Session-K1
Professor of Physical Chemistry at the Ecole Polytechnique Fédérale de Lausanne (EPFL) Michael Graetzel, PhD, directs there the Laboratory of Photonics and Interfaces. He pioneered research on energy and electron transfer reactions in mesoscopic systems and their use to generate electricity and fuels from sunlight. He invented mesoscopic injection solar cells, one key embodiment of which is the dye-sensitized solar cell (DSC). DSCs are meanwhile commercially produced at the multi-MW-scale and created a number of new applications in particular as lightweight power supplies for portable electronic devices and in photovoltaic glazings. They engendered the field of perovskite solar cells (PSCs) that turned our to be the most exciting break-through in the recent history of photovoltaics. He received a number of prestigious awards, of which the most recent ones include the RusNANO Prize, the Zewail Prize in Molecular Science, the Global Energy Prize, the Millennium Technology Grand Prize, the Samson Prime Minister’s Prize for Innovation in Alternative Fuels, the Marcel Benoist Prize, the King Faisal International Science Prize, the Einstein World Award of Science and the Balzan Prize. He is a Fellow of several learned societies and holds eleven honorary doctor’s degrees from European and Asian Universities. According to the ISI-Web of Science, his over 1500 publications have received some 230’000 citations with an h-factor of 219 demonstrating the strong impact of his scientific work.
Recently molecular photovoltaics, such as dye sensitized cells (DSCs) and perovskite solar cells (PSCs) have emerged as credible contenders to conventional p-n junction photovoltaics. Their certified power conversion efficiency currently attains 25.5 %, exceeding that of the market leader polycrystalline silicon. This lecture covers the genesis and recent evolution of DSCs and PSCs, describing their operational principles and current performance. DSCs have meanwhile found commercial applications for ambient light harvesting and glazing producing electric power from sunlight. The scale up and pilot production of PSCs are progressing rapidly but there remain challenges that still need to be met to implement PSCs on a large commercial scale. PSCs can produce high photovoltages rendering them attractive for applications in tandem cells, e.g. with silicon and as power source for the generation of fuels from sunlight. Examples to be presented are the solar generation of hydrogen from water and the conversion of CO2 to chemical feedstocks such as ethylene, mimicking natural photosynthesis.
1.1-I1
Sam Stranks is Professor of Energy Materials Optoelectronics in the Department of Chemical Engineering & Biotechnology and the Cavendish Laboratory, University of Cambridge. He obtained his DPhil (PhD) from the University of Oxford in 2012. From 2012-2014, he was a Junior Research Fellow at Worcester College Oxford and from 2014-2016 a Marie Curie Fellow at the Massachusetts Institute of Technology. He established his research group in 2017, with a focus on the optical and electronic properties of emerging semiconductors for low-cost electronics applications.
Sam received the 2016 IUPAP Young Scientist in Semiconductor Physics Prize, the 2017 Early Career Prize from the European Physical Society, the 2018 Henry Moseley Award and Medal from the Institute of Physics, the 2019 Marlow Award from the Royal Society of Chemistry, the 2021 IEEE Stuart Wenham Award and the 2021 Philip Leverhulme Prize in Physics. Sam is also a co-founder of Swift Solar, a startup developing lightweight perovskite PV panels, and an Associate Editor at Science Advances.
Halide perovskite semiconductors are generating enormous exciting for light-harvesting and light-emission technologies. One of the keys steps to maximising optoelectronic performance is through eliminating non-radiative recombination mechanisms and hence maximising luminescence yields. Here, I will detail recent work in our group exploring how to best make each photon count in absorbers, emitters and full device stacks. I will present a combination of modelling and experimental results investigating how photons interact within and between layers in device stacks such as tandems, including photon recycling effects and light coupling between layers -- leading to key device design parameters. Furthermore, I will present results on both solar cells and light-emitting diodes (LEDs) exploring routes towards suppressing non-radiative recombination and therefore maximising performance.
1.1-I2
Eva Herzig’s research interest focuses on the possibilities and limitations in the characterization of nanostructures in functional materials as well as how such nanostructures form and change as functions of external parameters. The examined materials range from organic molecules to nanostructured hybrid and inorganic systems. We examine processing-property relationships and the influence of external fields to investigate how the fundamental self-assembly processes influence the final material performance. To this end we exploit various scattering techniques to observe and control structure and function relationships in the examined materials in-situ. Using grazing incidence x-ray scattering we are particularly sensitive to nanostructures on flat surfaces and within thin films.
Starting from solutions, the formation of the perovskite structure for hybrid organic-inorganic components used for solar cells depends strongly on a multitude of parameters during processing. Similar to other known structure formation processes, also the perovskite formation can be divided into different stages. Additives can for example be used to suppress structure formation in a certain stage [1]. Equally the timing available of the various stages is relevant for the final structure as can be systematically shown by changing the processing atmosphere [ACS Appl. Mater. Interfaces, accepted] or processing method [2] and even individual processing parameters. Exploring the relevance of the individual steps in structure formation will help us understand how to achieve reproducible nanostructures and hence reliable material properties.
1.1-I3
Dr. Tze-Chien Sum is an Associate Professor at the Division of Physics and Applied Physics, School of Physical and Mathematical Sciences (SPMS), Nanyang Technological University (NTU) where he leads the Femtosecond Dynamics Laboratory. He is presently the Associate Dean (Research) at the College of Science. Tze-Chien received his Ph.D. in Physics from the National University of Singapore (NUS) in 2005, for the work in proton beam writing and ion-beam spectroscopy. His present research focuses on investigating light matter interactions; energy and charge transfer mechanisms; and probing carrier and quasi-particle dynamics in a broad range of emergent nanoscale and light harvesting systems. Tze-Chien received a total of 11 teaching awards from NUS and NTU, including the coveted Nanyang Award for Excellence in Teaching in 2006 and the 2010 SPMS Teaching Excellence Honour Roll Award. Most recently, he received the 2013 SPMS Young Researcher Award; the Institute of Physics Singapore 2014 World Scientific Medal and Prize for Outstanding Physics Research; the 2014 Nanyang Award for Research Excellence (Team); and the 2015 Chemical Society of Japan Asian International Symposium Distinguished Lectureship Award. More information can be found at http://www.ntu.edu.sg/home/tzechien/spms/index.html
Halide perovskites have demonstrated exceptional potential for both light harvesting and light emission applications spanning photovoltaics, light-emitting devices, photodetectors, radiation detectors, memory devices etc. In particular, brightly luminescent perovskite colloidal nanocrystals with their fascinating tunable optical and electronic properties have garnered significant attention across broad disciplines. In this talk, I will focus on their exceptional photophysics such as hot carrier properties, long exciton diffusion lengths as well as nonlinear properties. I will distill their underlying mechanisms and highlight our group’s efforts in some of these areas. A succinct overview of the state-of-the-art as well as the prospective outlook will also be presented.
Keywords: halide perovskite, colloidal nanocrystals, photophysics, nonlinear, carrier dynamics
References
M. J. Li et. al., “Slow Cooling and Highly Efficient Extraction of Hot Carriers in Colloidal Perovskite Nanocrystals”, Nature Communications 8:14350 (2017)
M. Righetto et al., “Hot carriers perspective on the nature of traps in perovskites”, Nature Communications 11: 2712 (2020),
J. Fu et. al., “Hot carrier cooling mechanisms in halide perovskites”, Nature Commununications 8:1300 (2017)
D. Giovanni et. al., “Origins of the long-range exciton diffusion in perovskite nanocrystal films: photon recycling vs exciton hopping”, Light: Science & Applications 10: 2 (2021)
W. Chen et. al., “Giant five-photon absorption from multidimensional core-shell halide perovskite colloidal nanocrystals”, Nature Communications 8: 15198 (2017)
Huajun He and Tze Chien Sum, “Halide perovskite nanocrystals for multiphoton applications”, Dalton Trans., 49, 15149-15160 (2020)
1.2-T1
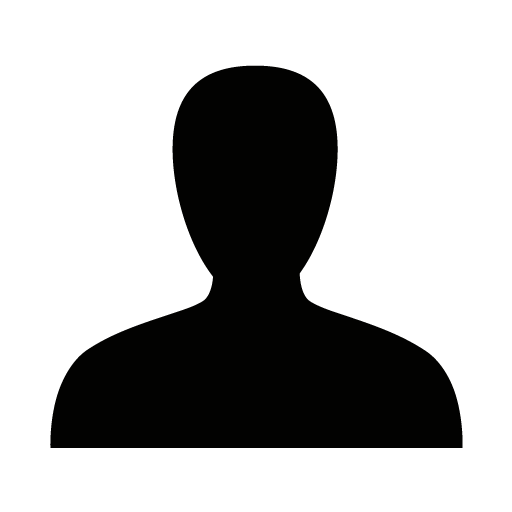
Inverted p-i-n perovskite solar cells (PSCs) are pivotal for fabricating perovskite-based tandem photovoltaics with the highest performance. However, severe non-radiative recombination at the perovskite/electron transport layer and at the grain boundaries (GBs) still limits their open-circuit voltage (VOC) and fill factor (FF) as compared to their n-i-p counterparts. We introduce a novel dual passivation approach using phenethylammonium chloride (PEACl) to simultaneuosly passivate the GBs and the perovskite/C60 interface by using PEACl:PbCl2 as the additive and PEACl for surface treatment, respectively. [1] Employing the self-assembled monolayer [2-(9H-carbazol-9-yl)ethyl]phosphonic acid (2PACz) as hole-transporting layer and a methylammonium-free Cs0.18FA0.82PbI3 perovskite absorber (bandgap ~1.57 eV), we achieve a substantially enhanced charge carrier lifetime and quasi-Fermi level splitting (~63 mV enhancement) compared to reference devices. By analyzing X-ray diffraction, cathodoluminescence, scanning electron microscopy, X-ray/ultraviolet photoelectron spectroscopy and Kelvin probe force microscopy measurements, we attribute the positive effects to the formation of a thin heterogeneous 2D Ruddlesden Popper (PEA)2(Cs1−xFAx)n−1Pbn(I1−yCly)3n+1 phase with n ~1-2 at the GBs and film surface. This leads to efficient chemical passivation of GB and surface defects as well as additional electronic passivation at the perovskite/C60 interface due to hole-blocking. Our dual passivation strategy results in one of the highest reported PCEs for p-i-n PSCs of 22.7% with a remarkable VOC and FF of 1.162 V and 83.2%, respectively. In addition, the dual passivated PSCs exhibit a strongly enhanced activation energy for ion migration together with an enhanced stability under light illumnation and prolonged heat treatment.
1.2-T2
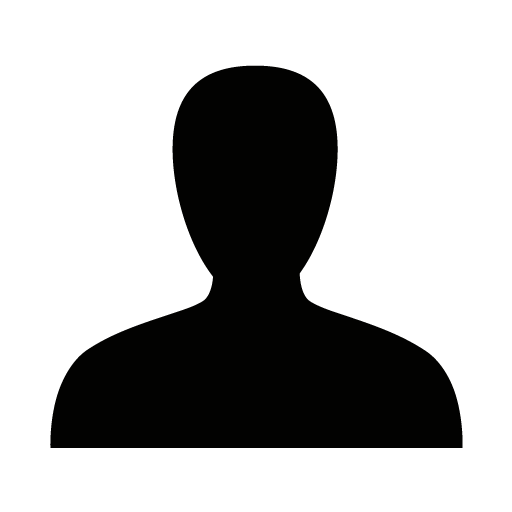
I am a enthusiasm and active learner who wishes to travel in the knowledge ocean. I had my bachlor and master diplomats in Nanchang Hankong Univ. and Huazhong Sicence & Technology Univ. in China respectively, and then got my phD diplomat in Aix-marseille Univ. in France. Right now I am working in INSA Rennes at FOTON lab, mainly focusing on the surface and interface enginnering of lead-free perovskites together with different charge transport materials by using DFT calculation.
Ever since organic-inorganic halide perovskites have been employed in electronics and optoelectronics, their unique and excellent photophysical and electrical properties[1–3] have guaranteed their versatile applications in solar cells, LEDs, lasers, photodetectors and beyond[4,5]. Sn-based perovskites along with their surface and interface functionalization after assembling with charge transport layers (CTLs) are considered promising ways to achieve a win-win between environmental friendliness and cell performance[6–10]. In view of the sophisticated chemical and physical properties of Sn-based perovskites, the theoretical calculation based on density functional theory (DFT) has been employed to feasibly and flexibly model the interplay between absorbers and CTLs. In order to understand the fundamental physico-chemical mechanisms of that interplay, the influence of surface termination on structural and electronic properties at FASnI3/C60 (as ETL) interfaces, in particular, the dependence of vacuum level (and by that work function) within surface alone and interface integrated frameworks, thereupon constructing the heterostructure energy level alignment, has been investigated thoroughly. The resulting type-II heterostructure as well as the increasing surface dipole and charge carrier mobility tell an effective charge transport from FASnI3 to C60. Our findings contribute to discovering other promising alternatives as ETLs for lead-free perovskites in the light of surface and interface engineering.
1.2-T3
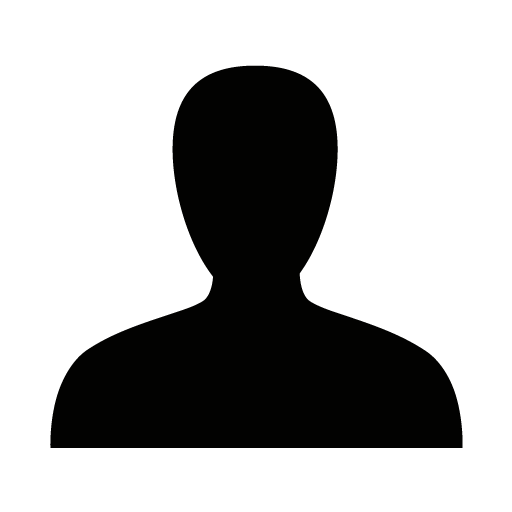
The spectacular rise of the photovoltaic power conversion efficiency of hybrid organic-inorganic perovskites to over 25% has conferred them a promising position in the field of photovoltaics and optoelectronics[1]. To further improve their device performance, there is a considerable ongoing research effort on tuning their interfacial energetics with charge selective contacts along with passivating and/or functionalizing their surfaces and interfaces[2]. Among possible charge selective contact materials, nickel oxide (NiO) is commonly used as a hole transporting layer in thin-film optoelectronic technologies based on organic or hybrid materials. Here[3], we computationally scrutinize the interfacial properties of the prototype MAPbI3/NiO heterostructure, which has shown excellent photovoltaic performance and in particular a large open-circuit voltage[4]. We study the valence band energy level alignment between MAPbI3 and NiO considering i) the defect-free system ii) the role of defects and iii) doping. To further highlight the influence of surface dipoles on work functions and absolute valence energy alignment, we present a theoretical methodology, bridging classical electromagnetism and modern atomistic approaches, to show their intimate connection[5]. We demonstrate the potentials of the methodology, through a variety of cases such as surface termination and passivation and/or functionalization. Our approaches to inspect the properties of heterojunctions, interfaces and surface dipoles transcend the limits of halide perovskites and provide computational strategies to fine-tune energy level alignments for optimizing the performance of broader families of optoelectronic devices.
1.3-T1
To develop a detailed understanding about halide perovskite processing from solution, the crystallization processes are investigated during spin coating and slot-die coating of MAPbI3 at different evaporation rates by simultaneous in situ photoluminescence, light scattering, and absorption measurements. Based on the time evolution of the optical parameters it is found that for both processing methods initially solvent-complex-structures form, followed by perovskite crystallization. The latter proceeds in two stages for spin coating, while for slot-die coating only one perovskite crystallization phase occurs. For both processing methods, it is found that with increasing evaporation rates, the crystallization kinetics of the solvent-complex structure and the perovskite crystallization remain constant on a relative time scale, whereas the duration of the second perovskite crystallization in spin coating increases. This second perovskite crystallization appears restricted due to differences in solvent-complex phase morphologies from which the perovskite forms. The work emphasizes the importance of the exact precursor state properties on the perovskite formation. It further demonstrates that detailed analyses of multimodal optical in situ spectroscopy allows gaining a fundamental understanding of the crystallization processes that take place during solution processing of halide perovskites, independent from the specific processing method.
1.3-T2
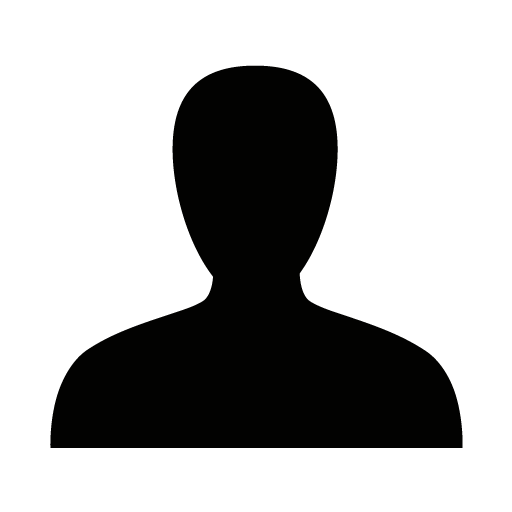
Highly efficient perovskite-based optoelectronic devices require the preparation of high-quality perovskite thin films. However, detailed understanding and rationalization of the solution-based perovskite processing are still lacking. Detailed process rationalization will lead to reliability and reproducibility in perovskite thin-film preparation, also on a large scale. Thus, here we rationalize the influence of the precursor solution concentration on the formation kinetics, which determines, e.g., the process window during the preparation process.
To rationalize the influence of the precursor solution concentration on the formation kinetics, the series of 0.5 M, 0.8 M, and 1.2 M Cs0.05FA0.85MA0.10PbBr3 (3CatPbBr3) solutions is spin-coated. A home-built optical in-situ setup[1] is utilized to follow the formation process of this series via in-situ UV-vis and PL measurements. A bromide-based perovskite composition is chosen since those form directly from solution without intermediate steps.[2]
In-situ UV-vis measurements show an increase in the transflectance signal over the entire wavelength range instead of a clear absorption edge expected to form during spin-coating. However, during in-situ PL measurements, a defined PL peak increases fast in intensity while the peak position shifts to a longer wavelength. Over time the PL signal vanishes. For each concentration, the signal onsets correlate in time for both in-situ measurements, and their appearance is delayed increasing the concentration. Thus, complementary in-situ UV-vis and PL measurements reveal a strong concentration dependence of the 3CatPbBr3 perovskite formation kinetics. Namely, a delayed crystallization onset accompanied by slowed-down crystallite growth is determined for higher concentrated solutions.
In addition, the concentration-dependent chemical characteristics of the solution concentration series are investigated by SAXS, NMR, and UV-Vis measurements. Concentration-dependent changes in the solution chemistry reason those slowed-down formation kinetics. Namely, the colloidal size, their interaction, and the individual chemical surrounding of the Pb2+ ion modify with the solution concentration.
Thus, this application example demonstrates the significance and importance of in-situ measurements during film formation. Overall, this connected study investigating the precursor solution chemistry and the corresponding formation kinetics identifies that slight modifications in the precursor solution impact the perovskite formation dramatically. Hence, even for slight variations in the precursor solution, preparation procedures need to be adjusted to achieve high-quality thin films. Thus, for complete process control, each detail influencing the perovskite formation, such as the precursor solution concentration, needs to be understood to fabricate high-quality thin-film by, e.g., aimed induction of the crystallization during an open process window.
1.3-T3
Hybrid perovskite photovoltaics is a very promising, emerging thin-film technology that has not only rapidly increased in power conversion efficiencies of perovskite solar cells featuring a large range of bandgaps, but also promises low-cost industrial-scale fabrication due to its compatibility with solution processing and the abundancy of the precursor materials. However, the fabrication of perovskite modules on the industrial scale still faces major challenges. Besides the material’s toxicity due to lead incorporation and its instability with respect to stress factors like humidity, temperature and light (which could be addressed in the future by employing suitable encapsulation and product cycle strategies), the scalability and reproducibility of large-scale solution processing does not yet meet industrial standards. For one thing, morphological defects are very likely to occur in large-area deposition techniques such as slot-die coating, spray coating and inkjet printing. For another thing, the opto-electronic functionality of solution processed perovskite thin-films varies from batch to batch due to their sensitivity to the processing parameters such as temperature, humidity, lighting, etc.
In response to these issues, we propose a combined toolkit of modeling and monitoring. First, we in situ characterize the perovskite formation process starting from the precursor solution thin-films with high spatial and temporal resolution, that is, we evaluate multidimensional series of reflectance and (spectral) photoluminescence signals. Second, we correlate these properties with sophisticated models of the perovskite thin-film drying and crystallization. That is to say, we vary the processing parameters over a wide range and evaluate the respective response signals from the in situ characterization techniques in reference to the predictions of our models. In this way, we show that the drying, as measured by reflectance oscillations, is mainly controlled by the temperature and the local mass transfer coefficient (as well as the choice of solvents). Likewise, we demonstrate that the crystallization dynamics, as correlated with the photoluminescence response, depend on the drying rate and temperature (as well as the precursor materials). To sum up, we manage to understand and surveil the morphology formation in perovskite thin-films both on the lateral and on the temporal scale. This methodology could enable direct process control in the future, meaning that both spatial and temporal non-homogeneities in solution processing can be directly identified and corrected instantly by feedback controlling the processing parameters.
1.3-T4
The key point in fabricating homogenous and pinhole-free metal halide perovskite films is controlling the nucleation and crystal growth process. For scalable coating and printing techniques, vacuum and gas flow-assisted drying processes turn out to be the most promising methods to induce favorable nucleation and crystallization. But, the exact interplay and nature of these processes are mostly unclear.
Our work provides the first deep insights into the crystallization dynamics of inkjet-printed metal halide perovskite thin films by optical in-situ monitoring [1,2]. We shed light on the so-called vacuum drying process, which enabled us to identify a novel process to print high-quality perovskite thin films. Contrary to the previously accepted notion that vacuum drying is the main contributor to perovskite crystallization, a decisive crystallization process is induced by the additional flow of gas over the sample. Moreover, we can show that this gas flow induces oriented crystallization during layer formation employing grazing incidence x-ray diffraction. The controlled preferential layer formation provides an effective route to fabricate high-efficiency perovskite solar cells. Utilizing this gas flow-assisted vacuum drying process, we find that regular, optically dense and pinhole-free MHP layers can be fabricated via inkjet printing, which yields solar cells with a power conversion efficiency of 16%, as compared to 4.5% for vacuum drying.
Nevertheless, further detailed analysis is necessary to fully understand the formation and nucleation process, and with this, establish the process to achieve performing larger area solar cells.
1.4-T1
The high-efficiency perovskite solar cells (PSCs) are mostly fabricated in a glovebox under controlled inert environments.[1] Even though there are many advancements have been made in the PSCs, it still limits the operational longevity under ambient atmospheric conditions. The formamidinium lead iodide (FAPI) perovskite, is the most appealing Pb-based 3D halide perovskite for solar cell application because of its narrow bandgap.[1,2] However, the FAPI perovskite a-black phase is not stable at room temperature and is challenging to stabilize in an ambient environment due to its thermodynamic stability limitation.[2] We show that pure FAPI PSCs show significantly higher stability when prepared under ambient air compared to FAPI PSCs fabricated under nitrogen. The concomitant use of the N-methylpyrrolidone (NMP) and the preparation under air conditions is demonstrated as an effective combination to structurally stabilize the a-phase of the material and to improve the film quality, which is handled in the air for the fabrication of the solar cell. However, the most dramatic change observed among cells fabricated in a different environment is in the long term stability of unencapsulated devices where the T80 parameter, increases from 21 (in N2) to 112 days (in ambient) to 145 days if PbS quantum dots (QDs) are introduced as additives in air-prepared FAPI PSCs.[3] Furthermore, by adding methylammonium chloride (MACl) the PCE reaches 19.4% and devices maintain 100% of the original performance during two months of storage at ambient conditions. The increase of stability for air fabricated FAPI PSCs is correlated to the presence of Pb-O bonds only in the FAPI films prepared in ambient conditions, thus opening the way to a new strategy for the stabilization in the air towards perovskite solar cells commercialization.
1.4-T2
Iván Mora-Seró (1974, M. Sc. Physics 1997, Ph. D. Physics 2004) is researcher at Universitat Jaume I de Castelló (Spain). His research during the Ph.D. at Universitat de València (Spain) was centered in the crystal growth of semiconductors II-VI with narrow gap. On February 2002 he joined the University Jaume I. From this date until nowadays his research work has been developed in: electronic transport in nanostructured devices, photovoltaics, photocatalysis, making both experimental and theoretical work. Currently he is associate professor at University Jaume I and he is Principal Researcher (Research Division F4) of the Institute of Advanced Materials (INAM). Recent research activity was focused on new concepts for photovoltaic conversion and light emission based on nanoscaled devices and semiconductor materials following two mean lines: quantum dot solar cells with especial attention to sensitized devices and lead halide perovskite solar cells and LEDs, been this last line probably the current hottest topic in the development of new solar cells.
Halide perovskite solar cells have revolutionized the photovoltaic field in the last decade. In a decade of intensive research it has been a huge improvement in the performance of these devices, however, the two main drawbacks of this system, the use of hazardous Pb and the long term stability, still to be open questions that have not been fully addressed. The photoconversion performance of perovskite solar cells containing alternative metals to Pb is significantly lower than the reported for devices containing Pb, where Sn-based perovskite solar cells is the alternative reporting higher photovoltaic performance close to 14%. Nevertheless, Sn-based perovskite solar cells exhibit a long term stability lower than their Pb containing counterparts, making stability their main problem. In this talk, we highlight how the use of proper additives can increase significantly the stability of formamidinium tin iodide (FASnI3) solar cells, and discuss about the different mechanism affecting this stability, beyond the oxidation of Sn2+, and how they can be countered.
1.4-T3
mmantsae Diale
Progress in perovskites solar cells has shown that internal conversion efficiency can be as high as 26% in 2021. The commercialization of these solar cells is hampered by stability, reproducibility, and scalability. Through sequential physical vapor deposition (SPVD), we have demonstrated reproducible and stable halide perovskites thin films for solar cells. Thin films such as methylammonium lead tri-iodide (MAPbI3), methylammonium lead tri-bromide (MAPbBr3), methylammonium lead iodide-bromide (MAPb(I1-xBrx)3), and cesium lead tri-iodide (CsPbI3) have been synthesized. The MAPbBr3 films were found to be more durable on certain metal surfaces than on others [1]. Gold-zinc (Au-Zn) substrates exhibit the highest degree of film stability, while aluminum (Al) substrates display the lowest degree of film stability [1]. Besides, previous results revealed that MAPbI3 produced a more efficient solar cell on zinc oxide (ZnO) electron transport layer (ETL) than on titanium dioxide [2]. However, MAPbI3 rapidly degrades to lead iodide during post-annealing [3]. By SPVD, no post-annealing step was required to promote the degradation of the MAPbI3. Thus, SPVD presents a promise towards large-scale, reproducible, and stable halide perovskite thin-film materials for solar cells.
Keywords: methylammonium lead tri-iodide, methylammonium lead tri-bromide, sequential physical vapor deposition, methylammonium lead iodide-bromide, cesium lead tri-iodide, stability, perovskite solar cells.
1.4-T4
The operational stability of perovskite solar cells (PSCs) is imperative for their commercialization. Although PSCs have almost reached the climax of device performance, their long-term operational stability remains a primary challenge for real-world applications. Despite significant progress through the engineering of the bulk and the interface layer in device configuration, the mechanisms underlying the degradation of HaPSCs under continuous illumination and heat stresses are still ambiguous. We report on the operational stability of devices made with PTAA; (PCE ≈19.32%) or sputtered NiOx (PCE≈15.60%) as a hole-transport layer (HTL) under light (for >1000 h) at 20, 60, and 85 °C to unravel the degradation mechanisms. Our work suggests that degradation is initiated mainly by the deterioration of the HaP bulk at columnar inter-grains and the interfacial junction with the release of I2 gas, which worsens the interface quality. Degradation of the PTAA device was accelerated by the interface deterioration and bulk decomposition initiated by the formation of voids and PbI2 via iodine migration from defective regions at the columnar grain boundaries with the release of I2 gas. The NiOx devices significantly improved the device stability with suppression of the HaP bulk degradation by alleviating internal defect dynamics. Capacitance–voltage analysis showed that the PTAA device develops a much wider defective interface layer than the NiOx device, driven mainly by the chemical reaction of iodine with the interfacial layer. Thus, our results reveal that although the cracking of columnar inter-grains and defective spots in the perovskite bulk is the main origin of device degradation, the nature of the HTL also partly contributes catalysing bulk degradation.
1.5-I1
Wolfgang Tress is currently working as a scientist at LPI, EPFL in Switzerland, with general interests in developing and studying novel photovoltaic concepts and technologies. His research focuses on the device physics of perovskite solar cells; most recently, investigating recombination and hysteresis phenomena in this emerging material system. Previously, he was analyzing and modeling performance limiting processes in organic solar cells.
Metal-halide perovskite semiconductors have received tremendous attention in research due to their excellent optoelectronic properties, making them interesting materials for solar cells and light emitting diodes (LEDs). It is fascinating that these perovskites are highly tolerant against electronic defects and at the same time show pronounced ionic conductivity mediated through mobile lattice defects.
In this talk an overview on the effect of mobile ions on solar cells and LEDs operated in pulsed mode is given. It is commented on the consequences, the interplay between electronic and ionic charges has on the interpretation of common characterization techniques such as photoluminescence and impedance spectroscopy.
An example discussed in more detail are lead-free Cs2AgBiBr6 double perovskite solar cells, whose efficiency-limiting processes remain a matter of research.
1.5-I2
Hybrid organic-inorganic halide perovskite materials are promising for light emitting applications. In this talk, I will discuss our recent work on perovskite-based LEDs, where we have established a general protocol for preparing ultrathin, smooth, passivated, and pinhole free films of metal halide perovskites with various compositions, by incorporating bulky organoammonium halide additives to the stoichiometric 3D perovskite precursors. In addition, we have found that a major factor contributing to roll-off of perovskite LEDs is heating. By avoiding heating through multiple strategies, we are able to reduce roll-off and report record-bright perovskite LEDs, pushing toward display, lighting, and even lasing-relevant current densities.
2.1-I1
Paulina Plochocka, Directrice de recherché de 2e classe (DR2) in Laboratoire National des Champs Magnétiques Intenses (LNCMI), CNRS in Toulouse.
P. Plochocka obtained her PhD cum-laude in 2004 at the University of Warsaw working on the dynamics of many-body interactions between carriers in doped semi-magnetic quantum wells (QW). During her first post doc at Weizmann Institute of science, she started working on the electronic properties of a high mobility 2D electron gas in the fractional and integer quantum Hall Effect regime. She continued this topic during second post doc in LNCMI Grenoble, where she was holding individual Marie Curie scholarship. At the same time, she enlarged her interest of 2D materials towards graphene and other layered materials as TMDCs or black phosphorus. In 2012 she obtained permanent position in LNCMI Toulouse, where she created the Quantum Electronics group, which investigates the electronic and optical properties of emerging materials under extreme conditions of high magnetic field and low temperatures. Examples include semiconducting layer materials such as transition metal dichalcogenides, GaAs/AlAs core shell nanowires and organic inorganic hybrid perovskites.
High environmental stability and surprisingly high efficiency of solar cells based on 2D perovskites have renewed interest in these materials. These natural quantum wells consist of planes of metal-halide octahedra, separated by organic spacers. Remarkably the organic spacers play crucial role in optoelectronic properties of these compounds. The characteristic for ionic crystal coupling of excitonic species to lattice vibration became particularly important in case of soft perovskite lattice. The nontrivial mutual dependencies between lattice dynamics, organic spacers and electronic excitation manifest in a complex absorption and emission spectrum which detailed origin is subject of ongoing controversy. First, I will discuss electronic properties of 2D perovskites with different thicknesses of the octahedral layers and two types of organic spacer. I will demonstrate that the energy spacing of excitonic features depends on organic spacer but very weakly depends on octahedral layer thickness. This indicates the vibrionic progression scenario which is confirmed by high magnetic fields studies up to 67T. Finally, I will show that in 2D perovskites, the distortion imposed by the organic spacers governs the effective mass of the carriers. As a result, and unlike in any other semiconductor, the effective mass in 2D perovskites can be easily tailored.
2.1-I2
I am an energetic, creative, female scientist with a solid expertise in Material Science and Technology. I have successfully implemented an engineering approach to guide the development of functional nanohybrids through general and simple routes. Throughout my work, I have introduced important mechanisms on the cooperative coupling of dissimilar materials in single structures, which represents a fundamental knowledge for the creation of a new-generation of nano and macro hybrid materials.
Layered perovskites are promising materials for applications beyond solar cells in view of their extreme versatile architecture and simple processing.1 The self-intercalation of organic moieties and inorganic slabs opens a door toward the engineering of efficient emitters from a single material.2 In this work, we rationalize the design of the organic layer made by cations from the amine family and study how the accommodation of the functional group at the anchor side, as well as, the length of the tail affect the material lattice dynamic and light emission.3 We discover a new set of single layer white and blue-emitting platelets and we demonstrate that the careful selection of the organic cations allows tuning of the colour emission of the resulting materials, from blue to warm white and pure white colours. Our work provides experimental guidelines for the selection of organic cations in these materials that can be extended to other organic molecules and unravel new functionalities.
References:
Avi Mathur, Hua Fan, and Vivek Maheshwari. Mater. Adv., 2021,2, 5274-5299.
Xiaotong Li, Justin M. Hoffman, and Mercouri G. Kanatzidis. Chem. Rev. 2021 121 (4), 2230-2291
Dhanabalan, B., Biffi, G., Moliterni, A., Olieric, V., Giannini, C., Saleh, G., Ponet, L., Prato, M., Imran, M., Manna, L., Krahne, R., Artyukhin, S., Arciniegas, M. P., Adv. Mater. 2021, 33, 2008004.
2.1-I3
Emil List-Kratochvil graduated from the Napier University Edinburgh with a first class BSc (Hons) in Applied Physics in 1996, followed by a first class Master Degree in 1998 and a first class degree of a Doctor Technicae in 2000, both from Graz University of Technology (TU Graz).
He received his Habilitation (Venia Docendi) in Solid State Physics in 2003 at TU Graz. At that time, he was a Christian-Doppler-Society funded Research Associate (2000-2007), directing a laboratory for “Advanced Functional Materials” focusing on an applied research agenda in collaboration with Industry. In 2004 he was appointed Associate Professor in Solid State Physics at TU Graz. In 2004 Professor List-Kratochvil was awarded the Fritz Kohlrauschpreis (ÖPG) and the Basic Research Nanotechnology Award (Province of Styria).
In 2006 he got the offer to found the NanoTecCenter Weiz Forschungsgesellschaft mbH, which he directed as Scientific Managing Director until 2015, in parallel to his appointment at TU Graz.
In 2015 he joined Humboldt-Universität zu Berlin as full professor (W3) at the Departments of Physics and Chemistry as well as a member of the Integrative Research Institute for the Sciences (IRIS Adlershof).
In August 2018 he also accepted the offer of Helmholtz-Zentrum Berlin to found and lead a Joint Laboratory as well as Joint Helmholtz Research Group on “Generative Manufacturing Processes for Hybrid Devices”.
Beyond the use in home and office-based printers, inkjet printing (IJP) has become a popular structuring and selective deposition technique across many industrial sectors. More recently great interest also exists in new industrial areas like in the manufacturing of printed circuit boards, solar cells, flexible organic electronic and medical products. In all these cases IJP allows for a flexible (digital), additive, selective and cost-efficient material deposition, which can be used in an in-line production process. Due to these advantages, there is the prospect that up to now used standard processes can be replaced through this low-cost innovative material deposition technique. However, using IJP as a production process in manufacturing, beyond the use in research laboratories, still requires rigorous development of cost and performance optimised functional electronic inks and processes, in particular those allowing for the fabrication on low-cost flexible substrates polyethylene terephthalate. By this means this important aspect also addresses the trend in industry for high-throughput, roll-to-roll device processing, where the use of common plastic substrates instead of glass poses problems concerning the thermal stability of the substrate and the mechanical stability of the deposited device layers, including the transparent conductive electrode (TCEs) against damages caused by substrate bending during the production and operation lifetime of the flexible devices. In this contribution we report on the design, realisation and characterization of printed TCEs as well printing processes for solution-processable metal halide perovskites in perovskite light emitting diodes and solar cells.
2.2-T1
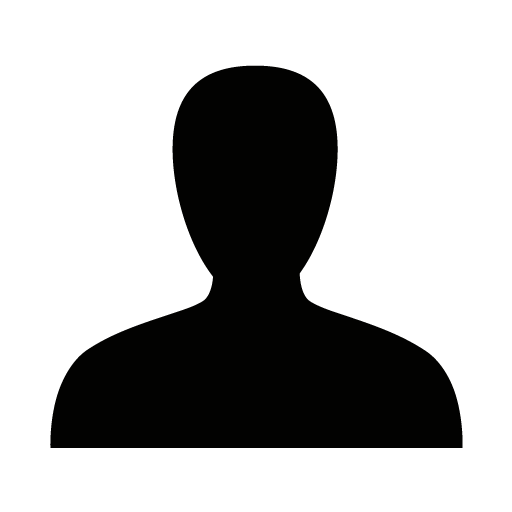
Light emitting materials are nowadays important in various applications and for many of them the development of graftable POOH emissive organic molecules combined with inorganic nanoparticle have opened new opportunities for future technologies. A possible way to enhance the emission of organic fluorophores and to control the size of solution-processable nanoparticles at the same time is their co-grafting with Oleic acid onto inorganic nanoparticle surfaces. In order to avoid the classical aggregation-caused quenching effect when high concentrations of organic ligands are present on nanoparticle surface, the opposite effect, namely aggregation-induced emission is a promising approach to generate strong light emission. Indeed, in this case, the high concentration helps to freeze the motion of the molecules to reduce the non-radiative deactivations. However, even though this new nanohybrid materials show strong emission, their solution-processing remains challenging and morphology control as nanoparticles suffer from large distributions over size and shape. Therefore to go towards efficient reproductible devices the transport and morphology limitations related to nanohybrids have to be controled. In this work we thus present the incorporation of nanohybrids in polymer host matrix PVK and oxadiazole. In order to have efficient LED devices, but also to control the emitted light by changing the organic part. By the co-grafting of different organic emitters on the nanoparticle surface we target to reach the white display on LED device.
2.2-T2
Dr. M. E. Calvo is Doctor in Chemistry by the University of Buenos Aires, Argentina. He is member of the permanent staff of the Spanish National Research Council (CSIC). He is part of the Multifunctional Optical Materials Group at the Institute of Materials Science of Seville where he has been working for 15 years. Nowadays, his main research interest is focused on the solution process of optical porous hybrid nanostructured materials for different applications like solar protection, optoelectronic devices and sensors. He is author of more than 70 publications and 7 patents and he is/was supervisor in several PhD theses. More information at http://mom.icmse.csic.es/group-members/mauricio-calvo/
Nanoscale perovskite materials present substantial advantages over large-grain perovskite thin films or bulk, such as high photoluminescence quantum yield, multi-exciton generation or enhanced defect tolerance. The synthetic colloidal approach is one of the most used methods to obtain ABX3 nanocrystals. However, there are many drawbacks in their processing as thin film (purification and deposition steps, ligand interchange) that require special careful in order to preserve the excellent optoelectronic properties of the colloidal suspension. Here, we prepare ligand-free ABX3 perovskite q-dots using a pore network of insulating porous matrices as nanoreactors. [1] The infiltration with perovskite precursors followed with a mild thermal treatment leads to nanocrystals embedded in the porous structure. From that way, we achieved strict perovskite size control in the sub 10 nm range that enables the observation of quantum confinement effects in their optical response.[2] Furthermore, the strong optical absorption besides the connectivity of the NCs leads us to integrate them in a solar cell device that reach 9% of photo-conversion efficiency in an alternative configuration to that employed in previously developed QD solar cells.[3] In addition, we demonstrate that the efficient charge transport between q-dots is due to a percolation mechanism and the stability of this type of cell is higher than standard bulk devices.
2.2-T3
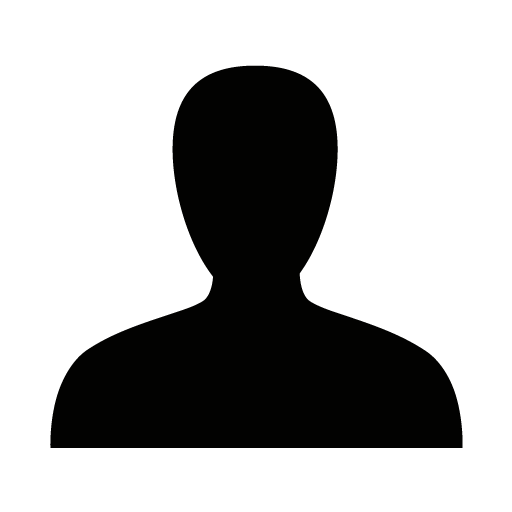
Continuous-wave optically pumped lasing from quasi-two-dimensional (2D) perovskite has been demonstrated recently. Understanding the key factors limiting the lasing performance is highly relevant for pursuing electrically driven lasing based on such solution-processed quasi-2D perovskite materials. In this work, we compare the photoluminescence (PL) and amplified spontaneous emission (ASE) of quasi-2D perovskite (CsPbBr3 with 80% butylammonium bromide (BABr) spacer) and its 3D counterpart formed by thermal removing the organic spacers. Surprisingly, the superior PL (at low excitation fluence) of quasi-2D perovskite does not translate into a lower ASE threshold (~600 μJ cm-2). Annealing the quasi-2D perovskite into the 3D perovskite results in a lower ASE threshold (~130 μJ cm-2) despite the much poorer PL efficiency at low excitation fluence of the 3D perovskite.
Power-dependent time-resolved PL revealed that the excited-state population of the quasi-2D perovskite based on the 80% BA spacer is dominated by excitons. This accounts for the superior PL at low fluence, as the excitonic emission is efficient at low excited-state densities, whereas the free-carrier-recombination-based PL of the 3D materials is a second-order process and needs higher excited-state densities to become efficient. However, at the rather low excited-state density of 1016 cm-3, exciton–exciton annihilation sets in for the quasi-2D sample and results in a decreasing radiative efficiency at high excitation power. In contrast, the free-carrier radiative recombination leads to a high radiative efficiency steadily increasing to the transparency carrier density (1018 cm-3), which explains its lower ASE threshold.
We further systematically investigate the ASE of a series of quasi-2D perovskites through varying the spacer type and concentration. The experimental results show that the optimum ASE threshold of quasi-2D perovskite films can be achieved by minimizing the surface roughness and thin QWs volume fraction through decreasing the spacer contents or utilizing 1-naphthylmethylamine bromide spacer. Time-resolved photophysical studies manifested that the increase of thick QWs volume fraction correlates with an increased contribution of free carrier radiative recombination to the total emission process of the quasi-2D perovskites. Our work suggests a detailed understanding of the excited-state population(s) in quasi-2D perovskites, and their dynamics is essential for engineering their gain performance. The concrete guidance for material development that our results suggest is that quasi-2D perovskite gain materials should target fast free carrier recombination by engineering the thickness and size of QW, but not maximum PL quantum yields under low power excitation.
2.2-T4
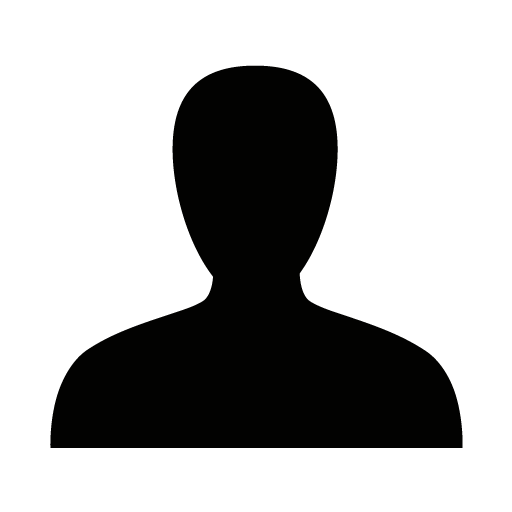
Recently, owing to their excellent optoelectronic properties combined with an enhanced material stability, two-dimensional (2D) hybrid halide perovskites have emerged as an attractive alternative to 3D perovskites for photovoltaics [1][2] More, their ability to emit white-light at room temperature with a good color rendering projected this family of materials into the spotlight of potential future low-cost white LED components[3]. However, the underlying physical mechanisms behind these emissive properties are not fully understood. Herein we propose to study basic optoelectronic properties that might be linked to the broad-band emission observed at different temperatures in a series of (C6H11NH3)2PbX4 2D Ruddlesden Popper perovskites (X= Br or I) [4] [5]. We rely on a coupling between advanced structural measurements and an atomistic approach based on the density functional theory (DFT). Indeed, as the temperature is decreased, phase transitions between undistorted and distorted structures were observed for the two compounds of the series by means of temperature dependent X-ray diffraction. While this crystallographic phase transition goes along with a measured polarization-electric field hysteresis for the bromide compound, more complex apparition of satellite incommensurate reflections was instead obtained for the Iodine one. We believe that these satellite reflections might also be connected to a low temperature ferroelectric ordering. In order to set an interplay between the structural distortions and the observed optoelectronic properties, theoretical studies intend to provide insights of the electronic band structures and the polarization characteristics for these compounds.
2.3-T1
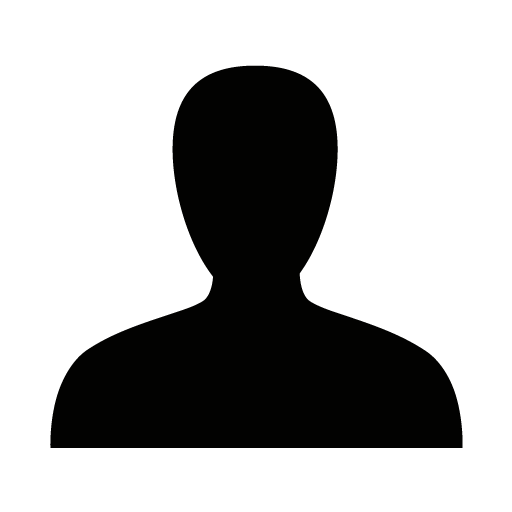
Despite that perovskite light emitting diodes (PeLEDs) have witnessed remarkable progress in their external quantum efficiency (EQE) reaching values > 20%, and half-lifetimes > 100 hours, a clear understanding of the role of ion migration on the optoelectronics characteristics of the PeLED and its stability is still lacking. Here, we demonstrate that ion migration processes which occur on timescales spanning different orders of magnitude, from milliseconds to hours, dictate PeLED performance. These processes are either reversible such that they are repeated each time the PeLED is measured, or irreversible where the PeLED is permanently activated or degraded compared to its initial state.
we show how the current and electroluminescence (EL) signals vary temporally in response to electrical bias and the relation between the extracted PeLED parameters and those transients.[1] Then, by combining several experimental techniques such as transient photoluminescence, transient EL, electro-absorption, etc., with theoretical modelling, we were able to reach a simple physical model which explains the transient characteristics of the PeLED on different timescales, and relates them to migrating ionic species, most notable among them are the halides. Moreover, we show how ion migration plays an important role in PeLED degradation. By using several mitigation mechanisms such as using transport layers with good blocking properties, reducing Joule heating and device active area scaling, we demonstrate an operationally stable PeLED with half-lifetimes > 500 hours at 50 mA/cm2 and a peak EQE of 11.4% at 330 mA/cm2.[2, 3]
[1] Elkhouly, K., Gehlhaar, R., Genoe, J., Heremans, P., Qiu, W., Perovskite Light Emitting Diode Characteristics: The Effects of Electroluminescence Transient and Hysteresis. Adv. Optical Mater. 2020, 8, 2000941.
[2] Elkhouly, K., Goldberg, I., Boyen, H.-G., Franquet, A., Spampinato, V., Ke, T.-H., Gehlhaar, R., Genoe, J., Hofkens, J., Heremans, P., Qiu, W., Operationally Stable Perovskite Light Emitting Diodes with High Radiance. Adv. Optical Mater. 2021, 9, 2100586.
[3] Goldberg, I., Qiu, W., Elkhouly, K., Annavarapu, N., Mehta, A.N., Rolin, C., Ke, T.H., Gehlhaar, R., Genoe, J. and Heremans, P., Active area dependence of optoelectronic characteristics of perovskite LEDs. Journal of Materials Chemistry C. 2021.
2.3-T2
In n-i-p type perovskite solar cells, during the oxidation of Spiro-OMeTAD layer, on the one hand, the diffusion of Li+ towards surface of Ag electrode completes the electrochemical cycle and increases conductivity of hole-transporting layer. On the other hand, the migration of Li+ through the perovskite layer into SnO2, which supposedly leads to increase of the built-in voltage. [1] This type solar cells suffer an unpredictable catastrophic failure under operation, which is a barrier for commercialization. The fluorescence enhancement at Ag electrode edge and performance recovery after cutting the Ag electrode edge off proved that the shunting position is mainly located at the edge of device. SEM and TOF-SIMS analyses proved the corrosion of the Ag electrode and the diffusion of Ag+ ions on the edge for the aged cells. Moreover, much condensed and larger Ag clusters formed on the MoO3 layer. Such a contrast was also observed while comparing the central and the edge of the Ag/Spiro-OMeTAD film. Hence, the catastrophic failure mechanism can be concluded as: photon-induced decomposition of the perovskite film causes the formation of reactive iodide species, which diffuse and react with the loose Ag clusters on the edge of the cell. The corrosion of Ag electrode and the migration of Ag+ ions into Spiro-OMeTAD and perovskite films leads to the forming of conducting filament that shunts the cell. The more condensed Ag cluster on the MoO3 surface as well as the blocking of holes within the Spiro-OMeTAD/MoO3 interface successfully prevent the oxidation of Ag electrode and suppress the catastrophic failure.[2]
2.3-T3
Cost-efficient, lightweight solar foils with high power-weight (W/g) values are the dream power source for private driven space exploration, planned satellite mega-constellations, and future habitats on Moon and Mars. Any application outside the earth’s protective atmosphere, however, places enormous demands on material and device stability. While shortwave UV light, atomic oxygen (AtOx), and low-energetic e-, p+ radiation can be shielded easily, high energetic irradiation will damage used semiconductors.
In this presentation, we discuss all-perovskite tandem solar cells that offer low-weight, high-efficiency, and high power-weight attributes, about five times larger than commercially available, industry-standard III-V semiconductor on Ge triple-junction space solar cells. We show that all-perovskite tandem PV possesses a remarkable radiation tolerance. Our tests under 68 MeV proton irradiation revealed negligible degradation (< 6 %) at a dose of 1013p+cm2. Their resilience thus exceeds not only previously tested perovskite/CIGS tandem PV1 but also commercially available radiation-hardened space PV (> 22%) that we tested under identical conditions.
Using sub-cell selective high-spatial-resolution PL microscopy & intensity dependant absolute PL measurements, we then bring to light the fundamentally different origin of radiation damage in traditional III-V semiconductor-based PV systems compared to halide perovskite-based tandem PV. Pseudo-JV measurements constructed from optically measured quasi-Fermi level (QFLS) splitting of high-and low-gap perovskite absorbers prior to and after proton irradiation reveal no degradation, suggesting that further improvements of their radiation resilience are possible with optimized contact layers.
2.4-I1
Angus Hin-Lap Yip joined the Department of Materials Science and Engineering (MSE) and the School of Energy and Environment (SEE) at the City University of Hong Kong as Professor in 2021. He has been the associate director of the Hong Kong Institute for Clean Energy (HKICE) since 2022. He was also elected as a member of the Hong Kong Young Academy of Sciences. From 2013-2020, he was a Professor at the State Key Laboratory of Luminescent Materials and Devices (SKLLMD) at the South China University of Technology (SCUT). He got his BSc (2001) and MPhil (2003) degrees in Materials Science from the Chinese University of Hong Kong (CUHK) and completed his PhD degree in MSE in 2008 at the University of Washington (UW), Seattle. His research combines materials, interface, and device engineering to improve polymer and perovskite solar cells and other optoelectronic devices. He has published more than 270 scientific papers with citations over 36000 and an H-index of 99. He was also honoured as ESI“Highly Cited Researcher” in Materials Science 9 times from 2014-2022.
Metal halide perovskite light-emitting diodes (PeLEDs) show great potentials to be the next-generation lighting technology, with external quantum efficiencies (EQEs) exceeding 20% for infrared, red and green LEDs. However, the efficiencies of blue and white devices severely lag behind. To improve the performance of blue PeLEDs, we employed an integrated strategy combining dimensional engineering of perovskite film and recombination zone modulation in the LED device to obtain an EQE up to 5%.[1] While further incorporating the strategy of interfacial engineering, highly efficient blue PeLEDs with EQEs over 10% have been successfully realized in our group, establishing an excellent platform for white-light emission. In our latest work, we demonstrated efficient white PeLEDs by optically coupling a blue PeLED with a red emitting perovskite nanocrystal layer in an advanced device structure, which allows to extract the trapped optical modes (waveguide and SPP modes) of blue photons in the device to the red perovskite layer via near-field effects. As a result, a white PeLEDs with EQE over 12% is achieved, which represents the state-of-the-art performance for white PeLEDs.[2]
2.4-I2
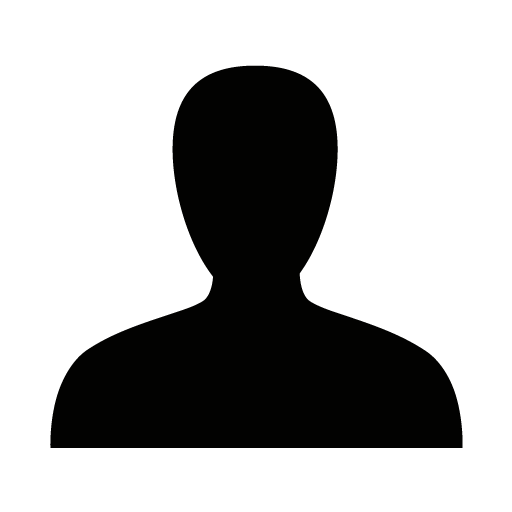
Solution-processed metal halide perovskites have emerged as highly promising materials for light-emitting devices such as LEDs and lasers. The external quantum efficiency (EQE) of perovskite LEDs (PeLEDs) has increased rapidly since its first demonstration, and optically pumped perovskite lasers under continuous-wave operation at room temperature has been achieved. Although much research has been devoted to improving the EQE of PeLEDs, their efficiency starts to drop significantly under high injection current density (J). Suppressing efficiency roll-off at high J is required for achieving ultra-bright LEDs, and ultimately, electrically driven lasers since the key parameter of J×EQE or luminance needs to be large enough to exceed a threshold for lasing. The detrimental behavior of efficiency roll-off is likely caused by a combination of charge injection imbalance, Auger-induced luminescence quenching and Joule heating. This phenomenon, also known as efficiency droop, can be quantified by the critical current density (Jc) at which the EQE reduces to half of its peak value. In this talk, we discuss our work in improving Jc and maximum luminance of PeLEDs. We first optimize the hole transport layers for balancing charge injection in quasi-2D PeLEDs, and achieved simultaneously high EQE (16.2%) and luminance (~31,000 cd/m2). Nevertheless, we ran into a limit in increasing Jc of quasi-2D PeLEDs, likely due to the poor charge transport with the insulating long-chain PEABr layer and increased non-radiative Auger recombination rate originated from the enhanced local charge carrier density in the multiple quantum well structures. These motivated us to further contrast the performance of quasi-2D and 3D perovskites. 3D perovskites generally can withstand higher J; however, their photoluminescence quantum yield (PLQY) is usually lower due to small exciton binding energy. We explored surface passivation to improve the PLQY of 3D perovskites by introducing excess KBr during CsPbBr3 synthesis. The resulting luminance improves by about 4-fold to ~120,000 cd/m2 and Jc increases by 20-fold to ~800 mA/cm2. Subsequently, we investigated thermal-induced luminescence quenching and fabricated current-focusing devices with nanoscale current injection areas. Using pulsed current injection, we increased Jc up to ~60 A/cm2 while operating the device at J values up to ~1 KA/cm2 without measurable damage to the device, ultimately leading to a luminance of 7.6 Mcd/m2. We further explored the effect of substrate heat conductivity by comparing glass and sapphire substrate based PeLEDs, and showed that the device performance can be further improved using sapphire substrates.
2.5-S1
FOM Technologies is a Danish provider of premium slot die coating equipment for lab- and pilot-scale thin film research. We emphasize precision, scalability and usability to meet the needs of modern materials researchers around the world.
Slot-die technology for scalable solution-processed PV devices.
2.5-S2
Assaf co- founded Materials Zone with Prof. Zaban Zaban (currently the President of Bar-Ilan University). Prior, he co-founded and Headed the combinatorial lab at Bar Ilan’s Nano Center. Assaf received his PhD (2011) from Imperial College London (UK), majoring in photochemistry and nanodevices. MSc (Magna Cum Laude, 2008) Chemistry from Bar Ilan University (Israel), majoring in materials science and nanotechnology. He also programmed the brain-research student-laboratory at Bar Ilan. Assaf has extensive experience in the Israeli hi-tech industry: DealTime (acquired by eBay), Dmatek (acquired by 3M), and Cyota (acquired by RSA).
Materials Zone is a scientific AI/ML collaborative platform. By harnessing data, innovative tools, and extensive know-how, the platform funnels and normalizes data into an interoperable and structured database, provides meaningful AI/ML insights, and enables collaboration and sharing. Thus, the Materials Zone platform accelerates Research and Development and manufacturing processes, improves performance and results, and reduces costs across industries. In addition, the Materials Zone platform enables to share and collaborate, achieve meaningful AI/ML insights, and drive better decision-making based on data. Materials Zone already serves paying customers and has a track record in several domains, including perovskites, energy storage, semiconductors, green construction, and more.
2.5-S3
CV
Diploma in Physics (Universities Frankfurt a. M., Saint Louis, Hamburg)
2004: Diploma Thesis at TU Munich (Prof. Stimming; electromchemistry)
2008: PhD thesis about Raman Spectroscopy and CuInS2 solar cells (Prof. Lux-Steiner; HZB, FU Berlin)
2009-2012: R&D Scientist at various silicon (PV Crystalox) and CIGS (Solyndra, Soltecture) PV companies in Germany and USA
2013-2015: Product line manager in the field of thin film metrology (LayTec)
2015-2018: Head of PV systems group at ZAE Bayern in Erlangen
Since 2019: Product manager large area devices @ LayTec
Since 2020: CMO @ LayTec
Multinary semiconconducting / hybrid materials like perovskites are usually deposited in wet-chemical or evaporation processes. Growth kinetics and reaction paths of these deposition methods generally exhibit a high degree of complexity and are influenced by effects such as diffusion, phase transitions and numerous chemical reactions. Due to this complexity, process optimization for these materials usually takes place in a very empirical instead of analytical, knowledge-based manner. Accordingly, the learning curve bears a great potential for further acceleration. Visualizing and modelling the effects underlying growth and deposition is key for an analytical way of optimization, as it provides the base data for modelling and obtaining in-depth understanding of the processes. At the same time, such data, if obtained “in-situ”, can also be used for controlling and automating the deposition process. Optical metrology methods like in-situ reflectance spectroscopy are ideally suited for these purposes. They are directly linked with the absorption properties of the material, which again directly depend on composition, crystallinity and roughness, and they do not influence the deposition process. In order to obtain the best possible in-situ data for a given process, the metrology system needs to be engineered for optimum performance, (choosing the appropriate optical design, wavelength range, resolution and acquisition speed). In this talk we will demonstrate how, with an appropriately designed system, in-situ metrology can be used for visualizing and analyzing the growth kinetics of wet-chemical and evaporation processes.
1.1-I1
Transmission electron microscopy is an excellent tool to investigate the structure and composition of a wide variety of nanomaterials. Unfortunately, metal halide perovskites are extremely sensitive to electron beam irradiation. For example, Pb clusters are easily formed, which hamper a further reliable analysis of the structure. In this talk, I will discuss recent progress in the field of transmission electron microscopy, enabling one to investigate these materials with reduced damage. Moreover, I will demonstrate how these results can be interpreted in a quantitative manner, transforming images into numbers. Finally, the possibilities of extending these studies into 3 dimensions will be discussed.
1.1-I2
Blinking nanoscale emitters, typically single molecules, are employed in single-molecule localization microscopy (SMLM) to overcome Abbe's diffraction limit, offering spatial resolution of few tens of nanometers. Colloidal quantum dots (QDs) feature high photostability, ultrahigh absorption cross-sections and brightness, as well as wide tunability of the emission properties, making them a compelling alternative to organic molecules. Here, CsPbBr3 nanocrystals, the latest addition to the QD family, are explored as probes in SMLM. Because of the strongly suppressed QD photoluminescence blinking (ON/OFF occurrence higher than 90%), it is difficult to resolve emitters with overlapping point-spread functions by standard dSTORM methods due to false localizations. A new workflow based on ellipticity filtering efficiently identifies false localizations and allows the precise localization of QDs with subwavelength spatial resolution. Aided by Monte-Carlo simulations, the optimal QD blinking dynamics for dSTORM applications is identified, harnessing the benefits of higher QD absorption cross-section and the enhanced QD photostability to further expand the field of QD super-resolution microscopy toward sub-nanometer spatial resolution.
References
[1] Advanced Optical Materials, https://doi.org/10.1002/adom.202100620
1.1-I3
Haizheng Zhong is a professor of photonic materials in the school of materials science and Engineering at Beijing Institute of Technology (BIT). He obtained his B.E. degree in 2003 from Jilin University, and then undertook his Ph.D. studies at the Institute of Chemistry, Chinese Academy of Sciences (ICCAS) from 2003 to 2008. During 2017/04-2017/10, he spent 6 months in UCLA as a visiting student. After that, he worked as postdoc in the University of Toronto during 2008–2010. He joined School of Materials Science & Engineering at Beijing Institute of Technology (BIT) as an associate professor in 2010 and was promoted to full professor in 2013. His current research interests are in the area of colloidal quantum dots for photonics and optoelectronics. His recent awards include Xu-Rong Xu Luminescence Best Paper Award (2013), the National Science Foundation for Excellent Young Scholars (2017), Beijing Science and Technology Award (2018, 2/10), 2019 IDW best paper award. Since 2019, he serves as senior editor for The Journal of Physical Chemistry Letters and moved to executive editor in 2020.
Perovskite quantum dots (PQDs) are now emerging as functional materials for many photonic applications due to their superior optical properties and easy fabrication. Recently, we developed the in-situ fabrication of hybrid perovskite PQDs in polymeric films (PQDF) with high transparency, superior photoluminescence emission and additional processing benefits for down-shifting applications. The potential use of PQDF as color converters in LCD backlights was successfully demonstrated, showing bright potential in display technology. Very recently, we developed the in-situ patterning technology of PQD for Micro LED and other down conversion applications. Moreover, we further explored the electroluminescence devices based in-situ fabricated PQDs. We illustrated the enhancing role of ligand-assisted reprecipitation (LARP) process and obtained uniform FAPbX3 (X=Br, Cl, I) PQDs films with photoluminescence quantum yield up to 78%. The electroluminescence devices with a maximum external quantum efficiency (EQE) of 16.3%, 15.8% and 8.8% were achieved for green, red and blue devices, showing the promising to achieve high efficiency. In all, the in-situ fabrication strategy provides very convenient route for display technology. In this talk, I will introduce the progress on the development of in-situ fabricated perovskite quantum dots toward photonic and optoelectronic applications.
1.2-T1
Solution processable semiconducting perovskites hold great promise for demanding applications involving light emission, such as printable lasers or quantum light sources. A case example is that of fully inorganic CsPbBr3quantum wells (CQWs) which display high quantum yield at room temperature. Less studied than their organic-inorganic counterparts, these CQWs sustain high single exciton binding energies and luminescence quantum yields, yet little is known on the nature of the exciton and multi-excitons states required for advanced applications. Here, we show that charge carriers in fully inorganic 2D perovskites exist as stable exciton - polarons, a complex between a charge neutral exciton and a lattice deformation. Next, we show that these unique species can fuse together to form a hereto unexplored bi-exciton polaron state, i.e. a two-particle complex bound by attractive Coulomb attraction whilst simultaneously being strongly coupled to the lattice. Finally, we show that net stimulated emission occurs through radiative recombination from this unique bi-exciton polaron state to a single free exciton polaron, showing the stability of the newly found species. Consequences of the polaronic character are identified as a low threshold for stimulated emission but with limited optical gain coefficients, both of which we can fully reproduce using a thermodynamic gain model. As such, our results provide a general framework to understand and predict the behavior of not only single, but also multi-exciton polaron states in perovskite materials.
1.2-T2
The metastable alpha phase of pure formamidinium lead halide perovskite has been obtained from controlled thermal growth. We have measured the activation energy of the heating rates able to kinetically activating the alpha phase. By using the flash infrared annealing method [1, 2], we have screened a large amount of sequential data. This data was compiled into a database to use as a reliable guide for fundamental studies of halide perovskites. Therefore, the correlation of thermal growth with morphology, structure, and photophysical behavior of the films under different growth conditions has been investigated. Thus, we manufactured highly stable perovskite solar cells with photonic sintering (antisolvent-free) for more than 500 hours.
1.2-T3
Relevant alternatives to decrease the density of surface defects such as ligand passivation, doping, and synthetic protocols have allowed to obtain high-quality perovskite nanocrystals (PNCs). However, the impact of the purity of the precursors involved during the PNCs synthesis to hinder the emergence of defects has not been widely explored. In this work, we analyzed the use of different crystallization processes of PbX2 to purify the chemicals and produce highly luminescent and stable blue- and red-emitting PNCs. The use of a hydrothermal (Hyd) process to improve the quality of the as-prepared PbCl2 provides CsPbCl3-xBrx PNCs with efficient ligand surface passivation, maximum PLQY ~88% and improved photocatalytic activity to oxidize organic compounds such as Benzyl alcohol. Then, the hot-recrystallization of PbI2 prior to Hyd treatment led to the formation of CsPbI3 PNCs with PLQY up to 100%, long-term stability around 4 months under ambient air and relative humidity of 50-60%. Thus, CsPbI3 LEDs were fabricated to provide a maximum external quantum efficiency up to 13.6%. We claim the improvement of the PbX2 crystallinity offers a suitable stoichiometry in the PNCs structure, reducing non-radiative carrier traps and so maximizing the radiative recombination dynamics. [1,2] This contribution gives an insight about how the manipulation of the PbX2 precursor is a profitable and potential alternative to synthesize PNCs with improved photophysical features, by making use of defect-engineering.
1.2-T5
Dr. Modestos Athanasiou received his bachelor’s degree in Electrical and Electronic Engineering from the University of Sheffield in 2011.He then pursue his doctoral studies in the field of engineering, applied physics and material science, with emphasis on nanofabrication and spectroscopical characterization of inorganic semiconductor material and devices, for optoelectronics applications at the University of Sheffield and the Center for GaN Materials and Devices. After the completion of his doctoral studies in 2015, he continued his post-doctoral studies at the University of Sheffield, focused on developing advanced nano-fabrication processes for gallium nitride and related materials. He was involved in the activities of Joint Centre of Wide Bandgap Semiconductors Optoelectronics (JCWSO) between Nanjing University and University of Sheffield and played a major role in the establishment of a joint collaborative research center between the two universities. He has co-supervised more than 10 Ph.D students and final undergraduate students, providing support to their projects. In January 2018, he joined industry based in Cyprus/China, serving the role of the Product Development Manager for architectural lighting fixtures. In January 2019, he was awarded an Advanced Research Fellowship at the University of Cyprus in the Department of Physics and Experimental Condensed Matter Physics Laboratory, under the supervision of Associated Professor Grigoris Itskos. His main research interests are the development of next generation of hybrid III-nitrides devices, for solid-state lighting and lasing applications. He has been awarded the prestigious Marie-Curie Fellowship for the development of ultrafast white light sources based on III-nitrides and lead halide perovskites nanocrystals for visible light communications purposes (VLC), also known as Lifi. He has published more than 25 peer-reviewed publications in respected high-impact factor journals (ACS journals, Nature family etc.) and presented his work in more than 50 national and international conferences, where in two of them his work was highlighted via best-presentation awards.
Solution-processed lasers have emerged as versatile, light sources for a variety of low-cost applications. Among the various resonator-gain media combinations, vertical cavity surface emitting lasers (VCSELs) based on lead halide perovskite nanocrystals (LHP NCs) appear particular attractive as they combine the impressive optical amplification properties of the LHP NCs with facile fabrication of relatively high Q-factor microcavities (MCs) suitable for low threshold optical amplification and lasing with stable intensity and small beam divergence. Yet, there are currently limited reports on such systems in the literature.
Herein, we demonstrate the fabrication of monolithic, all-solution processed MCs based on green-emitting CsPbBr3 and red-emitting CsPbI3 NCs combined with polymeric distributed Bragg reflectors (DBRs) produced out of alternate layers of cellulose acetate (CA) and polyvinylcarbazole (PVK) materials. Steady state and time-resolved photoluminescence (PL) experiments along with angle dependent reflectivity and PL are implemented for the characterization of the MCs. The photonic structures exhibit high Q-factors exceeding ~100 allowing the continuous wave excitation of amplified spontaneous emission (ASE) with low threshold of the order of ~100 mW/cm2. The ASE onset is evidenced by the: (i) threshold-type behavior and spectral narrowing on the excitation variable PL, (ii) amplification of the output intensity by up to one order of magnitude in the vicinity of the cavity mode, (iii) quenching of the PL lifetime when the cavity resonance overlaps with the NC emission. The aforementioned results, demonstrate the high potential of LHP NC photonic structures for practical, scalable, low cost laser applications.
1.2-T4
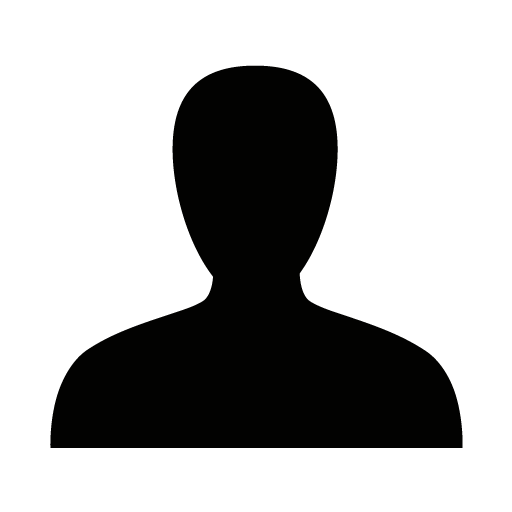
The problem of instability in halide perovskite nanocrystals (NCs) remains a major deterrent towards their rational development in different applications. This instability can be largely attributed to the dynamic nature of both the organic ligands and the inorganic core of the NCs. Thus, the search for a better stabilizing ligand has generated a lot of interest among different researchers. For example, quaternary alkylammonium ligands such as didodecyldimethylammonium bromide (DDAB) have been used in attempts to stabilize the surface of CsPbBr3 nanocrystals but often with contradictory results reported in literature. While some researchers have reported improvement in colloidal stability, quantum yield, and optoelectronic properties of CsPbBr3 NCs using DDAB,1 others have reported it to cause a phase transformation to poorly fluorescent 2D CsPb2Br5.2 Thus, the impact of quaternary alkylammonium ligands on the surface of CsPbBr3 NCs and its role in driving phase transformation have remained unclear. Here, we investigated the thermodynamics of ligand exchange and subsequent processes as DDAB is introduced to CsPbBr3 NCs coated with oleate/oleylammonium native ligands. Using isothermal titration calorimetry (ITC) combined with complementary spectroscopy analysis, we were able to resolve the processes that occur upon introduction of DDAB to natively capped CsPbBr3 nanocrystals. The first step, involving ligand exchange proceeds readily at low DDAB concentration and is endothermic (𝜟H ~30 kJ/mol) with an exchange equilibrium constant Kex >100 indicating that the ligand exchange is entropically driven. On the other hand, larger equivalencies of DDAB bring about a second, exothermic process which corresponds to the displacement of PbBrx complexes from the NCs surface. This process ultimately leads to the formation of 2D phases. Resolving these processes through direct thermal measurements helps to reconcile contradictory reports in prior studies of the surface passivation with quaternary ammonium ligands and also showed that ITC could be a viable technique in understanding the dynamics of the surface ligands on perovskite NCs that can be geared towards a better understanding of the passivation process for improved stability. In addition to this, we compared the ITC profile of DDAB exchange with other analogous ion pairs such as tetraoctylammonium bromide (TOAB), tetraoctylammonium hexafluorophosphate (TOA-PF6), and didodecyldimethylammonium hexafluorophosphate (DDA-PF6), revealing the ability of DDA cation to occupy Cs vacancy sites promoting strong interaction of the ligand with NC surface.
1.3-I1
Patanjali Kambhampati. BA Carleton College USA (1992), PHD University of Texas (USA) 1998, PDF University of Texas (USA) 1999 - 2001. Professor of Chemistry McGill University (2003 - present). Research focus of semiconductor nanostructures and femtosecond laser spectroscopy.
Semiconductors of the perovskite form have attracted considerable attention in recent years, most notably for their performance in photovoltaics. One key puzzle was how a disordered film could support such performance. A number of investigations have led to a current picture of defect tolerance due to a soft, ionic lattice giving rise to dynamic disorder. Moreover, the presence of polarons may be connected to this defect tolerance and ultimately in the device performance, whether photovoltaics or light emitting diodes. How do these ionic semiconductors relate to their covalent cousins, for which defect-free perfection is a main goal? In this Perspective, the question of ionicity as it gives rise to glassy structural dynamics is considered. How do these glassy structural dynamics control the optical response and thus the opto-electronic properties of the system? The recent literature is reviewed with a focus on ultrafast structural dynamics. Highlighted in the discussion of structure / function relation is our recent work which maps the chronology of events from electronic coherence to photon emission using a suite of three time-resolved spectroscopies: Two-Dimensional Electronic Spectroscopy, State-Resolved Pump/Probe Spectroscopy, and time resolved Photoluminescence Spectroscopy. These works collectively advance our understanding of liquid-solid duality in these defect tolerant ionic semiconductors. With the connection to liquid-like dynamics made, a brief discussion of ultrafast solvation dynamics is presented. The specific connection between excess charges in liquids and solids can be found in the solvated electron, which once again serves as a prototype for electronic structure and dynamics in the condensed phase. We hope that finding isomorphisms between phases can shed insight into these new materials.
1.3-I2
Brian A. Korgel is the Rashid Engineering Regents Chair Professor of Chemical Engineering at the University of Texas at Austin (USA) and works in the field of nanomaterials chemistry and complex fluids. He is Director of the Energy Institute at UT Austin. He received his PhD from UCLA in 1997 and was a post-doctoral fellow at University College Dublin, Ireland until 1998 before joining the faculty at UT Austin. He has been Visiting Professor at the University of Alicante in Spain as a Senior Fulbright Fellow, Visiting Professor at the Université Josef Fourier in France and Distinguished Visiting Professor at the Chinese Academy of Sciences in Beijing. He directs the Industry/University Cooperative Research Center for a Solar Powered Future, has co-founded two companies, Innovalight and Piñon Technologies, and serves as an Associate Editor for Chemistry of Materials. He has published more than 220 papers and has received various honors including the Professional Progress Award from the American Institute of Chemical Engineers (AIChE) and the ISHA Roy-Somiya Medal, and is a Fellow of the American Association for the Advancement of Science (AAAS).
Isothermal phase transformations in lead iodide perovskite nanocrystals of different A-site compositions were studied by tracking the black-to-yellow color change in nanocrystal films and modeling the transformation kinetics. At 210 oC, CsPbI3 turned completely yellow in 25 min, while it took MAPbI3 and FAPbI3 longer time to complete the color change. CsPbI3 transformed from the black perovskite phase to the yellow non-perovskite phase, while MAPbI3 and FAPbI3 chemically decomposed and formed PbI2 as the new phase. Mixed A-site perovskites CsMAPbI3 and CsFAPbI3 showed slower rate of transformation than single A-site perovskites, and their degradation products were coexisting yellow phase CsPbI3 and PbI2. Different capping ligands also played a role in changing the transformation kinetics.
1.3-I3
Halide perovskite nanocrystals are a promising platform for optoelectronic devices, including display light sources and single-photon emitters, owing to the fast, efficient radiative decay in these materials. Two-dimensional (2D) halide perovskite quantum dots or nanoplatelets would be particularly promising for bright, fast radiative recombination, since confinement will lead to large exciton binding energies and giant oscillator transition strength. In this work, we explore the thickness-dependent fine structure of excitons in 2D halide perovskite nanoplatelets. We build a quasi-2D effective-mass model of excitons which are strongly confined in the out-of-plane direction but weakly confined in-plane. The model includes the effect of shape anisotropy on the long-range exchange corrections to the fine structure and introduces the important effect of confinement on the band-edge Bloch functions. Applying the model to colloidal CsPbBr3 nanoplatelets, where electron-hole exchange splitting is expected to dominate the exciton fine structure, we predict a thickness-dependent level order of the in-plane-polarized and out-of-plane-polarized bright exciton states. The calculated fine structure is consistent with observed thickness-dependent spectral shifts [1]. We extend the model to 2D hybrid perovskite materials, for which large Rashba coefficients have been either measured or predicted, and describe the dispersion, level structure, and optical properties of the 2D “Rashba exciton”. The analysis reveals unusual features, notably a dispersion minimum at non-zero exciton wave-vector. Consequences of the dispersion to level structure and oscillator transition strengths of Rashba excitons confined in a 2D cylindrical QDs are developed and used to establish criteria under which a bright ground exciton state could be realized [2].
[1] "Dark and Bright Excitons in Halide Perovskite Nanoplatelets", M. Gramlich, M. W. Swift, C. Lampe, J. L. Lyons, M. Doblinger, Al. L.Efros, P C. Sercel, A. S. Urban, To be published.
[2] "Rashba exciton in a 2D perovskite quantum dot", M W. Swift, J. L. Lyons, Al. L. Efros, and P. C. Sercel, To be published.
2.1-I1
The urgency for affordable and reliable detectors for ionizing radiation in medical diagnostics, nuclear control and particle physics is generating growing demand for innovative scintillator devices combining efficient scintillation, fast emission lifetime, high interaction probability with ionizing radiation, as well as mitigated reabsorption to suppress losses in large volume/high-density detectors. Prized for their solution processability, strong light-matter interaction, large electron-hole diffusion length and tunable, intense radioluminescence at visible wavelengths, lead halide perovskite nanocrystals (LHP-NCs) are attracting growing attention as high-Z materials for next generation scintillators and photoconductors for ionizing radiation detection. Nonetheless, several key aspects, such as the trapping and detrapping mechanisms to/from shallow and deep trap states involved in the scintillation process and the radiation hardness of LHP NCs under high doses of ionizing radiation are still not fully understood, leaving scientists without clear indications of the suitability of LHP-NCs in real world radiation detectors or design strategies for materials optimization. In this talk I will focus on recent strategies for high performance radiation detection schemes based on LHP-NCs[1] and will report recent spectroscopic results of the scintillation process and its competitive phenomena[2], ultimately offering a possible path to the realization of highly efficient and extremely radiation hard LHP-NCs.
2.1-I2
Over the last few years, halide perovskite nanocrystals (NCs) have received great attention in many fields ranging from chemistry to physics and engineering owing to their extremely interesting properties such as defect tolerance, high photoluminescence quantum yield (PLQY), tunable optical bandgap not only by their dimensions but also by composition, enhanced stability compared to thin-film perovskites, and ease of synthesis.1 The morphology of perovskite NCs can be easily controlled from 3D nanocubes to quasi-0D nanodots, 2D nanoplates, and nanowires by means of simple chemistry such as precursor ratios, ligand concentration, and crystallization temperature.1-4 In addition, their chemical composition is easily tunable by in situ synthesis or post-synthetic ion exchange reactions. In this talk, different ways of shape and composition tunability in both inorganic and organic-inorganic hybrid perovskite NCs will be discussed.
2.1-I3
Colloidal lead halide perovskite nanocrystals (LHP NCs, NCs, A=Cs+, FA+, FA=formamidinium; X=Cl, Br, I) have become a research spotlight owing to their spectrally narrow (<100 meV) fluorescence, tunable over the entire visible spectral region of 400-800 nm, as well as facile colloidal synthesis. These NCs are attractive single-photon emitters as well as make for an attractive building block for creating controlled, aggregated states exhibiting collective luminescence phenomena. Attaining of such states through the spontaneous self-assembly into long-range ordered superlattices (SLs) is a particularly attractive avenue. In this regard also the atomically-flat, sharp cuboic shape of LHP NCs is of interest, because vast majority of prior work had invoked NCs of rather spherical shape. Long-range ordered SLs with the simple cubic packing of cubic perovskite NCs exhibit sharp red-shifted lines in their emission spectra and superfluorescence (a fast collective emission resulting from coherent multi-NCs excited states). When combined with spherical dielectric NCs, perovskite-type ABO3 binary NC SLs form, wherein CsPbBr3 nanocubes occupy B- and/or O-sites, while with spherical dielectric Fe3O4 or NaGdF4 NCs reside on A-sites. When truncated-cuboid PbS NCs are added to these systems, ternary ABO3-phase form (PbS NCs occuoy B-sites). Such ABO3 SLs, as well as other newly obtained SL structures (binary NaCl- and AlB2-types, columnar assemblies with disks etc.), exhibit a high degree of orientational ordering of CsPbBr3 nanocubes. These mesostructures exhibit superfluorescence as well, characterized, at high excitation density, by emission pulses with ultrafast (22 ps) radiative decay and Burnham-Chiao ringing behaviour with a strongly accelerated build-up time.
2.2-I1
Dr. Francesco Di Stasio obtained a Ph.D. in Physics at University College London (UK) in 2012. He then worked as a research Scientist at Cambridge Display Technology (Sumitomo Chemical group, UK) until he undertook postdoctoral research at the Istituto Italiano di Tecnologia (IIT, Italy). In 2015 he was awarded a Marie Skłodowska-Curie Individual Fellowship at the Institute of Photonic Sciences (ICFO, Spain). Since 2020 he is Principal Investigator of the Photonic Nanomaterials group at IIT after being awarded an ERC Starting grant. Francesco is a materials scientist with more than 10 years of research experience in optoelectronics.
Current research interests and methodology: Nanomaterials for classical and non-classical light-sources: This research activity focuses on the investigation of synthetic routes to obtain highly luminescent semiconductor colloidal nanocrystals and exploit such material in light-emitting diodes (LEDs). Here, we study how chemical treatments of colloidal nanocrystals can promote enhanced performance in devices, and physico-chemical properties of nanocrystals (e.g. self-assembly and surface chemistry) can be exploited to fabricate optoelectronic devices with innovative architectures. Novel methods and materials for light-emitting diodes: The group applies materials science to optoelectronics by determining which fabrication parameter lead to enhanced performance in LEDs. In order to transition from classical to non-classical light-sources based on colloidal nanocrystals, the group is developing novel methods for controlling the deposition and positioning of an individual nanocrystals in the device. Both “top-down” and “bottom-up” approaches are investigated.
Emission of single photons from an isolated colloidal semiconductor nanocrystal is a well-studied phenomenon. In fact, nowadays we can design nanocrystals with engineered shape and/or composition to optimize single-photon emission and prevent detrimental processes such as blinking or slow photon emission.
All the knowledge gathered on single-photon emission processes in colloidal semiconductor nanocrystals is based on the use of light as excitation source, and rightly so, as this allows to carefully study the photophysical processes governing photons generation via exciton recombination. Yet, for colloidal semiconductor nanocrystals to be appealing from an application point of view, single-photon emission from nanocrystals must be triggered via electrical injection of an electron and hole pair. Currently, electrical generation of single photons in nanocrystals has only been marginally explored; to reach such objective, electrode design, nanocrystals deposition control and other device fabrication aspects must be addressed.
In this seminar, I will present some initial results on how to position a single nanocrystal on an electrode or other venues to fabricate light-emitting diodes based on a single and isolated colloidal semiconductor nanocrystal. The methods employed exploit the properties of the two of the most studied types of nanocrystals: CdSe/CdS core-shell systems and perovskites ones.
2.2-I2
Jacky Even was born in Rennes, France, in 1964. He received the Ph.D. degree from the University of Paris VI, Paris, France, in 1992. He was a Research and Teaching Assistant with the University of Rennes I, Rennes, from 1992 to 1999. He has been a Full Professor of optoelectronics with the Institut National des Sciences Appliquées, Rennes,since 1999. He was the head of the Materials and Nanotechnology from 2006 to 2009, and Director of Education of Insa Rennes from 2010 to 2012. He created the FOTON Laboratory Simulation Group in 1999. His main field of activity is the theoretical study of the electronic, optical, and nonlinear properties of semiconductor QW and QD structures, hybrid perovskite materials, and the simulation of optoelectronic and photovoltaic devices. He is a senior member of Institut Universitaire de France (IUF).
Halide perovskite nanocrystals have been introduced in 2015 as a promising route to efficient light emitting devices, and more recently as potential single photon emitters. Their radiative recombinations are governed by excitons, but the bright or dark character of the ground state is debated. A Rashba effect correlated with a local polarization was proposed in 2018 as a general mechanism for inversion of bright and dark level ordering, but direct spectroscopic signatures of the dark exciton emission in the low-temperature photoluminescence of single FAPbBr3 and CsPbI3 nanocrystals under magnetic fields rather yield a dark singlet below the bright triplet. The dark-bright splitting values are in fair agreement with numerical estimations of the long-range electron–hole exchange interaction. The intense luminescence of perovskite nanocrystals is alternatively attributed to a reduced bright-to-dark phonon-assisted relaxation. The phonon side bands in nanocrystals match neutron scattering phonon spectroscopy results obtained at low temperature. Finally, a recent classification of 3D perovskites into ferroelectric or hyperferroelectric materials, points toward the role of the depolarization effect for the reduction of a spontaneous polarization in nanocrystals.
2.2-I3
Alex earned his Ph.D. in physics of semiconductors from Chernivtsi National University, Ukraine for his work on electronic properties of nitride semiconductor alloys.
In 2004 he joined the Quantum Semiconductors and Bionanophotonics lab at University of Sherbrooke as a postdoc, working on theoretical modeling of laser-assisted quantum well intermixing and self-assembly processes of organic monolayers on metal and semiconductor surfaces for applications in bio-sensing.
In 2008 he moved to Quantum Theory Group at National Research Council of Canada in Ottawa, where he worked on many-body problems in epitaxial and colloidal semiconductor and graphene quantum dots; in particular, simulations of multi-exciton generation, Auger processes and optical properties of nanocrystals used in hybrid polymer-semiconductor solar cells.
Alex joined Ted Sargent’s Nanomaterials for Energy Group in 2011 and worked on characterization and modeling of the semiconductor nanocrystal surfaces and developing the synthesis methods for nanomaterials with improved optical and transport properties for photovoltaics.
In 2018, Alex joined the Department of Physical and Environmental Sciences at the University of Toronto, Scarborough as an Assistant Professor in Clean Energy. His topics of interest are materials for energy storage and novel materials discovery using high-throughput experiments and machine learning.
Cesium lead halide perovskite nanocrystals are a highly attractive class of materials for coherent light emission, with implications for lasing, light-emitting diodes, and quantum computing. Fine-tuning their properties for the above applications requires an exact understanding of their exciton fine structure, in particular, spacing and polarization of their triplet and singlet states. Experimental reports have been controversial, implicating that the Rashba effect may be inducing an inversion in the order of bright and dark states.
To aid in the resolution of this debate, we performed investigation of the fine structure of the triplet emission properties in these materials. Using the wave functions generated via DFT calculations including spin-orbit coupling for cubic, orthorhombic and tetragonal caesium lead halide perovskite nanocrystals of ~3 nm in diameter, we further augmented them with Coulomb coupling between the exciton configurations, to resolve the absorption and emission fine structure in a configuration interaction method.
We anticipate our work will aid in the resolution of the debated emission fine structure of CsPbBr3 nanocrystals and thereafter allow for the development of bright materials for optoelectronics.
1.1-I1
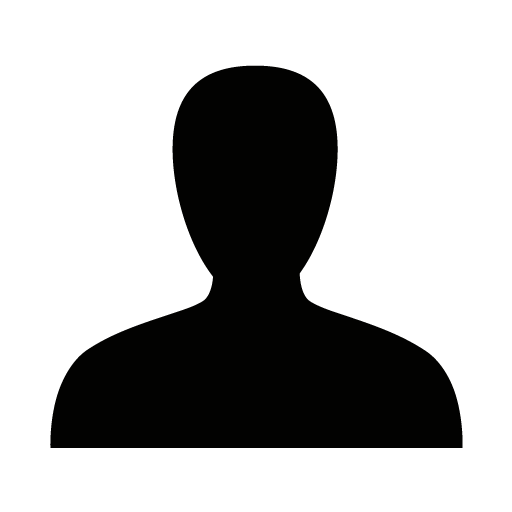
The first part of the presentation introduces the phenomenon of mixed ionic-electronic conductivity in solids and discusses defect-chemical implications and measuring techniques.
The second part of the presentation summarizes parts of our work on bulk ion conductivity in halide perovskites. It discusses contributions from halide ions and methylammonium cations, and their relevance for polarization phenomena and stability [1]. The finding that light enhances the ion conductivity in the iodides [2] is of broad interest and has the potential to lead to novel “opto-ionic” devices [3]. The fact that bromides do not show this effect can offer a simple explanation for the light-induced demixing [4].
The third part of the presentation refers to interfaces. It is shown that mobility of ions and the significant fraction of ionic charge carriers gives rise to ionic built-in potentials, a point that had not been addressed in the photovoltaic literature and might lead to a paradigm change in the understanding of photoactive interfaces [5].
The presentation includes conventional hybrid perovskites, but also “hollow perovskites” [6] and 2D perovskites [7].
1.1-I2
Further breakthroughs in perovskite solar cells require advances in new compositions and underpinning materials science. Indeed, a deeper understanding of these complex hybrid perovskites requires atomic-scale characterization of their transport, electronic and stability behaviour. This presentation will describe combined atomistic modelling and experimental studies on hybrid perovskites [1-5] in two related areas: (i) pathways and energetics of iodide ion transport and the effect of incorporating different sized A-cations including guanidinium-doped and ‘hollow’ type systems; (ii) structure–electronic property relationships and degradation mechanisms in Pb- and Sn-based iodide perovskites including 2D Ruddlesden-Popper type structures.
[1] L. Lanzetta et al., Nature Commun. 12, 2853 (2021); [2] A. Senocrate et al., Chem. Mater. 33, 719 (2021); [3] N. Zibouche, M.S. Islam, ACS Appl. Mater. Interfaces, 12, 15328 (2020); [4] D.W. Ferdani et al., Energy Env. Sci., 12, 2264 (2019); [5] N. Aristidou et al., Nature Commun., 8, 15218 (2017).
1.1-I3
Jacky Even was born in Rennes, France, in 1964. He received the Ph.D. degree from the University of Paris VI, Paris, France, in 1992. He was a Research and Teaching Assistant with the University of Rennes I, Rennes, from 1992 to 1999. He has been a Full Professor of optoelectronics with the Institut National des Sciences Appliquées, Rennes,since 1999. He was the head of the Materials and Nanotechnology from 2006 to 2009, and Director of Education of Insa Rennes from 2010 to 2012. He created the FOTON Laboratory Simulation Group in 1999. His main field of activity is the theoretical study of the electronic, optical, and nonlinear properties of semiconductor QW and QD structures, hybrid perovskite materials, and the simulation of optoelectronic and photovoltaic devices. He is a senior member of Institut Universitaire de France (IUF).
New results on the interplay between structural and optoelectronic properties of multilayered perovskites
The presentation will give an insight into the interplay between structural and optoelectronic properties in 2D multilayered halide perovskites, either in Ruddlesden Popper or Dion Jacobson structure. The results will particularly describe specific mechanisms for the interaction of hot carriers with a 2D lattice, including a discussion on the electron-phonon coupling in layered perovskites and a comparison with previous results on 3D perovskites. Moreover the funneling of electronic carriers to the 2D crystal edges, important for ionization of electron-hole pairs in 2D perovskite solar cells, is shown to be generalized as well to bromide materials. It is including a relaxation of collective polar ordering observed in the bulk at room temperature. Finally the impact of light soaking on the lattice relaxation and carrier trapping, is shown to have a strong influence on carrier transport in operating devices and performances of photovoltaic devices.
1.2-T1
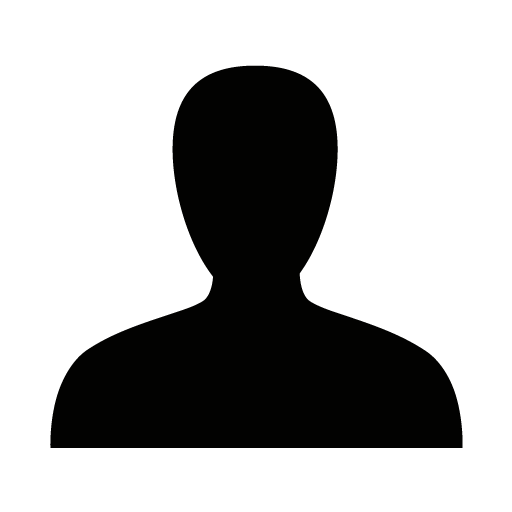
Lead halide perovskites have gained much attention in recent years for their remarkable optoelectronic properties as they can be applied in various devices as highly efficient active semiconductor material e.g., in solar cells, x-ray detectors or LEDs. One obstacle to commercialization is the migration of halide ions, which leads to efficiency losses and degradation. Ion migration via point defects also contributes significantly to the total electrical conductivity of halide perovskites. Thus, defect chemical investigations are a key to understand and engineer the electrical properties of halide perovskites.
In this work, we present a setup to measure the electrical conductivity of the model halide perovskite Methylammonium Lead Iodide over a wide range of iodine partial pressures. We find the electrical conductivity to change with different slopes in dependence of the iodine partial pressure in a double-logarithmic representation. This indicates changes in the perovskite’s conduction mechanism, i.e., changes in point defect concentrations. Considering differences in the mobilities of the various defect species, we discuss the dependence of the total conductivity of the perovskite on the iodine partial pressure. Our work will allow to develop a more fundamental understanding about the electrical properties of halide perovskites.
1.2-T2
Photo-induced halide segregation is critical to the stability of multijunction photovoltaic-compatible mixed-halide (iodide-bromide) 3D perovskites. However, the effect is not well understood in mixed-halide lower-dimensional (2D or quasi-2D) perovskites whose superior environmental stability can improve long-term performance. In this work, we study phenethylammonium-based mixed-halide 2D (PEA2Pb(I1-xBrx)4) and quasi-2D (PEA2MAn-1Pbn(I1-xBrx)3n+1) perovskite thin films and characterize the slow occurrence of halide segregation under illumination and its reversal in the dark. Time-dependent photoluminescence spectroscopy shows that a system’s propensity to undergo halide demixing strongly depends on its structural nature. While a pure-2D (n=1) system is largely immune to the light-induced demixing of halides, other structural phases (n>1) comprised of conjoined lead halide octahedral sheets show a lower tolerance to such stressors and consequently form low-energy iodide-rich traps. In multi-dimensional (nominally n=4) thin film systems, the distribution of these phases (n=1, 2…∞), and consequently the stability, are shown to be regulated through solvent-engineering strategies. Differences in ion migration behaviour between structural phases also influences entropy-driven ionic remixing in the dark, which successfully restores the statistical mixed-halide composition in higher-dimensional phases but not in lower-dimensional analogs. These observations therefore establish perovskite dimensionality as a key determinant to the photo-stability of lower-dimensional perovskite optoelectronic devices.
1.2-T3
Loreta Angela Muscarella was born in Palermo, Italy. In 2012, she moved to Rome where she started a bachelor in chemistry at Sapienza - University of Rome. During her Master’s studies, she spent seven months at the University of Amsterdam (UvA) under the supervision of Dr. René Williams to write her thesis on the effect of metallic ions in mixed-halide perovskites to improve the stability and optoelectronic properties. She received her MSc degree in inorganic and physical chemistry cum laude (with honors). In 2018, Loreta joined the group of Prof. Dr. Bruno Ehrler at AMOLF as a PhD student. Here, she investigated the relation between structure and optoelectronic properties of 3D and layered 2D lead-halide perovskites by monitoring the optoelectronic properties of mechanically compressed perovskites. In 2022, she joined the group of Dr. Eline Hutter (Utrecht University) as a postdoc to study photochemistry processes using lead-free perovskites. Since January 2024, she is assistant professor at the Vrije Universiteit Amsterdam where her group will combine spectroscopy and compositional engineering of perovskite-based materials to investigate on the external stimuli response of the emerging perovskite-based materials.
Given the vast chemical space of layered hybrid perovskites, the systematic analysis of structure-property relationship in this type of materials is of high importance. Understanding the differences between Dion-Jacobson and Ruddlesden-Popper (RP) hybrid halide perovskites is limited. Thus, in this study we analyze compositions based on iodide or bromide for the halide and on phenylenedimethanammonium (PDMA) and benzylammonium (BN) as the spacers, which are structurally comparable. We use hydrostatic pressure as a tool to investigate the structure-property relationship. We probe optical, structural properties in the range of 0-0.35 GPa, which effects might be comparable to the processes occurring in the optoelectronic devices (polaron induced strain, lattice mismatch, chemical strain). At this pressure levels we observe optical bandgap reduction in all the compositions. The most significant shift (-54.9 meV) is recorded in (BN)2PbBr4 composition under 0.35 GPa pressure. Structural analysis reveals that lattice of all compositions monotonically shrinks but (BN)2PbBr4 experience an abrupt change in the lattice parameters lenght at 0.14 GPa. In addition, though the compression along all axes is comparable, there is consistent trend in all the systems showing slightly larger compression along the c axis with a more pronounced trend in the (BN)2PbBr4. Bulk modulus calculations reveal a much softer perovskite lattice in the latter composition, in agreement with the much larger shift of the excitonic peak observed as a function of pressure. Similarly, the other three compositions show a comparable bulk modulus as expected from the trend of the excitonic peak under pressure. Molecular dynamics provide additional insights into structure-property relationship. With this work we extend the knowledge about the layered hybrid perovskites based on aromatic spacers providing important insights into the mechanochromic properties of layered hybrid perovskites. Finally, the unique reversibility of their mechanochromic response in this mild pressure range opens new perspective towards the utility of layered hybrid perovskites as platforms for amphidynamic materials and mechanophores, which expands the perspectives for their future applications.
1.3-T1
Rhiannon Kennard investigates structure-property relationships in novel functional materials. She completed her PhD in the group of Prof. Michael Chabinyc at UC Santa Barbara, where she investigated strain, light emission, ferroic properties and ion mobility in 2D and 3D perovskites. Following this, she moved to the University of Sheffield, to work on novel cathodes for Na batteries with Prof. Serena Cussen
The structural tunability of perovskites has paved the way for applications such as solar cells and resistive switching memory. Low-dimensional hybrid perovskites can be combined with 3D hybrid perovskites to make more stable solar cells. Interestingly, some low-dimensional hybrid perovskites also exhibit ferroelectricity, or the formation of electrically-polarized domains, making such materials attractive for resistive switching memory. For optoelectronic device applications, we must understand the behavior of the charge carriers, which in low-dimensional perovskites, is very diverse: free excitons, self-trapped excitons, phonon replicas, etc. In addition, because devices are primarily made from thin films, we must establish how the structural and optoelectronic properties of low dimensional perovskites might change with growth method.
Here, we investigate growth of the ferroelectric perovskite (EA)4Pb3Br10. [1] We find that changing the growth method can be used funnel charge carriers into the free exciton or phonon-coupled states. Indeed, strains acquired from spin-coating turn off phonon-coupled emission. However, slower film growth methods preserve this phonon-coupled emission, and also increase domain size. Photothermal deflection spectroscopy shows that strain increases electronic disorder near the free exciton absorbance, which is undesired for solar cell applications. In addition, the challenge of making phase-pure films in low-dimensional Ruddlesden-Popper structure ((A’)2(A)n−1BnX3n+1) is overcome by using a single A/A’-site cation, ethylammonium (EA), whose optimal size also prohibits formation of off-target phases. This suggests that molecular design of the A/A’ sites might be used to favor phase purity in low-dimensional films. These results help extend the utility of 2D perovskites by providing design rules for how to grow films with the targeted phase and optoelectronic properties.
[1] Kennard, R.M., Dahlman, C.J., Chung, J., Cotts, B.L., Mikhailovsky, A.A, Mao, L., DeCrescent, R.A., Stone, K.H., Venkatesan, N.R., Mohtashami, Y., Assadi, S., Salleo, A., Schuller, J.A., Seshadri, R. and Chabinyc, M.L. Growth-Controlled Broad Emission in Phase-Pure Two-Dimensional Hybrid Perovskite Films. Accepted to Chemistry of Materials, 2021.
1.3-T2
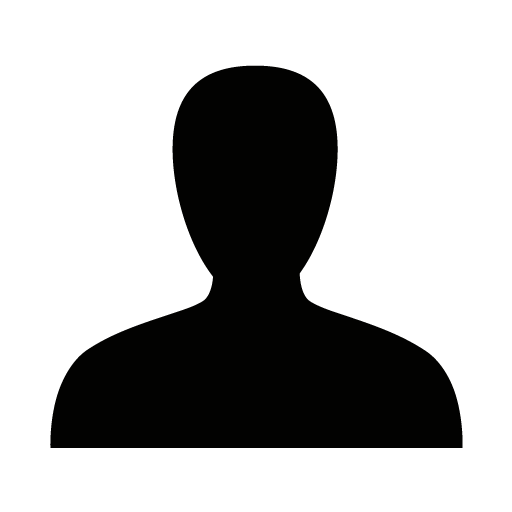
Ferroelastic twin domains are a crystallography related mechanism that affects the charge carrier Dynamics in methylammonium lead iodide (MAPI) crystals. In this work, we have found a chemical route to manipulate the ferroelastic twin domain structure in MAPI thin films by changing the Pb(Ac)2 / PbCl2 ratio in the perovskite precursor solution. The ferroelastic twin domain structure of each sample was monitored via piezoresponse force microscopy (PFM) and x-ray diffraction spectroscopy (XRD). Furthermore, we have shown that the changes in ferroelastic twin domains directly affects the charge carrier lifetimes via time-resolved photoluminescence (TRPL). Therefore, this chemical engineering route we have discovered offers a new way of strain engineering in perovskite research.
1.3-T3
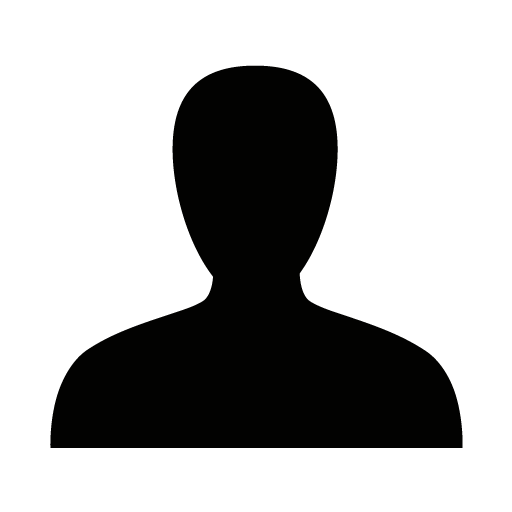
Along the past few years, the vacancy-ordered halide double perovskites (VOHDP) have been extensively investigated as promising candidates for various optoelectronic applications [1]. Among these compounds, Cs2TiBr6 and Cs2TiI6 are VOHDP that have been successfully synthesised, and are reported to be interesting photo-active materials with band-gaps well within the visible range (1.02-2.00 eV) and potentially good carrier transport properties [2–4]. Photovoltaic devices using Cs2TiBr6 achieved a 3.3% power-conversion efficiency [3], though their actual potential has been recently questioned [5]. In this work, we perform a thorough computational analysis of the electronic structure, the mechanical and chemical stability, and the optical properties, of the entire family of VOHDP that have the same B’-site electronic valence as Ti4+ (i.e., d10). By doing so, we probe the potential tuning limits of their opto-electronic properties, extend the existing understanding on the well-established Ti-based materials, and propose the less-known Zr-based compounds (i.e., Cs2ZrX6; X=Br, I) as potential prominent alternatives. In particular, we employ three levels of calculations: DFT-PBE, hybrid functionals, and state-of-the-art GW, to calculate their electronic structure, and perform a complete symmetry analysis of the electronic bands to reveal its consequences on the exhibited band-gaps and charge carrier effective masses. We also show the importance of spin-orbit coupling effects, highlight the limitations when calculating quasi-particle corrections, and demonstrate how a simple crystal field theory can be used to describe the observed electronic structure. Furthermore, we investigate all possible decomposition reaction pathways to competing compounds, and further assess their mechanical stability by means of phonon calculations. Overall, our findings unveil the fundamental electronic and optical properties of Cs2TiBr6, establish the stability and tunability limits of electronic band-gaps and effective mass within the family of VOHDP materials with a d10 electronic configuration, but most importantly propose a novel composition for the next generation of optoelectronic devices, namely Cs2ZrI6 that has been practically unexplored to date.
1.4-I1
Since 2019, Yana Vaynzof holds the Chair for Emerging Electronic Technologies at the Technical University of Dresden. Prior to that (2014-2019), she was a juniorprofessor in the Department of Physics and Astronomy, Heidelberg University (Germany). She received a B.Sc degree (summa cum laude) in electrical engineering from the Technion - Israel Institute of Technology (Israel) in 2006, and a M.Sc. degree in electrical engineering from Princeton University, (USA) in 2008. She pursued a Ph.D. degree in physics under the supervision of Prof. Sir. Richard Friend at the Optoelectronics Group, Cavendish Laboratory, University of Cambridge (UK), and investigated the development of hybrid polymer solar cells and the improvement of their efficiency and stability. Upon completing her PhD in 2011, she joined the Microelectronics group at the University of Cambridge as a Postdoctoral Research Associate focusing on the research of surfaces and interfaces in organic and hybrid optoelectronics. Yana Vaynzof was the recipient of a number of fellowships and awards, including the ERC Starting Grant, Gordon Y. Wu Fellowship, Henry Kressel Fellowship, Fulbright-Cottrell Award and the Walter Kalkhof-Rose Memorial Prize.
Despite remarkable progress, the performance of lead halide perovskite solar cells fabricated in an inverted structure lags behind that of standard architecture devices. In this talk, I will present several strategies for increasing the performance of inverted architecture devices. First, I will describe a general approach to the fabrication of efficient perovskite solar cells from any antisolvent. Next, I will show that the performance of inverted architecture solar cells can be enhanced by modifying the method of antisolvent deposition. Finally, I will present a novel strategy for modifying both the bottom and top interfaces in inverted architecture perovskite solar cells that leads to a simultaneous enhancement in all the photovoltaic parameters. Using this strategy results in a champion device with an efficiency of 23.7%.
1.4-I2
Sam Stranks is Professor of Energy Materials Optoelectronics in the Department of Chemical Engineering & Biotechnology and the Cavendish Laboratory, University of Cambridge. He obtained his DPhil (PhD) from the University of Oxford in 2012. From 2012-2014, he was a Junior Research Fellow at Worcester College Oxford and from 2014-2016 a Marie Curie Fellow at the Massachusetts Institute of Technology. He established his research group in 2017, with a focus on the optical and electronic properties of emerging semiconductors for low-cost electronics applications.
Sam received the 2016 IUPAP Young Scientist in Semiconductor Physics Prize, the 2017 Early Career Prize from the European Physical Society, the 2018 Henry Moseley Award and Medal from the Institute of Physics, the 2019 Marlow Award from the Royal Society of Chemistry, the 2021 IEEE Stuart Wenham Award and the 2021 Philip Leverhulme Prize in Physics. Sam is also a co-founder of Swift Solar, a startup developing lightweight perovskite PV panels, and an Associate Editor at Science Advances.
Halide perovskite semiconductors are extremely promising materials for next-generation photovoltaics and other devices. However, even in spite of their strong performance in devices, these materials exhibit heterogeneity in their chemical, structural, optoelectronic and morphological properties on multiple length scales -- from the macroscale down to the nanoscale. Here, I will present our group's recent work exploring these different length scales of heterogeneity, and how the heterogeneity impacts performance. In particular, I will present multimodal microscopy measurements in which we simultaneously correlate local strucural and chemical properties with local performance. We identify that local, nanoscale trap clusters are responsible for non-radiative power losses but also are the sites that seed degradation -- and these clusters should be the target of elimination to improve device performance and longevity. We show how the local chemical heterogeneity can provide pathways for carriers to avoid these problematic trap clusters, explaining the strong performance of alloyed perovskite compositions. These results highlight the large impact the nanoscale landscape has on performance and device stability -- and, although far from benign, offers a lever to further control device behaviour.
1.4-I3
The carrier transportation in perovskites materials is complicated by the presence of mobile ions, and the charges also affect ion migration. Defects in perovskites play a major role in changing the dynamics process of ion conduction, while light can change ion migration which in return introduce defects in perovskites. I will present our recent progress how defects of different type affect the ion migration as well as carrier transport in metal halide perovskites, including both three dimensional perovskites and low dimensional ones. The application of ion migration in perovskites for synapse devices will be briefed. Several methods of removing surface defects will be presented to alleviate the issues induced by ion migration for more stable perovskite solar cells.
2.1-T1
Relatively large organic cations, which on their own cannot stabilize the 3D corner-sharing perovskite framework, can be incorporated in perovskites by appropriate mixing with smaller 3D perovskite-forming cations. Here we utilize the solvent acidolysis crystallization (SAC) technique to grow mixed dimethylammonium/methylammonium (DMA/MAPbBr3) lead tribromide crystals with the highest, as of yet, dimethylammonium (DMA) incorporation of 44% while maintaining the 3D cubic phase. We then employ different temperature-dependent measurements to explore the implications of such mixed organic cations composition on the structural and optoelectronic properties at low temperatures. We confirm the suppression of the orthorhombic phase upon the incorporation of DMA and a lower tetragonal-cubic phase transition temperature. The anomalous enhancement in the integrated PL observed in the two crystals is due to the different organic cation dynamics. By fabricating charge extraction layer-free photodetectors, we observed that the mixed crystal devices showed higher detectivity and responsivity compared to MAPbBr3 crystals due to the observed structural compression and reduced surface trap density in the mixed crystals. Fascinatingly, the mixed crystal photodetectors, at 200 K, exhibit a large improvement in their characteristics compared to room temperature, while MAPbBr3 crystals demonstrate much lesser enhancement due to their higher degree of distortion at lower temperatures.
2.1-T2
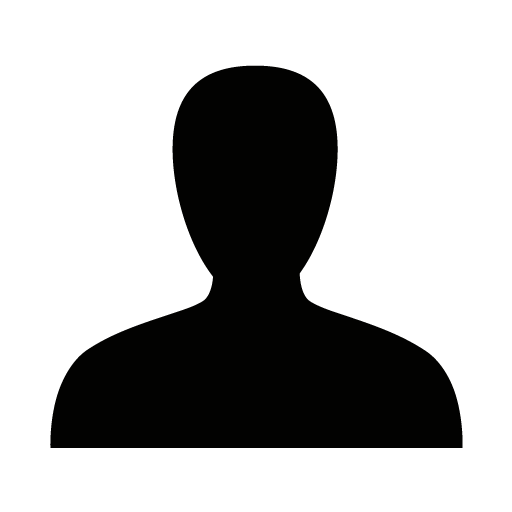
Organic-inorganic halide perovskites (OIHPs) has emerged as the most promising light-absorber materials in the photovoltaic community due to their near-ideal bandgaps. However, the low formation energies of OIHPs render them unstable. While significant progress has been made in improving the stability of OIHPs, perovskite solar cells (PSCs) will also need to be mechanically reliable if they are to service satisfactorily for decades. In this context, we study the fracture behavior of PSCs by measuring their cohesion energies (Gc) using double cantilever beam method and report a novel approach to strategically enhance the interfacial adhesion and performance of PSCs using self-assembled monolayers (SAMs), where we find that the perovskite solar cell stability is closely intertwined with its mechanical reliability. This work points to a new route for designing mechanically robust PSCs with long-term durability.
2.1-T3
“I’m a grad student at the Indian Institute of Technology Madras. Here, I am conducting fundamental research on Perovskites & Metal-Organic Frameworks (MOFs) materials for energy conversion.
Halide double perovskites (A2B’B”X6) have recently shown broadband emission in a single component and attracted various light-emitting device applications. Generally, in these double perovskites, valance and conduction bands are predominately obtained by mono (B’), trivalent (B”) cations, and halogen(X). In this work, we carried out systematically band engineering by distorted octahedra by substituted with mono (Na+), trivalent (In3+) cations, and halide anion (Cl-) and explored their structural, optical properties behavior of the cubic double perovskites Cs2AgBiBr6. In the undoped parent compound, only very weak photoluminescence is observed, but in substitution of Na, In, Cl samples broadband emission peak centered at around 620nm. In addition to this, the substitution of In3+ (25%) and Cl- (100%) showed increased lifetime compared to host material, and comparison of excitation and absorption spectra indicates that all the doped and undoped materials have shown indirect bandgap materials.
2.2-T1
I have a background in Electronics and Communication Engineering. I recently completed M.S. by Research in Energy Science and Engineering from Indian Institute of Technology-Bombay. Currently, I am pursuing Ph.D. at Karlsruhe Institute of Technology. My area of focus is in the 'Light and elevated temperature induced degradation of perovskite solar cells'.
Spectral conversion tailors the incident solar spectrum such that it is better suited for particular photovoltaic absorber material; in our work, an organometal halide perovskite semiconductor. In double cation perovskite solar cells (bandgap 1.57eV), 41% of the incident spectrum is wasted by sub-bandgap losses. In order to reduce these losses in the future, we research up-conversion (UC) crystal BaF2: Yb3+, Er3+ for the annihilation of two low-energy photons to form a high-energy photon. The low phonon energy of BaF2 crystal (240 cm−1) results in reduced non-radiative losses.1 Hence, BaF2: Yb3+, Er3+ UC crystal exhibits a high UC quantum yield of ~10%, comparable to the best available fluoride materials. When tested under terrestrial sunlight representing one sun above the perovskite’s bandgap and sub-bandgap illumination at 980 nm (via a laser), the BaF2: Yb3+, Er3+ crystal emits usable upconverted photons in the spectral range of 520 to 700 nm. In our bifacial PSC with the UC crystal beneath, these upconverted photons contribute to 0.38 mA/cm2 enhancement in short-circuit current density at a laser intensity equivalent to 120 suns. We demonstrate that UC scales non-linearly with incident intensity, with an intensity equivalent to 880 suns resulting in a 2.09 mA/cm2 enhancement in current. Our study validates that UC is a potentially viable process to extend the response of PSC to a wider spectral range.
2.2-T2
Lead-free hybrid perovskites have emerged as an attractive green and efficient alternative to be used in solar cells. In this work, we have prepared bismuth-based hybrid perovskites and studied their dehydration-hydration process. The BzImH[BiI]4·H2O structure (IEF-4 RT-phase) consists in one-dimensional iodobismuthates and the benzimidazolium cation (BzlmH)+ containing one hydration water molecule per formula unit [1]. The transformation from IEF-4 RT-phase to the dehydrated HT-phase (BzImH[BiI]4) occurs at around 100 °C, being a fully reversible process. Besides, stable and homogenous IEF-4 films have been successfully obtained by spin-coating method exhibiting an excellent absorption coefficient (1.5·105 cm-1), similar to that reported for lead perovskite materials. Both their good stability under working conditions (temperature and humidity) and excellent optical properties make them suitable for its use in optoelectronic devices. In addition, we have found a reversible dehydration-hydration process in other Bi-based perovskites.
Referencias
[1] A. A. Babaryk, Y. Pérez, M. Martínez, M. E. G. Mosquera, M. H. Zehender, S. A. Svatek, E. Antolín, P. Horcajada. J. Mater. Chem. C 2021, 9, 11358.
2.2-T3
Lead-halide perovskite (LHP) nanocrystals (NCs) have been broadly studied over the past few years as active material for several optoelectronic devices from LEDs to solar cells. Alternative approaches to colloidal synthesis have been actively explored where the absence of ligands can improve charge injection/transport without compromising material stability.
Among them, the direct synthesis of LHC NCs within metaloxide nanoporous matrices [1] has demonstrated to be a route with potential to grow stable NCs with good optoelectronic properties amenable to be incorporated into devices. [2]
In this work we use ligand-free MAPbBr3 NCs grown within SiO2 nanoporous matrices as a test-ground to study the interaction of light with LHP at the nanoscale in the presence of different atmospheres. The optical response of our system is fitted with a model that points to the simultaneous presence of light-induced activation/degradation processes strongly affected by the surrounding atmosphere. [3] The reversibility of both processes and their prevalence at the bulk/surface of the NCs is also discussed.
2.3-I1
Annamaria Petrozza received her PhD in Physics from the University of Cambridge (UK) in 2008 with a thesis on the study of optoelectronic processes at organic and hybrid semiconductors interfaces under the supervision of Dr. J.S. Kim and Prof Sir R.H. Friend. From July 2008 to December 2009 she worked as research scientist at the Sharp Laboratories of Europe, Ltd on the development of new market competitive solar cell technologies (Dye Sensitized Solar cells/Colloidal Quantum Dots Sensitized Solar cells). Since January 2010 she has a Team Leader position at the Center for Nano Science and Technology -IIT@POLIMI. She is in charge of the development of photovoltaic devices and their characterization by time-resolved and cw Photoinduced Absorption Spectroscopy, Time-resolved Photoluminescence and electrical measurements. Her research work mainly aims to shed light on interfacial optoelectronic mechanisms, which are fundamental for the optimization of operational processes, with the goal of improving device efficiency and stability.
Metal halide perovskites (MHPs) have demonstrated huge potential to build a rich library of materials for a new disruptive optoelectronic technology. The main strength comes from the possibility of easily tune the semiconductor bandgap in order to integrate it in devices with different functionalities – in principle. In reality, this cannot be achieved yet. In fact, while defect tolerance is claimed for MHPs with a bandgap of about 1.6 eV, the model system object of intense investigations, MHPs with lower and higher bandgaps are far from this claim. They show various forms of instabilities which are mainly driven by a strong defect activity.
Here I will discuss our studies on the nature of defects and their photo-chemistry as a function of the semiconductor bandgap and chemical composition in order to identify their role in defining the semiconductor electronic properties and stability.
2.3-I2
Organic-inorganic halide perovskites are record-breaking materials with a wide range of applications spanning photovoltaics, lighting, and detectors. These hybrid materials combine key properties of traditional inorganic semiconductors such as high carrier mobilities, with properties of organic semiconductors such as facile and low-cost synthesis. Single perovskites with stoichiometry ABX3 like the prototypical solar cell absorber CH3NH3PbI3 (MAPI) have excellent optoelectronic properties, but are hampered by instability, the toxicity of lead and scarcity of iodine. Furthermore, the ABX3 structural motif limits functionalization because only very few organic molecules fit into the A site cavity. New hybrid materials with alternative perovskite-like structural motifs vastly extend the chemical and structural diversity of this family of materials resulting in unusual and widely tuneable optoelectronic properties.
In this contribution I will demonstrate that it is the chemical and structural heterogeneity of such hybrid materials that determines their electronic and excited state properties. I will showcase first principles calculations - based on density functional theory and many-body perturbation theory - of several perovskite-type materials with optoelectronic properties significantly deviating from those of MAPI. Our calculations allow us to explore the atomistic origin of the unusual excited state properties of these materials and suggest routes for tuning them for tailored applications.
2.3-I3
A number of empirical additive and surface passivation treatments have been reported to improve the performance and stability of halide perovskite semiconductors. However, our mechanistic understanding of how these treatments affect the perovskite structures if often lagging, in part due to the wide range of perovskite formulations and growth conditions in use. We explore the effects of difference treatments on several different perovskite formulations, from the role of large A-site cations, to Lewis bases, and protonated forms of basic species. Using hyperspectral photoluminescence and nano-infrared microscopy, we show that compositional heterogeneity is ubiquitous in many perovskite formulations and that this compositional heterogeneity is in turn influenced by multiple surface treatment routes. Ultimately, we demonstrate surface recombination velocities < 10 cm/s and show that we can preserve high quality interfaces even in contact with electron and hole extraction layers.
2.4-I1
Cs2AgBiBr6 (CABB) has been proposed as a less toxic and more stable alternative to lead halide perovskites, and was successfully implemented as photoactive layer in optoelectronic devices. However, power conversion efficiencies of CABB-based devices remain much lower than compared to their lead-based analogues, suggesting poor charge transport. On the other hand, microsecond lifetimes have been reported for CABB. In this talk, I will elaborate on the dynamics of charge transport, localization and recombination in CABB thin films, studied using time-resolved photoconductivity and transient absorption/reflectance measurements. On comparing the temperature-dependent photoconductivity measurements of CABB with lead-based perovskites, we find a similar band-like charge transport mechanism.1 We also find that replacing Bi with Sb shifts the absorption onset, while preserving the charge transport mechanism. In contrast to state-of-the-art lead-based perovskites however, charges in CABB lose mobility within tens of nanoseconds, so that diffusion lengths remain relatively short (~100 nm). Using microsecond transient absorption measurements, we observe that these non-mobile charges have a lifetime of several microseconds, suggesting a long-lived localized state. In contrast with the nanosecond-lifetime observed for mobile charges, we observe microsecond-long lifetimes in transient absorption measurements. The observation that the diffusion length is similar to the grain size suggests that charge localization occurs at the surfaces. Therefore, improving surface quality could be a strategy to optimize performance of CABB-based optoelectronic devices.
Finally, we performed a toxicity study of methylammonium lead iodide perovskites on A. Thaliana plants, and found that the iodide was actually much more toxic than the lead. Altogether, these results stress the importance of further understanding which perovskites are most harmful to the environment, while optimizing the optoelectronic quality of materials with the lowest toxicity.
2.4-I2
Halide perovskites offer a flexible platform for tuning optoelectronic properties through changes in composition, connectivity, and dimensionality. In prior work, we have explored the dramatic consequences of stoichiometric and substoichiometric substitutions at the octahedral metal site in halide double perovskites. Our work on halide double perovskites has prompted us to explore still more complex perovskites. I will present our recent studies on further increasing the accessible electronic structures of this versatile family of materials by synthesizing mixed-metal perovskites with three different octahedral metal sites and on combining inorganic sublattices of different dimensionality in a single material. These new halide perovskites show unprecedented crystal structures and unusual electronic structures.
1.1-I1
Dr. Yadong XU received his PhD in School of Materials Science & Engineering, Northwestern Polytechnical University in 2010 and is currently a Professor in State Key Laboratory of Solidification Processing and Key Laboratory of Radiation Detection Materials and Devices, Northwestern Polytechnical University, China. Dr. XU has received many prestigious awards including “Second-class of National Technological Invention”, P.R. China, 2013, “First- class of Scientific and Technical Awards”, Shaanxi Province,2012, “Youth outstanding talent support program" in Shaanxi, China, (2017), Excellent Talents project in Shaanxi Province, China, 2016. His research interests cover development of new semiconductor materials for X/γ-ray detectors, growth of electro-optical crystals for THz application, optical and electrical properties of the semiconductor materials and defect engineering. Dr XU has published more than 70 SCI papers and documented 16 patents.
Here, we report the progress of detector-grade metal halide perovskite and device fabrication for X-ray and gamma-ray detection. For the 3D perovskite, the all-inorganic perovskite CsPbBr3 grown by either solution or melt method demonstrates an energy resolution of ~7% for 5.49 MeV 241Am α particles, while exhibits a good peak discrimination for 59.5 keV 241Am γ-rays. In terms of organic-inorganic hybrid perovskite, solution grown FAPbBr3 resolves 59.5 keV 241Am γ-rays with an energy resolution of 40.1% at room temperature for the first time. To further improve the perovskite stability resulted from the ion migration, dimensional reduction engineering was employed on the 3D perovskite. The 2D hybrid perovskite by incorporating large organic cations into CsPbBr3, the resulting (BDA)CsPb2Br7 detector shows a capability for detecting 5.49 MeV 241Am α-particles with an energy resolution of 37%, while possesses high X-ray sensitivity up to 725.5 μC∙Gy-1∙cm-2 with excellent working stability. Furthermore, we also demonstrate a potential candidate the 0-D perovskite material Cs2TeI6 grown by electrostatic assisted spray (E-spray) deposition, as a sensitive all-inorganic X-ray photoconductor for direct photon-to-current conversion X-Ray detectors. The electrospray apparatus can be readily automated and fully integrated with the existing display systems based on TFT or CMOS, which will help to implement and scale up this device for manufacturing next generation of flat panel X-ray imagers.
1.1-I2
Metal halide perovskites have fascinated the research community over the past decade, and demonstrated unprecedented success in the field of photovoltaics, light emitting devices and photodetection. In particular, perovskites have emerged as excellent candidates to detect ionizing radiation, mainly including direct detection and indirect devices with an additional scintillation process. Compared with visible photons, ionizing radiations have higher energy and long penetration depth, and require much thicker absorbing layers, i.e. a few hundreds of micrometers. Hence, we have to fabricate thick junction devices to effectively absorb X-ray or g-ray. On the other hand, charge transport and recombination losses are another issue for direct detection of ionizing radiations, limited by the moderate mobility-lifetime product. In this talk, I mainly introduce three strategies to prepare thick-junctions, which are based on perovskite /polymer composite films, perovksite single crystals and co-evaporated perovskite thick films. The balance between absorption and charge transport will be discussed, which is also correlated with the device performance, e.g., dark and noise current, photogeneration, response time and linear dynamic range.
1.2-T1
Advances in medical imaging relay equally on enhancements in computing power required by the increasing demand of machine learning and further material and technology improvements which will lead to better image quality and medical outcomes. Hybrid inorganic-organic perovskite materials like methylammonium lead triiodide (MAPbI3) promise direct conversion detectors with higher image quality, owing to their strong X-ray absorption, high electron and hole diffusion lengths with high charge carrier mobility and long carrier lifetime. However, their incorporation into pixelated sensing arrays remains challenging. Here we show a novel manufacturing process that decouples the fabrication of the several micrometre thick absorber layer and its integration onto an amorphous Silicon transistor backplane. Since no consideration of the limitations of the backplane electronics is necessary, the options for the production parameters of the wafers are not restricted. We chose a so-called mechanical soft-sintering approach for the fabrication of the 230 µm thick and freestanding MAPbI3 wafer. A photoresist grid functions as a mechanical anchor and recrystallized MAPbI3 acts as an adhesion promoter for the soft-sintered absorber layer. The resulting X-ray imaging detector with 508 pixels per inch combines a low detection limit of 0.22 nGyair frame-1, a sensitivity of 1060 µCGyair-1cm-2 with a high spatial resolution of 6 line-pairs mm-1. In conclusion, polycrystalline MAPbI3 X-ray imaging detectors provide a very high potential to stable and excellent detection over the whole energy range of X-ray application. Our fabrication technology offers the possibility of simply scale-up to large detector areas.
1.2-T2
Dr Juvet Nche Fru is a Post-doctoral Fellow and a Teaching Assistant in the Department of Physics at the University of Pretoria, South Africa.
Juvet's research focuses on the physical vapor deposition of 2D and 3D halide perovskites materials for solar cells. He is a member of the South African Institute of Physics and participated at the nanoGe International Conference on Perovskite Solar Cells, Photonics and Optoelectronics (NIPHO20) from 23rd to 25th of February 2020.
He received a PhD in Physics from the University of Pretoria in May 2021. The subject of his thesis was "Optimisation of halide perovskite thin films sequential physical vapour deposition for solar cell applications". He authored four articles in reputable international journals from his thesis.
Prior to his doctoral studies in physics, he obtained an MSc in Materials Science and Engineering from the African University of Science and Technology (AUST), Abuja, Nigeria. Juvet received an award, in the form of a medal, as the overall best graduating student from from AUST in 2016.
He is a highly motivated early career scientist with exceptional ability to solve problems under challenging circumstances and meeting deadlines. Excellent verbal and written research communicational skills to a variety of audiences. Expertize in vapor and solution deposition of thin films. Eager to learn from superiors and colleagues, while paying close attention to details. High emotional maturity with excellent interpersonal and organizational skills. Diligent teacher and researcher, with 4+ years of experience contributing to developing state-of-the-art halide perovskite materials for light absorption in the fastest emerging solar cell technology. Interested in building a career in research and teaching.
Our sequential physical vapor deposited methylammonium lead tri-iodide (MAPbI3) thin films and solar cells have shown remarkable resilience (hardness) under high energy (5.5 MeV) alpha radiation beams from americium 241 (241Am). Analyses of the structural, optical, morphological, and photoelectric properties of the perovskite thin films and solar cells with and without irradiation with alpha particles (He2+) were performed. An increase in crystallinity and a decrease in compression micro-strain of tetragonal MAPbI3 with an increased flux of He2+ were observed after XRD analysis. In the UV-Vis absorption spectrum, there was little change in absorbance up to a flux of 7.36 x 1012 He2+ cm-2. The decrease in bandgap for the irradiated films paralleled the non-irradiated up to a fluence of 3.07 x 1012 He2+cm-2, after that, there was a large difference. Photographs taken with a digital camera showed visible changes on the thin irradiated films at fluence levels exceeding 3.07 x 1012 He2+ cm-2 and electron micrographs confirmed that the changes are a result of pits forming in grains. The performances of irradiated and non-irradiated hole transport layer-free solar cells were monitored for the same period. A reduction in power conversion efficiency (PCE) from 6.56% to 6.42%, which is not significant, was observed up to a radiation dose of 1.23 x 1012 He2+ cm-2, thereafter, a substantial drop from 6.42% to 4.75% as the flux increased to 7.36 x 1012 He2+ cm-2. In contrast, the non-irradiated solar cell experienced a reduction in PCE from 6.39% to 6.23% within 48 hours, and as the time interval increased to 288 hours, there was a gradual decline from 6.23% to 5.70%. Based on what we found, MAPbI3 thin films can withstand He2+doses up to 3.07 x 1012 He2+ cm-2, and MAPbI3 solar cells can withstand doses up to 1.23 x 1012 He2+ cm-2 in space and He2+ environments.
Keywords: Methylammonium lead tri-iodide perovskite, sequential physical vapor deposition, He2+irradiation, perovskite solar cells, remarkable resilience, space environment, and He2+ environments.
1.2-T3
Metal halide perovskite materials are being explored as functional materials for a variety of optoelectronic applications but a general uncertainty exists about the relevant mechanisms governing the electronic operation. The presence of mobile ions and how these species alter the internal electrical field and interact with the contact materials or modulate electronic properties is still a challenging subject. In order to understand their working mechanisms, here, ionic current and electronic impedance in two different perovskite-based devices were independently monitored, showing self-consistent patterns. Firstly, analyzing the bias and time dependence of bulk resistance informs about the accumulation and relaxation dynamics of the moving ionic species in high-quality thick MAPbBr3 single crystals. Secondly, the change of the electronic doping profile within the bulk, determined by the ion inner distribution, induced a time dependence in the electronic conductivity and reproduces time patterns of the type ∝ t1/2, a clear fingerprint of diffusive transport in MAPI microcrystalline-pellets. Our findings point to a coupling of ionic and electronic properties as a dynamic doping effect caused by moving ions that act as mobile dopants. In conclusion, this research provides a connection between ionic and electronic properties that allow us progressing into the halide perovskite device physics and operating modes.
1.3-I1
Ionizing radiation, wherever present, e.g., in medicine, nuclear environment, or homeland security, due to its strong impact on biological matter, should be closely monitored. Availability of semiconductor materials with distinctive characteristics required for an efficient high-energy photon detection, especially with high atomic numbers (high Z), in sufficiently large, single-crystalline forms, which would also be both chemically and mechanically robust, is still very limited.
Metal halide perovskite were indeed found to meet all aforementioned key requirements, at an extremely low cost. In this work γ-ray detectors based on crystals of methylammonium lead tribromide (MAPbBr3) equipped with carbon electrodes were fabricated, allowing radiation detection by photocurrent measurements at room temperatures with record sensitivities (333.8 μC Gy-1 cm-2 ). Importantly, the devices operated at low bias voltages (<1.0 V), which may enable future low-power operation in energy-sparse environments, including space. The detector prototypes were exposed to radiation from a 60Co source at dose rates up to 2.3 Gy h-1 under ambient and operational conditions for over 100 h, without any sign of degradation. We postulate that the excellent radiation tolerance stems from the intrinsic structural plasticity of the organic-inorganic halide perovskites, which can be attributed to a defect-healing process by fast ion migration at the nanoscale level.
Furthermore, since the sensitivity of the γ-ray detectors is proportional to the volume of the employed MAPbBr3 crystals, a unique crystal growth technique is introduced, baptized as the “oriented crystal-crystal intergrowth” or OC2G method, yielding crystal specimens with volume and mass of over 1000 cm3 and 3.8 kg, respectively. Large-volume specimens have a clear advantage for radiation detection; however, the demonstrated kilogram-scale crystallogenesis coupled with future cutting and slicing technologies may have additional benefits, for instance, enable the development for the first time of crystalline perovskite wafers, which may challenge the status quo of present and future performance limitations in all optoelectronic applications.
1.3-I2
Perovskite solar cells and sensors. Defects in semiconduciors and charge recombiantion.
Here I will give insight into the uniqueness and challenges of charge transport in halide perovskites. Despite the tremendous efforts in the study of their electric properties, the free carriers - defects interactions and some critical defect properties are still unclear in methylammonium lead halide perovskites (MHPs). Here we use a multi-method approach to quantify and characterize defects in single crystal MAPbI3 giving a cross-checked overview of their properties. Time of Flight current waveform spectroscopy (ToF CWF) reveals the interaction of carriers with five shallow and deep defects. Photo Hall (PHES) and Thermoelectric effect spectroscopy (TEES) assess the defect density, cross-section, and relative (to the valence band) energy. The detailed reconstruction of free carrier relaxation through Monte Carlo (MC) simulation allow quantifying the lifetime, mobility, and diffusion length of hole and electron separately. We demonstrate that the dominant part of defects releases free carriers after trapping; this happens without non-radiative recombination with consequent positive effects on the photoconversion and charge transport properties. On the other hand, shallow traps decrease the drift mobility sensibly. Our results provide a trustworthy picture for future consideration on the defect properties in MAPbI3 thanks to the verification of our statements with multiple methods. Our results will be key for the optimization of the charge transport properties and defects in MHP and will contribute to the research aiming to improve their stability[1].
1.3-I3
Dr. Ge Yang is an Associate Professor at the Department of Nuclear Engineering of North Carolina State University (NCSU). His research interests have revolved around the opportunities at the intersection of nuclear engineering, materials science and engineering and electrical engineering. Special emphasis is placed on developing new materials and devices for improving radiation detection and imaging technologies, which are widely needed in medical imaging, nonproliferation, nuclear security, industrial process monitoring, environmental safety survey and remediation, astronomical observation instrumentation and high energy physics R&D. Dr. Yang’s research has yielded 7 patent disclosures, 151 publications in top-ranked scientific journals and conference proceedings, and numerous invited presentations at various professional conferences. He is a two-time recipient of the prestigious R&D 100 Award together with his collaborators for developing various compact sensors to detect and image radiation. Dr. Ge Yang is also the inaugural recipient of Goodnight Early Career Innovators Award.
Sensitive detection of X-rays, gamma rays, and energetic particles plays a key role in medical imaging, nonproliferation, homeland security, environmental monitoring, and fundamental scientific research. In this presentation, we will summarize the latest progress in the development of perovskite single crystal detectors and report our efforts in developing double perovskites for improved radiation detection. Detailed material characterization and detector tests will be presented and discussed. Examples include Cs2AgBiBr6 single crystals with different stoichiometry. These crystals have some desired characteristics, such as suitable bandgap energy, low density of trap states, low dark carrier concentration, and high mu-tau (μ-τ) product, for ionizing radiation detection applications. The measurement results show that perovskite crystals could serve as an emerging class of radiation detector materials for ionizing radiation detection.
1.1-I1
Utilizing solar energy and achieving artificial photosynthesis are vital process to building a sustainable society and an effective way to achieve the goal of carbon neutrality. This report presents our research progress in the construction of bias-free water splitting systems and the optimization of CO2 reduction systems. We have proposed a strategy to spatially decouple the light absorption site from the surface reaction site and developed a method to regulate the bending degree of the energy band, thus constructing an efficient charier transport channel to achieve efficient conversion of solar energy to hydrogen energy. In addition, we have combined theoretical calculations and in-situ spectroscopic techniques to clarify the relationship between active site structures and CO2 conversion pathways, leading to the development of a series of site-controlled construction methods. By introducing the basic roles of hydrodynamics into the design of reaction systems, we have elucidated the matching principle between solar energy and electrolyzer power. Through modulating the temperature, velocity and concentration fields in the CO2 reduction system, we have initially achieved the stable production of multi-carbon products under scaling-up conditions.
1.1-I2
The electrochemical reduction of CO2 to value-added products (e.g., CO, HCOO−)1 is increasingly regarded as an appealing strategy to valorize CO2 while decreasing its emissions. Driven by the sluggish kinetics of the CO2-reduction reaction (CO2RR), an ever-increasing body of work is being devoted to the development of new CO2RR- electrocatalysts.2 However, such studies are preponderantly performed in liquid electrolytes that suffer from a limited CO2-solubility (≈ 30 mM) that restricts the attainable currents to values well below the > 200 mA·cm−2 relevant for industrial applications. Those high currents can be attained in so-called co-electrolysis cells in which the CO2 is supplied as a humidified gas, and gas diffusion electrodes and ion-exchange membrane electrolytes are implemented to minimize mass-transport and ohmic losses, respectively.3 However, the development of such co-electrolyzers remains at an early stage in which elementary aspects like the choice of ion exchange membrane remain unclear. In this regard, the proton-exchange membranes used in polymer electrolyte water electrolyzers are not applicable, since they entail an acidic reaction environment (pH ≈ 0) that would favor H2-evolution over the CO2RR. Alternatively, recent studies have implemented anion exchange membranes (AEMs) featuring promising performances,4,5 but in which the (bi)carbonate formed at the cathode is transported to the anode and oxidized back to carbon dioxide, lowering the net CO2-utilization.6,7
This contribution will summarize our efforts from catalysts, component and cell development including a techno-economic analysis of technical scale-up feasibility.
[1] J. Durst, A. Rudnev, A. Dutta, Y. Fu, J. Herranz, V. Kaliginedi, A. Kuzume, A. A. Permyakova, Yohan Paratcha, P. Broekmann, and Thomas J. Schmidt, Chimia, 69, 769 (2015).
[2] J. Herranz, J. Durst, E. Fabbri, A. Patru, X. Cheng, A. A. Permyakova, and T. J.Schmidt, Nano Energy, 29, 4 (2016).
[3] J. Herranz, A. Patru, E. Fabbri, and T. J. Schmidt, Curr. Opin. Electrochem., 23, 89 (2020).
[4] K. Wu, E. Birgersson, B. Kim, and P. J. A. Kenis, J. Electrochem. Soc., 162, F23 (2014).
[5] J. J. Kaczur, H. Yang, Z. Liu, S. D. Sajjad, and R. I. Masel, Frontiers Chem., 6, 263 (2018)
1.1-I3
Continuous-flow electrolyzers allow CO2 reduction at industrially relevant rates, but long-term operation is still challenging. In this talk, I am going to present some interesting findings on the role of different ions crossing the anion exchange membrane in zero-gap electrolyzer cells, contributing to unstable operation.
In the first part of my talk, I will show that while precipitate formation in the cathode gas diffusion electrode is detrimental for the long-term stability, the presence of alkali metal cations at the cathode improves performance. To overcome this contradiction, we develop an operando activation and regeneration process, where the cathode of a zero-gap electrolyzer cell is periodically infused with alkali cation-containing solutions. [1] This enables deionized water-fed electrolyzers to operate at a CO2 reduction rate matching that of those using alkaline electrolytes (CO partial current density of 420 ± 50 mA cm−2 for over 200 hours). We deconvolute the complex effects of activation and validate the concept with five different electrolytes and three different commercial membranes. Finally, we demonstrate the scalability of this approach on a multi-cell electrolyzer stack, with a 100 cm2 / cell active area.
In the second part of the presentation, I will discuss the role of anode catalyst in CO2R cells. The urge to substitute Ir is driven by its high- and steeply rising market price as well as its limited stability in alkaline media. Although Ni is a ~ten thousand times cheaper, active, and stable oxygen evolution reaction (OER) catalyst in alkaline media, I will demonstrate that there are factors, which hinder its application in CO2 electrolysis. While Ni is a suitable OER catalyst in short experiments, the cell voltage increases, and the measured total Faradaic efficiency decreases continuously during prolonged electrolysis. This is caused by the local acidic pH at the anode surface, the crossing CO32- ions and by the gradual change in the anolyte composition, leading to Ni dissolution. The catalyst loss is only a minor part of the problem; the dissolving metal ions also penetrate into the anion exchange membrane, where precipitate forms due to the high local carbonate ion concentration, inducing cell failure.
1.2-T1
Federico Dattila is an Italian physicist interested in renewable energies and environmental sustainability. Though having a humanistic high school background, he got a Bachelor of Science (2015) and a Master of Science (2017) degree in Physics at University of Turin (Italy). The master thesis project was developed in collaboration with Chalmers University of Technology through the ERASMUS programme (Gothenburg, Sweden, spring 2017). In addition, he actively promoted sustainability in his university through the foundation of greenTO, a student association (2016). He is currently an Early Stage Researcher (ESR) within the ELCoREL Innovative Training Network at the Institute of Chemical Research of Catalonia (ICIQ, Tarragona, Spain) under the supervision of Prof. Núria López.
Electrochemical CO2 reduction (eCO2R) is a promising process to store renewable energy into chemical bonds and close the carbon cycle. However, to date the industrial exploitation of this technology is limited to CO and HCOO–, whilst production of desirable C2+ chemicals is possible only at laboratory scale and on copper-based catalysts. Dynamic phenomena, such as surface reconstruction during operation [1] or specific electrolyte effects,[2] can promote selectivity toward multi-carbon compounds and long-term stability of the device, thus calling for computational modeling to unveil this complexity.
Here, by means of ab initio molecular dynamics (AIMD) simulations, we modeled surface reconstruction on an oxide-derived copper material,[3] and we confirmed experimental observations on the crucial role of metal cations in electrochemical CO2 reduction.[4]
In the first study, we identified the main ensembles which control the catalytic performance of seven oxygen-depleted oxide-derived copper models. Generally, copper differentiates in three classes: metallic Cu0, polarized Cuð+, and oxidic Cu+, respectively coordinated to 0, 1, and 2 oxygens. These three species form 14 ensembles. Low coordinated Cu adatoms and polarized sites are responsible for binding CO2 and thus improving eCO2R activity. Metastable oxygens and metallic fcc-(111) or (100)-like Cu facets promote CO-CO dimerization step via a deprotonated glyoxylate species. In the second study, we rationalized sound experimental evidences on the absence of eCO2R on gold, silver, and copper without a metal cation in solution. By applying AIMD on a large Au supercell with 72 explicit water molecules and 1 cation, we demonstrated that cations steadily coordinate with adsorbed CO2, with a coordination rate which increases with the ionic cation radius. Overall, such coordination accounts for a short-range electrostatic interaction which enables eCO2R by stabilizing CO2 activation by 0.5 eV and enhancing the first electron transfer from the surface. Specific eCO2R activity trends are solely due to larger accumulation at the Outer Helmholtz layer for weakly solvated cations. Both studies provide new sets of concepts for modeling dynamic processes driven by high surface polarization and electrolyte species characteristic of CO2 reduction conditions.
1.2-T2
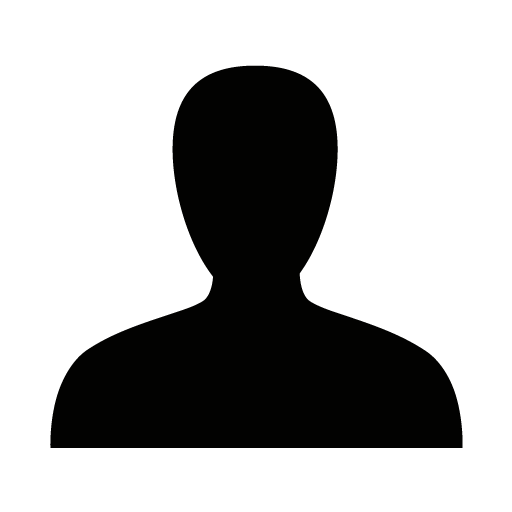
Electrochemical reduction of CO2 into valuable fuels has entered a pre-industrialization stage with more and more studies oriented toward competitive and scaled designs and processes. This trend is mostly visible in the aim for large current density operation and the use of gas diffusion electrode (GDE) based device approaches. To provide guidelines for optimization, multi-physics modeling of CO2 electrochemical reduction process, from the molecular to the device scale has become a necessary tool.[1], [2]
To reach a comprehensive and accurate model, each parameters requires to be established and validated independently as a first step. This sub-task is challenging for the determination of electrochemical kinetic parameters as mass transport and kinetics phenomena are always happening concomitantly and are convoluted.
In the present work, we investigate experimental conditions for which the mass transport characteristics can be modeled in detail allowing for the isolated determination of kinetic parameters. Such requirments are meet under certain conditions at rotating disk electrode (RDE). At a silver rotating disk, we investigate the electrochemical reduction of CO2 into CO and the competitive reduction of water into hydrogen and use the fully modelized convective flow to post determine the exact electrode concentration of reactant and product at steady state. We will show how this strategy enables the determination of finer kinetic models. Such parameters would eventually be implemented into more complex, complete electrode and device models to optimize state of the art silver-based gas diffusion electrode design.[3]
1.2-T3
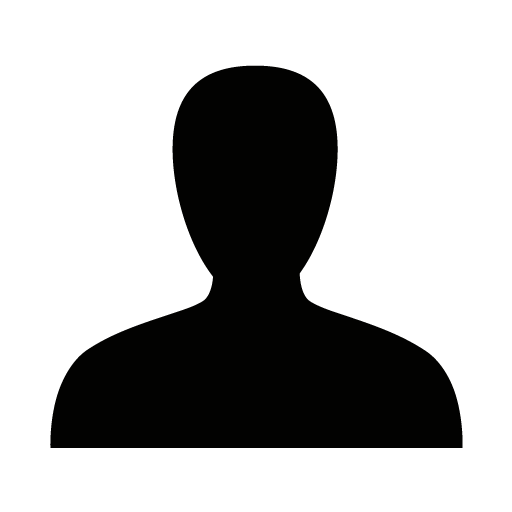
The production of cheap energy from renewable sources, like solar energy, provides the opportunity to use electrochemistry for the synthesis of added-value products in a cost-effective manner. The reduction half-reaction has typically been used for the production of H2, due to its high-density energy per weight unit. Additionally, the reduction of CO2, to energy-rich chemicals (CO, formic acid, etc.) is gaining increasing attention these days. In this line, there are other alternative chemical routes, such as the synthesis of products for the chemical industry, which despite being much less developed, may present a good intrinsic economical interest.
The production of aniline by reduction of nitrobenzene is a very useful transformation, as these species are widely employed as building blocks for the production of aniline-based dyes, explosives, pesticides and drugs. This is a 3-steps mechanism, involving 6 electron and 6 proton processes. Each of these steps involves the insertion of 2e- and 2H+ species in the NO2 group of the molecule. This organic transformation requires the formation (and stabilization) of H* in the electrode surface to reach an effective reduction and hydrogenation. This requirement highlights the importance of developing materials capable of producing H* in order to succeed in this electro-transformation.
Electrodes made of Cu and Cu based compounds have efficiently been used for the electro-reduction of nitrobenzene in aqueous media due to their high energy of activation for the competing hydrogen evolution reaction (HER), thus enhancing the reactivity of the hydrogen radical in the organic reduction and increasing the coulombic efficiency for the organic transformation. Compared with copper, palladium shows high activity for the hydrogenation of organic compounds, mainly due to their affinity for the adsorption and storage of H* species.
In this work, decoration of Cu foil surface with Pd by galvanic replacement technique was used to improve the catalytic properties of this material. Using bare Cu, the reduction of concentrated solutions of nitrobenzene has been tested. The introduction of Pd in the Cu surface enhanced the performance and selectivity of the electrode, achieving a complete reduction of a 30 mM nitrobenzene solution in 12 h at -0.8 V (vs Ag/AgCl), obtaining aniline with 70% yield. Finally, a detailed analysis using Impedance Spectroscopy has revealed the improvement in the catalytic performance of Cu with Pd electrodes by increasing the adsorption of hydrogen in the electrode surface, favoring the selectivity in the reduction of nitrobenzene to aniline.
1.2-T4
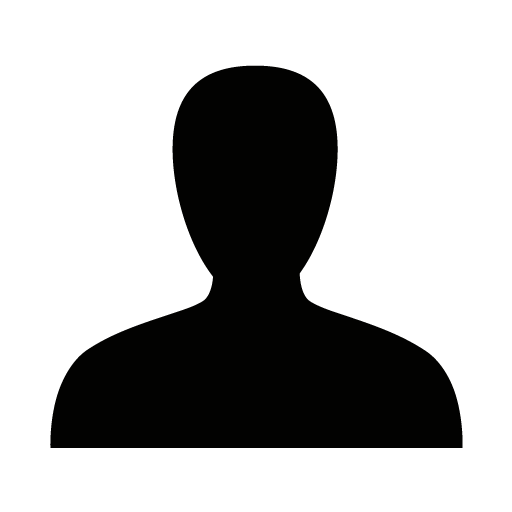
Photoelectrochemical (PEC) oxidation of alcohol to the value-added aldehyde using semiconductor materials has been one of the promising approaches for green chemistry synthesis. It offers a low-temperature and low-pressure reaction environment to replace high pressure and high energy consumption of the industrial approach using silver catalysis. However, such reaction has been overlooked in the PEC research since alcohol is often used as hole scavengers and the product of this oxidation has not been attracted great attention. In addition, the aldehyde product, as the result of the highly selective oxidation product from alcohol PEC oxidation[1], is still highly reductive. Therefore, the reason that the oxidative photogenerated holes in hematite could not further oxidize aldehyde still remains unclear.
My presentation will primarily focus upon the acetaldehyde formation from PEC ethanol oxidation and its over-oxidation by the highly oxidative photogenerated holes in hematite photoanodes. The kinetic competition of alcohol oxidation and aldehyde oxidation will be primarily elucidated based on operando-spectroelectrochemical measurements[2]. In addition, the Ahhrenius relationship will also be established to elucidate how we can achieve highly selective aldehyde formation by linking thermodynamic and kinetic information learnt from such optical spectroscopies. On the other hand, the challenges in photo-oxidation of organics for the application of water purification will be discussed in detail in terms of the thermodynamic driving force and the reaction kinetics of the photocatalysts.
1.3-T1
Hydrogen, when produced by electrochemical water splitting, powered by renewable and clean energy resources such as solar energy, tidal and wind, has emerged as a promising energy vector to respond to this increasing energy demand and to decarbonize transportation, heating and fine chemicals sectors.Traditionally, Pt and Ru based catalysts are considered the most efficient materials for the HER, however, they are expensive and scarce. More recently, electrocatalysts based on earth-abundant and cost-effective materials have been reported with remarkably efficient HER performance.
In this work, we report a (i) cost-effective and (ii) facile to synthetize reproducible Cu2S electrocatalyst with (iii) current densities close to 400 mA cm-2 and Faradaic efficiencies near 100% for HER. With this electrocatalyst we achive long stabilities never before reported.
1.3-T2
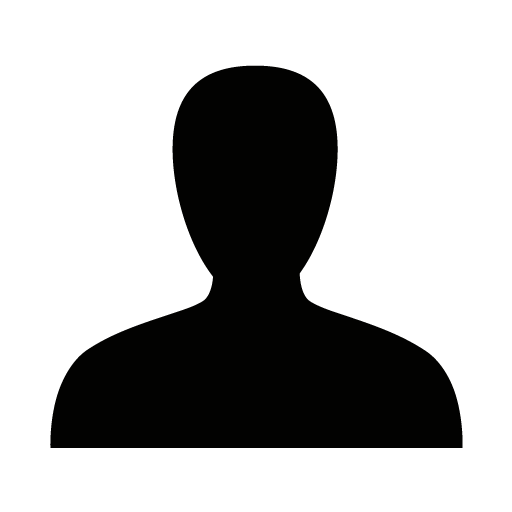
In the search for efficient electrocatalytic materials and devices, parameters such as current density, potential, selectivity and stability have played key roles due to the their links to future scale-up costs. These direct indicators, however, treat both small and large cells as a black box system where spatial variations across a catalyst are averaged into a singular measured output. Within this work we introduce a secondary method to visually compare electrocatalytic activity across a catalyst's surface using time-resolved infrared imaging. Specifically, the spatial temperature of a catalyst's surface is measured with 10 mK accuracy, which we show provides a link to the overpotential and activity of the catalyst at a given location. Such techniques can be applied to multiple material surfaces for combinatorial catalyst testing, as well as using a singular material to observe current density distributions across a gas-diffusion layer.
In general, heat generation within electrochemical systems is poorly studied, and the disambiguation of various heating effects (overpotential, ohmic, gas-diffusion layer resistivity) is useful for improving not only catalyst development, but scale-up of electrochemical systems. As a demonstration of the proposed infrared measurement method, we show the vast temperature difference occurring between a Pt and Ag catalyst for water-splitting, as well as the effects of CO2 dissolution on the temperature rise during CO2 electrolysis in an alkaline environment. Such observations provide a means of detecting premature gas-diffusion layer flooding, spatial variations, temperatures for reaction-diffusion modelling systems and flow imbalances. The thermal imaging method then has broad applications for H2O, CO2, CO and N2 reduction applications which all contain different phenomena and challenges.
1.3-T3
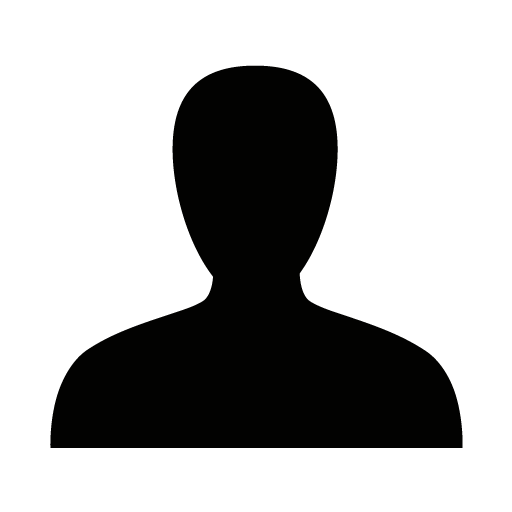
Increasing the structural complexity of (photo)electrodes is a possible approach to obtain higher activities and selectivities in – for example – CO2 reduction or oxygen evolution. Many synthesis methods produce random, porous morphologies in which the chemical and physical conditions may vary significantly with respect to the bulk, affecting activity and selectivity towards the desired product.
Here, we use a combined experimental-numerical technique to obtain, digitalize and quantitatively characterize morphologically complex (photo)electrodes. The material is digitally reconstructed starting from a physical sample investigated by means of the FIB-SEM nanotomography technique, with resolution of up to 4 nm in the three dimensions. The obtained image stacks are processed to obtain a digitalized representation of the morphology, which subsequently can be quantitatively characterized and used in pore-level transport simulations.
We present quantitative morphological characterization of the catalyst layer of five CO2 reduction gas diffusion electrodes. We calculated and compared roughness factor, specific area, connected volume, volume fraction profile, opening size distribution, chord length distribution, and two points correlation, providing a range of parameters difficult or impossible to obtain through experimental methods giving quantitative insight on electrode morphologies.
The digitalized morphologies can be meshed and used as geometrical domain in simulations for the characterization of transport properties and the extraction of effective physical properties (e.g. effective diffusivity or absorption coefficient) or in direct coupled pore-scale simulations for the characterization of operando physical and chemical conditions within the pores. The presented methodology is widely applicable, and will allow to shine some light on the link between morphology and physical properties of materials.
1.3-T4
Longfei Wu performed his PhD research at Eindhoven University of Technology in the Inorganic Materials and Catalysis group under the supervision of dr. Jan Philipp Hofmann and prof. dr. Emiel Hensen, working on transition metal chalcogenides for a variety of electrocatalytic reactions. During his PhD, he focused on spectroscopic methods such as X-ray absorption spectroscopy, X-ray Photoelectron Spectroscopy as well as FTIR to understand the active sites of electrocatalysts. After his graduation in February 2019, he started as a postdoc in ID01 at ESRF and a guest researcher at TU Eindhoven under the supervision of Prof. dr. Marie-Ingrid Richard and dr. Jan Philipp Hofmann, where he worked on Coherent Diffraction Imaging (CDI) of nanoparticles under operando conditions. As of November 2019, Longfei started as a postdoc in the Inorganic Chemistry and Catalysis (ICC) group of prof.dr.ir. Bert Weckhuysen at Utrecht University.
Electrocatalysts play an important role in the transition towards a sustainable society. Nanomaterials are used as catalysts for a range of electrochemical reactions, and the activity and selectivity of nanocatalysts depend on many factors.1 While extensive efforts have been devoted in optimizing electrode performance with high activity and selectivity, the poor stability of nanomaterials becomes a critical issue. The dynamic nature of nanoparticles under electrochemical conditions results in structural variations that determine the selectivity and activity on the single nanoparticle level. Therefore, thorough understanding of electrocatalysts requires both bulk electrode and single nanoparticle analysis.
TOC. a. Schematic drawing of in situ electrochemical cell for X-ray diffraction studies. b, c. Measured diffraction intensity of Cu nanoparticle in absence (b) and presence (c) of CO2 saturated 0.1 M KHCO3. d. Top isosurface view (top) of the reconstructed modulus. Side isosurface view (bottom) of the reconstructed modulus. The missing electron density corresponding to a part with a different crystallographic orientation (twin) is clearly visible. The tick spacing corresponds to 50 nm. e, f. 2D cross-sections of displacement field of Cu nanoparticle in absence (b) and presence (c) of CO2 saturated 0.1 M KHCO3.
In this work, we demonstrate a versatile in situ X-ray diffraction-based methodology which enables the structure and phase analysis of bulk electrodes and lattice strain analysis of single nanoparticles. A novel in situ electrochemical cell configured in back-illumination geometry has been fabricated to perform X-ray diffraction (XRD) and Bragg Coherent Diffraction Imaging (BCDI) with unfocused and focused beam respectively. In situ XRD has been collected during stepped cyclic voltammetry CO2 saturated 0.1 M KHCO3 to trace the phase evolution of Cu electrode, while ex situ BCDI has been performed on single Cu nanoparticle in absence and presence of CO2 saturated electrolyte. BCDI results demonstrate that that displacement appears mostly at the twin boundary and edges even without the presence of electrolyte. After introducing electrolyte, the displacement field at the twin boundary and the surface change significantly, which can be caused by adsorbed species or surface oxidation. With the strain and displacement field information, we observed the influence of electrolyte on the surface and boundary structure, which can help to identify the active sites and holds great promise for establishing the structure-performance relationship.
1.4-I1
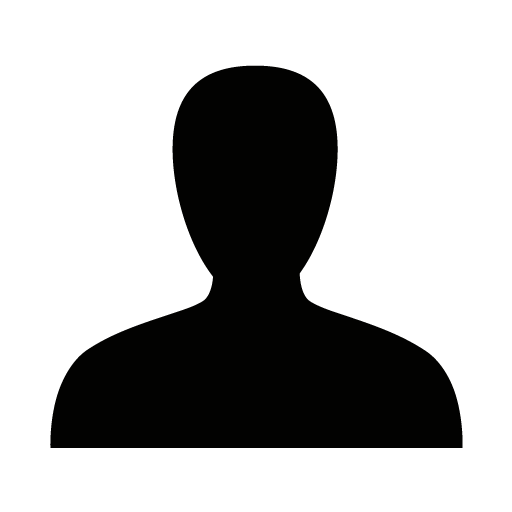
The massive quantities of fossil fuels used by our society have led to unprecedented atmospheric CO2 levels with widespread climate impacts. Carbon capture, utilization, and storage (CCUS) technologies are being developed to mitigate CO2 emission issues. Large-scale CO2 capture and sequestration facilities, such as Petra Nova, have been built to store thousands of tons of CO2 per day. However, the typical capital investment for centralized CCUS facilities is at a billion-dollar scale, making it challenging to finance. Sequestering the captured CO2 in geological repositories often requires additional investment in CO2 pipelines and infrastructure, which further increases the financial challenge to the rapid deployment of highly centralized facilities rapidly. More importantly, the carbon capture and sequestration process itself is not profitable without subsidies or a carbon tax. As a critical component in CCUS, carbon utilization holds the key to generating revenues that can offset the capture cost. It enables the captured CO2 to be converted into valuable materials, such as concrete, building materials, and platform molecules for fuel and chemical productions.
Recently, significant progress has been made in low-temperature CO2 electrolysis to carbon monoxide, formic acid, ethylene, and ethanol, which raised the demand for systematic techno-economic assessments (TEA) to evaluate its feasibility as a CO2 utilization process. In this talk, we will present our recent TEA of four major products and prioritizes the technological development with systematic guidelines to facilitate the market deployment of low-temperature CO2 electrolysis. We will first discuss the present state-of-the-art electrolyzer performance and parameterize figures of merit. Then, we will discuss a detailed roadmap to make C2 product production economically viable; an improvement in an energetic efficiency to ~50% and a reduction in electricity price to 0.01 USD/kWh. We also propose industrially relevant benchmarks: the 5-year stability of the electrolyzer components and the single-pass conversion of 30% for C1 and 15% for C2 products. In addition to the TEA work, we will present our latest experimental efforts to establish a two-step tandem electrolysis process to convert CO2 into ethylene and acetate with a high selectivity. We will show how operating conditions affect the performance of the tandem system and challenges associated with this system. Finally, we will discuss potential approaches to further improve the efficiency of the overall system.
1.4-I2
Prof. Aimy Bazylak is the Canada Research Chair in Thermofluids for Clean Energy and Professor in the Department of Mechanical and Industrial Engineering at the U of T. In 2011, she was awarded the I.W. Smith Award from the Canadian Society for Mechanical Engineering, and she received the Ontario Early Researcher Award in 2012. From 2015-2018, she served as the Director of the U of T Institute for Sustainable Energy. In 2015 she was named an Alexander Von Humboldt Fellow (Germany), and in 2019 she was named a Fellow of the American Society of Mechanical Engineers. In 2020, she was named a Helmholtz International Fellow (Germany), was awarded the U of T McLean Award, and was elected to the Royal Society of Canada College of New Scholars, Artists and Scientists.
A sustainable future requires that we harness renewable but intermittent sources of energy and transmit or store it to address real world patterns of use. Renewable energy can be used to sequester CO2 into a variety of products, such as carbon-neutral fuels and chemical feedstocks, thereby reducing atmospheric CO2. Reducing atmospheric CO2 levels requires the substitution of clean power for carbon-intensive fuels as well as CO2 conversion processes that transform emissions into useful chemical products. To reach cost targets for widespread commercial adoption, materials must enable more effective multiphase flow phenomena than what currently exists. This talk will discuss our latest work on performing in operando imaging of carbon dioxide electrolyzers to understand the role of mass transport losses on overall performance. Designing these materials requires the a priori knowledge of how the heterogeneous properties of the porous materials and their interfacial contacts influence electrochemical performance. I will discuss critical design factors and how they influence the flow and mass transport behaviour in carbon dioxide electrolyzers.
1.4-I3
Recently, carbon-based catalyst materials doped with nitrogen and transition metals (MNC) have emerged as a selective and cost-efficient alternative to metal catalysts for the of CO2 electrochemical reduction (CO2RR). [1] Usually, these solid catalysts are prepared using heat treatment of a carbon precursor, nitrogen containing compounds and inorganic salts to form different nitrogen functionalities, which coordinated to the metal centers resulting catalytically active MNx single sites.These different functionalities tend to be non uniformly distributed making it challenging to establish the correlations between the structure and the catalytic activity
To address these challenges, we have prepared polyanilline derived MNC catalysts and observed that the catalytic performance of MNCs toward the CO2RR is affected by the structure and composition of the catalyst and by the conditions of the catalytic process. In particular, we observe that the metal center plays a crucial role in activity and selectivity of the process. While N functionalities do have some activity, MNx moieties play a predominant role and a higher concentration of FeNx correlates with higher partial current densities towards CO production. [2]
In addition to the catalyst structure and composition the reaction conditions also play a crucial role in determining the reaction’s selectivity. In particular, the pH can be used to tune the ratio of the different products. [3] For a polyaniline derived FeNC catalyst it was observed that hydrogen formation was strongly dependent on pH, while the CO production was not affected, resulting in high selectivities toward CO at high local pH values. Nevertheless, working in the absence of protons (in a non-protic electrolyte) resulted in the suppression of both the HER and the CO2RR, as both reactions require protons. We found that adding small amounts of water enhances the CO2RR while keeping the HER low, showing that there is an optimal proton concentration to ensure a high activity and selectivity.
2.1-I1
CO2 conversion to valuable products has been recently highlighted and made significant progress in terms of current density as well as product selectivity. For instance, CO or formate can be achieved more than 95 % of Faradaic efficiency from electrochemical CO2 reduction reaction (CO2RR). The next technical challenges in the catalyst development is achieving long-term stability for the practical application. During electrochemical CO2 reduction reaction, various factors can affect the stability of the catalyst, and metal impurities (ions) in the electrolyte can be especially detrimental for CO2RR because the transition metal impurities can be depostied on the catalyst surface and activate hydrogen evolution reaction (HER). In this talk, I will compare Ag nanoparticle electrocatalyst with N-doped carbon based electrocatalyst for the electrochemical CO2 reduction reaction to CO production. We found that N-doped carbon catalysts have high resistance to the impurity metal attack in the electrolyte, and long-term durability was achieved1-2. In addition, for the practical point of view, low concentration of CO2 gas feed has to be considered as well because the flue gas contains diluted CO2 concentration not pure CO2. As decreasing the concentration of CO2 feed, CO2RR selectivity over hydrogen evolution reaction (HER) can be senstively affected. We also find that at low concentration of CO2, Ag nanoparticle showed decreased CO Faradaic efficiency due to increased HER, and modifying the operational condition in the gas-diffusion electrode (GDE) based membrane electrode assembly (MEA) electrolyzer can affect the water supply to the catalyst layer and thus contribution to suppressing HER. We propose that the CO selectivity can be adjustable both of intrinsic catalyst activity as well as extrinsic properties in MEA at low CO2 concentration condition.
2.1-I2
I willrevise the modelling advances fro. Our group trying to improve our understanding of CO2 reduction with particular atttention to cations and C3 formation.
2.1-I3
Raffaella Buonsanti obtained her PhD in Nanochemistry in 2010 at the National Nanotechnology Laboratory, University of Salento. Then, she moved to the US where she spent over five years at the Lawrence Berkeley National Laboratory, first as a postdoc and project scientist at the Molecular Foundry and after as a tenure-track staff scientist in the Joint Center for Artificial Photosynthesis. In October 2015 she started as a tenure-track Assistant Professor in the Institute of Chemical Sciences and Engineering at EPFL. She is passionate about materials chemistry, nanocrystals, understanding nucleation and growth mechanisms, energy, chemical transformations.
In the electrochemical CO2 reduction reaction (CO2RR) selectivity still remains an important issue.
In this talk, I will showcase a few examples which highlight how shape-controlled nanocrystals can contribute to address this challenge. First of all, I will discuss how size control of Cu nanocubes (Cucub) and Cu octahedra (Cuoh) has revealed the importance of facet-ratio to maximize the selectivity towards ethylene and methane, respectively. Second, I will present our recent computational-experimental efforts towards using well-defined NCs to elucidate selectivity rules at the hydrocarbons/alcohols branching nodes in the CO2RR pathway. The formation of ethanol via *CHx-*CO coupling or *CO-*CO coupling is an open debate in the literature. As a platform to address this question, we have used CH4 favoring (i.e. *CHx populated) Cuoh and C2H4 favoring (i.e. *CO-*CO populated) Cucub under enhanced *CO coverage induced by the presence of Ag NCs. The selective promotion of ethanol on the Cuoh provided evidence for *CHx-*CO coupling being the preferred pathway. Following theoretical predictions, we have also demonstrated that such pathway is favored on Cu(110) edge sites compared to Cu(100) terraces by studying the size-dependent edge/face ratio of the Cucub always in the presence of the CO-generating Ag domains. Indeed, we found that smaller cubes are more selective for ethanol than bigger sizes. Generally, these examples encourage the application of well-defined nano catalysts as a bridge between theory and experiments in electrocatalysis.
2.2-T1
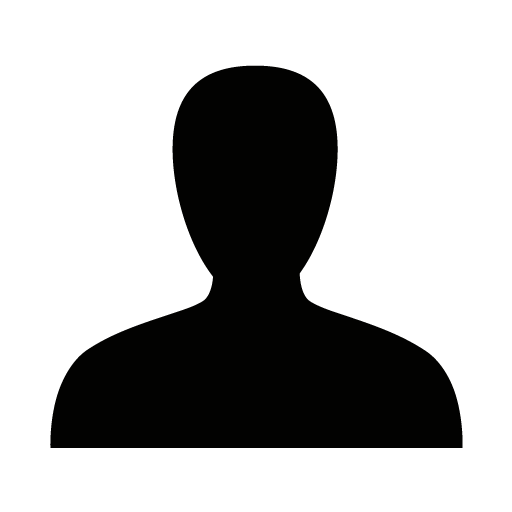
The electrochemical reduction of carbon dioxide requires access to ample CO2 gas and liquid water to fuel reactions at a high rate for industrially relevant applications. The application of gas-diffusion electrodes has notably improved the current densities by over an order of magnitude by positioning the catalyst at gas/liquid interfaces. Such configuration also adds complexity to the electrode that no longer consists of the only catalyst but also a multi-scale network of the gas-liquid-solid interfaces. Therefore, electrode wettability serves a vital role in determining the electrochemical surface area and gas pathways in the three-dimensional electrode structure and thus has a profound impact on the electrode activity, product selectivity, and long-term stability. Based on the content of our recent review paper[1], this presentation will provide an overview of the possible mechanisms underlying the phenomena such as electrowetting and salt precipitation commonly observed in the field of CO2 electrochemical reduction and discuss the inevitable critical issues in balancing electrochemical surface area and gas pathways. At last, the presentation will conclude with some recent attempts and future outlooks to address these critical issues and redesign the next generation of gas-diffusion electrodes.
2.2-T2
In order to address the threat of climate change, the electrochemical conversion of CO2 represents a viable solution[1]. Herein, the electroreduction of CO2 under atmospheric conditions has been performed in a continuous flow cell over gas diffusion electrodes (GDEs). A porous and conductive support has been employed to this end, where a Cu-based[2] catalyst has been manually deposited on the substrate by means of an airbrusher. Several variables of the investigated system have been assessed, with the aim to enhance the production of CO2 reduction liquid products. The most promising conditions have been explored among the cathodic applied potential, catalyst loading, binder content, electrolyte concentration and the presence of metal oxides in the catalytic material, like ZnO or/and Al2O3. In particular, it has been found that the binder content (Nafion) has affected the selectivity toward CO, leading to syngas with a H2/CO ratio of ⁓1 at the lowest Nafion content (15%). On the contrary, the highest Nafion content of 45% has led to a rise in C2+ products formation and a decrease of CO selectivity by 80%. The study undertaken revealed that liquid crossover, linked to electro-wetting, affects the GDE performance by severely compromising the CO2 transport to the active sites of the catalyst, and thus reducing the efficiency of CO2 conversion. A mathematical model[3] confirmed the role of a high local pH in promoting the formation of bi-carbonate species: beside the undesired consumption of CO2 with OH- ions, salts formation may cause the catalyst deactivation and hinder the mechanisms for C2+ liquid products. The ultimate intent of this work is to shift the attention of the scientific community toward other players of the CO2 reduction process, which can impact on both kinetics and mass transport and consequently on carbon efficiency of this systems[4], rather than narrowing the focus on the sole catalytic activity of the materials.
2.2-T3
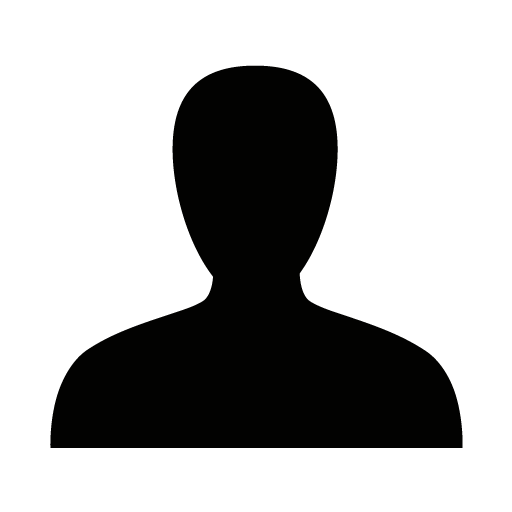
The production of value added compounds within CO2 electrolyzers has reached sufficient catalytic performance that system and process performance such as CO2 utilization have come more into consideration. Efforts to assess the limitations of CO2 conversion and crossover within electrochemical systems have been performed, providing valuable information to position CO2 electrolyzers within a larger process. Currently missing, however, is a clear elucidation of the inevitable trade-offs that exist between CO2 utilization and electrolyzer performance, specifically how the Faradaic Efficiency of a system varies with CO2 availability. Such information is needed to properly assess the viability of the technology. In this work, we provide a combined experimental and 3D modelling assessment of the trade-offs between CO2 utilization and selectivity at 200 mA/cm2 within a membrane-electrode assembly CO2 electrolyzer. Using silver gas diffusion electrode and varying inlet flow rates, we demonstrate that the variation in spatial concentration of CO2 leads to spatial variations in Faradaic Efficiency that cannot be captured using common ‘black box’ measurement procedures. Specifically, losses of Faradaic efficiency are observed to occur even at incomplete CO2 consumption (80%). Modelling of the gas channel and diffusion layers indicated at least a portion of the H2 generated is considered as avoidable by proper flow field design and modification. The combined work allows for a spatially resolved interpretation of product selectivity occurring inside the reactor, providing the foundation for design rules in balancing CO2 utilization and device performance in both lab and scaled applications.
2.3-T1
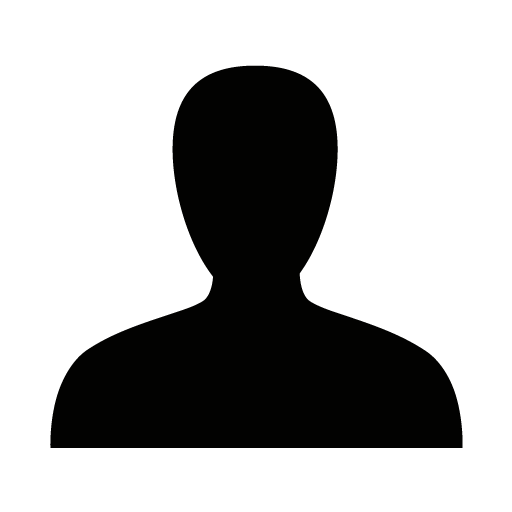
The boost in current density is a major achievement in the past decade for electrochemical CO2 reduction (ECO2R) process. Nevertheless, low CO2 utilization coming along with the high performance has been a setback for commercializing CO2 electrolyzers. The maximum utilization efficiency of CO2 in a device is plateaued at 50% under common operating conditions due to the parasitic loss of CO2 in the system. A promising approach is to introduce excess protons near the reaction domain. H+ can be provided either directly from an acidic catholyte or via a membrane, both of which will either neutralize the OH- produced directly, or regenerate CO2 from (bi)carbonates. Alternatively to acidic catholyte, protons provided by ion exchange membranes can be in a direct relation to the applied current density. Bipolar membranes (BPMs) operated under reversed bias can internally splits water to protons and hydroxide ions, thus protons are sent to the cathode and hydroxide to the anode. In addition, a BPM further allows for the use of an alkaline anolyte and non-noble metal-based anodes. Unfortunately, the use of BPMs reported in membrane electrode assemblies (MEAs) has shown low ECO2R activity, with H2 remaining a dominant product. In this work, we firstly succeeded to increase the performance of BPMEA by increasing the cation (K+) concentration on the catalyst surface, which achieved CO faradaic efficiency of 68%. We then compared the CO2 conversion and consumption efficiency in above BPMEA with traditional anion exchange membrane in a MEA cell (AEMEA). Results show a 5-fold reduction in lost CO2, thus 2-3 times higher CO2 utilization efficiency in a BPMEA than AEMEA at similar current densities. This work addresses the direct importance of alkali cation concentrations when using BPMs in MEA cells, providing an approach for tackling low CO2 utilization issue in common CO2 electrolyzers at present.
2.3-T2
The electrocatalytic reduction of CO2 (CO2R) presents a sustainable pathway for producing fuels and chemicals. Cations have a strong influence on the reaction activity and selectivity, and there are currently three theories regarding their main role: (1) to modify the local electric field, (2) to buffer the interfacial pH and (3) to stabilize reaction intermediates. Here, we tested these theories, and were able to define the main mechanism that explains how cations interact in the CO2R reaction. Cyclic voltammetry and Scanning Electrochemical Microscopy (SECM) measurements were performed without metal cations and in the presence of Cs+. On gold, CO2R does not take place at all in pure 1 mM H2SO4, unless Cs+ ions are added to the electrolyte (Fig. a). We used SECM to locally probe if CO is produced on other common CO2R catalysts, namely copper and silver. Without a cation in solution, CO2R does not take place at all, regardless the metal surface. These remarkable observations allow us to define the main role of cations in the CO2R reaction, and to propose a new reaction mechanism in which the cation is actually what enables the reaction to happen, by forming a complex with CO2 and allowing the first reaction intermediate to be formed (Fig b). We supported this model with Density functional theory (DFT) and ab initio molecular dynamics (AIMD) simulations, which confirmed that CO2R happens only if the CO2– intermediate is stabilized via a cation-oxygen chemical bond, which occurs depending on alkali cation solvation shell and, thus, on radius. From the system design point-of-view, it is desired to find charged species that have an even larger stabilizing effect on CO2–(ads) than Cs+. Based on that, we have also performed CO2R in acidic media in electrolytes containing: Li+, Cs+, Be2+, Mg2+, Ca2+, Ba2+, Al3+, Nd3+ and Ce3+. We find via experiments and DFT/AIMD that acidic cations with a moderate hydration radius, promote CO2R in acidic media/low overpotentials, while the non-acidic weakly hydrated Cs+ is the cation that leads to more CO in alkaline media/high overpotentials. These differences come from the promotional effects acidic cations have on water reduction, only allowing these species to favor the selectivity towards CO2R before the onset of this reaction. In this talk, we elucidate through experiments and simulations the main role of cations on CO2R, and also which cation properties are important when designing an optimal electrolyte for this system.
2.3-T3
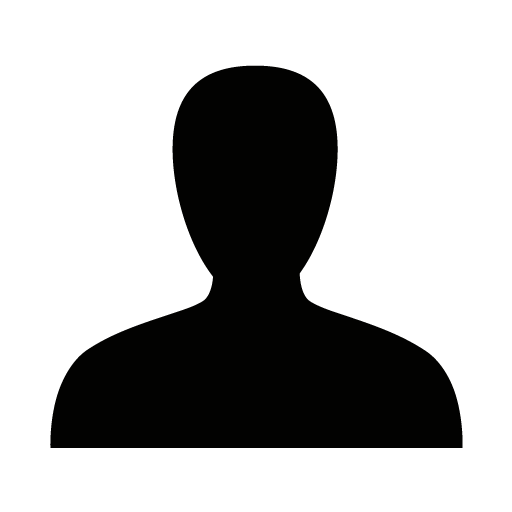
Electrochemical CO2 reduction has the potential to contribute to a closed carbon cycle by recycling CO2 from various sources and converting it into sustainable chemicals. The reaction environment is crucial to achieve high activity, conversion and selectivity. Hydrogen evolution is typically dominating in acidic environments (due to the sufficient presence of protons) leading to low Faradaic efficiency. Recently, it has been shown that the hydrogen evolution reaction could be suppressed by adding extra non-reactive cations, such as potassium ions in the electrolyte. We developed an electric double layer model, solving for the transport of ionic species in the diffusion layer and charge distribution in Stern layer, to describe the potential and concentration distribution in close vicinity of the electrode. We showed that the potassium cations can migrate to the electrode surface and modulate the electric field strength, and therefore, reduce the migration of protons towards the electrode. The concentration of all ionic species, the potential profile, and the hydrogen evolution current density can be quantitively obtained. This model explained the mechanism of hydrogen evolution suppression by potassium ions which was also observed and convinced in the experiment. The model reveals the possibility of electrochemical CO2 reduction in acidic environment and proposes a possible way to overcome the depletion of CO2 in alkaline environments.
2.4-T1
Electrocatalytic CO2 reduction (eCO2R) to valuable chemicals and fuels is of fundamental scientific and technological interest. However, it remains a huge challenge to control the selectivity of eCO2R toward certain products with near unity efficiency. Bimetallic electrocatalysts have been regarded as one of the most promising strategies to improve the selectivity of eCO2R, since the introduction of a second metal tunes the adsorption energy of key intermediates (e.g. CO and hydrogen). Here, we fabricated a bimetallic system of Sn-doped CuO nanoparticles for electrochemical CO2 conversion. Electrochemical measurements demonstrate that introduction of a trace amount (0.4 %) of Sn enhances the Faradaic Efficiency (FE) of CO up to 98% at –0.75 V vs. RHE cathodic bias, with a stable performance over the course of 15 h. A volcano-shape relationship is found between the CO enhancement and the percentage of Sn (0-0.8%), with maximum FE for 0.4% Sn doping. In situ Raman spectroscopy measurements reveal the subsequent reduction of CuOx and SnO2 domains, which tunes the adsorption of CO at the catalyst surface. Our work showcases the potential of bimetallic electrocatalysts to boost the eCO2R selectivity, and reveals the dynamic restructuring of the electrocatalyst under operating conditions through time-dependent Raman spectroscopy.
2.4-T2
I am 30 years old. I am Venezuelan. I have completed my master’s degree and bachelor’s degree at Politecnico di Torino (Italy), in the framework of a double degree between Politecnico di Torino (Italy) and Universidad Central de Venezuela (Venezuela). Currently, I am a research fellow focused on the conversion of CO2 via the electrocatalytic route. I work with professionalism and responsibility, respecting deadlines and demonstrating problem-solving skills.
Anthropogenic activities have impacted the planet’s carbon cycle by the emissions of large amounts of greenhouse gases (GHGs), shifting the equilibrium of human history since the industrial revolution. The electrocatalytic CO2 reduction (EC CO2R) is an interesting technology because renewable energy sources could drive it; as well, it can be used to store both renewable electricity and CO2 in added-value products such as liquid fuels (ethanol and other high-octane alcohols (>C2).[1,2] To date, researchers have focused on observing the effects of surface modification (e.g., nano-structuring and surface tailoring) on catalysts selectivity and activity to produce C2+ products. Nonetheless, it remains an ongoing challenge due to high C-C coupling barriers. Among these studies, incorporating light heteroatoms such as boron (B) into the Cu catalyst has been reported to induce the formation and stabilization of Cu+1/Cu0 interfaces and reduce the *CO dimerization barrier, promoting a high activity for the EC CO2R towards C2 products.[3] Herein, we proposed B-doped Cu oxide catalysts for the EC CO2R process. Briefly, Cu-based powder was prepared by ultrasound-assisted co-precipitation method. Then, the Cu-based powder was impregnated in a solution of B2O3 and dried. The obtained material was calcined under air or N2. The catalyst materials have been characterized by different physico-chemical methods like X-ray diffraction, BET, porosimetry, and filed-emission scanning electron microscopy (FESEM). Incorporating both B and N heteroatoms led to greater selectivity for reducing CO2 to products of interest: that is, a total FE of 64% to CO2 reduction products with 49% of liquid products (i.e. 77% selectivity towards formate and alcohols) at a total current density of 23 mA cm-2(see Figure 1). This behaviour is attributed to the fact that these elements belong to the p-block of the periodic table, which stabilize the carboxyl group by promoting the dimerization of the *CO intermediate. The physical and chemical properties of the synthesized materials can be manipulated to tune the performance of the electrochemical reaction. These interesting results could help find a suitable electrocatalyst to establish this technology at the industrial level.
2.4-T3
The electrocatalytic (EC) CO2 reduction (CO2R) can be exploited for the energy transition and to store C into valuable products like syngas (H2/CO mixtures), organic acids (formic acid) and chemicals/fuels (C1+ alcohols).1 A big challenge for the industrialization of this technology is to find low-cost electrocatalyst, efficient reactors and process conditions. Noble metals like Ag and Au are the most used ones for syngas production.2 To reduce the catalyst cost, we developed electrodes made of Ag nanoparticles (NPs) on TiO2 nanotubes (NTs),3 showing a higher electrochemical surface area and electrons transport than a bare Ag. Titania was used as an efficient support, for enhancing the stability of key CO2- radical intermediate and decreasing the EC CO2R overpotential. We are also exploiting the current knowledge of the thermocatalytic CO2 hydrogenation to develop noble-metal-free CO2R electrocatalysts.4–6 For instance, Cu/Zn/Al-based catalysts producing methanol and CO from the CO2 thermocatalytic (TC) hydrogenation (at H2 pressure (P) of 30 bar and temperature (T) > 200 oC) generates H2, CO and other C1 to C3 liquid products during the electrochemical CO2R in a gas-diffusion-electrode system; while operating in the liquid phase, the same catalyst produces syngas with a tunable composition (depending on the applied potential) and other liquid C2+ products (in both cases at ambient T,P). Our results open a promising path for the prospective implementation of metal-oxides nanostructures for the CO2 conversion to the chemicals and fuels of the future. From an environmental and technoeconomic analysis applied to the case of study of the scaled-up methanol production, including downstream purification processes, we determined that optimized EC process conditions could lead to a practical implementation of this technology; that is, recirculation of the not reacted CO2, achievement of current densities in the range of 100-200 mA/cm2 with faradaic efficiencies (> 90%), and use of renewable electricity sources (i.e. 30% of the total energy).4 Hence, considering the effective allocation of methanol on a real market scenario, the EC process results to be economically advantageous over the TC one at productivity scales as low as 19.1 kg/h, and could lead to a carbon footprint that is comparable to that of current industrial technologies for methanol production (climate change impact of 2.72 kgCO2/kgCH3OH). Moreover, in a scenario with a 100% renewable energy such as photovoltaic, it is possible to reach savings in that carbon footprint of up to 62%.
2.5-I1
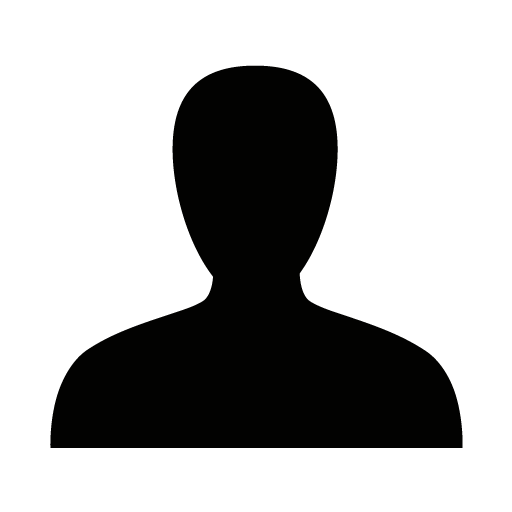
Disorder in real materials influences their properties and the chemical processes that occur at their interfaces. In order to unravel, and ultimately control processes at these interfaces, it is essential to gain a molecular-level understanding of the underlying physical manifestations caused by disordered materials. To accomplish this, measurement techniques through which disorder can be detected, quantified, and monitored are needed. However, such quantitative measurements are notoriously difficult, as effects often average out in ensemble measurements. In our lab, we have employed a combination of electrochemical and spatially resolved surface spectroscopy measurements to illuminate a molecular-level picture of disorder in materials. We study amorphous carbon which is an intrinsically disordered material. To the amorphous carbon, we covalently attached a monolayer of ferrocene. Interfacial electron transfer across the amorphous carbon–ferrocene interface is highly sensitive to the local microenvironment felt by the ferrocene, and thus to disruptions of order. By systematically varying linker properties and surface loadings, the influence of lateral interactions between nonuniformly distributed ferrocene headgroups on ensemble electrochemical measurements is gleaned.
2.5-I2
Marc T.M. Koper is Professor of Surface Chemistry and Catalysis at Leiden University, The Netherlands. He received his PhD degree (1994) from Utrecht University (The Netherlands) in the field of electrochemistry. He was an EU Marie Curie postdoctoral fellow at the University of Ulm (Germany) and a Fellow of Royal Netherlands Academy of Arts and Sciences (KNAW) at Eindhoven University of Technology, before moving to Leiden University in 2005. His main research interests are in fundamental aspects of electrocatalysis, proton-coupled electron transfer, theoretical electrochemistry, and electrochemical surface science.
The electrocatalytic reduction of carbon dioxide is a promising approach for storing (excess) renewable electricity as chemicalenergy in fuels. Here, I will discuss recent advances and challenges in the understanding of electrochemical CO2 reduction. I will summarize existing models for the initial activation of CO2 on the electrocatalyst and their importance for understanding selectivity. Carbon–carbon bond formation is also a key mechanistic step in CO2 electroreduction to high-density and high-value fuels. I will show that both the initial CO2 activation and C–C bond formation are influenced by an intricate interplay between surface structure (both on the nano- and on the mesoscale), electrolyte effects (pH, buffer strength, ion effects) and mass transport conditions. This complex interplay is currently still far from being completely understood.
2.5-I3
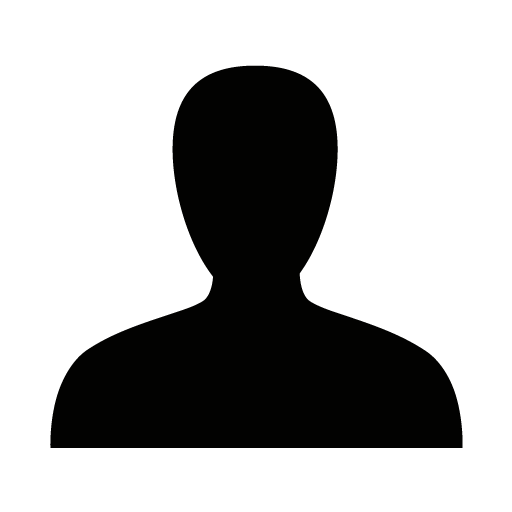
Many technical challenges remain for implementing CO2 electrolysis as a practical means for CO2 utilization. A key challenge for CO2 electrolysis is developing heterogeneous catalysts that can steer complex reaction networks and selectively convert CO2 into the desired product. First, I will describe how epitaxially grown Cu thin films can be used to probe CO2 electrolysis structure-reactivity relationships. We demonstrate that undercoordinated sites are selective motifs for oxygenates and C-C coupling using a combination of electrocatalysis experiments and in situ surface probe microscopy. Next, I will discuss how the same growth methods can be used to controllably deposit noble or base metal atoms onto well-defined Cu electrocatalysts and systematically investigate atomic-scale bimetallic effects. The metal atoms tend to suppress CO reduction to oxygenates and hydrocarbons while promoting competing pathways to CO, formate, and hydrogen, suggesting that the metal atoms segregate to undercoordinated Cu sites during physical vapor deposition. Finally, I will provide some perspectives on how to improve the intrinsic activity and selectivity of Cu through bimetallic effects, showing specific examples of enhancing C-C coupling through tandem catalysis and CO production through alloying with Zn.
Session-K1
Professor of Physical Chemistry at the Ecole Polytechnique Fédérale de Lausanne (EPFL) Michael Graetzel, PhD, directs there the Laboratory of Photonics and Interfaces. He pioneered research on energy and electron transfer reactions in mesoscopic systems and their use to generate electricity and fuels from sunlight. He invented mesoscopic injection solar cells, one key embodiment of which is the dye-sensitized solar cell (DSC). DSCs are meanwhile commercially produced at the multi-MW-scale and created a number of new applications in particular as lightweight power supplies for portable electronic devices and in photovoltaic glazings. They engendered the field of perovskite solar cells (PSCs) that turned our to be the most exciting break-through in the recent history of photovoltaics. He received a number of prestigious awards, of which the most recent ones include the RusNANO Prize, the Zewail Prize in Molecular Science, the Global Energy Prize, the Millennium Technology Grand Prize, the Samson Prime Minister’s Prize for Innovation in Alternative Fuels, the Marcel Benoist Prize, the King Faisal International Science Prize, the Einstein World Award of Science and the Balzan Prize. He is a Fellow of several learned societies and holds eleven honorary doctor’s degrees from European and Asian Universities. According to the ISI-Web of Science, his over 1500 publications have received some 230’000 citations with an h-factor of 219 demonstrating the strong impact of his scientific work.
Recently molecular photovoltaics, such as dye sensitized cells (DSCs) and perovskite solar cells (PSCs) have emerged as credible contenders to conventional p-n junction photovoltaics. Their certified power conversion efficiency currently attains 25.5 %, exceeding that of the market leader polycrystalline silicon. This lecture covers the genesis and recent evolution of DSCs and PSCs, describing their operational principles and current performance. DSCs have meanwhile found commercial applications for ambient light harvesting and glazing producing electric power from sunlight. The scale up and pilot production of PSCs are progressing rapidly but there remain challenges that still need to be met to implement PSCs on a large commercial scale. PSCs can produce high photovoltages rendering them attractive for applications in tandem cells, e.g. with silicon and as power source for the generation of fuels from sunlight. Examples to be presented are the solar generation of hydrogen from water and the conversion of CO2 to chemical feedstocks such as ethylene, mimicking natural photosynthesis.
1.1-I1
Prof. Beatriz Roldán Cuenya is currently Director of the Interface Science Department at the Fritz Haber Institute of the Max Planck Society in Berlin (Germany). She is an Honorary Professor at the Technische Universität Berlin, at the Freie Universität Berlin, and at the Ruhr-University Bochum, all in Germany. Also, she serves as a Distinguished Research Professor at the University of Central Florida (USA).
Prof. Roldán Cuenya began her academic career by completing her M.S./B.S. in Physics with a minor in Materials Science at the University of Oviedo, Spain in 1998. Afterwards she moved to Germany and obtained her Ph.D. from the Department of Physics of the University of Duisburg-Essen with summa cum laude in 2001. Subsequently, she carried out her postdoctoral research in the Department of Chemical Engineering at the University of California Santa Barbara (USA) until 2003.
In 2004, she joined the Department of Physics at the University of Central Florida (UCF) as Assistant Professor where she moved through the ranks to become a full professor in 2012. In 2013 Prof. Roldan Cuenya, moved to Germany to become Chair Professor of Solid State Physics in the Department of Physics at Ruhr-University Bochum until 2017.
During her academic career, Prof. Roldan Cuenya received an Early CAREER Award from the US National Science Foundation (2005) and the international Peter Mark Memorial award from the American Vacuum Society (2009). In 2016 she became Fellow of the Max Planck Society in Germany and also received the prestigious Consolidator Award from the European Research Council. In 2020, she became a member of the Academia Europaea (Academy of Europe). She received the AVS Fellow Award (2021), the International Society of Electrochemistry-Elsevier Prize for Experimental Electrochemistry (2021), the 2022 Paul H. Emmet Award of the North American Catalysis Society, and the Röntgen Medal of the City of Remscheid (2022).
Prof. Dr. Beatriz Roldan Cuenya is the author of 245 peer-reviewed publications, 6 book chapters and 6 patents. She has been supervising 74 postdoctoral fellows and 36 PhD students. She has given 245 invited talks, with 13 plenary talks and 33 keynote lectures since 2017. Her H-factor is 74 (Google Scholar) and her work has received over 21,500 citations.
She presently serves on the editorial advisory boards of the Journal of Catalysis and Chemical Reviews. In addition, she also contributes to a number of advisory committees, including the Liquid Sunlight Alliance (USA), the Advanced Research Center Chemical Building Blocks Consortium (Utrecht, the Netherlands), the Spanish Synchrotron Facility ALBA (Barcelona, Spain), the German Synchrotron DESY (Hamburg, Germany), the Helmholtz-Zentrum Berlin for the strategic development of BESSY II (Berlin, Germany), the Institute of Chemical Research of Catalonia (ICIQ in Tarragona, Spain), the UK Catalysis Hub and the Ertl Center for Electrochemistry & Catalysis (South Korea).
Prof. Roldan Cuenya’s research program explores physical and chemical properties of nanostructures, with emphasis on advancements in nanocatalysis based on operando microscopic and spectroscopic characterization.
The electrochemical CO2 reduction (CO2RR) driven by electrical energy from renewable sources has attracted attention as an environmentally friendly path to convert the undesired greenhouse gas into feedstock chemicals and fuels. Among the metal catalysts used for CO2RR, copper-based catalysts are of particular interest due to their unique capability to transform CO2 into various hydrocarbons and alcohols with high energy density. However, controlling the selectivity toward a specific product remains a challenge in this field. Interestingly, some of the critical parameters affecting the catalyst’s properties, including its structure and oxidation state, as well as the local pH near the active sites, may be tuned by applying potential pulses during CO2RR.
In this talk, I will discuss the impact of applying potential pulses to well-defined Cu-based materials (e.g. Cu(100), Cu2O nanocubes). In particular, I will establish structure/composition-selectivity correlations based on in depth in situ/operando microscopic (EC-AFM, EC-TEM), spectroscopic (XAS, XPS) and diffraction (XRD) characterization under realistic reaction conditions, including high current densities in flow cell electrolyzers. Thus, this work is expected to set up the ground for steering catalyst selectivity through dynamically-controlled structural and chemical transformations.
1.1-I2
Beyond surface reaction energetics, the structure and composition of the electric double layer exerts an influence on the activity and selectivity of electrochemical reactions. These phenomena often manifest themselves as so-called “pH effects”, i.e. deviations in the dependence of activity on a potential vs. RHE scale. In this talk, I first discuss how adsorbate dipole-field interactions give rise to higher activity for CO2R in alkaline conditions, and how these interactions are tuned by cations and in supported single site catalysts. I then discuss the impact of solution phase reactions and mass transport on the activity and selectivity towards acetate on nanostructured Cu at various pH. I discuss the implications of the mechanistic insights on catalyst design.
1.1-I3
A very important parameter for the electrocatalytic performance of a material is its potential of zero free charge (pzfc). For Cu(111) at pH 13 it was identified at −0.73 VSHE in the apparent double layer region.1,2 It shifts by (88 ± 4) mV to more positive potentials per decreasing pH unit.2 At the pzfc, Cu(111) starts to restructure. At higher potentials, full reconstruction and electric field dependent OH adsorption occur, causing a remarkable decrease in the atomic density of the first Cu layer.2
It is this restructuring that leads to the ability of Cu(111) to efficiently oxidize CO and to reduce water. Therefore, knowledge of the surface structure and the position of the pzfc is of paramount importance for the understanding of copper’s catalytic properties and for the rational design of electrocatalysts, in general.
CO is a key intermediate in the electro-oxidation of energy carrying fuels and known to act as a catalyst poison. Single-crystal Cu(111) model catalysts can efficiently electro-oxidize CO in alkaline media,3 where strong surface structural changes are observed under reaction conditions with electrochemical scanning tunneling microscopy (EC-STM). Supported by first-principles microkinetic modelling, we have shown that the concomitant presence of high-energy undercoordinated Cu structures at the surface is a prerequisite for the high activity. In water electrolyzers, it is possible to produce H2 in the course of the hydrogen evolution reaction (HER), which was studied with Ni(OH)2 modified Cu(111) electrodes in alkaline media.4 Strong morphological changes upon adatom modification lead to a significant HER rate enhancement. Intriguingly, this is induced through a decrease of the electric field strength negative of the potential of zero charge. This implies an easier reorganization of the interfacial water molecules facilitating charge transfer through the double layer, and thus enhancing the efficiency of electrocatalytic reactions.
References
[1] P. Sebastián-Pascual, F.J. Sarabia, V. Climent, J. Feliu, M. Escudero-Escribano, J. Phys. Chem. C 124 (2020) 23253.
[2] A. Auer, X. Ding, A. S. Bandarenka, J. Kunze-Liebhäuser, J. Phys. Chem. C 125 (2021) 5020.
[3] A. Auer, M. Andersen, E.M. Wernig, N.G. Hörmann, N. Buller, K. Reuter, J. Kunze-Liebhäuser, Nat. Catal. 3 (2020) 797.
[4] A. Auer, F.J. Sarabia, D. Winkler, C. Griesser, V. Climent, J.M. Feliu, J. Kunze-Liebhäuser, ACS Catal. 11 (2021) 10324.
1.2-T1
The electroreduction reaction of CO2 (eCO2RR) into hydrocarbons over copper electrodes has been studied extensively for the past few decades.[1] However, the eCO2RR mechanism, the activation of CO2 and the exact surface structure of the copper electrode are still heavily debated.[2] Raman spectroscopy is suitable for in situ characterization of CO2RR mechanisms, but the low signal intensity and resulting poor time resolution (often up to minutes) hampers the application of conventional Raman spectroscopy for the study of the reaction dynamics, which requires sub-second time resolution. In this presentation, I will discuss the development of time-resolved Raman spectroscopy (TR-Raman) with (sub) second time resolution, which allows us to investigate the electrocatalytic activation of CO2and the dynamic chemical structure of the electrode surface.[3] By subsequently measuring different Raman regions during a cyclic voltammetry scan, we are able to collect a broad range of vibrational modes related to adsorbed species (i.e. carbonate & CO) or the active surface (i.e. copper oxide and hydroxide) with sub-second time resolution. Our TR-Raman measurements reveal that the electrode surface is dynamic in the first seven seconds of cathodic bias onset due to stripping of surface Cu oxide species, after which the surface stabilizes as copper hydroxide due to local alkaline conditions.[3] After that, dynamic coupling of surface-bound carbon monoxide (CO) intermediates into ethylene is observed at an applied potential of -0.9 V vs. RHE, whereas lower cathodic bias (−0.7 V vs. RHE) results in gaseous CO production from isolated and static CO surface species. Furthermore, we observe the potential- and time-dependent adsorption of carbonate and bicarbonate electrolyte ions, which is a measure for variations in local alkaline conditions. Our combined electrochemical and TR-Raman measurements provide detailed information about the chemical structure of and the chemical landscape at the copper surface under reaction conditions.
1.2-T2
I am currently an Full Professor at the University of Bonn (Germany), Department of Chemistry. My overarching motivation is to discover and implement the chemistry necessary to transition to a sustainable energy-based society. Specifically, I am developing materials to convert electrical energy to fuels and chemicals.
Electrochemical conversion of abundant feedstocks to fuels and value-added chemicals is rapidly gaining significance as a promising method to harness renewable electricity. To this end, research group develops heterogeneous catalysts (e.g. nanomaterials, MOFs) geared for the reduction of CO2 and oxidation of biomass platforms to fuels and value-added chemicals. Further, we establish strategies in emergent areas of electrocatalysis such as electrochemically forming and cleaving C-N bonds. Because the design of new catalytic systems is inherently linked to a precise understanding of how these reactions proceed on heterogeneous surfaces, we put considerable efforts in developing methodology for operando probing of these systems with vibrational spectroscopy. In all, I show how using these experiments provides key mechanistic information on surface reaction mechanisms that enhance our understanding of functional interfaces and how the research provides avenues for future materials design within the context of renewable energy electrocatalysis.
1.2-T3
Freddy E Oropeza is a Marie Skłodowska-Curie fellow at IMDEA Energy. His research interests focus on the understanding and control of the structural and electronic properties that define the functionality of metal oxide thin films and interfaces for applications in electronics and (photo)electrochemical technologies. He obtained his PhD in Chemistry from the University of Oxford in 2012, followed by working as postdoc at the Ruhr University Bochum (2012-2013), Imperial College London (2013-2016) and the Eindhoven University of technology (2016-2019).
A range of transition metal-based materials such as metal chalcogenides, carbides, and phosphides as well as their alloys has emerged as earth-abundant electrocatalysts alternative to Pt for HER in either acidic or alkaline in recent years.[1,2,3] Despite the high electrochemical activity obtained with these types of materials, a fundamental understanding of the “real” active sites and the reaction mechanism is usually a challenge due to their characteristic high reactivity,[4] which makes it difficult to rely on ex-situ characterisation to elucidate the species and structure responsible for the performance.
We have been recently working on series of transition metal sulphides and selenides which exhibit high HER activity. Interestingly, we often observed that these materials also require a period of activation at the beginning of the electrochemical test in order to achieve high HER activity. In this contribution, we will discuss the activation process of α-NiS electrodes during the alkaline HER process. Based on operando X-ray absorption and Raman spectroscopies on α-NiS catalyst, we elucidate a complete phase transition from α-NiS to a mixed phase of Ni3S2 and NiO during HER operation. The synergistic effect of Ni3S2/NiO boosts a highly active HER with an overpotential of 85 for 10 mA cm-2 current density. Combined NAP-XPS and density functional theory (DFT) calculation reveal that Ni in NiO was identified as sites for OH* adsorption and S in Ni3S2 as sites for H* adsorption. The phase transition identified in our work highlights the significance of identifying the intrinsic active sites under realistic reaction conditions. Therefore, our study opens a new avenue for designing transition metal sulfide catalysts via the promotion of a phase transition with high activity.
1.2-T4
Born in Canada, Ronen Gottesman received his B.Sc. in Biophysics from the Department of Physics at Bar-Ilan University (BIU), Israel. He later conducted his Ph.D. at BIU in Physical Chemistry on the study of fundamental working mechanisms in photovoltaic systems which are based on nanoporous electrodes and hybrid perovskite absorbers. Currently, as a postdoc in the Institute for Solar Fuels at Helmholtz-Zentrum Berlin (HZB) his research topic is new complex metal oxides and oxynitrides photoabsorbers for solar fuels production, and the development of syntheses methods based on PLD+RTP and combinatorial approaches.
We will present the unique potential and effectiveness of rapid thermal processing (RTP) for overcoming two significant challenges in the development of oxide thin-film photoelectrodes. The first challenge is the need to bypass the normal temperature limit for the most commonly used glass-based F:SnO2 substrates (FTO, ~ 550 °C) in photoelectrochemistry and photovoltaics research. This limit is a problem since many multinary metal oxides require high processing temperatures (to at least ≈ 850 – 1000 °C in ambient pressure) to obtain the desired high density, high crystallinity, and low defect density.1 The second challenge is synthesizing multinary metal oxides that require a narrow range of optimal processing conditions (e.g., temperatures, heating times, and gas environment) in order to avoid undesired phase transformations and impurities segregation. We will show that RTP can overcome the first challenge by flash-heating Ta2O5, TiO2, and WO3 photoelectrodes to 850 °C without damaging the FTO, and the second challenge with the fabrication of ternary α-SnWO4, which recently attracted attention as a potential candidate for solar water oxidation. In both established and promising materials, heating by RTP resulted in superior crystallinity, electronic properties, and performance when compared with conventional furnace heating of similar photoelectrodes, culminating in a new performance record for α-SnWO4 for sulfite oxidation.
1.2-T5
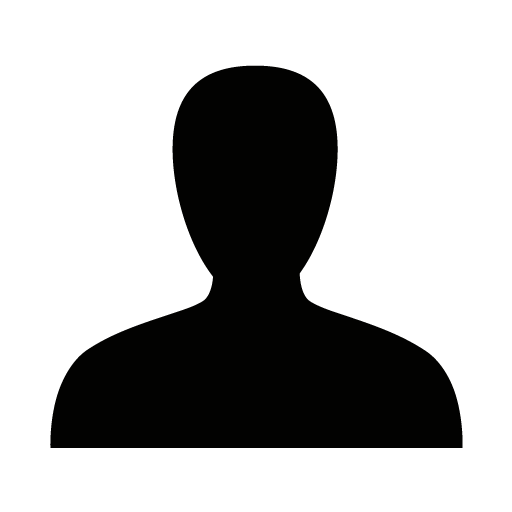
For photoelectrochemical energy conversion, metal nitride semiconductors have the potential to overcome several limitations associated with the more intensively investigated class of metal oxides. Among these materials, Ta3N5 is especially promising. However, it is commonly synthesized by nitridation of Ta2O5 films in ammonia atmosphere at high temperatures, which results in high concentrations of residual oxygen, nitrogen vacancies, and low-valent Ta cations within the Ta3N5 lattice. These defects often dominate the (opto)electronic properties of Ta3N5 photoelectrodes, impeding fundamental studies of its electronic structure, chemical stability, and photocarrier transport mechanisms. Here, we deposit tantalum nitride thin films by reactive magnetron sputtering and explore the role of subsequent NH3 annealing.[1] This synthesis process leads to thin films with near-ideal stoichiometry, as well as significantly reduced native defect and oxygen impurity concentrations compared to the commonly used nitridation of Ta2O5. By analyzing structural, optical, and photoelectrochemical properties as a function of NH3 annealing temperature, we provide new insights into the basic semiconductor properties of Ta3N5, as well as the role of defects on its optoelectronic characteristics. For example, the high material quality enables us to unambiguously identify the nature of the Ta3N5 bandgap as indirect, thereby resolving a long-standing controversy regarding the most fundamental characteristic of this material as a semiconductor. Improved understanding of not only the basic properties of this material, but also of how defect concentrations can be optimized, provides a path to high efficiency photoelectrodes.
1.3-I1
Boettcher is a Professor in the Department of Chemistry and Biochemistry at the University of Oregon. His research is at the intersection of materials science and electrochemistry, with a focus on fundamental aspects of energy conversion and storage. He has been named a DuPont Young Professor, a Cottrell Scholar, a Sloan Fellow, and a Camille-Dreyfus Teacher-Scholar. He was included as an ISI highly cited researcher (top 0.1% over past decade) over the past two years. In 2019, he founded the Oregon Center for Electrochemistry and in 2020 launched the nation’s first targeted graduate program in electrochemical technology.
Heterogeneous electrochemical processes, including photoelectrochemical water splitting to evolve hydrogen using electrocatalyst-coated semiconductors, are driven by the accumulation of charge carriers and thus the interfacial electrochemical potential gradients that promote charge transfer. Conventional electrochemical techniques measure/control potentials at the conductive substrate or semiconductor ohmic contact, but are unable to isolate processes and electrochemical potentials at the surface during operation. I will present our recent work demonstrating that the nanoelectrode tip of an atomic-force-microscope cantilever can effectively sense the surface electrochemical potential of electrocatalysts coating semiconductor photoelectrodes during operation. This technique allowed us to unambiguously show that metal (oxy)hydroxide layers act as both hole collectors and oxygen-evolution catalysts on metal-oxide photoanodes such as Fe2O3 and BiVO4. We also discovered the critical role that heterogeneous interfacial barrier heights, and a related nanoscale pinch-off effect, play in building carrier-selective interfaces in semiconductor photoelectrodes for generating fuel from sunlight. Recent results on Si, InP, and oxide based electrocatalyzed semiconductors will be discussed.
1.3-I2
Dr. Kelsey A. Stoerzinger joined Oregon State University as an Assistant Professor and Callahan Faculty Scholar in the School of Chemical, Biological and Environmental Engineering in the Fall of 2018. She holds a joint appointment at Pacific Northwest National Laboratory, where she was a Linus Pauling Distinguished Postdoctoral Fellow. Kelsey completed her doctoral studies in Materials Science and Engineering in 2016 from the Massachusetts Institute of Technology, supported by a National Science Foundation Graduate Research Fellowship. She received an M.Phil. in Physics from the University of Cambridge as a Churchill Scholar and a B.S. from Northwestern University. Prof. Stoerzinger is the recipient of the NSF CAREER Award (2020) and the Doctoral New Investigator Award of the ACS-PRF (2019), in addition to recognition for her contributions as a teacher and advisor.
Perovskite oxides (ABO3) are highly active for the oxygen evolution reaction (OER). Activity is observed to correlate with changes in bulk electronic structure parameters, such as metal-oxygen covalency and transition metal oxidation state. We employ spectroscopic approaches to consider such descriptors at the surface of perovskite oxides in situ, as well as their implications on the formation of surface adsorbates proposed to act as reaction intermediates during the OER.
Many surface science techniques, such as X-ray photoelectron spectroscopy (XPS), collect information from inherently surface-sensitive low-energy processes, requiring operation in ultrahigh vacuum. This constraint is lifted for ambient pressure XPS, which can probe the surface in equilibrium with the gas phase at pressures up to ~a few Torr, or with thin liquid layers using a higher incident photon energy. This presentation will discuss the insights obtained with this technique regarding the electronic structure of oxide electrocatalysts in an oxidizing or humid environment, as well as the reaction intermediates of relevance to electrocatalysis.1 We will then extend the technique to probe electrocatalysts in operando,2 driving current through a thin layer of liquid electrolyte and employing a tender X-ray source.
1.3-I3
This paper will focus on two key areas in solar fuels: (1) the development and application of advanced in-situ methods to achieve new fundamental insights, and (2) the design and development of new catalysts and chemical processes driven by renewables.
There are many challenges in the synthesis of solar fuels. This paper will describe efforts towards two reactions of interest, the hydrogen evolution reaction (HER) and the CO2 reduction reaction (CO2RR). First, we will cover research activities aimed at developing synchrotron-based methods aimed at shedding light onto catalysts that drive these important reactions, including Pd and Cu. Next, we will discuss efforts to design and develop advanced catalysts and their translation towards devices and technology in the solar fuels sector. The goal is ultimately to develop high performance systems with the desired efficiency, selectivity, and reaction rates for new technologies that can contribute to the fuels and chemicals sector. The role of theory and experiment collaboration will be highlighted.
2.1-I1
Many industrial chemical processes involve a high-energy demand (often still derived from fossil fuels), toxic reactants, and the production of high amounts of waste. Therefore, the development of more efficient, less hazardous technologies, based on renewable energies, has become one of the most challenging topics for chemical synthesis. For achieving these goals, the combination of catalysis with electrochemical methods, that is, electrocatalysis, can play a very important role [1]. With electrochemical methods, toxic and dangerous chemicals can be replaced with clean electrons, the efficiency and selectivity of the reactions can be tuned by choosing the applied potential, and more importantly, the energy used can come from renewable sources like wind or solar.
In this talk, I will focus on how electrocatalysis can help on the knowledge generation, reaction improvement and development of alternative industrial processes. I will give examples from studies of the electrosynthesis of high value chemicals such as organic carbonates, fuels and energy dense carriers.
2.1-I2
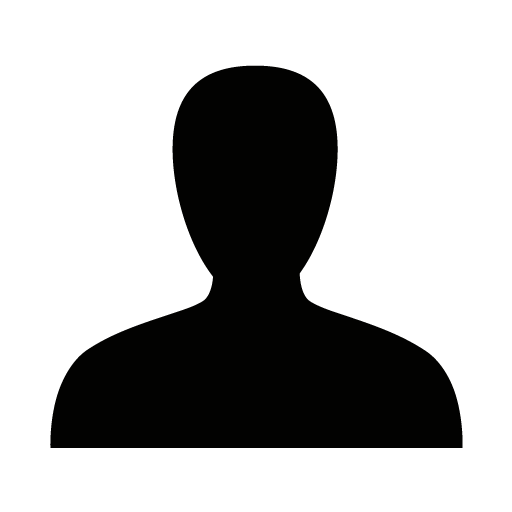
Ambient pressure X-ray photoelectron spectroscopy is a valuable tool for investigating surfaces and interfaces in elevated pressure conditions. We have recently constructed and commissioned a new end-station dedicated to Spectroscopic Analysis with Tender X-rays (SpAnTeX). The SpAnTeX end-station focuses on X-ray photoelectron spectroscopy measurements of solid-liquid interfaces. It is able to operate at pressures up to 30 mbar and photoelectron kinetic energies up to 10 keV. At the heart of the SpAnTeX end-station is a SPECS PHOIBOS 150 HV NAP electron spectrometer. This new spectrometer contains two additional features that allow for measurements with lateral resolution better than 30 μm and time resolved measurements with 100 ns or less time resolution. The SpAnTeX end-station is based on a modular concept which allows for the rapid exchange of sample environment modules. To date, we have constructed two modules. One module, the dip-and-pull module, is used for investigating solid-liquid interfaces under applied bias and illumination. The second module incorporates a droplet train which facilitates investigation of liquid phase processes with time resolution ranging from the μs to ms regimes. After a technical introduction to the SpAnTeX end-station and the experimental modules, results obtained using SpAnTeX will be presented. We will present results for Electrochemically Mediated Amine Regeneration (EMAR) for CO2 capture and sequestration processes, and light induced changes at the bismuth vanadate- and silicon-aqueous electrolyte interfaces.
2.1-I3
James Durrant is Professor of Photochemistry in the Department of Chemistry, Imperial College London and Ser Cymru Solar Professor, University of Swansea. His research addresses the photochemistry of new materials for solar energy conversion targeting both solar cells (photovoltaics) and solar to fuel (i.e.: artificial photosynthesis. It is based around employing transient optical and optoelectronic techniques to address materials function, and thereby elucidate design principles which enable technological development. His group is currently addressing the development and functional characterisation of organic and perovskite solar cells and photoelectrodes for solar fuel generation. More widely, he leads Imperial's Centre for Processable Electronics, founded the UK�s Solar Fuels Network and led the Welsh government funded S�r Cymru Solar initiative. He has published over 500 research papers and 5 patents, and was recently elected a Fellow of the Royal Society
The kinetics of electrochemical reactions are typically analysed through Butler-Volmer analyses of current – voltage data. Such analyses have been very effective at determining electrochemical kinetics on metal electrodes. However their application to the kinetics of (photo)electrocatalytic water oxidation / reduction on metal oxides can be more challenging, due to multiple redox transitions observed in such metal oxides, the localised nature of these transitions and the complexity of the water oxidation / reduction reactions. In my talk I will address the potential of operando spectrochemistry to determine redox state population densities in metal oxides electrodes and photoelectrodes, and the use of such data to undertake rate law analyses of water oxidation / reduction. These studies will primarily be applied to Ni/Fe oxyhydroxide electrocatalysts and hematite photoanodes for water oxidation, as well as comparison with other metal oxide for both water oxidation and reduction. These studies will address the nature of the states driving water oxidation / reduction and the reaction kinetics and dependence upon population density. For example for Ni(M)OOH electrocatalysts, these studies will address the impact of metal (M) substitution on both the population densities and reaction rate constants, and how these together impact upon the overall current / voltage behaviour. A key conclusion of my talk will be that for the systems studied the kinetics of water / oxidation appear to be primarily driven by the population of states driving these reactions – and as such it more appropriate to employ rate law rather than Butler-Volmer models in analysing these kinetics.
2.2-I1
Systematic computational materials modeling strategies using first-principles methods allow one to describe and understand chemistries of already known materials, and, importantly, they can be used to predict new materials through a careful analysis of the surface chemistry at the atomic level. In this talk, I demonstrate how we have been able to computationally predict oxide catalyst materials for the oxygen evolution reaction (OER) — that have been experimentally synthesized, characterized and tested. I then share recent theoretical insights on the stability-activity conundrum of oxide materials and present a computationally predicted nanostructured OER catalyst-systems that exhibit a new surface activation phenomenon leveraging our theoretical understanding. I conclude with some of our efforts to understand more complex nanostructured oxide materials.
2.2-I2
Associate Professor, Chemistry Department, University of Colorado, Boulder
Adjunct Professor, National Renewable Energy Laboratory
Catalytic mechanisms at electrode surfaces guide the development of electrochemically-controlled energy storing reactions and chemical synthesis. The intermediate steps of these mechanisms are challenging to identify experimentally, but are critical to understanding the speed, stability, and selectivity of product evolution. In the laboratory group, we employ photo-triggered vibrational and electronic spectroscopy to time-resolve the catalytic cycle at a surface, identifying meta-stable intermediates and critical transition states which connect one to another. The focus is on the highly selective water oxidation reaction at the semiconductor (SrTiO3)-aqueous interface, triggered by an ultrafast light pulse in an electrochemical cell. Here, I will summarize the work done to date by the group: the structure and kinetics of forming the initial intermediates that trap charge (Ti-OH*) through the next event at microseconds, suggested to be the formation of the first O-O bond of O2 evolution. There will be a focus on how time-resolving the intermediates leads to experimental identification of theoretical descriptors of oxygen evolution, one of which is the free energy difference to create the first meta-stable intermediate (DG1(OH*)). In so doing, reaction conditions that shift equilibria become an important, independent axis to the time & energy axes of the spectroscopy. While many open questions remain, these experiments provide and benchmark the opportunity to quantify intermediates at an electrode surface and follow a heterogeneous catalytic cycle in time.
2.2-I3
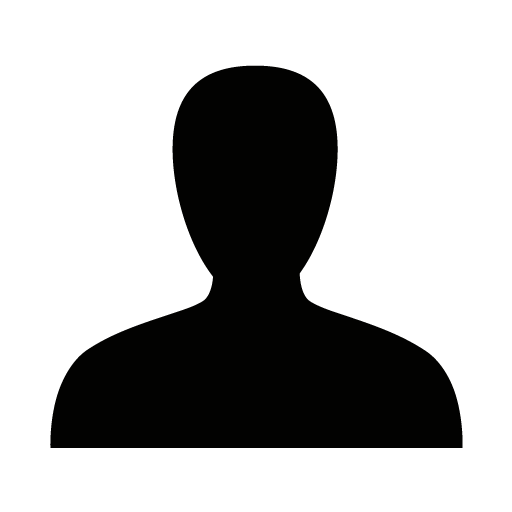
Electrified interfaces, such as electrodes for electrochemical CO2 reduction, can change their morphology as a reaction progresses and when the potential is changed. Likewise, the species in the electrolyte can change significantly while a reaction occurs. This makes operando probing important for understanding how reactions occur as these surfaces. Here, we have used electrochemical atomic force microscopy (EC-AFM) [1] and scanning electrochemical microscopy (AFM-SECM) to probe the cathode topography and the gradient in electrolyte pH near the cathode. I will discuss the experimental techniques, as well as the implications of the results on electrochemical CO2R reactors. I will focus on solvent (H2O) and solute (CO2(aq), CO(aq), OH-) activities [2], the nature of the triple-phase region in gas-diffusion electrodes (GDEs) [3], and how to optimize GDEs for selective and high current density CO2R.
1.1-I1
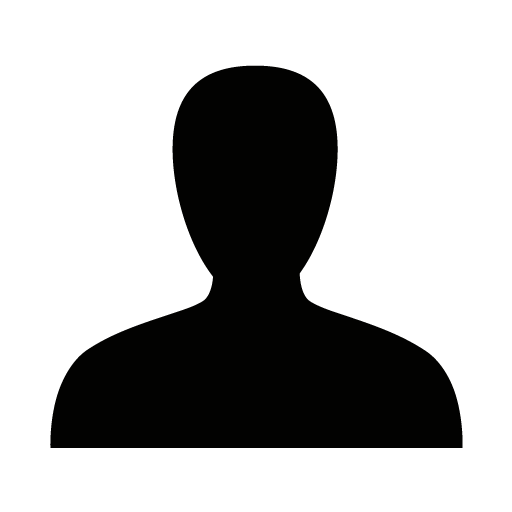
Taeghwan Hyeon received his B. S. (1987) and M. S. (1989) in Chemistry from Seoul National University (SNU), Korea. He obtained his Ph.D. in Chemistry from U. Illinois at Urbana-Champaign (1996), and conducted one-year postdoctoral research at the Catalysis Center of Northwestern University. Since he joined the faculty of the School of Chemical and Biological Engineering of Seoul National University in 1997, he has focused on the synthesis and applications of uniform-sized nanoparticles and related nanostructured materials, and published > 400 papers in prominent international journals (> 61,000 citations and h-index of > 125). He is a SNU Distinguished Professor. In September 2020, he was selected as 2020 Citation Laureate (known as Nobel Prize watch list) in Chemistry by Clarivate Analytics/Web of Science. In 2011, he was selected as “Top 100 Chemists” of the decade by UNESCO&IUPAC. Since 2014, he has been chosen as “Highly Cited Researcher” in Chemistry and Materials Science areas by Clarivate Analytics. Since 2012, he has been serving as a Director of Center for Nanoparticle Research of Institute for Basic Science (IBS). He is Fellow of Royal Society of Chemistry (RSC) and Materials Research Society (MRS). He received many awards including the Korea S&T Award from the Korean President (2016), Hoam Prize (2012, Samsung Hoam Foundation), POSCO-T. J. Park Award (2008), and the IUVSTA Prize for Technology (International Union for Vacuum Science, Technique and Applications, 2016). From 2010 to 2020, he served as an Associate Editor of Journal of the American Chemical Society. He has been serving as editorial (advisory) board members of ACS Central Science, Advanced Materials, Nano Today, and Small.
We present a synthesis of highly durable and active electrocatalysts based on ordered fct-PtFe nanoparticles and FeP nanoparticles coated with N-doped carbon shell (J. Am. Chem. Soc. 2015, 137, 15478; J. Am. Chem. Soc., 2020, 142, 14190; J. Am. Chem. Soc. 2017, 139, 6669). We report on the design and synthesis of highly active and stable Co-N4(O) moiety incorporated in nitrogen-doped graphene (Co1-NG(O)) that exhibits a record-high kinetic current density (2.84 mA cm-2 at 0.65 V vs. RHE) and mass activity (277.3 A g-1 at 0.65 V vs. RHE) with unprecedented stability (>110 h) for electrochemical hydrogen peroxide (H2O2) production (Nature Mater. 2020, 19, 436). We report on the design and synthesis of highly active TiO2 photocatalysts incorporated with site-specific single copper atoms (Cu/TiO2) that exhibit reversible & cooperative photoactivation process, and enhancement of photocatalytic hydrogen generation activity (Nature Mater. 2019, 18, 620). We synthesized multigrain nanocrystals consisting of Co3O4 nanocube cores and Mn3O4 shells. At the sharp edges of the Co3O4 nanocubes, we observed that tilt boundaries of the Mn3O4 grains exist in the form of disclinations, and we obtained a correlation between the defects and the resulting electrocatalytic behavior for the oxygen reduction reaction (Nature 2020, 359, 577).
We fabricated ultraflexible and/or stretchable soft-electronic and optoelectronic devices integrated with various functional nanomaterials and their applications to wearable and implantable medical and healthcare devices. We introduced electromechanical cardioplasty using an epicardial mesh made of electrically conductive and elastic Ag/Au nanowire-rubber composite material to resemble the innate cardiac tissue and confer cardiac conduction system function (Science Transl. Med. 2016, 8, 344ra86; Nature Nanotech. 2018, 13, 1048). We fabricated highly conductive and elastic nanomembrane for skin electronics (Science 2021, abh4357). We reported graphene-hybrid electrochemical devices integrated with thermo-responsive micro-needles for the sweat-based diabetes monitoring and feedback therapy (Nature Nanotech. 2016, 11, 566).
1.1-I2
ICREA Prof. Jordi Arbiol was born in Molins de Rei (Catalonia) in 1975. Having graduated in physics from the Universitat de Barcelona (UB) in 1997, he went on to obtain his PhD in transmission electron microscopy as applied to nanostructured materials from this same university in 2001, earning the “European Doctorate” label in recognition of the project’s European dimension, as well as the university’s extraordinary doctorate award. He then held the position of assistant professor at the UB, before becoming a group leader at the Institut de Ciència de Materials de Barcelona in 2009, as well as the scientific supervisor of this institute’s electron microscopy facility. It was here that he began his personal and professional mission to improve Barcelona’s baseline electron microscopy infrastructure, an endeavour he has continued to pursue at the ICN2, which he joined in 2015 as the leader of the Advanced Electron Nanoscopy Group.
He was President of the Spanish Microscopy Society (SME) (2017-2021), Vice-president (2013-2017) and member of its Executive Board (2009-2021). In 2019 he became a Member of the Executive Board of the International Federation of Societies for Microscopy (IFSM) (2019-2027). He is member of the Research Committee at the Barcelona Institute of Science and Technology (BIST) and scientific supervisor of Electron Microscopy at ICN2 and the ALBA Synchrotron EM Center.
Other recognitions include the FWO Commemorative Medal in 2021, the BIST Ignite Award in 2018, the 2014 EU40 Materials Prize (E-MRS), the 2014 EMS Outstanding Paper Award and being listed in the Top 40 under 40 Power List (2014) by The Analytical Scientist. He currently has more than 410 peer-reviewed publications, h-index 87 GoS (76 WoS), with more than 24,400 GoS (19,000 WoS) citations.
Technology at the nanoscale has become one of the main challenges in science as new physical effects appear and can be modulated at will. Especially 2D nanomaterials can be designed and engineered in order to improve their performance and efficiency for energy and environmental applications. In this way, a proper selection of defects, grain boundaries and surfaces, or the right selection of dopants allow a major increase on the properties of a new generation of (photo)electrocatalysts.
In the present work, by using powerful advanced electron microscopy related techniques, we will move to the atomic scale in order to visualize the beauty of such nanostructures. Modified nanostructures as support for single atom catalysts with a great enhancement on lithium-sulphur batteries stability and performance or CO2 Reduction will be shown [1]. Nanoengineered atom-thin transition metal dichalcogenides (MoS2 and WS2) showing a high density of grain boundaries acting as efficient active sites for the hydrogen evolution reaction (HER) will be also studied at the atomic scale [2]. A glimpse on the latest single atom catalysts developed in the group for CO2 Reduction (CO2RR) will be also shown [3]. Atomic resolution electron microscopy analyses will help us to visualize such fancy nanostructures and allow us to create 3D atomic models in order to understand not only the growth mechanisms implied, but also to be used as input models for further DFT simulations, which will allow us to gain knowledge on the novel catalytic mechanisms achieved.
We will show our latest results on direct visualization and modelling of nanomaterials at atomic scale, which will help to understand their growth mechanisms (sometimes complex) and also correlate their chemical properties ((photo)electrocatalytic) at sub-nanometer scale with their atomic scale structure.
1.1-I3
Dr. Pramod Pillai is an Associate Professor and a Physical Chemist in the Department of Chemistry at Indian Institute of Science Education and Research (IISER) Pune, India. Dr. Pillai obtained his Ph.D. in Chemistry in 2008 under the supervision of Prof. K. George Thomas at National Institute for Interdisciplinary Science and Technology (NIIST) Trivandrum, India. Prior to joining IISER Pune in June 2014, Dr. Pillai was a postdoctoral fellow in the group of Prof. Bartosz A. Grzybowski at Northwestern University, Evanston, USA (2011-2014), and an Alexander von Humboldt postdoctoral Fellow at Technische Universität in Dortmund, Germany with Prof. Christof M. Niemeyer (2008-2010). Currently Dr. Pillai’s research at IISER Pune is focused on controlling the interplay of forces to improve and impart newer properties at the nanoscale. Some of the properties of interest includes light harvesting, catalysis and self-assembly in hybrid nanomaterials.
The ability to move energy and electrons, under the influence of light, in an efficient manner is one of the fundamental challenges in the area of energy research. Our group is interested in designing principles based on the interplay of forces at the nanoscale, to improve the light harvesting properties of hybrid nanomaterials. For instance, the potency of electrostatic forces was elegantly explored to demonstrate efficient light induced energy and electron transfer processes in cationic quantum dots (QDs), in water. Further, a fine control over such interaction driven photophysical processes helped in the creation of high-contrast multicolor luminescent patterns from a single QD nanohybrid film. For this, the energy and electron transfer processes in QD thin films were regulated through the selective and controlled photodegradation of organic acceptor molecules. Similar control over interparticle interactions helped in outplaying the ligand poisoning effect in nanoparticle catalyzed photochemical transformations, and some of these aspects will be covered in the presentation. Such advancements in the existing light harvesting properties of nanomaterials, through the fine control of interactions at the nanoscale, can expand the scope of nanoscience in energy research.
1.2-T1
My research is focused on synthesis of (2D) two-dimensional materials and in surface-characterizations. I specialized in synthesis of 2D heterostructures with both "bottom-up" and "top-down" approaches. I use the surface science tools as main characterization of the materials, in particular I analyse their electronic properties by photoemission.
So far, I am coauthor of 48 peer-rewied international publications. In 2018, I published a review paper about the use of micro-angle resolved photoemission spectroscopy (µ-ARPES) for 2D materials [M. Cattelan*, N. A. Fox, Nanomaterials, 2018, 8, 284]. In 2020 I was part of the development of an angle resolved secondary electron spectroscopy [G. Wan ... M. Cattelan*, Advanced Functional Materials, 2021, 31, 2007319].
I gained my PhD in 2016 in Materials Science and Engineering at Surface Science and Catalysis Group of University of Padova (Italy).
My research focused on the growth and chemical-structural characterization of graphene, doped graphene and other 2D materials. I focused on “bottom-up” approach by ultra-high vacuum (UHV) in-situ preparation and chemical/physical characterization of 2D layers to analyse materials without air exposure and contamination. During the period in the Surface Science and Catalysis Group, I also acquired experience in synchrotron techniques.
From 2016 - 2020 I was NanoESCA Specialist Senior Research Associate of University of Bristol (UK).
I was the specialist and facility manager of the Bristol NanoESCA Facility, a state-of-the-art laboratory-based UK strategic equipment for surface analysis. The facility hosts a photoemission electron microscopy (PEEM) (NanoESCA II) which allows performing µ-ARPES, micro-ultraviolet photoelectron spectroscopy (µ-UPS) and local work function measurements.
From 2020, I am Research Associate of Spectromicroscopy beamline at Elettra synchrotron (Italy).
This beamline hosts a unique setup for µ-ARPES which allows the investigation of the band structure in a submicron spot. The scanning photoemission microscopy (SPEM) allows also acquiring micro- x-ray photoemission spectroscopy (µ-XPS) and dark-field SPEM. The quality and efficiency of Spectromicroscopy attract the best solid-state physics research groups, analysing several different materials including graphene, 2D semiconductors devices, high-temperature superconductors etc. I am focusing my efforts on innovative 2D materials heterostructures. I am working on a setup to fabricate complex structures of these materials using a “top-down” approach, by exfoliation of bulk crystals and dry transfer techniques.
In this talk I would like to present a perspective on the application of micro- Angle-Resolved PhotoEmission Spectroscopy (µ-ARPES) to study two-dimensional (2D) materials heterostructures. [1]
ARPES allows the direct measurement of the electronic band structure of solid-state materials, generating extremely useful insights into their electronic properties. The possibility to apply it to 2D materials is of paramount importance because these ultrathin layers are considered fundamental for future electronic, photonic and spintronic devices.
Moreover, recent technical developments of ARPES allow to visualize the entire band structure (including empty states) [2] and to obtain spin, real-space, many-body effects and electron-dynamics information. Direct measurements of k-space position, binding energy, effective mass, spin and dynamics of electrons at Valence Band Maximum and Conduction Band Minimum, are making ARPES an unmissable tool of investigation for 2D materials.
In this context, µ-ARPES has been developed for micro-area analysis, allowing the measurements of small samples and detecting variation of their properties in the micro-scale. It is routinely applied for exfoliated 2D materials and can complete other µ-investigations such as µ-Raman, µ-photoluminescence and scanning probe microscopies. µ-ARPES is very useful to understand electronic, spintronic and optoelectronic measurements for 2D heterostructures.
Because lateral dimensions and quality of sample are crucial for µ-ARPES, I would also briefly explain the preparation methods of 2D materials and heterostructures, presenting pros and cons of “bottom-up” [3] and “top-down” methods.
Some of the most interesting results obtained by ARPES for 2D heterostructures will be reported.
1.2-T2
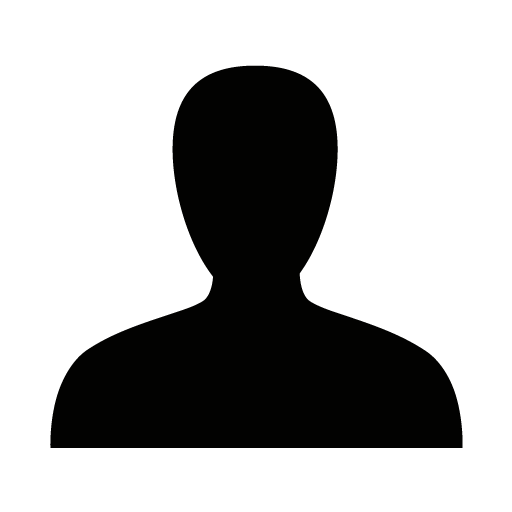
Transition metal dichalcogenide (TMD) nanosheets have become an intensively investigated topic in the field of 2D nanomaterials, due to their semiconductor nature, the direct band gap transition and the broken inversion symmetry going from bulk to monolayer. These properties makes TMDs suitable for different technological applications such as photovoltaics, valleytronics, or hydrogen evolution reactions (HER), or transistors. Among them, MoX2 (X = S, Se) are only direct-gap semiconductors when their thickness is reduced to a monolayer, hence an important effort is devoted to obtain single layer TMDs. Colloidal synthesis of TMDs has been developed in recent years as it provides a cost-efficient and scalable way to produce few-layer TMDs having homogenous size and thickness, yet obtaining a monolayer has proved challenging. Here we present a general method for the colloidal synthesis of mono- and few-layer MoX2 (X = S, Se) using elemental chalcogenide and metal chloride as precursors. Using a synthesis with slow injection of the MoCl5 precursor under nitrogen atmosphere, and optimizing the synthesis parameters with a Design Of Experiments (DOE) approach, we obtained a monolayer MoX2 sample with the required semiconducting (2H) phase, a band gap of 1.96 eV for 2H-MoS2 and 1.67 eV for 2H-MoSe2, respectively, both displaying fluorescence at cryogenic and elevated temperatures. A correlation between the blue shifted absorption spectrum and the spectral difference between the Raman modes was established and confirmed that a single-layer thickness was obtained.
1.2-T3
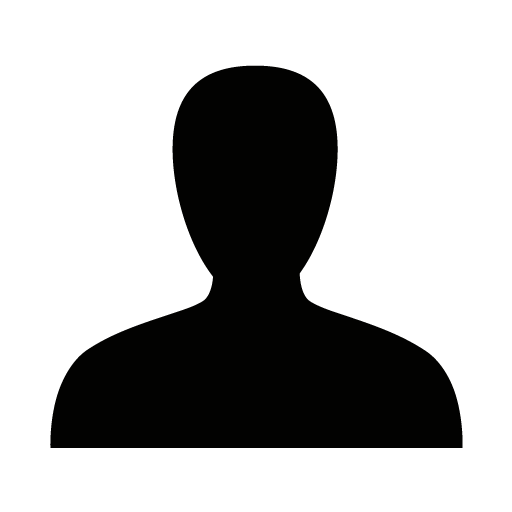
Liquid phase exfoliation (LPE) offers a scalable method to prepare colloids of two-dimensional (2D) Van der Waals (VdW) solids. Over the years, LPE has been rationalized within the context of solution thermodynamics. We outline this approach by introducing a method for the LPE of rhenium disulfide (ReS2), a promising novel semiconducting 2D material. By screening LPE in various solvents, we retrieve optimal conditions for high yield ReS2 exfoliation in solvents characterized by similar Hildebrand and Hansen solubility parameters – a result in agreement with the solution thermodynamics approach. Besides, our thermodynamic analysis points out that the current method to describe LPE fails to account for the considerable tolerance on the solubility parameter mismatch. In other words, the window for solvent exfoliation is much broader than solution thermodynamics allows for based on the size of the colloid.
We combine our experimental dataset on ReS2 with an extensive survey of literature studies to reconcile the too-broad exfoliation window observed for 2D VdW solids. Starting from standard Flory-Huggins theory, we address inconsistencies in the current line of thought and propose a more coherent way of working based on the critical exchange parameter χc – an idea inspired by polymer physics. In such a framework, suitable exfoliation solvents result in exchange parameters below a critical value χc. Notably, the predicted solubility parameter window for suitable exfoliation solvents based on such a criterium agrees well with the experimental data available for a wide range of 2D VdW solids. Therefore, we conclude that solution thermodynamics describes LPE, provided that the solubility parameter window is linked to the critical exchange parameter for solvent/solute immiscibility.
1.2-T4
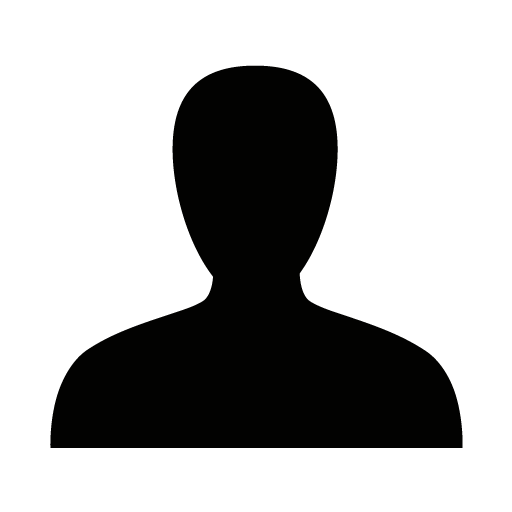
We investigate the charge carrier mobility in 1D and 2D II-VI semiconductor nanoparticles. Based on a quantum mechanical modeling of CdSe Nanorods and -platelets, we provide a microscopic understanding of the frequency-dependent charge carrier transport in structures of finite lateral size. In contrast to Drude-type terahertz conductivity models, which imply a quasi-continuous density of states, the domain size of 1D or 2D nanoparticles strongly affects the frequency-dependent mobility and strong confinement can further result in oscillations for sub-resonant THz-probing, as seen in experiments. In 2D systems the mobility is further governed by transitions in the two orthogonal x-
and y-directions and depends nontrivially on the THz polarization, as well as the quantum well lateral aspect ratio, defining the energetic detuning of the lowest THz-photon transitions in both directions.
1.3-T1
Indium Phosphide Quantum Dots (InP QDs) are promising non-toxic alternatives to the more established cadmium-based QDs for applications such as light-emitting diodes and display technologies. However, as-synthesized InP QDs exhibit low photoluminescent efficiencies and broad emission linewidths, hindering their successful implementation in commercial products. Those opto-electronic characteristics are strongly influenced by the surface chemistry of the QDs. Most importantly, surface defects such as under-coordinated atoms lead to localized trap states, strongly decreasing the emission efficiencies. To improve the properties of InP QDs, it is thus of uppermost importance to understand the termination of the inorganic QD cores, the binding modes of organic ligands, and to explore ways of tweaking the surface chemistry on demand.
The InP QDs studied in this work are synthesized via the aminophosphine-route, resulting in tetrahedrally shaped QDs, colloidally stabilized by oleylamine (OLA), and co-passivated by halide anions. In contrast to the traditional carboxylate-passivated InP QDs, the surface chemistry of the halide-amine InP QDs is still unexplored, and strategies to improve their emission qualities by surface treatments are limited.
To address this, we first performed ligand titration experiments with thiols and carboxylic acids to investigate the binding mode of OLA. Monitoring by quantitative nuclear magnetic resonance (NMR) spectroscopy and elemental analysis, we confirmed that OLA binds as a neutral L-type ligand, and can be replaced in an acid-base mediated ligand exchange. We further point out that surface-bound thiolates and carboxylates respectively decrease and increase the band edge emission of the InP QDs. Density functional theory (DFT) calculations confirm our finding, suggesting that thiolates easily form localized surface trap states.
In a second step, we added ZnCl2 as Z-type ligand to further increase the PLQY of the halide-amine co-passivated InP QDs. Most importantly, we find that pure metal chlorides strip OLA from the surface in a Z-type mediated L-type ligand replacement reaction, thus destabilizing the QDs. Interestingly, the complexation of ZnCl2 with OLA prevents spurious aggregation. In this case, the binding of ZnCl2 to the surface triggers band edge luminescence. DFT calculations support these findings, and show how the adsorption of ZnCl2 to under-coordinated P atoms can remove hole trap states.
1.3-T2
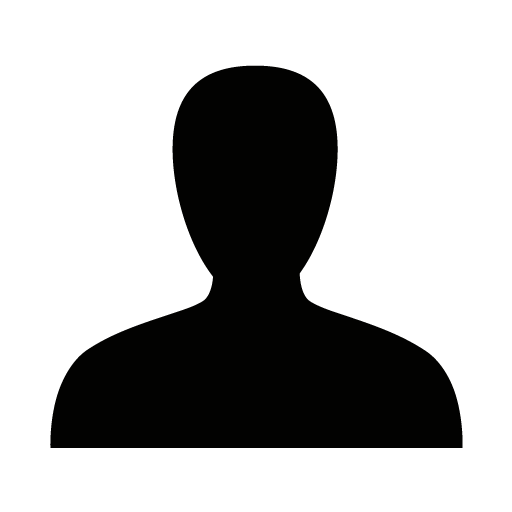
Colloidal InAs quantum dots are ideal candidates for QD based technologies in the near infrared region of the electromagnetic spectrum. Despite significant effort, scalable synthesis methods that produce large InAs nanocrystals absorbing beyond 1400 nm are scarce, rely on harsh precursors, require long reaction times and typically yield poorly luminescing QDs. In this work we overcome these challenges and report a 1-step colloidal synthesis of tetrahedrally shaped InAs QDs with tunable edge lengths between 4.5 and 9.5nm, relative size dispersions of 10% and excitonic absorption features with maxima as far as 1550 nm. With tris(diethylamino)phosphine as a mild reducing agent to synthesize InAs from InCl3 and tris(trimethylamino)arsine, we identify that the growth of InAs is triggered only at high temperatures. As a consequence, dilution and a steep temperature ramp can be used to suppress nucleation and promote growth, respectively. With these simple strategies, different sizes of InAs QDs can be synthesized in a reaction time of just 1 hour. Furthermore, after ligand exchange reactions with alkanethiols or zinc oleate the already luminescent InAs core is passivated better to yield PLQYs of 9 and 52% for the respective ligands. This work demonstrates the value of identifying and optimizing fundamental nanocrystal synthesis conditions, which culminates here in a meticulous control over InAs QDs with application ready optical properties.
1.3-T3
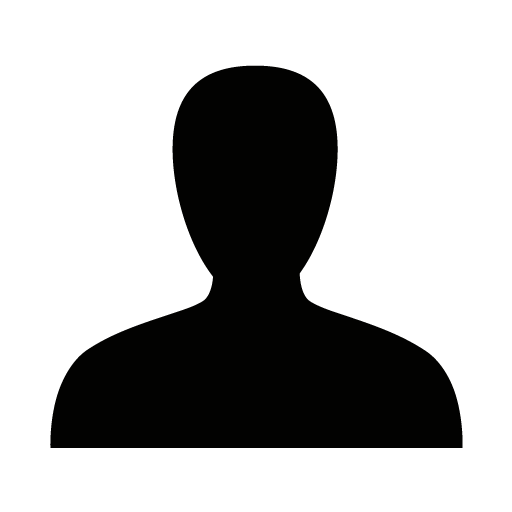
Quantum dots (QDs) are novel, nano-sized semiconductors with a size-tunable bandgap due to the quantum confinement effect [1]. Over the past few decades, the surface passivation of Cd- and Pb-based QDs has been studied using both experimental and computational methods, leading to significant advancements in the preparation of high-quality Cd- and Pb-based QDs [2,3]. Unfortunately, the toxicity of Cd- and Pb-based QDs often limits the utility of these QDs in consumer devices [4]. In recent years, indium phosphide (InP) has rapidly gained attention as a non-toxic alternative to Cd-based QDs [5]. However, as-synthesized InP QDs have a large density of trap states originating from unsaturated surface atoms, resulting in low PLQYs [5,6].
We report post-synthetic surface passivation utilizing benzoic acid (BZA) as an X-type surface ligand. To understand how BZA impacts their electronic structure, we conducted spectroscopic studies on InP QDs with various surface modifications, including in-situ fluorination and post-synthetic BZA treatment. Comparison of a variety of time-resolved spectroscopic techniques reveals that BZA can selectively remove electron trap states in InP QDs by passivating unsaturated indium atoms at the QD surface. When the BZA treatment is used in combination with a well-established fluoride treatment, the photoluminescence quantum yield of these unshelled InP QDs exceeds 20%. Compared with previous post-synthetic methods to increase the performance of InP QDs, including treatment with Z-type ligands and HF etching, the BZA treatment is green, safe, and easy to use. This research advances our understanding of the function of X-type ligands as passivants for unsaturated indium atoms for the post-synthetic treatment of InP QDs.
1.3-T4
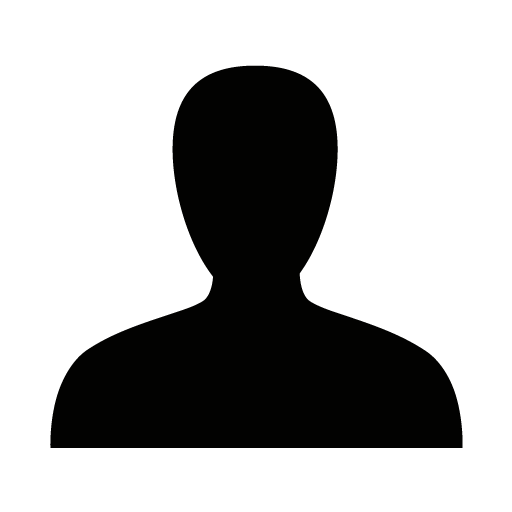
Photo-induced charge transfer is at the heart of many solar harvesting technologies including ones that involve semiconductor nanocrystals. To increase the efficacy of these technologies, it is imperative that the energetic barriers to charge separation and transfer are reduced. We have examined the unexplored role of the redox acceptor’s internal reorganization energy in effecting the charge transfer rates from quantum dots. The charge transfer rate to cobalt complexes having an electrochemical reduction potential difference of only 350 mV, but having nearly 2 eV difference in the reorganization energy, was experimentally studied and modelled with Marcus Theory. While driving force is important to the electron transfer rates, the difference in reorganization energy proved to have a more profound effect altering charge transfer rates by several orders of magnitude. The design of redox mediators to minimize reorganization energy rather than focusing solely on driving force would seem to be a previously ignored method to increase the efficiency of charge transfer from quantum dot applications.
1.4-I1
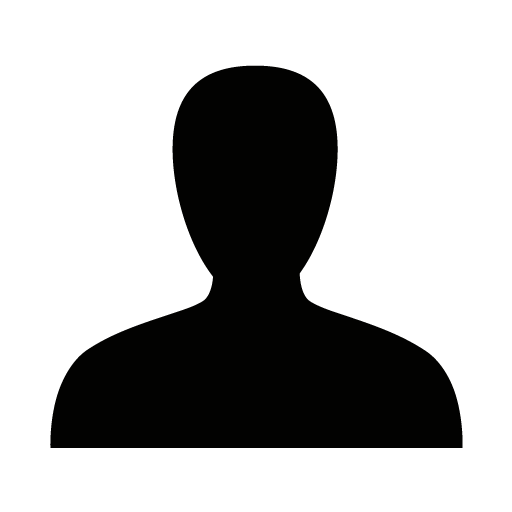
Sorting through nanoparticles and nanoplatelets with first principles calculations
Using first principles molecular dynamics and electronic structure calculations, we investigate fundamental properties of complex semiconducting nanoparticles and nanoplatelets and their relevance to the design of electronic and solar energy conversion devices. In particular, we discuss electronic properties [1] and structure-function relationships [2], including ensemble of nanoparticles [3-5].
[1] Stoichiometry of the Core Determines the Electronic Structure of Core-Shell III–V/II–VI Nanoparticles, Mariami Rusishvili, Stefan Wippermann, Dmitri Talapin and Giulia Galli, Chem. Mater., 32, 22, 9798-9804 (2020).
[2] Determining the Structure–Property Relationships of Quasi-Two-Dimensional Semiconductor Nanoplatelets, Arin Greenwood, Sergio Mazzotti, David Norris and Giulia Galli, J. Phys. Chem. C, 125, 8, 4820 (2021).
[3] Modelling Superlattices of Dipolar and Polarizable Semiconducting Nanoparticles, Sergio Mazzotti, Federico Giberti and Giulia Galli, Nano Letters 19(6), 3912-3917 (2019).
[4] Surface Chemistry and Buried Interfaces in All-Inorganic Nanocrystalline Solids, Emilio Scalise, Vishwas Srivastava, Eric M. Janke, Dmitri Talapin, Giulia Galli, and Stefan Wipperman, Nature Nanotechnology, 13, 841-848 (2018).
[5] Optical Absorbance Enhancement in PbS QD/Cinnamate Ligand Complexes, Daniel Kroupa, Márton Vörös, Nicholas Brawand, Noah Bronstein, Brett McNichols, Chloe Castaneda, Arthur Nozik, Alan Sellinger, Giulia Galli and Matthew Beard, J. Phys. Chem. Lett., 9(12), 3425-3433 (2018).
1.4-I2
The surface structure of nanocrystals (NC) strongly impacts their chemical and optolectronic properties because of their large surface area to volume ratios. However, techniques that can provide experimental atomic-level surface structures of NC are lacking. Here, we show how magic angle spinning (MAS) dynamic nuclear polarization (DNP) solid-state NMR spectroscopy can be used to determine the surface structures of some of the most widely investigated nanocrystals, zinc blende CdSe NCs with spheroidal and plate morphologies.[1] 1D 113Cd and 77Se cross-polarization magic angle spinning (CPMAS) NMR spectra reveal distinct signals from Cd and Se atoms on the surface of the nanoparticle, and those residing in bulk-like environments below the surface. 113Cd magic-angle-turning NMR experiments identifies CdSe3O and CdSeO3 coordination environments from {111} facets and CdSe2O2 coordination environments from {100} facets, where the oxygen atoms are from coordinated oleate ligands. The sensitivity gains from DNP enables acquisition of natural isotopic abundance 2D homonuclear 113Cd and 77Se and heteronuclear 113Cd-77Se correlation solid-state NMR experiments. 2D homonuclear 113Cd and 77Se correlation spectra reveal the connectivity of the surface and core Cd and Se atoms. Importantly, 77Se{113Cd} scalar heteronuclear multiple quantum coherence (J-HMQC) experiments illustrate the connection between the various Cd and Se environments and can be used to selectively measure one-bond 113Cd-77Se scalar coupling constants (1JSe-Cd). With knowledge of 1JSe-Cd, 77Se{113Cd} heteronuclear spin echo (J-resolved) NMR experiments are then used to determine the number of Se atoms bonded to Cd atoms, and vice versa. The J-resolved experiments directly confirm that major Cd and Se surface species have CdSe2O2 and SeCd4 stoichiometries, respectively. Considering the crystal structure of zinc blende CdSe and the NMR data obtained from NC with spheroidal and platelet morphologies, we conclude that the surface of the spheroidal CdSe nanocrystals is primarily composed of {100} and {111} facets. We will also present preliminary data showing how these methods can be used to probe the surface structure of ligand exchanged CdSe nanocrystals that are passivated with phosphine, amine and chloride ligands. The methods outlined here will be generally applicable to obtain detailed surface structures of a wide variety of main group semiconductors.
1.4-I3
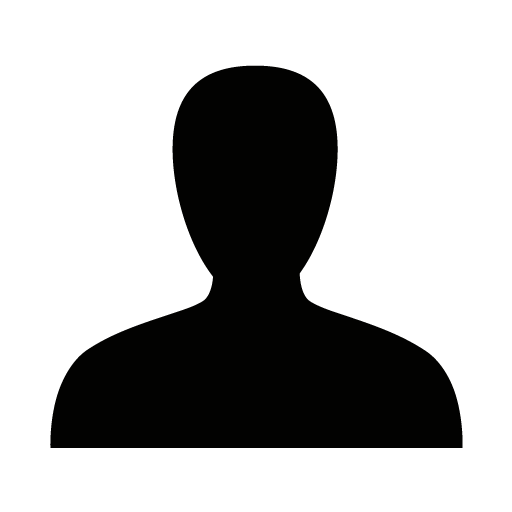
Metal oxide nanocrystals doped with a few percent of aliovalent dopants become electronically conducting and support strong light-matter interactions in the infrared due to localized surface plasmon resonance (LSPR). In the prototypical material tin-doped indium oxide (ITO), we explored the influence of the spatial distribution of electrons on optical and electronic properties. In as-synthesized nanocrystals, electrons are largely confined to a plasmonic core, surrounded by an electron-depleted shell governed by the electronic states at the nanocrystal surface. Deliberately sequestering dopants in either the core or shell of the nanocrystals modulates the electrostatic landscape and shapes the plasmonic volume, including compression or expansion of the depletion layer. Core- or shell-doped nanocrystals exhibit multimodal optical spectra that respond strongly to changes in the dielectric environment. In thin films of nanocrystals, electronic conductivity is greatly enhanced in shell-doped nanocrystals wherein the barrier to nanocrystal-nanocrystal electron transfer is minimized.
2.1-I1
The Kraus groups studies the basic mechanisms of the formation and the properties of colloidal particles and their interfaces. At INM - Leibniz Institute for New Materials, it uses such mechanisms to join molecules, polymers, and colloidal particles in order to form materials. (Link)
We study how the properties of composite and hybrid materials depend on their microstructures and how to change them. To this end, we systematically vary size, geometry, chemical composition, and arrangement of the materials’ constituents. We observe how microstructure and interfaces form and affect material properties to create transparent conductive layers of metal nanoparticles for electronics, composites of conductive polymers with optically active particles for sensors and supraparticles that contain optically active nanoparticles, for example. We see particles as the basis of future “active nanocomposites” that can interface with electronics and change their properties whenever required.
We study liquid inks that form hybrid functional electronic films when drying. The interplay between organic and inorganic components affects structure and electronic properties. In this talk, I will focus on the case of metal or semiconductor particles and discuss how non-polar organic shells change their agglomeration and assembly into dielectric films [1].
Experiments that monitor the agglomeration and self-assembly in non-polar dispersions allow us to follow the film formation of spherical and anisometric particles. Systematic variation of the geometry of spherical particles with metal or semiconductor cores and dense alkyl shells indicate a transition between core- and shell-dominated assembly of non-polar dispersions that I will outline [2]. The comparison of spherical particles with ultrathin wires indicates that entropic effects strongly depend on solvent molecule geometry and that they can induce nanowire agglomeration or “bundling” [3]. Comparatively little is known on the assembly of highly concentrated dispersions. I will introduce new types of experiments that probe this regime using small, evaporating droplets of dispersions that we bring into the X-ray beam of a small-angle beamline [4].
Complexity increases when using electronically conductive organic shells on inorganic particles. I will briefly discuss hybrids of gold spheres that are coated with polythiophenes. The molecular arrangement of the monomer units on the metal surface depends on the core geometry and affects bulk conductivity [5].
2.1-I2
Maria Ibáñez was born in La Sénia (Spain). She graduated in physics at the University of Barcelona, where she also obtained her PhD in 2013, under the supervision of Prof. Dr. Cabot and Prof. Dr. Morante. Her PhD thesis was qualified Excellent Cum Laude and awarded with the Honors Doctorate by the University of Barcelona. Her PhD research was funded by a Spanish competitive grant (FPU) which supported her to conduct short-term research stays in cutting-edge laboratories. In particular she worked at CEA Grenoble (2009), the University of Chicago (2010), the California Institute of Technology (2011), the Cornell University (2012) and the Northwestern University (2013). In 2014, she joined the group of Prof. Dr. Kovalenko at ETH Zürich and EMPA as a research fellow where in 2017 she received the Ružička Prize. In September 2018 she became an Assistant Professor (tenure-track) at IST Austria and started the Functional Nanomaterials group.
Conforming thermal energy to electricity and vice versa through solid-state thermoelectric devices is appealing for many applications. Not only because thermal waste energy is generated in many of our most common industrial and domestic processes but also because thermoelectric devices can be used for temperature sensing, refrigeration, etc. However, their extended use has been seriously hampered by the relatively high production cost and low efficiency of thermoelectric materials. The problem is that thermoelectric materials require high electrical conductivity, high Seebeck coefficient, and low thermal conductivity, three strongly interrelated properties.1
Thermoelectric materials are often dense, polycrystalline inorganic semiconductors. Usually, the processing of such materials has two steps: preparing the semiconductor in powder form and consolidating the powder into a dense sample. The most common route to prepare powders among the thermoelectric community is through high-temperature reactions and ball milling. Alternatively, solution methods to produce powders with much less demanding conditions (e.g. lower reagent purity, lower temperatures, shorter reaction times) have been explored to reduce the production costs. These methods also provide opportunities to produce particles with better-controlled features, such as crystallite size, shape, composition, and crystal phase, which allow modifying the properties of the consolidated material. However, when dealing with powders produced in solution, one should pay special attention to potential undesired elements coming from the reactants. Those elements may not affect the crystal structure and bulk composition of the powder but can be present as surface adsorbates. The composition, chemical stability, and bonding nature of surface species can influence the sintering process, and reaction byproducts can determine the final properties of the consolidated material.
Herein, we will demonstrate the importance of surface species in the use of solution-processed particles as precursors for bulk thermoelectric materials. In particular, we will provide examples in which surface species are used to deliberate control of the type and density of major carriers, engineer the electronic band structure, define composite microstructure, and hence charge carrier mobility and phonon transport.
2.1-I3
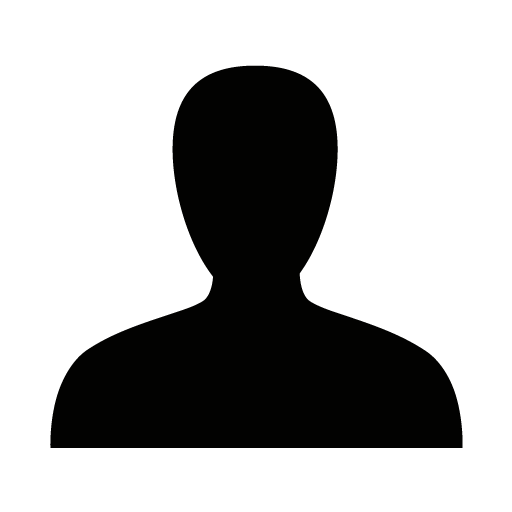
In the past few decades, there have been many advances in the development of plasmonic nanomaterials and their application in chemical sensing, photothermal therapy, enhancement of photovoltaic device performance, catalysis, solar-driven desalination, among others. To date, nano-sized metals such as gold, silver, and copper have been well-explored for plasmonic applications, however these can suffer from poor thermal and/or chemical stability. Gold is also expensive making it non-ideal material for large scale applications. In a quest to develop plasmonic nanomaterials that are low cost and chemically stable, transition metal nitrides have emerged as strong contenders based on computational studies performed in the last decade. However, the experimental studies on these materials remain scarce. This presentation will highlight some of the recent results from our group on the solid-state synthesis of free-standing plasmonic transition metal nitride nanoparticles [1], their chemical and thermal stability, and photothermal properties [2,3].
2.2-T1
Colloidal CdSe quantum rings (QRs) are a new class of nanomaterials synthetized via thermo-chemical edge reconfiguration of thinner CdSe nanoplatelets [1],[2]. In the latter, the photo-physics is consistently dominated by strongly bound electron-hole pairs, so-called excitons, that can merge to form excitonic molecules (biexcitons), giving rise to net stimulated emission along the molecule-to-exciton recombination pathway.[3] On the other hand, little is known on the nature of elementary excitations in thicker CdSe QRs - whether they are excitons or free electron-hole pairs- and their behavior at high density regime. Here, we show that charge carriers in QRs condense into a hot uncorrelated electron-plasma at high density opposed to the stable exciton gas found in thinner nanoplatelets. Through strong band gap renormalization, this plasma state is able to produce sizable optical gain with a broadband spectrum. Next, we show that the typical signatures of excitonic transitions are indeed absent in QRs. The gain is limited by a second order radiative recombination process and the buildup is counteracted by a typical charge cooling bottleneck. Overall, our results show that weakly confined QRs are a unique system to study uncorrelated electron-hole dynamics in nanoscale materials.
2.2-T2
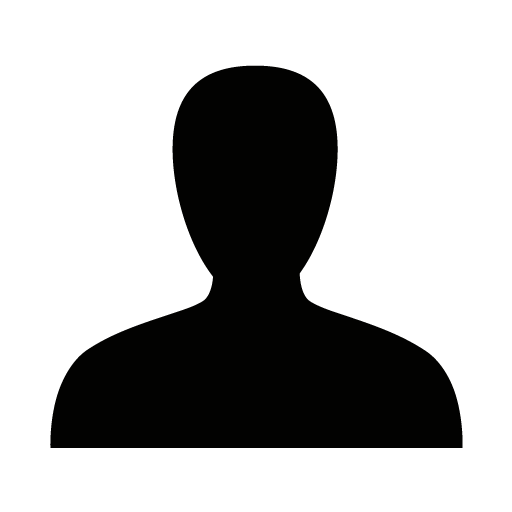
Based on synthesis and the sample preparation procedure, the surface of colloidal nanocrystal is being covered by organic ligands on a regular manner. The ligand structure and the way of ligand-surface interaction can be crucial for the physicochemical and optical properties of nanocrystal as a system. In this work, we investigate the binding of zinc oleate to ZnSe nanocrystals from the perspective of its physicochemical properties. ZnSe nanocrystals have been used as narrow blue/violet emitters, as hosts for luminescent ions, or as large bandgap shell material, for example to form InP/ZnSe core/shell QDs [1]. In this study, we focus on neat ZnSe nanocrystals synthesized by injecting black selenium powder in a solution of zinc oleate in octadecene. By use of quantitative solution Nuclear Magnetic Resonance spectroscopy, we confirm that the ZnSe nanocrystals, obtained through described synthesis, are capped by zinc oleate. Following resembling work on CdSe quantum dots and nanoplatelets, we have shown that the addition of butylamine, an organic Lewis base or L-type ligands, leads to the displacement of zinc oleate from the ZnSe surface. By gradually increasing the concentration of butylamine, we record a displacement isotherm to represent the dynamic displacement equilibrium between bound zinc oleate and the desorbed zinc oleate butylamine complex. Going through quantitative analysis of these displacement isotherms and compare it with similar II-VI material we can conclude that zinc oleate is more prone to displacement from ZnSe as, for example, cadmium oleate ligand on the surface of CdSe quantum dots [2]. The estimation of the binding energy and the distribution of binding energies of zinc oleate over the surface of ZnSe nanocrystals can be done by using DFT-calculations on ZnSe model of the same crystal size.
(1) Tessier, M.D.; Dupont, D.; De Nolf, K.; De Roo, J.; and Hens, Z. Economic and Size-Tunable Synthesis of InP/ZnE (E = S, Se) Colloidal Quantum Dots. Chem. Mater. 2015, 27, 13, 4893–4898.
(2) Drijvers, E.; De Roo, J.; Martins, J.C.; Infante, I,; Hens, Z. Ligand Displacement Exposes Binding Site Heterogeneity on CdSe Nanocrystal Surfaces. Chem. Mater. 2018, 30, 3, 1178–1186.
2.2-T3
Phd researcher in the department of inorganic and physical chemistry at Ghent university.
Experience in inorganic chemistry, environmental chemistry and supramolecular chemistry with +5 years teaching experience in university and secondary school. Research at Barcelona university in inorganic and organic chemistry department and research at Damascus University in Faculty of Sciences, Chemistry Department.
… Read more
The optoelectronic and chemical properties of semiconductor nanocrystals depend on their composition, size, shape and surface functionality. Cadmium telluride nanoplatelets (CdTe NPLs) are one of these semiconductor nanocrystals which, because of their relatively small bandgap (Eg = 1.44 eV) in bulk CdTe[1], are considered a promising material for photonic applications across the visible and near-infrared spectral range. Colloidal synthesis is a powerful strategy to alter the NPL optoelectronic and chemical properties, yet most efforts have been dedicated to CdSe NPLs[2]. In this work, we focused on the synthesis of size-controlled CdTe NPLs, and show that the chemical reactivity of the tellurium precursor plays a role in controlling the area of 3.5 monolayer (ML) CdTe NPLs. We built a novel 3.5 ML CdTe NPLs model based on a definitive screening design (DSD) to optimize the 3.5 ML CdTe NPLs synthesis and investigate the effects of additives on Cd precursor reactivity, such as oleic acid [OA], acetic acid [AcAc] and water [H2O]. Our results show that there is an optimal combination of additives that reduces the NPL area to a minimum. This benefits the fluorescence quantum efficiency (PL QE), as we observed an inverse relationship between the NPL area and the PL QE. Finally, we obtained 3.5 ML CdTe NPLs with up to 5% PL QE, and a reduced trap band emission.
2.3-I1
Colloidal nanocrystals of different metals, semiconductors, magnets, and other functional materials can self-assemble into long-range ordered crystalline and quasicrystalline phases. However, insulating organic ligands present at nanocrytal surfaces prevent the development of extended electronic states (minibands) in ordered supercrystalline materials. Here we report self-assembly of nanocrystals with compact and conductive inorganic ligands,6 with optical and electronic measurements confirming strong electronic coupling between neighboring nanocrystals and showing evidence of metallic transport. Structural characterization of the resulting all-inorganic assemblies reveals faceted supercrystalline structures with a high degree of internal crystalline order. The assembly of charge-stabilized nanocrystals can be rationalized and navigated using phase diagrams computed for spherical particles interacting through short range attractive potentials. nanocrystals with large static dielectric constants have a unique propensity to form long-range ordered structures because of image-charge induced ion structuring and short-range repulsive forces that prevent gelation and glass formation. The assembly occurs in proximity to a binodal line separating two metastable colloidal fluids, and the conditions can be tuned to enable either one-step nucleation of supercrystalline solids or non-classical two-step nucleation. We envision that the ability to grow all-inorganic long range ordered assemblies of strongly coupled nanoscale building blocks demonstrated in this work, combined with already available synthesis toolset for engineering nanocrystal size, shape, and functionality, will offer endless possibilities for engineering hierarchical solids.
2.3-I2
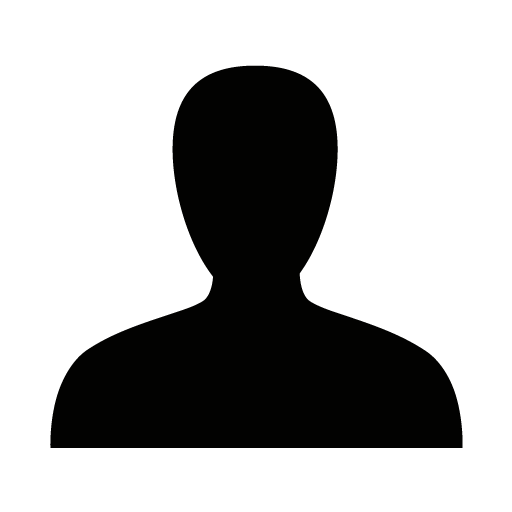
A “Holy Grail” of particle science has been to be able to predict and control particle average size and particle size distribution (PSD) for the myriad of particles formed synthetically, or naturally, throughout nature. We have recently been able to make a significant advance on this problem following (i) 25 years of developing the minimum mechanisms for particle formation and agglomeration—now a total of ≥96 distinct mechanism when including A. Karim’s important ligand-based pseudoelementary steps; and most recently (ii) the combination of those experimentally based, Ockham’s razor obeying, hence deliberately minimum mechanisms with classic population balance modeling, what we have termed Mechanism-Enabled Population Balance Modeling (ME-PBM). The talk will strive to present the essential features of ME-PBM and its main implications. Time permitting, the highlights of two recent comprehensive reviews of the ~2000 papers in the literature citing LaMer’s model of particle formation, as well as a review of the use of synchrotron radiation for XAFS and SAXS studies of particle formation, will be briefly summarized.
1.1-I1
One of the most promising future sources of alternative energy involves water-splitting photoelectrochemical cells (PECs) – a technology that could potentially convert sunlight and water directly to a clean, environmentally friendly, and cheap hydrogen fuel. Practical PEC- mediated hydrogen production requires robust and highly efficient semiconductors, which should possess good light-harvesting properties, a suitable energy band position, stability in harsh conditions, and a low price. Despite significant progress in this field, new semiconductors that entail such stringent requirements are still sought after.
Over the past few years, graphitic carbon nitride (CN) has attracted widespread attention due to its outstanding electronic properties, which have been exploited in various applications, including photo- and electro-catalysis, heterogeneous catalysis, CO2 reduction, water splitting, light-emitting diodes, and PV cells. CN comprises only carbon and nitrogen, and it can be synthesized by several routes. Its unique and tunable optical, chemical, and catalytic properties, alongside its low price and remarkably high stability to oxidation (up to 500 °C), make it a very attractive material for photoelectrochemical applications. However, only a few reports regarded the utilization of CN in PECs due to the difficulty in acquiring a homogenous CN layer on a conductive substrate and to our lack of basic understanding of the intrinsic layer properties of CN.
In this talk, I will introduce new approaches to grow CN layers with altered properties on conductive substrates for photoelectrochemical application1-4. The growth mechanism and their chemical, photophysical, electronic, and charge transfer properties will be discussed.
1.1-I2
Dr. Minghao Yu, PI, holds an independent research group (Electrochemistry for Sustainable Energy Storage) at Technische Universität Dresden. His research interest includes 1) the development of novel organic and inorganic 2D layered materials, 2) the investigation of advanced artificial interphases and electrolytes for next-generation batteries, 3) fundamental charge and ion dynamics during electrochemical energy storage processes, and 4) sustainable energy storage device fabrication, including supercapacitors, hybrid-ion capacitors, aqueous batteries, dual-ion batteries, and multivalent metal (Zn, Mg, Al) batteries. He has published more than 120 scientific articles which have attracted more than 20,000 citations with an H-index of 69 (Web of Science). Besides, he is also an associated member of the Center for Advancing Electronics Dresden (cfaed), an associated group leader at Max-Planck-Institut für Mikrostrukturphysik, a highly cited researcher (Clarivate Analytics, 2018-now), 2023 ERC Starting Grant winner, and a Fellow of the Young Academy of Europe.
Electrochemical energy storage technologies have been brought into the spotlight as they provide elegant and efficient approaches to store, transport, and deliver energy harvested from sustainable energy resources.1, 2 Typically, supercapacitors and batteries differ in electrochemical mechanisms, hence featuring almost opposite energy and power characteristics. However, the demand for power and energy supply is equally imperative in actual use and is keen to expand in the future. Thus it is highly desirable to design new electrode materials or rationally re-construct the recognized electrode materials for energy storage devices to mitigate the power-energy tradeoff.
2D layered materials are a class of materials with strong atom bonding in the basal plane and weak van der Waals (vdW) interaction between layers. These materials are equipped with versatile physical, chemical, electronic properties, as well as broad structural diversity. Importantly, the weak vdW interaction between the stacked layers enables layered materials with diverse possibilities for rational structure engineering, such as exfoliation into 2D nanoflakes, interlayer expansion with guest molecules, and hybrid structure construction. These structure engineering strategies are highly desired for layered materials to tailor their intrinsic properties (e.g., electronic structure, conductivity, and redox capability) and electrochemical behaviours (e.g., ion desolvation energy, solid-state ion diffusion kinetics, charge-storage mechanism) for diverse energy storage devices.3
Here, we will present our recent efforts in exploring 2D layered organic/inorganic materials for high-power energy storage applications.3, 4 We will show 2D redox-active carbon-rich frameworks as promising electrode alternatives for high-power energy storage devices by demonstrating 2D polyarylimide covalent organic framework (COF) as the first COF anode for Zn-ion aqueous batteries5 and dual-redox-site 2D conjugated metal-organic framework as a high-capacitance and wide-potential-window pseudocapacitive electrode. Moreover, we have demonstrated several interlayer engineering strategies for inorganic 2D layered materials to regulate the ion transport behaviors and boost the power-energy performance of the assembled energy storage devices.6, 7
1.1-I3
Prof. Zdenek Sofer is tenured professor at the University of Chemistry and Technology Prague since 2019. He received his PhD also at University of Chemistry and Technology Prague, Czech Republic, in 2008. During his PhD he spent one year in Forschungszentrum Julich (Peter Grünberg Institute, Germany) and also one postdoctoral stay at University Duisburg-Essen, Germany. Research interests of prof. Sofer concerning on 2D materials, its crystal growth, chemical modifications and derivatisation. His research covers various applications of 2D materials including energy storage and conversion, electronic, catalysis and sensing devices. He is an associated editor of FlatChem journal. He has published over 460 articles, which received over 15000 citations (h-index of 61).
Two-dimensional materials are currently in the forefront of material research. The graphene and other 2D materials play key role in electrocatalysis as well as energy storage and related field. In this presentation will be discussed the influence of impurities present in graphene and other 2D nanomaterials nanomaterials on electrocatalysis. The synthesis procedures typically introduce various impurities of metallic and non-metallic ions. Their presence can dominate electrocatalytic properties like for hydrogen evolution reaction and oxygen reduction reaction. The other factor influencing electrocatalytic activity like structure, particle size and edge vs basal planes of two-dimensional materials will be discussed. The experiments using single crystal electrodes shows dominant effect of edges for the electrocatalytic properties.
1.2-I1
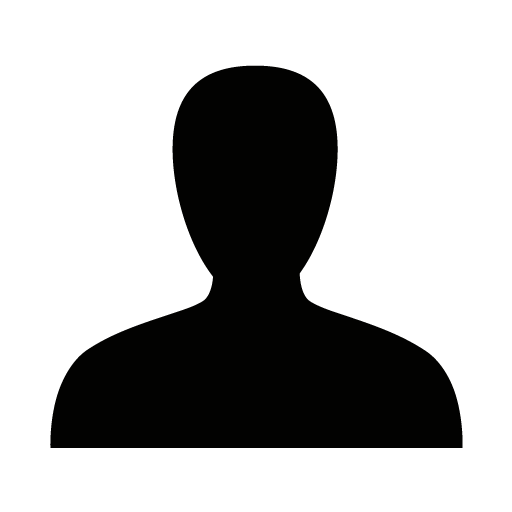
Metallic transition metal dichalcogenides (MTMDCs) have manifested many intriguing properties in their bulk states, such as magnetism, charge density wave, and superconductivity. To propel the related applications, our group have also realized the direct syntheses of high-quality VX2 and TaX2 related MTMDCs materials on both conducting Au foils and insulating substrates.[1-2]. Particularly, we have obtained thickness-tunable 2H-TaS2 flakes and centimeter-size ultrathin films on an electrode material of Au foils. Extra high hydrogen evolution reaction (HER) efficiency was demonstrated on the CVD-grown 2H-TaS2/Au foils.[3] The first synthesis of vertically oriented 1T-TaS2 nanosheets were also reported on nanoporous gold (NPG) substrates. For scalable production, a universal synthetic route was also developed for achieving high-quality 2D MTMDC (e.g., TaS2, V5S8, and NbS2) nanosheets using microcrystalline NaCl crystals as templates via a facile CVD route. [4] This synthetic route is perfectly compatible with a facile water dissolution−filtration process for obtaining high-purity MTMDC nanosheets powders towards high-performance energy-related applications.
It is well-known that, the as-prepared 2D MTMDCs are mostly environmentally unstable, and finding more stable 2D TMDCs has become a very critical issue. Herein, thickness-tunable and large-domain (∼1.5 mm) 1T-NiTe2 were also achieved on the mica substrate. [5] Significantly, this 2D material presents ultrahigh conductivity (∼1.15 × 106 S m−1) and high catalytic activity in pH-universal HER, and more interestingly robust environmental stability. We further uncover that, the CVD-derived 1T-VTe2 nanosheets can serve as a high-performance electrode material thanks to its ultrahigh conductivity, and act as electro-catalysts with excellent electrocatalytic activity in HER. All these work should provide brand new insights into the direct syntheses and property investigations of nano-thick metallic 2D TMDCs crystals.
References
[1] Yanfeng Zhang*, et al., Chem. Soc. Rev. 44(2015), pp. 2587; Adv. Mater. 28(2016), pp. 10664; Coordin. Chem. Rev. 376(2018), pp. 1.; Nature Commun. 9(2018), pp. 979; ACS Nano 13 (2019), pp. 3649; Nano Lett. 17(2017), pp. 4908; Phys. Rev. B. 96(2017), pp075402.
[2] Yanfeng Zhang*, et al., Adv. Mater. 2017, pp. 1702359; ACS Nano 13 (2019), pp.885.
[3] Yanfeng Zhang*, et al., Nature Commun. 8(2017), pp. 958; Adv. Mater. 2018, pp. 1705916.
[4] Yanfeng Zhang*, et al., J. Am. Chem. Soc. 141(2019), pp 18694.
[5] Yanfeng Zhang*, et al., ACS Nano 15 (2021), pp.1858; ACS Nano 14 (2020), pp.9011.
1.2-I2
Prof. Michal Otyepka, Ph.D. (*1975) is professor of physical chemistry and Head of CATRIN-RCPTM research division under roof of Palacký UniversityOlomouc and Head of Nanolab at IT4I Supercomputer center at Ostrava. His research interests cover physical-chemical properties and reactivity of graphene derivatives and 2D materials, non-covalent interactions to 2D materials. He has been developing chemistry of fluorographene (2D chemistry, 2Dchem.org) toward graphene derivatives, which can be applied in (bio)sensing, catalysis and energy storage. He specializes also in molecular dynamics of biomolecules, nanomaterials, and complex molecular systems, force field development and multiscale methods and their applications. He is principal investigator of ERC – Consolidator and Proof of Concept projects. He is the author or co-author of more than 300 papers in international journals, three book chapters and one book.
Covalent functionalization of graphene leads to graphene derivatives with significantly modulated electronic, magnetic and surface properties with respect to pristine graphene. The graphene derivatives can be applied in various application including catalysis. A wide range of various approaches have been developed for covalent graphene functionalization so far. Despite the progress in direct covalent functionalization of graphene, this approach suffers from a low reactivity of graphene. Recently, we developed alternative route toward graphene derivatives based on chemistry of fluorographene (FG). FG is a stoichiometric graphene derivative (having ~C1F1 composition), which can be prepared by chemical delamination of graphite fluoride in a large scale. FG undergoes various chemical reactions at rather mild conditions [1], which lead to graphene derivatives. FG is susceptible for reductive defluorination, nucleophilic attack, Grignard [2], Bingel-Hirsch [3], photo Diels-Alder [4] and Sonogashira [5] reactions. The reactions result in homogeneously and densely surface functionalized graphene derivatives. Such materials can be utilized in a broad spectrum of applications. Hydroxyfluorographenes bear room-temperature antiferromagnetic or ferromagnetic ordering based on their composition [6, 7]. Cyanographene, i.e., graphene functionalized by nitrile groups, and graphene acid bearing carboxyl groups are well biocompatible materials suitable for further functionalization [8]. Conjugating graphene acid with redox active centers, e.g., ferrocene, leads to redox active heterogenous catalyst for arene CH insertion [9]. Pd nanoparticles with controllable size can be grown on graphene acid. The prepared nanohybrids were highly active catalysts in the Suzuki–Miyaura cross coupling reaction [10]. Anchoring Cu ions to cyanographene resulted in a mixed valence single-atom catalyst (SAC) very active in oxidative amine coupling reactions [11]. Graphene acid was covalently conjugated with dehydrogenase enzymes to a nano-bio catalyst exhibiting good performance in electrocatalytic reduction of CO2 [12], also due to conductivity of graphene acid. It is worth noting that graphene acid shows metal free catalysis for alcohol oxidation, posing a new limit in carbocatalysis [13]. These examples demonstrate versatility of graphene derivatives in catalytic application.
ERC Consolidator grant (H2020, ID: 683024) 2D-Chem is gratefully acknowledged.
References
[1] Zbořil R. et al., Small, 6 (2010) 2885; Dubecký M. et al., J. Phys. Chem. Lett., 6 (2015) 1430; Medveď M. et al., Nanoscale (2018), 4696; Chronopoulos D. et al., Appl. Mat. Today 9 (2017) 60.
[2] Chronopoulos D. et al., Chem. Mater 29 (2017) 926.
[3] Bakandritsos A. et al., Adv. Funct. Mater 28 (2018) 1801111.
[4] Bares H. et al., Carbon 145 (2019) 251.
[5] Chronopoulos D. et al., ChemComm 55 (2019) 1088.
[6] Tuček J. et al., Nat. Commun. 8 (2017) 14525.
[7] Tuček J. et al., ACS Nano 12 (2018), 12847.
[8] Bakandritsos A. et al., ACS Nano 11 (2017) 2982.
[9] Mosconi D. et al., Carbon 143 (2019) 318.
[10] Blanco M. et al., Green Chem. 21 (2019) 5238.
[11] Bakandritsos A. et al., Adv. Mater. 31 (2019) 1900323.
[12] Seelajaroen H. et al., ACS Appl. Mater. Interfaces, 12 (2020) 250.
[13] Blanco M. et al., Chem. Sci. 10 (2019) 9438.
1.2-I3
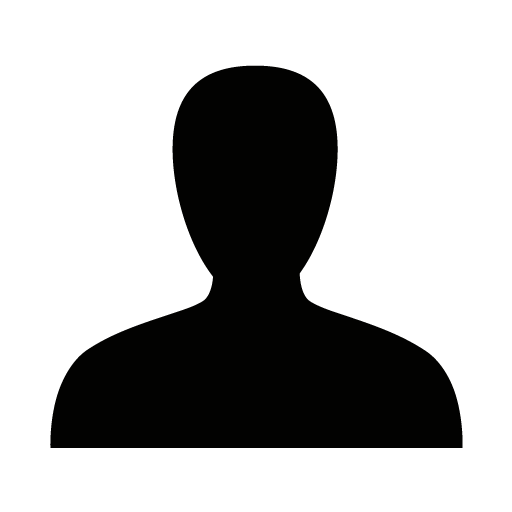
The development of industrial-scale, reliable, inexpensive production processes of graphene and related two-dimensional materials (GRMs)[1,2] is a key requirement for their widespread use in several application areas,[1-6] providing a balance between ease of fabrication and final product quality. In particular, in the energy sector, the production of GRMs in liquid phase [2,6] represents a simple and cost-effective pathway towards the development of GRMs-based energy devices, presenting huge integration flexibility compared to other production methods.
In this presentation, I will first briefly introduce the key properties of GRMs. Then, I will present the strategy of BeDimensional in the production of GRMs by wet-jet milling [7] and the Industrial scale up. Afterward, I will provide a brief overview on some key applications of the as-produced GRMs, for anticorrosion coatings and energy conversion and storage devices. [3,8-15]
REFERENCES
[1] F. Bonaccorso, et. al., Adv. Mater. 28, 6136-6166 (2016).
[2] F. Bonaccorso, et al., Materials Today, 15, 564-589, (2012).
[3] F. Bonaccorso, et. al., Nature Photonics 4, 611-622, (2010).
[4] E. Pomerantseva, F. Bonaccorso, et al., Science 366 (6468) eaan8285 (2019).
[5] G. Iannaccone F. Bonaccorso, et al., Nature Nanotech 13, 183, (2018).
[6] A. C. Ferrari, F. Bonaccorso, et al., Nanoscale, 7, 4598-4810 (2015).
[7] A. E. Del Rio Castillo et. al., Mater. Horiz. 5, 890 (2018).
[8] F. Bonaccorso, et. al., Science, 347, 1246501 (2015).
[9] M. Garakani, et al. Energy Storage Materials 34, 1-11 (2020).
[10] S. Bellani, et al. Nano Lett. 18, 7155-7164 (2018).
[11] A. E. Del Rio Castillo, et al., Chem. Mater. 30, 506-516 (2018).
[12] S. Bellani, et al. Nanoscale Horizons 4, 1077 (2019).
[13] S. Bellani, et al. Adv. Funct. Mater. 29, 1807659 (2019).
[14] L. Najafi et al., Advanced Energy Materials 8 (16), 1703212 (2018).
[15] E. Lamanna et al., Joule 4, 865-881 (2020).
1.2-I4
Although nanostructures contain tenths of thousands of atoms, the atoms at the interfaces make most of the impact on the functionality, especially regarding the catalytic activity. It is therefore an important mission to control the formation of interfaces at the atomic scale, but that is easier said than done. First, it is hard to characterize and understand what was obtained in the synthesis. Then, relating the specific structures to the macroscopic properties requires overcoming issues of adequate sampling and statistics, reproducibility to the atomic scale features and abundance of similar structures within a synthetic batch. Last, there are confusing cases where we synthesize a certain structure to best of our knowledge and spontaneous rearrangements produce other active particles. Here, I will present a few such cases.
1.3-I1
Since the last decade, electrolysis of water to produce green and renewable hydrogen fuel was one of the main interests in clean energy field. While water molecules are decomposed to hydrogen and oxygen, the latter serves as a limiting factor because of its sluggish kinetics and various catalysts that can mend this impediment are known. However, catalytic materials under electrochemical operation are subject to harsh chemical environments since they are located in solution, and as a result mechanical may appear in the material. The big challenge is to understand the correlation between the mechanical characteristics of materials and their catalytic performance. In this research we use theoretical methods in the field of computational materials science in order to explore the catalytic performance of NiOOH, one of the best catalysts existed for oxygen evolution reaction (OER), at different interlayer arrangements. NiOOH is a material with inner-layers and outstanding catalytic performance. The ability to lower the overpotential of NiOOH even further could give exceptional results in increasing the efficiency of the OER.
1.3-I2
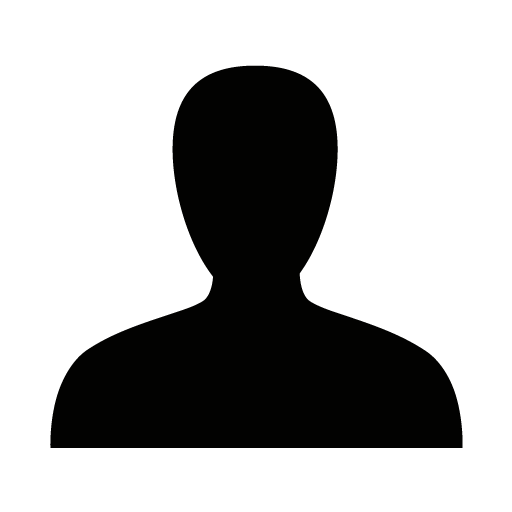
Transition-metal dichalcogenides (TMDs) have garnered much current interest as inexpensive, earth-abundant alternatives to platinum-group metals for electrocatalysis. However, TMD-based catalysts have yet to demonstrate the levels of activity and selectivity required for practical applications. Here, we employ density functional theory calculations to understand the role of transition-metal dopants in enhancing the activity and selectivity of TMDs towards specific catalytic reactions. Specifically, we present examples of dopant engineering of MoSe2 for the hydrogen evolution reaction (HER) and MoS2 for the nitrogen reduction reaction (NRR), and show how dopants can not only favorably modify the electronic structures of these TMDs for the chosen reactions but also act as promoters of highly active defect sites. We also examine the effects of co-adsorbates on the thermodynamics of NRR and show how these may assist the reaction pathways.
1.3-I3
The ability of transition metal atoms to reversibly change the oxidation state enables redox energy storage. Catalytic properties of some of the transition metals lead to use of their oxides in catalysis and electrocatalysis. A few oxides that are conductive find applications in transparent conductive layers in solar cells, thus contributing to energy harvesting. However, the majority of oxides have a low conductivity, limiting their applications in electrocatalysis, electrochemical energy storage, harvesting, and conversion. Two-dimensional (2D) carbides and nitrides of transition metals known as MXenes, with a thickness of a nanometer or less, have their surfaces terminated by oxygen or OH, forming compounds like Mo2CO2, Ti3C2(O,OH)x, or Nb4C3O2 with the surfaces resembling that of oxides or hydroxides. They are hydrophilic, form stable colloidal solutions in water, and work well with aqueous, ionic liquid or polar organic electrolytes. They chemically behave very much like the corresponding oxides/hydroxides; however, the fundamental difference is that electrons of the transition metal give them metallic conductivity, thus compensating for the key limitation of oxides and eliminating the need for conductive carbon additives. Moreover, the conductivity of titanium carbide MXene with O, OH, and/or F terminated surface is outstanding and can exceed 20,000 S/cm. A combination of high electronic conductivity with hydrophilicity and 2D structure facilitates electronic and ionic transport, allowing extremely fast charge/discharge with the electron transfer. Naturally, this opens new opportunities in energy storage, electrocatalysis, and other fields where a combination or surface redox with electrical conductivity is required. The family of 2D transition metal carbides and nitrides (MXenes) has been expanding rapidly since the discovery of Ti3C2Tx (T stands for surface terminations) in 2011 [1]. Approximately 30 different stoichiometric MXenes have been synthesized, and the structures and properties of numerous other MXenes have been predicted using density functional theory (DFT) calculations [2]. Furthermore, the availability of solid solutions on M and X sites, control of surface terminations, and the discovery of in-plane and out-of-plane ordered double-M MXenes (e.g., Mo2TiC2Tx) offer a potential for synthesis of dozens if not hundreds of new materials. The versatile chemistry of the MXene family renders their properties (conductivity, work function, surface charge, etc.) tunable for a large variety of catalytic and energy-related applications. Particularly, they are very promising candidates for energy storage [2], but applications in electrocatalysis, transparent conducting layers, biosensors, capacitive water desalination, and other fields are equally exciting [3].
1.1-I1
Organic photodetectors (OPDs) with a performance comparable to that of conventional inorganic ones have been demonstrated for the visible regime. However, near-infrared (NIR) photodetection has proven to be challenging and, to date, the true potential of organic semiconductors in this spectral range (800–2500 nm) remains largely unexplored. In this talk, I will introduce a new device concept for organic NIR detectors, based on charge-transfer absorption, enhanced by a resonant optical cavity device architecture. Design rules and optimization strategies will be discussed, yielding wavelength selective devices (20 nm resolution) with a tunability of the detection wavelength over several hundreds of nanometers, allowing the printing of miniature NIR spectrometers. In a second part of the talk, we explore the performance limitations of organic NIR detectors: A relation between open-circuit voltage, dark current, and noise current is demonstrated for OPDs with detection wavelengths beyond 1100 nm. Based on these findings we estimate an upper limit of achievable specific detectivity values for organic photodiodes as a function of their longest NIR detection wavelength.
1.1-I2
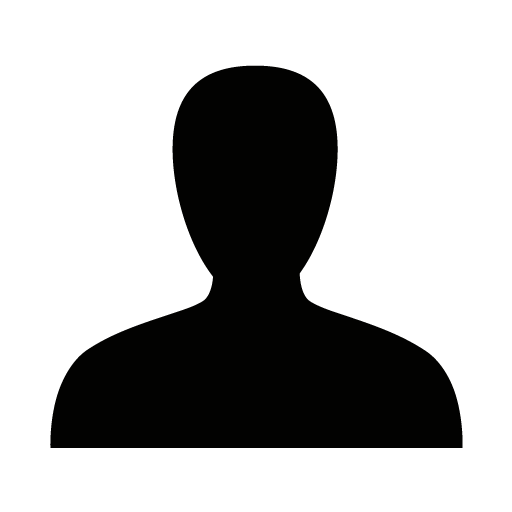
Near-infrared photodetectors based on narrow-gap organic semiconductor blends are attractive candidates for light-sensitive applications in industry and consumer electronics. However, these photodetectors currently suffer from large dark current densities, strongly limiting their sensitivity. Organic photodiodes typically show dark saturation current levels that are several orders of magnitude higher than expected from radiative band-to-band transitions only, suggesting the presence of a large non-radiative recombination channel – the origin of which is still debated. In this work we conduct ultra-sensitive external quantum efficiency and temperature dependent dark current measurements on organic photodiodes based on narrow-gap organic semiconductors. The thermal activation energy of the dark current at small reverse bias voltages is found to equal half of the effective bandgap energy revealing that the dominant recombination channel is trap-mediated via mid-gap states. By taking Shockley-Read-Hall statistics into account, we derive an analytical expression which accurately describes the dark current in the reverse bias, allowing for the upper limit of the specific detectivity in organic photodiodes to be calculated. Finally, upon comparing the light-to-dark current ratio of a large number of reported organic photodiodes from the literature, we find that the dark current is universally limited by trap-assisted recombination via mid-gap states in narrow gap systems. Our findings shed new light on the origin of noise in organic light-harvesting applications fundamentally limiting the low light performance and sensitivity in photodiodes and detectors.
1.1-I3
A strategy to artificially widen the linear dynamic range (LDR) of an organic photodiode (OPD) by introducing a light-intensity-dependent transition of its operation mode, such that a low saturation photocurrent can be overcome by additional operation mechanism, is suggested. The active layer of OPD is doped with a strategically designed and synthesized molecular switch (1,2-bis-(2-methyl-5-(4-cyanobiphenyl)-3-thienyl)tetrafluorobenzene; DAB), exhibiting typical OPD performances with an EQE < 100% under weak light and photoconductive behaviors with an EQE > 100% under strong light, which leads to an artificially extended LDR up to 225 dB. Such unique and reversible transition of the operation mode by light intensity self-recognition of molecular-switch-embedded OPDs can be explained by the unbalanced quantum yield of the cyclization-cycloreversion of the molecular switch. Details of the operation mechanism are discussed in conjunction with various photophysical analyses. A prototype image sensor based on wide-LDR OPDs is suggested to demonstrate superior sensitivity against strong light illumination.
1.2-I1
Organic semiconductors have attracted tremendous attention in the past few years, thanks to their excellent flexibility, solution-processability, low-cost and chemical versatility. In particular, organic photodetectors (OPDs) have demonstrated state-of-the-art device performance [1,2]. More recently, photomultiplication-type OPDs have merged as promising candidates for next-generation solution-processed photodetectors, thanks to the ultrahigh responsivity and low-dark current and noise. [3] However, this photomultiplication effect was mainly characterized with enhanced device performance, the underlying mechanism was attributed to the trap-mediated charge transport, and lacks of detail analysis. In this work, we characterized the devices with multiple transient techniques; the charge transport and accumulation of photo-generated carriers were fully analyzed, and we found that ultra-long carrier lifetime and imbalanced charge transport are required for the photomultiplication process.
1.2-I2
Inherited retinal dystrophies and late-stage age-related macular degeneration, for which treatments remain limited, are among the most prevalent causes of legal blindness. Retinal prostheses have been developed to stimulate the inner retinal network; however, lack of sensitivity and resolution, and the need for wiring or external cameras, have limited their application. Here I report on the use of conjugated polymer nanoparticles (P3HT NPs), showing that they mediate light-evoked stimulation of retinal neurons and persistently rescue visual functions when subretinally injected in a rat model of retinitis pigmentosa. I will then discuss the Photophysics of the NPs and the open questions related on their functioning in vivo.
1.3-I1
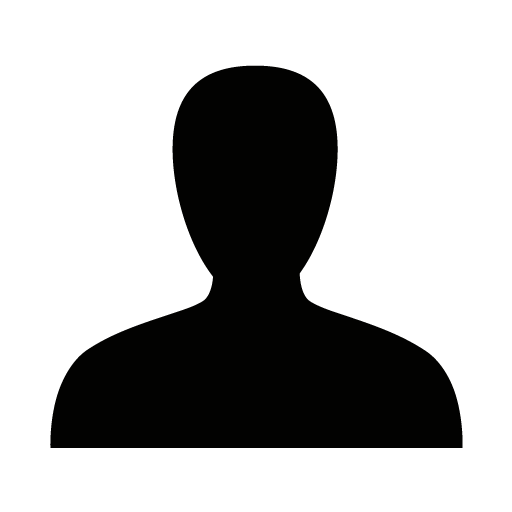
The ability to three-dimensionally pattern semiconducting electronic and optoelectronic materials could provide a transformative approach to creating active electronic devices without the need for a cleanroom or conventional microfabrication facility. This could enable the generation of active electronics on-the-fly, using only source inks and a portable 3D printer to enable electronics anywhere, including directly on the body. Indeed, interfacing active devices with biology in 3D could impact a variety of fields, including biomedical devices, regenerative biomedicines, bioelectronics, smart prosthetics, and human-machine interfaces. Indeed, developing the ability to 3D print various classes of materials possessing distinct properties could enable the freeform generation of active electronics in unique functional, interwoven architectures. Achieving seamless integration of diverse materials with 3D printing is a significant challenge which requires overcoming discrepancies in material properties in addition to ensuring that all the materials are compatible with the 3D printing process. We will present a strategy for three-dimensionally integrating diverse classes of materials using a custom-built 3D printer to fully create fully integrated device components with active properties. As a proof of concept for demonstrating the integrated functionality of these materials, we 3D printed quantum dot-based light-emitting diodes (QD-LEDs) that exhibit pure and tunable color emission properties, and polymer-based photodiodes for bionic eye applications, which are conformally printed onto curvilinear surfaces. These results represent a critical initial step toward the large-scale 3D printing of multifunctional materials and devices, without the need for conventional microfabrication processes or facilities.
1.3-I2
Philippe Guyot-Sionnest is a professor of Physics and Chemistry at the University of Chicago since 1991. His group developed original aspects of colloidal quantum dots and nanoparticles, including single dot PL microscopy, the luminescent core/shell CdSe/ZnS, intraband spectroscopy, charge transfer doping, electrochemical and conductivity studies, the "solid state ligand exchange", and mid-infrared quantum dots. Other significant works are the development of surface infrared-visible sum-frequency generation, and interfacial time resolved vibrational spectroscopy of adsorbates.
The first demonstration of mid-infrared photodetection with colloidal quantum dots took place in 2011 and used HgTe. The promise of wafer scale processing of infrared imaging devices was exciting, but the performances were 2-3 orders of magnitude below those provided by commercial single crystal materials or superlattices. There has been progress, with novel quantum dot synthesis, new spectroscopy results, vastly improved electrical transport properties, and more performant device structures. The ease of deposition of the quantum dot inks has also allowed to demonstrate potentially useful functions, such as dual band, dual polarization, curved arrays, hyperspectral, etc. At the same time, focal plane arrays are being developed. Nevertheless, performances at 5 microns are close but slightly less than microbolometers at room temperature, as well as commercial HgCdTe, and they are still about 10-fold inferior to state of the art HgCdTe single crystal detectors at any temperatures. In this contribution, I will discuss the present limitations and basic research avenues to overcome them. I will also briefly discuss intraband detection and alternative materials.
2.1-I1
In this talk I will present an overview of our activities at ICFO on the development of hybrid 2D-CQD photodetectors. I will start by the first demonstration of a highly sensitive graphene-PbS CQD photodetector, the advances made in increasing external quantum efficiency transforming PbS QDs from a passive sensitizer to an active CQD Photodiode and then showcase some use case examples in its operation in image sensors and wearable applications. Then I will discuss briefly the implications of replacing graphene with semiconducting TMD 2D materials and an overview of our activities in this class of hybrid detectors. In the second part of the talk I will discuss recent progress on efficient SWIR QD LEDs and their use in conjunction with a CQD photodiode to demonstrate an optical transmission link at the eye-safe 1550 nm based entirely on CQD optoelectronics (LEDs and photodetectors). In the last part of my talk, I will discuss recent efforts in extending the spectral coverage of PbS QD photodetectors towards the mid and long wave infrared exploiting intersubband transitions in heavily doped QD films.
2.1-I2
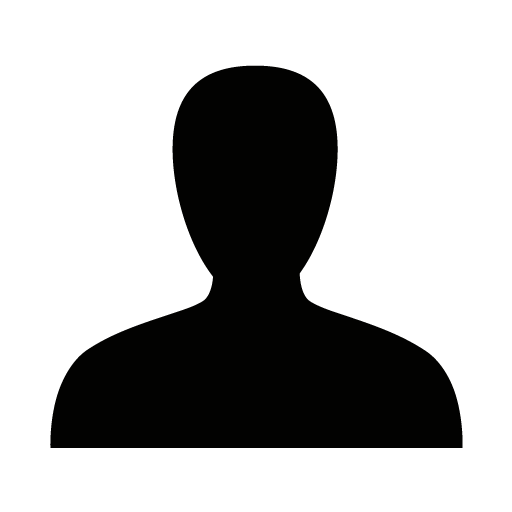
Semiconductor nanocrystals are now well established as high-performance photonic materials. The majority of applications are focused on their emissive properties, for example as exploited in bioassay and display applications. However, it is also well-known that these properties lend themselves to photodetection (and photovoltaic) technologies with a number of high performance device demonstrators such as those based on PbS reported within the literature [1]. In this talk I will provide a brief review of the use of semiconductor nanocrystals in photodetector applications. Key principles of materials design, device design and operation, and device characterisation will be reviewed based on existing work. Following this the use of hybrid systems to obtain improved performance will be discussed along with alternative nanocrystal systems that move away from those based on cadmium and lead.
2.1-I3
The wide spectral tunability of colloidal quantum dot (CQD) absorption, combined with good mobility of photogenerated charge carriers, make them competitive candidates for sensing wavelengths beyond the Si bandgap. I will present an overview of the latest achievements in CQD infrared photodetection fueled by advances in chemistry, materials science and device architectures. I will then touch on some of the remaining challenges, such as the realization of efficient infrared photodetectors that do not rely on heavy metals. I will present recent results on the use of InAs CQDs for infrared optoelectronics, showing how crucial surface management is in this materials family to achieve sufficient mobility, prevent heavy doping, and minimize surface defects – all aspects needed to enable photodetectors that combine high sensitivity (low dark current and noise, and high photoresponse) and fast operation.
2.2-T1
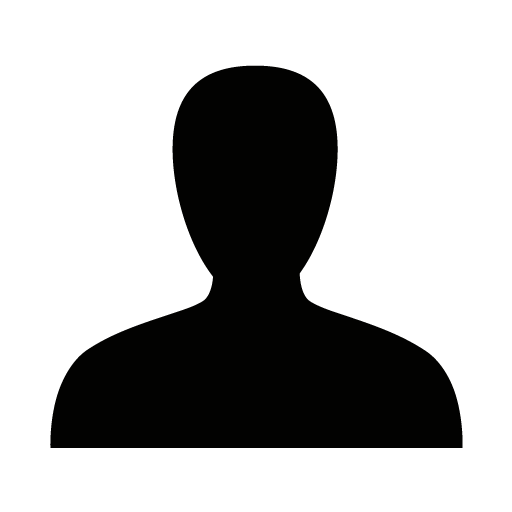
I will start with a brief introduction about recent results relative to HgTe nanocrystals (NCs) used to design short wave focal plane arrays (FPAs). I will, in particular, demonstrate an all nanocrystal based active imaging setup where both light emission (from electroluminescence) and light detection are obtained from HgTe NCs.
Then, I will discuss a strategy to design improved sensors based on phototransistors, where the gate enables carrier density tuning and thus dark current reduction. I will discuss two type of gating methods: (i) ionic glass based on LaF3 and (ii) paraelectric gating using SrTiO3 (STO). I will show that LaF3 ionic gates can be used to generate specific operating points leading to the formation of a p-n junction within the transistor channel. The latter is used to enhance charge dissociation. On the other hand, SrTiO3 is specifically designed for low temperature operation where low noise operations are required. In this regime, conventional high capacitance gating based electrolyte and ionic glass become ineffective due to the freezing of the ions, while STO thanks to the divergence of its dielectric constant is well suited for operation below 100 K which remains typical for mid wave infrared applications. Finally, I will show that phototransistor geometry can be coupled to a light trapping strategy. Here, using a guided mode resonator, I will demonstrate a broad band (>500 nm) enhancement of the light absorption through several resonances.
2.2-T2
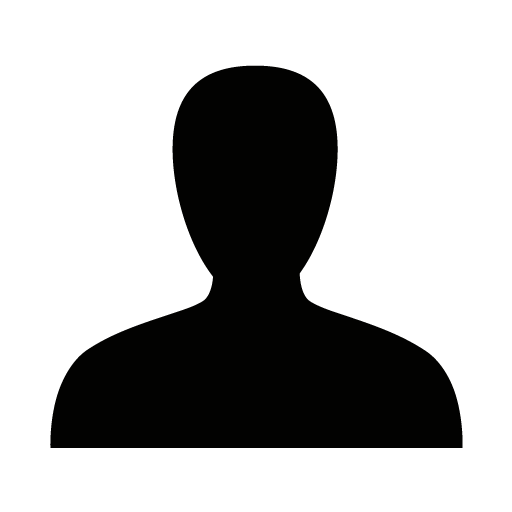
One of the great interests for colloidal nanocrystals (NCs) is their optical tunability controlled by the synthesis processes. Nonetheless, for NC-based devices, post-fabrication tunability remains very limited.1 Here, we show a NC-based active photonic device where the optical tunability is achieved thanks to an inhomogeneous absorption obtained by coupling a HgTe nanocrystal2 array to a plasmonic cavity. Bias tunability results from hopping transport which enables tuning the charge collection from low-field regions (in the very vicinity of the electrodes) to high-field ones (away from the electrodes).3 As a result, we observe a 15 meV blueshift of the photocurrent spectrum for a device working in the extended short-wave infrared under the application of 3 V bias voltage.3 This shift has the opposite sign with respect to the redshift expected from Stark effect while occurring under much weaker applied electric fields. We show that hopping transport, often seen as a bottleneck to achieve high-performing optoelectronic devices, can play an essential role in the operation of active photonic devices.
2.3-I1
Perovskite based on metal halide perovskites is a most promising material system for photovoltaics as well as for photodetectors. However, further advance demands improved device stability. Here we show the importance of perovskite composition and interface engineering by demonstrating long-term stable perovskite composites and devices. Most interestingly, the perovskite composite appears to be significantly more stable than the corresponding device, which is a strong argument that a major part of the instabilities are introduced from the interface. A high-throughput robotic approach is used to screen 160 mixed-cation mixed-halide perovskites based on several optical characterizations, such as UV-vis absorption and photoluminescence spectra. Such automated big data approaches allow to uniquely identify the most photo-thermal-stable perovskites under elevated temperature and illumination. Most interestingly, while several perovskite compositions are found to be stable against high temperatures of up to 85 C, the situation is more complex in working devices, as a stable device requires both - a stable semiconductor layer and stable interfaces. A p-type interface consisting of polymeric multilayers with variable doping is introduced as a most robust anode interface at elevated temperatures of 65 degrees Celsius, but still shows degradation at temperatures beyond 85 C. This work further introduces into the fundamentals how to accelerate the screening for stable perovskites layer and devices for long term operation.
2.3-I2
René Janssen is university professor at the Eindhoven University of Technology (TU/e). He received his Ph.D. in 1987 from the TU/e for a thesis on electron spin resonance and quantum chemical calculations of organic radicals in single crystals. He was lecturer at the TU/e since 1984, and a senior lecturer in physical organic chemistry since 1991. In 1993 and 1994 he joined the group of Professor Alan J. Heeger (Nobel laureate in 2000) at the University of California Santa Barbara as associate researcher to work on the photophysical properties of conjugated polymers. Presently the research of his group focuses on functional conjugated molecules and macromolecules as well as hybrid semiconductor materials that may find application in advanced technological applications. The synthesis of new materials is combined with time-resolved optical spectroscopy, electrochemistry, morphological characterization and the preparation of prototype devices to accomplish these goals. René Janssen has co-authored more than 600 scientific papers. He is co-recipient of the René Descartes Prize from the European Commission for outstanding collaborative research, and received the Research Prize of The Royal Institute of Engineers and in The Netherlands for his work. In 2015 René Janssen was awarded with the Spinoza Prize of The Dutch Research Council.
Narrow bandgap Pb-Sn-based perovskite photodiodes offer the possibility of spectral sensitivity from the visible to the near infrared but often suffer from relatively high dark current densities under a reverse bias where they are generally operated. Among the common strategies to reduce dark current density, the inclusion of charge-blocking layers between the electrodes and the perovskite layer has become popular. While these blocking layers are successful in increasing the energy barrier for charge injection, the lower limits of dark current density reached experimentally remain typically orders of magnitude higher than the expected intrinsic bulk thermal-generated dark current density. Hence, another process than bulk thermal generation is responsible for the remaining dark current. To find its origin we carefully analyzed the activation energy of the dark current by studying its temperature dependence in optimized Pb-Sn perovskite photodiodes with different bandgaps, employing a series of electron-blocking layers. The results demonstrate that the dark current activation energy corresponds to the energy offset between the highest occupied molecular orbital of the electron blocking layer and the conduction band minimum of the perovskite. As a conseqeunce, thermal charge generation at that interface is the main cause for the dark current and it is governed by the interfacial energy offset at the that interface. By increasing this energy offset by using an appropriate blocking layer, a perovskite photodiode was fabricated that has a wavelength sensitivity up to 1050 nm, combined with an ultralow dark current density of 5 × 10−8 mA cm−2 and noise current of 2 × 10−14 A Hz−1/2.
2.3-I3
The excellent optoelectronic properties of metal halide perovskites, such as strong light absorption, slow charge recombination, defect tolerance, and large carrier mobility, make them good candidates not only for solar cells but also for photodetectors. Their tunable bandgap allows light detection of near-infrared and visible light, which can be extended with combination of low-bandgap organic light absorbers. High-performance photodetectors with sensitivity superior to silicon ones have been quickly demonstrated with the capability to resolve weak light of sub-picowatt per square centimeter and short response speed of sub-nanosecond. In addition, new functionalities, such as wavelength selectivity, can easily be achieved without using a filter. In this talk, I will present the progress in understanding the noise, gain, sensitivity and color selectivity of perovskite photodetectors. The challenges, particularly stability, will be briefed as well, for applications such as X-ray imaging.
1.1-I1
Thomas D. Anthopoulos is a Professor of Emerging Electronics at the University of Manchester in the UK. Following the award of his BEng and PhD degrees, he spent two years at the University of St. Andrews (UK), where he worked on organic semiconductors for application in light-emitting diodes before joining Philips Research Laboratories in The Netherlands to focus on printable microelectronics. From 2006 to 2017, he held faculty positions at Imperial College London (UK), first as an EPSRC Advanced Fellow and later as a Reader and full Professor of Experimental Physics. From 2017 to 2023, he was a Professor of Material Science at King Abdullah University of Science and Technology (KAUST) in Saudi Arabia.
Organic photovoltaics (OPVs) represent a promising emerging technology for clean and renewable energy generation. Recent developments in the field of small molecule acceptors combined with an improved understanding of the device operation have propelled the power conversion efficiency (PCE) of OPVs to over 18 percent. However, further improvement in materials, processing and microstructural engineering of the photoactive layer, charge transport interlayers and cell architecture, would be required in order to attain the maximum theoretically predicted level of performance. In this talk I will discuss key recent developments in the field of OPVs with focus on practical strategies for boosting the overall cell performance. Particular emphasis will be placed on the use of electronic dopants and advanced interlayer technologies for improving the cell’s efficiency and operational stability.
1.1-I2
Christoph J. Brabec is holding the chair “materials for electronics and energy technology (i-MEET)” at the materials science of the Friedrich Alexander University Erlangen-Nürnberg. Further, he is the scientific director of the Erlangen division of the Bavarian research institute for renewable energy (ZAE Bayern, Erlangen).
He received his PhD (1995) in physical chemistry from Linz university, joined the group of Prof Alan Heeger at UCSB for a sabbatical, and continued to work on all aspects of organic semiconductor spectroscopy as assistant professor at Linz university with Prof. Serdar Sariciftci. He joined the SIEMENS research labs as project leader for organic semiconductor devices in 2001 and joined Konarka in 2004, where he was holding the position of the CTO before joining university.
He is author and co-author of more than 150 papers and 200 patents and patent applications, and finished his habilitation in physical chemistry in 2003.
OPV modules have a proven record efficiency of 12.6 % but a typical product efficiency of 5 – 7%. The first generation of OPV modules showed lifetimes of 5 - 10 years under outdoor conditions and product costs have come down from 10 €/Wp and are currently moving towards the 1 €/Wp regime. Forecasts anticipating the OPV technology at the GW level are predicting costs as low as 5 €ct/Wp [1]. This is the reason why organic modules were designed from the beginning of their product history to complement the classical PV portfolio. Applications such as power plants or roof top integration are of little relevance for OPV as long as the technology is still under development. Therefore, applications that are difficult to access for classical photovoltaic technologies are of high relevance. These make use of product properties such as transparency, integrability in surfaces, good indoor performance as well as high flexibility and low weight, but also flexible or digital production processes that allow the economic production of small production quantities or single-lot special designs. In summary, a central element of the OPV product is the design of flexible, colourful and semi-transparent products, which can be integrated into existing structures and fulfil requirements to operate applications with power requirements reaching from µWs up to MWs.
A significant improvement in operational stability would issue the most significant acceleration of OPV as a technology platform. Understanding lifetime limiting mechanisms in OPV is still at the beginning and is dominantly lacking reliable methods to uniquely identify degradation mechanisms within shortest time. Frist concepts to predict operational stability based on machine learning (ML) will be introduced in the 2nd part of the talk.
1.1-I3
Reducing non-radiative recombination of charge transfer (CT) state - appearing at the donor-acceptor interfaces in organic solar cells - is essential to achieve high fill factor (FF) and open circuit voltage (VOC) in organic solar cells. Whereas the role of energetic disorder in such devices for charge generation is discussed extensively, the impact on non-radiative recombination is largely unexplored. The present study introduces a new understanding of organic solar cells with reduced non-radiative recombination losses. We show that combining high FF and VOC is possible when non-radiative recombination of the CT state is supressed by reducing the energetic disorder. These findings open the route for the next prospect in the design of materials. This prospect asserts that intrinsic limit for the VOC and FF of organic solar cells can be reduced compared with other photovoltaic technologies. This study provides a rationale to explain and further improve the VOC and FF of organic solar cells, which is an essential step towards their commercialisation.
1.2-T1
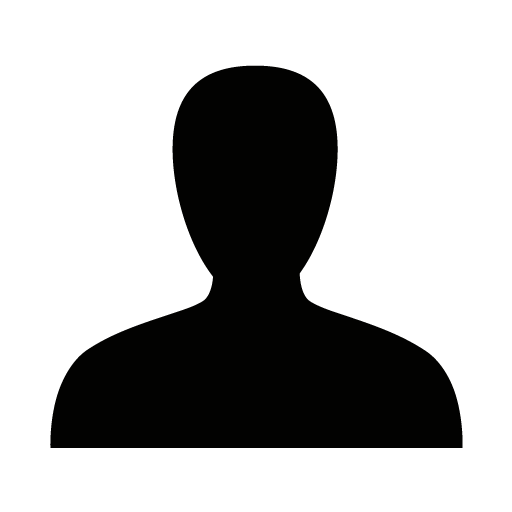
The high-performance OSCs with the optimal thickness of 100 nm are strongly related to the nanoscale formation of BHJ during and after the processing. However, upscaling of such devices from the laboratory to the industrial environment demands to increase the thickness of the photoactive layer to gain robust printing processes. However, this may lead to serious losses in the efficiency of NFA-based solar cells [1] due to enhanced recombination induced by longer transport paths towards the electrodes in combination with reduced electric fields inside the device. Nevertheless, under low light conditions, thick photoactive blends exhibit less space charge effects, leading to less recombination of the charge carriers [2] which is simply connected to less photon flux produced by low-intensity indoor illumination. Furthermore, transfer to the less toxic processing is an essential requirement for scalable deposition techniques such as roll-to-roll or other printing techniques usually combined with photoactive layers with more than 200 nm thickness [3,4]. Previously, we showed that the highly efficient solar cells with thick photoactive layers can be obtained by inkjet printing [5]. In this work, the non-halogenated ink formulation was applied to doctor-blade in air PM6:ITIC-4F-based blends. In order to further approach the industrial relevant processing conditions, another promising wide band-gap polymer PTQ-10[6] was exploited due to the low cost, solubility in non-halogenated solvents [7], high efficiency, and thick layer potential processing of this material [6,8]. Identical ink formulations using o-xylene: tetralin mixture were applied to PTQ-10:ITIC-4F blends allowing to compare the performance of corresponding solar cells using thick blends as a function of donor polymer as well as thermal post-treatment. The photovoltaic properties of the solar cells were studied under two light sources, i.e. simulated AM 1.5G and indoor light at 200 Lux and 1000 Lux, to learn more about recombination losses in OSC using thick blends. Under optimal processing conditions, PM6:ITIC-4F blends produce solar cells with a PCE of 9.3 % for 300 nm thick layer under 1 sun illumination, respectively, while the use of thicker blend layers leads to lower efficiencies. In contrast, solar cells based on PTQ-10:ITIC-4F blends showed the highest PCE for 500 nm thick layer with a PCE of 11.3 % under 1 sun illumination and 15.71% under indoor light at 200 Lux.
1.2-T2
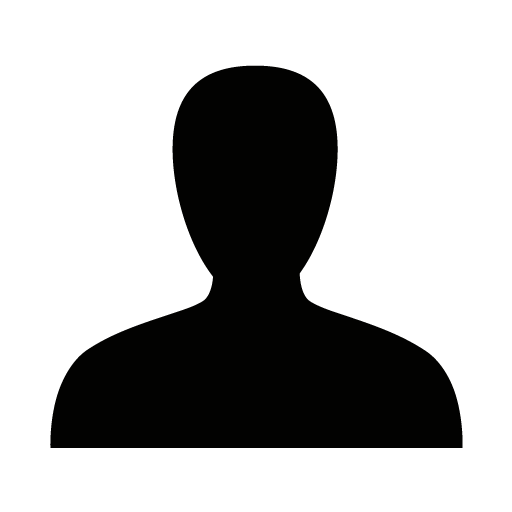
We present an approach to predictive modeling of organic photovoltaic systems that connects quantum mechanical treatments of electronic structure with the classical physics required to describe mesoscopic spatiotemporal scales.
The approach leverages the Martini coarse-grained model for the modeling of the morphology, thus incorporating chemical specificity while providing access to mesoscopic scales [1]. Martini-based coarse-grained molecular dynamics simulations are used to generate morphologies taking into account the processing conditions, such as solution processing and thermal annealing [2]. Given that such coarse-grained models retain a sizable degree of chemical specificity, the morphologies can be directly back-mapped to atomistic resolution. This allows not only to probe the impact of chemical modifications of the molecular structure on the resulting morphology, but also to gain access to electronic structure information while taking into account the large-scale self-organization process of the thin film [2,3].
As an application, we show how the approach can be used to probe the impact of polar side chains on electronic and structural properties of organic photovoltaic blends [3]. We find that the introduction of polar side chains on a similar molecular scaffold does not affect molecular orientations at interfaces. Such orientations are instead found to be strongly affected by processing conditions (i.e., thermal annealing) and polymer molecular weight. We find that polar side chains, instead, impact significantly the energy levels of the organic blend, causing broadening of these levels by electrostatic disorder.
Finally, we will conclude with a brief discussion of extensions of the approach, including coupling to machine learning techniques to enable higher-throughput characterization of the simulations, one aspect that is paramount to enable computer-aided materials design.
1.2-T3
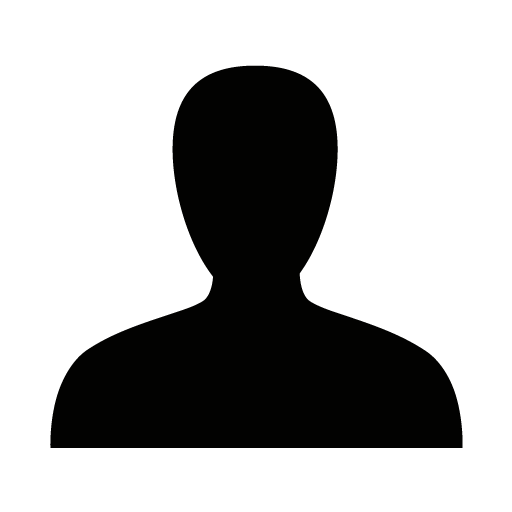
The record efficiencies for organic solar cells were increased strongly over the past few years due to significant advances in organic semiconductor materials, especially the replacement of fullerenes with mostly linear molecular acceptor materials, known as Non-Fullerene Acceptors. The improvements stem from broadening the absorption spectrum by strong and complementary absorption of donor and acceptor and simultaneously reducing the voltage loss due to charge carrier transfer between donor and acceptor significantly. Using the material combination D18:Y6, an independently confirmed efficiency of >18% was achieved [1]. After the materials were made available by several companies, we achieved the certified world record on 1cm² using this material at 15.24% efficiency [2, 3]. Recently we also fabricated a minimodule with laser ablation for the patterning, achieving 13.94% certified efficiency, which is as well slightly higher than the value of 13.6% which had set very recently the new record in the last version of the efficiency tables [4]. We will report on how this record was achieved and further optimization potential. These record values are important to highlight the potential of the OPV technology and closing in towards 20% efficiency will undoubtedly justify further and intensified work. Besides this, we as well develop device stacks and module concepts which aim at low-cost, high-volume production of efficient and longterm-stable OPV using roll-to-roll coating and printing. We will report on our ITO-free approach based on a metallized barrier substrate, where we achieved efficiencies up to 12% using PM6:DT-Y6 from non-chlorinated solvents on lab scale devices and up to 6.2% efficiency for fully R2R coated and printed ITO-free OPV-modules with an area of nearly 800cm².
1.2-T4
Non-fullerene acceptor-based ternary organic solar cells have demonstrated better photo charge generation than their binary counterparts.[1] But, underlying photophysics and the trade-off between complementary absorption and the energy offset between donor and acceptor(s) on the charge generation is not fully understood. This understanding is critical for material synthesis and device design.[2] In this work, we utilize ultrafast laser spectroscopy technique to study the charge generation in several NFA-based ternary blends with the polymer donor PM6. The selective excitation of PM6 leads to ultrafast singlet exciton energy transfer to the NFAs, outcompeting electron transfer. Subsequently, singlet excitons in the NFA undergo hole transfer to the donor, resulting in free charge generation. This requires sufficient ionization energy offset between donor and acceptors to ensure efficient free charge generation. While broad absorption spectrum is important for photon collection, but having sufficient ionization energy offset is crucial for charge generation and hence the quantum efficiency in ternary organic solar cells.
1.3-I1
Morten Madsen, Professor wsr at the University of Southern Denmark, SDU NanoSYD.
My field of expertise is thin-film growth, integration and devices for energy conversion and storage applications. In 2010-2011, I worked with high performance transistors from III-V nanoscale membranes at the Javey research lab, UC Berkeley, California. In 2011, I established the OPV group at SDU NanoSYD, where we work on improving the performance and stability of organic and hybrid solar cells, including thin film synthesis, metal oxide interlayers and interfaces, organic and hybrid active layers as well as film and device degradation. Since 2016, we also have a focus on device up-scaling through Roll-to-Roll (R2R) printing technology at the SDU R2R facility. Vist out site for more details:
https://www.sdu.dk/en/om_sdu/institutter_centre/c_nanosyd/forskningsomrader/organic+solar+cells
Organic photovoltaic (OPV) devices have recently experienced a fast rise in power conversion efficiency (PCE) with the introduction of non-fullerene acceptor (NFA) molecules, reaching above 18 % PCE today, placing device stability as the main focus area for this technology. Transition metal oxides have been demonstrated as an important class of materials for OPV devices, where they serve as charge carrier selective interlayers for efficient electron and hole extraction. In organic photovoltaics (OPV), the introduction of the high-performing non-fullerene acceptors has set new requirements on the embedded interlayers, as e.g. new interlayer related instabilities have recently been reported, making a thorough understanding of such interface effects highly important for the further development of this field and technology.
In this presentation, recent progress made within sputtered metal oxide electron [1,2] and hole [3] transport interlayers for thin film organic photovoltaics is presented. Supported by a variety of surface science characterization techniques, the role of e.g. microstructure, work function, oxygen vacancies and energy band alignment, on the performance of such interlayers in organic photovoltaic devices is discussed. This includes a focus on their positive impact on non-fullerene acceptor based OPV device stability, as compared to conventional metal oxide interlayers. Here, new results focusing on the integration of 2D materials in NFA OPV will also be elaborated on. Finally, an outlook addressing up-scaling of such metal oxides for new OPV applications will be presented.
M. Mirsafaei, P. B. Jensen, M. Ahmadpour, H. Lakhotiya, J. L. Hansen, B. Julsgaard, H.-G. Rubahn, R. Lazzari, N. Witkowski, P. Balling and M. Madsen, “Sputter-deposited titanium oxide layers as efficient electron selective contacts in organic photovoltaic devices” ACS Appl. Energy Mater., 3, 253 (2020)
D. Amelot, M. Ahmadpour, Q. Ros, H. Cruguel, N. Casaretto, A. Cossaro, L. Floreano, M. Madsen and N. Witkowski, “Deciphering electron interplay at the fullerene/sputtered-TiOx interface: a barrier-free electron extraction for organic solar cells” ACS Appl. Mater. Interfaces, 13, 19460 (2021)
M. Ahmadpour, A. L. F. Cauduro, C. Méthivier, B. Kunert, C. Labanti, R. Resel, V Turkovic, H.-G. Rubahn, N. Witkowski, A. K. Schmid and M. Madsen “Crystalline molybdenum oxide layers as efficient and stable hole contacts in organic photovoltaic devices” ACS Appl. Energy Mater. 2, 420 (2019)
1.3-I2
Alessandro Mattoni, received a master degree in physics at the University of Perugia and a PhD in solid state physics at the University of Padova. He is staff researcher of the Italian National Research Council (CNR) and in charge of the unit of Cagliari of the Istituto Officina dei Materiali, where he coordinates the theory group on the multiscale modeling of nanomaterials. A. Mattoni is author of more than 100 papers on international journals and coordinator of several projects on hybrid materials for photovoltaics and energy; he has been the principal investigator of several high-performance computing projects. A.M. developed the first interatomic force-field for classical molecular dynamics of hybrid perovskites.
Research Interests: Theoretical and computational methods for atomistic and multi-scale modeling of functional hybrid nanomaterials. Classical molecular dynamics, electronic structure methods including semi-empiricial tight binding and ab initio methods.
Polymer solar cells (PSCs) are among the most promising approaches toward low-cost environmentally sustainable and renewable photovoltaic devices. The insufficient stability is still a limiting factor for commercialization of PSCs and one of the key challenges of PSC technology. In PSCs based on fullerene acceptors, the thermal stability at high temperatures is affected by the diffusion of fullerenes inside the donor and the phase separation via Ostwald ripening mechanisms. The nanoscale morphology and thermodynamic stability in polymer blends are ultimately governed by miscibility of donor and acceptor (in the selected solvents and processing conditions). Predicting miscibility and stability is a formidable challenge that requires deeper fundamental understanding on polymer blends thermodynamics.
In this talk we discuss the application of large-scale atomistic methods for the modeling of polymer blends and for the prediction of their stability through the application of the Flory-Huggins theory[1]. We focus on the interesting case of PTB7 small band gap polymers that have been used to synthesize ultrastable PTB7:PC70BM blends for efficient PSCs[2]. The observed thermodynamic stability with the suppression of the thermally activated fullerene diffusion, is explained in terms local minima of the mixing enthalpy versus fullerene concentration. [2].
We also discuss results related to ternary blends with the sensitizer Si-PCPDTBT added to blends of PTB7 (or PTB7-th) donor polymers and fullerene acceptor PC71BM[3]. In addition to the dependence on fullerene concentration we find that the mixing enthalpy is strongly affected by the blend density. This outcome is relevant to model out-of-equilibrium conditions during which density fluctuations are expected and provides information on the preferable morphology of the sensitizer in the blend. We finally discuss perspectives and theoretical challenges for the atomistic modeling of polymer blends and for the reliable prediction of stability and thermodynamic properties.
1.4-T1
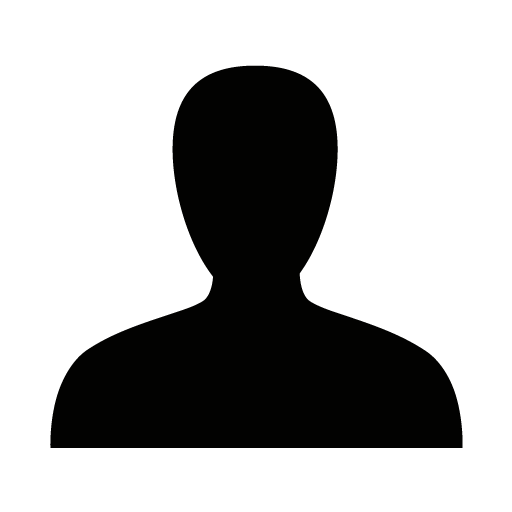
With the emergence of indoor photovoltaics as a possible application for organic solar cells, new design criteria are developed to facilitate optimum performance under indoor light sources such as light emitting diodes and low light intensities.[1] For instance, a high parallel resistance is more relevant than under 1 sun illumination. Such a high parallel resistance is typically realized by a thick absorber layer to avoid leakage currents.[2] However, organic solar cells with thick active layers are often limited in their performance by space-charge effects caused by doping, asymmetric carrier mobilities or charged trap states.[3, 4] The latter effect is not well understood yet even though shallow defects are commonly found in organic solar cells due to their intrinsic energetic disorder. Thus, we present a theoretical analysis of the influence of photo-induced space charge evoked by energetic disorder on the performance of organic solar cells under indoor conditions.[5] Drift-diffusion simulations enable us to watch space charge build up in shallow defects with increasing light intensity. Also, we observe that the performance of organic solar cells is less sensitive to high levels of energetic disorder under low light operation than under solar irradiation since the density of space charge increases with light intensity. As a consequence, experimentalists should not quickly discard material systems that perform badly under standard test conditions due to energetic disorder as they still could be viable candidates for indoor application.
1.4-T2
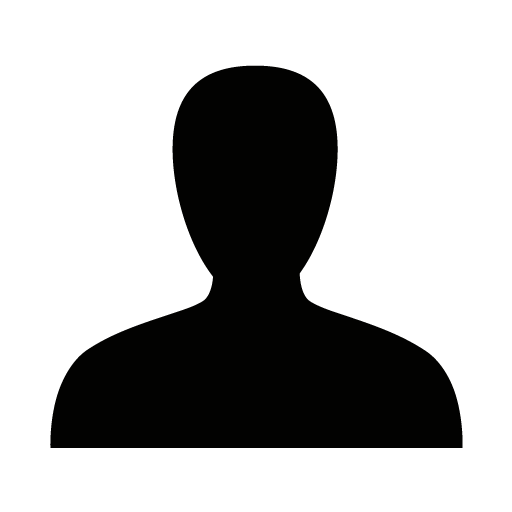
In recent years the development of new NFA molecules has been increasing performances and competitiveness. The new generations of NFAs offers tremendous advantages, like extended absorption ranges, better complementarity with polymers absorption, optimized blend morphologies and miscibility, along with better charge transport and exciton splitting properties. Despite showing efficiencies above 18% (1) the industrial transfer of OPV devices and their penetration to the market remains low, principally due to the need of improving industrial processes and long-term stability. On these days some of the new families of molecules seems to be very promising for industrial transfer. New generation of NFAs focus more on enhance efficiency, the Y6 molecule for example, shows an impressive efficiency of 15% (3), but this molecule is only soluble in halogenated solvents. Subsequent studies succeeded in solubilizing the molecule in non-halogenated solvents with 16% efficiency and similar results for blade coating fabrication.(4) However, stability tests and industrial transfer are still missing for this type of molecules. On this regard, it appears crucial to develop materials compatible with industrial requirements and intrinsically stables in parallel with their performances.
Today, a large library of building blocks is available to prepare NFAs with tunable optical and electronic properties. For the optimization of the performances in solar cells, it is necessary to focus on the development of narrow band gaps NFAs with a broad and intense absorption in the visible range and suitable LUMO energy level to maximize the open-circuit voltage (Voc), but additionally, they should demonstrate high stability and non-halogenated solvents compatibility for industrial use. Keeping in mind this requirement, we propose a molecular design inspired by ITIC molecules with an extended electron-donating core and functionalized with solubilizing groups. We synthesized and characterized a series of four NFAs called BITIC-C8, BITIC-PhC6, BITIC-PhC6F4 and BITIC-C8F4 based on an 4,9-dihydro-s-indaceno[1,2-b:5,6-b']dithiophene (IDT) central core.(5) The influence of the nature of the solubilizing groups and the presence of fluorine atoms on properties and performance of the molecules, was investigated. Interestingly, we observe increased photo-stability of the molecules in thin films, compare to reference ITIC. The photovoltaic performances of the four NFAs were assessed in binary blends using PM6 as the donating polymer and in ternary blends with ITIC-4F. Solar cells show power conversion efficiencies of up to 11.1% in ternary blends processed from non-halogenated solvents and without any thermal post-treatment, making this process more compatible with industrial requirements.(5)
1.4-T3
In 2023, Drew earned his PhD Sêr SAM group at Swansea University. His focus is on the disentangling of various relaxation pathways in disordered semiconductor systems including organics and perovskites. He is the resident Ultrafast expert and has been involved in the construction of many apparatuses the group currently uses. Drew’s interests lie in Ultrafast and Semiconductor Physics, specifically the relaxation mechanisms in disordered semiconductors. Throughout his career, Drew has worked with start-up companies, lectured to undergraduate students, tutored and taught at the undergraduate level, and volunteered with various science outreach groups. Before completing his eduction, Drew worked as a pastry chef. He is an avid musician, surfer, and traveller.
Understanding the role that exciton diffusion plays in organic solar cells is a crucial to understanding the recent rise in power conversion efficiencies brought about by non-fullerene
acceptors (NFAs) [1]. In this presentation I will discuss the role that exciton diffusion plays in efficient charge generation.
Firstly, I will outline how one can measure the exciton diffusion lengths through quenching experiments using time resolved photoluminescence, and through exciton-exciton annihilation (EEA) using high resolution TRPL [2]. I will then introduce a novel technique, coined pulsed-PLQY, to measure the diffusion length through EEA without the need for any temporal measurements, greatly increasing the speed and ease of the measurement while reducing the operational costs.
Traditional and pulsed-PLQY EEA techniques are simulated using a Kinetic Monte-Carlo approach and the limits of both techniques are discussed. It is found that pulsed-PLQY is less sensitive to the choice of excitation density and has increasing confidence with increasing densities measured. The simulations are validated by performing both experimental techniques on organic thin films, reproducing the predicted trends. Pulsed-PLQY is then used to measure the diffusion length in a range of organic semiconductors, including technologically relevant NFAs. We find that the NFAs show an increase in diffusion length, driven by an increase in diffusivity, compared to a benchmark fullerene acceptor [3].
1.4-T4
The globally growing market of smart applications and devices in a society which aims for sustainability and eco-friendliness leads to the question of powering all these systems. Powering and enabling their long-term self-sufficiency results in an increasing interest in (micro) energy harvesting solutions, from sources such as temperature differences, vibrations and light. In the special case of powering indoor devices with artificial (narrow) light spectra organic solar cells and modules have emerged as a promising photovoltaic technology which combines low fabrication cost, lightweight and mechanical flexibility. Most of the work focused on the development of new and specially tailored semiconductor materials (optimal bandgap of ~1.8eV) on the classical Indium Tin Oxide (ITO)-based architecture with the known issues related to that (mechanical brittleness, rare material/environmental impact, stability, roll-to-roll (R2R) compatibility). [1]
Herein we present ITO-free organic photovoltaic (OPV) cells and modules processed from non-halogenated solvents with high parallel resistance which enables their usage at extremely low intensities as 100 lux. The upscaling process from 0.1 cm2 cells to 7.3 cm2 modules (8 cells in series) was successfully done with performance losses less than 15 % relative. Under 500 lux our modules show an efficiency of 14 %. This is, to the best of our knowledge, the highest value reported for ITO-free non-fullerene acceptor OPV modules processed from green solvents. We can show that the ITO-free, R2R compatible electrode system is applicable to a wide range of modern organic semiconductors used in OPV technology. Furthermore, the presented ITO-free electrode system and cells stack work on rigid as on flexible substrates which increases the versatility of use cases. Surprisingly, novel donor:acceptor systems designed for sunlight application yield similar results under dim indoor light because of their outstanding external quantum efficiency, resulting in higher currents thus compensating for their typically lower band gap. However, these absorber materials are inferior in terms of their theoretical maximum performance. We also will address the influence of different light colours (warm white to cool white) on the efficiency.
2.1-I1
Dr. Qun LUO received her Bachelor degree in Material Science and Engineering in 2006 from Zhengzhou University, and Ph. D degree in Materials Physics and Chemistry in 2011 from Zhejiang University in China. She had a research experience in the field of photoluminescence properties of rare earth materials. During Jan, 2011 to July, 2011, she did research work in Rennes-1 University of France as a joint Ph.D student in the field of photoelectrochemical properties of sulfide. From Nov. 2011, she started research work of printable electronic inks and printing organic and perovskite solar cells as a post-doctor in Suzhou Institute of Nano-Tech and Nano-Bionics, Chinese Academy of Sciences. From May, 2015, she jointed Suzhou Institute of Nano-Tech and Nano-Bionics, Chinese Academy of Sciences as an associate professor. Now, her research interests are printable metal oxides semiconductor inks & printing thin films photovoltaics. She has published more than 60 papers on organic/perovskite photovoltaics and photovoltaic interface engineering.
Organic solar cells have great potential application in wearable electronics due to the advantages of flexible, light-weight, colorful and roll-to-roll compatible. Printable materials and inks are the materials basics regarding the printing fabrication of the organic solar cells. Large-area homogenous and low-thickness dependent printed films would ensure high reproducibility of the large-area devices. On the bases of these two points, a series of printable interface materials from functional grafting modification, organic-inorganic hybrid modification, crystalline and amorphous hybrid modification were developed, which showed high work thickness up to 200 nm, large thickness tolerance, excellent thin films quality, and as well as good photoelectronic properties. In addition, we developed large-area electrodes and interface layers using gravure printing. With these, the efficiency of 1 and 9 cm2 flexible organic solar cells has reached above 16% and 14%.
2.1-I2
Organic photovoltaics have a huge potential to decrease the environmental impact of electricity generation and over time enable lower cost PV. As a first step on the technology and market development of OPV, the Swedish company Epishine is focusing on the indoor PV market (IPV). The demand for connected sensors is increasing and the cost for battery replacements are a limiting factor. A credit card sized organic IPV module can replace the need for batteries or extend the battery life time. In this talk co-founder and CTO Jonas Bergqvist will give a brief introduction to IPV, give an update of the present status of Epishine and discuss the technology development at Epishine and what is important for further improvment of organic IPV.
2.1-I3
Mariano Campoy Quiles´s research is devoted to the understanding and development of solution processed semiconductors for energy and optoelectronic applications. He and his team have built substantial research efforts in two application areas, solar photovoltaic (light to electric) and thermoelectric (heat to electric) energy conversion based on organic and hybrid materials. He studied physics at the Univesity of Santiago de Compostela, obtained his PhD in experimental physics from Imperial College London, and since 2008 he leads his team at the Institute of Materials Science of Barcelona.
X. Rodríguez-Martínez1, E. Pascual-San José1, Zhuping Fei2, A. Sanchez-Díaz1, A. Harillo1, M. Heeney2, R. Gimerà3, M. Campoy Quiles1
1Institut de Ciència de Materials de Barcelona (ICMAB-CSIC), Spain
2Chemistry Department, Imperial College London, UK
3Universitat Rovira I Virgili, Spain
While the strong performance increase in organic photovoltaics is driven by the synthesis of improved materials, the highly entangled thickness-composition-morphology parameter space makes almost impossible the prediction of performance for new systems. One notable exception is the thickness dependence of the active layer, which was formulated in a seminal paper from 1999 [1]. On the other hand, the dependence of the device performance on donor/acceptor ratio has thus far been more elusive; despite composition being one of the parameters that affects efficiency more strongly. In order to address this complex problem, in this talk we will present the results of feeding high-throughput experimental methods into artificial intelligence algorithms in order to predict the composition-performance landscape.
We will first describe a novel methodology for the fast evaluation of donor/acceptor systems for photovoltaics. The new approach is based on the fabrication of samples with gradients in the relevant parameters of interest that represent a large fraction of the corresponding parameter space. In particular, we fabricate gradients in thickness, microstructure, composition and apply hyperspectral imaging to correlate material and device properties. The method is up to 100 faster than conventional optimization protocols, uses less than 50 mg of each active layer material and generates hundreds to millions of data points per system [2,3].
Then we show how this machinery can be used to find design rules for the optimum composition in non-fullerene acceptors based devices. For this, the combinatorial evaluation of more than 15 donor/acceptor systems -which generates thousands of data points in the thickness/composition parameter space-, is coupled to machine learning algorithms. Trained algorithms can predict the composition for maximum efficiency, and even reproduce multi-maximum efficiency vs composition diagrams, from basic material inputs, such as energy levels and optical and electrical traits [4].
2.2-T1
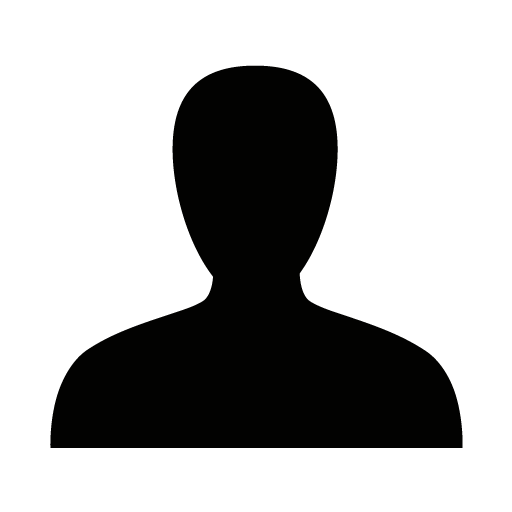
Photoluminescence originating from the recombination of electrons and holes within the absorber layers in solar cells is an important characterization technique for many photovoltaic technologies as it provides direct information about the separation of the quasi Fermi levels (QFL) inside the device. The photoluminescence (PL) in organic donor/acceptor (D/A) systems is typically dominated by local excitons which decay radiatively before dissociation into free charge carriers at the D/A interface. Thus, this (major) part of the PL signal neither correlates with the separation of the QFL nor with the operational state of the device. In contrast, during electroluminescence (EL) experiments electrons and holes are injected in their respective material phases directly and recombine pairwise at the D/A interface (partially radiatively). Therefore, the EL radiation is proportional to the product of electron and hole concentrations and thus directly to the QFL separation. In this work, we determine the time resolved QFL separation during charge carrier decay from transient EL within the absorber of high-efficiency organic solar cells, compare it to the externally measured voltage at the contacts and demonstrate the applicability of this method for a wide range of injection currents. We further show the potential of this measurement technique to get direct insights in the recombination dynamics of electrons and holes within organic D/A materials.
2.2-T2
The device performance of organic bulk heterojunction (BHJ) solar cells is ultimately derived from processes taking place between excitons, charge-transfer (CT) states, and free charge carriers. In fullerene-acceptor-based BHJ systems, characterized by large donor-acceptor energy level offsets, the photovoltage is typically limited by the energetics and kinetics of CT states.[1] However, this picture has been challenged with the emergence of nonfullerene-acceptor-based systems with low energy offsets,[2] where the role of excitons becomes important. In this work, the interplay between excitons, CT states and free charge carriers is investigated.[3] Based on detailed balance considerations, we derive an analytical framework describing the photocurrent quantum yield, the charge-carrier recombination rate constant, and the open-circuit voltage in low-offset BHJ systems. Furthermore, the essential conditions for mutual equilibrium between the different species to occur are clarified. Finally, we find that the photovoltaic parameters are not only limited by the energetics but also critically determined by the relative kinetics between the different species. These findings provide insights into the operation of state-of-art non-fullerene organic solar cells with low offsets, paving the way for power conversion efficiencies exceeding 20%.
2.2-T3
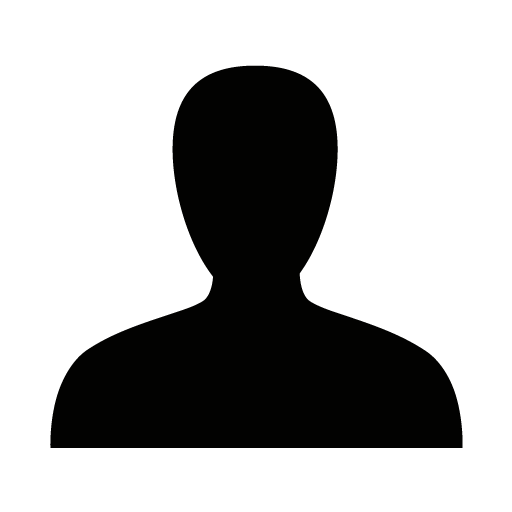
With the fast development of efficiency of organic solar cerlls, performance decay dynamics and detailed degradation mechanism of polymer solar cells become the next most important research topic for organic solar cells. Several research groups have been intensively worked on the degradation and stability of polymer:fullerene solar cells, and various degradation pathways were clarified, including: photon induced dimerization of fullerene molecules[1,2] and interfacial degradations.[3] Unlike fullerene based solar cells, our recent research results revealed that photon induced interfacial degradation of the non-fullerene molecules is the main pathway for the performance decay of polymer:non-fullerene soalr cells.[4-5] In this presentaion, we will present the latest resutls in this topics.
2.2-T4
Scaling effects of OPV devices for large-area solar cells and indoor applications
Gregory Burwell1, Wei Li1, Oskar J. Sandberg, Paul Meredith1, Ardalan Armin1
1Sustainable Advanced Materials (Sêr-SAM), Department of Physics, Swansea University, Singleton Park, Swansea SA2 8PP, United Kingdom
Abstract
Organic photovoltaic (OPV) devices have demonstrated steadily improving figures in merit in recent years, principally due to the development of non-fullerene acceptor (NFA)-based materials. However, superlative OPV devices are invariably lab-scale and small (< ~ 0.1 cm2) and it has proven challenging to replicate high power conversion efficiencies at practical sizes. At light intensities close to 1 Sun, the sheet resistance of available monolithic transparent conductive oxide (TCO) materials limits the performance of these larger devices. However, indoor OPV (IOPV) devices are required to operate at low light intensities, and thus demonstrate different area-scaling behavior. In contrast to 1 Sun operation, the performance of large-area IOPV devices are much less affected by the sheet resistances of transparent conductive electrodes, but instead by the shunt resistance, at low light intensities. In this work, we examine key parameters for improving the efficiency of large-area devices under different illumination regimes using drift-diffusion and finite element modeling. We examine application-specific device optimization of OPV devices for IOPV and standard 1 Sun operation reviewing aspects such as the transparent conductive electrode, material selection, and fabrication considerations.
Session-K1
Professor of Physical Chemistry at the Ecole Polytechnique Fédérale de Lausanne (EPFL) Michael Graetzel, PhD, directs there the Laboratory of Photonics and Interfaces. He pioneered research on energy and electron transfer reactions in mesoscopic systems and their use to generate electricity and fuels from sunlight. He invented mesoscopic injection solar cells, one key embodiment of which is the dye-sensitized solar cell (DSC). DSCs are meanwhile commercially produced at the multi-MW-scale and created a number of new applications in particular as lightweight power supplies for portable electronic devices and in photovoltaic glazings. They engendered the field of perovskite solar cells (PSCs) that turned our to be the most exciting break-through in the recent history of photovoltaics. He received a number of prestigious awards, of which the most recent ones include the RusNANO Prize, the Zewail Prize in Molecular Science, the Global Energy Prize, the Millennium Technology Grand Prize, the Samson Prime Minister’s Prize for Innovation in Alternative Fuels, the Marcel Benoist Prize, the King Faisal International Science Prize, the Einstein World Award of Science and the Balzan Prize. He is a Fellow of several learned societies and holds eleven honorary doctor’s degrees from European and Asian Universities. According to the ISI-Web of Science, his over 1500 publications have received some 230’000 citations with an h-factor of 219 demonstrating the strong impact of his scientific work.
Recently molecular photovoltaics, such as dye sensitized cells (DSCs) and perovskite solar cells (PSCs) have emerged as credible contenders to conventional p-n junction photovoltaics. Their certified power conversion efficiency currently attains 25.5 %, exceeding that of the market leader polycrystalline silicon. This lecture covers the genesis and recent evolution of DSCs and PSCs, describing their operational principles and current performance. DSCs have meanwhile found commercial applications for ambient light harvesting and glazing producing electric power from sunlight. The scale up and pilot production of PSCs are progressing rapidly but there remain challenges that still need to be met to implement PSCs on a large commercial scale. PSCs can produce high photovoltages rendering them attractive for applications in tandem cells, e.g. with silicon and as power source for the generation of fuels from sunlight. Examples to be presented are the solar generation of hydrogen from water and the conversion of CO2 to chemical feedstocks such as ethylene, mimicking natural photosynthesis.
1.1-I1
Ligand-functionalised gold nanoparticles, AuNPs, are promising conductive ink materials for printed electronics since they provide good dispersion in organic solvents, long shelf-life at ambient conditions, good printability and electrical conductivity after sintering. However, their mechanical and electrical performance degradation under external mechanical deformation often occurs due to the failure of the stress redistribution at microcracks and pores formed within printed structures, which limits their application for flexible electronics. Here, we employ a thiol-terminated additive as a cohesion enhancer in a gold conductive ink formulation to prevent the formation of microcracks and pores arising from the increased surface energy of nanoparticles during drying and sintering processes. The gold ink formulation with the cohesion enhancer exhibits high electrical conductivity of 5.3´106 S/m and electrical performance stability under mechanical deformation for a single printed layer due to the enhanced cohesion compared to the formulation without the cohesion enhancer, which offers exciting potential for their application in flexible electronics.
1.1-I2
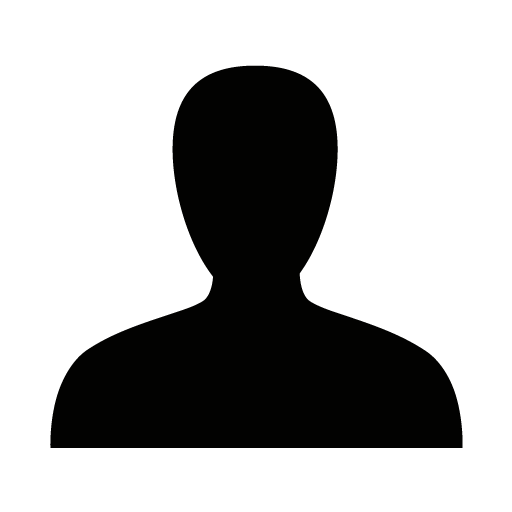
The development of water treatment technology has been spurred by the increasing threat of water scarcity faced by the global population. Various sensors such as pH sensors, dissolved oxygen sensors, biosensors, just to name a few have been developed to aid in water treatment processes such as monitoring and assessing the water quality. The advents of nanomaterial and printed electronic technology have opened up new opportunities for printed flexible electrodes in many fields, one of them is the water treatment technology. One example is the use of printed electrodes and electrochemical impedance spectroscopy (EIS) technique to monitor the health of the water filtration membrane that is used for treating seawater. Electrode is one of the most essential components for analytes detection applications with EIS. However, it remains a challenge to fabricate a compact and functional sensing electrode that can survive under such harsh conditions as seawater is known to be corrosive. With the advances in printing technology, the fabrication of advanced printed electrode systems with miniature designs and improved sensitivity for electrochemical sensing is made possible. This presentation aims to discuss the several aspects of printed electrodes, namely the (1) design consideration, (2) the fabrication consideration, and (3) measurement consideration for the printed electrodes. The challenges and considerations that will be explained include the material options, multi material printing, adhesion between interfaces, and compatibility of different post-processing techniques.
1.1-I3
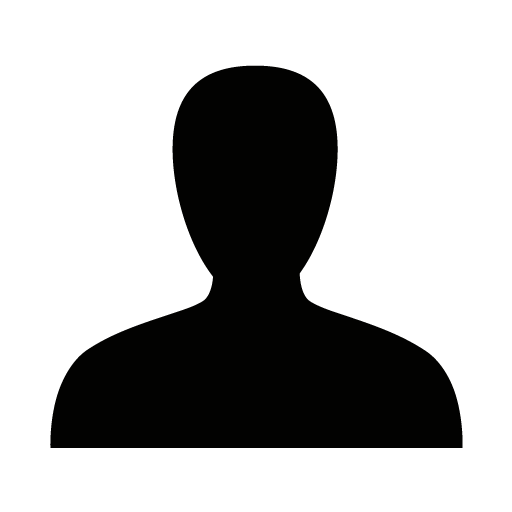
Inspired by plants systems, such as the Venus flytrap that closes in a few milliseconds under mechanical trigger, and the seedpods that open up under hydration, we developed methods to create multifunctional morphing composites. To achieve morphing and functionality, we control the microstructure of composite materials using magnetic orientation or shear-induced 3D printing. Supported by finite element modelling and experimental tests, the materials created can morph into predicted shapes. Including electrical particles inside the composite, at concentrations close to their percolation, the changes in the electrical conductivity can inform about the change in shape and the triggers that caused it. Indeed, morphing create local compression or extension that modifies the interparticle distance in the electrically conductive network. Specifically, 2 examples will be discussed. In the first one, stiff epoxy laminates are made to snap reversibly under mechanical or magnetic trigger. In the other, stiff 3D printed epoxy composites are able to morph reversibly into several preprogrammed configurations, in response to temperature and mechanical force. Combining the electrical response with the morphing, this strategy can create new kinds of computational materials for applications as sensors or actuators in robotics or aerospace.Inspired by plants systems, such as the Venus flytrap that closes in a few milliseconds under mechanical trigger, and the seedpods that open up under hydration, we developed methods to create multifunctional morphing composites. To achieve morphing and functionality, we control the microstructure of composite materials using magnetic orientation or shear-induced 3D printing. Supported by finite element modelling and experimental tests, the materials created can morph into predicted shapes. Including electrical particles inside the composite, at concentrations close to their percolation, the changes in the electrical conductivity can inform about the change in shape and the triggers that caused it. Indeed, morphing create local compression or extension that modifies the interparticle distance in the electrically conductive network. Specifically, 2 examples will be discussed. In the first one, stiff epoxy laminates are made to snap reversibly under mechanical or magnetic trigger. In the other, stiff 3D printed epoxy composites are able to morph reversibly into several preprogrammed configurations, in response to temperature and mechanical force. Combining the electrical response with the morphing, this strategy can create new kinds of computational materials for applications as sensors or actuators in robotics or aerospace.
1.2-T1
Printed electronics has opened up plethora of new opportunities towards low-cost and fast fabrication of flexible electronic components. So far, variety of printing techniques have been used to create different passive electronic components, among which inkjet technique demonstrates promising features like computer-controlled pattern reproduction and high resolution print. Recently, printing of piezoelectric materials has gained interest in both academic and industrial area to fabricate variety of components like sensors, actuators, transducers, and energy harvesting devices. Polyvinylidene difluoride (PVDF) and its copolymers like PVDF-TrFE are a class of piezomatrials that feature both flexibility and good piezoelectric performance. These polymers have semicrystalline nature, which means they are consist of small crystalline domains surrounded by amorphous matrix. PVDF based polymers can crystallize at five different crystalline phases, namely α, β, γ, δ, and ε; however, ferro-, piezo-, and pyroelectric properties of theses polymers only originate from polar phases of β and γ[1]. In this study we investigate how graphene oxide (GO) can increase polar crystalline content of PVDF – TrFE and its influence on piezoelectric, ferroelectric, and dielectric properties. After optimizaton of electronic properties, inkjet printable ink was fabricated from PVDF-TrFE and GO.
1.2-T2
I am an Industrial Designer specialized in Biomedical Engineering. In 2016, I first stepped into the area of modeling and 3D printing of healthcare products. In 2018, I started as a research technician working on the design and prototyping of microfluidic devices. Currently, I am in the second year of my Ph.D. degree, working on 4D printing of photosensitive polymers.
Photo-responsive materials are increasingly attracting attention as they can transduce light energy, which allows no contact stimulation with high spatial and temporal control, into mechanical energy1. Within these materials, Liquid Crystal Elastomers (LCEs) have shown the ability to accomplish large and reversible mechanical actuation under external stimuli. By four-dimensional (4D) printing, the precise programming of the morphology of azobenzene-containing liquid crystalline elastomers (LCEs) that respond to light is possible, achieving high control over the mechanical response. Printing of LCE elements is based on the extrusion of the LCEs precursor through a needle creating forces that align the mesogens along a preferential axis called “director” defined by the needle’s moving direction. In a second phase, the orientation of the mesogens is fixed by photo-polymerization.
Fast mechanical responses have been observed in these 4D printed LCE elements when excited with ultraviolet (UV) light, lifting weights heavier than the LCE element and performing significant work. Both photo-chemical and photo-thermal contributions are recognized, generating forces that can be released by blue light excitation2. In this regard, the ability to print light-responsive elements with programmed morphology and mechanical response, and capable of producing considerable work, open future opportunities for implementing remotely triggered mechanical functions in multiple fields, such as soft robotics and engineering.
1.3-I1
The expanding market of optoelectronic devices is ever-growing, from LEDs and displays to photovoltaic devices and photodetectors. Conventional device manufacturing requires several physical and/or chemical thin film deposition techniques, which typically require high vacuum and high temperature processes. In addition, masking or photolithography are necessary to define the desired device geometry. In contrast to conventional device manufacturing technologies, innovative fabrication approaches have gained great attention. Technologies such as screen printing and inkjet printing (IJP), which have been used extensively in the graphics art industry, are being used to deposit functional materials. Semiconductor material inks like halide perovskites can be prepared to deposit a wide array of functional materials in the form of precursor inks or nanoparticle colloidal suspensions. IJP is a digital material deposition technology, which means that material can be deposited in any pattern with precision without need for masks. It also allows great control of process parameters and is ideal for fast prototyping while having the potential for scalability. Thus, mass scale and cost-effective production of electronic devices is made possible by leveraging the maturity of IJP. However, despite the great potential of IJP, most applications to functional material deposition have involved organic materials, which are known to be less stable than their inorganic counterparts.
Ub this contribution, IJP is used for the fabrication of halide perovskite layers with and without lead and we also present the architecture of fully inorganic functional devices. The printing and characterization of halide perovskites is presented first, and that of transport layers is presented later. Characterization, including XRD, SEM, and profilometry and optical analysis will proceed layer. Afterwards, the fabrication and device characterization of a heterojunction diode and a full device with perovskite is introduced. Its dark I-V characteristics are presented and compared to their counterpart under illumination.
1.3-I2
Thanks to their low-temperature processing on large-area flexible substrates thin-film organic photodetectors (OPDs) are very attractive for a range of large area imaging and sensing applications. The presentation will consist of two parts. In the first part I will focus on better understanding the physical mechanisms that determine the intrinsic limits of the detectivity of OPDs, in particular the dark current in relation to dark injection and charge generation-recombination currents. In the second part will present some recent developments in realizing curved X-ray detectors and -upon integration with OLED displays - biometric scanners that can accurately image finger-, palm and vein patterns and simultaneously record PPG signals that can be used to monitor health parameters such as heart beat, oxygen saturation and blood pressure, thus demonstrating the advantages of organic photodiodes in high-resolution, flexible large-area photodetectors.
1.4-I1
Carlos Sánchez-Somolinos holds a CSIC Research Scientist position at INMA where he leads the Advanced Manufacturing Laboratory. His expertise and scientific objectives are focused on the development of polymers and their processing through advanced manufacturing techniques (direct laser writing, inkjet and 3D printing) in the search of polymeric surfaces or functional systems of interest in the areas of optics, biomedicine and soft robotics. He has recently developed at INMA the 4D printing of liquid crystal elastomers, a technique that introduces intelligent character to 3D printed structures, programing,though additive manufacturing, material response to external stimuli. He has published more than 80 papers in internationally recognized journals, and 5 book chapters. He is coinventor in 18 patents, almost all of them with Industry as co-proprietary and one of them leading to the formation of a spin-off company. Very much focused on the transfer of technology, he has undertaken lines of research in the functionalization of surfaces in direct collaboration with Industries (Bosch -BSH Spain- and Dupont Lightstone). He has previously participated in five EU projects, one of them as a PI at CSIC (FP7-SME-2013, ID.: 605934), and has led several National and Regional Research projects and contracts, some of them fully financed by Industry. Currently, he is coordinator of the PRIME FET-OPEN H2020 project, dedicated to the development of a platform of materials and advanced manufacturing techniques to create active and easy-to-operate microfluidic devices (ID: 829010). He is also coordinator of the STORM-BOTS ITN H2020 project on soft robotics of liquid cryistal elastomers (ID: 956150). In 2017 he was awarded with the Prize of the Royal Academy of Sciences of Zaragoza (Physics Section).
The introduction of new processing technologies and the parallel development of new functional (macro)molecules have provided access to well-defined polymer structures and morphologies at different length scales. These materials, organized from the submicron to the macroscopic, are the basis for the implementation of functional systems and devices with new properties and improved performance, necessary to address the challenges that Society faces.[1,2] In this lecture we will try to show, through different examples developed at the Advanced Manufacturing Laboratory at INMA, how advanced processing of polymeric materials, with emphasis in inkjet printing, help us to walk the way that goes from the molecule to the application.[3-6]
1.4-I2
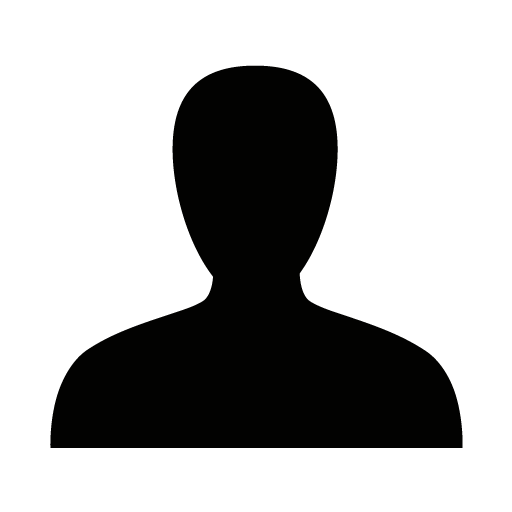
Inkjet printing of metal nanoparticles provides design flexibility, rapid processing and enables the 3D printing of functional electronic devices through co-deposition of multiple materials. A single step multifunctional AM of 3D electronic circuitry is demonstrated using a combination of conductive ink with silver nanoparticles (AgNPs) and dielectric materials within a multi-material jetting-based AM system. However, a lower and anisotropic electrical conductivity of printed AgNPs is observed compared to bulk materials. We employ a combination of electrical resistivity tests, morphological analysis and 3D nanoscale chemical analysis of printed devices using silver nanoparticles to show that the polymer stabiliser tends to concentrate between vertically stacked nanoparticle layers as well as at dielectric/conductive interfaces. Understanding the behaviour of organic residues in printed nanoparticles reveals potential new strategies to improve nanomaterial ink formulations and post-process techniques for functional printed electronics.
1.1-I1
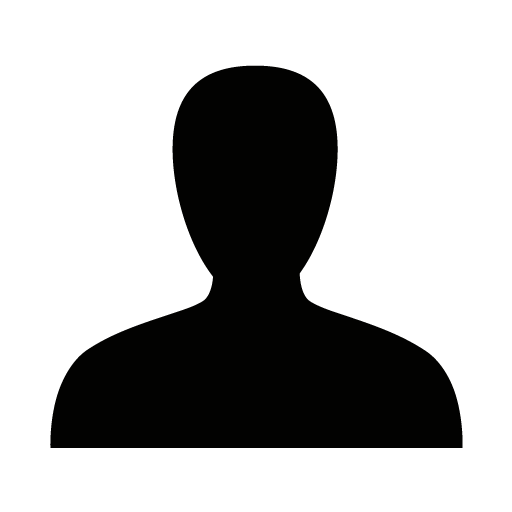
The functionality of electronic devices to a large extent is governed by the interfaces of semiconductors with contact materials. In solar cells, thermodynamic equilibration of the electrochemical potential of electrons in the absorber and in the contact phases leads to built in electric fields, which under solar excitation give rise to the photo-potential. In this talk it is demonstrated, how photoelectron spectroscopy can be applied to visualize potential variations in space charge regions of thin film solar cells and how these change under illumination.
As photoelectron spectroscopy is a surface analytical method the band structure of buried interfaces classically is determined by step by step experiments using vacuum integrated deposition and PES systems. In order to analyze the electronic structure of ex-situ solution processed devices, we developed tapered cross section photoelectron spectroscopy (TCS-PES) [1]. Here we use a shallow angle wedge to project the nm scale of the normal cross section to the mm scale on the TCS. Line scan PES with local resolution in the 10µm range now gives access to band bending in space charge regions in the dark and operating under illumination. In addition we use in vacuo prepared interfaces and ex situ prepared device stacks of classical and inverted solar cell structures to corroborate the TCS-PES findings [2]. Perovskite absorbers of different compositions are demonstrated to be doped n-type. Simulations of the potential distribution on TCS shows the importance of strong p-doping of the hole extraction layer to obtain large photopotentials.
In contrast to the current believe, we demonstrate that the photoactive interface of efficient MAPI3 and (FAPbI3)0.85(MAPbBr3)0.15 solar cells is a n-p heterojunction i.e. the working principle is n-n-p and not n-i-p.
1.1-I2
Ivan Scheblykin obtained Ph.D. in 1999 from Moscow Institute of Physics and Technology and Lebedev Physical Institute of Russian Academy of Sciences on exciton dynamics in J-aggregates. After a postdoctoral stay in the KU Leuven, Belgium, he moved to Sweden to start the single molecule spectroscopy group at the Division of Chemical Physics in Lund University where he became a full professor in 2014. His interests cover fundamental photophysics of organic and inorganic semiconductors and, in particular, energy transfer, charge migration and trapping. The general direction of his research is to comprehend fundamental physical and chemical processes beyond ensemble averaging in material science and chemical physics using techniques inspired by single molecule fluorescence spectroscopy and single particle imaging.
Many nanostructured materials when observed in a fluorescence microscope demonstrate fluctuations of local photoluminescence intensity. The fluctuations vary from drastic ON/OFF blinking of individual semiconductor nanocrystals to rather mild PL intensity flickering of micrometre-sized grains in metal halide perovskite films. The following necessary conditions for this phenomenon to occur can be formulated:
1) the material must contain some very strong metastable PL quenching sites (or efficient non-radiative (NR) recombination centres sometimes called super traps[1]) which switch from their active to passive state at the time scale of the experiment,
2) just one such NR centre should be able to substantially decrease PL quantum yield of the whole region over which photogenerated excitations (electrons, holes, excitons) can diffuse,[2,3]
3) these regions must be isolated from each other in such a way that luminescence microscope resolves them.
Luminescence micro-spectroscopy is an ideal tool study local PL intensity fluctuations and correlate them with local PL spectroscopic properties with spatial resolution limited by light diffraction. Moreover, naturally fluctuating PL allows for application of super-resolution methods based on PL blinking to increase the spatial resolution of imaging and local spectroscopy.[1] In addition activation/de-activation of a super trap in a nanocrystal drastically changes the local energy relaxation pathways and can reveal local energy inhomogeneities via PL spectral fluctuations couples with fluctuations of PL intensity.[4]
In my lecture I will illustrate the general ideas described above on MAPbI3 perovskite semiconductor where a very pronounced PL blinking effect allows for studying of local photophysics and photochemistry of this semiconductor.[5]
1.1-I3
Sascha is a Tenure-Track Assistant Professor in Physical Chemistry and Head of the Laboratory for Energy Materials at EPFL (Switzerland), while he is also maintaining strong ties with the Harvard community and in particular Winthrop House which he regularly visits as NRT and SCR member.
His team employs light-matter interactions to understand the next generation of soft semiconductors with the overarching goal of maximizing energy efficiency for a sustainable future by unlocking applications ranging from flexible light-weight solar cells & displays all the way to entirely new applications in quantum information processing.
Previously, he was a research group leader and Rowland Fellow at Harvard University. Before starting his lab at Harvard, Sascha studied Chemistry at Heidelberg University (Germany) and completed a PhD in Physics at the University of Cambridge (UK), where he subsequently worked as EPSRC Doctoral Prize Fellow.
Halide perovskites have emerged as high-performance semiconductors for efficient optoelectronic devices, not least because of their bandgap tunability using mixtures of different halide ions. In this talk, I will give an overview of our recent work on understanding why these materials show such unexpectedly high photoluminescence (PL) yields.
We show that spatially varying energetic disorder in the electronic states of such mixed-halide films causes local charge accumulation, creating photodoped regions, which unearths a strategy for efficient light emission at low charge-injection in solar cells and light-emitting diodes [1].
Combining temperature-dependent PL microscopy with computational modelling we quantify the impact of local bandgap variations from disordered halide distributions on the global PL yield in mixed-halide perovskite films [2]. We find that fabrication temperature, surface energy, and charge recombination constants are key for describing local bandgap variations and charge carrier funneling processes that control the PL quantum efficiency. Finally, we show that further luminescence efficiency gains are possible through tailored bandgap modulation in the future, even for materials that have already demonstrated high luminescence yields.
[1] Feldmann et al., Nature Photonics 14, 123 (2020).
[2] Feldmann et al., Advanced Optical Materials 18, 2100635 (2021).
1.2-I1
Libai Huang is currently a Professor of Chemistry at Purdue University. She received her B.S. from Peking University in 2001 and her Ph.D. from University of Rochester in 2006. She joined the Purdue faculty in 2014. Her research program is aimed at directly imaging energy and charge transport with femtosecond time resolution and nanometer spatial resolution to elucidate energy and charge transfer mechanisms. www.chem.purdue.edu/huang
Long-range propagation of energy and information is highly desirable for solar energy harvesting and quantum information applications. However, there currently lacks experimental tools to investigate transport with high temporal and spatial resolutions to directly elucidate coherent and incoherent regimes. To address this challenge, my research group has developed ultrafast microscopy tools to image energy transport in molecular and nanostructured materials with simultaneously high spatial and temporal resolutions.
In my talk, I will focus on our recent progress on the visualization of exciton and charge transport in the nonequilibrium and coherent regimes. One example is the quasi-ballistic transport of hot carriers in hybrid perovskite materials, which leads to 230 nanometers transport distance in 300 fs. These results suggest potential applications of hot carrier devices based on hybrid perovskites. Another example is the transport of delocalized excitons in molecular aggregates. Our measurements demonstrated that delocalization can greatly enhance exciton diffusion, even when the excitons are only weakly delocalized (< 10 molecules). Finally, I will discuss nonequilibrium transport resulting from many-body exciton interactions. We have shown that the migration of interlayer excitons in WS2-WSe2 heterobilayers is controlled by the interplay between the moiré potentials and strong many-body interactions, leading to exciton-density- and twist-angle-dependent transport length that deviates significantly from normal diffusion.
1.2-I2
Dr. Bryan D. Huey is a Professor and the Department Head of Materials Science and Engineering at the University of Connecticut (arrived 2004). This followed 18 months as an NRC fellow at NIST, 3 years as a postdoc at Oxford, a PhD at UPenn (1999), and a BS at Stanford, all working in the field of materials science emphasizing nanoscale materials property measurements. His research focuses on the development and application of advanced variations of AFM especially for measuring and mapping electronic, piezoelectric, photovoltaic, and mechanical properties at the nanoscale. He has nearly 150 publications, including articles in Science, Nature, and PNAS over the past 5 years. Huey was one of 5 co-organizers for the ~7000 attendee Fall 2019 MRS annual meeting, one of 3 co-organizers for the 2017 US-Japan Piezoelectrics and Dielectrics Symposium, and previously served as the Chair of the 1200+ member Basic Science Division of the American Ceramic Society. Huey is presently the 2nd Vice-Chair for the University Materials Council, the organization of MSE department heads in North America, in line to become the Chair in 2023.
Nano- and meso- scale materials properties are crucial to the macroscopic performance of a wide range of functional and photovoltaic devices. Photoconductivity, ferroelectricity, and coupled effects have been microscopically investigated for decades, especially in 2-dimensions using continuously evolving variations of Atomic Force Microscopy. Our work, along with many others, reveals how these properties are frequently mediated by strain, orientation, grain boundaries, and other microstructural defects or heterogeneities. However, practical devices are often sensitive to, or even controlled by, otherwise inaccessible sub-surface effects or thickness dependencies related to microstructure and concentration, polarization, and/or field gradients. Therefore, we are advancing Tomographic AFM for volumetric materials property mapping, with voxels of properties on the order of ~10 nm3. With polycrystalline photovoltaics such as MAPbI3 and CdTe, tomographic photoconductive AFM literally uncovers new pathways to improve carrier separation via inter- and intra- granular defects. For BiFeO3, Tomographic piezo-force microscopy confirms Kay-Dunn thickness scaling, LGD behavior with a minimum switchable thickness of <5 nm, and even co-located domain and current maps which together directly reveal sub-surface topological defects. In multiferroic CoFe2O4-BiFeO3 vertically aligned nanocomposites, TAFM directly visualizes lateral strain coupling, while superlattices demonstrate depth resolution on the few nanometer scale. Such volumetric insight is increasingly important for engineering optimal performance and reliability of real-world, 3-Dimensional materials devices for energy production and/or efficiency.
2.1-I1
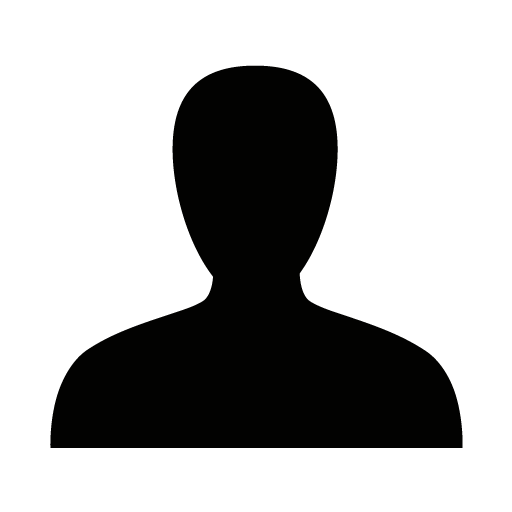
The functional properties of halide perovskite semiconductors at the mirco and nanoscales are heterogeneous,1 and there is a need to understand how these properties (electrical, chemical, etc.)2 affect overall photovoltaic (PV) performance. In this presentation, we begin by showing a variety of nanoscale microscopy methods that have been applied to perovskite solar cells to investigate the local properties of single-junction devices. These results reveal that the charge carrier dynamics in perovskites depend on the incident photon energy 3 and passivated perovskite films can exhibit chemical heterogeneities due to the inclusion of additives that subsequently affect the electrical response in three dimensions.2
Not only are perovskites complex on their own (as a thin film or single-junction device), but when embedded within a textured multi-junction solar cell, the interplay between device architecture and material performance introduces new challenges. It is not well understood how the local light-matter interactions of the perovskite material behave when incorporated into this type multijunction configuration. Therefore, we present optoelectronic microscopy measurements correlated with optical simulations of perovskite on textured c-Si solar cells. We measure the photon out-coupling of the perovskite material and find both a spectral and spatial dependence on the geometrical patterning which dominates any grain-to-grain variation.4 Such heterogeneity reveals the importance of the underlying c-Si texture design, suggesting that tuning the surface morphology could lead to a homogenization of the perovskite’s PL emission.
2.1-I2
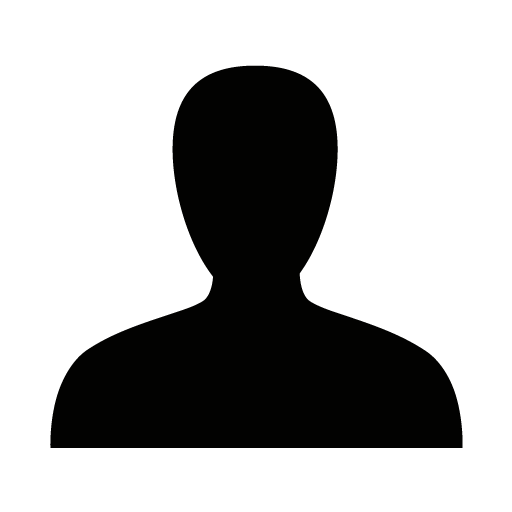
Kelvin-probe force microscopy (KPFM) has been widely used as a nanoscale surface potential measurement technique for investigating electrical properties of various materials and devices. However, this method cannot be used in an electrolyte since the application of a dc bias voltage between a tip and a sample often induces uncontrolled electrochemical reactions and/or redistribution of ions. To overcome this limitation, we have developed open-loop electric potential microscopy (OL-EPM) [1, 2], where we apply only a high-frequency ac bias voltage and detect the first and second harmonic vibration amplitude and phase of the cantilever to calculate the local potential near the sample surface. So far, we demonstrated that this method allows us to visualize nanoscale distribution of corrosion reaction activities at the surface of duplex stainless steel and cupper fine wires in electrolyte [3]. However, it has not been applied to the investigations on a specific important question in corrosion science.
In this study, we apply OL-EPM to the studies on the nanoscale corrosion mechanisms of aluminum alloys around Al-Fe intermetallic particles (IMPs) or grain boundaries in electrolyte. Aluminum alloys have attracted growing attention due to the increasing demands for reducing the weight and fuel consumption of cars, aircraft, and robots. To this end, tremendous efforts have been made for understanding their corrosion mechanisms and improving their corrosion resistance. However, this has been impeded by the difficulties in visualizing nanoscale distribution of local corrosion cells during the corrosion process. Here we use OL-EPM to visualize the dynamic changes in the surface potential around Al-Fe IMPs and grain boundaries and present the model to explain the corrosion mechanisms. These results provide important guidelines for the future improvements in the corrosion resistance of aluminum alloys, and also demonstrate the applicability of OL-EPM to the nanoscale studies on the corrosion mechanisms.
2.1-I3
Rechargeable battery technology, for example Li ion batteries (LIBs), has attracted much attention as demand for their use in portable electronics, electric vehicles, and so on has increased. To further improve the device performance, a deeper understanding of their working mechanisms is essential to gain valuable insights into design guidelines. In general, evaluations of a battery are performed by electrochemical measurements, including charge/discharge tests and cyclic voltammetry (CV). These macroscopic characterizations are the first necessary steps for grasping the battery performance but for investigating device working principles from fundamental aspects, additional direct microscopic analyses are desired, which clarifies how local electrochemical reactions proceed during the electrochemical measurements.
In this work, we have developed a method based on Kelvin probe force microscopy (KPFM) that enables dynamically imaging the change of potential distribution in an operating electrochemical device and used it for characterizing an all-solid-state Li ion battery (ASS-LIB)[1,2]. Experiments were conducted on the cross-section of the ASS-LIB with the KPFM setup equipped with an electrochemical measurement system. We continuously obtained CPD images at the cathode composite region during a CV operation of the ASS-LIB.
Our results clarified that electrochemical reactions proceeded non-uniformly from the outer electrode side in the charging process while those in the discharging process progressed uniformly over the composite electrode. Furthermore, from a direct comparison of the variation in the internal potential distribution with the CV characteristic, we demonstrated that the difference in the local electrochemical reactions can be explained by the change in the condition of the electronic conductive path network in the composite electrode, arising from the change of the electronic conductivity of the cathode active material due to charging/discharging reactions (Li-ion extraction and insertion).
2.2-T1
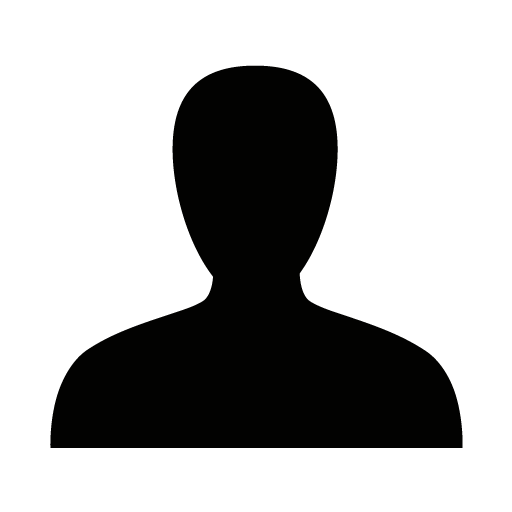
Perovkites have been shown as potential candidates for next generation optoelectronic applications. Therefore, their basic crystallographic features need to be understood deeply. Ferroelastic twin domains are crystallography related structures that affect the charge carrier dynamics in methylammonium lead iodide (MAPI) crystals. They form in MAPI crystals due to its tetragonal crystal structure with low symmetry. In this presentation, the methods such as piezoresponse force microscopy (PFM), polarized optical microscopy (POM), and x-ray diffraction (XRD) to monitor the ferroelastic twin domains in MAPI and strain engineering procedures to change the domain will be discussed. In addition, characterization techniques like time-resolved photoluminescence (TRPL) and PL microscopy on twin domain effect on charge carrier diffusion will be presented.
2.2-T2
Ferry Prins is a tenure-Track Group leader at the Condesed Matter Physics Center (IFIMAC) of the Universidad Autonoma de Madrid. Ferry obtained an MSc in Chemistry from Leiden University (2007) and a PhD in Physics from the Kavli Institute of Nanoscience at Delft University of Technology (2011). After completion of his PhD, he joined the the group of Prof. Will Tisdale at Massachusetts Institute of Technology (MIT). There, he started exploring the optical properties of nanomaterial assemblies with an emphasis on excitonic energy-transfer interactions. In 2014 he moved to ETH Zurich for a postdoc with Prof. David Norris at the Optical Materials Engineering Laboratory. With support from the Swiss National Science Foundation, he started an independent group at ETH in 2015. In Spring 2017 he joined he Condensed Matter Physics Center (IFIMAC) at the Autonoma University of Madrid where he directs the Photonic Nanomaterials and Devices Lab. His group specializes in the development of light-management strategies for semiconductor nanomaterials.
Metal-halide perovskites are a versatile material platform for light-harvesting and light-emitting applications as their variable chemical composition allows the optoelectronic properties to be tailored to specific applications. Halide mixing is one of the most powerful techniques to tune the optical bandgap of metal-halide perovskites across wide spectral ranges. However, halide mixing has commonly been observed to result in phase segregation, which reduces excited-state transport and limits device performance. While the current emphasis lies on the development of strategies to prevent phase segregation, it remains unclear how halide mixing may affect excited-state transport even if phase purity is maintained.
In this work, we use transient photoluminescence microscopy to study excitonic excited-state transport in phase pure mixed-halide 2D perovskites. We show that, despite phase purity, halide mixing inhibits exciton transport in these materials. We find a significant reduction even for relatively low alloying concentrations, with bromide-rich perovskites being particularly sensitive to the introduction of iodide ions. Performing Brownian dynamics simulations, we are able to reproduce our experimental results and attribute the decrease in diffusivity to the energetically disordered potential landscape that arises due to the intrinsic random distribution of alloying sites. Our results suggest that even in the absence of phase segregation, halide mixing may still impact carrier transport due to the local intrinsic inhomogeneities in the energy landscape.
2.2-T3
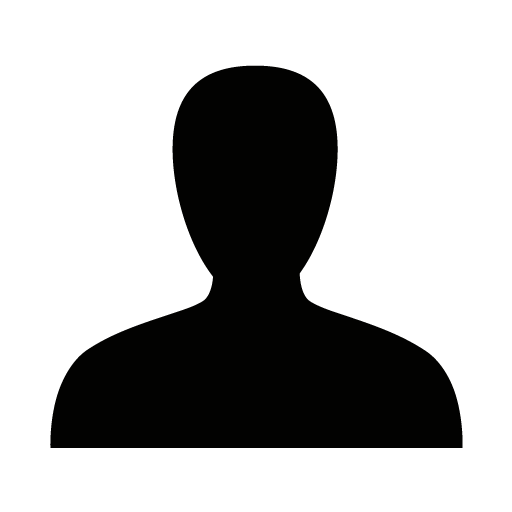
Employing 2D/3D heterostructures has become a prominent passivation strategy to enhance the open-circuit voltage (VOC) and fill factor (FF) of perovskite solar cells (PSC). The most widely employed 2D/3D interfaces are based on Ruddlesden Popper (RP) perovskites via treating the perovskite film surface with long chain alkylammonium salts. However, the detailed microscopic and electronic structure at the surface of such passivated films and the mechanisms governing the observed enhancements in device performance are still poorly understood. Here, we analyze methylammonium-free Cs0.18FA0.82PbI3 perovskite films that are passivated employing a recently developed passivation strategy using phenethylammonium chloride, that was shown to form 2D RP (PEA)2(Cs1−xFAx)n−1Pbn(I1−yCly)3n+1 phase with n ~1-2 at the GBs and film surface. [1] We perform cathodoluminescence (CL) in conjunction with Kelvin probe force microscopy (KPFM) on unpassivated reference films, grain boundary passivated films and grain boundary & surface passivated films with the aim to correlate the observations from these complementary techniques. We discuss the challenges and limitations of the measurements. Our results thereby improve the understanding of perovskite films passivated employing 2D RP perovskite phases.
2.3-I1
Plasmonic resonances can concentrate light into exceptionally small volumes, approaching the molecular scale. The extreme light confinement provides an advantageous pathway to probe molecules at the surface of plasmonic nanostructures with highly sensitive spectroscopies, such as surface-enhanced Raman scattering. Unavoidable energy losses associated with metals, which are usually seen as a nuisance, carry invaluable information on energy transfer to the adsorbed molecules through the resonance linewidth. We measured a thousand single nanocavities with sharp gap plasmon resonances that spanned the red to near-infrared spectral range and used changes in their linewidth, peak energy and surface-enhanced Raman scattering spectra to monitor the energy transfer and plasmon-driven chemical reactions at their surface. Using methylene blue as a model system, we measured shifts in the absorption spectrum of molecules on surface adsorption and revealed a rich plasmon-driven reactivity landscape that consists of distinct reaction pathways that occur in separate resonance energy windows.
2.3-I2
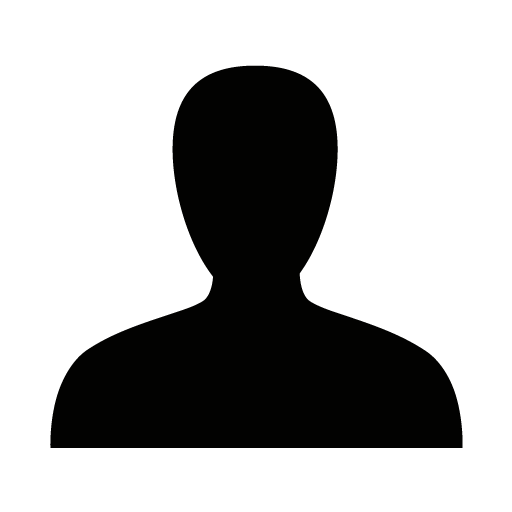
The family of layered thio- and seleno-phosphates has gained attention as possible control dielectrics for the rapidly growing family of 2D and quasi-2D electronic materials. Ferrielectric CuInP2S6 has been discovered in the 80’s but only recently it was revealed that this material exhibits a broad spectrum of unusual and even anomalous dielectric properties including negative electrostriction. Here we report a combination of density-functional-theory (DFT) calculations, DFT-based molecular-dynamics (MD) simulations, and variable-temperature, -pressure, and -bias piezoresponse force microscopy (PFM) data to predict and verify the existence of an unusual ferroelectric property – a uniaxial quadruple potential well for Cu displacements – enabled by the van-der-Waals (vdW) gap in ferrielectric CuInP2S6. Dependent on the position of Cu atoms, the polarization is either ±5 µC/cm2 when Cu resides within the layers or ±11 µC/cm2 when Cu displaces into the vdW gaps and forms ionic bonds with adjacent layers. Theoretical calculations provide values for the longitudinal piezoelectric coefficient of each of those four polarization states. Properly calibrated PFM amplitude and phase response can be related to the piezoelectric coefficient, which allows to identify these states. Consequently, we are able to track phase transitions as well as polarization switching in response to external stimuli. The calculated potential-energy landscape for Cu displacements is strongly influenced by strain, accounting for the origin of the giant negative piezoelectric coefficient. The combined theory-experiment approach also allows to map strain and stress in the material on the length scales of 10’s of nm using PFM. These phenomena offer new opportunities for both fundamental studies and applications in data storage and electronics.
2.3-I3
Joseph M. Luther obtained B.S. degrees in Electrical and Computer Engineering from North Carolina State University in 2001. At NCSU he began his research career under the direction of Salah Bedair, who was the first to fabricate a tandem junction solar cell. Luther worked on growth and characterization high-efficiency III-V materials including GaN and GaAsN. His interest in photovoltaics sent him to the National Renewable Energy Laboratory (NREL) to pursue graduate work. He obtained a Masters of Science in Electrical Engineering from the University of Colorado while researching effects of defects in bulk semiconductors in NREL�s Measurements and Characterization Division. In 2005, He joined Art Nozik�s group at NREL and studied semiconductor nanocrystals for multiple exciton generation for which he was awarded a Ph.D. in Physics from Colorado School of Mines. As a postdoctoral fellow, he studied fundamental synthesis and novel properties of nanomaterials under the direction Paul Alivisatos at the University of California and Lawrence Berkeley National Laboratory. In 2009, he rejoined NREL as a senior research scientist. His research interests lie in the growth, electronic coupling and optical properties of colloidal nanocrystals and quantum dots.
Halide perovskite semiconductors are making waves for their exceptional performance as photovoltaic materials, but since they are such profound optoelectronic materials, discovery is underway to elucidate additional breakthrough applications. In this talk, we will show how forming heterojunctions containing perovskites enable unconventional control over spin, charge and light.
In traditional optoelectronic approaches, control over spin, charge, and light requires the use of both electrical and magnetic fields. In a spin-polarized light-emitting diode (spin-LED), charges are injected, and circularly polarized light is emitted from spin-polarized carrier pairs. Typically, the injection of carriers occurs with the application of an electric field, whereas spin polarization can be achieved using an applied magnetic field or polarized ferromagnetic contacts. Here, we used chiral-induced spin selectivity (CISS) to produce spin-polarized carriers and demonstrate a spin-LED that operates at room temperature without magnetic fields or ferromagnetic contacts. The CISS layer consists of oriented, self-assembled small chiral molecules within a layered organic-inorganic metal-halide hybrid semiconductor framework. The spin-LED achieves ±2.6% circularly polarized electroluminescence at room temperature.[1]
Long-lived photon-stimulated conductance changes in solid-state materials can enable optical memory and brain-inspired neuromorphic information processing. It remains challenging to realize optical switching with low-energy consumption, and new mechanisms and design principles giving rise to persistent photoconductivity (PPC) can help overcome an important technological hurdle. Here, we demonstrate versatile heterojunctions between metal-halide perovskite nanocrystals and semiconducting single-walled carbon nanotubes that enable room-temperature, long-lived (thousands of seconds), writable, and erasable PPC. Optical switching and basic neuromorphic functions can be stimulated at low operating voltages with femto- to pico-joule energies per spiking event, and detailed analysis demonstrates that PPC in this nanoscale interface arises from field-assisted control of ion migration within the nanocrystal array. Contactless optical measurements also suggest these systems as potential candidates for photonic synapses that are stimulated and read in the optical domain. The tunability of PPC shown here holds promise for neuromorphic computing and other technologies that use optical memory.[2]
[1] Kim et al., Science 371, 1129–1133 (2021) 10.1126/science.abf5291
[2] Hao et al., Sci Adv. 2021 Apr; 7(18): eabf1959. 10.1126/sciadv.abf1959
Session-K1
Professor of Physical Chemistry at the Ecole Polytechnique Fédérale de Lausanne (EPFL) Michael Graetzel, PhD, directs there the Laboratory of Photonics and Interfaces. He pioneered research on energy and electron transfer reactions in mesoscopic systems and their use to generate electricity and fuels from sunlight. He invented mesoscopic injection solar cells, one key embodiment of which is the dye-sensitized solar cell (DSC). DSCs are meanwhile commercially produced at the multi-MW-scale and created a number of new applications in particular as lightweight power supplies for portable electronic devices and in photovoltaic glazings. They engendered the field of perovskite solar cells (PSCs) that turned our to be the most exciting break-through in the recent history of photovoltaics. He received a number of prestigious awards, of which the most recent ones include the RusNANO Prize, the Zewail Prize in Molecular Science, the Global Energy Prize, the Millennium Technology Grand Prize, the Samson Prime Minister’s Prize for Innovation in Alternative Fuels, the Marcel Benoist Prize, the King Faisal International Science Prize, the Einstein World Award of Science and the Balzan Prize. He is a Fellow of several learned societies and holds eleven honorary doctor’s degrees from European and Asian Universities. According to the ISI-Web of Science, his over 1500 publications have received some 230’000 citations with an h-factor of 219 demonstrating the strong impact of his scientific work.
Recently molecular photovoltaics, such as dye sensitized cells (DSCs) and perovskite solar cells (PSCs) have emerged as credible contenders to conventional p-n junction photovoltaics. Their certified power conversion efficiency currently attains 25.5 %, exceeding that of the market leader polycrystalline silicon. This lecture covers the genesis and recent evolution of DSCs and PSCs, describing their operational principles and current performance. DSCs have meanwhile found commercial applications for ambient light harvesting and glazing producing electric power from sunlight. The scale up and pilot production of PSCs are progressing rapidly but there remain challenges that still need to be met to implement PSCs on a large commercial scale. PSCs can produce high photovoltages rendering them attractive for applications in tandem cells, e.g. with silicon and as power source for the generation of fuels from sunlight. Examples to be presented are the solar generation of hydrogen from water and the conversion of CO2 to chemical feedstocks such as ethylene, mimicking natural photosynthesis.
1.1-I1
Laura Herz is a Professor of Physics at the University of Oxford. She received her PhD in Physics from the University of Cambridge in 2002 and was a Research Fellow at St John's College Cambridge from 2001 - 2003 after which she moved to Oxford. Her research interests lie in the area of organic and organic/inorganic hybrid semiconductors including aspects such as self-assembly, nano-scale effects, energy-transfer and light-harvesting for solar energy conversion.
Organic-inorganic metal halide perovskites have emerged as attractive materials for solar cells with power-conversion efficiencies now exceeding 25%. However, challenges and opportunities remain relating to material microstructure, ionic migration and toxicity.
We have recently investigated ultrafast charge-carrier dynamics in lead-free silver-bismuth semiconductors[1-3] which promise lower toxicity and potentially higher barriers against ion migration than their more prominent lead-halide counterparts. We examined the evolution of photoexcited charge carriers in the double perovskite Cs2AgBiBr6 using a combination of temperature-dependent photoluminescence, absorption and optical pump−terahertz probe spectroscopy.[1] We observe rapid decays in terahertz photoconductivity transients that reveal an ultrafast, barrier-free localization of free carriers on the time scale of 1.0 ps to an intrinsic small polaronic state. While the initially photogenerated delocalized charge carriers show bandlike transport, the self-trapped, small polaronic state exhibits temperature-activated mobilities, allowing the mobilities of both to still exceed 1 cm2V−1s−1 at room temperature. Self-trapped charge carriers subsequently diffuse to color centers, causing broad emission that is strongly red-shifted from a direct band edge. Overall, our observations suggest that strong electron−phonon coupling in this material induces rapid charge-carrier localization which may inhibit the use of this material as an efficient light harvester in photovoltaic devices.
We further demonstrate the novel lead-free semiconductor Cu2AgBiI6 which exhibits several advantages over Cs2AgBiBr6, namely a low exciton binding energy of ~29 meV and a lower and direct band gap near 2.1 eV,[2,3] making it a significantly more attractive lead-free material for photovoltaic applications. However, charge carriers in Cu2AgBiI6 are found to exhibit similarly strong charge-lattice coupling strength[3] to that in Cs2AgBiBr6, suggesting a link with the presence of AgBi. Tuning such charge-lattice interactions therefore emerges as a serious challenge for this class of materials.
Finally, we show that lead halide perovskites can exhibit intrinsic quantum confinement, apparent through surprising oscillatory features in the absorption spectrum.[4] Such materials may thus offer the sought-after target of bottom-up nanostructuring.
1.1-I2
Halide perovskite (HaP) materials have attracted great scientific interest in the past years, which is in part because of their unique combination of properties. Specifically, these systems show various fascinating physical properties revolving around their apparent electronic-structure and optical characteristics that are concurrent with finite-temperature lattice-dynamical properties that are very unusual for technologically useful semiconductors. Here, I will present our most recent theoretical findings obtained by means of molecular dynamics based on density-functional theory of the all-inorganic HaP material CsPbBr3. It will be shown that at finite temperature, the HaP structure favors atomic displacements perpendicular to the inorganic network, so-called transversal displacements, over longitudinal ones. The resulting high degree of transversality in HaP materials provides them with favorable optoelectronic properties at finite temperature, such as narrow energy distributions of electronic states, which has important implications for optical, transport and defect characteristics. These findings are contrasted with results obtained for the case of PbTe, a material that shares certain key properties with CsPbBr3, but due to its structure cannot allow for transversality, and thus is shown to exhibit less favorable optoelectronic properties at finite temperature. It is concluded that the simple concept of transversality may guide and support material design strategies for alternative compounds with favorable optoelectronic properties.
1.1-I3
Paulina Plochocka, Directrice de recherché de 2e classe (DR2) in Laboratoire National des Champs Magnétiques Intenses (LNCMI), CNRS in Toulouse.
P. Plochocka obtained her PhD cum-laude in 2004 at the University of Warsaw working on the dynamics of many-body interactions between carriers in doped semi-magnetic quantum wells (QW). During her first post doc at Weizmann Institute of science, she started working on the electronic properties of a high mobility 2D electron gas in the fractional and integer quantum Hall Effect regime. She continued this topic during second post doc in LNCMI Grenoble, where she was holding individual Marie Curie scholarship. At the same time, she enlarged her interest of 2D materials towards graphene and other layered materials as TMDCs or black phosphorus. In 2012 she obtained permanent position in LNCMI Toulouse, where she created the Quantum Electronics group, which investigates the electronic and optical properties of emerging materials under extreme conditions of high magnetic field and low temperatures. Examples include semiconducting layer materials such as transition metal dichalcogenides, GaAs/AlAs core shell nanowires and organic inorganic hybrid perovskites.
In 2D perovskites, as in all semiconductors, the degeneracy of the dark and bright exciton states is lifted by the exchange interaction between the electron and hole. This exciton fine structure is essential to understand the interaction of matter with light, and reflects the underlying symmetry of the system. The bright-dark splitting is also of paramount importance for light emitters which rely on the radiative recombination of excitons, since the excitons usually relax to the lowest lying dark state, which is detrimental for the device efficiency. It is expected that the enhanced Coulomb interaction in the 2D limit strongly increases the splitting of dark and bright excitonic states, however, such a splitting has never been measured directly in 2D perovskites. The only available report, based on photoluminescence (PL) studies, provides only a rough estimate of the splitting, since PL can be affected by trap states, and the complex exciton dynamics. For the particular case of the soft perovskites lattice, a direct comparison of the bright-dark splitting with the energy of phonons is crucial to understand the thermal mixing of the two excitonic states which drives radiative recombination in this material system. For the future development of 2D perovskites it is therefore of paramount importance to understand the exciton fine structure in 2D perovskites. I will discuss our optical spectroscopy measurements with an applied in-plane magnetic
field to mix the bright and dark excitonic states of (PEA)2PbI4, providing the first direct measurement of the bright-dark splitting. The induced brightening of the dark state allows us
to directly observe an enhancement of the absorption at the low-energy side of the spectrum related to the dark state. With the signature of dark state in the optical spectra we are able precisely determine the bright-dark exciton state splitting of 8 meV for (PEA)2PbI4at B= 0 T. The brightening of the dark state was also visible in photoluminescence, dominating the emission already at a moderate magnetic field of 6T. The evolution of the PL signal in the magnetic field, suggests that at low temperatures (4.5K) the exciton population is not fully thermalized due to the existence of a phonon bottle-neck, which occurs due to the specific nature of the exciton-phonon coupling in soft perovskite materials.
1.2-T1
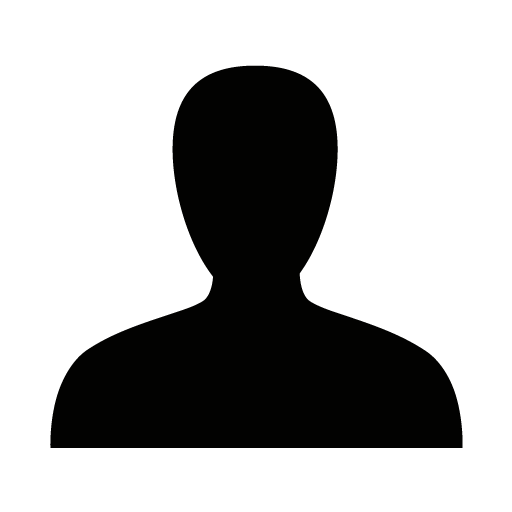
Lead-halide perovskite thin films have successfully been incorporated as light-absorbing and -emitting layers in a wide range of optoelectronic applications.[1,2] Cs2AgBiBr6 (CABB) has been proposed as a promising non-toxic alternative to lead-based perovskites. However, the indirect bandgap and low charge carrier collection efficiencies remain an obstacle for the incorporation of CABB in optoelectronic applications.[3] The limited charge carrier extraction has been ascribed to the high trap density and strong electron-phonon coupling, compared to its lead-containing analogues, resulting in fast charge carrier localization and low charge carrier mobilities. On the other hand, transient absorption experiments show a long-lived charge carrier lifetime ranging over several microseconds.[4] Moreover, at elevated temperatures mobile carriers are observed for microseconds after photoexcitation, highlighting CABB as a potential alternative for lead-halide analogues.[5]
To study the (free) charge-carrier dynamics we have performed transient absorption (TA) spectroscopy and time-resolved microwave conductivity (TRMC) experiments on CABB thin films on nanosecond to microsecond timescales. TA spectroscopy revealed that the charge carrier density decays on a 40-nanosecond timescale but a fraction of the photogenerated holes near the valence band maximum have a lifetime ranging over several microseconds. TRMC measurements showed that these long-lived carriers are, however, not mobile. Comparison of the TRMC and TA traces shows that the conductivity loss is the result of a combined effect of charge carrier loss and localization. Finally, we estimate that the charge carrier diffusion length is ca. 100 nm. This is of the same order of magnitude as the grain size, suggesting that grain boundaries are an important contributor to charge-carrier loss.
1.2-T2
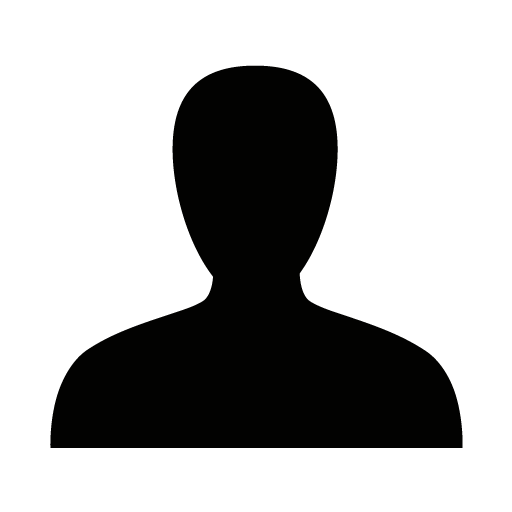
Halide double perovskites are an emerging class of materials with considerable structural and electronic diversity [1, 2, 3] and reliable stability towards heat and moisture under ambient conditions. We have recently shown that silver-pnictogen double perovskites, e.g. Cs2AgBiBr6, a material with promising optoelectronic properties, exhibit non-hydrogenic and strongly localized resonant excitons. This finding can be traced back to their chemical heterogeneity that leads to anisotropic effective masses and local field effects [4].
In this contribution, we systematically investigate how the electronic and optical excitations in halide double perovskites are impacted by the band edge orbital character. We use ab initio many-body perturbation theory within the GW approximation [6] and the Bethe-Salpeter equation [5] to compute excitations of a group of double perovskites with different valence and conduction band orbital character (Cs2AgBiCl6, Cs2AgInCl6, Cs2BiInCl6). We find that contrary to Cs2AgBiCl6, Cs2AgInCl6 and Cs2BiInCl6 exhibit delocalized excitonic states with low binding energy. Furthermore, carrier effective masses are highly isotropic and local field effects small, which we attribute to the orbital character at the band edges of these direct band gap semiconductors. Our results contribute to a detailed atomistic understanding of the light-matter interactions in chemically heterogeneous double perovskites and highlight the potential of these materials for light harvesting applications.
1.2-T3
Tom van der Pol is a Marie Curie fellow at the Laboratory of Organic Electronics within the Linköping University in Sweden. His research interests revolve around characterization of novel semiconductors, currently focused on organic mixed ion-electron conductors. He conducted his PhD research at the group of René Janssen studying optical characterization of thin film organic and perovskite semiconductors for solar cell applications.
Photoluminescence is an ubiquitous technique featuring in fields ranging from bio-diagnostics, organic electronics to perovskite solar cells. This accessible characterization tool provides researchers a window into the optoelectronic properties of a semiconductor. What many don’t realize, however, is the extent to which the optical environment plays a role in the recorded spectrum, especially in thin films. Effects extrinsic to the emitting dipole obscure the intrinsic spectrum, making interpretation of experimental spectra non-trivial.
In our work we have developed a model that takes into account the effect of self-absorption and thin film interference of emitted light [1]. We demonstrate the impact of these on the photoluminescence lineshape of an organic semiconductor as a function of film thickness and incident angle. The experimentally determined spectral characteristics are found to differ as much as 60% from their intrinsic material value. The differences in lineshape are calculated to be especially sensitive to film thickness.
We experimentally verified the model by reversely applying it to a measured spectrum. Further, we retrieve the intrinsic spectrum of two non-fullerene acceptor films, allowing for a correct analysis of the impact of their two different fabrication methods on the optoelectronics.
Finally, we provide a chart for the general experimentalist to assess the extent of extrinsic influences on the measured spectrum as function of film thickness, bandgap and Stokes shift.
Taken together, our work aims to bridge the gap between experimentalist and optical modeler to aid in the correct analysis of spectra. We find there is a clear need to take into account the influence of extrinsic effects on the measured spectrum, and provide estimates on the relative impact for various film parameters.
1.3-I1
Excitons dominate the optics of atomically-thin transition metal dichalcogenides and 2D van der Waals heterostructures. Interlayer 2D excitons, with an electron and a hole residing in different layers, form rapidly in heterostructures either via direct charge transfer or via Coulomb interactions that exchange energy between layers. Here I will discuss our recent work on the light-matter interaction on quasi-1D van der Waals heterostructures consisting of C/BN/MoS2 core/shell/skin nanotubes. I will describe how strong intertube excitonic coupling effects between excitons in CNTs and MoS2 NTs are evident under pulsed infrared excitation of excitons in the semiconducting CNTs. We observed a rapid (sub-picosecond) excitonic response in the visible range from the MoS2 skin following IR excitation, which we attribute to intertube biexcitons mediated by dipole-dipole Coulomb interactions in the coherent regime. On longer (>100ps) timescales hole transfer from the CNT core to the MoS2 skin further modified the MoS2's absorption. Our direct demonstration of intertube excitonic interactions and charge transfer in 1D van der Waals heterostructures suggests future applications in infrared and visible optoelectronics using these radial heterojunctions.
1.3-I2
Theoretical predictions of excited-state phenomena in emerging semiconductor systems can lead to a better understanding of nanoscale energy conversion mechanisms and of the excitonic properties and dynamical effects dominating these processes. In this talk, I will discuss the connection between exciton dynamics, lifetimes, and scattering to exciton dispersion. I will present our recent computational study within many-body perturbation theory, showing that nonanalytical discontinuities are arising from the exchange scattering of electron−hole pairs. These discontinuities are material-specific and stem from its symmetry and dimensionality, with jump discontinuities occurring in 3D and different orders of removable discontinuities in 2D and 1D. I will discuss a general theory to explain these unique features in the exciton bandstructure, combining ab initio and model Hamiltonian approaches. These features are directly connected to ultrafast ballistic transport and can be used to approximate exciton radiative lifetimes and diffusion coefficients, in good correspondence with recent experimental observations.
1.4-I1
I will describe a new suite of ultrafast optical microscopies capable of monitoring exciton and phonon dynamics on femtosecond and nanometer spatiotemporal scales in semiconductors and metals. I will focus on two-dimensional van der Waals materials whose properties are radically modified by strong interactions between light, excitons and phonons. Specifically, we find that in semiconductors whose excitons strongly hybridize with photons (forming exciton-polaritons whose wavefunctions are macroscopically delocalized), both polariton-polariton and polariton-exciton interactions overwhelmingly dictate energy flow and the semiconductors’ response to external electromagnetic fields. I will also show that acoustic phonons that strongly couple to localized excitons can be used to coherently manipulate the latter over macroscopic length scales. Directly imaging these quasiparticle dynamics in real space and time allows us to identify the key inter-particle interactions responsible for governing how materials respond to light and how light can be used to steer electronic transport and induce exotic phases of matter.
1.4-I2
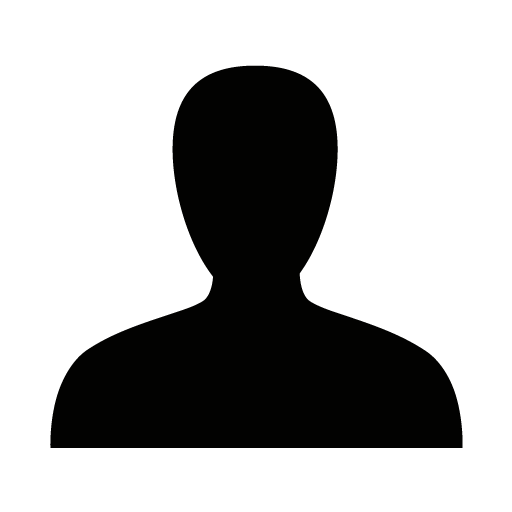
The GW approximation to the self-energy and the corresponding Bethe-Salpeter equation (BSE) together provide a state-of-the-art computational framework for the study of charged and neutral excitations in molecules and materials. However, their computational cost can be prohibitive for the description of certain electronic phenomena or large systems. In this talk, I'll describe two new directions that aim to lower the cost of GW/BSE calculations. The first direction addresses full-frequency implementations of GW/BSE including dynamical screening. I'll show that the requisite frequency-dependent eigenvalue problems of GW and BSE calculations can be exactly recast as frequency-independent eigenvalue problems in an enlarged space. Combined with the density fitting approximation to electron repulsion integrals, this reformulation leads to reduced scaling implementations of both methods. Moreover, it allows us to quantitatively study double excitations, including their energies and wavefunction character. In a second direction, I'll consider GW/BSE calculations on very large systems, with thousands of electrons or more. Here, we have used a simplifying approximation to the electron repulsion integrals with the same structure as in methods based on tensor hypercontraction. This approximation leads to GW/BSE calculations with storage and execution times that scale quadratically and cubically with the system size, respectively, and typically exhibit errors of only about 0.5 eV with respect to fully ab initio calculations. These methods can be applied to study systems containing thousands of electrons in only a few hours on commodity hardware.
1.4-I3
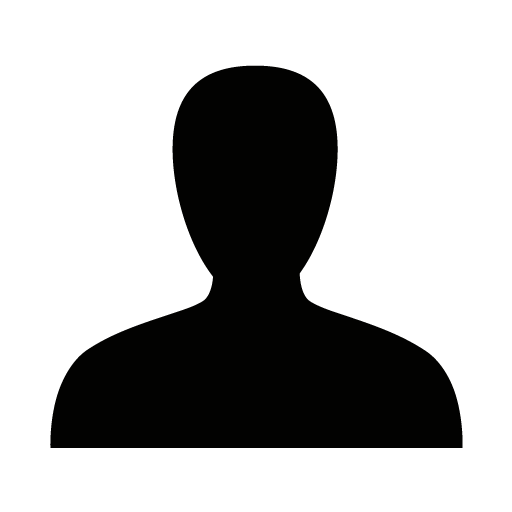
Photoinduced charge transfer in van der Waals heterostructures occurs on ultrafast timescales of order 100 fs, despite the weak interlayer coupling and momentum mismatch. However, little is understood about the microscopic mechanism behind it and role of the lattice in mediating this process. Here, we use ultrafast electron diffraction to directly visualize lattice dynamics in photoexcited heterostructures of WSe2/WS2 monolayers. Following selective optical excitation of WSe2, we measure surprisingly concurrent heating of both WSe2 and WS2 layers on ~1 ps timescales, two orders of magnitude faster than would be expected from the thermal phononic coupling between the layers alone. Using first-principles density functional theory calculations, we identify a fast channel, involving an electronic state hybridized across the heterostructure, for interlayer phonon-assisted transfer of photoexcited electrons between the two layers. Our calculations demonstrate that, via this channel, phonons are emitted in both layers on femtosecond timescales, consistent with the simultaneous lattice heating observed experimentally. Taken together, our work indicates strong electron-phonon coupling via layer-hybridized electronic states – a possible novel route to control thermal transport across van der Waals heterostructures.
2.1-I1
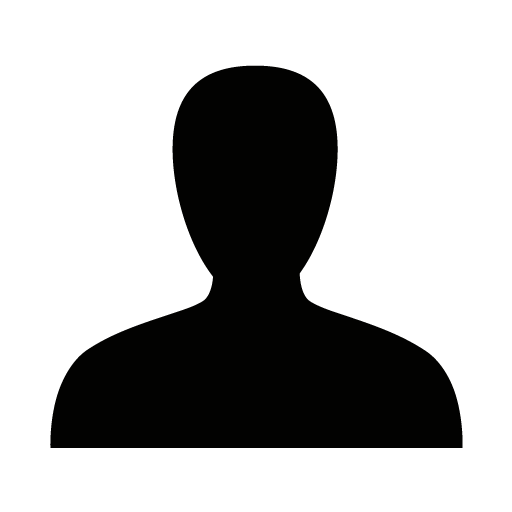
The fundamental band gap is a decisive observable when characterizing energy converting materials. Being able to predict it reliably at affordable numerical cost is a prerequisite for the large-scale computational search for new materials. Density Functional Theory (DFT) is often the method of choice for calculating a material's electronic structure. The band gap, however, has been a notoriously difficult observable for DFT. Inexpensive exchange-correlation approximations such as LDA and GGAs systematically underestimate the gap because they lack a derivative discontinuity. Hybrid functionals often yield more realistic results, but at a sharply increased computational cost. In any case, many-body perturbation theory methods, such as the GW approach, have often been required for a reliable prediction of the gap, adding a further layer of methodological complexity and cost. We here show that band gaps can be predicted accurately within DFT, without any empirical parameters, from the TASK meta-generalized gradient approximation (meta-GGA) functional. The latter has been derived from first principles by fulfilling known constraints and by taking into account the derivative discontinuity. Unlike some other meta-GGAs, the TASK functional leads to well converging calculations and predicts band gaps with reasonable accuracy for a large range of systems at the same order of computational expense as a GGA calculation.
2.1-I2
The concept of optimal tuning of range-separated hybrid functionals has become an important tool for overcoming the fundamental gap problem and the charge transfer excitation problem in molecular systems. Here, this concept is extended to the solid state by introducing dielectric screening into the functional form. This approach, couched rigorously within the generalized Kohn-Sham formalism of density functional theory, can produce quantitatively the same one and two quasi-particle excitation picture given by many-body perturbation theory (MBPT), without any empiricism. Specifically, for covalent/ionic semiconductors and insulators, accurate band structures and optical absorption spectra, which agree well with those obtained from MBPT, are obtained. For molecular solids, the approach predicts the correct gap renormalization - even from single molecule calculations if a polarizable continuum model is used in an electrostatically consistent manner – and also predicts absorption spectra well.
2.2-T1
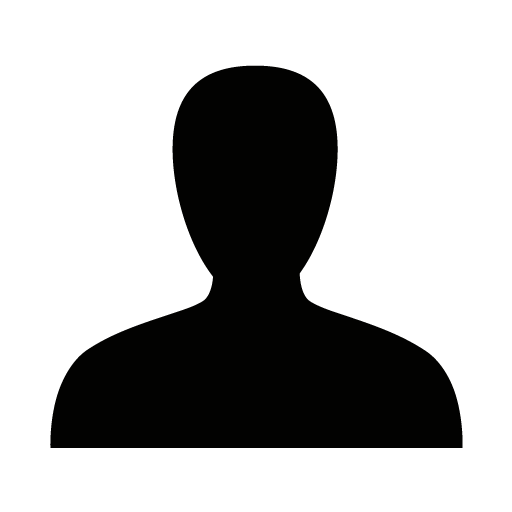
Exciton transport and separation processes across organic-inorganic interfaces are key ingredients in emerging applications in energy conversion. In particular, heterostructures consisting of molecular crystals are widely explored. While advances in experimental methods allow direct observation and detection of exciton properties across such junctions, a detailed understanding of the exciton nature and its relation to the interface structure and composition is still largely lacking. In this talk I will present a computational assessment of the many-body interactions dominating the excitonic nature at the interface between the perylene diimide (PDI) molecular crystal and an Au substrate, using many-body perturbation theory within the GW and Bethe-Salpeter equation (BSE) approach. We study the effect of structural modifications on the dielectric screening, manifested through changes in the quasiparticle and excitation energy gaps, as well as the electron-hole binding. Our findings suggest a close look into local and non-local interactions dominating the excitation energies and the exciton binding and nature in the examined PDI molecular crystals, and shed light on the change in exciton nature and properties upon their adsorption on an inorganic, weakly-interacting substrate, offering structural design principles for excitonic tuning at organic-inorganic interfaces.
2.2-T2
Two-dimensional (2D) semiconductors are primed as excellent optoelectronic materials to realize a variety of photonic devices which rely on the absorption of light and the consequent transient properties of photo-generated charges and excitons. Surprisingly, very little is known about the changes in the 2D material's refractive index upon excitation. The associated optical phase changes when light propagates through the 2D material, can be beneficial or undesired depending on the application at hand, but clearly require proper quantification. Measuring optical phase modulation of dilute 2D materials is however not trivial with common ultrafast methods. In this work, we first demonstrate that 2D colloidal CdSe quantum wells, a useful model system, can modulate the phase of light across a broad spectrum using an experimental ultrafast interferometry method. Next, we proceed to develop a toolbox to calculate the time-dependent refractive index of colloidal 2D materials from more widely available broadband transient absorption data using a modified effective medium algorithm. We confirm the experiments and show that the pronounced room temperature excitonic features found in 2D materials result in broadband, ultrafast and sizable phase modulation, even extending sub-band gap to the near-infrared where modulation is associated with well defined intraband transitions.
2.2-T3
Light-matter interaction between a molecular transition and a confined electromagnetic field of an absorber inside a resonator can modify the optical and electrical properties of molecules, which is relevant to improve solar devices.[1] When strong coupling regime is reached, two new hybrid states separated in energy, so-called polaritonic states, are formed instead of independent eigenstates, with the energy separation between them being proportional to the coupling strength, which is known as Rabi splitting. This modification of the energy spectra of the system, which have been already demonstrated in many configurations, offers new possibilities for controlled impact on various fundamental properties of coupled matter (rate of chemical reactions, conductivity of organic semiconductors).[2]
Recently, Subphthalocyanines (SubPcs)-based molecules have been integrated in a polaritonic organic solar cell that behaves as an optical resonator in order to modify device’s absorption onset and tune optoelectronic properties of the devices.[3] Therefore, understanding how the different electronic transitions of an absorber can couple to the resonant modes of a solar polaritonic device is an important issue that deserves to be addressed.
In view of this, we investigate how two electronically different transitions, namely charge transfer (CT)-band and excitonic Q-band, of a subphthalocyanine derivative (F12-SubPc-TCBD-aniline) within an optical cavity, respond to variations in the resonant modes of such cavity. By coupling the cavity resonance with one of the two electronic transitions we observe different coupling regimes, which give rise to very different spectral and directional light harvesting features. Also, by modelling the system under analysis, we can discriminate the productive absorption occurring in the SubPc layer from the parasitic one due to the presence of metallic films in the structure, which allow us to estimate the potential optical losses that may occur in a light harvesting device devised as an optical resonator. [4]
Session-K1
Prof. Kyoung-Shin Choi is a professor of chemistry at University of Wisconsin-Madison. She received her B.S. and M.S. degrees from Seoul National University in South Korea in 1993 and 1995, respectively. She received a Ph.D. degree from Michigan State University in 2000, and then spent two years at the University of California, Santa Barbara as a postdoctoral researcher. She initiated her independent research career as an assistant professor at Purdue University in 2002 and joined the chemistry faculty at University of Wisconsin-Madison as a full professor in 2012. She was a visiting scholar at the National Renewable Energy Laboratory (NREL) in 2008.
Her research combines solid state chemistry, electrochemistry, and materials chemistry in order to address materials-related issues of electrode materials for use in photoelectrochemical and electrochemical applications She was a recipient of a 2006 Alfred P. Sloan Research Fellowship, the 2007 ACS ExxonMobil Faculty Fellowship in Solid-State Chemistry, and the 2010 Iota Sigma Pi Agnes Fay Morgan Research Award. She also received the 2008 Purdue College of Science Outstanding Undergraduate Teaching Award and the 2015 Wisconsin Alumni Research Foundation Innovation Award. She has organized numerous symposia for the American Chemical Society (ACS) meetings and Materials Research Society (MRS) meetings as well as for the Gordon Research Conference. She is currently serving as an Associate Editor for Chemistry of Materials and a member of the Board of Directors for Materials Research Society.
Photoelectrochemical water splitting presents an attractive strategy to produce hydrogen gas as an alternative clean fuel in an environmentally benign and sustainable manner. The key component of a photoelectrochemical cell is a semiconductor electrode (photoelectrode) that absorbs solar light to generate, separate, and transport charge carriers to the semiconductor/electrolyte interface to participate in desired chemical reactions. The electron-hole separation and interfacial charge transfer of the photoelectrode are considerably affected by the interfacial energetics between the photoelectrode and the electrolyte and/or between the photoelectrode and the buffer, protection, or catalyst layers; hence, the interfacial properties of a photoelectrode are as important as the bulk properties of the photoelectrode.
To date, strategies for altering the atomic arrangement at the photoelectrode surface that do not involve extrinsic doping have mainly involved changing the semiconductor surface facets. However, for ternary oxide photoelectrodes with a formula of AxByOz, there exist numerous ways to terminate the surface even for the same facet. For example, the surface can be terminated with A-O or B-O, and the surface A:B ratio may be different from the bulk A:B ratio. In fact, if not grown as single crystals, AxByOz photoelectrodes can have an A-rich or B-rich surface depending on the synthesis method, which can affect their photoelectrochemical properties. However, despite being important and ubiquitous, the effects of surface termination/composition on a ternary oxide photoelectrode have not been systematically studied, and the atomic origin of their effects on interfacial energetics and photoelectrochemical properties have not been elucidated.
In this presentation, we will discuss the effects of surface termination/composition on the interfacial energetics and photoelectrochemical properties of photoanodes using BiVO4 as an example. We will compare epitaxially grown BiVO4photoelectrodes with V-rich and Bi-rich (010) exposed facets and demonstrate that the surface Bi:V ratio has a considerable effect on the surface energetics and photocurrent generation of BiVO4 even for the same (010) facet.
Session-K1
Professor of Physical Chemistry at the Ecole Polytechnique Fédérale de Lausanne (EPFL) Michael Graetzel, PhD, directs there the Laboratory of Photonics and Interfaces. He pioneered research on energy and electron transfer reactions in mesoscopic systems and their use to generate electricity and fuels from sunlight. He invented mesoscopic injection solar cells, one key embodiment of which is the dye-sensitized solar cell (DSC). DSCs are meanwhile commercially produced at the multi-MW-scale and created a number of new applications in particular as lightweight power supplies for portable electronic devices and in photovoltaic glazings. They engendered the field of perovskite solar cells (PSCs) that turned our to be the most exciting break-through in the recent history of photovoltaics. He received a number of prestigious awards, of which the most recent ones include the RusNANO Prize, the Zewail Prize in Molecular Science, the Global Energy Prize, the Millennium Technology Grand Prize, the Samson Prime Minister’s Prize for Innovation in Alternative Fuels, the Marcel Benoist Prize, the King Faisal International Science Prize, the Einstein World Award of Science and the Balzan Prize. He is a Fellow of several learned societies and holds eleven honorary doctor’s degrees from European and Asian Universities. According to the ISI-Web of Science, his over 1500 publications have received some 230’000 citations with an h-factor of 219 demonstrating the strong impact of his scientific work.
Recently molecular photovoltaics, such as dye sensitized cells (DSCs) and perovskite solar cells (PSCs) have emerged as credible contenders to conventional p-n junction photovoltaics. Their certified power conversion efficiency currently attains 25.5 %, exceeding that of the market leader polycrystalline silicon. This lecture covers the genesis and recent evolution of DSCs and PSCs, describing their operational principles and current performance. DSCs have meanwhile found commercial applications for ambient light harvesting and glazing producing electric power from sunlight. The scale up and pilot production of PSCs are progressing rapidly but there remain challenges that still need to be met to implement PSCs on a large commercial scale. PSCs can produce high photovoltages rendering them attractive for applications in tandem cells, e.g. with silicon and as power source for the generation of fuels from sunlight. Examples to be presented are the solar generation of hydrogen from water and the conversion of CO2 to chemical feedstocks such as ethylene, mimicking natural photosynthesis.
1.1-I1
In this contribution, I will first report on our recent studies on solution-based n-type doping of polymer semiconductors, aimed at understanding current limits in molecular doping schemes. I will then focus on the development of printed organic micro thermoelectric generators (µTEG), as interesting energy harvesters candidates for distributed low power electronics and sensors networks. In particular, I will on describe an architecture where 128 thermocouples are directly embedded in a plastic substrate to improve the thermal coupling and simplify the fabrication process. Such proof-of-principle µTEG allows to envisage future efficient devices delivering µW/cm2 close to room temperature and at low temperature differences.
1.1-I2
Mariano Campoy Quiles´s research is devoted to the understanding and development of solution processed semiconductors for energy and optoelectronic applications. He and his team have built substantial research efforts in two application areas, solar photovoltaic (light to electric) and thermoelectric (heat to electric) energy conversion based on organic and hybrid materials. He studied physics at the Univesity of Santiago de Compostela, obtained his PhD in experimental physics from Imperial College London, and since 2008 he leads his team at the Institute of Materials Science of Barcelona.
X. Rodríguez-Martínez1, F. Saiz1, S. Marina2, H. Chen3, O. Zapata-Arteaga1, B. Dorling1, J. Martin2,4,5, I. McCulloch3,6, R. Rurali1, J. S. Reparaz1, M. Campoy-Quiles1,*
1 Institut de Ciència de Materials de Barcelona, ICMAB-CSIC, Campus UAB, 08193, Bellaterra, Spain
2 POLYMAT and Polymer Science and Technology Department, Faculty of Chemistry, University of the Basque Country UPV/EHU, Manuel de Lardizabal 3, 20018, Donostia- San Sebastián, Spain
3 King Abdullah University of Science and Technology (KAUST) Solar Center (KSC), Thuwal, 23955-6900, Saudi Arabia
4 Ikerbasque, Basque Foundation for Science, 48011 Bilbao, Spain
5 Centro de Investigacións Tecnolóxicas, Universidade da Coruña, Campus de Esteiro, 15403, Ferrol, Spain
6 Department of Chemistry, University of Oxford, Oxford, OX1 3TA, United Kingdom
The thermal conductivity is the main parameter defining how heat propagates in a solid. It is of paramount importance for heat dissipation in electronics and transmission lines, but also in thermoelectrics. Despite its importance, little attention has been paid to its actual value, in part due to the general belief that the thermal conductivity in organic semiconductors “should be” small. Literature shows, however, values spanning more than two orders of magnitude.
In this invited talk, we will show a combined experimental/theoretical study, in which we have measured the thermal conductivity for a large polymer library and discovered the coexistence of two fundamentally distinct regimes. Semicrystalline conjugated polymers behave as conventional theory predicts, with increasing order leading to an increase in thermal conductivity, and this correlates also with an increase in charge carrier mobility. In other words, thermal and electrical transport go hand in hand. On the other hand, materials that do not show long range order in GIWAXS behave very differently, not following the same classic theory. As a consequence, for the latter, charge carrier mobility and thermal conductivity appear to be anticorrelated. We rationalize our results using Spearman statistics as well as theoretical calculations, which allow us to provide simple and exploitable design rules for materials that are able to decouple thermal and electronic transport, namely, texture/orientation and monomer/sidechain weight. Our results open a new avenue for highly efficient organic thermoelectrics.
1.1-I3
Millimeter thick bulk materials are needed to facilitate the design of thermoelectric devices. While submicrometer-thin films of organic semiconductors are needed for fundamental spectroscopic studies, they are not ideal for the construction of devices, unless folded or wrinkled. In my talk, I will discuss our recent work on the preparation of conducting bulk materials, from foams to fibers, and explore the interplay of electrical and mechanical properties. Further, I will discuss some of the challenges related to doping of bulk materials, as well as the (in)stability of the doped state. Finally, I will present prototype textile and 3D-printed devices that can be manufactured with bulk materials.
1.2-T1
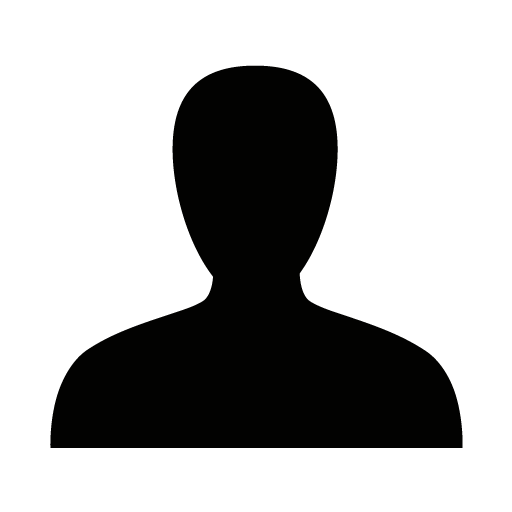
Organic semiconductors are excellent candidates for low temperature thermoelectric generators. However, such thermoelectric applications require that the materials be doped and highly conductive.
Here, we show how doping affects the Seebeck coefficient in organic semiconductors using kinetic Monte-Carlo simulations. Employing a hopping transport approach we demonstrate that at high dopant loading, carrier-carrier interactions can reduce the Seebeck coefficient. This results in systems with intrinsic disorder still following Heike’s formula for thermopower at high dopant density. Reducing these carrier-carrier interactions results in an increased Seebeck coefficient and power factor. Specifically a realistic reduction in carrier-carrier interactions can increase the power factor by more than a factor 15, increasing ZT above 1 for organic thermoelectrics.
1.2-T2
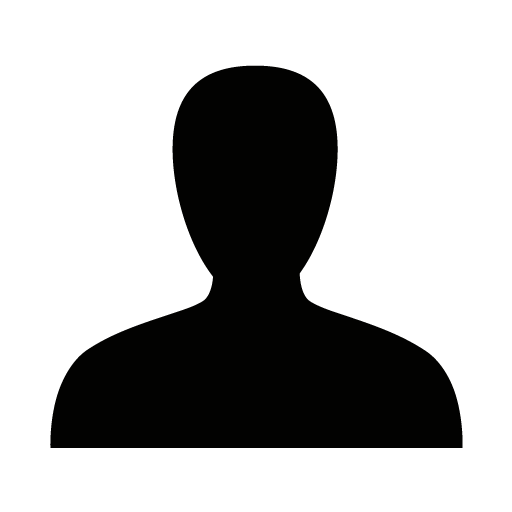
The lack of highly conducting n-type materials greatly hampers the realization of performing organic thermoelectric generators (OTEGs); molecular doping (e.g. blending with electron-donating compounds) has stemmed as one of the possible solutions to enhance charge transport. Despite the actual interaction mechanism has not yet been fully disclosed [1, 2], benzimidazoles derivatives have so far emerged has one of the most promising class of organic n-type dopants due to their solution-processability, generality and relatively good shelf-life when stored in air [3].
Here we report on a modification of the widely used and commercially available 4-(2,3-Dihydro-1,3-dimethyl-1H-benzimidazol-2-yl)-N,N-dimethylbenzenamine (N-DMBI) obtained by replacing the two methyl end-groups with an iminostilbene moiety, creating an innovative derivative named IStBI. The introduction of a bulkier and more sterically hindered substituent bears significant changes in the crystallinity (shown through DSC and Thermogravimetric analysis) and packing in the dopant-host matrix, with only a marginal effect on the compound electronic structure, therefore preserving its electron-donating character. The study is conducted using the widely spread n-type polymer P(NDI2OD-T2), ensuring a large platform of comparable results available in the literature [4, 5, 6]. All the processing was carried out in solution and under N2-atmosphere due to the lack of air and moisture stability of the obtained semiconducting blends. Thin films, casted through spin-coating technique, have been extensively characterized in their electrical conductivity and Seebeck coefficient. After optimizing the processing conditions (i.e. solvent, dopant concentration and mixing temperature) and post-deposition thermal treatment, we obtained an electrical conductivity of 1.14 x 10-2 S cm-1, which is to the best of our knowledge among the highest values ever reported for doped P(NDI2OD-T2) [7]. This resulted in also an improved power factor of 8 x 10-3 mW/mK2. To support these findings structural and morphological analysis have been performed through GIWAXS analysis and AFM imaging techniques respectively, suggesting reduced disruption of the pristine polymer crystalline structure compared to other reported dopant molecules leading to improved charge transport properties.
1.2-T3
Molecular doping is a powerful tool that is widely used for controlling the electrical properties of conjugated polymers. To design and develop polymer:dopant systems with improved ionization and/or dissociation efficiency, it is essential to measure the number of charges that are created on the backbone of the conjugated polymer. Here, it will be shown how a combination of UV-vis-NIR spectroscopy, spectro-electrochemistry and electron paramagnetic resonance (EPR) spectroscopy can be used to determine the number of polarons. P-doping of polythiophenes with alkyl or oligoether side chains will be compared in terms of the oxidation level and electrical properties. The strongest polaron density that can be achieved with oxidants such as F4TCNQ and Magic Blue is on the order of 5x1026 m-3, corresponding to an oxidation level of about 15%. Dopants with a high electron affinity, in combination with polymers that feature a low ionization energy of less than 5 eV give rise to double doping. The resulting exchange of two electrons between polymer and dopant allows to double the ionization efficiency and hence half the number of dopant molecules that are needed to achieve a certain oxidation level.
1.3-T1
Three lactone-based rigid semiconducting polymers were deliberately designed to tackle one the major limitations in the development of n-type organic thermoelectrics, such as electrical conductivity and air stability. Here, we show that phenyl core maximization along the backbone can play a major role in optimizing the thermoelectric performance. Especially when combined with the rigid locked conformation imposed by aldol condensation. Experimental and theoretical investigations demonstrated that increasing the phenyl acene content from 0% phenyl (P-0), to 50% (P-50), and 75% (P-75) resulted in progressively i) larger electron affinities up to -4.37 eV due to the increased density of electron withdrawing groups, thereby suggesting a more favorable doping process when employing (N-DMBI) as the dopant. ii) Larger polaron delocalization due to the more planarized conformation, which ultimately led to lower hopping energy barrier. As a consequence, the electrical conductivity increased by three orders of magnitude to achieve values of up to 12 S/cm, which is one the scarcely reported n-type polymers with electrical conductivities over 10 S/cm. Thereby, enabling power factors of 13.2 μWm−1 K−2 , and is among the highest reported in literature for n-type polymers. Importantly, the electrical conductivity of the doped P-75 maintained a high value of 1.2 S/cm after 18 days of exposure to air. These findings further highlight the benefits of phenyl core maximization to the electronic performance of the materials and suggest this approach as an effective design strategy to optimize the thermoelectric performance, thus also presenting new insights into material design guidelines for the future development of air stable n-type organic thermoelectrics.
1.3-T2
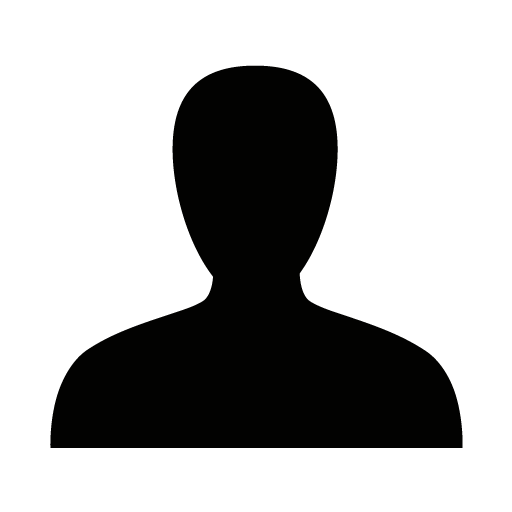
Organic semiconductors are exciting candidates for organic thermoelectrics due to their low cost, abundant constituting elements, and, intriguingly, an assumed and overlooked low thermal conductivity. Therefore, research focuses primarily on improving the electronic contribution of the thermoelectric figure of merit ZT through processes like electrical doping and modification of the structural order. However, it is not clear how these processes affect thermal conductivity, and a mere handful of publications have explored this subject. Here we present a high-throughput methodology based on annealing- and doping-gradients to analyze and correlate the electrical and thermal conductivity. We can obtain data equivalent of > 100 samples using a single polymer film through this approach. As a testbed for our experiments, we employ poly(2,5- bis(3-alkylthiophen-2-yl)thieno[3,2-b]thiophene) (PBTTT) and the dopant 2,3,5,6-tetrafluoro-7,7,8,8-tetra-cyanoquinodimethane (F4TCNQ). We show that doping in PBTTT does not deteriorate the crystalline quality of the film but reduces thermal conductivity by a factor of two, even at relatively low doping levels. Our results indicate that the lattice contribution of the system dominates thermal transport and that impurities within the polymer network can have a drastic effect on the total thermal conductivity.
1.3-T3
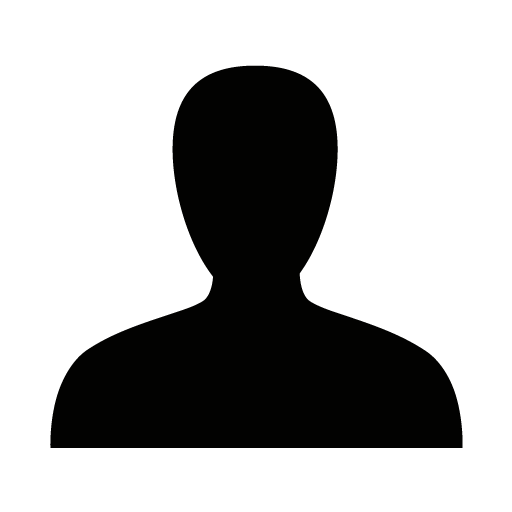
With the increased attention to alternative energy sources in the last decades, there has been a renewed interest in developing thermoelectric materials and devices. In this regard, organic semiconductors (OS) have emerged as a suitable alternative for low-power/low-cost thermoelectric devices. OS can be processed at room temperature using large-area printing techniques. They are potentially biocompatible and have intrinsically low thermal conductivity. Combined with the high abundance of their atomic elements, these features make them the ideal candidates for low-power electronic applications, such as wearable electronics, smart sensors, and the Internet of Things (IoT). Most of the printing technologies developed to date are suitable for thin-film patterning, which is not ideal for thermoelectric generators (TEGs), where the active material thickness should exceed at least 100 µm to sustain a temperature gradient properly. New geometries have been employed to solve this issue, exploiting flexible substrates to switch from a 2D to a 3D configuration through the folding of the flexible support. However, such devices still suffer from intrinsic limitations in the thermocouples density, and the addition of folding steps increases the chance of device failures due to the risks of open/short circuit in the generator. Here, we show the possibility of using 3D printing, a digital direct writing technique, to fabricate TEG with a vertical structure easily. The device, printed on top of a 2-µm-thick free-standing parylene substrate, is fully organic. The p-type and n-type legs are developed by printing PEDOT:PSS and BBL:PEI formulations, respectively. Finally, both the TEG scaffold and capping are realized using a 3D printable PDMS ink. Here we present the first 3D printed all-organic TEG, consisting of 8 thermocouples and capable of delivering 50 nW with a temperature gradient between its edges below 15 K.
1.4-I1
-
Chemical doping is crucial for the operation of organic thermoelectric generators. This is typically achieved by adding dopant molecules to the polymer bulk, enabling high electrical conductivities. However, this process can result in poor stability and performance due to nonoptimal charge transfer and thin-film morphologies. Besides, once the dopant molecules are incorporated, they tend to diffuse through the free volume between polymer chains or escape during the heating steps, degrading both the electrical and mechanical properties of the semiconductor. In addition, the diffusion of dopant molecules could pose a risk when these materials are placed in contact with the human body like, e.g., in the case of wearable devices. Thus, molecular doping of organic semiconductors significantly limits their implementation into novel thermoelectric applications. In this presentation, we will report on our recent efforts to develop stable and highly conductive polymer blends based on mutual electrical doping. First, we will discuss the case of non-conjugated polymeric dopants and then move toward a new generation of conjugated polymer-donor/polymer-acceptor blends based on the effect of ground-state electron transfer in all-polymer heterojunctions. These molecular dopant-free systems hold promise for developing next-generation thermoelectric generators, particularly targeting high stability, efficiency, and power performance.
1.4-I2
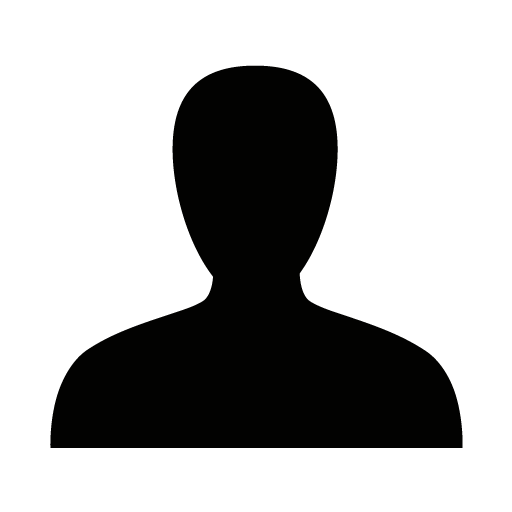
Chemically doped semiconducting polymers exhibit thermoelectric characteristics that range from localized (or hopping-like) transport to delocalized (or metal-like) transport. While a multitude of electronic transport models have been proposed, none of them capture the full spectrum from localized to delocalized transport.[1] Additionally, existing models do not quantitatively capture the dependency on charge carrier density (or carrier concentration or carrier ratio) that manifests through the measured temperature-dependent electrical conductivity and Seebeck coefficient. Recently, we developed a semi-localized transport (SLoT) model, building upon past insight, that can describe the full spectrum from localized to delocalized transport. This new model provides quantitative insight into charge carrier localization that is capable of more accurately describing electronic transport in a broad spectrum of organic thermoelectric semiconducting polymers.
This invited talk will discuss our recent publication[2] where I will first present motivation showing the previous short comings of our collective understanding with existing models. Next, I will briefly discuss the development of the SLoT model in the context of the organic thermoelectric field. I will then discuss the utility and prospects of deeper insight that the SLoT model affords. Then I will validate the model using the prototypical P3HT polymer doped with FeCl3 and show its broad applicability in accurately describing other polymers/organic materials (namely, PBTTT, PA, PEDOT, SWCNT, and N2200) that were not previously well described by other models. I will then extend this process to new polymers and describe the deeper insight gained from this model. I will then conclude my talk describing the relevant experimental measurements that research groups should undertake in characterization of their polymers to be able to use the SLoT model, in hopes of encouraging uniform material characterization internationally.
The future implications of the SLoT model in developing thermoelectric polymers could be profound. When coupled with chemical and structural characterization, the SLoT model connects the chemistry and structure to the macroscopic transport properties. Once the SLoT model parameters are calculated, we can quantify fundamental limits to a polymer’s potential (e.g., ability to achieve high electrical conductivity or high Seebeck coefficient). Ultimately, this allows us to accelerate the rational development of chemically doped organic thermoelectrics affording new functionality (e.g., thermal or electronic switching, thermoelectric cooling or power generation, etc.).
1.4-I3
Thuc-Quyen Nguyen is a professor in the Center for Polymers and Organic Solids and the Chemistry & Biochemistry Department at University of California, Santa Barbara (UCSB). She received her Ph.D. degree in physical chemistry from the University of California, Los Angeles, in 2001 under the supervision of Professor Benjamin Schwartz. Her thesis focused on photophysics of conducting polymers. She was a research associate in the Department of Chemistry and the Nanocenter at Columbia University working with Professors Louis Brus and Colin Nuckolls on molecular self-assembly, nanoscale characterization and molecular electronics. She also spent time at IBM Research Center at T. J. Watson (Yorktown Heights, NY) working with Richard Martel and Phaedon Avouris. Her current research interests are structure-function-property relationships in organic semiconductors, sustainable semiconductors, doping in organic semiconductors, interfaces in optoelectronic devices, bioelectronics, and device physics of OPVs, photodetectors, and electrochemical transistors. Recognition for her research includes 2005 Office of Naval Research Young Investigator Award, 2006 NSF CAREER Award, 2007 Harold Plous Award, 2008 Camille Dreyfus Teacher Scholar Award, the 2009 Alfred Sloan Research Fellows, 2010 National Science Foundation American Competitiveness and Innovation Fellows, 2015 Alexander von Humboldt Senior Research Award, 2016 Fellow of the Royal Society of Chemistry, 2015-2019 World’s Most InfluentialScientific Minds; Top 1% Highly Cited Researchers in Materials Science by Thomson Reuters and Clarivate Analytics, 2019 Fellow of the American Association for the Advancement of Science (AAAS), 2023 Wilhelm Exner Medal from Austria, 2023 Fellow of the US National Academy of Inventors, 2023 de Gennes Prize in Materials Chemistry from the Royal Society of Chemistry, 2023 Elected Member of the US National Academy of Engineering, 2024 Fellow of the European Academy of Sciences, and 2025 ACS Henry H. Storch Award in Energy Chemistry.
The ability to precisely control the equilibrium carrier concentration in organic semiconducting devices is of great interest. Solution processed doped layers are of extreme importance for high throughput production of organic electronic devices via roll-to-roll or ink-jet printing. The conventional picture of doping organic semiconductors involves full electron transfer from the semiconductor to the dopant (p-doping) or from the dopant to the semiconductor (n-doping) and the formation of species (polarons or bipolarons) that are the itinerant charge carriers. This process is known as “molecular doping”, which requires matching of the energy levels between the semiconductor and the dopant. In this talk, I will discuss unconventional doping methods that do not require energy level matching such as neutral polymers in non-aqueous solvents doped by Lewis acid-water complexes (Brønsted acids) and “self-doped” charged polymers in aqueous solution.
2.1-I1
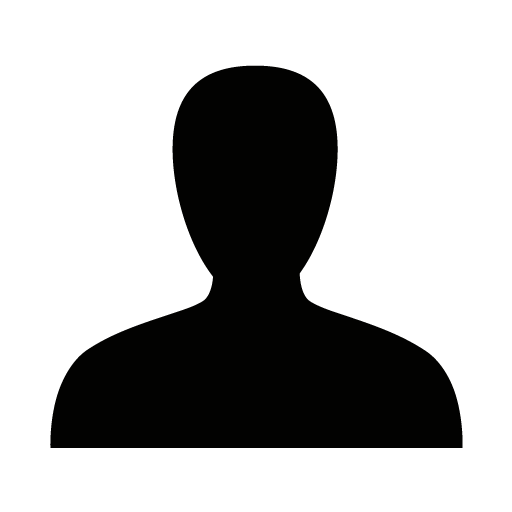
High performance flexible thermoelectric polymer-based material requires a high Seebeck coefficient, high electrical conductivity and low thermal conductivity. It is still challenging to meet all these requirements. Here we report the design and synthesis pi-conjugated polyelectrolytes with different degree of naphthalenediimide (NDI) core with covalently tethered quaternized ammonium cations. They are then incorporated with multi-valent oxalate anion which is a powerful latent electron donor when dispersed as small ion clusters to give in-situ n-dopable NDI polymers. We further optimised their morphologies using good-to-borderline solvent, and achieved both high seebeck coefficient and conductivity. Their doping level and electron mobility are also characterised.
Tang, C.G; Ang, M.C.Y.; Choo, K.K.; Keerthi, V.; Tan, J.K. ; Nur Syafiqah, M. ; Kugler, T. ; Burroughes, J.H.; Png, R.Q.; Chua, L.L.; Ho, P.K.H., Nature, 2016 , 539, 536 DOI: 10.1038/nature20133
Tang, C.G; Nur Syafiqah, M.; Koh, Q.M.; Zhao, C.; Zaini, J.; Seah, Q.J.; Humphries, MJ. Burroughes, J.H.; Png, R.Q.; Chua, L.L.; Ho, P.K.H. Nature, 2019, 573, 519; DOI: 10.1038/s41586-019-1575-7
2.1-I2
Developing highly conducting and air-stable n-type semiconductors for organic thermoelectrics have proven challenging, despite intensive research efforts in recent years. Thermoelectric generators convert heat into usable electricity, due to the Seebeck effect. Constructing an efficient thermoelectric generator based on organic semiconductors is difficult, however, primarily due to a significant shortage of suitable organic materials. While organic p-type semiconductors are abundant and relatively easy to dope, there is a shortage of suitable n-type materials and appropriate dopants. One popular approach is to extrinsically dope conjugated polymers. By mixing molecular dopants into a polymer matrix, it is possible to enhance the overall conductivity of the semiconductor to a certain degree, before morphological instabilities take over and cause microscopic phase separation of the dopant and polymer matrix, significantly limiting the doping efficiency.
In this paper, we will present the synthesis of intrinsic organic conductors, based on organometallic coordination polymers. We will discuss in detail how the choice of the ligand is crucial to control the electrical and thermoelectric properties. We will then take a more detailed look at the nature of the metal cation linking the organic ligands, and investigate their influence on charge carrier polarity and magnetic properties. Contrary to extrinsically doped semiconducting polymers, the organometallic coordination polymers exhibit excellent ambient and morphological stability and could pave the way to a new class of robust organic conductors for plastic electronic applications.
2.2-I1
Halide perovskites are well known as promising candidates for photovoltaics and light-emitting diodes. Additionally, promising thermoelectric performance has been reported for a small number a halide perovskites, with this class of materials offering ultralow thermal conductivity, good Seebeck coefficients and potential advantages in processing and sustainability. However, there is not yet a good understanding of how thermoelectric performance of halide perovskites can be optimised. This presentation will report the thermoelectric figure of merit (ZT) for hybrid and inorganic perovskites [1,2]. It will discuss the origins of ultralow thermal conductivity and quanitfy both the Lorenz number and the thermal boundary resistance [3] in polycrystalline films. Extrinsic doping and self-doping will be discussed as methods to optimise the thermoelectric figure of merit zT, with values of zT reaching 0.14 in CsSnI3 [2]. The case of self-doping by Sn-oxidation in CsSnI3 will be examined in detail and strategies to improve performance and control the rate of oxidation by modification of deposition procedures, or by using mixed halide and mixed metal stoichiometries will be presented.
2.2-I2
Electrochemical doping of organic conjugated polymers can be investigated in OECTs (Organic Electrochemical Transistors), which are sensitive sensors used for example in biological applications. They can be described using two circuits: an ionic circuit and an electronic circuit. The former arises from ions penetrating an organic channel due to switching of the gate, while the latter arises form source-drain electron flow across the organic channel. In the last years, advancing our knowledge of OECTs has allowed a drastic increase of their performance, however a complete picture of the two circuits and how they interact is still lacking. Here, we will introduce two new spectroscopic techniques to study the ionic and electronic transport processes in OECTs: 1) Time-resolved spectro-electrochemistry measures the kinetics of the ionic circuit. This measurement monitors the ion penetration into the organic channel and the doping processes with millisecond temporal resolution. 2) In-situ THz absorption spectroscopy, which investigates the electronic circuit and transport at different doping levels. It probes the nature of the electronic charges and the nanoscale conductivity of the organic channel. Results on PEDOT:PSS based OECTs and sandwish electrochemical devices will be presented. A deep understanding of the internal mechanisms occurring in OECTs is an essential step towards further interfacing with elaborate biological systems.