1.1-I1
The toxicity and bioavailability of lead (Pb) in halide perovskites motivates the search of Pb-free perovskite-inspired materials (PIMs), which would replicate the great optoelectronic properties of the traditional Pb-based counterparts. PIMs employing pnictogen cations from Group VA of the periodic table (e.g., antimony (III) (Sb3+) and bismuth (III) (Bi3+)) have recently emerged as low-toxicity alternatives for Pb-based halide perovskites, with applications in photovoltaics and beyond [1]. In particular, Bi-based PIMs are interesting because can lead to shallow traps and high defect tolerance in some compositions, while being eco-friendly. This field of research is, however, still in its early infancy with a growing number of efforts to improve the device efficiencies and lifetimes, with the majority of the studies focusing on the shelf-life rather than the operational stability.
In this talk, I will summarize key recent examples of bismuth-based PIMs studied by us for a range of applications, from photovoltaics for outdoor and indoor, non-linear optics, photocatalysis. In particular, I will summarize our recent findings on CU2AgBiI6 PIM and the compositional engineering efforts to improve its morphology and charge carrier transport [2,3]. I will also highlight novel Bi-based compositions for solar cells and indoor photovoltaics.
1.1-O1
Solar cells are among the most developed technologies in the renewable energy sector, not only academically, but also commercially. In this context, thin-film photovoltaics (based on emerging absorbers like perovskites, Sb2Se3, CZTS etc.) provide an excellent opportunity to expand the reach of solar energy production, thanks to the promising efficiencies combined with light-weight and flexibility. However, for many of these emerging materials, moderate/high temperature annealing (150-300 °C) is required, and the necessary temperature often limits the usable substrates. Photonic curing (also known as flash annealing) could represent a way to overcome this limitation and really unlock the potential of thin film photovoltaics. In this technique, heat is created by the absorption of strong and ultrarapid light flashes that permits to reach very high temperature on the top layer while keeping cold the bottom of the substrate. While this technique has widely been used in silicon,[1] very few reports are present for these emerging materials (mainly on perovskites), [2-4], and fundamental investigation is still missing.
In this talk I will present the most recent results collected on the use of photonic curing on different thin film absorbers, analyzing the structural, morphological and optoelectronic properties and comparing it with traditional thermal annealing.
1.1-O2
Perovskite solar cells in which methylammonium lead iodide (MAPbI3) is used as a solar absorber material, have reached maturity in the last years owing to a concerted effort to optimize material synthesis, stability, and device architectures and performance. However, the halide perovskite family features thousands of stable members beyond MAPbI3, with broad compositional tunabilty and a wide range of optoelectronic properties. With this talk, I will provide an overview of our current understanding of the electronic and excited-state structure of several classes of perovskites and perovskite-like structures beyond ABX3 featuring double perovskites, extended perovskites, and chalcohalide materials. Our first-principles calculations based on the GW and Bethe-Salpeter Equation approaches, allow us to map the complex landscape of electronic properties and excitons, understand the impact of chemical and structural heterogeneity, dimensionality, and temperature effects, and provide chemically intuitive rules for when to trust canonical textbook models for excitons in these materials.
2.1-I1
Metal halide semiconductors have emerged as attractive materials for solar cells with power-conversion efficiencies now exceeding 26%, however, these record efficiencies have all relied on incorporation of lead as the metal. The search for less toxic ingredients has led to the emergence of a plethora of new bismuth-based semiconductors, including bismuth halides and chalcogenides. Power conversion efficiencies around 6% have been realised for such materials, triggering new research efforts to explore and eliminate current limitations to performance.
Here, we show that an ultrafast charge-carrier self-trapping process limits long-range charge-carrier transport in most bismuth-based semiconductors.[1-7] We have examined the evolution of photoexcited charge carriers in the double perovskite Cs2AgBiBr6 using a combination of temperature-dependent photoluminescence, absorption and optical pump−terahertz probe spectroscopy.[1] We observe rapid decays in terahertz photoconductivity transients that reveal an ultrafast, barrier-free localization of free carriers on the time scale of 1.0 ps to an intrinsic self-trapped small polaronic state. Alloying Cs2AgBiBr6 with Cs2AgSbBr6 on the trivalent metal site interestingly leads to significantly stronger self-localisation,[2] which we attribute to self-localised charge carriers probing the energetic landscape more locally thus turning an alloy’s low-energy sites (here, Sb sites) into traps, which dramatically deteriorates transport properties. We further demonstrate the novel lead-free semiconductor Cu2AgBiI6 which exhibits a low exciton binding energy of ~29 meV and a lower and direct band gap near 2.1 eV,[3,4] making it a significantly more attractive lead-free material for photovoltaic applications. However, charge carriers in Cu2AgBiI6 are found to exhibit similarly strong charge-lattice interactions[4,5]. Further work examining five compositions along the AgBiI4–CuI solid solution line (stoichiometry Cu4x(AgBi)1−xI4) shows that increased Cu+ content enhances the band curvature around the valence band maximum, resulting in lower charge-carrier effective masses, reduced exciton binding energies, and higher mobilities, as well as partly mitigating the extent of such ultrafast self-localisation.[5] Interestingly, we show that thin films of BiOI lack of such self-trapping, with good charge-carrier mobility maintained over longer time scales, reaching ∼3 cm2 V–1 s–1 at 295 K and increasing gradually to ∼13 cm2 V–1 s–1 at 5 K, indicative of prevailing bandlike transport.[6] Finally, we examine thin films of AgBiS2 nanocrystals as a function of Ag and Bi cation-ordering,[7] which is modified via thermal-annealing. We show that homogeneous cation disorder reduces charge-carrier localization, most likely because cation-disorder engineering flattens the disordered electronic landscape, removing tail states that would otherwise exacerbate Anderson localization of small polaronic states.[7]
Overall, self-trapping of charge carriers therefore emerges as a clear challenge for this class of materials. Our findings explore the parameter space governing such self-localization, highlighting the effects of local energetic disorder as an exacerbating factor that may pose new challenges to alloying strategies. In addition, or findings show that cation-disorder engineering may partly mitigate such effects through flattening of the local energy landscape.
2.1-O1
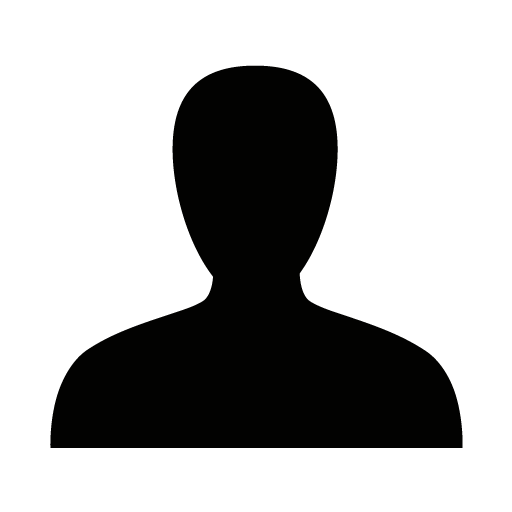
Following the emergence of lead halide perovskites (LHPs) as materials for efficient solar cells, research has progressed to explore stable, abundant and non-toxic alternatives. However, the performance of such lead-free perovskite-inspired materials (PIMs) still lags significantly behind that of their LHP counterparts. For bismuth (Bi)-based PIMs, one significant reason is a frequently observed ultrafast charge-carrier localization (or self-trapping). It has been suggested that self-trapping in Cs2AgBiBr6 originates from strong lattice deformation potential imparted by bismuth along with the presence of soft silver-halide bonds. Given that self-trapping places a fundamental limit on the performance of materials, investigating the influence of chemical composition on the charge-carrier dynamics is highly topical.
This work aims to understand whether the presence of Bi or Ag-halide bonds is in fact crucial to the emergence of self-trapped states. This was done through a dual study of charge-carrier dynamics in BiOI and (AgI)x(BiI3)y thin films. Our investigation reveals that despite possessing a low and strong electron-phonon coupling, there is no ultrafast localisation in BiOI. Instead, by unravelling the early and long-time charge-carrier dynamics in BiOI, we find that the material performance is limited by the presence of multi-phonon emission mediated non-radiative channels in the material.
On the other hand, ultrafast localisation is persistent across both mixed Ag-Bi iodides and BiI3. Thus, the presence of Bi and/or Ag-halide bonds alone cannot account for self-trapping in these materials. We find that a delicate interplay between chemical composition and crystal and band structures determine the charge-carrier dynamics in (AgI)x(BiI3)y. Overall, our dual study addresses crucial gaps in understanding the limitations of Bi-based PIMs and educate future material design.
2.1-O2
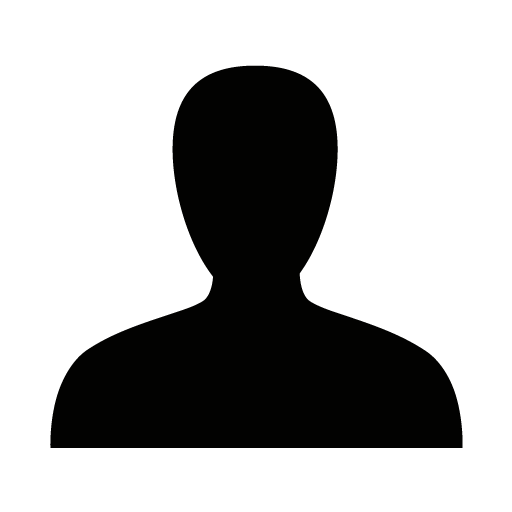
Halide double perovskites, such as Cs2AgBiX6 (X = Br, Cl), are emerging semiconductors for optoelectronic applications due to their lower toxicity.[1] Although exhibiting an indirect bandgap, colloidal Cs2AgBiCl6 nanocrystals (NCs) show bright dual-peak photoluminescence spectra.[2] However, the origin of both emission bands is still under debate. Here, we show that silver (Ag) plays a crucial role in both emissions of these double perovskite NCs. The trapping of holes in Ag vacancies leads to a spatial localization of the hole wave function on the scale of the lattice constant. This provides k-values for the hole wave function at all boundaries of the Brillouin zone, resulting in localized bound excitons with a red emission at 650 nm with an activated temperature dependence. The blue emission (425 nm) stems from the lecithin ligands of the NCs spectrally overlapping with the plasmon resonance of the surface-attached Ag nanoclusters. This work is of importance for the surface chemistry of Cs2AgBiCl6 double perovskites and their optoelectronic applications.
2.1-I2
Metal halide perovskites emerged as outstanding materials for optoelectronics due to their excellent optoelectronic properties, such as direct and tunable band gaps, large absorption cross sections and long lifetimes and diffusion paths of the charge carriers.[1] So far, the field has been dominated by lead-based perovskites, but issues concerning lead toxicity and stability have led to explore new possible perovskite candidates. In this context tin and double perovskites of different dimensionalities have attracted increasing interest.[2,3] The optoelectronic features, as well as the efficiencies of these materials in optoelectronic devices, is largely influenced by the nature of the chemical bond, the defects activity and the coupling of photogenerated charges with the lattice.
In this presentation a theoretical perspective is provided about the influence of chemical composition and dimensionality on the defect activity and the charge carrier photophysics in perovskites beyond the APbX3 composition, by focusing on less-toxic Sn and Bi/Ag double perovskites. The defect chemistry and photophysics of tin and double perovskites will be discussed, by keeping a parallelism between 3D and 2D, i.e. MASnI3 vs PEA2SnI4 and Cs2AgBiBr6 vs (BA)4AgBiBr8 phases, as test cases.[4,5] The analysis aims to highlight the effects of chemical composition and quantum confinement (QC) on several key properties of these materials. The origin of the self p-doping, strongly limiting the efficiency of tin perovskites, will be discussed, as well as computationally designed doping strategies aimed to reduce it. Hence, discussion will move to analyze the charge carrier photophysics in tin and double perovskites, by focusing on the processes possibly originating the sub-gap emissive features in these materials. Specifically, the competition between the exciton self-trapping and trapping/emission at defect centers vs the chemical composition will be discussed.
This contribution aims to provide a theoretical framework guiding experimentalists in the design of stable and efficient lead-free perovskite materials.
2.2-I1
In this talk I will discuss recent progress in our group on the development of a new class of thin film solar cells employing Silver Bismuth Sulfide as an emerging absorber for solution processed eco-friendly solar cells. I will first introduce our first report on AgBiS2 colloidal nanocrystal solar cells reporting power conversion efficiency of ~6% [1]. Then I will discuss on the opportunities of tuning the optical properties of this ternary compound via controlling cation disorder homogenization. We demonstrated that by homogenizing cation disorder in this compound we can drastically increase the absorption coefficient of this material as one with the high absorption amongst the semiconductors considered for photovoltaics. This taken together with advances on the device architecture led us to reach power conversion efficiencies of ~9% albeit using an absorber of only 35 nm [2]. In the last part of my talk I will describe our initial efforts on developing AgBiS2 nanocrystal inks and their use with environmentally friendly solvents that led us to achieve efficiencies in excess of 7% [3]. I will conclude my talk with our most recent findings towards an improved passivation strategy of AgBiS2 nanocrystal inks along with the formation of a double heterojunction in the device stack that led to power conversion efficiencies in excess of 10% with Voc of 0.5V, FF of 0.75 and Jsc of 28 mA/cm2.
[1] Solution-processed solar cells based on environmentally friendly AgBiS2 nanocrystals, M Bernechea, N Cates, G Xercavins, D So, A Stavrinadis, G Konstantatos, Nature Photonics 10 (8), 521-525, 2016
[2] Cation disorder engineering yields AgBiS2 nanocrystals with enhanced optical absorption for efficient ultrathin solar cells, Y Wang, SR Kavanagh, I Burgués-Ceballos, A Walsh, DO Scanlon, G Konstantatos, Nature Photonics 16 (3), 235-241, 2022
[3] Environmentally Friendly AgBiS2 Nanocrystal Inks for Efficient Solar Cells Employing Green Solvent Processing Y Wang, L Peng, Z Wang, G Konstantatos, Advanced Energy Materials 12 (21), 2200700, 2022
2.2-O1
Non-toxic, all-inorganic perovskite light absorbers received great attention in recent years due to their potential to replace lead-containing perovskite materials in photovoltaics. Regardless of the potential of lead-based halide perovskites, their application on large scale is challenging, mainly due to the toxicity of lead and stability issues. Lead-free absorbers, such as the double perovskite Cs2AgBiBr6 as a prominent representative, offer high stability and auspicious optoelectronic properties. Cs2AgBiBr6 has been studied intensively in the past few years. Various researchers pre-synthesize this absorber as powder by a synthesis method first suggested by Slavney et al. in 2016 [1] and the resulting absorber powder is further dissolved to render a thin film deposition. Although this method is well reproducible, it is only suitable for the lab-scale synthesis of Cs2AgBiBr6, with yields usually in the range of a few grams. Besides this rather small yield, the synthesis requires exceptional safety precautions.
Within this work, we present an alternative synthesis approach for Cs2AgBiBr6 powder, namely via spray-drying [2]. During spray-drying, the perovskite phase forms by in-situ crystallization upon drying of the atomized precursor solution. The product is compared to the conventionally synthesized absorber. XRD and Raman analyses confirm the product’s phase-purity, whereas UV-Vis spectroscopy confirms the desired bandgap. Furthermore, absorber films in single-junction perovskite solar cells are successfully produced from both absorber powders, clearly demonstrating the suitability of spray-drying as an alternative synthesis method.
Moreover, we investigated the Bi-substitution by Sb to reduce the perovskite’s original bandgap of ~2.2 eV achieving better suitability as top-cell absorber in perovskite-silicon tandem devices [3]. Spray-drying enabled a fast screening and identification of the substitution limit, and could overcome limitations of the conventional synthesis for the Sb-substituted Cs2AgBiBr6.
As a side notice, we can tell that we additionally investigated the lead-free perovskites Cs3Bi2Br9 and Cs3Bi2I9. Besides these, lead-based halide perovskites have been synthesized successfully via spray-drying, too. With the prospect of an industrial scale photovoltaic industry, the demand for high-quality perovskite materials will most likely increase in future. Therefore, spray-drying could pave the way for an easy adaptation to increasing production volumes of perovskite powders in general owing to its suitability as screening method and its capability of producing large amounts of powders.
2.2-I2
Thin-film PV is a key technology for low cost and low environmental impact solar energy conversion. Metal halide perovskites are leading among thin film PV technologies, but challenges remain regarding toxicity of Pb and stability. Motivated by the search for low cost, non-toxic and Earth abundant materials solutions for thin film PV, new inorganic semiconductors with complex compositions are being explored. One of the challenges with material discovery and new material compositions, is their fabrication in thin film form. Challenges such as volatility incompatibility or solvent incompatibility, hinders progress in either high quality material demonstration or functionality via device integration.
In this presentation, we will discuss how the combination of mechanochemical synthesis and pulsed laser deposition (PLD), allows the exploration of novel Earth abundant and non-toxic semiconductors materials. We will explore the development, growth and understanding of semiconductor materials such as Pb-free double perovskites (e.g. Cs2AgBiBr6), new photoactive light-absorbers such as Ag3SI and p-type transparent electrodes such as S-doped and Cs-doped CuI.
In summary, the presentation will highlight how controlled synthesis and material design can make a significant contribution to the emerging generation of efficient and sustainable thin film-based solar cell devices.
2.2-O2
In the field of perovskite solar cells, explorations of new lead-free all-inorganic perovskite materials are of great interest to address the instability and toxicity issues of lead-based hybrid perovskites. Recently, copper-antimony-based double perovskite materials have been reported with ideal band gaps, which possess great potential as absorbers for photovoltaic applications.1-2 Here, we synthesize Cs2CuSbCl6 double perovskite nanocrystals (DPNCs) at ambient conditions by a facile and fast synthesis method, namely, a modified ligand-assisted reprecipitation (LARP) method. We choose methanol as solvent for precursor salts as it is less toxic and easily removed in contrast to widely-used dimethylformamide. Our computational structure search shows that the Cs2CuSbCl6 structure containing alternating [CuCl6]5- and [SbCl6]3- octahedral units is a metastable phase that is 30 meV/atom higher in energy compared to the ground state structure with [CuCl3]2- and [SbCl6]3- polyhedra. However, this metastable Cs2CuSbCl6 double perovskite structure can be stabilized through the solution-based nanocrystal synthesis. Using an anion-exchange method, Cs2CuSbBr6 DPNCs are obtained for the first time, featuring a narrow band gap of 0.9 eV. Finally, taking advantage of the solution processability of DPNCs, smooth and dense Cs2CuSbCl6 and Cs2CuSbBr6 DPNC films are successfully fabricated.
2.3-O1
Ultrafast transient spectroscopy is a vital tool to investigate the photophysical processes taking place in energy harvesting materials. In particular, the findings provide valuable insights into the efficiency limiting processes in solar cells. The focus of this talk will be on solution-processed metal-halide perovskite solar cells as they have received immense attention in the field of photovoltaic research due to their outstanding power conversion efficiency, which has surpassed 26% in a relatively short time. Understanding carrier losses at metal halide perovskite/charge transport layer interfaces is a pre-requisite to bring the efficiency closer to the Shockley-Queisser limit. Interfacial recombination can to some extent be accessed through time resolved techniques, however identifying this is often a challenge due to the complexity associated to the interpretation and modelling of the extracted charge carrier transients.
approach is to utilize complementary transient spectroscopic techniques, namely transient absorption spectroscopy and transient photoluminescence spectroscopy, not only to unravel the processes limiting the solar cell’s short circuit density and the open circuit voltage but also to evaluate different charge recombination channels and extraction. Herein, we limit our focus to examine half-stacks comprised of the photoactive layer and the respective hole transport layers. Several different hole transport materials (PTAA, NiOx,,and 4PACz) are adjacent to the photoactive layer with a bandgap of 1.53 eV. We report on challenges faced during performing complementary spectroscopic techniques and interpretation of the extracted transients. Specifically, differentiation between photophysical processes such as charge extraction and interfacial charge recombination.
2.3-O2
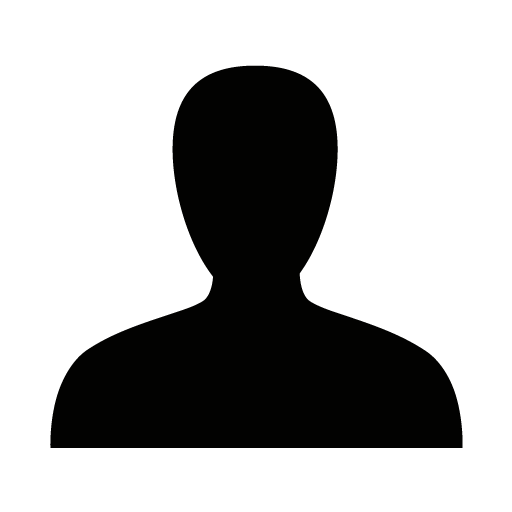
BiOI is a nontoxic, stable and polar semiconductor, which shows high conversion efficiencies in photocatalytic water splitting. This is due to an ultrafast and effective charge separation also launching coherent phonons after short laser pulse excitation [1]. The coupling of electronic excitations and phonons should also manifest itself in polaronic effects. To gain unambiguous experimental evidence for polaronic effects we have carried out time-resolved photoemission electron microscopy (TR-PEEM) experiments. This technique offers the unique possibility to learn about the dispersion E(k) of the conduction band (CB) and its occupation with electrons as a function of time after pulsed optical excitation. In order to interpret our data it is not sufficient to describe merely the temporal evolution of the electron distributions in a static CB. We observe that the dispersion of the CB changes itself in time. This is in particular monitored around the Gamma point of the CB. This combined temporal change in band-structure and electron distribution can be explained by the formation of a polaronic excitation.
2.3-O3
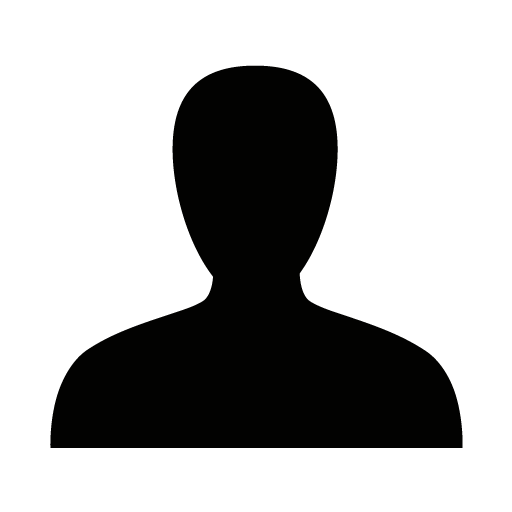
Two-dimensional transition metal carbides (MXenes) are of great interest for a range
of applications in electronics, including solar cells due to their tunable optoelectronic
characteristics, strong metallic conductivity, and attractive solution processability. In this study,
we used photo-conductive atomic force microscopy (pcAFM) to map the local (nanoscale)
photovoltaic performances of the Ti 3 C 2 T x MXene integrated TiO 2 electron transport layer (ETL)
based perovskite solar cells (PSCs) to determine the treatment's impact on the microscopic
charge flow inside the devices. The nanoscale photovoltaic performances of the devices with
MXene integrated ETLs is first studied by using the pcAFM technique. The underlying PV
mechanisms and their localized dependency on the interfacial modification across the layers
must be understood through the investigation of these photoresponses at the nanoscale. The
morphology and photocurrent maps with different applied voltages have been simultaneously
measured with nanoscale resolution from the top surfaces of the devices without back contacts.
Compared to the as-deposited samples, Ti 3 C 2 T x MXene based PSCs show a more uniform and
improved current flow throughout the film. Average local photocurrent for MXene-induced
PSCs is significantly larger than that of as-deposited PSCs at zero applied bias and steadily drops
as positive bias is raised until it reaches the open circuit voltage. Large variations in short-circuit
current were also observed at different locations across the film that appeared identical in
topography images. Our study reveals that MXene-integrated ETLs have the potential to improve
the polycrystalline photovoltaic devices' performance by enhancing the active layers' intrinsic
properties and nanoscale photoconduction.
2.3-O4
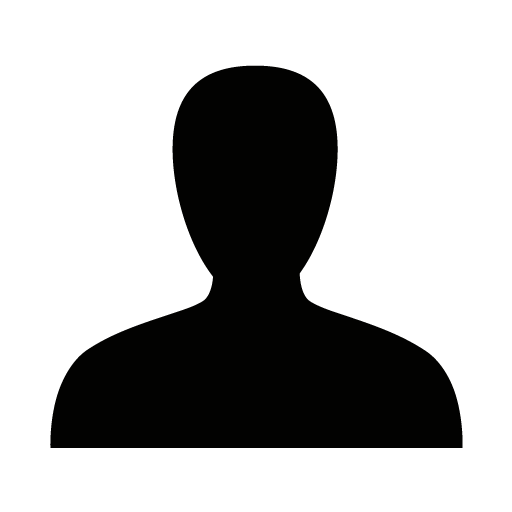
Metal halide perovskite (MHPs) solar cells represent a promising newcomer in the front of emerging photovoltaic technologies to address the dramatic energy crisis and climate change that we are facing. The exceptional properties of MHPs derive from their hybrid organic-inorganic nature, which allows also for low-cost and straightforward processing. Solar cells containing MHPs as absorbing layer have already achieved a power conversion efficiency of about 25,7 %, close to the efficiency of silicon-based devices. Nevertheless, a major limitation, still preventing the uptake of the technology, is related to the reduced stability of these materials when exposed to operative conditions, namely temperature, light, and moisture. Herein, an effective defect passivation of MHP surfaces is a key strategy to tackle both the stability and the enhancement of solar cell performances. Although many solution-based approaches 1 have been tested, we propose here an innovative use of plasma, as a solvent-free, scalable, and non-invasive promising strategy to boost MHP solar cells performances 2. As benchmark material we used Methylammonium Lead Iodide perovskite; thus we have exposed the surface of polycrystalline thin films made of this material to different plasma conditions implying the variation of power, gas, and treatment time, both for low-pressure (LP) and atmospheric pressure plasmas (APPs). The impact of Ar, N2, H2 and O2 LPPs on MAPbI3 optochemical properties and morphology was correlated to the performance of the photovoltaic devices and rationalized by density functional theory calculations3. An interesting improvement in photoluminescence was observed for the Ar and H2 treated films, while an improvement in PCE was observed only for the Ar treated device. This result was ascribed to the efficient removal of the superficial organic component, revealed through X-ray photoelectron spectroscopy (XPS), following suitable surface passivation by the electron transporting layer deposition. APP treatments, fed with He gas, were tested on the surface of MAPbI3, too. A milder morphological modification than LPP treatments was observed in this case, but withstanding good surface passivation, as confirmed through the improved photoluminescence intensity, while the effect in terms of photovoltaic devices is still under investigations. Moreover, starting from these encouraging results, new plasma surface processes are now object of evaluation, such as the plasma-deposition of thiophene-like films and the study of LPP treatments applied to tin-based perovskite solar cells, approaches which already show the great versatility and potential of plasma-based techniques.
2.3-O5
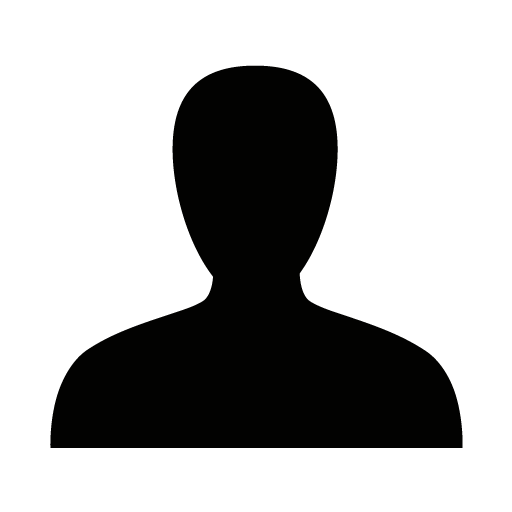
Recently, inorganic anti-perovskites with the formula X3AN (X = Ba, Sr, Ca, Mg; A = As, Sb) have been reported to exhibit excellent optoelectronic properties like small carrier effective masses, suitable direct bandgaps, high optical absorption coefficients as well as allowed optical transitions at band edges. These properties can be tuned depending on the X and A site. Extending the composition to quaternary anti-perovskites (X6AA′N2) enables the synthetic possibilities for new materials.[1,2]
Herein we report on the ammonothermal synthesis of EA5Pn2(NH)2. The three newly synthesized compounds Ca5AsSb(NH)2, Ca5AsBi(NH)2 and Sr5AsBi(NH)2 crystallize in the tetragonal space group P4/mmm. Their crystal structure was solved and refined by scXRD. Raman spectroscopy was used to further determine structural elements and verify the presence of imide-groups. Further investigations were carried out using powder X-ray diffraction, UV/Vis-spectroscopy, and density functional theory calculations.
The materials exhibit direct bandgaps in the range between 1.90 eV and 1.14 eV. By composition variation of the A-site elements as well as the X-site elements, the effective band gaps can be tuned. Hybrid density functional theory calculations verify the direct nature of the band gap, indicating large band dispersions through the enhanced covalency of the pnictides, benefiting the carrier transport. In summary, these new Imide-anti-perovskite materials exhibit interesting properties as efficient light harvesting materials for single junction solar cells.
1.1-I1
The shift towards an energy mix with more renewable sources have resulted in massive quantities of solar photovoltaics being installed over the worls. This is a growing electronic waste stream that needs to be planned for. Present appropaches to solar recycling involve high-temperature burning for separation and release of the PV cells for metal recovery processes. However, such processes can release gaseous by-products that can cause serious health and environmental issues as well as deprioritises the recovery of pure silicon. This talk will cover our group's efforts in developing new extraction techniques that eschew burning as well as incorporate green solvents. Through an incorporation of such novel techniques along with hydrometallurgy, we demonstrate separation of glass, extraction of the various metallic elements such as Ag, Cu and Al as well as silicon at good purity levels. Our efforts in upcycling the extracted elements into other products such as thermoelectrics as well as battery anodes will also be described.
1.1-I2
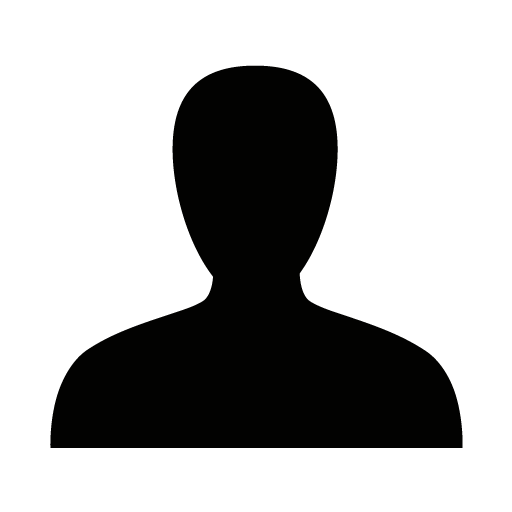
The current models of production and consumption are no longer deemed adequate because of the inefficient use of resources (especially energy) and its environmental consequences. To sustainably meet the growing energy demands of the world, a substantial increase in the deployment of photovoltaic and energy storage technologies is needed. Dye-sensitized solar cells (DSCs) have the potential to provide a viable source of renewable energy to help decarbonize our economy and power wearable devices. With their exceptional performance in diffused light under indoor conditions, DSCs remain competitive for powering the next digital revolution forming the internet of things (IoT). They can also be interfaced with storage materials to enable a new generation of energy storable solar cells. While this is all encouraging, with the increase in the number of deployed devices arises the need to evaluate their end-of-life, transition to a resource efficient circular economy. In this talk, I will evaluate each component of a dye-solar cell from an environmental point of view. I will present the various material design routes being developed to enable a sustainable approach both during fabrication and as readily ‘refurbishable/upgradable’ devices, leveraging the ability to replace dyes and electrolytes repeatedly with no observable loss in functionality over many product generations. My talk will identify features which are conducive to circular economy and identify barriers to resource efficiency for these technologies and suggest some potential solutions and priority areas for future research.
References: 1. R. G. Charles, M. L. Davies and P. Douglas, "Third generation photovoltaics — Early intervention for circular economy and a sustainable future," 2016 Electronics Goes Green 2016+ (EGG), Berlin, Germany, 2016, pp. 1-8, doi: 10.1109/EGG.2016.7829820.
1.1-I3
IRENA estimates that 70 million tonnes PV waste will have been generated by 2050. Furthermore, we are already seeing challenges in the supply chains of critical materials for renewable energy and electronics. Emerging solar technologies involve printable devices which have low embedded energy and are fully recyclable at the end of their life. Using recycled materials in these devices could lead to further enery savings and reduced environmental impact, as well as providing a versatile, low cost technology for clean energy. We present the outcomes of a proof-of-concept to provide data to assess the potential for printable electronic, electrochemical and photovoltaic devices using waste materials. Carbon black has numerous applications, but production leads to 2.4 kg CO2 emissions per kg virgin carbon black. Our project seeks to identify opportunities for the recycled carbon black as a higher-value product in electronic and electrochemical devices, such as printable photovoltaics. Most current research into printable solar cells using the triple mesoscopic stack configuration uses commercial carbon inks which is based on virgin carbon black. We have developed conductive inks from recovered carbon black from waste tyres, tested them in devices (indoors and outdoors) and then shown that the device itself can be regenerated and re-used.
1.2-I1
In this talk I will present our latest discoveries in converting bio and plastc waste into high value chemicals and H2 via electrooxidation reactions including catalyst design, mechanism and upscale . We will target prescursors such as ethylene glycol or glycerol. I will also talk about upgrading plastic into carbon composites for Na ion batteries. In particular I will talk about some high energy density C/Sn cpmpsotes and the alloying mechanism of Na with Sn.
In both cases i will talk about in operando techiques development such as XAS or XRD-PDF. Initial results on LCA will also be presented from the perspective of economic viability and environmental footprint.
Givcen the importance of net zero technologies, this talk will provide insights into waste utilisation towads high value added products. Products such as glycolic acid or lactic acid deriving from such processes can be used in cosmentic and renwable plastic . H2 represents a huge market and technology, where I still have a lot to learn
1.2-O1
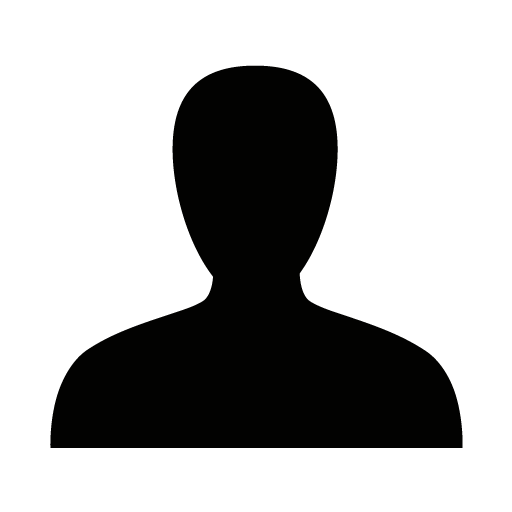
Recycling processes for lithium-ion batteries (LIBs) have been developed to remove chemical compounds from these batteries for secondary uses, as well as resynthesizing cathode material using direct or short-loop recycling, for use in cathodes of fresh batteries. The reason for extracting compounds from the cathode of discarded LIBs is that they are composed of high value and critical minerals such as Li, Co, Ni and Mn. In particular the cathodes with compositions such as LiCoO2, LiNiyMnzCoxO2, and LiMn2O4 are important to evaluate for reclamation of these metals and re-use. It is known from the literature that end-of-life LiNiyMnzCoxO2 contains around 15 to 20% less lithium than fresh cathode and therefore cannot be directly reuse without further lithiation. It is a challenge to return this end of life and recovered black mass, containing NMC materials to form that can be reused in a new cell. In this work we describe a direct recycling route for the discarded NMC532 using a low cost, and facile relithiation process. The cathode material was extracted from a disassembled end of life or scrap NMC vs graphite pouch cell, and then delaminated from aluminium using a 3.5M NaOH solution that selectively dissolved the Al foil. The recovered powder was analyzed by XRF, XRD, CHNS and ICP-OES techniques. After characterization, solid state relithiation was carried out with the lithium source LiOH at 700 °C and the product of this reaction was analyzed again using the above techniques and evaluated electrochemically.
1.2-I2
The aviation industry is responsible for 2% of the total GHG emissions and 10% of the fuel consumption worldwide, with a predicted market growth of 3.7% a year. It is expected that a large portion of the current global fleet will continue to be operational until 2040-2050 calling for the development of drop-in alternatives based on sustainable resources [1].
Significant research efforts have been devoted to identifying routes to produce liquid fuels from CO2 and H2 leveraging the Fisher-Tropsch (FT) synthesis. One option is to feed CO2 and H2 in a reverse water gas shift reactor (RWGS) and use the resulting syngas in a traditional FT process [2]. The limitations of this approach are: (i) the RWGS reactor operates at high temperatures (600-1000 °C); and (ii) the FT reactor produces a significant amount of wax, a mixture of heavy hydrocarbons that requires additional upgrading units, such as a hydrocracking (HC) reactor to improve the yield of liquid fuels. The direct hydrogenation of CO2 to liquid fuels in a single step is more appealing, but usually the formation of short chain hydrocarbons is favoured [3]. Yao et al. (2020) [4] synthesized a novel Mn-Fe-K based catalyst capable of converting CO2 and H2 with excellent yield and selectivity towards liquid hydrocarbons in the jet fuel range (C8–C16) and minimal wax production.
In this work, we carry out a techno-economic analysis and life-cycle assessment (LCA) of two SAF production processes: a one-step process (1sFT) based on the above-mentioned catalyst; and a two-step process (2sFT) based on the work of [2]. Both processes are fed with CO2 from direct air capture (DAC) and green H2. 1sFT and 2sFT are simulated at scale using Aspen HYSYS to evaluate their economic performances and obtain inventories for the LCA. The environmental assessment is conducted in SIMAPRO 9.3, using Ecoinvent 3.8 Cut-Off database for the background process inventories. The fuel obtained from 1sFT and 2sFT is compared against the fossil-based alternative considering different environmental KPIs, as for SAF to be truly sustainable the reduction is GHG emission should not be associated with high collateral ecological damage, a problem often referred to as burden shifting. ReCiPe2016 is used as life-cycle impact assessment method [5] and GWP100 is evaluated alongside the monetized endpoint impacts to human health, ecosystem quality and resource scarcity.
Our results show that the one-step process is superior both in economic and environmental terms to the two steps process, due to a lower capital cost, higher selectivity towards liquid hydrocarbons and lower energy requirements. On a well-to-wake basis the 1sFT process is predicted to reduce GHG emissions by 75% and the 2sFT process by 58%. The analysis of the endpoint environmental impacts confirms a better environmental performance of the synthetic fuels compared to the fossil counterpart, with the 1sFT outperforming the 2sFT by a larger extent, with 1sFT and 2sFT having a total externality cost 44% and 22% lower that the fossil fuel, respectively. At current CO2 and H2 prices the productions cost of low-carbon synthetic fuels is predicted to be 6-8 times higher than fossil-based aviation fuels, which is in agreement with the literature, and a combination of feedstock cost reduction and policy intervention (e.g. carbon taxation) is necessary for synthetic fuels to become cost competitive.
1.1-I1
The Internet of Things (IoT) has recently emerged as a technology with immense potential societal impacts. However, powering IoT devices presents a key challenge. Indoor photovoltaics (IPV), offering the potential for self-powered and low-maintenance IoT devices, may be a promising solution. Concurrently, driven by the need for tandem structure, wide-gap perovskite semiconductors are emerging. The optimal bandgap for IPV devices (1.8 – 1.9 eV) is considerably wider than most traditional solar materials but in accordance with existing wide-gap perovskites.
In this talk we discuss the performance of various wide-gap perovskite IPVs as model systems under spectrophotometrically calibrated indoor light conditions and compares them to thermodynamic performance predictions. Computational simulations indicate trap-assisted recombination as a primary loss mechanism in current perovskite IPVs. To support further advancement in the field, a computational tool is provided to extrapolate calibrated solar (AM1.5G) measurements for benchmark performance estimates at a range of indoor conditions. This work highlights the need for standardized characterization methods and suggests pathways for device optimization to unlock the full potential of perovskite IPVs for a future of sustainable, self-powered IoT devices
1.1-O1
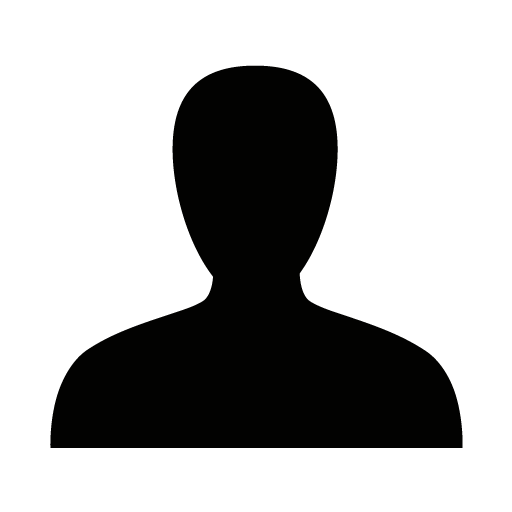
Metal halide perovskite semiconductors hold great promise for future photovoltaic applications. The efficiency of metal halide perovskites solar cells (PSCs) is determined by several parameters, one of them is the open circuit voltage (VOC). In order to achieve high efficiency solar cells, it is important to reach a high VOC. Consequently, we want to understand the loss mechanisms limiting the VOC. It is known that the VOC loss is dominated by non-radiative recombination occurring e.g. in the bulk of the perovskite, or at the interfaces. Measuring the VOC of a PSC in order to understand what exactly causes the non-radiative recombination and where in PSC, will not provide the answer, because it is just the overall result. Therefore, we try to understand the VOC loss in perovskite solar cells via absolute photoluminescence intensity analysis. With this analysis, the quasi Fermi level splitting (QFLS) of different components in a PSC can be determined. Since the QFLS is related to the maximum VOC achievable for a photovoltaic device, this relation can be used for predicting device properties.
In this work, the effect of passivation on defects and the non-radiative recombination of charge carriers in mid to wide bandgap perovskite solar cells at the perovskite and electron transport layer interface is examined with absolute PL to understand the detrimental processes happening in the PSCs. We begin by analyzing the voltage loss for p-i-n devices and by describing the passivation of surface defects at the interface of the mid-bandgap perovskite with the electron transport layer using a solution-processed quaternary ammonium halide (choline chloride, CCl) or an evaporated ultrathin lithium fluoride (LiF) layer, that translates to solar cells with a high VOC. We extend this passivation evaluation to an array of wide-bandgap perovskite devices. After this analysis, we demonstrate that non-radiative recombination is primarily located in the film and that it increases with increasing bandgap. The other prominent loss is located near the interface of the perovskite with the electron transport layer and is passivated equally for both passivation techniques and for all bandgaps investigated in this study. This implies that the surface treatment with two different materials result in the same gain. To further understand the working principle of the passivation strategies, we extend the passivation study by increasing the amount of passivation material in between the perovskite-ETL interface. It is found that upon application of increasing thicknesses of LiF or increasing concentration of CCl in between the perovskite/ETL interface, the gain in QFLS and VOC starts plateauing and an optimum thickness or concentration should be used.
In summary, by combining the absolute photoluminescence spectroscopy results, it is found that by using a LiF or CCl a significant reduction of the non-radiative recombination losses that currently limit wide-bandgap PSCs is achieved. And that this gain is primarily due to minimizing the loss at the perovskite-ETL interface. These results contribute to solving the main challenges of the wide-bandgap perovskite in achieving a high VOC.
1.1-O2
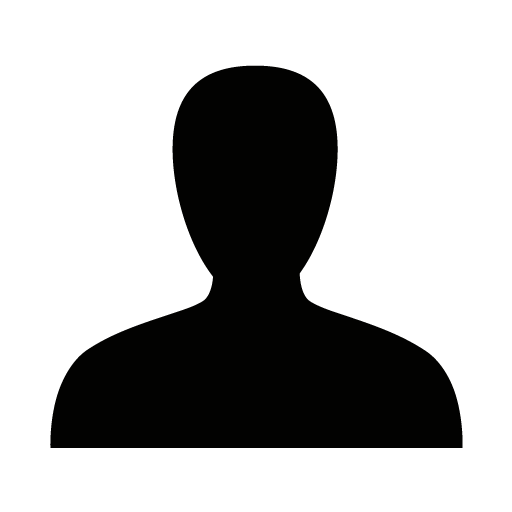
In conventional flexible perovskite solar cells (FPSCs) produced on plastic substrates such as polyethylene terephthalate (PET) and featuring a conductive transparent oxide layer like indium-doped tin oxide (ITO), the ITO and perovskite layers are susceptible to cracking when the devices are moderately bent. While changes in device series resistance can be indicative of cracks in the ITO layer, characterizing cracks in the perovskite layer is more complex.
At a microscopic level, perovskite layer cracking implies an upsurge in the density of bulk and surface defects, leading to an increase in trap states within the layer. This, in turn, can amplify the loss of photo-generated charge carriers through trap-assisted carrier recombination processes. However, comprehending and quantifying the impact of applied mechanical stress (MS) on device properties and behavior necessitates a sophisticated analysis of charge carrier generation, recombination, and extraction processes both before and after MS application [1].
This study is dedicated to examining the properties and behavior of FPSCs pre- and post-application of MS to unravel the dynamics of recombination losses induced by MS, providing insights into the mechanisms contributing to performance degradation in perovskite-based solar cells. The investigation involves a comprehensive analysis of device properties and behavior through experimental and theoretical approaches. The degradation of photoelectrical parameters in fabricated PFPSCs due to applied MS is elucidated by meticulously scrutinizing various recombination pathways within the perovskite layer.
1.1-I2
Interfacial charge carrier recombination is currently one of the major performance bottlenecks in single- and multi-junction metal halide perovskite (MHP) solar cells. In our work, we investigate interfacial charge carrier recombination processes in state-of-the-art MHP thin films and device structures by transient spectroscopies including transient reflection, transient absorption, time-resolved photoluminescence, and time-domain terahertz spectroscopy across a wide dynamic range from femto- to microseconds. The MHPs investigated are multi-cation (mixed) halide perovskites as neat MHP thin films, passivated MHP thin films, perovskite films adjacent to charge transport layers (CTLs), MHP films adjacent to CTLs with additional interlayers (ITLs) and in the presence of electrodes, as well as MHP films on transparent conductive oxides (TCOs) with and without common self-assembled monolayers (SAMs). Organic CTLs are also used, since they allow direct probing of the carrier dynamics in the CTL (not only in the perovskite film), thereby allowing to distinguish between carrier extraction and interfacial recombination. Our spectroscopic experiments are supported by computational studies providing insight into the role of (bulk and surface/interface) defects on carrier recombination, the chemistry of defect passivation at interfaces, and interfacial carrier extraction and recombination dynamics. Our studies provide in-depth insight into interfacial charge carrier recombination processes at various types of interfaces in perovskite devices and reveal pathways to mitigate those losses to enhance the device Voc and quantum efficiency in both single- and multijunction photovoltaic solar cells.
1.2-I1
Dr. Annalisa Bruno is an Associate Professor Nanyang Technological University (ERI@N), coordinating a team working on perovskite solar cells and modules by thermal evaporation. Annalisa is also a tenured Scientist at the Italian National Agency for New Technologies, Energy, and Sustainable Economic Development (ENEA). Previously, Annalisa was a Post-Doctoral Research Associate at Imperial College London. Annalisa received her B.S., M.S., and Ph.D. Degrees in Physics from the University of Naples Federico II. Her research interests include perovskite light-harvesting and charge generation properties and their implementation in solar cells and optoelectronic devices.
Metal-halide perovskites (MHP) stand out as highly promising and cost-effective optoelectronic materials due to their exceptional optoelectronic properties and versatile fabrication methods [1-6]. These materials find applications in diverse fields such as solar cells, light-emitting diodes, photodetectors, and even quantum emitters. Quantum confinement can unveil unexpected and advantageous characteristics, leading to the development of high-performance devices.
One approach to induce quantum confinement involves creating layers of quantum-confined materials through the deposition of multiple thin films. Thermal evaporation emerges as a particularly promising technique for fabricating halide perovskite films, offering precise control over layer thickness, fine-tuning of composition, stress-free film deposition, and the ability to modify surface properties. Thermal evaporation in perovskite fabrication has expanded the possibilities of thin film production, showcasing its capability to generate ultrathin perovskite films that serve as the foundation for multi-quantum well structures.
This method enables the manipulation of growth properties, influencing the optoelectronic characteristics of nanoscale thin films and inducing quantum confinement effects within the structure. The precise control over photoluminescence through quantum confinement opens up a wide array of possibilities for unconventional optoelectronic properties and novel applications of perovskites [7-10].
1. J. Li et al; , Joule 2020, 4, 1035
2. H.A. Dewi et al., Sust. Energy & Fuels. 2022, 6, 2428
3. E. Erdenebileg, et al Solar RRL, 2022, 6, 2100842
4. HA Dewi, et al., Adv. Funct. Mater. 2021, 11, 2100557
5. J.Li et al., Adv. Funct. Mater. 2021, 11, 2103252
6. E. Erdenebileg et al., Material Today Chemistry, 2023, 30, 101575
7. E. Parrott et al. Nanoscale, 2019, 11, 14276
8. KJ Lee et al., Nano Letters, 2019, 19, 3535
9. KJ Lee et al., Advanced Materials, 2021, 33, 2005166
10. T. Antrack et al., Adv. Sci. 2022, 9, 2200379
1.2-I2
Perovskite photovoltaics stand out as a top contender in the quest for new solar energy harvesting technologies. However, the presence of toxic lead in perovskite devices necessitates exploration of alternatives. The most popular of which has been tin-perovskites, known for their extremely fast and difficult to control crystallization. In this talk, a novel method of printing of tin-perovskite films will be presented, involving a crystallization trigger and subsequent control of nucleation and growth through solvent engineering. It was developed using an in-line optical spectroscopy characterization equipment providing analysis of the crystallization process in-situ. Photoluminescence, Raman and reflectance probes were installed and probed the corresponding signals to give information of film changes after printing and during post-printing treatment. The new method for printing tin-perovskite films enabled the fabrication of the first slot-die coated solar cell device, opening avenues for the development of fully printed, lead-free perovskite photovoltaics at an industrial scale.
1.2-I3
Hybrid perovskites are one of the most promising materials for next-generation optoelectronic properties, including solar cells. However, the solution-based fabrication process brings to the formation of polycrystalline films, resulting in defects, which have a detrimental effect on the perovskite solar cell performance because they are expected to trap charge carriers, facilitating nonradiative electron-hole recombination. Hence, the investigation of the impact of defects on fundamental photo-physical properties becomes crucial for the optimization of the device.
In this framework, I have investigated the optoelectronic properties of three differently defective materials. More in details, I employed different amount of antisolvent and the passivation procedure to finely tune the defects concentration. In order to accurately evaluate defects concentration, I performed transient absorption measurement, and I analyzed the results considering the Burnstein-Moss effect. With the aim of investigating the correlation between defects and fluence regime, I used time resolved techniques, i.e. time resolved photoluminescence and transient absorption spectroscopy. In particular, time resolved photoluminescence highlighted that, by increasing the trap states concentration in the material, a shift in the onset for radiative regime arises at progressively higher fluences. On the other hand, transient absorption spectroscopy evidenced the strong correlation between the organic cation used for the passivation and the occurrence of the Auger regime. Therefore, for the material with lower trap states concentration, the range in which radiative recombination dominates is enlarged. Ultimately, this is reflected in the solar cell efficiency which is enhanced by 22% with respect to the higher defective material.
1.2-O1
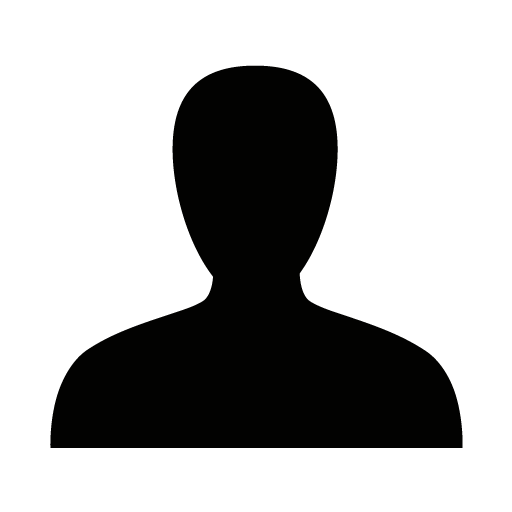
In organic solar cells, the charge-transfer (CT) electronic states are crucial in the charge-generation process, significantly influencing the overall device performance. They are formed at the interface between the electron-donor (D) and electron-acceptor (A) material, which usually exhibit significant electric fields.
In this study, we use a dedicated D-A system to tune intrinsic and extrinsic interface electric fields. It consists of two doped organic layers on top of each other, forming a planar organic p-n junction. By applying increasing voltages up to 6 V and introducing different thicknesses of intrinsic layers between 0 nm and 20 nm at the interface, the electric field at the interface can be deliberately varied. We observe substantial shifts in the CT-state peak emission, approximately 0.5 eV (150 nm), causing a transition from red to green color. This effect can be explained in a classical electrostatic picture, as the interface electric field superimposes the Coulomb interaction between the electron-hole pair. Our investigations illustrate the extent to which CT-state energies are influenced by their immediate electric environment. While CT state energy is often referred to as a fixed quantity, we want to emphasize that this understanding needs to be revised, especially for planar systems with a high degree of interfacial dipole alignment.
1.1-I1
Agustín is experienced in the optical design, fabrication and characterization of large area photonic architectures that can be easily implemented in emerging optoelectronic devices to improve their performance. His group specializes in soft nanoimprinting lithography, which offers an inexpensive and simple pathway to exploit the optical properties of nanophotonic structures with unconventional materials and devices.
Photonic architectures offer a promising avenue for optimizing the performance of various optoelectronic devices. Nevertheless, the future of optoelectronic devices hinges on cost-effective and large-scale manufacturing techniques that can reduce expenses and boost production efficiency. To harness the remarkable attributes of photonic structures for enhancing these devices, they must undergo a processing approach akin to that of the devices themselves.
In our research group, we employ soft nanoimprinting lithography, a versatile, rapid, and cost-efficient method for crafting nanostructures from a diverse variety of materials. In soft nanoimprinting lithography, we make use of pre-patterned soft elastomeric stamps to corrugate materials such as resists, biopolymers, colloids and nanomaterials in general. In all cases, the resulting photonic architectures can exhibit a resolution below 100 nm while covering areas up to 1 cm2. During this presentation, I will demonstrate our utilization of pre-patterned stamps to induce the long-range alignment of different colloids, including gold colloids and perovskite nanocrystals, to attain distinct optical properties, such as lattice resonances with high Q-factors. Moreover, I will showcase how elastomeric molds pre-patterned with chiral motifs can lead to chiral metasurfaces, exhibiting strong circular dichroism and intense circularly polarized photoluminescence (CPL), making them seamlessly applicable in various practical applications.
1.1-I2
Chiral plasmonic nanostructures have attracted much interest in recent years as new platforms for modulating the polarization of light. Other than photonic devices, chiral plasmonic nanostructures facilitate the ultrasensitive detection of chiral biological materials, e.g. DNA and proteins. To synthesize chiral plasmonic nanostructures, the most well-established method is chirality transfer from biological materials to inorganic nanostructures. Alternatively, circularly polarized light can be used to control the formation of the plasmonic enantiomeric nanostructures, where the chirality of the product solely defined by the polarization of the light. This synthetic method may be more cost-effective while allowing the wavelength and intensity of light to be used as tunable parameters. Here we investigate the use of circularly polarized light to create chiral gold nano bipyramids. Hot charge carriers can be generated with light and consumed to fabricate chiral plasmonic structures. Scanning electron microscopy (SEM) coupled with cathodoluminescence measurements comparing the nano bipyramids before and after circularly polarized light illumination is used for characterization. We will show that both dielectric or plasmonic chiral additions can be deposited depending on the identity of reagents. Circular dichroism (CD) spectra of the chiral plasmonic nanostructures further demonstrate the polarization dependent light-matter interactions of the materials synthesized.
1.1-I3
Alessandro Stroppa (July 14th 1976) is a Research Director of the CNR-SPIN Institute (Italy) and deputy director of the research unit in L’Aquila (Italy). He received his PhD in Theoretical Condensed Matter Physics from University of Trieste (Italy) in 2006 and he continued his research in computational materials science at University of Vienna in the group of Prof. Georg Kresse (VASP Team). After 2009, he joined the CNR in Italy where he became permanent staff in 2012. He is contract professor at University of L’Aquila (Italy), and invited professor at Shanghai and South East University (China).
His current research areas deal with solid-state physics and materials science. Specifically, he is interested in 3D and 2D hybrid inorganic-organic perovskites, non-magnetic and magnetic 2D systems with special focus on photo-ferroic, multiferroic, magnetoelectric, twistronic, topological, magneto-optical and non-linear optical properties, skyrmions, etc. He has great experience with Density Functional Theory (DFT) methods for the study of the structural, electronic and magnetic properties using all-electrons as well as pseudopotential approaches implemented in numerical codes. He has published about 138 peer-reviewed papers (h-index=43, Total citations 6744) in theoretical condensed matter also in collaboration with experimentalists. In 2017, 5 of his papers were Highly Cited (Source: Web of Science). He is on the World’s top 2% scientists lists published by Stanford University since 2019. He received honors such as the ‘Best 2008 New Journal of Physics Collection’; Research Highlight talk at EUROMAT 2013; Best oral talks at Italian Physical Society conferences in 2005 and 2011; Certificate of appreciation for “his important contributions to the theoretical understanding of microscopic mechanisms of multiferroicity and magnetoelectricity in perovskite metal-organic frameworks” by Nature Conference (Nankai University, 2019). He is carrying out an intense outreach activity for primary schools. [Last update Sept 04th 2023]
Selected papers
1. A. Stroppa, et al.“Electric Control of Magnetization and Interplay between Orbital Ordering and Ferroelectricity in a Multiferroic Metal-Organic Framework”, Angew. Chem. Int. Ed. Engl., 2011, 50, 5847-5850. Times cited:192.
2. A. Stroppa, et al. “Hybrid Improper Ferroelectricity in a Multiferroic and Magnetoelectric Metal-Organic Framework”, Adv. Mat., 2013, 25, 2284-2290. Times cited:215.
3. A. Stroppa, et al. “Tuning the Ferroelectric Polarization in a Multiferroic Metal-Organic Framework”, J. Am. Chem. Soc. 2013, 135, 18126-18130. Times cited:190.
4. A. Stroppa, et al. “Electric-Magneto-Optical Kerr Effect in a Hybrid Organic-Inorganic Perovskite”, J. Am. Chem. Soc. 2017, 139, 12883-12886. Times cited:23.
5. A. Stroppa, et al.”Tunable ferroelectric polarization and its interplay with spin-orbit coupling in tin iodide perovskites”, Nat. Commun., 2014, 5, 5900. Times cited:175 (Highly Cited Paper)
6. A. Stroppa, “Cross coupling between electric and magnetic orders in a multiferroic metal-organic framework”, Sci. Rep., 2014, 4, 6062. Times cited:134.
7. A. Stroppa, et al. “Magneto-Optical Kerr Switching Properties of (CrI3)2 and (CrBr3/CrI3) Bilayers”, ACS Appl. Electron. Mater. 2020, 2, 5, 1380-1373. Times cited:1.
8. A. Stroppa et al. “Activating magnetoelectric optical properties by twisting antiferromagnetic bilayers”, Phys. Rev. B, 106, 184408 (2022). Times cited: 0
Selected links (Outreach)
https://www.spin.cnr.it/outreach-and-t-t/events/item/240-spin-at-maker-faire-2023
https://outreach.cnr.it/risorsa/231/giocando-con-la-geometria
https://outreach.cnr.it/risorsa/79/dalla-geometria-alla-geo-materia-un-affascinante-percorso-didattico
Can we generate and transfer chirality?
Chirality is an important structural property: right-handed and left-handed chiral materials have identical chemical composition and connectivity, but they are related by mirror transformation, forming a couple of enantiomers.
Chiral materials show unique features: the intrinsic non-centrosymmetry leads to optical rotation, circular dichroism (CD), second-harmonic generation (SHG), piezoelectricity, pyroelectricity, ferroelectricity, and topological quantum properties.
In this talk, I will discuss the intriguing interplay between chirality and physical properties in innovative materials, ranging from twisted bilayers (non-magnetic as well magnetic) to chiral hybrid organic-inorganic perovskites.
About Figure 1: Generation of chirality by twisting in bilayer graphene and corresponding spin-
texture switching.
References:
[ 1 ] K. Yananose, G. Cantele, P. Lucignano, S.-W. Cheong, J. Yu, and A. Stroppa, Phys. Rev. B. 104
(2021) 075407.
[ 2 ] K. Yananose, P. G. Radaelli, M. Cuoco, J. Yu, and A. Stroppa, Phys. Rev. B. 106 (2022) 184408.
2.1-O1
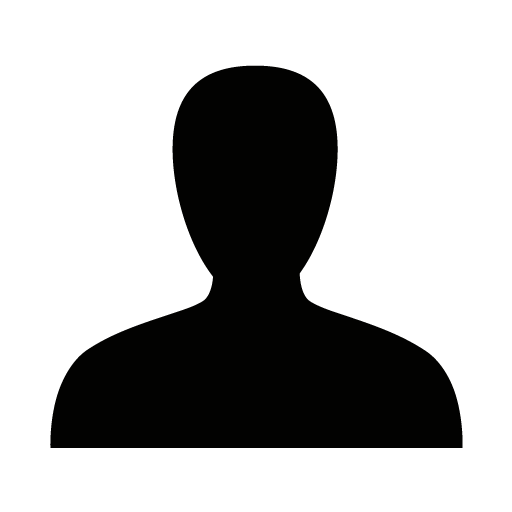
Hybrid semiconductor materials are predicted to lock chirality into place and encode asymmetry into their electronic states, while the softness of their crystal lattice accommodates lattice strain and maintains high crystal quality with the low defect densities necessary for high luminescence yields. The realization of chiral bulk emitters with bright circularly-polarized luminescence from such materials is desired for the design of chiroptical photonic and opto-spintronic applications. Here, we report the fabrication of novel chiral layered hybrid lead-halide perovskites with high photoluminescence quantum efficiencies and large degrees of circularly polarized photoluminescence at room temperature.[1] Using state-of-the-art transient chiroptical spectroscopy, we rationalize the excellent photoluminescence yields from suppression of non-radiative loss channels and very high rates of radiative recombination. We further find that photo-excitations sustain polarization lifetimes that exceed the timescales of radiative decays, which rationalize the high degrees of polarized luminescence. We postulate that the superior optoelectronic properties of the layered hybrid perovskites arise from their special tolerance to crystal structure chirality, which we carefully designed by cation engineering. We demonstrate that our materials can be used in electroluminescent devices and polarized-light detectors. Our findings pave the way towards high-performance solution-processed photonic systems for chiroptical applications and chiral-spintronic logic at room temperature.
2.1-O2
Dr. Beatriz Martín-García received her Ph.D. in Chemical Physics (Cum Laude) from University of Salamanca (Spain) in 2013. Then, she joined Istituto Italiano di Tecnologia (Italy) under the Graphene Flagship project working during almost 6 years on the modulation of optoelectronic properties of different materials (nanocrystals, 2D materials and hybrid metal-halide perovskites) by chemical-design and surface-functionalization strategies for their integration in solar cells, photodetectors and memories. She is currently an Ikerbasque researcher and Ramón y Cajal fellow at CIC nanoGUNE BRTA, leading a research line developing tailor-made low-dimensional materials and studying them by Raman and photoluminescence spectroscopy techniques to drive the selection of desired properties for their integration in optoelectronic and spintronic devices.
The significant growth, development, and evolution of technologies such as optoelectronics and spintronics have always been accompanied by access to materials with specific and extraordinary properties. Among these materials, recently, chiral trigonal tellurium (Te) stands out since it exhibits electrical magneto-chiral anisotropy and spin polarization,[1-3] electrical conductivity anisotropy and intrinsic polarized photoresponse,[3-5 tunable Rashba spin-orbit coupling,[6] optical activity[7,8] and bulk photovoltaic effect (BPVE)[9]. However, since its properties depend on its crystal structure, for its successful integration into devices and the development of new applications, it is key to determine its crystallographic orientation and handedness and its interaction with light. In this work, using bulk single crystals, we show how the response of Te to polarized light depends on the crystal orientation which has implications for optical and electrical transport studies. By linearly polarized Raman spectroscopy we identify different crystal faces (1 0 0), (1 1 0) and (0 0 1) and the orientation of the trigonal axis corresponding to the helical Te chains. Moreover, we correlate the angle-resolved experimental patterns derived from the data analysis with the symmetry of the crystal. Furthermore, by circularly polarized measurements, we highlight that only for incidence parallel to the trigonal axis, i.e. in the (0 0 1) face, is possible to determine the handedness. In this case, we observe different peaks shift for left- and right-handed crystals in the corresponding cross-helicity Raman spectra. We support our findings with X-ray diffraction and chirality- and orientation-sensitive chemical etching, providing robust insights for the analysis of chiral and low-dimensional materials.[10]
2.1-I1
In recent years, photoactive chiral materials are attracting considerable interest owing to relevant applications in optoelectronics as well as high resolution imaging [1]. In these regards, hybrid materials are skyrocketing the field of material science for optoelectronics because they can tune the properties of soft and inorganic assemblies [2]. A recent interesting class of luminescent chiral materials is represented by chiral hybrid perovskites, since they are showing prominent circularly polarized emissions without any need of expensive ferromagnets or extremely low temperatures [3]. Indeed, the chiral source impacts specific non-covalent interactions occurring within the chiral scaffold, which in turn affect the efficiency of the chiral emissions [4]. Modern multiscale modeling and simulations nowadays have an unprecedented level of accuracy, enabling an efficient chiral design of luminescent materials. The chiral design concepts of low-dimensional perovskites herein discussed are based on enhanced sampling simulations and TD-DFT calculations [5] from the predicted free-energy basins. This simulation strategy enables to consider a variety of contributions including molecular rotations within the chiral framework, that may affect the generated chiroptical properties.
2.1-I2
Sascha is a Tenure-Track Assistant Professor in Physical Chemistry and Head of the Laboratory for Energy Materials at EPFL (Switzerland), while he is also maintaining strong ties with the Harvard community and in particular Winthrop House which he regularly visits as NRT and SCR member.
His team employs light-matter interactions to understand the next generation of soft semiconductors with the overarching goal of maximizing energy efficiency for a sustainable future by unlocking applications ranging from flexible light-weight solar cells & displays all the way to entirely new applications in quantum information processing.
Previously, he was a research group leader and Rowland Fellow at Harvard University. Before starting his lab at Harvard, Sascha studied Chemistry at Heidelberg University (Germany) and completed a PhD in Physics at the University of Cambridge (UK), where he subsequently worked as EPSRC Doctoral Prize Fellow.
Chiral solution-processable semiconductors based, for example, on small molecules, polymers or halide perovskites offer an exciting new avenue to simultaneously control charge, spin and light using a single material. This could enable efficient spin-optoelectronic devices ranging from displays and holography to detectors, and even applications in quantum information technology.[1] In this talk, I will give an overview of our recent efforts to understand the underlying mechanisms by developing novel time-resolved chiroptical spectroscopy techniques.
By pushing broadband circular dichroism to diffraction-limited spatial and 15 fs time resolution, we create a spin cinematography technique to witness the ultrafast formation of spin domains in halide perovskite films due to local symmetry breaking and spin-momentum locking.[2]
In terms of circularly polarized photoluminescence (CPL), I will first explain the fundamentals and artifacts involved in measuring CPL reliably and introduce an open-access methodology and code to do so [3].
I will conclude by showing our most recent development of a transient broadband full Stokes vector polarimetry with unprecedented time and polarization resolution to track the emergence of chiral light emission [to be submitted].
[1] Nature Reviews Materials 8, 365 (2023)
[2] Nature Materials 22, 977 (2023)
[3] Advanced Materials 44, 2302279 (2023)
2.2-I1
Prof. R. Robinson received his PhD in Applied Physics from Columbia University. After his PhD, Prof. Robinson was awarded a postdoctoral fellowship at University of California, Berkeley/LBNL in the research group of Paul Alivisatos. There, he worked on nanoparticle synthesis, chemical transformations of nanoparticles, and advanced property characterizations of nanoparticles. In 2008 Richard began a faculty position at Cornell University in the Materials Science Department, and is currently an associate professor. His primary research interests are: (I) Synthesis and chemical transformations in nanocrystals, (II) Nanocrystals in energy applications, and (III) Synchrotron x-ray characterization of nanomaterials.
therobinsongroup.org/
Magic-sized clusters (MSC) are identical inorganic cores that maintain a closed-shell stability, inhibiting conventional growth processes. Because MSCs are smaller than nanoparticles, they can mimic molecular-level processes, and because of their small size and high organic-ligand/core ratio, MSCs have “softer” inter-particle interactions, with access to a richer phase diagram beyond the classical close packed structures seen with larger particles. These MSCs display a surprising ability to self-organize into films with hierarchical assembly that spans over seven orders of magnitude in length scale. The films are optically active with g-factors among the highest reported for all semiconductor particles. In this talk I will highlight some remarkable behavior we have recently found in magic sized clusters, with a focus on the symmetry breaking and chirality transfer that occurs during their self-organization into thin films. And I will discuss our method for extracting the chiroptic-CD signal from the raw data that contains highly linear anisotropic effects, derived using Mueller matrix and Stokes vector conventions, and our extension of that expression using a more accurate third order Taylor series expansion to reveal "pairwise interference" contributions to CD spectra that, unlike LDLB contributions, cannot be averaged out of the signal.
2.2-I2
Alicia Forment-Aliaga (Valencia, 1976) is a researcher at the Molecular Science Institute (ICMol) and a senior lecturer in the School of Chemistry at the University of Valencia (UVEG), Spain. She graduated in Chemistry and carried out her PhD on molecular magnetism at the UVEG, supervised by Prof. E. Coronado and Prof. F.M. Romero. Between 2004-2008 she joined Prof. K. Kern’s group as a postdoctoral researcher at Max-Planck Institute for Solid Sate Research in Stuttgart, Germany. During this period, she was awarded with different competitive postdoctoral grants for developing her research on molecular electronics. Since July 2008 she works at ICMol at the UVEG, in Prof. E. Coronado’s group. This period comprises a postdoctoral Juan de la Cierva contract and a tenure-track Ramón y Cajal contract, both competitive contracts granted by the Spanish Government, and her current position as senior lecturer. At the ICMol she has developed a line of research in molecular surface engineering and in the last years, she has also started working on 2D materials. Particularly, she has driven her research into four specific goals: (1) Non-conventional lithographies for the organization of molecular systems; (2) formation of self-assembled monolayers for molecular spintronics; (3) scanning force microscopies for surface modification and characterization and (4) 2D materials, targeting their exfoliation, molecular functionalization and application in different areas.
Spin-crossover (SCO) complexes are promising building blocks for spintronic and high-density memory devices as they can be reversibly switched between two distinct spin states, low-spin (LS) and high-spin (HS) using a variety of external inputs (i.e. temperature, light, pressure, etc…). Additionally, the spin transition goes with change in other properties like volume, conductivity, or color. One step forward, chiral SCO compounds have been proposed as active components of magneto-optical devices.[1 Interestingly, when electrons are injected through chiral molecules, one electronic spin is preferably transmitted, working as spin filters at room temperature. This fascinating outcome is called Chiral Induced Spin Selectivity (CISS) effect.[2] Few years ago, we observed a CISS enhancement when adding paramagnetic centers in chiral self-assembled monolayers (SAMs).[3] Now, we want to push the field further by taking benefit from SCO bistability to modulate the magnitude of CISS effect. In this scenario, we pursue using chiral SCO compounds self-assembled on surfaces to further elucidate the role of the metallic center in the CISS performance. To reach this final goal, we have synthetized chiral SCO complexes and prepared self-assembled monolayers (SAMs) by a solution approach (preliminary step to have enhanced CISS effect). Specifically, in this project we have prepared chiral SCO complexes with tetradentate chiral Schiff base ligands (L) of formula [Fe(L)(NCX)2] (X = S, Se).
2.2-I3
In this presentation I will discuss our studies of controlling the spin currents using chiral metal-halide hybrid semiconductors thru the chiral induced spin-selectivity (CISS) effect. Chirality is introduced through an enantiomerically pure organic ammine as the A-site in layered 2D Ruddlesden-Popper type structures. The inorganic component make up the layers and are either lead or tin halide. Chirality is introduced into the inorganic component thru structural distortions and chiral arrangement of the organic components. We have studied this chiral transfer in the 2D layered systems, 0D dimer inorganic structures, and in metal-halide semiconductor nanocrystals with chiral ligands attached.
These systems exhibit CISS whereby only one spin sense can transport across the chiral layer and the other spin sense is blocked for one handedness of the chiral perovskite layer. We show that chiral perovskite layers are able to achieve > 80% spin-current polarization using magnetic conductive probe AFM. We have demonstrated the CISS effect in a half spin-valve where only one of the contacts is a ferromagnet and these results agree with the magnetic conductive probe AFM measurements. However, to enable a broader range of opto-electronic functionality achieving spin accumulation in traditional semiconductor structures at room temperature and without magnetic fields is key. Current efforts that employ ferromagnet/semiconductor interfaces is limited due to the conductivity mismatch. We have demonstrated spin injection across chiral halide perovskite/III-V interfaces achieving spin accumulation in a standard semiconductor III-V [MH1] (AlxGa1-x)0.5In0.5P multiple quantum well (MQW) light emitting diode (LED). The MQW is recieved as a working LED and it's internal structure was not modified. The spin accumulation in the MQW is detected via emission of circularly polarized light with a degree of polarization of up to ~15%. Characterization of the chiral perovskite/III-V interface demonstrates a clean conformal semiconductor/semiconductor electrical contact where the fermi-level can equilibrate. Our findings demonstrate chiral perovskite semiconductors can transform well-developed semiconductor platforms to ones that can also control spin at room temperature.
1.1-I1
Organic or perovskite photovoltaics poses a multi-objective optimization problem in a
large multi-dimensional parameter space. Massive progress was achieved in developing
methods to accelerate solving such complex optimization tasks. We have demonstrated
for both types of semiconductors, that the combination of Gaussian Process Regression
(GPR) and Bayesian Optimization (BO) are most efficient in predicting new materials,
identify optimized processing conditions or invent alternative device architectures in
larger parameter rooms. For a 4 dim space (solvent, donor-acceptor ratio, spin speed,
concentration) with about 1000 variations in a 10 % grid space, 30 samples are sufficient
to find the optimum. For 6 dimensional spaces, the possible variations go into the
millions and billions. Nevertheless, our automated lines, being operated in an
autonomous optimization mode, were able to identify globalized optima within several
hundred´s of experiments. In the outlook we discuss whether these autonomously
operated research lines can as well handle unorthodox optimization problems such as
the recycling of organic or perovskite solar cells
1.1-O1
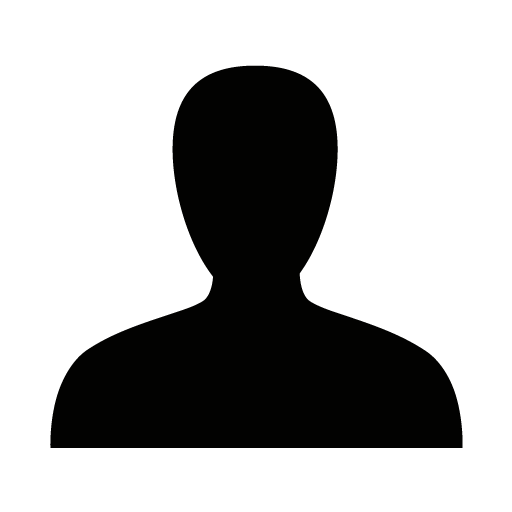
Narrow bandgap non-fullerene acceptors (NFAs) offer the semitransparency feature that makes organic photovoltaic (OPV) technology attractive for application in niche markets, such as building-integrated photovoltaic and agrivoltaics. However, evaluating each photoactive material system following classical experimentation methodologies and advancing it towards mass production presents a complex multiparametric challenge that requires intensive time and resources. In this talk, we propose the use of high-throughput lateral gradient methodology to evaluate the photovoltaic potential and semitransparency of narrow bandgap material systems. More than two thousand devices with different narrow bandgap material systems were manufactured using a platform that combines doctor blade coating technique with controllable velocity profiles to create thickness gradients of photoactive layer. As a result, the power conversion efficiency of the blade-coated devices varied between 0.06 to 10.45% in the champion devices of the screened material system. The large data pool collected for all samples was employed to perform statistical analysis to reveal the most influential parameters for narrow bandgap devices. We have found that the electronic descriptors (i.e. electron affinity, ionization energy, and optical band gap) had a more prominent impact on the final device performance than the processing conditions. Finally, we have shown the potential application of each narrow bandgap blend system for semitransparent devices by calculating its average visible transmittance as a function of photoactive layer thickness. Our study paves the way for high-throughput experimentation to accelerate the mass production and materials screening of narrow bandgap OPVs for semitransparent photovoltaic applications.
1.1-O2
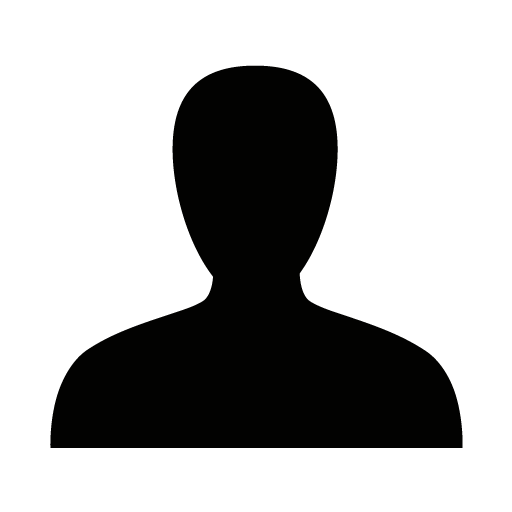
Semi-transparent organic photovoltaics are potential candidates for making smart windows in buildings and for agrivoltaics. The challenge here is to achieve high light utilization efficiency (LUE) without compromising on average visible transmittance (AVT). In this work, we designed and fabricated selective spectral tuned Distributed Bragg Reflectors (DBR) using alternating layers of TiO2 and SiO2, high and low refractive index oxide materials. The reactive sputtered layers on the back side of a pre-coated ITO glass exhibited close to 90% reflection between 650nm to 900nm wavelength range (near infra-red) with an outstanding AVT of around 90%. To test the superiority of these DBR integrated substrates for organic photovoltaics, we first optimized fully slot die coated organic solar cells to achieve above 14% PCE with blend PM6:Y7 systems using an opaque device configuration. Following that, the DBR integrated semi-transparent devices are made with blend PM6:Y7 and AgNWs top transparent electrodes, fully slot die coated. The resulting solar cells show 20% improvement in current density Jsc from the DBR substrates, compared to devices without integrated DBR, and LUE values above 3%, with an AVT around 40% and PCE around 8.3%. These results show the potential of achieving improved semi-transparent photovoltaics with industrial compatible printing processes.
1.2-O1
Device modeling is extensively used in solar cell research going from simple models such as the Shockley diode equation or partial differential equations (PDE) to more complex models like Monte-Carlo or drift-diffusion (DD). These models are often used as a diagnostic tool to understand and quantify the main losses for a given device by reproducing experimental measurements.
However, one of the main criticisms about using complex models as a means to quantify material properties is the many fitting (10 to 40) parameters that need to be estimated. Many argue that with so many fitting parameters one could fit almost any model to the experimental data.
In this presentation, the challenges of using high-dimensional physical models to quantify material properties accurately will be addressed. As well as, how machine learning methods such as Bayesian optimization (BO) combined with high-throughput (HT) experimental data can be leveraged to study material properties at scale.
The potential of using modeling, BO and HT data will be illustrated by several case studies using different physical models (PDE or DD) and experimental methods such as (i) transient absorption spectroscopy, (ii) transient photoluminescence and microwave conductivity, (iii) light-intensity-dependent current-voltage characteristics.
The open-source package BOAR (Bayesian Optimization for Automated Research) will be introduced here as a flexible package to address many challenges of solar cell research such as smart experimental planning or fitting of high-dimensional models to experimental data. This package combined with PDE solvers and open-source DD package SIMsalabim provides an easy-to-use and flexible toolbox for solar cell research.
1.2-O2
The need of scalable fabrication of high-efficiency organic photovoltaic cells and modules has gradually emerged. In particular, indoor organic photovoltaics (IOPV) constitutes an attractive energy harvesting solution to power IoT devices, given its deployability, reliability, and power density. A substantial portion of the billions of new IoT devices that will be installed in the coming years are expected to be located inside buildings. Such devices like environmental sensors, can have several shapes and sizes, hence the need to develop custom-made conformable photovoltaic devices to facilitate their integration into the final product. In this context, inkjet printing has become a very attracted printing technology for large-scale printed flexible cells and modules with freedom of shapes and designs.
Herein we demonstrate the challenge to go from lab-scale to industrial scale to achieve highly efficient fully inkjet printed IOPV cells and module. To prove the great advantage of inkjet printing as a digital technology allowing freedom of forms and designs, particular OPV modules with different shapes are demonstrated and integrated into different IoT devices to operate autonomously without using batteries or connections to the grid to ensure sufficient flexibility in their placement.
1.2-I1
Stelios A. Choulis is Professor of Material Science and Engineering at the Cyprus University of Technology (2008-present). He was the Organic Photovoltaic Device group leader of Konarka Technologies (2006-2008) and research and development (R&D) engineer of the Osram Opto-Semiconductors Inc, Organic Light Emitting Diode R&D team (2004-2006). During his PhD and first post-doc research associate (PDRA) position at Advanced Technology Institute (1999-2002, University of Surrey) he investigated the optical properties of quantum electronic materials and opto-electronic devices. In 2002 he joined the center of electronic materials and devices (Imperial College London, UK) as PDRA and work on the transport and recombination dynamics properties of molecular semiconductors (2002-2004). His current research interest focuses on the development of functional materials and devices for advanced optoelectronic applications.
Inverted Organic Photovoltaics (OPVs) allowed more flexibility on designing the roll-to-roll (R2R) production process and thus provided technological opportunities to OPV technology [1,2]. In addition, inverted OPVs are exhibiting significantly longer lifetime performance compared to normal structure OPVs, another important parameter for OPV commercialization [3]. The presentation will cover recent advances in developing high-performance metal oxides carrier selective contacts and Indium-tin-oxide (ITO)-free electrodes for non-fullerene acceptors (NFA) based Inverted OPVs. High-performance NFA inverted OPVs incorporating solution processed metal oxide hole selective contact (HSC) providing similar PCE and light stability performance to that achieved with the commonly used thermally evaporated MoO3 HSC will be presented [4]. Furthermore, the presentation will highlight the systematic understanding of the relationship between doped metal-oxide and Ag nanowires (NWs) interfaces that allowed the development of Ag-NWs based bottom transparent electrodes for inverted configuration OPVs with simplified processing [5]. Finally, laser printed nanoparticle-based metal grids for the development of efficient ITO-free Inverted OPVs with up-scalability perspectives will also be presented [6].
1.2-O3
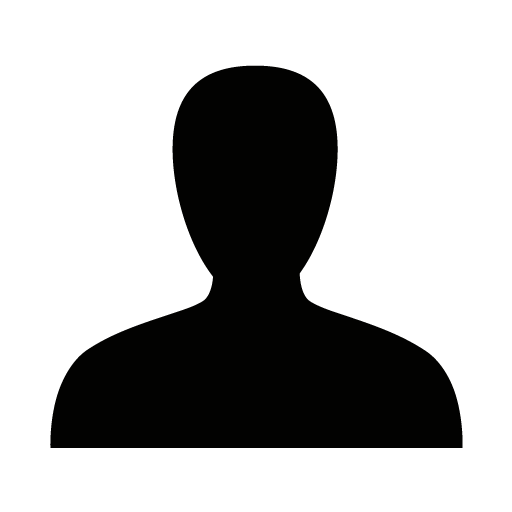
ZnO has been widely used as an electron transport layer (ETL) in organic photovoltaics (OPVs) to facilitate photo-generated electrons extraction due to its appropriate band alignment with various active absorbing layers. Typically, ZnO ETLs are prepared by solution-based techniques such as spin coating. However, with spin coating, it is difficult to control the oxygen stoichiometry, which has great impacts on the defects formation and hence materials properties of ZnO. For example, carrier mobility and optical transparency would affect charge transport and light utilization of the active layer. Moreover, surface defects at the interface between ZnO and the active layer could have great impacts on the stability of the devices.
In this work, we deposited ZnO thin films by radio frequency magnetron sputtering and studied the effects of deposition conditions on the materials properties of the ZnO. Furthermore, the sputtered ZnO were integrated in OPVs with a non-fullerene acceptor-based absorber, PM6:Y7. Our results show that sputtered ZnO on indium tin oxide (ITO) coated glass are polycrystalline and have high optical transparency of >85% in the visible region, which is comparable to a bare ITO glass substrate. Substrate temperature, oxygen flow rate ratio of the sputtering gas ([O2]/[Ar+O2]) and thickness of the ZnO all have significant effects on the OPV device performance. The roles of oxygen stoichiometry on OPV device performance were investigated by photoemission spectroscopy and J-V measurements. Our results indicate that a higher [O2]/[Ar+O2] effectively reduces the concentration of oxygen-related defects, resulting in improved device performance. Utilizing a two-step sputtering process to suppress interfacial defects, solar cells using optimized sputtered ZnO deliver a higher power conversion efficiency than using spin-coated ZnO. Lifetime measurements under controlled environments were carried out to evaluate the stability of the devices. The difference in stability is explained by the ZnO/PM6:Y7 interfacial quality, and can be related to the difference in defect concentration at the ZnO surface.
In summary, we have investigated the effects of different sputtering conditions of ZnO ETLs on PM6:Y7 device performance. Devices using optimized sputtered ZnO ETLs outperform those using spin-coated ZnO ETLs. Moreover, our results suggest that the tuning of ZnO surface defects through the manipulation of oxygen stoichiometry plays a crucial role on the interfacial quality between ZnO ETL and the active layer, which in turn can be used to optimize the interface stability and device lifetime. Our findings highlight the potential of sputtered ZnO as a promising ETL in highly efficient non-fullerene acceptor based organic photovoltaics.
1.2-O4
This research involved the creation via the coaxial electrospinning process of flexible core/shell nanofibrous mats with high porosity that can serve as alternative porous structures to the brittle porous TiO2-based photoelectrodes currently in use. The core was made from polyethylene terephthalate (PET), while the shell consisted of a PET-Titanium dioxide (PET-TiO2) nanocomposite. Two different shapes of TiO2 (nanobars and nanorhombics) were synthesized via the solvothermal method and then incorporated at various concentrations (0-20 wt.%) into the shell layer of the nanofibers to enhance their optical, thermal, and mechanical properties. The study investigated the surface morphology, porosity, specific surface area, thermal stability, dye adsorption, optical, and mechanical properties of the developed nanofibrous mats. Particular emphasis was placed on understanding the impact of solvent miscibility and solvent composition on the morphology of the fibrous mats. Various characterization techniques confirmed the successful incorporation of TiO2 nanoparticles into the shell layer of the nanofibers. It was observed that adding up to 15 wt.% TiO2 within the shell layer enhanced light harvesting and shifted the UV-Visible absorption edge of the nanofibrous mats to larger wavelengths. Moreover, PET/(PET-TiO2) core/shell nanofibrous mats obtained with nanobars TiO2 showed higher thermal stability due to a better TiO2/PET interfacial interaction. Photoluminescence results confirmed the efficient charge transfer between TiO2 nanoparticles and PET with a minimum recombination of the photogenerated charges. Furthermore, the high porosity of the coaxial electrospun mats, ranging from 75% to 81%, had a positive impact on the diffusion of dye molecules and their subsequent adsorption on the surface of the mats.
Finally, we believe that this detailed study on PET-TiO2 core-shell nanofibrous structures provides new insight for further development of optimum DSSC photoanode microstructure at low temperature and contribute towards achieving efficient flexible DSSCs.
2.1-I1
Jie Min obtained his PhD degree from the Friedrich-Alexander University Erlangen-Nurnberg in 2015. After obtaining his PhD degree, he worked as a Postdoctoral Fellow at the Institute of Materials for Electronics and Energy Technology, Erlangen, Germany (2016–2017). In 2017, he joined Wuhan University as a full professor. His current research interests relate to the reduction of the efficiency-stability-cost gap of organic photovoltaics. He also aimed to explore the emerging applications of building integrated photovoltaics. For more information, please see the lab website: http://jie min.whu.edu.cn/.
The performance optimization of organic solar cells is influenced not only by the design of the molecular structures but also by the regulation of the blend morphologies. Currently, there is a well-established understanding of how material design can regulate energy levels, film absorption, carrier mobility, and molecular crystallinity, leading to efficient device performance through the aggregation regulation of active layers. However, there is a lack of effective methods and investigations on the regulation of the metastable state of the active layer, the analysis of degradation mechanisms, and the improvement of operational stability. At present, organic solar cells are not only facing the basic problem of unclear metastable decay mechanism, but also facing the important challenge of synergistic development of "efficiency and stability". The development of physical and chemical methods of metastable regulation is an important breakthrough point for future work. On the basis of levelized cost of electricity analysis, this report will briefly indicate the performance requirements of organic photovoltaic materials and a brief analysis of performance improvement strategies and introduce the metastable morphology characteristics of active layer. By analyzing and revealing the attenuation mechanism of metastable morphology, combined with the intrinsic characteristics of small molecule materials, this report is used to understand the morphologic changes of the active layer under light/thermal stress, and explore the physical attenuation dynamics. Finally, the recent progress made by our research group in regulating the metastable state through physical and chemical methods, as well as the coupling strategies employed. This report aims to provide theoretical support for a deeper understanding of the mechanisms behind metastable morphology attenuation, and to offer valuable references for fellow researchers in the field.
2.1-O1
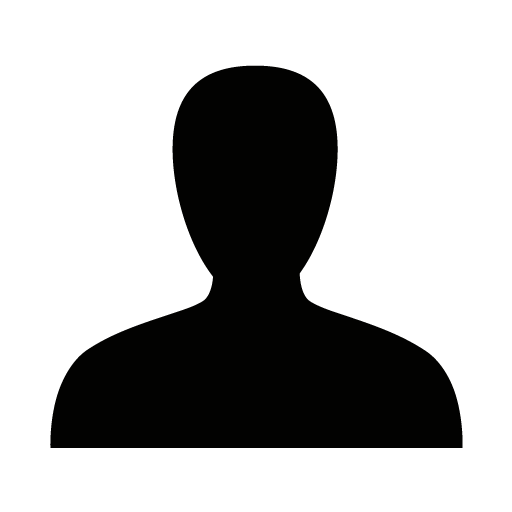
Abstract
Recently, non-fullerene acceptor based organic solar cells show a significant improvement in power conversion efficiency. However, rapid degradation occurs under illumination, particularly when photocatalytic metal oxide electron transport layers are used in these devices.[1,2] We introduced vitamin C (ascorbic acid) into the organic solar cells as a photostabilizer and systematically studied its photostabilizing effect on inverted PBDB-T:IT-4F devices. The presence of vitamin C as an antioxidant layer between the ZnO electron transport layer and the photoactive layer strongly suppressed the photocatalytic effect of ZnO that induces NFA photodegradation. Upon 96 h of exposure to AM 1.5G 1 Sun irradiation, the reference devices lost 64% of their initial efficiency, while those containing vitamin C lost only 38%. The UV–visible absorption, impedance spectroscopy, and light-dependent voltage and current measurements reveal that vitamin C reduces the photobleaching of NFA molecules and suppresses the charge recombination. This simple approach using a low-cost, naturally occurring antioxidant, provides an efficient strategy for improving photostability of organic semiconductor-based devices.
2.1-O2
The synthesis of carbon nano-rings and related structures opens a new field in the chemistry of cyclic conjugated systems with structural, dynamic and optical properties different from their counterparts, the linear conjugated systems.They possess unique zise-dependent optoelectronic properties that make them ideal candidates for use in nanoelectronics and photovoltaics. For this reason, the simulation of photoinduced processes is a substantial and complementary contribution to a better understanding and control of these systems.
I will present the results of our study of the structure-dynamics-optical properties relationship in carbon nano-rings and other cyclic conjugation systems. We performed the simulation of photoinduced dynamics in them. It was possible to find quantum confinements, electronic delocalization, thermal fluctuations, structural deformations and vibrational couplings during the non-radiative relaxation process, which are responsible for the changes observed in the optical properties.
The correct description of these processes requires atomistic simulations of non-adiabatic molecular dynamics (i.e., more than the Born-Oppenheimer approximation) considering multiple coupled excited electronic states. Using the NEXMD package it is possible to perform the calculation of the electronic structure, “on the fly”, i.e., the energies, energy gradients and non-adiabatic
couplings of the different excited electronic states are calculated at each step of the dynamics. In addition, nuclear propagation is performed using the hybrid classical-quantum hybrid method of quantum jumps. This method identifies the electric couplings during nuclear propagation.
2.1-I2
Morten Madsen, Professor wsr at the University of Southern Denmark, SDU NanoSYD.
My field of expertise is thin-film growth, integration and devices for energy conversion and storage applications. In 2010-2011, I worked with high performance transistors from III-V nanoscale membranes at the Javey research lab, UC Berkeley, California. In 2011, I established the OPV group at SDU NanoSYD, where we work on improving the performance and stability of organic and hybrid solar cells, including thin film synthesis, metal oxide interlayers and interfaces, organic and hybrid active layers as well as film and device degradation. Since 2016, we also have a focus on device up-scaling through Roll-to-Roll (R2R) printing technology at the SDU R2R facility. Vist out site for more details:
https://www.sdu.dk/en/om_sdu/institutter_centre/c_nanosyd/forskningsomrader/organic+solar+cells
tbc
2.1-O3
The efficiency of poly(3-hexylthiophene) (P3HT) – nonfullerene acceptor (NFA) based bulk heterojunction (BHJ) solar cells lacks considerably behind many other polymer donor:NFA systems. For reasons which are yet incomprehensible. Here, we report on a series of P3HT:NFA solar cells, and elucidate the origin of performance losses in terms of the photophysical processes. It is a matter of fact that the interfacial ionization energy (IE) offset is a critical parameter in NFA-based blends in determining the efficiency of the exciton-to-charge transfer (CT) state conversion. We show that while large IE offsets in excess of >0.9 eV still facilitate complete exciton quenching, the device internal quantum efficiency (IQE) is limited by geminate and / or non-geminate recombination processes in P3HT-based photoactive blends. Our finding shows a drop in IQE when the diagonal bandgap of the photoactive blend i.e the difference between the IE of the donor and the electron affinity (EA) of the acceptor is small irrespective of the IE offset. Understanding the relationship between the IE offsets, EA offsets at the interface of donor and acceptor materials, and the performance of organic solar cells (OSC) could improve the charge generation efficiency. Thus, enables us to understand the relation between small diagonal bandgap and the decrease of the IQE in energy gap law framework.
3.1-I1
Mariano Campoy Quiles´s research is devoted to the understanding and development of solution processed semiconductors for energy and optoelectronic applications. He and his team have built substantial research efforts in two application areas, solar photovoltaic (light to electric) and thermoelectric (heat to electric) energy conversion based on organic and hybrid materials. He studied physics at the Univesity of Santiago de Compostela, obtained his PhD in experimental physics from Imperial College London, and since 2008 he leads his team at the Institute of Materials Science of Barcelona.
Compared to single junction solar cells, multi-junction architectures can harvest a wider range of the sun´s spectrum while reducing thermalization losses, thus potentially leading to larger efficiencies [1, 2]. Regardless of the specific geometry, i.e. stack, spectral splitting or rainbow, material systems with band gaps wider and narrower than the single junction optimum should be developed to fully exploit the multi-junction concepts [2]. Importantly, the specific materials combination, as well as device parameters (e.g. thickness) are strongly correlated in a multiparametric optimization problem.
In this contribution, we will show our current strategy to tackle this challenge: combining high throughput material screening methods [3] with a spectrum on demand light source [4]. In particular, we focus on materials with narrow bandgap, for which we have screened more than 15 systems, and wide bandgap for which we investigated 9 material combinations and two solvents. Then, we use the best systems to build Rainbow solar cells, i.e. an in-plane spectral splitting geometry in which a series of sub-cells are placed next to each other laterally, and illuminated through an optical component that splits the incoming white beam into its spectral components, thus matching local spectrum and absorption for each sub-cell [5].
[1] D. CarloRasi and R. A. J. Janssen, “Advances in Solution-Processed Multijunction Organic Solar Cells,” Advanced Materials, 31 (2019) 1806499.
[2] I. M. Peters et al, “Practical limits of multijunction solar cells”, Prog. In Photovolt. 31 (2023) 1006.
[3] A. Harillo-Baños et al, “Efficient Exploration of the Composition Space in Ternary Organic Solar Cells by Combining High-Throughput Material Libraries and Hyperspectral Imaging”, Adv. Ener. Mater., 10 (2020) 1902417.
[4] M. Casademont-Viñas et al “Spectrum on demand light source (SOLS) for advanced photovoltaic characterization”, Rev. Sci. Instrum. 94 (2023) 103907.
[5] M. Gibert-Roca et al, “RAINBOW Organic Solar Cells: Implementing Spectral Splitting in Lateral Multi-Junction Architectures” Adv. Mater (2023) 2212226.
3.1-I2
Technological deployment of organic photovoltaics (OPV) requires improvements in device light-conversion efficiency and stability while keeping material costs feasible. Non-fullerene small molecule acceptors emerged as a new horizon pushing the boundaries of OPV and bring them back to competition with other solution processed photovoltaics with unique properties such as semitransparency and power per weight. The power conversion efficiency of OPV has reached 19% in just a couple of years after replacing fullerene alternatives. Non fullerene acceptors are inherently more stable than fullerene derivatives. However, they do still suffer from long term photo and thermal stabilities. There is an understanding gap where we look into acceptors and donot structure-proprty relationships to understand the performance-stability relationship.
In this talk, I will talk how NFAs exceeded the limits of fullerenes and share approaches to overcome photo and thermal stability issues. Finally, I will share state-of-the-art activities on transparent OPV and their scale-up and deployment in potential areas such as greenhouses.
3.1-O1
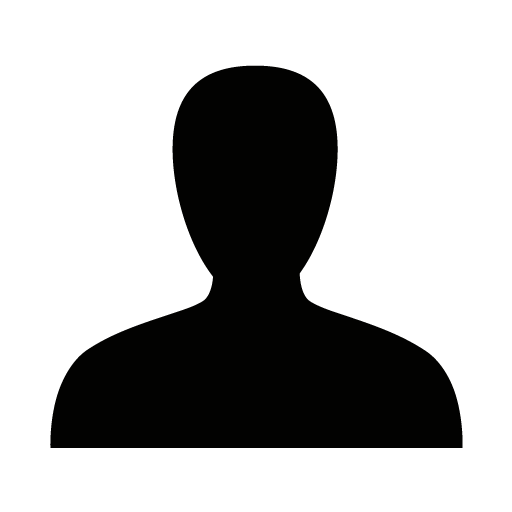
Recent advancements in Organic Solar Cells (OSCs) have led to peak efficiencies of up to 19%, primarily due to the development of novel organic molecules for active and transport layers. Despite this progress, OSC technology still encounters commercialization challenges. These are largely attributed to the extensive range of potential materials and the intricate interplay between their structures and properties, which complicates efficiency predictions. Addressing this, the materials science field is increasingly utilizing machine learning (ML) to decipher structure-property correlations.
Our research addresses this challenge by exploring the relationship between the electrical properties of OSCs, such as Power Conversion Efficiency (PCE) and Fill Factor (FF), and their structural features, including band center position and crystallinity. These are determined through the deconvolution of UV-Visible (UV-VIS) spectroscopy data. By employing Gaussian Process Regression, we have devised a method that predicts solar cell efficiency using only UV-VIS spectra. This approach potentially eliminates the need for evaporation and IV measurements, streamlining the process and reducing costs.
To ensure predictive accuracy, we compiled a dataset of 25 donor-acceptor combinations, yielding a total of 200 cells with intentional variations in film thickness and crystallinity. We ensured exceptional homogeneity in layer preparation using the Autonomous Materials and Device Application Platform (Amanda) 1. Analysis of this dataset enables us to foresee the performance of new material combinations. This significant breakthrough in OSC research could expedite the development of efficient and commercially viable solar cell materials.
3.1-O2
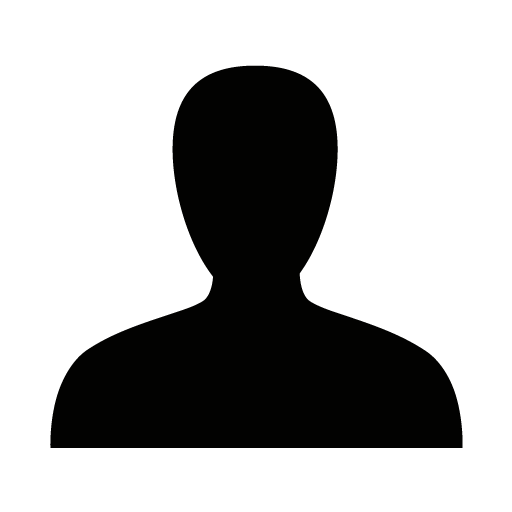
Implementation of 2D materials in organic photovoltaics (OPV) is one of the promising routes to modify fundamental device properties, and potentially improve device efficiency and stability due to their unique properties such as tunable electronic structure, high charge carrier mobility and high optical transparency. For MXenes, a family of 2D transition metal carbides and nitrides, the ability to tune work function via surfaces termination routes have made it especially promising as contact layer material in solar cell devices. In this work, we employ such 2D MXene, Ti3C2Tx, in electron transport layers (ETL) to develop composite 2D based ETL, and demonstrated their high performance for non-fullerene acceptor (NFA) based OPV. The composite 2D-ETL based OPV resulted in robust ETL-organic interfaces with efficient electron extraction and transport properties for high device performance (~14% using green solvents), and importantly, significantly prolonged device lifetime when compared to conventional 2D-free ETL based OPV. The integration of the 2D MXene interlayers in OPV is explored in terms of morphological, optical, and electrical properties along with ISOS-L device lifetime measurements. Importantly, photoelectron spectroscopy results provide insights into the modified surface defect state concentration on the ETL surface, which can directly be related to the improved device stability. The work demonstrates the unique potential of MXene as contact layer material in high performance organic photovoltaics with prolonged device lifetimes.
3.2-O1
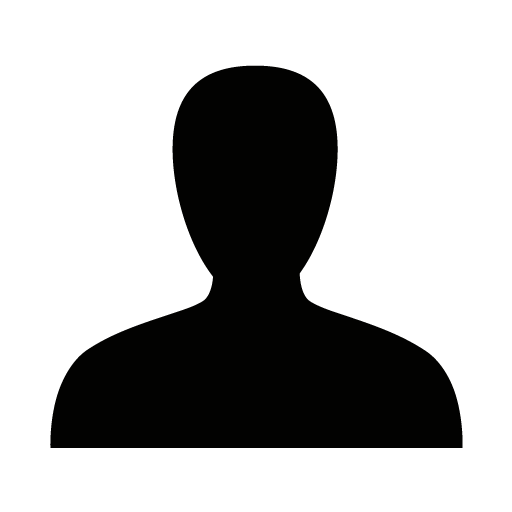
While multi-junction geometries have the potential to boost the efficiency of organic solar cells, the experimental gains yet obtained are still very modest.[1,2] This work proposes an alternative spectral splitting device concept in which various individual semiconducting junctions with cascading bandgaps are laid side by side, thus the name RAINBOW. Each lateral sub-cell receives a fraction of the spectrum that closely matches the main absorption band of the given semiconductor. Here, simulations are used to identify the important material and device properties of each RAINBOW sub-cell. Using the resulting design rules, three systems are selected, with narrow, medium, and wide effective bandgaps, and their potential as sub-cells in this geometry is experimentally investigated. With the aid of a custom-built setup that generates spectrally spread sunlight on demand, the simulations are experimentally validated, showing that this geometry can lead to a reduction in thermalization losses and an improvement in light harvesting, which results in a relative improvement in efficiency of 46.6% with respect to the best sub-cell. Finally, a working proof-of-concept monolithic device consisting of two sub-cells deposited from solution on the same substrate is fabricated, thus demonstrating the feasibility and the potential of the RAINBOW solar cell concept.
3.2-O2
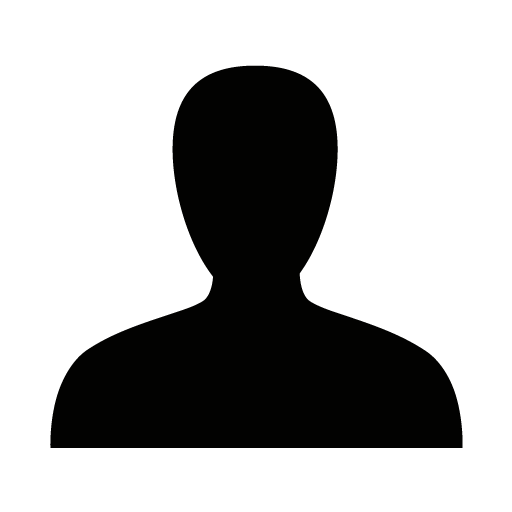
Organic Photovoltaics have seen a significant increase in power conversion efficiency (PCE) recently, approaching 20% on small lab cells. However, the record efficiencies on module level are still substantially lower (~30%, relatively). Hence, it is necessary to perform focused upscaling research to reduce the gap between small-area cells and large-area modules. In this work, we present successful upscaling of devices based on PM6:Y6-C12:PC61BM, processed in air from non-halogenated solvents, from cells (0.1 cm2) to large-area modules (>200 cm2) with barely any performance losses. High PCEs of >14% on total module area (>15% with respect to active area) are achieved by utilizing accelerated blade coating to eliminate thickness gradients in the layers. Inactive (interconnect) areas are significantly reduced by high-resolution short-pulse (nano- and femtosecond) laser patterning to achieve high geometric fill factors exceeding 97%. The results are confirmed by independent certified measurements.Organic Photovoltaics have seen a significant increase in power conversion efficiency (PCE) recently, approaching 20% on small lab cells. However, the record efficiencies on module level are still substantially lower (~30%, relatively). Hence, it is necessary to perform focused upscaling research to reduce the gap between small-area cells and large-area modules. In this work, we present successful upscaling of devices based on PM6:Y6-C12:PC61BM, processed in air from non-halogenated solvents, from cells (0.1 cm2) to large-area modules (>200 cm2) with barely any performance losses. High PCEs of >14% on total module area (>15% with respect to active area) are achieved by utilizing accelerated blade coating to eliminate thickness gradients in the layers. Inactive (interconnect) areas are significantly reduced by high-resolution short-pulse (nano- and femtosecond) laser patterning to achieve high geometric fill factors exceeding 97%. The results are confirmed by independent certified measurements.
3.2-I1
RnD manager at Epishine AB
www.epishine.com
The pursuit of more efficient buildings is driving digitalization, leading to a rapidly growing need for connected sensors. These sensors typically rely on batteries, necessitating costly maintenance to replace them every few years and contributing to a significant environmental impact.
By harnessing ambient energy, the lifespan of batteries can be extended, and in many cases, batteries may become unnecessary. Circumventing the issues with batteries will enable much easier deployment of distributed sensing, facilitating data collection that, in turn, yields a more energy-efficient society. Light is a primary source of ambient energy in buildings, and organic solar cells can be tuned to harvest that light energy with high efficiency. Examples of applications for connected devices suitable for light energy harvesting include electronic shelf labels, asset tracking labels, and consumer electronics such as remote controls.
Epishine, an innovative Swedish company, has developed a scalable manufacturing process for organic solar cells using roll-to-roll printing and lamination technologies. This technology puts Epishine in a favorable position to address the increasing demand for high-performance indoor solar cells. In this presentation, I will provide an overview of Epishine's technology and manufacturing process, as well as present some of Epishine’s internal research efforts based on our unique approach to producing OPV [1]
1.1-I1
Excited triplets of organic molecules have the interesting features of long excitation lifetime and spin polarization. Among various functions of excited triplets, our recent works on the development of chromophore assemblies exhibiting photon upconversion and singlet fission will be presented.
Triplet-triplet annihilation based photon upconversion (TTA-UC) from visible light to UV light is useful for various photocatalysts including artificial photosynthesis, but its low efficiency (<10%) has been a problem for several years. We have developed a chromophore pair that exhibits a high TTA-UC efficiency of over 20%, which is two times higher than the previous record for Vis-to-UV TTA-UC. The Ir coumarin complex shows strong absorption in the visible range and relatively weak absorption in the UV range and does not quench the upconverted UV emission. By combining the Ir coumarin complex with TIPS-naphthalene, we have achieved a high TTA-UC efficiency of over 20% and a very low threshold excitation intensity Ith below solar irradiance.1,2 Furthermore, we have recently developed bicontinuous porous monoliths showing visible to UV TTA-UC and will introduce the details in the talk.
We are also interested in the spin degree of freedom of singlet fission in the context of quantum information science (QIS).3 We developed chromophore assemblies and dimers exhibiting singlet fission, and successfully observed quantum coherence of quintet triplet dimer state at room temperature for the first time. We found that the suppressed chromophore dynamics is crucial for achieving both of quintet formation and quantum coherence.
1.1-I2
Photon upconversion offers the prospect of combining low energy near-infrared radiation to make high energy photons in the visible wavelengths. The goal is to improve photovoltaics and introduce new bioimaging techniques. In order to harness the intrinsic ability of colloidal semiconductor nanocrystals to couple strongly with light, it is important to efficiently outcouple energy from photoexcited quantum dots (QDs), much like how nature uses molecular antennas to direct light during photosynthesis. This talk focuses on the organic-inorganic interface for triplet-fusion based photon upconversion, where orbital overlap between the QD donor and molecular acceptor is critical for efficient energy transduction. In the past 8 years, we have learnt a great deal about how the electronic interactions between chalcogenide nanocrystals and acene conjugated molecules affect the photon upconversion quantum yield. We apply the lessons learnt to extending photon upconversion to silicon nanocrystal light absorbers, addressing synthetic challenges with invaluable insight from time-resolved spectroscopy.
1.1-I3
Quantum dot-organic hybrids have become popular as triplet-photon converting systems, for example as triplet sensitizers for triplet-triplet annihilation photon upconversion and photon multiplication based on singlet fission.[1] The organic ligand in such hybrid structures plays a crucial role in mediating triplet exciton transfer between the organic molecules and inorganic quantum dots in solution, and many studies have studied the intricate details of triplet mediation via the ligand.[2] Here I will higlight another aspect of the ligand, namely as a directing agent in bulk organic-semiconductor/quantum-dot blend films. These blends, comprising organic semiconductors and inorganic quantum dots are relevant for many optoelectronic applications and devices. However, the individual components in organic-QD blends have a strong tendency to aggregate and phase separate during processing to form films, compromising both their structural and electronic properties. Here, I will explain how a suitable organic ligand can achieve well-dispersed inorganic-organic blend films, as characterised by X-ray and neutron scattering and electron microscopy. As proof-of-concept I will show two examples of these films applied to singlet fission based photon multiplication[3] and triplet-triplet annihilation based photon upconversion. Due to the optimal blend morphology triplet excitons can be transferred with near unity efficiently across the organic-inorganic interface, while the TIPS-tetracene films maintain efficient SF (190% yield) in the organic phase, resulting in 95% of the triplet excitons generated via SF in the organic phase being harvested by the QDs. By changing the relative energy between organic and inorganic components yellow upconverted emission is observed upon 790 nm NIR excitation. Overall, this talk will exemplify a highly versatile approach to overcome long-standing challenges in the blending of organic semiconductors with QDs with relevance for many optical and optoelectronic applications.
2.1-I3
From 2019, A.M. serves as Associate Professor in Condensed Matter Physics at Department of Materials Science. His research is focused on the development of advanced hybrid functional nanomaterials for applications in photonics and theranostics in collaboration with several national and international universities and research institutes. He started his research by working on hybrid organic/inorganic light NIR emitters based on lanthanides ions and photonic crystals for lighting and telecom, in the framework of several national and international project and networks. The topic of the current research is the design and study of advanced materials and nanostructured materials for photon managing and scintillation applications. The experimental activity is centered on CW and ultrafast TRPL photoluminescence spectroscopy, transient absorption spectroscopy, confocal imaging, IR and FT-IR spectroscopy to tackle both fundamental and applicative aspects aimed at the development of materials to implemented real-world technologies.
The conversion of low-energy light into photons of higher energy based on sensitized triplet–triplet annihilation (sTTA) upconversion in bicomponent systems is emerging as the most promising wavelength-shifting methodology to recover sub-bandgap solar photons, because it operates efficiently at excitation powers as low as the solar irradiance. Excellent efficiencies have been obtained in liquid environments as well as in prototype upconversion enhanced solar cells, but the research is still focused on the realization of affordable solid states upconverters suitable to be implemented in current solar technologies. We show here that controlled confinement of the upconverting materials in nanostructured or nanosized materials can improve the material performance at low powers. [1] [2] [3] [4] The result presented will show demonstrate how this strategy can represent a crucial guideline for the future development of upconverting photonic devices operating at subsolar irradiances suitable for technological implementation.
[1] Mattiello et al. Diffusion-free intramolecular triplet–triplet annihilation in engineered conjugated chromophores for sensitized photon upconversion. ACS Energy Letters 7 (8), 2435-2442 (2022)
[2] A.Ronchi & A. Monguzzi; Developing solid-state photon upconverters based on sensitized triplet–triplet annihilation. J. Appl. Phys. 129 (5), 050901 (2021)
[3] Saenz et al. Nanostructured Polymers Enable Stable and Efficient Low‐Power Photon Upconversion, Adv. Funct. Mater. 31, 1, 2004495 (2021)
[4] Meinardi et al. Quasi-thresholdless photon upconversion in metal–organic framework nanocrystals, Nano Letters 19 (3), 2169-2177 (2019)
2.1-I1
The upconversion of light has numerous potential applications ranging from photocatalysis, photovoltaics, imaging, and advanced manufacturing. The strategies being investigated to achieve this include energy transfer upconversion in lanthanide nanoparticles, and sensitized triplet fusion, also known as photochemical upconversion or triplet-triplet annihilation upconversion (TTA-UC). The latter involves the generation of triplet excited states by a sensitizer and their subsequent transfer to an emitter. Emitter triplets annihilate (fuse) to generate an emissive singlet state from which a higher energy photon is emitted. This strategy is spectrally adaptable, and high efficiencies are routinely achieved in solution, approaching the quantum yield ceiling of 50%.
Recently, there has been progress in translating triplet fusion upconversion into the solid state, which is desirable from a device engineering standpoint.[1] There are many possible solid-state device architectures. Broadly these can be categorized into materials wherein the sensitizer and emitter are evenly distributed, and heterogeneous devices where triplet generation is spatially separated from triplet fusion. The latter strategy is attractive, as the upconverted singlet state is protected to an extent from Förster resonance energy transfer back to the sensitizer. A simple concept would be to lay down a monolayer of sensitizer overlaid with a thin film of emitter. However, such devices suffer from poor ligh absorption.
In dye-sensitized solar cells (DSSCs), the problem of poor light absorption by a monolayer of chromophores is addressed with a nanostructured metal oxide support, such that incoming light passes through a multitude of chromophore-bearing interfaces. Indeed, DSSCs incorporating TTA have been demonstrated.[2] As there are many steps in photochemical upconversion, it is desirable to investigate certain aspects in isolation. To concentrate on the solid-state sensitization process, here we present a strategy where the sensitizer is chemisorbed on a nanoporous Al2O3 support, but the emitter remains in solution.[3]
Using time-resolved spectroscopies, we show that the sensitizer triplets are long-lived, do not suffer significant aggregation-induced quenching and can be efficiently transferred to the emitter chromophores. We demonstrate devices with internal upconversion quantum yields as high as 9.4%, which is 19% of the maximum achievable value and 38% of what can be achieved with the diphenylanthracene emitter. This shows that using dyes bound to nanoporous Al2O3 films is a viable strategy to sensitize upconversion within a solid state material, and bodes well for future development with solid state emitters. Initial work on liquid-chromophore and fully solid state systems based on the same Al2O3 films will also be presented.
2.1-I2
Seiichiro Izawa is an associate professor in Tokyo Institute of Technology in Japan, since 2023. He received his Ph.D. from Department of Applied Chemistry in the University of Tokyo in 2015 and worked at RIKEN in Japan and University of California, Santa Barbara as a postdoctoral fellow, and Institute for Molecular Science in Japan as an assistant professor. His research interests are optoelectronic properties at organic semiconductor interface for efficient organic electronics devices such as photovoltaics and light-emitting diodes.
Among the three primary colors, blue emission in organic light-emitting diodes (OLEDs) are highly important but very difficult to develop. OLEDs have already been commercialized; however, blue OLEDs have the problem of requiring a high applied voltage due to the high-energy of blue emission. Herein, an ultralow voltage turn-on at 1.47 V for blue emission with a peak wavelength at 462 nm (2.68 eV) is demonstrated in an OLED device. This OLED reaches 100 cd/m2, which is equivalent to the luminance of a typical commercial display, at 1.97 V. Blue emission from the OLED is achieved by the selective excitation of the low-energy triplet states at a low applied voltage by using the charge transfer (CT) state as a precursor and the triplet-triplet annihilation, which forms one emissive singlet from two triplet excitons. We found that the essential component for efficient blue emission is a smaller energy difference between the CT state and triplet exciton, accelerating the energy transfer between the two states and achieving the optimal performance by avoiding direct decay from the CT state to the ground state. Our study demonstrates that the developed OLED allows for a much longer operation lifetime than that from a typical blue phosphorescent OLED because the blue emission originates from a stable low-energy triplet exciton that avoids degrading the constituent materials.
1.1-I1
Haowei Huang graduated and received his Ph.D. in Bioscience Engineering from the Centre for Membrane Separations, Adsorption, Catalysis and Spectroscopy for Sustainable Solutions, KU Leuven (Belgium), in 2020, where he studied metal halide perovskite photocatalysis. After that, he continued his postdoctoral research with financial support from the Belgium government (FWO) with Prof.Maarten Roeffaers at KU Leuven and Prof. Peidong Yang at UC Berkeley. His research focuses on the development of optically active materials and their application on photo(electro)catalysis.
The development of green, sustainable, and economical chemical processes represents a cornerstone challenge within chemistry today. Semiconductor heterogeneous photocatalysis is currently utilized within a wide variety of societally impactful processes, spanning reactions such as hydrogen production and CO2 conversion, to the organic transformation of raw materials for value-added chemicals. Metal halide perovskites (MHPs) have recently emerged as a new promising class of cheap and easy to make photocatalytic semiconductors, though their unstable ionically bound crystal structure has thus far restricted widespread application. Recently years, we examine the issues hampering MHP-based photocatalysis and proposal a general approach being taken to achieve promising and stable photocatalytic reaction environments. Specifically, we utilize relatively low-polarity solvents such toluene and trifluorotoluene for CO2 photoreduction and organic transformations over MHPs. However, the effectiveness of single MHP photocatalysts is relatively poor. We further introduction of a second component to form a heterojunction, including Schottky, type II, and Z-scheme heterojunction, to accelerate carrier migration and boost reaction rates, thus increasing the photoactivity.
1.1-O1
Lead halide perovskites (LHPs) have demonstrated enormous potentials in a wide range of application including optoelectronic devices and photocatalysis due to their exceptional optical and electronic properties. However, long-term stability and toxicity of LHPs are the main limiting factor towards their practical application. In this view, lead-free halide double perovskites (DPs), are under spotlight due to their superior material stability and attractive optoelectronic properties. Nevertheless, relatively larger band gap (> 4eV) of Cs2AInCl6 (A = Ag Na) DPs materials are the main bottlenecks towards their light harvesting application including photocatalysis.[1,2] Moreover, the severe charge recombination and presence of fewer catalytic active sites are considered as main limiting factor towards efficient photocatalytic CO2 reduction.[3] The doping and compositional engineering of Cs2AInCl6 (A = Ag, Na) DPs are under spotlight due to their dopant-induced extended light harvesting abilities and enhanced self-trapped excitons (STEs) emission for a wide range of applications including optoelectronic devices and photocatalysis.[1,2,4,5] Furthermore, the catalytic performances of lead-free DP NCs can be further improve by coupling them with two-dimensional (2D) nanosheet (NSs) with desirable energy offset. The type II heterojunction between DP NCs and 2D NSs can enhance the charge separation and inhibit the charge recombination. It is well established that 2D ultrathin g-C3N4 NSs possess large surface area, rich density of catalytic active sites, and superior charge transport. All these features are advantageous for photocatalytic CO2 reduction. In particular, the interface between Cs2AgBiBr6 DP NCs/ g-C3N4 2D NSs would significantly facilitate the charge transfer and separation of photogenerated excitons.
In this talk, I will present the colloidal synthesis of iron-doped Cs2AInCl6 (A = Ag, Na) DP NCs, which resulted in a significant extension of absorption edge into the visible part of spectrum. Furthermore, I will discuss how iron doping allows precise tuning of the optical band gap and electronic band structures of the resulting DP NCs and their application in photocatalytic CO2 reduction. Next, I will also explore our recent results on multifacet spheroidal Cs2AgBiBr6 DP NCs/g-C3N4 NSs heterostructure, including colloidal synthesis, optical properties and their application in visible-light driven photocatalytic CO2 reduction.
1.1-O2
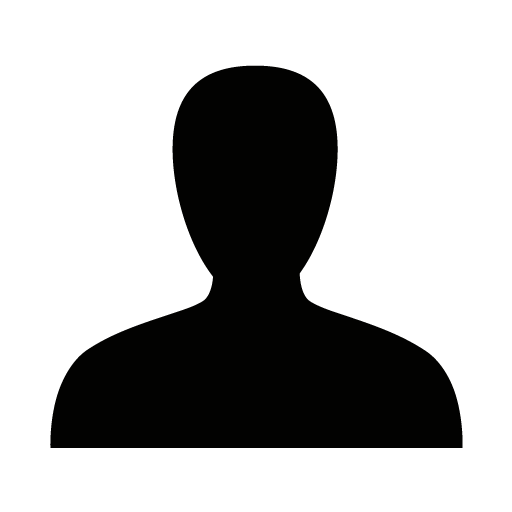
Lead halide perovskite nanocrystals (LHP NCs) are a state-of-the-art light-harvesting material, prized for their outstanding optoelectronic properties—high absorption coefficient, extensive charge carrier diffusion lengths, and low exciton binding energy. Despite achieving remarkable photovoltaic performance with a photoconversion efficiency exceeding 25% within a decade, the transition of LHP to industrial-scale development encounters obstacles due to moisture intolerance and ambient instability [1].
The inorganic/hybrid core of LHP NCs contributes to their unique photophysical properties, while surface ligands play a crucial role in stabilizing and influencing charge transfer dynamics in diverse environments [2,3]. Therefore, understanding the complex NC-ligand interfacial chemistry is vital for achieving colloidal stability in aqueous and polar media.
Our solution involves optimizing hydrophilic and hydrophobic interactions on the LHP NC surface using engineered multidentate ligands. We developed NKE-12, a bolaamphiphilic ligand derived from lysine (K) and glutamic acid (E), featuring multiple cationic (NH3+) and anionic (COO-) anchoring groups. Applied in a ligand exchange treatment on CsPbBr3 NCs, NKE-12 achieved effective surface passivation and water molecule localization away from the inorganic core through hydrophilic interactions [4]. Confirmation of successful ligand exchange was obtained through Fourier transform infrared and X-ray photoelectron spectroscopy. Structural integrity was preserved and validated by transmission electron microscopy and X-ray diffraction patterns. NKE-12 binding interactions on the NC surface were elucidated through multidimensional nuclear magnetic resonance ligand spectroscopy [5].
Deconstructing NKE-12 into NK-12 and NE-12 fragments provided insights into the individual roles of cationic and anionic terminal ends in passivation and solvent phase transfer. The synergistic effect of robust ligand binding and increased hydrophilicity resulted in significant two-week-long water stability for CsPbBr3/NKE-12 NCs compared to oleylamine/oleic acid capped NCs. Enhanced charge separation and transport capabilities observed through electrochemical measurements prompted the exploration of photocatalytic attributes. Water-stabilized CsPbBr3/NKE-12 NCs exhibited excellent photocatalytic activity for acrylamide polymerization, showcasing their potential for diverse applications.
Presently, we are expanding ligand engineering, exploring different multi-ionic ligands to further extend water stability and deepen our understanding of photogenerated charge extraction processes. Our findings on ligand design principles for developing moisture-resistant perovskite NCs hold significant promise for applications in photovoltaics and photocatalysis.
1.1-I2
Over the past decade, metal halide perovskites (MHP, CsPbX3: X = Cl, Br, I) have been widely investigated as promising materials for optoelectronics, achieving a record-breaking efficiency in solar cells.[1] From a fundamental point of view, MHP could be excellent candidates for photocatalysis due to their high photogenerated charge-carrier production and mobility as well as their narrow and tunable bandgap energy.[2] MHP with tuneable bang-gap energy could be obtained through fast substitution of bromide by iodine or chloride (CsPbBr3-yXy : X = Cl, Br, I).[3]
In this presentation, the electronic properties of MHPs were encapsulated by TiO2, and their electronic properties were tuned by anion substitution at room temperature. Then, charge-carrier lifetime and dynamics were investigated in CsPbBr3-yXy along with interfacial electron transfer from CsPbBr3-yXy to TiO2 by means of time-resolved photoluminescence (TRPL) and transient absorption spectroscopy (TAS). The results show that the bandgap engineering and the position of the conduction band and valence band level in both materials are detrimental for optimal interfacial charge transfer. In addition, the optimal bandgap configuration for the most efficient charge injection positively affects the photocatalytic activity. The encapsulation of MHPs by TiO2 did improve the stability for hydrogen generation from aqueous solution.
1.2-I1
Metal halide perovskites (MHPs) are extremely appealing emerging materials for photocatalytically active heterojunctions, thanks to their ability to promote light-driven reactions. However, a comprehensive understanding of photoexcitation and charge transfer mechanisms, as well as predictive models for photocatalytic performances are still missing. We present here a robust method to investigate photocatalytic activity of MHP heterojunctions in a variety of reactions, that combines in synergy a wide array of experimental techniques with advanced computational modelling. For all the studied compounds, we were able to assess a clear link between the photocatalytic activity and optoelectronic properties of the materials, in particular by exploiting ultrafast spectroscopy techniques to study available transitions and carrier dynamics as a function of the perovskite loading and finding a connection with the performance of the material.
More in detail, we studied heterojunctions obtained by combining graphitic carbon nitride (g-C3N4) with different kinds of lead-free perovskites to enhance selected features. We focused first on double perovskite Cs3Bi2Br9, where hydrogen photogeneration rate was successfully controlled by tuning the perovskite loading in the Cs3Bi2Br9/g-C3N4 mixture.[1] We also studied Ge-based layered 2D perovskite and g-C3N4 for light-induced hydrogen evolution: we showed how, thanks to organic cation engineering, perovskites based on 4-phenylbenzilammonium (PhBz), such as PhBz2GeBr4 and PhBz2GeI4, result in hydrogen production with promising air and water stability.[2] Recently, we have extended our study to a double perovskite mixture, namely Cs2AgBiCl6/g-C3N4, used both for solar-driven hydrogen generation and nitrogen reduction, with an activity strongly depending on the perovskite loading.[3] Through advanced spectroscopic investigation and density function theory (DFT) modelling we have identified the perovskite loading that allows the best performance of the heterojunction and, most importantly, accounted for the microscopic processes responsible for the photocatalytic performance. Our results are showing a systematic approach of MHP-based heterojunctions that is of crucial importance to get the ability to engineer and optimize novel materials for photocatalysis.
1.2-O1
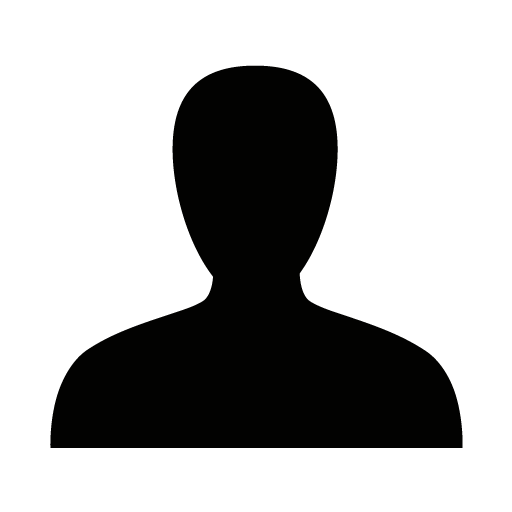
Metal halide perovskites are arising a great interest of the scientific community because of their opto-electronic properties, as the high extinction coefficient, the low exciton binding energy, the excellent charge transport properties and optimal band gap in the visible range that make them good candidates for photocatalytic applications. Among the different perovskite materials, Cs3Bi2Br9 (CBB) is a particularly interesting lead-free halide perovskite that shows excellent stability and has already been used as a visible light-driven photocatalyst in dye degradation experiments. However, the understanding of the interaction between dyes and the CBB and the mechanism underlying the behavior of the CBB in the photocatalytic system still needs to be fully elucidated [1]. Here, the chemical co-precipitation method was successfully applied to synthesize CBB powder that was then used as photocatalyst for the dye degradation. XRD investigation showed that the prepared Cs3Bi2Br9 powder materials undergoes to structural evolution when exposed to different environment (water, isopropanol, air). Namely, we detected a systematic structural evolution of Cs3Bi2Br9 to the kinetically stable non-perovskite BiOBr phase. In addition, such a transformation was found faster in water, where the conversion in BiOBr was already complete within 24 hours, than in isopropanol and in ambient air [2]. On the basis of the proved CBB higher stability in isopropanol, such a solvent has been selected as reaction medium for the heterogenous photocatalytic experiments carried out monitoring the decoloration of structurally different azodyes, cationic Methylene Blue (MB) and anionic Methyl Orange (MO), at different concentration. Preliminarily, the adsorption of each dye on the CBB under dark condition has been spectroscopically monitored to clarify the interaction between dyes and CBB powder samples. Finally, the photocatalytic experiments have been performed monitoring the dyes decoloration as a function of the catalyst conversion of the Cs3Bi2Br9 in BiOBr, finally proving the beneficial effect of BiOBr in the degradation of both types of cationic MB and anionic MO dyes [3].
1.2-O2
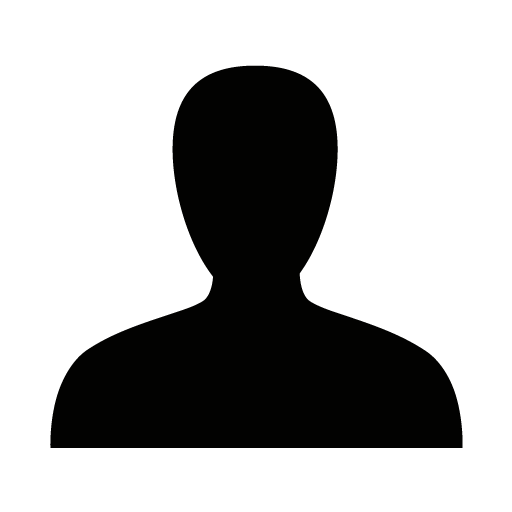
Photocatalytically active heterojunctions based on metal halide perovskites (MHPs) are drawing significant interest for their chameleon ability to foster several redox reactions. The lack of mechanistic insights into their performance, however, limits the ability of engineering novel and optimized materials. Herein, we report on a composite system including a double perovskite, Cs2AgBiCl6/g-C3N4, used in parallel for solar-driven hydrogen generation and nitrogen reduction. The composite efficiently promotes the two reactions, but its activity strongly depends on the perovskite/carbon nitride relative amounts. Through advanced spectroscopic investigation and density function theory modelling we studied the H2 and NH3 production reaction mechanisms, finding perovskite halide vacancies as the primary reactive sites for hydrogen generation, withstanding a positive contribution of low loaded g-C3N4, in reducing carrier recombination. For nitrogen reduction, instead, the active sites are g-C3N4 nitrogen vacancies, and the heterojunction best performs at low perovskites loadings, as the composites maximizes light absorption and reduced carrier losses. We believe these insights are important add-ons towards universal exploitation of MHPs in contemporary photocatalysis.
1.2-I2
Photocatalytic conversion of water and carbon dioxide using solar energy offers a clean solution to the world energy requirements of a sustainable future. Achieving its full potential depends on developing inexpensive photocatalysts that can efficiently absorb solar light and drive separated photoinduced charges to react with water and carbon dioxide. In this talk, I will present recent developments we have achieved in the preparation of inexpensive photocatalyst composites with enhanced charge transfer separation and photocatalytic performance. For example, I will present fast and convenient mechanochemical syntheses of halide perovskite nanocrystals of CsPbBr3 and Cs2AgBiBr6, together with copper-loaded reduced graphene oxide. I will also present our developed antisolvent crystallizations to prepare composites such as Cs2AgBiBr3/bismuthene and Cs3Bi2Br9/g-C3N4, all of which are active in the reduction of CO2 to CO and CH4. Extensive characterizations of these materials have enabled us to gain insights into their physical and charge-transfer properties and relate them to their photocatalytic activity. These characterizations underline the importance of surface area, reactant adsorption, and charge separation to achieve best performances. Some of these composites such as Cs3Bi2Br9/g-C3N4 separate charges via a direct Z-scheme heterojunction, boosting charge separation and photocatalytic conversions. Altogether, these results will contribute to the rational design and application of halide perovskites for solar fuels and chemicals.
1.3-O1
Iván Mora-Seró (1974, M. Sc. Physics 1997, Ph. D. Physics 2004) is researcher at Universitat Jaume I de Castelló (Spain). His research during the Ph.D. at Universitat de València (Spain) was centered in the crystal growth of semiconductors II-VI with narrow gap. On February 2002 he joined the University Jaume I. From this date until nowadays his research work has been developed in: electronic transport in nanostructured devices, photovoltaics, photocatalysis, making both experimental and theoretical work. Currently he is associate professor at University Jaume I and he is Principal Researcher (Research Division F4) of the Institute of Advanced Materials (INAM). Recent research activity was focused on new concepts for photovoltaic conversion and light emission based on nanoscaled devices and semiconductor materials following two mean lines: quantum dot solar cells with especial attention to sensitized devices and lead halide perovskite solar cells and LEDs, been this last line probably the current hottest topic in the development of new solar cells.
Halide perovskite solar cells have revolutionized the photovoltaic field in the last decade. Nevertheless, the two main drawbacks of this system, the use of hazardous Pb and the long term stability, still to be open questions that have not been fully addressed. Beyond perovskite solar cells halide perovskite are outstanding for the development of other optoelectronic device, causing that currently the research with these materials widespread to different optoelectronic fields and photocatalytic applications, where again stability and reduction Pb content still at the center of research effort. In the present talk, we will describe our efforts towards the application of these materials for solar-driven hydrogen production coupled to other processes like organic transformations and waste valorization. We will discuss on the rational design of halide perovskites containing non-critical raw materials towards photoelectrochemical processes, and the importance of extracting basic electronic and optical information to understand the carrier dynamics to maximize the performance and stability of these materials. Moreover, proper interrogation tools are needed to validate their photo(electro)catalytic activity and selectivity. The need for integration of the developed materials into tailored photoelectrochemical devices highlight the urgent need for stabilization strategies to move beyond the proof-of-concept stage to relevant technological developments.
1.3-O2
Dr. Galian received her Ph.D in Chemistry at the National University of Cordoba, Argentina in 2001. Then, she was a postdoc researcher at the Polythecnic University of Valencia, University of Valencia and University of Ottawa. During those years, she has studied photosensibilization processes by aromatic ketones using laser flash photolysis techniques and was involved in photonic crystal fiber/semiconductor nanocrystal interaction projects. In 2007, Dr. Galian came back to Spain with a Ramon y Cajal contract to study the surface chemistry of quantum dots and since 2017 she has a permanent position as Scientist Researcher at the University of Valencia. Her main interest is the design, synthesis and characterization of photoactive nanoparticles and multifunctional nanosystems for sensing, electroluminescent applications and photocatalysis.
Metal halide perovskites nanocrystas (NCs) have promising applications as heterogeneous photocatalysts due to their strong visible-light absorption, band-gap engineering, charge transfer ability and easy recovery. There are several factors that influence the photocatalytic performance, such as NCs sizes, co-catalyst nature, solvent, and atmosphere. Although there are several examples in their use as photocatalyst for the CO2 reduction and H2 generation a more limited information is available for organic chemical transformations. Here, the photocatalytic activity of colloidal CsPbBr3 NCs and Cs3Sb2Br9 NCs for the photoreduction of p-substituted benzyl bromides under visible-light excitation, using a sacrificial electron donor (N,N-diisopropylethylamine or methanol), will be discussed. Interesting the electron donating and electron withdrawing ability of the substituent determines the selectivity of the reaction towards C-C formation or reductive dehalogenation. The role of the organic ligands to drive forward the C-C coupling and the affinity of the benzyl radicals to the metal halide NC surface will be illustrated.
1.3-O3
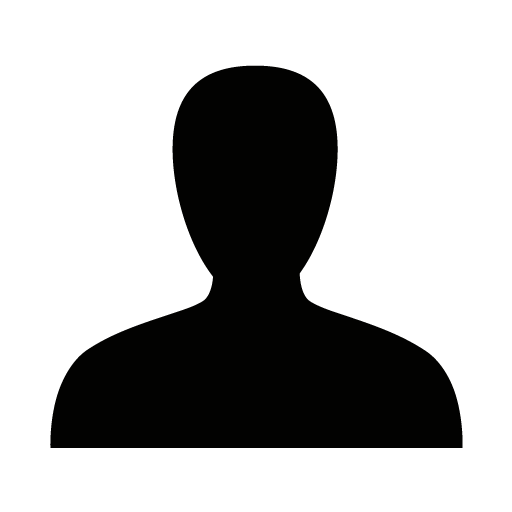
The chemical instability of halide perovskites (HaP) in protic solvents remains the main obstacle to their utilization in photoelectrochemical devices. Despite the implementation of protective strategies for PEC applications[1], [2], systematic studies of (photo)electrochemical processes and the potential corrosion of perovskite/electrolyte interfaces and their impact on energy conversion and stability are still lacking [3].
In this study, we examined the photoelectrochemical behavior of 2D di-phenylethylammonium lead tetra iodide (PEAH2PbI4) thin films as a model system to study fundamental photoelectrochemical processes of metal halide perovskites. We found the use of excess PEAI in solution necessary to stabilize the thin films like by chemical equilibrium and a surfactant effect. Introducing the Fe(CN)6 3-/4- redox couple electroactive species and considering PEAI a supporting electrolyte and stabilization agent [4],[5], we studied photo-electrochemical processes at the semiconductor/electrolyte interface by monitoring the open-circuit potential (OCP). Under illumination, a potential inversion was observed for different equilibrium concentrations of the redox probe. In-situ UV-Vis and XRD analyses provided compelling evidence indicating that the potential inversion, indicating either electron or hole accumulation on the PEAH2PbI4, leads to different photo-corrosion phenomena. Lead iodide, PbI2, as well as a phenylethylamine-lead iodide intercalation compound, (PEA)-PbI2 , are observed as degradation products. Surface photovoltage (SPV) studies revealed that the redox probe ratio determines carrier separation dynamics at the 2D perovskite / electrolyte interface. Based on the SPV results, we conclude that electron transfer to the redox couple is a critical step in suppressing photodegradation of the material in aqueous solutions. These findings shed light on the intricate interplay between carrier dynamics and chemical changes in the context of semiconductor/electrolyte interfaces, providing valuable insights for the development of design strategies to effectively utilize halide perovskites as photoelectrodes. In addition, our findings provide fundamental insight into the photo-corrosion processes of HaPs, which are currently of high interest for many applications foremost solar cells.
1.3-I1
Hybrid AMX3 perovskites (A=Cs, CH3NH3; M=Sn, Pb; X=halide) have in the last years revolutionized the scenario of photovoltaic technologies. Despite the extremely fast progress, the materials electronic properties which are key to the performance are relatively little understood. We developed an effective GW method incorporating spin-orbit coupling [1] which allows us to accurately model the electronic, optical and transport properties of halide perovskites, opening the way to new materials design. In parallel, a series of computational simulation carried out using Car-Parrinello molecular dynamics have been performed investigating the nature of the perovskites/TiO2 interface, the role of moisture in the perovskite degradation process and the effect of the defect on the device working mechanism. Finally, a series of different strategies will be reported to increase the device stability and efficiency.[2] While instability in aqueous environment has long impeded employment of metal halide perovskites for heterogeneous photocatalysis, recent reports have shown that some particular tin halide perovskites (THPs) can be water-stable and active in photocatalytic hydrogen production. To unravel the mechanistic details underlying the photocatalytic activity of THPs, we compare the reactivity of the water-stable and active DMASnBr3 (DMA = dimethylammonium) perovskite against prototypical MASnI3 and MASnBr3 compounds (MA = methylammonium), employing advanced electronic–structure calculations. We find that the binding energy of electron polarons at the surface of THPs, driven by the conduction band energetics, is cardinal for photocatalytic hydrogen reduction.[3] In this framework, the interplay between the A-site cation and halogen is found to play a key role in defining the photoreactivity of the material by tuning the perovskite electronic energy levels. Our study, by elucidating the key steps of the reaction, may assist in development of more stable and efficient materials for photocatalytic hydrogen reduction. The overall picture of our theoretical investigations underlines a crucial role of computational investigation, casting the possibility of performing predictive modeling simulations, in which the properties of a given system are simulated even before the materials laboratory synthesis and characterization. At the same time, computer simulations are shown to offer the required atomistic insight into hitherto inaccessible experimental observables.
1.1-I1
Two-dimensional hybrid perovskites are a highly intriguing class of materials, composed of alternating inorganic and organic molecular layers. Their reduced dimensionality combined with weak dielectric screening leads to the formation of tightly bound excitons that efficiently absorb and emit radiation. A central questions for excitons in perovskites from the perspectives of both fundamental physics and applications is their mobility. In addition, the external control of their optical response has proven difficult due to challenges to introduce electrical doping into this class of materials.
In this talk I will focus on studies of optically detected exciton transport in 2D perovskites via ultrafast microscopy, discussing different regimes of propagation including free and localized states. I will show how the excitons behave at the structural phase transition and how their transport is determined by strong interactions with the lattice vibrations. In the second part, I will illustrate how the formation of electrically tunable trions, bound states of excitons in free carriers can be achieved in these hybrid semiconductors to optically detect doping. The trions in 2D perovskites are stable for both n- and p- doped regimes, feature unusually large binding energies and a substantial mobility at elevated temperatures.
1.1-O1
Two-dimensional (2D) metal halide perovskite semiconductors have promising prospects for enhancing the stability of perovskite-based photovoltaic devices. In addition, these low-dimensional materials with electronic confinement offer further opportunities in light emission and quantum technologies. However, their technological applications still require a comprehensive understanding of the nature of charge carriers and their transport mechanisms.
In this work, we employ ultrafast optical and terahertz spectroscopy to investigate the exciton formation dynamics, the charge-carrier mobilities, and the charge-phonon couplings of 2D Ruddlesden-Popper perovskites (PEA)2PbI4 and (BA)2PbI4 (where PEA is phenylethylammonium and BA is butylammonium). We reveal band transport with high in-plane mobilities that give rise to efficient long-range conductivity. We show how the organic cation moderates the coupling of charge carriers to optical phonon modes, impacting the charge-carrier mobilities. Furthermore, we demonstrate a new experiment for simultaneously recording the terahertz and optical transmission transients, thus allowing us to monitor the exciton formation dynamics over the picosecond timescale. The observed dynamics reveal a long-living population of free charge-carriers that greatly surpasses the theoretical predictions of the Saha equation even at temperatures as low as 4K.
Our findings provide new insights into the temperature-dependent interplay of exciton and free charge carriers in 2D Ruddlesden-Popper perovskites. Furthermore, the sustained free charge-carrier population and high mobilities revealed by this work demonstrate the potential of these semiconductors for applications that require efficient charge transport, such as solar cells, transistors, and electrically driven light sources.
1.1-O2
Metal-halide perovskite materials demonstrated extraordinary performance in solar cells and light emission in recent years, and their layered low-dimensional counterparts promise even greater tunability due to the huge variety of molecules available for the organic phase. [1, 2] Single octahedra-layer structures act as two-dimensional quantum wells, showing strong confinement and large exciton binding energies. Band gap and light emission can be designed by the choice of the organic cations.[3-5]
We investigate the emission from single plates of two-dimensional Ruddlesden-Popper metal-halide perovskites with different organic cations by angle-resolved polarized photoluminescence (PL) spectroscopy. The choice of the organic cation strongly influences the number of PL peaks that we observe in the band edge emission, and the different PL peaks manifest a strong angle dependence in their linear polarization, manifesting an angle-dependent intensity maximum along one of the principal axes of the octahedra lattice. Such a marked intensity maximum along one in-plane direction is surprising, since theory predicts isotropic in-plane exciton wave function distributions [6]. Therefore, electron-phonon coupling could be at the origin of the distinct anisotropy of the emission polarization, since a large number of phonon bands in such materials show strong directionality. [7, 8] We investigate the angle resolved phonon response together with the emission polarisation of the single plates with resonant and non-resonant Raman spectroscopy, which allows for a detailed correlation of the directionality of the emission with the phonons. The combined angle-resolved emission and Raman spectroscopy allows for a detailed investigation of the exciton fine structure and assignment of confined exciton states and phonon side bands.
1.1-O3
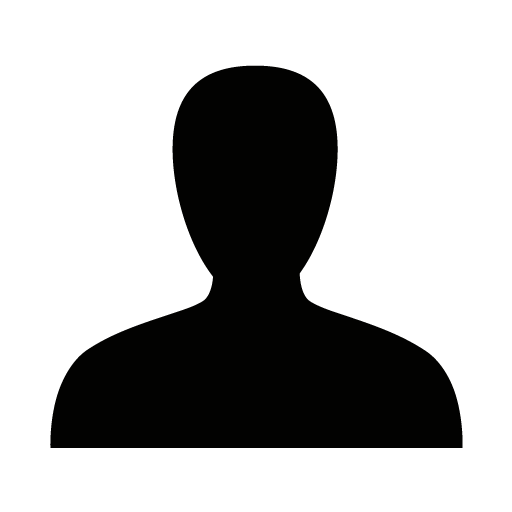
Layered perovskites are quasi-twodimensional crystals in which n layers of metal-halide octahedra are spatially separated by organic molecular layers. A wide variety of organic molecules can be used in the fabrication of these materials, allowing for variation of the interlayer distance and orientation, as well as functionalization, for example by introduction of chiral or electroactive organic molecules. Furthermore, chemical substitution at the metal and halide sites can drastically change the band gap size and symmetry in these materials. In this talk, I will discuss how chemical substitution affects the exciton fine structure of layered perovskites, focusing on metal site substitution and different organic interlayers. Our first-principles calculations based on the GW and Bethe-Salpeter Equation approaches demonstrate the importance of chemical, structural, and dielectric heterogeneity for controlling the nature and binding energy of excitons and the need for first-principles calculations to understand the electronic and excited state structure of this complex material class.
1.1-O4
Gustavo de Miguel graduated in Chemistry in 2002 by the University of Cordoba, Spain. He completed his PhD Thesis in the Physical Chemistry Department of the same University in 2007 studying the molecular organization of thin films prepared at the air-water interface. After several post-doc positions in the Friedrich-Alexander University of Erlangen-Nuremberg, University of Castilla-La Mancha and the Italian Institute of Technology, he moved back to the University of Cordoba with a Ramón y Cajal five-year tenure track position, becoming Associate Professor in 2020.
Dr. de Miguel is a physical chemist with an expertise in absorption and photoluminescence spectroscopy (steady-state and time-resolved) applied to elucidate the photophysics and photochemistry of organic compounds with application in photovoltaics. In the last years, he has added a good knowledge of structural characterization of hybrid materials (perovskites) through different X-ray diffraction techniques.
He participates in National and European projects focusing on how to enhance the stability of metal halide perovskite materials for photovoltaics (SUNREY, Ref:101084422). He has contributed with about 100 publications in international peer-reviewed journals.
Perovskite-derived two-dimensional (2D) materials are emerging as an excellent combination to the three-dimensional (3D) metal halide perovskites for enhancing its stability.[1] In this 2D/3D approach, bulky ammonium organic cations are deposited on top of the 3D perovskite active layer forming a very thin coating of a low dimensional 2D perovskite.[2] 2D/3D inverted perovskite solar cells (PSCs) have achieved to retain >95% of their initial value after >1000 hours at damp-heat test conditions (85 ºC and 85% RH), while power conversion efficiencies (PCE) above 25% have been recently reported in 2D/3D PSCs.[3]
In this work, we investigate the deposition of two diammonium spacers with similar chemical composition (4,4′-Dithiodianiline, 2S, and 4,4’-ethylenedianiline, ET) but with a totally different molecular geometry on top of a 3D perovskite with Cs0.09FA0.91PbI3 formula to manipulate the efficiency and stability of the PSCs. Our results demonstrate an improved PCE of 21% when using the 2S spacer in opposition to an inferior PCE of 16% in the cells covered with the ET spacer. The stability tests display no loss in the PCE upon constant illumination at RT at the MPPT when using the 2S spacer vs. a significant drop in the PCE for the cells with the ET spacer. This divergent behavior is ascribed to the formation of a parallel oriented layer of a 2D perovskite with the 2S spacer that facilitates the charge extraction in the PSC in opposition to the isotropically layer of a 1D perovskite detected upon addition the ET spacer.
1.2-O1
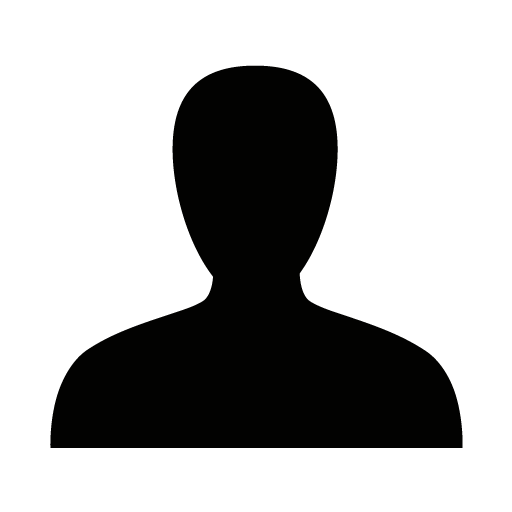
Perovskite materials have gained attention in the field of photovoltaics due to their high conversion efficiency, tunable bandgaps, high charge carrier mobility, low exciton binding energy, and ease of synthesis [1]. Perovskite Solar Cells (PSCs) have demonstrated promising Power Conversion Efficiencies (PCE) of up to 25\%, comparable to the widely commercialized crystalline silicon counterparts. However, achieving highly efficient PSCs requires careful optimization of the perovskite absorbing layer and interfaces with charge-selective contacts.
This study focuses on interface passivation strategies, with a particular emphasis on the use of organic molecules for surface treatment. Organic molecules, especially those containing ammonium (NH3$^+$), show promise in passivating surface trap states [2-3]. The introduction of ammonium-based organic molecules in post-surface treatments leads to the formation of low-dimensional 2D perovskite structures, impacting the quantum confinement regime.
The investigation approaches the correlation between molecular flexibility and the formation of 2D/3D perovskite heterointerfaces, exploring their subsequent effects on solar cell performance. The study synthesizes two variations of a well-studied phenylethylammonium iodide (PEAI): a more rigid trans-2-phenylcyclopropylammonium iodide (PCPEAI) and a more flexible cyclohexylethylammonium iodide (CHEAI).
Detailed analyses, including SEM-Cathodoluminescence [4], reveal that the 2D phases present a heterogeneous distribution over the 3D surface. The study also highlights that the absence of $\pi$-$\pi$ stacking interactions and a higher degree of freedom in the more flexible CHEAI molecule prevent undesirable aggregation, resulting in improved device efficiency. The CHEAI-based devices exhibit a commendable efficiency of close to 21\%. The results indicate that molecular flexibility plays a crucial role in facilitating the formation of 2D phases.
This research contributes valuable insights into the intricate relationship between molecular flexibility, 2D/3D perovskite heterointerfaces, and PSC performance. The findings offer guidance for designing more efficient and stable perovskite solar cells, making progress in next-generation photovoltaic technologies.
1.2-O2
Junke Jiang obtained his doctoral degree at Eindhoven University of Technology, in the Netherlands. His PhD research focuses on understanding the optoelectronic properties and stability of lead halide perovskite and lead-free perovskites. He was a postdoc researcher at Institut des Sciences Chimiques de Rennes (ISCR) - UMR CNRS 6226. Currently, he is a postdoc researcher at Institut National des Sciences Appliquées de Rennes (Institut FOTON - INSA Rennes) - UMR CNRS 6082, focusing on developing and implementing the semiempirical DFTB method for studying the physical properties of 3D and 2D metal halide perovskites.
Halide perovskites have garnered significant attention due to their unique properties and potential applications in optoelectronics and energy-related fields. However, the reduction in crystal dimension of perovskite from 3D to low dimensional structures introduces challenges, particularly regarding quantum and dielectric confinements, resulting in larger band gaps and exciton-binding energies. The low dimensional structures imply intrinsically bigger unit cells which makes challenging the application of standard density functional theory (DFT) calculation in terms of computational cost. Furthermore, DFT tends to significantly underestimate band gaps, which is extremely problematic for optoelectronic materials. Density Functional Tight-Binding (DFTB) is a flexible, semi-empirical method based on DFT which capable of simulating large system sizes and offers the possibility of accurate band gap prediction with low computational cost.1,2
In this work, we highlight the application of DFTB methodology for studying the electronic structure, effective masses, and charge density localization in low-dimensional perovskite materials. By employing empirical fitting and parameterization, the DFTB method captures the electronic band structures of model 2D halide perovskites (e.g., Cs2PbI4, BA2PbI4, PEA2PbI4, and BA2PbBr4). We show good agreement between DFTB results and experimental electronic band gaps, as well as reduced effective masses. This first attempt is promising for further applications to other low-dimensional (1D, 0D, hollow) perovskite nanostructures or 2D/3D perovskite heterostructures with large sizes and complexity, which demonstrated excellent operational stability in solar cell architectures.
1.2-I1
Julian obtained his B.S. in chemical engineering from the University of New Mexico and his M.Phil. in chemistry from the University of Cambridge, where he studied electrocatalytic materials. He recieved his Ph.D. in chemical engineering from Stanford University under the supervision of Profs. Hemamala Karunadasa and Michael Toney, focusing on synthesis, defect chemistry, and X-ray characterization of halide perovskite semiconductors. Julian is currently a Schmidt Science Fellow at the Unviersity of California, Berkeley; his present research concerns applying chemical design principles to next-generation electronics.
: A wide range of physical properties associated with layered (two-dimensional) perovskites has been realized due to the vast composition space of the inorganic layer and molecular diversity in organoammonium cations. A new class of layered halide perovskites recently discovered by our group, termed “mosaic” perovskites, form through alloying of single and double layered perovskites and feature three distinct metal ions. The first example of these disordered alloys comprised Cu(I), Cu(II), and In(III) in the perovskite layer, leading to emergent optoelectronic properties owing to intervalence charge transfer between Cu ions.
Here, we discuss facile synthetic methods to produce mosaic layered perovskites, the role of metal-cation ordering in optoelectronic and magnetic properties, and the interplay between stability and disorder in these complex mixtures. Moreover, we expand the diversity of mosaic layered perovskite alloys through exploration of transition-metal ion pairs that engender strong intra-layer coupling. We demonstrate that features of the known Cu(I)-Cu(II)-In(III) mosaic alloy can be used to systematically evaluate candidates and experimentally realize new examples of mosaic alloys.
Overall, these mosaic halide-perovskite alloys represent a platform for rational design of intra-layer coupling interactions within layered halides beyond the conventional compositional limitations of single or double perovskites.
1.2-O3
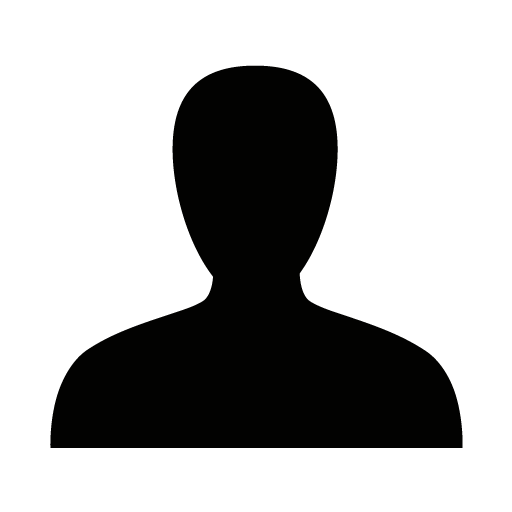
The development of efficient solar cells based on perovskite materials has emerged as a promising path for advancing photovoltaic technology. Specifically, 2D perovskites (2D-PVKs) have been found to exhibit higher stability properties against their 3D counterparts [1]. However, many factors are essential for an effective construction of these devices. Solvent engineering plays a critical role in controlling the crystalline structure and optoelectronic properties of 2D perovskites [2]. However, the existing and research-dominant perovskite solar cells (PSCs) base their formulations on highly toxic solvents such as DMF, increasing potential health risks for researchers and the environment [3].
Herein, we mainly focused on the development of novel solvent formulations, tailored to the specific requirements of 2D perovskite fabrication. Following solvent’s solubility parameters, we predict novel and green solvents that significantly enable the formation and growth of perovskite crystals. These new solvents along with the state-of-the-art fabrication processes aim to provide an enhanced control over crystal growth, minimizing defects, while meeting existing efficiencies of solar cells. All while prioritizing sustainable and environmentally green practices.
Our research extends beyond solvent considerations, seeking to use these new formulations to not only enhance efficiencies but also to contribute to the long-term stability that 2D PVKs offer. The strategic selection of solvents aims to mitigate degradation mechanisms, such as ion migration and moisture-induced instabilities, which are well-known challenges in perovskite solar cell technology.
In conclusion, this project underscores the role of solvent engineering and the selection of non-hazardous solvents in the fabrication of 2D perovskite films, and its further use in solar devices. The ongoing efforts to develop novel solvent formulations represent a stride towards a deeper understanding of perovskite crystallization and heighten the potential of these materials for its widespread adoption in energy conversion technologies.
1.2-O4
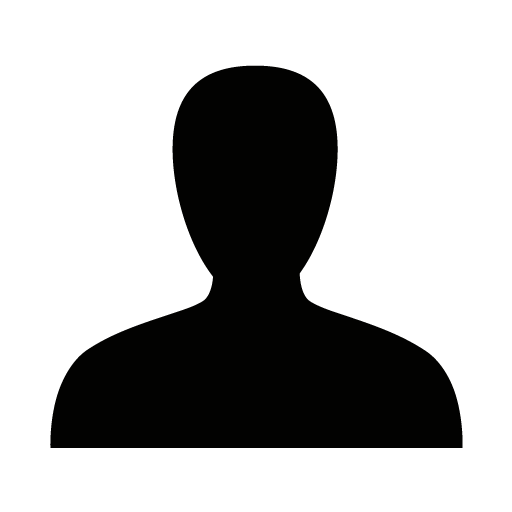
Two-dimensional (2D) organic-inorganic metal halide perovskites are persistently emerging as a promising class of semiconductors, not only due to their superior intrinsic and extrinsic stability but also due to their virtually endless compositional possibilities. Especially the versatile organic cations offer many options to study the underlying physics of these materials as well as to modify key properties on a device and application oriented research path. Looking for more stable and less toxic alternatives to 2D Pb-based perovskites, double perovskites based on Ag, Cu, Bi and Sb are at the focus of the most recent research efforts.
We synthesized 2D n=1 Ruddlesden-Popper double perovskite based on large, conjugated cations, creating different types of band gap alignments to break the quantum confinement of the 2D layered crystal structure. We discuss the structural and optical properties, the photoluminescence, the electronic structure, the charge-carrier mobilities and the mixed- and out-of-plane conductivities for 8 new materials. We isolate the most promising organic cation and successfully incorporate it into the first pure n=1, parallel oriented, 2D RP double perovskite solar cell.
1.3-O1
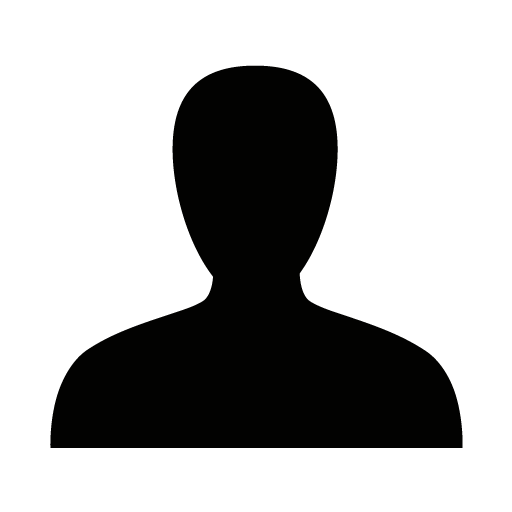
Two-dimensional layered metal-halide perovskites (2DLPs) represent an emerging class of materials where a semiconducting metal-halide octahedral layer is sandwiched between two layers of bulky organic cations. The distinctive structural characteristic of these materials results in high in-plane mobility of excitons and charge carriers, but at the same time, hinders the out-of-plane mobility due to the presence of mostly isolating organic cations [1], [2]. This limits the possibility of studying charge and energy transfer processes in vertical heterostructures of this material class. Instead, lateral heterostructures, wherein the composition changes along the in-plane direction, offer an intriguing approach for investigating potential transfer processes at the junction region.
We studied the formation of lateral heterostructures in the system PEA2PbBr4-PEA2PbI4 by developing a facile room-temperature anion exchange method in solution. Ion exchange methods are a common tool used to tune the optical properties in 3D metal-halide perovskites but are underrepresented in the case of 2DLPs [3]. The approach takes advantage of the highly anisotropic structure of 2DLPs, where the bulky organic cations suppress the vertical diffusion of the ions while lateral diffusion is preferred [4]. We observed that 2DLPs can be stabilized in polar solvents such as octanol by exposing them to the corresponding halide salt of the organic cation. Introducing a different halide salt of the cation compared to the parent 2DLP leads to the formation of lateral heterostructures, which initiate at the edges of the microcrystals and propagate toward the center. This process ultimately results in a core-crown-like microstructure containing 2DLPs of two different halides, each contributing to a distinct emission profile. We demonstrate the influence of different processing parameters like the type of ion source and the solvent on the microstructure and optical properties of the resulting heterostructure. Furthermore, we observe a strong dependency on the direction of the exchange process. Treating PEA2PbBr4 with an iodine source results in the predominant formation of nearly phase-pure PEA2PbI4 along the microcrystal peripheries. Conversely, subjecting PEA2PbI4 to a bromide source consistently yields an alloyed phase, with no discernible presence of a pure bromide phase. This behavior stands in stark contrast to that of 3D perovskites and is attributed to the preferential occupancy of halides in specific positions within the [PbX6]4- octahedral [5].
The formation of such a heterojunction in the in-plane direction of the semiconducting layer provides an opportunity to control the directionality of the charge carrier or energy flow toward the edges or the center of these microstructures. This, in turn, contributes significantly to a better understanding of the optoelectronic properties in heterostructured 2DLPs.
1.3-O2
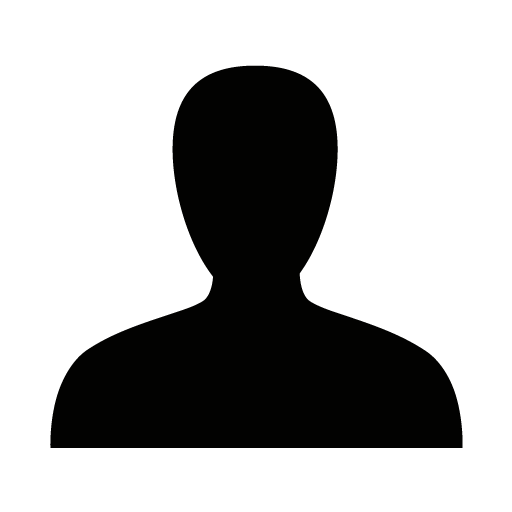
Halogen perovskites represent a family of semiconductors with optical and transport properties that can be widely modified through element substitutions or crystal structure distortions. They are processable in solution and find application in solar cells, LEDs, lasers and detectors. Despite the high efficiency of 3D perovskites, 2D halide perovskites are more stable, durable, and chemically versatile. These materials, characterized by a two-dimensional crystal structure, are composed of thin layers of perovskite separated by organic cations and specifically the single crystal atomic configuration confers several advantageous properties and characteristics. Indeed, in 2D single crystals the absence of grain boundaries enhances electronic properties, such as charge transport.
Achieving the right crystal thickness is crucial for device performance too, preventing carrier loss, and ensuring effective light absorption to unlock the full potential of carrier transport. This can be attained by a successful space-confined method, which allows to control the thickness during perovskite preparation. The last one is crucial for 2D and quasi-2D perovskites, since it is essential for perovskites in solar cells to align their crystallography orientation with the carrier collection direction into the device, to permit the conduction. Indeed, 2D perovskites have to deal with their preferential orientation growth, which is typically parallel to the substrate with which they are in touch, insulating the carriers into the layers and causing transport carrier problems into the device. To avoid this problem, the task is to modify the orientation growth from parallel to vertical to the substrate.
In this contribution my work related to space-confined and orientation-growth techniques will be explained, in which the details of the processes and results obtained will be explored. Specifically, by confining the growth within specific spaces introducing modifications to confinement surfaces, it was possible to dictate the size and shape of the resulting single crystal. Moreover, to change the crystallography orientation, we worked with the addition of additives and using functionalized cations as spacers, in order to favor the vertical layer disposition. The samples obtained have been analyzed by different instruments, such as AFM, confocal microscope, XRD and others, investigating their thickness, morphology and orientation.
1.3-I1
Giulia is Associate Professor at Physical Chemistry Unit at University of Pavia, leading the PVsquared2 team, and running the European Grant ERCStG Project “HYNANO”aiming at the development of advanced hybrid perovskites materials and innovative functional interfaces for efficient, cheap and stable photovoltaics. Within this field, Giulia contributed to reveal the fundamental lightinduced dynamical processes underlying the operation of such advanced optoelectronic devices whose understanding is paramount for a smart device development and for contributing to the transition of a green economy.
Giulia received an MS in Physical Engineering in 2008 and obtained her PhD in Physics cum laude in 2012 at the Politecnico of Milan. Her experimental thesis focused on the realisation of a new femtosecond-microscope for mapping the ultrafast phenomena at organic interfaces. During her PhD, she worked for one year at the Physics Department of Oxford University where she pioneered new concepts within polymer/oxide solar cell technology. From 2012-2015, she was a post-doctoral researcher at the Italian Institute of Technology in Milan. In 2015, she joined the Ecole Polytechnique Fédérale de Lausanne (EPFL) with a Co-Funded Marie Skłodowska-Curie Fellowship. From 2016 to 2019, she has been awarded by the Swiss Ambizione Energy Grant providing a platform to lead her independent research group at EPFL focused on the developemnt of new generation hybrid perovskite solar cells.
She is author of 90 peer-reviewed scientific papers bringing her h-index to 44 (>13’000 citations), focused on developement and understanding of the interface physics which governs the operation of new generation solar cells.
Recently, she received the USERN prize in Physical Science, the Swiss Physical Society Award in 2018 for Young Researcher and the IUPAP Young Scientist Prize in Optics. She is currently USERN Ambassador for Italy and board member of the Young Academy of Europe.
More can be found at https://pvsquared2.unipv.it.
Weblink: https://people.epfl.ch/giulia.grancini?lang=en
Two dimensional perovskites 2DPs – intended to be single/few inorganic layers (typically <3) spaced by large organic cations – have attracted a wide interest for their modular wide band gap and proved stability, but their application in solar cells has been so far unsuccessful. Lack of knowledge on how to manipulate the orientation of the inorganic sheets during solution-based film deposition only enabling a parallel orientation to the substrate is the actual bottleneck. This limits vertical charge percolation and efficiency, ultimately making them uncompetitive in the solar cells arena.
Here I will discuss a novel effective strategy to break such efficiency limit by controlling the orientation of the inorganic backbone of thiophene-based 2DPs (with n<3) inducing a vertical growth of the inorganic framework with respect to the substrate. Integrated in a solar cell, we enable vertical charge percolation, reaching a “record” efficiency of 9.4 %. Such proof of concept intimately demonstrates the potential of controlling the crystalline orientation of 2DPs providing an essential strategy for boosting their performances. Beyond such demonstration and its validation monitoring the crystal orientation at the nanometric scale, we reveal the fundamental mechanism behind. Two key ingredients play a concomitant role: 1) inducing the formation of nanometer-size crystallites already in the precursor solution and 2) creating a Cl-rich 2DP phase at the bottom interface where Chlorine – differently from what commonly happens in 3D perovskite- substitutes Iodine entering in the structure and ultimately inducing the vertical growth.
2.1-I1
Volker Blum is an Associate Professor in the Thomas Lord Department of Mechanical Engineering and Materials Science at Duke University, Durham, NC. He obtained his doctoral degree from University of Erlangen, Germany in 2001 and then pursued his post-doctoral research at National Renewable Energy Laboratory in Golden, CO, from 2002-2004. From 2004-2013, he was a scientist and group leader at the Fritz Haber Institute in Berlin, Germany. He develops computational methods and software for electronic structure simulations, data analysis and data sharing in materials science and in computational chemistry, including as the lead developer of the FHI-aims electronic structure code. His current applied research focuses on novel semiconductor materials as well as molecular spectroscopy. In particular, his group is working on hybrid perovskite materials and chalcogenide semiconductors.
This talk summarizes recent advances in tuning layered perovskite materials from a first-principles perspective, covering energy level alignment, spin character of energy levels, manipulating carrier concentrations by heteroatom doping as well as by coherent stimulation of charge oscillations between the organic and inorganic components. Energy level alignments between the organic and inorganic components are satisfactorily covered by large-scale, spin-orbit coupled hybrid density functional theory (HSE06) calculations, with simulation sizes up to several thousand atoms enabled by the FHI-aims code in an accurate all-electron approach. Using chiral organic cations, chirality is imparted to the inorganic part of the system via structural asymmetries and hydrogen bonds, leading to conduction band spin splitting that is characterized by a simple structural descriptor. In order to utilize this and similar effects in devices, manipulating carrier concentrations in the conduction bands and valence bands is an important prerequisite. We study Bi as a potential n-dopant in Pb based layered perovskites, as well as Sn as an effective p-dopant. Direct, large supercell calculations of these substitutions show that n-doping by Bi should be weak but attainable (with 0.55 eV offset to the conduction band edges in phenethylammonium lead iodide, Bi-induced doping levels are not shallow). However, a population of compensating deeper defects appears to limit the experimentally attainable doping efficiency. For Sn, we show that an enhanced tendency of forming Pb vacancies nearby is likely responsible for experimental observations of slight p doping. As a final point, we rationalize experimental observations of laser-controlled coherent charge oscillations in a layered perovskite in terms of electron-phonon coupling, offering a potential model for direct, picosecond control of carrier populations in perovskite materials.
2.1-O1
Wouter Van Gompel is an assistant professor in hybrid materials chemistry within the Institute for Materials Research (imo-imomec) of Hasselt University in Belgium. With his research group Hybrid Materials Design (HyMaD), he does research into the design, synthesis and characterization of novel hybrid materials for optoelectronics.
The family of hybrid organic-inorganic lead-halide perovskites are the subject of intense interest for optoelectronic applications, including solar cells, light-emitting diodes and detectors. Interest in the sub-class of 2D layered hybrid perovskites has been increasing due to their structural and compositional versatility. This versatility allows for the incorporation of spacer cations with an extended conjugated system that are electronically active, as opposed to the electronically inactive alkylammonium- and phenylethylammonium-derived spacer cations that are most often used.[1]
I will present our work on 2D layered perovskites containing optically and electronically active carbazole-based Cz-Ci molecules [2-3], where Ci indicates an alkylammonium chain and i indicates the number of CH2 units in the chain, varying from 3−5. These 2D perovskites, with a formula of (Cz-Ci)2PbI4, show a tunable electronic coupling between the inorganic lead-halide and organic layers. The strongest interlayer electronic coupling was found for (Cz-C3)2PbI4, containing the carbazole spacer with the shortest alkyl chain length. Using ultrafast spectroscopy, we measure ultrafast hole transfer from the photoexcited lead-halide layer to the Cz-Ci molecules, the efficiency of which increases by varying the chain length from i=5 to i=3. The charge transfer results in long-lived carriers (10 – 100 ns) and quenched emission. Electrical charge transport measurements using single-carrier devices show markedly increased out-of-plane carrier mobilities compared to the reference 2D perovskite (PEA)2PbI4, with carrier mobility increasing from i=5 to i=3.[4]
2.1-O2
Muhammad Umair Ali is a Postdoctoral Fellow working with Prof. Aleksandra B. Djurišić in the Department of Physics at The University of Hong Kong. He attained his Ph.D. in Advanced Materials & Mechanics, specializing in Semiconductor Physics, from Peking University in 2020. Following his Ph.D., he pursued a Postdoctoral Fellowship at the Tsinghua-Berkeley Shenzhen Institute (TBSI). His research primarily centers around the advancement of high-performance perovskite optoelectronic devices.
Muhammad can be contacted via email at muali@hku.hk or reached through his Twitter handle, @UmairAliSabir.
Quasi-2D perovskites with Ruddlesden-Popper structure, with the formula C2An-1PbnBr3n+1 , where C is a bulky spacer cation, A is a small organic cation (methylammonium (MA), or formamidinium (FA)) or Cs+, and n is the number of octahedral layers between the spacer cation bilayers, have been attracting increasing attention for applications in light emission in blue and green spectral ranges. Quasi-2D perovskites with different spacer cations exhibit vastly different crystallization, photoluminescence, and stability. One of the methods to adjust crystallization and achieve favourable energy landscape for efficient funnelling to result in bright sky-blue or green emissions is to use a mixture of spacer cations. In this work, we investigated the use of different carboxylic group containing spacers, including 5-ammonium valeric acid (5AVA), 4-ammonium butyric acid (4ABA), 3-ammonium propionic acid (3APA) and their mixtures, for preparation of n=2 and n=3 quasi-2D perovskites with MA and FA small cations. The films generally exhibited bright emission, which could be further increased with different additives, and the cations used affected the ratios of different n phases present in the film, and ultimately emission colour. However, the films exhibited strong tendency toward disproportionation upon exposure to elevated temperature or ambient. Thus, we explored mixing the carboxylic group containing spacers (5AVA was selected as it produces very bright films) with a spacer cation forming films with exceptional stability, 2-thiopheneethylammonium, namely TEA. For the optimal 5AVA-TEA ratio, we can obtain films with predominantly n=2 phase even after ambient exposure for n=2 solution stoichiometry, very different from pure 5AVA-based films (entirely dominated by n=1 phase after 3 min ambient exposure), as well as pure TEA-based films (showing prominent presence of n=1 and n=2 phases with no significant change with ambient exposure). For n=3, pure TEA-based films again show negligible change with atmosphere exposure, while for pure 5AVA there is a significant presence of 3D perovskite. In addition, for the same solution stoichiometry, changing the small cation affects the crystallization and consequently light emission, with sky blue emission achievable only for MA small cation and n=2 solution stoichiometry. The effect of solution composition (spacer cations used and their ratios to small cations) on the phase composition of resulting film and consequently light emission is discussed.
2.1-I2
Dr. Jovana V. Milić obtained her PhD in the Department of Chemistry and Applied Biosciences at ETH Zurich in July 2017. Her research interests encompass (supra)molecular engineering of bioinspired organic materials with the aim of developing functional nanotechnologies. Since October 2017, she has worked as a scientist with Prof. Michael Graetzel in the Laboratory for Photonics and Interfaces at EPFL in Switzerland on the development of novel photovoltaic materials, with the focus on dye-sensitized and hybrid perovskite solar cells. In September 2020, she has taken on a position of a Group Leader in the Soft Matter Physics Group of the Adolphe Merkle Institute at the University of Fribourg in Switzerland. For more information, refer to her LinkedIn profile (linkedin.com/in/jovanavmilic), ORCID 0000-0002-9965-3460, and Twitter (@jovana_v_milic).
Hybrid organic-inorganic perovskites have become one of the leading materials in
photovoltaics, although their instability under operating conditions interferes with practical
applications. This can be overcome by using tailored organic systems that interact with hybrid
perovskite frameworks through various noncovalent interactions, which predominantly involve
aromatic π-based systems as interfacial modulators or spacers forming layered hybrid
perovskites. We demonstrate the unique capacity to broaden this material space by relying on
unorthodox σ-hole-based interactions that can enhance the functionality of hybrid perovskites,
improving their operational stability without compromising photovoltaic performances,
providing a versatile supramolecular strategy for advancing hybrid photovoltaics.
1. J. V. Milić*, Chimia 2022, 76, 784.
2. W. Luo, G. AlSabeh, J. V. Milić*, Photochemistry 2022, 50, 342.
3. J. V. Milić, J. Phys. Chem. Lett. 2022, 13, 9869.
4. M. A. Ruiz Preciado, D. J. Kubicki, A. Hofstetter, L. McGovern, M. H. Futscher, A.
Ummadisingu, R. Gershoni-Poranne, S. M. Zakeeruddin, B. Ehrler, L. Emsley, J. V. Milić, M.
Grätzel, J. Am. Chem. Soc. 2020, 142, 1645.
5. W. Luo, J. V. Milić*, et al. 2023, manuscript submitted
1.1-I1
Tae-Woo Lee is an associate professor in Materials Science and Engineering at the Seoul National University, Korea. He received his Ph.D. in Chemical Engineering from the KAIST, Korea in 2002. He joined Bell Laboratories, USA as a postdoctoral researcher and worked at Samsung Advanced Institute of Technology as (2003-2008). He was an associate professor in Materials Science and Engineering at the Pohang University of Science and Technology (POSTECH), Korea until August 2016. His research focuses on printed flexible electronics based on organic, carbon, and organic-inorganic hybrid perovskite materials for displays, solar cells, and bio-inspired neuromorphic electronics.
Biological nervous systems possess versatile attributes, serving diverse functions; for instance, the central nervous system (CNS) governs learning and memory, while the peripheral nervous system (PNS) is responsible for sensory perception. Consequently, there is a need to engineer artificial synapses tailored to adapt to performance requirements in various applications. Brain-inspired neuromorphic computing aims to emulate the learning and memory capabilities of the CNS, being inspired from the long-term potentiation (LTP) observed in biological synapses. The application of artificial synapses in the fields of nervetronics and neuroprosthetics requires the emulation of the short-term plasticity (STP), enabling the rapid signal transmission and fast responses akin to those in the biological PNS. To demonstrate the broad applicability, spanning areas including neuromorphic computing and bio-inspired nervetronics, our study has explored the modulation of STP and LTP using ion-gel gated polymer synaptic transistors (IGPSTs). We have modulated the polymer semiconductor (PSC) film’s crystallinity through post-deposition film annealing, self-assembly monolayer treatment, and the introduction of various sidechain length, leading to the conversion between STP and LTP properties in IGPSTs. Moreover, we have utilized a straightforward yet effective approach by blending two PSCs with the same backbone but different sidechains. This blend strategy has led to a substantial improvement in LTP characteristics, whereas IGPSTs employing each PSC individually show only STP properties. IGPSTs with enhanced LTP properties demonstrate their potential as neuromorphic computing devices, effectively simulating learning processes in artificial neural networks. Concurrently, IGPSTs featuring STP capabilities are used to demonstrate various artificial nervous systems, such as artificial reflex arcs, neuromuscular systems, and neuro-prosthetic nerves incorporating artificial proprioceptors. These pioneering studies on neuromorphic devices have expanded the scope of applications for artificial synapses and validate the feasibility of these innovative technologies.
1.1-O1
Electrochemical random access memory (ECRAM) and organic electrochemical transistors (OECT) are gaining significant attention due to the unique properties introduced via the mobile ions. Despite the progress in device fabrication, there is very little in terms of device models.[1, 2] Here, we will describe results obtained using a 2D semiconductor device model that incorporates ions and reactions in a self-consistent manner.
For example, although OECTs electrolytes contain both cations and anions, it is common to consider only the primary ion (as a cation in a normally-off p-type transistor). We simulate normally-on and normally-off transistors showing that the normally-on is highly affected by the counter ions (anions) and field-screening at the contacts.
Inorganic ECRAM transistor devices have a structure similar to OECT, where the memory (ion retention) is provided by the diffusion's very high electric field activation. Comparing measured multi-level potentiation [3] to detailed device simulation, we reveal the role of electrolyte polarisation. We also show that sublinear potentiation response can be explained by an electrochemical reaction similar to that of lithium plating in batteries. Namely, electrochemical reactions must be considered when dealing with electrochemical devices.
1.1-I2
Joon Hak OH is a professor of School of Chemical and Biological Engineering at Seoul National University, Korea. He received his B.S, M.S, Ph.D degrees from Seoul National University. He worked as a senior engineer at Samsung Electronics. He then continued his postdoctoral research at Stanford University. He was a faculty at Ulsan National Institute of Science and Technology (UNIST, 2010-2014) and Pohang University of Science and Technology (POSTECH, 2014-2018), before moving to Seoul National University in 2018. His research focuses on synthesis of organic and polymeric nanomaterials and carbon nanomaterials, enhancement of their electrical and optical functions by controlling the physical and chemical features, and applications to flexible electronic devices and energy devices, such as organic field-effect transistors, chemical/bio/physical sensors, and organic solar cells.
Neuromorphic sensors, designed to emulate natural sensory systems, hold the promise of revolutionizing data extraction by facilitating rapid and energy-efficient analysis of extensive datasets. However, a significant challenge lies in accurately distinguishing specific analytes within mixtures of chemically similar compounds using existing neuromorphic chemical sensors. In this study, we present an artificial olfactory system (AOS), which is developed through the integration of human olfactory receptors (hORs) and artificial synapses, for the first time. This AOS is sophisticatedly engineered by interfacing an hOR-functionalized extended-gate with an organic synaptic device. The AOS generates clearly distinct patterns for odorants and mixtures thereof, at the molecular chain length level, attributed to specific hOR-odorant binding affinities. This approach enables precise pattern recognition via training and inference simulations. These findings establish a solid foundation for the development of high-performance sensor platforms and artificial sensory systems, which are ideal for applications in wearable and implantable devices.
1.1-I3
Yong-Young Noh is Chair Professor in the Department of Chemical Engineering, Pohang University of Science and Technology (POSTECH), Pohang, Republic of Korea. He received his PhD in 2005 from GIST, Republic of Korea, and then worked at the Cavendish Laboratory in Cambridge, UK, as a postdoctoral associate. Afterwards, he worked at ETRI as a senior researcher, at Hanbat National University as an assistant professor, Dongguk University-Seoul as an associate professor. He has won Merck Young Scientist Award (2013), Korea President Award (2014), IEEE George E. Smith Award (2014), as selected this month as a Scientist from Korea Government (September. 2016), and the Order of Science and Technology Merit, Science Medal from Korea Government (April 2024). He is a fellow of Fellow of Korean Academy of Science and Technology and a Fellow of The National Academy of Engineering of Korea. He has published over 360 papers in international journals in the field of materials for electronics and optoelectric devices, in particular, OFETs, OLEDs, Metal Halide, perovskites, carbon nanotube 2D layered materials, and oxide TFTs. Selected Recent Papers: Selenium alloyed tellurium oxide for amorphous p-channel transistors, Ao Liu, Yong-Sung Kim, Min Gyu Kim, Youjin Reo, Taoyu Zou, Taesu Choi, Sai Bai, Huihui Zhu, Yong-Young Noh, Nature, 629, pages798–802 (2024), Tin perovskite transistors and complementary circuits based on A-site cation engineering, Huihui Zhu, Wonryeol Yang, Youjin Reo, Guanhaojie Zheng, Sai Bai, Ao Liu, Yong‐Young Noh, Nature Electronics, 6(9), 650-657 (2023), High-performance metal halide perovskite transistors, Ao Liu, Huihui Zhu, Sai Bai, Youjin Reo, Mario Caironi, Annamaria Petrozza, Letian Dou, Yong-Young Noh, Nature Electronics, 6(8), 559-571 (2023), High-Performance Inorganic Metal Halide Perovskite Transistors, Ao Liu, Huihui Zhu, Sai Bai, Youjin Reo, Taoyu Zou, Myung-Gil Kim, Yong-Young Noh*, Nature Electronics 5(2), 78-83 (2022).
Enable GingerCannot connect to Ginger Check your internet connection
or reload the browserDisable GingerRephraseRephrase with Ginger (Ctrl+Alt+E)0Edit in GingerGinger is checking your text for mistakes...Disable Ginger in this text fieldDisable Ginger on this website×
Developing high-mobility p-type semiconductors that can be grown using cost-effective scalable methods at low temperatures, has remained challenging in the electronics community for the integration of complementary electronics with the well-developed n-type metal oxide counterparts. Tin (Sn2+) halide perovskites emerge as promising p-type candidates but suffer from low crystallisation controllability and high film defect density, which result in uncompetitive device performance (1). In this talk, I would like to introduce a general overview and recent progress of our group of p-type Sn-based metal halide perovskites for the application of field-effect transistors (FETs). In the first part of the talk, I will mainly address inorganic perovskite thin-film transistors with exceptional performance using high-crystallinity and uniform cesium-tin-triiodide-based semiconducting layers with moderate hole concentrations and superior Hall mobilities, which are enabled by the judicious engineering of film composition and crystallization. The optimized devices exhibit high field-effect hole mobilities of over 50 cm2 V−1 s−1, large current modulation greater than 108, and high operational stability and reproducibility (2). In the second part of the talk, I will introduce A-site cation engineering method to achieve high-performance pure-Sn perovskite thin-film transistors (TFTs). We explore triple A-cations of caesium-formamidinium-phenethylammonium to create high-quality cascaded Sn perovskite channel films, especially with low-defect phase-pure perovskite/dielectric interface. As such, the optimized TFTs show record hole mobilities of over 70 cm2 V−1 s−1 and on/off current ratios of over 108, comparable to the commercial low-temperature polysilicon technique level (3). The p-channel perovskite TFTs also show high processability and compatibility with the n-type metal oxides, enabling the integration of high-gain complementary inverters and rail-to-rail logic gates.
1.1-O2
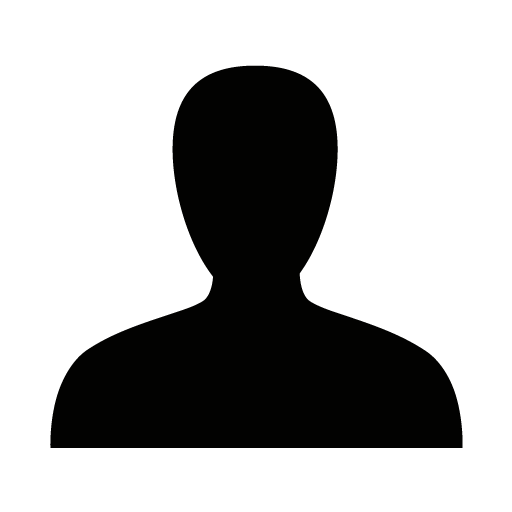
Halide perovskites have been attracted as potential candidates in various electronic devices such as memristors and neuromorphic devices with superior resistive switching properties such as low power consumption, scalability, and compositional and mechanical flexibility as well as photovoltaics, LED, and so on. Among them, we report here low-dimensional lead-free halide perovskites for the resistive switching memory devices and propose their potential applicability in multi-functional electronic devices using the resistive switching characteristics. Although most studies have been focused on the lead-based halide perovskites, it is necessary to focus the research on lead-free halide perovskites without toxicity of lead for various viable electronic applications such as tin, copper, antimony, or bismuth-based materials. Here we found that copper (Cu2+)- and bismuth (Bi3+)-based halide perovskites can be suitable candidates for a variety of applications, especially memristor devices due to their visible characteristic electrical traits and improved stability. With these reports, we also suggest materials and devices design perspectives for multi-functional electronic devices including next generation memristors. For copper(II)-based halide perovskites, 2-dimensional (2D) (BzA)2CuBr4 (BzA = benzylammonium) perovskite was synthesized on Pt substrate, which retained a higher ON/OFF ratio over 108 even at lower operational voltage around +0.2 - -0.3 V. Longer endurance over 2,000 cycles was observed with Schottky conduction at HRS. [1] For bismuth (III)-based halide perovskites, layered double perovskite with chemical formula of BA2CsAgBiBr7 was synthesized on Pt substrate by phase conversion of 3D Cs2AgBiBr6, which showed endurance of 1,000 cycles and retention time over 2×104 s at operational voltage as low as 0.5 V, along with ON/OFF ratio as high as ~107. [2] Both the previously reported 3D counterparts on ITO substrate [3] and 2D Ag-Bi system [2] here showed excellent memristor properties, but especially the ON/OFF ratio of 2D Ag-Bi system was five orders of magnitude larger than those of 3D counterparts. Modification of Schottky conduction at high resistance state (HRS) is found to be responsible for high ON/OFF ratio in low-dimensional structure. In addition, as another study of bismuth-based materials, we fabricated a low-dimensional Cs3Bi2Br9-based memristor with a thin film less than 1 micrometer, where synthetic Cs3Bi2Br9 powder precursor enabled uniform thin film formation.[4] Thin film also exhibited low operating voltages as +0.44 V with gradual current increase at the SET process with reliable cell-to-cell and device-to-device resistive switching behavior. It was also found that the 2D low-dimensional copper and bismuth-based halide perovskites studied here showed much better stability under high humidity and at elevated temperature than the 3D and/or lead-based counterparts, which is beneficial for practical applications. Therefore, it was confirmed that low-dimensional lead-free halide perovskite materials are promising candidates for resistive switching memory devices, and we also expect that various halide perovskites can be derived and applied to multi-functional electronic devices including memristors, neuronic or synaptic devices, and so on.
2.1-I1
Doping of organic semiconductor films enhances their conductivity for applications in organic electronics, thermoelectrics and bioelectronics. However, much remains to be learnt about the properties of the conductive charges in order to optimize the design of the materials. Electrochemical doping is important for organic electrochemical transistors (OECTs) used in neuromorphic systems. Benefits of doping via electrochemistry include controllable doping levels, reversibility and high achievable carrier densities. We introduce a new technique, applying in-situ terahertz (THz) spectroscopy directly to electrochemically doped polymers in combination with time-resolved spectro-electrochemistry, chronoamperometry and OECT device measurements. We evaluate the intrinsic short-range transport properties of the polymers (without the effects of long-range disorder, grain boundaries and contacts), while precisely tuning the doping level via the applied oxidation voltage. Moreover, temperature-dependent measurements allow to extract the thermodynamic and activation parameters of the electrochemical processes. Results will be shown for a variety of polymers based on polythiophene backbones with different sidechains, aligned polymer chains and novel 2D organic films.
2.1-I2
Juan Bisquert (pHD Universitat de València, 1991) is a Professor of applied physics at Universitat Jaume I de Castelló, Spain. He is the director of the Institute of Advanced Materials at UJI. He authored 360 peer reviewed papers, and a series of books including . Physics of Solar Cells: Perovskites, Organics, and Photovoltaics Fundamentals (CRC Press). His h-index 95, and is currently a Senior Editor of the Journal of Physical Chemistry Letters. He conducts experimental and theoretical research on materials and devices for production and storage of clean energies. His main topics of interest are materials and processes in perovskite solar cells and solar fuel production. He has developed the application of measurement techniques and physical modeling of nanostructured energy devices, that relate the device operation with the elementary steps that take place at the nanoscale dimension: charge transfer, carrier transport, chemical reaction, etc., especially in the field of impedance spectroscopy, as well as general device models. He has been distinguished in the 2014-2019 list of ISI Highly Cited Researchers.
Organic electrochemical transistors (OECTs) prove to be effective devices in various applications such as neuromorphic functionalities, bioelectronics, and sensors. Analyzing these mixed ionic-electronic devices is often complex due to the coupling of hole transport along the channel with ion insertion from the electrolyte. Numerous literature reports highlight persistent dynamical hysteresis effects in current-voltage curves, attributed to the gradual ionic charging of the channel under applied gate voltage.
We introduce a model that takes into account the primary electrical and electrochemical operational aspects of the device. This model is based on a thermodynamic function of ion insertion, allowing for the convenient classification of hysteresis effects. Such hysteresis can be categorized as capacitive or inductive. We specifically identify the volume capacitance as the derivative of the thermodynamic function, linked to the chemical capacitance of the ionic-electronic film.
Our findings reveal that the inductor response observed in impedance spectroscopy is associated with ionic diffusion from the surface, filling the channel up to the equilibrium value. The model uncovers multiple dynamical features tied to specific kinetic relaxations that govern the transient and impedance response of the OECT.
2.1-I3
In biological tactile somatosensory system, the cooperation of mechanoreceptors, neurons and synapses allows human to efficiently detect, transmit and process the tactile information. Emulation of the tactile sensory nerve to achieve advanced sensory functions in robotics with artificial intelligence is of great interest. Here, we report an artificial organic afferent nerve (AOAN) by integrating novel pressure-activated organic electrochemical synaptic transistor (OEST) and artificial mechanoreceptors. Owing to the effect of electrochemical ion doping and ion trapping in bulk conjugated semiconductor, external mechanical stimulation enables activation and modulation of OEST, endowing the system with the recognition/sensation of spatiotemporal tactile information and a low retention loss during signal transmission. Dendritic integration function for neurorobotics is achieved to perceive directional movement of object. An intelligent robot with our system, coupling with a closed-loop feedback program is demonstrated for slip detection and prevent slippage of objects. This work provides a promising approach towards next-generation intelligent neurorobotics and low power biomimetic electronics.
2.2-O1
Halide perovskites have been widely explored for numerous optoelectronic applications among which phototransistors appeared as one of the most promising light signal detectors. However, it is still a great challenge to endow halide perovskites with both mobility and high photosensitivity because of their high sensitivity to moisture in ambient atmosphere, which limits the efficiency of transporting and collecting charge carrier. Here, we explore FAPbBr3 perovskite quantum dots (QDs) phototransistor with band-like charge transport and measure a dark hole mobility of 14.2 cm2V-1s-1 at ambient atmosphere which is about an order of magnitude higher than solution processed perovskite QDs devices. Attaining both high mobility and good optical figures of merit, including photoresponsivity and detectivity, a detectivity of ~1016 Jones is achieved, which is a record for halide perovskite nanocrystals. Simple A-site salt (FABr) treatments offer a mechanism for connecting between perovskite QDs for better charge transfer in high-quality devices. All these important properties are superior to most advanced inorganic semiconductor phototransistor, indicating a promising future in optoelectronic applications.
2.2-I1
-
Future brain-computer interfaces will necessitate electronic circuits capable of processing signals in a localized and highly individualized manner within the nervous system and other living tissues. However, traditional neuromorphic implementations based on silicon have limitations in bio-integration due to poor biocompatibility, circuit complexity, and low energy efficiency. Organic mixed ionic-electronic conductors (OMIECs) are an emerging technology that has the potential to overcome these limitations. OMIECs enable efficient signal transduction by tightly coupling ions and electrons, making them ideal for interfacing electronics with biological systems. Here, we explore the use of OMIECs to develop organic electrochemical neurons and synapses that can be modulated using different types of chemical signals. These soft and flexible organic electrochemical neurons and synapses operate at low voltage and respond to multiple stimuli, signaling a new era for printed organic electronics [1]. We will discuss their ease of integration with biological nerves [2] and demonstrate the potential for OMIECs to enable highly localized and precise signal processing in brain-computer interfaces.
2.2-I2
Do Hwan Kim is currently a Distinguished Professor in the Department of Chemical Engineering at Hanyang University, South Korea. He received his PhD in Chemical Engineering from Pohang University of Science and Technology in 2005. From 2006 to 2010, he worked at the Samsung Advanced Institute of Technology as a senior researcher. He also worked at Stanford University, United States, as a postdoctoral fellow in the Department of Chemical Engineering (2011–2012) and worked as an Assistant Professor at Soongsil University, South Korea (2012–2017). His research interests are in the field of organic optoelectronics, electronic skins, and multimodal synaptic devices.
An iontronic-based artificial tactile nerve is a promising technology for emulating the tactile recognition and learning of human skin with low power consumption. However, its weak tactile memory and complex integration structure remain challenging. We present an ion trap and release dynamics (iTRD)-driven, neuro-inspired monolithic artificial tactile neuron (NeuroMAT) that can achieve tactile perception and memory consolidation in a single device. Through tactile-driven release of ions initially trapped within iTRD-iongel, NeuroMAT only generates non-intrusive synaptic memory signals when mechanical stress is applied under voltage stimulation. The induced tactile memory is augmented by auxiliary voltage pulses independent of tactile sensing signals. We integrate NeuroMAT with an anthropomorphic robotic hand system to imitate memory-based human motion; the robust tactile memory of NeuroMAT enables the hand to consistently perform reliable gripping motion. We utilized the augmented tactile memory of NeuroMAT to develop a NeuroMATICS-based robotic hand system, which consistently and reliably emulated tactile-memory-driven human motion. Our theoretical study and practical demonstration of the iTRD behavior can provide an inspiration with artificial nerve disciplines and can be extended to in the field of neuromorphic electronic skin and machine learning.
2.2-I3
Antonio Guerrero is Associate Professor in Applied Physics at the Institute of Advanced Materials (Spain). His background includes synthesis of organic and inorganic materials (PhD in Chemistry). He worked 4 years at Cambridge Dispaly Technology fabricating materiales for organic light emitting diodes and joined University Jaume I in 2010 to lead the fabrication laboratory of electronic devices. His expertise includes chemical and electrical characterization of several types of electronic devices. In the last years he has focused in solar cells, memristors, electrochemical cells and batteries.
Halide perovskite materials are mixed electronic and ionic conductors that find use in several applications. The ionic conductivity is responsible for a memory effect that leads to undesirable hysteresis in the solar cell configuration but it is a requirement in their use as a resistive memory. In this presentation, it is shown how conductive and insulating states are formed via migration of halide vacancy and electrochemically active metals halide perovskite useful as memristors. We show that the working mechanism and performance of the memory devices can be tuned and improved by a careful selection of each structural layer. Several configurations are evaluated in which structural layers are modified systematically: formulation of the perovskite,1 the nature of the buffer layer2,3 and the nature of the metal contact4. We show that in order to efficiently promote migration of metal contact the use of pre-oxidized metals greatly enhance the performance of the memristor and reduces the energy requirements. Overall, we provide solid understanding on the operational mechanism of halide perovskite memristors that has enabled increased stabilities approaching 105 cycles with well separated states of current and further improvements expected.5
1.1-I1
The escalating demand for solar energy highlights the critical need for advancing more efficient next-generation photovoltaic (PV) technologies. Currently, perovskite-based single junction and tandem solar cells are reaching or even exceeding the performance of established technologies like three-five tandem and GaAs solar cells. In the case of perovskite versus GaAs, employing nanophotonics to fine-tune the PV bandgap emerges as a viable strategy, especially when reaching the limits of composition engineering of FAPbI3. Tandem solar cells present another practical approach to surpass the single-junction Shockley-Queisser limit. Our initial efforts concentrated on solution-coating thick perovskite layers onto small, textured silicon substrates, successfully proving the concept's viability. In Singapore, we are actively working to shift this technology from laboratory research to manufacturing, focusing on applying perovskite layers to industrial Czochralski (CZ) silicon wafers. With cooperative efforts, our goal is to propel this technology from lab-scale to full-scale fabrication, aiming to surpass the performance of three-five PVs in full module size, thereby addressing the growing global energy demands with more efficient solar solutions.
1.1-I2
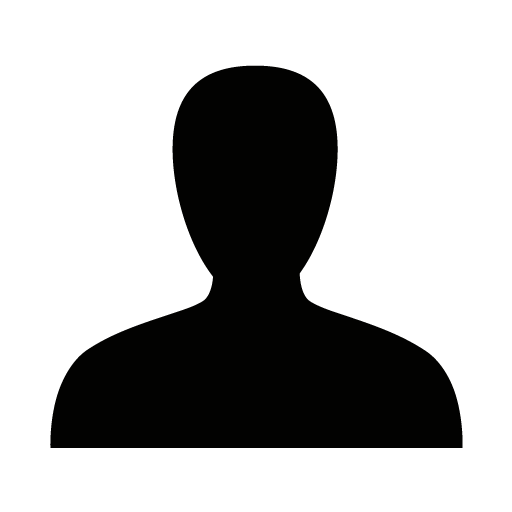
Integrating metal halide perovskite top cells with bottom cells formed by crystalline silicon or low band gap perovskites into monolithic tandem devices has recently attracted increased attention due to the high efficiency potential and application relevance of these cell architectures. Here we present our recent results on monolithic tandem combinations of perovskite top-cells with crystalline silicon, and Sn-Pb perovskites as well as tandem relevant aspects of perovskite single junction solar cells.
In 2020, we have shown that self-assembled monolayers (SAM) could be implemented as appropriate hole selective contacts. The implementation of new generation SAM molecules enabled further reduction of non-radiative recombination losses with high open circuit voltages and fill factor. By fine-tuning the SAM molecular structure even further, the photostability of perovskite composition with tandem-ideal band gaps of 1.68 eV could be enhanced by reduction of defect density and fast hole extraction. That enabled a certified efficiency for perovskite/silicon tandems at 29.15% [1].
By optical optimizations, we could further improve this value to 29.80% in 2021. Periodic nanotextures were used that show a reduction in reflection losses in comparison to planar tandems, with the new devices being less sensitive to deviations from optimum layer thicknesses. The nanotextures also enable a greatly increased fabrication yield from 50% to 95%. Moreover, the open-circuit voltage is improved by 15 mV due to the enhanced optoelectronic properties of the perovskite top cell on top of the nanotexture [2].
In the end of 2022, we enabled a new world record for perovskite/silicon tandem solar cells at 32.5% efficiency. We demonstrated that an additional surface treatment strongly reduces interface recombination and improves the band alignment with the C60 electron transporting material. With these modifications, single junction solar cells show high open circuit voltages of up to 1.28 V in a p-i-n configuration, and we achieve 2.00 V in monolithic tandem solar cells [3]. A comparable surface treatment was also applied to 1.80 eV band gap perovskites to enable Voc values of 1.35 V and these were integrated into monolithic all-perovskite tandem solar cells enabling a certified efficiency of 27.5% [4].
In addition to the experimental material and device development, also main scientific and technological challenges and empirical efficiency limits as well as advanced analysis methods will be discussed for perovskite based tandem solar cells. In addition, results on upscaling and stability of these industrial relevant tandem solar cells by thermal evaporation will be shown.
1.1-O1
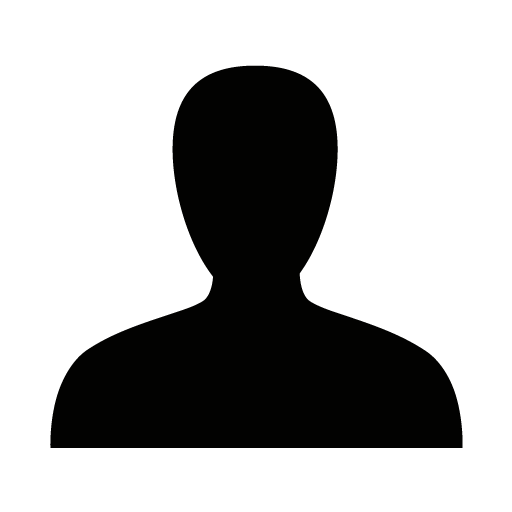
Perovskite-based tandem solar cells, with their compelling performance and cost-effective fabrication, stand at the forefront of photovoltaic technologies. Nevertheless, reduction in energy loss and enhancements in robustness of the front wide-bandgap perovskite sub-cell remain crucial, aiming for an efficient and stable perovskite-based tandem device. Surface passivation using large organic spacer cations on the top of perovskite films is a promising strategy to address the issues mentioned above, as it can effectively diminish the defect density. Simultaneously, the introduction of low-dimensional phases into perovskites has been demonstrated to decrease the minority concentration at the interface, effectively reducing nonradiative recombination and mitigating the mismatch between the open-circuit voltage (VOC) and the quasi-Fermi level splitting (QFLS), particularly for wide-bandgap-based perovskites.[1-4] In some other instances, the enhancement in VOC following surface passivation was attributed to the dipole moment of specific spacer cations that did not give rise to low-dimensional phases.[5,6] Moreover, the reduction in energy offsets of conduction bands (CBs) between the perovskite layer and the transporting layers also directly results in a VOC enhancement.[6,7] Uniquely separating passivation from energy alignment remains challenging, especially for multifunctional cations that can form 2D phases but also generate a dipole moment. Therefore, to achieve further enhancements in VOC and fill factor (FF) for wide bandgap perovskite sub-cells, it is essential to develop a comprehensive understanding of the underlying mechanisms.
As it will be discussed at the conference, the impacts of surface treatments with guanidinium bromide (GABr), 4-fluorophenylammonium iodide (F-PEAI) and their mixture on the formation of low-dimensional phases, device performances, as well as underlying loss mechanisms. Based on a hybrid experimental (QFLS) - theoretical (drift-diffusion simulation) approach, it reveals that the reductions in surface recombination velocity and energy level offsets between perovskite and electron transporting layer are the major contributors to a record VOC × FF production for 1.80 eV perovskite solar cell, which exhibited notably reproducibility transferring to another laboratory. After integrating with a narrow-bandgap perovskite rear cell, we demonstrated an efficient all-perovskite tandem solar cell with stable output of 27.2% under maximum power point.
2.1-I1
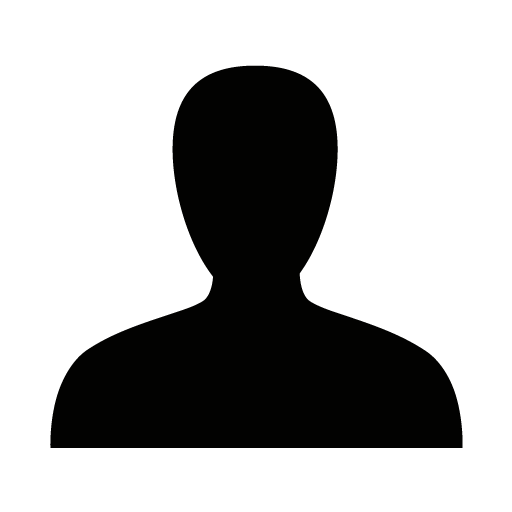
Tandem technology is the most promising route to further enhance the power conversion efficiency of solar cells. In recent years perovskite/silicon tandem solar cells have demonstrated an unprecedented rise of efficiencies far beyond the silicon single-junction efficiency limit. Further, all-perovskite multijunction solar cells gained increasing interest due to its compatibility with a fully printable, flexible, and lightweight technology platform.
In this contribution, optical aspects of perovskite tandem solar cells are discussed. This comprises 1) “classical” light management issues such as the minimization of reflection losses and the improvement of light trapping in thin absorber layers for the full spectral range. To achieve this, usually nano- and or micro-textures are implemented into the device coming along with special technological challenges concerning the compatibility of the textures with the respective perovskite deposition method. Further, we discuss 2) current-matching constraints in monolithic perovskite tandem devices. While under standard texting conditions there is little tolerance concerning the engineering of the perovskite bandgap(s), there is evidence that outdoor weather conditions, bifacial illumination as well as luminescent coupling can strongly relax current-matching constraints. Finally, we will give an outline how 3) optical engineering of luminescence and re-absorption processes can not only influence the short-circuit current density but also the electronic performance of the tandem solar cell device.
2.1-I2
Halide perovskites overwhelmed the field of photovoltaics with unprecedented progress in efficiency. Their facile bandgap tunability renders perovskite solar cells excellent building-blocks for multi-junction architectures, that provide the prospect to overcome fundamental efficiency limits of single-junctions. Combinations of perovskite wide-gap cells exist with a large variety of narrow-gap technologies, such as silicon or CIGS. Furthermore, low-cost tandem technologies are particularly interesting, such as all-perovskite tandems. However, stability concerns exist especially for narrow-gap perovskite cells, that typically contain large amounts of tin (instead of lead). The notorious oxidation of the Sn2+ to Sn4+ infers a detrimental self-doping [1]. Since this issue might impose a fundamental stability limit, organic solar cells are an attractive alternative as a narrow-gap subcell to form perovskite/organic tandems. Since the introduction of non-fullerene acceptors has revived the field, organic solar cells now reach efficiencies >19% and express absorption spectra extending well into the infrared. Organic and perovskite semiconductors share similar processing technologies, which makes them attractive partners in multi-junction architectures. I will discuss the prospects and challenges of perovskite-organic tandem solar cells by highlighting the key aspects of the individual building blocks and their interplay in the tandem. Specifically, the role of non-fullerene acceptors in efficient narrow-gap organic solar cells with high operational stability is discussed. A further focus is the wide-gap perovskite solar cell, where long term stability is the most pressing issue that needs attention. Eventually, the design and functionality of high-quality interconnects are outlined along with a view on its impacts on the characteristics of the tandem. I will present a specific example of a perovskite/organic tandem using an ALD grown InOx interconnect yielding a very promising efficiency of 24% with prospects reaching well beyond 30%.[2] In the end, I will benchmark perovskite-organic tandem solar cells against other emerging tandem solar cell technologies.
2.1-O1
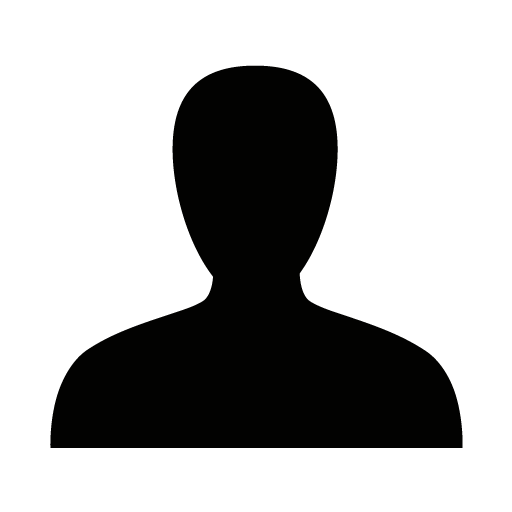
Tandem photovoltaic with complementary absorbing sub-cells is a promising pathway to overcome the thermalization loss and surpass the detailed balance limit of single junctions, stimulating intense interest. In recent years, the integration of wide-bandgap perovskite solar cells with narrow-bandgap organic solar cells represents a more prospective route in constructing tandem solar cells. However, for n-i-p type perovskite/organic tandems, the crucial interconnection layer (ICL) has been limited to the MoOx/metal nanoparticles/ZnO structure. As is well-known, this ICL has several limitations, leading to undesired optical and energy losses within the tandem's ICL, as well as high costs and time consumption from a fabrication perspective. In our study, we introduced a solution-processed hole transporting material to replace the traditional thermally evaporated MoOx in building the ICL for inorganic-perovskite/organic tandems. The optimized ICL exhibited significantly improved transmittance, particularly in the near-infrared region, which was conducive to enhancing the efficiency of the rear organic cell in harnessing light and achieved an increase in short-circuit current density (JSC) of approximately 1.5 mA/cm2. Additionally, owing to the favorable energy level alignment in the optimized ICL, the tandem device achieved a recorded open-circuit voltage of up to 2.3 V, along with an efficiency of 21% and enhanced stability.
2.1-O2
The efficiency of perovskite solar cells has surpassed 26%, approaching the fundamental efficiency limits for single-junction cells. Tandem technology, combining two solar cells with different bandgaps, offers a solution to overcome detailed-balance limits by reducing thermalization loss. Although the best perovskite/silicon and all-perovskite tandem devices have exceeded 33% and 29% efficiencies respectively, the high energy consumption of silicon wafers leads to significant carbon emissions, and the self-doping oxidation effect (Sn2+ to Sn4+) in narrow-bandgap perovskites poses an instability issue. These challenges can be addressed by changing the narrow bandgap cells to non-fullerene organic photovoltaics (OPVs). In such structures, solution-processed OPVs are energy-friendly and do not have stability concerns related to self-doping oxidation. The recent emergence of near-infrared acceptor Y6 further positions perovskite/organic tandem solar cells as a promising technology.
In previous work, we integrated PM6:Y6:PC61BM ternary OPVs into a perovskite/organic tandem devices, yielding a record efficiency of 24%. Notably, OPVs maintained approximately 95% original efficiency after 5000 hours of continuous operation under irradiation with low-energy photons (850 nm), but degraded rapidly when illuminated with a white light-emitting diode (LED), indicating that the visible spectral region could be responsible for device degradation[1]. In this work, we utilize monochromatic LED sources covering ultraviolet, visible, and near-infrared spectral region to systematically explore the photostability of Y6 ternary OPVs. Our results reveal that under continuous operation in the maximum-power point under irradiation with low-energy photons (λ > 590 nm), the devices show long-term stability (>1000 hours), while high-energy photons (λ < 530 nm) infer degradation, with the device's T90/T80 lifetime strongly correlated with the photon energy. Additionally, the distinct degradation behaviors of single polymers or NFAs under monochromatic light exposure elucidate their respective contributions to degradation in bulk heterojunction devices.
Interestingly, ternary OPVs featuring other Y-family NFAs with various energy gaps (Y18 (1.31 eV), CH1007 (1.30 eV), mBzS-4F (1.25 eV)) exhibit similar degradation behaviors under the same illumination conditions, verifying that the photo-degradation behavior evidenced above is generally valid. The distinctive photostable property of OPVs under low-energy photons can eliminate concerns regarding the stability of the rear cell in perovskite-based tandem structures, because the front perovskite cells function as spectral low-pass filters. We integrates these OPVs as sub-cells in a tandem structure with a wide-bandgap perovskite cells, the corresponding photostability of perovskite/organic tandem devices strongly depends on that of the wide-bandgap perovskite sub-cells, highlighting the advantage of organic rear cells and directing community focus more towards the stability of wide-bandgap perovskite in the future.
2.2-I1
Dr. Fan Fu is a group leader at Empa-Swiss Federal Laboratories for Materials Science and Technology. He received his bachelor's and master's degrees in materials science from the Wuhan University of Technology in 2010 and 2013, respectively. He joined Prof. Ayodhya N. Tiwari's group as a Ph.D. student in 2014 and earned his Ph.D. degree from ETH Zürich with distinction in 2017. His doctoral thesis on perovskite-CIGS thin-film tandem solar cells was awarded ETH Medal. From January 2018 to May 2019, he worked as a postdoc researcher in Prof. Christophe Ballif's group at EPFL. In June 2019, he joined Empa as a group leader. He is currently leading a research team investigating novel perovskite semiconductors for energy and optoelectronics applications. In particular, his group's recent research efforts focus on upscaling high-performance perovskite-based tandem solar cells and mini-modules on flexible substrates.
Stacking two perovskite layers with complementary bandgap in tandem device promise to exceed the efficiency limit of single-junction solar cells. In addition to high-efficiency potential, perovskite-perovskite (all-perovskite) thin-film tandems can be fabricated on flexible and lightweight substrates with very high power-to-weight ratios, thus opening numerous applications where flexibility and lightweight are important considerations. Leveraging low-temperature coating methods and high throughput roll-to-roll manufacturing, perovskite-based thin-film tandems promise very low manufacturing costs and CO2 footprint. Working towards our vision of roll-to-roll (R2R) manufacturing of flexible all-perovskite tandem solar modules, we present our latest progress on the development of highly efficient flexible all-perovskite tandem devices (Fig. 1). With interface and additive engineering, we demonstrate 25.4 % power conversion efficiency in a flexible 2-terminal all-perovskite tandem solar cell. Furthermore, we will present the first flexible all-perovskite tandem mini-modules and discuss the challenges of homogenous coating of perovskite on large areas using both scalable solution processes and vapor processes. Finally, we will discuss the potential and future challenges of developing highly efficient, lightweight, and stable all-perovskite tandem photovoltaics.
2.2-I2
René Janssen is university professor at the Eindhoven University of Technology (TU/e). He received his Ph.D. in 1987 from the TU/e for a thesis on electron spin resonance and quantum chemical calculations of organic radicals in single crystals. He was lecturer at the TU/e since 1984, and a senior lecturer in physical organic chemistry since 1991. In 1993 and 1994 he joined the group of Professor Alan J. Heeger (Nobel laureate in 2000) at the University of California Santa Barbara as associate researcher to work on the photophysical properties of conjugated polymers. Presently the research of his group focuses on functional conjugated molecules and macromolecules as well as hybrid semiconductor materials that may find application in advanced technological applications. The synthesis of new materials is combined with time-resolved optical spectroscopy, electrochemistry, morphological characterization and the preparation of prototype devices to accomplish these goals. René Janssen has co-authored more than 600 scientific papers. He is co-recipient of the René Descartes Prize from the European Commission for outstanding collaborative research, and received the Research Prize of The Royal Institute of Engineers and in The Netherlands for his work. In 2015 René Janssen was awarded with the Spinoza Prize of The Dutch Research Council.
Metal halide perovskite solar cells have advanced into a viable option for future renewable energy. Record single and tandem junction all-perovskite solar cells already provide power efficiencies of over ~26% and ~28%, respectively. A next target in photovoltaic energy conversion can possibly be met by developing perovskite triple or even quadruple junction solar cells. While these hold a promise to afford higher efficiencies, they require developing stable perovskite sub cells with bandgaps in the range of 1.8 to 2.3 eV, i.e., a range that has not received much attention so far. These wide-bandgap perovskites often suffer from more pronounced voltage losses due to non-radiative bulk and interfacial charge recombination. In developing new perovskite sub cells, photocurrent spectroscopy and absolute photoluminescence spectroscopy are used in combination with bulk and interface passivation strategies to eliminate these losses. This has enabled to reduce the voltage deficit over a wide range of bandgap. Guided by optical modeling, monolithic multi-junction solar cells have been fabricated by stacking two and three different bandgap perovskite sub cells in series using recombination junctions designed to provide near-zero electrical and optical losses. Collectively, these strategies enable monolithic tandem and triple junction solar cells with a power-conversion efficiency of over 26%.
2.2-O1
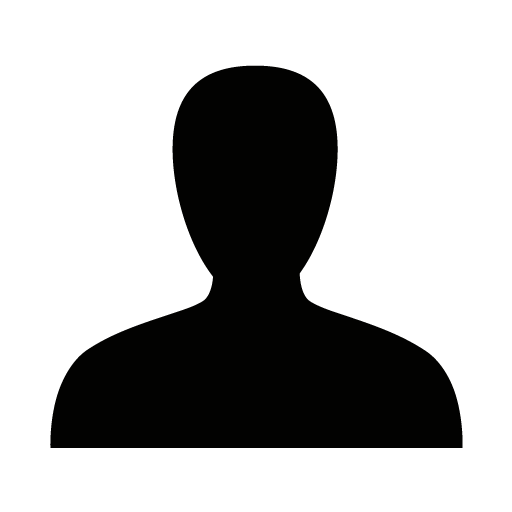
Understanding the performance limiting factors of organic solar cells (OSCs) with very small optical bandgaps is crucial for the development of novel tandem photovoltaics (PVs), such as OSC/OSC or perovskite/OSC tandems. For example, by combining a low-bandgap OSC ~ 1.1 eV with a high-bandgap absorber ~ 1.7 eV into a tandem solar cell, a power conversion efficiency (PCE) as high as 31.0% can be theoretically achieved, according to the detailed balance (DB) limit.
In this study, we investigate the loss mechanisms of ultra-low-bandgap OSCs based on the blend of the donor polymer PTB7-Th with the non-fullerene acceptor (NFA) COTIC-4F, with a bandgap of 1.15 eV, a promising candidate for tandem PV. In a conventional device structure, we reach a record PCE value of 8.55% with an enhanced short-circuit current density (JSC) and fill factor (FF) compared to previous records that relied on an inverted device geometry. While setting a record for this system, our PCE metrics still fall behind higher-bandgap OSC systems.
To guide further improvements, we investigate the various loss mechanisms and recombination processes using photoluminescence (PL) measurements, bias-dependent time-delayed collection field (TDCF) measurements, bias-assisted charge extraction (BACE), fluence-dependent photoinduced absorption (PIA), as well as light intensity-dependent open-circuit voltage (VOC). Complemented with optical and electrical device simulations, we show that JSC loss can be largely attributed to inefficient exciton dissociation in combination with geminate recombination of the charge transfer state. Further, we identify the fairly high bimolecular recombination coefficient as the main reason for the poor performance, while surface recombination is shown to mainly affect VOC. Finally, our simulations show that the simultaneous reduction of bimolecular recombination (e.g. by ternary blends), surface recombination (e.g. by self-assembled monolayers), exciton and charge transfer recombination (e.g. by vapor annealing) would enable efficiencies of >15% in the PTB7-Th:COTIC-4F system.
2.2-I3
Mobile ions play a significant role in perovskite photovoltaics (PV), yet their impact on the overall performance and stability of tandem solar cells (TSCs) remains largely unexplored. Moreover, the effects of hysteresis in the current-voltage (JV) characteristic and ionic field screening are usually not considered to be a major problem anymore in high-performance tandem cells due to the introduction of comparatively stable and well-performing pin-type perovskite cells. This conclusion is based on the established practice of using a relatively slow JV scanning rate for the characterization of these devices. Here, based on recent work,[1-4] I will present a comprehensive study that combines an experimental analysis of ionic losses in Si/perovskite and all-perovskite TSCs during device aging with drift-diffusion simulations. Our findings demonstrate that mobile ions have a significant influence on the hysteresis of both tandem cells at high JV scan speeds (e.g. 400 V/s) as well as on performance degradation due to field screening. Additionally, subcell-dominated “fast-hysteresis” measurements on all-perovskite tandems reveal more pronounced ionic losses in the wide-bandgap subcell during aging, which we attribute to its tendency for halide segregation. Drift-diffusion simulations fully corroborate the results. Finally, I will discuss how we can use the obtained ionic properties as an early fingerprint to predict the long-term stability of perovskite cells. Overall, our research provides valuable insights into how ion migration influences the energy-lifetime yield of perovskite PV and highlights new strategies to improve the stability of all perovskite-based single- and multi-junction cells.
1.1-I1
Bio Professional Preparation M.S. in Chemistry, with Honours, University of Bari, Italy, 1996 Ph.D. in Chemistry, University of Bari, Italy, 2001 Research interests Prof. L. Manna is an expert of synthesis and assembly of colloidal nanocrystals. His research interests span the advanced synthesis, structural characterization and assembly of inorganic nanostructures for applications in energy-related areas, in photonics, electronics and biology.
Halide perovskite semiconductors can merge the highly efficient operational principles of conventional inorganic semiconductors with the low‑temperature solution processability of emerging organic and hybrid materials, offering a promising route towards cheaply generating electricity as well as light. Following a surge of interest in this class of materials, research on halide perovskite nanocrystals (NCs) has gathered momentum in the last years. This talk will highlight several recent findings of our group on how the growth of NCs can be influenced by various exogenous cations and acid-based equilibria. We will also discuss our current understanding of halide exchange reactions involving perovskite nanocrystals as well as their heterostructures with other materials. We will outline new synthesis routes to strongly confined perovskite nanocrystals and their optical properties. Also, while most of the emphasis has been put on CsPbX3 perovskite NCs, more recently the so-called double perovskite NCs, having chemical formula A+2B+B3+X6, have been identified as possible alternative materials, together with various other metal halides structures and compositions, often doped with different elements. This talk will also discuss the research efforts of our group on these materials. I will highlight how for example halide double perovskite NCs are less surface tolerant than the corresponding Pb-based perovskites.
1.1-O1
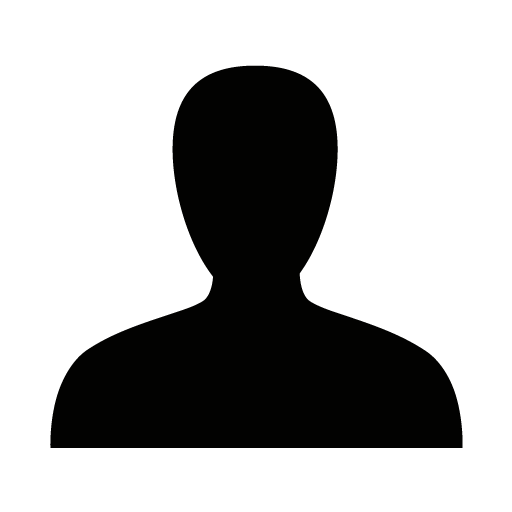
Over the last ten years, the role of hybrid metal halide perovskites has received significant attention as suitable materials for various electronic applications. Indeed, their outstanding optoelectronic properties, such as high-power conversion efficiency, tunable bandgap, and high absorption coefficient, make them suited for several applications in different devices as photovoltaic cells, photodetectors, light emitting diodes, and sensors [2]. Starting from the hybrid organic-inorganic perovskites (HOIPs), the introduction of a chiral molecule as organic cation leads to the breaking of the spatial inversion symmetry providing novel design tools by combining polarity and chirality [1,3]. This in turn open the way to explore several appealing chiroptoelectronic properties such as circular dichroism, circularly polarized emission, chiral-induced spin selectivity and so on. To extend the actual knowledge of these chiral systems and being able to properly engineer optimized phases it is important to carefully investigate the parameters affecting the chiral-induced response. From a materials chemistry point of view, this implies the ability of properties tuning by cation(s) and halide modulation/substitution. In this contribution we will present the results of the investigation of the role of the central metal and chiral cation, showing the modulation of the optoelectronic properties in two different approaches. On one side, we explored the preparation of a series of novel chiral metal halides including the same chiral cation, namely (4-Chlorophenyl)ethylenimine (Cl-MBA), but with a different central metal, namely Pb, Sn and Ge. This led to the discovery of the (R/S/rac-ClMBA)2SnI4 [4] and (R/S/rac-ClMBA)2GeI4 compositions showing also another structural 1D topology in presence of Ge, namely (R/S/rac-ClMBA)3GeI5 [5]. A comparison between these systems in terms of crystal structure and optical properties, coupled to computational modelling, sheds light on the tuning effect of central metal on the chiroptical properties. Another approach explore, relies on the properties modulation by including ad hoc synthesized bifunctional non-commercial chiral cations such as ((R)-1-amminobutan-2-ol) [6], thus exploring the impact of amine chemistry on the structural and optical properties. Final aim is to understand the impact of chemical degrees of freedom on the chirality transfer between the organic cation and the inorganic framework in order to provide tuning strategies for materials engineering.
1.1-O2
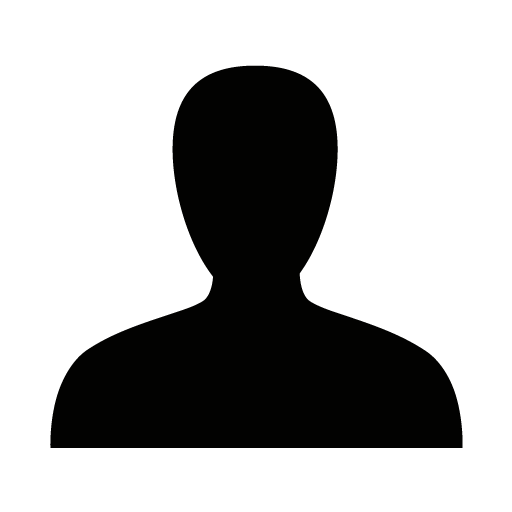
Future quantum technologies promise to deliver unprecedented computing power, secure communications and ultra-high precision measurements. A very promising way for realising these applications is via quantum optics, and single photon emitters are the key element of quantum optical technologies [1]. Among the various known methods for generating single photons, the use of optical transitions between quantum confined and isolated electronic states in semiconductor quantum dots (QDs) is one of the most promising approaches. Nowadays, after more than two decades of intensive development, epitaxial QDs have reached high fidelity performance; however, it appears that the best performance of these materials as quantum light sources is only achieved when operated at cryogenic temperatures. Instead, colloidal QDs have been much less explored as single photon emitters, but have the potential for operation beyond cryogenic temperatures (>4 K) due to stronger quantum confinement of carriers. Colloidal QDs can be synthesized in a variety of compositions, in particular metal chalcogenides such as CdSe, CdS, ZnSe, etc. and perovskite QDs are the most promising in terms of single photon emission properties. One of the fundamental technological difficulties for the realisation of single photon sources based on colloidal QDs is their slow decay rate at room temperature, since core-shell cadmium chalcogenide QDs have a typical lifetime in the range of tens of ns and perovskite QDs in the order of a few ns [2]. However, these lifetimes are too long to achieve ultrafast emission rates in the range of 100 Mph/s [1]. So far, various methods have been tried to increase the decay rate of colloidal QDs, e.g. by using a nanophotonic approach; experimental studies have shown an increase of the decay rate by a factor of 6 for a QD encapsulated in a plasmonic shell [3] and about 80 in plasmonic nanogaps [4] and patch antennas [5]. Another interesting method is based on exciton charging to enhance the QD emission rate itself, which can be realised by electrochemical or photochemical charge injection. Recent work by Morozov et al. [6] has shown a dramatic increase in the recombination rate for CdSe/CdS QDs using an electrochemical cell, from an initial lifetime of about 100-200 ns down to 1 ns at the highest applied voltage bias. Our study aims to exploit these results to further increase the room temperature decay rate of colloidal QDs by applying an exciton charging approach to CsPbBr3 perovskite QDs. Since perovskite QDs are not stable in polar solvents, an electrochemical method as used by Morozov et al. [6] cannot be applied here. For this reason, we investigate a novel approach based on a solid state device where an external electric field is applied directly to the perovskite QDs using a parent structure of a field effect transistor (FET) to generate highly charged excitons with a fast decay rate. Two alternative structures will be explored: one with an indium tin oxide (ITO) electrode to inject holes into the QDs, and the other with an aluminium layer to introduce electrons into the QDs. This will allow us to study the recombination of excitons in two different scenarios, the first with an excess of holes and the second with an excess of electrons. So far, we have investigated the performance of the device structure using a thin film of CsPbBr3 perovskite QDs. By applying a voltage bias to the device up to 40 V, we have observed a decrease in the lifetime of about 20% of the perovskite QDs that is reversible by returning to 0 V. These preliminary results are promising for moving on to the study of individual QDs.
1.2-I1
In this presentation I will discuss our studies of controlling spin populations in metal-halide organic/inorganic hybrid systems. Lower dimensional perovskites are of particular interest since the lower degree of symmetry of the metal-halide connected octahedra and the large spin-orbit coupling can potentially lift the spin degeneracy. To achieve their full application potential, an understanding of spin-relaxation in these systems are needed. Here, we report an intriguing spin-selective excitation of excitons in a series of 2D lead iodide perovskite (n = 1) single crystals by using time- and polarization-resolved transient reflection spectroscopy as well as transient circular dichroism. Exciton spin relaxation times are studied at room temperature for a range of 2D lead iodide perovskite systems and correlated to the exciton binding energy and degree of distortion in the metal halide framework. We also studied the spin-dynamics using THz emission spectroscopy and report on the circular photogalvanic and spin photogalvanic effect. Finally we are also investigating a novel class of chiral hybrid semiconductors based upon layered metal-halide perovskite 2D Ruddlesden-Popper type structures. These systems exhibit chiral induced spin selectivity (CISS) whereby only one spin sense can transport across the chiral layer and the other spin sense is blocked for one handedness of the chiral perovskite layer. We show that chiral perovskite layers are able to achieve > 80% spin-current polarization. I will discuss studies of spin-injection into a chiral-layer.
1.2-O1
Maria Chamarro is Professor in Physics at Sorbonne University, France and member of the Paris Institute of Nanosciences (INSP). She received her PhD in Physics (Optics speciality) form Zaragoza University, Spain, in 1989. Since 2021 she is a member of the French Committee for Scientific Research (five years) a position that she already occupied in the previous years (1995-2000). From 2012 to 2014 she was member of ‘Directory of Research’ at Pierre and Marie Curie University (now Sorbonne University). Her area of expertise is the experimental study of condensed matter electronic properties. In particular, she was interested in the spectroscopy of glasses doped with transition metals or rare earths, and the optical properties and relaxation dynamics of electronic excitations in semiconductor nanostructures. She was co-head of the "Spin Dynamics" team at INSP where she worked in the optical orientation and the all-optical manipulation of electron spin confined in a semiconductor quantum dot. In this framework, she developped ultrafast optical spectroscopies based on the photo-induced Faraday and Kerr effects. Now she coordinates a research project centred on the study of perovskite nanocrystals for nanophotonics applications.
In the last years, the hybrid metal-halide perovskites have attracted a great interest in the scientific community due to their excellent photo-physical properties and their outstanding optoelectronic and photovoltaic properties. In particular, formamidinium lead triiodide (FAPbI3) is the best candidate for single-junction HMP photovoltaics. It shows the highest theoretical photoconversion efficiency due to his energy bandgap, that is the lowest in the family of Pb-based halide perovskites (HPs). The very promising a-phase of FAPbI3 is however difficult to stabilize at room temperature. Recently, different groups work to implement a strategy to make it stable and some of them have obtained promising results [1]. The difficulties to stabilize the material have so far prevented exploring experimentally the whole peculiarities and characteristics of FAPbI3 beyond photovoltaic applications. However, FAPbI3, as other member of HP family, are highly promising material in the spintronic domain due to their large spin-orbit coupling and its spin-dependent optical selection rules.
In this work, we have studied, at 2K, the coherent evolution of the electronic spins in a polycrystalline film of FAPbI3 by using a picosecond pump-probe experimental set-up and measuring the photo-induced Faraday rotation technique (PFR) in transverse magnetic field. We identified two contributions to the PFR signal that we associated to localized electrons and holes respectively. We measured long spin coherence times of localized electrons (holes) of 3.1ns (1.6 ns) at 1.49 eV. These values are shorter than the values obtained in FA0.9Cs0.1PbI2.8Br0.2 [2], MAPI [3, 4,5] and CsPbBr3 [6] but are of the same order of magnitude. We have also obtained the value of g Landé factors for electrons 3.479 and for holes 1.137 that compares very well with previous results on FA0.9Cs0.1PbI2.8Br0.2 [2]. From the measured hole g factors and ellipsometry results on FAPbI3 we are able to obtain the Kane energy parameter of FAPbI3, Ep=13.5 eV, that is a central parameter to describe the band-structure and optical properties in the framework of the effective mass approximation [7]. This experimentally estimated value is smaller than the theoretical value [8]. All this results are of prime importance to evaluate the potentialities of the FAPbI3 as future actor in engineered HP spin-optronic devices.
1.2-O2
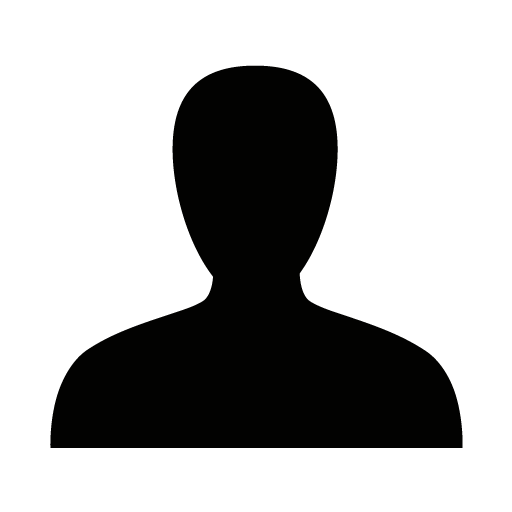
Lead-free double perovskites are emerging as an alternative to toxic lead-based perovskites for both light-emitting and light harvesting applications. Owing to the soft and polar nature, lattice deformations in metal halide perovksites can strongly couple to charge carriers upon photoexcitation. In contrast to lead-based perovskites, double perovskites exhibit intrinsic defects (Ag-vacancies), resulting in bound excitons with giant oscillator strengths. We utilize femtosecond pump-probe spectroscopy to investigate the dynamics of bulk and localized coherent phonons in spherical Cs2AgBiBr6 double perovskite nanocrystals at cryogenic temperatures. In this study, we observe that optically launched bulk lattice vibrations modify the spectral position of the bound excitonic resonance. Surprisingly, we found that this Ag-vacancy bound exciton is modulated in its giant oscillator strength. We argue that this is a consequence of an optically generated vibrational wave-packet localized at this very Ag-vacancy. This optically induced and spatially localized lattice shaking could become potentially useful for initiating photochemical or photocatalytic reactions with atomic precision.
1.2-I2
We provide a survey of our experimental studies of coherent spin dynamics of electrons and holes in lead halide perovskite crystals, nanocrystals and 2D materials. Time-resolved Faraday/Kerr rotation is used as technique in combination with magnetic fields up to 10 T and cryogenic temperatures. We measure spin relaxation and spin dephasing times, evaluate electron and hole Lande g-factors, demonstrate the hyperfine interaction of carrier spins with nuclear spin system. In perovskite nanocrystals grown both by colloidal chemistry and synthesized in glass matrix the electron spin coherence is detected even at room temperature and unusual temperature dependence of the electron g-factor is found. We establish experimentally and approve theoretically universal dependences of the electron, hole and exciton Lande g-factors on the bandgap energy, which in nanocrystals are further modified by carrier confinement. Spin mode locking effect based on spin synchronization under periodic laser excitation is found in nanocrystals in glass. Experimental approaches of spin physics give reach information about these materials.
1.3-I1
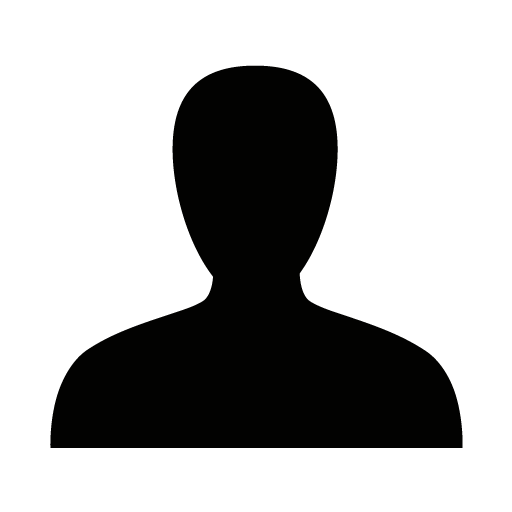
Novel growth and fabrication technologies allow for an unprecedented level of control over
nanoscale semiconductor quantum devices. Recently, several groups have demonstrated
samples where multiple quantum dots can be tuned into resonance [1,2]. When multiple
quantum emitters become indistinguishable, collective quantum effects like superradiance
and measurement-induced cooperative emission [2] emerge due to entanglement between
the emitters.
A detailed understanding of the signatures of cooperative emission is challenging for
several reasons: First, close-to-identical emitters operate in a regime where non-degerate
perturbation theory breaks down. Second, real-world quantum devices typically strongly
interact with local phonon baths as well as with a global photonic environment, and
the different environments generally influence each other. Moreover, the presence of ad-
ditional imperfections like spectral wandering further obfuscates the physical picture.
Nevertheless, for few emitters, the problem of cooperative emission in the presence of
multiple environments can be solved numerically exactly using the process tensor (PT)
formalism [3,4].
Here, I report on recent theoretical and experimental findings and summarize the
current understanding of cooperative emission in semiconductor nanostructures. This in-
cludes the discussion of how genuine superradiance can be distinguished from measurement-
induced cooperative emission, how to interpret peaks in g(2) photon correlation experi-
ments, and why inter-emitter correlations remain strong for long times despite strong
interactions with phonons.
1.3-I2
Lead halide perovskites have attracted tremendous attention, initially for their performance in thin film photovoltaics, and more recently for a variety of remarkable optical properties. Defect tolerance through polaron formation within the ionic lattice is a key aspect of these materials. In recent studies [1,2], some of us have applied two-dimensional electronic spectroscopy (2DES) to probe the timescale and amplitude of the electronic gap correlations in lead halide perovskite nanocrystals via homogeneous lineshape dynamics. The 2DES data reveal irreversible, diffusive dynamics that are consistent with liquid-like structural dynamics on the 100 femtosecond timescale. These dynamics were assigned to the optical signature of polaron formation, the conceptual solid-state analogue of solvation.
To complement the 2DES data, we have employed the method of femtosecond electron diffraction (FED) to directly measure the sub-picosecond lattice dynamics of weakly confined CsPbBr3 nanocrystals following above-gap photoexcitation. The data reveal a light-induced structural distortion appearing on a time scale varying between 380 and 1200 fs depending on the excitation fluence. We attribute these dynamics to the effect of exciton–polarons on the lattice and the slower dynamics at high fluences to slower sub-picosecond hot-carrier cooling, which slows down the establishment of the exciton–polaron population. Further analysis and simulations show that the distortion is consistent with motions of the [PbBr3]− octahedral ionic cage, and closest agreement with the data is obtained for Pb–Br bond lengthening.
Together, the 2DES and FED data offer a unique and complementary view of exciton-polaron dynamics in lead halide perovskite nanocrystals.
1.3-I3
Jacky Even was born in Rennes, France, in 1964. He received the Ph.D. degree from the University of Paris VI, Paris, France, in 1992. He was a Research and Teaching Assistant with the University of Rennes I, Rennes, from 1992 to 1999. He has been a Full Professor of optoelectronics with the Institut National des Sciences Appliquées, Rennes,since 1999. He was the head of the Materials and Nanotechnology from 2006 to 2009, and Director of Education of Insa Rennes from 2010 to 2012. He created the FOTON Laboratory Simulation Group in 1999. His main field of activity is the theoretical study of the electronic, optical, and nonlinear properties of semiconductor QW and QD structures, hybrid perovskite materials, and the simulation of optoelectronic and photovoltaic devices. He is a senior member of Institut Universitaire de France (IUF).
3D halide perovskites are direct band gap semiconductors, with low effective masses, sizeable optical oscillator strenghts. In relation with an original crystallographic structure for a semiconductor, electronic band edges at the R point define 3D halide perovskites as a novel semiconductor class characterized by Sz=1/2 for the top of the valence band and Jz=1/2 for a split-off band at the bottom of the conduction band due to a giant spin-orbit coupling [1,2]. Excitonic properties have been at the center of discussions since the beginning of the hype on photovoltaic in 2012. Following a prediction in 2014 [2], the direct measurement of the exciton binding energy in 2015 [3], led to values strongly reduced with respect to accepted values so far reported. Perovskite photovoltaics is since considered closer to the field of classical semiconductor solar cells than initially expected. The understanding of the excitonic properties, especially the exciton fine structure and the nature of exciton complexes, was further questioned with the advent of perovskite nanostructures leading to prospects for quantum light emission. A debate between an initial proposition of a fine structure related to a singlet dark ground state [4,5] and an inverted dark-bright ordering [6] , was closed recently in favour of the initial proposition [7,8,9]. Several open questions are remaining, especially about the nature of the coupling between the charge carriers and the lattice dynamics, which is unusual with respect to classical semiconductors [10]. Direct experimental investigations on the low energy lattice dynamics including neutron scattering are in progress [11]. State of the art abinitio simulations of the lattice dynamics and electron-phonon coupling will be shown [12]. The regime of low-temperature Fröhlich polaronic coupling in bulk 3D perovskites and quantum dots is also explored.
2.1-I1
Barbara Piętka received a doctorate at the University of Warsaw in Poland and at the Université Joseph Fourier in Grenoble, France as part of international co-tutelle. She gained professional experience working in France, Switzerland and Germany. She has built a research group focused on the study of non-equilibrium Bose-Einstein condensates of exciton-polaritons at the Faculty of Physics, University of Warsaw in Poland, where she has been working since 2010.
The main topic of her interests are quantum phenomena occurring in the regime of nonlinear light-matter coupled systems. She is concentrated on semiconductor materials, two-dimensional layered materials, perovskites and dielectric structures. The most important recent success is the demonstration of a device composed of a dielectric cavity filled with liquid crystal and perovskite demonstrating a tunable non-zero Berry curvature and chiral lasing. She is looking for efficient, room-temperature solutions for non-linear information processing, single-photon computing, and photonic accelerators.
The search for material platforms that ensure low cost, ease of fabrication, usability (room temperature) and nonlinearity in the same device is a serious challenge for today's photonics. Our work presents a first step towards a major breakthrough in the field of perovskite integrated photonics. For this purpose, we developed a versatile, template-assisted method for fabricating perovskite microstructures of any arbitrary, pre-defined shape. Our structures demonstrate waveguiding capabilities and facilitate the formation of spatially extended condensates of coherent exciton-polaritons.
Our microwires, characterized by their ability to bend without compromising optical quality, are formed from CsPbBr3 crystals and can be deposited on any substrate, enhancing compatibility with existing photonic devices. Notably, our method overcomes the limitations of traditional waveguiding setups by eliminating the need for extrinsic cavity mirrors. Our approach significantly simplifies the fabrication process, making on-chip polaritonic devices more accessible and cost-effective.
We demonstrate polariton lasing from the interfaces and corners of the microwires, with large blueshifts observed with excitation power. The high mutual coherence between different edge and corner lasing signals, evidenced in the far-field photoluminescence and angle-resolved spectroscopy, indicates the formation of a coherent, extended over macroscopic distance polariton condensate. This condensate is capable of propagating over long distances within the wires and even coupling between neighbouring wires through air-gaps.
The simplicity and scalability of our platform, combined with its compatibility with standard photonic components, pave the way for future large-scale, integrated polaritonic circuitry. Our findings not only demonstrate the potential of CsPbBr3 perovskites in photonic applications but also provide a more accessible path for the development of advanced on-chip optical devices with build-in nonlinearities.
2.1-O1
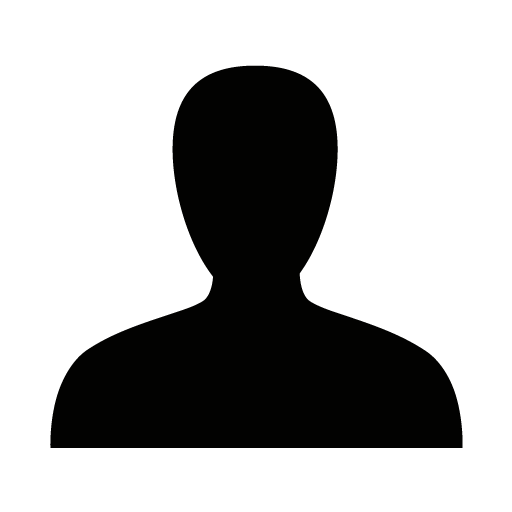
In recent years, lead halide perovskite (LHP) quantum dots (QDs) have attracted much attention for their unique optical properties. In contrast to conventional III-V and II-VI semiconductors with a rather complex valence band structure, LHPs are free of band degeneracies around the band gap. This reduces the complexity of their optical spectra around the band gap. Furthermore, the rather small effective electron and hole mass in LHPs generates a light exciton, which leads to significant confinement energies. The resulting well-separated energy levels simplify the assignment of electronic transitions, making LHP QDs an ideal model system to study quantum confinement effects.
We discuss highly monodisperse [1] spherical-like LHP QDs with diameters in the range of the exciton Bohr diameter, which feature a multitude of distinct resonances in their absorption spectra. By employing a simplified analysis within the envelope function approximation [2], we are able to assign the absorption resonances to center-of-mass motion confined excitons. Femtosecond transient absorption measurements on these QDs reveal bleaching of the exciton levels as well as a series of induced absorption features which we attribute to biexcitonic transitions [3]. In the context of light emitting devices, the emission and lasing properties of confined systems are of special interest. We therefore use our well-defined excitonic QDs to study their optical gain and amplified spontaneous emission characteristics. Our work shows the first insight into confined excitons in LHP QDs and provides a better understanding of their linear and nonlinear absorption and emission spectra as well as their polarization-dependent properties.
2.1-O2
Grigorios Itskos obtained a B.Sc. in Physics in 1997 from University of Thessaloniki, Greece and carried out his PhD studies at SUNY at Buffalo, USA (Ph.D. in Physics 2003), under the supervision of Prof. Athos Petrou within the newly-born field of semiconductor spintronics. He worked as postdoctoral researcher (Imperial College London, 2003-2007) under the supervision of Profs. Donal Bradely and Ray Murray, focusing on photophysical studies of hybrid organic-inorganic semiconductors. In September 2007 he was hired as a faculty member at the Department of Physics, University of Cyprus (Lecturer 2007-2011, Assistant Professor 2011- 2017, Associate Professor 2017- now). His group research activities focus on optical studies of inorganic, organic and hybrid solution-processed semiconductors, with recent emphasis on the characterization and optoelectronic applications of semiconductor nanocrystals.
Synthesis of tin (Sn) halide perovskite nanostructures has been challenging as the high surface to volume ratio promotes fast Sn2+ to Sn4+ oxidation and facilitates the production of polymorphs with different optoelectronic properties. Recently, an optimized synthetic route was developed, allowing the production of robust and monodisperse three-dimensional (3D) CsSnI3 nanocrystals (NCs) with sizes in the 6 to 10 nm range [1]. The synthetic process produces also small amounts of two-dimensional (2D) (R-NH3+)2SnI4, Ruddlesden–Popper nanosheets, that form stable colloidal mixtures with the 3D NCs [1].
Herein, the spontaneous and stimulated emission properties of thin films of the coexisting 3D-2D nanostructures are discussed. The films emission is dominated by the NC component, as transient spectroscopy indicates the presence of efficient energy funneling from the nanosheets to the nanocrystals. At cryogenic temperatures, two CsSnI3 NC luminescence species exist, identified as surface bound excitons and free excitons. The relative population of the two species depends on temperature, aging, thermal cycling and surface passivation.
Upon intense photoexcitation with nanosecond pulses, stable amplified spontaneous emission (ASE) is observed from the CsSnI3 NCs at temperature up to 150K. Fabrication of NC-polymer multilayer structures allows the reduction of the ASE threshold and the observation of ASE at temperatures approaching 300 K.
2.1-I2
Dr. Thilo Stöferle has been a permanent Research Staff Member at the IBM Research – Zurich Laboratory since August 2007. His current research interests are quantum simulation and quantum fluids, Bose-Einstein condensates with exciton-polaritons, integrated high Q/V cavities, nanophotonic lasers and switches. Another focus is on hybrid nanocomposite quantum materials for strong-light matter interaction and opto-electronic applications.
By embedding optically active materials with high oscillator strength in optical microcavities, the strong light-matter interaction regime can be reached where exciton-polariton quasiparticles are formed that are part photon and part exciton. At sufficiently high excitation density, eventually a non-equilibrium Bose-Einstein condensate can form, exhibiting nonlinear behavior, macroscopic coherence and quantum fluid properties. Bulk crystalline thin films of CsPbBr3 in microcavities have shown to allow room temperature polariton condensation. Nanocrystals have the promise of enhanced polariton interactions due to their spatial confinement.
Here we report on strong light-matter coupling of colloidal CsPbBr3 nanocrystals and polariton condensation at ambient conditions, as evidenced by nonlinearly increasing emission, line narrowing and coherence measurements. We use a tunable open microcavity based on distributed Bragg reflectors to tune the polariton energy. By precise nanofabrication, we create tiny indentations in the mirrors to effectively produce potential landscapes for the polariton condensate, which opens perspectives to use the polariton quantum fluid as analogue simulator for interesting hamiltonians.
2.2-I1
Luisa De Marco received her PhD in Nanoscience from Università del Salento in 2010 working on nanostructured semiconductors for photovoltaics. Since 2016 she is researcher at CNR NANOTEC leading a 6-person team working on the development of low-dimensional inorganic and hybrid nanomaterials. She is author of more than 70 papers that collectively have received more than 2600 citations, with an h-index of 31. Among the publications stand out Advanced Materials, Nature Nanotechnology, Energy & Environmental Science, ACS Nano and Science Advances.
Her research interests focus on the development and engineering of hybrid and inorganic low-dimensional semiconductors having specifically tailored functional properties and on design and fabrication of optoelectronic devices.
In recent years, hybrid organic-inorganic two-dimensional perovskites (2D PVKs) have attracted great interest due to the unique combination of intriguing properties at room temperature, making this class of semiconductors suitable for various optoelectronic applications. [1]
The alternating of organic and inorganic layers induces the formation of a natural multiple quantum well (MQW), offering the possibility to tailor the optical and structural properties of 2D PVKs acting directly on the chemical structure. In fact, by changing the elements of the metal-halide octahedra, it is possible to tune the band gap over a wide energy range, while varying the organic components enables a fine tuning of the MQW structure, introducing organic building blocks with new functionalities for the development of unexplored hybrid semiconductors. [2,4]
In this work, we modulate the structural distortion of novel 2D perovskites to design their optical characteristics and electrical band structure. We investigate the impact of the cation length and the ammonium binding group on the crystalline structure using interlayer cations with different characteristics. By doing so, we show that the exciton characteristics can be easily modified through careful organic cation design.
Furthermore, we integrate the developed PVKs single crystals into nanophotonic devices by synthesising microwires [5] that act as waveguides, in which strong light-matter coupling occurs between the optical modes of the crystal and the PVK exciton, with the generation of exciton−polaritons, the half-light half-matter quasi-particles that inherit unique properties from both excitons and photons.
The understanding of the structure-property relationship and the demonstration of new features can be exploited for the realization of compact, integrated optical devices with wide tunability.
2.2-O1
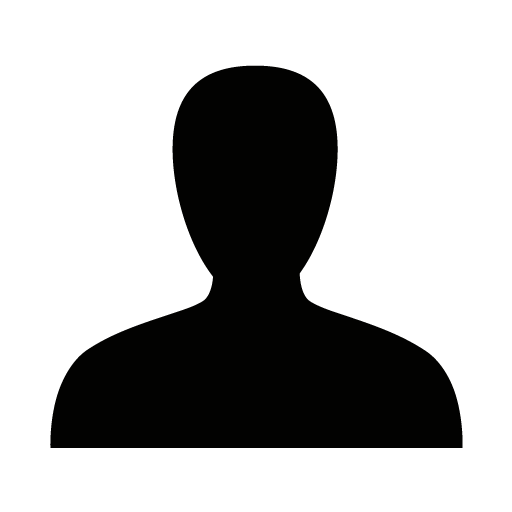
The production of single indistinguishable photons is important for linear optical operations, in the realization of photonic-based quantum computational circuits. The present work aims to investigate the use of the exciton recombination in single CsPbBr3 and CsPb(Br/CL)3 perovskite nanocrystals as a source of single indistinguishable photon emission. Using the HONG-OU-MANDEL(HOM) interference technique, two sequentially produced photons are overlapped to investigate the interfering visibility [1,2]. The nanocrystals are synthesized by the group of M. Kovalenko (ETH Zürich & EMPA Dübendorf) to have a rod-like symmetry. By breaking the symmetry of the confinement potential, the exciton emission spectra reduce to a pure singlet line. This eases the collection of the emitted photons, as filtering of orthogonal polarization states is not needed and a Bandpass filter can be employed (mainly to suppress biexciton light). The nanocrystals show ~50 ps lifetime [Fig.] and a narrow spectral line at cryogenic temperatures. I will present our HOM results and compare them with previous work[3]. We achieve low g2(0) values around 0.10 [Fig.] and uncorrected HOM interference visibility up to 0.44.
2.2-O2
Juan P. Martínez-Pastor, Full Prof. at the University of Valencia. PhD in Physics, 1990. Three years of postdoctoral experience at the European Laboratory of Non-Linear Spectroscopy (Florence, Italy) and at the École Normale Supérieure (Paris, France). Prof. Martínez-Pastor is expert in Semiconductor Physics, particularly optical properties and exciton recombination dynamics in quantum wells, wires and dots based on III-V semiconductors and other compounds since 1990. This research line continues nowadays focused on quantum light produced by quantum dot semiconductors and its management for quantum communications. After 2006 he has leaded/co-leaded several research lines in nanoscience and nanotechnology regarding the development of several types of nanomaterials (metal and quantum dots, multi-functional nanocomposites) and applications to photonics and plasmonics. In the last three years, he focuses his research in optical properties, exciton recombination dynamics and applications in photonics of two-dimensional semiconductors and metal halide perovskites. He has supervised 16 PhD theses and is author/co-author of 220 peer-reviewed publications, other than seven patents and promotor of a spin-off company.
Self-assembled nanocrystals (NCs) into superlattices (SLs) are alternative materials to polycrystalline films and single crystals, which can behave very differently from their constituents, especially when they interact coherently with each other, leading to collective emission, as superfluorescence [1]. In this work we concentrate on the Superradiance (SR) emission observed in SLs formed by CsPbX3 NCs synthesized by hot injection with synthesized with oleic acid and oleylamine (OA/OAM) and didodecyldimethylammonium bromide (DDAB) ligands. These SLs were studied by Photoluminescence (PL), Time Resolved PL (TRPL) and Micro-PL/TRPL spectroscopy at low and high temperatures to extract information about the SR states and uncoupled domains of NCs. In the case of CsPbBr3 SLs formed by large sized NCs we have measured very narrow (1-5 meV) emission lines on the low energy side of their µ-PL spectra, which can be considered near homogeneous, and characterized by lifetimes as short as 160 ps. The enhancement factor estimated for these low energy narrow lines can reach values as high as 10, like the value estimated from the inverse ratio of their lifetimes over that in a film, which is associated to domains of nearly identical NCs formed by 1000 to 40000 NCs within the micrometric SLs [2]. Therefore, the nature of these emission lines is consistent with a SR exciton state whose origin is due to the coupling of optical dipoles in perovskite NCs; this origin is consistent with our estimate of the correlation length for these SL domains with correlated NCs. The coherence of the SR exciton state has been studied in the temperature region dominated by a radiative recombination dynamics (from 4 to 70-80 K). The importance of thermal decoherence for the SR state is observed above 25 K and due to its coupling with an effective phonon energy of around 8 meV. The emission of high energy states in the SL spectra are also characterized by short lifetimes, because of Förster transfer of energy (from small to large NCs of the distribution or from high to low emission energies). More recent work on perovskite SLs is being directed to a better control of the SL growth and evaluating the effect of a solid-state anion state procedure [3] on initially formed CsPbBr3 SL and the optical properties of the SLs, including the superriant state.
2.2-I2
The brightness of an emitter is ultimately described by Fermi’s golden rule, with the decay rate of the emitter’s excited state proportional to the product of its oscillator strength and the local density of photonic states (LDOS). Since the former is an intrinsic material property and, hence, already set by the choice of the employed compound, the quest for ever brighter emission has mainly focused on enhancing the LDOS using dielectric or plasmonic resonators to achieve a large Purcell effect or strong light-matter interactions1,2. A much less explored avenue is to boost the oscillator strength, and hence the emission rate, via a collective behavior termed superradiance. Recently, it had been proposed that the latter can be realized via the giant oscillator-strength transitions of a weakly confined exciton in a quantum dot (QD) when its coherent motion extends over many unit cells. Here we demonstrate single-photon superradiance in perovskite quantum dots (QDs) with a record-low sub-100 ps radiative decay time, almost as short as the reported exciton coherence time3. The characteristic dependence of radiative rates on QD size, composition, and temperature suggest the formation of giant dipoles, as confirmed by effective-mass calculations. The results aid the development of ultrabright, coherent quantum-light sources and attest that quantum effects, e.g. single-photon emission, persist in nanoparticles ten times larger than the exciton Bohr radius.
1.1-I1
Perovskite solar cells have come to the forefront of solar research in the last decade with certified efficiencies of now >26%. This is approaching rapidly the Shockley-Queisser limit for single-junction solar cells, implying that the main breakthroughs for perovskites were achieved with relatively narrow bandgaps.[1a,1b] Less progress, however, was made for wider bandgap perovskites, which are of interest for multijunction photovoltaics, detector applications, or water splitting. These wide bandgap perovskites are often comprised of fully inorganic components, which are hard to dissolve in conventional solvent systems and require more sophisticated synthesis as well as crystallisation techniques.
In this talk, I will discuss strategies to address these challenges by providing a library of hitherto unexplored wider bandgap perovskites using combinatorics. Mechanosynthesis is then studied to attain otherwise inaccessible liquid precursors permitting the realization, e.g., of “triple cation” wide bandgap perovskites.[2]
Unfortunately, the newly formulated liquid precursors often exhibit complex crystallization behaviour struggling to expel the typically used DMSO solvent. To delay the crystallization time, two strategies are proposed to remove the strongly complexating DMSO molecules through a) modified processing of the liquid thin-film[3] and b) a coordination solvent with a high donicity and a low vapor-pressure[4] leading to a marked improvement in the overall film quality.
Lastly, interface manipulation, especially on top of the formed perovskite, is becoming a central topic to advance further. Typically, this involves chemical surface treatments with a complex interaction. Here, light annealing is introduced as a universal, non-chemical approach to modify the perovskite surface resulting in a reduced surface recombination.[5]
[1a] Saliba et al. Energy & Environmental Science (2016), [1b] Turren-Cruz, Hagfeldt, Saliba; Science (2018)
[2] Ferdowsi,…, Saliba ; Chemistry of Materials (2021)
[3] Byranvand,…, Saliba; One‐Step Thermal Gradient‐and Antisolvent‐Free Crystallization of All‐Inorganic Perovskites for Highly Efficient and Thermally Stable Solar Cells, Advanced Science (2022)
[4] Zuo,…, Saliba; Coordination Chemistry as a Universal Strategy for a Controlled Perovskite Crystallization, Advanced Materials (2023)
[5] Kedia,…, Saliba; Light Makes Right: Laser Polishing for Surface Modification of Perovskite Solar Cells, ACS Energy Letters (2023)
1.1-I2
Perovskite nanocrystals (NCs) such as those made from CsPbBr3 have numerous uses. Most involve exploiting their light emission and are motivated by their defect tolerance and large, as-made emission quantum yields (QYs). For the latter, near unity QYs are possible. This leads to ready applications as light emitters but also intriguingly to possible material platforms for demonstrating semiconductor-based optical refrigeration.
Absent, though, is a fundamental understanding of perovskite NC emitting states, central to these applications. Of particular note are observations of near-universal, size-, temperature-, and composition-dependent absorption/emission Stokes shifts where observed energy differences are those that separate absorbing and emitting states. Moreover, efficient (near-unity efficiency), photoluminescence up-conversion, induced by exciting NCs below gap, motivates better understanding the microscopic nature of perovskite band edge states.
I will explain work we have done recently within the context of attempts to optically refrigerate a semiconductor. These studies now provide new insight into the band edge states of CsPbBr3 (and possibly CsPbX3) NCs. Starting with a microscopic mechanism for explaining how it is possible to obtain efficient, near-unity efficiency photoluminescence up-conversion to we extend the conclusions of these measurements to explain the origin of observed, size-dependent absorption/emission Stokes shifts. Here, despite some initial studies we and others have conducted, very little is known about their true origin. This is to be contrasted to more conventional NCs such as CdSe where band edge exciton fine structure quantitatively accounts for both global and resonant Stokes shifts, with emission emerging from a dark exciton. Although similar perovskite NC fine structure might account for their shifts, both theory and experiment predict bright/dark fine structure splittings easily an order of magnitude too small to account for experiment.
We now propose that perovskite NC emitting states are polarons, which result from the lattice accommodation of photogenerated charges. Polaron binding energies and lifetimes are, in turn, suggested to be the origin of observed l-, T-, and composition-dependent absorption/emission Stokes shifts and excited state lifetimes. This represents a significant departure from more conventional descriptions of NC band edge states, which exclusively involve exciton fine structure and dark exciton emitting states.
1.1-I3
Halide Perovskite Photovoltaics are on the brink of commercialization and after having achieved high performance, the stability, scalability, and sustainability of the technology are now the most important technological challenges to overcome. Halide perovskites being versatile in terms of their processability, there are many options for the development of scalable processing technology. Solution processing based on slot-die coating and inkjet printing are two promising options with their respective design advantages and opportunities.
The development of precursor inks that allow reliable and sustainable processing is one of the most important challenges we are tackling. Our team's current efforts in this domain focus on three major aspects:
Accelerated ink and process optimization by combinatorial processing approaches: We are employing innovative combinatorial processing techniques based on inkjet printing and slot-die coating to expedite the optimization of precursor inks and associated manufacturing processes.
Exploration of Green Solvent Alternatives with Extended Ink Shelf-Life: In our commitment to sustainability, we are investigating environmentally friendly solvent alternatives. Our focus extends beyond performance considerations to include the development of green solvents that not only meet performance standards but also contribute to an extended ink shelf-life. This dual emphasis on performance and longevity is crucial for practical implementation and large-scale production.
Collaborative Data Management: Based on ongoing efforts of the PerovskiteData base Project, we are introducing Research Data Management Tools that can easily be adapted and multiplied by the entire research community. This collaborative approach facilitates efficient decision-making and accelerates the iterative optimization process.
By addressing these challenges head-on, our research aims to contribute to the acceleration of the deployment and utilization of Perovskite Photovoltaics for power generation.
1.1-I4
Hybrid organic-inorganic perovskite materials have gained emphasis in worldwide photovoltaic (PV) research, particularly due to their impressive power conversion efficiencies (PCEs) above 26% as well as their potential of becoming a candidate for inexpensive mass production1. Perovskite-based research today is mostly on thin layer solution based deposition techniques given their potential for integration into semitransparent. However, integration of this approach into windows and buildings is challenging as homogeneity, reproducibility, yield, and other aesthetic relevant characteristics required for building integration market have not been demonstrated to a satisfactory level. As an alternative, three-layered dielectric/metal/dielectric (DMD) thin film sequences have been successfully employed in perovskite-based research resulting in comparable PCEs up to 15%2, superior average visible transmittance (AVT), and good light utilization efficiency (LUE)3, defined as the product between AVT and PCE, highlighting the great promise of transparent electrodes. Here, we have studied semi-transparent DMD electrodes based on WO3 and Ag, to boost the PCE in semi-transparent formamidinium lead halide solar cells. A screening upon the mixed halide perovskite, from pure bromide to pure iodide has been made, demonstrating that the PCE increases with the semi-transparent electrode compare to the conventional semi-transparent Au. This work demonstrates the potential use of semi-transparent electrode to improve the light harvesting of the perovskite layer, by using optical methods to compensate chemical limitation, paving the way for application in building integration. It is proved herein that semitransparent electrodes with WO3 of 40 nm allows for the fabrication of solar cells covering a wide bandgap regime ranging from materials with suitable bandgaps for single-junction solar cells (FAPbI3, Eg = 1.5 eV) to wide bandgap materials (FAPbBr3, Eg = 2.3 eV). The solar cells prepared with this approach give promising results that compete well with more intricate fabrication approaches previously reported, while at the same time current and aesthetic parameter like Average Visible Transmittance (AVT) and Light Utilization Efficiency (LUE) are increased to real building relevant values.
2.1-I1
Halide perovskites have been under the focus for photovoltaic applications where their power conversion efficiencies have soared to efficiencies exceeding 26%. They have also garnered tremendous research interest over recent years for the development of next-generation light-emitting diodes and detectors. The spotlight on this class of semiconducting material stems from several of its enviable traits such as long carrier diffusion length, defect tolerance, high colour purity, and spectral bandgap tunability which spans across the visible and infrared spectrum. This talk will cover our recent efforts in utilising the ionic characteristics of halide perovskites to enable memristive devices that can be employed for neuromorphic applications. The talk will cover 2 and 3 terminal memristive devices as well as our efforts to utilise colour changing LEDs and photovoltaic devices as unconventional computing elements.
2.1-O1
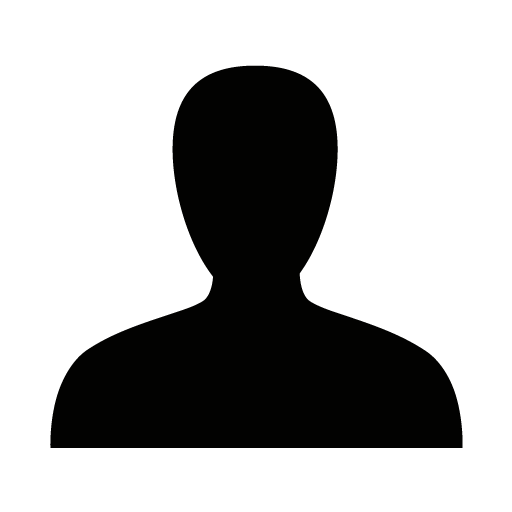
The family of Sn-perovskite has recently emerged as a nontoxic compound for a future ecofriendly photonic technology. With an extraordinary quantum yield of emission at room temperature, bandgap tunability with the composition, and straightforward incorporation in optical architectures by chemistry methods, the ASnX3 (A is an organic/inorganic anion and X is a halide) perovskite represents a suitable nontoxic material to implement lasers, optical amplifiers or light emitting diodes, among other devices. However, since Sn2+ is easily oxidized into Sn4+ losing its optoelectronic properties, there are few reports where a stable ASnX3 device is provided. In this work, high-quality FASnI3 (FA, formamidinium) polycrystalline thin films are successfully fabricated to demonstrate optical amplification and lasing. With an appropriate low temperature treatment and a polymethylmethacrylate(PMMA) clapping, the FASnI3 films exhibit a remarkable stability and an efficient generation of amplified spontaneous emission (ASE) under nanosecond optical pumping. First, these films are deposited on a SiO2 substrate to conform an optical waveguide whose geometrical parameters (i.e. thicknesses of the films) are properly designed to optimize the excitation and to enhance the generation of the photoluminescence. As a result, ASE is demonstrated with an extremely low threshold, about 100 nJ/cm2, and a strong polarization anisotropy preferable to the transverse electric (TE) polarization. Moreover, the waveguide exhibits narrow lasing lines (< 1 nm) caused by the formation of random cavity loops in the polycrystalline grains. Then, the same structure is implemented in a flexible device using polyethylene terephthalate (PET) substrates. Here, despite that the formation of films formation of films on a flexible platform is always challenging, the flexible waveguide also shows ASE and RL lines with only one fold higher threshold (1 µJ/cm2). Finally, the FASnI3 films are also incorporated as active medium in a Distributed Feedback Laser properly designed to achieve optical resonance at the ASE wavelength. This architecture conforms an optical cavity able to demonstrate the generation of lasing action with peaks narrower than 1 nm. The proposed devices represent an important step towards the development of future cheap and green photonic technology based on Sn perovskites.
2.1-O2
Marios Zacharias is currently an MSCA postdoctoral researcher at FOTON Institute, INSA, Rennes working with Prof. Jacky Even in the field of halide perovskites. He earned his Ph.D. in Materials Science at Oxford University, United Kingdom (2017) and held a post-doctoral appointment at Oxford University (2018), under the supervision of Prof. F. Giustino. In 2019, he joined the NOMAD laboratory of Prof. M. Scheffler at Fritz Haber Institute in Berlin. From 2020 to 2021, he moved to the Cyprus University of Technology and led the simulation group of RUNMS of Prof. P. C. Kelires. His research interests focus on electronic structure theory and the development of new first-principles techniques for the accurate and efficient description of anharmonicity, electron-phonon, and vibronic physics of condensed matter systems. He is the developer of the software package EPW/ZG in Quantum Espresso. He has developed the special displacement method (SDM) [1,2] for electronic structure calculations at finite temperatures. Marios has also introduced an approach for the calculation of multiphonon diffuse scattering allowing for the interpretation of thermal and ultrafast phenomena in solids [3,4]. He is currently working on the efficient treatment of anharmonicity and polymorphism in halide and oxide perovskites [5].
[1] Phys. Rev. Res. 2, 013357 (2020)
[2] Phys. Rev. B 108, 035155 (2023)
[3] Phys. Rev. Lett. 127, 207401 (2021)
[4] Nat. Mater. 23, 937 (2024)
[5] npj Comp. Mater. 9, 153 (2023)
Metal halide perovskites are of immense importance due to their excellent optoelectronic properties, holding great promise in the field of advanced and clean energy technologies. One of the fundamental mechanisms governing their physical properties is the interplay of electron-phonon coupling with the strong lattice anharmonicity and their inherent locally disordered structure [1,2]. To understand the consequences of these effects, we have recently introduced a powerful theoretical framework that allows to capture anharmonic electron-phonon coupling in these intrinsically polymorphous compounds [3,4]. In this talk, I will demonstrate our methodology for both inorganic and hybrid halide perovskites and show that (i) local disorder is at the origin of overdamped and strongly coupled anharmonic phonons, (ii) low-energy optical vibrations dominate electron–phonon renormalized band gaps, departing from a simplified picture of a Fröhlich interaction, and (iii) local disorder is the key to explain the monotonic increase of the band gap across phase transitions. Our work provides a new perspective for the interpretation of perovskite materials fundamentals as well as opens the way for efficient simulations of halide perovskites' key properties, like carrier mobilities, excitonic spectra, and polaron physics.
2.1-I2
Lead and tin halide perovskites are currently under intense investigation for their potential applications in optoelectronics, due to their favorable and adjustable semiconducting properties. One of the key advantages of perovskites lies in their ability to be deposited as thin films using energy-effective techniques, resulting in high-quality semiconductors. Despite the potential for widespread adoption in the industrial sector, vacuum deposition of perovskite films and devices remains a specialized area. Here we will examine the latest developments in the vacuum deposition of perovskite films, focusing on methods to manipulate their morphology and structure. Specifically, we will highlight the impact of factors such as composition, deposition rate, and substrate temperature on properties like luminescence quantum yield and recombination lifetime. Lastly, we will explore the use of multilayer semiconducting structures in single-junction solar cells and photodetectors.
2.2-I1
Juan Bisquert (pHD Universitat de València, 1991) is a Professor of applied physics at Universitat Jaume I de Castelló, Spain. He is the director of the Institute of Advanced Materials at UJI. He authored 360 peer reviewed papers, and a series of books including . Physics of Solar Cells: Perovskites, Organics, and Photovoltaics Fundamentals (CRC Press). His h-index 95, and is currently a Senior Editor of the Journal of Physical Chemistry Letters. He conducts experimental and theoretical research on materials and devices for production and storage of clean energies. His main topics of interest are materials and processes in perovskite solar cells and solar fuel production. He has developed the application of measurement techniques and physical modeling of nanostructured energy devices, that relate the device operation with the elementary steps that take place at the nanoscale dimension: charge transfer, carrier transport, chemical reaction, etc., especially in the field of impedance spectroscopy, as well as general device models. He has been distinguished in the 2014-2019 list of ISI Highly Cited Researchers.
The dynamic response of metal halide perovskite devices shows a variety of physical responses that need to be understood and classified for enhancing the performance and stability and for identifying physical behaviours that may lead to developing new applications. A multitude of chemical, biological, and material systems present an inductive behavior that is not electromagnetic in origin, termed a chemical inductor. We show that the structure of the chemical inductor consists of a two-dimensional system that couples a fast conduction mode and a slowing down element. The impedance spectra announce the type of hysteresis, either regular for capacitive response or inverted hysteresis for inductive response. We develop the methods to characterize time transient and photocurrent response to a voltage pulse. We can obtain important control of the time constant that determine hysteresis. We apply these insights in kinetics processes to the development of memristors and neurons. Overall we provide a method to determine important kinetic responses of the halide perovskites that can be aused in many different applications.
2.2-O2
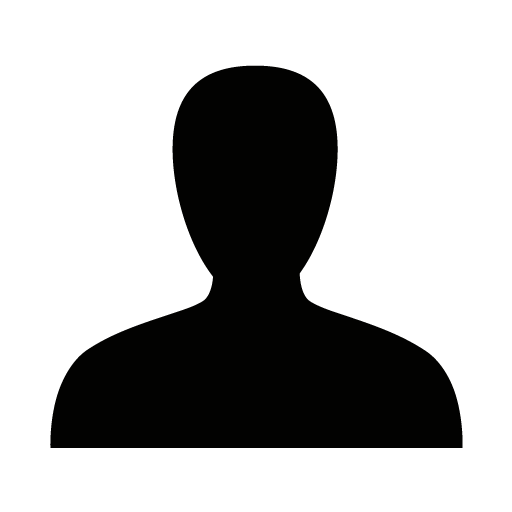
Perovskites, especially Pb-free tin halide perovskites, show promise for efficient optoelectronic/electronic microsystems and image sensors, fabricated through simple techniques like inkjet printing. This approach aims to reduce device costs globally, cater to various applications, and decrease reliance on current semiconductor technologies. In this work we anticipated innovations with 2D and 2D/3D tin halide perovskites which have the potential to become commercially viable products, addressing the evolving needs of a digital society while promoting sustainability and reducing dependence on existing semiconductor technologies.
Along the work we have proposed and tested experimentally (chemical synthesis and basic structural and optical properties) many perovskite and perovskite-like materials. From them, we focused on several of them: i) 2D/3D perovskite compounds (R0.5,BA0.5)2FA9Sn10I31 with R = PEA, TEA, DIP cations for allowing crystallization and stabilize the tin-perovskite phase for inkjet printing deposition techniques; ii) FASnI3 with additives and light soaking was further used for spin-coated films used in the optimization of vertical lasing structures; iii) PEA2SnI4SnI4 and TEA2SnI4 for red emitting LEDs and photonics (emitting devices and photodetectors); Cs3Cu2Cl5 and Rb3InCl6:Sb for blue and green LEDs.
In photovoltaic devices, important advances were achieved: world record efficiency for flexible lead-free perovskite solar modules of 5.7 % under 1-sun and an impressive 9.4% for 2000 lx, indicating their potential for indoor applications. This was possible through the in-situ synthesis of SnI2 from metallic tin and iodine in a DMF:DMSO solvent.
For lead-free LEDs: (i)-(ii) First inkjet-printed Pb-free perovskite LED emitting at red wavelengths and first on a flexible substrate with commom DMF and sustainable DMSO solvents.
In photonics applications several advances were achieved: (i) ASE/lasing action and near single-mode operation were observed over a threshold of 200 µJ/cm2 for inkjet-printed films of 2D/3D G-LFPs, which is close to that obtained with the counterpart device implemented with the 3D FASnI3 film deposited by spin-coating; (ii) This 2D/3D G-LFPs also exhibits good performances for low-level light photodetection: responsivity as high as 50 A/W was measured after one month at 450 nm in encapsulated devices, with an estimated (using dark current value) detectivity better than 8.1010 Jones; flexible photodetectors exhibit comparable performances. (iii) inkjet-printed films of 2D tin-perovskites (TEA2SnI4 among them) are exhibiting efficient two photon absorption mechanism at room temperature and also leading to efficient photodetectors in glass and PET substrates with very low dark currents.
The proposed eco-friendly devices should be taken into account in future advances for scalable flexible optoelectronic Sn-based applications.
2.2-O1
Perovskite solar cells and sensors. Defects in semiconduciors and charge recombiantion.
The pursuit of efficient and stable perovskite solar cells (PSCs) has propelled investigations into the nuanced realm of charge extraction dynamics. This presentation delves into the comprehensive exploration of charge extraction effects on the efficiency and stability of PSCs, encompassing the intricacies associated with mixed organic-inorganic, inorganic, and lead-free perovskite types. Special attention is devoted to the burgeoning topic of self-assembled monolayers (SAMs), with a focus on the critical role played by hole and electron-selective SAMs in tailoring charge extraction processes [1].
A focal point of our study involves the application of time-resolved surface photovoltage (TR-SPV) as the principal methodology. TR-SPV emerges as a novel and powerful technique, enabling the elucidation of charge dynamics within the remarkably short time scale of 1 nanosecond to 100 milliseconds. Through this method, we unravel the subtle intricacies governing charge extraction, shedding light on the temporal evolution of carrier behavior at the interface [1,2].
The discourse spans an investigation into the influence of various perovskite compositions and structures on charge extraction mechanisms. Our exploration includes a detailed analysis of mixed organic-inorganic, purely inorganic, and lead-free perovskite solar cells [3]. Additionally, we delve into the role of SAMs in modulating charge extraction dynamics, offering insights into their tailored design for enhanced PSC performance.
As a testament to the practical implications of our findings, this presentation culminates in the demonstration of a PSC device exhibiting exceptional stability. The device, subjected to rigorous testing, showcases remarkable resilience, maintaining its structural and functional integrity for an unprecedented 3000 hours without any discernible signs of degradation. This endeavor not only advances our understanding of charge extraction dynamics but also underscores the potential for achieving prolonged stability in perovskite solar cell technology.
2.2-I2
Lioz Etgar obtained his Ph.D. (2009) at the Technion–Israel Institute of Technology and completed post-doctoral research with Prof. Michael Grätzel at EPFL, Switzerland. In his post-doctoral research, he received a Marie Curie Fellowship and won the Wolf Prize for young scientists. Since 2012, he has been a senior lecturer in the Institute of Chemistry at the Hebrew University. On 2017 he received an Associate Professor position. Prof. Etgar was the first to demonstrate the possibility to work with the perovskite as light harvester and hole conductor in the solar cell which result in one of the pioneer publication in this field. Recently Prof. Etgar won the prestigious Krill prize by the Wolf foundation. Etgar’s research group focuses on the development of innovative solar cells. Prof. Etgar is researching new excitonic solar cells structures/architectures while designing and controlling the inorganic light harvester structure and properties to improve the photovoltaic parameters.
Recent discoveries have revealed a breakthrough in the field using inorganic-organic hybrid layers called perovskites as the light harvester in the solar cell. The inorganic-organic arrangement is self-assembled as alternate layers, being a simple, low cost procedure. These organic-inorganic hybrids promise several benefits not delivered by the separate constituents.
In this work we present a fully printable mesoporous indium tin oxide (ITO) perovskite solar cell. The solar cell structure consists of triple-oxide screen-printed mesoporous layers. In this structure, the perovskite is not forming a separate layer but fills the pores of the triple-oxide structure. The perovskite is utilized as both the light harvester and a hole transporting material. One of the advantageous of this solar cell structure is the transparent contact (mesoporous ITO) which permit the use of this cell structure in bifacial configuration without the need for additional layers or thinner counter electrode. We performed photovoltaic (PV) measurements on both sides (i.e. ITO-side and glass-side), where the glass side show 15.3% efficiency compare to 3.8% of the ITO-side. Further study of the mechanism shows that the dominant mechanism when illuminating from the glass-side is Shockley-Read-Hall recombination in the bulk, while illuminating from the ITO-side show recombination in multiple traps and inter gap defect distribution which explain the poor PV performance of the ITO-side. Electrochemical impedance spectroscopy shed more light on the resistance and capacitance. Finally, we demonstrate 18.3% efficiency in bifacial configuration. This work shows a fully printable solar cell structure which can function in bifacial configuration.
2.3-I1
Prof. Mónica Lira-Cantú is Group Leader of the Nanostructured Materials for Photovoltaic Energy Group at the Catalan Institute of Nanoscience and Nanotechnology (www.icn.cat located in Barcelona (Spain). She obtained a Bachelor in Chemistry at the Monterrey Institute of Technology and Higher Education, ITESM Mexico (1992), obtained a Master and PhD in Materials Science at the Materials Science Institute of Barcelona (ICMAB) & Autonoma University of Barcelona (1995/1997) and completed a postdoctoral work under a contract with the company Schneider Electric/ICMAB (1998). From 1999 to 2001 she worked as Senior Staff Chemist at ExxonMobil Research & Engineering (formerly Mobil Technology Co) in New Jersey (USA) initiating a laboratory on energy related applications (fuel cells and membranes). She moved back to ICMAB in Barcelona, Spain in 2002. She received different awards/fellowships as a visiting scientist to the following laboratories: University of Oslo, Norway (2003), Riso National Laboratory, Denmark (2004/2005) and the Center for Advanced Science and Innovation, Japan (2006). In parallel to her duties as Group Leader at ICN2 (Spain), she is currently visiting scientist at the École Polytechnique Fédérale de Lausanne (EPFL, CH). Her research interests are the synthesis and application of nanostructured materials for Next-generation solar cells: Dye sensitized, hybrid, organic, all-oxide and perovskite solar cells. Monica Lira-Cantu has more than 85 published papers, 8 patents and 10 book chapters and 1 edited book (in preparation).
Halide perovskite solar cells (PSCs) have already demonstrated power conversion efficiencies above 26%, which makes them one of the most attractive photovoltaic technologies. However, there are several challenges must be overcome for the technology to be competitive and commercially available. One of the main bottlenecks towards their commercialization is their long-term stability, which should exceed the 20-year mark. Several are the strategies currently employed to stabilize PSCs, for example, the use of complex metal oxides as transport layers, the passivation of defects in the halide perovskite layer through additive engineering, or the replacement of metal electrodes by carbon-based electrodes, etc. Among these, additive engineering is an effective pathway for the enhancement of device lifetime. Additives applied as organic or inorganic compounds, improve crystal grain growth enhancing power conversion efficiency. The interaction of their functional groups with the halide perovskite (HP) absorber, as well as with the transport layers, results in defect passivation and ion immobilization improving device performance and stability. In this talk, we will briefly summarize the different types of additives recently applied in PSC to enhance not only efficiency but also long-term operational stability. We discuss the different mechanism behind additive engineering and the role of the functional groups of these additives for defect passivation. Special emphasis is given to their effect on the stability of PSCs under environmental conditions such as humidity, atmosphere, light irradiation (UV, visible) or heat, taking into account the recently reported ISOS protocols. We also discuss the relation between deep-defect passivation, non-radiative recombination and device efficiency, as well as the possible relation between shallow-defect passivation, ion immobilization and device operational stability. We will also show our most recent results applying additives in PSC where we have been able to obtain efficiencies above 21 % and highly stable devices showing null degradation after more than 10000 h under continuous light irradiation of 1 sun.
REFERENCES
[1] M. V. Khenkin, E.A. Katz, et al., M. Lira-Cantu, Nat. Energy 5 (2020) 35–49.
[2] H. Xie, M. Lira-Cantu, J. Phys. Energy 2 (2020) 24008.
[3] A. Mingorance, et al., Adv. Mater. Interfaces 5 (2018).
[4] M. Lira-Cantú, Nat. Energy 2 (2017) 17115.
[5] A. Pérez-Tomas, Sustain. Energy Fuels 3 (2019) 382–389.
[6] H. Xie, et al., Submitted (2020).
[7] C. Pereyra, H. Xie and M. Lira-Cantu. J. Energ Chem. 2021
2.3-O1
Perovskite solar cells (PSC) are highly promising candidates for future space photovoltaics due to their high specific power potential. Yet, for successful application, devices need to resist several sets of extremes in space. In this presentation we will discuss their extraordinary radiation tolerance [1]–[3] and then move on to extreme temperatures and temperature cycles, two extremes that perovskites are well suited to. Atomic oxygen (AtOx), on the other hand, corrodes unencapsulated PSCs swiftly, as we identify. And while we find that this can be avoided using ultrathin evaporated 0.7 µm silicon oxide (SiOx) barriers, we set out to understand the AtOx-induced degradation mechanisms of phenethylammonium iodide (PEAI)-2D passivated and non-passivated devices. Surprisingly, degradation is more severe in 2D passivated PSCs. To disentangle damage mechanisms between 2D passivated and non-passivated devices we apply injection-current-dependent electroluminescence (EL) and intensity-dependent photoluminescence quantum yield (IPLQY) measurements. These allow us to derive pseudo-JV curves that are independent of parasitic resistance effect from damaged transport layers. We quantify an implied FF, that remains high after degradation suggesting that the perovskite bulk is not severely degraded. On the other hand, GIWAX studies reveal a degraded surface that limits charge extraction, and thus leads to lower performance metrics. This surface degradation is severely accelerated for 2D passivations and proceeds to areas that are covered by copper contacts due to lateral diffusion of AtOx though the 2D surface owing to the large interplanar distance of 2D perovskites.
[1] F. Lang et al., “Efficient minority carrier detrapping mediating the radiation hardness of triple-cation perovskite solar cells under proton irradiation,” Energy Environ. Sci., vol. 12, no. 5, pp. 1634–1647, 2019, doi: 10.1039/C9EE00077A.
[2] F. Lang et al., “Proton‐Radiation Tolerant All‐Perovskite Multijunction Solar Cells,” Adv. Energy Mater., vol. 11, no. 41, p. 2102246, Nov. 2021, doi: 10.1002/aenm.202102246.
[3] F. Lang et al., “Radiation Hardness and Self-Healing of Perovskite Solar Cells,” Adv. Mater., vol. 28, no. 39, pp. 8726–8731, Oct. 2016, doi: 10.1002/adma.201603326.
2.3-O2
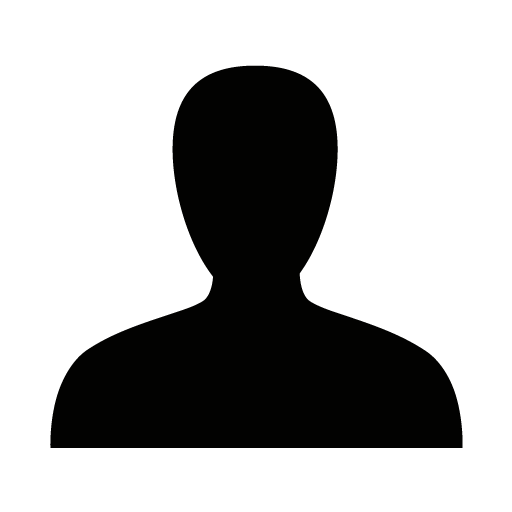
All-inorganic cesium lead triiodide (CsPbI3) perovskites show high potential for photovoltaic applications due to their excellent thermal stability and suitable bandgap (Eg≈1,72 eV) ideal for tandem device. However, the photovoltaic performances of CsPbI3 perovskite solar cells (PSCs) are significantly restricted by a scarcely controllable crystal quality leading to high nonradiative recombination processes [1]. Therefore, the potential of CsPbI3 is overshadowed by the black-phases (α, β, and γ) structural instability, prone to spontaneous evolution into the photoinactive δ-phase at room temperature. This transition can be accelerated by ambient moisture, resulting in diminished PCE. Consequently, stabilizing black CsPbI3 at room temperature has become a critical topic. The regulation of the nucleation and growth rates has been identified as an efficient strategy for enhanced film coverage [2]. CsPbI3 perovskite films are produced via a cost-effective and straightforward solution-based manufacturing process. However, the nucleation and growth processes are largely uncontrollable, leading to inevitable defects stemming from conventional coating methods. Understanding the fundamental mechanism underlying film formation, specifically in terms of nucleation and growth, is crucial. Identifying this mechanism is a necessary step for precisely adjusting film crystallization kinetics. The optimization of additive engineering has proven to be a successful and straightforward approach in developing Cs-based photovoltaic devices with enhanced efficiency and stability. Consequently, it has emerged as a significant focus in PSC (perovskite solar cell) research. The aim of this work is to obtain a stable CsPbI3 polycrystalline thin film modulating the different parameters involved in the solution processing of the films, spanning from the employed solvent to the use of additives modifying the solution chemistry, to the use of alternative Pb sources DMAPbI3 [3]. The chemistry involving additives currently revolves around the utilization of HI and DMAI [4,5] within the precursors salts solution. Experimental evidence in both scenarios substantiates the formation of the intermediate DMAPbI3 within the solution, HI reacts with DMF and DMAI with PbI2. By synthesizing DMAPbI3 through hydrolysis and utilizing it directly as a lead source in the solution, it's possible to achieve better stoichiometric control involving DMA+ and to produce higher-quality films.The formation of intermediate complexes during the crystallization process assumes a pivotal role. For instance, the inclusion of additives, such as Cl− ions in the precursor solution has a positive impact on the nucleation of perovskites. Importantly, these Cl− ions aid in the nucleation process without becoming part of the perovskite lattice structure itself. This, in turn, leads to the production of perovskite films with enhanced optoelectronic properties, suggesting an improvement in their ability to interact with and respond to light. The presented strategies allow to investigate the effect on the nucleation process and how the existence of solute–solvent crystalline intermediate phases have an impact on chemical reaction kinetics. Finally, the optimized films have been integrated and tested in solar cells.
2.3-I2
Silvia Colella is a researcher at the National research council, CNR-NANOTEC, in Bari, Italy. She received her PhD in “Nanoscience” at National Nanotechnology Laboratory in Lecce (Italy), in 2010. She has been visiting student in the group of professor Luisa De Cola at the Westfälische Wilhelms-Universität of Münster (Germany), where she dealt with the synthesis and photophysical characterization of electroluminescent metal complexes. In 2010 she joined BASF – The Chemical Company (Strasbourg) with a Marie Curie fellowship as experienced researcher in the frame of the EU project ITN SUPERIOR, working on Dye Sensitized Solar Cells. She continued as post-doc researcher at the Institut de science et ingénierie supramoléculaires (ISIS) in Strasbourg, France. In 2012 she started her independent research in Lecce (Italy) at the University of Salento in collaboration with CNR-NANOTEC, the team focused on the conception and optoelectronic characterization of innovative optoelectronic devices based on hybrid halide perovskites. Many high impact publication were produced in this time interval, among them one of the first report in halide perovskite for PV exploitation (Colella et al, Chemistry of Materials, 2013 25, 4613-4618).
Silvia Colella is author of >70 peer-reviewed publications in renowned international journals (including Energy and Environmental Science, Advanced Materials, ACS Energy Letters).
Her scientific production led to >3000 total citations and a h-index of 28 (https://scholar.google.it/citations?user=S2TZd_4AAAAJ&hl=it; https://www.scopus.com/authid/detail.uri?authorId=24170650100).
Metal halide perovskite (MHP) semiconductors are excellent candidates for contemporary optoelectronics innovation, particularly for photovoltaics.[1] The advantages of this class of materials derive from their hybrid nature, allowing for straightforward fabrication processes, and from their unique optoelectronic properties. A typical 3D organic-inorganic perovskite has a chemical formula of ABX3, where A is an organic cation (such as MA [methylammonium] or FA [formamidinium]), B is a metal cation (such as Pb2+), and X is a halogen anion (such as I or Br).[2] However, recent advances have also explored more complex compositions embedding diverse cations/anions.[3] These materials are prepared by simple and straightforward solution processing, the material precursors dissolved in a solvent undergoes self-assembly into a perovskite structure during spin-coating onto a substrate under mild thermal annealing.
As the technology continues to mature, this still is a key advantage, allowing for affordable and scalable processing. Understanding perovskite ink properties is therefore a fundamental requirement towards industrialization, with special regards to their evolution over time.
It has been demonstrated that even for the simplest system, the precursor solution is a complex – and dynamic – dispersion which contains not only solvated ions but also lead halide complexes, colloids and aggregates of different natures and dimensions. In these complex dispersions, multiple chemical species are present and can interact – or react – between each other or with the solvent.
We have proved the existence of a reactivity between two of the perovskite components – MA and FA – in the precursors solutions, that leads to the formation of a novel condensation product, methylformamidinium (MFA). We have studied different parameters that affects such reactions kinetics, therefore modifying the ink composition over time, and proposed solutions to overcome these issues.[4] With the aim of correlating the solution chemistry with the film structural properties, through the synergic use of solution Nuclear Magnetic Resonance (NMR) spectroscopy, X-ray Diffraction and Density Functional Theory (DFT) calculations, we have recognized and explained for the first time a correlation between the aging of perovskite precursor solutions, the presence of MFA species in solution and the emergence of photoinactive hexagonal polytypes (6H and 4H) [5]
Starting from the known reactivity of the chemical species present in ink solutions, we outline the directions towards which future research efforts should be directed.[6]
2.3-I3
Perovskite on silicon (Si) tandem solar cells are predicted by the ITRPV to be the first Si-based tandem solar cells to enter the market. Their superior power conversion efficiency promises a step change in module efficiency that is not possible with silicon only technologies, as shown by Oxford PV’s recent certified world record of 28.6% in a full area cell. With its integrated pilot line at Brandenburg, Germany, Oxford PV is currently ramping up to high-volume production of commercial M6 tandem wafer product.
Perovskite tandem commercialisation has taken place in a very short period of time compared to other photovoltaic technologies. This rapid progress has been possible due to the development of in-house protocols and the statistical analysis of the large datasets generated. Some of the advanced characterisation at Oxford PV and how they inform decision making on process and technology designs in view of cell efficiency and longevity targets will be presented. The measurements can be categorised according to their speed, the complexity of data analysis and the value of the measured parameters – increasing measurement speed and analysis allows for more insights on more samples more frequently, and so a key part of industrial solar R&D involves innovations on measurement protocols. In this talk, there will be examples of the advanced characterisation suite that have developed at Oxford PV to maximise the learning for as many samples as possible whilst focusing on the most important parameters, and eliminating redundancies. Upon generation of high quality datasets, machine learning tools are used to improve our statistical understanding of trends we see with development of the Oxford PV tandem cell technology. As well as cell efficiency enhancements, these complimentary research methods are just as suited for improving cell reliability, for which the perovskite field has made good steps in the last 12 months in aligning on predictive accelerated stressing protocols. The big data approach is also suitable for identifying process sensitivities in view of designing scalable processes, a critical part of R&D in an industrial setting.
Finally, sustainability, both in financial and ecological terms, is a crucial aspect of manufacturing at large scale. Leveraging advanced characterisation and machine learning can expedite from small scale proof of concepts towards achieving comparable KPIs with high-TRL production ready processes. Oxford PV is committed to bring to the market the most sustainable technology in the most sustainable way; examples of the initiatives Oxford PV has implemented to make that happen will be presented.
1.1-I1
Organic photodetectors (OPDs) with a performance comparable to that of conventional inorganic ones have been demonstrated for the visible wavelength regime. However, near-infrared (NIR) photodetection has proven to be challenging and, to date, the true potential of organic semiconductors in this spectral range (800–2500 nm) remains largely unexplored. In this talk, I will introduce new device concepts for organic NIR detectors, based on resonant optical cavities and doped photo-active layers. Design rules and optimization strategies will be discussed, yielding wavelength selective devices (20 nm resolution) with a tunability of the detection wavelength over several hundreds of nanometers, allowing the printing of miniature NIR spectrometers. In a second part of the talk, we explore the performance limitations of organic NIR detectors: A relation between open-circuit voltage, dark current, and noise current is demonstrated for OPDs with detection wavelengths beyond 1100 nm. Based on these findings we estimate an upper limit of achievable specific detectivity values for organic photodiodes as a function of their longest NIR detection wavelength.
1.1-O1
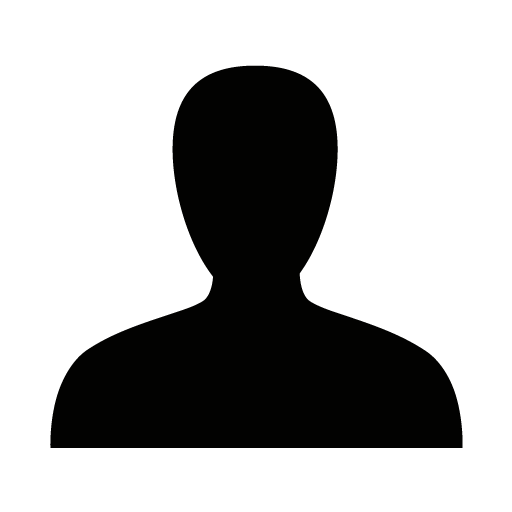
Near-infrared organic photodetectors (OPDs) experienced a fast growth in recent years owing to their advantages over traditional inorganic photodetectors. By fine-tuning the optical bandgap of the organic material, OPDs can be engineered for high and specific light detection. In particular, the advent of non-fullerene acceptors (NFAs), with tunable energy levels, allowed OPDs with light detection in the near-infrared (NIR) region.
Analyzing the structures of reported NFAs, many of the best performing contain thiophene-based fused aromatics within the donor core. A strategy to shift the absorption windows is to replace the thiophene moiety with selenophene. Selenophene has lower aromatic stabilization energy than thiophene and will increase the quinoidal character of the material.
Until now, few fused selenophene containing NFAs containing straight chain alkyl groups have been reported. The replacement of the alkyl aryl groups with straight chain alkyl groups will change the solid-state microstructure of thiophene-based NFAs and then enhance the power conversion efficiency (PCE). In this work, we report the first NFA containing a fused indacenodiselenophene with octyl sidechains at the bridgehead positions. This is the direct selenophene analogue of the previously indacenodithiophene acceptor known as IDIC, which has been widely reported in the OPV community.
Herein we show that the selenophene analogue IDSe exhibits a red-shifted absorption compared to the thiophene analogue IDIC, and when paired with a suitable wide band gap donor PTQ10, organic photodetector devices using a bulk heterojunction configuration have an ultralow dark current density (Jd) of 1.65×10-9 A cm-2 at -2V and a specific detectivity (D*) of 1012 Jones at -2V bias. With a combination of optoelectronic measurements, transient analyses and morphological investigations based on GIWAXS measurements, we attributed the low Jd of the PTQ10:IDSe blend to the higher and more balanced carrier mobilities compared to the thiophene benchmark. In addition, we fabricated large-area OPDs (5x5 cm substrates) using doctor blade coating in air and obtained extraordinarily low dark current, indicating its ability to be large-scaled processed in the future.
1.1-O2
Planner hetero-junction (PHJ) structure is one of the key device fabrication strategies to increase specific detectivity by reducing dark current in organic photodiodes (OPDs). We demonstrated that efficient free charge generation in partially chlorinated subphthalocyanine molecules (Cl6-SubPc) can assist PHJ OPDs to achieve detectivity up to 1013 Jones and dark current below 10-7 A cm-2 up to -5 V. The photophysical analysis of Cl6-SubPc based devices clearly show that device photocurrent is primarily generated through direct charge generation within the Cl6-SubPc layer, rather than exciton separation at layer interfaces. Molecular modelling suggests that direct charge generation is facilitated by Cl6-SubPc’s high octupole moment which generates a shift in molecular energetics [1,2] (Fig(1(a)). Also, lower trap densities analysed from capacitance-frequency response [3] in thick PHJ device helps in further minimising leakage currents as shown in Fig(1(b)). In summary, this study provides clear indication that Cl6-SubPc is promising material for single component OPD devices.
1.1-I2
Photodiodes based on organic semiconductors exhibit many advantageous properties including tailorable light absorption, low embodied energy manufacturing, structural conformality, and low material toxicity. These properties make organic photodiodes attractive for emerging photodetector applications, especially for novel applications requiring different optoelectronic and mechanical properties than provided by conventional photodiodes based on inorganic semiconductors.[1] However, thus far the specific detectivity of organic photodiodes has remained subpar relative to conventional photodiodes. A critical parameter limiting the specific detectivity of photodiodes is the dark saturation current. In organic photodiode devices, the dark saturation current is strongly limited by non-radiative processes resulting in dark saturation currents several orders of magnitude higher than expected for radiative band-to-band transitions; however, the origin of these non-radiative processes is still debated. In this work, we show that the dark saturation current, along with the specific detectivity, in organic photodiode devices is fundamentally limited by transitions via mid-gap trap states.[2] This new insight is generated by a universal trend observed for a large set of organic bulk heterojunction systems and substantiated by sensitive external quantum efficiency and temperature-dependent current measurements. These findings have important implications for organic photodiodes, providing new insight into the origin of non-radiative losses and the associated noise, establishing the performance limits for these devices.
1.2-I1
Solution-processed metal halide perovskite photodetectors (PPDs) have a great potential in visible and near-infrared light sensing and X-ray imaging applications, owing to their excellent optoelectronic properties. This presentation will consist of two parts. After a brief survey of the advantages of solution-processed perovskites in high-resolution, flexible large-area imagers we will focus on better understanding the physical mechanisms that determine the dark current density and detectivity of thin-film photodetectors in the first part [1].
We will demonstrate that extrinsic leakage current at the periphery of the bottom pixel electrode is greatly reduced by introducing a so-called pixel edge cover layer. When such an edge cover layer is inserted, the dark current density decreases to ~10−6 mA cm−2 at −0.5 V, which is among the lowest values reported for polycrystalline PPDs.1 Moreover, the dark current density becomes independent of pixel size, up to 1000 ppi. To reduce dark current density even further, we carefully investigated a range of charge blocking layers, effectively reducing the dark current density even further.
By combining the appropriate blocking layer with the edge cover, a perovskite thin-film photodiode was fabricated that has a spectral responsivity of 0.5 A/W at 950 nm (Fig. 1a), combined with an ultralow dark current density of 5 × 10−8 mA cm−2 (Fig. 1b) and noise current of 2 × 10−14 A Hz−1/2 .
In the second part we will present recent developments in realizing three prototypical applications, i.e. a paper-thin photo-imager [2], a direct conversion X-ray detector [3] and a novel narrowband optical sensor that can measure a person’s heartbeat and respiration rate from a distance of over one meter using NIR light [4].
Including thin-film encapsulation layers - required to guarantee a long lifetime - the photo-imager [2] is only ca. 100 mm thick and can be wrapped around a non-flat object (radius of curvature of 0.6 cm). The wrapping ensures the close proximity between sensor surface area and a curved object, effectively increasing the area of the object that can be imaged with high-resolution. This is relevant for instance for nail-to-nail fingerprint imaging. Biometric fingerprinting is demonstrated with liveness detection.
The resulting direct-conversion X-ray detector [3] has a low detection limit of 0.22 nGyair frame-1, a sensitivity of 1060 µCGyair-1cm-2 (at a bias of 0.03 V µm−1) with a high spatial resolution of 6 line-pairs mm-1.
Finally, we report an inherently-narrowband solution-processed, thin-film photodiode with ultrahigh and controllable NIR responsivity based on a tandem-like perovskite-organic architecture [4]. The device possesses low dark currents (< 10−6 mA cm−2), linear dynamic range > 150 dB, and operational stability over time (> 8 h). With a narrowband quantum efficiency that can exceed 200% at 850 nm and intrinsic filtering of other wavelengths to limit optical noise, the device exhibits higher tolerance to background light than optically-filtered silicon-based sensors.
1.2-O1
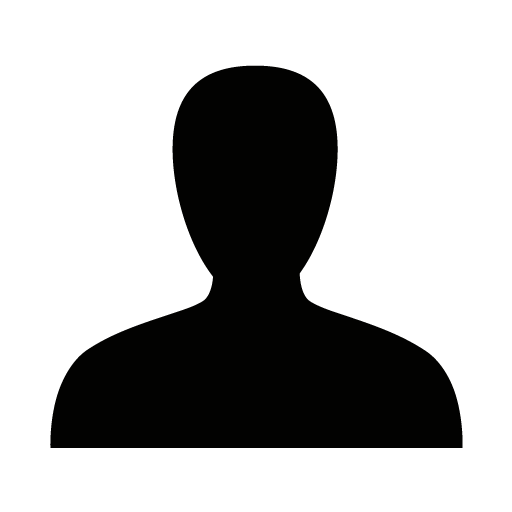
Formamidinium lead iodide (FAPbI3) has gained considerable interest as a promising photoactive layer for optoelectronic devices due to its broad spectrum of light absorption and increased thermal stability when compared to its conventional methylammonium counterpart (MAPbI3). Recent developments in substituting formamidinium (FA) with smaller Cs cations have further accelerated its growth in the photovoltaic (PV) community by enhancing phase stability and power conversion efficiency (PCE). However, only a few studies have been reported on perovskite photodetectors (PPDs). Here, we investigate the influence of Cs incorporation on PD performance in the CsXFA1-XPbI3 system (X = 0–0.25). We demonstrate that A-site substitution with Cs reduces microstrain within the crystal lattice and promotes the formation of a compact, well-ordered surface with improved morphology. To evaluate the performance of the CsXFA1-XPbI3 system, we have prepared p-i-n photodiode-type PDs using a one-step spin-coating method. We find that the lattice strain relaxation considerably reduces the Jd in the device, which may be partially due to the reduction in lattice strain which reduces trap density and improves charge transport. Particularly, the lowest Jd value of 3.3x10-9 A cm-2 is achieved for Cs0.05FA0.95PbI3 at -0.5 V, which also greatly contributes to its highest specific detectivity of 6.1x1011 A Hz-1/2 and widest linear dynamic range of 135 dB. Furthermore, when this mixed-cation perovskite material is integrated into a device, long-term stability without sacrificing performance is observed, which holds great promise for future optoelectronics applications.
1.2-O2
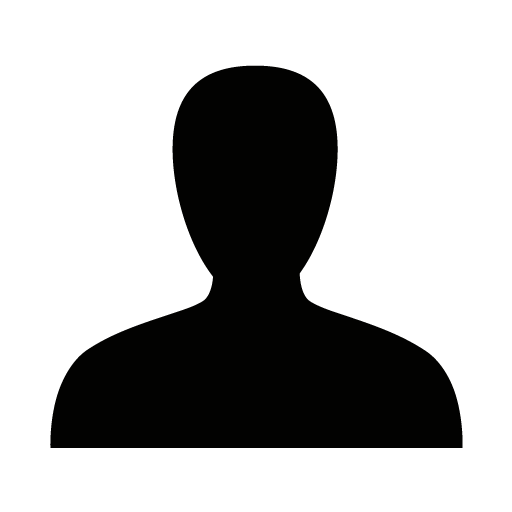
Lead halide perovskite and organic semiconductors have been promising classes of materials for photodetector (PD) applications. However, despite their high performances with low noise and specific detectivity (D*) values exceeding the one of silicon detectors, perovskite PDs working regime is limited to the visible and the first fraction of near-infrared (NIR) spectrum due to their bandgap.1,2 Differently, organic PDs have the advantage of tunable absorption thanks to their chemical design. In this work, we fabricated perovskite-organic heterojunction (POH) PDs with extended absorption up to 900 nm, thanks to the deposition of a donor:acceptor bulk-heterojunction (BHJ) layer on top of the perovskite. We systematically studied the effect of the energetic of the donor materials on the dark current (Jd) of the device by using polymers from the PBDB-T family (PCE12, PM6 and PM7). By using this approach, PM7-based POH delivered ultra-low noise of 2 x 10-14 A Hz-1/2 and high specific detectivity D* of 4.7 x 1012 Jones (-0.5 V) at 840 nm. This was achieved by increasing the energetic barrier between the HOMO of the donor and the LUMO of the acceptor as explained by our analytical model.3 We found that the donor sites effectively act as recombination centres at the perovskite/BHJ interface, affecting Jd. In particular, PM7 with a deeper HOMO level, formed a larger LUMO(Y6)-HOMO(D) gap compared to PM6. This resulted in a smaller contribution of the HOMO of the donor to the dark current, similar to the intrinsic traps introduced by Y6 at perovskite/BHJ interface.
1.2-I2
Printing technology is set to enable the high-throughput low-cost and customized fabrication of optoelectronic and sensors devices. For this goal to become a reality, this functional printing approach should encompass a material process development which enables high device performance and industrial compatibility, thus enabling a facile transfer of research results into consumer applications.
In this contribution, I will present the fabrication of fully printed organic photodiodes (OPDs) by digital printing techniques (e.g. aerosol and inkjet). The devices show mechanical flexibility, semitransparency and an excellent reproducibility. I will discuss the use of the ink formulation as way to access and tailor material optoelectronic properties. In this direction, we have focused on the control of the molecular order in organic semiconductors though an inkjet printing process. This enabled us to deposit functional polymers with a high degree of alignment and explore its use in polarization sensitive OPDs. Secondly, I will outline our recent efforts in the fabrication of inkjet printed OPDs based on novel non-fullerene acceptors (NFAs). The devices show a photo-response up to 800nm and reach record responsivities of 400mA/W as well as cut-off frequencies surpassing 2MHz. Furthermore, we demonstrate the successful decoupling of the optical and rheological properties by using a visibly transparent polymer donor and color-selective NFAs. This approach offers spectral flexibility without the need for a variation in process parameters. The choice of NFA enabled devices with color selectivity in the ranges of 400-600nm and 500-800nm enabling a proof of concept filterless visible-light communication system. Finally, I will present the fabrication printed passive and active matrix OPD arrays.
1.3-I1
Photodetectors that are sensitive in shortwave infrared (SWIR) range (1 µm - 2 µm) are of significant interest for applications in 3D, night and adverse weather imaging, machine vision, autonomous driving, among others. Currently available technologies in the SWIR rely on costly epitaxial semiconductors that are not monolithically integrated with CMOS electronics. Solution-processed quantum dots have been developed to address this challenge enabling low-cost manufacturing and facile monolithic integration on silicon in a back-end-of-line (BEOL) process. To date, colloidal quantum dot (CQD) materials to access the SWIR have been based on lead sulfide (PbS) and mercury telluride (HgTe) compounds imposing major regulatory concerns and potentially impeding their deployment in consumer electronics. In this talk I will present a novel synthetic method of environmentally friendly silver telluride quantum dots and their application in high-performance SWIR photodetectors. The CQD photodetector stack is entirely based on Restriction of Hazardous Substance (RoHS) compliant materials and exhibits a spectral range from 350 nm - 1600 nm, with room-temperature detectivity of the order 1012 Jones, 3dB bandwidth in excess of 100 KHz and linear dynamic range of over 118 dB. We further demonstrate, for the first time, a monolithically integrated SWIR imager that is based on solution processed, heavy-metal-free materials, paving the way of this technology to consumer electronics market [1]. In the second part of the talk I will turn to our recent activities on the development of III-V CQDs and their applicaiton in photodetectors aiming for speeds suited for gated imaging. I will dicuss the challenges on the synthesis of InSb CQDs in terms of surface passivation and environmental stability and present our approach to overcome those challenges reporting InSb CQD SWIR photodetector that exhibits external quantum efficiency (EQE) of 25% at 1240 nm, a wide linear dynamic range exceeding 128 dB, fast photoresponse time of 70 ns, and specific detectivity of 3.6 × 1012 Jones.
[1] Silver Telluride Colloidal Quantum Dot Infrared Photodetectors and Image Sensors, Yongjie Wang1, Lucheng Peng1, Julien Schreier2, Yu Bi2, Andres Black2, Aditya Malla1, Stijn Goosens2, Gerasimos Konstantatos1,3*, Nature Photonics, accepted.
1.3-O1
The ultraviolet (UV) part of spectrum is of paramount importance for a large range of applications from health and safety to process and environmental monitoring. Yet the complexity of integrating UV sensitive optoelectronics on standard complementary metal–oxide–semiconductor (CMOS) technology has curtailed to a large extent the use of UV spectrum, especially in consumer electronics. Currently UV photodetectors rely on III-V nitride semiconductors that are not monolithic to silicon and are typically fabricated off-die.
We overcome this challenge by developing RoHS compliant environmentally friendly colloidal quantum dots (QDs) (zinc magnesium oxide , ZnMgO) which has compositionally tunable absorption across UV and high photoluminescence quantum yield (> 90%) in the visible.[1] Herein, we will present a non-toxic, visible blind QD based down-converting thin-film technology that expands the spectral coverage of Si-CMOS-sensors into the UV, enabling efficient UV detection without affecting the sensor performance in the visible and NIR. A Si-photodetector (PD) integrated with the QDs results in a record 9-fold improvement (800% relative enhancement) in photoresponsivity from 0.83 to 7.5 mA W−1 at 260 nm. Leveraging the tunability of these QDs, we will show a simple UV-band identification scheme (a sensor), which uses two distinct-bandgap ZnMgO QDs stacked in a tandem architecture whose spectral emission color depends on the UV-band excitation light. The downconverting stack enables facile discrimination of UV light (different UV bands) using a standard CMOS image sensor (camera) or by the naked eye and avoids the use of complex optics.
1.3-O2
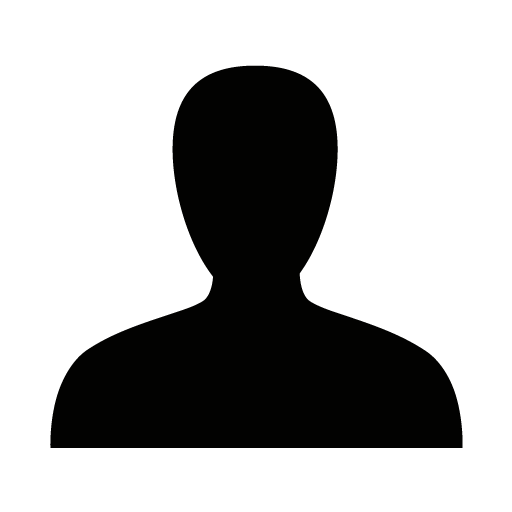
Colloidal infrared (IR) quantum dots (QDs) have been studied with many advantages, such as tunable absorption and fluorescence emission spectra, high molar extinction coefficients, high photoluminescence (PL) quantum yield, high stability, cost-efficient and scalable synthesis, and solution processability. Most of the reported IR-active QDs are based on materials containing toxic heavy metals, such as PbS and HgTe QDs. III- V semiconductors containing one group-13 and one group-15 element of the periodic table are highly promising Pb- and Hg-free alternative materials.[1] Colloidal InSb QDs have been considered as a low-toxic alternative to Pb or Hg chalcogenide QDs. The InSb has a large excitonic Bohr radius of ~60 nm, and it allows the band gap of InSb QDs to be widely tuned in the IR spectral range through size control. However, only a few chemical synthesis methods have been reported due to the limited precursors and the synthesized InSb QDs have featureless or broad excitonic absorption peaks showing extremely broad size distribution so far.
Here, we report the chemical synthesis and surface engineering of colloidal InSb QDs. In the synthesis part, various synthesis parameters such as precursors, temperature, time, and an introduction of additional ions are explored to produce InSb QDs with unprecedented optical quality. The optical properties of InSb QDs are studied mainly based on the wavelength and the width of the excitonic absorption features. We demonstrate full tunability of the first excitonic absorption peak in the IR range from 700 to 2000 nm and narrow line widths by virtue of an improved size homogeneity of the InSb QDs. The effect of each synthesis parameter on the optical properties of InSb QDs and their growth mechanism will be discussed. In the surface engineering part, the surface ligands of InSb QDs are altered from the original long-chain organic ligands to short-chain ligands to afford conductive InSb QDs films. Several ligands were tested and their optical/structural properties of InSb QDs were compared before and after surface modification. With the thus optimized experimental conditions, we obtain InSb QDs films with high stability, high conductivity, and suitable band energy levels for application as environmentally benign IR photodetectors.
1.3-O3
Photodetectors on the basis of transition metal dichalcogenides (TMDCs) have let to impressive performance numbers with regard to gain and responsivity.1 In contrast, their speed, parametrized by the response time and 3dB electrical bandwidth, has been mostly disappointing so far with response times often ranging in the milli- to microsecond regimes. The corresponding 3dB bandwidths seldomly exceed a few MHz, and this is too slow to be competitive with established, fast photodetection materials that typically operate with GHz speed.
This presentation details how rational tuning of the substrate material,2 the contact geometry,3 the photoresistance and the capacitance4 of lateral photodetectors based on mechanically exfoliated WSe2 layers affects the 3dB bandwidth and enables photodetection with a speed in excess of 230 MHz. We show that optical switching with such devices can be carried out at zero bias, requiring just 27 fJ per switching event. Reducing the detector thickness to mono- and bilayers of WSe2 only weakly decreases the response speed, rendering WSe2 advantageous over MoS2 for fast optical communication. Further miniaturizations of the device geometry have the potential for Gigahertz photodetection with such easily fabricated TMDC photodetectors which exhibit long-term stability under ambient conditions.
2.1-O1
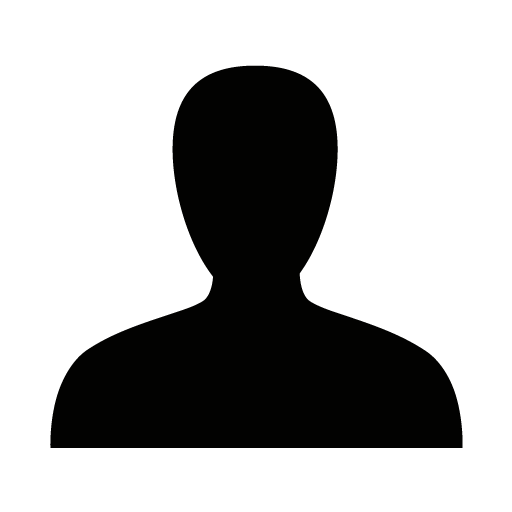
Organic semiconductors have raised much attention due to their many attractive properties, like low-cost, light weight, mechanical flexibility, and abundant availability. Despite that, they still lack high mobility compared to their inorganic counterparts as a consequence of their often amorphous nature.
An exception to this is rubrene, which can form highly ordered phases, demonstrating an extraordinarily high charge carrier mobility for holes (>10 cm2/(Vs)-1) even in thin films. Depending on the post-treatment of our vacuum-deposited films, we can control different crystalline phases, like the orthorhombic and triclinic ones.
For fast-response OPDs, the triclinic phase is a promising candidate since it exhibits high hole mobility in the vertical direction. However, the high surface roughness is a crucial reason why these devices fall short in specific detectivity up to now. In this work, we employ different strategies to reduce the impact of Ohmic shunts within the device to minimize the noise current of our devices. Optimizing the concentration of rubrene and C60 in the active layer and adjusting the electron transport material and its thickness are essential building blocks for achieving low noise currents. However, the most promising approach has been to establish a suppressing layer for the post-treatment of the film. This suppressing layer allows to reduce the dark current by four orders of magnitude. We characterize the morphology of our films with atomic force microscopy and grazing-incidence wide-angle X-ray scattering and investigate the performance parameters of fully working devices. In addition to a low noise current, a high external quantum efficiency is crucial for achieving high specific detectivity values. We can accomplish a competitive specific detectivity of 1013 with the employed strategies based on thermal noise domination at 0 V. Speed, noise, and linear dynamic range are investigated to complement the study.
2.1-O2
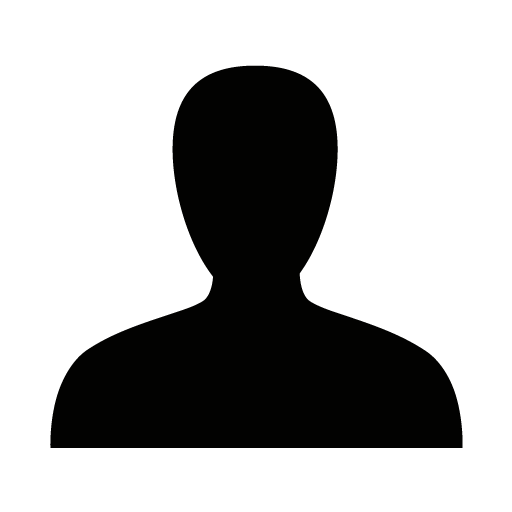
Metal halide perovskites (MHPs) have shown excellent results as X-ray scintillation detectors. X-ray tomography requires many projections and therefore scintillators with excellent stability, which is challenging for MHPs that often suffer from fast degradation under X-ray irradiation and ambient conditions. MHP nanowires could offer improved sensitivity and resolution by exploiting nanophotonic light guiding, and the stability could be improved by growing physically protected nanowires directly in anodized aluminum oxide (AAO) nanopore templates.
We have developed a one-step solution method to grow arrays of up to 30 μm long single-crystalline CsPbBr3 nanowires in an AAO template, with controlled diameters ranging from 30 to 360 nm [1,2]. The CsPbBr3 nanowires in AAO (CsPbBr3 NW/AAO) show increasing X-ray scintillation efficiency with decreasing nanowire diameter, with a maximum photon yield of ∼5 300 ph/MeV at 30 nm diameter. 2D X-ray images can distinguish line pairs with a spacing of 2 μm and slanted edge measurements show a spatial resolution of ∼160 lp/mm at modulation transfer function (MTF) = 0.1 [1]. The stability was tested over 2 weeks of X-ray exposure [3]. The X-ray scintillation unexpectedly correlated positively with room humidity, but showed no systematic degradation. The resolution was stable at (180 ± 20) , i.e., about 2.8 micron.
For 3D microscopy, a tomogram with 600 projections was taken with a Cu X-ray source over 41 h. The scintillation variations remained below 5% during the acquisition, which allowed a successful 3D reconstruction with high spatial resolution [3]. The combination of high spatial resolution, radiation stability, and easy fabrication makes these CsPbBr3 NW/AAO scintillators a promising candidate for high-resolution X-ray imaging applications.
2.1-I1
The development of detectors for high energy photons, protons and heavy particles is a long-lasting research topic not only for fundamental applications but also, more recently, for medical applications in radio and hadron therapy. There is an increasing demand for sensors able to provide, ideally in-situ and in real-time, an accurate recording and mapping of the dose delivered during a treatment plan. The development of novel high performing, thin and flexible sensors for the detection of ionizing radiation in real-time at affordable costs is rapidly increasing, as the technology currently available still fails to address the requirements of large-area, conformability and portability, lightweight and low power operation.
Organic small molecules and polymers are promising active layers for advanced dosimetry purposes, as their mechanical features allow the development of devices able to adapt to complex contoured surfaces with outstanding portability (low power operation) and lightweight. They also provide the unique possibility to develop human-tissue-equivalent detectors, thanks to their density and composition, which makes them ideal candidates for medical dosimetry applications. Their low average atomic number and density, also grants a low absorption of the incoming radiation, making them extremely radiation-tolerant. The physical process of radiation detection for organic thin- film based detectors will be discussed in two different configurations: 1) the direct one, based on a simple planar device with an organic thin film as active conversion layer, and 2) the indirect one, based on a polysiloxane-based scintillating layer effectively coupled to an organic phototransistor (OPT).
We report on their performance under exposure to intense photons and MeV protons radiation fields and will discuss how to detect and exploit the energy absorbed both by the organic semiconducting layer and by the plastic substrate, allowing to extrapolate information on the irradiation history of the detector. A new kinetic model has been developed to describe the detector response mechanism, able to precisely reproduce the dynamic response of the device under photon/proton irradiation and to provide further insight into the physical processes controlling its response.
2.1-I2
Organic semiconductors have proven to be a versatile technology platform and are compatible with high-scale mass production. Vacuum-deposited organic light-emitting diodes (OLEDs) dominate mobile display applications and are superior due to their mechanical flexibility, high contrast, low-cost, and form-free production. Recently, organic photodetectors have attracted much interest since they are directly compatible with the OLED display technology and have excellent potential to complement it. Furthermore, their physical properties, such as semitransparency, flexibility, narrowband detection, and, most importantly, high detectivity, offer new perspectives for optoelectronic sensing and will stimulate new consumer electronics applications.
Typically, organic solar cells and photodetectors are comprised of an electron donating and accepting material to facilitate efficient charge carrier generation. This approach has proven successful in achieving high-performance devices but has several drawbacks. For example, creating the proper donor-acceptor microstructure is critical to achieve the desired performance. However, this is challenging when it comes to upscaling and is considered one of the significant degradation pathways regarding the stability of these devices. Therefore, we investigate a vacuum-deposited oligothiophene A-D-A molecule in a single-component device and find that it can generate free charge carriers with an internal quantum efficiency of 20% already at zero bias. Optimizing the device structure, we achieve specific detectivities of 1013 Jones (based on noise measurements), high speed (f-3dB = 330 kHz), and linear dynamic range (190 dB). To unveil the charge carrier generation within this material system, we employ ultrafast transient absorption spectroscopy and quantum chemical calculations and find that free charge carriers are already formed at time scales below one picosecond. Exhibiting this excellent performance and using the simple device structure, such single-component devices are perfect candidates for application.
2.2-O1
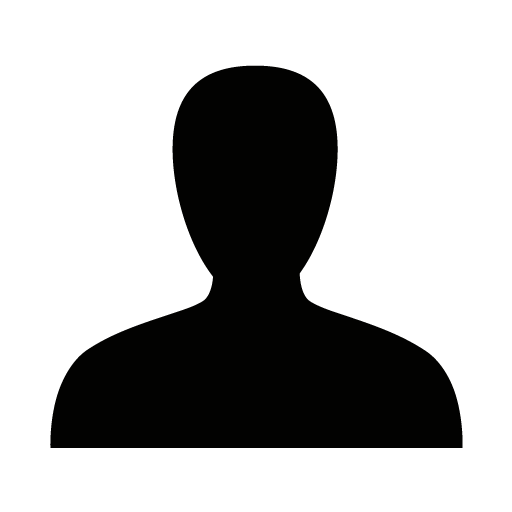
Methylammonium lead bromide (MAPbBr3) have emerged as the next-generation materials for self-powered photodetectors due to its easy fabrication protocol and better stability. However, there is still a lack of sufficient understanding of the effect of electrode distance, metal contacts, irradiation power and applied temperature on the performance of MAPbBr3 SC-based perovskite PDs. Here, we demonstrated the impact of different electrode materials, variable electrode distance,1 light intensities and temperatures on the performance of MAPbBr3 SC-based perovskite PDs and analyse them with the help of transient photoresponse and impedance spectroscopy.2 This study reveals that the key performance parameters of PD decrease with increasing irradiation intensity as well as increase in Schottky barrier height (SBH) between the metal and the semiconductor, due to changes in charge recombination and carrier lifetime.2,3 On the other hand, the temperature-dependent study revealed that the performance of PD was found to be related to the increasing scattering of impurities, temperature activated ion accumulation, and phonons, change in conductivity and band gap rather than the change in charge recombination. Next, we fabricated a planar-type PD based on metal doped p-type MAPbBr3/n-type MAPbBr3 SC junction photodiode using asymmetric electrode and use the combined effect of electric field due to asymmetric Schottky barrier formation and additional built-in electric field in the p-n junction depletion region demonstrate for the first time to achieve excellent self-powered photodetection properties.4 The p-n junction on the MAPbBr3 single crystal was formed by a controlled epitaxial grow of Ag+ doped MAPbBr3 SC (p-type) on the facet of Sb3+ doped MAPbBr3 SC (n-type). The as-integrated p-n junction device with asymmetric electrodes shows a typical photovoltaic behaviour with a high open circuit voltage of 0.95 V and great sensitivity to 530 nm illumination at zero bias with a responsivity of up to 0.41 A W-1 and a specific detectivity of 6.39 × 1011 Jones, which are among the highest values reported for MAPbBr3 single crystal based self-powered photodetectors. In addition, the p-n junction device maintained 80% of the initial performance after being exposed under continuous operation illumination for 12 h in atmospheric condition and restored 94% performance of its initial value after 36 h of storage in the dark. This work provides a new way for designing a high performance self-powered perovskite-based p-n junction photodiodes.
2.2-O2
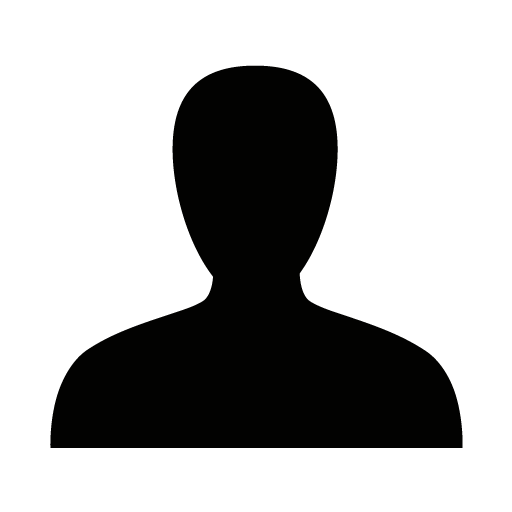
The rapid development of industry in field of machine vision systems, the Internet of Things (IoT) and smart houses rises the demand for scalable bifacial photodetectors [1-3]. Perovskite photodetectors (PPDs) hold a great potential to dominate photodetector market due to their high detectivity, fast response, and cost-effectiveness. However, unlocking the full potential of PPDs for the industry requires overcoming critical technological challenges, notably in scalability, robustness, weight, and semitransparency.
To bring PPDs towards commercialization, we have fabricated scalable self-powered PPDs using the industrially compatible slot-die coating technique on flexible plastic substrates. The integration of thin dielectric-metal-dielectric layers as transparent top contacts has granted the devices with an impressive bifaciality factor of 78% and light-to-dark current ratios reaching an order of magnitude of 104. Alongside their high detectivity, fabricated PPDs possess the capability to harness solar energy, exhibiting reasonable power conversion efficiencies (PCEs) from both, the bottom- and top-side illuminations.
In this presentation, we will showcase the advancements we made in scalable PPD technology. We will discuss the role of selection of top contacts to optimize the performance of bifacial optoelectronics and conclude the key aspects of fabrication of scalable devices.
2.2-I1
The very rapid growth of internet-connected devices brings two important challenges. The first is how will all these devices communicate? The second is how will they be powered? Optical communications can help, particularly in the form of “Li-Fi” which modulates indoor lighting to transmit information. It is important to supplement Wi-Fi because the radio frequency spectrum is now very crowded. Organic semiconductors have been shown to be useful as both transmitters and receivers for Li-Fi, and also for indoor power harvesting.
This talk will give an overview of Li-Fi using organic semiconductors and then focus on their use as receivers. In particular it will show how organic photovoltaics can be used to receive data and generate power simultaneously. By using an array of four detectors in a multiple input multiple -output (MIMO) setup data rates up to 363 megabits per second were achieved together with simultaneous harvesting of power. These results show that organic photovoltaics can be used for wireless data communication while prolonging the battery life of a mobile device.
1.1-I1
Sophia Haussener is a Professor heading the Laboratory of Renewable Energy Science and Engineering at the Ecole Polytechnique Federale de Lausanne (EPFL). Her current research is focused on providing design guidelines for thermal, thermochemical, and photoelectrochemical energy conversion reactors through multi-physics modelling and experimentation. Her research interests include: thermal sciences, fluid dynamics, charge transfer, electro-magnetism, and thermo/electro/photochemistry in complex multi-phase media on multiple scales. She received her MSc (2007) and PhD (2010) in Mechanical Engineering from ETH Zurich. She was a postdoctoral researcher at the Joint Center of Artificial Photosynthesis (JCAP) and the Lawrence Berkeley National Laboratory (LBNL) between 2011 and 2012. She has published over 70 articles in peer-reviewed journals and conference proceedings, and 2 books. She has been awarded the ETH medal (2011), the Dimitris N. Chorafas Foundation award (2011), the ABB Forschungspreis (2012), the Prix Zonta (2015), the Global Change Award (2017), and the Raymond Viskanta Award (2019), and is a recipient of a Starting Grant of the Swiss National Science Foundation (2014).
The design and implementation of complete, operational photo-electrochemical devices requires a careful consideration of material requirements and their interplay in an integrated device. Multi-physical, multi-dimensional and multi-scale modeling tools are essential for the investigation of feasibility, optimization and implementation of such devices. Modeling activities however always have to be fed and, ultimately, validated by experimental data of material and device characteristics. In this talk, I will discuss the development of predictive modeling tools for photo-electrochemical water and CO2 reduction. These models account for the atomistic-scale via density functional theory, molecular-scale by micro-kinetic and molecular dynamics models, nm-scale by double layer transport models, meso-scale by tomography-based direct numerical simulations, and device scale by volume-averaged device models. I will review each of these modeling steps and highlight the importance of interfacing them. I will then show what experimental data is needed to run these simulations and how these models can be validated. I will end with some device-level demonstrations of photo-electrochemical devices that follow the design guidelines developed by the multi-scale models.
1.1-O1
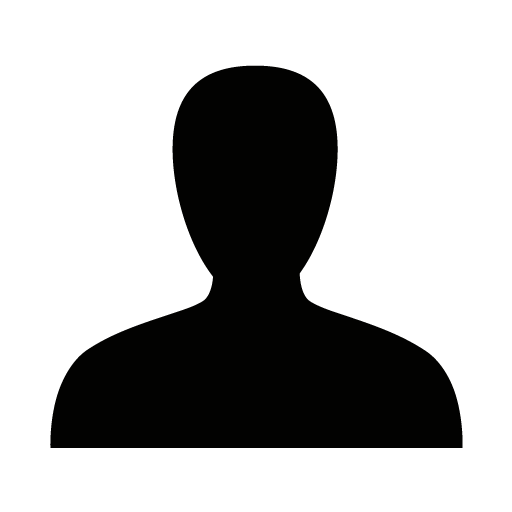
Unlimited access to clean, sustainable and renewable source of energy seems to be a dream scenario, however with an assistance of machine learning (ML) approach the experimental work which actually reveals the right descriptors may gain a powerful tool allowing to omit a time consuming trials path. As a matter of fact, the ML has already shown the potential to significantly reduce the time and effort required for developement of new and efficient photocatalysts.
However, precise definition of descriptors will require an identification of the bottleneck materials parameters, features and processes, thus different architectures and working arrangements will be shown and disscused in this presentation in order to test their usfulness for prediction of novel and better arrangements for hydrogen and other carbon based solar fuels production.
Over 400 samples based on the semiconductor oxides have been prepared and measured in order to bulid a pre-base for ML tests. Different materials and working systems, their compositions, geometry, properties will be disscused in view of their impact on the final prediction. In this presentation we will discuss the strengths and weaknesses of different architectures and working arrangements for hydrogen and carbon-based solar fuels production.
1.1-O2
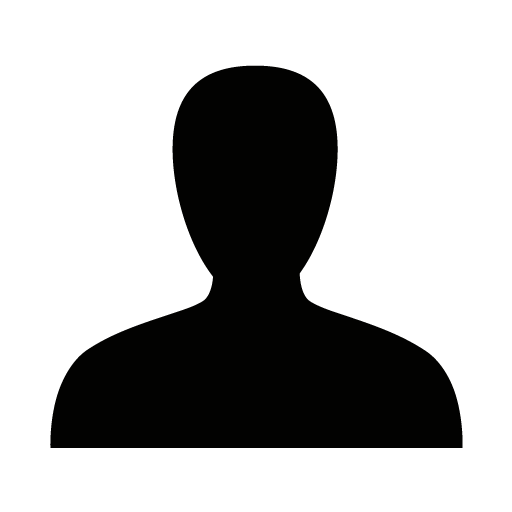
Nowadays, the existence of high entropy perovskite oxides is well established and, since their discovery, their fields of application have been continuously studied. The main advantage provided by these types of materials with general formula ABO3 is the high possibility of tuning their properties by acting on the chemical compositions. The study of stability, fields of existence, and solubility limits in a systematic way remains lacking in comparison with continuous application research, despite the presence of large datasets and computational studies.1 In our work, we investigated the structure and solubility limits using a chemometric approach for two families of perovskite oxides with the following compositions: on the site A we chose to keep lanthanum due to its stability, while, on the B site, we explored different cation mixtures based on Cr, Mn, Fe, Co, Ni and Zn, to determine an experimental domain by including various experimental data, i.e. crystal structure, oxygen vacancies amount and temperature-dependance, and composition.2 The synthesized samples were subjected to x-ray diffraction (XRD) analysis followed by Rietveld refinement to determine crystallographic parameters. Through multivariate analysis, elemental concentrations were correlated to phase stability and nature, and cell parameters. The structural study aims at highlight possible correlations between the crystal symmetry and the catalytic characteristics of the material. Finally, through thermogravimetric analysis, it is possible to study non-stoichiometry and phase transitions to be integrated within the previously designated experimental domains.3 The overall dataset will allow to define the best compositions to be used for the desired applications such as heterogeneous catalysis, solid-oxide fuel cells, and oxygen transport membranes.4
1.1-I2
Luminescent solar concentrators (LSCs) are a promising technology to help integrate photovoltaic (PV) cells as functional architecture in the urban environment.[1] LSCs typically consist of a waveguide slab, which is doped or coated with a luminescent species, or luminophore.[2] Incident light is absorbed by the luminophores and re-emitted at longer wavelengths through photoluminescence. A large portion of the emitted light is concentrated by the waveguide via total internal reflection to the edges of the LSC, where a strip of PV cells may be retrofitted attached to collect the spectrally converted light. While LSCs have traditionally been cuboidal in geometry, in recent years, new architectures have been explored for example, circular, curved, polygonal, wedged, and leaf-shaped designs.[3,4] These new
designs can help reduce optical losses, enable integration within existing infrastructure, or unlock new applications.
However, fabrication of complex LSC geometries can be both time- and resource-intensive. LSCs are commonly made using conventional manufacturing methods such as casting, which either limits possible architectures to the availability of suitable moulds or leads to material waste as designs are cut to shape. Moreover, it is not possible to introduce secondary internal structure within LSCs by casting. To tackle these limitations, in this talk the use of 3D printing (specifically fused deposition modelling) as a tool to fabricate complex prototype LSC architectures for rapid optical evaluation will be described. It will be shown that the print pattern (e.g. line thickness, build-up of layers) introduces internal structure within the LSCs, affecting the optical pathways for light absorption and emission. Moreover, we will demonstrate how we have implemented upgrades to the Monte Carlo ray-trace software pvtrace, to enable advance prediction of the optical efficiency of 3D-printed LSCs.[5] The more versatile computational workflow afforded by our upgrades, coupled with 3D-printed prototypes, will enable rapid screening of more intricate LSC architectures, while reducing experimental waste.
1.1-I3
The search for new photoactive materials able to efficiently produce solar fuels is a matter of growing interest due to the current global energetic crisis. In response to this situation, the generation of solar fuels has appeared as a sustainable alternative. In last years, extensive efforts have been made to develop efficient catalytic systems capable of harvesting light absorption and reducing CO2 especially when using water as the electron donor.
Herein, we report different strategies and modifications of photocatalysts to increase process performance. Among them, an interesting approach to improve charge separation in photocatalytic systems is the use of heterojunctions. In this line, the combination of different semiconductors with noble metal nanoparticles or organic semiconducting polymers leads to a separation of the photogenerated charge carriers to increasing their life time, facilitating charge transfer to adsorbed molecules.
Organo-inorganic hybrid materials show a dramatic reactivity improvement in CO2 photoreduction, enhancing methane selectivity. Reaction pathways are not well defined for this reaction and several uncertains are still unsolved. To explain this behavior a combination of in-situ NAP-XPS, FTIR, TAS spectroscopies and theoretical tools has been used, showing a more efficient light absorption and charge transfer in the hybrid photocatalyst compared with bare materials.
2.1-I1
James Durrant is Professor of Photochemistry in the Department of Chemistry, Imperial College London and Ser Cymru Solar Professor, University of Swansea. His research addresses the photochemistry of new materials for solar energy conversion targeting both solar cells (photovoltaics) and solar to fuel (i.e.: artificial photosynthesis. It is based around employing transient optical and optoelectronic techniques to address materials function, and thereby elucidate design principles which enable technological development. His group is currently addressing the development and functional characterisation of organic and perovskite solar cells and photoelectrodes for solar fuel generation. More widely, he leads Imperial's Centre for Processable Electronics, founded the UK�s Solar Fuels Network and led the Welsh government funded S�r Cymru Solar initiative. He has published over 500 research papers and 5 patents, and was recently elected a Fellow of the Royal Society
In photocatalytic systems for solar energy conversion, a key challenge for efficient operation is the efficient separation and stabilisation of photogenerated charges, and thereby the minimisation of undesired recombination losses. This challenge is particularly great for photocatalytic systems because of the long charge lifetimes required to drive catalysis A further consideration is to minimise the energy losses required to drive this charge separation. My talk will cover examples of recent work from my group addressing aspects of this challenge. I will start by considering the role of polaron localisation / relaxation in driving charge separation in metal oxides, and quantification of the energetic loss associated with this polaron formation. Kinetic competion between polaron formation and ligand field state mediated charge recombination will be highlighted as the key challenge limiting the performance of many visible light absorbing metal oxides. The roles of metal oxide dielectric constant, surface facet energies, traps, ‘photocharging’ and heterojunctions in aiding charge separation in metal oxides will be discussed, drawing on examples from BiVO4 and SrTiO3. I will then go on to discuss charge separation and stabilisation in alternative photocatalyst materials, including organic semiconductor nanoparticles and metal-organic frameworks, highlighting the observation of remarkably long lived charge photogeneration in both systems and their relevance to the efficiency photocatalytic performance.
2.1-O1
Hydrogen and other solar fuels have been highlighted as one of the future energy vectors. Having natural photosynthesis as inspiration, we can develop a device capable to split water using sunlight, obtaining oxygen and hydrogen. [1], [2] Different strategies can be used to achieve this: from separated light harvesting and catalytic systems to all integrated devices able to transform directly sunlight into fuels. These systems can be built with a variety of (photo)electrodes such as organic based material, chalcogenides or metal oxides. In most of them the addition of a co-catalyst layer it is pivotal to improve their efficiency. Although rapid progress is being made in the field, understanding of the limiting factors of these catalysts has allowed remarkable improvements in their performance.
In this talk I will focus on the use of organometallic method to prepare nanoparticles as catalysts for solar fuels production. This is a versatile method able to grow NPs in bulk or in the presence of a support.[3], [4] In here I will present the use of this method to prepare different metal NPs on different organic based light absorbers and their activity as photocatalysts for hydrogen production. Interestingly in this work the photocatalysts containing Ni NP present a similar performance than the Pt ones. In order to correlate the performance with the nature of each photocatalyst, we have characterised of the materials before and after catalysis. The latter is a key information to unravel the main deactivation pathways, and consequently, systematically designing new and more efficient photocatalytic systems.
2.1-O2
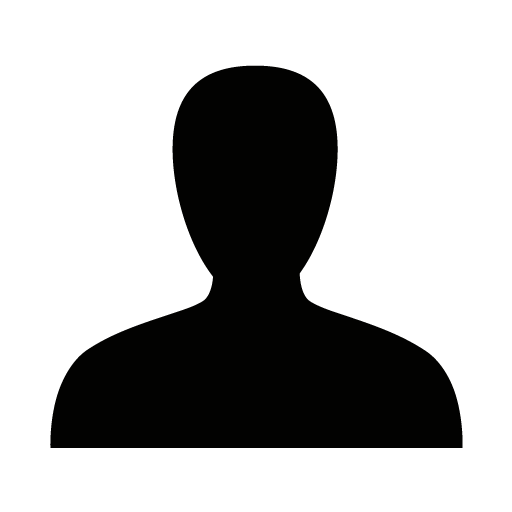
Bismuth vanadate (BiVO4) is a nontoxic and medium band gap (2.4 eV) n-type semiconductor that has attracted worldwide attention as a visible-light active photocatalyst. Particularly, scheelite monoclinic BiVO4 is one of the most active polymorphs in photocatalytic oxygen evolution. However, the oxidation capability of bare BiVO4 is hindered by weak charge carrier mobilities, short charge carrier diffusion lengths, and a high charge recombination rate at the surface. To circumvent these inconveniences, heterostructuring by contacting BiVO4 with other materials such as noble metals and metal oxides has been proposed.
Usually, contact materials are deposited in a random manner on particulate BiVO4-based photocatalysts. In this case, no distinct flow path for photogenerated holes and electrons can exist which makes bulk and surface recombination still prominent due to insufficient charge carrier separation. Since the pioneer works on anatase and rutile TiO2 particles which have revealed the key role of exposed facets in the separation of photoinduced electron–hole pairs, photodeposition is now considered as a sort of general route for synthesizing heterostructures. Indeed, photogenerated electrons and holes preferentially accumulate on different crystalline facets which renders cocatalyst deposition regioselective depending on whether the process involves an oxidation or reduction reaction. Systematic studies on single crystalline TiO2 (anatase) exposing different facets have shown that the different surfaces (100) vs (110) provide indeed different electronic surface properties including different work functions that promote charge carrier separation into different directions.
Anisotropic photodeposition on BiVO4 microcrystals has been extensively studied. It has been shown that metallic deposits were preferentially found on {010} facets after metal ion photoreduction, while metal oxide deposits were found on the {110} facets after metal ion photo-oxidation, evidencing that electrons and holes accumulate on different sites on anisotropic BiVO4 crystals. Besides providing proof of enhanced charge carrier separation, the preferential photodeposition was used to construct BiVO4 photocatalysts with even better photocatalytic oxygen evolution efficiencies by depositing reduction cocatalysts on the more reducing {010} facets and oxidation cocatalysts on the more oxidative {110} facets.
On the other hand, BiVO4 microcrystals generally reported in the literature present compromised colloidal stability and low surface activity due to size effects. Thus, dedicated experiments need to be tackled to control and decrease the size.
In this context, we herein report for the first time a universal photodeposition of noble metal [Au, Ag, Pd] and/or metal oxide [CoOx, MnOx, FeOx] cocatalysts, via the original use of laser light, on BiVO4 nanocrystals exposing well-defined {010} and {110} facets, being the main advantage of laser photodeposition the shorter procedure times due to high intensity effects. The monoclinic BiVO4 nanocrystals here exhibited are one of the smaller reported ever, showing more than an 8-fold edge length and 4-fold thickness reduction compared to the common microcrystals generally reported in the literature. Both the chemistry and regioselectivity of the deposition were investigated by employing X-ray photoelectron spectroscopy and various electron microscopy techniques. Then, the photocatalytic efficiencies of the structures prepared were tested for water oxidation reactions and were compared with previously studied systems.
2.1-I2
Roel van de Krol is head of the Insitute for Solar Fuels at the Helmholtz-Zentrum Berlin fuer Materialien und Energie (HZB), and professor at the Chemistry Department of TU Berlin. After earning his PhD from TU Delft in 2000 and a postdoctoral stay at M.I.T. (USA), he returned to TU Delft where he was an assistant professor until 2012. At HZB, his research focuses on the development of materials and devices for the photoelectrochemical conversion of sunlight to chemical fuels. Understanding how surface and bulk defects in thin films and nanomaterials affect light absorption, charge transport, recombination and catalytic activity is at the heart of these efforts.
Metal oxide photoelectrodes tend to be cheap, easy to fabricate, and show relatively good (photo)chemical stability in aqueous solutions. This makes them attractive candidates as light absorbers in a variety of photoelectrochemical and photocatalytic applications. However, the efficiencies of these absorbers are poor compared to photovoltaic-grade materials. This is usually attributed to polaron formation and recombination or trapping at defects. To determine the quality of metal oxide absorbers, time-resolved photoconductance measurements are often used as a convenient and contact-free method to determine the carrier diffusion length. Here, one of the main challenges is to determine whether the decay in photoconductivity is due to a decay in carrier concentration (recombination), a decay in carrier mobility (trapping, polaron formation), or both. I will present a general analysis method for determining the diffusion length which is valid for time-dependent mobilities as well as time-dependent lifetimes [1]. We have applied this method to a range of metal oxides and validated the method by applying it also to crystalline silicon and a halide perovskite. We find a carrier diffusion length of only 15 nm for BiVO4, which is significantly shorter than previously reported values by us and others. I will discuss how this value can be reconciled with the relatively high photocurrents that many groups have reported for this material. For several other oxides that we studied, we find evidence for nm-scale carrier localization [2], which is likely due to nano-sized crystallites or defect clusters. Mitigation of this strong localization may be possible and would offer a promising strategy for designing more efficient metal oxide-based photoelectrodes.
2.2-I1
Electro and photochemical CO2 reduction (CO2R) is the quintessence of modern-day sustainable research. We report our studies on the electro and photoinduced interfacial charge transfer occurring in nanocrystalline mesoporous TiO2 film and two TiO2/Iron porphyrin hybrid films (meso-aryl and b-pyrrole substituted porphyrins, respectively) under CO2R conditions. We used Transient Absorption Spectroscopy (TAS) to demonstrate that under 355 nm laser excitation and an applied voltage bias (0 to -0.8 V vs. Ag/AgCl), the TiO2 film exhibited a diminution in the transient absorption (at -0.5 V by 35%), as well as a reduction of the lifetime of the photogenerated electrons (at -0.5 V by 50%) when the experiments were conducted under CO2 atmosphere changing from inert N2. The TiO2/Iron porphyrin films showed faster charge recombination kinetics, featuring 100-fold more rapid transient signal decay than the TiO2 film. The electro, photo, and photoelectrochemical CO2R performance of the TiO2 and TiO2/Iron porphyrin films are evaluated within the bias range of -0.5 to -1.8 V vs. Ag/AgCl. The bare TiO2 film produced CO and CH4, as well as H2, depending on the applied voltage bias.
In contrast, the TiO2/Iron porphyrins films showed the exclusive formation of CO (100% selectivity) under identical conditions. During the CO2R, gain in the overpotential values is obtained under the light irradiation conditions. This finding indicated a direct transfer of the photogenerated electrons from the film to absorbed CO2 molecules and a decreased decay of the TAS signals. In the TiO2/Iron porphyrin films, we identified the interfacial charge recombination processes between the oxidized iron-porphyrin and the electrons of the TiO2 conduction band. These competitive processes are considered responsible for the diminution of direct charge transfer between the film and the adsorbed CO2 molecules, explaining the moderate performances of the hybrid films for the CO2R.
2.2-O1
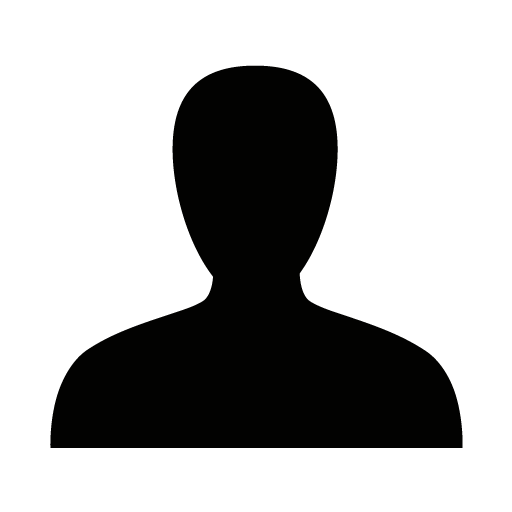
The increasing levels of carbon dioxide (CO2) in the atmosphere and the growing global need for energy necessitate the development of renewable methods for electricity production that can help reduce carbon emissions and of green energy-based CO2 utilization technologies. In this context, sustainable and clean energy sources have attracted a lot of attention. In particular, solar energy-based techniques can be used to convert CO2 into valuable chemicals and fuels, such as CO, formate or methanol [1]. Currently, one established way to utilize CO2 is to use the coenzyme-dependent activity of formic acid dehydrogenase (FDH) to catalyze the reduction of CO2 to formate [2]. Nevertheless, the high cost of the coenzymes (i.e., NADH and MV•+) have so far limited the application of this technology [3].
Therefore, efficient and inexpensive coenzymes regeneration methods using economic energy sources would provide a greener and sustainable pathway for CO2 reduction.
In recent years, different groups have been focused their activity on the improvement of visible-light-driven photoredox reactions to implement artificial photosynthesis systems for the efficient regeneration of enzymatic cofactors, in order to lower the cost of the CO2 reduction process [4-7]. These systems are usually formed by a photosensitizer, an electron donor, and an electron acceptor [4-7]. Upon illumination and light absorption, the photosensitizer is converted from the ground state to the excited state. The photo-excited electrons from the triplet state are then transferred to the electron acceptor (i.e., NAD+ or MV2+), and at the same time, the triplet state of the photosensitizer regains the electrons from the electron donor (usually, triethanolamine or TEOA). Moreover, for NADH regeneration, rhodium-based compounds are commonly added to the reaction and used as electron mediators to guarantee the regiospecific reduction of NAD+ to the enzyme active form of NADH (i.e., 1,4-NADH) [8, 9].
So far, different materials have been used as photosensitizers, from inorganic species [10] to organic dyes such as porphyrins and their derivatives [11]. Compared to the inorganic materials, the organic molecules are cheaper and easier to obtain and have a wide variety of chemical and physical properties [12]. Nevertheless, there are some practical limitations to their application, including instability, slightly high cost and difficulty of recovery [12].
Here we report about the use of conjugated-polymer-based water-processable nanoparticles (WPNPs) as photosensitizer in a photoredox system for the regeneration of both NADH and MV•+. Such materials show excellent thermo- and photo-stability, good visible absorption properties, low cost/low temperature solution processing and easy removal [13]. Moreover, the use of non-toxic solvents (i.e., water) makes them appealing in biomedical and biocompatible applications [14].
For this purpose, we synthetized a derivative of the semiconducting polymer P3HT, i.e., poly[2,2''''-bis[[(2-butyloctyl)oxy]carbonyl][2,2':5',2'':5'',2'''-quaterthiophene]-5,5'''-diyl] (PDCBT), and prepared the corresponding WPNPs through a miniemulsion approach [15-17]. Then, the PBDTTPD WPNPs preparation was employed in a visible-light-driven NADH/MV•+ regeneration system consisting of TEOA as electron donor and NAD+ or MV2+ as electron acceptors and enzyme-catalyzed redox reactions were used to validate the production of the regenerated cofactor.
Our results show a successful example of conjugated-polymer WPNPs used as a photosensitizer for selective coenzyme regeneration in an artificial photosynthesis system, which is easy to build and usable under mild conditions for the facile regeneration of different coenzymes. These results could pave the way for other photoredox reaction systems that enable the selective and sustainable production of chemicals and fuels through the use of solar light.
2.2-O2
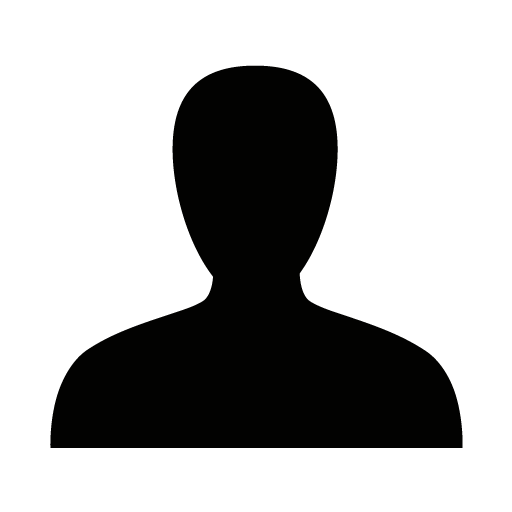
Carles Ros is currently working as senior postdoctoral researcher with the fellowships Juan de la Cierva (JdC-F) and Severo Ochoa Excellence Program in the Organic Nanostructured Photovoltaics group in ICFO research institute lead by Prof. Jordi Martorell in the fields of Photonics, Photovoltaics and Photoelectrochemistry.
He has research experience in the fields of advanced materials science, (photo)electrochemistry, nanostructured synthesis, semiconductor physics and photovoltaics. In particular, (photo)electrocatalysis and light management in metal-oxide-organic-perovskite tandems and protective metal-oxide layers for photoelectrodes for applications in H2, CO2RR, hydrogen storage and release in graphene, solar fuels and photobatteries. His highly interdisciplinary background based on applied physics has helped him find its place in a group that is applying solid light management physics into the field of renewable synthetic fuels.
This study demonstrates the disruptive potential of organic photoabsorbing blends in overcoming a critical limitation of metal oxide photoanodes: insufficient photogenerated current. Various organic blends, including PTB7-Th:FOIC, PTB7-Th:06T-4F, PM6:Y6, and PM6:FM, were systematically tested. When coupled with state-of-the-art electron transport layer (ETL) contacts, these blends exhibit exceptional charge separation and extraction, with PM6:Y6 achieving saturation photocurrents up to 17 mA/cm² at 1.23 VRHE (oxygen evolution thermodynamic potential). Employing a novel architecture, we highlight the remarkable attributes of PM6:Y6 blends, showcasing their ability to be fabricated as semitransparent organic photoanodes in tandem structures. The implementation in a double PM6:Y6 photoanode/photovoltaic structure resulted in photogenerated currents exceeding 7 mA/cm² at 0 VRHE (hydrogen evolution thermodynamic potential) and cathodic onset potentials as low as -0.5 VRHE.
To our knowledge, this work represents the first time a tandem structure utilizing organic photoanodes has been computationally designed and fabricated, achieving unprecedented performance levels for both the standalone photoanode and the tandem configuration. The structural design has been modeled using the transfer matrix method and experimentally tested, determining the optimal thickness of the first semitransparent light-absorbing element, the photoanode, to be 75 nm. This integrated approach holds promise for advancing the field of photoelectrochemical solar conversion to fuels and paves the way for further exploration of blend combinations and oxidative reactions targeting.
2.2-O3
Virgil Andrei obtained his Bachelor and Master of Science degrees in chemistry from Humboldt-Universität zu Berlin, where he studied thermoelectric polymer pastes and films in the group of Prof. Klaus Rademann (2014-2016). He then pursued a PhD in chemistry at the University of Cambridge (2016-2020), where he developed perovskite-based artificial leaves in the group of Prof. Erwin Reisner, working closely with the Optoelectronics group of Prof. Richard Friend at the Cavendish Laboratory. He was a visiting Winton fellow in the group of Prof. Peidong Yang at University of California, Berkeley (2022), and is currently a Title A Research Fellow at St John's College, Cambridge. His work places a strong focus on scalability, material design, complementary light harvesting and synthesis of added-value carbon products, introducing modern fabrication techniques towards low-cost, high-throughput solar fuel production.
Photoelectrochemical (PEC) artificial leaves can lower the costs of sustainable solar fuel production by integrating light harvesting and catalysis within a compact panel.[1] However, most prototypes can only perform water splitting over a few hours on a cm2 scale, whereas conventional light absorbers limit solar-to-fuel efficiencies and product rates.[2,3] Here, we explore alternative routes to expand the performance and functionality of PEC devices, by designing integrated systems which benefit from unconventional materials and complementary energy harvesting. To this end, we first demonstrate the fabrication of lightweight artificial leaves by employing thin, flexible substrates and carbonaceous protection layers,[4,5] which are compatible with modern fabrication techniques.[6] These materials allow 100 cm2 perovskite-BiVO4 artificial leaves to float along River Cam (UK), showcasing the potential of floating solar fuel farms.[5] The same carbonaceous protection layers can be employed to increase the moisture stability of an underexplored BiOI photocathode from minutes to >500 h, whereas a pixelated design provides the additional photovoltage required for unassisted water and CO2 splitting.[7] Product rates can be significantly boosted by integrating PEC devices onto thermoelectric (TE) generators, which harvest waste heat from thermalisation and IR photons.[8,9] Accordingly, a Pt-TE-BiVO4 device can already yield unassisted water splitting under 2 sun irradiation, while the photocurrent of a Pt-perovskite-TE-Fe2O3 device is boosted 30-fold under 5 sun irradiation.[9] Further up-scaling of PEC systems towards m2 areas can be performed by taking advantage of the modularity of artificial leaves,[10] however, manual fabrication must be replaced by high-throughput techniques for large scale applications.[6]
2.3-I1
Kevin Sivula obtained a PhD in chemical engineering from UC Berkeley in 2007. In 2011, after leading a research group in the Laboratory of Photonics and Interfaces at EPFL, he was appointed tenure track assistant professor. He now heads the Laboratory for Molecular Engineering of Optoelectronic Nanomaterials (http://limno.epfl.ch) at EPFL.
Organic semiconductors are gaining prominence as promising materials for heterogeneous photocatalytic (PC) solar fuel production due to their molecular tunability and scalable processability. Notably, bulk heterojunction (BHJ) nanoparticles (NPs), synthesized through a mini-emulsion approach, have proven to be high-performance and cost-effective photocatalysts for solar hydrogen production under sacrificial conditions. Despite their success, the factors crucial for optimizing the performance of these BHJ NPs remain poorly understood. This presentation delves into the intricacies of BHJ-based systems, drawing insights from photoelectrochemical measurements and model photocatalysts. It highlights the significance of co-catalyst loading and particle formation methods, unraveling their impact on the stability and overall water-splitting ability of BHJ NPs. In particular, the importance of controlling the nucleation and growth of the Pt co-catalyst on the BHJ surface is highlighted. By elucidating this critical factor and others, the presentation paves the way for a more comprehensive understanding of BHJ-based NP systems, aiming to enhance their efficiency and durability in the realm of solar fuel production.
2.3-O1
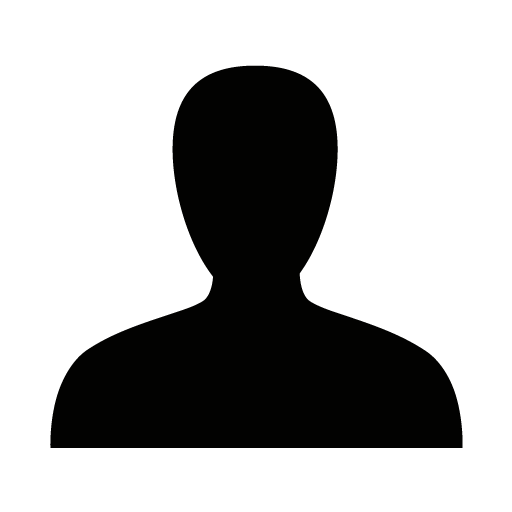
To fight against climate change, the production of carbon-neutral energy is critical. Among the solar fuels, hydrogen stands out, allowing the storage of the sun’s energy as chemical bonds, by the photoreduction of protons. Pinaud et al. [1] have shown that using particle slurries can be a competitive and green way to produce hydrogen (Fig. 1).
Figure 1 Scheme of the cycle of Photocatalytic Hydrogen Generation, from the harvesting of the photons to the use of the solar fuel
In 2016 Tian et al.[2] managed to produce hydrogen using organic semiconductor nanoparticles. A hydrogen evolution rate (HER) of 8 mmol.h-1.g-1 was achieved and showed how the surfactant is essential to increase the catalytic activity, by creating pdots.[2] Moreover, the surfactant’s charge can potentially increase the surface photon affinity and the surfactant itself can lead to nanoparticles with a more suitable morphology, as demonstrated by Kosco et al.[3]: by changing the surfactant from SDS to TEBS, the morphology of the nanoparticles shifted from core-shell to intermixed, which resulted in a higher HER. It appears clear that it is fundamental to understand the role of the surfactant, in order to find the best one to increase the hydrogen evolution rate.
In this communication, it will be discussed how changing the surfactant used for nanoparticle stability affects the HER. A first study on the system P3HT:PC61BM was conducted, as a reference, with nanoparticles prepared via nanoprecipitation. For instance, a Janus morphology was recently reported for this system, which can be of great interest in the case of photocatalytic applications.[4]. From this, a state-of-the-art donor:acceptor couple will be introduced, with a promising HER of 13 mmol.h-1.g-1. To characterize the particles before and after the hydrogen evolution Dynamic Light Scattering, Cryo-TEM, and UV-Visible spectroscopy were used.
2.3-O2
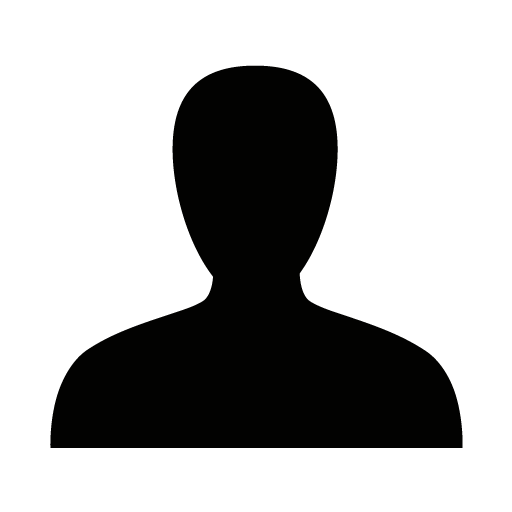
Photoelectrochemical (PEC) water reduction is one of the key reaction routes to harness solar energy for the production of green hydrogen (H2). Copper oxides are promising semiconductor materials for PEC applications as it possesses a narrow bandgap and favourable energy band positions. Owing to its severe photocorrosion, poor charge separation and rapid recombination of photogenerated electron-hole pairs, however, the use of conventional pristine Cu2O photocathodes is not technically feasible for PEC reduction of water. Moreover, it is widely known that the morphology and structure of copper oxide-based photocathodes are pivotal to their PEC performance as they heavily influence the intrinsic properties, such as the density of active sites and specific surface areas. Modulation of these properties is crucial in increasing the available active sites for surface redox reactions and improving the separation efficiency of photogenerated charge carriers. Therefore, it is highly desirable to construct an efficient nanostructured heterojunction of Cu2O-based photocathode to improve its photoconversion efficiency. Herein, we report a carefully engineered multi-component cuprous-cupric oxide heterostructure with distinct 2D-nanoflake morphologies (Cu2O-CuO-NF) to overcome the technical bottlenecks encountered in conventional Cu2O photocathodes. This was achieved through hot alkali treatment and subsequent thermal oxidation on the electrodeposited Cu2O/FTO, which partially oxidises Cu2O into 2D-CuO nanoflakes. Positive characteristics brought upon through this synthesis technique resulted in an enhanced specific surface area, which could facilitate a greater photocathode-electrolyte interfacial contact and higher water reduction activity during the PEC application. Enhanced charge separation efficiency is achieved as a result of the band bending at the Cu2O-CuO interface, which provides a strong driving force for electron-hole separation and therefore, reduces the electron-hole pairs’ recombination process. Through morphological and compositional analyses using FE-SEM, XRD, and XPS, the formation of CuO nanoflakes on the surface of the Cu2O photocathode was proven and validated. From the optical and electrochemical measurements, the charge carrier density was enhanced while the interfacial charge transfer resistance was reduced. This confirmed that the formation of heterojunction coupled with the surface nanoflake morphology of CuO plays a pivotal role in facilitating charge separation of photogenerated charge carriers. A high photocurrent density of -2.96 mA/cm2 at -0.6 V vs Ag/AgCl under AM 1.5 G solar irradiation (100 mW/cm2) was achieved, which was approximately 27 times, and 1.5 times higher than that of the pristine Cu2O (-0.11 mA/cm2) photocathode and Cu2O-CuO (-1.96 mA/cm2) photocathode without 2D surfaces nano-structuration, respectively. Ultimately, experimental-derived information and data were deployed to construct a theoretical band diagram that elucidates the heterojunction band alignment of Cu2O and CuO over the PEC water reduction mechanism.
2.3-I2
Transition metal nitride semiconductors are rapidly emerging as a promising class of materials that provide a combination of properties well suited for advanced optoelectronic and energy conversion applications. Compared to oxides, nitride semiconductors offer narrower bandgaps, stronger bond covalency, and improved carrier transport properties that make them well suited for harvesting sunlight in photovoltaic and photoelectrochemical systems. Despite this considerable promise, dramatically fewer nitrides than oxides have been experimentally investigated due to their synthetic complexity. Consequently, a broad range of new compounds remain to be explored as functional semiconductors. Furthermore, synthesis challenges have led to poorly controlled defect and impurity properties within this class of materials. In this work, we overcome these limitations using reactive co-sputtering as a non-equilibrium deposition approach to synthesize thin film nitride semiconductors with precisely controlled compositions, exploring both dopants and new compounds in the Ti-Ta-N and Zr-Ta-N composition spaces. Starting with orthorhombic Ta3N5, which stands as the best performing photoanode material within this class, we investigate the critical roles of nitrogen vacancy (vN), substitutional oxygen (ON), and reduced Ta centers (Ta3+) on thin film photoconversion efficiencies [1]. Using in situ photoelectrochemical transient absorption spectroscopy, we identify spectral signatures of photogenerated holes and assess the competition between recombination and chemical reaction at the interface, which is directly impacted by point defect concentrations. Armed with these insights, we show that substitutional Ti doping on Ta sites (TiTa) can be used to improving photoconversion efficiencies [2]. While the structural properties and optical bandgap are only minimally affected by the incorporation of Ti impurities, we observe substantial changes in surface photovoltage, band bending, and recombination dynamics with Ti content. At low Ti content, Ti doping substantially improves the photoelectrochemical performance, manifesting in increased photocurrent densities and favorably reduced onset potentials. While high Ti contents lead to the precipitation of a secondary TiN phase, different behavior is observed for the case of Zr incorporation. In particular, introduction of a 1:1 Zr:Ta ratio leads to formation of a new ternary nitride compound, bixbyite-type ZrTaN3. Comprehensive investigation of the properties of this material reveal that it is a strong visible light absorber and is an active photoanode material. Complementary DFT calculations indicate a direct bandgap that is tunable based on cation site occupancy. Thus, this material offers exciting prospects not only for solar energy conversion but also for optoelectronics applications. Overall, these results highlight the promise of both established and new transition metal nitride semiconductors for solar energy harvesting, as well as the importance of precise composition engineering to tune optoelectronic and charge transport characteristics.
3.1-I1
Photocatalytic systems for the reduction of CO2 into fuels and platform chemicals are multi-variable multi-metric systems. As such, long-established optimisation approaches are poorly suited maximising system performance because they aim to optimise one performance metric while sacrificing the others and thereby limit overall system performance. I will present work to address this multi-metric challenge by defining a metric for holistic system performance that takes all figures of merit into account, and employing a machine learning algorithm to efficiently guide our experiments through the large parameter matrix to make holistic system optimisation accessible for human experimentalists. The test platform is a five-component system that self-assembles into photocatalytic micelles for CO2-to-CO reduction, which we experimentally optimised to simultaneously improve yield, quantum yield, turnover number, and frequency while maintaining high selectivity. Leveraging the dataset with machine learning algorithms allows quantification of each parameter’s effect on overall system performance, revealing that the buffer concentration is the dominating parameter for optimal performance with nearly four times more importance than the catalyst concentration. The expanded use and standardisation of this methodology to define and optimise holistic performance will accelerate progress in different areas of catalysis by providing unprecedented insights into performance bottlenecks, enhancing comparability, and taking results beyond comparison of subjective figures of merit.
3.1-O1
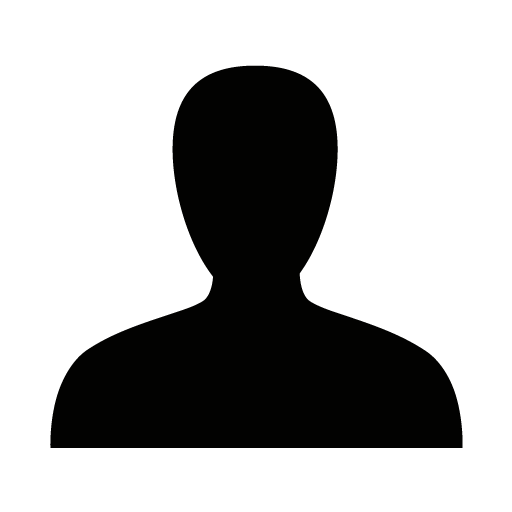
Simultaneously harvesting, converting and storing solar energy in a single device represents an appealing technological approach for the next generation of power sources. Herein, we propose a device consisting of a single piece of (rhenium, Re; niobium, Nb) doped WSe2 photocathode and zinc foil anode system capable of harvesting solar energy (and converting it into electricity) and a rechargeable aqueous zinc metal cell. The proposed cell demonstrated an improvement in the specific capacitance of around 45% under visible light irradiation. Also, Re doped WSe2 exhibited a loss of 4.44 % at its initial specific capacitance after 500 galvanostatic charge-discharge cycles at 50 mA g-1. As a result, the device delivered a specific energy density of 574.21 mWh Kg-1 at a power density of 5906 mW Kg-1 and at 0.015A g-1. Furthermore, the notable enhancement in capacitance achieved through the Re-WSe2 photo-zinc ion capacitor (photo-ZIC) offers a straightforward and constructive avenue to establish a cost-effective and highly efficient solar-electrochemical capacitor system.
3.1-O2
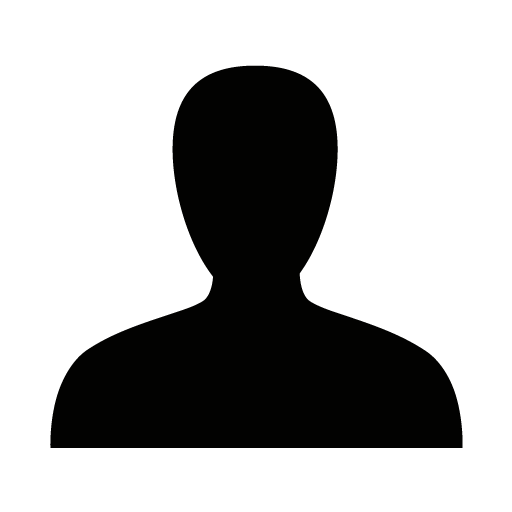
Post doctoral student at BGU, Israel
Polymeric carbon nitride (CN) has emerged as a promising photoanode material in photoelectrochemical cells (PEC) thanks to its cost-effectiveness, lack of toxic or rare elements, high chemical stability, and suitable band edge positions (for common redox reactions as water-splitting and a band gap in visible range.1–3 However, CN faces several challenges as an active photoanodic material due to its inherent moderate light-harvesting capabilities and inadequate exciton separation properties. These intrinsic properties, combined with the difficulty in establishing intimate contact with the substrate, hinder the successful application of CN in PEC systems. Controlling the properties of a CN layer during its in–situ growth on a conductive substrate, including its directionality, morphology, surface area, and defects, is challenging because of the high temperature of the reaction and the substrate properties. Here, we report the growth of defect–rich, porous 1D CN with enhanced optical properties and photocatalytic activity by utilizing sophisticated supramolecular assemblies composed of melamine and HCl as starting precursors. The supramolecular assembly composition directs the 1D growth, and the presence of protonated amines in the precursor leads to partial condensation and defect generation. The 1D configuration, high surface area, and abundance of defects of the photoanode result in high photoelectrochemical activity for water and benzylamine oxidation. The new design of the CN photoanode leads to very low overpotential, good photocurrent density of 183 ± 8 μA cm−2 at 1.23 V vs. RHE, with an incident photon to current efficiency (IPCE) of up to 10.5% and enhanced stability (retaining ~62% activity) for 10 h. Moreover, the conversion of benzylamine into benzaldehyde and imine N–benzylideneaniline in the presence of O2 reaches 56% compared to 16% for the reference CN photoanode.
3.1-O3
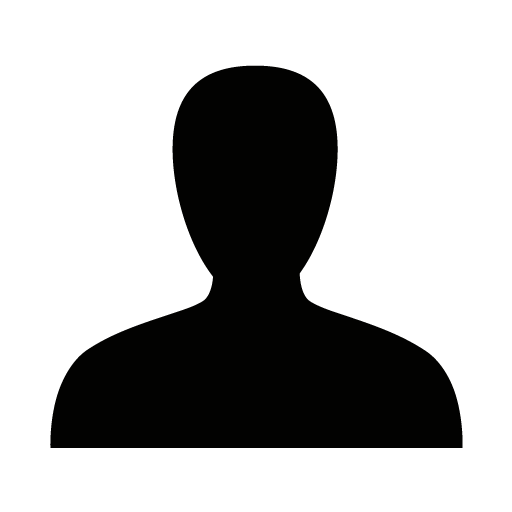
Graphitic carbon nitride (gC3N4) has been deployed in various applications, including photocatalysis. Among photocatalytic reactions studied, H2O splitting for the production for H2 is the most common one, and carbon nitride can serve as pure catalyst, cocatalyst, catalyst support, or part of a heterojunction. Photocatalytic CO2 reduction is another reaction of interest, combining utilisation of CO2 emissions and production of sustainable fuels and chemicals. Research on the use of carbon nitride for this purpose is less extensive, and almost always includes the use of dopant materials or heterojunctions. For instance, the role of boron (B) as dopant for gC3N4 has started to be explored but mostly for application in zinc batteries, photodegradation of organics, and photocatalytic H2O splitting/H2 production. Only a couple of studies have investigated the role of B-doping on CO2 photoreduction and B-gC3N4 seems superior to the pristine material, for all reactions studied. Yet, the relationship between the structure/chemistry of B-doped gC3N4 on its chemical, sorptive and optoelectronic properties, as well as CO2 photoreducing activity remains largely unknown. If understood, a greater control of and more efficient B-doped gC3N4 could be reached.
In our study, we aim to bridge this knowledge gap in (photo)chemistry of B-gC3N4. We produced two sets of B-doped gC3N4 samples through calcination of melamine mixture with varying amount of either amorphous boron, or boric acid. Once synthesised, we characterized our samples using: XPS, XRD, N2 sorption (77 K), CO2 sorption (288, 298, 308 K), DRS UV-Vis, steady-state PL, TAS and EPR. We confirmed the successful B-doping of gC3N4 using both B precursors (from 0.5 to 11 at% B). We could control better the amount of doping using boric acid, owing to its greater reactivity with melamine. Introducing B causes oxygen (O) to be also included in the structure in analogous amounts and B-O bonds. High B content results in increased BET area and enhanced CO2 adsorption. B-doping lowers the band edges, without changing the bandgap of the material. All samples show similar tri-s-triazine structure and light absorbance, however different relaxation patterns and creation of mid-gap states. The samples share similar charge carrier lifetimes and kinetics, even though B-doping up to 5 at% increases the amount of excitons. We noticed differences in the amount of unpaired electrons, which are potentially connected to the chemical structure changes caused by B integration from different precursors. Most of the samples show change in EPR signal intensity before and after irradiation, an indication of excited electrons. Our study provides for the first time a comparison between B precursors for B-doping of C3N4, and thorough investigation of their effect on the material’s sorptive and optoelectronic properties. We will next study the B-gC3N4 materials’ photocatalytic properties.
3.1-O4
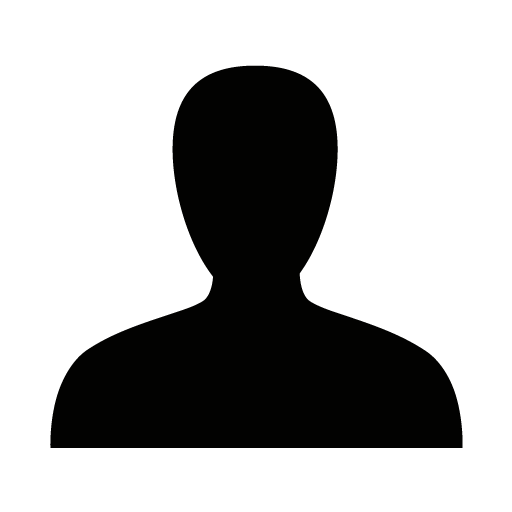
Wastewater generated in gold leaching processes, particularly in cyanidation treatments, still contains harmful amounts of cyanide (free and complexed with different heavy metals). In most cases, the cyanide content in these effluents exceeds the minimum concentration limits established for their discharge. Therefore, the highly toxic nature of these effluents requires adequate treatment prior to disposal. In this respect, a novel advanced oxidation technique was evaluated for the elimination of cyanide compounds, called here as photo-electrocatalytic-peroxone (PEPX), consisting of the coupling of two processes: photoelectrocatalysis (PEC) and electro-peroxone (EPX).
Different parameters and operating conditions were evaluated such as the electrogeneration of hydrogen peroxide, pH, anodic and cathodic overpotential, photon flux, the presence of substrates on the photocurrent and cathodic reduction of oxygen. To interpret the interfacial phenomena that were presented, electrochemical techniques such as linear and cyclic voltammetry, chronoamperometry, open-circuit chronopotentiometry, rotating disk electrode (RDE) and electrochemical impedance spectroscopy were used.
Likewise, through a response surface analysis, the effect of variables such as current density and initial substrate concentration on the free cyanide degradation capacity and on the specific energy consumption of the process was determined.
With the proposed process, 100% of the CN- ion was degraded using 0.086 mA/cm2 and 94 ppm initial concentration, with the additional degradation of CNO- (29.4%) and a specific energy consumption of 4.68 kW-h L-1. Furthermore, in the treatment of cyanide wastewater from mining activities, the complete degradation of the metal cyanide complexes (copper and iron) and the total precipitation of their metals as hydroxides and oxy-hydroxides were evident. Finally, it was evident that the PEPX process could not only degrade cyanide substrates but could also provide, through photovoltaic effects, part of the electrical energy necessary for the operation of the cell.
3.2-I1
Our group focus on physical chemistry, materials science, and the application of materials for energy production, studying the synthesis-structure-property relationship of functional materials for energy production. We emphasize developing novel syntheses for advanced materials and devices for solar energy into useful forms of sustainable energy & fuels. Our research lies at the intersection between innovative approaches, fundamental studies, and applying advanced materials for solar energy conversion.
Unique insights into the synthesis-structure-properties dependencies of α-SnWO4 photoanodes will be presented, enabled by synthesis conditions far from thermodynamic equilibrium (i.e., non-equilibrium, NE) based on plasma deposition processes combined with rapid thermal processing (RTP).
Recently, we have shown that a highly controlled NE synthesis approach based on pulsed laser deposition (PLD) combined with RTP had significant effects on the crystallinity, morphology, crystallographic orientation, and sulfite oxidation performances of α-SnWO4, culminating in photocurrents ~ 70 % higher than PLD-grown photoanodes annealed via conventional furnaces (furnace heating, FH).[1] In a follow-up study of the structural and electronic properties of α-SnWO4 films annealed via RTP and FH, we utilized X-ray diffraction texture analysis, electron backscatter diffraction in scanning electron microscopy, and atomic force microscopy modes of Kelvin probe, electrical conductivity, and tapping mode for surface morphology.
Our results show that the local (micrometer-scale) crystallographic orientation is very homogenous in the RTP-treated films, both in-plane and perpendicular to the substrate. Considering their reported higher crystallinity and the anisotropic charge transport nature of orthorhombic α-SnWO4,[1–3] the RTP-treated films demonstrate significant changes in contact potential difference (CPD) and conductivity. The CPD between α-SnWO4 and platinum-coated silicon tip was ~ 0.35 eV lower than the CPD of the FH-treated films, indicating increased band bending. This suggests that the RTP treatment strongly contributes to an increased driving force for charge injection. In addition, the local conductivity of the RTP-treated films was higher by more than two orders of magnitude than that of the FH-treated films.
Our results can lead toward broader tunable materials synthesis and design pathways and more scalable discovery and development of new chemical spaces of multinary metal-oxide photoelectrodes inaccessible through conventional solid-state reactions.[4]
3.2-O1
Wide bandgap semiconductors are well-known active materials in photo-driven processes. Howbeit, a specific class of nanocrystals (NCs) made of doped metal oxides stands out among the others for an additional degree of manipulation of the light–matter interaction.[1] Such nanomaterials can increase their charge density upon absorbing photons above the bandgap.[2] This photo-doping effect occurs primarily thanks to illumination and can induce an accumulation of electrons, as for the case of Tin doped Indium Oxide (ITO) nanoparticles.[3] The possibility to access such excess of charges can open the way for many different applications in light-based technologies like solar batteries or photo-electro-catalysis.[4] In order to promote such a phenomenon, the material must be able to delocalize the charges and neutralize those of opposite sign. In the work herein presented, we investigated the ITO NCs photo-doping with the aim of supporting and enhancing it with opportune counterparts acting as hole-scavenger.[5,6] By means of spectroscopic analysis we explored reversible charge transfer with redox mediators and demonstrated improved stability thanks to electron donating graphene quantum dots. We further studied the ITO NCs in the form of thin films and in particular the photo-electrochemical response under the influence of the light-driven charging of the ITO-based electrodes. With this paper, we give an overview over our recent achievements in the field.
3.2-O2
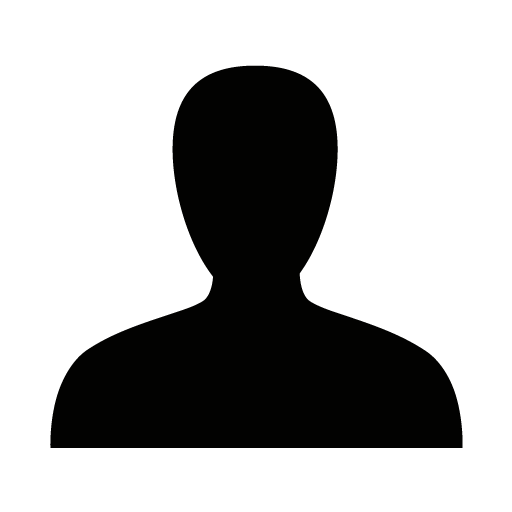
To master the energy transition, green hydrogen is heavily discussed as a supplement to fossil energy carriers such as oil and natural gas. Photocatalysis enables the direct conversion of solar power to hydrogen. In this work, the photocatalytic application of aqueous organic semiconductor dispersions is investigated for photocatalytic hydrogen generation. The nanoparticles comprise bulk-heterojunctions of donor/acceptor-materials and are synthesized by nanoprecipitation. Using colloidal particles warrants largest photoactive interfaces in the dispersion. To further maximize the photoactive interface, this work deliberately omits the use of surfactants for steric stabilization of the dispersion. Instead, for the first time, electrical dopants are used to charge the nanoparticles and thus to implement electrostatic repulsion of the nanoparticles. By attaching the co-catalyst platinum to the nanoparticle surface, the overpotential of the hydrogen evolution reaction is reduced. The result is an efficient generation of hydrogen. These aqueous dispersions exhibit great stability of more than 10 weeks on the shelf and are also stable during operation.
1.1-I1
Dr Jordi Jacas obtained his PhD in Materials at Sheffield University, working on the electrical and electrochemical characterisation of electrode materials for Li-ion batteries. During his postdoc at ISIS neutron spallation source and Stockholm University, he developed new tools to characterise batteries in-situ using neutron diffraction. Dr Jacas is currently a leading researcher for the battery section at the Catalonia Institute for Energy Research. His research aims to develop new electrode materials for next-generation batteries, including Co-free, Li-S and solid-state batteries. Since 2020, he has been the coordinator of the COBRA project (H2020-875568) devoted to fabricating generation 3b Li-ion batteries for electric vehicles.
A new cathode material, FeS2-decorated carbon nanofiber (CNF), is proposed for Li-S batteries. The structure and physicochemical properties of the material have been engineered to enhance the poor cycling stability typically displayed by sulfur composites. The composite material shows a complex architecture with a matrix of CNF hosting the sulfur and core-shell FeS2 nanoparticles acting as a catalyst for a solid phase conversion-type reaction. This cathode delivers high discharge capacities of 864, 798, 689, 595 and 455 mAhg−1 at C/10, C/5, C/2, 1C and 2C, respectively, with a stable capacity retention of 87% at 2C after 300 cycles. FeS2-decorated CNF has been characterised using several techniques, including in-situ battery measurements at the ALBA synchrotron facility and high-throughput microscopy, giving valuable insights into its charge/discharge reaction mechanism. The excellent performance obtained is combined with the use of just low-cost and abundant elements such as iron, sulfur and carbon, which makes this battery highly promising for the next generation of electrochemical energy storage devices.
1.1-I2
Lithium-sulfur (Li-S) batteries are increasingly pursued as an alternative to Li-ion batteries due to their potential for reduced environmental impact. The combination of abundant, low-cost sulfur and its high theoretical capacity positions it as a promising candidate. Typically, Li-S batteries employ a highly porous carbon-sulfur cathode with an organic electrolyte and a lithium metal anode. This setup facilitates the reversible cycling of sulfur to lithium sulfide (S/Li2S) via intermediate polysulfides, Li2Sx (where 2 < x < 8). However, several challenges hinder the practical realization of Li-S cells, including poor S/Li2S mass loadings, rapid capacity fading, low rate capabilities, and the irreversible reactions of polysulfides at the anode. These issues largely stem from an incomplete understanding of the conversion mechanism.
While the formation and dissolution mechanisms of solid Li2S are subjects of ongoing debate, numerous studies suggest that Li2S forms via direct electroreduction of Li2S2 or longer-chain polysulfides at the carbon-electrolyte interface. The observation that Li2S deposits are tens to hundreds of nanometers in size and porous indicates a solution-mediated formation process, potentially through the disproportionation of dissolved polysulfides or direct electroreduction of molecular Li2S2. Achieving a comprehensive understanding of Li2S formation mechanisms necessitates a detailed chemical and structural analysis at both atomic and nanometer scales [1], [2].
To extend the practical cycle life of Li-S batteries, integrating sulfur-infused microporous carbon with carbonate-based electrolytes [3] has shown promise. This approach, featuring the formation of a protective cathode-electrolyte interphase (CEI), helps mitigate polysulfide dissolution and reduce capacity fading. However, the mechanism, the factors limiting capacity, rate, and sulfur loadings are not fully understood.
In this context, we present an investigation aimed at deepening the understanding of solid-state sulfur conversion in classical Li-S cell and those with confined spaces. A variety of structure-sensitive and electrochemical techniques have been employed. These include electrochemical impedance spectroscopy (EIS), galvanostatic charge/discharge testing, operando X-ray diffraction, spectroscopy, electron microscopy, and small-angle scattering. Each technique has its limitations, but recent advancements in operando small and wide-angle X-ray scattering (SAXS/WAXS) and small-angle neutron scattering (SANS) have enabled simultaneous structural and chemical insights from atomic to sub-micrometer scales, with time resolutions as short as several seconds.
1.1-I3
Dr. Zhang Chaoqi graduated with a Ph.D. in Nanoscience from the University of Barcelona, Spain, and is currently an Associate Professor at the College of Materials Science and Engineering at Fuzhou University, China. His research interests are primarily in the electrochemical energy storage applications of functionalized nanomaterials. In his studies related to lithium-sulfur batteries, he has developed a range of functionalized nanomaterials to serve as cathode hosts in lithium-sulfur batteries, which have led to rapid lithium-sulfur reaction kinetics and suppression of the shuttle effect. To date, he has published over 30 academic papers in this field and has garnered more than 1,400 citations.
Two-dimensional (2D) superlattices are rising stars on the horizon of energy storage and conversion[1]. While preserving the distinctive characteristics of their 2D counterparts, they not only significantly enrich the family of 2D materials but also introduce novel functionalities such as adjustable interlayer spacing, entirely new physicochemical properties, and maximized heterojunction effects[2]. However, the intricate and low-yield synthesis procedures impede their large-scale production and application. In this context, we present a simple and high-yield solution-based approach for generating a series of 2D organic–inorganic hybrid superlattices. We demonstrate the substantial potential of these hybrid superlattices and their derivatives in the field of electrochemical energy storage, including applications in metal-sulfur batteries and metal-ion batteries.
Our synthesis primarily revolves around 2D selenide-based hybrid superlattices, exploring dozens of superlattice materials, including WSe2, MoSe2, VSe2-based superlattices. The synthesis route for these materials consistently exhibit remarkably high yields (exceeding 85%), enabling facile laboratory-scale production at the kilogram level. These superlattice materials exhibit physicochemical properties starkly distinct from those of the original selenide-based primitive units. For instance, the PVP-WSe2 superlattice allows for a continuously adjustable interlayer space ranging from 10.4 to 21 Å during annealing (compared to 6.5 Å for the original WSe2 crystal)[3]. This bears crucial significance for ion transport and volume changes during alkali metal ion charge-discharge processes. Simultaneously, the introduction of interlayer components is bound to alter the electronic structure in the bulk phase, causing not only changes in electron transport behavior but also exerting a significant tuning effect on catalytic active centers.
Overall, this work not only establishes a cost-effective strategy for producing new artificial superlattices but also pioneers their application in the field of electrochemical energy storage.
1.2-I1
High theoretical energy density and low cost make lithium-sulfur (Li-S) batteries a very promising system for next-generation energy storage. Li-S batteries (LSB) performance largely depends on the reversibility of elemental sulfur to Li2S conversion and the mitigation of the polysulfide shuttle effect. Well-designed sulfur host materials including Fe or Co single atoms embedded on N-doped carbon are proposed to tackle the LSB challenges and enhance its electrochemical performance [1]. The physiochemical characterization of these materials was performed with different accurate techniques such as XRD, XPS, BET, Raman and Mössbauer spectroscopies, HAADF-TEM and TEM. The atomic dispersion of Co and Fe was proved up to relatively large amount. The catalytic effect on the sulfur conversion reactions, using the different structures developed, could be highlighted, by measuring kinetic constants. When comparing these materials with sulfur loadings between 3 and 4 mg cm2 and electrolyte/sulfur (E/S) ratio higher than 8 ml.g-1, the performance in terms of capacity and cyclability are relatively similar. The differentiation of developed materials was only be observed, when the experimental conditions are less favorable, especially in lean electrolyte, ratio E/S equal or lower than 6 mL.g-1. In these conditions, the materials presenting a porous structure including mesopores with a specific surface at least equal to 300 m2 g-1 enable to obtain high performance, with capacity equivalent to those obtained with high E/S ratios. Moreover, the coulombic efficiency is improved by the limitation of the redox shuttle effect.
1.2-O1
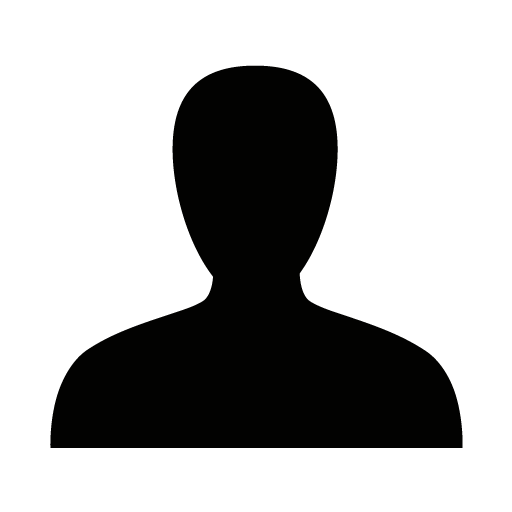
Lithium-sulfur (Li-S) batteries are a very promising technology to achieve high-energy rechargeable batteries thanks to its high specific capacity (1672 mAh g-1). Sulfur is an affordable material because of its abundance on the planet and its availability as a waste product of petroleum refinement[1]. However, the discharge mechanism of a conventional Li-S battery with liquid electrolyte based on the dissolution of lithium polysulfides in the electrolyte leads to numerous issues that impede the performance of the cells: the polysulfide shuttle effect and the corrosion of the lithium negative electrode are two major hurdles faced by Li-S technology. Most importantly, this conventional mechanism requires large volumes of electrolyte to dissolve the active material of the cathode. This results in a high electrolyte to sulfur (E/S) ratio, in which limits the energy density. To achieve a lower E/S ratio, one solution consists in decoupling the electrochemical mechanism from the amount of electrolyte needed.[2] To do so, some research teams have tuned the electrolyte or the cathode structure to prevent polysulfide dissolution and invoke a so-called “quasi-solid” (QS) reaction mechanism, which limits the dissolution of long polysulfide chains. [3] In order to obtain this QS mechanism, one can modify the nature of the electrolyte (e.g. sparingly solvating electrolytes) or the structure of the cathode (e.g. confinement of the sulfur in carbon nanotubes).[4]
Initially, we started by fabricating state-of-the-art Li-S coin cells using a standard electrolyte (1.0 M LiTFSI and 0.25 M LiNO3 in DOL:DME (v:v/1:1)). Although they were unoptimized, our batteries showed promising results (800 mAh cm-1 after 50 cycles, 2.80 ± 0.16 mgs cm-1). These batteries will serve as a foundational benchmark throughout the remainder of the thesis, providing a basis for comparison with future results obtained using QS mechanism batteries. The choice of electrolyte is crucial as it significantly influences the discharge mechanism. Therefore, we also initiated a screening of various electrolytes with limited polysulfide solubility, by measuring their ionic conductivity. Additional experiments will be conducted, such as polysulfide solubility and cycling performance.
To reduce the environmental impact of our battery cathode, we are planning to use waste rubber from used tires as a starting material.[5] Tire rubber already contains the main components of a sulfur cathode: sulfur, carbon and a polymeric binder, but in proportions that are not suitable for a battery. One objective of this work will be to increase the sulfur content in the composition of the waste rubber to make it a viable cathode for lithium-sulfur cells. The initial phase of this thesis involved a comprehensive analysis of tire samples (elemental analysis, XRD, FTIR-ATR, ICP-MS). The strategy involves employing inverse vulcanization of sulfur with rubber, aiming to impede sulfur dissolution in the process. Our most advanced results will be shown.
1.2-I2
Marc Walter received his PhD in Chemistry from ETH Zurich in 2016. From 2016 to 2021, he worked as R&D and Application Manager at Wacker Chemie, mostly focusing on silicon-based anode materials for Li-ion batteries. Since 2021 he is a Senior Manager for Technology at BASF Stationary Energy Storage pursuing the joint development of sodium-sulfur (NAS®) batteries with NGK Insulators.
Global demand for power generated from renewable sources, such as wind or solar, is growing. Stationary energy storage is one of the key technologies to ensure stable and reliable power supply despite the intermittent nature of these sources. Stationary storage helps not only to balance short but frequent fluctuations, it can also store large amounts of excess energy for many hours or days and discharge it at time of high demand. High-temperature sodium-sulfur (NAS®) batteries are well suited for such long-duration stationary storage applications.
NAS® battery cells consist of sodium as the negative electrode and sulfur as the positive one. A beta-alumina ceramic tube functions as electrolyte, which allows only sodium-ions to pass through. When discharging, sodium is oxidized and sulfur is reduced to form sodium-polysulfide (Na2SX). The charging step recovers again metallic sodium and elemental sulfur. This technology is based on abundant, non-toxic materials and provides high energy density with an optimum discharge window of 6 – 8 hours. The NAS® battery has a design life of 20 years, high capacity retention and an elaborated safety concept.
Since the first NAS® batteries have been commercialized more than 20 years ago, the total installed capacity is now approaching 5 GWh. NGK Insulators Ltd. and BASF Stationary Energy Storage cooperate to deploy NAS® batteries worldwide and to develop the next generation of batteries. This presentation will give an overview over NAS® battery technology and the features it offers for stationary energy storage.
1.2-I3
Field effect (Electric, Magnetic) enhancement of catalytic performance refers to improving the efficiency and activity of catalytic reactions through the application of external fields. The field effect can introduce an electric field or magnetic field on the surface of the catalyst to regulate the energy barrier, electron transfer rate and catalytic activity of the catalytic reaction. This method can provide an effective ways to enhance the performance of the catalyst and promote the catalytic reaction. Metal-sulfur batteries(Li, Na) have been widely studied by researchers due to their high theoretical specific capacity (1675 mA h g-1) and energy density (2600 Wh kg-1). Due to the "shuttle effect" caused by the solubility of its intermediate product polysulfide, battery failure is caused. In this context, we extend the field effect to the field of metal-sulfur batteries to reduce the polysulfide redox reaction kinetics barrier to achieve high-performance in metal-sulfur batteries.
1. Through external heat-assisted electric polarization, the traditional α-phase PVDF binder without ferroelectric effect is converted into β-phase PVDF with ferroelectric enhancement to anchor polysulfide through electrostatic interaction force while enhancing the lithium ion diffusion resistance. The structure of the PVDF film before and after polarization was analyzed through XRD, Raman and FTIR, and it was found that as polarization increased, PVDF gradually transformed from the α phase to the β phase. The modified electrode can still maintain stable capacity after 1000 cycles at 1 C, and the decay rate of specific capacity is only 0.038%.[1]
2. Apply a constant magnetic field outside the battery and use CoSx as the catalytic additive.[2] By exploring the impact of the magnetic field generated by the external magnet on the electrochemical performance of the battery, we found that the addition of the magnetic field can significantly improve the adsorption ability of polysulfides and the Li-S reaction kinetics. The CNF/CoSx/S electrode exhibits excellent electrochemical performance. In the presence of a magnetic field, even at a high current density of 2 C, CNF/CoSx/S dropped to 315 mA h g-1 after 8150 cycles, with a decay rate of only 0.0084% per cycle.
3. CoFe2O4 catalyst under an external magnetic field assists sodium-sulfur battery to realize high electrochemical performance.[3] This work details the process of growing CoFe2O4, VO2 and Co3O4 particles on the surface of CNF using a combination of electrospinning and hydrothermal processes. At the same time, this work systematically explores the process of the prepared cathode material catalyzing sodium polysulfides (SPSs) through ferromagnetic and paramagnetic catalysts under an external magnetic field. DFT calculations show that under a magnetic field, the adsorption energy of SPS on the ferromagnetic catalyst applying a magnetic field increases, and the kinetic barrier for SPS conversion decreases. Experimental results show that the spin-polarized Co ions in CoFe2O4 improve the ability to adsorb SPS and the electrocatalytic activity of SPS conversion. Experiments and theory have proven that the magnetic field can polarize the electrons of Co ions, thereby enhancing the adsorption of SPS and its catalytic conversion. The CNF/CoFe2O4/S cathode with spin polarization can last for 2700 cycles at 1 C, with a decay rate as low as 0.0039% per cycle.
In summary, these works not only establish a new catalytic relationship and strategy for the field effect in the redox reaction kinetics of polysulfides, but also provide new ideas for applications in the field of efficient electrochemical energy storage.
1.3-I1
The Nanotechnology Research Group at the Bernal Institute is led by Professor Kevin M. Ryan who holds a Personal Chair in Chemical Nanotechnology and is Course Director of the Pharmaceutical and Industrial Chemistry Degree at the Department of Chemical and Environmental Sciences (CES), University of Limerick. Previous affiliations included Marie Curie Fellowship positions at the University of California, Berkeley, USA and Merck Chemicals Southampton, UK following BSc and PhD degrees at University College Cork. The group research Interests are in Semiconductor Nanocrystals and Nanowires with emphasis on Synthesis, Assembly and Device Applications in Energy Storage and Energy Conversion Applications. The group also studies nucleation and growth in both hard (metal, semiconductor) and soft (pharmaceutical) nanocrystal materials with emphasis on size, shape and crystal phase control.
TBC TBC TBC TBC TBC TBC TBC TBC TBC TBC TBC TBC TBC TBC TBC TBC TBC TBC TBC TBC TBC TBC TBC TBC TBC TBC TBC TBC TBC TBC TBC TBC TBC TBC TBC TBC TBC TBC TBC TBC TBC TBC TBC TBC TBC TBC TBC TBC TBC TBC TBC TBC TBC TBC TBC TBC TBC TBC TBC TBC TBC TBC TBC TBC TBC TBC TBC TBC TBC TBC TBC TBC TBC TBC TBC TBC TBC TBC TBC TBC TBC TBC TBC TBC TBC TBC TBC TBC TBC TBC TBC TBC TBC TBC TBC TBC
1.3-O1
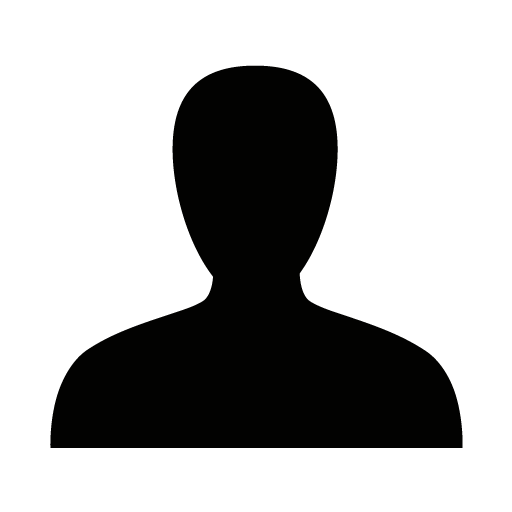
As the demand for high energy density storage increases, lithium-sulfur batteries (LSBs) are being considered as a promising solution. However, before commercialization, they must overcome several limitations, including instability of sulfur cathodes and lithium metal anodes. Considering this, silicon nanowires (SiNWs), with a high theoretical capacity and low discharge potential, represent an interesting alternative anode material capable of meeting this demand. Nonetheless, their synthesis requires a cost reduction to achieve industrial relevance. Our study introduces low-cost catalysts, tin sulfide/or oxide, that allow growing silicon nanowire directly on graphite, producing Si-rich anode composites (over 30%wt of Si). The non-uniform distribution of self-confined conductive silicon nanowires on the surface of graphite takes advantage of their matrix to effectively pad volume changes and significantly reduce the phenomenon of sputtering and capacity fading during lithium insertion and extraction, as well as maintaining the SiNWs' integrity.
Within the ERA-MIN3 project “2BoSS” (2boss.eu), we recently inquired how to improve this process towards a more sustainable material to be included in lithium-sulfur batteries. First we developed a post-synthesis treatment to remove the tin growth catalyst from the composite. Second, we turned to recycled graphite to include this material in a circular strategy in the battery sector. Third, we used porous carbon obtained from biowaste to synthesize composites with very high silicon content (over 40%wt).
We also optimized a formulation of electrolyte able both to stabilize the SEI of Si-rich anodes and the cycling of sulfur cathodes. Assembly of full cells is under way.
1.3-I2
Lithium–sulfur (Li–S) batteries stand out as promising next-generation power systems due to their high energy density, cost-effectiveness, and environmental compatibility [1]. Nevertheless, these batteries are known to suffer from several drawbacks that impede their widespread use in commercial devices, such as the low conductivity of the sulfur cathode, irreversible polysulfide formation and shuttling, and sluggish kinetics [2]. Recently, it has been demonstrated that using binary metallic Prussian Blue Analogues (PBAs) as chemical precursors for obtaining transition-metal chalcogenophosphates (MPSx) is an interesting strategy to address many of the issues that sulfur batteries face. The electronic configuration of the metals in the precursor PBA and final MPSx proves pivotal in enhancing the interaction between the cathode and sulfide species, resulting in improved kinetics and reduced shuttling, accompanied by high sulfur utilization [3]. In the talk, I will delve into the application of the newly discovered high-entropy PBAs (HE-PBAs) [4] as chemical precursors for the preparation of MPSx materials, opening innovative pathways to materials with high electronic disorder. I will underscore the significance of varying degrees of disorder, achievable through meticulous control of the chemical composition of HE-PBAs, in the production of highly stable cathodes (and even anodes) with enhanced electrical performance. Additionally, I will demonstrate how the manipulation of particle properties, such as size, can have a role in shaping the kinetics of the battery.
1.3-I1
Christophe Aucher holds a doctorate in Energy and Material Sciences from both the University of Québec at Montréal (Canada, UQAM) and the Material Institute of Nantes (France, IMN). He is developing his career in the LEITAT R&D department since 2011. LEITAT is a private Technological Centre based in Barcelona and dedicated to R&D activities in the areas of biomedicine, biotechnologies, environment, surface treatments, material science, nanotechnology and energies with deep knowledge and experience in technological transfers to several industrial sectors. Christophe is leading the Energy Storage team working on solid state, lithium sulfur, metal air and lithium recovery. His team is currently involved in National and European initiatives for electrical mobility, stationary, printed electronic and batteries recycling.
BATRAW main objective is to develop and demonstrate two innovative pilot systems for sustainable recycling and end of life management of EV batteries, domestic batteries, and battery scraps contributing to the generation of secondary streams of strategically important CRMs and battery RMs. The first pilot will deliver innovative technologies and processes for dismantling of battery packs achieving recovery of 95% of battery pack components and separating waste streams including cells and modules by semi-automated processes for recycling. BATRAW's second pilot will scale and demonstrate efficient pre-treatment and continuous hydrometallurgical recycling of battery cells and modules including innovative steps for C-graphite, Al and Cu separation from black mass and Mn extraction, achieving a recovery of the full range of battery RMs (Co, Ni, Mn, Li, C-graphite, Al and Cu) at selectivity of 90-98%. Innovations wil be scaled and demonstrated in a pilot systems with recycling capacity of 1 ton lithium-ion battery (LIB) packs dismantled per shift (8 hours) and treat 300 kg BM per day. BATRAW outcomes are of strategic importance within the prospects of the exponentially growing EU battery market and reducing EU import dependency of CRMs. The project will further promote the overall sustainability and circularity of battery products and raw materials by developing new procedures for battery repair and reuse, enabling faster diagnostics and conversion of EV packs into second life batteries, delivering eco-design guidelines for battery manufacturing, demonstrating blockchain platform for raw material tracking and supply chain transparency (Battery Passport) and delivering guidelines for safe transports and handling of battery waste. The project aims to maximize market uptake and impact through ambitious C&D&E plan including circular business models, innovations workshops, dissemination in EU platforms, policy briefs and other strategies to reach markets and stakeholders.
1.3-I2
Giulia Pezzin graduated from Politecnico di Torino, Italy, with a Master’s Degree in Environmental and Land Engineering (2022).
Currently, she is a Research Fellow at Politecnico di Torino (Turin, Italy), where she is part of the Life Cycle Assessment (LCA) research group.
Conducting a Life Cycle Assessment (LCA) is crucial for developing Li-S batteries to identify key impact phases and to prevent impact shifting. However, a significant challenge arises from the scarcity of primary data, especially about the use phase and EOL, given the current laboratory-scale status of these technologies. Anticipating improved precision in environmental impact data and the emergence of more reliable and comparable studies with the implementation of the new European Commission regulatory framework, this study presents a prospective LCA on Li-S batteries. The analysis focuses on the technology developed within the 2BOSS project, offering insights into challenges faced and lessons learned.
1.3-I3
The consumption of rechargeable batteries has exponentially grown since their first commercialization. Of interest, lithium-ion batteries (LIBs) are currently dominating the market, both for stationary and mobile applications. From one LIB to power a cellphone to a pack of six cells in a laptop or thousands in an electric vehicle (E.V.), the number of LIBs currently in use and in need for end-of-life management in the coming years is tremendous. Furthermore, considering the new regulations following commitments to allow for the energy transition, the E.V. market is expected to continue to grow, which implies a surge both in the international battery demand and their end-of-life management. As a sustainable solution, the secondary use of spent E.V. batteries as stationary application may appear as interesting and profitable. However, such a repurposing of spent E.V. batteries for a less demanding application is a short-term answer, as the end-of-life issue remains unsolved as soon as the second life of this battery reaches its end. In addition, the important increase of demand puts pressure on the stock and on the availability of the valuable elements composing the battery (e.g. Lithium, Cobalt, Nickel and Graphite). These minerals currently extracted from mines have recently been classified as “critical” by Canada. For both these reasons, the LIBs recycling became a necessity as it offers several advantages, including: (i) providing a sustainable feedstock of battery components; (ii) avoiding mining of raw limited minerals; (iii) adding value to a system (i.e. the battery pack) that was meant to be discarded; (iv) avoiding the creation of waste.
The focus here will be placed on the recovery and regeneration of the critical minerals that compose the cathode (28% wt) and the anode (22%wt) of a LIB, accounting for 50% of its total mass. LIBs are primarily powered by layered oxides materials composing the cathode (e.g. Li(Ni,Mn,Co)O2 and Li(Ni,Co,Al)O2) and graphite composing the anode. At the end of life, the LIBs are usually crushed to obtain a “black mass” from which minerals must be extracted. Nowadays, most of the spent batteries actually end their life in China, where pyrometallurgy is used (i.e. heating up the batteries to high temperature (e.g. 1000°C)) to recover cobalt, and nickel. However, lithium can not be recovered using the pyro-metallurgical process as it will be lost in the slag, as well as aluminium. Graphite is also destroyed in this process. Another technique is hydrometallurgy to recover the valuable metals in solution as well as the graphite as a solid. Moreover, lithium can also be recovered in this recycling process, by precipitation of lithium carbonate but it generates sodium sulfate Na2SO4 as an undesired by-product. The latter is costly to eliminate and as no value on the market. This issue, as well as the large acidic wastewater generated by hydrometallurgy will be tackled during this presentation. On one hand, we will highlight our patented sustainable and economical recycling process developed for the recovery of lithium from spent lithium-ion batteries using a new electrochemical technology. On the other hand, we will explain our developments for the recovery of both valuable metals and graphite through a gentle hydrometallurgy process, all towards pushing forward the concept of circular economy. A perspective regarding post-lithium battery technologies will also be given.
1.1-I1
i
II-VI semiconductor nanoplatelets have attracted much interest due to their narrow optical properties controlled by the thickness of these 2D particles. Thanks to the synthesis of heterostructures, it is possible to grow NPLs with more than one emission thus breaking the Kasha’s rule. With core/crown/crown CdSe/CdTe/CdSe NPLs composed at 95% of CdSe, a dual emission is obtained where a green emission arises from the recombination of the exciton through the band edge in 4 ML CdSe NPLs while a red emission arises from the type II band alignment between CdSe and CdTe. To improve their quantum yield a shell needs to be grown however this also leads to a red shift of their optical features. So, we propose a new heterostructure with blue-shifted dual emission which shell growth induces two emissions in a range with potential applications in displays. These NPLs can be introduced in light emitting diodes which exhibit dual NPLs as in the active materials.
1.1-O1
The direct wet-chemical synthesis of two-dimensional (2D) lead chalcogenide semiconductors yields photoluminescent materials with strong excitonic contribution at room temperature. [1,2,3] In the spirit of the recent Nobel prize for the discovery and synthesis of quantum dots (QDs) we report herein on our studies of strongly confined wet-chemically synthesized flat lead selenide (PbSe) QDs. These 2D nanocrystals possess dimensions of e.g. 6 x 5 x 0.8 nm3 and exhibit PL in the near-infrared region between 860 – 1510 nm with a PL quantum yield of up to 60 %, which is mainly determined by their lateral dimensions. [1,2] Their highly efficient photoluminescence (PL) at fiber-optics-relevant telecommunication wavelengths renders colloidal lead chalcogenide 2D semiconductors intriguing materials for future solution-processable optics.
Scanning tunnelling spectroscopy of single PbSe NCs reveals a conduction and valence band density of states that is typical for QDs rather than a steplike function linked to 2D nanoplatelets and substantiates the strong confinement in the flat PbSe QDs. These experimental observations are substantiated by theoretical calculations of the electronic band structure using the tight-binding approach.
Our results paint a comprehensive picture of the optical and electronic properties of near-infrared active 2D PbSe QDs.
1.1-O2
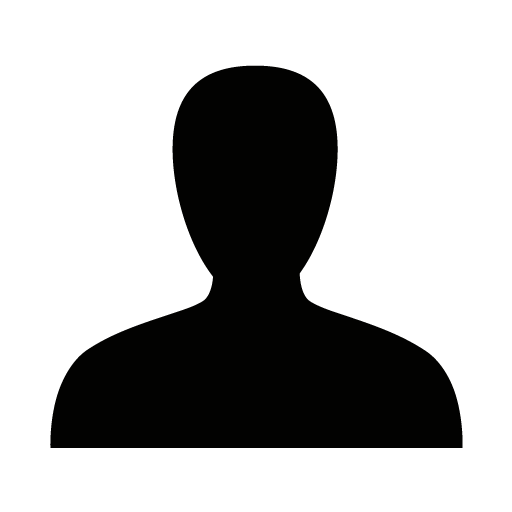
The discovery of the atomically flat two-dimensional cadmium chalcogenide nanoplatelets (NPLs) is considered to be one of the notable milestones in the field of semiconductor nanocrystals, due to their remarkable electronic and optical features and anisotropic shape. One of the important synthetic aspects of such NPLs is the control over their thickness and lateral dimensions, since they determine NPLs’ optical properties and surface area, which, in turn, are crucial parameters for photovoltaic devices. At the same time, despite great progress in new synthesis methods, the production of NPLs with a thickness larger than 5 monolayers (MLs) still remains a challenging task and thus the optical range covered by absorption and emission bands remains limited, which hinders the wider use of planar semiconductor NPLs.
In this work, we demonstrate straightforward methods to prepare six and seven ML-thick zinc-blende CdSe nanoplatelets (NPLs). The synthesized laterally small CdSe NPLs show emission maxima at 582 nm (6 ML) and 596 nm (7 ML), respectively. Moreover, our study shows that CdS crowns can be grown on both six and seven ML-thick CdSe NPLs, as evidenced by the increase of their lateral size and the appearance of new absorption features that correlate with the absorption features of CdS NPLs of the same thickness. Our novel and optimized synthesis procedures and post-synthetic crown growth demonstrate the direct fabrication of 6+ ML thick CdSe NPLs and their core/crown alternatives, allowing for further investigation of these mostly unstudied two-dimensional (2D) nanomaterials.
1.1-I2
Vanmaekelbergh's research started in the field of semiconductor electrochemistry in the 1980s; this later evolved into the electrochemical fabrication of macroporous semiconductors as the strongest light scatterers for visible light, and the study of electron transport in disordered (particulate) semiconductors. In the last decade, Vanmaekelbergh's interest shifted to the field of nanoscience: the synthesis of colloidal semiconductor quantum dots and self-assembled quantum-dot solids, the study of their opto-electronic properties with optical spectroscopy and UHV cryogenic Scanning Tunneling Microscopy and Spectroscopy, and electron transport in electrochemically-gated quantum-dot solids. Scanning tunnelling spectroscopy is also used to study the electronic states in graphene quantum dots. More recently, the focus of the research has shifted to 2-D nano structured semiconductors, e.g. honeycomb semiconductors with Dirac-type electronic bands.
Macroscopic Bi2Se3 is a layered material consisting of a high number (>10) Se/Bi/Se/Bi/Se quintuple layers, separated by van der Waals gaps. The material has been characterized as a topological quantum spin Hall insulator, with an inverted bandgap of 300 meV, and protected helical surface states. Now we consider the high-energy electron-hole excitations involving bands below - and further above theFermi-level: In bulk Bi2Se3, the absorption spectrum shows intriguing optical transitions in the 0.5 – 3.5 eV energy region: a strong optical transition at 2.8 eV located at the surface, followed by luminescence in the region of 2.3 eV, preserving chirality (1,2).
We used colloidal two-dimensional Bi2Se3 nanoplatelets as a model system to monitor the transition from bulk Bi2Se3 to two-dimensional Bi2Se3, to finally a single quintuple layer. We studied the optical transitions in the 1-3 eV region with absorption and transient absorption quenching spectroscopy (3). We observe more than 10 strong optical transitions. Comparison with DFT-GW theory allowed us to identify these transitions, group them as surface and interior transitions, and locate them in the two-dimensional Brillouin zone. In the time region below 10 ps, the electron and hole cooling and recombination dynamics are very specific for the bands involved, with in some cases signatures of electron-hole separation in momentum space by cooling.
1. Observation of chiral surface excitons in a topological insulator Bi2Se3. Proceedings of the National Academy of Sciences of the United States of America 116, 4006-4011 (2021)
2. Complex optical conductivity of Bi2Se3 thin film: Approaching two-dimensional limit Applied Physics Letters 118, DOI: 10.1063/5.0049170 (2021)
3. Identification of high-energy excitations in two-dimensional topological Bi2Se3 platelets. Jara Vliem, Ricardo Reho, Servet Ataberk Cayan*, Pieter Geiregat*, Zeila Zanolli, and D. Vanmaekelbergh
1.1-I3
Quasi-two-dimensional (2D) semiconductor nanocrystals (NCs) are captivating due to their improved optical properties, as compared to their spherical counterparts, such as extremely narrow emission line widths, suppressed Auger recombination, and giant oscillator strength. However, procedures for the direct synthesis of 2D nanoplatelets (NPLs) are not yet developed for a broad range of materials, and even well understood systems, such as CdSe, are still limited due to limited thicknesses that are readily available. At the same time, extending the optical properties into the near-infrared region (NIR) is desirable for applications in telecommunications, photovoltaics, and photodetectors, where light sources with photoluminescence (PL) inside the optical windows of glass fibers, as well as active light-absorbing and charge-transporting layers are necessary to produce high-performance components at the low cost associated with solution-processing techniques. To capitalize on the research success of the CdSe system, one can employ postsynthetic cation exchange reactions to obtain narrow band-gap materials such as lead and mercury chalcogenides, which show optical activity in the NIR. Control of the chemical composition as well as the size of the NPLs allows for broad and fine tuning of their absorption and emission spectra.
In this presentation, our recent results on the synthesis, characterisation, and application of NIR NPLs will be summarized. Two main cation exchange reactions Cd2+-to-Hg2+ and -to-Pb2+ have been employed to synthesize corresponding CdxHg1–xSe and PbSe NPLs. Already small inclusions of mercury ions into template CdSe NPLs result in a pronounced shift of their PL to longer wavelengths.[1] A more extended partial Cd2+-to-Hg2+ cation exchange yields CdxHg1–xSe NPLs emitting in the range of 700–1100 nm with quantum yields reaching 55%.[2] The resulting NPLs possess broad PL spectra due to inhomogeneous distribution of HgSe domains within CdSe cores. By optimizing the synthesis conditions, we achieved much narrower PL tunable from 1300 to 1500 nm with bandwidths down to 102 meV for CdxHg1–xSe/ZnyCd1–yS core/shell NPLs, thus, covering the first and the second telecommunication windows.[3] A further shift of the PL can be realised by complete Cd2+-to-Pb2+ cation exchange, depending on the thickness of initial CdSe NPLs.[4] Resulting PbSe NPLs exhibit extremely narrow PL spectra with bandwidths down to 80 meV with quantum yields reaching 15%. Thus synthesized NPLs were successfully applied in photodetectors [5] and efficient NIR LEDs [3].
2.1-I1
David J. Norris received his B.S. and Ph.D. degrees in Chemistry from the University of Chicago (1990) and Massachusetts Institute of Technology (1995), respectively. After an NSF postdoctoral fellowship with W. E. Moerner at the University of California, San Diego, he led a small independent research group at the NEC Research Institute in Princeton (1997). He then became an Associate Professor (2001–2006) and Professor (2006–2010) of Chemical Engineering and Materials Science at the University of Minnesota, where he also served as Director of Graduate Studies in Chemical Engineering (2004–2010). In 2010, he moved to ETH Zurich where he is currently Professor of Materials Engineering. From 2016 to 2019 he served as the Head of the Department of Mechanical and Process Engineering. He has received the Credit Suisse Award for Best Teacher at ETH, twice the Golden Owl Award for Best Teacher in his department, the Max Rössler Research Prize, an ERC Advanced Grant, and the ACS Nano Lectureship Award. He is a Fellow of the American Physical Society and the American Association for the Advancement of Science, and an editorial board member for ACS Photonics and Nano Letters. His research focuses on how materials can be engineered to create new and useful optical properties.
Quantum dots are nanometer-sized crystallites of semiconductor that have a roughly spherical shape. Due to extensive research, quantum dots are now commercially used as a robust fluorescent material in displays and lighting. However, even with our best procedures, state-of-the-art samples still contain particles with a distribution in size and shape. Because this causes variations in their optical properties, their performance for applications is reduced. This leads to a fundamental question: can we achieve a sample of semiconductor nanocrystals in which all the particles are exactly the same? In this talk we will discuss this possibility by examining two classes of nanomaterials. First, we will consider thin rectangular particles known as semiconductor nanoplatelets. Amazingly, nanoplatelet samples can be synthesized in which all crystallites have the same atomic-scale thickness (e.g., 4 monolayers). This uniformity in one dimension suggests that routes to monodisperse samples might exist. After describing the underlying growth mechanism for nanoplatelets, we will then move to a much older nanomaterial—magic-sized clusters (MSCs). Such species are believed to be molecular-scale arrangements (i.e., clusters) of semiconductor atoms with a specific (“magic”) structure with enhanced stability compared to particles slightly smaller or larger. Their existence implies that MSC samples can in principle be the same size and shape. Unfortunately, despite three decades of research, the formation mechanism of MSCs remains unclear, especially considering recent experiments that track the evolution of MSCs to sizes well beyond the “cluster” regime. Again, we will discuss the underlying growth mechanism and its implications for nanocrystal synthesis. Finally, we will present an outlook if perfect nanomaterials can be obtained.
2.1-O1
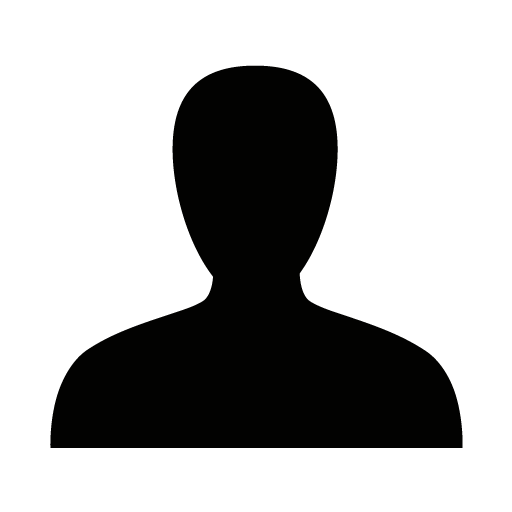
Semiconductor nanocrystals (SNCs) have become the most important material for colloidal nanophotonics – a rapidly advancing research field that evolves into a powerful technological platform for lighting, biomedicine, lasing, photovoltaics, etc. Over the years major strides in this field were made for II-VI and IV-VI SNCs, due to the availability of precursors and relative simplicity of synthetic protocols for obtaining high-quality SNCs with varying shape, tunable composition and structure, and surface properties. However recently their attractiveness was severely undercut by tightening restrictions on the use of elements comprising such crystals (e.g. Cd, Pb and Hg) due to their toxicity. This highlights the importance of alternative materials among which III-V semiconductors stand out: The nature of their composing elements is relatively benign and they exhibit improved chemical stability due to a higher covalent bond share in the materials.
Although the first syntheses of III-V colloidal NCs can be traced back to the early 90s, their development was much slower than that of their II-VI and IV-VI counterparts, and many unresolved challenges still exist. One such attractive research avenue is the realization of shape control III-V NCs, in particular the synthesis of two-dimensional (2D) nanoplatelets (NPls). The interest in such nanocrystals is mainly driven by the studies of cadmium chalcogenide NPls which showed that they exhibit much superior properties to their counterparts with other shapes, i.e., among others, narrow emission and absorbance bands, high absorption coefficients directed emission, etc. Regardless of the recent advancements in understanding the driving forces of the directed growth of cadmium chalcogenide NPls [1,2], the proposed models have limited predictive power and currently, it is impossible to straightforwardly use or adapt existing protocols to the direct synthesis of III-V NPls.
In this work, we investigate a more general indirect approach consisting in the Cu-for-In cation exchange in pre-synthesised Cu3-xP NPls. This approach benefits from the inherent structural anisotropy of Cu3-xP driving their 2D growth into NPls, which can subsequently serve as templates for InP NPls. In addition, this approach can be potentially further expanded to other indium pnictides as well as a broad range of heterostructured NPls, which are hard or impossible to obtain using direct synthesis. A few reports have already demonstrated the possibility of cation exchange in Cu3-xP, however, they mostly focused on the process itself and have not yielded InP NPls with pronounced absorbance features and noticeable emission, which was related to the excessive amounts of the residual copper atoms. [3,4] In this work, we further optimize the cation exchange conditions, such as the effect of ligands, and complexing additives to tune the balance between incoming and outgoing cations, which is important to avoid a considerable amount of vacancies and domains that act as centers for nonradiative recombination of charge carriers.
2.1-O2
Husam Alshareef is a Professor of Materials Science and Engineering at King Abdullah University of Science and Technology (KAUST). He is also the Director of the newly-established Center of Excellence in Renewable Energy and Storage Technologies at KAUST. He obtained his Ph.D. at NC State University followed by a post-doctoral Fellowship at Sandia National Laboratories, USA.
He spent over 10 years in the semiconductor industry where he implemented processes in volume production for chip manufacturing. He joined KAUST in 2009, where he initiated an active research group focusing on the development of nanomaterials for energy and electronics applications. His work has been recognized by over 25 awards including the SEMATECH Corporate Excellence Award, two Dow Sustainability Awards, the Kuwait Prize for Sustainable and Clean Technologies, and the KAUST Distinguished Teaching Award. He has published over 600 papers and 80 issued patents. He is a Fellow of several prestigious societies including the American Physical Society (APS), Institute of Electrical and Electronics Engineers (IEEE), US National Academy of Inventors (NAI), Institute of Physics (IoP), Royal Society of Chemistry (RSC), and the Institute of Materials, Minerals and Mining. He has been a Clarivate Analytics Highly-cited Researcher in Materials Science for several years.
MXenes have recently shown promising properties in a variety of device applications. In this talk, I will present recent results on using MXenes as gate materials in thin film transistors using MoS2 and GaN semiconductor channels. Gate controllability is a key factor that determines the performance of GaN high electron mobility transistors (HEMTs). However, at the traditional metal-GaN interface, the direct chemical interaction between metal and GaN can result in fixed charges and traps, which can significantly deteriorate the gate controllability. We show that Ti3C2Tx MXene films integrated into GaN HEMTs as the gate contact induce van der Waals heterojunctions between MXene films and GaN without direct chemical bonding. The GaN HEMTs with enhanced gate controllability exhibited an extremely low off-state current (IOFF) of 10−7 mA/mm, a record high ION/IOFF current ratio of ~1013 (which is six orders of magnitude higher than conventional Ni/Au contact), a high off-state drain breakdown voltage of 1085 V, and a near-ideal subthreshold swing of 61 mV/dec. However, MXenes do not fare as well when used as contacts in MoS2 transistors. The origin in this difference will be discussed in the context of the interface between MXene and GaN and MoS2 channel materials.
2.1-I2
Laurens Siebbeles (1963) is leader of the Opto-Electronic Materials Section and deputy head of the Dept. of Chemical Engineering at the Delft University of Technology in The Netherlands. His research involves studies of the motion of electrons in novel nanostructured materials that have potential applications in e.g. solar cells, light-emitting diodes and nanoelectronics. Materials of interest include organic nanostructured materials, semiconductor quantum dots, nanorods and two-dimensional materials. Studies on charge and exciton dynamics are carried out using ultrafast time-resolved laser techniques and high-energy electron pulses in combination with quantum theoretical modeling.
We studied excitons, charge carriers and many-body complexes thereof in CdSe nanoplatelets with thickness of a few atomic layers and lateral sizes of tens of nanometers. Excitons and charge carriers were generated by photoexcitation with ultrashort laser pulses and detected by time-resolved optical absorption and terahertz conductivity measurements [1].
The shape of photoluminescence and absorption spectra varies with the lateral sizes of the nanoplatelets. We attribute this to quantum-confinement effects on the center-of-mass motion of excitons in the plane of the nanoplatelets. The spectra can be reproduced by a theoretical description of excitons based on the quantum mechanical particle-in-a-box model [2].
The initial photogeneration quantum yields of free charge carriers versus excitons were found to increase with photon energy [3]. Biexcitons and trions were observed due to formation of an exciton by a probe photon near an already present exciton or charge carrier left after the pump laser pulse. Initially hot excitons and charges were found to relax to the same energy distribution in about one picosecond for different pump photon energies. We found that excitons are stable even at high densities where they start to exhibit spatial overlap. A crossover to an electron-hole plasma of uncorrelated free electrons and holes was not observed. This counter intuitive result can be understood theoretically from the fact that the Coulomb screening length, and thus the exciton binding energy, remain non-zero even at high density [4,5].
References
[1] R. Tomar et al., J. Phys. Chem. C 123, 9640 (2019).
[2] M. Failla et al., Phys. Rev. B., 102, 195405 (2020).
[3] M. Failla et al., J. Phys. Chem. C, 127, 1899 (2023).
[4] F. García Flórez et al., Phys. Rev. B 100, 245302 (2019).
[5] F. García Flórez et al., Phys. Rev. B 102, 115302 (2020).
2.2-O1
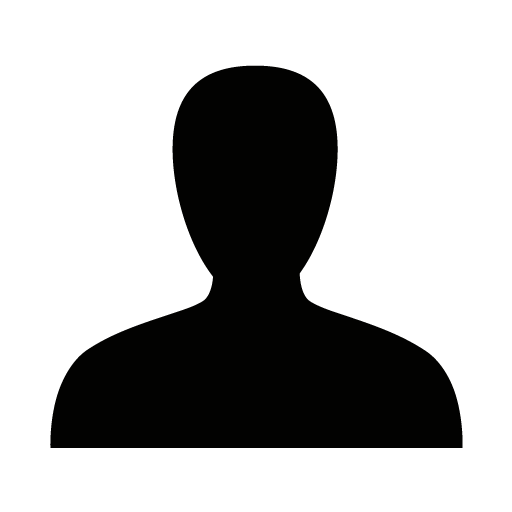
Transition metal dichalcogenides (TMDCs) such as MoS2 and WS2 are well studied van der Waals materials forming stable layers without dangling bonds.
Recently, we obtained colloidal, phase-pure mono- and few-layered semiconducting MoS2 and WS2 nanoplatelets (NPLs).[1] The wet-chemical approach allows for the formation of scalable amounts of TMDC NPLs with different lateral sizes, each with a highly monodisperse size distribution.[1,2] By confining the 2D NPLs not only in z-direction but also in the lateral dimension, the excitonic features can be shifted to higher energy, allowing a limited extend of band tuning.[1] Expanding on these findings, we show, that besides changing the morphology of the NPLs, it is possible to further tune the band structure of colloidal TMDCs via the composition of MoS2 and WS2 content in the structure. The study of the optical transitions shifting as a function of the elemental composition is supported by microscopic and macroscopic structural information from scanning transmission electron spectroscopy (STEM) and Raman spectroscopy. This wet chemical synthesis protocol is designed to be adapted also for other combinations of TMDCs. Alloying is the next step for tailoring colloidal TMDCs towards optoelectronics, catalysis and sensing.[3]
[1] Niebur, A.; Söll, A.; Haizmann, P.; Strolka, O.; Rudolph, D.; Tran, K.; Renz, F.; Frauendorf, A.; Hübner, J.; Peisert, H.; Scheele, M.; Lauth, J. Untangling the intertwined: metallic to semiconducting phase transition of colloidal MoS2 nanoplatelets and nanosheets. Nanoscale, 2023,15, 5679-5688.
[2] Frauendorf, A.; Niebur, A.; Harms, L.; Shree, S.; Urbaszek, B.; Oestreich, M.; Hübner, J.; Lauth, J. Room Temperature Micro-Photoluminescence Studies of Colloidal WS2 Nanosheets. J. Phys. Chem. C 2021, 125, 34, 18841–18848.
[3] Xie, L. Two-dimensional transition metal dichalcogenide alloys: preparation, characterization and applications. Nanoscale, 2015,7, 18392-18401.
2.2-I1
Optical studies of colloidal CdSe nanoplatelets at cryogenic temperatures (typically 1.7–4 K) and in high magnetic fields (typically above 10 T) provide valuable information about their emission properties. Importantly, magnetooptics allows for distinguishing the emission from excitons and trions (charged excitons). These particles have very different photoluminescence kinetics, additionally, their emission lifetimes are affected differently by magnetic fields and temperatures [1]. The exciton fine structure can be visualized by time-resolved polarized photoluminescence and fluorescence line narrowing, and exciton binding energy can be obtained by measuring one- and two-photon absorption [2]. The trion charge, positive or negative, can be identified by studying the photoluminescence polarization in magnetic fields [1].
Interestingly, the surface spins in CdSe nanoplatelets at cryogenic temperatures can be detected by optical techniques [3]. In applied magnetic field, the surface spins orientation results in large Zeeman splitting for the exciton interacting with these spins, similar to the known effect of the giant Zeeman splitting in diluted magnetic semiconductors, like (Cd,Mn)Se. The circular polarization degree about 50% in a magnetic field of 3 T is observed in bare CdSe nanoplatelets.
2.2-I2
Christian Klinke studied physics at the University of Karlsruhe (Germany) where he also obtained his diploma degree in the group of Thomas Schimmel. In March 2000 he joined the group of Klaus Kern at the Institute of Experimental Physics of the EPFL (Lausanne, Switzerland). Then from 2003 on he worked as Post-Doc at the IBM TJ Watson Research Center (Yorktown Heights, USA) in the group of Phaedon Avouris. In 2006 then he became member of the Horst Weller group at the Universitiy of Hamburg (Germany). In 2007 he started as assistant professor at the University of Hamburg. In 2009 he received the German Nanotech Prize (Nanowissenschaftspreis, AGeNT-D/BMBF). His research was supported by an ERC Starting Grant and a Heisenberg fellowship of the German Funding Agency DFG. Since 2017 he is an associate professor at the Swansea University and since 2019 full professor at the University of Rostock.
Dopant emission in colloidal ZnX nanoplatelets
Ultrathin, atomically flat, two-dimensional semiconductor nanocrystals receive a rapidly increasing attention due to their unique physicochemical properties. I will show that ZnS and ZnSe nanoplatelets with low toxicity exhibit sharp excitonic absorption and narrow excitonic emission. Further, I will demonstrate that direct manganese doping leads to tunable emission and photoluminescence lifetime. The initially low dopant PL quantum yield can be dramatically enhanced by passivating the surface trap states of the samples. Using time-resolved PL spectroscopy and density functional theory calculations, a connection between (magnetic) coupling and PL kinetics of Mn ions can be established. Using a descriptive mathematical model, we recognized the dominant role of the Mn2+ nonradiative relaxation channels in the energy-transfer route from the matrix absorption to the luminescence of Mn2+ ions.
We believe that the presented doping strategy and simulation methodology of the Mn-doped ZnS system is a universal platform to study dopant location- and concentration-dependent properties also in other semiconductors and might be an interesting system for catalysis.
1.1-I1
Since 2019, Yana Vaynzof holds the Chair for Emerging Electronic Technologies at the Technical University of Dresden. Prior to that (2014-2019), she was a juniorprofessor in the Department of Physics and Astronomy, Heidelberg University (Germany). She received a B.Sc degree (summa cum laude) in electrical engineering from the Technion - Israel Institute of Technology (Israel) in 2006, and a M.Sc. degree in electrical engineering from Princeton University, (USA) in 2008. She pursued a Ph.D. degree in physics under the supervision of Prof. Sir. Richard Friend at the Optoelectronics Group, Cavendish Laboratory, University of Cambridge (UK), and investigated the development of hybrid polymer solar cells and the improvement of their efficiency and stability. Upon completing her PhD in 2011, she joined the Microelectronics group at the University of Cambridge as a Postdoctoral Research Associate focusing on the research of surfaces and interfaces in organic and hybrid optoelectronics. Yana Vaynzof was the recipient of a number of fellowships and awards, including the ERC Starting Grant, Gordon Y. Wu Fellowship, Henry Kressel Fellowship, Fulbright-Cottrell Award and the Walter Kalkhof-Rose Memorial Prize.
In recent years, remarkable progress has been made in the field of perovskite solar cells, resulting in power conversion efficiencies (PCE) surpassing 26%. The vast majority of research efforts are dedicated to the processing of perovskites from solution, often from highly toxic solvents.[1] While this is manageable for lab-scale fabrication, the use of such solvents raises considerable concerns regarding the possibility to fabricate perovskite devices on the mass scale. In this talk, I will present a strategy towards the selection of more sustainable solvent alternatives for the deposition of perovskite layers. The strategy is based on the utilization of Hansen solubility parameter space for the prediction of suitable solvents and solvent-antisolvent systems.[2] I will also present alternative deposition methods which are solvent free, and can be more easily scaled up in a sustainable manner.[3]
[1] “Sustainability in Perovskite Solar Cells”, K. P. Goetz, A. D. Taylor, Y. J. Hofstetter and Y. Vaynzof,
ACS Appl. Mater. Interfaces 13, 1, 1 (2021).
[2] “Solvent–antisolvent interactions in metal halide perovskites”, J. R. Bautista-Quijano, O. Telschow, F. Paulus, Y. Vaynzof, Chem. Comm. 59 (71), 10588-10603 (2023).
[3] “The Future of Perovskite Photovoltaics – Thermal Evaporation or Solution Processing?”, Y. Vaynzof,
Adv. Energy Mater. 10, 48, 2003073 (2020).
1.1-O1
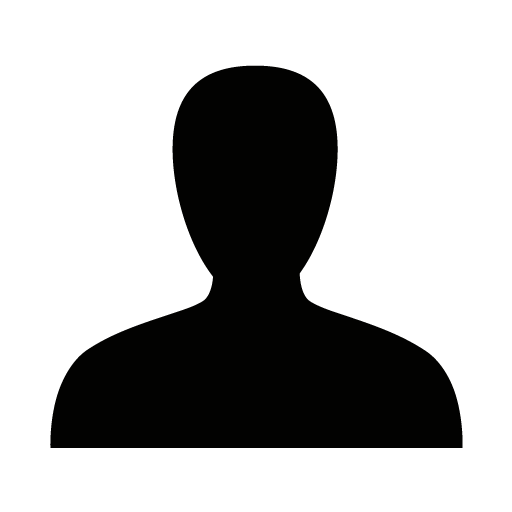
Recently, flexible Perovskite Solar Cells (f-PSC) have been a growing niche within the research on PSC with the aim of broadening its range of applications toward light-weight Building Integrated Photovoltaics (BIPV), Internet of Things (IoT), portable electronics and even space applications. [1] This is mainly related to the higher power-to-weight (PWR) ratio of flexible devices compared to glass-based counterparts. [2] However, to unlock f-PSC full potential, research must focus on boosting the sustainability of the cell, besides its performance and stability. Sustainability concerns mainly deal with the use of organic Hole Transport Materials, whose synthesis has a huge impact on the overall score of the final device, especially when polymeric HTMs are considered.
Indeed, state of the art polymeric HTMs, such as poly(triarylamine) (PTAA), are still processed with toxic solvents such as chlorobenzene (CB), dichlorobenzene (DCB) or toluene, [3] which pose an obvious barrier to the upscaling of this technology. As the literature in green-solvent-deposition charge transport layers is still scarce, we present a new set of HTMs processed using Tetrahydrofuran (THF) that is a non-aromatic, non-halogenated, cheap low environmental risk solvent and has low human health toxicity. [4] We find that the choice of green solvent processable HTM’s is a defining parameter for polymers when compared to small molecules, due to their limited solubility resulting from prioritising extended 𝜋-𝜋 conjugation frameworks, [5] yielding this study even more promising to the sustainable upscaling of f-PSCs.
Throughout this work, the four newly synthetized HTMs incorporate additional scaffolds to modify the conventional triphenylamine moiety characterizing the PTAA, with the scope of improving their solubility in THF. Aiming at this, the phenothiazine moiety was opted due to its good solubility in common organic solvents, high chemical stability, high tunability, high hole mobilities and extremely low cost. [6] The methyl substitution of the TPA phenyl unit was also altered to evaluate the trade-off effect on solubility and polymeric chain packing. As an alternative to PTZ-PTAA polymers, we also considered the benzothiadiazole unit due to its electron deficient system, [7] giving rise to the ability to tune the electronic properties of the final HTM. To the best of our knowledge, the coupling of these three scaffolds in a polymeric system has never been reported.
The novel polymers P1-4 were successfully synthesized using specially optimized protocols, adhering to Green Chemistry Principles, [8] and thoroughly characterized in terms of structural, optoelectronic, and thermal properties. All the polymers have appropriate band gaps and a HOMO energy levels aligned with the valence band of the active layer, assuring fast and efficient charge extraction. Additionally, they are thermally stable well above operating standard temperatures and could withstand high annealing temperatures.
After their characterization, P1-4 were implemented as HTMs on flexible n-i-p devices using PTAA as the reference. Results reveal that P1-4 can achieve competitive efficiencies compared to PTAA when the latter is processed with toluene, and even outperform the reference processed with THF.
This work will serve as a baseline for the pursuit of increasing environmentally friendly, solution processable materials (e.g BioRenewable sources, 2-MeTHF), paving the way toward highly efficient, stable, and sustainable f-PSCs to enter the market.
1.1-O2
After her PhD degree in Telecommunications and Microelectronics Engineering on flexible dye solar cells, awarded by University of Rome ‘Tor Vergata’ in 2014, Dr De Rossi spent nearly 4 years abroad, working as a Technology Transfer Fellow in SPECIFIC Innovation and Knowledge Centre at Swansea University (UK). She was part of the PV team led by Prof T.M. Watson, focusing on the upscaling of printable perovskite solar cells, and lead of the stability activity within his group.
She is currently a fixed term researcher (RTDa) in the group led by Prof F. Brunetti, working on smart designed, fully printed flexible perovskite solar cells and photocapacitors.
Perovskite solar cells (PSC) have experienced a dramatic improvement of power conversion efficiency (PCE) in just one decade, from ~10% to the current 26.1% [1]. The high efficiency and the device manufacturing based on low-cost solution processing, which spares high temperature and vacuum, have attracted the interest of academia and industry and substantial funding from governments and supranational institutions.Generally fabricated on rigid glass, PSC can be also made on flexible foils, achieving record PCE of 24.6% on small area and opening up to a wide range of applications, where heavy and fragile rigid glass would be less suitable: IoT sensors, retrofitting of existing buildings, i.e. building-applied PV, space. Flexible perovskite solar cells (f-PSCs) have been demonstrated on large area substrates, reaching up to 15.5% PCE on 100 cm<sup>2</sup> modules [3], with the perovskite layer only deposited via blade coating and the remaining layers by spin coating.</p> <p>To demonstrate f-PSC on large scale and make the technology appealing to industry, reliable and sustainable fabrication routes, compatible with high throughput roll-to-roll manufacture, must be developed. Thus far, most studies of f-PSCs are focusing on small-scale methods and hazardous solvents such as DMF, NMP, and 2-ME. Here, we present f-PSC with device architecture of PET/ITO/SnO 0.1 FA 0.9Pb(I 0.94 Br 0.06 ) 3 / PTAA/Au, in which both the electron transport layer and the absorber are deposited by air-flow assisted blade coating method on 5×7 cm2 flexible substrates. Notably, the perovskite layer is deposited in ambient air via a double-step method, starting from our previous work[4] and changing solvent system, using a DMF-free solvent system, i.e. DMSO only. By fine-tuning the coating parameters, we obtained promising results in terms of PCE reaching 12.7% for 2.5×2.5 cm 2 cells obtained from cutting large-area substrates. In addition, to demonstrate the scalability of this double-step perovskite deposition method, we successfully deposited films on flexible substrates up to 10×10 cm
1.1-I2
Senol Öz obtained his diploma in chemistry in 2013 at the University of Cologne
(Germany). Completing his PhD under supervision of Prof. Sanjay Mathur in 2018 at
University of Cologne (Merck KGaA PhD scholarship). In 2019 he joined Prof.
Tsutomu Miyasaka`s group as a post-doctoral fellow at Toin University of Yokohama
under a JSPS scholarship. His research interests include the synthesis, chemical
engineering, and solution processing of inorganic-organic hybrid metal halide
perovskite materials for photovoltaic application. He is currently a senior R&D project
leader at Saule Technologies and managing director of Solaveni GmbH.
Perovskite solar technology has rapidly demonstrated its commercial potential within just over ten years of intense research efforts worldwide. Its remarkable attributes, including high specific power, cost-effective production, and superior performance in low-light conditions, have significantly enhanced its value across various applications, distinguishing it from other photovoltaic technologies. Hybrid organic-inorganic perovskites merge the benefits from both realms: the ease of solution processing inherent in organic small molecules or polymeric semiconductors, as seen in organic polymer solar cells, and the exceptional physical characteristics of high-performance (poly or single)-crystalline inorganic semiconductors. This fusion creates a single material class that encompasses the advantages of both.
Sustainable, safe and industry compatible processing routes are paramount to enable large scale production of perovskite photovoltaics. Green and sustainable perovskite photovoltaics are not only enabled by the choice of processing, but sustainability starts already at the material level!
Sustainable perovskite precursor synthesis is enabled by novel and innovative green halide chemistry developed by Solaveni in Germany.[1] In this talk, the advantages of the novel synthesis routes for organic alkylammonium halides as well as metal halides (PbX2, SnX2; X= halide) are highlighted and benchmarked from an LCA perspective against the conventional production routes. The LCA analysis delves into the specific environmental impacts of these precursor materials, assessing factors such as resource consumption, energy use, and emissions, offering critical insights into their sustainability profiles and contributing to the overall understanding of the ecological footprint associated with such materials.
Furthermore, we present the latest developments in lead-free tin halide perovskite solar modules obtained via industry compatible coating techniques using Solaveni’s custom made SnI2 inks.[2]
1.2-O1
Organic-inorganic Pb halide perovskite (HaP)-based solar cells have shown great promise as a sustainable and economic way of electricity generation as its power conversion efficiencies skyrocketed to over 25% [1] within a decade of its introduction. To date, all the best-performing HaPs contain Pb and are fairly soluble in water. Pb is considered a hazardous heavy metal with major health concerns affirmed by various international organizations with recommended restricted levels of Pb in potable water (10-15 µg/L) [2]. One of the challenges of this technology is its potential environmental hazard of accidental Pb leached from broken cells/modules due to failure of encapsulation/packaging, vandalism, hail, storm, and subsequent rain. Quantifying the environmental hazard of accidental Pb2+ leaching, i.e., the reactivity and mobility of Pb2+ leached from HaP-based solar cells into the soil and from there to groundwater, was the goal of this research. Pb2+ originated from inorganic salts was previously found to remain in the upper soil layers due to adsorption. However, Pb-HaPs contain additional organic and inorganic cations, and competitive cation adsorption may affect Pb2+ retention in soils. We measured, analyzed and simulated the penetration depth profile of Pb2+ from HaPs and non-HaP sources into 3 types of agricultural soil under typical rain conditions, and its adsorption mechanism to the soil was described [2]. Most of the leached Pb2+ was found to be retained already in the first cm of the soil columns, and subsequent rain events do not induce Pb2+ penetration below the first few cm of soil surface irrespective of its sources and types of soil [3]. At conditions relevant to outdoor damage to HaP-based solar cells, it was found that the Pb2+ content retained by the topsoil is at least 100 times smaller than that of their maximum adsorption capacity. Surprisingly, organic co-cations from the dissolved HaP are found to enhance the Pb2+ adsorption capacity, compared to non-HaP-based Pb2+ sources. Our findings suggest that Pb2+ from damaged HaP-based solar cells is unlikely to contaminate groundwater, hence mass-scale utilization does not imply an environmental hazard. Panel installation over soil types with improved Pb2+ adsorption, regular inspection and removal of only the contaminated topsoil, are sufficient means to make the technology innocuous.
1.2-O2
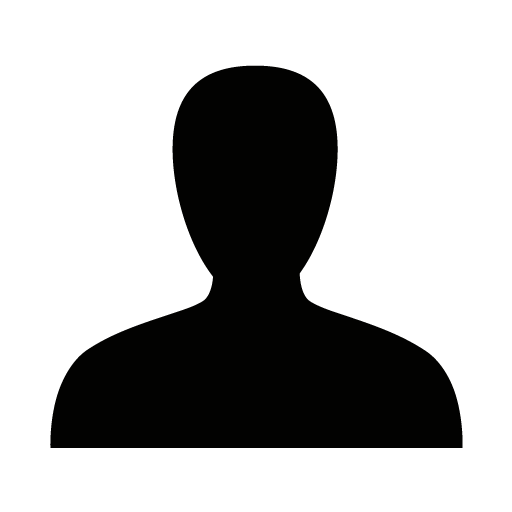
Although tin-based perovskite solar cells (PSCs) are regarded as a good replacement for lead-based PSCs due to their environmental friendliness and a suitable band gap, tin-based PSCs still have stability and efficiency issues to overcome related to their fabrication process. Specifically, it is well known that DMSO, the most popular solvent used in the fabrication of hybrid perovskites, triggers the oxidation of Sn2+ to Sn4+. Additionally, tin-based perovskite materials suffer from uncontrolled crystallization, leading to a high density of defect states and non-radiative recombination sites. Here, we explore solvent alternatives to DMSO with the aim of preventing Sn2+ oxidation and reducing the density of defect states and unnecessary non-radiative recombination sites. Moreover, we test the photovoltaic performance and stability of the devices, as compared to those prepared with a DMSO-containing solvent system and correlate it to the optoelectronic and charge carrier dynamics properties of the tin-based perovskite materials.
Keywords: tin-based PSCs, solvent engineering, DMSO-free.
1.2-O3
Tin halide perovskites are the main candidate for developing successful non-toxic alternatives to Pb-based materials, with great potential for a variety of applications. However, tin perovskites face serious challenges that originate from their intrinsic chemical characteristics. Apart from the oxidation of Sn(II) component, these materials are known to have limited processability: Their thin films are of lower quality, have a lower defect tolerance, and on top of that their fabrication conditions are strongly restricted to a few substrates and solvents. These aspects are accountable for the stagnation of the efficiency values around 15% in the particular case of tin perovskite solar cells. However, the fundamental properties determining their fast and ineffective crystallization remain essentially unexplored.
In this work, we have defined the factors influencing tin perovskite precursor solution properties the extent of their impact in crystallization. We carried out a comprehensive characterization of solutions in a wide range of solvents, concentrations and compositions, using a novel combination of solution and film characterization techniques, with particular interest on nuclear magnetic resonance and synchrotron-based small-angle X-ray scattering. This study does not only provide detailed instructions for the efficient preparation and processing of tin perovskite solutions, but also introduces critical fundamental information applicable to other metal halide perovskite materials, including other Pb-free ones.
1.2-I1
The author was graduated from Osaka University in 1978 and received Ph.D from Osaka University in 1983. He joined R&D Center in Toshiba from 1978 to 2000, during which the author was engaged in development of ULSI lithography, solar cells direct methanol fuel cells, and polysilane. He joined polysilane research in Robert West group of Wisconsin University (US) from 1988 to 1990. He was a professor of Kyushu Institute of Technology (National Institute) since 2001. From 2019, the author is a professor in The University of Electro-Communications in Japan. His research interest is printable solar cells.
Sn-based perovskite solar cells (Sn-based PVK-PV) are attracting attentions, because these bandgaps (1.2 -1.4 eV) are narrower than that of Pb-PVK-PV (wider than 1.55 eV). All-perovskite tandem cells are composed of the top cell having 1.6-1.8 eV band gap (Pb-PVK-PV) and the bottom cell having 1.1-1.3 eV band gap (Sn-based PVK-PV). 28.2 % has been reported as the highest efficiency of the tandem cell [1]. To improve the tandem solar cell efficiency, optimization of the Pb-PVK-PV for the top cell, the Sn-based PVK-PV for the bottom cell, and the interconnecting layer are needed. We have reported Pb-free Sn-PVK-PV with 14.6% efficiency [2,3] and SnPb-PVK-PV with 23.3% efficiency [4]. In this presentation, recent progresses of these two Sn-based PVK-PVs in our Lab and those of all-perovskite solar cells are reported. PEDOT-PSS is commonly employed as the hole transport layer (HTL) for the Sn-based PVK-PV. However, it has been reported that low stability is caused by the PEDOT-PSS. This prompted us to develop PEDPT-PSS-free Sn-based PVK-PV. Recently, we found that SnOx was effective for the HTL of the Sn-PVK-PV, even though SnO2 family is well-known as the electron transport layer. The basic structure is FTO glass/SnOx/Sn-PVK/C60/BCP/Ag. Efficiency over 14% and the carrier dynamics are reported. The direct Sn metal deposition on the perovskite to reduce Sn4+ concentration is included in the presentation[2,3]. As for SnPb-perovskite solar cells, the relationship between the high temperature stability and the cell structures are discussed. The stable SnPb-PVK-PV at 85 ℃ is reported. Finally, All-perovskite tandem solar cells (26-27 % efficiency) consisting of Pb-PVK PV (top cell) and PbSn-PVK PV (bottom cells) are reported [5].
1.3-I1
Metal halide perovskites constitute a very attractive class of materials for optoelectronic applications, including solar cells, light-emitting diodes, lasers, and photodetectors. Most notably, solid-state photovoltaic devices have reached a power conversion efficiency (PCE) of 26% within only a decade of academic research.
In my talk I will discuss the special case of perovskite PV technology, focusing on devices made on flexible substrates, outlining industrial opportunities of this technology, with new value propositions and market versatility. One of the exceptional features of metal halide perovskites is bandgap tunability in a broad spectral range, which enables the facile fabrication of multijunction all-perovskite PV systems. I will address the topic of all-perovskite tandems, discussing the challenges of scalable production.
Moreover, I will provide insight into physical processes occurring during solar cell operation, especially at the interfaces with charge selective contacts, where most of the performance losses take place. Importantly, these non-optimal operations have a major influence on the long-term device reliability. Systematic interfacial engineering, combined with spectroscopic characterization of recombination losses, can lead to significant improvements in performance and stability. I will demonstrate flexible perovskite solar cells successfully passing IEC-based accelerated aging tests, where device engineering was complemented with robust flexible packaging.
1.3-O1
Samyuktha earned a Master's in Mechanical Engineering from the University of Cassino, Italy, in October 2022. Currently a Ph.D. student at the University of Turin. Her research is carried out at CHOSE, University of Rome Tor Vergata, collaborating with Great cell Solar Italy. The research activity is mainly focussed on flexible carbon-based perovskite solar cells, its environmental and economic impacts. Passionate about sustainable energy, she combines academic rigor with real-world applications in shaping the future of emerging solar technology.
Perovskite solar cell (PSC) technology [1] [2] is very promising for the generation of solar energy. However, the main impediment toward the large-scale commercial utilization of PSCs lies in their sensitivity to moisture, with the metal top electrode being one of the primary sources of degradation [3]. As a result, the development of effective solutions to prevent metal-electrode-induced degradation is critical to enable the full potential of PSCs. To prevent device degradation, several methods are being used, including optimizing the hole transporting layer (HTL), which is crucial in device operation since it acts as a protective layer for the perovskite absorber layer against environmental influences such as humidity [4].[MB1] To address the problem, this work explores a low-temperature n-i-p device architecture on flexible substrate in which a hydrophobic carbon-based electrode deposited by blade coating replaces the conventional thermally evaporated gold top electrode. Carbon electrodes are considered as a greener alternative compared to gold counterparts, due to the low-cost of the starting material that can be easily processed using simple fabrication techniques. Moreover, they could be derived from natural sources or wastes enabling a circular route [5]. Additionally, the low-temperature carbon-based PSC devices show numerous advantages, such as the ability to integrate a planar hole transport layer (HTL), a better control over perovskite crystallization, and a good compatibility with flexible substrates, roll-to-roll fabrication by using scalable deposition techniques (e.g. screen printing, inkjet printing and doctor blade coating). However, the power conversion efficiency of carbon-based PSCs still lower than that of conventional gold-based counterparts due to the inefficient charge transport and collection and poor perovskite/carbon interfacial contact.
Throughout this work, a screening of the different hole transporting materials (HTM) is carried out aiming at finding the most promising candidate to improve the performance and stability of the Carbon-based PSCs. In doing this, copper(I) thiocyanate (CuSCN), was employed as HTL since it combines intrinsic hole-transport (p-type) characteristics with wide band gaps larger than 3.5 eV [6]. At the optimized concentration, and without using any dopants, a power conversion efficiency (PCE) of 8.4% was achieved on a 1 cm2 active area device. The obtained results were compared with the performance of both HTL-free devices (as internal reference) and of gold-based devices using PTAA as HTM (as state-of-art reference).
The optimization of the HTL, allowed for the demonstration of a significant improvement in the performance of the device, which could pave the way for the large-scale commercialization of PSCs with low environmental impact and promising cost-effectiveness.
1.3-I2
Perovskite Solar cells are skyrocketing in the scientific PV scenario recently exceeding a power conversion efficiency of 26% in single junction configuration and approaching 34% in tandem configuration under standard AM1.5G illumination. Despite the high efficiencies reached to date, to close the performances gap with Silicon PV, an efficient upscaling process need to be demonstrated at industrial level, whilst long-term stability (>20 years lifetime) under outdoor conditions still needs to be proven. On the other side, Perovskite technology recently attracted attention as the most suited marketable solution for low power PV, working in diffused or low-light condition (indoor) for which the theoretical PCE limit is larger than 50%. Innovative low-power applications, such as IoT, low-power electronics, sensors, domotics and various devices working indoor, have a lifetime reduced to few years, thus representing a ready solution as launching pad of this technology. The combination of sustainable and low-cost fabrication routes for high-efficient devices to power low-energy consumption devices is a key for a first stage commercialization. Here we present possible approaches to fabricate efficient and low-cost devices avoiding the utilization of expensive benchmark materials, such as organic HTMs and metallic electrodes (i.e. Ag or Au), substituted with printed Low-Temperature carbon electrode. The combination of interface engineering and the integration of commercial low-Temperature Carbon pastes will lead to efficient HTM-free large area single cells and minimodules capable to power small electronics under indoor illumination conditions. We demonstrated to achieve efficiency above 23% and 20% respectively for low-cost single cells and minimodules. In addition, the full low-temperature processes pave the way to large scale flexible perovskite PV achieving high power-per-weight innovative application and devices.
1.3-O2
If Earth’s economical systems continue to rely on linearity, by 2060 we will need at least two planets to meet the current materials demand. This renders the circular design of new technologies a crucial strategy to achieve sustainable production and consumption, as required by the 12th Sustainable Development Goal of the Agenda 2030.
Within the photovoltaic field, perovskite solar cells (PSCs) have become promising alternatives to more energy-demanding, mainstream technologies, due to their low levelized cost of electricity and carbon footprint.[1,2] With the aim of approaching circularity in the emerging photovoltaic industry, it is compelling to forsee a recycling strategy for PSCs.
In this work, we perform the recycling of all critical components of n-i-p PSCs, i.e. indium tin oxide/tin oxide (ITO/SnO2) substrate, lead iodide (PbI2) and N2,N2,N2′,N2′,N7,N7,N7′,N7′-octakis(4-methoxyphenyl)−9,9′-spirobi[9H-fluorene]−2,2′,7,7′-tetramine (SpiroOMeTAD). Our approach involves the sequential and selective dissolution of the hole-transporting material (SpiroOMeTAD) and active material (perovskite) with green solvents, such as ethyl acetate and distilled water. Then, the collected materials are purified: SpiroOMeTAD is washed and extracted with distilled water, while PbI2 is separated from the organic components of the perovskite and re-crystallized employing ethanol. Finally, the as-obtained SnO2/ITO substrates are cleaned with water, acetone and isopropyl alcohol and re-used, together with refurbished PbI2 and SpiroOMeTAD, to fabricate recycled PSCs. Since our aim is to establish a sustainable and circular method, we recover the employed solvents by distillation and we reuse them.
By analyzing the purity of recovered materials and by comparing the photovoltaic performances of PSCs fabricated with fresh and recycled materials, we demonstrate the efficacy of our recycling protocol.
2.1-I2
Photovoltaic (PV) module production in the multi-TW scale is required for cost-efficient climate change mitigation. In the coming decades, a substantial fraction of this market may be provided by perovskite solar cells (PSC) and perovskite-based tandem concepts. Although the thin-film perovskite PV concepts require minute layer thicknesses, the huge required annual PV production rates of thousands square kilometers imply material demands in the range of kilotons. This raises the questions which materials and processes chemicals are sufficiently abundant, what is the impact of the CO2e emissions, and how the accumulating waste streams can be recycled.
First, we discuss the CO2e emissions of state-of-the art silicon as well as perovskite PV technologies. We show that, although the carbon footprint of silicon PV technologies is much lower than that of fossil fuel-based energy technologies, even with continued technological learning, the CO2e emissions of the PV industry may require 4 to 11% of the remaining carbon budget to limit global warming to 1.5°C. With perovskite PV technologies, the CO2e emissions can be further reduced to the lower boundary represented by the glass substrate.
Moreover, we present a comprehensive quantitative assessment of the material demand for multi-TW scale perovskite PV production. The supply criticality is assessed by comparing inorganic and synthetic material demands with current production rates and by estimating the scalability of material production. We find that scaling perovskite PV production is feasible from a material perspective and that the it is valid to claim that PSC are “made from abundant materials”. However, a range of materials which are commonly applied in highest-efficient PSC have been identified as critical in supply: Indium and gold used in transparent and opaque electrodes and interconnection layers must be replaced. Current cesium mining is several orders of magnitudes below the required production. This finding is especially critical for the research on stable high-bandgap inorganic perovskites. Furthermore, with the exception of PEDOT:PSS, the synthesis routes of the organic hole transport materials are currently incompatible with industrial upscaling. In contrast, the industrial production of most synthetic nanoparticulate materials has sufficient maturity. The supply of organic solvents is also not critical.
Finally, the foreseeable massive material waste streams mandate that researchers adapt a design-for-recycling thinking already in early stages of technology development. Possibilities to realize this are demonstrated by an approach to remanufacture fully encapsulated perovskite solar mini-modules.
Overall, we show that besides the improvement of efficiency and stability, the perovskite PV research community should also focus on the various aspects of long-term sustainability considerations.
2.1-I3
In last decades halide perovskites have emerged as strong candidates for widespread applications in the field of optoelectronics and solar cells [1]. Due to the significant progresses obtained in photoconversion yield during last years [2], metal halide perovskite-based devices have enabled competitive technical performance compared with that of commercialized rivals. In the context of the technological lab and semi-industrial development of an emerging technology, such innovative materials might greatly benefit from environmental sustainability assessment in an eco-design perspective. The use of inorganic-based sensitizers, the consumption of organic solvents during the synthesis of perovskite, the use of critical raw materials and the energy and resources efficiencies of processes used during the manufacturing of cells and modules are some of the main issues that need to be considered to have an exhaustive evaluation of the environmental profile of these innovative devices [3]. Stemming from the research output on sustainability of halide perovskite solar cells [4-7], in this work we present the advantages and insightful perspectives that life cycle assessment can provide to support the eco-design of innovative devices.
2.1-I1
Future societies must rely on clean energy sources based on sustainable technologies, and any sustainable technology must: a) generate enough power with reduced space usage (efficiency); b) be cost-effective and c) not be detrimental to the environment or society. In addition, if the goal of that technology is generating green energy to fight climate change, d) durability is to be added to these main requirements. In the global scenario of technological implementation of renewable energies, metal halide perovskites (HaP) have the potential to fulfill these criteria. However, they require a radically new approach, diverging from the roadmap followed by previous technologies. The stability of HaP devices still trails standard silicon solar cells. Long-term stability appears to be restrained by the soft nature of HaPs.
To address the present instability issues and ensure long-term effectiveness, new approaches based on remanufacturing are imperative. Remanufacturing aims to recover value from used perovskite modules by reusing glass and other components, restoring the module to a like-new condition. Additionally, recycling lead and electrodes is crucial to avoiding environmental burdens.
Remanufacturing leverages the low cost of new photovoltaic materials, their minimal environmental impacts, and the innovative design possibilities offered by new encapsulation systems. In this context, the sustainability assessment of remanufacturing is more influenced by life cycle costs than environmental impacts. Compared with a passivated emitter rear cell (PERC) module with the same photoconversion efficiency (PCE), even after 10 remanufactures, the carbon footprint and energy payback time of HaP are lower than those of PERC. However, economic studies indicate that up to three remanufactures within a 25-year lifespan, with a 5% discount rate and an average PCE of 15%, are feasible to match the same levelized cost of electricity (LCOE) as silicon crystalline modules.
The feasibility of remanufactures is influenced by various parameters, introducing uncertainty at this early technology readiness level. Some parameters are inherent to HaP, such as PCE for large areas, the cost of delamination, and the final minimum sustainable price. However, certain economic parameters, such as the economic discount rate or the price of PERC, are external to HaP.
We find ourselves at a pivotal juncture, wherein we possess the opportunity to steer the trajectory of solar energy's future toward enhanced sustainability through the strategic application of design principles aimed at facilitating recycling. This endeavor involves drawing valuable lessons from past experiences with silicon, acknowledging its challenges in the realm of recycling.
1.1-I1
Marc T.M. Koper is Professor of Surface Chemistry and Catalysis at Leiden University, The Netherlands. He received his PhD degree (1994) from Utrecht University (The Netherlands) in the field of electrochemistry. He was an EU Marie Curie postdoctoral fellow at the University of Ulm (Germany) and a Fellow of Royal Netherlands Academy of Arts and Sciences (KNAW) at Eindhoven University of Technology, before moving to Leiden University in 2005. His main research interests are in fundamental aspects of electrocatalysis, proton-coupled electron transfer, theoretical electrochemistry, and electrochemical surface science.
The electrocatalytic hydrogen and oxygen evolution reactions are the cornerstone reactions of water electrolysis. Here, I will discuss recent advances from my group in how the electrolyte composition determines the rate of these reactions. This complex interplay between electrode and electrolyte is currently still far from being completely understood. For the hydrogen evolution reaction (HER), cations play a key role in alkaline media in promoting or inhibiting the rate-determining step of HER. For the oxygen evolution reaction (OER) on NiFe-oxyhydroxide catalysts, cations have a strong effect on the non-kinetic contribution to the OER rate, suggesting that cations influence the accessibility of the active sites inside the layered oxyhydroxide. Finally, the electrolyte composition can have a major influence on bubble dynamics at high current densities, for both reactions. If time permits, I will also include examples on the effect of different electrolyte conditions on the electrocatalytic CO2 reduction in conjuction with local pH measurements.
1.1-O1
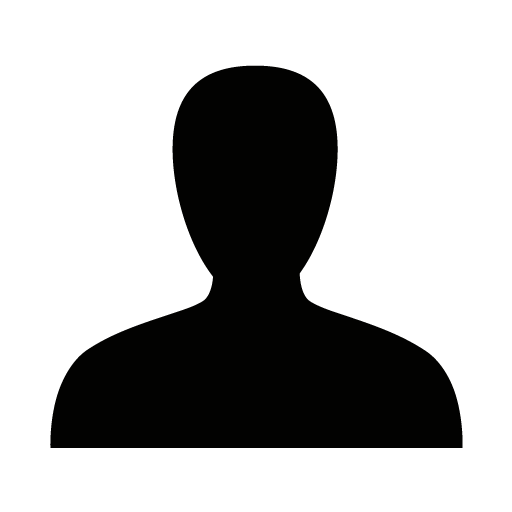
Carbon dioxide (CO2) exhibits a great potential as the raw reactant for the formation of various carbon-based compounds. Using copper (Cu) as a cathode catalyst in a CO2 electrolyser offers a unique feature of producing multi-carbon products (C2+-products) [1]. The scale up of the CO2 electrolyser technology does require the catalyst activity for these products to remain stable for a high number of operational hours. Despite of this, copper experiences structural changes and , as a result, loss of catalytic activity with time towards ethylene when exposed to a negative potential [2]. Implementing periodic oxidation phases induces formation of cuprite (Cu2O), with other works showing its benefit to the stability of CO2 electrolysis towards C2 products [3]. In this work, different criteria for the (electro)chemical oxidation of copper in a PEEK- flow cell as method of lengthening the catalytic activity are formulated. Using different ‘off’ potential values and different times spend at the open circuit potential (OCP) after a reduction period at 100 mA⋅cm-2, the process of electrochemical and chemical oxidation were studied, respectively. The results show the degree of oxidation during both chemical and electrochemical oxidation to be a determining factor for lengthening the catalyst activity towards ethylene. The latter did show an oxidation period of less than 30 seconds to be sufficient to reach similar effects if an adequate off potential is implemented, whereas chemical oxidation requires 15 minutes to demonstrate the benefits on the stabilization of ethylene selectivity with time. With the oxygen reduction reaction being the cathodic reaction during the chemical oxidation at OCP, additional supply of oxygen into the catholyte’s headspace did result in an accelerated chemical oxidation, reducing the required OCP time from 15 minutes to 5 minutes. In-situ Raman spectroscopy and ex-situ SEM supported the claims made. The gained insights were utilized to conduct a stability test, reaching 15 hours without significant selectivity loss of ethylene.
1.1-O2
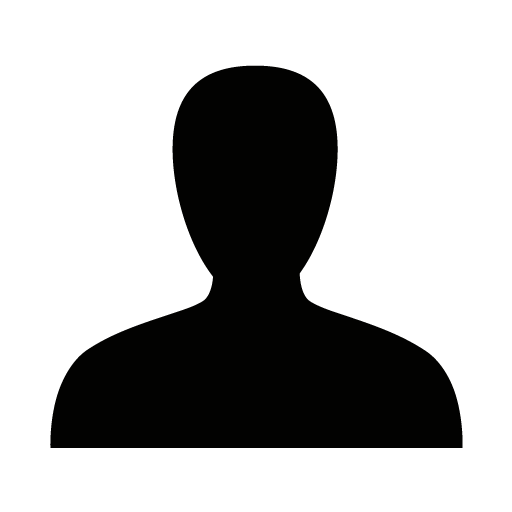
CO2 electroreduction (CO2RR) using renewable electricity is a versatile technology capable of replacing fossil resources in the flexible production of fuels and valuable chemicals. Among the various products generated, CO is notable because of its role in numerous industrial processes, including its application in syngas production along with H2.
Appropriate electrolyte selection is crucial to ensure good selectivity and efficiency in the electroreduction process. Halides have emerged as compelling candidates, offering stabilizing effects on the reaction intermediates in the CO2RR and localized pH control at the CO2-electrode interface, preventing the formation of species that could compromise reaction selectivity, electrode stability, and recyclability. [1]
Au catalysts exhibit high CO-faradaic efficiencies and selectivity at low overpotentials; however, their widespread use is hindered by their associated high cost. Thus, bimetallic AuX alloys have been proposed. Au-Cu is particularly intriguing, as Cu is a unique metal capable of generating high-value C1–C4 products (e.g., CO, alcohols, and other hydrocarbons) along with the well-known favourable Cu-halide interactions in CO2RR. [2]
Despite the apparent convergence of Au-based catalysts and halide electrolytes in CO production, few strategies have been reported for functionalizing Au-Cu alloys with halides, especially after Cu–Cl– interactions. Although studies have been conducted on halide adsorption on Au electrodes, CO2RR conditions are far from optimal for their application.
This work demonstrates high Faradaic efficiencies in C1 products, especially CO, using Au-Cu alloy electrodeposited onto a gas diffusion electrode with chloride as a catholyte in a CO2-flow cell. As a result, a positive effect of chloride on gold in the presence of copper was observed, which encourages further exploration of new electrode-electrolyte configurations that may, in some cases, enhance Cu performance in obtaining C1 products.
1.1-I2
Corina Andronescu received her B.Sc. and M.Sc. from the University Politehnica of Bucharest (Romania) in 2009 and 2011, respectively. Her Ph.D. title she received from the same university in 2014. In 2016 she joined the group of Prof. W. Schuhmann (Ruhr University Bochum, Germany) first as postdoctoral researcher and later as group leader. December 2018, she was appointed Junior Professor at the University of Duisburg-Essen, where she is currently leading the group of Electrochemical Catalysis in the Faculty of Chemistry. Her research interests include development of hybrid electrocatalysts for the CO2 electroreduction reaction, alcohol electrooxidation as well as investigation of electrocatalysts at nanoscale using Scanning Electrochemical Cell Microscopy.
The electrochemical CO2 reduction reaction (CO2RR) driven by renewable electricity sources represents an attractive approach for converting CO2 into energy-dense fuels and synthetic chemicals, promoting sustainable chemistry and carbon neutrality.1 The reaction involves the transfer of multiple protons coupled to electrons which enables the synthesis of multiple chemicals, but up to date, controlling the reaction selectivity towards multicarbon product formation still poses challenges to be overcome. Besides controlling the reaction selectivity, another challenge is associated with suppressing the hydrogen evolution reaction (HER), which occurs in the same potential window as CO2RR when aqueous-based electrolytes are used. While catalysts can potentially be used to control reaction selectivity, up to date, this was not been fully achieved in the CO2RR field, where all known CO2RR catalysts also catalyze the H2 formation.[2]
Different strategies to increase the multicarbon formation at industrially relevant current densities (> 500 mA cm-2) on Cu and Ni -based electrocatalysts will be discussed in the present communication. This includes synthesizing catalysts having different active centers or developing different catalyst layers in gas diffusion electrodes. The influence of the gas diffusion layer [3] and reactor design will also be highlighted. In addition, strategies to employ high-throughput experimentation for the discovery of new CO2RR electrocatalyst materials will be presented.
1.2-I2
Dr. Samira Siahrostami is an Associate Professor and Canada Research Chair in the Department of Chemistry at Simon Fraser University in Canada. Prior to that, she was an associate professor (2022-2023) and assistant professor (2018-2022) in the Department of Chemistry at the University of Calgary. Prior to that, she was a research engineer (2016–2018) and postdoctoral researcher (2014–2016) at Stanford University's Department of Chemical Engineering. She also worked as a postdoctoral researcher at the Technical University of Denmark from 2011 to 2013. Her work uses computational techniques such as density functional theory to model reactions at (electro)catalyst surfaces. Her goal is to develop more efficient catalysts for fuel cells, electrolyzers, and batteries by comprehending the kinetics and thermodynamics of reactions occurring at the surface of (electro)catalysts. Dr. Siahrostami has written more than 100 peer-reviewed articles with an h-index of 47 and over 13,000 citations. She has received numerous invitations to give talks at universities, conferences, and workshops around the world on various topics related to catalysis science and technology. Dr. Siahrostami is the recipient of the Environmental, Sustainability, and Energy Division Horizon Prize: John Jeyes Award from the Royal Society of Chemistry (RSC) in 2021. She received the Tom Zeigler Award and the Waterloo Institute in Nanotechnology Rising Star award in 2023. She has been named as an emerging investigator by the RSC in 2020, 2021 and 2022. Dr. Siahrostami's contribution to energy research was recognized in the most recent Virtual Issue of ACS Energy Letters as one of the Women at the forefront of energy research in 2023. She is currently the board member of the Canadian Catalysis Foundation and editor of Chemical Engineering Journal (CEJ) and APL Energy journal (AIP Publishing).
Electrocatalysis is at the heart of emerging renewable energy technologies such as fuel cells, electrolyzes, and rechargeable metal-air batteries, all of which are expected to play a significant role in transitioning to a more sustainable future. Two-dimensional materials have emerged as promising electrocatalysts with a wide range of application in electrocatalysis involving oxygen, carbon and nitrogen reactions. In this talk, I will present our recent progress on atomic scale design of two-dimensional materials for various electrocatalysis reactions. More specifically, I focus on computational catalyst design for 1) 2e- oxygen reduction reaction (ORR) for hydrogen peroxide (H2O2) synthesis1-4, and 2) CO2 reduction reaction (CO2RR)5-7. In the first part, I show various strategies that we have applied to tune the activity and selectivity of carbon-based materials for 2e-ORR to enhance the production of H2O2. The main drawback of carbon-based materials is related to their limited performance under acidic conditions which is desired for storage and transportation of H2O2. Extensive experimental work demonstrates that the majority of carbon-based materials are highly active in alkaline conditions and moderately active in neutral conditions. I discuss the computational efforts in understanding the pH effect on the activity of carbon-based structures as well as the insight we can gain from them. In the second part, I describe how we can tune carbon-based materials and two-dimensional metal organic frameworks for CO2RR as well as the insights we acquired from computational analysis.
1.2-O1
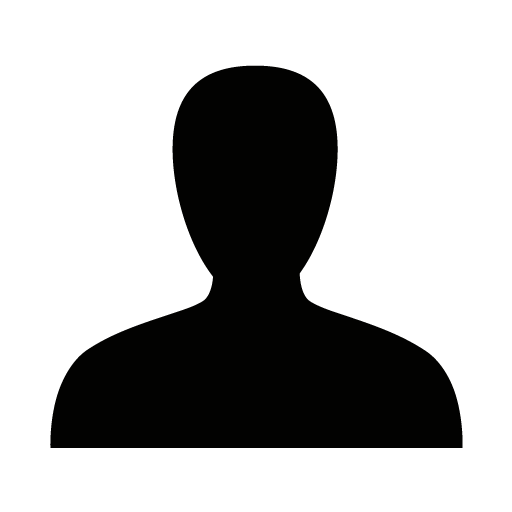
Herein we describe a novel type of hybrid material for the reduction of CO2 under high reaction rates. It consists of nanocrystalline organometallic clusters whose core comprises several covalently linked silver or copper atoms, with organic ‘ligands’ orderly distributed in the outer shell. Known as silver acetylides, these molecules involve stable alkyne-metal covalent bonds, allowing for the direct modulation of the metal clusters’ electronic properties by tailoring the chemical structure of the organic moiety. The versatility of these catalysts is further enhanced by the fact that their synthesis involves only one highly selective synthetic step from an alkyne precursor and a silver salt and can be carried out on a multigram scale without any purification step. The metal acetylides form readily from mixing aqueous and methanolic solutions of the latter precursors as highly insoluble species. Therefore, large libraries of catalysts can be synthesised from a vast selection of commercially available alkyne precursors. The catalytic properties of such entities were discovered only very recently, with only three alkynyl clusters (silver and gold) reported for the reduction of CO2 in laboratory-scale electrolysis systems. We took the field a significant leap further from reported literature studies, demonstrating the ability of alkynyl silver clusters to efficiently catalyse the CO2 reduction reaction under high reaction rates in a flow cell electrolyser. Electrolysis currents well above 200 mA/cm2, a widely accepted benchmark, were achieved with high selectivity towards CO (>95% FE) a remarkable stability
1.2-O2
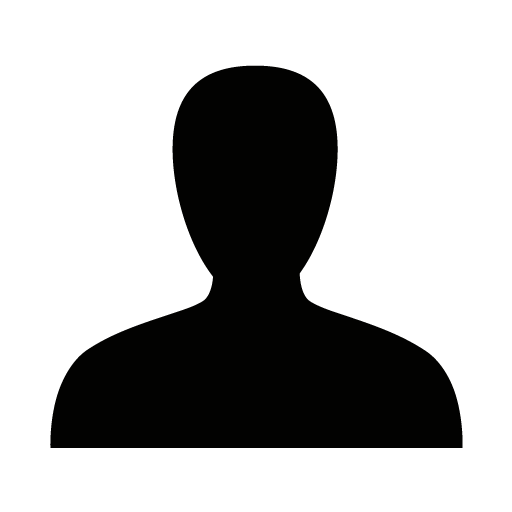
Electrocatalytic hydrogenation processes (ECH) allow the sustainable direct conversion of organic substances using electric current on a catalytically active electrode without the necessity of hydrogen gas, elevated temperatures, or pressures. In a previous project, our group developed a zero-gap electrolyzer that allowed Faraday efficiencies (FE) of 75% for the semi-hydrogenation of 2-methyl-3-butyne-2-ol (MBY) to the Vitamin E-synthon 2-methyl-3-butene-2-ol (MBE) at current densities and run times significantly surpassing those of established protocols.[1]
Herein, we illustrate the electrochemical deposition of silver, copper and nickel on different carbon carrier materials on a time scale of 5 – 15 min per electrode in an electrochemical flow cell. Depending on the applied current density, the time of deposition and the flow rate of the metal electrolyte, entirely different morphologies, sizes and crystallinities of the metal particles were obtained featuring characteristic current efficiencies in the ECH of MBY. Among other things, this makes the configuration of this work stand out against state-of-the-art systems, in which electrode preparation is mostly carried out upon application of specifically prepared catalyst inks on a carrier material e.g. by spray coating or drop-casting, which means a significant additional time and resource expense for the whole process. Since the electrodes were applied in a zero-gap flow reactor similar to the flow cell that was used for electrode preparation, the presented electrode configuration allows the preparation and use of the materials in one process step without intermediate processing or purification.
The prepared silver-plated air-stable carbon electrodes enable the ECH of MBY at 93% current efficiency at 80 mA cm-2. The concept features extraordinary resource efficiency, resistance towards errors in preparation and an outstanding performance of 76% FE and a cell voltage of 2.7 V at 240 mA cm-2 current density and a loading of 0.2 mg cm‑2, corresponding to a production rate of 1465 gMBE gcat-1 h-1. These numbers are reproducible even upon single-pass operation which is widely applied in industrial protocols. With this, it surpasses the performance of state-of-the-art electrocatalytic systems[1,2] for MBE-generation and even approaches that of the best reported thermocatalytic ones.[3]
Remarkably, the electrode concept is applicable to a range of 17 C-C‑, C-O- and N-O-unsaturated compounds among which seven could be converted with a current efficiency >45% with MBY, benzaldehyde, 2-butynol and nitromethane even allowing their hydrogenation in neat form without the addition of solvent or electrolyte salts.
This work provides a valuable contribution to the transfer of electrocatalytic hydrogenation processes onto an industrially relevant platform and addresses the room for improvement of established processes from a wide point of view by considering the whole electrode life cycle.
1.2-O3
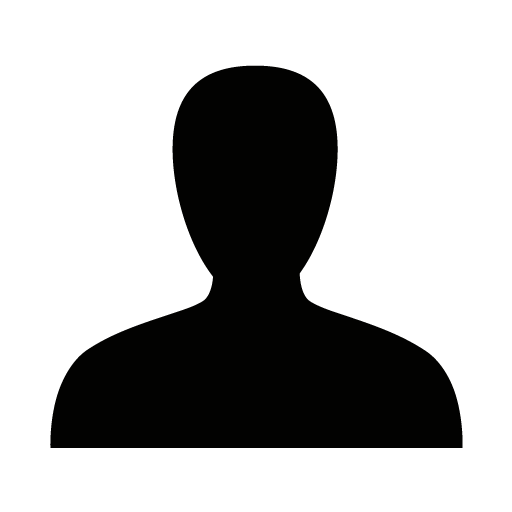
The urgent need for large-scale renewable energy storage and carbon mitigation strategies calls for efficient methods of converting carbon dioxide (CO2) into valuable hydrocarbon fuels1. Among these, methane (CH4) holds particular promise due to its high energy density and compatibility with existing infrastructure. Electrochemical CO2 reduction (CO2R) to CH4 offers a direct pathway to decarbonize natural gas, but practical applications require high current densities, selectivity, and energy efficiency [1,2].
In this study, we present a novel approach to enhance CH4 production via CO2R in aqueous bicarbonate systems [3]. Our research addresses the limitations of previous systems and introduces a paradigm shift in CO2 electroreduction. We leverage the benefits of large-pore Cu electrodes, which facilitate the transport of dissolved CO2 and promote efficient bicarbonate conversion into CO2. This architectural innovation results in high local CO2 concentrations crucial for CH4 selectivity.
Furthermore, we introduce an in-situ Cu activation strategy achieved through alternating current operation. This activation method not only generates, but also maintains a highly selective Cu catalyst surface, favoring CH4 production over hydrogen evolution. Our aqueous-fed system achieves remarkable CH4 Faradaic efficiencies, exceeding 70% across a wide current density range (100–750 mA cm-2), and maintains stability for at least 12 hours at 500 mA cm-2.
Importantly, our system also demonstrates the highest CH4 product concentration, compared to previous CO2-to-CH4 systems. These findings open new avenues for the large-scale production of CH4 via CO2R, with implications for renewable energy storage and the reduction of greenhouse gas emissions. We believe our innovative approach paves the way for practical and sustainable CH4 production from CO2, contributing to the global effort to combat climate change.
1.2-I1
In this talk, I will present some recent results from my group on CO2 and CO electroreduction on transition metals and compare them to NO electroreduction.
First, I will show that the choice of CO2 or CO as feedstocks for electrolysis ought to be made depending on the morphology of Cu electrodes, as the structural sensitivity of those two species is markedly different [1].
Moreover, I will discuss pathway bifurcation in the CO2 reduction pathway to CH4 upon hydrogenation of adsorbed CO in two different ways: from a joint experimental-computational perspective on single-crystal and polycrystalline Cu electrodes [2], and from a purely computational perspective on various transition metal electrodes [3].
Finally, I will introduce the concept of “catalytic matrix” [3, 4], a computational tool that will help me illustrate the overall similarities and differences between CO hydrogenation and NO hydrogenation on a wide number of active sites at transition metal electrodes [4, 5].
1.3-I1
Electrolyte effect in electrochemical reduction of CO2 on Cu electrode.
Amanda C. Garcia
a.c.garcia@uva.nl
This lecture delves into the core principles underlying the electrochemical reduction of carbon dioxide (CO2RR) and its interaction with the hydrogen evolution reaction (HER). Our focus centers on our group's extensive exploration of CO2RR on copper electrodes, especially in organic solvents.
Employing online gas chromatography techniques, our findings highlight the profound influence of electrode morphology and solvent composition on this reaction. Notably, in organic solvents such as acetonitrile, our in situ FTIR measurements elucidate the critical role of water content at the solid-liquid interface in shaping reaction selectivity. Moreover, we unveil that the CO2 reduction reaction impedes HER in these conditions.
Extending prior observations in aqueous solvents, our investigation reveals the decisive impact of alkyl cation length—commonly employed as an electrolyte in organic solvents—on dictating the reaction mechanism. Specifically, our research showcases that smaller cations (TEA) promote oxalic acid formation, while the largest cation (TBA) favors carbon monoxide production.
This lecture will leverage in situ spectroscopic techniques alongside DFT calculations to elucidate the intricate mechanism behind these reactions and unravel the pivotal role of the cation in determining product distribution.
1.3-I2
The influence of the reaction environment on the rate and selectivity of electrocatalytic reactions has received increasing attention in the last years. With that, many insights were gained on how to optimize electrolyte compositions. In practice, however, ideal conditions cannot always directly be applied due to solubility or conductivity limitations. This is the case for, e.g., multivalent cations which significantly improve the reactivity of H2O – a key reactant in various electrocatalytic reduction and oxidation reactions. Such species are only soluble in acidic media (at pH below the ion´s pKa of hydrolysis), which limits their applicability under reducing conditions, which are often associated with an increase in local alkalinity. Therefore, new strategies are desired to ensure the presence of these reaction-boosting multivalent cations in the double layer under (local) alkaline conditions. Here, I discuss the modification of different electrocatalysts with porous amphoteric hydroxide layers. This strategy is depicted in Fig. 1, using as example the hydrogen (HER, 2H2O + 2e– à H2 + 2OH–) and oxygen (OER, 2OH− → ½O2 + H2O + 2e−) evolution reactions taking place in alkaline media (0.1 M KOH), and Al(OH)3. The Al(OH)3 is initially insoluble, but once either HER or OER start taking place, strong alkalinization or acidification of the interface, respectively, lead to the partial dissolution of the hydroxide locally forming Al(OH)–4 or M3+ ions. Here, I present new insights on the synthesis and characterization of various of these layers and the impact they have on the rate of different reactions in which H2O participates as a H+ or OH– donor.
1.3-O1
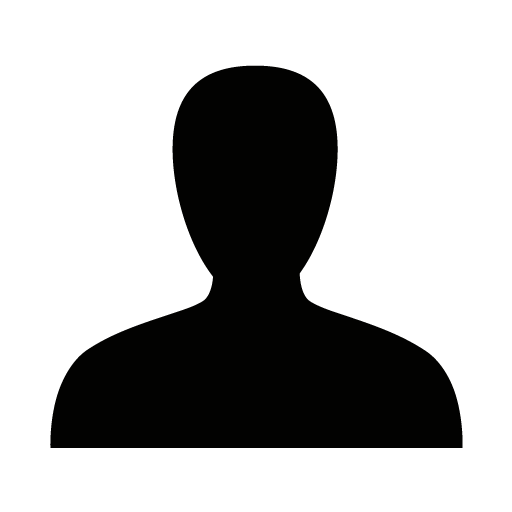
Water splitting in acidic media is a promising way for the production of hydrogen fuel. The limiting process of such electrochemical splitting in proton exchange membrane water electrolyzers (PEMWE) is the oxygen evolution reaction (OER). Among tested materials, Ir-based electrocatalysts currently achieve the best ratio between efficiency in the acidic environment. Despite many studies, some questions regarding the splitting mechanisms still remain open [1]. This means that, in addition to the more frequently used research techniques, it is also necessary to use other approaches. Raman spectroscopy and spectroelectrochemistry can give us additional information to support or disprove the current state-of-the-art regarding the Ir-based electrocatalyst performance.
In this work, we compared the performance of commercial IrO2 (i) and Ir nanoparticles (ii). Their characteristics were first studied using X-ray diffraction (XRD) and energy dispersive X-ray spectroscopy (EDS). XRD clearly revealed the presence of rutile type IrO2 in the first sample (i) and metallic Ir in the second one (ii). For the latter sample (ii), EDS showed that some surface oxidation of Ir nanoparticles occurred which was confirmed by Raman spectroscopy through the presence of the broad bands of amorphous IrO2. Rutile IrO2 is characterized by four active Raman modes, which appeared in our Raman spectra of the IrO2 sample (i) at 548 cm-1 (Eg), 719 cm-1 (overlapping B2g and A1g modes) while the low-intensity B1g mode at 145 cm-1 [2] could not be identified. Our in situ Raman spectroelectrochemical studies showed the presence of broad bands that were ascribed to different phases in rare previous works [3,4]. However, these works were performed in various electrolytes and conditions and, for electrochemically deposited Ir nanoparticles. Our aim is to identify and compare changes in in situ Raman spectra in both types of samples, in different acidic electrolytes (HClO4 and H2SO4) and in different potential ranges. According to current results, it is expected to obtain important insights into the mechanisms of water splitting.
Spectroelectrochemistry has just recently paved its way into the studies of electrocatalysts, although being a well-established technique in the investigation of electrochromic thin film materials. Extending the potential into the OER region was shown to offer important conclusions about the oxidized states of Ni-based electrocatalysts [5]. Such experiments have not yet been reported for Ir-based electrocatalysts but have already been made in our laboratory for drop-casted Ir-based electrocatalysts during cyclovoltammetric cycling.
1.3-O2
Professor Min Hyung Lee received pH.D. in chemistry from Northwestern University in 2010. He performed postdoctoral researches from 2010 to 2012 in University of California at Berkeley and Joint Center for Artificial Photosynthesis (JCAP) at Lawrence Berkeley National Lab. Since 2012, he joined as a faculty of the Department of Applied Chemistry, Kyung Hee Univeristy, Yongin, Korea. His researches have focused on developing new type of nanostructures and applying in plasmonics, solar-to-fuel conversion, energy storage, and piezoelectric and triboelectric energy harvesting devices.
Carbon materials, such as graphene and carbon nanotubes (CNTs), are frequently incorporated to develop multifunctional electrocatalysts for energy conversion reactions, such as water oxidation and reduction, and oxygen reduction reactions, etc. Many studies have reported that the enhanced catalytic performance resulting from the introduction of carbon materials is primarily attributed to their conductivity, which improves charge transfer kinetics compared to pristine electrocatalysts. In this presentation, we synthesized nanosheet-shaped Ni-based catalysts on CNTs and achieved comparable or even superior catalytic performance to those of the well-develpoed noble catalysts for the oxygen evolution reaction (OER) and oxygen reduction reaction (ORR). Through systematic analyses and DFT calculations, we discovered that the distortion of Ni active sites induced by the CNT support is the main factor contributing to improved OER and ORR performance. Utilizing this bifunctional catalyst, we also successfully achieved Zn-air batteries with high capacity and extended cyclability. The local structural distortion induced by interfacial charge transfer contributes to tunable catalytic activity that opens an avenue for design of low-cost and multifunctional catalysts and extends its applications in the fields of clean and renewable energy.
1.3-O3
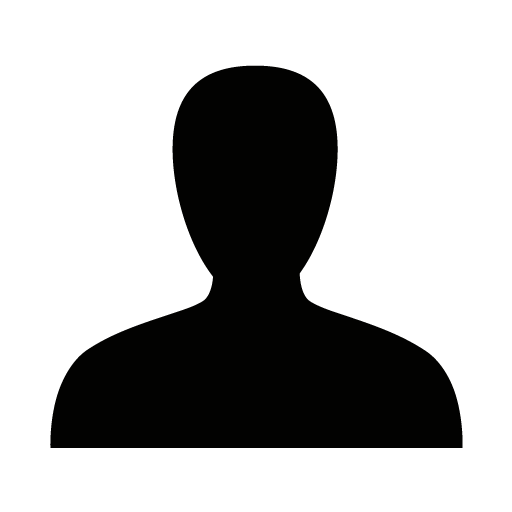
The oxygen evolution reaction (OER) represents an important bottleneck in water electrolysis systems. Currently, one important limitation of the OER is that the best catalysts for efficient (active and stable) operation in acidic media rely on Iridium (Ir) oxide – a rare, scarce metal. Alternative ruthenium (Ru) based systems have the prospect of high intrinsic activity, resulting from the optimum binding energy with the reaction intermediate [1], but suffer from limited stability compared to Ir catalysts.
Identifying the origin of stability limitations in Ru-based systems and using this information to improve the activity and stability performance is thus of interest. Strategies to do so comprise lowering the oxidation state of Ru centres and thereby lowering the dissolution chances [2-5]. To favour the OER, the active sites can be structurally and (or) electronically modified by the dopants.
In our work, we explore the role of metal dopants introduced in the Ruthenium oxide structure through a sol-gel synthesis protocol. We find, for selected metal dopants, a pre-catalyst reconstruction that favours the exposure of active facets with a different oxide network compared to Ru control samples, at increasing current densities. This leads to improved OER activity and stability metrics: the reconstructed catalysts show an overpotential of 171 mV at 10 mAcm-2 and 306 mV and 100 mAcm-2, versus a reversible hydrogen electrode. X-Ray Diffraction and Raman spectroscopy informs on the differences in the pre-catalyst and constant current traces show the effect of reconstruction on performance.
1.3-O4
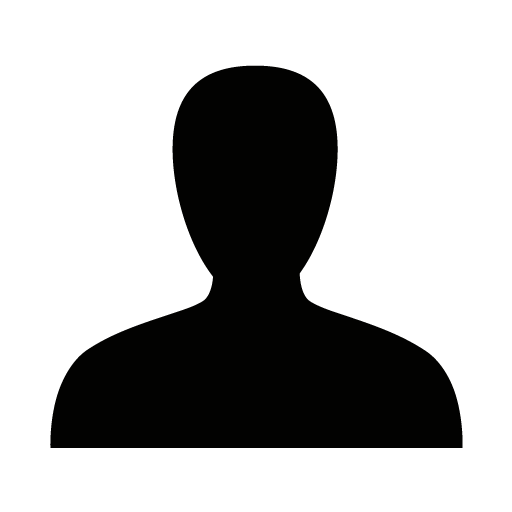
Catalytic conversion of solar energy into energy vectors is prospected to play a key role in the decarbonization of future societies. The process consists in the use of an intermittent renewable energy source to catalytically synthetize added-value molecules, which can later be used as fuels. Thus, rendering the stored renewable energy available on all occasion and available on demand. The large availability and high gravimetric energy density of hydrogen make it a promising molecule to be used as a chemical storage of renewable energy. Indeed, hydrogen can be obtained as the product of the Hydrogen Evolution Reaction (HER), taking place at the cathode of a water splitting electrochemical cell. Hydrogen production is not the only energy-relevant electrochemical cathodic process that can be exploited in the optic of carbon emissions: CO2 can also be reduced into e-fuels or added value chemicals through a series of cathodic reactions (CO2RR). At the present technological state, the main bottleneck that hinders a faster electrochemical production of some of the mentioned energy vectors is the slow kinetics of the Oxygen Evolution Reaction (OER). This reaction takes place on the anode of an electrochemical cell and provides the electrons required by HER or CO2RR.
Ni and Ni-functionalized materials have been showing good results as catalysts for OER [1,2], acting to increase the kinetics of the anodic processes. The Ni nanostructures presented in this work are produced via the organometallic approach [3]. This synthetic method consists in the decomposition of an organometallic precursor of the metal under mild reducing conditions and in the presence of a stabilizing agent (i.e., a ligand). This synthetic approach allows great control on the surface properties of the final product as well as facilitating the functionalization and the characterization of the produced catalyst (since it avoids the presence of possible contaminants). This communication will center on the synthesis, characterization, and testing of a family of nanostructured Ni catalysts for OER. The advantages provided by the unique synthetic method, yielding fast and durable systems, will be related to the catalyst morphology and surface-functionalization [4]. Moreover, some of the reported structures are expected to act as catalysts also for CO2RR [5]. If proved, this might open the way to the use of just a single catalyst in both compartments of an electrochemical cell for e-fuels production.
2.1-I1
The electrification of chemical processes for the sustainable production of fuels and chemicals has garnered substantial interest in recent years. Three particularly intriguing opportunities are green H2 production by water electrolysis, the electrochemical reduction of CO2 to carbon-based fuels and chemicals, as well as ammonia production through electrochemical N2 conversion. In all of these cases, understanding interfaces with greater molecular detail is key to advancing activity, selectivity, and durability, important metrics that need to be improved for broader-scale implementation and commercialization of these technologies. This paper will describe efforts to design and develop new methods to understand relevant interfaces in these reactions, with a focus on in-situ, operando, and/or on-line techniques. This includes attenuated total reflection Fourier transform infrared absorption spectroscopy (ATR-SEIRAS), X-ray Absorption Spectroscopy (XAS), and inductively coupled plasma mass spectrometry (ICP-MS). The focus is to understand molecular-level processes at interfaces that govern performance and durability, with examples ranging from model surfaces to applied, high-performance systems.
2.1-O1
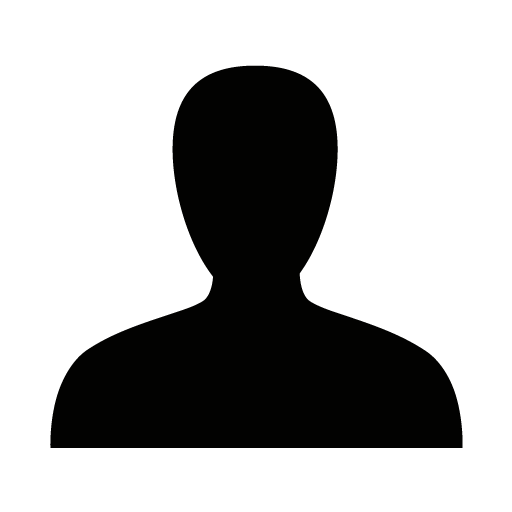
Transitioning from fossil to renewable resources is crucial for advancing clean energy conversion and fostering a sustainable chemical industry. The current dependence on fossil resources for production of carbon-based platform chemicals poses a significant challenge. Utilizing inedible biomass as a renewable carbon source is a promising alternative. Electrochemistry offers an innovative route to transform biomass into valuable chemical building blocks, leveraging green chemistry principles such as compatibility with aqueous media, generation of benign redox environments, and operability under near-ambient conditions. Despite these advantages, the electrochemical valorisation of many biomass-derived chemicals remains largely unexplored, particularly in understanding electrocatalytic interfaces for these reactions. This gap presents a significant opportunity for pioneering advances in sustainable chemical (electro)synthesis.
In this contribution, I will showcase our research on the electrocatalytic conversion of a series of biomass-derived organic acids.[1-3] Our investigation bridges fundamental aspects from model compounds to practical applications with industrial biomass streams. I will discuss the multifaceted challenges and prospects of electrocatalytic conversion of these chemicals, including revealing structure-activity relationships, and proposing strategies to enhance activity, counteract catalyst deactivation and enhance reaction selectivity. The opportunities to integrate paired biomass electrolysis for energy-efficient green hydrogen production will also be considered. Our work provides further understanding on the sustainable electrosynthesis of chemicals from renewable biomass resources, opening new avenues for new developments in this field.
2.1-O2
Chemical Engineer & Master's Degree in Chemical Engineering
Predoctoral Researcher of Catalonia Institute for Energy Research (IREC)
The global transition to sustainable energy sources and the evolution toward a hydrogen-based economy demand energy formats that enable prolonged storage and efficient transportation from remote, renewable energy-rich locations. Liquefied energy forms, notably ammonia (NH3), are gaining favor as viable alternatives, serving not only as a prominent energy carrier but also playing a crucial role as feedstock for the chemical and fertilizer industries.
In this context, highly efficient nitrate electroreduction (NO3-RR) emerges as a pivotal process for sustainable NH3 production, promising to overcome limitations associated with the current Haber-Bosch process. However, existing electrocatalysts face significant drawbacks in productivity yield, energy efficiency, and stability, especially under industrial conditions.
The NO3-RR process involves an eight-electron transfer assisted by protons, generating multiple intermediates that can diminish overall efficiency, particularly when NH3 is the desired end product. Several studies recognize the reduction of NO3- to nitrite (NO2-) as the rate-determining step (RDE), with subsequent chemical and electrochemical steps occurring after the formation of NO2-. Consequently, at low overpotentials with negligible competition from the Hydrogen Evolution Reaction (HER), the deoxygenation of adsorbed NO2- determines the overall NO3-RR, accentuating the importance of targeting this step to maximize NH3 generation. The accumulation of NO2- intermediate byproducts in the electrolyte necessitates the simultaneous acceleration of NO3-RR and NO2-RR to NH3, presenting a challenging yet promising approach for efficient ammonia generation.
This study introduces a tandem NO3-RR process, involving sequential electrochemical processes converting NO3- to NO2- and then NO2- to NH3. An employed composite electrocatalyst of Titanium Dioxide with oxygen vacancies (TiO2-x) deposited on a Copper oxide (I)-copper (Cu2O-Cu) surface, coupled with an optimized flow-cell configuration, produces compelling results toward NH3: a Faradaic Efficiency (FENH3) of 97%, selectivity (SENH3) of 80%, and a productivity yield of 0.45 mmol h-1 cm-2. The cooperative synergy of intrinsic properties of the electrode composition and cell configuration enables high Half-Cell (EENH3) and full-cell (EECELL) energy efficiencies (52% and 43%, respectively).
In summary, our tandem NO3-RR process represents a significant advancement in addressing the challenges of sustainable ammonia production, providing a promising and efficient approach for environmentally friendly energy applications.
2.1-I2
Marta Hatzell is an Associate Professor of Mechanical Engineering at Georgia Institute of Technology. Prior to starting at Georgia Tech in August of 2015, she was a Post-Doctoral researcher in the Department of Material Science and Engineering at the University of Illinois - Urbana-Campaign. During her post doc, she worked in the Braun Research group on research at the interface between colloid science and electrochemistry. She completed her PhD at Penn state University in the Logan Research Group. Her PhD explored environmental technology for energy generation and water treatment. During graduate school she was an NSF and PEO Graduate Research Fellow.
Currently her research group focuses on exploring the role photochemistry and electrochemistry may play in future sustainable systems. She is an active member of the American Chemical Society, the Electrochemical Society, ASEEP, AICHE, and ASME. Dr. Hatzell has also been awarded the NSF Early CAREER award in 2019, the Alfred P. Sloan Fellowship in Chemistry in 2020, the ONR Young Investigator Award in 2020, the ECS Toyota Young Investigator award in 2021, and the Moore Inventor Fellow in 2021.
The synthesis of ammonia for fertilizer use has the highest energy consumption (2.5 EJ / year) and carbon emissions (340 Mt CO2 eq/year) of any commodity chemical. The high environmental impact is attributed to the use of steam reforming to produce hydrogen, the production scale (200 MMtons NH3/year), and the energy intensity required to maintain reactor operating conditions (~100 bar and ~700 K). Interestingly, most of this ammonia is used for the production of carbon-nitrogen-based fertilizer (urea). The direct production of ammonia and/or urea using electrons is of growing interest to decarbonize fertilizer production. The chief challenge with these approaches remains the low catalytic selectivity. Low selectivity is attributed often to the the more facile hydrogen evolution reaction. However, during reactions with multiple reactants (co-reactants), low selectivity is also due to an increase in products that can form on the catalyst. For instance, during electrocatalytic urea synthesis, dinitrogen gas, nitrate, and carbon dioxide often serve as reactants, while ammonia, nitrite, and a range of C1+ and C2+ carbon products can be formed. Here, we aim to discuss recent progress made in understanding the role co-reactants play during the production of nitrogen-based fertilizers (e.g. ammonia and urea). We will highlight through bulk product analyses and in situ spectroscopic investigations the critical adsorbates that inhibit and augment the production of urea.
2.2-I1
I am currently an Full Professor at the University of Bonn (Germany), Department of Chemistry. My overarching motivation is to discover and implement the chemistry necessary to transition to a sustainable energy-based society. Specifically, I am developing materials to convert electrical energy to fuels and chemicals.
Energy-intensive thermochemical processes within chemical manufacturing are a major contributor to global CO2 emissions. With the increasing push for sustainability, the scientific community is striving to develop renewable energy-powered electrochemical technologies in lieu of CO2-emitting fossil-fuel-driven methods. However, to fully electrify chemical manufacturing, it is imperative to expand the scope of electrosynthetic technologies, particularly through the innovation of reactions involving nitrogen- and sulfur- based reactants as products from water/CO2 electrolysis do not cover the full scope of industrial needs.
To this end, this talk focuses on my lab’s efforts not only in CO2 conversion in the use of this molecule as a building block. This entails the co-electrolysis of CO2 with additional small molecule reactants (N2, NO3-, SO32-…) in generating products with C-N and C-S bonds like amides, urea, and sulfonates that are important as commodity and fine chemicals in the chemical industry. In particular, I will discuss several new reaction pathways discovered towards several of the above-mentioned products and the application of operando techniques to understand the key C-N/C-S coupling steps in the reaction process.
2.2-S1
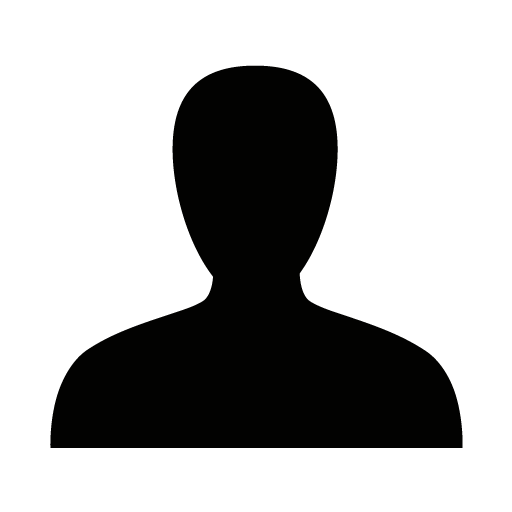
A (very) short introduction to the RSC’s portfolio of journals covering the breadth of energy, materials, nanoscience and catalysis.
2.2-O1
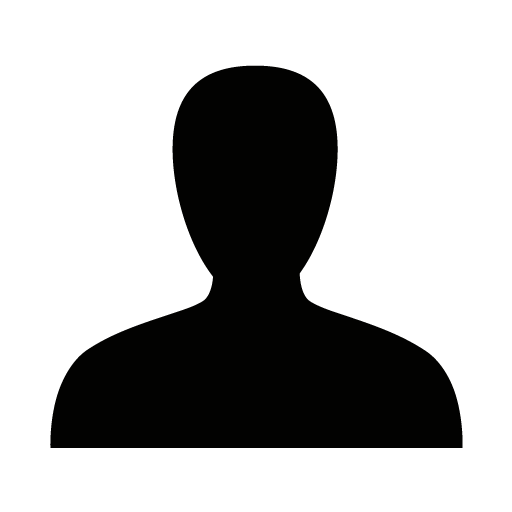
Low-temperature electrochemical CO2 reduction (ECO2R) is proven to be one of several promising strategies to valorise CO2 through its conversion into value-added products and fuels. In fact, it is the only alternative for the direct conversion of CO2 into ethylene, with Cu being the only metal exhibiting any selectivity and activity towards the conversion of CO2 to C2 products. However, developing functional materials as electrocatalysts and electrodes is imperative to achieve industrially relevant performances and efficiencies. In this sense, besides the necessary improvements in terms of decreased energy consumption at high current densities, it is important to improve the faradaic efficiencies towards desired carbon products, while hindering the competitive hydrogen evolution reaction (HER). Gas-diffusion electrodes (GDE) based on carbon paper and cloth are the standard approach used in ECO2R; however, during operation, the surface properties (e.g. wettability) of such carbon-based GDEs might change, ultimately favouring the HER and, thus, affecting the efficiency of the process. As alternative, few examples proposing the use of PTFE-based porous structures on which metallic Cu layers are used as both conductive support and catalyst[1], have demonstrated to be a promising approach to avoid the use of carbon-based electrodes. In the context of SolDAC HE project, we have developed a systematic study on the deposition conditions of sputtered Cu on PTFE structures. Therefore, we have observed that these physical features have a significant effect not only on the electrical conductivity of the electrodes, but also on the ECO2R performance towards specific products. As observed, different pressure conditions during sputtering modify the texture of the surface and induce crack formation. This variation leads to different operation cathode potentials, that can decrease up to 400 mV at 200 mA·cm-2 in the electrodes with smoother surfaces, while the product distribution is also significantly affected by the current density on electrodes with varied physical properties. Faradaic efficiencies to ethylene >70% are attained with as-prepared GDEs based on Cu-PTFE, reaching excellent performance in electrodes of geometric areas of 10 cm2, after tunning the interface by modifying the electrode surface.
2.2-O2
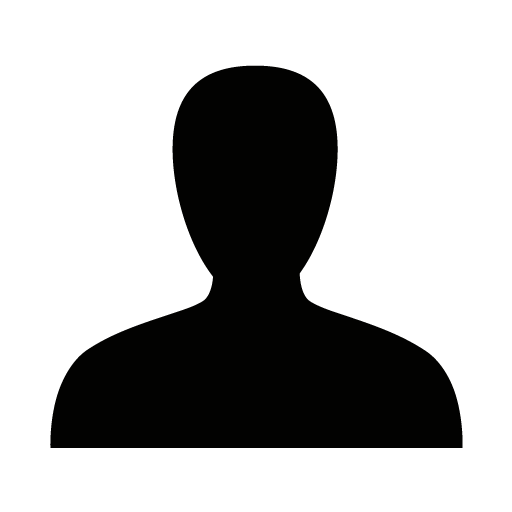
It is well known that climate change is one of the main challenges of our generation. The large CO2 emissions generated by anthropogenic activities are the main contributor to the so-called greenhouse effect. Therefore, the decarbonisation of industries and energy sources is of utmost importance. In this context, the electrochemical CO2 reduction reaction (CO2RR) is a promising way of reutilising the emitted CO2 to form value-added chemicals. Amongst the broad spectrum of species that can be obtained, CO is arguably one of the most industrially relevant products.[1] In addition, CO is a more reactive molecule than CO2 and can therefore be activated to form more reduced carbon products, such as formate or ethanol. Hence, it can be employed in a tandem-catalyst system to convert CO2 into C2+ species in a more controlled manner.[2] Regarding the selective conversion of CO2 into CO, there are only a few candidates. Au-based catalysts, and in particular Au nanoparticles (NPs), have been shown to achieve high efficiencies and current densities at low overpotentials for this reaction. Furthermore, it has been demonstrated in a number of studies how the functionalisation of the surface of these NPs with different ligands, such as amines or thiols, can enhance the activity and selectivity of Au catalysts for the CO2RR.[3] In this work, ligand-capped Au NPs have been synthesised by a novel organometallic approach using hexadecylamine (HDA) and tetradecanethiol (TDT) as stabilisers. The NPs obtained with both ligands present the same size around 1 nm and show no significant aggregation in TEM micrographs. However, electrochemical studies showed that the TDT-capped Au NPs had a poor catalytic performance in comparison with its HDA-capped analogue. Interestingly, these two systems of identical size, showed a shift in their plasmon resonance peaks as seen by in the UV-vis spectroscopy. Hence, this unusual behaviour was explored in order to get to the root of the surprising differences in their catalytic activities.
2.2-O3
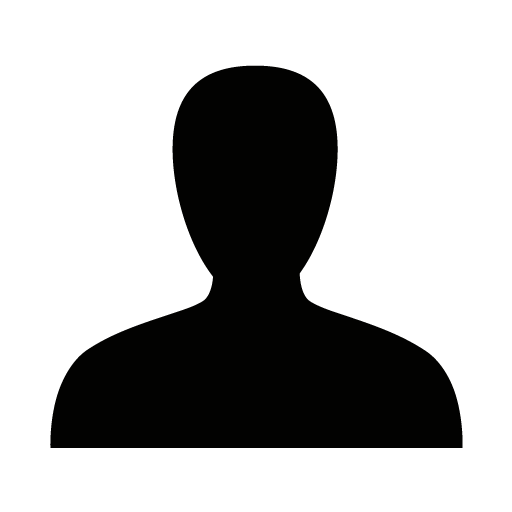
To date 80% of worldwide energy is derived from fossil fuels, which is unsustainable in the mid-long term. Solar fuels are promising candidates for replacing fossil fuels as energy sources.[1] However, its renewable and clean generation is still a great challenge. A particularly attractive solution is artificial photosynthesis, which follows the blueprint of the photosynthetic processes found in plants or phototrophic microorganisms in which carbohydrates are made from H2O and CO2 using solar energy. Various reactions are under study, including water splitting, where water is converted into hydrogen fuel and oxygen, and CO2 reduction, where CO2 and electrons are converted into carbon-based fuels (e.g.CH4, CH3OH, etc.) and/or useful chemical feedstocks (e.g. CO, ethylene, etc.). However, the practical development of these technologies is still hampered by the lack of selective, cost-efficient catalysts able to speed up the redox areactions involved, namely oxygen evolution, CO2 reduction and hydrogen evolution reactions (OER, CO2RR and HER, respectively).
Both molecular and metal/metal-oxide species are plausible candidates for efficiently catalyze these processes. If durability and the attainment of high current densities are the main challenge for molecular HER/OER/CO2RR catalysts, slow reaction rates are common when their counterparts at the nanoscale are employed. Furthermore, the reduced mechanistic knowledge and lack of well-controlled synthetic strategies to fine-tune these species prevents the rational development of more selective nanocatalysts for these transformations.[2]
This contribution deals with the work carried out in our laboratories to unravel the key factors able to change these last general trends by means of tunable surface-functionalized nanocatalysts.[3] This strategy allowed obtaining stable and efficient OER (photo)catalysts based on 1st-row transition metal oxide species,[4],[5] fast and rugged Ru-based (photo)cathodes[6],[7],[8],[9] superior to state-of-the-art Pt/C for HER, and selective photocatalyst for the CO2RR.[10]
2.2-I2
Ifan is a Professor in Electrochemistry at the Department of Materials at Imperial College: he leads the Interfacial Electrochemistry Group there and is also Atoms to Devices Research Area Lead at the Henry Royce Institute.
Ifan joined Imperial College in July 2017. Prior to Imperial, he was at the Department of Physics at the Technical University of Denmark (DTU); he was first employed as a postdoctoral researcher, then as assistant professor and finally as associate professor and leader of the Electrocatalysis Group there. In 2015, Massachusetts Institute of Technology (MIT) appointed Ifan as the Peabody Visiting Associate Professor. He taught and conducted research at the Department of Mechanical Engineering at MIT for a whole semester.
Ifan’s research aims to enable the large-scale electrochemical conversion of renewable energy to fuels and valuable chemicals and vice versa. Such processes will be critical in order to allow the increased uptake of renewable energy. His focus is on the catalyst at the electrode, i.e. the electrocatalyst. It turns out that the electrocatalyst material defines the efficiency of several important electrochemical processes, including:(i) electrolysis for the storage of renewable electricity — which is inherently intermittent — in the form of fuels, such as hydrogen or alcohols.(ii) fuel cells as a potentially zero emission source of power for automotive vehicles. (iii) the green synthesis of valuable chemicals, such as ammonia and H2O2. (iv) batteries, which tend to degrade by gas evolution at the electrode-electrolyte interface. Hence the reactions that need to be accelerated in electrolysers and fuel cells — such as CO2, CO, O2 and H2 evolution — are precisely those that need to be inhibited in batteries.
Ifan has discovered or co-discovered several new catalysts for the oxygen reduction reaction, which exhibited significant improvements in performance over the prior state-of-the-art. In particular, his research on hydrogen peroxide production led to the establishment of the spinout company, HP Now.
Ifan is the recipient of RSC's Geoffrey Barker Medal (2024), the RSC's John Jeyes Award (2021). He also currently holds an European Resarch Council Consolidator Grant (2021-2025). Since 2022, he has been a Clarivate Highly Cited Researcher.
There is a burgeoning interest in the development of a green method of ammonia synthesis; ammonia, already critical for fertilisers in the agricultural industry, is also being touted as a possible future energy vector or carbon-free fuel. The current method of production - the Haber Bosch process - is environmentally damaging and energy intensive but to date no viable alternative has been demonstrated. An electrochemical method operating under ambient conditions would be particularly attractive, as it would enable ammonia to be produced on a decentralised basis on-site and on-demand.
Thus far, amongst solid electrodes, only lithium based electrodes in organic electrolytes can unequivocally reduce nitrogen to ammonia.Even so, at present, the lithium based system is too inefficient for practical uses; moreover, it is highly unstable.
To investigate this reaction, we use a combination of electrochemical experiments, cryo-microscopy, infrared spectroscopy, electrochemistry mass spectrometry, time-of-flight secondary ion mass spectrometry, X-ray photoelectron spectroscopy and density functional theory. By drawing from the adjacent fields of enzymatic nitrogen reduction and battery science, I will aim to build a holistic picture of the factors controlling nitrogen reduction.
In the current contribution, I will explore (a) the underlying reasons why lithium can reduce nitrogen to ammonia and (b) how to optimise lithium mediated nitrogen reduction and (c) propose new avenues towards going beyond lithium in electrochemical nitrogen fixation.
2.3-I1
Water electrolysis and carbon dioxide electroreduction are examples of electrochemical technologies with promise to aid in the energy transition, for example for the seasonal storage of renewable electricity, or carbon sequestration, thereby aiding in the abatement of anthropogenic climate change. Such reactions are often even more difficult to characterize “at work” with spectroscopy than traditional thermo- or heterogeneous catalysis[1-3], as they bring at least one additional complicating variable; the electrolyte which, particularly for reactions in aqueous environments dramatically attenuates the electromagnetic radiation with which we set out to probe the reaction. Ideally, one would be able to speciate the kinetic regime where e.g., charge-transfer, and adsorption/desorption reactions happen, and be able to separate (speciate) these events from for example those which relate to capacitance, e.g., charging and discharging of the electric double layer, and mass transfer phenomena all happening on a different time scale. To fully understand the morphology of the electrode, the effect of local pH gradient, and the resistance of the electrolyte, one should develop a method able to combine the study of both the electrode, and the electrolyte and reactants preferably simultaneously. Through the development of a methodology that includes bespoke reactor and setup design, along with potential-modulated excitation experimentation, operando high time resolution FT-IR and operando sub-second time resolution X-ray absorption spectroscopy (quick-XAS), and supervised regression, we are able to speciate signals from the diffusion, and Helmholtz layers, to the catalyst surface, and reaction intermediates of electrocatalytic reactions in aqueous media. We thereby establish, for example, structure-performance relationships of electrocatalytic oxidation and reduction reactions (e.g., ammonia, and urea oxidation, oxygen evolution reaction and CO2 reduction) over several different metals and electrode geometries.
This supervised-pulsed-speciation operando spectroscopic approach is a powerful tool to overcome some of the common issues dealt with in operando spectroscopy of electrocatalysis, such as weak reaction intermediate signals which are clouded by electrolyte rearrangement in for example double layer formation, thereby elucidating several details of mechanisms not yet described in literature, such as the contribution of ice-like water layers, and nanoparticle geometry-dependent adsorption events.
2.3-I2
A promising approach to store energy is the use of excess electricity to drive the production of fuels and chemicals in electrochemical reactors. However, the lack of understanding of the relation between transport phenomena (e.g. mass, heat, charge), intrinsic kinetics and electrocatalytic performance is currently limiting the scale-up of electrocatalytic technologies particularly for transformations where transport phenomena play a role in determining product selectivity and production rate [1],[2].
In this presentation, I will discuss our novel approach of combining reactors design, multi-scale modeling and dimensionless analysis to understand and decouple mass transfer effects and intrinsic electrode kinetics particularly for transformations that involve dissolved gas substrates such as CO2 and diluted protons in near neutral pH.
In this talk, the development of a multi-scale first-principles reaction-transport model is presented for the electrochemical reduction of CO2 to fuels and chemicals on polycrystalline copper electrodes. The model utilizes a continuous stirred-tank reactor (CSTR)-volume approximation that captures the relative timescales for mesoscale stochastic processes at the electrode/electrolyte interface that determine product selectivity. The model is built starting from a large experimental dataset obtained under a broad range of well-defined transport regimes in a gastight rotating cylinder electrode cell[1]. Product distributions under different conditions of transport, applied potential, bulk electrolyte concentration, temperature, and catalyst porosity are rationalized by introducing dimensionless numbers that reduce complexity and capture relative timescales for mesoscopic and microscopic dynamics of electrocatalytic reactions on copper electrodes. This work demonstrates that one CO2 reduction mechanism can explain differences in selectivity reported for copper-based electrocatalysts when mass transport, concentration polarization effects, and primary and secondary current distributions are taken into account. The reaction-transport model presented here should enable the rational design of CO2 electrolyzers.
2.3-O1
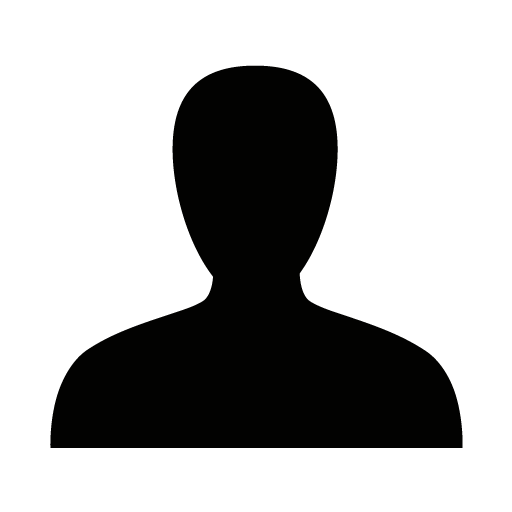
Electrochemical reduction of CO2 presents an attractive way to store renewable energy in chemical bonds in a potentially carbon-neutral way. Novel catalyst screening for this electrochemical process is crucial in improving selectivity, stability and required mass loadings. To date, however, catalyst testing occurs mainly on a 1-by-1 basis that strains quick identification of potential candidates. While advances in combinatorial screening have been presented, most of these rely on system designs that do not resemble representative conditions in lab-scale or industrial scales. To bridge this gap in benchmarking, we developed a lab-scale electrolyzer to screen 16 combinations of catalysts at the same time, using infrared thermography as the proxy for electrochemical activity. To showcase the effectivity of this method, we perform test runs on blank ink and sputtered catalyst samples, and subsequently analyse the spatial effects in our system. Subsequently, we study different catalyst loadings of copper for hydrocarbon production. Finally, we compare different catalyst precursors for the catalyst particles. The method shows an improved accuracy over existing solutions while employing a much more adequate system architecture.
2.3-O2
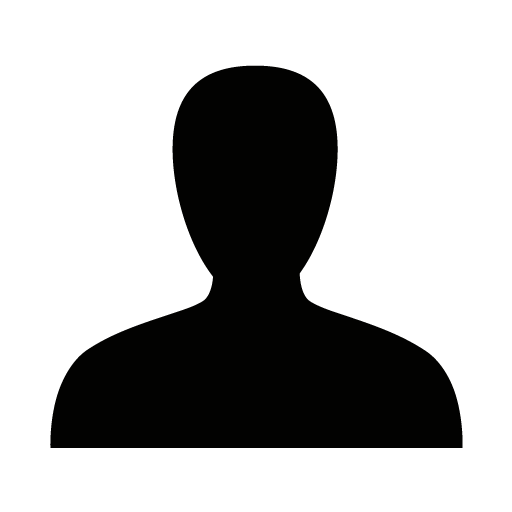
Tailoring the properties of the catalytic layer (CL) alongside its architecture is a key development towards ensuring both improve efficiency and selectivity for CO2 electrolyzers. Traditionally, CLs for the CO2R consist of a single binder-material or a combination of them overtaking both the ion-conductance and maintenance of a hydrophobic environment.[1], [2], [3]
We herein decoupled these processes into two individual, stacked catalyst-containing layers. Specifically, a hydrophobic catalytic layer was herein placed on the GDL aiming to improve water management within the CL during CO2R in zero-gap electrolyzers, while a second catalytic layer bound by an ion-conducting binder allows for the conduction of OH- and HCO3-/CO32- during CO2R, improving both the ionic conductivity between the GDE and AEM as well as the mechanical adhesion between the different interfaces. Notably, we present the complete stepwise CL-optimization pathway, regarding both the single and segmented-CLs towards the CO2-CO conversion at current densities ≥ 300 mA cm‑2, highlighting the role of the operational parameters regarding scalability in different cell sizes and long-term stability > 100 h.
2.3-O3
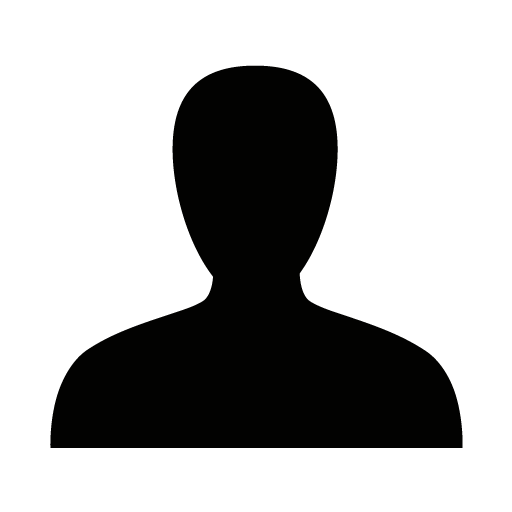
The oxygen evolution reaction (OER) is the predominant overall reaction bottleneck in water electrolysis towards achieving the large-scale production of sustainable H2. A proton exchange membrane-based water electrolyzer (PEMWE) shows advantages in operating at higher current densities, low H2 cross-over, and improved energy efficiency; over diaphragm and anion transport-based water electrolysers [1]. However, today´s OER catalysts in acid rely on rare and expensive critical raw materials such as Ir, Pt, or Ru. This limits the broad-scale implementation of PEMWE technology. Hence, the development of new platinum-group metal (PGM)-free electrocatalysts for the OER that combine efficiency and robustness in acidic conditions is paramount for clean H2 production from water [2–3]. We present new Co-based anode catalysts that achieve high activity and stable operation. Our synthetic strategy triggers a structural reconstruction of Co-based oxides that enables a different OER pathway by controlling the local oxide structure. The catalyst achieves 1.8 A‧cm−2 of current density at 2.0 V in PEMWE system at 80˚C using milli-Q water as electrolyte. It exhibited 278 h and 250 h of durability at 0.2 and 1.0 A‧cm−2 of current density, respectively, in industrial relevant conditions. Our study outperforms the durability of benchmark non-PGM catalysts over eight-times in the PEMWE system [2–3].
2.3-O4
Electrochemical carbon dioxide (CO2) conversion to multi-carbon (C2+) gas/liquid chemicals, such as ethylene (C2H4), offers a promising solution for the long-term and large-scale storage of sustainable energy. The viability of this approach requires further progress in terms of combined high selectivity at a high current density, and carbon efficiency. Today, this is challenged by the favorable conversion of CO2 into carbonates in the OH- rich environments associated to high current density operation. Here, we present an heterostructured core-shell structured Cu-based catalyst that enables control over the local OH- and carbonate balance, and improved *CO and *CO2·- coverage – as revealed by a suite operando Raman spectroscopy, pre and postmortem characterization, and electrochemical studies. The resulting gas diffusion cathode electrodes, implemented in a bipolar membrane configuration, achieve a 40% Faradic Efficiency towards C2H4 at a 1-A×cm-2 current density. The robustness of the catalyst is proved by 30 hours continuous electrochemical stability measurement performance.
1.1-I1
A scaffold designed for tissue engineering should feature biocompatibility, tissue-like mechanical properties, and a hydrated and porous environment. To this end, hydrogels that are 3-dimensional crosslinked polymeric networks are considered excellent scaffolds for tissue engineering [1], with optimal physical and biological characteristics. Further, added functionalities can be incorporated in hydrogels such as electroconductivity, a property critical for electro-responsive tissues such as neural and cardiac tissue [2], self-healing providing the scaffold with mechanical and electronic stability under strain [3], and self-adhesion enabling the placement of the scaffold at the targeted tissue site in a minimally invasive approach [4] . Here, we report the fabrication and characterisation of an adhesive, self-healing, and conductive hydrogel. We show that the hydrogel is porous, has high swelling ratio and is physically stable. We demonstrate its rapid self-healing, and its electroactivity in physiological buffer with both ionic and electronic conduction. Further, its self-adhesion indicates a facile placement on tissues. Coupled together, these properties indicate that the hydrogel with its individual biocompatible components is a suitable scaffold for cell growth and differentiation.
1.1-I2
Understanding and controlling the morphology of conjugated polymers is crucial for optimizing device performance in various applications such as stretchable electronics, wearable sensors, and flexible displays. Manipulating the morphology of such polymers into specific nanostructures like nanofibers or nanowires can not only maintain their electrical properties but also enhance mechanical robustness, enabling them to withstand mechanical deformations such as stretching, bending, or twisting without compromising their electrical functionality. While the majority of research on controlling conjugated polymers has focused on p-type materials, establishing an approach to precisely control diverse conjugated polymers, especially for n-type conjugated polymers, into such morphology remains a significant and elusive challenge in the field. In this work, we design a new n-type semiconducting polymer and present a pre-aggregation controlling approach that enables the morphology of the novel n-type conjugated polymer to transition from fibril into nanofibers. Utilizing the Hansen solubility parameter, we systematically screen thousands of solvents to refine our selection, thereby providing a comprehensive guideline for selecting solvents conducive to nanofiber formation. We visualize a shape change in polymer pre-aggregation within the solution state upon the addition of different anti-solvents, driven by their interaction affinities with the polymer's backbone or alkyl chain. We further establish a self-assembly nanofiber formation mechanism, discussing the effects of solvent/anti-solvent interaction and the influence of boiling points on this process and draw correlations between the pre-aggregation in the solution state and the morphology of the solid state. By extending our rules to p-type conjugated polymers, we successfully tune them into nanofibers, thereby validating our mechanism and demonstrating the universality of our guideline. The demonstrated n-type semiconductor nanofibers will offer deeper insights into the development of complementary circuits and sensors. The ability to control the morphology of conjugated polymers offers enhanced avenues for advancements in flexible and stretchable electronics and paves the way for the development and design of materials with tailored properties for next-generation electronic applications.
1.1-I3
We demonstrate electroadhesion, i.e., adhesion induced by an electric field, between cationic hydrogels and animal tissues [1, 2]. When gel and tissue are placed under an electric field (DC, 10 V) for 20 s, the pair strongly adhere, and the adhesion persists indefinitely thereafter. Applying the DC field with reversed polarity reverses the adhesion. Electroadhesion works with tissues of many mammals (cow, pig, chicken, and mouse), and is especially strong in the case of the aorta, cornea, lung, and cartilage. Only cationic gels can be electroadhered to tissues, which suggests that the tissues have anionic character.
We then show the use of electroadhesion to seal cuts or tears in tissues or model anionic gels. Electroadhered gel-patches provide a robust seal over openings in bovine aorta. Moreover, a gel sleeve is able to rejoin pieces of a severed tube (this is the equivalent of a surgery called an anastomosis). These studies raise the possibility of using electroadhesion in surgery while obviating the need for sutures or staples [1, 2]. Advantages include the ability to achieve adhesion on-command, and moreover the ability to reverse this adhesion in case of error.
1.2-I1
Conjugated polymers with features of flexibility, stretchability, and printability have huge potential applications in soft robotics, health monitoring, and human-machine interface. Here, I will present our group's efforts in developing various materials for developing these functional polymers. For instance, we have developed a highly stretchable and autonomic self-healable conducting film, which exhibits stretchability as high as 630% and high electrical conductivity of 320 S cm−1, while possessing the ability to repair both mechanical and electrical breakdowns when under-going severe damage at ambient conditions.This polymer composite film is further utilized in a tactile sensor, which exhibits good pressure sensitivity of 164.5 kPa−1, near hysteresis-free, an ultrafast response time of 19 ms, and excellent endurance over 1500 consecutive presses. Additionally, an integrated 5 × 4 stretchable and self-healable organic electrochemical transistor (OECT) array with great device performance is successfully demonstrated. The developed stretchable and autonomic self-healable conducting film significantly increases the practicality and shelf life of wearable electronics, which in turn, reduces maintenance costs and build-up of electronic waste.
1.2-I2
Material degradation stands as a paramount concern for both material scientists and engineers. The repercussions of degradation extend beyond mere failure, often necessitating costly repair efforts. Consequently, there exists a keen interest in the development of self-healing materials to render maintenance obsolete. Unlike their inorganic semiconductor counterparts, organic semiconducting materials exhibit notably low Young's moduli, rendering them exceptionally suited for deployment in wearable electronic devices that can be directly applied to human skin [1]. However, wearable electronics are particularly vulnerable to a host of environmental stressors, including mechanical wear, chemical exposure, temperature fluctuations, and radiation. The relentless impact of these stressors can lead to the deterioration of the chemical structure, resulting in the degradation and eventual loss of the material's physical properties.
In this context, we will delve into our strategy for mitigating the loss of physical properties through the development of intrinsically self-healing polymers. This accomplishment was made possible by harnessing the principles of supramolecular chemistry, particularly the utilization of intramolecular hydrogen bonds [2-4]. To gain a comprehensive understanding of the impact of hydrogen bonding on the viscoelastic and electrical characteristics of organic semiconductors, we formulated two distinct material sets: Firstly, a conjugated polymer with incorporated hydrogen bonding functionality and seondly a composite material comprising a conjugated polymer embedded within a self-healing polysiloxane matrix.
Our discussion will encompass the effects of these diverse approaches on charge transport and self-healing capabilities. Furthermore, we will outline how we can exploit the observed disparities to tailor not only the electronic properties but also the self-healing and mechanical attributes of the material to suit specific applications and their unique demands.
1.2-I3
Materials able to regenerate after damage have attracted a great deal of attention since the ancient times. For instance, self-healing concretes, able to resist earthquakes, aging, weather, and seawater are known since the times of ancient Rome and are still the object of research.
While several mechanically healable materials have been reported, self-healing conductors are still relatively rare, and are attracting enormous interest for applications in electronic skin, wearable and stretchable sensors, actuators, transistors, energy harvesting, and storage devices, such as batteries and supercapacitors.1 Self-healable and recyclable conducting materials have the potential to reduce electronic waste by enabling the repair and reuse of electronic components, which can extend the lifespan of electronic devices. Furthermore, they can be used for wearable electronic and biomedical devices, which are often subject to mechanical stress causing damage to their components.
Conducting polymers exhibit attractive properties that makes them ideal materials for bioelectronics and stretchable electronics, such as mixed ionic-electronic conductivity, leading to low interfacial impedance, tunability by chemical synthesis, ease of process via solution process and printing, and biomechanical compatibility with living tissues. However, they show typically poor mechanical properties and are therefore not suitable as self-healing materials.
In our group, we produced several self-healing and stretchable conductors by mixing aqueous suspensions of the conducting polymer poly(3,4-ethylenedioxythiophene) polystyrene sulfonate (PEDOT:PSS) with other materials providing the mechanical characteristics leading to self-healing, like for instance polyvinyl alcohol (PVA), polyethylene glycol, polyurethanes and tannic acid. 2-10 In this talk, various types of self-healing will be presented and correlated with the electrical ad mechanical properties of the materials. The use of the self-healing gels and films as epidermal electrodes and other devices will be also discussed.
REFERENCES
Y. Li, X. Zhou, B. Sarkar, F. Cicoira et al., Adv. Mater. 2108932, 2022.
Y. Li, X. Li, S. Zhang, F. Cicoira et al., Adv. Funct. Mater. 30, 2002853, 2020.
Y. Li, X. Li, R. N., S. Zhang, F. Cicoira, et al. Flexible and Printed Electronics 4, 044004, 2019.
N. Rossetti, F. Cicoira et al., ACS Appl. Bio Mater. 2, 5154-5163, 2019.
C. Bodart, N. Rossetti, F. Cicoira et al. ACS Appl. Mater. Interfaces,11, 17226-17233, 2019.
S. Zhang, Y. Li, F. Cicoira et al. Adv. Electron. Mater 1900191, 2019.
S. Zhang, F. Cicoira, Adv. Mater. 29, 1703098, 2017.
X. Zhou, G. A. Lodygensky, F. Cicoira et al., Acta Biomaterialia 139, 296-306, 2022.
X. Zhou et al, Advanced Sensor Research, in press, 2023
P. Kateb et al., Flexible and Printed Electronics, in press.
1.1-I1
Recent advances in electrochemical atomic force microscopy will be presented, with a focus on deciphering in situ an electrocatalyst’s morphological, structural, electrical and chemical properties at nanometer- and atomic-scale resolution. Selected results include unraveling the surface structure of state-of-the-art electrocatalysts during CO2RR, COR, and OER, as well as probing ion-induced viscosity variations in nanoconfined electrolytes alongside with a material’s local electrical conductivity. Complementary spectroscopic approaches, including in situ ATR-SEIRAS and on-line DEMS, provide direct molecular-level insights into reaction intermediates and interfacial hydration layer structures during CO2RR. A perspective will be given on multimodal approaches for nanoscale catalysis and rational innovation of energy conversion materials.
1.1-I2
There has been a growing recognition that not only electrocatalysts but electrolytes influence the activity of surface electrocatalytic reactions. Therefore, understanding the electrolyte-electrode interface under realistic operating conditions is critical to designing active and stable electrochemical devices. The past few decades of research have focused on developing a range of spectroscopic and imagining tools to enable the study of the chemical and structural changes at the electrolyte-electrode interface. Here, we introduce operando surface-enhanced infrared absorption spectroscopy (SEIRAS), a promising lab-based tool for gaining a molecular-level understanding of interfacial electrochemical processes.
This talk will bring the most recent understanding of the electrochemical interface during the operation of energy storage (ex., lithium-ion battery) and electrolysis (ex., electrochemical CO2 reduction reaction) applications using operando SEIRAS. We will highlight how the holistic information about the nature of the electrolyte-electrode interface can provide additional knobs to tune the reactions, leading to further improvements in activity and stability for energy applications. Also, fundamentals and experimental tips for operando SEIRAS will be explained, which is essential for those interested in introducing operando SEIRAS to probe your electrochemical system.
1.1-O1
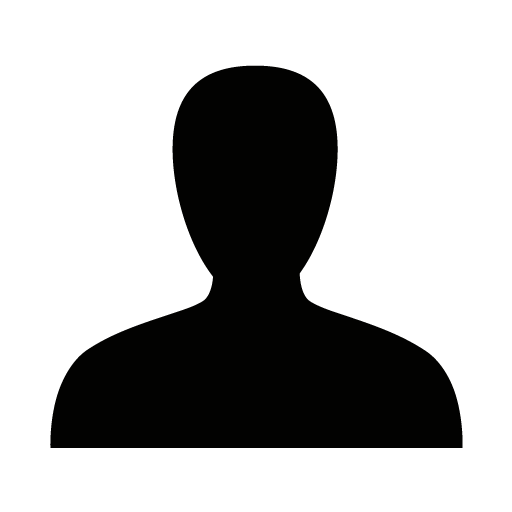
Abstract: In the relentless pursuit of advancing electrochemical technologies, gaining a profound understanding of the intricate dynamics governing nano alloy catalysts during reduction reactions, such as the electrochemical CO2 reduction reaction (CO2RR) or oxygen reduction reaction (ORR), emerges as a pivotal endeavor. This presentation delves into a pioneering approach employing direct imaging techniques, specifically focused on copper-based/platinum-based alloys, utilizing in situ electrochemical transmission electron microscopy (TEM). The objective is to illuminate the evolving nanostructures of these catalysts in real-time under the dynamic conditions of electrochemical reduction reactions.
Through the application of this cutting-edge technique, we aim to unravel the intricate interplay between catalyst morphology and electrochemical performance. This comprehensive exploration provides crucial insights that extend beyond mere observation, offering a deeper understanding of the structural transformations occurring during dynamic electrochemical processes. The outcomes of this study are anticipated to offer valuable guidance for optimizing catalyst design strategies, ultimately leading to the enhancement of overall electrochemical performance and stability.
1.1-O2
Electrocatalysis, whose reaction venue locates at the electrode-electrolyte interface, is controlled by electron transfer across the electric double layer, envisaging a mechanistic link between the electrocatalytic property and the EDL structure. One of the most intriguing questions is the mechanistic role of the alkali metal cation at the electrode-electrolyte interface, often referred to as the “cation effect”. In this presentation, we show that the identity of the alkali metal cation not only influences catalytic activity and selectivity, but can also directly affect electrode stability using an online inductively coupled plasma mass spectrometer coupled to an electrochemical flow cell. An increasing amount of Pt dissolution during cyclic voltammetry was found as the atomic number of alkali metal cations decreased. To explain this observation, various control experiments and computational studies were performed to explore potential scenarios such as changes in oxophilicity, electric field strength, and ion pairing. With a comprehensive understanding of alkali metal identity-dependent Pt dissolution, we propose a conceptual strategy for better electrocatalysis with prolonged catalytic durability.
1.1-O3
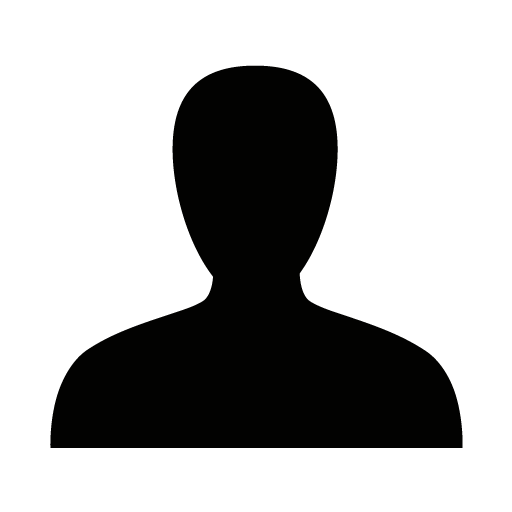
Electrochemical solid-liquid interfaces (SLI) are extremely complex and dynamic. SLI plays a significant role in dictating both charge transfer dynamics and selectivity of reaction pathways at any given electrochemical interface. Probing the structure and arrangement of interfacial ion and solvent molecules in-situ with nanoscale resolution is crucial to understand the SLI and develop efficient electrocatalysts.
Electrochemical atomic force microscopy (EC-AFM) technique offers in-situ/operando correlative measurements that can probe SLI with nanometer spatial resolution, providing access to nanoscale heterogeneities. In this contribution, I will highlight the use of EC-AFM as an interfacial force sensor to map the interfacial adhesion forces in addition to the local topography of the electrode under study. As the electrode surface charge is tuned by external applied bias, the local SLI structure is modulated, which leads to quantifiable changes in the adhesion forces mapped by the EC-AFM. Adhesion forces extracted from the AFM force curves (retract) provides direct insights on the interfacial energy associated with the molecular arrangement of the SLI.
I will discuss the presence of adhesion force inhomogeneities on the SLI when a (111) textured polycrystalline gold electrode is immersed in 10mM of sodium sulphate solution. We observe the presence of potential-induced hysteresis of adhesion forces as the potentials are cycled from low to high values and back. Additionally, increasing the ionic concentration of the electrolyte, reduces the adhesion hysteresis. Under applied anodic potentials the sulphate anions in the electrolyte adsorb on gold electrode, which leads to a substantial increase in the interfacial adhesion forces. A force minimum is observed before the onset of sulphate adsorption, which we attribute to the point of zero charge as the surface charge switches from negative to positive.
We also identify a negative correlation of electrode grain curvature and its mean adhesion force, highlighting the role of solid electrode asperities in influencing the interfacial energy of the SLI. The grain curvature also dictates the potential-dependent adhesion response of any individual grain at applied potentials; the flatter grain the higher is their adhesion response. Furthermore, we observe adhesion force inhomogeneities at the single grain level, characterized by two distinct surface states that exhibit similar potential-dependent responses.
Our work demonstrates the use of EC-AFM as a multimodal technique that can probe SLI arrangement in-operando with nanoscale resolution. This work opens the door to future work probing of the solid-liquid interface in the presence of different types of (non-)specifically adsorbing anions and cations, as well as studying the dynamics of EDL formation via measuring adhesion forces at applied potential pulses.
2.1-I1
Doing my BSc/MSc in Physics and PhD in an interdisciplinary program crossing the disciplines like Chemical Engineering, Nanotechnology, and Electrochemistry made me who I am today – a scientist who enjoys the challenge of multifaceted research.
I enjoy doing basic research in order to solve applied tasks. This explains my research interest in fundamental physical chemistry, e.g. oxidation and dissolution of metals and semiconductors, electrocatalysis, and electrochemistry at modified interfaces but also electrochemical engineering, e.g. development and optimization of catalyst layers in fuel cells and water electrolyzes.
Progress in basic research is often a direct outcome of previous achievements in experimental instrumentation. Hence, a significant part of my interest is in the development of new tools, e.g. electrochemical on-line mass spectrometry, gas diffusion electrode approaches, and high-throughput screening methods.
Fuel cells and electrolyzers require electrocatalysts to minimize losses during energy conversion processes. It is common practice that researchers rely solely on electrochemical methods to test stability in search of novel electrocatalysts. While degradation can be tracked using such methods, they fail when one aims to understand governing degradation mechanisms responsible for the losses in catalyst performance. Complementary physicochemical techniques are required. One such technique is inductively coupled plasma mass spectrometry (ICP-MS) – the main topic of my talk.
ICP-MS is commonly used as a quality control tool, e.g., to confirm if a desired alloy composition is obtained during synthesis. Recently, ICP-MS has been increasingly involved in electrocatalysis research, e.g., checking electrolytes from batch cells on the presence of dissolved species. However, this technique's potential is revealed when ICP-MS is directly connected to an electrochemical cell – online or in-situ ICP-MS. This tool provides time- and potential-resolved analysis of electrocatalyst corrosion during electrochemical tests.
Since ICP-MS is still a somewhat less known technique in catalysis research, my talk will start with a brief introduction to this analytical chemistry tool. Main operational principles and their importance when coupling ICP-MS to an electrochemical cell will be discussed. Existing approaches to couple electrochemistry to ICP-MS will be briefly overviewed. To demonstrate how scientists can benefit from employing online ICP-MS in their research, representative examples from the author's works will be presented, including the dissolution of Pt in fuel cells and Ir in water electrolyzers. The talk will be summarized by showing how in-situ ICP-MS can be implemented in modern automated and autonomous electrocatalysis research.
2.1-O1
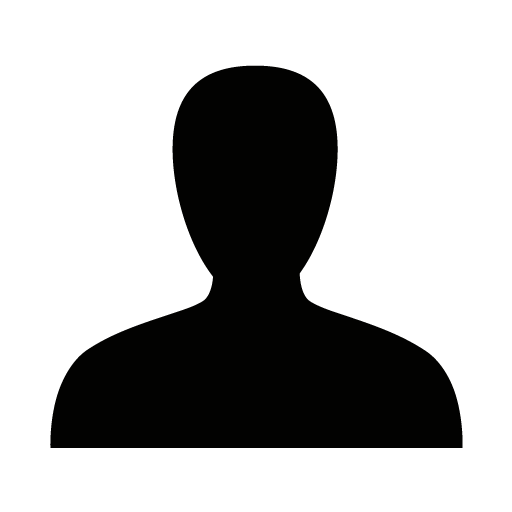
The distribution of active sites is ubiquitous in many natural and industrial processes, due to the diverse physical or chemical features on an interface discernible only at the local level. However, a comprehensive understanding remains elusive due to the challenge of probing micro/nanoscopic regions and extracting and adequate statistical interpretation of the interfacial phenomenon. Moreover, the electrochemical response is generally recorded taken from macroscopic areas, which are then correlated with surface characterization obtained on a vastly different scale (~μm2), which is not necessarily representative of the macroscopic surface. This discrepancy may arise from the inadequate assessment of active site distributions, further complicating the interpretation of electrochemical data. Furthermore, it has been shown that the electrochemical magnitudes are also distributed at the micro/nanoscopic scale, contingent on the nature of the substrate [1,2]. Consequently, a more robust approach is warranted, utilizing techniques like scanning probe methods, which offer high spatial-temporal resolution, or in-situ techniques providing real-time insights into dynamic nanoscale processes.
In this work, we have employed Scanning Electrochemical Cell Microscopy (SECCM) as a powerful and versatile scanning probe technique, capable of surveying the surface with high spatial-temporal resolution, by independently probing hundreds of different surfaces, providing a novel perspective and insights into the activity distribution and role of the substrate state for the synthesis of nanostructures. Instead of relying on the response of a single region for interpretation, which may not offer a comprehensive representation, we emphasize the significance of acknowledging the diversity at the microscopic scale by summarizing the electrochemical profiles with statistical measures and observing their temporal- or potential-dependent evolution. This local approach offers a new perspective on interfacial phenomena, justifying the need for high-throughput experimentation and data-driven analysis. By combining the local electrochemical information with other imaging methods, such as Scanning Electron Microscopy (SEM), that can be co-located with the same area on the same scale, we can establish unambiguous correlations and a more complete understanding of the electrochemical response at the local scale. This so-called multi-microscopy approach facilitates studying the relationship between physicochemical features of a substrate and nanostructures, fundamental for relating microscopic events to macroscopic properties of new deposits. This understanding is the bridge connecting the microscopic world to macroscopic outcomes, shedding light on how surface heterogeneities influence reaction rates and nanostructure properties. Our multi-microscopy strategy, using data-driven high throughput local investigation to bring a new perspective to old phenomena, could be easily replicated for different interfacial phenomena such as electrocatalysis, phase growth, or corrosion [3,4].
2.1-O2
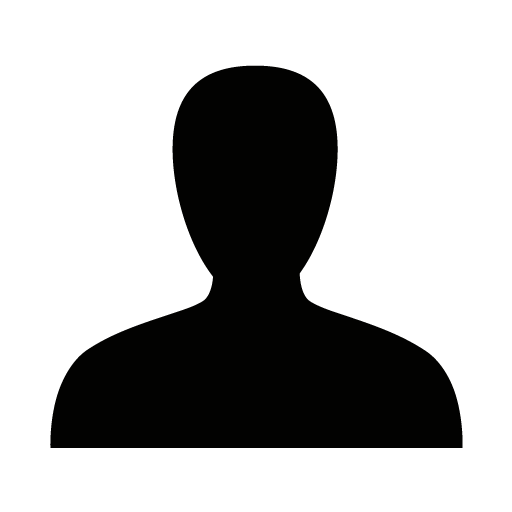
In-situ techniques play an important role in gaining real-time insights into dynamic processes at the nanoscale. Employing a specialized TEM holder and chips, the electrochemical TEM (EC-TEM) setup allows for the direct observation of (nano)materials undergoing electrochemical reactions within their confined environments. This dynamic characterization enables the tracking of structural changes, such as phase transformations and morphological evolution.[1,2] In our research, we studied the electrochemical growth of Cu on a GC electrode, as a model system.
While the in-situ characterization offers a new avenue of opportunities, it also comes with specific challenges that we need to overcome, such as the confined environment of the EC-TEM holder, and the beam-related effect. Moreover, nucleation and growth are especially sensible to the surface state, which can end up in the formation of different structures. To consider this, we conducted a detailed analysis of the GC working electrode situated on the specialized TEM chip surface using a scanning probe technique, Scanning Electrochemical Cell Microscopy (SECCM). This technique allowed us to characterize the GC electrochemical activity within a few micrometers, which, for the case of GC, have been shown before to be heterogenous.[1,3] Overall, performing an extensive characterization of the electrochemical response of the TEM chips, in both in-situ and ex-situ conditions, allows us to better understand the electrochemical processes under TEM imaging conditions. In the context of our work, this is of special interest for the better understanding the fundamentals of metal nucleation, growth and dissolution on/from glassy carbon electrodes. This knowledge is essential for the rationalized design of active and durable nanostructured electrocatalysts for a wide range of electrochemical energy conversion and storage technologies.
2.1-I2
Prof. Magalí Lingenfelder is a PI with an excellent track record and a passion for atomically controlled interfaces. Her work contributes to the design of new materials by elucidating chemical processes by Scanning Probe Microscopies and Surface Sensitive Spectroscopies, including dynamic (bio) molecular recognition processes at the liquid/solid interface.
She created and led for over 10 years the Max Planck-EPFL laboratory for Molecular Nanoscience at EPFL campus in Switzerland, and is currently leading the Helvetia Institute for Science and Innovation.
She studied physical and biological chemistry at the National University of Córdoba in Argentina. In 2003, she finished her MSc thesis at the Max Planck Institute for the Solid State Research (MPI-FKF in Stuttgart, Germany) with seminal contributions to the field of metal-organic coordination networks on solid surfaces. She continued with her doctoral studies in Physics, and received the Otto Hahn medal of the Max Planck Society in 2008 for the microscopic understanding of the chiral recognition process with submolecular resolution. In her quest to study molecular recognition going from 2D to 3D complex systems, she made postdoctoral stays at the Institute of Materials Sciences in Barcelona, and at the Molecular Foundry of the Lawrence Berkeley National Lab in the US.
She is a committed mentor who directed 4 MSc theses, 5 PhD theses, and 5 postdocs. She advocates for problem-oriented interdisciplinary research, by pioneering the emerging field of BioNanoarchitectonics. She led 5 international research consortiums, delivered over 50 invited presentations, and organized 9 conferences and 4 doctoral schools. She and her team had received multiple awards and international recognitions for their creative and rigurous work on molecular recognition, chirality and operando studies at catalytic interfaces. In 2018, the Royal Society of Chemistry included her work in the first collection “Celebrating Excellence in Research: 100 Women of Chemistry”.
Ensuring a sustainable future and reducing carbon emissions heavily relies on renewable energy sources. These sources allow us to harness electrons to drive chemical reactions and store energy in the form of chemical bonds. However, the performance of current electrocatalytic processes needs significant improvement.
Magnetic field effects on electrocatalysis have recently gained attention due to the substantial enhancement of the oxygen evolution reaction on ferromagnetic catalysts. In this context, it is crucial to carefully characterize how magnetic fields affect mass transfer of charged reactants and products at the interfacial level, a phenomenon that occurs even on non-magnetic electrodes and is often overlooked.
In this talk, I will highlight the use of unconventional strategies to achieve spin control of reaction pathways on the surface of a catalyst. By decoupling the effects involved in global (interfacial) vs local magnetic enhancement using in situ techniques, I will provide a versatile strategy that can be easily implemented to boost electrocatalytic reactions across diverse materials.
2.2-I1
Structural characterization of an electrocatalyst under operando conditions is a prerequisite for fully understanding its activity and stability. Surface X-ray Diffraction (SXRD) is a powerful tool to obtain such information due to its high surface sensitivity and the possibility to probe the electrode-electrolyte interface in a noninvasive manner, especially when employing high energy photons. Furthermore, SXRD can easily be combined with other, e.g. spectroscopy or microscopy, techniques to aqcuire complementary information within the same experiment. Here, I will show the application of SXRD for atomic scale structural characterization of single crystal model electrocatalysts. First, I present the evolution of a Pt(111) surface under oxidizing (oxygen evolution as well as oxygen reduction reaction) conditions in a combined rotating disk electrode (RDE) SXRD experiment [1]. These experiments resolve the complete oxidation of the surface layer near the onset of the oxygen evolution reaction and the surface stability during oxygen reduction. Similar studies were performed under hydrogen evolution conditions in strong alkaline media to study the electrode restructuring due to cathodic corrosion under operando conditions. I further show how coupling SXRD with other techniques such as infrared reflection absorption spectroscopy (IRRAS) or surface optical reflectance (SOR) provides insights in the oxidation of CO on Pt(111) [2] and the reconstruction of Au(111) [3], respectively. Finally, I will discuss the applicability of SXRD for studying model electrocatalysts beyond bulk single crystals. This is exemplified by the characterization of epitaxial Cu thin films under CO2 electroreduction conditions.
2.2-I2
Electrochemical nucleation and growth (EN&G) are the cornerstone for many (nano)material growth routes and the main factor limiting battery durability [1]. The in-depth experimental assessment of the process is very challenging due to the random nature of initiation events (nucleation), the heterogeneity of surfaces and the (very) fast kinetics across several length scales. For all that, our understanding of the mechanisms involved is inaccurate and incomplete [2]. On the other hand, electrochemical dissolution (ED) of nanostructured interfaces is the main cause of material degradation in exposed environments (corrosion) or energy conversion and storage devices. Dissolution is also affected by random initiation events, fast kinetics and surface heterogeneity. Hence, a complete picture of the (combination of) dissolution pathways taking place is also elusive.
To address these challenges, we combine Scanning Electrochemical Cell Microscopy (SECCM) and in-situ Electrochemical Transmission Electron Microscopy (EC-TEM).
On the one hand, we performed thousands of high-throughput local electrochemical measurements for the nucleation of Au and Cu on different surfaces such as glassy carbon (GC), ITO, and Pt [3-4], which we analyzed with a data-centric approach. In addition, the spatially resolved electrochemical characterization enables a one-to-one correlation between the electrochemical data and the local surface properties, which are evaluated by SEM in identical locations to where the local electrochemical measurements have been performed.
On the other hand, we use SECCM as a high throughput tool to collect ED activity from randomly probed locations where metal nanoparticles (NPs) are pre-deposited by different methods (electrodeposition, sputtering, drop casting, etc.). Taking gold NPs as a case study, we show that, in the presence of chloride and at certain potentials, NP dissolution events are separated in time and can be therefore monitored by SECCM as single NP dissolution events [3, 5]. Data science methods, previously applied in the group for the study of pitting corrosion in stainless steel [6, 7] have been here deployed to explore the very large datasets containing information of the dissolution of several thousands of NPs.
The analysis of the SECCM EN&G and ED datasets is leveraged to discuss mechanistic aspects of noble metal nucleation, growth and dissolution processes. In addition, we show how EC-TEM allows the study of the early-stage growth/dissolution dynamics of the metallic phase. Interestingly, these measurements corroborate that the nature of the events resolved by SECCM corresponds to the dissolution of individual NPs. The combination of high-throughput SECCM, EC-TEM and data-centric analysis opens new opportunities for the rational design (electrodeposition) of functional nanostructured materials and the evaluation of their durability under electrochemical polarization (resistance to electrodissolution).
2.2-O1
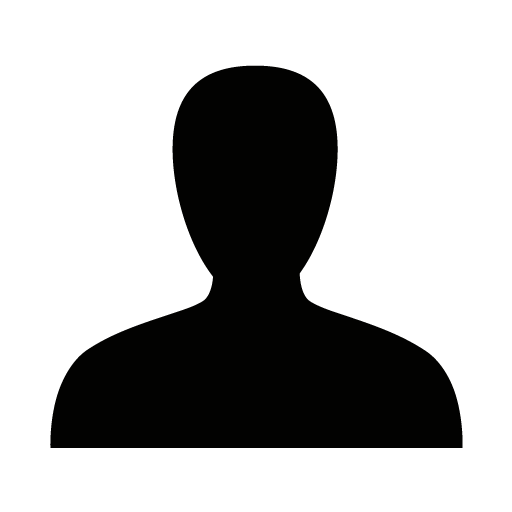
CO2 reduction reaction (CO2R) in acidic media is one of the alternatives to overcome low carbon utilization efficiencies and energy penalties due to the carbonate formation in an alkaline/neutral environment. In acid electrolytes the hydrogen evolution reaction (HER) is kinetically more favorable, limiting the conversion of CO2 in C2+ products. Modulating the reaction microenvironment at the catalyst-electrolyte interface with inorganic and organic components is a strategy to promote the reaction activity, selectivity, and stability [1-3]. Here, we report a CO2R reaction environment modulation strategy consisting of a polymer heterojunction coating that (1) increases local CO2 availability; (2) stabilizes CO2 reaction intermediates, leading to a high *CO coverage and improved C-C coupling in acid; and (3) regulates local proton activity with dedicated functional groups – as demonstrated by a combination of operando Raman spectroscopy and electrochemical characterizations. The polymer-coated PTFE/copper electrodes achieve a multicarbon Faradaic efficiency of 60% at 300 mA cm−2 in acidic media.
2.2-O2
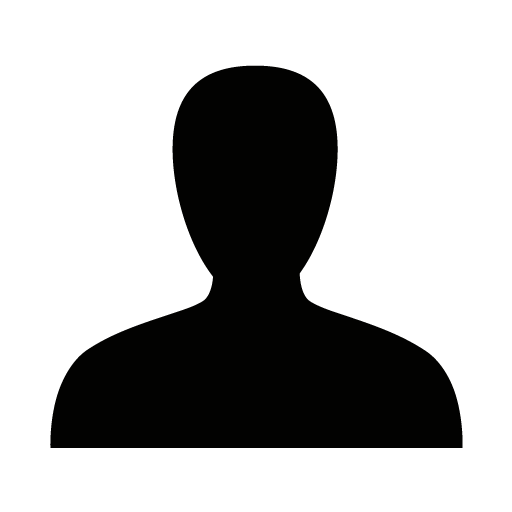
CO2 electroreduction (CO2E) offers a path generate widely used chemicals using CO2 waste and renewable electricity. The viability of CO2E requires further progress in combined performance metrics such as selectivity, current density, and carbon utilization (among others). This is challenged by the loss of CO2 into carbonates in high pH systems. CO2E operation in acid is one strategy to overcome this loss process, as carbonate species would get locally regenerated into CO2 in a high-density H+ environment. Unfortunately, this approach also promotes the undesired hydrogen evolution reaction (HER). Control over the local CO2E reaction environment, and ion species in the double layer, has been shown as a promising direction to address this challenge.1–5 Here, we present a strategy that uses different ionomer coatings to modulate the local reaction environment. We perform mechanistic studies, including operando spectroscopies, to study how these affect the dynamics of key ion species and reaction intermediates over the catalyst surface, and correlate this with performance trends. The ionomer coated PTFE/Cu electrodes achieve a Faradaic Efficiency of 73±6% for CO2 to C2+ at 400 mA/cm2 using an acidic electrolyte. This approach opens new possibilities for optimizing CO2 electroreduction processes and achieving higher selectivity for value-added products, contributing to the advancement of sustainable and efficient energy conversion technologies.
2.2-O3
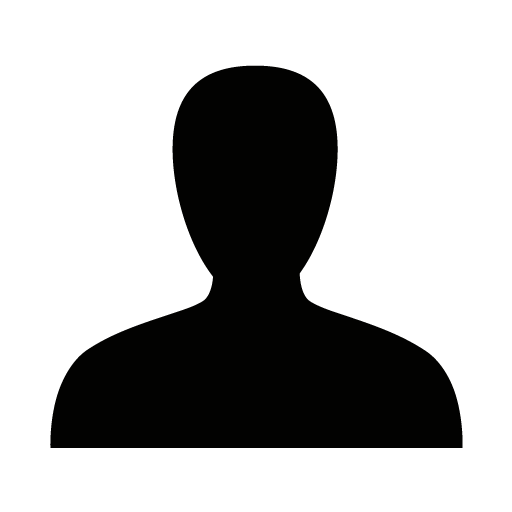
Renewable-powered electrochemical carbon dioxide (CO2) reduction reaction (CO2R) offers an attractive approach for the generation of low-carbon footprint chemicals. CO2R in acid has emerged as a promising solution to overcome drawbacks of traditional CO2R alkaline electrolyzers, such as limited carbon efficiency, which can lead to low energy efficiencies. Ionic species in the electrolyte have proven crucial in CO2R.[1,2] Especially in acid, cations have been shown to be essential to enable CO2R.[3] The role of anions, on the other hand, has received less attention in this context. Further advances in the field rely on resolving the CO2R environment and the role of ionic species at increasing current densities, where CO2R onset takes place in acid (> 50 mA·cm-2).[4] Herein, we study the role of anionic species by assessing the local CO2R reaction environment at high current densities (> 200 mA·cm-2), using operando Surface Enhanced Raman Spectroscopy (SERS). We have done so by investigating the different adsorbed intermediates and anions at varying pHs using a copper catalyst and H2SO4/K2SO4 electrolyte. We correlated changes in selectivity with presence and stabilization of adsorbed species and CO2R intermediates, revealing the key dynamic role that anions play in the competition between CO2R, carbonate-loss-regeneration pathways, and the suppression of HER in acid. Our study shows the paramount importance of measuring these processes at high current densities, offering crucial insights for advancing in acid CO2R.
2.3-O1
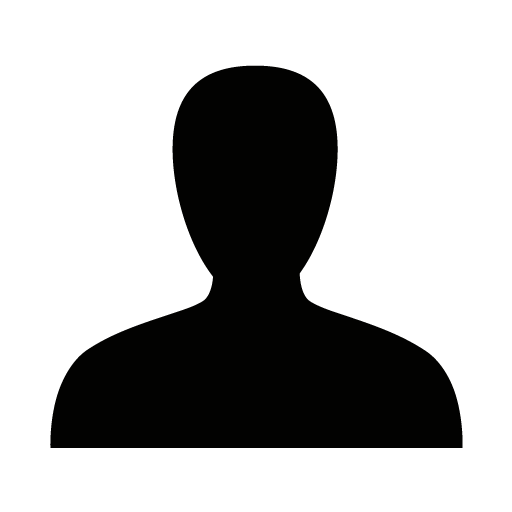
The recent IPCC report emphasizes the necessity of scenarios with negative carbon dioxide emissions to meet the 1.5°C Paris Agreement goal. This underscores the vital role of negative emission technologies (NETs) in complementing comprehensive decarbonization efforts. Various NETs, such as afforestation, biochar, bioenergy with carbon capture and storage, direct air carbon capture and storage (DACS), and soil carbon sequestration, have been proposed. However, achieving COP27 goals requires a rational combination of technologies, as no single NET alone is likely to suffice. Among NETs, electrical approaches have garnered attention for their convenience, efficiency, and safety, although the electrochemical route is still in an early stage of development.
Capturing CO2 from dilute sources, including flue gas and the atmosphere, is crucial for managing global emissions and advancing downstream storage and utilization efforts. Electrochemical carbon capture methods stand out for their high energy efficiency, decentralized operation, ambient reaction conditions, and compatibility with renewable electricity.
In this context, a robust electroDAC approach with switchable electroactive adsorption materials is urgently needed. Cu-based electrochemically mediated amine regeneration (EMAR) has shown promise for controlled, reversible complexation with CO2 capture amines in aqueous solutions based on stability and performance. However, uncertainties persist regarding the precise mechanisms of Cu2+/Cu interfacial chemistry, dynamic competitive interactions at the surface and in bulk, and potential degradation routes or parasitic faradaic currents.
To address these uncertainties, we propose a reversible system using an electrostatic charge transfer mechanism at the electrode interface for energy-efficient CO2 capture and release. Our study focuses on investigating the stability and reactivity of various Cu-based catalysts under different conditions, employing spectroelectrochemical analysis to elucidate in-situ changes and interactions between adsorbed species and CO2 at the electrode interface.
2.3-O2
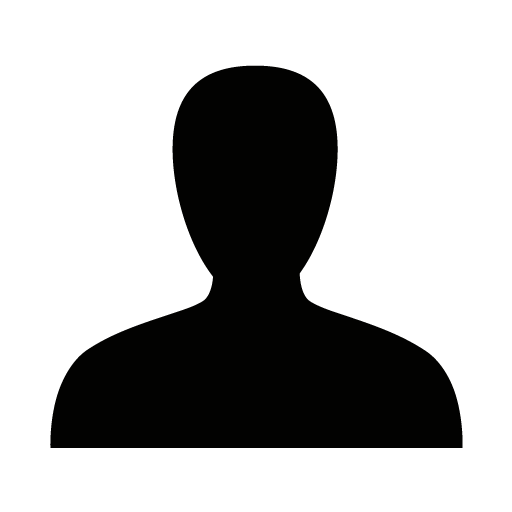
Tuning the electrode-electrolyte interfaces via cations, pH, and concentrations is a widely accepted method for tuning the product selectivity of electrochemical carbon dioxide reduction (CO2R) reaction in aqueous media. However, the role of the interfacial water remains an unsolved puzzle to date. Being a polar molecule, the water interacts either via the hydrogen end for cathodic reactions or via the oxygen end for anodic reactions by H-bonding or other chemical interactions to the electrode surface. In the electrode-electrolyte interface, water remains in mainly three forms: four H- H-bonded water (4-HB‧ H2O), three H-bonded water (3-HB‧ H2O), and free water (0-HB‧ H2O). Depending on the no of H-bonded water, the activation energy trend is 4-HB‧ H2O > 3-HB‧ H2O > 0-HB‧ H2O.[1,2] Presence of more rigid water (4-HB‧ H2O) in the electrode-electrolyte interface decreases the competing hydrogen evolution reaction (HER) [3] at the same time decreases the accessibility of CO2 to the catalytic surfaces impeding CO2R. [4] To explain this anomaly, we have employed in situ surface-enhanced Raman spectroscopy (SERS) to correlate the interfacial water structure with CO2RR product selectivity under higher current density using Cu as model catalyst varying the pH, concentration, cations, and anions of the electrolyte. The role of the interfacial pH, intermediate species specially adsorbed CO2, competition between carbonate/bicarbonate formation, and adsorbed hydroxide (*OH) are also explored.
2.3-I1
Stability and catalytic activity of metal oxides for the water oxidation at an electrolyzer anode are linked through the reactivity of oxygen atoms: metal–oxygen bonds are formed and broken during the electrocatalytic cycle, and metal–oxygen bonds are also what holds the material together. One way to probe this interplay is by isotope labelling of the oxygen in the electrocatalyst with 18O and determining by electrochemistry – mass spectrometry (EC-MS) whether this labelled oxygen is incorporated into the O2 released. Through our work with chip EC-MS on sputter-deposited 18O-labelled oxides of Ru and Ir, we show the importance of quantitative and transparent data analysis in such oxygen-tracking experiments and their limitations. We then expand on the concept using CO oxidation, showing how the incorporation of labelled oxygen into the product CO2 probes the reactivity of pre-catalytic surface states. A more direct probe of these states is to observe them spectroscopically. We show through fitting the UV-vis spectra of iridium oxide as a function of potential that the pre-catalytic redox transitions follow Frumkin isotherms, and propose a way of quantifying the relative importance of chemical vs electrochemical driving force in the rate-determining step.
2.3-I2
The electrocatalytic reduction of CO2 (CO2RR) into valuable base chemicals and fuels is a very complex reaction that depends on the intimate relation between catalyst structure and external reaction conditions.[1] Despite considerable progress over the past few years, it is evident that the precise identification of the active sites of the electrocatalyst under operation remains a challenge, which hinders the rational design and industrial application of advanced electrocatalysts for eCO2RR. For this purpose, in situ characterization techniques are required that probe the catalyst structure, from bulk to surface, with improved time and space resolution.
In this presentation, I will discuss how we deploy in situ time-resolved Raman spectroscopy (TR-SERS) and advanced in situsynchrotron-based X-ray scattering and spectroscopy techniques to investigate the electrocatalytic activation of CO2 and the dynamic chemical structure of the electrode surface.[2,3] We have combined TR-SERS with cyclic voltammetry, chronoamperometry and pulsed electrolysis to study the time- & potential-dependent behavior of the electrode surface and the adsorbed species.[4] Furthermore, we deployed TR-SERS mapping to elucidate spatiotemporal heterogeneities of copper electrodes at work.[5] Finally, we used in situ small- and wide-angle X-ray scattering to couple the dynamic structure of the electrocatalyst to the performance for well-defined copper oxide nanoparticle electrodes.
References
[1] S. Nitopi et al. Chem. Rev. 2019, 119, 7610.
[2] H. An et al. Angew. Chem. Int. Ed. 2021, 60, 16576.
[3] S. Yang et al. Nat. Catal 2023, 6, 796.
[4] J. de Ruiter et al. J. Am. Chem. Soc. 2022, 144, 15047.
[5] H. An, J. de Ruiter et al. JACS Au 2023, 3, 1890.
1.1-I1
Photoelectrochemical cells (PECs) hold great promise as an environmentally friendly method of converting sunlight into energy-dense chemicals. For example, the PEC approach can find application in the synthesis of multi-carbon-based hydrocarbons (C2+) by solar driven reduction of carbon dioxide (CO2R), and in the generation of hydrogen by solar water splitting. However, the electrochemical environment poses significant challenges to the performance and stability of semiconductor based photoelectrodes. Tackling these challenges requires careful understanding of the behaviour of photoelectrode interfaces at the microscopic scale.
Here we show examples of characterization techniques that can help to quantify the performance and stability of photoelectrode surfaces with nanometer resolution, using, as a model system, thin films of TiO2 deposited for corrosion protection by atomic layer deposition (ALD). A detailed analysis of Kelvin probe force microscopy (KPFM) measurements under intermittent illumination allows us to analyze the evolution of surface potential over time and extract localized time constants for carrier dynamic processes on the surface. Furthermore, using operando spectroscopic ellipsometry (SE), we can directly quantify the intrinsic stability of these protective overlayers under PEC water splitting conditions, particularly as a function of the degree of crystallinity of the TiO2 film. In addition, we show how rationally designed catalytic environments can significantly improve the stability and performance of photoelectrodes for PEC CO2R. We demonstrate that by tuning microenvironmental factors such as pH, wettability, and CO2 mass transport, we can enhance C2+ selectivity on halide perovskite photoelectrodes. Understanding photoelectrode interfaces at the micro and nanoscale allows the systematic improvement of photoelectrode stability and performance, opening avenues for the implementation of PEC technologies towards sustainable energy production and climate change mitigation.
1.1-I2
Roel van de Krol is head of the Insitute for Solar Fuels at the Helmholtz-Zentrum Berlin fuer Materialien und Energie (HZB), and professor at the Chemistry Department of TU Berlin. After earning his PhD from TU Delft in 2000 and a postdoctoral stay at M.I.T. (USA), he returned to TU Delft where he was an assistant professor until 2012. At HZB, his research focuses on the development of materials and devices for the photoelectrochemical conversion of sunlight to chemical fuels. Understanding how surface and bulk defects in thin films and nanomaterials affect light absorption, charge transport, recombination and catalytic activity is at the heart of these efforts.
Efficient charge separation is a crucial step in any photoelectrochemical (PEC) or photocatalytic (PC) process. The role of the electric field is, however, often misunderstood in the PEC and PC communities [1]. While it is often stated that the charges are separated by the electric field, this is not the case for a system without externally applied bias; instead, charge separation requires the presence of selective contacts. Another commonly encountered statement is that by increasing the externally applied potential, one can increase the field strength and thereby improve charge separation. Under normal conditions, however, the applied potential only affects the width of the space charge layer and has zero effect on the actual strength of the electric field within the photoelectrode. In this talk I will discuss these misconceptions and offer a tentative explanation of where they originate from. I will discuss the true driving force for charge separation and outline various strategies for making selective contacts, with a focus on metal oxide photoelectrodes. Most of these strategies originate from the field of photovoltaics, in which the concept of selective concepts has been well established. Some approaches are relatively easy to implement for metal oxide absorbers and several examples will be shown. Others strategies may be too complex and costly for scaleup to large areas but can still offer valuable insights and help us to design efficient PEC and PC systems.
1.1-I3
The accelerated consumption of fossil fuels and the concomitant rise in greenhouse gas emissions emphasize the need for transitioning towards renewable “green” resources, and environmentally sustainable processes. Photocatalysis has the potential to simultaneously mitigate the energy and environmental concerns.[1] However, the development of economically and environmentally sustainable processes creates the pressing need for new materials of low cost and toxicity, photoabsorbers and catalysts, which are robust and maintain good performances under outdoor conditions.
Carbon dots (CDs) and carbon nitride (CNx) can efficiently serve as photoabsorbers for this purpose since they fulfil these requirements.[2-6] In particular, they are hydrophilic materials of low toxicity which are chemically and photochemically robust, can be synthesized at low cost, and show good photocatalytic properties upon pre-designed synthesis. In this work, we describe the synthesis of CNx and CDs from low-cost organics and/or Earth abundant waste (i.e., circular economy), the structure of which bestows the derived photoabsorbers with distinctive photocatalytic performances. These light harvesters, when combined with noble-metal free catalysts in aqueous photocatalytic systems, not only facilitate “green” fuel synthesis but also waste/water pollutant utilization. The use of waste and aqueous pollutants, eliminates the need for additional sacrificial reagents traditionally used in great excess, which add to the overall cost of the process, and result in toxic by-products.[7] We anticipate that this approach could be a breakthrough in the development of robust, scalable, economically, and environmentally sustainable systems, which can efficiently serve energy and environmental applications.
References
[1] Kamat, P. V.; Bisquert, J., J. Phys. Chem. C 2013, 117, 14873-14875.
[2] Achilleos, D. S.; Kasap, H.; Reisner, E., Green Chem. 2020, 22, 2831-2839.
[3] Achilleos, D. S.; Yang, W.; Kasap, H.; Savateev, A.; Markushyna, Y.; Durrant, J. R.; Reisner, E., Angew. Chem. Int. Ed. 2020, 59, 18184-18188.
[4] Kasap, H.; Achilleos, D. S.; Huang, A.; Reisner, E., J. Am. Chem. Soc. 2018, 140, 11604-11607.
[5] Kasap, H.; Godin, R.; Jeay-Bizot, C.; Achilleos, D. S.; Fang, X.; Durrant, J. R.; Reisner, E., ACS Catalysis 2018, 8, 6914-6926.
[6] Ren, J.; Achilleos, D. S.; Golnak, R.; Yuzawa, H.; Xiao, J.; Nagasaka, M.; Reisner, E.; Petit, T., J. Phys. Chem. Lett 2019, 10, 3843-3848.
[7] Pellegrin, Y.; Odobel, F., C. R. Chim. 2017, 20, 283-295.
1.2-O1
Halide perovskites have gained wide interest for their application in photovoltaics, sensors, photocatalysis, and photoelectrochemistry, due to their excellent optoelectronic properties. However, their development in photoelectrochemistry for solar fuels and chemicals is hampered by their instability in aqueous environments. In this talk, I will present our recent progress in the protection of halide perovskite-based photoanodes with different sheets of carbon allotropes, namely mesoporous carbon, graphite, glassy carbon, and boron-doped diamond, decorated with electrocatalysts. The protection of a CsPbBr3 photoabsorber film with a mesoporous carbon layer results in photoanodes that last a few hours [1]. Addition of porous graphite sheets increases the stability from hours to days, especially with the addition of water-oxidation NiFeOOH electrocatalyst on the surface of the porous graphite sheet [2]. Longer stabilities of weeks can be achieved by creating porosity gradients with different graphite sheets and by replacing the top graphite sheet upon signs of deterioration. Finally, the best stabilities, projected to be of months, can be realized with more chemically and mechanically stable glassy carbon and boron-doped diamond sheets [3]. These achieve world-record stability: 97% of initial photocurrent close to 8 mA cm-2 is preserved for 210 h under harsh +1.23 V vs RHE of applied bias (stability projection = 10 months). All these carbon allotropes provide the halide perovskites with durable protection, efficient charge separation and transport, electrocatalyst support, and, importantly, photothermal properties for enhanced thermal management, which are invaluable additions for their future development and application of halide perovskite photoelectrochemical devices.
1.2-O2
Photoelectrochemical (PEC) water splitting is one of the few truly renewable pathways toward “green” H2 production. All PEC water splitting devices demonstrated thus far operate at atmospheric pressure. However, most applications and processes that use H2 require it to be supplied at elevated pressure. Although PEC-generated H2 can be pressurized afterward with e.g. mechanical compression, operating the PEC water-splitting device itself at elevated pressure offers an intriguing alternative and several possible advantages. For example, bubble formation is strongly reduced at elevated pressure, which lowers bubble-induced electrode deactivation and bubble-induced product crossover.[1,2] In addition, the optical reflection and diffraction losses induced by bubbles can be minimized.[3] Finally, the electrochemical production of hydrogen at higher pressure requires only a small (though not insignificant) increase in the thermodynamic cell voltage (29 mV for a 10-fold increase in pressure).[4] To quantitatively evaluate these pros and cons, a device that can be used for PEC water splitting at higher pressure is required.
In this work, we demonstrate a membrane-free, laminar flow cell that enables PEC water splitting at elevated pressure (see the TOC graphic for the schematic of the cell). In such a cell, a continuous, laminar liquid flow is essential for adequate product separation. Therefore, the flow velocity profile between the two parallel electrodes was examined using particle image velocimetry (PIV). A nearly parabolic velocity profile was obtained. This observation agrees well with the finite element simulation results. Shadowgraph images also show that O2 bubbles are restricted to the vicinity of the anode due to the laminar liquid flow. The design of our PEC flow cell also allows operation at moderate pressure elevation, up to 5 barg, without any observed leakage. More importantly, the pressure elevation significantly diminished the bubble curtain, potentially leading to a higher photoelectrochemical performance due to smaller bubble-induced drawbacks. Finally, we will discuss a quantitative comparison of the benefits and drawbacks of performing PEC water splitting at elevated pressure using our laminar flow cell.
1.2-O3
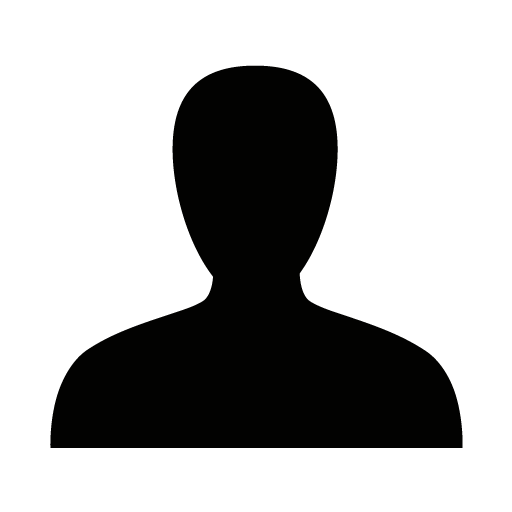
Electrochemical reduction of CO2 into valuable chemicals from renewable electricity is envisioned as a means to avoid fossil resources in many applications. As the field is urged to increase its technology readiness, numerical models are necessary tools to accelerate device development.[1], [2] Nevertheless, fully reliable and accurate models have yet to be developed. The challenges lie in the complexity of the reaction and the convolution of many effects including mass-transport, microkinetics, and kinetics. In this study, we aim at deconvoluting these aspects by studying the reduction of CO2 into carbon monoxide at a flat silver electrode, one of the most studied catalyst material under controlled mass-transport conditions. We hypothesize that one of the major gap between previous model and experiments is the carbon monoxide affinity for some active sites that prevent other electrochemical reactions to take place. In order to model this effect independently, we conducted a series of measurement in presence of CO and determined equilibrium constants to be fed into a microkinetic model. These experiments confirmed our hypothesis that the produced CO significantly hinders the competitive hydrogen evolution reaction. We then developed a computational model and show that the consideration of the CO effect on hydrogen evolution reaction allows for improved accuracy in predicting experimental observations.[3] The study also provides unexpected insights in the different nature of active sites responsible for CO and H2 evolution at Ag electrodes.
1.2-I1
High conversion efficiencies are key for solar fuel technologies to compete with natural photosynthesis. Here, photoelectrochemical approaches should be in a similar efficiency range as the combination of photovoltaics with electrolysis. So far, the highest photoelectrochemical solar-to-hydrogen efficiencies (STH) are achieved using multi-junction solar absorbers based on the III-V semiconductor material class. Reported areas, however, only lie in the range of up to 0.3 cm2 as surface defects quickly lead to corrosion and failure of the device. I will present our latest results where we demonstrate significantly higher areas at >18% STH using a photoelectrochemical surface functionalisation approach in a Schlenk cell. The higher level of interface quality and reproducibility is a prerequisite for scale-up and optical in situ control with electrochemical reflection anisotropy spectroscopy contributes to this development [1,2]. Furthermore, I will discuss some of the challenges and potential pitfalls related to the benchmarking of multi-junction solar water splitting devices which are still present in the literature.
1.3-I1
Vincent Artero was born in 1973. He is a graduate of the Ecole Normale Supérieure (Ulm; D/S 93) and of the University Pierre et Marie Curie (Paris 6). He received the Ph.D. degree in 2000 under the supervision of Prof. A. Proust. His doctoral work dealt with organometallic derivatives of polyoxometalates. After a postdoctoral stay at the University of Aachen (Aix la Chapelle) with Prof. U. Kölle, he joined in 2001 the group of Prof. M. Fontecave in Grenoble with a junior scientist position in the Life Science Division of CEA. Since 2016, he is Research Director at CEA and leads the SolHyCat group. His current research interests are in bio-inspired chemistry including catalysis related to hydrogen energy and artificial photosynthesis.
Vincent Artero received the "Grand Prix Mergier-Bourdeix de l'Académie des Sciences" in 2011 and has been granted with a Consolidator Grant from the European Research Council (ERC, photocatH2ode project 2012-2017). He's a member of the Young academy of Europe (YAE). He currently acts as Chair of the Scientific Advisory Board of the ARCANE Excellence Laboratory Network (LABEX) for bio-driven chemistry in Grenoble and as co-head of the French network (CNRS-Groupement de recherche) on Solar Fuels. Since 2016, Vincent Artero is associate editor of the Royal Society of Chemistry journal "Sustainable Energy and Fuels". From January 2018 onward, he actsas associate editor of the Royal Society of Chemistry flagship journal "Chemical Science"
Mimicking photosynthesis and producing solar fuels is an appealing way to store the huge amount of renewable energy from the sun in a durable and sustainable way. Various technologies exist with different readiness levels [1]. A variety of solar fuels can also be produced, including hydrogen but also syngas or methane. In that context, we will exploit data from our recent contributions regarding the preparation of photoelectrode materials and the design of tandem photoelectrochemical cells [2], but also artificial leaves [3] and integrated PV-EC cells to benchmark these approaches and propose a path forward for solar fuels technologies.
1.3-I2
Sophia Haussener is a Professor heading the Laboratory of Renewable Energy Science and Engineering at the Ecole Polytechnique Federale de Lausanne (EPFL). Her current research is focused on providing design guidelines for thermal, thermochemical, and photoelectrochemical energy conversion reactors through multi-physics modelling and experimentation. Her research interests include: thermal sciences, fluid dynamics, charge transfer, electro-magnetism, and thermo/electro/photochemistry in complex multi-phase media on multiple scales. She received her MSc (2007) and PhD (2010) in Mechanical Engineering from ETH Zurich. She was a postdoctoral researcher at the Joint Center of Artificial Photosynthesis (JCAP) and the Lawrence Berkeley National Laboratory (LBNL) between 2011 and 2012. She has published over 70 articles in peer-reviewed journals and conference proceedings, and 2 books. She has been awarded the ETH medal (2011), the Dimitris N. Chorafas Foundation award (2011), the ABB Forschungspreis (2012), the Prix Zonta (2015), the Global Change Award (2017), and the Raymond Viskanta Award (2019), and is a recipient of a Starting Grant of the Swiss National Science Foundation (2014).
High power density devices are required for economically competitive and sustainable solar fuel devices. I will review our solar hydrogen demonstrations at high current density and based on thermally integrated photo-electrochemical approaches. I will show how the design evolved when moving from lab-scale, to system-scale, and to industrial scale [1]. I will comment on the indoor experimental conditions compared to outdoor experiments. I will show how the thermal-integration principle can be extended for CO2 reduction devices [2] and for reversibly operated devices (forward mode for hydrogen generation, backward mode for power generation). I will show how indoor experiments with high-flux solar simulators can be used for the performance assessment of such devices, including the assessment of longevity and degradation [3]. I will end with the introduction of a novel design concept for photocatalytic solar fuel generation based on droplets. I will show how such drop-based system con provide a continuously operating system with high throughput and competitive production rates.
1.3-O1
While hydrogen production via photoelectrochemical (PEC) water splitting has been demonstrated on a small scale, developing an industrial scale device is a challenge that intrigues and brings together researchers from a range of disciplines. I will present our prototype PV-PEC device, with a photo-absorbing area >100 cm2, which operates with a photoanode comprising of a WO3/BiVO4 heterojunction on FTO. A key bottleneck in the scalability of PEC devices remains the development of scalable photocatalyst materials for the water splitting reaction. Our photoanodes are produced by conformally coating BiVO4 onto WO3 nanoneedles using low-cost and scalable chemical vapour deposition methods. With the band gap of BiVO4 enabling light absorption up to 517 nm and a theoretical STH of up to 9.2%, the WO3/BiVO4 heterojunction system is one of the most promising in terms of performance, cost and durability. Combined with a Ni mesh cathode and externally connected homojunction silicon PVs this creates a cost-effective and scalable photoelectrochemical-photovoltaic (PV-PEC) device with a commercially viable fabrication method. I will discuss how the arrangement of the PVs, either behind (in tandem) or next to (side-by-side) the photoanode, affects the operation and performance of the device. Many photoelectrodes are produced as thin films on transparent conducting oxides, such as fluorine-doped tin oxide (FTO) or indium-doped tin oxide (ITO). However, one difficulty to overcome is the resistivity of FTO glass, which can result in severe resistance losses in scale-up. Without mitigation, this can lead to reductions in photocurrent of over 80%. I will present steps we have taken to mitigate this issue, with minimal performance losses between 1 cm2 and 36 cm2 electrodes.
Considering heat and mass transfer, as well as fluid dynamics, is not only critical when optimising the efficiency of scaled-up devices, but also to address safety challenges associated with increasingly larger systems. I will therefore discuss our work to optimise the temperature and electrolyte flowrates for long-term, stable operation. To deliver stable operating conditions, the PEC stability of materials must also be addressed. I will present work to improve the PEC stability of BiVO4 photoanodes, through the use of co-catalysts, doping, different electrolytes and varying fabrication conditions. In preliminary results, WO3/BiVO4 electrodes with NiOx co-catalyst layers have shown stability for 24 hours with an applied potential of 1.23 VRHE in pH 9 potassium borate buffer.
This research seeks to elucidate challenges of developing up-scaled materials for water splitting, to facilitate the pathway to commercially viable photoelectrochemical hydrogen production.
2.1-I1
The Liquid Sunlight Alliance (LiSA) has demonstrated two photochemical architectures that combine a light absorber and a multi-catalyst cascade to achieve conversion of CO2 and water into liquid fuels using sunlight. Both are complete systems including a light absorber and a multi-catalyst cascade to achieve CO2 reduction to carbon-based fuels. Realization required careful management of light absorption and conversion and supply of electrons to multiple catalyst sites, while at the same time controlling reactant, intermediate, and product fluxes. I will describe the work of two task forces within LiSA that designed the systems, synthesized, and integrated the components, and evaluated their performance.
The first architecture is a three-terminal tandem (3TT) system which uses a monolithic semiconductor photoelectrode using sunlight to drive chemical reactions in a cascade that produces a liquid fuel. The semiconductor architecture, based on a 3TT solar cell, absorbs light and generates electrons that are used for two separate reduction reactions at two of the terminals. The product of the CO2 reduction reaction in the first location, CO, moves from where it is formed to the second location where it is reduced further to form methanol. The protons needed for the reduction reaction are generated from water at the third terminal. The photoelectrochemical cascade has two steps: (1) two-electron reduction of CO2 to CO (driven by the GaInP subcel) and subsequent four-electron reduction of CO to methanol (driven by the GaInP/GaAs tandem subcell).
The second architecture combines photoelectrochemical and solar-driven thermocatalytic environments. A photoelectrochemical (PEC) reactor is used to reduce CO2 to ethylene (with minimal coproduction of H2, CO, and CH4). Its design minimizes losses due to crossover between the electrodes and can achieve efficient conversion of CO2 as well as high selectivity. The PEC cell can achieve an ethylene Faradaic efficiency (FE) of ~30% and a single pass concentration of > 0.5 vol.% to ethylene. Ethylene is then oligomerized in a second reactor using a supported Ni catalyst. This PEC – solar thermal tandem system has successfully produced butene and hexene. Notably also, the thermocatalytic reactor operating by itself in batch mode can produce C7 – C24 products from a pure ethylene feed using 1 Sun illumination.
2.1-I2
The establishment of so-called artificial photosynthetic reactions that use solar energy to drive the synthesis of organic matter using H2O and CO2 as raw materials is significant for reducing CO2 emissions and realizing carriers of abundant solar energy. 1, 2 3 Our primary approach to artificial photosynthetic reactions is the combinatorial technologies that effectively utilize the excellent properties of solid semiconductor photosensitizers and molecular metal complex catalysts. One important progress is the electrochemical and photocatalytic CO2 reduction reaction (CO2RR) over metal complex catalysts at a low reaction overpotential approaching the theoretical lower limit in aqueous solution. The essential point of the low overpotential CO2RR is the operation of the metal complex catalysts with coexisting carbon support and metal cations such as K+. 3, 4 In addition, we have realized the low overpotential CO2RR combined the H2O oxidation reaction (WOR), which is a pair reaction for artificial photosynthesis, in a single aqueous solution at near neutral pH. 5 Simultaneous operation of the CO2RR and the WOR in a single solution is one of the concepts necessary to construct a new simplified form of an artificial photosynthetic system that does not require a setup for separation of sites for CO2RR and WOR like the thylakoid membrane existing in natural photosynthesis.
In this presentation, I will explain systems that make effective use of semiconductors and metal-complex catalysts driven in a single aqueous solution under simulated sunlight or visible light irradiation: a photocatalytic system functioning by a two-step photoexcitation (Z-scheme) mechanism in a self-organized manner using a combination of particulate (CuGa)0.3Zn1.4S2, BiVO4, and Co-bipyridine complex 6, 7, a so-called artificial leaf with a thickness of less than 1 mm consisting of Ru-bipyridine complex, IrOx, and an amorphous Si-Ge triple junction 5, and a 1m2-sized cell composed of Ru-bipyridine complex, IrOx, and a crystalline Si solar cell. 8-10
2.1-I3
The most challenging deal we face today is the need to lower greenhouse gas (GHG) emissions and tackle climate change. Though calls to reduce it are growing louder yearly, emissions remain unsustainably high. CO2 is the key contributor to global climate change in the atmosphere. Electrochemical CO2 reduction (EC CO2R) into chemicals or fuels holds great research interest as a promising approach to mitigate CO2 emissions and reach a carbon-neutral future.[1] In this regard, an extraordinary effort has been made to discover new efficient and sustainable catalysts at the laboratory level over recent years. High-performance electrocatalysts in aqueous electrolytes often rely on noble metals, which may hinder their industrial applications. Herein, we successfully synthesized core-shell Cu2O/SnO2 nanoparticles functionalized with a silane group, using a simple and versatile methodology based on a three-step scalable synthesis method involving wet precipitation followed by salinization and, finally, a rhenium-based complex has been assembled by electro-polymerization. The carbon paper-supported Cu2O/SnO2-Re electrocatalyst was characterized at 10 cm2 scale, demonstrating a steady-state production of syngas at -20 mA·cm-2 up to 24 hours, achieving a CO:H2 ratio from 3 to 9. To translate those developments from the laboratory level to a higher TRL towards the practical application for CO2 capture and utilization[2], an additional chamber was added to the system for continuous CO2 capture and electrochemical conversion, increasing the electrode area from 10 cm2 to 100 cm2. Captured CO2 co-electrolysis to syngas (H2:CO ratio of 5) in one step was demonstrated with a high CO2 conversion at a current up to -2 A, indicating the scale-up potential of this intensified system. The technology is under validation in a TRL4 reactor composed of an array of 5 modules (i.e., 5 x 4 cells x 100 cm2 or 0.2m2) for direct CO2 conversion from simulated anthropogenic sources. The design ensures a self-bias operation by integrating low-cost perovskite photovoltaic (PV) cells to provide any required additional bias to drive the reaction with Perovskite PV panels with a cost of up to 5 times lower (10 €/m2) than Si PV cells. Besides, to further enhance the performance, ionic liquids (ILs), which have unique properties, have been proposed to perform CO2 capture and to boost CO2-derived products. Some of us have identified as role of the anions of imidazolium based ILs the tuning of the CO/H2 ratio over Ag-based catalyst in aprotic media.[3] In this work, for the first time, a Cu2O/SnO2-Re-based electrode has been used within a continuous flow cell and in the presence of ILs-based solutions. However, we have observed different stability issues, such as the blackening of typical carbon-based gas diffusion layers (GDLs) and the degradation/colour changes of ILs-electrolyte. Field Emission Scanning Electron Microscopy (FESEM) and Electrochemical Impedance Spectroscopy (EIS) techniques have been employed to carry out the physicochemical characterization of the electrodes and to assess the electrochemical interfaces within the system, respectively. The observed findings offer openings for large-scale carbon capture and CO2 reduction technology deployment. The TRL5 demonstration our most stable developed technology is planned in 2024 at the facilities of IIF Spain with real flue gas emissions.
1.1-I1
The unproven durability of perovskite photovoltaics (PVs) is likely to pose a significant technical hurdle in the path towards the widespread deployment of this burgeoning thin-film PV technology. The overall durability of perovskite PVs, which includes operational stability, is directly affected by the mechanical reliability of metal-halide perovskite materials, cells, and modules, but this issue has been largely overlooked. Thus, there is a sense of urgency for addressing the mechanical reliability issue comprehensively, and help perovskite PVs reach their full potential. This presents many challenges, but it also offers vast research opportunities for making meaningful progress towards more durable perovskite PVs. Here I will highlight the important challenges and opportunities, together with best practices, pertaining to the three key interrelated elements that determine the mechanical reliability of perovskite PVs: (i) driving stresses, (ii) mechanical properties, and (iii) mechanical failure. I will also present examples of approaches to mitigate failure and extend the durability of perovskite PVs.
1.1-O1
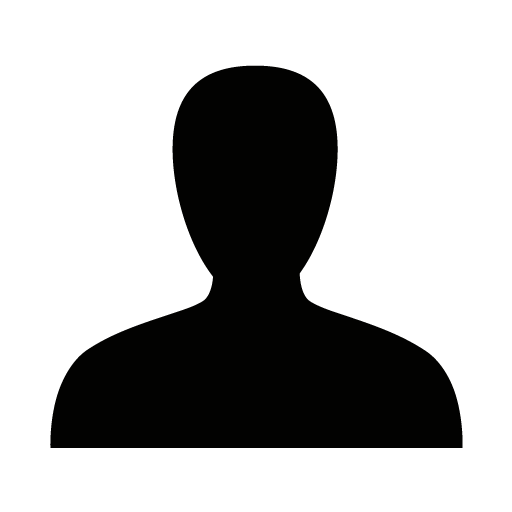
Mixed tin–lead perovskite materials encounter multiple degradation pathways, limiting their efficacy in tandem photovoltaic technologies. Overcoming these challenges necessitates addressing the thermal instability of the methylammonium cation within the perovskite composition and concurrently enhancing the oxidation resistance of the tin-based component. This study introduces a comprehensive approach to address these limitations, resulting in methylammonium-free tin–lead perovskite solar cells with heightened efficiency and stability. Our methodology involves a multi-component strategy, targeting each constraint. By incorporating cations capable of fine-tuning precursor solution properties, we achieve significantly improved film quality in methylammonium-free perovskites. Concurrently, the integration of reducing agents and surface engineering techniques substantially enhances the robustness and carrier dynamics of the perovskite films. As a testament to the success of our approach, the methylammonium-free perovskite solar cells exhibit an exceptional efficiency exceeding 22%, coupled with significantly enhanced device stability. Remarkably, these devices maintain negligible losses even after over 700 hours of continuous operation under 1 sun illumination. This work underscores the potential of comprehensive strategies in processing delicate materials like tin-containing perovskites, elevating their quality and positioning them for broader, successful applications.
1.1-O2
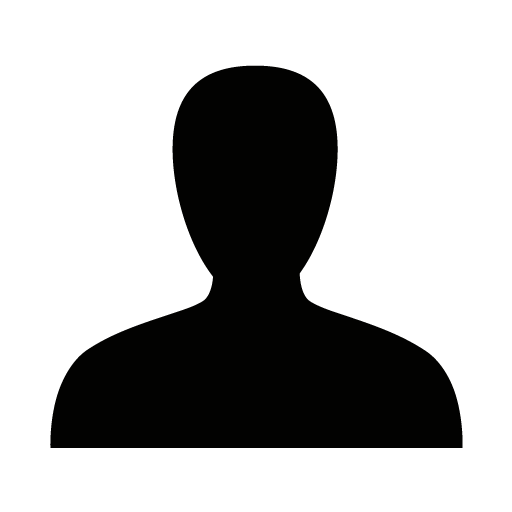
Metal-halide perovskites have been under intense research, for their capability to outperform the single-junction detailed-balance limit through the tandem device architecture by tuning the bandgap via compositional engineering. Mixed lead-tin halide perovskites offer the ideal low bandgap as the bottom subcell for such tandem photovoltaic devices. However, they suffer from various instabilities when exposed to ambient air, comparable to tin-halide perovskites. Mixed lead-tin halide perovskites have been considered to degrade in a manner akin to that of tin-halide perovskites, through formation of tin vacancies and self hole-doping. The differences in the way optoelectronic properties worsen and how trap states develop between FA0.75Cs0.25Pb0.5Sn0.5I3 and FA0.75Cs0.25SnI3 in ambient air were studied and compared in our work, which we present. When exposed to ambient air, both perovskite compositions are subject to optoelectronic degradation through development of trap states, evidenced by the reduction of charge-carrier diffusion lengths. However, it was revealed that deep trap states are formed in lead-tin perovskites during degradation, which deteriorates charge-carrier lifetimes but does not considerably affect charge-carrier sum mobilities. Tin-only perovskites, however, undergo formation of energetically shallow tin vacancy trap states and valence band doping. We also compare the structural degradation in these perovskites. The urge for specific passivation methods for mixed lead-tin iodide perovskites is emphasised in this work. We anticipate such passivation will lead to air stability enhancement, expediting the commercialisation of all-perovskite tandem devices.
Lim, V. J.-Y. et al., Air-Degradation Mechanisms in Mixed Lead-Tin Halide Perovskites for Solar Cells, Adv. Energy Mater. 2023, 13, 2200847
1.1-I2
Iván Mora-Seró (1974, M. Sc. Physics 1997, Ph. D. Physics 2004) is researcher at Universitat Jaume I de Castelló (Spain). His research during the Ph.D. at Universitat de València (Spain) was centered in the crystal growth of semiconductors II-VI with narrow gap. On February 2002 he joined the University Jaume I. From this date until nowadays his research work has been developed in: electronic transport in nanostructured devices, photovoltaics, photocatalysis, making both experimental and theoretical work. Currently he is associate professor at University Jaume I and he is Principal Researcher (Research Division F4) of the Institute of Advanced Materials (INAM). Recent research activity was focused on new concepts for photovoltaic conversion and light emission based on nanoscaled devices and semiconductor materials following two mean lines: quantum dot solar cells with especial attention to sensitized devices and lead halide perovskite solar cells and LEDs, been this last line probably the current hottest topic in the development of new solar cells.
Halide perovskite solar cells have revolutionized the photovoltaic field in the last decade. In a decade of intensive research it has been a huge improvement in the performance of these devices, however, the two main drawbacks of this system, the use of hazardous Pb and the long term stability, still to be open questions that have not been fully addressed. Sn-based perovskite solar cells are the devices presenting the highest performance after Pb-based but significantly below them. In addition, Sn-based perovskite solar cells exhibit a long term stability lower than their Pb containing counterparts, making stability their main problem. In this talk, we highlight how the use of proper additives and light soaking for defect engineering can increase significantly the stability of formamidinium tin iodide (FASnI3) solar cells, and discuss about the different mechanism affecting this stability, beyond the oxidation of Sn2+, and how they can be countered, analyzing specially the light soaking treatment. In addition, the up-scaling of these devices by the use of blade coating introduces new challenges that will be analyzed in this talk. Beyond perovskite solar cells halide perovskite are outstanding for the development of other optoelectronic device, causing that currently the research with these materials widespread to different optoelectronic fields. Preparation and optimization of Sn-based LEDs will be discussed as well as the possibilities of fabrication with industrially friendly methods as inkjet printing.
1.2-I1
Organic-inorganic hybrid perovskites (OIHPs) are a fascinating class of semiconductors that can be low-temperature synthesized, crystallized and processed on a large variety of substrates, and at the same time offering outstanding optical and electronic properties, such as broadband spectral tunability, high defect tolerance, high absorption/emission efficiency, room-temperature excitons, etc. These advantages allow OIHPs to complement conventional inorganic semiconductors (e.g., Si and GaAs) in photonics applications that require low-cost, large active area, wide spectral or polarization tunability or flexibility in substrate selection. In this talk I will talk about two OIHP-based photonic devices – photodetector and optically pumped laser. First, I will focus on the commonly seen gain – bandwidth trade-off problem of photodetectors and introduce a monolithically integrated photovoltaic transistor (PVT) design to solve this dilemma. The PVT exploiting a lead halide perovskite as the photoactive layer achieved a record high gain – bandwidth product of ~ 1011. Secondly, I will talk about our recent exploration of using quasi-two-dimensional Dion-Jacobson (DJ) phase perovskites for laser application. With properly selected organic spacers, DJ phase OIHPs offer excellent chemical resistance and thermal processability. These properties allow us to achieve optically pumped perovskite lasers with record-high quality factor, record-low lasing threshold and excellent operational stability.
1.2-O1
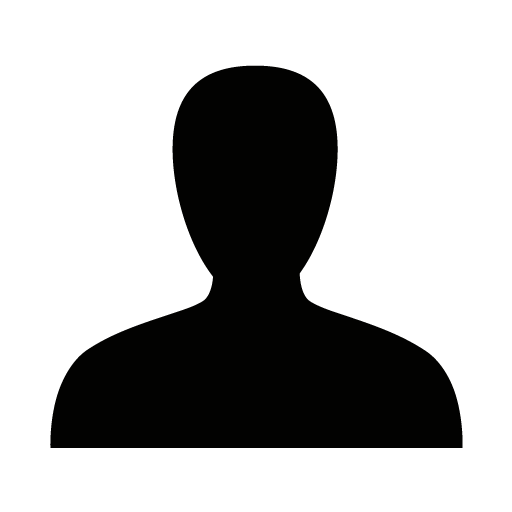
Nanoparticle supercrystals extend the fascinating properties of colloidal solutions of microscopic quantum dots to the macroscopic realm and are therefore of significant interest for various optoelectronic device applications such as solar cells, LEDs, electro-optic modulators, etc. [1]
We recently reported a study on defect/strain-related optical properties in self-assembled supercrystals of lead halide perovskites. Specifically, reproducibly observed spatial gradients in the photoluminescence energies and lifetimes of CsPbBr2Cl and CsPbBr3 supercrystals were shown to result from a combination of compressive strain, a loss of structural coherence, and an increasing atomic misalignment between adjacent nanocrystals. These findings expand on the idea of quantum dots functioning as quasi-atomic building blocks in the formation of macroscopic superstructures. [2]
The presentation will detail the spatially and temporally resolved optical measurements and present current efforts towards understanding and achieving control over the defect-related optical properties through adjustment of parameters both in the synthesis (particle size distribution, surfactant concentration) and the self-assembly (evaporation-method, -time, -temperature) of the nanoparticular building blocks. Furthermore we show that precise and quantifiable mechanical deformations and compressive strain induced by micromanipulators may be used to further explore the relationship between structural and optical properties of these superstructures.
1.2-I2
Jacky Even was born in Rennes, France, in 1964. He received the Ph.D. degree from the University of Paris VI, Paris, France, in 1992. He was a Research and Teaching Assistant with the University of Rennes I, Rennes, from 1992 to 1999. He has been a Full Professor of optoelectronics with the Institut National des Sciences Appliquées, Rennes,since 1999. He was the head of the Materials and Nanotechnology from 2006 to 2009, and Director of Education of Insa Rennes from 2010 to 2012. He created the FOTON Laboratory Simulation Group in 1999. His main field of activity is the theoretical study of the electronic, optical, and nonlinear properties of semiconductor QW and QD structures, hybrid perovskite materials, and the simulation of optoelectronic and photovoltaic devices. He is a senior member of Institut Universitaire de France (IUF).
2D multilayered perovskites share similarities with 3D perovskites including direct electronic band gap, sizeable optical absorption, small effective masses, Rashba-like effects. A recent classification of multilayered perovskites as Ruddlesden-Popper, Dion-Jacobson and "Alternative cations in the interlayer" was introduced in relation with the chemistry of the compounds or the crystallographic order along the stacking axis. Interestingly, they exhibit other attractive features related to tunable quantum and dielectric confinements, strong lattice anisotropy, more complex combinations of atomic orbitals and lattice dynamics, extensive chemical engineering possibilities. This will be illustrated by recent combined experimental and theoretical studies on excitons, formation of edge states, hot carrier effects and carrier localization. 2D multilayered perovskites have exhibited improved device stability under operation. More, combined in 2D/3D bilayer structures using new versatile growth methods, excellent solar cell device stability can be achieved. Band alignment calculations nicely explain the difference of performances for ni-p or p-i-n devices. The lattice mismatch concept can provide further guidance for the choice of the proper 2D/3D combination, leading to enhanced stability for 3D-based solar cells.
1.3-I1
Our group focus on physical chemistry, materials science, and the application of materials for energy production, studying the synthesis-structure-property relationship of functional materials for energy production. We emphasize developing novel syntheses for advanced materials and devices for solar energy into useful forms of sustainable energy & fuels. Our research lies at the intersection between innovative approaches, fundamental studies, and applying advanced materials for solar energy conversion.
A perspective into synthesis methods far from thermodynamic equilibrium (i.e., non-equilibrium, NE) and their dependencies with the structure and properties of light-absorbing semiconductors and their devices will be presented. The talk will focus mainly on multinary metal oxides as case study materials using plasma deposition processes combined with rapid thermal processing (RTP).[1,2] However, the insights are also strongly transferable into metal halide perovskites.[3,4]
In the research and development of metal oxide semiconductors and a few metal halide perovskites for solar energy conversion, scientists are confronted with two significant challenges: i) the need to exceed normal temperature limits for glass-based F:SnO2 substrates (FTO, ∼550°C) to achieve the desired density, crystallinity, and low defect concentrations, and ii) avoiding the formation of structural defects, trap states, grain boundaries, and phase impurities, which can be particularly difficult in multinary materials which may contain ions that vary widely in size, oxidation state, and vapor pressure under heating treatment conditions. The unique possibilities of NE synthesis methods can be utilized to form a holistic approach that will overcome these challenges and also provide a broad array of synthesis "tuning knobs" under highly controlled synthesis conditions.
I will demonstrate, using the emerging metal oxide semiconductors for photoelectrochemical water-splitting α-SnWO4 and CuBi2O4, that even subtle changes in synthesis significantly impact material properties, physical working mechanisms, and performances. These materials ' challenges were greatly overcome using the NE synthesis conditions, which were inaccessible through conventional solid-state reactions, with increased crystallinity, conductivity, and device performance.[1,2,5]
The NE synthesis methods can successfully address a primary need to focus on novel syntheses and design approaches of disruptive and innovative materials and NextGen devices that meet the chemical and physical requirements for reducing global warming through sustainable development.
1.3-O1
Metal halide perovskites have achieved impressive energy conversion efficiencies (PCEs)in both single junction and tandem solar cells. However, a key challenge for their practical applications lies in the lower stability of the devices, which is determined not only by the perovskite materials but also by the charge transport layers. Currently, all high-performance perovskite solar cells (PSCs) with > 24% PCE are based on the bench-mark hole transport layer Spiro-OMeTAD, which is doped with lithium bis(trifluoromethane)sulfonimide (LiTFSI) and 4-tert-butylpyridine (tBP), which has a negative impact on the stability of the devices. Moreover, due to the complex in situ oxidation processes, it is difficult to understand the mechanism of conventional spiro-OMeTAD doping, which further limits the development of stable HTLs with high PCEs.
We have developed a clean and free post-oxidation doping for spiro-OMeTAD by using stable organic radicals as the dopant and ionic salts as the dopant modulator (termed ion-modulated (IM) radical doping).1 The doped Spiro-OMeTAD based on our IM radical doping strategy provided high PCE of over 25% and excellent stability (T80 for ~ 1200 h under 70±5% relative humidity and T80 for ~ 800 h under 70±3 °C without encapsulation), minimizing the trade-off between efficiency and stability of PSCs. In this doping strategy, the radicals provide hole polarons that immediately increase the conductivity and work function, and the ionic salts further modulate the work function by affecting the energetics of the hole polarons. The IM radical doping strategy provides a simple and effective approach to separately optimize the conductivity and WF of organic semiconductors for a variety of optoelectronic applications.
1.3-O2
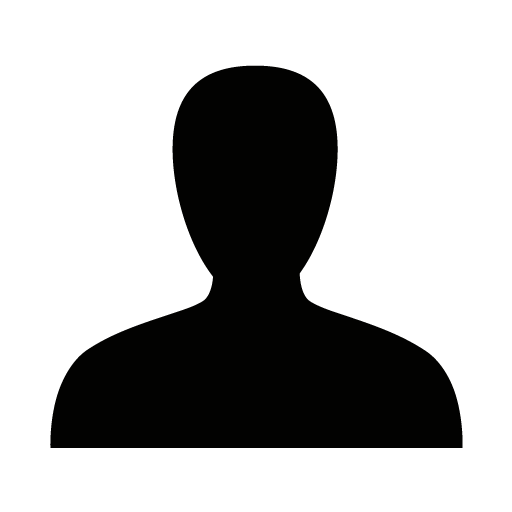
Perovskite Solar Cells (PSCs) have reached impressive performances above 25% in just few years of research.[1] However, there are several factors leading to the limited long-term stability of the PSCs [2], which is still hampering their industrialization. Among them, the highly hygroscopic doping agents, frequently used to increase the conductivity of the hole transporting layer (HTL), accelerate the Perovskite degradation. Hence, the hole transporting materials (HTMs) are now being developed to get high efficiency in the absence or low concentration of these dopants. [3]
In this context, starting from the low-cost phenothiazine and carbazole scaffolds, which are characterized by easily tuneable energetic levels, we synthesised small molecules and polymers through a Suzuki coupling, eventually employing microwaves to promote the reaction yield and selectivity. In the meanwhile, greener synthetic routes have been studied. Once characterized, the materials were implemented in inverted PSCs, which are normally cheaper and more stable than the direct devices. [4] Their performances were studied: the most promising results were obtained with the small molecule A (figure 1), which was able to reach up to 10.96% of Power Conversion Efficiency (PCE) in the optimized conditions (vs 13.07% with the PTAA reference), attesting a high charge extraction. The applied thermal treatments allowed to increase the stability of the devices during the time.
1.3-O3
Perovskite solar cells (PSCs) are the most promising PV technology in recent years. Efficiency rocketed from 3.1% to 26% in the last decade. The highest-performing PSCs are lead-based, which increases concerns about the environmental impact of this type of solar cell. Consequently, interest is growing in tin-based PSCs as an environmentally friendly alternative to the lead counterpart. However, tin perovskites are hindered by the tin (II) oxidation to tin (IV), which leads to self-doping and devastating cell performance. DMSO, the universal solvent for tin perovskites, was found to oxidize tin [1]. In our previous work, we introduced a group of 15 different solvents that can form 1 Molar solution of FASnI3 [2]. However, the film formation dynamics were challenging due to unsuitable coordination between the solvent molecules and the metal, leading to bad micro-structured films. In this work, we introduce a high-throughput screening of 73 antisolvents against the previously introduced solvents to engineer the crystallization dynamics in tin perovskites. Then, we feed the resultant data into a machine learning algorithm with the solvents and the antisolvents parameters to conclude the most related parameters that control the solvent-antisolvent interaction in tin halide perovskites. In addition, the algorithm predicts the most effective solvent-antisolvent pairs that can form a perovskite film based on the film darkness prediction. Furthermore, we use Hansen parameters spheres to explain the relationship between the solvents and the antisolvents that make the highest-performing perovskite film. Finally, we test the most promising tin perovskite films for their efficiency and report a PCE of around 9%, the highest DMSO-free tin halide PSC as far as we know.
2.1-O1
Metallic lead is easily formed in films due to decomposition of residual PbI2 in excess lead iodide-incorporated perovskites under light or X-ray irradiation. Pb0 is a typical intrinsic defect which limits mainly the stability of this type of solar cell [1]. A common strategy to heal the defective perovskite is passivation with thiol (-SH) containing ligands which can bind strongly to Pb0 or Pb2+ [2], and reduce directly -or indirectly- Pb0 impurities. Even though a large number of multifunctional compounds, bearing thiol groups, has been tested successfully, there is a lack of consensus on the origins of Pb0 elimination through this approach [3,4].
In this work, we used a model compound, 2-diethylaminoethanethiol (DEAET) in a very low concentration, as a top surface passivator of 10% PbI2 excessive-FAPbI3 and confirmed the enhanced radiative recombination through photoluminescence spectroscopy. XPS analysis has shown a total elimination of Pb0. When depositing DEAT ontop of PbI2 as a reference experiment, XPS revealed a simultaneous down shift for both Pb and I peaks, pointing to a clear chemical interaction of DEAT with PbI2; this interaction causes the disappearance of metallic lead from FAPI films. On the other hand, liquid 1H and 13C NMR, upon titration of DEAT with PbI2, has shown that the starting thiol disappears over time and a disulfide (oxidized form of the thiol) appears; finally, only the complex of PbI2 with the disulfide exists. The findings of this study point to the necessity of understanding the redox chemistry of thiol-based salts that dictates the passivation of PbI2-excessive FAPI perovskite.
2.1-O2
Perovskite solar cells currently enter a stage, where market introduction is within reach. For serious upscaling, control over the quality of the perovskite material is most critical. To this end, the community currently relies heavily on laboratory experience, engineering, and fine-tuning approaches. A key challenge is the controlled growth of perovskite crystallites while processing thin films. Several strategies are currently in use, such as like anti-solvent or additive engineering. While the impact of these strategies is well-evidenced in the resulting layers, the underlying mechanisms that govern the crystallization process are still subject to a vigorous debate. A frequently cited theory is that the nuclei for the perovskite crystallization evolve from intermediate Pb2+-MA+-I--solvate clusters. [1] A dominant role of solvate clusters implies a strong impact of complexing and coordination in the precursor solution on the crystallization process. Reports of the colloidal structures and Lewis-base-acid interactions in the perovskite precursor ink seemingly support this theory. [2] As of yet, however, insights that unambiguously link the complex formation in the precursor inks to the perovskite formation are lacking.
We present a holistic approach in which we study the entire course of perovskite formation. We begin with as study of lead complexation in the precursor stage (using NMR and electrical conductivity measurements in the solution) and proceed with in-situ GIWAXS investigations during thin film deposition and along the way to thin film formation (bare layers and solar cells). We systematically study the impact of common solvents like DMF, DMSO and NMP. As an exemplary additive, to study the influence of Lewis-base additives, we chose thiourea, which is a strong sulphur donor and an effective crystallization mediator. With 207Pb NMR and conductivity studies, we found a strong and systematic impact of the choice of solvents on the formation of lead complexes in the precursor solution, as well as indications, that suggest the presence of 3D corner sharing structures already in the precursor ink. Importantly, the differences in lead complexation depending on the solvent apparently diminish with increasing the concentration of the precursor ink; the final grain sizes remained largely unaffected by even strong variations found in the diluted precursors. On the other hand, the addition of thiourea did not affect the nature of lead complexes in the precursor solution. By in-situ GIWAXS, we are finally able to identify the annealing step as the decisive stage, where the presence of the additive affects the formation of perovskite grains and their crystallographic orientation. We could further substantiate our interpretation by FTIR studies. As such, for the first time, we provide a convincing link (or the lack thereof) between precursor chemistry and final thin film formation.
2.1-I1
Pablo P. Boix, Ph.D. in Nanoscience, is a Research Scientist at Instituto de Tecnologia Química (CSIC). He led a pioneer perovskite research team at Nanyang Technological University (NTU), Singapore (2012-2016) with relevant contributions to materials and devices’ development (such as the first use of formamidinium cation in perovskite solar cells). His track record has more than 100 publications, which resulted in his selection as a Highly Cited Researcher in 2020 (Cross-Field) by Clarivate Web of Science, with an h index of 57. Dr. Boix is the co-inventor of 3 patents in the field of perovskite optoelectronics. Prior to his current position, he worked as a research group leader in a perovskite solar cell company (Dyesol Ltd, Switzerland), focusing on product R&D, and at Universitat de València. Currently, he is the PI of 2 research projects and the coPI of 3, including regional, national, and European funding.
Metal halide perovskite crystals in the macro and nano scales can leverage the huge versatility of the perovskite family. They present exceptional optoelectronic properties that make them attractive for a variety of applications. However, these structures usually depend on complex synthesis processes, where the ligands play a dominating role, or are limited by growth form factor and surface losses.
We present crystallization insights that enable less demanding synthesis processes, such as a promising sol-gel approach [1] for humidity-triggered nanoparticle crystallization or a contactless surface passivation technique for large crystals. The particularity of these systems can be adapted to specific applications such as memristive devices or downconversion films with efficiencies >95%, which also serve as a platform to characterize the fundamental working mechanisms of these materials.
The combination of the crystallization control with additive engineering also opens new routes for lead-free perovskite photovoltaics, improving their ambient and operational stabilities.
2.2-I2
We are a multidisciplinary and collaborative research team with the overarching goal to establish structure-function relationships by understanding and advancing the fundamental knowledge rooted in the physics, chemistry and engineering of next generation materials for optoelectronics, sustainable, energy conversion, quantum computing, sensing and environmental preservation. Our philosophy is to develop creative and out-of-the-box approaches to solve fundamental scientific problems and apply this knowledge to demonstrate technologically relevant performance in devices.
Three-dimensional (3D) organic–inorganic lead halide perovskites have emerged in the past few years as a promising material for low-cost, high-efficiency optoelectronic devices. Spurred by this recent interest, several subclasses of halide perovskites such as two-dimensional (2D) halide perovskites have begun to play a significant role in advancing the fundamental understanding of the structural, chemical, and physical properties of halide perovskites, which are technologically relevant. While the chemistry of these 2D materials is similar to that of the 3D halide perovskites, their layered structure with a hybrid organic–inorganic interface induces new emergent properties that can significantly or sometimes subtly be important. Synergistic properties can be realized in systems that combine different materials exhibiting different dimensionalities by exploiting their intrinsic compatibility. In many cases, the weaknesses of each material can be alleviated in heterostructures. For example, 3D-2D halide perovskites can demonstrate novel behavior that neither material would be capable of separately.
In this talk, I will describe several examples of how synergistic properties between 3D and 2D give rise to emergent materials properties. I will describe the impact of deterministically combining 3D and 2D heterostructures on long-term durability, and stabilizing FAPbI3 without MA, Cs or Br, with >24% photovoltaic efficiency in a p-i-n architecture and also exceptional durability under 85 C, continuous AM1.5 illumination at MPPT.
2.2-O1
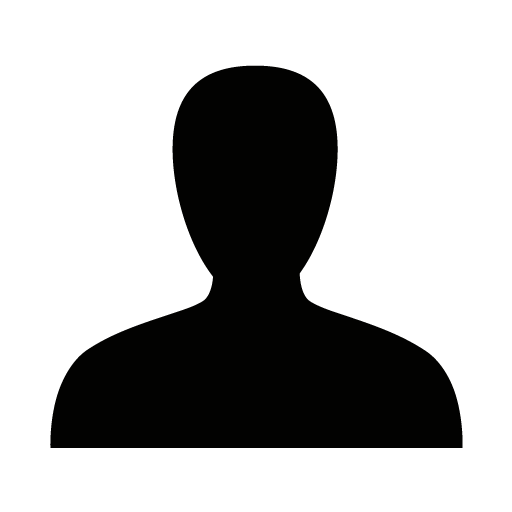
Recent advancements in halide perovskite photovoltaics have brought power conversion efficiencies to levels suitable for commercial use. Despite these advances, the durability of these devices is a major challenge that needs further improvement. A notable development in this area is the use of Ruddlesden-Popper (RP) two-dimensional (2D) perovskite layers on top of traditional three-dimensional (3D) perovskite active layers, enhancing the stability of perovskite solar cells (PSCs) [1-2]. While there have been several reports of efficient and stable PSCs using 2D/3D heterojunctions [3-4], the detailed interactions at the 2D/3D interface are not fully understood. Understanding the relationship between the atomic structure at this interface and electron behavior is essential to comprehend the effectiveness of these methods and to optimize the benefits of interface engineering. In this work, we used density-functional theory (DFT) calculations to explore how the atomic arrangement at interfaces influences the characteristics of 2D/3D halide perovskite heterojunctions. Our findings indicate that the thermodynamic stability and band alignment of these heterojunctions are significantly influenced by the arrangement of Cs/PEA cations at the interfaces.
2.2-O2
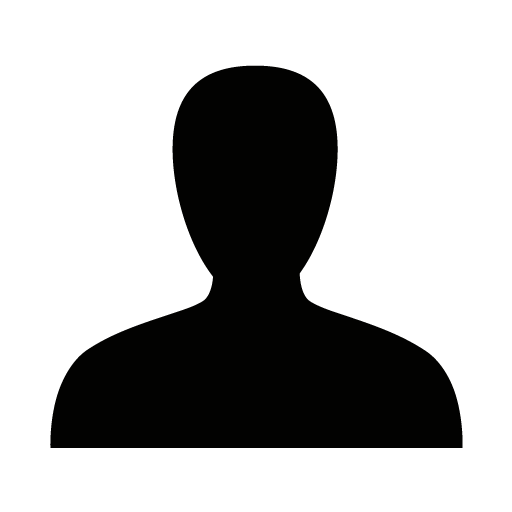
Two-dimensional (2D)-based passivation on three-dimensional (3D) perovskite layers has made great progress in enhancing the efficiency of perovskite solar cells, though 2D/3D has worse operational stability than 2D or 3D alone at elevated temperatures due to the severe ion migration and enhanced reactivity with formamidinium (FA+) [1-3]. It still remains mysterious in terms of how 2D/3D worsens device performance by altering device physics under heat and light soaking conditions. In this work, 11 kinds of ligands are chosen to passivate perovskite films with different concentrations and degraded under 3 ageing conditions (25℃-light, 85℃-dark and 85℃-light). Most of the 2D perovskite signals disappear after 85℃-light degradation. An unexpected absorbance upshift occurs for most of the passivated perovskite films after light soaking, which is attributed to light scattering by an undesirable phase of metallic lead (Pb0) decomposed continuously from lead iodide (PbI2) during light soaking. We find that it is ligands such as phenethylammonium (PEA+), which form the Ruddlesden-Popper (RP) phase, that accelerate the production of PbI2, which in turn produces more Pb0 and iodine (I2) in the presence of light or heat-light. Dion-Jacobson (DJ) and alternating-cation-interlayer (ACI) phases, in contrast, decelerate the production of Pb0 and I2. By choosing the proper ligand and passivation method, we have successfully fabricated ultra-stable solar cell, retaining 80% of its initial performance after 550 hours under 85℃ and two-sun illumination.
2.2-I1
Giulia is Associate Professor at Physical Chemistry Unit at University of Pavia, leading the PVsquared2 team, and running the European Grant ERCStG Project “HYNANO”aiming at the development of advanced hybrid perovskites materials and innovative functional interfaces for efficient, cheap and stable photovoltaics. Within this field, Giulia contributed to reveal the fundamental lightinduced dynamical processes underlying the operation of such advanced optoelectronic devices whose understanding is paramount for a smart device development and for contributing to the transition of a green economy.
Giulia received an MS in Physical Engineering in 2008 and obtained her PhD in Physics cum laude in 2012 at the Politecnico of Milan. Her experimental thesis focused on the realisation of a new femtosecond-microscope for mapping the ultrafast phenomena at organic interfaces. During her PhD, she worked for one year at the Physics Department of Oxford University where she pioneered new concepts within polymer/oxide solar cell technology. From 2012-2015, she was a post-doctoral researcher at the Italian Institute of Technology in Milan. In 2015, she joined the Ecole Polytechnique Fédérale de Lausanne (EPFL) with a Co-Funded Marie Skłodowska-Curie Fellowship. From 2016 to 2019, she has been awarded by the Swiss Ambizione Energy Grant providing a platform to lead her independent research group at EPFL focused on the developemnt of new generation hybrid perovskite solar cells.
She is author of 90 peer-reviewed scientific papers bringing her h-index to 44 (>13’000 citations), focused on developement and understanding of the interface physics which governs the operation of new generation solar cells.
Recently, she received the USERN prize in Physical Science, the Swiss Physical Society Award in 2018 for Young Researcher and the IUPAP Young Scientist Prize in Optics. She is currently USERN Ambassador for Italy and board member of the Young Academy of Europe.
More can be found at https://pvsquared2.unipv.it.
Weblink: https://people.epfl.ch/giulia.grancini?lang=en
Three-dimensional (3D)/low-dimensional (LD) perovskite solar cells (PSCs) offer an effective strategy to overcome the trade-off between perovskite solar cells device performance and stability. Improving the device longevity, while concomitantly simultaneously enhancing increasing the solar cell open circuit voltage and fill factor of the solar cell is the current challenge. Despite being one of the most popular and effective way processing techniques, whether the presence of this surface LDP layer would be - or not-represents the winning crucial path for the future of this technology remains elusive. In particular, atomic layer combined surface and bulk passivation using surface modifiers such as organic dopants, casts the doubts on the effective need for of having an homogeneous LDP cover capping layer. In this talk, I will discuss the interfacial passivation with different cations such as M-PEAI, M-PEABr, and M-PEACl, showing the role of the passivation and how different halides affect the device performance of the devices. In addition, I will compare recent results obtained in the 3D/LDP configuration with the surface cation passivation strategy, which can still pushes produce the performances to values comparable to the LDP/3D bilayers. I will providing a comprehensive perspective on the benefits from of the two different strategies, but also presenting how LDP interfaces can play a role in alternative new configurations, such as tandem solar cells.
2.3-I1
Inter alia, the application of 2D phenylethylammonium lead quaternary iodide (PEA2PbI4)/three-dimensional (3D) metal halide perovskite (MHP) interfaces has improved various optoelectronic devices, where a staggered type-II energy level alignment was often assumed. However, a type-II heterojunction seems to contradict the enhanced photoluminescence observed for 2D PEA2PbI4/3D MHP interfaces, which raises fundamental questions about the electronic properties of such junctions. Using direct and inverse photoelectron spectroscopy, we reveal that a straddling type-I energy level alignment is present at 2D PEA2PbI4/3D methylammonium lead triiodide (MAPbI3) interfaces, thus explaining that the photoluminescence enhancement of the 3D perovskite is induced by energy transfer from the 2D perovskite.
On another note, formamidinium lead triiodide (FAPbI3) is gaining attention as a perovskite solar absorber due to its close to optimal bandgap for single-junction solar cells and enhanced thermal stability compared to many other MHPs. However, in order to achieve the stable black-phase FAPbI3, a relatively high temperature annealing (150 °C) is required. Recent studies have highlighted the impact of high-temperature annealing on FAPbI3 crystal structure originating from lattice distortion and volume expansion. Nonetheless, the fundamental understanding of its electronic properties remained unclear. Here, we show that intrinsic iodine vacancies lead to n-type character, and that in-diffusing oxygen can fill iodine vacancies, leading to a Fermi level shift towards the valence band by about 0.5 eV. Furthermore, we demonstrate how the energy levels at interface to charge selective contacts can be controlled by doping, and how this influences the quasi-Fermi level splitting under illumination due to charge accumulation at the interface.
2.3-O1
In typical stability studies, perovskite solar cells (PSC) are tracked at its maximum power point (MPP) under continuous or cycled illumination at elevated temperature to simulate outdoor conditions. MPP decay curves evolve differently on different device configurations, depending on the perovskite composition, the selective contact materials or even batch-to-batch variations. Having a better understanding of the complex mechanisms that cause the performance decay at different timeframes is key for the future development of PSCs. In this work we propose a non-destructive method that can be used quasi in-situ along the MPP to gain additional information on the evolution of various mechanisms that govern the device efficiency, beyond merely measure JV curves. We analyzed several PSC devices with electrochemical impedance spectroscopy (EIS) and steady-state- and time-resolved- photoluminescence (PL/TRPL) before and after different stages of its MPP tracking and show the consecutive changes of multiple parameters including shunt resistances, recombination, ionic density/kinetics and formation of barriers and propose a model on the evolution of the degradation. We also show the importance of analyzing statistically-significant number of samples to account for the intrinsic –uncontrolled- variability existing within a batch.
2.3-I2
This talk will explore the use of carbon electrodes in the fabrication of perovskite solar cells via a high-volume continuous slot-die roll-to-roll (R2R) method, discussing both the opportunities and challenges this technology presents.
A key aspect of the presentation will be the development and application of a specialized carbon ink in the R2R process. This ink is crucial for creating an efficient and compatible electrode layer in perovskite solar cells. However, the integration of carbon into the tightly controlled sequence of the R2R process presents significant challenges. Achieving uniform deposition of the carbon layer at the same processing speeds as other layers requires precise control over the rheology and drying behavior of the ink. These factors are critical to maintain the efficiency and consistency of the solar cells.
One of the major hurdles in this process is ensuring that the carbon ink can be deposited seamlessly within the high-speed, continuous R2R process without compromising the quality of the perovskite layer. Achieving this requires an understanding of the material properties and the interaction between the different layers of the solar cell.
The talk will also address the broader vision of this technology. The ultimate goal is to realize the concept of "liquid in, solar cell out," where the entire solar cell, from the transport layers to the perovskite layer to the carbon electrode, is fabricated in a single, continuous process. This approach promises not only a reduction in production costs but also a significant increase in the scalability and accessibility of perovskite technology.
1.1-I1
Guillaume Wantz graduated from the School of Chemistry and Physics of Bordeaux (ENSCPB) in 2001 including a thesis work at Philips Research (Eindhoven, NL) on ink-jet printing. He received his Ph.D. in Electronics Engineering from the University of Bordeaux in 2004 working on Polymer Light Emitting Diodes. He was Assistant Professor at the University of Bordeaux working on Organic Field Effect Transistors with research stays at Queen’s University (Kingston, Canada). In 2006, he was appointed as tenure Associate Professor at the Bordeaux Institute of Technology (Bordeaux INP). He is Professeur des Universités since 2021. His research interest is on Organic Electronics with a focus on polymer photovoltaic solar cells (OPV). He was invited-professor at Queen’s University (Kingston, Ontario, Canada) in Spring 2012 and at Univ. of Massachusetts (Amherst, USA) in Fall 2014. He has been appointed at the “Institut Universitaire de France” (IUF Paris) in 2016. Since 2017, he is Associate Editor for the journal “Materials Chemistry Frontiers” (RSC). He is co-founder of Héole, a company developing flexible OPV products including solar-powered sails for yachting, a solar zeppelin and some BIPV flexible OPV products. To date, he has published 125 research papers in peer-reviewed international journals and issued 7 patents (h = 37 – 6000 citations – source Google Scholar).
Printable organic photovoltaic solar cells (OPV), i.e. polymer solar cells, have now reached impressive power conversion efficiencies at lab scale up to 19%. It is one crucial milestone towards the deployment of OPV products in real life. OPV holds many promisses including potential low cost, large scale, low temperature processing, low energy payback time, low carbon footprint for the production of photovoltaic modules exempt of critical raw materials. However, today, not all are yet scientifically achieved. For example, commercially available OPV modules suffer from low PCE, from 3 to 5 % (30-50 Wp/m2) and are made with still costly raw materials mostly processed from organic solvents. It is a matter of time for the industrial players to catch up with recent academic research to push industrial OPV performances further. This presentation will focuss on three of our recent results: (1) a doping strategy to enable a homojunction hole exctration layer for improved efficiency and stability of OPV, (2) the processing of OPV active layer from water based inks as the ultimate non-toxic, responsible printing with record efficiencies thanks to nanoparticules control and surface energy matching, (3) the investigation on the impact of synthesis impurities, such as metal catalyst residues, on the performances of OPV to design a strategy for cost-effective purification of raw materials. The presentation will close on some real life outdoor OPV energy yield considerations. Will be presented the results on recent products from Héole for marine decarbonation, such as the first OPV sail designed full-sized (92 m2) on a 52” catamaran, released in spring 2022, under testing since then.
1.1-I2
Stefania Zappia is a researcher at the "Istituto di Scienze e Tecnologie Chimiche “Giulio Natta” (SCITEC)" of "Consiglio Nazionale delle Ricerche (CNR)" in Milano (taly) since 2018. She obtained her Ph.D. in Chemical Sciences and Technologies from the University of Genova (Italy) in 2013, working on synthesis and characterization of organic and metal-organic hybrid materials for sensoric and photovoltaic applications. She then worked as a Research Fellow at "Istituto per lo Studio delle Macromolecole" of CNR in Milan from 2013 to 2018. She works on the synthesis of low band gap conjugated polymers for optoelectronic applications through bulk and flow synthesis, and on the synthesis of advanced polymeric and hybrid materials for CO2 recovery. She developed a strong expertise in the design and synthesis of amphiphilic rod-coil block copolymers for the functionalization of inorganic nanoparticles and/or for the preparation of polymeric nanoparticles processable from aqueous media for photovoltaic and/or biological applications.
The fabrication of the active layers in optoelectronic devices involves the use of large amounts of chlorinated organic solvents on the laboratory scale (i.e., chloroform, chlorobenzene, dichlorobenzene) in order to obtain morphology with an optimized interpenetrating network between electron donor and acceptor materials [1]. The ideal industrial production should be highly sustainable dramatically reducing the use of chlorinated solvents, then the environmental impact and the manufacturing cost of the devices [2,3].
Water-processable organic nanoparticles (WPNPs) of semiconducting polymers recently received wide attention for optoelectronic applications due to their simple fabrication and tunable properties. The WPNP-based approach could be appealing to control active layer morphology, and considerably reducing halogenated solvent use.[4]
Here we will report about a series of amphiphilic low band gap rod-coil block copolymers (BCPs), constituted by semiconducting electron donor polymers as the rigid segment (PCPDTBT and PTB7), and differing for the poly-4-vinylpyridine (P4VP)-based flexible blocks with different length and chemical composition.[5-8] These materials were designed in order to prepare blend WPNPs in aqueous suspensions with surfactant-free miniemulsion approach exploiting the interaction of the P4VP-based flexible segments with the non-solvent aqueous phase. Thus, we were able to prepare working OPV devices, exhibiting high short-circuit current density (Jsc=11.5 mA·cm−2, PCE 2.5%), with a sustainable fabrication process [9].
It is important to underline that the phase separation between the electron acceptor and donor materials leads to complex internal structures in the nanostructures. This is controlled by the inherent material properties, as long as the solvent evaporates. As in standard OPV devices, the surface energy plays a crucial role in the improvement of the miscibility of two components of the blend. Moreover, the surface energy affects the quality of the active layer’s morphology, enhancing the interfacial area between the materials with an efficient charge generation and dissociation, until the efficiency of the device is increased [10]. We prepared semiconducting blend WPNPs by combining the BCPs with suitable fullerene derivatives, showing higher surface energy with respect to the semiconducting polymers. The high surface energy leads to core–shell blend WPNPs with fullerene derivatives in the core and the electron donor polymers in the shell [11].
In order to clarify how the morphology of the nanodomains into the blend WPNPs is related to the features of the different coil molecular structures in the BCPs, and in turn how they led to different device performances, we achieved a complete spectroscopical, electrical and morphological WPNP characterization [12-14].
1.1-I3
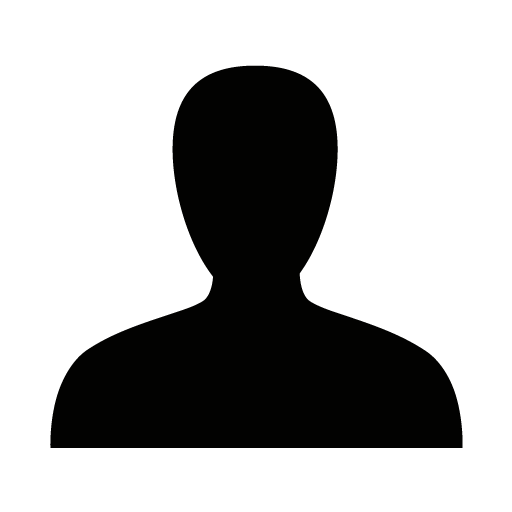
Organic photovoltaics (OPVs) have emerged as a promising technology for sustainable energy harvesting due to their lightweight, flexibility, and cost-effectiveness. However, realizing their full potential relies heavily on understanding and optimizing the intricate interplay of material properties, especially in the context of optical characteristics.
The optical properties of organic materials, such as absorption and emission spectra, exciton dynamics, and light scattering behavior, play a pivotal role in dictating the overall performance of OPVs. Accurate characterization of these properties provides insights into fundamental processes like exciton generation, dissociation, and charge transport within the active layer. Precise knowledge of absorption spectra enables the selection and design of materials with optimal light-harvesting capabilities, ensuring an absorption range compatible with the source spectrum, whether it is the sun or an artificial source.
Optical characterization techniques provide exceptional sensitivity and precision in probing material properties. Spectroscopic methods, including UV-Vis, Ellipsometry, Photoluminescence and Raman spectroscopy, enable the identification of molecular structures, chemical compositions, and electronic transitions with high accuracy. One of the primary advantages of optical characterization lies in its non-destructive nature. These techniques allow for the examination of materials without altering their intrinsic properties. This non-invasive quality facilitates the continuous monitoring of dynamic processes, making it possible to study real-time changes in materials under varying conditions. Also, as these techniques are contactless and do not require special preparation of the samples in most of the cases are particularly adapted for inline and online quality control on industrial environments.
In this work we present some examples of how the use of techniques such as Ellipsometry, Photoluminescence, optical spectroscopy or raman spectroscopy can help us to better understand the optical properties of the materials that are part of an organic photovoltaic cell, and lead to improvements in the performance on the final device, unveil the mechanism of degradation process es or develop special features on our final device.
1.2-I1
Solution processing from nanoparticle dispersions allows the use of eco-friendly processing agents for the deposition of organic semiconductor thin-films for photovoltaic and other optoelectronic applications. Omitting surfactants to stabilize the dispersions is essential to not jeopardize the solar cell performance. So far, solar cells could only be fabricated from surfactant-free P3HT dispersions which show some intrinsic self-stabilization. In this work, the self-stabilization of P3HT nanoparticle dispersions is demystified, and electrostatic effects are identified as the origin of self-stabilization. By application of this gained knowledge, novel surfactant-free nanoparticle dispersions from other, high-performance organic semiconductors are synthesized by nanoprecipitation. Design criteria will be discussed how to select the components of the dispersion. Electrical doping warrants the electrostatic stabilization of the dispersions. The role of the ionization potential of donors, the miscibility of donors and acceptors as well as the properties of the dispersion medium are elucidated. For the first time, the corresponding solar cells achieved power conversion efficiencies of up to 10.6%, demonstrating the general feasibility of this alternate, all-eco-friendly processing route.
1.2-O1
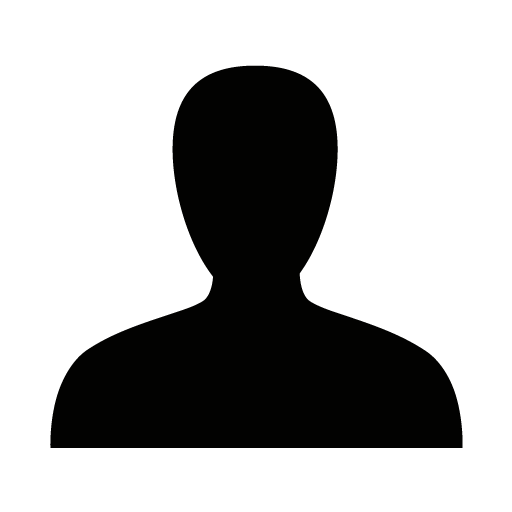
Organic photovoltaic (OPV) devices require active layers comprised of molecular heterojunctions to split excitons into free charges. These molecular heterojunctions are comprised of a binary blend of electron donor and electron acceptor material, where the highest occupied molecular orbital (HOMO) and lowest unoccupied molecular orbital (LUMO) offsets are sufficient for exciton dissociation. While optimising the morphology of organic photovoltaic active layers is increasingly important, measuring the morphology accurately has for some time been a challenge for researchers in the discipline. Here we report, for the first time, sub-4 nm mapping of donor : acceptor nanoparticle composition in eco-friendly colloidal dispersions for organic photovoltaics.1 Low energy scanning transmission electron microscopy (STEM) energy dispersive X-ray spectroscopy (EDX) mapping has revealed the internal morphology of organic semiconductor donor : acceptor blend nanoparticles at the sub-4 nm level. A unique element was available for utilisation as a fingerprint element to differentiate donor from acceptor material in each blend system. Si was used to map the location of donor polymer PTzBI-Si in PTzBI-Si:N2200 nanoparticles, and S (in addition to N) was used to map donor polymer TQ1 in TQ1:PC71BM nanoparticles. For select material blends, synchrotron-based scanning transmission X-ray microscopy (STXM), was demonstrated to remain as the superior chemical contrast technique for mapping organic donor : acceptor morphology, including for material combinations lacking a unique fingerprint element, or systems where the unique element is in a terminal functional group and hence can be easily damaged under the electron beam, e.g. F on PTQ10 donor polymer in the PTQ10:IDIC donor : acceptor blend. We provide both qualitative and quantitative compositional mapping of organic semiconductor nanoparticles with STEM EDX, with sub-domains resolved in nanoparticles as small as 30 nm in diameter. The sub-4 nm mapping technology presented shows great promise for the optimisation of organic semiconductor blends for applications in organic electronics (solar cells and bioelectronics) and photocatalysis, and has further applications in organic core–shell nanomedicines.
1.2-O2
The recent developments in organic photovoltaic (OPV) field make this technology highly promising with record power conversion efficiencies (PCE) of 19% in single junction solar cells achieved.1 This printed and low temperature processes technology is very attractive due to several advantages such as a low energy payback time, light weight, flexibility and transparency. However, one of the drawbacks of OPV is the high toxicity of the solvents used to process the active layer which are mainly aromatic and/or chlorinated.
In order to render this PV technology more environmentally friendly, we have been working on the development of water-based organic semiconductor colloidal dispersions.2 In this communication, I propose to present our recent developments in this field, on the control of the size and the morphology during the nanoparticle synthesis to fabricated highly efficiency OPV devices. Two strategies have been investigated. On the one hand, attempts to fabricated pure donor or acceptor nanoparticles with diameter below 30 nm have been done. To do so, we have been working on millifluidic systems to control precisely the size of the nanoparticles synthesized by nanoprecipitation. By increasing the flow rate of water anti-solvent, turbulent regimes were achieved in the mixing chambers, leading to smaller nanoparticles size. Using such kind of continuous flow devices, the effect of different additives such as surfactant on the nanoparticle size have been investigated. On the other hand, composite donor/acceptor nanoparticles were fabricated and control of the nanoparticle internal morphology was targeted. To this aim, we have been studying the influence of the surface energies of the donor and acceptor materials on the morphology of donor/acceptor composite NPs. We showed that matching the surface energies of the donor and the acceptor plays a major role to control the internal morphology of the NPs: a large interfacial energy between the donor and the acceptor leads to core-shell structure while a small one tends to give intermixed morphology. Organic photovoltaic devices were fabricated from water-based inks with varying donor/acceptor combination. Optimal performances were with PTQ10:Y6 NP, a donor/acceptor system presenting low interfacial energy. As a result, a NP-based active layer with optimal intermixed morphology was achieved and high efficiency devices with up to 9.98% PCE were fabricated.3 This work highlights the importance of selecting donor/acceptor combination with matching surface energy to ensure an optimal nanoparticle morphology in miniemulsion processes and, ultimately, reach highly efficient water-processed organic photovoltaic devices.
1.3-I2
Benjamin Watts is a beamline scientist at the PolLux scanning transmission soft X-ray spectro-microscope (STXM) specialising in the materials analysis of soft matter. He obtained a BSc (Professional) in Physics, with Honours, and a PhD in Physics from the University of Newcastle, Australia, before working as a post-doctoral researcher with North Carolina State University (permanently stationed at the Advanced Light Source, Berkeley) in the USA and then moving to PSI. Details of the interactions between soft X-ray light and organic materials has been a continuing theme throughout both his university research and later career.
The scanning transmission soft X-ray spectromicroscopy (STXM) technique allows detailed characterisation of nanoscale materials with strong natural contrast mechanisms. STXM scans a sample across a focused X-ray beam and sequentially measures the intensity of the transmitted beam to produce high resolution images (typically about 30 nm) and scans the photon energy of the incident X-ray beam to produce near-edge X-ray absorption fine structure (NEXAFS) spectra of nanoscale objects.[1] While the nanoscale imaging resolution is advantageous, the real power of the technique comes from using spectroscopic effects to achieve strong natural contrast based on molecular structure (and orientation via linear dichroism), elemental composition or oxidation state, and/or magnetisation (via XMCD).[2] The STXM hyperspectral datasets can be quantitatively analysed (i.e. produce percentage composition maps) either by comparing to the known spectra of the component materials, or by grouping sets of similar pixels (i.e. principal component analysis) to identify the materials present and quantify their proportion in each image pixel.
STXM is an excellent tool for characterising nanoengineered polymer films. The molecular structures present in the materials correspond to resonance peaks in the C K-edge NEXAFS spectra (280 – 350 eV), and so STXM images recorded at these photon energies provide strong, natural contrast to differentiate organic materials according to their molecular structures. This works especially well with polymeric materials since the molecular structures are repeated many times and the resulting resonance peaks tend to be intense, providing very clear contrast between the component materials. Here, we will present the operation principles of STXM and discuss some illustrative STXM characterisations of nanoengineered conjugated polymer materials.
1.3-O1
The sun’s photons provide the largest source of energy on earth and its exploitation could help solve the energy crisis without being detrimental for the environment. Organic semiconductors, either polymers (usually electron donor) or small molecules (fullerene or non-fullerene acceptors), can be dispersed in water to provide human and environmentally-friendly processes for photovoltaics as alternative to classical options typically using toxic organic solvents. Concerning solar fuel production, Pinaud et al. have demonstrated by life cycle analysis that a colloidal system, in which the photocatalysts are in the form of nanoparticles dispersed in water, would be the cheapest technology to the production of H2.[1] Therefore there is an important need to study the formation of organic semiconducting nanoparticles and develop their use in these solar-technologies.[2]
In this communication, I will show what we have developed the 4 last years on this topic and more precisely the use of the Nanoprecipitation methodology to prepare the nanoparticles.[3] I will discuss its advantages and drawbacks and the Donnor/Acceptor morphologies that can be expected from nanoprecipitation. Through two systems, P3HT/PCBM and PTQ10/Y6 I will describe the incorporation of these dispersions as the medium for photocatalyzed hydrogen generation and as the active layer of photovoltaics cells.
1.3-O2
Anna Maria Ferretti is Senior Research Scientist and Branch manager at SCITEC-CNR Branch of Via G. Fantoli at CNR-SCITEC Milano Italy. She received her PhD in Chemistry from University of Milano (Italy) in 2002. Her research topic is focused on synthesis, design and optimization of inorganic, organic and mixed inorganic organic based nanomaterials for applications in nanomedicine, organic photovoltaic and catalysis. Moreover, she is expert in Transmission Electron Microscopy (TEM) and its related analytical technique (EELS, ESI, STEM/EDX). In the last years, she focused on the TEM characterization of polymeric nanoparticles for sustainable OPV from morphological and structural point of view. She has co-authored over 75 JCR papers, 3 book chapters.
Assembling hydrophobic conjugated polymers into water-treatable nanoparticles is an innovative technology with many potential applications in optoelectronics, biology, and medicine. [1][2]
We demonstrate that it is possible to prepare stable water-processable nanoparticles (WPNPs) with good control of the morphology and the domain shape and distribution, exploiting amphiphilic low band gap rod-coil block copolymers (LBG-BCPs). The WPNPs were synthesized by an adapted miniemulsion approach, without surfactants. [3]
The LBG-BCPs consist of a rigid hydrophobic p-type semiconductor polymer, like PCPDTBT or PTB7, and 4-vinylpyridine(4VP)-based coil blocks. The obtained WPNPs are stable in water thanks to the presence of the 4VP-based coil, which improve the WPNPs stability in water without the presence of surfactants. The used copolymers have different molecular structure and length for each BCP used. To make the WPNPs a good material for OPV device active layer, we mixed the LBG-BCPs with an electron acceptor fullerene derivative ([6,6]-phenyl-C61-butyricacid methyl ester, PC61BM) to achieve blend WPNPs (b-WPNPs). The WPNPs and b-WPNPs were fully characterized by TEM, STEM-EDX, EFTEM, AFM, DLS, and z-potential. [4] [5] [6]
The Transmission Electron Microscopy and the micro-analytical technique associated provide information about morphology, and elemental intraparticle distribution with nanometric resolution (STEM-EDX analysis), which allows us to understand how the LBG-BCPs auto-assemble to give WPNPs. In fact, using the sulfur as a maker of the rod components in the LBG-BCPs, we were able to identify that the rods, the most hydrophobic components of the LBG-BCP, prefer staying in the inner part of each WPNP and the coils, which are more hydrophilic, lay at the surface. Moreover, by exploiting the correlation between the plasmon peak position and the variation of the electron density [7], we were able to identify the b-WPNPs-rich and the PCBM-rich areas and the shape of domains by the EFTEM, allowing us to correlate the domain shape and distribution to the material efficiency. [6][8][9]
1.3-I1
Dr. Cheng Wang is a Physicist Staff Scientist at the ALS, LBNL. He obtained his bachelor’s degree in physics from Jilin University, China in 2002, and received his Ph.D. in physics in North Carolina State University advised by Prof. Harald Ade in 2008. After graduation, he joined the ALS, LBNL where he led the development of Resonant Soft X-ray Scattering for soft materials and led the construction of the world’s first RSoXS beamline at ALS beamline 11.0.1.2. He is a leading expert on the development of soft X-ray metrology and utilize advanced synchrotron x-ray probes such as X-ray scattering, microscopy and spectroscopy to elucidate the morphology, chemistry, and interfacial structure of broad range of complex materials.
An improved understanding of the fundamental chemistry, morphology, and dynamics of polymers and soft materials necessitates advanced characterization techniques that are suitable for in situ and in operando studies. Small angle scattering methodologies have evolved rapidly over the past few decades in response to the ever-increasing demand for more detailed information on the complex nanostructures of multiphase and multicomponent soft materials, such as polymer assemblies and biomaterials. Currently, element-specific and contrast variation techniques—such as resonant (elastic) soft/tender X-ray scattering, anomalous small angle X-ray scattering, and contrast-matching small angle neutron scattering, or a combination thereof—are routinely employed to extract the chemical composition and spatial arrangement of constituent elements at multiple length scales and to examine electronic ordering phenomena. This presentation will discuss the recent development of resonant soft X-ray scattering (RSoXS) at the Advanced Light Source (ALS), which has enabled its application to various critical areas of materials research. RSoXS, by integrating conventional X-ray scattering with soft X-ray absorption spectroscopy, has emerged as a chemically sensitive structural probe that provides a novel method for unambiguously resolving the complex morphologies of mesoscale materials. Tuning the X-ray photon energies to match the absorption spectra of different chemical components allows for the selective enhancement of the scattering contributions from these components, thus offering a detailed view of their complex morphologies. The applications of RSoXS have broadened to include structured polymer assemblies, organic electronics, functional nanocomposites, liquid crystals, and bio/bio-hybrid materials. The advancement in correlative analysis through multimodality, combined with high-throughput and autonomous experiments, is opening a new paradigm in materials research. The further development of resonant X-ray scattering instrumentation with cross-platform sample environments will facilitate multimodal in-situ and in-operando characterization of system dynamics with significantly improved spatial and temporal resolution.
1.1-I1
This talk will consider practical approaches for fabricating perovskite solar modules that use commonly available machinery rather than relying on novel or highly industrialized equipment. For example, the use of screen printing methods significantly reduces financial barriers, making advanced solar technology more accessible, especially in under-resourced areas. By focusing on machinery and tools that are widespread and easily obtainable globally, this approach democratizes the production of perovskite modules, ensuring that even communities in less affluent economies can participate in renewable energy advancements. The strategy intentionally avoids the use of overly expensive materials such as Spiro OMeTAD or gold, further contributing to the cost-effectiveness of the production process. If the factory cost entry-point can be driven down without overly compromising on performance then the manufacturing of perovskite solar modules can represent a key advancement in distributing solar energy solutions more equitably across different economic landscapes. The overall aim is to foster a more inclusive approach to renewable energy technology, making it a feasible option for a broader range of global communities.
1.1-O1
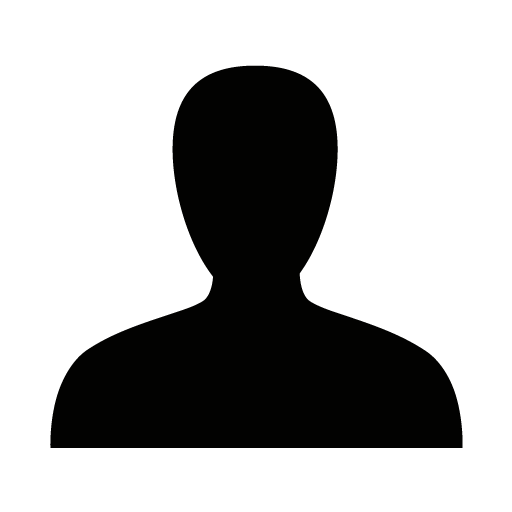
Despite its current predominance in the photovoltaics (PV) energy market, silicon is being gradually complemented with other photovoltaic materials, able to simplify the production processes, lower the costs, as well as improve the overall efficiency performance. Among these new materials, perovskites can lead to solar cells with a very high efficiency (33.7% ESTI certified efficiency in May 2023[1]), These results have attracted major interest and significant investments toward their industrial scale-up.
Nevertheless, as perovskite solar cells (PSC) electrical properties vary over time due to various meta-stability related factors (material diffusion, chemical imbalance as well as temperature, measurement set-up, light and/or voltage bias triggers..), repeatability of the I-V curve is not always granted, and many uncertainties related to the PSC characterization process are still under scrutiny. In fact, for PSC at present there is not one recognized evaluation technique grounded on international standards yet.
Additionally, PSC contain compounds that require very accurate handling ways, either due to their tangible health-hazard nature or because they can still be considered valuable for the new production chain. These observations raise the need to develop an effective material recovery/recycle strategy to reduce the life cycle impact of this technology before it hits industrial scale production.
The Joint Research Center (JRC) of the European Commission is currently carrying on the “Recycle-PSC” project (Evaluation, assessment and improvement of Process for Recycling and Reusing Innovative). Recycle PV focuses on both aspects of the measurement and characterization of PSC, as well as on the assessment and improvement of certain recycling processes to recover and reuse the materials comprising a PSC, with the aim of developing a safe and sustainable protocol to measure and handle these devices.
From the characterization side, we are exploring ways to work around the Maximum Power Point Tracking routine currently applied at ESTI [2], in order to optimize measurement time and measurement accuracy. The rationale behind it, is that the highly time consuming measurement procedure currently applied, is considered to not be feasible in the long term, as an accurate up-scaled screening protocol of PSC. From the recycling perspective instead, we concentrated on the glass substrate as the most promising and suitable component to recover, thanks to its high market value, strong physio-chemical stability and ease of processing. Compared to the common results reported so far in the specific literature of the field, which focused on the use of hazardous solvents like DMF, i here we report the use of acetone as a non-hazardous and inexpensive solvent to recover the substrate from PSC, thus potentially reducing the environmental impact of the whole process.
Results achieved at the JRC aim to contribute to the development of future policies regarding the large-scale implementation of PSC as high-efficiency and low-cost PVs.
[1] https://www.pv-magazine.com/2023/05/30/kaust-claims-33-7-efficiency-for-perovskite-silicon-tandem-solar-cell/
[2] Giorgio Bardizza et al 2021 J. Phys. Energy 3 021001
1.1-O2
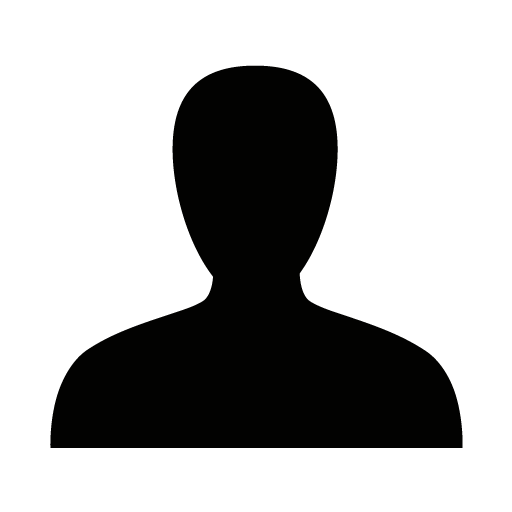
Perovskite solar cells (PSCs) are emerging as a cornerstone in the pursuit of efficient and sustainable solar energy. Their exceptional laboratory-scale performance has sparked considerable interest, yet transitioning these high-efficiency cells to scalable, commercial applications presents a myriad of challenges. Central to these challenges is the reliance on high-toxicity solvents, such as DMF/DMSO for the perovskite layer and Chlorobenzene/ Chloroform for the hole transporting layer (HTL). These substances, while effective in controlled settings, pose significant health and environmental hazards, particularly when considered for large-scale manufacturing. The exposure limits and handling complexities of such chemicals demand urgent attention and alternative solutions.
Moreover, the long-term stability of PSCs under real-world environmental conditions, especially humidity, has been a persistent obstacle. Despite their impressive initial performance, these cells often face degradation over time when exposed to varying humidity levels, questioning their practicality for long-term use. The current production methods and materials, optimized for highly efficient in PSCs, often result in increased production costs, making it challenging to compete with established solar technologies in the market.
In response to these limitations, our study delves into the development of roll-to-roll (R2R) coated perovskite solar cells including the top electrode using low-toxicity solvents, marking a significant shift from traditional methods. This approach not only seeks to reduce the ecological footprint of PSC production but also to enhance the safety and feasibility of large-scale manufacturing. We strategically replaced the conventional solvents with safer alternatives: DI water was used for the tin oxide layer, for the critical perovskite and hole transporting layers, we opted for Acetonitrile (ACN) and Oxylene, respectively, significantly mitigating the environmental and health hazards. Furthermore, 2-Methylanisole was chosen for the carbon ink, reinforcing our commitment to safer and much cheaper production processes.
The results of our approach showed that the R2R coated PSCs, incorporating carbon electrodes, not only achieved a power conversion efficiency (PCE) of over 10% but also demonstrated extraordinary stability (D1- ISOS). Unencapsulated devices maintained 85% of their initial PCE after 90 days, a testament to the durability that can be achieved alongside environmental consciousness.
These findings do more than just validate the effectiveness of low-toxicity solvents in the fabrication of PSCs. They represent a crucial advancement in making PSC technology a realistic, sustainable option for large-scale solar energy production. By addressing the pivotal issues of toxicity, stability, and cost, our study paves the way for PSCs to transition from laboratory breakthroughs to robust, environmentally responsible, and commercially viable energy solutions, setting a new precedent in the solar energy landscape.
1.1-I2
Dr. Anurag Krishna is an R&D Project Leader at Interuniversity Microelectronics Centre (IMEC) and EnergyVille, Belgium, where his research activities focus on developing perovskite module technology. Previously, he has been a Marie Skłodowska-Curie fellow in the laboratory of Prof. Anders Hagfeldt and Prof. Michael Graetzel at Ecole Polytechnique Fédérale de Lausanne, Switzerland. He obtained Ph.D. from Nanyang Technological University, Singapore. The noble mission of his research is to facilitate sustainable and affordable low-carbon and green technology solutions for the world. On the fundamental side, his research interests focus on developing hybrid materials suitable for photovoltaic, optoelectronic, and nanoelectronic devices
Metal Halide perovskites have emerged as highly promising candidates for photovoltaics with the certified record power conversion efficiency (PCE) reaching 26.1% for single-junction perovskite solar cells (PSCs)1. However, to date, most of the reported highly efficient PSCs were obtained based on the regular n-i-p architectures at the laboratory scale, i.e., typically ~0.1 cm2 2-5, which are not suitable for upscaling. Inverted p-i-n cells, on the other hand, are attractive for upscaling due to their architecture simplicity at relatively low material cost and potentially high stability, however, their PCE still lags behind the n-i-p counterparts6,7. Therefore, our work has been focused on improving the efficiency of p-i-n cells and scaling them to produce efficient and stable modules. To push the PCE of cells, we developed a dual interface passivation strategy which led to a champion PCE of 24.3% for small-area cells and a champion PCE of 22.6% for a 3.63 cm2 mini-module. Next, we developed a bladed-coated interlayer to passivate the NiOx/perovskite interface. As a result, PCEs of 21.8% and 20.5% are demonstrated for cells of 0.13 cm2 and 1 cm2, respectively. The scalability of this p-i-n architecture is successfully demonstrated, achieving aperture area module efficiencies of 19.7%, 17.5%, and 15.5% for minimodules of 4 cm2, 16 cm2, and 100 cm2, respectively. Furthermore, we have upscaled up our baseline process and device stack to large-area modules. We fabricated bi-facial (781 cm2) perovskite solar modules exhibiting a power conversion efficiency of 16.3%, respectively. Moreover, the bi-facial mini-module retained ~ 92% of initial PCE after 1000 h of standard IEC 61215-based damp heat (85 °C, 85% relative humidity) test.
References
1NREL Best Research-Cell Efficiencies Chart. Accessed on May 10, 2023.
2 Min, H. et al. Perovskite solar cells with atomically coherent interlayers on SnO2 electrodes. Nature 598, 444–450 (2021).
3 Zhao, Y. et al. Inactive (PbI2)2RbCl stabilizes perovskite films for efficient solar cells. Science 377, 531–534 (2022).
4 Zhang, T. et al. Ion-modulated radical doping of spiro-OMeTAD for more efficient and stable perovskite solar cells. Science 377, 495–501 (2022).
5 Kim, M. et al. Conformal quantum dot–SnO2 layers as electron transporters for efficient perovskite solar cells. Science 375, 302–306 (2022).
6 Jiang, Q. et al., Surface reaction for efficient and stable inverted perovskite solar cells, Nature 611, 278–283 (2022).
7 Li, Z et al., Organometallic-functionalized interfaces for highly efficient inverted perovskite solar cells. Science 376, 416–420 (2022).
1.2-I1
Vapor phase deposition of organic-inorganic perovskite solar cells is raising increasing interest in both academia and industry, holding great promise for the commercialization of perovskite-based photovoltaics. Despite the dominance of vapor phase deposition processes in commercial thin film manufacturing of photovoltaics and other optoelectronic applications, research on vapor phase processed PSCs is still underrepresented compared to their solution-based counterparts. Solution-processed PSCs still dominate the field, benefiting from fast optimization feedback and straightforward integration in modern research laboratories. This contribution will present a recent perspective that coveys a balanced viewpoint of industry and academics on the prospects of vapor phase deposition of perovskite photovoltaics. The perspective highlights strategic opportunities of vapor phase deposition for the commercialization of perovskite-based PV. In addition, the latest developments at the Karlsruhe Institute of Technology on vapor phase deposited perovskite thin films for perovskite solar cells and perovskite/Si tandem solar cells will be presented.
1.2-I2
Dr. Quentin Jeangros received a PhD in Materials Science from EPFL in 2014 for his work on solid oxide fuel cells degradation pathways. After a postdoc between the University of Basel and the Photovoltaics and Thin Film Electronics Laboratory (PV-Lab) of EPFL on transparent conductive oxides, Quentin has overseen the "Perovskite Cells for Tandem Applications" activities at EPFL PV-Lab since early 2018. Within the laboratory headed by Prof. C. Ballif, his team consists of 6 PhD students and postdocs dedicated to the development of high-efficiency perovskite/silicon solar cells. His research activities focus on the use and development of advanced electron microscopy characterisation methods to understand and optimise the nanostructure of solar materials materials, with the aim of improving efficiency and reliability.
The addition of a perovskite thin-film solar cell on the front side of a commercial silicon solar cell promises power conversion efficiencies beyond the theoretical efficiency limit of silicon photovoltaics, which sits at around 29.5%. Advantageously, the cost of adding this perovskite solar cell to form a tandem device should be diluted at the system level by balance-of-system components, making the approach attractive from a commercial perspective.
This contribution will review perovskite/silicon tandem solar cells developments ongoing at CSEM and EPFL PV-Lab, which closely collaborate on the topic. Focusing first on 1-cm2 tandem solar cell prototypes, different approaches to reduce losses occurring at the interfaces of the perovskite top-cell absorber will be discussed. For example, the use of phosphonic acid compounds, as hole transport layer and as additive in the perovskite ink, has enabled the demonstration of tandem solar cells reaching certified power conversion efficiencies >30% with both planar and textured Si wafers.1,2 Furthermore, the development of process flows compatible with industrial requirements will be discussed. Notably, a Ag paste screen-printing process compatible with the low thermal budget of the perovskite top cell has been developed, yielding for example 25-cm2 tandem cells with a certified power conversion efficiency of 29.6%. Also, progress on perovskite absorber deposition processes compatible with larger, industrial-sized tandems (M6 and above, see Figure) will be discussed and challenges ahead highlighted. Finally, the presentation will review the results of various stability testing procedures, including damp heat, thermal cycling, light soaking and other accelerated aging conditions set up to reveal the failure modes of perovskite solar cells.3
1.3-O1
Dr. Hadjipanayi is a research scientist at the Photovoltaic Technology group in the Department of Electrical and Computer Engineering of the University of Cyprus working on the investigation of the optoelectronic characteristics and photovoltaic performance of novel solar cell devices and her latest work focuses on the characterization of perovskite-based PV and measurement protocol development.
She has received her BSc in Physics (2001) from the University of Cyprus and her DPhil (PhD) in Condensed Matter Physics (2006) from the University of Oxford. Her employment record includes a Post-Doctoral Research Associate position at the Quantum Information Processing Interdisciplinary Research Collaboration (QIP IRC), Department of Physics, University of Oxford (2006-2009) and an Associate Research Scientist post at the Energy, Environment and Water Research Centre of the Cyprus Institute (2009-2012). Her research interests lie within the area of fundamental and applied physics of novel materials which are promising for future energy-efficient technological applications, especially in the field of solar energy. More specifically and more recently, these include: Investigation of optoelectronic properties and degradation mechanisms of novel solar cell devices including multi-junction solar cells, nanostructured silicon cells, perovskites; Development of accurate standardized and non-standardised testing protocols for new solar cell technologies.
Maria has over 10 years’ experience in national and European research projects as a partner and as a Coordinator covering the full project life-cycle involvement: from initiation to implementation, monitoring and reporting. She led the efforts to attract funds and develop a new strategic infrastructure unit at the University of Cyprus, the DegradationLab, which focuses in the accurate characterization of new and emerging solar cells, and is currently the Head of this new lab (https://fosscy.eu/laboratories/degradation-lab/).
Perovskite solar cells have been attracting increasing attention in recent years due to their rapid progress with record efficiency of 26.1% for single junction and 33.9% for tandem devices respectively [1]. The biggest concern that impedes the application of perovskites and poses tremendous challenges for their commercialization is their long-term stability under operation [2]. Even if now perovskites are passing standardized protocols (IEC 61215) and ISOS procedures, those tests cannot resemble the outdoor operational conditions with day-night cycles and continuous change in temperature and irradiance levels. Although outdoor stability testing has been reported for different types of perovskite devices so far [3], [4] there is still a lack of quantitatively precise information about the diurnal trend in long-term performance of perovskites under outdoor conditions.
In this work, several perovskite and perovskite on Silicon tandem mini-modules were extensively investigated outdoors at real environmental conditions for a duration of up to two years and indoors using a range of advanced optoelectronic methods to set-up a complete optical and electrical characterization of perovskite devices. Characterization methods of spatially - resolved Electroluminescence/Photoluminescence, Dark Lock-In Thermography, Raman and Ultrafast spectroscopies have been utilized for this purpose. Outdoor testing for several months in the field demonstrated the impact of irradiance and temperature on the major electrical parameters of the devices. Interplay of metastability and temperature effects were detected in the output power temperature coefficients results while agreement was found between indoor laboratory tests and outdoor results for voltage temperature coefficients.
Diurnal performance degradation and recovery overnight were calculated for the first time outdoors. Seasonality effects in the diurnal changes are discussed demonstrating the values of performance recovery overnight and diurnal performance degradation as well as the degradation-to-recovery ratio at different environmental conditions. The diurnal changes in performance, current and voltage have been calculated and facilitated on the understanding of irradiance effects on the major electrical parameters of the perovskite samples. A data-driven predictive model has been utilized for predicting the output power time-series of perovskites.
Moreover, several optoelectronic and spectroscopic methods such as Ultrafast and Raman spectroscopies and Dark Lock-In-Thermography methods have been employed to understand changes in carrier relaxation dynamics and chemical properties after outdoor exposure as well as hotspot evolution in the devices under test. Results demonstrated FA decomposition products and changes in carrier relaxation mechanisms in Raman and Ultrafast methods respectively. Finally, Dark Lock-In Thermography method revealed hotspot evolution in both perovskite active and interconnection areas of the cell.
1.3-I1
Metal-halide perovskite (MHP) photovoltaic (PV) modules are progressing towards commercialization. One major hurdle that remains is establishing confidence in long-term field performance and durability of MHP modules. A lot of progress has been made in addressing many reliability issues in MHP cells and modules; however, there is still work to be done to understand degradation and demonstrate real world operation of this technology. In addition to establishing field performance and reliability, in the absence of established test protocols for MHPs, many reliability studies have been conducted using the International Electrotechnical Commissions 61215-series (IEC 61215) of standardized tests, which do not yet have specifications for MHP modules. While some of the existing IEC 61215 protocols may be partially relevant to degradation mechanisms incurred by MHPs, a set of testing protocols specifically relevant to this technology is likely needed to ensure that accelerated testing can accurately assess the reliability of MHPs. Here, I will present a brief overview of an initial field demonstration of MHP modules as part of the Perovskite PV Accelerator for Commercializing Technologies, PACT, program. In addition, I will discuss accelerated testing results in the development of a test procedure for the qualification of commercial MHP modules. Particular attention will be given to light and elevated temperature testing, which has shown a particularly large impact on MHP performance but is not thoroughly covered in IEC 61215.
1.3-O2
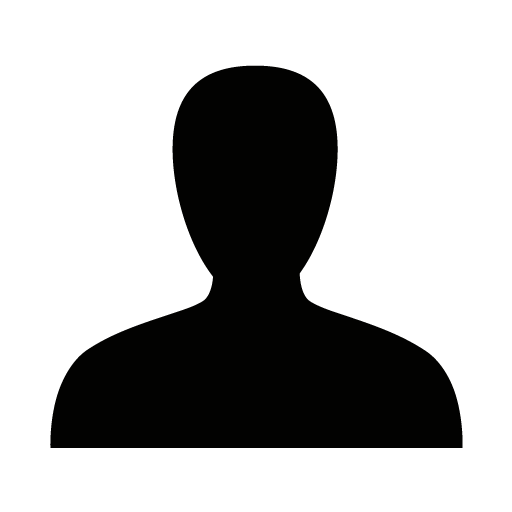
Degradation of perovskite solar cells (PSCs) is often the result of exposure to extrinsic environmental factors: humidity, oxygen, and thermal stress. However, intrinsic factors, notably ion migration, are also linked to stability issues, such as via lattice strain[1] or phase segregation[2,3]. Being intrinsic, these factors could limit stability and energy yield in commercial devices even if they manage to achieve excellent isolation from the atmosphere.
The most extreme conditions under which ion migration could trigger degradation is under day-night cycling, where swings in the PSC’s internal electric fields will trigger large shifts in the distribution of ionic defects in the perovskite absorber. This challenge is recognised by the proposed day-night cycling stability testing protocol presented in the consensus statement for perovskite solar cell stability testing[4].
In this context, our work assesses the extent of the stress placed on PSCs by day-night cycling, relative to more conventional maximum power point tracking. We consider the influence of the perovskite composition, notably the number of different A-site cations and X-site halides (Double and Triple). Simultaneous with the day-night cycling, we capture photoluminescence (PL) intensity images to determine the spatial homogeneity of the degradation triggered by the cycling effects, and thus whether the degradation mechanisms are intrinsic to the film or precipitated by initial regions of heterogeneity.
Our analysis focuses on an inverted ITO/PolyTPD/PFN-Br/Perovskite absorber/C60/BCP/Au structure, fabricated using an anti-solvent method. The perovskite composition was based on triple cation and triple halide. Moreover, to examine the effect of perovskite composition and film fabrication route, triple and double halide-based perovskite was further formulated with the anti-solvent and vacuum zig method.
References:
1. Tsai, H. et al. Light-induced lattice expansion leads to high-efficiency perovskite solar cells. Science 360, 67–70 (2018).
2. Duong, T. et al. Light and Electrically Induced Phase Segregation and Its Impact on the Stability of Quadruple Cation High Bandgap Perovskite Solar Cells. ACS Appl. Mater. Interfaces 9, 26859–26866 (2017).
3. A. Jacobs, D. et al. Lateral ion migration accelerates degradation in halide perovskite devices. Energy Environ. Sci. 15, 5324–5339 (2022).
4. Khenkin, M. V. et al. Consensus statement for stability assessment and reporting for perovskite photovoltaics based on ISOS procedures. Nat. Energy 5, 35–49 (2020).
1.3-I2
Sjoerd Veenstra - Program Manager Perovskite Solar Cells and Modules at TNO, partner in Solliance.
Sjoerd has a passion for photovoltaics (PV). He received his PhD from the University of Groningen (2002). Sjoerd stayed at UCSB (intern) and Cornell University (visiting scientist). He started as a researcher working on organic solar cells at the Energy research Center of the Netherlands (ECN, 2002). In 2011 he moved to Eindhoven (NL) when ECN joined the thin film PV activities of Solliance. He started working on perovskite solar cells in 2014. In 2018 ECN and TNO merged and since he works for TNO and leads the perovskite team.
The scalable slot-die coating methods adopted within TNO enable fabrication of efficient single junction perovskite solar devices with intrinsic stability using various material and layer combinations. Furthermore, several different encapsulation strategies are investigated to define a low-cost route to guarantee long term stable modules. Demonstration of a stable opaque and semi-transparent bifacial flexible perovskite module is a step forward on various applications, such as building- and vehicle-integrated PV (BIPV & VIPV) and noise barriers on highways. In this talk, we will give an overview of our story towards realizing a stable, efficient, and bifacial perovskite processed via roll-to-roll slot-die coating technique.
As an outlook, we show recent work on realizing multi-junction perovskite solar cells and modules. Furthermore, we reveal a method how flexible modules may be integrated in bespoke photovoltaic modules as enabling process for integrated PV and an important step towards commercializing this emerging PV technology.
The graphical abstract shows an example of roll-to-roll coated perovskite.
1.1-I1
Water splitting is emerging as a promising route for generating hydrogen in a more sustainable way compared to conventional production methods. Finding affordable and scalable catalysts for the anodic half-reaction, the oxygen evolution reaction (OER), could help with its industrial widespread implementation. Nonetheless, the electrocatalysts’ near-surface structure during OER is still largely unknown, which hampers knowledge-driven optimization. Here, we provide quantitative near-surface structural insights into oxygen-evolving CoOX(OH)Y clusters and nanoparticles by tracking their size-dependent catalytic activity from 4 atom clusters to 50 nm particles and their structural adaptation to OER conditions combining operando X-ray absorption spectroscopy (XAS) and density functional theory calculations. We uncover a superior intrinsic OER activity of 1-5 nm nanoparticles and a size-dependent oxidation leading to a near-surface Co-O bond contraction and charge redistribution during OER.
Moreover, I will also report on the role of Fe in the performance of Co- and Ni-oxide-based electrocatalysts. I will discuss the catalytic performance of epitaxial Co3O4, Co1+δFe2-δO4 (|δ|<=0.2), and Fe3O4 thin film electrocatalysts with (111) and (100) surface orientations. Under OER conditions, all three oxides are covered by oxyhydroxide, but the characteristics of this overlayer, including its thickness, stability, structural and chemical evolution were found to strongly depend on the Fe content and the initial surface structure of the pre-catalyst. Similar investigations carried out for epitaxial NiO(001) and Ni0.75Fe0.25Ox(001) thin films with operando Raman and grazing Incidence XAS will also be presented to demonstrate the generality of the findings obtained for the CoOx thin films. Finally, the observed compositional, structural, and electronic properties of each system will be correlated with their electrochemical performance.
1.1-O1
ALBA Synchrotron Light Source [1] is a 3rd generation synchrotron with up to 11 operative beamlines, and 5 others under commissioning, construction, or design, open to the scientific user community since 2012.
In this contribution, we will give an overview of ALBA focusing on some of the beamlines that allow measuring under more realistic conditions. One of these tools is the CIRCE-NAPP [2], dedicated to Near Ambient Pressure X-ray Photoemission Spectroscopy (NAP-XPS) NAP-XPS. This technique has experienced a boost in the last two decades as a powerful tool to investigate surface chemical processes minimizing the pressure gap with respect to the UHV conditions traditionally applied. Other beamlines are: CLÆSS [3], which provides simultaneous and unified access to two complementary techniques, X-ray absorption (XAS) and emission spectroscopies (XES); MSPD [4], the Materials Science and Powder Diffraction beamline; and NCD-SWEET [5], that allows Small and Wide angle X-ray scattering measurements (SAXS & WAXS) both in transmission and also in grazing incidence (GISAXS & GIWAXS).
We will put special emphasis on the NOTOS beamline [6], which is the latest beamline from the ALBA Synchrotron that was opened to external users in April 2022. This beamline is devoted to XAS, X-ray diffraction (XRD), and metrology applications in the energy range of 4.5 – 30 keV with the capability to perform XAS and XRD investigations in a quasi-simultaneous way. NOTOS, as a new tool open for the scientific community, allows to study the electronic structure and short- and long-range order within a wide range of scientific disciplines: chemistry, catalysis, energy-related science, nanomaterials, condensed matter and environmental science. In particular, NOTOS has been further developed to focus on in situ and operando measurements on heterogeneous catalysis, electrocatalysis and electrochemistry in general.
[1] https://www.cells.es/en/
[2] BL24 – CIRCE, https://www.cells.es/en/beamlines/bl24-circe
[3] BL22 – CLÆSS, https://www.cells.es/en/beamlines/bl22-claess
[4] BL-NOTOS, https://www.cells.es/en/beamlines/bl16-notos
[5] BL11 - NCD-SWEET, https://www.cells.es/en/beamlines/bl11-ncd
[6] BL16 - NOTOS, https://www.cells.es/en/beamlines/bl16-notos
1.1-O2
Dr. Minghao Yu, PI, holds an independent research group (Electrochemistry for Sustainable Energy Storage) at Technische Universität Dresden. His research interest includes 1) the development of novel organic and inorganic 2D layered materials, 2) the investigation of advanced artificial interphases and electrolytes for next-generation batteries, 3) fundamental charge and ion dynamics during electrochemical energy storage processes, and 4) sustainable energy storage device fabrication, including supercapacitors, hybrid-ion capacitors, aqueous batteries, dual-ion batteries, and multivalent metal (Zn, Mg, Al) batteries. He has published more than 120 scientific articles which have attracted more than 20,000 citations with an H-index of 69 (Web of Science). Besides, he is also an associated member of the Center for Advancing Electronics Dresden (cfaed), an associated group leader at Max-Planck-Institut für Mikrostrukturphysik, a highly cited researcher (Clarivate Analytics, 2018-now), 2023 ERC Starting Grant winner, and a Fellow of the Young Academy of Europe.
Electrochemical energy storage technologies have gained prominence due to their efficient means of storing, transporting, and delivering energy harnessed from sustainable resources.[1-3] However, the safety, resource, and cost issues associated with state-of-the-art Li-ion batteries have prompted the development of next-generation batteries. This has given rise to innovative battery concepts like dual-ion batteries, aqueous batteries, and multivalent metal batteries. Nonetheless, the creation of these next-generation batteries is not as straightforward as substituting Li-ion with alternative ion charge carriers. Challenges persist, especially at the electrode/electrolyte interface, where electrochemical reactions encounter obstacles such as high energy barriers linked to ion desolvation/dissociation, electrolyte decomposition, and the co-insertion of solvent/ion aggregates. In this presentation, I will present our recent endeavors in exploring 2D crystalline polymers as effective interfacial coatings for batteries.[4-5] These coatings facilitate efficient interfacial ion transport and charge transfer. Through in-situ/operando X-ray techniques (XRD & XAS), we have unveiled the pivotal role of 2D crystalline polymer membranes in enhancing the overall electrochemical reaction kinetics, reversibility, and durability.
References:
[1] Yu et al., Chem. Soc. Rev. 2021, 50, 2388-2443.
[2] Yu et al., Joule 2019, 3, 338-360.
[3] Yu et al., J. Am. Chem. Soc. 2020, 142, 12903-12915.
[4] Yu et al., Small 2022, 18, 2107971.
[5] Yu et al., Nat. Commun. 2023, 14, 760.
1.1-I2
Freddy E Oropeza is a Marie Skłodowska-Curie fellow at IMDEA Energy. His research interests focus on the understanding and control of the structural and electronic properties that define the functionality of metal oxide thin films and interfaces for applications in electronics and (photo)electrochemical technologies. He obtained his PhD in Chemistry from the University of Oxford in 2012, followed by working as postdoc at the Ruhr University Bochum (2012-2013), Imperial College London (2013-2016) and the Eindhoven University of technology (2016-2019).
The development of solar technologies for production of renewable fuels are interesting processes not only a way to reduce and reuse CO2 emissions, but also a way of storing solar energy by obtaining so-called solar fuels. Among, these technologies several processes have been included such as: H2 production, CO2 reduction or NH3 production, among others.
One of the main challenges is the elucidation of the reaction mechanisms that take place in these processes. In this sense, the development of new in-situ and operational characterization tools has become a powerful tool to understand these complex processes in depth. In this talk some examples will be given on different solar fuel production technologies.
Here, we will revise some examples of (photo)electro catalysts for H2 production. As example, the synthesis of well-controlled vertically aligned Ni/NiO nanocomposites consisting of Ni nanoclusters embedded in NiO, which result in highly efficient electrocatalysts for overall water splitting. We show that such a high catalytic efficiency toward both the hydrogen evolution reaction (HER) and the oxygen evolution reaction (OER) originates from a synergetic effect at Ni/NiO interfaces that significantly reduces the energy barrier for water dissociation, and favours the formation of reactive H* intermediates on the Ni side of the interface, and OHads on the NiO side of the interface.
On the other hand, also will show the non-innocent role of the vanadium dopant in TiO2 in the photoelectrochemical properties. In situ XAS measurements of the V K-edge revealed that the vanadium ions, beyond improving the redox behavior of the host, also actively participate in the reduction process. The significant changes in the V K-edge XANES and EXAFS spectra observed under reduction conditions can be ascribed to a change in the structure and oxidation state of the vanadium ions during the electrochemical reaction.
1.2-I1
Dr. Marine Reynaud is a Chemical Engineer from Chimie ParisTech (France) and Doctor in Materials Sciences. She completed her PhD in 2013 under the direction of Prof. Tarascon, Dr. Chotard and Dr. Rousse. Then, she joined the group of Dr. Montse Casas-Cabanas at CIC energiGUNE, where she is has recently been appointed Research Team Leader. Her research is focused on the design and development of electrode materials for Li-ion and Na-ion batteries. She is expert in inorganic syntheses and materials characterizations, looking for determining correlation between compositions, (micro)structure and electrochemical properties. For the last few years, she has been developing innovative strategies to accelerate the discovery of new battery materials.
She is author of c.a. 40 scientific publications in peer reviewed journals. She has been PI of several industrial projects and competitive national and European research projects. She has supervised 5 PhD students and currently leads a team of 12 researchers. She has recently received the first BRTA award from the Basque Research and Technology Alliance, recognizing young researchers’ passion, talent and ambition.
Lithium-ion (Li-ion) and Sodium-ion (Na-ion) batteries function through reversible intercalation reactions involving an intercalant cation (A = Li+ or Na+) into a host compound (AxH), which serves as the active material in the positive electrode (often referred to as the 'cathode'). These intercalation reactions generally occur through two distinct mechanisms:
(i) Homogeneous reactions, characterized by a single phase or solid solution, involves the formation of a non-stoichiometric compound (AxH) where the insertion content 'x' continuously varies within the intercalation domain xmin ≥ x ≥ xmax.
(ii) Heterogeneous reactions involve multi-phase processes, leading to the nucleation and growth of a second phase (AyH, where y ≠ x) as a results of the compositional changes within the electrode.
In single-phase reactions, the host structure undergoes minimal structural changes, primarily a continuous volume variation to accommodate the compositional shift. This is generally considered more favorable for fast diffusion compared to the moving interfaces found in two-phase reactions. However, the rate of intercalation reactions, (driven by the imposed rate of the charge and/or discharge processes) can lead electrodes away from equilibrium conditions. This departure can induce significant variations in reaction mechanisms, including changes in phase transition sequences.
This presentation will showcase how operando powder X-ray diffraction (XRD) and absorption spectroscopy (XAS) experiments offer valuable insights into the understanding of reaction mechanisms in electrode materials. Examples include Li-rich layered oxides [1], the high-voltage spinel LiNi0.5Mn1.5O4 [2], and Na-ion triphylite (olivine-type) cathode materials such as NaFePO4 and NaFe0.8Mn0.2PO4 [3,4].
1.2-I2
In context of the growing need for a more sustainable energy sector, the efficient storage of excess energy from intermittent renewable sources is of paramount interest and significant efforts have been devoted to the quest for more efficient electrocatalyst materials for energy conversion and storage devices such as water electrolyzers, fuel cells and batteries. Thus, in-situ studies of promising energy materials in conditions close to real operation are of crucial importance for understanding of the performance-limiting mechanisms occurring at the electrochemical interfaces
Photon-in/photon-out x-ray absorption spectroscopy (XAS) is an established tool to probe the chemical and electronic structure of solid, liquid, and gaseous samples, providing insights into the local density of states of the studied material, e.g. its oxidation state and local geometry around the probed atom. Due to the short attenuation length of x-ray photons, application-tailored sample environments bridging the technical requirements of the method and the electrochemical devices are required to monitor real-world materials in liquids under operating conditions.
In this work, we show operando XAS studies of relevant energy materials in the field of electrocatalysis and battery research, showcasing the opportunities and challenges arising from the use of photons energies ranging from the high to the soft x-ray regime.
1.2-O1
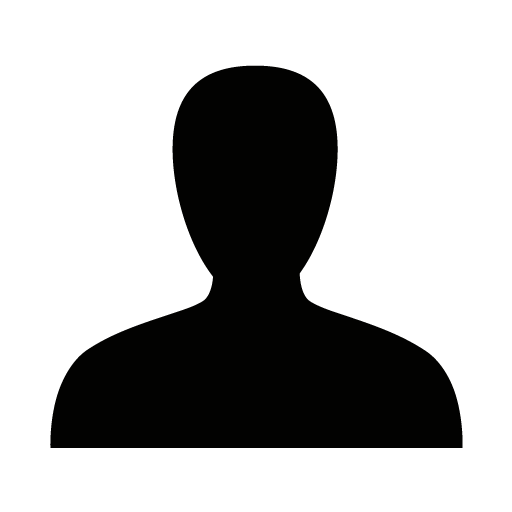
The reaction processes in Li-ion batteries can be highly heterogeneous at the electrode scale, leading to local deviations in the lithium content or local degradation phenomena. To access the distribution of lithiated phases throughout a high energy density silicon-graphite composite anode, we apply correlative operando small- and wide-angle X-ray scattering (SAXS and WAXS) tomography.
In-plane and out-of-plane inhomogeneities are resolved during cycling at moderate rates, as well as during relaxation steps performed at open circuit voltage (OCV) at given states of charge. Lithium concentration gradients in the silicon phase are formed during cycling, with regions close to the current collector being less lithiated when charging.
In relaxing conditions, the multi-phase and multi-scale heterogeneities vanish to equilibrate the chemical potential. In particular, Li-poor silicon regions pump lithium ions from both lithiated graphite and Li-rich silicon regions.
This charge redistribution between active materials is governed by distinct potential homogenization throughout the electrode and hysteretic behaviours. Such intrinsic concentration gradients and out-of-equilibrium charge dynamics, which depend on electrode and cell state of charge, must be considered to model the durability of high capacity Li-ion batteries.
1.2-O2
An in depth understanding of the atomistic mechanism underlying different electrochemical processes requires recording large sets of data under operando conditions yielding key information of the electrified interface. Thus, the desired parameters to be known include the chemical composition at the interface, chemical states of the atoms and their variation as a result of the electrochemical reactions as well as the structural evolution. Unfortunately the analytical techniques able to provide interface information are very limited and hardly compatible with liquids allowing usually only ex situ characterizations leading to a loss of important information as in many cases the intermediates and electrocatalytic actives species cannot be “quenched” in post process analysis. X-ray spectroscopy techniques are able to provide relevant information of the electronic structure in an element specific manner but real electrochemical interfaces are buried and most of the time in presence of liquid electrolytes being inaccessible directly to the common electron based surface sensitive techniques. Recently new approaches were developed allowing the investigation of the electronic structure variation of the electrocatalysts under reaction conditions using photoelectron spectroscopy, from gas phase up to bulk aqueous electrolyte. These experimental setups based in photoelectron spectroscopy were used, among others, to investigated the not kinetically favored oxygen evolution reaction onto IrOx catalysts, which is the example used in this talk.
1.2-I3
Ambient pressure XPS is vital in unraveling the intricacies of surfaces under reaction condition, yet its efficacy is capped at 50 mbar. The pressure limit has inhibited its applicability, most notably for ammonia synthesis, where pressures of at least 1 bar are needed for appreciable ammonia production. Without the detailed knowledge from XPS there is no complete understanding of the Haber-Bosch process. In response, a new type of XPS has been developed overcoming the barrier and reach pressures of over 1 bar, a true High pressure XPS.
The innovation relies on changing the way the gas is used, making a virtual pressure cell between the sample and the entrance to the XPS analyzer. The new style has proven to be highly productive yielding detailed understanding of many vital catalytic systems like, carbon monoxide oxidation, methanol synthesis, and the Haber-Bosch process.
While the technique utilized well there are many issues that have been overcome, some new but many old. This presentation will cover many of the problems solved along the way along with several example systems. Finally, the discussion will focus on a new beamline at designed to advance this technique to new frontiers.
1.1-I1
Professor Maria Forsyth AM is an elected Fellow of the Australian Academy of Sciences and the Australian Academy of Technology and Engineering. She is an Alfred Deakin Professo at Deakin University and an Ikerbasque Visiting Professorial Fellow at the University of the Basque Country, Spain. She has worked at the forefront of energy materials research since her Fulbright Research Fellowship in 1990 and has consistently made breakthrough discoveries in next-generation lithium and sodium battery technologies. Recognising a critical need for facilities to test new energy materials, Forsyth led the establishment of Deakin University’s Battery Research and Innovation Hub, a unique pre-commercial battery prototyping facility that supports over $20M R&D across a range of local and international industries. She has supervised over 100 PhD students and is a co-author of over 900 journal and conference publications that attracted more than 40000 citations.
The electrolyte is arguably one of the most critical components of a battery. It controls the available operating voltage through its electrochemical stability window, it supports the flow of the target ion for any given battery chemistry (eg. Li+, Na+, Zn2+, Al3+ etc….) between electrodes, it limits temperature of operation depending on its phase behaviour and - probably a key function - it can react on the electrodes to form an interphase, known as the solid electrolyte interphase (SEI) whose properties govern the stability and cycle life of the battery.
Traditional electrolytes currently used for Li-ion and Na-ion devices are not compatible with higher energy-density anodes required for next generation devices, such as Li metal and Na metal anodes. In addition, there is now a recognition that operation at elevated temperatures is desirable for some applications. Therefore, new electrolyte materials are currently actively being investigated for beyond Li-ion technologies. It has recently been shown that, by using an ultra-high concentration of lithium or sodium salt in an ionic liquid (or indeed some organic solvents), it is possible to achieve stable cycling of several high capacity anodes including Li and Na metal anodes as well as silicon and hard carbon anodes. These electrolytes indicate a decoupling of the alkali metal ion dynamics from the bulk with tLi+ or tNa+ transport numbers approaching or even exceeding 0.5. Interestingly, the nature of the ionic liquid cation can have a significant influence on the electrochemical performance, apparently related to the interfacial structuring and the influence on the SEI layer. In this work we will discuss these non-traditional electrolyte materials in terms of their physicochemical properties, electrochemical behavior and performance in devices.
1.1-O1
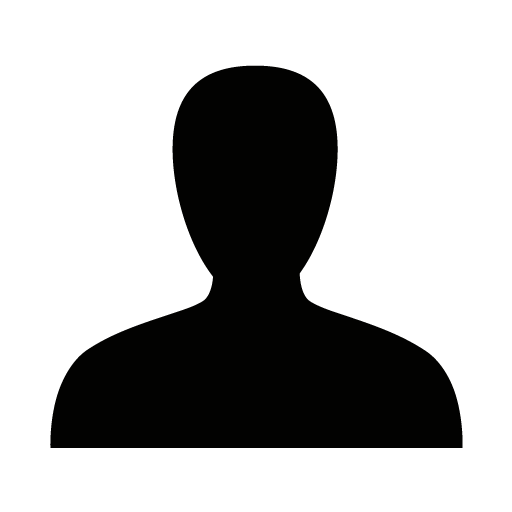
Emerging sodium-ion batteries (NIBs) and potassium-ion batteries (KIBs) show promise in complementing lithium-ion battery (LIB) technology and diversifying the battery market. Hard carbon is a potential anode candidate for LIBs, NIBs and KIBs due to its high capacity, sustainability, wide availability, and stable physicochemical properties. Herein, a series of hard carbons are synthesized by hydrothermal carbonization and subsequent pyrolysis at different temperatures to finely tune their structural properties. When tested as anodes, the hard carbons exhibit differing ion storage trends for Li, Na and K, with NIBs achieving the highest reversible capacity. Extensive materials and electrochemical characterizations are carried out to study the correlation of structural features with electrochemical performance, and to explain the specific mechanisms of alkali-ion storage in hard carbons. In addition, the best-performing hard carbon is tested against a sodium cathode Na3V2(PO4)3 in a Na-ion pouch cell, displaying a high power density of 2172 W kg-1 at an energy density of 181.5 Wh kg-1 (based on the total weight of active materials in both anode and cathode). The Na-ion pouch cell also shows stable ultralong-term cycling (9000 hours or 5142 cycles) and demonstrates the promising potential of such materials as sustainable, scalable anodes for beyond Li-batteries.
1.1-O2
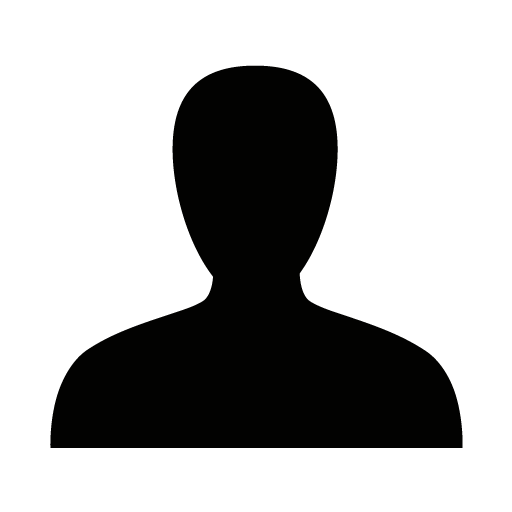
The demand for versatile and sustainable energy materials is on rise given the importance of developing novel clean technologies to transition to a net zero economy. Here, we present the synthesis, characterization, and application of lignin-derived ordered mesoporous carbons with various pore sizes (from 5 nm to approx. 50 nm) as anodes in sodium-ion batteries. We have varied the pore size using self-synthesized PEOn-b-PHAm block copolymers with different PEO and PHA chain lengths applying the “soft templating” approach to introduce isolated closed spherical pores of 20 nm to 50 nm in diameters. The pore structure was evaluated by transmission electron microscopy (TEM), nitrogen physisorption, and small-angle X-ray scattering (SAXS). We report microstructure analysis of such mesoporous lignin-based carbons using Raman spectroscopy and wide-angle X-ray scattering (WAXS). In comparison with non-templated carbon and carbons templated employing commercial Pluronic® F-127 and PIB50-b-PEO45, which created accessible channels and spherical pores up to approx. 10 nm in diameter, the carbon microstructure analysis revealed that templating with all applied polymers significantly impede the graphitization upon thermal treatment. Furthermore, the gained knowledge of similar carbon microstructures regardless of the type of template allowed the investigation of the influence of different pore morphologies in carbon applied as anode material in sodium-ion batteries supporting the previous theories in the literature that closed pores are beneficial for sodium storage while providing insights into the importance of pore size.
1.1-I2
Robert Dominko is a Research Professor at the National Institute of Chemistry and a Professor at the University of Ljubljana. He is the head of the battery group at the National Institute of Chemistry and deputy director of the ALISTORE-ERI network. He obtained his Ph.D. in Materials sciences in 2002 from the University of Ljubljana. Since his Ph.D. study, his research interests are in the field of materials science and electrochemical systems for energy storage, with main activities in the field of modern battery systems. Between 2009 and 2010 he worked in UPJV, Amiens, where he started the development of Li-S batteries. He was the coordinator of two large-scale EU projects focused on the development of Li-S batteries. His current research interests are focused on different types of multivalent batteries and the implementation of smart functionalities in battery cells. He is strongly connected with the Battery 2030+ initiative and with Batteries Europe, where he is one of the co-leaders of the task force preparing a strategy on the education level. He is involved in the MESC master program (https://mesc-plus.eu/) and in the doctoral school DESTINY (https://www.destiny-phd.eu/). He is a member of the Slovenian Academy of Engineering.
Magnesium and calcium batteries are considered promising post-Li battery technologies due to the high gravimetric and volumetric densities of metal anodes, their low redox potentials (–2.37 V and –2.87 V vs. SHE, respectively), and natural abundance, which is an important factor in terms of price and sustainability. The main challenge is the development of suitable electrolytes that enable reversible plating/stripping of Mg/Ca with high Coulombic efficiency in a wide potential window, while also being compatible with the metal anode and cathode materials.
In our recent work, we explore the field of advanced electrolytes based on alkoxyborates and alkoxyaluminates. Different salts were synthesized by exchanging hfip ligands for a variety of alcohols with different sterical properties, number of binding groups, and degree of fluorination. A variety of electrolytes for both systems are studied by physicochemical and electrochemical characterization.
The application of new salts along with some additives will be discussed along with the importance of proper electrochemical wiring of cathode composite containing polymerized redox active organic moieties.
1. Pavčnik, T. et al. Batter. Supercaps (2021)
2. Pavčnik, T. et al. ACS Appl. Mater. Interfaces (2022)
3. Lužanin, O., et al. Batter. Supercaps, 6(2), e202200437 (2023)
4. Lužanin, O., et al. J. Mater. Chem. A, 11, 21553–21560 (2023)
1.2-I1
Since the 1st of October 2023, Sonia Dsoke holds a Professorship for “Electrochemical Energy Carriers and Storage” at the Department of Sustainable Systems Engineering (INATECH), University of Freiburg, she leads a group “Innovative Battery Materials” at Fraunhofer Institute for Solar Energy Systems (ISE) and she is member of the Freiburger material center (FMF).
At the international level, she is the chair of Division 3 (electrochemical energy conversion and storage) of the International Society of Electrochemistry (ISE), one of the largest electrochemical communities in the world.
From 2017 until September 2023 Sonia Dsoke was the leader of a multidisciplinary group at the Institute for Applied Materials – Energy Storage Systems (KIT, Germany). In the same period, she was the deputy director of the platform CELEST and a spokesperson for Research Unit A (electrode materials) in the Cluster of Excellence POLiS dealing with “post-lithium” battery research. Previously she led an independent young research group focused on designing novel electrodes for Hydrid Battery-Supercapacitors at ZSW-Ulm (Germany). She also had industrial experience at an Italian battery manufacturing company FAAM (in 2009) and she was a researcher at the University of Camerino (Italy), where she also obtained her PhD in the field of Li-ion batteries.
Sonia Dsoke was honored with the Brigitte-Schlieben-Lange Programm Grant (2017-2019, Ministry of Science and Culture, Baden-Württemberg) and a Young Investigator Group Grant (2012-2016, Federal Ministry of Education and Research) within the framework “Energy Storage Initiative”.
Her actual main research subjects are the development of novel advanced functional materials for supercapacitors, lithium, and post-lithium ion batteries, with a special focus on tackling challenges of novel battery concepts such as Na, K, Mg, Ca, and Al batteries.
Can aluminum batteries promise enhanced energy density compared to other technologies? The key to high energy density lies not only in the transport of three charges per ion and in the design of a suitable cathode material but also in the (efficient) use of metallic aluminum as the negative electrode.
Currently used electrolytes for aluminum batteries (AlCl3/EMimCl acidic mixtures) can enable plating and stripping but they are extremely corrosive [1]. This drawback opens serious challenges for the choice of components (current collectors, binders, separators, active materials) that are stable in such a corrosive environment. This crucial issue urges to be overcome to advance Al batteries from simple scientific curiosity to real competitors in the realm of energy storage systems. On the one hand, one strategy is to identify corrosive-resistant components, and on the other hand to develop non-corrosive and benign electrolyte compositions [2]. This presentation will give an overview of the current challenges and propose some solutions in terms of materials and combinations to advance the Aluminum battery technology.
1.2-I2
Diversification of battery technologies is crucial both to reduce dependence of specific raw materials and, most important, to adapt to specific use requirements including not only performance figures of merit (energy density, power, lifetime etc.,) but also economic and environmental considerations. Among the different possible rechargeable battery concepts, those based on multivalent charge carrier ions, such as Mg2+, Ca2+, Zn2+ or Al3+, have attracted great attention, especially coupled to the use of the corresponding metal as negative electrode. Aside from the case of Zn, which is the most electropositive element that can be plated using aqueous electrolytes, most attention has been placed in Mg based batteries, for which proof-of-concept was achieved already in 2000 while exploration of the analogous systems is much more recent. None of such concepts has yet reached the market, as significant hurdles remain, affecting not only electrolyte and negative electrode (efficiency of plating/stripping process) but also the positive electrode. [1] In this regard, migration of multivalent charge carrier ions in inorganic hosts has been proved to be sluggish due to strong coulombic interactions which can be diminished by enhancing the covalency of the bonding and moving from oxides to sulfides, which penalizes the operation potential.
Open framework structures have also recently attracted attention, amongst which Prussian Blue analogues (PBAs) AxM[M’(CN)6]y · zH2O (A = alkaline metal (mostly K+ or Na+); M and M’ = transition metals; 0 ≤ x ≤ 2; y ≤ 1) represent an interesting alternative, which has proved excellent performances in Na and K based batteries. [2] Their structure oconsists of a double perovskite framework with (C≡N)− anions bridging MN6 and M’C6 octahedra. A+ and H2O occupy the cubes defined by the transition metal framework. These have shown electrochemical activity in aqueous electrolytes containing multivalent cations but controversies remain as to whether the redox response observed being related to the intercalation of protons rather than divalent ions.
The study of these compounds in non-aqueous electrolytes is appealing, especially if both M and M’ are redox active (e.g. Fe, Mn), and they have shown excellent performance in K+ and Na+ batteries with very good kinetics and cycle life. The presentation will deal with analogous studies carried out in Mg2+ and Ca2+ containing electrolytes. [3] Electrochemical performance, possible side reactions, and operando diffraction studies aimed at unravelling the redox mechanism will be discussed.
1.2-O1
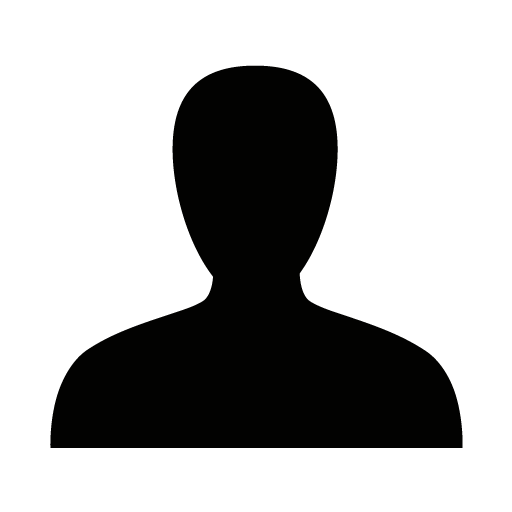
The growing interest in room-temperature sodium metal rechargeable batteries (SMBs) for large-scale energy storage applications stems from their potential to serve as a cost-effective alternative to lithium-based batteries. This is attributed to the natural abundance of sodium and its low redox potential (-2.71 vs. SHE).[1] However, the use of metallic sodium as an anode entails drawbacks such as the generation of a low conductive solid electrolyte interface (SEI), together with dendrites formation during the sodium plating/striping process, which implies short-circuit and safety hazards.[2] Therefore, the development of advanced electrolytes that enable the use of metallic sodium as an anode is crucial for the overall good performance battery.
On the other hand, the commercialization of SMBs also requires the development of practical cathodes that provide satisfactory rate performance, long cycling life, and high areal capacities. During several years, inorganic active materials such as layered transition metal oxide (LTMO), polyanionic compounds, or Prussian blue analogs (PBA) have been widely studied as cathodes in sodium-based batteries. However, these inorganic compounds present low insertion kinetic, their theoretical capacity is commonly limited to one-electron transfer, and exhibit severe volume alterations during intercalation–deintercalation cycling leading to poor cycling stability. On the contrary, redox-active organic compounds can offer multielectron reactions, present high theoretical capacity, and are able to better accommodate volume changes.[3,4] However, the low electrical conductivity and the partial dissolution of organic materials in the electrolyte often limit the areal capacity and the long-term cyclability of the battery.
In this communication, it is described the use of a highly concentrated electrolyte (> 7M) based on liquid ammonia, whose formula is NaI·3.3NH3,[5] which can effectively stabilize the metallic sodium delivering a coulombic efficiency of 99.8 % during the plating/stripping process, even at very high currents. This electrolyte is utilized in a sodium metal battery (SMB) where the metallic sodium anode is combined with a hybrid anthraquinone-based conjugated microporous polymer as cathode.[6] Due to the intrinsic textural properties of this compound it is possible to prepare thick electrodes maintaining excellent electrochemical performance.
All in all, the battery depicted here shows an outstanding areal capacity of 7 mAh·cm-2, never reported before for SMBs. In addition, this battery has demonstrated excellent rate capability up to 250C (37.3 A g-1, near 70% capacity retention) and stable cycling (80% capacity retention after 4000 cycles with coulombic efficiency close to 100 %). In summary, the combination of a hybrid conjugated porous polymer with an ammoniate-based electrolyte results in a sodium metal battery characterized by high energy and power density, excellent areal capacity and long-term cyclability. This combination proves useful in designing practical Sodium Metal Batteries (SMBs).
1.2-O2
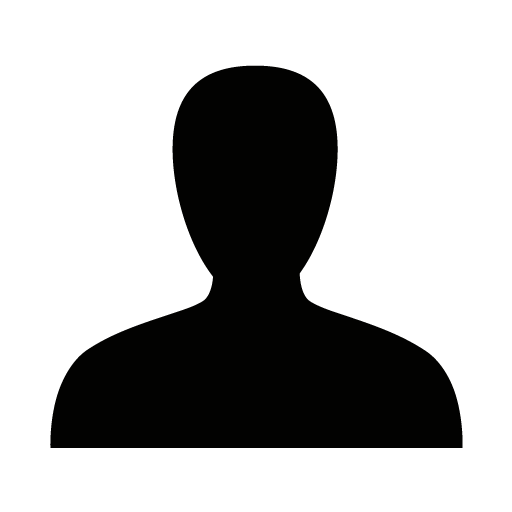
The rapidly growing need for energy storage exceeds the energy density of the currently dominant commercial lithium-ion batteries (~200 Wh/kg). Therefore, there is an exhaustive search to find a viable alternative to lithium-ion batteries. Thus, metal-sulfur technology is strongly emerging to become the next-generation of rechargeable batteries. Lithium-sulfur (Li-S) and sodium-sulfur (Na-S) batteries are gaining attention because of their high theoretical gravimetric energy densities, 2615 and 1673 Wh/kg, respectively, as well as the low cost and non-toxicity of sulfur [1][2].
Nevertheless, sulfur has a few drawbacks that negatively affect battery life, being the shuttle effect and its sluggish reaction kinetics the most studied. Among the different solutions proposed to alleviate these problems, it is worth highlighting the trapping of polysulfides through chemical interaction [3]. Recently, it has been demonstrated that the application of a magnetic field to materials with paramagnetic properties can improve cycling performance [4].
One of the materials showing these properties is ZnFe2O4 [5]. As a novelty, we propose the use of recycled ZnFe2O4 from display wires. Detailed study on the effect of applying a magnetic field on the electrochemical behavior has been carried out. Tests have shown that this sustainable material can act as an efficient sulfur matrix in electrodes with high sulfur content >70% S and high sulfur loading of up to 10 mg/cm2. This excellent performance has also been demonstrated for high-rate capability up to 10C. These results overperform those previously published for ZnFe2O4 based sulfur cathodes, demonstrating higher specific charge values when applying a permanent magnetic field, especially in long-term tests.
1.2-O3
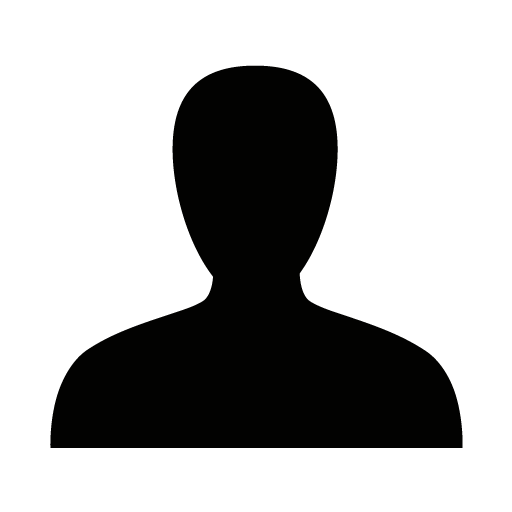
Flexible lithium-ion batteries (LIBs) have garnered significant attention as essential power sources for wearable and flexible electronic devices [1-6]. Despite the increasing interest, achieving optimal flexibility, mechanical stability, and high energy density in flexible LIBs remains a formidable challenge. This study explores the potential of molybdenum carbide (Mo2C) [7] and vertically-oriented graphene nanowalls (VGNWs) as anode materials for Lithium-Ion batteries.
A bottom-up synthesis approach is employed, involving the deposition of Mo carbide nanostructures on VGNWs using chemical vapor deposition, magnetron sputtering, and thermal annealing processes, followed by in-situ carburization via thermal annealing, resulting in binder-free hybrid electrodes. The resulting Mo2C/VGNWs hybrids exhibit exceptional structural durability, small particle size, and a porous configuration, promoting enhanced electron and ion accessibility at the electrode-electrolyte interface. SEM results demonstrate varied Mo carbide morphologies based on annealing time, while TEM analyses reveal uniformly anchored Mo2C nanoparticles on VGNWs.
Electrochemical tests reveal that Mo2C/VGNWs hybrids outperform VGNWs/Papyex® electrodes in lithium storage behavior. Evaluation of the Mo2C/VGNW hybrid electrode as a LIB anode material demonstrates superior electrochemical performance compared to VGNWs/Papyex® electrodes. The Mo2C/VGNW hybrid electrode exhibits a higher first discharge capacity (0.23 mA·h·cm−2) compared to VGNWs/Papyex® (0.11 mA·h·cm−2) at 1C scan rate. Furthermore, the Mo2C/VGNW electrode maintains a reversible specific capacity of 0.005 mA·h·cm−2 after 200 cycles, while the VGNWs/Papyex® electrode shows a significantly lower capacity of 0.001 mAh cm−2 after the same cycles. The synergistic effects of Mo2C nanoparticles and highly conductive VGNWs contribute to the superior electrochemical characteristics, positioning the Mo2C/VGNWs hybrid structure as a promising candidate for high-performance and flexible energy storage devices.
Notably, the Mo2C/VGNW hybrid electrode demonstrates a progressively increasing coulombic efficiency from 30% to 90.4% in the first 50 cycles, followed by stable performance. The dynamic interactions between Mo2C nanoparticles and the highly conductive VGNW support contribute to the superior electrochemical performance. This study presents a promising synthesis approach for developing highly efficient and flexible energy storage devices through other carbide/VGNW hybrids.
1.3-O1
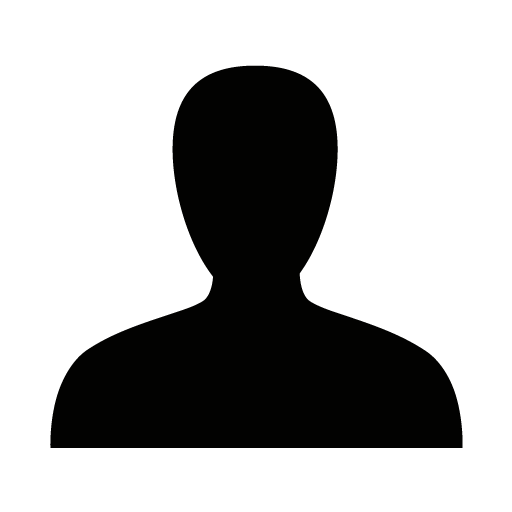
Lithium–ion batteries (LiBs) are currently leading the portable electronic market due to their great cyclability. However, the current energy demand is pushing the limits of LiBs in terms of energy density (100 – 265 Wh kg−1), cycle life (1000 cycles at > 80% of capacity) and charge/discharge rate capabilities (1C). Aprotic sodium-oxygen batteries (Na-O2) are promising devices to tackle such energy demands due to their much higher theoretical energy density (1086 Wh kg-1 based on sodium superoxide discharge product, NaO2). These conversion batteries reduce oxygen gas to form solid NaO2, which is deposited on the surface of the cathode during discharge. Rechargeability depends on an efficient charge, where complete redissolution of the superoxide in the organic electrolyte is required to further release all the oxygen back. The size, the distribution, the morphology and the chemical nature of the solid deposits are crucial parameters for the battery performance, due to the electronically insulating nature of NaO2. Thus, the passivation of the cathode surface by NaO2 generally leads to a premature death of the battery. Electrolyte formulation has demonstrated to have a profound effect on the morphology of the NaO2 cubes by affecting their solvation and crystallization processes [1,2]. It is believed that small micrometric particles form a film and promote passivation while large cubes enhance the electron conduction towards the discharge product. However, recent works have demonstrated the importance of the cathode surface chemistry on the nucleation and growth of the discharge products [3-5]. A controlled surface-mediated mechanism enabling the deposition of defined and homogeneously distributed nanometric particles is, in fact, beneficial for battery performance as; i) the discharge product will not insulate the cathode surface by an effective utilization of its surface area and ii) the oxidation of nanosized NaO2 during charge will be facilitated by nanometric particles at a still conductive electrode.
In this work, edge-defected graphene nanoplatelets will be discussed as cathode in Na-O2 batteries which act as nucleation points, promoting homogeneous nucleation of nanosized and well-defined NaO2 cubes at the surface of the conductive graphene air-cathode.
1.3-I1
Lee Johnson received his first degree from Newcastle University, after which he completed a PhD and post-PhD Fellowship in physical chemistry and electrochemistry at the University of Nottingham. He then joined the research group of Prof Sir P.G. Bruce FRS at the University of Oxford, where he studied the elementary processes taking place within the lithium-O2 battery. In 2017, he was awarded a Nottingham Research Fellowship, University of Nottingham, followed by an EPSRC Fellowship in 2018, both to support study of next-generation batteries. In 2019 he was promoted to Associate Professor in the School of Chemistry. His current research interests focus on understanding interfacial reactions, degradation, and charge transfer, in electrochemical energy devices.
Lithium-ion batteries have delivered a revolution in portable electronics and have begun to unlock electrification of the automotive industry. However, intrinsic performance limitations mean that many applications will be out of reach for lithium-ion technology. We must explore alternatives if we are to have any hope of meeting the long-term needs for energy storage. The Johnson group is focused on tackling the underpinning chemical and materials challenges within next-generation energy devices and in particular batteries.
One such alternative is the Li-air (O2) battery; its theoretical specific energy exceeds that of Li-ion, but many hurdles hinder its realization. Early cell death, resulting in low capacity and limited rate capability, is one of the most significant problems. Studies of the processes at the positive electrode have shown that this is a result of passivating Li2O2 at the electrode surface, poor utilisation of the electrode structure, and degradation of the cell components. Here, we discuss recent advances made in our labs to understand the chemistry within the lithium-air battery and to combat the problems limiting its performance. For example, we have identified a redox mediator able to promote longer discharge and electrode compositions able to facilitate O2 transport throughout the positive electrode. New understanding of the degradation processes have been identified, shedding further light on the failure mechanisms within the cells. Finally the impact of operating in air with the inevitable introduction of H2O will be considered and we will showcase a demonstrator gas handling system and cell designed to explore these effects.
1.3-I2
Dr. Nagore Ortiz-Vitoriano (https://cicenergigune.com/en/nagore-ortiz-vitoriano) is an Ikerbasque Research Associate, who has been spearheading metal-air research at CIC energiGUNE (Spain) since 2016, of which she became research line manager in 2018.
She obtained her doctorate in 2011 for her work on solid oxide fuel cells (University of the Basque Country, UPV/EHU, Spain), during the course of which she undertook research stays at Risø DTU (Denmark) and Imperial College London (UK). In 2013 she was awarded a Marie Curie International Outgoing Fellowship from the European Union, enabling her to join the Department of Mechanical Engineering at the Massachusetts Institute of Technology (MIT) in Cambridge (USA) where she worked with both lithium and sodium-air batteries. In 2015, she continued this fellowship at CIC energiGUNE, where she conducted research stays at Oak Ridge National Laboratory (USA), Deakin University (Australia) and Chalmers University (Sweden). Recently, she has been promoted to Ikerbasque Research Associate and granted the Ramon y Cajal fellowship financed by the European Commission's European Social Fund through the Spanish Ministry of Science and Innovation.
Dr. Ortiz-Vitoriano has focused on both rational design of electrode and electrolyte materials for energy storage (e.g., solid oxide fuel cells, electrocatalysis, Na-ion and metal-air batteries), as well as fundamental research focused on elucidating key processes (by establishing relevant physiochemical models) in order to facilitate rapid future developments at both the material and system levels.
Energy storage plays, undoubtedly, a fundamental role in the process of total decarbonization of the global economy that is expected to take place in the coming decades. The energy transition to a renewable and sustainable generation is the solution to reduce greenhouse gas emissions and thus achieve the European Commission´s goal of becoming the world´s first decarbonized economy. In this transition, beyond li-ion technologies will play a key role to meet the increasing energy demand that cannot be covered by Li-ion batteries solely. In this scenario, sodium-oxygen have become an attractive alternative due to their high gravimetric energy densities (1605 or 1108 Wh/kg based on Na2O2 or NaO2, respectively) resulting from the use of an oxygen-based phase-change reaction (potentially reducing the weight and freeing up space for other components).
These batteries have been considered as the holy grail of battery research due to their high theoretical energy densities; however, several challenges remain to be solved before commercialization. In this talk, novel approaches on cathode materials and electrolytes will be covered with special focus on sustainability. One of the bottlenecks in this type of device is the kinetic limitations related to oxygen reduction reactions (ORR) and oxygen evolution reactions (OER). Therefore, we have proposed to mimic nucleotides role in cellular respiration by using them as electrocatalysts in metal-air batteries. Regarding electrolytes, we aim to identify an eco-friendly solid membrane electrolyte to achieve stable and high-performing solid-state NaBs by overcoming oxygen crossover from the cathode to the anode. Moreover, the main challenges remaining in this field will be highlighted, along with the future steps required to advance Na-O2 batteries.
2.1-I2
The development of next generation batteries depends heavily on the capability of electrolytes to quickly and selectively transport alkali and alkaline earth metal cations, and form stable electrochemical interfaces. In high energy density metal anode batteries, issues such as dendrite and continuous solid electrolyte interphase (SEI) growth can be addressed by suitable interfacial and electrolyte chemistry.
In the first part of my talk, current understanding of ion transport mechanisms and related electrochemical measurement techniques (impedance spectroscopy, galvanostatic polarization) in soft matter battery electrolytes including liquids, polymers and hybrid (e.g. liquid/oxide and polymer/solid state electrolyte) materials will be discussed. According to this discussion, I will give guidelines and examples of improvements of the relevant electrochemical properties including ionic conductivity and the cationic transference number.
In the second part of my talk, I will show recent findings related to the electrochemical and chemical growth and transport in SEIs on several alkali and alkaline earth metal anodes in contact with liquid and solid-state electrolytes. The multitechnique approach involving the measurement of activation energy for ion transport showed that such SEIs are complex composite liquid/solid materials, with sometimes predominant ionic pathways in the liquid phase. The relevance of the native passive layer on alkali and alkaline earth metals, possibility of forming artificial SEIs (e.g. sulfides and Al2O3) and electrodeposition through porous SEI will be discussed. Finally, I will show a new modelling approach for treatment of impedance spectroscopy data of symmetric alkaline and alkaline earth metal cells.
2.1-O2
Doctor Min Zhou. Lectuer in School of Electrical and electronic engineering, Huazhong University of Science and Technology (HUST).
Graduate from Wuhan University in 2014, getting the doctor’s degree. Postdoctoral research in HUST from 2014 to 2016. Research interest in advanced energy storage systems and novel energy storage materials for grid scale energy storage applications. Research work has been published on Advanced Materials, Advanced Energy Materials, Energy & Environmental Science et al.
Grid-scale energy storage technologies is important and in urgent need for the Chinese National strategy of “carbon peak and carbon neutrality ”. Among the most energy storage technologies, electrochemical energy storage technologies, such as batteries, shows the great advantages of simple structure and high efficiency, which is developing quickly and widely applicated for varied fields of EES. For the electrochemical energy storage technologies, the electrodes play the key role for the battery performances. Current batteries suffer from low energy/power densities, poor cycling stability and safety issues, it is important to tune the molecule and electronic structure of the electrodes, constructing stable electrode/electrolyte interface, in order to provide more redox active sites, accelerate the ion/electron transfer kinetics as well as guarantee the stable and reversible redox reactions.
Electrodes with special composition and structure always show attracting electrochemical performances, but are difficult to be synthesized through conventional chemical methods. Multi-physical fields, such as electric, magnetic, plasma, for the precise regulation of the electrodes. Firstly, supercritical condition is introduced for the preparation of heteroatoms doped carbon with ultrahigh doping levels. P doped carbon with an ultra-high doping level (30%) is successfully synthesized and demonstrate an ultrahigh reversible capacity with low potential of 0. 54 V vs Na/Na+. Secondly, electronic and thermal fields are coupled for the precisely control of metal valence in the transition metal compounds. Compared to TiO2, low valence Ti oxides, such as TiO, Ti2O show higher electronic conductivity and electrochemical reactivity due to metal-like properties. In this work. Ti-O compounds with low Ti valence is prepared through the molten-salts electrosynthesis and deliver a high reversible capacity of 484 mAh g-1 through the multi-electron conversion reactions. In addition, plasma was introduced for the surface modification of the electrodes for the construction of stable artificial SEI layers with certain species. Artificial SEI layers with certain species of LiF, Li2C2 and polythiophene are prepared through the plasma treatment. Benefiting from the high mechanical strength of LiF, low Li+ diffusion barrier of Li2C2 and flexible structure of the polythiophene, modified Li anodes exhibit an long-term cycling stability of 8000 h with dendrite free structure. When coupling with LiFePO4, the full cell demonstrate longer cycling performances.
2.1-O1
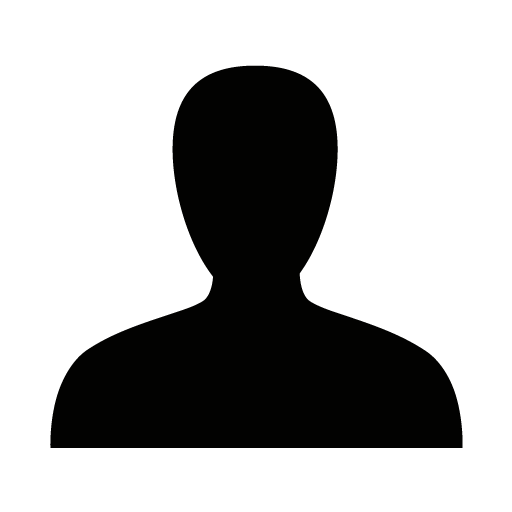
Research and development of lithium-ion batteries (LIBs) has gathered a great deal of momentum in the last two decades due to their potential promising applications in portable electronics and electric vehicles. This is partly due to rapid advancement of inorganic intercalation compounds and carbon-involved composite electrode materials. However, albeit their substantial success, in present commercial LIBs, the urgent need for cost-effective, large-scale, safe, and sustainable battery technologies feeds an ever-growing quest for alternative energy related materials. In this context, substituting traditional metal oxide-based intercalation compounds with organic electrode materials (OEMs) with electroactive organic redox functionalities has attracted the attention of researchers due to the greater abundance of organic compounds, huge synthetic possibilities, lower cost, and safety and sustainability aspects.[1]
Among the different types of OEMs, redox-active polymers (RAPs) are materials of choice due to their high structural diversity, rich and tunable electrochemistry, etc.[2] However, their high electrochemical performance is primarily linked to the use of low polymer mass-loading electrodes (typically below 2 mg cm−2) with a high carbon-additives content (20–80 wt%) that together hinders their practicability in real batteries.[3–5] In my oral presentation, I will present an interesting strategy to develop high performance and practical naphthalene-tetracarboxylic dianhydride-derived polyimide (PI) electrodes that are processed into buckypaper electrodes without binder and current collector. This effective electrode fabrication method enables high-mass-loading composite electrodes (up to 55 mg cm−2) with low carbon-additive content (20 wt %), which attained high gravimetric (170 mAh g−1), areal (8.5 mAh cm−2) and volumetric (205 mAh cm−3) capacities with good rate capability (1.6 mAh cm−2 at 5C; 25 mA cm−2) in Li-ion half-cells. To the best of our knowledge, these are most probably the highest values reported for an organic electrode in Li-ion batteries, constituting a great leap forward in the development of practical organic batteries. As a proof of concept, semi-organic full cells were assembled in Li_Graphite||PI, Li_LTO||PI, PI||LFP, and PI||NMC configurations in both coin-type and pouch-type cell prototypes to assess the practicality of these high-mass-loading PI electrodes. Electrochemical performance metrics in terms of capacity, energy and power density (gravimetric, volumetric, and areal) of these full cells were evaluated, along with preliminary cost prediction of practical Li ion batteries for e-mobility application.[6] Both performance metrics (32 mWh cm−2) and cost factors (98$ kWh−1) for the presented redox polymer were favourable that could have great prospects for future practical (semi)organic batteries. I believe that this study may be of broad interest to the audience of the conference, providing insights for the development of practical and sustainable energy storage solutions.
2.1-I1
Sodium ion batteries (SIBs) are a potential alternative to diversify the energy landscape, beyond Lithium-ion batteries (LIBs), due to their similar storage mechanism and easy technology transfer. Currently, the benchmark anodes for SIBs are hard carbons (HCs), since sodium ions do not intercalate into graphite, the current anode of choice for LIBs. HCs can be produced from a variety of waste precursors that are more sustainable and less geopolitically compromised than natural graphite, mainly concentrated in China.
The electrochemical degradation of SIBs occurs at a higher rate than in LIBs. This can be attributed to the larger electrochemical reactivity of the HC anodes. A deeper operando understanding of the degradation mechanisms in SIBs, coupled with engineering of the materials and electrolyte to ensure that a better and more protective solid electrolyte interface (SEI) is formed, is needed for an accelerated scale up of this technology. In this talk I will show you some of the strategies we have developed for these aims.
2.2-I2
Dr. Fellinger is Head of the Division 3.6 Electrochemical Energy Materials at the German Federal Institute for Materials Research and Testing (BAM). He is a nanostructure and molecular scientist by training (diploma at University of Kassel, DE), who received his PhD in colloid chemistry (with summa cum laude) at the University of Potsdam/DE under the direct supervision of Prof. Markus Antonietti in 2011. After a short postdoctoral stays at the Tokyo Institute of Technology (Prof. Ichiro Yamanaka) he was a research group leader at the Max Planck Institute for Colloids and Interfaces in Potsdam-Golm (2012-2017). In 2016/17 he was an awarded Researcher-in-Residence at Chalmers Institute of Technology in Gothenburg (Prof. Anders Palmqvist), followed by one term as W2-substitute professor for inorganic chemistry at the University of Applied Science Zittau/Görlitz. Afterwards until 2020 he joined Prof. Hubert Gasteiger´s Chair for Technical Electrochemistry (Technical University Munich) with a fuel cell project. In 2020 Dr. Fellinger´s group joined the Federal Institute for Materials Research and Testing (BAM) in Berlin. Dr. Fellinger received the Donald-Ulrich Award 2017 of the International Sol-Gel Society and the Ernst-Haage Award for Chemistry of the Max-Planck Institute for Chemical Energy Conversion. His research interests are the synthetic chemistry of novel materials and their usage in energy-related applications with a focus on different carbon-based materials like nitrogen-doped carbons, M-N-C catalysts or hard carbon anodes. He has published ~60 articles in peer-reviewed journals (>6000 citations, H-index: 41).
The current strong interest in electromotive mobility and the need to transition to an energy grid with sustainable storage devices has led to a renewed interest in sodium ion batteries (SIBs). Amorphous disordered carbons such as hard carbons (HCs) are promising candidates for high-capacity negative electrode materials in SIBs. Their high capacities, however, are often accompanied with high irreversible capacity losses during the initial cycles,[1] while low initial losses are accompanied with moderate capacities.[2] In our research we are aiming morphologically and chemically functional carbons. Morphologically, our target is a local separation of reversible sodium storage and irreversible losses in novel synthetic carbon anodes, using a core-shell concept. On chemical side, we aim at ion-binding functional groups.
We investigated different methods to obtain core-shell structures to restrict SEI formation to the external particle surface, while leveraging the Na storage potential of porous carbon core materials. Moreover, we apply active-site imprinting to realise disting ion-binding features into the core carbons. The electrochemical performance of those materials can be rather easily altered upon removing/exchanging the ions bound to the functional group. Herein, we will focus on the synthesis of zeolitic imidazolate framework (ZIF) based NDCs. Different analytical methods, e.g., physisorption (N2, Ar, CO2), XPS, XAS, and NMR, will be used to understand the alteration of morphological and chemical features upon ion exchange.
2.2-I1
Dr. Minghao Yu, PI, holds an independent research group (Electrochemistry for Sustainable Energy Storage) at Technische Universität Dresden. His research interest includes 1) the development of novel organic and inorganic 2D layered materials, 2) the investigation of advanced artificial interphases and electrolytes for next-generation batteries, 3) fundamental charge and ion dynamics during electrochemical energy storage processes, and 4) sustainable energy storage device fabrication, including supercapacitors, hybrid-ion capacitors, aqueous batteries, dual-ion batteries, and multivalent metal (Zn, Mg, Al) batteries. He has published more than 120 scientific articles which have attracted more than 20,000 citations with an H-index of 69 (Web of Science). Besides, he is also an associated member of the Center for Advancing Electronics Dresden (cfaed), an associated group leader at Max-Planck-Institut für Mikrostrukturphysik, a highly cited researcher (Clarivate Analytics, 2018-now), 2023 ERC Starting Grant winner, and a Fellow of the Young Academy of Europe.
Two-dimensional (2D) porous frameworks have drawn growing research interest as a new generation of multifunctional materials. Typical 2D porous frameworks include 2D covalent organic frameworks and 2D conjugated metal-organic frameworks, which are characterized by regular porosities, large specific surface areas, and superior chemical stability. In addition, 2D porous frameworks exhibit certain notable properties (e.g., designable topologies and defined redox-active sites), which have motivated increasing efforts to explore 2D porous frameworks for electrochemical energy storage applications.[1] In this talk, I will show 2D porous frameworks as promising electrode alternatives for next-generation energy storage devices by demonstrating 2D polyarylimide covalent organic frameworks for multivalent metal batteries[2-3] and 2D conjugated metal-organic frameworks as attractive pseudocapacitive electrodes[4-5].
[1] Yu et al., J. Am. Chem. Soc. 2020, 142, 12903-12915.
[2] Yu et al., J. Am. Chem. Soc. 2020, 142, 19570-19578.
[3] Yu et al., J. Am. Chem. Soc. 2023, 145, 6247-6256.
[4] Yu et al., J. Am. Chem. Soc. 2021, 143, 10168-10176.
[5] Yu et al., Angew. Chem. Int. Ed. 2023, e202306091.
2.2-O3
Husam Alshareef is a Professor of Materials Science and Engineering at King Abdullah University of Science and Technology (KAUST). He is also the Director of the newly-established Center of Excellence in Renewable Energy and Storage Technologies at KAUST. He obtained his Ph.D. at NC State University followed by a post-doctoral Fellowship at Sandia National Laboratories, USA.
He spent over 10 years in the semiconductor industry where he implemented processes in volume production for chip manufacturing. He joined KAUST in 2009, where he initiated an active research group focusing on the development of nanomaterials for energy and electronics applications. His work has been recognized by over 25 awards including the SEMATECH Corporate Excellence Award, two Dow Sustainability Awards, the Kuwait Prize for Sustainable and Clean Technologies, and the KAUST Distinguished Teaching Award. He has published over 600 papers and 80 issued patents. He is a Fellow of several prestigious societies including the American Physical Society (APS), Institute of Electrical and Electronics Engineers (IEEE), US National Academy of Inventors (NAI), Institute of Physics (IoP), Royal Society of Chemistry (RSC), and the Institute of Materials, Minerals and Mining. He has been a Clarivate Analytics Highly-cited Researcher in Materials Science for several years.
Aqueous batteries using non-metallic charge carriers, especially of protons, have recently received significant attention. The proton is the smallest and lightest charge carrier among all cations, which contributes to fast transport kinetics and relatively small structural strain during proton (de)intercalation, leading to promising cycling performance. In recent years, several electrodehost materials with high-rate capability were explored using acidic electrolytes in terms of the proton’s tiny size and unique hopping transport through the hydrogen bond network. For instance, defective Prussian blue analog with high-rate performance (4000 C) and excellent cycling stability were observed. More proton anode materials were explored in metal oxides, mainly including WO3·xH2O, MoO3, H2W2O7, H2Ti3O7, and anatase TiO2. The α-MoO3 has attracted much attention for proton storage owing to its easily modified bilayer structure, fast proton insertion kinetics, and high theoretical-specific capacity. However, the fundamental science of the proton insertion mechanism in α-MoO3 has not been fully understood. Herein, we have uncovered a three-proton intercalation mechanism in α-MoO3 using a specially designed phosphoric acid surfactant lyotropic liquid crystalline electrolyte. The semiconductor-to-metal transition behavior and the expansion of the lattice interlayers of α-MoO3 after trapping one mole of protons were verified experimentally and theoretically. Further investigation of the morphology of α-MoO3 indicated its fracture behavior upon the proton (de)intercalation process, which created ultrafast diffusion channels for hydronium ions. Notably, the first observation of an additional redox behavior at low potential regimes endows a significantly enhanced specific capacity of 362 mAh g-1.
2.2-O2
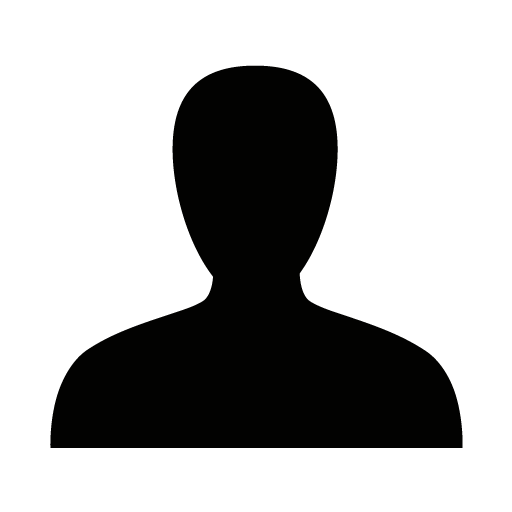
Nowadays, aqueous rechargeable batteries (ARBs) are emerging as highly promising energy storage alternatives to current Li-ion batteries for stationary applications. This is attributed to their significant advantages in terms of safety and cost-effectiveness, in addition to high performance. Especially, acid-based ARBs stand out as a compelling substitute due to the accelerated proton kinetics resulting from their minimal ionic mass and diminutive radius,[1] distinguishing them from their neutral[2] and alkaline[3][4] counterparts. Moreover, of paramount importance is the proton's ability to achieve exceptionally rapid ionic conduction in aqueous electrolytes, due to its advantageous utilization of the Grotthuss mechanism.[5]
Conventional acid-based ARBs are based on inorganic electrode materials and face significant drawbacks, including high costs of both anode and cathode material (based on rare elements) and severe active-material corrosion and/or dissolution. Moreover, using a metal anode (e.g., Pb) results in dendrite formation caused by uneven and irregular metal plating on the anode side, in addition to the formation of a thick lead (II) sulphate (PbSO4) passivation layer.[2] As a consequence, battery cycle stability is seriously impeded, possibly with short-circuit risks and safety compromises.
Recently, organic electrode materials (OEMs) are re-emerging as green and sustainable alternatives over traditional inorganic materials as they offer distinct advantages. First, they are composed of readily available and cost-effective elements (C, O, N, H). Moreover, by their distinctive ion-coordination mechanism, they can interact with different charge carriers (Li+, Na+, H+, Zn2+, etc), finding application in different battery technologies.[2] Furthermore, under acidic conditions these materials are less prone to the dendrites formation, corrosion, and/or dissolution, common issues faced by their inorganic counterparts.
Recently, we demonstrated the rapid kinetics, good electrochemical performance and excellent robustness of a new conjugated microporous polymer based on phenazine (named IEP-27-SR) in 1 M H2SO4 electrolyte.[6] Here, I will present our recent results on the use of this anode (IEP-27-SR) in combination with an electrodeposited MnO2-based cathode in a full acid battery. The full battery not only reached an impressive number of cycles (20000 at 30 C with 83% retention) but also could withstand high current densities (100 C, yet achieving 40 mAh g-1) using 2 mg cm-2 polymer mass loading anode. Moreover, in this study we could increase the polymer mass loading up to 30 mg cm-2, while keeping its content high (80 wt%) in the electrode. This enhancement contributed to a significant increase in the areal capacity of the aqueous battery up to 2.8 mAh cm–2, while maintaining a noteworthy value of 1 mAh cm- 2 even under the extreme high current of 79.6 mA cm-2. This porous polymer//MnO2 battery offers a sustainable and cost-effective alternative to the conventional acidic battery (e.g., PbO2 / / Pb), without compromising its performance, paving the way toward practical and sustainable energy storage solutions.
2.2-O1
Large-scale energy storage is a key technology for efficient integration of renewable energy generation into the grid and the development of smart grids. Among various energy storage technologies, electrochemical energy storage stands out as a flexible and efficient option, making it an important direction for the development of utility-scale power storage. Liquid metal batteries based on liquid metal electrodes and molten salts electrolytes, with the merits of long-lifespan, low-cost, and high-safety, shows … in large-scale energy storage applications. This article focuses on the key materials and technologies of liquid metal batteries, demonstrating our team's recent research progress at three aspects: materials, devices and energy systems. A novel strategy of alloying liquid electrode for liquid metal batteries was proposed, and a series of high-performance electrode material systems were developed that achieve a high battery energy density of 156 Wh/kg. Moreover, a multi-physics coupling model of liquid metal battery was constructed and revealing the mechanisms and migration laws governing mass transfer at liquid-liquid interfaces. Furthermore, we have put forward a strategy for stabilizing large-scale liquid-liquid interfaces through internal field restriction combined with external active regulation, which enabled the cycle life of Li-Sb-Sn liquid metal battery exceeded 15000 cycles. Based on those strategies, 600 Ah high-capacity liquid metal batteries were constructed. Moreover, we have proposed control strategies for electro-thermal coupled energy storage systems and built a 5 kW liquid metal battery energy storage system.
2.3-I1
Professor Emma Kendrick, CChem FIMMM FRSC FIMMM - Chair of Energy Materials, School of Metallurgy and Materials, University of Birmingham.
Prof Kendrick’s career to date has included industrial and academic roles leading to her current role as Chair of Energy Materials, where in addition to group lead of the energy materials group (EMG), she is co-director of the Centre for Energy Storage (BCES) and part of Birmingham Energy institute (BEI) and Birmingham Centre for Strategic Elements and Critical Materials (BCSECM). The EMG investigates sustainability in novel battery technologies from materials, manufacturing, performance and parameterisation, and recycling. Her recent work has led to a 2021 joint UoB - Imperial College London (ICL) spin out company, based around the methods of experimental parameterisation of applied multi-physics cell models, called About:Energy, for which she is founder and director.
Prior to UoB, she spent two years as Reader in WMG, University of Warwick. Before academia, she led innovations in the battery industry, latterly as Chief Technologist in Energy Storage at SHARP Laboratories of Europe Ltd (SLE) and prior to that for two lithium-ion battery SMEs, Fife Batteries Ltd and Surion Energy Ltd.
She is fellow of the Royal Society of chemistry (RSC) and Institute of Metals, Mining and Materials (IoM3). Recently, she has been recognised through several awards; 2021 Faraday Institution (FI) Researcher Development Champion, RSC 2021 Environment, Sustainability and Energy Division Mid-Career Award, and the 2019 Hothersall Memorial Award for outstanding services to Metal Finishing.
Prof Kendrick holds a PhD from Keele University, obtained as part of a postgraduate transfer partnership (PTP) scheme with CERAM Research, a MSc in new materials from the University of Aberdeen and a BSc in chemistry from the University of Manchester.
Critical materials are those which have a supply chain risk and are of high economic importance, this means that criticality is unique to each area of the globe, due to the sourcing of these different materials. In Europe, the critical materials list published in 2023 shows lithium, graphite, phosphate and phosphorous, silicon, cobalt and manganese, all classified as critical. Nickel is although, a strategic material, and with carcinogenic implications is not currently classified as critical. This assessment is dynamic due to changes in the sourcing, supply and also recycling levels of these important elements and materials. With the new battery passport and EU battery directive regulations, 50% of lithium recovery by 2027 and 80% by 2031 from spent lithium-ion batteries. SLI and EV batteries will require a recycled content of 16% for Co, 6% Li and 6% Ni, and recycling efficiency is 50% by 2025. Environmental and social impact needs to be understood throughout the life cycle of the battery, with supply chain due diligence. By 1st of Feb 2028, a full impact assessment of battery life cycle, or LCA must be supplied, including the recycled content sources.
In this work we look at optimisation of lithium-ion and sodium-ion technologies and the investigation of cathodes and anodes at low and elevated temperatures. In particular a co-doped lithium nickelate is discussed, and its performance in a full cell. By co-doping the layered nickelate with boron and tin, the cathode material has improved low temperature performance properties. In addition, the performance of hard carbon in a sodium-ion system is also discussed, and its performance at low and elevated temperatures.
2.3-O1
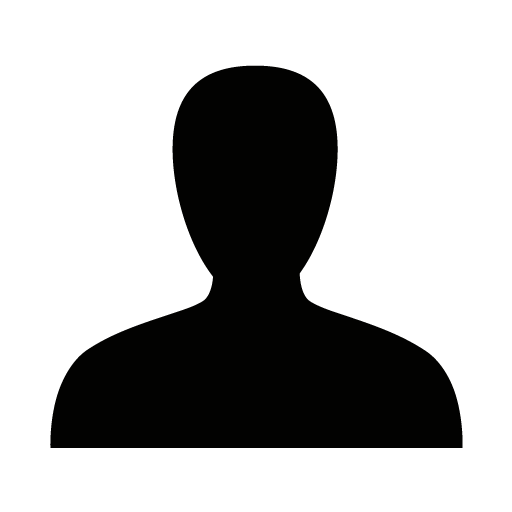
The transition to “green energy” relies heavily on energy storage devices, especially lithium-ion batteries (LIBs). Even though new battery chemistries are emerging and pose advantages, waste associated with all batteries requires adequate treatment. Just in this decade, about 11 million metric tonnes of spent LIBs will be disposed, being a source of refined, critical materials such as Li, Co and Ni.1 Hydro- and solvometallurgy are recycling strategies that are showing industrial potential for a more efficient and greener process.2 However, the latter is often dictated by the amount of corrosive reagents, waste streams and downstream processing required. Hydroxylated solvents such as glycerol or gallol- and cathecol-based polyphenols can coordinate with metals. Thus, their application, individually or as components of other systems can occur.
In this work, the versatility of these solvents for metal recovery from spent LIBs is expanded, by using them in both liquid and solid state.
As liquids, these solvents were combined with low HCl concentrations and high water content to achieve leaching of Co, Ni and Mn from NMC materials. The increased chloride activity, a promoting ligand for metal dissolution, and direct coordination by the hydroxylated solvents were relevant factors for metal dissolution.
As solids, when combined with [2-(methacryloyloxy) ethyl] trimethylammonium chloride, these solvents could be photopolymerized into absorbing polymers. These could be used in a simple and cyclic process to extract metal ions such as Co, Ni and Li from solutions containing a complex mixture of metals present in spent batteries. Results showed that the hydroxylated solvent, especially glycerol, contributed to maintain the mechanical and structural integrity of the polymers, as well as improving the overall metal absorption capacity of the material.
1.1-I1
I am an energetic, creative, female scientist with a solid expertise in Material Science and Technology. I have successfully implemented an engineering approach to guide the development of functional nanohybrids through general and simple routes. Throughout my work, I have introduced important mechanisms on the cooperative coupling of dissimilar materials in single structures, which represents a fundamental knowledge for the creation of a new-generation of nano and macro hybrid materials.
The self-intercalation of organic and inorganic components in two-dimensional (2D) layered perovskites brings up new avenues for the structural engineering of efficient white light emitters from a single material. Recent studies focused on the impact of organic cations on the material’s dimensionality, bandgap, and crystallographic structure.[1,2] Yet, their effects on the emission properties have only been investigated using random selection.[3] Here, we show an experimental approach based on molecular descriptors of organic cations to study their role in the emission characteristics of organic-inorganic layered perovskites. To this aim, we collected experimental data in real-time and uploaded it to a digital platform for data interaction, analysis, and preservation. We first carefully selected primary and secondary amines and established a robust synthetic protocol that is performed at relatively low temperatures and through simple steps.[4] Up to date, we have prepared 47 different layered structures, and established seven digital protocols that allow straightforward correlation between structural and optical. Initial correlations between amine molecular descriptors and the collected synthesis and optical data indicate that the use of short organic cations with heteroatoms and with a low number of valence electrons allows broadband emitting layered structures, and the heteroatom position might lead to tunable emission from blue to white. Such data integration and analysis in real-time, including sorting, interactive exploration, and data graphical representation, open alternative avenues to fabricate efficient white-emitting structures from a single material, with a broad perspective for other functionalities.
1.1-I2
Accelerating materials discovery as well as green and sustainable ways to manufacture them will have a profound impact on the global challenges in energy, sustainability, and healthcare. The current human-dependent paradigm of experimental research in chemical and materials sciences fails to identify technological solutions for worldwide challenges in a short timeframe. This limitation necessitates the development and implementation of new strategies to accelerate the pace of materials discovery. Recent advances in reaction miniaturization, automated experimentation, and data science provide an exciting opportunity to reshape the discovery, development, and manufacturing of new advanced functional materials related to energy transition and sustainability. In this talk, I will present a Self-Driving Fluidic Lab for accelerated discovery, optimization, and manufacturing of emerging advanced functional materials with multi-step chemistries, through the integration of flow chemistry, online characterization, and artificial intelligence (AI).1-3 I will discuss how modularization of different synthesis and processing stages in tandem with a constantly evolving AI-assisted modeling and decision-making under uncertainty can enable resource-efficient navigation through high dimensional experimental design spaces. Example applications of SDFL for the autonomous synthesis of clean energy nanomaterials will be presented to illustrate the potential of autonomous labs in reducing materials discovery timeframe from +10 years to a few months. Finally, I will present the unique reconfigurability aspect of self-driving fluidic labs to close the scale gap in clean energy materials research through on-demand switching from reaction exploration/exploitation to smart manufacturing mode.
1.1-O1
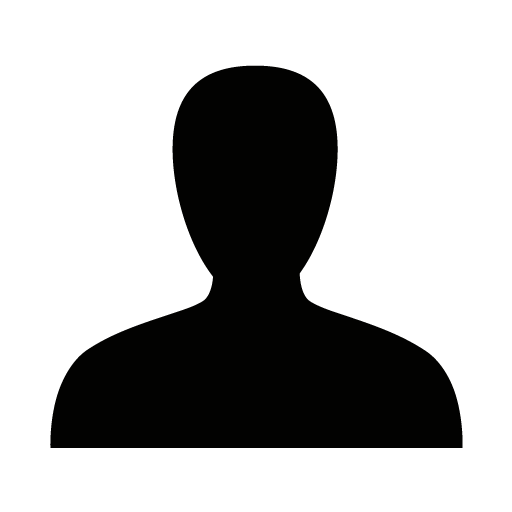
Perovskite solar cells (PSCs) hold great potential as a technology for the next generation of thin-film photovoltaics. For PSCs to be commercially viable, scalable fabrication processes must be improved based on state-of-the-art techniques used for small-area devices. Hence, there is a need to investigate and optimize the formation of perovskite thin films fabricated through scalable deposition methods to facilitate large-scale production of solution-based, large-area PSCs. However, the formation of perovskite thin films from precursor solution is complex and involves the interrelated phases of drying, nucleation, and crystal growth. Therefore, in-depth understanding and precise control of these stages are vital to consistently fabricate high-quality optoelectronic films.
The application of in situ multi-channel photoluminescence (PL) imaging allows recording the temporal evolution of the perovskite thin-film formation, while also providing spatial resolution and spectral information. We have generated and made publicly available unique experimental data that comprises in situ PL data of blade-coated PSCs and corresponding quality measures, such as power conversion efficiency and perovskite layer thickness. Exploring this in situ PL data has the potential to reveal important variations in the thin-film formation process, however, the limits of human analysis are exceeded due to the high dimensionality. Here, machine learning (ML) methods are a promising route to investigate such complex, multi-dimensional PL data to elucidate the large-area formation of blade-coated perovskite thin-films during vacuum quenching.
To accelerate empirical-based traditional, incremental scientific progress, we employ deep learning and explainable artificial intelligence (XAI) techniques to identify correlations between the in situ PL data collected during the perovskite thin-film fabrication and resulting solar cell performance metrics, while making these correlations humanly understandable. Our analysis shows that variations in the quality of PSCs can be understood by examining the thin-film formation process with ML and XAI. By applying various XAI methods we explain not only which data features are important but also provide a data-driven explanation why they are important. Additionally, our research illustrates how these insights can be translated into practical guidelines for optimizing perovskite thin-film fabrication by giving actionable recommendations to experimental scientists. Remarkably, we are able to generate these insights just by analyzing the given dataset without having to perform additional laborious and expensive trial-and-error experiments.
Our research improves the understanding of the complex large-area formation of perovskite thin-films and highlights the pivotal role of ML, and XAI methodologies in particular, in accelerating energy materials research. As XAI methods are not limited to the investigated dataset, this study is a prime example of how similar analyses can be performed to interpret and improve processes in numerous other areas of sustainable materials research.
For an in-depth analysis and further discussion of these findings, see our full research paper “Discovering Process Dynamics for Scalable Perovskite Solar Cell Manufacturing with Explainable AI”, L. Klein*, S. Ziegler*, F. Laufer et al., Adv. Mater., 202307160 (2023), doi: 10.1002/adma.202307160.
2.1-I1
Optoelectronic properties of ultrasmall semiconductor nanocrystals are strongly related to their structural and microstructural features. However, due to the complexity of these materials, this intermingled relationship remains mostly elusive.
Over the past decades, total scattering methods, in particular the ones based on the Debye Scattering Equation (DSE) and operating in reciprocal space, have been established as essential tools for characterizing the structure, microstructure, and morphology of nanocrystals, including ultrasmall Quantum Dots (QDs).[1–5]
Although wide-angle scattering-based techniques are primarily sensitive to the atomic-scale structures of materials, reciprocal space total scattering methods provide robust information on multiple length scales, in particular if nanocrystalline materials are considered.
Nevertheless, constructing reliable, material-oriented atomistic models, to be optimized against the experimental data in order to extract structural and microstructural parameters remains a highly challenging task and often poses a bottleneck for scattering-based methods.[6–9]
To overcome this limitation, we tackle the challenge of developing reliable, efficient, and user-friendly methods for determining the average size of colloidal QDs, with a combination of reciprocal space total scattering methods based on DSE and an all-convolutional neural network (all-CNN) that provides physically interpretable results.
In this talk, I will present the development and first application of this novel tool to a selected class of lead-chalcogenide binary QDs that serves as a benchmark system. Indeed, they have been extensively characterized within the DSE approach, which has provided well-established knowledge about their structural and morphological features.[1]
The presented automated tool can be readily employed for real-time size classification of PbS QDs, even from diluted colloidal suspensions, within the limitations of the Q-range and signal-to-noise ratio typically encountered in in-situ and in-operando diffraction experiments.[10]
Additionally, it may serve as a rapid screening tool for the optimization of synthetic protocols.
Furthermore, the proposed method can be easily extended to other classes of nanocrystals, allowing non-experts in crystallography and X-ray diffraction to utilize the automated workflow for creating DSE pattern libraries used for training the all-CNN.
2.1-O1
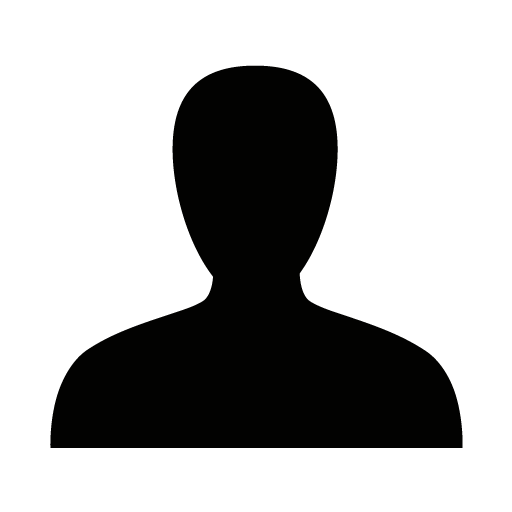
Colloidal quantum dots (QDs) and in particular perovskite QDs have attracted broad interest for their highly efficient, spectrally tunable and narrowband photoluminescence (PL). Their potential implementation in a plethora of nanomaterials technologies spanning efficient lighting and light harvesting to bioimaging, demand a thorough understanding of the effects of QD structure and surface structure dynamics on PL characteristics. In principle, low-cost simulations with classical force fields (FFs) reveal no electronic structure information and thus provide restricted insight into PL features. Enabling molecular dynamics (MD) simulations in both the ground and excited states with electronic structure information up to the nanosecond timescale is a desirable advance.
For this purpose, we propose a customized platform that helps constructing machine learning FFs for colloidal QDs, expanding the already existing platform dedicated to the parameterization of classical FF parameters for QDs. By considering quantum mechanical properties (such as potential energies and bandgaps) previously computed at a high-level of theory like density functional theory (DFT), along with position and forces, this platform automatically trains a neural network to generate the so-called machine learning FFs (MLFF). These MLFFs not only allow to expand the timescale of the MD simulations from picoseconds to nanosecond, but will also enhance sampling efficiency and simulation accuracy by including also information on the electronic density of states at each point of the trajectory. The machine learning platform has been tested for CsPbBr3 QDs obtaining promising results.
2.1-O2
Quantum Dots (QDs) combine inorganic semiconductors scaffolds with organic surface ligands. A key role played by the ligands in these systems is the stabilization of the inorganic region in organic solvents to prevent its dissolution. The suitability of a certain ligand depends on several factors such as magnitude of the QD-ligand binding strength, ligand-ligand packing, and ligand-solvent interactions. In practice, each QD type has its best ligand, however finding such ligand is a tedious work if one considers the labor and material costs involved in the experiments. In this context, computational screening provides an attractive alternative to browse the largely unexplored space of potentially interesting ligands. In this work, we introduce a public database of organic molecules, derived by an advanced filtering of the PUBCHEM database,[1] with the aim of building a subset of surface ligands candidates that are potentially suitable to passivate the surface of any inorganic semiconductor substrate. For each ligand in the database, we additionally provide relevant chemical and physical properties, from the boiling and melting points to more specific properties that account for the interactions with the solvent. These ligand properties are material-independent and can therefore be used as an orientation tool for ligand-capped semiconductor materials research in general.
2.1-I2
Born June 23, 1966 in Meppel, The Netherlands
Professor in Theoretical Chemistry, Vrije Universiteit Amsterdam, The Netherlands
Ph. D. (cum laude) University of Groningen (1993), postdoctoral stays at NASA Ames (1994-1995) and at the University of Odense (1996-1997). Professor at Vrije Universiteit Amsterdam (1998-present). Visiting professor stays at University of Strasbourg and at Pacific Northwest Laboratories. Awards: KNCV Clemens Roothaan Prize (1996), NWO vici (2005), WATOC Dirac Medal (2006).
Main research Interests
1. Subsystem electronic structure methods
2. Reducing the time-to-solution of computational models
3. Development and application of relativistic computational chemistry techniques
In this presentation I will report on our progress on developing quantum chemistry methods and workflows for nano-sized materials.
For the quantum chemical methods I will mostly focus on (approximate) density functional theory [1,2], but will also briefly mention our recent work on higher-level methods[3,4] that can be used to benchmark density functional approximations. I will thereby in particular focus on the efficient description of electronically excited states.
Two different approaches to deal with electronically excited states in large systems will be discussed. In the subsystem approach we first solve for local excitations and a limited number of charge-transfer states, before proceeding to the treatment of the full system. For systems with inherently delocalized (plasmonic) excitations and a high density of states in the absorption spectrum, we need to take a different approach however. For this purpose, we have developed techniques in which the most time-consuming steps of the calculations are identified and approximated. I will discuss the effect of these approximations in terms of computational efficiency and adequacy to retain the quality of the original method.
Concerning workflows, I will discuss examples of our work related to (solar) energy conversion by dye-sensitized solar cells[5]. Here I would like to discuss in particular also the design of the workflow software[6] for which deployment on a parallel compute architecture is a key requirement.
2.2-O1
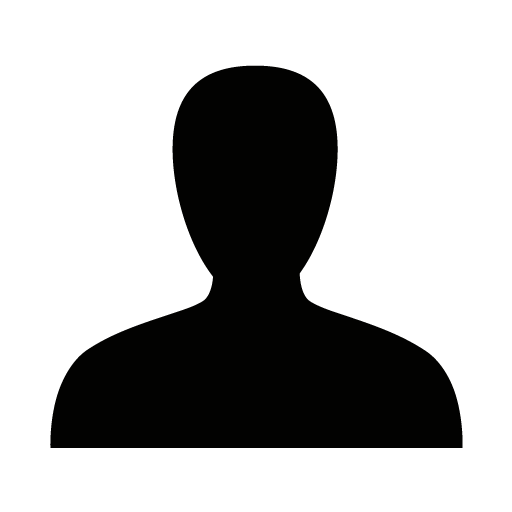
Data science and artificial intelligence are bringing about a revolution in our society, and materials science is no exception. In the future, it will be possible to design materials with tailored properties by relying almost completely on existing data. Indeed, combining data mining with cleverly designed machine learning (ML) algorithms can overcome the need to rely on large experimental and/or computational facilities for discovering new materials[i]. One promising field of application for these types of approaches is that of semiconductors. On the one hand, these materials are central to many technological applications. On the other hand, there is a constant need for improvement, for example for replacing toxic or rare elements while fulfilling optoelectronic and stability criteria.
In this contribution, we present our platform for the discovery of new chalcohalides, an emerging class of semiconducting materials which display the well-known excellent optoelectronic properties of metal halide perovskites while also being considerably more stable[ii]. Our approach consists of two steps. First, the platform connects to databases (AFLOW[iii], NOMAD[iv], and Materials Project[v]) to automatically find all the materials with user-defined constituting elements and properties. For chalcohalides, a key property for optical applications is the direct character of the band gap. Then, ML algorithms are trained to predict the stability, and the width and character of the band gap. The ML model is then applied to predict new compounds, and the prediction is then verified by quantum chemical simulations. We present representative examples of the application of our approach. We note that this protocol can be easily extended to the data mining of other semiconductors (an example on perovskites will be presented).
References:
[i] Keith J.A. et al. "Combining machine learning and computational chemistry for predictive insights into chemical systems." Chemical reviews 121.16 (2021): 9816-9872.
[ii] Ghorpade U.V. et al. "Emerging chalcohalide materials for energy applications." Chemical Reviews 123.1 (2022): 327-378.
[iii] Curtarolo S. et al. "AFLOW: An automatic framework for high-throughput materials discovery." Computational Materials Science 58 (2012): 218-226.
[iv] Draxl C. et al. "NOMAD: The FAIR concept for big data-driven materials science." Mrs Bulletin 43.9 (2018): 676-682.
[v] Jain A. et al. "Commentary: The Materials Project: A materials genome approach to accelerating materials innovation." APL materials 1.1 (2013).
2.2-O2
Lanthanum strontium-based perovskites (ABO3) are among the state-of-the-art cathode materials for solid oxide fuel cell operating at intermediate and low temperatures (<800 ºC).(1,2) However, the effects of the composition on the nanostructure and the intrinsic properties of the materials and on the electrochemical performance are typically non-linear and hard to generalize.(3,4) Machine learning techniques have emerged as an unprecedented tool to identify complex patterns in large datasets, also in heterogeneous electrocatalysis (5,6). Herein, we have applied these techniques to delve deeper in the composition-property-performance relationships of La0.8Sr0.2(Mn,Co,Fe)O3±𝞭 and predict performance maps that can help optimize these materials. High-throughput characterization of a compositional map of La0.8Sr0.2(Mn,Co,Fe)O3±𝞭 has been carried out: information on the metal stoichiometry, the crystallinity, electrochemical performance, the structural symmetry, and the electronic configuration was obtained from X-ray diffraction (XRD), X-ray fluorescence (XRF), electrochemical impedance spectroscopy (EIS), Raman spectroscopy and ellipsometry, respectively. We processed the raw data to derive characteristic features and match the samples from different measurements. Then, a variety of supervised and unsupervised modern machine learning methods were utilized to build highly generalizable models correlating experimental features relative to the composition, the optical properties and the electrochemical pperformance of the materials, and to identify the most relevant ones. Experimental data from Raman and ellipsometry and XRD measurements was demonstrated to model the material composition and the electrochemical performance with R2 of 0.913 ± 0.002 and 0.900 ± 0.003, and mean absolute errors of 0.053 ± 0.001 and 0.189 ± 0.005, respectively, with 5-fold cross-validation.
2.2-I1
Dr. Samira Siahrostami is an Associate Professor and Canada Research Chair in the Department of Chemistry at Simon Fraser University in Canada. Prior to that, she was an associate professor (2022-2023) and assistant professor (2018-2022) in the Department of Chemistry at the University of Calgary. Prior to that, she was a research engineer (2016–2018) and postdoctoral researcher (2014–2016) at Stanford University's Department of Chemical Engineering. She also worked as a postdoctoral researcher at the Technical University of Denmark from 2011 to 2013. Her work uses computational techniques such as density functional theory to model reactions at (electro)catalyst surfaces. Her goal is to develop more efficient catalysts for fuel cells, electrolyzers, and batteries by comprehending the kinetics and thermodynamics of reactions occurring at the surface of (electro)catalysts. Dr. Siahrostami has written more than 100 peer-reviewed articles with an h-index of 47 and over 13,000 citations. She has received numerous invitations to give talks at universities, conferences, and workshops around the world on various topics related to catalysis science and technology. Dr. Siahrostami is the recipient of the Environmental, Sustainability, and Energy Division Horizon Prize: John Jeyes Award from the Royal Society of Chemistry (RSC) in 2021. She received the Tom Zeigler Award and the Waterloo Institute in Nanotechnology Rising Star award in 2023. She has been named as an emerging investigator by the RSC in 2020, 2021 and 2022. Dr. Siahrostami's contribution to energy research was recognized in the most recent Virtual Issue of ACS Energy Letters as one of the Women at the forefront of energy research in 2023. She is currently the board member of the Canadian Catalysis Foundation and editor of Chemical Engineering Journal (CEJ) and APL Energy journal (AIP Publishing).
Currently, researchers are exploring copper-based catalysts as the most effective agents for electrochemical CO2 reduction reactions (CO2RR) that convert carbon dioxide into valuable hydrocarbons like methane, ethylene, and ethanol. However, copper catalysts are susceptible to restructuring and degradation, prompting the quest for alternative catalyst families not based on copper that facilitate CO2RR. Our approach involves employing high-throughput Density Functional Theory (DFT) calculations to systematically explore a diverse materials space, expediting the identification of catalyst materials that outperform copper in CO2RR. In this presentation, I will elaborate on the application of robust high-throughput DFT calculations to screen an extensive library of unconventional materials, including perovskites, transition metal nitrides, and metal-organic frameworks. In the computational screening of perovskites, we place a particular emphasis on screening a massive library of ABO3 Perovskites based on their electrochemical and thermochemical stability to minimize the restructuring and degradation under CO2RR operating pH and electrode potentials. I will discuss the valuable insights gained from these investigations.
2.2-I2
Sustainable global human existence requires a shift in the management and utilization of research data across all domains. In the realm of energy conversion materials, the intense exploration of halide perovskite materials since 2012 for potential use in optoelectronics has led to numerous startups actively pursuing commercialization efforts. However, the escalating volume of globally generated research data presents a critical challenge: the traditional dissemination strategies reliant on the publication of peer-reviewed articles. This causes big issues leading to the underutilization of information and research results generated. On the one hand, the spreading of information across numerous scientific journals results in an overwhelming, disparate dataset, impossible for any individual researcher to navigate. On the other hand, only a fraction of the experimental data gathered is being disseminated, with a bias toward positive outcomes, creating an incomplete and skewed dataset.
In response to this growing issue and “out of despair”, we initiated a collaborative data collection campaign in 2019 to consolidate research data from the sub-domain of perovskite solar cell test-cell development into a unified database [1]. While this dataset is currently one of the largest and most comprehensive in its field, it requires modernization to adhere to FAIR data principles and facilitate integration with machine learning tools, thereby making research more sustainable.
This presentation outlines our recent strides in expanding the Perovskite "literature" database on www.perovskitedatabase.com into an experimental database utilizing the NOMAD data infrastructure and management tools. Alongside the technical implementation, we are standardizing the data ontology for experimental samples and datasets, promoting easy replication and linkage to collect and utilize data generated by the global research community.
As a collaborative group of idealists, we dedicate our time and effort to this initiative, seeking to connect with like-minded researchers—especially those with advanced abilities and experience in research data management and AI. At this stage, input from AI experts is crucial to ensure the research data infrastructure we are building for experimental PV scientists fulfills the needs of the research community while enabling the efficient use of AI tools to make information exploitation faster and more sustainable.
2.3-I1
Supervised machine learning (ML) has proven to be an incredibly powerful enabling technology for electronic structure calculations. We now routinely see highly accurate predictions at a fraction of the computational cost of traditional methods. Machine learning has enabled electronic structure simulation at length-scales which previously seemed out-of-reach. The number of papers which use machine learning is increasing exponentially, with no signs of slowing down.
Unfortunately, these advantages have come at a high price - ML models such as deep neural networks provide no intuitive explaination about how they arrived at a particular prediction. They also offer limited generalization capabilities: each problem is treated as a new one. Unlike simple models that appear in textbooks (and inform our intuition), machine learning models are often treated as black boxes by practitioners. Even popular explainability tools fall short - they highlight correlations rather than causation.
I will discuss some of the failures and limitations of machine learning and provide examples which attempt to provide generalized insight and intutition.
2.3-I2
Kangming Li is a post-doctoral fellow in the Department of Materials Science and Engineering at University of Toronto. He received his PhD in Physics from Université Paris-Saclay, where he was a CEA-NUMERICS Fellow funded under the Marie Curie Actions. He was awarded the Dalla Torre Medal by the French Society for Metallurgy and Materials for his PhD work on finite-temperature magnetic effects in concentrated alloys. Currently he is using machine learning and high-throughput first principles calculations to accelerate the discovery of novel inorganic materials.
The rapid growth of big data in materials science has led to significant advancements in materials property prediction by machine learning (ML) models. However, big data does not necessarily lead to robust prediction performance of ML models. In addition, the issue of information redundancy in materials data has been largely overlook. This talk intends to present an examination of these two correlated challenges related to materials data: prediction robustness and data redundancy.
First, we will discuss the challenges in ensuring the prediction robustness of ML models, by showcasing the severe performance degradation when the models are trained on the Materials Project 2018 dataset and tested on the Materials Project 2021 dataset. We will demonstrate the impact of distribution shifts and use tools such as UMAP and query-by-committee to foresee performance degradation and to improve prediction accuracy. Next, we will delve into the issue of data redundancy across large materials datasets, revealing that up to 95% of materials data can be safely removed with little impact on the model performance. We will highlight the application of uncertainty-based active learning algorithms to create smaller but informative datasets, leading to more efficient data acquisition and ML training. By examining these challenges, this talk aims to provide insights into building more efficient and robust materials databases and ML models for accurate and reliable predictions in materials science.
2.3-O1
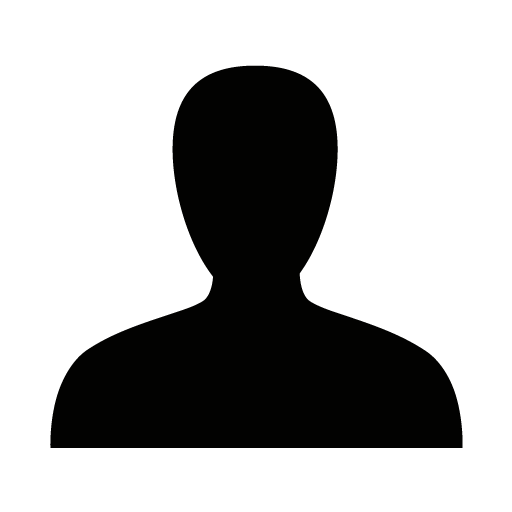
Machine-learning force fields (MLFF) show high accuracy and efficiency for modeling the potential energy surfaces of molecules, materials, and interfaces. However, the performance of MLFFs greatly depends upon incorporating the physical symmetries. Finding all relevant symmetries becomes a challenging task for large system sizes. Here, we develop a data-driven method based on molecular graphs to reveal relevant permutational symmetries and distinguish atoms with different chemical environments in molecules and materials. The kernel-based model architecture of BIGDML and the message-passing neural network architecture of MACE were enhanced to demonstrate the applicability of the developed method to the most widely used MLFF architectures. The BIGDML model, enhanced with extracted symmetries, demonstrates superior accuracy and performance, enabling comprehensive investigations of complex systems like the 1,8-naphthyridine/graphene interface and its behavior at finite temperatures. MACE was enhanced by expanding atomic species using the extracted distinctive chemical environments, resulting in improved accuracy for CsPbI3 slab systems, particularly notable with larger training sets. Overall, this research underscores the critical role of symmetries in advancing MLFFs for complex systems with broad implications in various research fields.
1.1-I1
1.1-S1
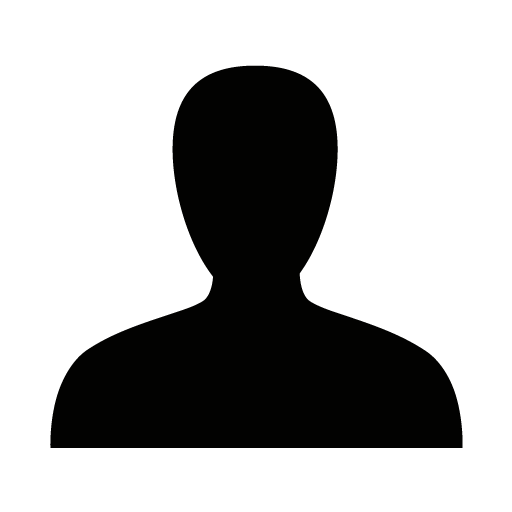
A brief introduction to the RSC’s portfolio of journals covering the breadth of energy, materials, nanoscience and catalysis, and our initiatives to share and highlight research with the community.
1.1-O1
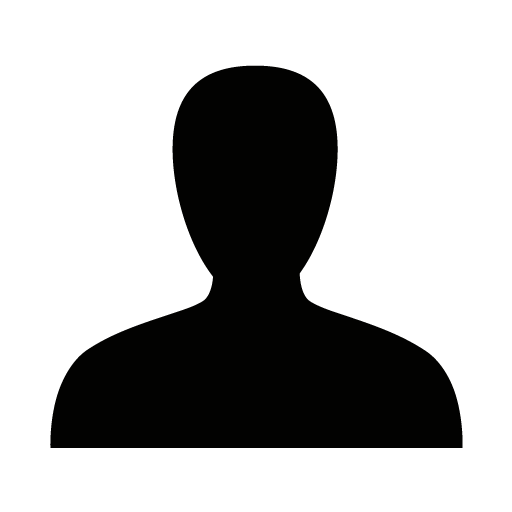
Third generation solar cells have the potential to provide a viable source of renewable energy to help decarbonize our economy and power wearable devices. With their exceptional performance in diffused light under indoor conditions, dye-sensitized solar cells (DSCs) remain competitive for powering the next digital revolution forming the internet of things.[1] When integrated with an energy storage system such as an electric double layer capacitor (EDLC), DSCs can offer reliable uninterrupted energy output as a photocapacitor. However, conventional DSCs use liquid electrolyte as the redox mediator, which limits the suitability of the technology for scale up and commercialization. Joining the quest for stable, reproducible solid-state hole transport materials (HTM), we are exploring nanostructured metal-organic frameworks (MOFs). These emerging materials possess the highly ordered structure of inorganic materials combined with the chemically tailorable properties and low cost of organics, and have outperformed their precursors in a wide range of applications.[2]
In my talk, I will introduce copper benzenetetrathiol (CuBTT) system with highly efficient redox conductivity as a solid state HTM. We demonstrate that creation of highly interconnected networks of these polymeric nanowires improves the conductivity and when epitaxially assembled, these nanowire architectures infiltrate and form better contact with the dye molecules. We also show that modulator assisted bottom-up synthesis gives stacked 2D layers penetrated by 1D cylindrical channels for ion conduction. This can act as a suitable interface when the DSC is coupled with an EDLC in a photocapacitor architecture. This work establishes MOF nanosheets as efficient interface materials for solar capture and storage devices.
1.1-O2
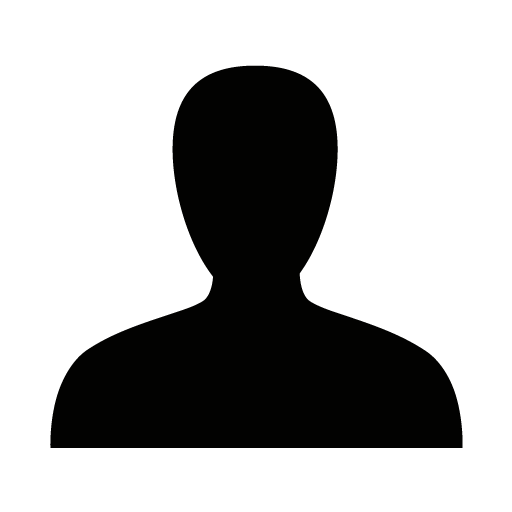
The optimization of various mechanisms is essential to reach the efficiency limit in solar cells, as highlighted by Shockley and Queisser[1]. One critical aspect involves enhancing the transparent conductive oxide (TCO) positioned at the front of the device. The TCO plays a crucial role in extracting photogenerated carriers and acts as a window for the Sun's light. Additionally, introducing periodic micro- and nanostructures on TCO surfaces has the potential to increase transmittance and surface area, leading to improved efficiency[2–5]. In this research, direct laser interference patterning (DLIP) was employed to modify thin films of fluorine-doped tin oxide (FTO) and indium tin oxide (ITO), which were subsequently utilized as TCOs for perovskite solar cells.
Results reveal dot-like periodic sub-microstructures with an approximate 700 nm spatial period, increasing incident light spread and total transmittance. These structured films improved perovskite solar cell performance, demonstrating the positive impact of the generated patterning in the light-trapping capabilities. Additionally, the increase in effective surface area might lead to a more efficient charge transfer in the interface between FTO/ITO and SnO2.
1.1-I2
Ludmilla is an Associate Professor of Inorganic Chemistry at the Univeristy of Oxford. She obtained her B.Sc and M.Sc. degrees from the University of Siegen (Germany). During her undergraduate studies she developed an interest in electrochemistry and semiconductor physics driving her to pursue a M.Sc. project on dye-sensitized solar cells in the group of Professor Michael Grätzel at the École Polytechnique Fédérale de Lausanne (EPFL, Switzerland). Staying in the same group, Ludmilla worked on oxide thin film photoelectrodes applied in photoelectrochemical water splitting and perovskite solar cells during her Ph.D. degree which she obtained in 2016. She then joined the group of Professor James Durrant at Imperial College London to study photochemical and photophysical processes in semiconductors using time-resolved spectroscopy and shortly after was awarded the Marie Skłodowska-Curie Fellowship (2017-2019). Ludmilla began her independent research career as Imperial College Research Fellow (2019-2021) before moving to Oxford in October 2021. Her research at Oxford aims at the design of atomically defined photo- and electrocatalysts that convert CO2, water and other “waste products” to energy-rich fuels and chemicals with high conversion efficiency, selectivity and long operational stability.
Doping or alloying strategies are heavily employed in the design of new catalysts for photoelectrochemical, photochemical and electrochemical conversion reactions with enhanced solar energy harvesting efficiencies and/or desired surface chemistry. In the electrochemical reduction of CO2 for example, efforts are focused on the development of electrocatalysts with high activity and selectivity towards C2+ products ideally breaking the scaling relations currently observed for metal surfaces.[1] In photoelectrochemical and photocatalytic devices research has focused on developing catalysts with light harvesting in the visible, strong surface electric fields and long charge carrier lifetimes.[2,3] In both, electro- and photocatalytic systems, perovskite oxides have been employed heavily, offering a more versatile defect chemistry platform.
Here, I will showcase some of our studies on oxide perovskite materials employed in photo- and electrocatalysis discussing links between defect chemistry and catalytic performance. One example will be the visible light absorber (La,Sr)(Rh,Ti)O3 employed in the Z-scheme photocatalyst sheet device from Profs. Wang and Domen which led to a record 1% solar-to-hydrogen efficiency.[4,5] Taking inspiration from this material, we have been investigating the performance of other dopants and compositions in photo- and electrocatalytic conversion of CO2 leading us to look very closely into the material surface compositions and the ways of reporting of catalytic activity.
1.2-I1
Portable applications powered by solar energy face challenges with both sunlight's intermittency and illumination variability from outdoor to indoor transitions necessitating the development of efficient energy storage systems to ensure consistent power availability. In this context, photosupercapacitor could represent a viable solution toward an efficient energy supply. In this presentation, after introducing the concept of photosupercapacitor I will report on the realization of flefible hybrid photosupercapacitor based on the combination of an ultra-flexible carbon screen printed interdigitated supercapacitor on paper and a flexible perovskite solar module (PSM) on PET.
This all-flexible device functions as a self-powering unit for both energy conversion and storage. Impressively, the supercapacitor showcases remarkable stability and a coulombic efficiency of 100%. Through a hybrid bifurcated structure designed to enhance their applicability, the supercapacitors were connected in series. They were neatly layered on a paper substrate with the solar module strategically placed on top.
The device quickly reached saturated voltage value with exposure to various light intensities such as 1 sun, 1000 lx, 500 lx and 200 lx and displayed a self-discharge beaviour which lasted more than two minutes. With peak overall and storage
efficiencies determined to be 2.8% and 23% respectively. The integrated hybrid photosupercapacitor exhibited an extensive potential window of 3.8 V making it an prominent choice for real time application in electronic systems.
1.2-I2
M. Ibrahim Dar is a Royal Society University Research Fellow in the Cavendish Laboratory at the University of Cambridge. From 2018 to 2020, he was an Advanced Swiss National Science Foundation Post-Doctoral Fellow in the group of Professor Sir Richard Friend, University of Cambridge. Prior to this, he worked as a Post-Doctoral Scientist with Professor Michael Graetzel at Ecole Polytechnique Fédérale de Lausanne (EPFL) Switzerland (2014-2018). For his postdoctoral research at EPFL, he was awarded the prestigious Zeno Karl Schindler-EPFL Prize for particular excellence in the field of sustainability and was twice awarded a special prize by the School of Basic Sciences, EPFL, Switzerland. During his PhD, he was awarded the Swiss Government Excellence Research Scholarships for two consecutive years (2012-2014), which allowed him to work in Professor Graetzel’s group as a guest PhD student. Ibrahim’s interdisciplinary research combines solid-state chemistry, physics, and materials science to design and understand new functional materials with desired structural and optoelectronic properties for energy-oriented applications.
Solution processability of high-quality metal halide perovskites (MHPs) with a wide range of compositions has enabled the unprecedented evolution of perovskite solar cells (PSCs) and perovskite light-emitting devices (PLEDs), which has fascinated researchers from academia and industry. Due to the ionicity and antibonding nature of valence and conduction band states, highly luminescent crystalline structures ranging from nanocrystals to thin films are produced in the case of metal halide perovskites using solution-based strategies. The vulnerability of the lattice of metal halide perovskites towards distortions is increased due to their ionic nature when exposed to bias and illumination both in materials and devices, which further amplifies ionic motions under operational conditions. Although the development in terms of efficiencies and stability has been phenomenal, the mixed conduction of electrons and ions has proven to be an "Achilles heel" for perovskite devices thus far. Mixed conduction behaviour allows migration and accumulation of ions in PSCs and PLEDs, which induce detrimental chemical and structural changes both in the bulk of MHP layer and at the interfaces under external stimuli. Interestingly, the nature of chemical bonding in other solution-processable molecular and quantum dot semiconductors has also provided us with adequate insights regarding their performance and stability issues, especially in a device configuration.
In my presentation, I will discuss some intriguing examples illustrating the significance of chemical bonding both in the active perovskite layer and charge conductors in a solar cell configuration.
1.2-I3
The Internet of Things (IoT) underpins our future smart world where various electronic devices will be integrated with, and controlled by, wireless communication.[1] Many of these devices will be standalone or portable, creating an urgent demand for off-grid power sources. Solar photovoltaic (PV) cells are viable alternatives to batteries as perpetual power sources for IoT devices. However, crystalline silicon (c-Si) PV cells (which currently account for 95% of the global PV market) are not designed to work with diffuse, artificial indoor light-emitting diode (LED) lighting and perform poorly under these conditions.[2]
Luminescent waveguide-encoded lattices (LWELs) are a new class of photonic material that have recently been proposed to compensate for the limitations of c-Si PV cells for indoor PV.[3,4] LWELs consist of a thin (ca. 1 mm) luminescent polymer film encoded with a patterned array of discrete waveguides. The waveguide array is formed through the self-trapping of incident beams of light within a photopolymerisable matrix. This leads to the permanent inscription of polychromatic cylindrical waveguide channels within the polymer matrix, which impart LWELs with an exceptionally wide field-of-view (80% enhancement shown previously[5]). The LWEL is retrofitted to the top surface of a PV cell to enhance light collection.
While the optical and materials properties of non-emissive WELs are reasonably well-understood [5,6], the inclusion of a luminophore can complicate the self-trapping processes that led formation of the waveguide channels. In this talk, the relationship between the photopolymerization kinetics, materials composition and optical properties of LWELs will be discussed, with a view to understanding the design rules that underpin efficient performance upon integration with PV cells.
1.2-I4
Tremendous advances have been made in photovoltaics (PV) in recent years, including in the development of emerging technologies such as perovskite PV, organic PV and dye-sensitised solar cells (DSSCs). These technologies can be applied to areas where silicon PV is not well suited, including power for consumer electronics, electromobility, building-integrated PV with attractive appearance, flexible, light-weight and semi-transparent PV, indoor energy scavenging and more. In some of these applications areas, simplicity and low-cost for the devices can become as important as efficiency to achieve practical systems. DSSCs offer a promising choice for applications requiring low-cost indoor power generation for powering the emerging internet-of-things. However, they currently include complex and costly sensitizers which require long synthesis procedures, alongside electrolytes that can be detrimental to stability and/or ease of fabrication. The presentation will focus on our recent work on DSSCs aiming to simplify the dye [1, 2] and the electrolyte components [3, 4] towards more-readily fabricated and readily-scaled technology.
2.1-I1
Juan A. Anta is Full Professor of Physical Chemistry at the University Pablo de Olavide, Seville, Spain. He obtained a BA in Chemistry in the Universidad Complutense of Madrid (Spain) and carried out his PhD research at the Physical Chemistry Institut of the National Research Council of Spain. His research focuses on fundamental studies of energy photoconversion processes, especially on dye and perovskite solar cells, using numerical simulation and modelling tools, as well as advanced optoelectronic characterization techniques such as impedance spectroscopy and other small perturbation techniques.
Electron collection in dye-sensitized solar cells (DSCs) is determined by a delicate equilibrium between electronic transport within the mesoporous oxide layers and recombination losses. While state-of-the-art DSC devices have shown quantitative collection under standard 1-sun conditions, the nonlinear nature of the trapping/detrapping dynamics in the oxide layer in relation to the overall electron density may result in suboptimal performance under the lower illumination levels commonly encountered in indoor settings. In this study, we thoroughly examine the key factors influencing electron collection with respect to light intensity using impedance spectroscopy and numerical analysis
2.1-I2
Mary Pryce is a Professor at the School of Cehmical Sciences at Dublin City Universirty, Ireland. Prior to joining DCU in 1997, she was employed as a postdoctoral Fellow at the University of Milan, Italy. She obtained her PhD from Dublin City University in the area of organometallic photochemistry (in 1995). Current research projects within her research group focus on designing new materials (polymers, organometallic compounds or organic dyes) for energy applications such as hydrogen generation, or CO2 conversion. Another aspect of research focuses on antimicrobial materials. Central to both of these research areas is understanding the photophysical properties using time resolved techniques.
The development of renewable energy sources is a global challenge, as we seek new routes to address increasing CO2 levels, climate change and energy security. Many solutions have been suggested, including hydrogen generation. Photocatalytic water splitting using photosensitisers (PS) together with catalytic centres (Cat) (artificial photosynthesis) have been extensively studied by us and others.[1-4] The ultimate goal is to incorporate these assemblies into dye-sensitised photoelectrochemical cells. We have developed a range of intramolecular assemblies (inorganic and organic systems) containing various bridges such as terpyridine, triazole, or imines linking the PS to the catalytic centre to enhance hydrogen generation. We have also modified these systems and used them for CO2 reduction. We have performed in depth time-resolved studies to probe the excited states of the complexes to investigate the nature of the electron transfer processes, and carried out spectroelectrochemistry to aid in design of superior photocatalysts for both reduction processes. Our studies indicate that many parameters must be considered when designing such assemblies.
2.1-I3
In nature, solar energy is captured and stored in chemical bonds by activating small molecules such as CO2 and H2O. This requires catalysts capable of carrying out CO2 reduction and water oxidation catalysis. In this presentation, molecular catalysts and mechanisms related to these two processes will be discussed. CO2 reduction to C1 products has received considerable attention as part of many efforts to try to address the devastating effects of climate change. Among various C1 reduction products, liquid fuels such as formic acid and methanol are particularly important to achieve carbon-neutral cycles. But the underlying chemistry to generate liquid fuels from CO2 efficiently and selectively has yet to be developed, particularly in the case of methanol. Cascade strategies using a combination of catalysts that can carry out some of the required multistep reactions are being pursued because achieving this goal with a single catalyst is difficult. One of these strategies involves CO2 reduction to CO by one catalyst and further reduction of CO to a liquid fuel by another catalyst or a combination of catalysts. In this presentation, a strategy for the generation of methanol efficiently and selectively from CO is discussed, with a transition metal-organic hydride combination. Using NMR, IR and mass spectrometry, together with labeling studies, chemical synthesis of authentic samples of intermediates and theoretical calculations, selective and efficient conversion of CO to methanol is demonstrated. The key to the high selectivity is the use of organic hydride donors that are inactive towards direct CO2 reduction and hydrogen generation. These organic hydride donors are inexpensive and renewable and are a promising platform for solar fuels generation from CO2. In the water oxidation front, a new mechanism for water oxidation catalysis with molecular catalysts will be presented. In this mechanism, the transition metal participates in the redox chemistry but not in the thermally activated processes, which take place on the ligands. In the oxygen evolution step, the ligands are returned to their original state and, as a result, these catalysts are very robust because they follow a “self-healing” mechanism. In addition, because the mechanism is ligand-based, no open coordination site is required and this enables the development of highly active molecular catalysts for water oxidation with first-row transition metals.
1.1-I3
Heterogeneous molecular catalysts based on transition metal complexes supported on conductive substrate have received increasing attention for their potential application in electrocatalysis and electrosynthesis. In this talk I will report some exemplary work on tuning the electrocatalytic activity of such heterogeneous molecular catalysts. The heterogenization of molecular catalyst was first demonstrated by anchoring molecular Co2+ ions onto N/S/O doped graphene. The S dopant of graphene is found to be most effective in improving the intrinsic activity of the Co sites. In another demonstration based on Ni molecular catalyst, we found for the first time that the presence of Fe3+ ions in the solution could bond at the vicinity of the Ni sites, generating heterogeneous Ni-Fe dual sites anchored on doped graphene. These Ni-Fe sites exhibited drastically improved oxygen evolution activity. Using CO2 reduction on well-defined molecular catalysts as a probe, we also demonstrated the importance of axial ligand, relay molecule and microenvironment in improving interfacial electron transfer and subsequent electrocatalytic activity.
1.1-O1
Electrode interface is the important place of electrochemical reactions inside batteries, properties of which has an influence on performance and states of batteries. To obtain and precisely evaluate state of charge (SoC) and state of health (SoH) of the energy storage batteries, exploring reliable In-situ characterization techniques to monitor the interfacial electrochemical dynamics and properties variation should have significant importance. At present, applied interface characterization techniques are used to analysis the components and morphology, Scanning Electrochemical Microscope (SECM) is the only detection technique that can provide spatially resolved information of the kinetics of interfacial reactions [1,2], and ultrasonics is a nondestructive technique to locate interface based on differentiate acoustic impedance of polyphase system, which has been widely used in medical examination and diagnose. Some attempts of ultrasonics detection for batteries have also made recently [3,4].
Here in this work, SECM and an ultrasonic longitudinal wave reflection nondestructive testing technology was used for different mainstreamed secondary batteries like lithium-ion batteries, sodium-ion batteries, liquid metal batteries and aqueous zinc metal batteries.
1.1-O2
Ungyu Paik is a distinguished HYU professor of Department of Energy Engineering at Hanyang University, Korea. He received his Ph.D. degree from Department of Ceramic Engineering at Clemson University in 1991. Prior to starting his professor position at Hanyang University in 1999, he conducted postdoctoral research at the National Institute of Standards and Technology, USA. His research interest is the synthesis and engineering of nanomaterials for the applications in energy devices. He has abundant academic achievement with more than 390 SCI papers and hold 88 patents. With his knowledge, expertise, and insight, he served as a minister in the ministry of trade, industry, and energy of Korea from 2017 to 2018. Now he is back on an academic career. He was selected in highly cited researchers as part of the “Crossfield” arena in 2020 ~ 2022 by Clarivate Analytics.
In the current growth of the electric vehicle (EV) market, the roll-to-roll dry coating process, enabling mass production of high-thick electrode (≥10mAh cm-2), stands out as an innovative and practical approach to fabricate the low-cost and high-energy-density Li-ion batteries (LIBs). However, as the thickness of the electrode fabricated by dry coating process becomes greater (≥10 mAh cm-2), Li-ion migration resistance (Rion) and charge-transfer resistance (Rct) in the electrode dramatically increase due to long diffusion lengths for Li-ion and electron. This is primarily associated with the non-uniform distribution of electrode components and severe fractures of cathode active material during the dry coating process. Therefore, it is crucial to reduce diffusion lengths in the thick electrode to achieve high energy density LIBs. In this study, we identified the factors associated with the increase in ionic resistance through electrochemical analysis. Then, manipulation of material and process parameters was conducted to optimize the microstructure of the dry electrode. Finally, we successfully developed a 10mAh cm-2 dry electrode with low ionic resistances, exhibiting the enhanced of Li+/e- kinetics and improved high C-rate capability.
1.1-I1
Prof. Zijian Zheng is currently Chair Professor of Soft Materials and Devices at the Department of Applied Biology and Chemical Technology, Associate Director of Research Institute for Intelligent Wearable Systems, Lead Investigator of Research Institute for Smart Energy at The Hong Kong Polytechnic University (PolyU). His research interests include surface and polymer science, nanofabrication, flexible and wearable electronics, energy conversion and storage. Prof. Zheng received his B. Eng. in Chemical Engineering at Tsinghua University in 2003, PhD in Chemistry at University of Cambridge in 2007, and postdoctoral training at Northwestern University in 2008-2009. He joined PolyU as Assistant Professor in 2009, and was promoted to tenured Associate Professor in 2013 and then Professor in 2017. He has published more than 200 papers in journals such as Science, Nat. Mater., Nat. Comm., Adv. Mate., JACS, Angew. Chem.. He also files more than 40 patents and is recipient of more than 15 academic awards. He serves as Editor-in-Chief of EcoMat (impact factor: 14.6), a flagship open-access journal in green energy and environment published by Wiley. He is Founding Member of The Young Academy of Sciences of Hong Kong (2018), Chang Jiang Chair Professor by the Ministry of Education of China (2020), Senior Research Fellow of the University Grant Commission of Hong Kong (2021), Fellow of International Association of Advanced Materials (FIAAM, 2021), Fellow of the Royal Society of Chemistry (FRSC, 2022). He is awardee of the inaugural Hong Kong Engineering Science and Technology Award.
Wearable energy storage devices are indispensable corner stones for future wearable electronics. Current energy storage technologies are based on materials and devices that are rigid, bulky, and heavy, making them difficult to wear. On the other hand, fibers are flexible and lightweight materials that can be assembled into different textiles and have been worn by human beings thousands of years. Different from conventional two-dimensional thin films and foils, the three-dimensional fibre and textile structures not only provide superior wearing ability, but also much larger surface areas. This talk will introduce how our research group makes use of the attributes of fibres for high-performance wearable energy storage devices. We develop textile composite electrodes that not only address the flexibility challenge, but also provide additonal benefit to the electrochemical properties of batteries. We will demonstrate the strategies and discuss the perspectives to modify fibers and textiles for making wearable capacitators and batteries with excellent mechanical durability, electrochemical stability, and high energy/power density.
1.1-I2
All-solid-state batteries (ASSBs) with sulfide-based solid electrolytes with high ionic conductivity are regarded as the ultimate next-generation energy storage systems due to their enhanced safety and energy density by enabling the use of metallic anodes. Li metal is considered a promising anode material for ASSBs because of its high theoretical specific capacity (3860 mAh/g) and the lowest electrochemical potential (-3.04 V versus standard hydrogen electrode). However, its practical use has been hindered by several issues related to the interface, such as contact loss during cycling, which accelerates Li dendrite growth, and chemical instability between Li and sulfide-based solid electrolytes. In this talk, the fundamental degradation mechanisms of the ASSBs underlying electrochemical and mechanical aspects are introduced first. Subsequently, we introduce our strategies to stabilize the Li metal and sulfide-based solid electrolytes interface. The designed ASSBs could effectively retard the Li dendrite growth and unwanted side reaction and shows much enhanced electrochemical performance.
2.1-O1
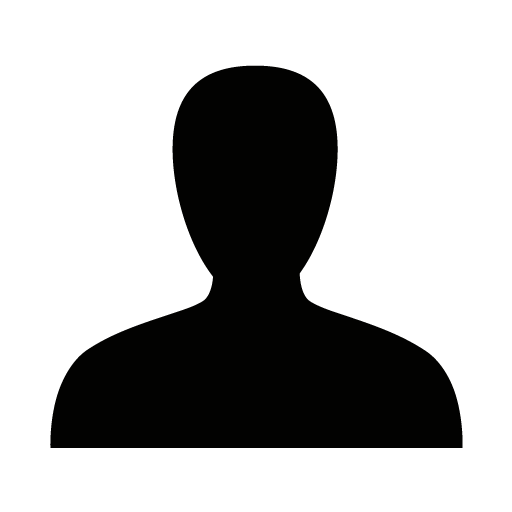
Sustainable energy storage is the bottleneck for the integration of high-ratio renewable energy to the grid. The all-liquid-structure and membrane-free liquid metal batteries (LMBs), with the merits of low-cost, long-lifespan and easy-scale-up, are promising for large-scale energy storage applications. Previously reported lithium LMBs exhibit excellent electrochemical performance, however, the practical application of lithium LMBs was hindered by the sealing issue of highly corrosive lithium vapor, along with the very limited reserve of lithium.
Instead of lithium, due to the weaker corrosion and much higher abundance, sodium LMBs show great potential for long-term and large-capacity energy storage applications. However, sodium has a high dissolution in single-cationic molten sodium halide mixtures salt electrolytes, which causes severe self-discharge and low coulombic efficiency of batteries. Here, for the first time, high-performance molten salt electrolyte and alloy electrodes are designed for the realization of practical sodium LMBs. A multi-cationic ternary chloride (LiCl-NaCl-KCl) with a low melting point (~390 ℃) and low sodium halide content (5 mol% NaCl) is rationally designed as the electrolyte, which significantly inhibits the dissolution of sodium in the molten salt electrolyte. Based on the designed cationic electrolyte, cooperating with a dual-active Bi9Sb alloy positive electrode, a sodium LMB is constructed and the battery stably cycles over 700 cycles with a coulombic efficiency of 97% at 100 mA cm-2 and 450 ℃, indicating the design of coupled cation electrolyte and alloy electrode is feasible to inhibit the self-discharge of batteries. Meanwhile, the adoption of electrolytes with low active substance content in this work also provides new directions for the design of electrolytes for high-performance electrochemical cells based on molten salts for high-efficiency energy conversion and storage. The calculation based on MW energy storage system indicates that the estimated Levelized Cost of Storage (LCOS) of the sodium LMB is lower than 0.029 $/kWh. To further reduce the cost, LiCl-NaCl-CaCl2 (28:32:40 mol%) and NaCl-CaCl2 (50:50 mol%) have been investigated for their potential as electrolytes for sodium LMBs. The significant reduction of Li+ content resulted in batteries with lower cost (reduced by 32%), but the interaction of the anode (Na) with the electrolyte led to difficulties in releasing the capacity of the battery and poor stability. To address this issue, the structure and composition of the anode were optimized, which achieved the thermodynamic compatibility between the anode and the electrolyte and the stable operation of the battery. The results demonstrate that sodium LMB is a promising technology for grid-scale energy storage applications.
2.1-O2
Dr. Minghao Yu, PI, holds an independent research group (Electrochemistry for Sustainable Energy Storage) at Technische Universität Dresden. His research interest includes 1) the development of novel organic and inorganic 2D layered materials, 2) the investigation of advanced artificial interphases and electrolytes for next-generation batteries, 3) fundamental charge and ion dynamics during electrochemical energy storage processes, and 4) sustainable energy storage device fabrication, including supercapacitors, hybrid-ion capacitors, aqueous batteries, dual-ion batteries, and multivalent metal (Zn, Mg, Al) batteries. He has published more than 120 scientific articles which have attracted more than 20,000 citations with an H-index of 69 (Web of Science). Besides, he is also an associated member of the Center for Advancing Electronics Dresden (cfaed), an associated group leader at Max-Planck-Institut für Mikrostrukturphysik, a highly cited researcher (Clarivate Analytics, 2018-now), 2023 ERC Starting Grant winner, and a Fellow of the Young Academy of Europe.
Electrochemical energy storage technologies have been brought into the spotlight as they provide elegant and efficient approaches to storing, transporting, and delivering energy harvested from sustainable energy resources.[1-2] The demand for power and energy supply is equally imperative in actual use and is keen to expand in the future. Thus, it is highly desirable to design new electrode materials or rationally re-construct the recognized electrode materials for energy storage devices to mitigate the power-energy tradeoff. In this talk, I will present our recent efforts in exploring 2D layered materials for sustainable energy storage applications.[3] Specifically, I will present several interlayer engineering strategies for inorganic 2D layered materials to regulate the ion transport behaviors and boost the power-energy performance of the assembled energy storage devices.[4-7]
References:
[1] Yu et al., Chem. Soc. Rev. 2021, 50, 2388-2443.
[2] Yu et al., Joule 2019, 3, 338-360.
[3] Yu et al., J. Am. Chem. Soc. 2020, 142, 12903-12915.
[4] Yu et al., Angew. Chem. Int. Ed. 2021, 60, 896-903.
[5] Yu et al., Nat. Commun. 2020, 11, 1348.
[6] Yu et al., Adv. Mater. 2022, 34, e2108682.
[7] Yu et al., Adv. Energy Mater. 2023, accepted.
2.1-O3
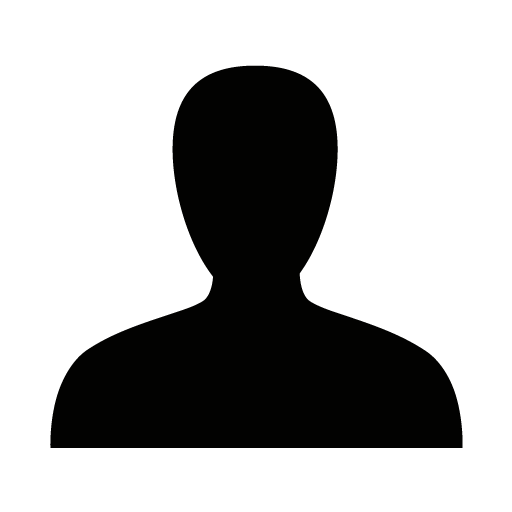
Supercapacitors are considered optimal storage devices for electric vehicles and wireless technology due to their superior power density, cycle stability, and charge-discharge efficiency in comparison to traditional batteries [1]. Their inability to act as primary energy sources, however, is hindered by their low energy density. Research efforts are thus concentrated on nanostructured materials that exhibit enhanced specific capacitance, with a focus on carbon-based materials such as activated carbon, carbon nanotubes (CNTs), carbon aerogels and graphene [2]. Supercapacitors operate via electrochemical double-layer capacitors (EDLCs) and pseudocapacitors. EDLCs store energy at the electrode/electrolyte interface through ion adsorption, relying on carbon-based electrodes [3]. Although graphene has superior electrical and thermal conductivity, its theoretical capacitance leads to limitations, despite showing promise. Adjusting the alignment of graphene from horizontal to vertical, as discovered in previous studies, leads to a 38% increase in capacitance, thereby overcoming some of the limitations [4]. Vertical graphene nanowalls (GNWs), manufactured via plasma-enhanced chemical vapor deposition (PECVD), provide superior electrical conductivity and a three-dimensional structure that makes them ideal scaffolds for supercapacitor electrodes [5], [6]. Pseudocapacitors, comprising of metal oxides and polymers, demonstrate a higher specific capacitance in comparison to EDLCs but necessitate a carbon substrate for better charge transfer [7]. The amalgamation of carbon materials and metal oxides manifests a synergistic effect, fulfilling vital requisites for novel energy storage devices. Nevertheless, the elevated cost and toxicity linked with most metal oxides present obstacles. Zinc oxide (ZnO) is a promising electrode material for supercapacitors due to its natural abundance, eco-friendliness, cost-effectiveness, and long cycling life [8]. The specific capacitance of ZnO can be improved by preserving oxygen vacancies, which can be attained by annealing in inert atmospheres [9]. Although integrating ZnO with graphene-based structures has exhibited notable advancements, it remains a complicated and expensive process.
In this study, GNWs represent a promising porous structure, offering a substantial surface area for active sites and facilitating rapid ion diffusion. To enhance their specific capacitance, we present a supercapacitive improvement through a hierarchical arrangement of defect-engineered ZnO nanorods (ZNRs) anchored onto the GNWs. This hierarchical structure is synthesized through a multi-step process involving inductively coupled plasma-chemical vapor deposition, magnetron sputtering, and hydrothermal methods. The presence of oxygen vacancy (OV) defects within the ZNRs, induced by argon annealing, has been characterized utilizing X-ray photoelectron spectroscopy. The emergence of OV defects below the conduction band of the ZNRs results in a narrowing of the bandgap within the hybrid structure, thereby enhancing its conductivity and increasing the reaction sites. In the capacity of supercapacitor electrodes, the ZNRs/GNWs hybrids were evaluated in an aqueous KOH electrolyte solution, operating within a voltage range of 0.5 V and at a current density of 0.1 mA cm-2. This assessment yielded an area capacitance of 21.45 mF cm-2, signifying a 1.5-fold increase in capacitance compared to GNWs grown on graphite sheets. The ZNRs/GNWs hybrid demonstrates remarkable electrochemical performance and exhibits substantial potential for energy storage applications. Our work is expected to offer valuable insights for the enhancement of electrochemical properties in various composite and hybrid materials.
2.1-I1
Low temperature fuel cells are electrochemical devices that convert chemical energy directly to electricity. They have great potential for both stationary and transportation applications and are expected to help address the energy and environmental problems that have become prevalent in our society. Despite their great promise, commercialization has been hindered by lower than predicted efficiencies and high loading of Pt-based electrocatalysts in the electrodes. For more than five decades, extensive work has been focused on the development of novel electrocatalysts for fuel cell reactions. In this talk, I will present recent progress in developing advanced electrocatalysts and their fuel cell performance in my group, with an emphasis on core-shell and non-precious metal materials. The results demonstrate that core-shell structure not only enhances the mass activity of Pt, but also its stabilit in a real fuel cell environment. Our hydrbid catalysts consiting of Pt-Fe nanoparticles and Fe-N-C support don't decacy even after 100,000 potential cycles in a fuel cell testing.
1.1-K1
1.1-K2
Greg Scholes is the William S. Tod Professor of Chemistry at Princeton University. Originally from Melbourne, Australia, he later undertook postdoctoral training at Imperial College London and University of California Berkeley. He started his independent career at the University of Toronto (2000-2014) where he was the D.J. LeRoy Distinguished Professor. He was appointed Editor-in-Chief of the Journal of Physical Chemistry Letters in 2019. Dr. Scholes was elected a Fellow of the Royal Society (London) in 2019 and a Fellow of the Royal Society of Canada in 2009. He served as Chair of Department at Princeton 2020-2023 and Director of an Energy Frontier Research Center (BioLEC) since 2018.
1.2-K1
Prof. Beatriz Roldán Cuenya is currently Director of the Interface Science Department at the Fritz Haber Institute of the Max Planck Society in Berlin (Germany). She is an Honorary Professor at the Technische Universität Berlin, at the Freie Universität Berlin, and at the Ruhr-University Bochum, all in Germany. Also, she serves as a Distinguished Research Professor at the University of Central Florida (USA).
Prof. Roldán Cuenya began her academic career by completing her M.S./B.S. in Physics with a minor in Materials Science at the University of Oviedo, Spain in 1998. Afterwards she moved to Germany and obtained her Ph.D. from the Department of Physics of the University of Duisburg-Essen with summa cum laude in 2001. Subsequently, she carried out her postdoctoral research in the Department of Chemical Engineering at the University of California Santa Barbara (USA) until 2003.
In 2004, she joined the Department of Physics at the University of Central Florida (UCF) as Assistant Professor where she moved through the ranks to become a full professor in 2012. In 2013 Prof. Roldan Cuenya, moved to Germany to become Chair Professor of Solid State Physics in the Department of Physics at Ruhr-University Bochum until 2017.
During her academic career, Prof. Roldan Cuenya received an Early CAREER Award from the US National Science Foundation (2005) and the international Peter Mark Memorial award from the American Vacuum Society (2009). In 2016 she became Fellow of the Max Planck Society in Germany and also received the prestigious Consolidator Award from the European Research Council. In 2020, she became a member of the Academia Europaea (Academy of Europe). She received the AVS Fellow Award (2021), the International Society of Electrochemistry-Elsevier Prize for Experimental Electrochemistry (2021), the 2022 Paul H. Emmet Award of the North American Catalysis Society, and the Röntgen Medal of the City of Remscheid (2022).
Prof. Dr. Beatriz Roldan Cuenya is the author of 245 peer-reviewed publications, 6 book chapters and 6 patents. She has been supervising 74 postdoctoral fellows and 36 PhD students. She has given 245 invited talks, with 13 plenary talks and 33 keynote lectures since 2017. Her H-factor is 74 (Google Scholar) and her work has received over 21,500 citations.
She presently serves on the editorial advisory boards of the Journal of Catalysis and Chemical Reviews. In addition, she also contributes to a number of advisory committees, including the Liquid Sunlight Alliance (USA), the Advanced Research Center Chemical Building Blocks Consortium (Utrecht, the Netherlands), the Spanish Synchrotron Facility ALBA (Barcelona, Spain), the German Synchrotron DESY (Hamburg, Germany), the Helmholtz-Zentrum Berlin for the strategic development of BESSY II (Berlin, Germany), the Institute of Chemical Research of Catalonia (ICIQ in Tarragona, Spain), the UK Catalysis Hub and the Ertl Center for Electrochemistry & Catalysis (South Korea).
Prof. Roldan Cuenya’s research program explores physical and chemical properties of nanostructures, with emphasis on advancements in nanocatalysis based on operando microscopic and spectroscopic characterization.
1.2-K2
Laura Herz is a Professor of Physics at the University of Oxford. She received her PhD in Physics from the University of Cambridge in 2002 and was a Research Fellow at St John's College Cambridge from 2001 - 2003 after which she moved to Oxford. Her research interests lie in the area of organic and organic/inorganic hybrid semiconductors including aspects such as self-assembly, nano-scale effects, energy-transfer and light-harvesting for solar energy conversion.