard Ertl. In the same year, he was awarded the �Otto-Hahn Research Medal� by the Max-Planck Society. In 1996, Dr. Strasser was visiting scientist with Sony Central Research, Yokohama, Japan. He studied chemistry at Stanford University, the University of Tuebingen, and the University of Pisa, Italy. Professor Strasser is interested in the fundamental Materials Science and Catalysis of electrified liquid solid interfaces, in particular for renewable energy conversion, energy storage, production of fuels and chemicals.
The rising share of renewable electricity is testament to the increasing importance of solar/wind-electric routes to harvest sun light in form of potential differences and free electrons. While some electricity is used directly or stored capacitively, an increasing portion calls for direct conversion into valuable molecular solar fuels or chemicals. This “dark” e-conversion is made possible by heterogeneous electrocatalysis on the surface of solid electrodes coupled to mass and charge transport processes. Sustainable materials synthesis pathways coupled to novel advanced characterization techniques result in a deeper understanding of the origin of reaction kinetic barriers and the origin of transport limitations. This is critically needed for the design of more efficient, electrochemical materials, interfaces, and electrodes for practical electrolytic devices for the production of e-fuels and e-chemicals.
In this presentation, I will report on recent advances in our design and molecular understanding of carbon-embedded, N-coordinated single metal atom (MNC) catalyst materials. MNC have long been attracted attention as porphyrin-inspired O2-activating reduction catalysts for hydrogen fuel cell cathodes, yet have recently been proposed by our group as highly efficient and stable CO2 – activating reduction catalysts for use at cathodes of CO2 electrolyzers. I will describe ways to characterize MNC active sites and their reactivity, and will compare and contrast their reactivity and reaction mechanisms compared to metal surfaces.
2.1-I1
The development of cheap and environmentally friendly fuels and chemicals whose production will be based on abundant and renewable resources appears as one of the main challenges in our society. Among different approaches, the direct use of sunlight for the valorisation of abundant resources, such as H2O, N2 or CO2, has been proposed as an appealing approach for the environmentally friendly and sustainable production of high added-value fuels and chemicals, such as H2, NH3, CH3OH or CH2CH2, among others, the so-called “solar fuels”. In this context, the photochemical solar fuels production is currently limited by the low light-to-chemical efficiency and the fact that in semiconductor-based photocatalysts light harvesting is usually restricted to the UV and visible blue light, and typically is affected by low charge separation efficiency and high recombination rates. In an alternative mechanism, photons from the visible and near infra-red (NIR) regions may interact with metal nanoparticles supported on thermally insulating materials by localized metal surface plasmon resonance (LSPR). In this pathway, much higher production rates and conversions have been reported since photon absorption promotes a localized heating in the active sites due to photon energy thermalization and generation of “hot carriers”, which influence the electronic structure of the species involved in the reaction.
In this presentation, it will be shown the design and fabrication of advanced multifunctional materials for the photothermal CO2 hydrogenation and NH3 production reactions in continuous flow. Moreover, mechanistic studies aimed to unravel which are the actual acfive sites and operational mode will be commented in detail. These research has the potential to advance the field and pave the way for the sustainalbe production of fuels and chemicals.
2.1-I2
Roughly 95 % of the hydrogen consumed in Europe and over 95% of organic chemicals still rely on fossil resources. Therefore, finding alternative resources and clean technologies to mine those chemicals is instrumental to secure a sustainable chemical industry. The prospects of sourcing hydrogen from water and upcycling carbon dioxide and lignocellulosic biomass into chemical commodities and fine chemicals has paved the way for the advent of a new generation of technologies and concepts in this chemical space. Among them, (photo)electrochemical and photocatalytic approaches have gained in recent years capitalizing on their scalability, competitive performance and low-cost. To date, however, these technologies still remain at an early stage of development displaying conversion efficiencies below the expectations. A better understanding of the fundamental processes that govern the catalytic transformations at the reactive interface, together with innovative manufacturing protocols to engineer the materials holds the key to rationally advance these promising technologies.
In this talk, we will discuss our most recent progress in the fields of photoelectrochemical (PEC) water splitting and carbon dioxide conversion, as well as new electrochemical and photocatalytic platforms for biomass valorization. Firstly, we will describe various methodologies to improve the performance of photoelectrodes (chalcogenides, metal oxides) for solar water splitting while identifying the key processes that limit the catalytic response. Likewise, we will show the prospects of driving the PEC CO2 conversion directly at the semiconductor-liquid interface when using chalcopyrite electrodes and how the nature of the solvent dictates the response. Secondly, we will present various photocatalytic reactors, based on semiconductor nanocrystals and photoredox systems, capable of driving the selective fragmentation of lignocellulosic biomass into simple aromatics. In addition, electrochemical reactors for lignin valorization will be presented including a detailed mapping of the catalytic activity – composition relationships of the electrodes.
2.1-I3
Photoelectrochemical and photocatalytic conversion of water and carbon dioxide using solar energy offers a clean solution to the world energy requirements of a sustainable future. Achieving its full potential depends on developing inexpensive photoelectrodes and photocatalysts that can efficiently absorb solar light and drive the photoinduced charges to react with water and carbon dioxide. In this talk, I will present recent developments we have achieved in the group in the preparation of inexpensive photoanodes, photocathodes and photocatalyst composites. For example, we have achieved nanostructured BiVO4 functionalized with bismuthene and NiFeOx that influence surface states and boost 6 times their photocurrent performance, as well as BiVO4 with Ni and Co phosphide co-catalysts that offer a useful series of samples to understand semiconductor-electrocatalyst synergies. We have also achieved halide perovskites CsPbBr3 photoanodes protected with printed carbon layers and graphite sheets and functionalized with NiFeOx water-oxidation electrocatalyst, achieving photoanodes of low onset potential of +0.4 V vs. RHE and photocurrents around 8 mA cm-2 at 1.23 V vs. RHE for water oxidation. These, moreover, achieve operational stabilities for oxygen evolution above 100 h, inexpensively extended to weeks by replacement of the graphite protection. We will also briefly present photocathodes made of oxide perovskites and composites of halide perovskites, such as Cs2AgBiBr3/bismuthene and Cs3Bi2Br9/g-C3N4, for their use in the photocatalytic reduction of CO2. An extended characterization helps us relate their physical and charge-transfer properties to their performance, guiding us in their rational design for their optimization and future application.
2.2-I1
Prof. Dr. Matthias Driess
Born: 1961 – Eisenach, Germany
Affiliation: Department of Chemistry, TU Berlin, Straße des 17 Juni 135, 10623 Berlin
Telephone: +49 (0) 30 314–22265
Email: matthias.driess@tu-berlin.de
https://www.tu.berlin/en/metallorganik
Scientific vita:
2005– Professor of Inorganic Chemistry (Metalorganics and Inorganic Materials), TU Berlin 1996–2004 Professor of Inorganic Chemistry (Cluster and Coordination Chemistry), U Bochum 1996 Professor at the Institute of Inorganic Chemistry, U Freiburg
1993 Habilitation in Inorganic Chemistry, U Heidelberg
1990–1996 Junior Scientist at the Institute of Inorganic Chemistry, U Heidelberg 1988–1989 Postdoc, Department of Chemistry (R. West), Madison, WI, USA
1988 PhD Chemistry (W. Siebert), Metalorganic Chemistry, Boron Chemistry 1985 Diploma in Chemistry, U Heidelberg
Fields of interest:
Molecular models of heterogeneous catalysts and bioinspired homogeneous catalysts; Molecular approach to heterogeneous catalysts for efficient light-driven and electrocatalytic energy conversion (e.g., overall water-splitting); Organometallic precursors for low-temperature synthesis of nanoscaled metal oxides; Coordination chemistry for activation of small molecules and homogeneous catalysis; Development of multifunctional, low-valent silicon-based strong s-donor ligands in homogeneous catalysis
Awards (selection):
2016 Davison Lecture of the Inorganic Chemistry Division of the MIT (USA) 2016 Visiting Professor, ETH Zürich (Switzerland)
2014 Member of the Berlin-Brandenburg Academy of Sciences and Humanities 2013 Member of the German National Academy of Sciences, Leopoldina
2011 WACKER Silicone Award
2010 Alfred-Stock-Memorial Award of the German Chemical Society
Industry cooperations:
BASF SE; Wacker AG
Organizational activities (selection):
2016– Vice coordinator of the Einstein Center of Catalysis 2012– Scientific Director of the UniCat-BASF Jointlab (BasCat) 2007–2018 Spokesperson of the Cluster of Excellence UniCat
2017- Scientific Director of the Chemical Invention Factory (CIF, John Warner Center for start-ups in Green Chemistry)
2019- Deputy of the Cluster of Excellence UniSysCat
Publications (selection):
N. J. Lindenmaier, S. Wahlefeld, E. Bill, T. Szilvási, C. Eberle, S. Yao, P. Hildebrandt, M. Horch, I. Zebger, M. Driess, An S-oxygenated [NiFe] complex modelling sulfenate intermediates of an O2- tolerant hydrogenase, Angewandte Chemie International Edition 2017, 56, 2208–2211.
Y. Wang, A. Kostenko, S. Yao, M. Driess, Divalent Silicon-Assisted Activation of Dihydrogen in a Bis(N-heterocyclic silylene)xanthene Nickel(0) Complex for Efficient Catalytic Hydrogenation of Olefins, Journal of the American Chemical Society 2017, 139, 13499-13506.
A. Indra, P. W. Menezes, K. Kailasam, D. Hollmann, P. StrasserM. Schröder, A. Thomas, A. Brückner, M. Driess, Nickel as a co-catalyst for photocatalytic hydrogen evolution on graphitic-carbon nitride (sg-CN): what is the nature of the active species?, Chem. Commun. 2016, 52, 104-107.
Yao, F. Meier, N. Lindenmaier, R. Rudolph, B. Blom, M. Adelhardt, J. Sutter, S. Mebs, M. Haumann, K. Meyer, M. Kaupp, M. Driess, Biomimetic [2Fe-2S] clusters with extensively delocalized mixed-valence iron centers, Angewandte Chemie International Edition 2015, 53, 12185.
T. Mätsenen, D. Gallego, T. Szilvasi, M. Driess, M. Oestreich, Peripheral mechanism of a carbonyl hydrosilylation catalysed by an SiNSi iron pincer complex, Chemical Science 2015, 6, 7143–7149.
P. W. Menezes, A. Indra, N. R. Sahraie, A. Bergmann, P. Strasser, M. Driess, Cobalt–manganese- based spinels as multifunctional materials that unify catalytic water oxidation and oxygen reduction reactions, ChemSusChem 2015, 8, 164–171.
P. W. Menezes, A. Indra, O. Levy, K. Kailasam, V. Gutkin, J. Pfrommer, M. Driess, Using nickel manganese oxide catalysts for efficient water oxidation, Chemical Communications 2015, 51, 5005– 5008.
P. W. Menezes, A. Indra, D. González-Flores, N. R. Sahraie, I. Zaharieva, M. Schwarze, P. Strasser, H. Dau, M. Driess, High-performance oxygen redox catalysis with multifunctional cobalt oxide nanochains: Morphology-dependent activity, ACS Catalysis 2015, 5, 2017–2027.
G. Tan, T. Szilvási, S. Inoue, B. Blom, M. Driess, An elusive hydridoaluminum(I) complex for facile C–H and C–O bond activation of ethers and access to its isolable hydridogallium(I) analogue: Syntheses, structures, and theoretical studies, Journal of the American Chemical Society 2014, 136, 9732.
B. L. Tran, B. Li, M. Driess, J. F. Hartwig, Copper-catalyzed intermolecular amidation and imidation of unactivated alkanes, Journal of the American Chemical Society 2014, 136, 2555.
The carbon dioxide reduction reaction (CO2RR), in particular electrochemically, to produce carbonaceous fuels is considered as a viable approach to store energy and to enable a CO2-neutral carbon management. Besides CO2RR, there is an additional strong demand for benign electrochemical reduction of other important heavy nonmetal oxo species (e.g., SiO2, phosphine oxides, SO2) with thermodynamically stable E-O bonds, which accrue in large quantities in industry. In this respect, the energy-intense deoxygenation of oxo compounds of silicon, phosphorus and sulfur is of particular technological importance because they represent one of the main feedstocks to produce important molecules and functional materials. For example, the release of elemental silicon, phosphorus (P4) and sulfur (S8) from naturally occurring minerals (e.g., silicate, phosphate, sulfate) follows energy-intensive chemical routes.
Thus, the established chemical reduction routes to deoxygenate such oxo precursors produce tons of reagent waste or, in the case of carbothermal treatment of minerals, afford a lot of CO2. On the contrary, electrochemical strategies developed for the selective deoxygenation of E-O compounds remain as a feasible alternative powered by renewable electricity instead of fossil energy. Moderate reaction conditions, a large scope in experiment design for selective reactions, easy product isolation and zero reagent waste by applying electrochemical methods offer a promising solution to overcome the drawbacks of chemical reduction routes. This talk summarizes the emergence of electrochemical strategies developed for the reduction of selected examples of E-O/E=O compounds with E = silicon, phosphorus and sulfur in the past few decades and highlights opportunities and future challenges. [1]
2.2-I2
Photocatalytic conversion of CO2 and H2O is an interesting route to produce fuels and chemicals; this process is also known as Artificial Photosynthesis (AP). In last years, extensive efforts have been made to develop efficient catalytic systems capable of harvesting light absorption and reducing CO2 especially when using water as the electron donor.
Herein, we report different strategies and modifications of photocatalysts to increase process performance. Among them, an interesting approach to improve charge separation in photocatalytic systems is the use of heterojunctions. In this line, the combination of different semiconductors with noble metal nanoparticles or organic semiconducting polymers leads to a separation of the photogenerated charge carriers to increasing their life time, facilitating charge transfer to adsorbed molecules.
The main products, using bare TiO2, were CO and H2, with low concentrations of CH4. The deposition of surface plasmon nanoparticles (SP-NPs) leads to changes in the selectivity to higher electron-demanding products, such as CH4. TAS measurements confirm that this behavior is due to the electron scavenging ability of SP-NPs [2].
Organo-inorganic hybrid materials show a dramatic reactivity improvement in CO2 photoreduction, enhancing methane selectivity. Reaction pathways are not well defined for this reaction and several uncertains are still unsolved [3]. To explain this behavior a combination of in-situ NAP-XPS, FTIR, TAS spectroscopies and theoretical tools has been used, showing a more efficient light absorption and charge transfer in the hybrid photocatalyst compared with bare materials.
1.1-O2
Sodium ion batteries (SIBs) are a potential alternative to diversify the energy landscape, beyond Lithium-ion batteries (LIBs), due to their similar storage mechanism and easy technology transfer. Currently, the benchmark anodes for SIBs are hard carbons (HCs), since sodium ions do not intercalate into graphite. HCs can be produced from a variety of waste precursors and therefore are more sustainable and less geopolitically compromised than natural graphite, mainly concentrated in China.
The electrochemical degradation of SIBs occurs at a higher rate than in LIBs. This can be attributed to the larger electrochemical reactivity of the HC anodes. A deeper operando understanding of the degradation mechanisms in SIBs, coupled with engineering of the materials and electrolyte to ensure that a better and more protective solid electrolyte interface (SEI) is formed, is needed for an accelerated scale up of this technology. In this talk I will show you some of the strategies we have developed for these aims.
1.1-O1
Increasing the operating voltage of batteries brings additional challenges to the electrolyte, as it should be stable at higher potentials. Therefore, investigating the electrochemical stability window (ESW) of electrolytes is extremely important. While this could be considered an easy task with liquid electrolytes because mass transport limitation is seldom an issue, it is more complicated with solid polymer electrolytes. Common techniques used are cyclic voltammetry (CV) and linear sweep voltammetry (LSV). However, the main drawback of these methods is that the electrochemical stability of electrolytes is often arbitrarily determined and using conditions far from realistic. Therefore, alternative or complementary methods are required.
Herein, we investigate alternative techniques to determine the oxidative stability of solid polymer electrolytes. Staircase voltammetry (SV) is used to avoid the mass transport limitation and it is combined with electrochemical impedance spectroscopy to simultaneously detect changes in resistance and interfacial degradation. Synthetic charge–discharge profile voltammetry (SCPV) is used to apply the real voltage profile of the active material of interest. Finally, to include the effect of the active material, cut-off increase cell cycling method (CICC) has been developed where the upper cut-off voltage is gradually increased up to 5 V. Monitoring the voltage profile and the Coulombic efficiency can provide information of side reactions occurring at different voltages. The feasibility of these different methods has been investigated with two model solid polymer electrolytes: poly(ethylene oxide) and poly(trimethylene carbonate).[1]
1.1-I1
Ozlem Sel joined “Centre national de la recherche scientifique (CNRS)” as a principle investigator in 2011, and currently working at the “Solid-State Chemistry and Energy Lab (CSE)” at College de France, Paris. Her research interests include piezoelectric sensors employed in junction with electrochemical analysis, for a real-time monitoring of the interfacial processes occurring in energy storage devices.
She has obtained her Ph.D. degree in materials chemistry at the Max Planck Institute of Colloids and Interfaces, in Germany (2007), on the synthesis, characterization and applications of hierarchically porous metal oxides, under the supervision of Prof. Markus Antonietti. Following her Ph.D. degree, she moved to France, joined the group of Prof. Clement Sanchez (LCMCP, Sorbonne University-UPMC, 2007-2009) as a post-doctoral researcher, focusing on the nanostructured hybrid materials and their characterization for energy conversion devices. In the period of 2010-2011, she was at the UC-Davis (group of Prof. Alexandra Navrotsky), USA within a framework of Energy Frontier Research Centers (EFRCs) program. Prior joining the CSE lab at the Collège de France, she has worked at “Laboratory of Interfaces and Electrochemical Systems, CNRS” and obtained her research habilitation at the Sorbonne University, Paris.
Through these years, she has developed an expertise in nanostructured energy materials and electrochemistry, as well as operando methods for surface and interface characterization. She is particularly interested in EQCM and its coupling with EIS, and has been actively working on, already more than 15 years.
As the world's need for energy storage grows at an accelerated pace, researchers are looking for new strategies to take batteries to the next level of performance, both in terms of capacity and durability, while improving safety. The focus has been on the discovery of new materials but also on the development of a wide range of sophisticated diagnostic techniques. This allows on the one hand to anticipate their behavior in the presence of electrolytes in various electrochemical devices and on the other hand to study the processes leading to the evolution of interfaces. Mastering the interface processes is a real challenge in electrochemistry, and it is crucial for the development of electrochemical devices.
One strategy to overcome this challenge is the implementation of sensing technologies (optical1, acoustic2...) providing a real-time monitoring of the interfacial processes occurring in a battery. In this regard, interest in piezoelectric sensors employed in junction with electrochemical analysis, has also been growing steadily in the energy storage community.3,4 In our group, we aim at describing this complex interface by operando piezoelectric sensors, during electrochemical cycling of battery electrodes. This methodology, commonly known as Electrochemical Quartz Crystal Microbalance (EQCM) based interface analysis,4-6 is able to provide vital understanding of the positional cohabitation of ions and solvent molecules within the electrical double layer (EDL), which itself conveniently falls in the penetration depth of the acoustic shear wave emanating from the QCM sensor.
In this contribution, we will describe our piezoelectric sensing strategy to investigate the impact of electrolyte composition on the electrochemical performance. The objective is to establish an interface model for different electrolyte compositions, via a study of the various solvent/salt mixtures, differing in their dipole moment and size/weight, respectively. Our experimental methodology lifts the challenge in the probing the EDL’s composition and structuration: specifically, accessing to the identity and the repartition profile of the charge carriers, as well as the role of solvation. Our results exemplified for both Li and Na ion cells,5,6 will be complemented by the recent fiber optic based sensing methodology,6,7 which provides the information on the chemical evolution of this interface.
1.1-I2
Operando measurements are a powerful approach to understanding the performance and degradation of batteries. Nuclear magnetic resonance (NMR) spectroscopy is well-suited to such measurements due to its non-invasive, non-destructive nature and its sensitivity to both structure and dynamics. However, it does not come without challenges. Among them are technical aspects such as achieving realistic battery cycling performance with a minimum amount of metal in the operando cell, as well as reducing the interference between the battery and NMR electrical circuits. Moreover, the resolution of operando NMR spectra is much lower than in conventional solid-state NMR due to the absence of magic-angle spinning and the signal overlap from different cell components.
In this contribution, we demonstrate how the above challenges can be addressed and that operando NMR can provide information that may be difficult to obtain with other analytical techniques. We will focus on Li-ion batteries, presenting 7Li operando NMR data obtained on NMC811/graphite and LNO/graphite cells. Two aspects will be discussed in detail: Unwanted Li metal plating on graphite and the changes of structure and Li dynamics in the cathode materials.
Li metal deposition on graphite is an unwanted process which does not only accelerate capacity fade, but also poses a serious safety hazard. Since NMR spectroscopy is an excellent technique for the unambiguous detection of Li metal, we employ it here for studying the Li plating process in operando under different cycling conditions, with varying charging current, voltage and temperature.[1]
The Ni-rich cathode materials LiNi0.8Mn0.1Co0.1O2 (NMC811)[1] and LiNiO2 (LNO) are studied during charge and discharge, illustrating their structural changes as well as the changes of Li-ion mobility with state-of-charge. For the first time, we present well-resolved operando NMR data of LNO/graphite cells. We use this data to refine the description of phase transformations in LNO during (de)lithiation, in particular with respect to changes of Li mobility. Open questions regarding the 7Li solid-state NMR spectra of LixNiO2 are discussed as well.
1.2-I1
In this invited talk I will present our latest advances in Na and Al based batteries. In particular I will discuss advances in anodes for Na ion batteries from atomistic understanding to upscale at pauch cell level. These will be based on both hard carbons from biomass as well as Sn/C composites. I will pay particular atnetion to the SEI development in Na-ion batteries using different electrolytes and aditives based on analysis using electrochemistry coupled with mass spectroscopy to detect the gases evolved as well as ToF-SIMS and XPS on the electrodes to understand the chemical composition of the SEI.I will also discuss Al dual ion batteries and in particular the interface on the Al metal due to corosion because of the electrolytes as well as the role of the curent colectors and the stainless steel casing of coin cells.
Various in operando characterisation btechniquyes such as solid state NMR as well as Raman will be discussed for both na and al systems.
1.2-I2
Dr. Fellinger is Head of the Division 3.6 Electrochemical Energy Materials at the German Federal Institute for Materials Research and Testing (BAM). He is a nanostructure and molecular scientist by training (diploma at University of Kassel, DE), who received his PhD in colloid chemistry (with summa cum laude) at the University of Potsdam/DE under the direct supervision of Prof. Markus Antonietti in 2011. After a short postdoctoral stays at the Tokyo Institute of Technology (Prof. Ichiro Yamanaka) he was a research group leader at the Max Planck Institute for Colloids and Interfaces in Potsdam-Golm (2012-2017). In 2016/17 he was an awarded Researcher-in-Residence at Chalmers Institute of Technology in Gothenburg (Prof. Anders Palmqvist), followed by one term as W2-substitute professor for inorganic chemistry at the University of Applied Science Zittau/Görlitz. Afterwards until 2020 he joined Prof. Hubert Gasteiger´s Chair for Technical Electrochemistry (Technical University Munich) with a fuel cell project. In 2020 Dr. Fellinger´s group joined the Federal Institute for Materials Research and Testing (BAM) in Berlin. Dr. Fellinger received the Donald-Ulrich Award 2017 of the International Sol-Gel Society and the Ernst-Haage Award for Chemistry of the Max-Planck Institute for Chemical Energy Conversion. His research interests are the synthetic chemistry of novel materials and their usage in energy-related applications with a focus on different carbon-based materials like nitrogen-doped carbons, M-N-C catalysts or hard carbon anodes. He has published ~60 articles in peer-reviewed journals (>6000 citations, H-index: 41).
The current strong interest in electromotive mobility and the need to transition to an energy grid with sustainable storage devices has led to a renewed interest in sodium ion batteries (SIBs). Hard carbons (HCs) are promising candidates for high-capacity negative electrode materials in SIBs. Their high capacities, however, are often accompanied with high irreversible capacity losses during the initial cycles,[1] while low initial losses are accompanied with moderate capacities.[2] In the DialySorb project, which is funded by the German Ministry for Research and Education, we are aiming at a local separation of reversible sodium storage and irreversible losses in novel synthetic carbon anodes, using a core-shell concept.
Advanced analytical techniques are employed to identify structure-performance relation of the core-shell anodes and will be presented in the present presntation.
Literature:
[1] Mehmood A., Ali G., Koyutürk B., Pampel J., Chung K. Y., Fellinger, T.-P., Nanoporous nitrogen doped carbons with enhanced capacity for sodium ion battery anodes, Energy Storage Materials, 2020, 28, 101-111.
[2] Matsukawa, Y.; Linsenmann, F.; Plass, M. A.; Hasegawa, G.; Hayashi, K.; Fellinger, T.-P. Gas sorption porosimetry for the evaluation of hard carbons as anodes for Li-and Na-ion batteries, Beilstein J. Nanotechnol. 2020, 11, 1217–1229.
1.2-O1
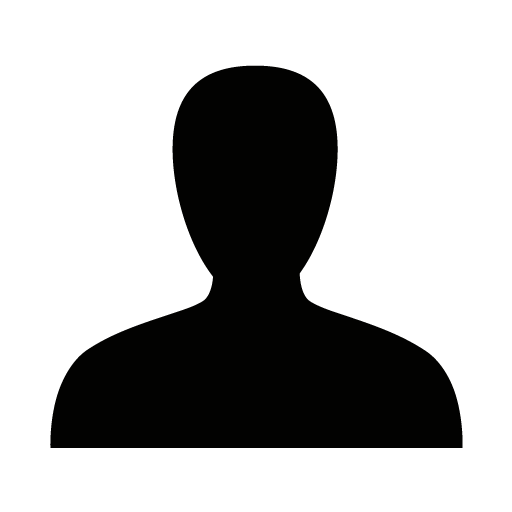
Silicon is a promising active material for anodes in lithium-ion batteries owing to its high theoretical capacity (3579 mAh/g)1. However, it is well known that the compound contends with large volume changes during cycling, creating cracks in the material and therefore limiting the lifetime and capacity of the cell. To cope with these volume changes, this project aims to develop self-healing binders to improve the cycling stability of lithium-ion batteries.
Both hydrogen bonds and dynamic covalent bonds have previously been used to modify binder systems to get self-healing properties through reversible cross-linking with the binder in the electrode. While the hydrogen bonds have eminent reversibility, the dynamic covalent bonds provide high mechanical stability2–4. Both of which is desirable properties to implement in the polymeric binder system.
Specifically, the focus of this work has been to utilize the reversibility of borate ester bonds and couple them with the polymer binder poly(vinyl alcohol) (PVA). The electrochemical performance of the cells has been investigated, where the borate ester-based binders have shown an increased capacity compared to the PVA binder alone. Besides the performance, understanding the self-healing mechanism of these binders and possible degradation reactions from these functionalities is key to further improving the system and cycle life. Therefore, the mechanism behind the self-healing was investigated through FTIR measurements at room and elevated temperatures. Furthermore, the electrochemical stability of the functional groups was investigated in order to compare the impact of dynamic covalent bonding, especially at the low operating voltages of silicon. This purposes that the self-healing functionalities are electrochemically stable and do not contribute to increased degradation in the cell.
1.2-O2
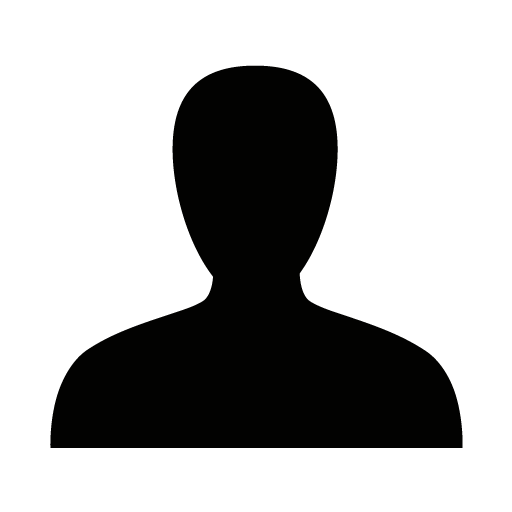
For increasing the energy density, lithium metal is considered the "holy grail" anode material due to its high theoretical capacity (3860 mA h g-1) and lowest electrochemical potential (-3.04 V vs the normal hydrogen electrode) [1]. However, the commercialisation of a lithium metal anode is hindered by several issues including safety concerns and rapid lithium inventory loss [2]. To address these challenges, research has focused on electrolyte modification strategies and, more recently, 3D current collectors [3]. Here we propose using free-standing, electro-spun, lignin-derived carbon fibre as a sustainable, high performance lithium anode host structure to replace the conventional current collector.
Optimisation of the fibre fabrication method, in particular the carbonisation process, resulted in an average coulombic efficiency greater than 99% over 100 cycles, demonstrating low lithium inventory loss. In addition, these fibres promote cycling stability and inhibit dendrite formation as demonstrated by extended lifetimes, and a low and stable voltage hysteresis.
Increasing the carbonisation temperature from 700°C to 1000°C decreases the amount of solid-electrolyte interface (SEI) formed initially on the fibre surface, with electrochemical impedance spectroscopy (EIS) confirming a decrease in SEI resistance as well as an improvement in SEI stability for fibres carbonised at higher carbonisation temperatures. Increasing the carbonisation temperature from 700°C to 1000°C further increases the pre-plating plateau capacity, defined as the capacity in the plateau region of the voltage curve before the lithium metal nucleation point is reached. Ex-situ nuclear magnetic resonance spectroscopy (NMR) indicates that, in this region, lithium intercalates in ordered crystal regions, while small lithium clusters form in the closed porosity. An increased pre-plating plateau capacity appears to have a positive effect on the fibre lithiophilicity.
Overall, an improvement in cycling performance at increased carbonisation temperatures has been attributed to a combination of decreased SEI resistance and increased fibre lithiophilicity. In detail, the reduced SEI resistance ensures homogeneous lithium-ion flux and low deposition overpotential, which favours large, uniform and low surface area lithium deposits. The increased lithiophilicity further homogenises the lithium-ion flux by electrostatic interaction [4].
Finally, the lithium deposition behaviour and cycling performance at different carbonisation temperatures were studied in relation to the varying fibre properties such as heteroatom content, structural order, as well as open and closed porosity [5]. This knowledge will help us to tune the fibre structure to optimise performance, and ultimately minimise the amount of excess lithium needed in the electrode for improved safety and sustainability.
1.2-O3
The metallic lithium (Li) represents the most promising anode material among the next generation of solid-state lithium batteries [1]. An efficient strategy to achieve durable and effective Li-anode batteries is by engineering the solid-electrolyte interphase (SEI) with purposely designed molecules. To this aim, the vinylene carbonate (VC) is one of the most used additives in conventional electrolytes. Some recent experiments proved that the VC promotes the formation of a stable and protective SEI layer between Li metal and electrolyte [2, 3]. Unless the well-known SEI composition, it is difficult to control the VC reactivity, that involves dissociation and polymerization at the electrode surface. Therefore, to dissect these tangled processes, here we present new atomistic insights on VC-Lithium SEI formation via first-principles calculations by Density Functional Embedding Theory (DFET) [4,5], see Fig. 1. Such approach has potentialities for modeling complex reactions at hybrid interfaces in electrocatalysis: it is well suited to combine the best feasible approaches for molecular species (in this case, hybrid HF-DFT for VC molecules and derivatives) and for Li metal electrode (semi-local GGA density functional). Our results highlight different VC dissociation pathways, with formation of reactive radical species and localized cluster of Li2O and Li2CO3. The use of hybrid-DFT-in-DFT embedding is crucial for obtaning energy barriers and qualtitative results in agreement with experiments [3,6]. Overall, the energetics and structural features of these intermediates improve the current understanding of SEI formation process and can be exploited to drive the reactions toward the desired interfacial properties.
1.2-O4
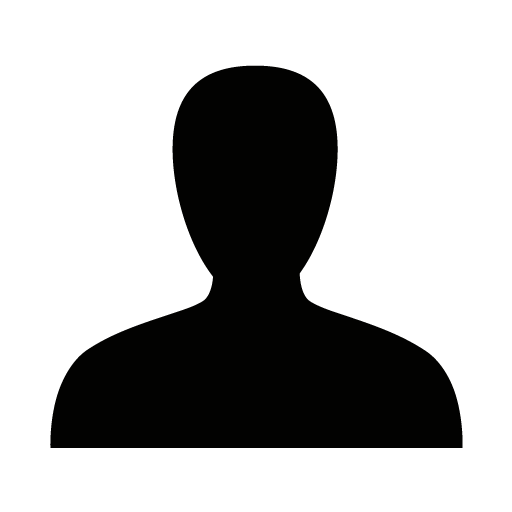
Transition-metal based layered compounds are considered as promising cathode materials in sodium-ion batteries (SIBs), in which sodium ions (Na+) can occupy the interstitial sites. [1] In addition to the typical transition-metal (cationic) redox activities, the anions in layered compounds, such as the lattice oxygen or sulfur, may also participate in the electrochemical redox reactions during sodiation/desodiation process. [2] Although layered oxides are very promising cathode materials for SIBs, there are still limitations such as voltage fade due to ion migration and loss of molecular oxygen during overcharging. [3,4] Therefore, replacing oxygen with sulfur and designing layered sulfide compounds with reversible anionic redox activities are promising for next generation of cathode for SIBs.
Titanium (Ti), as a transition metal element with abundant resources, has attracted considerable attention. In our study, we synthesized a series of Ti-based layered sulfide compounds and investigated them as cathode materials in SIBs. Through the utilization of several synchrotron radiation techniques, phase transitions and redox processes during sodiation and desodiation are comprehensively explored. Furthermore, electrolyte-dependent electrochemical behaviors of layered sulfide compounds are also investigated. These investigations can offer valuable insights into the working mechanisms of Ti-based sodium layered sulfide compounds, providing reference information for the design of layered sulfide cathode materials for SIBs in the future.
2.1-I1
Professor Emma Kendrick, CChem FIMMM FRSC FIMMM - Chair of Energy Materials, School of Metallurgy and Materials, University of Birmingham.
Prof Kendrick’s career to date has included industrial and academic roles leading to her current role as Chair of Energy Materials, where in addition to group lead of the energy materials group (EMG), she is co-director of the Centre for Energy Storage (BCES) and part of Birmingham Energy institute (BEI) and Birmingham Centre for Strategic Elements and Critical Materials (BCSECM). The EMG investigates sustainability in novel battery technologies from materials, manufacturing, performance and parameterisation, and recycling. Her recent work has led to a 2021 joint UoB - Imperial College London (ICL) spin out company, based around the methods of experimental parameterisation of applied multi-physics cell models, called About:Energy, for which she is founder and director.
Prior to UoB, she spent two years as Reader in WMG, University of Warwick. Before academia, she led innovations in the battery industry, latterly as Chief Technologist in Energy Storage at SHARP Laboratories of Europe Ltd (SLE) and prior to that for two lithium-ion battery SMEs, Fife Batteries Ltd and Surion Energy Ltd.
She is fellow of the Royal Society of chemistry (RSC) and Institute of Metals, Mining and Materials (IoM3). Recently, she has been recognised through several awards; 2021 Faraday Institution (FI) Researcher Development Champion, RSC 2021 Environment, Sustainability and Energy Division Mid-Career Award, and the 2019 Hothersall Memorial Award for outstanding services to Metal Finishing.
Prof Kendrick holds a PhD from Keele University, obtained as part of a postgraduate transfer partnership (PTP) scheme with CERAM Research, a MSc in new materials from the University of Aberdeen and a BSc in chemistry from the University of Manchester.
Materials play a crucial role in the circular economy, and their selection and supply are currently driven primarily by cost. However, considering that different minerals and materials feature on various global critical materials lists, alternatives may be necessary. Lithium, graphite, phosphate, silicon, and cobalt, for example, are listed as critical materials by the EU and the UK, highlighting supply chain risks and their economic importance. By embracing the principles of sustainability, including reduce, reuse, recover, and recycle, we can gradually reduce our reliance on these materials. Sodium-ion batteries are being explored as a viable alternative, allowing for the substitution of lithium, cobalt, and graphite with sodium, iron, and hard carbon, respectively. This substitution significantly reduces material costs. However, it is important to consider other sustainability factors, such as recyclability and duration of use, in-life phases and additionally the current higher embedded carbon emissions per kilowatt-hour (kWh) reported for sodium-ion batteries.
Furthermore, this study examines design considerations for disassembly in sodium-ion batteries. We discuss recycling routes, materials recovery, and potential reuse cases for these batteries. The recovery rates are often influenced by the design of the cells and electrodes. To maximize the performance and recovery rates of materials, we propose specific routes for electrode manufacturing, compositions, and design considerations. By implementing these strategies, we aim to enhance the overall sustainability of sodium-ion battery technologies and promote effective recycling and reuse.
2.1-I2
The development of high voltage and high capacity Na-ion cathodes is one of the key challenges for the successful realization of high energy density sodium ion batteries.1 Among the Na-ion cathodes explored so far, NASICON-Na3V2(PO4)3 cathode is attractive because of its high intercalation voltage (3.45 V vs. Na+/Na0), moderate capacity (~120 mAh g-1) and excellent rate capability. Further, other cations are substituted in the place of vanadium of the NVP lattice to seek the participation multiple redox centres, thereby enhancing intercalation capacities. Recently, our group has developed a series of Na3V2(PO4)3-type cathode in which vanadium cations are partially replaced by different cations such as Mn2+, Mg2+, Al3+ and In3+.2-3 The Mn-rich Na3.75V1.25Mn0.25(PO4)3 cathode has displayed highest capacity and rate performances (100 and 89 mAh g-1 at 1 and 5C rates, respectively) due to its modulated V- and Mn-redox centers and optimum bottleneck size. The substitution of Mg2+ and Al3+ into the Na3VMn(PO4)3-type cathode has enhanced its rate performances and cycling stability. The In-substituted Na3VIn(PO4)3 cathode has exhibited asymmetric multi-redox V5+/V4+/V3+ operation during Na-ion (de)intercalation reaction. During the talk, we will highlight the importance of chemical tunning to enhance the electrochemical performances of NASICON cathodes.
2.1-I3
Dr. Gustav Graeber is the principal investigator of the Graeber Lab for Energy Research (GER) at Humboldt-Universität zu Berlin in the Department of Chemistry. He earned his B.Sc. degree in Mechanical Engineering from TU Berlin; his M.Sc. in Mechanical Engineering from RWTH Aachen University; and his PhD from ETH Zurich. He was a postdoctoral researcher at the Swiss Federal Laboratories for Materials Science and Technology (Empa) from 2019-2021 and a postdoctoral researcher at the Massachusetts Institute of Technology (MIT) from 2021-2023. He joined Humboldt-Universität zu Berlin in March 2023 as the principal investigator of GER. His research interests range from thermodynamics, to functional materials and electrochemistry with the goal to increase performance of energy conversion processes.
The transition from fossil fuels to renewable energy sources requires economic and sustainable electrochemical energy storage solutions with high energy and power density. With the aim of providing a promising alternative to the voluminous intercalation anodes (negative electrode) of state-of-the-art Li-ion batteries, we propose anode-free cells with alkali-metal anodes and solid electrolytes. Such cells require no intercalation material in the anode and no addition of alkali metal during cell assembly. This increases the energy density by up to 35% and reduces production costs [1].
Depending on the operating temperature and the materials used, the alkali-metal anodes can be in a solid or a liquid state. Solid alkali-metal anodes suffer from dendrite formation and limited power capabilities. In contrast, liquid alkali-metal anodes, when combined with solid electrolytes, allow for high power charging and discharging, as well as high areal capacities [2,3]. However, a major challenge with liquid anodes is alkali-metal management, which involves storing and releasing the liquid alkali metal within the anode compartment during cell cycling, accommodating the volumetric changes within the anode, and ensuring good electrical contact between the alkali metal and the solid electrolyte [4]. Effective alkali-metal management is crucial to achieving cycling stability and efficient utilization of all active materials at low overpotentials and low operating temperatures [5]. Currently, wetting and phase change phenomena of alkali metals under battery-relevant conditions are not sufficiently understood to guide the design of coatings and capillary structures for effective alkali-metal management. Therefore, here we propose a series of dedicated experiments on the wetting behavior of alkali metals on a variety of materials.
2.2-I1
Heather is a Royal Society University Research Fellow in the Department of Chemical Engineering at Imperial College London.
She obtained her PhD in 2017 from Imperial College developing covalent modification strategies on carbon nanomaterials. She was a postdoctoral research associate at Queen Mary University of London and Imperial College, where her research interests shifted to investigating charge storage mechanisms in sodium-ion battery anodes, and later a Faraday Institution Research Fellow, working on the development of engineered carbon hosts for sulfur cathodes in lithium-sulfur batteries.
Heather was awarded a Royal Society University Research Fellowship in 2023, allowing her to establish an independent research team exploring sustainable materials for structural energy storage.
The development of next generation battery chemistries beyond Li-ion is of crucial importance to achieving a zero emissions economy by 2050, and batteries are expected to play a pivotal role in the electrification of a range of sectors, including transport, aerospace and grid-scale storage. Li-S batteries are a particularly attractive option, due to their projected high energy density, low cost, operating temperature range, and safety; however, sulfur, on its own, is a poor electrode material due to its extremely low conductivity (5 x 10‑30 S cm‑1). Various forms of carbon have been explored as the host material for sulfur cathodes because they are conductive, lightweight and mechanically robust; furthermore, depending on the structure and functionality, employing carbon hosts can address other performance degradation mechanisms arising from volume expansion, polysulfide shuttling, sluggish kinetics and poor contact during cycling. In this work, free-standing carbon fibre electrodes were prepared via electrospinning of biomass precursors followed by further heat treatment. The porosity, functionality and conductivity can be tailored by varying the precursor and carefully tuning the treatment conditions, to enable free-standing electrodes with high sulfur loadings and improved redox kinetics. In addition, enhanced polysulfide interactions result in the suppression of shuttling and sulfur inventory loss, leading to greater capacity retention over long-term cycling. The ability to tailor these hierarchical nanostructures therefore makes this process a promising route to achieving new cathode materials for Li‑S technologies.
2.2-I2
Solid-state batteries have undergone significant progress in the recent years, mainly driven by the development of fast solid conductors. Fulfilling the ambitious promise of high gravimetric and volumetric energy density still represents several challenges, covering the processing of materials into components, cell integration and performance. Even fast ionic conductors such as oxides, sulfides or halides dare with resistive and dynamic solid-solid interfaces which are still the main bottleneck to develop high-performance devices.
Such interfaces appear naturally when processing the materials into components or even when stacking components during cell assembly. Some examples are the active material/solid electrolyte interface present at the positive electrode, the solid electrolyte/Li metal anode interface and even between electrolyte particles after densification. Such type of resistive interfaces can be minimized to a great extent from the careful choice of materials chemistry, optimization of microstructure and processing strategies.
Interfaces in SSBs can also be dynamic as a result of the different processes taking place during cell cycling. This leads to even more complex challenges, such as volume expansion and void formation at the anode during lithium electro-platting and dissolution, lithium dendrite propagation across the solid electrolyte, cracking of the positive material from continuous redox processes and resistive interfaces formed from poor electrochemical stability between solid electrolyte and electrodes. These complex interfaces may adversely affect the Li transport, often leading to a fast decrease on the cell performance.
In this talk we will analyze key solid-solid interfaces of different electrolytes present within the cell and how they affect to the performance and ionic transport; from redox processes at the positive electrode and electrochemical stability with Li metal electrodes to dendrite propagation. Besides, we will discuss on how the engineering of interfaces may improve cell performance and paves the way towards the desired anode-less concept.
2.2-I3
The need for application specific materials is ever increasing. Materials design plays a pivotal role in this, and the future technologies cannot be realised without efficient materials. The development and optimization of high-performance anode materials for alternative and complementary battery technologies to lithium ion is a crucial challenge for the sustainable energy revolution. 1–3 Atomic scale computational modeling using density functional theory (DFT) allows for efficient and targeted materials design from the nanoscale up, and is increasingly used by the scientific community as an integral part of material development.4–6 Computational modeling does not only provide fundamental insight, but also direct input for experimental synthesis, often where quantities cannot readily be obtained by other means. In this talk, I will give an overview of the work we conducted on carbon materials for sodium and potassium batteries using an intelligent materials design framework combing computational and experimental methods. 7–11 Through DFT simulations, we identified performance limiting and enhancing defect structures, and probed the effect of morphology on battery performance. By simulating the metal ion behaviour at different carbon motifs, the role of the atomic scale structure on the anode performance could be shown.
2.2-O1
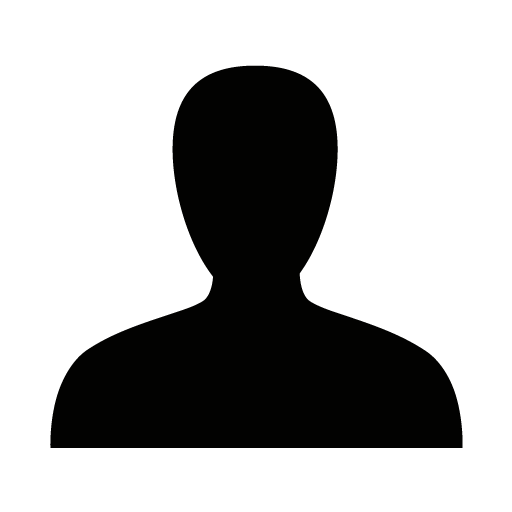
High capacity lithium-ion electrodes made from earth-abundant materials are highly desirable for large-scale energy storage applications. High entropy materials are a relatively new class of materials that have only recently started to be explored in applications ranging from battery electrodes[1],[2] to solid electrolytes[3] and electrochemical catalysts[4]. High entropy materials comprise of five or more elements randomly arranged in the lattice, with equal probability of occupying the same sites.[5] This disorder, or high configurational entropy, endows the materials with unusual chemical and electronic properties that are still being uncovered.[6] In this study, we present two novel high entropy sulfides including wurtzite Cu7Mg2Sn2ZnGeS13 and zinc-blende Cu3AlGaInZnS7, as conversion-type lithium-ion cathode materials with extremely high capacities.
Our results show high capacities of ~1000 mAh g-1 at 50 mAh g-1 which is ca. 15% higher than the theoretical capacity of the binary sulfide CoS2, an extensively studied material as a lithium-ion conversion material.[7] The plateaus in the discharge curves of both materials at ~ 1.7 V and ~0.8 V can be attributed to the reduction of the high entropy sulfide to metal and Li2S, while in the charging curve, the anodic peaks at ~2.0 V and ~ 2.4 V are assigned to a two-step resulfidation process.[8] These processes are largely reversible, with a variance of not more than +/- 10 mA g-1 between the charge and discharge capacities . At higher current densities, from 100 – 500 mA g-1, the specific capacity degrades quickly, but unexpectedly recovers in the last five cycles when the current density returns to 50 mA g-1. The capacity fade can be attributed to lithium-aluminium alloy formation which occurs at 0.23 V – visible as a low-voltage plateau in the final stage of discharge, as well as SEI formation. Current work is focused on replacing the aluminium current collector with copper foil and carrying out galvanostatic charge-discharge tests over a narrower potential window. Further work will focus on characterising the phases being formed and experimenting with different carbon and electrolyte additives to stabilise the SEI.
This study demonstrates versatile novel high entropy materials with high capacities that could lead to the development of more energy dense lithium-ion batteries using earth abundant transition metals. The findings of this study may be applied to a variety of unexplored high-entropy materials for a range of electrochemical energy conversion/storage applications thanks to their desirable properties, tunability and easy synthesis methods.
2.2-O2
Molybdenum disulphide (MoS2) is seeking to replace graphite as the negative electrode in lithium-ion batteries (LIB), due to the natural abundance of bulk 2H MoS2 and its high theoretical capacity (670 mAh/g). However, bulk MoS2 suffers from rapid loss of performance during the first 50 cycles when fully discharged (0.01 V). Aiming to understand the degradation mechanism at play in bulk MoS2 electrodes, coin cells with electrode material coatings of 10 μm, 40 μm, and 80 μm were opened after lithiation to 0.80 V, 0.40 V, and 0.01 V. Cells lithiated to 0.01 V and then delithiated to 3.00 V were also studied. The cycled electrodes revealed a tri-colored inhomogeneous concentric ring pattern, which remains present during initial delithiation and further cycling. Thicker electrode coatings resulted in a more noticeable ring pattern, which was mimicked by surface corrugation on the Li foil counter electrode. Ex-situ characterization involving scanning electron microscopy (SEM), X-ray diffraction (XRD), Raman spectroscopy, and X-ray photoelectron spectroscopy (XPS) was carried out on the 40 μm electrodes to analyze the different regions of the ring pattern. All three segments were covered by a solid electrolyte interphase (SEI) made up of fluoride, carbon, and oxygen. The central segment of the electrode is made up of 2H MoS2, largely unaltered by the lithiation process. The middle segment is composed of predominantly 1T phase MoS2 and a small presence of 2H phase MoS2. The outer segment has a thick SEI cover, with MoS2 underneath whose phase could not be confirmed. Overall, it is postulated that the ring pattern occurs due to poor contact between the bulk MoS2 electrode, separator, and Li counter electrode within the coin cell configuration. Thus, a significant fraction of the bulk MoS2 material is unavailable for lithiation, especially in thicker electrodes.
1.1-I1
Bruno Ehrler is leading the Hybrid Solar Cells group at AMOLF in Amsterdam since 2014 and is also a honorary professor at the University of Groningen since 2020. His group focuses on perovskite materials science, both on the fundamental level, and for device applications. He is recipient of an ERC Starting Grant and an NWO Vidi grant, advisory board member of the Dutch Chemistry Council, recipient of the WIN Rising Star award, and senior conference editor for nanoGe.
Before moving to Amsterdam, he was a research fellow in the Optoelectronics Group at Cambridge University following post-doctoral work with Professor Sir Richard Friend. During this period, he worked on quantum dots, doped metal oxides and singlet fission photovoltaics. He obtained his PhD from the University of Cambridge under the supervision of Professor Neil Greenham, studying hybrid solar cells from organic semiconductors and inorganic quantum dots. He received his MSci from the University of London (Queen Mary) studying micro-mechanics in the group of Professor David Dunstan.
2022 Science Board member Netherlands Energy Research Alliance (NERA)
2021 Member steering committee National Growth fund application Duurzame MaterialenNL
2021 Member advisory board Dutch Chemistry Council
2020 Honorary professor Universty of Groningen for new hybrid material systems for solar-cell applications
2020 ERC starting Grant for work on aritifical synapses from halide perovskite
2019 Senior conference editor nanoGe
2018 WIN Rising Star award
2017 NWO Vidi Grant for work on metal halide perovskites
since 2014 Group Leader, Hybrid Solar Cell Group, Institute AMOLF, Amsterdam
2013 – 2014 Trevelyan Research Fellow, Selwyn College, University of Cambridge
2012-2013 Postdoctoral Work, University of Cambridge, Professor Sir Richard Friend
2009-2012 PhD in Physics, University of Cambridge, Professor Neil Greenham
2005 – 2009 Study of physics at RWTH Aachen and University of London, Queen Mary College, MSci University of London
Perovskite solar cells are very efficient, but mobile ions currently hamper their long-term stability. Mobile ions drift and diffuse through the device during operation, driven for example by a light or voltage bias. Since perovskites are intrinsic semiconductors, these ions determine the electric field distribution in a perovskite-based device. However, it is not clear what this means for device operation. I will discuss the influence of the field screening on capacitance and photoluminescence measurements.
The capacitance value measured for a perovskite device depends on the doping level of the perovskite and the adjacent transport layers, and the ion distribution within the perovskite layer. The latter changes with voltage, temperature, and time, so that mobile ions influence all kinds of voltage, temperature, and time-dependent measurements in non-trivial ways. With capacitance measurements, many properties of mobile ions can be accessed, but only if we understand the way the transport layers and doping levels influence these measurements. I will present drift-diffusion simulations that explore this parameter space.
Photoluminescence measurements are also influenced by the local electric field. The presence of a field can lead to drift out of the PL collection zone, or change the local trap filling. Again, mobile ions determine the field, and the local photoluminescence is hence strongly influenced by their presence. I will show lateral device measurements that we use to attempt an understanding of the changes in photoluminescence in the presence of mobile ions.
1.1-I2
Antonio Guerrero is Associate Professor in Applied Physics at the Institute of Advanced Materials (Spain). His background includes synthesis of organic and inorganic materials (PhD in Chemistry). He worked 4 years at Cambridge Dispaly Technology fabricating materiales for organic light emitting diodes and joined University Jaume I in 2010 to lead the fabrication laboratory of electronic devices. His expertise includes chemical and electrical characterization of several types of electronic devices. In the last years he has focused in solar cells, memristors, electrochemical cells and batteries.
Halide perovskite materials are mixed electronic and ionic conductors that find use in several applications. The ionic conductivity is responsible for a memory effect that leads to undesirable hysteresis in the solar cell configuration. Alternatively, a he resistive memory configuration (memristor) this hysteresis is required and needs to be well understood to offer a good control of the conductive states. In this presentation, it is shown how conductive and insulating states are formed via migration of halide vacancy and electrochemically active metals making them useful as memristors. We show that the working mechanism and performance of the memory devices can be tuned and improved by a careful selection of each structural layer. Several configurations are evaluated in which structural layers are modified systematically: formulation of the perovskite,1 the nature of the buffer layer2,3 and the nature of the metal contact4. Overall, we provide solid understanding on the operational mechanism of halide perovskite memristors that has enabled increased stabilities approaching 105 cycles with well separated states of current and further improvements expected.5
1.1-O1
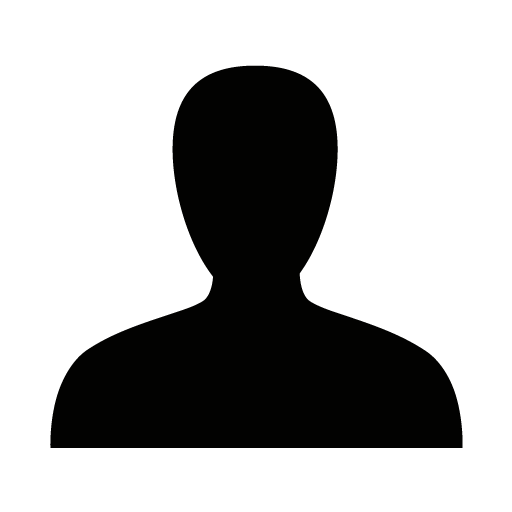
Halide perovskites have been established as one of the most promising semiconductors for a myriad of optoelectronic applications. Besides their exceptional optical properties, perovskites have been demonstrated to behave as mixed ionic-electronic conductors. While ion migration is typically detrimental for conventional perovskite applications (such as solar cells or LEDs), this particular property has proven useful in devices such as memristors, electrochemical transistors, or bolometers. Therefore, it is essential to develop a deep fundamental understanding of the mixed ionic-electronic conduction properties in this class of materials to enable further advances in multiple perovskite-based technologies.
Amongst the vast number of perovskite compositions, Sn-based perovskites have received great attention due to their reduced toxicity and narrow bandgaps compatible with NIR absorption. However, mixed conduction in this class of materials has been underexplored relative to their Pb-based analogues. While a few reports have recently unveiled a smaller degree of ion migration in a limited number of Sn-based compositions, the use of standard electrical characterization techniques to quantify this phenomenon in a wider range of Sn perovskites is still required.
In this work, we used the galvanostatic polarization technique to obtain the ionic and electronic conductivities of various Sn-based perovskite compositions (ASnxPb1-xI3, where A=methylammonium and formamidinium). This technique has been established as one of the most standardized methods to measure the mixed conduction properties of several semiconducting materials. In particular, we shed light on the role of the Sn content (x=0, 0.25, 0.5, 0.75, and 1), the A-site composition, and the concentration of Sn vacancies (tuned by adjusting the content of SnF2 vacancy modulator additive) on the ionic and electronic conductivities. We found ionic conductivity to be intimately correlated to electronic conductivity, suggesting that the electronic carrier concentration is proportional to the number of mobile ions in the perovskite and/or their ionic mobility. These findings provide key design rules to harness ion migration in Sn-based perovskites via compositional engineering.
1.1-O2
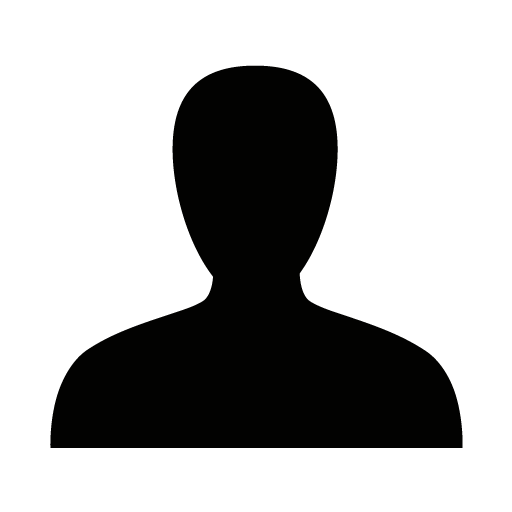
Lead halide perovskites are highly promising materials for a wide range of optoelectronic applications, such as photovoltaics and LEDs. However, perovskite optoelectronic devices typically display unwanted, large hysteresis effects under mild operating conditions, which is commonly attributed to the efficient conduction of mobile ions. Here we utilize this efficient ion conduction to make energy-efficient artificial synapses that change their conductive state when a bias voltage is applied. We fabricate low-energy consumption artificial synapses by downscaling of the device in a way that prevents degradation of the perovskite layer during the lithography procedure. The devices demonstrate large resistance changes with energy consumptions in the femtojoule range, among the lowest reported for artificial synapses and comparable to that of biological synapses. Networks of these artificial synapses could potentially emulate the analog and parallel way that information is processed in the brain to achieve orders of magnitude lower energy consumption computation compared to digital computers.
1.2-I1
The replication of neural information processing in electrical devices has been extensively studied over the years. The paradigm of parallel computing, which allows information to be simultaneously detected, processed and stored, is required for numerous applications in many fields. In the case of brain-computer interfaces, another important requirement is the suitability of the device for communication with cells. Organic electrochemical transistors (OECTs) based on PEDOT:PSS are used for this purpose due to their ionic-to-electronic signal transduction and biocompatibility [1]. Many works have demonstrated the reproduction of neural plasticity mechanisms, such as short-term facilitation and long-term potentiation. In each device, the physical mechanism of transduction may be different, but it is known that the electrolyte plays a key role in the functioning of these devices, as it provides the ions responsible for the chemical transmission of information. Focusing on long-term memory, this can be reproduced in the OECTs with the oxidation of the neurotransmitter, as in the case of the biohybrid synapse [2]. It is crucial to understand the influence of the material chemistry and the electrolyte composition on the memory effect of the device, as long-term modulation is based on a change in the ionic balance between the electrolyte and the organic polymer.
This electrolyte-dependency plasticity will be discussed as it should be considered when the OECT is used in a biological environment in which a large number of molecules of a different nature are present in addition to neurotransmitters. Furthermore, I will discuss how conjugated polymers can be engineered with azopolymers (opto-sensitive polymers which switch from cis to trans conformation upon certain light exposure) to feature diverse optoelectronic short and long term plasticity, enabling the use of such platforms as neurohybrid devices as building blocks of retina-inspired devices.
References
1 Bernard et al. (2007). In: Advanced Functional Materials 17.17, pp. 3538–3544.
2 Keene, Scott T et al. (2020). In: Nature Materials 19.9, pp. 969–973
1.2-I2
Juan Carlos Gonzalez-Rosillo obtained holds a M.Sc. in Materials Science and Nanotechnology and a PhD in Materials Science from the University Autonomous of Barcelona. He performed his MSc and PhD research (2011-2017) at the Materials Science Institute of Barcelona (ICMAB-CSIC), where he studied the relation of the resistive switching properties of metallic perovskite oxides with their intrinsic metal-insulator transitions for memristive devices and novel computation paradigms. He also was a visiting researcher at the University of Geneva (CH) and Forschungszentrum Jülich (DE). Then he joined the Massachusetts Institute of Technology (USA) for a postdoctoral position (2017-2020) working on the memristive properties of lithium-based oxides for neuromorphic computing and processing of next-generation solid-state electrolyte thin films for All-Solid-State Batteries and Microbatteries. Juan Carlos has been awarded with a Tecniospring postdoctoral fellowship to join IREC and to develop thin film microbattery architectures to power micro- and nanodevices for the Internet of Things revolution
Title: Unlocking Extra Functionalities: Exploring Energy Storage Materials for Iontronic Applications with Li-Based Materials
Deep learning has demonstrated remarkable success in various applications, but the energy consumption of conventional CMOS circuitry remains a challenge, limiting their implementation to large data centers rather than end-user devices. In response, neuromorphic computing has emerged as a promising solution for highly efficient computation, drawing inspiration from the human brain.
At the core of neuromorphic computing architectures are artificial synapses that mimic the behavior of biological synapses. These synapses must modulate their resistance in an analog manner with minimal energy consumption to adjust synaptic weights. To achieve this, two- and three-terminal devices that utilize ions instead of electrons have been proposed, aiming to reduce energy consumption while improving linearity and symmetry of conductance changes.
Significant progress has been made at the materials level, exploring various ions and material systems, including lithium (Li+), oxygen (O2-), and hydrogen (H+). Li-based materials offer several advantages, such as typically higher diffusivities at room temperature, low-energy intercalation potentials, and a rich library of electrochemically diverse materials already developed for Li-ion batteries. Additionally, the growing industry involving the thin-film processing of Li-based materials for microbattery applications demonstrates the industrial viability of fabricating these materials.
This presentation will provide a historical perspective on the development of Li-based iontronics and highlight recent advancements in two-terminal -memristive or resistive switching devices- and three-terminal -ionic transistors- approaches for electrochemical synapses in neuromorphic computing. This presentation will address as well the challenges faced in this field and discuss future research directions aimed at improving device performance and reliability. The integration of energy storage materials not only enhances the energy efficiency of these architectures but also opens new avenues for their applications in diverse fields, ranging from artificial intelligence to robotics and edge computing in which both their main energy source and the active neuromorphic elements are made with the material class.
1.2-O1
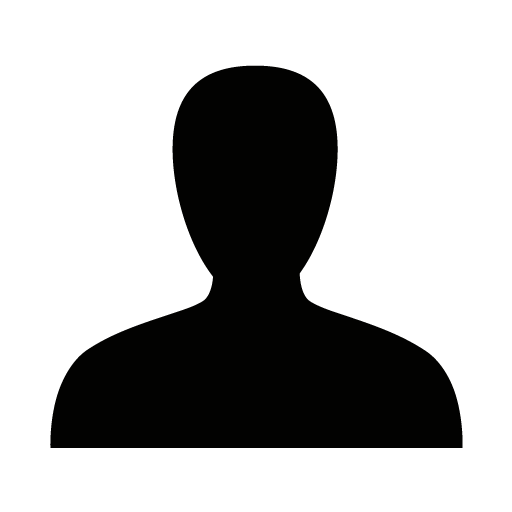
Gaining a deep understanding of the factors governing electrocatalytic reactions requires reliable measurement of the identity and amount of the products generated at the electrode as a function of electrochemical parameters. Detecting these products in real-time enables the observation of otherwise inaccessible, fast and transient phenomena. Coupling mass spectrometry (MS) with electrochemistry (EC) allows for real-time detection with outstanding sensitivity. Several methods have been employed in the monitoring of volatile products: Differential Electrochemical Mass Spectrometry (DEMS) [1] and On-line Electrochemical Mass Spectrometry (OLEMS) [2] have been used successfully to study reaction mechanisms of aqueous systems. Similarly, Online Electrochemical Mass Spectrometry (OEMS) [3] has helped improve our understanding of gas evolution in batteries.
To fully exploit the potential of coupled EC-MS, it is of importance to be able to quantify the amounts of products evolved to accurately relate these amounts to the electrochemical charge passed. To this end, calibration of the MS signals is required. In aqueous systems, MS calibration for some important analytes can be carried out by generating the analyte electrochemically on a catalyst yielding this analyte at close to 100 % faradaic efficiency. Nonetheless, this approach is limited to few analytes, and is especially challenging in non-aqueous systems.
In this contribution, we show how a simple gas-based procedure using chip-based Electrochemistry-Mass Spectrometry (EC-MS) can be used for calibration of electrochemically accessible analytes such as hydrogen and oxygen in aqueous systems. We then demonstrate how we can extend this procedure to analytes not accessible via electrochemical calibration, as well as to non-aqueous electrolytes. We illustrate how quantitative chip-based EC-MS can be used for the identification of surface adsorbates in electrochemical oxidation reactions, and consequently aid in elucidating the role of these species in steering selectivity. [4,5] Finally, we attest the method’s usefulness in non-aqueous systems for studying electrocatalytic reactions such as nitrogen reduction, as well as gas evolution in Li-ion batteries.
1.2-O2
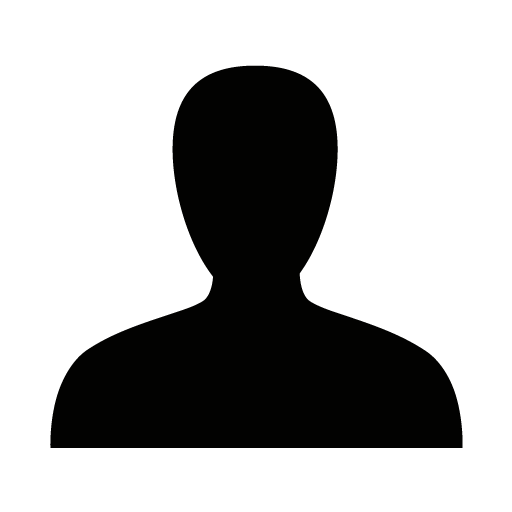
orcid: 0000-0001-7246-2149
The intermittent nature of solar energy is one of the main causes that is delaying the implementation of this renewable energy. In fact, to cover the energy mismatch between production and consumption, it is common for solar cells to be connected to a storage system, mainly batteries. However, having two connected devices is not very practical for portable electronic systems where it is important to keep volume and weight small and require low power consumption. In this context, in recent years research on solar-electrochemical energy storage in a single technology, particularly based on Li-ion batteries, is increasing (LIBs)1, 2.
In this study we present the fabrication of a lithium battery in an adapted coin cell capable of being directly recharged with light energy thanks to the design of a photoelectrode based on a heterostructured Cu2O-TiO2 film3. The electrodeposited Cu2O semiconductor layer is the light harvester component of the photoelectrode, and the TiO2 nanoparticle layer acts as the host of Li+ ions. The semiconductor energy bands and the electrochemical redox potential of the processes involved were determined by UV-vis and UPS spectroscopy and cyclic voltammetry (CV) of the Cu2O and TiO2 films individually. The fabricated half-cell photobattery can be charged in open circuit using only light energy (1 Sun), so that when discharged in darkness at 0.1 C it provides ~150 mAh g-1. The overall system efficiency is 0.29%, calculated as the ratio of output electric energy to input light energy. This value is about an order of magnitude higher than the values reported for the photocharge of lithium-based batteries 1, 4, and is similar to that of zinc-based batteries 5.
1.1-I1
Tao Wang is Professor of Materials Science in the School of Materials Science & Engineering, Wuhan University of Technology, China. He received his B.S. in Polymer Materials and Engineering (2002) and M.Sc. in Materials Science (2005). He obtained his Ph.D. in Soft Condensed Matter Physics from the University of Surrey (UK) in Feb. 2009. Subsequently, he moved to the Department of Physics and Astronomy at the University of Sheffield (UK), where he worked with Prof. Richard Jones (FRS) and Prof. David Lidzey on organic solar cells. He became a professor in Wuhan University of Technology (China) in 2014. He is admitted as Fellow of Royal Society of Chemistry in 2019, and is an Editoral Board Member of Reports on Progress in Physics. His current research interests are organic and hybrid optoelectronic devices. He has published over 100 journal papers in Joule, Advanced Materials, Advanced Energy Materials and so on.
The emergence of new organic semiconductors has driven the continuous development of organic solar cells, with the power conversion efficiency of single-junction devices having passed 19%. The strong intermolecular interactions between organic semiconductors lead to the self-assembly of them into hierarchical aggregates, which exhibits vastly different optoelectronic properties compared to those of the single molecules. Revealing and controlling the complex aggregation structure of organic semiconductors, and establishing the key relationship between structure and the power conversion process, is vitally important toward high performance organic solar cells, but remains as a grand challenge.
Dedicating to this field, we have developed a number of physical and chemical approaches to tune the hierarchical aggregates of organic photovoltaic molecules: We developed the heating-induced aggregation strategy to suppress the large-size aggregation of crystalline semiconductors, realizing the conversion from large-size aggregation to small aggregation, which broadens the light absorption range and enhances exciton splitting; we developed the solution-induced aggregation strategy, realizing the conversion from random aggregation to ordered aggregation, which increases the light absorption intensity and improves charge transport; we also developed the small-molecular fibrillization strategy, realizing the conversion from short-range aggregation to long-range aggregation, which resolves the serious charge recombination issue during charge hoping and achieves a device efficiency of over 19%. The correlation between aggregates and light absorption, exciton dissociation and charge transport processes is eventually established to direct the future development of organic solar cells.
1.1-I2
Organic solar cells (OSCs) are one of the most promising cost-effective options for utilizing solar energy in high energy-per-weight or semi-transparent applications. Recently, the OSC field has been revolutionized through synthesis and processing advances, primarily through the development of numerous novel non-fullerene small molecular acceptors (NFA) with efficiencies now reaching >19% when paired with suitable donor polymers. The device stability and mechanical durability of these non-fullerene OSCs have received less attention and developing devices with high performance, long-term morphological stability, and mechanical robustness remains challenging, particularly if the material choice is restricted by roll-to-roll and benign solvent processing requirements and desirable ductility requirements. Yet, morphological and mechanical stability is a prerequisite for OSC commercialization. Here, we discuss our current understanding of the phase behavior of OSC donor:acceptor mixtures and the relation of phase behavior and the underlying hetero- and homo-molecule interactions to performance, processing needs (e.g., kinetic quenches), and morphological and mechanical stability. Characterization methods range from SIMS and DSC measurements to delineate phase diagrams and miscibility to x-ray scattering to determine critical morphology parameters and molecule packing and dynamic mechanical analysis (DMA) to assess specifically the hetero-interactions. The results presented and its ongoing evolution are intended to uncover fundamental molecular structure-function relationships that would allow predictive guidance on how desired properties can be targeted by specific chemical design. Comparative studies show that the molecular hetero-interactions between the donor and NFA are not always the geometric mean of the homo-interactions. This underscores the limited success often encountered when Hanson Solubility Parameters and surface energies are used to estimate molecular interactions. – We will also present a vignette detailing some work at NCSU regarding the integration of OPV into greenhouses [1-4].
[1] “Achieving net zero energy greenhouses by integrating semitransparent organic solar cells”, E Ravishankar, RE Booth, C Saravitz, H Sederoff, HW Ade, BT O’Connor, Joule 4, 490-506 (2020)
[2] “Balancing crop production and energy harvesting in organic solar-powered greenhouses”, E Ravishankar, M Charles, Y Xiong, R Henry, J Swift, J Rech, J Calero, et al. Cell Reports Physical Science 2, 100381 (2021)
[3] “Organic solar powered greenhouse performance optimization and global economic opportunity”, E Ravishankar, RE Booth, JA Hollingsworth, H Ade, H Sederoff, et al. Energy & Environmental Science 15, 1659-1671(2021)
[4] “Beyond energy balance in agrivoltaic food production: Emergent crop traits from color selective solar cells”, M Charles, B Edwards, E Ravishankar, J Calero, R Henry, J Rech, et al, bioRxiv (2022) doi: https://doi.org/10.1101/2022.03.10.482833
1.1-I3
The rapid growth of the Internet of Things (IoT) has created a demand for energy-efficient, autonomous devices capable of operating over extended periods. Indoor photovoltaic (PV) systems offer a promising solution to power such IoT devices, providing a sustainable and renewable energy source. However, accurate evaluation of the performance of indoor PV systems in real-world conditions is essential to optimize their design and ensure reliable long-term operation.
We have conducted a comprehensive study on the in-situ evaluation of indoor PV performance for autonomous, long-range IoT applications. The objective is to assess the feasibility and effectiveness of indoor PV systems as a reliable power source for IoT devices, considering the unique challenges posed by indoor environments.
The study employs a practical approach, involving the deployment of a prototype indoor PV system in a real-world setting. The system is designed to capture and convert ambient light into electrical energy, which is then utilized to power an array of autonomous, long-range IoT devices. By measuring and analyzing the system's performance metrics, including power generation, energy storage, and device autonomy, we gain valuable insights into the capabilities and limitations of indoor PV systems.
To ensure accurate evaluation, the study also investigates the impact of different indoor lighting conditions on the PV system's performance. This includes analyzing the effects of artificial lighting sources, variations in light spectra, and dynamic light levels encountered in typical indoor environments. The data collected is used to develop predictive models and optimization algorithms that enable efficient energy harvesting and utilization.
The findings from this research contribute to the understanding of indoor PV system performance, enabling the design and implementation of sustainable, autonomous IoT solutions. By evaluating the system's energy generation, storage, and usage in real-world conditions.
1.2-I1
René Janssen is university professor at the Eindhoven University of Technology (TU/e). He received his Ph.D. in 1987 from the TU/e for a thesis on electron spin resonance and quantum chemical calculations of organic radicals in single crystals. He was lecturer at the TU/e since 1984, and a senior lecturer in physical organic chemistry since 1991. In 1993 and 1994 he joined the group of Professor Alan J. Heeger (Nobel laureate in 2000) at the University of California Santa Barbara as associate researcher to work on the photophysical properties of conjugated polymers. Presently the research of his group focuses on functional conjugated molecules and macromolecules as well as hybrid semiconductor materials that may find application in advanced technological applications. The synthesis of new materials is combined with time-resolved optical spectroscopy, electrochemistry, morphological characterization and the preparation of prototype devices to accomplish these goals. René Janssen has co-authored more than 600 scientific papers. He is co-recipient of the René Descartes Prize from the European Commission for outstanding collaborative research, and received the Research Prize of The Royal Institute of Engineers and in The Netherlands for his work. In 2015 René Janssen was awarded with the Spinoza Prize of The Dutch Research Council.
The wide variations in bandgaps offered by metal halide perovskites provide a unique opportunity to develop multijunction thin-film solar cells with an efficiency that surpass the limits of a single-absorber photovoltaic devices. Additionally, perovskite-based multijunction solar cells enable lower the levelized cost of energy. Recent results on perovskite-perovskite and perovskite-copper indium gallium selenide (CIGS) multijunction cells will be presented. Often wide-bandgap mixed-halide perovskites and narrow-bandgap mixed-metal perovskites suffer from non-radiative recombination due to bulk traps and interfacial recombination centers that limit the open-circuit voltage in wide-bandgap sub-cells and restrict photocurrent for narrow-bandgap materials. We studied the origin of these traps with photocurrent spectroscopy and absolute photoluminescence spectroscopy and employ passivation strategies to eliminate losses and increase stability. Combined with reducing parasitic absorption losses using optical simulations as guide, this allows fabricating efficient multi-junction cells in all-perovskite configurations and in combination with other thin-film semiconductors such as CIGS. Collectively, these strategies enable monolithic tandem solar cells with a power-conversion efficiency of 26%.
1.2-I2
Several different theoretical concepts promise to allow going beyond the efficiency limitations of single junction solar cells, tandem devices being arguably the most promising approach, in many cases already exceeding the best performance of the constituting single junction devices. Among the many different possible combinations of semiconductors, halide perovskites in conjunction with crystalline silicon in a monolithic tandem configuration are currently the most advanced contender in the race for the technology that will most likely gain market maturity in the near future. In this contribution, I’ll share several of our recent key developments that led us to exceed 30% efficiency on a small area for different types of monolithically integrated two-terminal perovskite-silicon tandem solar cells. Beyond that, I will discuss which obstacles will have to be overcome to further enhance the efficiency and critically the operational stability under accelerated indoor and prolonged outdoor conditions along several of our most recent findings.
1.2-O1
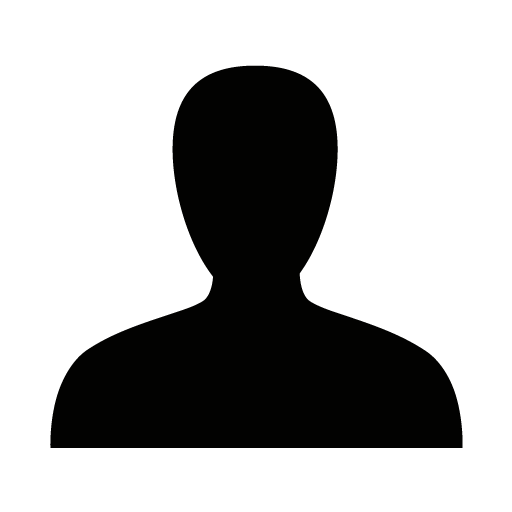
A tremendous increase in the efficiency of organic and perovskite solar cells has been observed over the past few years, where single-junction OPVs (organic photovoltaics) and wide bandgap PSCs (perovskite solar cells) demonstrate efficiencies of over 19% and 20%, respectively.1–3 This has enabled the fabrication of perovskite-organic tandem solar cells where it is possible to overcome the fundamental efficiency limits of single-junction devices. However, several challenges related to the choices of recombination layers remain. Most of the perovskite-perovskite and perovskite-organic tandem solar cells in the literature utilize metallic recombination junctions consisting of an ultrathin layer of Ag or Au. However, despite their ultrathin nature, such metallic layers can significantly reduce the light transmission to the back subcell, minimizing the device's performance.4 Here, we report the fabrication of perovskite-organic tandem solar cells with a remarkable power conversion efficiency (PCE) of 23.6% in a 2T (two-terminal) configuration, utilizing different combinations of material systems for the recombination layer. We show that using a bilayer recombination layer composed of an ultrathin Ag and a subsequently deposited PEDOT:PSS layer exhibits significant parasitic absorption and reduces the device's overall performance. Replacing the hybrid Ag/PEDOT:PSS recombination layer with an inorganic bilayer consisting of a thin indium zinc oxide (IZO) layer and conformal thin molybdenum oxide (MoOx), leads to significant cell performance improvements with the ensuing tandem solar cells exhibiting a Voc of 2.1 V, Jsc of 14.56 mA/cm2, and a FF of 77% leading to an overall PCE of 23.6%. Our work highlights the critical role of recombination layers in tandem perovskite-organic solar cells while expands the range of interlayer systems that could lead to further performance improvements.
1.2-O2
Hendrik (Henk) Bolink obtained his PhD in Materials Science at the University of Groningen in 1997 under the supervision of Prof. Hadziioannou. After that he worked at DSM as a materials scientist and project manager in the central research and new business development department, respectively. In 2001 he joined Philips, to lead the materials development activity of Philips´s PolyLED project.
Since 2003 he is at the Instituto de Ciencia Molecular (ICMol )of the University of Valencia where he initiated a research line on molecular opto-eletronic devices. His current research interests encompass: inorganic/organic hybrid materials such as transition metal complexes and perovskites and their integration in LEDs and solar cells.
Vacuum-based technologies have proven to be a promising route for industrial scale production of perovskite photovoltaics, but performance is still lagging behind its solution-based counterparts. Currently, vapor-based methods such as co-evaporation struggle to grow complex perovskites (6 or more elements), being limited by the number of sources available and the ability to control them. This further limits the ability of many vacuum-based processes to introduce passivating or stabilizing agents. In particular, these are needed for the synthesis of high-efficiency low-bandgap materials (e.g. Sn/Pb) needed for perovskite-perovskite tandems or silicon-perovskite tandems.We will report on the progress on vapor phase deposited perovskites, including novel low vacuum based deposition methods such as close space sublimation. Using substrate configuration we optimize the incoupling of sunlight which leads to current densities very close to the detailed balance limit. We have prepared semi-transparent with varying perovskite thickness allowing for their use in building integrated PV as well as for bi-facial solar cells.
1.3-I1
Indoor photovoltaics (IPV) offer a range of potential applications, from powering the Internet-of-Things to providing clean, renewable energy for residential and commercial settings. These devices can be realized through a variety of materials such as organics and perovskites, both of which demonstrate impressive power conversion efficiencies, due in part to their spectral tunability. The theoretical thermodynamic power conversion efficiency limit for single-junction PV devices under typical indoor spectra is over 50%, significantly higher than the detailed balance limit of 33.7% under 1 Sun, and the optimal bandgap shifts to ∼1.8 eV. However, accurate characterization of IPV devices is essential for enabling device optimization and thus unlocking their full potential. The IPV performance is measured using a bespoke spectrophotometrically calibrated test setup to simulate realistic indoor lighting conditions. In this work, we explore parameters for improving the performance of large-area IPV devices, focusing on organic and perovskite-based devices. We experimentally and theoretically probe and demonstrate the performance of organic and perovskite IPV devices at low light intensities, which are verified by their implementation in IPV-powered wireless sensor nodes (WSNs).
1.3-I2
The strive for more efficient buildings is pushing digitalization. With that the need for connected sensors is growing rapidly. These sensors are commonly powered by batteries and require costly maintainace to change batteries with a few years intervall and generate a large amount of waste batteries.
By harvesting ambient energy the battery life time can be extended and in many cases the battery become redundant. In buildings the main source of ambient energy is light that can be harvested by solar cells. Other examples of applications for connected devices suitable for light energy harvesting include electronic shelf labels and asset tracking labels.
Epishine is a Swedish innovative company that has developed a scalable manufacturing process to produce organic solar cells by roll to roll printing and lamination technologies, to meet an increasing demand for high performance indoor solar cells.
In this talk I will give an overview of Epishine´s technology and manufacturing process, together with potential and requirements for indoor solar cells in selected market segments and applications. I will also discuss our view on the future of scaling indoor solar powered products and manufacturing.
1.3-O1
Our in-house developed ITO-free organic photovoltaics (OPV) device stack proved to be well suited both for upscaling to larger area modules as well as for low-light applications as power source for the internet of things. It is based on a reflective (electron) metal back contact, followed by the absorber layer and a transparent (hole) front contact based on PEDOT:PSS with or without an Ag grid, depending on the requirements for the conductivity. With such devices, we were able to achieve efficiencies >15% under '1 sun' and up to more than 20% under cold white LED illumination with 500 lux. The solar cells designed for indoor usage were found to be extremely stable, with lifetimes deduced from accelerated ageing experiments of more than 100 years. Also under full sunlight the stability is very promising if a UV-filter is employed.
In order to achieve high visual transmission with our OPV devices we only had to replace the back electrode by thin Ag layers sandwiched between metal oxides. This type of electrode offers both high visual transparency and strong near infrared reflectivity and thus we were able to achieve promising values of 8.65% power conversion efficiency (PCE) at an average visual transmission (AVT) of 46.3%, giving rise to a light utilization efficiency (i.e., the product of PCE and AVT) of 4.0. This is amongst the highest values for semitransparent devices processed from non-halogenated solvents.
The optimization of these devices is based on thorough characterization complemented by optical and electrical simulations which shall be discussed in the talk.
1.3-O2
Due to a number of desirable attributes, including tailorable optical properties and scalable, low-embodied energy fabrication techniques, next-generation photovoltaics based on organic semiconductors and perovskites show great potential for a variety of niche applications. Indoor photovoltaics (IPVs) are one such application; in the coming decades, IPVs promise to reduce the carbon footprint associated with networked Internet-of-Things devices by recycling low-intensity, artificial light (generated by, e.g., LEDs and fluorescent lamps) to power them.[1] Due to the relative infancy of the field, however, the performance limits of organic semiconductors and perovskites in indoor applications are poorly understood, and the most suitable photo-active materials are yet to be identified. In the work presented here, we therefore step beyond the conventional Shockley-Queisser model to present a thermodynamic limit of IPV performance that accounts for the effects of intrinsic material characteristics, including sub-gap absorption, energetic disorder, and non-radiative open-circuit voltage loss.[2-4] Following this, we present a methodology that utilizes a device’s photovoltaic external quantum efficiency spectrum and its open-circuit voltage under one-Sun conditions to predict its performance under illumination by any spectrum, at any intensity.[5] We apply this methodology to current state-of-the-art photovoltaics (including inorganics, organics, and perovskites) to predict which could perform best under typical indoor light sources.
2.1-O6
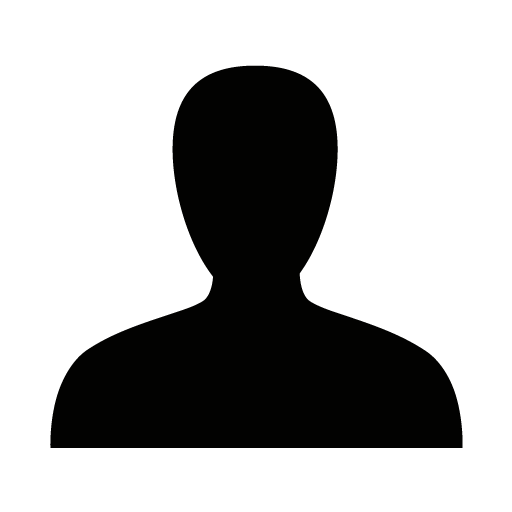
Despite recent developments in organic photovoltaic (OPV) devices at lab scales (< 1 cm2), commercially viable OPV devices suffer performance losses at scale (> 10 cm2).[1,2] This is largely due to the large series resistance exhibited by transparent conducting electrodes (TCEs), even when using current state-of-the-art transparent conductive oxides (TCOs) such as indium tin oxide. To reduce the series resistance of TCEs, one solution is to use the TCO in conjunction with a metallic grid to form gridded-TCEs (g-TCEs),[3–5] which essentially divides a cell into an array of smaller sub-cells.[6] Although TCO materials exhibit a trade-off between average visible transmittance (AVT) and conductivity, the use of TCOs with metallic grids allows the electro-optical properties of high-performance TCEs to be optimised. In this work, the scaling-related performance barrier is presented for OPVs. The performance impact of critical grid geometry is studied with varying light intensity for different OPV applications. At high irradiances, smaller grid critical dimensions (CD) result in improved OPV device performance and can be combined with TCOs with high AVT. At low irradiances, the requirement for a highly conductive TCE reduces, because of the reduced photocurrent, allowing coarser (greater CD) grid structures to be utilised. As grid structures with high topographies result in challenging surface morphologies for scalable printing techniques, a method to recess metallic grids into substrates is demonstrated through exemplary planar (± 30 nm) 5 cm2 g-TCEs, demonstrated with silver nanowires and aluminium-doped zinc oxide (AZO). The AZO g-TCEs were shown to provide a sheet resistance of 0.5 Ω/□, with AVT greater than 77 %. These results show that high-performance g-TCE structures are a highly versatile approach for utilising OPV in various applications.
2.1-O1
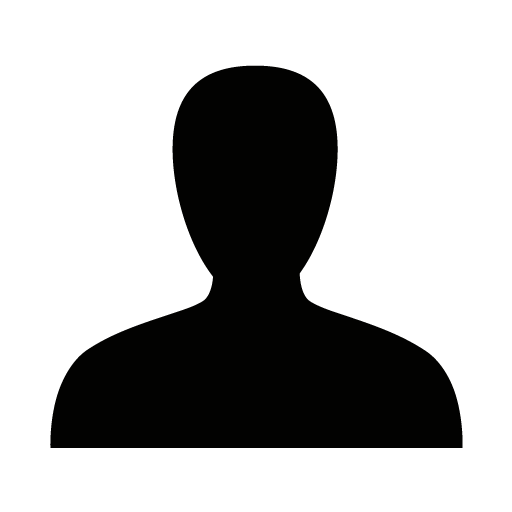
Organic solar cells (OSCs) have garnered significant interest for potential commercial uses because of their light weight, mechanical flexibility, semitransparency, and large-area manufacturing properties. In the past few years, the efficiency of organic solar cells (OSCs) has greatly improved owing to the emergence of Y-series non-fullerene acceptors (Y-NFAs) and the advancements in polymer donors. Given the rapid progress in efficiency, it becomes crucial to prioritize the examination of the stability of photovoltaic materials. These materials play an important role in determining the lifetime of OSCs under real-world conditions, ensuring they meet the necessary criteria for future commercialization. However, the connection between the molecular structure and the outdoor stability of their devices remains elusive. A comprehensive operational outdoor study paired with photo- and thermo-induced degradation has yet to be documented.
In this study, we examine the stability of various Y-NFAs when paired with a common polymer donor, and vice versa. For Y-NFAs, we establish a connection between the molecular structure, specifically the end-group and side-chain, and their photostability. Through the combination of density functional theory (DFT) calculations on the energy barrier of photoisomerization and the analysis of device photostability, we have discovered that suppressing light-induced vinyl rotation can significantly improve the lifetime of the devices. Shifting our focus to polymer donors, we delve into the significance of various building blocks in determining the device lifetime. In order to evaluate the potential occurrence of side-chain breakage, we introduce a molecular descriptor for predicting the photostability of polymer donors and validate its effectiveness by testing it on 28 different polymeric materials. Under illumination, the chemical changes occurring in both Y-NFAs and polymer donors result in a noticeable increase in trap-assisted recombination, leading to a degradation in device performance when exposed to outdoor conditions. Furthermore, we conduct a systematic comparison of the photostability, thermostability, and outdoor stability of devices based on state-of-the-art materials. This comprehensive analysis enables us to gain a thorough understanding of how the photoactive layers impact the long-term performance, particularly in the hot and sunny climate of Saudi Arabia. Our findings provide valuable insights into the design and synthesis of photoactive materials, with the goal of attaining high efficiency and long-term stability in OSCs for outdoor applications.
2.1-O2
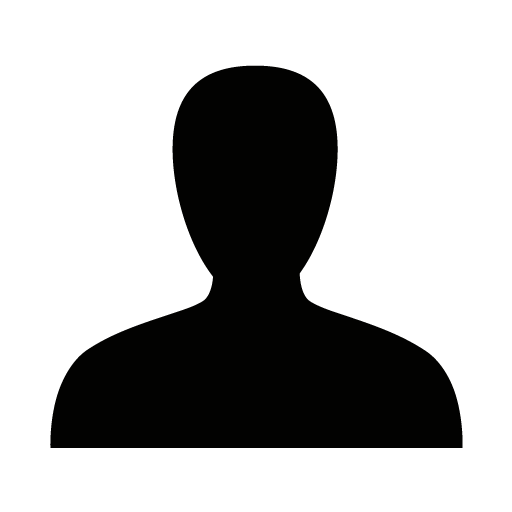
Low power high voltage sources can be used to drive many interesting devices, for example, dielectric elastomer actuators (DEAs)[1], electroaerodynamic propulsion (EAD thruster)[2], micro-electromechanical systems (MEMS)[3], among others. These devices show great potential for smart life applications in the future. However, one common bottleneck is power supply, which usually comes from cables or converters via a battery. The cables limit working space, while batteries restrict working time. To overcome these drawbacks, some groups developed triboelectric nanogenerators to drive DEAs[4]. However, this type of energy supply is based on motion and the performance relies on vibration frequency which is confined for real applications. Developing a solid state, high efficiency and environmentally compatible high voltage source is therefore timely. Harvesting light energy from environment by photovoltaics (solar cells) is a common way to realize energy autonomy. One single junction solar cell usually can generate a voltage of 0.5-1.5 V, depending on the active material and the illumination intensity. To achieve high voltage output, interconnecting single solar cells in series to a module is an efficient way. A lot has been done to meet the need of MEMS in the range from dozens to hundreds of Volts[5,6,7]. But this is not high enough for DEAs and EAD thrusters that usually need thousands of Volts. To the best of our knowledge, no mini solar module with an open circuit voltage (VOC) higher than 1000 V has been reported thus far. Here we report a mini organic solar module with 1640 individual solar cells interconnected in series on 3.6 × 3.7 cm2 realized by laser patterning. We used two different active materials, namely PV-X plus and PM6:GS-ISO. Under 100 k lux warm white LED illumination, the PV-X plus gives a VOC of 1362 V, with a short circuit current (ISC) of 97.2 µA, fill factor (FF) of 0.67 and a maximum power (Pmax) of 89.3 mW, while the PM6:GS-ISO gives a VOC of 1640 V, ISC of 25.2 µA, FF of 0.59 and Pmax of 24.4 mW. Noteworthy, the PV-X plus device can still provide a VOC of 1141 V under an illuminance as low as 1000 lux. This means that the solar modules can work excellently under normal indoor environment even without any additional illumination sources. As a power source, stability is also important for real applications. We kept the devices near the VOC point to test the stability of the organic solar modules under 100 k lux illumination. The performance of PV-X plus shows almost no degradation at all within 30 min, while the PM6:GS-ISO degrades slightly, but still keeps 89.6% of the initial value. To proof the usefulness of the high voltage modules, we combined several modules to get a higher voltage output and could this way successfully drive different types of DEAs. In addition, we also powered a DEA thruster. In a hanging mode, the DEA thruster moved about 20 mm in 0.5 s. The thrust force is measured as 136 µN. Future work on the solar modules will include the use of flexible substrates and enhancing VOC values by further minimizing the individual cell size and thus the number of series interconnected cells hence providing more potential for integration with DEAs and EAD thrusters.
2.1-O3
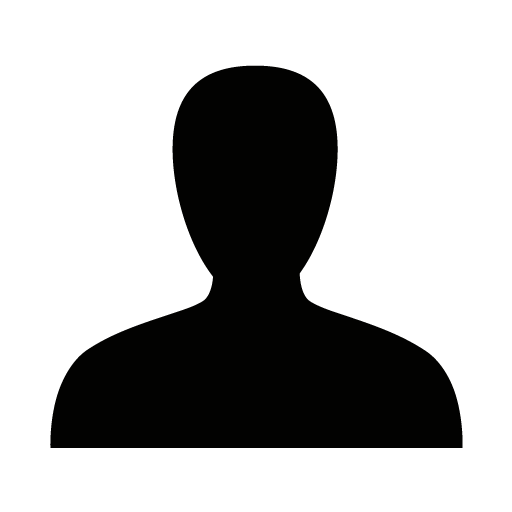
Solution processing from nanoparticle dispersions allows the use of eco-friendly processing agents for the deposition of organic semiconductor thin-films for photovoltaic and other optoelectronic applications. Omitting surfactants, which are commonly used to stabilize dispersions, is essential to not jeopardize the solar cell performance. So far, solar cells could only be fabricated from surfactant-free P3HT dispersions which show some intrinsic self-stabilization. In this work, the self-stabilization of P3HT nanoparticle dispersions is demystified, and electrostatic effects are identified as the origin of self-stabilization. By application of this gained knowledge, novel surfactant-free nanoparticle dispersions from other, high-performance organic semiconductors are synthesized by nanoprecipitation. Electrical doping via oxidation of the polymer by iodine promotes the electrostatic repulsion of the nanoparticles and hence the colloidal stability of the respective dispersions. For the first time, the corresponding solar cells achieved power conversion efficiencies of up to 10.6%, demonstrating the general feasibility of this alternate, all-eco-friendly processing route.
2.1-O4
For the consolidation of organic photovoltaics (OPV), it is crucial to create market pull through the identification and target of strategic niches, where this technology can exploit its fundamental differentiators.[1] For instance, materials engineering has enabled wavelength-selective harvesting with transparent OPV for power-generating windows[2] and building-integrated photovoltaics.[3] Therein, a simultaneous high efficiency and high transparency are needed. While the community has made relevant developments to maximize the optoelectronic properties of OPV devices, little attention has been paid to their structural properties. High-volume manufacturing technologies such as plastic thermoforming and injection moulding can help expand the opportunities, the capabilities, and the seamless integration of OPV.
In this work we demonstrate, for the first time, the feasibility of fabricating OPV cells and modules embedded into structural plastic parts through injection molding. This process yields lightweight OPV devices with enhanced device robustness and durability, thanks to the hermetical and conformable encapsulation resulting from the plastic injection. We discuss the interplay between the plastic processing conditions and the OPV device performance and stability, as well as highlight relevant optomechanical and physico-chemical material properties, including recyclable thermoplastic polymeric materials that might facilitate material reuse. Finally, we also show how plastic processing can be used to fabricate low-cost, three‑level hierarchically organized micro/nanometric surface textures that provide additional functionalities, such as light management or self-cleaning. [4]
2.1-O5
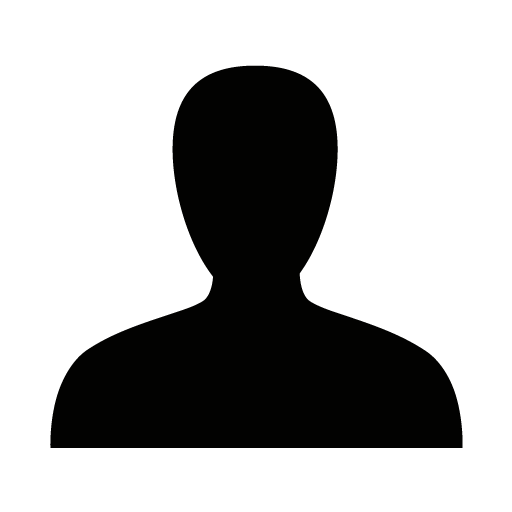
The active layer of Organic Solar Cells typically comprises a mixture of donor and acceptor molecules, forming a so-called bulk-heterojunction (BHJ), whose optimal nanostructure is highly material specific. The nanostructure of a BHJ blend comprises both mixed regions where the donor and acceptor molecules are in intimate contact as well as relatively pure domains of either blend component, which facilitate exciton dissociation and charge extraction, respectively. The poor intrinsic stability arises because the optimal nanostructure of a best performing BHJ tends to be far away from thermodynamic equilibrium1. As a result, the initial BHJ nanostructure can evolve with time through short- and/or long-range diffusion of either of the blend components resulting in a decrease in device performance2,3.
Here, we explore whether the use of acceptor mixtures comprising more than two components can substantially increase the active layer's thermal stability. The use of acceptor mixtures with more than two components is motivated by our recent observation that blending of up to eight perylene derivatives can lead to mixtures with an unprecedented ability to form a molecular glass, driven by the formation of a high-entropy ordered liquid composed of perylene aggregates4. In the current work, up to five Y-series (ITIC-derivative) acceptors are mixed, in analogy to bulk metallic glasses, which tend to comprise up to five elements5,6,7. The combination of several acceptors has a minimal effect on their electronic disorder and blending with the widely used donor polymer PM6 results in hexanary blends with best device efficiencies of 17.6 %. The hexanary blends display a high degree of thermal stability, independent of the film thickness (up to 390 nm), resulting in an unaltered photovoltaic performance upon annealing at 130 oC for 23 days (552 hours) in the dark and under inert conditions.
2.2-I1
Hybrid halide perovskite has established its credibility as high performance thin film photovoltaic technology. In only one-decade, the hybrid organic-inorganic halide perovskite solar cell achieved to compete with all mature crystalline technologies, by reaching a certified 25.7 % power conversion efficiency (PCE) on cells and 17.9 % PCE on small modules.[1] Perovskite’s strength stem from their remarkable opto-electronic properties. However, the technology still requires significant considerations regarding stability. Rapid structural and electronic degradation can be engendered when exposed under various external stressors (temperature [2-3], humidity [4-6], light [7-8], electrical bias [9]).
To cope with the stability issue, it is mandatory to precisely understand the multiple degradation pathways of the perovskite. In situ or operando characterization techniques are central characterization tools in order to clarify the different degradation pathways. In this communication, we will be discussing the degradation of different perovskite composition on the basis of humidity or temperature-controlled in situ x-ray diffraction and corroborated with in situ electron spin resonance spectroscopy and in situ transmission electron microscopy. For example, one key finding which we will discuss is that α-FAPbI3 degradation is substantially accelerated when temperature is combined to illumination and when it is interfaced with the extraction layers, and, second the existence of a temperature gap region which takes place only under illumination involving an intermediate stage between the thermal-induced perovskite degradation and the formation of PbI2 by-product. [10]
2.2-I2
Dr. Monika Rai is a senior researcher and group leader at IMO-IMOMEC, University of Hasselt, Belgium. She received her doctoral degree from the Banaras Hindu University (BHU), India in 2017. Before she joined IMOMEC, she worked as an Alexander von Humboldt research fellow at the University of Stuttgart from 2021 to 2022, and a post doctoral fellow at the Nanyang Technological University (NTU), Singapore from 2017 to 2021. She was also a Visiting Researcher at the Hebrew University Jerusalem, Israel in 2018. Dr. Monika Rai has worked on different projects including perovskite solar cells and modules, transparent conducting oxides and printing technologies with expertise in solar cell devices and their optoelectronic characterizations. Her current research interests include strectchable electronics and energy harvesting devices.
The staggering rise in perovskite solar cells (PSCs) efficiency in past years has led to multi-fold advancements. They are promising candidate for aesthetically appealing, electrically and optically efficient building integrated photovoltaics, BIPVs. However, the losses in power conversion efficiency are limited due to the spectral mismatch between the incident solar spectra and absorption range of the active perovskite layer. Besides PSCs’ performance, the photoinduced degradation/instability is also a major concern. This talk is about three cross-dependent aspects: band-gap engineering vs “thinning” for semi-transparent perovskite solar cells, transparent front contact and its effect on PCE and, photon management strategy by down-converting (DC) fluorophores to enhance carrier generation.
The discussion further points out the advantages of perovskites for diffuse light irradiance. It is important for indoor light applications where the incident power is already too low for power generation. The talk will also emphasize on the true challenge in the realization of DC effect. The flux and wavelength dependent PCE study shows the real promise of DC effect in PSCs. Further research is imperative for in-depth mechanistic understanding of the semi-transparent PSCs operations.
2.2-I3
Jenny Nelson is a Professor of Physics at Imperial College London, where she has researched novel varieties of material for use in solar cells since 1989. Her current research is focussed on understanding the properties of molecular semiconductor materials and their application to organic solar cells. This work combines fundamental electrical, spectroscopic and structural studies of molecular electronic materials with numerical modelling and device studies, with the aim of optimising the performance of plastic solar cells. She has published around 200 articles in peer reviewed journals, several book chapters and a book on the physics of solar cells.
Fully decarbonising our energy system will require increased implementation of renewable energy technologies such as photovoltaics, but also more efficient utilisation of the energy that is generated. This can be achieved through design of systems where the photovoltaic generation is integrated into the overall system to maximise efficiency of energy use, or by combining photovoltaic generation with other functions such as production of fuels, energy storage, heat management, clean water, or other uses of space. Optimising the integrated system requires knowledge of the optical and electrical response of the solar module in different conditions and control of those properties as well models of the whole system performance, resource availability and demand. The choice of PV technology may not be the most important factor. We have developed a flexible energy system modelling tool [1], that can be applied to such optimisations. We will present examples of the integration of PV in different systems, and consider how system design and operation influence overall performance.
2.2-I4
Impedance spectroscopy (IS) and current-voltage measurement (jV) form the most useful tandem-techniques to characterise in depth interfacial processes taking place in perovskite solar cells (PSCs). Among these processes, hysteresis is one of the most remarkable, due to its relationship with the instability of these devices. This phenomenon refers to a difference between forward scan (from short circuit to open circuit) and reverse scan (open circuit to short circuit) during jV measures. Normal hysteresis (NH) is considered when the reverse scan (RS) performs with better photovoltaic response. This type of behaviour has been associated with the lower-frequency capacitance in the IS spectra. However, the opposite effect is also usually found: forward scan (FS) with improved fill factor (FF) and photovoltage (Voc), known as inverted hysteresis (IH). In addition, a funny feature known as loop or inductance in some impedance spectra, is also frequently reported for perovskite devices. The capacitance versus frequency of this feature, leads to a negative capacitance (NC). We have demonstrated experimentally how both phenomena, IH and NC, are governed by the same process. Using the surface polarization model (SPM), obtaining the time constant, τkin, associated. The kinetics obtained have been associated with surface interactions involving ions/vacancies at the ESL/perovskite interface. These interactions lead to a decrease in the overall recombination resistance, modifying the low frequency perovskite response and yielding a flattening of the cyclic voltammetry. We have proved these findings in different types of perovskites (bromide and iodide) and under different treatments such as cation addition, which limits NC and thus IH. The shared origin between inverted hysteresis (IH) and negative capacitance (NC) is crucial for understanding perovskite devices. In the context of perovskite solar cells (PSCs), detecting IH and NC helps to study recombination pathways that impact photovoltage losses. In the case of emerging devices like perovskite-based memristors, the presence of both IH and NC indicates the occurrence of ionic motion required for encoding information. This presentation will show how ionic motion can be reduced to minimize photovoltage losses in PSCs, and how, instead, can be favoured to obtain high-performance memristors with outstanding endurance, allowing to uncover the underlying mechanisms through the negative capacitance analysis.
1.1-I1
Colloidal lead halide perovskite nanocrystals (LHP NCs, APbX3, where A=Cs+, FA+, FA=formamidinium; X=Cl, Br, I) have become a research spotlight owing to their spectrally narrow (~100 meV) fluorescence, tunable over the entire visible spectral region of 400-800 nm, as well as facile colloidal synthesis. These NCs are attractive single-photon emitters and building blocks for creating controlled, aggregated states exhibiting collective luminescence phenomena. Attaining such states through the spontaneous self-assembly into long-range ordered superlattices (SLs) is a particularly attractive avenue. The atomically flat, sharp cubic shape of LHP NCs is also of interest because the vast majority of prior work had invoked NCs of spherical shape. Long-range ordered SLs with the simple cubic packing of cubic perovskite NCs exhibit sharp red-shifted lines in their emission spectra and superfluorescence (a fast collective emission resulting from coherent multi-NCs excited states).
When CsPbBr3 NCs are combined with spherical dielectric NCs, perovskite-type ABO3 binary NC SLs form, wherein CsPbBr3 nanocubes occupy B- and/or O-sites, while spherical dielectric Fe3O4 or NaGdF4 NCs reside on A-sites. When truncated-cuboid PbS NCs are added to these systems, ternary ABO3-phase form (PbS NCs occupy B-sites). Such ABO3 SLs, as well as other newly obtained SL structures (binary NaCl, AlB2- and ABO6 types, columnar assemblies with disks, etc.), exhibit a high degree of orientational ordering of CsPbBr3 nanocubes. These mesostructures also exhibit superfluorescence, characterized, at high excitation density, by emission pulses with ultrafast (22 ps) radiative decay and Burnham-Chiao ringing behavior with a strongly accelerated build-up time. Co-assembly of steric-stabilized CsPbBr3 nanocubes with disk-shaped LaF3 NCs yields six columnar structures with AB, AB2, AB4, and AB6 stoichiometry, not observed before with NC systems comprising spheres and disks. Combining CsPbBr3 nanocubes with large and thick NaGdF4 nanodisks results in the orthorhombic SL resembling CaC2 structure with pairs of CsPbBr3 NCs on one lattice site. Additionally, we have also implemented two substrate-free methods of SL formation. The first method involves oil-in-oil templated assembly that leads to the formation of binary supraparticles, while the second method utilizes self-assembly at the liquid-air interface.
1.1-I2
Semiconductor colloidal nanoplatelets are quasi-2D nanocrystals, which are often considered to be the
wet chemistry analogous of epitaxial quantum wells. They show however a few distinct features that lead to
characteristic properties: (i) the presence of weak, but finite lateral confinement; (ii) the presence of strong
dielectric confinement posed by organic ligands surrounding the inorganic platelets; (iii) the possibility of
building radial heterostructures.
In this presentation we give an overview on our group’s attempts to provide insight into the photophysics
of these systems using effective mass Hamiltonians. The electronic structure of CdSe-based nanoplatelets
(homo and heterostructures) is reported for excitons, charged excitons and biexcitons. It is found that
Coulomb interactions, boosted by dielectric confinement, play a prominent role in determining the physical
response of these systems.[1-5]
Excitons in metal halide perovskite platelets are also investigated. Here, the soft lattice leads to a sizable
polaron radius (~1 nm), which we incorporate to our model by means of Haken's potential. We find that
dielectric screening is weakened in quasi-2D structures because the exciton radius becomes comparable
to the polaron one. The resulting exciton binding energies and self-energy potentials agree well with
experiments and atomistic calculations for layered perovskites.[6]
REFERENCES:
[1] F. Rajadell et al. Phys. Rev. B 2017, 96, 035307.
[2] D. Macias-Pinilla et al. J. Phys. Chem. C 2021, 125, 15614.
[3] J. Llusar et al. J. Phys. Chem. C 2022, 126, 7152.
[4] J. Llusar et al. Phys. Rev. Lett. 2022, 129, 066404.
[5] D. Macias-Pinilla et al. Nanoscale 2022, 14, 8493.
[6] J.L. Movilla et al. Nanoscale Adv. 2023, DOI: 10.1039/D3NA00592E.
1.1-I3
The coupling of elementary excitations to phonons in a material plays a critical role in determining its excited state properties and rates of dynamical processes. Performance metrics of applications utilizing a material are therefore intimately linked with the strength of Electron-Phonon Coupling (EPC). Stronger EPC increases the rate of non-radiative recombination of charge carriers, ultimately limiting the efficiency of semiconductor devices. For applications utilizing luminescence, stronger EPC leads to broadening of the emission spectrum reducing its spectral purity, and drives decoherence and loss of information in single coherent photon sources. Strong electron-phonon coupling, however, is not always detrimental, as exemplified by the case of superconductivity. To understand the intrinsic limitations or aptitude of a material for a given application, precise determination of its phonons and how they couple to transitions is required. Additionally, through material engineering, one can aim to tune EPC in a material system in order to improve performance metrics. To achieve this, detailed understanding of the mechanistic origins of EPC in the material is required.
In this talk I will review recent studies we have performed to quantify EPC in lead-halide perovskites. Ab-initio calculations indicate strong deformation potential type coupling to low energy optical phonons, vibrational modes which drive octahedral tilting in lead-halide perovskites. Optical-pump diffraction-probe, single dot luminescence, and time resolved emission measurements on lead-halide perovskite nanocrystals corroborate this finding, and confirm that deformation potential coupling to octahedral tilting is the dominant coupling to the excitation/recombination of excitons in LHPs. The dependence of strength of this EPC on temperature and on the LHP composition (and phase) point to strong enhancement of the EPC as a result of dynamic structural disorder.
1.2-O1
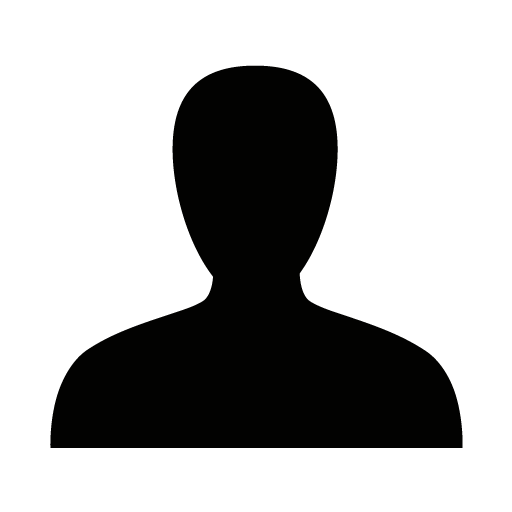
Our recent works,1,2 along with the reports of few other groups from Columbia University3 and MIT4–6 shed a new light on a hybrid quantum well material platform based on metal organic chalcogenides (MOCs) that allow the manipulation of stable 2D Wannier-type excitons at room temperature, in a bulk solid.
Layered metal organic chalcogenides (MOCs) with molecular formula [MEPh]∞ (with M metal, and E chalcogen) form hybrid multi-quantum-well nanostructures. These materials can host tightly bound 2D excitons in a 3D crystal. MOCs show high optoelectronic quality, they are chemically tunable, and may therefore offer an air-stable alternative to the moisture-sensitive layered (so-called “2D”) metal halide perovskites in future optoelectronic applications.
A key aspect of hybrid inorganic-organic material systems is that their “soft” lattice structures render the excitonic properties particularly susceptible to exciton-phonon interactions. In this talk, I will present an investigation of the complex optical transitions of the prototypical MOC [AgSePh]∞. We visualized the excitons dynamics with transient absorption spectroscopy whose temporal oscillations reveal coherent exciton-phonon coupling. Steady state absorption and Raman spectroscopies revealed a strong exciton-phonon coupling and its anharmonicity, manifested as a nontrivial temperature-dependent Stokes shift. Our work highlight the general importance of exciton coupling to optical phonons hybrid quantum wells and it finally suggests that the peculiar spatial symmetry of the excitonic states and phonon modes needs to be accounted for in a proper treatment of exciton−phonon coupling.7
1.2-O2
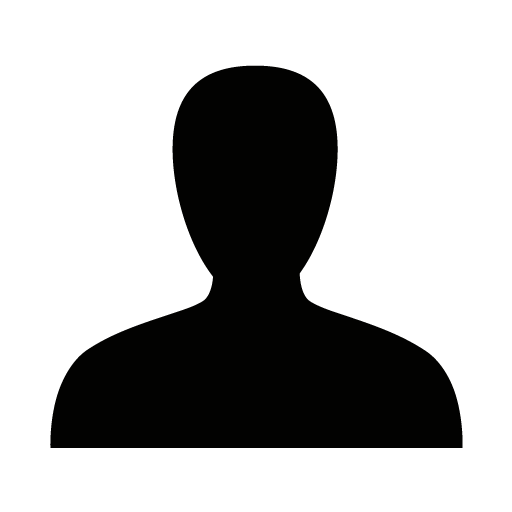
Transparent conductive oxides (TCOs) are materials of particular interest in the electronics industry. For instance, in the manufacture of electronic devices such as high-resolution flat screens, electrochromic windows, solar cells and gas sensors. These materials simultaneously present optimal electrical (resistivity lower than 10-3 Ohm-cm) and optical properties (average transmittance greater than 80 % in the visible spectral range and a bandgap greater than 3.3 eV) [1-2]. The structural, optoelectronic and electrical properties of TCOs can be tuned by the incorporation of transition metal ions as dopants. In particular, Al-doped ZnO (ZnO:Al or AZO) is a material that has generated interest in recent years due to its potential to replace ITO, the most widely used conductive transparent oxide.
This work aims to identify the effects of aluminum doping and the post-deposition annealing treatment conditions on the structural, optical, and electrical properties of ZnO sputtered thin films. The films were deposited on silicon and fused silica (FS) substrates by radiofrequency magnetron sputtering using AZO targets (ZnO doped with 2 wt.% and 3 wt.% Al). For both Al concentrations, active cooling and non-cooling conditions were used independently for each set of substrates during the deposition process. Subsequently, post-deposition shock thermal treatments were carried out at 100, 200, 300, 400, 500 and 600 °C in an inert atmosphere of ultra-high purity argon.
Optical properties of the films were investigated through variable angle spectroscopic ellipsometry (VASE) and Ultraviolet-Visible-Near-Infrared (UV/VIS/NIR) spectrophotometry. The thickness, extinction coefficient (k), refractive index (n) and optical bandgap (Eg) of the films were determined using Drude and Tauc-Lorentz models. Point by point analysis was also used as an alternative method to determine n and k. Charge carrier density and mobility were estimated from the optical analysis.
Electrical resistivity, mobility, and charge carrier density of the films were estimated by the Van der Pauw method and Hall effect. The experimental and optically estimated values for the charge carrier density and mobility exhibit good agreement.
1.2-O3
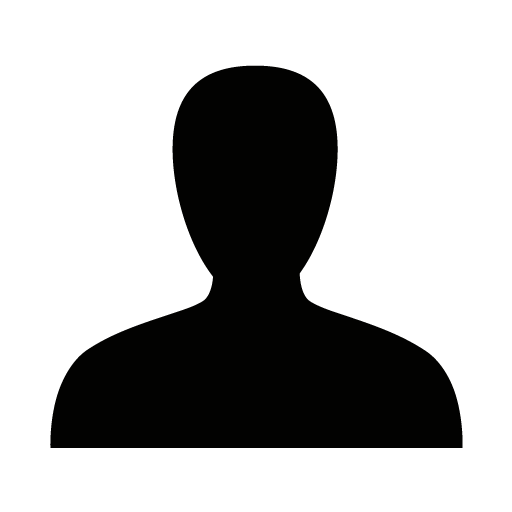
Colloidal nanoparticles are of growing importance in a broad range of practical applications, from medicine and biodiagnostics through to energy and environmental science. However, the fact that they are non-discreet reaction products, and their properties vary extremely sensitively as a function of size, shape and composition, makes materials discovery, characterization and optimization a daunting task in the face of an extensive reaction parameter space.
Here we present our experience in the development and application of segmented-flow microfluidic systems for the advanced synthesis, analysis and optimization of colloidal nanoparticles [1]. These systems allow rapid and efficient exploration of the mentioned extensive reaction parameter space. Intrinsic advantages include rapid heat and mass transfer in micro-isolated reaction volumes, leading to highly uniform and reproducible reaction conditions. Incorporation of in-line analytics (e.g. photoluminescence and absorption spectroscopy) allows real-time characterization and data feedback for powerful real-time reaction optimization. We discuss how this approach interfaces with new opportunities in data science, including machine learning, and how we are pushing to move materials chemistry into a new ‘big data’ regime. We present examples from our lab of the application of such systems in nanomaterials investigations, with a particular focus on the synthesis and characterization of cesium lead bromide perovskite nanocrystals. Here, we have used multiparametric reaction mapping to elucidate how nanocrystal optical and morphological properties vary with ligand type, concentrations, and blend ratios.
There are many opportunities and challenges in the use of automated microfluidic systems in the future of colloidal nanocrystal synthesis, and there is vast potential for such systems to be developed and applied by a variety of research groups for wide impact.
1.2-O4
Perovskite nanocrystal superlattices (NC SLs), made from millions of ordered crystals, support collective optoelectronic phenomena such as superfluorescent (SF) emission. In these SL collectives, the coupled NC emitters are highly sensitive to structural and spectral inhomogeneities of the NC ensemble. We use Free electrons in scanning electron microscopy (SEM) to probe the cathodoluminescence (CL) properties of CsPbBr3 SLs with a ~20nm spatial resolution. Correlated CL-SEM measurements allow simultaneous characterization of structural and spectral heterogeneities of the SLs. Hyperspectral CL mapping, in which a full CL spectrum is acquired from every pixel, shows multipole emissive domains within a single SL. Consistently, the edges of the SLs are blue-shifted relative to the central domain by up to 65meV. Residual uniaxial compressive strains, indicated by structural characterizations of the SLs, accompanying SL formation are contributors to these emission shifts. We discover a relation between NC building block colloidal softness and the extent of the CL shift. In SLs made from smaller NC building blocks, the CL shift is much higher due to the high colloidal softness which influence the residual strains in the SL from the assembly process. Therefore, precise control over the NC building blocks colloidal softness is critical for SL engineering.
1.2-O5
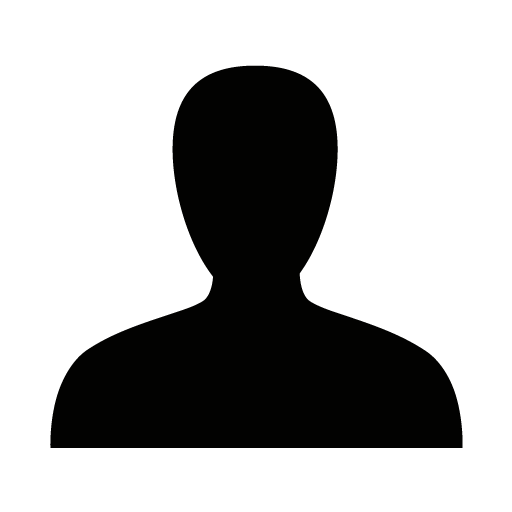
Colloidal quantum dots (QDs) are promising photoredox catalysts that offer broadly tunable potentials, high absorption coefficients and regenerability. These properties have prompted QDs to be examined for various photocatalytic reactions, from water splitting and CO2 reduction to various organic transformations [1]. An even wider parameter space emerges upon coupling QDs with other homogeneous catalysts – transition metal complexes or organic dyes – in hybrid nanoassemblies exploiting energy transfer (ET). Such nanoassemblies could significantly exceed the performance of the individual constituents thanks to the very large absorption cross section of QDs combined with the long-lived triplet states of co-catalysts [2]. However, understanding the complex behavior arising in hybrid nanoassemblies requires methods with high spatio-temporal resolution [3]. Here, we probe ET from single lead halide perovskite QDs to organic dye molecules employing single-particle photoluminescence spectroscopy with single-photon resolution. We identify ET by spatial, temporal, and photon-photon correlations in the QD and dye emission. Exploiting the high temporal resolution of our experiment as well as the discrete quenching steps due to photobleaching of individual organic dyes, we observe a characteristic Förster-type ET, with efficiencies higher than 70% in the sole case of a strong donor-acceptor spectral overlap. Our work sheds light on the processes occurring at the QD/molecule interface and demonstrates the feasibility of sensitizing organic catalysts with QDs.
1.2-O6
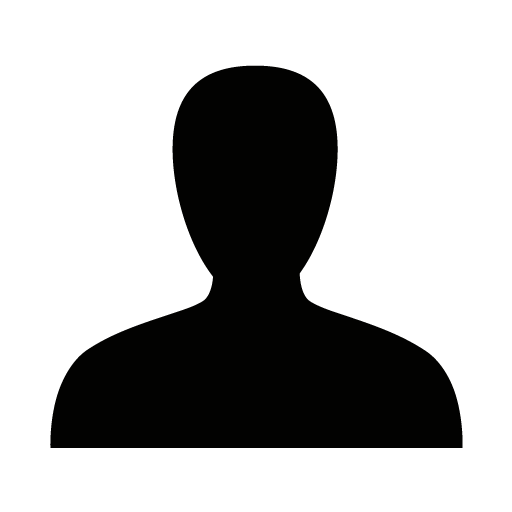
Metal halide perovskite (MHP) nanocrystals (NCs) are newcomer materials with excellent features to be exploited as luminescent labels in immunochemistry and biosensing, such as high emission yields (~100%), easy synthesis, narrow emission bands, and wide color gamut. They also exhibit giant multiphoton absorption, which makes them promising NIR-label biosensing. Thus, we envision that shortly perovskite NCs will enable the manufacture of high-throughput analytical platforms for the simultaneous and sensitive detection of multiple analytes, reducing the costs of the current detection kits. However, their future relies on addressing several challenges, such as their stabilization in water, the media of serological and biological samples, the obtention of monodispersed colloidal NCs, and their size reduction. [1]
This work presents different strategies to prepare metal halide perovskite NCs stable in water and with suitable properties as luminescent tags. The approaches tackled include (A) an adaptation of the sol-gel process consisting of a post-synthetic chemical transformation of pre-synthesized NCs, either CsPbBr3 or Cs4PbX6, in the presence of silica precursors, [2-3] (B) in situ growth of perovskite NCs in silica mesoporous nanoparticles, (C) polymeric nanobeads produced by coprecipitation methods [4] and (D) adaptation of liquid atomization methods. The stabilized materials were successfully probed as fluorescent agents for staining mouse cell cultures and in immunosensing assays. Finally, we discuss open research challenges and future directions toward their final use as a luminescent tag.
References
[1] C. Collantes, W. Teixeira; et al. Appl. Mater. Today. 2023, 31, 101775
[2] C. Collantes, V. González-Pedro, et al. ACS Appl. Nano Mater. 2021, 4, 2011−2018.
[3] C. Collantes, W. Teixeira et al. J. Mater. Chem. B. Submitted.
[4] Avugadda, S. K., Castelli A. et al. ACS Nano. 2022, 16(9), 13657-13666.
1.2-O7
Thais Caroline de Almeida da Silva obtained her B.Sc. degree in Chemistry in 2019 and the M.Sc. degree in 2021, both from the Chemistry Institute - São Paulo State University - UNESP. She joined Universitat Jaume I in January of 2022 where she is currently Doctoral Candidate (Santiago Grisolia Program) at Inorganic and Organic Chemistry Department and active member of the Institute of Advanced Materials (INAM). She has experience in inorganic chemistry, materials chemistry, synthesis and characterization of functional materials, sol-gel process and heterogeneous photocatalysis. Her current interests are focused on the development of metallic perovskites doped with lanthanide ions for application in optics, energy, ceramics and catalysis.
Climate change and environmental degradation, mainly associated to the use of fossil fuels, are existential threats to the world. So, new and more advanced systems with reduced energy consumption have to be developed in order to allow real energy savings. In this context, the fabrication of low-cost, environmentally-friendly and high-efficiency photovoltaic and optoelectronic devices such as solar cells, LEDs, photodetectors, sensors or luminescent solar concentrators, is now more than ever a need to be addressed. Lead-halide perovskites have emerged as promising candidates for solid-state lighting applications. Perovskite light-emitting diodes (PeLEDs), akin to solar cells, have witnessed significant advancements.
This study presents a novel approach to address this challenge by utilizing CsPbBr3 colloidal perovskite nanocrystals (PeNCs) synthesized through a microwave-assisted method. The PeNCs exhibit a photoluminescence emission peak centered at 514 nm, with a narrow full width at half-maximum of 20.4 nm, resulting in an impressive photoluminescence quantum yield of 66.8%. Consequently, these PeLEDs demonstrate exceptional external quantum efficiency of 23.0% and power luminance levels surpassing 40000 cd m-2, meeting the requirements for various applications. These findings highlight the tremendous promise of PeNCs for LED lighting and displays, offering feasible levels of device performance.
1.3-I1
Maria Ibáñez was born in La Sénia (Spain). She graduated in physics at the University of Barcelona, where she also obtained her PhD in 2013, under the supervision of Prof. Dr. Cabot and Prof. Dr. Morante. Her PhD thesis was qualified Excellent Cum Laude and awarded with the Honors Doctorate by the University of Barcelona. Her PhD research was funded by a Spanish competitive grant (FPU) which supported her to conduct short-term research stays in cutting-edge laboratories. In particular she worked at CEA Grenoble (2009), the University of Chicago (2010), the California Institute of Technology (2011), the Cornell University (2012) and the Northwestern University (2013). In 2014, she joined the group of Prof. Dr. Kovalenko at ETH Zürich and EMPA as a research fellow where in 2017 she received the Ružička Prize. In September 2018 she became an Assistant Professor (tenure-track) at IST Austria and started the Functional Nanomaterials group.
Thermoelectricity is the phenomenon of converting heat directly into electricity and vice versa, offering a sustainable path to produce electricity from waste heat. To maximize the efficiency of this process, complex materials where not only the crystal structure but also other structural features such as defects, grain size, orientation, and interfaces must be controlled. To date, conventional solid-state techniques cannot provide this level of control. Herein, we present a synthetic approach in which dense inorganic thermoelectric materials are produced by the consolidation of nanoparticle powders produced in solution. This synthetic approach can provide a significant degree of control over the nanoparticle features and hence a unique opportunity to control the final characteristics of the inorganic solid through nanoparticle design.
In particular, in this talk, we will focus on Ag2Se, an important thermoelectric material for the use of thermoelectricity near room temperature, where the library of high-performing materials is minimal. Despite Ag2Se being a promising candidate, the main problems are the large discrepancy in the reported thermoelectric properties and the struggles to reproduce the high performance achieved. Such divergence appears to arise from the difficulty of controlling the defects present in the material, such as vacancies, interstitial atoms, dislocations, grain boundaries, precipitates, etc. We will show that our solution synthesis allows for precise control of such defects, especially avoiding fluctuations in stoichiometry. Furthermore, we show how we can tune microstructural defects, such as strain, dislocations, and grain boundary density, utilizing the characteristic phase transition of Ag2Se during the sintering process. Overall, our results will highlight that besides stoichiometry, the microstructure is crucial for tuning Ag2Se transport properties and how this control can be provided by our novel synthetic route.
1.3-I2
In the last decade, metal halide perovskites (MHPs) have rapidly emerged as one of the most interesting classes of materials, with strong light-matter interactions, tunable luminescence over the entire visible spectrum and large electron-hole diffusion lengths, which have proven extremely useful in a wide range of scientific and technological disciplines. The subsequent development of material preparation using low-temperature solution-based strategies has allowed the same material to be obtained as nanocrystals (NCs) with high control over size and shape and further increase their optical properties tunability via dimensional confinement. Also importantly, this novel material preparation approach further extended LHPs applicability thanks to the possibility of processing directly from solution to realize functional thin films, nanocomposites or simple solution-based devices. In their NCs form, LHP have been widely applied as efficient emitters in artificial light sources, overcoming the limits of resolution and color purity of single crystals, or as high-Z materials efficiently interacting with ionizing radiation for scintillators, showing performances comparable or even higher than their large macroscopic crystal counterparts. In this talk, I will focus on our recently developed strategies for engineering LHP-NCs for different application: from high-power direct and down-converting light emitting devices (LEDs) to potential biomedical applications. I will present the different approaches that have been adopted to specifically tune the spectroscopical, chemical and physical properties of LHP NCs for these application, pursued via chemical modification (compositional tuning, post-synthesis surface treatments) of physical confinement (encapsulation into mesoporous silica nanoparticles).
1.3-I3
Kaifeng Wu obtained his B.S. degree in materials physics from University of Science and Technology of China (2010) and his PhD degree in physical chemistry from Emory University (2015). After his postdoc training at Los Alamos National Laboratory, he moved to China to start his independent research in 2017. His current work focuses on the ultrafast spectroscopy of carrier and spin dynamics in low-dimensional optoelectronic materials, as well as relevant applications in quantum information and energy conversion technologies. He is the winner of the 2022 Distinguished Lectureship Award by the Chemical Society of Japan, 2021 Future of Chemical Physics Lectureship Award by the American Physical Society, 2020 Chinese Chemical Society Prize for Young Scientists, 2019 Robin Hochstrasser Young Investigator Award by the Chemical Physics journal, and 2018 Victor K. LaMer Award by the American Chemical Society. He also serves as the Editorial Advisory Board of J. Phys. Chem. Lett.
Near-infrared to visible photon upconversion holds great promise for a diverse range of applications. Current photosensitizers for triplet-fusion upconversion across this spectral window often contain either precious or toxic elements, and have relatively low efficiencies. Although colloidal nanocrystals have emerged as versatile photosensitizers, the only family of nanocrystals discovered for near-infrared upconversion is the highly-toxic lead chalcogenides. Here we report zinc-doped CuInSe2 nanocrystals as a low-cost and lead-free alternate, allowing for near-infrared to yellow upconversion with an external quantum efficiency reaching 16.7%. When directly merged with photoredox catalysis, this system enables efficient near-infrared-driven photoreducation, oxidation, C-O couplong and polymerization, which in turn solves the issue of reabsorption loss for nanocrystal-sensitized upconversion. Moreover, the broadband light capturing of these nanocrystals allows for very rapid reactions under indoor sunlight, an especially remarkable example among which is the polymerization of acrylates within just 30 seconds. Extending the reach of "solar synthesis" into the near-infrared may realize the century-long dream of conducting high added-value chemical transformations using sunlight.
2.1-I1
Mercury is a peculiar metal, which can be found as a liquid in its native form in the nature. Its distinct properties arise from the strong relativistic effects and the ensuing lanthanide contraction.[1] Mercury chalcogenides, such as HgTe, manifest the characteristic physics of mercury by having the Gamma6 band (arising from Hg 6s orbitals) lower than the Gamma8 one (arising from 5p orbitals of Te). This is contrary to other metal chalcogenides such as CdSe, and makes HgTe have a so-called negative band gap (i.e. a semi-metal). Because quantum confinement restores the usual band order, it has been used to open gaps controllably from the THz regime up to the near infrared, which makes HgTe nanocrystals the most widely tunable colloidal material.[2]
In this presentation we explain the electronic structure and optical properties of HgTe nanoplatelets.[3,4] Optical measurements carried out on these systems reveal scarce qualitative differences with respect to their CdTe counterparts. We show this is because the small thickness (3.5 monolayers) suppresses any
trace of band inversion.[4] Next we investigate, by means of an eight-band k·p Hamiltonian and configuration interaction methods, how the response should change when increasing the thickness.
We predict that for thicknesses beyond 2 nm, the physics of HgTe starts being governed by the Gamma6-Gamma8 band mixing, which leads to important departures with respect to the expectations of quantum confinement. A prominent example is the formation of hybrid states in the conduction band, where the charge density is not localized in the volume, but partly migrates towards the surface of the platelet. Because this does not happen to the valence band states, we foresee the possibility of building indirect excitons without the need of using type-II heterostructures.
Around 6 nm the Gamma6-Gamma8 band inversion takes place, which is indeed close to the critical thickness reported in epitaxial HgTe/CdTe quantum wells.[5] At this point a quantum phase transition from regular to topological insulator takes place. A few hints are given on the topological magnetoelectric effect that could be achieved in this regime. Namely, we show through axion electrodynamics equations that electrical charges could induce magnetic fields in the nanostructure. These could be modulated through the vertical and lateral confinement of the platelet.[6]
2.1-I2
We provide a survey of our experimental studies of spin phenomena involving electrons, holes and nuclei in lead halide perovskite nanocrystals [1-6] and 2D structures [7,8]. Several experimental techniques are used: polarized emission in strong magnetic fields, time-resolved Faraday/Kerr rotation and spin-flip Raman scattering. Perovskite nanocrystals for these studies are grown by colloidal chemistry and synthesized in glass matrix. We measure spin relaxation and spin coherence times, evaluate electron and hole Lande g-factors, analyze the effects of the quantum confinement and perovskite composition, which tune the band gap, on the g-factors and compare that with model predictions. Electron spin coherence is detected even at room temperature, and unusual temperature dependence of the electron g-factor is found. Combination of the pump-probe Faraday rotation with radiofrequency allow us to use this spin resonance technique for detecting spin relaxation times of hundreds microseconds. Spin mode locking effect based on spin synchronization under periodic laser excitation is found in nanocrystals in glass. Experimental approaches of spin physics give reach information about these materials.
References
[1] D. Canneson, et al., Negatively charged and dark excitons in CsPbBr3 perovskite nanocrystals revealed by high magnetic fields, Nano Letters 17, 6177 (2017).
[2] P. S. Grigoryev, et al., Coherent spin dynamics of electrons and holes in CsPbBr3 colloidal nanocrystals, Nano Letters 21, 8481 (2021).
[3] V. V. Belykh, et al., Submillisecond spin relaxation in CsPb(Cl,Br)3 perovskite nanocrystals in a glass matrix, Nano Letters 22, 4583 (2022).
[4] E. Kirstein, et al., Mode locking of hole spin coherences in CsPb(Cl,Br)3 perovskite nanocrystals, Nature Communications 14, 699 (2023).
[5] M. O. Nestoklon, et al., Tailoring the electron and hole Lande factors in lead halide perovskite nanocrystals by quantum confinement and halide exchange, Nano Letters 23, 8218 (2023).
[6] S. R. Meliakov, et al., Coherent spin dynamics of electrons in CsPbBr3 perovskite nanocrystals at room temperature, Nanomaterials 13, 2454 (2023).
[7] E. Kirstein, et al., Coherent spin dynamics of electrons in two-dimensional (PEA)2PbI4 perovskites, Nano Letters 23, 205 (2023).
[8] C. Harkort, et al., Spin-flip Raman scattering on electrons and holes in two-dimensional (PEA)2PbI4 perovskites, Small 2300988 (2023).
2.1-I3
Vanmaekelbergh's research started in the field of semiconductor electrochemistry in the 1980s; this later evolved into the electrochemical fabrication of macroporous semiconductors as the strongest light scatterers for visible light, and the study of electron transport in disordered (particulate) semiconductors. In the last decade, Vanmaekelbergh's interest shifted to the field of nanoscience: the synthesis of colloidal semiconductor quantum dots and self-assembled quantum-dot solids, the study of their opto-electronic properties with optical spectroscopy and UHV cryogenic Scanning Tunneling Microscopy and Spectroscopy, and electron transport in electrochemically-gated quantum-dot solids. Scanning tunnelling spectroscopy is also used to study the electronic states in graphene quantum dots. More recently, the focus of the research has shifted to 2-D nano structured semiconductors, e.g. honeycomb semiconductors with Dirac-type electronic bands.
Colloidal nanocrystals underwent a tremendous development with full control over dimensions and surface chemistry, resulting in vast opto-electronic applications. Can they also form a platform for quantum materials, in which electronic coherence is key? We use colloidal, two-dimensional Bi2Se3 crystals, uniform in thickness and with limited lateral dimensions, as a model system to study the evolution of a three-dimensional topological insulator to the technologically important case of two-dimensions and limited crystal domains.
Individual Bi2Se3 platelets with diameter in the 100-200 nm range and well-defined thickness (1-6 quintuple layers) with cryogenic scanning tunneling microscopy and spectroscopy. For 4-6 Bi2Se3 quintuple layers, we observe an edge state, 8 nm wide, around the entire crystal. The edge state is faint or absent for thinner (1-2 QLs) Bi2Se3 platelets. The edge states are resilient under a perpendicular magnetic field. Ab-initio calculations confirm that crystals with 3 QLs or more have a non-trivial band structure with a one-dimensional quantum channel at the edge. The quantum channel consists of 2 counter propagating states with momentum-spin locking, key for non-dissipative information transfer and quantum computing.
We've performed optical spectroscopy in the high energy region (1-3 eV). We coud classify the optical transitions as (1) transitions due to the surface (outer QLs) or (2) due to the inner QLs. By comparison with GW simulations, we identified all transitions in a (energy, momentum in x, momentum in y) two-dimensional Brillouin zone frame. Some transitions show electron and hole cooling in which the carriers separate in momentum space.
Colloidal Bi2Se3 platelets are not only a model system for a two-dimensional toplogical insulator, but also a layer semi-metal with exotic optical transitions. The processability and dimensional control of topological insulator colloidal nanocrystals opens a unique window to devices with a large density of addressable quantum states.
REFERENCES
J. Moes et al., manuscript in preparation
Swart, I., Liljeroth, P. & Vanmaekelbergh, D. Scanning probe microscopy and spectroscopy of colloidal semiconductor nanocrystals and assembled structures. Chem. Rev. 116, 11181-11219 (2016).
Kane, C. L. & Mele, E. J. Z(2) topological order and the quantum spin Hall effect. Physical Review Letters 95 (2005).
Zhang, H. J. et al. Topological insulators in Bi2Se3, Bi2Te3 and Sb2Te3 with a single Dirac cone on the surface. Nature Physics 5, 438-442 (2009).
Yazyev, O. V. et al. Spin Polarization and Transport of Surface States in the Topological Insulators Bi2Se3 and Bi2Te3 from First Principles. Physical Review Letters 105 (2010).
Zhang, Y. et al. Crossover of the three-dimensional topological insulator Bi2Se3 to the two-dimensional limit. Nature Physics 6, 584-588 (2010).
Liu, C. X. et al. Oscillatory crossover from two-dimensional to three-dimensional topological insulators. Physical Review B 81 (2010).
Neupane, M. et al. Observation of quantum-tunnelling-modulated spin texture in ultrathin topological insulator Bi2Se3 films. Nature Communications 5 (2014).
Chiatti, O. et al. 2D layered transport properties from topological insulator Bi2Se3 single crystals and micro flakes. Sci Rep 6 (2016).
2.2-I1
Alexander S. Urban studied Physics at the University of Karlsruhe (Germany) obtaining an equivalent to an M.Sc. degree (German: Dipl. Phys.) at the University of Karlsruhe (Germany) in 2006. During his studies he spent a year at Heriot Watt University (UK), where he obtained an M.Phys. in Optoelectronics and Lasers in 2005. He then joined the Photonics and Optoelectronics Chair of Jochen Feldmann at the Ludwig-Maximilians-University (LMU) Munich (Germany) in 2007 where he worked on the optothermal manipulation of plasmonic nanoparticles, earning his Ph.D. summa cum laude in 2010. He expanded his expertise in the fields of plasmonics and nanophotonics in the group of Naomi J. Halas at the Laboratory for Nanophotonics at Rice University (Houston, TX, USA), beginning in 2011. He returned to the LMU in 2014 to become a junior group leader with Jochen Feldmann, where he led the research thrusts on optical spectroscopy, focusing on hybrid nanomaterials such as halide perovskite nanocrystals and carbon dots. In 2017 he was awarded a prestigious Starting Grant from the European Research Council and shortly after that in 2018 he received a call as a Full Professor of Physics (W2) at the LMU. Here, he now leads his own research group working on nanospectroscopy in novel hybrid nanomaterials.
Halide perovskite nanocrystals (NCs) have emerged as an intriguing material for optoelectronic applications, most notably for light-emitting diodes (LEDs), lasers, and solar cells. Despite impressive advances, halide perovskite nanocrystals have not yet been commercialized due to certain inherent limitations. Importantly, they are highly prone to environmentally induced degradation. Additionally, the incorporation of chloride, necessary to achieve blue emission, renders the perovskite nanocrystals defect intolerant.
In this talk, I will explore our recent results on specifically tailoring halide perovskite nanocrystals to improve their overall stability and performance in the blue spectral region. To achieve the latter we produce anisotropically quantum confined nanocrystals, in the form of two-dimensional (2D) nanoplatelets (NPLs). These colloidal quantum wells have additional appeal for light emission, as the one-dimensional quantum confinement enhances their radiative rates and enables directional outcoupling. On top of this, due to a monolayer-precise control over their thickness, they constitute an intriguing system for spectroscopic studies on their fundamental optical, phononic, and energetic properties. To achieve a high degree of stability, we introduce block copolymer micelles, which serve as nanoreactors and shield the encapsulated nanocrystals from the environment. Importantly, the hydrophobic shell prevents moisture from degrading the nanocrystals and prohibits ion migration. It is thus possible to fabricate halide perovskite heterolayers.
2.2-I2
The generation, identification, and utilization of excited states is of paramount importance for optimizing semiconductor nanocrystals for optoelectronic applications. Of particular interest is characterizing the number and type of excitations present in a nanocrystal to understand the efficiency of processes such as Auger recombination, carrier multiplication, or charge transfer. While charged (trion) and multiexciton states are functionally quite different from single excitons states with respect to their excited state lifetime, they are spectrally quite similar, resulting in challenges in conclusively identifying these states. Through power dependent transient absorption measurements and globally fitting the exciton and multiexciton component spectra, we directly identify both the recombination dynamics, spectral form, and relative intensities affiliated with each state in CdSe quantum dots. Through these measurements we illustrate that the biexciton transient absorption spectra is < 1.6 times as intense as the exciton spectrum at the band-edge across a range of CdSe quantum dot sizes. This is in contrast to the oft-assumed factor of 2 between the exciton and biexciton absorption due to the degeneracy of the state. We identify that differences in the spectral intensity between samples is most sensitive to the difference in peak energies, which is correlated with the surface termination rather than quantum dot size. Surface effects also have a large impact on the excited state properties of CdS nanocrystals. One such example is surface/ligand mediated photoreduction which we identify through both steady-state absorption and photoluminescence measurements [1]. Of particular importance is the ubiquitous nature of this ligand-mediated photoreduction, which can have an enormous impact on optical measurements and device functionality for a variety of applications of CdS nanocrystals. While problematic for spectroscopic measurements of exciton properties, these photogenerated charged states can be long-lived (minutes to hours), opening avenues for highly efficient charge transfer to enable photocatalytic applications.
2.2-I3
The advent of SPAD-array based parallelized detection of time-stamped single photons opens a pathway for extracting previously inaccessible spectroscopic information from dim sources of quantum light. In particular, it enables to multiplex detection both in time and in additional dimensions such as space, frequency or spatial frequency. We use this to study the interactions between pairs of excitons in doubly excited colloidal semiconductor nanocrystals, revealing weak multielectron effects at room temperature against a strong temperature broadened background.
We present heralded spectroscopy [1], the unique identification and post-selection of pair emission events from single nanocrystals while performing a spectral measurement (using a line SPAD array [1]) or a defocused imaging measurement (using a 2D SPAD array [2]). Using this method we can characterize subtle differences between the first emitted photon (representing emission from the doubly excited state) and the second emitted photon (representing the singly excited state). Several examples for this will be given, including interaction effects on emission anisotropy from semiconductor nanorods [2] and identification of multiexcitonic states in quantum dot molecules. The utility of our method for study of higher excited states via three-photon correlation will also be discussed.
1.1-O1
Despite the incredible progress made, the highest efficiency perovskite solar cells are still restricted to small areas (<1 cm2). In large part, this stems from a poor understanding of the widespread spatial heterogeneity in devices. Conventional techniques to assess heterogeneities can be time consuming, operate only at microscopic length scales, and demand specialized equipment. We overcome these limitations by using luminescence imaging to reveal large, millimeter-scale heterogeneities in the inferred electronic properties. We determine spatially resolved maps of “charge collection quality”, measured using the ratio of photoluminescence intensity at open and short circuit. We apply these methods to quantify the inhomogeneities introduced by a wide range of transport layers, thereby ranking them by suitability for upscaling. We reveal that top-contacting transport layers are the dominant source of heterogeneity in the multilayer material stack. We suggest that this methodology can be used to accelerate the development of highly efficient, large-area modules, especially through high-throughput experimentation.
1.1-O2
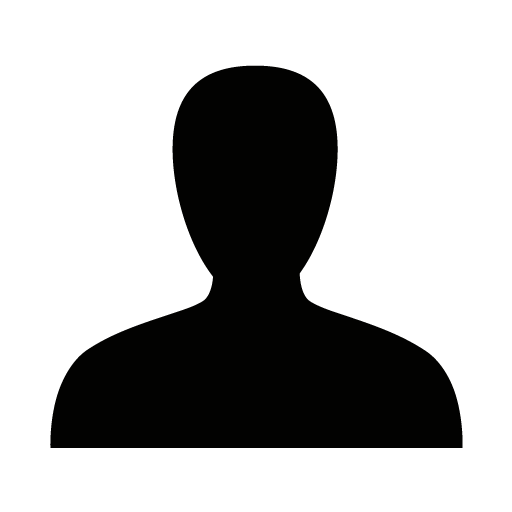
Two-dimensional Ruddlesden-Popper perovskites (2D-LRPPs) are an interesting material class with potential applications in the field of optoelectronics [1]. The 2D-LRPP semiconductor includes a metal-halide inorganic octahedral layer separated by large organic cations. This configuration provides quantum confinement and strong dielectric effects [2]. In 2D-LRPPs, the decreased mobility of halide anions in contrast to 3D counterparts limits the interdiffusion across heterostructures, which ultimately could lead to more stable heterostructures even during external perturbations like light and heat [3]. This report presents a scalable solution-based two-step methodology to fabricate microcrystalline PEA2PbX4-PEA2PbX4 (X = Br, I) lateral heterojunctions at room temperature. The fabrication of the high band gap PEA2PbBr4 semiconductor at the edges of the low band gap PEA2PbI4 semiconductor, and vice versa, lead to a band alignment that could enable a directional energy and charge flow from the high band gap material toward the low band gap material. Different anion sources, solvent mixtures, and anion concentrations influence the quality of the formed heterojunction. The results indicate that the formation of the lateral heterojunction is based on an anion exchange for PEA2PbI4-PEA2PbBr4 and dissolution-recrystallization processes for PEA2PbBr4-PEA2PbI4. The fabrications of such 2D-LRPPs heterostructure offer an opportunity to investigate charge- or energy-transfer phenomena at the heterojunction and the interchange between these materials, which could lead to their applications in optoelectronics, and among others.
1.1-O3
Despite the meteoric rise in the development of a variety of perovskite electronic and optoelectronic devices, the phenomenon of ion migration remains a common and longstanding Achilles’ heel limiting their performance and operational stability. In particular, ionic screening of the applied gate potential especially near room temperature reduces the gate modulation of carriers in the semiconducting channel of lead (Pb) perovskite thin film transistors (PeTFTs), resulting in inferior carrier mobilities and non-idealities in device characteristics [1]. Similarly, ionic movements under light and/or bias have been shown to result in current-voltage hysteresis [2], open circuit voltage gain [3] and short circuit current losses [4] in operating Pb perovskite solar cells (PSCs). Despite several efforts in the literature to mitigate such effects through compositional and additive engineering, there appears no clarity on the impact of tin (Sn) substitution on the resulting ion dynamics of Pb halide perovskites.
In this talk, I will share our research on understanding the ionic transport in methylammonium (MA)-free mixed Pb-Sn perovskites using PeTFTs and PSCs as two different platforms. Firstly, we demonstrate that mixed Pb-Sn based PeTFTs do not suffer from ion migration effects as significantly as their pure-Pb counterparts, thereby reliably exhibiting hysteresis-free p-type transport with high mobility reaching 5.4 cm2/Vs, which is one of the highest reported TFT mobilities for hybrid perovskite thin films [5]. The reduced ionic migration in mixed Pb-Sn PeTFTs is manifested in the relative invariance of the current-voltage hysteresis as well as ON-current for a range of scan rates and improved temporal stability of the ON-current with time. Moreover, the observation of an activated temperature dependence of the field-effect mobility with low activation energy (< 50 meV for T > 200 K) in the case of mixed Pb-Sn compositions (as opposed to the negative temperature coefficient resulting from ionic screening effects in the pure-Pb composition) is consistent with the presence of shallow defects present in these materials, thereby reinforcing the suppressed role of ionic transport in dictating the charge transport in these materials. In fact, to the best of our knowledge, such activated temperature dependence of FET mobility is also reported for the first time in the field of 3D halide perovskites. Furthermore, by performing photoluminescence microscopy under bias on lateral two-terminal devices, we visualize the suppressed in-plane ionic migration in Sn-containing perovskite compositions compared to their pure-Pb counterparts.
Next, we performed scan-rate dependent current-voltage (and hysteresis) measurements and temperature dependent impedance spectroscopy measurements on optimized MA-free Pb and Pb-Sn perovskite solar cells, which demonstrate the suppressed motion of ions in Pb-Sn devices as compared to their Pb-only analogues, thereby generalizing our earlier observations from PeFETs [6]. We also obtain mechanistic insights into these experimental observations by conducting first-principles calculations, which reveal the key role played by Sn vacancies (with low formation energy) in increasing the migration barrier for iodides due to severe local structural distortion in the lattice.
All in all, our results emphasize the relatively unexplored bright prospect of tin substitution in obtaining improved lateral charge transport and suppressed ionic migration effects in Pb halide perovskite devices.
References
* denotes equal-contributing first author.
[1] Senanayak, S. P. et al. Sci. Adv. 2017, 3, e1601935.
[2] Snaith, H.J. et al. J. Phys. Chem. Lett. 2014, 5, 1511-1515.
[3] Herterich, J. et al. Energy Technol. 2021, 9, 2001104.
[4] Thiesbrummel, J. et al. Adv. Energy Mater. 2021, 11, 2101447.
[5] Senanayak, S.P.* and Dey, K.* et al. Nat. Mater. 2023, 22, 216–224.
[6] Dey, K. et al. arXiv 2023.
1.1-O4
Nanocrystals (NCs) have become a key component in lead halide perovskite-based devices due to their outstanding optoelectronic properties. While colloidal synthesis has reached an impressive level of maturity in less than a decade allowing for the fabrication of stable NCs with near unity quantum yield (QY), other fabrication approaches have emerged over the past few years. Among them, the synthesis within nanoporous matrices allows obtaining stable and ligand-free pNCs within thin films with optical quality perfectly suited for integration into devices. [1] Beyond the possibility of employing these films as active layers for solar cells with good efficiency [2] they represent an ideal test bench for exploring light matter interaction at the nanoscale in lead halide perovskites without ligand or solvent induced effects characteristic of colloidal NCs. [3]
In this work, we present a study on charge carrier recombination in FAPbBr3 NCs assemblies synthesized within metal-oxide porous matrices where interparticle distance is controlled via changes in the pore filling fraction. A broad photophysical characterization comprising time resolved absorption and emission spectroscopies as a function of temperature and fluence is employed to study the different recombination pathways of photogenerated carriers. We observe a transition from isolated emitters to interconnected NCs that preserve confinement effects while allowing for long range electronic transport. We show how interconnectivity determines charge carrier dynamics as it affects trap filling, radiative recombination and multiparticle interactions. These results are discussed in terms of recombination and diffusion models for both the isolated and interconnected case and study the transition between these scenarios. These findings provide an insight into the photophysical properties of an exciting nanostructured material in a configuration suited to be incorporated into a device.
1.2-I1
The need for self-powered electronics is progressively growing in parallel to the flourishing of the Internet of Things (IoT). Although batteries are dominating as powering devices, other small systems are attracting attention, such as piezoelectrics, thermoelectrics and photovoltaics. These last ones can be adapted from their classical outdoor configuration to work preferentially under indoor illumination, i.e. through the harvesting of the spectrum emitted by LEDs and/or fluorescent lamps. However, crystalline silicon, the classical photovoltaic material for solar panels, has a bad gap not suitable for ensuring good efficiency with such spectra. Other semiconductors, with wider band gaps, can come into play for this task. Still, the materials of choice, having to be integrated within households, should also satisfy the criterion of non-toxicity, as well as maintain low-costs of production. While lead- based halide perovskites cannot represent a valuable solution for this scope, due to the strong environmental and health concerns associated to the presence of Pb, analogous compounds based on the heaviest pnictogen, i.e. bismuth, could work as sustainable light-harvesters for indoor photovoltaic devices.
In this contribution, we will show our most recent results obtained from the integration of the double perovskite Cs2AgBiBr6 in carbon-based perovskite solar cells, devices characterized by a high degree of sustanaibility, also due to the use of recycled materials within the carbon eletrodes. Similar technologies might have interesting applications for powering the IoT within private households or other indoor environments.
We will also show our studies on the use of a perovskite-inspired material, the oxyhalide BiOI, produced from a fully aqueous-based deposition method, as photoanodes for the oxygen reduction reaction as well as for the light-driven charging of liquid electrolytes for flow cell batteries.
1.2-I2
Growing demand for ultralow detection limits in medical radiography, high-energy physics, and security screening has spurred extensive research on X-ray imaging scintillators and detectors. Current market offerings of high-performance ceramic scintillators require harsh and costly fabrication techniques. In contrast, perovskite nanosheets and copper nanoclusters offer unique optical properties and high X-ray absorption cross-sections, making them promising alternatives. This presentation will discuss the room-temperature synthesis of colloidal CsPbBr3 nanosheets, exhibiting superior scintillation performance due to efficient energy transfer processes between stacked thin and thick nanosheets. Moreover, by combining CsPbBr3 perovskite nanosheets with thermally activated delayed fluorescence (TADF), reabsorption-free organic X-ray imaging scintillators were developed, offering a low detection limit of 38.7 nGy/s and exceptional imaging resolution. Cu-based halide nanoclusters composed of Cu4I6 nanoparticles and nanorods exhibit ultrahigh photoluminescence quantum yields, low detection limits of 96.4 and 102.1 nGy/s - about 55 times lower than standard medical diagnosis doses (5.5 μGy s–1) - and extraordinary X-ray imaging resolutions exceeding 30 lp/mm, more than doubled compared to conventional CsI:Tl and Ga2O2S:Tb scintillators. This research paves new avenues for developing high-resolution X-ray imaging screens based on Cu-based halide nanoclusters for applications in medical radiography and non-destructive detection.
1.2-I3
Perovskite semiconductors are emerging low-cost materials for photovoltaics, light emitting devices and detectors. Because of the inclusion of high atomic numbered elements, perovskites are promising candidates for high efficiency X-ray sensing. In this talk, I will discuss the properties of perovskite semiconductors for X-ray and visible photon sensing. Firstly, we report a long carrier diffusion length in 2D perovskite single crystals, assisted by the shallow trap and de-trapping process. Next, we show that such a long diffusion length ensures a full charge collection after charge ionization, which is beneficial for detectors for X-ray and other photons. In addition, we have found the shallow trap also extend the carrier transport lifetime that facilitate a charge multiplication in the detector driven under high voltages. Such a process introduces a photo conductivity gain, leading to an unusually high X-ray and visible photon sensing efficiency. A high gain can be also achieved by building a hetero-structured device. Interfacing perovskites with a high mobility graphene channel can also multiplicate the photo-generated carriers. With a hetero-structured device, we show a high X-ray sensitivity over 108 µCGy-1cm-2.
1.3-O1
Organic–inorganic Pb-free layered perovskites are promising green solution for lighting applications. In particular, Sn-based layered structures are highly efficient photoluminescent materials, but their emission color is limited to the yellow-orange range of the visible spectrum and their synthesis requires a controlled atmosphere and long preparation time. Here, we developed a synthesis protocol, which is performed in three key steps under ambient conditions and at low temperatures (4 °C). This simple synthesis approach enabled to investigate of a set of organic cations with different molecular structures, including both conventional alkylammonium and cyclic cations with heteroatoms, which resulted in stable structures showing different color coordinates, from yellowish-orange to orange-red, to blue-green. The observed variations in color chromaticity are attributed to the distinct connectivity of Sn–Br octahedra, ranging from fully disconnected to face-sharing, while retaining the intercalation of organic-inorganic layers, as confirmed by X-ray and 3D electron diffraction analyses. Our findings can inspire further research into the tunability of the emission of Pb-free layered perovskites via the use of organic cations to promote their use in solid-state lighting.[1]
1.3-O2
Halide perovskite (HaP) semiconductors, commonly synthesized from room temperature solutions, possess exceptional optoelectronic properties that rival those achieved by more complex fabrication methods used for conventional semiconductors. The absence of detrimental defects in HaPs is a topic of debate, often attributed to their defect tolerance or self-healing ability.
To contribute to this discussion, we conducted an experimental investigation focused on determining the absolute volume deformation potential (AVDP) of CsPbBr3. The AVDP is a crucial physical parameter that characterizes the energy level shift of a semiconductor in response to volume changes. It therefore allows to quantify the amount of energy necessary to for example rearrange a crystal lattice locally to efficiently screen the electrical energy barrier of defects. Furthermore, it provides insights into the inherent molecular orbital bonding nature of a semiconductor material.
In our study, synchrotron radiation-based X-ray photoelectron spectroscopy was employed to measure the VBM (valence band maximum) energy of CsPbBr3 across a temperature range from room temperature to 125 K. Our experimental findings demonstrate that the AVDP of CsPbBr3 is negative and relatively small compared to conventional semiconductors. This observation suggests that electronic defects can be easily screened through lattice rearrangement. Moreover, the negative sign indicates that the valence band maximum primarily consists of anti-bonding type molecular orbitals. The disruption of these bonds typically generates defect energy levels near or within the bands. Both the magnitude and sign of the AVDP support the notion of defect tolerance in Halide perovskites.
Additionally, we conducted measurements of transient photoluminescence and employed a comprehensive interpretation based on a kinetic rate equation encompassing various recombination processes of different orders. This allowed us to quantify the defect density in CsPbBr3 at similar temperatures, providing a deeper understanding of the evolution of defect properties under volumetric changes.
Our results offer valuable insights into the origins of defect tolerance in Halide perovskites, shedding light on their unique optoelectronic characteristics.
1.3-O3
Narrow bandgap perovskite solar cells based on mixed lead-tin perovskites tend to suffer from poor stability under operating conditions. This impedes the successful development of all-perovskite tandems. We explore the causes of this instability under extended periods of combined 65°C thermal and 1 sun illumination stressing, using a range of structural, optical, and electronic characterization techniques on lead-tin perovskite films, half-stacks and devices.
We show that the phase, absorbance, morphology and mobility of lead-tin perovskite films are stable on timescales that exceed those of device degradation, although we reveal an interesting pattern of phase segregation after stressing for much longer amounts of time. Additionally, we observe only a slight increase in background carrier density and a moderate decrease in charge lifetime over the first few hundred hours of stressing. We argue that these changes likely only partially account for the observed device degradation.
Investigating the EQE and J-V characteristics of devices reveals the formation of a charge extraction barrier in aged devices. We find that the impact of this barrier is hugely decreased in very fast J-V scans, suggesting that mobile ions contribute significantly to device degradation. Device simulations enable us to weigh the impact of all of these changes on photovoltaic performance. We are able to identify more closely the various processes that limit the stability of lead-tin perovskite solar cells. Finally, we propose solutions related to both bulk perovskite composition and device architecture to overcome these challenges.
1.3-O4
Dr Luis Lanzetta is a Postdoctoral Fellow at King Abdullah University of Science and Technology (KAUST, KSA). He obtained his PhD in Chemistry at Imperial College London (UK) in 2020, where he focused on developing eco-friendly, tin-based perovskites for photovoltaic and light-emitting applications. His research focuses on next-generation materials for energy harvesting. Specifically, his expertise lies in the chemical degradation and stabilisation mechanisms of halide perovskite solar cells, aiming to provide design rules towards more efficient and stable technologies. He is additionally interested in molecular doping approaches for narrow-bandgap perovskites, as well as the spectroscopic and surface characterisation of this class of materials.
Google Scholar: https://scholar.google.com/citations?user=OcCV1VUAAAAJ&hl=es
Tin-lead (Sn-Pb) halide perovskites stand out as top candidates for future near-infrared (NIR) optoelectronics, being particularly promising in all-perovskite tandem solar cells and NIR photodetectors. However, their facile degradation under operational conditions (e.g., ambient-mediated oxidation of Sn2+) remains the main impediment towards their widespread deployment. To enable the design of Sn-Pb perovskite compositions with higher innate stability, it is critical to unravel how the constituent ions of perovskite besides Sn2+ participate in the degradation of these materials. Specifically, the inconspicuous role of A-site cations in the decomposition of technologically relevant ASn0.5Pb0.5I3 perovskite structures has been largely overlooked.
In this talk, I will describe the effect that A-site cation tuning has on the degradation mechanism under ambient air of Sn-Pb perovskite compositions commonly employed in solar cells, i.e., CsxMA0.3-xFA0.7Sn0.5Pb0.5I3 (where x = 0, 0.15 and 0.3; MA = methylammnonium; FA = formamidinium). By employing thermogravimetric and spectroscopic techniques, we track the formation of molecular iodine (I2) and tin(IV) iodide (SnI4) versus time as indicators of perovskite degradation caused by exogenous O2 and endogenous I2, respectively. We detect the rate of I2 and SnI4 generation to be approximately one order of magnitude lower for Cs-rich compositions relative to their MA-rich counterparts, clearly indicating that MA replacement by Cs leads to enhanced resilience against oxidative stress. Consequently, this translates into higher stability of optical, electrical and structural properties in Cs-rich perovskite thin films upon air exposure. The talk will conclude with details on the origin of the lower stability of MA-rich compositions, which we ascribe to stronger I2 adsorption at the surface of perovskite mediated by the polarising power of the MA cation. This work provides key insights on the role of A-site choice on perovskite degradation that serve as valuable guidelines for the design of Sn-Pb perovskite optoelectronics with enhanced stability.
2.1-I1
Bismuth-based semiconductors have gained increasing attention as potential nontoxic alternatives to lead-halide perovksites [1]. Whilst early works focussed on the role of defects (particularly defect tolerance) in these materials, recent work has emphasized the important role of electron-phonon coupling [2]. This talk examines electron-phonon coupling in two emerging bismuth-based perovskite-inspired materials: NaBiS2 and BiOI.
NaBiS2 is part of a growing family of ternary chalcogenides. We show NaBiS2 to be phase-stable in ambient air for 11 months, with high absorption coefficients >105 cm-1 reached from its optical bandgap of 1.4 eV. As a result, a 30 nm thick film has a spectroscopic limited maximum efficiency of 26%, higher than lead-halide perovksites or established thin film solar absorbers. However, we show through ultrafast spectroscopy that the photogenerated charge-carriers in NaBiS2 slowly decay on a microsecond timescale, and yet the photoconductivity decays within 1 ps. This arises due to carrier localization, and we rationalize this as due to inhomogeneous cation disorder, leading to the formation of S 3p states just above the valence band maximum that facilitate the formation of small hole polarons [3].
The second part of the talk examines BiOI. We show through detailed spectroscopic measurements and state-of-the-art computations that this material is an exception to recent Bi-based perovskite-inspired materials and avoid carrier localization. As a result, mobilities exceeding 80 cm2 V-1 s-1 are achieved, along with high mobility-lifetime products exceeding 10-2 cm2 V-1 in single crystals. We show that BiOI photoconductors are highly promising radiation detectors, capable of resolving dose rates down to 22 nGyair s-1, which is well below the current medical standard of 5500 nGyair s-1.
2.1-I2
Layered double perovskites (LDPs) have emerged as a highly promising alternative to traditional single-metal perovskites. Their distinct advantages include improved stability, reduced toxicity, and unexplored chemical space, making them an exciting area of research.
This talk aims to delve into our extensive investigations on the chemistry and diversity of LDPs, also referred to as double metal vacancy-order perovskites. We will discuss our efforts to comprehend their unique properties and photophysics. The scope of our studies encompasses various aspects such as crystal structure analysis, examination of optical properties, evaluation of stability, and exploration of the photoluminescence mechanism.
Furthermore, we will shed light on the current and potential applications of LDPs in the fields of photovoltaics and optoelectronics. The multifaceted nature of LDPs makes them a compelling choice for advancing these technologies. By uncovering their fundamental characteristics and harnessing their unique features, we can pave the way for innovative and sustainable solutions in the realm of energy conversion and light-based applications.
2.1-I3
Atsushi Wakamiya received his Ph.D. degree from Kyoto University in 2003. He began his academic carreer at Nagoya University as an assistant professor in 2003. In 2010, he moved to Kyoto University as an associate professor and was promoted to full professor in 2018. He received several awards: The Chemical Society of Japan Award for Creative Work (2020), Commendation for Science and Technology by MEXT Japan: Award for Science and Technology Research Category (2022), etc. He is a project leader of the Green Innovation Program (NEDO) and JST-Mirai Program. He is a co-founder and a director (as Chief Scientific Officer, CSO) of “EneCoat Technologies, Co. Ltd.”, a startup company for perovskite solar modules. His research interests include physical organic chemistry, elemental chemistry and materials chemistry.
Perovskite solar cells attract attention as promising cost-effective next generation printable photovoltaics.
The power conversion efficiencies (PCEs) have been substantially increased in a short period, based on the improvements of the fabrication protocols for the perovskite layer, and the development of new materials for passivation of the surface or efficient charge collection, etc.
Our research approaches for lead and lead-free perovskite solar cells are as follows.
1) Development of highly purified perovskite precursor materials: We synthesis a series of complexes of lead halides or tin halides as purified precursor for perovskite materials.
2) Development of fabrication methods for perovskite layer by solution process: We focus on the intermediates formed during the solution process and develop efficient fabrication methods [1-3] including surface passivation based on the mechanism.[4-7]
3) Development of organic semiconductors as efficient charge-collection materials from perovskite layer: We design and synthesis novel pi-conjugated materials, including PATAT,[8] in terms of control of the energy level of frontier orbitals, molecular orientation, and interface between perovskite layer.
In this talk, our recent progress on Sn-based perovskite solar cells as well as Pb-based perovskites will be introduced.
2.2-I1
Iván Mora-Seró (1974, M. Sc. Physics 1997, Ph. D. Physics 2004) is researcher at Universitat Jaume I de Castelló (Spain). His research during the Ph.D. at Universitat de València (Spain) was centered in the crystal growth of semiconductors II-VI with narrow gap. On February 2002 he joined the University Jaume I. From this date until nowadays his research work has been developed in: electronic transport in nanostructured devices, photovoltaics, photocatalysis, making both experimental and theoretical work. Currently he is associate professor at University Jaume I and he is Principal Researcher (Research Division F4) of the Institute of Advanced Materials (INAM). Recent research activity was focused on new concepts for photovoltaic conversion and light emission based on nanoscaled devices and semiconductor materials following two mean lines: quantum dot solar cells with especial attention to sensitized devices and lead halide perovskite solar cells and LEDs, been this last line probably the current hottest topic in the development of new solar cells.
Halide perovskite solar cells have revolutionized the photovoltaic field in the last decade. Among other properties direct and tunable bandgap as well as low non-radiative recombination have been a key factors in this success. Moreover, these properties are also fundamental for the development of other optoelectronic devices, which has also extended the application of halide perovskites to other fields such as LEDs, lasers or photocatalytic systems. Nevertheless beyond the interest of this family of materials, and despite a decade of intensive research with improvement in the performance of perovskite devices, the two main drawbacks of this system, the use of hazardous Pb and the long term stability, still to be open questions that have not been fully addressed. Sn-based perovskite solar cells are the devices presenting the highest performance after Pb-based but significantly below them. In addition, Sn-based perovskite solar cells exhibit a long term stability lower than their Pb containing counterparts, making stability their main problem. In this talk, we highlight how the use of proper additives and light soaking for defect engineering can increase significantly the stability of formamidinium tin iodide (FASnI3) solar cells, and discuss about the different mechanism affecting this stability, beyond the oxidation of Sn2+, and how they can be countered. As a second topic of the talk, the use of halide perovskite nanocrystals for the preparation of reproducible perovskite LEDs (PeLEDs) will be analyzed as well as the fabrication of Pb-free Sn-based LEDs by inkjet printing. Improvement of both efficiency and stability of the fabricated devices will be analyzed.
2.2-I2
The development of high performance lead-free perovskite solar cells (PSCs) is important to address the environmental concern of heavy metal lead. In recent years, tin perovskite solar cell (TPSCs) is developing quickly and emerging as a promising candidate for high efficiency lead-free PSCs. Meanwhile, the narrow bandgap of tin perovskite solar cells enables it to be used for the fabrication of tandem solar cells. In this presentation, I will summarize recent work of our group about quasi-2D tin halide perovskite materials and devices. I will introduce the basic properties of low dimensional tin perovskite, crystal growth kinetic control to manipulate its nanostructure and orientation, as well as device structural engineering to reduce interface carriers recombination. Based on these efforts, the highest efficiency of tin perovskite solar cells is up to 14.6% that is certified in an independent agency. In addition, the quasi-2D structure is also applied for the fabrication of inorganic tin perovskite solar cells. Meanwhile, quasi-2D structure is applied for surface treatment of tin-lead mixed perovskite solar cells to reduce surface defects and ensure effective interface carrier transport. This interface design brings effectively enhanced device performance for both perovskite single junction and tandem solar cells.
2.2-I3
Solar energy conversion is a fundamental pillar in the ongoing ecological transition towards a sustainable economy and society. Emerging photovoltaic (PV) technologies such as perovskite solar cells (PSCs) inspire a vast scientific community due to their intrinsic advantages with respect to entrenched Si-based PVs: high efficiencies, low materials cost, easy manufacturing, and low energy/mass requirements, all resulting in a lighter environmental footprint. In these systems, the conversion of light into electricity requires charge transport (CT) across several materials. Due to the inherent complexity of the functional interfaces involved, CT key features are difficult to understand holistically solely from experimental outcomes, which hinders a rational design of new devices with better performances. For this reason, the application of computational modeling tools with atomistic resolution represents an ongoing revolution in materials design and optimization for PSCs.
This contribution will discuss how DFT-based approaches are able to assess the CT features across several interfaces between optically active materials and charge-collector layers in different electrochemical environments, which are the core events for PV functioning. In particular, we will discuss heterogenous interfaces between lead halide perovskites and inorganic and organic hole transport materials [1, 2], from the analysis of electronic structures to the determination of CT dynamics and rates [3] and comment on the composition-properties relationships in attractive lead-free perovskite-inspired absorbers [4] targeting indoor PV devices.
[1] Pecoraro, A.; De Maria, A.; Delli Veneri, P; Pavone, M.; Muñoz-García, A. B. Interfacial electronic features in methyl-ammonium lead iodide and p-type oxide heterostructures: new insights for inverted perovskite solar cells. Phys. Chem. Chem. Phys. 2020, 22, 28401-28413.
[2] Mäkinen, P.; Fasulo, F.; Liu, M,; Grandhi, G. K.; Conelli, D.; Al-Anesi, B.; Ali-Löytty, H.; Lahtonen, K.; Toikkonen, S.; Suranna, G. P.; Muñoz-García, A. B.; Pavone, M.; Grisorio, R.; Vivo, P. Less Is More: Simplified Fluorene-Based Dopant-Free Hole Transport Materials Promote the Long-Term Ambient Stability of Perovskite Solar Cells. Chem. Mater. 2023, 35, 2975-2987.
[3] Pecoraro, A.; Fasulo, F.; Pavone, M.; Muñoz-García, A. B. First-principles study of interfacial features and charge dynamics between spiro-MeOTAD and photoactive lead halide perovskites. Chem. Commun. 2023, 59, 5055-5058.
[4] Lamminen, N.; Grandhi, G. K.; Fasulo, F.; Hiltunen, A.; Pasanen, H.; Liu, M.; Al-Anesi, B.; Efimov, A.; Ali-Löytty, H.; Lahtonen, K.; Mäkinen, P.; Matuhina, A.; Muñoz-García, A. B.; Pavone, M.; Vivo, P. Triple A-Site Cation Mixing in 2D Perovskite-Inspired Antimony Halide Absorbers for Efficient Indoor Photovoltaics. Adv. Energy Mater. 2023, 13, 2203175.
2.3-O1
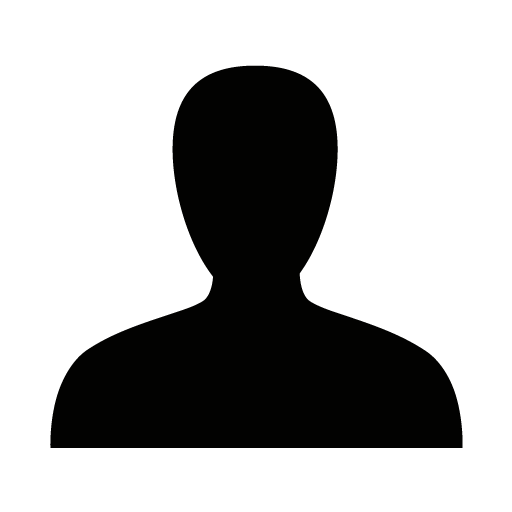
Pure black FAPbI3 (FAPI) perovskites have recently attained record photovoltaic efficiencies over 26% [1]. To stabilize the a-phase of FAPbI3 without the incorporation of methylammonium, cesium or bromide in the cubic lattice, methylammonium chloride and (often) excess of PbI2 are always added in the precursor solution to increase the crystallinity and induce preferred orientation for the perovskite layer. However, the presence of these additives can increase defects and reduce long-term stability of the film [2]. To mitigate defects and enhance the performance of the devices, surface passivation is a common strategy to heal the defective perovskite surface with a large variety of molecules bearing functional groups.
In this work, we have investigated the use of 2-diethylaminoethanethiol hydrochloride (DEAET) as a surface passivator of FAPI. The motivation behind this experiment was the fact that the -SH (thiol) group can bind strongly to Pb2+ [3], thus excess PbI2 could be mitigated and/or undercoordinated Pb2+ could be passivated. Simultaneously, the hydrophobic character of the thiols can create a barrier that prevents the infiltration of moisture and oxygen into the perovskite layer [4]. To ensure effective coverage of the FAPbI3 perovskite film with DEAET, different concentrations and solvents were tested. Upon optimization, we were able to decrease the roughness of the perovskite films by 7 nm and diminished the presence of PbI2 in the final film after annealing. With these features in hand, the films became more photoluminescent (PL) at the bandgap (1.54 eV), simultaneously increasing the PL lifetimes from 140 to over 190 ns. The enhanced non-radiative recombination led to increased open-circuit potential (from 1.04 to 1.06 V) and efficiencies (from 18.5 to 18.9%) in n-i-p solar cells, accompanied by better reproducibility. Accordingly, DEAET-treated FAPI devices did not lose any efficiency after storage in a desiccator for 1 month. The findings of this study lay the foundation for the utilization of thiol-based salts as efficient agents for interface engineering in pure FAPI devices.
2.3-O2
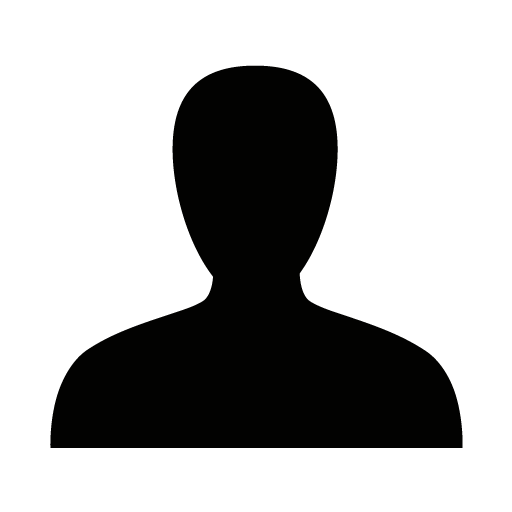
Since their appearance, perovskite solar cells (PSCs) were a promising technology due to their low synthesis temperature and potentially cheap manufacturing process. Besides high potential for conventional photovoltaic applications perovskite solar cells have suitable properties for indoor PV energy harvesting especially under modern LED lighting. Thin, light weight and low-cost energy harvesters are of interest for the “Internet of Things” (IoT) devices and smart home indoor devices requiring uninterrupted operation.
Optimal operation under low intensity and short wavelength LED spectra can be achieved with higher bandgap of the absorber material than it is usually expected for the solar spectrum. In terms of device parameters this implies development of the cells with higher VOC [1]. Current record efficiency of 40.1% at 0.3W/m2 of “White LED” light with 2700K color temperature was achieved by the cell with high VOC of 1V at this irradiance [2]. Although increasing the band gap of Perovskite absorber layer is required to produce a solar cell with high VOC, the proper energy alignment between absorber and charge transport layers together with low non-radiation recombination rate are vital [3].
In this work we evaluate the performance of recently developed high band gap lead halide Perovskite solar cells with fullerene electron transport layer (ETL) under low light LED illumination. These cells were reported [4] to achieve 1.35V VOC under 1 Sun illumination, so they have high potential under low light LED conditions. Unlike for field applications, characterization procedures for indoor solar cells are still in development and application scenarios vary significantly. Therefore, the cell performance can not be characterized under one specific irradiance. In our work we evaluate the performance of high band gap PSC with CH3NH3Pb(I0.8,Br0.2)3 absorber layer and CMC:ICBA (Here CMC is C60fused NmethylpyrrolidinemC12phenyl and ICBA is the indeneC60 bisadduct) fullerene ETL under wide range of LED lamp (Cree XLamp CXA3050 LED with 3000K color temperature) illumination conditions (200-10000 lx). These perovskite solar cells achieve VOC of 1.33V, FF of 70.93% and efficiency of 16.4% at 1 Sun, while their performance under 533 lux LED light was outstanding with 1.03V VOC and 28.6% efficiency. To validate the guidelines of increasing VOC value for better indoor performance, we compare these high band gap solar cells with CH3NH3Pb(I0.8,Br0.2)3 absorber layer and PSCs with CH3NH3PbI3 absorber layer, [6,6]-phenyl-C61-butyric acid methyl ester (PCBM) ETL and a VOC of 1.17V at 1Sun.
2.3-O3
Interest in pre-crystallisation of metal halide perovskite has increased in recent years because it allows for easy production of large quantities of powder perovskite. Perovskite thin film devices subsequently made from the powder exhibit high performance [1,2]. In this study we compare methanol, ethanol, 2-propanol and pentanol as solvents for precipitation reactions forming methyl ammonium lead iodide MAPbI3 and methyl ammonium formamidinium lead iodide MA0.5FA0.5PbI3 perovskites. Using time resolved in-situ small and wide X-ray scattering (SAXS and WAXS), we were able to follow the reaction of the dissolved methylammonium iodide and formamidinium iodide when a suspension of lead iodide was added to the system. The evolution of the diffraction pattern with time was determined whereby the structural assembly from precursors to perovskite could be followed. The perovskite formation was discovered to be, not surprisingly, initially fast on the surface and during small grain formation. However, the rate of total perovskite formation of the bulk was slower and clearly dependent on the polarity of the solvents and progressed via intermediate phases. The measurements were supported with scanning electron microscopy and optical spectroscopy techniques to correlate grain formation with functionality. Our results led to a deeper understanding of the intermediate steps during the reaction. The precipitation method to produce metal halide perovskite described in this work is cost-effective and has fewer safety issues due to the low solvent toxicity. Furthermore, the stoichiometric homogeneity achieved by the pre-crystallisation process in alcohol subsequently simplifies thin film deposition and therefore improves photovoltaic device performance over large areas. This work outlines an alternative processing route for stable photovoltaics based on perovskite powder.
2.3-O4
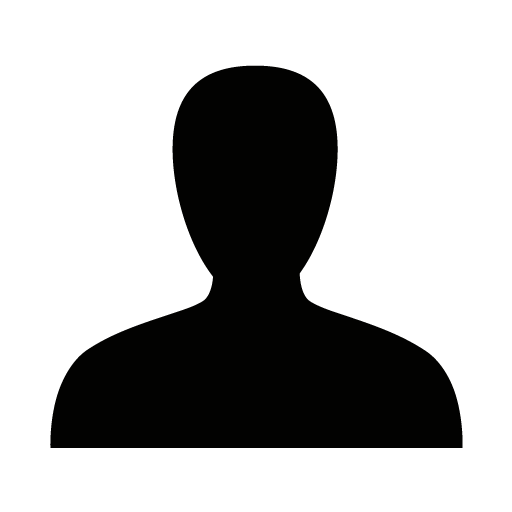
Mixed lead-tin (Pb:Sn) halide perovskites are promising absorbers with narrow bandgaps (1.2-1.3 eV) suitable for high-efficiency all-perovskite tandem solar cells. Currently, the highest efficiency Pb:Sn perovskite solar cells (PSCs) employ methylammonium (MA) as an A-site cation. However, MA is known to be thermally and chemically less stable than formamidinium (FA), therefore it would be favourable to have an MA-free Pb:Sn PSCs that could also deliver high efficiency. Additionally, the solution processing of thick Pb:Sn perovskite films is notoriously difficult in comparison with their neat-Pb counterparts. This is partly due to the rapid crystallization of Sn-based perovskites, resulting in films that have a high degree of roughness. It is more difficult to coat conformal subsequent layers using solution-based techniques on top of rougher films, leading to contact between the absorber and the top metal electrode in completed devices, resulting in a loss of VOC, fill factor, efficiency and stability. Here, we investigate the impact of adding a non-continuous thin layer of alumina nanoparticles inserted in between the thick, rough Pb:Sn perovskite films and the electron transport layers (ETL) in a 'p-i-n' device configuration. This approach leads to enhanced conformality of the subsequent ETL. As a result, devices that employ the thin alumina nanoparticles layer achieved a champion maximum power point tracked efficiency of 15.0% versus 10.3% for the champion control device and the steady-state open-circuit voltage was improved from 0.65 V to 0.75 V. Application of the alumina nanoparticles as an interfacial buffer layer also results in highly reproducible Pb:Sn solar cell devices whilst simultaneously improving device stability at 65 °C under 1 sun illumination. Aged devices showed a 6‑fold improvement in stability over pristine Pb:Sn devices, increasing their lifetime to 120 hours.
2.3-O5
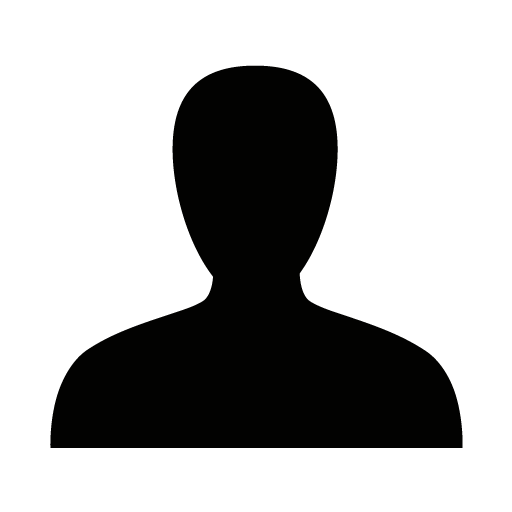
One of the major challenges in perovskite research today is the fabrication of stable perovskite materials for optoelectronic applications without performance loss.
To improve the stability of metal halide perovskite films and devices, layered (2D) perovskites are frequently used as a passivating layer. This improved long-term stability, however, often comes at the expense of device performance. More fundamental understanding of the perovskite material is needed to understand this effect. In a recent study, we therefore used pulsed, transient photoconductivity, to estimate the long-range mobility of phase-pure 2D perovskites.[1,2] To our surprise, we discovered that PEA2PbI4, a well-studied 2D material, has an 8 times higher long-range mobility than FA0.9Cs0.1PbI3, a typical three-dimensional perovskite. To gain a better understanding, we used optical probe terahertz spectroscopy to measure short-range mobility and found an identical value for the mobility of the 2D material, indicating superior material quality. We also hypothesize that the main reasons for the underperformance of perovskite device stacks with 2D passivation are the high exciton fraction and the anisotropy of charge carrier transport.
Using our newly acquired knowledge, we attempted to find an improved 2D passivation layer. We begin by screening a variety of candidates using similar methods to those used in previous studies to find one with improved optoelectronic properties as compared to PEA2PbI4. Most importantly, we are attempting to modify the exciton binding energy and structural properties of the materials. Our findings show that multiple parameters influence the formation of 2D perovskites, which governs the optoelectronic properties of the final thin films.
2.3-O6
MAPbI3 amongst other OMH perovskites is undergoing tetragonal-to-cubic phase transitions within the operational temperature range of solar cells. Many properties of these hybrid perovskite materials, such as piezoelectricity, pyroelectricity, ferroelectricity and ionic conductivity are directly linked to the crystal phase and temperature of the sample. Notably, polar domains that form in MAPbI3 thin-films during the solar cell fabrication have been observed,[1,2,3] We have previously shown that poling of these domains can be achieved in an external E-field that is similar to built-in fields in solar cells during operation.[4]
In this work, we use Piezoresponse Force Microscopy to monitor an evolution of these domains that is triggered by common thermal treatment of perovskite solar cell at 100°C.[5] Our findings suggest that control over the domain structure in these light absorbing, semiconducting compounds may be essential in order to maximize performance and stability of hybrid perovskite solar cells.
Misaligned domains can hamper charge carrier transport in a solar cell and temperature cycling during operation will have an immediate impact on degradation processes. In particular, microstructural changes due to stress and strain when the thin-films undergo a tetragonal/cubic phase transition are an often overlooked factor contributing to the instability of perovskite solar cells. We show that local crystal defects can form and vanish both at ferroic domain walls and crystal grains and in turn modulate ionic conductivity and diffusion. Finally, we extend our investigations towards triple-cation perovskites in order to identify design rules for new compositions such as lead-free perovskites and more stable perovskite-inspired solar cell absorber materials.[6]
3.1-O1
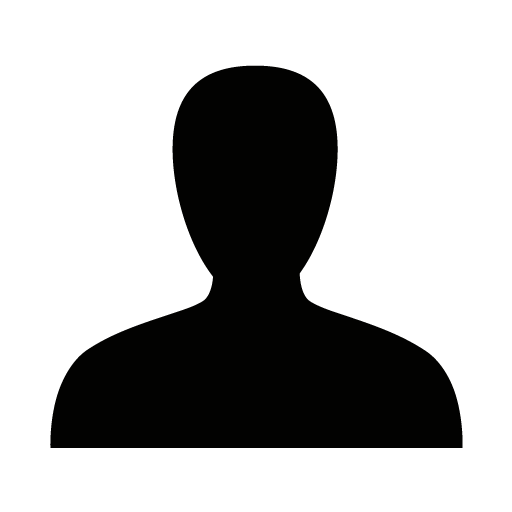
Metal-halide perovskites (MHPs) are a type of materials that have the potential to greatly impact optoelectronic devices. A significant material’s science challenge has been the presence of electronic trap states, which are difficult to characterize and mitigate. Various attempts have been made to use electronic spectroscopies to study the defect states in bulk crystals of MHPs. However, due to the mixed nature of electronic and ionic conductivity in MHPs, the results of these experiments often have a high level of uncertainty in distinguishing between electronic and ionic charge contributions.
In this study, we employ a method called photo-induced current transient spectroscopy (PICTS), which was previously used in highly resistive inorganic semiconductors, to analyze single crystals of two types of MHPs: lead bromide 2D-like ((PEA)2PbBr4) and standard "3D" (MAPbBr3) perovskites. By applying PICTS, we are able to obtain two distinct outcomes that allow us to differentiate between the electronic and ionic contributions to the photocurrents. This differentiation is based on the ion mobility of the two different materials. Our experimental findings reveal the presence of deep level trap states in the 2D perovskite ((PEA)2PbBr4), which has limited ion mobility. Furthermore, our results establish new boundaries for the use of PICTS in studying 3D perovskites, which exhibit greater ionic diffusion.
3.1-O2
With the increasing demand for artificially intelligent hardware systems for brain-inspired in-memory and neuromorphic computing, perovskite-based memristors has emerged as a promising candidate for resistive random-access memory (ReRAM) devices [1]. Metal halide perovskite semiconductors exhibit mixed ionic-electronic conduction resulting to intrinsic memory effects (hysteresis) in the current-voltage (I-V ) response [2-4]. In order to meet the necessary demands in various complex computing frameworks, understanding the underlying mechanisms governing the resistive switching of these perovskite-based memristor devices is of paramount importance. Here, we present the dynamic impedance spectral evolution of the state transition in perovskite-based memristor devices exhibiting significant transformation of the low frequency capacitance to inductance near the threshold voltage [5]. This transition implies that the interfacial reactivity between the migrating ions with the thin Ag metal contact results to the further gradual decrease in the device resistance indicative of a non-filamentary switching mechanism. Moreover, the incorporation of a thin undoped interfacial buffer layer exhibits an abrupt state transition in the characteristic I-V response [6]. This abrupt state transition is part of a two-step SET process where both drift-related halide migration and diffusion-related filamentary formation is observed. Furthermore, we develop a dynamical model that helps untangle and quantify the switching regimes consistent with the experimental memristive response. This further insight on the complex interplay among mobile ions, vacancies, and metal provides another degree of freedom in device design for versatile applications with varying levels of complexity.
3.1-O3
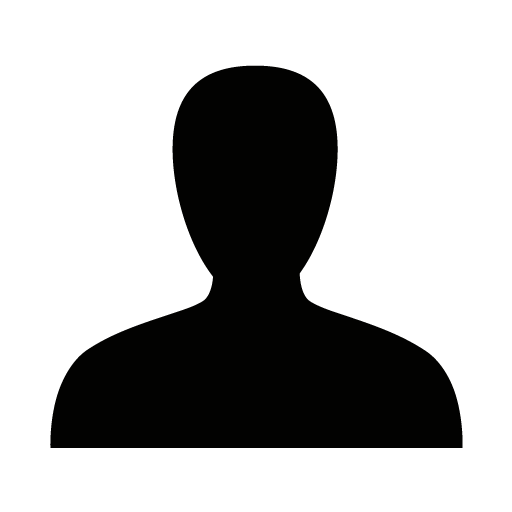
Over the past decade, perovskite-based solar cell efficiency has dramatically increased from 10% to over 25%, but their instability remains a significant challenge. Environmental factors such as humidity, oxygen, light, and heat have been shown to have a negative impact on these devices. In our previous work, we used drift-diffusion device simulations to demonstrate the role of ion penetration in transport layers toward contacts [2, 3]. This study expands on that model by including chemical reactions within or at connections. By using a drift-diffusion-reaction device model, we can account for the efficiency degradation of perovskite solar cells.
In this study, we simulate a MAPbI3 perovskite solar cell with free iodine anions and investigate how these ions affect cell performance. Our study indicates that chemical reactions involving iodine [4] can alter the charge distribution within the device and moreover lead to the formation of recombination centers for the charge carriers participating in these reactions. We also investigate the possibility of iodine ions penetrating the soft organic blocking layers and affecting the device contacts. To gain a deeper understanding of the interplay between different mechanisms, we analyze the impact of light and dark stress conditions. Finally, we examine irreversible processes such as the release of iodine molecules as gas and the immobilization of iodine species by reactive contacts.
3.1-O4
Huge compositional space of perovskites make this class of compounds a fertile ground for search of exotic properties that can lead to new technological developments. Especially, the limit of channel length minimization in traditional semiconductor electronics has led to the focus on spintronics and other such efforts where the spin of electrons can be controlled by virtue of the special properties of the host material. One such manifestation of spin control can be seen in Rashba-Dresselhaus effect discovered around 60 years ago in non-magnetic insulators with broken inversion symmetry [1]. This phenomenon of spin splitting in the momentum space if it occurs in the band edges can help in realising the spin field effect transistor proposed by Datta and Das [2]. To achieve the spin control via switching of gate voltage, existence of ferroelectric polarisation in the material is necessary. This has led to a lot of research on ferroelectric Rashba semiconductors (FERSC) [3,4] and formulation of design principles necessary to search for such materials [5].
In this work, we look into Iodate perovskites in non-centrosymmetric rhombohedral phases with Iodine as a heavy element giving spin orbit coupling effects. We study the effect of A cation on the Rashba spin splitting in these iodates by comparing the Rashba parameters of (A)IO3 in R3m phases where A=K, Rb, Cs, and Tl. Through ab-initio density functional theory calculations, electronic band structure of these compounds are obtained and the energy splitting near the conduction and valence band edges are noted. Our study reveals that the Rashba splitting is inversely proportional to the ionic radii of the cation while the band gap is directly proportional. KIO3 with a band gap of 2.29 eV and Rashba splitting of ~1 eVÅ is the most suitable candidate for FERSC applications.
3.1-O5
Intragap states are among the critical factors that limit the performance and stability of perovskite solar cells (PeSCs). They not only serve as trapping sites for photogenerated carriers and open the dominant non-radiative loss mechanism in PeSCs under sunlight illumination conditions, but also become original sites (such as defects) where degradation starts from. Further PeSCs development requires a comprehensive understanding of trap state properties, including how they are filled and depopulated in a working device. Conventional spectroscopic techniques are not sufficiently selective to specifically follow the dynamics of trapped carriers, particularly at actual PeSC working conditions. Here we apply novel infrared optical activation spectroscopy [i.e., optical pump-IR push-photocurrent (PPPc)], to observe in real time the evolution and properties of trapped carriers in operando PeSCs. In these techniques, band-edge carriers are generated by an optical visible “pump” beam, followed by the carrier trapping processes. Then the photons of IR “push” beam absorbed by the trapped carriers excite them back to the band states. The IR de-trapped carriers contribute to the device photocurrent, therefore the amplitude and behaviour of IR-induced current help to evaluate the concentration and dynamics of trapped carriers in the device.
We compared the behaviour difference due to trapped holes in pristine and surface-passivated FA0.99Cs0.01PbI3 PeSCs using a combination of temperature-dependent steady-state PPPc, ns time-resolved PPPc, and kinetic models. We found that the trap-filling process occurred in two steps: first, in a few-ns timescale, low-concentration trap states are filled in the bulk of perovskite material; then, in a much longer (~100 ns) timescale, high density of traps at material interfaces is populated. Surface passivation by n-octylammonium iodide dramatically reduces the number of trap states (~10 times) and hence substantially improves the device performance. The activation energy of the dominant hole traps was measured to be in the order of ~280 meV and was not affected by the surface-passivation process.
Our results successfully demonstrate that PPPc techniques are powerful and highly sensitive to reveal the dynamic, concentration, and activation energy of trapped carriers, facilitating a comprehensive understanding of the role of trap states in PeSCs. We expect that the in-situ measuring of PPPc signals in working PeSCs under different ageing conditions (e.g., heat, illumination, humidity) allows us to trace the change of trapped carriers’ dynamics and properties. Therefore, it becomes another starting point for material scientists to further develop the existing materials and devices for the next-generation PeSCs with excellent stabilities.
3.2-I1
Perovskite photophysics in the past decade has been uprooted and revisited from the grounds several times. Initially, Metal halide perovskites were considered as excitonic semiconductors, due to their pronounced excitonic absorption and narrow-band efficient optical emission. Then ultrafast spectroscopy studies have revealed bimolecular recombination dynamics, proving that excitons are dissociated into opposite charge carriers, with beneficial effects for charge separation in solar cells. Perovskite photophysics seemed to have been rationalized: free carriers are favored over exciton by Saha equilibrium, radiative recombination is bimolecular and is the inverse process of optical absorption.
Yet several issues with such a picture started appearing, again from ultrafast spectroscopy measurements. I will review how radiometric time-resolved photoluminescence reveals that the radiative recombination rate is much lower than what expected from the absorption rate. Furthermore, the combination of transient absorption and time resolved photoluminescence in a tandem setup demonstrates that free carriers are majority even at low temperature and in 2D perovskites, when Saha equilibrium predicts instead that bound excitons should prevail.
An extensive debate on the exciton binding energy has ensued, resulting in the realization that the exciton binding energy measured in absorption, when perovskites are in their ground state, is significantly different from the binding energy after excitons have been created.
Clearly, a sound description of photophysics of halide perovskites needs additional ingredients describe the dissociation of excitons in the excited state. Phonon coherences, ultrafast electron diffraction, XAS and XRD are among the techniques that have evidenced a significant distortion of the perovskite lattice upon optical absorption, a phenomenon also known as the formation of a new quasiparticle, the polaron.
I will present a picture of perovskite photophysics that applies to both 3D and 2D materials and consists in the creation of excitons upon optical absorption, their spontaneous dissociation into opposite charge polarons and, finally optical emission by bimolecular recombination of polarons into excitons.
3.2-I2
We provide a survey of our experimental studies of coherent spin dynamics of electrons and holes in lead halide perovskite semiconductors: bulk crystals [1-3], nanocrystals [4-5] and 2D structures [6]. Time-resolved Faraday/Kerr rotation techniques was used for that, measurements were performed at cryogenic temperatures and in strong magnetic fields. We measure spin relaxation and spin coherence times, evaluate electron and hole Lande g-factors for a representative set of perovskites with the band gap energy varying from 1.5 to 3.2 eV. We establish universal trends for the g-factors on the band gap energy in bulk materials and show that they are modified by the quantum confinement in perovskite nanocrystals. Strong interaction of hole spins with nuclear spins is found, which is considerably stronger that the one for the electron. Optically detected nuclear magnetic resonance highlights the dominating role of Pb ions in interaction with electrons and holes. Spin mode locking effect based on spin synchronization under periodic laser excitation is found in nanocrystals in glass. Experimental approaches of spin physics give reach information about these materials.
1.1-I1
Light is ubiquitous in the urban environment – from the sun that shines down upon us to the artificial sources that light-up our devices and homes. While some of this light is used very effectively, for example by plants in the process of photosynthesis, much is wasted, either due to inefficient capture or poor recycling of the broad spectrum of photon energies available. Photon conversion materials can help bridge the energy mismatch between a light source and the collector (e.g. a solar photovoltaic (PV) cell or fibre optic) through use of a photoluminescence process to convert the incident photon energy.[1] For ease of processing and eventual integration with (opto)electronic devices, the photon converter must usually be integrated within a solid-state host. Judicious consideration of the host material is essential to ensure that host-emitter interactions enhance rather than diminish the optical performance.
In this talk, recent highlights from our research into the bottom-up design of photon conversion materials utilising organic-inorganic hybrid hosts will be presented. It will be shown that materials chemistry strategies can be used to control the packing, orientation and placement of emitters, which provides a means of modulating the optical properties – from enhanced photoluminescence quantum yields[2], to tunable photon energies via Förster resonance energy transfer[3,4] or triplet-triplet annihilation upconversion (TTA-UC). These characteristics can be exploited to improve light-harvesting and trapping, which can be used to develop highly efficient luminescent solar concentrators,[4] optical amplifiers for visible light communications[5], and sensor platforms for bioimaging.
1.1-I2
Organic semiconductors are an emerging class of materials with various optoelectronic applications. To enable faster commercialisation of this technology, there are two requirements: (i) improve their performance and (ii) follow eco-friendly manufacturing strategies.
Improving their performance is a key requirement to enable faster commercialisation. Charge carrier mobility is one crucial parameter for electronic applications, particularly organic thin film transistors (OTFTs). A generic approach to achieve this is through the addition of molecular dopants in the organic semiconductor layer. Here, we report the use of novel materials as molecular dopants for both p1,2 and n-type3,4 organic semiconductors in OTFT devices. We show that key device parameters such as charge carrier mobility, contact resistance and threshold voltage improve dramatically upon adding the dopant. The effect of the dopant was analysed with Electron Paramagnetic Resonance (EPR) and by extracting the activation energy (EA) from low-temperature electrical characterisation. The impact of the dopant on the morphology of the OSCs will also be discussed as studied from Atomic Force Microscopy (AFM) and X-Ray diffraction (XRD). Overall, this work highlights that controlled doping of organic semiconductor materials is the key to enhanced electronic devices.
In the second part of the talk, I will introduce sustainable routes to manufacture solution-processed organic electronics. Particular focus will be given to organic solar cells, which recently attracted immense attention due to the development of a new family of semiconductors that allows highly efficient light harvesting in indoor and outdoor conditions. One current limitation, though, is the use of not eco-friendly solvents and materials during the device development stages. Most organic electronic devices require halogenated and non-halogenated aromatic solvents during their fabrication. For large-scale production and further commercialisation, this is a key limitation. This arises from the fact that organic semiconductors are highly soluble in this category of solvents, which are often carcinogenic or toxic to the human reproductive systems and negatively impact the environment. Here I will show high-performing organic solar cells fabricated from biomass-based solvents.5. Overall, this work highlights the importance of replacing harmful chemicals and materials in the organic electronics fabrication stages, resulting in faster and wider commercialisation and new market opportunities.
1.1-I3
Solution-processed nanocomposite films comprising small molecule organic semiconductors (OSCs) and inorganic colloidal quantum dots (QDs) are promising systems for low-cost, high efficiency, solar energy harvesting technologies [1]. In these systems, OSCs capable of singlet fission (SF) offer a mechanism to surpass the radiative efficiency limits of single-junction photovoltaics (PV). The SF-generated triplet excitons can be harvested by the inorganic QDs, where they radiatively recombine to achieve photon multiplication, converting a single high-energy photon into two low-energy photons. Such a SF photon multiplication film (SF-PMF) has the potential to enhance the efficiency of the best Si-PV from 26.7% to 32.5%, a substantial gain [2]. For efficient SF-PMF, the ideal film nanomorphology consists of QDs that are highly dispersed throughout the OSC phase at a length scale comparable to the triplet exciton diffusion length. However, controlling QD dispersibility in OSC:QD blends is challenging given the strong tendency of QDs to aggregate and phase-separate due to the mismatch of their size, shape and surface energies. Understanding the self-assembly mechanisms of the organic and inorganic components during large-scale, high throughput film coating methods is therefore crucial for precise control of film nanomorphology [3]. This talk will demonstrate how in-situ grazing incidence X-ray scattering (GIXS) offers direct insights into the self-assembly of OSC:QD blends during blade coating. It will outline some of our latest strategies to control structure formation in nanocomposite films via subtle changes in composition and processing conditions. The results provide routes for the structural design and optimization of solution-processed nanocomposites that are compatible with large-scale coating techniques, essential for driving the commercialisation of SF-PMF architectures for solar energy harvesting applications.
1.2-I1
Optoelectronics devices based on closed-shell organic small molecules and polymers have proven themselves as disruptive technology in display applications. Their ability to interchange light absorption or emission with electrical generation or excitation has allowed for the development of organic light emitting diodes (OLED), organic photovoltaics (OPV) and other devices with commercially relevant performance and costs. However, the fundamental electronic structure of these closed-shell materials imposes limitations on their use. The excitonic nature of these materials means that for every bright, optically accessible (singlet) state there is a corresponding dark (triplet) state at a lower energy. The presence of dark triplet states is the cause for both the lower efficiency and lower stability in current generation organic optoelectronic devices relative to their inorganic counterparts. We propose to generate an entirely new set of optoelectronic materials based on open-shell (radical) organic materials where the lowest energetically excited state is an optically bright state, thus eliminating the major loss mechanism in current organic optoelectronic devices. In addition, the proposed materials can have high-spin which can be optically (or otherwise) manipulated opening up the possibility of using organic materials in next generation quantum technologies.
1.2-I2
Masers, the microwave version of lasers, are a promising emerging class of ultralow-noise microwave amplifiers with the potential as quantum sensors in a range of commercial applications.[1] These devices rely on the photogeneration of electron spin-polarized triplet states in organic crystals of acene-doped-p-terphenyl or nitrogen-vacancy (NV) diamond. The stimulated collapse of these states by injected microwaves results in microwave emission and amplification which is highly sensitive to magnetic fields. However, due to inefficient electronic processes and triplet spin dynamics, these materials require large crystals with strong light pump sources to overcome the maser threshold, increasing their bulk and expense and damaging the gain media. Therefore, before masers can be widely applied, we must develop new materials with enhanced spin dynamics to ease their operation while increasing their power.
To tackle these issues, we have designed novel approaches to tune the properties of maser candidate molecular systems.[2,3] We have synthesized several new triplet and radical-based materials capable of producing extremely strong and long-lived electron spin polarisation through intersystem crossing, singlet fission, and triplet-radical interactions. We employed transient photoluminescence and absorption spectroscopy alongside electron paramagnetic resonance to understand the link between their spin dynamics and their merit as maser devices. Our new materials demonstrate the capacity to operate at various resonant frequencies and can be optically pumped at more easily generated wavelengths. Furthermore, their triplet spin dynamics suggest that similar systems could exhibit enhanced maser cooperativity. These results pave the way for the synthesis of more efficient and applicable maser technologies.
References:
[1] D. M. Arroo, N. M. Alford, J. D. Breeze, Appl. Phys. Lett. 2021, 119, 140502.
[2] W. Ng, X. Xu, M. Attwood, H. Wu, Z. Meng, X. Chen, M. Oxborrow, Adv. Mater. 2023, 35, 2300441.
[3] M. Attwood, X. Xu, M. Newns, Z. Meng, R. A. Ingle, H. Wu, X. Chen, W. Xu, W. Ng, T. T. Abiola, V. G. Stavros, M. Oxborrow, Chem. Mater. 2023, 35, 4498–4509.
1.2-I3
Quantum sensors can harness the sensitivity of the entangled electron spin states to external stimuli to probe physical properties such as temperature, electric and magnetic fields. However, improvements are still required for efficient and reliable read-out of the stored quantum information to expand the applicability of devices. Molecular systems are the most recent candidates for quantum device applications as these systems can be tuned to have specific magnetic properties and much work is being done to achieve optical readout, analogous to NV centers in diamond. Here, we investigate the possibility of a digital state readout by combining a molecular spin system to a valleytronic material.
Coordination compounds use structural tuning to provide precise tailoring of magnetic properties, with phthalocyanines established as a versatile system. Specifically, vanadyl phthalocyanine (VOPc) is considered, a spin ½ system with long (μs) coherence times that can be easily and predictably deposited on a range of substrates. Films consisting of a few layers of transition metal dichalcogenides (TMDC) have demonstrated circular polarized photoluminescence from the transition to a direct band gap semiconductor. Chiral emission is relevant to spin-selective optoelectronics and combining the molecular spin qubit with a TMDC substrate could lead to interesting opportunities in quantum sensing. However, at this stage little is known of the electronic and spin interactions at such an interface and the extent of spin-valley cooperativity. We therefore present an optical study of the VOPc thermally evaporated on WSe2. Transient absorption (TA) spectroscopy and time resolved circular dichroism (TRCD) are performed to extract spin-valley relaxation parameters and correlate these to the molecular orientation on the 2D layer. This provides critical information to design new architectures for quantum sensors.
1.3-I1
In recent years, the investigation of strong light-matter coupling has emerged as a powerful avenue to manipulate and control the excitonic properties of organic materials. Strong light-matter coupling leads to the formation of hybrid light-matter states called exciton-polaritons, which have the potential to revolutionise the future of molecular electronics owing to their promise to induce long-range energy transfer [1]. One intriguing aspect of strong coupling is its potential impact on processes involving excited triplet states, such as triplet-triplet annihilation (TTA), which plays a significant role in organic photophysics and optoelectronic devices through upconversion.
This talk discusses the intricate interplay between strong light-matter coupling and triplet-triplet annihilation in organic materials. We explore how the formation of exciton-polaritons modifies the TTA process, potentially affecting the rate of triplet-state quenching and singlet exciton generation which influences the delayed-emission dynamics [2]. Through a combination of advanced spectroscopic techniques, we explore the underlying mechanisms governing the interactions between exciton-polaritons and triplet excitons.
Our findings provide valuable insights into the fundamental photophysical processes in the strong-coupling regime and pave the way for novel strategies to enhance the efficiency of organic optoelectronic devices. By elucidating the intricate dynamics of triplet-triplet annihilation under strong light-matter coupling, we contribute to the broader understanding of excitonic interactions and offer a new perspective on designing advanced organic materials for next-generation optoelectronics.
1.3-I2
Jeanne Crassous studied at the “Ecole Normale Supérieure de Lyon” (ENS Lyon, France). In 1992, she passed the national exam “Concours de l’Agrégation de Sciences Physiques, option Chimie”. In1993, she obtained a DEA (“Diplôme d’Etudes Approfondies”, Master degree) in Organic Chemistry from the University of Lyon 1. She received her PhD in 1996, prepared under the supervision of Prof. André collet (ENS Lyon, France), on the Absolute Configuration of Bromochlorofluoromethane (CHFClBr). After a one-year postdoctoral period studying the Chirality of Fullerenes in Prof. François Diederich’s group (ETH Zurich, Switzerland), she received a CNRS researcher position at the ENS Lyon in 1998 and then she joined the Institut des Sciences Chimiques de Rennes (University of Rennes, France) in 2005. She became Director of Research in 2010.
Her group is dealing with many fields of chirality (metal-based helicene derivatives, chiral π-conjugated assemblies, fundamental aspects of chirality such as parity violation effects) and chiroptical activity (electronic and vibrational circular dichroism, circularly polarized luminescence) with potential applications in optoelectronics, spintronics and chirality-coded systems.
She is co-author of more than 170 articles and book chapters and has presented her work in more than 60 invited lectures and 65 seminars in laboratories. She is co-author/co-editor of two monographs: « Molécules Chirales : Stéréochimie et Propriétés », Editions du CNRS, 2006 and « Helicenes - Synthesis, Properties and Applications », Wiley, 2022.
She is currently coordinating a French national network (GDR CHIRAFUN, Chirality and multifunctionality) and a European ITN Project (HEL4CHIROLEDs, Helical molecules for Chiral OLEDs). She is also currently an elected member of the Executive Board of the DCO-SCF (Division of Organic Chemistry of the French Chemical Society) and member of the Editorial Boards of Chirality and ChemPhysChem (Wiley journals).
In 2013, she was elected distinguished junior member of the French Chemical Society (SCF). In 2020, she received the National Prize of the Organic Chemistry Division of the French Chemical Society (DCO-SCF). In 2021, she was elected Member of the European Academy of Science (EurASc) and Fellow of Chemistry Europe (Class 2020/2021). In 2023, she was awarded the CNRS Silver Medal in molecular chemistry (CNRS Talent).
Ortho-fused aromatic rings form helically shaped chiral molecules such as carbo[6]helicenes, that wind in a left-handed (M) or a right-handed (P) sense.[1-3] The helical topology combined with extended pi-conjugation provides helicenes with peculiar properties such as strong photophysical and chiroptical properties (high optical rotation values, intense electronic circular dichroism - CD, and circularly polarized emission - CPL). The molecular engineering of helicenes using organometallic and heteroaromatic chemistries offers a convenient way to tune the properties of these helically shaped ligands. Indeed, their combination with metallic or organic assembling units leads to chiral materials with appealing properties (circularly polarized phosphorescence, magnetochirality, spin selectivity). Applications in materials science (Circularly Polarized OLEDs, Chiroptical Switches, Spintronics) are targetd. A set of representative examples will be presented while emphasizing the specific features of each system. When possible, theoretical calculations enable to shed light on the phenomena (exciton coupling chirality, singlet or triplet excited state features, molecular orbitals involvement, dissymmetry factors improvement, ...).[4-6]