1.1-I1
Vincent Artero was born in 1973. He is a graduate of the Ecole Normale Supérieure (Ulm; D/S 93) and of the University Pierre et Marie Curie (Paris 6). He received the Ph.D. degree in 2000 under the supervision of Prof. A. Proust. His doctoral work dealt with organometallic derivatives of polyoxometalates. After a postdoctoral stay at the University of Aachen (Aix la Chapelle) with Prof. U. Kölle, he joined in 2001 the group of Prof. M. Fontecave in Grenoble with a junior scientist position in the Life Science Division of CEA. Since 2016, he is Research Director at CEA and leads the SolHyCat group. His current research interests are in bio-inspired chemistry including catalysis related to hydrogen energy and artificial photosynthesis.
Vincent Artero received the "Grand Prix Mergier-Bourdeix de l'Académie des Sciences" in 2011 and has been granted with a Consolidator Grant from the European Research Council (ERC, photocatH2ode project 2012-2017). He's a member of the Young academy of Europe (YAE). He currently acts as Chair of the Scientific Advisory Board of the ARCANE Excellence Laboratory Network (LABEX) for bio-driven chemistry in Grenoble and as co-head of the French network (CNRS-Groupement de recherche) on Solar Fuels. Since 2016, Vincent Artero is associate editor of the Royal Society of Chemistry journal "Sustainable Energy and Fuels". From January 2018 onward, he actsas associate editor of the Royal Society of Chemistry flagship journal "Chemical Science"
Hydrogen is now confirmed as a key component of a CO2-neutral economy, we need to transition towards. The production of large quantities of hydrogen now requires breakthroughs in finding new catalysts that are efficient, stable and cheap, i.e. based on abundant elements. Indeed fuel formation involves multi-electron multi-proton reactions that are inherently kinetically sluggish. Efficient catalysts can be found in living micro-organisms producing or metabolizing hydrogen thanks to hydrogenases. Catalysis in these enzymes only requires Earth-abundant metal centers, the reactivity of which is enhanced thanks to the presence of basic sites acting as proton relays [1] at their vicinity. Such active sites have been used as an inspiration to design new synthetic catalysts for H2 evolution [2-4] and oxidation [5-6]. Specification, catalytic platforms with installed proton relays display bidirectional [7] and, in rare cases, reversible catalysis [5]. In this presentation we will show how a detailed molecular electrochemistry study can help understanding and quantifying the role of the protons relays related to these remarkable behaviors.
1.1-I2
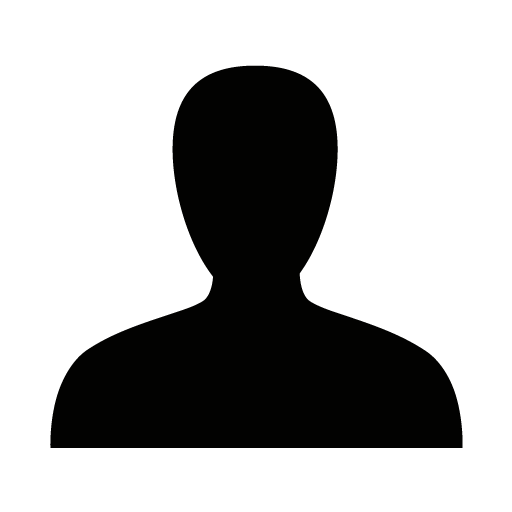
Hydrogenation reactions are fundamental functional group transformations in chemical synthesis. Initially, research on hydrogenation reactions focused on the use of noble metal complexes based on Ru, Rh, Ir, Pd, Pt and remarkable achievements have been made using such complexes. However, recently the focus shifted towards the use of base metal catalysts, as these metals exhibit a higher availability in earth’s crust.[1] In the first instance, the direct reduction of C=O bonds with H2 seems to be a very atom and redox economic approach. However, the reaction protocols for base-metal catalyzed hydrogenation reactions require often harsh reaction conditions and high H2 pressure to activate H2 and each ton of H2 that is formed via steam reforming produces about 12 tons of CO2.[2]
I will present an electrochemical approach utilizing electrons and protons for the catalytic hydrogenation of polar double bonds, such as C=O in CO2, ketones, and aldehydes.[3] In a proof of principle study we demonstrated that in-situ generated Mn hydride species hydrogenate CO2 forming formic acid. This protocol was later expanded to aromatic, and aliphatic ketones as well as aldehydes. The method is selective for C=O bonds over the thermodynamically favored hydrogenation of C=C bonds. The competing hydrogen evolution reaction was suppressed successfully, and the reactions proceed with high Faraday efficiencies. A large variety of substrates was accessible by this method and in-depth mechanistic studies gave valuable insights for catalyst improvement.
1.1-I3
With a two million ton global production hydrogen peroxide is a valuable product.[1] Yet it is produced via a non-catalytic and energy intensive anthraquinone process rather than via a sustainable electrochemical procedure.
We have shown that Cu-tmpa can produce hydrogen peroxide upon electrochemical reduction of dioxygen at rates of 1.8 x 106 s–1 and in significant amounts providing that no mass transport limitations in O2 occur.[2] When H2O2 starts to accumulate and O2 concentrations drop overreduction of peroxide to water becomes significant.
We have shown that the rate determining step for O2 reduction is binding of dioxygen to Cu(I), in line with studies by the Karlin group,[3] while reduction of H2O2 appears to proceed via Fenton-like chemistry, showing similarities with lytic polysaccharide monooxygenase chemistry.[4] Despite that strongly oxidative reactive oxygen species are likely to be involved turnover numbers exceed 1000 without any sign of catalyst deactivation.
Detailed mechanistic studies, structure-correlation studies, comparisons with Cu-monooxygenase chemistry, DFT calculations, and experiments to boost the hydrogen peroxide production employing flow cell chemistry and gas diffusion electrodes are in progress.
1.1-I4
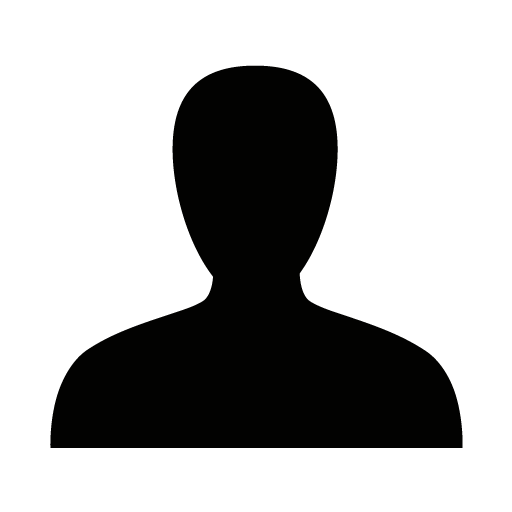
In the early studies, on Oxygenic Photosynthesis, the “quantasome hypothesis” led to seminal discoveries correlating the structure of natural photosystems with their complementary photo-redox functions.[1,2] Indeed, and despite the vast bio-diversity footprint, just one protein complex is used by Nature as the H2O-photolyzer: photosystem II (PSII). Man-made systems are still far from replicating the complexity of PSII, showing the ideal co-localization of Light Harvesting antennas with the functional Reaction Center (LH-RC). Here we report the design of multi-perylenebisimide (PBI) networks shaped to function by interaction with a polyoxometalate water oxidation catalyst (Ru4POM).[3-5] Our results point to overcome the classical “photo-dyad” model, based on a donor-acceptor binary combination, with integrated artificial “quantasomes” formed both in solution and on photoelectrodes, showing a: (i) red-shifted, light harvesting efficiency (LHE>40%), (ii) favorable exciton accumulation and negligible excimeric loss; (iii) a robust amphiphilic structure; (iv) dynamic aggregation into large 2D-paracrystalline domains.[5] Photoexcitation of the PBI-quantasome triggers one of the highest driving force for photo-induced electron transfer applied so far.[5-7]
[1] Scheuring, S., Sturgis, J. N. Chromatic Adaptation of Photosynthetic Membranes. Science 309, 484–487 (2005);
[2] Sartorel, A., Carraro, M., Toma, F. M., Prato, M., Bonchio, M. Shaping the beating heart of artificial photosynthesis: oxygenic metal oxide nano-clusters. Energy Environ. Sci. 5, 5592 (2012);
[3] Piccinin, S.; Sartorel, A.; Aquilanti, G.; Goldoni, A.; Bonchio, M.; Fabris, S. Water oxidation surface mechanisms replicated by a totally inorganic tetraruthenium-oxo molecular complex. Proc. Natl. Acad. Sci. 110, 4917–4922 (2013)
[4] Toma, F. M.; Prato, M.; Bonchio, M. et al. Efficient water oxidation at carbon nanotube–polyoxometalate electrocatalytic interfaces. Nature Chemistry 2, 826-831 (2010).
[5] Bonchio, M.; Sartorel, A.; Prato, M. et al. Hierarchical organization of perylene bisimides and polyoxometalates for photo-assisted water oxidation. Nature Chemistry 11, 146-153 (2019).
[6] Gobbo, P.; Bonchio, M.; Mann, S. et al. Catalytic processing in ruthenium-based polyoxometalate coacervate protocells Nature Commun 11, 41 (2020).
[7] GobbatoT.; Rigodanza F.; Benazzi, E.; Prato, M.; Bonchio M. et al. J. Am. Chem. Soc.144,14021-14025 (2022).
2.1-I1
Tailoring and understanding the structure of the electrochemical interface at the atomic level is key to elucidating the design principles for the discovery of new electrocatalysts for renewable energy conversion. This talk will focus on our recent work on new catalyst (nano)materials and engineered interfaces for electrochemical energy conversion and storage. In particular, I will discuss structure sensitivity and electrolyte effects for oxygen and carbon dioxide/carbon monoxide electrocatalysis.
First, I will present our work toward understanding and tuning the structure-activity-stability relations for oxygen reduction [1] and oxygen evolution [2], for applications in fuel cells and water electrolysers, respectively. Then, I will discuss our model studies on Cu-based surfaces to understand structure-properties relations for CO2 reduction [3-4]. We have investigated the effect of pH, specific anion adsorption, and potential dependence for CO reduction on Cu single crystals. We show how well-defined studies are essential to design efficient electrocatalysts to produce renewable fuels.
2.1-I2
Catalysis is key in the future development of green chemistry and in the renewable production of chemicals and fuels. However, this transition requires a new approach to catalysis, as central chemical reactions cannot be catalyzed with existing materials. The grand challenge is to discover new catalyst materials, which are both stable and active. We identify three energy conversion reactions, which are corner stones for the green transition and in urgent need of new catalysts.
High Entropy Alloys are solid solutions where five or more elements are mixed randomly together. The realization of HEAs has opened for a vast composition space with a practically infinite number of new not yet explored catalyst materials. We can tune their properties by smoothly change their composition. This has led to the statement that: HEA is a shift of paradigm “from using the materials we have, to engineer the materials we need”. The hypothesis is that among the HEAs there are catalysts with superior stability and activity for the important green reactions.
will present the challenges regarding simulations and prediction of the relation between surface structure and catalytic activity on HEA surfaces. Further, I will show studies where the flexibility of the vast chemical space of HEAs is utilized to predict new catalysts.
2.1-I3
Hydrogen and other solar fuels have been highlighted as one of the future energy vectors. Having natural photosynthesis as inspiration, we can develop a device capable to split water using sunlight, obtaining oxygen and hydrogen. [1], [2] Different strategies can be used to achieve this: from separated light harvesting and catalytic systems to all integrated devices able to transform directly sunlight into fuels. In both approaches the catalyst is pivotal to improve the system efficiency. Although rapid progress is being made in the field, understanding of the limiting factors of these catalysts has allowed remarkable improvements in their performance.
In this talk I will discuss different approaches to modify the activity of metal/metal oxide nanoparticles towards redox catalytic processes for water splitting. Nanoparticles are excellent catalysts due to their high surface/volume ratio and electronic and chemical surface properties.[3],[4] Consequently, several strategies can be used to modulate the activity of NP towards catalytic processes. In this talk I will discuss how doping, NP size and surface functionalization effects the catalytic activity of Ni/NiOOH NPs for oxygen evolution in the first case and Ru NP for hydrogen in the other two.[5] I will discuss these effects in terms of electrocatalytic activity and thoughtful characterisation of the system: spectroelectrochemical experiments, XPS, TEM, SEM, TOF-Sims, ATP, etc. All this acquired knowledge will help to systematically improve the next generation of catalysts for water splitting.
2.2-O1
The construction of carbonyl compounds via carbonylation reaction using a safe CO source remains a long-standing challenge to synthetic chemists.[1] In this work, we explored the electrosynthetic approach using CO2 as a CO surrogate in the carbonylation of benzyl chlorides, utilizing a combination of two earth-abundant metal catalysts. As proof of concept, the protocols allow the synthesis of symmetric ketones from good to excellent yields in an undivided cell with non-sacrificial electrodes. The mechanistic studies suggested a synergistic effect between the two metal complexes. Based on the mechanistic studies, we propose the coupling of the electrocatalytic CO formed from CO2 reduction with the benzyl chlorides activated by the Ni-catalyzed. Our approach that has proven more challenging is the use of an undivided cell to perform tandem CO2 reduction to CO, followed by CO utilization in carbonylation reactions. While this approach has the potential to offer several benefits such as simple experimental setups, straightforward synthetic protocols, direct utilization of the CO formed, decrease of the employed toxic CO, and facilitation of the formation of labeled compounds. This is complex due to the need to balance all required catalytic cycles. Few methods used CO2 as a CO source because of its inertness but also due to its inherent reactivity that delivers preferably carboxylation products. In this line, Skrydstrup, Daasbjerg and co-workers developed a set of classical Pd-catalyzed carbonylation reactions using the CO evolved from the electrochemical CO2 reduction in a two-compartment setup.[2] Before that, Perichon and co-workers reported a stepwise electrochemical/chemical method for the stoichiometric synthesis of ketones.[3]
2.2-O2
Porphyrins have been actively investigated as oxygen evolution reaction (OER) catalysts, yet only a few studies refer to their integration into electrode devices as heterogeneous catalysts [1-2], mainly due to processability constraints. Moreover, the catalytic activity of porphyrin films can be substantially improved by their modification in the form of conjugated polymers. The enhanced conductivity of metalloporphyrin conjugated polymers, resulting from the extended delocalization of π-electrons in the conjugated system, may promote a faster charge transfer to the active sites, improving the catalytic performance. Recently, our group developed a methodology allowing the straightforward preparation of fused metalloporphyrins conjugated polymers thin films over a wide variety of substrates, based on the oxidative chemical vapour deposition (oCVD) [3,4]. In this way, a new venue for the study of the electrocatalytic activity of fused metalloporphyrin conjugated polymers bearing different metal centres and substituents was opened. Consequently, we have investigated the electrocatalytic activity of Ni(II) and Co(II) fused porphyrins conjugated polymers on FTO substrates towards the OER. We found out that there is a significant role of the direct fusion reaction to form organized electrocatalysts able to operate at lower overpotentials than the monomeric counterparts, resulting in enhanced electrocatalytic performance. Nonetheless, previous reports on Ni and Co complexes, including porphyrins, have demonstrated the decomposition of the molecular matrix to form a material thin film[2,5], possibly a metal oxide specie, acting as the true catalyst for water oxidation at the electrode surface. Therefore, we have conducted a deep-through investigation of the possible conversion of fused metalloporphyrin polymers into a material catalyst, using the combination of structural and compositional analysis with theoretical studies. We pay special attention to the role of polymerization and the presence of substituents of different natures on the transformation kinetics and resulting electrocatalytic activity.
2.2-O3
Electrochemical water oxidation (2e- WOR) to hydrogen peroxide (H2O2) is a topic of growing interest. Still material development, mechanistic understanding and process conditions are yet to be defined to allow for efficient and durable hydrogen peroxide production at industrially-relevant current densities [1]. Carbonate ions (CO32−) are suggested to enable indirect water oxidation to H2O2 and alkali metal cations (Li+, Na+, and K+) are known to influence electrochemical activity of various electrochemical reactions including the oxygen evolution reaction [2,3]. Also, in electrocatalysis the electrolyte composition is known to influence electrode stability. Material stability in the context of anodic H2O2 production is barely addressed in recent literature. Moreover, the influence of the electrolyte used for selective electrochemical water oxidation is yet to be fully disclosed [4].
Herein, we discuss the effect of different electrolytes (K2CO3 and Na2CO3) on the H2O2 production and electrode stability using commercially available fluorine-doped tin oxide (FTO) anodes frequently used as active material and support. The determined faradaic efficiencies (FE) suggest that the use of K2CO3 over Na2CO3 electrolyte (FEH2O2 = 34.8 % in K2CO3 and FEH2O2 = 25.3 % in Na2CO3 at 5 mA/cm2) is preferred. We observe a maximum production rates of 0.158 mmol min−1cm−2 and 0.118 min−1cm−2 at a current density of 100 mA cm−2 in K2CO3 and Na2CO3, respectively highlighting the influence of alkali metal cations on the H2O2 formation. Moreover, we will highlight the influence of electrolyte composition on the stability of the FTO electrodes using ICP-MS and SEM analysis. Overall, in this contribution we will disclose our understanding of the role of carbonate on the H2O2 formation in the context of the reaction parameter space.
2.2-O4
Dr. Samira Siahrostami is an Associate Professor and Canada Research Chair in the Department of Chemistry at Simon Fraser University in Canada. Prior to that, she was an associate professor (2022-2023) and assistant professor (2018-2022) in the Department of Chemistry at the University of Calgary. Prior to that, she was a research engineer (2016–2018) and postdoctoral researcher (2014–2016) at Stanford University's Department of Chemical Engineering. She also worked as a postdoctoral researcher at the Technical University of Denmark from 2011 to 2013. Her work uses computational techniques such as density functional theory to model reactions at (electro)catalyst surfaces. Her goal is to develop more efficient catalysts for fuel cells, electrolyzers, and batteries by comprehending the kinetics and thermodynamics of reactions occurring at the surface of (electro)catalysts. Dr. Siahrostami has written more than 100 peer-reviewed articles with an h-index of 47 and over 13,000 citations. She has received numerous invitations to give talks at universities, conferences, and workshops around the world on various topics related to catalysis science and technology. Dr. Siahrostami is the recipient of the Environmental, Sustainability, and Energy Division Horizon Prize: John Jeyes Award from the Royal Society of Chemistry (RSC) in 2021. She received the Tom Zeigler Award and the Waterloo Institute in Nanotechnology Rising Star award in 2023. She has been named as an emerging investigator by the RSC in 2020, 2021 and 2022. Dr. Siahrostami's contribution to energy research was recognized in the most recent Virtual Issue of ACS Energy Letters as one of the Women at the forefront of energy research in 2023. She is currently the board member of the Canadian Catalysis Foundation and editor of Chemical Engineering Journal (CEJ) and APL Energy journal (AIP Publishing).
Hydrogen peroxide is known as an essential green chemical oxidant for advanced oxidation processes such as wastewater treatment, sterilization (which includes the pathogenic SARS-CoV-2), decolorization of waste dyes, and as an oxidant in chemical reactions. Besides its oxidizing ability, hydrogen peroxide is considered an emerging green fuel and energy career. Because of these properties, hydrogen peroxide is an ideal green energy carrier comparable to that of compressed hydrogen gas and a medium for energy storage. It is also set to play an important role in energy conversion technologies such as fuel cells and batteries. These emergent energy aspects promise an even higher global demand for this valuable chemical. In this talk, I will discuss our efforts in electrosynthesis of hydrogen peroxide, as well as the potential applications of hydrogen peroxide in battery technologies, and how computational material design can lead to the design and discovery of novel materials for a revolutionary new application.
2.2-O5
Transition-metal phosphides are of high interest for electrochemical applications, owing to their chemical stability, composition and phase tunability, and economic potential.
In this talk, I will discuss our new route for metal phosphides synthesis, utilizing a direct reaction of transition-metal nitrate salts with triphenylphosphine (PPh3) in molten-state. This method was found straightforward, benign and scalable. We demonstrated this method for nanoscale transition-metal phosphides synthesis, as an attractive alternative to common solvothermal or gas-solid phosphidation synthetic methods, allowing tunable phase composition of the produced nanoparticles, along with significant simplification of procedures and equipment.
We initially demonstrated the new method for nickel-phosphides molten-state synthesis from Ni(NO3)2∙6H2O and triphenylphosphine (PPh3)[1]. By combining analytical and computational methods, we elucidated the reaction mechanism, indicating that the reaction propagates dominantly through favored Ni-P bonding and consecutive cleavage of phenyl–P bonds of PPh3. Interestingly, we found both by DFT and XRD that an intermediate formation of metallic nickel nanoparticles, originating from ligand-to-metal charge transfer at the Ni-P bond, is essential for in-situ production of phosphides.
Furthermore, we exemplified the advantages of this simple and controllable method for the investigation of phase/composition-activity correlation of different Ni/P products for hydrogen evolution reaction (HER) and as anode-materials for Li-ion batteries.
We found a clear composition-activity trend for both applications, providing high performance which is comparable to nickel phosphides produced by other common methods. We found Ni3P products favorable as HER electrocatalysts, with 145 mV overpotential at 10 mA/cm2, and Ni2P was found favorable as LIBs anode materials, with 206 mAh g-1 capacity; both with high morphological and electrochemical stability.
This new simple synthetic path offers new possibilities for low-cost synthesis and design of other transition-metal based materials. I will present and discuss our latest results of an improved and generalized synthesis method based on the above detailed molten-state method, allowing the production of a variety of single and multi-component transition-metal phosphides for electrochemical applications.
2.2-O6
Perovskite oxides are commonly viewed as excellent electrocatalysts for oxygen evolution reaction (OER). However, OER conditions can substantially damage the perovskite structure and can lead to a wide range of phenomena, including cation leaching, dissolution, oxygen intercalation and modification of electronic structure.
In this talk, using single-crystalline perovskite electrocatalysts, I will demonstrate how operando electrochemical atomic force microscopy (EC-AFM) can be used to record nanoscale changes in the surface morphology during anodic corrosion of the perovskite surface. I will also show that perovskite surfaces can drive a spontaneous ORR in an aqueous environment and, through this reaction, become substantially oxidized, reaching potentials close to the OER, which results in cation leaching, dissolution and oxygen intercalation. Finally, I will discuss the effect of oxygen intercalation and how it can lead to extreme oxidation of a perovskite oxide, which we observe for the first time using resonant inelastic X-ray scattering and rationalize using DFT and molecular dynamics.
Ultimately, our results highlight the complexity of the electrochemical and structural behavior of perovskites and the importance of taking their structural evolution into account when considering elementary reaction steps on such surfaces.
2.3-I1
Professor Erwin Reisner received his education and professional training at the University of Vienna (PhD in 2005), the Massachusetts Institute of Technology (postdoc from 2005-2007) and the University of Oxford (postdoc from 2008-2009). He joined the University of Cambridge as a University Lecturer in the Department of Chemistry in 2010, became a Fellow of St. John’s College in 2011, was appointed to Reader in 2015 and to his current position of Professor of Energy and Sustainability in 2017. He started his independent research programme on artificial photosynthesis (solar fuels) with the support of an EPSRC Career Acceleration Fellowship (2009-2015), which also received substantial early support by the Christian Doppler Laboratory for Sustainable SynGas Chemistry (2012-2019). In 2016, he received a European Research Council (ERC) Consolidator Grant to develop the field of semi-artificial photosynthesis (biohybrid systems for solar fuel synthesis) and has recently been awarded an ERC Advanced Grant (now funded by the UKRI underwrite scheme) on semi-biological domino catalysis for solar chemical production. He is the academic lead (PI) of the Cambridge Circular Plastics Centre (CirPlas; since 2019), where his team develops solar-powered valorisation technologies for the conversion of solid waste streams (biomass and plastics) to fuels and chemicals. He has acted as the academic lead of the UK Solar Fuels Network, which coordinates the national activities in artificial photosynthesis (2017-2021) and is currently a co-director of the Centre for Doctoral Training in Integrated Functional Nano (nanoCDT) in Cambridge as well as a member of the European research consortia ‘Sofia’ and ‘solar2chem'.
Solar panels can not only produce electricity, but are also in early-stage development for the production of sustainable fuels and chemicals. They can therefore mimic plant leaves in shape and function as demonstrated for overall solar water splitting for green H2 production by the laboratories of Nocera and Domen.[1,2] This presentation will give an overview of our recent progress to construct prototype solar panel devices for the conversion of carbon dioxide and solid waste streams into fuels and higher-value chemicals through molecular surface-engineering of solar panels with suitable electrocatalysts. Specifically, a standalone ‘photoelectrochemical leaf’ based on an integrated lead halide perovskite-BiVO4 tandem light absorber architecture has been built for the solar CO2 reduction to produce syngas using an integrated Co porphyrin catalyst.[3] Recent advances in the manufacturing have enabled the reduction of material requirements to fabricate such devices and make the leaves sufficiently light weight to even float on water, thereby enabling application on open water sources.[4] The tandem design also allows for the integration of biocatalysts as ideal model catalysts and the selective and bias-free conversion of CO2-to-formate has been demonstrated using enzymes.[5] The versatility of the integrated leaf design has been demonstrated by replacing the perovskite light absorber by BiOI for solar water and CO2 splitting.[6] An alternative solar carbon capture and utilization technology is based on co-deposited semiconductor powders on a conducting substrate.[2] Modification of these immobilized powders with a molecular Co bis-terpyridine catalyst provides us with a photocatalyst sheet that can cleanly produce formic acid from aqueous CO2.[7] CO2-fixing bacteria grown on the photocatalyst sheet enable the production of multicarbon products through clean CO2-to-acetate conversion.[8] The deposition of a single semiconductor material on glass gives panels for the sunlight-powered conversion plastic and biomass waste into H2 and organic products, thereby allowing for simultaneous waste remediation and fuel production.[9] The concept and prospect behind these integrated systems for solar energy conversion, related approaches,[10] and their reliance on suitable transition metal catalysts will be discussed.
References
[1] Reece et al., Science, 2011, 334, 645–648.
[2] Wang et al., Nat. Mater., 2016, 15, 611–615.
[3] Andrei et al., Nat. Mater., 2020, 19, 189–194.
[4] Andrei et al., Nature, 2022, 608, 518–522.
[5] Moore et al., Angew. Chem. Int. Ed. 2021, 60, 26303–26307.
[6] Andrei et al., Nat. Mater., 2022, 21, 864–868.
[7] Wang et al., Nat. Energy, 2020, 5, 703–710.
[8] Wang et al., Nat. Catal., 2022, 5, 633–641.
[9] Uekert et al., Nat. Sustain., 2021, 4, 383–391.
[10] Wang et al., Nat. Energy, 2022, 7, 13-24.
2.3-I2
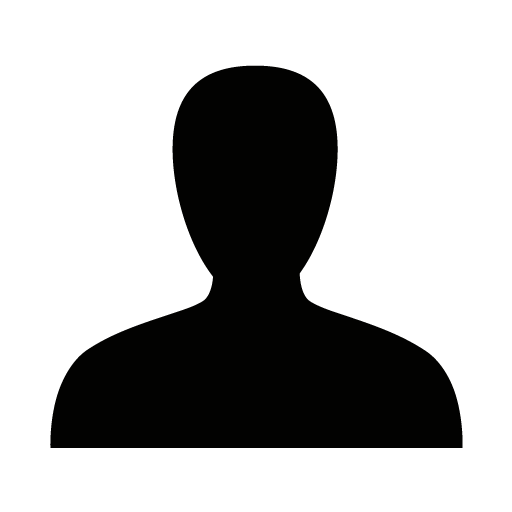
Molecular electrocatalysts have received a renewed interest due to their capabilities towards sustainable and energy–efficient redox chemical transformations.1 Particularly, those involved in new strategies towards energy storage application.1a, 2 Along these lines, I will present our first means of molecular cooperativity under electrochemical reduction conditions targeting carbon dioxide reduction.3
First, I will provide direct experimental evidence on the correlation of remote interactions between a synthesized MnI-complex and different alkali cations with redox potential tuning. Additionally, the electrochemical behavior of the Mn-complex towards carbon dioxide will be discuss, including the effects of added alkali salts using cyclic voltammetry.3a
Later, I will present the formation, characterization and use of a dinuclear cobalt complex bearing a pyrazole-based ligand substituted with terpyridine groups at the 3 and 5 positions, for the electrocatalytic reduction carbon dioxide to carbon monoxide in the presence of Brönsted acids (94 % selectivity towards CO formation, at –1.35 V vs Saturated Calomel Electrode in DMF/TFE mixtures).3b Chemical, electrochemical and UV-vis spectro-electrochemical studies under inert atmosphere of this complex indicate pairwise reduction processes. And, infrared spectro-electrochemical studies under carbon dioxide and carbon monoxdie atmosphere are consistent with a reduced CO-containing dicobalt complex which results from the electroreduction of carbon dioxide. Additionally, our theoretical results indicate the cooperativity of the ligand platform during the reduction process, delocalizing the electron density at the ligand and reducing the overpotential of the reduction reaction.
References
(1) a) N. W. Kinzel, C. Werlé, W. Leitner, Angew. Chem. Int. Ed. 2021, 60, 11628–11686; b) N. Wolff, O. Rivada‐Wheelaghan, D. Tocqueville, ChemElectroChem 2021, 8, 4019–4027.
(2) a) T. R. Cook, D. K. Dogutan, S. Y. Reece, Y. Surendranath, T. S. Teets, D. G. Nocera, Chem. Rev. 2010, 110, 6474–6502.
(3) a) A. Srinivasan, J. Campos, N. Giraud, M. Robert, O. Rivada–Wheelaghan, Dalton Trans. 2020, 49, 16623–16626; b) A. Bohn, J. J. Moreno, P. Thuéry, M. Robert, O. Rivada-Wheelaghan, 2022, 10.1002/chem.202202361.
2.3-S1
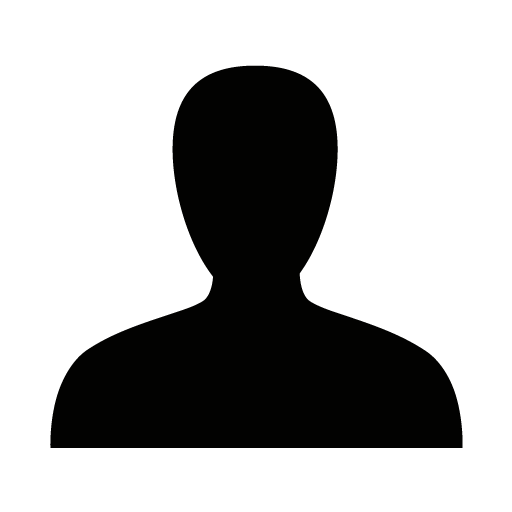
RSC Industry Talk RSC Industry Talk RSC Industry Talk RSC Industry Talk RSC Industry Talk RSC Industry Talk RSC Industry Talk RSC Industry Talk RSC Industry Talk RSC Industry Talk RSC Industry Talk RSC Industry Talk RSC Industry Talk RSC Industry Talk RSC Industry Talk RSC Industry Talk RSC Industry Talk RSC Industry Talk RSC Industry Talk RSC Industry Talk RSC Industry Talk RSC Industry Talk RSC Industry Talk RSC Industry Talk RSC Industry Talk RSC Industry Talk RSC Industry Talk RSC Industry Talk RSC Industry Talk RSC Industry Talk Talk RSC Industry Talk RSC Industry Talk RSC Industry Talk RSC Industry Talk RSC Industry Talk RSC Industry Talk RSC Industry Talk RSC Industry Talk RSC Industry Talk RSC Industry Talk RSC Industry Talk RSC Industry Talk RSC Industry Talk RSC Industry Talk RSC Industry Talk RSC Industry Talk RSC Industry Talk RSC Industry Talk RSC Industry Talk RSC Industry Talk RSC Industry Talk RSC Industry Talk RSC Industry Talk Talk RSC Industry Talk RSC Industry Talk
2.3-I3
Victor Mougel completed his Bachelor's and Master's degree in Chemistry at the ENS of Lyon, and obtained his PhD at the University of Grenoble under the supervision of Prof. Marinella Mazzanti. He then joined ETH Zürich as an ETH/Marie Skłodowska-Curie Fellow before starting his independent career as a CNRS associate researcher at Collège de France in 2016. Since December 2018, he is a tenure track assistant professor at the Department of Chemistry and Applied Biosciences at ETH Zürich.
Enzymatic systems have evolved complex strategies to maximize the efficiency and product selectivity in small molecule activation, among which CO2 reduction. Beside unique active sites containing by definition earth-abundant elements, enzyme further control catalytic activity through second sphere interactions and a fine control of electron transfer chains. In this talk, we will introduce a series of bio-inspired strategies for the design of electrocatalytic systems for CO2 reduction. We will highlight a series of earth-abundant metal based molecular catalysts inspired by the active sites of enzymatic systems and will introduce a new strategy for the electrocatalytic metal hydride generation using synthetic Fe4S4 clusters acting as concerted proton electron transfer (CPET) mediators. We will demonstrate that the combination of synthetic Fe4S4 clusters with the CO2 electroreduction catalyst [MnI(bpy)(CO)3Br] (bpy = 2,2’-bipyridine) allows the preparation of a benchmark catalytic system for HCOOH generation. We will finally introduce the preparation of the first complete redox series of Fe4S4 complexes and their use to generate potent strong reducing agents via entatic activation strategies. References: 4. L. Grunwald, M. Clémancey, D. Klose, L. Dubois, S. Gambarelli, G. Jeschke, M. Wörle, G. Blondin, V. Mougel* A complete biomimetic iron-sulfur cubane redox series PNAS, 2022, 119 (31), e2122677119 3. S. Dey, F. Masero, E. Brack, M. Fontecave, V. Mougel* Electrocatalytic metal hydride generation using CPET mediators Nature, 2022, 607 (7919), 499-506 2. S. Dey, T. Todorova, M. Fontecave*, V. Mougel* Electroreduction of CO2 to Formate with low overpotential using Cobalt Pyridine Thiolate Complexes Angew. Chem. Int. Ed., 2020, 59(36), 15726-15733 1. A. Mouchfiq, T. Todorova, S. Dey , M. Fontecave*, V. Mougel* A Bioinspired Molybdenum-Copper Molecular Catalyst for CO2 Electroreduction Chem. Sci., 2020, 11, 5503-5510
2.3-I4
Marc Robert was educated at the Ecole Normale Supérieure (Cachan, France) and gained his Ph.D. in 1995 from Paris Diderot University under the guidance of Claude Andrieux and Jean-Michel Savéant. After one year as a postdoctoral fellow at Ohio State University (USA), working alongside Matt Platz, he joined the faculty at Paris Diderot University as Associate Professor. He was promoted to full Professor in 2004, and distinguished Professor in 2019 at Université de Paris. He became a junior Fellow of the University Institute of France (IUF) in 2007 and a senior Fellow in 2017. He was a JSPS (Japan Society for the Promotion of Science) research Fellow (2015). Among various distinctions, Marc Robert received the first International Prize Essential Molecules Challenge from Air Liquide (2016) and the Chemistry and Energy Research Prize from the French Chemical Society (2019). His interests include electrochemical, photochemical, and theoretical approaches of electron transfer reactions and reactivity in chemistry, as well as catalytic activation of small molecules, mainly CO2 and N2.
A current chemistry challenge is the (photo)electrochemical production of ammonia (NH3) from atmospheric N2 without using H2 or emitting CO2, as an alternative to the current Haber-Bosch process which consumes 1% of the world energy and emits nearly 1.5% of the CO2. The nitrogen reduction reaction (N2 + 6H+ + 6e- -> 2NH3) implies a cascade of elementary steps that require a delicate balance of reactivity at the metal active site M (cleavage of the N-N triple bond without creating a too strong, poisoning M-N interaction; avoiding competitive reduction of protons to H2 …) [1,2]. To date, examples of molecular electrocatalysts including abundant metals remain scarce, although recent spectacular progress has been made, notably with Fe and Mo based catalysts [3,4]. In this presentation, we will present and discuss reactivity and mechanistic aspects of a Mn catalyst able to reduce dinitrogen to ammonia in homogeneous as well as in electrochemical conditions [5].
3.1-I1
Prof. Beatriz Roldán Cuenya is currently Director of the Interface Science Department at the Fritz Haber Institute of the Max Planck Society in Berlin (Germany). She is an Honorary Professor at the Technische Universität Berlin, at the Freie Universität Berlin, and at the Ruhr-University Bochum, all in Germany. Also, she serves as a Distinguished Research Professor at the University of Central Florida (USA).
Prof. Roldán Cuenya began her academic career by completing her M.S./B.S. in Physics with a minor in Materials Science at the University of Oviedo, Spain in 1998. Afterwards she moved to Germany and obtained her Ph.D. from the Department of Physics of the University of Duisburg-Essen with summa cum laude in 2001. Subsequently, she carried out her postdoctoral research in the Department of Chemical Engineering at the University of California Santa Barbara (USA) until 2003.
In 2004, she joined the Department of Physics at the University of Central Florida (UCF) as Assistant Professor where she moved through the ranks to become a full professor in 2012. In 2013 Prof. Roldan Cuenya, moved to Germany to become Chair Professor of Solid State Physics in the Department of Physics at Ruhr-University Bochum until 2017.
During her academic career, Prof. Roldan Cuenya received an Early CAREER Award from the US National Science Foundation (2005) and the international Peter Mark Memorial award from the American Vacuum Society (2009). In 2016 she became Fellow of the Max Planck Society in Germany and also received the prestigious Consolidator Award from the European Research Council. In 2020, she became a member of the Academia Europaea (Academy of Europe). She received the AVS Fellow Award (2021), the International Society of Electrochemistry-Elsevier Prize for Experimental Electrochemistry (2021), the 2022 Paul H. Emmet Award of the North American Catalysis Society, and the Röntgen Medal of the City of Remscheid (2022).
Prof. Dr. Beatriz Roldan Cuenya is the author of 245 peer-reviewed publications, 6 book chapters and 6 patents. She has been supervising 74 postdoctoral fellows and 36 PhD students. She has given 245 invited talks, with 13 plenary talks and 33 keynote lectures since 2017. Her H-factor is 74 (Google Scholar) and her work has received over 21,500 citations.
She presently serves on the editorial advisory boards of the Journal of Catalysis and Chemical Reviews. In addition, she also contributes to a number of advisory committees, including the Liquid Sunlight Alliance (USA), the Advanced Research Center Chemical Building Blocks Consortium (Utrecht, the Netherlands), the Spanish Synchrotron Facility ALBA (Barcelona, Spain), the German Synchrotron DESY (Hamburg, Germany), the Helmholtz-Zentrum Berlin for the strategic development of BESSY II (Berlin, Germany), the Institute of Chemical Research of Catalonia (ICIQ in Tarragona, Spain), the UK Catalysis Hub and the Ertl Center for Electrochemistry & Catalysis (South Korea).
Prof. Roldan Cuenya’s research program explores physical and chemical properties of nanostructures, with emphasis on advancements in nanocatalysis based on operando microscopic and spectroscopic characterization.
Climate change concerns have spurred a growing interest in developing environmentally friendly technologies for energy generation, including green H2 production from water splitting. Moreover, concerted attempts are also being made to re-utilize CO2 by electrocatalytically converting it into value-added chemicals and fuels, offering the possibility to store renewable energy into chemical bonds. Thus, efficient, selective and durable electrocatalysts that can operate under mild reaction conditions (atmospheric pressure and low overpotential) are urgently needed. However, the latter challenging goal can only be achieved when fundamental understanding of the electrocatalyst structure and surface composition under reaction conditions becomes available. It should also be kept in mind that even morphologically and chemically well-defined pre-catalysts are commonly susceptible to drastic modifications under operando conditions, especially when the reaction conditions themselves change dynamically. Here, a synergistic experimental approach taking advantage of a variety of cutting-edge microscopy (EC-AFM, EC-TEM), spectroscopy (NAP-XPS, XAS, Raman Spect., GC/MS) and diffraction (XRD) has been employed to unveil the complexity of energy conversion electrocatalysts.
In particular, I will provide new insights into the electrocatalytic reduction of CO2 as well as the oxygen evolution reaction using operando characterization methods while targeting model pre-catalyst systems ranging from single crystals to thin films and metal nanoparticles. Some of the aspects that will be discussed include: (i) the design of size- and shape-controlled catalytically active nanoparticle pre-catalysts (Cu, Cu2O cubes, ZnO@Cu2O cubic NPs, CoOx NPs), (ii) the understanding of the active state formation and (iii) the correlation between the dynamically evolving structure and composition of these electrocatalysts under operando reaction conditions and their activity, selectivity and durability.
Our results are expected to open up new routes for the reutilization of CO2 through its direct conversion into industrially valuable chemicals such as ethylene and ethanol and the generation of H2 through water splitting.
[1] Grosse, P.; Yoon, A.; Rettenmaier, C.; Herzog, A.;. Chee, S.W.; and Roldan Cuenya, B., Nature Comm. 2021, 12, 6736
[2] Timoshenko, J.; Bergmann, A.; Rettenmaier, C.; Herzog, A.; Aran Ais, R.; Jeon, H.; Haase, F.; Hejral, U.; Grosse, P.; Kühl, S.; Davis, E.; Tian, J.; Magnussen, O.; Roldan Cuenya, B.; Nature Catalysis 2022, 5, 259.
[1] Haase, F.; Bergmann, A.; Jones, T.; Timoshenko, J.; Herzog, A.; Jeon, H.; Rettenmaier, C.; Roldan Cuenya, B., Nature Energy (2022) 7, 765.
3.1-I2
Raffaella Buonsanti obtained her PhD in Nanochemistry in 2010 at the National Nanotechnology Laboratory, University of Salento. Then, she moved to the US where she spent over five years at the Lawrence Berkeley National Laboratory, first as a postdoc and project scientist at the Molecular Foundry and after as a tenure-track staff scientist in the Joint Center for Artificial Photosynthesis. In October 2015 she started as a tenure-track Assistant Professor in the Institute of Chemical Sciences and Engineering at EPFL. She is passionate about materials chemistry, nanocrystals, understanding nucleation and growth mechanisms, energy, chemical transformations.
Correlating activity, selectivity and stability with the structure and composition of catalysts is crucial to advancing the knowledge in chemical transformations which are essential to move towards a more sustainable economy. Among these, the electrochemical CO2 reduction reaction (CO2RR) holds the promise to close the carbon cycle by storing renewable energies into chemical feedstocks. Yet it still suffers from the lack of efficient, selective and stable catalysts.
In this talk, I will showcase a few examples which highlight how shape-controlled nanocrystals can contribute to address the selectivity challenge in CO2RR. First of all, I will discuss how size control of Cu nanocubes and Cu octahedra has revealed the importance of facet-ratio to maximize the selectivity towards ethylene and methane, respectively. Second, I will describe our recent efforts towards using shape-controlled NCs beyond pure Cu, in the framework of tandem schemes and bimetallics. Finally, I will present our strategies to enhance the stability of these Cu catalysts during operation.
3.1-I3
CO2 reduction to long-chain compounds has the potential to close the carbon cycle and if using excess renewables be a route to sustainability. However, most of the materials we have only produce C2. I will analyze theoretically some of the aspects behind these difficulties in going higher in the C-C bond forming strategies. On one side I will describe why easy one material one orientation do not provide enough flexibility to generate large chains and why the role of the microenvironment and the materials complexity is so intrinsically crucial to the improvement of the electrocatalytic activity. At the same time I will demonstrate how mastering the microenvironment properties improvements can be introduced following different strategies. The work presented will illustrate how much complexity both at the electrocatalytic site but also in the solution and with different operational modes are needed and how the integration of different methodologies is crucial.
3.2-O1
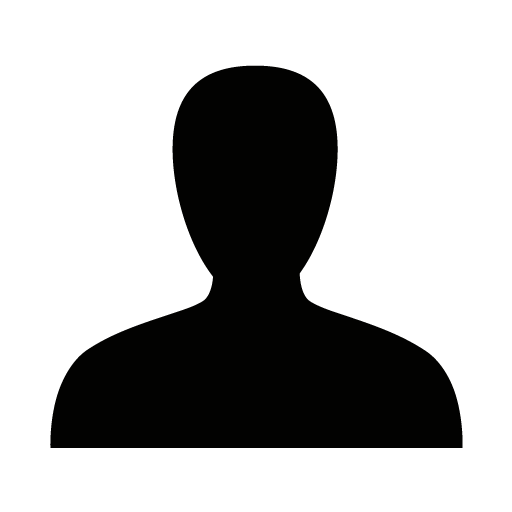
The electrocatalytic CO2 reduction reaction (CO2RR) powered by renewable energy and promoted by transition metal catalysts, will play an important role in the global decarbonization process of the chemical industry, since represents a sustainable route for the production of value-added chemicals using CO2 as a feedstock. [1]-[2] However, despite the significant progress made in the field, selectivity, durability and intrinsic activity of the catalysts are still key challenges to achieve an efficient CO2RR. For organometallic molecular systems, characterized by a well-defined chemical environment of the active site, a rational tuning of the CO2RR efficiency and selectivity can be precisely controlled through a rational modification of the ligand scaffold. [3]-[5] Moreover, the encapsulation of molecularly defined active units into reticular frameworks was recently shown as an effective strategy to boost the CO2RR performances of molecular catalysts, but also to alter their redox behavior. [6]
In recent years, the synergy between molecules and nanostructured materials has been proposed as a promising approach to design efficient heterogeneous catalysts for CO2 electroreduction. For instance, the presence of organic modifiers on metallic surfaces was found to tune the stability of key reaction intermediates or the local surface microenvironment, thus altering the product selectivity. [7]-[8] In this contribution, we will discuss novel strategies and approaches to form hybrid electrocatalysts based on the utilization of reticular or molecular chemistry as tools to steer the CO2RR selectivity and activity of transition metal-based nanostructured catalysts. The discussion will focus on providing a molecular perspective towards a rational design of heterogeneous catalysts.
3.2-O2
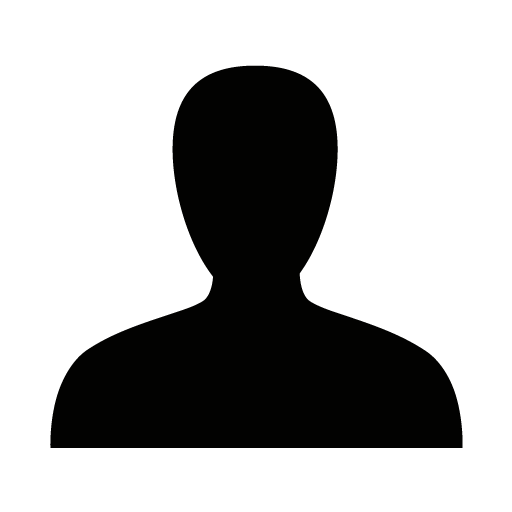
Global warming, climate change and our over-dependence on non-renewable fossil fuels demand long-term solutions to reduce CO2 emissions and develop sustainable energy technologies. The electrochemical CO2 reduction has the potential to accomplish a “carbon-neutral energy cycle”, which incorporates CO2 as the unlimited carbon source for the production of high-density fuels, and renewable energy as the driving force behind the process.[1] However, for an industrial application, there are still challenges to overcome, such as low selectivity, short durability and low current densities along with high overpotentials. New sustainable, modular, robust and efficient catalytic platforms are needed. In this regard, this work entails a fundamental understanding of the CO2 mechanisms using Single Atom Catalyst (SAC) within Covalent Organic Frameworks (COFs). This work focuses on the investigation of the electrochemical CO2 reduction (CO2RR) in water using new Manganese based-Covalent Organic Frameworks with emphasis on understanding the relationship between structure and electrocatalytic activity. The initial center of interest was to accomplish active performance and durability for CO2RR using highly-organized {Mn(CO)3} active sites within COFs. The catalytic activity of the materials was benchmarked against other molecular supported catalysts reported in the literature. Compared to equivalent Mn derivates, COFs exhibited higher selectivity and activity towards CO2 reduction [2]. Additionally, mechanistic studies based on in situ / in operando spectroelectrochemical techniques (ATR-IR, UV-vis, SEIRA, EPR) together with DFT calculations were used to detect key catalytic intermediates and correlate the catalytic activity with the mechanical constraints impose to the {Mn(CO)3} active sites by reticular framework. Of particular note is the detection of a radical intermediate within a Mn based COFs avoiding the detrimental formation of a dimeric specie determined as a resting state in the catalytic cycle. In addition, the study of the Mn centers within the COF was expanded and focused on the understanding of the mechanism and dynamic processes at the electrode interface. This study show case the richness and complexity of reticular materials and can serve as a guide to investigate the dynamics of these organic frameworks.
3.2-O3
Copper (Cu) is an excellent catalyst capable to convert CO2 beyond CO and produces fuels and hydrocarbons. However, it is low product-selective.(1) One strategy to improve product-selectivity on Cu is tuning the geometry of the surface active sites positions, i.e., the group of atoms in the catalyst surface that catalyse the CO2 conversion. In this communication, I show an easy and feasible strategy to tune and quantify the number and geometry of the surface active sites on Cu nanostructures. (2) At first, we have assessed the reversible adsorption/desorption of lead (Pb), or Pb under potential deposition (UPD), on different well-ordered Cu single facets (Figure 1). The lead adsorption/desorption on Cu, is monitored by a cyclic voltammetry technique (CV), which provides distinguishable peaks on each single facet, i.e., it is highly sensitive to the active site´s geometry (Figure 1). Then, we have nanostructured a Cu electrode using and electroplating method. We have recorded the Pb UPD on the nanostructured Cu surface, which voltammetric shape has several peak-contributions. Using the Pb UPD voltammetric data recorded on Cu single facets, we decouple the different facet contributions on our nanostructured Cu surfaces. This voltammetric analysis can be used to rationally design nanostructured copper catalysts with tailored geometry at the active sites positions, which is relevant to improve the CO2 reduction reaction.
Figure 1 shows the voltammetric Pb UPD profiles recorded on Cu single facets at pH 3 and from a 0.1 M KClO4 + 1mM NaCl solution.
3.2-O4
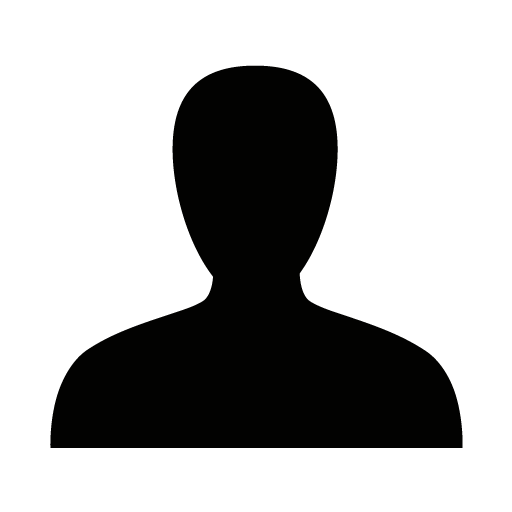
In recent years, two dimensional metal organic frameworks (2D-MOF) have attract much interest not only for the ease of their synthesis, but also for their semiconducting properties and catalytic activities. The appeal of these materials is that they are layered and can be easily exfoliated to obtain a mono (or few) layer material with interesting optoelectronic properties. Moreover, they have great potential for CO2 reduction to obtain solar fuels with more than one carbon atom, such as ethylene and ethanol, in addition to methane and methanol. The production of ethylene has been reported in a recent communication, but its mechanism of action is still unknown.
In this talk, I will explore how a particular class of 2D-MOF based on a phthalocyanine core can be the reactive center for the production of ethylene and ethanol, focusing on the mechanism of action by mean of a novel computational method called 'Gran Canonical Potential Kinetic', in which an applied potential is explicitly considered to obtain accurate results which are directly comparable with experimental electrocatalytic measurements.
We observed that not only methane and ethylene can be formed, along with methanol, acetylene, ethanol and other different alcohols, but also that the key reaction step -the insertion of CO- occur without the presence of a CO-CO dimerization, as commonly observed on metal surfaces, but with a novel method which can still lead to the formation of C2 products, making the 2D-MOF behave like a single atom catalyst.
3.2-O5
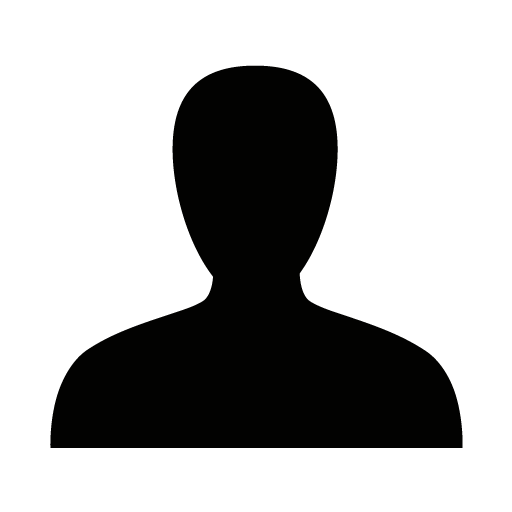
The electrochemical reduction reaction of CO2 (CO2RR) into valuable chemical products is foreseen as a promising lever to move from fossil to renewable energy with the benefit of closing the carbon look. Copper is a unique catalyst for this reaction as it generates products beyond CO, such as CH4 or C2H4. While polycrystalline copper is unselective, faceting copper at the nanoscale has proven to improve its selectivity while retaining attractive activity.1,2 However, its severe lack of stability during operation dramatically hampers its further use on the industrial scale. Indeed, Cu surfaces suffer from drastic restructuring which often results in a performance loss.3,4
Herein, we propose the encapsulation of Cu nanocatalysts with a thin amorphous oxide coating as one promising solution against their structural changes during CO2RR. Specifically, we utilize colloidal atomic layer deposition (c-ALD) to grow an alumina (AlOx) shell with tunable thickness around 7 nm copper spheres to form well-defined Cu@AlOx core@shell structures. We find that the improved morphological stability of the Cu@AlOx is reached when full encapsulation is achieved. To understand the mechanisms behind the observed behavior, we correlate the shift in product distribution with observations from ex situ electron microscopy and operando X-ray absorption spectroscopy. Overall, this work offers the big opportunity to create stable Cu catalysts during CO2RR which is currently one of the most important challenges in the field.
3.3-O1
Electrochemical CO2 reduction is an appealing approach to diminish CO2 emissions, while obtaining valuable chemicals and fuels from renewable electricity. However, efficient electrocatalysts exhibiting high selectivity and low operating potentials are still needed. Herein , we present a preparation method for the obtention of Cu and Fe nanoparticles supported on porous N-doped graphitic carbon matrix as efficient and selective electrocatalysts for CO2 reduction to CO at low overpotentials. XRD and Raman spectroscopy confirmed independent Cu and Fe metals as the main phases. HRSEM and HRTEM images show the coral-like morphology of the porous N-doped graphitic carbon matrix supporting Cu and Fe metal nanoparticles (about 10 wt. %) homogeneously distributed with an average size of 1.5 nm and narrow size distribution. At the optimum Fe/Cu ratio of 2, this material present high activity for CO2 reduction to CO at -0.3 V vs RHE with a Faradaic efficiency of 96 %. Moreover, at -0.5 V vs RHE this electrocatalyst produces 27.8 mmol of CO x gcat-1 x h-1, the production rate being stable for 17 h. A synergy between Cu and Fe nanoparticles due to their close proximity in comparison with independent Cu or Fe electrocatalysts is discussed.
3.3-O2
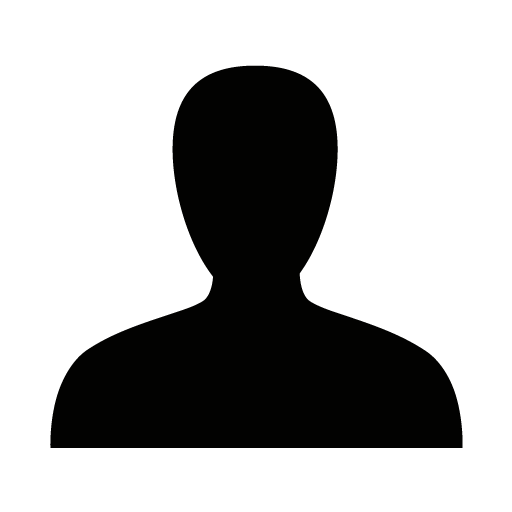
Artificial photosynthesis requires a hybrid assembly that integrates light absorption, electron transfer, and catalysis. Covalent anchoring of a molecular catalysts to a light harvesting semiconductor results in a photocathode that is able to perform selective photoelectrocatalytic CO2 reduction (PEC-CO2RR). In hybrid materials, the molecular catalyst provides with selective catalytic activity whereas the photoactive semiconductor material is used as support and for light harvesting [1]. In these systems, charge accumulation and kinetics of photoinduced electron transfer from the photocathode to the molecular catalyst and to the CO2 are crucial for an efficient CO2 conversion [2, 3]. In this work, a mesoporous anatase TiO2 layer is used as a solid photosensitizer support to anchor iron porphyrin-based molecular catalysts for CO2 to CO obtention. Micro to mili second Transient absorption spectroscopy (TAS) is employed to investigate the accumulation and lifetime of photogenerated electrons in TiO2 by controlling the applied bias and in the presence of CO2. By probing the transient signal of TiO2 conduction band electrons, we observe a decrease in the accumulation of charges in non-functionalized TiO2 under applied bias when CO2 is present (at -0.5 V vs. Ag/AgCl, by 35%), which proves the transference of electrons from the photocathode to the CO2. Furthermore, we observe a shorter lifetime of photogenerated electrons when the TiO2 is functionalized with iron porphyrins (10-fold decrease) derived from a fast electron transfer from the photocathode to the molecular catalyst. Ultimately, we correlate the results to the potentiostatic electrocatalysis performance of TiO2 and TiO2-Iron porphyrin hybrid materials under CO2; with bare TiO2, CO and CH4 along with H2 are produced, whereas TiO2-Iron porphyrin shows 100% selective CO formation. However, we identify moderate CO2RR performances caused by interfacial charge recombination between the oxidized iron porphyrin and conduction band electrons of TiO2 which decrease the charge transfer process to the CO2 molecule.
3.3-O3
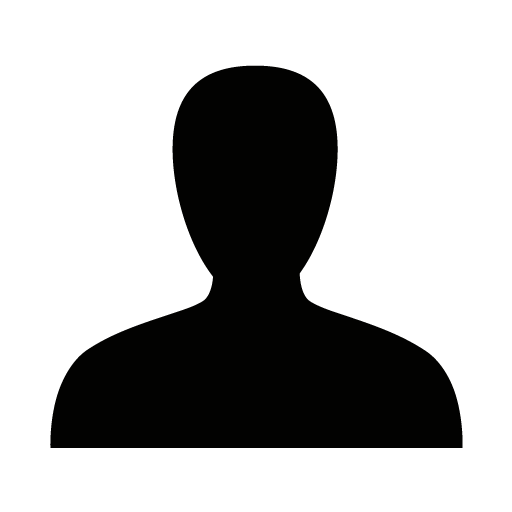
Global warming, as a consequence of the dependence on the use of fossil fuels, is one of the major problems that our current society is facing. For this reason, the production of energy by renewable sources has become a great challenge for the scientific community. Thus, researchers have recently focused their efforts on the development of new technologies that guarantee the production of high amounts of energy with low to minimal carbon footprint. Intermittence of renewable energies is a present challenge that requires to develop technologies able to store huge amounts of energy to be easily supplied at periods of low or zero energy production. In this scenario, (photo)electrochemical technologies have become an attractive candidate with the purpose of producing green fuels in order to store energy at the moments of excess of production. Research in the field of (photo) electrocatalysis has mainly been focused on the generation of green H2, from the oxygen evolution reaction (OER) through the oxidation of H2O; and the reduction of CO2 (CO2RR) to obtain green fuels.[1] in these studies, notable advances have been made in recent years, both in terms of understanding the mechanisms of reactivity, as well as the selectivity of the processes activated by sunlight.[2,3] In both cases, O2 is obtained as by-product at the anode, with a very low price at the market, thus reducing the economic viability and the interest in this technology.[4] With the aim to store energy at the same time that obtaining compounds with added value, several organic transformations have been pointed out as alternative to the OER.at the anode and H2 production or CO2RR at the cathode. [5,6]
One very interesting alternative anodic reaction is based on the oxidation of lignin or compounds from biomass, since these species also allow reducing overpotentials while obtaining valuable products for the chemical industry, with the subsequent biomass revalorization. 5-hydroxymethylfurfural (HMF) is a biomass derived species, which can be easily obtained from C6 natural sugars. The selective oxidation of one of the hydroxyl groups of HMF leads to 2,5-furandicarbox-aldehyde (DFF), which can be used as a monomer in the synthesis of various polymers based in furan; [7,8] while further oxidation of HMF gives rise to 2,5-furandicarboxylic acid (FDCA), a very important component in the pharmaceutical and polymer industry, which can replace the monomers traditionally used in the synthesis of ethylene terephthalate (PET).[9] Besides, the reduction of HMF can lead to 2,5-bi(hydroxymethyl)furan (BHMF), a species that can be used as a precursor for the synthesis of many bio-based polymers, [10] consequently making this process very interesting as alternative to H2 production or CO2RR in photoelectrochemical processes.
In our project we have performed the transformation of HMF to added value products by means of electrochemistry. The electrochemical oxidation of HMF to 2,5 FDCA has been achieved using easily made and inexpensive NiO-OH electrodes, obtained by Ni electrodeposition on pencil graphite rods (Ni/PGR). We have optimized the reaction conditions to prevent HMF degradation, leading to a nearly complete conversion of HMF into FDCA, with 88% Faradaic Efficiency (FE) and very low degradation in less than 2 hours. The electrochemical reduction of HMF to BHMF has been achieved using Cu foils electrodes decorated with Ag deposition using the galvanic replacement technique. BHMF has been obtained with 89% yield and 87% FE at pH 9 media in less than 3 hours. Impedance Spectroscopy (IS) has been analysed in both electrochemical processes to elucidate the electron transfer mechanism and the adsorption of reactants and intermediate species on the surface of the electrodes.
3.3-O4
Photocatalytic liposomes are a promising supramolecular platform for artificial photosynthesis,[1] but the understanding of reaction mechanisms and surface dynamics between membrane-bound molecular components still remain a challenge. In this presentation, we will report the photocatalytic CO2 reduction performance of a series of 3d transition metal terpyridine and porphyrin catalysts immobilised on liposomes,[2-4] and briefly about their use in (photo)electrocatalytic CO2 reduction.[5] Time-resolved spectroscopy was utilised to provide new insights into electron transfer processes taking place between the electron donor (sodium ascorbate) and membrane-bound photosensitiser and catalyst molecules. The most active molecular photocatalyst system, containing a cobalt porphyrin, was studied by (spectro)electrochemistry and density functional theory to gain a better understand of its enhanced performance compared to the other studied assemblies, and to propose a possible reaction mechanism for CO2 reduction.[4] Furthermore, the same cobalt porphyrin catalyst is successfully utilised in a (photo)electrochemical device that can simultaneously reduce CO2 and reform plastic waste.[5] Therefore, our findings demonstrate the great potential of liposomes as versatile photocatalytic scaffolds. We also show how the same molecular catalyst can be utilised successfully in photocatalytic and (photo)electrocatalytic systems, and illustrate the power of combining time-resolved and in situ spectroscopic techniques to understand molecule-based systems.
References:
[1] Pannwitz, A.; Klein, D. M.; Rodríguez-Jiménez, S.; Casadevall, C.; Song, H.; Reisner, E.; Hammarström, L.; Bonnet, S., Roadmap towards solar fuel synthesis at the water interface of liposome membranes. Chem. Soc. Rev. 2021, 50, 4833-4855.
[2] Wang, Q.; Warnan, J.; Rodríguez-Jiménez, S.; Leung, J. J.; Kalathil, S.; Andrei, V.; Domen, K.; Reisner, E., Molecularly engineered photocatalyst sheet for scalable solar formate production from carbon dioxide and water. Nat. Energy 2020, 5, 703–710.
[3] Zhang, X.; Cibian, M.; Call, A.; Yamauchi, K.; Sakai, K., Photochemical CO2 Reduction Driven by Water-Soluble Copper(I) Photosensitizer with the Catalysis Accelerated by Multi-Electron Chargeable Cobalt Porphyrin. ACS Catal. 2019, 9, 11263-11273.
[4] Rodríguez-Jiménez, S.; Song, H.; Lam, E.; Wright, D.;Pannwitz, A.; Bonke, S. A.; Baumberg, J. J.; Bonnet, S.; Hammarström, L.; Reisner E., Self-Assembled Liposomes Enhanced Electron Transfer for Efficient Photocatalytic CO2 Reduction. J. Am. Chem. Soc. 2022, 144, 9399-9412.
[5] Bhattacharjee, S.; Rahaman, M.; Andrei, V.; Miller, M. Rodríguez-Jiménez, S.; Lam, E.; Pornrungroj C.; Reisner, E., Photoelectrochemical CO2-to-fuel conversion with simultaneous plastic reforming. Nat. Synth (2023). in print (https://doi.org/10.1038/s44160-022-00196-0).
3.3-O5
Working on Electrocatalytic CO2 Reduction to alternative fuels as a Marie S Curie DOC FAM COFUND Early Stage Researcher (Marie Curie Doctoral Researcher) in the group of Advanced Materials for Energy Area led by Prof. Joan Ramon Morante, Director and Head, Catalonia Institute for Energy Research (IREC), Barcelona, Spain. Interested in Travelling and Photography.
Electrocatalytic CO2 reduction to renewable fuels has drawn increasing attention due to its great potential in addressing the issues of the greenhouse effect, energy crisis, and industrial transformation thus having the utmost importance in attaining a carbon-neutral society. Nevertheless, there are general practical limitations such as poor selectivity for multi-carbon products and most importantly the electrocatalysts suffer from high overpotentials while directly converting the CO2 to C2+ products. To overcome these challenges and enhance the ECO2R, a cascade mechanistic approach is explored as an alternative to the direct conversion of CO2 to C2+ products. A two-step tandem set-up is employed where CO2 is converted to CO in the first step, enabled with a highly efficient Ni single-atom catalyst on activated carbon black, and sequentially CO is converted to multi-carbon products in the second step. The single-atomic catalytic sites yield a nearly 100 % faradaic efficiency towards CO at an average current density of over -40 mA·cm-2. The as-produced product stream is directly supplied as the reactant stream to a second electrolyzer that is equipped with oxide-derived copper supported on carbon black. Initially, it is noticed that the presence of unreacted CO2 in the second cell has an adverse effect and results in poor performance. When the unreacted CO2 is trapped by using an absorption chamber in between these two electrolyzers, a surge in the faradaic efficiency towards ethylene, ethanol, and n-propanol thus accounting for more than 80% total faradaic efficiency at an average current density of over 120-130 mA·cm-2 and the unwanted yet competitive H2 is limited to just 10-12 % faradaic efficiency. The aforementioned approach is found to be highly efficient not only in terms of faradaic efficiency but also energy efficiency. It is evident that multi-step electrolysis yields a highly promising conversion of CO2 to multi-carbon products. On the flip side, direct or indirect routes are also explored to electrocatalytically reduce the carbonated solvent stream placed in between the electrolyzers. The observed results clearly are imperative by enabling a direct cascade approach and exclusive separation of unreacted feed gas aid in attaining higher electro-conversion of CO2 to alternative fuels.
3.3-O6
Electrocatalytic hydrogen production or C-based fuels synthesis via water splitting and CO2 reduction, respectively, is considered a promising technology to store renewable energy into chemical bonds when using Earth-abundant electrocatalysts. The efficiency of these catalysts does not only depend on the nature of the metal centre, but also on the morphology and crystalline structure. However, chemical and morphological changes in the catalysts take place in operando conditions that are poorly understood.
In this poster, I will present recent advances on the understanding of morphological transformations of Cu-based electrocatalysts for the hydrogen evolution and CO2 oxidation reactions during catalysis and the effect of such transformations in the reactions kinetics. In particular, the effect of morphological transformations on increasing the electrochemically active surface area (ECSA) will be discussed. In this poster, I will show that such increase in the ECSA is found to increase the efficiency of the catalyst noting this work is performed in mild pH conditions.
1.1-I1
Dr. Nagore Ortiz-Vitoriano (https://cicenergigune.com/en/nagore-ortiz-vitoriano) is an Ikerbasque Research Associate, who has been spearheading metal-air research at CIC energiGUNE (Spain) since 2016, of which she became research line manager in 2018.
She obtained her doctorate in 2011 for her work on solid oxide fuel cells (University of the Basque Country, UPV/EHU, Spain), during the course of which she undertook research stays at Risø DTU (Denmark) and Imperial College London (UK). In 2013 she was awarded a Marie Curie International Outgoing Fellowship from the European Union, enabling her to join the Department of Mechanical Engineering at the Massachusetts Institute of Technology (MIT) in Cambridge (USA) where she worked with both lithium and sodium-air batteries. In 2015, she continued this fellowship at CIC energiGUNE, where she conducted research stays at Oak Ridge National Laboratory (USA), Deakin University (Australia) and Chalmers University (Sweden). Recently, she has been promoted to Ikerbasque Research Associate and granted the Ramon y Cajal fellowship financed by the European Commission's European Social Fund through the Spanish Ministry of Science and Innovation.
Dr. Ortiz-Vitoriano has focused on both rational design of electrode and electrolyte materials for energy storage (e.g., solid oxide fuel cells, electrocatalysis, Na-ion and metal-air batteries), as well as fundamental research focused on elucidating key processes (by establishing relevant physiochemical models) in order to facilitate rapid future developments at both the material and system levels.
Energy storage plays, undoubtedly, a fundamental role in the process of total decarbonization of the global economy that is expected to take place in the coming decades. The energy transition to a renewable and sustainable generation is the solution to reduce greenhouse gas emissions and thus achieve the European Commission´s goal of becoming the world´s first decarbonized economy. In this transition, beyond li-ion technologies will play a key role to meet the increasing energy demand that cannot be covered by Li-ion batteries solely. In this scenario, sodium-oxygen and zinc air batteries have become an attractive alternative due to their high gravimetric energy densities (1605 or 1108 Wh/kg based on Na2O2 or NaO2, respectively; and 1086 Wh/kg based on ZnO discharge products) resulting from the use of an oxygen-based phase-change reaction (potentially reducing the weight and freeing up space for other components).
In this talk, a general overview of these systems will be given along with the materials requirement and system performance. These batteries have been considered as the holy grail of battery research due to their high theoretical energy densities; however, several challenges remain to be solved before commercialization.
1.1-I2
Renewables are essential for the energy transition to a sustainable and eco-friendly energy system that avoids the use of fossil fuels. One of the major limitations of renewables is their intermittent character which depends on climate conditions. This climate dependence leads to a complete disconnection between energy production and demand. Electrochemistry, fed by renewables, has emerged as a smart procedure that can pave the way for the energy transition. The main chemical procedures studied in electrochemistry are water splitting and CO2 reduction. In both cases, the oxygen evolution reaction (OER) takes place at the anode, providing the electrons and protons needed in the cathode for the generation of H2 in water splitting or the conversion of CO2 into valuable species, such as CH4 or CH3OH. The OER produces O2 that despite being a very important compound, its price in the market is very low, which limits the economic viability of the process and ultimately reduces the interest in this technology.1 Furthermore, this reaction exhibits large overpotentials when using catalysts based on earth abundant materials, limiting energy conversion efficiency.2 For these two reasons, there is a growing interest to find alternative reactions to OER at the anode that, by one side, reduce the overpotentials needed and by the other, produce compounds with higher added-value and interest for the chemical industry.1,3 In this framework biomass valorization, has emerged as an attractive substitute to the oxidation of H2O.4 Herein we present our more recent results in the electrochemical transformation of biomass, as well as the use of electrochemistry for energy storage.
1.1-I3
Solid oxide fuel cells (SOFCs) are capable of providing high energy efficiency and fuel flexibility leading to use of hydrogen and hydrocarbons due to high operation temperature (600–800°C). Recently, ammonia (NH3) is considered as one of the most promising hydrogen carriers because of great advantages of zero-carbon emission, a high hydrogen content and worldwide infrastructures established for production, transport and storage. Thus, much effort has been given to directly utilize ammonia as a fuel for SOFCs, so called direct ammonia (DA)-SOFCs in which the fuel is decomposed hydrogen and nitrogen, and the released hydrogen is electrochemically oxidized in the anode consecutively. Thus, DA-SOFCs are believed to accomplish a CO2-free power generation with high efficiency. In this study, the current status on the development of SOFCs fed with ammonia, and strategical approaches to overcome technical issues limiting the development of DA-SOFCs will be introduced. In addition, results on the application of an exsolution catalyst to decompose the fuel in the anode of DA-SOFCs are present, which has proven the feasibility of ammonia fuel for high performance and reliable SOFCs.
1.1-O1
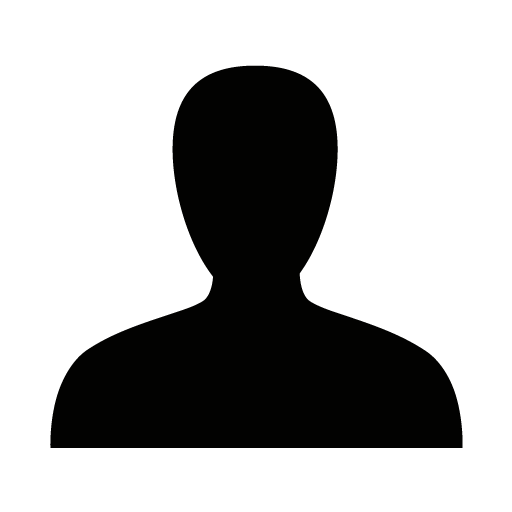
Abstract
One of the challenges faced by photoelectrochemical (PEC) water splitting technology is the relatively high levelized cost of hydrogen (LCOH) compared with other hydrogen generation methods. A potential strategy to increase its competitiveness is to couple PEC water splitting with hydrogenation reactions that produce valorized chemicals[1][2]. In this study, we evaluate the economic potential of co-producing hydrogen and methylsuccinic acid (MSA) by coupling the hydrogenation of itaconic acid (IA) inside a PEC water splitting device based on BiVO4 and silicon heterojunction (SHJ) absorbers[3][4].
This study examines the economics of a 1000 kg H2/day rated capacity PEC plant with the coupled co-production feature by conducting a techno-economic assessment (TEA) under a base case scenario where PEC devices with solar-to-hydrogen (STH) efficiency of 10% and longevity of 20 years are considered. When H2 is the only product, the obtained cradle-to-gate LCOH is 22.3 €/kg. However, with the coupled hydrogenation reaction, the LCOH can be reduced to 2.8 €/kg when only 3.9% of the generated H2 molecules are converted to MSA. This LCOH value is already competitive with the benchmark H2 derived from steam methane reforming (SMR)[5]. This means that the technical advancements based on currently demonstrated coupled catalyst PEC technology can provide sufficient cost reductions to allow solar hydrogen to directly compete on a levelized cost basis with hydrogen produced from fossil energy.
Our sensitivity analysis further indicates that the H2-to-MSA conversion efficiency and MSA sales price have the greatest impact on the reduction of LCOH. Therefore, the system can take advantage of its flexibility to maximize benefits by adjusting the conversion rates accordingly to market conditions. Further optimization is suggested regarding the substitution of expensive system components, i.e., PV absorber and membrane, with envisioned cheaper alternatives, which might substantially lower the overall system cost.
Key words: water splitting, (photo)electrochemistry, technoeconomic analysis, coupled catalysis, hydrogenation
1.1-O2
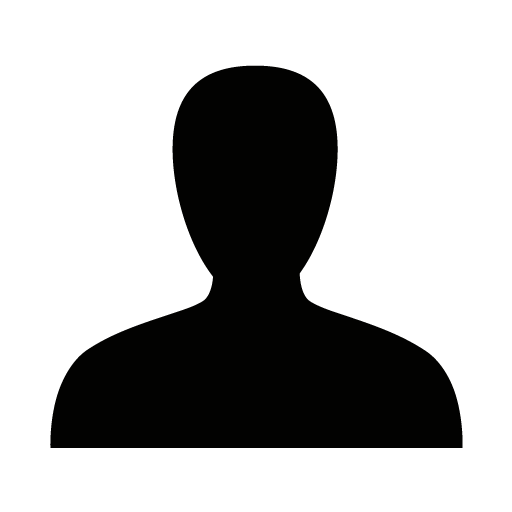
Oxygen reduction reaction activity is governed by the oxygen adsorption/dissociation, proton conduction, and electron transfer kinetics in protonic ceramic fuel cells (PCFCs). Various strategies have been explored to enhance the proton and electron conductivity via tuning oxygen vacancy concentration in the electrode materials and introducing electronic conducting agents. However, there are few studies on improving surface exchange reaction (oxygen adsorption/dissociation) kinetics in protonic ceramic fuel cells. In this study, we report uniformly distributed nickel oxide (NiO) nanoparticles as a catalyst to enhance the electrochemical performance of BaCo0.4Fe0.4Zr0.1Y0.1O3-δ-BaZr0.1Ce0.7Y0.1Yb0.1O3 (BCFZY-BZCYYb) composite cathode by improving surface exchange reaction kinetics. The 0D NiO nanoparticles with high adsorption and fast dissociation ability of oxygen could enlarge the active sites for surface exchange reaction without fading the BCFZY surface and triple-phase boundaries where H2O formation reaction occurs. The cathode employing NiO nanoparticles exhibits largely reduced polarization resistance and superior power density of 780 mW/cm2 at 600 °C. In addition, the water-stable characteristic of NiO and reduced polarization resistance of composite cathode drastically improved the cell stability.
2.1-I1
Much research has shown that crystal structure and dimensionality in materials play an important role in determining their functional properties besides material composition itself. From this aspect, attention to two-dimensional (2D) materials with different three-dimensional (3D) crystallography has been given. The initial boost in research of 2D materials is largely attributed to graphene, which shows interesting phenomena such as super-electrical conductivity and high flexibility with good strength that are absent in bulk graphite. After the discovery of graphene, relevant research on single layers of transition metal dichalcogenides (TMDCs) with lamellar structures similar to that of graphite generated a second wave of interest. Accordingly, many unusual physical, chemical, or electronic properties compared to their bulk counter-parts have been reported. On the other hand, whereas much research effort has been devoted to graphene and TMDC, to date, relatively little research has been conducted in the area of 2D oxide nanosheets because most oxides have 3D crystal structures (it is noted that graphene and TMDC are inherently 2D structures, which are strongly bonded in plane but weakly bonded out of plane with van der Waals force). However, oxides have advantages such as chemical stability and low cost compared to the above materials, and thus, if synthesized to 2D structures, they can be another potential group with valuable functionality. In this presentation, the synthesis of 2D materials based on oxides and, further, their usage for various applications such as energy, sensor, and display will be dealt with.
2.1-I2
Characterization of multi-component slurry such as cathode and anode slurries of Li-ion battery is important, however, it is very difficult task. Thus, we investigated both the flow ability and the packing (settling) ability to discuss the particles dispersion and aggregation state for the cathode slurry of Li-ion battery. The slurries were prepared by changing the mixing condition of acetylene black particles and then the prepared slurry was casted and dried to fabricate the cathode layer. The flow curve of the slurry was measured by a rheometer and the time change of settling hydrostatic pressure was measured by a hydrostatic pressure analyzer. The density and electric resistance of the fabricated cathode layer were measured as well. It was found that a slurry in which acetylene black particles forms a network structure, with sufficient strength and the ability to rapidly recover after breaking by an external force, yields a cathode with comparatively high density and comparatively low volume resistivity [1]. It was also found that the normalized settling time of a cathode slurry determined from its change in hydrostatic pressure over time correlates well with both the density and volume resistivity of a resulting as-cast cathode [1].
2.1-I3
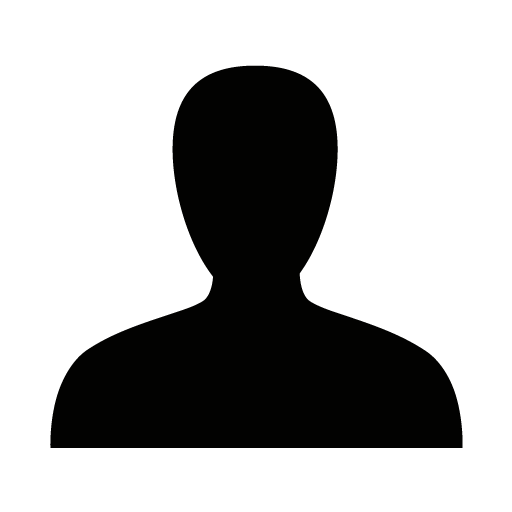
The demand for rechargeable Li batteries having more safety and higher energy density has been increased to meet the strong demand of novel applications such as electric vehicles and energy storage system. In this aspect, Li ion batteries containing typical liquid electrolytes have fundamental limitations because liquid electrolytes can act as fuels in thermal runaway behavior leading to a fire or an explosion of battery and can be decomposed at high potential (> 4.5V) leading to the restricted use of high potential cathodes. To address these problems, there are several approaches. One of promising approaches is to apply proper oxide-based solid electrolytes (SEs) instead of liquid electrolytes because they can enable to deliver superior safety with Li metal and to achieve high energy density simultaneously.
In this talk, I will discuss about the progress of the development of the oxide-based SEs, especially, focusing on the newly developed garnet-type SE that has superior electrochemical/chemical properties with respect to the wettability with Li metal, ionic conductivity, and chemical stability with air, and the revisited Lisicon-type SE that has superior compatibility of most of active materials. Also, I will talk about the efforts in our group to build up all solid-state battery by using the developed oxide-based SEs.
2.2-I1
Solid oxide regenerative fuel cells (SORFCs), which perform the dual functions of power generation and energy storage at high temperatures, offer one of the most efficient and environmentally friendly options for future energy management systems. Although the functionality of SORFC electrodes could be significantly improved by reducing the feature size of electrode to nanoscale, the practical use of nanomaterials has been limited due to the lack of stability and controllability at high temperatures. Herein, we demonstrate an advanced infiltration technique that allows nanoscale control of highly active and stable catalysts at elevated temperatures. Homogeneous precipitation in chemical solution, which is induced by urea decomposition, allows the precise tailoring of the phase purity and geometric properties. Particularly, effective complexing followed by instantaneous precipitation enables the atomic-scale dispersion of active catalysts on support. Controlling the key characteristics of nanocatalysts yields an electrode that is very close to the ideal electrode structure. Consequently, outstanding performance and durability are demonstrated in both fuel cell and electrolysis modes [1, 2].
References
[1] K.J. Yoon, M. Biswas, H.-J. Kim, M. Park, J. Hong, H. Kim, J.-W. Son, J.-H. Lee, B.-K. Kim, H.-W. Lee, Nano Energy 2017, 9.
[2] J. Shin, Y.J. Lee, A. Jan, S.M. Choi, M.Y. Park, S. Choi, J.Y. Hwang, S. Hong, S.G. Park, H.J. Chang, M.K. Cho, J.P. Singh, K.H. Chae, S. Yang, H.-I. Ji, H. Kim, J.-W. Son, J.-H. Lee, B.-K. Kim, H.-W. Lee, J. Hong, Y.J. Lee, K.J. Yoon, Energy & Environmental Science 2020 13 4903
2.2-I2
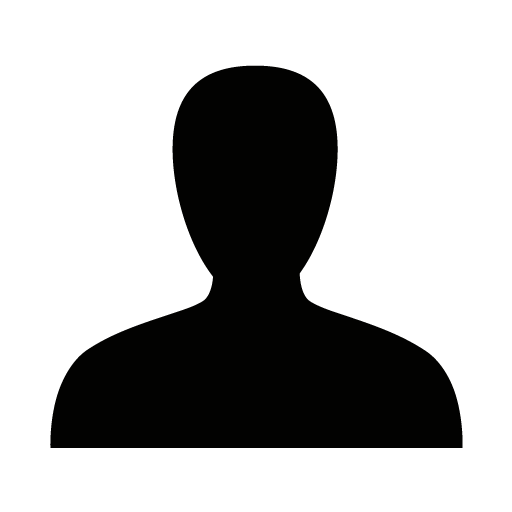
Irreversible phase transformations of layered oxide cathodes during charging have been detrimental for most of them. Even if a lot of efforts have been made to relieve this highly irreversible phase transformation, there have been just a few successful results, which definitely limit the amount of extracted alkali ions and therey the available capacitie of the layered oxides. So, this presentation will suggest two strategies to get over the limitation of previous researches.
As an inverse conceptual strategy, we first observed the possibility to make this irreversible phase transformation extremely reversible by utilizing crystal water as a pillar. Although we found a few cyrstal structures working with this reversible phase transition, the works using Na-birnessite (NaxMnO2•yH2O; Na-bir) or Li-birnessite (Li-bir) as basic structural units will be highlighted here. The crystal water in the structure contributes to generating metastable spinel-like phase, which is the key factor for making this unusual reversibility happen. The reversible structural rearrangement between layered and spinel-like phases during electrochemical reaction could activate new cation sites and enhance ion diffusion with higher structural stability. This unprecedented reversible phase transformation between spinel and layered structure was maximized through modulating the steric coordination or amount of cyrstal water in the lattice resultantly optimizing the electrochemical performances of the birnessite layered oxides.
Pseudo Jahn-Teller effect (Pseudo JTE) will be also stressed out as a fundamental reason behind lattice distortion of layered oxide cathodes. Even though Jahn-Teller effect (JTE) has been regarded as one of the most important determinators of how much stress layered cathode materials undergo during charge and discharge, there have been many reports that traces of superstructure exist in pristine layered materials and irreversible phase transitions occur even after eliminating the JTE. A careful consideration of the energy of cationic distortion using a Taylor expansion indicated that second-order JTE (Pseudo JTE) is more widespread than the aforementioned JTE because of the various bonding states that occur between bonding and anti-bonding molecular orbitals in transition metal octahedra. As a model case, some of layered oxide cathodes including P2-type Mn-rich cathode (Na3/4MnO2) will be dealt with in this presentation
The two new insights provide deep insight into novel class of intercalating materials which can deal with highly reversible framework changes, and thus it can break up some typical prejudices which we have about the layered cathodes for Li or Na secondary batteries.
2.2-O1
I am working at the Institute of Advanced Materials of the University Jaume I. My research is focused in the development of advanced hybrid materials for energy conversion and storage based on catalytic transformations. The hybrid materials are developed from well-defined organometallic complexes. The approach for such applications is divided on three different research lines: i) Organometallic chemistry: design, characterisation and properties of new catalysts ii) Catalytic applications in processes related to hydrogenation and dehydrogenation. iii) New materials: study of the properties and applications of organometallic compounds and metal nanoparticles supported in graphene derivatives for energy conversion and storage. Research Lines:
Ad. Mat. for catalysis
Rationale design of catalytic materials derived from organometallic complexes.
Development of stable metal nanoparticles as improved catalytic systems.
Ligand design for the immobilization of metal complexes and nanoparticles.
Fundamental and applied study of catalytic hydrogenation and dehydrogenation processes.
Ad. Mat. for energy storage
Development of systems for the storage of hydrogen in the liquid form using “Liquid Organic Hydrogen Carriers (LOHCs).
Development of Hydrogen Storage technologies for transport and uses of hydrogen.
Industrial Innovation and Technology Transfer
The group is involved in industrial projects on Hydrogen Storage, depolymerization processes and catalyst development in connection with a regional funding program (AVI).
The intermittent nature of renewable energies inevitably leads to energy surpluses to maintain energy demand at peak times. Consequently, the storage of energy surpluses from renewables becomes a necessity. To tackle this problem, in this work we propose an efficient way of energy storage based on using green hydrogen as an energy vector. Green hydrogen can be store using different technologies but among them, the storage forming chemical bonds represents an unexplored technology that will have an important impact in handling, management and transporting hydrogen. Our aim is to develop efficient systems for hydrogen storage in the form of chemical bonds based on Liquid Organic Hydrogen Carriers (LOHCs) [1].
The key point of efficient LOHCs lies in the design of suitable catalysts and processes for the conversion and reconversion of hydrogen into chemical bonds. In this work, we describe the state of the art in the field [2] [3] and our last results in for hydrogen storage in the form LOHCs.
2.2-O2
Sixto Giménez (M. Sc. Physics 1996, Ph. D. Physics 2002) is Associate Professor at Universitat Jaume I de Castelló (Spain). His professional career has been focused on the study of micro and nanostructured materials for different applications spanning from structural components to optoelectronic devices. During his PhD thesis at the University of Navarra, he studied the relationship between processing of metallic and ceramic powders, their sintering behavior and mechanical properties. He took a Post-Doc position at the Katholiek Universiteit Leuven where he focused on the development of non-destructive and in-situ characterization techniques of the sintering behavior of metallic porous materials. In January 2008, he joined the Group of Photovoltaic and Optoelectronic Devices of University Jaume I where he is involved in the development of new concepts for photovoltaic and photoelectrochemical devices based on nanoscaled materials, particularly studying the optoelectronic and electrochemical responses of the devices by electrical impedance spectroscopy. He has co-authored more than 80 scientific papers in international journals and has received more than 5000 citations. His current h-index is 31.
All-Inorganic Halide Perovskite Nanocrystals (QDs) have emerged as a new class of fascinating nanomaterials with outstanding optoelectronic properties, with promise to revolutionize different fields like photovoltaics, lasing and emission. In the present talk, we will describe our efforts towards the application of these materials for solar-driven hydrogen production coupled to other processes like organic transformations and waste valorization.[1-2] We will discuss on the rational design of these fascinating materials towards photoelectrochemical processes, and the importance of extracting basic electronic and optical information to understand the carrier dynamics,[3] the influence of trap states and to define adequate defect passivation strategies to maximize the performance and stability of these materials.[4] Moreover, proper interrogation tools are needed to validate their photoelectrocatalytic activity and selectivity. The need for integration of the developed materials into tailored photoelectrochemical devices highlight the urgent need for stabilization strategies to move beyond the proof-of-concept stage to relevant technological developments.
2.2-O3
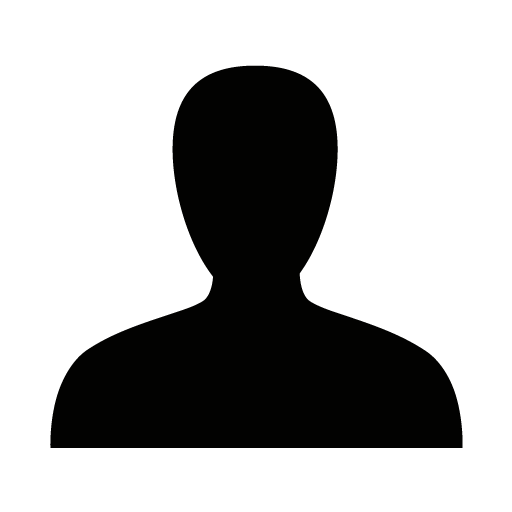
All-solid-state batteries (ASSBs) with sulfide-based solid electrolytes (SEs) have been considered the most promising next-generation energy storage system due to their high energy density. Silicon (Si) has been intensively studied as an anode material due to the high theoretical capacity (3572 mAh g-1, Li15Si4) and a relatively low working voltage (~0.5 V vs Li/Li+) in ASSBs. However, poor electrochemical properties caused by the large volume change (~ 300%) and low electronic conductivity of Si have limited its practical use. Here, we report Si nanoparticles embedded in carbon nanofiber (CNF) coated with solid electrolyte (LPSCl) (Si/CNF@LPSCl) as anode material to achieve high energy density and cycle stability for ASSBs. The CNF is a desirable host matrix for the Si nanoparticles due to its good mechanical property and high electronic conductivity. The conformal coating of SE on the surface of Si/CNF composite enhances the interfacial stability between the active material and the SE, which leads to the improvement in electrochemical properties by suppressing the contact loss. The Si/CNF@LPSCl composite electrode exhibits a reversible capacity of ~ 1172 mAh g-1 at 0.1C and stable cyclability of ~ 84.3% at 0.5C after 50 cycles.
1.1-I1
Maksym Kovalenko has been a tenure-track Assistant Professor of Inorganic Chemistry at ETH Zurich since July 2011 and Associate professor from January 2017. His group is also partially hosted by EMPA (Swiss Federal Laboratories for Materials Science and Technology) to support his highly interdisciplinary research program. He completed graduate studies at Johannes Kepler University Linz (Austria, 2004-2007, with Prof. Wolfgang Heiss), followed by postdoctoral training at the University of Chicago (USA, 2008-2011, with Prof. Dmitri Talapin). His present scientific focus is on the development of new synthesis methods for inorganic nanomaterials, their surface chemistry engineering, and assembly into macroscopically large solids. His ultimate, practical goal is to provide novel inorganic materials for optoelectronics, rechargeable Li-ion batteries, post-Li-battery materials, and catalysis. He is the recipient of an ERC Consolidator Grant 2018, ERC Starting Grant 2012, Ruzicka Preis 2013 and Werner Prize 2016. He is also a Highly Cited Researcher 2018 (by Clarivate Analytics).
Colloidal lead halide perovskite (LHP) nanocrystals (NCs), with bright and spectrally narrow photoluminescence (PL) tunable over the entire visible spectral range, are of immense interest as classical and quantum light sources. Attaining pure single-photon emission is key for many quantum technologies, from optical quantum computing to quantum key distribution and quantum imaging. Across single CsPbX3 NCs (X: Br and I) of different sizes and compositions, we find that increasing quantum confinement is an effective strategy for maximizing single-photon purity due to the suppressed biexciton quantum yield. We achieve 98% single-photon purity (g(2) (0) as low as 2%) from a cavity-free, nonresonantly excited single 6.6 nm CsPbI3 NCs, showcasing the great potential of CsPbX3 NCs as room-temperature highly pure single-photon sources for quantum technologies [1]. In another study, we address the linewidth of the single-photon emission from perovskite NCs at room temperature. By using ab-initio molecular dynamics for simulating exciton-surface-phonon interactions in structurally dynamic CsPbBr3 NCs, followed by single quantum dot optical spectroscopy, we demonstrate that emission line-broadening in these quantum dots is primarily governed by the coupling of excitons to low-energy surface phonons. Mild adjustments of the surface chemical composition allow for attaining much smaller emission linewidths of 35−65 meV (vs. initial values of 70–120 meV), which are on par with the best values known for structurally rigid, colloidal II-VI quantum dots (20−60 meV) [2]. NC self-assembly is a versatile platform for materials engineering, particularly for attaining collective phenomena with perovskite NCs, such as superfluorescence [3, 4, 5]. The NC shape anisotropy leads to structures not observed with spherical NCs. We present a broad structural diversity in multicomponent, long-range ordered superlattices (SLs) comprising highly luminescent cubic CsPbBr3 NCs (and FAPbBr3 NCs) co-assembled with the spherical, truncated cuboid, and disk-shaped NC building blocks. CsPbBr3 nanocubes combined with Fe3O4 or NaGdF4 spheres and truncated cuboid PbS NCs form binary SLs of six structure types with high packing density; namely, AB2, quasi-ternary ABO3, and ABO6 types as well as structures already known in all-spheres systems [NaCl, AlB2, and CuAu types]. In these structures, nanocubes preserve orientational coherence. Combining nanocubes with large and thick NaGdF4 nanodisks results in the orthorhombic SL resembling CaC2 structure with pairs of CsPbBr3 NCs on one lattice site. Also, we implement two substrate-free methods of SL formation. Collective electronic states arise at low temperatures from the dense, periodic packing of NCs, observed as sharp red-shifted bands at 6 K in the photoluminescence and absorption spectra and persisting up to 200 K. Co-assembly of CsPbBr3 nanocubes with very thin disk LaF3 nanodisks (9.2–28.4 nm in diameter, 1.6 nm in thickness) yields six columnar structures with AB, AB2, AB4, and AB6 stoichiometry, not observed before and in our reference experiments with NC systems comprising spheres and disks. Perovskite SLs exhibit superfluorescence, characterized, at high excitation density, by emission pulses with ultrafast (22 ps) radiative decay and Burnham-Chiao ringing behaviour with a strongly accelerated build-up time.
[1] Chenglial Zhu et al. Nano Lett. 2022, 22, 3751−3760
[2] Gabriele Raino et al. Nat. Commun., 2022, 13, 2587
[3] Ihor Cherniukh et al. Nature, 2021, 593, 535–542.
[4] Ihor Cherniukh et al. ACS Nano, 2021, 15, 10, 16488–16500
[5] Ihor Cherniukh et al. ACS Nano, 2022, 16, 5, 7210–7232
1.1-I2
We present Pulsed Laser Deposition (PLD) as a physical vapor deposition of halide perovskites, allowing near stoichiometric transfer and multi-element film formation independent of the relative volatility of the elements from a single solid target. We will discuss the effects of deposition pressure, deposition rate and PLD target composition on the formation of stoichiometric and phase-pure films of CsSnI3, MAPbI3, MA1-xFAxPbI3 and Cs2AgBiBr6. Microstructural, compositional and optoelectronic characterization of the films confirmed that PLD allows control on polymorph formation, optical properties, thickness, and conformal coating on textured substrates [1,2,3]. At the device level, we discuss the influence of the bottom contact layers on the first stages of the perovskite film growth, final film morphology and solar cell performance. Proof-of-concept n-i-p and p-i-n solar cells with > 14 % efficiency are demonstrated with as-deposited room-temperature grown PLD-MA1-xFAxPbI3 films. All these are important steps forward in the controlled growth and future scalability of vapor-deposition methods for perovskite solar cells.[4]
1.2-O1
Antonio Guerrero is Associate Professor in Applied Physics at the Institute of Advanced Materials (Spain). His background includes synthesis of organic and inorganic materials (PhD in Chemistry). He worked 4 years at Cambridge Dispaly Technology fabricating materiales for organic light emitting diodes and joined University Jaume I in 2010 to lead the fabrication laboratory of electronic devices. His expertise includes chemical and electrical characterization of several types of electronic devices. In the last years he has focused in solar cells, memristors, electrochemical cells and batteries.
Halide perovskite materials find applications in solar cells, light emitting diodes or memory storage devices. This family of materials are mixed electronic and ionic conductors and the ionic conductivity is responsible for the hysteresis observed in the electrical characterization.1 Here we explain how this ion migration can be used to our advantage to promote formation of conductive and insulating states making them useful as resistive memories (memristors). We show that the working mechanism and performance of the memory devices can be tuned and improved by a careful selection of each structural layer. Several configurations are evaluated in which structural layers are modified systematically: formulation of the perovskite2, the nature of the buffer layer3 and the nature of the metal contact4. In addition, we develop an electrical model to account for the observed j-V response.5 Overall, we provide solid understanding on the operational mechanism of halide perovskite memristors that unveils the connection between electronic and ionic conduction.
1.2-O2
Besides the outstanding properties such as high absorption coefficient, bandgap tunability and long diffusion length, organometal halide perovskite devices (MHPs) continue to suffer from the consequences of their dual ionic/electronic nature. Hysteresis behaviour during IV measures is the direct sentinel of the ion migration or defect drift occurring in these devices. But this defect drift or ionic migration in MHPs can be positively utilized to design a novel memory device. In fact, perovskites are emerging as promising candidates for the next generation of resistive random-access memory (ReRAM) devices or memristors. ReRAM have been developed as promising next-generation of nonvolatile memory devices due to the simple device architecture, fast operation, and low power consumption[1]. Perovskite memristors are being reported lately with remarkable success due to their good electrical performances, meeting the requirements for memory technology; i.e: non-destructive reading process, nonvolatility, high ON/OFF ratio, and fast switching speed. [2]. Due to their solution processability, perovskite memristor devices have been fabricated both in their polycrystalline and monocrystalline forms, as thin-film and bulk configurations respectively. Outstanding results have been obtained for both.[3] However, the mechanisms behind their good performance and how their crystalline nature affects them are still under discussion. In this work, we report the first memristor device based on MAPbBr3 thin-film single crystal. The synergy between its thin-film configuration and its monocrystalline nature, allows displaying very high stability during operation conditions and massive operative currents, exhibiting an outstanding ON/OFF ratio of 106. The mechanisms governing the operation of this device are analysed in depth by impedance spectroscopy measures, contributing to a better understanding of the physics governing these devices.
1.2-O3
Matteo Zaffalon is a Postdoctoral researcher at the Department of Materials Science of the University of Milano-Bicocca (IT), where he earned his Ph.D. in Materials Science and Nanotechnology in 2022. In 2020 he collaborated with the Nanotechnology & Advanced Spectroscopy group at the Los Alamos National Laboratory (NM, USA) working on the spectroscopic investigation of solution grown functional nanostructures for application in photonic and optoelectronic devices. His research is now focused on the spectroscopic investigation and development of novel nanomaterials for the ultrafast detection and conversion of ionising radiation for energy and medical imaging applications.
Radiation detection is of utmost importance in fundamental scientific research, as well as medical diagnostics, homeland security, environmental monitoring and industrial control [1]. Lead halide perovskites (LHPs) are attracting growing attention as high-atomic-number materials for next-generation scintillators and photoconductors for ionizing radiation detection. To unlock their full potential as reliable and cost-effective alternatives to conventional materials [2], it is necessary for LHPs to conjugate high scintillation yields with emission stability under high doses of ionizing radiation. To date, no definitive solution has been devised to optimize the scintillation efficiency and kinetics of LHPs and nothing is known of their radiation hardness for doses above a few kiloGrays, to the best of our knowledge. Here we demonstrate that CsPbBr3 nanocrystals exhibit exceptional radiation hardness for γ-radiation doses as high as 1 MGy [3]. Spectroscopic and radiometric experiments highlight that despite their defect tolerance, standard CsPbBr3 nanocrystals suffer from electron trapping in dense surface effects that are eliminated by post-synthesis fluorination. This results in >500% enhancement in scintillation efficiency, which becomes comparable to commercial scintillators, and still retaining exceptional levels of radiation hardness. These results have important implications for the widespread use of LHPs in ultrastable and efficient radiation detectors for strategic applications such as national and industrial security, high-energy physics and nuclear power plant control, from space exploration to medical imaging diagnostics such as CT and PET.
1.2-O4
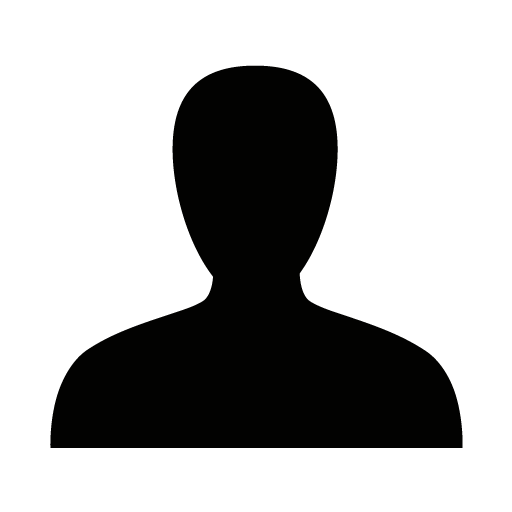
The detection of ionising radiation is the focus of many strategic applications in both science and technology. Typically, the detection is carried out by scintillator materials that emit light following interaction with ionising radiation, which is detected by highly sensitive photodetectors. The fundamental characteristics of scintillators are the probability of interaction with ionising radiation, scintillation yield, scintillation rate, and stability to high doses of absorbed radiation, commonly referred to radiation hardness. For large scale applications, it is essential that scintillators can be manufactured in large sizes and massive quantities with cost-effective techniques in terms of both energy and raw material consumption.
Recently, lead halide perovskite nanocrystals (LHP-NCs)[1] have emerged as promising nanoscintillators prized for their tuneable optical properties, radiation stability, high average Z-number and defect tolerance that enables high light yields and resistance to radiation damage up to extreme radiation levels[2]. However, hot-injection synthesis methods used for high-optical-quality LHP NCs are not suitable for mass production and scalable LARP approaches are limited by concentration gradients in the reaction environment resulting in poorer optical performance. LHP-NCs are also susceptible to the fabrication protocols for polymeric matrices mostly due to the polarity of monomers, the presence of initiators and the polymerization temperature that lead to surface damage, NC dissolution and/or phase shift to non-emissive allotropes.
In this talk, I will report the fabrication of new ultrafast and radiation hard plastic scintillators based on CsPbBr3 NCs formed within a polyacrylate matrix from precursor nanoparticles synthesized by a high-throughput, large scale turbo-emulsion method. Importantly, the formation of CsPbBr3 NCs is accompanied by the gradual curing of quenching defects, resulting in large size nanocomposites with photoluminescence quantum yield of ~90% and light yield of about 1000 ph/MeV for NC loading of only 0.2wt%. Radiometry experiments after as much as 1 MGy of certified γ-ray dose from 60Co source reveal perfect radiation hardness. Also fundamentally, pulsed X-ray scintillation measurements demonstrate ultrafast <60 ps scintillation due to multi-excitonic radiative decay as further confirmed by transient absorption measurements, indicating that CsPbBr3 NCs are efficient scintillators despite the negative effects of nonradiative Auger decay.
The combination of fabrication scalability with ultrafast and stable scintillation under extreme operational conditions represents offers a valid platform for future advancements in radiation detection schemes, in particular for time-of-flight radiometry applications in high-energy physics and medical diagnostics.
1.2-O5
In the last decades, the interest in nanoparticles in the biomedical field experienced a rapid growth because of the tunability of their physical and chemical properties and their rich surface chemistry that enables specific functionalization by design. Different classes of functional nanoparticles, including metals, semiconductors, metal/lanthanide oxides and organic or hybrid systems have found successful application in several medical branches, such as nano-therapy, diagnostics and imaging. Today, one of the most advanced biomedical uses of nanoparticles is offered by their strong interaction with ionizing radiation, which makes it possible to improve the effectiveness of conventional cancer treatments, imaging techniques and radiation dosimetry [1]. In oncological therapies, one of the most adopted medical treatment is radiotherapy (ca. 50% of total cases), a non-invasive technique typically consisting in the local release of the energy of X-rays to stop tumor cells proliferation, either by directly damaging their DNA, or indirectly by forming cytotoxic free radicals [2]. Among the different material classes with potential application in this field, metal halide nanocrystals (NCs) have recently attracted substantial attention for ionizing radiation detection, prized for their high average atomic number (Z) that enhances the interaction probability with ionizing radiation and strong robustness to prolonged exposure to ionizing radiation [3][4][5]. However, despite such promise, very few examples of medical diagnostic and therapeutic strategies based on metal halide NCs have been proposed mainly due to their low stability in aqueous environment resulting in their rapid dissolution and further consequent release of potentially harmful Pb2+ ions.
In this talk, we show that multicomponent systems consisting of lead halide perovskite NCs (CsPbX3-NCs, X=Br, I) grown inside mesoporous silica nanospheres (NSs) with selectively sealed pores are potentially promising candidates for enhanced radiotherapy and radio-imaging strategies, yhanks to the intense scintillation and strong interaction with ionizing radiation of CsPbX3 NCs with the chemical robustness in aqueous environment of silica particles. We demonstrate that CsPbX3 NCs boost the generation of singlet oxygen species (1O2) in water under X-ray irradiation and the encapsulation into sealed SiO2 NSs warrants perfect preservation of the inner NCs after prolonged storage in harsh conditions. We find that the 1O2 production is triggered by the electromagnetic shower released by the CsPbX3 NCs with a striking correlation with the halide composition (I3>I3-xBrx>Br3), without quenching their radioluminescence. This opens to the possibility of designing multifunctional radio-sensitizers able to reduce the local delivered dose and the undesired collateral effects in the surrounding healthy tissues by improving a localized cytotoxic effect of therapeutic treatments and concomitantly enabling optical diagnostics by radio imaging.
1.2-O6
One of the main thrusts of medical X-ray imaging is to minimize the X-ray dose acquired by the patient, down to the fundamental limit set by the Poisson photon statistics. Such low-dose X-ray detection characteristics have been demonstrated with only a few direct-detection semiconductor materials such as Si and CdTe; however, their industrial deployment in medical diagnostics is still impeded by elaborate and costly fabrication processes. Hybrid metal halide perovskites – newcomer semiconductors -– make for a viable alternative owing to their scalable, inexpensive, robust, and versatile solution growth and recent demonstrations of single gamma-photon counting under high applied bias voltages. The major hurdle with perovskites as mixed electronic-ionic conductors, however, arises from the rapid material's degradation under high electric field, thus far used in perovskite X-ray detectors. Here we discuss the negative effects of the ion migration on X-ray detection performance and demonstrate the mitigation path by utilizing the perovskite X-ray detectors in the photovoltaic mode of operation at zero-voltage bias. We show both countings of almost every single incoming photon and long-term stable performance, by employing thick and uniform methylammonium lead iodide single crystal films, solution-grown directly on hole-transporting electrodes The operational device stability is equivalent to the intrinsic chemical shelf lifetime of MAPbI3, being at least one year in the studied case. Furthermore, direct readout array integration of detectors is demonstrated as well. A high spatial resolution of 11 lp mm-1 is obtained with a linear detector array. We also comment the lack of a clear commonly accepted X-ray detection characterization, approach, particularly in material research community, often leads to a misguiding assessment of performance. We propose the guidelines for the determination of figures of merit for the low-dose X-ray imagers. These findings pave the path for the implementation of hybrid perovskites in low-cost and low-dose commercial detector arrays for X-ray imaging.
1.2-O7
Metal halide perovskites have now achieved impressive power conversion efficiencies (PCEs) in both single junction and tandem solar cells, making them promising candidates to solve energy and environment crisis. Record efficiencies of perovskite solar cells (PSCs) are obtained with doped spiro-OMeTAD as the hole transport layer (HTL). Conventionally, spiro-OMeTAD is doped by hygroscopic lithium salts with the assistance of volatile 4-tert-butylpyridine, which, however, brings a time-consuming doping process as well as poor device stability. To exclude the aforementioned disadvantages, researchers have tried to replace the LiTFSI with other dopants, like other metallic salts, F4TCNQ or organic radicals, which could also help to generate radicals improving the conductivity and get rid of the moisture sensitive byproduct LixOy. However, all the recipes with varied dopants can only achieve high PCE with tBP, but the mechanism behind have not been fully discussed to the point. Here, we develop an instantly effective (without post oxidation) doping strategy for spiro-OMeTAD, by employing stable organic radicals and ionic salts (referred to as ion-modulated (IM) radical doping). We achieve high power conversion efficiencies (PCEs) over 25% and much improved device stability under harsh conditions. In addition, the IM doping mechanism was revealed through advanced characterizations and theoretical analysis: electron transfer from neutral spiro-OMeTAD to radicals provide free holes to instantly increase the conductivity and work function (WF); the electrostatic interactions from ionic salts further modulate the WF by affecting the electron transfer activation energy. Our IM radical doping strategy proves effective with a variety of ionic salts, and the doped spiro-OMeTAD demonstrates universal applicability in different PSCs. The understandings on the doping mechanism also solve the mystery of tBP in conventional spiro-OMeTAD doping. Last but not least, the IM doping strategy addressed the importance of localized electrostatic environment in organic doping process, offering new insights for organic semiconductor doping by decoupling the conductivity and WF tunability, and can find applications in a number of optoelectronic devices.
1.3-I1
Senol Öz obtained his diploma in chemistry in 2013 at the University of Cologne
(Germany). Completing his PhD under supervision of Prof. Sanjay Mathur in 2018 at
University of Cologne (Merck KGaA PhD scholarship). In 2019 he joined Prof.
Tsutomu Miyasaka`s group as a post-doctoral fellow at Toin University of Yokohama
under a JSPS scholarship. His research interests include the synthesis, chemical
engineering, and solution processing of inorganic-organic hybrid metal halide
perovskite materials for photovoltaic application. He is currently a senior R&D project
leader at Saule Technologies and managing director of Solaveni GmbH.
Since the emergence of hybrid perovskite photovoltaics in 2009 the scientific community has witnessed disruptive progress of the technology in terms of solar to electric power conversion efficiency (25.7%) [1] accompanied by advancement in addressing stability issues and developing a deeper understanding of fundamental structure-processing-property relationships. Merely a decade after discovery, the commercialization of organic-inorganic metal halide perovskites photovoltaic devices is just around the corner! Many issues had to be tackled to meet industrialization, among them large area deposition techniques, safety standards and material wastage without compromising efficiency and stability. Saule technologies has been developing a fully scalable inkjet printing process of perovskite solar cell modules on lightweight flexible substrates. This talk will focus on recent advancements in the technology and the product development process required to bring inkjet printed perovskite modules closer to market entry. Moreover, it will underline the unique properties of the inkjet printing technique, which allow scalable solution-based fabrication of high-quality perovskite films and devices, processed in ambient atmosphere. [1] https://www.nrel.gov/pv/cell-efficiency.html
1.3-I2
Recent experiments involving a new carbon electrode demonstrate a true fully roll to roll coated perovskite solar cell via a continous slot die coating method. All previous reports of roll to roll coated perovskite solar cells have completed the device off-line with an evaporated metal contact. The application of a wet carbon film continuously and compatibly with an underlying perovskite device stack in a moving web at manufacturing speeds is complex but game-changing. The ability to sequentially deposit all layers of the device stack culminating in a fully working device entirely in-line means that the promise of high volume “liquid in/solar cell out” can be realised.
This multifunctional carbon electrode can be safely deposited on top of a layered solar cell without any deformation or dissolution of the underlying layers. The new contact material overcomes issues of solvent incompatibility, interface incompatibility and narrow rheology and heating process windows.
In this talk we will present the development journey of this new electrode material and the recent succesful pilot run of the material. In particular we will show how we formulate a new carbon ink with solvent compatible with the perovskite stack that crucially has suitable boiling point for low temperature, high speed processing coupled with very low toxicity (no work place exposure limit). The solid loading of the ink is optimised for a rheological profile suitable for slot die and we demonstrate the roll to roll slot die coating of the electrode sequentially following the roll to roll coating of the NIP device stack incorporating a low temperature processed p type interlayer. The carbon ink is formulated with solvent system orthogonal with the device stack and with no detrimental action on the perovskite active layer as shown through X-ray diffraction analysis. Electrochemical impedance spectroscopy and steady state photoluminescence analysis reveal that charge transfer at the interface is equivalent to evaporated gold electrodes. Further, the device stack is demonstrated to have no detrimental effect on stability, unencapsulated cells retained 90% of original PCE at atmospheric temperature for 1000 hours and outperform gold electrode cells at elevated temperature.
This work introduces the very first entirely roll to roll devices achieving efficiency matching evaporated gold electrodes. This first fully roll-to-roll coated perovskite prototype promises the possibility of transferring to industrially efficient PV production in the near future.
1.3-I3
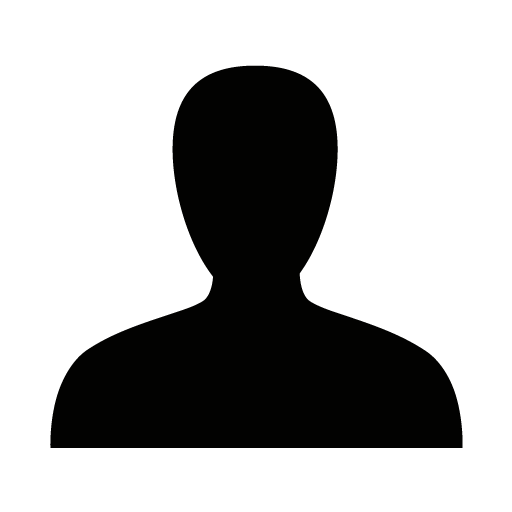
Since 2014, Oxford PV has been focused on the integration of perovskite PV in tandem configuration with silicon, holding the world record in large area at 26.8%. Less than a decade later, perovskite-on-silicon modules are close to enter the market. This achievement is the result of an accelerated effort to address efficiencies, technology fundamentals, manufacturing processes and supply chain, all under a sustainable perspective.
Energy security concerns have accelerated the deployment of solar, this opportunity for a faster transition to clean energies, comes with the added responsibility to making sure it considers the needs of generations to come. In this presentation, I will show Oxford PV’s sustainable journey, where environmental considerations go in parallel with the selection of materials, product designs and manufacturing processes, together with operational factors. Perovskite PV market integration comes with many challenges, but also the great opportunity to be the most efficient and sustainable solar energy source
1.3-O1
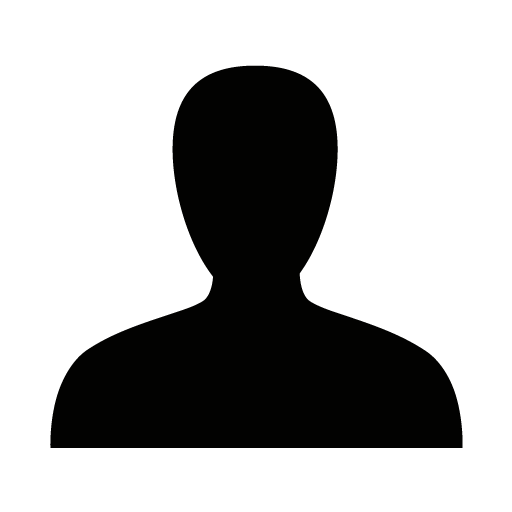
Abstract:
Wide bandgap perovskite materials show promising potential as tandem top cells to pair with silicon bottom cells and achieve power conversion efficiencies (PCEs) over 32%, while the fabrication costs are likely to stay low. To date, most of the efficient wide bandgap perovskite layers are fabricated by spin coating, which is difficult to scale up to large area and the crystallization mechanism remains unknown. In this work, we report on slot-die coating for an efficient, wide bandgap triple-halide perovskite, (Cs0.22FA0.78)Pb(I0.85Br0.15)3 + 5 mol% MAPbCl31 2. A suitable solvent system was designed and optimized specifically for the slot-die coating technique. We demonstrate that with this perovskite, our fabrication route enables a bandgap of 1.68 eV which is suitable for tandem solar cells, and without phase segregation typically observed for high Br loadings. The slot-die coated wet perovskite film was dried using a stream of nitrogen (N2) from an ''N2 knife'' with high reproducibility, and avoiding the need to use antisolvents.
We explored varying drying and annealing conditions from 100°C to 170°C and measured absolute as well as transient photoluminescence (PL) to extract information about the perovskite bandgap, quasi Fermi level splitting (QFLS) and charge carrier lifetimes. We find parameters allowing to crystallize the perovskite film into large grains reducing charge collection losses and thus enabling higher current density in solar cells (Fig. 1B). With annealing at 150 °C, an optimized tradeoff between crystallization and the detrimental formation of PbI2 aggregates on the film’s top surface is found. Insitu Grazing-Incidence Wide-Angle X-ray Scattering (GIWAXS) investigation of the solution intermediate and film annealing at various stages has also unveiled the perovskite crystallization and PbI2 formation processes. With the optimized annealing conditions, we improve the cell stability and performance of perovskite single junction cells towards a stabilized power output of up to 19.4 %.
By integrating the optimized perovskite fabrication with commercial saw damage etched Czochralski silicon bottom cells, a two-terminal monolithic tandem solar cell with a PCE of 25.2 % on 1 cm² active area is demonstrated with fully scalable processes. Note that this is reached for a wafer thickness of around 120 µm, not enabling the full photocurrent potential in the NIR wavelength regime compared to thicker wafers (> 250 µm) that are typically used in literature. Furthermore, a 4 cm² tandem solar cell has been developed with this fabrication route and using screen printed silver front grids, yielding PCEs up to 24 %.
Finally, we show a detailed comparison between spin coated and slot-die coated perovskite films. For the solar cells, we present the loss mechanisms as well as guidelines for further improving the printed films. With that, we highlight the high potential for slot-die coating as fabrication route for scalable and industrially relevant perovskite/silicon tandem solar cells.
2.1-O1
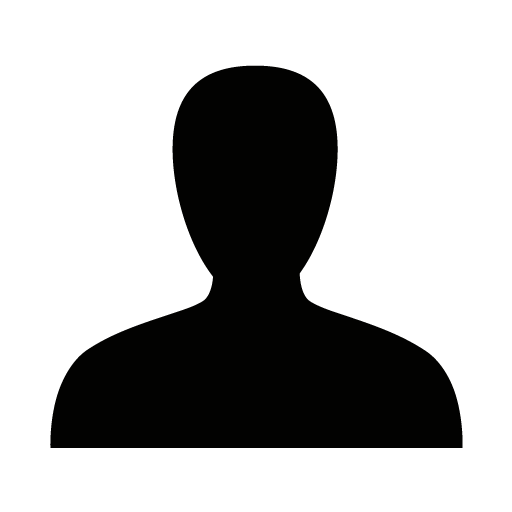
While light-emitting diodes (LEDs) made from lead halide perovskites have demonstrated external quantum efficiencies (EQEs) well over 20% [1]–[4], their electrical stability must be addressed before they are seriously considered for commercial applications [5]–[8]. In an effort to improve the optoelectronic properties of lead halide perovskites for light emission, many researchers have investigated introducing both alkaline-earth metal ions [9], [10] (e.g., Ba2+ and Sr2+) and transition metal ions [11]–[13] (e.g., Mn2+, Zn2+, Cd2+, and Ni2+) into the B-site of the perovskite’s ABX3 structure. Additionally, the factors that limit the electrical stability of perovskite LEDs remain under investigation [5]–[7], [14]–[16]. In this work, we dope Mn2+ ions into an organic-inorganic hybrid quasi-bulk 3D perovskite resulting into (PEABr)0.2Cs0.4MA0.6Pb0.7Mn0.3Br3 thin films with the addition of tris(4-fluorophenyl)phosphine oxide (TFPPO) dissolved in a chloroform antisolvent to achieve an EQE of 13.4% and a peak luminance of 95,400 cd/m2. While the inclusion of TFPPO into the chloroform antisolvent dramatically increases the EQE of perovskite LEDs, the electrical stability is severely compromised. At an electrical bias of 5 mA/cm2, our perovskite LED fabricated with a pure chloroform antisolvent (2.5% EQE) decays to half of its initial luminance in 90.68 minutes. Alternatively, our perovskite LED fabricated with TFPPO (13.4% EQE) decays to half of its initial luminance in 2.07 min. In order to investigate this trade-off in EQE and electrical stability, we study both photophysical and electronic characteristics before and after electrical degradation of the perovskite LEDs. We find that given identical electrical degradation conditions, the TFPPO-based device’s turn on voltage and overall electrical resistance increases in a much larger fashion as compared to the pure chloroform-based device. While the EQE characteristics of this Mn2+-doped perovskite LED show promise for B-site engineered perovskites, there is still large concern to simultaneously achieve both energy-efficient and electrically stable perovskite-enabled lighting. Uncovering the effects from the TFPPO additive on perovskite LEDs will reveal pathways on how to mitigate their negative consequences on electrical stability while retaining their energy-efficiency boosting properties.
2.1-O2
Rafael Sánchez (M.Sc. degree in Chemistry in 2006 and Ph.D. degree in 2011, both from the Universitat Autònoma de Barcelona, Spain). To date, he has worked without interruptions in several international research institutions: Universitat Jaume I (2012-2017), University of Liverpool (2017-2018), Henkel Ibérica-UAB (2018-2019) and Université de Bordeaux (2019-2020). The main research topics he has developed are based on the synthesis and electro-optical characterization of functional materials and/or semiconductors for light generation, photovoltaics and water splitting applications. His current interests are focused on the chemical design and synthesis of quaternary diazaaromatic dications for the development of novel 2D metal halide perovskite semiconductors suitable for the preparation low-cost, highly efficient and durable optoelectronic devices. He is the author of 1 book chapter and 27 publications in peer-review international journals (27 publications in Q1 journals, 18 of which in D1 journals with impact factor > 6.9 in different areas) with 2733 citations and a h-index of 21 (https://scholar.google.es/citations?user=kzbjcFQAAAAJ&hl=es).
After establishing themselves as promising active materials in the field of solar cells, perovskites are currently being explored for fabrication of low-cost, easy processable and highly efficient light emitting diodes (LEDs). Even though higher efficiencies are reported for perovskite-based LEDs (PeLEDs), the fabrication technique used is spin coated or vacuum evaporation. Herein, we show a successful incorporation of air stable inkjet-printed (IJP) NiOX as an electron blocking layer (EBL) in addition to poly(3,4‑ethylenedioxythiophene): polystyrene sulfonate (PEDOT:PSS) as hole injection layer. 2,4,6-tris[3(diphenylphosphinyl)phenyl]-1,3,5-triazine (POT2T) or inkjet-printed (IJP) SnO2 as hole blocking layer (HBL). Individual layer properties and the morphology of IJP NiOX were analysed through X-ray diffraction (XRD), scanning electron microscopy (SEM) and UV-Vis spectroscopy. Comparing p-n junction diodes with and without IJP NiOX as EBL shows a low leakage current and good rectification behaviour. Owing to its higher valence band levels, high hole mobility, low trap density at its interface with perovskite, PeLEDs with IJP NiOX and POT2T achieved higher luminance values of 19230 cd m-2 and an EQE of ~ 2.5 % using IJP of high purity CsPbBr3 layers. Furthermore, all inkjet printed PeLEDs replacing IJP SnO2 reached a luminance of 324 cd/m2 with an EQE of 0.017 %. Thus, an ambient processed PeLED with inorganic perovskite sandwiched between inorganic charge injection layers (CILs) which are also inkjet-printed is demonstrated. The results potentially lay a route towards ambient processed air stable all inorganic fully inkjet-printed PeLEDs.
2.1-O3
Engineering the chemical composition of metal-halide perovskites via halide mixing allows a facile bandgap modulation but renders perovskite materials prone to photoinduced halide segregation.[1] Triple-halide alloys containing Cl, I,
and Br were recently reported as a means to stabilize CsyFA1–yPb(BrxI1–x)3 perovskite under illumination.[2] Herein, these triple-halide alloys are found to be intrinsically less stable with respect to the reference I-Br in ambient conditions. By exploiting the influence of low-molecular-weight organic gelators on the crystallization of the perovskite material, a triple-halide alloy with improved moisture tolerance and thermal stability at temperatures as high as 120 °C is demonstrated.
The hydroxyl-terminated organic gelators are found to aggregate into nanoscale fibers and promote the gelation of the solvent inducing the formation of a 3D network, positively interfering with perovskite solidification. The addition
of a tiny amount of organic gelators imparts a more compact morphology, higher crystallinity, and compositional stability to the resulting triple-halide polycrystalline films, making them more robust over time without compromising the
photovoltaic performance.[3] Overall, this approach offers a solution toward fabrication of active perovskite materials with higher energy gap and improved stability, making these triple-halide alloys truly exploitable in solar cells.
2.1-O4
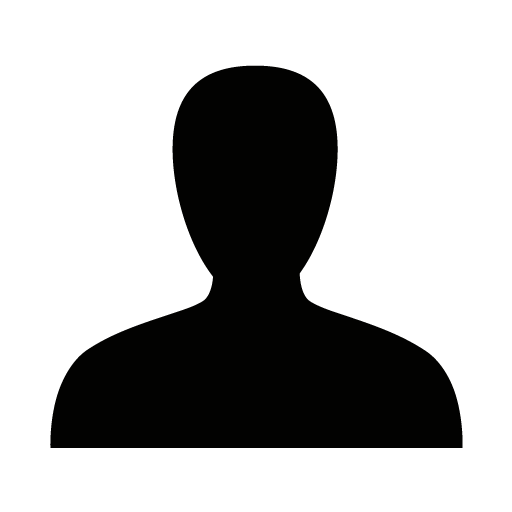
Inorganic cesium lead iodide (CsPbI3) perovskite solar cells (PSCs) have attracted enormous attention due to their promising thermal stability and optical bandgap (~1.73 eV), making them particularly well-suited for tandem device applications. Although the performance of CsPbI3 PSCs has surged to over 20%, most high-performance devices are fabricated using the dimethylammoniumiodide (DMAI)-assisted method, which hinders the options for mass production. Furthermore, the potential presence of the organic component DMA in the final CsPbI3 films remains under debate. Therefore, it is imperative to develop a plethora of methods to fabricate highly phase-pure CsPbI3 thin films. However, many methods of fabricating such CsPbI3 films require processing at high-temperatures (~340 ℃), which limits their applicability on flexible substrates. Thus, it is still challenging to achieve high-performing photovoltaic devices processed at low temperatures.
Here we reported a new method to fabricate high-efficiency and stable γ-CsPbI3 PSCs at low temperatures (~180 ℃) by introducing long-chain organic cation salt ethane-1,2-diammonium iodide (EDAI2) and regulating the content of lead acetate (Pb(OAc)2) in the perovskite precursor solution. By optimizing the excess amount of Pb(OAc)2 in the precursor solution, we demonstrate improved crystallinity, morphology and reduced carrier recombination are observed in the EDAI2 and Pb(OAc)2 synergistically stabilized CsPbI3 films. By optimizing the hole transport layer of CsPbI3 inverted architecture solar cells, we demonstrate efficiencies of up to 16.6%, which surpass previous reports examining γ-CsPbI3 in inverted PSCs. Notably, the encapsulated solar cells maintain 97% of their initial efficiency at room temperature and dim light for 25 days, demonstrating the synergistic effect of EDAI2 and Pb(OAc)2 in stabilizing γ-CsPbI3 PSCs.
2.1-O5
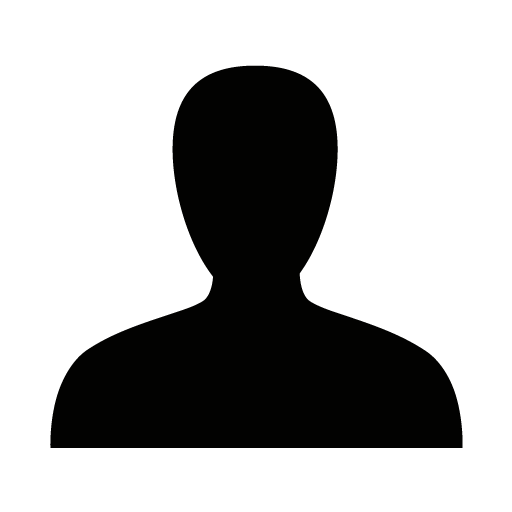
Metal halide perovskite photovoltaics (MHP) faces fundamental challenges which hinder their tangible impact on the final applications, with performance and stability being critical limiting parameters. Adjusting the perovskite grain boundaries and the crystalline surface are the primary tools to overcome these issues. Additionally, the research in new selective transport materials also plays a key role in improving optoelectrical devices' stability and performance to their theoretical limit to extend the perovskite application range.
Beyond efficiency and stability issues, perovskite photovoltaics must rely on sustainable technology for a feasible and fast green transition. Sustainability simultaneously considers environmental, economic, and social dimensions. Any sustainable technology must: a) generate enough power with reduced space usage (efficiency), b) be cost-effective, and c) not be detrimental to the environment or society. The environmental impact of the device fabrication is seriously affected not only by harmful materials (heavy metals and solvents) but also by the energy consumption of the synthetic and deposition routes used at the laboratory scale, which are not realistic for scaled-up manufacturing. Therefore, developing low-demanding synthetic routes is compulsory for a fast green transition.
Here, we present the research already performed by our team towards the synthesis and passivation methods of device-oriented metal halide perovskites (monocrystals at the macro and nanoscale) and metal oxides as selective transport materials. Our synthetic routes are mainly based on the in-situ synthesis approach. This one-step, low-cost, and low-demanding approach offers the possibility of modifying the precursor solution formulation very easily to adapt them to commercial-available printing techniques, one of the reasons behind MHP's success. Regarding sustainability, the in-situ synthesis approach is a low-carbon footprint synthetic route compared to traditional wet chemistry and colloidal routes. Following the global tendency to develop solution-processed materials, we have been involved for years in the in-situ synthesis approach of different materials, from MHP [1,2] to metal oxides [3] and conducting polymers [4]. We will show our work with solution-processed MHP and transparent metal oxides formulated as precursor inks compatible with roll-to-roll printing for large-scale production. The working principle analysis, including electrical and optical techniques, displays how the optoelectrical response can be adjusted by controlling the crystal growth and synthesis conditions and surface passivation, which can be linked to fundamental variations in the surface and bulk structure and composition.
1. Low-demanding in situ crystallization method for tunable and stable perovskite nanoparticle thin films
J. Noguera-Gómez, I. Fernández-Guillen, P. F. Betancur, V. S. Chirvony, P. P. Boix, R. Abargues.
Matter. 2022, 5, 3541-3552
https://doi.org/10.1016/j.matt.2022.07.017
2. Spray-driven halide exchange in solid-state CsPbX3 nanocrystal films
RI Sánchez-Alarcón, J Noguera-Gomez, VS Chirvony, H Pashaei Adl, Pablo P Boix, G Alarcón-Flores, JP Martínez-Pastor, R Abargues.
Nanoscale. 2022, 14 (36), 13214-13226
https://doi.org/10.1039/D2NR03262G
3. Solution-processed Ni-based nanocomposite electrocatalysts: an approach to highly efficient electrochemical water splitting.
J. Noguera-Gómez, M. García-Tecedor, J. F. Sánchez-Royo, L. M. Valencia Liñán, M. Herrera-Collado, S. I. Molina, R. Abargues, and S. Giménez.
ACS Appl. Energy Mater. 2021, 4, 5255-5264
https://doi.org/10.1021/acsaem.1c00776
4. In Situ Synthesis of Polythiophene and Silver Nanoparticles within a PMMA Matrix: A Nanocomposite Approach to Thermoelectrics
J F Serrano-Claumarchirant, A Seijas-Da Silva, J F Sanchez-Royo, M Culebras, A Cantarero, C M Gómez, R Abargues
ACS Applied Energy Materials 2022, 5 (9), 11067-11076
2.1-O6
The mixture of inorganic quantum dots and hybrid perovskite in one nanocomposite is considered a favorable approach to overcome restrictions of metastable perovskite. However, to date only few examples of improved opto-electronic perovskites have been realized with such materials. Here, we show a systematic approach to characterize the standard methylammonium lead iodide (MAPbI3) perovskite system by: (i) the substitution of some MA by guanidinium (Gu); (ii) the incorporation of PbS quantum dot (QD) additives and (iii) addition of both Gu and PbS at the same time. We studied the effect of the incorporations of the big cation on the film strain and crystal cell unit volume, and on the solar cell device efficiency and stability. With the control of Gu and PbS QD content, higher performance and longer solar cell stability are obtained. PbS QDs aid Gu incorporation, resulting in an expected more stable material and devices with high amount of guanidnium. From the optical point view, the tuning of the energy levels of perovskite nanocomposite with PbS with different ligands will be also exemplified, to show that a slight shift of the energy level leads to improved efficiencies especially in the case of formamidinium/PbI2 capping ligand. With this study, we demonstrated the reason why the solar cells performances are improved up to 20%, even when the optical properties are detrimental.
2.2-I1
Nam-Gyu Park is professor and SKKU-Fellow at School of Chemical Engineering and adjunct professor at Department of Energy Science, Sungkyunkwan University. He got Ph.D. in Inorganic Solid State Chemistry from Seoul National University in 1995. He worked at ICMCB-CNRS, France, from 1996 to 1997 and at National Renewable Energy Laboratory, USA, from 1997 to 1999 as postdoctoral researchers. He worked as Director of Solar Cell Research Center at Korea Institute of Science and Technology from 2005 to 2009 and as a principal scientist at Electronics and Telecommunications Research Institute from 2000 to 2005 before joining Sungkyunkwan University in 2009. He has been doing researches on high efficiency mesoscopic solar cells including perovskite solar cell and dye-sensitized solar cell since 1997. He is pioneer in solid state perovskite solar cell, which was first developed in 2012. He received awards, including Scientist Award of the Month (MEST, Korea), KyungHyang Electricity and Energy Award (KEPCO, Korea), KIST Award of the Year (KIST, Korea) and Dupont Science and Technology Award (Dupont Korea), SKKU fellowship, and MRS Outstanding Research Award (MRS, Boston) and WCPEC Paper Award (Kyoto, Japan). He published over 230 scientific papers, including Science, Nature Materials, Nature Nanotechnology, Nature Energy and Nature Communications, 80 patent applications and 8 book chapters. He received H-index of 67 as of May, 2017.
Since the seminal report on the 9.7% efficient and 500 h-stable solid-state perovskite solar cell (PSC) in 2012 based on methylammonium lead iodide, power conversion efficiency (PCE) was swiftly increased to 25.7% due to unique photophysical property of halide perovskite. According to Web of Science, number of publications on PSCs increases exponentially since 2012, leading to the accumulated publications of more than 30,900 as of December 2022. PSC is regarded as a game changer in photovoltaics because of low-cost and high efficiency surpassing the conventional high efficiency thin film technologies. High photovoltaic performance was realized by compositional engineering, device architecture and fabrication methodologies for a decade. Toward theoretical efficiency over 30% along with long-term stability, exquisite control of light management and photo-excited charges are highly required, along with thermodynamic phase stability. In this talk, facet engineering of perovskite films is reported. A specific crystal facet was found to have strong interaction with photon, leading to high photocurrent. Furthermore, a certain facet was found to be quite stable under moisture and PSC based on a perovskite film with abundant moisture-tolerant facet was remarkably stable in humid atmosphere even without encapsulation.
2.2-I2
Iván Mora-Seró (1974, M. Sc. Physics 1997, Ph. D. Physics 2004) is researcher at Universitat Jaume I de Castelló (Spain). His research during the Ph.D. at Universitat de València (Spain) was centered in the crystal growth of semiconductors II-VI with narrow gap. On February 2002 he joined the University Jaume I. From this date until nowadays his research work has been developed in: electronic transport in nanostructured devices, photovoltaics, photocatalysis, making both experimental and theoretical work. Currently he is associate professor at University Jaume I and he is Principal Researcher (Research Division F4) of the Institute of Advanced Materials (INAM). Recent research activity was focused on new concepts for photovoltaic conversion and light emission based on nanoscaled devices and semiconductor materials following two mean lines: quantum dot solar cells with especial attention to sensitized devices and lead halide perovskite solar cells and LEDs, been this last line probably the current hottest topic in the development of new solar cells.
Halide perovskites constitute a fascinating family of materials that has revolutionized the optoelectronic field in the last decade. Halide perovskite are soft materials presenting a benign defect physics that can be prepared a relative low temperature. This appealing property, from the point of view of industrialization, also makes of material and device stability the Achiles heel of the optoelectronic halide perovskite technology. Consequently, the development of stabilization procedures is probably the current main topic of these systems. The soft nature that limits stability also allows the easy combination of halide perovskites with other materials. In this talk we show as an accurate choice of additives can promote interesting synergies boosting the long term stability of halide perovskites devices. We show how the use of PbS quantum dots increases significantly the stability of FAPbI3. Incorporation of PbS QDs allows the dramatic decrease of the annealing temperature for the formation of black FAPbI3 phase perovskite thin film, from the 170ºC required without QDs to 85ºC when QDs are present. We have also verified the synergic combination of different additives for a significant increase of device stability of Pb free Sn-based solar cells, LEDs and lasers. Eventually, stabilization of halide perovskite nanoparticles is a necessary step for the development of high performance Halide Perovskite LEDs. Control of post synthetic washing processes as well as nanoparticle capping allows the preparation of LEDs with enhanced performance and stability.
2.2-I3
Maria Antonietta Loi studied physics at the University of Cagliari in Italy where she received the PhD in 2001. In the same year she joined the Linz Institute for Organic Solar cells, of the University of Linz, Austria as a post doctoral fellow. Later she worked as researcher at the Institute for Nanostructured Materials of the Italian National Research Council in Bologna Italy. In 2006 she became assistant professor and Rosalind Franklin Fellow at the Zernike Institute for Advanced Materials of the University of Groningen, The Netherlands. She is now full professor in the same institution and chair of the Photophysics and OptoElectronics group. She has published more than 130 peer review articles in photophysics and optoelectronics of nanomaterials. In 2012 she has received an ERC starting grant.
Mixed Tin/Lead (Sn/Pb) perovskites have the potential to achieve higher performances in single junction solar cells than Pb-based compounds. The best Sn/Pb based devices are fabricated in the p-i-n structure and frequently PEDOT: PSS is utilized as hole transport layer, even if there are many doubts on a possible detrimental role of this conductive polymer. Here, we propose the use of [2-(9H-Carbazol-9-yl)ethyl]phosphonic acid (2PACz) and the functionalized [2-(3, 6-dibromo-9H-carbazol-9-yl) ethyl] phosphonic acid (Br-2PACz) version, as substitutes for PEDOT: PSS. By using Br-2PACz as HTL we achieve record efficiency (19.51%) with the perovskite composition Cs0.25FA0.75Sn0.5Pb0.5I3 without any anti-reflective coating. The halogen functionalization of the SAMs is an efficient way to improve both the device performances and stability. Several factors seem to determine these improvements. The two carbazole-based molecules are able to form a self-assembled monolayer which show minimal parasitic absorption and low charge recombination when compared to PEDOT: PSS films. Additionally, the perovskite layer deposited on SAMs show an higher crystallinity, with reduced pinhole density and larger grains. The defect density for the perovskite films was also reduced when deposited on Br-2PACz or 2PACz compared to PEDOT: PSS. PL and TRPL measurements further confirmed the better perovskite film quality when deposited on SAMs, with reduced charge recombination.
Finally, the wettability of the perovskite precursor solution on top of the SAMs is a problem which limits SAMs application in Sn- or mixed Sn/Pb- perovskite solar cells on a large scale, limiting enormously the yield of device fabrication. I will present a solution for this issue, that may allow to improve both the scalability and reproducibility of the SAMs-based perovskite solar cell.
2.3-I1
Metal-Halide Perovskite (MHP) photovoltaic (PV) modules are at the cusp of commercialization. One major hurdle that remains is establishing confidence in long-term field performance and durability of MHP modules. A lot of progress has been made in addressing many performance stability issues in MHP cells and modules; however, there is still work to be done to understand degradation and demonstrate real world operation of this technology. In this talk I will share examples of both lab-scale and field studies to improve our understanding of MHP solar cell and module degradation mechanisms. First, I will discuss an application of X-ray scattering methods to probe the nanoscale heterogeneity of metal halide perovskite absorber layers and couple these results to device level stability studies to understand the role heterogeneity plays in device performance. I will then present a brief overview of an initial field demonstration of MHP modules as part of the Perovskite PV Accelerator for Commercializing Technologies (PACT) program. Together this work aims to improve our confidence in real world MHP module performance.
2.3-I2
Lioz Etgar obtained his Ph.D. (2009) at the Technion–Israel Institute of Technology and completed post-doctoral research with Prof. Michael Grätzel at EPFL, Switzerland. In his post-doctoral research, he received a Marie Curie Fellowship and won the Wolf Prize for young scientists. Since 2012, he has been a senior lecturer in the Institute of Chemistry at the Hebrew University. On 2017 he received an Associate Professor position. Prof. Etgar was the first to demonstrate the possibility to work with the perovskite as light harvester and hole conductor in the solar cell which result in one of the pioneer publication in this field. Recently Prof. Etgar won the prestigious Krill prize by the Wolf foundation. Etgar’s research group focuses on the development of innovative solar cells. Prof. Etgar is researching new excitonic solar cells structures/architectures while designing and controlling the inorganic light harvester structure and properties to improve the photovoltaic parameters.
In this work I will present two new concepts related to hybrid perovskite synthesis and devices.
(i) Chiral molecules were implemented into hybrid perovskite forming 2D hybrid perovskite with chirality properties. We used the two enantiomers R)-(+)-α-Methylbenzylamine (R-MBA) and, (S)-(-)-α-Methylbenzylamine (S-MBA). The chirality is manifested at low n values and pure 2D structure measured by circular dichroism (CD). The anisotropy factor (gabs) decreased by an order of magnitude when decreasing the n value achieving 0.0062 for pure 2D. Ab initio many-body perturbation theory successfully describes the band gaps, absorbance and CD measurements. For the first time these quasi 2D chiral perovskites were integrated into the solar cell. Using circular polarization (CP) and cut off filter we were able to distinguish the chirality effect from the solar cells photovoltaic response.
(ii) In the second part we developed unique fully printable mesoporous indium tin oxide (ITO) perovskite solar cell. In this structure, the perovskite is not forming a separate layer but fills the pores of the triple-oxide structure. One of the advantageous of this solar cell structure is the transparent contact (mesoporous ITO) which permit the use of this cell structure in bifacial configuration without the need for additional layers or thinner counter electrode. We performed full characterizations on both sides (i.e. ITO-side and glass-side) and elucidate the solar cell mechanism, where the glass side show 15.3% efficiency compare to 3.8% of the ITO-side. Further study of the mechanism shows that the dominant mechanism when illuminating from the glass-side is Shockley-Read-Hall recombination in the bulk, while illuminating from the ITO-side show recombination in multiple traps and inter gap defect distribution which explain the poor PV performance of the ITO-side. Electrochemical impedance spectroscopy shed more light on the resistance and capacitance. Finally, we demonstrate 18.3% efficiency in bifacial configuration. This work shows a fully printable solar cell structure which can function in bifacial configuration.
2.3-O1
We fabricate and characterize carbon-based lead halide perovskite solar cells composed of a mesoscopic scaffold of metal oxides that is screen printed and infiltrated with a lead halide perovskite precursor solution with a methylammonium cation.(MAPbI3) We characterize the cells over time to investigate degradation pathways and improve fabrication methods. We measure the current produced by the cells under various illumination conditions as a function of applied bias voltage (IV curves) as well as spatially mapping both the structure and the photovoltaic performance of our cells to track and classify defects using both optical micrographs and Light Beam Induced Current (LBIC) imaging. Observations of the spectral response of the cells enables us to determine the External Quantum Efficiency (EQE) of our devices as well.
In an undergraduate laboratory environment we fabricate and characterize Screen-Printed Mesoporous Carbon Perovskite Solar Cells (CPSCs). The fabrication is based on pioneering work by Hongwei Han's research group.[1] We adapt our methods from those developed by Trystan Watson's group [2]. We start with FTO coated glass substrates and laser engrave isolation lines. We spay coat a compact titania layer followed by screen printing a mesoporous layers of titania (for electron transport), zirconia (for a spacer), and Carbon (for hole transport and a back contact). A Methylammoina Lead Iodide (MAPbI) perovskite precursor is then inflitrated into the mesoporous layers crystalizing to form the perovskite semiconductor structure. Silver contact electrodes are added to complete the devices. The fabrication is performed in an ambient environment and no encapsulations are added to the devices. Each substrate has 36 devices each with an active area of 0.49 sq. cm. We have produced over a thousand devices with 4 generations of students in the lab.
We characterize our devices with a wide range of techniques. Current Voltage characteristics are measured for all devices. Hero cells have power conversion efficiencies over 12%. The devices show negligible hysteresis, and are limited in performance by moderate shunt and series resistances. New higher conductivity Carbon and Silver ink formualations have been tested with significantly better conductivities that have improved Fill Factors and reduced series resistance. We observe a variety of spatial defects by both LBIC and optical micrographs in the printing process and do statistical analysis of yields on every run to optimize our initial device performance. We measure EQEs with peaks approaching 80% for freshly made devcies.
We have performed dark storage shelf life measurements over two years. While devices still work after two years in dark storage, the performance decreases and defects clearly evolve as seen in our spatial imaging over time. We also perform light soaking studies of both individual cells and modules of cells in series. We record not only the IV characteristics and the IV curve evolution, but also the changes in EQE and spatial imaging as a function of light soaking under 1 sun conditions with no encapsulation or UV filtering. We will present these data and discuss what this says about the degradation of these CPSCs.
1.1-I1
Will Tisdale joined the Department of Chemical Engineering at MIT in January, 2012, where he holds the rank of Associate Professor and is currently the ARCO Career Development Professor in Energy Studies. He earned his B.S. in Chemical Engineering from the University of Delaware in 2005, his Ph.D. in Chemical Engineering from the University of Minnesota in 2010, and was a postdoc in the Research Laboratory of Electronics at MIT before joining the faculty in 2012. Will is a recipient of the Presidential Early Career Award for Scientists and Engineers (PECASE), the DOE Early Career Award, the NSF CAREER Award, an Alfred P. Sloan Fellowship, the Camille Dreyfus Teacher-Scholar Award, the AIChE Nanoscale Science & Engineering Forum Young Investigator Award, and MIT’s Everett Moore Baker Award for Excellence in Undergraduate Teaching.
In semiconductors, exciton or charge carrier diffusivity is typically described as an inherent material property. Here, we show that the transport of excitons (i.e., bound electron-hole pairs) in CsPbBr3 perovskite nanocrystals (NCs) depends markedly on how recently those NCs were occupied by a previous exciton. Using fluence- and repetition-rate-dependent transient photoluminescence microscopy, we visualize the effect of excitation frequency on exciton transport in CsPbBr3 NC solids. Surprisingly, we observe a striking dependence of the apparent exciton diffusivity on excitation laser power that does not arise from nonlinear exciton-exciton interactions nor from thermal heating of the sample. We interpret our observations with a model in which excitons cause NCs to undergo a transition to a metastable configuration that admits faster exciton transport by roughly an order of magnitude. This metastable configuration persists for ~microseconds at room temperature, and does not depend on the identity of surface ligands or presence of an oxide shell, suggesting that it is an intrinsic response of the perovskite lattice to electronic excitation. The exciton diffusivity observed here (>0.15 cm2/s) is considerably higher than that observed in other NC systems on similar timescales, revealing unusually strong excitonic coupling in a NC material. The finding of a persistent enhancement in excitonic coupling between NCs may help explain other extraordinary photophysical behaviors observed in CsPbBr3 NC arrays, such as superfluorescence. Additionally, faster exciton diffusivity under higher photoexcitation intensity is likely to provide practical insights for optoelectronic device engineering.
1.1-I2
Sub-nanosecond radiative decay time was first observed for weakly bound excitons in bulk semiconductors and was understood in terms of the phenomenon known as giant oscillator strength (GOS).1 GOS is a quantum phenomenon connected with coherent excitation of excitons over the entire volume of exciton localization, and is counterintuitive because the radiative decay time is inversely proportional to this volume. Consequently, it was predicted theoretically2 that an exciton weakly confined in a nanocrystal (NC), with radius a much larger than the exciton radius aex, is characterized by GOS with magnitude fNC = f0(a/aex)3 >> f0, where f0 is the exciton oscillator strength. Indeed ~100 ps radiative decay times were observed in large-size CsPbX3 (X = Cl, Br, I) perovskite NCs.3
The development of organic-inorganic perovskite photovoltaics (PV) and radiation detectors (RD) has been particularly impressive. In the past 10 years, perovskite-based PV cells have reached a certified efficiency of 25.2% and perovskite-based RDs have demonstrated comparable progress by combining remarkable defect tolerance, large mobility-lifetime products, tunable band gaps, crystal growth from low-cost solution processes, and strong stopping power from Pb. We connect this progress with suppressed recombination in this material. Our analysis of the best PV cells, including perovskites, shows that their efficiencies are very close to ultimate PV limits in the absence of carrier recombination.4 The recent data on perovskite RDs5 also indicate, from our point of view, the advent of ultimate collection efficiency. For such RDs, we provide a theory that describes current collection efficiency in the absence of the carrier recombination.5
1 E. I. Rashba and G. E. Gurgenishvili, Edge absorption theory in semiconductors. Sov. Phys. Solid State, 4, 759-760 (1962).
2 Al. L Efros and A. L. Efros, Interband absorption of light in a semiconductor sphere, Sov. Phys.
3 M. A. Becker, et al. “Bright triplet excitons in caesium lead halide perovskites,” Nature, 553, 189-193 (2018).
4Al. L. Efros and V. G. Karpov “Electric power and current collection in semiconductor devices with suppressed electron−hole recombination”, ACS Energy Lett. 2022, 7, 3557−3563
5 M. Kovalenko, et al. Stable Near-to-Ideal Performance of a Solution grown Single-Crystal Perovskite X-Ray Detector. 2022, https://doi.org/10.21203/rs.3.rs-1117933/v1
1.1-I3
Sam Stranks is Professor of Energy Materials Optoelectronics in the Department of Chemical Engineering & Biotechnology and the Cavendish Laboratory, University of Cambridge. He obtained his DPhil (PhD) from the University of Oxford in 2012. From 2012-2014, he was a Junior Research Fellow at Worcester College Oxford and from 2014-2016 a Marie Curie Fellow at the Massachusetts Institute of Technology. He established his research group in 2017, with a focus on the optical and electronic properties of emerging semiconductors for low-cost electronics applications.
Sam received the 2016 IUPAP Young Scientist in Semiconductor Physics Prize, the 2017 Early Career Prize from the European Physical Society, the 2018 Henry Moseley Award and Medal from the Institute of Physics, the 2019 Marlow Award from the Royal Society of Chemistry, the 2021 IEEE Stuart Wenham Award and the 2021 Philip Leverhulme Prize in Physics. Sam is also a co-founder of Swift Solar, a startup developing lightweight perovskite PV panels, and an Associate Editor at Science Advances.
Halide perovskites are exciting materials ushering in a new wave of photovoltaic and light-emitting technologies, with efficiencies already comparable to or beating more traditional semiconducting systems. However, a full understanding of carrier recombination and how it relates to performance losses and device operation in various device structures remains elusive. Here I will present results where we exploit luminescence from these materials to understand carrier recombination. I will give examples of different dimentionality systems and where carrier/exciton funnelling is critical for device operation. The luminescence approaches also allow tracking of device operation over time, and a decoupling of the impact of the active layer and contacts in understanding performance losses. These results allow us to draw generalised conclusions about carrier recombination and device performance and instability pathways driven by carrier trapping. Finally, I will show how we are exploiting this understanding to develop new solar cell and lighting devices, further pushing performances up.
1.2-O1
Quantum dots (QDs) offer unique physical properties and novel application possibilities like single-photon emitters for quantum technologies.1,2 While strongly confined III–V and II–VI QDs have been studied extensively, their complex valence band structure often limits clear observations of individual transitions. In recently emerged lead-halide perovskites, band degeneracies are absent around the bandgap reducing the complexity of optical spectra. We show that for spherical-like CsPbBr3 QDs with diameters >6 nm,3 excitons confine with respect to their center-of-mass motion leading to well-pronounced resonances in their absorption spectra. Optical pumping of the lowest-confined exciton with femtosecond laser pulses not only bleaches all excitons but also reveals a series of distinct induced absorption resonances which we attribute to exciton-to-biexciton transitions and are red-shifted by the biexciton binding energy (∼40 meV).5 The temporal dynamics of the bleached excitons further support our exciton confinement model. Our study provides the first insight into confined excitons in CsPbBr3 QDs and gives a detailed understanding of their linear and nonlinear optical spectra.
1.2-O2
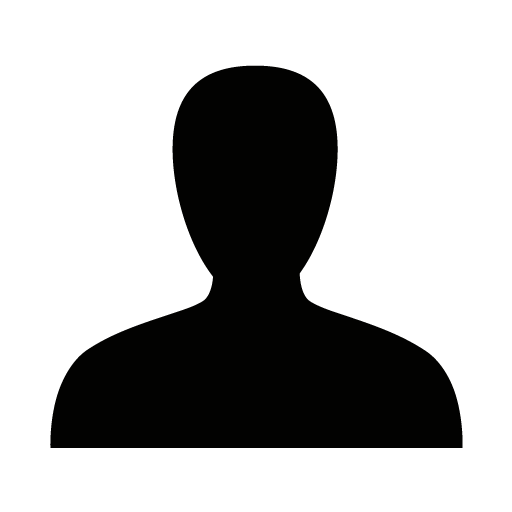
All-inorganic lead-halide perovskite (CsPbX3, X = Cl, Br, I) quantum dots (QDs) have emerged as a competitive platform for classical light emitting devices (in the weak light-matter interaction regime, e.g., LEDs and laser)[1], as well as for devices exploiting strong light-matter interaction and operated at room-temperature.[2] Many-body interactions and quantum correlations among photogenerated exciton complexes play an essential role, e.g., by determining the laser threshold, the overall brightness of LEDs, and the single-photon purity[3, 4] in quantum light sources. Here, by combining single-QD optical spectroscopy performed at cryogenic temperatures in combination with configuration interaction (CI) calculations, we address the trion and biexciton binding energies and unveil their peculiar size dependence. We find that trion binding energies increase from 7 meV to 17 meV for QD sizes decreasing from 30 nm to 9 nm, while the biexciton binding energies increase from 15 meV to 30 meV, respectively. CI calculations quantitatively corroborate the experimental results and suggest that the effective dielectric constant for biexcitons slightly deviates from the one of the single excitons, potentially as a result of coupling to the lattice in the multiexciton regime. Our findings provide a deep insight into the multiexciton properties in all-inorganic lead-halide perovskite QDs, essential for classical and quantum optoelectronic devices.
1.2-O3
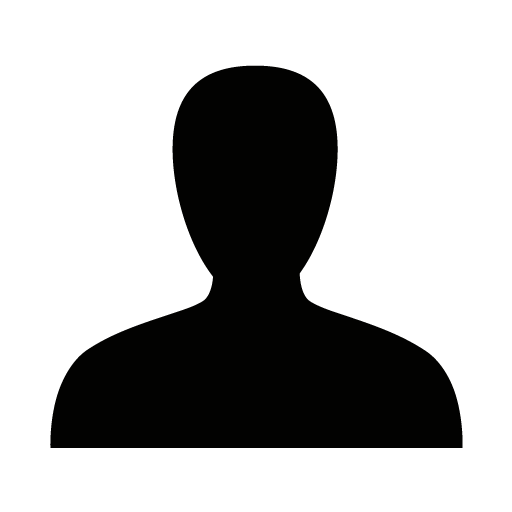
Lead-based semiconductors are among the most explored compounds for the synthesis of colloidal nanomaterials, mainly due to the appealing optoelectronic properties demonstrated by lead halide perovskites in the UV-VIS and by lead chalcogenides in the IR spectral ranges. The mature research on both classes of materials has recently led to the exploration of systems where such properties can coexist and interact. One promising direction is that of heterostructures, which are composite materials formed by intimately connected domains of two different compounds. They are generally challenging to obtain, mostly because of the poor compatibility in terms of required synthetic conditions and crystal structures of the two materials. Another direction to investigate is represented by compounds that are chemically related to both lead halides and lead chalcogenides, namely the lead chalcohalides. These materials, with general formula PbaEbXc ,(E=S, Se, Te, X=F, Cl, Br) are expected to show intermediate properties in between those of lead halides and chalcogenides, and more importantly might feature the chemical and structural compatibility needed to interface with both. We pioneered the investigation of lead chalcohalides at the nanoscale, discovering additional colloidal nanocrystals (NCs): the new compounds are semiconductors with a band gap in between those of lead halide perovskites and of lead sulfide, were obtained in relatively mild reaction conditions and feature a remarkable chemical stability. Moreover, we achieved the synthesis of colloidal heterostructures formed by epitaxially connected domains of Pb4S3Br2 sulfobromide and CsPbX3 perovskite, thus demonstrating a synthetic and structural compatibility between lead halide perovskites and lead chalcohalides. Stability of the system is highly increased respect to CsPbX3 NCs We take advantage of the domains? compatibility and exploit CsPbCl3 perovskite NCs as disposable and phase-selective epitaxial templates to drive the synthesis of lead sulfochloride NCs. As a result, we expand the family of lead chalcohalides with two new phases: Pb3S2Cl2 and Pb4S3Cl2.The perovskite domain is called a disposable template because, at a later stage, it can be etched from the heterostructures by exploiting the solubility of CsPbCl3 in polar solvents, while leaving the Pb4S3Cl2 domains intact. Hence, the full procedure delivered colloidally stable Pb4S3Cl2 NCs that could not be obtained by direct synthesis due to the competitive nucleation. Our use of perovskite NCs as disposable and phase-selective epitaxial templates parallels that of reaction-directing groups in traditional organic chemistry and catalysis. Such an approach to a deterministic synthesis of NCs might be extended to other pairs of materials with known or predictable epitaxial relations, taking advantage of the vast library of already reported nanomaterials as starting templates. This approach could open new routes for the colloidal syntheses of materials which are now hindered by an excessive activation energy for the homogeneous nucleation, or by the competitive formation of undesired phases
1.2-O4
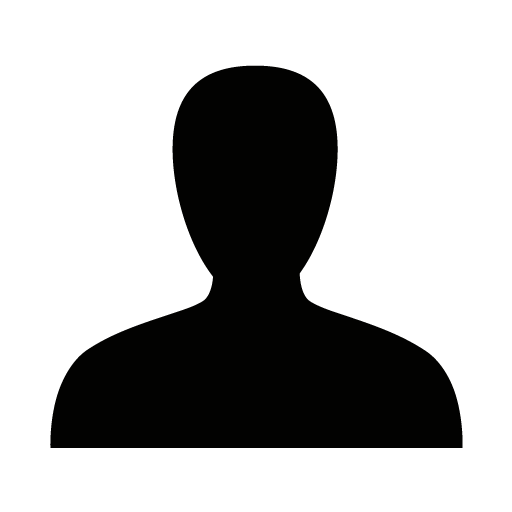
Perovskite nanocrystals are highly advantageous semiconductor materials for tailored light applications [1]. Large quantum yields, narrow emission and broad spectral tunability are only some of the unique optoelectronic properties at the center of their beneficial performance. On the other hand, flat patterned microstructures have emerged as powerful platforms for controlled light-matter interactions [2]. When asymmetrically shaped nano-objects are chosen as the unit cell of the array structure, these near-field interactions get dependent on the circular polarization state. Particularly nonlinear optoelectronic devices governed by multi-photon processes could benefit from such a near-field control.
With the aim to equip perovskite nanocrystals with chiral effects, we have built a hybrid perovskite-metasurface system [3]. A suitable chiral z-shaped Si nanoantenna array was coated with a monolayer of cubic all-inorganic lead halide perovskite nanocrystals. The nanoantenna array exhibits pronounced chiral resonances in the visible to IR region which allow to confine the excitation light in the fabricated nanostructures. We demonstrate that the chiral near-field interactions can serve to induce polarization effects in the two-photon absorption process of the perovskite nanocrystals. By tuning the thickness of the perovskite film down to one monolayer, we restricted the interactions exclusively to the near-field regime. We show that the layer’s two-photon excited luminescence is enhanced by up to one order of magnitude in this configuration. In particular, the enhancement is controllable by the excitation wavelength and by its polarization, revealing a pronounced fluorescence detected circular dichroism of the hybrid emitter-antenna system. Altogether, our findings present a pathway to control perovskite light emission via polarization sensitive near-field interactions, highlighting the potential of this hybrid system for sensing applications and display technologies.
1.2-O5
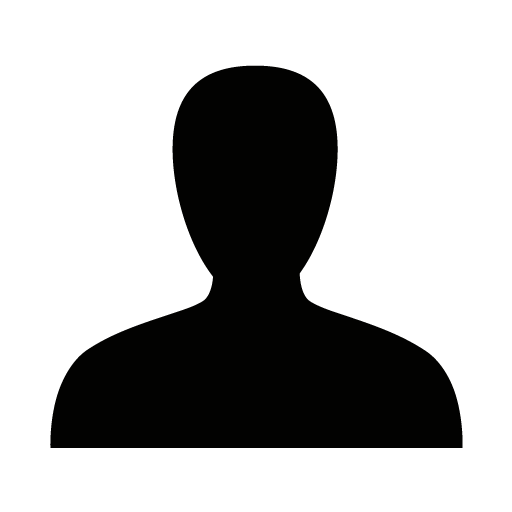
In recent years, scintillating nanoparticles have been recognized as valid potential alternatives to inorganic and organic bulk scintillators, since they meet the needs and the demanding requirements of cutting-edge applications, such as nuclear and homeland security technologies, clinical and imaging devices. Nanoscintillators feature adaptable luminescence and scintillation properties, tuned by their physical and chemical characteristics, like the electronic structure, the dimensionality, and the defectiveness [1]. Most importantly, nanoscintillators can be embedded in suitable polymeric hosts to create composite materials and produce fast, efficient, and more sensitive detectors in a cost-effective way, thanks to reduced time-consuming synthesis procedures, to meet the specific demands of all up-to-date technological and medical applications [2].
The modern research is considering scintillating nanocomposites based on lead halide perovskite (LHP) nanocrystals (NCs) for the next generation of scintillation detectors [3,4]; when embedded in polymeric matrices, LHP NCs favors the enhancement of the interaction cross-section with ionizing radiation, thanks to their high atomic number [5], and retain exceptional levels of radiation hardness [6]. The implementation of this novel class of scintillating composites is imperative and aims at the achievements of scintillators with improved performances. This goal is essentially linked to the fundamental understanding of the correlation between the physical-chemical properties and the luminescence features and to the comprehension of the scintillation mechanism in nanosystems, from the primary interaction with the ionizing radiation, through energy transfer and trapping processes, to the emission of light. A fundamental stage in the scintillation process is the transport of free carriers generated upon the interaction between ionizing radiation and the scintillating material: it is often largely affected by the presence of trapping sites, which can capture migrating charge carriers and either delay their radiative recombination or decrease the overall scintillation efficiency, according to the characteristics of the traps involved.
In this work, we disclose the role of trapping defects and their interplay with the scintillation properties of LHP NCs and nanocomposites. Specifically, we present a thorough investigation of the tight correlation existing between delayed scintillation processes and defects acting as carrier traps, as well as of the competition between trapping sites and luminescent centers in free carrier capture. To these purposes, steady-state radio-luminescence as a function of both temperature and cumulated X-ray dose is combined with time-resolved photo-luminescence measurements and wavelength-resolved thermally stimulated luminescence (TSL) at cryogenic temperatures. The performances and defectiveness of CsPbBr3 NCs prepared by hot-injection method are investigated and compared with those of CsPbBr3 NCs produced by ligand-assisted reprecipitation synthetic approach. Our results suggest that shallow trap states, likely related to bromine vacancies, capture and slowly release electrons via a-thermal tunnelling to spatially correlated emissive centers responsible for delayed emission: because of the relatively large concentration of such defects, electron trapping in shallow defects is the main competitive channel to radiative exciton decay. The presence of surface-related defects is common in this class of materials, although advanced strategies for their passivation are continuously improved to enhance the emission efficiency. In addition, we prove that CsPbBr3 NCs can be effectively embedded into polymethylmethacrylate matrix to obtain a high optical quality flexible and smooth scintillating nanocomposite film, whose defectiveness resembles that of LHP bulk crystals [7], featuring isolated energetically deep defects states that trap carriers which, upon heating, recombine in a specific intragap emission center, as revealed by TSL measurements. The effectiveness of this investigation approach coupling scintillation and TSL measurements, traditionally exploited only for classical single component bulk scintillators, is therefore here demonstrated also for nanosized materials.
1.2-O6
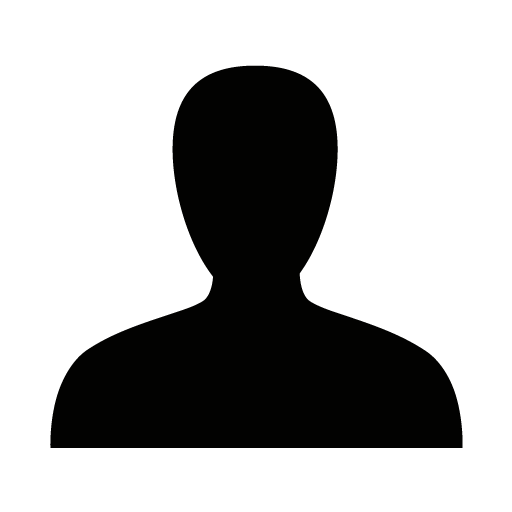
Lead halide perovskite nanocrystals (LHP NCs) are an emerging class of light-emitting semiconductors owing to their remarkable optoelectronic properties such as their high photoluminescence quantum yield (PLQY), tunable emission across the visible spectrum emission, and facile synthesis. The optical properties of LHP NCs are not only tunable by their halide composition, but also through doping with metal cations. Generally, doping not only improves the stability of LHP NCs but also reduces Pb-related toxicity by replacing them with non-toxic dopants. Among all, Mn2+-doped LHP NCs have received significant interest to understand the exciton-to-dopant energy or electron transfer process.1-4 The Mn2+-doping in CsPbCl3 NCs results in the transfer of the exciton energy from CsPbCl3 to the dopants leading to orange emission from a spin-forbidden Mn d–d transition. However, the energy transfer efficiency not only depends on the amount of the dopant and band alignment of the dopants with respect to the excitons but also on the surface traps. In this presentation, I will discuss our findings on the enhanced exciton-to-dopant energy transfer by post-synthetic surface treatment with didodecyldimethylammonium chloride (DDAC), a tightly binding ligand. The DDAC ligands have the ability to replace the weakly bound oleyalammonium cations and reduce the density of chlorine vacancies on the surface, resulting in an increase in the PLQY of Mn2+ ions. Ultrafast time pump-probe studies and time-resolved luminescence of dopants revealed that the DDAC ligands remove the surface traps and thus promote energy transfer as well as reduce the quenching of Mn2+ emission by the surface traps.
1.2-O7
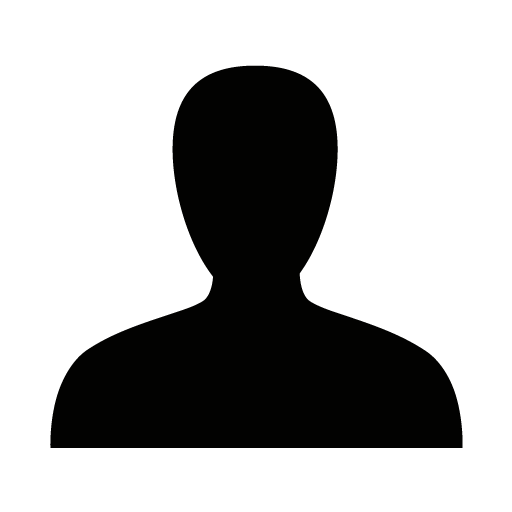
PhD student at University of Notre Dame, Indiana, USA and exchange visitor student at IIT, Genova, Italy. I work on colloidal synthesis and optoelectronic properties of perovskite nanocrystals.
Perovskite material appeared to be perspective and promising in photovoltaic application, such as solar cells, photodetectors, light emitting diodes and etc. due to outrageous properties like high carrier mobility, effective light absorption, mechanical flexibility, cheapness and simplicity of production makes them worthy opponent to crystalline silicon in creation less environment polluted world for our children. Although perovskites are already started to be used in solar cell fabrication on the market level, the extrinsic and intrinsic stability of them can be greatly improved. The most discussed issue nowadays is halide movement under continuous light illumination for I-rich and Br-rich domains. I-rich domain act as recombination centers in halide perovskites what impedes the carrier generation in the bromine-rich domain and blocks the electron flow through the device and reduces the efficiency of solar cells.
Although broad consensus exists that photoirradiation of mixed-halide lead perovskites leads to anion segregation, no model today fully rationalizes all aspects of this near ubiquitous phenomenon. In this work, we quantitatively compare experimentally the variety of dimensionality materials (such as bulk thin films, 2D Ruddlesden-Popper and 0D nanocrystals (NCs)) terminal anion photosegregation stoichiometries and excitation intensity thresholds to a band gap-based, thermodynamic model of mixed-halide perovskite photosegregation. Mixed-halide NCs offer strict tests of theory given physical sizes, which dictate carrier diffusion lengths. Further highlighting the importance of these studies are prior results, which suggest increased stabilities of mixed-anion perovskite NCs to irradiation. Observed qualitative and quantitative agreement with theory support a band gap-based model for anion photosegregation. More importantly, they suggest that mixed-halide perovskite photostabilities can be predicted using local gradients of (empirical) Vegard’s law expressions of composition-dependent band gaps. We have recently tested this alternative photostability metric on a mixed-cation/mixed-anion system, MA0.5Cs0.5Pb(I1−xBrx)3 compared to MAPb(I1−xBrx)3 and predicted that smaller local band gap gradients indeed correspond to improved anion photostabilities. Thus, not only is the developed band gap-based photosegregation model predictive but future extensions may rationalize remaining unexplained phenomena in lead halide perovskites such as halide remixing under large excitation intensities.
1.3-I1
Paulina Plochocka, Directrice de recherché de 2e classe (DR2) in Laboratoire National des Champs Magnétiques Intenses (LNCMI), CNRS in Toulouse.
P. Plochocka obtained her PhD cum-laude in 2004 at the University of Warsaw working on the dynamics of many-body interactions between carriers in doped semi-magnetic quantum wells (QW). During her first post doc at Weizmann Institute of science, she started working on the electronic properties of a high mobility 2D electron gas in the fractional and integer quantum Hall Effect regime. She continued this topic during second post doc in LNCMI Grenoble, where she was holding individual Marie Curie scholarship. At the same time, she enlarged her interest of 2D materials towards graphene and other layered materials as TMDCs or black phosphorus. In 2012 she obtained permanent position in LNCMI Toulouse, where she created the Quantum Electronics group, which investigates the electronic and optical properties of emerging materials under extreme conditions of high magnetic field and low temperatures. Examples include semiconducting layer materials such as transition metal dichalcogenides, GaAs/AlAs core shell nanowires and organic inorganic hybrid perovskites.
High environmental stability and surprisingly high efficiency of solar cells based on 2D perovskites have renewed interest in these materials. These natural quantum wells consist of planes of metal-halide octahedra, separated by organic spacers. Remarkably the organic spacers play crucial role in optoelectronic properties of these compounds. The characteristic for ionic crystal coupling of excitonic species to lattice vibration became particularly important in case of soft perovskite lattice. The nontrivial mutual dependencies between lattice dynamics, organic spacers and electronic excitation manifest in a complex absorption and emission spectrum which detailed origin is subject of ongoing controversy. First, I will discuss electronic properties of 2D perovskites with different thicknesses of the octahedral layers and two types of organic spacer. I will demonstrate that the energy spacing of excitonic features depends on organic spacer but very weakly depends on octahedral layer thickness. This indicates the vibrionic progression scenario which is confirmed by high magnetic fields studies up to 67T. Next, I will show that in 2D perovskites, the distortion imposed by the organic spacers governs the effective mass of the carriers. As a result, and unlike in any other semiconductor, the effective mass in 2D perovskites can be easily tailored. In the end, I will discuss exciton fine structure. The bright-dark splitting is also of paramount importance for light emitters which rely on the radiative recombination of excitons, since the excitons usually relax to the lowest lying dark state, which is detrimental for the device efficiency. I will discuss our optical spectroscopy measurements with an applied in-plane magnetic field to mix the bright and dark excitonic states of (PEA)2PbI4, providing the first direct measurement of the bright-dark splitting. The induced brightening of the dark state allows us to directly observe an enhancement of the absorption at the low-energy side of the spectrum related to the dark state. The evolution of the PL signal in the magnetic field, suggests that at low temperatures the exciton population is not fully thermalized due to the existence of a phonon bottleneck, which occurs due to the specific nature of the exciton-phonon coupling in soft perovskite materials.
1.3-I2
Dr. Tze-Chien Sum is an Associate Professor at the Division of Physics and Applied Physics, School of Physical and Mathematical Sciences (SPMS), Nanyang Technological University (NTU) where he leads the Femtosecond Dynamics Laboratory. He is presently the Associate Dean (Research) at the College of Science. Tze-Chien received his Ph.D. in Physics from the National University of Singapore (NUS) in 2005, for the work in proton beam writing and ion-beam spectroscopy. His present research focuses on investigating light matter interactions; energy and charge transfer mechanisms; and probing carrier and quasi-particle dynamics in a broad range of emergent nanoscale and light harvesting systems. Tze-Chien received a total of 11 teaching awards from NUS and NTU, including the coveted Nanyang Award for Excellence in Teaching in 2006 and the 2010 SPMS Teaching Excellence Honour Roll Award. Most recently, he received the 2013 SPMS Young Researcher Award; the Institute of Physics Singapore 2014 World Scientific Medal and Prize for Outstanding Physics Research; the 2014 Nanyang Award for Research Excellence (Team); and the 2015 Chemical Society of Japan Asian International Symposium Distinguished Lectureship Award. More information can be found at http://www.ntu.edu.sg/home/tzechien/spms/index.html
Halide perovskites possess outstanding optoelectronic properties favorable for applications as highly efficient absorbers in perovskite photovoltaics and as bright emitters in perovskite light emitting devices, lasers as well as quantum emitters. Underpinning this spectacular rise are their exceptional properties such as large absorption cross-sections, defect tolerance, large spin-orbit coupling, long balanced charge diffusion lengths, slow hot carrier cooling, ion migration, radiation tolerance etc. Hence, there has been a rapid proliferation of their applications beyond solar cells and light-emitting devices to fields such as spintronics, radiation detectors, memristors, bioimaging etc. Their versatile structures and diverse dimensionalities afford new levers for tunning their photophysical properties. For instance, the vast library of large organic cations allows one to tune the energy landscape in layered perovskites. In this talk, I will distill some of the underpinning photophysical mechanisms in low-dimensional emitters such as layered Ruddlesden Popper perovskites and colloidal perovskite nanocrystals. I will also take the opportunity to highlight some of our latest works on this topic.
1.3-I3
Laura Herz is a Professor of Physics at the University of Oxford. She received her PhD in Physics from the University of Cambridge in 2002 and was a Research Fellow at St John's College Cambridge from 2001 - 2003 after which she moved to Oxford. Her research interests lie in the area of organic and organic/inorganic hybrid semiconductors including aspects such as self-assembly, nano-scale effects, energy-transfer and light-harvesting for solar energy conversion.
Organic-inorganic metal halide perovskites have emerged as attractive materials for solar cells with power-conversion efficiencies of single-junction devices now exceeding 25%.
On issue that still limits the implementation of silicon-perovskite tandem cells in particular, is the peculiar mechanisms underlying detrimental halide segregation in mixed iodide-bromide lead perovskites with desirable electronic band gaps near 1.75eV.[1,2,3,4] We reveal that, surprisingly, halide segregation results in negligible impact to the THz charge-carrier mobilities.[2] However, remarkably fast, picosecond charge funnelling into the narrow-bandgap I-rich domains leads to enhanced radiative recombination.[3] Performance losses in photovoltaic devices may therefore potentially be mitigated by deployment of careful light management strategies. We further demonstrate[3] how a combination of simultaneous in-situ photoluminescence and X-ray diffraction measurements is able to demonstrate clear differences in compositional and optoelectronic changes associated with halide segregation in MAPb(Br0.5I0.5)3 and FA0.83Cs0.17Pb(Br0.4I0.6)3 films. While MAPb(Br0.5I0.5)3 exhibits rearrangement of halide ions only in localized volumes of perovskite, FA0.83Cs0.17Pb(Br0.4I0.6)3 lacks such low-barrier ionic pathways and is, consequently, more stable against halide segregation. However, under prolonged illumination, it exhibits a considerable ionic rearrangement throughout the bulk material, which may be triggered by an initial demixing of A-site cations, altering the composition of the bulk perovskite and reducing its stability against halide segregation.[4] We further explore the influence of a hole-transport layer, necessary for a full device. We show that top coating FA0.83Cs0.17Pb(Br0.4I0.6)3 perovskite films with a poly(triaryl)amine (PTAA) hole-extraction layer surprisingly leads to suppression of halide segregation because photogenerated charge carriers are rapidly trapped at interfacial defects that do not drive halide segregation.[4]
We also discuss the charge-carrier dynamics in layered, 2D perovskites that have been found to improve the stability of metal halide perovskite thin films and devices.[5] We show that the 2D perovskite PEA2PbI4 exhibits an excellent long-range mobility of 8.0 cm2 (V s)–1, ten times greater than the long-range mobility determined for a comparable 3D material FA0.9Cs0.1PbI3. These values shows that the polycrystalline 2D thin films already have single-crystal-like qualities. We further demonstrate that these materials exhibit unexpectedly high densities of sustained populations of free charge carriers.
[1] A. J. Knight and L. M. Herz, Energy Environmental Science 13, 2024 (2020).
[2] S. G. Motti, J. B. Patel, R. D. J. Oliver, H. J. Snaith, M. B. Johnston, L. M. Herz, Nature Communications 12, 6955 (2021).
[3] A. J. Knight, J. Borchert, R. D. J. Oliver, J. B. Patel, P. G. Radaelli, H. J. Snaith, M. B. Johnston, and L. M. Herz, ACS Energy Letters 6, 799 (2021).
[4] Impact of hole-transport layer and interface passivation on halide segregation in mixed-halide perovskites, V. J.-Y. Lim, A. J. Knight, R. D. J. Oliver, H. J. Snaith, M. B. Johnston, and L. M. Herz, Advanced Functional Materials 32, 2204825 (2022).
[5] Excellent long-range charge-carrier mobility in 2D perovskites, M. Kober-Czerny, S. G. Motti, P. Holzhey, B. Wenger, J. Lim, L. M. Herz, and H. J. Snaith, Advanced Functional Materials 32, 2203064 (2022).
1.3-I4
Lead halide perovskite nanocrystals are promising candidates for applications in light-emitting devices due to their high exciton binding energy, exceptionally high quantum efficiency, and good stability. Depending on crystal symmetry and shape anisotropy, a distinct exciton fine structure controls their emission properties [1]. Here, we demonstrate the strength of polarization-resolved micro-photoluminescence (PL) spectroscopy on a single nanocrystal level for getting insight into exciton fine structure states and their relation to crystal symmetry and shape anisotropy.
In nearly cubic FAPbBr3 nanocrystals, the degeneracy of the bright exciton triplet is lifted leading to three bright states with transition dipoles oriented along the orthorhombic crystal symmetry at cryogenic temperatures. Depending on the orientation of the nanocrystals with respect to the optical axis between one and three polarized emission lines are visible [2]. Magneto-PL and time-resolved-PL experiments on single nanocrystals demonstrate that the dark singlet exciton is energetically below the bright one, shifted by about 2.6 meV.
In highly anisotropic CsPbBr3 nanoplatelets (NPLs) either one, two or three resolvable emission lines (case I, II and III, respectively) with significantly different polarization patterns are found. In case a single peak is observed, the emission is mostly unpolarized and linewidths generally exceed 1 meV. In contrast, the polarization of the two emission lines of case II NPLs are oriented orthogonally with respect to each other. In case III NPLs, the lowest and highest energy peaks are polarized collinearly, while the central emission line is polarized in a direction orthogonal to the former two. This quite characteristic polarization pattern can be explained by the occurrence of orthorhombic CsPbBr3 NPLs with two different orientations of the crystal axes [3]. In case II NPLs, one of the orthorhombic crystal axes is aligned with the NPL thickness and the observation direction and the radiation emitted by the corresponding dipole cannot be detected. In case III NPLs, in contrast, all orthorhombic crystal axes have a finite projection perpendicular to the observation direction allowing the observation of all emission lines of the fine structure split triplet. The negligible fine structure splitting in case I NPLs is consistent with the coexistence of these different lattice polymorphs within a single NPL. Our findings not only allow the unambiguous identification of the crystal configuration of individual CsPbBr3 nanoplatelets from pure optical measurements, but in addition facilitate the determination of a nanoplatelets’ absolute spatial orientation with respect to the lab coordinates via its characteristic polarization pattern.[4]
2.1-I2
Bruno Ehrler is leading the Hybrid Solar Cells group at AMOLF in Amsterdam since 2014 and is also a honorary professor at the University of Groningen since 2020. His group focuses on perovskite materials science, both on the fundamental level, and for device applications. He is recipient of an ERC Starting Grant and an NWO Vidi grant, advisory board member of the Dutch Chemistry Council, recipient of the WIN Rising Star award, and senior conference editor for nanoGe.
Before moving to Amsterdam, he was a research fellow in the Optoelectronics Group at Cambridge University following post-doctoral work with Professor Sir Richard Friend. During this period, he worked on quantum dots, doped metal oxides and singlet fission photovoltaics. He obtained his PhD from the University of Cambridge under the supervision of Professor Neil Greenham, studying hybrid solar cells from organic semiconductors and inorganic quantum dots. He received his MSci from the University of London (Queen Mary) studying micro-mechanics in the group of Professor David Dunstan.
2022 Science Board member Netherlands Energy Research Alliance (NERA)
2021 Member steering committee National Growth fund application Duurzame MaterialenNL
2021 Member advisory board Dutch Chemistry Council
2020 Honorary professor Universty of Groningen for new hybrid material systems for solar-cell applications
2020 ERC starting Grant for work on aritifical synapses from halide perovskite
2019 Senior conference editor nanoGe
2018 WIN Rising Star award
2017 NWO Vidi Grant for work on metal halide perovskites
since 2014 Group Leader, Hybrid Solar Cell Group, Institute AMOLF, Amsterdam
2013 – 2014 Trevelyan Research Fellow, Selwyn College, University of Cambridge
2012-2013 Postdoctoral Work, University of Cambridge, Professor Sir Richard Friend
2009-2012 PhD in Physics, University of Cambridge, Professor Neil Greenham
2005 – 2009 Study of physics at RWTH Aachen and University of London, Queen Mary College, MSci University of London
2D perovskites are often used to stabilize perovskite solar cells. By adding a thin layer of 2D perovskite on top of a 3D bulk solar cell, stability can be massively improved. This effect has lead to the impression that 2D perovskites are stable materials. However, we show that these materials are extremely unstable under illumination, especially when combined with air.
We quantify the decrease in photoluminescence after illumination. The photoluminescence first increases (photobrightens) and then decays dramatically. We show that this decay in luminescence coincides with a loss of material and a decomposition into gasses and precursors. We also show some initial mechanistic explanation for a better stability under nitrogen atmosphere.
Our results indicate that 2D perovskites are not intrinsically stable, but rather protect the underlying 3D perovskite from ambient influences, presumably by forming a hydrophobic barrier. Our results further illustrate the caution required when studying 2D perovskites because they continuously change during the measurement.
2.1-I1
Dr. Qihua Xiong from Tsinghua University is a leading expert in semiconductor optics. His research interest is particularly driven by the light-matter interactions in quantum materials in reduced dimensionality by optical spectroscopy. He has published more than 290 papers, which attracted more than 23000 citations with an H-index of 84. He was elected Fellow of American Physical Society in 2018, Academician of Asian Pacific Academy of Materials in 2019, Fellow of Optical Society of America in 2020 and Fellow of Materials Research Society in 2022. His outstanding achievement has been recognized by a few prestigious awards, such as IPS Nanotechnology Physics Award (2015), Nanyang Award for Research Excellence by NTU (2014) and Singapore National Research Foundation Inaugural Investigatorship (NRFI) Award (2014), Highly cited researcher in cross-field by Clarivate Analytics (2019-2022). He currently serves as Associate Editor for Nano Letters and serves as international advisory board for many prestigious international journals, such as ACS Photonics, Science China Materials, Nano Research, eScience, Science Bulletin, etc.
Recently, microcavity exciton polariton research has attracted considerable interests in a number of excellent optical gain materials that demonstrate unique properties compared with conventional III-V or II-VI semiconductor quantum wells and organic semiconductors. Those materials include transition metal dichalcogenides (TMDs), and certain halide perovskite semiconductors. Particularly, those materials exhibit large exciton binding energies (much larger than thermal fluctuation energy ~ 26 meV), large oscillator strength and peculiar electronic band structures such as valley polarization or encoded chiroptical responses. In this talk, we will discuss our recent effort in manipulating exciton polariton condensates in halide perovskite semiconductors microcavities, for instance using artificial lattices to engineer the strong optical responses including topological properties, and their ultrafast propagation. Finally, we will briefly discuss the nonlinear optical properties in polariton condensate trapped in artificial potential landscapes, by a pump-probe transient spectroscopy at momentum space. Our results demonstrate a promising perspective of polaritonics in a wide range of ultrafast optical and photonic applications at room temperature.
- S. Ghosh, R. Su*, J.X. Zhao, A. Fieramosca, J.Q. Wu, T.F. Li, Q. Zhang, F. Li, Z.H. Chen, T.C.H. Liew, D. Sanvitto, Qihua Xiong*, “Microcavity exciton polaritons at room temperature”, Photonics Insights 1, R04 (2022) (review)
- J.Q. Wu#, S. Ghosh#, R. Su*, A. Fieramosca, T.C.H. Liew*, and Qihua Xiong*, “Nonlinear parametric scattering of exciton polaritons in perovskite microcavities”, Nano Lett. 21, 3120–3126 (2021)
- J.G. Feng et al., “All-optical switching based on interacting exciton polaritons in self-assembled perovskite microwires”, Science Advances 7, eabj6627 (2021)
- R. Su et al., “Optical control of topological polariton phase in a perovskite lattice”, Science Advances 7, eabf8049 (2021)
- R. Su et al., “Observation of exciton polariton condensation in a perovskite lattice at room temperature”, Nature Physics 16, 301-306 (2020)
- R. Su et al., “Room temperature long-range coherent exciton polariton condensate flow in lead halide perovskites”, Science Advances 4, eaau0244 (2018)
- R. Su et al., “Room-temperature polariton lasing in all-inorganic perovskite nanoplatelets”, Nano Lett. 17, 3982–3988 (2017)
2.2-O1
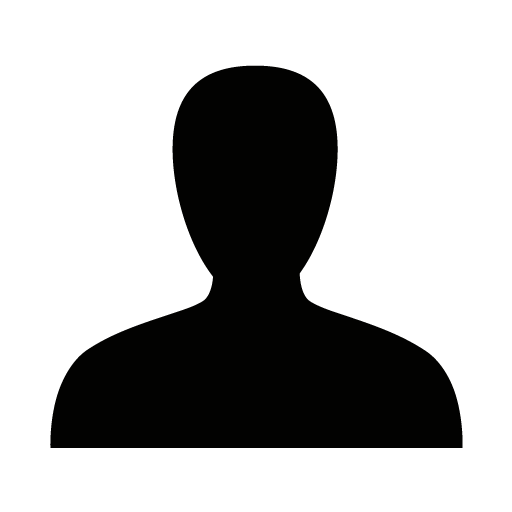
Increasing the efficiency of light-emitting devices is currently a hot topic in the field of semiconductor research. Here, perovskites are of special interest due to their excellent optical properties. Additionally, the possibility of sample preparation by vacuum deposition makes this class of material suitable for future large-scale industrial production. It is known that light-emission efficiency can benefit from an island-type active layer structure. If the lateral dimension of the structures is sufficiently small, excitons are confined and thus are far less likely to dissociate prior to radiative recombination.
In this work, we investigate the impact of the environment on the island formation in thermally deposited bilayers of CsPbBr3/LiBr. We demonstrate that the island formation occurs only in humid environments, leading to an enhancement of the photoluminescence quantum yield by a factor of 350. Time-resolved grazing-incidence wide-angle X-ray scattering (GIWAXS) experiments document the island growth process and reveal that the LiBr has already changed the perovskite crystal orientation before the island formation.
2.2-O2
Rafael Sánchez (M.Sc. degree in Chemistry in 2006 and Ph.D. degree in 2011, both from the Universitat Autònoma de Barcelona, Spain). To date, he has worked without interruptions in several international research institutions: Universitat Jaume I (2012-2017), University of Liverpool (2017-2018), Henkel Ibérica-UAB (2018-2019) and Université de Bordeaux (2019-2020). The main research topics he has developed are based on the synthesis and electro-optical characterization of functional materials and/or semiconductors for light generation, photovoltaics and water splitting applications. His current interests are focused on the chemical design and synthesis of quaternary diazaaromatic dications for the development of novel 2D metal halide perovskite semiconductors suitable for the preparation low-cost, highly efficient and durable optoelectronic devices. He is the author of 1 book chapter and 27 publications in peer-review international journals (27 publications in Q1 journals, 18 of which in D1 journals with impact factor > 6.9 in different areas) with 2733 citations and a h-index of 21 (https://scholar.google.es/citations?user=kzbjcFQAAAAJ&hl=es).
Despite the relatively early level of development, Perovskite light-emitting diodes (PeLEDs) have reached outstanding luminance and radiative efficiency levels that roughly graze the maximum theoretical efficiency limits. Unfortunately, the complete understanding of the working principles and the photo-electrochemical mechanisms involved in the charge carrier generation/recombination dynamics is still a conundrum. Additionally, the strong ionic character of MHPs enables the migration of ions and the gradual formation of crystalline defects upon exposing to light and/or to an external electric field, which aggravate the complexity of these systems. In fact, these ionic processes are apparently coupled with those electrical involved in the generation of light, and seem to be connected with the widely reported limited long-term stability of the devices. Here, I will discuss on the exploitation of a new methodology based on the combination of two frequency-domain modulated techniques, i.e. electrochemical impedance spectroscopy (EIS) and light emission voltage-modulated spectroscopy (LEVS), aimed at reaching a full understanding of the working principles of perovskite LEDs. Particularly important is the deconvolution of the electrical, optical and ionic processes that are involved in the current-to-photon conversion, heat generation and/or degradation of the materials employed. We propose a new theoretical model and an equivalent circuit that considers both the non-radiative and radiative contributions, as a new tool for the advanced characterization of perovskite-based optoelectronic devices.
2.2-O3
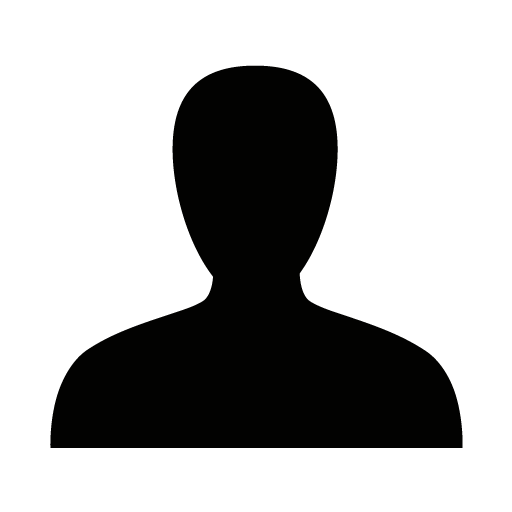
Metal-Halide perovskite materials are known to be of high potential for solar and LED applications thanks to their very high absorption coefficients, remarkably long carrier-lifetime and high photoluminescence quantum yield in comparison to traditional semiconductor absorbers [1], [2]. This is particularly remarkable given that high materials quality can be obtained even when samples are grown from solutions. It seems that not only their defect tolerance but, even more so, the self-healing ability of halide perovskites are a fundamental reason for their excellent opto-electronic properties. Nevertheless, dynamic effects also give rise to a number of meta-stabilities. Materials can be degraded and temporarily perturbed under external stressors, such as light and bias [3],[4],[5]. In this work, we focus on methyl-ammonium lead tri-bromide microplatelets, prepared following the work from Mao et. al. [6]. We use these microplatelets to study fundamental dynamics of charge carriers interacting with physical or light-induced defects generated in these crystals by external perturbation. The samples can be considered an idealized model-system that exclude typical intra-grain boundaries of polycrystalline thin film samples, thus removing the different phenomena that could occur at these local sites.
Within these model systems, we show a correlation between time-resolved photoluminescence decay dynamics and sample properties, perturbed by the history of the micro-sized sample to illumination, generating light-induced defects; or mechanical stress, generating structural defects. We utilized excitation-intensity and repetition rate-dependent photoluminescence measurements [7] to provide a "finger print" of samples exposed to different degrading conditions to investigate the characteristic difference between light- and mechanically-induced defects affecting the charge carrier dynamics within the crystal.
Finally, we refined a numerical model to fit photoluminescence lifetime data based on population rate equations, including trapped populations [8],[9]. A fit of the presented data is used to see how material properties, such as trap densities, are affected by illumination intensity and/or repetition rate.
2.2-O4
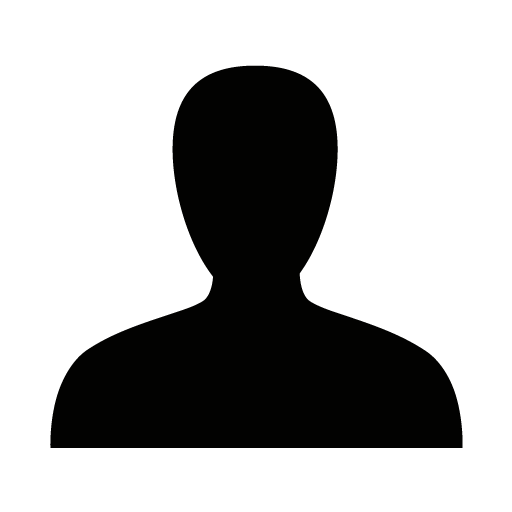
Photoexcitation of semiconductors by photons of higher energy than the bandgap creates ‘hot-charge carriers’ (i.e. different thermal energy w.r.t. lattice), which further cool down to the band-edge state by releasing their excess energy as a waste form of heat. Harvesting the excess energy of these hot carriers (HC) could eventually boost the performance of solar cells by manifold, while practical realization of this is still limited owing to the rapid cooling of these HC [1, 2]. On the contrary, in light-emitting applications, rapid HC cooling is highly desired to enable efficient radiative recombination by preventing carrier trapping. Therefore, detailed understanding of the HC cooling mechanisms and further controlling their dynamical pathways is prerequisite for engineering the semiconductor optoelectronics.
Herein I will explore this key area on CsPbX3 perovskite nanostructures of various dimensions and compositions by employing novel pump-push-probe based transient spectroscopy [3], which provides direct access to selectively control the hot carrier density and study their influence. This experimental finding unravels the role of carrier-carrier, carrier-phonon interactions to carrier-impurity (defect) scatterings in tailoring the hot carrier cooling dynamics in perovskite nanosystems. Our results of HC cooling dynamics among halide-composition space with controlled defect densities reveals that this dynamics is defect-tolerant for pure-iodide based perovskites unlike pure-Br and mixed (Br/I)-systems. Importantly, we also examine the slowing down pattern of HC cooling dynamics under high HC-density (termed as hot-phonon bottleneck effect), whose effect is significantly suppressed with the increase in quantum confinement (from 3D to 2D systems) due to reduced screening by phonons and enhanced Coulombic interaction between electron and holes for the latter systems. Although halide-vacancy related defects accelerate the HC cooling dynamics for pure Br-and mixed (Br/I)-systems, but they do not induce any such effect on the hot-phonon bottleneck process possibly due to saturation of the trap-states. This detailed understanding of the key routes that control the HC cooling dynamics would be instrumental for prospective applications of the next-generation perovskite optoelectronics.
2.2-O5
Matteo Zaffalon is a Postdoctoral researcher at the Department of Materials Science of the University of Milano-Bicocca (IT), where he earned his Ph.D. in Materials Science and Nanotechnology in 2022. In 2020 he collaborated with the Nanotechnology & Advanced Spectroscopy group at the Los Alamos National Laboratory (NM, USA) working on the spectroscopic investigation of solution grown functional nanostructures for application in photonic and optoelectronic devices. His research is now focused on the spectroscopic investigation and development of novel nanomaterials for the ultrafast detection and conversion of ionising radiation for energy and medical imaging applications.
Lead halide perovskites (LHP) are rapidly emerging as efficient, low-cost, solution-processable scintillators for radiation detection [1], [2]. Carrier trapping is arguably the most critical limitation to the scintillation performance [3], [4]. Nonetheless, no clear picture of the trapping and detrapping mechanisms to/from shallow and deep trap states involved in the scintillation process has been reported to date, as well as on the role of the material dimensionality. In this talk, this issue is addressed by performing, for the first time, a comprehensive study using radioluminescence and photoluminescence measurements side-by-side to thermally-stimulated luminescence (TSL) and afterglow experiments on CsPbBr3 with increasing dimensionality, namely nanocubes, nanowires, nanosheets, and bulk crystals. All systems are found to be affected by shallow defects resulting in delayed intragap emission following detrapping via a-thermal tunneling. TSL further reveals the existence of additional temperature-activated detrapping pathways from deeper trap states, whose effect grows with the material dimensionality, becoming the dominant process in bulk crystals. These results highlight that, compared to massive solids where the suppression of both deep and shallow defects is critical, low dimensional nanostructures are more promising active materials for LHP scintillators, provided that their integration in functional devices meets efficient surface engineering.
2.2-O6
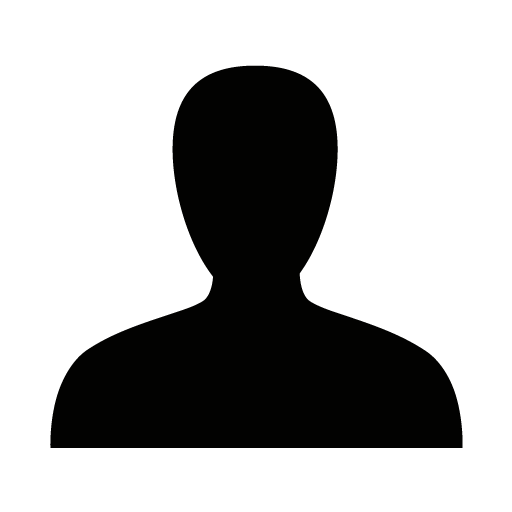
Organic-inorganic hybrid low-dimensional perovskites are attracting significant attention for optoelectronic applications due to their higher stability, uncomplicated manufacturing process, and wide tunability of the optical and electronic properties. The use of various organic cations leads to the formation of two-dimensional (2D) layers, one-dimensional (1D) chains, or isolated zero-dimensional (0D) clusters depending on how the metal halide octahedra are connected. Even minimal differences in the structure of the organic cations can cause remarkable changes in the structural arrangement and, as a consequence, a modification of the optoelectronic properties of the perovskite system.
The incorporation of functionalized ammonium cations in perovskites allows the design of a variety of new organic-inorganic hybrid materials, in which functionalized and even semiconducting organic layers are assembled with a semiconducting inorganic layer at the molecular scale. In recent works devoted to low-dimensional inorganic-organic halide perovskites much attention has been paid to the broadband emission properties primarily originating from the self-trapped excitons (STEs) [1,2]. High structural distortion of low-dimensional perovskite structures induces electron-phonon coupling and enhances the STE process, resulting in an enhanced broadband emission. In addition, it has been demonstrated that perovskites grown in a form of atomically thin sheets possess unique features compared to their bulk counterparts [3]. For example, hybrid perovskite sheets exhibit an unusual structural relaxation, which leads to a band gap shift. Consequently, the controllable synthesis of ultrathin perovskite sheets has gained a great research interest for implementation in high-performance optoelectronic devices.
In this work, a series of low-dimensional hybrid organic-inorganic metal halides, based on multiple-ring aromatic ammonium cations and lead iodide were fabricated and their structures and properties were investigated. Examination of the influence of organic cations on the structural properties reveals that the number and position of amino groups at the aromatic ring affect the dimensionality of the perovskite, resulting in 2D and 1D corner- and face-sharing structures with different optical behavior. Highly distorted 1D perovskites show broadband emissions originating from STEs. Theoretical results reveal, that the organic cations in the 1D compounds strongly contribute to the band structure resulting in strong orbitals hybridization. Using a facile and fast crystallization method we also synthesized organic-inorganic hybrid perovskite few-layer free-standing nanosheets containing the naphthalene diammonium-based linker. Varying the synthetic conditions, we can modulate the thickness and lateral sizes of the nanosheets.
2.2-O7
The success of the colloidal semiconductor quantum dots (QDs) field is rooted in the precise synthetic control of QD size, shape, and composition, enabling electronically well-defined functional nanomaterials that foster fundamental science and motivate diverse fields of applications. While the exploitation of the strong confinement regime has been driving commercial and scientific interest in InP or CdSe QDs, such a regime has still not been thoroughly explored and exploited for lead-halide perovskite QDs, mainly due to a so far insufficient chemical stability and size monodispersity of perovskite QDs smaller than about 7 nm. Here, we demonstrate chemically stable strongly confined 5 nm CsPbBr3 colloidal quantum dots via a post-synthetic treatment employing didodecyldimethylammonium bromide ligands. The achieved high size monodispersity (7.5%±2.0%) and shape-uniformity enables the self-assembly of QD superlattices with exceptional long-range order, uniform thickness, an unusual rhombic packing with an obtuse angle of 104º, and narrow-band cyan emission. The enhanced chemical stability indicates the promise of strongly confined perovskite QDs for solution-processed single-photon sources, with single QDs showcasing a high single-photon purity of 73% and minimal blinking (78% “on” fraction), both at room temperature.
1.1-O1
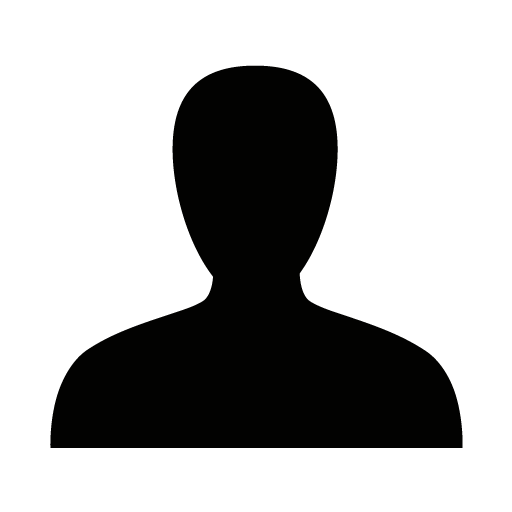
Hybrid perovskites have recently emerged as a promising material for optoelectronic applications due to their unique combination of properties. In particular, their 2D crystalline form is of great interest since the alternating inorganic and organic layers naturally form a multiple quantum-well structure, leading to the formation of stable excitonic resonances at room temperature. However, a controlled modulation of the quantum well width, which is defined by the number of inorganic layers (n) between two organic ones, is not straightforward and represents the main synthetic challenge in the field. This study presents a novel approach to easily tune the number of inorganic layers (n) in lead iodide perovskite single-crystalline flakes. This approach exploits an iodide salt as an additive that acts as an additional source of I−, inducing the generation of iodoplumbate species and resulting in an increase of the monomer density, resulting in the fine-tuning of the n value. Unlike other methods, our protocol allows us to fix the molar ratio of precursors and to control the "n" value by simply modulating the amount of potassium iodide (KI) added to the solution. The excellent optical quality of the synthesized flakes enables an in-depth analysis by Fourier-space microscopy, which reveals that the excitons orientation can be manipulated by modifying the number of inorganic layers. It is found that the excitonic out-of-plane component is enhanced when n is increased. This combined advance in synthesis and optical characterization provides a better understanding of the exciton behavior in low-dimensional perovskites, opening up possibilities for the design of materials with improved optoelectronic characteristics.
1.1-O2
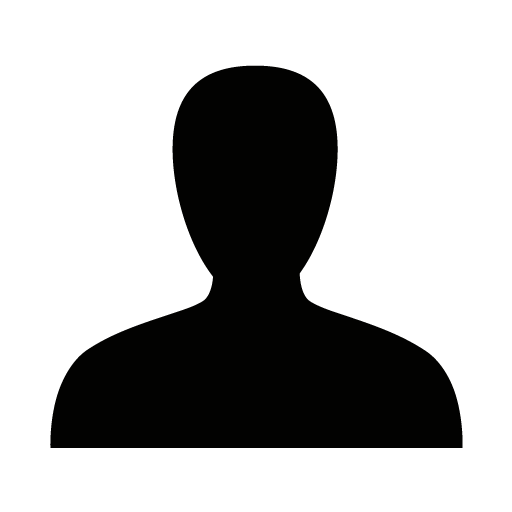
Tin halide perovskites display similar or even superior electronic and optical properties when compared to well-established Pb-based perovskites. Despite their favorable optoelectronic properties, the tin perovskite solar cells still exhibit power conversion efficiency (PCE) much lower than that of their lead analogs. This is mainly attributed to the fact that metastable Sn(II) in perovskite lattice is prone to the oxidation. The presence of Sn(IV) in the material results in self-doping and formation of non-radiative recombination centers. One of the strategies to overcome this obstacle is addition of bulky A-site cations which stabilize the resulting 3D films in a quasi-2D fashion or preparation of 2D tin halide perovskites. Especially in recent years, single crystal 2D-tin halide perovskites have attracted significant attention. However, the synthesis and growth of such materials high-quality remain very challenging. In this work, we report a comprehensive study of the synthesis and the growth of single-crystal 2D tin halide perovskites (Ruddlesden-Popper), which is still poorly explored (excluding extensively studied materials containing PEA derivatives acting as an A-site cations). By careful control the growth conditions (temperature, and composition), we obtained mm-sized (up to 10 mm), high-quality single crystals of 2D tin halide perovskites. The single crystals were characterized structurally and optically by means of inter alia X-ray diffraction, steady-state photoluminescence, and optical absorption.
1.1-O3
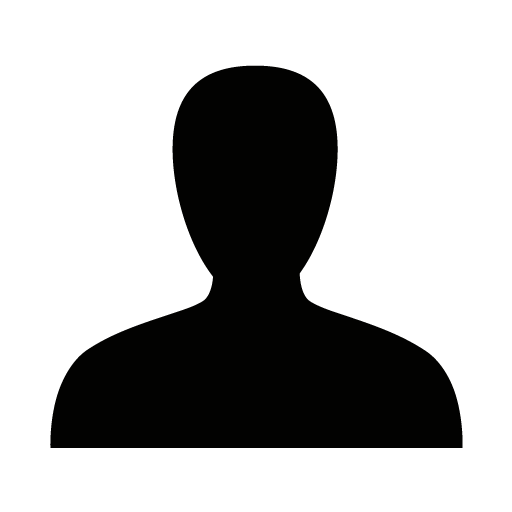
The introduction of chiral organic spacers in 2D and 1D perovskites breaks the inversion symmetry operation in the crystal, due to the asymmetric hydrogen bonding between the H atom of the ammonium group in the organic cation and the halide atom (Cl, Br, or I) in the lead octahedra. [1,2] The absence of inversion symmetry operation results in the emergence of Rashba/Dresselhaus spin-splitting, i.e. the splitting of the double degenerated electronic band into two bands with the same energy, but shifted in k-space,[3,4] triggering spin-dependent properties even in the absence of magnetic field. Such a feature has been utilized for applications in polarized light detectors,[5] spin-dependent charge transport,[6] and polarized light-emitting diodes.[7] In this work, we investigate how the processing of chiral 2D perovskites impacts their optical properties, microstructure, and phase purity. Specifically, we synthesized the R-, S-, and rac-MBA2PbI4 (MBA: methylbenzylammonium) and varied the solvent used for film deposition (dimethylformamide, DMF, or acetonitrile, ACN), and the subsequent thermal annealing procedure. We found that the processing conditions strongly impact the optical properties and microstructure of the chiral 2D perovskites. Moreover, we identified the anisotropic emergence of a 1D phase, which is dependent on the molecule's chirality and seems more prone to occur with pure enantiomers than in the racemic mixture. We demonstrate that its formation is suppressed when the 2D perovskite is processed from DMF, due to its higher boiling point and a stronger interaction between the solvent and Pb2+ ions, thus enabling a higher phase purity. These observations give fundamental insights into the film formation processes of chiral 2D perovskites and offer processing strategies to control anisotropic growth in 2D perovskite and to tune their chiroptical response.
1.1-O4
Wouter Van Gompel is an assistant professor in hybrid materials chemistry within the Institute for Materials Research (imo-imomec) of Hasselt University in Belgium. With his research group Hybrid Materials Design (HyMaD), he does research into the design, synthesis and characterization of novel hybrid materials for optoelectronics.
Hybrid organic-inorganic perovskites have received tremendous research attention over the past decade for use in optoelectronic applications such as solar cells and photodetectors. Despite their excellent optoelectronic properties, their commercialization is hindered by their limited intrinsic stability. A prime route to obtain more stable perovskite materials is the addition of a large organic ammonium cation to form quasi-2D perovskites.[1-2]
We employ a functionalized benzothienobenzothiophene (BTBT) organic cation either as an additive in the precursor solution to obtain a quasi-2D perovskite for use in photodetectors[1], or as an interlayer in perovskite solar cells.
The BTBT cation was added to a CsPbI3 precursor solution to obtain a n = 2 quasi-2D perovskite film. We compared the stability and performance of this film to that of a state-of-the-art (BA)2CsPb2I7 (n = 2) film. While the (BA)2CsPb2I7 film starts to degrade at 130 °C, the thermal stability of the film containing the BTBT cation is significantly enhanced to 230 °C. Furthermore, while the (BA)2CsPb2I7 film degrades into (BA)2PbI4 and other compounds after 1 day of storage in air at 77% relative humidity, the film containing the BTBT cation shows excellent resistance against humidity, with no apparent degradation after 1 year of storage at high relative humidity. We fabricated planar photodetectors using both films. The specific detectivity of the photodetectors with films containing our tailored cation is similar in magnitude compared to those containing the state-of-the-art (BA)2CsPb2I7 films. In summary, we prepared a quasi-2D perovskite with a similar optoelectronic performance as the literature reference but with significantly enhanced stability.
The same BTBT cation was also used as an interlayer in a solar cell between a NiOx hole-transporting layer and a triple-cation 3D perovskite leading to an enhanced lifetime of photo-generated charge carriers based on TRPL measurements. Furthermore, a significant VOC improvement is achieved in solar cells leading to a higher power conversion efficiency. The moisture stability of the solar cells was enhanced with the presence of the interlayer.
1.1-O5
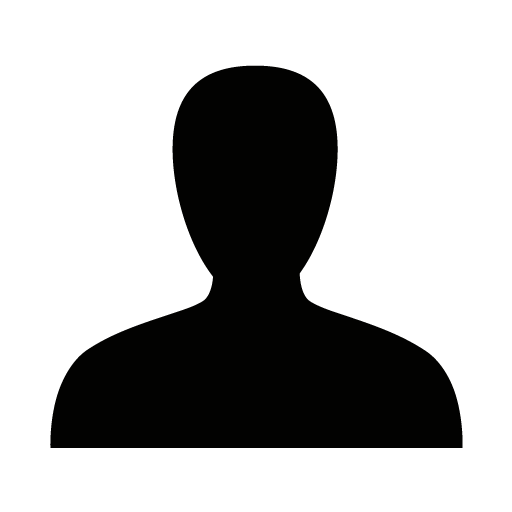
Starting from the well-studied ABX3 (A = CH3NH3+, Cs+; B = bivalent metal cation, X = Cl-, Br-, I-) perovskite, new derivates can be obtained with the partial or full substitution of the A and B cations with different metal ions [1]. Besides a change in the elemental composition, the dimension of the ion in the A site enlarges the formation of polymorphs of a lower dimensionality (2D or 0D). Indeed, the use of an organic cation, usually bigger than the inorganic metal ion, is one of the most promising strategies to tune and confer new optoelectronic properties in metal halide compounds [2]. For example, the 2D layered lead halides have excellent emission comparable with the 3D counterpart but with better electronic conductivity and quantum confinement effect observable even in the bulk materials [3].
Here, we present our recent results on the preparation of emissive 2D (Pb,Mn)-based hybrid metal halide as single crystals. Starting from the reported synthesis of Bz2PbBr4 (Bz+ = (C6H5CH2NH3)+), the Pb2+ was progressively replaced by Mn2+, even if the percentage of Pb substitution strongly depends on the metal ratio in the starting materials. The presence of Bz cation in the structure is confirmed by FTIR analyses. The emission varies from the light blue of Bz2PbBr4 to the typical orange one of the Mn. The PL spectrum exhibits a weak emission for the excitonic peak at 410 nm and a strong Mn emission, centered at 610 nm, ascribable to the spin-forbidden Mn2+ d−d transition (4T1 → 6A1). The ABS spectra demonstrate that even at low Pb concentrations it is observable an energy-transfer process from the Pb2+ to Mn2+ emitter. Interestingly, the emission intensity reaches the maximum for the experimental Mn composition of 8.0% (PLQY » 47.9%), even if a notable value is measurable also for the Mn-rich composition (PLQY » 37.2% for Mn = 66.5%).
1.1-O6
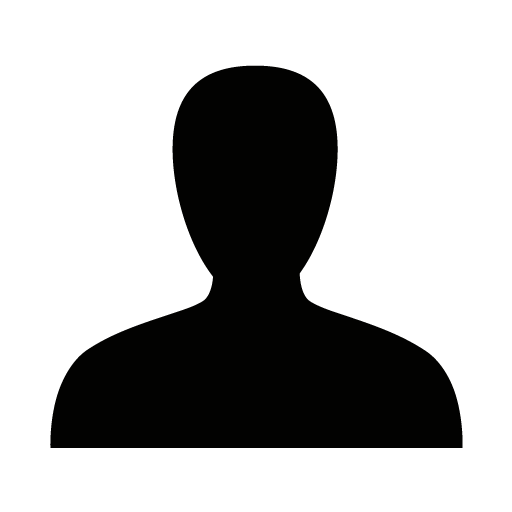
Incorporating organic semiconductor (OS) spacer cations into layered lead halide perovskites provides a powerful approach to tune the optoelectronic properties of the resulting organic-inorganic hybrid materials. Forming type-II nano heterostructures using OS spacers, for example, allows the partitioning of charge carriers either in the organic or inorganic layer and provides a potential strategy to overcome intrinsically high exciton binding energies in layered perovskites by facilitating charge separation.[1] However, large OS cations with suitable energy levels remain challenging to incorporate into a layered perovskite structure resulting in relatively few systems reported and characterized.
In this regard, our lab developed novel OS cations and optimized processing conditions to afford incorporation in layered perovskites.[2,3] In this presentation, we elucidate novel OS cations based on rylene dyes chromophores incorporation into a layered perovskite and elaborate the size limitations of the chromophore when it comes to the formation of (2D) layered perovskite structure. Using transient absorption (TA) and time resolved photoluminescent spectroscopy (TRPL) we indicate the formation of charge-transfer excitons at the perovskite-OS interface and further investigate the effect of the distance of the chromophore to the perovskite layer on the electron-transfer process from the perovskite layer to the OS cation. Time resolved microwave conductivity (TRMC) measurements and terahertz spectroscopy was used to elucidate the effect of the type-II nano heterostructure on the photogenerated free charge-carrier showing enhanced carrier lifetimes compared to layered perovskites incorporating aliphatic spacers cations. The presented work implies the possibility to mitigate the inefficient charge-separation process due to the high exciton-binding energy in lead-halide layered perovskite by forming OS-perovskite nano heterostructures opening the door for a new class of material.
1.2-I1
Double perovskites are promising candidates for less toxic and highly stable metal halide perovskites, but their optoelectronic performances still lag behind those of the lead halide counterpart, due to the indirect nature of the bandgap and the strong electron-phonon coupling. Reducing the dimensionality of Cs2AgBiBr6 down to a 2D layered form is strategic in order to tune the band gap from indirect to direct and provides new insights into the structure-property relationships of double perovskites. Herein, we report on a series of monolayer 2D hybrid double perovskites of formula (RA)4AgBiBr8, where RA represents different primary ammonium large cations with alkyl- and aryl-based functionalities.[1] An in-depth experimental characterization of structure, film morphology and optical properties of these perovskites is carried out. Interestingly, the variation of the ammonium cation and the inter-planar distance between adjacent inorganic monolayers has peculiar effects on the film-forming ability and light emission properties of the perovskites. Experiments have been combined with DFT calculations in order to understand the possible origin of the different emissive features. Our study provides a toolbox for future rational developments of 2D double perovskites, with the aim of narrowing the gap with lead halide perovskite optoelectronic properties. We further discuss on the achievement of a bathochromic shift in the absorption features of a butylammonium-based silver-bismuth bromide monolayer double perovskite through doping with iodide and study the optical properties and stability of the resulting thin films in environmental conditions.[2] Finally, we report on recent, on-going work on the 2D/3D interface engineering to tune the efficiency of solar cells based on the double perovskite and carbon electrode charge extracting layers.[3]
1.2-I2
Epitaxial heterostructures based on oxide perovskites and III–V, II–VI and transition metal dichalcogenide semiconductors form the foundation of modern electronics and optoelectronics. Halide perovskites—an emerging family of tunable semiconductors with desirable properties—are attractive for applications such as solution-processed solar cells, light-emitting diodes, detectors and lasers. Their inherently soft crystal lattice allows greater tolerance to lattice mismatch, making them promising for heterostructure formation and semiconductor integration. Atomically sharp epitaxial interfaces are necessary to improve performance and for device miniaturization. However, epitaxial growth of atomically sharp heterostructures of halide perovskites has not yet been achieved, owing to their high intrinsic ion mobility and their poor chemical stability. Therefore, understanding the origins of this instability and identifying effective approaches to suppress ion diffusion are of great importance. In this talk I will present an effective strategy to substantially inhibit in-plane ion diffusion in two-dimensional halide perovskites by incorporating rigid π-conjugated organic ligands. Highly stable and tunable lateral and vertical epitaxial heterostructures, multiheterostructures and superlattices will be demonstrated. Furthermore, using these 2D heterostructures as a new platform, I will present our recent efforts in 1) quantitatively understanding the anion inter-diffusions and migrations, and 2) controlling and manipulating exciton transport and light-emission in halide perovskites.
2.1-I1
Metal halide perovskites are emerging materials for optoelectronics due to their excellent optoelectronic properties, such as direct and tunable band gaps, large absorption cross section, long lifetimes and diffusion paths of the charge carriers, coupled to an elevated defects tolerance.[1] The band gaps and the exciton binding energies of these materials can be tuned by varying the chemical composition of the inorganics or by reducing dimensionality by introducing large cations. Interestingly, reduction in dimensionality leads to the emergence of novel optical features, such as the sub-gap broad emission (BE) in 2D perovskites, whose origin is hotly debated.[2] Contrasting hypotheses assign BEs to the recombination of intrinsic self-trapped excitons (STEs) or to emission from native defects.[3-4]
In this presentation the defect chemistry and photophysics of PEA2MX4 (PEA=phenethylammonium; M=Pb, Sn; X=I, Br, Cl) <100> 2D perovskites is discussed by a computational perspective, in order to provide a microscopic picture of the defects processes taking place in these quantum confined materials. The trends in the defects chemistry moving from 3D to 2D and the effects of the metal by replacing lead with tin will be discussed. Hence, by comparing predictions with experiments, the diverse hypothesis about the origin of the BE in this class of materials will be analyzed. DFT results show that sensibly lower defect densities are expected in 2D perovskites compared to 3D analogues, but a deepening of defect charge transitions in the band gap is reported due to QC. Despite the low calculated defect densities, emission from halide vacancies is compatible with the experimentally observed sub-gap features in the relatively large set of compounds considered in the study, suggesting that the density of these optically active centers is modulated by the crystallization kinetics. On the other hand, the simulation of STEs indicates that the self-trapping of holes and electrons is a feasible process only in the wide band gap Br- and Cl-based 2D perovskites, even though the process is thermodynamically hindered by the shallow nature of the transition.[5]
Our work provides useful insights into the intrinsic and extrinsic mechanisms generating BEs in 2D perovskites and it demonstrates that a control of the crystallization process, e.g. by tuning PEA-halide stoichiometry in the precursors, is key to selectively control the optical features of these materials.
2.1-I2
Ferry Prins is a tenure-Track Group leader at the Condesed Matter Physics Center (IFIMAC) of the Universidad Autonoma de Madrid. Ferry obtained an MSc in Chemistry from Leiden University (2007) and a PhD in Physics from the Kavli Institute of Nanoscience at Delft University of Technology (2011). After completion of his PhD, he joined the the group of Prof. Will Tisdale at Massachusetts Institute of Technology (MIT). There, he started exploring the optical properties of nanomaterial assemblies with an emphasis on excitonic energy-transfer interactions. In 2014 he moved to ETH Zurich for a postdoc with Prof. David Norris at the Optical Materials Engineering Laboratory. With support from the Swiss National Science Foundation, he started an independent group at ETH in 2015. In Spring 2017 he joined he Condensed Matter Physics Center (IFIMAC) at the Autonoma University of Madrid where he directs the Photonic Nanomaterials and Devices Lab. His group specializes in the development of light-management strategies for semiconductor nanomaterials.
2D perovskites have emerged as more stable analogues for photovoltaic applications as their 3D counterparts. However, in contrast to the free charge carriers in 3D perovskites, the confined space of the layered 2D perovskites leads to the formation of excitonic excited states. To harvest the energy of the excitonic excited state in a solar cell, transport of the excitons to charge-separating interfaces is required. Understanding the transport of excitons in 2D perovskites is therefore a crucial step for the development of perovskite photovoltaics containing 2D phases which benefit from both improved stability and maximized efficiency.
In this talk I will present our recent efforts to visualize exciton diffusion dynamics in a variety of 2D perovskite materials using Transient Photoluminescence Microscopy (TPLM). [1-4] TPLM combines diffraction limited excitation with time and spatially resolved detection of excitonic emission to reconstruct a movie-like representation of exciton transport with sub-nanosecond and few-nanometer resolution. Using this technique, a number of important structure-property relationships for exciton transport in 2D perovskites have been revealed, including the importance of lattice rigidity [1], the influence of trap states [3] and halide mixing [4], as well as the influence of doping strategies. I will highlight the importance of our use of Brownian dynamics simulations to explain the various anomalous transport regimes that can be encountered in these materials.
2.1-I3
In recent years, the interest in 2D materials and low-dimensional perovskites (LDP) as building blocks for photovoltaic devices has flourished. This is due to their chemical and electronic properties, which enable them to minimise performance losses and improve the stability of the devices. In this talk, it is presented an innovative strategy to improve the performances of inverted perovskite solar cells (PSC) by incorporating high-quality graphene flakes (GF) in the fullerene-based electron transporting layer (ETL) [1]. It has been proved that the so-formed ETL can be processed as a composite (Graphene:Fullerene) ultra-thin film atop the perovskite, reducing the defect-mediated recombination while creating preferential paths for the extraction of the electrons towards the current collector. Moreover, the properties and the synthesis of LDP based on thiophene cations will be discussed. At first, it will be shown how to control the properties of LDP: the optical and structural ones, and the segregation of different phases upon the modification of the halide composition. Indeed, the rational substitution of halides led to a spatial distribution of phases in the thin film, where the minor phase was responsible for the light emission while the major one gave a human-eye transparent appearance to the material [2]. Lastly, it will be discussed the implementation of this LDP in highly efficient PSC, giving rise to multidimensional 2D/3D perovskite heterostructure by means of two different passivation approaches.
[1] A.Zanetta, I.Bulfaro, F.Faini, M.Manzi, G.Pica, M.De Bastiani, S.Bellani, M.I.Zappia, G.Bianca, L.Gabatel, J.K.Panda, A.E.Del Rio Castillo, M.Prato, S.Lauciello, F.Bonaccorso, G.Grancini; Journal of Materials Chemistry A, 2023.
[2] A.Zanetta, Z.Andaji‐Garmaroudi, V.Pirota, G.Pica, F.U.Kosasih, L.Gouda, K.Frohna, C.Ducati, F.Doria, S.D.Stranks, G.Grancini; Advanced Materials, 34 (1), 2105942.
2.2-I1
Maria Antonietta Loi studied physics at the University of Cagliari in Italy where she received the PhD in 2001. In the same year she joined the Linz Institute for Organic Solar cells, of the University of Linz, Austria as a post doctoral fellow. Later she worked as researcher at the Institute for Nanostructured Materials of the Italian National Research Council in Bologna Italy. In 2006 she became assistant professor and Rosalind Franklin Fellow at the Zernike Institute for Advanced Materials of the University of Groningen, The Netherlands. She is now full professor in the same institution and chair of the Photophysics and OptoElectronics group. She has published more than 130 peer review articles in photophysics and optoelectronics of nanomaterials. In 2012 she has received an ERC starting grant.
Metal halide perovskites are hot contenders as next generation of light emitters. Bright and colour-pure light-emitting diodes (LEDs) were demonstrated based on bulk 3D, nanocrystals, and quasi-2D structures (Ruddlesden-Popper phases) with targeted compositions that allow for accessing different spectral regions. Green and red LEDs have reached external quantum efficiencies exceeding 20%, but success in the blue spectral region has so far been limited.
Here we demonstrate blue LEDs with a peak wavelength of 481 nm, a colour purity of up to 88 % (CIE coordinates (0.1092, 0.1738)), an external quantum yield of 5.2 % and a luminance of 8260 cd m-2. These devices are based on quasi-2D PEA2(Cs0.75MA0.25)Pb2Br7, which is cast from solutions containing isopropylammonium (iPAm). The iPAm-modified sample when deposited on a PEDOT:PSS coated substrate, displays an exceptional PLQY as high as 64%. Cross-correlation of the optical and structural investigations indicate that the RP phase is composed of domains of n=3 phases surrounded by higher dimensionality phases, which allow the efficient transport of charge carriers towards the low dimensional domains. Interestingly, the energy transfer of the photoexcitations towards the low dimensional phases is blocked in samples using the iPAm additive, mostly due to the random orientation of very small crystalline domains. These interesting features present in our iPAm-modified system allowed us to fabricate bright blue-emitting PeLEDs with an average wavelength of 483 nm and FWHM of the electroluminescent of 25 nm for our champion device.
Our work demonstrates the great potential to tailor the composition and the structure of thin films based on Ruddlesden-Popper phases to boost performance of optoelectronic devices – specifically for blue-LEDs.
2.2-O1
Low-dimensional perovskite structures are at the forefront of light emitting applications, mainly owing to high carrier and exciton confinement. The ability to tune the emission wavelength through halide alloying and addition of organic cations promoting formation of 2D structure enables fabrication of light emitting diodes (LEDs) with external quantum efficiency comparable with solutions based on organic semiconductors, with the benefit of high colour purity. One of the challenges in the development of perovskite LEDs is their fundamental absorption-related optical characterisation, including assessing the influence of possible processes at the interfaces between electrodes and the active layer compared to the pristine material. Photocurrent spectroscopy remains the standard high-sensitivity technique for probing defect-related absorption and material disorder, applicable but limited to fully contacted devices, using LEDs in reverse as photodetectors. Here we demonstrate a complementary approach of incorporating modulated photocurrent and photothermal deflection spectroscopy (PDS) experiments for characterisation of 2D perovskite LEDs on different fabrication stages: bare perovskite thin films, the same deposited on top of transparent transport layers from the substrate-side (half-devices), and full LED structures. The results confirm that in optimised device preparation arrangements the sandwiched perovskite layer can fully preserve its optical quality, confirmed by quantifying absorption related to sub-gap trap states and sharpness of the absorption edge (the Urbach energy), revealing a crucial information for future development of LEDs.
2.2-O2
Among hybrid perovskites (HP), 2D are playing a major role in many optoelectronics applications including solar cells, thanks also to their stabilizing effect. Despite their huge success, the dynamics of photogenerated carriers in these materials still needs to be fully assessed. In this work we report the result of ultrafast optical spectroscopy measurement in “tandem” configuration, combining transient absorption and time resolved photoluminescence in the same excitation conditions, as already applied to 3D and 2D HP thin films [1]. To get clearer information about intrinsic property, for the first time we apply our technique on single crystals of 2D HP (phenethylammonium (PEA)2PbI4), comparing nonresonant excitation, generating hot carriers, with resonant excitation, directly injecting cold excitons in the material. The results of our measurements show that both in the tihn film and in the crystal the photophysics consists in an initial massive formation of excitons, followed by a spontaneous ultrafast exciton splitting, occuring on a sub ps timescale. The evidences of this phenomenon are shown both in thin film and crystals wiht different excitation condition, eventually at low temperature, demonstrating that is an intrinsic effect. We believe that this results provide a better understanding in the photophysics laying under the exceptional optoelectronic properties of 2D HPs.
2.2-O3
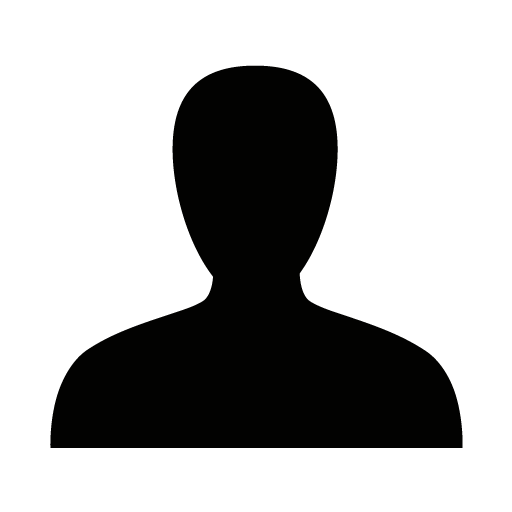
Layered metal-halide perovskites have shown great promise for applications in optoelectronic devices, where a large number of suitable organic cations give the opportunity to tune their structural and optical properties. However, especially for Sn-based perovskites, a detailed understanding of the impact of the cation on the crystalline structure is still missing. By employing two cations, 2,2’-oxybis(ethylammonium) (OBE) and 2,2’-(ethylenedioxy)bis(ethylammonium) (EDBE), we obtain a planar <100> and a corrugated <110>-oriented perovskite, respectively, where the hydrogen bonding between the EDBE cations stabilises the corrugated structure. We will show that OBESnI4 exhibits a relatively narrow band gap and photoluminescence bands compared to EDBESnI4. An in-depth analysis shows that the markedly different optical properties of the two compounds have an extrinsic origin. Interestingly, thin films of OBESnI4 can be obtained both in black and red colours. This effect is attributed to a second crystalline phase that can be obtained by processing the thin films at 100 °C. Our work highlights that the design of the crystal structure as obtained by ligand chemistry can be used to obtain the desired optical properties, whereas thin film engineering can result in multiple crystalline phases unique to Sn-based perovskites.
1.1-I1
He studied electrical engineering in Stuttgart and started working on Si solar cells in 2004 under the guidance of Uwe Rau at the Institute for Physical Electronics (ipe) in Stuttgart. After finishing his undergraduate studies in 2006, he continued working with Uwe Rau first in Stuttgart and later in Juelich on simulations and electroluminescence spectroscopy of solar cells. After finishing his PhD in 2009 and 1.5 years of postdoc work in Juelich, Thomas Kirchartz started a three year fellowship at Imperial College London working on recombination mechanisms in organic solar cells with Jenny Nelson. In 2013, he returned to Germany and accepted a position as head of a new activity on hybrid and organic solar cells in Juelich and simultaneously as Professor for Photovoltaics with Nanostructured Materials in the department of Electrical Engineering and Information Technology at the University Duisburg-Essen. Kirchartz has published >100 isi-listed papers, has co-edited one book on characterization of thin-film solar cells whose second edition was published in 2016 and currently has an h-index of 38.
In order to further improve the efficiencies and in particular the open-circuit voltages of perovskite solar cells it is important to understand non-radiative recombination and their relation to defect densities. Here, the charge-carrier lifetime is frequently measured using transient spectroscopic techniques to provide an assay of charge carrier recombination. Frequently, the expectation is that at sufficiently low excitation conditions the experimentally observed lifetime should be a constant value that scales inversely with the defect density. We show that in (our) triple-cation perovskite films, layer stacks and devices, recombination is actually dominated by shallow defects, implying that the lifetime becomes a quantity that is continuously changing with carrier density. We thereby show that (i) it is not the low density of deep defects that limits the performance in typically triple-cation perovskites but a quite high density of shallow defects. Furthermore, we show that lifetime cannot be used anymore as a figure of merit if it is not plotted vs. carrier density, Fermi level splitting or voltage [1].
1.1-I2
Dr. Evelyne Knapp is a research associate at the Institute of Computational Physics at the Zurich University of Applied Sciences in Winterthur, Switzerland. She holds a Diploma and Ph.D. degree in Computational Science and Engineering from ETH Zurich.
For the continuous improvement of optoelectronic devices such as organic light-emitting diodes and solar cells in terms of e.g. stability and efficiency, a comprehensive model including all major physical processes is crucial. Further, the combined analysis of measurement and simulation paves the way for the understanding of novel devices such as perovskite solar cells. In this work, an up-scaled R&D solar cell is investigated. Once a model and material & device parameter set describing the cell is found, the optimization of the cell performance can start. The initial challenge, however, is to determine such a set of parameters since the availability of e.g. material parameters is limited and is usually obtained by tailored measurements or taken from literature. The values for certain material parameters can vary depending on the literature source or the sequence of layers in the experimental stack. A way to determine the parameters is fitting. In least-square algorithms the sum of the squared differences between the measurement and simulation is minimized by varying the material parameters until an optimal set is found. This task however can be cumbersome, especially with a large number of unknown parameters. Often the parameters are also correlated which again increases the complexity of the problem. In such situations, domain knowledge is required to facilitate the search for the minimal error.
In this contribution, we discuss traditional and machine-learning assisted approaches [1] to determine the model parameters for perovskite solar cells based on electroluminescence images and current-voltage measurements.
1.1-I3
Organic semiconductor-based photovoltaic (OPV) devices have many advantageous properties which makes them attractive for future energy harvesting technologies [1]. Owing to the low charge carrier mobilities in OPVs, a substantial built-in voltage is generally required within the active layer to ensure efficient charge carrier extraction [2,3]. Nevertheless, reliable methods to determine the built-in voltage in thin film OPVs are currently lacking. Standard Mott-Schottky analysis of capacitance-voltage (C-V) characteristics has been frequently used in the past to determine the built-in voltage in organic semiconductor diode devices. However, since OPVs are typically dominated by contact-induced background carriers with highly non-uniform charge distributions [4], this technique is generally not applicable for thin film OPVs [5]. In this work, we present an alternative method based on C-V characteristics, which accounts for the influence of injected carriers. Guided by device simulations, we derive a theoretical framework which describes the relationship between the capacitance and the built-in voltage in thin diode devices. We further use numerical drift-diffusion simulations to validate the theoretical framework. Finally, we substantiate the method experimentally on organic solar cells. Based on these findings, we clarify the role of ohmic contacts and the meaning of the built-in voltage in OPVs and related devices.
1.1-O1
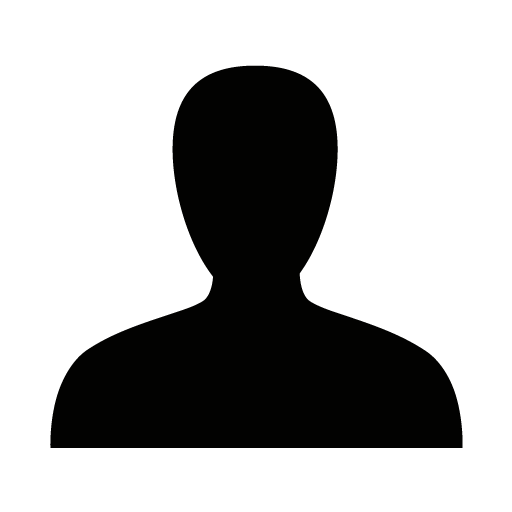
Halide perovskite semiconductors have captured significant research attention for application in optoelectronic devices, in particular solar cells, due to their outstanding properties and straightforward material synthesis. Amongst these favourable properties, the seemingly “slow” cooling of hot (nonequilibrium, high energy) carriers—which distinguishes halide perovskites as candidate materials for the light-absorbing layer in hot carrier solar architectures—is the subject of an increasing share of the community’s focus. The dynamics of hot carriers are commonly studied experimentally via transient absorption spectroscopy (TA), which allows for observation of the cooling of hot carriers following photoexcitation by an ultrashort laser pulse, and the advanced two-dimensional electronic spectroscopy technique (2DES), which can resolve shorter timescales and, thus, additionally enables scrutiny of carrier thermalization. These techniques are powerful, but there is generally a limit to the strength of the conclusions that can be drawn concerning the physical processes underlying the carrier dynamics in the probed material by virtue of these experimental measurements alone. Consequently, the origin of observations of long hot carrier cooling times in halide perovskite materials remains debated, despite the increase in traction of the “hot phonon bottleneck” hypothesis. Here, we present semiclassical simulation of semiconductor charge carrier dynamics—using the ensemble Monte Carlo method to solve the Boltzmann transport equation for non-equilibrium carrier distributions—as a valuable partner to experiment in elucidating the transient carrier dynamics in halide perovskites and other semiconductors. We include results from such simulations and draw qualitative inferences about the carrier dynamics in halide perovskites, also highlighting the care that must be taken in analysing TA experiments. We discuss remaining challenges and opportunities to develop our approach to allow experimental measurements to be reproduced.
1.1-O2
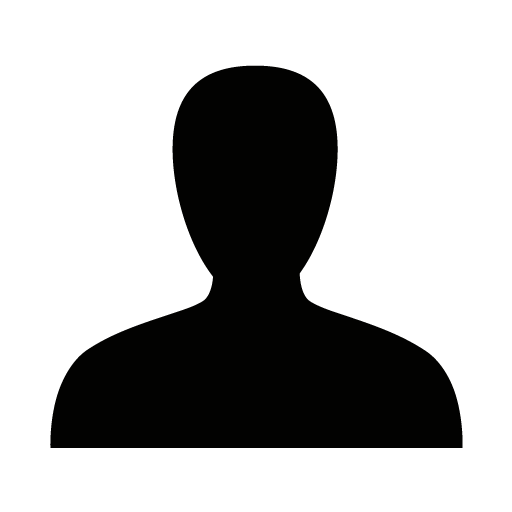
Semitransparent organic solar cells (STOSC) exhibit promising applications as power-generating windows in buildings and agricultural greenhouses. Due to their widely adjustable optical band gap, organic semiconducting materials have the potential to efficiently utilize near infrared light while maintaining semitransparency in the visible light range. One challenge in improving the overall performance of STOSCs is maximizing both power conversion efficiency (PCE) and average visible transmittance (AVT) simultaneously, since intrinsically they are often in a trade-off relationship.[1] A key factor in optimizing both values, is choosing the right material for each intermediate layer of the cell. One well-established material used for electrodes in semitransparent cells is Indium tin oxide (ITO). Although known for its high transparency and good conductivity, it is considered brittle, and its production is cost- and energy-intensive. Furthermore, Indium is a metal that rarely occurs in nature.[2] Lastly ITO exhibits a comparably low reflection in the infrared region.[3] One alternative for these problems is using multiple interlayers of silver and Al-doped ZnO (AZO) as a distributed Bragg reflector on the backside of the solar cell. By varying the layer thicknesses, the optical properties of this electrode can be modified to maximize reflection in the infrared range. This way the current generation of the photoactive layer can be improved while simultaneously maintaining a high transparency for the cell.
Herein we report recent progress in ITO-free semitransparent organic solar cells by implementing a multilayer silver back electrode as an infrared mirror, achieving a power conversion efficiency of 10.2% for a cell stack based on PV-X Plus with an average visible transmittance of 33.1%. Both optical modelling and experimental findings were used to maximize the photocurrent generation and the average visible transmission of the solar cell. The back electrode consists of alternating layers of Al-doped ZnO and thin silver. As the reflected part of the light passes the photoactive layer a second time, an increase in absorption is achieved. Optical simulations show that the distance between the two silver layers has a strong influence on the wavelength range of the reflected light and thus determines the generation of the photocurrent in the cell. By optimizing the spacing between the two silver layers, it was possible to increase the photocurrent for a solar cell based on PV-X Plus by 9.8% (from 19.3 mA/cm2 to 21.2 mA/cm2). At the same time, it was found that the fill factor decreases with an increase in the thickness of the interlayer. When using a single silver layer of 14 nm, the fill factor could be kept reasonably high (71.3%) that despite a comparably lower current generation (17.0 mA/cm2) a LUE (PCE x AVT) of 3.38% was achieved.
2.1-I1
Thermally Activated Delayed Fluorescence (TADF) process has appeared as the most popular design strategy towards reaching 100% internal quantum efficiency for Organic Light-Emitting Diodes (OLEDs). TADF consists in promoting upconversion of triplet excited states into emissive singlet ones through Reverse InterSystem Crossing (RISC), a process driven by spin-orbit coupling (SOC) and requiring a small singlet-triplet gap dEST. The advancement of the TADF field occurred essentially through materials design, the first strategy, as proposed by C. Adachi and co, consisting in connecting electron donating and accepting units to decrease the dEST. However, in doing so, the lower-lying singlet and triplet excited states bear a dominant charge transfer character that translates into a broad emission spectrum.
In this contribution, we will discuss based on computational considerations how doped triangle-shaped molecules can lead to (i) concomitant narrow emission, high quantum yield of emission and small dEST resulting in a whole new generation of TADF emitters, the multi-resonant TADF emitters and to (ii) a new family of compounds with an inverted singlet-triplet gap and potentially, a downwards energy RISC. To do so, we rely on high level quantum chemical calculations and show that an accurate description of electron correlation effects is key to correctly predict the excited states ordering as well as the optical properties of these compounds.
2.1-I2
1993-1997 PhD, U Texas at Austin 1997-1998 Postdoc, Yale U 1998-2010 Prof, U Washington at Seattle 2010-2014 Prof, U Rochester, NY 2014-current Prof. U. Southern California
Excited state dynamics play key roles in numerous molecular and nanoscale materials designed for energy conversion. Controlling these far-from-equilibrium processes and steering them in desired directions require understanding of material’s dynamical response on the nanometer scale and with fine time resolution. We couple real-time time-dependent density functional theory for the evolution of electrons with non-adiabatic molecular dynamics for atomic motions to model such non-equilibrium response in the time-domain and at the atomistic level. The talk will introduce the simulation methodology [1] and discuss several exciting applications among the broad variety of systems and processes studied in our group [2,3], including metal halide perovskites, transition metal dichalcogenides, semiconducting and metallic quantum dots, metallic and semiconducting films, polymers, molecular crystals, graphene, carbon nanotubes, etc. Photo-induced charge and energy transfer, plasmonic excitations, Auger-type processes, energy losses and charge recombination create many challenges due to qualitative differences between molecular and periodic, and organic and inorganic matter. Our simulations provide a unifying description of quantum dynamics on the nanoscale, characterize the timescales and branching ratios of competing processes, resolve debated issues, and generate theoretical guidelines for development of novel systems.
2.1-I3
Shuxia Tao is a compuational materials scientist and she studies how photons, electrons and ions interact with each other and how such interactions determine the formation, function and degradation of materials. Currently, she leads the Computational Materials Physics group at the department of Applied Physics, Eindhoven University of Technology, the Netherlands.
Tao's group focuses on multiscale modelling of energy and optoelectronic materials, studying the growth of nanomaterials and developing theory of light-matter interactions. The ultimate goal is perfecting the quality of these materials and maximizing their efficiency for converting and storing energy and information. Her recent contribution to PV materials focuses on halide perovskites, where she made important contribution in the understanding of the electronic structure, the defect chemistry/physics and the nucleation and growth of halide perovskites. Recently, she also expanded the research to the interactions of perovskites with other contact materials in devices and novel optoelectronic properties, such as optical chirality and chiral induced spin selevetivity.
Perovskites is a class of materials with notable crystal structure of ABX3, exceptionally wide tunability in chemical compositions and dimensionality in crystal-structures. Due to their exceptional optoelectronic properties, they are widely used for converting and storing (solar) energy, e.g. oxide perovskites as photocatalysts, halide perovskites as absorbers in solar cells, nitride perovskites as
mechanical energy harvesters. In these applications, the understanding of optoelectronic properties, chemical stability and their changes upon external stimuli (light excitation, mechanical, thermal and chemical stress) are paramount.
In this talk, I will show how our research group investigate these properties using atomistic multiscale modelling by combing electronic structure calculations with reactive molecular dynamics simulations. Her focus is on halide perovskites and the impact of defects on the efficiency and the stability of perovskite solar cells. We identify harmful defects which lead to either recombination losses and/or chemical degradations and show several strategies to mitigate and passivate these defects. These include engineering the composition of perovskite absorbers, optimizing interfaces with contact materials, and finetuning growth conditions. The atomistic insights provide a basis for further improving the efficiency and stability of perovskite materials and devices. The multiscale computational framework can be enhanced with The emerging data-driven approach and straightforwardly applied to other materials and applications.
2.2-I1
In this talk, I will discuss the employment of state-of-the-art electronic structure theory for the modelling of novel perovskite-like materials within the Ag/Bi double salt and the vacancy ordered double perovskite (VODP) lattices. I will first discuss the case of halide double perovskites, and explore the link between the type and position of the atomic orbitals in the crystal and their electronic structure, and connect this established structure with the so-called rudorffites or Ag/Bi double salts. I will demonstrate the necessity of proper modelling of these, and present a symmetry-based method we developed [1], which correctly describes the exhibited electronic and optical properties of AgBiI4, a prototypical material. I will employ this to probe other materials within the same family of double-salts, and further use the model to investigate the ideal (i.e., maximum limits) photovoltaic properties that these materials could achieve. In the second part, I will thoroughly analyze the properties of the VODP family of materials by employing state-of-art ab initio calculations and unveil key details of the electronic structure and the effects of electron-hole coupling on the optical properties [2]. I will discuss prototypical VODP structures by selecting members based on the electronic configuration of the tetravalent metal at the B-site (e.g. Sn, Te, Zr). I will go through their structural properties, and show that the size of the vacant site, can be tuned solely by the halogens. The electronic structures are investigated within the GW many-body green’s function method, and we obtain band-gaps spanning a range of 1.5-5.0 eV at G0W0. More importantly, the optical and excitonic properties of these compounds are probed within the independent particle approach, and by solving the Bethe-Salpeter equation (BSE). The exciton binding energies and dark-bright exciton exchange splitting are calculated for each type of VODP. Finally, the excitonic fine structure is unveiled by performing a complete symmetry analysis of the compound’s band structure and excitonic wavefunctions, on which a direct link between these and the metal site species is established. Overall, here I comment on the suitability and the prospect of the application in opto-electronics of Ag/Bi double salts, like AgBiI4, and VODP, like Cs2TeBr6, might exhibit. I will identify the most and least promising materials that could act as photo-active materials or for selective charge transport layers, for light-emitting and solar-cell applications.
2.2-I2
Lead halide perovskites have revolutionized the scenario of photovoltaics due to their excellent and tunable optoelectronic properties leading to solar cell efficiencies comparable to traditional high-quality silicon materials.[1] Issues concerning the presence of lead, however, have led researchers to find less toxic candidates possibly replacing lead in the perovskite, particularly tin. The performance of tin halide perovskites, however, is strongly limited by an enhanced self p-doping and the easy oxidation of Sn(II) to Sn(IV).[2] To guide the development of lead-free materials withenhanced efficiency and stability, a deep understanding of the defect chemistry and photophysics of these materials is needed. Ab-initio simulations represent a powerful tool to investigate defect properties and processes at the atomistic level. In this presentation a theoretical tour of the defect activity in lead- and tin-based perovskites is provided. We will show how DFT calculations may be applied to study defects in materials, the useful quantities obtained by simulations and the best practices in the case of metal halide perovskites. Hence, the trend in defect activity by moving from lead to tin will be discussed.[3] The nature of most abundant defects, their trapping activity and the impact on the doping of the materials will be illustrated. Defect driven mechanisms possibly activating the degradation of these perovskites will be discussed based on the defect analysis.[4] Furthermore, the effects of quantum confinement on the optoelectronic features and defect properties moving to low dimensional 2D perovskites will be showed, also in relation to emission features emerging in these quantum confined systems, such as broad emission, whose origin is hotly debated.[5] This work aims to provide a unified picture of defect chemistry of metal halide perovskites with emphasis on the factors governing the stability and the efficiency of these materials. It also aims to stimulate the community to a synergistic use of computer simulations to support the design of more
efficient and long-term stable devices.
2.3-I1
Device modeling and energy yield (EY) calculations are essential tools to optimize solar cell architectures. Device modeling through opto-electrical simulations allows to analyze device performance under standard conditions. In perovskite solar cells (PSC); the energy alignment at the interfaces, ion migration and potential distribution along the device play a critical role on device performance. To investigate them, we have characterized horizontal PSC microstructures[1]. We were able to draw the potential distribution along the solar cell structure by coupling X‐ray photoelectron spectroscopy (XPS) and drift-diffusion modeling[1]. Furthermore, in this work we considered the role of ion migration for the analysis of the band device structure. On the other hand, EY calculations estimate the total output generated energy of a solar cell after one year in a specific place. EY calculations also allow the analysis of device stability which is highly affected by device temperature[2]. Therefore, it is crucial to determine accurately the device temperature. To estimate cell temperature, we propose a thermal model which is a function of device parameters, environmental variables, and is strongly linked with the experimental optical-electrical-thermal performance[2]. We studied the effect of realistic temperature conditions on the performance of PSCs and their transient response to environmental external changes using a theoretical-experimental combined approach. Linking the experimental results and our model, we were able to evaluate the most sensible device layers that increment device temperature affecting device stability.
2.3-I2
Juan Bisquert (pHD Universitat de València, 1991) is a Professor of applied physics at Universitat Jaume I de Castelló, Spain. He is the director of the Institute of Advanced Materials at UJI. He authored 360 peer reviewed papers, and a series of books including . Physics of Solar Cells: Perovskites, Organics, and Photovoltaics Fundamentals (CRC Press). His h-index 95, and is currently a Senior Editor of the Journal of Physical Chemistry Letters. He conducts experimental and theoretical research on materials and devices for production and storage of clean energies. His main topics of interest are materials and processes in perovskite solar cells and solar fuel production. He has developed the application of measurement techniques and physical modeling of nanostructured energy devices, that relate the device operation with the elementary steps that take place at the nanoscale dimension: charge transfer, carrier transport, chemical reaction, etc., especially in the field of impedance spectroscopy, as well as general device models. He has been distinguished in the 2014-2019 list of ISI Highly Cited Researchers.
The dynamic response of metal halide perovskite devices shows a variety of physical responses that need to be understood and classified for enhancing the performance and stability and for identifying physical behaviours that may lead to developing new applications. Beyond the well-established characteristics of regular impedance arcs, we address the appearance of inductor effect at high voltage in perovskite solar cell. We present a physical model in terms of delayed recombination current that explains the evolution of impedance spectra and the evolution of current-voltage curves. A multitude of chemical, biological, and material systems present an inductive behavior that is not electromagnetic in origin. Here, it is termed a chemical inductor. We show that the structure of the chemical inductor consists of a two-dimensional system that couples a fast conduction mode and a slowing down element. Therefore, it is generally defined in dynamical terms rather than by a specific physicochemical mechanism. The impedance spectra announce the type of hysteresis, either regular for capacitive response or inverted hysteresis for inductive response. We apply the dynamic picture based on a few neuron-like equations to the characterization of halide perovskite memristors. Memristors show an intense hysteresis that can be characterized in terms of the emergence of inductive components. We address the characterization of electron diffusion and radiative emission in halide perovskites using a range of light stimulated techniques as IMPS, IMVS, and voltage controlled light emission technique (LEVS).
2.3-O1
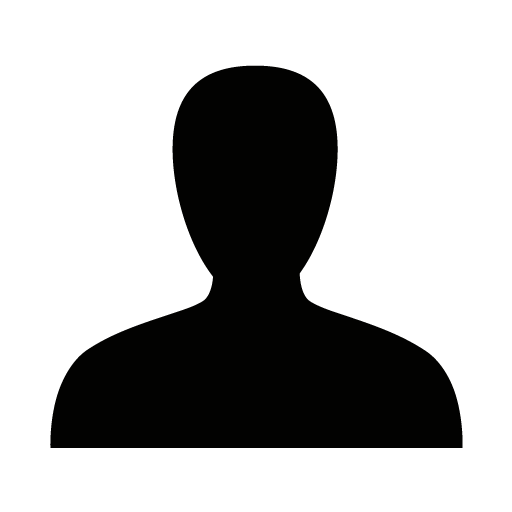
Laser-induced transient grating (LITG) spectroscopy has been used to measure the carrier transport properties of perovskite materials and devices, including the diffusion coefficient and carrier lifetime [1]. These measurements are important for understanding the electronic properties of materials and how they can be optimized for different applications. LITG has the advantage of being a non-destructive method, which can be important for protecting the integrity of the material during measurement.
LITG method itself was realized several decades ago [2, 3]. The principle scheme of a LITG measurement is depicted in Fig.1. A pair of ultrashort pulses are overlapped both spatially and temporally to create an interference pattern on the sample – a transient grating with a spatial period Λ that depends on the pump wavelength and the beam intersection angle. Excitation by periodic pattern excites a spatially-modulated carrier distribution and, effectively, a periodic modulation of the refractive index, thus, diffracting the delayed probe beam.
Over time, the laser-induced grating decays due to carrier recombination (electronic decay with the rate of τR) and carrier diffusion (spatial decay with the rate of τD). The diffusion term depends on the transient grating period: fine gratings diffuse faster than coarser ones. Accordingly, if we measure the temporal behavior of the diffracted signal over a series of different periods Λ, we can determine the carrier diffusion coefficient D [2].
The device presented in this paper allows automated continuous tuning of the excitation grating period Λ and selection of excitation wavelength from 340 to 560 nm, which allows measuring carrier diffusion in the range from 0.1 to 50 cm2/s and carrier lifetime in the range from 1 ps to ca. 80 ns.
Exemplary carrier diffusion and lifetime measurements were performed with a thin film of metal-halide perovskite Cs0.05(FA0.85MA0.15)Pb(I0.85Br0.15)3 with Et2NH additive in a wide range of excitation densities. The results showed that the charge carriers of the material are in a trap-filling and de-trapping diffusion regime, from which carriers easily escape with increasing excitation density.
The extensive experience in optomechanical engineering and high-level automation enabled the all-optical measurement for carrier diffusion and carrier lifetime. It was extensively tested with different samples, including but not limited to the aforementioned perovskites. The results will be presented in detail during the conference.
2.3-O2
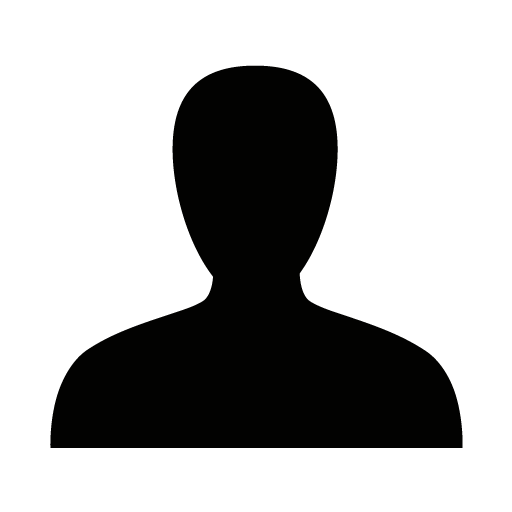
The photovoltaic (PV) effect provides the most efficient means of converting the overwhelming amount of freely available energy from the sun (ca. 1000 times greater than the total energy consumption worldwide) into electricity. Emerging PV technologies based on materials such as organic, transparent oxides, kesterites, quantum dots and hybrid halide perovskites are increasingly gaining in importance nowadays.[1] Within the emerging PV technologies, many of the breakthroughs achieved in the power conversion efficiency and in device lifetime have been obtained through intensive materials research.[1, 2, 3] Current trends in PV research focus on the search for higher efficiencies through multi-junction concept and the expansion of the range of applications beyond standard solar farms. In this context, material screening and characterization requires many different pieces of equipment (one solar simulator, another light source for indoor, another set for EQE measurements, etc.). In cases like lateral tandem devices, the required setups are simply not commercially available.
We report a multi-purpose spectrum-on-demand light source (SOLS), conceived, primarily, but not exclusively, for the multiple and advanced characterization of photovoltaic (PV) materials and devices. The apparatus is a spectral shaper illumination device, providing a tunable, spectrally shaped and focused light beam, modulated in intensity and/or in a wavelength range with respect to a primary light source. SOLS stands out from the state of the art because it produces almost any spectrum on demand and delivers two types of output: a spectrally shaped and spatially homogeneous beam over its cross section for areal illumination; or a spatially and spectrally split beam into its wavelength components, a unique capability suited to characterize lateral-tandem (Rainbow) solar cells. The tuneability from broadband to narrow band illumination enables two characterization devices into one, namely, a solar simulator for the determination of the power conversion efficiency and an external quantum efficiency measuring system. We expect the SOLS setup to accelerate material screening, enabling the discovery and optimization of novel multi-component materials and devices, in particular, for emergent PV technologies like organic or metal halide perovskites PV, indoors and building integrated PV, agrovoltaics, multi-junction, etc.
1.1-I1
Raffaella Buonsanti obtained her PhD in Nanochemistry in 2010 at the National Nanotechnology Laboratory, University of Salento. Then, she moved to the US where she spent over five years at the Lawrence Berkeley National Laboratory, first as a postdoc and project scientist at the Molecular Foundry and after as a tenure-track staff scientist in the Joint Center for Artificial Photosynthesis. In October 2015 she started as a tenure-track Assistant Professor in the Institute of Chemical Sciences and Engineering at EPFL. She is passionate about materials chemistry, nanocrystals, understanding nucleation and growth mechanisms, energy, chemical transformations.
Over the past 40 years, scientists have learned to synthesize colloidal nanocrystals of different composition with tunable size and shape. These features dictate the properties of these nanomaterials. Thus, their control is crucial for the discovery of phenomena, many of which contribute to technological advances. One example of the societal impact of this class of materials is the use of semiconductor nanocrystals as the active component in displays with the best color purity on the market. A second example is their use as materials platforms to advance catalyst development. Yet, the synthesis of colloidal nanocrystals still proceeds by trial and error. The search for the reaction conditions to obtain the nanocrystals with the desired composition, size and shape is time consuming and can fail in delivering the target product.
In this talk, I will highlight the importance of identifying the reaction intermediates during the formation of colloidal nanocrystals for the development of a more predictive synthesis to these nanomaterials. By discussing specific examples, I will illustrate that the chemical nature of these intermediates is diverse and that state-of-the-art in-situ techniques combined with theory are often needed to capture those transient species during the synthesis of nanocrystals. Nevertheless, I will use concrete examples to demonstrate that such efforts are worth it as the discovered mechanistic pathways pinpoint the critical steps to enhance the tunability of existing materials and to accelerate the discover of new ones.
1.1-I2
Maria Ibáñez was born in La Sénia (Spain). She graduated in physics at the University of Barcelona, where she also obtained her PhD in 2013, under the supervision of Prof. Dr. Cabot and Prof. Dr. Morante. Her PhD thesis was qualified Excellent Cum Laude and awarded with the Honors Doctorate by the University of Barcelona. Her PhD research was funded by a Spanish competitive grant (FPU) which supported her to conduct short-term research stays in cutting-edge laboratories. In particular she worked at CEA Grenoble (2009), the University of Chicago (2010), the California Institute of Technology (2011), the Cornell University (2012) and the Northwestern University (2013). In 2014, she joined the group of Prof. Dr. Kovalenko at ETH Zürich and EMPA as a research fellow where in 2017 she received the Ružička Prize. In September 2018 she became an Assistant Professor (tenure-track) at IST Austria and started the Functional Nanomaterials group.
In the same way metal complexes are converted into well-defined nanoparticles, nanoparticles can be used as tunable precursors capable of evolving into macroscopic solids with specific structural features. A consolidation step is usually required to produce the macroscopic solid from nanoparticles. The consolidation process defines the density and microstructure of the solids, which directly correlate with the electronic, thermal, and mechanical properties of the resulting material. In order to provide the material with densities as close as possible to the respective theoretical density, pressure-assisted sintering techniques are preferred, such as hot pressing or spark plasma sintering.
Characteristics of the particles such as size, shape, composition, and surface chemistry determine the sintering process and therefore dictate densification, grain growth, and materials' final microstructure. Consequently, using carefully-curated nanoparticles provides a unique opportunity to control material microstructure. In our framework, a nanoparticle is a multi-structured system consisting of an inorganic nanocrystalline domain, named the inorganic core, surrounded by surface species. Both the inorganic and surface species are tunable parameters in the design of nanoparticle-based precursors. Herein, we will employ solution-based synthesis procedures to produce precisely defined nanoparticles and explore their surface chemistry to adjust nanoparticles' reactivity during sintering to achieve a solid with specific targeted features.
Two very different approaches to controlling particles' surface chemistry need to be separated. One refers to the particle termination atoms, the other to the connected adsorbates that can be covalently bonded molecules or electrostatically adsorbed ionic groups. In this talk, we will show how surface adsorbates, intentionally or unintentionally introduced, are critical to controlling densification, grain growth, and materials' final microstructure. Furthermore, we will present different surface functionalization strategies that allow defining: atomic defects, grain boundary complexions, crystalline domain size, and the formation of secondary phase inclusions in the macroscopic solid.
Finally, we will discuss the effect of the different structural properties introduced in the macroscopic solids on their electrical and thermal transport properties and evaluate their potential as thermoelectric materials.
1.1-I3
Photocatalytic sheets have disruptive potential in the field of solar hydrogen production from water. Recent demonstrations have shown the feasibility of their safe operation on a large scale.[1] The key challenge is the development of visible light absorbing photocatalytic particles that are efficient and stable for at least one year. In this talk, I will discuss recent efforts in our laboratory towards adapting our thin film semiconductor water splitting materials towards stable, photocatalytic particles. The methods for the synthesis of the particles and particle sheets will be highlighted, as well as the challenges for the stringent stability requirements (in water, under illumination). Proof-of-principle systems involving hydrogen evolution coupled with the oxidation of various organic molecules (so-called "value added oxidations") will be highlighted. Initial efforts with BiVO4 will be discussed, as this is a well-studied particle for the oxygen evolution reaction. Subsequently, copper oxide and antimony selenide-based systems will be discussed.
1.1-I4
PROFESSIONAL EXPERIENCE
Since 2022University of Lincoln (UK)Visiting Professor of Functional Materials
Since 2019BCMaterials (Spain)Ikerbasque Research Professor
2017 – 2019University of Lincoln (UK)Senior Lecturer
2011 – 2017University of Munich (Germany)Junior group leader (Habilitand, Chair: Prof. T. Bein)
ACADEMIC EDUCATION & TRAINING
2009 – 2010Institute Lavoisier Versailles & ENSICAEN (France)Postdoctoral fellow with Prof. G. Férey, Prof. C. Serre, Prof. M. Daturi
2006 – 2009Humboldt-Universität zu Berlin (Germany)Ph.D. thesis with Prof. E. Kemnitz
2001 – 2005Humboldt-Universität zu Berlin (Germany) and University of Glasgow (UK)Master student in Chemistry (Diplom)
FELLOWSHIPS & AWARDS
2022CeNS publication award (Best Interdisciplinary Publication)
2022Visiting Professor of Functional Materials
2020CeNS publication award (Best junior scientist publication)
2019Prize of the City of Poznań and Polish Chemical Society for the most prominent international representatives of science and art
2019Visiting Professor at Adam Mickiewicz University in Poznań
2019CeNS publication award (Best junior scientist publication)
2018CeNS publication award (Best junior scientist publication)
2018Editor Advisory Board of Advanced Functional Materials
2017CeNS publication award (Best junior scientist publication)
2017Publication selection for the first JACS Young Investigator Virtual Issue
2011Feodor Lynen-return research fellowship
2009Feodor Lynen-postoctoral fellowship
2008Young Scientist Award (14th International Congress on Catalysis)
2005Socrates-Erasmus Scholarship
The chemistry of metal-organic and covalent organic frameworks (MOFs and COFs) is perhaps the most diverse and inclusive among the chemical sciences, and yet it can be radically expanded by blending it with nanotechnology. The result is reticular nanoscience, an area of reticular chemistry that has an immense potential in virtually any technological field.
In this talk, we explore the extension of such an interdisciplinary reach by surveying the explored and unexplored possibilities that framework nanoparticles can offer. We localize these unique nanosized reticular materials at the juncture between the molecular and the macroscopic worlds, and describe the resulting synthetic and analytical chemistry, which is fundamentally different from conventional frameworks. Such differences are mirrored in the properties that reticular nanoparticles exhibit, which we described while referring to the present state-of-the-art and future promising applications in medicine, catalysis, energy-related applications, and sensors. Finally, the bottom-up approach of reticular nanoscience, inspired by nature, is brought to its full extension by introducing the concept of augmented reticular chemistry. Its approach departs from a single-particle scale to reach higher mesoscopic and even macroscopic dimensions, where framework nanoparticles become building units themselves and the resulting super-materials approach new levels of sophistication of structures and properties.
2.1-O1
Aliovalent I-V-VI semiconductor nanocrystals represent promising candidates for non-toxic and earth-abundant thermoelectric and infrared optoelectronic applications. Among them, famatite Cu3SbSe4 material stands out due to high absorption coefficients and narrow band gap in the mid-infrared spectral range. In this presentation we combine experiments and theory calculations to study the effects of composition and defects on optical properties of Cu3SbSe4 nanocrystals. Using fast and modular hot-injection colloidal synthesis approach, we achieve size-uniform and stoichiometric Cu3SbSe4 nanocrystals and study the influence of reaction parameters such as reaction temperature, growth time, and precursor concentrations. While a reduced activity of the Cu precursor induces the formation of Cu-deficient Cu3SbSe4 nanocrystals, an increased anion concentration or a balancing of cation precursor concentrations result in stoichiometric Cu3SbSe4 products with an absorption onset of 0.2 eV. Characterizing the products, we discover a broad solid solution for nanocrystals (nominally, CuxSbSe4, where x is 2.4–3.0), which is significantly larger than for the bulk Cu3SbSe4 phase. Within this CuxSbSe4 solid solution, the optical band gap widens to 0.35 eV as the amount of Cu decreases. Tight-binding simulations point to the existence of large amount of Cu vacancies, which are non-harmful for the optical performance of CuxSbSe4 nanocrystals. In contrast, even a small amount of CuSb and SbCu antisite defects creates intrinsic doping states, which are detrimental for Cu3SbSe4 properties. We affirm tunable band gaps by infrared spectroscopy and observe a good agreement to the theory calculations. We further report the energy-resolved photoelectric response of Cu3SbSe4 nanocrystals and show their excellent resilience towards oxidation. Our results will provide a launch platform for Cu3SbSe4 nanocrystals as one of very few non-toxic alternatives for mid-infrared applications.
2.1-O2
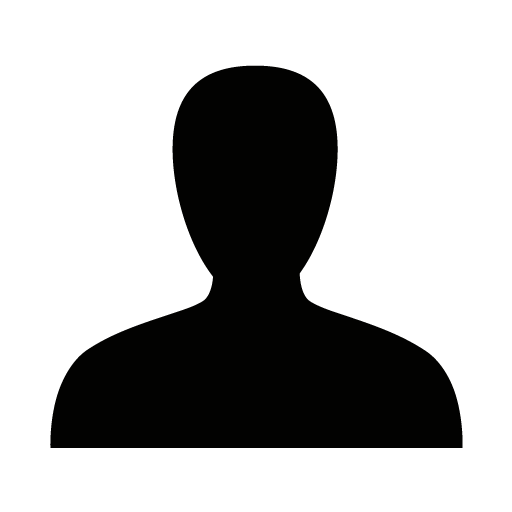
Nickel boride NixBy has shown potential as an efficacious catalyst for a broad range of systems (hydrogenations, hydrogen, and oxygen evolution reactions) under challenging conditions (such as high pH or high temperatures). Preparing the nanoscale analogues of nickel borides in an attractive prospect as the increased surface area and creation of more active sites will enhance their catalytic activity. However, the nanostructure of NixBy remains underdeveloped mainly due to the notoriously difficult and energy demanging synthetic process which is not industrially compatible.[1-3] Recent works have shown that synthetic methods at lower temperatures (<400 ºC) yield amorphous polydisperse nanocrystals (NCs), while phase purity remains an issue at higher temperatures. Here, a simple, scalable synthesis is demonstrated to obtain a phase-pure nanocrystalline NixBy. Through the solid-state reaction of either metallic Ni0 or NiCl2 with NaBH4 at a relatively low temperature (400oC) under atmospheric pressure, crystalline nanosized Ni2B and Ni3B could be obtained with high yield in pure phase and with narrow size distribution (15-30 nm). Through extensive mechanistic studies, we show that Ni nanoclusters (approx. 1 nm) are intermediate in the boriding process, while the halide precursors lower the decomposition temperature of the NaBH4 (used as a reducing agent and B source). We further explore the size control using reaction mediators, and we probe the differential nucleation and growth of Ni (clusters) or NixBy NCs while using L (amine, phosphine) and X-type (carboxylate) mediators. The synthesized Ni3B nanopowder can undergo surface functionalization with inorganic ligands in polar solvents, forming a stable ink. Furthermore, the Ni3B nanocrystals ink can be ligand-exchanged with organic ligands in a non-polar low-boiling point solvent. This work opens the door for the large-scale production of NixBy nanocrystals solution-processable inks to make it a commercially viable alternative catalyst to noble-metals and pave the way for other metal borides colloidal nanostructures.
2.1-O3
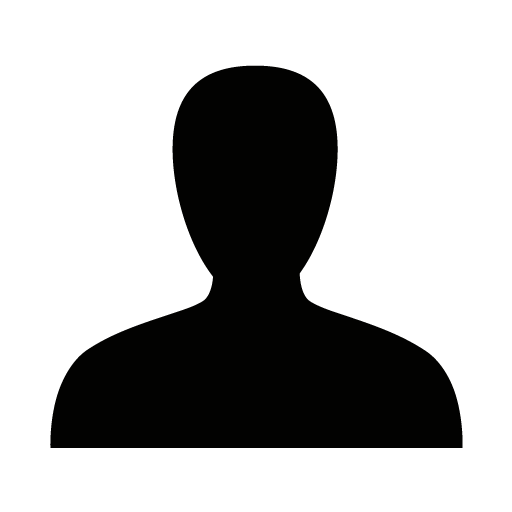
Colloidal semiconductor nanocrystals are opening new routes for a wide range of optoelectronic applications, spanning from lighting to photodetection, as their electronic properties can be tuned by changing their size and shape[1]. Core-shell nanocrystals further widen the tunability of their optoelectronic properties, due to the various energy alignments between the core and shell [2].
In this contribution, we show how the interplay between density functional theory (DFT) simulations and various experimental techniques can provide fundamental insights into the atomistic and electronic structures of core-shell InAs@ZnSe semiconductor nanocrystals. In particular, we carry out a systematic comparison between various structural models simulated with DFT and the results of photoluminescence as well as x-ray diffraction and electron microscopy. This detailed comparison unveils information that is not available to either theory or experiment alone. We demonstrate that the composition of the core-shell structure does not switch abruptly between the core and the shell, but rather displays an In-Zn concentration gradient. Moreover, only by considering atomistic reconstructions on the surface of the nanocrystal as well as on the external core layer is it possible to obtain an electronic structure that matches the experimentally observed optical properties. Finally, we derived general insights to explain the observed boost in the photoluminescence quantum yield when the InAs particles are coated with the ZnSe shell.
2.1-O4
Cardiovascular diseases are the leading cause of death worldwide. New treatments are continuously developed but require an in-depth understanding of cardiovascular morphology. Vascular corrosion casting provides 3D knowledge of anatomical structures by injecting a polymer resin and subsequently removing the surrounding tissue via chemical maceration, i.e. corrosion. [1] This process often leads to deformations or fragmentation of the fragile cast, resulting in a loss of information. In-situ high-resolution computed tomography (micro-CT) scans could provide detailed information on the vascular architecture without corroding the tissue. Unfortunately, distinguishing the polymer cast from the animals’ surrounding soft tissue is impossible due to a lack of CT contrast. To improve this, we introduce hafnium oxide nanocrystals (HfO2 NCs) as contrast agents to the polymer resin. Here we communicate our insights on HfO2 NC synthesis, their surface chemistry and their application as CT contrast agents.
We synthesize 5 – 10 nm HfO2 NCs starting from HfCl4.2THF in benzyl alcohol. Initially identified as a purely nonaqueous sol-gel route [2], we find the in-situ water formation to be responsible for gelation of the reaction mixture prior to particle crystallization. Through mechanistic investigation using in-situ Pair Distribution Function (PDF) analysis, Nuclear Magnetic Resonance (NMR), Extended X-ray Absorption Fine Structure (EXAFS) and rheology measurements we study this rapid precursor-to-gel conversion and subsequent crystallization. Using our new-found insight we gain better reaction control over the reaction and scale-up the synthesis, yielding gram-scale HfO2 NCs with narrow size distribution.
To obtain a stable and homogeneous dispersion of the synthesized NCs in the casting resin, we optimized the particle’s surface chemistry. The ideal ligand is found to be a combination of a strong binding group (phosphonate), while matching the resin’s polarity via its organic tail (ethylene glycol oligomers). We demonstrate that the NCs remain stable during resin curing and homogeneously improve the contrast with concentrations as low as 5 m% of NCs.
Finally, we perform ex-vivo injections of both zebrafish and mouse models with the NC-doped resin and obtain high-quality cast visualization via segmentation of the obtained scans without having to adapt the existing injection methods. We are able to differentiate even µm scale details. This confirms the application potential of HfO2 NCs as CT contrast agents in corrosion casting, while paving the way to obtain full cardiovascular information from casts without the need for tissue corrosion. Our results emphasize that through mechanistic insight and control over the nanocrystal synthesis, combined with an optimized surface chemistry, we can create high quality stable nanocomposites.
2.1-O5
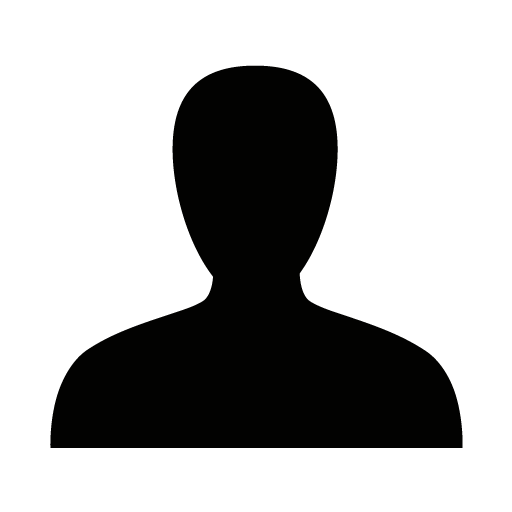
I started studying Chemistry at Ghent University, Faculty of Science, Chemistry Department, in 2015 and got my Master of Science in Chemistry in 2020. At this moment I'm doing a PhD at Physics and Chemistry of Nanostructures (PCN) at Ghent University under supervision of Prof. Dr. Ir. Zeger Hens.
AECs are composed of a IIa metal (Mg, Ca, Sr) and S or Se (X), feature large bandgaps positioned in the UV and are commonly used as hosts for emissive ions. AEC nanocrystals could therefore be used as UV emitters, or scatter-free emitters based on lanthanide ions. Moreover, while most AEC crystallize in the rocksalt structure, MgX can also be grown as zinc blende crystals with lattice parameters that come close to those of commonly examined and used IIb-VI chalcogenides or III-V pnictides, such as CdSe and InP. Hence, AECs could extend the range of materials to form core/shell heterostructures out of these compounds. However, such implementations of AECs are hampered by the limited knowledge of the colloidal synthesis and the surface chemistry of these compounds. Here, we report on possible routes to synthesize these materials and the resulting surface termination of AECs by organic ligands, for which we take the formation of CaS as a starting point. We show that ~12 nm large CaS nanocubes can be formed by reacting calciumacetate and diphenylthiourea in a mixture of oleylamine (OLA), trioctylamine and oleic acid (OA)[1]. Using Nuclear Magnetic Resonance (NMR) and infrared (IR) spectroscopy, we demonstrate that such as-synthesized CaS nanocubes are terminated by a dense shell of oleate ligands, which are unequally packed at the surface, and small traces of OLA. Addition of a carboxylic acid shows the dynamic behavior of this dense shell and induces a slow reorganization of the ligands by breaking up the dense packing and replacing hydroxides at the surface by oleates. Apart from providing detailed insight in the surface chemistry of CaS NCs, this work shows that known approaches and concepts to analyze and rationalize the interaction between colloidal nanocrystals and surface-active ligands can be extended to AEC nanocrystals.
2.1-O6
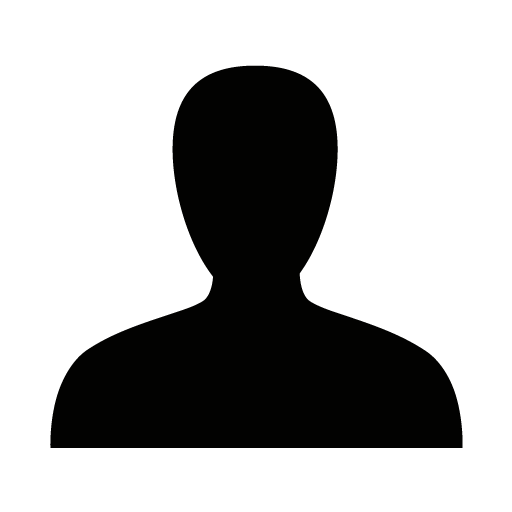
The pulmonary administration of nanomedicines such as viral vaccines is a promising delivery route because it is direct, noninvasive, and it can be used for both systemic delivery and lung targeting. However, the same mechanisms that protect us from pathogens entering via the inhalation route lead to the rapid elimination or degradation of nanomedicines. Most nanomedicines designed for pulmonary administration do not reach clinical practice and this is reflected in the low number of newly approved inhalation drugs.[1] This reality contrasts with the increase in lung diseases due to global ageing and new infectious diseases.[2] Since one of the advantages of pulmonary administration is the direct targeting of the lung, the challenges of improving this route of administration are worthy of being addressed towards overcoming unmet clinical needs. In this context, we will demonstrate that it will be key to study and understand the nano-bio interaction with lung barriers to design better drug nanovectors. To this end, we will give examples of how multifunctional nanoparticles capable of encapsulating drugs and being labeled with contrast agents to perform multiscale studies will be an essential tool to characterize these interactions.[3] We will provide examples of how different coating agents determine lung retention time, clearance by alveolar macrophages, penetration into the lung, etc. Finally, we will show how this may impact the therapeutic efficiency of some of these nanomedicines.
2.2-I1
Maksym Kovalenko has been a tenure-track Assistant Professor of Inorganic Chemistry at ETH Zurich since July 2011 and Associate professor from January 2017. His group is also partially hosted by EMPA (Swiss Federal Laboratories for Materials Science and Technology) to support his highly interdisciplinary research program. He completed graduate studies at Johannes Kepler University Linz (Austria, 2004-2007, with Prof. Wolfgang Heiss), followed by postdoctoral training at the University of Chicago (USA, 2008-2011, with Prof. Dmitri Talapin). His present scientific focus is on the development of new synthesis methods for inorganic nanomaterials, their surface chemistry engineering, and assembly into macroscopically large solids. His ultimate, practical goal is to provide novel inorganic materials for optoelectronics, rechargeable Li-ion batteries, post-Li-battery materials, and catalysis. He is the recipient of an ERC Consolidator Grant 2018, ERC Starting Grant 2012, Ruzicka Preis 2013 and Werner Prize 2016. He is also a Highly Cited Researcher 2018 (by Clarivate Analytics).
Colloidal lead halide perovskite (LHP) nanocrystals (NCs), with bright and spectrally narrow photoluminescence (PL) tunable over the entire visible spectral range, are of immense interest as classical and quantum light sources. Severe challenges LHP NCs form by sub-second fast and hence hard-to-control ionic metathesis reactions, which severely limits the access to size-uniform and shape-regular NCs in the sub-10 nm range. We show that a synthesis path comprising an intricate equilibrium between the precursor (TOPO-PbBr2 complex) and the [PbBr3-] solute for the NC nucleation may circumvent this challenge [1]. This results in a scalable, room-temperature synthesis of monodisperse and isolable CsPbBr3 NCs, size-tunable in the 3-13 nm range. The kinetics of both nucleation and therefrom temporally separated growth are drastically slowed, resulting in total reaction times of up to 30 minutes. The methodology is then extended to FAPbBr3 (FA = formamidinium) and MAPbBr3 (MA = methylammonium), allowing for thorough experimental comparison and modeling of their physical properties under intermediate quantum confinement. In particular, NCs of all these compositions exhibit up to four excitonic transitions in their linear absorption spectra, and we demonstrate that the size-dependent confinement energy for all transitions is independent of the A-site cation. We then show that this synthesis – relying on the labile ligand capping with TOPO-phosphinic acid mixture – makes for a convenient platform for the subsequent surface functionalization with diverse capping ligands [2]. Robust surface functionalization of highly ionic surfaces, as is the case of LHP NCs, has remained a formidable challenge due to the inherently non-covalent weak surface bonding. Leveraging the vast and facile molecular engineering of phospholipids, we present their efficacy as surface capping ligands for LHP NCs. Molecular dynamics simulations and solid-state NMR confirm that the surface affinity of these zwitterionic molecules is primarily governed by the geometric fitness of their anionic and cationic moieties. Judicious selection of the ligands yielded colloidally robust FAPbBr3 and MAPbBr3 NCs and enabled colloids in a variety of solvents, from n-hexane to acetone. Robustness of the surface capping is also reflected in optical properties: NCs exhibit PL quantum yield (QY) above 96% after numerous purifications. NCs are essentially blinking-free at a single particle level.
We will discuss the atomistic details of the ligand surface binding, ranging from conventional alkylammonium ligands [3] to diverse zwitterionic moeities. In particular, we show that solution and solid-state NMR readily captures the surface binding motifs and the impact of the ligands on the surface structure of CsPbBr3 NCs [4].
Q. Akkerman et al. Science 2022, 377, 1406-1412
V. Morad et al. submitted
A. Stelmakh et al. Chem. Mater. 2021, 33, 5962−5973
M. Aebli et al. submitted
2.2-I2
Andreu Cabot received his PhD from the University of Barcelona in 2003. From 2004 to 2007, he worked as a postdoctoral researcher in Prof. A. Paul Alivisatos group in the University of California at Berkeley and the Lawrence Berkeley National Laboratory. In 2009 he joined the Catalonia Institute for Energy Research – IREC, where he is currently ICREA Research Professor. His research interests include the design and preparation of nanomaterials, the characterization of their functional properties and their use in energy technologies.
Colloidal synthesis routes allow precise control over material parameters at the nanometer scale with moderate capital or operating costs and with high-throughput production and material yields. Stimulated by its simplicity and huge potential, countless groups all around the world have developed an extensive library of synthetic strategies and routes to produce nanocrystals with almost any composition, size, and shape. However, for such control over material parameters at the nanoscale to truly impact real applications, colloidal nanocrystals need to be arranged into functional patterns, thin films, porous nanomaterials, or highly dense nanocomposites, depending on the application. Additive manufacturing technologies based on layer-by-layer deposition of material ejected from a nozzle in the form of an ink are well-suited to produce macroscopic structures from colloids but are limited in terms of printing speed and resolution. Electrohydrodynamic (EHD) jetting uniquely allows the generation of submicrometer jets that can reach speeds above 1 m s-1, but such jets cannot be precisely collected by too slow mechanical stages. In this talk, I will present our progress in the control of the jet trajectory in EHD jetting technologies through a voltage applied to electrodes located around the jet. This method allows to continuous adjust the jet trajectory with lateral accelerations up to 106 m s-2. Through electrostatically deflecting the jet, 3D objects with submicrometer features can be printed by stacking material layers on top of each other at layer-by-layer frequencies as high as 2000 Hz. The fast jet speed and large layer-by-layer frequencies achieved translate into printing speed up to 0.5 m s-1 in-plane and 0.4 mm s-1 in the vertical direction, three to four orders of magnitude faster than techniques providing equivalent feature sizes. This technique is applied to the production of electrode materials for energy conversion and storage.
2.2-I3
Epitaxial growth methods usually need dedicated equipment, high energy consumption to maintain pure vacuum conditions and evaporation of source materials, and elevated substrate temperatures. Solution epitaxial growth requires nothing of that but is rarely used because the achieved microstructures are of low quality, not homogeneous, and finally exhibit worse performances in devices. We have introduced several growth methods including ink-jet printing [1], drop-casting [2] or antisolvent-vapor-assisted-crystallization [3] to obtain epitaxial perovskite micro-crystallites whose shapes and sizes can be controlled by the synthetic conditions. These micro-crystallites are not only oriented on the substrates such as mica or PbS single crystals, but also exhibit smooth side walls, which coincide with crystalline facets. Thus, they are acting as optical micro-resonators, supporting lasing under optical excitation. The obtained threshold powers as well as the environmental stability are competitive to those obtained by vapor deposition methods. The solution epitaxial growth can also be performed to obtain closed epitaxial films, instead of arrays of ordered micro-crystals, representing a common goal for epitaxial growth. Thus, solution epitaxy applied to metal-halide perovskites and performed in almost ambient conditions is rivaling with vapor deposition methods, even though it is achieved by cost-effective and simple methods and on cheap and commercially available substrates.
2.3-I1
Prof. Z. Hens received his PhD in applied physics from Ghent University in 2000, worked as a postdoctoral fellow at Utrecht University and was appointed professor at the Ghent University department of inorganic and physical chemistry in 2002. His research concerns the synthesis, processing and characterization of colloidal nanocrystals.
III-V quantum dots are printable semiconductors free of hazardous substances. In this talk, recent progress in III-V QDs synthesis at Ghent University and the device implementation of these materials is discussed. First, we show that the formation of InP-based QDs with near-unity photoluminescence quantum yield across the visible spectrum is now possible. This result is achieved by implementing a core/shell/shell structure, in which the composition of the inner shell is gradually changed from Se-rich to S-rich Zn(Se,S) with decreasing core QD size. We demonstrate that such application grade QDs can be used as color convertors to make on-chip QD-LEDs with +50% colo conversion efficiency. Second, we discuss how rational adaptations to the synthesis of In(As,P) QDs result in one-batch-one-size protocols yielding In(As,P) QD batches with a band-edge absorption up to 1600 nm, and we demonstrate the formation of QD photodiodes sensitive up to 1400 nm from these QDs. Both examples highlight how III-V QDs are evolving from a material for lab-scale proof-of-principle to devices ready of the consumer-market
2.3-I2
Operando characterization techniques are indispensable for understanding the catalysts evolution during operation. Electrochemical liquid-phase transmission electron microscopy (ec-LPTEM) grants the capability of real-time imaging of electrochemically-induced processes in liquid media. Herein, we describe the advancements of ec-LPTEM towards studying the solid-liquid-gas interfacial processes occurring on Co-based oxide oxygen evolving catalysts. By performing real-time measurements, we can associate the potential-dependent variation of the local contrast to the effects that precede the electrocatalytic reaction such as electrowetting and redox-induced reactions. Further, through optimization of the microcell, we report on the direct probing of the evolution of molecular oxygen by operando electron energy loss spectroscopy (EELS). Similar experiments on IrO2 particles indicate the capability to separate the contribution of different components in the EEL spectra, providing qualitative maps of O2 and liquid electrolyte. Our work on catalytic particles exemplifies the crucial role that surface sensitive and electrochemical microcell TEM techniques can play in detailing the mechanism of electrocatalytic processes and can provide a new characterization framework aiding development of novel design routes for targeted nanocatalyst preparation.
2.3-O1
Lead halide perovskites successfully advance towards applications in solar cells, light-emitting devices, and high-energy radiation detectors. Recent progress in understanding their uniqueness highlights the role of optoelectronic tolerance to intrinsic defects, particularly long diffusion lengths of carriers, and highly dynamic 3d inorganic framework. This picture indicates that finding an analogous material among non-group-14 metal halides can be very challenging, if possible at all. On the other hand, a judicious choice of chemistry made it possible to noticeably increase the performance of formamidinium tin iodide perovskites when integrated into thin-film photovoltaic devices. The main challenge with this material originates from the easiness of the trap states generation, which are typically ascribed to the oxidation of Sn(II) to Sn(IV). In this work, we describe the synthesis of colloidal monodisperse FASnI3 NCs, whereby thorough control of the purity and redox chemistry of the precursors allows the concentration of Sn(IV) to be reduced to an insignificant level, to probe the intrinsic structural and optical properties of these NCs. Intrinsic FASnI3 NCs exhibit unusually low absorption coefficients of 4•103 cm-1 at the first excitonic transition, a 190 meV increase of the bandgap as compared to the bulk material, and a lack of excitonic resonances. These features are attributed to a highly disordered lattice, distinct from the bulk FASnI3 as supported by structural characterizations and first-principles calculations.
2.3-O2
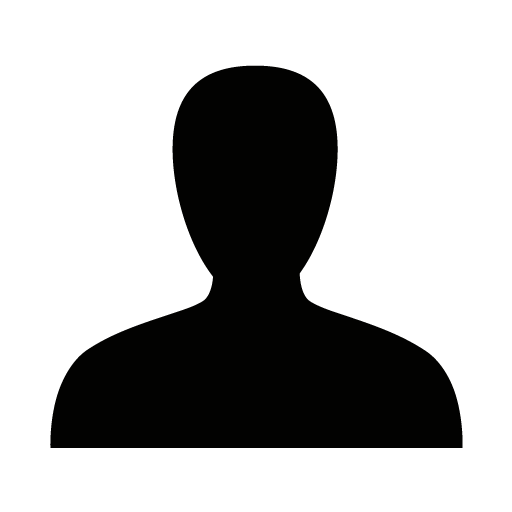
Self-assembly of colloidal nanocrystals into long-range-ordered structures through a bottom-up approach is highly promising for creating metamaterials with programmable functionalities arising from various synergistic effects and emergent interactions between neighboring NCs. Both single-component and binary superlattices reported so far were mainly composed of spherical NCs limiting the variety of obtained structures to the ones isostructural with the known atomic lattices [1]. A far greater structural diversity is accessible via assembling NCs of different shapes, e.g. nanoplates, nanorods, nanocubes, or nanodiscs. Notably, lead halide perovskite nanocrystals are characterized by remarkable optical properties together with being synthetically available in the monodisperse form making them attractive candidates as building blocks for self-assembly. A new step toward unique positional and orientational order would be to combine perovskite NCs with dielectric NCs of anisotropic shape. To this end, we have chosen LaF3 and NaGdF4 NCs as a second component for SLs due to their synthetic accessibility and well-defined, ensemble-uniform morphology.
Co-assembly of cesium lead halide nanocubes with disc-shaped LaF3 NCs (9.2-28.4 nm in diameter) leads to the formation of six columnar structures with AB, AB2, AB4, and AB6 stoichiometry as well as to noncolumnar lamellar and ReO3-type SLs by employing larger perovskite NCs [2]. The latter two SLs proved to exhibit characteristic features of the collective ultrafast emission – superfluorescence. Intending to broaden the variety of building blocks for NC SLs, we utilized organic-inorganic perovskite NCs, namely FAPbBr3 NCs, for the formation of binary SLs with spherical NaGdF4 NCs (b-ABO3-, AlB2-, AB2-, NaCl-type structures) and LaF3 nanodiscs (columnar AB-type and lamellar SLs). Combining larger and thicker NaGdF4 nanodiscs (18.5 nm in thickness) with cesium lead halide NCs resulted in the CaC2-like and AB3-type SLs. For CaC2- and ABO3-type structures, we expanded the scope of the available dimensionalities of SLs by employing microemulsion-templated self-assembly which allowed for the formation of three-dimensional binary supraparticles [3].
1.1-I1
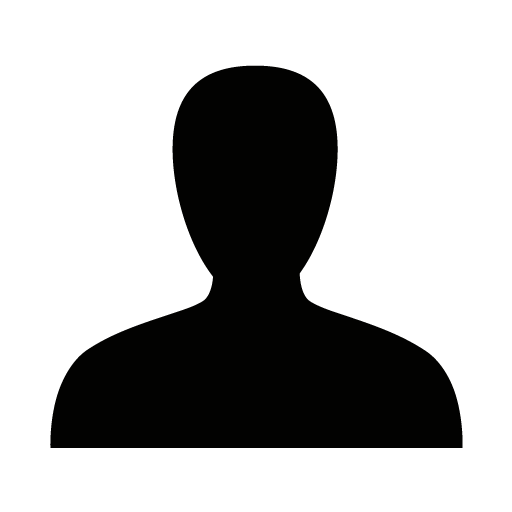
2D materials shows great promise for use in flexible electronics because their atomic thickness allows for maximum electrostatic control, optical transparency, sensitivity and mechanical flexibility. In addition, different 2D crystals can be easily combined in one stack, offering unprecedented control on the performance and functionalities of the resulting heterostructure device [3]. Solution processing of 2D materials allows simple and low-cost techniques, such as ink-jet printing, to be used for fabrication of heterostructure-based devices of arbitrary complexity.
Our group has developed highly concentrated, defect-free, biocompatible and inkjet printable and water-based 2D crystal formulations, by exploiting a supramolecular approach based on non-covalent functionalization of 2D materials with pyrene derivatives [1]. Examples of printed heterostructures, such as arrays of photosensors, programmable logic memories, transistors and memristors will be discussed [2-3]. I will show that inkjet printing can be easily combined with semiconducting 2D materials produced by chemical vapor deposition, allowing simple and quick fabrication of complex circuits on paper, compatible with CMOS technology [4-5] and also offers a simple way to integrate 2D materials into Si-based technology [6].
1.1-I2
In contrast to the tremendous efforts dedicated to the exploration of graphene and inorganic 2D crystals such as metal dichalcogenides, boron nitride, black phosphorus, metal oxides, and nitrides, there has been much less development in organic 2D crystalline materials, including the bottom-up organic/polymer synthesis of graphene nanoribbons, 2D metal-organic frameworks, 2D polymers/supramolecular polymers, as well as the supramolecular approach to 2D organic nanostructures. One of the central chemical challenges is to realize a controlled polymerization in two distinct dimensions under thermodynamic/kinetic control in solution and at the surface/interface. In this talk, we will present our recent efforts in bottom-up synthetic approaches toward novel organic 2D crystals with structural control at the atomic/molecular level. On-water surface synthesis provides a powerful synthetic platform by exploiting surface confinement and enhanced chemical reactivity and selectivity. We will particularly present a surfactant-monolayer assisted interfacial synthesis (SMAIS) method that is highly efficient in promoting the programmable assembly of precursor monomers on the water surface and subsequent 2D polymerization in a controlled manner. 2D conjugated polymers and coordination polymers belong to such material classes. The unique 2D crystal structures with possible tailoring of conjugated building blocks and conjugation lengths, tunable pore sizes and thicknesses, as well as impressive electronic structures, make them highly promising for a range of applications in electronics, optoelectronics, and spintronics. Other physicochemical phenomena and application potential of organic 2D crystals, such as in membranes, will also be discussed.
1.1-I3
Research Scientist at the CNR- Institute for Organic Synthesis and Photoreactivity, (ISOF-CNR, Bologna).
MM research focuses on the design and synthesis of functional molecular materials, graphene related materials and composites.
Target applications of the newly developped materials are thin film devices (i.e. OFET), sensors for biosensing and water monitoring, membranes and sorbents for water purification from emerging contaminants.
MM is currently Leader of the Advanced Materials Synthesis group of ISOF,
Member of the Body of Knowledge of the EIT-Climate KIC- Water scarcity and pollution,
Deputy Leader of the spearhead project of the Graphene Flagship ‘Graphil- graphene enhanced filters for water purification’,
Coordinator of the Flagera project GO-FOR-WATER and PI of the project LIFE-Remembrance.
She is also Scientific manager of industrial collaboration aimed at market exploitation graphene materials (i.e. Tetrapak, Culligan, Medica, Hera)
The last few years have seen the increasing occurrence of water contamination by pharmaceuticals, personal care products, plastic and their additives among the other,1-5 that are not satisfyingly removed by conventional drinking water treatment technologies.6, 7 In some cases, as for the endocrine disrupting agents such as per and polyfluoroalkyl substances (PFAS) and bisphenol A (BPA), the eco and human toxicity has been demonstrated 8-11 calling for the urgent development of new technologies for detection, early warning and remediation of such emerging contaminants.
Due to its commercial availability at good standard quality and processability in composite or membranes, graphene is one of the most promising nanomaterials for the realization of new technologies for water purification. In this talk, I will present a selection of case studies of graphene-and chemically modified based materials and devices realized at CNR to detect and remove emerging contaminants including pharmaceuticals, personal care products and PFAS), from drinking water.[1,2,3] I will discuss about performance, sustainability issues in comparison with standard technologies and future challenges to respond to the sustainable development goal n6 of the UN ‘ensuring clean water and sanitation to all’.
1.2-I1
The synthesis of different hydrophilic polymeric networks, by in situ radical polymerization in the presence of 2D materials, gives rise to three-dimensional soft structures. The role of the nanomaterial within the polymeric network is mainly intended for reinforcement (i.e. stiffness and toughness enhancement). However, we have shown that the presence of 2D materials, such as graphene, can also enhance features such as biocompatibility [1], sensing [2] or self-healing capability [3], giving rise to truly hybrid composites [4]. Moreover, the ability of these soft materials to respond to different stimuli, such as electric and magnetic fields, to behave as sensors, and the possibility to prepare them following 3D printing methodologies, open the way to applications in soft robotics [5]. The preparation of these smart soft systems requires the production of large quantities of 2D materials easily dispersible in water; ball milling methods developed in our laboratories have proven to be both sustainable and easily scalable technologies. [6], [7].
1.2-I2
This talk aims to show the latest advances we have made on two-dimensional (2D) materials for energy storage and conversion. Specifically, we will show the great promise of a disruptive family of 2D transition metal layered hydroxides (LH), which exhibit a wide chemical tunability and can be produced in large scale. Indeed, a series of single and layered double hydroxides will be discussed including the Simonkolleite phases of cobalt-LH or the NiFe-LDHs of great interest in electrocatalysis, or the hybrid organic-inorganic LDHs of interest as precursors for the preparation of electrodes for rechargable alkaline batteries. Along with their synthesis and characterization, we will show the performance of these wonderful materials in the development of new battery prototypes or better electrocatalysts for the production of green hydrogen. Last but not least, we will show a new family of hybrid 2D-LHs with great potential as electrode materials: the so-called "meta-layered hydroxides".
1.2-I3
A precise knowledge of the optical and electrical properties of 2D nanomaterials, is essential for pushing forward these materials in energy applications. In this regard, synthesis and studies of nanohybrids at single-crystal scale is a promising alternative to study the intrinsic properties of a photoactive material, since it is avoided the effect of the grain boundaries and amorphous domains present in polycrystalline films using for that purpose a homemade setup. [1, 2]
In this communication, we present the optical study of different microstructured materials such as hybrid perovskites for the development materials with applications in the field of energy conversion, optoelectronics and lighting. For instance, the incorporation of SubPc in the interlayer space of 2D–3D perovskites expands the photoresponse in the visible region [3]. SubPc incorporation was achieved by selectively controlling the composition during the synthesis. Photocurrent spectra on isolated single crystals indicates that SubPc molecules are participating in the charge generation process upon illumination, and acts as light harvester for long wavelength radiations. All these studies provide relevant information for device manufacturing in the development of high-efficiency solar cells, photocatalysts and optoelectronic devices. [1-4]
1.2-I4
Heterogeneous photocatalysis by readily available, metal-free catalysts is of great appeal in view of the increasing pressure on industry to move towards sustainable schemes of chemical production. Graphitic carbon nitride (g-CN) is a versatile semiconductor nanomaterial, well known for applications in energy, such as H2 photocatalytic production and CO2 reduction. We recently highlighted the key role that g-CN could play in the realm of photocatalytic organic synthesis, [1] and showed that tailoring the structure of g-CN by means of minimally invasive post-synthetic protocols could be the solution to tackle challenging coupling reactions with great efficiency.[2,3] Through international collaborations, our group embarked in the in depth investigation of the structure/activity relationship of new g-CN derivatives related to photocatalysis, by means of a combination of advanced spectroscopic techniques [4] and computational methods.[5] The final goal is aims at establishing an approach based on rational design of modified CN materials, with properties tailored to the specific photocatalytic process. Apart from the strict metal-free photocatalysis, an additional possibility arises from the inclusion of single metal atoms into the CN scaffold, whereby the opportunely adjusted CN structure can interact and be in synergy with the metal site. This can be of high relevance both in dual photoredox catalysis for organic synthesis and in energy conversion processes.
1.3-O1
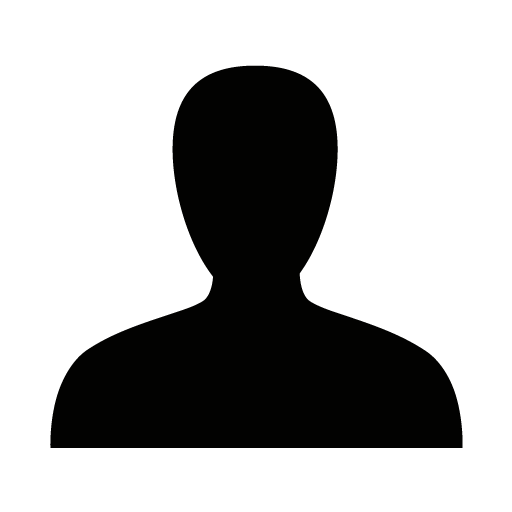
In recent years, a plethora of material systems have been designed and prepared to increase the performance of light harvesting and light-emitting technologies, and to develop new and attractive applications. Limitations of state-of-the-art devices based on organics (both conjugated polymers or small molecules/oligomers) derive largely from material stability issues after prolonged operation. This challenge could be tackled by leveraging the enhanced stability of carbon nanostructures (CNSs, including carbon nanotubes and the large family of graphenebased materials) in carefully designed nano-hybrid or nano-composite architectures to be integrated within photo-active layers, paving the way to the exploitation of these materials in contexts in which their potential has not been yet fully revealed. In this talk, I will discuss the theoretical background behind CNSs hybridization with other materials such as graphene with donor-acceptor molecules, for the establishment of novel optoelectronic properties and provide an overview of new optoelectronic and transfer properties of twisted graphene nanoribbons with controlled peripheral size that allow to forecast interesting future perspectives for use in real devices.
1.3-O2
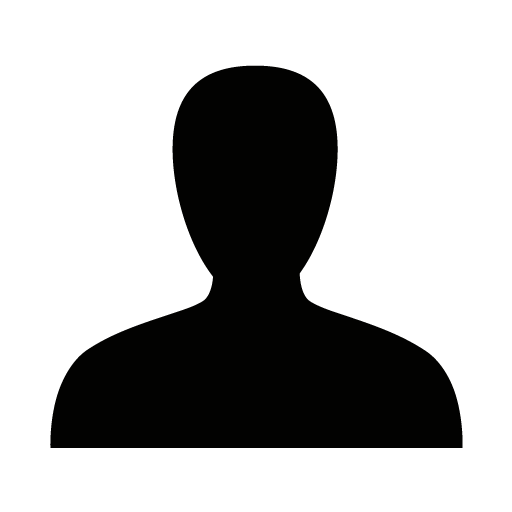
Since the first discovery of graphene by Novoselov in 2004, graphene and in general 2D materials showed an increasing interest in the scientific community. Specifically Transition Metal Dichalcogenides (TMDC) showed a surge in interest, due to their different properties and flexibility due to the large amount of variability in the group. Not only this, but they can be easily engineered in different ways to tune their properties, examples are doping, functionalization and hybridation with other material1. In this last context, Conductive Polymers (CP)2 show complementary electrochemical properties to the 2D TMDC materials and can be exploited to further improve their performances. Here, we report on the synthesis of hybrids based polyaniline (PANI) and 2D transition metal dichalcogenides (TMDCs), employing different methodologies and formulations. In particular, we resort to liquid phase exfoliation (LPE)3 to produce 2D TMDCs in both the 2H and 1T phase and to in-situ polymerization to produce PANI chains directly on the surface of these nanomaterials to further amplify the electrochemical properties of the 2D material. Then the as obtained material can be further characterized electrochemically and can be also used in conjunction with flexible polymer scaffold in order to obtain piezo- active hydrogels. The as-obtained hydrogels are characterized through a combination of techniques and their swelling behaviour and mechanical properties are investigated.
1.3-O3
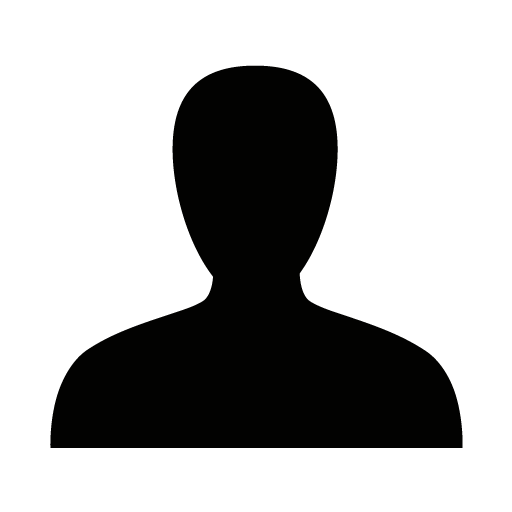
Due to their outstanding electronic, thermal, optical, chemical, and mechanical properties, 0/1/2D carbon nanostructures (CNSs) have attracted great interest in the last two decades.[1] Among CNSs, different procedures have been developed to synthesize carbon nanodots (CNDs) starting from a large pool of small molecules through a bottom-up approach, and several applications for these nanomaterials have been investigated.[2,3] CNDs can be further decorated through chemical functionalization for sensing, catalysis, and optoelectronic applications. For example, light-conversion processes in covalently functionalized CNDs with donor-acceptor organic dyes can be investigated.[4] Therefore, it is important to analyze the reactivity and accessibility of the surface functional groups, as well as their total quantities. This contribution discusses the characterization and quantification of the terminal functional groups of four different bottom-up synthesized CNDs.[5] We resorted to pH back-titrations, Kaiser tests, X-ray photoelectron spectroscopy (XPS), and quantitative 19F-NMR of incorporated fluorine atoms to quantify the terminal amine content in the prepared CND samples. XPS provides both the surface functional group content and, together with elemental analysis (EA), the elemental composition of the synthesized CNDs. The amount of ethylene diamine used as starting material governs the fraction of functional groups on the surface. This quantification, before and after functionalization, provides useful information about the reactivity and accessibility of the terminal functional groups. Hereby, the yields of reactions can subsequently be determined. Based on the different amounts of terminal amino groups of these four CNDs, it is possible to determine which is most suitable for further functionalization.
1.3-O4
Matteo Zaffalon is a Postdoctoral researcher at the Department of Materials Science of the University of Milano-Bicocca (IT), where he earned his Ph.D. in Materials Science and Nanotechnology in 2022. In 2020 he collaborated with the Nanotechnology & Advanced Spectroscopy group at the Los Alamos National Laboratory (NM, USA) working on the spectroscopic investigation of solution grown functional nanostructures for application in photonic and optoelectronic devices. His research is now focused on the spectroscopic investigation and development of novel nanomaterials for the ultrafast detection and conversion of ionising radiation for energy and medical imaging applications.
Colloidal CdTe nanoplatelets featuring a large absorption coefficient and ultrafast tunable luminescence coupled with heavy-metal-based composition present themselves as highly desirable candidates for radiation detection technologies [1]. Historically, however, these nanoplatelets have suffered from poor emission efficiency, hindering progress in exploring their technological potential [2], [3]. In this talk, we report the synthesis of CdTe nanoplatelets possessing a record emission efficiency of 9%. This enables us to investigate their fundamental photophysics using ultrafast transient absorption, temperature-controlled photoluminescence, and radioluminescence measurements, elucidating the origins of exciton- and defect-related phenomena under both optical and ionizing excitation. For the first time in CdTe nanoplatelets, in this talk is reported the cumulative effects of a giant oscillator strength transition (GOST) and exciton fine structure. Simultaneously, thermally stimulated luminescence measurements reveal the presence of both shallow and deep trap states and allow us to disclose the trapping and detrapping dynamics and their influence on the scintillation properties [4].
1.3-O5
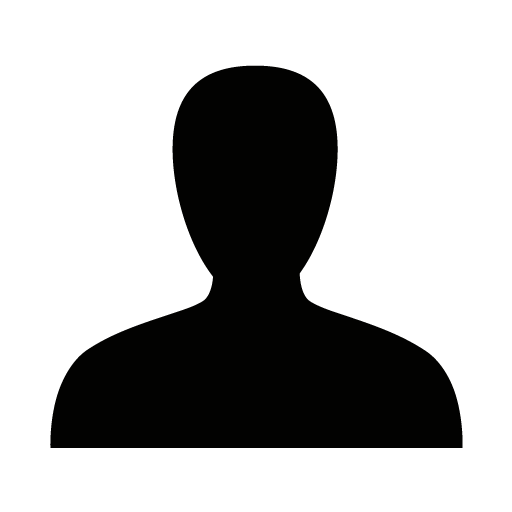
Silver phenylselenolate (AgSePh) and silver phenyltellurolate (AgTePh) are novel two-dimensional (2D) van der Waals semiconductors. In contrast to 2D layered perovskites and transition metal dichalcogenides, AgSePh and AgTePh have strong covalent interaction between organic and inorganic components, becoming truly hybrid organic-inorganic semiconductors. However, despite having the similar crystal structure, composition and absorption characteristics, AgSePh and AgTePh exhibit strikingly different light emission characteristics. Whereas AgSePh exhibit narrow, fast luminescence with a minimal Stokes shift that tracks the temperature-dependent shifting of the lowest-energy excitonic absorption resonance, AgTePh exhibits comparatively slow, significantly broadened luminescence with large Stokes shift that does not track the shifting of excitonic absorption resonance peak with changing temperature. In this presentation, we will present the synthesis, structure and excitonic optical properties of AgSePh and AgTePh films. Furthermore, we will discuss different physical mechanisms underlying light emission in AgSePh and AgTePh. Using time-resolved and temperature-dependent optical spectroscopy, combined with sub-gap photoexcitation spectroscopy, we will show that exciton dynamics in AgTePh are dominated by intrinsic self-trapping behavior, whereas dynamics in AgTePh are dominated by interaction of band edge excitons with extrinsic defect states. Finally, we will show tunable excitonic properties in AgSe1-xTexPh alloys depending on composition.
2.1-I1
Two-dimensional (2D) materials can provide a suitable platform to develop new technologies for energy applications. In my group we are combining functional molecules with 2D materials with the idea of tuning/improving the properties of the “all surface” 2D material via an active control of the hybrid interface. This concept, which goes much beyond the conventional chemical functionalization of a 2D material, can provide an entire new class of hybrid molecular / 2D heterostructures, which may be at the origin of a novel generation of materials and devices of direct application in electronics, spintronics, molecular sensing and energy. Here this concept will be presented and used in the design of low energy consumption spintronic devices as well as in energy storage and conversion. In the first part I will show that 2D magnetic materials as well as hybrid molecular /2D antiferromagnets can be used to store and transport information with extremely low energy disipation and tunability. In the second part, hybrid materials formed by electroactive inorganic layers will be used as electrodes in supercapacitive devices and as electrocatalysts.
2.1-I2
Photovoltaic and solar fuels technologies rely upon the intentional movement of charge carriers (i.e. electrons and holes) and/or energy (i.e. excitons) in prescribed directions to convert sunlight into electricity or fuels. Two-dimensional semiconductors have several advantages for PV and (photo)catalytic technologies, due to their large absorption coefficients, high mobilities for charge carriers and excitons, and catalytic activity for important fuel-forming reactions. To realize the full potential of 2D nanomaterials and related heterostructures for sustainable energy technologies, fundamental studies are needed to probe the key photochemical processes that occur upon photon absorption, including exciton diffusion and dissociation, interfacial charge and energy transfer, and charge recombination. In this presentation, I will highlight our ongoing studies probing charge and energy transfer across heterojunctions formed between monolayer transition metal dichalcogenides (TMDCs) and other nanoscale semiconductors such as semiconducting single-walled carbon nanotubes, small molecules, and nanocrystals. Appropriate tuning of the interfacial band alignment can enable rapid exciton dissociation and exceptionally long-lived charge-separated states that are essential for PV and catalytic applications. Tuning the binding motif of molecular species between van der Waals association and covalent bonds can also influence the mechanisms of interfacial charge/energy transfer. If time permits, I will also discuss how certain 2D nanomaterials can facilitate charge/energy transfer while simultaneously blocking undesired ion movement across the heterojunction.
2.1-I3
Associate Professor of Physical Chemistry at the University of Malaga (UMA) since 2017. Bachelor in Chemical Engineering at UMA in 2001, graduating with Honors. PhD summa cum laude in Chemistry (J.T. López Navarrete´s group: vibrational spectroscopy of conjugated materials). In April 2007, she joined Prof. Jean-Luc Brédas group at Georgia Tech (Atlanta, USA), as a MEC/Fulbright Postdoctoral Research. Her postdoctoral research dealt with the computational study of the impact of the intermolecular interactions on the charge transport properties of conjugated organic nanomaterials. She was awarded with the “AACP Postdoctoral Award” of the Georgia Tech and Emory University for excellence during her postdoctoral research. In 2010, she returned to the University of Malaga as a Ramón y Cajal researcher, where she has implemented a new line of research focused on the computational study of organic materials. This field of research is intended to guide synthetic chemists to create new molecules and materials with more efficient, reliable and environmentally friendly properties and applications, and it is a highly interdisciplinary field. She then combines theoretical calculations (postdoc expertise) with experimental physico-chemical analyses (predoc expertise) for a proper analysis of the structure/properties relationships of molecular materials. In 2010, she was honored with the award of the “2010 Spanish Royal Society of Chemistry” to the most talented young people. In recent years, she has carried out research stays at the University of Stuttgart (2016, 2017, 2019), the Polytechnic Institute of Milan (2015) and at the University of Cergy-Pontoise (2016-2022) as a visiting professor.
She has published > 105 JCR articles and one book chapter, attracting > 5500 citations (WoS). In the last 5 years, she published 27 scientific articles with 11 articles as a corresponding author. Her H index is 32 (WoS). In the last five years, three of her papers has been granted with artistic journal covers and two others were selected as hot papers in high impact factor journals. She has presented more than 85 contributions in national and international conferences, including 22 oral communications and delivered 8 invited talks in national and international Universities. She has participated in 12 research projects funded with public funds from the Spanish Ministry (5), Junta de Andalucía (4), European project (1) and USA projects (2),being IP of one national project and 1 regional project. She has coadvised 2 PhD Thesis, along with 5 PhD currently in progress. She has also supervised the research stays of 3 PhD students from the Politechnico di Milano, University of Stuttgart and University of Brasilia. She has taught more than 1600 hours in different physico-chemical courses in Chemistry and Chemical Engineering bachelors and Master degree. In addition, she frequently participates in dissemination activities aim to make visible the scientific results to the society and/or to support the gender equlity and women´s empowerment, such as “La Noche Europea de los Investigadores” or “Female Leaders in Science” since 2016. Since 2017 she is Adjunct Vice-Rector for Research Staff at UMA.
2D organic semiconducting polymers have emerged as an important class of materials that offer high potential for a variety of applications, such as energy storage, sensing or organic electronics. However, the design of novel materials generally involves an expensive and environmentally unfriendly methodology that strongly contrasts with the ecological transition spirit. In this sense, computational design offers a green alternative to experimental laboratory research. On the other hand, Raman spectroscopy is a fast and nondestructive characterization tool widely used to evaluate the structural and electronic properties of pi-conjugated materials. In this study, we combine an experimental and theoretical approach that links DFT calculations with Raman spectroscopy aiming to control the electronic and structural properties of conjugated organic materials Overall, our findings open the door to the control of the degree of the π-conjugation of 2D organic polymers for their subsequent synthesis and real applications ranging from sensing to electronics.
1.1-I1
Electron tomography enables one to measure the morphology and composition of nanostructures in three dimensions (3D), even at atomic resolution. However, an emerging challenge is to fully understand the connection between the 3D structure and properties under realistic conditions, including high temperatures as well as in the presence of liquids and gases. Our recent experiments demonstrate the progress that can be obtained by accelerating both the acquisition and reconstruction during electron tomography [1,2]. In this manner, we were able to perform a dynamic characterisation of shape transformations of metal nanoparticles at high temperatures [1,3]. Moreover, we measured the elemental diffusion dynamics of individual anisotropic bimetallic nanoparticles in 3D and determined the effect of parameters such as type of interfacial facets, aspect ratio, shape and presence of defects [4]. Finally, by combining aberration corrected electron microscopy with a quantitative interpretation, we can provide quantitative measurements of the coordination numbers of the surface atoms of catalytic nanoparticles at high temperatures and in gaseous environments [5].
1.1-I2
i
II-VI semiconductor nanoplatelets are colloidal nanoparticles confined in one dimension which gives them exceptionally narrow optical properties. We are showing that through the design of core/crown/crown heterostructures, it is possible to synthesize nanoplatelets with green and red emissions from a single population. In the CdSe/CdTe/CdSe core/crown/crown NPLs, the exciton either recombine at the interface between 2 semiconductors due to the type II band alignment or in the CdSe area. The ratio of the two emissions can be tuned by the incident power. With increased power, the red emission saturates due to non-radiative Auger recombination thus affecting its emission much stronger than the green one. These NPLs can be introduced in LED. So, the design of the NPLs inorganic core enables to tune their optical properties but their shape can be further modified through the surface chemistry. NPLs with their large lateral dimensions can be considered as flexible substrate for the self-assembly of ligands which originally ensure the colloidal stability of NPLs. The stress brought by surface ligands and the specific zinc blende crystal structure of the NPLs induce a folding of NPLs as helices or twists. We show that an exchange from carboxylate ligands to halides ligands and thiolate ligands enable to unfold CdSe NPLs and to invert the chirality of the helices. Finally, this surface chemistry also enables to control the kinetic of a cation exchange.
1.1-I3
Celso de Mello Donega is an Associate Professor in the Chemistry Department of the Faculty of Sciences at Utrecht University in the Netherlands. His expertise is in the field of synthesis and optical spectroscopy of luminescent materials. His research is focused on the chemistry and optoelectronic properties of nanomaterials, with particular emphasis on colloidal nanocrystals and heteronanocrystals.
Colloidal semiconductor heteronanocrystals (HNCs) exhibit unique optoelectronic properties that are inaccessible to single-component NCs, making them promising materials for a wide range of applications. The optoelectronic properties of HNCs are determined not only by the bandgap and band alignment of its constituent materials but also by their morphology and heteroarchitecture.1 Applications requiring efficient charge separation (e.g., photovoltaics and photocatalysis) and polarized emission greatly benefit from anisotropic morphologies, such as heteronanorods. Most of the work on heteronanorods has focused on Cd-chalcogenide-based HNCs. However, given the toxicity of Cd, the potential of these materials for large scale applications is limited.
Copper-chalcogenide based NCs have attracted increasing attention as promising alternatives for Cd- and Pb-chalcogenide NCs due to their low toxicity, large absorption cross-sections across a broad spectral range, composition-dependent band gaps in the 1 to 2.5 eV range, and photoluminescence tunability, spanning a spectral window that extends from the UV to the NIR depending on the NC size and composition.2,3
Several synthetic strategies have been used in the quest for high-quality colloidal copper chalcogenide based HNCs. The most promising ones are based on the multistage approach, which allows the combination of different synthesis techniques (e.g., cation exchange4,5 or seeded growth6-9) in a sequential manner in order to achieve the targeted preparation of colloidal HNCs. In this talk, I will discuss a selection of recent examples, chosen to illustrate specific synthesis strategies: CuInSe2/CuInS2 dot core/rod shell heteronanorods,4 Cu1.8S-based multicomponent axially segmented heteronanorods,5 CuInS2/ZnS dot core/rod shell heteronanorods,6 and Janus-type Cu2‒xS/CuInS2 and Cu2−xS/ZnS heteronanorods.8,9 I will show that by combining the design principles of post-synthetic heteroepitaxial seeded growth and nanoscale cation exchange into multistage synthesis strategies one can potentially gain access to a plethora of Cu-chalcogenide-based multicomponent heteronanorods with diameters in the quantum confinement regime.
1.2-O1
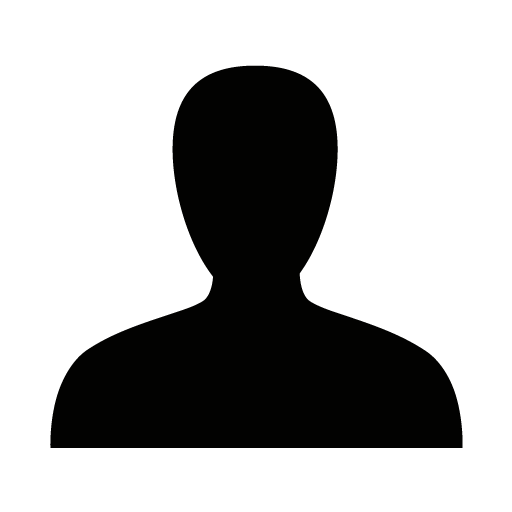
Discovering and developing novel nanomaterials to improve the sustainability of optoelectronic devices, while maintaining reasonable efficiency, is of key importance to drive future research and make commercialization possible. With the criteria of earth-abundant, stable, and low-toxicity elements for nanocrystal (NC) synthesis, we focus on ABX3 chalcogenides, where A is an alkaline earth metal and B a group four transition metal. We seek to exploit the unique properties of NCs and the perovskite crystal structure while shifting from lead (Pb) and cadmium (Cd) containing materials to more sustainable ternary chalcogenides of low toxicity. Specifically, in this class of materials, BaTiS3 (in the hexagonal phase) and BaZrS3 (in the perovskite structure) are promising. A flexible method for controlling the physical and chemical properties of these materials is through colloidal synthesis with organometallic precursors. Synthetic routes using different sulfur and barium sources are investigated. Characterization by TEM, XRD, and absorbance measurements reveal how the shape of the nanocrystals can be tuned from isotropic to anisotropic with aspect ratios ranging from 1:1 to 1:100 by changing the barium precursor from a methylamino compound to a halide salt. Alternatively, adjusting the sulfur source between various thioureas alters the crystallinity and reaction temperature needed to achieve NCs. Transient absorption (TA) spectroscopy shows long excitation lifetime (ca. 1 µs) and clear spectral features resembling PbS quantum dots with respect to spectral width of the band-edge bleach and photoinduced absorption signal. For the application in optoelectronic devices, it is necessary to exchange the long insulating ligands used during synthesis for shorter or more conductive ones. We have developed a ligand exchange procedure to cap the NCs with halides and process the NC solutions as thin films. With the gained knowledge from TA and film formation strategies, first work on the usage of BaTiS3 NCs in photovoltaic devices and field-effect transistors will be presented.
1.2-O2
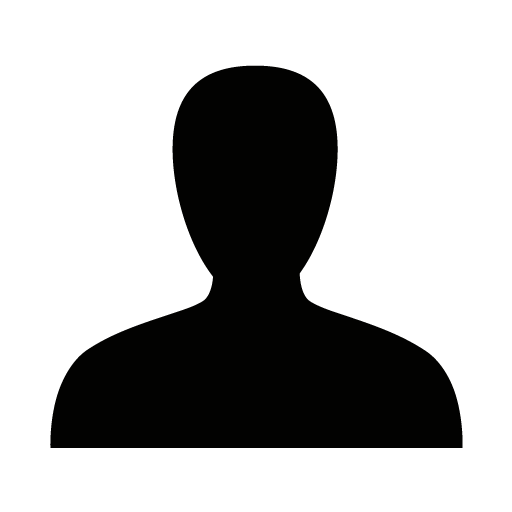
Ternary alkali-metal dichalcogenides {AMeE (A = Li, Na, K, Rb, Cs, Cu, Ag, Tl; Me= Metals E = S, Se, Te} are an intriguing class of semiconductors with tremendous technological potential.[1,2] For more than a decade, the compounds of these structural types have been explored as potent thermoelectric materials.[3] High-temperature solid-state synthesis is typically employed to synthesize alkali metal-based chemicals. However, it naturally forms highly aggregated, polydisperse particles with little control over different phases and understanding in terms of mechanistic insights. In this case, solution phase synthesis can be advantageous to form uniform nanocrystals. Herein, we develop a facile colloidal hot-injection strategy to synthesize shape and size-tunable caesium copper selenide nanocrystals comprising earth-abundant and non-toxic elements. Ex-situ mechanistic investigation reveals that the NCs formation is driven by the dissolution of binary Cu2-xSe, followed by the incorporation of Cs+ to form the ternary CsCu5Se3. A thorough case study on the addition of alkyl acid and amine ligands indicates that the involvement of both acid and amine functionality is required to synthesize pure-phase NCs. The current study also reveals that variation in the alkyl chain length of amines influences the size, shape, and formation of distinct phases. Furthermore, the nanocrystals were consolidated as pellets to study thermoelectric transport properties showing ultra-low thermal conductivity of 0.65 W/mK at 728 K, promising for improved thermoelectric applications. Altogether, the present study provides a mechanistic understanding of the factors that impact the synthesis of materials based on alkali metal chalcogenides and exhibit their promising thermoelectric properties.
1.2-O3
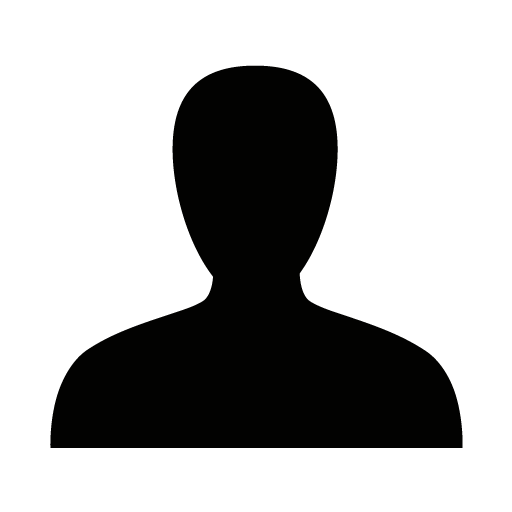
The activity of electrocatalysts during water electrolysis is controlled by their surface chemistry. The control of the surface structure and composition of an electrocatalyst requires understanding of the solid state reactions that govern the formation of the surface and bulk during synthesis. Probing these reactions requires characterization that can cover multiple length scales, from the nanoscale near-surface layer to the mesoscale phase behavior.
In my talk, I will discuss how the phase evolution of oxide electrocatalysts across different length scales can be probed using a combination of scanning transmission X-ray microscopy (STXM) and transmission electron microscopy (TEM). Specifically, my talk will address the formation of (La,Sr)CoO3 nano- and meso-scale particles that are often used as electrocatalysts for oxygen evolution reaction. The impact of the size and phase state of precursors as well as the time-temperature heat treatment profile on the resulting surface and bulk chemistry of the nanoparticles will be discussed.
1.2-O4
Dr. Galian received her Ph.D in Chemistry at the National University of Cordoba, Argentina in 2001. Then, she was a postdoc researcher at the Polythecnic University of Valencia, University of Valencia and University of Ottawa. During those years, she has studied photosensibilization processes by aromatic ketones using laser flash photolysis techniques and was involved in photonic crystal fiber/semiconductor nanocrystal interaction projects. In 2007, Dr. Galian came back to Spain with a Ramon y Cajal contract to study the surface chemistry of quantum dots and since 2017 she has a permanent position as Scientist Researcher at the University of Valencia. Her main interest is the design, synthesis and characterization of photoactive nanoparticles and multifunctional nanosystems for sensing, electroluminescent applications and photocatalysis.
Colloidal semiconductor nanocrystals (NCs) such as chalcogenides and perovskites have outstanding optical and electronic properties that make them interesting for multiple applications that involve, sensing, solar cells, light-emitting device technology, and more recently photocatalysis. 1
Tailoring the surface chemistry of colloidal metal halide perovskite NCs (AMX3; A= monovalent metal such as CH3NH3+, or Cs+; M= Pb2+, Sn2+; and X= Cl-, Br-, I-) is very challenging and crucial to determine their final properties. Therefore, the nature of the organic capping agents and their interaction with the surface atoms will be relevant to control the photophysical properties of the nanocrystals and prepare novel assemblies. The most common ligands, according to the covalent bond classification, used to obtain highly emissive and stable colloidal perovskite NCs are X-type and L-type binding ligands (Figure 1).
Several examples of the role of those types of ligands in i) the emissive properties of the NCs2; ii) the formation of blue emissive 2D low-dimensional materials3; iii) the photocatalytic ability of the metal halide perovskite NCs,4 and iv) the preparation of nano-heterostructures for NIR light-harvesting applications 5 will be discussed.
1.2-O5
Ligands play a crucial role in the synthesis and/or stabilization of colloidal nanocrystals. Nevertheless, only a handful molecules are currently used, of which oleic acid being the most typical example. Here we show that monoalkyl phosphinic acids are another ligand class.
We put forward monoalkyl phosphinic acids as an alternative ligand class, similar to carboxylic acids and phosphonic acids. After presenting the ligand synthesis for several selected substrates, we proceed to show the intermediate reactivity of the phosphinic acids in CdSe quantum dot syntheses. The nanocrystals synthesized with phosphinic acids are also easier to purify since there is no gel formation. Very small (2–3 nm) CdSe quantum dots with low polydispersity and high photoluminescence quantum yields can be easily accessed with phosphinic acid ligands. CdSe and CdS nanorods were also synthesized using phosphinic acids, whereby the rods showed high purity an d uniformity.
In addition, we investigate the ligand-NC interaction on both metal oxide (i.e., HfO2) as metal chalcogenide (i.e., CdSe and ZnS) NCs. Given their intermediate acidity, phosphinic acids (pKa ≈ 3.08) bind to the surface with an affinity between carboxylic and phosphonic acids. Using solution NMR, we quantify the X-for-X ligand exchange via the alkene resonance of the oleyl chain (carboxylic and phosphonic acid), and the ether resonance of the 6-(hexyloxy)hexyl phosphinic acid. We conclude that the monoalkyl phosphinic acids quantitatively displace carboxylate ligands and are in equilibrium with phosphonates (although phosphonate binding is favored). This results show that monoalkyl phosphinic acids are suitable reagents to efficiently functionalize nanocrystal surfaces.
In conclusion, by careful design new type of ligands can be created, and can tailored towards specific functionalities such as solubility matching or intermediate reactivity and binding strength.
1.2-O6
The detection of high-energy radiation has gained relevant interest thanks to its application in technologic-relevant fields, such as particle physics, astronomy, geology, medical diagnostics, nuclear monitoring and space exploration [1][2]. In all these areas, the most widely used detectors are scintillating materials that convert the energy deposited by incoming ionizing radiation into visible photons which is finally revealed by coupled photodiodes [3]. Beside typical inorganic materials based on high-Z elements, an alternative class of scintillators which further widens their applicability is plastic scintillators, which exhibit the advantages of a large sizes production, low weight and affordable costs making them particularly adapt for radiation monitors in border and industrial control[4]. Despite these advantages, the low density of plastic materials compared to inorganic crystals limits their interaction with ionizing radiation and typically requires doping these systems with high-Z components, such as organometallic complexes, perovskite or heavy metal chalcogenides nanocrystals (NCs). However, the intrinsic small Stokes shift of these materials represents an issue when used as nanoscintillators in highly dense or large volume detectors because of the strong reabsorption of the scintillation light along its path to the waveguide edges. We aim to takle this issue by developing a new strategy of scintillator consisting in a polymeric scintillating matrix incorporating reabsorption free Cd0.5Zn0.5S/ZnS core/shell NCs doped with manganese ions. Doping NCs with Mn is an established approach to activate efficient luminescence at intragap energy arising from the 4T1 →6A1 optical transition, yielding the characteristic Mn-emission at ~ 580-600 nm. Crucially, since such a transition is spin-forbidden, the corresponding optical absorption features negligible oscillation strength, resulting in an apparent Stokes shift between the band-edge absorption of the NCs host and the Mn-related luminescence. In our approach, we further adopted a synergic strategy in which both the plastic matrix waveguide and the NCs interact with incoming ionizing radiation, while the propagating emission is generated by the sole NCs, whose optical properties have been properly engineered to efficiently down-convert matrix emission. The emission efficiency and compatibility of the NCs with the polymer host have been optimized resulting in high optical quality nanocomposites completely transparent in the spectral region of their own emission, with scintillation efficiency comparable to commercial plastic scintillators.
1.2-O7
Matteo Zaffalon is a Postdoctoral researcher at the Department of Materials Science of the University of Milano-Bicocca (IT), where he earned his Ph.D. in Materials Science and Nanotechnology in 2022. In 2020 he collaborated with the Nanotechnology & Advanced Spectroscopy group at the Los Alamos National Laboratory (NM, USA) working on the spectroscopic investigation of solution grown functional nanostructures for application in photonic and optoelectronic devices. His research is now focused on the spectroscopic investigation and development of novel nanomaterials for the ultrafast detection and conversion of ionising radiation for energy and medical imaging applications.
Lead halide perovskites are emerging materials that can be synthesised at low cost on a large scale and have potentially disruptive optical performances in photonic and scintillation applications [1]. On the other hand, these materials are inherently toxic, due to the presence of Pb, and generally unstable, especially when exposed to heat, air and moisture. Therefore, there is vivid research aimed to replace Pb-based perovskites with non-toxic metal halide alternatives that may exhibit similar optical properties and, ideally, greater stability. In this context, the broad family of double perovskites (DPs) is particularly promising and offers fertile ground for new discoveries. Among the various structures, Sb3+-doped DPs are attracting increasing interest due to their bright and exceptionally large (more than 1.2eV) Stokes-Shifted PL [2], [3]. Here, we present colloidal nanocrystals (NCs) of Rb3InCl6, composed of isolated metal halide octahedra ("0D"), and dual perovskites of Cs2NaInCl6 and Cs2KInCl6, in which all octahedra share angles and are interconnected ("3D"), with the aim of elucidating and comparing their optical characteristics once doped with Sb3+ ions [4]. Our optical and computational analyses demonstrate the photophysical mechanism underlying PL in these systems, and that it is possible to double the quantum yield through localisation of the exciton in 0D structures by preventing its migration to trap states at the surface. Scintillation properties are evaluated by means of radioluminescence experiments and waveguide performance without resorption in large-area plastic scintillators is assessed by means of Monte Carlo ray-tracing simulations.
1.3-I1
Victor I. Klimov is a Fellow of Los Alamos National Laboratory and the Director of the Center for Advanced Solar Photophysics of the U.S. Department of Energy. He received his M.S. (1978), Ph.D. (1981), and D.Sc. (1993) degrees from Moscow State University. He is a Fellow of both the American Physical Society and the Optical Society of America, and a recipient of the Humboldt Research Award. His research interests include optical spectroscopy of semiconductor and metal nanostructures, carrier relaxation processes, strongly confined multiexcitons, energy and charge transfer, and fundamental aspects of photovoltaics.
Electrically pumped lasers or laser diodes based on solution-processable materials have been long-desired devices for their compatibility with virtually any substrate, scalability, and ease of integration with on-chip photonics and electronics. Such devices have been pursued across a wide range of materials including polymers, small molecules, perovskites, and colloidal quantum dots (QDs). The latter materials are especially attractive for implementing laser diodes as in addition to being compatible with inexpensive and easily scalable chemical techniques, they offer multiple advantages derived from a zero-dimensional character of their electronic states. These include a size-tunable emission wavelength, a low optical-gain threshold, and high temperature stability of lasing characteristics stemming from a wide energy separation between their atomic-like discrete energy levels.
Several challenges complicate the realization of colloidal QD laser diodes (QLDs). These include extremely fast nonradiative Auger recombination of optical-gain-active multicarrier states, poor stability of QD solids under high current densities required to achieve lasing, and unfavorable balance between optical gain and optical losses in electroluminescent (EL) devices wherein a gain-active QD medium is a small fraction of the overall device stack comprising multiple optically lossy charge-transport layers.
Here we resolve these challenges and achieve electrically driven laser action due to amplified spontaneous emission (ASE) in a colloidal-QD optical-gain medium. To demonstrate this effect, we employ compact, continuously graded QDs with strongly suppressed Auger recombination incorporated into a low-loss photonic waveguide integrated into a pulsed, high-current density light-emitting diode. These prototype QLDs exhibit strong, broad-band optical gain and demonstrate low-threshold, room-temperature laser action which leads to intense edge-emitted EL with intensity of more than 100 microwatts.
1.3-S1
Development Editor for the Royal Society of Chemistry (www.rsc.org) working on Nanoscale Horizons (https://rsc.li/nanoscale-horizons) and Nanoscale (https://rsc.li/nanoscale).
RSC Industry Talk RSC Industry Talk RSC Industry Talk RSC Industry Talk RSC Industry Talk RSC Industry Talk RSC Industry Talk RSC Industry Talk RSC Industry Talk RSC Industry Talk RSC Industry Talk RSC Industry Talk RSC Industry Talk RSC Industry Talk RSC Industry Talk RSC Industry Talk RSC Industry Talk RSC Industry Talk RSC Industry Talk RSC Industry Talk RSC Industry Talk RSC Industry Talk RSC Industry Talk RSC Industry Talk RSC Industry Talk RSC Industry Talk RSC Industry Talk RSC Industry Talk RSC Industry Talk RSC Industry Talk RSC Industry Talk RSC Industry Talk RSC Industry Talk RSC Industry Talk RSC Industry Talk RSC Industry Talk RSC Industry Talk RSC Industry Talk RSC Industry Talk RSC Industry Talk RSC Industry Talk RSC Industry Talk RSC Industry Talk RSC Industry Talk RSC Industry Talk RSC Industry Talk RSC Industry Talk RSC Industry Talk RSC Industry Talk RSC Industry Talk RSC Industry Talk RSC Industry Talk RSC Industry Talk
1.3-I2
Nanostructured semiconductors, or quantum dots (QDs), are heavily investigated for their applications in light emission such as light emitting diodes and lasers. The premise of cost-effective solution processing of such devices based on nanocrystals has recently driven research towards electrically pumped population inversion in laser diode structures. Challenges however remain to achieve net light amplification in the cavity due to a balance between limited material gains and lossy electrical contacts. Further reductions in threshold current densities, mainly limited by the non-radiative cap of ca. 1 nanosecond on the gain lifetime, are also required to achieve stable operation. Finally, color tunability is limited to the red by the gain bandwidth of the red-emitting CdSe/CdS QDs or even core/shell nanoplatelets used.
Here, we show that weakly confined charge carriers in giant CdS quantum dots display disruptive optical gain metrics that could alleviate these remaining issues. Being active in the green part of the spectrum, their properties match and even outcompeting state-of-the-art colloidal materials in the red. Material gain coefficients up to 50.000 cm-1 combined with a broad gain window of 160 nm are found. Also, a very promising gain lifetime close to 3 ns is found. Invoking a model of stimulated emission based on bulk semiconductor physics, we are able to explain all of these remarkable gain metrics, yet only if a large band gap renormalization effect is invoked. Based on these unique materials, we demonstrate amplified spontaneous emission and lasing action under nanosecond optical excitation. Our results show that weakly confined nanomaterials are excellent gain materials, combining straightforward wet chemical synthesis and the promise of solution processability with beyond state-of-the-art gain metrics.
1.3-O1
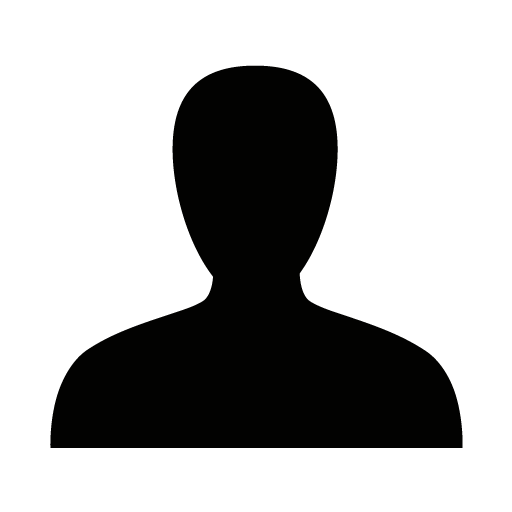
In condensed matter physics, a stepwise reduction of the dimensionality from bulk to the nanoscale is known to lead to striking electronic and optical properties such as the giant oscillator strength transition for two- dimensional (2D) semiconductor quantum wells and the spin-charge separation in one-dimensional (1D) systems. However, in contrast to metals, the lateral confinement in semiconductor materials competes with the exciton Coulomb interaction, which can be magnified by dielectric mismatch. Due to the complexity of these interactions, the degree of freedom in the electron motion remains poorly understood, whereas its clear circumscription could significantly improve the optoelectronic performances of quasi-2D systems such as CdSe colloidal nanoplatelets (NPLs).
Since the distribution of the quantized states, the so-called density of states (DOS), reflects the restrictions of the electron motion with dimensionality, it can serve as a genuine fingerprint to unambiguously disclose the confinement experienced by the charge carriers. A unique way to probe the DOS consists in measuring single-particle excitation spectra with scanning tunnelling spectroscopy (STS). Ji et al. have demonstrated that the hole DOS is consistent with a free in-plane motion, while the electrons have a more complex DOS that deviates from the simple 2D model. When the width (W) or/and the length (L) are smaller than ten to five times the exciton Bohr radius, the electron behavior, which has a smaller effective mass than the hole, becomes affected, with a significant impact on its interaction with the hole and the dielectric image charges. The already present complexity in understanding electron−hole correlation in these box-shaped nanostructures is further enhanced with the existence of electrical surface traps, which are caused by the sporadic absence of ligands. It makes the electron confinement in the NPLs still unknown, leading to intense debates about their true dimensionality.
Here we used this technique to study electron confinement in colloidal CdSe nanoplatelets (NPLs), which have a quantized thickness d due to a discrete number of monolayers (MLs) and a rectangular shape with finite length L and width W. The observation of Van Hove singularities in the conduction band implies a paradigm shift on the electronic structure of typical CdSe NPLs considered in the literature. As the electron DOS exhibits a striking modulation that is directly related to the length of the NPLs, delineating the in-plane electron motion at low temperature has important conconsequences for a deeper understanding of the exciton dissociation, diffusive transport, and annihilation in NPLs.
Moreover, it is shown that the side facets of NPLs host electronic deep trap states, which cause a Coulomb blockade in the tunnelling current. As they could be fully removed by the formation of core-crown NPL, our results anticipate a genuine boost for NPL-based lateral heterostructures that will notably allow for the mixing of the dimensionalities to favor specific electronic or optical properties.[1]
1.3-O2
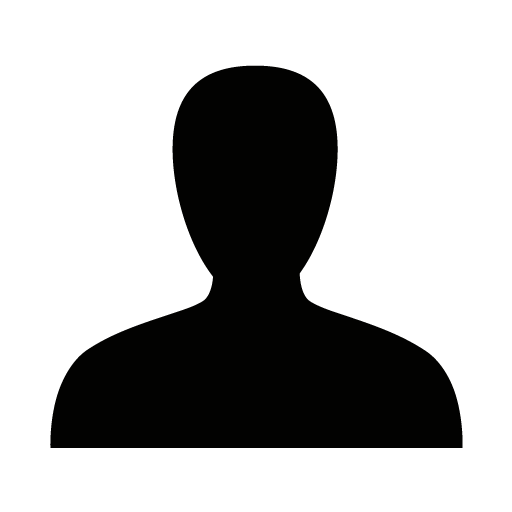
When studying the opto-electronic properties of (nanostructured) semiconductors, Transient Absorption (TA) or pump-probe spectroscopy is often used to measure ultrafast changes optical properties due to photo-excitation. Besides making direct conclusions on carrier recombination processes, quantification of net optical gain or studying photo-induced absorption, a typical analysis that is done on such datasets is a Boltzmann fit on the high-energy tail from which the temperature of the photo-excited charge carriers can be extracted. Such information is vital in understanding carrier cooling bottlenecks which are detrimental for optical gain applications, but potentially very useful in solar energy conversion. Usually, this extraction of temperature is done for different pump-probe delays, excitation energies and different excitation powers to see the impact on the thermalization of the carriers.1 In this work, we take a more detailed look at what this high-energy tail actually entices for a direct gap semiconductor. We show that within this simple fitting approach, one gravely overestimates carrier temperatures and propose a more consistent modelling to find the temperature. Next, we compare this approach to Ultrafast Photoluminescence (UFPL) measurements on the same timescales as TA. We show that extracting the temperature is a more straightforward process for this approach.
2.2-O1
Colloidal lead halide perovskites LHP (LHP) nanocrystals (NCs) have recently become popular light-emissive materials, of practical interest for LEDs, LCDs, lasers, as well as single photon light sources.1,2 Most studies on LHP NCs focus on relatively large cuboidal NC exceeding 10 nm in size, pointing out the inherent challenge of producing small (sub 10 nm), stable and monodisperse LHP quantum dots (QDs). This problem directly originates from the highly ionic lattice of LHPs, generally resulting in sub second reaction dynamics, making it very challenging to control their growth on an atomic level. Consequently, the current generation of LHP QDs (especially the hybrid organic-inorganic ones) show significantly less excitonic absorption landscapes compared to conventional QDs such as CdSe, even though LHPs have more simplistic band structure. This thus hinders studies into the size-quantization of excitons in LHPs (and possible practical use) as well as understanding of the mechanism of LHP QD formation, which still significantly lacks behind compared to conventional CdSe and PbS QDs. To solve this, we developed a room-temperature synthesis, in which the overall QD formation occurred on a time scale of up to 30 min, slowing down the reaction kinetics by several orders of magnitudes compared conventional LHP QD syntheses.3 The size of these QDs were tunable between 3 and 13 nm range and exhibited a rhombicuboctahedral (spheroidal) shape.3,4 These CsPbBr3 QDs, as well as FAPbBr3 and MAPbBr3 exhibited up to four well-resolved excitonic transitions, finally bringing them on par with the highly excitonic absorption landscapes of CdSe and PbS QDs. This slow growth method also allowed for the first time to direct in-situ study the illusive reaction mechanism of LHP QDs, demonstrating the effective separation of the nucleation and growth stages due to the self-limiting formation of an Cs[PbBr3] intermediate precursors. The slow growth approach was further extended by using an additional in-situ anion exchange step, resulting also in spheroidal CsPb(Cl:Br)3 QDs with any Cl:Br ration and sizes from 4-10 nm.3,5 These quaternary QDs still exhibited up to five sharp excitonic absorption transitions, further demonstrating the versatility of the slow growth method.
2.2-O2
Research interest in all inorganic lead halide perovskite nanocrystals (LHP-NCs), featuring general chemical formula of CsPbX3, has recently grown fast thanks to their outstanding chemical and physical properties that make them optimal candidates for a wide range of technological applications such as photovoltaics1, light emitting devices2 and photodetectors3. In this fast-growing and heterogeneous playground, we report a robust, reproducible, and easy scalable synthetic method allowing the production up to the unprecedented scale of 8g of high quality CsPbBr3 NCs for either fundamental studies or in-solution and device applications. To this aim, we modified the synthetic procedure reported by Akkerman et al. 4 by introducing, for the first time, the use of a turbo emulsifier (Ultra Turrax Homogeneizer) usually employed for the preparation of large batches of formulates, to improve the reaction mixture homogenization and overcome concentration gradients and reproducibility issues that usually affect LHP-NCs liter-scale reaction volumes. We also introduced tetrabutylammonium bromide (TBAB) as extra bromide precursor: working in halogen rich environment is known to help reducing defectivity and this specific quaternary ammonium salt is too bulky to be competitive with other cations in the perovskite crystal lattice. We demonstrated that the amount of recovered solid material is proportional to the volume of solution used at every scale, suggesting that the process is well controlled up to the biggest scale. There are also evidences that increasing the scale, magnetic stirring becomes insufficient where turbo-emulsifier remains reliable. Moreover, the procedure is easy extendable to CsPbCl3 and mixed phase and other acid and amine ligands. We further pushed the limit by demonstrating that, with our approach, the low-boiling solvents and the excess reactants, in particular lead bromide, can be recovered and reused, thus reducing the environmental impact connected to waste production and moving a step towards final industrial application. We finally preliminary tested radioluminescence properties both in solution and in polymer matrix to apply them to scintillation field: the big amount that can be easily produced, in one pot, with low waste favors many applications, especially the production of wide scintillating windows even with at high concentration.
2.2-O3
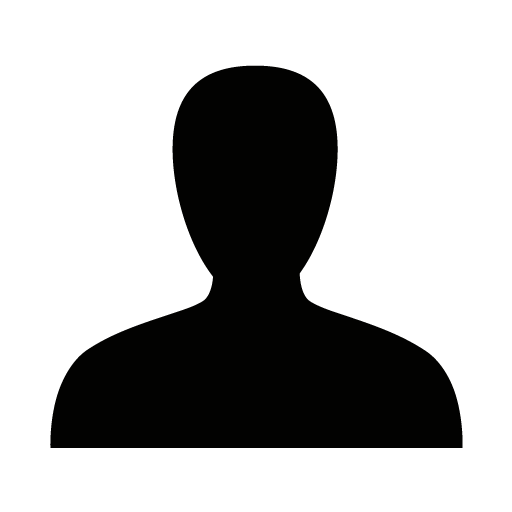
Recently, lead halide perovskite nanocrystals (LHP NCs) have emerged as a highly promising class of luminescent materials. The optical properties of LHP NCs are easily tunable by their dimensions as well as chemical composition, and they exhibit extremely high photoluminescence quantum yield (PLQY).1, 2 Despite great progress in the synthesis and application of LHP NCs, they still suffer from poor surface stability and thus leading to a drastic reduction of PLQY upon washing them.3, 4 Interestingly, we find that the PLQY and surface stability of inorganic LHP NCs significantly improves upon replacing some of the inorganic A-cations (Cs+) with organic cations (FA+ or MA+) by cation exchange. Recently, we demonstrated the preparation of mixed A-cation NC systems with a range of compositions by simple room-temperature cation exchange.5 Unlike halide segregation, the A-cations in the lattice do not undergo phase segregation under light illumination.5 We then systematically studied the surface stability as well as the optical properties of mixed A-cation LHP NCs by washing them several times with an antisolvent. Surprisingly, the mixed A-cation compositions with a small percentage of organic cation exhibit superior stability and PLQY as compared with inorganic LHP NCs upon washing them with antisolvent. In this presentation, I will discuss the synthesis, optical properties, and stability of mixed A-cation LHP NCs in comparison with individual counterparts.
2.2-O4
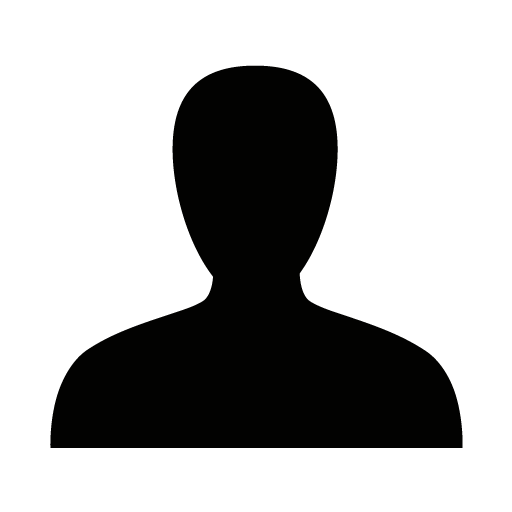
Lead halide perovskite (LHP) nanocrystals (NCs) have gained research interest due to their exceptional optoelectronic properties including high photoluminescence (PL) quantum yield and narrow PL linewidth suggesting a promising future in light-emitting technologies [1]–[3]. Emission tunability in colloidal LHP NCs can be achieved by either size [4] or compositional modifications [5]. Since the breakthrough of the LHP NCs family, it has been well-established that the NCs΄ emission can be finely tuned covering the whole visible spectrum by controlling the ratio of the halides (Br/Cl) or (Br/I) forming homogeneous alloys. Particularly, the ionic and labile character of the lattice promotes the facile post-synthesis bandgap engineering via partial or full anion exchange from Br- to either Cl- or I- while preserving the NCs’ original morphology and the 3D perovskite structure [6], [7]. In this work, a systematic study of anion exchange reactions on oleate-capped CsPbCl3 colloidal NCs is presented. We will introduce novel insights into selective anion exchange manifested by partial exchange presenting either positive cooperativity (all-or-nothing exchange behavior) or segmented nanoheterostructures. We will also address the kinetics of the reaction by means of in-situ spectroscopy and energy-dispersive X-Ray high-resolution scanning transmission electron microscopy.
2.2-O5
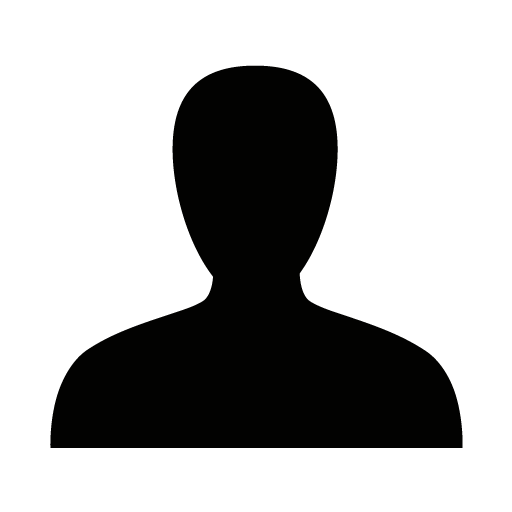
Colloidal metal halide perovskite nanocrystals (NCs) are regarded as candidates for a variety of applications – spanning from optoelectronics[1,2] to biotechnology[3,4] – on account of their many tunable optoelectronic properties[5–7]. The construction of theoretical models and the investigation of the fundamental atomistic processes influencing their features are paramount for tuning the overall performance of these materials, especially under realistic reaction conditions. An effective tool in the investigation of the dynamical behavior and properties of NC models is provided by classical molecular dynamics (MD) simulations[8–11].
In this presentation, we will gain insight into anion-exchange reactions occurring in CsPbX3 (X = Cl, Br) perovskite structures. This multi-step process is composed a series of intermediate states, each one associated to a potential energy barrier[12]. We employed enhanced sampling methods[13] to overcome the free-energy barriers associated to the individual reaction steps for the Br-to-Cl anion-exchange starting from an oleate-capped CsPbBr3 perovskite NC model. We believe that this investigation will shed light on the mechanisms and kinetic rates of this process and on the nature of its intermediate steps, allowing for an appropriate tuning of the most suitable reaction conditions of the process.
2.2-O6
Versatile surface functionalization of highly ionic surfaces, ubiquitous among inorganic nanomaterials, remains a formidable challenge in view of inherently non-covalent surface bonding. Colloidal lead halide perovskite nanocrystals (NCs), which are of interest for classical and quantum light generation,[1,2] are one of the examples. Despite some recent empirical progress in surface chemistry of lead halide perovskite NCs, the general strategy towards their robust surface functionalization still remains a challenge.[3] One of the reasons is a limited atomistic understanding of the NC-ligand-solvent interface. Here we would like to present how classical molecular dynamics (MD) simulations can be used in combination with experimental techniques to aid in understanding the surface chemistry of ionic nanomaterials and to guide experimental discovery of new better capping ligands. In particular, we would like to present the first structural investigation of perovskite NC surfaces capped with zwitterionic phospholipid molecules. Combined computational and experimental evidence suggests that the phospholipid ligands bind to the surface of the NCs with both head-groups by displacing native ions of the perovskite. The ligand head-group affinity to the surface is primarily governed by a geometric fitness of its cationic and anionic moieties into the crystal lattice. As a result, stable and colloidally robust nanocrystals of inherently soft and chemically labile lead halide perovskites – FAPbX3 and MAPbX3 (X – Br, I) – can be obtained for the first time with a lattice-matched phosphoethanolamine head-group. Stable surface passivation enables excellent optical performance of the NCs. As an example, alkylphospholipid-capped FAPbBr3 NCs display stable emission with a near-unity photoluminescence quantum yield in a broad concentration range, as well as in thick films. Ligand tail engineering, on the other hand, allows diverse surface functionalization of the NCs, broadening the scope of their potential applications.
2.2-I1
Bio Professional Preparation M.S. in Chemistry, with Honours, University of Bari, Italy, 1996 Ph.D. in Chemistry, University of Bari, Italy, 2001 Research interests Prof. L. Manna is an expert of synthesis and assembly of colloidal nanocrystals. His research interests span the advanced synthesis, structural characterization and assembly of inorganic nanostructures for applications in energy-related areas, in photonics, electronics and biology.
Halide perovskite semiconductors can merge the highly efficient operational principles of conventional inorganic semiconductors with the low‑temperature solution processability of emerging organic and hybrid materials, offering a promising route towards cheaply generating electricity as well as light. Following a surge of interest in this class of materials, research on halide perovskite nanocrystals (NCs) has gathered momentum in the last years. While most of the emphasis has been put on CsPbX3 perovskite NCs, more recently the so-called double perovskite NCs, having chemical formula A+2B+B3+X6, have been identified as possible alternative materials, together with various other metal halides structures and compositions, often doped with different elements. This talk will also discuss the research efforts of our group on these materials. I will highlight how for example halide double perovskite NCs are less surface tolerant than the corresponding Pb-based perovskites. Other topics that will be covered are the role of surface ligands on stabilizing the NCs (including those with alloy compositions), doping, and our ongoing research on various other metal halides (for example the Mn-based ones).
2.2-I2
The urgency for affordable and reliable detectors for ionizing radiation in medical diagnostics, nuclear control and particle physics is generating growing demand for innovative scintillator devices combining efficient scintillation, fast emission lifetime, high interaction probability with ionizing radiation, as well as mitigated reabsorption to suppress losses in large volume/high-density detectors. Prized for their solution processability, strong light-matter interaction, large electron-hole diffusion length and tunable, intense luminescence at visible wavelengths, lead halide perovskite nanocrystals (LHP-NCs) are attracting growing attention as highly efficient emitters in artificial light sources and as high-Z materials for next generation scintillators and photoconductors for ionizing radiation detection. Nonetheless, several key aspects, such as the trapping and detrapping mechanisms to/from shallow and deep trap states involved in the scintillation process and the radiation hardness of LHP NCs and LHP NC-based plastic nanocomposites under high doses of ionizing radiation are still not fully understood, leaving scientists without clear indications of the suitability of LHP-NCs in real world radiation detectors or design strategies for materials optimization. In this talk, I will present on our recent strategies for high performance radiation detection schemes1,3 and will report recent spectroscopic results of the scintillation process and its competitive phenomena, ultimately offering a possible path to the realization of highly efficient and extremely radiation hard LHP-NCs.
2.2-I3
Long-range coherence and correlations between atoms and electrons in solids are the cornerstones for developing future quantum materials and devices. In 1954, Dicke described correlated spontaneous emission from closely packed quantum emitters, forming the theoretical basis of superradiance and superfluorescence. It has remained an open challenge to observe such phenomena with nanometer spatial resolution, precisely the critical scale at which the collective correlations occur. In my talk, I will demonstrate superfluorescence triggered by ultra-fast free electrons pulses for the first time using superlattices of lead-halide perovskite quantum dots. These new materials have a significant interaction cross-section with electrons, efficient emission, and fast radiative rates that allow the buildup of correlation between the exciton before dephasing. The emission is toggled between spontaneous to superfluorescence by controlling the excitation area. The correlated emission is faster, brighter, and narrower than the spontaneous emission. On-demand correlated emission has applications in non-classical light sources, superfluorescence biomarkers for electron microscopy, and enhanced detectors.
2.2-O1
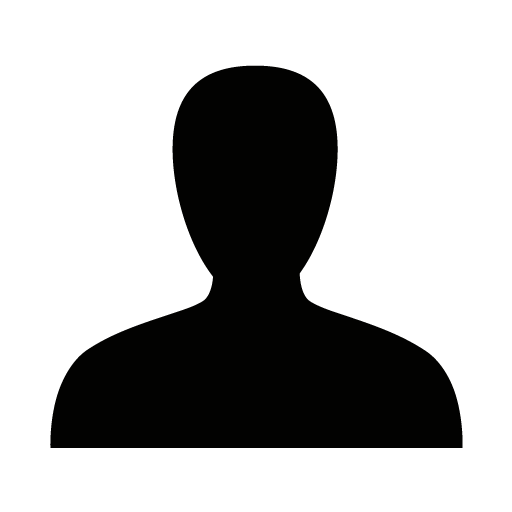
Lead-halide perovskite nanocrystals have been the subject of countless publications. Most of these works report XRD patterns featuring Bragg peaks with unusual shapes, which appear composed of two or more overlapping contributions. These however are too narrow for a nanocrystal, and the material’s structure does not account for their formation. The quest to understand these observations led us to the (re)discovery of Multilayer Diffraction, an intriguing collective interference effect known for epitaxial thin films but never investigated for colloidal nanocrystals before.[1]
Multilayer Diffraction occurs when X-rays are diffracted by nanocrystals packed with a periodic arrangement: here, the X-rays scattered by each particle interfere with those diffracted by neighbors, creating fringes of constructive interference.[2] Since the interfering radiation comes from nanocrystals, the fringes are visible only in correspondence with their Bragg peaks, thus explaining the unusual peak shape. Being a collective interference phenomenon, Multilayer Diffraction is highly sensitive to disorder, and requires precise stacking of nanocrystals to be observed. This condition is typical of self-assembled nanocrystal superlattices or stacks of nanoplatelets. Nevertheless, the Bragg peak split is routinely observed in a variety of samples, and not just in highly ordered superlattices. Why is that so?
This is only one of the many questions we will answer. Together, we will discuss why the peak split affects only some of the Bragg peaks, we will explore the influence of morphology (i.e., nanocrystals vs nanoplatelets) on Multilayer Diffraction,[3] and we will explain why this effect was not reported for popular materials like Au or CdSe, despite the extensive investigations on their superlattices. We will present models for the quantitative description of Multilayer Diffraction, which can be exploited to extract structural information hardly accessible from other techniques, first and foremost the degree of structural disorder in nanocrystal assemblies. Finally, we will demonstrate that Multilayer Diffraction is often observed yet unrecognized in a variety of nanomaterials beyond metal halide perovskites. Your sample might be next!
2.2-O2
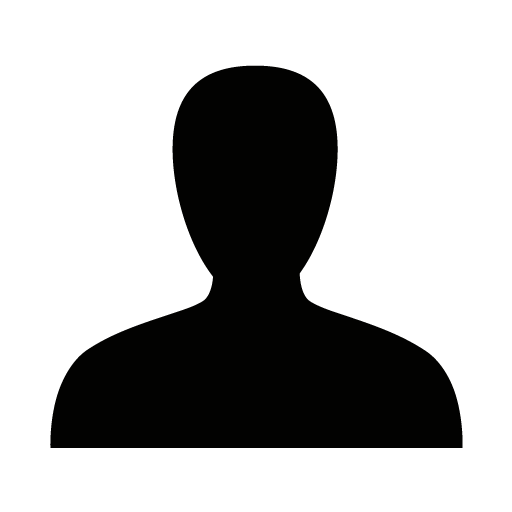
Cesium lead halide perovskite nanocrystals, owing to their outstanding optoelectronic properties (high oscillator strength of bright triplet excitons, slow dephasing, minimal inhomogeneous broadening of emission lines), are promising building blocks for creating superlattice structures that exhibit collective phenomena in their optical spectra. Thus far, only single-component superlattices with the simple cubic packing have been devised from these nanocrystals, which have been shown to exhibit superfluorescence – a collective emission resulting in a burst of photons with ultrafast radiative decay (ca. 20 ps) that could be tailored for use in ultrabright (quantum) light sources [1]. However, far broader structural engineerability of superlattices, required for programmable tuning of the collective emission and for building a theoretical framework can be envisioned from the recent advancements in colloidal science. We show that the co-assembly of cubic and spherical steric-stabilized nanocrystals is experimentally possible and that the cubic shape of perovskite nanocrystals leads to a vastly different outcome compared to all-spherical systems. Five superlattice structures have been obtained: novel AB2, ABO3, ABO6, besides expected NaCl or common to all-sphere assemblies AlB2 superlattices [2, 3]. In binary ABO3 and ABO6 superlattices, larger spherical nanocrystals occupy the A sites and smaller cubic CsPbBr3 nanocrystals reside on the B and O sites. Targeted substitution of B-site nanocubes by truncated cuboid PbS nanocrystals leads to the exclusive formation of ternary perovskite-type ABO3 superlattice. Truncated cuboid PbS NCs can occupy A-sites in binary ABO3, NaCl, and AlB2 SLs with smaller CsPbBr3 nanocubes. All synthesized superlattices exhibit a high degree of orientational ordering of the CsPbBr3 nanocubes. We also demonstrate the effect of superlattice structure on the collective optical properties. In addition, we explore the on-liquid assembly method that allows for obtaining free-floating SL films comprising perovskite nanocrystals.
2.3-I1
Prof. R. Robinson received his PhD in Applied Physics from Columbia University. After his PhD, Prof. Robinson was awarded a postdoctoral fellowship at University of California, Berkeley/LBNL in the research group of Paul Alivisatos. There, he worked on nanoparticle synthesis, chemical transformations of nanoparticles, and advanced property characterizations of nanoparticles. In 2008 Richard began a faculty position at Cornell University in the Materials Science Department, and is currently an associate professor. His primary research interests are: (I) Synthesis and chemical transformations in nanocrystals, (II) Nanocrystals in energy applications, and (III) Synchrotron x-ray characterization of nanomaterials.
therobinsongroup.org/
Magic-sized clusters (MSC) are identical inorganic cores that maintain a closed-shell stability, inhibiting conventional growth processes. Because MSCs are smaller than nanoparticles they can mimic molecular-level processes, and because of their high organic-ligand/core ratio they have “soft” inter-particle interactions, with access to a richer phase diagram beyond the classical close packed structures. In this talk I will highlight some remarkable behavior we have recently found in both of these areas. MSCs have the ability to undergo a chemically-induced, reversible isomeric transformation between two discrete states. The diffusionless reconfiguration of the inorganic core follows a first order kinetic rate driven by a distortion of the ligand binding motifs. These MSCs also display a surprising ability to self-organize into hierarchical assemblies which span over six orders of magnitude in length scale. The films are optically active with g-factors among the highest reported for semiconductor particles. Since the physical origin of the chirality for highly-structured films is challenging, we developed a method for extracting the true chiroptic-CD signal from the raw data, derived using Mueller matrix and Stokes vector conventions, and we find that the origin of the chirality is from exciton coupling between adjacent MSCs. Beyond optical properties, the multiscale self-organization behavior of these MSCs provides a new platform for the design and study of complex materials.
References
JACS 140, 3652 (2018) DOI: 10.1021/jacs.5b10006
Science 363, 731 (2019) DOI: 10.1126/science.aau9464
Nat. Mat. 21, 518 (2022) https://doi.org/10.1038/s41563-022-01223-3
ACS Nano (2022 accepted https://doi.org/10.1021/acsnano.2c06730)
2.3-I2
Crystal structure plays a central role in the properties exhibited by a material. As such, intense research efforts are focused on polymorph selectivity during materials synthesis in the nano and bulk scales. The MnS system, having three known polymorphs, has received considerable attention towards developing a better understanding of factors that control crystal structure selectivity. The three polymorphs are metastable zincblende- and wurtzite-MnS, with rock salt-MnS as the thermodynamic product. In addition, MnS is an Earth-abundant semiconductor with potential applications that include photovoltaics, batteries, and opto-electronics. This talk focuses on the halide-driven polymorph selectivity of MnS nanoparticles. Specifically, we show that the product can be tuned between the wurtzite and rock salt structures by varying the Mn halide precursor, under otherwise identical conditions. Temperature and aliquot studies were employed to obtain insights into the reaction pathway. The synthesized particles were characterized with powder X-ray diffraction, Transmission Electron Microscopy (TEM) and high-resolution TEM. This halide-driven selectivity can also be extended to the MnSe system.
2.3-I3
Colloidal semiconductor Quantum Dots (QDs) of the III-V family (InP and InAs) are characterized by a large surface-to-volume ratio that renders them extremely sensible to surface processes. Passivating ligands, employed to stabilize QDs in organic solvents, play a pivotal role in influencing the structure and the optoelectronic properties of these materials. Despite major progresses attained in the last years to model the QD surfaces, there are still several key questions to be answered on the nature of the QD-ligand interactions and how trap states, which are deleterious to optical efficiency, develop on the surface.
A leap forward in solving the above issues is to analyze the surface using first principle simulations, such as Density Functional Theory (DFT). Until now some of the major drawbacks of this approach have been: (i) the size of the system that can be handled that in the best cases is restrained to a few hundredths atoms (i.e. a small sized QD surrounded by short ligands), and (ii) the description of static properties with the absence of dynamic effects.
Here, I will present a tool to automatically parametrize the force-field of nanoscale semiconductor crystallites, that we can then use to perform multiscale modeling of real sized InP and InAs QDs passivated with chlorine and primary ammine ligands with a simulation box containing up to a million of atoms including the solvent. Molecular dynamics simulations, carried out up to the nanosecond timescale, provide crucial insights on the surface dynamics, and the role of the ligands in influencing the properties of these materials.
2.3-O1
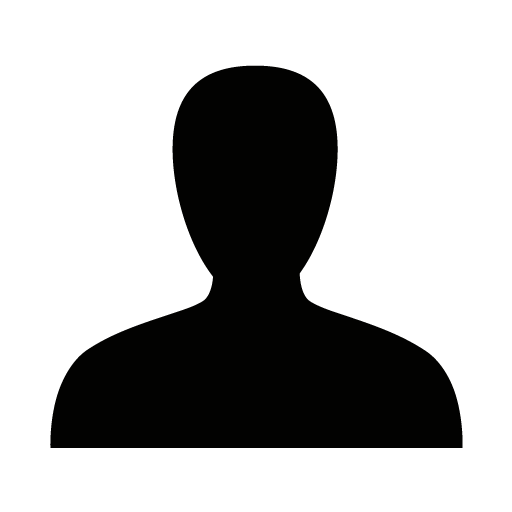
In the short-wavelength infrared range, there is poor or no availability of cheap and optically tunable bulk semiconductors for optoelectronic applications like telecommunications and sensing in automated transport. Semiconducting colloidal quantum dots like lead chalcogenides instead, display bandgap tunability (800-3000 nm) with size and are cheaply synthesized in solution. Unfortunately, due to their deposition techniques, thin films of such materials are disordered and therefore display poor transport properties. Self-assembled arrays of colloidal quantum dots, the so called superlattices, are expected to lead to coherent transport through minibands therefore approaching the electronic properties of the bulk counterparts. Nevertheless, the poor control on the ordering, on the electronic coupling and on surface trap passivation has led to the observation of disappointing charge transport properties.
Here [1] we report the self-assembly of highly ordered 3D superlattices with tunable structure and thickness with single layer precision. The superior quality of such superlattices is demonstrated by a combination of advanced structural characterization techniques like atomic resolution electron microscopy and grazing incidence X-Ray scattering. We measure in-plane coherence lengths up to 100 nm but most noticeably, the superlattices are fully coherent throughout their thickness. This outstanding ordering results in record electron mobilities up to 278 cm2/Vs as measured in a field effect transistor using ionic gel as gate dielectric. This value is not only approaching the bulk mobility, but it is a record among any self-assembled superlattice with fully quantum-confined nanocrystals as building blocks. Such findings demonstrate that we were able to obtain an optoelectronic metamaterial and this could pave the way for a new generation of optoelectronic devices with highly tunable properties.
2.3-O2
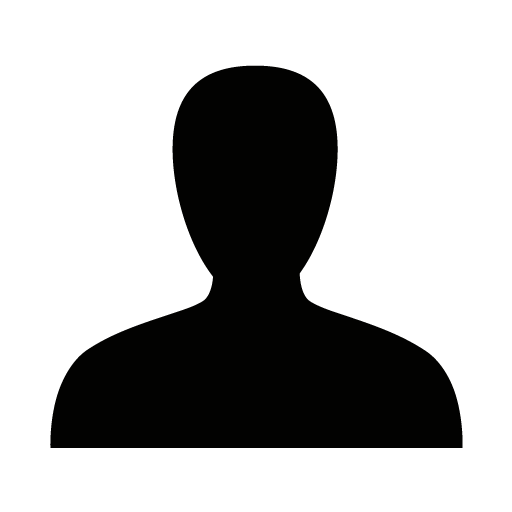
Solar cells fabricated from metal sulfide quantum dots require facile and reproducible synthetic methods that determine the particle size, distribution, shape and faceting of the quantum dots. These properties will affect the energetic landscape of the nanocrystal thin films, by modifying the bandgap, trap density and surface composition. So far, an established hot injection synthesis route has been adapted for most metal sulfide quantum dots, resulting in solar cells with record efficiencies such as 15.5% for PbS dots (Advanced Energy Materials 2022, 12 (35), 2201676) and 9.2% for environmentally-friendly AgBiS2 (Nature Photonics 2022, 16, 235). The synthesis of most metal sulfide quantum dot relies on the use of a strongly reactive sulfur source: bis(trimethylsilyl) sulfide (TMS)2S. This sulfur compound is known to hydrolyze in the presence of water, creating toxic and foul smelling H2S. Consequently, its storage and handling are problematic even for a small-scale laboratory facility. Moreover, this hydrolyzation causes the synthesis to be irreproducible, since seemingly the same synthetic conditions end up leading to large variations in the quantum dot average size and distribution. These disadvantages, together with its high cost, make (TMS)2S unsuitable for large scale applications and support the motivation to find alternative sulfur precursors for metal sulfide quantum dot synthesis.
In this study we demonstrate that bis(stearoyl) sulfide (St2S) is an excellent alternative to (TMS)2S and illustrate this via the synthesis of PbS and AgBiS2 quantum dots and their application in photovoltaics. St2S is a solid, odor-free, air-stable sulfur compound with a low melting point of 60°C, which can be used instead of (TMS)2S with minimal changes to the synthesis procedure. We characterize the quantum dots by transmission electron microscopy, and confirm the chemical composition by diverse spectroscopic techniques. Furthermore, we prove that without further optimization, PbS quantum dot solar cells made using St2S are equally efficient as those made using (TMS)2S. In the case of AgBiS2, the performance with St2S is slightly lower than that of the referenced devices, due to a smaller overall size of the nanoparticles. Importantly, photovoltaic cells fabricated using St2S precursors are more stable because the dots are less prone to surface oxidation. To summarize, the substitution of the unstable (TMS)2S with the air-stable St2S is highly promising for the synthesis of metal sulfide quantum dots for a broad range of applications.
1.1-I1
Twisted van der Waals materials have risen as a powerful platform to engineer artificial quantum matter. Artificial moire heterostructures, in general, display two length scales, the original lattice constant and the emergent moire length. Here we reveal a microscopic mechanism to engineer van der Waals multiferroics at those two length scales from the interplay of non-collinear magnetism and spin–orbit coupling, both in van der Waals monolayers [1] and twisted multilayers [2]. First, focusing on the recently isolated NiI2 multiferroic monolayer, we reveal the origin of the helimagnetic order, and the critical role of halide spin-orbit coupling in driving a ferroelectric distortion. We demonstrate that the electronic reconstruction accounting for the ferroelectric order emerges from the interplay of such a non-collinear magnetism and spin-orbit coupling. Second, we show the emergence of multiferroic order in twisted chromium trihalide bilayers, an order fully driven by the moiré pattern and absent in aligned multilayers. We show that a spin texture is generated in the moiré supercell of the twisted system as a consequence of the competition between stacking-dependent interlayer magnetic exchange and magnetic anisotropy. An electric polarization arises associated with such a non-collinear magnetic state due to the spin-orbit coupling, leading to the emergence of a local ferroelectric order following the moiré. Among the stochiometric trihalides, our results show that twisted CrBr3 bilayers give rise to the strongest multiferroic order. We further show the emergence of a strong magnetoelectric coupling, which allows the electric generation and control of magnetic skyrmions. Our results put forward van der Waals materials as a powerful platform to engineer artificial multiferroic order and electrically control exotic magnetic textures.
[1] Adolfo O Fumega and J L Lado 2022 2D Mater. 9 025010 (2022)
[2] Adolfo O. Fumega, Jose L. Lado, arXiv:2207.01416 (2022)
1.1-I2
Progress of light-based technology has led to a paradigm shift in materials design: light, which has been commonly relegated to a mere probe of material properties, can be instead exploited to alter material properties altogether [1,2]. Central in the paradigm shift is the possibility of generating strong coupling between light and matter and in turn induce the formation of light-matter hybrid states with properties which can be controlled on demand. An intriguing route to achieve strong light-matter coupling is to embed materials inside cavities [3, 4], where the coupling is enhanced by the confinement of light in a small region of space [4]. In the talk, I will present theoretical results based on first-principles methods on three different hybrid light-matter designs. I will demonstrate the formation of exciton-light hybrid states in 2D crystals embedded in a cavity and the tunability over their energetics and brightness [5]. I will then show how the strong light-matter coupling allows for the design of a three-way exciton-phonon-photon quasiparticle which is characterized by unique features in optical response [6]. Finally, I will introduce the concept of a photo-groundstate by demonstrating that the vacuum fluctuations of light can induce a change of the collective phase from paraelectric to ferroelectric in the groundstate of SrTiO3 (see Fig. adapted from [7]), which has thus far only been achieved in out-of equilibrium strongly excited conditions [1]. These findings demonstrate the potential of cavity material engineering as a new paradigm for material manipulation.
1.1-I3
Nicolò holds a tenure-track professorship and is the head of the ‘Ultrafast Nanophotonics and Advanced Functional Materials’ Group at the Department of Physics, Umeå University (Sweden) supported by the Swedish Research Council, the European Innovation Council, the Faculty of Science and Technology and the Kempe Foundations. Currently, he is also a visiting researcher and group leader at the Department of Physics and Materials Science, University of Luxembourg. He is author of more than 50 scientific papers in renowned international journals (including Physical Review Letters, Nature Communications, Advanced Optical Materials and Nano Letters) and co-author of an international patent on metamaterials for nanophotonic applications. He has more than 100 contributions at international conferences, international symposia, and colloquia, more than 25 as invited speaker.
Nicolò studied Physics at the University of Ferrara (Italy) from 2007 to 2012, and earned his PhD in Physics of Nanostructures and Advanced Materials (grade: outstanding cum laude) from the University of the Basque Country and CIC nanoGUNE (Spain) in 2016. As Predoctoral researcher in the Nanomagnetism Group at CIC nanoGUNE, he studied the optical properties of nanostructured magnetic materials, under the supervision of Prof. Paolo Vavassori. In 2015, he received the “Piero Brovetto” Award from the Italian Physical Society for “his contributions in the fields of nanomagnetism and nanooptics and the study of the physical properties of magnetoplasmonic nanoantennas and their application in bio-sensing”. During his PhD, he was Visiting Scientist at the Department of Applied Physics at Aalto School of Science (Finland) in 2014, and at the Department of Physics at Chalmers University of Technology (Sweden) in 2015.
From 2017 to 2018, he was Research Associate at the Italian Institute of Technology in Genoa (Italy) in the Plasmon Nanotechnologies Unit led by Dr. Francesco De Angelis, working on plasmonic nanostructures to control fundamental light-matter interactions, such as absorption and scattering of light, at the nanoscale. Concurrently, he contributed to the design and development of plasmonic nanoarchitectures for single protein sequencing through fluorescence and energy transfer mechanisms enhancement in collaboration with Dr. Denis Garoli, as well as for cell’s membrane and nucleus investigations through surface enhanced spectroscopy in collaboration with Dr. De Angelis.
From 2019 to 2021, he was a Junior Group Leader at the University of Luxembourg in the Ultrafast Phenomena in Condensed Matter Group led by Prof. Daniele Brida, leading a research team working on the FNR CORE Project ‘Ultrafast coherent hybridization of photons and spins in multi-functional magnetoplasmonic metamaterials’ (2020-2022) and the European H2020 FET-Open Project ‘Ultrafast Raman Technologies for Protein Identification and Sequencing’ (2020-2024). Here, he also developed a research line focused on the study of ultrafast phenomena in magnetoplasmonic nanostructures, and on the development of an ultrafast magneto-optical pump-probe spectroscopy setup working in different configurations (e.g., Faraday effect, polar and longitudinal Kerr effect, etc..), in a broad spectral range (from the visible to the mid infrared) and based on the use of few-optical cycle light pulses. In 2019 he was also Visiting Scientist in the Group of Prof. Alfred Leitenstorfer at the University of Konstanz.
In December 2021, he moved to Umeå University (Sweden) to start his own research group. In November 2021 he was awarded a prestigious Starting Grant from the Swedish Research Council aiming at studying nonthermal charge and spin dynamics in magnetoplasmonic nanostructures with sub-10 fs time resolution, and a Horizon Europe EIC-Pathfinder Project aiming at studying molecular structure by using advanced computational tools and ultrafast spectroscopy techniques together with international partners from Germany, Italy, France, Luxembourg and Sweden. Until now, he was able to collect more than 2.5 M€ (>25M SEK, including start-up packages) to fund his own research as Principal Investigator. In 2022, he became a Fellow of the Young Academy of Europe, an independent pan-European initiative of top young scientists for networking, scientific exchange and science policy.
Nicolò’s research span a broad range of fundamental and applied aspects of natural sciences, with a special focus on both the fundamental and applied aspects of light-matter interactions in advanced and multifunctional nano- and meta-materials for opto-electronics and information processing, photochemistry and biotechnology, by using frequency- and time-resolved (magneto-)optical spectroscopy, finite-element computational methods and bottom-up/top-down nanofabrication techniques (for a brief introduction on nano- and meta-materials, you can see his TEDx talk entitled Metamaterials matter: smart material of future).
From 2018 to 2021 Nicolò has been an Early Career Editorial Board Member of 'Nano Letters' (published by the American Chemical Society). Currently, he is an Associate Editor of 'Advanced Photonics Nexus' (published by SPIE and Chinese Laser Press) and a Young Editorial Board Member of 'Ultrafast Science', a Science partner journal published by the Chinese Academy of Sciences and distributed by the American Associations for Advances in Science (AAAS).
Finally, Nicolò is a certified Research Integrity trainer in the framework of the European Project VIRT2UE, aiming to provide the knowledge and skills to conduct virtue-based ethical research and to foster reflection on scientific virtues in researchers, in line with the European Code of Conduct for Research Integrity. From 2019 until 2021, he was a Research Coach and a pro-bono consultant at the Luxembourg Agency for Research Integrity.
Ultrafast control of light-matter interactions is fundamental in view of new technological frontiers, for instance in light-driven information processing and nanoscale photochemistry [1]. In this framework, we explore metal-dielectric nanocavities to achieve all-optical modulation of the light reflectance at a specific wavelength. Without the need of driving higher order effects, our system is based on linear absorption, provides large relative modulation exceeding 100% and switching bandwidths of few hundred GHz at moderate excitation fluence [2]. This archetypical system becomes even more interesting if the “gain medium” is an inorganic van der Waals bonded semiconductor, like a transition metal dichalcogenide (TMD). TMDs are subject of intense research due to their electronic and optical properties which are promising for next generation optoelectronic devices. In this context, understanding the ultrafast carrier dynamics, as well as charge and energy transfer at the interface between metals and semiconductors is crucial and yet quite unexplored. By employing a pump-push-probe scheme, we experimentally study how thermally induced ultrafast charge carrier injection affects the exciton formation dynamics in bulk WS2 [3], opening up excellent opportunities also in nano-chemistry. In fact, if an electronic transition strongly interacts with the light modes of a resonator, we can tailor the energetics and the morphology of a molecular state. By combining quantum mechanical modelling and pump-probe spectroscopy, we shed light on the ultrafast dynamics of a hybrid system composed of photo-switchable dye molecules coupled with
anisotropic plasmonic nanoantennas, which allow us to selectively switch between two regimes where the light-matter interaction is either weak or strong [4]. Our synergistic approach is instrumental to devise new strategies for tailoring electronic states by using plasmons for applications in polaritonic chemistry on femtosecond timescales.
[1] A. N. Koya, M. Romanelli, J. Kuttruff, N. Henriksson, A. Stefancu, G. Grinblat, A. De Andres, F. Schnur, M. Vanzan, M. Marsili, M. Rahaman, A. Viejo Rodríguez, T. Tapani, H. Lin, B. D. Dana, J. Lin, G. Barbillon, R. Proietti Zaccaria, D. Brida, D. Jariwala, L. Veisz, E. Cortes, S. Corni, D. Garoli, and N. Maccaferri, “Advances in ultrafast plasmonics”, arXiv:2211.08241.
[2] J. Kuttruff, D. Garoli, J. Allerbeck, R. Krahne, A. De Luca, D. Brida, V. Caligiuri, and N. Maccaferri. “Ultrafast all-optical switching enabled by epsilon-near-zero-tailored absorption in metal-insulator nanocavities”, Commun. Phys. 3, 114, 2020.
[3] K. R. Keller, R. Rojas-Aedo, H. Zhang, P. Schweizer, J. Allerbeck, D. Brida, D. Jariwala, and N. Maccaferri.
“Sub-ps thermionic electron injection effects on exciton-formation dynamics at a van der Waals semiconductor/metal interface”, ACS Photonics 9, 2683-2690, 2022.
[4] J. Kuttruff, M. Romanelli, E. Pedrueza-Villalmanzo, J. Allerbeck, J. Fregoni, V. Saavedra-Becerril, J. Andreasson, D. Brida, A. Dmitriev, S. Corni, and N. Maccaferri. “Ultrafast dynamics of a hybrid plasmon polariton-molecular system at the weak and strong coupling regimes”, arXiv:2205.06358.
1.2-I1
Agustín is experienced in the optical design, fabrication and characterization of large area photonic architectures that can be easily implemented in emerging optoelectronic devices to improve their performance. His group specializes in soft nanoimprinting lithography, which offers an inexpensive and simple pathway to exploit the optical properties of nanophotonic structures with unconventional materials and devices.
Photonic architectures provide means to manage light which can greatly improve the performance of many optoelectronic devices. These nanostructures bring novel pathways to couple more sunlight into solar cells or they can help light escape the bulk of an LED. However, traditional nanofabrication routes are costly, cumbersome and incompatible with high throughput processes. This is why in our group we work with soft nanoimprinting lithography, a scalable, fast and inexpensive way to produce nanostructures from a variety of materials. In this presentation I will show how we use our pre-patterned stamps to induce the long-range ordering of different types of colloids (gold colloids and perovskite nanocrystals) and exploit the optical properties of the resulting metasurface. The combination of templated induced assembly and metal colloids [1],enables 2D plasmonic crystals exhibiting surface plasmon lattice resonances in the visible with high quality factors (> 60), which can be applied to enable lasing from an organic dye. Similarly, nanoimprinting lithography can be applied to cesium lead halide perovskite nanocrystals [2,3] (CsPbBr3 NCs) to produce a photonic structure that improves photoluminescence from the material and even enables a reduced threshold for ASE (amplified spontaneous emission). In addition, the use of stamps with pre-patterned chiral motifs results in the seamless production of chiral perovskite metasurfaces exhibiting high circularly polarized photoluminescence with dissymmetry factors (glum) as high as 0.3 for unmodified green or red emitting NCs.
1.2-I2
The full blossoming of quantum technologies requires the availability of easy-to-prepare materials where quantum coherences can be effectively initiated, controlled, and exploited, preferably at ambient conditions. Solid-state multilayers of colloidally grown quantum dots (QDs) are highly promising for this task because of the possibility of assembling networks of electronically coupled QDs through the modulation of sizes, inter-dot linkers, and distances. [1–3]
In this work, the coherent dynamics of solid-state multilayers of electronically coupled colloidally grown CdSe QDs are explored by two-dimensional electronic spectroscopy (2DES). 2DES techniques have been developed starting from the beginning of 2000, and historically they have been mainly exploited for the investigation of subtle dynamic mechanisms of energy and charge transport in biological complexes. Only later, these techniques have been recognized to be particularly valuable also for the study of transport processes in artificial nanomaterials and nanodevices. [4] In particular, recently proposed ‘action-based techniques’ appear particularly suited to address coherent dynamics in real functioning devices. [5]
In this work, we present the study of semiconductor nanocrystals (‘quantum dots’) in solid-state devices by different variants of 2DES: (i) the fully noncollinear optically detected BOXCARS configuration and (ii) the fully collinear photocurrent detected setup. The time evolution of coherent superposition of states was captured at ambient conditions in both cases. We thus provide important evidence for inter-dot coherences in such solid-state materials, opening up new avenues for the effective application of these materials in
quantum technologies. [6]
1.3-I1
MemComputing is a new physics-based approach to computation that employs time non-locality (memory) to both process and store information on the same physical location [1]. Its digital version is designed to solve combinatorial optimization problems. A practical realization of digital memcomputing machines (DMMs) can be accomplished via circuits of non-linear dynamical systems with memory engineered so that periodic orbits and chaos can be avoided. A given logic (or algebraic) problem is first mapped into this type of dynamical system whose point attractors represent the solutions of the original problem. A DMM then finds the solution via a succession of elementary avalanches (instantons) whose role is to eliminate configurations of logical inconsistency (‘‘logical defects’’) from the circuit. I will discuss the physics behind MemComputing and show many examples of its applicability to various combinatorial optimization problems, Machine Learning, and Quantum Mechanics, demonstrating its advantages over traditional approaches and even quantum computing.
1.3-O1
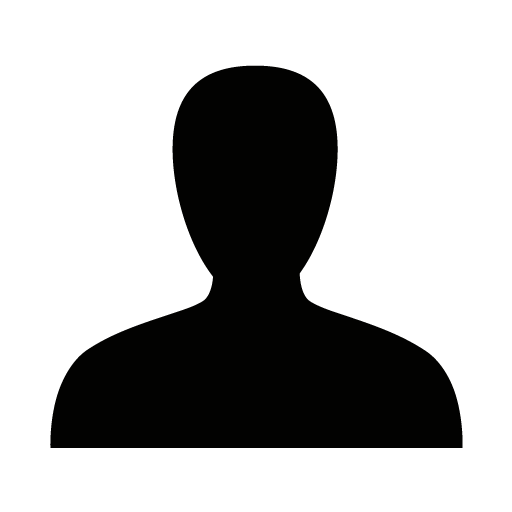
Two-dimensional magnetic materials —being the true limit of miniaturization—provide extensive control over theirs structural and magnetic properties and open prodigious opportunities in spintronics and magnonics [1,2]. Thus, the need for theoretical modelling of the large system at the atomic scale arises. Taking the robust and air-stable layered A-type antiferromagnet CrSBr [3] with high Curie temperature [4], which exhibits highly anisotropic electronic transport [5] and magnetic [6,7] properties as an example, we demonstrate an efficient computational approach for modelling of thermodynamic properties and spin dynamics under applied static strain. Starting from the full spin Hamiltonian we evince high sensibility of the critical temperature to the applied strain (40K increase at 5% strain) in the framework of renormalized spin wave theory. By the means of dynamic simulation, we display that the magnetic monolayer exhibit pronounced anisotropy of magnon propagation velocity with respect to the applied strain direction. Thus, such an anisotropic behavior makes this system a promising platform to study magnons in 2D materials.
1.3-O2
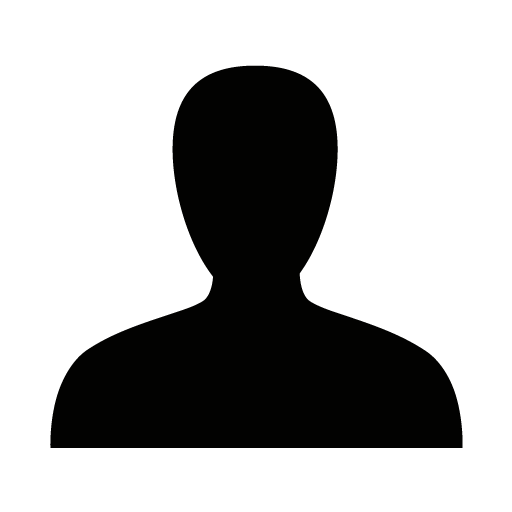
Transition metal dichalcogenides (TMDs), such as WSe2 or MoS2, are van der Waals materials with exceptional properties, such as chemical stability and mechanical flexibility[1], high binding energies[2], [3], high oscillator strengths and narrow photoluminescence linewidths[4]. However, by changing the crystal thickness (from bulk to monolayer), they show a transition from indirect to direct bandgap and so the monolayer is strictly required. On the other hand, Rhenium disulfide (ReS2) is a group-VII TMDs, with attractive properties, such as exceptionally high refractive index, significant oscillator strength and optical birefringence[5]. This envolves the formation of two orthogonally polarized in–plane excitons[6] induced by ReS2 crystallization in a distorted single-layer trigonal structure of triclinic symmetry. It is worth nothing that these exceptional optical properties persist from bulk to monolayer, making possible to exploit the high refractive index of this material and to tune the characteristics of the nanophotonic devices by changing crystal thickness. In this work, we observed that for crystals with a thickness higher than 50 nm, the excitonic transitions show a fine structure observed in previous works in literature [6]–[9], but whose nature is widely debated. With our work we clearly demonstrate that this fine structure is due to the longitudinal and transverse exciton, which becomes evident in ReS2 crystals thanks to the unique combination of its exceptional high refractive index and large exciton oscillator strength that ReS2 possesses.
By transferring ReS2 flake on a Distributed Bragg Reflector (DBR), we also observed a polarization-dependence strong coupling between Fabry-Perot modes and both excitons in the fundamental and Rydberg excited states, resulting in the formation of middle polariton branches. These show a strong polariton-polariton interaction which results in a blueshift of the polariton mode when the polariton density inside the system increases, that clearly reflect an outstanding interest of ReS2 as a solid-state counterpart of Rydberg atomic systems. This study offers a complete interpretation of ReS2 optical features, making this material interesting for a plethora of photonic applications and a novel platform for the exploration of topological properties.
1.3-O3
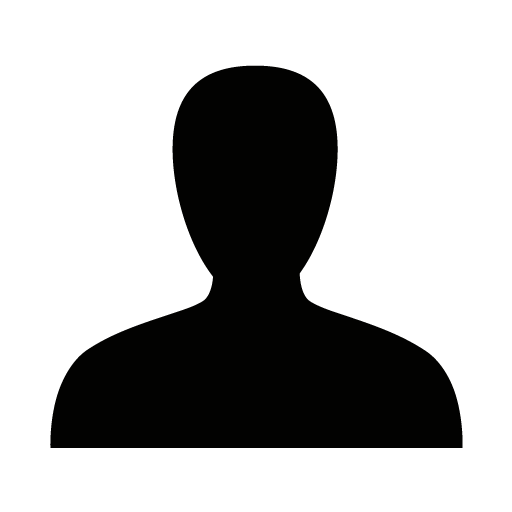
Perovskite nanocrystal superlattices are three-dimensional solid-state ordered ensembles of monodispersed semiconductor nanoparticles. Upon interaction with light pulses, these highly ordered systems can give rise to unexpected phenomena such as superfluorescence, a recently reported many-body quantum state in which the excitons generated in each single perovskite nanocrystal are coherently locked [1]. The possibility to realize macroscopic quantum states with high exciton densities, low energy broadening and long dephasing time, makes these systems promising platform to develop entangled multi-photon quantum light devices or quantum information storage systems [2, 3]. Among the halide perovskite nanocrystals, hybrid organic-inorganic compositions have attracted attention owing to high conversion efficiencies in solar cells [4] and the promise to maintain quantum coherence [5, 6]. For further progress in this field, relationship between cooperative phenomena, nanocrystal composition and excitonic features still need to be established.
In this work, we compare all-inorganic CsPbX3 (X = Cl, Br or I) and hybrid FAPbX3 (FA = formamidinium; X = Br or I) superlattices assembled from nanocrystals derived from either a direct synthesis or an aging-assisted size-selection. By combining halides and A-cation alloying, we demonstrate exciton tunability over all the visible spectrum. We employ an ultrashort resonant optical pumping scheme to study the collective states that can arise from an ordered system of quantum confined excitons interacting with laser pulses. Starting from superlattices of CsPbBr3 nanocrystals, we demonstrate that these systems can be driven across different quantum phases by tuning the laser intensity: from the excitonic Mott insulating phase to the collective superradiant state and the metallic electron-hole liquid phase. The behavior of CsPbBr3 is compared with that of FAPbI3 nanocrystal superlattices to understand structure-property relationships.
1.3-O4
The direct wet chemical synthesis of lead chalcogenide based 2D semiconductors, so-called nanoplatelets (NPLs), yields photoluminescent materials with strong excitonic effects at room temperature. [1,2,3] Here, we report a direct wet chemical approach [1] followed by a subsequent surface passivation step [2] toward bright colloidal PbSe NPLs with high emission efficiency. The NPLs exhibit excitonic features in the range of 800 - 1000 nm and photoluminescence in the range of 860 - 1510 nm, with the respective positions being seamlessly tunable by adjusting the NPLs lateral size via the reaction parameters. [2] Upon surface passivation with metal halides the NPLs exhibit a photoluminescence quantum yield of up to 60 % depending on the PL position as well as a reduced full width at half maximum of the photoluminescence by 10 %. [1] The enhanced optical properties are ascribed to a combined passivating role of both X- and Z-type binding halides and metal halides, providing an additional tool for tailoring the optical properties, colloidal stability and photostability of the PbSe NPLs. [1]
Efficient and narrow photoluminescence in the technologically desirable near-infrared and shortwave-infrared regions are vital requirements for single photon emission and applicability in quantum optics, rendering colloidal PbSe NPLs promising candidates compatible with integrated and fiber-based technology.
1.3-O5
Photonic quantum technologies like quantum key distribution for safe data exchange use single photons as information carrier, provided by a single photon source. Among others, semiconductor nanomaterials have shown their capability in single photon emission.[1]
Ultrathin colloidal two-dimensional (2D) semiconductors, so-called nanoplatelets, represent a highly topical material class with optical properties including very narrow absorption and emission.[2] They exhibit strong excitonic effects caused by a few-atom layer thickness of the structures.
Lead chalcogenide-based colloidal 2D nanoplatelets are of special interest, since their band gap can be tailored over a broad near-infrared spectral range.[3,4] For example, colloidal chemistry methods yield 2D PbS nanoplatelets with an optical absorption onset at 680 nm, a thickness of only 1-2 nm and a lateral dimension of ~10 nm2. Photoluminescence quantum yields of 20 % at 720 nm are obtained. However, at room temperature, exciton-phonon coupling contributes to photoluminescence linewidth broadening in the structures.[5]
Here, we show single PbS nanoplatelet photoluminescence measurements at cryogenic temperatures, revealing an extremely narrow full-width at half maximum of 0.6 meV. Due to the 2D shape of the nanoplatelet, a polarization anisotropy unknown from spherical quantum dots is observed. We will discuss the prospects of PbS nanoplatelets as interesting colloidal candidates for single photon emission.
2.1-I1
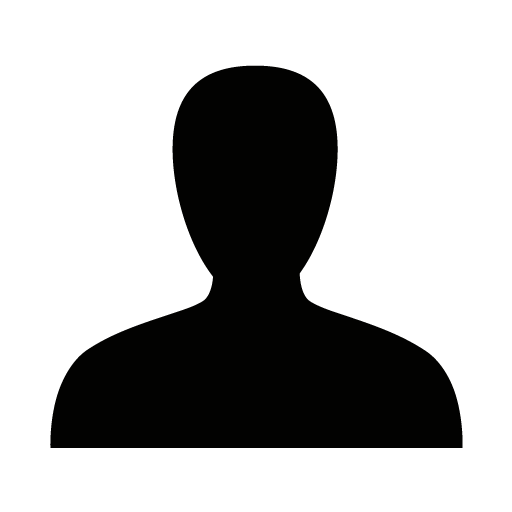
In recent years, the study of charge transfer and excitonic properties of van der Waals matter has been a rapidly growing area of research due to their importance for ultrathin optoelectronic, photovoltaic and photocatalytic components. In such applications, quasi-particles and excitons, often act as carriers in charge, spin and energy transfer processes. These transport processes can be significantly impacted by structural complexity, reduced dimensionality, interface composition or the presence of impurities and adatoms. In this talk, I will address some of these complexities from first principles, focusing on transition-metal dichalcogenides (TMDC) and TMDC-graphene interfaces. I will discuss the role of state localization due to atomic-size defects on the optical and magnetic properties [1, 2]. Using many-body perturbation theory within the GW and Bethe-Salpeter equation approach, I will show how the excitonic picture associated with the presence of defects, which result in a reduced valley and spin selectivity due to hybridized electron-hole transitions, can lead to structurally controllable exciton magnetic response. Subsequently, I will delve into the role of symmetries in charge transfer and excitonic properties on TMDC-graphene heterobilayers containing monoatomic chalcogen vacancies [3]. I will analyze the impact of the subgap defect-based features on the microscopic dynamics and absorption features, including the interplay between the spatial and the spin degrees of freedom through the spin-orbit interaction. Finally, I will show how defects become a slow coherent transport channel for interlayer charge transfer while simultaneously stronly altering the exciton properties of the TMDC-graphene interface due to a combination of folding, screening and mixing of the optical transitions.
[1] E. Mitterreiter, B. Schuler, A. Micevic, D. Hernangómez-Pérez, et al. Nat. Comm. 12, 3822 (2021).
[2] T. Amit, D. Hernangómez-Pérez, G. Cohen, D. Y. Qiu, and S. Refaely-Abramson, Phys. Rev. B 106, L161407 (2022).
[3] D. Hernangómez-Pérez, A. Donarini, and S. Refaely-Abramson (Phys. Rev. B, accepted, Editor's Suggestion).
2.1-I2
Large-scale, distributed quantum states are the basis for novel applications in quantum communication, quantum remote sensing or distributed quantum computing. The necessary infrastructure will be provided by distributed quantum networks [1], allowing for quantum bit transmission, processing and storage at single nodes. Sources of single photons and entangled photon states are important constituents of such networks. Epitaxially grown quantum dots (QDs) show great potential for the deterministic generation of such photon states. Here we utilize the emerging family of epitaxially grown GaAs/AlGaAs quantum dots obtained by droplet etching and nanohole infilling. They are outstanding sources of indistinguishable single photons and polarization-entangled photon pairs, with a unique combination of metrics (brightness, purity, coherence, emission rate) [2,3]. Under pulsed resonant two-photon excitation, we observe emission of polarization-entangled photon pairs with high purity of 0.99 and high entanglement fidelities up to 0.94. Bright entangled photon emission is realized by tailoring the dielectric environment around the QDs, allowing for the first implementation of a core element in quantum communication: Entanglement swapping between two pairs of photons emitted by a single quantum dot is demonstrated with a fidelity up to 0.81 [2].
Coupling these emitters to quantum memories with long coherence times enables the development of hybrid nanophotonic devices for quantum communication applications. GaAs/AlGaAs QDs exhibit very narrow wavelength distributions at rubidium based quantum memory transitions. We apply strain tuning via piezoelectric actuators to allow for reversible fine-tuning of the emission frequency. Employing active feedback then allows to stabilize the frequency of single photons to an atomic rubidium standard [4]. Another promising quantum memory candidate is Silicon-vacancy centers in diamond, as they show strong interactions with single photons via their zero phonon line at around 737nm. We report the first GaAs/AlGaAs quantum dots emitting single photons at this wavelength. Polarization entangled photons are generated via the biexciton-exciton cascade decay with a fidelity of 0.73. High single photon purity above 89% is maintained up to 80K (above liquid nitrogen temperature), rendering this system attractive for real-world implementations.
To ensure excellent quantum optical characteristics, epitaxial QDs have to be buried under thick barrier layers to maintain a distance from detrimental surface states. However, to unlock their full potential, QDs need to be integrated into scalable (hybrid) nanophotonic devices that require a vanishing distance to the surface. For instance, investigating strong coupling to optical cavity fields requires ultra-small mode volumes, and efficient interaction with plasmonic or dielectric nanoparticles can only be achieved within a few nanometers, therefore requiring surface proximity of QDs. Although single epitaxial QDs very close to the surface have been studied for over two decades. Weak and broad emissions were the result, rendering advanced quantum optical experiments infeasible. We show the complete restoration of optical properties from quantum dots grown directly on a semiconductor surface, leading to bright, ultra-stable, coherent and blinking-free single photon emission [5]. Under quasi-resonant excitation, single photons are generated with 98.8% purity, 77% indistinguishability, linewidths down to 4µeV and 99.7% persistency across 11 orders of magnitude in time. The emission is stable even after two years and when being subjected to nanomanufacturing processes. Some long-standing stumbling blocks for surface-dominated quantum dots are thereby removed, unveiling new possibilities for hybrid nano-devices and applications in quantum communication or sensing.
2.1-I3
Dr. Francesco Di Stasio obtained a Ph.D. in Physics at University College London (UK) in 2012. He then worked as a research Scientist at Cambridge Display Technology (Sumitomo Chemical group, UK) until he undertook postdoctoral research at the Istituto Italiano di Tecnologia (IIT, Italy). In 2015 he was awarded a Marie Skłodowska-Curie Individual Fellowship at the Institute of Photonic Sciences (ICFO, Spain). Since 2020 he is Principal Investigator of the Photonic Nanomaterials group at IIT after being awarded an ERC Starting grant. Francesco is a materials scientist with more than 10 years of research experience in optoelectronics.
Current research interests and methodology: Nanomaterials for classical and non-classical light-sources: This research activity focuses on the investigation of synthetic routes to obtain highly luminescent semiconductor colloidal nanocrystals and exploit such material in light-emitting diodes (LEDs). Here, we study how chemical treatments of colloidal nanocrystals can promote enhanced performance in devices, and physico-chemical properties of nanocrystals (e.g. self-assembly and surface chemistry) can be exploited to fabricate optoelectronic devices with innovative architectures. Novel methods and materials for light-emitting diodes: The group applies materials science to optoelectronics by determining which fabrication parameter lead to enhanced performance in LEDs. In order to transition from classical to non-classical light-sources based on colloidal nanocrystals, the group is developing novel methods for controlling the deposition and positioning of an individual nanocrystals in the device. Both “top-down” and “bottom-up” approaches are investigated.
Since their discovery in 1981 by Alexey I. Ekimov, semiconductor nanocrystals (NCs) have seen a tremendous development and they are now exploited in the consumer electronics market. Colloidal semiconductor NCs present remarkable properties for the fabrication of a variety of photonic applications such as: light-sources, solar cells, X-rays scintillators and detectors. Colloidal semiconductor NCs are synthetized via wet chemistry approaches that offer an endless amount of freedom on the parameters that determine their optoelectronic properties such as crystal size, shape, crystallinity, chemical composition and architectures.
In this talk, I will present how we are focusing on the development of near-infrared (NIR) emitting NCs[1-4], NIR-LEDs[1] and single photon emitters (SPEs) based on isolated NC. NIR emitting NCs and their respective LEDs are of interest for a variety of optoelectronic applications such as hyperspectral and biomedical imaging, night vision and telecommunication systems. Up until now, all efficient NIR NCs are based on toxic heavy metals compositions while The European Union’s “Restriction of Hazardous Substances” (RoHS) directive limits the use of such compounds. Colloidal indium arsenide and lead-free perovskite NCs are emerging as promising candidate for NIR applications thanks to their low toxicity and recent progresses in material synthesis leading to stable and highly efficient NCs. We exploited InAs/ZnSe core-shell NCs to produce NIR-LEDs emitting in the range of 800-1000 nm,[1] while we developed cesium manganese bromide NCs that can be employed as sensitizers for broadband Vis-to-NIR downshifting.[3] Last but not least, these NCs are of interest for the fabrication of SPEs operating in the NIR; yet, study of their emission properties has just begun.
Another important property of NCs is their capability to operate as a SPE. Yet, complex small-footprint devices based on single NCs require controlled placement of isolated NCs on a planar surface to exploit NCs in the emerging fields of quantum communication, computing, and encryption, where optically pumped quantum dots are quickly gathering attention. A whole range of techniques have been explored to control the placing of colloidal NCs from solution on a solid substrate, exploiting e.g., chemical/wettability contrast between different areas of the substrate or other chemical physical interactions. We have applied capillary assembly (a method that exploits the force exerted on NCs at the interface between the moving meniscus of the solution solvent and a solid substrate) to entrap a NC in pre-patterned holes on the surface, typically realized by means of lithographic methods such as electron beam lithography. The large area arrays we fabricate contain hundreds of single NCs, and we correlated the morphological data collected by scanning electron microscopy with optical data to unveil how the effective density of SPEs depends on an interplay between NC photoluminescence quantum yield and Poisson statistics of the nanohole filling process.
2.2-I1
Saül Vélez pursed his master’s (2008) and PhD studies (2012) at the University of Barcelona under the supervision of Prof. Tejada, receiving in both the Extraordinary award for his results on quantum magnetism. In April 2013, Saül joined the nanodevices group at CIC nanoGUNE to work with Prof. Hueso with the purpose to transition towards spintronics and nanodevices, areas in which he is now a reference. From September 2017 to May 2021, Saül also hold a senior postdoctoral position in the groups of Prof. Gambardella and Prof. Fiebig at ETH Zürich.
With interest in spintronics, magnetotransport, and optoelectronics phenomena, his recent research focused on exploring magnetoresistive effects and magnetic dynamic phenomena in metal/oxide heterostructure devices. Among his recent works, he has demonstrated that interfacial interactions and spin currents can be used for probing and manipulating the magnetic moments of electrically insulating materials, opening a new research field with profound fundamental and technological impacts. His contributions to polaritonics and optoelectronics in low dimensional materials and heterostructures are also multiple.
Saül joined IFIMAC in June 2021 as Junior Group Leader and found the Spintronics and Nanodevices group. In his lab, he aims at exploring non-conventional materials and new device concepts for spintronic applications.
The use of the spin of the electrons is having a tremendous impact on our electronics and computing technologies. Tell-tale examples are found in the controlled switching and reading of thin film ferromagnets, which enabled the realization of technologies such as the hard disk read head and the magnetic random-access memory. Despite the enormous progress that has been made, current devices are still based on metallic ferromagnets, which typically suffer from high-losses and are limited in frequency.
Magnetic insulators (MIs), such as rare earth garnets (R3Fe5O12; R=Y,Tm,..), have attracted a lot of interest because of their low Gilbert damping and high-frequency dynamics. Interestingly, unlike charge currents, spin currents can couple and propagate through MIs, making possible to realize spintronic devices based on these materials.
In this talk, we will discuss how we can exploit the charge to spin conversion phenomena in heavy metals for reading and writing the magnetic state of MIs [1,2], for electrically injecting and detecting magnons carrying spin information in MIs [3,4,5], and for stabilizing and driving topological magnetic textures (Néel chiral domain walls and skyrmions) in thin film magnetic oxides [6,7]. We will discuss the key role of antisymmetric chiral interactions on the emergence of non-reciprocal phenomena in both magnon transport and the dynamics of magnetic textures. We will see that the performance of garnet devices is better than their metallic counterparts and demonstrate novel device functionalities enabled by chirality that could be exploited in future memory and spin-logic applications.
*saul.velez@uam.es
2.2-I2
Dr. Beatriz Martín-García received her Ph.D. in Chemical Physics (Cum Laude) from University of Salamanca (Spain) in 2013. Then, she joined Istituto Italiano di Tecnologia (Italy) under the Graphene Flagship project working during almost 6 years on the modulation of optoelectronic properties of different materials (nanocrystals, 2D materials and hybrid metal-halide perovskites) by chemical-design and surface-functionalization strategies for their integration in solar cells, photodetectors and memories. She is currently an Ikerbasque researcher and Ramón y Cajal fellow at CIC nanoGUNE BRTA, leading a research line developing tailor-made low-dimensional materials and studying them by Raman and photoluminescence spectroscopy techniques to drive the selection of desired properties for their integration in optoelectronic and spintronic devices.
The development of new technologies has been always accompanied by the access to functional materials with targeted and exceptional properties. Among these materials and looking towards the future, layered materials and metal halide perovskites outline a prospective path for their potential application in optoelectronic, spintronic and quantum technologies.[1–4] However, for their successful integration into devices and the development of new applications, it is key to understand the relationship between composition, crystal structure and optical/magnetic properties and how to control them.
Layered hybrid organic-inorganic metal halide perovskites (HOIPs) have emerged as promising materials for optoelectronic and spintronic applications, namely due to their tunable bandgap, high carrier mobility, strong spin-orbit coupling and magnetic ordering.[1,2] Indeed, HOIPs are an ideal platform for optical (photons) and magnetic (spins) tunability due to their chemical and structural versatility.[5,6] In this line, two case studies are presented. The first one is focused on modulating the optical properties, concretely the photoluminescence (PL), by strain engineering. We report the tuning of the micro-PL emission of 2D lead-bromide HOIP flakes subject to biaxial strain. To generate the mechanical strain, we placed the flakes by viscoelastic stamping on a rigid SiO2 ring platform, leading to the formation of domes. At low temperatures, we found that a strain < 1% can change the PL emission spectrum from a single peak (unstrained) to three well-resolved peaks. Combining temperature-dependent micro-PL and Raman spectroscopy mapping and reverse mechanical engineering strain modeling, we confirm that the emergence of the two new PL peaks is related to tensile and compressive thermo-mechanically generated strain coexisting along the flake surface and thickness.[7] Our findings provide new insight into strain-based optoelectronic and sensing devices using 2D HOIPs, leveraging on the material composition selection and substrate platform design. The second case deals with the control of the magnetic properties by varying the transition metal (Cu2+, Mn2+ and Co2+), organic spacer (alkyl- and aryl-ammonium) and perovskite phase (Ruddlesden-Popper and Dion-Jacobson). We show that for Cu2+ HOIPs, an increase of in-plane anisotropy and a reduction of the interlayer distance lead to a change in their magnetic behavior from a 2D ferromagnet to a quasi-3D antiferromagnet. In contrast, the magnetism of Mn2+ HOIPs is intrinsically characterized by antiferromagnetic intralayer interactions. Finally, Co2+ crystals with a non-perovskite structure present a dominant paramagnetic behavior. Therefore, our results demonstrate that the chemical flexibility of HOIPs can be exploited to develop novel layered magnetic materials with tailored magnetic properties.[8]
2.2-I3
Dr. Amilcar Bedoya-Pinto completed his undergraduate studies in Physics at the Technical University of Munich (TUM), carrying out his Master thesis at Walther-Meissner-Institute (WMI) on the growth and characterization of ZnO-based magnetic semiconductors. He received his PhD in Condensed-matter Physics at the University of Goettingen, focusing on charge and spin transport studies of epitaxial metal-semiconductor heterostructures, and being awarded with the Dr. Berliner-Ungewitter Prize for outstanding PhD theses (2011). He started his Postdoctoral work at CiC nanoGUNE research center in San Sebastian (Spain), focusing on molecular-based spintronics and hybrid metal-molecule functional interfaces. After that, he moved to the Max-Planck Insitute of Microstructure Physics (Director: Stuart Parkin) as a Research Associate, leading projects on two-dimensional materials and Weyl semimetal-based thin films and heterostructures. In 2022, he got appointed as a Distinguished Researcher and Principal Investigator at the Institute of Molecular Science, University of Valencia, where he is building up a research group working on molecular beam epitaxy of 2D ferroic heterostructures.
How physical properties change in solids with reduced dimensionalities and symmetry-breaking elements, is a scientific question that has fascinated researchers for decades. However, only the recent advances in material synthesis and nano-characterization have triggered an atomistic study of low-dimensional and topological phenomena. In this talk, I will discuss how the ultra-high-vacuum growth of two-dimensional and topological materials allows the realization of exotic physical model systems and, at the same time, grants the flexibility to perform atomic engineering to achieve functional material properties.
In the first part, I will present the long-sought thin-film realization of an inversion-symmetry breaking Weyl Semimetal [1], a recently discovered topological material class featuring a linear electronic dispersion and degeneracy (Weyl) points that lead to peculiar open-loop surface states (Fermi-Arcs). In particular, I will show how topology and electronic structure are connected to epitaxy-dependent parameters such as strain, doping and surface termination [2]. With a careful preparation of in-situ heterostructures, I will discuss how these topological properties of Weyl Semimetal thin films can be exploited for applications in spin-orbitronics and topological superconductivity.
In the second part, I will focus on the van-der-Waals epitaxy of a nearly-ideal two-dimensional magnet, a CrCl3 monolayer grown on Graphene/6H-SiC(0001). In-situ X-ray magnetic circular dichroism reveals intrinsic ferromagnetic order with easy-plane anisotropy and a 2DXY universality class [3], suggesting the first realization of a Berezinskii-Kosterlitz-Thouless (BKT) phase transition in a quasi-freestanding monolayer magnet. The important role of the van der Waals substrate interaction and the underlying crystal symmetry will be discussed, thereby highlighting routes on how to control the anisotropy of 2D magnets via growth engineering. Finally, taking advantage of our all-in-situ approach, perspectives to interface 2D magnets with other two-dimensional ferroic materials -such as ferroelectrics- will be outlined to study novel collective phenomena in van der Waals heterostructures.
1.1-I1
Organic solar cell (OSC) bulk heterojunctions (BHJ) typically feature a rich phase morphology with the phase composition and distribution significantly affecting processes such as charge generation, recombination and extraction, and, in turn, device performance. After deposition, the active layer is in a metastable state including several phases that vary in composition (from pure to mixed) and degree of order (from crystalline to amorphous). This metastable morphology is generally prone to changes that lead to cell degradation. To stall this degradation, it is necessary to identify the different phases and follow their dynamics as a function of composition, processing conditions and temperature. Recently we developed a new staining methodology that offers imaging of the BHJ in electron microscopy. Using this technique we were able to study the temperature-induced morphology evolution of a high efficiency fullerene:polymer blend, as a model system. Based on the results we developed a unified thermodynamic and kinetic mechanism that allowed us to correlate the morphology evolution with OSC degradation during thermal annealing of fullerene based OSCs. We then turned to donor:non-fullerene acceptor (NFA) blends that are more challenging to elucidate because they often display different polymorphs. Moreover, their glassy states can be more complex. Using the developed electron microscopy analysis, we followed the dynamics of the phases as a function of composition and temperature. We identify multiple glassy phases and complex NFA-based BHJ morphology emphasizing the need for a deeper understanding of the phase behavior of such systems.
1.1-I2
Christoph J. Brabec is holding the chair “materials for electronics and energy technology (i-MEET)” at the materials science of the Friedrich Alexander University Erlangen-Nürnberg. Further, he is the scientific director of the Erlangen division of the Bavarian research institute for renewable energy (ZAE Bayern, Erlangen).
He received his PhD (1995) in physical chemistry from Linz university, joined the group of Prof Alan Heeger at UCSB for a sabbatical, and continued to work on all aspects of organic semiconductor spectroscopy as assistant professor at Linz university with Prof. Serdar Sariciftci. He joined the SIEMENS research labs as project leader for organic semiconductor devices in 2001 and joined Konarka in 2004, where he was holding the position of the CTO before joining university.
He is author and co-author of more than 150 papers and 200 patents and patent applications, and finished his habilitation in physical chemistry in 2003.
OPV cells have a proven efficiency of over 19 % while OPV modules have a proven record efficiency
of 13.5 %. Both values are still increasing, towards > 20 % for small area cells and > 15 % for large
scale modules. With these performance values, solution processed emerging photovoltaic
technologies are reaching out to applications that are going beyond the typical niche markets. The
first generation of commercially available printed PV modules showed a lifespan in the order of
beyond 5 years and more under outdoor conditions (OPV). Interestingly, several experiments are
strongly suggesting that solution processed semiconductors like organics can be stable under light
and, to some extent, under oxygen as well. Despite these impressive numbers, one should not forget
that these are “best you can do” lifetime values.
On the other hand, the community did not progress significantly in overcoming the fundamental limitations of OPV. The energy gap law for excitonic materials, the precise microstructure control of binary or ternary composites, the design principles for environmentally stable materials or the Kirchhoff law for multi-junction cells continue to be major barriers for this technology. We briefly introduce into these long-time challenges for excitonic absorbers and then discuss concepts and strategies how to resolve them. Among them, the development of a digital twin for OPV which has inverse predictive power is a most promising concept. “Solar FAU”, an alliance of research partners in the Erlangen-Nürnberg region that is headed by Friedrich Alexander University, is exploring the basic concepts and methodologies how to build a digital twin for emerging-PV technologies. The central and most desired element of the digital twin is the power of inverse design, e.g., inventing molecules, device architectures and processes with tailored properties. Insight from first pieces (agents) of the digital twin strongly supports the assumption that inverse design capability is possible, even in the case of considerable experimental uncertainty. Coupling the digital twin to Material Acceleration Platforms (MAP) reduces experimental uncertainty and allows to learn predictions which otherwise would be impossible. We have recently demonstrated the power of of such coupled systems and demonstrated correlations which were previously unthinkable, like the prediction of performance and lifetime of OPV cells from simple absorption data or the identification of molecular features that determine the environmental operational stability of OPV.
1.1-I3
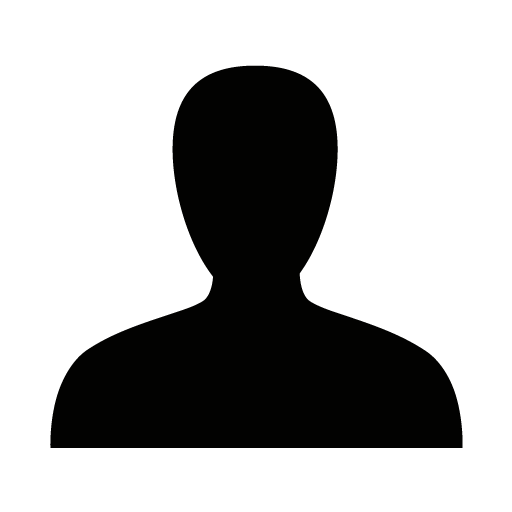
Organic solar cells have witnessed a rapid improvement in device performance over the past few years, now achieving an exceptional power conversion efficiency of over 19% under standard solar irradiation (over 30% under indoor illumination) in a single junction device, rapidly closing the performance gap with competing technologies such as crystalline silicon. This upsurge in performance is primarily driven by the emergence of non-fullerene organic small molecular and polymeric acceptors, surpassing conventional fullerene acceptors due to stronger optical absorption, optimal energy levels and potentially lower cost in synthesis and purification. This is further coupled with major advances in device design (e.g. ternary bulk-heterojunction blends, novel device interlayers), placing organic solar cells in an unprecedentedly promising position for potential large scale commercialisation in multiple application areas.
A further key factor to realise the full commercialisation potential of organic solar cells is stability, that is, a PV device must have a sufficiently long lifespan that exceeds the required operational period for a particular application. For example, it is desirable for a PV device lifespan of >20 years for building integrated applications, whereas a shorter lifespan of ~5-10 years may be sufficient for powering indoor autonomous sensors. The modest device stability has been a widely-recognised and long-standing challenge for conventional fullerene-based organic solar cells, with multiple degradation mechanisms already identified that result in rapid losses of device performance under illumination, ambient air and thermal stress conditions. Nevertheless, the recent transition of organic solar cells from fullerene acceptors to non-fullerene acceptors, as well as the major advances in their molecular and device design, has brought exciting opportunities to fully overcome this challenge.
In this talk I will give a summary of the recent research progress of my group in understanding the degradation mechanisms of non-fullerene organic solar cells. I will highlight the distinct roles of several molecular and device design factors in the resulting degradation behaviour, and propose potential strategies to overcome these degradation mechanisms toward achieving long-term stability of organic solar cells.
1.2-I1
Professor of Materials Physics at Karlstad University, Sweden, since 2011. Research interests: morphology of conjugated polymer thin films, photodegradation of OPV materials, energy level allignment in organic and perovskite multilayer structures. Employed at Karlstad university since 2000. Previously Research Scientist at Cambridge Display Technology in Cambridge,UK, and Research Assistant at University of Cambridge. Post-doc at EPFL Lausanne (1996-98) and TU Delft (1995-1996). PhD degree from the Weizmann Institute of Science in Rehovot, Israel.
The organic photovoltaics (OPV) field has seen a boost in performance with the introduction of small-molecule electron acceptors, such as Y6, and their copolymer derivatives, leading to power conversion efficiencies above 18% [1]. The device operational lifetime depends critically on the photochemical stability of the materials and the film morphology. Here we report the evolution of optical properties, composition, and energy levels, during one-sun (AM1.5) illumination in air of thin films of the donor polymer PBDB-T, the small-molecule acceptor Y5 [2], its copolymer counterpart PF5-Y5 [3], as well as their blends. UV-Vis spectroscopy, Fourier transform infrared spectroscopy (FTIR), X-ray photoelectron spectroscopy (XPS), Near-edge X-ray Absorption Fine Structure (NEXAFS) spectroscopy, and atomic force microscopy (AFM) were used to monitor the thin film properties. The absorption spectra show that the PBDB-T polymer and the copolymer PF5-Y5 undergo rapid photobleaching, while the Y5 film spectrum remains, surprisingly, almost intact even after 30 hours of light exposure in air. The corresponding blend films of PBDB-T with Y5 and PF5-Y5 show similar losses in absorption as their components. New carbonyl peaks emerge in the FTIR spectra of PBDBT, PF5-Y5 and blend films, but are absent in those of Y5, indicating that the bulk of the Y5 film is resistant to photooxidation, while the copolymer containing a Y5-moiety undergoes photochemical degradation reactions. The faster photodegradation of PF5-Y5 compared to Y5 raises the question about the role of the copolymer’s BDT moiety in the photooxidation. However, the effect of film packing on the rate of degradation should also be considered. Angle-resolved NEXAFS spectra reveal a stronger linear dichroism in the spin-coated PF5-Y5 films compared to Y5 films, confirming a preferred orientation of the PF5-Y5 polymer backbone, while the random average orientation of Y5 molecules suggests a multi-crystalline Y5 film. Surface analysis by core level XPS indicates, moreover, that the surface of blend films is donor-enriched. These new insights on the effects of intentional photodegradation on donor and acceptor materials properties are expected to contribute to the design of stable acceptors as well as the development long-lived OPV devices.
1.2-I2
Our research group is focused on the development of functional polymer interlayers for various kinds of optoelectronic devices, including thin-film transistor (TFT), (photo-)memory, light-emitting diode (LED), and solar cell devices. We are particularly interested in exploring the structure-performance relationships of polymers. In addition to advances in the controlled synthesis of organic semiconductors, we also explore innovative interfacial and device engineering to optimize the device performance. Herein, we highlight our recent works on interface engineering for polymer solar cells. In this presentation, an integrated study of combining material synthesis, interface engineering, and morphology analyses will be introduced and discussed to explore the full promise of the devices, with a particular focus on long-term device stability, including thermal stability, photostabiltiy, and underwater-stability.[1,2] We first present robust interface engineering of graphene oxide nanosheets (GNS) to improve the thermal and photostability of non-fullerene bulk-heterojunction (NFA BHJ) OPVs to practical levels. Afterwards, we demonstrate the great potential of all-polymer blends and np-TiO2 ETL to improve the durability of unencapsulated OPVs in high humidity environments and even underwater immersion.
1.2-I3
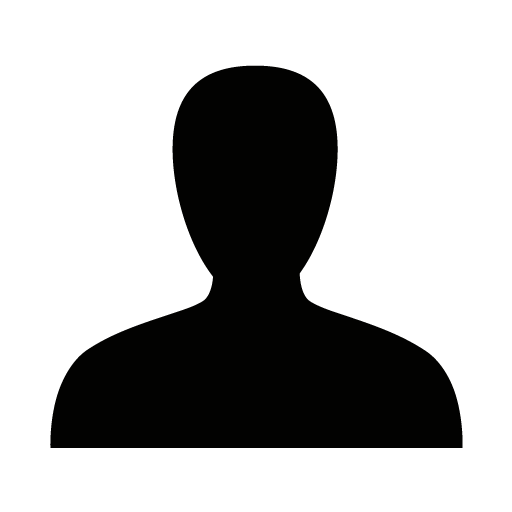
Non-fullerene acceptors (NFAs) have attracted a lot of attention within the organic PV community. Several NFAs are available today outperforming fullerene acceptors in bulk heterojunction solar cells and power conversion efficiencies > 18% have been reported for several different donor acceptor systems. In addition, NFA-based solar cells show also very promising device stabilities under standard operation conditions. In this contribution, I will review the status and the potential of non-fullerene acceptors. I will discuss the charge generation and recombination processes and the role of triple states in NFA-based absorbers. Photoluminescence experiments performed at low temperatures and high magnetic fields reveal the efficient generation of triplet states in non-fullerene acceptors like Y6. Different recombination processes in bulk-heterojunctions containing fullerene or non-fullerene acceptors are found. The role to triplet states in NFA-based bulk-heterojunction blends will be discussed. Especially the role of triplet-triplet annihilation and singlet fission processes will be considered in detail.
1.3-O1
Enthusiastic and disciplined individual, willing to contribute for scientific research in the field of low-cost, eco-friendly energy production. Gained extensive experience in the field of organic solar cells (OSCs), particularly in various device fabrication and characterization techniques with an aim of contributing the findings regarding OSCs, related to new materials, improved morphology device engineering, new device structure, optimization, low- cost/ambient air processing, and enhanced stability as well as improved fundamental OSC mechanisms such as charge carrier mobility/transfer, recombination, and transport.
Recent advancements in material design and related power conversion efficiency (PCE) improvements; organic photovoltaics (OPVs) are positioned as a very promising technology for the needed green energy transition. Particularly, the development of non-fullerene acceptors (NFAs) is continuously raising the bar for PCE and device stability. However, translating these findings to industrially applicable techniques, while maintaining high performance and stability parameters, remains a significant challenge. The aim of this study is to facilitate a paradigm shift in the development of OPV by introducing a device structure which can maintain its morphology over a longer period of time. Sequentially depositing the donor and acceptor materials to create bilayer architecture, where the morphology governing nano interfaces throughout the bulk is eliminated, provides an efficient method to develop morphologically robust OPVs. Here investigation on different active layer thicknesses using PM6 and Y7 materials have been carried out to see how varied donor and acceptor layer thicknessess effect charge transport and recombination behavior, and how the device's performance can be tweaked further. Combined with the electrical and morphological characterizations, the device performance of bilayers has also been compared with the conventional bulk-heterojunctions (BHJs). To further illustrate the significant advantages of bilayer structure over BHJs, the photo and thermal degradation measurements have not only been conducted for PM6 ̶ Y7, but for a number of other NFA systems like PM6 ̶ IT4F, PM6 ̶ Y6, PM6 ̶ N3, PBDB-T ̶ ITIC, TPD-3F ̶ IT4F, PCE10 ̶ O-IDTBR, and more demonstrating the robustness of the structure.
1.3-O2
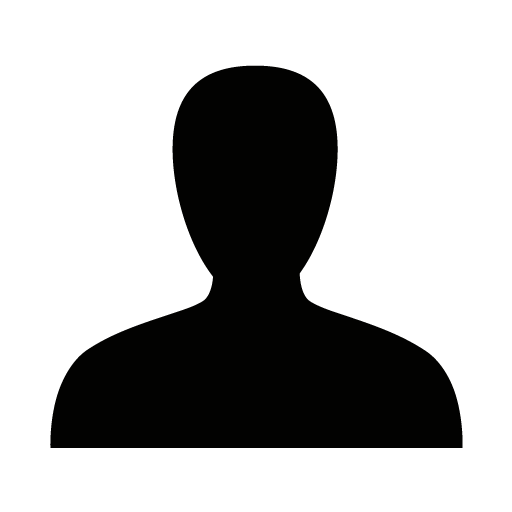
Lately, non-fullerene acceptors (NFA’s) have received increasing attention for use in polymer-based bulk-heterojunction organic solar cells, as improved photovoltaic performance compared to classical polymer-fullerene blends could be demonstrated. In this study, polymer solar cells based on high-efficiency donor polymers are used in combination with a number of different electron-accepting materials. Both donors and acceptors are either fluorinated and/or chlorinated (or, in more general terms, halogenation) and compared with the non-halogenated version. To see the influence of end-group fluorination of ITIC in a photovoltaic application, the well-known donor polymers PBDB-T (PCE12) and its fluorinated and chlorinated representative, PBDB-T-2F (PM6), PBDB-T-2Cl (PM7) were used to process bulk heterojunction solar cells. Different thermal annealing during device processing is investigated from their photovoltaics parameters. Charge generation and recombination mechanism upon annealing were investigated via several optoelectronic characterization methods. Especially, Time-Delayed Collection Field (TDCF) measurements demonstrate that field-dependent charge generation and bimolecular recombination processes affect the fill factor and, thus, the efficiency of devices.
1.3-O3
Agrivoltaics is an encouraging application area for organic photovoltaics (OPVs) that merges solar power generation with the energy demands of crop growth, thus providing dual functionality to dedicated farmland. When integrated on greenhouses, semitransparent OPV technologies are expected to improve the sustainability of crop growth and turn net zero energy greenhouses a reality. In this work, we screen all-polymer and polymer:small-molecule, donor:acceptor blends for their use in organic agrivoltaics. The optical requirements of the blends are benchmarked according to a novel greenhouse figure-of-merit (gh-FoM) to account for the -simultaneous- transparency requirements of crops and humans. Modeling of the gh-FoM reveals that photoactive layer thicknesses between 25-125 nm and acceptor-enriched ratios (such as 1:4, w:w, with the acceptor being the lower band gap material) are more suitable for the optical constraints imposed by most types of crops. Nevertheless, such ratios are found to be less thermally stable, thus imposing an undesirable trade-off between the required device semitransparency and its long-term stability.
The optimized blends are then upscaled to form 25 cm2 active area laminated modules processed entirely from solution, on flexible substrates, via roll-to-roll compatible methods (slot-die and blade coating) and in ambient conditions. These modules are installed on a domestic greenhouse in Sweden, where their outdoor stability is tracked 24 hours per day over the spring and summer periods while in compliance with the ISOS-O-2 protocol. We accordingly design an autonomous, Arduino-based IV-tracing setup that automatically collects, analyses and uploads relevant device data to the cloud for remote tracking worldwide, at very low cost. Our outdoor stability study shows degradation modes undetectable under laboratory conditions such as decreased ideality factors and detrimental module delamination, which accounts for 10-20% loss in active area as per photocurrent imaging. Harsh thermal and humidity cycling conditions are correspondingly tracked during the day-night cycles, which are found to contribute to the amplified degradation pace of the agrivoltaic modules. Among the active layers tested in this work in the form of air-processed laminated modular architectures, polymer:small-molecule blends are the most stable and position as prominent photoactive layers toward sustainable organic agrivoltaic technologies.
1.3-O4
My inner attitude towards the inter-connection of all the various fields of my life is what truly keeps me motivated and enthusiastic.
Currently I am involved in a PhD aimed to study energy transfer in photosynthetic systems, combining in a new way my B.Sc. computational background in energy transfer and my M.Sc. experimental experience in photo-chemistry and super-resolution microscopy.
Understanding the spatial dynamics of nanoscale exciton energy transport beyond the temporal decay is at the core of photosynthesis and essential to provide a better framework for further improvements of nanostructured optoelectronic devices, such as solar cells. The diffusion coefficient (D) of photovoltaic films has so far only been determined indirectly, from transient singlet-singlet annihilation (SSA) experiments. Here, we present the full picture of the exciton distribution dynamics, adding the spatial domain to the temporal one, by spatio-temporally resolved photo-luminescence microscopy. In this way, we directly track diffusion and we are able to decouple the real spatial broadening from its overestimation given by SSA. We measured the diffusion coefficient, D = 0.017 ≈ 0.002 cm2/s, of the non-fullerene electron acceptor Y6, which combined with an exciton lifetime of τ = 840 ps, gives a Y6 film diffusion length of L = √ Dτ ≈ 35 nm. Thus, we provide an essential tool that enables a direct and free-of-artifacts determination of diffusion coefficients, which we expect to be at the core of further methodical studies on exciton dynamics in energy materials.
1.3-O5
The world’s energy consumption is expected to grow vastly over the next few decades. Contributing to this growing energy demand is the advent of the Internet-of-Things (IoT), through which many devices and sensors may one day share immense quantities of information to benefit society as a whole.[1] Indoor photovoltaics (IPVs) based on easy-to-process, earth-abundant organic semiconductors have recently gained considerable traction as a potential source of power for the IoT.[2] These IPVs would recycle low-intensity, artificial light generated by LEDs to power small devices and IoT nodes, and they are fast approaching commercial viability. Organic semiconductors, however, are not yet optimised for IPV applications – they are plagued by detrimental non-radiative open-circuit voltage losses, and the majority of previous research has actually been channelled towards outdoor solar cell applications instead.[3] In this work, a realistic model is used to demonstrate the limiting effects of an intrinsic material property of organic semiconductors – the energetic disorder.[4, 5] The thermodynamic constraints imposed on the power conversion efficiency of IPVs by energetic disorder are explored, alongside the effects of sub-optical gap absorption, non-radiative recombination losses, and the incident light intensity. Finally, a methodology that takes a photovoltaic external quantum efficiency spectrum and one measurement of the open-circuit voltage (under one-sun conditions) is presented for estimating the performance of organic semiconductor-based IPVs under illumination by any spectrum, at any intensity.
1.3-O6
The first part shall provide an overview of the development of roll-to-roll processed organic solar modules at Fraunhofer ISE.
The focus is on slot-die coated, laser-processed modules with ITO-free electrode systems.
Results will be presented both from opaque devices as well as semitransparent ones based on visibly transparent electrodes with high NIR reflection.
The second part is on photoluminescence measurements of organic solar cells. These are far more complicated compared to all other PV technologies as the signal is strongly dominated by the radiative decay of photogenerated excitons in the donor and/or acceptor phase [1]. The underlying luminescence signal related to the free charge carriers is therefore hidden and can not be determined quantitatively. I will present a newly developed method that enables separating the contribution of photogenerated excitons from the one related to the radiative recombination of free charge carriers. This way the quasi Fermi level separation within the absorber can be determined. An excellent agreement between the latter and the electrical voltage measured between the terminals of the solar cells is observed for devices with selective electrodes.
This newly developed method enables new optimization strategies, including the quantitative comparison of pure absorber films or half cells with complete solar cells.
2.1-O1
Throughout his career, Jens has worked with successfully designing and developing complex experiments for in situ structural studies in the fields of polymer solar cells and functional organic materials, catalysis and hydrogen storage using synchrotron radiation, in fact since the beginning of his PhD studies. From 2001-2010 his focus was mainly on synchrotron radiation scattering techniques and their use for in situ experiments and for determining structure-property relations in functional thin films.
In 2011, Jens shifted his focus towards synchrotron-based 3D imaging of energy materials, particularly the very demanding case of polymer solar cells, where the low-contrast soft matter constitute a specific challenge. Through a dedicated effort of synchrotron experiments this led from 3D ptychographic imaging of roll to roll coated polymer solar cell active layers to 3D imaging of a complete polymer tandem solar cell. The scope of applications is all the time broadening, and Jens is now developing new tools for 3D imaging and organizing training and teaching in these.
In the same period, he developed a new setup for fast mapping of nano-structure, crystallinity and texture in R2R coated thin-films, which is still being improved and extended. Most recently, he has taken up research in ultrafast X-ray scattering and spectroscopy applied to solar energy materials, using X-ray free electron lasers.
In parallel with the studies of nano-structure in energy materials, Jens is running a small group that works with upscaling of organic solar cells, in order to maintain research into the entire development of this technology, from the fundamental understanding of charge generation and transport, over mesoscale structure formation to the performance of the final devices.
We demonstrate a method to manipulate the morphology of thin film semiconducting polymers. The manipulation is achieved by optical excitation of the polymer during roll-to-roll slot-die coating, providing a technique that is viable for large-scale production. Along with establishing the technique, the entire knowledge chain from fundamental insight in polymer physics and structure to application is presented. By correlating X-ray and neutron scattering techniques with density functional theory and molecular dynamics simulations of solvent evaporation, we successfully determine the packing of the polymers in the ground state and excited state. The findings are coupled with measurements of dynamical physical properties and solar cell device performance to pinpoint the structure-property relationship.
Different processing was applied to match energies below and above the excitation energy levels of P3HT in solution and in thin film [1]. Specifically, either no illumination (i.e., dark), or LEDs emitting red, green, or blue light illuminated the films while being coated. It is known from density functional theory (DFT) calculations [2] that, when exciting P3HT with visible light, i.e. going from the ground state to the excited state, the electron density changes to form double bonds between neighbouring thiophene rings. This change in the bonding pattern planarizes the polymer backbone. Consequently, the final morphology is affected when exciting the polymer during coating. To probe the morphological changes caused by the four treatments, we use Grazing-Incidence Wide-Angle X- ray Scattering (GIWAXS) on the coated films. To elucidate the physical origin of the changes, DFT and solvent evaporation molecular dynamics (MD) are used to simulate the effect of excitation on single polymer chains and thin films. The full picture of morphological changes is followed by a thorough investigation of the change of physical properties in the thin film. Here, the UV-VIS absorption spectra are analysed, followed by THz spectroscopy measuring the thin-film photo- conductivity. Finally, the consequence of light treating a complete OPV device (P3HT:O-IDTBR) is discussed.
The experimental and computational results demonstrate that exciting P3HT with visible light during deposition serves as a tool for manipulating the packing behaviour of P3HT and can be used to modify the final thin film morphology. It can be considered a new processing parameter for achieving the desired performance of organic thin films. Particularly, the capability to increase the out-of-plane mobility by light treatment can be used for transistor applications where directional mobility and patterning is essential [3]. Application in OPV devices will require an optimization process for the right crystal packing and domain size of donor and acceptor constituents. For the specific combination P3HT:O- IDTBR, the domain size decreases with light treatment and still results in a decrease in power conversion efficiency. Understanding how light treatment during fabrication influences the final morphology of a film can enable major improvements for specific materials systems or other technologies in flexible electronics and photovoltaics.
In conclusion, we report a method to manipulate the morphology of P3HT thin films through illumination with visible LED light during roll-to-roll slot-die coating. Optical polymer excitation temporarily constrains large sections of the chains into a planar geometry that is the minimum of the excited state potential energy surface. This structural effect is strong enough to affect the aggregation behaviour and, thereby, the final morphology of the P3HT film. The light-treated films are less crystalline overall, display a higher degree of face-on orientation, shorter conjugation length, and a change of the unit cell dimensions with less efficient packing. Consequently, the in-plane photoconductivity decreases and the out-of-plane conductivity increases drastically with light treatment.
2.1-O2
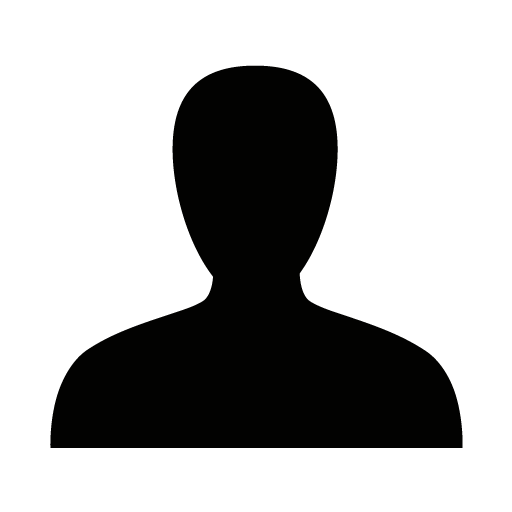
The global trend towards automatization and miniaturization of smart devices has triggered the development of reliable off-grid power sources with low economic and environmental impact. Such autonomy can be provided when a photovoltaic cell is integrated with an electrochemical storage device in one monolithic device. This work demonstrates a reliable and straightforward approach to monolithically integrate high-performance single and multijunction organic solar cells with mesoporous nitrogen doped carbon nanosphere-based supercapacitors or lithium-organic batteries in a single device with a three-electrode configuration. To assess the efficiency of these devices, a novel approach is presented that relies on the direct monitoring of both integrating parts during illuminated and dark phases and accounts for possible losses. This versatile approach is applicable for all kinds of integrated multifunctional photoconversion-storage systems. For the photosupercapacitors, the evaluation with the standard literature approach showed an outstanding performance with a peak photoelectrochemical energy conversion efficiency of 17 %. However, in our opinion this type of efficiency does not properly represent the real overall device efficiency. Based on our newly developed efficiency determination, a more modest overall cycle efficiency of 2 % is obtained. For battery-based devices, a higher output voltage is achieved, but at the cost of a lower, 0.3% cycle efficiency. In our view these values represent the real overall performance of the integrated device in a precise manner and will thus enable meaningful direct comparisons among different photoelectrochemical storage systems.
2.1-O3
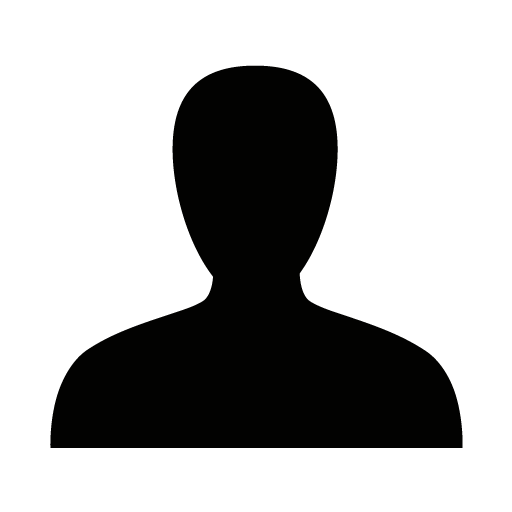
Organic bulk heterojunction photodiodes (OPDs) attract wide attention for light sensing and imaging but their detectivity is typically limited by a substantial reverse bias dark current density Jd. Recently, the Jd in OPDs was attributed to thermal charge generation mediated by mid-gap states. The evidence of such trap states, however, comes from rather indirect techniques such as thermal admittance and/or photocurrent measurements. In this work, we study the temperature dependence of Jd in state-of-the-art OPDs that have Jd values down to 10−9 mA cm−2. For a variety of donor-acceptor bulk-heterojunction (BHJ) blends we find that the activation energy of Jd is lower than the effective bandgap of the blends, by ca. 0.3 to 0.5 eV. Ultra-sensitive sub-bandgap photocurrent spectroscopy on these diodes show evidence for sub-bandgap states. The minimum energy for optical charge generation from these sub-bandgap states correlates very well with the dark current thermal activation energy. From this, we conclude that the dark current in these OPDs is caused by thermal charge generation at the donor-acceptor interface mediated by sub-bandgap states that create an activation energy that is 0.3-0.5 eV below the bandgap energy. By studying donor- and acceptor-only diodes, we find that such sub-bandgap states are prevalent in polymer (donor) semiconductor materials, and in the fullerene acceptor PCBM, but not in the non-fullerene acceptor materials studied.
2.1-O4
The extraordinary increase in efficiency of organic solar cells has rushed the interest towards an industrial scale-up. However, for a realistic lab-to-fab transition, strategies to improve simultaneously the performance and the solar cell lifetime need to be explored. In this aspect, the interface between the photoactive layer and the buffer interlayers is considered critical. As an example, ZnO triggers photodegradation of non-fullerene acceptor molecules at the interface, while surprisingly it is still the benchmark buffer layer in organic solar cells [1]. Recently, SnO2 nanoparticles have emerged as a high performing, scalable and low-cost alternative to ZnO, and it has already proven its potential for perovskite solar cells. However, although several reports point at SnO2 as a promising layer also for organic solar cells, most of the well performing systems are still reported with ZnO, suggesting SnO2 has intrinsic limitations in organic solar cells. On the other hand, several reports indicate a need for surface modification via chemical treatments to improve the interfacial properties [2]. Driven by these subject, in our work we make use of water-based SnO2 nanoparticles in n-i-p organic solar cells. We prove that the presence of surface cations produce an inherent defective interface with several organic blends, leading to s-shaped J/V curves and we amend it via a very simple an entirely environmentally-safe method. More strikingly, we demonstrate that the device stability is strongly correlated with the concentration of surface ions, and our method raises the operational stability factor T80 under continuous illumination from 8.4h to 244h. Finally, the power conversion efficiency and its standard deviation improve universally for several systems, showing its potential to be systematically applied in both lab scale but also industrial scale.
2.1-O5
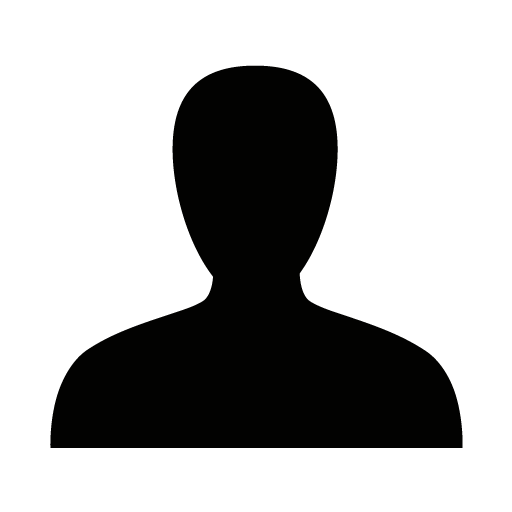
The inverted (n-i-p) structure is considered more suitable for the industrial production of organic solar cells (OSCs), due to the superior stability of the materials used in this configuration. Transparent conductive oxides are usually employed as electron transport layer (ETL) in OSCs with the inverted structure. Among them, zinc oxide (ZnO) is the most studied and adopted, since it is easy to obtain with relative good crystalline quality and it provides a good band alignment. Tin oxide (SnO2) could represent a valid alternative to ZnO as ETL, with superior transparency to the visible light and higher electron mobility. Most importantly, unlike ZnO, SnO2 is reported to have an excellent ambient stability and to not cause any photocatalytic degradation of the organic materials [1].
The main drawback that hinder an extended use of SnO2 as ETL in OSCs is the difficulty to fabricate films of the material with high crystalline quality. SnO2 films are often fabricated by solution processing, starting from a nanoparticle colloidal dispersion. This method is lacking in control over the material quality, giving rise to films with high density of defects, especially on the surface. Moreover, organic ligands, used to stabilize the nanoparticle dispersion, often leave residuals on the deposited film, which can compromise the interface with the active layer. As a result, the overall performance of the OSCs with SnO2 as ETL is often inferior to that of devices with ZnO, due to substantially lower values of fill factor (FF).
An alternative method to fabricate SnO2 films, with the desired crystalline quality, is the use of atomic layer deposition (ALD). ALD is a deposition technique widely used in the fabrication of electronic devices, since it is scalable and allows obtaining compact layers the material with exceptional quality. SnO2 deposited by ALD is attracting attention in the research field of photovoltaic and it is currently used in perovskite [2] and tandem solar cells [3]. Nonetheless, its use in OSCs is scarcely reported and its potential in these devices still need to be evaluated.
In this work, we fabricated OSCs with SnO2 deposited by ALD as ETL. We compared the performance of the solar cells with those of devices with either SnO2 or ZnO deposited from solution. The scalability of the performance with the device active area and device stability have also been investigated. Our results show the higher efficiency of the OSCs with SnO2 deposited by ALD, thanks to a superior FF. The improvement of FF has been verified on OSCs with different composition of the active layer. Values of FF close to 80% have been achieved, an exceptional result for OSCs with the inverted structure, which demonstrate the great potential of SnO2 deposited by ALD as ETL for high-performance OSCs.
2.1-O6
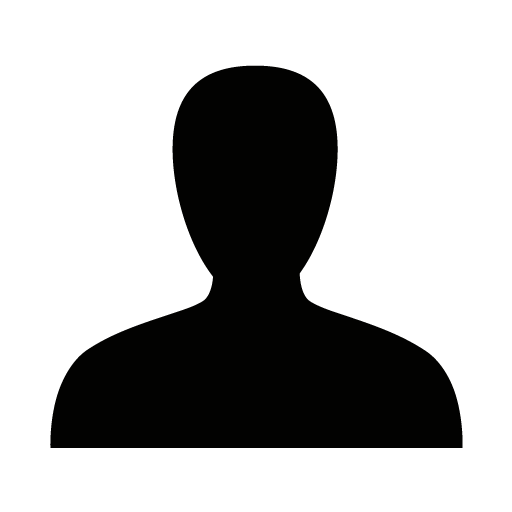
Organic solar cells are showing great potential to be employed in indoor and outdoor applications. The utilization of portable electronics, internet of things (IoT), and sensors for wearable and healthcare applications that are used in both indoor and 1-sun conditions highlight the importance of the development of photovoltaic devices that can efficiently perform under different light intensities. In this paper, we fabricated OSCs with polyethyleneimine ethoxylated (PEIE) and a water-based material Poly-lysine as interfacial layers (IFL) and investigated the device's performance under 1-sun and indoor light intensity. Our results show that the choice of the interfacial layer is important and affect significantly the performance of devices. Under 1 sun illumination devices with poly-Lysine as an interfacial layer show the highest average efficiency of 7.72% whereas PEIE-based devices displayed lower average efficiency of 7.13%. Under the low light intensity of 1000 lux Poly-Lysine-based devices due to their low shunt resistance causing leakage current resulting in lower average efficiency of 7.09% while on the contrary PEIE-based devices exhibited increased average efficiency of 12.25%. Our study paves the way toward OPV devices for portable and wearable applications.
2.2-I1
The discovery of several new classes of electron-accepting molecule that work well as electron acceptors at molecular heterojunctions had led to a strong increase in the power-conversion efficiency in organic solar cells, from 11 to 19% in the last 5 years. The impressive performance of the new materials and the range of chemical structures has led to interest in whether alternatives to the traditional ‘bulk heterojunction’ architecture for an organic solar cell can be found.
One approach concerns structures where, through chemical design, electron-rich and electron-poor components of a single macromolecular material are constrained to have a particular geometry relative to each other, being electronically coupled either through space or through bond. In a second intriguing development, devices based on a single molecular material have been reported deliver relatively high charge-generation efficiency in the absence of a donor-acceptor interface. This latter example challenges the current understanding of how free charges are generated in organic semiconductors. Achieving efficient energy conversion with a single macromolecular component would be both interesting and practically useful.
In this work, we report on studies of the design, characterisation and modelling of both types of structure, including a variety of non-fullerene acceptors based on alternating donor and acceptor units, and bonded macromolecular compounds with donor and acceptor type domains. By combining experimental characterisation under varying conditions (field, temperature, excitation) with molecular and device-level calculations, we endeavour to relate exciton and charge dissociation efficiency in single-component devices to molecular parameters.
2.2-I2
Despite an impressive increase over the last decade, experimentally determined power conversion efficiencies of organic photovoltaic (OPV) cells still fall considerably below the theoretical upper bound for near-equilibrium solar cells, set by the Shockley-Queisser limit. Even in otherwise optimized devices, a prominent yet incompletely understood loss channel is the thermalization of photogenerated charge carriers in the density of states that is broadened by energetic disorder. First, we will show by a detailed comparison between numerical modeling and experiments that the slowness of the mentioned thermalization leads to a 0.1-0.2 eV higher open circuit voltage (Voc) in OPV devices than would be expected for instantaneous thermalization.[1] The latter is commonly assumed when analyzing Voc of OPV devices. Second, we demonstrate by extensive numerical modelling how this loss channel can be mitigated in carefully designed morphologies. Specifically, we show how funnel-shaped donor- and acceptor-rich domains in the phase-separated morphology that is characteristic of organic bulk heterojunction solar cells can promote directed transport of positive and negative charge carriers towards the anode and cathode, respectively.[2] We demonstrate that in optimized funnel morphologies this kinetic, non-equilibrium effect, which is boosted by the said slow thermalization of photogenerated charges, allows to surpass the Shockley-Queisser limit for the same material in absence of gradients and under near-equilibrium conditions. Methodologies by which the proposed morphologies can be realized are discussed, along with the relevance to already published results.
2.2-I3
Natalie Stingelin (Stutzmann) FRSC is a Full Professor of Organic Functional Materials at the Georgia Institute of Technology, with prior positions at Imperial College London; the Cavendish Laboratory, University of Cambridge; the Philips Research Laboratories, Eindhoven; and ETH Zürich. She was an External Senior Fellow at the Freiburg Institute for Advanced Studies and is Associate Editor of the RSC journal ‘Journal of Materials Chemistry C’. She was awarded the Institute of Materials, Minerals & Mining's Rosenhain Medal and Prize (2014) and the Chinese Academy of Sciences (CAS) President's International Fellowship Initiative (PIFI) Award for Visiting Scientists (2015); she was the Chair of the 2016 Gordon Conference on 'Electronic Processes in Organic Materials' as well as the Zing conference on ‘Organic Semiconductors’. She has published >160 papers and 6 issued patents. Her research interests encompass organic electronics & photonics, bioelectronics, physical chemistry of organic functional materials, and smart inorganic/organic hybrid systems.
We address here the long-standing quest to gain broadly applicable understanding of the structure formation of donor:acceptor blends that are used in organic solar cells, leading generally to a rich phase morphology where intermixed and neat phases of the donor and acceptor material co-exist. This is especially true for blends with non-fullerene acceptors because NFAs often display different polymorphs; moreover, their glassy states can be highly complex. Here we show that some donor polymer:non-fullerene acceptor blends comprise two different populations of glassy phases: a molecularly intermixed and an ITIC-rich one. This picture assists to understand the performance of some of these systems when used in solar cells; it also allows us to establish a tentative picture of the complex correlation of structure and electronic landscape for the understanding of organic photovoltaic cells. Our works furthermore highlights the challenges that are faced when using next generation materials, especially if long-term stability is sought.
2.3-I1
The energetic offset between the highest occupied molecular orbital (HOMO) levels of the donor and acceptor components of the organic photovoltaic (OPV) blends is well-known to affect the efficiency of the singlet exciton (S1) dissociation into separated charges (CS) via the chargetransfer (CT) state, however the impact of this offset on bimolecular recombination of free charge carriers has not been explored. In this study, using different non-fullerene acceptors blended with the same donor polymer PM6, we demonstrate that, apart from reducing the driving force for charge generation, diminishing HOMO-HOMO energy offset also activates faster bimolecular recombination of free charges either through the CT state or through exciton reformation as a new channel. Using the comparison between PM6:ITIC and PM6:o-IDBTR, we show that neither morphology, nor carrier mobilities can on their own explain the observed difference in performance, signaling the importance of the energy landscape in controlling the OPV device efficiency, both through generation and recombination of charge carriers.
2.3-I2
Triplet formation is generally regarded as an energy loss process in organic photovoltaics. Understanding charge photogeneration and triplet formation mechanisms in non-fullerene acceptor blends is essential for deepening understanding of photophysics in these important organic photovoltaic materials. Here, we present a comprehensive spectroscopy and morphology study on non-fullerene acceptors ITIC, ITIC-Th, ITIC-2F and Y6, both pristine and blended with reference polymer PffBT4T-C9C13. Atomic force microscopy and grazing-incidence X-ray diffraction provided information regarding the morphology of the films while spectroelectrochemistry combined with microsecond transient absorption spectroscopy allowed triplets and charge carriers to be investigated in detail. Crucially, we used triplet sensitisation to determine molar extinction coefficients of the non-fullerene acceptor triplets (2.7 – 6.5 ´ 104 mol L-1 cm-1), allowing triplet populations to be quantified in the blends. Intriguingly, no consistent trends were found in the photophysics of the studied blend systems, with each presenting its own unique mechanism. PffBT4T-C9C13:Y6 showed no triplet formation, only charge carriers that decayed rapidly in a relatively crystalline environment, consistent with the observed highly segregated morphology. In contrast, all blends in the ITIC series produced evidence of considerable triplet formation in addition to charge carriers. PffBT4T-C9C13:ITIC-Th blend produced acceptor triplets irrespective of excitation wavelength, and these were formed via intersystem crossing and/or energy transfer. Conversely, both ITIC and ITIC-2F blends displayed triplet formation via non-geminate recombination of charge carriers, with both NFA and polymer triplets observed. However, PffBT4T-C9C13:ITIC-2F produced a substantially higher charge carrier population than the ITIC blend. Because its triplet formation mechanism relies on the presence of charge carriers, PffBT4T-C9C13:ITIC-2F, with the highest charge carrier population, also had the highest triplet population. These results exemplify the prevalence of triplet states across a range of NFA blend systems, despite the varying formation mechanisms. Furthermore, they showcase that triplet populations can reach very high levels, particularly in cases of concomitantly high charge populations. Since high charge carrier densities correlate with large short circuit currents, this has significant ramifications for organic photovoltaic performance.
2.3-I3
Impedance Spectroscopy (IS) is a non-destructive characterization technique that has been traditionally applied to inorganic optoelectronic devices, such as standard diodes, LEDs, LASERs and Solar Cells. This technique consists in applying a small sinusoidal voltage superimposed on a bias voltage and measuring the current response. This technique allows to distinguish between the different dynamical mechanisms occurring inside the device at different time scales, from ms to s, such as carrier recombination, carrier diffusion and/or ionic related phenomena. In our research group, we use this technique as a complementary tool to identify the main physical mechanisms responsible of organic solar cells degradation, under different ISOS protocols. In addition, this technique sheds light about where the governing degradation process is taking place, either at the active layer or at the intermediate layers and/or contacts. Here, we will present a degradation study in non-fullerene OSC with layer structure Ag /ZnO /PBDBT-ITIC /PEDOT, using IS. In addition, we will compare devices with and without the processing additive DIO in terms of pristine efficiency and devices stability.
1.1-I1
Rechargeable aqueous Zinc (Zn) batteries are one of the emerging beyond lithium-ion batteries that could fulfil the requirements of cost-effective, safe, and reliable large-scale storage systems.[1,2] Zn metal is abundant, globally available, non-toxic and stable under air and humid atmosphere, with a promising theoretical capacity of 820 mA h/g.[3] However, aqueous Zn batteries encounter several degradations upon cycling due to the dendrite formation, poor Coulombic efficiency and hydrogen evolution reaction in dilute electrolytes.[4]
In this work, we report eutectic aqueous electrolytes based on Zn and lithium /or sodium salts at different ratios with very low water content. The cost-effective and fluorine-free electrolyte shows a high electrochemical stability window (~3V), which enables reversible and uniform Zn electrodeposition during long-term cycling. The eutectic aqueous electrolytes also demonstrate a low glass transition temperature allowing a wide temperature-range battery operation. This work significantly provides an effective strategy for high voltage and low-temperature aqueous Zn metal batteries.
1.1-I2
Husam Alshareef is a Professor of Materials Science and Engineering at King Abdullah University of Science and Technology (KAUST). He is also the Director of the newly-established Center of Excellence in Renewable Energy and Storage Technologies at KAUST. He obtained his Ph.D. at NC State University followed by a post-doctoral Fellowship at Sandia National Laboratories, USA.
He spent over 10 years in the semiconductor industry where he implemented processes in volume production for chip manufacturing. He joined KAUST in 2009, where he initiated an active research group focusing on the development of nanomaterials for energy and electronics applications. His work has been recognized by over 25 awards including the SEMATECH Corporate Excellence Award, two Dow Sustainability Awards, the Kuwait Prize for Sustainable and Clean Technologies, and the KAUST Distinguished Teaching Award. He has published over 600 papers and 80 issued patents. He is a Fellow of several prestigious societies including the American Physical Society (APS), Institute of Electrical and Electronics Engineers (IEEE), US National Academy of Inventors (NAI), Institute of Physics (IoP), Royal Society of Chemistry (RSC), and the Institute of Materials, Minerals and Mining. He has been a Clarivate Analytics Highly-cited Researcher in Materials Science for several years.
Despite its resounding success, lithium ion battery technology has some drawbacks that has motivated researchers around the world to look for future alternative battery technologies. These include safety issues, material abundance and cost, and geographical distribution of lithium. Aqueous zinc ion batteries are currently one of the most actively investigated battery technologies in the hope that it can one day replace lithium ion batteries. This is because aqueous zinc ion batteries are safe, environmentally friendly, use more abundant and cheaper materials, have somewhat suitable redox potential, which can minimize side reactions in aqueous electrolyte, and divalent charge which increases energy density. Despite these promises, aqueous zinc batteries suffer from several side reactions that degrade their stability and Coulombic efficiency. We have been developing strategies to mitigate these effects, including cathode material design, anode material surface treatments and passivation, and electrolyte and solvation structure engineering. In this talk, I will discuss some of the recent results from our group aiming to address these issues in zinc metal batteries.
HN Alshareef et al, Advanced Materials 2022, 34, 2106937
HN Alshareef et al, J. Am. Chem. Soc. 2022, 144, 16, 7160–7170
HN Alshareef et al, Energy Environ. Sci., 2021, 14, 4463–4473
HN Alshareef et al, ACS Energy Letters 2022, 7, 1, 197–203
1.2-O1
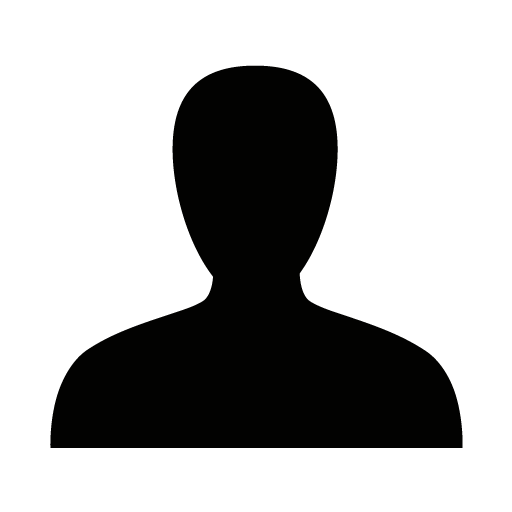
Currently, the only metal-air technology developed at high TRL is represented by the zinc-air primary battery (ZAB)[1], for a small number of applications, such as portable hearing aids, railway signalling, electric fence, and generally low-power/low-consumption long term uses. Although presenting many green advantages, like the intrinsic safety and non-toxicity of the aqueous electrolyte, use of abundant and recyclable Zn, absence of expensive or precious metals, ZABs’ barrier to larger and stationary applications (to compete with the already mature Li-ion, Ni-Zn, or lead-acid technologies)[2] is mainly represented by electrical rechargeability (i.e., developing a bifunctional cathode) and durability of the liquid electrolyte (i.e., leakage and/or evaporation of the KOH-based liquid solution)[3].
In this work, we survey the actual developments in electrically rechargeable ZAB (ERZAB) and analyse the selection criteria of materials by general and specific constrains, suggesting emerging directions for industrial development, guided by a sustainable point of view. ZABs should switch from conventional liquid electrolytes to gelled electrolytes, based on naturally occurring biopolymers; for the cathode, engineered mixes of specialized catalysts for oxygen reduction and evolution reactions (ORR/OER), not based on critical raw materials (CRMs), should be investigated, pursuing tuneable wettability and long-lasting durability of the cathode. There is an extensive literature about bifunctional cathodes for ERZABs[4,5], but performance validation should go more towards longer cycles and higher depth-of-discharge (DoD). Finally, interesting electrolyte and cathode materials are tested, and preliminary results are discussed to establish the next steps for future research and developments.
1.2-O2
In this presentation, we report the impact of electrolyte solvents (EC:DEC and diglyme) with NaPF6 on the cycling stability and rate capability of Na2Ti3O7 (NTO). This material has been widely investigated as an anode for SIBs because of its reasonably high specific capacity (177 mAh g-1) and low insertion potential (0.2 V vs. Na+/Na, outside the stability window of electrolytes). However, its rapid capacity decay and rate capability have been highly linked to the formation of an unstable and thick SEI layer. Yet, many studies have focused on the improvement of NTO properties and to date no alternative electrolytes have been proposed. Overall, we observed that the electrochemical performance of Na/NTO half-cells in DiG outperformed that in the EC:DEC solvent in terms of specific capacity, rate capability and cycling stability. Furthermore, we provide a detailed interfacial analysis of both the SEI layer and charge-transfer processes using PES, electrochemical impedance spectroscopy and operando electrochemical AFM under different cycling regimes to explain the enhanced electrochemical behaviour observed in DiG, which results from the formation of a stable, thin and compact SEI.
1.2-O3
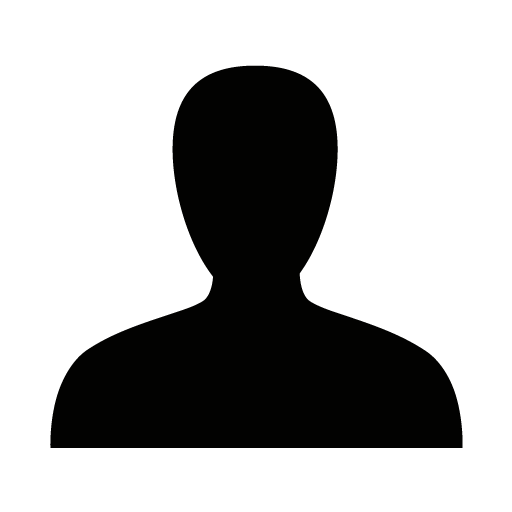
Rechargeable lithium-ion batteries (LIBs) have been recognized as the most successful energy storage devices with an energy density increase of about 7-8 Wh kg-1 per year, now reaching between 260 and 270 Wh kg-1. However, the massive growing market of portable electronic devices, electric vehicles, stationary grid storage, etc. and the safety problems resulting from this technology involve demand for alternatives. Among the intensely studied technologies (i.e., Na-ion batteries, K-ion batteries, Li-sulphur batteries, solid state batteries, Li/Na-air batteries, etc.), Na-ion batteries (NIBs) represent a promising alternative to Li-ion batteries because sodium is widely available and exhibits similar chemistry to that of LIBs.
However, a major challenge of this technology lies in the development of anode materials because graphite, the reference anode used in Li-ion batteries, intercalates only small amounts of Na ions. Hard carbon (HC) has been found to be more suitable anode candidates, being able to reach specific capacities of around 300 mAh g-1. This is possible due to its disordered complex structure combining graphitic domains and micropores, being recognized as a non-graphitizable carbon. Moreover, they can be synthesized from a large number of bio-sourced precursors (i.e., cellulose, carbohydrates, bio-wastes, plants, etc.), offering thus the possibility to develop eco-friendly anodes.
In this context, our team proposed to develop hard carbons from a series of bio-sourced precursors: natural polyphenols1 and biopolymers. More precisely, five natural tannin based-polyphenols and five biopolymers were selected to synthesize hard carbons, following a single pyrolysis process at 1500°C, under Ar. Their carbon content, morphology, structure and texture were studied by several techniques. These complementary analyzes confirmed the formation of typical hard carbon structure but also the presence of some residual inorganic compounds, inherently induced by the parent precursor.
HCs electrochemical performance were evaluated versus Na metal, in coin cells. Reversible capacities of around 300 mAh g-1 were obtained for most of the materials with an initial Coulombic efficiency between 81% and 88%.
Finally, the electrochemical performance was plotted versus different physico-chemical properties of the hard carbon materials and several strong correlations could be found.
This simple approach provides new possibilities for the use of bio-sourced precursors as electrode materials for NIBs, as they provide a reliable, natural, renewable, nontoxic, and low-cost resource for hard carbon production.
1.2-O4
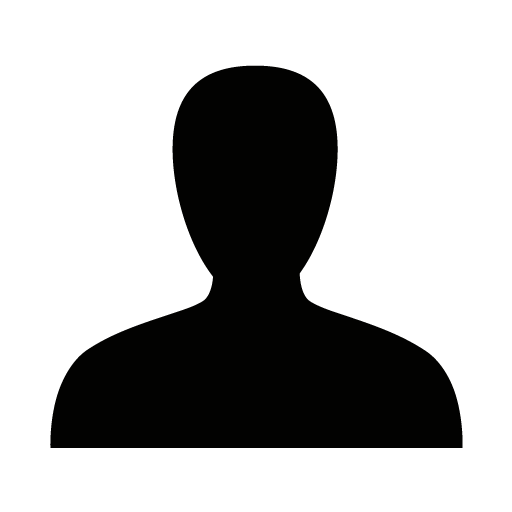
The omnipresent Li-ion batteries are reaching their energy density limitations. At the same time, energy storage demands are drastically increasing, motivating researchers to pursue new battery systems. Due to the bivalent nature of ions, low redox potentials, high gravimetric capacities, and natural abundance, Mg and Ca metal anode batteries are considered as promising post-Li energy storage systems. One of the main challenges of both systems is the development of electrolytes.
Fluorinated alkoxyborate-based electrolytes were introduced into Mg and Ca batteries a few years ago and presented significant progress over the previous generations of electrolytes in terms of overpotentials, stability, and Cl-free character.1 Specifically, Mg[B(hfip)4]2 and Ca[B(hfip)4]2 (hfip = 1,1,1,3,3,3–hexafluoroisopropoxy) served as model compounds to show that weakly coordinated anion with a high degree of fluorination enables large charge delocalization, decreases the anion–cation interaction, and consequently reduces the ion-pair formation.2 Additional improvement for Mg batteries has been reached with the introduction of Mg fluorinated alkoxyaluminate electrolytes. Among those, Mg[Al(hfip)4]2 with Coulombic efficiency over 99.5% and overpotentials below 60 mV is considered a state-of-the-art Mg electrolyte.3
In our research, we explore the field of fluorinated alkoxy electrolytes by synthesizing Mg alkoxyborates with exchanged hfip ligand for various alcohols with different sterical properties, number of binding groups, and degree of fluorination. Additionally, a synthesis of a novel Ca[Al(hfip)4]2 salt is developed. All electrolytes are studied by extensive physicochemical and electrochemical characterization. Our study shows that changing the anion structure allows the development of electrolytes offering improved electrochemical performance compared to the model [B(hfip)4]2– – based electrolytes and exposes the most promising candidates for further studies for practical Mg and Ca rechargeable batteries.
1.2-O5
Luisa De Marco received her PhD in Nanoscience from Università del Salento in 2010 working on nanostructured semiconductors for photovoltaics. Since 2016 she is researcher at CNR NANOTEC leading a 6-person team working on the development of low-dimensional inorganic and hybrid nanomaterials. She is author of more than 70 papers that collectively have received more than 2600 citations, with an h-index of 31. Among the publications stand out Advanced Materials, Nature Nanotechnology, Energy & Environmental Science, ACS Nano and Science Advances.
Her research interests focus on the development and engineering of hybrid and inorganic low-dimensional semiconductors having specifically tailored functional properties and on design and fabrication of optoelectronic devices.
Energy storage devices play a key role in the clean energy transition, enabling the use of energy from renewable sources and electric mobility. Although Lithium-Ion Batteries (LIBs) have several desirable characteristics (such as pollution-free operation, high efficiency, long lifetime), [1] the rapid expansion of their market has raised concerns about the availability of the raw materials used, particularly Lithium and Cobalt, with projections predicting a shortage in less than ten years. [2]
The aim of the project HYNANOSTORE - Hybrid Nanostructured Systems for Sustainable Energy Storage - recently funded by an ERC Consolidator Grant, is to revolutionize the conventional battery electrode, based on Li insertion, and develop a new architecture, in which redox organic molecules anchored to a nanostructured electrode are the active part of the battery and are able to reversibly uptake and release electrons.
The nanostructured conductive scaffold, with tailored features and an extended surface area, immobilizes redox-active molecules, facilitates charge transport, and enhances interactions between active material and electrolyte. Preliminary results are presented in order to demonstrate the working mechanism of the proposed bio-inspired engineering system, which will lead to the introduction of a new concept for the realization of economically and environmentally sustainable energy storage devices, characterized by great versatility in terms of electrode materials and electrolytes.
1.2-O6
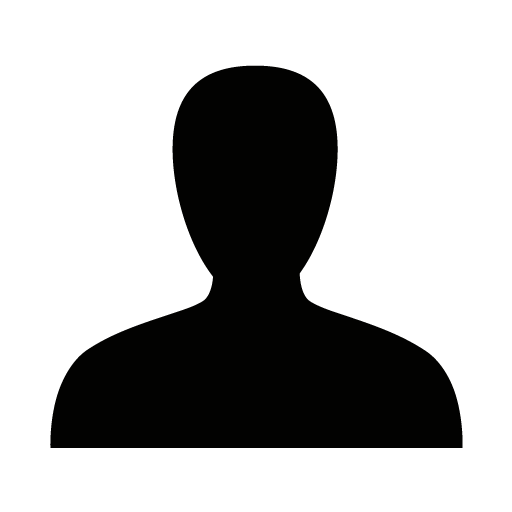
Rechargeable batteries that use energy-dense multivalent metals as anodes (such as magnesium and calcium) could represent a major step forward in the transition to a renewable energy economy. When combined with sustainable and pliable organic cathodes, multivalent metal batteries can realize their full potential.[1] While offering good reversibility, organic electrodes continue to underperform in multivalent electrolytes, especially in terms of achievable capacities. Therefore, to improve existing and design better organic materials for Mg and Ca batteries, a deeper understanding of their kinetic constraints must be obtained.
A major obstacle to detailed kinetic studies of organic materials in multivalent electrolytes is the lack of reliable electrochemical setups that would allow both galvanostatic cycling and electrochemical impedance spectroscopy measurements. This is a direct consequence of large overpotentials of multivalent metal anodes, which can lead to the impedance response of organic cathode being completely concealed by large anode response. A stable reference electrode for multivalent systems has yet to be found, and the use of alternative counter electrodes, such as activated carbon, sometimes introduces more problems for electrochemical characterization than it solves. Therefore, we propose the use of cyclable symmetric cells, where two organic electrodes are used, with one electrode fully charged and the other fully discharged.[2] By using a model compound poly(anthraquinonyl sulfide) we demonstrate the feasibility of the approach in lithium batteries. We further show that, in multivalent electrolytes, this type of symmetric cell allows access to the true performance of organic electrodes, by successfully eliminating counter-electrode contribution and enabling both rate capability and long-term cycling experiments. On top of that, cyclable symmetric cells allow reliable impedance measurements. We show how the impedance response differs in shape and magnitude when organic polymers interact with Li, Mg, and Ca species. Besides opening the doors for discovering the true limits of organic materials coupled with multivalent charge carriers, cyclable symmetric cells could improve the comparability of the results obtained in the field of multivalent-metal organic batteries by eliminating the role of the anode/electrolyte interface in the electrochemical tests.
1.3-I1
Today, when a real expansion of electrode materials is happening, plenty of anode and cathode structures for alkaline-ion batteries have been developed. Mixed polyoxyanion cathode materials are becoming interesting as electrode materials for sodium-ion batteries [1]. Among them, the specific family of sodium mixed polyanionic phase, with combined phosphate and pyrophosphate units, has attracted attention as cathodes for Na-ion batteries [2]. Herein, the talk will focus on sol-gel synthesis of Fe-based mixed polyanionic phase such as Na4Fe3(PO4)2P2O7 and its interrelation with the secondary polyanion pyrophosphate phase (Na2FeP2O7). It will be shown how the synthesis conditions control the thermal PO43- - P2O74- phase transition and consequently the amount of P2O74- structural units in the synthesized powder. The special attention will be paid to assignation of phosphate/pyrophosphates vibrational modes and correlation of the polyanionic phase composition with electrochemical properties in Na-based aqueous electrolyte. Also, the difference in the electrochemical behavior of mixed polyanionic phase, between typical aqueous and water-in-salt electrolytes, will be presented and discussed in terms of new directions towards development of a new generation of more sustainable energy storage devices.
1.3-I2
Integrating a lithium-metal anode and a high-voltage cathode into a solid-state battery remains a formidable challenge, especially when the battery is charged beyond 4 V. Most electrolytes genuinely do not possess such a wide electrochemical stability window, but have to rely on the formation of a passivating solid electrolyte interphase, require protective electrode coatings, or have to be combined with a secondary electrolyte to achieve stable dis-/charge cycling, adding complexity.
This is also the case for prototypical polyethylene oxide-based polymer electrolytes, which form a relatively stable interface to lithium metal [1], but start oxidizing already at 3.2 V vs Li/Li+ via deprotonation of the terminal O-H group, as we have recently shown combining electrochemical impedance spectroscopy, infrared spectroscopy, and differential electrochemical mass spectrometry [2].
We recently employed a polymer electrolyte based on a polymerized ionic liquid to demonstrate a 4 V class solid-state battery with a lithium metal anode and a LiNi0.8Mn0.1Co0.1 cathode operating at room temperature and delivering an initial capacity of 162 mAh/g and a capacity retention of 72% after 600 cycles to 4.4 V [3]. The polymer matrix consists of poly(diallyldimethylammonium) bis(fluorosulfonyl)imide (PDADMAFSI) and N-butyl-N-methylpyrrolidinium bis(trifluoromethylsulfonyl) imide (PYR13FSI) is employed as plasticizer in combination with lithium bis(fluorosulfonyl)imide (LiFSI) as lithium salt. PDADMAFSI and PYR13FSI were selected because of their outstanding chemical stability and wide electrochemical stability window. Comparing to typical lithium-ion-coordinating polymer matrices, the positively charged PDADMA+ chains reduce lithium-ion coordination with the polymer promoting high lithium-ion mobility. LiFSI has low binding energy between Li+ and FSI− and the ability to form stable interphases in contact with lithium metal. To confirm the high oxidative stability of this electrolyte, we also assembled a solid-state lithium-metal cell with a high-voltage spinel LiMn1.5Ni0.5O4 cathode reaching an initial capacity of 132 mAh/g and a capacity retention of 76% after 300 cycles to an upper cut-off voltage of 5 V at room temperature.
2.1-I1
Dr. Rebeca Marcilla received her PhD in Chemistry in 2006 from the University of the Basque Country (UPV/EHU) in the field of ionic liquids and polymers with application in electrochemistry and nanotechnology. After a postdoctoral stay at the University College London she joined CIDETEC (Centro de Tecnologías Electroquímicas, Donostia-San Sebastian). In 2010 she moved to the Unit of Electrochemical Processes IMDEA Energy (Madrid) and in 2015 she became Senior Researcher. During her fruitful scientific career, she has acquired proven experience in advanced materials for energy storage (eg. ionic liquids, polymer electrolytes, redox-active polymers, etc) and in next-generation batteries including organic batteries and redox flow batteries). As a result, she has co-authored 7 patents (1 of them licensed to a private company) and published more around 120 articles, achieving an h-index of 46. She has supervised 12 PhD thesis (6 presented+6 on-going) and several postdoctoral researchers. In 2017, she was awarded with a ERC Consolidator Grant to develop Membrane-free Redox Flow Batteries. Dr. Marcilla is member of the Governing Board of the Electrochemistry Group of the Spanish Royal Society of Chemistry (GEE-RSEQ) and Editor of Journal Power Sources.
The expected growth over the next few years in the battery sector is huge, with approximately 7 million tons of new batteries manufactured per year. This growth is mainly triggered by the deployment of the electric vehicle and by the energy storage coupled to wind and photovoltaic generation. However, the massive development of the sector could become an environmental problem since most commercial batteries are based on inorganic materials such as lithium, nickel and cobalt in lithium-ion batteries or vanadium in flow batteries. These materials are scarce, their production in some cases is not sustainable and some are even toxic. In this context, the replacement of these materials by organic compounds based on elements as abundant as C-H-O-N has become a very promising alternative [1].
Here I will present a wide overview of our research lines moving from organic aqueous redox flow batteries (OARFBs) to static batteries using redox-active polymer electrodes. In the case of RFBs, I will give a flavour on the multiple requirements of immiscible electrolyte including organic active species to develop a new concept of membrane-free RFB. In the second part, I will introduce the different polymer structures (linear, porous, hyperbranched, nanoparticles) containing redox active functionalities (quinones, phenazynes, etc) that we have developed in the last years. I will be focussed on their electrochemical properties in different electrolytes and their application in several battery technologies including Li-ion [2], multivalent [3], aqueous [4], all-polymer [5], etc).
2.1-I2
Manuel Souto Salom (Valencia, 1988) is an Oportunius Research Professor and Principal Investigator at CIQUS (University of Santiago de Compostela). He is also a Guest/Visiting Professor at the University of Aveiro. He holds a double degree in Chemistry and Chemical Engineering from the University of Valencia (Spain) and from the École de Chimie, Polymères et Matériaux (ECPM) de Strasbourg (France), respectively, doing a research internship at PLAPIQUI (Argentina). He also earned a Master’s degree in Molecular and Supramolecular Chemistry (2011) from the University of Strasbourg conducting his Master thesis at Instituto Superior Técnico (IST, Lisbon). He obtained his PhD in Materials Science at Institut de Ciència de Materials de Barcelona (ICMAB-CSIC) with Prof. Jaume Veciana in 2016 conducting two research stays at the National University of Singapore (NUS) and at the University of Antwerp. In 2017, he started to work as a postdoctoral researcher at the Institute of Molecular Science (ICMol-UV) with a Juan de la Cierva fellowship. In 2019, he started his independent research career as an Assistant Professor at the Chemistry Department of the University of Aveiro and CICECO-Aveiro Institute of Materials. In 2022 he was promoted to Principal Researcher (tenure, Permanent Researcher/Assoc. Prof.) at the same institution. His research interests encompass molecular electronics, electroactive polymers and organic batteries. His main current research interest is the design and synthesis of new functional electroactive porous frameworks (e.g., COFs & MOFs) based on redox-active organic building blocks for energy storage applications. In 2021, he was awarded an ERC Starting Grant with the project ELECTROCOFS, which aims to design new redox-active COF-based electrodes for rechargeable batteries. He received, among other distinctions, the NanoMatMol PhD award, the PhD Extraordinary award, and the European Award on Molecular Magnetism Doctoral Thesis. He is member of the RSEQ (GENAM) and SPQ chemical societies and Fellow of the Young Academy of Europe.
A major breakthrough in chemistry and materials science has been the development of Lithium-Ion Batteries (LIBs), which show great potential for storing energy from renewable sources and as the power source for electric cars [1]. However, commercially available LIBs are based on transition metal oxide cathodes, presenting limited energy density and raising relevant environmental concerns. Organic materials have received much attention as alternative electrodes because of their high theoretical capacity, resources availability and sustainability [2,3]. In particular, Covalent Organic Frameworks (COFs), crystalline porous polymers based on organic building blocks linked by strong covalent bonds, have emerged in the past few years as promising organic electrode materials due to their high stability, high ionic conductivity and outstanding chemical and structural versatility [4]. In this presentation, I will show some different examples of redox-active COFs used as cathode materials for coin-cell type lithium batteries highlighting some of its advantages and challenges in comparison with other organic electrodes. I will also focus on the COFs processing optimization to improve the batteries performance. Finally, I will highlight some strategies to improve the charge transport in COFs, one of the main bottlenecks of these organic electrode materials [5].
2.1-I3
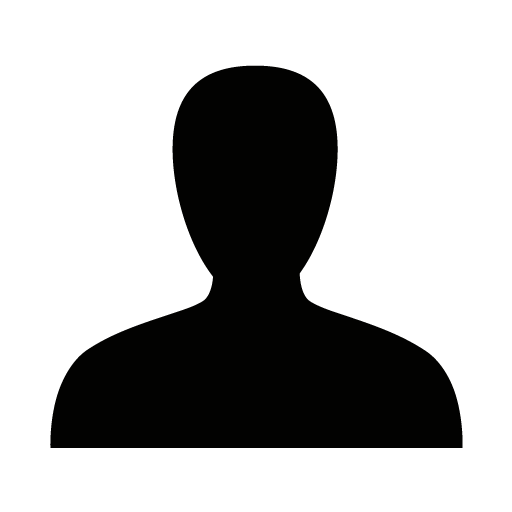
Controlling and mastering electrode/electrolyte interfaces is a central goal in energy storage applications to improve the performance, the lifetime and the safety of Metal-ion batteries. This implies understanding the microscopic mechanisms taking place at these interfaces during the battery operation. The large electric fields developing at these interfaces may alter the local properties of the solvent in the vicinity of the electrode surface. To account for the potential dependence of the interface reactivity, ab initio molecular dynamic simulations or classical MD with reactive force-fields should be the appropriate methods but are still far prohibitive for the complexity of these electrochemical interface. A Grand canonical DFT approach was then develop to elucidate the mechanisms at play in these complex system. The methodology was applied to several challenging issues of electrode/electrolyte interfaces to shed light on undesired phenomena such as electrolyte degradation/ageing or metal-dendrites growth. [1,2] The perspective of this work on the development of new electrolytes for post-Li technologies and/or on the functionalization of electrode surfaces will be discussed. [3,4]
[1]
[2] A. Hagopian et al. Energy Environ. Sci., 2020, 13, 5186-5197
[3]
[4] L. H. B. Nguyen et al. Phys. Chem. Chem. Phys. 2022 Accepted
2.2-I1
Renewable energy production is characterized by intermittent power output and requires large-scale applications to improve energy storage capability (currently, less than 1% of the electrical energy production can be stored). Developing low-cost and environmentally friendly electrochemical storage systems characterized by high performance is of fundamental importance for a sustainable energy economy. The currently most mature battery technology is the lithium-ion battery, considered one of the most appealing candidates as a power source for electric vehicle applications. However, the large-scale application of lithium-ion batteries is currently under discussion due to the limited lithium and certain transition metals such as Co and Ni resources. Several other metallic anodic materials such as sodium, potassium, calcium, magnesium and aluminium [1-3], characterized by a higher abundance of lithium, have been considered suitable candidates for electrochemical storage devices in replacing lithium systems. Notably, significant efforts are being devoted to understanding and addressing key challenges in developing these so-called “beyond Li-ion” chemistries, and substantial insights into their storage and failure mechanisms have been obtained over the past few years thanks to advanced characterization and computation/modelling techniques. An overview of our activity in “beyond Li-ion” batteries field and an evaluation of the capability of this technology will be presented. [4–8]
References
[1] G. A. Elia, K. Marquardt, K. Hoeppner, S. Fantini, R. Lin, E. Knipping, W. Peters, J.-F. Drillet, S. Passerini, R. Hahn, Adv. Mater. 2016, 28, 7564.
[2] G. A. Elia, K. V Kravchyk, M. V Kovalenko, J. Chacón, A. Holland, R. G. A. Wills, J. Power Sources 2021, 481, 228870.
[3] G. G. Eshetu, G. A. Elia, M. Armand, M. Forsyth, S. Komaba, T. Rojo, S. Passerini, Adv. Energy Mater. 2020, 10, DOI 10.1002/aenm.202000093.
[4] F. Colò, F. Bella, J. R. Nair, C. Gerbaldi, J. Power Sources 2017, 365, 293.
[5] G. A. Elia, G. Greco, P. H. Kamm, F. García‐Moreno, S. Raoux, R. Hahn, Adv. Funct. Mater. 2020, 30, 2003913.
[6] X. Liu, G. A. Elia, S. Passerini, J. Power Sources Adv. 2020, 2, 100008.
[7] X. Liu, H. Euchner, M. Zarrabeitia, X. Gao, G. A. Elia, A. Groß, S. Passerini, ACS Energy Lett. 2020, DOI 10.1021/acsenergylett.0c01767.
[8] X. Liu, G. A. Elia, B. Qin, H. Zhang, P. Ruschhaupt, S. Fang, A. Varzi, S. Passerini, ACS Energy Lett. 2019, DOI 10.1021/acsenergylett.9b01675.
2.2-I2
All-solid-state lithium metal batteries (ASSLMBs) with sulfide-based solid electrolytes with high ionic conductivity are regarded as the ultimate next-generation energy storage systems due to their enhanced safety and energy density by enabling the use of metallic anodes. Li metal is considered the holy grail anode material because of its high theoretical specific capacity (3860 mAh/g) and the lowest electrochemical potential (-3.06 V versus standard hydrogen electrode). However, its practical use has been hindered by several issues related to the interface, such as contact loss during cycling, which accelerates Li dendrite growth, and chemical instability between Li metal and sulfide-based solid electrolyte. To construct safe and high energy density ASSLBs, understanding the degradation mechanism of the ASSLBs is imperative. In this talk, the fundamental degradation mechanisms of the ASSLMBs underlying electrochemical and mechanical aspects are introduced first. Subsequently, we briefly review the current research on the ASSLMSs. Finally, this presentation introduces our strategies for developing high-performance ASSLMBs by stabilizing the Li metal and sulfide-based solid electrolytes interface. The designed ASSLMBs could effectively retard the Li dendrite growth and unwanted side reaction and shows much enhanced electrochemical performance.
2.3-O1
Claudio Gerbaldi got his PhD in Material Science and Technology in 2006 at the Politecnico di Torino, where he is now Full Professor, Chair of Chemistry for Applied Technologies. He leads the Group for Applied Materials and Electrochemistry, developing innovative electrochemical energy storage/conversion systems and related materials, with strong collaboration with academia, industry, and EU. He is co-author of > 175 research articles in ISI journals (h-index 67). He is the President of GISEL, the Italian Group for Electrochemical Energy Storage. Among others, he received the International “Roberto Piontelli” Award by the President of Italian Republic for outstanding contributions in the field of electrochemistry for energy-related applications.
The transformation from liquid- to solid-state architecture is expected to improve safety, fabrication, and temperature stability of energy storage devices, particularly if constraints of low ionic conductivity, low cation transport properties and stringent processing conditions are overcome [1].
Here, an overview is offered of the recent developments in our labs on innovative polymer-based electrolytes allowing high ionic mobility, particularly attractive for Li-metal batteries, and obtained by different techniques, including solvent-free UV-induced photopolymerization. Electrochemical performances in lab-scale devices can be readily improved using different kind of RTILs or other specific low-volatile additives. Cyclic voltammetry and galvanostatic charge/discharge cycling coupled with electrochemical impedance spectroscopy exploiting different electrode materials (e.g., LFP, Li-rich NMC, Si/C) demonstrate specific capacities approaching theoretical values even at high C-rates and stable operation for hundreds of cycles at ambient temperature [2,3]. Direct polymerization procedures on top of the electrode films are also used to obtain an intimate electrode/electrolyte interface and full active material utilization in both half and full cell architectures. In addition, results of composite hybrid polymer electrolytes, as well as new single-ion conducting polymers are shown [4,5], which are specifically developed to attain improved ion transport and high oxidation stability for safe operation with high voltage electrodes even at ambient conditions.
2.3-O2
The rapid technological evolution and population growth demand more sustainable materials compatible with the circular economy paradigm. Natural polymers, a class of materials produced by biological and renewable resources, represent an important oportunity for the development of more sustainable technologies for their application in energy storage systems in general and batteries in particular [1].
Silk fibroin (SF), obtained from Bombyx mori silkworm cocoons, carrageenan, that are sulphated polysaccharides that occur as matrix material in several species of red seaweeds of the class Rhodophyceae and poly(L-lactic acid) (PLLA), an enantiomeric polyester produced from lactic acid synthesis are one of those materials due to their mechanical and thermal properties, non-toxicity biodegradability and biocompatibility.
In order to improve the battery performance it is usual to add different inorganic fillers to the polymeric separators. Metal-organic frameworks (MOFs) are a class of porous crystalline solids that impregnated with ionic liquids (ILs) can substantially improve the conductivity values of the separator [2].
The present work presents the development of separator membranes for lithium-ion batteries (LIBs) applications based on those natural polymers and preliminary results based on the MOF-74 impregnated with the [EMIm][TFSI] ionic liquid.
The samples were evaluated in terms of morphology, physical-chemical characteristics, thermal behavior, mechanical and electric and ionic proprieties in order to evaluate their applicability for separator membranes in LIBs. The half-cells prepared with silk fibroin, carrageenan and poly(L-lactic acid) show good cyclability and a discharge capacity of 131,1 mAh.g-1 at C/8-rate, 145 mAh.g-1 at C/10-rate and 93 mAh.g-1 at 1C-rate, respectivelly, demonstrating excellent battery performance.
2.3-O3
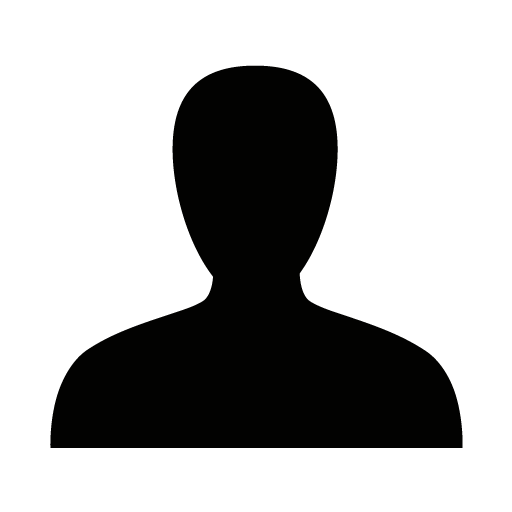
Cobalt is a key material for the production of Li-ion batteries. Today already more than 60% of mined cobalt is destinated for battery cathodes. Additionally, 60% of the worldwide cobalt resources are in the politically unstable Congo (DRC) and are extracted in many cases by child labour that implies an ethical and health concern due to its toxicity[1]. LiNi1.5Mn0.5O4 spinels are between the most promising alternatives as they present a long high voltage plateau, very fast lithium insertion and extraction, which yield to high energy densities of 650 Wh/kg at cell level (vs the cathode mass). However, among the main challenges of these batteries, are the oxidation stability of the electrolyte when cycling at high voltage and the cycle life when practical electrode loadings are employed [2,3]. Within the CoFBAT project, gel electrodes and electrolytes based on the new Solef® PVdF copolymer have been developed. The current results at full cell level shows over 250 cycles up to 80% state of health when the LNMO cathode electrode is jellified. Still a ten-fold increase of the cycle life is needed to outperform commercial Li-ion batteries. For better understanding the degradation mechanisms of these batteries, new post-mortem protocols for gel-based Li-ion batteries need to be developed. The use of gel-based electrodes and electrolytes difficult the disassembly and characterization process, especially the analysis of the solid-electrolyte interphases. In this work we summarized the main challenges of LNMO to outperform current commercial Li-ion batteries as well as strategies proposed to overcome them. Finally, we present a multi-technique approach for the post-mortem analysis of gel-based Li-ion batteries that allow to identify the main driving source of capacity fade.
2.3-O4
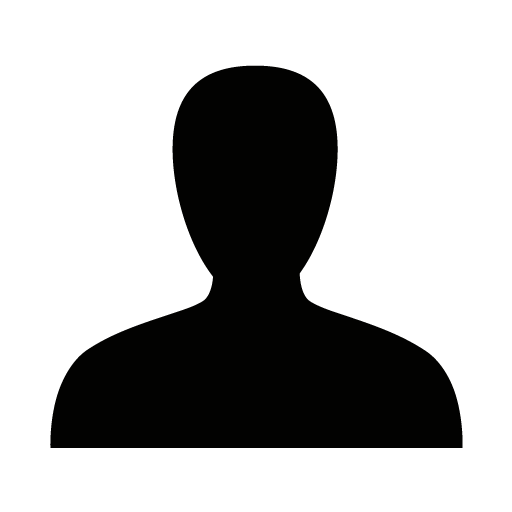
High-throughput approaches in computational materials discovery often yield a combinatorial explosion that makes the exhaustive rendering of complete structural and chemical spaces impractical. A common bottleneck when screening new compounds with archetypal crystal structures is the lack of fast and reliable decision-making schemes to quantitatively classify the computed candidates as inliers or outliers (too distorted structures). Machine learning-aided workflows can solve this problem and make geometrical optimization procedures more efficient. However, for this to occur, there is still a lack of appropriate combinations of suitable geometrical descriptors and accurate unsupervised models which are capable of accurately differentiating between systems with subtle structural changes. Here, considering as a case study the compositional screening of cubic Li-argyrodites solid electrolytes, we tackle this problem head on. We find that Steinhardt order parameters are very accurate descriptors of the cubic argyrodite structure to train a range of common unsupervised outlier detection models. And, most importantly, the approach enables us to automatically classify crystal structures with uncertainty control. The resulting models can then be used to screen computed structures with respect to an user-defined error threshold and discard too distorted structures during geometrical optimization procedures. Implemented as a decision node in computer-aided materials discovery workflows, this approach can be employed to perform autonomous high-throughput screening methods and make the use of computational and data storage resources more efficient.
2.3-O5
Recent advances in wearable and IoT technologies has led to a strong demand for embedded Li-ion microbatteries. There is also a need for microbatteries with superior mechanical characteristics such as flexibility, stretchability while maintaining strong electrochemical performances.
In this work, we present micro/nano-structuration of microbatteries electrodes surface [1]. Patterning of electrodes materials allows improvement of both mechanical resistance and electrochemical performance of these microbatteries [2]. 3D surface enhancement owing to the design of micropillar electrodes permits to fulfil this need. Lithium nickel manganese oxide (positive electrode) and Lithium titanate oxide (negative electrode) micropillars with different sizes are fabricated on aluminium foils by laser ablation technique and are then separated by a polymer electrolyte to form flexible lithium-ion microbatteries [3].
We have demonstrated that the micropillar size has an influence on the electrochemical performance of Li-ion microbatteries. Optimized pillar dimensions drastically enhance areal capacity. For micropillar size of 25 µm*25 µm the areal capacity is 822 μAh.cm–2 at 1C (1×2 cm electrodes) [4].
In addition, a treatment of electrolyte-coated electrodes under vacuum improves electrochemical properties due to a better filling of the interpillar space by the electrolyte polymer. Under optimized conditions, the capacity delivered by the microbattery is four times higher than the planar system counterpart. Higher areal discharge capacity is obtained when the electrodes follow a treatment in vacuum: 1049 μAh.cm–2 at 1C for micropillar size of 25 µm*25 µm. This vacuum treatment can be easily done at room temperature. Figure 1 shows the increase of areal capacity at 1C versus the time vacuum treatment.
Thanks to low thickness below 1 mm and high flexibility, these optimized microbatteries can be used in applications such as powering smart contact lens [5].
1.1-I1
Wolfram Jaegermann, born 1954, studied Chemistry at the University of Dortmund and got his Ph.-D. in Inorganic Chemistry from the University of Bielefeld, Germany. Afterwards he started his scientifc career as a Post-Doc at the Hahn-Meitner-Institute in Berlin in Photoelectrochemistry. He spent one year as DuPont Guest Scientist in Wilmington, Deleware, before he got his Habilitation in Physical Chemistry at the Free University of Berlin. Afterwards he was appointed Head of Department of Interfaces at the Hahn-Meitner-Institute. In 1997 he became Full Professor with the chair of Surface Science, in the newly founded Department of Materials Science, TU Darmstadt. His main research fields are: Surface Science, Photovoltaic Converters, Intercalation Batteries, Inorganic/Organic Composites, Semiconductor Interfaces, Photoelectrochemistry.
For an effective conversion of solar energy to electric power or a chemical fuel a lot of different materials as well as device structures have been suggested but only very few provide technological competitive conversion efficiencies so far. Especially thin film devices originally considered to provide the most cost competitive solution have not fulfilled their promises. Limitations and loss processes can be deduced from a detailed consideration of the involved photovoltaic and electrochemical elementary steps. Optimized performance can only be reached when the photovoltaic and electrolytic boundary conditions of integrated systems are comparable to those of separated devices with no extra loss due to the coupling process.
Feasible solutions seem to be possible for thin film PV and water splitting generation, as we will show with a number of investigations performed recently combining thin film synthesis, electrical or electrochemical investigations with surface science studies. Especially photoemission results on the demands on the bulk and surface electronic structure provide clear boundary conditions on the material’s and surface properties: i) the semiconductors must provide a wide splitting of quasi Fermi levels which will be provided by multi-junctions of classical semiconductors but are not to be expected for semiconductors with localized electron states as e. g. oxides as e. g. hematite due to polaron formation. ii) The growth of the materials must provide compact, grain boundary poor absorber layers to avoid charge recombination and/or transport barriers to adjust the diffusion lenghts to the absorption lengths. Iii) The interfaces between the semiconductor absorber material and the contact layers also including the electrocatalyst must be prepared avoiding Fermi level pinning and non-adjusted electron transfer states by using proper aligned passivation layers. As a consequence a buried junction is needed. We will compare PV and/or PEC cells based on Si, GaInP, and SnS to illustrate our conceptual considerations.
In summary, we do believe that effective thin film PV and PEC devices need the input and coupled scientific approach from different disciplines and expertise ranging from solid state physics, surface science to electrochemistry. Promising advanced technological solutions seem possible based on improved materials science approaches in correlating material’s engineering, manufacturing and resulting properties to the involved physical boundary conditions of the elementary steps of energy conversion.
1.1-I2
Photoelectrochemical (PEC) water splitting with Earth abundant and/or low-cost materials can potentially lead to low-cost green hydrogen fuel. One material that will be discussed is copper oxide (Cu2O)–fabricated by thermal oxidation of copper foils–for use as a photocathode for solar hydrogen production. Capacitance-based methods are used to identify the origin of the improvement of an etching treatment on the cuprous oxide and a performance record is achieved. The orientation of the millimeter-sized single crystal grains, which can be seen with the naked eye due to selective etching and the resulting optical effects on the different facets, enable the impact of crystal orientation on the photocathodes to be assessed. It is found that the high index crystal orientations yield statistically higher performance than low index facets, and the origins of this improvement will be discussed. Another material under investigation in our lab is Sb2Se3, and I will discuss recent developments. I will close with an outlook on how thin film studies can inform the development of photocatalytic particles, which have disruptive potential in the green hydrogen space.
1.1-I3
Dr. Edgardo Saucedo studied Chemical Engineering at the University of the Republic, Montevideo, Uruguay, and received his PhD in Materials Physic at the Universidad Autónoma de Madrid, Madrid, Spain in 2007 with a FPU fellowship. In 2007, he joined the Institut de Recherche et Développement sur l’Énergie Photovoltaïque IRDEP (Paris, France), with a CNRS associated Researcher fellowship, working in the development and optoelectronic characterization of CIGS low cost based solar cells. In 2009, he joined NEXCIS, a spin-off created from IRDEP, to further pursue their training in photovoltaic technology. In 2010, he joined the Solar Energy Materials and SystemsGroup at the Catalonia Institute for Energy Research (IREC) under a Juan de la Cierva Fellowship first (2010-2011) and a Ramon y Cajal Fellowship afterwards (2012-2016), with the aim to develop new low cost materials and processes for thin film photovoltaic devices. In 2020 he joined the Polytechnic University of Catalonia (UPC) to continuous his scientific and professorhip career.
He holds five patents and has authored or co-authored more than 215 papers in recognized international journals, including: Energy and Environmental Science, Advanced Materials, Adv. Energy Materials, Journal of the American Chemical Society, Chemistry of Materials, Progress in Photovoltaics: Research and Applications, Solar Energy Materials and Solar Cells, NanoEnergy, J. Mater. Chem. A, J. Phys. Chem. C, etc. He has more than 350 contributions to the most important Congresses in Physics, Chemistry and Materials, and more than 35 invited talks around the world. He has been involved in more than 25 European and Spanish Projects (Scalenano, Inducis, Pvicokest, KestPV, Larcis, etc.), and he was the Coordinator of the ITN Marie Curie network Kestcell (www.kestcells.eu), the research and innovation project STARCELL (www.starcell.eu), and the RISE project INFINITE-CELL (www.infinite-cell.eu), three of the most important initiatives in Europe for the development of Kesterites. In 2019 he was granted with an ERC-Consolidator Grant by the European Research Council (SENSATE, 866018, 2020-2025), for the development of low dimensional materials for solar harvesting applications to be developed at UPC. Currently he is also the scientific coordinator of the European project SUSTOM-ART (952982), for the industrialization of kesterite for BIPV/PIPV applications.
He is frequently chairman and invited speakers in the most relevant Conferences in Photovoltaic (E-MRS, MRS, IEEE-PVSC, EUPVSEC, European Kesterite Workshop, etc.). He has supervised 11 PhD Thesis and is currently supervising 5 more. He has an h factor of 38 and more than 5000 citations. In 2020 he has been awarded with the ASEVA-Toyota Award for his contribution to the development of sustainable photovoltaic technologies using vacuum techniques (https://aseva.es/resolucion-de-los-primeros-premios-nacionales-de-ciencia-y-tecnologia-de-vacio-aseva-toyota/).
Quasi one-dimensional (Q1-D) structures based on van der Waals materials such as (Sb,Bi)2(S,Se)3 have demonstrated impressive progresses over the past 5 years. These types of materials typically form nano/micro-ribbons with strong covalent bonding in one direction, and at the same time, weak van der Waals bonding in the others two. This fingerprint feature confers unusual optoelectronic properties to these materials when properly oriented, and has allowed fast progresses in terms of conversion efficiency of solar cell devices, with a current record exceeding 10%. Beyond Sb and Bi-chalcogenide compounds, there is a limited body of knowledge on other Q1-D systems such as mixed chalco-halide van der Waals compounds, that combine chalcogens (S,Se) with halogens (Br,I) in the same structure. In fact, recent theoretical studies suggests that mixed chalco-halides can simultaneously achieve the robustness and stability of chalcogenide materials, along with the excellent optical and electrical properties of halides, and in particular showing high defect tolerance similarly to halide-perovskites.
This presentation will introduce a novel family of materials based on mixed Sb and Bi chalco-halides [(Sb,Bi)(S,Se)(Br,I)]. The first part will be devoted to reviewing the most relevant results reported so far for the few examples available in the literature, that have reached encouraging conversion efficiencies around 5% within a very limited timeframe, with a meso-porous solar cell configuration. In addition, the fundamental properties of these compounds obtained by DFT modelling will be discussed. The calculation of the bandgaps, band structures, optical and transport properties will be presented, discussing the trends of these properties depending on the chalcogen or the halogen introduced into the structure. The results will show that all these compounds can be relevant for different thin film photovoltaic applications, with bandgaps ranging from 1.6 eV for SbSeBr up to 2.3 eV for BiSBr.
In the second part of the presentation, the complexity of the synthesis of mixed chalco-halides will be discussed, and a new methodology for their synthesis, developed by the authors of this work, and based on the combination of co-evaporation of chalcogenides and high-pressure reactive annealing under halogen atmosphere, will be presented. This methodology allowed for the first time the demonstration of working solar cells with absorbers produced by vapor deposition techniques with these compounds. The tunability of the Q1-D structures by changing the synthesis temperature and pressure will be demonstrated. It will be shown that the synthesis of bromine-based compounds requires higher temperatures and pressures than iodine ones, due to the thermodynamics associated with the incorporation of Br and I in the chalcogenide phase. A detailed analysis of the mechanisms behind the formation of the different compounds will be presented by implementing interrupted-synthesis processes, and supported by the combination of thermo-gravimetric analysis, differential scanning calorimetry, and advanced structural/compositional characterizations.
The last part of the presentation will be devoted to the challenges and possible technological solutions for the fabrication of planar-heterojunction solar cell devices with these innovative photovoltaic absorbers. The use of different electrons and holes transport layers will be discussed, demonstrating for the first-time conversion efficiencies with these architectures between 1-5% and with very encouraging Voc values above 600 mV in some cases. Finally, the perspective of these materials and the possible advantages with respect to current chalcogenide and halide technologies, will be presented and discussed.
1.2-I1
Although lead-halide perovskites have demonstrated astonishing increases in efficiency in solar cells, they contain lead in a soluble form and are processed with toxic solvents. Bismuth-based compounds have gained increasing attention as a non-toxic alternative because bismuth is a heavy-metal cation that can replicate many of the features of lead thought to enable defect-tolerance. Defect-tolerance is the ability of materials to achieve long charge-carrier transport lengths despite the presence of defects, potentially enabling efficient performance to be achieved in materials made by low-cost methods. This talk explores our recent work on two such materials, bismuth oxyiodide (BiOI) and sodium bismuth sulfide (NaBiS2). We demonstrate BiOI to be tolerant towards its most common point defects, and develop an all-inorganic device structure to achieve external quantum efficiencies up to 80% in photovoltaics [1]. Next, we develop this into a photocathode, making use of a graphite epoxy to further protect the devices from degradation. In doing so, we show that we can improve the stability of the devices from a couple of minutes (BiOI in direct contact with the electrolyte) to a couple of months of operation for water splitting [2]. We further show that integrating these BiOI photocathodes in tandem with BiVO4 photoanodes leads to fully oxide-based photoelectrochemical tandems capable of self-driven water splitting and syngas production [2]. Finally, we cover our recent work on NaBiS2, demonstrating this material to be stable in air for at least 11 months, and a stronger light harvester than established thin-film absorbers, such that a 30 nm thick film has a spectroscopic limited maximum efficiency of 26% [3]. Through detailed computations, we rationalise the cause behind this strong light absorption as resulting from cation disorder. We further show that cation disorder has significant consequences on charge-carrier transport by enabling the formation of small hole polarons.
1.2-I2
Fatwa Abdi is an Associate Professor at the School of Energy and Environment, City University of Hong Kong. Until July 2023, he was a group leader and the deputy head of the Institute for Solar Fuels, Helmholtz-Zentrum Berlin (HZB). He obtained his PhD (cum laude) in Chemical Engineering from TU Delft, the Netherlands, in 2013. He was the recipient of the Martinus van Marum prize from the Royal Dutch Society of Sciences and Humanities. His research focusses on the development of novel (photo)electrode materials as well as engineering and scale-up of devices for solar fuels and chemicals conversion.
The generation of chemical fuels (e.g., hydrogen, hydrocarbons) using sunlight offers a carbon-neutral route to meet the ever-increasing world’s energy needs. In recent years, significant progress has been reported, especially in solar water splitting. Compared to indirectly coupling photovoltaic cells with water electrolyzers, direct photoelectrochemical (PEC) water splitting offers potential advantages in terms of system and thermal integration. Nevertheless, the demonstrated solar-to-hydrogen (STH) efficiencies are still insufficient to obtain technological competitiveness. Efforts in increasing efficiency are, unfortunately, faced with a classic material science dilemma: high STH efficiencies (~20%) are achieved in devices employing high quality semiconductors that are cost-prohibitive,[1-2] while devices based on low-cost metal oxide semiconductors have only demonstrated modest STH efficiencies (<10%).[3-4] These metal oxide-based PEC water splitting devices typically utilize BiVO4 as the absorber, and further increase of the efficiency is hindered by the optical absorption limit of BiVO4 that has a ~2.4 eV bandgap. Novel metal oxide semiconductors with a bandgap of 1.7-1.9 eV, which are stable and efficient, are therefore desired. In this talk, our recent efforts in developing two complex metal oxides will be presented. The first metal oxide is alpha-SnWO4 photoanode with a bandgap of ~1.9 eV. We deposited alpha-SnWO4 thin films using pulsed laser deposition and investigated the pH stability window using a combination of inductively coupled plasma optical emission spectroscopy, x-ray photoelectron spectroscopy and in situ spectro(photo)electrochemistry measurements.[5] We found that photocorrosion occurs in alkaline pH electrolytes, while at acidic to neutral pH, a self-passivating oxide layer is formed on the surface of alpha-SnWO4 that blocks the transfer of photogenerated holes to the electrolyte. The latter could be overcome by depositing NiOx as a protection layer, but it is also accompanied by the reduction of the photovoltage. A thorough interface investigation using synchrotron-based hard x-ray photoelectron spectroscopy (HAXPES) and surface photovoltage spectroscopy (SPV) reveals the presence of an interfacial SnO2 layer,[6] which must be avoided to overcome the photovoltage limitation. The second metal oxide is modified BaSnO3. While pristine BaSnO3 has a large bandgap (> 3 eV), the introduction of defects, such as oxygen vacancy and sulfur substitution, extends the optical absorption onset to ~1.7 eV and increases the charge carrier mobility and photocurrent by a factor of ~20.[7] By combining analyses from photoluminescence spectroscopy, modulated SPV, and wavelength-dependent time-resolved microwave conductivity, these defects can be attributed to the generation of delocalized mid-bandgap states through which charge transport via a long-lived carrier-hopping mechanism is enabled. Further outlook on these two promising complex metal oxides, alpha-SnWO4 and modified BaSnO3, will be discussed.
1.2-I3
Electron transport layers (ETLs) have been used in photovoltaics (PV) cells particularly for silicon PV for efficient charge collection resulting in significantly improving its power conversion efficiency. However, when employing ETLs for Si photoelectrochemical cells particularly for CO2 reduction (CO2R) there are additional constraints to consider. First the ETLs must be stable to the reduction reaction (for example CO2R) under operation. Second, the ETLs when interfaced with a catalyst layer needs to be selective to the reduction reaction of interest (CO2R) and inert to other reactions (like hydrogen evolution reaction (HER)). Design and exploration of new ETLs for CO2R photocathodes must take into account these considerations and in this work, we show that TaOx satisfies all of the above criteria. TaOx films were synthesized by both pulsed laser deposition (PLD) and RF sputtering. In both cases, careful control of the oxygen partial pressure during growth was required to produce ETLs with acceptable electron conductivity. p-Si/TaOx photocathodes were interfaced with ca. 10 nm of a CO2R catalyst: Cu or Ag. Under front illumination with simulated AM 1.5G in CO2-saturated bicarbonate buffer, we observed, for both metals, faradaic efficiencies for CO2R products of ~50% and ~ 30% for PLD TaOx and RF sputtered TaOx, respectively, at photocurrent densities up to 8 mA cm-2. p-Si/TiO2/Cu photocathodes were also evaluated but produced mostly H2 (> 97%) due to reduction of the TiO2 to Ti metal under CO2R conditions. In contrast, a dual ETL photocathode (p-Si/TiO2/TaOx/Cu) was selective for CO2R, which suggests a strategy for separately optimizing selective charge collection and the stability of the ETL/water interface. The RF sputtered TaOx ETL based Si photocathode was also found to be stable for CO2R for about 240 mins, with metal crossover from the counter electrode being a limiting factor. Our techno-economic analysis shows that the reported system, if scaled, could allow for an economically viable production of feedstocks for chemical synthesis under the adoption of specific CO2 credit schemes, thus become a significant component to carbon-neutral manufacturing. Further evidence of efficient metal oxide catalyst support for CO2R is unravelled by ambient pressure x-ray photoelectron spectroscopy.
1.3-I1
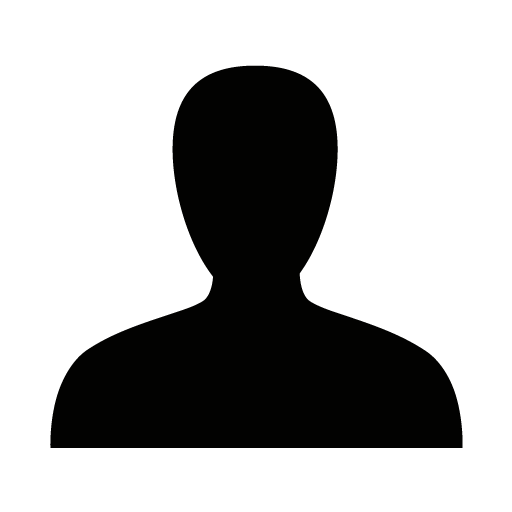
Cu-Ga-Se chalcopyrite structures with a band gap of 1.68 eV (CuGaSe2) to 1.85 eV (CuGa3Se5) are considered to be promising materials to be used as the photocathode in a tandem photoelectrochemical (PEC) water splitting configuration. Therefore, we prepared polycrystalline Cu-Ga-Se films with different compositions ranging from Cu-poor CuGaSe2 (Cu/Ga = 0.85) to extremely Cu-poor CuGa3Se5 (Cu/Ga = 0.33) and investigated the effect of the Cu/Ga ratio on the crystal structure, morphology and PEC performance of the films. Without any surface treatment or formation of a p-n junction, we report remarkable saturated photocurrent densities of -19.0 and -12.1 mA/cm2 (measured at -0.40 V vs. RHE) for our films with Cu/Ga = 0.85 and Cu/Ga = 0.33, respectively, using an LED-based solar simulator. These outstanding results cover 86% and 68% of the maximum theoretical photocurrents for materials with a band gap of 1.68 eV and 1.85 eV, respectively. Furthermore, we were able to obtain and validate a realistic equivalent circuit via potentiodynamic electrochemical impedance spectroscopy (P-EIS), which among others confirmed that the obtained difference in onset potential (270 mV) between these two films was in agreement with the obtained difference in flat-band potential (290 mV).
1.3-O1
Our group focus on physical chemistry, materials science, and the application of materials for energy production, studying the synthesis-structure-property relationship of functional materials for energy production. We emphasize developing novel syntheses for advanced materials and devices for solar energy into useful forms of sustainable energy & fuels. Our research lies at the intersection between innovative approaches, fundamental studies, and applying advanced materials for solar energy conversion.
A unique approach to exploring non-equilibrium synthesis parameter spaces of oxide thin film photoelectrodes will be presented, broadening the pathway toward discovering new chemical spaces inaccessible through conventional solid-state reactions.
There is an increasingly urgent need for disruptive and innovative materials that satisfy the chemical and physical requirements to reduce global warming through sustainable development. Fortunately, only a fraction of the possible combinations were studied, making it likely that the best materials are still awaiting discovery. Unfortunately, designing controlled synthesis routes of single-phase oxides with low defects concentration will become more difficult as the number of elements increases;1,2 and there are currently no robust and proven strategies for identifying promising multi-elemental systems.
These challenges demand an initial focus on synthesis parameters of novel non-equilibrium synthesis approaches rather than chemical composition parameters by high-throughput combinatorial investigations of synthesis-parameter spaces, opening new avenues for stabilizing metastable materials, discovering new chemical spaces, and obtaining light-absorbers with enhanced properties to study their physical working mechanisms in photoelectrochemical energy conversion.
Using two non-equilibrium synthesis tools: pulsed laser deposition and flash photonic sintering, can form gradients in synthesis parameters without modifying composition parameters, which enables reproducible, high-throughput combinatorial synthesis over large-area substrates and high-resolution observation and analysis. Even minor changes in synthesis significantly impact material properties, physical working mechanisms, and performances, demonstrated by the relationship between synthesis conditions, crystal structures of α-SnWO4,3 and properties over a range of thicknesses of CuBi2O4,1 both emerging light-absorbers for photoelectrochemical water-splitting used as model multinary oxides. Our approach addresses an immediate need by focusing on novel non-equilibrium synthesis approaches of disruptive and innovative materials that meet the chemical and physical requirements for reducing global warming through sustainable development.
1.3-O2
Solar driven photoelectrochemical (PEC) reduction to produce chemical fuel is a promising strategy for solar energy harvesting, which could address the intermittent and dispersed supply of solar energy and provide a high-density solar energy storage method in the form of chemical bonds. PEC CO2 reduction, converting CO2 into value-added chemical, has attracted significant attention because of its potential to capture and reduce the massive emission of CO2. Another desirable PEC application is NH3 synthesis from NOx reduction reaction (NOxRR), which provides not only a promising alternative to the conventional energy-intensive NH3 production process, but a sustainable solution for balancing the global nitrogen cycle by restoring ammonia from wastewater.
Kesterite Cu2ZnSnS4 (CZTS)-based photocathodes have attracted increasing research interest due to its excellent light harvesting capability, earth-abundance, and environmental-friendliness. After constructing p–n junction with CdS buffer layer, CZTS photocathode exhibits robust charge separation efficiency, which can enable an energetic photoinduced reaction driving force to power the PEC reactions. This work will present the strategies we applied to achieve high PEC reduction performance and controllable PEC reduction selectivity in both CO2 and NOx reduction.
In CO2 reduction, by introducing heat treatment (HT) strategy on CZTS/CdS photocathodes, interface charge transfer optimization and surficial S vacancy engineering have been simultaneously realized (Figure 1). HT improves the CZTS/CdS heterojunction interface by introducing elemental inter-diffusion between Cd in CdS and Cu/Zn in CZTS, leading to a more favorable p-n junction with enlarged built-in potential, prolonged carrier lifetime and suppressed charge recombination.
In NOx reduction for NH3 production, the uniquely designed CZTS photocathode by loading defect engineered TiOx cocatalyst enables selective NH3 production from NOxRR, yielding up to 89.1% Faradaic efficiency (FE) with a remarkable positive onset potential (Figure 2). By tailoring the amount of surface defective Ti3+ species, the adsorption of reactant NO3− and NO2 intermediate is significantly promoted while the full coverage of TiOx also suppresses NO2− liberation as a by-product, contributing to high NH3 selectivity.
1.3-O3
Kesterite materials are promising alternatives to conventional Cu(In,Ga)Se2 and CdTe compounds for thin-film photovoltaics (PV) based on abundant and non-toxic elements. However, the efficiency of kesterite solar cells is still lagging due to large open-circuit voltage (Voc) losses that are mainly ascribed to electrostatic fluctuations and high density of point defects, related to their typical off-stoichiometric composition. Counteracting such losses can be achieved to a certain extent by substituting Ge to Sn in Cu2Zn(Sn1-x,Gex)Se4 (CZTGSe) compounds, while also allowing Sn-Ge bandgap tuning through the x=Ge/(Ge+Sn) ratio, which is the focus of this work.
We implement a sequential process involving a physically evaporated Ge/Zn/Sn/Cu/Sn/Zn/Ge metallic precursor stack. This stack is pre-annealed in N2 to favour crystallization, and afterwards annealed with an optimized recipe in a Se-containing environment to activate and enhance grain growth. Polycrystalline CZTGSe absorbers with the expected composition, low surface roughness and close to micron-size crystalline grains are obtained, according to SEM-EDS and Raman data. TOF-SIMS measurements calibrated by EDX results demonstrate that a Sn-Ge back gradient is reached, with an average Ge/(Ge+Sn) ratio around 40%, corresponding to about 60% at the back and 20% at the front. This bandgap gradient likely has its minimum bandgap at the top surface, estimated to be 1.04eV from the PL spectrum. So as to attempt solving the TRPL-measured limited carrier lifetime, front surface sulfurization is investigated but still requires optimization.
Using these CZTGSe absorbers, complete solar cells are processed and their IV characteristics are measured under AM1.5G illumination, leading to values of 487 mV and 28.1 mA/cm² for Voc and the short-circuit current density (Jsc), respectively. These encouraging values beyond 60% of the Shockley-Queisser limits are possibly the result of enhanced carrier collection by the Sn-Ge bandgap gradient, supporting the potential of Ge inclusion to boost the efficiency of kesterite solar cells. However, these devices are affected by a large fill factor deficit, which cannot be counteracted without a deeper understanding of the mainly responsible loss mechanisms.
This work was recently submitted for peer-review as a Feature Paper in the “Materials for Energy Applications 2022-2023” Special Issue of the Crystals journal.
2.1-I1
G.-M. Rignanese is Professor at the Ecole Polytechnique de Louvain (EPL) and Research Director at the F.R.S.-FNRS. He received his Engineering degree from the Université catholique de Louvain in 1994 and Ph.D. in Applied Sciences from the Université catholique de Louvain in 1998.
During his Ph.D., he also worked as a Software Development Consultant for the PATP (Parallel Application Technology Project), collaboration between CRAY RESEARCH and Ecole Polytechnique Fédérale de Lausanne (EPFL) in the group of Prof. Roberto Car. He carried his postdoctoral research at the University of California at Berkeley in the group of Prof. Steven Louie. In 2003, he obtained a permanent position at the Université catholique de Louvain. In 2022, he was appointed as Adjunct Professor at the Northwestern Polytechnical University in Xi'an (China).
In 2019, he was named APS Fellow for original efforts developing free license software in the field of electronic structure calculations, and high-throughput calculations in a broad range of materials types.
Identifying new photovoltaic absorbers purely experimentally is a very time-consuming and expensive process involving complex synthesis and characterization. Recently, the progress in first-principles simulation codes and supercomputing capabilities have given birth to the so-called high-throughput ab initio approach, thus allowing for the identification of many new compounds.
In this talk, I will present the materials screening that was performed including for the first time an estimate of carrier lifetime based on defect computations. Among 7000 known copper-based compounds, a few unsuspected solar absorber
candidates have been identified which combine a potential for high efficiency and earth-abundance. Further analysis of the data highlights two challenges in discovering Cu-based solar absorbers: deep anti-site defects lowering the carrier lifetime and low formation-energy copper vacancies leading to metallic behavior. The alkali copper phosphides and pnictides offer unique chemistries that tackle these two issues.
The high-throughput ab initio approach offers a new avenue to search rapidly for new solar absorbers and
highlights new promising chemistries especially alkali-based phosphides to be targeted by future solar cell research.
2.1-I2
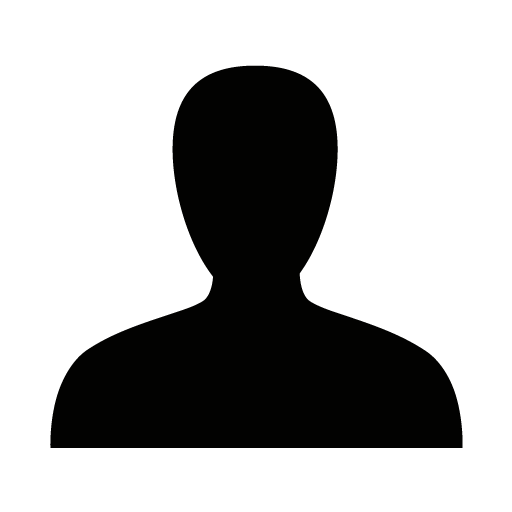
Lone pair containing solar absorbers are of interest due to their beneficial charge and defect screening properties which are similar to those of the exceptionally efficient lead halide hybrid perovskites. Both bismuth and antimony absorbers are composed of earth-abundant materials and experience the same beneficial relativistic effects that act to increase the width of the conduction band. Unlike lead, however, bismuth and antimony are non-toxic and non-bioaccumulating, meaning the impact of environmental contamination is greatly reduced. In this talk, we outline general design rules for emerging earth-abundant chalcogenide photovoltaics. We demonstrate how these rules apply to the most promising PV absorbers. Using a data driven approach, we identify a new class of layered lone-pair materials that have not yet been tested as photovoltaics but which possess promising optoelectronic and charge transport properties. We highlight the potential of this materials class as PV absorbers using relativistic hybrid density functional theory and simulations of device performance.
2.1-I3
Photocatalytic technologies are growing at higher technology readiness levels to mediate the reduction of CO2 to generate alternatives for fossil fuels with the assistance of outdoor solar light. Good designed photocatalytic structures have a great potential in splitting of water for the production of hydrogen and reduction of carbon dioxide to generate hydrocarbon fuels. Among all the photocatalytic structures that can mediate this process the Z-scheme equivalent to photosynthesis looks the most promising. Such a structure can optimize the photocatalytic activity due to the effectiveness of separating the electron hole pair compared to a single photocatalyst. The efficiency of the generation of carriers by this dual n-type photocatalysts as in the Z-scheme that combine into a heterojunction, supporting the oxidation and reduction reaction are studied. In this work a theory is presented for the electronic characteristics of a direct Z-scheme semiconductor heterojunction under solar irradiation. Based on the idealization of this theory we predicted the maximal efficiency for the conversion of solar energy to chemical energy of such a direct Z-scheme to be 11.4 %.
2.2-I1
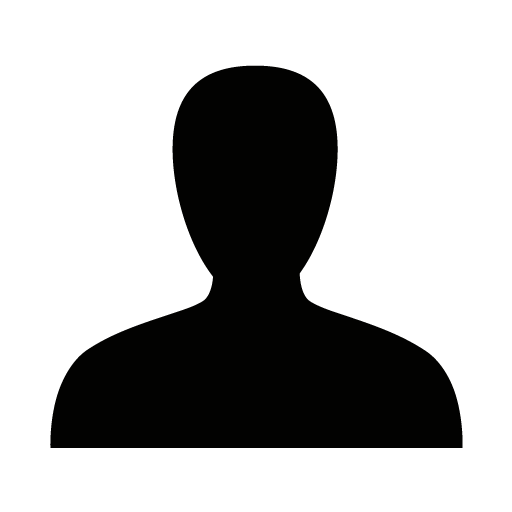
A tandem photoelectrochemical (PEC) water-splitting device for solar hydrogen production consists of two light absorbers with different bandgaps. It is important to enhance the performance of both cells to achieve high solar-to-hydrogen (STH) conversion efficiency. In this regard, silicon photoelectrodes have been widely investigated because of their bandgap (1.12 eV), which is suitable for the low bandgap bottom cell of a tandem device. Herein, we apply a tunnel oxide passivated contact (TOPCon) on the front and back sides of a Si wafer to prepare a TOPCon Si PEC device. Photocathodes and photoanodes based on TOPCon Si are demonstrated over a broad pH range (0–14), and they produce photovoltages of 640–650 mV under 1 sun illumination, which are the highest values obtained from crystalline Si photoelectrodes. TOPCon Si demonstrates excellent thermal stability, enduring a high processing temperature of up to 600 °C for 1 h in air. These advantages of TOPCon Si provide high efficiency and great design flexibility for monolithic tandem cells. TOPCon Si was coupled with BiVO4, a large bandgap top cell consisting of earth-abundant and non-toxic elements, in monolithic construction, i.e., a wireless PEC tandem cell. A photovoltage exceeding 1.7 V was produced by the BiVO4/Si with a successful demonstration of unbiased PEC water splitting. I will discuss details of the challenges and our solutions in the monolithic integration of BiVO4 and Si.
2.2-I2
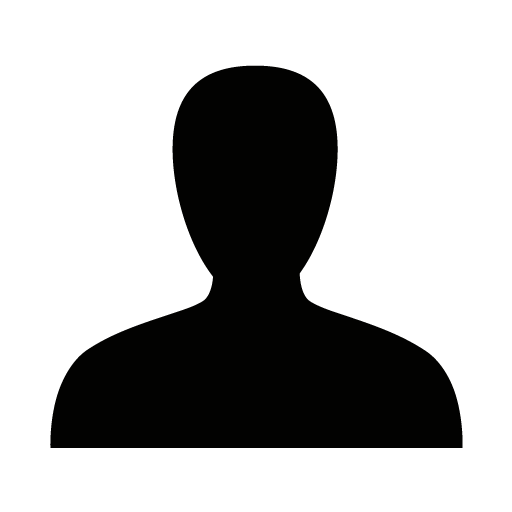
Thomas Hannappel is W3 full professor (physics) at Ilmenau University of Technology, Germany, department ‘Photovoltaics’, since 2011. Before, he was provisional head of the Institute “Materials for Photovoltaics” at the Helmholtz-Zentrum Berlin and lecturer at the Free University Berlin, where he received his state doctorate in 2005. At Technical University Berlin he obtained his PhD in Physics with studies on ultrafast dynamics of photo-induced charge carrier separation in dye solar cells, he performed at Fritz-Haber-Institute Berlin of the Max-Planck-Society. In 2003/04 he conducted research on silicon/III-V-interfaces at National Renewable Energy Laboratory, Colorado. His current investigations are focused on high-performance solar cells and critical interfaces and he is a key player in the fields solar energy conversion and reactions of critical semiconductor interfaces including silicon/ and germanium/III-V-interfaces, and nano- and quantum-structures.
Epitaxial semiconductors involving III-V compounds, germanium, and silicon provide the highest performance levels in optoelectronic applications such as in solar cells and photoelectrochemical cells [1-3]. However, highest performance in solar energy conversion can only be achieved, when using optimum absorber layers and sophisticated contact formation for electronic and chemical passivation, i.e. for the protection of the solid-liquid interface against corrosion as well as preventing interfacial non-radiative recombination. In order to address the surface and interface properties of III-V semiconductor layer structures in relation to their performance, we present the synthesis, theoretical modelling and properties of critical and well-defined interfaces such as GaInP/AlInP. Here, lattice matched n-type AlInP(100) charge selective contacts are commonly grown on n-p GaInP(100) top absorbers in highest-efficiency III–V multijunction solar or photoelectrochemical cells, where the cell performance can be greatly limited by missing electron selectivity and detrimental valance band offsets. Hence, understanding of the atomic and electronic properties of the GaInP/AlInP heterointerface, for instance, is crucial for the reduction of photocurrent losses in III–V multijunction devices [4]. We discuss the essential considerations on the properties of critical interfaces in relation to photoelectrochemical cells from a conceptual and from a theoretical modeling point of view assuming mostly idealized surface conditions. We also address latest progress on the important III-V/Si interface, modifications by fine-tuning of the preparation and describe experimental model experiments on the surface reactivity of III-phosphide surfaces to H2O exposure. These different surface science approaches are then related to photoelectrochemical cells for H2 evolution and CO2 reduction using different III-V based tandem cells and providing highest conversion yields.
References
[1] M.M. May, H.-J. Lewerenz, D. Lackner, F. Dimroth, T. Hannappel, Nature Communications 6 (2015) 8256;
[2] W.-H. Cheng, M.H. Richter, M. M. May, J. Ohlmann, D. Lackner, F. Dimroth, T. Hannappel, H.A. Atwater, H.-J. Lewerenz; Monolithic Photoelectrochemical Device for Direct Water Splitting with 19% Efficiency, ACS Energy Letters 3 (2018) 1795
[3] W.H. Cheng, M.H. Richter, R. Müller, M. Kelzenberg, S. Yalamanchili, P.R. Jahelka, A.N. Perry, P.C. Wu, R. Saive, F. Dimroth, B.S. Brunschwig, T. Hannappel, H.A. Atwater, Advanced Energy Materials 12 (36) (2022) 2201062
[4] O. Romanyuk, A. Paszuk, I. Gordeev, R.G. Wilks, S. Ueda, C. Hartmann, R. Felix, M. Bär, C. Schlueter, A. Gloskovskii, I. Bartoš, M. Nandy, J. Houdková, P. Jiříček, W. Jaegermann, J.P. Hofmann, T. Hannappel;
Applied Surface Science 605 (2022) 154630
2.2-I3
Green hydrogen will play an important role in the energy transition as a renewable energy vector for long-duration energy storage and as feedstock chemical for the industry. To reduce the price below 1.5 €/ kg H2, competitive to production from fossil fuels, PV-powered efficient anion exchange membrane (AEM) water electrolysis is a promising combination. Practical implementation of such a PV-EC technology requires standard area-sized solar cells and electrolyzers operated at large current densities (>400mA/cm2). Nonetheless, state-of-the-art research often employs <10cm2 PV devices and electrolyzers operated at current densities <10mA/cm2.
In this work a commercially relevant PV-EC system that couples shingled standard silicon technology with AEM electrolysis based on high surface area nickel nanomesh electrodes is presented. AEM water electrolysis combines the advantage of using low-cost materials such as nickel electrodes with the high operation current densities shown in proton-exchange membrane (PEM) water electrolysis. The nanomesh electrodes developed in our group consist of a regular 3D-network of interconnected nanowires with a large surface area of 26m2/cm3 and a porosity of 70%. [1] These exceptional properties result in >100x current enhancement for the hydrogen evolution reaction compared to planar nickel electrodes due to the accessibility of a high number of active surface sites and outperforms commercial nickel foams. The photovoltaic module and the electrolyzer were wire-connected over a custom-build monitoring unit that in-situ measures the amount of produced H2, operating current & voltage of the system over the entire operation time. We demonstrate stable solar-to-hydrogen efficiency (ηSTH) of 10% at highest electrolyzer current densities seen in literature (58mA/cm2) over >20h.
Based on the measured PV-EC system data best practices to accurately determine the ηSTH for PV-powered and (photo)electrocatalytic water splitting devices and the validation of this benchmark against important component parameters for practical technology implementation are discussed.
2.3-I1
Barium zirconium sulfide (BaZrS3) is an earth-abundant and environmentally friendly chalcogenide perovskite with promising properties for various energy conversion applications. Recently, sulfurization of oxide precursors has been suggested as a viable solution for effective synthesis, especially from the perspective of circumventing the difficulty of handling alkali earth metals. In this work, we explore in detail the synthesis of BaZrS3 from Ba-Zr-O oxide precursor films sulfurized at temperatures ranging from 700 ∘C to 1000 ∘C. We propose a formation mechanism of BaZrS3 based on a two-step reaction involving an intermediate amorphization step of the BaZrO3 crystalline phase. We show how the diffusion of sulfur (S) species in the film is the rate-limiting step of this reaction. The processing temperature plays a key role in determining the total fraction of conversion from oxide to sulfide phase at a constant flow rate of the sulfur-containing H2S gas used as a reactant. Finally, we observe the formation of stoichiometric BaZrS3 (1:1:3), even under Zr-rich precursor conditions, with the formation of ZrO2 as a secondary phase. This marks BaZrS3 quite unique among the other types of chalcogenides, such as chalcopyrites and kesterites, which can instead accommodate quite a large range of non-stoichiometric compositions. This work opens up a pathway for further optimization of the BaZrS3 synthesis process, straightening the route towards future applications of this material.
2.3-I2
Julien Bachmann studied chemistry at the University of Lausanne, Switzerland, and graduated with a Diplôme de chimiste in 2001 (with Carlo Floriani). He then joined the Massachusetts Institute of Technology in the USA to obtain his Ph. D. in inorganic chemistry (with Dan Nocera, 2006). He moved with a Humboldt Fellowship to the Max Planck Institute of Microstructure Physics in Germany to learn the chemistry and physics of solids (with Ulrich Gösele) and worked as a postdoc at the University of Hamburg (with Kornelius Nielsch). He started as an Assistant Professor position (‘Juniorprofessur’, W1) in physics and chemistry in Hamburg in 2009 and was appointed as an Associate Professor (W2) of Inorganic Chemistry at the Friedrich Alexander University of Erlangen-Nürnberg in 2012. In 2017, he was promoted to the Full Professor (W3) status in the framework of the Cluster of Excellence ‘Engineering of Advanced Materials’, and he now leads the Chair for ‘Chemistry of Thin Film Materials’ at FAU.
Prof. Bachmann obtained several teaching prizes. He was awarded by the European Research Council an ERC Consolidator Grant (2015) and a Proof of Concept Grant (2022). He used to hold an adjunct position as a Full Professor at Saint Petersburg State University (2017 to February 2022) and was invited to the Danish Technical University as an Otto Mønsted Guest Professor (2021). He is also a co-founder and director of the company Atlant 3D Nanosystems.
Atomic layer deposition (ALD) and variants of the technique are ideally suited to the generation of ‘extremely thin absorber’ (ETA) solar cells, in which three distinct semiconductors are combined as electron transport layer (SnO2, TiO2, ZnO), light absorption layer (Sb2S3, Sb2Se3), and hole transport layer (V2O5 or spin-coated organics). We have demonstrated six main advantages.
(1) ALD can deliver a material quality significantly improved with respect to solution processing techniques, with crystals of 10 µm lateral size obtained in planar films of 50 nm thickness.
(2) It enables the experimentalist to vary the thickness of layers within the semiconductor stack systematically and identify the geometric parameters that optimize the overall energy conversion efficiency.
(3) It offers the opportunity to engineer interfaces with the use of interfacial layers providing chemical or physical functions (adhesion layers, tunnel barriers) with extreme thickness sensitivity, on the length scale of 0.5 to 1.5 nm.
(4) It provides the capability, unique among the deposition techniques from the gas phase, to deliver conformal coatings of non-planar substrates. Parallel arrays of cylindrical p-i-n heterojunctions in a coaxial geometry allow for decoupling the path lengths for light absorption and charge separation. Ordered monolayers of nanospheres can be exploited to scatter light of near-bandgap energy, enhancing the conversion of light incident in the red spectral range and/or under oblique angle.
(5) Our extension of ALD to the use of precursors dissolved in the liquid phase, instead of classically delivered from the gas phase (‘solution ALD’ or sALD) expands the range of materials accessible by ALD. It also provides additional experimental tools to adjust the deposit’s morphology, and it provides an inexpensive access to ALD.
(6) The recent invention of ‘atomic-layer additive manufacturing’ (ALAM) circumvents the limitations associated with traditional blanket layering methods. It opens the door to rapid prototyping approaches in the photovoltaics field that mirror the use of 3D printing in manufacturing.
In summary, each major material family exploited in photovoltaics has been associated with a certain set of processing techniques, and our research indicates that atomic-layer processing provides all ingredients required for the success of the group 5 chalcogenides and similar light absorbers.
2.3-I3
Diego Colombara is Associate Professor of Inorganic Chemistry at the University of Genova (Italy).
Educational background: Master studies in Italy and UK focused on solid state chemistry and metallurgy, with emphasis on phase equilibria, crystallography and alloys microstructure. Associate researcher at an SME (Fabbricazioni Nucleari Spa, Italy) dealing with semi-industrial scale synthesis of ceramic materials for molten carbonate fuel cells. Doctoral studies in the UK devoted to the synthesis and photoelectrochemical characterization of Cu-Sb, Cu-Bi, Cu-Zn-Sn and Sn chalcogenides for photovoltaic (PV) solar cell applications by electroplating and reactive annealing, as well as by chemical vapour transport, as part of the EPSRC-funded project SUPERGEN (2008-2012). The PhD was pursued at the University of Bath under the supervision of Prof. Laurie M. Peter, pioneer of semiconductor (photo)electrochemistry.
Professional experience before his faculty appointment: 5 years of postdoctoral research, teaching and supervision within the Physics department at the Unversity of Luxembourg (UniLu), including 3 years as researcher in FP7-funded project SCALENANO and 2 years as the Principal Investigator of GALDOCHS (a 453 kEUR research project funded by the FNR), where he discovered, studied and developed a novel methodology for extrinsic doping of PV semiconductors that made it to the news, by refuting a 20-years old assumption on CIGS PV technology. 2 years of Marie Curie cofund fellowship at INL (Portugal), where he is associated researcher, and he appears as the inventor of one patent on template-free microfabrication.
He is currently the coordinator of REMAP consortium (Reusable mask patterning, EIC Pathfinder Open grant no. 101046909; total budget: ca. 4 M€). https://re-map.eu/ https://cordis.europa.eu/project/id/101046909
The photovoltaic (PV) market has long been dominated by crystalline silicon-based technologies. Their success is rooted also in the laboratory, with record efficiencies ultimately approaching the theoretical limit for monocrystalline silicon [1]. Market competitors are Cu(In,Ga)(S,Se)2 [2,3,4] (CIGS aka chalcopyrite) and CdTe [5,6,7,8] thin films. Currently, the commercial attractiveness of CIGS is curtailed by the module efficiency gap and by the supply of critical raw materials, with respect to silicon.
Overcoming the Shockley-Queisser efficiency gap requires the pursuit of strategic approaches, leaving plenty of room for research at both industrial and laboratory scale. Likewise, the availability of critical raw materials may constrain the future deployment of CIGS at GW scales needed for the ecological transition [9]. The progress on both fronts poses compelling scientific challenges.
This contribution starts with outlining the large opportunities offered by a concerted effort on wide-gap chalcopyrite. It then reveals a novel strategy making use of ferrofluids to explore the large scale fabrication of microconcentrator solar cells, promising to reduce critical raw materials needs by one to two orders of magnitude. Here, insights are drawn from recent and older literature on narrow-gap chalcopyrite with the ambition to fully unlock the potential of the material [10–12] and afford efficient dual junction chalcopyrite PV devices through a paradigm shift in microfabrication [13].